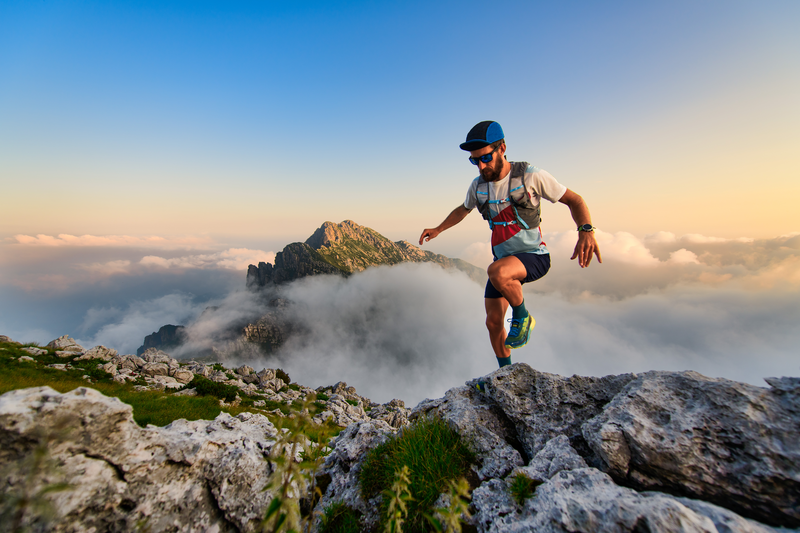
94% of researchers rate our articles as excellent or good
Learn more about the work of our research integrity team to safeguard the quality of each article we publish.
Find out more
ORIGINAL RESEARCH article
Front. Microbiol. , 18 September 2024
Sec. Microbial Symbioses
Volume 15 - 2024 | https://doi.org/10.3389/fmicb.2024.1465992
This article is part of the Research Topic Unveiling the Potential of Microbiome in Semi-Wild and Wildlife Animals: Exploring Opportunities for Disease Mitigation and Animal Health across Ecological Zones View all 3 articles
Introduction: Limited information exists regarding the microbiome composition of yak calves of varying weights. Therefore, this study aimed to investigate the microbiomes of mother-calf pairs with different weight profiles.
Methods: Fecal and blood samples were collected from both lower-weight (CB) and higher-weight (HB) yak calves, along with their corresponding female yaks (CA, HA).
Results: The results revealed significantly higher levels of T-AOC (total antioxidant capacity) and GSH-Px (glutathione peroxidase) in HB animals (p < 0.001). Sequencing yielded 652,181 and 643,369 filtered reads in female and calf yaks, respectively. Alpha diversity analysis indicated that Chao1, Faith_pd, and Observed species were significantly higher in CA compared to HA (p < 0.01). Furthermore, nine genera were notably different between HA and CA yaks, including Avispirillum, Fimenecus, CAG-1031, Odoribacter 865974, and Jeotgalicoccus A 310962. Compared to CB yaks, CA animals exhibited significant differences in one phylum and six genera, including CAG-485 (p < 0.05), CAG-83 (p < 0.01), Copromorpha (p < 0.01), Phocaeicola A 858004 (p < 0.05), and UBA2253 (p < 0.05).
Conclusion: In summary, higher-weight yak calves demonstrated increased oxidative resistance, and weight profiles were linked to the microbiomes of both female yaks and their calves. These findings offer valuable insights for optimizing yak breeding practices in high-altitude regions.
The Qinghai-Tibet Plateau, often referred to as the “Third Pole” of the world, spans the geographic coordinates of approximately 26°00′ to 39°47′ north latitude and 73°19′ to 104°47′ east longitude. This region is characterized by its oxygen-deficient atmosphere, extreme cold temperatures, and intense ultraviolet radiation exposure (Liu et al., 2022). The yak is a ruminant bovine species primarily inhabiting in plateau regions. This ancient breed has inhabited the Tibetan Plateau for thousands of years and has developed a strong adaptability to the region’s harsh ecological conditions (Wan et al., 2021; Ding et al., 2023), which provide nourishing meat and milk products, serve as transportation, and produce valuable resources such as fueling dung, fur, and medicinal materials, with their horns being utilized by plateau herdsmen (Li et al., 2020). Over 16 million of these economically significant animals reside in China’s western regions, comprising 90% of the global population of this species (Dong et al., 2023; Shang et al., 2022). Yak breeding serves as a primary industry in the remote plateau regions, making efficient farming practices crucial and significant for local communities.
The gut microbial communities consist of billions of microorganisms, including viruses, bacteria, fungi, and protozoa (McCallum and Tropini, 2024; Xi et al., 2021), which are also considered microbial organs making contributions to the digestion and metabolism, gut barrier development and immune functions. The microbiome, a complex microbial organ in the host’s gastrointestinal tract, significantly influences the host’s metabolic system (Islam et al., 2023; Xie et al., 2024). Previous studies have reported that microbiome was highly associated with child growth, and become a devasting global health issue with long-lasting consequences such as stunted growth and abnormal development and animal growth (Blanton et al., 2016; Wang et al., 2023). In many animals, the microbiome is crucial for survival, as it supports essential functions such as digestion, immunity, and behavior through mutualistic relationships (Perlman et al., 2022; Ren et al., 2023). The mother’s microbiota significantly influences the establishment of an infant’s microbiome in early life during delivery, maternal environmental exposures, and subsequent environmental factors, all of which play a crucial role in shaping the infant’s future health (Bogaert et al., 2023; Browne et al., 2022). Furthermore, previous experiments have also confirmed that microbiota enhances the growth performance of chicks and young mice (Pronovost et al., 2023; Zhang et al., 2022). However, there is limited information available regarding the microbiome of yak calves with varying weights. Therefore, we conducted this research to investigate the mother-calf microbiome of yaks with different weights.
This study is crucial as it sheds light on the previously unexplored relationship between weight profiles and microbiomes in yaks, especially between dams and calves. This study also provides valuable insights into the intricate relationship between weight profiles and microbiomes in yak dams and calves. The study also aims to correlate the levels of T-AOC and GSH-Px with the weight and microbiome profiles of the calves, to elucidate the potential mechanisms through which weight variation influences oxidative resistance and overall health in livestock. By examining this connection, the research contributes to a deeper understanding of how maternal and offspring microbiomes influence growth and health outcomes in livestock. The findings of this study could have significant implications for improving breeding strategies, optimizing livestock management, and potentially informing interventions to enhance the health and productivity of yaks and other similar species in the future.
Fecal and blood samples were obtained from 1 month-old yak (Figure 1) calves with lower (CB) and higher (HB) weights, along with their corresponding female yaks (CA, HA), from a local breeding company in Lhasa, China, in December 2023.
Each blood sample underwent centrifugation at 3,800 g for 15 min to isolate serum, which was then stored at −20°C for subsequent analysis. The antioxidant capacity (T-AOC) of yak calves was determined using a commercial Assay kit using a colorimetric method to assess antioxidant levels in biological samples. Samples were diluted and mixed with standard antioxidants provided in the kit. After incubation and the addition of a chromogenic reagent, absorbance was measured at 405 nm. Results were calculated using a standard curve and expressed as mM of Trolox equivalents per sample volume. Oxidative resistance was assessed by measuring the malondialdehyde (MDA), superoxide dismutase (SOD), and glutathione peroxidase (GSH-Px) levels. Malondialdehyde levels were determined spectrophotometrically, superoxide dismutase activity was assessed using a commercially available assay kit, and glutathione peroxidase levels were measured via colorimetric methods. All kits were procured from Jiancheng Bioengineering Research Institute Co., Ltd. (China).
The total DNA of mother yaks (HA, HB) and calf yaks (CA, CB) was extracted using a soil DNA kit (Omega Bio-Tek, United States). Subsequently, the quality of the yak DNA was assessed using a NanoDrop eight spectrophotometer (Thermo Scientific, United States) and 0.8% agarose gel electrophoresis (He et al., 2023). The 16S rRNA region V3–V4 of mother and calf yaks were amplified by employing a universal primer pair (338F: 5’-ACTCCTACGGGAGGCAGCA-3; 806R: 5’-GGACTACHVGGGTWTCTAAT-3′) (Zeng and An, 2021). The amplified products were purified via GeneJET (Thermo Scientific, United States) for constructing sequencing library through Hieff NGS® OnePot kit (Yeasen, China). At last, sequencing of yak microbiomes was performed on Illlumina MiSeq platform at Bioyi Biotechnology Co., Ltd. (Wuhan, China).
The raw data from mother and calf yaks was filtered to create the feature form of amplicon sequence variants by QIIME2 (Mohsen et al., 2022). The ASVs were aligned using MAFFT to generate a systematic form (Nakamura et al., 2018), and Venn diagram was constructed using the R package to illustrate the shared ASVs between HA and CA, as well as HB and CB, respectively (Xiao et al., 2022). Richness and evenness comparisons of ASVs between HA and CA, as well as HB and CB yaks, were conducted by plotting ranked abundance curves. Alpha diversity metrics, including Chao1, Faith_pd, Shannon, Goods_coverage, Observed_species, Pielou_e, and Simpson, were analyzed using QIIME2. To explore the configurational variation between HA and CA, and HB and CB ruminants, we performed beta diversity analysis of principal coordinate analysis (Shi et al., 2020) and nonmetric multidimensional scaling (Feng et al., 2023). The differential abundance of taxa between the HA and CA groups, as well as the HB and CB groups, was assessed using Linear Discriminant Analysis Effect Size (LEfSe) and t-tests (Wang et al., 2024). Microbial functions of HA and CA, as well as HB and CB yaks, were analyzed using PICRUSt2 with reference to the MetaCyc and KEGG databases.
Results of HA, CA, HB and CB ruminants were examined through student’s t-test utilizing SPSS (IBM, 26.0), and presented as average value ± SD, and significance is considered when p < 0.05.
The average weight of CB yaks (29.6 kg) was significantly lower than that of HB yaks (40.2 kg) (Figure 1A). Serum analysis revealed significantly higher levels of T-AOC (p < 0.001) and GSH-Px (p < 0.001) in HB animals (Figure 1B).
In female and calf yaks (Table 1), there were over 67,000 (CA), 49,000 (HA), 63,000 (CB), and 64,000 (HB) raw sequences, and 62,000 (CA), 46,000 (HA), 60,000 (CB), and 59,000 (HB) filtered sequences. Alpha diversity analysis revealed that Chao1, Faith_pd, and Observed_species were significantly higher (p < 0.01) in CA yaks compared to HA yaks (Table 2; Figure 2A). However, no significant differences were observed between HB and CB yaks in terms of these indices (Figure 3A). The rarefaction curves in all ruminant groups appeared as nearly horizontal lines (Figures 2B, 3B), indicating that the current sequencing depth was adequate. Furthermore, the rank abundance curves in HA, CA, HB, and CB groups all displayed smooth horizontal curves (Figures 2C, 3C), suggesting a high uniformity in the current flora composition of yaks. At the phylum level of female yaks, Firmicutes_A (52.77%), Bacteroidota (20.57%), and Proteobacteria (11.86%) were the dominating phyla in HA, while Firmicutes_A (63.98%), Bacteroidota (24.98%) and Firmicutes_D (4.95%) were the main phyla in CA (Figure 4A). At the class level, Clostridia_258483 (52.74%), Bacteroidia (20.58%), and Gammaproteobacteria (11.67%) were the staple classes in HA, while Clostridia_258483 (63.94%), Bacteroidia (24.98%) and Bacilli (4.95%) were the staple classes in CA (Figure 4B). At the order level, Oscillospirales (23.73%), Bacteroidales (20.37%) and Pseudomonadales_660879 (11.91%) were the prime orders in HA, while Oscillospirales (34.14%), Bacteroidales (24.58%) and Christensenellales (13.49%) were the prime orders in CA (Figure 4C). At the family level, Oscillospiraceae_88309 (14.11%), UBA932 (12.33%) and Moraxellaceae (12.06%) were mainly examined in HA, while Oscillospiraceae_88309 (19.43%), UBA932 (14.24%) and CAG-74 (12.87%) were mainly examined in CA (Figure 4D). At the genus level, Faecousia (15.02%), Cryptobacteroides (15.31%) and Psychrobacter (14.16%) were the stable genera in HA, while Faecousia (20.64%), Cryptobacteroides (17.41%) and UBA737 (3.93%) were the stable genera in CA (Figure 4E).
Figure 2. Alpha diversity analysis of female yaks in different groups. (A) Indexes, (B) rarefaction curve, (C) rank abundance curve.
Figure 3. Alpha diversity analysis of yak calves in different groups. (A) Indexes, (B) rarefaction curve, (C) rank abundance curve.
Figure 4. Analyzing the intestinal flora structure female yaks in different taxa. (A) Phylum, (B) class, (C) order, (D) family, (E) genus.
At the phylum level of yak calves, Firmicutes_A (60.64%), Bacteroidota (20.56%), and Firmicutes_D (8.74%) were the primary phyla in HB, while Firmicutes_A (36.32%), Proteobacteria (23.88%) and Firmicutes_D (18.11%) were the primary phyla in CB (Figure 5A). At the class level, Clostridia_258483 (60.64%), Bacteroidia (20.56%), and Bacilli (8.74%) were the dominating classes in HB, while Clostridia_258483 (36.31%), Gammaproteobacteria (23.82%) and Bacilli (18.11%) were the dominating classes in CB (Figure 5B). At the order level, Oscillospirales (26.51%), Bacteroidales (20.31%), and Peptostreptococcales (11.52%) were mainly detected in HB, while Pseudomonadales_660879 (24.31%), Oscillospirales (16.17%) and Lactobacillales (15.11%) were mainly detected in CB (Figure 5C). At the family level, Oscillospiraceae_88309 (17.52%), CAG-74 (8.94%) and UBA932 (8.26%) were the prime families in HB, while Moraxellaceae (24.59%), Oscillospiraceae_88309 (10.82%) and CAG-74 (7.05%) were the prime families in CB (Figure 5D). At the genus level, Faecousia (18.61%), Cryptobacteroides (9.85%), and Romboutsia_B (4.22%) were the staple genera in HB, while Psychrobacter (27.30%), Faecousia (10.15%) and Streptococcus (8.45%) were the staple genera in CB (Figure 5E).
Figure 5. Analyzing the intestinal flora structure yak calves in different taxa. (A) Phylum, (B) class, (C) order, (D) family, (E) genus.
Analysis of beta diversity using PCoA and NMDS revealed that the variation between CA and HA animals, as well as CB and HB yaks, was minimal. This similarity in distance suggested a close resemblance in the microbiome composition between female yaks in CA, HA, and between yak calves in CB and HB (Figure 6). LEfSe analysis revealed significant differences in genera abundance. In CA, the genera g__UBA5905 (LDA = 4.05, p < 0.05) and g__UBA2658 (LDA = 3.92, p < 0.05) were notably elevated (Figure 7A). Conversely, in HB, Clostridia_258483 (LDA = 5.13, p < 0.05), Firmicutes_A (LDA = 5.13, p < 0.05), o__Bacteroidales (LDA = 4.75, p < 0.05), f__Acutalibacteraceae (LDA = 4.23, p < 0.05), and g__CAG_83 (LDA = 3.87, p < 0.05) exhibited significantly higher levels (Figure 7). At the genera level, Avispirillum, Fimenecus, CAG-1031, and Odoribacter_865,974 were considerably higher (p < 0.01) in CA yaks, while Jeotgalicoccus_A_310,962 (p < 0.05), Fundicoccus (p < 0.05), Paraclostridium (p < 0.01), Sphingomonas_L_486704 (p < 0.01) and SIO2C1 (p < 0.05) were markedly higher in HA ruminants (Figure 8A). Compared with CB yaks, Firmicutes_A was significantly higher (p < 0.01) in HB animals (Figure 8B). CAG-485 (p < 0.05), CAG-83 (p < 0.01), Copromorpha (p < 0.01), Phocaeicola_A_858,004 (p < 0.05) and UBA2253 (p < 0.05) were significantly higher in HB (Figure 8C).
Figure 6. Beta diversity analysis of female yaks ((A) PCoA, (B) NMDS) and yak calves ((C) PCoA, (D) NMDS).
Figure 7. Revealing the markedly different species between different groups via LEfSe. (A) Phylum (HA and CA), (B) genus (HA and CA), (C) phylum (HB and CB), (D) genus (HB and CB).
Figure 8. Revealing the markedly different species between different yaks via t-test. (A) Genus (CA and HA), (B) phylum (CB and HB), (C) genus (CB and HB).
The MetaCyc pathways analysis highlighted distinct patterns in female yaks. In CA yaks, pathways including P161-PWY, PWY-6588, and PWY0-1298 exhibited notably elevated levels (p < 0.05). Likewise, in HA animals, PWY-7539 and PYRIDNUCSAL-PWY showed significantly higher (p < 0.05) activity (Figure 9A). KEGG pathways of female yaks showed that pathways of chloroalkane and chloroalkene degradation (p < 0.05), D-Arginine and D-ornithine metabolism (p < 0.05), Inositol phosphate metabolism (p < 0.05), Toluene degradation (p < 0.05) and Tropane, piperidine and pyridine alkaloid biosynthesis (p < 0.05) were memorably higher in HA, while Vibrio cholerae pathogenic cycle (p < 0.05) was memorably higher in CA (Figure 9B). The MetaCyc pathways analysis of yak calves unveiled significant differences between HB and CB. In HB, pathways such as COBALSYN-PWY, FUC-RHAMCAT-PWY, GALACT-GLUCUROCAT-P, GALACTUROCAT-PWY, GLUCARDEG-PWY, GLYCOCAT-PWY, and numerous others (p < 0.05 or p < 0.01) were notably elevated. Conversely, in CB, pathways including FOLSYN-PWY, POLYISOPRENSYN-PWY, PWY-6125, and others (p < 0.05) exhibited substantially higher levels (Figure 9C). KEGG pathways of yak calves showed that pathways of arginine and proline metabolism, bacterial chemotaxis, epithelial cell signaling in Helicobacter pylori infection, Flagellar assembly, Glycosaminoglycan degradation, Other glycan degradation, Pentose and glucuronate interconversions, Polyketide sugar unit biosynthesis and Vibrio cholerae pathogenic cycle were observably higher (p < 0.05) in HB, while Chloroalkane and chloroalkene degradation, Inositol phosphate metabolism, Protein export, Purine metabolism, synthesis and degradation of ketone bodies and tyrosine metabolism were dramatically higher (p < 0.05) in CB (Figure 9D).
Figure 9. Analyzing the microbiota function between female yaks and yak calves. (A) MetaCyc pathways of female yaks, (B) KEGG pathways of female yaks, (C) MetaCyc pathways of yak calves, (D) KEGG pathways of yak calves.
The yak holds significant economic and religious importance in the plateau regions of western China. Efficient propagation and breeding of yaks are pressing issues that require urgent attention. In this study, we specifically selected young yak calves exhibiting notable weight differences. The study revealed a clear association between weight variation in yak calves and significant differences in both microbiome composition and oxidative stress markers. Specifically, higher-weight calves were characterized by enhanced microbial richness and phylogenetic diversity, coupled with increased levels of oxidative resistance markers (T-AOC and GSH-Px). These findings suggest a multifaceted relationship where weight profiles may influence gut microbiota, which in turn could impact the calves’ oxidative stress responses and overall health. Reactive oxygen species, such as superoxide and peroxynitrite, are produced during physiological processes. Excessive ROS levels can lead to inflammatory responses and diseases by damaging biomacromolecules. However, the excessive activation of the inflammatory response inevitably harms the organism and leads to severe damage (Liu et al., 2023; Mendonça et al., 2022; Kazmi et al., 2023). T-AOC and GSH-Px are two vital antioxidant enzymes (Xie et al., 2022), the higher levels of these enzymes indicate that the HB yak calves had better oxidation resistance.
Subsequently, we conducted microbiota analysis on female yaks in HA and CA, as well as yak calves in HB and CB. Our analysis yielded 652,181 filtered reads for female yaks and 643,369 filtered reads for yak calves, respectively. Alpha diversity analysis revealed significant differences between CA and HA. The Chao1 index, a richness estimator, was employed to assess the number of observed species, providing insights into the microbial diversity within each group. The Chao1 index revealed significant differences in microbial richness between the high-weight and low-weight calves, indicating a greater number of unique species present in the microbiomes of the high-weight calves. Chao1, Faith_pd, and Observed_species were notably higher (p < 0.01) in CA than in HA, indicating a higher species richness in CA (Galvão et al., 2019). At different taxa, the microbiota structure of female yaks (HA, CA) and yak calves (HB, CB) were different. Firmicutes and Bacteroidota are the dominating phyla in healthy host (Jandhyala, 2015), and the ratio of Firmicutes/Bacteroidota in HA (2.34) was lower than CA (3.40), while HB (3.40) was higher than CB (2.68). The higher Firmicutes/Bacteroidota values in HB yaks were in line with obese people (Asha and Sharma, 2020; Koliada et al., 2017).
Additionally, we conducted a comparison of the yak microbiota at the phylum and genus levels. Our analysis revealed significant differences between HA and CA yaks, with 9 genera (Avispirillum, Fimenecus, CAG-1031, Odoribacter_865,974, and Jeotgalicoccus_A_310,962) showing distinctive patterns. Due to the limited available information on these genera, we can infer their potential relevance to intestinal flora homeostasis in yaks. Contrasting with CB yaks, HB animals exhibited significant differences in one phylum and six genera. Genus of CAG-485 was related to host disease resistance (Bowerman et al., 2020), and a higher abundance of Phocaeicola was found in healthy people (Al Bataineh et al., 2023), which may reveal that the higher weight of yak calves was related to those genera.
The microbiota difference also affected its function in yaks. Through MetaCyc and KEGG pathways analysis, we identified five and six significantly distinct pathways in female yaks, respectively. Among them, inositol phosphate is an important coordinator in nutrition metabolism (Tu-Sekine and Kim, 2022), the higher abundance of inositol phosphate HA yaks may promote the growth of its calves. Similarly, thirty-three MetaCyc pathways and fifteen KEGG pathways were observably different in yak calves, among them, Glycosaminoglycan and other glycan were considered the primary energy and nutrition for the intestinal flora (Ndeh and Gilbert, 2018), which may favor the growth of yak calves.
The positive correlation between higher weight and oxidative resistance observed in this study may be indicative of a protective mechanism facilitated by a diverse microbiome. A richer microbiota could contribute to the synthesis of metabolites that enhance antioxidant defenses, thereby reducing oxidative stress. This potential mechanism warrants further investigation, as it could reveal novel strategies for improving livestock health through microbiome modulation. To build on the current study, future research should explore the mechanistic pathways that link microbiome diversity with oxidative stress and health outcomes in yak calves. Additionally, studies that investigate these relationships under different environmental and dietary conditions would provide a more comprehensive understanding of how to optimize livestock management practices.
In our study, we observed that yak calves with greater weights exhibited increased oxidation resistance, and we noted an association between yak calf weights and the microbiomes of both female yaks and yak calves. These results underscore the critical role of weight profiles in modulating the gut microbiome and oxidative stress responses, which are vital for the overall health and resilience of the calves. The findings not only contribute to our understanding of the microbiome-health axis in livestock but also offer practical implications for improving animal management practices. Future research should explore the mechanistic pathways underlying these associations and evaluate their potential impact on livestock productivity and welfare.
All raw sequence data from female yaks and yak calves were deposited in the NCBI Sequence Read Archive database under accession number PRJNA1104563.
The animal study was approved by all procedures performed in this research were approved by the Laboratory Animal Welfare and Ethics Committee of Institute of Animal Husbandry and Veterinary Medicine, Tibet Academy of Agriculture and Animal Husbandry Science. The study was conducted in accordance with the local legislation and institutional requirements.
HW: Conceptualization, Investigation, Writing – original draft, Writing – review & editing. WB: Investigation, Writing – original draft. ZP: Investigation, Writing – original draft. NJ: Investigation, Writing – original draft. GS: Investigation, Writing – original draft. YC: Investigation, Writing – original draft. YL: Investigation, Writing – original draft. YZ: Investigation, Writing – original draft. DL: Funding acquisition, Investigation, Resources, Supervision, Validation, Visualization, Writing – original draft, Writing – review & editing. SN: Writing – original draft, Writing – review & editing.
The author(s) declare that financial support was received for the research, authorship, and/or publication of this article. The study was partially supported by the research on environmental control and development of facilities and equipment for yak breeding in Tibet (ZX2021ZD0002N-04), the Natural Science Foundation of Tibet Autonomous Region (XZ202401ZR0122), and Ministry of Agriculture and Rural Affairs of China (CARS-37).
The authors declare that the research was conducted in the absence of any commercial or financial relationships that could be construed as a potential conflict of interest.
All claims expressed in this article are solely those of the authors and do not necessarily represent those of their affiliated organizations, or those of the publisher, the editors and the reviewers. Any product that may be evaluated in this article, or claim that may be made by its manufacturer, is not guaranteed or endorsed by the publisher.
Al Bataineh, M. T., Künstner, A., Dash, N. R., Alsafar, H. S., Ragab, M., Schmelter, F., et al. (2023). Uncovering the relationship between gut microbial dysbiosis, metabolomics, and dietary intake in type 2 diabetes mellitus and in healthy volunteers: a multi-omics analysis. Sci. Rep. 13:45066. doi: 10.1038/s41598-023-45066-7
Asha, S. A., and Sharma, K. K. (2020). Gut-organ axis: a microbial outreach and networking. Lett. Appl. Microbiol. 72, 636–668. doi: 10.1111/lam.13333
Blanton, L. V., Charbonneau, M. R., Salih, T., Barratt, M. J., Venkatesh, S., Ilkaveya, O., et al. (2016). Gut bacteria that prevent growth impairments transmitted by microbiota from malnourished children. Science 351:3311. doi: 10.1126/science.aad3311
Bogaert, D., van Beveren, G. J., de Koff, E. M., Lusarreta Parga, P., Balcazar Lopez, C. E., Koppensteiner, L., et al. (2023). Mother-to-infant microbiota transmission and infant microbiota development across multiple body sites. Cell Host Microbe 31, 447–460.e6. doi: 10.1016/j.chom.2023.01.018
Bowerman, K. L., Varelias, A., Lachner, N., Kuns, R. D., Hill, G. R., and Hugenholtz, P. (2020). Continuous pre-and post-transplant exposure to a disease-associated gut microbiome promotes hyper-acute graft-versus-host disease in wild-type mice. Gut Microbes 11, 754–770. doi: 10.1080/19490976.2019.1705729
Browne, H. P., Shao, Y., and Lawley, T. D. (2022). Mother–infant transmission of human microbiota. Curr. Opin. Microbiol. 69:102173. doi: 10.1016/j.mib.2022.102173
Ding, X., Jiang, H., Zhang, R., Chen, X., Yu, H., Zu, Y., et al. (2023). Comparative analysis of nasal microbial community between Tibetan sheep with different ages. Pak. Vet. J. 43, 723–731. doi: 10.29261/pakvetj/2023.075
Dong, H., Chen, X., Zhao, X., Zhao, C., Mehmood, K., Kulyar, M. F., et al. (2023). Intestine microbiota and scfas response in naturally cryptosporidium-infected plateau yaks. Front. Cell. Infect. Microbiol. 13:5126. doi: 10.3389/fcimb.2023.1105126
Feng, J., Gong, Z., Sun, Z., Li, J., Xu, N., Thorne, R. F., et al. (2023). Microbiome and metabolic features of tissues and feces reveal diagnostic biomarkers for colorectal cancer. Front. Microbiol. 14:34325. doi: 10.3389/fmicb.2023.1034325
Galvão, K. N., Higgins, C. H., Zinicola, M., Jeon, S. J., Korzec, H., and Bicalho, R. C. (2019). Effect of pegbovigrastim administration on the microbiome found in the vagina of cows postpartum. J. Dairy Sci. 102, 3439–3451. doi: 10.3168/jds.2018-15783
He, X., Hao, P., Wang, Y., Wu, C., Yin, W., Shahid, M. A., et al. (2023). Swertia bimaculata moderated liver damage in mice by regulating intestine microbiota. Ecotoxicol. Environ. Saf. 263:115223. doi: 10.1016/j.ecoenv.2023.115223
Islam, M. M., Islam, M. M., Rahman, M. A., Ripon, M. A. R., and Hossain, M. S. (2023). Gut microbiota in obesity and related complications: unveiling the complex interplay. Life Sci. 334:122211. doi: 10.1016/j.lfs.2023.122211
Jandhyala, S. M. (2015). Role of the normal gut microbiota. World J. Gastroenterol. 21:8787. doi: 10.3748/wjg.v21.i29.8787
Kazmi, S. A. H., Iqbal, R., Al-Doaiss, A. A., Ali, M., Hussain, R., Latif, F., et al. (2023). Azoxystrobin-induced oxidative stress in gills, hematological biomarkers, and histopathological ailments in freshwater fish. Pak. Vet. J. 43, 321–326. doi: 10.29261/pakvetj/2023.025
Koliada, A., Syzenko, G., Moseiko, V., Budovska, L., Puchkov, K., Perederiy, V., et al. (2017). Association between body mass index and firmicutes/bacteroidetes ratio in an adult ukrainian population. BMC Microbiol. 17:120. doi: 10.1186/s12866-017-1027-1
Li, K., Liu, J., Zeng, Z., Kulyar, M. F., Wang, Y., Li, A., et al. (2020). The complete genome of probiotic lactobacillus sakei derived from plateau yak feces. Genes. 11:1527. doi: 10.3390/genes11121527
Liu, J., Han, X., Zhang, T., Tian, K., Li, Z., and Luo, F. (2023). Reactive oxygen species (ros) scavenging biomaterials for anti-inflammatory diseases: from mechanism to therapy. J. Hematol. Oncol. 16:1512. doi: 10.1186/s13045-023-01512-7
Liu, J., Xin, Z., Huang, Y., and Yu, J. (2022). Climate suitability assessment on the Qinghai-Tibet plateau. Sci. Total Environ. 816:151653. doi: 10.1016/j.scitotenv.2021.151653
McCallum, G., and Tropini, C. (2024). The gut microbiota and its biogeography. Nat. Rev. Microbiol. 22, 105–118. doi: 10.1038/s41579-023-00969-0
Mendonça, J. D. S., Guimarães, R. D. C. A., Zorgetto-Pinheiro, V. A., Fernandes, C. D. P., Marcelino, G., Bogo, D., et al. (2022). Natural antioxidant evaluation: a review of detection methods. Molecules 27:3563. doi: 10.3390/molecules27113563
Mohsen, A., Chen, Y., Allendes Osorio, R. S., Higuchi, C., and Mizuguchi, K. (2022). Snaq: a dynamic snakemake pipeline for microbiome data analysis with qiime2. Front. Bioinf. 2:893933. doi: 10.3389/fbinf.2022.893933
Nakamura, T., Yamada, K. D., Tomii, K., and Katoh, K. (2018). Parallelization of mafft for large-scale multiple sequence alignments. Bioinformatics 34, 2490–2492. doi: 10.1093/bioinformatics/bty121
Ndeh, D., and Gilbert, H. J. (2018). Biochemistry of complex glycan depolymerisation by the human gut microbiota. FEMS Microbiol. Rev. 42, 146–164. doi: 10.1093/femsre/fuy002
Perlman, D., Martinez-Alvaro, M., Morais, S., Altshuler, I., Hagen, L. H., Jami, E., et al. (2022). Concepts and consequences of a core gut microbiota for animal growth and development. Annu. Rev. Anim. Biosci. 10, 177–201. doi: 10.1146/annurev-animal-013020-020412
Pronovost, G. N., Yu, K. B., Coley-O’Rourke, E. J. L., Telang, S. S., Chen, A. S., Vuong, H. E., et al. (2023). The maternal microbiome promotes placental development in mice. Sci. Adv. 9:eadk1887. doi: 10.1126/SCIADV.ADK1887
Ren, Y., Zhaxi, Y., Liu, M., Idrees, A., and Li, K. (2023). Revealing the fungi microbiome difference of Suffolk cross with Tibetan sheep on plateau. Pak. Vet. J. 43, 748–756. doi: 10.29261/pakvetj/2023.112
Shang, Z., Wang, Y., An, M., Chen, X., Kulyar, M. F., Tan, Z., et al. (2022). The successional trajectory of bacterial and fungal communities in soil are fabricated by yaks’ excrement contamination in plateau, China. Front. Microbiol. 13:16852. doi: 10.3389/fmicb.2022.1016852
Shi, Y., Zhang, L., Do, K., Peterson, C. B., and Jenq, R. R. (2020). Apcoa: covariate adjusted principal coordinates analysis. Bioinformatics 36, 4099–4101. doi: 10.1093/bioinformatics/btaa276
Tu-Sekine, B., and Kim, S. F. (2022). The inositol phosphate system—a coordinator of metabolic adaptability. Int. J. Mol. Sci. 23:6747. doi: 10.3390/ijms23126747
Wan, R., Zhao, Z., Zhao, M., Hu, K., Zhai, J., Yu, H., et al. (2021). Characteristics of pulmonary microvascular structure in postnatal yaks. Sci. Rep. 11:18265. doi: 10.1038/s41598-021-97760-z
Wang, S., Cao, Z., Wu, Q., Idrees, A., Almutairi, M. H., and Dong, H. (2023). A comparative analysis and verification of differentially expressed miRNAs could provide new insights for the treatment of endometritis in yaks. Pak. Vet. J. 43, 486–492. doi: 10.29261/pakvetj/2023.061
Wang, X., Liu, Y., Qing, C., Zeng, J., Dong, J., and Xia, P. (2024). Analysis of diversity and function of epiphytic bacterial communities associated with macrophytes using a metagenomic approach. Microb. Ecol. 87:37. doi: 10.1007/s00248-024-02346-7
Xi, L., Song, Y., Han, J., and Qin, X. (2021). Microbiome analysis reveals the significant changes in gut microbiota of diarrheic baer’s pochards (aythya baeri). Microb. Pathog. 157:105015. doi: 10.1016/j.micpath.2021.105015
Xiao, C., Wang, Y., and Fan, Y. (2022). Bioinformatics analysis identifies potential related genes in the pathogenesis of intrauterine fetal growth retardation. Evol. Bioinformatics Online 18:1556601161. doi: 10.1177/11769343221112780
Xie, Q., Zhang, Y., Zhang, Z., Gong, S., Mo, Q., and Li, J. (2024). Characteristics and dynamic changes of gut microbiota in cats with colitis. Pak. Vet. J. 44, 414–422. doi: 10.29261/pakvetj/2024.175
Xie, Y., Zhu, G., Yi, J., Ji, Y., Xia, Y., Zheng, Y., et al. (2022). A new product of multi-plant extracts improved skin photoaging: an oral intake in vivo study. J. Cosmet. Dermatol. 21, 3406–3415. doi: 10.1111/jocd.14620
Zeng, Q., and An, S. (2021). Identifying the biogeographic patterns of rare and abundant bacterial communities using different primer sets on the loess plateau. Microorganisms. 9:139. doi: 10.3390/microorganisms9010139
Keywords: yak, calf, weight, microbiota, oxidative resistance
Citation: Wang H, Basang W, Pingcuo Z, Jiang N, Sun G, Nawaz S, Cidan Y, Liu Y, Zhu Y and Luosang D (2024) Impact of weight variation on the microbiome of yak dams and calves. Front. Microbiol. 15:1465992. doi: 10.3389/fmicb.2024.1465992
Received: 17 July 2024; Accepted: 26 August 2024;
Published: 18 September 2024.
Edited by:
Aoyun Li, Huazhong Agricultural University, ChinaReviewed by:
Cuncai Guo, Washington University in St. Louis, United StatesCopyright © 2024 Wang, Basang, Pingcuo, Jiang, Sun, Nawaz, Cidan, Liu, Zhu and Luosang. This is an open-access article distributed under the terms of the Creative Commons Attribution License (CC BY). The use, distribution or reproduction in other forums is permitted, provided the original author(s) and the copyright owner(s) are credited and that the original publication in this journal is cited, in accordance with accepted academic practice. No use, distribution or reproduction is permitted which does not comply with these terms.
*Correspondence: Dunzhu Luosang, c29udHNhNzZAMTYzLmNvbQ==
Disclaimer: All claims expressed in this article are solely those of the authors and do not necessarily represent those of their affiliated organizations, or those of the publisher, the editors and the reviewers. Any product that may be evaluated in this article or claim that may be made by its manufacturer is not guaranteed or endorsed by the publisher.
Research integrity at Frontiers
Learn more about the work of our research integrity team to safeguard the quality of each article we publish.