- 1Centro Experimental Yanayacu, Dirección de Supervisión y Monitoreo en las Estaciones Experimentales Agrarias (DSME), Instituto Nacional de Innovación Agraria (INIA), Lima, Peru
- 2Corporación para la Investigación de la Corrosión (CIC), Piedecuesta, Colombia
- 3Grupo de Investigación en Compuestos Orgánicos de Interés Medicinal (CODEIM), Universidad Industrial de Santander (UIS), Bucaramanga, Colombia
The increasing use of plastics globally has generated serious environmental and human health problems, particularly in the agricultural sector where low-density polyethylene (LDPE) and other plastics are widely used. Due to its low recycling rate and slow degradation process, LDPE is a major source of pollution. This paper addresses the problem of plastic accumulation in agriculture, focusing on LDPE biodegradation strategies. The studies reviewed include recent data and the methodologies used include state-of-the-art technologies and others that have been used for decades, to monitor and measure the degree of biodegradation that each treatment applied can have, including SEM, GCMS, HPLC, and microscopy. The countries investigating these biodegradation methodologies are identified, and while some countries have been developing them for some years, others have only begun to address this problem in recent years. The use of microorganisms such as bacteria, fungi, algae, and insect larvae that influence its decomposition is highlighted. A workflow is proposed to carry out this type of research. Despite the advances, challenges remain, such as optimizing environmental conditions to accelerate the process and the need for further research that delves into microbial interactions in various environmental contexts.
1 Introduction
Plastic production worldwide has increased since 1950, the time when large-scale production started, reaching more than 368 million tons reported in 2019 and 2020 (Ekanayaka et al., 2022; Plastics-Europe, 2021; Temporiti et al., 2022). Global consumption of plastics will continue to increase, so much so that by 2050 it is estimated that there will be up to 5.3 gigatons of plastics discarded in the environment (Schwarz et al., 2023). More than 90% of discarded plastic is not pretreated and goes into the sea and landfills (UNDP, 2023). This accumulation is evidenced by macro and microplastics, representing an environmental and public health problem, with high accumulation capacity in different ecosystems. This affects agricultural and livestock development and human health, causing various pathologies and in some cases even the death of different living beings (Hale et al., 2020; Skariyachan et al., 2021; Yee et al., 2021; Welden, 2020). Most of these materials are non-biodegradable, highly efficient, and versatile, exhibiting a range of applications, due to their distinctive physical, chemical, mechanical, and thermal properties with high resistance to atmospheric and corrosive agents, obtained from petroleum derivatives and other hydrocarbons (Ali et al., 2018). The most widely applied and therefore the main plastic wastes are low-density polyethylene (LDPE), high-density polyethylene (HDPE), polyethylene terephthalate (PET), polypropylene (PP), polyvinyl chloride (PVC), and polystyrene (PS) (Temporiti et al., 2022; Lear et al., 2021; Matjašič et al., 2021; Zeghal et al., 2021). After their use, plastics become solid waste, which depending on the treatment in each country can follow three processes: recycling, incineration as a source of energy, or accumulation in landfills (Temporiti et al., 2022; Lear et al., 2021; Buchholz et al., 2022). When accumulated, these wastes require long periods for their natural degradation. The condition associated with the inherent characteristics of the plastic, such as high molecular weight, crystallinity, hydrophobicity, and the additives used to improve the quality of the final product, favoring resistance to atmospheric and corrosive agents (Temporiti et al., 2022; Lear et al., 2021; Matjašič et al., 2021; Harshvardhan and Jha, 2013).
Modern agriculture in some countries of the world has become dependent on single-use and extended-use plastic products for crop production. These materials have facilitated increased productivity and efficiency in agriculture (Lakhiar et al., 2024). The most frequent uses of plastics are oriented to soil covering, greenhouses, irrigation systems, seed germination, production, and packaging of products (Hofmann et al., 2023). The massive use of these polymers has been generating several environmental problems because they take hundreds of years to degrade; this problem is aggravated by the low recycling rate of agricultural plastics, partly due to contamination with soil, crop residues, and agricultural chemicals (Lakhiar et al., 2024; Hofmann et al., 2023; Correa-Cano et al., 2023). The proper management of these wastes is related to Sustainable Development Goals 3, 12, and 15 (FAO, 2023).
In the search for green alternatives for managing plastic waste, biodegradation mechanisms are highlighted as a possible solution to mitigate this problem, particularly in the agricultural context. This biodegradation mechanism is a physicochemical and biological process mediated by microorganisms, such as bacteria, fungi, algae, and other organisms or substances of biological origin (Koh and Khor, et al., 2022; Jacquin et al., 2019; Rogers et al., 2020). The biodegradation mecanism has four main stages (see Figure 1): biodeterioration (colonization), biofragmentation, assimilation, and mineralization (Lucas et al., 2008), each influenced by a combination of environmental and microbial factors. In the biodeterioration phase, abiotic conditions such as temperature, humidity, UV radiation, and mechanical forces weaken the polymer matrix, creating favorable sites for microbial colonization (Nag et al., 2021; Islami et al., 2019; Chaudhary et al., 2021).
Since plastisphere, as plastics have come to be called on the planet, linger in nature after disposal, causing damage with consequences for wildlife and human life (Žuna Pfeiffer et al., 2022). Furthermore, being a developing research topic, methodologies for biodegrading and measuring the biodegradation of these plastics are still under investigation. This review article examines various organisms and methods employed in the biodegradation of plastics, with a particular focus on LDPE due to its widespread use in agriculture. Differences and similarities between methods are discussed, elucidating procedures that allow biodegradation to be measured accurately and reproducibly. In addition, the review discusses the challenges and opportunities associated with the implementation of these technologies in agriculture and reviews the organisms used for this purpose. The analysis presented in this manuscript can contribute to the development of a more sustainable and resilient agriculture in the face of global environmental challenges.
The term “plastisphere,” coined to describe the accumulation of plastics in the environment, has gained prominence in recent years. These persistent pollutants, often referred to as “plastics” on Earth, have been linked to adverse effects on wildlife and human health (Žuna Pfeiffer et al., 2022). Moreover, as this is a developing research area, these plastics’ biodegradation and measurement methodologies are still under investigation. This is the pioneering study to explore this topic. For Peru that examines the various organisms and methods utilized in the biodegradation of plastics, with a particular focus on LDPE due to its pervasive usage in agricultural applications, and discusses the differences and similarities between methods and procedures to accurately and reproducibly measure LDPE biodegradation. Furthermore, the review addresses the challenges and opportunities associated with the implementation of these technologies in agriculture, as well as the organisms utilized for this purpose. The analysis presented in this manuscript can contribute to the awareness of the responsible use of plastics in the agricultural sector, to generate more sustainable and resilient agriculture in the context of global environmental challenges, while always prioritizing the welfare of human health.
2 Literature retrieval
The bibliographic review began with the search protocol, establishing the terms and criteria. The databases selected were: Scopus, Nature, Web of Science, and the Google Scholar search engine due to their recognition and high impact (Zárate-Rueda et al., 2021). The search equation [“Biodegradation” OR “Biological degradation” OR “Biomineralization” AND (“Low-density polyethylene OR LDPE”)] was applied and the filters of the scientific tools were used with the application of inclusion and exclusion criteria. The inclusion criteria were keywords such as “Polyethylene” and “Biodegradation,” in original research articles and conference papers, published in the period from 2019 to 2024, in the English language. Subsequently, a review of article titles and abstracts was performed. A total of 118 articles were obtained, of these 116 original research articles and 2 conference articles. Those articles that aligned with the objectives of the present study were selected and managed in the free license software Mendeley desktop v1.19.8. These were downloaded, analyzed, and diagrammed using computer tools such as Microsoft Excel, Word, QGis, VOSviewer, and Python as programming languages through the: Os, Pandas, Matplotlib, Seaborn, WordCloud, and PyPDF2 libraries. To generate the distribution map of the studies that are part of the analysis of this manuscript, information on the year of publication, country of the institution of the first author was used, the base shape of the map was downloaded from the UN page and worked in the QGis v2.0.2 tool. Then the extracted information from the papers was analyzed and diagrammed, and finally, a work flowchart was proposed.
2.1 Geographical analysis
The geographic distribution of articles published between 2019 and 2024, and focused on the biodegradation of plastic used in agriculture are presented in Figure 2. The data indicate a significant concentration of studies in Asia, particularly in China and India, which together account for 68 studies; these countries with the highest number of research also stand out for their substantial production of plastics. Between May 2021 to May 2024 alone, China produced 6.52 million metric tons of plastic products (Statista, 2024a). Similar is the case with India, which in 2022 produced a volume of 1.7 million metric tons of plastics (Statista, 2024b). Continents such as Oceania and South America present few studies related to the subject, while Oceania presents only one study during the period analyzed. This disparity highlights the low commitment to research on the biodegradation of plastics and underlines the need for a broader commitment to address plastic pollution fully. In Europe, a more balanced distribution of studies is observed, with countries such as Germany, Spain, and the UK contributing notably (Figure 2). This trend may be related to stringent environmental policies and research funding in the European Union, which promotes sustainable solutions for plastic waste management. In Canada, research activity is evident, aligning with its national policies to reduce plastic waste and promote a circular economy (CEPA, 1999). In addition, the map in Figure 2 reveals a growing contribution from developing countries, particularly in Africa and Latin America. In this case, countries such as Brazil and South Africa are beginning to emerge in the field of plastic biodegradation research, possibly driven by the need to manage large amounts of plastic waste and the implementation of stricter environmental policies; they also receive foreign funding as part of biodiversity conservation efforts driven by plastic-producing countries.
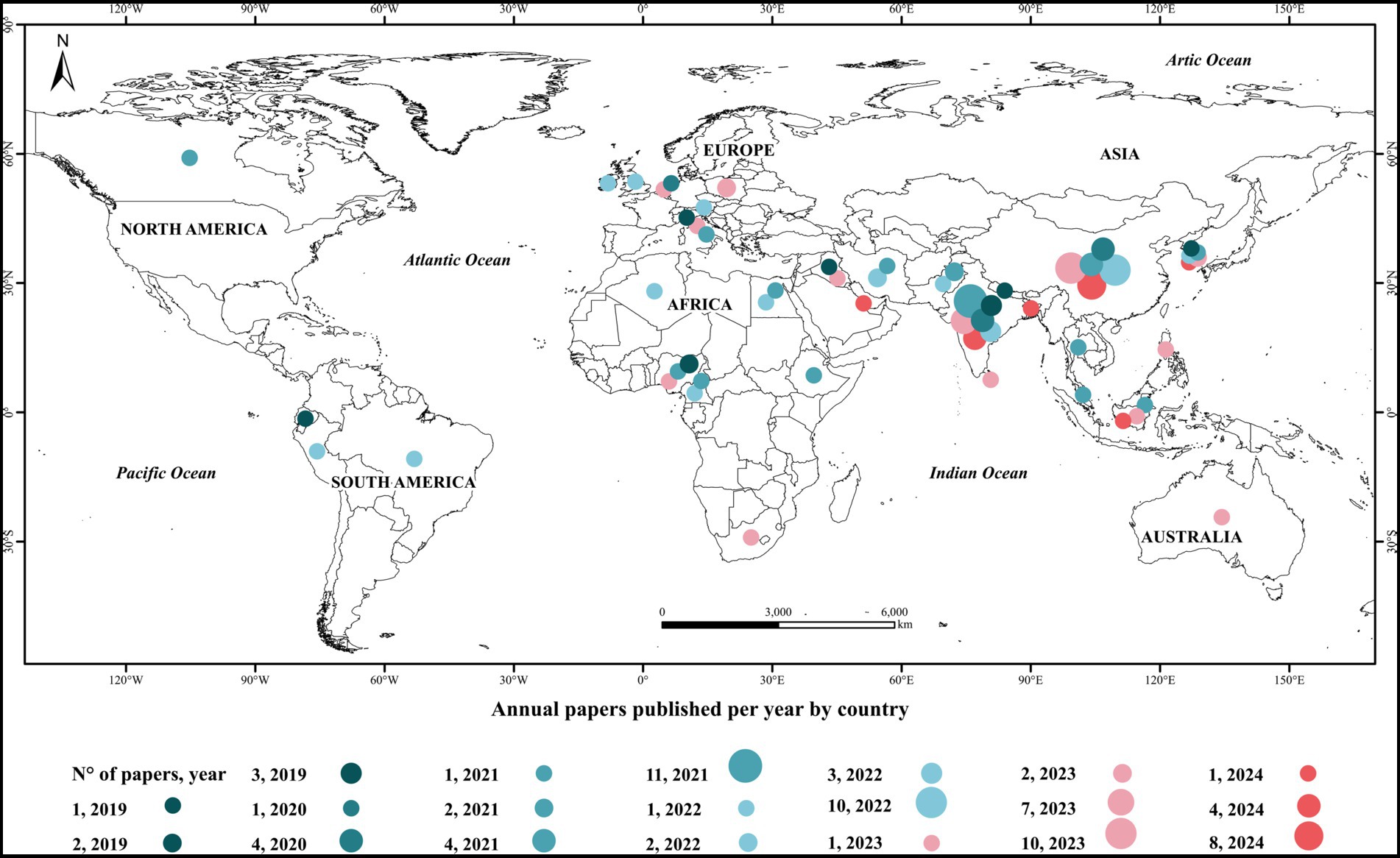
Figure 2. World distribution map of polyethylene biodegradation articles published from 2019 to 2024.
The uneven distribution of research also reflects differences in the technological capacity and resources available for research in different regions. While developed countries have access to more funding and advanced technologies (Hsu et al., 2022), developing countries face challenges related to infrastructure and funding, which limits their ability to conduct high-quality research (Orona-Návar et al., 2022). In this context, it is essential to continue fostering international collaboration to share knowledge and strengthen human talent and technologies that can accelerate progress in plastics biodegradation research. Cooperative programs and joint funding can help to balance regional management inequalities and promote global solutions to plastic pollution.
2.2 Keywords
The graph generated in VOSviewer represents a co-occurrence map of terms used in titles and keywords related to the biodegradation of LDPE and other plastics in articles published between 2019 and 2024. The largest and most central nodes, such as “Biodegradation,” “Polyethylene” and “LDPE,” indicate that these terms are the most mentioned and relevant in the analyzed articles. The connections between the nodes reflect the frequency with which these terms appear together in the same studies, suggesting a strong interrelationship between the concepts. Likewise, terms in the green cluster, such as “FTIR,” “GC–MS,” “SEM” and “Fungi,” indicate a focus on analytical techniques and organisms associated with biodegradation. Their prominence is related to methodologies used to assess plastic degradation after various treatments. The blue cluster, which includes terms such as “Microplastics,” “Polypropylene” and “Isolated,” highlights research focused on the degradation of different plastics and microorganisms. The pink group, which contains terms such as “Polystyrene,” “Tenebrio molitor” and “Depolymerization,” reflects an interest in the degradation of specific plastics and associated degradation mechanisms (Figure 3).
3 Industrial biodegradation
Industrial biodegradation offers a critical solution for managing polyethylene (PE) waste by utilizing controlled environments to optimize the activity of microbial consortia and enzymatic systems. This approach capitalizes on advanced methodologies to address the limitations of natural biodegradation in uncontrolled settings. Constant developments highlight the use of enzyme-facilitated processes, such as those employing oxidoreductases and laccases, which break down complex polymers into simpler, more manageable compounds. These enzymes, often derived from marine microorganisms, exhibit remarkable stability under industrial conditions, including variable temperatures, pH, and salinity, making them highly suitable for large-scale applications (Ayilara and Babalola, 2023; Sivaperumal et al., 2017). Additionally, specialized bioreactors have been developed to enhance microbial activity, ensuring consistent degradation rates and efficient conversion of plastic into non-toxic byproducts, such as carbon dioxide and water (Verma and Jaiswal, 2016). The effectiveness of industrial biodegradation is further amplified by leveraging microbial consortia capable of synergistic actions. Bacterial and fungal strains have demonstrated the ability to depolymerize PE and similar materials, transforming them into oligomers that are subsequently mineralized. The controlled nature of industrial processes also allows for the use of genetically modified organisms, tailored to target specific types of plastic waste, thereby maximizing efficiency and minimizing residual contamination. Moreover, advancements in bioprocess technology, including the integration of co-metabolism strategies, have shown significant promise in enhancing degradation rates, particularly for recalcitrant plastics (Rüthi et al., 2023).
4 Polyethylene biodegradation
In this section, the principal findings are described and summarized in Figure 4. In addition, the organization of data to make this section in Supplementary Table S1 which contains details of various organisms or substances and their origins, as well as corresponding pre-treatments, conditions, treatments, controls, evaluation times, and techniques used to measure degradation and identification. For each entry, the purpose of the techniques and the results are provided, with their references included for sources of data.
4.1 Organisms involved in the biodegradation of plastics
The degraders reported in the analysis period include bacteria (Table 1), fungi (Table 2), microalgae, microbial consortia, and insect larvae (Table 2), among others. Some of these microorganisms have been employed continuously while others only in specific years (Supplementary Figure S1). In the case of bacteria, they have been used consistently every year, reflecting their sustained effectiveness in plastic biodegradation. Fungi, although less frequent, have shown intermittent use, while microbial consortia, which combine bacteria and fungi, have recently gained interest because they combine the metabolic capabilities of several species to achieve more efficient biodegradation. These consortia can produce complementary enzymes that fragment plastic polymers faster and more effectively than individual microorganisms. Their ability to form biofilms improves the adhesion and decomposition of plastics, especially when their activity is stimulated with oxidizing agents such as H2O2, which enhances the production of key enzymes such as peroxidases (Skariyachan et al., 2021; Fachrul et al., 2024; Mohammadi et al., 2022) On the other hand, larvae have been occasionally employed, highlighting their potential in certain specific contexts (He et al., 2024; Burd et al., 2023; Yang et al., 2021) and algae (Sanniyasi et al., 2021) which represent an innovative strategy in LDPE biodegradation have also been used sporadically. This temporal distribution highlights the diversification of the methodologies applied to biodegradation in recent years, with an increasing trend toward the use of microbial combinations, as well as the exploration of new degraders such as larvae and algae.
4.2 Comparison between different agents
Studies on LDPE biodegradation demonstrate varying efficiencies depending on the organism and conditions. Weight loss percentages range from 1.50 to 88.50%, with Zophobas atratus larvae achieving the highest reduction (88.50% in 45 days) (Wang et al., 2022). Fungi such as Aspergillus niger (Sáenz et al., 2019), and bacteria like Alcaligenes faecalis (Nag et al., 2021), also show promising results, with weight loss exceeding 20% in specific setups. These results highlight the diverse potential of biological agents in plastic degradation and the differences in percentages of weight loss (Table 3).
Each type of organism has unique degradation capabilities that are influenced by its origin, environmental conditions, and previous treatments. Table 4 presents some advantages and disadvantages of the different organisms in the process of biodegradation of plastics. In the case of degrading bacteria, they commonly belong to genera such as Pseudomonas, Bacillus, Streptomyces and Rhodococcus. Pseudomonas putida (Li et al., 2024), is known for its ability to degrade polyesters, whereas Bacillus subtilis (Kumar Gupta and Devi, 2019), has shown successful degradation of polystyrene. Fungi have a significant advantage due to their ability to secrete large amounts of enzymes such as; peroxidase, and lignin peroxidase enzymes outside their cells, which allows the degradation of complex polymers in the immediate environment (Wang et al., 2019); this characteristic has enabled multiple assays in plastic degradation. One example is Rhodotorula mucilaginosa, a fungus isolated from marine sediments.
In recent studies, this fungus was exposed to polyethylene treated with ultraviolet (UV) radiation, a pretreatment that favors plastic biodegradation (Giyahchi and Moghimi, 2023). Therefore, the ability of fungi to degrade plastics is not limited to only R. mucilaginosa species, but also to other fungi, such as the genera Aspergillus, Penicillium, and Fusarium, as they have also shown potential in the biodegradation of different types of plastics. For example, Aspergillus niger (Fachrul et al., 2024), has been reported for its ability to degrade polyurethane, while Penicillium chrysogenum (Khan et al., 2023), and Fusarium solani (Wróbel et al., 2023), have shown effectiveness in the degradation of polyethylene and polypropylene. Certain microorganisms can produce compounds that facilitate the decomposition of plastic by other organisms in the consortium, this is due to the release of compounds such as organic acids, peroxides, and other secondary metabolites (Tiwari et al., 2024; Wang et al., 2023), which modify the structure of the plastic and make it more susceptible to enzymatic degradation.
As for the enzymes, laccase, peroxidase, and cutinase (Gao et al., 2022; Tamnou et al., 2022), are some of the most studied in this context. These enzymes can break the chemical bonds of plastic polymers, facilitating their decomposition into monomers and other simpler compounds, such as carbon and carbon dioxide (CO2). Often, this material is integrated into the organism as part of its carbon source. However, the production of these enzymes may be limited by factors such as nutrient availability, pH, temperature, and the presence of specific inducers, such as plastid-related substrates and cofactors necessary for enzymatic activity (Khandare et al., 2022; Li et al., 2023).
The biodegradation of plastics using larvae has emerged as a novel and promising approach. Various insect species, in particular the larvae of certain beetles and flies, have demonstrated the ability to degrade plastics through their digestive processes. This approach not only offers an environmentally friendly alternative for plastic waste management but can also be integrated with sustainable agricultural practices. One documented case is the beetle Tenebrio molitor, commonly known as the mealworm. Larvae of T. molitor can degrade plastics such as PS and PE (Wang et al., 2023; Wang et al., 2024). This process is facilitated by the presence of symbiotic microorganisms in the larval digestive system, which produces enzymes such as alkane monooxygenase (Zaman et al., 2024), and laccase (Kundungal et al., 2021), that are capable of breaking down these polymers into smaller carbon chains and sometimes into CO2 and water. T. molitor larvae can consume approximately 34–39 mg of EPS, a plastic widely used in containers and packaging (Wang et al., 2022). As a result, EPS is oxidized and fragmented, with a significant reduction in its molecular weight. However, many times plastic can be integrated into the same organism of larvae in the form of microplastics and nanoplastics, and although it may seem insignificant this can be a problem since the end of these larvae can be food for other larger organisms and indirectly can expand and integrate into their organism (Yang et al., 2021).
Another species, that has shown potential in the biodegradation of plastics, is the black soldier fly (Hermetia illucens). The larvae of H. illucens are known for their ability to break down organic matter and have been studied for their ability to degrade bioplastics such as polyhydroxyalkanoate (PHA) and polylactate (PLA), breaking down PLA into simpler compounds such as lactic acid and CO2 (Wang et al., 2024). Therefore, this helps us to understand that the efficiency of larvae in biodegrading plastics is enhanced by the synergy between insects and the microorganisms present in their gut. Symbiotic bacteria and fungi produce a variety of hydrolytic enzymes, such as esterase, cutinase, and lipase, which are essential for the degradation of plastic polymers. In addition, the mechanical action of chewing and the intestinal movement of the larvae facilitates the fragmentation of the plastic, increasing the surface area exposed to enzymatic action, this process although not a decomposition is an important part of subsequent biodegradation processes (Ma et al., 2023).
The integration of plastic-degrading larvae in agricultural systems has multiple benefits. Not only does it contribute to reducing plastic waste in the agricultural environment, but it also produces valuable by-products such as frass, the so-called “insect excrement,” which can be used as an organic fertilizer. The resulting frass is applied to crops as fertilizer, and although the mature larvae can be fed for poultry or fish, creating a more sustainable and circular agricultural system, (Peng et al., 2022). However, the effect of possible micro and nano plastics on larvae should be studied in detail. Therefore, an integrated system model in on-farm plastic waste management could include the use of T. molitor larvae, where agricultural plastics such as PE mulch can be degraded in situ. Despite the promising potential, using larvae for biodegradation of plastics faces several challenges. One of the main ones is optimizing culture conditions to maximize degradation efficiency. In addition, it is necessary to ensure that micro-and nanoplastics do not enter the food chain, due to their negative effects such as drug resistance and new diseases (Shi et al., 2024).
Supplementary Figure S2 presents a comparative analysis of the average evaluation time for different organisms or substances used in the biodegradation of polyethylene. A great variability in the evaluation times is observed, which reflects both the diversity of the organisms studied and the complexity of the biodegradation processes. Some microorganisms such as Brevibacillus brevis (Tiwari et al., 2023), and the soil microbiome (Ma et al., 2023; Jayan et al., 2023; Maddison et al., 2023; Peng et al., 2023; Yuan et al., 2023; Zhang et al., 2023), stand out as having significantly long evaluation times, exceeding 450 days. This suggests that these microorganisms may have a slow biodegradation process, or that studies conducted with them have required a prolonged time to observe significant results. In contrast, the fungus Rhizopus sp. (Gupta and Devi, 2020), and the beetle Zophobas atratus (He et al., 2024; Yang et al., 2021; Lou et al., 2021; Sanluis-Verdes et al., 2022), show evaluation times in the range of 20–60 days, which could indicate higher biodegradation efficiency or studies designed for faster results. Different strains of Z. atratus and their combinations with other organisms such as Tenebrio molitor show a wide range of evaluation times, suggesting that the interaction between species and the specificity of the organisms used have a significant impact on biodegradation efficiency and the conditions they require.
Another notable aspect is the case of the bacterium Gordonia polyisoprenivorans (Wang et al., 2023), which has an intermediate evaluation time, which could be related to its specialized capabilities in the degradation of complex polymers but requires considerable time to demonstrate its effectiveness. The use of microbial consortia, such as Bacterial-Fungal Consortium (BFC) (Li et al., 2024; Adithama et al., 2023; Bao et al., 2023; Gong et al., 2023), seems to offer a robust strategy, with more efficient assessment times compared to some individual microorganisms, suggesting that cooperation between different types of microorganisms could accelerate the biodegradation process, so the outlook for such studies is encouraging, although interactions between different taxonomic groups could result in antagonism and competition, requiring careful observations to create partnerships that can work successfully. A deducible rule of thumb is that more specialized organisms, such as certain fungi and bacteria, tend to require less time for evaluation, whereas microbial consortia and microorganisms working in more complex environments may need longer times to show results. However, this may also indicate that, in the future, the development of optimized consortia and genetic engineering techniques could significantly reduce these times, making biodegradation faster and more efficient. It is worth mentioning that for practicality a single study that evaluated Soil microbiome and climatic conditions for 2,556 days was not represented in Supplementary Figure S2, more details in Supplementary Table S1.
5 Aspects of LDPE in biodegradation processes
5.1 Mechanism of biodegradation
Microorganisms, including bacteria and fungi, attach to the polymer surface using biofilm-forming mechanisms and secrete compounds like exopolysaccharides and surfactants to further degrade the structure (Sáenz et al., 2019; Elsamahy et al., 2023). During biofragmentation, extracellular enzymes such as hydrolases, laccases, and esterases break down long polymer chains into smaller oligomers and monomers (Li et al., 2024). The efficiency of this stage depends on the chemical composition, crystallinity, and hydrophobicity of the polymers, as well as the availability of cofactors like oxygen and nutrients. Once fragmented, the smaller compounds are taken up by microbial cells in the assimilation stage, where they undergo intracellular metabolic processes to produce energy, biomass, and intermediate metabolites (He et al., 2024). Factors like microbial metabolic diversity, the availability of electron donors and acceptors, and community interactions significantly influence this stage (Sunny and Saleena, 2020). The final phase, mineralization, involves the complete degradation of organic matter into inorganic molecules such as carbon dioxide, water, and methane (El-Sayed et al., 2021; Zahari et al., 2021; Khan et al., 2023). This stage is heavily influenced by environmental parameters, including pH, redox potential, and oxygen availability, which dictate whether the process occurs under aerobic or anaerobic conditions (Obaid and AL-Jawhari, 2023). In addition to these environmental factors, microbial interactions play a critical role in enhancing biodegradation efficiency. Cooperative relationships, such as cross-feeding and enzyme complementation, improve substrate utilization, while competitive interactions can reduce efficiency by limiting resource availability (Pinto et al., 2022). Operational parameters, such as temperature, moisture content, agitation, and nutrient supplementation, are also key drivers of biodegradation outcomes. These factors, extensively studied in the literature, underline the complexity of the process, demonstrating how microbial and environmental conditions synergistically dictate the transformation of polymers into simpler, environmentally benign products, such as carbon dioxide, water, and biomass (Temporiti et al., 2022; Thomas Zumstein et al., 2018; He et al., 2024; Figure 1).
5.2 Parameters affecting biodegradation efficiency
The parameters influencing biodegradation efficiency in the reviewed studies are diverse and often interdependent, highlighting the complexity of this process. Key variables include microbial interactions, operating conditions, and substrate-specific characteristics. For instance, Rhodotorula mucilaginosa demonstrated varied efficiency depending on the UV pre-treatment duration and the addition of 13C-PE as a sole carbon source (Vaksmaa et al., 2023). Similarly, the inclusion of enriched media, such as mineral salt broth inoculated with Gordonia polyisoprenivorans, enhanced LDPE degradation over 35 days, emphasizing the role of nutrient availability and sterilization methods (Tiwari et al., 2023) incorporating microbial consortia, such as Bacillus licheniformis and Achromobacter xylosoxidans, further underscore the significance of synergistic microbial interactions, which enhance degradation pathways (Fachrul et al., 2024). Environmental conditions also critically impact biodegradation outcomes. Temperature, pH, and agitation significantly influenced results, as seen with Bacillus subtilis, which required optimized incubation parameters to achieve notable LDPE degradation (Mohammadi et al., 2022). Furthermore, pre-treatment processes, such as UV irradiation or chemical oxidation, were consistently noted to enhance microbial attachment and enzymatic activity, as demonstrated in Microbulbifer hydrolyticus experiments with LLDPE particles (Li et al., 2020), in the S1 the columns “Organism or substance and its origin,” “Type of degrader,” “Pre-treatment condition and initial size,” “Treatments,” and “Evaluation Time” can give an extent analysis about this point.
5.3 Forms of LDPE undergoing biodegradation
In LDPE biodegradation studies, the physical form of the plastic plays an important role in the interaction with the degrading organisms. The different presentations of the plastic, such as films, powder, sheets, beads, granules, and foams, among others (Supplementary Table S2), affect the surface area available for adhesion and subsequent degradation. In the publications analyzed, 46% used plastics in the form of films (Supplementary Figure S3), this form offers a larger surface area in contact, which favors adhesion and colonization (Skariyachan et al., 2021; Huang et al., 2022; Jeon et al., 2021; Kong et al., 2024; Puglisi et al., 2019). Their advantage lies in their similarity to agricultural plastics, such as mulching films, making them relevant models in these studies. Their disadvantage is their thickness and low resistance to aggressive pretreatment, which may disintegrate them before completing biodegradation. The plastic powder was 18.4%, and the reduced particle size positively influences the rapid interaction with microorganisms (Tiwari et al., 2023; Bernat et al., 2023; Montazer et al., 2021; Pathak and Navneet, 2023; Yang et al., 2022). Their limitation is the additional mechanical pretreatment for their production, hindering complexity in the practical application of the biodegradation process in the agricultural sector. 16.1% report the use of sheets, which are more manageable and have greater durability compared to pretreatments. In some cases, they may take longer to degrade due to their thickness (Pinto et al., 2022; Zaman et al., 2024; Yuan et al., 2023; Hou et al., 2022; Joshi et al., 2022; Mishra et al., 2024), Bags with 6.9% represent a high surface-to-volume ratio, which favors biodegradation. The main difficulty of this form is the uniformity of the experimental conditions and their respective comparisons with other studies, taking into account the different sizes and thicknesses (Jayan et al., 2023; Gerritse et al., 2020; Mohy Eldin et al., 2022; Nademo et al., 2023; Nnaji et al., 2021; Poma et al., 2022) LDPE foams with 5.75% present a porous structure that increases the interaction with microorganisms, allowing better penetration of nutrients and microbial enzymes, and accelerating the biodegradation process. Its limitation is the difficulty of the precise quantification of the degraded material (Yang et al., 2021; Peng et al., 2022; Lou et al., 2021; Wang et al., 2022), Granules (4.6%) and beads (2.3%), being more compact in shape, reducing the surface area available for microbial colonization, reducing biodegradation efficiency. However, as they are easy to manipulate and quantify, they are useful in small-scale studies (Khandare et al., 2022; Adithama et al., 2023; Dey et al., 2020; Kunlere et al., 2019; Zadjelovic et al., 2022).
5.4 Pretreatment of LDPE under biodegradation
Most studies report the pretreatment of the plastic in the biodegradation process, taking into account that this increases its susceptibility to microbial degradation. One of the most common treatments is exposure to UV radiation, a process that partially degrades the plastic, facilitating its decomposition by modifying its physical and chemical properties (Yuan et al., 2023; Chaudhary et al., 2023; Min et al., 2020; Montazer et al., 2020). To mention an example, LDPE treated with UV radiation for 48 h develops carbonyl groups and peroxides on its surface, which enhances the adhesion and activity of bacteria isolated from landfills, resulting in higher production of degrading enzymes and a higher biodegradation rate compared to untreated plastics (Soleimani et al., 2021). In the case of polyester, chemical treatment with nitric acid facilitates its decomposition by bacteria of the genus Pseudomonas. Culture conditions also play a key role in biodegradation, as factors such as optimum temperature (30°C for HDPE), pH (7.0 for polystyrene), and the availability of nutrients, such as glucose, influence microbial activity (Ji et al., 2023).
5.5 Growing conditions
By using plastic as the sole or main carbon source, a selective pressure is induced that forces the organism to consume the polymer, which allows observing its behavior and evolution under these challenging conditions. Culture conditions, such as temperature, pH, and nutrient availability, are critical factors affecting microbial activity and the efficiency of plastic biodegradation. Most studies maintain controlled conditions to optimize the activity of the degrading organisms, varying these conditions according to the type of organism and plastic to be degraded. Aderiye et al. (2019), in their study published in 2023 found that the optimum temperature for the biodegradation of HDPE by bacteria isolated from a waste landfill was 30°C. On the other hand, Chaudhary et al. (2023), found that a pH of 7 was optimal for the enzymatic activity of Pseudomonas fluorescens in the biodegradation of polystyrene. DSouza et al. (2021), showed that the addition of glucose in the experimental setups as an additional carbon source increased the rate of polypropylene biodegradation by Aspergillus niger. Oxygen concentration is another crucial factor that can influence the biodegradation of plastics. In one study, bacteria of the genus Bacillus were found to be more effective in the degradation of polyethylene under aerobic conditions, while bacteria of the genus Clostridium showed higher efficiency under anaerobic conditions (Islami et al., 2019; Fibriarti et al., 2021).
5.6 Limitations and considerations in the biodegradation of polyethylene in the agricultural system
Biodegradation of polyethylene in agricultural systems faces limitations due to the chemical nature of this polymer and the specific conditions of agricultural environments. PE, widely used in agricultural plastics such as mulching and coverings, has a molecular structure with highly stable carbon–carbon bonds, which makes its biological decomposition difficult (Hou et al., 2022). In these systems, soil organisms, such as larvae, and microorganisms such as fungi and bacteria, must compete with other more accessible carbon sources, which reduces the metabolic priority of PE and slows down its biodegradation (Yang et al., 2021; Montazer et al., 2020). Environmental factors such as temperature, pH, humidity, and nutrient availability vary considerably in the soil, limiting the enzymatic activity necessary for polymer decomposition (Montazer et al., 2020). In many cases, PE requires pretreatments to increase its bioavailability to microorganisms. Exposure to UV radiation or the use of oxidizing agents can partially break the polymer chains, increasing the susceptibility of the plastic to biodegradation (Yuan et al., 2023). However, these pretreatments not only increase operational costs but can also generate byproducts that alter soil microbial dynamics or negatively impact the quality of the agricultural ecosystem. Smaller fragments, such as microplastics generated by partial degradation of mulch, can be integrated into the soil, which presents a double challenge: on the one hand, they increase the contact surface for microorganisms, but on the other, they pose ecological risks by being transported to other parts of the ecosystem and incorporated into food chains. Intermediate products generated during biodegradation, such as low molecular weight compounds, must be assessed at a toxicological level, to ensure that they do not interfere with soil health or crops (Nelson et al., 2022).
6 Biodegradation measurement techniques
Table 5 presents a summary of the main techniques used in the measurement of biodegradation of plastics, describing the purpose and expected results of each. These techniques range from FTIR, which can identify changes in the functional groups and chemical structure of the material, to SEM, which focuses on observing physical changes and the formation of biofilms on the surface of plastics. In addition, techniques such as EDS and XPS are used to analyze elemental composition and chemical properties, while TGA and DSC evaluate the thermal stability of materials undergoing biodegradation. HPLC and GC–MS allow the identification of specific degradation products, such as organic acids and complex compounds, while the use of XRD and PY-GC–MS focuses on changes in crystallinity and differences in the chemical composition of treated polymers. The evolution of CO2 is also measured to assess the amount of gas released as an indicator of biodegradation, carbon conversion, and mineralization.
7 Considerations for control of biodegradation test results
To develop a consolidated methodology to maximize the efficiency of plastic biodegradation in agriculture, it is essential to consider several key factors: proper selection of degrading organisms, pretreatment of the plastic, and the use of accurate analytical techniques. A roadmap for the plastic biodegradation process is proposed in Figure 5. The selection of degrading organisms should be based on their ability to produce specific enzymes that can degrade the type of plastic in question. The combination of fungi and bacteria isolated from marine and terrestrial environments is effective in many studies (Fachrul et al., 2024; Wróbel et al., 2023; Mohy Eldin et al., 2022; Chigwada et al., 2023; Maheswaran et al., 2023). The use of microbial consortia, which include multiple species with complementary capabilities, can significantly improve biodegradation efficiency, or a specific strain or organism that needs to be tested as a plastic biodegrader must also be chosen. Pretreatment of plastic, such as UV exposure, thermal oxidation, and chemical treatment, can significantly increase its susceptibility to biodegradation. These treatments should be optimized for different types of plastics and experimental conditions to maximize their effectiveness. Further research should evaluate the effectiveness of these pretreatments in combination with microbial biodegraders to develop integrated and efficient processes.
Culture conditions must be controlled to optimize microbial activity and the production of degradative enzymes. Factors such as temperature, pH, nutrient availability, and oxygen concentration must be adjusted to maximize biodegradation efficiency. Continued research on optimizing these conditions for different microorganisms and types of plastics is crucial. The use of advanced analytical techniques is essential to assess the efficiency and extent of biodegradation. Techniques such as GC quadrupole mass spectrometry, GC flame ionization detection, FTIR, and SEM should be used to analyze changes in the chemical structure and morphology of the plastic during biodegradation. Despite significant advances in LDPE biodegradation, several areas require further research to improve the efficiency and applicability of these technologies. It is crucial to conduct comprehensive studies on the environmental impact and economic feasibility of LDPE biodegradation processes on an industrial scale. This includes assessing the toxicity of degradation products and the costs associated with the implementation of biodegradation technologies in different industrial contexts.
8 Perspectives in plastics biodegradation
The future of plastic biodegradation depends on the advancement of biotechnology, genetic engineering, and the implementation of sustainable solutions on a large scale. It is not enough to optimize degradation processes; it is essential to design, develop, and apply more efficient and cost-effective strategies that are applicable in various sectors such as agriculture, industry, and urban waste management. The application of genetically improved microorganisms may be an option for the development of more efficient, specific, and stable enzymes for the degradation of polymers such as polyethylene. The immobilization of enzymes on solid supports offers opportunities for their reuse and for continuous biodegradation processes. Biodegradation could be integrated with physical–chemical recycling procedures, within a holistic circular economy approach. Biodegradation derivatives, such as carbon dioxide, biomass, and/or recovered monomers, could be reused as inputs in production chains, thus ending the plastics cycle. For plastic biodegradation to be implemented, obstacles related to production costs and government regulations must be overcome.
9 Final reflections
From this review, it is established that it is essential to consider several measurements to assess biodegradation, covering different aspects: in the physical area, it is necessary to control the mass by measuring the percentage of weight lost from the plastic, make direct observations using SEM to monitor physical changes, and evaluate the level of crystallinity using TGA; in the chemical aspect, it is essential to use chromatography techniques such as HPLC and GC–MS to identify and quantify new compounds generated during biodegradation; and in the biological area, metabolic tests and identification of metabolic pathways should be carried out, applying omics sciences to study the genes and enzymes involved, as well as the interactions that are crucial, especially in the case of larvae whose microbiome plays a key role in biodegradation, since it was observed that there was a decrease in degradation capacity when antibiotics were applied to the larvae before subjecting them to the plastic biodegradation test. On the other hand, it is also important to evaluate the long-term sustainability of the consortia when trials with more than one microorganism are proposed. This integrated approach will allow a deeper and more accurate understanding of the biodegradation process, encompassing both the physical and chemical changes as well as the biological and microbial aspects involved.
According to the analysis of the methodologies currently used the most used organisms in recent years have been listed. Despite the progress made in the biodegradation of LDPE and other plastics used in everyday life including agriculture, fundamental aspects remain to be explored. Current research has identified promising organisms and methods, but it is clear that more in-depth and diversified studies are required to fully understand microbial interactions and their effectiveness under different environmental conditions. In addition, the disparity in the geographic distribution of research suggests an urgent need for more inclusive approaches that consider the global applicability of these technologies, especially in regions with fragile ecosystems. Furthermore, studies on microplastics and nanoplastics, i.e., on material undergoing biodegradation, are essential, since their effects can still be harmful and further treatments must be found for their complete mineralization. Therefore, this work lays a solid foundation but also leaves open several questions that future research will need to address to develop more effective biodegradable solutions adapted to various contexts. The remaining challenges include the need to deepen the understanding of the complex interactions between different microbial consortia, as well as their relationships with the environment, the enzymes involved, and their long-term efficacy under varying environmental conditions.
Detail of abbreviations in Supplementary Table S3.
Author contributions
JM: Conceptualization, Data curation, Formal analysis, Funding acquisition, Investigation, Methodology, Project administration, Resources, Software, Validation, Visualization, Writing – original draft, Writing – review & editing. DT: Data curation, Supervision, Writing – review & editing. BC-C: Data curation, Writing – review & editing. NA-M: Data curation, Visualization, Writing – review & editing. VT-M: Resources, Supervision, Writing – review & editing. JT-C: Data curation, Writing – review & editing. ET: Data curation, Writing – review & editing. DG-F: Data curation, Writing – review & editing. MG: Supervision, Writing – review & editing. MR-R: Conceptualization, Data curation, Formal analysis, Funding acquisition, Investigation, Methodology, Project administration, Resources, Software, Validation, Visualization, Writing – original draft, Writing – review & editing.
Funding
The author(s) declare that financial support was received for the research, authorship, and/or publication of this article. This research was funded by the Instituto Nacional de Innovación Agraria (INIA) through an Investment Project with CUI No. 2472675 entitled: “Mejoramiento de los servicios de investigación y transferencia de tecnología agraria en la estación agraria experimental Baños del Inca en la localidad de Baños del Inca del distrito de Baños del Inca - provincia de Cajamarca - departamento de Cajamarca”.
Conflict of interest
The authors declare that the research was conducted in the absence of any commercial or financial relationships that could be construed as a potential conflict of interest.
Generative AI statement
The authors declare that no Gen AI was used in the creation of this manuscript.
Publisher’s note
All claims expressed in this article are solely those of the authors and do not necessarily represent those of their affiliated organizations, or those of the publisher, the editors and the reviewers. Any product that may be evaluated in this article, or claim that may be made by its manufacturer, is not guaranteed or endorsed by the publisher.
Supplementary material
The Supplementary material for this article can be found online at: https://www.frontiersin.org/articles/10.3389/fmicb.2024.1510817/full#supplementary-material
References
Aderiye, B. I., Akinyeye, R. O., Sulaimon, A., Oluwole, O. A., Kehinde, F. J., Ojo, O. E., et al. (2019). Monitoring fungal biodegradation of low-density polyethylene [LDPE] from plastic wastes dump sites using FT-IR spectra. Microbiol. Res. J. Int. 26, 1–15. doi: 10.9734/MRJI/2018/44851
Adithama, R. M., Munifah, I., Yanto, D. H. Y., and Meryandini, A. (2023). Biodegradation of low-density polyethylene microplastic by new halotolerant bacteria isolated from saline mud in Bledug Kuwu, Indonesia. Bioresour. Technol. Rep. 22:101466. doi: 10.1016/j.biteb.2023.101466
Ali, A., Bahadar, A., Khan, A., and Sanaullah, K. (2018). “Role of agricultural waste in recycled plastic biocomposites” in Recycled plastic biocomposites (Elsevier), 165–194. Available at: https://www.sciencedirect.com/science/article/pii/B978032388653600002X
Ali, S. S., Elsamahy, T., Zhu, D., and Sun, J. (2023). Biodegradability of polyethylene by efficient bacteria from the guts of plastic-eating waxworms and investigation of its degradation mechanism. J. Hazard. Mater 443:130287. doi: 10.1016/j.jhazmat.2022.130287
Ayilara, M. S., and Babalola, O. O. (2023). Bioremediation of environmental wastes: the role of microorganisms. Front. Agron. 5, 1–15. doi: 10.3389/fagro.2023.1183691
Bao, T., Qian, Y., Xin, Y., Collins, J. J., and Lu, T. (2023). Engineering microbial division of labor for plastic upcycling. Nat. Commun. 14:5712. doi: 10.1038/s41467-023-40777-x
Bernat, P., Jasińska, A., Niedziałkowska, K., Słaba, M., Różalska, S., Paraszkiewicz, K., et al. (2023). Adaptation of the metolachlor-degrading fungus Trichoderma harzianum to the simultaneous presence of low-density polyethylene (LDPE) microplastics. Ecotoxicol. Environ. Saf. 267:115656. doi: 10.1016/j.ecoenv.2023.115656
Buchholz, P. C. F., Feuerriegel, G., Zhang, H., Perez-Garcia, P., Nover, L. L., Chow, J., et al. (2022). Plastics degradation by hydrolytic enzymes: the plastics-active enzymes database—PAZy. Proteins Struct. Funct. Bioinf. 90, 1443–1456. doi: 10.1002/prot.26325
Burd, B., Mussagy, C., de, J., Tanaka, J., Scontri, M., Brasil, G., et al. (2023). Galleria Mellonella larvae as an alternative to low-density polyethylene and polystyrene biodegradation. J. Polym. Environ. 31, 1232–1241. doi: 10.1007/s10924-022-02696-8
CEPA (1999). Canadian Environmental Protection Act : Government of Canada, Canadá Available at: https://laws-lois.justice.gc.ca/eng/acts/c-15.31/ (Accessed September 17, 2024).
Chaudhary, A. K., Chaitanya, K., Dalmia, R., and Vijayakumar, R. P. (2021). Synergistic effect of UV, thermal, and chemical treatment on biological degradation of low-density polyethylene (LDPE) by Thermomyces lanuginosus. Environ. Monit. Assess. 193, 1–11. doi: 10.1007/s10661-021-09296-4
Chaudhary, A. K., Chitriv, S. P., Chaitanya, K., and Vijayakumar, R. P. (2023). Influence of ultraviolet and chemical treatment on the biodegradation of low-density polyethylene and high-density polyethylene by Cephalosporium strain. Environ. Monit. Assess. 195:395. doi: 10.1007/s10661-023-10982-8
Chigwada, A. D., Ogola, H. J. O., and Tekere, M. (2023). Multivariate analysis of enriched landfill soil consortia provide insight on the community structural perturbation and functioning during low-density polyethylene degradation. Microbiol. Res. 274:127425. doi: 10.1016/j.micres.2023.127425
Correa-Cano, M. E., Burton, K., Mueller, M., Kouloumpis, V., and Yan, X. (2023). Quantification of plastics in agriculture and fisheries at a regional scale: a case study of south West England. Recycling 8:99. doi: 10.3390/recycling8060099
Dey, A. S., Bose, H., Mohapatra, B., and Sar, P. (2020). Biodegradation of Unpretreated low-density polyethylene (LDPE) by Stenotrophomonas sp. and Achromobacter sp., isolated from waste dumpsite and drilling fluid. Front. Microbiol. 11, 1–15. doi: 10.3389/fmicb.2020.603210
Dimassi, S. N., Hahladakis, J. N., Chamkha, M., Ahmad, M. I., Al-Ghouti, M. A., and Sayadi, S. (2024). Investigation on the effect of several parameters involved in the biodegradation of polyethylene (PE) and low-density polyethylene (LDPE) under various seawater environments. Sci. Total. Environ. 912:168870. doi: 10.1016/j.scitotenv.2023.168870
Ekanayaka, A. H., Tibpromma, S., Dai, D., Xu, R., Suwannarach, N., Stephenson, S. L., et al. (2022). A review of the Fungi that degrade plastic. J Fungi 8:772. doi: 10.3390/jof8080772
Elsamahy, T., Sun, J., Elsilk, S. E., and Ali, S. S. (2023). Biodegradation of low-density polyethylene plastic waste by a constructed tri-culture yeast consortium from wood-feeding termite: degradation mechanism and pathway. J. Hazard Mater. 448:130944. doi: 10.1016/j.jhazmat.2023.130944
El-Sayed, M. T., Rabie, G. H., and Hamed, E. A. (2021). Biodegradation of low-density polyethylene (LDPE) using the mixed culture of aspergillus carbonarius and A. Fumigates. Environ. Dev. Sustain. 23, 14556–14584. doi: 10.1007/s10668-021-01258-7
Fachrul, M. F., Fulazzaky, M. A., Rinanti, A., Muda, K., Tazkiaturrizki, T., and Sunaryo, T. (2024). Mass transfer kinetics of polyethylene degradation by bacterial-fungal consortium. Clean. Waste Syst. 7:100136. doi: 10.1016/j.clwas.2024.100136
FAO. Breaking the plastic cycle in agriculture. (2023). Available at: https://www.fao.org/newsroom/story/breaking-the-plastic-cycle-in-agriculture/en (Accessed June 28, 2024).
Ferrero, P., Attallah, O. A., Valera, M. Á., Aleksic, I., Azeem, M., Nikodinovic-Runic, J., et al. (2022). Rendering bio-inert low-density polyethylene amenable for biodegradation via fast high throughput reactive extrusion assisted oxidation. J. Polym. Environ. 30, 2837–2846. doi: 10.1007/s10924-022-02400-w
Fibriarti, B. L., Feliatra,, Amin, B., and Darwis,. (2021). Biodegradation of ldpe plastic by local strain of Bacillus sp. isolated from dump soil of Pekanbaru, Indonesia. Biodiversitas 22, 5484–5490. doi: 10.13057/biodiv/d221232
Gao, R., Liu, R., and Sun, C. (2022). A marine fungus Alternaria alternata FB1 efficiently degrades polyethylene. J. Hazard. Mater. 431:128617. doi: 10.1016/j.jhazmat.2022.128617
DSouza, G. C., Sheriff, R. S., Ullanat, V., Shrikrishna, A., Joshi, A. V., Hiremath, L., et al. (2021). Fungal biodegradation of low-density polyethylene using consortium of aspergillus species under controlled conditions. Heliyon 7:e07008. doi: 10.1016/j.heliyon.2021.e07008
Gerritse, J., Leslie, H. A., de Tender, C. A., Devriese, L. I., and Vethaak, A. D. (2020). Fragmentation of plastic objects in a laboratory seawater microcosm. Sci. Rep. 10, 1–16. doi: 10.1038/s41598-020-67927-1
Giyahchi, M., and Moghimi, H. (2023). Aerobic biodegradation of untreated polyester–polyether urethanes by newly isolated yeast strains Exophilia sp. NS-7 and Rhodotorula sp. NS-12. Sci. Rep. 13, 1–10. doi: 10.1038/s41598-023-31639-z
Gong, Z., Jin, L., Yu, X., Wang, B., Hu, S., Ruan, H., et al. (2023). Biodegradation of low density polyethylene by the fungus Cladosporium sp. recovered from a landfill site. J. Fungi 9:605. doi: 10.3390/jof9060605
Gupta, K. K., and Devi, D. (2020). Characteristics investigation on biofilm formation and biodegradation activities of Pseudomonas aeruginosa strain ISJ14 colonizing low density polyethylene (LDPE) surface. Heliyon 6:e04398. doi: 10.1016/j.heliyon.2020.e04398
Hale, R. C., Seeley, M. E., La Guardia, M. J., Mai, L., and Zeng, E. Y. (2020). A global perspective on microplastics. J. Geophys. Res. 125. doi: 10.1029/2018JC014719
Harrat, R., Bourzama, G., Ouled-Haddar, H., and Soumati, B. (2022). In vitro and ex situ biodegradation of low-density polyethylene by a Rhizopus sp. strain isolated from a local dumpsite in north-East Algeria. Environ. Nat. Resour. J. 20, 1–10. doi: 10.32526/ennrj/20/202200026
Harshvardhan, K., and Jha, B. (2013). Biodegradation of low-density polyethylene by marine bacteria from pelagic waters, arabian sea, India. Mar. Pollut. Bull. 77, 100–106. doi: 10.1016/j.marpolbul.2013.10.025
He, Y., Deng, X., Jiang, L., Hao, L., Shi, Y., Lyu, M., et al. (2024). Current advances, challenges and strategies for enhancing the biodegradation of plastic waste. Sci. Total Environ. 906:167850. doi: 10.1016/j.scitotenv.2023.167850
He, L., Yang, S. S., Ding, J., Chen, C. X., Yang, F., He, Z. L., et al. (2024). Biodegradation of polyethylene terephthalate by Tenebrio molitor: insights for polymer chain size, gut metabolome and host genes. J. Hazard. Mater. 465:133446. doi: 10.1016/j.jhazmat.2024.133446
Hofmann, T., Ghoshal, S., Tufenkji, N., Adamowski, J. F., Bayen, S., Chen, Q., et al. (2023). Plastics can be used more sustainably in agriculture. Commun. Earth Environ. 4, 1–11. doi: 10.1038/s43247-023-00982-4
Hou, L., Xi, J., Liu, J., Wang, P., Xu, T., Liu, T., et al. (2022). Biodegradability of polyethylene mulching film by two Pseudomonas bacteria and their potential degradation mechanism. Chem. Int. 286:131758. doi: 10.1016/j.chemosphere.2021.131758
Hsu, W. T., Domenech, T., and McDowall, W. (2022). Closing the loop on plastics in Europe: the role of data, information and knowledge. Sustain. Prod. Consum. 33, 942–951. doi: 10.1016/j.spc.2022.08.019
Huang, F., Liu, Z., Li, Z., Wang, B., Zhang, P., and Jia, Z. K. (2022). Is biodegradable film an alternative to polyethylene plastic film for improving maize productivity in rainfed agricultural areas?—Evidence from field experiments. Agric. Water Manag. 272:107868. doi: 10.1016/j.agwat.2022.107868
Islami, A. N., Tazkiaturrizki, T., and Rinanti, A. (2019). The effect of pH-temperature on plastic allowance for low-density polyethylene (LDPE) by Thiobacillus sp. and Clostridium sp. J. Phys. Conf. Ser. 1402:033003. doi: 10.1088/1742-6596/1402/3/033003
Jacquin, J., Cheng, J., Odobel, C., Pandin, C., Conan, P., Pujo-Pay, M., et al. (2019). Microbial ecotoxicology of marine plastic debris: a review on colonization and biodegradation by the “plastisphere.”. Front. Microbiol. 10, 1–16. doi: 10.3389/fmicb.2019.00865
Jayan, N., Skariyachan, S., and Sebastian, D. (2023). The escalated potential of the novel isolate Bacillus cereus NJD1 for effective biodegradation of LDPE films without pre-treatment. J. Hazard. Mater. 455:131623. doi: 10.1016/j.jhazmat.2023.131623
Jebashalomi, V., Emmanuel Charles, P., and Rajaram, R. (2024). Microbial degradation of low-density polyethylene (LDPE) and polystyrene using Bacillus cereus (OR268710) isolated from plastic-polluted tropical coastal environment. Sci. Total Environ. 924:171580. doi: 10.1016/j.scitotenv.2024.171580
Jeon, J. M., Park, S. J., Choi, T. R., Park, J. H., Yang, Y. H., and Yoon, J. J. (2021). Biodegradation of polyethylene and polypropylene by Lysinibacillus species JJY0216 isolated from soil grove. Polym. Degrad. Stab. 191:109662. doi: 10.1016/j.polymdegradstab.2021.109662
Ji, S. H., Seok, D. C., and Yoo, S. (2023). Improved biodegradability of low-density polyethylene using plasma pretreatment and plastic-degrading bacteria. Environ. Technol. Innov. 32:103449. doi: 10.1016/j.eti.2023.103449
Joshi, G., Goswami, P., Verma, P., Prakash, G., Simon, P., Vinithkumar, N. V., et al. (2022). Unraveling the plastic degradation potentials of the plastisphere-associated marine bacterial consortium as a key player for the low-density polyethylene degradation. J. Hazard. Mater. October 2021:128005. doi: 10.1016/j.jhazmat.2021.128005
Khan, S., Ali, S. A., and Ali, A. S. (2023). Biodegradation of low density polyethylene (LDPE) by mesophilic fungus ‘Penicillium citrinum’ isolated from soils of plastic waste dump yard, Bhopal, India. Environ. Technol. 44, 2300–2314. doi: 10.1080/09593330.2022.2027025
Khan, M. S., Dhavan, P. P., Ratna, D., Sonawane, S. S., and Shimpi, N. G. (2021). LDPE:PLA and LDPE:PLA:OMMT polymer composites: preparation, characterization, and its biodegradation using Bacillus species isolated from dumping yard. Polym. Adv. Technol. 32, 3724–3739. doi: 10.1002/pat.5392
Khandare, S. D., Agrawal, D., Mehru, N., and Chaudhary, D. R. (2022). Marine bacterial based enzymatic degradation of low-density polyethylene (LDPE) plastic. J. Environ. Chem. Eng. 10:107437. doi: 10.1016/j.jece.2022.107437
Khandare, S. D., Chaudhary, D. R., and Jha, B. (2021). Marine bacterial biodegradation of low-density polyethylene (LDPE) plastic. Biodegradation 32, 127–143. doi: 10.1007/s10532-021-09927-0
Khruengsai, S., Sripahco, T., and Pripdeevech, P. (2021). Low-density polyethylene film biodegradation potential by fungal species from Thailand. J. Fungi 7:594. doi: 10.3390/jof7080594
Koh, L. M., and Khor, S. M. (2022). Biodegradation process: Basics, factors affecting, and industrial applications. In: Handbook of Biodegradable Materials. Eds. G. A. M. Ali and A. S. H. Makhlouf. Cham: Springer.
Kong, D., Zhang, H., Yuan, Y., Wu, J., Liu, Z., Chen, S., et al. (2024). Enhanced biodegradation activity toward polyethylene by fusion protein of anchor peptide and Streptomyces sp. strain K30 latex clearing protein. Int. J. Biol. Macromol. 264:130378. doi: 10.1016/j.ijbiomac.2024.130378
Kumar Gupta, K., and Devi, D. (2019). Biodegradation of low density polyethylene by selected Bacillus sp. Gazi. Univ. J. Sci. 32, 802–813. doi: 10.35378/gujs.496392
Kumar Shrestha, J., Raj Joshi, D., Regmi, P., Badahit, G., and Author, C. (2019). Isolation and identification of low density polyethylene (LDPE) degrading Bacillus spp. from a soil of landfill site identification of functional marker for the gene coding brittleness trait in rice view project isolation and identification of low density. Acta Sci. Microbiol. 2, 30–34.
Kundungal, H., Gangarapu, M., Sarangapani, S., Patchaiyappan, A., and Devipriya, S. P. (2021). Role of pretreatment and evidence for the enhanced biodegradation and mineralization of low-density polyethylene films by greater waxworm. Environ. Technol. 42, 717–730. doi: 10.1080/09593330.2019.1643925
Kunlere, I. O., Fagade, O. E., and Nwadike, B. I. (2019). Biodegradation of low density polyethylene (LDPE) by certain indigenous bacteria and fungi. Int. J. Environ. Stud. 76, 428–440. doi: 10.1080/00207233.2019.1579586
Lakhiar, I. A., Yan, H., Zhang, J., Wang, G., Deng, S., Bao, R., et al. (2024). Plastic pollution in agriculture as a threat to food security, the ecosystem, and the environment: An overview. Agronomy 14:548. doi: 10.3390/agronomy14030548
Lear, G., Kingsbury, J. M., Franchini, S., Gambarini, V., Maday, S. D. M., Wallbank, J. A., et al. (2021). Plastics and the microbiome: impacts and solutions. Environ. Microb. 16:2. doi: 10.1186/s40793-020-00371-w
Li, X., Li, G., Wang, J., Li, X., Yang, Y., and Song, D. (2024). Elucidating polyethylene microplastic degradation mechanisms and metabolic pathways via iron-enhanced microbiota dynamics in marine sediments. J. Hazard. Mater. 466:133655. doi: 10.1016/j.jhazmat.2024.133655
Li, A., Sheng, Y., Cui, H., Wang, M., Wu, L., Song, Y., et al. (2023). Discovery and mechanism-guided engineering of BHET hydrolases for improved PET recycling and upcycling. Nat. Commun. 14:4169. doi: 10.1038/s41467-023-44289-6
Li, Z., Wei, R., Gao, M., Ren, Y., Yu, B., Nie, K., et al. (2020). Biodegradation of low-density polyethylene by Microbulbifer hydrolyticus IRE-31. J. Environ. Manag. 263:110402. doi: 10.1016/j.jenvman.2020.110402
Liu, X., Zhang, Y., Sun, Q., Liu, Z., Zhao, Y., Fan, A., et al. (2022). Rapid colonization and biodegradation of untreated commercial polyethylene wrap by a new strain of Bacillus velezensis C5. J. Environ. Manag. 301:113848. doi: 10.1016/j.jenvman.2021.113848
Lou, H., Fu, R., Long, T., Fan, B., Guo, C., Li, L., et al. (2022). Biodegradation of polyethylene by Meyerozyma guilliermondii and Serratia marcescens isolated from the gut of waxworms (larvae of Plodia interpunctella). Sci. Total Environ. 853:158604. doi: 10.1016/j.scitotenv.2022.158604
Lou, Y., Li, Y., Lu, B., Liu, Q., Yang, S. S., Liu, B., et al. (2021). Response of the yellow mealworm (Tenebrio molitor) gut microbiome to diet shifts during polystyrene and polyethylene biodegradation. J. Hazard. Mater. 416:126222. doi: 10.1016/j.jhazmat.2021.126222
Lucas, N., Bienaime, C., Belloy, C., Queneudec, M., Silvestre, F., and Nava-Saucedo, J. E. (2008). Polymer biodegradation: mechanisms and estimation techniques - a review. Chemosphere 73, 429–442. doi: 10.1016/j.chemosphere.2008.06.064
Ma, Y., Wu, S., Xu, Y., Zhou, X., and Ruan, A. (2023). Degradation characteristics of polyethylene film by microorganisms from lake sediments. Environ. Pollut. 333:122115. doi: 10.1016/j.envpol.2023.122115
Maddison, C., Sathish, C. I., Lakshmi, D., Wayne, O. C., and Palanisami, T. (2023). An advanced analytical approach to assess the long-term degradation of microplastics in the marine environment. Npj Mater Degrad. 7, 1–11. doi: 10.1038/s41529-023-00377-y
Maheswaran, B., Al-Ansari, M., Al-Humaid, L., Sebastin Raj, J., Kim, W., Karmegam, N., et al. (2023). In vivo degradation of polyethylene terephthalate using microbial isolates from plastic polluted environment. Chem. Int. 310:136757. doi: 10.1016/j.chemosphere.2022.136757
Maleki Rad, M., Moghimi, H., and Azin, E. (2022). Biodegradation of thermo-oxidative pretreated low-density polyethylene (LDPE) and polyvinyl chloride (PVC) microplastics by Achromobacter denitrificans Ebl13. Mar. Pollut Bull. 181:113830. doi: 10.1016/j.marpolbul.2022.113830
Maroof, L., Khan, I., Yoo, H. S., Kim, S., Park, H. T., Ahmad, B., et al. (2021). Identification and characterization of low density polyethylenedegrading bacteria isolated from soils of waste disposal sites. Environ. Eng. Res. 26:200167. doi: 10.4491/eer.2020.167
Matjašič, T., Simčič, T., Medvešček, N., Bajt, O., Dreo, T., and Mori, N. (2021). Critical evaluation of biodegradation studies on synthetic plastics through a systematic literature review. Sci. Total Environ. 752:141959. doi: 10.1016/j.scitotenv.2020.141959
Min, K., Cuiffi, J. D., and Mathers, R. T. (2020). Ranking environmental degradation trends of plastic marine debris based on physical properties and molecular structure. Nat. Commun. 11:727. doi: 10.1038/s41467-020-14538-z
Mishra, R., Chavda, P., Kumar, R., Pandit, R., Joshi, M., Kumar, M., et al. (2024). Exploring genetic landscape of low-density polyethylene degradation for sustainable troubleshooting of plastic pollution at landfills. Sci. Total. Environ. 912:168882. doi: 10.1016/j.scitotenv.2023.168882
Mohammadi, S., Moussavi, G., and Rezaei, M. (2022). Enhanced peroxidase-mediated biodegradation of polyethylene using the bacterial consortia under H2O2-biostimulation. Polymer 240:124508. doi: 10.1016/j.polymer.2021.124508
Mohy Eldin, A., Al-Sharnouby, S. F. S., ElGabry, K. I. M., and Ramadan, A. I. (2022). Aspergillus terreus, Penicillium sp. and Bacillus sp. isolated from mangrove soil having laccase and peroxidase role in depolymerization of polyethylene bags. Process. Biochem. 118, 215–226. doi: 10.1016/j.procbio.2022.04.030
Montazer, Z., Habibi Najafi, M. B., and Levin, D. B. (2021). In vitro degradation of low-density polyethylene by new bacteria from larvae of the greater wax moth, galleria mellonella. Can. J. Microbiol. 67, 249–258. doi: 10.1139/cjm-2020-0208
Montazer, Z., Najafi, M. B. H., and Levin, D. B. (2020). Challenges with verifying microbial degradation of polyethylene. Polymers 12:123. doi: 10.3390/polym12010123
Mouafo Tamnou, E. B., Tamsa Arfao, A., Nougang, M. E., Metsopkeng, C. S., Noah Ewoti, O. V., Moungang, L. M., et al. (2021). Biodegradation of polyethylene by the bacterium Pseudomonas aeruginosa in acidic aquatic microcosm and effect of the environmental temperature. Environ. Challenges 3:100056. doi: 10.1016/j.envc.2021.100056
Nadeem, H., Alia, K. B., Muneer, F., Rasul, I., Siddique, M. H., Azeem, F., et al. (2021). Isolation and identification of low-density polyethylene degrading novel bacterial strains. Arch. Microbiol. 203, 5417–5423. doi: 10.1007/s00203-021-02521-1
Nademo, Z. M., Shibeshi, N. T., and Gemeda, M. T. (2023). Isolation and screening of low-density polyethylene (LDPE) bags degrading bacteria from Addis Ababa municipal solid waste disposal site “Koshe.”. Ann. Microbiol. 73:6. doi: 10.1186/s13213-023-01711-0
Nag, M., Lahiri, D., Dutta, B., Jadav, G., and Ray, R. R. (2021). Biodegradation of used polyethylene bags by a new marine strain of Alcaligenes faecalis LNDR-1. Environ. Sci. Pollut. Res. 28, 41365–41379. doi: 10.1007/s11356-021-13704-0
Nanthini Devi, K., Raju, P., Santhanam, P., Dinesh Kumar, S., Krishnaveni, N., Roopavathy, J., et al. (2021). Biodegradation of low-density polyethylene and polypropylene by microbes isolated from Vaigai River, Madurai, India. Arch. Microbiol. 203, 6253–6265. doi: 10.1007/s00203-021-02592-0
Nelson, T. F., Baumgartner, R., Jaggi, M., Bernasconi, S. M., Battagliarin, G., Sinkel, C., et al. (2022). Biodegradation of poly(butylene succinate) in soil laboratory incubations assessed by stable carbon isotope labelling. Nat. Commun. 13, 1–16. doi: 10.1038/s41467-022-33064-8
Nie, H., Nie, M., Diwu, Z., Wang, L., Yan, H., and Bai, X. (2021). Immobilization of Rhodococcus qingshengii strain FF on the surface of polyethylene and its adsorption and biodegradation of mimic produced water. J. Hazard. Mater. 403:124075. doi: 10.1016/j.jhazmat.2020.124075
Nnaji, C. F., Ogu, E. C., and Akpor, O. B. (2021). Biosurfactants as facilitators in biodegradation of low-density polyethylene (LDPE). IOP Conf. Ser. Mater. Sci. Eng. 1107:012135. doi: 10.1088/1757-899X/1107/1/012135
Obaid, T. G., and AL-Jawhari, I. F. H. (2023). Biodegradation of low-density polyethylene (LDPE) by aspergillus Niger. GSC Adv. Res. Rev. 17, 101–108.
Orona-Návar, C., García-Morales, R., Loge, F. J., Mahlknecht, J., Aguilar-Hernández, I., and Ornelas-Soto, N. (2022). Microplastics in Latin America and the Caribbean: a review on current status and perspectives. J. Environ. Manag. 309:114698. doi: 10.1016/j.jenvman.2022.114698
Pathak, V. M., and Navneet,. (2023). Exploitation of bacterial strains for microplastics (LDPE) biodegradation. Chem. Int. 316:137845. doi: 10.1016/j.chemosphere.2023.137845
Peng, B. Y., Li, Y., Fan, R., Chen, Z., Chen, J., Brandon, A. M., et al. (2020). Biodegradation of low-density polyethylene and polystyrene in superworms, larvae of Zophobas atratus (Coleoptera: Tenebrionidae): broad and limited extent depolymerization. Environ. Pollut. 266:115206. doi: 10.1016/j.envpol.2020.115206
Peng, B. Y., Sun, Y., Wu, Z., Chen, J., Shen, Z., Zhou, X., et al. (2022). Biodegradation of polystyrene and low-density polyethylene by Zophobas atratus larvae: fragmentation into microplastics, gut microbiota shift, and microbial functional enzymes. J. Clean. Prod. 367:132987. doi: 10.1016/j.jclepro.2022.132987
Peng, B. Y., Sun, Y., Zhang, X., Sun, J., Xu, Y., Xiao, S., et al. (2023). Unveiling the residual plastics and produced toxicity during biodegradation of polyethylene (PE), polystyrene (PS), and polyvinyl chloride (PVC) microplastics by mealworms (larvae of Tenebrio molitor), J Hazard Mater 452:131326. doi: 10.1016/j.jhazmat.2023.131326
Perera, P., Herath, H., Paranagama, P. A., Wijesinghe, P., and Attanayake, R. N. (2023). Wood decay fungi show enhanced biodeterioration of low-density polyethylene in the absence of wood in culture media. PLoS One 18:e0288133. doi: 10.1371/journal.pone.0288133
Pinto, M., Zhao, Z., Klun, K., Libowitzky, E., and Herndl, G. J. (2022). Microbial consortiums of putative degraders of low-density polyethylene-associated compounds in the ocean. mSystems 7:e0141521. doi: 10.1128/msystems.01415-21
Plastics-Europe. An analysis of European plastics production, demand and waste data. 2021 Plastics Europe. (2021). Available at: https://plasticseurope.org/knowledge-hub/plastics-the-facts-2021/ (Accessed September 05, 2024).
Poma, O., Ricce, B., Beraún, J., Perez Carpio, J. E., Fernandez, H., and Soria, J. (2022). Biodegradation of low-density polyethylene—LDPE by the lepidopteran galleria Mellonella reusing beekeeping waste. Front. Bioeng. Biotechnol. 10, 1–8. doi: 10.3389/fbioe.2022.915331
Puglisi, E., Romaniello, F., Galletti, S., Boccaleri, E., Frache, A., and Cocconcelli, P. S. (2019). Selective bacterial colonization processes on polyethylene waste samples in an abandoned landfill site. Sci. Rep. 9, 1–13. doi: 10.1038/s41598-019-50740-w
Rani, R., Rathee, J., Kumari, P., Singh, N. P., and Santal, A. R. (2022). Biodegradation and detoxification of low-density polyethylene by an indigenous strain Bacillus licheniformis SARR1. J. Appl. Biol. Biotechnol. 10, 9–21. doi: 10.7324/JABB.2021.100102
Rogers, K. L., Carreres-Calabuig, J. A., Gorokhova, E., and Posth, N. R. (2020). Rogers 2020 - icro-by-micro interactions_ how microorganisms influence the fate of marine microplastics.Pdf, Limnol. Oceanogr. Lett. 5, 18–36. doi: 10.1002/lol2.10136
Rong, Z., Ding, Z. H., Wu, Y. H., and Xu, X. W. (2024). Degradation of low-density polyethylene by the bacterium Rhodococcus sp. C-2 isolated from seawater. Sci. Total Environ. 907:167993. doi: 10.1016/j.scitotenv.2023.167993
Rüthi, J., Cerri, M., Brunner, I., Stierli, B., Sander, M., and Frey, B. (2023). Discovery of plastic-degrading microbial strains isolated from the alpine and Arctic terrestrial plastisphere. Front. Microbiol. 14, 1–16. doi: 10.3389/fmicb.2023.1178474
Sáenz, M., Borodulina, T., Diaz, L., and Banchon, C. (2019). Minimal conditions to degrade low density polyethylene by aspergillus terreus and Niger. J. Ecol. Eng. 20, 44–51. doi: 10.12911/22998993/108699
Saira, A., Abdullah,, Maroof, L., Iqbal, M., Farman, S., Lubna,, et al. (2022). Biodegradation of low-density polyethylene (LDPE) bags by fungi isolated from waste disposal soil. Appl. Environ. Soil Sci. 2022, 1–7. doi: 10.1155/2022/8286344
Samanta, S., Datta, D., and Halder, G. (2020). Biodegradation efficacy of soil inherent novel sp. Bacillus tropicus (MK318648) onto low density polyethylene matrix. J. Polym. Res. 27, 1–16. doi: 10.1007/s10965-020-02296-x
Sanluis-Verdes, A., Colomer-Vidal, P., Rodriguez-Ventura, F., Bello-Villarino, M., Spinola-Amilibia, M., Ruiz-Lopez, E., et al. (2022). Wax worm saliva and the enzymes therein are the key to polyethylene degradation by galleria mellonella. Nat. Commun. 13:5568. doi: 10.1038/s41467-022-33127-w
Sanniyasi, E., Gopal, R. K., Gunasekar, D. K., and Raj, P. P. (2021). Biodegradation of low-density polyethylene (LDPE) sheet by microalga, Uronema africanum Borge. Sci Rep. 11, 1–33. doi: 10.1038/s41598-021-96315-6
Sarmah, P., and Rout, J. (2019). Cyanobacterial degradation of low-density polyethylene (LDPE) by Nostoc carneum isolated from submerged polyethylene surface in domestic sewage water. Energy Ecol. Environ. 4, 240–252. doi: 10.1007/s40974-019-00133-6
Sathiyabama, M., Boomija, R. V., Sathiyamoorthy, T., Mathivanan, N., and Balaji, R. (2024). Mycodegradation of low-density polyethylene by Cladosporium sphaerospermum, isolated from platisphere. Sci. Rep. 14, 1–13. doi: 10.1038/s41598-024-59032-4
Schwarz, A. E., Lensen, S. M. C., Langeveld, E., Parker, L. A., and Urbanus, J. H. (2023). Plastics in the global environment assessed through material flow analysis, degradation and environmental transportation. Sci. Total Environ. 875:162644. doi: 10.1016/j.scitotenv.2023.162644
Shi, J., Sun, C., An, T., Jiang, C., Mei, S., and Lv, B. (2024). Unraveling the effect of micro/nanoplastics on the occurrence and horizontal transfer of environmental antibiotic resistance genes: advances, mechanisms and future prospects. Sci. Total Environ. 947:174466. doi: 10.1016/j.scitotenv.2024.174466
Sivaperumal, P., Kamala, K., and Rajaram, R. (2017). Bioremediation of industrial waste through enzyme producing marine microorganisms. Adv. Food Nutr. Res. 80, 165–179. doi: 10.1016/bs.afnr.2016.10.006
Skariyachan, S., Taskeen, N., Kishore, A. P., Krishna, B. V., and Naidu, G. (2021). Novel consortia of enterobacter and pseudomonas formulated from cow dung exhibited enhanced biodegradation of polyethylene and polypropylene. J. Environ. Manag. 284:112030. doi: 10.1016/j.jenvman.2021.112030
Soleimani, Z., Gharavi, S., Soudi, M., and Moosavi-Nejad, Z. (2021). A survey of intact low-density polyethylene film biodegradation by terrestrial Actinobacterial species. Int. Microbiol. 24, 65–73. doi: 10.1007/s10123-020-00142-0
Soud, S. A. (2019). Biodegradation of polyethylene LDPE plastic waste using locally isolated Streptomyces sp. J. Pharmeceutical. Sci. Res. 11, 1333–1339.
Statista. Production of plastic products in China from May 2021 to May 2024. (2024a). Available at: https://www.statista.com/statistics/226239/production-of-plastic-products-in-china-by-month/ (Accessed June 28, 2024).
Statista. Production volume of performance plastics in India from financial year 2015 to 2022. (2024b). Available at: https://www.statista.com/statistics/1067510/india-performance-plastics-production-volume/#:~:text=Infiscalyear2022%2Cthe,ofchemicalsintheworld (Accessed June 28, 2024).
Sunny, J. S., and Saleena, L. M. (2020). “Metagenomics: a vital source of information for modeling interaction networks in bacterial communities” in Recent advancements in microbial diversity (Academic Press), 507–535.
Tamnou, E. B. M., Nougang, M. E., Metsopkeng, C. S., Awawou, M. N., Eheth, J. S., Masse, P. S. M., et al. (2022). Comparison of the biodegradation of polypropylene (PP) and low density polyethylene (LDPE) by Pseudomonas aeruginosa and Staphylococcus aureus at different pH ranges under mesophilic condition. J. Adv. Microbiol. 22, 60–76. doi: 10.9734/jamb/2022/v22i930489
Temporiti, M. E. E., Nicola, L., Nielsen, E., and Tosi, S. (2022). Fungal enzymes involved in plastics biodegradation. Microorganisms 10:1180. doi: 10.3390/microorganisms10061180
Thomas Zumstein, M, Schintlmeister, A, Nelson, TF, Baumgartner, R, Woebken, D, Wagner, M, et al. Biodegradation of synthetic polymers in soils: tracking carbon into CO2 and microbial biomass. (2018). Available at: https://www.science.org
Tiwari, N., Santhiya, D., and Sharma, J. G. (2023). Degradation of polyethylene microplastics through microbial action by a soil isolate of Brevibacillus brevis. Polym. Degrad. Stab. 215:110436. doi: 10.1016/j.polymdegradstab.2023.110436
Tiwari, N., Santhiya, D., and Sharma, J. G. (2024). Significance of landfill microbial communities in biodegradation of polyethylene and nylon 6,6 microplastics. J. Hazard Mater. 462:132786. doi: 10.1016/j.jhazmat.2023.132786
UNDP. Why aren’t we recycling more plastic? (2023). Available at: https://stories.undp.org/why-arent-we-recycling-more-plastic (Accessed June 28, 2024).
Vaksmaa, A., Polerecky, L., Dombrowski, N., Kienhuis, M. V. M., Posthuma, I., Gerritse, J., et al. (2023). Polyethylene degradation and assimilation by the marine Yeastrhodotorula mucilaginosa. ISME Commun. 3, 1–8. doi: 10.1038/s43705-023-00267-z
Verma, J. P., and Jaiswal, D. K. (2016). Book review: advances in biodegradation and bioremediation of industrial waste. Front. Microbiol. 6:1555. doi: 10.3389/fmicb.2015.01555
Wang, H., Guan, F., Zhu, Y., Pan, Y., Liu, Q., Liu, Q., et al. (2023). Biofilm formation promoted biodegradation of polyethylene in Gordonia polyisoprenivorans B251 isolated from bacterial enrichment acclimated by hexadecane for two years. Chem. Int. 344:140383. doi: 10.1016/j.chemosphere.2023.140383
Wang, J., Liu, C., Cao, Q., Li, Y., Chen, L., Qin, Y., et al. (2024). Enhanced biodegradation of microplastic and phthalic acid ester plasticizer: the role of gut microorganisms in black soldier fly larvae. Sci. Total Environ. 924:171674. doi: 10.1016/j.scitotenv.2024.171674
Wang, J., Liu, X., Li, Y., Powell, T., Wang, X., Wang, G., et al. (2019). Microplastics as contaminants in the soil environment: a mini-review. Sci. Total Environ. 691, 848–857. doi: 10.1016/j.scitotenv.2019.07.209
Wang, M., Rivenbark, K. J., and Phillips, T. D. (2024). Kinetics of glyphosate and aminomethylphosphonic acid sorption onto montmorillonite clays in soil and their translocation to genetically modified corn. J. Environ. Sci. 135, 669–680. doi: 10.1016/j.jes.2023.02.006
Wang, P., Song, T., Bu, J., Zhang, Y., Liu, J., Zhao, J., et al. (2022). Does bacterial community succession within the polyethylene mulching film plastisphere drive biodegradation? Sci. Total Environ. 824:153884. doi: 10.1016/j.scitotenv.2022.153884
Wang, J., Wang, Y., Li, X., Weng, Y., Wang, Y., Han, X., et al. (2022). Different performances in polyethylene or polystyrene plastics long-term feeding and biodegradation by Zophobas atratus and Tenebrio molitor larvae, and core gut bacterial-and fungal-microbiome responses. J. Environ. Chem. Eng. 10:108957. doi: 10.1016/j.jece.2022.108957
Welden, N. A. (2020). “Chapter 8 - the environmental impacts of plastic pollution” in Plastic waste and recycling (Elsevier), 195–222. Available at: https://www.sciencedirect.com/science/article/abs/pii/B9780128178805000086 (Accessed June 28, 2024).
Wróbel, M., Szymańska, S., Kowalkowski, T., and Hrynkiewicz, K. (2023). Selection of microorganisms capable of polyethylene (PE) and polypropylene (PP) degradation. Microbiol. Res. 267:127251. doi: 10.1016/j.micres.2022.127251
Wu, H., Liu, Q., Sun, W., Lu, Y., Qi, Y., and Zhang, H. (2023). Biodegradability of polyethylene mulch film by Bacillus paramycoides. Chem. Int. 311:136978. doi: 10.1016/j.chemosphere.2022.136978
Yang, S. S., Ding, M. Q., Ren, X. R., Zhang, Z. R., Li, M. X., Zhang, L. L., et al. (2022). Impacts of physical-chemical property of polyethylene on depolymerization and biodegradation in yellow and dark mealworms with high purity microplastics. Sci. Total Environ. 828:154458. doi: 10.1016/j.scitotenv.2022.154458
Yang, S. S., Ding, M. Q., Zhang, Z. R., Ding, J., Bai, S. W., Cao, G. L., et al. (2021). Confirmation of biodegradation of low-density polyethylene in dark-versus yellow-mealworms (larvae of Tenebrio obscurus versus Tenebrio molitor) via. Gut microbe-independent depolymerization. Sci. Total Environ. 789:147915. doi: 10.1016/j.scitotenv.2021.147915
Yang, L., Gao, J., Liu, Y., Zhuang, G., Peng, X., Wu, W. M., et al. (2021). Biodegradation of expanded polystyrene and low-density polyethylene foams in larvae of Tenebrio molitor Linnaeus (Coleoptera: Tenebrionidae): broad versus limited extent depolymerization and microbe-dependence versus independence. Chem. Int. 262:127818. doi: 10.1016/j.chemosphere.2020.127818
Yao, Z., Seong, H. J., and Jang, Y. S. (2022). Degradation of low density polyethylene by Bacillus species. Appl. Biol. Chem. 65:84. doi: 10.1186/s13765-022-00753-3
Yee, M. S. L., Hii, L. W., Looi, C. K., Lim, W. M., Wong, S. F., Kok, Y. Y., et al. (2021). Impact of microplastics and nanoplastics on human health. Nano 11, 1–23. doi: 10.3390/nano11020496
Yin, C. F., Xu, Y., and Zhou, N. Y. (2020). Biodegradation of polyethylene mulching films by a co-culture of Acinetobacter sp. strain NyZ450 and Bacillus sp. strain NyZ451 isolated from Tenebrio molitor larvae. Int. Biodeterior. Biodegrad. 155:105089. doi: 10.1016/j.ibiod.2020.105089
Yuan, Y., Liu, P., Zheng, Y., Li, Q., Bian, J., Liang, Q., et al. (2023). Unique Raoultella species isolated from petroleum contaminated soil degrades polystyrene and polyethylene. Ecotoxicol. Environ. Saf. 263:115232. doi: 10.1016/j.ecoenv.2023.115232
Zadjelovic, V., Erni-Cassola, G., Obrador-Viel, T., Lester, D., Eley, Y., Gibson, M. I., et al. (2022). A mechanistic understanding of polyethylene biodegradation by the marine bacterium Alcanivorax. J. Hazard. Mater. 436:129278. doi: 10.1016/j.jhazmat.2022.129278
Zahari, N. Z., Abdullah, S. N., Tuah, P. M., and Cleophas, F. N. (2021). Biodegradation of low-density polyethylene (LDPE) and starch – based plastic (SBP) by thermophiles Bacillus subtilis and Candida tropicalis. IOP Conf. Ser. Mater. Sci. Eng. 1173:012035. doi: 10.1088/1757-899X/1173/1/012035
Zaman, I., Turjya, R. R., Shakil, M. S., Al Shahariar, M., Emu, M. R. R. H., Ahmed, A., et al. (2024). Biodegradation of polyethylene and polystyrene by Zophobas atratus larvae from Bangladeshi source and isolation of two plastic-degrading gut bacteria. Environ. Pollut. 345:123446. doi: 10.1016/j.envpol.2024.123446
Zampolli, J., Mangiagalli, M., Vezzini, D., Lasagni, M., Ami, D., Natalello, A., et al. (2023). Oxidative degradation of polyethylene by two novel laccase-like multicopper oxidases from Rhodococcus opacus R7. Environ. Technol. Innov. 32:103273. doi: 10.1016/j.eti.2023.103273
Zampolli, J., Orro, A., Manconi, A., Ami, D., Natalello, A., and Di Gennaro, P. (2021). Transcriptomic analysis of Rhodococcus opacus R7 grown on polyethylene by RNA-seq. Sci. Rep. 11, 1–14. doi: 10.1038/s41598-021-00525-x
Zárate-Rueda, R., López-Gualdrón, C. I., Ortega-Zambrano, C., Zárate-Rueda, R., López-Gualdrón, C. I., and Ortega-Zambrano, C. (2021). Revisión sistemática sobre modelos de rehabilitación con víctimas de Minas Antipersonal (MAP), en el marco de conflictos armados y el enfoque comunitario. El Ágora USB 21, 771–793. doi: 10.21500/16578031.5882
Zeghal, E., Vaksmaa, A., Vielfaure, H., Boekhout, T., and Niemann, H. (2021). The potential role of marine Fungi in plastic degradation – a review. Front. Mar. Sci. 8:1783. doi: 10.3389/fmars.2021.738877
Zhang, X., Feng, X., Lin, Y., Gou, H., Zhang, Y., and Yang, L. (2023). Degradation of polyethylene by Klebsiella pneumoniae Mk-1 isolated from soil. Ecotoxicol. Environ. Saf. 258:114965. doi: 10.1016/j.ecoenv.2023.114965
Zhang, T., Li, X., Rao, X., Peng, Y., Zhao, C., Xu, Y., et al. (2024). Biodegradation of polystyrene and polyethylene by Microbacterium esteraromaticum SW3 isolated from soil. Ecotoxicol. Environ. Saf. 274:116207. doi: 10.1016/j.ecoenv.2024.116207
Zhang, H., Liu, Q., Wu, H., Sun, W., Yang, F., Ma, Y., et al. (2023). Biodegradation of polyethylene film by the Bacillus sp. PELW2042 from the guts of Tenebrio molitor (mealworm larvae). Process. Biochem. 130, 236–244. doi: 10.1016/j.procbio.2023.04.006
Keywords: microbial consortia, plastic degradation pathways, HPLC, enzymatic biodegradation, metagenomic, SEM, FTIR, biological waste management
Citation: Mendoza JE, Tineo D, Chuquibala-Checan B, Atalaya-Marin N, Taboada-Mitma VH, Tafur-Culqui J, Tarrillo E, Gómez-Fernández D, Goñas M and Reyes-Reyes MA (2025) Global perspectives on the biodegradation of LDPE in agricultural systems. Front. Microbiol. 15:1510817. doi: 10.3389/fmicb.2024.1510817
Edited by:
Bo Li, Guangdong University of Technology, ChinaReviewed by:
Azadeh Babaei, Islamic Azad University of Karaj, IranHuoqing Wang, Guangdong University of Technology, China
Copyright © 2025 Mendoza, Tineo, Chuquibala-Checan, Atalaya-Marin, Taboada-Mitma, Tafur-Culqui, Tarrillo, Gómez-Fernández, Goñas and Reyes-Reyes. This is an open-access article distributed under the terms of the Creative Commons Attribution License (CC BY). The use, distribution or reproduction in other forums is permitted, provided the original author(s) and the copyright owner(s) are credited and that the original publication in this journal is cited, in accordance with accepted academic practice. No use, distribution or reproduction is permitted which does not comply with these terms.
*Correspondence: Jani E. Mendoza, ZWxpc2FiZXRtZW5kb3phamFuaUBnbWFpbC5jb20=
†These authors have contributed equally to this work