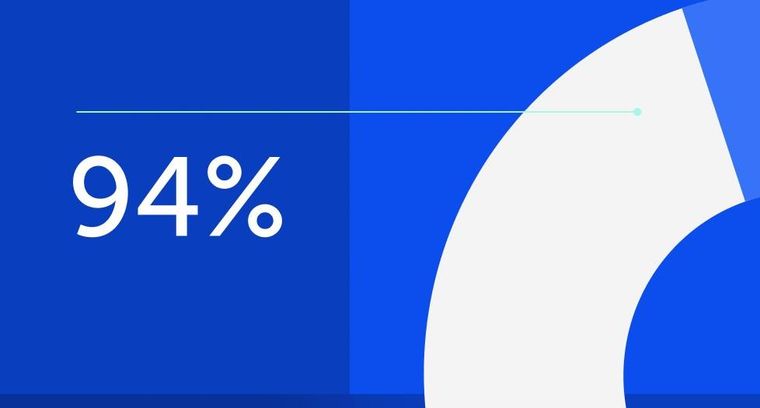
94% of researchers rate our articles as excellent or good
Learn more about the work of our research integrity team to safeguard the quality of each article we publish.
Find out more
ORIGINAL RESEARCH article
Front. Microbiol., 29 November 2024
Sec. Microorganisms in Vertebrate Digestive Systems
Volume 15 - 2024 | https://doi.org/10.3389/fmicb.2024.1492035
Introduction: This study aimed to assess the protective efficacy of Bacillus coagulans MF-06 as a potential alternative to antibiotics in mitigating intestinal mucosal damage in chicks infected with Salmonella pullorum.
Methods: A total of 150 one-day-old SPF chicks were selected and randomly divided into five groups: control group (CK), probiotics group (EM), probiotics treatment group (PT), antibiotic treatment group (AT), Salmonella pullorum group (SI), CK, AT and SI groups were fed a basal diet, EM and PT groups were fed a basal diet supplemented with 1.0 × 108 CFU/g Bacillus coagulans; PT, AT and SI groups were gavaged with 1.0 × 109 CFU/0.5 mL Salmonella pullorum at 7 days of age; AT group were fed with 0.375 g/kg neomycin sulfate in the basal diet from days 7–14.
Results: Subsequently, the study evaluated alterations in growth performance, the integrity of the intestinal mucosal barrier, cytokines associated with the Wnt/β-catenin signaling pathway, and gut microbiota composition. The results revealed that the administration of Bacillus coagulans MF-06 significantly reduced the feed conversion ratio of chicks (p < 0.05), and significantly increased the average daily weight gain and average daily feed intake in chicks challenged with Salmonella Pullorum (p < 0.05). Furthermore, Bacillus coagulans MF-06 treatment diminished the presence of Salmonella pullorum colonies in the intestinal tract. Additionally, the administration of Bacillus coagulans MF-06 restored levels of (Diamine oxidase) DAO and (D-lactic acid) D-LA levels, as well as the levels of tight junction protein, including TJP1, CLDN1, CLDN2, Occludin, and MUC2 (p < 0.05). The study noted a significant decrease in cell apoptosis (p < 0.05) and a significant increase in the expression of Proliferating Cell Nuclear Antigen (PCNA) and v-myc avian myelocytomatosis viral oncogene homolog (C-MYC) (p < 0.05), which activated the Wnt/β-catenin signaling pathway. Analysis through 16S rRNA sequencing revealed that the intake of Bacillus coagulans MF-06 led to a significant decrease in the relative abundance of Lachnoclostridium, Shuttleworthia, and unidentified-Eggerthellaceae (p < 0.05).
Discussion: Collectively, the Bacillus coagulans MF-06 may provide a protective effect against Salmonella pullorum infection in chicks by enhancing growth performance, strengthening the integrity of the intestinal mucosal barrier, and stabilizing the gut microbiota.
Salmonella pullorum (S. pullorum) is the causative agent of Pullorum disease (PD), which primarily affects chicks, turkeys, and other domestic poultry at 1–2 weeks of age. Clinical manifestations of chicks include anorexia, white diarrhea, weakness, and increased mortality, causing severe economic losses to the poultry industry in developing countries (Shivaprasad, 2000; Barrow and Neto, 2011; Jiaqi et al., 2022; Shen et al., 2022). S. pullorum is transmitted by the vertical and horizontal routes, the vertical route facilitates the transmission of S. pullorum from hens to chicks via eggs, while the horizontal route is considered the main transmission strategy of this pathogen in which chicks are infected by ingesting the contaminated feed and water (Cheng et al., 2020). For the horizontal route of S. pullorum, it initially colonization and attachment to the intestinal mucosal epithelium, causing severe damage to the intestinal function and microbiota of chicks, and subsequently spreads to host tissues and organs, weakening immunity, causing sepsis, and impairing immune responses. Therefore, the first step of S. pullorum infection is invasion of the intestinal mucosal epithelium (Wang et al., 2019; Wang et al., 2021b; Zou et al., 2024).
The production of various epithelial cells driven by ISCs is integral to the maintenance of the intestinal epithelial barrier, and the proliferation and apoptosis of ISCs are essential for maintaining intestinal homeostasis (Ding et al., 2024). Various signaling pathways, including the Wnt/β-catenin pathway, exert significant influence on intestinal homeostasis. Wnt/β-catenin pathway-related proteins are abundant in the gut microenvironment, and they play a crucial role in intestinal epithelial renewal (Hao et al., 2024). The Wnt ligand is released by Paneth, stromal, and endothelial cells located below the epithelial surface, including macrophages. Upon release, Wnt activates low-density lipoprotein receptor-associated protein 5/6 (LRP5/6) and Frizzled co-receptor, promoting β-catenin nuclear translocation, where it interacts with transcription factor 4 (TCF4) to maintain stem cell and epithelial cell proliferation and differentiation (Lee et al., 2018). Therefore, Enhancement of the intestinal mucosal barrier is essential for defense against S. pullorum in chicks (Deng et al., 2021; Liangyu et al., 2022).
Traditionally, antibiotic therapy has been considered the main prevention and treatment strategy for S. pullorum, but the persistent and widespread use of antibiotics can lead to the emergence of antibiotic-resistant strains, drug residues, and microbial dysbiosis in animals, posing a potential threat to human health (Song et al., 2020). Since 2006, the European Union (EU) has banned the application of antimicrobial growth promoters in animal feed and water (Martin et al., 2015). Subsequently, China and the United States have also banned the use of antibiotics as feed additives (Wang et al., 2022; Attia et al., 2023). Therefore, safer and more effective alternatives to antibiotics need to be developed or discovered.
It is reported that probiotics can enhance food safety and promote the health of animal gastrointestinal tracts by generating organic acids, stimulating the host immune response, and producing antimicrobial substances (Wang et al., 2021a). Among the various probiotics, Bacillus coagulans (B. coagulans), as a facultative anaerobic, Gram-positive, and non-pathogenic bacterium, can form spores and produce lactic acid (Hou et al., 2023). Spore-forming B. coagulans exhibits strong resistance to extreme environmental conditions, which allows it to adapt to the acidic and hypoxic environment of the gastrointestinal tract and survive in the intestine, where it exerts the functions of lactic acid bacteria, B. coagulans can also diminish intestinal pH, facilitate the proliferation of advantageous bacteria, and impede the growth of detrimental microorganisms, which has potential application value of alternative antibiotics (Hung et al., 2012; Zhang et al., 2021). At present, B. coagulans is widely used in aquaculture, livestock, and poultry (Zhou et al., 2020; Liu et al., 2022; Hu et al., 2024). For example, Dietary B. coagulans supplementation attenuated inflammation by modulating Th cell function and T cell responses, and restored intestinal microflora by enriching beneficial bacteria and suppressing harmful flora (Guo et al., 2021); Addition of 1.0 × 108 CFU/mL B. coagulans to the water can improves growth performance, enhances intestinal innate immunity and improves intestinal microbial communities of broilers (Liu et al., 2022); Addition of 2.0 × 108 CFU/g B. coagulans to the feed could considerably improve the growth performance of broilers (Hou et al., 2023); Dietary supplementation of B. coagulans in piglets infected by Enterotoxigenic Escherichia coli (ETEC) K88 can prevent the decrease in average daily feed intake by alleviating intestinal damage and modulating the gut microbiota (Zhang et al., 2023). However, there have been few reports about the effects of avian autochthonous probiotics on the intestinal mucosal barrier and gut microbiota of chicks infected with S. pullorum. In view of those, this study explored the effects of B. coagulans MF-06 on the growth performance, intestinal mucosal barrier, and gut microbiota of chicks infected with S. pullorum.
The experiments were approved by the Institutional Animal Care and Use Committee of Yangtze University (Approval No. 202401003).
Bacillus coagulans strain MF-06 was previously isolated and identified in our laboratory. Briefly, the strain was isolated from the intestinal contents of chicks and then identified through physiological, biochemical, and molecular biological analyses. The dominant B. coagulans strains MF-06 were selected based on the preliminary results of physiological, biochemical, and in vitro antibacterial experiments (Lee et al., 2014). The isolated probiotics were prepared into freeze-dried powder, which was modified according to Chen et al. (2020) the research method. The glycerin storage tubes containing strains were inoculated with 2% inoculate into YPD medium and activated for three generations. Strains were activated with 2% inoculum to 100 mL YPD medium at 37°C for 16 h. Then, 2% of the activated strains were inoculated into 2.5 L YPD medium and incubated in a 5 L bioreactor (FR-P series; Shanghai Jichen Biotechnology Co., LTD., Shanghai) in the high-density fermentation, 37°C for 16 h. The bacterial sludge was centrifuged at 4°C for 20 min at 8000 rpm and collected. The bacteria pellet was resuspended in 250 mL of sterilized degreased milk powder protectant, mixed, and frozen at −80°C for 12—14 h. The frozen samples were then freeze-dried under vacuum (ZLGJ-18 multi-manifold model, Huachen Instrument Co., LTD., Zhengzhou, China) at −50°C for 48 h. This freeze-dried powder was the final probiotic. Lyophilized powder was prepared and viable counts per gram of B. coagulans were determined by the plate coating method. The samples were then stored at 4°C until use. Neomycin sulfate-soluble powder was purchased from Inner Mongolia Huatian Pharmaceutical Co. Ltd. (Inner Mongolia, China).
The S. pullorum strain (CVCC No. 534) was obtained from the China Veterinary Microbial Strain Preservation and Management Center in Beijing, China. The species of bacteria were preserved in 20% glycerol at −80°C. Bacterial viability was measured by the plate smear method, and the corresponding Optical density (OD) values were measured to plot the growth curve of S. pullorum. Bacterial suspensions were prepared after determined the concentrations.
A total of 150 one-day-old SPF chicks (Jinghong No.1) were Hubei Yukou Poultry Industry Co., Ltd., Hubei. They selected and randomly divided into five groups: CK group: control group, which was fed with basic diet with no administration of antibiotics or probiotics. EM group: probiotics group, whose basal diet was added with 1.0 × 108 CFU/g B. coagulans MF-06; PT group: probiotics treatment group, whose basal diet was added with 1.0 × 108 CFU/g B. coagulans MF-06 from days 1 to 14, and when chicks were seven-day-old, 1.0 × 109 CFU/0.5 mL of S. pullorum was administered by gavage; AT group: antibiotic treatment group, for whom basal diet was provided for the first 7 days, and when chicks were seven-day-old, they administered with 1.0 × 109 CFU/0.5 mL of S. pullorum by gavage, and the basal diet supplemented with 0.375 g/kg neomycin sulfate from days 7 to 14. SI group: S. pullorum infection group, which was fed with basic diet, and when chicks were seven-day-old, they were administered with 1.0 × 109 CFU/0.5 mL of S. pullorum by gavage (Deng et al., 2021); Each group comprised six replicates, with each replicate consisting of five chicks. The added dose of B. coagulans was obtained from a preliminary dose exploration experiment. The trial design is shown in Supplementary Figure S1, the chicks in the CK and EM groups were accommodated in an independent house in case of cross-contamination and humidity, and chicks in each group were housed in wire cages (110 × 80 × 45 cm). All chicks tested negative for pathogens before the experiment and the chicken house was thoroughly sterilized before the experiment. During the 14-day experiment, all chicks were allowed unrestricted access to water and fed with a starter feed devoid of antibiotics. All feeds were ground into powders and stored in a low-temperature dry environment, the basal diet composition and basic feed composition are presented in Table 1. At 9 am, the time of the first feeding and water change, chickens were observed for any abnormalities. The second feeding and water change was at 3:00 pm, when the chickens were observed again. The amount of feed remaining was recorded daily and the enclosure was cleaned regularly. The same rearing conditions were used, and the chick chambers were maintained at 34–36°C and a 60% relative humidity from day 1 to day 3. The temperature was decreased by 1°C each day from day 4 to day 14 and maintained at 31–33°C. The chambers were illuminated for 24 h on days 1 to day 3, for 22 h on days 4 to day 7, and for 20 h on days 8 to day 14. The growth status, body weight, survival rate, and clinical symptoms were recorded daily.
On the 14th day, two chicks were randomly selected from each replicate group for a total of six chicks per group. Two mL of blood sample was collected from the vein under the wing and stored in procoagulant tubes, followed by incubation at room temperature for 2 h and centrifugation at 3,000 g and 4°C for 15 min for separation of the serum, which was then collected and stored at −80°C for subsequent analysis. The chicks were euthanized under anesthesia by cervical dislocation, and then sample was aseptically collected from the duodenum, jejunum, ileum, and cecum and stored at 4°C for quantifying the bacterial load. To perform 16S rRNA sequencing analysis, 0.5 g of the remaining cecal content was collected, frozen in liquid nitrogen, and stored at −80°C. The jejunum (50 mg) was aseptically collected and immersed in an RNA sample preservation solution, flash-frozen with liquid nitrogen, and stored at −80°C for total RNA isolation. Additionally, Midsections (3—5 cm) of the jejunum were collected and fixed in a 4% paraformaldehyde solution for subsequent immunohistochemical analysis.
The D-lactic acid (D-LA) level in the serum was assessed with a commercially available ELISA kit (Shanghai Enzyme Linked Biology Co., Ltd., Shanghai, China); The diamine oxidase (DAO) level in the serum was measured with a commercially available kit (Nanjing Jiancheng Bioengineering Institute, Nanjing, China).
The bacterial load in tissues was determined according to the previous method with some modifications (Li et al., 2024), the tissue specimens of duodenum, jejunum, ileum, and cecum (100 mg) after sterile collection were placed in 2 mL sterile centrifuge tube. Subsequently, 900 μL of PBS buffer was added, and the mixture was thoroughly homogenized. Following gradient dilution (10−2–10−8), all samples were inoculated on bismuth sulfite agar; the process six experiments were repeated, with each replicate conducted three times, and then the samples were cultured at 37°C for 18–24 h. The morphological characteristics of the colonies were used to quantify S. pullorum in the intestinal tissue. S. pullorum counts, expressed as logarithmic transformation (log10), were reported as colony-forming units per 0.1 g (CFU/0.1 g).
Before the chicks were dissected, body weight was recorded, duodenum, jejunum, and ileum were isolated, and the lengths of the corresponding parts were measured. Three parallel biological replicates were used for all procedures and measurements.
Small segments of mid-jejunum were fixed with 4% paraformaldehyde solution and dehydrated through an ascending ethanol gradient. The segments were cleaned with xylene and embedded in paraffin. Next, the samples were sectioned at 5 μm for immunohistochemical analysis of Proliferating Cell Nuclear Antigen (PCNA) and v-myc avian myelocytomatosis viral oncogene homolog (C-MYC), the immunohistochemistry was conducted following the method by previous research (Shen et al., 2024). The sections were dewaxed with xylene, anhydrous ethanol, distilled water and incubated with 3% H2O2 for 20 min at room temperature (25 ± 2°C) to restrict the endogenous peroxidase activity. Then, the citric acid buffer (G1202 Servicbio Co., Ltd., Wuhan, China) was used to restore antigen activity for 10 min. Next, the sections were immersed in 3% Bovine serum BSA (GC305010, Servicbio Co., Ltd., Wuhan, China) for 30 min at room temperature. Gently shake off the sealing solution, add PBS to the section in 1:500 proportion of primary antibody (Anti -PCNA Rabbit pAb and Anti -C-MYC Rabbit pAb), and the section is placed flat in a wet box at 4°C for overnight incubation. The slide was placed in PBS (pH 7.4) and washed by shaking on the decolorizing shaker for three times, 5 min each time. Next, the sections were incubated with biotinylated goat anti-rabbit antibody (1:200, GB23303 Servicbio Co., Ltd., Wuhan, China) at room temperature for 50 min, and washed with PBS (pH 7.4) for three times. After the sections were slightly dried, the freshly prepared DAB color developing solution (G1212, Servicbio Co., Ltd., Wuhan, China) was added into the circle, the color developing time was controlled under the microscope. The positive color was brown and yellow, and the sections were rinsed with tap water to terminate the color development. Next, hematoxylin restaining for about 3 min, washing with tap water, hematoxylin differentiation solution for a few seconds, rinse with tap water, hematoxylin return to blue solution, and rinse with running water. Then were conventionally dehydrated to transparency and sealed using neutral balsam. Four sections were randomly selected for each group and chose three different regions of each section. The average optical density of immune positive substances in the intestine was measured using ImageJ software (version 1.8).
Total RNA was extracted from jejunum samples using the TRIzol™ Reagent Kit (Thermo Fisher Scientific, Waltham, Massachusetts, USA) according to the manufacturer’s protocol, and the RNA concentration was measured using a microspectrophotometer (nanodrop2000, Thermo Fisher Scientific, Massachusetts). cDNA was synthesized from the RNA using the Color Reverse Transcription Kit with gDNA Remover (EZBioscience, USA). All gene expression levels were normalized with β-actin as the internal standard. PCR primer sequences (Table 2) were designed and synthesized by Shanghai SANGON bio (Shanghai, China). SYBR Green fast qPCR mix (RK21203; Abclonal, Wuhan, China) was used with the following program: 95°C for 3 min, 30 cycles of 95°C for 5 s, 60°C for 30 s. This experiment was performed in six biological replicates with three replicates each. The quantitative RT-PCR (RT-qPCR) assay was performed to analyze the relative mRNA levels of the targeted genes using the 2-△△CT method as previously described (Livak and Schmittgen, 2001).
For each group, samples of cecal content were randomly selected for full-length 16S rRNA sequencing. Total genome DNA from samples was extracted using CTAB method. DNA concentration and purity was monitored on 1% agarose gels. According to the concentration, DNA was diluted to 1 ng/L using sterile water (Jiali et al., 2024). 16S rRNA genes of regions (V3-V4/16S) were amplified used specific primer (F: 5′-CCTAYGGGRBGCASCAG-3′, R: 5’-GGACTACNNGGGTATCTAAT-3′). All PCR reactions were carried out with 15 L of Phusion® High-Fidelity PCR Master Mix (New England Biolabs); 2 M of forward and reverse primers, and about 10 ng template DNA. Thermal cycling consisted of initial denaturation at 98°C for 1 min, followed by 30 cycles of denaturation at 98°C for 10 s, annealing at 50°C for 30 s, and elongation at 72°C for 30 s. Finally, 72°C for 5 min. Mix same volume of 1X loading buffer (contained SYB green) with PCR products and operate electrophoresis on 2% agarose gel for detection. PCR products was mixed in equidensity ratios. Then, mixture PCR products was purified with Qiagen Gel Extraction Kit (Qiagen, Germany). Then, Sequencing libraries were generated using TruSeq® DNA PCR-Free Sample Preparation Kit (Illumina, USA) following manufacturer’s recommendations and index codes were added. The library quality was assessed on the Qubit@ 2.0 Fluorometer (Thermo Scientific) and Agilent Bioanalyzer 2,100 system. At last, the library was sequenced on an Illumina NovaSeq platform and 250 bp paired-end reads were generated.
Paired-end reads was assigned to samples based on their unique barcode and truncated by cutting off the barcode and primer sequence. Quality filtering on the raw tags were performed under specific filtering conditions to obtain the high-quality clean tags according to the fast (v0.22.0).1 Paired-end reads were merged using FLASH (v1.2.11),2 The tags were compared with the reference database3 using UCHIME Algorithm4 to detect chimera sequences, and then the chimera sequences were removed. Then the Effective Tags finally obtained. Sequences analysis was performed by Uparse software (Uparse v7.0.1001).5 Sequences with 97% similarity were assigned to the same OTUs. Representative sequence for each OTU was screened for further annotation. Amplicon sequence variant (ASV) was analyzed by Deblur, which uses error profiles to obtain putative error-free sequences from Illumina MiSeq and HiSeq sequencing platforms. For each representative sequence, the Silva Database6 was used based on Mothur algorithm to annotate taxonomic information. OTUs abundance information were normalized using a standard of sequence number corresponding to the sample with the least sequences. Alpha diversity is applied in analyzing complexity of species diversity for a sample through 6 indices, including Observed-species, Chao1, Shannon, Simpson, ACE, Good-coverage. All these indices in our samples were calculated with QIIME and displayed with R software (Version 4.1.2). To further investigate the differences in community structure and variations in species composition among grouped samples, we performed Student t-tests and LEfSe analysis.
In this study, Kruskal-Wallis test was used to compare the differences of different microbial communities in each group, Because the data did not conform to the assumption of normal distribution, we chose to use nonparametric test methods to analyze differences in microbial diversity or abundance between groups. If the test results showed a significant difference (p < 0.05), a further Dunn’s multiple comparison test was performed to determine which groups were significantly different. Growth performance was analyzed by a Mixed Effects Model using SPSS software (version 25.0, SPSS Inc., Chicago, IL, USA), and other data, such as bacterial load, serum indicators and gene expression, were analyzed by one-way ANOVA significant differences between means were compared using Duncan’s multiple range test. Statistical significance of differences was expressed by the presence of a-d letters within a column; different letters within a column signify a significant difference between means (p < 0.05). Data visualization was achieved using GraphPad Prism 9.0 (GraphPad Inc., La Jolla, CA), and the ImageJ software (version 1.8) was employed to measure the data. All experimental data have at least three independent replicates, and the results are expressed as mean ± standard deviation (SD).
Table 3 presents the impact of B. coagulans MF-06 on the growth performance of chickens infected with S. pullorum. On day 7, the body weight of the EM group (62.85 g) was significantly greater than that of the other groups (p < 0.05), while no significant differences were observed among the remaining four groups (p > 0.05). By day 14, the body weight of the SI group had decreased by 5.39% in comparison to the CK group (p < 0.05), the body weights of the PT and AT groups increased by 4.75 and 5.68%, respectively, when compared to the SI group (p < 0.05), the EM group exhibited the highest body weight at 126.50 g on day 14. During the experimental period (1–14 days), the SI group experienced reductions in ADG and ADFI of 7.30 and 2.6%, respectively, compared to the CK group, with an increase in FCR of 4.73% (p < 0.05). When compared to the SI group, the ADG in the PT and AT groups increased by 6.50 and 7.30%, respectively (p < 0.05), while ADFI increased by 2.54 and 3.20% (p < 0.05), additionally, FCR decreased by 3.51 and 4.02% in the PT and AT groups, respectively (p < 0.05). The average daily gain (ADG) in the EM group (6.70 g) was significantly higher than that of the other groups (p < 0.05), while the feed conversion ratio (FCR) was lower than that of the other groups (p < 0.05).
Table 3. Body weight (BW), average daily gain (ADG), average daily feed intake (ADFI), and feed conversion ratio (FCR) under different treatments.1
The number of S. pullorum colonizing the duodenum, jejunum, ileum, and cecum in each group is shown in Figure 1. S. pullorum was not detected in the tissues from the CK and EM groups. The colonization levels in the duodenum, jejunum, ileum, and cecum of the SI group were 4.76 × 106, 1.33 × 106, 1.18 × 107, and 6.73 × 106 CFU/0.1 g, respectively, which were higher than those recorded in other groups (p < 0.05). For the PT and AT groups, the colonization number of S. pullorum was 1.62 × 106 and 1.40 × 106 CFU/0.1 g in duodenum, 6.41 × 104 and 5.25 × 105 CFU/0.1 g in jejunum, 6.31 × 105 and 2.76 × 106 CFU/0.1 g in ileum, and 2.72 × 106 and 2.28 × 106 CFU/0.1 g in cecum, respectively. These values were lower than those found in the corresponding parts of the SI group (p < 0.05).
Figure 1. Logarithmic colony counts of S. pullorum in the duodenum, jejunum, ileum, and cecum of various treatment groups. Different lowercase letters indicate significant differences between groups. Data are presented as the mean ± SD, n = 6. CK, control group; EM, B. coagulans group; PT, probiotics treatment group; AT, antibiotic treatment group; SI, S. pullorum infection group.
The effects of B. coagulans MF-06 on intestinal index of chicks infected with S. pullorum are shown in Table 4. There was no significant difference in duodenal length index among the groups (p > 0.05). The jejunum length index was similar among the SI, PT, and AT groups (21.9, 20.89, 20.87%), and the values were significantly lower than that of the CK group (23.9%) (p < 0.05). The ileum length index in the SI group (17.29%) was lower than that in the CK group (22.51%) and PT group (20.54%) (p < 0.05), whereas showed no significant difference from that in the AT group (17.82%) (p > 0.05).
To assess the intestinal barrier function across the five groups, the mRNA expression levels of TJP1, CLDN1, CLDN2, Occludin, and MUC2 genes in the jejunum were analyzed by RT-qPCR. As shown in Figure 2, the expression levels of these five genes were up-regulated in the EM group relative to that in the CK group (p < 0.05). In contrast, the TJP1, CLDN1, Occludin, and MUC2 genes were down-regulated in the SI group compared with those in the CK group (p < 0.05). The CLDN1, Occludin, and MUC2 genes were up-regulated in the PT group relative to those in the SI group (p < 0.05). Additionally, all these five genes were up-regulated in the AT group compared with those in the SI group (p < 0.05).
Figure 2. Expression of jejunal tight junction-related genes. (A) Relative expression of the mRNAs of TJP1, (B) CLDN1, (C) CLDN2, (D) Occludin, and (E) MUC2. Different lowercase letters indicate differences between groups. Values are presented as the mean ± SD, n = 6. CK, control group; EM, B. coagulans group; PT, probiotics treatment group; AT, antibiotic treatment group; SI, S. pullorum infection group.
The levels of D-LA and DAO in serum samples were determined to assess the intestinal permeability of the five groups. As shown in Figure 3, there were no significant differences in D-LA and DAO levels between the CK and EM groups (p > 0.05). However, the SI group had higher DAO and D-LA levels than the CK group, which reached 20.48 U/L and 76.40 nmol/L, respectively. Additionally, the PT and AT groups showed lower DAO (17.85 U/L and 17.38 U/L) and D-LA (62.82 nmol/L and 63.34 nmol/L) than the SI group (p < 0.05).
Figure 3. Effect of B. coagulans treatments on the intestinal permeability of chicks. (A) Serum levels of DAO and (B) D-LA. Differences between groups are indicated by different lowercase letters. Values are presented as the mean ± SD, n = 6. CK, control group; EM, B. coagulans group; PT, probiotics treatment group; AT, antibiotic treatment group; SI, S. pullorum infection group.
The expression levels of apoptosis-related genes (BAX, BCL2, CASP8, and CASP3) in the jejunum are shown in Figure 4. The pro-apoptotic gene CASP8 was down-regulated and the anti-apoptotic gene BCL2 was up-regulated in the EM group compared with those in the CK group (p < 0.05). The expression of BAX, CASP8, and CASP3 was up-regulated (p < 0.05), and that of BCL2 was down-regulated in the SI group (p < 0.05). Moreover, BAX, CASP8, and CASP3 were down-regulated (p < 0.05) and BCL2 was up-regulated in the PT group compared with those in the SI group (p < 0.05). Compared with the SI group, the AT group showed significant down-regulation of BAX and CASP8 (p < 0.05), while significant up-regulation of BCL2 (p < 0.05).
Figure 4. Effect of B. coagulans on cell apoptosis. (A) Relative expression of the mRNAs of CASP3, (B) CASP8, (C) BAX, and (D) BCL2 is shown. Different lowercase letters indicate differences between groups. Values are presented as the mean ± SD, n = 6. CK, control group; EM, B. coagulans group; PT, probiotics treatment group; AT, antibiotic treatment group; SI, S. pullorum infection group.
The effect of B. coagulans MF-06 on expression of proliferating cell nuclear antigen (PCNA) in jejunum is shown in Figure 5. The expression of PCNA showed no significant difference between the EM group and the CK group (p > 0.05). In contrast, the PCNA expression in the SI group decreased (p < 0.05). Moreover, the PT and AT groups had higher expression of PCNA than the SI group (p < 0.05).
Figure 5. Expression of proliferating cell nuclear antigen (PCNA) in the jejunum. (A) PCNA was detected by immunohistochemical staining and (B) expression of PCNA in the jejunum (AOD) was measured. The arrow in the figure refers to a part of the stained PCNA-positive cells. Different lowercase letters indicate differences between groups. All values are presented as the mean ± SD, n = 6. CK, control group; EM, B. coagulans group; PT, probiotics treatment group; AT, antibiotic treatment group; SI, S. pullorum infection group.
As shown in Figure 6, The EM group had higher expression of Wnt3, C-MYC, β-catenin, and LGR5 than the CK group (p < 0.05). In contrast, the SI group had lower expression of all the genes related to the Wnt/β-catenin signaling pathway (p < 0.05) compared with the CK and PT group, and lower expression of Wnt3, TCF4, C-MYC, and LGR5 (p < 0.05), but non-significant increase in the expression of β-catenin and BMI1 (p > 0.05) compared with the AT group. Immunohistochemistry (Figures 7A,B) showed that compared with that of the CK group, the expression of C-MYC protein in jejunum was higher (p < 0.05) in the EM group, but lower in the SI group (p < 0.05). Moreover, the PT and AT groups showed higher expression of the C-MYC protein than the SI group (p < 0.05).
Figure 6. Effect of B. coagulans on the Wnt/β-catenin signaling pathway. (A) Relative expression of the mRNAs of Wnt3, (B) TCF4, (C) β-catenin, (D) C-MYC, (E) LARG5, and (F) BMI1. The arrow in the figure refers to a part of the stained C-MYC-positive cells. All values are presented as the mean ± SD, n = 6. CK, control group; EM, B. coagulans group; PT, probiotics treatment group; AT, antibiotic treatment group; SI, S. pullorum infection group.
Figure 7. Effect of B. coagulans on the Wnt/β-catenin signaling pathway. (A) C-MYC was detected by immunohistochemical staining and (B) expression of C-MYC in the jejunum (AOD) was measured. Different lowercase letters indicate differences between groups. All values are presented as the mean ± SD, n = 6. CK, control group; EM, B. coagulans group; PT, probiotics treatment group; AT, antibiotic treatment group; SI, S. pullorum infection group.
We evaluated the cecal microbial richness through 16S rRNA sequencing. The results are presented in Figure 8. The petal diagram for the structure of the gut microbiota demonstrates the common and unique ASVs across different groups: there were 99 common ASVs, and the unique ASVs included 649 ASVs in CK, 531 ASVs in EM, 624 ASVs in PT, 986 ASVs in AT, and 687 ASVs in SI (Figure 8J). The α-diversity, which reflects species abundance and diversity in a single sample, showed no significant difference in the Chao, Ace, and Simpson indices (p > 0.05), but Shannon showed a distinction trend among 5 groups (p = 0.06), with a significant difference between the AT group and the CK group (p < 0.05) (Table 5). According to β-diversity results, principal component analysis (PCA) showed a distinction trend (p < 0.05), which implied that the structure of intestinal microbiota was pronounced altered by B. coagulans MF-06 supplementation.
Figure 8. Changes in the microbial community in the chick gastrointestinal tract. (A) A comparison of the distribution of the top 10 bacterial communities among different treatment groups at the phylum level. Distribution of (B) Actinobacteriota, (C) Proteobacteria, and (D) Firmicutes in the five groups. (E) A comparison of the distribution of the top 10 bacterial communities among various treatment groups at the genus level. Distribution of (F) Lachnoclostridium, (G) Shuttleworthia, (H) Unidentified Eggerthellaceae, and (I) Coriobacteriaceae bacterium CHKCI002 in the five groups. (J) Venn diagram of the distribution of ASVs in the five groups. (K) Differentially enriched taxa were identified in the five groups by LEfSe analysis. Different lowercase letters indicate differences between groups. All values are presented as the mean ± SD, n = 4. CK, control group; EM, B. coagulans group; PT, probiotics treatment group; AT, antibiotic treatment group; SI, S. pullorum infection group.
We then conducted a taxonomic analysis to compare the relative abundance at the phylum, genus, and species levels. In terms of different phyla, Actinobacteriota, Proteobacteria, and Firmicutes were predominant (Figure 8A). The EM group showed no significant differences in the relative abundance of these three phyla from the CK group (p > 0.05). The SI group exhibited 4.46 times more abundant Actinobacteriota than the CK group (p < 0.05) (Figure 8B), but no significant difference in the relative abundance of Proteobacteria and Firmicutes (p > 0.05) (Figures 8C,D). Compared with the SI group, the PT group exhibited a 0.82-fold decrease in the relative abundance of Actinobacteriota (p < 0.05) (Figure 8B), but no significant difference in Proteobacteria and Firmicutes (p > 0.05) (Figures 8C,D), while the AT group showed no significant differences in the relative abundance of all the three phyla (p > 0.05).
At the genus level, there were statistically significant differences in the relative abundance of Lachnoclostridium, Shuttleworthia, and Unidentified_Eggerthellaceae among groups (p < 0.05) (Figure 8E). The SI group had higher relative abundance of Shuttleworthia and Unidentified_Eggerthellaceae than the CK group and the PT group (p < 0.05), but no significant differences in the relative abundance of these genera from the AT groups (p > 0.05) (Figures 8F–H). At the species level, the SI group showed higher relative abundance of Coriobacteriaceae bacterium CHKCI002 than the CK and PT groups (p < 0.05), but no significant difference from the AT group (p > 0.05) (Figure 8I). Based on the linear discriminant analysis (LDA), we identified 18 species with significant differences in relative abundance across all taxonomic levels (Figure 8K). The results indicated that B. coagulans MF-06 can maintain intestinal health and mitigate S. pullorum-induced inflammation by modulating cecal microbial composition.
The results of Spearman correlation analysis for microbiome and production performance, Wnt/β-catenin signaling pathway-related genes, and intestinal barrier indices are presented in Figures 9A–C. The relative abundance of Actinobacteriota, Unidentified_Eggerthellaceae, and Coriobacteriaceae bacterium CHKCI002 was negatively correlated with ADG and the mRNA expression of Wnt3, TCF4, C-MYC, β-catenin, BMI1, LGR5, BCL2, TJP1, CLDN1 and MUC2, but positively correlated with FCR, the mRNA expression of BAX, CASP, CASP8, and DAO content.
Figure 9. Correlation analysis of growth performance, serum permeability indicators, gene expression, and microbiome in chicks. Correlation between (A) performance and microbiome, (C) serum permeability indicators, tight junction-related gene expression, and microbiome, and (B) Wnt/β-catenin-related gene expression, apoptosis-related gene expression, and microbiome in the five groups. The names of microbiota are provided on the right of the graph; and the bottom presents the growth performance indices in (A) and the names of gene or serum indicators in (B,C). The red and blue squares represent positive and negative correlations, respectively. Statistically significant correlations are represented by *p < 0.05, **p < 0.01, and ***p < 0. 001. CK, control group; EM, B. coagulans group; PT, probiotics treatment group; AT, antibiotic treatment group; SI, S. pullorum infection group.
Pullorum disease (PD) caused by S. pullorum is an avian-specific septicemic disease responsible for significant economic losses in the poultry industry (Barrow and Neto, 2011). Antibiotics can be used to control S. pullorum infection. However, prolonged use of antibiotics can lead to the emergence of antibiotic-resistant strains and disrupt the balance of the intestinal microbial ecosystem. Probiotics have been shown to maintain the stability of intestinal barrier (Liu et al., 2023). Probiotics have different types of effects and action mechanisms, such as competition with pathogenic bacteria, secretion of antimicrobial substances, and modulation of intestinal pH (Kocot et al., 2022). These probiotics are promising alternatives to antibiotics in the poultry industry (Zhou et al., 2024). However, the specific mechanisms for the effects of probiotics on the intestine of chicks infected with S. pullorum remain unclear.
In this study, we isolated B. coagulans MF-06 from the intestines of healthy adult chickens. Treatment of chicks with B. coagulans MF-06 alleviated symptoms such as drowsiness, rapid breathing, depressed mood, poor appetite, poor growth, and sticky white or pale-yellow feces excretion. These symptoms were similar to those reported in previous studies (Barrow and Neto, 2011; Chen et al., 2020; El-Sharkawy et al., 2020; Peng et al., 2022), confirming that B. coagulans MF-06 can prevent and treat infections caused by S. pullorum in chicks.
As B. coagulans MF-06 showed therapeutic effects, we evaluated the growth performance of chicks in different treatment groups. Chicks infected with S. pullorum showed a decrease in BW compared to the CK group. However, treatment with B. coagulans MF-06 and antibiotics increased BW and decreased FCR. Some studies have shown that dietary supplementation with B. coagulans can increase BW and ADG of broiler chicks (Fitzpatrick, 2013; Zhen et al., 2018). For example, It has been shown that infection with Clostridium perfringens decreased the BW (344.7 g) in broilers, whereas dietary supplementation with B. coagulans decreased FCR (1.74) and increased BW (377.2 g) (Wu et al., 2018). Our results are consistent with previous studies, suggesting that B. coagulans MF-06 supplementation improves the growth performance of chicks, probably because the bacteria secrete beneficial enzymes and growth-promoting factors (Al-Otaibi et al., 2023), which improve the digestive and absorptive capacity and reduce the colonization rate of pathogens, thus protecting the intestine (El-Moneim et al., 2020; Kai, 2021).
S. pullorum colonizes the intestinal wall of chicks, invades intestinal mucosal cells, and migrates through the bloodstream, causing systemic infection (Barrow and Neto, 2011). To determine the effect of B. coagulans on the colonization of pathogenic bacteria in chick intestine, we measured the bacterial load in the chick intestines. The results showed that B. coagulans MF-06 reduced the colonization of S. pullorum in the duodenum, jejunum, ileum, and cecum of chicks. Thus, we speculate that the B. coagulans MF-06 used in this study may decrease S. pullorum colonization in the gut, and further lower the infection rate of S. pullorum in chicks.
To evaluate the effect of B. coagulans MF-06 on S. pullorum colonization in chicks at the physiological level, we examined the intestinal physical barrier, including physical connection, cell proliferation, and apoptosis after S. pullorum infection. Intestinal epithelial cells (IECs) are important for the establishment of physical connections and preservation of the intestinal physical barrier integrity. These connections, which are involved in tight junctions (TJs), adherens junctions (AJs), and desmosomes (Buckley and Turner, 2018), can effectively prevent the leakage of substances from the intestinal lumen (Vancamelbeke and Vermeire, 2018). TJs are generally constituted by transmembrane proteins, including Claudins, occludins, peripheral membrane proteins (ZO), and regulatory proteins (Buckley and Turner, 2018). They can greatly restrict pathogen invasion from the intestinal lumen into deeper tissues, thus contributing to the defense system in the body. We found that diet supplementation with B. coagulans MF-06 enhanced the transcription level of TJP1, CLDN1, CLDN2, MUC2, and Occludin genes.
DAO, an enzyme secreted by intestinal epithelial cells, and D-LA, a metabolic product of intestinal bacteria, are released into the bloodstream when the intestinal mucosal barrier. Thus, intestinal permeability and integrity can be monitored by assessing blood levels of D-LA and DAO (Wu et al., 2022). It has been reported that S. typhimurium infection induced high serum DAO levels in chicks (Wang et al., 2023). Similarly, our results showed that the serum DAO and D-LA levels increased in chicks after being challenged with S. pullorum. Treatment of S. pullorum-infected chicks with B. coagulans MF-06 effectively reduced the D-LA and DAO levels in the serum, confirming that B. coagulans MF-06 can enhance the integrity of intestinal mucosal barrier.
To further elucidate the mechanisms underlying the effects of B. coagulans MF-06 on the intestinal barrier, we performed qRT-PCR to analyze the transcription levels of genes associated with the proliferation of the IECs. The Wnt/β-catenin signaling pathway facilitates the regeneration of the intestinal epithelium, particularly the proliferation of intestinal stem cells (ISCs) (Tong et al., 2023). The proliferation of IECs is regulated by β-catenin. LGR5 enhances Wnt/β-catenin signaling pathway, and BMI1 mediates the generation of proliferative ISCs (Liu and Chen, 2020; Li et al., 2022). When the Wnt/β-catenin signaling pathway is activated, active β-catenin will be translocated from the cytoplasm to the nucleus and interacts with T cell-specific factor/lymphoid enhancer factor, thereby enhancing the expression of target genes such as C-MYC and CF/LEF target genes (Zhang and Wang, 2020). In this study, there was a significant increase in the transcription levels of genes associated with the Wnt/β-catenin signaling pathway, including Wnt3, β-catenin, TCF4, C-MYC, LGR5, and BMI1. Our immunohistochemical analysis showed that the expression level of C-MYC was increased in the intestinal epithelium of S. pullorum-infected chicks treated with B. coagulans MF-06, further confirming that B. coagulans MF-06 inhibits S. pullorum infection by regulating the Wnt/β-catenin signaling pathway.
To preliminarily explore the effect of B. coagulans MF-06 on intestinal cell proliferation, we assessed the expression levels of intestinal PCNA, a cell proliferation marker associated with IECs and the reparative capacity of the intestinal mucosal epithelium. The results showed a significant increase in the expression of PCNA, suggesting that B. coagulans MF-06 may promote IECs proliferation within crypts, followed by migration along the crypt-villus axis (Sanden and Olsvik, 2009). An increase in PCNA level indicates an increase in cell division rate, which further contributes to the positive effect of B. coagulans MF-06 on the intestinal mucosal barrier integrity.
The apoptosis of IECs is critical for the regeneration of intestinal tissue and maintenance of normal structure. An imbalance between apoptosis and regeneration can severely impair the intestinal structure and function (Zhu et al., 2014). In eukaryotes, the BAX protein induces cell apoptosis (Westphal et al., 2011). Our qRT-PCR analysis showed increased transcription of the BAX gene in the intestinal tissues of S. pullorum-infected chicks. However, supplementation with B. coagulans MF-06 decreased the expression of BAX, indicating that B. coagulans MF-06 can alleviate cell apoptosis and maintain a balance between cell regeneration and apoptosis.
Microbiota is an integral part of the gut with important roles in nutrition, physiology, and intestinal morphology. Moreover, it is involved in the host immune defense against pathogens (Sugiharto and Ranjitkar, 2019). Some studies have shown that gut microbiota may influence the proliferation of epithelial cells. Here, we assessed the composition of the gut microbiota by high-throughput 16S rRNA sequencing. The results showed that S. pullorum increased the ASV number and altered the community structure. However, although B. coagulans MF-06 influenced the cecal microbiota structure, it led to no significant change in the α-diversity of bacterial community. At the phylum level, S. pullorum infection increased the relative abundance of Actinobacteriota in the cecum. Actinobacteriota is reported to improve feed utilization by producing extracellular enzymes, and to decompose undigested components in feed through secreting endogenous enzymes (Lee et al., 2014). In addition, Actinobacteriota is helpful for the maintenance of overall microbial structure. Due to the production of bacteriocins and the ability to convert feed into fermentable microbial biomass (Samson et al., 2020). Studies have shown that intestinal injury can result in an increased content of ethanolamine, and all kinds of non-pathogenic and pathogenic Actinobacteriota use ethanolamine as a source of carbon or nitrogen (Zeng et al., 2017). In this study, the abundance of Actinobacteriota increased in the SI group, suggesting inflammation and injury in the intestine. The effects of intestinal injury, Actinobacteriota, and ethanolamine need to be further studied. However, the PT group showed a less dramatic increase in the abundance of Actinobacteriota in the intestine than the SI group, the reduction of Actinobacteriota may be related to the fact that B. coagulans can produce core metabolites such as organic acids and amino acids, promote the metabolism and growth of beneficial bacteria in the cecum, reduce intestinal pH value, prevent the growth and proliferation of pathogenic bacteria, regulate the balance of intestinal flora, improve the intestinal environment, and maintain the integrity of intestinal epithelium (Li et al., 2021; Xiong et al., 2024). Some studies have found that heat stress impairs the antioxidant system and intestinal barrier function, resulting in a significant increase in Actinobacteria at the phylum level in the gut of broilers, while dietary Ellagic Acid significantly reduces their abundance (Yang et al., 2022), which is similar to the results of the present study. In addition, our results showed that the relative abundances of Lachnoclostridium, Shuttleworthia, and unidentified_Eggerthellaceae were dramatically increased in SI groups compared to PT group at the genus level. unidentified_Eggerthellaceae is a bacterial group that is positively correlated with inflammation, may promote intestinal inflammation, and is widely involved in the etiology of gastrointestinal diseases (Aparicio et al., 2020; Xia et al., 2023). Therefore, the addition of B. coagulans to the diet may cause competitive exclusion with pathogenic bacteria, reduce the colonization of pathogenic bacteria, and have a positive effect on intestinal health. Surprisingly, some studies have found that species of Lachnoclostridium and Shuttleworthia has produce butyrate with anti-inflammatory properties and enhance the intestinal barrier by upregulating the tight junction protein (Dai et al., 2023), which was controversial with our study. The possible reason may be due to the activation of the host immune system by S. pullorum infection, triggering an immune response. Flora with antioxidant or antimicrobial capabilities (including Lachnoclostridium) may gain a proliferative advantage to help suppress excessive growth of harmful bacteria.
Our correlation analysis showed that Actinobacteriota, Shuttleworthia, and unidentified_Eggerthellaceae had positive correlation with the DAO content in serum, but were negatively correlated with ADG, tight junction genes, and genes related to the Wnt/β-catenin signaling pathway. DAO, ADG, tight junction genes, and the Wnt/β-catenin signaling pathway are important indicators to evaluate the integrity, function, and health of the intestine. Therefore, we speculate that Actinobacteriota, Shuttleworthia and unidentified_Eggerthellaceae may have certain effects on the jejunal mucosa, but the specific mechanisms need to be further studied.
In summary, we elucidated the effects of probiotics on growth performance, intestinal mucosal barrier and gut microbiota of chicks challenged with S. pullorum. Our results demonstrated that probiotics are promising alternatives to address some challenges associated with the use of antibiotics in the poultry industry. However, this study also has some limitations. For instance, although we found that B. coagulans MF-06 can restrain S. pullorum colonization, improve the protective function of the intestinal mucosal barrier, and modulate the microbial community to prevent S. pullorum infection, the relevant metabolites produced by B. coagulans MF-06 need to be further investigated.
In conclusion, this study showed that avian autochthonous B. coagulation MF-06 can resist S. pullorum infection, mainly through the activation of the Wnt/β-catenin signaling pathways, regulation of apoptosis cycle, improvement of intestinal mucosal barrier and regulation of microbiota. A combined analysis of growth performance, intestinal integrity, and microbiota demonstrated the probiotic effect of B. coagulation MF-06. Our results will help to understand the protective mechanism of B. coagulation MF-06 against S. pullorum infection, and develop new alternative candidate strains or treatments for traditional antibiotic therapies. In addition, our study has some limitations, the present study only observed the effects of B. coagulans within 14 days, and the specific core metabolites of probiotics and their long-term effects on gut microbiota are still unclear and need to be further studied. In view of the findings in this study, future metabolomics studies or trials in larger animal populations will be conducted to provide more comprehensive information about probiotics on animal gut health.
The data presented in the study are deposited in the NCBI repository (https://www.ncbi.nlm.nih.gov/), accession number PRJNA1150955.
The animal study was approved by Institutional Animal Care and Use Committee of Yangtze University. The study was conducted in accordance with the local legislation and institutional requirements.
LM: Visualization, Writing – original draft, Writing – review & editing. GT: Conceptualization, Funding acquisition, Project administration, Writing – review & editing. YP: Resources, Writing – review & editing. XQ: Resources, Writing – review & editing. YZ: Writing – review & editing. HJW: Writing – review & editing. LY: Writing – review & editing. GZ: Visualization, Writing – review & editing. CF: Writing – review & editing. XL: Methodology, Writing – review & editing. HBW: Writing – review & editing. LT: Writing – review & editing. LJ: Visualization, Writing – review & editing.
The author(s) declare that financial support was received for the research, authorship, and/or publication of this article. The research was supported by the 4th modern agricultural industry technology system of Hubei Province, poultry and egg industry technology system (2023HBSTX4-04), Open Project Funding of the State Key Laboratory of Biocatalysis and Enzyme Engineering (SKLBEE2021022), Key laboratory of prevention and control agents for animal bacteriosis (Ministry of Agriculture and Rural Affairs) and Hubei provincial key laboratory of animal pathogenic microbiology (KLPCAAB-2022-03). This study was also partially supported by the unveiled project of “Advanced Technology Integration Demonstration Base Construction and Targeted Research” in the pilot County of Shishou (SS202310).
We thank the teachers of the School of Animal Science and Technology and the members of the Microecologics Laboratory for their assistance in this experiment, and Mavey Metabolic Biotechnology Co., Ltd. for their assistance in 16S sequencing of cecal microorganisms.
The authors declare that the research was conducted in the absence of any commercial or financial relationships that could be construed as a potential conflict of interest.
All claims expressed in this article are solely those of the authors and do not necessarily represent those of their affiliated organizations, or those of the publisher, the editors and the reviewers. Any product that may be evaluated in this article, or claim that may be made by its manufacturer, is not guaranteed or endorsed by the publisher.
The Supplementary material for this article can be found online at: https://www.frontiersin.org/articles/10.3389/fmicb.2024.1492035/full#supplementary-material
1. ^https://github.com/OpenGene/fastp
2. ^http://ccb.jhu.edu/software/FLASH/
Al-Otaibi, A. M., Abd El-Hack, M. E., Dmour, S. M., Alsowayeh, N., Khafaga, A. F., Ashour, E. A., et al. (2023). A narrative review on the beneficial impacts of probiotics on poultry: an updated knowledge. Ann. Anim. Sci. 23, 405–418. doi: 10.2478/aoas-2023-0001
Aparicio, M., Alba, C., Proctocolitis Study Group Of Cam Public Health AreaRodríguez, J. M., and Fernández, L. (2020). Microbiological and immunological markers in Milk and infant feces for common gastrointestinal disorders: a pilot study. Nutrients 12:634. doi: 10.3390/nu12030634
Attia, Y. A., Basiouni, S., Abdulsalam, N. M., Bovera, F., Aboshok, A. A., Shehata, A. A., et al. (2023). Alternative to antibiotic growth promoters: beneficial effects of Saccharomyces cerevisiae and/or Lactobacillus acidophilus supplementation on the growth performance and sustainability of broilers’ production. Front. Veter. Sci. 10:1259426. doi: 10.3389/fvets.2023.1259426
Barrow, P. A., and Neto, O. C. F. (2011). Pullorum disease and fowl typhoid—new thoughts on old diseases: a review. Avian Pathol. 40, 1–13. doi: 10.1080/03079457.2010.542575
Buckley, A., and Turner, J. R. (2018). Cell biology of tight junction barrier regulation and mucosal disease. Cold Spring Harb Perspect Biol. 10:a029314. doi: 10.1101/cshperspect.a029314
Chen, C., Li, J., Zhang, H., Xie, Y., Xiong, L., Liu, H., et al. (2020). Effects of a probiotic on the growth performance, intestinal flora, and immune function of chicks infected with Salmonella pullorum. Poult. Sci. 99, 5316–5323. doi: 10.1016/j.psj.2020.07.017
Cheng, Y., Sihua, Z., Lu, Q., Zhang, W., Wen, G., Luo, Q., et al. (2020). Evaluation of young chickens challenged with aerosolized Salmonella Pullorum. Avian Pathol. 49, 507–514. doi: 10.1080/03079457.2020.1783433
Dai, W., Cai, D., Zhou, S., Li, A., Xie, J., and Zhang, J. (2023). Uncovering a causal connection between the Lachnoclostridium genus in fecal microbiota and non-alcoholic fatty liver disease: a two-sample Mendelian randomization analysis. Front. Microbiol. 14:1276790. doi: 10.3389/fmicb.2023.1276790
Deng, Z., Han, D., Wang, Y., Wang, Q., Yan, X., Wang, S., et al. (2021). Lactobacillus casei protects intestinal mucosa from damage in chicks caused by Salmonella pullorum via regulating immunity and the Wnt signaling pathway and maintaining the abundance of gut microbiota. Poult. Sci. 100:101283. doi: 10.1016/j.psj.2021.101283
Ding, X., Tang, R., Zhao, J., Xu, Y., Fu, A., and Zhan, X. (2024). Lactobacillus reuteri alleviates LPS-induced intestinal mucosal damage by stimulating the expansion of intestinal stem cells via activation of the Wnt/β-catenin signaling pathway in broilers. Poult. Sci. 103:104072. doi: 10.1016/j.psj.2024.104072
El-Moneim, A. E.-M. E. A., El-Wardany, I., Abu-Taleb, A. M., Wakwak, M. M., Ebeid, T. A., and Saleh, A. A. (2020). Assessment of in Ovo Administration of Bifidobacterium bifidum and Bifidobacterium longum on performance, Ileal Histomorphometry, blood hematological, and biochemical parameters of broilers. Probiotics Antimicrobial Proteins 12, 439–450. doi: 10.1007/s12602-019-09549-2
El-Sharkawy, H., Tahoun, A., Rizk, A. M., Suzuki, T., Elmonir, W., Nassef, E., et al. (2020). Evaluation of Bifidobacteria and Lactobacillus probiotics as alternative therapy for Salmonella typhimurium infection in broiler chickens. Animals 10:1023. doi: 10.3390/ani10061023
Fitzpatrick, L. R. (2013). Probiotics for the treatment of Clostridium difficile associated disease. World J. Gastrointest. Pathophysiol. 4, 47–52. doi: 10.4291/wjgp.v4.i3.47
Guo, S., Xi, Y., Xia, Y., Wu, T., Zhao, D., Zhang, Z., et al. (2021). Dietary Lactobacillus fermentum and Bacillus coagulans supplementation modulates intestinal immunity and microbiota of broiler chickens challenged by Clostridium perfringens. Front. Vet. Sci. 8:680742. doi: 10.3389/fvets.2021.680742
Hao, J., Liu, C., Gu, Z., Yang, X., Lan, X., and Guo, X. (2024). Dysregulation of Wnt/β-catenin signaling contributes to intestinal inflammation through regulation of group 3 innate lymphoid cells. Nat. Commun. 15:2820. doi: 10.1038/s41467-024-45616-1
Hou, J., Lian, L., Lu, L., Gu, T., Zeng, T., Chen, L., et al. (2023). Effects of dietary Bacillus coagulans and Tributyrin on growth performance, serum antioxidants, intestinal morphology, and Cecal microbiota of growing yellow-feathered broilers. Animals 13:3534. doi: 10.3390/ani13223534
Hu, R.-G., Yang, B.-T., Zheng, Z.-Y., Liang, Z.-L., Kang, Y.-H., and Cong, W. (2024). Improvement of non-specific immunity, intestinal health and microbiota of crucian carp (Carassius auratus) juvenile with dietary supplementation of Bacillus coagulans BC1. Aquaculture 580:740327. doi: 10.1016/j.aquaculture.2023.740327
Hung, A. T., Lin, S.-Y., Yang, T.-Y., Chou, C.-K., Liu, H.-C., Lu, J.-J., et al. (2012). Effects of Bacillus coagulans ATCC 7050 on growth performance, intestinal morphology, and microflora composition in broiler chickens. Anim. Prod. Sci. 52, 874–879. doi: 10.1071/AN11332
Jiali, L., Pupu, Y., Yana, L., Jie, Y., Yongxi, H., Ruonan, B., et al. (2024). Gut microbiota and serum metabolome reveal the mechanism by which TCM polysaccharides alleviate salpingitis in laying hens challenged by bacteria. Poult. Sci. 103:103288. doi: 10.1016/j.psj.2023.103288
Jiaqi, H., Lu, L., Ketong, C., Peiyong, L., Guijuan, H., and Shuhong, S. (2022). Salmonella phage CKT1 significantly relieves the body weight loss of chicks by normalizing the abnormal intestinal microbiome caused by hypervirulent Salmonella pullorum. Poult. Sci. 101:101668. doi: 10.1016/j.psj.2021.101668
Kai, Y. (2021). Intestinal villus structure contributes to even shedding of epithelial cells. Biophys. J. 120, 699–710. doi: 10.1016/j.bpj.2021.01.003
Kocot, A. M., Jarocka-Cyrta, E., and Drabińska, N. (2022). Overview of the importance of biotics in gut barrier integrity. Int. J. Mol. Sci. 23:2896. doi: 10.3390/ijms23052896
Lee, J. P., Eric, D., Patrick, R., Martina, H., and Neufeld, J. D. (2014). Recovering glycoside hydrolase genes from active tundra cellulolytic bacteria. Can. J. Microbiol. 60, 469–476. doi: 10.1139/cjm-2014-0193
Lee, Y.-S., Kim, T.-Y., Kim, Y., Lee, S.-H., Kim, S., Kang, S. W., et al. (2018). Microbiota-derived lactate accelerates intestinal stem-cell-mediated epithelial development. Cell Host Microbe 24, 833–846.e6. doi: 10.1016/j.chom.2018.11.002
Li, Y., Chen, J., Sun, D., Liu, J., Wang, Z., and Li, A. (2022). Lactobacillus GG regulates the Wnt/β-catenin pathway to reinforce intestinal barrier function and alleviate necrotizing enterocolitis. J. Funct. Foods 97:105243. doi: 10.1016/j.jff.2022.105243
Li, W., Zeng, Z., Zhou, D., Wang, G., Wang, Z., Li, Y., et al. (2024). Effect of oral administration of microcin Y on growth performance, intestinal barrier function and gut microbiota of chicks challenged with Salmonella pullorum. Vet. Res. 55:66. doi: 10.1186/s13567-024-01321-x
Li, H.-Y., Zhou, D.-D., Gan, R.-Y., Huang, S.-Y., Zhao, C.-N., Shang, A., et al. (2021). Effects and mechanisms of probiotics, prebiotics, Synbiotics, and Postbiotics on metabolic diseases targeting gut microbiota: a narrative review. Nutrients 13:3211. doi: 10.3390/nu13093211
Liangyu, Y., Yuanhong, C., Qian, B., Xi, C., Yunteng, S., Ronghai, W., et al. (2022). Protective effect of Bifidobacterium lactis JYBR-190 on intestinal mucosal damage in chicks infected with Salmonella pullorum. Front. Vet. Sci. 9:879805. doi: 10.3389/fvets.2022.879805
Liu, Y., and Chen, Y.-G. (2020). Intestinal epithelial plasticity and regeneration via cell dedifferentiation. Cell Regeneration 9:14. doi: 10.1186/s13619-020-00053-5
Liu, C., Qi, X., Li, D., Zhao, L., Li, Q., Mao, K., et al. (2023). Limosilactobacillus fermentum HF06-derived paraprobiotic and postbiotic alleviate intestinal barrier damage and gut microbiota disruption in mice with ulcerative colitis. J. Sci. Food Agric. 104, 1702–1712. doi: 10.1002/jsfa.13057
Liu, C., Radebe, S. M., Zhang, H., Jia, J., Xie, S., Shi, M., et al. (2022). Effect of Bacillus coagulans on maintaining the integrity intestinal mucosal barrier in broilers. Vet. Microbiol. 266:109357. doi: 10.1016/j.vetmic.2022.109357
Livak, K. J., and Schmittgen, T. D. (2001). Analysis of relative gene expression data using real-time quantitative PCR and the 2−ΔΔCT method. Methods 25, 402–408. doi: 10.1006/meth.2001.1262
Martin, M. J., Thottathil, S. E., and Newman, T. B. (2015). Antibiotics overuse in animal agriculture: a call to action for health care providers. Am. J. Public Health 105, 2409–2410. doi: 10.2105/AJPH.2015.302870
Peng, X., Ed-Dra, A., Song, Y., Elbediwi, M., Nambiar, R. B., Zhou, X., et al. (2022). Lacticaseibacillus rhamnosus alleviates intestinal inflammation and promotes microbiota-mediated protection against Salmonella fatal infections. Front. Immunol. 13, 1–17. doi: 10.3389/fimmu.2022.973224
Samson, O., Alyssa, K., Janice, M., Ibeagha-Awemu, E. M., and Adewole, D. I. (2020). Bacillus subtilis delivery route: effect on growth performance, intestinal morphology, cecal short-chain fatty acid concentration and cecal microbiota in broiler chickens. Poult. Sci. 100, –100809. doi: 10.1016/j.psj.2020.10.063
Sanden, M., and Olsvik, P. A. (2009). Intestinal cellular localization of PCNA protein and CYP1A mRNA in Atlantic salmon Salmo salar L. exposed to a model toxicant. BMC Physiol. 9, 3–13. doi: 10.1186/1472-6793-9-3
Shen, J., Liu, T., Qian, Y., Yan, S., Liu, Z., and Jia, F. (2024). Therapeutic effect of probiotic-fermented herbal blend as antibiotic alternative on salmonellosis by multi-drug resistant Salmonella pullorum. Food Biosci. 57:103585. doi: 10.1016/j.fbio.2024.103585
Shen, X., Zhang, A., Gu, J., Zhao, R., Pan, X., Dai, Y., et al. (2022). Evaluating Salmonella pullorum dissemination and shedding patterns and antibody production in infected chickens. BMC Vet. Res. 18:240. doi: 10.1186/s12917-022-03335-z
Shivaprasad, H. L. (2000). Fowl typhoid and pullorum disease. Rev. Sci. Tech. 19, 405–424. doi: 10.20506/rst.19.2.1222
Song, Y., Wang, F., Liu, Y., Song, Y., Zhang, L., Zhang, F., et al. (2020). Occurrence and characterization of Salmonella isolated from chicken breeder flocks in nine Chinese provinces. Front. Vet. Sci. 7:479. doi: 10.3389/fvets.2020.00479
Sugiharto, S., and Ranjitkar, S. (2019). Recent advances in fermented feeds towards improved broiler chicken performance, gastrointestinal tract microecology and immune responses: a review. Anim. Nutr. 5, 1–10. doi: 10.1016/j.aninu.2018.11.001
Tong, D. Q., Lu, Z. J., Zeng, N., Wang, X. Q., Yan, H. C., and Gao, C. Q. (2023). Dietary supplementation with probiotics increases growth performance, improves the intestinal mucosal barrier and activates the Wnt/β-catenin pathway activity in chicks. J. Sci. Food Agric. 103, 4649–4659. doi: 10.1002/jsfa.12562
Vancamelbeke, M., and Vermeire, S. (2018). The intestinal barrier: a fundamental role in health and disease. Expert Rev. Gastroenterol. Hepatol. 11, 821–834. doi: 10.1080/17474124.2017.1343143
Wang, J., Ishfaq, M., Miao, Y., Liu, Z., Hao, M., Wang, C., et al. (2022). Dietary administration of Bacillus subtilis KC1 improves growth performance, immune response, heat stress tolerance, and disease resistance of broiler chickens. Poult. Sci. 101:101693. doi: 10.1016/j.psj.2021.101693
Wang, Y., Li, J., Xie, Y., Zhang, H., Jin, J., Xiong, L., et al. (2021b). Effects of a probiotic-fermented herbal blend on the growth performance, intestinal flora and immune function of chicks infected with Salmonella pullorum. Poult. Sci. 100:101196. doi: 10.1016/j.psj.2021.101196
Wang, W., Ou, J., Ye, H., Cao, Q., Zhang, C., Dong, Z., et al. (2023). Supplemental N-acyl homoserine lactonase alleviates intestinal disruption and improves gut microbiota in broilers challenged by Salmonella Typhimurium. J. Anim. Sci. Biotechnol. 14:7. doi: 10.1186/s40104-022-00801-4
Wang, Y., Yan, X., Han, D., Liu, Y., Song, W., Tong, T., et al. (2019). Lactobacillus casei DBN023 protects against jejunal mucosal injury in chicks infected with Salmonella pullorum CMCC-533. Res. Vet. Sci. 127, 33–41. doi: 10.1016/j.rvsc.2019.09.010
Wang, X., Zhang, P., and Zhang, X. (2021a). Probiotics regulate gut microbiota: an effective method to improve immunity. Molecules 26:6076. doi: 10.3390/molecules26196076
Westphal, D., Dewson, G., Czabotar, P. E., and Kluck, R. M. (2011). Molecular biology of Bax and Bak activation and action. Biochimica et Biophysica Acta (BBA) - molecular. Cell Res. 1813, 521–531. doi: 10.1016/j.bbamcr.2010.12.019
Wu, Y., Shao, Y., Song, B., Zhen, W., Wang, Z., Guo, Y., et al. (2018). Effects of Bacillus coagulans supplementation on the growth performance and gut health of broiler chickens with Clostridium perfringens-induced necrotic enteritis. J. Anim. Sci. Biotechnol. 9:9. doi: 10.1186/s40104-017-0220-2
Wu, Y., Wang, W., Kim, I. H., and Yang, Y. (2022). Dietary hydrolyzed wheat gluten supplementation ameliorated intestinal barrier dysfunctions of broilers challenged with Escherichia coli O78. Poult. Sci. 101:101615. doi: 10.1016/j.psj.2021.101615
Xia, L., Li, R., Tao, T., Zhong, R., Du, H., Liao, Z., et al. (2023). Therapeutic potential of Litsea cubeba essential oil in modulating inflammation and the gut microbiome. Front. Microbiol. 14, 1–12. doi: 10.3389/fmicb.2023.1233934
Xiong, S., Zhang, K., Wang, J., Bai, S., Zeng, Q., Liu, Y., et al. (2024). Effects of xylo-oligosaccharide supplementation on the production performance, intestinal morphology, cecal short-chain fatty acid levels, and gut microbiota of laying hens. Poult. Sci. 103:104371. doi: 10.1016/j.psj.2024.104371
Yang, T., Liu, B., Wang, Y., Huang, X., Yan, Z., Jiang, Q., et al. (2022). Ellagic acid improves antioxidant capacity and intestinal barrier function of heat-stressed broilers via regulating gut microbiota. Animals 12:1180. doi: 10.3390/ani12091180
Zeng, M. Y., Inohara, N., and Nuñez, G. (2017). Mechanisms of inflammation-driven bacterial dysbiosis in the gut. Mucosal Immunol. 10, 18–26. doi: 10.1038/mi.2016.75
Zhang, Y., Tian, X., Dong, Y., Li, R., Shen, M., Yi, D., et al. (2023). Bacillus coagulans prevents the decline in average daily feed intake in young piglets infected with enterotoxigenic Escherichia coli K88 by reducing intestinal injury and regulating the gut microbiota. Front. Cell. Infect. Microbiol. 13:1284166. doi: 10.3389/fcimb.2023.1284166
Zhang, Y., and Wang, X. (2020). Targeting the Wnt/β-catenin signaling pathway in cancer. J. Hematol. Oncol. 13:165. doi: 10.1186/s13045-020-00990-3
Zhang, B., Zhang, H., Yu, Y., Zhang, R., Wu, Y., Yue, M., et al. (2021). Effects of Bacillus Coagulans on growth performance, antioxidant capacity, immunity function, and gut health in broilers. Poult. Sci. 100:101168. doi: 10.1016/j.psj.2021.101168
Zhen, W., Shao, Y., Gong, X., Wu, Y., Geng, Y., Wang, Z., et al. (2018). Effect of dietary Bacillus coagulans supplementation on growth performance and immune responses of broiler chickens challenged by Salmonella enteritidis. Poult. Sci. 97, 2654–2666. doi: 10.3382/ps/pey119
Zhou, L., Abouelezz, K., Momenah, M. A., Bajaber, M. A., Baazaoui, N., Taha, T. F., et al. (2024). Dietary Paenibacillus polymyxa AM20 as a new probiotic: improving effects on IR broiler growth performance, hepatosomatic index, thyroid hormones, lipid profile, immune response, antioxidant parameters, and caecal microorganisms. Poult. Sci. 103:103239. doi: 10.1016/j.psj.2023.103239
Zhou, Y., Zeng, Z., Xu, Y., Ying, J., Wang, B., Majeed, M., et al. (2020). Application of Bacillus coagulans in animal husbandry and its underlying mechanisms. Animals 10:454. doi: 10.3390/ani10030454
Zhu, L. H., Xu, J. X., Zhu, S. W., Cai, X., Yang, S. F., Chen, X. L., et al. (2014). Gene expression profiling analysis reveals weaning-induced cell cycle arrest and apoptosis in the small intestine of pigs. J. Anim. Sci. 92, 996–1006. doi: 10.2527/jas.2013-7551
Keywords: Bacillus coagulans , Salmonella pullorum , chick, intestinal mucosal barrier, Wnt/β-catenin pathway, gut microbiota
Citation: Ma L, Tian G, Pu Y, Qin X, Zhang Y, Wang H, You L, Zhang G, Fang C, Liang X, Wei H, Tan L and Jiang L (2024) Bacillus coagulans MF-06 alleviates intestinal mucosal barrier from damage in chicks infected with Salmonella pullorum via activating the Wnt/β-catenin pathway. Front. Microbiol. 15:1492035. doi: 10.3389/fmicb.2024.1492035
Received: 06 September 2024; Accepted: 13 November 2024;
Published: 29 November 2024.
Edited by:
Juan D. Latorre, University of Arkansas, United StatesReviewed by:
Doaa Ibrahim, Zagazig University, EgyptCopyright © 2024 Ma, Tian, Pu, Qin, Zhang, Wang, You, Zhang, Fang, Liang, Wei, Tan and Jiang. This is an open-access article distributed under the terms of the Creative Commons Attribution License (CC BY). The use, distribution or reproduction in other forums is permitted, provided the original author(s) and the copyright owner(s) are credited and that the original publication in this journal is cited, in accordance with accepted academic practice. No use, distribution or reproduction is permitted which does not comply with these terms.
*Correspondence: Guangming Tian, Zy50aWFuQHlhbmd0emV1LmVkdS5jbg==; Lei You, NDUzNjc4NzVAcXEuY29t
†These authors have contributed equally to this work
Disclaimer: All claims expressed in this article are solely those of the authors and do not necessarily represent those of their affiliated organizations, or those of the publisher, the editors and the reviewers. Any product that may be evaluated in this article or claim that may be made by its manufacturer is not guaranteed or endorsed by the publisher.
Research integrity at Frontiers
Learn more about the work of our research integrity team to safeguard the quality of each article we publish.