- Department of Microbiological Diagnostics and Infectious Immunology, Medical University of Bialystok, Bialystok, Poland
Enterobacterales (especially carbapenem-resistant) are considered an urgent threat to public health. The available antibiotic therapy is limited due to the increase of multidrug-resistant (MDR) strains. Tigecycline, a minocycline derivative, has emerged as a potential key agent in the treatment of MDR isolates. The aim of the study was to evaluate the synergistic effect of tigecycline in combination with nine antimicrobial agents—ceftazidime/avibactam, colistin, ertapenem, gentamicin, imipenem, levofloxacin, meropenem/vaborbactam, polymyxin B, and rifampicin. Eighty clinical Enterobacter cloacae strains were obtained from patients of two University Hospitals in Bialystok, Poland. The E-test method was used to determine synergistic interactions. Among all combinations, synergy was reported in 61% of cases, addition in 32%, and indifference in 7%. The highest synergy rates were observed in tigecycline combinations with: ceftazidime/avibactam (60/80; 75%), imipenem (60/80; 75%), polymyxin B (55/80; 68.75%) and rifampicin (55/80; 68.75%), while the lowest synergy rate was noted in tigecycline-levofloxacin (26/80; 32.5%). The tigecycline-gentamicin showed the highest rate of indifference; antagonism, was not observed in any combination. In conclusion, tigecycline appears more suitable for use in combination therapy rather than as monotherapy and can be effectively paired with various antimicrobial agents against MDR E. cloacae. Further research will be necessary to confirm these results.
Introduction
Enterobacter cloacae is a gram-negative pathogen causing nosocomial infections and outbreaks in hospital settings. Infections can affect immunocompromised patients, such as neonates and premature infants; patients with chronic diseases, such as diabetes mellitus; and patients who are undergoing immunosuppressive therapy, such as injured or burned patients. Therefore, extended hospitalization in intensive care units (ICU) (>14 days) can also be an important risk factor (Bazaid et al., 2022; Qureshi et al., 2011). Gram-negative bacteria such as E. cloacae can develop multiple mechanisms of antibiotic resistance, including efflux pumps, pump regulators, enzymatic inactivation [via tet(X) genes], or heteroresistance (Ruppé et al., 2015; Zhong et al., 2014; Stojowska-Swedrzyńska et al., 2021). The phenomenon of efflux pumps can lead to development of the MDR phenotype in bacterial strains (Li et al., 2019). Interestingly, the number of MDR strains is still increasing and is a major threat to public health (Catalano et al., 2022).
Tigecycline (a 9-t-butyl glycol amide derivative of minocycline) is becoming a key therapeutic option in the development of multidrug resistance. Multidrug resistance is defined as non-susceptibility to ≥1 agent in ≥3 antimicrobial categories (Cosentino et al., 2023). The mechanism of action is associated with inhibition of bacterial protein translation toward bacterial ribosome 30S subunit (Yaghoubi et al., 2022). Tigecycline exhibits a broad spectrum of antimicrobial action including gram-positive pathogens (Staphylococcus aureus, methicillin-resistant S. aureus, Streptococcus pneumoniae, Enterococcus spp., vancomycin-resistant strains), gram-negative microorganisms [Enterobacterales, extended-spectrum-beta-lactamase (ESBL) producers and carbapenem-resistant (CRE) strains], anaerobes, and atypical microorganisms (Yaghoubi et al., 2022). Tigecycline is used for the treatment of complicated abdominal infections, complicated skin infections, and community-acquired pneumonia as a monotherapy (Yaghoubi et al., 2022). Due to the fact that tigecycline can overcome major mechanisms of resistance, tigecycline-resistant strains are still developing (Goodarzi et al., 2021). Combination therapy may be a solution when resistance involves broad-spectrum antibiotics.
In the era of multidrug resistance, studying antimicrobial combinations in vitro is becoming increasingly important. Interestingly physicians prefer combination therapy over monotherapy, due to in vitro experiments showing synergistic effects of combinations of various antibiotics (Tumbarello et al., 2018). Moreover, combination therapy exhibited lower mortality rates vs. monotherapy, especially in bloodstream infections and carbapenemase-producing Enterobacterales (CPE) (Schmid et al., 2019). Enterobacterales is a large group of gram-negative organisms including E. cloacae. Commonly used antibiotic combinations against Enterobacterales include tigecycline with polymyxin, tigecycline with aminoglycoside, and tigecycline with rifampicin or fosfomycin (Papst et al., 2018).
In vitro synergy testing can be assessed with different methodologies: the checkerboard method, time-kill assays (TKA), the multiple-combination bactericidal test (MCBT), and the E-test method (Doern, 2014). The checkerboard method evaluates the effectiveness of antimicrobial combinations tested in serial 2-fold dilutions at clinically relevant concentrations, typically including antibiotics from different classes. Limitations of that method include the requirement for a large amount of reagents and resources to evaluate different combinations. Moreover, only two antimicrobials can be tested simultaneously; combinations of three or four antimicrobial agents are not possible (Saiman, 2007). MCBT is preferred to test combinations of more than two antibiotics. The concentration used in MCBT is determined by what can be assessed in the patient's serum. Unlike checkerboard synergy testing, MCBT only evaluates fixed concentrations (Aaron et al., 2000). TKAs use the principle of MCBT by assessing activity over 48 h instead of a single point. Unlike MCBT, TKA evaluates the rate of killing, providing more a relevant outcome for patients (Doern, 2014). E-test methods are based on the diffusion of a continuous concentration gradient of antibiotic from an impregnated strip on a solid agar. E-tests are placed on an agar medium inoculated with the tested microorganism and incubated overnight. Then minimum inhibitory concentration (MIC) is determined by the point, where the no-growth zone touches the strip. There are two modifications of the E-test method. In the first modification, two strips are placed perpendicularly, intersecting at the MIC for each antimicrobial when tested individually. The second modification involves placing the first strip with antibiotic on the agar inoculated with the tested microorganism. After 60 min, the strip is removed and the second strip is placed in the same position (Doern, 2014; Lewis et al., 2002).
The aim of the study was to assess the synergistic effect of tigecycline in vitro combined with colistin, ceftazidime/avibactam, ertapenem, gentamicin, imipenem, levofloxacin, meropenem/vaborbactam, polymyxin B, and rifampicin among clinical E. cloacae strains.
Materials and methods
Clinical strains, media preparation, and antimicrobial agents
Eighty non-duplicate E. cloacae strains collected from patients of two hospitals—University Clinical Hospital and University Children's Clinical Hospital in Bialystok, Poland, between 2012 and 2023 were analyzed in this study. The origins of the strains and their sequence types (ST) and types of clinical materials are collected in Table 1. All isolates were preserved at −80°C in Tryptone Soya Broth (TSB) with 30% glycerol until further research. For biochemical identification of isolates and initial determination of the MIC, the VITEK 2® system (bioMérieux SSC, France) was used. Strains were cultured on previously prepared MacConkey agar (OXOID LTD., Basingstoke, UK), a selective medium to culture gram-negative microorganisms. Determination of MIC for each antimicrobial agent was done on Mueller-Hinton agar (OXOID LTD., Basingstoke, UK). All E-tests (ceftazidime/avibactam, colistin, ertapenem, gentamicin, imipenem, levofloxacin, meropenem/vaborbactam, polymyxin B, rifampicin, tigecycline) were purchased from Liofilchem (Liofilchem Inc., Waltham, MA, USA).
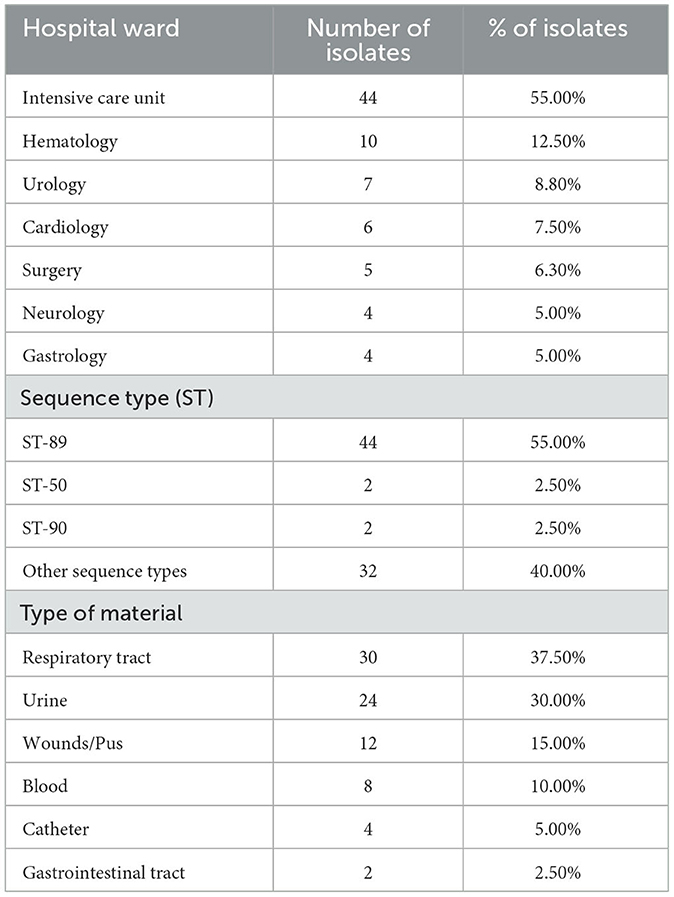
Table 1. The origin of strains examined in the study, their sequence types and types of clinical material from which the strains originated.
Antimicrobial susceptibility testing (MIC determination)
The MIC of each antibiotic was assessed using the E-test method according to the manufacturer's instructions. To perform AST, the bacterial inoculum was prepared to a density of 0.5 on the McFarland scale measured by the turbidimetric method. Strains were then plated onto Mueller-Hinton agar and E-test strips were placed on the medium. Plates were incubated at 35°C for 18–20 h. Interpretation of AST was performed according to guidelines of the European Committee on Antimicrobial Susceptibility Testing (EUCAST 2024, version 14.0) (The European Society of Clinical Microbiology Infectious Diseases, 2024). In order to maintain consistency and ensure an appropriate methodology in our study, we decided to determine the MIC values for colistin and its combinations with tigecycline using E-test method, despite EUCAST and CLSI recommendations for using the microdilution method. This decision was made after reviewing the literature, which indicated a high level of agreement between the microdilution and E-test methods. The concordance between the two methods ranged from 86 to 98% (García-Meniño et al., 2020; Arroyo et al., 2005; Reese et al., 2018).
AST was performed using the E-test MIC:MIC cross-formation method described by White et al. (1996). E-test strips were placed on the Mueller-Hinton agar in a cross formation, intersecting at a 90° angle at their MICs for the tested strain. Plates were incubated at 35–37°C for 18–20 h. After this time the zones of inhibition were read as described previously (Doern, 2014). The principle of method is illustrated in Figure 1. Moreover the fractional inhibitory concentration (FIC) index was calculated for each antibiotic in each combination. The formula to calculate the FIC index is as follows: FIC INDEX = (MIC of drug A in combination/MIC of drug A alone) + (MIC of drug B in combination/MIC of drug B alone).Synergy was defined as FIC index of ≤ 0.5; additive effect was defined as FIC index >0.5 to ≤ 1.0; indifference was defined as FIC index >1.0 to ≤ 4.0; and antagonism was defined as FIC index > 4.0 (Doern, 2014).
Detection of AmpC β-lactamases and New Delhi metallo-β-lactamases
All tested strains were analyzed for the presence of AmpC β-lactamases and New Delhi metallo-β-lactamases. The presence of AmpC was confirmed using cloxacillin-supplemented agar with an evaluation of the differences in the inhibition zones between cefotaxime and imipenem discs. New Delhi metallo-β-lactamases were detected using O.K.N.V.I. RESIST-5 -in vitro rapid diagnostic tests for detection in bacterial culture. Tests were purchased from Coris BioConcept (Coris BioConcept, Gembloux, Belgium).
Basic statistical tools
Basic statistical tools (such as median, mean, minimum, maximum and standard deviation) were used to calculate MIC and FIC values for antibiotics alone and in combination, as well as the median and mean FIC values to obtain a more complete picture of the results and a better understanding of its characteristics. STATA 17 (StataCorp, 2021) was used to assess these data.
Results and discussion
Eighty isolates were examined in vitro, among which thirty-six (45%) were found to be susceptible to tigecycline. MICs for tigecycline ranged from 0.19 to 8 mg/L. Among the eighty isolates, forty-four of them were non susceptible to tigecycline (55%). For certain bacterial strains the MIC values exceeded the maximum value of the E-test strip. All examined MIC ranges for antimicrobial agents are presented in Table 2. During AST the phenomenon of heteroresistance was observed (subpopulation of bacterial cells exhibited a higher MIC value than the majority of the population). In that case higher MIC values were recorded. Sixty strains were chromosomal AmpC β-lactamases hyperproducers, and two of the tested isolates were New Delhi metallo-β-lactamase (NDM)-producing bacteria.
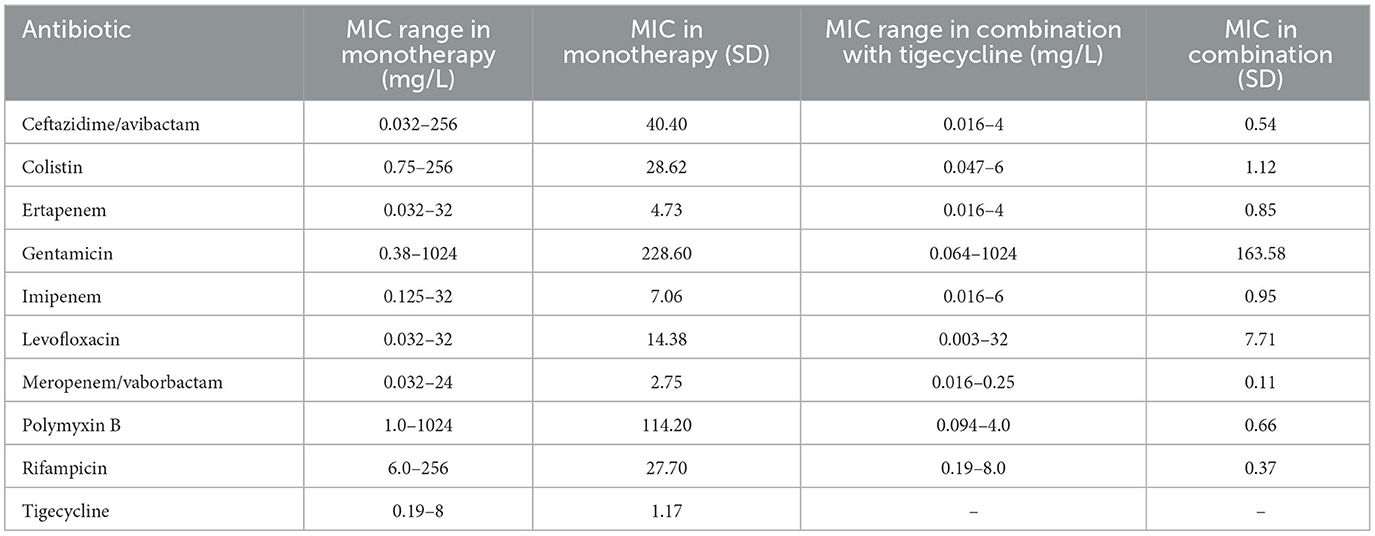
Table 2. The MIC ranges of all antimicrobial agents in monotherapy and in combination with tigecycline and their standard deviations.
Among all nine combinations tested against eighty bacterial strains (total 720 samples for analysis) of antimicrobial agents used, synergy was observed in 440 samples (61%), addition in 230 cases (32%), and indifference in fifty cases (7%). Antagonism was not observed during research. The exact MIC values of antibiotics alone, MIC values in combination, and FICI values are presented in Supplementary Tables S1–S4. In tigecycline-ceftazidime/avibactam, 60/80 (75%) strains exhibited synergy. In tigecycline-colistin there were 52/80 (65%) synergistic strains. In tigecycline-ertapenem, 54/80 (67.5%) isolates exhibited synergy, while in tigecycline-gentamicin there were only 35/80 (43.75%) synergistic strains. Synergy was observed in 60/80 (75%) isolates in tigecycline-imipenem and 26/80 (32.50%) in tigecycline-levofloxacin. In tigecycline-meropenem/vaborbactam there were 43/80 (53.75%) synergistic strains, while tigecycline-polymyxin B exhibited 55/80 (68.75%) synergistic strains. In tigecycline-rifampicin there were 55/80 (68.75%) strains exhibiting synergy. Among nine combinations with tigecycline, the highest synergy rate was observed in tigecycline with imipenem (75%; 60/80) and tigecycline with ceftazidime/avibactam (75%; 60/80). The lowest synergy rate was observed in combination of tigecycline with levofloxacin (32.5%; 26/80). The highest rate of additive interaction was noted in tigecycline with levofloxacin (53.75%; 43/80), while the lowest was in tigecycline-imipenem and tigecycline-ceftazidime/avibactam (23.75%; 19/80). The highest indifference rate was observed in the tigecycline-gentamicin combination (18.75% 15/80), and the lowest rate in tigecycline-imipenem and tigecycline-ceftazidime/avibactam (1.25%; 1/80). All rates are presented in Figure 2. The most significant MIC reduction was noted in the levofloxacin combination; the tested strain's MIC was reduced from 32 mg/L in monotherapy to 0.064 mg/L in combination with tigecycline (500-fold reduction). Among all antibiotic combinations tested, there were fifteen cases where there was no reduction of MIC values in the combination compared to monotherapy. There were only two cases when MIC values in combination were higher compared to monotherapy. The most significant reduction in the average MIC value for tigecycline was observed in combination with ceftazidime/avibactam (7-fold reduction), whereas the poorest reduction was noted in tigecycline-gentamicin (3-fold reduction). The mean FIC index of all antibiotics tested was 0.5, while mean median was 0.44. A higher standard deviations in monotherapy indicates greater variability in the results. Lower standard deviations in combinations with tigecycline may suggest a more consistent and potentially more effective response to antibiotics than in monotherapy. All means, medians and standard deviations are presented in Table 3. Some of the resistant strains became susceptible during combination therapy with tigecycline. Among these, the highest amount of strains was observed in the tigecycline combinations with imipenem, meropenem/vaborbactam and polymyxin B (25/28, 11/11, 35/39, respectively). The lowest percentage of susceptible strains was observed in the combinations of gentamicin-tigecycline and levofloxacin-tigecycline (7/61, 6/59, respectively). All tested combinations and percentage rates are listed in Table 4.
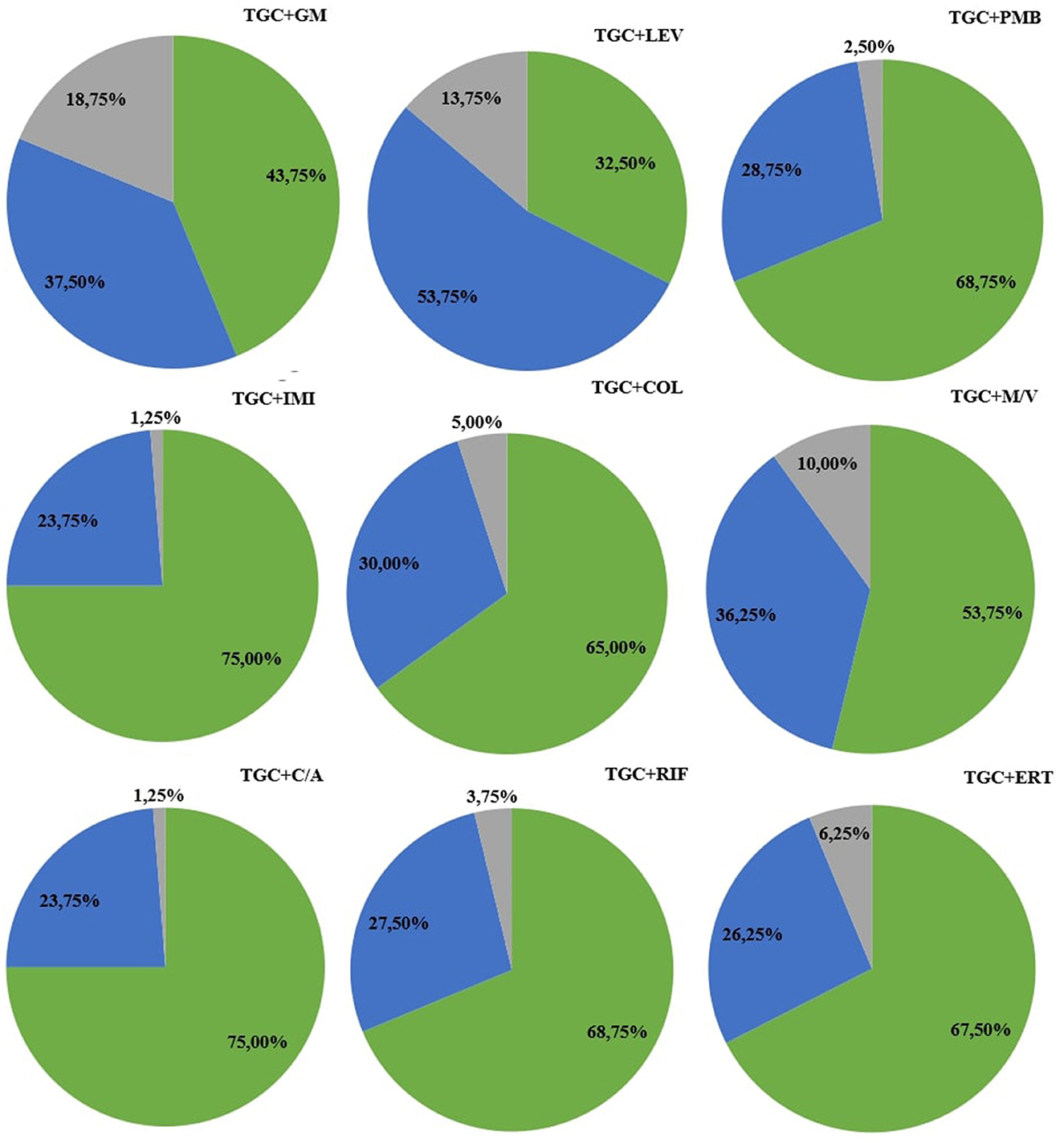
Figure 2. Percentage rates of antimicrobial synergy testing in combination with tigecycline. The green color represents synergy, blue indicates an additive effect, and gray represents indifference. Antimicrobial agents' abbreviations: C/A, ceftazidime/avibactam; COL, colistin; ERT, ertapenem; GM, gentamicin; IMI, imipenem; LEV, levofloxacin; M/V, meropenem/vaborbactam; PB, polymyxin B; RIF, rifampicin; TGC, tigecycline.
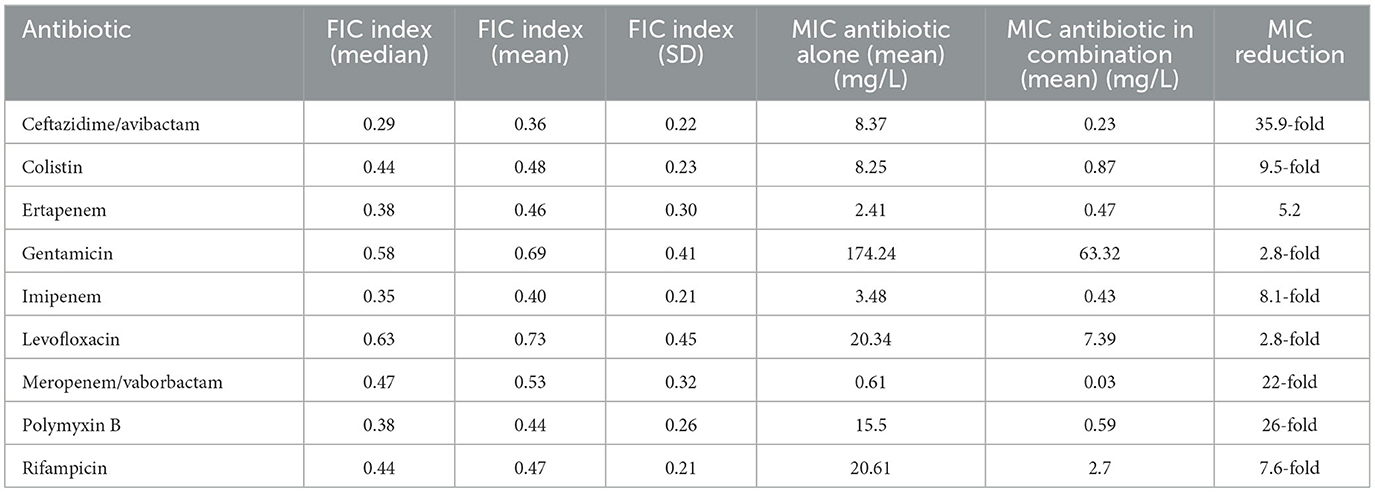
Table 3. The FIC median, mean, standard deviation and MIC means with MIC reduction of all tested antimicrobial agents.
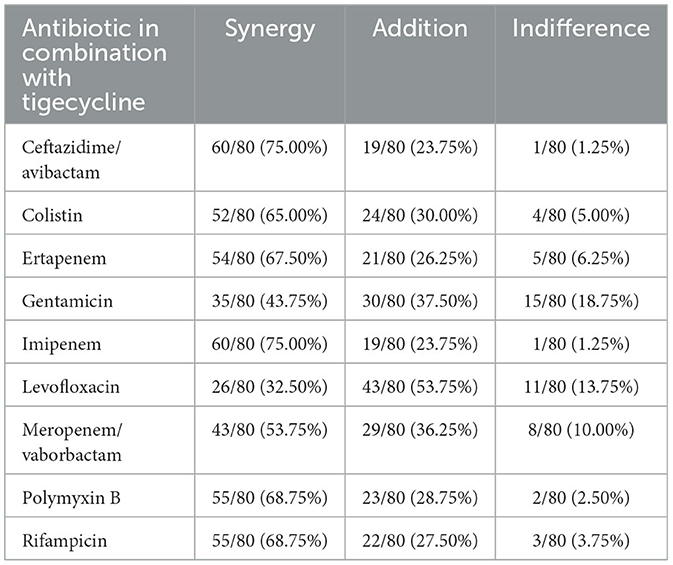
Table 4. The synergy, addition, and indifference rates of isolated strains in all combinations with tigecycline.
In the edge of post-antibiotic era combination therapy is becoming increasingly important. The TKA is regarded as the reference method for antibiotic synergy testing; however, it is time challenging and, therefore, it is not routinely used in microbiological laboratories. Interestingly, the E-test method appears to be more straightforward to perform and more cost efficient (Doern, 2014). Despite the simplicity of the E-test method, it is challenging to definitively determine which method is superior. Lewis et al. concluded that the E-test method was more accurate than the checkerboard method when comparing synergy methods in Enterobacterales. Surprisingly Papoutsaki et al. (2020) demonstrated poor correlation among all three methods (checkerboard, TKA, and E-test) particularly with tigecycline combinations in Klebsiella pneumoniae isolates (White et al., 1996). However, many studies reported a good agreement between E-test and checkerboard methods (Aktas, 2020; Orhan et al., 2005) or E-test with other synergy testing methods (White et al., 1996; Bonapace et al., 2000). White et al. conducted a comparative analysis of the three synergy testing methods to examine an efficacy of four antibiotic combinations against Enterobacterales family members (P. aeruginosa, E. coli, E. cloacae) and S. aureus. The study reported an agreement of 63–75% between the time-kill and E-test methods. Moreover, a 75% agreement rate was observed between checkerboard and E-test methods (White et al., 1996). Commonly used antibiotic combinations against Enterobacterales include tigecycline with polymyxin, tigecycline with aminoglycoside, and tigecycline with rifampicin or fosfomycin (Papst et al., 2018).
In vitro synergy testing can be assessed with different methodologies: the checkerboard method, time-kill assays (TKA), the multiple-combination bactericidal test (MCBT), and the E-test method (Doern, 2014). The checkerboard method evaluates the effectiveness of antimicrobial combinations tested in serial 2-fold dilutions at clinically relevant concentrations, typically including antibiotics from different classes. Limitations of that method include the requirement for a large amount of reagents and resources to evaluate different combinations. Moreover, only two antimicrobials can be tested simultaneously; combinations of three or four antimicrobial agents are not possible (Saiman, 2007). MCBT is preferred to test combinations of more than two antibiotics. The concentration used in MCBT is determined by what can be assessed in the patient's serum. Unlike checkerboard synergy testing, MCBT only evaluates fixed concentrations (Aaron et al., 2000). TKAs use the principle of MCBT by assessing activity over 48 h instead of a single point. Unlike MCBT, TKA evaluates the rate of killing, providing more a relevant outcome for patients (Doern, 2014). E-test methods are based on the diffusion of a continuous concentration gradient of antibiotic from an impregnated strip on a solid agar. E-tests are placed on an agar medium inoculated with the tested microorganism and incubated overnight. Then minimum inhibitory concentration (MIC) is determined by the point, where the no-growth zone touches the strip. There are two modifications of the E-test method. In the first modification, two strips are placed perpendicularly, intersecting at the MIC for each antimicrobial when tested individually. During our research we decided to analyze various E. cloacae isolates, particularly MDR/XDR strains. The E-test method was employed in our research owing to its simplicity and the promising results it has demonstrated. In this study, the antibiotic synergy testing was performed for tigecycline with nine antimicrobial agents (ceftazidime/avibactam, colistin, ertapenem, gentamicin, imipenem, levofloxacin, meropenem/vaborbactam, polymyxin B, and rifampicin). The rate of tigecycline susceptibility in monotherapy was 45% (36/80 isolates). Interestingly the susceptibility rates in the previous studies ranged from 58 to 86% (Tang et al., 2019; Evren et al., 2013; Lutgring et al., 2020). However, Nulsopapon et al. (2021) demonstrated that only 20% of strains were susceptible to tigecycline. This may be related to prior antibiotic use or horizontal gene transfer (HGT) and the dissemination of tigecycline resistance genes (Korczak et al., 2024).
The highest synergy rate was observed in tigecycline-ceftazidime/avibactam and tigecycline-imipenem combinations (75% of strains). In prior studies synergy rates of tigecycline-ceftazidime/avibactam ranged from 3.0 to 12.5% among Enterobacterales. Moreover, indifference rates were significantly higher in prior studies (67–87.5%) in comparison to our results (1.25%) (Wang et al., 2021; Ojdana et al., 2019). The tigecycline-imipenem synergy ratios varied from 9 to 88% in previous studies. Indifference rates were significantly higher than reported in this study (50–91% vs. 1.5%) (Evren et al., 2013; Goel et al., 2021; Dundar et al., 2018). Tigecycline-polymyxin B (TGC-PMB) and tigecycline-rifampicin (TGC-RIF) synergy rates were also high (68.75%). Prior studies revealed synergy rates at 29–35% for TGC-PMB and 22.6–60% for TGC-RIF (Lim et al., 2011; Huang et al., 2024). As the data in Table 2 suggest, due to the highest MIC values of polymyxin B and rifampicin in monotherapy, a combination therapy should be considered in patients treated with these antimicrobial agents. Moreover, TGC-RIF combination could suppress the expressions of efflux pump genes, like rarA and acrB, and might inhibit development of resistance in carbapenem-resistant Klebsiella pneumoniae strains in comparison to monotherapy (Shi et al., 2023).
The lowest synergy ratios were 32.50 and 43.75% for tigecycline-levofloxacin (TGC-LEV) and tigecycline-gentamicin (TGC-GM), respectively. Prior studies' synergy rates range from 20 to 80% for TGC-LEV and 8.2% for TGC-GM (Nulsopapon et al., 2021; Petersen et al., 2006). The indifference rate in the TGC-GM combination was similar (22.4% in prior study vs. 18.75% in our study) (Nulsopapon et al., 2021; Zhang et al., 2019).
In our study the tigecycline-colistin (TGC-COL) combination exhibited the synergy rate of 65%, while addition and indifference rates were 30 and 5%, respectively. None of the strains displayed antagonism. Dundar et al. reported the synergy of TGC-COL in 36% of strains. Additionally 8% of isolates exhibited antagonism. The remaining strains (56%) were indifferent or additive (Dundar et al., 2018). Betts et al. (2014) reported 47% of synergy and 53% of indifference and addition. Interestingly they also reported that the TGC-COL combination was definitely more effective against E. coli, E. cloacae, and K. pneumoniae than monotherapy.
Carbapenem-resistant Enterobacterales (CRE) is recognized as a critical group in the WHO Bacterial Priority Pathogens List (World Health Organization, 2024). Currently, the therapeutic options for treatment of CRE strains are limited. Even the effectiveness of newer beta-lactamase inhibitors like meropenem/vaborbactam depends on the specific types of carbapenemases produced by CRE isolates, which may depend on the region. Moreover, combination therapy is favored over monotherapy for treatment of CRE infections (Gutiérrez-Gutiérrez et al., 2017; Ni et al., 2016). Tigecycline-meropenem/vaborbactam synergy needs to be further investigated. Our research showed 53.75, 36.25, and 10% for synergy, addition, and indifference rates, respectively. Zhang et al. (2019) reported the tigecycline-meropenem combination with 50, 40, and 10% for synergy, addition, and indifference rates, respectively. Our results seem to be comparable to this prior study. Tigecycline-ertapenem synergy rate appeared to be higher (67.5%) in comparison to other studies with other carbapenem combinations (20–60%) (Petersen et al., 2006; Entenza and Moreillon, 2009).
This study has limitations: this research was performed only in in vitro settings, with only one method to assess antimicrobial synergy, and we did not evaluate patients' outcomes. However tigecycline combinations with almost all of the tested antimicrobial agents yielded promising results with high synergy ratings in the treatment of resistant E. cloacae isolates. Finally, our results may vary as our study included a larger group of isolates, whereas previous studies included only a few strains. Further research is needed for the evaluation of safety and efficacy of tigecycline combinations and to determine whether the E-test method can be considered as the reference method.
Data availability statement
The original contributions presented in the study are included in the article/Supplementary material, further inquiries can be directed to the corresponding author.
Ethics statement
This investigation uses strains obtained from a collection of strains deposited in the Department of Microbiological Diagnostics and Infectious Immunology, Medical University of Bialystok, Poland. The Bioethics Commission of the Medical University of Bialystok did not require the study to be reviewed or approved by an ethics committee because, apart from the strains from the department's collection, no data enabling patient identification were used in the study.
Author contributions
LK: Formal analysis, Investigation, Methodology, Validation, Visualization, Writing – original draft. PM: Conceptualization, Data curation, Funding acquisition, Investigation, Methodology, Project administration, Resources, Supervision, Validation, Writing – original draft, Writing – review & editing. KR: Investigation, Methodology, Resources, Writing – original draft. DI: Investigation, Methodology, Resources, Writing – original draft. PS: Investigation, Methodology, Conceptualization, Writing – review & editing. MM: Investigation, Methodology, Resources, Writing – original draft. ET: Conceptualization, Data curation, Project administration, Resources, Validation, Writing – review & editing.
Funding
The author(s) declare that no financial support was received for the research, authorship, and/or publication of this article.
Acknowledgments
We thank Steven J. Snodgrass for editorial assistance.
Conflict of interest
The authors declare that the research was conducted in the absence of any commercial or financial relationships that could be construed as a potential conflict of interest.
Publisher's note
All claims expressed in this article are solely those of the authors and do not necessarily represent those of their affiliated organizations, or those of the publisher, the editors and the reviewers. Any product that may be evaluated in this article, or claim that may be made by its manufacturer, is not guaranteed or endorsed by the publisher.
Supplementary material
The Supplementary Material for this article can be found online at: https://www.frontiersin.org/articles/10.3389/fmicb.2024.1490032/full#supplementary-material
References
Aaron, S. D., Ferris, W., Henry, D. A., Speert, D. P., and Macdonald, N. E. (2000). Multiple combination bactericidal antibiotic testing for patients with cystic fibrosis infected with Burkholderia cepacia. Am. J. Respir. Crit. Care Med. 161, 1206–1212. doi: 10.1164/ajrccm.161.4.9907147
Aktas, G. (2020). Activity of vancomycin combined with linezolid against clinical vancomycin-resistant Enterococcus strains. Arch. Med. Sci. 19, 189–193. doi: 10.5114/aoms.2020.96400
Arroyo, L. A., García-Curiel, A., Pachón-Ibañez, M. E., Llanos, A. C., Ruiz, M., Pachón, J., et al. (2005). Reliability of the E-test method for detection of colistin resistance in clinical isolates of Acinetobacter baumannii. J. Clin. Microbiol. 43, 903–905. doi: 10.1128/JCM.43.2.903-905.2005
Bazaid, A. S., Punjabi, A. A., Aldarhami, A., Qanash, H., Alsaif, G., Gattan, H., et al. (2022). Bacterial infections among patients with chronic diseases at a tertiary care hospital in Saudi Arabia. Microorganisms 10:1907. doi: 10.3390/microorganisms10101907
Betts, J. W., Phee, L. M., Hornsey, M., Woodford, N., and Wareham, D. W. (2014). In vitro and in vivo activities of tigecycline-colistin combination therapies against carbapenem-resistant Enterobacteriaceae. Antimicrob. Agents Chemother. 58, 3541–3546. doi: 10.1128/AAC.02449-14
Bonapace, C. R., White, R. L., Friedrich, L. V., and Bosso, J. A. (2000). Evaluation of antibiotic synergy against Acinetobacter baumannii: a comparison with Etest, time-kill, and checkerboard methods. Diagn. Microbiol. Infect. Dis. 38, 43–50. doi: 10.1016/S0732-8893(00)00163-2
Catalano, A., Iacopetta, D., Ceramella, J., Scumaci, D., Giuzio, F., Saturnino, C., et al. (2022). Multidrug resistance (MDR): a widespread phenomenon in pharmacological therapies. Molecules 27:616. doi: 10.3390/molecules27030616
Cosentino, F., Viale, P., and Giannella, M. (2023). MDR/XDR/PDR or DTR? Which definition best fits the resistance profile of Pseudomonas aeruginosa? Curr. Opin. Infect. Dis. 36, 564–571. doi: 10.1097/QCO.0000000000000966
Doern, C. D. (2014). When does 2 plus 2 equal 5? A review of antimicrobial synergy testing. J. Clin. Microbiol. 52, 4124–4128. doi: 10.1128/JCM.01121-14
Dundar, D., Duymaz, Z., Genc, S., Er, D. K., Irvem, A., Kandemir, N., et al. (2018). In-vitro activities of imipenem-colistin, imipenem-tigecycline, and tigecycline-colistin combinations against carbapenem-resistant Enterobacteriaceae. J. Chemother. 30, 342–347. doi: 10.1080/1120009X.2018.1516270
Entenza, J. M., and Moreillon, P. (2009). Tigecycline in combination with other antimicrobials: a review of in vitro, animal and case report studies. Int. J. Antimicrob. Agents 34:8.e1–9. doi: 10.1016/j.ijantimicag.2008.11.006
Evren, E., Azap, O. K., Çolakoglu, S., and Arslan, H. (2013). In vitro activity of fosfomycin in combination with imipenem, meropenem, colistin and tigecycline against OXA 48-positive Klebsiella pneumoniae strains. Diagn. Microbiol. Infect. Dis. 76, 335–338. doi: 10.1016/j.diagmicrobio.2013.04.004
García-Meniño I. Lumbreras P. Valledor P. Díaz-Jiménez D. Lestón L. Fernández J. Comprehensive statistical evaluation of etest. Antibiotics. (2020) 9:861. 10.3390/antibiotics9120861 .
Goel, A., Gupta, V., Singhal, L., Palta, S., and Chander, J. (2021). evaluation of antibiotic synergy for carbapenem-resistant. Indian J. Med. Res. 154, 520–526. doi: 10.4103/ijmr.IJMR_760_19
Goodarzi, R., Arabestani, M., Alikhani, M. Y., Keramat, F., and Asghari, B. (2021). Emergence of tigecycline-resistant Klebsiella pneumoniae ST11 clone in patients without exposure to tigecycline. J. Infect. Dev. Ctries. 15, 1677–1684. doi: 10.3855/jidc.15157
Gutiérrez-Gutiérrez, B., Salamanca, E., de Cueto, M., Hsueh, P. R., Viale, P., Paño-Pardo, J. R., et al. (2017). Effect of appropriate combination therapy on mortality of patients with bloodstream infections due to carbapenemase-producing Enterobacteriaceae (INCREMENT): a retrospective cohort study. Lancet Infect. Dis. 17, 726–734. doi: 10.1016/S1473-3099(17)30228-1
Huang, Y. S., Yang, J. L., Wang, J. T., Sheng, W. H., Yang, C. J., Chuang, Y. C., et al. (2024). Evaluation of the synergistic effect of eravacycline and tigecycline against carbapenemase-producing carbapenem-resistant Klebsiella pneumoniae. J. Infect. Public Health 17, 929–937. doi: 10.1016/j.jiph.2024.03.027
Korczak, L., Majewski, P., Iwaniuk, D., Sacha, P., Matulewicz, M., Wieczorek, P., et al. (2024). Molecular mechanisms of tigecycline-resistance among. Front. Cell. Infect. Microbiol. 14:1289396. doi: 10.3389/fcimb.2024.1289396
Lewis, R. E., Diekema, D. J., Messer, S. A., Pfaller, M. A., and Klepser, M. E. (2002). Comparison of Etest, chequerboard dilution and time-kill studies for the detection of synergy or antagonism between antifungal agents tested against Candida species. J. Antimicrob. Chemother. 49, 345–351. doi: 10.1093/jac/49.2.345
Li, J., Zhang, H., Ning, J., Sajid, A., Cheng, G., Yuan, Z., et al. (2019). The nature and epidemiology of OqxAB, a multidrug efflux pump. Antimicrob. Resist. Infect. Control. 8:44. doi: 10.1186/s13756-019-0489-3
Lim, T. P., Tan, T. Y., Lee, W., Sasikala, S., Tan, T. T., Hsu, L. Y., et al. (2011). In-vitro activity of polymyxin B, rifampicin, tigecycline alone and in combination against carbapenem-resistant Acinetobacter baumannii in Singapore. PLoS ONE 6:e18485. doi: 10.1371/journal.pone.0018485
Lutgring, J. D., Balbuena, R., Reese, N., Gilbert, S. E., Ansari, U., Bhatnagar, A., et al. (2020). Antibiotic susceptibility of NDM-producing. Antimicrob Agents Chemother. (2020) 64:e00499-20. doi: 10.1128/AAC.00499-20
Ni, W., Han, Y., Liu, J., Wei, C., Zhao, J., Cui, J., et al. (2016). Tigecycline treatment for carbapenem-resistant enterobacteriaceae infections: a systematic review and meta-analysis. Medicine 95:e3126. doi: 10.1097/MD.0000000000003126
Nulsopapon, P., Nasomsong, W., Pongchaidecha, M., Changpradub, D., Juntanawiwat, P., Santimaleeworagun, W., et al. (2021). The synergistic activity and optimizing doses of tigecycline in combination with aminoglycosides against clinical carbapenem-resistant. Antibiotics. 10:736. doi: 10.3390/antibiotics10060736
Ojdana, D., Gutowska, A., Sacha, P., Majewski, P., Wieczorek, P., Tryniszewska, E., et al. (2019). Activity of ceftazidime-avibactam alone and in combination with ertapenem, fosfomycin, and tigecycline against carbapenemase-producing. Microb. Drug Resist. 25, 1357–1364. doi: 10.1089/mdr.2018.0234
Orhan, G., Bayram, A., Zer, Y., and Balci, I. (2005). Synergy tests by E test and checkerboard methods of antimicrobial combinations against Brucella melitensis. J. Clin. Microbiol. 43, 140–143. doi: 10.1128/JCM.43.1.140-143.2005
Papoutsaki, V., Galani, I., Papadimitriou, E., Karantani, I., Karaiskos, I., Giamarellou, H., et al. (2020). Evaluation of in vitro methods for testing tigecycline combinations against carbapenemase-producing Klebsiella pneumoniae isolates. J. Glob. Antimicrob. Resist. 20, 98–104. doi: 10.1016/j.jgar.2019.07.028
Papst, L., Beovi,ć, B., Pulcini, C., Durante-Mangoni, E., Rodríguez-Baño, J., Kaye, K. S., et al. (2018). Antibiotic treatment of infections caused by carbapenem-resistant Gram-negative bacilli: an international ESCMID cross-sectional survey among infectious diseases specialists practicing in large hospitals. Clin. Microbiol. Infect. 24, 1070–1076. doi: 10.1016/j.cmi.2018.01.015
Petersen, P. J., Labthavikul, P., Jones, C. H., and Bradford, P. A. (2006). In vitro antibacterial activities of tigecycline in combination with other antimicrobial agents determined by chequerboard and time-kill kinetic analysis. J. Antimicrob. Chemother. 57, 573–576. doi: 10.1093/jac/dki477
Qureshi, Z. A., Paterson, D. L., Pakstis, D. L., Adams-Haduch, J. M., Sandkovsky, G., Sordillo, E., et al. (2011). Risk factors and outcome of extended-spectrum β-lactamase-producing Enterobacter cloacae bloodstream infections. Int. J. Antimicrob. Agents. 37, 26–32. doi: 10.1016/j.ijantimicag.2010.09.009
Reese, N., Lonsway, D., Rasheed, J., and Karlsson, M. (2018). 322 Correlation Between Etest and broth microdilution for colistin antimicrobial susceptibility testing of enterobacteriaceae. Am. J. Clin. Pathol. 149(suppl_1):S138–S9. doi: 10.1093/ajcp/aqx126.321
Ruppé, É., Woerther, P. L., and Barbier, F. (2015). Mechanisms of antimicrobial resistance in Gram-negative bacilli. Ann. Intensive Care 5:61. doi: 10.1186/s13613-015-0061-0
Saiman, L. (2007). Clinical utility of synergy testing for multidrug-resistant Pseudomonas aeruginosa isolated from patients with cystic fibrosis: ‘the motion for'. Paediatr. Respir. Rev. 8, 249–255. doi: 10.1016/j.prrv.2007.04.006
Schmid, A., Wolfensberger, A., Nemeth, J., Schreiber, P. W., Sax, H., Kuster, S. P., et al. (2019). Monotherapy versus combination therapy for multidrug-resistant Gram-negative infections: systematic review and meta-analysis. Sci. Rep. 9:15290. doi: 10.1038/s41598-019-51711-x
Shi, S., Xu, M., Zhao, Y., Feng, L., Liu, Q., Yao, Z., et al. (2023). Tigecycline-rifampicin restrains resistance development in carbapenem-resistant. ACS Infect. Dis. 9, 1858–1866. doi: 10.1021/acsinfecdis.3c00186
Stojowska-Swedrzyńska, K., Łupkowska, A., Kuczyńska-Wiśnik, D., and Laskowska, E. (2021). Antibiotic heteroresistance in Klebsiella pneumoniae. Int. J. Mol. Sci. 2:449. doi: 10.3390/ijms23010449
Tang, H. J., Lai, C. C., Chen, C. C., Zhang, C. C., Weng, T. C., Chiu, Y. H., et al. (2019). Colistin-sparing regimens against Klebsiella pneumoniae carbapenemase-producing K. pneumoniae isolates: combination of tigecycline or doxycycline and gentamicin or amikacin. J. Microbiol. Immunol. Infect. 52, 273–281. doi: 10.1016/j.jmii.2016.03.003
The European Committee on Antimicrobial Susceptibility Testing (2024). Breakpoint Tables for Interpretation of MICs and Zone Diameters. Sweden, Version 14.0. Available at: http://www.eucast.org
Tumbarello, M., Losito, A. R., and Giamarellou, H. (2018). Optimizing therapy in carbapenem-resistant Enterobacteriaceae infections. Curr. Opin. Infect. Dis. 31, 566–577. doi: 10.1097/QCO.0000000000000493
Wang, F., Zhou, Q., Yang, X., Bai, Y., and Cui, J. (2021). Evaluation of ceftazidime/avibactam alone and in combination with amikacin, colistin and tigecycline against Klebsiella pneumoniae carbapenemase-producing K. pneumoniae by in vitro time-kill experiment. PLoS ONE 16:e0258426. doi: 10.1371/journal.pone.0258426
White, R. L., Burgess, D. S., Manduru, M., and Bosso, J. A. (1996). Comparison of three different in vitro methods of detecting synergy: time-kill, checkerboard, and E test. Antimicrob. Agents Chemother. 40, 1914–1918. doi: 10.1128/AAC.40.8.1914
World Health Organization (2024). WHO Bacterial Priority Pathogens List, 2024: Bacterial Pathogens of Public Health Importance to Guide Research, Development and Strategies to Prevent and Control Antimicrobial Resistance. Geneva: World Health Organization.
Yaghoubi, S., Zekiy, A. O., Krutova, M., Gholami, M., Kouhsari, E., Sholeh, M., et al. (2022). Tigecycline antibacterial activity, clinical effectiveness, and mechanisms and epidemiology of resistance: narrative review. Eur. J. Clin. Microbiol. Infect. Dis. 41, 1003–1022. doi: 10.1007/s10096-020-04121-1
Zhang, J., Yu, L., Fu, Y., Zhao, Y., Wang, Y., Zhao, J., et al. (2019). Tigecycline in combination with other antibiotics against clinical isolates of carbapenem-resistant Klebsiella pneumoniae in vitro. Ann. Palliat. Med. 8, 622–631. doi: 10.21037/apm.2019.09.11
Keywords: Enterobacter cloacae, E-test method, multidrug-resistant, synergy testing, tigecycline
Citation: Korczak L, Majewski P, Rombel K, Iwaniuk D, Sacha P, Modzelewski M and Tryniszewska E (2024) In vitro evaluation of tigecycline synergy testing with nine antimicrobial agents against Enterobacter cloacae clinical strains. Front. Microbiol. 15:1490032. doi: 10.3389/fmicb.2024.1490032
Received: 02 September 2024; Accepted: 27 September 2024;
Published: 18 October 2024.
Edited by:
Juan A. Ayala, Autonomous University of Madrid, SpainReviewed by:
Tales Fernando Da Silva, Federal University of Minas Gerais, BrazilDhirendra Kumar, National Centre for Disease Control (NCDC), India
Copyright © 2024 Korczak, Majewski, Rombel, Iwaniuk, Sacha, Modzelewski and Tryniszewska. This is an open-access article distributed under the terms of the Creative Commons Attribution License (CC BY). The use, distribution or reproduction in other forums is permitted, provided the original author(s) and the copyright owner(s) are credited and that the original publication in this journal is cited, in accordance with accepted academic practice. No use, distribution or reproduction is permitted which does not comply with these terms.
*Correspondence: Piotr Majewski, cGlvdHIubWFqZXdza2kmI3gwMDA0MDt1bWIuZWR1LnBs