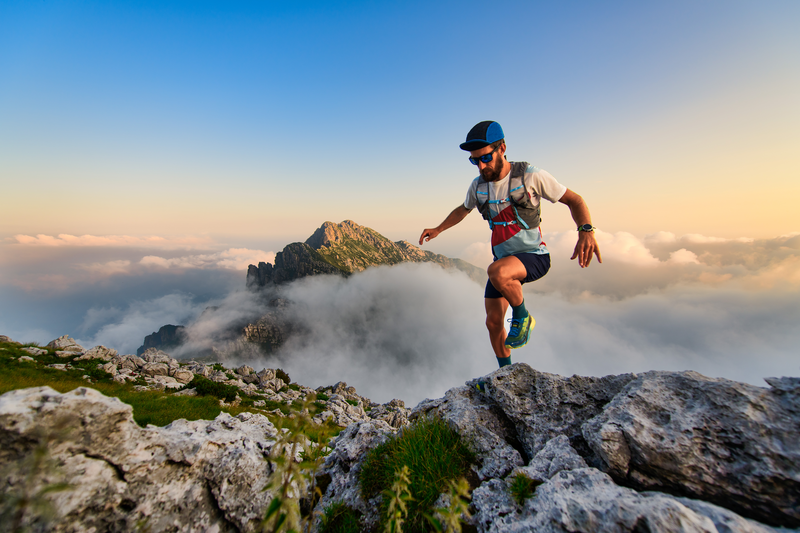
94% of researchers rate our articles as excellent or good
Learn more about the work of our research integrity team to safeguard the quality of each article we publish.
Find out more
MINI REVIEW article
Front. Microbiol. , 05 September 2024
Sec. Antimicrobials, Resistance and Chemotherapy
Volume 15 - 2024 | https://doi.org/10.3389/fmicb.2024.1468383
This article is part of the Research Topic Benefit and Risk on Drug-drug Interactions in Infections View all 3 articles
Non-tuberculous mycobacterial pulmonary disease (NTM-PD) is a refractory chronic respiratory infectious disease and its prevalence is increasing globally. The standard treatment regimen for NTM-PD involves long-term multidrug therapy including macrolides. The incidence of adverse events is high given the advanced age of many NTM-PD patients. In addition, drug–drug interactions under coexisting conditions add additional complexity. Despite guidelines advocating multidrug therapy for NTM-PD, low adherence rates probably owing to the relatively frequent adverse events and drug interactions. An appropriate treatment regimen can improve the bacteriological response rates, reduce the development of macrolide resistance, and mitigate adverse events. Of particular concern are the interactions arising from new complications that develop with NTM-PD. Notably, chronic pulmonary aspergillosis occasionally co-infects NTM-PD, which can lead to poor prognosis. The primary therapeutic modality for chronic pulmonary aspergillosis is the azoles. However, the interaction with rifamycin is problematic, making it challenging to continue standard treatment for NTM-PD and requiring drug adjustments. The implications of rifamycin extend beyond chronic pulmonary aspergillosis, impacting various other diseases such as those requiring immunosuppressive agents and AIDS patients requiring antiretroviral therapy. Hence, a comprehensive consideration of drug interactions is imperative for the initiation of NTM-PD treatment. This mini-review focuses on drug–drug interactions in a multidrug regimen for NTM-PD and discusses the essential points to be considered in the treatment of NTM.
Non-tuberculous mycobacterial pulmonary disease (NTM-PD) is a refractory chronic respiratory infectious disease in patients with pulmonary structural abnormalities such as bronchiectasis, cystic pulmonary fibrosis, and chronic obstructive pulmonary disease (COPD), as well as in those with mild immunosuppression caused by the use of oral steroids, immunosuppressants, and biological agents. The incidence of NTM-PD is rising, and the mortality rates associated with the disease are increasing globally (Adjemian et al., 2012; Namkoong et al., 2016; Harada et al., 2021; Dahl et al., 2022). Long-term multidrug therapy, which includes macrolides, is the standard treatment for NTM-PD, but this treatment can sometimes cause problematic drug–drug interactions. The treatment duration for NTM-PD is longer than that for pulmonary tuberculosis, and the frequency of adverse events is higher because many of the treated patients are elderly. In Japan, the continuation rates of standard treatment at six and 12 months are 59 and 41%, respectively (Morimoto et al., 2019). Although guidelines recommend multidrug therapy, low compliance rates have been reported, possibly because of the high frequency of adverse events (Adjemian et al., 2014; van Ingen et al., 2017). Patients with macrolide-resistant Mycobacterium avium intracellulare (MAI) PD have poor prognosis; therefore, it is crucial to select a treatment regimen that does not induce macrolide resistance (Kobashi et al., 2006; Griffith et al., 2006). This mini-review provides an overview of drug–drug interactions that can occur in NTM-PD multidrug therapy.
The drug–drug interactions between the NTM-PD drugs are presented in Table 1. Based on the results of a randomized controlled trial (RCT) that showed the efficacy of multidrug therapy, including clarithromycin (CAM), in treating AIDS-MAI bacteremia, CAM has become the standard treatment for MAI disease (Shafran et al., 1996). Subsequently, RCT were conducted to evaluate the effects of CAM and clofazimine (CFZ) with and without ethambutol (EB) on AIDS-MAI bacteremia. The results showed that regimens that included EB significantly reduced the relapse rate and emergence of CAM-resistant strains (Dube et al., 1997). In another RCT, the addition of rifabutin (RBT) to CAM and EB did not improve the bacteriological response rate in AIDS patients with disseminated MAI infection, but improved survival and inhibited the development of macrolide resistance (2% RBT, 14% placebo; Gordin et al., 1999). These findings indicate that both EB and RBT can inhibit macrolide resistance in AIDS patients. However, a prospective trial comparing CAM and EB with and without CFZ resulted in significantly higher mortality in the CFZ group (61% CFZ, 38% placebo; Chaisson et al., 1997). Nevertheless, a recent retrospective study of non-AIDS MAI-PD patients showed a higher rate of culture conversion in the CFZ group than in the rifampicin (RFP) group (100% vs. 71%), which differs significantly from the results of AIDS-MAI bacteremia (Jarand et al., 2016).
Several studies have been conducted on MAI-PD in non-AIDS patients, following the results of a prospective RCT on AIDS-MAI bacteremia. A retrospective study of macrolide-resistant MAI-PD found macrolide monotherapy and macrolide and quinolone dual therapy to be risk factors for macrolide resistance (Griffith et al., 2006). Furthermore, macrolide resistance is inhibited in patients treated with macrolides, EB, and rifamycin. In another retrospective study of macrolide-resistant MAI disease, 64.4% of the patients were treated with a regimen that did not include EB prior to therapy (Morimoto et al., 2016). The addition of EB to CAM has been shown to suppress CAM resistance in MAI in vitro and in vivo (Kent et al., 1992; Bermudez et al., 1996). These results suggest that EB plays a crucial role in the suppression of the emergence of macrolide resistance. This may be due to the fact that EB has additive and synergistic effects with many of the antimicrobial agents (Kent et al., 1992). Since macrolide monotherapy easily induces macrolide resistance, guidelines in the United States, United Kingdom, and Japan recommend macrolide as the key drug and a combination of EB and RFP as the standard regimen (Daley et al., 2020; Haworth et al., 2017). In a randomized, double-blind, comparative study of three-drug therapy plus streptomycin (SM), the culture conversion rate in the SM group was 71.2%, which was higher than that in the placebo group (50.7%; Kobashi et al., 2007). Based on these results, guidelines recommend the use of concomitant aminoglycosides such as SM in severe cases (Daley et al., 2020). In an RCT that included amikacin liposome inhalation suspension (ALIS) in guideline-based therapy (GBT) for refractory MAI-PD, ALIS significantly improved the culture conversion rate after 6 months of treatment (Griffith et al., 2018).
Many drugs commonly used in standard therapies exhibit drug–drug interactions. For instance, RFP and RBT are potent CYP3A4 inducers that significantly decrease blood CAM concentrations (van Ingen et al., 2012a; Miwa et al., 2014). Azithromycin (AZM) is less affected by RFP than CAM, exhibiting a 23% reduction in Cmax (van Ingen et al., 2012a). Although RBT also induces CYP3A4-inducing effects, its impact is less than that of RFP, with a limited reduction in CAM and AZM concentrations (van Ingen et al., 2012a; Hafner et al., 1998). In contrast, CAM significantly increased RBT concentrations, and the concomitant use of CAM considerably increased the incidence of uveitis (Hafner et al., 1998; Benson et al., 2000). In a retrospective study comparing CAM and EB with and without RFP, the rate of treatment interruption due to adverse events was significantly lower in the group without RFP (Miwa et al., 2014). Conversely, the culture conversion rate was higher in the RFP-free group, although the difference was not statistically significant, and the macrolide resistance was not substantially different between the two groups. RFP has not demonstrated efficacy against MAI in vitro or in vivo, raising questions regarding its significance in NTM treatment (van Ingen et al., 2024). Currently, a prospective study (NCT 03672630) is underway in the United States to compare the efficacy of AZM and EB with and without RFP, which may clarify the significance of adding rifamycins to standard therapy. Additionally, an ongoing RCT compared RFP and CFZ as concomitant drugs with AZM and EB; however, the results await (Zweijpfenning et al., 2024). A global clinical trial (NCT04630145) using bedaquiline (BDQ) as a concomitant drug has recently been initiated. Although BDQ has no significant drug interactions, potential concerns regarding QT prolongation may arise from its interaction with macrolides. Therefore, it is crucial to closely monitor the results of safety evaluation trials.
Preventing the interruption of EB caused by adverse events helps to prevent macrolide resistance in MAI. In a retrospective study comparing daily and intermittent dosing for non-cavitary MAI-PD, there was a significant decrease in EB interruption owing to adverse events in the intermittent group (24% vs. 1%; Jeong et al., 2015). However, there was no significant difference in the culture conversion rate between the two groups (76% vs. 67%), and the rate of macrolide resistance was lower in the intermittent group. Another retrospective study comparing EB ≥ 12.5 mg/kg/day to <12.5 mg/kg/day showed a significant reduction in the incidence of EB-induced ocular neuropathy at lower dose group (20% vs. 3%), with similar rates of macrolide resistance and culture conversion (Ando et al., 2021). Lower-dose EB administration is also a treatment option, although the extent to which reducing EB will help prevent macrolide resistance remains unclear. The exact mechanism how EB suppresses the emergence of macrolide resistance remains unclear; however, it is thought that EB exerts its effects on mycobacterial cell walls, thereby increasing the penetrability of combination agents (Källenius et al., 1989; Hoffner et al., 1989).
Quinolones are recommended in the guidelines for RFP-resistant M. kansasii and M. xenopi but not for MAI-PD (Daley et al., 2020). A retrospective study was conducted in which moxifloxacin (MFLX) was added to patients with MAI-PD who failed to achieve negative conversion after 6 months of standard therapy. In this study, 29% of the patients who received additional MFLX achieved culture conversion (Koh et al., 2013). Another retrospective study adding sitafloxacin (STFX) to MAI-PD patients who did not achieve culture conversion after 1 year of standard therapy reported culture conversion in 44% of patients after 6 months (Fujita et al., 2016). Quinolones such as MFLX and STFX may be effective agents for refractory MAI-PD.
M. abscessus requires a multidrug therapeutic approach, including injectable drugs, at treatment initiation, and maintenance treatment requires a prolonged duration. According to these guidelines, macrolide-resistant strains should receive two or more drugs during maintenance therapy. However, selection of an effective antimicrobial agent remains challenging (Daley et al., 2020). CFZ has demonstrated synergistic effects against M. abscessus and MAI in vitro when combined with AMK and CAM, making it a promising agent (van Ingen et al., 2012b; Ferro et al., 2016; Gangadharam et al., 1988). CFZ is known to cause adverse effects including QT prolongation, skin pigmentation changes, and gastrointestinal symptoms. RBT has also been reported to synergize with CAM and Tigecycline (TGC) in vitro (Pryjma et al., 2018). M. abscessus develops macrolide-induced resistance via whiB7 and erm (Bich Hanh et al., 2021) gene expression, but RBT suppresses these genes in vitro and displays synergistic effects with macrolides (Aziz et al., 2020). This synergistic effect was not observed in the erm41-negative strain, suggesting that it may be an effective treatment for macrolide-resistant M. abscessus. In a clinical trial conducted to evaluate the efficacy of adding TGC to the initial treatment phase, the culture conversion rate was significantly higher in the TGC-treated group (89%) than in the control group (50%) after 1 month of treatment. However, no significant difference was observed between the two groups at 12 months (Kim S. R. et al., 2022). Prolonged use of TGC is complicated by its poor tolerability and the occurrence of adverse events. In an open-label prospective trial using ALIS for M. abscessus infection, 50% of cases achieved culture conversion within 1 year (Siegel et al., 2023). Omadacycline (OMD) has also shown synergistic effects with CAM both in vitro and in vivo (Bich Hanh et al., 2021; Fujiwara et al., 2023). In drug susceptibility studies, STFX exhibited a lower MIC than MFLX, suggesting that it may be effective (Kamada et al., 2021; He et al., 2021; Fujiwara et al., 2021). The use of a β-lactam antibiotic in combination with an appropriate β-lactamase inhibitor has been proposed as a potential treatment for M. abscessus infections. M. abscessus expresses a chromosome-encoded Ambler class A β-lactamase (BlaMab), which confers resistance to various β-lactam antibiotics. The combination of avibactam and amoxicillin showed synergistic effects in vitro and improved survival in a zebrafish model of M. abscessus infection (Dubee et al., 2015). Other combinations, such as amoxicillin-imipenem-relebactam and vaborbactam in combination with carbapenems, have also demonstrated efficacy against M. abscessus (Lopeman et al., 2020; Kaushik et al., 2019). Although the efficacy of β-lactamase inhibitors has not yet been established in clinical trials, these findings might provide promising results for future treatment options. Given the limited number of effective agents against M. abscessus, continued development of new agents is anticipated.
The interactions between NTM-PD treatment and medication for comorbidities are shown in Table 2. RFP and RBT are potent inducers of CYP3A4, and can affect the efficacy of other therapeutic agents. Approximately 10% of NTM-PD cases are complicated by CPA, with fibrocavitary NTM-PD, COPD, and systemic steroid administration being recognized as risk factors for CPA development (Takeda et al., 2016; Phoompoung and Chayakulkeeree, 2020; Fukushima and Kida, 2021). It has been reported as an independent prognostic factor for NTM-PD (Takeda et al., 2016; Fukushima and Kida, 2021). CPA treatment in patients with NTM-PD is typically prolonged, and oral azoles are the primary therapeutic option. However, rifamycin cannot be used in combination with azoles, because it significantly reduces the concentration of azoles (Jaruratanasirikul and Sriwiriyajan, 1998; Moon et al., 2017; Geist et al., 2007; Townsend et al., 2017). The role of RFP in the treatment of NTM-PD has been questioned, and the impact of discontinuing RFP on treatment efficacy may be limited (van Ingen et al., 2024). CAM and AZM increase the blood concentration of voriconazole (VRCZ); therefore, dosage adjustment based on therapeutic drug monitoring (TDM) is required. CAM cannot be used in combination because of its significant increase in isavuconazole (ISCZ) concentration (Mushtaq et al., 2023; Purkins et al., 2003). Meta-analyses reported no difference in microbial outcomes between AZM and CAM; hence, AZM is recommended for patients receiving ISCZ (Pasipanodya et al., 2017). Given these interactions, it is recommended that rifamycin be discontinued while using azoles when NTM-PD develops with CPA, and that NTM-PD and CPA be treated simultaneously (Phoompoung and Chayakulkeeree, 2020). If rifamycin cannot be discontinued, echinocandin is an alternative, although its administration via intravenous infusion makes its long-term use difficult. Currently, global clinical trials are being conducted on Fosmanogepix and Olorofim, which can be used as alternatives to azoles. In a phase one study examining the interaction between Fosmanogepix and RFP, the addition of RFP resulted in a slight decrease in the AUC of Fosmanogepix, although the effect was limited and could be managed with dose adjustment (Hodges et al., 2024). Olorofim is a weak inhibitor of CYP3A4 that may interact with rifamycin, although the degree of interaction is lower than that observed with azoles (Rauseo et al., 2020).
Table 2. Drug–drug interactions between non-tuberculous mycobacterial infections and coexisting diseases.
An elevated incidence of NTM and increased mortality rates have been reported in patients with rheumatoid arthritis and those using immunosuppressants (Brode et al., 2015; Marras et al., 2018; Uno et al., 2020; Yeh et al., 2014). Additionally, increased mortality due to NTM-PD caused by rapidly growing mycobacteria has been documented in patients receiving anti-TNF-α inhibitors (Winthrop et al., 2009; Mori and Sugimoto, 2012). It is crucial for patients using these immunosuppressive agents to be aware of drug–drug interactions, as they are being treated for NTM-PD concurrently with their underlying diseases. RFP lowers the AUC of prednisolone by 66%, necessitating an increase in prednisolone dose (McAllister et al., 1983). Rifamycin decreased the blood concentration of tacrolimus, whereas CAM enhanced its AUC by approximately 4-fold. Therefore, TDM should be performed and adjusted to maintain appropriate blood levels (Hebert et al., 1999; Kim O. H. et al., 2022; Wen et al., 2023).
Mycobacterial infections are prevalent in patients with AIDS. In a large cohort study of HIV-positive individuals in North America, disseminated MAI infection was reported to be the third most prevalent opportunistic infection following Pneumocystis jirovecii pneumonia and esophageal candida (Buchacz et al., 2016). Disseminated MAI infection tends to develop within 90 days of initiating antiretroviral therapy (ART; Yen et al., 2019). Some AIDS patients may require treatment for NTM disease while on ART, and rifamycin may interact with antiviral drugs because of its strong CYP3A4 induction. Although RFP is not recommended for certain combinations, RBT can be used in certain cases. Thus, the reference of the Department of Health and Human Services, 2024 guidelines is recommended.
RFP is a potent inducer of CYP3A4 that can interact with various drugs. A significant proportion of patients with NTM-PD are elderly and may have various lifestyle comorbidities. The tuberculosis guidelines provide information on the potential interactions between RFP and lifestyle medications (Nahid et al., 2016). Warfarin, for example, necessitates a dose increase due to its diminished concentration, while calcium blockers exhibit reduced efficacy. Similarly, the diminished efficacy of digoxin necessitates TDM to adjust its dosage. Theophylline also demonstrates reduced efficacy, which necessitates TDM to adjust its dosage. The reduced blood levels of statins resulting from RFP necessitate blood tests to evaluate their effectiveness. If statins appear ineffective, switching to another antihyperlipidemic drug may be required.
Macrolides are crucial medications for the treatment of NTM-PD, and EB is an essential drug for suppressing macrolide resistance. To prevent interruptions in EB treatment due to adverse events, adjustments such as intermittent dosing in non-cavitary NB-type cases and low-dose EB administration should be considered (Jeong et al., 2015; Ando et al., 2021). The impact of RFP in non-HIV MAI-PD patients may be limited, and discontinuation may be considered when concomitant use is difficult (van Ingen et al., 2024). The significance of RFP administration awaits the outcomes of the ongoing RCT. In contrast, CFZ regimens may be effective; however, RCT results are limited. ALIS has demonstrated efficacy in refractory MAI-PD, but AMK has been reported to be resistant in 10% of cases after ALIS administration (Griffith et al., 2018). Additionally, the knowledge regarding which patients are likely to respond to treatment and the appropriate treatment duration is limited. In refractory MAI-PD, the addition of BDQ, CFZ, or STFX may be considered as an alternative treatment option in cases where ALIS is less effective. Effective agents for the maintenance treatment of M. abscessus are currently limited, and there is a need for new agents such as OMD and STFX.
Higher complication rates have been reported for CPA in patients with cavities as well as in those with COPD and immunosuppressive drug use (Phoompoung and Chayakulkeeree, 2020). Given the poor prognosis associated with CPA, prioritizing treatment is crucial (Takeda et al., 2016; Phoompoung and Chayakulkeeree, 2020; Fukushima and Kida, 2021). In some cases of CPA development, RFP must be discontinued because of its interaction with azoles. Fosmanogepix and Olorofim, which are currently in clinical trials and exhibit reduced susceptibility to CYP3A4 compared with azoles, are promising CPA treatments that have developed into NTM-PD (Hodges et al., 2024; Rauseo et al., 2020). For patients receiving immunosuppressants, TDM should be performed to adjust the optimal dose or RFP should be discontinued. Additionally, reviewing the latest HIV guidelines can help determine the appropriate concomitant medications. Considering the long-term nature of NTM disease treatment, which often requires multidrug therapy, it is essential to understand the characteristics of each drug and be aware of the potential drug interactions.
This mini-review has several limitations. First, there is a lack of information regarding drug–drug interactions related to newly developed drugs under clinical trials; therefore, it has not been described. Second, there is no discussion on the interactions of NTM-PD drugs with lifestyle-related diseases such as hypertension and dyslipidemia. Third, owing to the extensive number of medications used in ART for HIV, we limited the description to the table.
This mini-review provided an overview of the synergistic effects associated with conventional therapies for NTM-PD, and their interactions with medications used to treat other conditions. Since many NTM-PD patients are elderly, the frequency of treatment-related adverse events is high, and interactions with medications to treat complications can be problematic. It is essential to carefully evaluate drug–drug interactions and the potential for adverse events when selecting appropriate treatment regimens.
KT: Investigation, Writing – original draft. TT: Conceptualization, Investigation, Project administration, Supervision, Writing – review & editing. HM: Supervision, Writing – review & editing.
The author(s) declare that no financial support was received for the research, authorship, and/or publication of this article.
The authors declare that the research was conducted in the absence of any commercial or financial relationships that could be construed as a potential conflict of interest.
All claims expressed in this article are solely those of the authors and do not necessarily represent those of their affiliated organizations, or those of the publisher, the editors and the reviewers. Any product that may be evaluated in this article, or claim that may be made by its manufacturer, is not guaranteed or endorsed by the publisher.
Adjemian, J., Olivier, K. N., Seitz, A. E., Holland, S. M., and Prevots, D. R. (2012). Prevalence of nontuberculous mycobacterial lung disease in U.S. Medicare beneficiaries. Am. J. Respir. Crit. Care Med. 185, 881–886. doi: 10.1164/rccm.201111-2016OC
Adjemian, J., Prevots, D. R., Gallagher, J., Heap, K., Gupta, R., and Griffith, D. (2014). Lack of adherence to evidence-based treatment guidelines for nontuberculous mycobacterial lung disease. Ann. Am. Thorac. Soc. 11, 9–16. doi: 10.1513/AnnalsATS.201304-085OC
Ando, T., Kage, H., Matsumoto, Y., Zokumasu, K., and Nagase, T. (2021). Lower dose of ethambutol may reduce ocular toxicity without radiological deterioration for Mycobacterium avium complex pulmonary disease. Respir. Investig. 59, 777–782. doi: 10.1016/j.resinv.2021.06.010
Aziz, D. B., Go, M. L., and Dick, T. (2020). Rifabutin suppresses inducible clarithromycin resistance in Mycobacterium abscessus by blocking induction of whiB7 and erm41. Antibiotics (Basel). 9:72. doi: 10.3390/antibiotics9020072
Benson, C. A., Williams, P. L., Cohn, D. L., Becker, S., Hojczyk, P., Nevin, T., et al. (2000). Clarithromycin or rifabutin alone or in combination for primary prophylaxis of Mycobacterium avium complex disease in patients with AIDS: a randomized, double-blind, placebo-controlled trial. The AIDS Clinical Trials Group 196/Terry Beirn community programs for clinical research on AIDS 009 protocol team. J. Infect. Dis. 181, 1289–1297. doi: 10.1086/315380
Bermudez, L. E., Nash, K. A., Petrofsky, M., Young, L. S., and Inderlied, C. B. (1996). Effect of ethambutol on emergence of clarithromycin-resistant Mycobacterium avium complex in the beige mouse model. J. Infect. Dis. 174, 1218–1222.
Bich Hanh, B. T., Quang, N. T., Park, Y., Heo, B. E., Jeon, S., Park, J. W., et al. (2021). Omadacycline potentiates clarithromycin activity against Mycobacterium abscessus. Front. Pharmacol. 12:790767. doi: 10.3389/fphar.2021.790767
Brode, S. K., Jamieson, F. B., Ng, R., Campitelli, M. A., Kwong, J. C., Paterson, J. M., et al. (2015). Increased risk of mycobacterial infections associated with anti-rheumatic medications. Thorax 70, 677–682. doi: 10.1136/thoraxjnl-2014-206470
Buchacz, K., Lau, B., Jing, Y., Bosch, R., Abraham, A. G., Gill, M. J., et al. (2016). Incidence of AIDS-defining opportunistic infections in a multicohort analysis of HIV-infected persons in the United States and Canada, 2000-2010. J. Infect. Dis. 214, 862–872. doi: 10.1093/infdis/jiw085
Chaisson, R. E., Keiser, P., Pierce, M., Fessel, W. J., Ruskin, J., Lahart, C., et al. (1997). Clarithromycin and ethambutol with or without clofazimine for the treatment of bacteremic Mycobacterium avium complex disease in patients with HIV infection. AIDS 11, 311–317. doi: 10.1097/00002030-199703110-00008
Dahl, V. N., Molhave, M., Floe, A., van Ingen, J., Schon, T., Lillebaek, T., et al. (2022). Global trends of pulmonary infections with nontuberculous mycobacteria: a systematic review. Int. J. Infect. Dis. 125, 120–131. doi: 10.1016/j.ijid.2022.10.013
Daley, C. L., Iaccarino, J. M., Lange, C., Cambau, E., Wallace, R. J. Jr., Andrejak, C., et al. (2020). Treatment of nontuberculous mycobacterial pulmonary disease: an official ATS/ERS/ESCMID/IDSA clinical practice guideline. Clin. Infect. Dis. 71, e1–e36. doi: 10.1093/cid/ciaa241
Department of Health and Human Services (2024). Panel on antiretroviral guidelines for adults and adolescents. Guidelines for the use of antiretroviral agents in adults and adolescents with HIV. Available at: https://clinicalinfo.hiv.gov/en/guidelines/adult-and-adolescent-arv (Accessed August 30, 2024).
Dube, M. P., Sattler, F. R., Torriani, F. J., See, D., Havlir, D. V., Kemper, C. A., et al. (1997). A randomized evaluation of ethambutol for prevention of relapse and drug resistance during treatment of Mycobacterium avium complex bacteremia with clarithromycin-based combination therapy. California collaborative treatment group. J. Infect. Dis. 176, 1225–1232. doi: 10.1086/514116
Dubee, V., Bernut, A., Cortes, M., Lesne, T., Dorchene, D., Lefebvre, A. L., et al. (2015). beta-lactamase inhibition by avibactam in Mycobacterium abscessus. J. Antimicrob. Chemother. 70, 1051–1058. doi: 10.1093/jac/dku510
Ferro, B. E., Meletiadis, J., Wattenberg, M., de Jong, A., van Soolingen, D., Mouton, J. W., et al. (2016). Clofazimine prevents the regrowth of Mycobacterium abscessus and Mycobacterium avium type strains exposed to amikacin and clarithromycin. Antimicrob. Agents Chemother. 60, 1097–1105. doi: 10.1128/AAC.02615-15
Fujita, K., Fujita, M., Ito, Y., Hirai, T., Mio, T., Watanabe, K., et al. (2016). Preliminary evaluation of a Sitafloxacin-containing regimen for relapsed or refractory pulmonary Mycobacterium avium complex disease. Open Forum Infect Dis. 3:ofw147. doi: 10.1093/ofid/ofw147
Fujiwara, K., Aono, A., Asami, T., Morimoto, K., Kamada, K., Morishige, Y., et al. (2023). In vitro synergistic effects of Omadacycline with other antimicrobial agents against Mycobacterium abscessus. Antimicrob. Agents Chemother. 67:e0157922. doi: 10.1128/aac.01579-22
Fujiwara, K., Uesugi, F., Furuuchi, K., Tanaka, Y., Yoshiyama, T., Saotome, M., et al. (2021). Minimum inhibitory concentrations before and after antibacterial treatment in patients with Mycobacterium abscessus pulmonary disease. Microbiol Spectr. 9:e0192821. doi: 10.1128/Spectrum.01928-21
Fukushima, K., and Kida, H. (2021). New/different look at the presence of aspergillus in mycobacterial pulmonary diseases. Long-term retrospective cohort study. Microorganisms 9:270. doi: 10.3390/microorganisms9020270
Gangadharam, P. R., Perumal, V. K., Podapati, N. R., Kesavalu, L., and Iseman, M. D. (1988). In vivo activity of amikacin alone or in combination with clofazimine or rifabutin or both against acute experimental Mycobacterium avium complex infections in beige mice. Antimicrob. Agents Chemother. 32, 1400–1403. doi: 10.1128/AAC.32.9.1400
Geist, M. J., Egerer, G., Burhenne, J., Riedel, K. D., and Mikus, G. (2007). Induction of voriconazole metabolism by rifampin in a patient with acute myeloid leukemia: importance of interdisciplinary communication to prevent treatment errors with complex medications. Antimicrob. Agents Chemother. 51, 3455–3456. doi: 10.1128/AAC.00579-07
Gordin, F. M., Sullam, P. M., Shafran, S. D., Cohn, D. L., Wynne, B., Paxton, L., et al. (1999). A randomized, placebo-controlled study of rifabutin added to a regimen of clarithromycin and ethambutol for treatment of disseminated infection with Mycobacterium avium complex. Clin. Infect. Dis. 28, 1080–1085. doi: 10.1086/514748
Griffith, D. E., Brown-Elliott, B. A., Langsjoen, B., Zhang, Y., Pan, X., Girard, W., et al. (2006). Clinical and molecular analysis of macrolide resistance in Mycobacterium avium complex lung disease. Am. J. Respir. Crit. Care Med. 174, 928–934. doi: 10.1164/rccm.200603-450OC
Griffith, D. E., Eagle, G., Thomson, R., Aksamit, T. R., Hasegawa, N., Morimoto, K., et al. (2018). Amikacin liposome inhalation suspension for treatment-refractory lung disease caused by Mycobacterium avium complex (CONVERT). A prospective, open-label, randomized study. Am. J. Respir. Crit. Care Med. 198, 1559–1569. doi: 10.1164/rccm.201807-1318OC
Hafner, R., Bethel, J., Power, M., Landry, B., Banach, M., Mole, L., et al. (1998). Tolerance and pharmacokinetic interactions of rifabutin and clarithromycin in human immunodeficiency virus-infected volunteers. Antimicrob. Agents Chemother. 42, 631–639. doi: 10.1128/AAC.42.3.631
Harada, K., Hagiya, H., Funahashi, T., Koyama, T., Kano, M. R., and Otsuka, F. (2021). Trends in the nontuberculous mycobacterial disease mortality rate in Japan: a Nationwide observational study, 1997-2016. Clin. Infect. Dis. 73, e321–e326. doi: 10.1093/cid/ciaa810
Haworth, C. S., Banks, J., Capstick, T., Fisher, A. J., Gorsuch, T., Laurenson, I. F., et al. (2017). British Thoracic Society guidelines for the management of non-tuberculous mycobacterial pulmonary disease (NTM-PD). Thorax 72, ii1–ii64. doi: 10.1136/thoraxjnl-2017-210927
He, S., Guo, Q., Zhao, L., Xu, L., Fan, J., Wu, W., et al. (2021). Sitafloxacin expresses potent anti-Mycobacterium abscessus activity. Front. Microbiol. 12:779531. doi: 10.3389/fmicb.2021.779531
Hebert, M. F., Fisher, R. M., Marsh, C. L., Dressler, D., and Bekersky, I. (1999). Effects of rifampin on tacrolimus pharmacokinetics in healthy volunteers. J. Clin. Pharmacol. 39, 91–96. doi: 10.1177/00912709922007499
Hodges, M. R., van Marle, S., Kramer, W. G., Ople, E., Tawadrous, M., and Jakate, A. (2024). Phase 1 drug-drug interaction study to assess the effect of CYP3A4 inhibition and pan-CYP induction on the pharmacokinetics and safety of fosmanogepix in healthy participants. Antimicrob. Agents Chemother. 17:e0165023. doi: 10.1128/aac.01650-23
Hoffner, S. E., Källenius, G., Beezer, A. E., and Svenson, S. B. (1989). Studies on the mechanisms of the synergistic effects of ethambutol and other antibacterial drugs on Mycobacterium avium complex. Acta Leprol. 7, 195–199.
Jarand, J., Davis, J. P., Cowie, R. L., Field, S. K., and Fisher, D. A. (2016). Long-term follow-up of Mycobacterium avium complex lung disease in patients treated with regimens including Clofazimine and/or rifampin. Chest 149, 1285–1293. doi: 10.1378/chest.15-0543
Jaruratanasirikul, S., and Sriwiriyajan, S. (1998). Effect of rifampicin on the pharmacokinetics of itraconazole in normal volunteers and AIDS patients. Eur. J. Clin. Pharmacol. 54, 155–158. doi: 10.1007/s002280050437
Jeong, B. H., Jeon, K., Park, H. Y., Kim, S. Y., Lee, K. S., Huh, H. J., et al. (2015). Intermittent antibiotic therapy for nodular bronchiectatic Mycobacterium avium complex lung disease. Am. J. Respir. Crit. Care Med. 191, 96–103. doi: 10.1164/rccm.201408-1545OC
Källenius, G., Svenson, S. B., and Hoffner, S. E. (1989). Ethambutol: a key for Mycobacterium avium complex chemotherapy? Am. Rev. Respir. Dis. 140:264.
Kamada, K., Yoshida, A., Iguchi, S., Arai, Y., Uzawa, Y., Konno, S., et al. (2021). Nationwide surveillance of antimicrobial susceptibility of 509 rapidly growing mycobacteria strains isolated from clinical specimens in Japan. Sci. Rep. 11:12208. doi: 10.1038/s41598-021-91757-4
Kaushik, A., Ammerman, N. C., Lee, J., Martins, O., Kreiswirth, B. N., Lamichhane, G., et al. (2019). In vitro activity of the new beta-lactamase inhibitors Relebactam and Vaborbactam in combination with beta-lactams against Mycobacterium abscessus complex clinical isolates. Antimicrob. Agents Chemother. 63:e02623-18. doi: 10.1128/AAC.02623-18
Kent, R. J., Bakhtiar, M., and Shanson, D. C. (1992). The in-vitro bactericidal activities of combinations of antimicrobial agents against clinical isolates of Mycobacterium avium-intracellulare. J. Antimicrob. Chemother. 30, 643–650. doi: 10.1093/jac/30.5.643
Kim, S. R., Jang, M., Kim, S. Y., Kim, D. H., and Jhun, B. W. (2022). Outcomes of short-term Tigecycline-containing regimens for Mycobacterium abscessus pulmonary disease. Antimicrob. Agents Chemother. 66:e0077422. doi: 10.1128/aac.00774-22
Kim, O. H., Shim, T. S., and Jo, K. W. (2022). Drug-level change and optimal dose adjustment of tacrolimus with the use of rifabutin for treating mycobacterial disease in solid organ transplant recipients. Transpl. Infect. Dis. 24:e13893. doi: 10.1111/tid.13893
Kobashi, Y., Matsushima, T., and Oka, M. (2007). A double-blind randomized study of aminoglycoside infusion with combined therapy for pulmonary Mycobacterium avium complex disease. Respir. Med. 101, 130–138. doi: 10.1016/j.rmed.2006.04.002
Kobashi, Y., Yoshida, K., Miyashita, N., Niki, Y., and Oka, M. (2006). Relationship between clinical efficacy of treatment of pulmonary Mycobacterium avium complex disease and drug-sensitivity testing of Mycobacterium avium complex isolates. J. Infect. Chemother. 12, 195–202. doi: 10.1007/s10156-006-0457-8
Koh, W. J., Hong, G., Kim, S. Y., Jeong, B. H., Park, H. Y., Jeon, K., et al. (2013). Treatment of refractory Mycobacterium avium complex lung disease with a moxifloxacin-containing regimen. Antimicrob. Agents Chemother. 57, 2281–2285. doi: 10.1128/AAC.02281-12
Lopeman, R. C., Harrison, J., Rathbone, D. L., Desai, M., Lambert, P. A., and Cox, J. A. G. (2020). Effect of amoxicillin in combination with Imipenem-Relebactam against Mycobacterium abscessus. Sci. Rep. 10:928. doi: 10.1038/s41598-020-57844-8
Marras, T. K., Vinnard, C., Zhang, Q., Hamilton, K., Adjemian, J., Eagle, G., et al. (2018). Relative risk of all-cause mortality in patients with nontuberculous mycobacterial lung disease in a US managed care population. Respir. Med. 145, 80–88. doi: 10.1016/j.rmed.2018.10.022
McAllister, W. A., Thompson, P. J., Al-Habet, S. M., and Rogers, H. J. (1983). Rifampicin reduces effectiveness and bioavailability of prednisolone. Br. Med. J. (Clin. Res. Ed.) 286, 923–925.
Miwa, S., Shirai, M., Toyoshima, M., Shirai, T., Yasuda, K., Yokomura, K., et al. (2014). Efficacy of clarithromycin and ethambutol for Mycobacterium avium complex pulmonary disease. A preliminary study. Ann. Am. Thorac. Soc. 11, 23–29. doi: 10.1513/AnnalsATS.201308-266OC
Moon, S. M., Jhun, B. W., Lee, H., Park, H. Y., Jeon, K., Lee, S. Y., et al. (2017). Effect of a 150 mg dose of rifabutin on serum itraconazole levels in patients with coexisting chronic pulmonary aspergillosis and Mycobacterium avium complex lung disease. J. Infect. Chemother. 23, 658–660. doi: 10.1016/j.jiac.2017.02.004
Mori, S., and Sugimoto, M. (2012). Is continuation of anti-tumor necrosis factor-alpha therapy a safe option for patients who have developed pulmonary mycobacterial infection?: Case presentation and literature review. Clin. Rheumatol. 31, 203–210. doi: 10.1007/s10067-011-1902-3
Morimoto, K., Izumi, K., Ato, M., Hasegawa, N., and Mitarai, S. (2019). Actual practice of standard treatment for pulmonary nontuberculous mycobacteriosis in Japan. Respir. Med. 158, 67–69. doi: 10.1016/j.rmed.2019.10.002
Morimoto, K., Namkoong, H., Hasegawa, N., Nakagawa, T., Morino, E., Shiraishi, Y., et al. (2016). Macrolide-resistant Mycobacterium avium complex lung disease: analysis of 102 consecutive cases. Ann. Am. Thorac. Soc. 13, 1904–1911. doi: 10.1513/AnnalsATS.201604-246OC
Mushtaq, M., Fatima, K., Ahmad, A., Mohamed Ibrahim, O., Faheem, M., and Shah, Y. (2023). Pharmacokinetic interaction of voriconazole and clarithromycin in Pakistani healthy male volunteers: a single dose, randomized, crossover, open-label study. Front. Pharmacol. 14:1134803. doi: 10.3389/fphar.2023.1134803
Nahid, P., Dorman, S. E., Alipanah, N., Barry, P. M., Brozek, J. L., Cattamanchi, A., et al. (2016). Official American Thoracic Society/Centers for Disease Control and Prevention/Infectious Diseases Society of America clinical practice guidelines: treatment of drug-susceptible tuberculosis. Clin. Infect. Dis. 63, e147–e195. doi: 10.1093/cid/ciw376
Namkoong, H., Kurashima, A., Morimoto, K., Hoshino, Y., Hasegawa, N., Ato, M., et al. (2016). Epidemiology of pulmonary nontuberculous mycobacterial disease, Japan. Emerg. Infect. Dis. 22, 1116–1117. doi: 10.3201/eid2206.151086
Pasipanodya, J. G., Ogbonna, D., Deshpande, D., Srivastava, S., and Gumbo, T. (2017). Meta-analyses and the evidence base for microbial outcomes in the treatment of pulmonary Mycobacterium avium-intracellulare complex disease. J. Antimicrob. Chemother. 72, i3–i19. doi: 10.1093/jac/dkx311
Phoompoung, P., and Chayakulkeeree, M. (2020). Chronic pulmonary Aspergillosis following nontuberculous mycobacterial infections: an emerging disease. J Fungi (Basel). 6:346. doi: 10.3390/jof6040346
Pryjma, M., Burian, J., and Thompson, C. J. (2018). Rifabutin acts in synergy and is bactericidal with frontline Mycobacterium abscessus antibiotics clarithromycin and Tigecycline, suggesting a potent treatment combination. Antimicrob. Agents Chemother. 62:e00283-18. doi: 10.1128/AAC.00283-18
Purkins, L., Wood, N., Ghahramani, P., Kleinermans, D., Layton, G., and Nichols, D. (2003). No clinically significant effect of erythromycin or azithromycin on the pharmacokinetics of voriconazole in healthy male volunteers. Br. J. Clin. Pharmacol. 56, 30–36. doi: 10.1046/j.1365-2125.2003.01996.x
Rauseo, A. M., Coler-Reilly, A., Larson, L., and Spec, A. (2020). Hope on the horizon: novel fungal treatments in development. Open Forum Infect. Dis. 7:ofaa016. doi: 10.1093/ofid/ofaa016
Shafran, S. D., Singer, J., Zarowny, D. P., Phillips, P., Salit, I., Walmsley, S. L., et al. (1996). A comparison of two regimens for the treatment of Mycobacterium avium complex bacteremia in AIDS: rifabutin, ethambutol, and clarithromycin versus rifampin, ethambutol, clofazimine, and ciprofloxacin. Canadian HIV trials network protocol 010 study group. N. Engl. J. Med. 335, 377–384. doi: 10.1056/NEJM199608083350602
Siegel, S. A. R., Griffith, D. E., Philley, J. V., Brown-Elliott, B. A., Brunton, A. E., Sullivan, P. E., et al. (2023). Open-label trial of amikacin liposome inhalation suspension in Mycobacterium abscessus lung disease. Chest 164, 846–859. doi: 10.1016/j.chest.2023.05.036
Takeda, K., Imamura, Y., Takazono, T., Yoshida, M., Ide, S., Hirano, K., et al. (2016). The risk factors for developing of chronic pulmonary aspergillosis in nontuberculous mycobacteria patients and clinical characteristics and outcomes in chronic pulmonary aspergillosis patients coinfected with nontuberculous mycobacteria. Med. Mycol. 54, 120–127. doi: 10.1093/mmy/myv093
Townsend, R., Dietz, A., Hale, C., Akhtar, S., Kowalski, D., Lademacher, C., et al. (2017). Pharmacokinetic evaluation of CYP3A4-mediated drug-drug interactions of Isavuconazole with rifampin, ketoconazole, midazolam, and Ethinyl estradiol/Norethindrone in healthy adults. Clin Pharmacol Drug Dev. 6, 44–53. doi: 10.1002/cpdd.285
Uno, S., Asakura, T., Morimoto, K., Yoshimura, K., Uwamino, Y., Nishimura, T., et al. (2020). Comorbidities associated with nontuberculous mycobacterial disease in Japanese adults: a claims-data analysis. BMC Pulm. Med. 20:262. doi: 10.1186/s12890-020-01304-6
van Ingen, J., Egelund, E. F., Levin, A., Totten, S. E., Boeree, M. J., Mouton, J. W., et al. (2012a). The pharmacokinetics and pharmacodynamics of pulmonary Mycobacterium avium complex disease treatment. Am. J. Respir. Crit. Care Med. 186, 559–565. doi: 10.1164/rccm.201204-0682OC
van Ingen, J., Hoefsloot, W., Dartois, V., and Dick, T. (2024). Rifampicin has no role in treatment of Mycobacterium avium complex pulmonary disease and bactericidal sterilising drugs are needed: a viewpoint. Eur. Respir. J. 63:2302210. doi: 10.1183/13993003.02210-2023
van Ingen, J., Totten, S. E., Helstrom, N. K., Heifets, L. B., Boeree, M. J., and Daley, C. L. (2012b). In vitro synergy between clofazimine and amikacin in treatment of nontuberculous mycobacterial disease. Antimicrob. Agents Chemother. 56, 6324–6327. doi: 10.1128/AAC.01505-12
van Ingen, J., Wagner, D., Gallagher, J., Morimoto, K., Lange, C., Haworth, C. S., et al. (2017). Poor adherence to management guidelines in nontuberculous mycobacterial pulmonary diseases. Eur. Respir. J. 49:1601855. doi: 10.1183/13993003.01855-2016
Wen, J., ChenYang, Z. M., Hu, W., and Xiao, Y. W. (2023). Effects of clarithromycin on the pharmacokinetics of tacrolimus and expression of CYP3A4 and P-glycoprotein in rats. Fundam. Clin. Pharmacol. 37, 843–848. doi: 10.1111/fcp.12882
Winthrop, K. L., Chang, E., Yamashita, S., Iademarco, M. F., and LoBue, P. A. (2009). Nontuberculous mycobacteria infections and anti-tumor necrosis factor-alpha therapy. Emerg. Infect. Dis. 15, 1556–1561. doi: 10.3201/eid1510.090310
Yeh, J. J., Wang, Y. C., Sung, F. C., and Kao, C. H. (2014). Rheumatoid arthritis increases the risk of nontuberculosis mycobacterial disease and active pulmonary tuberculosis. PLoS One 9:e110922. doi: 10.1371/journal.pone.0110922
Yen, Y. F., Chen, M., Jen, I. A., Chuang, P. H., Lee, C. Y., Lin, S. I., et al. (2019). Short- and long-term risks of highly active antiretroviral treatment with incident opportunistic infections among people living with HIV/AIDS. Sci. Rep. 9:3476. doi: 10.1038/s41598-019-39665-6
Zweijpfenning, S. M. H., Aarnoutse, R., Boeree, M. J., Magis-Escurra, C., Stemkens, R., Geurts, B., et al. (2024). Safety and efficacy of Clofazimine as an alternative for rifampicin in Mycobacterium avium complex pulmonary disease treatment: outcomes of a randomized trial. Chest 165, 1082–1092. doi: 10.1016/j.chest.2023.11.038
Keywords: non-tuberculous mycobacterial infection, drug interaction, chronic pulmonary aspergillosis, antiretroviral therapy, adverse event
Citation: Takeda K, Takazono T and Mukae H (2024) Drug–drug interactions in the management of non-tuberculous mycobacterial infections. Front. Microbiol. 15:1468383. doi: 10.3389/fmicb.2024.1468383
Received: 22 July 2024; Accepted: 28 August 2024;
Published: 05 September 2024.
Edited by:
Hideo Kato, Mie University Graduate School of Medicine, JapanReviewed by:
Satoshi Mitarai, Japan Anti-tuberculosis Association, JapanCopyright © 2024 Takeda, Takazono and Mukae. This is an open-access article distributed under the terms of the Creative Commons Attribution License (CC BY). The use, distribution or reproduction in other forums is permitted, provided the original author(s) and the copyright owner(s) are credited and that the original publication in this journal is cited, in accordance with accepted academic practice. No use, distribution or reproduction is permitted which does not comply with these terms.
*Correspondence: Takahiro Takazono, dGFrYWhpcm8tdGFrYXpvbm9AbmFnYXNha2ktdS5hYy5qcA==
Disclaimer: All claims expressed in this article are solely those of the authors and do not necessarily represent those of their affiliated organizations, or those of the publisher, the editors and the reviewers. Any product that may be evaluated in this article or claim that may be made by its manufacturer is not guaranteed or endorsed by the publisher.
Research integrity at Frontiers
Learn more about the work of our research integrity team to safeguard the quality of each article we publish.