- 1International Centre for Advanced Mediterranean Agronomic Studies (CIHEAM of Bari), Valenzano, Italy
- 2National Research Council of Italy (CNR), Institute for Sustainable Plant Protection (IPSP), University of Bari, Bari, Italy
- 3National Research Council of Italy (CNR), Institute for Sustainable Plant Protection (IPSP), Piazzale Enrico Fermi, Portici, Italy
Black rot, caused by Xanthomonas campestris pv. campestris (Xcc), is considered the most destructive disease affecting cruciferous vegetables, resulting in significant losses worldwide. The need for biocontrol agents against Xcc that can reduce reliance on chemical pesticides, enhance sustainability, and ensure crops and environmental health is crucial. Combining phages with other antibacterial agents (i.e., antibiotics and bacteriocins) to treat bacterial infections is gaining increased attention due to the frequently observed synergistic effects. This study introduces for the first time the combination of a lytic phage, i.e., Xylella phage MATE 2 (MATE 2) with nisin-producing Lactococcus lactis subsp. lactis (L. lactis) bacterium as an eco-friendly, cost-effective, and practical strategy for controlling Xcc in cruciferous vegetables. The antibacterial efficacy of MATE 2 and L. lactis, individually and in combination, against Xcc was investigated through a series of in vitro assays and in planta experiments conducted on broccoli plants. The time-killing curves results showed that under conditions of reduced Xcc population concentration (103 CFU/mL), MATE 2 at 108 PFU/mL exerted a persistent inhibitory effect on Xcc growth for 7 days. The Spot assays and v-qPCR analysis showed that both L. lactis and its bacteriocin nisin have significant antibacterial potential to contrast Xcc. Furthermore, combined application of MATE 2 and L. lactis in broccoli plants by foliar spraying generated significant synergistic efficacy in preventing Xcc infections, achieving a 71% reduction in symptoms, compared with 64 and 38% for single applications, respectively. In this study, the positive synergistic effect of the combined application of phage and beneficial bacteria in preventing black rot disease underscores this eco-friendly and cost-effective approach as a promising control measure against plant bacterial diseases.
1 Introduction
Xanthomonas campestris pv. campestris (Xcc) is a Gram-negative bacterium belonging to the family Xanthomonadaceae, which includes the most devastating plant pathogens that continually threaten food security (Dimkić et al., 2023). Xcc is an economically important phytopathogen that causes black rot disease in many Brassicaceae species (Alvarez, 2000; Papaianni et al., 2020). Black rot is considered the main yield-limiting and destructive disease of brassica vegetable crops worldwide, including cabbage, radish, cauliflower, broccoli, kale, and Brussels sprouts (Vicente and Holub, 2013; Hakalová et al., 2022). Characteristic symptoms of black rot are V-shaped yellow lesions starting from the leaf margins and blackening of the veins that results from Xcc movement in the vascular system (Vega-Álvarez et al., 2021). The affected leaves can fall prematurely, and the disease can cause stunted growth and the death of young plants, significantly affecting crop biomass and quality (Vicente and Holub, 2013). The pathogen can live epiphytically on leaf surfaces or as a saprophyte in necrotic tissues and generally enters the plant through hydathodes on leaf margins or through wounds and colonizes the plant’s vascular system (Danhorn and Fuqua, 2007; Boulanger et al., 2014).
Historically, the control of Xcc has been challenging and primarily relies on the use of Xcc-free planting material (seeds or transplants), crop rotation, and the elimination of other potential inoculum sources such as residues of infected crops and cruciferous weeds (Iglesias-Bernabé et al., 2019). The development and use of resistant cultivars has had limited success due to the scarcity of resistance sources and the existence of nine races of the pathogen (Vicente and Holub, 2013; Iglesias-Bernabé et al., 2019). Additionally, chemical treatments and the use of antibiotics in crop production are being hampered by the emergence of resistant bacterial strains, soil accumulation, as well as growing concerns about their environmental and health implications (Behlau et al., 2020; Holtappels et al., 2022). Nowadays, bacteriophages (viruses that specifically infect and lyse bacteria) are being evaluated as alternative biocontrol agents to be integrated into the management of plant diseases (Anyaegbunam et al., 2022). Lytic bacteriophages are responsible for the destruction of the host bacterial population by infecting and replicating within the bacteria, causing lysis and release of new phage particles, which can then infect other bacterial cells (Greer et al., 2024). In the case of black rot disease, numerous studies have highlighted the potential usefulness of implementing phage therapy to combat Xcc infections (Papaianni et al., 2020; Holtappels et al., 2022).
Phage therapy is currently one of the recommended therapeutic options, preferably in combination with another antibacterial agent, a practice thought to improve efficacy (Bhargava et al., 2021). Several studies have reported the potential of bacteriophages to act synergistically with other antimicrobial agents such as antibiotics and bacteriocins, reinforcing the concept that when combined, they are more efficient (Gu Liu et al., 2020; Bhargava et al., 2021; Rendueles et al., 2022). Among antibacterial agents, Lactococcus lactis subsp. lactis (L. lactis) represents one of the most extensively studied lactic acid bacteria (LAB) due to its aptitude to produce nisin, a bacteriocin that exhibits potent antibacterial activity against both Gram-positive and Gram-negative disease-associated pathogens (Shin et al., 2016; Bukvicki et al., 2020). This LAB and its cell-free supernatant have been shown to exhibit antibacterial activity against Xanthomonas spp. strains (Mačionienė et al., 2022). Additionally, Serna-Cock et al. (2013) had also showed that L. lactis exerts an in vitro antimicrobial activity against Xanthomonas albilineans. Nevertheless, research on the efficacy of L. lactis against Xcc infections is still lacking.
Nisin, recognized as an FDA-approved bacteriocin, is widely used in food preservation and veterinary medicine for the treatment of dermatological conditions and intramammary infections in animals (Greer et al., 2024). It exerts an antibacterial activity by generating pores in the cell membrane and interrupting cell wall biosynthesis through specific lipid II interaction (Prince et al., 2016). In the field of plant protection, nisin has been demonstrated to be effective in vitro and in planta against plant pathogenic bacteria belonging to the Xanthomonadaceae family, namely Xylella fastidiosa (Xf) (Sabri et al., 2024a). Additionally, the combination of nisin with lytic phages has demonstrated high potential to simultaneously control Salmonella typhimurium, Salmonella enteritidis, and Escherichia coli (Duc et al., 2023). This encourages the exploration of the potential use of L. lactis in combination with Xcc lytic phages as a cost-efficient biocontrol strategy for combating Xcc infections in planta. Accordingly, this study offers a viable alternative to conventional pesticides by integrating the Xylella phage MATE 2 (Sabri et al., 2024b) with L. lactis and explores for the first time their antibacterial activities against Xcc in vitro and in planta.
2 Materials and methods
2.1 Bacteriophage, bacterial strains, and culture conditions
Xcc strain CFBP 1710 and L. lactis strain ATCC 11454 were grown either in liquid yeast extract peptone glucose broth (YPG) (5.0 g/L yeast extract, 5.0 g/L peptone and 10.0 g/L glucose) or on yeast extract peptone glucose agar (YPGA, i.e., YPG supplemented with 1.5% agar). The strains were stored at −80°C in 25% glycerol in YPG broth. Before use, the strains were plated from glycerol stocks onto YPGA agar plates and incubated at 28°C for 24 h. The bacteriophage named Xylella phage MATE 2 (MATE 2) from our collection, which possessed a broad spectrum of antibacterial activity against various phytobacteria of the Xanthomonadaceae family (Sabri et al., 2024b), including Xcc, was used in this study.
Nisin (ITSISLCTPGCKTGALMGCNMKTATCHCSIHVSK), extracted and purified from L. lactis, was purchased from Sigma-Aldrich (Merck KGaA, Darmstadt, Germany). According to the manufacturer, the formulation contains 2.5% (w/w) pure nisin with potency ≥900 IU/mg. Lyophilized nisin was solubilized in sterile Milli-Q water to a stock concentration of 12.5 mg/mL and filter sterilized through a 0.22 μm nylon Acrodisc® syringe filter (Merck, Rome, Italy).
2.2 Spot assay
The lytic activity of MATE 2 against Xcc was assessed using a spot assay as follows: 200 μL of Xcc suspension (OD600 = 0.2) were mixed with 6 mL of YPG soft agar (i.e., YPG supplemented with 0.7% agar), poured into YPGA plates, and allowed to dry. Subsequently, drops of 10 μL of phage solution at 108, 107, 106, 105, 104, 103, 102, and 10 PFU/mL were spotted onto the surface of the plates. Spots were dried at room temperature and the plates cultured for 24 h at 28°C.
2.3 MATE 2 inhibitory effect on Xcc growth
To evaluate the lysis potential of MATE 2 in suppressing the growth of Xcc in liquid culture over a 7-day growth period, a bacterial growth reduction assay was performed. Briefly, 100 μL of MATE 2 suspensions (108 and 104 PFU/mL) were separately mixed with 100 μL of each concentration of Xcc (ranging from 108 to 102 CFU/mL) in triplicates. The mixture was then inoculated into 2 mL of YPG broth in a 10 mL centrifuge tube and incubated at 28°C for 7 days. During incubation, 11 optical density measures (0, 4, 8, 18, 24, 48, 72, 96, 120, 144, and 168 h) at OD600 were obtained using the UV spectrophotometer (UV -1800, Shimadzu Corporation, Kyoto, Japan). All the data are presented as mean ± standard deviation (SD) of three independent experiments.
2.4 In-vitro investigation for antagonism between Lactococcus lactis and Xcc
The antagonistic potential of L. lactis ATCC 11454 against Xcc was assessed through a spot assay as follows: 300 μL of Xcc suspension (OD600 = 0.1) were equally spread on YPGA plates and allowed to dry under the laminar flow hood. Subsequently, drops of 10 μL of L. lactis suspension (OD600 = 0.2) were spotted onto the surface of the plates. Spots were dried at room temperature and the plates were incubated for up to 2 days at 28°C. Sterile distilled water served as the negative control. The antagonistic activity of L. lactis was perceived as an inhibition zone of Xcc growth, which was measured using a digital caliper. This experiment was conducted in triplicates.
2.5 Antibacterial activity of nisin against Xcc
L. lactis ATCC 11454 is well known as one of the main nisin producers (Penna et al., 2005). In this regard, viable-quantitative PCR (v-qPCR), a suitable method for estimating the viability of bacterial cells (Baró et al., 2020), and spot assay were performed to explore whether nisin was involved in the antagonistic activity exhibited by L. lactis ATCC 11454 against Xcc. For v-qPCR assay, 50 μL of Xcc suspension (OD600 = 0.2) were treated with 50 μL of nisin at 6, 4, 3, 2, 1, 0.8, 0.6, 0.4, 0.2, and 0.1 mg/mL and incubated for 3 h at 28°C. Viable and dead Xcc cells (OD600 = 0.2) treated at 95°C for 15 min were served as controls. After incubation, samples were treated with PMAxx™ (Biotium, Rome, Italy) at a final concentration of 7.5 μM, incubated in the dark at room temperature for 8 min, and followed by photoactivation for 15 min using the PMA-Lite™ LED Photolysis Device (Biotium, Fremont, United States). Genomic DNA of all samples was extracted following the cetyltrimethyl ammonium bromide (CTAB) protocol (Wilson, 2001). V-qPCR was carried out in a thermocycler apparatus (Bio-Rad CFX96, BioRad, Milan, Italy), using the primers HrcCF2 (5′-CGTGTGGATGTGCAGACC-3′) and HrcCR2 (5′-CAGATCTGTCTGATCGGTGTCG-3′) (Papaianni et al., 2020). For spot assay, 300 μL of Xcc suspension (OD600 = 0.2) were equally spread on YPGA plates and allowed to dry under the laminar flow hood. Subsequently, drops of 10 μL of nisin at 6, 4, 3, 2, 1, 0.8, 0.6, 0.4, 0.2, and 0.1 mg/mL were spotted onto the surface of the plates as described earlier.
2.6 Fluorescence microscopy
To further evaluate the bactericidal activity of nisin against Xcc cells, 100 μL of Xcc suspension (OD600 = 0.2) was mixed with 100 μL of nisin at 2 mg/mL and incubated at room temperature for 3 h. The LIVE/DEAD® BacLight™ viability kit (ThermoFisher Scientific, Milan, Italy) was used according to the manufacturer’s instructions to assess the viability of Xcc cells treated with nisin at 15 min, 30 min, 1 h, 2 h and 3 h post-incubation. Photomicrographs were taken using a Nikon E800 microscope equipped with fluorescein isothiocyanate (480/30 excitation filter, DM505 dichroic mirror, 535/40 emission filter) and tetramethyl rhodamine isothiocyanate (546/10 excitation filter, DM575 dichroic mirror, 590 emission filter) fluorescence filter sets.
2.7 Transmission electron microscopy
The antibacterial effect of nisin against Xcc cells at the ultrastructural level was assessed using a TEM. Briefly, 100 μL of Xcc suspension (OD600 = 0.2) was treated with 100 μL of nisin at 2 mg/mL for 3 h at room temperature. Micrographs were taken after 3 h via TEM (FEI MORGAGNI 282D, United States) using the dip method. Briefly, carbon-coated copper/rhodium grids were immersed in both the nisin-treated and untreated bacterial suspensions for 5 min, followed by a rinse with 200 μL of distilled water. To achieve negative staining, the grids were floated on 200 μL of a 0.5% w/v UA-zero EM stain solution (Agar-Scientific Ltd., Stansted, United Kingdom), and observed under an accelerating voltage of 80 kV.
2.8 Nisin-MATE 2 combination against Xcc
To study the synergistic effects, 50 μL of phage suspension (108 PFU/mL) was added to 50 μL of nisin at the sublethal concentration (1 mg/mL) and mixed with 100 μL of Xcc suspension (OD600 = 0.2). Additionally, 100 μL of MATE 2 (108 PFU/mL) and 100 μL of nisin (1 mg/mL) were individually mixed with 100 μL of Xcc suspension (OD600 = 0.2) and served as controls. The mixtures were then inoculated into 2 mL of YPG broth in a 10 mL centrifuge tube and incubated at 28°C for 3 days. Optical density measures were determined using the UV spectrophotometer and compared to the controls.
2.9 In planta antagonistic effects of Lactococcus lactis and MATE 2 against Xcc
To evaluate the efficacy of L. lactis and MATE 2, individually and in combination, in contrasting Xcc infections within plant tissues, an in-planta assay was carried out on one-month-old broccoli plants (Brassica oleracea var. italica) in a greenhouse under controlled conditions (25 ± 2°C and ~ 70% humidity). Three leaves per plant were wounded by multi-needle prickers and then sprayed with the Xcc suspension (0.66 mL/plant; OD600 = 0.2) and the biocontrol agents. L. lactis (2.5 mL/plant; OD600 = 0.7) and MATE 2 (2.5 mL/plant; 108 PFU/mL), individually and in combination, were applied preventively, i.e., 24 h prior to Xcc inoculation, and curatively (24 h after Xcc inoculation). Ten broccoli plants were used for each treatment. Untreated plants inoculated with sterile water or with Xcc only were included as negative and positive controls, respectively. Fourteen days post-infection (dpi), disease symptoms were quantified by measuring the area of necrosis on the treated leaf according to an arbitrary six-level disease scale whereby: 0 = no symptoms, 1 = symptoms begin to appear, 2 = ¼ of leaf area showing symptoms, 3 = ⅓ of leaf area showing symptoms, 4 = ½ or more of leaf area showing symptoms, 5 = the entire leaf showing symptoms. The efficacy of each treatment in reducing Xcc infections was calculated as follows:
Where P = severity score, T = number of treated leaves having the same score, and C = number of infected leaves (positive control) having the same score.
2.10 Statistical data analysis
To test the antagonistic effect of L. lactis and MATE 2 on Xcc infections in broccoli plants, a one-way analysis of variance (ANOVA) was performed using the IBM SPSS Statistics 26 software. The significance of differences was calculated by Duncan’s post-hoc test and a p-value less than 0.05 was considered statistically significant. All data are presented as mean ± standard deviation (SD) of two independent experiments.
2.11 Persistence of Lactococcus lactis and MATE 2 in broccoli plants
To investigate the persistence of L. lactis and MATE 2 within broccoli plants, the treated leaves were analyzed at the end of the in-planta experiment (14 dpi). Briefly, two leaves from each treatment were ground in 4 mL of sterile water and centrifuged at 1,000 g for 5 min to remove plant debris. In the case of L. lactis samples, the resultant supernatant was diluted six times using a 10-fold serial dilution and 50 μL were plated on YPGA plates. The plates were incubated at 28°C for 24 h and L. lactis-like colonies were purified on YPGA plates and subjected to PCR testing using the L. lactis subsp. lactis-specific primers Nis-F: 5′-CGGCTCTGATTAAATTCTGAAG-3′ and Nis-R: 5′-GGATTAGCTAGTAGTAACTGTTC-3′ reported in Alegría et al. (2010). For MATE 2 samples, the macerate was filtered through 0.22-μm filter and 500 μL of the filtrate was added to 200 μL of Xcc suspension (OD600 = 0.2). The mixture was then inoculated into 2 mL of YPG broth in a 10 mL centrifuge tube and incubated for 24 h at 28°C for phage enrichment. The phage was then tested against Xcc using a spot assay and subjected to PCR using MATE 2-specific primers MATE 2-F: 5′-CCCGCCCAGGATCGCAAGCCGT-3′ and MATE 2-R: 5′-TCGTGCTCTCAGATTGTTGACGC-3′ designed in this study. The PCR cycles consisted of an initial denaturation step at 94°C for 5 min; 35 cycles of 94°C for 30 s, 60°C for 30 s, and 72°C for 30 s; and a final elongation step at 72°C for 5 min.
3 Results
3.1 Spot assay
The lytic activity of MATE 2 against Xcc was initially examined through a spot assay. The results showed that MATE 2 produced clear lysis zones on the Xcc lawn at 108, 107, 106, and 105 PFU/mL (Figure 1), indicating complete lysis of Xcc bacteria. At 104 PFU/mL, the lysis zone turned semi-translucent, demonstrating reduced antibacterial activity. Beyond this titer, no distinct lysis zones were observed, indicating that the phage titer was insufficient to induce distinct effect on Xcc lawn. These results highlight the lytic effectiveness of MATE 2 against Xcc and shed light on phage concentration requirements for combating Xcc infections.
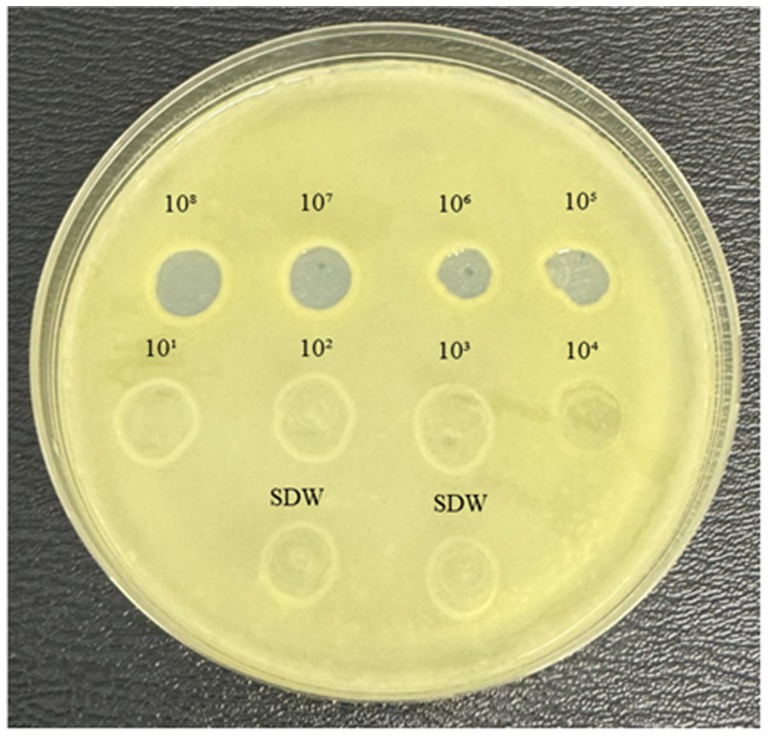
Figure 1. YPGA agar plate showing the lytic activity of MATE 2 at different titers (108–101 PFU/mL) against Xcc. SDW: sterile distilled water used as a negative control.
3.2 Bacteriolytic effect of MATE 2 on Xcc growth
To examine the dynamics of MATE 2 virulence, killing curves were generated by infecting Xcc at concentrations of 108, 107, 106, 105, 104, 103, and 102 CFU/mL with MATE 2 at two titers of 108 and 104 PFU/mL. These latter were selected based on the results of the spot assay, wherein a titer of 104 PFU/mL was identified as the minimum titer of MATE 2 capable of inducing a lytic effect on Xcc lawn. The results showed that both phage titers effectively inhibited the growth of all Xcc cultures for 24 h (Figure 2). However, after 24 h, MATE 2-infected bacteria at high concentrations (108, 107, and 106 CFU/mL) started to display an increase in OD reading, indicating the emergence of phage resistance (Figure 2). Despite the resistance development, MATE 2 showed significant antibacterial activity against Xcc growth, with an inhibition rate of 60%, 7 dpi. Xcc at 105 and 104 CFU/mL concentrations developed resistance to both phage titers after 48 h, similar to Xcc concentrations (103 and 102 CFU/mL) treated with MATE 2 at 104 PFU/mL (Figure 2). Most importantly, the infection of Xcc at 103 and 102 CFU/mL with MATE 2 at 108 PFU/mL resulted in a persisting inhibitory effect for 7 days, leading to the complete inhibition of Xcc growth (OD600 = 0) (Figure 2). At the end of the experiments, MATE 2-resistant mutants were isolated on YPGA agar plates and resistance was confirmed by spot assay. These results provide insights into the Xcc-MATE 2 interaction, revealing that a 108 PFU/mL titer appears to be suitable for phage preparation to reduce the development of bacterial resistance. The results also indicated that at controversial concentrations, i.e., low Xcc population and high MATE 2 titer, Xcc is unable to develop resistance to MATE 2 during 7 days of co-incubation. This suggests that application of MATE 2 at high concentrations could significantly contrast Xcc infections and delay the emergence of phage resistance.
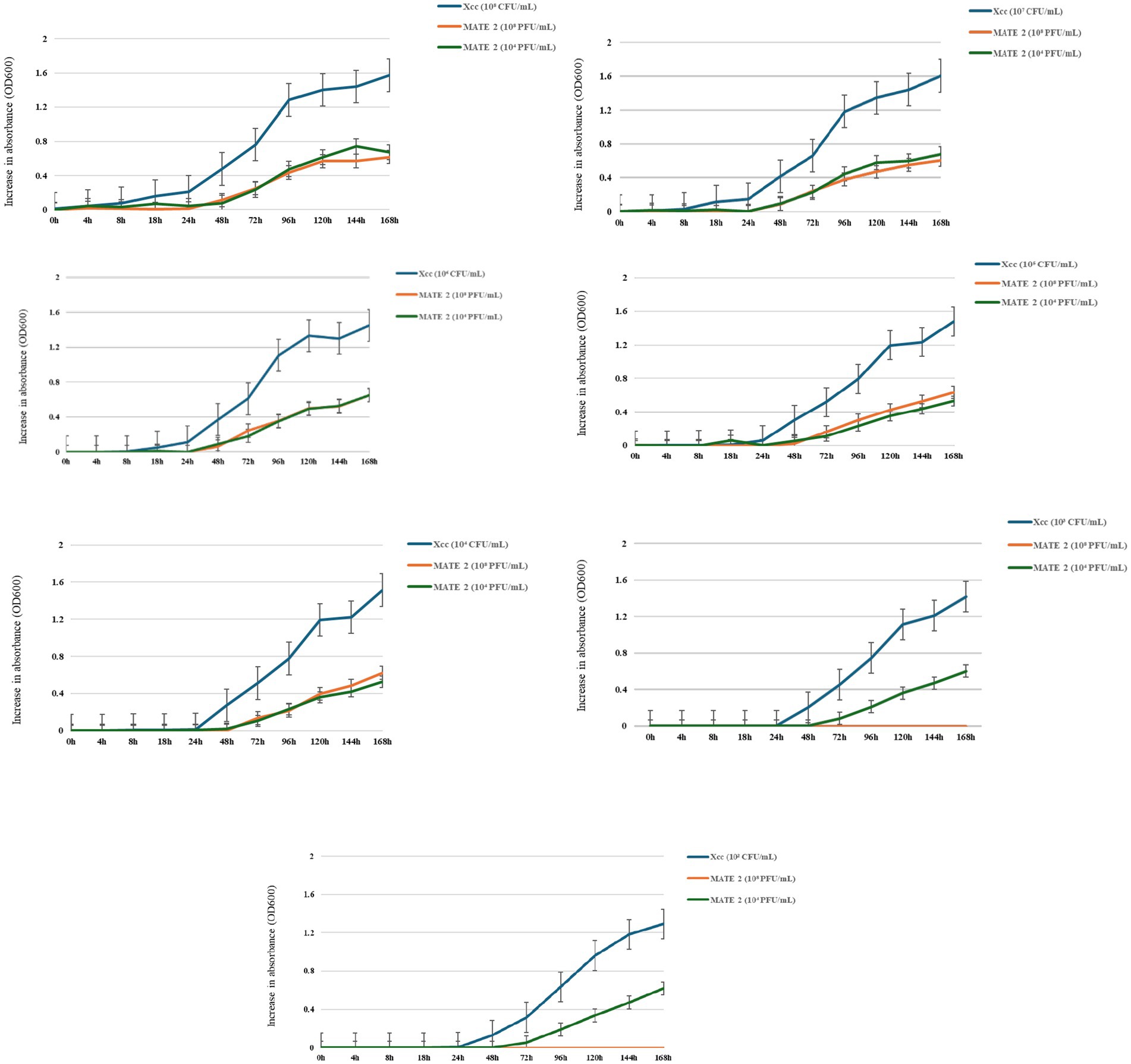
Figure 2. Time-killing curves showing the bacteriolytic effect of MATE 2 at 108 and 104 PFU/mL on Xcc growth at various concentrations (108, 107, 106, 105, 104, 103, and 102 CFU/mL). The optical density of treated and untreated-bacterial culture with MATE 2 at 24-h interval for 7 days is compared. The bars indicate the error means for three replicates.
3.3 Antibacterial activities of Lactococcus lactis and nisin against Xcc
The antagonistic activity of L. lactis on Xcc cells was examined through in-vitro culture assay, resulting in an inhibition zone diameter of 22.5 mm for Xcc growth (Figure 3). This inhibitory effect highlights the efficacy of L. lactis in contrasting Xcc, suggesting its potential use as a biocontrol agent or a source of antimicrobial compounds targeting Xcc in agricultural applications.
The bactericidal activity of nisin against Xcc was also determined using v-qPCR and spot assays. Results of the v-qPCR showed that nisin exerted antibacterial activity against Xcc in a dose-dependent manner (Figure 4). Xcc bacteria treated with nisin at 6, 4, 3, and 2 mg/mL exhibited no amplification, similarly to dead Xcc cells used as control (Figure 4), indicating complete bacterial lysis. Below these concentrations, nisin showed reduced activity against Xcc, with 1 mg/mL as the sublethal concentration. These results were consistent with the spot assay results, which showed clear lysis zones on the Xcc lawn induced by nisin at 6, 4, 3, and 2 mg/mL, while 1 mg/mL produced a clouded lysis zone (Figure 5). These findings provide valuable insight into the bactericidal activity of nisin against Xcc and suggest that nisin is involved in the antagonistic activity exhibited by L. lactis against Xcc.
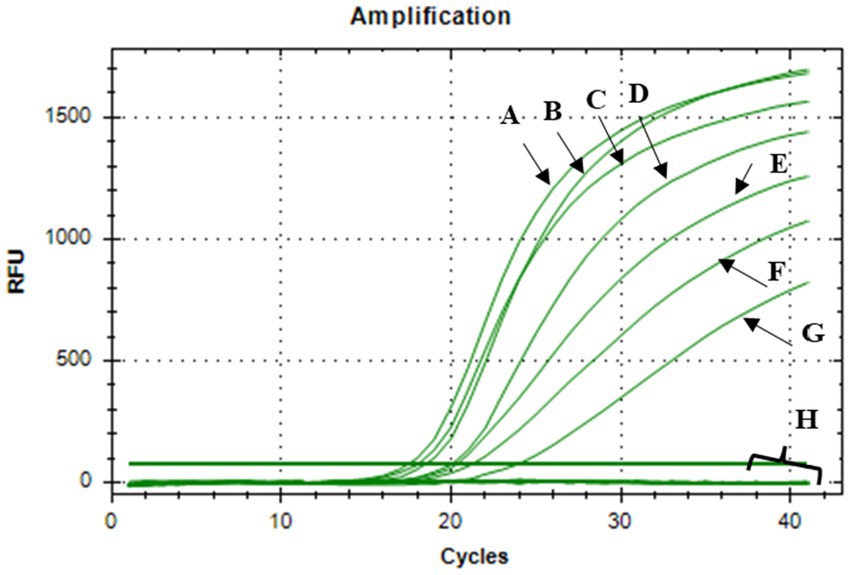
Figure 4. V-qPCR assay showing DNA amplification curves obtained from: (A) untreated Xcc, (B) nisin-treated Xcc at 0.1 mg/mL, (C) at 0.2 mg/mL, (D) at 0.4 mg/mL, (E) at 0.6 mg/mL, (F) at 0.8 mg/mL, (G) at 1 mg/ mL, (H) DNA amplification curves obtained from nisin-treated Xcc at 2, 3, 4, 6 mg/mL, dead Xcc cells, and sterile distilled water used as negative control.
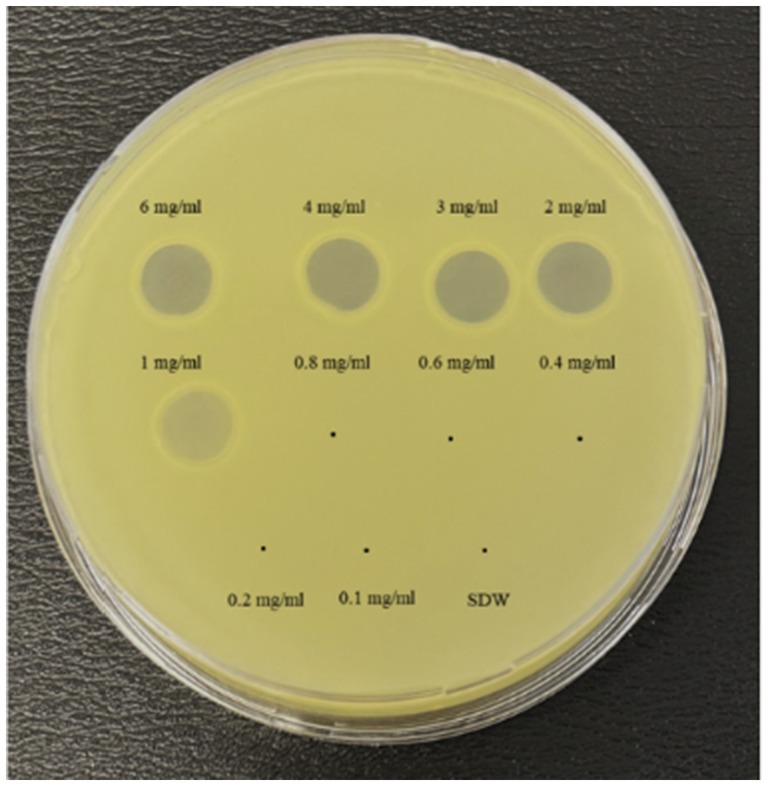
Figure 5. Spot assay showing the antibacterial activity of nisin at various concentrations against Xcc. SDW: sterile distilled water used as a negative control.
3.4 Nisin activity against Xcc cells under electron and fluorescence microscopy
FM and TEM analyses were performed to visually evaluate the bactericidal activity of nisin against Xcc. FM micrographs showed that the staining of nisin-treated Xcc cells at 2 mg/mL shifted progressively from green (live bacterial cells) to red (dead bacterial cells), indicating damages in the cell membrane (Figure 6). Three hours after contact, intense red channels were observed (Figure 6), indicating robust lysis of Xcc and confirming the spot assay and v-qPCR results. At the ultrastructural level, TEM analyses revealed that nisin treatment causes significant structural changes in Xcc cells compared to untreated cells (Figure 7A). Electron microscope analysis highlighted the disrupting action of nisin on the bacterial double membrane (Figure 7C, arrows) and the cytoplasm alterations through the production of vesicles (Figure 7B, asterisks), which impair bacterial viability.
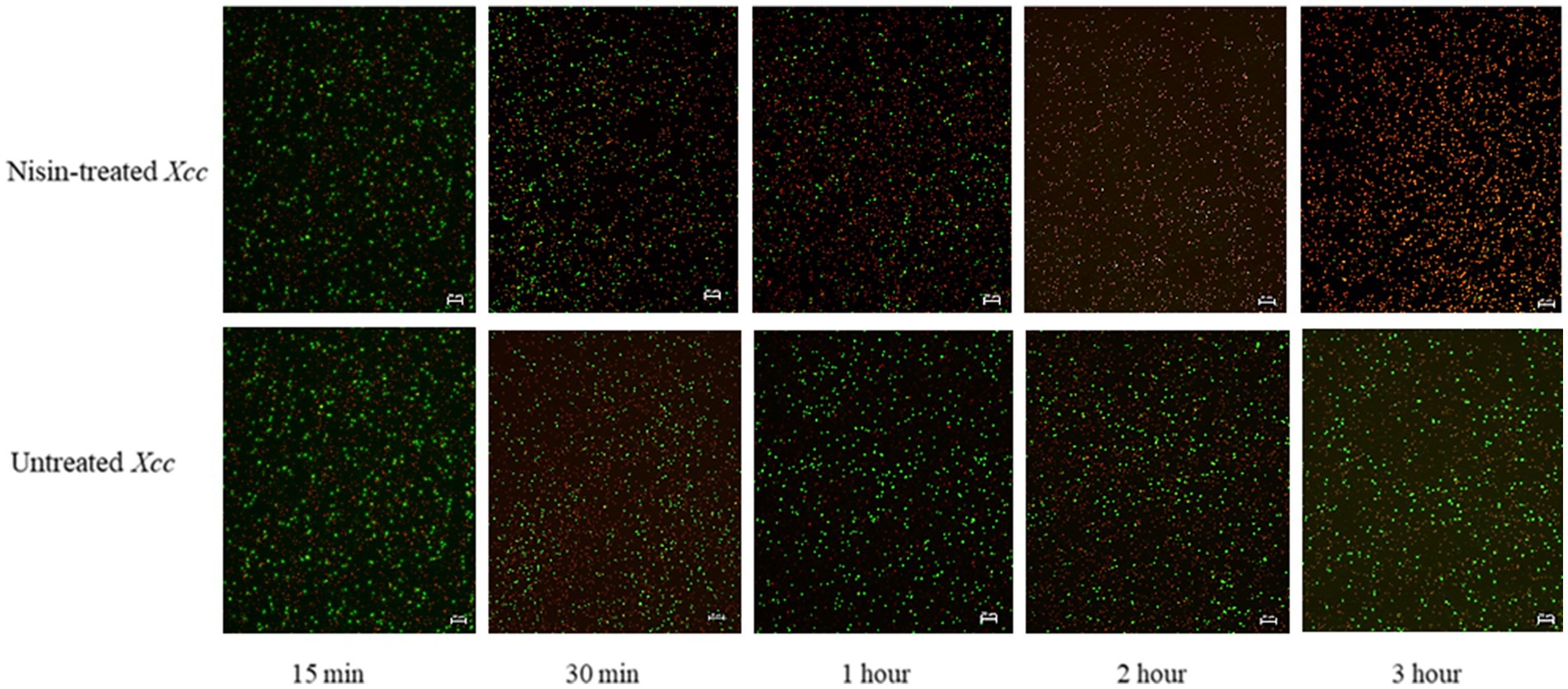
Figure 6. Fluorescent micrographs showing nisin-treated Xcc cells at 2 mg/mL. Green and red fluorescence represent live and dead cells, respectively. Magnification 20X, bar 20 μm.
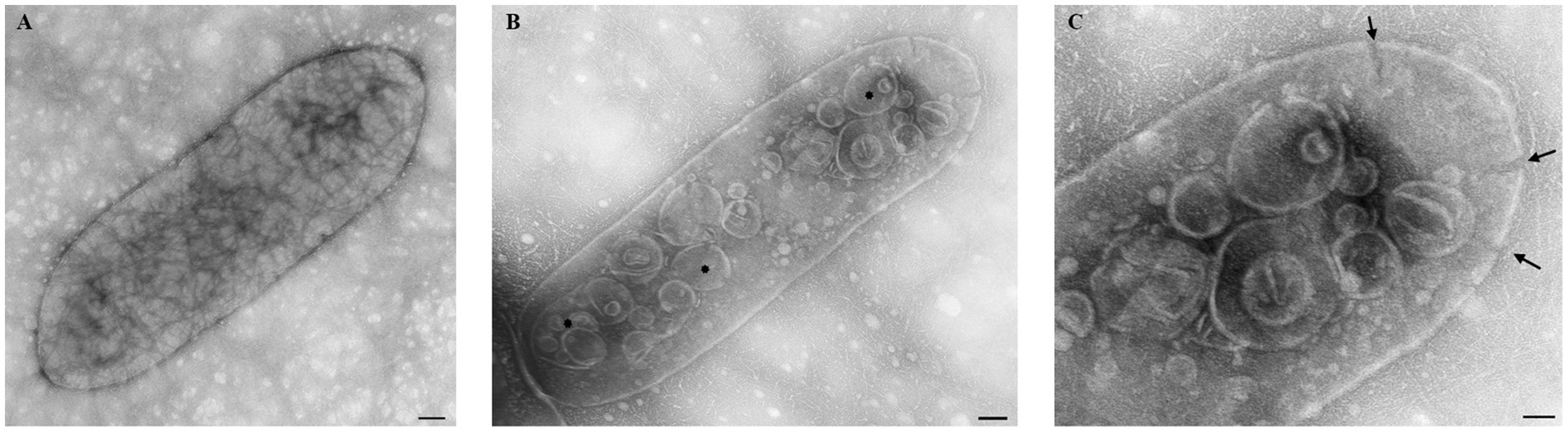
Figure 7. Transmission electron micrographs showing the bactericidal effect of nisin at 2 mg/mL on Xcc cells. (A) untreated Xcc cells, used as control. (B,C) Nisin-treated Xcc cells showing structural, cell wall, and cytoplasm alterations. Bar (A,B) 100 nm; (C) 50 nm.
3.5 Combined effect of nisin and MATE 2 against Xcc
The combined effect of nisin and MATE 2 on Xcc growth was determined by measuring variations in optical density at OD600 over a 3-day incubation period. The results showed that the nisin alone at its sublethal concentration (1 mg/mL) was less effective against Xcc growth than MATE 2, resulting in a 45% reduction in Xcc growth after 72 h of treatment (Figure 8). Additionally, as previously demonstrated, MATE 2 alone maintained high inhibitory efficacy against Xcc for 24 h, after which bacterial growth slightly resumed, resulting in an inhibition rate of 67% after 72 h of co-incubation (Figure 8). Remarkably, the combination of MATE 2 with nisin was more effective against Xcc than single treatments, achieving a 76% reduction in bacterial growth (Figure 8). This suggested a synergistic inhibitory effect against Xcc and the potential use of MATE 2 and nisin-producing L. lactis bacteria to cost-efficiently tackle Xcc infections in cruciferous vegetables.
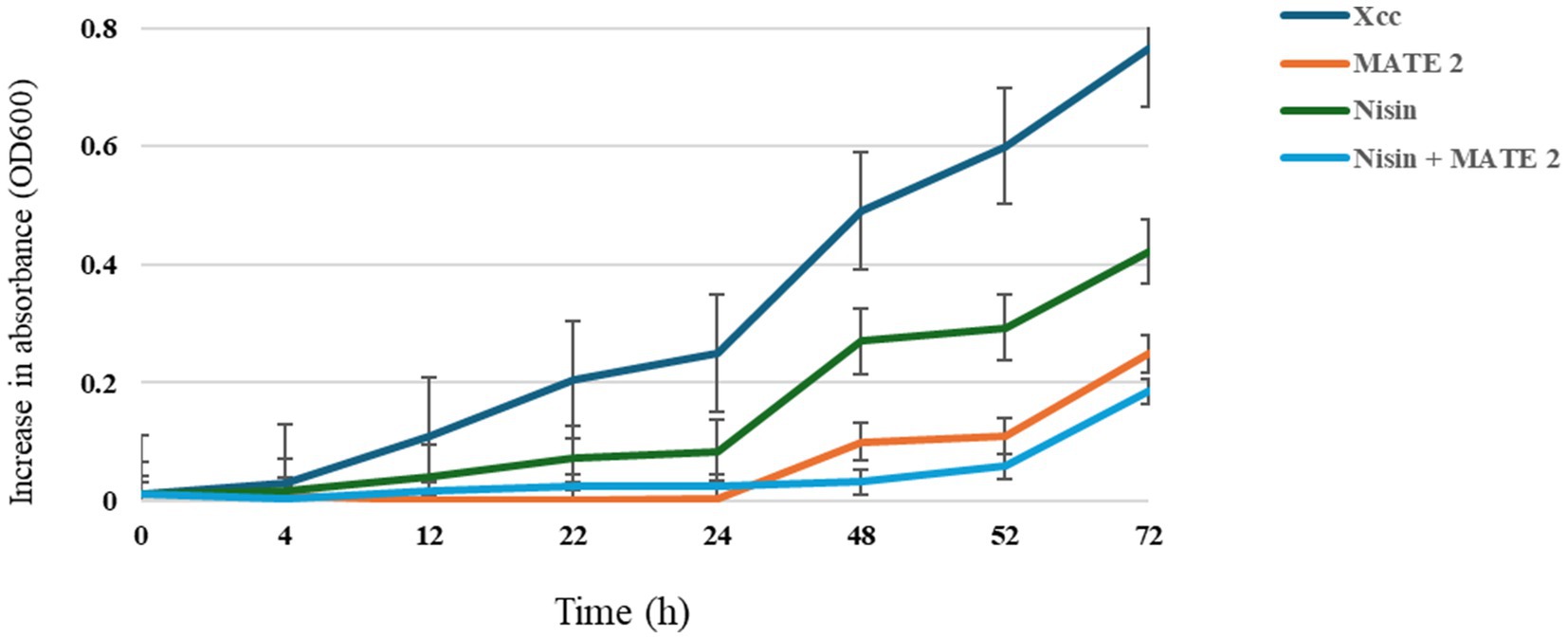
Figure 8. In-vitro killing curves displaying the antibacterial activity of MATE 2 and nisin, individually and in combination, against Xcc. The bars show the average for three replicates.
3.6 Efficiency of Lactococcus lactis and MATE 2 as individual and combined applications in controlling Xcc infections in broccoli
The in-planta antibacterial potentials of MATE 2 and L. lactis, individually and in combination, against Xcc were evaluated in broccoli plants. The untreated Xcc-infected plants (positive control) displayed severe infection symptoms 14 dpi, namely V-shaped yellow lesions on leaf margins, chlorosis, blackening of veins, and loss of leaves, while uninfected plants (negative control) showed no symptoms of Xcc (Figure 9). Interestingly, plants treated with MATE 2 and L. lactis, individually and in combination, showed reduced Xcc symptoms compared to the positive control (Figure 9). In terms of the efficacy of each treatment in reducing Xcc symptoms, which was calculated based on the symptom severity scores as described in section 2.9, analysis of our data revealed a very highly significant difference between the treatments (p = 0.000) (Figure 10). Once applied preventively, the combination of MATE 2 and L. lactis significantly decreased disease symptoms on broccoli leaves by 71% 14 dpi, while their individual applications reduced disease by 64 and 38%, respectively. Curatively, MATE 2 and L. lactis exhibited modest efficacy in mitigating Xcc symptoms in broccoli plants, achieving respective efficacy rates of 30 and 15%, while their combination resulted in a 31% disease reduction (Figure 10). At the end of the experiment, MATE 2 and L. lactis were successfully reisolated from treated broccoli plants and their identity was confirmed by PCR analyses (Figure 11). Furthermore, their persistent antibacterial activity against Xcc was validated afterward by spot assays (data not shown). These results clearly indicated the suitability of MATE 2 and L. lactis for controlling Xcc in cruciferous vegetables and demonstrated their effective combined application in preventing black rot disease.
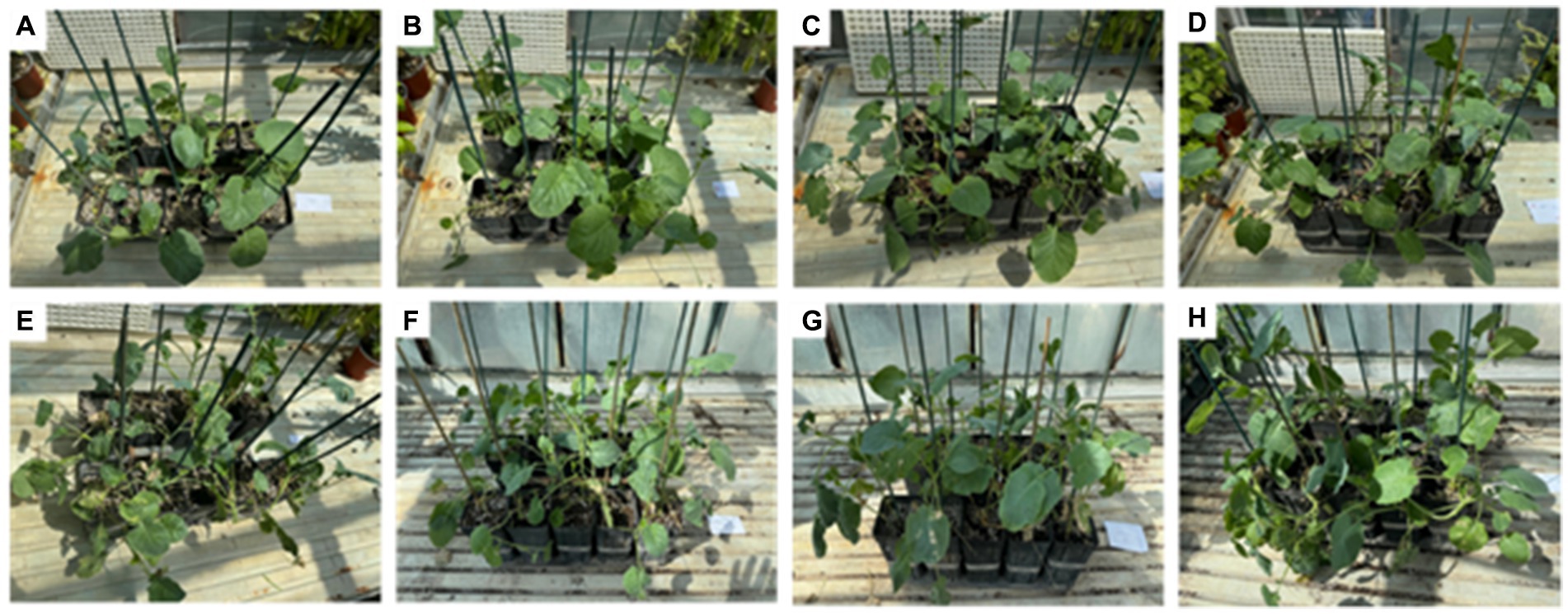
Figure 9. In-planta assays showing the antibacterial effects of L. lactis and MATE 2, individually and in combination, against Xcc infections in broccoli plants 14 dpi. (A) Healthy plants used as negative control; (B) preventive MATE 2-treated Xcc-infected plants; (C) preventive L. lactis-treated Xcc-infected plants; (D) preventive MATE 2 + L. lactis-treated Xcc-infected plants; (E) untreated Xcc-infected plants used as positive control; (F) curative MATE 2-treated Xcc-infected plants; (G) curative L. lactis-treated Xcc-infected plants; (H) curative MATE 2 + L. lactis-treated Xcc-infected plants.
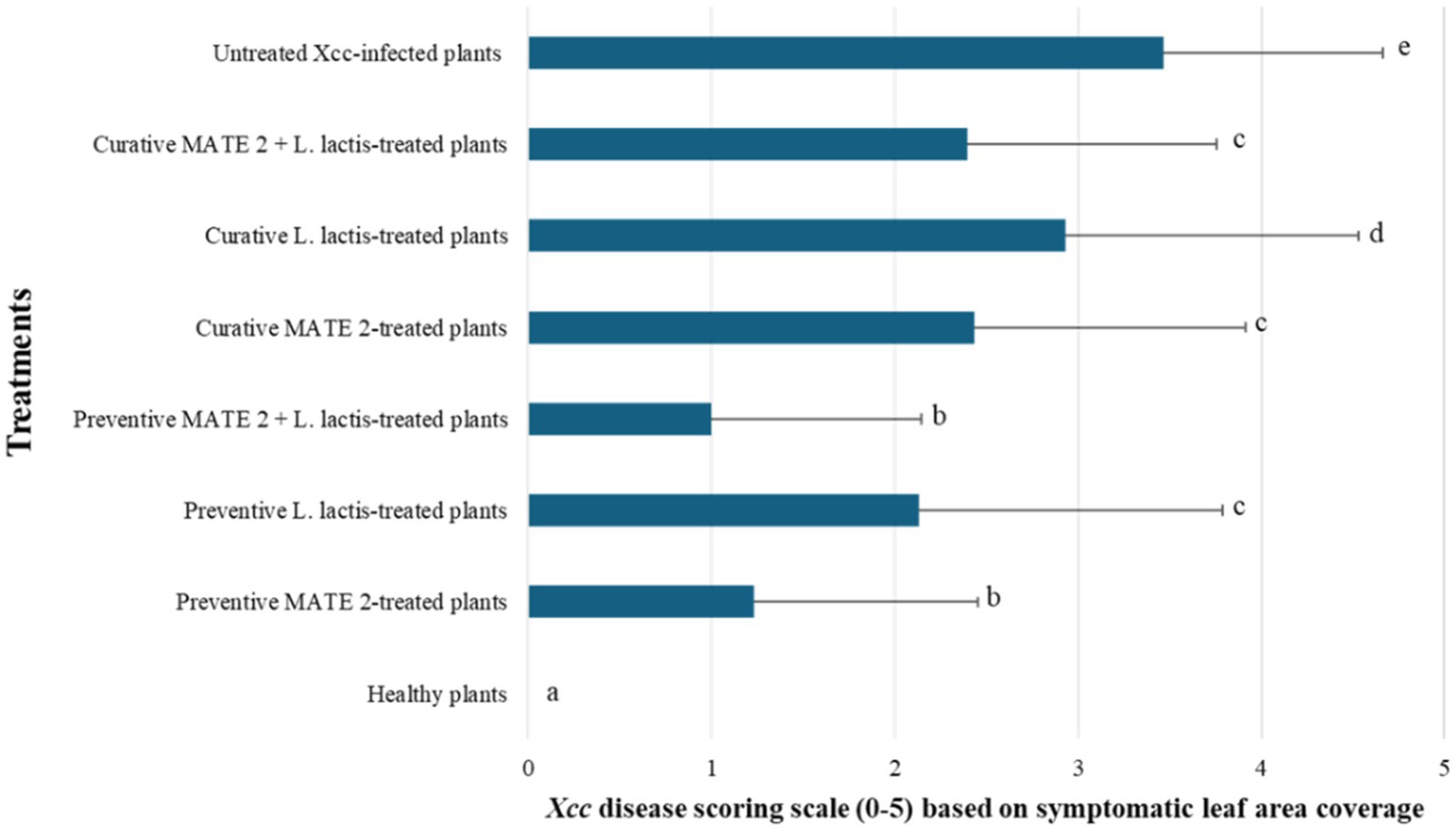
Figure 10. Histograms showing the severity of Xcc symptoms on broccoli plants treated preventively and curatively with MATE 2 and L. lactis, individually and in combination.
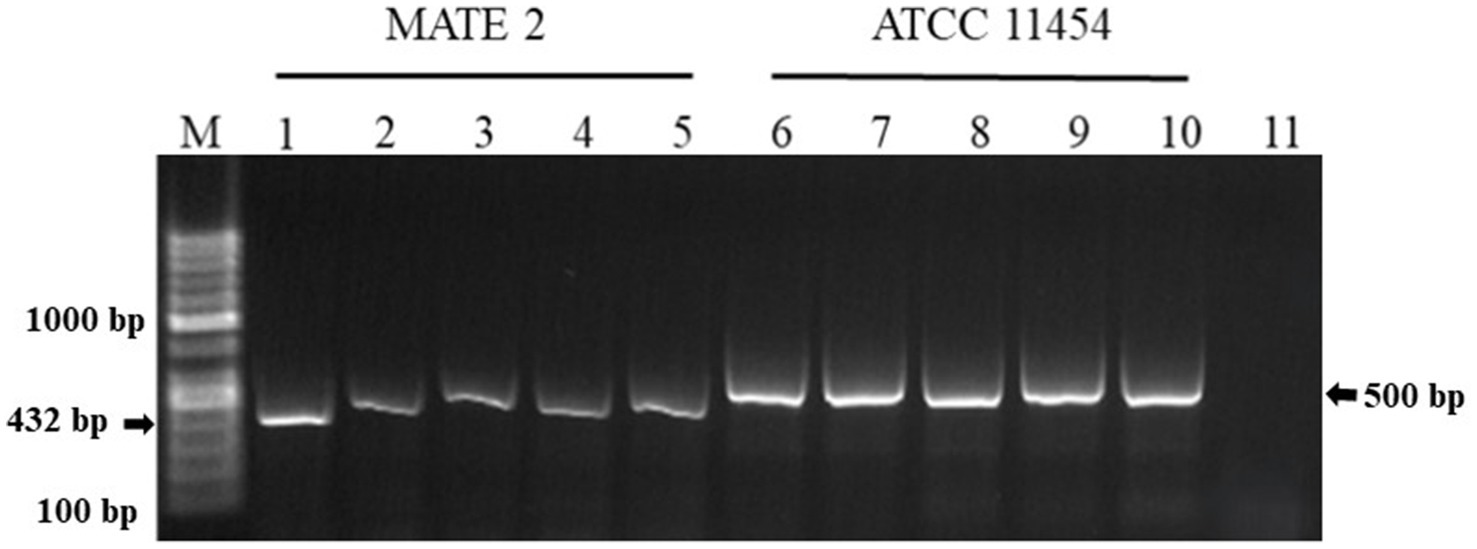
Figure 11. Agarose gel electrophoresis showing PCR products amplified from genomic DNA of L. lactis ATCC 11454 and MATE 2 reisolated from broccoli plants 14 dpi. Lane M: 100 bp DNA ladder. Lanes 1–4: MATE 2 reisolated from broccoli plants. Lane 5: MATE 2 used as positive control. Lanes 6–9: L. lactis ATCC 11454 reisolated from broccoli plants. Lane 10: L. lactis ATCC 11454 used as positive control. Lane 11: sterile water used as negative control reaction.
4 Discussion
In agriculture, the use of phages and LAB offers significant advantages, characterized by cost-effectiveness, sustainability, and ease of application (Costa et al., 2023; Ojo and de Smidt, 2023). Phages provide targeted antimicrobial activity against specific bacterial pathogens, thereby lowering the need for broad-spectrum antimicrobials and limiting environmental impacts (Strathdee et al., 2023). Their capacity to multiply inside bacterial hosts enables self-renewal and scalability, adding to cost efficiency in production and application (Ling et al., 2022). Furthermore, phages can be applied in diverse forms, including sprays, drenching, seed treatment and irrigation, facilitating their adoption in agricultural settings (Holtappels et al., 2021). LAB, known for their safety use and production of bacteriocins, offer natural antibacterial properties that improve food preservation and agricultural safety (Ayivi et al., 2020). Their ease of application through fermentation processes and incorporation into probiotic formulations supports practical integration into food production (Timothy et al., 2021). Together, phages and LAB provide cost-effective solutions that support sustainable practices by decreasing the use of chemicals, improving food quality, and managing risks related to pathogens; thus, promoting and building resilience in agricultural and food sectors. To this end, this study reports for the first time the successful use of phage MATE 2 and L. lactis in combination to prevent black rot in broccoli plants.
The dynamic of MATE 2 virulence indicated that a high titer of this phage is more efficacious against Xcc, maintaining a persistent inhibitory effect on low bacterial populations for 7 days. Similarly, in our previous study we found that MATE 2 was able to significantly inhibit the growth of Xf over 7 days with a titer of 108 PFU/mL (Sabri et al., 2024b). However, the emergence of bacterial resistance is a common challenge in phage therapy that is conditioned by many aspects, i.e., phage and bacterial concentrations, lysis potential, etc. Therefore, employing MATE 2 in conjunction with other antimicrobial compounds (bacteriocins) would help target bacteria more effectively, preserving the therapeutic potential of MATE 2, and reducing the risk of resistance development. These findings emphasize the importance of conducting a preliminary evaluation of bacterial concentration to intervene with accurate phage concentration, thereby ensuring an effective biocontrol. On the other hand, L. lactis showed for the first time an antagonistic activity against Xcc, effectively inhibiting its growth on YPGA plates. This aligns with previous reports demonstrating the antibacterial effect of L. lactis on both Gram-negative and Gram-positive bacteria, strengthening its role as a possible biocontrol agent in agricultural practices (Settier-Ramírez et al., 2021; Sabri et al., 2024a). Subsequent in vitro tests revealed that nisin exerts bactericidal activity against Xcc cells, suggesting its involvement in the antagonistic effect of L. lactis. The TEM analyses showed that nisin induces severe structural changes in Xcc cells, including alterations in the outer membrane structure and cell cytoplasm, compromising the viability. This activity against Xcc can be added to the long list of nisin’s antimicrobial effects against pathogenic bacteria (Villarreal et al., 2024). Notably, the MATE 2-nisin combination resulted in over 76% reduction in Xcc growth after 72 h of treatment, whereas phage and nisin alone showed a 67 and 45% reduction, respectively, underscoring the enhanced effectiveness of the antimicrobial combination. Indeed, the synergistic effect of phage-nisin combination has been previously reported, demonstrating that it is based on the complementary action of phages, which specifically lyse bacterial cells, and of nisin, which disrupts resistance mechanisms and bacterial physiology, resulting in a significant increase in their individual actions (Rendueles et al., 2022). This is in addition to a possible role of phages in constraining bacterial defense mechanisms against bacteriocins (Backman et al., 2024), making their combination of practical importance in ensuring more efficient antimicrobial activity, along with a reduced possibility of bacterial resistance.
For biocontrol purposes, the stability and the safety of biocontrol agents are mandatory. In this context, MATE 2 has already demonstrated stability over a wide range of pH and temperatures, and its genome is devoid of genes encoding virulence factors, antibiotic resistance, toxins, or genes related to lysogenic action (Sabri et al., 2024b). Additionally, L. lactis has “generally recognized as safe” (GRAS) status and is found in a wide range of environments and a large variety of food products (de Lacerda et al., 2016). L. lactis strains have already been isolated from stems of Eucalyptus, from the interior of aquatic plants, and from the inner tissues and leaves of sugarcane (de Lacerda et al., 2016). Furthermore, Golomb and Marco (2015) showed that certain strains of L. lactis are well adapted for growth on plants. Likewise, in our in-planta experiments, MATE 2 and L. lactis successfully remained in broccoli leaves for 14 dpi and their persistent antibacterial activity against Xcc was confirmed, indicating their suitability in controlling black rot disease.
In the management of black rot disease in broccoli plants, preventive applications of MATE 2 and L. lactis were more effective in reducing disease symptoms than their curative applications. These findings align with those of a prior study, which demonstrated that applying phages as a preventative agent could reduce bacterial infections more effectively than post-challenge application (Ahmadi et al., 2016). The results also showed that MATE 2 was more effective than L. lactis in controlling black rot. This differential efficacy could be attributed to their behaviors and interactions within the host plant. Phages, unlike other types of antimicrobial compounds, their post-application levels will increase at the expense of bacterial host survival. Additionally, their replication within bacterial cells enables them to maintain proximity to Xcc throughout the plant, which may allow them to effectively track and lyse Xcc cells within the plant. Consistent with the in-vitro results, the combined application of MATE 2 and L. lactis preventively against Xcc resulted in increased effectiveness, successfully preventing black rot symptoms by 71% in broccoli plants, while their individual applications reduced disease by 64 and 38%, respectively. These findings validate the potential of MATE 2 and L. lactis to act synergistically and boost significantly their individual actions for enhanced black rot control. In addition to nisin production, L. lactis can produce various antimicrobial compounds such as organic acids, hydrogen peroxide, diacetyl, reuterin, acetaldehyde, acetoin, carbon dioxide, and other bacteriocins (Siroli et al., 2020), which may in our case be involved in the antibacterial activity against Xcc and may positively interact with MATE 2 against Xcc infections in plant tissues. Whether this is the case, is yet to be determined.
Beyond their antimicrobial activities, LAB and phages have also demonstrated synergistic benefits in enhancing plant production. Papaianni et al. (2020) highlighted the diverse potential advantages of phages in influencing plant metabolism and enhancing defense mechanisms. Their research underscores the role of phages in agricultural systems by potentially boosting plant resilience and response to stress factors. Furthermore, Jaffar et al. (2023) demonstrated the broad-spectrum activities of L. lactis and its bacteriocin nisin, which contribute positively to plant growth, soil health, and overall crop productivity. Additionally, Raman et al. (2022) reported that L. lactis significantly promoted the root and shoot length of rice, as well as cabbage growth and production. Integrating these properties, the combination of MATE 2 and L. lactis emerges as a pivotal strategy in integrated pest management of black rot disease. However, further research is needed to explore their potential applications in field conditions against Xcc and their effects on cruciferous vegetable production.
This study extends previous research on Xf, reaffirming the broad-spectrum of antibacterial efficacy of MATE 2 and nisin-producing L. lactis (Sabri et al., 2024a,b) and their suitability for being used in plant systems; thus, supporting their application in Xf-infected plants. These attributes, together with ease of production, make them a practical and cost-effective strategy for dealing with Xf and Xcc infections in plants. Such investigations will also be crucial to translating these promising results into viable solutions for farmers, thus contributing to more resilient and productive agricultural systems.
Data availability statement
The original contributions presented in the study are included in the article/supplementary material, further inquiries can be directed to the corresponding author.
Author contributions
MS: Conceptualization, Data curation, Formal analysis, Investigation, Methodology, Software, Validation, Visualization, Writing – original draft, Writing – review & editing. KH: Conceptualization, Data curation, Formal analysis, Investigation, Methodology, Software, Validation, Visualization, Writing – original draft, Writing – review & editing. AT: Data curation, Formal analysis, Investigation, Software, Writing – original draft. AS: Data curation, Formal analysis, Investigation, Methodology, Software, Validation, Visualization, Writing – review & editing. TE: Conceptualization, Data curation, Formal analysis, Funding acquisition, Investigation, Methodology, Project administration, Resources, Software, Supervision, Validation, Visualization, Writing – original draft, Writing – review & editing.
Funding
The author(s) declare that financial support was received for the research, authorship, and/or publication of this article. This research was financially supported by the Italian Ministry of Agriculture, Food Sovereignty and Forestry (MASAF), in the frame of project “Approcci Nanotecnologici per un Controllo Sostenibile e Innovativo di Xylella fastidiosa (ANCOSIX) CUP n. J83C22001990005.”
Acknowledgments
The authors would like to thank Franco Valentini from the IPM Department at CIHEAM Bari for generously providing the Xanthomonas campestris pv. campestris bacteria used in our experiments.
Conflict of interest
The authors declare that the research was conducted in the absence of any commercial or financial relationships that could be construed as a potential conflict of interest.
Publisher’s note
All claims expressed in this article are solely those of the authors and do not necessarily represent those of their affiliated organizations, or those of the publisher, the editors and the reviewers. Any product that may be evaluated in this article, or claim that may be made by its manufacturer, is not guaranteed or endorsed by the publisher.
References
Ahmadi, M., Karimi Torshizi, M. A., Rahimi, S., and Dennehy, J. J. (2016). Prophylactic bacteriophage administration more effective than post-infection Administration in Reducing Salmonella enterica serovar Enteritidis shedding in quail. Front. Microbiol. 7:1253. doi: 10.3389/fmicb.2016.01253
Alegría, Á., Delgado, S., Roces, C., López, B., and Mayo, B. (2010). Bacteriocins produced by wild Lactococcus lactis strains isolated from traditional, starter-free cheeses made of raw milk. Int. J. Food Microbiol. 143, 61–66. doi: 10.1016/j.ijfoodmicro.2010.07.029
Alvarez, A. M. (2000). “Black rot of crucifers” in Mechanisms of resistance to plant diseases. eds. A. J. Slusarenko, R. S. Fraser, and L. C. van Loon (Dordrecht: Springer), 21–52.
Anyaegbunam, N. J., Anekpo, C. C., Anyaegbunam, Z. K. G., Doowuese, Y., Chinaka, C. B., Odo, O. J., et al. (2022). The resurgence of phage-based therapy in the era of increasing antibiotic resistance: from research progress to challenges and prospects. Microbiol. Res. 264:127155. doi: 10.1016/j.micres.2022.127155
Ayivi, R. D., Gyawali, R., Krastanov, A., Aljaloud, S. O., Worku, M., Tahergorabi, R., et al. (2020). Lactic acid Bacteria: food safety and human health applications. Dairy 1, 202–232. doi: 10.3390/dairy1030015
Backman, T., Latorre, S. M., Symeonidi, E., Muszyński, A., Bleak, E., Eads, L., et al. (2024). A phage tail–like bacteriocin suppresses competitors in metapopulations of pathogenic bacteria. Science 384:eado0713. doi: 10.1126/science.ado0713
Baró, A., Badosa, E., Montesinos, L., Feliu, L., Planas, M., Montesinos, E., et al. (2020). Screening and identification of BP100 peptide conjugates active against Xylella fastidiosa using a viability-qPCR method. BMC Microbiol. 20:229. doi: 10.1186/s12866-020-01915-3
Behlau, F., Gochez, A. M., and Jones, J. B. (2020). Diversity and copper resistance of Xanthomonas affecting citrus. Trop. Plant Pathol. 45, 200–212. doi: 10.1007/s40858-020-00340-1
Bhargava, K., Nath, G., Bhargava, A., Aseri, G. K., and Jain, N. (2021). Phage therapeutics: from promises to practices and prospectives. Appl. Microbiol. Biotechnol. 105, 9047–9067. doi: 10.1007/s00253-021-11695-z
Boulanger, A., Zischek, C., Lautier, M., Jamet, S., Rival, P., Carrère, S., et al. (2014). The plant pathogen Xanthomonas campestris pv. Campestris exploits N-acetylglucosamine during infection. MBio 5, e01527–e01514. doi: 10.1128/mbio.01527-14
Bukvicki, D., Siroli, L., D’Alessandro, M., Cosentino, S., Fliss, I., Said, L. B., et al. (2020). Unravelling the potential of Lactococcus lactis strains to be used in cheesemaking production as biocontrol agents. Food Secur. 9:1815. doi: 10.3390/foods9121815
Costa, S. P., Nogueira, C. L., Cunha, A. P., Lisac, A., and Carvalho, C. M. (2023). Potential of bacteriophage proteins as recognition molecules for pathogen detection. Crit. Rev. Biotechnol. 43, 787–804. doi: 10.1080/07388551.2022.2071671
Danhorn, T., and Fuqua, C. (2007). Biofilm formation by plant-associated bacteria. Ann. Rev. Microbiol. 61, 401–422. doi: 10.1146/annurev.micro.61.080706.093316
de Lacerda, J. R. M., da Silva, T. F., Vollú, R. E., Marques, J. M., and Seldin, L. (2016). Generally recognized as safe (GRAS) Lactococcus lactis strains associated with Lippia sidoides Cham. Are able to solubilize/mineralize phosphate. Springerplus 5:828. doi: 10.1186/s40064-016-2596-4
Dimkić, I., Jelušić, A., Kruščić, K., and Janakiev, T. (2023). “Pathobiome and microbial community shifts associated with vegetable, fruit, and cereal crops” in Plant pathogen interaction. eds. P. K. Verma, S. Mishra, V. Srivastava, and S. Mehrotra (Singapore: Springer Nature), 237–258.
Duc, H. M., Zhang, Y., Hoang, S. M., Masuda, Y., Honjoh, K. I., and Miyamoto, T. (2023). The use of phage cocktail and various antibacterial agents in combination to prevent the emergence of phage resistance. Antibiotics 12:1077. doi: 10.3390/antibiotics12061077
Golomb, B. L., and Marco, M. L. (2015). Lactococcus lactis metabolism and gene expression during growth on plant tissues. J. Bacteriol. 197, 371–381. doi: 10.1128/JB.02193-14
Greer, S. F., Rabiey, M., Studholme, D. J., and Grant, M. (2024). The potential of bacteriocins and bacteriophages to control bacterial disease of crops with a focus on Xanthomonas spp. J. R. Soc. N. Z. 1–25, 1–25. doi: 10.1080/03036758.2024.2345315
Gu Liu, C., Green, S. I., Min, L., Clark, J. R., Salazar, K. C., Terwilliger, A. L., et al. (2020). Phage-antibiotic synergy is driven by a unique combination of antibacterial mechanism of action and stoichiometry. MBio 11, e01462–e01420. doi: 10.1128/mbio.01462-20
Hakalová, E., Čechová, J., Tekielska, D. A., Eichmeier, A., and Pothier, J. F. (2022). Combined effect of thyme and clove phenolic compounds on Xanthomonas campestris pv. campestris and biocontrol of black rot disease on cabbage seeds. Front. Microbiol. 13:1007988. doi: 10.3389/fmicb.2022.1007988
Holtappels, D., Fortuna, K., Lavigne, R., and Wagemans, J. (2021). The future of phage biocontrol in integrated plant protection for sustainable crop production. Curr. Opin. Biotechnol. Syst. Biol. Nanobiotechnol. 68, 60–71. doi: 10.1016/j.copbio.2020.08.016
Holtappels, D., Fortuna, K. J., Moons, L., Broeckaert, N., Bäcker, L. E., Venneman, S., et al. (2022). The potential of bacteriophages to control Xanthomonas campestris pv. Campestris at different stages of disease development. Microb. Biotechnol. 15, 1762–1782. doi: 10.1111/1751-7915.14004
Iglesias-Bernabé, L., Madloo, P., Rodríguez, V. M., Francisco, M., and Soengas, P. (2019). Dissecting quantitative resistance to Xanthomonas campestris pv. campestris in leaves of Brassica oleracea by QTL analysis. Sci. Rep. 9:2015. doi: 10.1038/s41598-019-38527-5
Jaffar, N. S., Jawan, R., and Chong, K. P. (2023). The potential of lactic acid bacteria in mediating the control of plant diseases and plant growth stimulation in crop production - a mini review. Front. Plant Sci. 13:1047945. doi: 10.3389/fpls.2022.1047945
Ling, H., Lou, X., Luo, Q., He, Z., Sun, M., and Sun, J. (2022). Recent advances in bacteriophage-based therapeutics: insight into the post-antibiotic era. Acta Pharm. Sin. B 12, 4348–4364. doi: 10.1016/j.apsb.2022.05.007
Mačionienė, I., Čepukoit, D., Šalomskienė, J., Černauskas, D., Burokienė, D., and Šalaševičienė, A. (2022). Effects of natural antimicrobials on Xanthomonas strains growth. Horticulturae 8:7. doi: 10.3390/horticulturae8010007
Ojo, A. O., and de Smidt, O. (2023). Lactic acid: a comprehensive review of production to purification. PRO 11:688. doi: 10.3390/pr11030688
Papaianni, M., Paris, D., Woo, S. L., Fulgione, A., Rigano, M. M., Parrilli, E., et al. (2020). Plant dynamic metabolic response to bacteriophage treatment after Xanthomonas campestris pv. campestris infection. Front. Microbiol. 11:732. doi: 10.3389/fmicb.2020.00732
Penna, T. C. V., Jozala, A. F., De Lencastre Novaes, L. C., Pessoa, A., and Cholewa, O. (2005). Production of nisin by Lactococcus lactis in media with skimmed milk. Appl. Biochem. Biotechnol. 122, 0619–0638. doi: 10.1385/ABAB:122:1-3:0619
Prince, A., Sandhu, P., Ror, P., Dash, E., Sharma, S., Arakha, M., et al. (2016). Lipid-II independent antimicrobial mechanism of Nisin depends on its crowding and degree of oligomerization. Sci. Rep. 6:37908. doi: 10.1038/srep37908
Raman, J., Kim, J.-S., Choi, K. R., Eun, H., Yang, D., Ko, Y.-J., et al. (2022). Application of lactic acid Bacteria (LAB) in sustainable agriculture: advantages and limitations. Int. J. Mol. Sci. 23:7784. doi: 10.3390/ijms23147784
Rendueles, C., Duarte, A. C., Escobedo, S., Fernández, L., Rodríguez, A., García, P., et al. (2022). Combined use of bacteriocins and bacteriophages as food biopreservatives. A review. Int. J. Food Microbiol. 368:109611. doi: 10.1016/j.ijfoodmicro.2022.109611
Sabri, M., El Handi, K., Cara, O., De Stradis, A., Valentini, F., and Elbeaino, T. (2024a). Xylella phage MATE 2: a novel bacteriophage with potent lytic activity against Xylella fastidiosa subsp. pauca. Front. Microbiol. 15:1412650. doi: 10.3389/fmicb.2024.1412650
Sabri, M., El Handi, K., Valentini, F., De Stradis, A., Cara, O., Calvano, C. D., et al. (2024b). Nisin-based therapy: a realistic and eco-friendly biocontrol strategy to contrast Xylella fastidiosa subsp. pauca infections in planta. Front. Microbiol. 15:1406672. doi: 10.3389/fmicb.2024.1406672
Serna-Cock, L., Camargo-Guarnizo, A. F., and Rengifo-Guerrero, C. A. (2013). Antimicrobial activity against Xanthomonas albilineans and fermentation kinetics of a lactic acid bacterium isolated from the sugar cane crop. Chilean J. Agricult. Res. 73, 250–258. doi: 10.4067/S0718-58392013000300007
Settier-Ramírez, L., López-Carballo, G., Gavara, R., and Hernández-Muñoz, P. (2021). Broadening the antimicrobial spectrum of nisin-producing Lactococcus lactis subsp. Lactis to gram-negative bacteria by means of active packaging. Int. J. Food Microbiol. 339:109007. doi: 10.1016/j.ijfoodmicro.2020.109007
Shin, J. M., Gwak, J. W., Kamarajan, P., Fenno, J. C., Rickard, A. H., and Kapila, Y. L. (2016). Biomedical applications of nisin. J. Appl. Microbiol. 120, 1449–1465. doi: 10.1111/jam.13033
Siroli, L., Patrignani, F., D’Alessandro, M., Salvetti, E., Torriani, S., and Lanciotti, R. (2020). Suitability of the Nisin Z-producer Lactococcus lactis subsp. lactis CBM 21 to be used as an adjunct culture for Squacquerone cheese production. Animals (Basel) 10:782. doi: 10.3390/ani10050782
Strathdee, S. A., Hatfull, G. F., Mutalik, V. K., and Schooley, R. T. (2023). Phage therapy: from biological mechanisms to future directions. Cell 186, 17–31. doi: 10.1016/j.cell.2022.11.017
Timothy, B., Iliyasu, A. H., and Anvikar, A. R. (2021). Bacteriocins of lactic acid Bacteria and their industrial application. Curr. Top. Lactic Acid Bacteria Probiot. 7, 1–13. doi: 10.35732/ctlabp.2021.7.1.1
Vega-Álvarez, C., Francisco, M., and Soengas, P. (2021). Black rot disease decreases young Brassica oleracea plants’ biomass but has no effect in adult plants. Agronomy 11:569. doi: 10.3390/agronomy11030569
Vicente, J. G., and Holub, E. B. (2013). Xanthomonas campestris pv. Campestris (cause of black rot of crucifers) in the genomic era is still a worldwide threat to brassica crops. Mol. Plant Pathol. 14, 2–18. doi: 10.1111/j.1364-3703.2012.00833.x
Villarreal, L. A., Ladero, V., Sarquis, A., Martinez, B., Del Rio, B., and Alvarez, M. A. (2024). Bacteriocins against biogenic amine-accumulating lactic acid bacteria in cheese: Nisin a shows the broadest antimicrobial spectrum and prevents the formation of biofilms. J. Dairy Sci. 107, 4277–4287. doi: 10.3168/jds.2023-24358
Keywords: phage-lactic acid bacteria synergy, bacteriocin, biocontrol, Xanthomonas campestris pv. campestris, cruciferous vegetables
Citation: Sabri M, El Handi K, El Tousy A, De Stradis A and Elbeaino T (2024) Synergistic antibacterial activity of Lactococcus lactis and Xylella phage MATE 2 for an effective biocontrol strategy against black rot disease in broccoli. Front. Microbiol. 15:1468792. doi: 10.3389/fmicb.2024.1468792
Edited by:
Atte Johannes Von Wright, University of Eastern Finland, FinlandReviewed by:
Dexi Li, Henan Agricultural University, ChinaNorma Margarita De La Fuente Salcido, Polytechnic University of Gomez Palacio, Mexico
Copyright © 2024 Sabri, El Handi, El Tousy, De Stradis and Elbeaino. This is an open-access article distributed under the terms of the Creative Commons Attribution License (CC BY). The use, distribution or reproduction in other forums is permitted, provided the original author(s) and the copyright owner(s) are credited and that the original publication in this journal is cited, in accordance with accepted academic practice. No use, distribution or reproduction is permitted which does not comply with these terms.
*Correspondence: Toufic Elbeaino, ZWxiZWFpbm9AaWFtYi5pdA==
†These authors share first authorship