- 1Department of Ultrasound Medicine, The Second Affiliated Hospital of Soochow University, Suzhou, China
- 2Department of Pharmacy, The 960th Hospital of PLA, Jinan, China
- 3Department of Obstetrics and Gynecology, The Second Affiliated Hospital of Soochow University, Suzhou, China
Cryptococcus neoformans, a critical priority pathogen designated by the World Health Organization, poses significant therapeutic challenges due to the limited availability of treatment options. The emergence of antifungal resistance, coupled with cross-resistance, further hampers treatment efficacy. Aneuploidy, known for its ability to induce diverse traits, including antifungal resistance, remains poorly understood in C. neoformans. We investigated the impact of tunicamycin, a well-established ER stress inducer, on aneuploidy formation in C. neoformans. Our findings show that both mild and severe ER stress induced by tunicamycin lead to the formation of aneuploid strains in C. neoformans. These aneuploid strains exhibit diverse karyotypes, with some conferring resistance or cross-resistance to antifungal drugs fluconazole and 5-flucytosine. Furthermore, these aneuploid strains display instability, spontaneously losing extra chromosomes in the absence of stress. Transcriptome analysis reveals the simultaneous upregulation of multiple drug resistance-associated genes in aneuploid strains. Our study reveals the genome plasticity of C. neoformans as a major mechanism contributing to non-antifungal-induced antifungal resistance.
Introduction
Cryptococcus species are significant contributors to opportunistic fungal infections in individuals infected with HIV worldwide. The incidence and mortality rates associated with these infections are particularly high in sub-Saharan Africa (ref). C. neoformans and C. gattii are the primary causative agents of cryptococcosis, which typically manifests as meningitis and pneumonia. The global annual estimated death toll from cryptococcal meningitis is approximately 181,100, with the majority of cases occurring in sub-Saharan Africa (Rajasingham et al., 2017). In 2022, the World Health Organization (WHO) released its inaugural list of priority fungal pathogens, categorizing Cryptococcus neoformans, Candida auris, Aspergillus fumigatus, and Candida albicans as “critical priority” pathogens (WHO, 2022).
Currently, treatment options for cryptococcosis are limited to three classes of antifungals: azoles, polyenes, and 5-flucytosine (5FC). Unfortunately, there has been a steady increase in antifungal resistance among clinical isolates of C. neoformans. For instance, studies of clinical isolates of C. neoformans collected from 134 sites across 40 countries indicated that resistance to fluconazole (FLC) increased from 7.3% between 1997 and 2000 to 11.7% between 2005 and 2007 (Pfaller et al., 2010). Today, FLC resistance is relatively common, particularly in relapse cases of cryptococcal meningitis (Bongomin et al., 2018).
Antifungal resistance often arises from genetic mutations that alter drug targets or increase drug efflux (Fisher et al., 2022). Notably, while point mutations in the ERG11 gene can induce FLC resistance, the primary mechanism of FLC resistance observed in vitro and in vivo involves increased ERG11 copy numbers and overexpression, facilitated by the formation of extra copies of Chromosome 1 (Sionov et al., 2010; Zafar et al., 2019; Yang et al., 2021a). Similarly, in over 50% of clinical fluconazole-resistant isolates of C. albicans, amplification of the left arm of Chromosome 5, which houses ERG11 and TAC1, has been detected. TAC1 encodes a transcription factor that regulates efflux pump genes (Selmecki et al., 2006; Selmecki et al., 2008). Thus, aneuploidy, which involves changes in gene copy numbers, is a common strategy used by human pathogens to rapidly adapt to stress (Tsai and Nelliat, 2019). Importantly, aneuploidy is considered as a significant mutation that can drive phenotypic changes by simultaneously altering the copy number and expression of hundreds of genes. This alteration can lead to the emergence of complex traits as a result of a single mutational event (Pavelka et al., 2010; Chen et al., 2012). Recent studies have highlighted the role of aneuploidy in mediating rapid adaptation to antifungal drugs and, in some cases, cross-adaptation to unrelated drugs in species such as C. albicans (Yang et al., 2019; Yang et al., 2021b), C. parapsilosis (Yang et al., 2021c; Sun et al., 2023a), and C. neoformans (Yang et al., 2021a).
The endoplasmic reticulum (ER) is a vital organelle in eukaryotic cells, responsible for protein folding and secretion. When the ER becomes overwhelmed with unfolded proteins, ER stress occurs, leading to a range of cellular responses (Luoma, 2013). Tunicamycin (TUN), a commonly used chemical, induces ER stress by inhibiting protein glycosylation, causing the accumulation of unfolded proteins (Wu et al., 2018). Recent studies have demonstrated that aneuploidy plays a crucial role in adapting to TUN-induced ER stress in yeast species. For example, in Saccharomyces cerevisiae and C. albicans, exposure to TUN primarily results in the formation of disomy of Chromosome II and trisomy of Chromosome 2, respectively. These aneuploidies are associated with specific genes that confer TUN resistance, including ALG7, PRE7, and YBR085C-A in S. cerevisiae, and ALG7, RTA2, and RTA3 in C. albicans (Beaupere et al., 2018; Yang et al., 2021b). Furthermore, TUN-induced trisomy of Chromosome 2 in C. albicans has been shown to confer cross-adaptation to caspofungin and hydroxyurea (Yang et al., 2021b). In contrast, the mechanisms by which C. neoformans adapts to ER stress remain poorly understood, including whether this adaptation is accompanied by the acquisition of new traits, such as antifungal resistance.
Reference strains are a crucial component of laboratory research, providing a standardized genotype that facilitates the comparison of scientific observations within a specific species across the research community. C. neoformans strain H99 (ATCC 208821) was originally isolated on February 14 1978 by Dr. John Perfect at Duke University Medical Center from a 28-year-old male with Hodgkin’s disease (Janbon et al., 2014), and has since established itself as the most commonly utilized reference strain for C. neoformans worldwide. While several distinct lineages of H99 have arisen, displaying diverse levels of virulence as a result of storage, passage, and subculturing across various laboratories, thorough sequencing analyses of these strains have uncovered a limited number of unique mutations among them. Overall, the strains exhibit significant similarity in both growth characteristics and antifungal susceptibility (Fernandes et al., 2022). However, although H99 serves as an excellent model for grasping the fundamental biology of C. neoformans, conducting comparative analyses of clinical isolates will significantly enhance our understanding (Jackson et al., 2023).
In this study, we exposed the C. neoformans laboratory strain H99 to both mild and severe ER stresses induced by TUN to investigate the effects on antifungal resistance. Our findings showed that TUN primarily induced aneuploidy in C. neoformans, resulting in diverse karyotypes with recurring amplifications of Chromosomes 4 and 6, either individually or in combination with amplifications of other chromosomes. Notably, specific aneuploid strains exhibited resistance to FLC or 5FC, and some displayed cross-resistance to both drugs. We also observed that these aneuploid strains were unstable, with spontaneous loss of extra chromosomes and reversion to euploidy when grown in the absence of stress. Importantly, exposure to TUN also triggered cross-resistance to FLC in one clinical isolate of C. neoformans. Furthermore, our results indicate that cross-adaptation to ER stress and FLC in aneuploid strains was attributed to the simultaneous upregulation of multiple genes across the genome. This study highlights the role of ER stress in inducing aneuploidy formation and underscores the potential of non-antifungal-induced genome plasticity in driving antifungal resistance in C. neoformans.
Materials and methods
Strains and growth conditions
The C. neoformans laboratory strain H99 served as the wild-type strain in this study. Stock cultures were preserved in a glycerol solution and stored at a temperature of −80°C. Cells were routinely cultured in a nutrient-rich medium called Yeast extract-Peptone-Dextrose (YPD) at a temperature of 30°C in a shaking incubator set to a moderate speed of 150–200 rpm. To solidify the medium, agar was added at a concentration of 2% (w/v). The drugs used in this study were dissolved in a solvent called dimethyl sulfoxide (DMSO) and stored at a temperature of −20°C.
Growth curves of H99 in the presence of tunicamycin
H99 was thawed from the −80°C freezer and streaked onto YPD-agar plates, which were then incubated at 30°C for 72 h. After incubation, cells were resuspended in YPD broth and their densities were adjusted to 2.5 × 103 cells/mL in YPD broth with or without TUN in a 96-well plate. The TUN concentrations were 0.03–0.125 μg/mL. The plate was then incubated at 30°C, and the optical density at 595 nm (OD595) was monitored using a Tecan plate reader (Infinite F200 PRO, Tecan, Switzerland) at 15-min intervals for 72 h. The data are presented as the mean ± standard deviation (SD) of three biological replicates.
Spot assay
Cells were re-suspended in distilled water and adjusted to a concentration of 1 × 107 cells/mL. Following this, 3 μL of 10-fold serial dilutions were spotted onto YPD agar plates with or without the presence of drugs. The petri dish plates were then incubated at 30°C and photographed after 72 h.
Broth microdilution assay
The broth microdilution method was performed in accordance with the Clinical and Laboratory Standards Institute (CLSI) M27-2017 guidelines (CLSI, 2017) with minor modifications.
Stock solutions of the drugs (TUN and FLC in YPD broth; 5FC in SD broth) were prepared and subjected to a twofold dilution process. A volume of 0.1 mL of each drug at varying concentrations was sequentially dispensed into U-shaped wells of 96-well microdilution plates (Thermo Fisher Scientific), resulting in final drug concentrations ranging from 0.125 to 128 μg/mL (FLC and 5FC) or 0.03–32 μg/mL (TUN).
To prepare the inoculum of test strains, 3–5 colonies were suspended in sterile saline and standardized to a turbidity of 0.5 McFarland using a spectrophotometer (NanoPhotometer® NP80, Implen, Germany). The inoculum was then diluted with either YPD broth (for TUN and FLC) or SD broth (for 5FC). Following this, 0.1 mL of the yeast suspension was added to each well of the 96-well microdilution plates, yielding a final inoculum concentration between 0.5 × 103 and 2.5 × 103 CFU/mL. The plates were subsequently incubated at 35°C. The minimum inhibitory concentration (MIC) was defined as the lowest drug concentration at which complete growth inhibition was observed. Three replicates were conducted for each drug concentration.
Isolating mutants using low dose of tunicamycin
To generate mutants, we inoculated approximately 2.5 × 103 cells/mL of the H99 strain into 1.5 mL of YPD broth containing a low concentration of 0.06 μg/mL of TUN. We performed three biological replicates. After 72 h of incubation with shaking, the cultures were washed and diluted with distilled water. We then spread approximately 300 cells onto YPD plates and incubated them at 30°C for 72 h. From each culture, we randomly selected 120 colonies and tested them for resistance to TUN using a spot assay.
Induction of mutations with high-dose tunicamycin
To generate mutants, we suspended cells in distilled water and adjusted the concentration to 1 × 107 cells/mL. We then spread 100 μL of this suspension onto YPD plates supplemented with a high concentration of 0.25 μg/mL TUN. The plates were incubated at 30°C for 5 days, allowing for the selection of resistant mutants. We randomly selected 30 mutants for further analysis and characterization. These 30 mutants were maintained in a −80°C freezer.
Assay for genome instability
To investigate the genome instability of the aneuploid strain with disomies of Chromosomes 4 and 6, we performed the following steps: First, the strain with Chrs (4,6) x2 was thawed from the-80°C freezer and streaked onto YPD-agar plates, which were then incubated at 30°C for 72 h. From the resulting colonies, we randomly selected one small colony and suspended it in distilled water. We then diluted the cells in distilled water and spread approximately 200 cells onto a YPD plate, which was incubated at 30°C for 72 h. After incubation, we observed small (S), medium (M), and large (L) colonies. We randomly selected four colonies from each size category for further analysis.
Next-generation sequencing
DNA extraction, library construction, and sequencing were performed using previously described methods (Yang et al., 2021d). The data was then visualized using Ymap (Abbey et al., 2014). The raw fastq files were uploaded to Ymap (version 1.0)1 and plotted as a function of chromosome position using the C. neoformans H99 reference genome (NCBI RefSeq assembly no. GCF_000149245.1).
RNA-seq
Comparative analysis of strains: To investigate the transcriptome profiles of the strains, we performed RNA-seq as described previously with minor modifications (Ke et al., 2024). We first streaked the strains onto YPD plates from the −80°C freezer and incubated them at 30°C for 72 h. We then selected colonies with similar sizes, suspended them in a solution with an optical density (OD) of 0.1, and grew them in a shaker at 30°C until they reached an OD of 1.0. The cells were then collected by centrifugation and frozen in liquid nitrogen.
Treatment of H99 with TUN: We grew H99 in YPD broth in a shaker at 30°C from an OD of 0.1 to 1.0, and then divided the culture into two batches. One batch was treated with TUN at a final concentration of 0.25 μg/mL, while the other batch was treated with an equal volume of DMSO. Three hours later, the cells were collected by centrifugation and frozen in liquid nitrogen.
Total RNA extraction, purification, library construction, and sequencing were performed as described previously (Ke et al., 2024). We obtained three biological replicates for each strain and used DESeq2 (Love et al., 2014) to analyze the differential gene expression profiles. We considered genes with a False Discovery Rate (FDR)-adjusted p value <0.05 and expression fold changes of more than 1.5 or less than −1.5 as differentially expressed.
Statistical analysis
Significance analysis of differences between growth curves was performed using Tukey HSD (Honestly Significant Difference) test.
Results
Tunicamycin impact on C. neoformans H99 growth
The sensitivity of the C. neoformans lab strain H99 to TUN was evaluated. In YPD broth, the minimum inhibitory concentration (MIC) of TUN was found to be 0.125 μg/mL. Additionally, TUN at a concentration of 0.06 μg/mL significantly inhibited the growth of H99 (p < 0.001, Tukey test), while TUN at a concentration of 0.03 μg/mL did not significantly inhibit growth (p > 0.05, Tukey test) (Figure 1A).
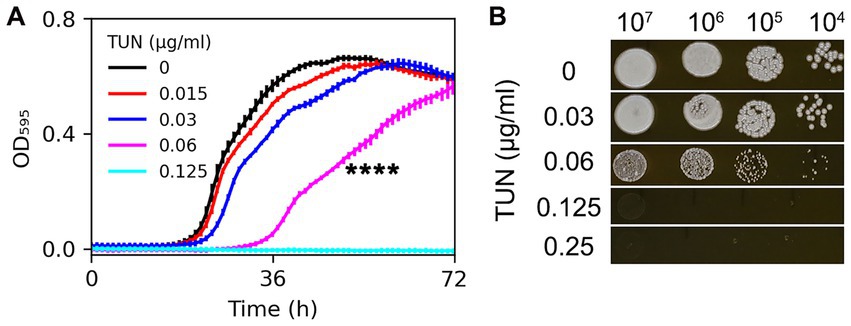
Figure 1. Assessment of H99 susceptibility to tunicamycin. Susceptibility was evaluated in both broth and solid medium using twofold increased concentrations of TUN. (A) Cells were cultured in a 96-well plate. Optical density at 595 nm (OD595) was monitored every hour for 72 h at 30°C using a Tecan plate reader. Data are presented as the mean ± SD of three biological replicates. (B) 3 μL of 10-fold serial dilutions of cell suspensions were spotted on YPD agar plates supplemented with TUN. The plates were then incubated at 30°C for 3 days and subsequently photographed.
On YPD agar plates, H99 was able to grow in the presence of TUN at a concentration of 0.06 μg/mL, although the resulting colonies were smaller than those on the control plate. TUN at concentrations of 0.125 μg/mL and 0.25 μg/mL completely inhibited the growth of H99 (Figure 1B).
Based on these results, TUN at a concentration of 0.06 μg/mL in YPD broth was found to be sub-inhibitory to H99, while TUN at a concentration of 0.125 μg/mL on YPD agar (and in YPD broth) was inhibitory to H99.
Selective pressure of sub-MIC tunicamycin leads to emergence of antifungal resistant mutants
We explored whether sub-MIC concentrations of TUN could select for adaptive mutants in the H99 strain. We cultured the strain in YPD broth supplemented with 0.06 μg/mL TUN, starting with a cell density of approximately 2.5 × 103 cells/mL. After 72 h, we randomly selected 120 colonies from each culture and tested them for TUN susceptibility, with three biological replicates. Among these colonies, we identified five mutants that outperformed the parent strain (Figure 2A). The broth microdilution assay revealed that the parent strain H99 exhibited a MIC of 0.125 μg/mL for TUN, whereas the five mutants displayed MICs ranging from 0.5 to 1 μg/mL. Consequently, the five mutants demonstrated greater resistance to TUN compared to the parent strain.
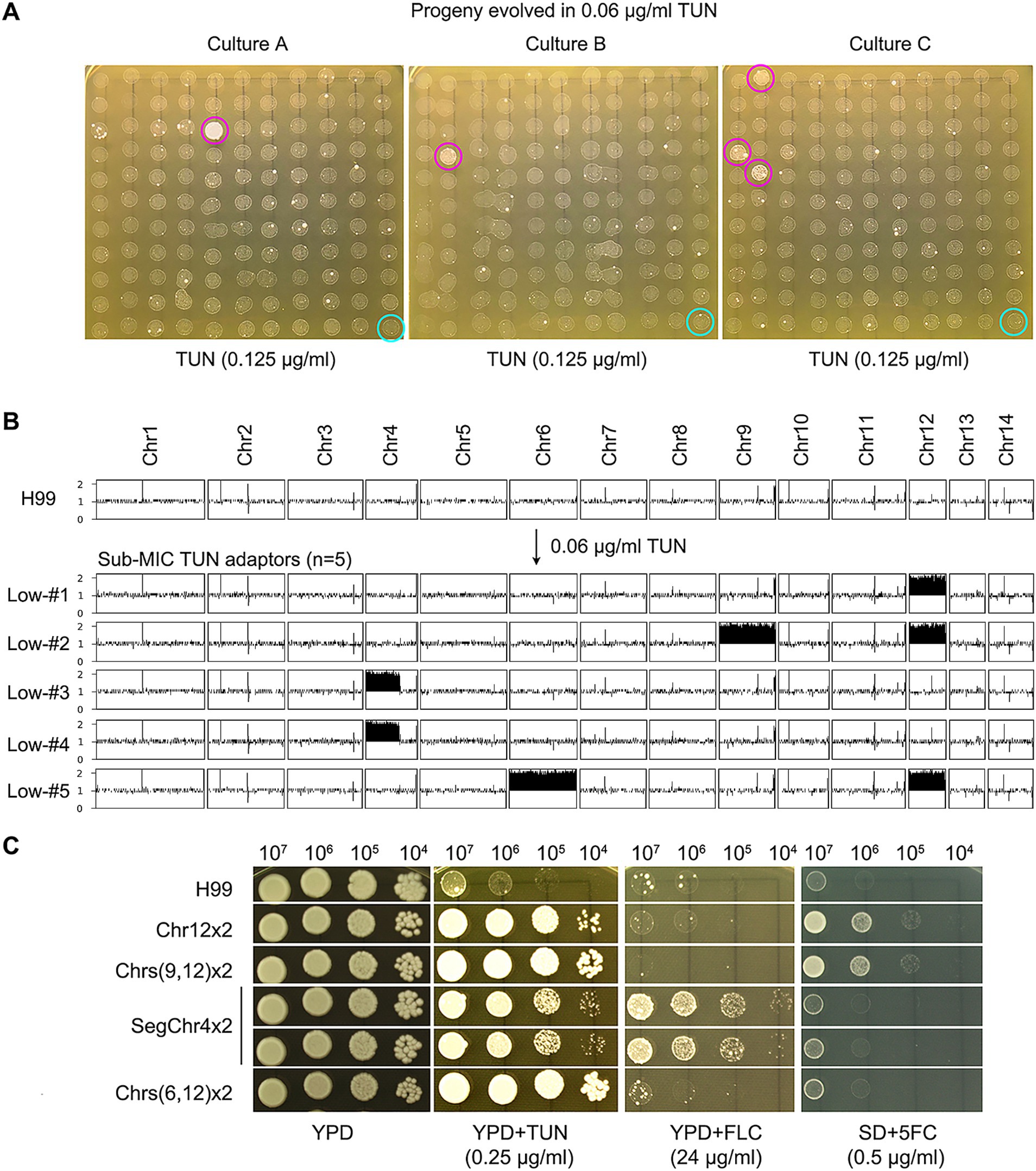
Figure 2. Selection of aneuploid mutants with antifungal resistance by low concentrations of tunicamycin. (A) The wild-type strain H99 was cultured in YPD broth supplemented with sub-minimal inhibitory concentration (sub-MIC) of TUN. Three biological replicates were conducted, and 120 colonies from each culture were randomly selected for testing their resistance to TUN (magenta circles) compared to the wild type (cyan circles). The five colonies displaying resistance were subjected to sequencing. (B) The karyotypes of the wild type and the five resistant colonies were visualized using Ymap. The y-axis represents copy number, while the x-axis indicates the location of the sequence reads on the chromosome. (C) The five mutants were subjected to spot assay to evaluate their resistance to TUN, fluconazole (FLC), and 5-flucytosine (5FC). Testing for 5FC was conducted on SD medium, while YPD medium was used for other tests.
Sequencing analysis of five selected mutants revealed diverse aneuploid karyotypes: Low-#1 had disomy of Chr12 (Chr12x2), Low-#2 had disomies of Chr9 and Chr12 (Chrs (9,12) x2), Low-#5 had disomies of Chr6 and Chr12 (Chrs (6,12) x2), and Low-#3 and Low-#4 had segmental disomy of the left arm of Chr4 (SegChr4x2) (Figure 2B). Thus, exposure to sub-inhibitory levels of TUN led to the selection of aneuploid TUN-resistant mutants with diverse karyotypes.
Variation analysis revealed few genetic mutations in the mutants (Supplementary Table S1). Low-#1 had a missense mutation in CNAG_06222, encoding the large subunit ribosomal protein L32e, while Low-#3 had a missense mutation in CNAG_00807, whose function is unknown.
We further examined whether the mutants displayed cross-resistance to antifungal drugs. Spot assays demonstrated that mutants with SegChr4x2 were more resistant to FLC than H99, while mutants with Chr12x2 and Chrs (9,12) x2 were more resistant to 5FC than H99 (Figure 2C). The broth microdilution assay indicated that the parent strain H99 had a MIC of 32 μg/mL for FLC, while the two mutants containing SegChr4x2 exhibited MICs greater than 128 μg/mL. For 5FC, H99 displayed an MIC of 0.5 μg/mL, whereas the mutants with Chr12x2 and Chrs (9,12) x2 had MICs of 4 μg/mL.
Thus, depending on the aneuploid chromosome, certain mutants exhibited cross-resistance to antifungals.
Exposure to high concentrations of tunicamycin selects for diverse aneuploid mutants
We plated approximately 1 million H99 cells on YPD plates containing 0.125 μg/mL and 0.25 μg/mL TUN, respectively. After 5 days, we observed lawn growth on the plate with 0.125 μg/mL TUN, while 92 colonies grew on the plate with 0.25 μg/mL TUN. We randomly selected 30 mutants from the latter plate for further analysis. Whole-genome sequencing revealed that 8 mutants had a normal euploid karyotype, while 22 mutants displayed diverse aneuploid karyotypes, consisting of 18 distinct patterns. Notably, 17 out of 22 aneuploids exhibited disomy or trisomy of Chromosomes 4 and 6, either alone or in combination with other chromosomes. Five aneuploids had either Chr4x2 or Chr6x2, but not both, along with disomy of 2–6 other chromosomes (Figure 3). This suggests that amplifications of Chr4 and Chr6, alone or in combination with other chromosomes, were the primary genomic changes among mutants selected under high TUN concentration.
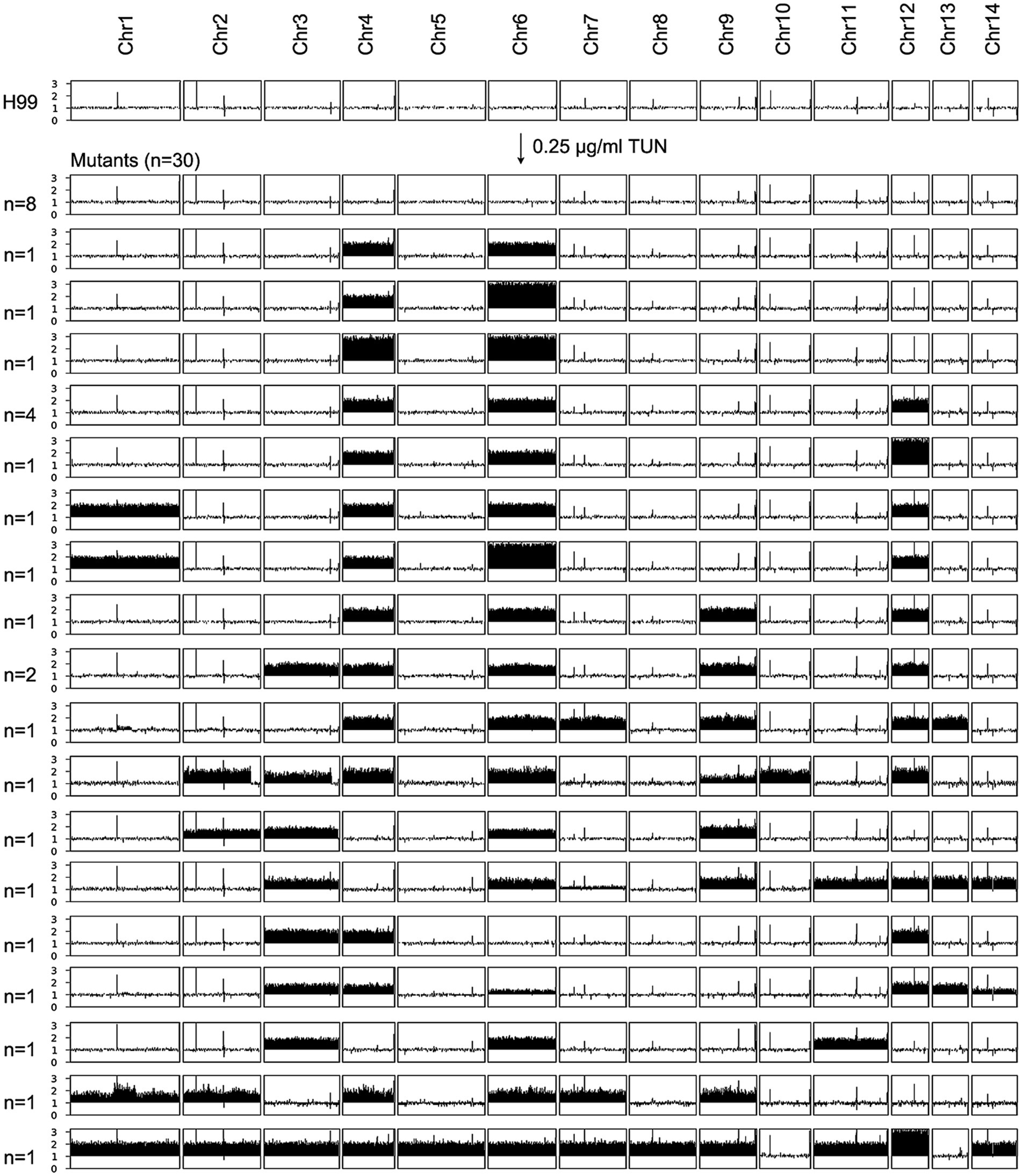
Figure 3. Karyotypes of mutants selected by high concentrations of tunicamycin. The wild-type strain H99 was exposed to supra-minimal inhibitory concentration (supra-MIC) of TUN. Thirty mutants were randomly selected for sequencing. The karyotypes of these mutants were visualized using Ymap, and the number of mutants bearing each karyotype is indicated in the figure.
We identified several genetic mutations in the mutants (Supplementary Table S1). For example, mutants high-#8 and high-#12 had the same frameshift mutation in gene CNAG_06130, while mutants high-#15, high-#17, and high-#26 had missense mutations in CNAG_05936, CNAG_01727, and CNAG_05396, respectively. Mutant high-#20 had a missense mutation in CNAG_04310 and a stop-gained mutation in CNAG_06382, while mutant high-#29 had missense mutations in CNAG_03862 and CNAG_03059. However, the functions of proteins encoded by CNAG_06130, CNAG_04310, CNAG_03862, and CNAG_03059 remain unknown.
Some supra-MIC mutants are cross-resistant to fluconazole and/or 5-flucytosine
We found that some mutants that grew in the presence of high concentrations of TUN also showed resistance to other antifungal drugs, including FLC and 5FC. To further investigate this, we tested the antifungal resistance of all these mutants using disk diffusion assays. Based on their resistance profiles, we categorized the mutants into four distinct groups (Figure 4).
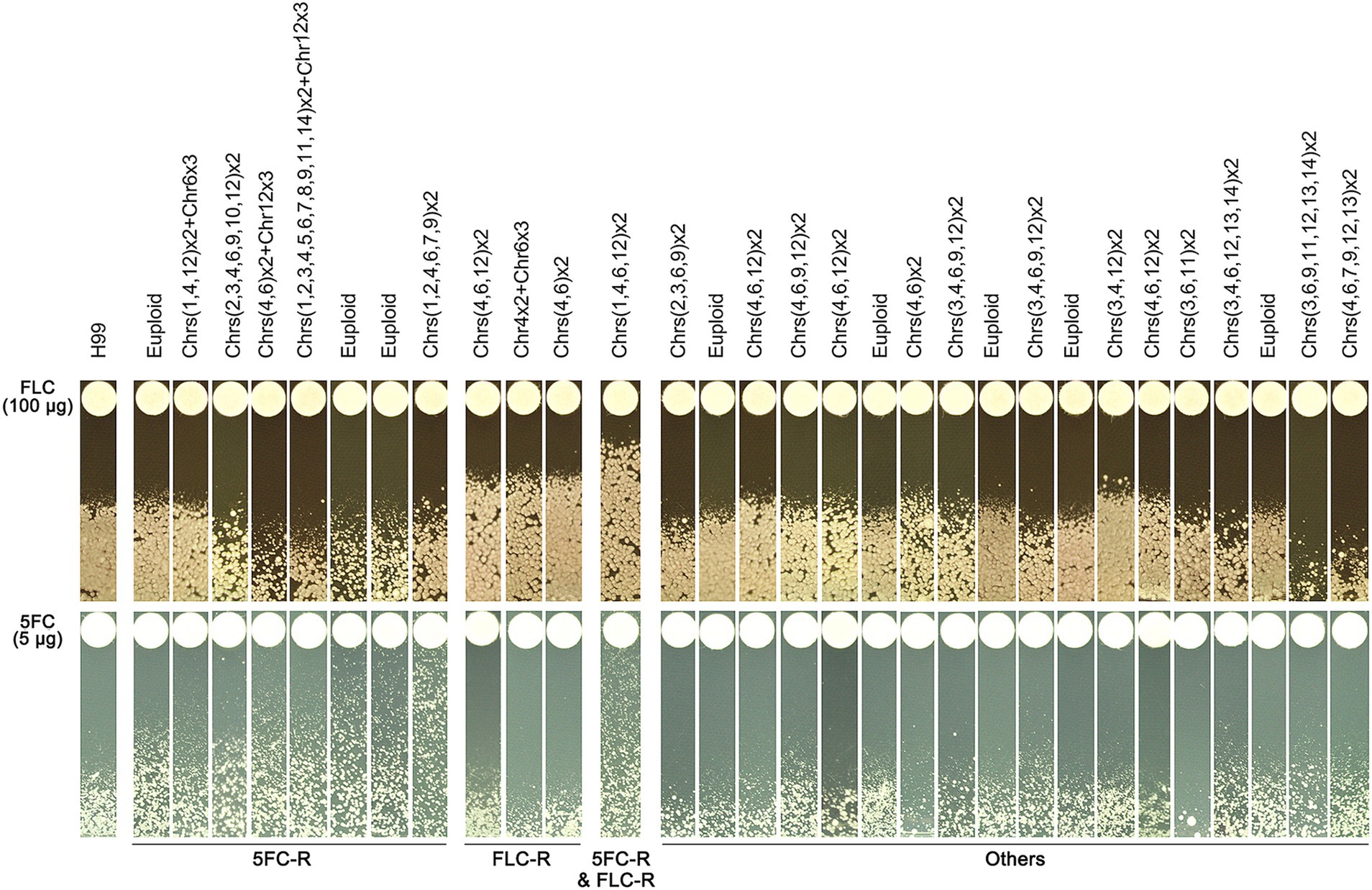
Figure 4. Profile of antifungal resistance in mutants induced by supra-MIC tunicamycin. Thirty mutants, induced by supra-minimal inhibitory concentration (supra-MIC) of TUN, were tested using disk diffusion assays. The top panel shows disks containing 200 μg of fluconazole (FLC), while the bottom panel shows disks containing 5 μg of 5-flucytosine (5FC). The diameter of the inhibition zone is inversely proportional to the level of drug resistance. The plates were incubated at 30°C for 3 days and then photographed. YPD plates were used for FLC testing, and SD plates were used for 5FC testing.
One group of mutants was resistant to 5FC only and consisted of 8 mutants, which had MICs for 5FC ranging from 1 to 8 μg/mL. Interestingly, three of these mutants had a normal euploid karyotype, while the remaining five had amplifications of at least three chromosomes.
Another group of mutants was resistant to FLC only and consisted of three mutants, each having an MIC of 64 μg/mL for FLC. Each of these mutants had distinct chromosomal changes, including disomy of Chromosomes 4 and 6, disomy of Chromosome 4 and trisomy of Chromosome 6, and disomy of Chromosomes 4, 6, and 12.
A third group of mutants showed cross-resistance to both 5FC and FLC, and consisted of only one mutant. The MICs for FLC and 5FC were 128 μg/mL and 8 μg/mL, respectively. This mutant had a complex karyotype, with disomy of Chromosomes 1, 4, 6, and 12.
The final group of mutants did not show obvious resistance to either 5FC or FLC, and consisted of 18 mutants. However, some of these mutants were hypersensitive to FLC, while others were hypersensitive to 5FC.
Aneuploidy is unstable
A mutant with a chromosomal abnormality, Chrs (4,6) x2, was analyzed for its physical characteristics and genetic stability. To do this, around 400 cells of the mutant were grown on a special agar plate (Figure 5A). After two days, differences in the size of the resulting colonies were observed (Figure 5A). The area of each colony was then measured (Figure 5B). Colonies that were smaller than 0.03 cm2 were classified as small, those between 0.035 cm2 and 0.04 cm2 as medium, and those larger than 0.045 cm2 as large (Figure 5A,B). Four colonies from each size group were randomly selected for further genetic analysis. The results showed that all four small colonies still had the Chrs (4,6) x2 abnormality, while the four medium colonies had a different abnormality, Chr4x2, and the four large colonies had a normal chromosome count (Figure 5C). This suggests that the Chrs (4,6) x2 mutant is unstable and may tend to lose the extra copy of chromosome 6 or all extra chromosomes.
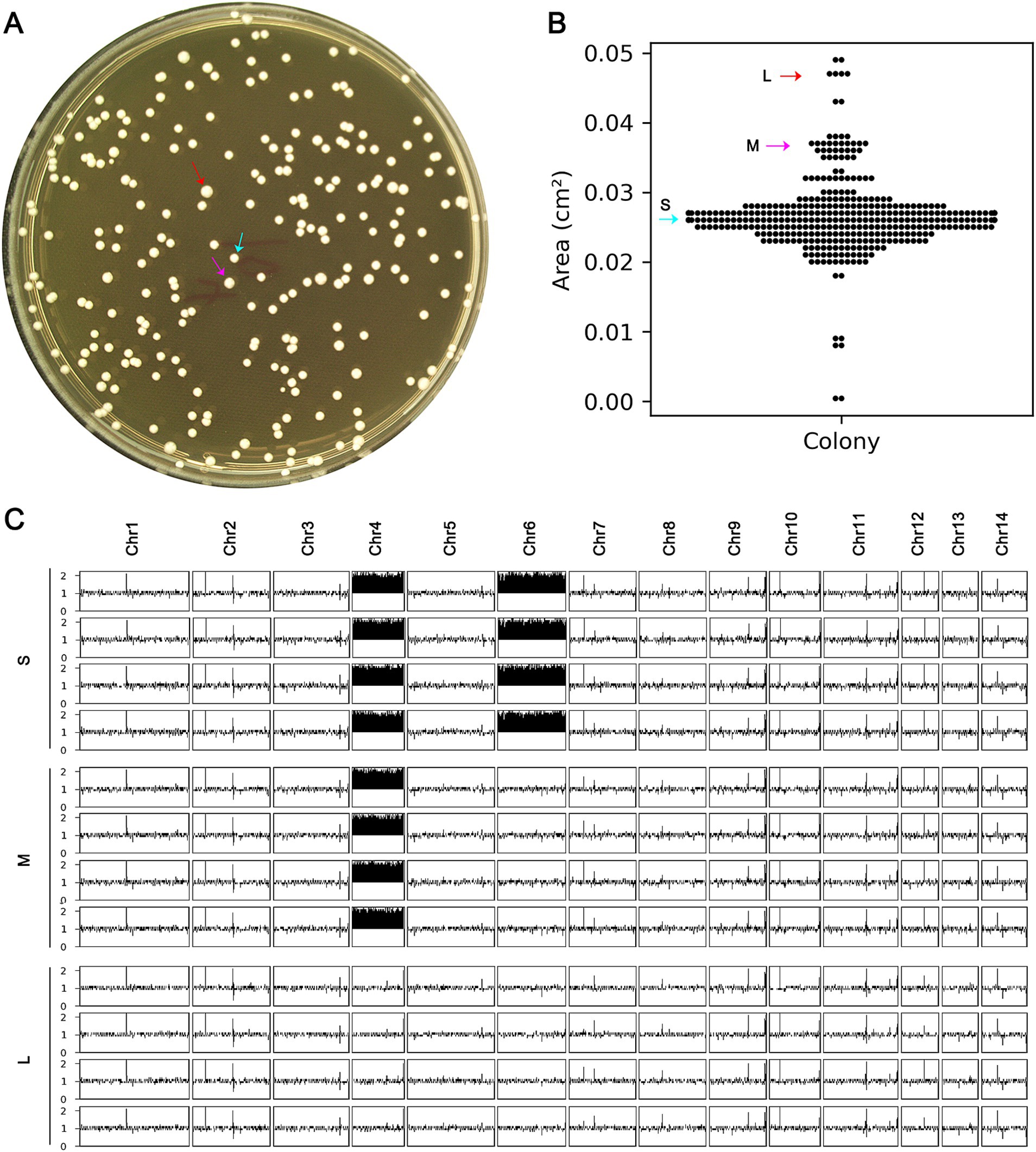
Figure 5. Instability of complex aneuploidy. (A) A mutant with disomies of Chr4 and Chr6 was spread on YPD plate. (B) After 48 h of growth, the areas of the colonies were measured. (C) Colonies were classified as small (S), medium (M), or large (L), indicated by cyan, magenta, and red arrows, respectively. Four colonies of each category were randomly selected for sequencing, and the karyotypes were visualized using Ymap.
Randomly selected S, M, and L colonies, one from each type, were compared to the H99 strain for resistance to TUN. The spot assay indicated that both S and M colonies exhibited resistance, while the L colony did not (Supplementary Figure S1). Thus, the presence of an additional copy of Chr4 alone was sufficient to confer resistance to TUN, and the loss of all extra copies of aneuploid chromosomes resulted in a loss of TUN resistance.
Aneuploidy causes cross-resistance to tunicamycin and fluconazole via regulating expression of multiple genes across the genome
Our study reveals that disomy of Chrs (4,6), either alone or in combination with disomy of other chromosomes, was the primary genomic alteration among the mutants. Additionally, Chrs (4,6) x2 conferred resistance not only to TUN but also to FLC. To elucidate why Chrs (4,6) x2 led to cross-resistance to both drugs, we compared the transcriptome of one Chrs (4,6) x2 mutant to that of the wild type (Figure 6).
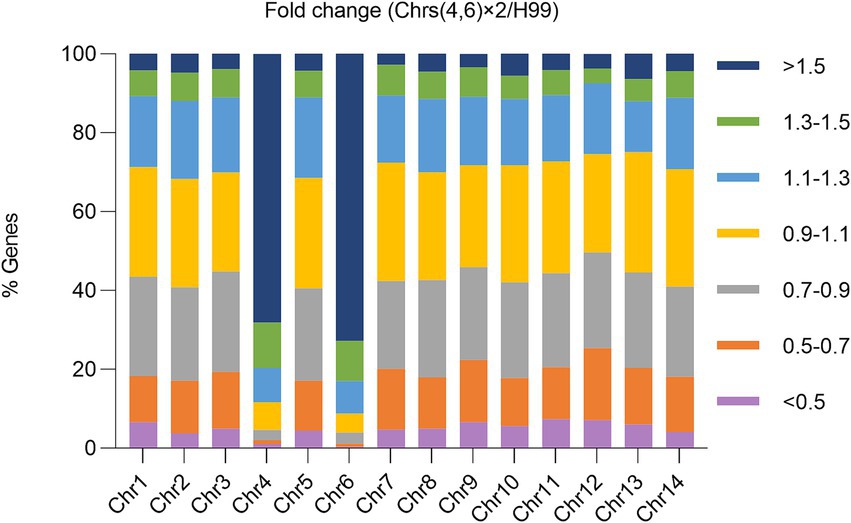
Figure 6. Impact of aneuploidy on transcription of genes across the genome. The transcriptome of a mutant with Chrs (4,6) x2 was compared to the haploid wild type H99. Relative expression was determined based on the expression ratio of a target gene in the aneuploid strain versus in H99. The graph displays the proportions of genes on each chromosome falling within specified relative expression ratio ranges.
Transcription of 392 genes was detected on Chr4, with 312 genes showing ratios higher than 1.3. Similarly, transcription of 533 genes was detected on Chr6, with 442 genes having ratios higher than 1.3. Consequently, 79.6 and 82.9% of transcribed genes on Chr4 and Chr6, respectively, were up-regulated at least 1.3 times in the Chrs (4,6) x2 strain compared to H99. In contrast, on euploid chromosomes, only 7.5–12.1% of genes were up-regulated to this extent.
Overall, 910 genes were up-regulated across the genome, with 267 and 388 genes on Chr4 and Chr6, respectively. Conversely, 990 genes were down-regulated, with only 8 and 4 on Chr4 and Chr6, respectively. Thus, 72.0% (655 out of 910) of the up-regulated and 1.2% (12 out of 990) of the down-regulated genes were located on aneuploid chromosomes. Consequently, disomy of Chr4 and Chr6 generally led to increased expression of genes on the aneuploid chromosomes and altered expression of genes on euploid chromosomes.
Gene ontology (GO) analysis revealed that 21 genes involved in response to TUN (GOID: 1904576) were significantly enriched among the up-regulated genes, with 14 of them located on Chr4 or Chr6 (Supplementary Table S2). Notably, among the genes encoding efflux pumps, AFR1/CNAG_00730, MDR1/CNAG_00796, and PDR16/CNAG_04984 were up-regulated, with AFR1 and MDR1 on Chr1 and PDR16 on Chr4. Among the ERG genes, only ERG19/CNAG_05125 and ERG20/CNAG_02084 were up-regulated, with ERG19 on Chr4 and ERG20 on Chr6. Conversely, ERG4/CNAG_02830 was down-regulated (Supplementary Table S2). However, other ERG genes, such as ERG11/CNAG_00040, ERG3/CNAG_00519, or ERG6/CNAG_03819, showed no differential expression in Chrs (4,6) x2 compared to H99. Thus, we propose that Chrs (4,6) x2 confers resistance to TUN and FLC by simultaneously up-regulating multiple genes on both euploid and aneuploid chromosomes.
Exposure to tunicamycin leads to cross-resistance to fluconazole in a clinical isolate of C. neoformans
We investigated whether TUN also induced antifungal resistance in clinical isolates. Approximately 1 million cells of the clinical isolate GLS#4458 were spread on YPD-agar plates supplemented with TUN. After 5 days, the plate with 0.125 μg/mL TUN showed lawn growth, while the plate with 0.25 μg/mL TUN displayed a few 100 visible colonies (Figure 7A). Sixteen mutants (#1–#16) were randomly selected. Spot assays revealed that all of them were able to grow in the presence of 0.25 μg/mL TUN, whereas growth of the wild-type GLS#4458 was completely inhibited (Figure 7B), indicating that all the mutants developed resistance to TUN. Disk assays were conducted using disks containing 200 μg FLC, and three mutants, #1, #9, and #12, exhibited smaller inhibition zones compared to the parent strain (Figure 7C), suggesting that these three mutants acquired resistance to FLC.
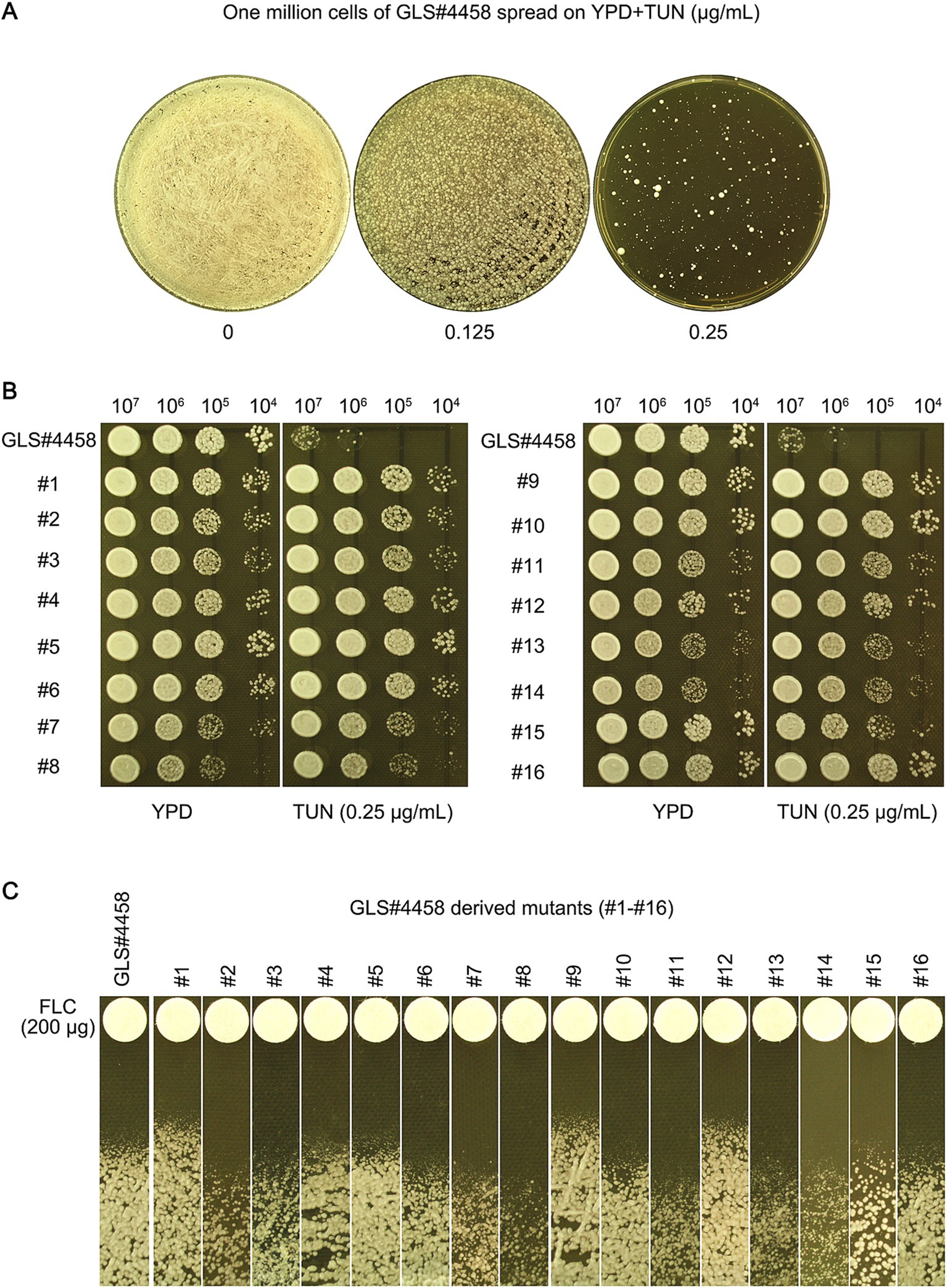
Figure 7. Tunicamycin induces fluconazole resistance in clinical isolate of C. neoformans. (A) GLS#4458 is a clinical isolate of C. neoformans. The cells of GLS#4458 were suspended in distilled water and adjusted to a concentration of 1.0 × 107 cells/mL. A volume of 100 μL of the cell suspension was spread onto YPD-agar medium supplemented with TUN, with drug concentrations indicated in the figure. The plates were incubated at 30°C for 5 days before being photographed. Sixteen colonies (#1–#16) were randomly selected from the plate containing 0.25 μg/mL for further analysis. (B) A spot assay was conducted to compare the resistance to TUN between the 16 mutants and the wild type. For each strain, 3 μL of 10-fold serial dilutions were spotted on YPD-agar plates with 0.25 μg/mL TUN or without TUN (as a control). The plates were then incubated at 30°C for 3 days and subsequently photographed. (C) In the disk diffusion assay, disks containing 200 μg FLC were used. The plates were incubated at 30°C for 3 days before being photographed.
Discussion
ER is a fundamental organelle found in eukaryotic cells. Traditionally, studies investigating ER stress induced by chemical agents have mainly focused on elucidating the associated signaling pathways. However, recent research has uncovered a connection between ER stress and the formation of aneuploidy in yeast species, with subsequent implications for the fortuitous development of antifungal resistance (Beaupere et al., 2018; Yang et al., 2021b). To our knowledge, our study represents the first direct link between ER stress and the emergence of antifungal resistance, including cross-resistance, in C. neoformans. It’s important to note that while aneuploidy itself generally does not confer resistance to various stresses, including antifungal drugs, it’s the specific aneuploidy of particular chromosome (s) that can alter susceptibility to specific stressors.
The mechanisms underlying TUN-induced ER stress and its subsequent role in aneuploidy formation in C. neoformans remain elusive. Aneuploidy provides a fitness advantage under stress conditions by directly regulating genes on the aneuploid chromosome or indirectly impacting genes on euploid chromosomes (Pavelka et al., 2010). In species like S. cerevisiae and C. albicans, TUN-induced amplification of specific chromosomes is attributed to the presence of particular genes, such as ALG7 (Beaupere et al., 2018; Yang et al., 2021b). However, in the genome of C. neoformans, ALG7/CNAG_06901 is located on Chr3, and only a minority of mutants exhibit amplification of Chr3. Instead, amplification of Chr4 and Chr6 emerges as the predominant genomic alteration among the mutants. Gene ontology (GO) analysis has identified at least 14 genes on Chr4 and Chr6 associated with resistance to TUN (refer to Supplementary Table S2). Future investigations will focus on determining whether amplification of these genes in the wild type is sufficient to confer resistance to TUN.
In the Chrs (4,6) x2 strain, which exhibited resistance to FLC, several genes encoding efflux pumps, such as AFR1 and MDR1 on Chr1, as well as PDR16 on Chr4, were up-regulated compared to the wild type. However, most of the ERG genes, including ERG11, showed no differential expression. We propose that Chrs (4,6) x2 induces cross-resistance to TUN and FLC by concurrently up-regulating multiple genes on both aneuploid and euploid chromosomes.
The TUN-selected mutants exhibit diverse genomic alterations, including increases in copy number across various chromosomes and to varying extents, while showing few genetic mutations, suggesting that TUN primarily induces genome instability rather than hypermutation in C. neoformans. ER stress and genome damage are interconnected, as ER stress can induce genome instability by impeding the repair of double-strand breaks (DSBs) (Yamamori et al., 2013). DSBs are particularly detrimental to genome integrity (Blackford and Jackson, 2017), and their unrepaired status contributes to genome instability, leading to chromosome missegregation and aneuploidy formation (Srivastava and Raghavan, 2015; Blackford and Jackson, 2017).
Our study illustrates that brief exposure to mild ER stress is adequate to trigger aneuploidy in C. neoformans, mirroring the previous findings in C. albicans (Yang et al., 2021b). Additionally, besides ER stress, short-term exposure to sub-inhibitory concentrations of FLC also prompts aneuploidy in C. neoformans (Yang et al., 2021a) and C. albicans (Sun et al., 2023b; Todd et al., 2023; Yang et al., 2023). Thus, we propose that the genome plasticity of fungal pathogens can be readily and swiftly induced by mild stresses.
Our study underscores the genome plasticity of C. neoformans as a swift and reversible mechanism for adapting to TUN-induced ER stress, offering fresh insights into the mechanism underlying the emergence of antifungal resistance.
Data availability statement
The datasets presented in this study can be found in online repositories. The names of the repository/repositories and accession number(s) can be found below: https://www.ebi.ac.uk/arrayexpress/, E-MTAB-12268, E-MTAB-12276, EMTAB- 12286 and E-MTAB-12275.
Author contributions
LZ: Data curation, Formal analysis, Investigation, Methodology, Validation, Visualization, Writing – review & editing. YX: Conceptualization, Data curation, Formal analysis, Funding acquisition, Investigation, Methodology, Validation, Visualization, Writing – review & editing. LG: Conceptualization, Funding acquisition, Project administration, Resources, Supervision, Writing – original draft, Writing – review & editing.
Funding
The author(s) declare that financial support was received for the research, authorship, and/or publication of this article. This study was supported by the Science and Technology Development Plan of Suzhou (SLJ2022018), Scientific Research Project of Suzhou Commission of Health (GSWS2020028) to Liang-sheng Guo, Natural Science Foundation of Shandong Province (ZR2023MH227) and the National Natural Science Foundation of China (81402978) to Yi Xu.
Acknowledgments
We thank Dr. Feng Yang of Shanghai Tenth People’s Hospital for assistance in analyzing NGS data and for critical reading of the manuscript.
Conflict of interest
The authors declare that the research was conducted in the absence of any commercial or financial relationships that could be construed as a potential conflict of interest.
Publisher’s note
All claims expressed in this article are solely those of the authors and do not necessarily represent those of their affiliated organizations, or those of the publisher, the editors and the reviewers. Any product that may be evaluated in this article, or claim that may be made by its manufacturer, is not guaranteed or endorsed by the publisher.
Supplementary material
The Supplementary material for this article can be found online at: https://www.frontiersin.org/articles/10.3389/fmicb.2024.1470454/full#supplementary-material
Footnotes
References
Abbey, D. A., Funt, J., Lurie-Weinberger, M. N., Thompson, D. A., Regev, A., Myers, C. L., et al. (2014). YMAP: a pipeline for visualization of copy number variation and loss of heterozygosity in eukaryotic pathogens. Genome Med. 6:100. doi: 10.1186/s13073-014-0100-8
Beaupere, C., Dinatto, L., Wasko, B. M., Chen, R. B., VanValkenburg, L., Kiflezghi, M. G., et al. (2018). Genetic screen identifies adaptive aneuploidy as a key mediator of ER stress resistance in yeast. Proc. Natl. Acad. Sci. USA 115, 9586–9591. doi: 10.1073/pnas.1804264115
Blackford, A. N., and Jackson, S. P. (2017). ATM, ATR, and DNA-PK: the trinity at the heart of the DNA damage response. Mol. Cell 66, 801–817. doi: 10.1016/j.molcel.2017.05.015
Bongomin, F., Oladele, R. O., Gago, S., Moore, C. B., and Richardson, M. D. (2018). A systematic review of fluconazole resistance in clinical isolates of cryptococcus species. Mycoses 61, 290–297. doi: 10.1111/myc.12747
Chen, G., Rubinstein, B., and Li, R. (2012). Whole chromosome aneuploidy: big mutations drive adaptation by phenotypic leap. BioEssays 34, 893–900. doi: 10.1002/bies.201200069
CLSI (Ed.) (2017). Reference method for broth. (Dilution) antifungal susceptibility testing of yeasts. 4th ed. CLSI standard M27. Wayne, PA: Clinical and Laboratory Standards Institute.
Fernandes, K. E., Fraser, J. A., and Carter, D. A. (2022). Lineages derived from Cryptococcus neoformans type strain H99 support a link between the capacity to be pleomorphic and virulence. MBio 13:e0028322. doi: 10.1128/mbio.00283-22
Fisher, M. C., Alastruey-Izquierdo, A., Berman, J., Bicanic, T., Bignell, E. M., Bowyer, P., et al. (2022). Tackling the emerging threat of antifungal resistance to human health. Nat. Rev. Microbiol. 20, 557–571. doi: 10.1038/s41579-022-00720-1
Jackson, K. M., Ding, M., and Nielsen, K. (2023). Importance of clinical isolates in Cryptococcus neoformans research. J Fungi (Basel) 9:364. doi: 10.3390/jof9030364
Janbon, G., Ormerod, K. L., Paulet, D., Byrnes, E. J. 3rd, Yadav, V., Chatterjee, G., et al. (2014). Analysis of the genome and transcriptome of Cryptococcus neoformans var. grubii reveals complex RNA expression and microevolution leading to virulence attenuation. PLoS Genet. 10:e1004261. doi: 10.1371/journal.pgen.1004261
Ke, W., Xie, Y., Chen, Y., Ding, H., Ye, L., Qiu, H., et al. (2024). Fungicide-tolerant persister formation during cryptococcal pulmonary infection. Cell Host Microbe 32, 276–289.e7. doi: 10.1016/j.chom.2023.12.012
Love, M. I., Huber, W., and Anders, S. (2014). Moderated estimation of fold change and dispersion for RNA-seq data with DESeq2. Genome Biol. 15:550. doi: 10.1186/s13059-014-0550-8
Luoma, P. V. (2013). Elimination of endoplasmic reticulum stress and cardiovascular, type 2 diabetic, and other metabolic diseases. Ann. Med. 45, 194–202. doi: 10.3109/07853890.2012.700116
Pavelka, N., Rancati, G., Zhu, J., Bradford, W. D., Saraf, A., Florens, L., et al. (2010). Aneuploidy confers quantitative proteome changes and phenotypic variation in budding yeast. Nature 468, 321–325. doi: 10.1038/nature09529
Pfaller, M. A., Diekema, D. J., Gibbs, D. L., Newell, V. A., Ellis, D., Tullio, V., et al. (2010). Results from the ARTEMIS DISK global antifungal surveillance study, 1997 to 2007: a 10.5-year analysis of susceptibilities of Candida species to fluconazole and voriconazole as determined by CLSI standardized disk diffusion. J. Clin. Microbiol. 48, 1366–1377. doi: 10.1128/JCM.02117-09
Rajasingham, R., Smith, R. M., Park, B. J., Jarvis, J. N., Govender, N. P., Chiller, T. M., et al. (2017). Global burden of disease of HIV-associated cryptococcal meningitis: an updated analysis. Lancet Infect. Dis. 17, 873–881. doi: 10.1016/S1473-3099(17)30243-8
Selmecki, A., Forche, A., and Berman, J. (2006). Aneuploidy and isochromosome formation in drug-resistant Candida albicans. Science 313, 367–370. doi: 10.1126/science.1128242
Selmecki, A., Gerami-Nejad, M., Paulson, C., Forche, A., and Berman, J. (2008). An isochromosome confers drug resistance in vivo by amplification of two genes, ERG11 and TAC1. Mol. Microbiol. 68, 624–641. doi: 10.1111/j.1365-2958.2008.06176.x
Sionov, E., Lee, H., Chang, Y. C., and Kwon-Chung, K. J. (2010). Cryptococcus neoformans overcomes stress of azole drugs by formation of disomy in specific multiple chromosomes. PLoS Pathog. 6:e1000848. doi: 10.1371/journal.ppat.1000848
Srivastava, M., and Raghavan, S. C. (2015). DNA double-strand break repair inhibitors as cancer therapeutics. Chem. Biol. 22, 17–29. doi: 10.1016/j.chembiol.2014.11.013
Sun, L. L., Li, H., Yan, T. H., Cao, Y. B., Jiang, Y. Y., and Yang, F. (2023a). Aneuploidy enables cross-tolerance to unrelated antifungal drugs in Candida parapsilosis. Front. Microbiol. 14:1137083. doi: 10.3389/fmicb.2023.1137083
Sun, L. L., Li, H., Yan, T. H., Fang, T., Wu, H., Cao, Y. B., et al. (2023b). Aneuploidy mediates rapid adaptation to a subinhibitory amount of fluconazole in Candida albicans. Microbiol. Spectr. 11, e0301622–e0303022. doi: 10.1128/spectrum.03016-22
Todd, R. T., Soisangwan, N., Peters, S., Kemp, B., Crooks, T., Gerstein, A., et al. (2023). Antifungal drug concentration impacts the Spectrum of adaptive mutations in Candida albicans. Mol. Biol. Evol. 40:msad009. doi: 10.1093/molbev/msad009
Tsai, H. J., and Nelliat, A. (2019). A double-edged sword: aneuploidy is a prevalent strategy in fungal adaptation. Genes (Basel) 10:787. doi: 10.3390/genes10100787
WHO (2022). WHO fungal priority pathogens list to guide research, development and public health action. Geneva: World Health Organization.
Wu, J., Chen, S., Liu, H., Zhang, Z., Ni, Z., Chen, J., et al. (2018). Tunicamycin specifically aggravates ER stress and overcomes chemoresistance in multidrug-resistant gastric cancer cells by inhibiting N-glycosylation. J. Exp. Clin. Cancer Res. 37:272. doi: 10.1186/s13046-018-0935-8
Yamamori, T., Meike, S., Nagane, M., Yasui, H., and Inanami, O. (2013). ER stress suppresses DNA double-strand break repair and sensitizes tumor cells to ionizing radiation by stimulating proteasomal degradation of Rad51. FEBS Lett. 587, 3348–3353. doi: 10.1016/j.febslet.2013.08.030
Yang, F., Gritsenko, V., Lu, H., Zhen, C., Gao, L., Berman, J., et al. (2021a). Adaptation to fluconazole via aneuploidy enables cross-adaptation to amphotericin B and flucytosine in Cryptococcus neoformans. Microbiol. Spectr. 9:e0072321. doi: 10.1128/Spectrum.00723-21
Yang, F., Gritsenko, V., Slor Futterman, Y., Gao, L., Zhen, C., Lu, H., et al. (2021b). Tunicamycin potentiates antifungal drug tolerance via aneuploidy in Candida albicans. MBio 12:e0227221. doi: 10.1128/mBio.02272-21
Yang, F., Lu, H., Wu, H., Fang, T., Berman, J., and Jiang, Y. Y. (2021c). Aneuploidy underlies tolerance and cross-tolerance to drugs in Candida parapsilosis. Microbiol. Spectr. 9:e0050821. doi: 10.1128/Spectrum.00508-21
Yang, F., Scopel, E. F. C., Li, H., Sun, L. L., Kawar, N., Cao, Y. B., et al. (2023). Antifungal tolerance and resistance emerge at distinct drug concentrations and rely upon different aneuploid chromosomes. MBio 14:e0022723. doi: 10.1128/mbio.00227-23
Yang, F., Teoh, F., Tan, A. S. M., Cao, Y., Pavelka, N., and Berman, J. (2019). Aneuploidy enables cross-adaptation to unrelated drugs. Mol. Biol. Evol. 36, 1768–1782. doi: 10.1093/molbev/msz104
Yang, F., Todd, R. T., Selmecki, A., Jiang, Y. Y., Cao, Y. B., and Berman, J. (2021d). The fitness costs and benefits of trisomy of each Candida albicans chromosome. Genetics 218:iyab056. doi: 10.1093/genetics/iyab056
Keywords: Cryptococcus neoformans, tunicamycin, aneuploidy, drug resistance, fluconazole
Citation: Zheng L, Xu Y and Guo L (2024) Unveiling genome plasticity as a mechanism of non-antifungal-induced antifungal resistance in Cryptococcus neoformans. Front. Microbiol. 15:1470454. doi: 10.3389/fmicb.2024.1470454
Edited by:
Octavio Luiz Franco, Catholic University of Brasilia (UCB), BrazilReviewed by:
Tanmay Dutta, Indian Institutes of Technology (IIT), IndiaSomanon Bhattacharya, Wuxi Advanced Therapeutics, Inc., United States
Camila Maurmann De Souza, Universidade Católica Dom Bosco, Brazil
Copyright © 2024 Zheng, Xu and Guo. This is an open-access article distributed under the terms of the Creative Commons Attribution License (CC BY). The use, distribution or reproduction in other forums is permitted, provided the original author(s) and the copyright owner(s) are credited and that the original publication in this journal is cited, in accordance with accepted academic practice. No use, distribution or reproduction is permitted which does not comply with these terms.
*Correspondence: Liangsheng Guo, Z2xzMjEzNUBzaW5hLmNvbQ==
†These authors have contributed equally to this work