- 1Shaanxi Key Laboratory of Research and Utilization of Resource Plants on the Loess Plateau, Yan’an University, Yan’an, China
- 2Pest and Pathogen Ecology, NIAB East Malling, West Malling, United Kingdom
Apple is an economically important fruit crop in northern Shaanxi Province in China. In recent years, a new type of apple black spot disease, “bagged apple black spot disease,” has occurred in the main apple production area of Yan’an City, Shaanxi Province, during the apple ripening season. It seriously affects the appearance quality and commercial value of apples. In this study, 120 isolates recovered from symptomatic apples were identified based on morphological characteristics, pathogenicity, and multilocus sequence analyses of the internal transcribed spacer of ribosomal DNA (rDNA-ITS), translation elongation factor 1-α (EF1-α), RNA polymerase II subunit B (RPB2), endopolygalacturonase (endo-PG), and anonymous region OPA1-3. Alternaria alternata was the most abundant species (64%), followed by Fusarium acuminatum (36%). Pathogenicity assays were conducted by inoculating them individually and together on detached apples (Venus Golden and Fuji varieties). The results showed that the two fungal species could infect apples individually and together. Co-infection enhanced the disease severity. F. acuminatum led to increased severity and speed of disease development compared to A. alternata. This is the first report of Fusarium and Alternaria co-infection causing apple black spot disease worldwide, and the first report of F. acuminatum affecting apples. The optimal growth of A. alternata occurred at 25–30°C and pH 7; the optimal growth of F. acuminatum occurred at 25°C and pH 7. The results of this study can provide a theoretical basis for exploring the occurrence and epidemiology of apple black spot disease and strategies for its control.
1 Introduction
Apple is one of the most widely grown and consumed fruits in the world, and China is the world’s largest apple producer, producing about half of the world’s apple supply (Liang et al., 2022). Shaanxi Province is the most important apple-producing area in China, and its cultivation area and yield rank first in the country. Apples are one of the most distinctive and advantageous agricultural products in Shaanxi, and they have strong international competitiveness (Zhou et al., 2021). The Loess Plateau in northern Shaanxi is recognized globally as a high-quality apple-producing area. The apple industry has become one of the fastest growing and most efficient agricultural industries in the region, as well as a major industry that farmers participate in to augment their income (Yan et al., 2019).
Apple bagging is an important technical measure in apple cultivation in China. Bagging fruits can reduce damage caused by diseases and insect pests, reduce pesticide residues on fruits, improve the appearance of fruits, and increase the economic gains. However, with the increased use of fruit bagging, “bagged apple black spot disease,” which causes spots (with various shapes, sizes, and colors) on apple surfaces, collectively referred to as black spot disease involving black necrotic spots (with various shapes, sizes, and colors) on apple surfaces, often occurs during apple harvesting. The disease can cause 5–30% yield losses each year, and up to 80% in severe cases (Li et al., 2013; Wang et al., 2015). The disease strongly affects apple quality and commodity value and has become a major obstacle to the development of China’s apple industry, seriously dampening fruit growers’ enthusiasm for growing apple trees (Hu et al., 2016). The disease is caused by changes in the temperature, humidity, air permeability, and other microecological environmental factors affecting bagged apples (Luan et al., 2019).
Due to the significant differences in recent years in climate conditions in the main apple cultivation areas in China, there are large differences in the pathogen species composition and pathogenicity characteristics of the disease among different areas, as evidenced by pathogen morphology and multi-gene phylogenetic analysis. From 2000 to 2023, a variety of causal fungal species have been reported throughout China. In Gansu Province in 2000, Cylindrosporium pomi Brooks and Phoma pomi Pass were reported to cause bagged apple black spot disease (based on traditional phytopathogenic fungi morphology plus pathogenicity methods) (Xu and Wei, 2000; Xu et al., 2002). In Shandong Province in 2014, bagged apple black spot disease was divided into four symptom types, which were reported to be caused by Acremonium sclerotigenum, Alternaria tenuissima, and Trichothecium roseum (Wang et al., 2014; Wang et al., 2015). In Guanzhong region of Shaanxi Province in 2016, bagged apple black spot disease was also divided into four symptom types, which were reported to be caused by eight fungal genera, including the taxa Acremonium spp., Alternaria alternata, and Trichothecium roseum (Hou, 2016). In Shaanxi Province in 2019, bagged apple black spot disease were reported to be caused by Acremonium mali, Sarocladium liquanensis, and Sarocladium mali (based on morphology plus phylogenetic analysis involving ITS, ACT, LSU and BT2) (Hou et al., 2019). In Shaanxi Province in 2018, Alternaria malicola sp. nov. was discovered as a new species affecting apple fruits (Dang et al., 2018). In Hebei Province in 2021, bagged apple black spot disease occurred widely and there were four symptom types. They were reported to be caused by five fungal genera, with Trichothecium roseum being dominant, followed by Acremonium sclerotigenum, and Alternaria tenuissima (based on morphology plus sequence analysis involving large subunit (LSU) and OPA10-2) (Meng et al., 2021). In conclusion, the pathogens of bagged apple black spot disease differ greatly by region in China.
From 2020 to 2023, the incidence of bagged apple black spot disease was >75% in some orchards in Baota District and Yichuan County of Yan’an City, northern Shaanxi. Alternaria spp. and Fusarium spp. were the putative causal agents. Due to the dramatic differences in climate conditions across the mountainous fruit cultivation areas of the Loess Plateau in northern Shaanxi, the symptoms and pathogen composition of the disease are very complex, and clarifying the dominant pathogens is key to effective scientific control of the disease. Therefore, it was necessary to identify and analyze the pathogens causing bagged apple black spot disease in Baota District and Yichuan County of Yan’an City, northern Shaanxi. The objectives of this study were (1) to investigate the incidence of bagged apple black spot disease in Baota District and Yichuan County of Yan’an City, northern Shaanxi, and to identify the pathogenic species by morphological and multi-gene sequence analysis; (2) to clarify the pathogenicity of the pathogen on the main cultivated apple varieties; and (3) to clarify the effects of temperature and pH on the mycelial growth of the main pathogens.
2 Materials and methods
2.1 Sample collection and fungal isolation
Bagged apples with black spot disease were collected from three orchards in (1) Baota District and (2) Yichuan County of Yan’an City, Shaanxi Province, during apple harvesting from September to October 2023. The main varieties planted in the orchards were Fuji and Venus Gold. Apples fruits with typical symptoms of bagged apple black spot disease were collected with plastic bags (labeled with the place, time, and number of samples), and brought back to the laboratory.
The apples were disinfected with 75% alcohol for 2 min, rinsed twice with sterile water, and naturally air-dried. They were then cut with a sterile blade to obtain infected apple tissue samples (0.5 × 0.5 × 0.5 cm) from the margins of black spot lesions, which were transferred to potato dextrose agar (PDA) plates supplemented in advance with 50 μg/mL streptomycin sulfate. The cultures were incubated for 3–5 days in an incubator at 25°C in the dark. Hyphal tips from the developing fresh mycelium were transferred to fresh PDA plates for purification. Based on the morphological characteristics of the colonies and conidia, the isolates were divided into two groups: Alternaria and Fusarium. All putative Alternaria and Fusarium isolates were subcultured on PDA plates for single spore purification. The representative isolates were transferred to PDA plates, and putative Fusarium isolates were also transferred to carnation leaf agar (CLA) medium plates. The colonies were cultured in a constant-temperature incubator at 25°C for 5–20 days. A total of 120 isolates were obtained and stored at 4°C. The isolates were identified to species level based on the morphology and molecular phylogenetic analysis.
2.2 Morphological characterization
The colony diameters were assessed daily, and the mean daily colony growth rate (mm/day) was calculated. The morphological characteristics of the colonies and conidia were observed and photographed under a microscope daily. Fifty conidia were randomly selected to measure their length and width. The isolates were identified based on the morphological characteristics of colonies and conidia in previous studies (Leslie and Summerell, 2006; Simmons, 2007).
2.3 DNA extraction, PCR amplification, and phylogenetic analysis
Representative four Alternaria isolates and seven Fusarium isolates in this study were inoculated on PDA medium and cultured at 26°C in the dark for 7 days. A sample of the mycelium (50 mg) of each isolate was collected from the surface of the PDA medium and then transferred to a 1.5-ml centrifuge tube. The genomic DNA of the mycelium was then extracted using the cetyltrimethylammonium bromide (CTAB) method (Zhou et al., 2011), dissolved in ddH2O, and stored at −20°C. Internal transcribed spacer of ribosomal DNA (rDNA-ITS), translation elongation factor 1-α (EF1-α), endopolygalacturonase (endo-PG), anonymous region OPA1-3, and RNA polymerase II subunit B (RPB2) gene fragments were PCR amplified and sequenced using the following primers (Table 1) (Shanghai Bioengineering Co., Ltd.): ITS1/ITS4, EF1-728F/EF1-986R, PG3/PG2b, OPA1-3 L/OPA1-3R, and RPB2-5F2/FRPB2-7cR. The 25 μL PCR system contained 12.5 μL 2× Taq enzyme, 1.5 μL DNA, 0.5 μL upstream primer, 0.5 μL downstream primer, and 10 μL ddH2O. The amplified PCR products were assessed by 1% agarose gel electrophoresis and sent to Sangon Biotech (Shanghai, China) for sequencing. Our ITS, EF1-α, RPB2, endo-PG, and OPA1-3 sequences were compared to related sequences in the NCBI database by BLAST, and our sequences were submitted to GenBank (accession numbers are listed in Table 2).
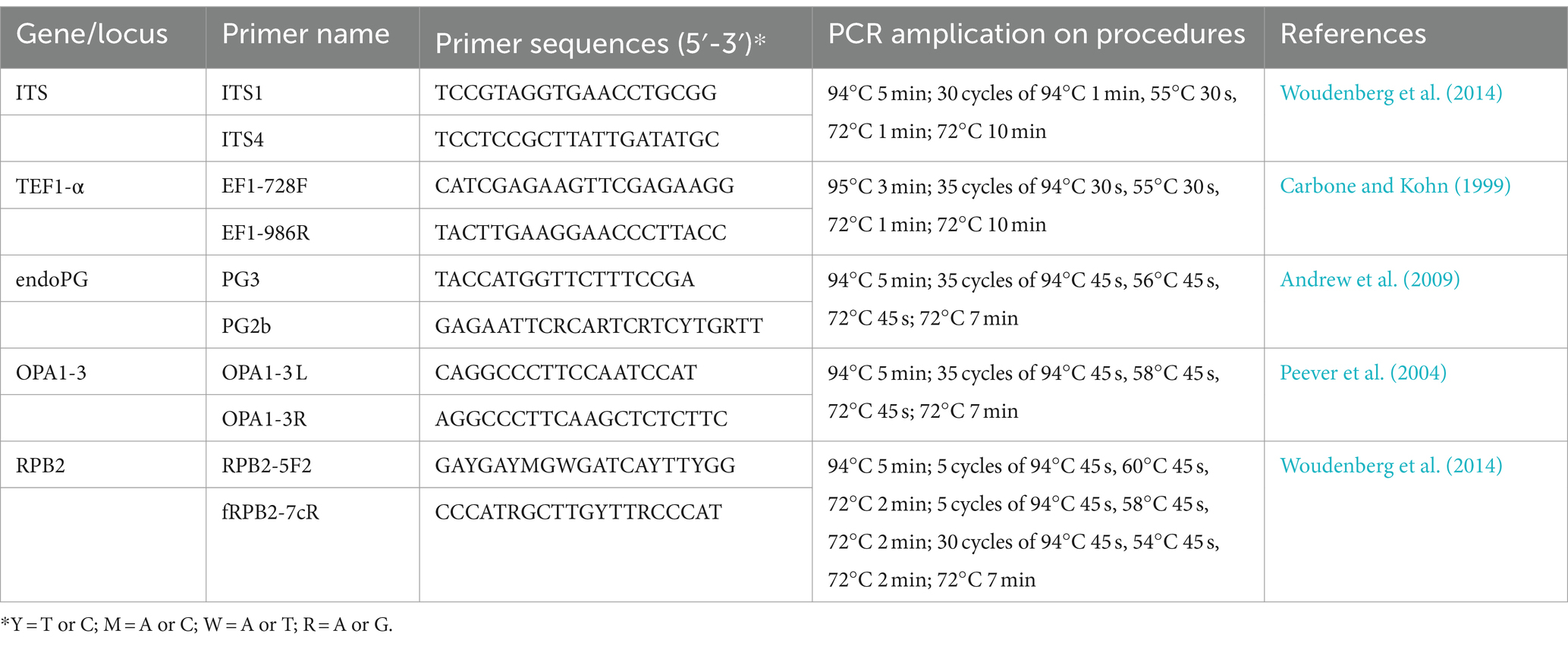
Table 1. Summary of published universal primers used for Fusarium and Alternaria species determination associated with bagged apple black spot in Shaanxi, China.
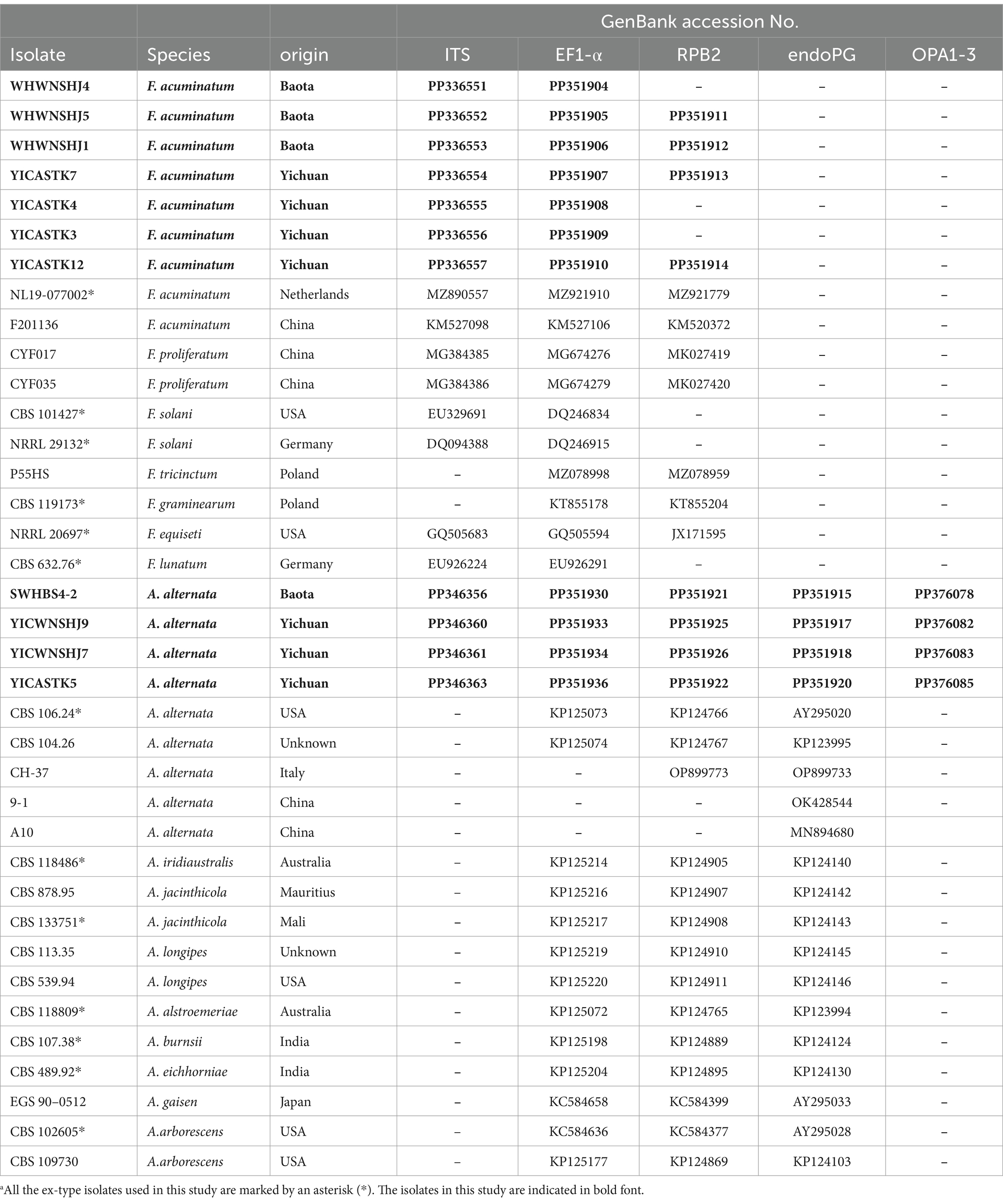
Table 2. Alternaria and Fusarium isolates obtained in this study and downloaded from GenBank with accession numbers used for phylogenetic analyses.a
Based on a report by Woudenberg et al., the EF1-α, RPB2, and endo-PG sequences of 6 standard Alternaria isolates were downloaded from the NCBI database (Woudenberg et al., 2015). Based on reports by Crous et al. and Zhou et al., rDNA-ITS, EF1-α, and RPB2 sequences of 2 standard Fusarium isolates were downloaded from the NCBI database (Zhou et al., 2016; Crous et al., 2021a). The obtained sequences were edited using Bio-Edit software, and multiple sequence alignment was performed using Clustal X 1.83. The sequences of our four representative Alternaria isolates and seven representative Fusarium isolates were compared to those of the standard isolates in MEGA 7.0. Maximum-likelihood phylogenetic trees were constructed, and confidence was evaluated using the bootstrap method with 1,000 replications. According to the results of phylogenetic tree analysis and sequence alignment, the species of the representative isolates obtained in this study were determined.
2.4 Temperature and pH experiments
As all the Alternaria and Fusarium isolates in the two respective groups exhibited similar growth characteristics, representative isolates A. alternata YICASTK5 and F. acuminatum WHWNSHJ1 were selected to evaluate the effects of temperature and pH on colony growth on PDA medium. A 5-mm-diameter mycelial plug of each isolate from the edge of a 7-day-old colony was placed in the center of a 90-mm-diameter PDA plate and incubated in the dark. Temperatures ranged from 5°C to 40°C at 5°C intervals (5°C, 10°C, 15°C, 20°C, 25°C, 30°C, 35°C, and 40°C). PDA medium was adjusted to 3.0, 4.0, 5.0, 6.0, 7.0, 8.0, 9.0, 10.0, and 11.0 using 0.1 mol/L sodium hydroxide (0.1 M NaOH) and 0.1 mol/L hydrochloric acid (0.1 M HCl). The colony diameters were measured daily. The experiments involved three replicates and were repeated twice. GraphPad Prism 9.0 was used for data analysis and visualization.
2.5 Pathogenicity assays
The representative isolates A. alternata YICASTK5 and F. acuminatum WHWNSHJ1 obtained in this study underwent pathogenicity assays. There were three treatments: (1) Alternaria inoculation; (2) Fusarium inoculation; and (3) co-inoculation with both fungi. Healthy, mature, detached Fuji and Venus Golden apples of the same size were surface sterilized with 75% alcohol for 2 min and then washed with sterile water twice. A 5-mm-diameter PDA plug containing mycelia was taken from a 5-day-old colony and used to inoculate an apple that had been punctured at the inoculation site with a sterile syringe needle to a depth of 2 mm. The co-inoculation method comprises the following steps: representative isolates A. alternata YICASTK5 and F. acuminatum WHWNSHJ1 were cultured in the same PDA petri dish at 25°C. When the mycelium of two strains grow together in the center of PDA petri dish, 5-mm-diameter PDA plug containing mycelia for both species were taken from the junction of mycelium for inoculation. The inoculated apples were placed in a plastic box (25 × 15 × 10 cm) and left for 10 days at 25°C and 100% relative humidity. There were three inoculation sites per apple and three apples per treatment, and the experiment was repeated three times per treatment. Pathogenicity was determined by the necrotic lesion diameter at 3 days after inoculation. To fulfill Koch’s postulates, small pieces of infected apple tissues were plated on PDA medium to reisolate the fungal isolates, which were reidentified based on morphological characterization and DNA sequences.
2.6 Statistical analyses
The statistical analysis was performed using GraphPad Prism 9.0 software. Lesion diameters measured in the pathogenicity test were subjected to the analysis of variance (ANOVA) and the mean values were separated by Tukey test (p = 0.05).
3 Results
3.1 Field disease symptoms and pathogen isolation
From the end of September to the beginning of October 2023, bagged apple black spot disease was investigated in three commercial apple orchards in (1) Baota District and (2) Yichuan County of Yan’an City, Shaanxi Province. The management level is medium and both Fuji and Venus Gold varieties are grown there. Black spots on the bagged apples in the three orchards occurred throughout the study period. The incidence was 30 and 75% for Fuji and Venus Golden apples, respectively.
The main symptoms of apple black spots are as follows: small black spots (approximately round, with a diameter of about 2–5 mm and a lenticel at the center) on the surface of the apple are formed as it reaches maturity. The pericarp around each spot is necrotic, forming a red or black halo (Figures 1A,B). The spots are unevenly distributed on the surface and the lesions do not expand, but the apples completely lose their commercial value.
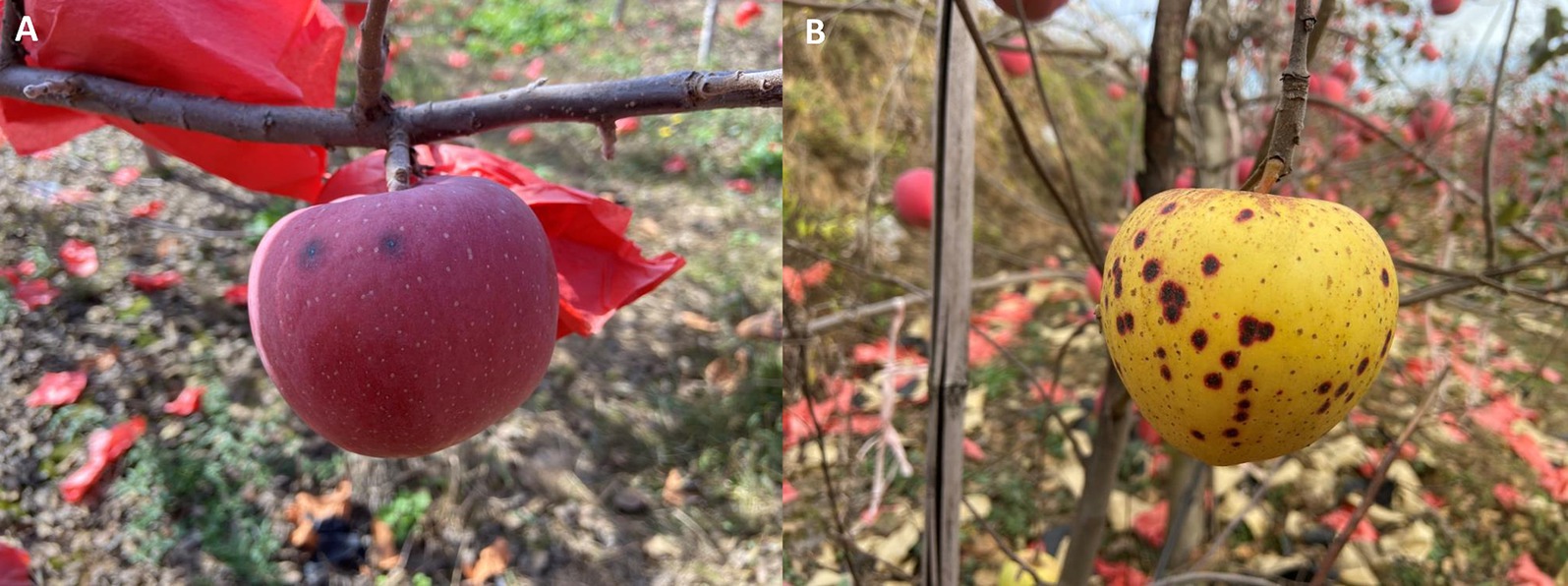
Figure 1. Field symptoms of bagged apple black spot disease caused by Alternaria alternata and Fusarium acuminatum in Shaanxi Province, China. Typical symptoms on (A) Fuji and (B) Venus Golden apples.
A total of 51 diseased apples (25 Fuji and 26 Venus Gold apples) were collected from the three orchards, with varying disease severity. A total of 120 suspected pathogenic isolates were obtained, involving 77 (64%) Alternaria isolates and 43 (36%) Fusarium isolates (Alternaria was the dominant genus). For the Fuji apples, 26 Alternaria isolates and 23 Fusarium isolates were isolated (Alternaria was the dominant genus). For the Venus Golden apples, 51 Alternaria isolates and 20 Fusarium isolates were isolated (Alternaria was the dominant genus). These isolates were stored at the Phytopathology Research Laboratory of Yan’an University.
3.2 Morphological characterization
Based on morphological characteristics (colony morphology and conidial characteristics) and phylogenetic analysis, the 120 isolates were divided into two groups: 77 isolates in group A (identified as Alternaria spp.) and 43 isolates in group B (identified as Fusarium spp.). The morphological characteristics of isolates in each group were consistent, so Alternaria isolate YICASTK5 and F. acuminatum WHWNSHJ1 were selected as the main representative isolate, inoculated on PDA medium, and cultured in the dark in a constant-temperature incubator at 25°C. The growth status of the isolates was observed and recorded daily.
Group A: On PDA medium, the colony is olive green to grayish brown with densely packed hyphae on the front side and a dark gray reverse side (Figure 2A), with a growth rate of 8.50 mm/day on PDA medium. The conidiophores are brown, either single or branched, and produce numerous short conidial chains. The conidia are ovoid or ellipsoid, with 1 to 5 transverse septa and 0 to 2 oblique septa, and a size of (6.58 to 27.73) μm × (5.13 to 16.18) μm (n = 50) (Figure 2B). Morphological characteristics were consistent with description of A. alternata (Simmons, 2007).
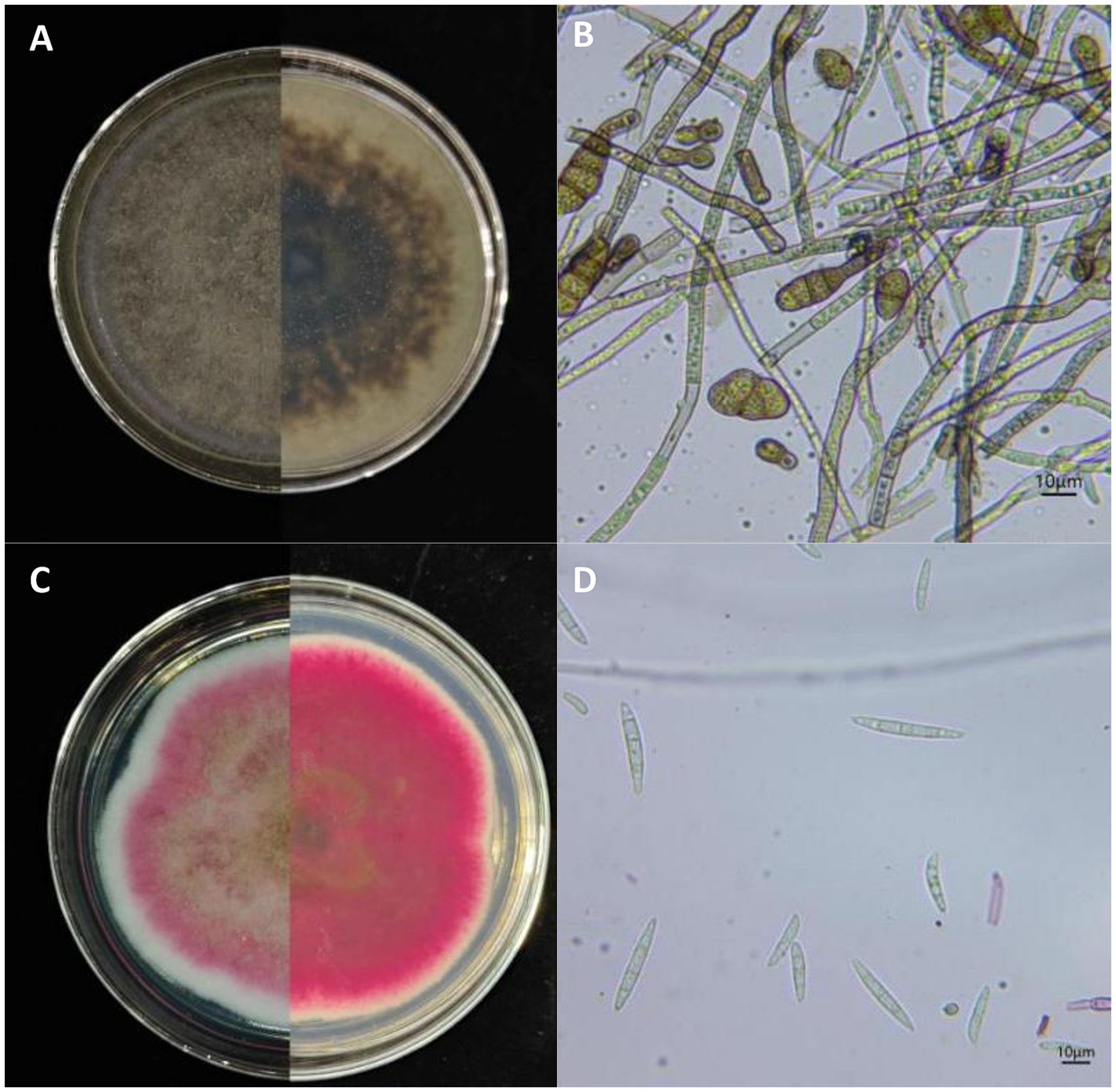
Figure 2. Morphological characteristics of Alternaria alternata and Fusarium acuminatum isolated from apples with bagged apple black spot disease in Shaanxi Province, China. (A) Front and reverse of colonies cultured on PDA medium for 7 days and (B) conidia of A. alternata YICASTK5 (representative isolate). Scale bar: D = 10 μm. (C) Front and reverse of colonies cultured on PDA for 15 days and (D) conidia of F. acuminatum WHWNSHJ1 (representative isolate). Scale bar: D = 10 μm.
Group B: On PDA medium, colony grew slowly, with a growth rate of 4.30 mm/day on PDA medium. Isolate formed abundant white aerial mycelium, then became floccose with rose pigmentation, and developed a brownish tinge in the center and grayish rose at the periphery on PDA after 15 days’ inoculation. The reverse side of the colony was red to burgundy (Figure 2C). On carnation leaf agar, macroconidia with 3 to 5 septa were abundant, relatively slender, curved to lunate, and measured (24.65 to 55.40) μm × (3.40 to 5.95) μm (n = 50) (Figure 2D). Microconidia were oval, with 0 to 1 septa. Chlamydospores were globose with a smooth outer wall in chains or single. Morphological characteristics were consistent with description of F. acuminatum (Leslie and Summerell, 2006).
3.3 Molecular characterization
Five gene fragments (ITS, EF1-α, RPB2, endo-PG, and OPA1-3) commonly used for Alternaria spp. and Fusarium spp. identification were selected for PCR and sequencing to identify the four representative Alternaria isolates and seven representative Fusarium isolates. The sequences were submitted to GenBank (accession numbers are listed in Table 2). Based on ITS, EF1-α, RPB2, endo-PG, and OPA1-3, our four representative Alternaria isolates exhibited 99–100% similarity to the nucleotide sequences of representative Alternaria alternata isolates from GenBank (using BLAST searches). Based on ITS, EF1-α, and RPB2, our seven representative Fusarium isolates exhibited 99–100% similarity to the nucleotide sequences of representative published Fusarium acuminatum isolates from GenBank (using BLAST searches).
Maximum-likelihood phylogenetic trees were constructed in MEGA 7.0 for our four Alternaria isolates (based on EF1-α, RPB2, and endo-PG) (Figure 3) and our seven Fusarium isolates (based on ITS, EF1-α, and RPB2) (Figure 4) plus standard Alternaria and Fusarium isolates sequences, respectively, from GenBank. Our Alternaria isolates clustered in the same branch as A. alternata CBS104.26, CBS106.24, CH-37, A10, and 9–1 (their phylogenetic relationships were the closest), and the branch support rate was 96%. Our Fusarium isolates clustered in the same branch as F. acuminatum NL19-077002 and F201136 (their phylogenetic relationships were the closest), and the branch support rate was 99%.
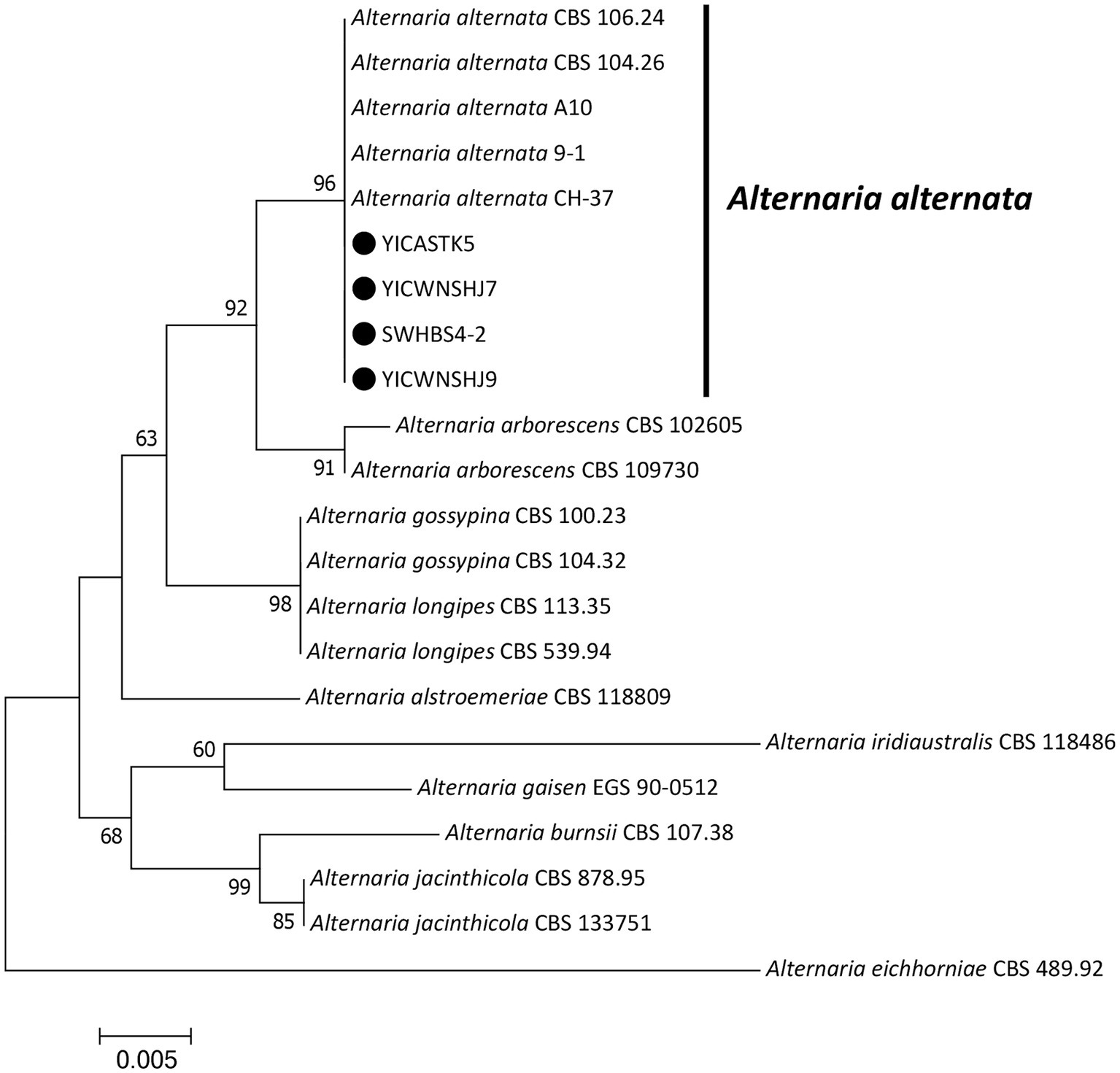
Figure 3. Maximum-likelihood phylogenetic tree based on translation elongation factor 1-α (EF1-α), RNA polymerase II subunit B (RPB2), and endopolygalacturonase (endo-PG) gene sequences of our four representative Alternaria alternata isolates associated with bagged apple black spot disease in China (indicated by ●). Related Alternaria species were downloaded from GenBank. Support values at nodes represent RAxML bootstrap percentages ≥60%.
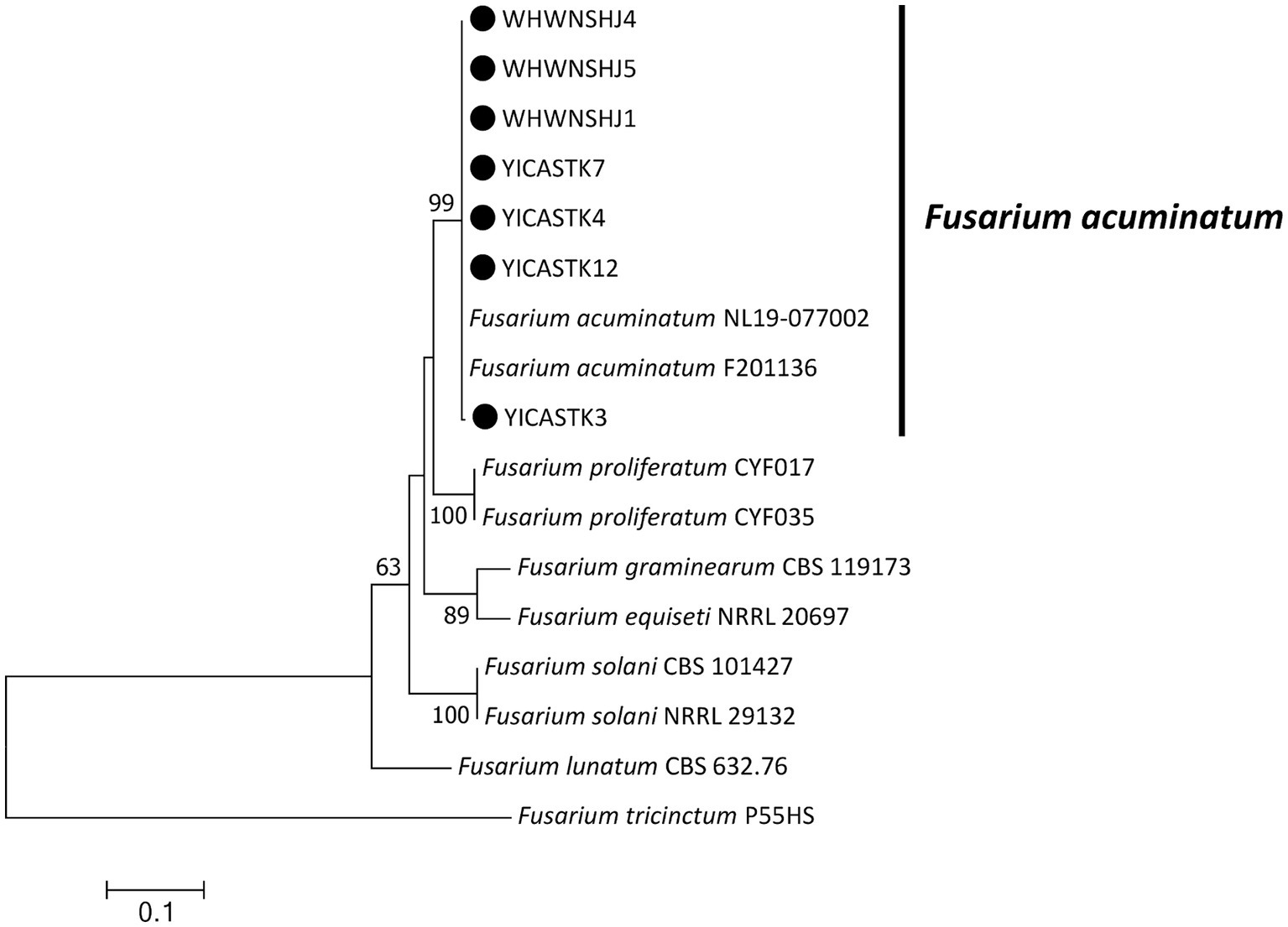
Figure 4. Maximum-likelihood phylogenetic tree based on internal transcribed spacer of ribosomal DNA (rDNA-ITS), translation elongation factor 1-α (EF1-α), and RNA polymerase II subunit B (RPB2) gene sequences of our seven representative Fusarium acuminatum isolates associated with bagged apple blackspot disease in China (indicated by ●). Related Fusarium species were downloaded from GenBank. Support values at nodes represent RAxML bootstrap percentages ≥60%.
According to the morphological and molecular characterization, the main associated isolates of bagged apple black spot disease in Baota District and Yichuan County of Yan’an City, Shaanxi Province, were Alternaria alternata and Fusarium acuminatum.
3.4 Effects of temperature and pH on mycelial growth
3.4.1 Effects of temperature
A. alternata YICASTK5 mycelia grew at 5–35°C but was strongly influenced by temperature (Figure 5A). It grew fastest at 25°C and 30°C, did not grow at 40°C, and grew optimally at 25–30°C.
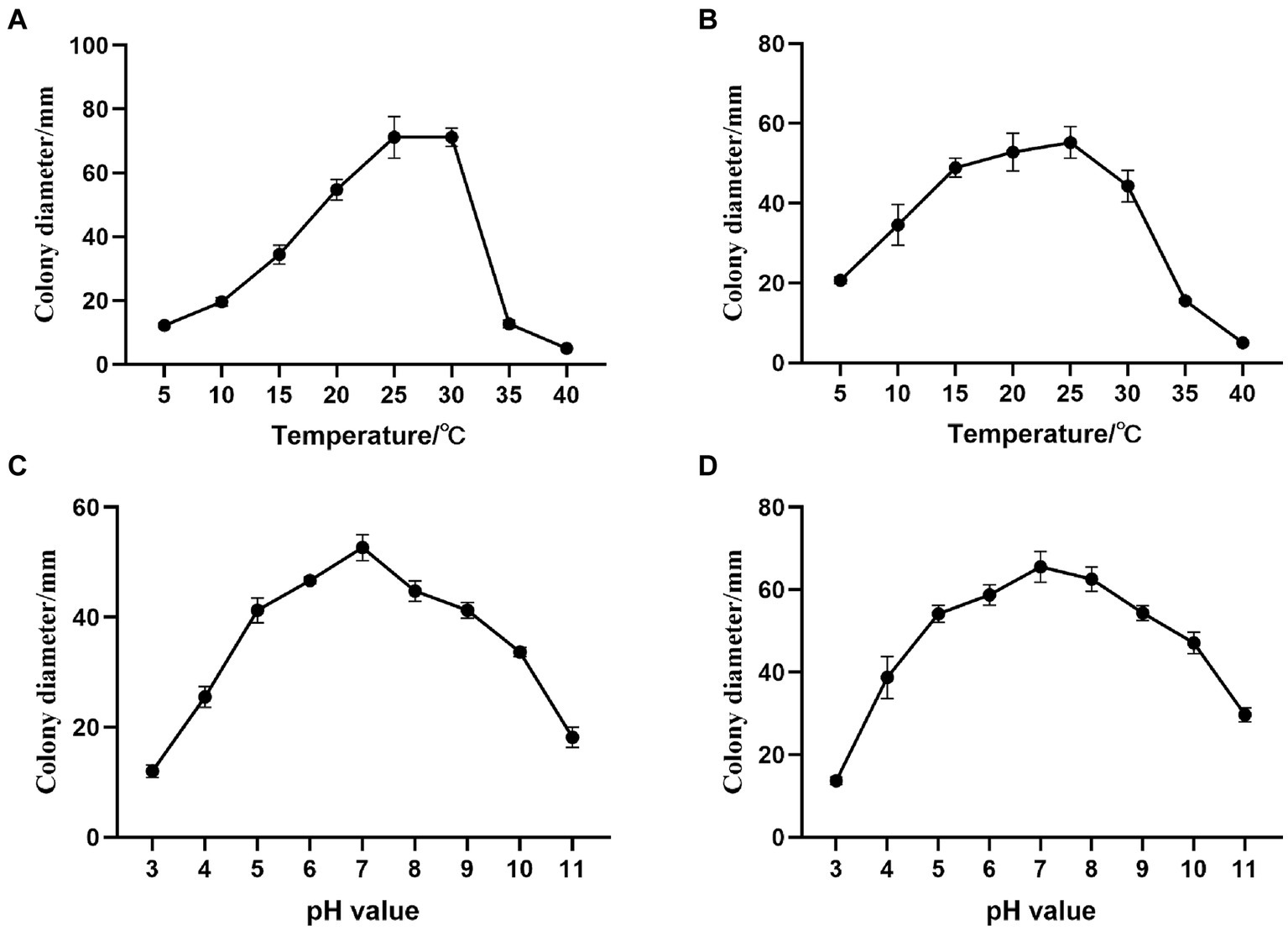
Figure 5. Effects of temperature and pH on Alternaria alternata and Fusarium acuminatum mycelial growth on PDA medium. Changes in mycelial growth of (A) A. alternata YICASTK5 with temperature, (B) F. acuminatum WHWNSHJ1 with temperature, (C) A. alternata YICASTK5 with pH, and (D) F. acuminatum WHWNSHJ1 with pH.
F. acuminatum WHWNSHJ1 mycelia grew at 5–35°C (Figure 5B). The mycelial growth rate gradually increased from 5°C to 25°C and rapidly decreased from 25°C to 35°C. It did not grow at 40°C and grew optimally at 25°C.
3.4.2 Effects of pH
A. alternata YICASTK5 mycelia grew at pH 3.0–11.0 (Figure 5C), but it varied significantly, with dense, vigorous. The mycelia grew fastest at pH 7 and slower at pH 3 and 11. Thus, it grew best in a neutral environment.
F. acuminatum WHWNSHJ1 mycelia grew at pH 3.0–11.0 (Figure 5D), but it varied significantly, with dense, vigorous, and fastest growth at pH 7, and slower growth at pH 3 and 11. Thus, it grew best in a neutral environment.
3.5 Pathogenicity assays
The representative isolates A. alternata YICASTK5 and F. acuminatum WHWNSHJ1 were inoculated into Fuji and Venus Golden apples. After 3 days, black spots appeared on the apple surfaces, which was basically consistent with the field disease symptoms. The incidence rate was 100%.
At 7 days after A. alternata inoculation, the mean lesion diameter was 1.5 mm in both apple varieties (it did not expand further after 7 days) (Figures 6A,B). At 7 days after F. acuminatum inoculation, the mean lesion diameter was 3.44 mm in Fuji apples and 9.44 mm in Venus Golden apples (Figures 6C,D). At 7 days after co-inoculation of A. alternata and F. acuminatum, the mean lesion diameter was 7 mm in Fuji apples and 13.5 mm in Venus Golden apples (Figures 6E,F). Co-inoculation had the strongest impact on both apple varieties, with the lesions expanding the fastest (Figures 6E,F). The control-inoculated apples were not diseased (Figures 6G,H). There were significant differences in lesion size among the three inoculation treatments, with co-inoculation having the strongest effect, F. acuminatum inoculation having a moderate effect, and A. alternata inoculation having the weakest effect (Figure 7).
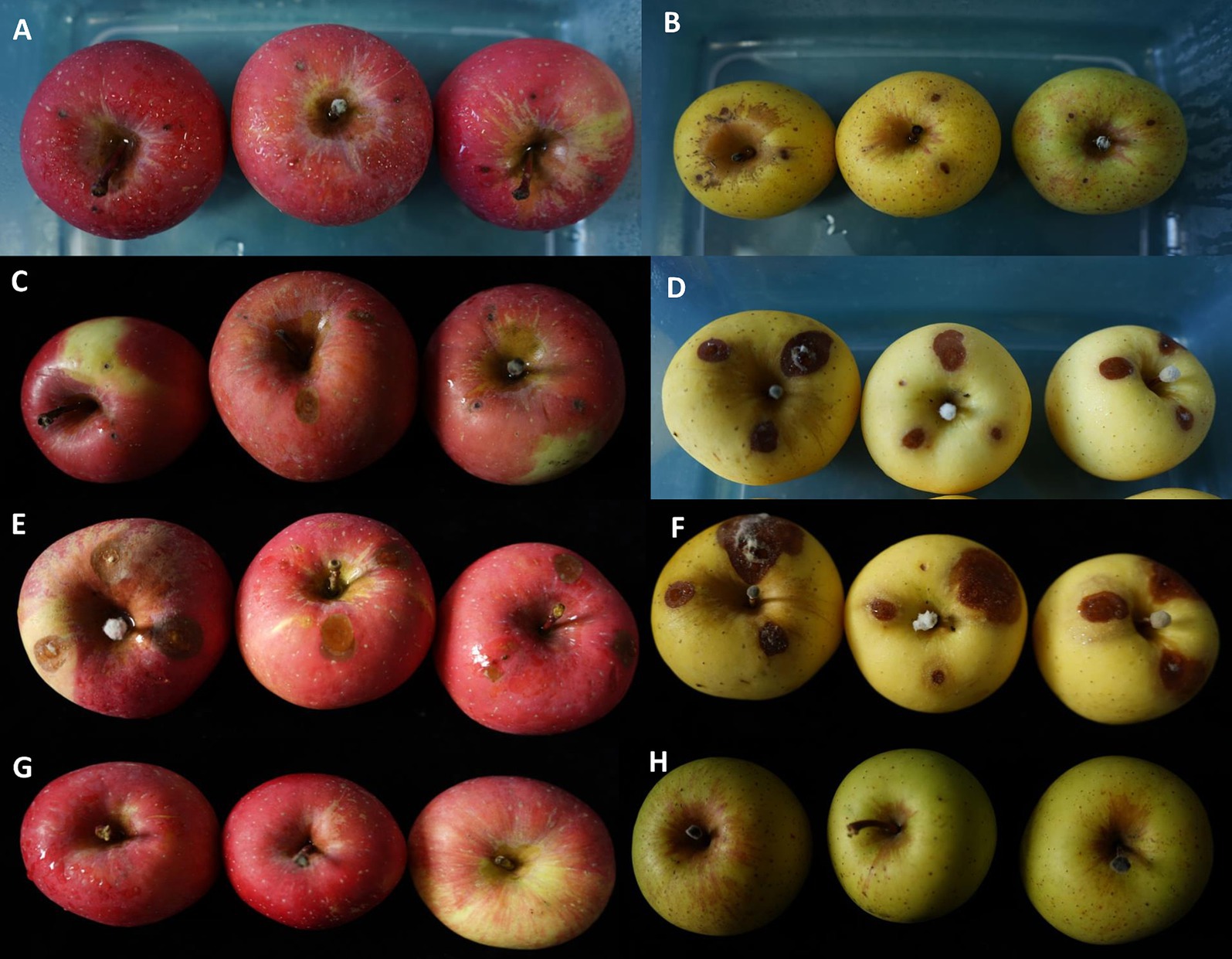
Figure 6. Symptoms on Fuji and Venus Golden apples inoculated with Alternaria alternata and Fusarium acuminatum at 7 dpi. A. alternata YICASTK5 on (A) Fuji and (B) Venus Golden apples. F. acuminatum WHWNSHJ1 on (C) Fuji and (D) Venus Golden apples. A. alternata and F. acuminatum co-inoculation on (E) Fuji and (F) Venus Golden apples. (G) Control Fuji apples. (H) Control Venus Golden apples.
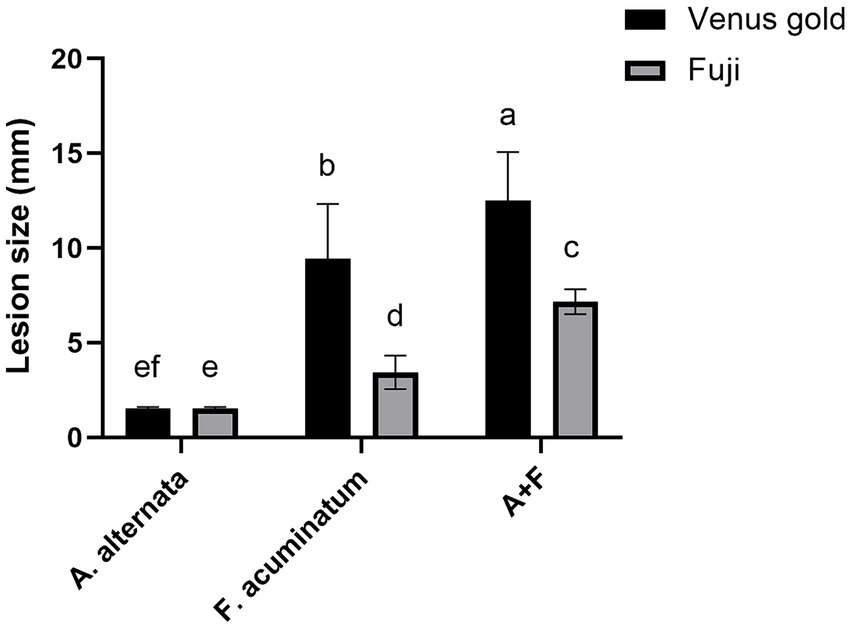
Figure 7. Alternaria alternata YICASTK5 and Fusarium acuminatum WHWNSHJ1 lesion sizes on Fuji and Venus Golden apples at 7 dpi. Different letters above error bars indicate a significant difference (p < 0.05).
Each pathogen was reisolated from apples inoculated with each pathogen by conventional tissue isolation methods. Based on morphological identification, the reisolated pathogens were the same species as the inoculated species. Thus, it was verified that A. alternata and F. acuminatum were the main pathogens causing bagged apple black spot disease.
4 Discussion
Bagged apple black spot disease is becoming an important disease in the Chinese apple industry. In particular, it is a serious problem that needs to be solved for apple growers in the Loess Plateau of northern Shaanxi Province. It has a large impact on the appearance of apples, seriously affecting the percentage of quality apples and commodity value. In this study, A. alternata and F. acuminatum were identified as causal agents of this disease in the northern areas of Shaanxi Province: (1) Baota District and (2) Yichuan County of Yan’an City. A. alternata was dominant, which may be related to the introduction and cultivation of Venus Golden apples (for the Venus Golden apples, 20 Fusarium isolates vs. 51 Alternaria isolates were isolated) and the arid climate in the Loess Plateau of northern Shaanxi. To our knowledge, this is the first report in the world on Alternaria and Fusarium co-infection of apples, and this is also the first report on F. acuminatum causing apple disease in the world.
Apple is an economically important fruit crop that is widely cultivated in China. Due to the differential effects of climate change in different regions, bagged apple black spot disease can be caused by a variety of pathogens. Acremonium sclerotigenum, Alternaria tenuissima, and Trichothecium roseum have been widely reported in Hebei provinces (Meng et al., 2021). A. tenuissima and T. roseum are the dominant pathogens causing bagged apple black spot disease in Shandong, Shaanxi, and western Liaoning (Hao et al., 2004; Zhou et al., 2012; Wang et al., 2014). The genus of Acremonium, Alternaria, Sarocladium, and Trichothecium exhibited weak pathogenicity after inoculation of punctured apples (Wang et al., 2014; Hou, 2016; Meng et al., 2021). Our results on A. alternata inoculation of punctured Fuji and Venus Golden apples were similar. Thus, these pathogens could be considered as opportunistic, weak pathogens, which can only infect the surface of apples under suitable conditions. However, most of the previous studies did not carry out inoculation tests on Venus Golden apples, while we studied both Fuji and Venus Golden apples. Our inoculation tests after puncturing the apples showed that both A. alternata and F. acuminatum are pathogenic to both Fuji and Venus Golden apples, though A. alternata was only weakly pathogenic to both varieties.
Pathogencity tests showed that A. alternata and F. acuminatum have variations in the disease progression on detached apple fruits. F. acuminatum infects fruits and develops symptoms more quickly than A. alternate. Additionally, compared to single infection of these two species, Alternaria and Fusarium co-inoculation significantly increased the pathogenicity. Based on the results above, it is evident that apple black spot disease is a complex disease that might be caused by different fungal species. These different fungal taxa may have varying impacts on apple fruits. Our findings suggest that multiple fungi causing a primary infection in an apple orchard during apple ripening can increase the disease complexity. Researchers in Australia and Israel have found that many Alternaria isolates can concurrently cause diseases on apple leaves and fruits, and cross-pathogenicity assays have shown that pathogenic Alternaria isolates do not exhibit specificity regarding apple leaves or fruits (Harteveld et al., 2014; Gur et al., 2017). Apple orchards are complex ecosystems and identifying the primary infection source of apple diseases is very important for effective scientific disease control. We speculate that the pathogens isolated from apple fruits surfaces in this study may originate from apple leaf fungal infections.
The genus Alternaria was established by Nees von Esenbeck in 1816. It contains many endophytic, saprophytic, and pathogenic species, and A. tenuis is the representative species. Traditional Alternaria taxonomic identification is mostly based on morphological characteristics (Simmons, 1967), but this is often affected by conditions such as medium composition, culture environment, and culture duration (Thomma, 2003). Therefore, molecular techniques have been widely used to aid morphological identification of Alternaria (Kusaba and Tsuge, 1995; Peever et al., 2004). Gene segments such as EF1-α, RPB2, endo-PG, OPA1-3, CAL, and TUB have been widely used in molecular phylogenetic analyses of Alternaria species (Andrew et al., 2009; Lawrence et al., 2013; Woudenberg et al., 2014; Woudenberg et al., 2015; Zheng et al., 2015). Recent studies have shown that multiple Alternaria microspore species can co-infect a variety of fruit trees, including apple, blueberry, and pomegranate (Gao et al., 2013; Ntasiou et al., 2015; Zhu and Xiao, 2015; Luo et al., 2017). In this study, three gene fragments combined phylogenetic analyses (EF1-α, RPB2 and endo-PG) accurately distinguished the Alternaria isolates.
The genus Fusarium Link is widely distributed in nature, and it is one of the most important phytopathogenic fungi. Symptoms (including rhizome rot, fruit rot, wilting, and leaf spotting) caused by Fusarium species on various plants can lead to severe economic losses (Leslie and Summerell, 2006; Wang et al., 2022). In recent years, disease caused by Fusarium has gradually become a severe problem in global apple cultivation. Fusarium proliferatum was confirmed as the dominant pathogen of apple replant disease in China (based on phylogenetic analysis involving six gene loci) (Duan et al., 2022). Fusarium tricinctum was first reported as a new pathogen causing apple dieback in Gansu, China (Zhang et al., 2022). Fusarium fujikuroi was first identified as the pathogen of apple heart rot in India (based on morphology, molecular analysis, and pathogenicity) (Watpade et al., 2024). Fusarium decemcellulare was first reported as an important pathogen causing fruit rot in Korean apples (Lee et al., 2017). This study is the first study in the world to report that Alternaria and Fusarium species can co-infect apples and cause black spot symptoms. There are 18 species complexes and more than 300 Fusarium species. The morphological characteristics of Fusarium species are very similar, though with a large amount of minor morphological variability. It has also been difficult to identify the sexual stages of most Fusarium species, which causes many challenges for taxonomic identification (Thomas et al., 2019; Crous et al., 2021b). Accurate identification of Fusarium-induced plant diseases is a critical factor for effective scientific disease control (Jayawardena et al., 2021; Manawasinghe et al., 2021). By far the most informative and frequently used genes include EF1-α, RPB1, and RPB2, and they often accurately distinguish between Fusarium species (Crous et al., 2021b; Wang et al., 2022; O’Donnell et al., 2022). Over the past 25 years, genealogical concordance phylogenetic species recognition (GCPSR)-driven delimitation and identification of Fusarium species has largely been dependent on the nucleotide sequences of parts of phylogenetically informative protein-coding genes. By far the most informative and frequently used genes are EF1-α, RPB1, and RPB2, and they often accurately distinguish between Fusarium species (O’Donnell et al., 2022). In this study, a phylogenetic analysis (combining ITS, EF1-α, and RPB2 gene sequences) showed that our seven representative Fusarium isolates from apples with bagged apple black spot disease clustered in the same branch as representative F. acuminatum isolates from GenBank (with 99% branch support rate).
5 Conclusion
In summary, this study revealed that bagged apple black spot disease during apple harvesting from September to October 2023 in northern Shaanxi is caused by A. alternata and F. acuminatum, with A. alternata being the dominant species. The pathogenicity of these two species was confirmed on detached apple fruits as single inoculations and co-inoculations. F. acuminatum is more pathogenic than A. alternate, while these two species infect together the disease severity is higher than a single infection. The results of this study provide an important foundation for understanding the epidemics of bagged apple black spot disease and formulating chemical control measures in northern Shaanxi Province. However, additional research is needed to clarify the roles of these fungi and their relationship with apple black spot disease during the whole apple-growing season.
Data availability statement
The datasets presented in this study can be found in online repositories. The names of the repository/repositories and accession number(s) can be found in the article/Supplementary material.
Author contributions
DD: Investigation, Methodology, Software, Writing – original draft. YS: Investigation, Methodology, Writing – review & editing. JZ: Investigation, Software, Writing – review & editing. JL: Formal analysis, Investigation, Methodology, Software, Writing – review & editing. XZ: Investigation, Writing – original draft. XW: Software, Writing – review & editing. XX: Formal analysis, Supervision, Writing – review & editing. CX: Funding acquisition, Investigation, Methodology, Project administration, Resources, Software, Writing – original draft.
Funding
The author(s) declare that financial support was received for the research, authorship, and/or publication of this article. This work was supported by the Youth Innovation Team Research Program of Shaanxi Education Department (No. 23JP194); the Key R&D Program in Shaanxi Province (No. 2021NY-065); Shaanxi Province “two chains” integration key project (No. 2023LLRH-01); Doctor Support Project of Yan’an University (No. YDBK2019-45); the Youth Innovation Team of Shaanxi Universities (2022); and the China Scholarship Council Program (No. 202308610211).
Acknowledgments
The authors sincerely thank the editors and reviewers for taking their valuable time to review this article. The authors thank The Charlesworth Group for linguistic improvement of the manuscript.
Conflict of interest
The authors declare that the research was conducted in the absence of any commercial or financial relationships that could be construed as a potential conflict of interest.
The author(s) declared that they were an editorial board member of Frontiers, at the time of submission. This had no impact on the peer review process and the final decision.
Publisher’s note
All claims expressed in this article are solely those of the authors and do not necessarily represent those of their affiliated organizations, or those of the publisher, the editors and the reviewers. Any product that may be evaluated in this article, or claim that may be made by its manufacturer, is not guaranteed or endorsed by the publisher.
Supplementary material
The Supplementary material for this article can be found online at: https://www.frontiersin.org/articles/10.3389/fmicb.2024.1457315/full#supplementary-material
References
Andrew, M., Peever, T. L., and Pryor, B. J. M. (2009). An expanded multilocus phylogeny does not resolve morphological species within the small-spored Alternaria species complex. Mycologia 101, 95–109. doi: 10.3852/08-135
Carbone, I., and Kohn, L. M. J. M. (1999). A method for designing primer sets for speciation studies in filamentous ascomycetes. Mycologia 91, 553–556. doi: 10.1080/00275514.1999.12061051
Crous, P. W., Hernández-Restrepo, M., Van Iperen, A. L., Starink-Willemse, M., Sandoval-Denis, M., and Groenewald, J. Z. (2021a). Citizen science project reveals novel fusarioid fungi (Nectriaceae, Sordariomycetes) from urban soils. Fungal Syst. Evol. 8, 101–127. doi: 10.3114/fuse.2021.08.09
Crous, P. W., Lombard, L., Sandoval-Denis, M., Seifert, K. A., Schroers, H.-J., Chaverri, P., et al. (2021b). Fusarium: more than a node or a foot-shaped basal cell. Stud. Mycol. 98:100116. doi: 10.1016/j.simyco.2021.100116
Dang, J. L., Gleason, M. L., Li, L. N., Wang, C., Niu, C. K., Zhang, R., et al. (2018). Alternaria malicola sp. nov., a new pathogen causing fruit spot on apple in China. Plant Dis. 102, 1273–1282. doi: 10.1094/PDIS-07-17-1027-RE
Duan, Y. N., Jiang, W. T., Zhang, R., Chen, R., Chen, X. S., Yin, C. M., et al. (2022). Discovery of fusarium proliferatum f. sp. Malus domestica causing apple replant disease in China. Plant Dis. 106, 2958–2966. doi: 10.1094/PDIS-12-21-2802-RE
Gao, L. L., Zhang, Q., Sun, X. Y., Jiang, L., Zhang, R., Sun, G. Y., et al. (2013). Etiology of moldy core, core browning, and core rot of Fuji apple in China. Plant Dis. 97, 510–516. doi: 10.1094/PDIS-01-12-0024-RE
Gur, L., Reuveni, M., and Cohen, Y. (2017). Occurrence and etiology of Alternaria leaf blotch and fruit spot of apple caused by Alternaria alternata f. sp. Mali on cv. Pink lady in Israel. Eur. J. Plant Pathol. 147, 695–708. doi: 10.1007/s10658-016-1037-0
Hao, X. A., Wu, Y. F., Zhou, X. M., Yang, Y., Wang, X. M., and Liu, S. B. (2004). Preliminary studies on the identification and occurrence of black-dot disease on bagged apple fruit in Shaanxi. Acta Agricult. Boreali-occidentalis Sin. 13, 54–57. doi: 10.3969/j.issn.1004-1389.2004.04.012
Harteveld, D. O. C., Akinsanmi, O. A., and Drenth, A. (2014). Pathogenic variation of Alternaria species associated with leaf blotch and fruit spot of apple in Australia. Eur. J. Plant Pathol. 139, 789–799. doi: 10.1007/s10658-014-0433-6
Hou, Y. M. (2016). Etiology of black spot disease on bagged apple fruit. Yangling, China: Northwest A&F University.
Hou, Y. M., Zhang, X., Zhang, N. N., Naklumpa, W., Zhao, W. Y., Liang, X. F., et al. (2019). Genera Acremonium and Sarocladium cause brown spot on bagged apple fruit in China. Plant Dis. 103, 1889–1901. doi: 10.1094/PDIS-10-18-1794-RE
Hu, Q. Y., Hu, T. L., Wang, Y. N., Wang, S. T., and Cao, K. Q. (2016). Survey on the occurrence and distribution of apple diseases in China. Plant Prot. 42, 175–179. doi: 10.3969/j.issn.0529-1542.2016.01.032
Jayawardena, R. S., Hyde, K. D., De Farias, A. R. G., Bhunjun, C. S., Ferdinandez, H. S., Manamgoda, D. S., et al. (2021). What is a species in fungal plant pathogens? Fungal Divers. 109, 239–266. doi: 10.1007/s13225-021-00484-8
Kusaba, M., and Tsuge, T. (1995). Phologeny of Alternaria fungi known to produce host-specific toxins on the basis of variation in internal transcribed spacers of ribosomal DNA. Curr. Genet. 28, 491–498. doi: 10.1007/BF00310821
Lawrence, D. P., Gannibal, P. B., Peever, T. L., and Pryor, B. M. (2013). The sections of Alternaria: formalizing species-group concepts. Mycologia 105, 530–546. doi: 10.3852/12-249
Lee, S. Y., Park, S. J., Lee, J. J., Back, C. G., Ten, L. N., Kang, I. K., et al. (2017). First report of fruit rot caused by fusarium decemcellulare in apples in Korea. Kor. J. Mycol. 45, 54–62. doi: 10.4489/KJM.20170006
Leslie, J. F., and Summerell, B. A. (2006). The fusarium laboratory manual. Ames: Blackwell Publishing.
Li, B. H., Wang, C. X., and Dong, X. L. (2013). Research progress in apple diseases and problems in the disease management in China. Plant Prot. 39, 46–54. doi: 10.3969/j.issn.0529-1542.2013.05.007
Liang, X. F., Zhang, R., Gleason, M. L., and Sun, G. Y. (2022). Sustainable apple disease management in China: challenges and future directions for a transforming industry. Plant Dis. 106, 786–799. doi: 10.1094/PDIS-06-21-1190-FE
Luan, M., Pan, T. T., Dong, X. L., Lian, S., Wang, C. X., and Li, B. H. (2019). Pathogen, mechanism and conditions causing black spot disease on bagged apple fruits. Acta Phytopathol. Sin. 4, 520–529. doi: 10.13926/j.cnki.apps.000452
Luo, Y., Hou, L., Förster, H., Pryor, B., and Adaskaveg, J. E. (2017). Identification of Alternaria species causing heart rot of pomegranates in California. Plant Dis. 101, 421–427. doi: 10.1094/PDIS-08-16-1176-RE
Manawasinghe, I. S., Phillips, A. J. L., Xu, J. P., Balasuriya, A., Hyde, K. D., Stępień, Ł., et al. (2021). Defining a species in fungal plant pathology: beyond the species level. Fungal Divers. 109, 267–282. doi: 10.1007/s13225-021-00481-x
Meng, X. L., Zhang, Q., Shi, C. Y., Wang, S. T., Wang, Y. N., Cao, K., et al. (2021). Primary study on the symptoms and pathogen of apple fruit black spot diseases in Hebei Province. Acta Phytopathol. Sin. 51, 496–506. doi: 10.13926/j.cnki.apps.000712
Ntasiou, P., Myresiotis, C., Konstantinou, S., Papadopoulou-Mourkidou, E., and Karaoglanidis, G. (2015). Identification, characterization and mycotoxigenic ability of Alternaria spp. causing core rot of apple fruit in Greece. Int. J. Food Microbiol. 197, 22–29. doi: 10.1016/j.ijfoodmicro.2014.12.008
O’Donnell, K., Whitaker, B. K., Laraba, I., Proctor, R. H., Brown, D. W., Broders, K., et al. (2022). DNA sequence-based identification of fusarium: a work in progress. Plant Dis. 106, 1597–1609. doi: 10.1094/PDIS-09-21-2035-SR
Peever, T. L., Su, G., Carpenter-Boggs, L., and Timmer, L. W. (2004). Molecular systematics of citrus-associated Alternaria species. Mycologia 96, 119–134. doi: 10.1080/15572536.2005.11833002
Simmons, E. G. (1967). Typification of Alternaria, Stemphylium, and Ulocladium. Mycologia 59, 67–92. doi: 10.1080/00275514.1967.12018396
Simmons, E. G. (2007). Alternaria: An Identification Manual. Utrecht: CBS Funal Biodiversity Centre.
Thomas, B., Audonneau, N. C., Machouart, M., and Debourgogne, A. (2019). Molecular identification of fusarium species complexes: which gene and which database to choose in clinical practice? J. Mycol. Med. 29, 56–58. doi: 10.1016/j.mycmed.2019.01.003
Thomma, B. P. H. J. (2003). Alternaria spp.: from general saprophyte to specific parasite. Mol. Plant Pathol. 4, 225–236. doi: 10.1046/j.1364-3703.2003.00173.x
Wang, M. M., Crous, P. W., Sandoval-Denis, M., Han, S. L., Liu, F., Liang, J. M., et al. (2022). Fusarium and allied genera from China: species diversity and distribution. Pers. Mol. Phylog. Evol. Fungi 48, 1–53. doi: 10.3767/persoonia.2022.48.01
Wang, C. C., Jin, J., Li, B. H., Wang, C. X., Dong, X. L., and Zhang, Z. F. (2014). Pathogens isolated from different symptoms of black spot disease and infection conditions. Acta Agricult. Boreali-Sin. 29, 136–144. doi: 10.7668/hbnxb.2014.06.024
Wang, C. C., Wang, C. X., Jin, J., Dong, X. L., and Li, B. H. (2015). Identification and pathogenicity of brown spot pathogen of bagged apples. Mycosystema 34, 1036–1045. doi: 10.13346/j.mycosystema.140097
Watpade, S., Lal, M. K., Tiwari, R. K., Kumar, R., Naga, K. C., Kumar, R., et al. (2024). Fusarium core rot disease affects physicochemical and pathophysiological attributes of apple (Malus× domestica Borkh.) fruit. Postharvest Biol. Technol. 208:112648. doi: 10.1016/j.postharvbio.2023.112648
Woudenberg, J. H. C., Seidl, M. F., Groenewald, J. Z., De Vries, M., Stielow, J. B., Thomma, B. P. H. J., et al. (2015). Alternaria section Alternaria: species, formae speciales or pathotypes? Stud. Mycol. 82, 1–21. doi: 10.1016/j.simyco.2015.07.001
Woudenberg, J. H. C., Truter, M., Groenewald, J. Z., and Crous, P. W. (2014). Large-spored Alternaria pathogens in section Porri disentangled. Stud. Mycol. 79, 1–47. doi: 10.1016/j.simyco.2014.07.003
Xu, B. L., and Wei, Z. Z. (2000). Symptoms and identification of black spot disease on apple fruit. Plant Prot. 26, 6–8. doi: 10.3969/j.issn.0529-1542.2000.05.003
Xu, B. L., Yu, J. H., and Wei, Z. Z. (2002). The host range and biological characteristics of pathogen of Cylindrosporium pomi. Acta Phytophyl. Sin. 29:129. doi: 10.3321/j.issn:0577-7518.2002.02.008
Yan, X. Y., Li, L., Li, J., and Wang, Y. F. (2019). Research starus of Shaanxi apple based on bibliometric analysis. J. Yanyan Univ. (Natural Science Edition) 38, 82–86. doi: 10.13876/J.cnki.ydnse.2019.01.082
Zhang, S. W., Chen, J. H., Ma, L. J., Li, E. C., Ji, B. L., Sun, C. X., et al. (2022). First report of a new disease caused by fusarium tricinctum on apple tree in China. Plant Dis. 106:1524. doi: 10.1094/PDIS-08-21-1789-PDN
Zheng, H. H., Zhao, J., Wang, T. Y., and Wu, X. H. (2015). Characterization of Alternaria species associated with potato foliar diseases in China. Plant Pathol. 64, 425–433. doi: 10.1111/ppa.12274
Zhou, X., Odonnell, K., Aoki, T., Smith, J. A., Kasson, M. T., and Cao, Z.-M. (2016). Two novel fusarium species that cause canker disease of prickly ash (Zanthoxylum bungeanum) in northern China form a novel clade with fusarium torreyae. Mycologia 108, 668–681. doi: 10.3852/15-189
Zhou, Z. S., Wu, Y. X., Xu, C. N., Chi, F. M., Cheng, C. G., Cao, K. Q., et al. (2012). Investigation of apple black spot disease in west region of Liaoning Province. China Fruits 1, 69–74. doi: 10.16626/j.cnki.issn1000-8047.2012.01.006
Zhou, Z. S., Xu, C. N., Wu, Y. X., Chi, F. M., Zhang, H. J., and Ding, Y. J. (2011). Identification of the pathogen causing anthracnose of Chinese dwarf cherry. Acta Phytopathol. Sin. 41, 538–541. doi: 10.13926/j.cnki.apps.2011.05.011
Zhou, J. T., Zhao, D. Y., Chen, Y. H., Kang, G. D., and Cheng, C. G. (2021). Analysis of apple producing area changes in China. J. Fruit Sci. 38, 372–384. doi: 10.13925/j.cnki.gsxb.20200406
Keywords: apple, black spot disease, Alternaria and Fusarium, co-infection, pathogenicity
Citation: Ding D, Shao Y, Zhao J, Lin J, Zhang X, Wang X, Xu X and Xu C (2024) Identification and pathogenicity of Alternaria and Fusarium species associated with bagged apple black spot disease in Shaanxi, China. Front. Microbiol. 15:1457315. doi: 10.3389/fmicb.2024.1457315
Edited by:
Xiao-qiong Zhu, China Agricultural University, ChinaReviewed by:
Laith Khalil Tawfeeq Al-Ani, Universiti Sains Malaysia, MalaysiaYing Zhang, Beijing Forestry University, China
Copyright © 2024 Ding, Shao, Zhao, Lin, Zhang, Wang, Xu and Xu. This is an open-access article distributed under the terms of the Creative Commons Attribution License (CC BY). The use, distribution or reproduction in other forums is permitted, provided the original author(s) and the copyright owner(s) are credited and that the original publication in this journal is cited, in accordance with accepted academic practice. No use, distribution or reproduction is permitted which does not comply with these terms.
*Correspondence: Chengnan Xu, eHVjaGVuZ25hbjE5ODFAc2luYS5jb20=