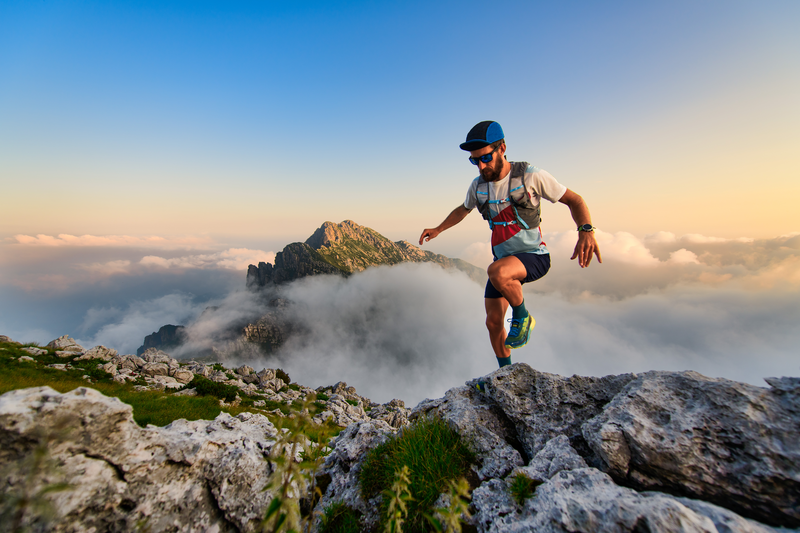
95% of researchers rate our articles as excellent or good
Learn more about the work of our research integrity team to safeguard the quality of each article we publish.
Find out more
REVIEW article
Front. Microbiol. , 24 December 2024
Sec. Systems Microbiology
Volume 15 - 2024 | https://doi.org/10.3389/fmicb.2024.1500890
The coronavirus disease 2019 (COVID-19), caused by severe acute respiratory syndrome coronavirus-2 (SARS-CoV-2), has led to major global health concern. However, the focus on immediate effects was assumed as the tip of iceberg due to the symptoms following acute infection, which was defined as post-acute COVID-19 syndrome (PACS). Gut microbiota alterations even after disease resolution and the gastrointestinal symptoms are the key features of PACS. Gut microbiota and derived metabolites disorders may play a crucial role in inflammatory and immune response after SARS-CoV-2 infection through the gut-lung axis. Diet is one of the modifiable factors closely related to gut microbiota and COVID-19. In this review, we described the reciprocal crosstalk between gut and lung, highlighting the participation of diet and gut microbiota in and after COVID-19 by destroying the gut barrier, perturbing the metabolism and regulating the immune system. Therefore, bolstering beneficial species by dietary supplements, probiotics or prebiotics and fecal microbiota transplantation (FMT) may be a novel avenue for COVID-19 and PACS prevention. This review provides a better understanding of the association between gut microbiota and the long-term consequences of COVID-19, which indicates modulating gut dysbiosis may be a potentiality for addressing this multifaceted condition.
Since the pandemic of coronavirus disease 2019 (COVID-19) caused by severe acute respiratory syndrome coronavirus 2 (SARS-CoV-2) (Sungnak et al., 2020; Zhu et al., 2020), multiple variants have been identified, which act as a threat to public health and have been speculated to coexist with humans for a long time (Kissler et al., 2020; Kim, 2024). The clinical manifestations of COVID-19 mostly focus on the respiratory systems, including fever, cough and dyspnea, pneumonia, acute respiratory distress syndrome (ARDS), and respiratory failure (Chen N. et al., 2020). However, the symptoms may be multisystem and have long-lasting effects on people termed long-COVID or post-acute COVID-19 syndrome (PACS), which persist beyond 4 weeks after infection up to several years and involve physical, cognitive, and mental health impairments (Parotto et al., 2023; Santos et al., 2024). The prevalence of PACS is approximately 10–30% and over 65 million individuals worldwide are plagued (Davis et al., 2023). More than one-fifth of COVID-19 adult patients could not recover within 3 months, especially for women and those with cardiovascular disease (Oelsner et al., 2024). Among hospitalized COVID-19 individuals, the risk of PACS declined over the 3 years but still contributed 90 disability-adjusted life years (DALYs) per 1,000 persons, suggesting the burden of health loss remains even in the third year after infection (Cai et al., 2024). Due to the heterogeneity of disparate symptoms in long-COVID patients, different mechanisms have been proposed, including viral persistence or reactivation, permanent inflammation, immune dysregulation, and alteration of gut microbiota, but there is not an adequate perception of this complex condition (Fernández-de-Las-Peñas et al., 2023).
Numerous studies have revealed that dysregulation of gut microbiota is common in acute to post-COVID and has a significant impact on this process through the gut-lung axis. Direct or indirect microbial pathways are thought to contribute to the interaction between SARS-CoV-2 and the gut. Substantial evidences indicate that symbiotic microbiota regulates invading viruses through various mechanisms, which in turn are regulated by viruses, thereby exerting stimulatory or inhibitory effects on virus infections (Karst, 2016). Baseline gut microbiota and metabolome can modulate the durability of immunity to the SARS-CoV-2 vaccine to overcome waning immune responses over time and potentially predict immunogenicity to vaccines for up to 6 months (Peng et al., 2023). Additionally, the disordered gut microbiota is associated with persistent inflammation even after COVID-19 resolution, which suggests the long-reaching effect of the gut microbiota and hints at the significance of microbial management in long-COVID. Several studies have reported the detection of viral RNA in the feces and gastrointestinal tract of COVID-19 patients (Xing et al., 2020; Wu et al., 2020; Guo M. et al., 2021). The median duration of viral RNA in feces was found to be 22 days and the viral load typically peaked 2–3 weeks after symptom onset during the later stages of the disease (Zheng et al., 2020; Walsh et al., 2020). In some patients, fecal samples remained positive for the virus even when respiratory and/or sputum samples were negative (Xu et al., 2020; Wölfel et al., 2020).
While the development of pharmaceutical treatments for COVID-19 is currently in progress, the long-term effects of COVID-19 demand greater attention and coordinated preventive strategies. Nirmatrelvir-ritonavir was found to reduce the risk of post-acute inpatient death as well as cardiovascular and respiratory complications among hospitalized patients with COVID-19 (Wang H. et al., 2024). Pre-infection vaccination was associated with reduced risk of post-COVID conditions, including sensor, circulatory, blood and hematologic, skin and subcutaneous, and non-specific COVID-19 related disorders (Malden et al., 2024). Surprisingly, healthy lifestyle behaviors may play an important role in maintaining public health in the COVID-19 pandemic (Murakami et al., 2023). The causes of long-COVID are unclear, but certain dietary interventions may manage symptoms and support overall recovery (Cheong et al., 2023). The proper diet and supplementation are postulated to maintain the host homeostasis in COVID-19 and post-COVID by altering the microbial configuration, affecting host metabolism, and regulating the immune response. Individuals with a diet high in fat could reduce the richness and diversity of microbiota (Kolodziejczyk et al., 2019), which are highly prone to COVID-19 and related adverse outcomes (Moallemian et al., 2021). On the contrary, diets rich in fruit and vegetables have anti-inflammatory properties, which could protect lung function and may be an auxiliary tool in COVID-19 (Singh et al., 2022). However, whether diet is a pivotal factor related to the susceptibility and persistence of COVID-19 deserves further discussion. Regulating the gut microbiome by dietary interventions may act as a preventative and alternative strategy to minimize the severity and long-term implications of SARS-CoV-2. In this review, we will highlight the dynamic evolution of gut microbiota in acute COVID-19 to PACS, and further discuss the perspectives of diet as one of the possible prophylactic and therapeutic measures.
The reciprocal communication between the gut and lung, which is mainly mediated by microbial metabolites and toxins translation through blood or lymphatic circulation, is known as the “gut-lung axis” (Synodinou et al., 2022). Gut microbiota dysbiosis may induce lung disorders and respiratory infections, by causing local or long-reaching immune, hormonal, and metabolic dysregulation (Dang and Marsland, 2019). Gut microbiota promotes granulocyte-macrophage colony-stimulating factor (GM-CSF) production, which activates extracellular signal-regulated kinase signaling in alveolar macrophages to enhance airway defenses (Brown et al., 2017). More specifically, the translocation of disordered microbial bacteria and derived metabolites may play a potential role in SARS-CoV-2 infection. The composition of gut microbiota was considered to be an important factor in regulating the expression of ACE2 in the gastrointestinal tract (Yang T. et al., 2020). Specific bacterial species, such as Bacteroides dorei, Bacteroides thetaiotaomicron, Bacteroides massiliensis, and Bacteroides ovatus (Geva-Zatorsky et al., 2017), were found to downregulate the expression of ACE2 and correlated inversely with fecal SARS-CoV-2 load (Zuo et al., 2020a). The intact intestinal epithelial cells could produce antiviral compounds that are hostile to viruses (Kalantar-Zadeh et al., 2020). The gut microbiome is also an important component of intestinal barrier function, which can defend against the invasion of potentially pathogenic bacteria (Bernard-Raichon et al., 2022). Epithelial barrier damage and gut microbiota disruption lead to gut pathogenic bacteria and their metabolites migrating to the lung (Chakradhar, 2017). COVID-19 patients were found to exhibit more occurrence of microbial translocation, especially for subjects admitted to the ICU (Oliva et al., 2021). Gut microbial dysbiosis could also influence the alteration of pulmonary flora during COVID-19. The translocation of microbiota and immune cells through the gut-lung axis may lead to more severe lung injury of COVID-19. Enrichment of gut bacteria in the lung microbiome was observed to be associated with sepsis and ARDS (Dickson et al., 2016).
The gut microbiome of patients with COVID-19 has impaired SCFA biosynthesis ability. SCFAs could move to the lung and promote the recruitment and maturation of immune cells locally (Anand and Mande, 2018). Treatment of gut epithelial organoids with butyrate could inhibit viral infection. A disintegrin and metalloproteinase 17 (ADAM17) serves as a metallopeptidase involved in ACE2 shedding and regulates its availability. Transmembrane protease serine 2 (TMPRSS2) facilitates virus-cell membrane fusion (Zlacká et al., 2021). SCFAs, especially butyrate, could downregulate the expression of the TMPRSS2 and ACE2 gene which is necessary for SARS-CoV-2 infection (Ohira et al., 2017), while upregulating the expression of ADAM17, thereby inhibiting viral infectivity to cells (Takabayashi et al., 2022). The kynurenine pathway of tryptophan metabolism was observed to activate in COVID-19 patients (Lionetto et al., 2021), which is involved in regulating inflammation and immune tolerance. Tryptophan metabolites can also cause the gut microbiota shift and indirectly regulate host intestinal inflammation and immune responses (Sonner et al., 2019). Furthermore, gut microbial metabolism of sphingolipid, lipopolysaccharide, and neutral amino acids was reported to increase in patients with COVID-19, which suggested the state of oxidative stress of gut microbiome in patients (Zhou et al., 2022). Thus, healthy gut microbiota and derived metabolites may enhance antiviral defenses, while inflammatory metabolism may exacerbate COVID-19.
Moreover, the increased severity of SARS-CoV-2 is associated with not only the virus but also an aggressive immune response. The concept of a common mucosal immunologic system has been proposed (McDermott and Bienenstock, 1979), and evidence is increasing in support of the hypothesis that microbiota may influence lung mucosal immune response by affecting intestinal mucosal immunity. Alteration of the gut microbiota or their metabolites can impair the immune activation against respiratory viruses including SARS-CoV-2. The damaged mucosa and shifted immune response cannot inhibit the overgrowth of pathogenic bacteria, further aggravating the pathophysiological state (Statovci et al., 2017). Interestingly, the interaction of the gut-lung axis is not restricted to a single way. The infection of the lungs can also indirectly increase the systemic pro-inflammatory factors and decrease oxygen levels, which induce intestinal inflammation, epithelial destruction, and antimicrobial peptide reduction, resulting in gut dysbiosis (Rastogi et al., 2022). The microbial dysbiosis of the respiratory tract could disrupt homeostasis and increase the load of gut microbiota due to bacterial translocation. Thus, the lung-gut axis is assumed as a bilateral loop, which would be regulated dynamically by the modulation of the lung or gut immune responses, and is perturbated in acute to post-acute COVID-19 syndrome (Figure 1).
Figure 1. The gut-lung axis crosstalk in COVID-19. The key crosstalk between the gut microbiota and lung was termed the “gut-lung axis.” SARS-CoV-2 infected the lung by binding with ACE2 and downregulated the expression of intestinal ACE2, decreasing the transportation of tryptophan and the production of antimicrobial peptides, which then disturb the gut microbes and facilitate pathogen growth. Cytokine storm induced by SARS-CoV-2 infection in the lung could promote systematic or intestinal inflammation and contribute to gut microbiota dysbiosis. Opportunistic pathogens (eg., Coprobacillus, Enterococcus, Parabacteroides) were enriched while beneficial bacteria (eg., Lachnospiraceae, Actinobacteria, Bifidobacterium) were decreased in the gut of patients, inducing the disorder of derived metabolites including SCFAs and LPS. Disordered pathogens could translocate to the lungs to impact the local microecology and the recruitment of immune cells in the gut and lungs, affecting the local mucosal immune and inflammation. ACE2, angiotensin-converting-enzyme 2; LPS, lipopolysaccharide; SARS-CoV-2, severe acute respiratory syndrome coronavirus-2; SCFAs, short chain fatty acids.
SARS-CoV-2 infection has been attested to negatively affect the composition of gut microbiota, characterized by the increase of opportunistic pathogens and the reduction of beneficial commensals. The potential mechanisms of the gut microbiota alteration in COVID-19 have not been fully elucidated. Cytokine storm induced by pulmonary SARS-CoV-2 infection or intestinal impairment caused by direct gut infection could promote systematic or intestinal inflammation and contribute to gut microbiota dysbiosis (Merad and Martin, 2020; Guo Y. et al., 2021). Angiotensin-converting enzyme 2 (ACE2), which has been confirmed to have a high affinity with the coronavirus spike protein, is expressed not merely in the lung but also in the extrapulmonary organs including the gastrointestinal (GI) tract (Shang et al., 2020; Yan et al., 2020). SARS-CoV-2 infection could downregulate the expression of intestinal ACE2, which performed tryptophan transport and decreased the production of antimicrobial peptides, disturbing the ecology of gut microbes and then facilitating pathogen growth (Lecarpentier and Vallée, 2021; Penninger et al., 2021).
The sequencing studies required COVID-19 patients and healthy individuals to refrain from using antibiotics, probiotics, or a combination of both within 4 to 8 weeks before enrollment (Wu et al., 2021; Li S. et al., 2021; Yeoh et al., 2021), indicating that the gut microbiota exhibited significant differences in diversity and composition between patients with COVID-19 and healthy cohorts (Wu et al., 2021; Gu et al., 2020). Opportunistic pathogens were enriched and short-chain fatty acid (SCFA)-producing bacteria were exhausted in the patients, suggesting the universality of gut microbiota dysbiosis in COVID-19 infection (Yeoh et al., 2021). At the phylum level, Bacteroides was significantly increased and Actinomycetes was decreased in COVID-19 patients (Ren et al., 2021). Faecalibacterium prausnitzii (F. prausnitzii), which produced SCFAs and was positively correlated with a higher quantity of blood neutrophils, was reduced in patients with COVID-19 (Zhang F. et al., 2022). Furthermore, Bacteroides stercoris, B. vulgatus, B. massiliensis, Streptococcus thermophilus (S. thermophilus) were enriched in COVID-19 patients, while Clostridium nexile, S. salivarius, Coprococcus catus, Eubacterium hallii, and Enterobacter aerogenes were decreased. Four microorganisms, including S. thermophilus, Bacteroides oleiciplenus, Fusobacterium ulcerans, and Prevotella bivia, were identified only in patients with COVID-19 (Li S. et al., 2021). Even asymptomatic infants exhibited changes in gut microbiota, notably by decreasing anti-inflammatory bacteria including Bifidobacterium bifidum (B. bifidum) and Akkermansia muciniphila (Nashed et al., 2022). High fecal SARS-CoV-2 infectivity, which suggested active gastrointestinal SARS-CoV-2 infection, was associated with a higher abundance of Collinsella aerofaciens, Collinsella tanakaei, Streptococcus infantis, Morganella morganii in the gut (Zuo et al., 2021). Opportunistic fungal pathogens were found to increase in patients with COVID-19 as well, such as Candida albicans, Candida auris, and Aspergillus flavus (Zuo et al., 2020b) (Table 1).
Surprisingly, the gut microbiome characteristics of COVID-19 were largely restored following the disease subsided but did not fully revert to normal. The microbial diversity gradually increased in the recovery process especially butyric acid-producing microbes and Bifidobacterium while LPS-producing microbes decreased (Cui et al., 2022). F. prausnitzii, Eubacterium rectale (E. rectale), and Bifidobacteria are less abundant in patients 1 month after the infection is resolved. However, patients with PACS still had significant microbial alterations at 6 months after acute infection, which was characterized by a higher abundance of Ruminococcus gnavus (R. gnavus), Bacteroides vulgatus and lower abundance of F. prausnitzii (Liu et al., 2022a). The alterations of gut microbiome linger beyond 1 year, showing enrichment of potentially pathogenic bacteria such as Erysipelatoclostridium ramosum and R. gnavus, while a depletion of beneficial bacteria such as B. adolescentis and B. pseudocatenulatum (Su et al., 2023). Enterobacteriaceae strains with antibiotic-resistance were found to dominate in the gut of post-COVID patients along with the reduced SCFAs in feces. Fecal microbiota transplantation (FMT) from post-COVID subjects to germ-free mice resulted in exacerbated lung inflammation and brain dysfunction. A. flavus and Aspergillus niger, as respiratory fungal pathogens, were still detected in the feces of COVID-19 patients after SARS-CoV-2 clearance and respiratory symptoms resolution (Zuo et al., 2020b). Therefore, gut microbiota may directly contribute to the prolonged sequelae of COVID-19, suggesting its potential as a therapeutic target (Mendes de Almeida et al., 2023) (Table 2).
Based on millions of confirmed cases worldwide, several risk factors have been identified to be associated with the disease severity of COVID-19, including older age, male sex, ethnic variations, and underlying comorbidities. In addition, laboratory indices, pro-inflammatory cytokine levels, and complications are also the predictive factors for the progression of COVID-19 into a severe and critical stage. In contrast, a healthy diet and sufficient nutrition, COVID-19 vaccination, and atopic conditions may avoid the disease progression and poor outcome (Zhang et al., 2023). However, numerous studies have found that gut microbiota dysbiosis could negatively impact the recruitment of immune cells and the development of immune responses in the lungs, which could contribute to respiratory tract infections (Baradaran Ghavami et al., 2021). It has been demonstrated that COVID-19 severity is indeed highly correlated with the characteristics of gut microbiota, which can be utilized as a predictive biomarker and provide potential treatment strategies (Cao et al., 2021). Even after the SARS-CoV-2 clearance, persistent disturbance of the gut microbiota may be a pivotal factor for protracted symptoms and multisystem inflammation syndromes (Yeoh et al., 2021).
The gut microbiome in SARS-CoV-2 infected patients was associated with a pro-inflammatory signature, and the connectivity of an anti-inflammatory bacterial network was observed to reduce in patients with severe COVID-19 (Reinold et al., 2021). A multicenter cross-sectional study has shown that the gut microbiota of moderate and severe COVID-19 patients tended to have decreased Firmicutes/Bacteroidetes ratio, higher abundance in Proteobacteria, lower abundance in butyrate-producing bacteria from the family Lachnospiraceae and the phylum Actinobacteria (Moreira-Rosário et al., 2021). In the gut, significantly fewer Bacteroides and increased Enterococcus are found in intensive care unit (ICU) patients (Shen et al., 2022). The baseline abundance of Coprobacillus, Clostridium ramosum, and Clostridium hathewayi was positively associated with the severity of COVID-19 while the abundance of F. prausnitzii and Alistipes onderdonkii showed an inverse correlation with the disease severity (Zuo et al., 2020a). Severe COVID-19 was significantly associated with the Turicibacter and Olsenella genera and potentially linked to Ruminococcus1, CandidatusSoleaferrea, and Parasutterella genus (Zhang and Zhou, 2023). Two bacterial species Oribacterium sp. GMB0313 and Ruminococcus sp. GMB0270 are both observed to associated with COVID-19 resistance, and the pair of which protect against SARS-CoV-2 infection by activating CD8+ T cell-mediated immunity (Wang M. et al., 2024). Surprisingly, gastrointestinal fungal dysbiosis is also present in COVID-19 patients. The overgrowth of intestinal Candida and the elevated levels of Candida albicans IgG antibodies symbolized the severity of the disease. The transcriptional level of antifungal immunity pathways and reprogramming of granulocyte myeloid progenitors were increased, suggesting that gut fungal pathobionts may activate the immune response during COVID-19 (Kusakabe et al., 2023).
Poor basic physical status and severe acute COVID-19 convey the increased risk for the development of post-acute COVID-19, but mild COVID-19 may also culminate in post-acute sequelae (Xie et al., 2021). Species of the gut microbiome associated with host immune response modulation, such as F. prausnitzii, E. rectale, and Bifidobacterial, were decreased in patients even in post-COVID. More significantly, these perturbed compositions represented stratification with disease severity, plasma concentrations of inflammatory factors, and blood markers of tissue damage (Yeoh et al., 2021). Furthermore, the composition of gut microbiota was also observed to be associated with complications and mortality in COVID-19 patients. The gut bacterial richness was decreased with the number of complications. Parabacteroides was increased in patients with ARDS and hemodialysis, which suggested a more complicated course and a positive correlation to mortality. On the contrary, butyrate-producing bacteria, such as F. prausnitzii, were significantly reduced in patients with multiple complications (Liu et al., 2022b; Schult et al., 2022). An observational prospective cohort study had shown that the increase of Enterococcus spp., Staphylococcus aureus and Candida spp. both in oropharyngeal and rectal samples were associated with a 17% or greater higher risk of death (Patrier et al., 2022). Mortality from COVID-19 was demonstrated to be associated with enriched Proteobacteria in the feces and decreased secondary bile acids (Stutz et al., 2022). Thus, a balanced and robust gut microbiome may propel a favorable outcome for COVID-19 individuals. The gut bacterial signatures can be used for disease diagnosis and mortality estimation.
Individuals with PACS may experience over 50 persistent symptoms, including fatigue, dyspnea, dry coughing, olfactory and gustatory dysfunctions, diarrhea, nausea, and abdominal pain, which may fluctuate or relapse over time. The exact mechanisms of PACS may be multiply overlapping, several hypotheses have been proposed, including persistent viral existence in tissues, dysfunction of vascular endothelium and nervous system, reactivation of latent pathogens and gut microbiota alteration, long-lasting inflammation, and immune dysregulation (Davis et al., 2023). The high abundance of ACE2 receptors on intestinal cells supports the persistent viral reservation, which may be a potential mechanism of PACS (Lee et al., 2020). Intestinal affinity to SARS-CoV-2 was correlated with gastrointestinal symptoms in the early stage and may increase the risk of developing GI disorders even after COVID-19. More importantly, disruptions in the intestinal barrier and imbalances in gut microbiota could further result in an increase of bacterial lipopolysaccharide and peptidoglycan at the systemic level to amplify systemic inflammation among patients with PACS persistently (Teixeira et al., 2021; Schultheiß et al., 2022). The toxins generated by leaky gut microbiota and uraemic solutes generated by the kidney with chronic inflammation, potentially lead to symptoms such as fatigue, disturbances in mineral bone metabolism, neurological issues, and compromised cardiovascular function (Adhikari et al., 2024). Recent studies have revealed that gut microbiota alterations, mucosal inflammation, and intestinal permeability were associated with metabolic disorders in PACS. SARS-CoV-2 can trigger specific metabolic conditions and can push a compromised system into a positive feedback loop, contributing to limited mitochondrial dysfunction, including down-regulation of core mitochondrial genes and unusual swelling of cristae (Guarnieri et al., 2023; Cortese et al., 2020). Mitochondrial dysfunction impedes the process of antioxidants and respiratory chain, which then affect the oxidative phosphorylation and cellular signaling cascades (Mandò et al., 2021). Similar mitochondrial dysfunction has been observed in myalgic encephalomyelitis/chronic fatigue syndrome (ME/CFS) of PACS, which may result from the reactivation of EBV (Vernon et al., 2006). However, the heterogeneity and complexity of PACS hindered the decryption of the pathophysiological mechanisms, which need further multidisciplinary studies to better understand and address these underlying health problems. The involvement of coexisting symptoms suggests the requirement to provide insights into different symptom clusters and identify potential targets for personalized therapeutic interventions. In this review, we highlighted the persistent respiratory and gastrointestinal symptoms in PACS and discussed underlying mechanisms involved in the gut-lung crosstalk (Figure 2).
Figure 2. Hypothesized mechanisms of respiratory and gastrointestinal post-acute COVID-19 syndrome. Shortness of breath, cough, dyspnea and sore throat are the common respiratory symptoms in patients with post-acute COVID-19 syndrome. GI symptoms of PACS, including diarrhea, constipation, loss of appetite are mainly presented. The exact mechanisms of PACS may be multiply overlapping, several hypothesized mechanisms mainly including persistent viral existence in tissues, gut microbiota alteration, long-lasting inflammation and immune dysregulation.
Shortness of breath and cough are the most common respiratory symptoms in patients with long-COVID at 7 months (Davis et al., 2021), and 43.4% of patients showed persisting dyspnea at 60 days (Carfì et al., 2020). Severe COVID-19 patients often present long-term pulmonary sequelae, which are accompanied by decreased lung function, systemic inflammation, and persistent respiratory immune imbalance (Littlefield et al., 2022). Specifically, dysregulated responses of potential pathogenic subsets of CD8+ T cells were associated with impaired lung function after acute COVID-19 (Cheon et al., 2021). SARS-CoV-2 specific CD8+ T cells in the nasal mucosa were found to persist 2 months after viral clearance, indicating the long-term effects of COVID-19 on immune reactions in the upper respiratory tract (Roukens et al., 2022). Respiratory dysfunction at 3 months after COVID-19 was related to the altered composition of gut microbiota, such as reduced Erysipelotrichaceae UCG-003 and increased Veillonella and Flavonifractor, which persistently elevated the plasma levels of gut barrier dysfunction marker lipopolysaccharide-binding protein (LBP) (Vestad et al., 2022). Thus, the gut-lung axis may be involved in the development of respiratory symptoms of PACS persistently.
Along with respiratory injury, about 15% of patients presented GI manifestations during acute infection, including nausea or vomiting, diarrhea, and loss of appetite (D'Amico et al., 2020; Mao et al., 2020), even as the onset symptom (Song et al., 2020). The feces of COVID-19 patients were detected to have more nutrients that should be metabolized or absorbed and harmful metabolites. Fecal sucrose content is increased and glucose content is decreased in patients with COVID-19 due to sucrase-isomaltase insufficiency, which may cause typically manifest, such as osmotic diarrhea with vomiting, flatulence, and abdominal pain (Lv et al., 2021). In patients with PACS, GI symptoms are increasingly recognized as an important public health concern (Meringer and Mehandru, 2022). Functional dyspepsia-like and irritable bowel syndrome (IBS)-like post-COVID GI symptoms were found in approximately 40% of patients (Vélez et al., 2022), which may be associated with the increased permeability of gut cells caused by gut microbiome dysbiosis, adverse effects of antiviral drugs, and inflammatory response to the virus. The altered gut microbiome activated the immune response and affected neuromuscular function, causing IBS-like symptoms in GI PACS (Freedberg and Chang, 2022). Anxiety after COVID-19 frequently occurred and was associated with an increased risk of GI symptoms, especially for patients with mental health symptoms before COVID-19 (Blackett et al., 2022). SARS-CoV-2 antigen persistence in the gut after resolution may play a potential role in this process. SARS-CoV-2 RNA was detected in the gut mucosa and viral nucleocapsid protein persisted in gut epithelium and CD8+ T cells after mild acute COVID-19 infection in inflammatory bowel diseases (IBD) patients. The majority of patients with persistent viral antigens reported sequelae of COVID-19, which indicated viral antigen persistence may be the basics of PACS (Zollner et al., 2022). Intestinal biopsies obtained from patients at 4 months after acute COVID-19 still revealed the persistence of SARS-CoV-2 nucleic acids and immunoreactivity (Gaebler et al., 2021). However, Park et al. (2021). demonstrated that SARS-CoV-2 RNA shedding in the feces of patients at 2 months was common but had no association with GI symptoms, and the virus was detected to clear from the gut by 6 months. Therefore, whether GI PACS is driven by sustained viral replication in the gut directly remains skeptical (Freedberg and Chang, 2022).
Almost all PACS were associated with the depletion of beneficial bacteria in gut microbiota such as Gemmiger formicilis and B. adolescentis, while the enrichment of potential pathogens such as R. gnavus, Clostridium bolteae, Flavonifractor plautii and E. ramosum were associated with the majority of the PACS. Depletion of several profitable bacteria, such as B. adolescentis, was associated with specific symptoms post-acute COVID-19 (Su et al., 2023). The gut microbiome was found to be associated with the phenotypic manifestations of PACS, which suggested the potential clinical utility for its prediction and diagnosis (Su et al., 2024). Moreover, fungal translocation, which was measured as β-glucan, was elevated in the plasma of long-COVID patients, possibly inducing higher NF-κB signaling and cytokine production, indicating a potential target linking fungal translocation and inflammation in PACS (Giron et al., 2022). Gut microbiota dysbiosis modifies the immune response and may affect the recovery from PACS among multiple systems far beyond the GI tract.
Ulteriorly, the mechanisms of PACS involve viral infection-induced inflammatory and immune responses (Ruf, 2024). Nucleocapsid IgG levels at 3 months post-infection and neutralizing capacity at 8 months were detected to elevate in long-COVID patients. Spike-specific and nucleocapsid-specific CD4+ T cells as well as TIM-3 expression on CD4+ and CD8+ T cells were observed to increase at 3 and 8 months, but return to comparable immune responses over 24 months (Phetsouphanh et al., 2024). Post-acute COVID-19 was related to hyperactivated innate immune cells and T and B cells, along with elevated expression of the proinflammatory cytokine, such as IFN-β and IFN-λ1 that remained persistently high at 8 months after infection (Phetsouphanh et al., 2022). The IL-1β, IL-6, and TNF cytokine triad was found to be associated with PACS and create self-sustaining feedback in pro-inflammatory macrophages (Schultheiß et al., 2022). C-reactive protein (CRP) levels were detected to increase persistently during SARS-CoV-2 infection to PACS, indicating the long-term symptoms may be related to a specific increase in the CD8+ T cell response. The peripheral immune system of COVID-19 convalescents has a subset of changes for at least 6 months post-infection, which were associated with long-COVID (Ryan et al., 2022). Notably, SARS-CoV-2-specific T cells are differently activated in distinct PACS. SARS-CoV-2 specific CD8+ T cells exhibited cytotoxic characteristics for GI PACS, while T cells followed the opposite trend in patients with respiratory symptoms. The cytotoxic T cells and newly emerging cytotoxic CD4+ T cells were enriched in patients with GI PACS, indicating its correlation with unique T cell clonal and transcriptome (Su et al., 2022). Specific inflammatory pathways and markers were observed to be implicated in subtypes of long-COVID, such as IL-1R2, MATN2 and COLEC12 were associated with cardiorespiratory symptoms, fatigue and anxiety (Liew et al., 2024). These findings indicate that managing long-COVID patients on account of the different subphenotypes may be more effective and support the use of immunomodulatory agents.
The composition of gut microbiota could be dynamically altered by numerous factors, including diet, lifestyle, disease, and aging (Zuo et al., 2020a; Zmora et al., 2019). It is well-established that diet impacts the gut microbiota structure and function rapidly but persistently. Short-term consumption of an animal-based diet consisting of meat, eggs, and cheese increased the abundance of bile-tolerant microorganisms (Alistipes, Bilophila, and Bacteroides) and reduced the levels of Firmicutes (Roseburia, E. rectale, and Ruminococcus bromii) that metabolize dietary plant polysaccharides (David et al., 2014). Although there is no specific dietary supplementation that has been shown to have exact benefits for COVID-19 treatment or prevention, dietary patterns have been shown to be associated with the infection risk, severity, and even consequences (Merino et al., 2021; Yue et al., 2022; Bell et al., 2023). Although the recovery of PACS is frequently complicated by sustained dysfunction, such as fatigue, dysphagia, appetite loss, and taste alterations, making the appropriate evaluation of dietary intake difficult management for these patients, personalized dietary recommendations represent one of the optimal strategies for PACS recovery (Barrea et al., 2022). Thus, encompassing the role of diet in gut microbiota modulation and being mindful of dietary patterns may reduce susceptibility to and long-term complications from COVID-19 (Butler and Barrientos, 2020) (Figure 3).
Figure 3. The effects of dietary patterns on the pathogenesis or prevention of COVID-19. WD intake significantly altered the gut microbial composition, opportunistic pathogens (eg., Desulfovibrio, Streptococcus, and Parabacteroides, Odoribacter) were increased while beneficial bacteria (eg., Prevotellaceae, Rikenellaceae, and Bifidobacterium) were decreased. WD could destroy the intestinal barrier integrity and promote the bacteria invasion and excessive production of metabolite toxins. The composition of gut microbiota was important in downregulating the expression of ACE2, reducing the absorption of tryptophan and increasing oxidative stress. Meanwhile, WD could reduce the abundance of SCFAs-producing bacteria and the production of SCFAs, thereby facilitating the secretion of pro-inflammatory cytokines and dysregulating immune function, which can increase the susceptibility of lungs to COVID-19 in various ways. A healthy diet such as MD and KD may be beneficial to COVID-19. MD balanced the gut microbiota, including the increase of beneficial bacteria (eg., Lactobaccillus and Bifidobacterium) and the decrease of opportunistic pathogens (eg., Bacteroides, Escherichia, and Bifidobacterium). MD can be metabolized by gut microbiota to produce SCFAs, which mediate systematic immunoregulation and anti-inflammatory response through IFN-I signaling. Ketone bodies such as BHB can balance the structure of the intestinal flora and restore the intestinal barrier. BHB not only induces M2 macrophage polarization to exert anti-inflammatory effects, but also promotes oxidative phosphorylation, reshaping the mitochondrial reoxidation-reduction balance and restoring the immune function of CD4+ T cells, thereby enhancing resistance against SARS-CoV-2 infection. WD could directly contribute to hyperglycemia and hyperinsulinemia, promoting the formation of lung inflammation. On the contrary, healthy diets are linked to lower glycemia and higher concentrations of adiponectin, which have an anti-inflammatory effect. ACE2, angiotensin-converting-enzyme 2; BHB, β-hydroxybutyrate; COVID-19, coronavirus disease 2019; IFN-1, interferon-1; KD, ketogenic diet; LPS, lipopolysaccharide; MD, Mediterranean diet; SARS-CoV-2, severe acute respiratory syndrome coronavirus-2; SCFAs, short chain fatty acids; WD, Western diet.
The Western diet (WD) is high in saturated fats, sugars and refined carbohydrates, which can lead to gut dysbiosis and profound implications (Reimer, 2019; Liu et al., 2020a). High-fat diet (HFD) increased the Firmicutes/Bacteroidetes ratio and opportunistic pathogens including Desulfovibrio, Streptococcus, Parabacteroides, and Odoribacter, while decreasing the beneficial bacteria, such as Prevotellaceae, Rikenellaceae and Bifidobacterium, which were negatively correlated with gut barrier function (Liu et al., 2020b; Kociszewska et al., 2021). HFD was demonstrated to reduce the abundance of Roseburia and Lachnospiraceae bacterium (Liu et al., 2020b), which were significantly altered in the fecal microbiome of COVID-19 patients (Zuo et al., 2020a). Furthermore, HFD-induced microbiota dysbiosis disrupted the intestinal barrier, increasing gut pathogenic bacteria and circulating endotoxin, which disequilibrated the local microenvironment and caused inflammation in the lung (Chakradhar, 2017). COVID-19 patients were found to exhibit more occurrence of microbial translocation, especially for subjects admitted to the ICU (Oliva et al., 2021). Diet intake or gut microbial dysbiosis could also influence the alteration of pulmonary flora. The translocation of microbiota and immune cells through the gut-lung axis may lead to more severe lung injury of COVID-19 (Kruglikov et al., 2020). Enrichment of gut bacteria in the lung microbiome was observed to be associated with sepsis and ARDS (Dickson et al., 2016). In addition to the effects of bacteria, the metabolites of gut microbiota can also transfer and activate the immune response. LPS produced by most Gram-negative bacteria could activate the NF-κB pathway and induce severe lung injury, which is a potentially critical determinant of the pathobiology in ARDS (Everhart et al., 2006). HFD could also promote the overgrowth of pathogenic bacteria in the intestine and induce the maturation of proinflammatory immune cells, leading to uncontrolled inflammation and mucosal damage indirectly, which were unable to inhibit the overgrowth of pathogenic bacteria, further aggravating the pathophysiological state (Statovci et al., 2017). Gut dysbiosis could impact the recruitment of immune cells in the lungs through the gut-lung axis. Mucosa-associated invariant T (MAIT) cells are antimicrobial T cells to recognize bacterial metabolites and play a part in antiviral responses, which were activated in the circulation and enriched in the airways of patients with COVID-19 (Parrot et al., 2020).
Long-term WD composition impaired insulin clearance through a small cluster of gut microbes or their metabolites during the progression of obesity and diabetes (Foley et al., 2020), which could place these populations at an increased risk for severe COVID-19 and PACS (Turnbaugh, 2017; Flint and Tahrani, 2020; Wang C. et al., 2023). Both obesity and diabetes can lead to lung function impairment by causing chronic inflammation and delaying ineffective immune response. The risk of hospitalization and death due to COVID-19 showed a linear increase at BMI above 23 kg/m2 and the risk of ICU admission increased linearly across the whole BMI range (Gao et al., 2021). Adipose tissue may be a major player in the spread of SARS-CoV-2 and systemic immune activation. The overexpression of inflammatory adipokines can impair chemotaxis, alter macrophage differentiation, and upregulate inflammatory cytokines such as IL-6 to contribute to the increased morbidity of COVID-19 (Dietz and Santos-Burgoa, 2020; Malavazos et al., 2020). Diet-induced obesity and non-alcoholic steatohepatitis (NASH) were found to impair the recovery from COVID-19 (Briand et al., 2022). Furthermore, the vaccine for SARS-CoV-2 was found to be less effective in obese individuals compared with healthy-weight individuals (Ealey et al., 2021). Remarkably, diabetes was found to be positively associated with COVID-19 severity and complications incidence post-COVID, which may be attributed to systemic inflammation, metabolite disorders (Heintz-Buschart et al., 2016), and the effects of innate immune deficiency (Bornstein et al., 2020). On the contrary, participants missing PACS were more likely to have lower BMI and be less likely to have type 2 diabetes, which may be related to less release of cytokines after infection and long-term complications of multiple organs subsequently (Wang S. et al., 2023).
Severe patients with COVID-19 more frequently had increased inflammatory monocytes and neutrophils and a sharp decrease in lymphocytes (Giamarellos-Bourboulis et al., 2020), with higher levels of IL-1β, IL-6, and TNF-α (Chen G. et al., 2020), leading to systemic organ failure and tissue damage. Diet may have a widespread role in regulating the immune response to SARS-CoV-2 infection and consequently modulate the disease severity (Morais et al., 2021). WD consumption could activate innate and inhibit adaptive immunity, inducing chronic inflammation and impairing the defense against viruses. HFD was observed to increase macrophage infiltration in alveoli, which is strongly associated with ARDS and poor outcomes in COVID-19 patients (Purbey et al., 2023). For the adaptive immune system, WD consumption induced oxidative stress to consume the number and inhibit the function of T and B lymphocytes, which led to immunodepression and was associated with more severe pathogenesis of COVID-19 (Butler and Barrientos, 2020). Excessive production of proinflammatory molecules, which is called the cytokine storm, may be aggravated by WD with high pro-inflammatory potential. Severe COVID-19 was found to have higher concentrations of inflammatory markers than mild patients (Ji et al., 2020). The pro-inflammatory blood markers were observed to be higher and the CD8+ T cell number was lower in patients with severe COVID-19 illness (Reinold et al., 2021). Moreover, peripheral inflammation post-COVID may have long-term consequences, such as dementia and neurodegenerative disease, which can be further promoted by an unhealthy diet (Butler and Barrientos, 2020).
On the contrary, the Mediterranean diet (MD) is rich in fiber, antioxidants, vegetable protein, vitamins, and minerals. A statistical analysis included a cohort of 5,194 participants and found that higher adherence to the MD may be associated with a lower subsequent risk of COVID-19 (Schwingshackl and Hoffmann, 2014). The increased MD score values reduced the rate of COVID-19 occurrence and were observed to have a negative association with both COVID-19 cases and related deaths (El Khoury and Julien, 2021; Greene et al., 2021). The severity of SARS-CoV-2 infection was associated with higher age and saturated fat intake, while lower MD and cereal consumption (Ponzo et al., 2021). A comparative study among vegetarians, vegans and omnivorous diet subjects showed that the abundance of Bacteroides, Escherichia coli and Enterobacteriaceae in vegetarians was significantly reduced compared with the omnivorous control group, while the subjects on vegetarians ranked between vegans and controls (Zimmer et al., 2012). Dietary fiber has been reported to increase the diversity of gut microbiota related to suppressive mucosal inflammation and promoted health, such as Bifidobacterium and Lactobaccillus (Carlson et al., 2018). Firmicutes and Proteobacteria were higher while Bacteroidetes was lower in mice fed by fiber deficiency diet, which eroded the colonic mucus barrier, decreased the level of SCFAs, and disrupted the mucosal barrier integrity (Neumann et al., 2021). Dietary fibers could be fermented by some species of gut microbiota and produce SCFAs, which provide energy to intestinal epithelium cells and strengthen the intestinal barrier, preventing inflammation induced by LPS (Seethaler et al., 2022). Increased concentrations of SCFAs in systemic circulation play an anti-inflammatory role far beyond the gut by immune modulation (Yao et al., 2022). Cao et al. (2021) found that severe COVID-19 cases showed depletion of butyrate-producing bacteria compared with mild to moderate cases. Butyrate increases the expression of TLRs which are involved in antiviral mechanisms and may also inhibit SARS-CoV-2 replication by downregulating high-mobility group box protein-1 (HMGB1) expression (Li J. et al., 2021). Dietary fiber intake is negatively correlated with the serum levels of inflammatory markers, including CRP, IL-6 and TNFα, which are massively released in COVID-19. Furthermore, MD can influence sex-specific responses to and the tiredness side effect of the SARS-CoV-2 vaccine, which suggests the significance of individual dietary recommendations on enhancing vaccine responses (Gualtieri et al., 2024).
Dietary recommendations for PACS include several foods with anti-inflammatory and immuno-stimulating activities, and MD might be a valuable strategy to achieve (Barrea et al., 2022). Patients were found to better recover from COVID-19 with a diet enriched in fiber, which is mediated by SCFAs in systematic immunoregulation and anti-inflammatory response through IFN-I signaling. Long-term sequelae of COVID-19 subjects had a remarkable predominance of Enterobacteriaceae strains in the gut with reduced SCFA levels in feces (Mendes de Almeida et al., 2023). These disorders persist even after disease recovery, which positively correlate with disease severity and increased plasma concentrations of CXCL-10, NT-proB-type natriuretic peptide, and CRP (Zhang F. et al., 2022). In addition, SCFAs could move to the lung and promote the recruitment and maturation of immune cells locally (Anand and Mande, 2018). Specifically, SCFAs exert immunomodulatory effects by engaging with receptors on immune cells, such as GPR41 and GPR43, inhibiting the generation of pro-inflammatory cytokines while enhancing anti-inflammatory cytokines like IL-10 and antioxidant enzymes (Sungnak et al., 2020; Yang W. et al., 2020). Furthermore, high-fiber diets are linked to lower glycemia and higher concentrations of adiponectin, which have an anti-inflammatory effect. Inhibition of dipeptidyl peptidase 4 (DPP4) could suppress T-cell proliferation and IL-6 and IL-10 secretion, which regulate the immune response to COVID-19 and reduce inflammation (Iacobellis, 2020), which has been shown to provide cardiovascular and cognitive benefits to the population.
The ketogenic diet (KD) is characterized by very low-carbohydrate and high-fat, which induces a metabolic shift and elevates circulating ketone bodies (Ang et al., 2020). KD can mitigate the metabolic reprogramming and systemic inflammation in SARS-CoV-2 infection, including reduced serum proinflammatory cytokines storm (eg., TNF-α, IL-15, IL-22, G-CSF, M-CSF, MCP-1) and restored amino acid and energy metabolism (Palermo et al., 2023). Indirectly, KD was shown to shed weight along with glycemic control, which protected against oxidative stress in individuals with obesity and type 2 diabetes mellitus (T2DM). KD may be conducive to reducing ventilatory requirements, showing a potential as an adjuvant therapy for obese COVID-19 patients (Gangitano et al., 2021). KD leads to the polarization of M2 macrophages and the reduction of M1 macrophage development, which displays anti-inflammatory effects in COVID-19 patients (Sukkar and Bassetti, 2020).
The synthesis of ketone bodies including β-hydroxybutyrate (BHB), and the immune function of CD4+ T cells are impaired in SARS-CoV-2-induced ARDS. BHB, as an alternative carbon source, promoted oxidative phosphorylation and produced bioenergy amino acids and glutathione, thus remodeling the mitochondrial reoxidation-reduction balance of CD4+ T cells and restoring the immune function of CD4+ T cells. KD or BHB supplementation restored mitochondrial metabolism and immune function of CD4+ T cells, thereby reducing severe illness and mortality in mice during severe SARS-CoV-2 infection (Karagiannis et al., 2022). SARS-CoV-2 encodes viroporines E and 3a to activate the NOD-like receptor family pyrin domain containing 3 (NLRP3) inflammasome, which could be inhibited by BHB through the reduction of K+ efflux from macrophages and the secretion of IL-1β and IL-18 secretion (Paoli et al., 2020). Consistently, KD was demonstrated to restrain aging-facilitated coronavirus infection in mice, showing activation of ketogenesis, enriched γδ T cells, reduced NLRP3 inflammasome and decreased pathogenic monocytes in the lungs (Ryu et al., 2021). Reduced muscle mass was shown to be related to the onset of complications from COVID-19, and KD represents a viable approach to preserving muscle mass in post-COVID (Schiaffino et al., 2021). Thus, healthy gut microbiota and diet-derived metabolites may enhance antiviral defenses, while the inflammatory metabolism may exacerbate COVID-19 and hinder the recovery of post-COVID-19.
Several countries have compiled guidelines for dietary recommendations by healthy organizations during the COVID-19 pandemic, which are mostly in agreement. They advocate the intake of whole grains, fruits, and vegetables, which supply essential vitamins, minerals and hydration (de Faria Coelho-Ravagnani et al., 2021). Fruits and vegetables may reduce the risk of high blood pressure, diabetes and obesity, all of which are important risk factors for COVID-19 complications (Maggini et al., 2018; Wang et al., 2020). A recent study indicated the antiviral effects of luteolin against SARS-CoV-2, which could be enhanced by vitamin C, magnesium and zinc (Ferreira et al., 2024). Vitamins A, B2, C, D, and E could modulate the microbial diversity and composition as well as increase the production of SCFAs in varying degrees. A dietary supplement of vitamin B2 for 14 days increased the number of butyrate producers, namely F. prausnitzii and Roseburia (Pham et al., 2021). Vitamin E supplementation enhanced the synthesis of SCFAs and the proportion of beneficial bacteria such as Akkermansia, Lactobacillus, Bifidobacterium, and Faecalibacterium (Choi et al., 2020). SCFAs reduce viral loads in the respiratory tract and gastrointestinal tract by suppressing the expression of the SARS-CoV-2 receptor ACE2 and boosting adaptive immunity (Brown et al., 2022). COVID-19 patients displayed impaired SCFA and L-isoleucine biosynthesis in their gut microbiome that persisted beyond 30 days post-recovery and was associated with the severity of disease and host immune responses. Strategies to supplement SCFA or L-isoleucine might be formulated to promote the prognosis of COVID-19 (Zhang F. et al., 2022).
The issuance of some guidelines on the management of long-term COVID guides certain appropriate patient rehabilitation but awaits clinical practice and individualized strategy (Koc et al., 2022). Dietary multivitamin supplements were demonstrated to improve the clinical symptoms of long COVID (Nurek et al., 2021). Diets rich in omega-3 fatty acids and low in trans fats and refined carbohydrates enhance mental health and aid in the recovery from PACS. Whether an anti-inflammatory diet can dysregulate immune response and be extended to PACS patients is currently being studied by clinical trials (Wang C. et al., 2023). Dietary supplements such as acetyl-L-carnitine, hydroxytyrosol, and vitamins B, C, and D have been shown to have significant potential in improving PACS characterized by chronic fatigue (Naureen et al., 2021). Furthermore, mast cell activation and histamine release may play a role in acute COVID-19 infection and chronic PACS, indicating a therapeutic and prognostic potential for an anti-histamine diet (Afrin et al., 2020). Histamine intolerance in PACS may be related to a reduction in diamine oxidase levels, resulting in mast cell activation syndrome. Dietary polysaccharides were excavated as promising management for PACS with multiple biological activities, including immunomodulatory, antioxidant, and antiviral activities (Cheong et al., 2023). A clinical study showed that fermented tropical fruits such as Morinda citrifolia and Carica papaya diminished the deteriorated heart and lung functions manifestations of long-COVID, via restoring the immune cells, generating energy, suppressing inflammation and balancing redox status (Kharaeva et al., 2022). Two trials examining the effects of nicotinamide riboside and low-dose naltrexone (LDN) are currently underway (NCT04809974, NCT04604704) to reduce cognitive symptoms and fatigue by regulating inflammatory responses (Omran and Almaliki, 2020). A healthy diet could be considered a safe and cost-effective way to improve post-COVID symptoms and possible future complications.
The supplement of probiotics has been suggested as an auxiliary treatment for COVID-19 (Conte and Toraldo, 2020; Stavropoulou and Bezirtzoglou, 2020; Patra et al., 2021; Santacroce et al., 2021). Probiotics may favor virus clearance by enhancing immune function, inhibiting the activation of NLRP3 inflammasome (Synodinou et al., 2022), protecting the gut barrier, and preventing the co-infection of COVID-19 related bacteria (Patra et al., 2021; Bottari et al., 2021). Several clinical trials have been initiated. Probiotics formulation (S. thermophilus DSM 32345, L. acidophilus DSM 32241, L. helveticus DSM 32242, L. paracasei DSM 32243, L. plantarum DSM 32244, L. brevis DSM 27961, B. lactis DSM 32246, B. lactis DSM 32247) supplementation to patients with COVID-19 could effectively alleviate diarrhea, fever, asthenia, headache and other symptoms. The risk of respiratory failure was estimated to be 8 times lower in patients treated with bacteriotherapy than in the control group (d'Ettorre et al., 2020). A single-center, quadruple-blinded randomized controlled trial evaluating the efficacy and safety of a probiotic formulation consisting of three strains of Lactiplantibacillus plantarum and Pediococcus acidilactici in symptomatic COVID-19 outpatients, showed significantly reduced viral load and lung abnormality scores and increased SARS-CoV-2 specific antibodies (Gutiérrez-Castrellón et al., 2022). Similar benefits were also observed in hospitalized patients, probiotics containing Lactobacillus and Bifidobacterium strains reduced overall symptoms and decreased hospitalization duration and recovery time, which suggested the potential of probiotics in COVID-19 (Xu et al., 2022). Lactobacilli may reduce the risk of gastrointestinal symptoms, respiratory failure and death by regulating the immune response, reducing inflammation, and exerting direct antiviral effects (Taufer et al., 2024).
Probiotics can not only resist the virus but also improve the effectiveness of the vaccine. The probiotic Lactobacillus plantarum GUANKE after SARS-CoV-2 vaccination was able to boost both effective humoral and cellular responses by enhancing interferon signaling and inhibiting apoptotic and inflammatory pathways against COVID-19 in mice. However, in another randomized, controlled, single-center, open-label trial (NCT04854941), probiotics (Lacticaseibacillus rhamnosus PDV 1705, B. bifidum PDV 0903, Bifidobacterium longum subsp. infantis PDV 1911, and B. longum subsp. longum PDV 2301) were effective in treating COVID-19 related diarrhea, but did not significantly reduce mortality (Ivashkin et al., 2023). Therefore, more investigations are warranted to investigate the safety and efficacy of probiotics in COVID-19 patients.
Recently, a synbiotic preparation (SIM01), which includes three bacterial strains (B. adolescentis, B. bifidum, and B. longum), has been demonstrated to hasten the SARS-CoV-2 antibody formation, reduce the nasopharyngeal viral load and pro-inflammatory markers, and restore gut dysbiosis in patients with COVID-19 (Zhang L. et al., 2022). Surprisingly, SIM01 was found to significantly alleviate multiple symptoms of PACS at 6 months after acute infection compared to a placebo, accompanied by beneficial changes in the gut microbiota (Lau et al., 2024a). A clinical trial is underway to evaluate the effectiveness of probiotic and prebiotics supplements in normalizing the gut microbiota composition and immune function in long-COVID (NCT04813718) (Crook et al., 2021). The supplementation with probiotics during hospitalization may prevent the development of chronic fatigue of COVID-19 by impacting metabolites involved in glucose utilization and energy metabolism, including increased levels of Arginine, Asparagine, Lactate and reduced levels of 3-Hydroxyisobutirate (Santinelli et al., 2021). The study provides a new perspective for treating post-acute COVID-19 syndrome by regulating the gut microbiota.
Prebiotics are non-digestible food components that promote the growth and activity of beneficial bacteria in the colon through fermentation (Salminen et al., 2021). By serving as substrates for probiotics, prebiotics suppress the proliferation of harmful bacteria and enhance the breakdown and assimilation of vital nutrients (Markowiak and Śliżewska, 2017). One study showed that inulin treatment increased the abundance of Bifidobacterium and Lactobacillus, thereby increasing SCFA production and thus regulating the gut microbiome (Vandeputte et al., 2017). Pectin and 1-kestose may induce the proliferation of F. prausnitzii with anti-inflammatory effects (Tochio et al., 2018). Phenolic compounds are also considered potential prebiotics as they interact with transcription factors such as NF-κB and Nrf2 exerting immunomodulatory effects. A randomized controlled trial showed that oral administration of curcumin significantly reduced morbidity, mortality and recovery time in patients with mild, moderate, and severe COVID-19 symptoms (Ahmadi et al., 2021; Batista et al., 2023). The metabolic product of Lactiplantibacillus plantarum, plantaricin BN, D, W, and JLA-9, presented antiviral activity by blocking the essential protein S during the SARS-CoV-2 life cycle (Anwar et al., 2021). In addition, the most common side effects of prebiotics are abdominal bloating and discomfort when consumed in large quantities (Hu et al., 2021). More researches on postbiotics and SARS-CoV-2 infection are needed, especially considering animal and human populations, to develop well-planned clinical trials and establish sufficient parameters to draw safe scientific conclusions and optimal dosage. Similarly, combined co-supplementation of both probiotics and prebiotics, in the form of synbiotics such as the blend of the Lactobacillus strain with inulin has been shown to improve health outcomes of individuals with long COVID, leading to reduced cough, fatigue and gut symptoms.
FMT is a treatment where fecal bacteria from healthy donors or complex microbial communities from in vitro culture or purification of fecal materials are inoculated into the intestines of patients. FMT was attested to partially restore gut dysbiosis, alleviate gastrointestinal symptoms, and modulate the immune status of peripheral lymphocyte subsets in discharged COVID-19 patients (Liu et al., 2021). FMT restores the imbalanced gut microbiome and may affect immune responses including the respiratory system through the gut-lung axis, which may contribute to pulmonary-epithelial resistance to SARS-COV-2. The application of FMT in COVID-19 is still in the early stages of research. Most studies related to FMT and COVID-19 focus on general microbiome modulation rather than specific donor criteria. Currently, there is limited detailed literature regarding donor selection for FMT in the context of COVID-19 prevention and treatment. Recently, Biliński et al. have been performing a clinical trial (NCT04824222) that attempted to validate the effectiveness of FMT with the progression of COVID-19 and its association with cytokine storms and inflammation escalation (FeMToCOVID). As the first clinical trial using FMT in COVID-19, FeMToCOVID inhibits the inflammatory process by reconstructing the right influence of the gut-lung axis could be fundamental meaning to the development of ARDS in COVID-19 patients. Notably, the gut microbiome of donors recovered from COVID-19 may be altered and miss critical microbiome, which compromises the efficacy of FMT for COVID-19 (Kazemian et al., 2021).
A pilot study containing 60 PACS patients with insomnia showed that FMT could be effective and safe in alleviating sleep disturbance in PACS, with the enrichment of bacteria such as Gemmiger formicilis and depletion of menaquinol derivatives production (NCT05556733) (Lau et al., 2024b). To prevent the transmission of SARS-CoV-2 in FMT, donors should be screened for travel history and symptoms of COVID-19, as well as exposure of patients before each stool donation. Donors who pass this screening should be tested for COVID-19 while those who fail are excluded from fecal donation (Khanna and Pardi, 2020). There is an urgent need for comprehensive and optimized fecal donor screening programs to ensure the safety and effectiveness of FMT during the COVID-19 pandemic. Uncovering the bidirectional effects of the gut-lung axis is critical to adapt traditional FMT strategies and novel microbiome-based therapeutic interventions for COVID-19 in the future.
SARS-CoV-2, as a highly infectious respiratory virus, induced the ongoing COVID-19 global pandemic. Even with the evolution of preventive vaccines and medicine, persistent and prolonged effects after acute COVID-19 have debilitated millions of subjects worldwide. SARS-CoV-2 infection could impact the complex gut community and drive immunological changes. Conversely, the alterations of gut microbiota have been demonstrated to have long-reaching and long-lasting potential in COVID-19 and PACS, which remains unexplored. The heterogeneity and complexity of PACS hindered the decryption of the pathophysiological mechanisms, which need further multidisciplinary studies to better understand and address these underlying health problems. Some predicting models have been used to identify individuals with a high risk of long-COVID to process education and rehabilitation in the early phase (Sudre et al., 2021). The involvement of coexisting symptoms suggests the requires to provide insights into different symptom clusters and identify potential targets for personalized therapeutic interventions. Current treatment options for PACS still primarily emphasize managing the symptoms but neglect the underlying cause, such as persistent inflammation, immune dysfunction, and tissue damage.
Multiple risk factors may predispose patients to worse consequences in acute infection and promote PACS through microbial and immune regulation. This review emphasized the gut microbiota dysbiosis in COVID-19 and the sequelae of PACS. Elucidating the underlying mechanisms may contribute to propose personalized and non-invasive strategies for patients with severe disease progression or complications post-acute infection. However, prospective studies are required to determine whether gut microbiota alterations contribute to or result from SARS-CoV-2 infection, and the effects of the drugs, especially the antibiotics in COVID-19 cannot be ignored. An incorporated dietary therapy or specialized diet, as one of the modifiable factors, is more inclined for patients to facilitate post-COVID-19 recovery. Thus, subjects should be mindful of dietary habits to reduce susceptibility to and long-term sequelae from COVID-19. Personalized nutrition which modulates the gut microbiota might be a crucial prophylactic factor to minimize the disturbance on COVID-19 patients. Reducing the risk of dietary habits in every phase of COVID-19 will play a crucial role in combatting its pandemic and postinfection effect with minimum financial loss and adverse outcomes. Regrettably, clinical outcomes of diet supplements in long-COVID therapy are still lacking. Moreover, the review also highlights the possibility of targeting the gut microbiota as a conceivable therapeutic management for COVID-19 and PACS. The role of microbiome modulation strategies, such as healthy diet, probiotics or prebiotics, and FMT in regulating PACS warrants investigation.
YA: Conceptualization, Data curation, Formal analysis, Writing – original draft, Writing – review & editing. LH: Conceptualization, Formal analysis, Methodology, Writing – original draft, Writing – review & editing. XX: Data curation, Investigation, Writing – original draft. MP: Project administration, Software, Writing – original draft. BW: Investigation, Supervision, Writing – review & editing. TL: Funding acquisition, Investigation, Resources, Validation, Writing – review & editing. HC: Conceptualization, Funding acquisition, Investigation, Supervision, Writing – review & editing.
The author(s) declare that financial support was received for the research, authorship, and/or publication of this article. This work was supported by the National Natural Science Foundation of China [grant numbers 82270574, 82070545, and 82100574]; Tianjin Medical University General Hospital Fund for Distinguished Young Scholars [grant number 22ZYYJQ02]; Tianjin Key Medical Discipline (Specialty) Construction Project [grant number TJYXZDXK-002A]; Diversified fund project of the Natural Science Foundation of Tianjin, China [grant number 21JCQNJC01240].
The authors declare that the research was conducted in the absence of any commercial or financial relationships that could be construed as a potential conflict of interest.
All claims expressed in this article are solely those of the authors and do not necessarily represent those of their affiliated organizations, or those of the publisher, the editors and the reviewers. Any product that may be evaluated in this article, or claim that may be made by its manufacturer, is not guaranteed or endorsed by the publisher.
Adhikari, A., Maddumage, J., Eriksson, E. M., Annesley, S. J., Lawson, V. A., Bryant, V. L., et al. (2024). Beyond acute infection: mechanisms underlying post‐acute sequelae ofCOVID‐19 (PASC). Med. J. Aust. 221, S40–S48. doi: 10.5694/mja2.52456
Afrin, L. B., Weinstock, L. B., and Molderings, G. J. (2020). Covid-19 hyperinflammation and post-Covid-19 illness may be rooted in mast cell activation syndrome. Int. J. Infect. Dis. 100, 327–332. doi: 10.1016/j.ijid.2020.09.016
Ahmadi, R., Salari, S., Sharifi, M. D., Reihani, H., Rostamiani, M. B., Behmadi, M., et al. (2021). Oral nano-curcumin formulation efficacy in the management of mild to moderate outpatient COVID-19: a randomized triple-blind placebo-controlled clinical trial. Food Sci. Nutr. 9, 4068–4075. doi: 10.1002/fsn3.2226
Anand, S., and Mande, S. S. (2018). Diet, microbiota and gut-lung connection. Front. Microbiol. 9:2147. doi: 10.3389/fmicb.2018.02147
Ang, Q. Y., Alexander, M., Newman, J. C., Tian, Y., Cai, J., Upadhyay, V., et al. (2020). Ketogenic diets Alter the gut microbiome resulting in decreased intestinal Th17 cells. Cell 181, 1263–1275.e16. doi: 10.1016/j.cell.2020.04.027
Anwar, F., Altayb, H. N., Al-Abbasi, F. A., Al-Malki, A. L., Kamal, M. A., and Kumar, V. (2021). Antiviral effects of probiotic metabolites on COVID-19. J. Biomol. Struct. Dyn. 39, 4175–4184. doi: 10.1080/07391102.2020.1775123
Baradaran Ghavami, S., Pourhamzeh, M., Farmani, M., Raftar, S. K. A., Shahrokh, S., Shpichka, A., et al. (2021). Cross-talk between immune system and microbiota in COVID-19. Expert Rev. Gastroenterol. Hepatol. 15, 1281–1294. doi: 10.1080/17474124.2021.1991311
Barrea, L., Grant, W. B., Frias-Toral, E., Vetrani, C., Verde, L., de Alteriis, G., et al. (2022). Dietary recommendations for Post-COVID-19 syndrome. Nutrients 14:1305. doi: 10.3390/nu14061305
Batista, K. S., de, J., Vasconcelos, M., Bezerra, M., da, M., Pinheiro, R., et al. (2023). Probiotics and prebiotics: potential prevention and therapeutic target for nutritional management of COVID-19. Nutr. Res. Rev. 36, 181–198. doi: 10.1017/S0954422421000317
Bell, M. G., Ganesh, R., and Bonnes, S. L. (2023). COVID-19, the gut, and nutritional implications. Curr. Nutr. Rep. 12, 263–269. doi: 10.1007/s13668-023-00465-0
Bernard-Raichon, L., Venzon, M., Klein, J., Axelrad, J. E., Zhang, C., Sullivan, A. P., et al. (2022). Gut microbiome dysbiosis in antibiotic-treated COVID-19 patients is associated with microbial translocation and bacteremia. Nat. Commun. 13:5926. doi: 10.1038/s41467-022-33395-6
Blackett, J. W., Wainberg, M., Elkind, M., and Freedberg, D. E. (2022). Potential Long coronavirus disease 2019 gastrointestinal symptoms 6 months after coronavirus infection are associated with mental health symptoms. Gastroenterology 162, 648–650.e2. doi: 10.1053/j.gastro.2021.10.040
Bornstein, S. R., Zimmet, P., Rubino, F., and Ludwig, B. (2020). Management of diabetes in patients with COVID-19 – Authors' reply. Lancet Diabetes Endocrinol. 8, 669–670. doi: 10.1016/S2213-8587(20)30223-0
Bottari, B., Castellone, V., and Neviani, E. (2021). Probiotics and Covid-19. Int. J. Food Sci. Nutr. 72, 293–299. doi: 10.1080/09637486.2020.1807475
Briand, F., Sencio, V., Robil, C., Heumel, S., Deruyter, L., Machelart, A., et al. (2022). Diet-induced obesity and NASH impair disease recovery in SARS-CoV-2-infected Golden hamsters. Viruses 14:2067. doi: 10.3390/v14092067
Brown, J. A., Sanidad, K. Z., Lucotti, S., Lieber, C. M., Cox, R. M., Ananthanarayanan, A., et al. (2022). Gut microbiota-derived metabolites confer protection against SARS-CoV-2 infection. Gut Microbes 14:2105609. doi: 10.1080/19490976.2022.2105609
Brown, R. L., Sequeira, R. P., and Clarke, T. B. (2017). The microbiota protects against respiratory infection via GM-CSF signaling. Nat. Commun. 8:1512. doi: 10.1038/s41467-017-01803-x
Butler, M. J., and Barrientos, R. M. (2020). The impact of nutrition on COVID-19 susceptibility and long-term consequences. Brain Behav. Immun. 87, 53–54. doi: 10.1016/j.bbi.2020.04.040
Cai, M., Xie, Y., Topol, E. J., and Al-Aly, Z. (2024). Three-year outcomes of post-acute sequelae of COVID-19. Nat. Med. 30, 1564–1573. doi: 10.1038/s41591-024-02987-8
Cao, J., Wang, C., Zhang, Y., Lei, G., Xu, K., Zhao, N., et al. (2021). Integrated gut virome and bacteriome dynamics in COVID-19 patients. Gut Microbes 13, 1–21. doi: 10.1080/19490976.2021.1887722
Carfì, A., Bernabei, R., and Landi, F. (2020). Persistent symptoms in patients after acute COVID-19. JAMA 324, 603–605. doi: 10.1001/jama.2020.12603
Carlson, J. L., Erickson, J. M., Lloyd, B. B., and Slavin, J. L. (2018). Health effects and sources of prebiotic dietary Fiber. Curr. Dev. Nutr. 2:nzy005. doi: 10.1093/cdn/nzy005
Chakradhar, S. (2017). A curious connection: teasing apart the link between gut microbes and lung disease. Nat. Med. 23, 402–404. doi: 10.1038/nm0417-402
Chen, G., Wu, D., Guo, W., Cao, Y., Huang, D., Wang, H., et al. (2020). Clinical and immunological features of severe and moderate coronavirus disease 2019. J. Clin. Invest. 130, 2620–2629. doi: 10.1172/JCI137244
Chen, N., Zhou, M., Dong, X., Qu, J., Gong, F., Han, Y., et al. (2020). Epidemiological and clinical characteristics of 99 cases of 2019 novel coronavirus pneumonia in Wuhan, China: a descriptive study. Lancet 395, 507–513. doi: 10.1016/S0140-6736(20)30211-7
Cheon, I. S., Li, C., Son, Y. M., Goplen, N. P., Wu, Y., Cassmann, T., et al. (2021). Immune signatures underlying post-acute COVID-19 lung sequelae. Sci. Immunol. 6:eabk1741. doi: 10.1126/sciimmunol.abk1741
Cheong, K. L., Yu, B., Teng, B., Veeraperumal, S., Xu, B., Zhong, S., et al. (2023). Post-COVID-19 syndrome management: utilizing the potential of dietary polysaccharides. Biomed. Pharmacother. 166:115320. doi: 10.1016/j.biopha.2023.115320
Choi, Y., Lee, S., Kim, S., Lee, J., Ha, J., Oh, H., et al. (2020). Vitamin E (α-tocopherol) consumption influences gut microbiota composition. Int. J. Food Sci. Nutr. 71, 221–225. doi: 10.1080/09637486.2019.1639637
Conte, L., and Toraldo, D. M. (2020). Targeting the gut-lung microbiota axis by means of a high-fibre diet and probiotics may have anti-inflammatory effects in COVID-19 infection. Ther. Adv. Respir. Dis. 14:1753466620937170. doi: 10.1177/1753466620937170
Cortese, M., Lee, J. Y., Cerikan, B., Neufeldt, C. J., Oorschot, V. M. J., Köhrer, S., et al. (2020). Integrative imaging reveals SARS-CoV-2-induced reshaping of subcellular morphologies. Cell Host Microbe 28, 853–866.e5. doi: 10.1016/j.chom.2020.11.003
Crook, H., Raza, S., Nowell, J., Young, M., and Edison, P. (2021). Long covid-mechanisms, risk factors, and management. BMJ 374:n1648. doi: 10.1136/bmj.n1648
Cui, G. Y., Rao, B. C., Zeng, Z. H., Wang, X. M., Ren, T., Wang, H. Y., et al. (2022). Characterization of oral and gut microbiome and plasma metabolomics in COVID-19 patients after 1-year follow-up. Mil. Med. Res. 9:32. doi: 10.1186/s40779-022-00387-y
D'Amico, F., Baumgart, D. C., Danese, S., and Peyrin-Biroulet, L. (2020). Diarrhea during COVID-19 infection: pathogenesis, epidemiology, prevention and management. Clin. Gastroenterol. Hepatol. 18, 1663–1672. doi: 10.1016/j.cgh.2020.04.001
Dang, A. T., and Marsland, B. J. (2019). Microbes, metabolites, and the gut-lung axis. Mucosal Immunol. 12, 843–850. doi: 10.1038/s41385-019-0160-6
David, L. A., Maurice, C. F., Carmody, R. N., Gootenberg, D. B., Button, J. E., Wolfe, B. E., et al. (2014). Diet rapidly and reproducibly alters the human gut microbiome. Nature 505, 559–563. doi: 10.1038/nature12820
Davis, H. E., Assaf, G. S., McCorkell, L., Wei, H., Low, R. J., Re'em, Y., et al. (2021). Characterizing long COVID in an international cohort: 7 months of symptoms and their impact. EClinicalMedicine 38:101019. doi: 10.1016/j.eclinm.2021.101019
Davis, H. E., McCorkell, L., Vogel, J. M., and Topol, E. J. (2023). Long COVID: major findings, mechanisms and recommendations. Nat. Rev. Microbiol. 21, 133–146. doi: 10.1038/s41579-022-00846-2
de Faria Coelho-Ravagnani, C., Corgosinho, F. C., Sanches, F., Prado, C., Laviano, A., and Mota, J. F. (2021). Dietary recommendations during the COVID-19 pandemic. Nutr. Rev. 79, 382–393. doi: 10.1093/nutrit/nuaa067
d'Ettorre, G., Ceccarelli, G., Marazzato, M., Campagna, G., Pinacchio, C., Alessandri, F., et al. (2020). Challenges in the management of SARS-CoV2 infection: the role of Oral Bacteriotherapy as complementary therapeutic strategy to avoid the progression of COVID-19. Front. Med. (Lausanne) 7:389. doi: 10.3389/fmed.2020.00389
Dickson, R. P., Singer, B. H., Newstead, M. W., Falkowski, N. R., Erb-Downward, J. R., Standiford, T. J., et al. (2016). Enrichment of the lung microbiome with gut bacteria in sepsis and the acute respiratory distress syndrome. Nat. Microbiol. 1:16113. doi: 10.1038/nmicrobiol.2016.113
Dietz, W., and Santos-Burgoa, C. (2020). Obesity and its implications for COVID-19 mortality. Obesity (Silver Spring) 28:1005. doi: 10.1002/oby.22818
Ealey, K. N., Phillips, J., and Sung, H. K. (2021). COVID-19 and obesity: fighting two pandemics with intermittent fasting. Trends Endocrinol. Metab. 32, 706–720. doi: 10.1016/j.tem.2021.06.004
El Khoury, C. N., and Julien, S. G. (2021). Inverse association between the Mediterranean diet and COVID-19 risk in Lebanon: a case-control study. Front. Nutr. 8:707359. doi: 10.3389/fnut.2021.707359
Everhart, M. B., Han, W., Sherrill, T. P., Arutiunov, M., Polosukhin, V. V., Burke, J. R., et al. (2006). Duration and intensity of NF-kappaB activity determine the severity of endotoxin-induced acute lung injury. J. Immunol. 176, 4995–5005. doi: 10.4049/jimmunol.176.8.4995
Fernández-de-Las-Peñas, C., Raveendran, A. V., Giordano, R., and Arendt-Nielsen, L. (2023). Long COVID or Post-COVID-19 condition: past, present and future research directions. Microorganisms 11:2959. doi: 10.3390/microorganisms11122959
Ferreira, J. C., Fadl, S., Cardoso, T., Andrade, B., Melo, T., Silva, E., et al. (2024). Boosting immunity: synergistic antiviral effects of Luteolin, vitamin C, magnesium, and zinc against SARS-CoV-2 3CLpro. Biosci. Rep. 44:BSR20240617. doi: 10.1042/BSR20240617
Flint, S. W., and Tahrani, A. A. (2020). COVID-19 and obesity-lack of clarity, guidance, and implications for care. Lancet Diabetes Endocrinol. 8, 474–475. doi: 10.1016/S2213-8587(20)30156-X
Foley, K. P., Zlitni, S., Duggan, B. M., Barra, N. G., Anhê, F. F., Cavallari, J. F., et al. (2020). Gut microbiota impairs insulin clearance in obese mice. Mol. Metab. 42:101067. doi: 10.1016/j.molmet.2020.101067
Freedberg, D. E., and Chang, L. (2022). Gastrointestinal symptoms in COVID-19: the long and the short of it. Curr. Opin. Gastroenterol. 38, 555–561. doi: 10.1097/MOG.0000000000000876
Gaebler, C., Wang, Z., Lorenzi, J., Muecksch, F., Finkin, S., Tokuyama, M., et al. (2021). Evolution of antibody immunity to SARS-CoV-2. Nature 591, 639–644. doi: 10.1038/s41586-021-03207-w
Gangitano, E., Tozzi, R., Mariani, S., Lenzi, A., Gnessi, L., and Lubrano, C. (2021). Ketogenic diet for obese COVID-19 patients: is respiratory disease a contraindication? A narrative review of the literature on ketogenic diet and respiratory function. Front. Nutr. 8:771047. doi: 10.3389/fnut.2021.771047
Gao, M., Piernas, C., Astbury, N. M., Hippisley-Cox, J., O'Rahilly, S., Aveyard, P., et al. (2021). Associations between body-mass index and COVID-19 severity in 6·9 million people in England: a prospective, community-based, cohort study. Lancet Diabetes Endocrinol. 9, 350–359. doi: 10.1016/S2213-8587(21)00089-9
Geva-Zatorsky, N., Sefik, E., Kua, L., Pasman, L., Tan, T. G., Ortiz-Lopez, A., et al. (2017). Mining the human gut microbiota for immunomodulatory organisms. Cell 168, 928–943.e11. doi: 10.1016/j.cell.2017.01.022
Giamarellos-Bourboulis, E. J., Netea, M. G., Rovina, N., Akinosoglou, K., Antoniadou, A., Antonakos, N., et al. (2020). Complex immune dysregulation in COVID-19 patients with severe respiratory failure. Cell Host Microbe 27, 992–1000.e3. doi: 10.1016/j.chom.2020.04.009
Giron, L. B., Peluso, M. J., Ding, J., Kenny, G., Zilberstein, N., Koshy, J., et al. (2022). Markers of fungal translocation are elevated during post-acute sequelae of SARS-CoV-2 and induce NF-κB signaling. JCI Insight 7:e164813. doi: 10.1172/jci.insight.164813
Greene, M. W., Roberts, A. P., and Frugé, A. D. (2021). Negative association between Mediterranean diet adherence and COVID-19 cases and related deaths in Spain and 23 OECD countries: an ecological study. Front. Nutr. 8:591964. doi: 10.3389/fnut.2021.591964
Gu, S., Chen, Y., Wu, Z., Chen, Y., Gao, H., Lv, L., et al. (2020). Alterations of the gut microbiota in patients with coronavirus disease 2019 or H1N1 influenza. Clin. Infect. Dis. 71, 2669–2678. doi: 10.1093/cid/ciaa709
Gualtieri, P., Frank, G., Cianci, R., Smeriglio, A., Alibrandi, A., di Renzo, L., et al. (2024). Mediterranean diet influence on SARS-CoV-2 vaccine adverse reaction: friend or foe. Nutrients 16:1846. doi: 10.3390/nu16121846
Guarnieri, J. W., Dybas, J. M., Fazelinia, H., Kim, M. S., Frere, J., Zhang, Y., et al. (2023). Core mitochondrial genes are down-regulated during SARS-CoV-2 infection of rodent and human hosts. Sci. Transl. Med. 15:eabq1533. doi: 10.1126/scitranslmed.abq1533
Guo, Y., Luo, R., Wang, Y., Deng, P., Song, T., Zhang, M., et al. (2021). SARS-CoV-2 induced intestinal responses with a biomimetic human gut-on-chip. Sci. Bull. (Beijing) 66, 783–793. doi: 10.1016/j.scib.2020.11.015
Guo, M., Tao, W., Flavell, R. A., and Zhu, S. (2021). Potential intestinal infection and faecal-oral transmission of SARS-CoV-2. Nat. Rev. Gastroenterol. Hepatol. 18, 269–283. doi: 10.1038/s41575-021-00416-6
Gutiérrez-Castrellón, P., Gandara-Martí, T., Abreu Y Abreu, A. T., Nieto-Rufino, C. D., López-Orduña, E., Jiménez-Escobar, I., et al. (2022). Probiotic improves symptomatic and viral clearance in Covid19 outpatients: a randomized, quadruple-blinded, placebo-controlled trial. Gut Microbes 14:2018899. doi: 10.1080/19490976.2021.2018899
Heintz-Buschart, A., May, P., Laczny, C. C., Lebrun, L. A., Bellora, C., Krishna, A., et al. (2016). Integrated multi-omics of the human gut microbiome in a case study of familial type 1 diabetes. Nat. Microbiol. 2:16180. doi: 10.1038/nmicrobiol.2016.180
Hu, J., Zhang, L., Lin, W., Tang, W., Chan, F., and Ng, S. C. (2021). Review article: probiotics, prebiotics and dietary approaches during COVID-19 pandemic. Trends Food Sci. Technol. 108, 187–196. doi: 10.1016/j.tifs.2020.12.009
Iacobellis, G. (2020). COVID-19 and diabetes: can DPP4 inhibition play a role. Diabetes Res. Clin. Pract. 162:108125. doi: 10.1016/j.diabres.2020.108125
Ivashkin, V., Fomin, V., Moiseev, S., Brovko, M., Maslennikov, R., Ulyanin, A., et al. (2023). Efficacy of a probiotic consisting of lacticaseibacillus rhamnosus PDV 1705, bifidobacterium bifidum PDV 0903, bifidobacterium longum subsp. Infantis PDV 1911, and bifidobacterium longum subsp. Longum PDV 2301 in the treatment of hospitalized patients with COVID-19: a randomized controlled trial. Probiotics Antimicrob Proteins 15, 460–468. doi: 10.1007/s12602-021-09858-5
Ji, P., Zhu, J., Zhong, Z., Li, H., Pang, J., Li, B., et al. (2020). Association of elevated inflammatory markers and severe COVID-19: a meta-analysis. Medicine (Baltimore) 99:e23315. doi: 10.1097/MD.0000000000023315
Kalantar-Zadeh, K., Ward, S. A., Kalantar-Zadeh, K., and El-Omar, E. M. (2020). Considering the effects of microbiome and diet on SARS-CoV-2 infection: nanotechnology roles. ACS Nano 14, 5179–5182. doi: 10.1021/acsnano.0c03402
Karagiannis, F., Peukert, K., Surace, L., Michla, M., Nikolka, F., Fox, M., et al. (2022). Impaired ketogenesis ties metabolism to T cell dysfunction in COVID-19. Nature 609, 801–807. doi: 10.1038/s41586-022-05128-8
Karst, S. M. (2016). The influence of commensal bacteria on infection with enteric viruses. Nat. Rev. Microbiol. 14, 197–204. doi: 10.1038/nrmicro.2015.25
Kazemian, N., Kao, D., and Pakpour, S. (2021). Fecal microbiota transplantation during and Post-COVID-19 pandemic. Int. J. Mol. Sci. 22:3004. doi: 10.3390/ijms22063004
Khanna, S., and Pardi, D. (2020). Fecal microbiota transplantation for recurrent Clostridioides difficile infection: the COVID-19 era. Am. J. Gastroenterol. 115, 971–974. doi: 10.14309/ajg.0000000000000689
Kharaeva, Z., Shokarova, A., Shomakhova, Z., Ibragimova, G., Trakhtman, P., Trakhtman, I., et al. (2022). Fermented Carica papaya and Morinda citrifolia as perspective food supplements for the treatment of Post-COVID symptoms: randomized placebo-controlled clinical laboratory study. Nutrients 14:2203. doi: 10.3390/nu14112203
Kim, S. E. (2024). Four years on: the career costs for scientists battling long COVID. Nature 627, 689–691. doi: 10.1038/d41586-024-00819-w
Kissler, S. M., Tedijanto, C., Goldstein, E., Grad, Y. H., and Lipsitch, M. (2020). Projecting the transmission dynamics of SARS-CoV-2 through the postpandemic period. Science 368, 860–868. doi: 10.1126/science.abb5793
Koc, H. C., Xiao, J., Liu, W., Li, Y., and Chen, G. (2022). Long COVID and its management. Int. J. Biol. Sci. 18, 4768–4780. doi: 10.7150/ijbs.75056
Kociszewska, D., Chan, J., Thorne, P. R., and Vlajkovic, S. M. (2021). The link between gut Dysbiosis caused by a high-fat diet and hearing loss. Int. J. Mol. Sci. 22:13177. doi: 10.3390/ijms222413177
Kolodziejczyk, A. A., Zheng, D., and Elinav, E. (2019). Diet–microbiota interactions and personalized nutrition. Nat. Rev. Microbiol. 17, 742–753. doi: 10.1038/s41579-019-0256-8
Kruglikov, I. L., Shah, M., and Scherer, P. (2020). Obesity and diabetes as comorbidities for COVID-19: underlying mechanisms and the role of viral–bacterial interactions. eLife 9:9. doi: 10.7554/eLife.61330
Kusakabe, T., Lin, W. Y., Cheong, J. G., Singh, G., Ravishankar, A., Yeung, S. T., et al. (2023). Fungal microbiota sustains lasting immune activation of neutrophils and their progenitors in severe COVID-19. Nat. Immunol. 24, 1879–1889. doi: 10.1038/s41590-023-01637-4
Lau, R. I., Su, Q., Ching, J. Y. L., Lui, R. N., Chan, T. T., Wong, M. T. L., et al. (2024b). Fecal microbiota transplantation for sleep disturbance in Postacute COVID-19 syndrome. Clin. Gastroenterol. Hepatol. 22, 2487–2496.e6. doi: 10.1016/j.cgh.2024.06.004
Lau, R. I., Su, Q., Lau, I., Ching, J., Wong, M., Lau, L., et al. (2024a). A synbiotic preparation (SIM01) for post-acute COVID-19 syndrome in Hong Kong (RECOVERY): a randomised, double-blind, placebo-controlled trial. Lancet Infect. Dis. 24, 256–265. doi: 10.1016/S1473-3099(23)00685-0
Lecarpentier, Y., and Vallée, A. (2021). The key role of the level of ACE2 gene expression in SARS-CoV-2 infection. Aging (Albany NY) 13, 14552–14556. doi: 10.18632/aging.203181
Lee, S., Yoon, G. Y., Myoung, J., Kim, S. J., and Ahn, D. G. (2020). Robust and persistent SARS-CoV-2 infection in the human intestinal brush border expressing cells. Emerg. Microbes Infect. 9, 2169–2179. doi: 10.1080/22221751.2020.1827985
Li, J., Richards, E. M., Handberg, E. M., Pepine, C. J., and Raizada, M. K. (2021). Butyrate regulates COVID-19-relevant genes in gut epithelial organoids from normotensive rats. Hypertension 77, e13–e16. doi: 10.1161/HYPERTENSIONAHA.120.16647
Li, S., Yang, S., Zhou, Y., Disoma, C., Dong, Z., du, A., et al. (2021). Microbiome profiling using shotgun metagenomic sequencing identified unique microorganisms in COVID-19 patients with altered gut microbiota. Front. Microbiol. 12:712081. doi: 10.3389/fmicb.2021.712081
Liew, F., Efstathiou, C., Fontanella, S., Richardson, M., Saunders, R., Swieboda, D., et al. (2024). Large-scale phenotyping of patients with long COVID post-hospitalization reveals mechanistic subtypes of disease. Nat. Immunol. 25, 607–621. doi: 10.1038/s41590-024-01778-0
Lionetto, L., Ulivieri, M., Capi, M., de Bernardini, D., Fazio, F., Petrucca, A., et al. (2021). Increased kynurenine-to-tryptophan ratio in the serum of patients infected with SARS-CoV2: an observational cohort study. Biochim. Biophys. Acta Mol. basis Dis. 1867:166042. doi: 10.1016/j.bbadis.2020.166042
Littlefield, K. M., Watson, R. O., Schneider, J. M., Neff, C. P., Yamada, E., Zhang, M., et al. (2022). SARS-CoV-2-specific T cells associate with inflammation and reduced lung function in pulmonary post-acute sequalae of SARS-CoV-2. PLoS Pathog. 18:e1010359. doi: 10.1371/journal.ppat.1010359
Liu, T., Guo, Z., Song, X., Liu, L., Dong, W., Wang, S., et al. (2020b). High-fat diet-induced dysbiosis mediates MCP-1/CCR2 axis-dependent M2 macrophage polarization and promotes intestinal adenoma-adenocarcinoma sequence. J. Cell. Mol. Med. 24, 2648–2662. doi: 10.1111/jcmm.14984
Liu, Q., Mak, J., Su, Q., Yeoh, Y., Lui, G., Ng, S., et al. (2022a). Gut microbiota dynamics in a prospective cohort of patients with post-acute COVID-19 syndrome. Gut 71, 544–552. doi: 10.1136/gutjnl-2021-325989
Liu, Q., Su, Q., Zhang, F., Tun, H. M., Mak, J. W. Y., Lui, G. C. Y., et al. (2022b). Multi-kingdom gut microbiota analyses define COVID-19 severity and post-acute COVID-19 syndrome. Nat. Commun. 13:6806. doi: 10.1038/s41467-022-34535-8
Liu, T., Wang, B., and Cao, H. (2020a). Effects of high-fat diet-induced gut microbiota dysbiosis: far beyond the gut. Gut 69:2259. doi: 10.1136/gutjnl-2020-320717
Liu, F., Ye, S., Zhu, X., He, X., Wang, S., Li, Y., et al. (2021). Gastrointestinal disturbance and effect of fecal microbiota transplantation in discharged COVID-19 patients. J. Med. Case Rep. 15:60. doi: 10.1186/s13256-020-02583-7
Lv, L., Jiang, H., Chen, Y., Gu, S., Xia, J., Zhang, H., et al. (2021). The faecal metabolome in COVID-19 patients is altered and associated with clinical features and gut microbes. Anal. Chim. Acta 1152:338267. doi: 10.1016/j.aca.2021.338267
Maggini, S., Pierre, A., and Calder, P. C. (2018). Immune function and micronutrient requirements change over the life course. Nutrients 10:1531. doi: 10.3390/nu10101531
Malavazos, A. E., Corsi Romanelli, M. M., Bandera, F., and Iacobellis, G. (2020). Targeting the adipose tissue in COVID-19. Obesity (Silver Spring) 28, 1178–1179. doi: 10.1002/oby.22844
Malden, D. E., Liu, I. L. A., Qian, L., Sy, L. S., Lewin, B. J., Asamura, D. T., et al. (2024). Post-COVID conditions following COVID-19 vaccination: a retrospective matched cohort study of patients with SARS-CoV-2 infection. Nat. Commun. 15:4101. doi: 10.1038/s41467-024-48022-9
Mandò, C., Savasi, V. M., Anelli, G. M., Corti, S., Serati, A., Lisso, F., et al. (2021). Mitochondrial and oxidative unbalance in placentas from mothers with SARS-CoV-2 infection. Antioxidants (Basel) 10:1517. doi: 10.3390/antiox10101517
Mao, R., Qiu, Y., He, J. S., Tan, J. Y., Li, X. H., Liang, J., et al. (2020). Manifestations and prognosis of gastrointestinal and liver involvement in patients with COVID-19: a systematic review and meta-analysis. Lancet Gastroenterol. Hepatol. 5, 667–678. doi: 10.1016/S2468-1253(20)30126-6
Markowiak, P., and Śliżewska, K. (2017). Effects of probiotics, prebiotics, and Synbiotics on human health. Nutrients 9:1021. doi: 10.3390/nu9091021
McDermott, M. R., and Bienenstock, J. (1979). Evidence for a common mucosal immunologic system. I. Migration of B immunoblasts into intestinal, respiratory, and genital tissues. J. Immunol. 122, 1892–1898. doi: 10.4049/jimmunol.122.5.1892
Mendes de Almeida, V., Engel, D. F., Ricci, M. F., Cruz, C. S., Lopes, Í. S., Alves, D. A., et al. (2023). Gut microbiota from patients with COVID-19 cause alterations in mice that resemble post-COVID symptoms. Gut Microbes 15:2249146. doi: 10.1080/19490976.2023.2249146
Merad, M., and Martin, J. C. (2020). Pathological inflammation in patients with COVID-19: a key role for monocytes and macrophages. Nat. Rev. Immunol. 20, 355–362. doi: 10.1038/s41577-020-0331-4
Meringer, H., and Mehandru, S. (2022). Gastrointestinal post-acute COVID-19 syndrome. Nat. Rev. Gastroenterol. Hepatol. 19, 345–346. doi: 10.1038/s41575-022-00611-z
Merino, J., Joshi, A. D., Nguyen, L. H., Leeming, E. R., Mazidi, M., Drew, D. A., et al. (2021). Diet quality and risk and severity of COVID-19: a prospective cohort study. Gut 70, 2096–2104. doi: 10.1136/gutjnl-2021-325353
Moallemian, I. M., Emam-Djomeh, Z., Rao, I. M., and Rezaei, N. (2021). Nutrition and immunity in COVID-19. Adv. Exp. Med. Biol. 1318, 485–497. doi: 10.1007/978-3-030-63761-3_28
Morais, A., Passos, T. S., de, S., da, J., and Maciel, B. (2021). Obesity and the increased risk for COVID-19: mechanisms and nutritional management. Nutr. Res. Rev. 34, 209–221. doi: 10.1017/S095442242000027X
Moreira-Rosário, A., Marques, C., Pinheiro, H., Araújo, J. R., Ribeiro, P., Rocha, R., et al. (2021). Gut microbiota diversity and C-reactive protein are predictors of disease severity in COVID-19 patients. Front. Microbiol. 12:705020. doi: 10.3389/fmicb.2021.705020
Murakami, K., Kuriyama, S., and Hashimoto, H. (2023). General health literacy, COVID-19-related health literacy, and protective behaviors: evidence from a population-based study in Japan. Front. Public Health 11:1208815. doi: 10.3389/fpubh.2023.1208815
Nashed, L., Mani, J., Hazrati, S., Stern, D. B., Subramanian, P., Mattei, L., et al. (2022). Gut microbiota changes are detected in asymptomatic very young children with SARS-CoV-2 infection. Gut 71, 2371–2373. doi: 10.1136/gutjnl-2021-326599
Naureen, Z., Dautaj, A., Nodari, S., Fioretti, F., Dhuli, K., Anpilogov, K., et al. (2021). Proposal of a food supplement for the management of post-COVID syndrome. Eur. Rev. Med. Pharmacol. Sci. 25, 67–73. doi: 10.26355/eurrev_202112_27335
Neumann, M., Steimle, A., Grant, E. T., Wolter, M., Parrish, A., Willieme, S., et al. (2021). Deprivation of dietary fiber in specific-pathogen-free mice promotes susceptibility to the intestinal mucosal pathogen Citrobacter rodentium. Gut Microbes 13:1966263. doi: 10.1080/19490976.2021.1966263
Nurek, M., Rayner, C., Freyer, A., Taylor, S., Järte, L., MacDermott, N., et al. (2021). Recommendations for the recognition, diagnosis, and management of long COVID: a Delphi study. Br. J. Gen. Pract. 71, e815–e825. doi: 10.3399/BJGP.2021.0265
Oelsner, E. C., Sun, Y., Balte, P. P., Allen, N. B., Andrews, H., Carson, A., et al. (2024). Epidemiologic features of recovery from SARS-CoV-2 infection. JAMA Netw. Open 7:e2417440. doi: 10.1001/jamanetworkopen.2024.17440
Ohira, H., Tsutsui, W., and Fujioka, Y. (2017). Are Short chain fatty acids in gut microbiota defensive players for inflammation and atherosclerosis. J. Atheroscler. Thromb. 24, 660–672. doi: 10.5551/jat.RV17006
Oliva, A., Miele, M. C., di, F., de, M., Mauro, V., Aronica, R., et al. (2021). Persistent systemic microbial translocation and intestinal damage during coronavirus Disease-19. Front. Immunol. 12:708149. doi: 10.3389/fimmu.2021.708149
Omran, H. M., and Almaliki, M. S. (2020). Influence of NAD+ as an ageing-related immunomodulator on COVID 19 infection: a hypothesis. J. Infect. Public Health 13, 1196–1201. doi: 10.1016/j.jiph.2020.06.004
Palermo, A., Li, S., ten, J., Chellappa, A., Morris, A., Dillon, B., et al. (2023). A ketogenic diet can mitigate SARS-CoV-2 induced systemic reprogramming and inflammation. Commun. Biol. 6:1115. doi: 10.1038/s42003-023-05478-7
Paoli, A., Gorini, S., and Caprio, M. (2020). The dark side of the spoon – glucose, ketones and COVID-19: a possible role for ketogenic diet. J. Transl. Med. 18:441. doi: 10.1186/s12967-020-02600-9
Park, S. K., Lee, C. W., Park, D. I., Woo, H. Y., Cheong, H. S., Shin, H. C., et al. (2021). Detection of SARS-CoV-2 in fecal samples from patients with asymptomatic and mild COVID-19 in Korea. Clin. Gastroenterol. Hepatol. 19, 1387–1394.e2. doi: 10.1016/j.cgh.2020.06.005
Parotto, M., Gyöngyösi, M., Howe, K., Myatra, S. N., Ranzani, O., Shankar-Hari, M., et al. (2023). Post-acute sequelae of COVID-19: understanding and addressing the burden of multisystem manifestations. Lancet Respir. Med. 11, 739–754. doi: 10.1016/S2213-2600(23)00239-4
Parrot, T., Gorin, J. B., Ponzetta, A., Maleki, K. T., Kammann, T., Emgård, J., et al. (2020). MAIT cell activation and dynamics associated with COVID-19 disease severity. Sci. Immunol. 5:eabe1670. doi: 10.1126/sciimmunol.abe1670
Patra, S., Saxena, S., Sahu, N., Pradhan, B., and Roychowdhury, A. (2021). Systematic network and Meta-analysis on the antiviral mechanisms of probiotics: a preventive and treatment strategy to mitigate SARS-CoV-2 infection. Probiotics Antimicrob. Proteins 13, 1138–1156. doi: 10.1007/s12602-021-09748-w
Patrier, J., Villageois-Tran, K., Szychowiak, P., Ruckly, S., Gschwind, R., Wicky, P. H., et al. (2022). Oropharyngeal and intestinal concentrations of opportunistic pathogens are independently associated with death of SARS-CoV-2 critically ill adults. Crit. Care 26:300. doi: 10.1186/s13054-022-04164-0
Peng, Y., Zhang, L., Mok, C., Ching, J., Zhao, S., Wong, M., et al. (2023). Baseline gut microbiota and metabolome predict durable immunogenicity to SARS-CoV-2 vaccines. Signal Transduct. Target. Ther. 8:373. doi: 10.1038/s41392-023-01629-8
Penninger, J. M., Grant, M. B., and Sung, J. (2021). The role of angiotensin converting enzyme 2 in modulating gut microbiota, intestinal inflammation, and coronavirus infection. Gastroenterology 160, 39–46. doi: 10.1053/j.gastro.2020.07.067
Pham, V. T., Fehlbaum, S., Seifert, N., Richard, N., Bruins, M. J., Sybesma, W., et al. (2021). Effects of colon-targeted vitamins on the composition and metabolic activity of the human gut microbiome-a pilot study. Gut Microbes 13, 1–20. doi: 10.1080/19490976.2021.1875774
Phetsouphanh, C., Darley, D. R., Wilson, D. B., Howe, A., Munier, C. M. L., Patel, S. K., et al. (2022). Immunological dysfunction persists for 8 months following initial mild-to-moderate SARS-CoV-2 infection. Nat. Immunol. 23, 210–216. doi: 10.1038/s41590-021-01113-x
Phetsouphanh, C., Jacka, B., Ballouz, S., Jackson, K. J. L., Wilson, D. B., Manandhar, B., et al. (2024). Improvement of immune dysregulation in individuals with long COVID at 24-months following SARS-CoV-2 infection. Nat. Commun. 15:3315. doi: 10.1038/s41467-024-47720-8
Ponzo, V., Pellegrini, M., D’Eusebio, C., Bioletto, F., Goitre, I., Buscemi, S., et al. (2021). Mediterranean diet and SARS-COV-2 infection: is there any association? A proof-of-concept study. Nutrients 13:1721. doi: 10.3390/nu13051721
Purbey, P. K., Roy, K., Gupta, S., and Paul, M. K. (2023). Mechanistic insight into the protective and pathogenic immune-responses against SARS-CoV-2. Mol. Immunol. 156, 111–126. doi: 10.1016/j.molimm.2023.03.009
Rastogi, S., Mohanty, S., Sharma, S., and Tripathi, P. (2022). Possible role of gut microbes and host's immune response in gut-lung homeostasis. Front. Immunol. 13:954339. doi: 10.3389/fimmu.2022.954339
Reimer, R. A. (2019). Establishing the role of diet in the microbiota-disease axis. Nat. Rev. Gastroenterol. Hepatol. 16, 86–87. doi: 10.1038/s41575-018-0093-7
Reinold, J., Farahpour, F., Fehring, C., Dolff, S., Konik, M., Korth, J., et al. (2021). A pro-inflammatory gut microbiome characterizes SARS-CoV-2 infected patients and a reduction in the connectivity of an anti-inflammatory bacterial network associates with severe COVID-19. Front. Cell. Infect. Microbiol. 11:747816. doi: 10.3389/fcimb.2021.747816
Ren, Z., Wang, H., Cui, G., Lu, H., Wang, L., Luo, H., et al. (2021). Alterations in the human oral and gut microbiomes and lipidomics in COVID-19. Gut 70, 1253–1265. doi: 10.1136/gutjnl-2020-323826
Roukens, A., Pothast, C. R., König, M., Huisman, W., Dalebout, T., Tak, T., et al. (2022). Prolonged activation of nasal immune cell populations and development of tissue-resident SARS-CoV-2-specific CD8(+) T cell responses following COVID-19. Nat. Immunol. 23, 23–32. doi: 10.1038/s41590-021-01095-w
Ryan, F. J., Hope, C. M., Masavuli, M. G., Lynn, M. A., Mekonnen, Z. A., Yeow, A. E. L., et al. (2022). Long-term perturbation of the peripheral immune system months after SARS-CoV-2 infection. BMC Med. 20:26. doi: 10.1186/s12916-021-02228-6
Ryu, S., Shchukina, I., Youm, Y. H., Qing, H., Hilliard, B., Dlugos, T., et al. (2021). Ketogenic diet restrains aging-induced exacerbation of coronavirus infection in mice. eLife 10:e66522. doi: 10.7554/eLife.66522
Salminen, S., Collado, M. C., Endo, A., Hill, C., Lebeer, S., Quigley, E. M. M., et al. (2021). The international scientific Association of Probiotics and Prebiotics (ISAPP) consensus statement on the definition and scope of postbiotics. Nat. Rev. Gastroenterol. Hepatol. 18, 649–667. doi: 10.1038/s41575-021-00440-6
Santacroce, L., Inchingolo, F., Topi, S., del Prete, R., di Cosola, M., Charitos, I. A., et al. (2021). Potential beneficial role of probiotics on the outcome of COVID-19 patients: an evolving perspective. Diabetes Metab. Syndr. 15, 295–301. doi: 10.1016/j.dsx.2020.12.040
Santinelli, L., Laghi, L., Innocenti, G. P., Pinacchio, C., Vassalini, P., Celani, L., et al. (2021). Oral Bacteriotherapy reduces the occurrence of chronic fatigue in COVID-19 patients. Front. Nutr. 8:756177. doi: 10.3389/fnut.2021.756177
Santos, M., Dorna, M., Franco, E., Geronutti, J., Brizola, L., Ishimoto, L., et al. (2024). Clinical and physiological variables in patients with Post-COVID-19 condition and persistent fatigue. J. Clin. Med. 13:13. doi: 10.3390/jcm13133876
Schiaffino, S., Albano, D., Cozzi, A., Messina, C., Arioli, R., Bnà, C., et al. (2021). CT-derived chest muscle metrics for outcome prediction in patients with COVID-19. Radiology 300, E328–E336. doi: 10.1148/radiol.2021204141
Schult, D., Reitmeier, S., Koyumdzhieva, P., Lahmer, T., Middelhoff, M., Erber, J., et al. (2022). Gut bacterial dysbiosis and instability is associated with the onset of complications and mortality in COVID-19. Gut Microbes 14:2031840. doi: 10.1080/19490976.2022.2031840
Schultheiß, C., Willscher, E., Paschold, L., Gottschick, C., Klee, B., Henkes, S. S., et al. (2022). The IL-1β, IL-6, and TNF cytokine triad is associated with post-acute sequelae of COVID-19. Cell Rep. Med. 3:100663. doi: 10.1016/j.xcrm.2022.100663
Schwingshackl, L., and Hoffmann, G. (2014). Mediterranean dietary pattern, inflammation and endothelial function: a systematic review and meta-analysis of intervention trials. Nutr. Metab. Cardiovasc. Dis. 24, 929–939. doi: 10.1016/j.numecd.2014.03.003
Seethaler, B., Nguyen, N. K., Basrai, M., Kiechle, M., Walter, J., Delzenne, N. M., et al. (2022). Short-chain fatty acids are key mediators of the favorable effects of the Mediterranean diet on intestinal barrier integrity: data from the randomized controlled LIBRE trial. Am. J. Clin. Nutr. 116, 928–942. doi: 10.1093/ajcn/nqac175
Shang, J., Ye, G., Shi, K., Wan, Y., Luo, C., Aihara, H., et al. (2020). Structural basis of receptor recognition by SARS-CoV-2. Nature 581, 221–224. doi: 10.1038/s41586-020-2179-y
Shen, Y., Yu, F., Zhang, D., Zou, Q., Xie, M., Chen, X., et al. (2022). Dynamic alterations in the respiratory tract microbiota of patients with COVID‐19 and its association with microbiota in the gut (Adv. Sci. 27/2022). Adv. Sci. 9:e2200956. doi: 10.1002/advs.202270173
Singh, B., Eshaghian, E., Chuang, J., and Covasa, M. (2022). Do diet and dietary supplements mitigate clinical outcomes in COVID-19. Nutrients 14:1909. doi: 10.3390/nu14091909
Song, Y., Liu, P., Shi, X. L., Chu, Y. L., Zhang, J., Xia, J., et al. (2020). SARS-CoV-2 induced diarrhoea as onset symptom in patient with COVID-19. Gut 69, 1143–1144. doi: 10.1136/gutjnl-2020-320891
Sonner, J. K., Keil, M., Falk-Paulsen, M., Mishra, N., Rehman, A., Kramer, M., et al. (2019). Dietary tryptophan links encephalogenicity of autoreactive T cells with gut microbial ecology. Nat. Commun. 10:4877. doi: 10.1038/s41467-019-12776-4
Statovci, D., Aguilera, M., MacSharry, J., and Melgar, S. (2017). The impact of Western diet and nutrients on the microbiota and immune response at mucosal interfaces. Front. Immunol. 8:838. doi: 10.3389/fimmu.2017.00838
Stavropoulou, E., and Bezirtzoglou, E. (2020). Probiotics as a weapon in the fight against COVID-19. Front. Nutr. 7:614986. doi: 10.3389/fnut.2020.614986
Stutz, M. R., Dylla, N. P., Pearson, S. D., Lecompte-Osorio, P., Nayak, R., Khalid, M., et al. (2022). Immunomodulatory fecal metabolites are associated with mortality in COVID-19 patients with respiratory failure. Nat. Commun. 13:6615. doi: 10.1038/s41467-022-34260-2
Su, Q., Lau, R. I., Liu, Q., Chan, F., and Ng, S. C. (2023). Post-acute COVID-19 syndrome and gut dysbiosis linger beyond 1 year after SARS-CoV-2 clearance. Gut 72, 1230–1232. doi: 10.1136/gutjnl-2022-328319
Su, Q., Lau, R. I., Liu, Q., Li, M. K. T., Yan Mak, J. W., Lu, W., et al. (2024). The gut microbiome associates with phenotypic manifestations of post-acute COVID-19 syndrome. Cell Host Microbe 32, 651–660.e4. doi: 10.1016/j.chom.2024.04.005
Su, Y., Yuan, D., Chen, D. G., Ng, R. H., Wang, K., Choi, J., et al. (2022). Multiple early factors anticipate post-acute COVID-19 sequelae. Cell 185, 881–895.e20. doi: 10.1016/j.cell.2022.01.014
Sudre, C. H., Murray, B., Varsavsky, T., Graham, M. S., Penfold, R. S., Bowyer, R. C., et al. (2021). Attributes and predictors of long COVID. Nat. Med. 27, 626–631. doi: 10.1038/s41591-021-01292-y
Sukkar, S. G., and Bassetti, M. (2020). Induction of ketosis as a potential therapeutic option to limit hyperglycemia and prevent cytokine storm in COVID-19. Nutrition 79–80:110967. doi: 10.1016/j.nut.2020.110967
Sungnak, W., Huang, N., Bécavin, C., Berg, M., Queen, R., Litvinukova, M., et al. (2020). SARS-CoV-2 entry factors are highly expressed in nasal epithelial cells together with innate immune genes. Nat. Med. 26, 681–687. doi: 10.1038/s41591-020-0868-6
Synodinou, K. D., Nikolaki, M. D., Triantafyllou, K., and Kasti, A. N. (2022). Immunomodulatory effects of probiotics on COVID-19 infection by targeting the gut-lung Axis microbial Cross-talk. Microorganisms 10:1764. doi: 10.3390/microorganisms10091764
Takabayashi, T., Yoshida, K., Imoto, Y., Schleimer, R. P., and Fujieda, S. (2022). Regulation of the expression of SARS-CoV-2 receptor angiotensin-converting enzyme 2 in nasal mucosa. Am. J. Rhinol. Allergy 36, 115–122. doi: 10.1177/19458924211027798
Taufer, C. R., da Silva, J., and Rampelotto, P. H. (2024). The influence of probiotic lactobacilli on COVID-19 and the microbiota. Nutrients 16:1350. doi: 10.3390/nu16091350
Teixeira, P. C., Dorneles, G. P., Santana Filho, P. C., da Silva, I. M., Schipper, L. L., Postiga, I. A. L., et al. (2021). Increased LPS levels coexist with systemic inflammation and result in monocyte activation in severe COVID-19 patients. Int. Immunopharmacol. 100:108125. doi: 10.1016/j.intimp.2021.108125
Tochio, T., Kadota, Y., Tanaka, T., and Koga, Y. (2018). 1-Kestose, the smallest Fructooligosaccharide component, which efficiently stimulates Faecalibacterium prausnitzii as well as Bifidobacteria in humans. Food Secur. 7:140. doi: 10.3390/foods7090140
Turnbaugh, P. J. (2017). Microbes and diet-induced obesity: fast, cheap, and out of control. Cell Host Microbe 21, 278–281. doi: 10.1016/j.chom.2017.02.021
Vandeputte, D., Falony, G., Vieira-Silva, S., Wang, J., Sailer, M., Theis, S., et al. (2017). Prebiotic inulin-type fructans induce specific changes in the human gut microbiota. Gut 66, 1968–1974. doi: 10.1136/gutjnl-2016-313271
Vélez, C., Paz, M., Silvernale, C., Stratton, L. W., Kuo, B., Staller, K., et al. (2022). Factors associated with chronic De novo Post-coronavirus disease gastrointestinal disorders in a metropolitan US County. Clin. Gastroenterol. Hepatol. 20, e1488–e1492. doi: 10.1016/j.cgh.2021.10.020
Vernon, S. D., Whistler, T., Cameron, B., Hickie, I. B., Reeves, W. C., and Lloyd, A. (2006). Preliminary evidence of mitochondrial dysfunction associated with post-infective fatigue after acute infection with Epstein Barr virus. BMC Infect. Dis. 6:15. doi: 10.1186/1471-2334-6-15
Vestad, B., Ueland, T., Lerum, T. V., Dahl, T. B., Holm, K., Barratt-Due, A., et al. (2022). Respiratory dysfunction three months after severe COVID-19 is associated with gut microbiota alterations. J. Intern. Med. 291, 801–812. doi: 10.1111/joim.13458
Walsh, K. A., Jordan, K., Clyne, B., Rohde, D., Drummond, L., Byrne, P., et al. (2020). SARS-CoV-2 detection, viral load and infectivity over the course of an infection. J. Infect. 81, 357–371. doi: 10.1016/j.jinf.2020.06.067
Wang, B., Li, R., Lu, Z., and Huang, Y. (2020). Does comorbidity increase the risk of patients with COVID-19: evidence from meta-analysis. Aging (Albany NY) 12, 6049–6057. doi: 10.18632/aging.103000
Wang, S., Li, Y., Yue, Y., Yuan, C., Kang, J. H., Chavarro, J. E., et al. (2023). Adherence to healthy lifestyle prior to infection and risk of Post-COVID-19 condition. JAMA Intern. Med. 183, 232–241. doi: 10.1001/jamainternmed.2022.6555
Wang, M., Lkhagva, E., Kim, S., Zhai, C., Islam, M. M., Kim, H. J., et al. (2024). The gut microbe pair of Oribacterium sp. GMB0313 and Ruminococcus sp. GMB0270 confers complete protection against SARS-CoV-2 infection by activating CD8+ T cell-mediated immunity. Gut Microbes 16:2342497. doi: 10.1080/19490976.2024.2342497
Wang, C., Ramasamy, A., Verduzco-Gutierrez, M., Brode, W. M., and Melamed, E. (2023). Acute and post-acute sequelae of SARS-CoV-2 infection: a review of risk factors and social determinants. Virol. J. 20:124. doi: 10.1186/s12985-023-02061-8
Wang, H., Wei, Y., Hung, C. T., Lin, G., Jiang, X., Li, C., et al. (2024). Association of nirmatrelvir-ritonavir with post-acute sequelae and mortality in patients admitted to hospital with COVID-19: a retrospective cohort study. Lancet Infect. Dis. 24, 1130–1140. doi: 10.1016/S1473-3099(24)00217-2
Wölfel, R., Corman, V. M., Guggemos, W., Seilmaier, M., Zange, S., Müller, M. A., et al. (2020). Virological assessment of hospitalized patients with COVID-2019. Nature 581, 465–469. doi: 10.1038/s41586-020-2196-x
Wu, Y., Cheng, X., Jiang, G., Tang, H., Ming, S., Tang, L., et al. (2021). Altered oral and gut microbiota and its association with SARS-CoV-2 viral load in COVID-19 patients during hospitalization. NPJ Biofilms Microbiomes 7:61. doi: 10.1038/s41522-021-00232-5
Wu, Y., Guo, C., Tang, L., Hong, Z., Zhou, J., Dong, X., et al. (2020). Prolonged presence of SARS-CoV-2 viral RNA in faecal samples. Lancet Gastroenterol. Hepatol. 5, 434–435. doi: 10.1016/S2468-1253(20)30083-2
Xie, Y., Bowe, B., and Al-Aly, Z. (2021). Burdens of post-acute sequelae of COVID-19 by severity of acute infection, demographics and health status. Nat. Commun. 12:6571. doi: 10.1038/s41467-021-26513-3
Xing, Y. H., Ni, W., Wu, Q., Li, W. J., Li, G. J., Wang, W. D., et al. (2020). Prolonged viral shedding in feces of pediatric patients with coronavirus disease 2019. J. Microbiol. Immunol. Infect. 53, 473–480. doi: 10.1016/j.jmii.2020.03.021
Xu, Y., Li, X., Zhu, B., Liang, H., Fang, C., Gong, Y., et al. (2020). Characteristics of pediatric SARS-CoV-2 infection and potential evidence for persistent fecal viral shedding. Nat. Med. 26, 502–505. doi: 10.1038/s41591-020-0817-4
Xu, L., Yang, C. S., Liu, Y., and Zhang, X. (2022). Effective regulation of gut microbiota with probiotics and prebiotics May prevent or alleviate COVID-19 through the gut-lung Axis. Front. Pharmacol. 13:895193. doi: 10.3389/fphar.2022.895193
Yan, R., Zhang, Y., Li, Y., Xia, L., Guo, Y., and Zhou, Q. (2020). Structural basis for the recognition of SARS-CoV-2 by full-length human ACE2. Science 367, 1444–1448. doi: 10.1126/science.abb2762
Yang, T., Chakraborty, S., Saha, P., Mell, B., Cheng, X., Yeo, J. Y., et al. (2020). Gnotobiotic rats reveal that gut microbiota regulates colonic mRNA of Ace2, the receptor for SARS-CoV-2 infectivity. Hypertension 76, e1–e3. doi: 10.1161/HYPERTENSIONAHA.120.15360
Yang, W., Yu, T., Huang, X., Bilotta, A. J., Xu, L., Lu, Y., et al. (2020). Intestinal microbiota-derived short-chain fatty acids regulation of immune cell IL-22 production and gut immunity. Nat. Commun. 11:4457. doi: 10.1038/s41467-020-18262-6
Yao, Y., Cai, X., Fei, W., Ye, Y., Zhao, M., and Zheng, C. (2022). The role of short-chain fatty acids in immunity, inflammation and metabolism. Crit. Rev. Food Sci. Nutr. 62, 1–12. doi: 10.1080/10408398.2020.1854675
Yeoh, Y. K., Zuo, T., Lui, G. C. Y., Zhang, F., Liu, Q., Li, A. Y. L., et al. (2021). Gut microbiota composition reflects disease severity and dysfunctional immune responses in patients with COVID-19. Gut 70, 698–706. doi: 10.1136/gutjnl-2020-323020
Yue, Y., Ma, W., Accorsi, E. K., Ding, M., Hu, F., Willett, W. C., et al. (2022). Long-term diet and risk of severe acute respiratory syndrome coronavirus 2 (SARS-CoV-2) infection and coronavirus disease 2019 (COVID-19) severity. Am. J. Clin. Nutr. 116, 1672–1681. doi: 10.1093/ajcn/nqac219
Zhang, J. J., Dong, X., Liu, G. H., and Gao, Y. D. (2023). Risk and protective factors for COVID-19 morbidity, severity, and mortality. Clin. Rev. Allergy Immunol. 64, 90–107. doi: 10.1007/s12016-022-08921-5
Zhang, F., Wan, Y., Zuo, T., Yeoh, Y. K., Liu, Q., Zhang, L., et al. (2022). Prolonged impairment of Short-chain fatty acid and L-isoleucine biosynthesis in gut microbiome in patients with COVID-19. Gastroenterology 162, 548–561.e4. doi: 10.1053/j.gastro.2021.10.013
Zhang, L., Xu, Z., Mak, J., Chow, K., Lui, G., Li, T., et al. (2022). Gut microbiota-derived synbiotic formula (SIM01) as a novel adjuvant therapy for COVID-19: an open-label pilot study. J. Gastroenterol. Hepatol. 37, 823–831. doi: 10.1111/jgh.15796
Zhang, H., and Zhou, Z. (2023). Association of gut microbiota and dietary component intake with COVID-19: a mendelian randomization study. Clin. Nutr. 42, 1308–1313. doi: 10.1016/j.clnu.2023.06.017
Zheng, S., Fan, J., Yu, F., Feng, B., Lou, B., Zou, Q., et al. (2020). Viral load dynamics and disease severity in patients infected with SARS-CoV-2 in Zhejiang province, China, January-March 2020: retrospective cohort study. BMJ 369:m1443. doi: 10.1136/bmj.m1443
Zhou, T., Wu, J., Zeng, Y., Li, J., Yan, J., Meng, W., et al. (2022). SARS-CoV-2 triggered oxidative stress and abnormal energy metabolism in gut microbiota. MedComm (2020) 3:e112. doi: 10.1002/mco2.112
Zhu, N., Zhang, D., Wang, W., Li, X., Yang, B., Song, J., et al. (2020). A novel coronavirus from patients with pneumonia in China, 2019. N. Engl. J. Med. 382, 727–733. doi: 10.1056/NEJMoa2001017
Zimmer, J., Lange, B., Frick, J. S., Sauer, H., Zimmermann, K., Schwiertz, A., et al. (2012). A vegan or vegetarian diet substantially alters the human colonic faecal microbiota. Eur. J. Clin. Nutr. 66, 53–60. doi: 10.1038/ejcn.2011.141
Zlacká, J., Stebelová, K., Zeman, M., and Herichová, I. (2021). Interactions of renin-angiotensin system and COVID-19: the importance of daily rhythms in ACE2, ADAM17 and TMPRSS2 expression. Physiol. Res. 70, S177–S194. doi: 10.33549/physiolres.934754
Zmora, N., Suez, J., and Elinav, E. (2019). You are what you eat: diet, health and the gut microbiota. Nat. Rev. Gastroenterol. Hepatol. 16, 35–56. doi: 10.1038/s41575-018-0061-2
Zollner, A., Koch, R., Jukic, A., Pfister, A., Meyer, M., Rössler, A., et al. (2022). Postacute COVID-19 is characterized by gut viral antigen persistence in inflammatory bowel diseases. Gastroenterology 163, 495–506.e8. doi: 10.1053/j.gastro.2022.04.037
Zuo, T., Liu, Q., Zhang, F., Lui, G. C. Y., Tso, E. Y. K., Yeoh, Y. K., et al. (2021). Depicting SARS-CoV-2 faecal viral activity in association with gut microbiota composition in patients with COVID-19. Gut 70, gutjnl-2020-322294–gutjnl-2020-322284. doi: 10.1136/gutjnl-2020-322294
Zuo, T., Zhan, H., Zhang, F., Liu, Q., Tso, E. Y. K., Lui, G. C. Y., et al. (2020b). Alterations in fecal fungal microbiome of patients with COVID-19 during time of hospitalization until discharge. Gastroenterology 159, 1302–1310.e5. doi: 10.1053/j.gastro.2020.06.048
Zuo, T., Zhang, F., Lui, G., Yeoh, Y., Li, A., Zhan, H., et al. (2020a). Alterations in gut microbiota of patients with COVID-19 during time of hospitalization. Gastroenterology 159, 944–955.e8. doi: 10.1053/j.gastro.2020.05.048
ACE2 - angiotensin-converting enzyme 2
ARDS - acute respiratory distress syndrome
BHB - β-hydroxybutyrate
CRP - C-reactive protein
COVID-19 - coronavirus disease 2019
DALYs - disability-adjusted life years
FMT - fecal microbiota transplantation
GM-CSF - granulocyte-macrophage colony-stimulating factor
HFD - High-fat diet
HMGB1 - high-mobility group box protein-1
IBD - inflammatory bowel diseases
IBS - irritable bowel syndrome
ICU - intensive care unit
KD - ketogenic diet
LBP - lipopolysaccharide-binding protein
LPS - lipopolysaccharide
MD - Mediterranean diet
NASH - non-alcoholic steatohepatitis
NLRP3 - NOD-like receptor family pyrin domain containing 3
PACS - post-acute COVID-19 syndrome
PRRs - pattern-recognition receptors
SARS-CoV-2 - severe acute respiratory syndrome coronavirus-2
SCFA - short-chain fatty acid
T2DM - type 2 diabetes mellitus
WD - Western diet
Keywords: coronavirus disease 2019, post-acute COVID-19 syndrome, severe acute respiratory syndrome coronavirus-2, gut microbiota, diet, gut-lung axis
Citation: An Y, He L, Xu X, Piao M, Wang B, Liu T and Cao H (2024) Gut microbiota in post-acute COVID-19 syndrome: not the end of the story. Front. Microbiol. 15:1500890. doi: 10.3389/fmicb.2024.1500890
Received: 24 September 2024; Accepted: 09 December 2024;
Published: 24 December 2024.
Edited by:
Ravindra Veeranna, Xavier University School of Medicine, NetherlandsCopyright © 2024 An, He, Xu, Piao, Wang, Liu and Cao. This is an open-access article distributed under the terms of the Creative Commons Attribution License (CC BY). The use, distribution or reproduction in other forums is permitted, provided the original author(s) and the copyright owner(s) are credited and that the original publication in this journal is cited, in accordance with accepted academic practice. No use, distribution or reproduction is permitted which does not comply with these terms.
*Correspondence: Hailong Cao, Y2FvaGFpbG9uZ0B0bXUuZWR1LmNu; Tianyu Liu, bGl1dGlhbnl1QHRtdS5lZHUuY24=
†These authors have contributed equally to this work
Disclaimer: All claims expressed in this article are solely those of the authors and do not necessarily represent those of their affiliated organizations, or those of the publisher, the editors and the reviewers. Any product that may be evaluated in this article or claim that may be made by its manufacturer is not guaranteed or endorsed by the publisher.
Research integrity at Frontiers
Learn more about the work of our research integrity team to safeguard the quality of each article we publish.