- 1St. Francis University College of Health and Allied Sciences, Ifakara, Tanzania
- 2Medical Research Center, Kantonsspital St. Gallen, St. Gallen, Switzerland
- 3Graduate School for Cellular and Biomedical Sciences, University of Bern, Bern, Switzerland
- 4Functional Genomics Center Zurich, University of Zürich/ETH Zürich, Zürich, Switzerland
- 5Division of Human Microbiology, Centre for Laboratory Medicine, St. Gallen, Switzerland
- 6Division of Infectious Diseases, Infection Prevention, and Travel Medicine, Kantonsspital St. Gallen, St. Gallen, Switzerland
- 7St. Francis Regional Referral Hospital, Ifakara, Tanzania
- 8Clinical Trials Unit, Kantonsspital St. Gallen, St. Gallen, Switzerland
- 9Division of Infectious Diseases, University Hospital Basel, Basel, Switzerland
- 10Swiss Tropical and Public Health Institute, Allschwil, Switzerland
- 11Ifakara Health Institute, Ifakara, Tanzania
- 12Department of Infectious Diseases, Inselspital, Bern University Hospital, University of Bern, Bern, Switzerland
Introduction: The global rise of extended-spectrum beta-lactamase-producing Enterobacterales (ESBL-PE) challenges resource-limited countries with insufficient laboratory infrastructure. This study investigates fecal carriage and risk factors for ESBL-PE and carbapenemase-producing organisms among patients with urinary tract infection (UTI) in rural Tanzania.
Methods: This cross-sectional study was conducted at St. Francis Regional Referral Hospital, Ifakara, Tanzania, from October 2021 to August 2023, involving 326 UTI patients. Demographic data and resistance risk factors were collected via structured questionnaires. Stool samples collected pre-antibiotic treatment were screened for ESBL-PE and carbapenemase locally. Positive samples underwent further analysis in Switzerland using MALDI-ToF, Vitek MS, and whole-genome sequencing. Multivariable analysis assessed predictors associated with ESBL-PE carriage for risk factors with p < 0.05.
Results: We enrolled 326 UTI patients (median age: 35.5 years, range: 25–52) and 189 (58.0%) were females. Fecal ESBL-PE colonization was detected in 70.9% of patients, predominantly E. coli (62.8%) and K. pneumoniae (33.0%). Whole-genome sequencing identified diverse phylogroups and sequence types, with CTX-M-15 being the most common ESBL gene. IncF plasmids were the primary carriers. Younger age (aOR: 0.98, 95% CI: 0.97–0.99; p = 0.0239) and inpatient status (aOR: 1.77, 95% CI: 1.08–2.91; p = 0.0036) were significant risk factors for ESBL-PE carriage.
Conclusion: The high prevalence of ESBL-PE fecal carriage in rural Tanzania highlights the need for improved infection control and further research into community transmission dynamics.
Background
Antimicrobial resistance (AMR) is a major global health challenge with significant consequences for public health and healthcare systems. In 2024, the Global Research on Antimicrobial Resistance (GRAM) project estimated that by 2050, there will be 1.91 million annual deaths globally attributable to AMR, with 6.63 million deaths attributable to AMR in sub-Saharan Africa. Without effective prevention measures, AMR will lead to a cumulative total of 39·1 million deaths attributable to AMR from 2025 to 2050 (Global Burden of Disease 2021 Antimicrobial Resistance Collaborators, 2024).
Reports indicate that the widespread fecal carriage of Extended-spectrum beta-lactamase producing Enterobacterales (ESBL-PE) is an emerging public health concern, contributing to infections in both community and hospital settings. Even in the absence of active infection, colonization with ESBL-PE remains a significant cause for concern (Godonou et al., 2022).
The high prevalence of fecal carriage of resistant pathogens facilitates the global transmission of resistance genes through travel, trade, and migration (Bokhary et al., 2021; Zamudio et al., 2024). In resource-limited settings with inadequate sanitation and hygiene infrastructure, the spread of resistance genes is exacerbated through contaminated food, water, and direct contact. This one health dynamic extends to animal populations via zoonotic spillover, cycling resistance genes back to humans through the environment or food chain (Aslam et al., 2021; Gebreyes et al., 2014). These mechanisms not only challenge effective treatment options but also contribute to increased morbidity and mortality due to limited therapeutic options (Iskandar et al., 2021).
The fecal carriage of ESBL-PE varies geographically, with prevalence ranging from 6 to 20% in Europe and North America, approximately 20% in the Eastern Mediterranean and Africa, and 24.5–27% in the Western Pacific and Southeast Asia in community and hospital settings (Bezabih et al., 2021; Pilmis et al., 2018; Islam et al., 2017).
In Africa, studies conducted in Chad and Rwanda have demonstrated a higher prevalence of ESBL-PE carriage in hospital settings than in the community (Ouchar Mahamat et al., 2019; Kurz et al., 2017). This pattern may be attributed to antibiotic use, antibiotic-induced gut dysbiosis, and reduced colonization resistance, which might promote ESBL-PE transmission through various routes, including person-to-person, contaminated food and water, and environmental exposure (Mai and Espinoza, 2023; Djuikoue et al., 2016).
In Eastern Africa specifically, ESBL-PE prevalence ranges from 6 to 17% in community settings and 38–83% in hospital environments (Storberg, 2014). A study conducted in East African hospitals has documented an overall pooled proportion of ESBL-PE at 42% with variation among countries: 61.7% in Uganda, 45.8% in Kenya, 38.8% in Tanzania and 30.9% in Ethiopia (Sonda et al., 2016).
Despite the growing concern about ESBL-PE in Tanzania, there is a notable lack of comprehensive studies on carriage of ESBL-PE across diverse age groups and clinical presentations (Silago et al., 2022; G et al., 2017; Ngowi et al., 2021; Mlugu et al., 2023). The available studies primarily focus on specific subpopulations or environments, leaving a fragmented understanding of the broader distribution of ESBL-PE. For instance, a study conducted among pregnant women reported a high prevalence of ESBL carriage rate of 64.3% (Mwandigha et al., 2020). Another study in urban informal settlements found that over 24% of ESBL-producing E. coli were isolated from private and shared latrines, indicating these facilities act as reservoirs for ESBL-PE transmission (Erb et al., 2018). Similarly, studies targeting hotel employees (Büdel et al., 2019) and healthcare settings (Manyahi et al., 2020) have provided valuable insights but are limited in scope. Most of these studies are urban-centric focus leaving rural communities underrepresented resulting in an incomplete picture of the epidemiology and dissemination of ESBL-PE in Tanzania. To address this knowledge gap, our study aims to evaluate the fecal carriage rate of and risk factors for multidrug-resistant Enterobacterales in patients presenting with symptoms of urinary tract infection at St. Francis Regional Referral Hospital (SFRRH) in Ifakara, a rural area in Tanzania. This research will contribute to the understanding of ESBL-PE epidemiology in the region and inform strategies for antimicrobial stewardship and infection control.
Methodology
Study design and study setting
The study was conducted at St. Francis Regional Referral Hospital (SFRRH) in Ifakara, Tanzania, from October 2021 to August 2023. SFRRH is a 371-bed hospital with approximately 16,480 in-patient admissions, and 100,000 out-patient visits yearly serving a population of close to 1 million people in the Kilombero Valley. It is the main teaching hospital in South-Central Tanzania, playing a key role in promoting health and training human resources in that area (Figure 1).
The map was created using Quantum GIS v3.26 open source (Congedo, 2021).
Patient recruitment
The study included in- and outpatients aged 18 years or older who presented with clinical evidence of urinary tract infection (UTI) according to the Infectious Diseases Society of America (IDSA) guidelines (Nicolle et al., 2019). All participants provided written informed consent. Ethical approval was granted by the National Health Research Committee of the Tanzania National Institute for Medical Research (certificate number NIMR/HQ/R.8a/Vol. IX/3759). Consent was obtained in Kiswahili. For illiterate individuals, clinicians or registered nurses provided detailed study explanations in the presence of a chosen witness, and willing participants indicated their consent with a thumbprint.
Sample collection
Patients were instructed by a clinician or registered nurse to collect stool (Stool container, Mshale, Dar-es-Salaam, Tanzania). The samples were taken to the Microbiology laboratory at SFRRH within 2 h.
Data collection and management
Structured questionnaires gathered socio-demographic and clinical data. Variables included age, gender, residence, history of past UTI, pregnancy status, history of diabetes, antibiotic use in the past 2 months, and companion animals. Data management and analysis plans were developed at SFRRH in Tanzania and Kantonsspital St. Gallen (KSSG) in Switzerland, respectively.
Laboratory procedures
In Tanzania
Mid-stream urine of all patients who fulfilled the definition of UTI were collected aseptically and streaked on Blood, Cystine Lactose Electrolyte Deficient agar (CLED), and/or MacConkey agar [Liofilchem, Roseto degli Abruzzi (Te), Italy] using a calibrated 0.01 mL inoculating loop. Cultures underwent incubation for 18–24 h at 37°C. Agar plates exhibiting pure growth equivalent to or exceeding 105 colony-forming units (CFU/mL) were categorized as having significant growth. Plates with no visible colonies were reported as having no bacterial growth. Results showing bacterial growth ≥102 CFU/mL but below the threshold for significant bacterial growth of 105 CFU/mL were considered insignificant. Cultures displaying more than two types of organisms were classified as mixed growth. The pure bacterial isolates underwent further identification based on colonial characteristics, microscopic features following Gram’s stain, and biochemical tests.
Stool samples underwent screening for extended-spectrum cephalosporin and carbapenem-resistant Enterobacterales. Briefly, the stool samples were enriched overnight, incubated for 18 h at 37°C, in Mueller Hinton broth containing cefuroxime (30 μg), and subsequently plated on CHROMagar™ ESBL and CHROMagar™ mSuperCARBA (CHROMagar, Paris-France). All colonies exhibiting variations in color and/or morphology were carefully selected and further inoculated on nutrient agar slants. These inoculated slants were then incubated for 18 h at 37°C and subsequently preserved in trypticase soy broth (TSB) containing 20% glycerol at −80°C.
For retrieval, bacteria were scraped from frozen bacterial glycerol stocks using a sterile loop. Subsequently, they were streaked onto nutrient agar plates and incubated for 24 h at 37°C. Following this incubation period, bacterial colonies were picked and inserted into a swab within the Stuart transport system (Trust lab, China). Tubes were labeled with specimen source and packed for shipment following the International Air Transport Association (IATA) regulations. A shipment was made after obtaining the Material Transfer Agreements (MTAs) and other shipping permits from the responsible authorities and were then shipped at room temperature to the Laboratory of Experimental Infectious Diseases at Kantonsspital St. Gallen (KSSG) in Switzerland for further analysis. Upon arrival at the laboratory, biosafety procedures for BSL-2 pathogens were followed. The isolates were processed in a Class II biosafety cabinet, workspaces and tools were disinfected before and after processing, and personal protective equipment gloves and lab coats were worn during handling. The isolates were kept frozen at −80°C for further use.
In Switzerland
Bacterial storage, identification, and susceptibility testing
The isolates were streaked on Luria Bertani agar (Sigma Aldrich, USA) and incubated at 37°C for 24 h. The following day, individual colonies were selected and then suspended in separate 1.5 mL tubes containing 1 mL of Luria Broth (Sigma Aldrich, USA) with 10% glycerol and stored in a − 80°C freezer for future experiments. The bacteria were retrieved for identification by using a sterile loop to scrape them off from a frozen bacterial glycerol stock.
The identification of isolates from urine was performed at the species level using MALDI-ToF mass spectrometry (MALDI Biotyper Smart System, Bruker Daltonics, Bremen, Germany). Based on the susceptibility test patterns obtained from the BD Phoenix™ M50 system (Becton Dickinson, Sparks, MD, USA), additional confirmation tests were performed E-test ESBL confirmation with specific E-test stripes, Carba-NP carbapenemase detection kit, all purchased from BioMérieux, Marcy l’Etoile, France and Carba-5 carbapenemase detection kit (NG Biotech, France).
For identification and antimicrobial susceptibility testing of isolates from stool, the VITEK® MS PRIME MALDI-TOF (Matrix Assisted Laser Desorption Ionization-Time of Flight) system was employed. Additionally, the VITEK MS VITEK® 2 COMPACT instrument, along with the VITEK® 2 PC software and ready-to-use bacteria identification (ID) cards, as well as antibiotic susceptibility testing (AST) VITEK® 2 ID/AST cards (N283, N240, P655), were utilized for the identification and antimicrobial susceptibility testing of the bacterial isolates (BioMérieux, Marcy L’ É toile, France). Additional susceptibility testing to confirm the presence of ESBL resistance mechanisms was conducted using the disk diffusion method in accordance with and interpreted according to European Committee on Antimicrobial Susceptibility Testing (EUCAST) guidelines (version 13.1, valid from 2023-06-29). Confirmation of carbapenemase producers was performed on a GeneXpert® System (Cepheid, CA 94089, USA), utilizing the Xpert® Carba-R test.
Nucleic acid extraction
Genomic DNA (gDNA) was extracted from all ESBL and carbapenemase-producing bacterial isolates derived from single colonies on plates. The QiaAMP Mini Kit (QIAGEN, Hilden, Germany) was employed for DNA extraction, following the manufacturer’s instructions, and the concentration was determined using the Nanodrop OneC spectrophotometer (Thermo Fisher Scientific, Massachusetts, United States). The extracted DNA was eluted in 50–100 μL of sterile water, and the DNA templates were stored at −20°C until further analysis.
Whole genome sequencing
Library preparation
A total 1 ng of DNA from each sample was tagmented using Illumina Nextera XT according to standard protocol. Nextera adapters containing Unique Dual Indices (UDI) were added by PCR. The libraries were double-sided size selected and the quality and quantity of the libraries were validated using the Fragment Analyzer (Agilent, Santa Clara, California, USA). The libraries were normalized to 10 nM in Tris-Cl 10 mM, pH8.5 with 0.1% Tween 20 and pooled equimolar.
Cluster generation and sequencing
After library quantification, libraries were prepared for loading according to the NovaSeq workflow with the NovaSeq6000 Reagent Kit (Illumina, Catalog no. 20012865). Cluster generation and sequencing were performed on a NovaSeq6000 System with a run configuration of paired-end at 2 × 150 bp.
Illumina paired-end (PE) reads underwent quality checks using FastQC (v0.11.9) (Chen et al., 2018; Wingett and Andrews, 2018). Adapter sequences and low-quality read ends (identified with a sliding window of 4 bp and a base quality lower than Q20) were trimmed away using Fastp (v0.20.0). Trimmed reads were quality (Q20) and length (18 bp) were filtered using the same tool. Trimmed and filtered reads were mapped to the reference genome (Ensembl Escherichia_coli K12 MG1655 ASM584v2) using bowtie2 (v2.4.2). Variants were identified using samtools (v1.11)/bcftools (v1.11) (Li, 2012). A hierarchical cluster dendrogram based on pairwise identity-by-state (IBS) values from SNP data for all samples was computed using SNPRelate (v1.30.1) (Zheng et al., 2012). A phylogeny tree from SNP data was constructed using mashtree (v1.4.6) and combined with metadata using itol (v6).
Trimmed and filtered reads were assembled using Spades (v3.15.5) (Bankevich et al., 2012). Assembled contig sequences were annotated using prodigal (v2.6.1) (Hyatt et al., 2010). Annotated protein sequences were compared to the Swiss-Prot (downloaded on 2023/02/10) (Bairoch and Apweiler, 2000; Altschul et al., 1990). Phylogroups of assembled genomes were determined using the Clermont Typing pipeline (v20.03) and Ridom SeqSphere (v10.0.2) (Jünemann et al., 2013).
E. coli Achtman MLST database was downloaded from pubmlst (Roer et al., 2017). Annotated gene sequences were compared to the MLST database (downloaded on 2023/03/15 and 2023/08/30) using blastn (ncbi-blast v2.12.0+). A customized perl script was used to identify the MLST profile, associated ST, and clonal complex. In silico serotyping was performed using SerotypeFinder (v2.0.1). Fim type was determined using FimTyper (v1.1) (Jolley et al., 2018) by comparing assembled contig sequences against the FimTyper database using blast (2.12.0+). Antibiotic-resistant genes were predicted using fARGene (Fragmented Antibiotic Resistance Gene iENntifiEr) (v0.1) (Berglund et al., 2019) and blastp (2.12.0+) comparison against the Comprehensive Antibiotic Resistance Database (CARD v3.2.6) (Alcock et al., 2023). Contigs of plasmid origin were predicted using plasmid finder (v2.1.6) and plaScope (v1.3.1) (Carattoli and Hasman, 2020).
Klebsiella pneumoniae isolates and their related species complex phylogroups, sequence type (ST) assignment, and antibiotic resistance gene detection were performed using Kleborate (Lam et al., 2021). Plasmid replicons were identified using the PathogenWatch platform,1 an online global database for genomic surveillance of K. pneumoniae isolates (Argimón et al., 2021). Enterobacter cloacae isolates were analysed using Ridom SeqSphere (v10.0.2) (Jünemann et al., 2013).
Data analysis
Categorical variables were summarized as frequencies with their percentages and compared between patients with ESBL and non-ESBL Enterobacterales using Fisher’s exact test.
Age was presented as mean ± standard deviation (SD) and median [interquartile range] and compared with the presence or absence of ESBL Enterobacterales using the student t-test. Results with p < 0.05 were considered statistically significant. Those risk factors that were statistically significant with p < 0.05 were included in a multivariable regression model assessing which predictors are associated with ESBL Enterobacterales when controlling for other factors. Odds ratios (ORs) and their 95% confidence intervals (95% CIs) of the unadjusted and adjusted models and their p-values were reported. Statistical analyses were performed using R (version 4.2.2) (R Core Team, 2022).
Results
We enrolled 326 eligible patients meeting IDSA criteria for UTI. Out of these, 166 (50.9%) were outpatients, 189 (58.0%) were female, and the median age was 35.5 years [IQR: 25–52]. The collective prevalence of ESBL-PE in stool was 70.9% (231/326 patients). A total of 860 phenotypically distinct bacterial isolates were obtained from the 326 stool samples. Among these, 347 (40.3%) were ESBL-producing Enterobacterales. E. coli accounted for the majority of all ESBL-producing Enterobacterales 219 (62.8%) followed by K. pneumoniae 115 (33.0%), details in Table 1.
Additionally, bacterial growth in urine was 3.7% (12 out of 326 patients). The bacterial isolates detected in urine included: four (Zamudio et al., 2024) E. coli, four (Zamudio et al., 2024) P. aeruginosa, three (Bokhary et al., 2021) K. pneumoniae and one (1) P. mirabilis. ESBL was only detected in one E. coli urinary isolate but was not identical to the concomitant ESBL E. coli detected in stool.
Geographical distribution of ESBL Enterobacterales at the patient level
The distribution of ESBL-PE carriers based on their geographical origin is illustrated in Supplementary Figure S1. The figure displays the number of patients who are carriers of ESBL in different villages.
Genomic diversity of ESBL Enterobacterales
a. Genomic diversity of ESBL E. coli (n = 219, Figure 2; Supplementary Table S1)
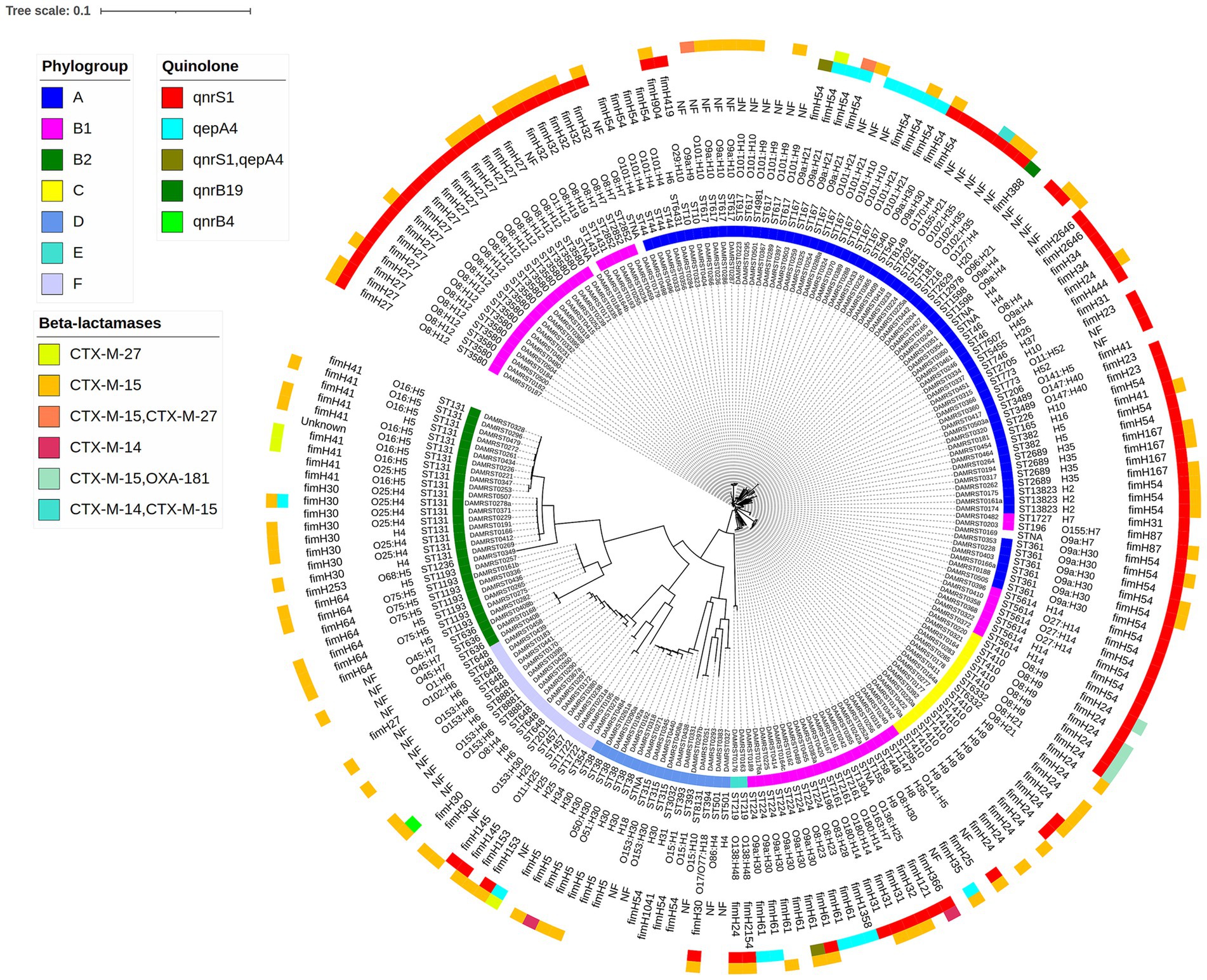
Figure 2. SNP-based phylogenetic tree of 219 ESBL-E. coli. From center to periphery, the layers correspond to the isolate name, phylogroup, sequence type (according to the Achtman scheme), serotype, the fimH allele, the quinolone resistance gene, and beta-lactamase genes.
The ESBL-E. coli mainly belonged to phylogroup A (79/219, 36.0%), followed by phylogroup B1 (50/219, 23.0%) and B2 (29/219, 13.0%).
Multilocus sequence typing (MLST) using the Achtmann scheme revealed 61 distinct sequence types (STs). The predominant STs identified were B2-ST131 (18/219, 8.2%), B1-ST 3580 (17/219, 7.7%), C-ST410 (14/219, 6.4%), A-ST167 (12/219, 5.5%), and A-ST 617 (12/219, 5.5%).
In silico serotyping revealed the presence of 76 distinct serotypes. The predominant serotypes were O8:H12 (17/219, 7.7%), O9a: H30 (15/219, 6.8%), H9 (9/219, 4.1%), and O16:H5 (8/219, 3.7%). Additionally, a total of 41 different fimH alleles were identified. The primary fimH alleles detected included fimH27 (18/219, 8.2%), fimH24 (17/219, 7.7%), fimH54 (16/219, 7.3%) and fimH30 (12/219, 5.5%).
Antimicrobial resistance and plasmid replicon content (Supplementary Table S2)
Among the 219 ESBL E. coli isolates, CTX-M-15 (189/219, 86.3%) emerged as the predominant ESBL gene, followed by CTX-M-27 (15/219, 6.8%). The quinolone resistance gene qnrS1 was predominant among isolates (97/219, 44.3%) 0.3.7% of the E. coli were carbapenemase producers, all harboring OXA-181 (8/219). Plasmid analysis in the 219 ESBL-E. coli isolates showed diversity in replicons, with IncFIB (AP001918) being the most common (122/219, 55.7%), followed by IncFIA (74/219, 33.8%).
a. Genomic diversity of ESBL K. pneumoniae and related species (n = 115, Supplementary Table S3)
Of 115 K. pneumoniae 90 isolates (78.2%) were identified as K. pneumoniae / phylogroup Kp1, (19/115, 16.5%) Klebsiella quasipneumoniae / phylogroup Kp2, (4/115, 3.5%) Klebsiella variicola / Kp3 and (2/115, 1.7%) Klebsiella quasivariicola/Kp6. Remarkably, among 115 K. pneumoniae isolates, MLST using Pasteur scheme highlighted 61 different STs. The top four most common STs identified were ST 110 (6/115, 5.2%), ST 334 (6/115, 5.2%), ST 3717 (6/115, 5.2%) and ST 17 (5/115, 4.3%).
Antimicrobial resistance and plasmid replicon content (Supplementary Tables S4, S5)
ESBL genes were identified among 115 K. pneumoniae and its related species with CTX-M-15 (106/115, 92.2%) being predominant. Carbapenemase KPC-2 was detected in 1.7% (2/115). The quinolone resistance gene qnrS1 was predominant among isolates 84 (73.0%) (84/115, 73.0%) followed by qnrB6 (9/115, 7.8%).
Plasmid analysis showed diversity in replicons, with IncFIB (K) being the most common (95/115, 82.6%), followed by IncY (44/115, 38.3%), IncR (42/115, 36.5%) and IncFII (K) (39/115, 33.9%).
a. Genomic diversity of ESBL E. cloacae (n = 5, Supplementary Table S6)
Out of five E. cloacae, three different ST were detected ST1, ST171, and ST 922. Four had CTX-M-15.
Factors associated with fecal carriage of ESBL-PE
Of the 326 patients, 312 (95.7%) indicated to not have used antibiotics in the past 2 months, 317 (97.2%) had history of UTI and 324 (99.4%) had no history of diabetes.
No statistically significant associations were found between a history of UTI, history of diabetes, past antibiotic use, pregnancy, animal interaction and ESBL carriage.
Following the results of the associations presented in Table 2, the following explanatory variables were selected to include in a multivariable model assessing ESBL-PE risk: age and patient status. In the multivariable analysis, the odds of ESBL-PE carriage decreased with increasing age (OR: 0.98, 95% CI: 0.97–0.99) and were higher in inpatients (OR: 1.77, 95% CI: 1.08–2.91) than in outpatients (Table 3).
Discussion
This study found that more than two-third (70.9%) of patients presenting with UTI were ESBL-PE carriers. This high prevalence of carriage in rural Tanzania serves as an important indicator of antibiotic resistance in the area. Prevalence rates for ESBL-PE colonization vary substantially among different populations in Tanzania (Manyahi et al., 2020). Geographical distribution data revealed potential hotspots of ESBL-PE carriage rates across villages, with some showing higher prevalence. These findings highlight areas with potentially elevated transmission risk, which could guide future studies and inform targeted intervention strategies.
Previous studies have reported lower carriage rates in the urban area of Dar es Salaam, with rates of 32.6% in HIV patients and 34.3% in children (Manyahi et al., 2020; Tellevik et al., 2016). Additionally, a rate of 59.7% has been observed in adult patients (Kibwana et al., 2020). In contrast, other research has found much higher (91.5%) colonization rates among hotel employees on the island of Zanzibar (Büdel et al., 2019). Furthermore, most studies have linked risk factors such as sharing of toilets (Erb et al., 2018), poor working environments and hygienic practices (Mwanginde et al., 2021), use of third-generation cephalosporins (Manyahi et al., 2020), history of antibiotic use (Moremi et al., 2018), old age (Kibwana et al., 2020) with increased ESBL fecal carriage.
These findings highlight the diverse distribution of ESBL-PE colonization within Tanzania, suggesting potential differences between urban and rural settings, as well as among various demographic groups.
Notably, this study highlights that younger individuals and inpatients were associated with an increased risk of being ESBL carriers. Inpatients exhibited a higher ESBL carriage rate than outpatients, consistent with literature identifying prolonged hospitalizations and extensive antibiotic treatments as key predictors for ESBL carriage (Mai and Espinoza, 2023; Moremi et al., 2021).
Our study did not reveal significant associations between ESBL carriage and factors such as previous antibiotic use, or animal contact. However, patients might not have reported previous antibiotic use on the one hand, on the other hand the relatively small sample size limits our ability to draw definitive conclusions. Further research with larger cohorts may be necessary to fully elucidate the relationships between these variables and ESBL carriage in this population.
Despite the high prevalence of patients with a history of UTI (97.2%), our study did not find an association between this factor and ESBL carriage. This disparity can be attributed to most individuals delaying seeking medical treatment, which may result in recurrent UTIs. Furthermore, patients frequently self-medicate using over-the-counter antibiotics (Torres et al., 2021; Maldonado-Barragán et al., 2024) or use traditional remedies, such as herbal medicines. This practice often results in a common history of UTIs among those who do not seek medical attention. In addition, in rural settings, poor sanitation, limited access to clean water, and inadequate hygiene infrastructure increase the risk of UTIs and their recurrence (Mwambete and Msigwa, 2017). We understand that the 97.2% figure might differ from patient populations in other settings. However, this difference highlights the challenges faced in rural health care due to the factors outlined earlier.
ESBL-producing E. coli emerged as the dominant colonizing species, followed by K. pneumoniae, with the CTX-M-15 enzyme being the most prevalent ESBL. The ubiquity of CTX-M-15 genes in both E. coli (86.3%) and K. pneumoniae (92.2%) aligns with global trends as well as with studies from Tanzania (Bevan et al., 2017; Moremi et al., 2017; Onduru et al., 2021; Slown et al., 2022; Sonda et al., 2018). Highly mobile genetic elements are responsible for carrying the predominant CTX-M genes between bacterial strains. The scenario is further supported by the established association between CTX-M enzymes and E. coli IncF resident plasmids, which are particularly well-adapted to E. coli strains and can readily transfer between them (Woerther et al., 2013).
Carbapenemases were only detected in a minority of cases, such as OXA-181 in 3.7% of E. coli and KPC-2 in 1.7% of K. pneumoniae. This low prevalence of carbapenemases suggests that carbapenems are likely to remain an effective treatment option for the time being. However, continued vigilance is essential to uphold infection control measures and prevent the potential rise of carbapenem-resistant isolates in our setting. We observed a phylogenetic diversity among the ESBL-producing E. coli, with the majority belonging to phylogenetic group A, followed by B1 and B2. ST-131 was the most common sequence type in the latter phylogroup. This finding aligns with earlier studies that have shown considerable phylogenetic diversity within ESBL-producing E. coli populations (Bevan et al., 2017). We also observed a notable diversity in sequence types, serotypes, and fimH alleles.
The diverse phylogroups and sequence types observed among ESBL-PE isolates, suggest complex transmission dynamics. Our research underscores the need for further research into community transmission patterns in resource-limited settings.
Limitations of the study include reliance on short-read sequencing data and the lack of environmental sampling, which restricted the comprehensive investigation of plasmid population structures and environmental transmission factors.
In addition, we were not able to investigate the relationship between fecal ESBL carriage and urinary tract infections, as most urinary cultures remained negative or showed only insignificant growth. The negative urine cultures could be due to (a) some symptoms may have been caused by conditions other than a UTI, such as sexually transmitted infections (STIs) which cannot be diagnosed by urine cultures, (b) medical incompliance, patients might be consuming antibiotics but hide this information from the clinician these consumed antibiotic agents may inhibit in-vitro culture growth of bacteria in the urine in this study we did not perform any inhibitory test to detect the presence of any previous antibiotic therapy in our urine samples (c) there may have been improper collection, handling and processing of urine samples in our setting. Our results challenge the assumption that all patients presenting with UTI symptoms will have positive urine cultures but it underscores the importance of including differential diagnoses in patients presenting with UTI-like symptoms. In future, further studies are needed to investigate the causes of these symptoms and the role of non-UTI infections and antibiotic stewardship in our settings. Without correlating fecal ESBL carriage to urine culture results, we cannot definitively establish the gut as a reservoir for invasive UTIs in this population.
In this study, we observed a low rate of history of antibiotic use. This might be because certain patients were unaware/ cannot accurately recall when last they used antibiotics. Additionally, we did not capture self-treatment with non-prescribed antibiotics or the use of traditional remedies in our setting. We recognize the potential for recall bias, given the reliance on self-reported information.
We recognize other ESBL risk factors such as history of surgery, hospitalization in the last 6 months, urinary catheterization, history of ESBL colonization, travel to other countries, and number of people living in the same house are under-recorded. In this study, due to limited access to patient records, we could not have details for these risk factors. Additionally, some factors, such as international travel history, may have been less applicable in our study population compared to urban settings.
Our study included all patients who met UTI IDSA criteria, which introduces selection bias and explains why we had a high proportion of patients with a history of UTI. The model selection for our multivariable model was prone to bias given that we used a p-value cutoff from the bivariate associations to determine which variables to include, however, this was chosen due to limited data availability and unbalanced groupings for other categorical factors. Statistical significance should be taken with caution due to the observational nature of the study.
In conclusion, this study reveals a high fecal carriage rate of ESBL Enterobacterales, especially among younger individuals in Tanzania, underscoring the need to investigate transmission dynamics beyond hospital settings. Although only one ESBL-positive isolate was identified as a cause of UTI, the high fecal carriage rate indicates a significant potential for infections with resistant organisms. This suggests a broader reservoir of resistant pathogens, particularly in areas with limited infection control measures.
Our findings stress the importance of understanding local epidemiology and highlight the need for healthcare interventions in rural areas to ensure proper treatments, appropriate antibiotic use, and education on personal hygiene. These results have significant implications for empirical treatment strategies and infection prevention policies in similar contexts.
Data availability statement
The datasets presented in this study can be found in online repositories. The names of the repository/repositories and accession number(s) can be found in the article/Supplementary material.
Ethics statement
The studies involving humans were approved by the National Health Research Committee of the Tanzania National Institute for Medical Research (certificate number NIMR/HQ/R.8a/Vol. IX/3759). The studies were conducted in accordance with the local legislation and institutional requirements. The participants provided their written informed consent to participate in this study.
Author contributions
MM: Conceptualization, Data curation, Writing – original draft. WQ: Formal analysis, Methodology, Writing – review & editing. SS: Formal analysis, Methodology, Writing – review & editing. AB: Writing – review & editing. PK: Writing – review & editing. HU: Project administration, Writing – review & editing. SH: Writing – review & editing. EW: Formal analysis, Methodology, Writing – review & editing. MR: Supervision, Writing – review & editing. BB: Conceptualization, Data curation, Funding acquisition, Methodology, Project administration, Supervision, Writing – review & editing.
Funding
The author(s) declare that financial support was received for the research, authorship, and/or publication of this article. This study was supported by the Federal Government (Swiss Government Excellence Scholarships to MEM-2021.0673), through a grant from the Research Committee of the Kantonsspital St. Gallen grant number (22/25), by the Vontobel Stiftung and Leading House Africa-Research Partnership Grant I by the Swiss Tropical and Public Health Institute and the University of Basel.
Acknowledgments
We thank Michael Ritzler and Flurina Buob from Labordiagnostic St. Gallen, for the bacterial identifications and resistance testing. In addition, we would like to thank the high-throughput sequencing team at the Functional Genomics Center Zurich for the Illumina sequencing service. We would like to express our gratitude to Winfrid Gingo, the Director of Clinical Services at SFRRH, for providing us with space to use the SFRRH laboratory. We are also thankful for the contributions of Paschael Mahenda and Anania Petro for their work in enrolling participants for the study. We are also grateful to all patients who consented to participate in this study. Additionally, we would like to thank Christopher Lekaleka and Nice Urassa for their efforts in performing the initial laboratory analyses of bacterial isolates at SFRRH and for handling the sample shipments to Switzerland. We would like to thank Alex Limwagu for creating the maps. The project was presented at the 34th European Society of Clinical Microbiology and Infectious Diseases (ESCMID) Global Congress from April 27 to 30, 2024 in Barcelona-Spain and at the American Society for Microbiology (ASM Microbe) Conference from June 13 to 17, 2024, in Atlanta, Georgia, United States.
Conflict of interest
The authors declare that the research was conducted in the absence of any commercial or financial relationships that could be construed as a potential conflict of interest.
Generative AI statement
The authors declare that no Gen AI was used in the creation of this manuscript.
Publisher’s note
All claims expressed in this article are solely those of the authors and do not necessarily represent those of their affiliated organizations, or those of the publisher, the editors and the reviewers. Any product that may be evaluated in this article, or claim that may be made by its manufacturer, is not guaranteed or endorsed by the publisher.
Supplementary material
The Supplementary material for this article can be found online at: https://www.frontiersin.org/articles/10.3389/fmicb.2024.1517182/full#supplementary-material
Footnotes
References
Alcock, B. P., Huynh, W., Chalil, R., Smith, K. W., Raphenya, A. R., Wlodarski, M. A., et al. (2023). CARD 2023: expanded curation, support for machine learning, and resistome prediction at the comprehensive antibiotic resistance database. Nucleic Acids Res. 51, D690–D699. doi: 10.1093/nar/gkac920
Altschul, S. F., Gish, W., Miller, W., Myers, E. W., and Lipman, D. J. (1990). Basic local alignment search tool. J. Mol. Biol. 215, 403–410. doi: 10.1016/S0022-2836(05)80360-2
Argimón, S., David, S., Underwood, A., Abrudan, M., Wheeler, N. E., Kekre, M., et al. (2021). Rapid genomic characterization and global surveillance of Klebsiella using Pathogenwatch. Clin. Infect. Dis. 73, S325–S335. doi: 10.1093/cid/ciab784
Aslam, B., Khurshid, M., Arshad, M. I., Muzammil, S., Rasool, M., Yasmeen, N., et al. (2021). Antibiotic resistance: one health one world outlook. Front. Cell. Infect. Microbiol. 11:771510. doi: 10.3389/fcimb.2021.771510
Bairoch, A., and Apweiler, R. (2000). The SWISS-PROT protein sequence database and its supplement TrEMBL in 2000. Nucleic Acids Res. 28, 45–48. doi: 10.1093/nar/28.1.45
Bankevich, A., Nurk, S., Antipov, D., Gurevich, A. A., Dvorkin, M., Kulikov, A. S., et al. (2012). SPAdes: a new genome assembly algorithm and its applications to single-cell sequencing. J. Comput. Biol. 19, 455–477. doi: 10.1089/cmb.2012.0021
Berglund, F., Österlund, T., Boulund, F., Marathe, N. P., Larsson, D. G. J., and Kristiansson, E. (2019). Identification and reconstruction of novel antibiotic resistance genes from metagenomes. Microbiome. 7:670. doi: 10.1186/s40168-019-0670-1
Bevan, E. R., Jones, A. M., and Hawkey, P. M. (2017). Global epidemiology of CTX-M β-lactamases: temporal and geographical shifts in genotype. J. Antimicrob. Chemother. 72, 2145–2155. doi: 10.1093/jac/dkx146
Bezabih, Y. M., Sabiiti, W., Alamneh, E., Bezabih, A., Peterson, G. M., Bezabhe, W. M., et al. (2021). The global prevalence and trend of human intestinal carriage of ESBL-producing Escherichia coli in the community. J. Antimicrob. Chemother. 76, 22–29. doi: 10.1093/jac/dkaa399
Bokhary, H., Pangesti, K. N. A., Rashid, H., Abd El Ghany, M., and Hill-Cawthorne, G. A. (2021). Travel-related antimicrobial resistance: a systematic review. Trop Med. Infect. Dis. 6:10011. doi: 10.3390/tropicalmed6010011
Büdel, T., Kuenzli, E., Clément, M., Bernasconi, O. J., Fehr, J., Mohammed, A. H., et al. (2019). Polyclonal gut colonization with extended-spectrum cephalosporin-and/or colistin-resistant Enterobacteriaceae: a normal status for hotel employees on the island of Zanzibar, Tanzania. J. Antimicrob. Chemother. 74, 2880–2890. doi: 10.1093/jac/dkz296
Carattoli, A., and Hasman, H. (2020). PlasmidFinder and in silico pMLST: identification and typing of plasmid replicons in whole-genome sequencing (WGS). Methods Mol. Biol. 2075, 285–294. doi: 10.1007/978-1-4939-9877-7_20
Chen, S., Zhou, Y., Chen, Y., and Gu, J. (2018). Fastp: an ultra-fast all-in-one FASTQ preprocessor. Bioinformatics 34, i884–i890. doi: 10.1093/bioinformatics/bty560
Congedo, L. (2021). Semi-automatic classification plugin: a Python tool for the download and processing of remote sensing images in QGIS. J. Open Source Softw. 6:3172. doi: 10.21105/joss.03172
Djuikoue, I. C., Woerther, P. L., Toukam, M., Burdet, C., Ruppé, E., Gonsu, K. H., et al. (2016). Intestinal carriage of extended spectrum beta-lactamase producing E. coli in women with urinary tract infections, Cameroon. J. Infect. Dev. Ctries. 10, 1135–1139. doi: 10.3855/jidc.7616
Erb, S., D’Mello-Guyett, L., Malebo, H. M., Njee, R. M., Matwewe, F., Ensink, J., et al. (2018). High prevalence of ESBL-Producing E. coli in private and shared latrines in an informal urban settlement in Dar Es Salaam, Tanzania. Antimicrob. Resist Infect. Control. 7:3. doi: 10.1186/s13756-017-0292-y
G, J., Philemon, R., S, M., M, A., Temu, T., Kunjumu, I., et al. (2017). Prevalence, Aetiology, and antimicrobial susceptibility patterns of urinary tract infection amongst children admitted at Kilimanjaro Christian medical Centre, Moshi, Tanzania. East African Heal Res. J. 1, 53–61. doi: 10.24248/eahrj.v1i1.388
Gebreyes, W. A., Dupouy-Camet, J., Newport, M. J., Oliveira, C. J. B., Schlesinger, L. S., Saif, Y. M., et al. (2014). The global one health paradigm: challenges and opportunities for tackling infectious diseases at the human, animal, and environment interface in low-resource settings. PLoS Negl. Trop. Dis. 8:e3257. doi: 10.1371/journal.pntd.0003257
Global Burden of Disease 2021 Antimicrobial Resistance Collaborators (2024). Global burden of bacterial antimicrobial resistance 1990-2021: a systematic analysis with forecasts to 2050. Lancet 404, 1172–1173. doi: 10.1016/S0140-6736(24)01885-3
Godonou, A. M., Lack, F., Gbeasor-Komlanvi, F. A., Konlani, L., Dossim, S., Ameyapoh, Y. A., et al. (2022). High faecal carriage of extended-spectrum beta-lactamase producing Enterobacteriaceae (ESBL-PE) among hospitalized patients at Sylvanus Olympio teaching hospital, Lomé, Togo in 2019. African J. Clin. Exp. Microbiol. 23, 40–48. doi: 10.4314/ajcem.v23i1.6
Hyatt, D., Chen, G.-L., LoCascio, P. F., Land, M. L., Larimer, F. W., and Hauser, L. J. (2010). Prodigal: prokaryotic gene recognition and translation initiation site identification. BMC Bioinf. 11:119. doi: 10.1016/B978-0-12-407863-5.00023
Iskandar, K., Molinier, L., Hallit, S., Sartelli, M., Hardcastle, T. C., Haque, M., et al. (2021). Surveillance of antimicrobial resistance in low- and middle-income countries: a scattered picture. Antimicrob. Resist. Infect. Control 10:63. doi: 10.1186/s13756-021-00931-w
Islam, S., Selvarangan, R., Kanwar, N., McHenry, R., Chappell, J. D., Halasa, N., et al. (2017). Intestinal carriage of third-generation cephalosporin-resistant and extended-Spectrum β-lactamase-producing Enterobacteriaceae in healthy US children. J. Pediatr. Infect Dis. Soc. 176, 139–148. doi: 10.1093/jpids/pix045
Jolley, K. A., Bray, J. E., and Maiden, M. C. J. (2018). Open-access bacterial population genomics: BIGSdb software, the PubMLST.org website and their applications. Wellcome Open Res. 3:124. doi: 10.12688/wellcomeopenres.14826.1
Jünemann, S., Sedlazeck, F. J., Prior, K., Albersmeier, A., John, U., Kalinowski, J., et al. (2013). Updating benchtop sequencing performance comparison. Nat. Biotechnol. 31, 294–296. doi: 10.1038/nbt.2522
Kibwana, U. O., Majigo, M., Kamori, D., and Manyahi, J. (2020). High fecal carriage of extended Beta lactamase producing Enterobacteriaceae among adult patients admitted in referral hospitals in Dar Es Salaam, Tanzania. BMC Infect Dis. 20, 1–7. doi: 10.1186/s12879-020-05272-4
Kurz, M. S. E., Bayingana, C., Ndoli, J. M., Sendegeya, A., Durst, A., Pfüller, R., et al. (2017). Intense pre-admission carriage and further acquisition of ESBL-producing Enterobacteriaceae among patients and their caregivers in a tertiary hospital in Rwanda. Trop Med. Int. Heal. 22, 210–220. doi: 10.1111/tmi.12824
Lam, M. M. C., Wick, R. R., Watts, S. C., Cerdeira, L. T., Wyres, K. L., and Holt, K. E. (2021). A genomic surveillance framework and genotyping tool for Klebsiella pneumoniae and its related species complex. Nat. Commun. 12:4188. doi: 10.1038/s41467-021-24448-3
Li, H. (2012). A statistical framework for SNP calling, mutation discovery, association mapping and population genetical parameter estimation from sequencing data. Bioinformatics 27, 2987–2993. doi: 10.1093/bioinformatics/btr509
Mai, H. T. T., and Espinoza, J. L. (2023). The impact of COVID-19 pandemic on ESBL-producing Enterobacterales infections: a scoping review. Antibiotics. 12:1064. doi: 10.3390/antibiotics12061064
Maldonado-Barragán, A., Mshana, S. E., Keenan, K., Ke, X., Gillespie, S. H., Stelling, J., et al. (2024). Predominance of multidrug-resistant bacteria causing urinary tract infections among symptomatic patients in East Africa: a call for action. JAC-Antimicrobial Resist. 6:019. doi: 10.1093/jacamr/dlae019
Manyahi, J., Moyo, S. J., Tellevik, M. G., Langeland, N., and Blomberg, B. (2020). High prevalence of fecal carriage of extended Spectrum β-lactamase-producing Enterobacteriaceae among newly HIV-diagnosed adults in a community setting in Tanzania. Microb. Drug Resist. 26, 1540–1545. doi: 10.1089/mdr.2020.0066
Mlugu, E. M., Mohamedi, J. A., Sangeda, R. Z., and Mwambete, K. D. (2023). Prevalence of urinary tract infection and antimicrobial resistance patterns of uropathogens with biofilm-forming capacity among outpatients in Morogoro, Tanzania: a cross-sectional study. BMC Infect. Dis. 23:8641. doi: 10.1186/s12879-023-08641-x
Moremi, N., Claus, H., Rutta, L., Frosch, M., Vogel, U., and Mshana, S. E. (2018). High carriage rate of extended-spectrum beta-lactamase-producing Enterobacteriaceae among patients admitted for surgery in Tanzanian hospitals with a low rate of endogenous surgical site infections. J. Hosp. Infect. 100, 47–53. doi: 10.1016/j.jhin.2018.05.017
Moremi, N., Claus, H., Vogel, U., and Mshana, S. E. (2017). Faecal carriage of CTX-M extended-spectrum beta-lactamase-producing Enterobacteriaceae among street children dwelling in Mwanza city, Tanzania. PLoS ONE 12, 1–11. doi: 10.1371/journal.pone.0184592
Moremi, N., Silago, V., Mselewa, E. G., Chifwaguzi, A. P., Mirambo, M. M., Mushi, M. F., et al. (2021). Extended-spectrum β-lactamase Bla CTX-M-1 group in gram-negative bacteria colonizing patients admitted at Mazimbu hospital and Morogoro regional Hospital in Morogoro, Tanzania. BMC Res. Notes. 14, 1–7. doi: 10.1186/s13104-021-05495-x
Mwambete, K. D., and Msigwa, M. (2017). Prevalence of asymptomatic urinary tract infections among pregnant women residing in a rural and an urban area in Tanzania. East Cent African J. Pharm. Sci. 20, 27–32. doi: 10.12688/wellcomeopenres.14826.1
Mwandigha, A. M., Kamori, D., Kibwana, U. O., Masoud, S., Manyahi, J., and Majigo, M. (2020). Fecal carriage and factors associated with extended-spectrum β-lactamase-producing Enterobacteriaceae among pregnant women at the tertiary referral hospital, Tanzania. Trop. Med. Health 48:271. doi: 10.1186/s41182-020-00271-2
Mwanginde, L. W., Majigo, M., Kajeguka, D. C., and Joachim, A. (2021). High carriage rate of extended-Spectrum β-lactamase-producing Escherichia coli and Klebsiella species among poultry meat vendors in Dar Es Salaam: the urgent need for intervention to prevent the spread of multidrug-resistant pathogens. Int. J. Microbiol. 2021, 1–6. doi: 10.1155/2021/6653993
Ngowi, B. N., Sunguya, B., Herman, A., Chacha, A., Maro, E., Rugarabamu, L. F., et al. (2021). Prevalence of multidrug-resistant UTI among people living with HIV in northern Tanzania. Infect Drug Resist. 14, 1623–1633. doi: 10.2147/IDR.S299776
Nicolle, L. E., Gupta, K., Bradley, S. F., Colgan, R., DeMuri, G. P., Drekonja, D., et al. (2019). Clinical practice guideline for the management of asymptomatic bacteriuria: 2019 update by the Infectious Diseases Society of America. Clin. Infect. Dis. 68, E83–e110. doi: 10.1093/cid/ciy1121
Onduru, O. G., Mkakosya, R. S., Aboud, S., and Rumisha, S. F. (2021). Genetic determinants of resistance among ESBL-producing Enterobacteriaceae in community and hospital settings in east, central, and southern Africa: a systematic review and Meta-analysis of prevalence. Can. J. Infect. Dis. Med. Microbiol. 2021, 5153237–5153239. doi: 10.1155/2021/5153237
Ouchar Mahamat, O., Tidjani, A., Lounnas, M., Hide, M., Benavides, J., Somasse, C., et al. (2019). Fecal carriage of extended-spectrum β-lactamase-producing Enterobacteriaceae in hospital and community settings in Chad. Antimicrob. Resist. Infect. Control 8, 1–7. doi: 10.1186/s13756-019-0626-z
Pilmis, B., Cattoir, V., Lecointe, D., Limelette, A., Grall, I., Mizrahi, A., et al. (2018). Carriage of ESBL-producing Enterobacteriaceae in French hospitals: the PORTABLSE study. J. Hosp. Infect. 98, 247–252. doi: 10.1016/j.jhin.2017.11.022
R Core Team (2022). R: A language and environment for statistical computing. R foundation for statistical computing. Vienna, Austria. Vienna, Austria: R Foundation for Statistical Computing.
Roer, L., Tchesnokova, V., Allesøe, R., Muradova, M., Chattopadhyay, S., Ahrenfeldt, J., et al. (2017). Development of a web tool for Escherichia coli subtyping based on fimH alleles. Clin. Microbiol. 55, 2538–2543. doi: 10.1128/JCM.00737-17
Silago, V., Moremi, N., Mtebe, M., Komba, E., Masoud, S., Mgaya, F. X., et al. (2022). Multidrug-resistant Uropathogens causing community-acquired urinary tract infections among patients attending health facilities in Mwanza and Dar Es Salaam, Tanzania. Antibiotics 11, 1–13. doi: 10.3390/antibiotics11121718
Slown, S., Walas, N., Amato, H. K., Lloyd, T., Varghese, V., Bender, M., et al. (2022). Clonal lineages and virulence factors of Carbapenem Resistant E. coli in Alameda County, California, 2017–2019. Antibiotics 11, 2017–2019. doi: 10.3390/antibiotics11121794
Sonda, T., Kumburu, H., van Zwetselaar, M., Alifrangis, M., Lund, O., Kibiki, G., et al. (2016). Meta-analysis of proportion estimates of extended-Spectrum-Beta-lactamase-producing Enterobacteriaceae in East Africa hospitals. Antimicrob. Resist. Infect. Control 5:18. doi: 10.1186/s13756-016-0117-4
Sonda, T., Kumburu, H., van Zwetselaar, M., Alifrangis, M., Mmbaga, B. T., Aarestrup, F. M., et al. (2018). Whole genome sequencing reveals high clonal diversity of Escherichia coli isolated from patients in a tertiary care hospital in Moshi, Tanzania. Antimicrob. Resist Infect. Control. 7, 1–12. doi: 10.1186/s13756-018-0361-x
Storberg, V. (2014). ESBL-producing Enterobacteriaceae in Africa – a non-systematic literature review of research published 2008–2012. Infect Ecol. Epidemiol. 4:20342. doi: 10.3402/iee.v4.20342
Tellevik, M. G., Blomberg, B., Kommedal, Ø., Maselle, S. Y., Langeland, N., and Moyo, S. J. (2016). High prevalence of faecal carriage of ESBL-producing Enterobacteriaceae among children in Dar Es Salaam, Tanzania. PLoS ONE 11, 1–13. doi: 10.1371/journal.pone.0168024
Torres, N. F., Chibi, B., Kuupiel, D., Solomon, V. P., Mashamba-Thompson, T. P., and Middleton, L. E. (2021). The use of non-prescribed antibiotics; prevalence estimates in low-and-middle-income countries. A systematic review and meta-analysis. Arch. Public Heal. 79:2. doi: 10.1186/s13690-020-00517-9
Wingett, S. W., and Andrews, S. (2018). FastQ screen: a tool for multi-genome mapping and quality control. F1000Research. 7:1338. doi: 10.12688/f1000research.15931.1
Woerther, P. L., Burdet, C., Chachaty, E., and Andremont, A. (2013). Trends in human fecal carriage of extended-spectrum β-lactamases in the community: toward the globalization of CTX-M. Clin. Microbiol. Rev. 26, 744–758. doi: 10.1128/CMR.00023-13
Zamudio, R., Boerlin, P., Mulvey, M. R., Haenni, M., Beyrouthy, R., Madec, J.-Y., et al. (2024). Global transmission of extended-spectrum cephalosporin resistance in Escherichia coli driven by epidemic plasmids. EBioMedicine 103:105097. doi: 10.1016/j.ebiom.2024.105097
Keywords: fecal carriage, extended-spectrum beta-lactamase, enterobacterales, urinary tract infections, rural Tanzania
Citation: Macha ME, Qi W, Seiffert SN, Bösch A, Kohler P, Urassa HM, Haller S, West E, Rohacek MW and Babouee Flury B (2025) High prevalence of fecal carriage of extended-spectrum beta-lactamase producing Enterobacterales among patients with urinary tract infections in rural Tanzania. Front. Microbiol. 15:1517182. doi: 10.3389/fmicb.2024.1517182
Edited by:
Miklos Fuzi, Independent Researcher, Seattle, WA, United StatesReviewed by:
Tungki Pratama Umar, University College London, United KingdomKevin Bouiller, Centre Hospitalier Universitaire de Besançon, France
Copyright © 2025 Macha, Qi, Seiffert, Bösch, Kohler, Urassa, Haller, West, Rohacek and Babouee Flury. This is an open-access article distributed under the terms of the Creative Commons Attribution License (CC BY). The use, distribution or reproduction in other forums is permitted, provided the original author(s) and the copyright owner(s) are credited and that the original publication in this journal is cited, in accordance with accepted academic practice. No use, distribution or reproduction is permitted which does not comply with these terms.
*Correspondence: Magreth Erick Macha, bWFncmV0aGVyaWNrQHlhaG9vLmNvbQ==