- 1School of Agriculture and Bioengineering, Longdong University, Qingyang, China
- 2Gansu Key Laboratory of Protection and Utilization for Biological Resources and Ecological Restoration, Qingyang, China
- 3Department of Plant Pathology, College of Agriculture, Guizhou University, Guiyang, China
- 4Institute of Plant Protection, MNS University of Agriculture, Multan, Pakistan
- 5National Key Laboratory of Green Pesticide, Key Laboratory of Green Pesticide and Agricultural Bioengineering, Ministry of Education, Center for R&D of Fine Chemicals of Guizhou University, Guiyang, China
Damping-off disease in chili (Capsicum annum L.) cultivation is a significant global issue, severely affecting seeds, seedlings, and young plants, regardless of the location of cultivation, whether in greenhouses or open fields. Despite chili being a widely popular vegetable used in various cuisines globally, farmers face challenges in meeting the growing demand due to the extensive damage caused by this disease, ranging from 20 to 85%. The shelf life and quality of mature pods are also severely affected. Damping-off disease is mainly caused by soil-borne fungus from the Pythium species, with additional contributions from Phytophthora, Fusarium, and Rhizoctonia species. These pathogens’ adaptability to diverse environmental conditions and resistance to synthetic fungicides make controlling damping-off on a commercial scale challenging. However, integrated disease management has shown promising results as a remedial approach. In this review, we discuss the current state of chili diseases, the nature of the pathogens causing damping-off, the epidemiology of the disease, and various control mechanisms. In this review, we broadly discuss the current state of chili diseases, the nature of the pathogens causing damping-off, the epidemiology of the disease, and various control mechanisms. Furthermore, we highlight the importance and efficacy of integrated disease management techniques, along with future prospects in unexplored areas, such as host–pathogen interaction and sustainable disease control measures. The information in this review aims to assist chili growers in understanding the epidemiology and management of damping-off in chili cultivation.
1 Introduction
Chili (Capsicum annum L.) is a member of the Solanaceae family and is considered a vital vegetable cum spice, which is one of the most extensively cultivated crops worldwide. Morphologically, this is a heavily branched herbaceous annual shrub that grows well in sandy, fertile soil. The flowers are terminal, pentamerous, solitary, and bisexual, with a structure that allows the corolla to rotate. The stamens have blue to purple anthers attached at the base of the corolla (Awang et al., 2013). Based on the fruit’s shape, color, pungency, size, flavor, and use, chili is classified as a perennial crop and can be grown throughout the year. Approximately 400 different cultivars of chilies are grown, and well-developed pods are used as food ingredients for spices, sauces, pickles (Zou and Zou, 2021), or beverages and medicine. In urban lifestyles, chili is grown as an ornamental plant in gardens. Chili contains a significant amount of minerals: potassium (K), iron (Fe), magnesium (Mg), and vitamins A and C, along with other significant nutrients (Sarkar et al., 2022).
Chili originated in the tropical and subtropical regions of Central and South America and was introduced to Zhejiang Province (China) in 1591. It is now distributed across northern, western, and southern China (Zou et al., 2020). China, Mexico, Indonesia, Turkey, and Spain are the leading green chili-producing countries, collectively responsible for approximately 75% of global production in 2013, according to FAOSTAT (2020). China, in particular, made the largest contribution, accounting for over half of the world’s chili production, with a cultivation area exceeding 21,474 km2 in 2019 (Zou and Zou, 2021). This surpasses the current land area allocated to versatile vegetables in the Chinese market. Moreover, more than 3,070 km2 is cultivated in Guizhou province (26.5982° N, 106.7072° E), which is higher than in any province in China.
Different crop genotypes are found in China, with productivity varying across provinces. The total chili yield in 2019 exceeded 64 million tons, which accounted for 7.76% of China’s total national vegetable output. The total pepper yield in China reached 250 billion CNY, accounting for 11.36% of the total output value of national vegetables and contributing to 1.14% of the total farm income (Zou and Zou, 2021). Though there is a rising demand for chili for versatile purposes, today, the supply chain has failed to cater to existing demand due to various challenges in chili cultivation. Among them, biotic stresses such as pest and disease attacks and abiotic stresses such as temperature fluctuations, water scarcity, and salinity are crucial. In addition, pre- and post-harvest losses due to poor management practices occurred on various scales.
Chili plant death in nurseries due to damping-off caused by pathogens has become a major issue in chili cultivation worldwide. The main causal organism is a soil-borne pathogenic fungus, Pythium spp., which affects chili plants in seedlings and younger roots. In addition, Rhizoctonia solani, Fusarium, and Phytophthora spp. are considered other pathogenic organisms that cause damping-off (Rini and Sulochana, 2006). In this study, we have thoroughly discussed the damping-off disease in chili cultivation, current yield losses, sustainable crop management practices, integrated pest control methods, advancements in disease control technology, and its prospects.
2 History and distribution of the host crop, Capsicum annuum L.
Capsicum annuum L. has been known for over 9,500 years. Chili is a native crop of Southern America and was first cultivated in Peru at approximately 7,500 BC (MacNeish, 1964). First, three species of chili, i.e., C. chinense, C. frutescens, and C. annuum, developed from common ancestors that grew widely in the north of the Amazon basin (NW-Brazil, Columbia), spreading to the other parts of America. Then, two more species, i.e., C. baccatum and C. pubescens, developed in different parts of America at the end of the eighteenth century (IBPGR, 1983). The introduction of chili to China is endorsed by the journey of Columbus, who took the chili seeds from Spain, introduced them to Europe, and spread them to the subcontinents of Asia and Africa (Heiser, 1976). Columbus confused the pungent fruits of Capsicum with black pepper Piper nigrum L., calling them red pepper owing to the red-colored fruits. Capsicum is not only related to the Piper genus. However, the terminology surrounding Capsicum is often confusing. Names such as chili, chili, aji, chile, pepper, and paprika denote the pungent fruits of Capsicum. The crop status spread quickly across Europe, moving to China, Japan, India, and Pakistan. Unlike other important cuisines, it became one of the most important cuisines in Asia and Europe (Zou and Zou, 2021).
While there are reports of 20 wild species of chili peppers, only five species are currently used for cultivation: C. annuum, C. baccatum, C. frutescens, C. chinense, and C. pubescens. Among these, Capsicum annuum var. annum is the most extensively cultivated and economically significant among domesticated chili peppers. Notably, it is the only species commercially grown outside America, in Asia and Africa. Particularly in China, the crop was introduced to Zhejiang Province in 1591, and then it became the most important ingredient in various cuisines throughout the country (Zou and Zou, 2021). The common name for chili in China is “Lajiao” in Chinese. The bell pepper is the non-pungent fruit of Capsicum and is known as “Tianjin or Caijiao” in Chinese. Over 95% of crops cultivated in China are C. frutescens L., C. annuum L., and C. chinense Jacq (Zou and Zou, 2021), which are mostly cultivated in the northern and southern parts of China.
3 Global production and Chinese contribution
Chili peppers are widely grown and consumed worldwide, with larger shares in Asia, Africa, America, and Europe. China is one of the major consumers, producers, and exporters of chili peppers (Sundari et al., 2023). China’s share of global chili pepper exports was approximately 13%. In 2020, global production was estimated at 37.9 million tons, with China accounting for approximately 36%. Mexico and Indonesia were the second and third largest green chili producers. According to the Guinness World Records and the Food and Agriculture Organization (FAO), China produces approximately 46% of the global chili supply to the market, as mentioned in Table 1 (FAOSTAT, 2020; Sachin et al., 2022).
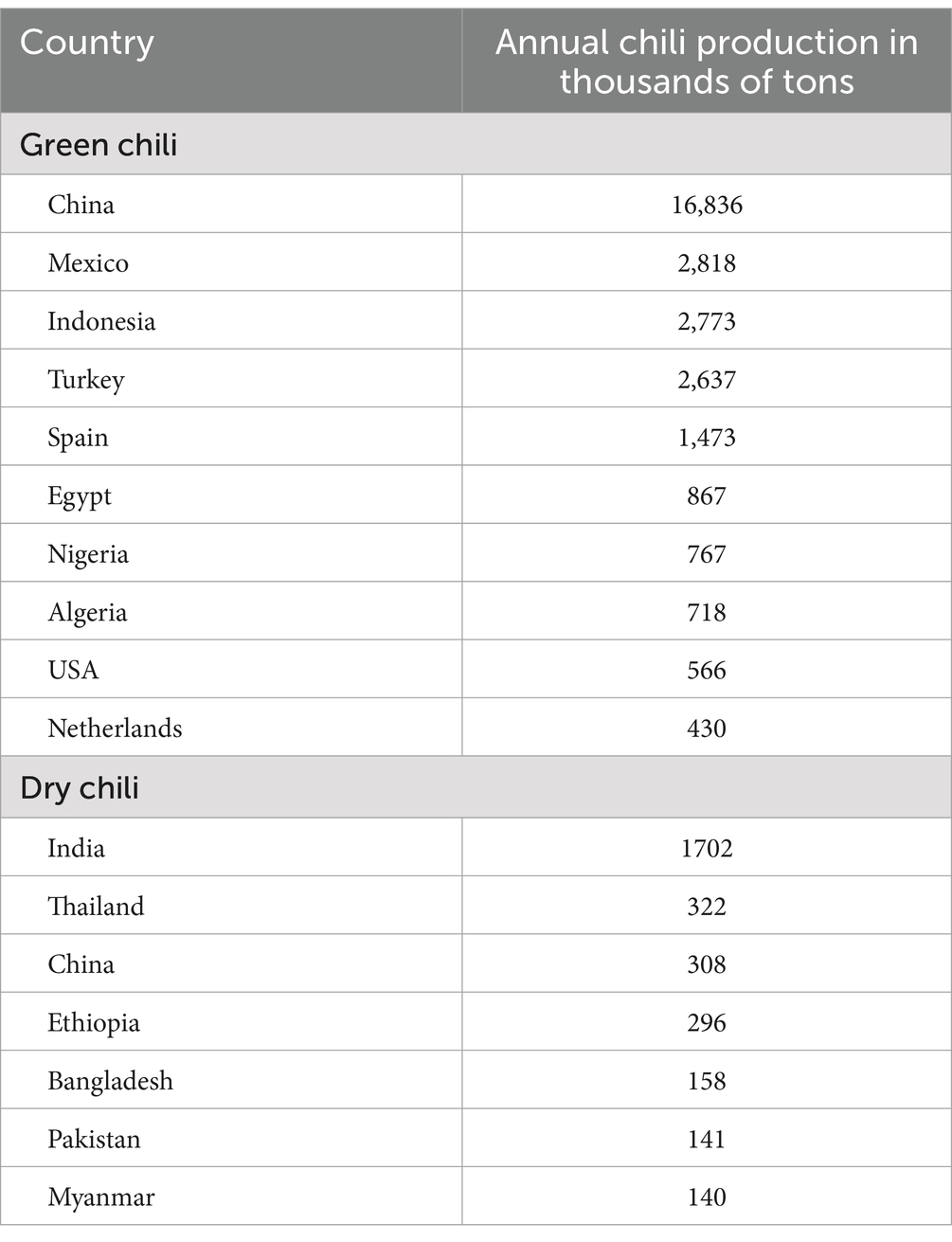
Table 1. Annual chili production by the world’s top producers in million tons (FAOSTAT, 2020).
The growth in chili exports worldwide has been driven by production surpluses and the significant potential of the global chili trade. Since 2004, the volume of chili exports has experienced consistent growth, with an average annual growth rate of 6.04% (Shimbov et al., 2019; Sundari et al., 2023). However, interpreting global chili production trends and China’s role in the industry is essential for policymakers, researchers, and stakeholders involved in the chili trade (Yin et al., 2022).
4 Uses and importance of chili
Chili is utilized in various forms, including fresh, green, or ripened fruits, along with dried and powdered forms. Fresh green pungent chili fruits are generally used in stuffing, salads, pickles, and as a flavoring agent in cooked meals, while fresh green non-pungent fruits are used as vegetables or processed with other food items for flavor. Additionally, highly pungent fruits are used as a spice to stimulate appetite and as a flavoring agent in ketchup. In addition to culinary uses, small quantities of chili are used in the cosmetic and garment industries (Saxena et al., 2016).
Chilies have the potential to alleviate micronutrient deficiencies by supplying vitamins A, C, E, and B, along with minerals such as molybdenum, manganese, folate, potassium, and copper. They also supply significant macronutrients, such as protein, carbohydrates, fats, and dietary fiber, which are essential for human health (Olatunji and Afolayan, 2018).
Peppers contain a wide range of phytochemicals, such as phenolics and flavonoids, which possess important antioxidant activities that help reduce the risk of degenerative diseases (Saleh et al., 2018). Additionally, capsaicin in chili improves digestive health and nutrient absorption (Marini et al., 2015; Rosca et al., 2020), provides relief from joint pain (Fattori et al., 2016), exhibits anti-inflammatory properties (Chung and Campbell, 2016; Fattori et al., 2016), and inhibits the growth of several types of fungi, including Candida albicans (Behbehani et al., 2023).
Hot chili peppers also have antiviral properties, which can help prevent colds and flu while boosting the immune system (Maurya and Sharma, 2023). Regular consumption of chili fruit is helpful against anorexia, varicose veins, hemorrhoids, and liver congestion. Chili extracts, both in pure and processed forms, are used externally as analgesic rubefacients for treating rheumatism, back pain, muscle pain, and articular and swollen feet, and are even used as antidotes for poisoning. Additionally, chilies have non-food and non-pharmacological uses, such as in the preparation of “pepper sprays” for self-defense and in making “natural and organic pesticides.”
5 Chili plant morphology
The chili plant displays unique morphological features. The chili plant is a vigorously branched herbaceous species with primary, secondary, and tertiary branching. Its height typically ranges from 50 to 100 cm (Pawaskar, 2023). Its solitary, often paired, bisexual flowers have bell-shaped, twisted corollas with 5–6 lobes. Notably, chili plants are primarily cross-pollinated by insects, with natural rates reaching up to 50% (Gao et al., 2023). Chili peppers are distinctive berries with seeds not embedded in the pericarp (Azlan et al., 2022). They change from green to red or purple upon ripening, with variations in shape, size, and pungency. Pungency is determined by capsaicin content and genetics, while the red color comes from capsanthin (Wang et al., 2023). Orbicular seeds are developed within the fruit, and their size is influenced by nutrition (Barboza et al., 2022). Chili plants, which are highly branched and have shallow roots, exhibit drought tolerance but struggle in waterlogged soil (Supplementary Figure S1; Guo et al., 2022).
5.1 Favorable climate for chili and chili diseases
Chilies thrive in tropical and subtropical regions up to 2000 m in altitude, excluding pungent varieties (Aashish et al., 2022). Optimal conditions include warm, humid climates that promote growth and fruit maturity, with an ideal annual rainfall of 850–1,200 mm. However, excessive rain and humidity can lead to poor fruit set and fruit rot. The ideal temperature for chilies ranges between 20°C and 25°C during the day and 15°C and 21°C at night (Arom, 2022). A day length of 9–10 h stimulates plant growth, boosting productivity by 21–24% and enhancing capsicum quality (Kramchote and Suwor, 2022).
Soil is the main substrate for chili in open field conditions, and various other solid substrates, such as grow bags, are used under greenhouse conditions. Chili prefers well-drained, aerated soils rich in organic matter. Ideal soils are light loamy or sandy loamy with lime and organic content, while light soils require irrigation and organic fertilization. The 6–7 pH range is ideal for optimum growth, and higher salinity hampers plant growth. Chili diseases have been a major reason for yield reductions in the world. Diseases caused by bacteria, viruses, fungi, and nematodes have badly affected chili crops worldwide (Su et al., 2023; Xie et al., 2023). Diseases associated with chili crops are summarized in Table 2. One such fatal disease is damping-off, which occurs by soil-borne fungi genera, whether occurring pre- or post-plant emergence, accounting for up to 90% plant death and 62% seedling viability loss in nurseries and fields, as originally documented by Majeed et al. (2018).
6 Damping-off disease on chili
Damping-off is a significant fungal disease affecting chili production worldwide, leading to substantial economic losses. The disease primarily targets seedlings, causing poor germination and plant death, which reduces overall crop yield. Farmers experience financial setbacks due to the need for replanting, increased use of fungicides, and lower productivity. In regions highly dependent on chili cultivation, such as China, India, and Mexico, the disease poses a significant threat to growers’ livelihoods, disrupting both local and global markets. The chili crop is infected by more than 100 different types of plant pathogens during its vegetative and reproductive stages (Jayapala et al., 2019). Damping-off disease is caused by soil-borne pathogens such as Pythium sp., Phytophthora sp., Rhizoctonia solani, and Fusarium spp. (Thakur and Singh, 2023).
Damping-off is one of the most critical diseases that leads to the decay of germinated seeds and young seedlings, which causes a huge economic loss for farmers in nurseries and fields. These fungal or fungal-like organisms cause seed death during germinating in nurseries, with losses of up to 90% under pathogen-favorable conditions (Arora et al., 2021). Damping-off on chili occurs in two stages: the pre-emergence and post-emergence phases. In the pre-emergence phase, disease typically begins during the seedling stage, where the fungi invade seeds or young plants in waterlogged or poorly drained soil. Initial symptoms include seed rot or germination failure, leading to poor crop emergence. Infected seedlings may develop water-soaked lesions at the base of the stem, which gradually turn brown, causing the stem tissue to collapse. This pre-emergence damping-off stage is particularly destructive, as the seedlings die before breaking the soil surface (Majeed et al., 2018). However, the stem tissue near the soil line becomes soft, sunken, and girdled during the post-emergence phase. The affected seedlings wilt, fall over, and die due to disrupted nutrient and water transport, a condition often referred to as “wire stem” (Lamichhane et al., 2017).
This deterioration often results in reduced seedling vigor, and the seedlings may die near the coleoptile, exposing whitish fungal growth on the surface. In the initial stages, affected plants exhibit wilting, eventually leading to a severe infestation. Surviving plants display stunted growth, and the affected areas often exhibit irregular and uneven development (Were et al., 2023). Damping-off symptoms can generally be observed from seeding until the 4th to 6th week post-sowing (Supplementary Figure S2). In this study, we focus mainly on Pythium spp. (Lamichhane et al., 2017) regarding damping-off. Several species of Pythium associated with chili to cause damping-off disease in different parts of the world are mentioned in Table 3.
6.1 The biology of different pathogens causing damping-off
Pythium species are a group of soil-borne pathogens known for causing damping-off and root rot in a wide range of plants, including chili. They thrive in wet, poorly drained soils and attack seedlings, leading to stunted growth and plant death. Some Phytophthora species can cause damping-off in seedlings. For instance, Phytophthora capsici is known to cause damping-off in seedlings of various crops, including peppers and other vegetables. The pathogen attacks young seedlings, leading to poor germination, stem collapse, and death, similar to other damping-off pathogens such as Pythium and Rhizoctonia. Rhizoctonia solani is a soil-borne fungal pathogen that affects chili seedlings by attacking their roots and stems, leading to poor germination, wilting, and eventual death of the young plants. Rhizoctonia thrives in warm, moist conditions and can lead to significant crop losses if not managed effectively. Fusarium species are soil-borne fungi that attack chili seedlings’ roots and lower stems, leading to poor emergence, wilting, and seedling death. Fusarium thrives in warm, moist soils and can cause significant damage if conditions are favorable.
6.2 Epidemiology of the disease
Environmental factors play a key role in spreading the disease (Ghorbani et al., 2024a). The susceptible host, the chili plant, and the virulent pathogen, Pythium spp., along with conducive environmental conditions, are key elements for disease establishment. Temperature, moisture, and organic matter (Hansen and Keinath, 2013) have been directly linked with the incidence and severity of the damping-off owing to better establishment of the Pythium spp. with respect to attachment, establishment, and penetration into host tissues. Other environmental factors such as soil type, clay soil with high moisture holding capacity, rainfall intensity and duration, irrigation, humidity, and seedling surface witness are key factors responsible for damping-off disease. The pre-emergence damping-off requires 12°C while post-emergence damping-off require18-30°C and the optimum range lies from 24 to 30°C for successful infection of Pythium spp. (Dubey et al., 2019). Furthermore, a high soil moisture (Martin and Loper, 1999; Majeed et al., 2019) supports the dissemination of propagules and increases the size of the spermosphere, while a pH of ˃5.8 supports successful infection. The relationship between environmental factors with inoculum and crop geometry spread also leads to the possible development of disease (Arora et al., 2021). The detection and diagnosis of Pythium spp. pathogenic to chili plants involves utilizing both morphological characteristics and the analysis of specific DNA marker regions from the isolated pathogen. Recent research on Pythium spp. has predominantly focused on advanced molecular techniques based on PCR, including real-time PCR, multiplex PCR, loop-mediated isothermal amplification (LAMP) (Sridapan and Krajaejun, 2023), and internal transcribed spacer (ITS) analysis (Gaikwad et al., 2023).
6.3 Infection stages and disease cycle
Pythium spp. are eukaryotic organisms composed of filamentous, non-septate hyphae. Cell walls are primarily composed of cellulose and glucan, with limited amounts of chitin (Kiselev, 2020). Pythium spp. in soil is attracted to the exudates released from germinating seeds and developing seedlings, leading to seed rot and seedling death (Wohor et al., 2022). There are two main stages of Pythium spp. When the environmental conditions are favorable in the first stage, pyrium produces sporangia that grow into hyphae and vesicles with motile zoospores asexually. They are attracted to plant roots or other organic matter in the soil. In the other stage, Pythium reproduces sexually by combining an antheridium and an oogonium to form oospores, mostly during unfavorable environments. Sporangia can be single-celled or multi-celled and can be motile or non-motile.
Furthermore, Pythium spp. can be heterothallic or homothallic depending on the type of reproduction (Figure 1). When spores come into contact with a susceptible host, they can adsorb onto the root surface, forming a germ tube. The germ tube then penetrates the host’s cells via wounds, root tips, or direct penetration, grows to branch mycelium through intracellular and intercellular spaces, and absorbs nutrients while exhibiting pathogenic symptoms externally (Kushwaha, 2020). Sexual spores can thrive dormant in the soil in harsh environmental conditions as saprophytes and become active again when conditions become favorable (Parveen et al., 2020).
7 Integrated management of damping-off
Chemical control methods, such as applying pesticides and other synthetic compounds, have been widely used for managing various pests and diseases. While initially effective, these methods often face significant challenges, including developing resistance in target species, environmental contamination, and negative impacts on non-target organisms, including beneficial species (Ranjbar et al., 2023; Ghorbani et al., 2024b). Over time, repeated use of chemical agents can lead to diminishing returns, requiring higher doses for the same effect and exacerbating ecological damage (Ghorbani et al., 2023; Nanehkaran et al., 2024). Additionally, concerns regarding human health risks and ecosystem disruption further highlight chemical control’s limitations. These factors underscore the necessity of an integrated approach, combining biological, physical, and chemical methods to achieve more sustainable and resilient pest management strategies. Seed treatments are conducted to improve germination and seedling vigor (Bhattarai et al., 2022). There are physical, chemical, and biological seed treatment methods in use aiming at seed disinfection, disinfestation, and seed protection. Chemical seed treatment includes soaking seeds in thiram, captan, and brassicol at the rate of 2.5 g/kg (Misra et al., 2022), while physical methods include hot air, hot water, and electron treatments, compressed moist heat (Saha and Kumari, 2023). Biological seed treatment methods are neem leaf extract, garlic clove extract, ginger extract, and allamonda extract for chili (Mishra et al., 2020). The promising microbial seed treatment is carried out by treating chili seeds with T. viride and P. fluorescence (Mondal et al., 2022). Using synthetic seed treatments leads to phytotoxicity and germination defects, ultimately affecting human and animal safety. This is a disease preventive measure that can be taken before infestation from soil (Volynchikova and Kim, 2022).
Good agricultural practices follow guidelines such as sterilizing nursery soil using a combination of physical and biological methods, including solarization and the application of Trichoderma as a soil drench (Kumhar et al., 2022). Additionally, measures like burning soil with rice husks and bran, selecting soil from areas with no prior Pythium infestation history, using pure water and clean implements, using clean polythene sheets and organic amendments as mulch, fertilizing with organic materials from trustworthy, uncontaminated sources, and preventing water runoff from external sources are vital to avoid soil pathogen contamination (Zamoum et al., 2022). If symptoms of damping-off arise, the immediate uprooting and isolation of affected seedlings from the rest of the plants is necessary to prevent further spread. These practices collectively contribute to the successful adoption of best cropping practices.
In situations where the aforementioned preventive measures prove insufficient due to the severity of the infestation, the use of chemical control becomes a viable option. It is crucial to first assess the extremity of the disease within the nursery to determine the appropriate dosage of fungicide required. Before moving to chemical intervention, it is imperative to consider the soil’s overall health and the purity of the seeds selected for cultivation. More importantly, an immediate foundation of research outcomes to elucidate the morphology and physiology of Pythium and its response to external factors is needed to draw decisions. Fungicides should only be employed when all other methods have been exhausted, and the disease cannot be effectively managed or eradicated through alternative measures.
7.1 Use of chemical fungicides
Chemical control via fungicides is often considered a quick and effective way to prevent pathogens from infecting plant systems, particularly when applied to young, growing tissues like leaves, fruits, and flowers. This method is widely adopted due to the immediate results it yields compared to the lengthy process of developing resistant cultivars (Sawant et al., 2022). Farmers have resorted to various chemical alternatives to methyl bromide for soil fumigation, as non-chemical methods can sometimes be labor-intensive and less effective against soilborne diseases (Duniway, 2002). However, the use of chemical fungicides is constrained by a limited number of products registered for use on various crops and the high costs associated with these products, limiting chemical management of damping-off to a few active ingredients (Garzón et al., 2011). Fungicides from groups such as captan, benzimidazole, triazole, and dicarboximide have been recognized for their efficacy in controlling damping-off diseases (Lamichhane et al., 2016). Specifically, metalaxyl, tridiazole, and captan are effective against Pythium and Phytophthora species, while maneb and mancozeb work against Pythium and Fusarium species, and these are commonly used by growers (Lamichhane et al., 2016). Using systemic fungicides like metalaxyl before sowing can reduce Phytophthora and Pythium populations in the soil (Russell et al., 1990). For seed treatments, Satija and Hooda found that benlate (0.1%) and dithane M-45 were the most effective against Pythium aphanidermatum and Fusarium solani (Ansari et al., 1990). Soil drench applications of captan (Khrieba, 2020), as well as soil treatments with methyl bromide, solarization, and metalaxyl soil drench, have been successful in preventing P. aphanidermatum infection (Hickman and Michailides, 1998).
7.2 Recommended cultural practices
Cultural practices are vital in ensuring disease-free chili production with optimum yield. In detail, proper site selection, avoiding areas with a history of damping-off infection, ensuring good drainage, applying organic amendments to enhance soil quality, adopting the best cropping systems, and using healthy seeds are best cultural practices to avoid damping-off disease (Magnee et al., 2022). Further measures include infected plant parts being removed as soon as possible from the fields (Manjunatha et al., 2022), timely and adequate fertilizing, irrigation, mulching to regulate soil temperature and suppress weeds, implementation strategies to protect crops from pests and diseases, sterilization through solarization or seed treatment (Arora et al., 2022), cover cropping, green manuring, crop rotation, tillage, and managing proper space between crops (Moura et al., 2022). Soil physiochemical properties management, such as pH, CEC, and moisture, is also important. Combining these practices is the most effective approach to managing damping-off disease in chili crops (Cook and Haglund, 1991; Cook, 2001). Furthermore, enhancing seed vigor is crucial for controlling damping-off, even in high pathogen density (Lamichhane et al., 2017).
7.3 Use of botanicals and biological control agents
The utilization of botanical extracts presents a highly promising approach for managing chili damping-off disease caused by Pythium spp. Plant extracts, including neem, garlic, ginger, turmeric, lemon, and pepper, have been shown to possess inherent antifungal properties. Consequently, these botanical extracts can be a viable and eco-friendly substitute (Postma et al., 2003) for conventional chemical fungicides (Islam and Faruq, 2012; Hyder et al., 2021; Arora et al., 2022). However, it is essential to recognize that the effectiveness of botanicals can show variability dependent upon the particular plant species, the specific plant part used, and the method that was used for extraction (Arora et al., 2022). Moreover, the utilization of plant growth-promoting rhizobacteria (PGPR) in disease management endeavors shows promising results in the biocontrol of Pythium spp., as well as their positive impact on promoting the growth of solanaceous crops (Kenawy et al., 2019), and many other major crop varieties. In many cases, it has been properly noted that imported bioformulations occasionally exhibit suboptimal performance owing to the influence of climate change (Compant et al., 2010) and limitations in nutrient availability (Kandeler et al., 2006). Therefore, the detection and characterization of PGPR endemic to chili rhizospheres capable of suppressing the inoculum of P. myriotylum and enhancing the growth of chilies in nurseries is in need.
7.4 Trichoderma and Bacillus as a biocontrol agent against Pythium spp
Trichoderma, a prominent genus of filamentous fungi within the phylum Ascomycota, demonstrates remarkable potential as a biocontrol agent (BCA) against Pythium pathogens (Thambugala et al., 2020; Yao et al., 2023). These beneficial fungi, particularly the avirulent strains, have proven effective in plant protection, biostimulation, and biofertilization. Their effectiveness in agricultural applications depends on their metabolic activity and interactions with plants and other microorganisms.
Trichoderma species can colonize the rhizoplane and plant roots (Poveda and Eugui, 2022). They produce an array of metabolites with antimicrobial properties, including cell wall-degrading enzymes, both volatile and non-volatile antibiotics (Jayaraj et al., 2006), as well as phytohormones and phytoregulators such as Indole Acetic Acid (IAA), cytokinin and ethylene that stimulate plant growth indirectly (Rahman et al., 2012). They also secrete chitinase, which enhances plant defense mechanisms (Subash et al., 2013) against fungal pathogens, and 1–3 glucanase, a protease contributing to fungal pathogen defense and improved nutrient supply (Mannai and Boughalleb-M’Hamdi, 2023). Conversely, Trichoderma stimulates plant resistance locally or systemically by releasing products known as elicitors (Majeed et al., 2019). These elicitors originate from the cell walls of both the host plant (endoelicitors) and the invading microorganism (exoelicitors). Pythium acidifies soil by secreting organic acids that can solubilize phosphate and other micronutrients supporting plant nutrition (Rahman et al., 2012).
Notably, Trichoderma can control Pythium spp. through mechanisms such as necrotrophic mycoparasitism, competition for nutrients and space, and the synthesis of antifungal metabolites (Tyśkiewicz et al., 2022). These fungi exhibit rapid growth, metabolic versatility, and resistance to various toxic chemicals, including fungicides and herbicides, as adaptations (El Enshasy et al., 2020; Jia et al., 2024; Yang et al., 2024).
Among Trichoderma species, T. harzianum strains stand out for their ability to protect plants by antagonizing Pythium pathogens in damping-off (Muthukumar et al., 2011b), showing more promising performance than T. viridae, B. subtilis, and T. asperellum, which exhibit synergistic effects against P. aphanidermatum in solanaceous crops (Kipngeno et al., 2015). Peroxidase activity was significantly higher in the roots of sugar beet seedlings treated with T. virens as biocontrol agents (Hanson and Howell, 2004).
Pseudomonas fluorescens is a beneficial bacterium widely used as a biocontrol agent against damping-off disease in chili via antibiosis, siderophore production, competition, induced systemic resistance, and enzyme production against damping-off disease in chili (Mehmood et al., 2023). Bacterial species such as Bacillus have been proven to control fungal diseases. B. subtilis showed high antagonistic activity against Colletotrichum gloeosporioides, which caused anthracnose disease of chili (Ashwini and Srividya, 2014). Bacillus has chitinolytic activity to control different fungal pathogens. Chitinolytic is a pathogenesis-related protein that increases the plant’s defense mechanism against fungal pathogens (Mabuchi et al., 2000; Huang et al., 2005). Bacillus species were found to colonize the root surface, increase plant growth, and cause the lysis of fungal mycelia (El-Bendary et al., 2016). As shown in previous reports, Bacillus spp. significantly control the damping-off chili caused by P. myriotylum (Jimtha et al., 2016). Similar results from Hyder et al. (2021) showed that B. cereus and B. megaterium significantly control damping off disease (Hyder et al., 2021).
7.5 Use of resistant varieties
Recent advances in plant breeding have significantly improved efforts to develop resistant chili cultivars to combat biotic and abiotic stresses. Marker-assisted selection (MAS) has accelerated the identification and incorporation of resistance genes into new cultivars, allowing breeders to develop varieties resistant to diseases such as powdery mildew, anthracnose, bacterial wilt, and viral diseases. Quantitative trait loci (QTL) mapping has been crucial in identifying resistance traits linked to these pathogens. Additionally, genomic selection and CRISPR-Cas9 gene editing technologies are revolutionizing breeding by enabling the precise modification of specific genes responsible for resistance. Traditional methods like germplasm screening and hybridization remain integral, but these are now supplemented with advanced genomic tools to improve efficiency and precision.
Host plants have evolved complex defense mechanisms that can either activate or suppress a range of genes when under attack by pathogens (Kumar et al., 2022). These induced resistance responses include various physiological processes such as the generation of reactive oxygen species, synthesis of phytohormones, and production of pathogenesis-related (PR) proteins. The utilization of host plant resistance as a management strategy encompasses two distinct approaches: (i) the utilization of plant varieties that possess resistance to pathogens, resulting in reduced pathogen populations or enhanced ability to resist pathogen-induced damage, and (ii) the integration of these resistant varieties with other management tactics as part of an integrated pest management (IPM) framework. However, a significant number of plant diseases, especially those responsible for damping-off diseases, lack any plant cultivar that exhibits quantifiable resistance (Babadoost and Islam, 2003). As a result, the optimal utilization of crop varieties possessing pathogen resistance can only be achieved by effectively integrating them with other strategies for disease management. However, there has been a lack of emphasis on incorporating plant resistance into other IPM strategies and a lack of research on quantifying the benefits of plant resistance within IPM programs that utilize various approaches (Davis, 2009).
The current breeding methodology employed thus far for the development of crop varieties that exhibit resistance should be reevaluated if the intent is to prioritize sustainable crop protection strategies based on IPM. This is especially valid because the majority of cultivated plant varieties developed thus far have been rooted in a market-oriented strategy emphasizing the cultivation of high-yielding and economically advantageous crop varieties. The current tendency has resulted in increased utilization of short rotations or monoculture methods while neglecting the potential benefits that minor crops may offer for IPM (Messéan et al., 2016). Crop diversification is hindered by the limited range of minor crop types, which limits beneficial activities like multiple cropping and intercropping (Benvenuto et al., 2020). Hence, in light of the pivotal role of breeding in enhancing crop competitiveness and facilitating adaptation to diverse cropping systems, it is imperative to use a distinct approach for breeding in IPM compared to conventional methods (Benvenuto et al., 2020). Detailed information about management strategies is described in Table 4.
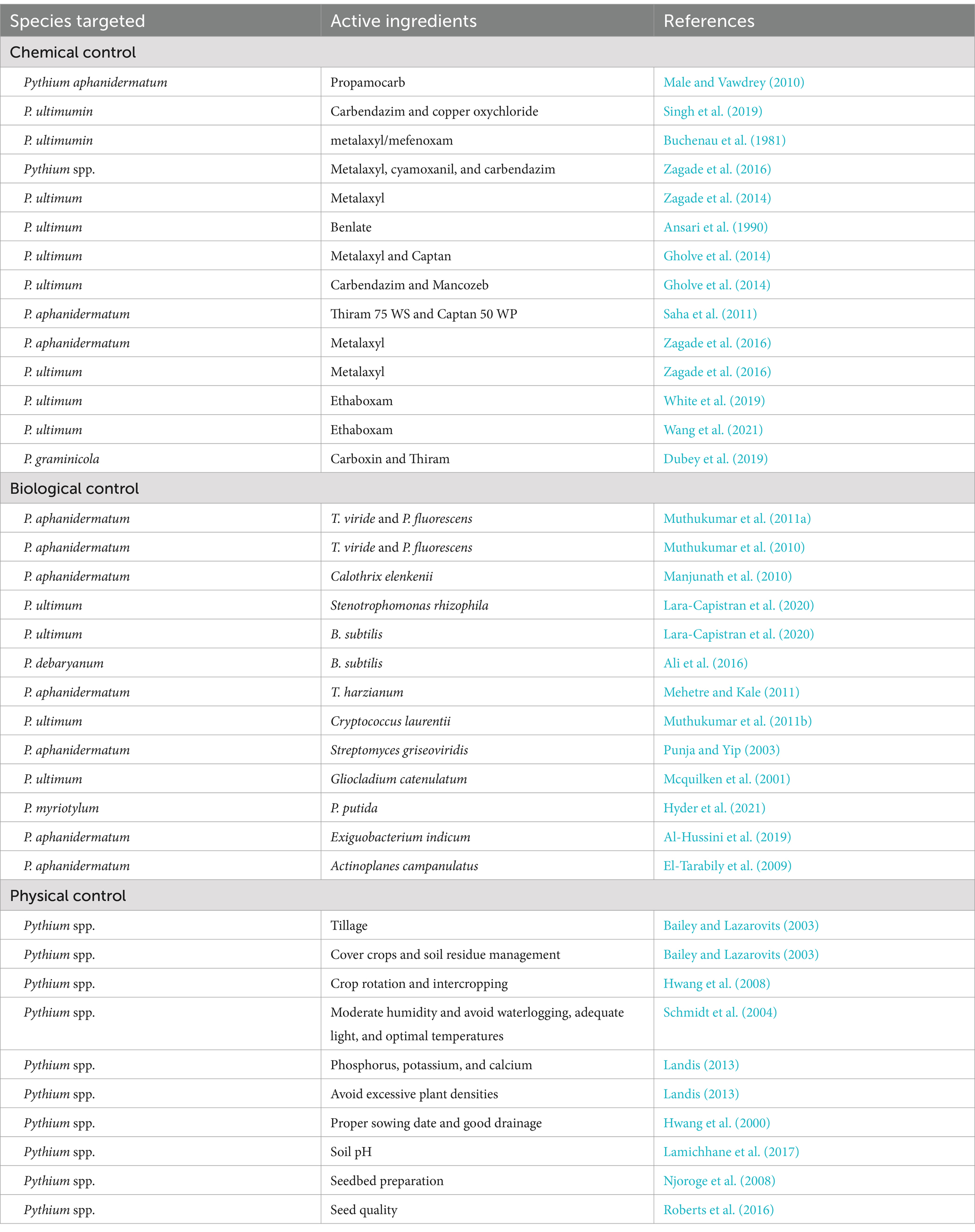
Table 4. Control measures for managing damping-off in chili reported from different parts of the world.
7.6 Detrimental effects of synthetic fungicides
Even though chemical fungicides are effective in rapidly controlling damping-off, they lead to human and ecotoxicity, particularly in developing nations (Voorrips et al., 2004). Human toxicity includes irritant dermal injuries, mucus membranes, and dermal sensitization. For animals, livestock poisoning and aquatic organisms, particularly fish, experience adverse impacts from fungicides. Furthermore, fungicides reduce the fungal and total microbial biomass in soil (Verdenelli et al., 2023), influencing the imbalance in soil microbiota, and another detrimental effect is phytotoxicity by fungicide residues (Kovačič et al., 2013). Different fungicides have different modes of action and differ in how long they last to suppress disease. At the same time, fungicide resistance occurs with the longer usage of the same fungicide type. For a greater chance of enhanced disease protection in the fields, it is strongly advised to rotate two or more distinct classes of fungicides to prevent the development of fungicide-resistant pathogens (Förster et al., 2007). It is hard to achieve sustainable disease management by using fungicides alone. Hence, the need for integrated disease management is discussed.
7.7 Advances in molecular aspects of detection as well as management of damping-off diseases
Recent advances in the molecular understanding of damping-off diseases have significantly enhanced detection and management strategies. Molecular diagnostics, such as polymerase chain reaction (PCR) and quantitative PCR (qPCR), now allow for rapid and precise identification of soil-borne pathogens like Pythium, Fusarium, and Rhizoctonia at early stages of infection. These techniques enable species-level identification and quantification of pathogen load in soil and plant tissues, which can help predict disease outbreaks. The advent of metagenomics and next-generation sequencing (NGS) has further revolutionized detection by allowing a comprehensive analysis of microbial communities, identifying emerging pathogens, and understanding the microbial shifts that occur during disease progression (Fu et al., 2024).
On the management side, molecular advances have improved the use of biological control agents and the development of resistant cultivars. Functional genomics, through tools like RNA interference (RNAi) and CRISPR-Cas9, is used to knock out or modify plant susceptibility genes, providing a novel method for increasing disease resistance. Furthermore, insights into the molecular interactions between pathogens and biocontrol agents, such as Trichoderma and Pseudomonas fluorescens, have led to enhanced formulations that can better suppress pathogen activity through targeted modes of action, such as mycoparasitism and antibiosis. These advances highlight the critical role of molecular tools in both early detection and integrated management approaches, offering a promising future for sustainable control of damping-off diseases.
8 Conclusion
Damping-off disease remains a formidable challenge in chili cultivation, further exacerbated by environmental changes and the advent of more aggressive pathogen strains. Our comprehensive examination reveals that integrated disease management is the key to addressing this issue. While chemical fungicides provide immediate control, their long-term usage is unsustainable due to environmental and human health concerns as well as the potential for resistant pathogen strains to develop. Sustainable management of damping-off requires a multifaceted approach, including the development of resistant chili varieties, the implementation of strategic cultural practices, and the adoption of novel technologies such as nanoparticles. These strategies must be adaptable to evolving climatic conditions and tailored to local agro-ecological contexts.
Moreover, there is a pressing need for further molecular studies to enhance our understanding of plant–microbe interactions, which could lead to more effective and targeted control measures. The innovative application of nanoparticles in the laboratory setting shows great promise and could pave the way for new biocontrol methods that are both effective and environmentally sustainable. Ultimately, a comprehensive integrated pest management framework that combines traditional practices with cutting-edge research is essential for the holistic and sustainable management of damping-off disease in chili cultivation. As we advance our scientific knowledge and integrate it into field-based applications, we move closer to safeguarding the future of chili production against the persistent challenges posed by this destructive disease.
8.1 Future prospects
The challenge of damping off disease remains as critical as ever, especially with its large-scale spread exacerbated by flooding and climate change. Despite extensive research on the epidemic nature of the disease, gaps still persist in our understanding of host–pathogen interactions, disease proliferation, and effective management strategies.
There is an urgent need to devise efficient integrated management strategies that consider the increasing incidence of flooding, environmental factors, and the variability of pathogenic races. Developing resistant varieties of chili appears to be one of the most promising approaches for the long-term management of damping-off disease. By breeding for resistance, we can reduce the vulnerability of crops to this disease under various environmental conditions.
In addition to developing resistant varieties, integrating cultural practices tailored to specific climatic changes is crucial for more effective disease management. As the climate continues to change, previously effective strategies may need to be adapted to remain effective under new conditions. Furthermore, there is a growing need for more molecular studies to deepen our understanding of plant–microbe interactions. Insights into the infection mechanisms of pathogens will be invaluable for creating targeted management strategies that can interrupt the infection process and mitigate the disease’s impact.
Additionally, our laboratory is exploring the potential of nanoparticles as a novel approach to combat Pythium spp. Nanoparticles represent an innovative frontier in managing damping-off disease in chili. These microscopic particles could offer new mechanisms of action against the pathogen, potentially enhancing the effectiveness of existing treatments or providing alternative management solutions. As this research advances, it could open new pathways for controlling this persistent and damaging disease.
Author contributions
CD: Writing – original draft. GM-U-D: Writing – original draft. RA: Writing – review & editing, Data curation. TT: Writing – review & editing, Formal analysis, Data curation. RL: Writing – review & editing, Data curation, Conceptualization. YX: Writing – review & editing, Data curation, Conceptualization. SM: Writing – review & editing, Software, Conceptualization. AG: Writing – review & editing.
Funding
The author(s) declare that financial support was received for the research, authorship, and/or publication of this article. We are thankful to the projects of the Longdong University Doctoral Fund Project (XYBYZK2108), Gansu Provincial Natural Science Foundation Project (22JR5RM207), and Gansu Provincial University Youth Doctoral Fund Project (2021QB-120).
Conflict of interest
The authors declare that the research was conducted in the absence of any commercial or financial relationships that could be construed as a potential conflict of interest.
Publisher’s note
All claims expressed in this article are solely those of the authors and do not necessarily represent those of their affiliated organizations, or those of the publisher, the editors and the reviewers. Any product that may be evaluated in this article, or claim that may be made by its manufacturer, is not guaranteed or endorsed by the publisher.
Supplementary material
The Supplementary material for this article can be found online at: https://www.frontiersin.org/articles/10.3389/fmicb.2024.1479957/full#supplementary-material
Supplementary Figure S1. | The botanicals characteristics of chilli plant. (a) Chilli plant. (b) Ripe red fruit. (c) Immature green fruits. (d) Ripe red fruit.
Supplementary Figure S2. | Characteristics symptoms of damping-off disease on young seedlings of chilli plants. (a) Symptoms on young seedlings. (b) Whitish growth on the stem near soil surface. (c) Diseased plants wilted after severe attack. (d) Healthy plants.
References
Aashish, K. C., Kumar, J., Silas, V. J., Giri, H., Khadka, H., and Yadav, M. K. (2022). Effect of foliar application of urea and naphthaleneacetic acid (NAA) on growth and yield of chili (Capsicum annum L.) Var Pusa Jwala. Pharma Innov. J. 11, 1187–1198.
Abbasi, P. A., Soltani, N., Cuppels, D. A., and Lazarovits, G. (2002). Reduction of bacterial spot disease severity on tomato and pepper plants with foliar applications of ammonium lignosulfonate and potassium phosphate. Plant Dis. 86, 1232–1236. doi: 10.1094/PDIS.2002.86.11.1232
Ahn, H. I., Yoon, J. Y., Hong, J. S., Yoon, H. I., Kim, M. J., Ha, J. H., et al. (2006). The complete genome sequence of pepper severe mosaic virus and comparison with other potyviruses. Arch. Virol. 151, 2037–2045. doi: 10.1007/s00705-006-0776-1
Al-Hussini, H. S., Al-Rawahi, A. Y., Al-Marhoon, A. A., Al-Abri, S. A., Al-Mahmooli, I. H., Al-Sadi, A. M., et al. (2019). Biological control of damping-off of tomato caused by Pythium aphanidermatum by using native antagonistic rhizobacteria isolated from Omani soil. J. Plant Pathol. 101, 315–322. doi: 10.1007/s42161-018-0184-x
Ali, M., Shahid, A. A., and Haider, M. S. (2016). Isolation and in-vitro screening of potential antagonistic. Pakistan J. Phytopathol. 28, 231–240.
Ansari, N. A., Khan, M. W., and Muheet, A. (1990). Evaluation of some fungicides for seed treatment and foliar application in management of damping-off of seedlings and blight of rapeseed caused by Alternaria brassicae. Mycopathologia 110, 163–167. doi: 10.1007/BF00437541
Arom, P. (2022). Influence of irrigation regimes on physiological traits, yield, and capsaicinoids production in chili. Doctoral dissertation, School of Crop Production Institute of Agricultural Technology Suranaree University of Technology.
Arora, H., Sharma, A., Poczai, P., Sharma, S., Haron, F. F., Gafur, A., et al. (2022). Plant-derived protectants in combating soil-borne fungal infections in tomato and chili. J. Fungi 8:213. doi: 10.3390/jof8020213
Arora, H., Sharma, A., Sharma, S., Haron, F. F., Gafur, A., Sayyed, R. Z., et al. (2021). Pythium damping-off and root rot of capsicum annuum l.: impacts, diagnosis, and management. Microorganisms 9:823. doi: 10.3390/microorganisms9040823
Ashwini, N., and Srividya, S. (2014). Potentiality of Bacillus subtilis as biocontrol agent for management of anthracnose disease of chilli caused by Colletotrichum gloeosporioides OGC1. Three biotech 4, 127–136. doi: 10.1007/s13205-013-0134-4
Awang, N. A. A., Islam, M. R., Ismail, M. R., Zulkarami, B., and Omar, D. (2013). Effectiveness of different elicitors in inducing resistance in chili (Capsicum annuum L.) against pathogen infection. Sci. Hortic. 164, 461–465. doi: 10.1016/j.scienta.2013.08.038
Azlan, A., Sultana, S., Huei, C. S., and Razman, M. R. (2022). Antioxidant, anti-obesity, nutritional and other beneficial effects of different chili pepper: A review. Molecules 27:898. doi: 10.3390/molecules27030898
Babadoost, M., and Islam, S. Z. (2003). Fungicide seed treatment effects on seedling damping-off of pumpkin caused by Phytophthora capsici. Plant Dis. 87, 63–68. doi: 10.1094/PDIS.2003.87.1.63
Bahari, A., Castillo, A. G., Safaie, N., Bejarano, E. R., Luna, A. P., and Shams-Bakhsh, M. (2022). Functional analysis of v2 protein of beet curly top Iran virus. Plan. Theory 11:3351. doi: 10.3390/plants11233351
Bailey, K. L., and Lazarovits, G. (2003). Suppressing soil-borne diseases with residue management and organic amendments. Soil Tillage Res. 72, 169–180. doi: 10.1016/S0167-1987(03)00086-2
Barboza, G. E., García, C. C., de Bem Bianchetti, L., Romero, M. V., and Scaldaferro, M. (2022). Monograph of wild and cultivated chili peppers (Capsicum L., Solanaceae). Phytokeys 200, 1–423. doi: 10.3897/phytokeys.200.71667
Behbehani, J. M., Irshad, M., Shreaz, S., and Karched, M. (2023). Anticandidal activity of capsaicin and its effect on ergosterol biosynthesis and membrane integrity of Candida albicans. Int. J. Mol. Sci. 24:1046. doi: 10.3390/ijms24021046
Benvenuto, D., Giovannetti, M., Ciccozzi, A., Spoto, S., Angeletti, S., and Ciccozzi, M. (2020). Advocating a need for suitable breeding approaches to boost integrated pest management: A European perspective. Pest Manag. Sci. 74, 1219–1227.
Bhattarai, A., Sharma, A., Yadav, R. K., and Wagle, P. (2022). Interacting effects of botanicals, biocontrol agents, and potting media on Rhizoctonia solani led damping-off of okra seedlings. J. Agric. Food Res. 10:100410. doi: 10.1016/j.jafr.2022.100410
Buchenau, G. W., Vigil, F. R., Mankin, C. J., and Wirth, C. W. (1981). Control of alfalfa damping-off with seed treatment. Phytopathology 71, 205–206.
Butt, M., Sattar, A., Abbas, T., Hussain, R., Ijaz, M., Sher, A., et al. (2021). Morpho-physiological and biochemical attributes of chili (Capsicum annum L.) genotypes grown under varying salinity levels. PLoS One 16:e0257893. doi: 10.1371/journal.pone.0257893
Chattopadhyay, B., Singh, A. K., Yadav, T., Fauquet, C. M., Sarin, N. B., and Chakraborty, S. (2008). Infectivity of the cloned components of a begomovirus: DNA beta complex causing chili leaf curl disease in India. Arch. Virol. 153, 533–539. doi: 10.1007/s00705-007-0017-2
Chen, X. R., Liu, B. B., Xing, Y. P., Cheng, B. P., Liu, M. L., Tong, Y. H., et al. (2016). Identification and characterization of Phytopythium helicoides causing stem rot of Shatangju mandarin seedlings in China. Eur. J. Plant Pathol. 146, 715–727. doi: 10.1007/s10658-016-0952-4
Chung, M. K., and Campbell, J. N. (2016). Use of capsaicin to treat pain: mechanistic and therapeutic considerations. Pharmaceuticals 9:66. doi: 10.3390/ph9040066
Compant, S., Van Der Heijden, M. G. A., and Sessitsch, A. (2010). Climate change effects on beneficial plant-microorganism interactions. FEMS Microbiol. Ecol. 73, 197–214. doi: 10.1111/j.1574-6941.2010.00900.x
Cook, R. J. (2001). Management of wheat and barley root diseases in modern farming systems. Australas. Plant Pathol. 30, 119–126. doi: 10.1071/AP01010
Cook, R. J., and Haglund, W. A. (1991). Wheat yield depression associated with conservation tillage caused by root pathogens in the soil not phytotoxins from the straw. Soil Biol. Biochem. 23, 1125–1132. doi: 10.1016/0038-0717(91)90024-E
Dai, T. T., Meng, J., Dong, S., Lu, C. C., Ye, W., Zheng, X., et al. (2013). A Phytophthora conserved transposon-like DNA element as a potential target for soyabean root rot disease diagnosis. Plant Pathol. 62, 719–726. doi: 10.1111/j.1365-3059.2012.02672.x
Davis, M. S. J. (2009). “Keys to the increased use of host plant resistance in integrated pest management” in Integr. Pest Manag. Innov. process. eds. R. Peshin and A. K. Dhawan, vol. 1, 1 (Dordrecht: Springer Netherlands), 163–181.
Dubey, M. K., Zehra, A., Aamir, M., Yadav, M., Samal, S., and Sanmukh, R. (2019). Isolation, identification, carbon utilization profile and control of Pythium graminicola, the causal agent of chili damping-off. J. Phytopathol., 1–15. doi: 10.1111/jph.12872
Duniway, J. M. (2002). Status of chemical alternatives to methyl bromide for pre-plant fumigation of soil. Phytopathology 92, 1337–1343. doi: 10.1094/PHYTO.2002.92.12.1337
El Enshasy, H. A., Ambehabati, K. K., El Baz, A. F., Ramchuran, S., Sayyed, R. Z., Amalin, D., et al. (2020). Trichoderma: biocontrol agents for promoting plant growth and soil health. Trichoderma 2, 239–259. doi: 10.1007/978-3-030-48474-3_8
El-Bendary, M. A., Hamed, H. A., and Moharam, M. E. (2016). Potential of Bacillus isolates as bio-control agents against some fungal phytopathogens. Biocatal. Agric. Biotechnol. 5, 173–178. doi: 10.1016/j.bcab.2016.02.001
El-Garhy, H. A. S., Abdel-Rahman, F., Sham, A. S., Osman, G., and Moustafa, M. M. A. (2020). Comparative analyses of four chemicals used to control black mold disease in tomato and its effects. Plan. Theory 9:808. doi: 10.3390/plants9070808
El-Tarabily, K. A., Nassar, A. H., Hardy, G. E. S. J., and Sivasithamparam, K. (2009). Plant growth promotion and biological control of Pythium aphanidermatum, a pathogen of cucumber, by endophytic actinomycetes. J. Appl. Microbiol. 106, 13–26. doi: 10.1111/j.1365-2672.2008.03926.x
FAOSTAT. (2020). Food and Agriculture Organization Corporate Statistical Database. Available online at: http://www.fao.org/faostat/en/
Fattori, V., Hohmann, M. S. N., Rossaneis, A. C., Pinho-Ribeiro, F. A., and Verri, W. A. (2016). Capsaicin: current understanding of its mechanisms and therapy of pain and other pre-clinical and clinical uses. Molecules 21:844. doi: 10.3390/molecules21070844
Figueiredo, J., Vieira, P., Abrantes, I., and Esteves, I. (2021). Detection of the root lesion nematode Pratylenchus penetrans in potato tubers. Plant Pathol. 70, 1960–1968. doi: 10.1111/ppa.13425
Fletcher, J. D. (1983). New plant disease records in New Zealand: additional hosts of alfalfa mosaic virus. New Zeal. J. Agric. Res. 26, 403–404. doi: 10.1080/00288233.1983.10427050
Förster, H., Driever, G. F., Thompson, D. C., and Adaskaveg, J. E. (2007). Postharvest decay management for stone fruit crops in California using the “reduced-risk” fungicides fludioxonil and fenhexamid. Plant Dis. 91, 209–215. doi: 10.1094/PDIS-91-2-0209
Fu, C. C., Huang, B. X., Wang, S. S., Song, Y. C., Metok, D., Tan, Y. X., et al. (2024). Deciphering the roles of bacterial and fungal communities in the formation and quality of agarwood. Stress Biology 4:40. doi: 10.1007/s44154-024-00179-5
Gaikwad, P. N., Sharma, V., Singh, J., Sidhu, G. S., Singh, H., and Omar, A. A. (2023). Biotechnological advancements in Phytophthora disease diagnosis, interaction and management in citrus. Sci. Hortic. 310:111739. doi: 10.1016/j.scienta.2022.111739
Galloway, B. A., Monks, D. W., and Schultheis, J. R. (2000). Effect of herbicides on pepper (Capsicum annuum) stand establishment and yield from transplants produced using various irrigation systems. Weed Technol. 14, 241–245. doi: 10.1614/0890-037X(2000)014[0241:EOHOPC]2.0.CO;2
Gao, R., Hu, B., Yuan, Y., He, M., Wang, R., Lou, Y., et al. (2023). Nitrogen addition affects floral and vegetative traits, reproduction, and pollinator performance in Capsicum annuum L. Ann. Bot. 132, 1131–1144. doi: 10.1093/aob/mcad121
Garzón, C. D., Molineros, J. E., Yánez, J. M., Flores, F. J., del Mar Jiménez-Gasco, M., and Moorman, G. W. (2011). Sublethal doses of mefenoxam enhance Pythium damping-off of geranium. Plant Dis. 95, 1233–1238. doi: 10.1094/PDIS-09-10-0693
Gholve, V. M., Tatikundalwar, V. R., Suryawanshi, A. P., and Dey, U. (2014). Effect of fungicides, plant extracts/botanicals and bioagents against damping off in brinjal. African J. Microbiol. Res. 8, 2835–2848. doi: 10.5897/ajmr2013.6336
Ghorbani, A., Emamverdian, A., Pehlivan, N., Zargar, M., Razavi, S. M., and Chen, M. X. (2024a). Nano-enabled agrochemicals: mitigating heavy metal toxicity and enhancing crop adaptability for sustainable crop production. J. Nanobiotechnol. 22:91. doi: 10.1186/s12951-024-02371-1
Ghorbani, A., Emamverdian, A., Pishkar, L., Chashmi, K. A., Salavati, J., Zargar, M., et al. (2023). Melatonin-mediated nitric oxide signaling enhances adaptation of tomato plants to aluminum stress. S. Afr. J. Bot. 162, 443–450. doi: 10.1016/j.sajb.2023.09.031
Ghorbani, A., Pehlivan, N., Zargar, M., and Chen, M. (2024b). Synergistic role of melatonin and hydrogen sulfide in modulating secondary metabolites and metal uptake/sequestration in arsenic-stressed tomato plants. Sci. Hortic. 331:113159. doi: 10.1016/j.scienta.2024.113159
Glawe, D. A., Barlow, T., Eggers, J. E., and Hamm, P. B. (2010). First report of powdery mildew caused by Leveillula taurica of field-grown sweet pepper in the pacific northwest. Plant Heal. Prog. 11, 2–3. doi: 10.1094/php-2007-0708-01-br
Gomathi, S., Ambikapathy, V., and Pannerselvam, A. (2012). Potential strain of Trichoderma spp. To control dampingoff disease in chili. Int. J. Appl. Biol. Pharm. Technol. 2, 64–69.
Guo, H., Tian, H., Wang, Y., Xiong, X., Tian, H., and Suqin Zhang, G. G. (2022). Comparative analysis of growth, physiological and transcriptomic response revealed mechanisms of waterlogging tolerance of hot pepper (Capsicum annuum var. Conoides). Plant Breed. 141, 807–819. doi: 10.1111/pbr.13050
Hansen, Z. R., and Keinath, A. P. (2013). Increased pepper yields following incorporation of biofumigation cover crops and the effects on soilborne pathogen populations and pepper diseases. Appl. Soil Ecol. 63, 67–77. doi: 10.1016/j.apsoil.2012.09.007
Hanson, L. E., and Howell, C. R. (2004). Elicitors of plant defense responses from biocontrol strains of Trichoderma virens. Phytopathology 94, 171–176. doi: 10.1094/PHYTO.2004.94.2.171
Heiser, C. B. (1976). “Peppers capsicum (Solanaceae)” in The evolution of crops plants. ed. S. W. Simmonds (London: Longman Press), 265–268.
Hickman, G. W., and Michailides, T. J. (1998). Control options for greenhouse cucumber damping-off disease. J. Veg. Crop. Prod. 4, 45–48. doi: 10.1300/J068v04n01_05
Huang, C. J., Wang, T. K., Chung, S. C., and Chen, C. Y. (2005). Identification of an antifungal chitinase from a potential biocontrol agent, Bacillus cereus 28-9. J. Biochem. Mol. Biol. 38, 82–88. doi: 10.5483/bmbrep.2005.38.1.082
Hwang, S. F., Ahmed, H., and Turnbull, G. D. (2008). Effect of crop rotation on canola seedling blight and soil pathogen population dynamics. Can. J. Plant Pathol. 30:369.
Hwang, S. F., Gossen, B. D., Turnbull, G. D., Chang, K. F., Howard, R. J., and Thomas, A. G. (2000). Seeding date, temperature, and seed treatment affect Pythium seedling blight of field pea. Can. J. Plant Pathol. 22, 392–399. doi: 10.1080/07060660009500458
Hyder, S., Gondal, A. S., Rizvi, Z. F., Atiq, R., Haider, M. I. S., Fatima, N., et al. (2021). Biological control of chili damping-off disease, caused by Pythium myriotylum. Front. Microbiol. 12:587431. doi: 10.3389/fmicb.2021.587431
IBPGR (1983). “Genetics resources of Capsicum” in The Capsicum Genome. Compendium of Plant Genomes. eds. N. Ramchiary and C. Kole (Cham: Springer).
Islam, M. T., and Faruq, A. N. (2012). Effect of some medicinal plant extracts on damping-off disease of winter vegetable. World Appl. Sci. J. 17, 1498–1503.
Jayapala, N., Mallikarjunaiah, N. H., Puttaswamy, H., Gavirangappa, H., and Ramachandrappa, N. S. (2019). Rhizobacteria Bacillus spp. induce resistance against anthracnose disease in chili (Capsicum annuum L.) through activating host defense response. Egypt. J. Biol. Pest Control 29, 1–19. doi: 10.1186/s41938-019-0148-2
Jayaraj, J., Radhakrishnan, N. V., and Velazhahan, R. (2006). Development of formulations of Trichoderma harzianum strain M1 for control of damping-off of tomato caused by Pythium aphanidermatum. Arch. Phytopathol. Plant Protect. 39, 1–8. doi: 10.1080/03235400500094720
Jia, Z. C., Yang, X., Wu, Y. K., Li, M., Das, D., Chen, M. X., et al. (2024). The art of finding the right drug target: emerging methods and strategies. Pharmacol. Rev. 76, 896–914. doi: 10.1124/pharmrev.123.001028
Jimtha, J. C., Jishma, P., Arathy, G. B., Anisha, C., and Radhakrishnan, E. K. (2016). Identification of plant growth promoting rhizosphere bacillus sp. WG4 antagonistic to Pythium myriotylum and its enhanced antifungal effect in association with Trichoderma. J. Soil Sci. Plant Nutr. 16, 578–590. doi: 10.4067/s0718-95162016005000026
Johari, A., Herlinda, S., Pujiastuti, Y., Irsan, C., and Sartiami, D. (2014). Morphological and genetic variation of thrips parvispinus (Thysanoptera: Thripidae) in chili plantation (Capsicum annuum L.) in the lowland and highland of Jambi Province. Am. J. Biosci. 2, 17–21. doi: 10.11648/j.ajbio.s.2014020601.14
Joukhadar, I., and Walker, S. (2020). Effect of stand reduction at different growth stages on yield of paprika-type Chile pepper. Horticulturae 6:16. doi: 10.3390/horticulturae6010016
Kandeler, E., Mosier, A. R., Morgan, J. A., Milchunas, D. G., King, J. Y., Rudolph, S., et al. (2006). Response of soil microbial biomass and enzyme activities to the transient elevation of carbon dioxide in a semi-arid grassland. Soil Biol. Biochem. 38, 2448–2460. doi: 10.1016/j.soilbio.2006.02.021
Kaur, S., Kang, S. S., Sharma, A., and Sharma, S. (2014). First report of pepper mottle virus infecting chili pepper in India. New Dis. Reports 30:14. doi: 10.5197/j.2044-0588.2014.030.014
Kenawy, A., Dailin, D. J., Abo-Zaid, G. A., Malek, R. A., Ambehabati, K. K., Zakaria, K. H. N., et al. (2019). “Biosynthesis of antibiotics by PGPR and their roles in biocontrol of plant diseases” in Plant growth promoting rhizobacteria for sustainable stress management. Volume 2: rhizobacteria in biotic stress management, 1–35.
Khaitov, B., Umurzokov, M., Cho, K.-M., Lee, Y.-J., Park, K. W., and Sung, J. (2019). Importance and production of chili pepper; heat tolerance and efficient nutrient use under climate change conditions. Korean J. Agric. Sci. 46, 769–779. doi: 10.7744/kjoas.20190059
Khrieba, M. I. (2020). “Damping-off caused by Pythium species: disease profile and management” in Pythium (Boca Raton, FL: CRC Press), 257–269.
Kipngeno, P., Losenge, T., Maina, N., Kahangi, E., and Juma, P. (2015). Efficacy of Bacillus subtilis and Trichoderma asperellum against Pythium aphanidermatum in tomatoes. Biol. Control 90, 92–95. doi: 10.1016/j.biocontrol.2015.05.017
Kiselev, A. (2020). Multiomics identification of effectors candidates from oomycete root plant pathogen Aphanomyces euteiches. Doctoral dissertation. Toulouse: Université Paul Sabatier-Toulouse III.
Kovačič, G. R., Lešnik, M., and Vršič, S. (2013). An overview of the copper situation and usage in viticulture. Bulg. J. Agric. Sci. 19, 50–59.
Kramchote, S., and Suwor, P. (2022). Preharvest chitosan effects on growth, yield and quality of ‘super hot’ and ‘num khao’ chili pepper (Capsicum annuum L.). Hortic. J. 91, 522–530. doi: 10.2503/hortj.UTD-360
Kumar, K., Mandal, S. N., Neelam, K., and de los Reyes, B. G. (2022). Microrna-mediated host defense mechanisms against pathogens and herbivores in rice: balancing gains from genetic resistance with trade-offs to productivity potential. BMC Plant Biol. 22:351. doi: 10.1186/s12870-022-03723-5
Kumhar, K. C., Kumar, K., Arora, I., Bhatia, A. K., Batra, V. K., and Raj, H. (2022). Management of tomato damping-off disease in the nursery using of Trichoderma asperellum. Int. J. Econ. Plants 9, 145–151. doi: 10.23910/2/2022.0427c
Lamichhane, J. R., Dachbrodt-Saaydeh, S., Kudsk, P., and Messéan, A. (2016). Toward a reduce reliance on conventional pesticides in agriculture. Plant Dis. 100, 10–24. doi: 10.1094/PDIS-05-15-0574-FE
Lamichhane, J. R., Dürr, C., Schwanck, A. A., Robin, M. H., Sarthou, J. P., Cellier, V., et al. (2017). Integrated management of damping-off diseases. A review. Agron. Sustain. Dev. 37:417. doi: 10.1007/s13593-017-0417-y
Lara-Capistran, L., Zulueta-Rodriguez, R., Castellanos-Cervantes, T., Reyes-Perez, J. J., Preciado-Rangel, P., and Hernandez-Montiel, L. G. (2020). Efficiency of marine bacteria and yeasts on the biocontrol activity of Pythium ultimum in ancho-type pepper seedlings. Agronomy 10:408. doi: 10.3390/agronomy10030408
Lewis Ivey, M. L., and Miller, S. A. (2000). First report of bacterial canker of pepper in Ohio. Plant Dis. 84:810. doi: 10.1094/PDIS.2000.84.7.810C
Li, X., Guo, K., Zhang, Y., and Yan, X. (2010). First report of the stubby root nematode, paratrichodorus minor, in mainland China. Plant Dis. 94:376. doi: 10.1094/PDIS-94-3-0376A
Long, Y. Y., Wei, J. G., Sun, X., He, Y. Q., Luo, J. T., and Guo, L. D. (2012). Two new Pythium species from China based on the morphology and DNA sequence data. Mycol. Prog. 11, 689–698. doi: 10.1007/s11557-011-0778-6
López-Marín, J., González, A., and Gálvez, A. (2011). Effect of shade on quality of greenhouse peppers. Acta Hortic. 893, 895–900. doi: 10.17660/actahortic.2011.893.99
Luo, X., and Yu, C. (2020). First report of damping-off disease caused by fusarium oxysporum in Pinus massoniana in China. J. Plant Dis. Prot. 127, 401–409. doi: 10.1007/s41348-020-00303-3
Mabuchi, N., Hashizume, I., and Araki, Y. (2000). Characterization of chitinases excreted by Bacillus cereus CH. Can. J. Microbiol. 46, 370–375. doi: 10.1139/w99-148
MacNeish, R. S. (1964). Ancient Mesoamerican civilization. Science 143, 531–537. doi: 10.1126/science.143.3606.531
Magnee, K. J. H., Postma, J., Groot, S. P. C., Gort, G., van Bueren, E. T. L., and Scholten, O. E. (2022). Evaluation of damping-off tolerance in spinach cultivars in field soils and in a standardized lab assay with Pythium ultimum. Plant Heal. Prog. 23, 174–187. doi: 10.1094/PHP-07-21-0107-RS
Maharijaya, A., Vosman, B., Steenhuis-Broers, G., Harpenas, A., Purwito, A., Visser, R. G. F., et al. (2011). Screening of pepper accessions for resistance against two thrips species (Frankliniella occidentalis and Thrips parvispinus). Euphytica 177, 401–410. doi: 10.1007/s10681-010-0277-x
Majeed, M., Hassan Mir, G., Hassan, M., Mohuiddin, F. A., Paswal, S., and Farooq, S. (2018). Damping off in Chilli and its biological management-A review. IntJCurrMicrobiolAppSci 7, 2175–2185. doi: 10.20546/ijcmas.2018.704.247
Majeed, M., Mir, G. H., Hassan, M., and Mohuiddin, F. A. (2019). Biological management of damping-off disease of chili (Capsicum annuum L.). Eco. Env. Cons. 25, 353–356.
Male, M. F., and Vawdrey, L. L. (2010). Efficacy of fungicides against damping-off in papaya seedlings caused by Pythium aphanidermatum. Aust Plant Dis Notes 5, 103–104. doi: 10.1071/DN10037
Manjunath, M., Prasanna, R., Nain, L., Dureja, P., Singh, R., Kumar, A., et al. (2010). Biocontrol potential of cyanobacterial metabolites against damping off disease caused by Pythium aphanidermatum in solanaceous vegetables. Arch. Phytopathol. Plant Protect. 43, 666–677. doi: 10.1080/03235400802075815
Manjunatha, V., and Bhattacharjee, D. And Flores, C. (2022). Disease management of faba beans. In Faba bean: Chemistry, properties and functionality. (pp. 357–394). Cham: Springer International Publishing.
Mannai, S., and Boughalleb-M’Hamdi, N. (2023). Evaluation of Trichoderma harzianum, Bacillus subtilis and aspergillus species efficacy in controlling Pythium ultimum associated with apple seedlings decline in nurseries and their growth promotion effect. Egypt. J. Biol. Pest Control 33:59. doi: 10.1186/s41938-023-00705-z
Marcelis, L. F. M., and Ho, L. C. (1999). Blossom-end rot in relation to growth rate and calcium content in fruits of sweet pepper (Capsicum annuum L.). J. Exp. Bot. 50, 357–363. doi: 10.1093/jxb/50.332.357
Marini, E., Magi, G., Mingoia, M., Pugnaloni, A., and Facinelli, B. (2015). Antimicrobial and anti-virulence activity of capsaicin against erythromycin-resistant, cell-invasive group A streptococci. Front. Microbiol. 6:1281. doi: 10.3389/fmicb.2015.01281
Martin, F. N., and Loper, J. E. (1999). Soilborne plant diseases caused by pythium spp.: ecology, epidemiology, and prospects for biological control. CRC. Crit. Rev. Plant Sci. 18, 111–181. doi: 10.1080/07352689991309216
Maurya, D. K., and Sharma, D. (2023). Culinary spices and herbs in managing early and long-COVID-19 complications: A comprehensive review. Phyther. Res. 37, 4908–4931. doi: 10.1002/ptr.7957
Mcquilken, M. P., Gemmell, J., and Lahdenperä, M. L. (2001). Gliocladium catenulatum as a potential biological control agent of damping-off in bedding plants. J. Phytopathol. 149, 171–178. doi: 10.1046/j.1439-0434.2001.00602.x
Mehetre, S. T., and Kale, S. P. (2011). Comparative efficacy of thermophilic bacterium, Bacillus licheniformis (NR1005) and antagonistic fungi, Trichoderma harzianum to control Pythium aphanidermatum-induced damping off in chili (Capsicum annuum L.). Arch. Phytopathol. Plant Protect. 44, 1068–1074. doi: 10.1080/03235401003755262
Mehmood, N., Saeed, M., Zafarullah, S., Hyder, S., Rizvi, Z. F., Gondal, A. S., et al. (2023). Multifaceted impacts of plant-beneficial pseudomonas spp. in managing various plant diseases and crop yield improvement. ACS omega 8, 22296–22315. doi: 10.1021/acsomega.3c00870
Messéan, A., Lamichhane, J. R., and Menard, J.-M. (2016). Role of crop diversification to boost IPM and implications for breeding. Breed. IPM Sustain. Low-input Agric. Syst :6.
Mishra, M., Singh, S. K., Shanker, R., and Sundaram, S. (2020). Design and simulation of diatom-based microcantilever immunobiosensor for the early detection of Karnal bunt. Three biotech 10, 201–213. doi: 10.1007/s13205-020-02191-8
Misra, V., Lal, R. J., Mall, A. K., and Srivastava, S. And Baitha, A. (2022). Integrated disease management in sugar beet for sustainable productivity. In Sugar beet cultivation, management and processing. (pp. 607–619). Singapore: Springer Nature Singapore.
Mondal, S., Panda, D., and Bose, B. (2022). “Seed biopriming with biopesticide: A key to sustainability of agriculture” in New and future developments in microbial biotechnology and bioengineering, 265–288.
Moura, A. B., Backhouse, D., de Souza Júnior, I. T., and Gomes, C. B. (2022). “Soilborne pathogens” in Subsoil constraints for crop production (Cham: Springer International Publishing), 199–224.
Moury, B., Palloix, A., Caranta, C., Gognalons, P., Souche, S., Selassie, K. G., et al. (2005). Serological, molecular, and pathotype diversity of pepper veinal mottle virus and chili veinal mottle virus. Phytopathology 95, 227–232. doi: 10.1094/PHYTO-95-0227
Mustafa, Z., Pervez, M. A., Ayyub, C. M., Matloob, A., Khaliq, A., Hussain, S., et al. (2014). Morpho-physiological characterization of chili genotypes under nacl salinity. Soil Environ. 33, 133–141.
Muthukumar, A., Eswaran, A., Nakkeeran, S., and Sangeetha, G. (2010). Efficacy of plant extracts and biocontrol agents against Pythium aphanidermatum inciting chili damping-off. Crop Prot. 29, 1483–1488. doi: 10.1016/j.cropro.2010.08.009
Muthukumar, A., Eswaran, A., and Sangeetha, G. (2011a). Induction of systemic resistance by mixtures of fungal and endophytic bacterial isolates against Pythium aphanidermatum. Acta Physiol. Plant. 33, 1933–1944. doi: 10.1007/s11738-011-0742-8
Muthukumar, A., Eswaran, A., and Sangeetha, G. (2018). Occurrence, virulence and pathogenicity of species of Pythium inciting damping-off disease in chili. J Mycol Pl Pathol 40, 67–71.
Muthukumar, A., Eswaran, A., and Sanjeevkumas, K. (2011b). Exploitation of Trichoderma species on the growth of Pythium aphanidermatum in chili. Brazilian J. Microbiol. 42, 1598–1607. doi: 10.1590/S1517-83822011000400047
Nanehkaran, F. M., Razavi, S. M., Ghasemian, A., Ghorbani, A., and Zargar, M. (2024). Foliar applied potassium nanoparticles (K-NPs) and potassium sulfate on growth, physiological, and phytochemical parameters in Melissa officinalis L. under salt stress. Environ. Sci. Pollut. Res. 31, 31108–31122. doi: 10.1007/s11356-024-33306-w
Nawaz, K., Shahid, A. A., Anwar, W., Iftikhar, S., and Nasir, M. (2017). Genetic diversity of the Pythium spp. associated with root rot of chili (Capsicum annum L.) in Pakistan and its management through plant extracts. In: Proceedings of the 5th international conference on food, agricultural, biological and medical science (FABMS-2017), 84–89.
Nawaz, K., Shahid, A. A., Subhani, M. N., Anwar, W., and Aslam, M. (2016). First report of Pythium spinosum causing root rot of chili (Capsicum annuum) in Pakistan. Plant Dis. 100:526. doi: 10.1094/PDIS-07-15-0745-PDN
Nguyen, M. T., and Ranamukhaarachchi, S. L. (2010). Soil-borne antagonists for biological control of bacterial wilt disease caused by Ralstonia solanacearum in tomato and pepper. J. Plant Pathol. 92, 395–406. doi: 10.4454/jpp.v92i2.183
Njoroge, S. M. C., Riley, M. B., and Keinath, A. P. (2008). Effect of incorporation of Brassica spp. residues on population densities of soilborne microorganisms and on damping-off and fusarium wilt of watermelon. Plant Dis. 92, 287–294. doi: 10.1094/PDIS-92-2-0287
Olatunji, T. L., and Afolayan, A. J. (2018). The suitability of chili pepper (Capsicum annuum L.) for alleviating human micronutrient dietary deficiencies: A review. Food Sci. Nutr. 6, 2239–2251. doi: 10.1002/fsn3.790
Parveen, T., Meena, M., Jain, T., Rathore, K., Mehta, S., and Sharma, K. (2020). “Pythium aphanidermatum and its control measures” in Pythium (Boca Raton, FL: CRC Press), 299–313.
Pawaskar, M. M. (2023). Evaluating the potential of hypersaline bacteria as a biocontrol agent against microbial pathogens affecting chili (Capsicum annuum L.) plant. Doctoral dissertation. Taleigão: Goa University.
Pohronezny, K., Hewitt, M., Infante, J., and Datnoff, L. (1992). Wind and wind-generated sand injury as factors in infection of pepper by Xanthomonas campestris pv. Vesicatoria. Plant Dis. 76, 1036–1039. doi: 10.1094/PD-76-1036
Postma, J., Montanari, M., and Van Den Boogert, P. H. J. F. (2003). Microbial enrichment to enhance the disease suppressive activity of compost. Eur. J. Soil Biol. 39, 157–163. doi: 10.1016/S1164-5563(03)00031-1
Poveda, J., and Eugui, D. (2022). Combined use of Trichoderma and beneficial bacteria (mainly Bacillus and Pseudomonas): development of microbial synergistic bio-inoculants in sustainable agriculture. Biol. Control 176:105100. doi: 10.1016/j.biocontrol.2022.105100
Punja, Z. K., and Yip, R. (2003). Biological control of damping-off and root rot caused by Pythium aphanidermatum on greenhouse cucumbers. Can. J. Plant Pathol. 25, 411–417. doi: 10.1080/07060660309507098
Rahman, M. A., Sultana, R., Begum, M. F., and Alam, M. F. (2012). Effect of culture filtrates of Trichoderma on seed germination and seedling growth in chili international journal of biosciences. Int. J. Biosci. 2, 46–55.
Rai, M., Abd-Elsalam, K. A., and Ingle, A. P. (2020). Pythium: Diagnosis, diseases and management. Boca Raton, FL: CRC Press.
Ranjbar, M., Khakdan, F., Ghorbani, A., Zargar, M., and Chen, M. X. (2023). The variations in gene expression of GAPDH in Ocimum basilicum cultivars under drought-induced stress conditions. Environ. Sci. Pollut. Res. 30, 119187–119203. doi: 10.1007/s11356-023-30549-x
Rini, C. R., and Sulochana, K. K. (2006). Management of seedling rot of chili (Capsicum annuum L.) Using Trichoderma spp. And fluorescent pseudomonads (Pseudomonas fluorescens). J. Trop. Agric. 44, 79–82.
Roberts, D. P., Lakshman, D. K., McKenna, L. F., Emche, S. E., Maul, J. E., and Bauchan, G. (2016). Seed treatment with ethanol extract of serratia marcescens is compatible with trichoderma isolates for control of damping-off of cucumber caused by pythium ultimum. Plant Dis. 100, 1278–1287. doi: 10.1094/PDIS-09-15-1039-RE
Rosca, A. E., Iesanu, M. I., Zahiu, C. D. M., Voiculescu, S. E., Paslaru, A. C., and Zagrean, A. M. (2020). Capsaicin and gut microbiota in health and disease. Molecules 25:5681. doi: 10.3390/molecules25235681
Russell, K., Hamm, P. B., Sutherland, J. R., Hansen, E. M., Littke, W. R., Kliejunas, J. T., et al. (1990). Growing healthy seedlings: Identification and management of pests in northwest forest nurseries. United States: Department of Agriculture.
Sachin, M., Warghane, A., and Patil, G. (2022). Chili leaf curl disease: an Indian scenario. Indian Phytopathol. 75, 627–637. doi: 10.1007/s42360-022-00531-7
Saha, A., and Kumari, P. (2023). Effect of alkaline treatment on physical, structural, mechanical and thermal properties of Bambusa tulda (northeast Indian species) based sustainable green composites. Polym. Compos. 44, 2449–2473. doi: 10.1002/pc.27256
Saha, S., Rai, A. B., and Pandey, S. (2011). Efficacy of seed dressing agents against damping-off disease of chili (Capsicum frutescens). Indian J. Agric. Sci. 81, 92–93.
Salam, R., Deen, P., Upadhyay, D., Author, C., Rk, K. N., Singh, I., et al. (2022). Studies on leaf spot of chili caused by Cercospora capsici and its management in Manipur from Imphal east district. Pharma Innov. 11, 4363–4374.
Saleh, B. K., Omer, A., and Teweldemedhin, B. (2018). Medicinal uses and health benefits of chili pepper (Capsicum spp.): a review. MOJ Food Process. Technol. 6, 325–328. doi: 10.15406/mojfpt.2018.06.00183
Sanogo, S. (2003). Chile pepper and the threat of wilt diseases. Plant Heal. Prog. 4:23. doi: 10.1094/PHP-2003-0430-01-RV
Sanogo, S., and Carpenter, J. (2006). Incidence of Phytophthora blight and Verticillium wilt within Chile pepper fields in New Mexico. Plant Dis. 90, 291–296. doi: 10.1094/PD-90-0291
Sarkar, A., Chakraborty, N., and Acharya, K. (2022). Chitosan nanoparticles mitigate Alternaria leaf spot disease of chili in nitric oxide dependent way. Plant Physiol. Biochem. 180, 64–73. doi: 10.1016/j.plaphy.2022.03.038
Sawant, K., Kumar, D., Chavan, S. S., Chavan, A., Reddy, S. K., Akhilesh, C., et al. (2022). Recent advances on Colletotrichum capsici causing anthracnose of chili: A review. Plant Sci. Today 10, 63–71. doi: 10.14719/pst.2196
Saxena, A., Raghuwanshi, R., Gupta, V. K., and Singh, H. B. (2016). Chili anthracnose: the epidemiology and management. Front. Microbiol. 7:1527. doi: 10.3389/fmicb.2016.01527
Saxena, A., Raghuwanshi, R., and Singh, H. B. (2014). Molecular, phenotypic and pathogenic variability in Colletotrichum isolates of subtropical region in North-Eastern India, causing fruit rot of chilies. J. Appl. Microbiol. 117, 1422–1434. doi: 10.1111/jam.12607
Schmidt, C. S., Agostini, F., Leifert, C., Killham, K., and Mullins, C. E. (2004). Influence of inoculum density of the antagonistic bacteria Pseudomonas fluorescens and Pseudomonas corrugata on sugar beet seedling colonisation and suppression of Pythium damping off. Phytopathology 265, 111–122. doi: 10.1007/s11104-005-8943-9
Shahid, A. A., Ali, M., and Ali, S. (2017). First report of Pythium debaryanum causing chili damping off in Pakistan. Plant Dis. 101:391. doi: 10.1094/PDIS-08-16-1209-PDN
Shaver, B. R., Agudelo, P., and Martin, S. B. (2017). Damage functions for sting nematode on bermudagrass turf. ITSRJ 13, 517–523. doi: 10.2134/itsrj2016.06.0478
Shimbov, B., Alguacil, M., and Suárez, C. (2019). Export structure upgrading and economic growth in the western Balkan countries. Emerg. Mark. Financ. Trade 55, 2185–2210. doi: 10.1080/1540496X.2018.1563538
Singh, R. N., Kumar, A., Sheohar, K., Kumar, I. P., Thakur, S. K., Kumar, P., et al. (2019). Management of damping off disease of papaya seedlings in nursery. J. Entomol. Zool. Stud. 7, 1657–1660.
Sridapan, T., and Krajaejun, T. (2023). Nucleic acid-based detection of Pythium insidiosum: A systematic review. J. Fungi 9:27. doi: 10.3390/jof9010027
Stommel, J. R., Goth, R. W., Haynes, K. G., and Kim, S. H. (1996). Pepper (Capsicum annuum) soft rot caused by Erwinia carotovora subsp. atroseptica. APS 81:305.
Su, F., Das, D., Aslam, M. M., Xie, Q., Li, Y., and Chen, X. (2023). Eukaryotic splicing machinery in the plant–virus battleground. Wiley Interdisciplinary Reviews RNA 14:e1793. doi: 10.1002/wrna.1793
Subash, N., Meenakshisundaram, M., Unnamalai, N., and Sasikumar, C. (2013). In vitro evaluation of different strains of Trichoderma harzainum as biocontrol agents of chili. Int. J. Biol. Pharm. Appl. Sci 2, 495–500.
Sundari, M. T., Darsono, D., Sutrisno, J., and Antriyandarti, E. (2023). Analysis of trade potential and factors influencing chili export in Indonesia. Open Agric. 8:20220205. doi: 10.1515/opag-2022-0205
Suryanto, D. W. I., Patonah, S. I. T. I., and Munir, E. (2010). Control of fusarium wilt of chili with chitinolytic bacteria. HAYATI J. Biosci. 17, 5–8. doi: 10.4308/hjb.17.1.5
Tapia, D. H., Troncoso, A. J., Vargas, R. R., Olivares-Donoso, R., and Niemeyer, H. M. (2008). Experimental evidence for competitive exclusion of Myzus persicae nicotianae by Myzus persicae s.s. (Hemiptera: Aphididae) on sweet pepper, Capsicum annuum (Solanaceae). Eur. J. Entomol. 105, 643–648. doi: 10.14411/eje.2008.088
Thakur, P., and Singh, I. (2023). “Impact of Fungi on the world economy and its sustainability: current status and potentials” in Fungal resources for sustainable economy. Current status and future perspectives (Singapore: Springer Nature Singapore), 3–37.
Thambugala, K. M., Daranagama, D. A., Phillips, A. J. L., Kannangara, S. D., and Promputtha, I. (2020). Fungi vs. Fungi in biocontrol: an overview of fungal antagonists applied against fungal plant pathogens. Front. Cell. Infect. Microbiol. 10:604923. doi: 10.3389/fcimb.2020.604923
Than, P. P., Jeewon, R., Hyde, K. D., Pongsupasamit, S., Mongkolporn, O., and Taylor, P. W. J. (2008). Characterization and pathogenicity of Colletotrichum species associated with anthracnose on chili (Capsicum spp.) in Thailand. Plant Pathol. 57, 562–572. doi: 10.1111/j.1365-3059.2007.01782.x
Thiyagarajan, S. S. (2014). Biological control of root knot nematodes in chilies through Pseudomonas fluorescens’s antagonistic mechanism. J. Plant Sci. 2:152. doi: 10.11648/j.jps.20140205.12
Tyśkiewicz, R., Nowak, A., Ozimek, E., and Jaroszuk-ściseł, J. (2022). Trichoderma: the current status of its application in agriculture for the biocontrol of fungal phytopathogens and stimulation of plant growth. Int. J. Mol. Sci. 23:2329. doi: 10.3390/ijms23042329
Varghese, T. S., and Mathew, T. B. (2013). Evaluation of newer insecticides against chili aphids and their effect on natural enemies. Pest Manag. Hortic. Ecosyst. 18, 114–117.
Vauterin, L., Rademaker, J., and Swings, J. (2000). Synopsis on the taxonomy of the genus Xanthomonas. Phytopathology 90, 677–682. doi: 10.1094/PHYTO.2000.90.7.677
Venzon, M., Rosado, M. C., Molina-Rugama, A. J., Duarte, V. S., Dias, R., and Pallini, A. (2008). Acaricidal efficacy of neem against Polyphagotarsonemus latus (banks) (Acari: Tarsonemidae). Crop Prot. 27, 869–872. doi: 10.1016/j.cropro.2007.10.001
Verdenelli, R. A., Dominchin, M. F., Barbero, F. M., Pérez-Brandán, C., Aoki, A., Vargas Gil, S., et al. (2023). Effect of two broad-spectrum fungicides on the microbial communities of a soil subjected to different degrees of water erosion. Appl. Soil Ecol. 190:104984. doi: 10.1016/j.apsoil.2023.104984
Volynchikova, E., and Kim, K. D. (2022). Biological control of oomycete soilborne diseases caused by Phytophthora capsici, Phytophthora infestans, and Phytophthora nicotianae in solanaceous crops. Mycobiol. 50, 269–293. doi: 10.1080/12298093.2022.2136333
Voorrips, R. E., Finkers, R., Sanjaya, L., and Groenwold, R. (2004). QTL mapping of anthracnose (Colletotrichum spp.) resistance in a cross between Capsicum annuum and C. chinense. Theor. Appl. Genet. 109, 1275–1282. doi: 10.1007/s00122-004-1738-1
Wang, M., van Vleet, S., Mcgee, R., Paulitz, T., Porter, L., Schroeder, K., et al. (2021). Chickpea seed rot and damping-off caused by metalaxyl-resistant Pythium ultimum and its management with ethaboxam. Plant Dis. 105, 1728–1737. doi: 10.1094/PDIS-08-20-1659-RE
Wang, L., Zhong, Y., Liu, J., Ma, R., Miao, Y., Chen, W., et al. (2023). Pigment biosynthesis and molecular genetics of fruit color in pepper. Plan. Theory 12:2156. doi: 10.3390/plants12112156
Were, E., Viljoen, A., and Rasche, F. (2023). Back to the roots: understanding banana below-ground interactions is crucial for effective management of fusarium wilt. Plant Pathol. 72, 19–38. doi: 10.1111/ppa.13641
White, D. J., Chen, W., and Schroeder, K. L. (2019). Assessing the contribution of ethaboxam in seed treatment cocktails for the management of metalaxyl-resistant Pythium ultimum var. Ultimum in Pacific northwest spring wheat production. Crop Prot. 115, 7–12. doi: 10.1016/j.cropro.2018.08.026
Wohor, O. Z., Rispail, N., Ojiewo, C. O., and Rubiales, D. (2022). Pea breeding for resistance to rhizospheric pathogens. Plan. Theory 11:2664. doi: 10.3390/plants11192664
Xie, J. Q., Zhou, X., Jia, Z. C., Su, C. F., Zhang, Y., Fernie, A. R., et al. (2023). Alternative splicing, an overlooked defense frontier of plants with respect to bacterial infection. J. Agric. Food Chem. 71, 16883–16901. doi: 10.1021/acs.jafc.3c04163
Yang, X., Li, M., Jia, Z. C., Liu, Y., Wu, S. F., Chen, M. X., et al. (2024). Unraveling the secrets: evolution of resistance mediated by membrane proteins. Drug Resist. Updat. 77:101140. doi: 10.1016/j.drup.2024.101140
Yao, S., Zhou, B., Duan, M., Cao, T., Wen, Z., Chen, X., et al. (2023). Combination of biochar and Trichoderma harzianum can improve the phytoremediation efficiency of Brassica juncea and the rhizosphere micro-ecology in cadmium and arsenic contaminated soil. Plan. Theory 12:2939. doi: 10.3390/plants12162939
Yin, Y., Zhang, Y., Li, F., Jiao, J., Lebailly, P., Zhang, Y., et al. (2022). Driving mechanism for farmers’ participation in improving farmland ecosystem: evidence from China. J. Clean. Prod. 380:134895. doi: 10.1016/j.jclepro.2022.134895
Zagade, S. N., Deshpande, G. D., Gawade, D. B., and Atnoorkar, A. A. (2016). Biocontrol agents and fungicides for management of damping off in chili. World J. Agric. Sci. 8, 590–597. doi: 10.5829/idosi.wjas.2012.8.6.1689
Zagade, S. N., Desphande, G. D., and Gawade, D. B. (2014). Effect of cultural practices and seed treatment against damping off (Pythium Ultimum) of chili. J. Plant Dis. Sci. 9, 55–59.
Zamoum, M., Allali, K., Benadjila, A., and Abdelghani Zitouni, Y. G. (2022). Formulation of biofungicides based on Streptomyces caeruleatus strain ZL-2 spores and efficacy against Rhizoctonia solani damping-off of tomato seedlings. Arch. Microbiol. 204:629. doi: 10.1007/s00203-022-03251-8
Zou, X. X., Ma, Y. Q., and Dai, X. Z. (2020). Spread and industry development of pepper in China. Acta Hortic. Sin. 47, 1715–1726.Li XF, Y. S
Keywords: biocontrol, chili cultivation, epidemiology, Fusarium spp., Pythium spp., resistant cultivars, sustainability
Citation: Delai C, Muhae-Ud-Din G, Abid R, Tian T, Liu R, Xiong Y, Ma S and Ghorbani A (2024) A comprehensive review of integrated management strategies for damping-off disease in chili. Front. Microbiol. 15:1479957. doi: 10.3389/fmicb.2024.1479957
Edited by:
Ravinder Kumar, Indian Agricultural Research Institute (ICAR), IndiaReviewed by:
Ashish Kumar Singh, ICAR-Vivekananda Parvatiya Krishi Anusandhan Sansthan, IndiaAbdul Gafur, SMF Corporate R&D Advisory Board, Indonesia
Copyright © 2024 Delai, Muhae-Ud-Din, Abid, Tian, Liu, Xiong, Ma and Ghorbani. This is an open-access article distributed under the terms of the Creative Commons Attribution License (CC BY). The use, distribution or reproduction in other forums is permitted, provided the original author(s) and the copyright owner(s) are credited and that the original publication in this journal is cited, in accordance with accepted academic practice. No use, distribution or reproduction is permitted which does not comply with these terms.
*Correspondence: Chen Delai, Y2RsODI5QDEyNi5jb20=; Ghulam Muhae-Ud-Din, Z20zMDg1cHBAb3V0bG9vay5jb20=
†These authors have contributed equally to this work