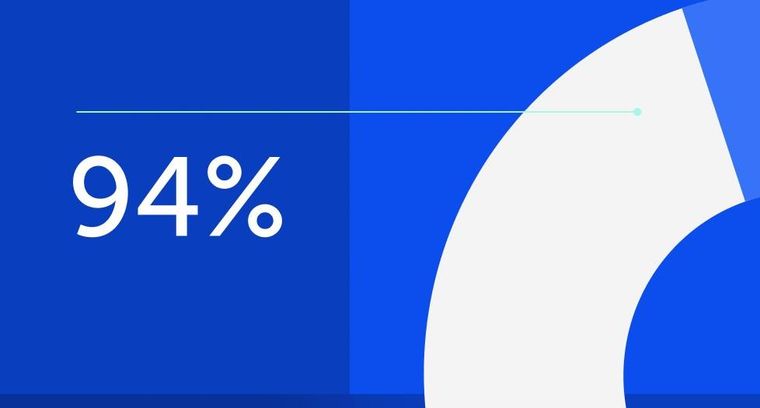
94% of researchers rate our articles as excellent or good
Learn more about the work of our research integrity team to safeguard the quality of each article we publish.
Find out more
ORIGINAL RESEARCH article
Front. Microbiol., 07 January 2025
Sec. Antimicrobials, Resistance and Chemotherapy
Volume 15 - 2024 | https://doi.org/10.3389/fmicb.2024.1502528
Background: Colistin is an antibiotic used as a last resort to treat multidrug-resistant Gram-negative bacterial infections. Plasmid-mediated mobile colistin-resistant (mcr) genes in Escherichia coli (E. coli) are disseminated globally and are considered to be a major public health threat. This study aimed to determine the molecular characteristics of colistin-resistant Escherichia coli isolates in clinical settings in Pakistan.
Methods: A total of 240 clinical E. coli strains isolated from urine and pus cultures were collected from two hospitals in Faisalabad and analyzed for phenotypic resistance to colistin by cultivation on CHROMagar plates supplemented with colistin 2 ug/ml. Molecular characteristics of colistin-resistant isolates were analyzed using conventional PCR, whole genome sequencing, and bioinformatics analysis.
Results: PCR and whole genome analysis confirmed the presence of the mcr-1 gene in 10 E. coli isolates. The minimum inhibitory concentration for colistin ranged from 4 ug/ml to 32 ug/ml. ResFinder analysis revealed the presence of multiple resistance determinants conferring co-resistance to β-lactams, aminoglycosides, trimethoprim, sulfonamides, tetracycline, quinolones, florfenicol, and macrolides. Hybrid genomic assembly indicated that mcr-1 is carried on IncI2 plasmids. Plasmid replicon typing indicated that IncI2-type plasmids (n = 10) were the most prevalent plasmids in these strains, followed by IncFIB (n = 8), IncFIC (n = 7), IncFIA (n = 6), IncFII (4), IncQ1 (n = 3), IncI1 (n = 1), IncY (n = 1), and IncN (n = 1). The Achtman MLST typing scheme revealed a large diversity of STs among the mcr-1-positive E. coli. VirulenceFinder analysis revealed the presence of numerous virulence factors ranging from 4 to 19.
Conclusion: Our study revealed the emergence and dissemination of colistin-resistant E. coli isolates carrying mcr-1 in hospital settings, posing a potential risk to anti-infective therapy. More efforts should be taken to monitor the prevalence of mcr-1-carrying bacteria in Pakistan.
Antimicrobial resistance (AMR) is a major global public health concern, which has made the effective treatment of an ever-increasing array of infectious diseases very challenging (Aljeldah, 2022). Among Gram-negative bacteria, Escherichia coli (E. coli) causes a wide range of clinical infections due to endemic gut carriage, multidrug resistance, and, most importantly, rapid acquisition/transfer of resistance traits by horizontal gene transfer (Sora et al., 2021). Globally, 4.95 million deaths were reported in 2019 due to multidrug-resistant infections, with 1.27 million directly attributed to AMR (Murray et al., 2022). Moreover, the emergence of extended-spectrum β-lactamase- and carbapenemase-producing E. coli has resulted in the reduced effectiveness of antimicrobial treatment (van Den Bunt et al., 2020), such that healthcare professionals are now considering alternative antimicrobials such as colistin.
Colistin (also known as polymyxin E) is a cationic polypeptide antibiotic that interacts with the outer membrane of the Gram-negative bacteria (El-Sayed Ahmed et al., 2020). Despite colistin once being avoided due to its nephro- and neurotoxicity, this drug has now become a last-resort antimicrobial agent for treating life-threatening infections caused by MDR Gram-negative bacteria (Chibabhai et al., 2023). A gradual increase in the prevalence of colistin resistance has been noted in the last few years. The situation gets exacerbated by the fact that colistin resistance mediated by the mcr genes gets rapidly disseminated across diverse bacteria by horizontal gene transfer (Shafiq et al., 2023). The predominant carriers of mcr-1 were IncX4, IncI2, and IncHI2 plasmids, which are transferable and adaptive plasmid types with broad host ranges. These plasmids contributed to the spread of mcr-1 across various sources and bacterial species (Anyanwu et al., 2023).
A comprehensive review revealed that the mcr gene has been identified in 47 countries/regions across 6 continents, with 95% of the cases attributed to the mcr-1 variant in 2019 (Feng et al., 2023). In Pakistan, the mcr-1 gene has been detected in E. coli isolated from wildlife, humans, poultry, chickens, dairy cows, and insects (Azam et al., 2020; Umair et al., 2023; Li et al., 2022). However, there remains a scarcity of data, especially from clinical settings in Pakistan. Although a number of studies exist, they primarily focus on phenotypic resistance patterns (Javed et al., 2020; Hameed et al., 2021; Bilal et al., 2020a), and data showing detailed genomic analyses are scarce (Li et al., 2021). The gap in literature underscores the need for comprehensive genomic studies to understand the prevalence, genetic diversity, and mechanisms of co-resistance in clinical E. coli isolates harboring the mcr gene. By addressing this gap, the aim of this study was to characterize a set of colistin-resistant E. coli clinical isolates recovered from hospital settings in Pakistan. The results presented here will contribute to updating the status of colistin resistance in the country and improve the monitoring and surveillance of MDR E. coli harboring mcr-1 gene within hospitals.
A total of 240 E. coli strains recovered from urine or pus culture were collected from laboratories of two tertiary care hospitals in Faisalabad in 2019 and 2020, as described previously (Abdullah et al., 2023). These isolates were subcultured on CHROMagar media plates supplemented with 2 μg/mL colistin and incubated overnight at 37°C to confirm the purity and isolation of colistin-resistant E. coli isolates.
Protein-based confirmation was carried out primarily to confirm the identity of bacterial species using a matrix-assisted laser desorption ionization-TOF (MALDI-TOF; Bruker Daltonics, Germany).
DNA of E. coli isolates was subjected to conventional PCR to screen the presence of the mcr-1 gene using primers and conditions, as described previously (Uddin et al., 2022).
The minimum inhibitory concentration (MIC) of E. coli isolates positive for the mcr-1 gene was determined using the broth microdilution. Antimicrobial susceptibility testing of all colistin-resistant E. coli isolates was performed by disk diffusion using the Mueller–Hinton agar plates against 12 antimicrobials. E. coli ATCC 25922 was used as a negative control strain. The interpretation of colistin susceptibility was based on the breakpoint value defined by EUCAST (v. 13.0; Satlin et al., 2020).
Total gDNA was extracted from an overnight culture (2 mL) on a QIAcube automated system (Qiagen). Following extraction, gDNA was quantified using fluorometric methods with a Qubit (Thermo Fisher Scientific). The quality ratios of gDNA (A260/280 and 260/230) were determined using a NanoDrop Spectrophotometer (Thermo Fisher Scientific). Genomic DNA libraries were prepared for whole genome sequencing using the Nextera XT kit (Illumina), as described by the manufacturer. Paired-end sequencing was performed using the Illumina MiSeq platform (MiSeq Reagent V3 Kit; 2 × 300 cycles). For each E. coli isolate, at least 80× coverage was generated. Raw sequence reads were trimmed using Trim Galore, and the genomes were de novo-assembled into contigs using SPAdes (3.9.0) with a pre-defined kmers set. Raw reads were also assembled using the Geneious (10.0.9; Biomatters Ltd.) de novo assembler, set at medium sensitivity for analysis of paired Illumina reads. Geneious was used to map both sets of contigs to reference genes identified using the closest BLAST homology and was also used to annotate genes from the closest homologs in the NCBI Genome database. Resistance genes were identified using Resfinder within CGE (Florensa et al., 2022), and wgMLST profiles were generated using the CGE platform coupled with the PubMLST.org database (Uelze et al., 2020). Plasmids were identified within the genome assembly and typed using Plasmidfinder (Carattoli and Hasman, 2020).
To reconstruct full circular plasmid sequences, isolates were also sequenced by MinION sequencing (Oxford Nanopore Technologies Ltd., Oxford Science Park, United Kingdom). Large-scale bacterial gDNA was extracted. The DNA library was prepared by pooling all barcoded samples to aim for a final DNA concentration of >500 ng/μL, and 1 μL of RAD was added to the DNA. A final mixture of 75 μL (34 μL sequencing buffer, 30 μL water, and 11 μL DNA library) was loaded into the flow cell. The MinION device was connected to the MinKNOW GUI to obtain the reads. The raw data in fast5 format were base-called with the high-accuracy mode and demultiplexed using Guppy 4.2.2 (Ulrich, 2023). Unicycler (0.4.4) was used to yield hybrid assembly using both Illumina short reads and MinION long reads. This process included assembling the long reads with Flye v2.8 (Hu et al., 2024) and following five rounds of polishing using Pilon (Vu et al., 2022) with the Illumina short reads of the same sample. The comparisons of the complete E. coli plasmids were visualized using BRIG (Darphorn et al., 2021).
The single-nucleotide polymorphism (SNP)-based phylogenetic tree was generated by calling and filtering SNPs, site validation, and phylogeny based on a concatenated alignment of the high-quality SNPs. For inferring phylogeny, the analysis was run with the standard parameters, and the EC958 strain genome (GenBank accession number HG941718.1) was used as a reference sequence. The number of SNPs exhibited among closely related isolates was calculated using a distance matrix file generated as a result of phylogeny (Kaas et al., 2014). The output core alignment file was used to construct the maximum-likelihood tree with 1,000 bootstrap replications using MEGA-X v 10.0.5 (Kumar et al., 2018).1 The phylogenetic tree of the alignments was visualized and edited using iTOL v 4.4.2 software (Letunic and Bork, 2019).2
Ten non-repetitive colistin-resistant E. coli strains were isolated from two hospitals over 1 year. These non-duplicated strains were mainly isolated from urine (70%) followed by pus (30%) cultures. Similarly, the percentage of colistin-resistant E. coli isolated from female and male samples was found to be 60 and 40%, respectively. The number of patients belonging to age ranges of 15–25 years, 35–45 years, 45–55 years, 55–65 years, and 65–75 years were 2, 3, 3, 1, and 1, respectively. All 10 colistin-resistant E. coli isolates were found to be mcr-1 producers using specific mcr-1 PCR.
All of the isolates exhibited an MIC of colistin between 4 μg/mL and 32 μg/mL (Supplementary file 1). Antimicrobial susceptibility testing revealed that all 10 E. coli isolates were MDR strains. MDR was defined as acquired non-susceptibility to at least one agent in three or more antimicrobial categories (Magiorakos et al., 2012). Isolates exhibited resistance to fluoroquinolones (100%), tetracyclines (100%), trimethoprim (80%), various types of penicillins (40–100%), and cephalosporins (10–60%). Moreover, 80% of isolates were susceptible to amoxiclav and aminoglycosides, while 100% susceptibility was observed for carbapenem antibiotics (Supplementary file 2).
Whole genome sequencing provided comprehensive information for the isolates and their phylogenetic relationship. Phylogenetic relationships among E. coli isolates were determined, and a maximum likelihood phylogenetic tree based on concatenated alignments of high-quality SNPs from the core genome was constructed. The genome of the sequenced isolates covered 72% of the EC958 reference genome.
We determined the SNP distance of the core genome. This method involves aligning the core genome sequences of the isolates, calling SNPs, and calculating SNP distances for comparing differences across E. coli isolates in the core genome, typically arising from whole-genome alignment and variant calling. The SNP matrix in the final dataset revealed a minimum of 27 SNPs and a maximum of 41,769 SNPs between all examined genomes. The core alignment showed that in ST-3902, isolates PK-5121 and PK-5139 differed from each other by 85 SNPs. These two isolates also differed by 277 and 256 SNPs, respectively, with the isolate PK-5235 from ST-167. Similarly, in ST-156, the isolates PK-5163 and PK-5199 differed by 45 SNPs (Figure 1; Supplementary file 3).
Figure 1. Phylogenetic tree of mcr-1-positive Escherichia coli isolates based on core genome SNP alignments.
Whole genome analysis confirmed the presence of the mcr-1 gene in 10 isolates. ResFinder analysis revealed the presence of multiple resistance genes for β-lactams, aminoglycosides, tetracycline, chloramphenicol, sulfonamides, trimethoprim, and quinolones, located on chromosomes and plasmids. Isolates carried antimicrobial resistance genes ranging from 5 to 14. PK-5185, containing 14 resistance genes, was the isolate with the largest number of resistance genes. The most prevalent resistance gene was floR, conferring resistance to florfenicol (n = 10). VirulenceFinder analysis showed the presence of various virulence factors (n = 22; cia, cib, etsC, fyuA, gad, hlyF, hra, iroN, irp2, iss, iucC, iutA, lpfA, ompT, papA_F19, papC, sitA, terC, traT, cvaC, cma, and tsh) in isolates ranging in number from 4 to 19 (Table 1).
Plasmid replicon typing exhibited 8 different Inc. types of plasmids, ranging from 1 to 10 per isolate. The incI2-type plasmid was observed to harbor the mcr-1 gene among all E. coli isolates (n = 10; Table 2). The most prevalent Inc. types of plasmids observed were IncFIB, IncFIC, and IncFIA in 7, 7, and 5 numbers of isolates, respectively. IncFII and IncQ1 types were detected in three isolates each. Furthermore, IncI1 and IncN plasmid types were observed in a single isolate each.
BLASTn analysis of plasmids harboring the mcr-1 gene, pPK-5073, pPK-5088, pPK-5090, pPK-5121, and pPK-5205 against the NCBI nr database, showed that they exhibited homology with plasmid pPK105 (Accession no. MG808035.1), an E. coli strain isolated from chicken of healthy broiler in 2020 in Faisalabad, Pakistan, with identities of 94.98, 87.84, 94.57, 93.87, and 95.12 at a coverage of 97, 97, 98, 97, and 98%, respectively (Figure 2A). BLASTn analysis of these plasmids adding pPK-5185 against the NCBI nr database also exhibited homology with plasmid pZJ3920-3 (Accession no. CP020548.1) of E. coli strain isolated from the human bile in 2015 in Hangzhou, China, with identities of 95.32, 90.53, 94.98, 93.74, 93.63, and 94.98 at a coverage of 98, 98, 99, 98, 99, and 99%, respectively (Figure 2B). E. coli plasmid pHLJ179-141 (Accession no. MN232210.1) isolated from the chicken gut in 2019 in Guangzhou, China, had a backbone structure similar to plasmid pPK-5139 with identities of 94.76% and at a coverage of 99% (Figure 2C). BLAST search of pPK-5163 and pPK-5199 exhibited identity 91.97 and 100% at coverage of 99 and 96.85% with plasmid pHeN867 (Accession no. KU934208.1) of E. coli strain HeN867 isolated from chicken samples in China in 2020 and plasmid pC2 (Accession no. CP042471.2) of E. coli strain A50 isolated from Gallus gallus domesticus ISA15 in Algeria in 2017 (Figure 2D). Plasmid pPK-5235 shared a coverage of 80% and an identity of >95% with plasmid pHLJ109-92 (Accession no. MN232203.1) in E. coli strain isolated from the chicken gut in China in 2020 and plasmid pBA76-MCR-1 (Accession no. KX013540.1) in E. coli strain BA76 isolated from sacral wound swab in Bahrain in 2015 (Figure 2E).
Figure 2. (A–E) Circular comparisons of IncI2 plasmid harboring the mcr-1 gene with other different plasmids.
Owing to the increasing incidence of carbapenemase-producing Enterobacteriaceae (Abdullah et al., 2023), colistin has become a last-resort antimicrobial, largely used for the treatment of severe bacterial infections. An additional challenge when it comes to polymyxin resistance is the rapid dissemination of MCR-producing Enterobacteriaceae. The emergence of mcr-1-producing E. coli isolates in hospital settings is a significant public health concern (Xie et al., 2022). Various approaches exist for detecting colistin resistance and mcr genes, each with unique strengths and limitations. Culture-based methods, such as broth microdilution, remain the gold standard for colistin susceptibility but are labor-intensive and costly. Rapid tests such as the colistin drop and colistin agar spot tests offer quicker alternatives but may suffer from accuracy issues, particularly with false-negative results. PCR-based and other nucleic acid amplification tests (NAATs) enable the rapid detection of the mcr genes directly from samples but rely on known gene variants, potentially missing novel or rare mutations. Whole genome sequencing (WGS) provides comprehensive resistance profiling, but its high cost and bioinformatics requirements may limit its use in routine diagnostics, especially in low-resource settings. The limited detection capabilities of many assays underscore the need for methodological advancements to improve the accuracy and accessibility of mcr-mediated colistin resistance screening (Zhang et al., 2022).
In this study, whole genome sequencing (WGS) was used to characterize clinical E. coli isolates harboring the mcr-1 gene, a plasmid-mediated colistin resistance determinant, collected from two tertiary care hospitals in Pakistan. This study revealed the presence of the mcr-1 gene in colistin-resistant E. coli isolates (n = 10/240, 4%). Other studies reported 2.6 and 2.8% prevalence of mcr genes in E. coli isolates in clinical settings in Pakistan (Bilal et al., 2020b; Ejaz et al., 2021). The findings of other studies performed in India and Iran also correspond to the results of our study and reported a 3.2 and 3% prevalence of mcr genes among clinical E. coli isolates (Wise et al., 2018; Aghapour et al., 2019). Several studies, particularly in Asia, documented a significant decrease in the emergence rate of isolates resistant to colistin due to the ban on its use to avoid the further emergence of isolates harboring the plasmid-mediated mcr-1 gene (Binsker et al., 2022; Saeed et al., 2021; Wang et al., 2020; Usui et al., 2021). The relatively low proportion of mcr-1-producing E. coli isolates in hospital settings in this and other studies may reflect the limited use of colistin in clinical practice as opposed to the higher use of colistin in agriculture with corresponding higher proportions of resistant isolates among food-producing animals and agricultural communities (Lencina et al., 2024; Li et al., 2023). Countries including the United States, the United Kingdom, and Canada also never approved the use of colistin in livestock farming and have constantly described lower rates of detection of colistin-resistant isolates (Anwar et al., 2022; Binsker et al., 2022). Nevertheless, no such regulation on the ban of veterinary use of colistin was implemented in Pakistan, where colistin is frequently used in different antimicrobial combinations in animal farming for prophylactic and therapeutic purposes. Despite the low prevalence, continuous surveillance and strict control measures are vital to prevent the rapid spread of mcr-mediated resistance through horizontal gene transfer, preserving colistin’s role as a last-resort antibiotic.
It is noteworthy that no isolates in this study showed colistin resistance due to chromosomal mutations, which often contribute more frequently to resistance than the plasmid-mediated mcr genes. Typically, colistin resistance can arise from mutations in chromosomal genes such as pmrA, pmrB, phoP, phoQ, or mgrB, which alter the bacterial outer membrane by modifying lipopolysaccharides. These mutations are often observed in clinical isolates and can confer resistance without the need for mcr genes (Tkadlec et al., 2021). This is in agreement with the majority of the studies in Pakistan (Umair et al., 2023; Li et al., 2022).
Surveillance of AMR rates is a valuable tool to aid physicians in understanding the local resistance trends and improving the prescribing of antimicrobials. This study showed the resistance pattern in E. coli strains carrying the mcr-1 gene isolated from pus (30%) or urine (70%) samples in hospital settings. The strains exhibited resistance to fluoroquinolones (100%), tetracyclines (100%), trimethoprim (80%), various types of penicillins (40–100%), and cephalosporins (10–60%). These findings are in agreement with the recent studies reporting increasing AMR in the region (Chaurasia et al., 2019; Browne et al., 2021). A recent study analyzed the AMR rates for GLASS-specified pathogen/antimicrobial combinations from Pakistan (2006–2018) and reported high resistance rates (>50%) to fluoroquinolones and third-generation cephalosporins among the E. coli and K. pneumoniae (Saeed et al., 2021). The emergence and spread of resistant pathogens are driven by factors such as high antibiotic selection pressure and poor infection control, often resulting in cross-transmission among patients (Castro-Sánchez et al., 2016). In this study, several mcr-1 isolates also carried genes conferring resistance to β-lactams, aminoglycosides, and fluoroquinolones, complicating treatment options and underscoring the adaptive potential of E. coli under antibiotic pressure. Although data here are limited, these findings highlight the importance of antimicrobial stewardship and the need to explore alternative treatments.
The AMR gene profiles largely correlated with the phenotypic AST results, demonstrating resistance in line with the detected genes. For example, mcr-1 correlated with colistin resistance, and the presence of β-lactamase genes (e.g., blaTEM-1 and blaEC-18) matched with β-lactam resistance in AST. Aminoglycoside and tetracycline resistance genes (e.g., aph(6)-Id and tet(A)) similarly corresponded to observed phenotypic resistance. However, a few isolates showed susceptibility to certain antibiotics (e.g., aminoglycosides and amoxiclav) despite carrying resistance genes, suggesting potential gene expression variability or regulatory influences affecting the phenotype.
Understanding the occurrence of specific virulence genes among colistin-resistant E. coli isolates in this study sheds light on their potential pathogenicity. Predominant genes identified, such as terC, gad, traT, and iss, are linked to immune evasion and stress resistance, while hlyF, iucC, and iroN enhance iron acquisition, critical for survival in host environments. The co-presence of these virulence traits with colistin resistance highlights the heightened risk posed by these isolates, suggesting a need for more focused monitoring and tailored infection control strategies in healthcare settings.
The present study also evaluated the prevalence of STs in colistin-resistant E. coli clinical isolates. The MLST typing analysis of colistin-resistant E. coli isolates reveals a significant genetic diversity, with the isolates distributed across six different sequence types (STs). While the presence of multiple isolates from the sequence type ST-3902 might hint at its resilience under selective pressures, such as colistin use. In addition, colistin use alone may not be sufficient to drive the expansion of this particular ST over others, as clonal spread can result from various ecological and clinical factors beyond antibiotic selection. The presence of isolates within ST-4388 and ST-156 further indicates the prevalence of these lineages, while the unique STs (ST-351, ST-10, and ST-167) highlight the genetic variability among colistin-resistant E. coli isolates. The most common ST among clinical isolates of colistin-resistant E. coli was ST10, with a frequency of 11.63% worldwide (Dadashi et al., 2022). A notable aspect of the study involves examining the links between the colistin-resistant E. coli strains found in environmental and agricultural settings, particularly poultry, and those identified in clinical settings (Zahra et al., 2018; Tang et al., 2019). It is crucial to highlight that specific sequence types such as ST10 and ST156 have been observed in both contexts. This overlap suggests a potential zoonotic transmission pathway, indicating that the use of colistin in agriculture may contribute to the emergence and spread of resistant strains in humans. The presence of shared STs between environmental, agricultural, and clinical settings highlights the urgent need for coordinated AMR surveillance and control strategies across both sectors.
Numerous studies have reported various Inc-type plasmids carrying mcr-1; however, IncI2, IncHI2, and IncX4 are the three major types found in different species of Enterobacteriaceae (Aworh et al., 2021; Binsker et al., 2023; Vu et al., 2022). Notably, this study found the IncI2 plasmid type harboring the mcr-1 gene among all isolates (n = 10), suggesting a strong association between IncI2 plasmids and the mcr-1 gene, which confers colistin resistance. The most prevalent plasmid types included IncFIB, IncFIC, and IncFIA, indicating that these plasmid types are common carriers of resistance genes. In addition, IncFII, IncQ1, IncI1, and IncN types were also found among isolates. This diversity of plasmid types, especially the prevalence of various IncF plasmids, underscores the complex mechanisms of horizontal gene transfer contributing to the spread of colistin resistance.
The whole genome-based characterization of mcr-1-producing clinical E. coli isolates in Pakistan provides critical insights into the genetic diversity, resistance mechanisms, and transmission dynamics of these pathogens. By identifying similarities in resistance genes and virulence factors, the findings provide indirect insights into how these pathogens may spread across different settings. Colistin, often considered a last-resort antibiotic for MDR infections, is rendered ineffective by the presence of the mcr-1 gene, leaving limited therapeutic options. The spread of such resistant pathogens could lead to increased morbidity, mortality, and healthcare costs. Based on our findings, we recommend implementing enhanced surveillance programs to monitor the prevalence and spread of mcr-1-producing E. coli and other resistant pathogens. Additionally, promoting the rational use of antibiotics through antimicrobial stewardship programs is essential to reduce selective pressure and prevent the emergence of resistance. We also advocate for increased investment in research to develop new antibiotics and alternative therapies, as well as rapid diagnostic tools for the timely detection of resistance genes.
The genome sequences generated in this study are available in the NCBI GenBank database under accession numbers JBGKBF000000000, JBGKBG000000000, JBGKBH000000000, JBGKBI000000000, JBGKBJ000000000, JBGKBK000000000, JBGKBL000000000, JBHEFN000000000, JBHEFO000000000, and JBHEFP000000000.
The studies involving humans were approved by the Institutional Biosafety Committee (IBC) D. No. 8025/ORIC of University of Agriculture, Faisalabad. The studies were conducted in accordance with the local legislation and institutional requirements. Written informed consent for participation was not required from the participants or the participants’ legal guardians/next of kin because the Escherichia coli strains isolated from clinical samples were collected from laboratories of hospitals.
SA: Writing – original draft, Writing – review & editing. MAM: Methodology, Writing – original draft. KU: Formal analysis, Writing – review & editing. BH: Data curation, Project administration, Writing – review & editing. MA: Investigation, Writing – review & editing. MZ: Data curation, Writing – review & editing. JW: Formal analysis, Writing – review & editing. JX: Formal analysis, Writing – review & editing, Investigation. MT: Writing – original draft, Writing – review & editing. MM: Writing – original draft, Writing – review & editing.
The author(s) declare that no financial support was received for the research, authorship, and/or publication of this article.
The authors declare that the research was conducted in the absence of any commercial or financial relationships that could be construed as a potential conflict of interest.
The authors declare that no Generative AI was used in the creation of this manuscript.
All claims expressed in this article are solely those of the authors and do not necessarily represent those of their affiliated organizations, or those of the publisher, the editors and the reviewers. Any product that may be evaluated in this article, or claim that may be made by its manufacturer, is not guaranteed or endorsed by the publisher.
The Supplementary material for this article can be found online at: https://www.frontiersin.org/articles/10.3389/fmicb.2024.1502528/full#supplementary-material
Abdullah, S., Almusallam, A., Li, M., Mahmood, M. S., Mushtaq, M. A., Eltai, N. O., et al. (2023). Whole genome-based genetic insights of Bla NDM producing clinical E. coli isolates in hospital settings of Pakistant. Microbiol Spectrum 11, e00584–e00523. doi: 10.1128/spectrum.00113-24
Aghapour, Z., Gholizadeh, P., Ganbarov, K., Bialvaei, A. Z., Mahmood, S. S., Tanomand, A., et al. (2019). Molecular mechanisms related to colistin resistance in Enterobacteriaceae. Infect. Drug Resist. 12, 965–975. doi: 10.2147/IDR.S199844
Aljeldah, M. M. (2022). Antimicrobial resistance and its spread is a global threat. Antibiotics 11:1082. doi: 10.3390/antibiotics11081082
Anwar, M. A., Aziz, S., Ashfaq, K., Aqib, A. I., and Shoaib, M. (2022). Trends in frequency, potential risks and antibiogram of E. coli isolated from semi-intensive dairy systems. Pak. Vet. J. 42, 167–172. doi: 10.29261/pakvetj/2022.018
Anyanwu, M. U., Jaja, I. F., Okpala, C. O. R., Njoga, E. O., Okafor, N. A., and Oguttu, J. W. (2023). Mobile colistin resistance (mcr) gene-containing organisms in poultry sector in low-and middle-income countries: epidemiology, characteristics, and one health control strategies. Antibiotics 12:1117. doi: 10.3390/antibiotics12071117
Aworh, M. K., Kwaga, J. K., Hendriksen, R. S., Okolocha, E. C., and Thakur, S. (2021). Genetic relatedness of multidrug resistant Escherichia coli isolated from humans, chickens and poultry environments. Antimicrob. Resist. Infect. Control 10, 1–13. doi: 10.1186/s13756-021-00930-x
Azam, M., Mohsin, M., Johnson, T. J., Smith, E. A., Johnson, A., Umair, M., et al. (2020). Genomic landscape of multi-drug resistant avian pathogenic Escherichia coli recovered from broilers. Vet. Microbiol. 247:108766. doi: 10.1016/j.vetmic.2020.108766
Bilal, H., Hameed, F., Khan, M. A., Khan, S., Yang, X., and Rehman, T. U. (2020a). Detection of mcr-1 gene in extended-spectrum β-lactamase-producing Klebsiella pneumoniae from human urine samples in Pakistan. Jundishapur J. Microbiol 13:646. doi: 10.5812/jjm.96646
Bilal, H., Tu, R., Khan, M., Hameed, F., and Zg, J. (2020b). Discovery of mcr-1 harboring Incl2 plasmids from clinical isolates of multiclonal E. coli prevalent in Pakistan. Research Square.
Binsker, U., Käsbohrer, A., and Hammerl, J. A. (2022). Global colistin use: a review of the emergence of resistant Enterobacterales and the impact on their genetic basis. FEMS Microbiol. Rev. 46:46. doi: 10.1093/femsre/fuab049
Binsker, U., Oelgeschläger, K., Neumann, B., Werner, G., Käsbohrer, A., and Hammerl, J. A. (2023). Genomic evidence of mcr-1.26 IncX4 plasmid transmission between poultry and humans. Microbiol Spectrum 11, e01015–e01023. doi: 10.1128/spectrum.01015-23
Browne, A. J., Chipeta, M. G., Haines-Woodhouse, G., Kumaran, E. P. A., Hamadani, B. H. K., Zaraa, S., et al. (2021). Global antibiotic consumption and usage in humans, 2000–18: a spatial modelling study. Lancet. Planet. Heal. 5:e893. doi: 10.1016/S2542-5196(21)00280-1
Carattoli, A., and Hasman, H. (2020). PlasmidFinder and in silico pMLST: identification and typing of plasmid replicons in whole-genome sequencing (WGS). Horizontal gene transfer: methods and protocols. 2075, 285–294. doi: 10.1007/978-1-4939-9877-7_20
Castro-Sánchez, E., Moore, L. S. P., Husson, F., and Holmes, A. H. (2016). What are the factors driving antimicrobial resistance? Perspectives from a public event in London. England. BMC Infect. Dis. 16, 1–5. doi: 10.1186/s12879-016-1810-x
Chaurasia, S., Sivanandan, S., Agarwal, R., Ellis, S., Sharland, M., and Sankar, M. J. (2019). Neonatal sepsis in South Asia: huge burden and spiralling antimicrobial resistance. BMJ 364:k5314. doi: 10.1136/bmj.k5314
Chibabhai, V., Bekker, A., Black, M., Demopoulos, D., Dramowski, A., du Plessis, N. M., et al. (2023). Appropriate use of colistin in neonates, infants and children: interim guidance. South. Afr. J. Infect. Dis. 38:555. doi: 10.4102/sajid.v38i1.555
Dadashi, M., Sameni, F., Bostanshirin, N., Yaslianifard, S., Khosravi-Dehaghi, N., Nasiri, M. J., et al. (2022). Global prevalence and molecular epidemiology of mcr-mediated colistin resistance in Escherichia coli clinical isolates: a systematic review. J. global antimicrobial resistance 29, 444–461. doi: 10.1016/j.jgar.2021.10.022
Darphorn, T. S., Bel, K., Koenders-van, B., Brul, S., and ter, B. (2021). Antibiotic resistance plasmid composition and architecture in Escherichia coli isolates from meat. Sci. Rep. 11:2136. doi: 10.1038/s41598-021-81683-w
Ejaz, H., Younas, S., Qamar, M. U., Junaid, K., Abdalla, A. E., Abosalif, K. O. A., et al. (2021). Molecular epidemiology of extensively drug-resistant mcr encoded Colistin-resistant bacterial strains co-expressing multifarious β-lactamases. Antibiot 10:467. doi: 10.3390/antibiotics10040467
El-Sayed Ahmed, M. A. E. G., Zhong, L. L., Shen, C., Yang, Y., Doi, Y., and Tian, G. B. (2020). Colistin and its role in the era of antibiotic resistance: an extended review (2000–2019). Emerg. microbes & infections 9, 868–885. doi: 10.1080/22221751.2020.1754133
Feng, J., Xu, Z., Zhuang, Y., Liu, M., Luo, J., Wu, Y., et al. (2023). The prevalence, diagnosis, and dissemination of mcr-1 in colistin resistance: progress and challenge. Decoding Infection and Transmission 1:100007. doi: 10.1016/j.dcit.2023.100007
Florensa, A. F., Kaas, R. S., Clausen, P. T. L. C., Aytan-Aktug, D., and Aarestrup, F. M. (2022). ResFinder–an open online resource for identification of antimicrobial resistance genes in next-generation sequencing data and prediction of phenotypes from genotypes. Microbial genomics 8:000748. doi: 10.1099/mgen.0.000748
Hameed, F., Khan, M. A., Bilal, H., Muhammad, H., and Rehman, T. U. (2021). Detection of MCR-1 gene in multiple drug resistant escherichia coli and klebsiella pneumoniae in human clinical samples from Peshawar, Pakistan. Comb. Chem. High Throughput Screen. 24, 737–742. doi: 10.2174/1386207323666200914100119
Hu, J., Wang, Z., Sun, Z., Hu, B., Ayoola, A. O., Liang, F., et al. (2024). NextDenovo: an efficient error correction and accurate assembly tool for noisy long reads. Genome. Biol. 25:107. doi: 10.1186/s13059-024-03252-4
Javed, H., Saleem, S., Zafar, A., Ghafoor, A., Shahzad, A. B., Ejaz, H., et al. (2020). Emergence of plasmid-mediated mcr genes from gram-negative bacteria at the human-animal interface. Gut Pathogens 12:54. doi: 10.1186/s13099-020-00392-3
Kaas, R. S., Leekitcharoenphon, P., Aarestrup, F. M., Lund, O., and Friedrich, A. (2014). Solving the problem of comparing whole bacterial genomes across differentsequencing platforms. PLoS One 9:e104984. doi: 10.1371/journal.pone.0104984
Kumar, S., Stecher, G., Li, M., Knyaz, C., and Tamura, K. (2018). MEGA X: molecular evolutionary genetics analysis across computing platforms. Mol. Biol. Evol. 35, 1547–1549. doi: 10.1093/molbev/msy096
Lencina, F. A., Bertona, M., Stegmayer, M. A., Olivero, C. R., Frizzo, L. S., Zimmermann, J. A., et al. (2024). Prevalence of colistin-resistant Escherichia coli in foods and food-producing animals through the food chain: a worldwide systematic review and meta-analysis. Heliyon. 10:e26579. doi: 10.1016/j.heliyon.2024.e26579
Letunic, I., and Bork, P. (2019). Interactive tree of life (iTOL) v4: recent updates and new developments. Nucleic Acids Res. 47, W256–W259. doi: 10.1093/nar/gkz239
Li, R., Lu, X., Munir, A., Abdullah, S., Liu, Y., Xiao, X., et al. (2022). Widespread prevalence and molecular epidemiology of tet (X4) and mcr-1 harboring Escherichia coli isolated from chickens in Pakistan. Sci. Total Environ. 806:150689. doi: 10.1016/j.scitotenv.2021.150689
Li, R., Mohsin, M., Lu, X., Abdullah, S., Munir, A., and Wang, Z. (2021). Emergence of plasmid-mediated resistance genes tet (X) and mcr-1 in Escherichia coli clinical isolates from Pakistan. Msphere 6, 10–1128. doi: 10.1128/mSphere.00695-21
Li, X., Zhu, X., and Xue, Y. (2023). Drug resistance and genetic relatedness of Escherichia coli from mink in Northeast China. Pak. Vet. J. 43, 824–827. doi: 10.29261/pakvetj/2023.062
Magiorakos, A. P., Srinivasan, A., Carey, R. B., Carmeli, Y., Falagas, M. E., Giske, C. G., et al. (2012). Multidrug-resistant, extensively drug-resistant and pandrug-resistant bacteria: an international expert proposal for interim standard definitions for acquired resistance. Clin. Microbiol. Infect. 18, 268–281. doi: 10.1111/j.1469-0691.2011.03570.x
Murray, C. J., Ikuta, K. S., Sharara, F., Swetschinski, L., Robles, G., Gray, A., et al. (2022). Global burden of bacterial antimicrobial resistance in 2019: a systematic analysis. Lancet 399, 629–655. doi: 10.1016/S0140-6736(21)02724-0
Saeed, D. K., Farooqi, J., Shakoor, S., and Hasan, R. (2021). Antimicrobial resistance among GLASS priority pathogens from Pakistan: 2006–2018. BMC Infect. Dis. 21, 1–16. doi: 10.1186/s12879-021-06795-0
Satlin, M. J., Lewis, J. S., Weinstein, M. P., Patel, J., Humphries, R. M., Kahlmeter, G., et al. (2020). Clinical and laboratory standards institute and European committee on antimicrobial susceptibility testing position statements on polymyxin B and colistin clinical breakpoints. Clin. Infect. Dis. 71, e523–e529. doi: 10.1093/cid/ciaa121
Shafiq, M., Bilal, H., Permana, B., Xu, D., Cai, G., Li, X., et al. (2023). Characterization of antibiotic resistance genes and mobile elements in extended-spectrum β-lactamase-producing Escherichia coli strains isolated from hospitalized patients in Guangdong, China. J. Appl. Microbiol. 134:lxad125. doi: 10.1093/jambio/lxad125
Sora, V. M., Meroni, G., Martino, P. A., Soggiu, A., Bonizzi, L., and Zecconi, A. (2021). Extraintestinal pathogenic Escherichia coli: virulence factors and antibiotic resistance. Pathogens 10:1355. doi: 10.3390/pathogens10111355
Tang, B., Chang, J., Cao, L., Luo, Q., Xu, H., Lyu, W., et al. (2019). Characterization of an NDM-5 carbapenemase-producing Escherichia coli ST156 isolate from a poultry farm in Zhejiang, China. BMC Microbiol. 19:82. doi: 10.1186/s12866-019-1454-2
Tkadlec, J., Kalova, A., Brajerova, M., Gelbicova, T., Karpiskova, R., Smelikova, E., et al. (2021). The intestinal carriage of plasmid-mediated colistin-resistant Enterobacteriaceae in tertiary care settings. Antibiotics 10:258. doi: 10.3390/antibiotics10030258
Uddin, M. B., Alam, M. N., Hasan, M., Hossain, S. B., Debnath, M., Begum, R., et al. (2022). Molecular detection of Colistin resistance mcr-1 gene in multidrug-resistant Escherichia coli isolated from chicken. Antibiotics 11:97. doi: 10.3390/antibiotics11010097
Uelze, L., Grützke, J., Borowiak, M., Hammerl, J. A., Juraschek, K., Deneke, C., et al. (2020). Typing methods based on whole genome sequencing data. One Health Outlook 2, 1–19. doi: 10.1186/s42522-020-0010-1
Ulrich, J. U. (2023). Advanced methods for real-time metagenomic analysis of Nanopore sequencing data (doctoral dissertation).
Umair, M., Hassan, B., Farzana, R., Ali, Q., Sands, K., Mathias, J., et al. (2023). International manufacturing and trade in colistin, its implications in colistin resistance and one health global policies: a microbiological, economic, and anthropological study. The Lancet Microbe 4, e264–e276. doi: 10.1016/S2666-5247(22)00387-1
Usui, M., Nozawa, Y., Fukuda, A., Sato, T., Yamada, M., Makita, K., et al. (2021). Decreased colistin resistance and mcr-1 prevalence in pig-derived Escherichia coli in Japan after banning colistin as a feed additive. J. Glob. Antimicrob. Resist. 24, 383–386. doi: 10.1016/j.jgar.2021.01.016
van Den Bunt, G., Fluit, A. C., Bootsma, M. C., van Duijkeren, E., Scharringa, J., van Pelt, W., et al. (2020). Dynamics of intestinal carriage of extended-Spectrum Beta-lactamase–producing Enterobacteriaceae in the Dutch general population, 2014–2016. Clin. Infect. Dis. 71, 1847–1855. doi: 10.1093/cid/ciz1091
Vu, B., le, T., Nguyen, M., Nguyen, T., Nguyen, D., le, D., et al. (2022). Characterization of genetic elements carrying mcr-1 gene in Escherichia coli from the community and hospital settings in Vietnam. Microbiology spectrum 10, e01356–e01321. doi: 10.1128/spectrum.01356-21
Wang, Y., Xu, C., Zhang, R., Chen, Y., Shen, Y., Hu, F., et al. (2020). Changes in colistin resistance and mcr-1 abundance in Escherichia coli of animal and human origins following the ban of colistin-positive additives in China: an epidemiological comparative study. Lancet Infect. Dis. 20, 1161–1171. doi: 10.1016/S1473-3099(20)30149-3
Wise, M. G., Estabrook, M. A., Sahm, D. F., Stone, G. G., and Kazmierczak, K. M. (2018). Prevalence of mcr-type genes among colistin-resistant Enterobacteriaceae collected in 2014-2016 as part of the INFORM global surveillance program. PLoS One 13:e0195281. doi: 10.1371/journal.pone.0195281
Xie, J., Liang, B., Xu, X., Yang, L., Li, H., Li, P., et al. (2022). Identification of mcr-1-positive multidrug-resistant Escherichia coli isolates from clinical samples in Shanghai, China. J. Global Antimicrobial Resistance 29, 88–96. doi: 10.1016/j.jgar.2022.02.008
Zahra, R., Javeed, S., Malala, B., Babenko, D., and Toleman, M. A. (2018). Analysis of Escherichia coli STs and resistance mechanisms in sewage from Islamabad, Pakistan indicates a difference in E. coli carriage types between South Asia and Europe. J. Antimicrob. Chemother. 73, 1781–1785. doi: 10.1093/jac/dky109
Keywords: Escherichia coli, Colistin, MCR-1, whole-genome sequencing, antibiotic resistance
Citation: Abdullah S, Mushtaq MA, Ullah K, Hassan B, Azam M, Zahoor MA, Wang J, Xu J, Toleman MA and Mohsin M (2025) Dissemination of clinical Escherichia coli harboring the mcr-1 gene in Pakistan. Front. Microbiol. 15:1502528. doi: 10.3389/fmicb.2024.1502528
Received: 27 September 2024; Accepted: 06 December 2024;
Published: 07 January 2025.
Edited by:
Miklos Fuzi, Independent researcher, Seattle, United StatesReviewed by:
Charity Wiafe Akenten, Kumasi Centre for Collaborative Research in Tropical Medicine (KCCR), GhanaCopyright © 2025 Abdullah, Mushtaq, Ullah, Hassan, Azam, Zahoor, Wang, Xu, Toleman and Mohsin. This is an open-access article distributed under the terms of the Creative Commons Attribution License (CC BY). The use, distribution or reproduction in other forums is permitted, provided the original author(s) and the copyright owner(s) are credited and that the original publication in this journal is cited, in accordance with accepted academic practice. No use, distribution or reproduction is permitted which does not comply with these terms.
*Correspondence: Brekhna Hassan, aGFzc2FuYjFAY2FyZGlmZi5hYy51aw==; Mark A. Toleman, dG9sZW1hbm1hQGNhcmRpZmYuYWMudWs=; Mashkoor Mohsin, bWFzaGtvb3Jtb2hzaW5AdWFmLmVkdS5waw==
Disclaimer: All claims expressed in this article are solely those of the authors and do not necessarily represent those of their affiliated organizations, or those of the publisher, the editors and the reviewers. Any product that may be evaluated in this article or claim that may be made by its manufacturer is not guaranteed or endorsed by the publisher.
Research integrity at Frontiers
Learn more about the work of our research integrity team to safeguard the quality of each article we publish.