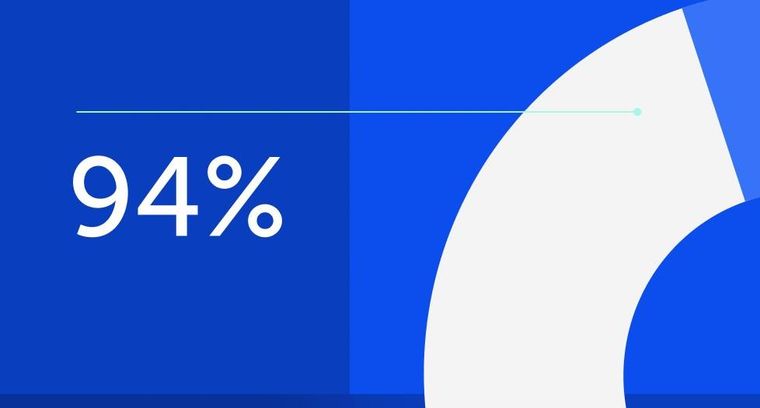
94% of researchers rate our articles as excellent or good
Learn more about the work of our research integrity team to safeguard the quality of each article we publish.
Find out more
SYSTEMATIC REVIEW article
Front. Microbiol., 08 January 2025
Sec. Infectious Agents and Disease
Volume 15 - 2024 | https://doi.org/10.3389/fmicb.2024.1514738
This article is part of the Research TopicEmerging Pathogens and Contaminants in the Environment: Human Health Risks, Exposure Pathways and Epidemiological OutcomesView all 4 articles
Background: Leptospirosis is an acute zoonotic disease caused by pathogenic Leptospira, primarily transmitted to humans through contact with water or soil contaminated by the bacteria. It is globally distributed, with heightened prevalence in tropical regions. While prior studies have examined the pathophysiology, epidemiology, and risk factors of leptospirosis, few have explored trends and emerging topics in the field. This study applies bibliometric analysis to generate a visual knowledge map, identifying research hotspots and forecasting future trends in leptospirosis investigations.
Methods: Data were extracted from the Web of Science Core Collection (WOSCC), encompassing all publications up to May 1, 2024. CiteSpace and VOSViewer software were used to analyze annual publication trends, as well as contributions from countries, institutions, journals, authors, references, and keywords, thereby pinpointing current research priorities and potential future directions.
Results: A total of 5,244 articles were included, sourced from 4,716 institutions, 955 journals, and 156 countries or regions. The United States led with 1,315 publications and had the most significant influence in the field. “PLOS Neglected Tropical Diseases” published the highest number of articles (166), while “Infection and Immunity” garnered the most citations (6,591). Prominent research areas included restriction endonucleases, monoclonal antibodies, outer membrane proteins, water environments, detection methods, and antimicrobial agents. Research focus has shifted from early genomic and antigenic studies to investigations into outer membrane protein functions and environmental persistence, culminating in recent advances in molecular mechanisms and diagnostic technology development.
Conclusion: This bibliometric analysis provides a comprehensive snapshot of leptospirosis research, emphasizing collaborations and impact among authors, countries, institutions, and journals. It offers valuable insights into ongoing trends and serves as a reference for future collaboration and research opportunities in the field.
Pathogenic Leptospira are primarily transmitted via rodents, pigs, cattle, and sheep, with infection primarily originating from urine excreted by these carrier animals into water sources (Dara, 2019). Humans become infected upon contact with contaminated water, particularly when the bacteria penetrate through the skin or mucous membranes, especially via broken skin. Once inside the body, Leptospira disseminates through the bloodstream, impacting various organs and leading to multi-organ dysfunction (Mathieu, 2017). Clinical manifestations of leptospirosis vary, ranging from mild influenza-like symptoms to severe impairment of vital organs such as the lungs, liver, and kidneys. In severe cases, patients may experience hypotensive shock, pulmonary hemorrhage, acute liver and kidney failure, and multi-organ dysfunction syndrome, with mortality rates reaching up to 50% (Ajay et al., 2003; Eddy et al., 2005).
Leptospirosis prevalence is influenced by a complex interaction of pathogen, host, and environmental factors, compounded by geographical, natural, and socio-economic conditions that heighten the risk of outbreaks and human transmission. Despite its life-threatening nature, the pathogenesis of leptospirosis remains incompletely understood, and global incidence and mortality estimates remain unclear (Federico et al., 2015). Furthermore, limited awareness among clinicians and public health professionals has hindered effective prevention and control measures.
Bibliometric analysis serves as a valuable tool for assessing the status of research fields, identifying scientific achievements, and pinpointing emerging hotspots (Wei et al., 2024). This approach has proven beneficial in various biomedical fields, including inflammation, immunity, and cancer (Peixiang et al., 2024; Qi et al., 2024; Xiang'an et al., 2024; Ziye et al., 2024). Although leptospirosis has gained attention in recent years, specific research trends and emerging focus areas remain unclear. To address this gap, a bibliometric analysis of leptospirosis-related literature from the WOSCC, spanning the last 30 years, was conducted. Specialized bibliometric software was used to assess contributions from countries, institutions, journals, authors, references, and keywords, identifying the most influential entities. Additionally, outbreak-related references were retrieved, and key thematic clusters in leptospirosis research were identified. The goal was to construct a visual model to evaluate current research trends, track the evolution of the research landscape, and predict future directions. This study provides a comprehensive visual knowledge map and valuable insights into leptospirosis, offering a critical reference for researchers and guiding future research initiatives.
The WOSCC was selected as the data source for this study due to its global influence, authority, and comprehensive nature, providing extensive citation data across multiple disciplines. This feature makes WOSCC particularly advantageous for multidisciplinary and international bibliometric analyses (Christian et al., 2024). Our data retrieval strategy used the following search terms: (TS = (Leptospirosis)) OR (TS = (Leptospira)), with a search period up to May 1, 2024. This search yielded a total of 6,181 relevant publications. Two authors independently screened the literature according to predefined inclusion and exclusion criteria, focusing on articles and reviews published in English. Initial screening involved examining titles and abstracts, which led to the exclusion of 523 documents, including conference papers, abstracts, letters, and other non-relevant document types. Additionally, 414 non-English publications were excluded. The final dataset consisted of 5,244 articles (Figure 1). The data was then exported in both full-record plain text and tab-delimited file formats, containing metadata such as titles, authors, abstracts, keywords, nationalities, research institutions, and citation information, preparing it for further analysis.
For the bibliometric analysis, we used VOSviewer 1.6.19 (Leiden University, Netherlands) to explore co-cited articles, keywords, countries/regions, institutions, journals, authors, and references. CiteSpace 6.1.R6 (Drexel University, Pennsylvania, USA) was employed for cluster analysis of co-occurring keywords and outbreak term analysis. The main parameters of our analysis included countries, institutions, journals, authors, references, and keywords. To visualize the trends in publication and citation numbers over time, we utilized Microsoft Office Excel 2013 to create line graphs.
The dataset, exported in RefWorks format, was initially named “download_.” We performed data de-duplication and conversion using CiteSpace 6.3.R1. Following data organization, the information was imported into bibliometric software for analysis. For institutional collaboration, network analysis, and keyword co-occurrence analysis, we employed VOSviewer 1.6.18. Further analysis using CiteSpace 6.3.R1 included author collaboration, network analysis, keyword clustering, and timeline analysis to provide a comprehensive overview of the current research landscape in leptospirosis. CiteSpace was configured with a time span from 1967 to 2024, setting “Years Per Slice” to one year. Additionally, synonymous keywords and institutions were merged to ensure consistency in the analysis. In VOSviewer, the “Co-occurrence” option was selected from the “Type of analysis” menu for in-depth keyword analysis.
Our analysis covered 5,244 papers published between 1967 and 2024, comprising 4,922 articles and 322 reviews. As illustrated in Figure 2, the annual number of publications and citations related to leptospirosis shows a consistent upward trend. Research on leptospirosis began in 1967, marked by E. Shenberg’s development of a protein-free medium for culturing pathogenic Leptospira (Shenberg, 1967). For the next 25 years, the field experienced slow growth, with an average of about 20 articles published annually. This rate accelerated significantly in 1991, peaking in 2022 with 342 articles. Predictive algorithms indicate a continuing increase in research output in the coming years, suggesting that leptospirosis is gaining attention as a research hotspot globally.
Figure 2. Number of Leptospira related articles published in Web of Science from 1967 to 2024. (A) Cumulative number of articles published; (B) annual number of articles published and article publication trends.
The analysis revealed that 4,716 institutions from 156 countries/regions have contributed literature on Leptospira. Visualizing countries with more than 20 published articles, we found that 53 countries meet this criterion (Figures 3A,B). Table 1 lists the top 10 countries and institutions based on publication volume. The United States leads with 1,315 publications and 47,695 citations, followed by Brazil (890 publications, 18,573 citations) and France (411 publications, 14,860 citations). India ranks fourth with 390 publications and 4,487 citations, while China is fifth with 311 publications and 6,137 citations. Together, these five countries contribute approximately 58.5% of the total publications. The strength and tone of network connections illustrate the degree of research collaboration between countries or regions. Nodes closer to red indicate stronger cooperation. The results indicate that the United States has the most robust international collaborations (total strength: 47,377), followed by Brazil (30,066) and France (23,174), forming a broad cooperative network. Although India and China are among the top five in terms of publication numbers, their influence in terms of total citations and Total Link Strength (TLS) rankings is comparatively lower, this indicates that India and China have limited influence in high-quality literature and hot research, and need to further enhance their academic value.
Figure 3. Visual maps created by VOSviewer. (A,B): countries/regions; (C,D): institutions; (E,F): authors; (G,H): co-cited authors; (I,J): journals; (K,L): co-cited journals. (A,C,E,G,I,K) Different color groups in the figure represent the cooperative relationship between various indicators. (B,D,F,H,J,L) Different color groups in the figure represent the time points at which various indicators are discovered.
A total of 4,716 institutions are engaged in leptospirosis research. According to VOSViewer results, 88 institutions have published more than 20 articles (Figures 3C,D; Table 2). The University of São Paulo leads with 256 papers, followed by the Pasteur Institute (230 papers) and Cornell University (137 papers). In terms of total citations, San Bortolo Hospital ranks first (2,073 citations), followed by the University of Pittsburgh (1,978 citations) and the University of Michigan (1,813 citations). The top ten institutions are primarily located in the United States (40%), Australia (20%), and Brazil (20%), highlighting their extensive research interest and strong influence in this field.
Our analysis identified contributions from 5,918 authors in leptospirosis research. Key scholars driving research trends were pinpointed using VOSViewer (Figures 3E–H; Table 3). Mathieu Picardeau from France led in both publication volume (117 papers, 4.61%) and citations, followed by Walter Lilenbaum (89 papers, 3.17%). The highest total link strength (TLS) was attributed to Walter Lilenbaum (5,969), followed by Albert I. Ko (4,074) and Ben. Adler (3,995). In terms of average article citations (AAC), Ben. Adler (71.78), Albert I. Ko (63.60), and David A. Haake (61.71) ranked highest. Table 3 presents a timeline of prominent authors, with larger circles denoting significant contributions at various time points. Albert I. Ko, David A. Haake, and Ben. Adler are identified as key pioneers, both as citing and co-citing authors, suggesting their teams as key potential collaborators in this study (Supplementary Table S1).
Since 1993, leptospirosis research has been published across 955 academic journals. Using VOSViewer, co-occurrence relationships between journals (Figures 3I,J) and co-citation relationships were visualized (Figures 3K,L). Table 4 and Supplementary Table S2 list the top 10 journals and co-cited journals. “PLOS Neglected Tropical Diseases” ranks first with 166 articles, followed by “Journal of Wildlife Diseases” (126 articles) and “PLOS One” (123 articles). Among the top ten journals, three belong to the Q2 quartile, with “Journal of Clinical Microbiology” having the highest impact factor (IF: 6.10), followed by “PLOS Neglected Tropical Diseases” (IF: 3.40), highlighting their academic significance in leptospirosis research. Notably, most of these journals are based in the United States, reflecting the country’s dominance in the field, consistent with earlier findings on country/region and institutional contributions.
Subsequent CiteSpace analysis included dual-map overlays (Figure 4A), linking cited journals on the left with citing journals on the right. This provided a visual representation of citation relationships, topic distributions, and interdisciplinary movement patterns. Ellipses on the map represent the number of publications and author-to-publication ratios. Citation trajectories, depicted by thicker Z-scores, indicate stronger citation links. Four main citation trajectories were identified: three yellow and one green. The first yellow trajectory, focused on veterinary and animal science, was influenced by veterinary, animal, and parasitology research (Z = 2.54, f = 3,567). The second yellow trajectory, driven by molecular biology and genetics, had a Z-score of 4.71 and 6,275 publications. The third yellow trajectory, which intersected molecular biology and immunology, had a Z-score of 6.99 with 9,133 publications. The green trajectory, representing medicine and clinical fields, was influenced by molecular biology and genetics (Z = 4.13, f = 5,549).
Figure 4. (A) Dual-map overlay of journals. The left nodes represent the included literature; the right represent the references in the literature. The labels represent the discipline. The link represents the cited path. (B) The timeline view of reference clusters by using CiteSpace software.
Using VOSviewer, 119 keywords appearing more than 50 times were identified, resulting in four distinct clusters (Figures 5A,B; Table 5). Core terms include “leptospirosis, ““Leptospira, ““diagnosis,” and “sepsis.” Cluster 1 (Red) consists of 45 terms, such as “activation,” “antigen,” “bacteria,” “binding,” “Borrelia burgdorferi,” “cells,” “Escherichia coli,” and “expression,” focusing primarily on the etiology and pathogenic mechanisms of Leptospira. Cluster 2 (Green) includes 42 terms related to the transmission routes of Leptospira in animals, such as “abortion,” “animals,” “antibodies,” “bovine,” “bovine leptospirosis,” and “Brazil.” Cluster 3 (Blue) features 19 terms like “assist,” “diagnosis,” “differentiation,” “DNA,” and “epidemic,” highlighting the role of DNA technology in diagnosing Leptospira. Cluster 4 (Yellow) comprises 13 terms, including “canine leptospirosis,” “disease,” and “ELISA,” focusing on various detection methods.
Figure 5. (A,B) Visual maps of keywords generated by VOSviewer. (A) Different color groups in the figure represent the relationship between keywords. (B) Different color groups in the graph represent the time points at which each keyword appears. (C) The top 25 keywords with the strong citation bursts. (D,E) Visual maps of highly cited References and co-cited References generated by VOSviewer (Different color groups in the figure represent the relationship between highly cited References and co-cited References). (C) The top 25 References with the strong citation bursts.
Keywords with sudden citation bursts indicate emerging areas of research. The top 25 keywords exhibiting the strongest citation bursts are shown in Figure 5C, with the red line marking the duration of the burst. The research hotspots can be categorized into three stages: the early stage (before 2004) included keywords like “restriction endonuclease analysis,” “monoclonal antibody,” “DNA,” “Hardjo,” “Interrogans serovar Hardjo,” “Escherichia coli,” “linked immunosorbent assay,” “Lyme disease,” “Borrelia burgdorferi treponema,” and “pallidum nucleotide sequence.” The mid-term period (2005–2017) highlighted keywords such as “outer membrane proteins,” “insights,” and “water.” The most recent stage (2018-present) saw citation bursts for “spp.,” “state,” and “One Health.” The analysis of keyword bursts reveals evolving research trends and reflects the dynamic nature of the field, helping researchers quickly identify emerging research directions. Figure 4B demonstrates that from 2014 to 2024, research in the seven clusters continued to intensify, with the following key keywords showing sustained attention: "outer membrane protein," "Toxoplasma gondii," "seroprevalence," "scrub typhus," "real-time PCR," "gene," "lipopolysaccharide," and "equine recurrent uveitis".
The top 10 highly cited studies on Leptospira are presented in Figures 5D,E. Noteworthy recent papers from the co-cited literature salience chart include Paul N. Levett’s seminal work, “Leptospirosis,” published in Clinical Microbiology Reviews in 2001, which has accumulated 3,988 citations and a TLS of 60. The second most cited study is Ajay R. Bharti’s “Leptospirosis: a zoonotic disease of global importance,” published in Lancet Infectious Diseases in 2003, with 2,828 citations and a TLS of 19. Ben Adler’s article, Leptospira and leptospirosis, ranks third, published in Veterinary Microbiology in 2010, with a TLS of 100 and 1983 citations.
CiteSpace analysis of citation bursts, which identifies articles with the strongest citation bursts, provides insight into future research directions. Figure 5F highlights the top 25 references with the most significant citation bursts, with the burst duration marked in red. The study Global Morbidity and Mortality of Leptospirosis: A Systematic Review has the highest burst intensity (intensity = 126.73), shedding light on the global incidence and mortality of leptospirosis. The review emphasizes leptospirosis as a leading zoonotic disease, with mortality rates comparable to or exceeding those of hemorrhagic fever, particularly in regions with limited research and statistical resources. Ben Adler’s 2010 paper, Leptospira and leptospirosis, ranks second in burst intensity (intensity = 110.84) and provides a comprehensive analysis of Leptospira’s biology and pathogenesis, forecasting significant progress through the interaction of the pathogen with its mammalian hosts and the environment. Additionally, two co-cited references emerged during the recent outbreak phase. The first is Leptospirosis: A Neglected Tropical Zoonotic Infection of Public Health Importance – An Updated Review, published in European Journal of Clinical Microbiology & Infectious Diseases by Krishnan Baby Karpagam et al. This review offers a detailed introduction to leptospirosis, covering its occurrence, transmission, clinical manifestations, diagnosis, treatment, preventive measures, and future prospects. The second is Kenneth Boey’s 2019 study, Leptospira Infection in Rats: A Literature Review of Global Pandemic and Distribution, published in PLOS Neglected Tropical Diseases. This paper summarizes global data on Leptospira infection in rats, comparing prevalence rates, geographic distribution, detection methods, and serogroup diversity, providing essential insights for global Leptospira research. These authoritative reviews are critical for guiding further research and addressing key gaps in understanding the disease’s global impact.
To accurately predict research hotspots and identify future directions in Leptospira research, a comprehensive bibliometric analysis of global literature from 1967 to 2024 was performed using CiteSpace and VOSViewer software. The United States, Brazil, and France have been key contributors to Leptospira research, with the United States and Brazil leading in publications. Numerous institutions in these countries have conducted extensive studies, benefiting from a strong commitment to Leptospira research. The publications from scholars in these nations, along with those from France, are highly cited, reflecting close international collaboration and high academic influence in the field. In terms of research institutions, TLS values with medical institutions in Brazil, the United States, and France are notably high, indicating significant exchanges and cooperation in the field. This cross-national and institutional synergy is pivotal for advancing research. Moving forward, China and other countries should foster the establishment of international and multi-regional research centers, ensuring stable, multi-center collaborative research and striving to produce high-quality, highly cited publications to enhance academic impact. Regarding author contributions, Albert I. Ko, David A. Haake, and Ben. Adler have emerged as prominent experts, consistently ranking high in citation volume and TLS for both citing and co-referencing literature. Their work has been instrumental in advancing Leptospira research, and their research teams represent valuable potential collaborators for future projects.
Among the top 10 journals, PLOS Neglected Tropical Diseases focuses on infectious diseases, critical care medicine, and related fields (José María et al., 2021). As the journal with the highest number of published articles, it is regarded as a leading international platform for tropical medicine research. Published by the Public Library of Science, it covers pathology, epidemiology, prevention, treatment, control of neglected tropical diseases, and associated public policies. The journal emphasizes human disease research in the fields of translational medicine and epidemiology, regularly publishing original research articles, brief communications, reviews, laboratory studies, and cutting-edge scientific developments in tropical medicine. Other journals in the top 10 also exert considerable influence on Leptospira research. In conclusion, these top journals will remain essential for accessing pivotal and groundbreaking findings in the field of Leptospira. By analyzing these key journals, researchers can identify the most suitable outlets for publishing their work on Leptospira and target high-impact journals for disseminating their significant results. The overlay map of journals and disciplines in this study reveals that leptospirosis research intersects with basic sciences, clinical practice, nursing, and social sciences, underscoring the importance of interdisciplinary and collaborative research in this field.
As a significant zoonotic pathogen, Leptospira has garnered considerable attention in recent years. Our quantitative analysis of Leptospira-related literature revealed substantial shifts in research directions and emerging hotspots over time. By analyzing the top 10 keywords, temporal overlay visualizations, and emerging trends, three major phases in Leptospira research were identified. Key developments and trends were discussed in relation to burst keywords at different time points, as shown in Figure 5C.
Before 2004, Leptospira research was primarily focused on understanding its basic biological characteristics and immunological properties. Keywords such as “restriction endonuclease analysis,” “monoclonal antibody,” “DNA,” “hardjo,” “serovar., “Escherichia coli, “linked immune assay,” “Lyme disease,” Borrelia burgdorferi, Treponema pallidum, and “nucleotide sequence” capture the research priorities of this period. During this phase, restriction enzyme analysis emerged as a pivotal tool for studying Leptospira’s genomic structure and diversity. Notable studies from this era include William A. Ellis’s 1991 work, which utilized 20 types of restriction endonucleases to analyze the DNA of various Leptospira strains, demonstrating the utility of this method as a classification tool for identifying pig isolates of the southern Leptospira serogroup (Ellis et al., 1991). In 1994, M. L. Savio highlighted the role of polymerase chain reaction (PCR) combined with DNA amplification and restriction endonuclease analysis in detecting and identifying Leptospira serum samples (Savio et al., 1994). Furthermore, in 1996, Carole A. Bolin et al. employed DNA restriction endonuclease analysis to investigate restriction fragment length polymorphism, establishing a correlation between this polymorphism and the host animal origin (Bolin and Zuerner, 1996). This analytical approach significantly advanced epidemiological studies of leptospirosis, aiding in the understanding of genetic variation among Leptospira strains across regions and their transmission routes among hosts.
The development and application of monoclonal antibodies also played a pivotal role in the identification of Leptospira antigens, providing foundational tools for immune diagnostics and vaccine development. In 1983, Ben. Adler et al. first identified species-and genus-specific antigens of Leptospira using monoclonal antibodies and enzyme immunoassays (Adler and Faine, 1983b). Later that year, they utilized monoclonal antibodies to detect a Pomona serogroup-specific agglutination antigen in Leptospira (Adler and Faine, 1983a). Similarly, in 1987, Rance B. Lefebvre introduced a DNA probe for diagnosing and classifying the Hardjo bovis genotype, a North American bovine pathogen, which proved specific to this genotype and did not cross-react with the genomic DNA of other common Leptospira pathogens in North America (LeFebvre, 1987). Additional advances included the application of monoclonal antibodies in China in 1989 for isolating Leptospira serotypes, significantly enhancing diagnostic accuracy (Zhang et al., 1989). In 1991, John V. Hookey et al. used monoclonal antibodies and DNA restriction endonucleases to identify jaundice hemorrhagic strains of Leptospira from various reference laboratories (Hookey and Palmer, 1991). These studies collectively established monoclonal antibody usage as a key method for Leptospira identification, laying the groundwork for further research in immune diagnostics and vaccine development.
In 1993, M. L. Savio et al. employed recombinant probes and serovar-specific monoclonal antibodies to identify 31 Leptospira strains from cattle and pigs. This study marked a pioneering integration of immunological and genetic approaches, combining monoclonal antibody-based identification with genomic analysis to assess strain variability (Savio et al., 1993). The early 2000s saw further advancements in diagnostic techniques. In 2005, Junpen Suwimonteerabutr utilized a monoclonal antibody-based dot blot Enzyme-Linked ImmunoSorbent Assay (ELISA) to detect Leptospira infection in blood and urine samples. This method was compared with dark-field microscopy, microbial culture, and PCR, highlighting its effectiveness in detecting Leptospira in bovine urine samples (Junpen et al., 2005).
Research during this period also explored the similarities between Leptospira and other spirochete pathogens, such as Borrelia burgdorferi (B. burgdorferi) and Treponema pallidum, suggesting potential cross-immune responses and shared evolutionary pathways. For example, in 1994, James L. Coleman identified escape variants of B. burgdorferi and used monoclonal antibody selection to express a truncated form of OspB. The resulting mutations provided insight into immune evasion mechanisms, with implications for Leptospira diagnosis and vaccine development (Coleman et al., 1994). The investigation of immune escape mechanisms in Leptospira continued, focusing on live pathogenic spirochete populations. For instance, Antonella et al. (2004) found that live Leptospira and T. pallidum stimulated Kupffer cells in vitro, triggering cellular responses and varying levels of tumor necrosis factor-alpha release (Antonella et al., 2004). Researchers developing moderately infectious, non-pathogenic live vaccines for Leptospira also discovered that complement-mediated selection in tissue co-cultures provided an innovative method for isolating pathogenic spirochete populations from attenuated strains. In 2005, Ece Sen demonstrated that complement resistance and pathogenic phenotype could be used to isolate B. burgdorferi in co-culture systems supported by tissue feeder layers from LEW/N rat tibial joints. This work confirmed that animal passage of complement escape variants is essential for isolating helicoids from highly passaged, non-arthrogenic, and attenuated cultures (Ece and Ronald, 2003). These studies provided key insights into the immune escape mechanisms of Leptospira, laying the foundation for subsequent research on pathogenesis and immune response. In summary, this period represents a shift from basic microbiological studies to more applied research focused on the prevention and treatment of Leptospira infections. By exploring the function of outer membrane proteins, researchers not only revealed the molecular mechanisms underlying Leptospira pathogenicity but also laid the groundwork for the development of novel prevention and therapeutic strategies.
From 2005 to 2017, research on Leptospira increasingly focused on the functional roles of outer membrane proteins and the organism’s environmental survival strategies. This shift in focus is reflected in keywords such as “outer membrane proteins,” “insights,” and “water.” During this period, outer membrane proteins were identified as key pathogenic determinants, influencing Leptospira’s interaction with host cells and its virulence. The adhesion and invasion of host mammalian cells in vitro—primarily observed in highly virulent strains—was found to be a key step in its pathogenicity. This adhesion, absent in cultured, attenuated, or saprophytic Leptospira strains, likely involves molecules secreted by the bacteria or present on their surface (Merien et al., 1997; Michele et al., 2002). Several surface proteins of Leptospira have been shown to bind to multiple extracellular matrix components in vitro (Stevenson et al., 2007; Merien et al., 2000). Cryo-electron microscopy revealed that highly virulent strains exhibit a significantly reduced number of protein particles on their outer membrane, with distinct protein and lipopolysaccharide (LPS) profiles compared to attenuated strains (David et al., 2008).
As with other spirochetes, the Leptospira genome contains a high number of lipoprotein genes. Genomic analysis of L. interrogans revealed approximately 145 lipoproteins, as well as several extracellular and outer membrane proteins (João et al., 2005; Wasna et al., 2008). Consistent with Leptospira’s ability to migrate through host tissues, its genome encodes a variety of hemolysins and proteases that likely contribute to this process. The L. interrogans genome contains nine genes encoding hemolysins, including the sphingomyelinase gene (Mathieu et al., 2008) and pore-forming protein gene (Seoung Hoon et al., 2001), absent in saprophytic L. biflexa. Additionally, L. interrogans possesses a microbial collagenase, implicated in host tissue degradation. However, very few proteins have been experimentally verified on the surface of hook bodies (Paul et al., 2004).
Current literature identifies 12 proteins as outer membrane components, including OmpL1 (Haake et al., 1993), LipL32 (Haake et al., 2000), LigB (James et al., 2003), and Loa22 (Paula et al., 2007). Loa22, exposed on the bacterial surface, is recognized by the serum of patients with leptospirosis (Marcia et al., 2005) and upregulated in acute infection models. While the binding of Loa22 to extracellular matrix components in vitro is relatively weak (Angela et al., 2006), LipL32, also known as Hap-1, a hemolysis-related protein (Lee et al., 2000), comprises 75% of the outer membrane proteome (Paul et al., 2002). The lipoproteins in pathogenic Leptospira are highly conserved (David et al., 2004), with homologs of LipL32 absent in L. biflexa. LipL32 has long been considered a potential virulence factor, with its expression in acute lethal infections higher than in vitro cultured Leptospira (Jarlath et al., 2006). Further advancing the understanding of Leptospira’s pathogenic mechanisms, high-molecular-weight proteins—LigA, LigB, and LigC—were identified as members of the bacterial immunoglobulin (BIg)-like protein superfamily (Nobuo and Haruo, 2004; Raghavan et al., 2002). These proteins, anchored on the outer membrane, contain 12 to 13 tandem repeat domains. Similar to LipL32, Lig proteins are found exclusively in pathogenic Leptospira. Recombinant Lig proteins bind to host extracellular matrix proteins, such as fibronectin, fibrinogen, collagen, and laminin (Choy et al., 2007; Lin and Chang, 2007). The repetitive domain of LigB also binds calcium, enhancing its adhesion to fibronectin (Lin et al., 2008). These proteins are upregulated under physiological osmotic pressure and are recognized by the serum of patients with leptospirosis (Julio et al., 2007; Srimanote et al., 2008). In addition to the aforementioned proteins, other outer membrane proteins, such as those from Leptospira serovar Shermani, have been shown to enhance extracellular matrix synthesis, suggesting a potential link between Leptospira-induced acute renal tubulointerstitial nephritis and renal tubulointerstitial fibrosis (Ya-Chung et al., 2006). James Matsunaga, in 2006, identified the novel surface-exposed lipoprotein LipL46, distinguishing it from the previously characterized P31 (LipL45). Through surface immunoprecipitation and whole-cell ELISA assays, his team demonstrated that LipL46 is displayed on the surface of Leptospira (James et al., 2006).
These proteins are pivotal in host cell adhesion and invasion, and they likely contribute to immune evasion. The extensive study of outer membrane proteins has revealed their potential as vaccine candidates, offering promising avenues for vaccine development. During this period, research also emphasized Leptospira’s remarkable persistence in aquatic environments, a survival capability influenced by factors such as temperature and pH (Emilie et al., 2020). Leptospirosis, widely distributed across the globe, exhibits a higher incidence in tropical regions compared to temperate ones. Outbreaks often correlate with floods and hurricanes, which wash Leptospira from urine-contaminated soil into water bodies (Smita Benjamin et al., 2010). Reports of leptospirosis outbreaks have been documented in Brazil, India, and other areas following heavy rainfall. Leptospira can be isolated from various water bodies, including ponds, rivers, lakes, puddles, dams, springs, ornamental fountains, sewage, farmland, and moist soil. Flood-induced rainwater runoff can create alkaline conditions that support the pathogen’s environmental survival. Leptospira can persist in freshwater and moist soil for extended periods, from weeks to years, particularly under slightly alkaline conditions (Andrew et al., 2018; Arnau et al., 2018).
While leptospirosis is predominantly a waterborne disease, the bacterial load in soil is often underestimated. Human infections typically occur through skin contact with contaminated water or soil, though transmission via ingestion, inhalation, animal bites, or human-to-human contact remains rare (Didier and Bernard, 2013; Krishna Kanchan and Usha, 2008). Risk factors for Leptospira infection include occupations such as agriculture, veterinary medicine, slaughterhouse work, fishing, and sewage treatment. Additionally, individuals traveling to endemic areas, participating in freshwater sports, or engaged in disaster relief are at increased risk (Desai et al., 2016; Tippawan et al., 2018). Urban areas with rodent infestations, inadequate sanitation, and poor drainage systems are also at higher risk for leptospirosis outbreaks (Federico et al., 2014; José et al., 2016; Norlan de Jesus et al., 2017). These findings not only enhance our understanding of Leptospira’s ecological behavior but also underscore the critical need for effective water pollution control to mitigate public health risks.
Since 2018, research on Leptospira has entered a new phase, driven largely by advancements in molecular biology. Current efforts are focused on elucidating molecular mechanisms and implementing precision medicine strategies. While further clarification of research trends is ongoing, emerging keywords highlight areas such as vaccine development, gene editing technologies, antimicrobial resistance, and novel diagnostic approaches. In vaccine development, studies have concentrated on outer membrane proteins of Leptospira, with initial findings indicating promising immune protection. DNA vaccines, a relatively recent advancement, have attracted significant attention due to their ability to sustain long-term expression of exogenous proteins in animal hosts, leading to prolonged antigen exposure. These vaccines offer several advantages, including cost-effective scalability, enhanced stability, and the capacity to encode multiple genes in a single construct (Marcelle Moura et al., 2017; Yingying et al., 2014). Furthermore, DNA vaccines can elicit both cellular and humoral immune responses, particularly when administered using prime-boost strategies. However, challenges persist, including relatively low immunogenicity in human clinical trials and potential tolerance development following prolonged antigen exposure (Garba and Abdul Rani, 2018).
To overcome these challenges, researchers are focusing on DNA vaccines targeting highly conserved proteins in pathogenic Leptospira, which are absent in saprophytic strains. One such protein, LipL32, is the most abundant in pathogenic strains and has been extensively studied as a potential antigen due to its strong immunogenicity in animal models (Guerreiro et al., 2001; Xiang-Yan et al., 2005). The first DNA-based Leptospira vaccine utilized the LipL32 gene, delivered via the mammalian expression vector pCDNA3.1. This vaccine conferred significant protection to mice against a lethal challenge with L. interrogans serovar Canicola, resulting in a 60% survival rate, compared to just 35% in the control group (Branger et al., 2005). Another DNA vaccine, using plasmid pTarget/LipL32, was evaluated alongside recombinant subunit vaccines and live recombinant BCG vaccines. All three vaccine platforms induced robust humoral immune responses, with antibodies capable of recognizing native proteins on the intact membrane of Leptospira (Fabiana Kömmling et al., 2007a).
Feng et al. further evaluated DNA vaccine efficacy using a prime-boost strategy that combined recombinant LipL32 with LipL41 and OmpL1 (LipL32-41-OmpL1). Mice immunized with these DNA constructs exhibited significantly higher levels of IL-2 and IFN-γ compared to those vaccinated with homologous proteins, indicating the enhanced immune responses facilitated by DNA vaccination. The prime-boost regimen also induced a more robust antibody response and a more pronounced cytokine profile than DNA vaccination alone (Feng et al., 2009). OmpL37, another surface-exposed protein in Leptospira, demonstrated a higher adhesion affinity for elastin tissue than other outer membrane proteins, suggesting its critical role in the infection process (Marija et al., 2010; Mariko et al., 2012). Studies investigating the protective immune response of an OmpL37 DNA vaccine using a prime-boost approach found no significant protection in groups receiving only the DNA vaccine, although notable IgG responses were observed in the prime-boost group (Thaís Larré et al., 2015).
The live vaccine strategy relies on attenuated self-replicating microorganisms with immune-stimulating properties, such as BCG (Fabiana Kömmling et al., 2007b) or adenovirus (Branger et al., 2001), to deliver exogenous antigens from pathogens. Seixas and colleagues employed various methods to screen for the immune-protective activity of LipL32, including a DNA vaccine in the pTarget vector, recombinant protein expression in E. coli using the pAE expression vector, and live recombinant BCG. These constructs were used to immunize mice, and the evaluation of humoral immune responses revealed that the rLipL32 antibody titer was the highest, while the LipL32 rBCG group showed a steady increase in antibodies (Fabiana Kömmling et al., 2007a). Although not directly evaluated, it was anticipated that rBCG would elicit a strong cellular immune response against heterologous antigens. Growth inhibition of Leptospira was observed in vitro in the presence of an anti-LipL32 monoclonal antibody, further highlighting LipL32’s immunogenicity. Seixas and colleagues also explored the capacity of rBCG to express LipL32 as an antigen against leptospirosis (Fabiana Kömmling et al., 2007b), revealing that immunization with various rBCG/LipL32 constructs promoted serum conversion against LipL32, with higher antibody titers than the control group. In a hamster model of leptospirosis, however, inconsistent data on the protective efficacy of rBCG/LipL32 immunization were observed. In subsequent work, the team developed a recombinant chimeric multi-epitope vaccine, r4R, consisting of four repeats of T and B cell binding epitopes from six identical proteins (OmpL1, LipL32, and LipL21). This vaccine was tested for its ability to induce protective immune responses in guinea pigs. Compared to the PBS control group, r4R immunization resulted in increased survival rates and reduced renal colonization following infection with pathogenic Leptospira (Xu'ai et al., 2016).
Gene-editing technologies, such as CRISPR/Cas9, have emerged as powerful tools for investigating Leptospira gene function and drug resistance mechanisms. Sirawit Jirawannaporn’s team developed an RPA CRISPR/Cas12a detection platform to identify the LipL32 gene in pathogenic Leptospira strains. This platform demonstrated impressive sensitivity (85.2%), specificity (100%), and accuracy (92.7%) in a study involving 110 patients across 15 hospitals in Thailand. In addition, a lateral flow detection test was developed to accurately distinguish known positive and negative clinical samples, highlighting the potential of RPA CRISPR/Cas12a technology in enhancing the speed and precision of spirochete diagnosis, particularly in resource-limited settings (Sirawit et al., 2022). Further research in 2023 confirmed the platform’s high specificity and accuracy, positioning it as a promising tool for early detection and effective treatment, especially in rural healthcare settings (Sirawit et al., 2023). These advancements in Leptospira research, particularly in vaccine development and diagnostic methods, reflect a comprehensive approach to combating this global public health challenge. Future efforts should continue to incorporate interdisciplinary methodologies to refine diagnostic accuracy and develop robust therapeutic strategies.
In terms of diagnosis, rapid detection methods for molecular markers—such as those based on the microscopic agglutination test (MAT), PCR, and next-generation sequencing (NGS)—have substantially improved diagnostic efficiency for Leptospira infections. MAT remains a standard diagnostic tool, offering high sensitivity during the early stages of infection. However, its sensitivity diminishes as antibody titers decrease over time. A positive diagnosis is typically confirmed when the titer value is ≥400, or the paired serum titer value is ≥100 (Jane et al., 2022). While MAT is considered the ‘gold standard, it has limitations, including lack of specificity for IgM or IgG antibodies, and the requirement for well-trained technicians to interpret results and maintain live Leptospira cultures in enriched EMJH medium (Krishna Kanchan and Usha, 2008).
PCR has become a critical molecular tool for Leptospira detection due to its high specificity and sensitivity, enabling the identification of even trace amounts of bacterial nucleic acid. C. Gravekamp et al. demonstrated that PCR could achieve 100% specificity under controlled conditions (Gravekamp et al., 1993). Kate Woods et al. later found that quantitative PCR (qPCR) performed upon patient admission is not only a reliable and rapid diagnostic method but also outperforms both MAT and culture techniques. This underscores its significance in clinical and epidemiological investigations of this neglected disease (Woods et al., 2017). Other PCR techniques, such as nested PCR and arbitrary primer PCR, are also employed for Leptospira detection (Senaka et al., 2015). However, PCR is not without its challenges, including the need for sophisticated laboratory setups, the risk of contamination, potential for false positives, and the requirement for skilled personnel and substantial financial investment. Real-time PCR (RT-PCR) has gained prominence due to its ability to deliver specific results quickly by monitoring DNA amplification rates. This method is capable of quantifying bacterial DNA load, providing valuable insights into infection severity. The LipL32 gene serves as the primary target for qPCR-based identification, known for its high specificity and sensitivity in the early stages of leptospirosis diagnosis (Daša et al., 2020). However, qPCR requires specialized, expensive equipment, making it impractical for low-resource or basic laboratory settings. To improve sensitivity and specificity across all stages of infection, various serological and molecular techniques have been developed. One such approach is immune capture polymerase chain reaction (IC-PCR), which combines ELISA with PCR. In 2018, Balassiano et al. introduced IC-PCR, focusing on serological rather than genotypic traits, thus offering a novel tool for early diagnosis and valuable epidemiological surveillance by characterizing infected sera or serum groups (Ilana Teruszkin et al., 2012).
Recently, metagenomic next-generation sequencing (mNGS) has gained attention for Leptospira detection, though it has primarily been documented in case reports. For example, Michael R. Wilson et al. reported the case of a 14-year-old boy with severe combined immunodeficiency, where second-generation sequencing of cerebrospinal fluid confirmed Leptospira infection, identifying Leptospira santarosai as the causative pathogen. This marked the first instance of Leptospira being detected via next-generation sequencing (Wilson et al., 2014). Another case involved a 75-year-old male diagnosed with pulmonary tuberculosis complicated by leptospirosis. Following delayed serological testing, mNGS of cerebrospinal fluid, urine, plasma, and sputum samples revealed both Mycobacterium tuberculosis complex and Leptospira, illustrating how mNGS can complement traditional diagnostic methods by providing comprehensive pathogen screening (Yunzhen et al., 2023). Ji et al. described the case of a 40-year-old pig farmer with severe pulmonary hemorrhagic leptospirosis and multiple organ failure, who was diagnosed with leptospirosis through mNGS, enabling timely antibiotic therapy adjustments. The patient recovered following intensive care unit treatment and extracorporeal membrane oxygenation support (Ji et al., 2023). As NGS technology continues to evolve, whole-genome sequencing has become increasingly integral to leptospirosis research. In 2014, Chou et al. completed the first whole-genome sequencing of Leptospira santarosai serovar Shermani, analyzing its transcriptional response and providing foundational insights into the pathogenic mechanisms of Leptospira. This work has helped inform the development of preventive and therapeutic strategies (Li-Fang et al., 2015). Similarly, Sérgio Jorge et al. employed next-generation sequencing to obtain the complete genome of a virulent Leptospira strain isolated from southern Brazil, enhancing understanding of the genetic characteristics of local isolates and aiding the identification of potential diagnostic and vaccine targets (Sérgio et al., 2017). With rapid advancements in molecular techniques, a variety of other methods have emerged, including DNA–DNA hybridization (Priscyla et al., 2023), restriction endonuclease analysis (REA) (Arent et al., 2017), random amplified polymorphic DNA (RAPD) fingerprinting (Rafael et al., 2019), and pulsed-field gel electrophoresis (PFGE) (Pejvak et al., 2024). These sophisticated methods, largely utilized in advanced laboratories in developed countries, have significantly expanded our understanding of genetic variation and genomic diversity within Leptospira.
The growing issue of antimicrobial resistance has become a major concern, particularly as Leptospira shows increasing resistance to traditional antibiotics, presenting new challenges for public health strategies. Early initiation of antibacterial therapy is crucial in preventing the progression of leptospirosis to more severe stages. For patients with severe leptospirosis requiring hospitalization, treatment typically involves intravenous administration of penicillin (1.5 million units every 6 h), ampicillin (0.5–1 gram every 6 h), ceftriaxone (1 gram every 24 h), or cefotaxime (1 gram every 6 h). In contrast, adult outpatients in the early stages of the disease are generally prescribed doxycycline (100 milligrams orally, twice daily) or azithromycin (500 milligrams orally, once daily). Pregnant women and children can also be treated with azithromycin or amoxicillin, with dosage adjustments based on body weight. These treatment guidelines are informed by in vitro susceptibility data, animal studies, and clinical experience, including a randomized, placebo-controlled, double-blind study demonstrating that doxycycline shortens the course of leptospirosis by two days and alleviates symptoms such as fever, discomfort, headache, and myalgia (Hospenthal and Murray, 2003; McClain et al., 1984). Additionally, doxycycline therapy can prevent the shedding of Leptospira in urine.
Despite the extensive research on in vitro antibiotic screening for Leptospira, studies on the in vivo efficacy of these treatments remain limited. Some research has even suggested that while antibiotics may show antibacterial effects in vitro, subtherapeutic dosing in vivo can worsen the clinical course of leptospirosis. Zhang et al. reported that treating golden hamsters with subtherapeutic doses of ciprofloxacin and norfloxacin increased mortality and aggravated pathological damage to the kidneys, liver, and lungs (Wenlong et al., 2014). Furthermore, although doxycycline demonstrates higher in vitro antibacterial potency compared to penicillin, in vivo studies have indicated its superior therapeutic effect, likely due to its immunomodulatory properties (Henrique et al., 2021). In late-stage leptospirosis, antibiotic administration becomes less effective. Jin et al. combined antibiotics with polyclonal antibodies, increasing the survival rate of golden hamsters infected with lethal leptospirosis to 50% (Xuemin et al., 2016), suggesting that a combination of antibiotics and antibody therapy could benefit patients with severe leptospirosis.
Antibiotics also play a significant role in influencing the prevalence of leptospirosis, particularly within the aquaculture industry. Pigs and mice are recognized as primary reservoirs for Leptospira, with L. interrogans serovar Pomona being common in pigs and Leptospira serovar Lai in mice. Interestingly, in recent years, the incidence of serovar Pomona in both humans and pigs has declined, while serovar Lai has become the dominant strain in human leptospirosis cases. A study found that adding oxytetracycline to pig feed effectively eliminated Leptospira infection at an early stage, leading to a reduction in the yearly incidence rate and a decreased risk of transmission to humans (Weilin et al., 2014). Recent research has also focused on utilizing immune modulators that target various host responses to enhance resistance to Leptospira infection. For example, Cao’s research group demonstrated protective effects in hamster models when pattern recognition receptor (PRR) agonists were administered during or one day before infection (Maria et al., 2017). Studies have shown that early expression of TLR2 occurs in tolerant Balb/c mice, whereas delayed expression is observed in more susceptible hamster models (Wenlong et al., 2016). This aligns with an earlier study by Matsui et al., which suggested that early activation of inflammatory mediators provides protection in Leptospira-infected mice, while a delayed response in hamsters may lead to a cytokine storm (Mariko et al., 2011). Co-injection of L. interrogans serovar Autumnalis and the synthetic TLR2 agonist Pam3cysSK4 in golden hamsters alleviated acute leptospirosis, reduced Leptospira load after three weeks, and mitigated pathological changes in organs (Wenlong et al., 2016). Furthermore, administering crude E. coli LPS to hamsters infected with Leptospira showed an increase in effectiveness, correlating with enhanced inflammation levels (Wenlong et al., 2020). Interestingly, co-injection of β-glucan (a fungal cell wall component that agonizes the Dectin-1 receptor and synergizes with TLR2) one day before infection also increased early inflammation and improved survival rates (Jiaqi et al., 2019).
Using Lactobacillus plantarum as a platform to express Leptospira proteins has shown unexpected protective effects in immune-sensitive C3H/HeJ mice when administered orally. After six weeks of intermittent gavage treatment, L. plantarum provided protection in these sensitive model mice (Hari-Hara et al., 2017). Although L. plantarum did not prevent kidney colonization, it reduced inflammation and fibrosis, suggesting the concept of ‘innate immune memory’ or ‘trained immunity, ‘where initial infection triggers metabolic and epigenetic reprogramming of macrophages and natural killer cells, leading to a heightened response to subsequent challenges. Further investigation by the team revealed that two intraperitoneal injections of CL429, a TLR2-NOD2 dual agonist, replicated the protective and anti-inflammatory effects observed in oral L. plantarum administration in viral lung infection models (Tyler et al., 2016). These results confirmed that CL429 pretreatment induces an innate memory effect, independent of B and T cells in the peritoneal cavity, as well as natural killer cells from distant sites like the bone marrow and spleen (Ignacio et al., 2019).
In conclusion, significant progress has been made in Leptospira research over recent decades. From early genomic and antigen characterization studies to mid-term investigations into the functions of outer membrane proteins and environmental survivability, and now to advanced explorations of molecular mechanisms and diagnostic technologies, the study of Leptospira is a dynamic and continuously evolving field. Future studies should continue integrating interdisciplinary approaches to develop more precise diagnostic tools and therapeutic strategies, addressing the global public health challenges posed by Leptospira.
This study is the first to analyze the evolution and research trends of Leptospira using bibliometric methods. While bibliometric analysis offers a comprehensive and objective overview, it does come with certain limitations. Firstly, our study relied solely on publications from the WOSCC database, which may not encompass all relevant research on Leptospira. Secondly, we focused exclusively on English-language publications, introducing a potential linguistic bias by excluding research in other languages. Additionally, we did not assess the quality of the publications, treating high-quality and low-quality studies equally. This is an inherent limitation that should be acknowledged when interpreting the results.
To the best of our knowledge, this is the first comprehensive bibliometric analysis of research on Leptospira. The study of leptospirosis has gradually shifted from the early basic biological exploration to the in-depth study of molecular mechanisms, and is closely integrated with global health strategies at the latest stage. By analyzing the research hotspots at different stages, it can be seen that the research in this field has expanded from the early stage of pathogen identification and diagnostic tool development to understanding its pathogenic mechanism and environmental impact, and finally developed into an interdisciplinary integrated prevention and control strategy. Future research will continue to focus on global control of leptospirosis, especially in improving diagnostic capabilities, developing effective vaccines and reducing the risk of environmental transmission, contributing to reducing the public health burden of the disease.
The datasets presented in this study can be found in online repositories. The names of the repository/repositories and accession number(s) can be found in the article/Supplementary material.
WW: Conceptualization, Data curation, Formal analysis, Methodology, Resources, Software, Supervision, Validation, Visualization, Writing – original draft, Writing – review & editing. YG: Conceptualization, Data curation, Formal analysis, Methodology, Resources, Software, Validation, Visualization, Writing – original draft, Writing – review & editing. JJ: Formal analysis, Investigation, Resources, Validation, Writing – review & editing. ZH: Funding acquisition, Investigation, Project administration, Resources, Supervision, Writing – review & editing. BX: Conceptualization, Data curation, Funding acquisition, Methodology, Project administration, Supervision, Writing – review & editing. SX: Conceptualization, Data curation, Funding acquisition, Methodology, Project administration, Supervision, Validation, Writing – original draft, Writing – review & editing.
The author(s) declare that financial support was received for the research, authorship, and/or publication of this article. This work was supported by Youth Science Fund Project of Guangxi Natural Science Foundation (No. 2023GXNSFBA026024), Guangxi Natural Science Foundation (Project No. 2023GXNSFAA026161), Guangxi Zhuang Autonomous Region Clinical Medical Research Center for Severe Treatment of Major Infectious Diseases (No. Guike AD22035101), and Guangxi Key Laboratory of Diagnosis and Treatment of Acute Respiratory Distress Syndrome (No. ZZH2020013).
The authors declare that the research was conducted in the absence of any commercial or financial relationships that could be construed as a potential conflict of interest.
The authors declare that no Gen AI was used in the creation of this manuscript.
All claims expressed in this article are solely those of the authors and do not necessarily represent those of their affiliated organizations, or those of the publisher, the editors and the reviewers. Any product that may be evaluated in this article, or claim that may be made by its manufacturer, is not guaranteed or endorsed by the publisher.
The Supplementary material for this article can be found online at: https://www.frontiersin.org/articles/10.3389/fmicb.2024.1514738/full#supplementary-material
Adler, B., and Faine, S. (1983a). A pomona serogroup-specific, agglutinating antigen in Leptospira, identified by monoclonal antibodies. Pathology 15, 247–250. doi: 10.3109/00313028309083501
Adler, B., and Faine, S. (1983b). Species-and genus-specific antigens in Leptospira, revealed by monoclonal antibodies and enzyme immunoassay. Zentralbl Bakteriol Mikrobiol Hyg A Med Mikrobiol Infekt Parasitol. 255, 317–322. doi: 10.1016/S0174-3031(83)80172-3
Ajay, R., Nally, J. E., Ricaldi, J. N., Matthias, M. A., Diaz, M. M., Lovett, M. A., et al. (2003). Leptospirosis: a zoonotic disease of global importance. Lancet Infect. Dis. 3, 757–771. doi: 10.1016/s1473-3099(03)00830-2
Andrew, G., Casanovas-Massana, A., Hacker, K. P., Wunder, E. A. Jr., Begon, M., Reis, M. G., et al. (2018). Quantification of pathogenic Leptospira in the soils of a Brazilian urban slum. PLoS Negl. Trop. Dis. 12:e0006415. doi: 10.1371/journal.pntd.0006415
Angela, S., Abreu, P. A. E., Neves, F. O., Atzingen, M. V., Watanabe, M. M., Vieira, M. L., et al. (2006). A newly identified leptospiral adhesin mediates attachment to laminin. Infect. Immun. 74, 6356–6364. doi: 10.1128/iai.00460-06
Antonella, M., Rita, A., Vittorio, S., Lorenzo, G., Korinne, D. L., and Roberto, C. (2004). Production of tumor necrosis factor alpha by Treponema pallidum, Borrelia burgdorferi s.l., and Leptospira interrogans in isolated rat Kupffer cells. FEMS Immunol. Med. Microbiol. 40, 187–191. doi: 10.1016/s0928-8244(03)00345-6
Arent, Z., Gilmore, C., San-Miguel Ayanz, J. M., Neyra, L. Q., and García-Peña, F. J. (2017). Molecular Epidemiology of Leptospira Serogroup Pomona Infections Among Wild and Domestic Animals in Spain. EcoHealth 14, 48–57. doi: 10.1007/s10393-017-1210-8
Arnau, C.-M., Gabriel Ghizzi, P., Elsio, A. Jr., Peter, J. D., Mike, B., and Albert, I. K. (2018). Quantification of Leptospira interrogans Survival in Soil and Water Microcosms. Appl. Environ. Microbiol. 84, e00507–e00518. doi: 10.1128/aem.00507-18
Bolin, C. A., and Zuerner, R. L. (1996). Correlation between DNA restriction fragment length polymorphisms in Leptospira interrogans serovar pomona type kennewicki and host animal source. J. Clin. Microbiol. 34, 424–425. doi: 10.1128/jcm.34.2.424-425.1996
Branger, C., Chatrenet, B., Gauvrit, A., Aviat, F., Aubert, A., Bach, J., et al. (2005). Protection against Leptospira interrogans sensu lato challenge by DNA immunization with the gene encoding hemolysin-associated protein 1. Infect. Immun. 73, 4062–4069. doi: 10.1128/iai.73.7.4062-4069.2005
Branger, C., Sonrier, C., Chatrenet, B., Klonjkowski, B., Ruvoen-Clouet, N., Aubert, A., et al. (2001). Identification of the hemolysis-associated protein 1 as a cross-protective immunogen of Leptospira interrogans by adenovirus-mediated vaccination. Infect. Immun. 69, 6831–6838. doi: 10.1128/iai.69.11.6831-6838.2001
Choy, H., Kelley, M., Chen, T., Møller, A., Matsunaga, J., and Haake, D. (2007). Physiological osmotic induction of Leptospira interrogans adhesion: LigA and Lig B bind extracellular matrix proteins and fibrinogen. Infect. Immun. 75, 2441–2450. doi: 10.1128/iai.01635-06
Christian, E., David, K., Rohin, K., Ardissino, M., Rogowski, M., Coles, B., et al. (2024). Risk Stratification in Nonischemic Dilated Cardiomyopathy Using CMR Imaging: a systematic review and meta-analysis. JAMA 332:1535. doi: 10.1001/jama.2024.13946
Coleman, J. L., Rogers, R. C., Rosa, P. A., and Benach, J. L. (1994). Variations in the osp B gene of Borrelia burgdorferi result in differences in monoclonal antibody reactivity and in production of escape variants. Infect. Immun. 62, 303–307. doi: 10.1128/iai.62.1.303-307.1994
Daša, P., Eva, R.-S., Mateja, L., Andrea, P., Tatjana, R., Zvonko, B., et al. (2020). Evaluation of real-time PCR targeting the lipL32 gene for diagnosis of Leptospira infection. BMC Microbiol. 20:59. doi: 10.1186/s12866-020-01744-4
David, A. H., Marc, A. S., Melissa, M. K., Manjula, D., David, P. A., and Richard, L. Z. (2004). Molecular evolution and mosaicism of leptospiral outer membrane proteins involves horizontal DNA transfer. J. Bacteriol. 186, 2818–2828. doi: 10.1128/jb.186.9.2818-2828.2004
David, E. H., Suhelen, E., Paul, A. C., and Ben, A. (2008). Lip L32 is an extracellular matrix-interacting protein of Leptospira spp. and Pseudoalteromonas tunicata. Infect. Immun. 76, 2063–2069. doi: 10.1128/iai.01643-07
Desai, K. T., Patel, F., Patel, P. B., Nayak, S., Patel, N. B., and Bansal, R. K. (2016). A case-control study of epidemiological factors associated with leptospirosis in South Gujarat region. J. Postgrad. Med. 62, 223–227. doi: 10.4103/0022-3859.188551
Didier, M., and Bernard, L. S. (2013). Laboratory diagnosis of leptospirosis: a challenge. J. Microbiol. Immunol. Infect. 46, 245–252. doi: 10.1016/j.jmii.2013.03.001
Ece, S., and Ronald, F. S. (2003). Isolation of moderately infectious Borrelia burgdorferi sensu stricto from attenuated cultures by using complement-mediated, antibody-dependent lysis selection technique in a mammalian tissue co-culture system. Microbes Infect. 5, 869–878. doi: 10.1016/s1286-4579(03)00178-3
Eddy, R., Ganoza, C. A., Campos, K., Ricaldi, J. N., Torres, S., Silva, H., et al. (2005). Clinical spectrum of pulmonary involvement in leptospirosis in a region of endemicity, with quantification of leptospiral burden. Clin. Infect. Dis. 40, 343–351. doi: 10.1086/427110
Ellis, W., Montgomery, J. M., and Thiermann, A. B. (1991). Restriction endonuclease analysis as a taxonomic tool in the study of pig isolates belonging to the Australis serogroup of Leptospira interrogans. J. Clin. Microbiol. 29, 957–961. doi: 10.1128/jcm.29.5.957-961.1991
Emilie, B., Roman, T., Dominique, G., Marie-Estelle, S-G., and Cyrille, G. (2020). A systematic review of Leptospira in water and soil environments. PLoS One. 15. doi: 10.1371/journal.pone.0227055
Fabiana Kömmling, S., Claudia Hartleben, F., Daiane Drawanz, H., Fabricio Rochedo, C., Guimarães, J. A., and Odir Antônio, D. (2007a). Evaluation of different ways of presenting Lip L32 to the immune system with the aim of developing a recombinant vaccine against leptospirosis. Can. J. Microbiol. 53, 472–479. doi: 10.1139/w06-138
Fabiana Kömmling, S., Fagonde, E., Daiane Drawanz, H., Gustavo Maia, C., Marta, A., Michel Quevedo, F., et al. (2007b). Recombinant Mycobacterium bovis BCG expressing the LipL32 antigen of Leptospira interrogans protects hamsters from challenge. Vaccine 26, 88–95. doi: 10.1016/j.vaccine.2007.10.052
Federico, C., Guilherme, S., Felzemburgh, R. D. M., Santos, N., Reis, R. B., Santos, A. C., et al. (2014). Influence of household rat infestation on leptospira transmission in the urban slum environment. PLoS Negl. Trop. Dis. 8:e3338. doi: 10.1371/journal.pntd.0003338
Federico, C., José, E. H., Juan, C., Michael, K., Paul, T., Martha, S. M.-S., et al. (2015). Global Morbidity and Mortality of Leptospirosis: a systematic review. PLoS Negl. Trop. Dis. 9:e0003898. doi: 10.1371/journal.pntd.0003898.eCollection2015
Feng, C. Y., Li, Q. T., Zhang, X. Y., Dong, K., Hu, B. Y., and Guo, X. K. (2009). Immune strategies using single-component LipL32 and multi-component recombinant LipL32-41-OmpL1 vaccines against leptospira. Braz. J. Med. Biol. Res. 42, 796–803. doi: 10.1590/s0100-879x2009005000013
Garba, B., and Abdul Rani, B. (2018). Advances & challenges in leptospiral vaccine development. Indian J. Med. Res. 147, 15–22. doi: 10.4103/ijmr.IJMR_1022_16
Gravekamp, C., Van de Kemp, H., Franzen, M., Carrington, D., Schoone, G., Van Eys, G. J., et al. (1993). Detection of seven species of pathogenic leptospires by PCR using two sets of primers. J. Gen. Microbiol. 139, 1691–1700. doi: 10.1099/00221287-139-8-1691
Guerreiro, H., Croda, J., Flannery, B., Mazel, M., Matsunaga, J., Galvão Reis, M., et al. (2001). Leptospiral proteins recognized during the humoral immune response to leptospirosis in humans. Infect. Immun. 69, 4958–4968. doi: 10.1128/iai.69.8.4958-4968.2001
Haake, D., Champion, C. I., Martinich, C., Shang, E. S., Blanco, D. R., Miller, J. N., et al. (1993). Molecular cloning and sequence analysis of the gene encoding OmpL1, a transmembrane outer membrane protein of pathogenic Leptospira spp. J. Bacteriol. 175, 4225–4234. doi: 10.1128/jb.175.13.4225-4234.1993
Haake, D., Chao, G., Zuerner, R. L., Barnett, J. K., Barnett, D., Mazel, M., et al. (2000). The leptospiral major outer membrane protein LipL32 is a lipoprotein expressed during mammalian infection. Infect. Immun. 68, 2276–2285. doi: 10.1128/iai.68.4.2276-2285.2000
Hari-Hara, P., Luciana, R., Catherine, W., and Maria, G.-S. (2017). Pre-treatment with Lactobacillus plantarum prevents severe pathogenesis in mice infected with Leptospira interrogans and may be associated with recruitment of myeloid cells. PLoS Negl. Trop. Dis. 11:e0005870. doi: 10.1371/journal.pntd.0005870
Henrique, G. C., André, B., Ana, N.-C., Elsa, S., Dora, P., Tiago, V., et al. (2021). Tetracycline Antibiotics Induce Host-Dependent Disease Tolerance to Infection. Immunity 54, 53–67. doi: 10.1016/j.immuni.2020.09.011
Hookey, J. V., and Palmer, M. F. (1991). A comparative investigation and identification of Leptospira interrogans serogroup icterohaemorrhagiae strains by monoclonal antibody and DNA fingerprint analyses. Zentralbl Bakteriol 275, 185–199. doi: 10.1016/s0934-8840(11)80065-4
Hospenthal, D. R., and Murray, C. K. (2003). In vitro susceptibilities of seven Leptospira species to traditional and newer antibiotics. Antimicrob. Agents Chemother. 47, 2646–2648. doi: 10.1128/aac.47.8.2646-2648.2003
Ignacio, S., Frédérique, V.-P., Orhan, R., Jessica, Q., Maria, G.-S., Ivo, G. B., et al. (2019). Innate immune memory through TLR2 and NOD2 contributes to the control of Leptospira interrogans infection. PLoS Pathog. 15:e1007811. doi: 10.1371/journal.ppat.1007811
Ilana Teruszkin, B., Juliana Magalhães, V.-B., and Martha Maria, P. (2012). Leptospirosis diagnosis by immunocapture polymerase chain reaction: a new tool for early diagnosis and epidemiologic surveillance. Diagn. Microbiol. Infect. Dis. 74, 11–15. doi: 10.1016/j.diagmicrobio.2012.05.028
James, M., Kristian, W., Richard, L., Frank, A., and Haake, D. A. (2006). LipL46 is a novel surface-exposed lipoprotein expressed during leptospiral dissemination in the mammalian host. Microbiology 152, 3777–3786. doi: 10.1099/mic.0.29162-0
James, M., Michele, A. B., Julio, C., Tracy, A. Y., Yolanda, S., Isadora, S., et al. (2003). Pathogenic Leptospira species express surface-exposed proteins belonging to the bacterial immunoglobulin superfamily. Mol. Microbiol. 49, 929–946. doi: 10.1046/j.1365-2958.2003.03619.x
Jane, E. S., Krystle, L. R., Jarlath, E. N., Renee, L. G., and David, A. H. (2022). Role of Diagnostics in Epidemiology, Management, Surveillance, and Control of Leptospirosis. Pathogens 11, 1–24. doi: 10.3390/pathogens11040395
Jarlath, E., Whitelegge, J. P., Bassilian, S., Blanco, D. R., and Lovett, M. A. (2006). Characterization of the outer membrane proteome of Leptospira interrogans expressed during acute lethal infection. Infect. Immun. 75, 766–773. doi: 10.1128/iai.00741-06
Ji, J., Wang, W., Xiang, S., Wei, X., Pang, G., Shi, H., et al. (2023). Diagnosis of leptospira by metagenomics next-generation sequencing with extracorporeal membrane oxygenation support: a case report. BMC Infect. Dis. 23:788. doi: 10.1186/s12879-023-08793-w
Jiaqi, W., Zhao, J., Wenlong, Z., Xufeng, X., Ning, S., Tianbao, L., et al. (2019). The preventable efficacy of β-glucan against leptospirosis. PLoS Negl. Trop. Dis. 13:e0007789. doi: 10.1371/journal.pntd.0007789
João, C. S., Marcelo, R., James, M., and David, A. H. (2005). Lipoprotein computational prediction in spirochaetal genomes. Microbiology 152, 113–121. doi: 10.1099/mic.0.28317-0
José María, G., Jean Philippe, C., and Geoffrey, K. I. (2021). PLOS Neglected Tropical Diseases broadens its coverage of envenomings caused by animal bites and stings. PLoS Negl. Trop. Dis. 15:e0009481. doi: 10.1371/journal.pntd.0009481
José, E., Moraga, P., Costa, F., Capian, N., Ribeiro, G. S., Wunder, E. A. Jr., et al. (2016). Spatiotemporal Determinants of Urban Leptospirosis Transmission: Four-Year Prospective Cohort Study of Slum Residents in Brazil. PLoS Negl. Trop. Dis. 10:e0004275. doi: 10.1371/journal.pntd.0004275
Julio, C., João, G. R., Matsunaga, J., Queiroz, A., Homma, A., Riley, L. W., et al. (2007). Leptospira immunoglobulin-like proteins as a serodiagnostic marker for acute leptospirosis. J. Clin. Microbiol. 45, 1528–1534. doi: 10.1128/jcm.02344-06
Junpen, S., Wanpen, C., Patcharin, S., Pramuan, T., Manas, C.-N., Thareerat, K., et al. (2005). Evaluation of a monoclonal antibody-based dot-blot ELISA for detection of Leptospira spp in bovine urine samples. Am. J. Vet. Res. 66, 762–766. doi: 10.2460/ajvr.2005.66.762
Krishna Kanchan, S., and Usha, K. (2008). Early diagnosis of leptospirosis by conventional methods: one-year prospective study. Indian J. Pathol. Microbiol. 51, 209–211. doi: 10.4103/0377-4929.41687
Lee, S., Kim, K. A., Park, Y. G., Seong, I. W., Kim, M. J., and Lee, Y. J. (2000). Identification and partial characterization of a novel hemolysin from Leptospira interrogans serovar lai. Gene 254, 19–28. doi: 10.1016/s0378-1119(00)00293-6
LeFebvre, R. B. (1987). DNA probe for detection of the Leptospira interrogans serovar hardjo genotype hardjo-bovis. J. Clin. Microbiol. 25, 2236–2238. doi: 10.1128/jcm.25.11.2236-2238.1987
Li-Fang, C., Ting-Wen, C., Yi-Ching, K., Ming-Jeng, P., Ya-Chung, T., Cheng-Hsun, C., et al. (2015). Potential impact on kidney infection: a whole-genome analysis of Leptospira santarosai serovar Shermani. Emerg Microbes Infect. 3:e82. doi: 10.1038/emi.2014.78
Lin, Y., and Chang, Y. (2007). A domain of the Leptospira Lig B contributes to high affinity binding of fibronectin. Biochem. Biophys. Res. Commun. 362, 443–448. doi: 10.1016/j.bbrc.2007.07.196
Lin, Y., Raman, R., Sharma, Y., and Chang, Y. (2008). Calcium binds to leptospiral immunoglobulin-like protein, Lig B, and modulates fibronectin binding. J. Biol. Chem. 283, 25140–25149. doi: 10.1074/jbc.M801350200
Marcelle Moura, S., Thaís Larré, O., Rodrigo Andrade, S., Alexander, A. J., Odir Antônio, D., and Daiane Drawanz, H. (2017). DNA vaccines against leptospirosis: A literature review. Vaccine 35, 5559–5567. doi: 10.1016/j.vaccine.2017.08.067
Marcia, G., Ricardo, M., Atzingen, M. V., Martins, E. A. L., Vasconcellos, S. A., Romero, E. C., et al. (2005). Whole-genome analysis of Leptospira interrogans to identify potential vaccine candidates against leptospirosis. FEMS Microbiol. Lett. 244, 305–313. doi: 10.1016/j.femsle.2005.02.004
Maria, G.-S., Ignacio, S., and Catherine, W. (2017). Animal Models of Leptospirosis: Of Mice and Hamsters. Front. Immunol. 8, 1–20. doi: 10.3389/fimmu.2017.00058
Marija, P., Henry, A. C., and David, A. H. (2010). The OmpL37 surface-exposed protein is expressed by pathogenic Leptospira during infection and binds skin and vascular elastin. PLoS Negl. Trop. Dis. 4:e815. doi: 10.1371/journal.pntd.0000815
Mariko, M., Marie-Estelle, S., Jérôme, B., and Cyrille, G. (2012). Differential in vivo gene expression of major Leptospira proteins in resistant or susceptible animal models. Appl. Environ. Microbiol. 78, 6372–6376. doi: 10.1128/aem.00911-12
Mariko, M., Vincent, R., Lilian, B.-O., and Cyrille, G. (2011). Gene expression profiles of immune mediators and histopathological findings in animal models of leptospirosis: comparison between susceptible hamsters and resistant mice. Infect. Immun. 79, 4480–4492. doi: 10.1128/iai.05727-11
Mathieu, P. (2017). Virulence of the zoonotic agent of leptospirosis: still terra incognita? Nat. Rev. Microbiol. 15, 297–307. doi: 10.1038/nrmicro.2017.5
Mathieu, P., Dieter, M., Bouchier, C., Zuerner, R. L., Zidane, N., Wilson, P. J., et al. (2008). Genome sequence of the saprophyte Leptospira biflexa provides insights into the evolution of Leptospira and the pathogenesis of leptospirosis. PLoS One 3:e 1607. doi: 10.1371/journal.pone.0001607
McClain, J. B., Ballou, W. R., Harrison, S. M., and Steinweg, D. L. (1984). Doxycycline therapy for leptospirosis. Ann. Intern. Med. 100, 696–698. doi: 10.7326/0003-4819-100-5-696
Merien, F., Baranton, G., and Perolat, P. (1997). Invasion of Vero cells and induction of apoptosis in macrophages by pathogenic Leptospira interrogans are correlated with virulence. Infect. Immun. 65, 729–738. doi: 10.1128/iai.65.2.729-738.1997
Merien, F., Truccolo, J., Baranton, G., and Perolat, P. (2000). Identification of a 36-kDa fibronectin-binding protein expressed by a virulent variant of Leptospira interrogans serovar icterohaemorrhagiae. FEMS Microbiol. Lett. 185, 17–22. doi: 10.1111/j.1574-6968.2000.tb09034.x
Michele, A., Albert, K. I, Reis, M. G., McDonald, K. L., and Riley, L. W. (2002). Rapid translocation of polarized MDCK cell monolayers by Leptospira interrogans, an invasive but nonintracellular pathogen. Infect. Immun. 70, 6926–6932. doi: 10.1128/iai.70.12.6926-6932.2002
Nobuo, K., and Haruo, W. (2004). Leptospiral immunoglobulin-like proteins elicit protective immunity. Vaccine 22, 1545–1552. doi: 10.1016/j.vaccine.2003.10.007
Norlan de Jesus, S., Erica, S., Mitermayer, G., Albert, I. K., and Federico, C. (2017). Rat infestation associated with environmental deficiencies in an urban slum community with high risk of leptospirosis transmission. Cad. Saude Publica 33:e00132115. doi: 10.1590/01021-311x000132115
Paul, A., Cordwell, S. J., Bulach, D. M., Haake, D. A., and Adler, B. (2002). Global analysis of outer membrane proteins from Leptospira interrogans serovar Lai. Infect. Immun. 70, 2311–2318. doi: 10.1128/iai.70.5.2311-2318.2002
Paul, A. C., David, A. H., and Ben, A. (2004). Outer membrane proteins of pathogenic spirochetes. FEMS Microbiol. Rev. 28, 291–318. doi: 10.1016/j.femsre.2003.10.004
Paula, R., Pascale, B., Weykamp, F., Figueira, C. P., Huerre, M., Ave, P., et al. (2007). The OmpA-like protein Loa22 is essential for leptospiral virulence. PLoS Pathog. 3:e97. doi: 10.1371/journal.ppat.0030097
Peixiang, W., Liping, Z., Lihua, J., and Li, W. (2024). Immune checkpoint inhibitors: A bibliometric analysis of research trends and hotspots based on the most frequently cited clinical trials (2013-2021). Int. J. Clin. Pharmacol. Ther., 1–5. doi: 10.5414/cp204629
Pejvak, K., Mohsen, B., Mehdi, G., Maryam, S. S., Fereshteh, S., and Vajihe, S. N. (2024). Application of pulsed-field gel electrophoresis for molecular identification of pathogenic Leptospira species in Iran: a rapid and reliable method. Iran J Microbiol. 16, 323–328. doi: 10.18502/ijm.v16i3.15763
Priscyla, D. S. R., Natália Barbosa, C., Flávia, A., Thiago, S., Graciete, V., Talita, G., et al. (2023). Environmental Biofilms from an Urban Community in Salvador, Brazil, Shelter Previously Uncharacterized Saprophytic Leptospira. Microb. Ecol. 86, 2488–2501. doi: 10.1007/s00248-023-02253-3
Qi, W., Lei, Y., Fei, W., and Fei, S. (2024). Global research trends and prospects on immune-related therapy in ischemic stroke: a bibliometric analysis. Front. Cell. Neurosci. 18, 1–12. doi: 10.3389/fncel.2024.1490607
Rafael, G.-G., Angélica, R.-T., María Del Rocío, R.-M., Esperanza, D.-E., María Guadalupe, F.-D.-L., Beatriz, R.-S., et al. (2019). Genotyping of Leptospira interrogans isolates from Mexican patients. Rev. Inst. Med. Trop. Sao Paulo 61:e26. doi: 10.1590/s1678-9946201961026
Raghavan, U. M., Chang, Y.-F., Jusuf, S. S. D., Artiushin, S., Timoney, J. F., et al. (2002). Cloning and molecular characterization of an immunogenic LigA protein of Leptospira interrogans. Infect. Immun. 70, 5924–5930. doi: 10.1128/iai.70.11.5924-5930.2002
Savio, M., Pacciarini, M. L., Cinco, M., and Tagliabue, S. (1993). Identification of Leptospira interrogans strains by monoclonal antibodies and genomic analysis. New Microbiol. 16, 315–321
Savio, M. L., Rossi, C., Fusi, P., Tagliabue, S., and Pacciarini, M. L. (1994). Detection and identification of Leptospira interrogans serovars by PCR coupled with restriction endonuclease analysis of amplified DNA. J. Clin. Microbiol. 32, 935–941. doi: 10.1128/jcm.32.4.935-941.1994
Senaka, R., Chaturaka, R., Shiroma, M., and Sumadhya Deepika, F. (2015). Current immunological and molecular tools for leptospirosis: diagnostics, vaccine design, and biomarkers for predicting severity. Ann. Clin. Microbiol. Antimicrob. 14, 2–8. doi: 10.1186/s12941-014-0060-2
Seoung Hoon, L., Sangduk, K., Seung Chul, P., and Min Ja, K. (2001). Cytotoxic activities of Leptospira interrogans hemolysin SphH as a pore-forming protein on mammalian cells. Infect. Immun. 70, 315–322. doi: 10.1128/iai.70.1.315-322.2002
Sérgio, J., Frederico Schmitt, K., Rodrigues, N., Navarro, O. S. V., Guimarães, A. M., Sanchez, C. D., et al. (2017). Whole-genome sequencing of Leptospira interrogans from southern Brazil: genetic features of a highly virulent strain. Mem. Inst. Oswaldo Cruz 113, 80–86. doi: 10.1590/0074-02760170130
Shenberg, E. (1967). Growth of pathogenic Leptospira in chemically defined media. J. Bacteriol. 93, 1598–1606. doi: 10.1128/jb.93.5.1598-1606.1967
Sirawit, J., Umaporn, L., Sasipha, T., Janejira, D., Patcharakorn, K., Watchadaporn, C., et al. (2022). Rapid and sensitive point-of-care detection of Leptospira by RPA-CRISPR/Cas12a targeting lipL32. PLoS Negl. Trop. Dis. 16:e0010112. doi: 10.1371/journal.pntd.0010112
Sirawit, J., Umaporn, L., Sasipha, T., Janejira, D., Patcharakorn, K., Watchadaporn, C., et al. (2023). The combination of RPA-CRISPR/Cas12a and Leptospira IgM RDT enhances the early detection of leptospirosis. PLoS Negl. Trop. Dis. 17:e0011596. doi: 10.1371/journal.pntd.0011596
Smita Benjamin, S., Belgode Narasimha, H., Godfred Antony, M., and Subhash Chandra, P. (2010). Clinical and serological evaluation of Leptospirosis in Puducherry, India. J Infect Dev Ctries. 4, 139–143. doi: 10.3855/jidc.384
Srimanote, P., Wongdeethai, N., Jieanampunkul, P., Samonkiert, S., Leepiyasakulchai, C., Kalambaheti, T., et al. (2008). Recombinant ligA for leptospirosis diagnosis and ligA among the Leptospira spp. clinical isolates. J. Microbiol. Methods 72, 73–81. doi: 10.1016/j.mimet.2007.10.012
Stevenson, B., Choy, H. A., Pinne, M., Rotondi, M. L., Miller, M. C., Demoll, E., et al. (2007). Leptospira interrogans endostatin-like outer membrane proteins bind host fibronectin, laminin and regulators of complement. PLoS One 2:e1188. doi: 10.1371/journal.pone.0001188
Thaís Larré, O., André Alex, G., Rodrigo Andrade, S., Pinto, A. C., Marcelo, M., Daiane Drawanz, H., et al. (2015). Evaluation of the Leptospira interrogans Outer Membrane Protein OmpL37 as a Vaccine Candidate. PLoS One 10, e0142821–e0142222. doi: 10.1371/journal.pone.0142821
Tippawan, P., Santi, M., Nonglucksanawan, R., Ngamphol, S., Onrapak, R., Poom, A., et al. (2018). Immunodominance of LipL32(93-272) peptides revealed by leptospirosis sera and therapeutic monoclonal antibodies. J. Microbiol. Immunol. Infect. 53, 11–22. doi: 10.1016/j.jmii.2017.12.006
Tyler, A., Brenner, T. A., Percopo, C. M., Ma, M., Keicher, J. D., Domachowske, J. B., et al. (2016). Signaling via pattern recognition receptors NOD2 and TLR2 contributes to immunomodulatory control of lethal pneumovirus infection. Antivir. Res. 132, 131–140. doi: 10.1016/j.antiviral.2016.06.002
Wasna, V., Supawadee, I., Eakasit, P., and Prasit, P. (2008). Genome-wide subcellular localization of putative outer membrane and extracellular proteins in Leptospira interrogans serovar Lai genome using bioinformatics approaches. BMC Genomics 9, 181–115. doi: 10.1186/1471-2164-9-181
Wei, W., Bin, X., Shulin, X., Jianyu, J., Jing, P., and Lin, H. (2024). Visual analysis of the research literature on extracorporeal membrane oxygenation-assisted support for respiratory failure based on Cite Space and VOSviewer: a 20-year study. J. Thorac. Dis. 16, 12–25. doi: 10.21037/jtd-23-1184
Weilin, H., Xu'ai, L., and Jie, Y. (2014). Leptospira and leptospirosis in China. Curr. Opin. Infect. Dis. 27, 432–436. doi: 10.1097/qco.0000000000000097
Wenlong, Z., Naisheng, Z., Wei, W., Fei, W., Yue, G., Haichao, J., et al. (2014). Efficacy of cefepime, ertapenem and norfloxacin against leptospirosis and for the clearance of pathogens in a hamster model. Microb. Pathog. 77, 78–83. doi: 10.1016/j.micpath.2014.11.006
Wenlong, Z., Naisheng, Z., Xufeng, X., Jian, G., Xuemin, J., Feng, X., et al. (2016). Toll-Like Receptor 2 Agonist Pam 3CSK4 Alleviates the Pathology of Leptospirosis in Hamster. Infect. Immun. 84, 3350–3357. doi: 10.1128/iai.00708-16
Wenlong, Z., Xufeng, X., Jiaqi, W., Ning, S., Tianbao, L., Dianjun, W., et al. (2020). Increased inflammation with crude E. coli LPS protects against acute leptospirosis in hamsters. Emerg Microbes Infect. 9, 140–147. doi: 10.1080/22221751.2019.1710435
Wilson, M., Naccache, S., Samayoa, E., Biagtan, M., Bashir, H., Yu, G., et al. (2014). Actionable diagnosis of neuroleptospirosis by next-generation sequencing. N. Engl. J. Med. 370, 2408–2417. doi: 10.1056/NEJMoa1401268
Woods, K., Nic-Fhogartaigh, C., Arnold, C., Boutthasavong, L., Phuklia, W., Lim, C., et al. (2017). A comparison of two molecular methods for diagnosing leptospirosis from three different sample types in patients presenting with fever in Laos. Clin. Microbiol. Infect. 24, 1017.e1–1017.e7. doi: 10.1016/j.cmi.2017.10.017
Xiang'an, W., Bao, J., Xiao, L., Yilei, M., Xueshuai, W., and Shunda, D. (2024). Research trends of cellular immunotherapy for primary liver cancer: A bibliometric analysis. Hum. Vaccin. Immunother. 20, 1–12. doi: 10.1080/21645515.2024.2426869
Xiang-Yan, Z., Yang, Y., Ping, H., Yi-Xuan, Z., Bao-Yu, H., Yang, Y., et al. (2005). Expression and comparative analysis of genes encoding outer membrane proteins LipL21, LipL32 and OmpL1 in epidemic leptospires. Acta Biochim. Biophys. Sin. 37, 649–656. doi: 10.1111/j.1745-7270.2005.00094.x
Xu'ai, L., Guohui, X., Dongjiao, L., Liangliang, K., Xu, C., Dexter, S., et al. (2016). Chimeric epitope vaccine against Leptospira interrogans infection and induced specific immunity in guinea pigs. BMC Microbiol. 16:2159. doi: 10.1186/s12866-016-0852-y
Xuemin, J., Wenlong, Z., Zhuang, D., Hai, W., Dianjun, W., Xufeng, X., et al. (2016). Efficacy of the Rabbit Polyclonal Anti-leptospira Antibody against Homotype or Heterotype Leptospira Infection in Hamster. PLoS Negl. Trop. Dis. 10:e0005191. doi: 10.1371/journal.pntd.0005191
Ya-Chung, T., Yung-Chang, C., Cheng-Chieh, H., Chiz-Tzung, C., Mai-Szu, W., Aled, O. P., et al. (2006). Leptospiral outer membrane protein induces extracellular matrix accumulation through a TGF-beta 1/Smad-dependent pathway. J. Am. Soc. Nephrol. 17, 2792–2798. doi: 10.1681/asn.2006020159
Yingying, X., Pak-Wai, Y., and Jenny Ka-Wing, L. (2014). Intranasal DNA Vaccine for Protection against Respiratory Infectious Diseases: The Delivery Perspectives. Pharmaceutics. 6, 378–415. doi: 10.3390/pharmaceutics6030378
Yunzhen, S., Wanru, G., Ming, H., Yuxuan, W., Jingnan, L., Wenjuan, H., et al. (2023). A case of severe leptospirosis with Jarisch-Herxheimer reaction presenting as respiratory failure. Front. Public Health 11, 1–4. doi: 10.3389/fpubh.2023.1125306
Zhang, W. H., Bai, X. F., Nie, D. K., and Deng, Q. D. (1989). An application of monoclonal antibodies to identification of leptospires of serogroup icterohaemorrhagiae found in China. Microbiol. Immunol. 33, 1039–1043
Keywords: Leptospira , bibliometric analysis, research hotspots, trends, Web of Science Core Collection
Citation: Wang W, Gao Y, Ji J, Huang Z, Xiong B and Xiang S (2025) Trends and advances in Leptospira, a bibliometric analysis. Front. Microbiol. 15:1514738. doi: 10.3389/fmicb.2024.1514738
Received: 29 October 2024; Accepted: 16 December 2024;
Published: 08 January 2025.
Edited by:
Olayinka Ayobami Aiyegoro, North-West University, South AfricaReviewed by:
Shih Keng Loong, University of Malaya, MalaysiaCopyright © 2025 Wang, Gao, Ji, Huang, Xiong and Xiang. This is an open-access article distributed under the terms of the Creative Commons Attribution License (CC BY). The use, distribution or reproduction in other forums is permitted, provided the original author(s) and the copyright owner(s) are credited and that the original publication in this journal is cited, in accordance with accepted academic practice. No use, distribution or reproduction is permitted which does not comply with these terms.
*Correspondence: Shulin Xiang, eGlhbmdzaHVsaW4yN0AxNjMuY29t
†These authors have contributed equally to this work
Disclaimer: All claims expressed in this article are solely those of the authors and do not necessarily represent those of their affiliated organizations, or those of the publisher, the editors and the reviewers. Any product that may be evaluated in this article or claim that may be made by its manufacturer is not guaranteed or endorsed by the publisher.
Research integrity at Frontiers
Learn more about the work of our research integrity team to safeguard the quality of each article we publish.