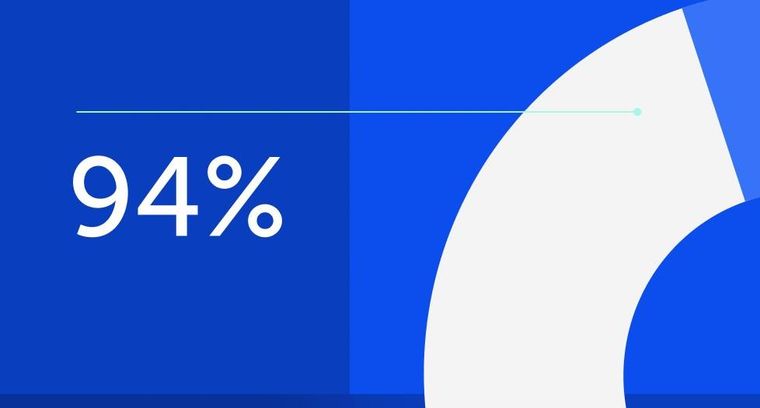
94% of researchers rate our articles as excellent or good
Learn more about the work of our research integrity team to safeguard the quality of each article we publish.
Find out more
ORIGINAL RESEARCH article
Front. Microbiol., 18 October 2024
Sec. Microbiotechnology
Volume 15 - 2024 | https://doi.org/10.3389/fmicb.2024.1455699
Gray mold, caused by Botrytis cinerea, is a significant threat to tomato production. Traditional chemical control methods have become increasingly ineffective because of the development of resistance. This study aimed to isolate methanol-utilizing bacteria from tomato leaves and evaluate their biocontrol potential against gray mold. To obtain bacterial suspensions, tomato leaf samples were collected and washed. We analyzed the microbial communities of these samples using 16S rRNA amplicon sequencing and identified several methylotrophic strains. Among these, 405 isolated strains were cultivated on a solid low-nutrient inorganic salt medium containing methanol, and 7 strains exhibiting considerable antifungal activity against B. cinerea were identified. Greenhouse tests revealed that two strains—SY163 and SY183—significantly reduced the severity of gray mold on tomato leaves. Disease index scores and the area under the disease progress curve values confirmed the efficacy of these strains as biocontrol agents. Statistical analysis indicated the effectiveness of pre- and co-application of these strains with B. cinerea. Phylogenetic analysis identified Serratia rubidaea as the inhibitory strain. The biocontrol activity is likely mediated through the production of antifungal compounds and suppression of B. cinerea sporulation. This study provides the basis for developing a technology of gray mold suppression by controlling the abundance of S. rubidaea in plant microbial communities.
Pathogens that cause significant damage to crop plants include fungi, bacteria, viruses, and nematodes. Crop losses pose a major threat to food production, with approximately 27–42% of the global food production being lost to plant diseases arising from these pathogens. Various biotic and abiotic factors contribute to economic yield losses in crops, with diseases caused by filamentous fungi being the most important factor (Singh, 2014).
Botrytis cinerea, the causal agent of gray mold, is considered an important pathogen worldwide. Although global expenditures related to the implementation of control measures against B. cinerea exceed €1 billion per year, product and quality losses are a major concern (Dean et al., 2012). Even in tomato, which accounts for approximately 16% of total vegetable production, gray mold is one of the most destructive rot diseases, posing a serious threat to its yield (Food and Agriculture Organization of the United Nations, 2023; Liu et al., 2021). The host can be infected by fungal mycelia, conidia, or sclerotia. Additionally, B. cinerea has a short life cycle and exhibits rapid genetic variation, complicating the management of gray mold in agricultural production (Breeze, 2019). Although benzimidazoles, dicarboximide fungicides, and anilinopyrimidine fungicides (Banno et al., 2008; Leroux et al., 1999; Leroux, 2007) have been used for B. cinerea control, resistance to these fungicides remains a challenge. Owing to its high genetic variability, B. cinerea is associated with an increased risk of fungicide resistance. In many countries, the long-term and frequent use of these fungicides has led to the development of B. cinerea resistance (Esterio et al., 2017; Liu et al., 2021).
The most common agents for the biocontrol of B. cinerea include filamentous fungi of the genera Trichoderma, Ulocladium, and Gliocladium; bacteria of the genera Bacillus and Pseudomonas; and yeasts of the genera Pichia and Candida (Elmer and Michailides, 2007); among them, Trichoderma fungi have been widely studied (Vinale et al., 2008; Dutta et al., 2023; Vos et al., 2015). Biocontrol is an alternative and environmentally friendly strategy for controlling plant diseases and reducing chemical use in agriculture. In addition to its biocontrol activity, Trichoderma is widely used for plant growth promotion (PGP) through the regulation of phytohormone formulation. Several studies have isolated Trichoderma strains from the rhizosphere and indicated their ability to control disease-causing bacteria (Vinale et al., 2008; Hajji-Hedfi et al., 2023). A previous study isolated Trichoderma strains from cucumber leaves and evaluated their ability to control gray mold in various plants (Sawant, 2014).
Most studies on plant growth-promoting bacteria (PGPB) have focused on their ability to enhance plant growth and suppress plant pathogens. For example, Bacillus and Pseudomonas species exhibit antagonistic activity against a wide range of plant pathogens through the production of antibiotics, enzymes, and other bioactive compounds (Kloepper et al., 2004; Lugtenberg and Kamilova, 2009). Similarly, the potential of Rhizobium and Azospirillum species in promoting plant growth and inducing systemic resistance against pathogens has been reported (Bashan and de-Bashan, 2010; Zamioudis and Pieterse, 2012). These PGPBs enhance plant health by improving nutrient uptake, producing phytohormones, and stimulating the plant’s immune system.
Methylotrophs are microorganisms that use one-carbon (C1) compounds, such as methanol and methylamine, as carbon sources. They settle on the leaves of some plants that release methanol, which is used as a carbon source for growth. Thus, a symbiotic relationship exists in which methylotrophs produce plant hormones that benefit the plants (Iguchi et al., 2015; Omer et al., 2004; Trotsenko et al., 2001). Methylotrophic bacteria can survive in various extreme environments, including low and high temperatures and drought conditions (Romanovskaia et al., 2005; Sapp et al., 2018; Amin et al., 2017; Kerry et al., 2018). Plants develop stress tolerance by receiving valuable nutrients from methylotrophs under stress. Additionally, PGP methylotrophic microbiota help plants grow and adapt to adverse environmental conditions. The rhizosphere, epiphytic, and endophytic microbiomes play an important role in plant growth and adaptation (Kumar et al., 2019). However, to the best of our knowledge, no studies have reported the use of C1-utilizing bacteria for controlling gray mold.
In this study, we collected symbiotic methylotrophic bacteria from plant leaves and searched for microorganisms that can control B. cinerea. We evaluated the ability of the identified microbes to control B. cinerea on plant foliage.
B. cinerea (Agricultural Biological Resources Gene bank MAFF No. 241680 Strain), a known cause of gray mold on tomato plants, was used in this study. Tomato leaves and stems (Reiki; Sakata Seed Corporation, Kanagawa, Japan) sampled for microbial collection were grown in plastic greenhouses at Yoshimura Farm (130°38′21.68′′E, 32°43′42.599′′N, Kumamoto, Japan). Grafted tomato saplings (Momotaro Haruka) were purchased from Kokkaen (Osaka, Japan) for use in biological control bioassays.
Phosphate-buffered saline (PBS), comprising 8.0 g NaCl, 0.2 g KCl, 1.15 g Na2HPO4, and 0.2 g KH2PO4 dissolved in 1.0 L distilled water, was prepared and used for the recovery of microbes from tomato leaf and stem surfaces.
The composition of the inorganic salt liquid medium containing methanol was as follows: 2.0 g KH2PO4, 2.4 g K2HPO4, 1.0 g (NH4)2SO4, 1.0 g KNO3, 0.2 g MgSO4, 1.0 g trace element mixture, 5 mL methanol, and 1.0 L distilled water (Meiberg and Harder, 1978; Aida and Nomoto, 1988). The medium composition was modified from that of a previously reported medium. The carbon source (methanol) was mixed to 0.5%. The inorganic solid medium containing methanol was prepared in 90- mm diameter petri dishes by adding 10.0 g gellan gum and 0.4 μg tunicamycin to the liquid medium. All components except for methanol and tunicamycin were dissolved in water and autoclaved at 120°C for 20 min. Methanol and tunicamycin were added to the medium via filter sterilization after autoclaving.
The nutrient broth (NB) liquid medium was prepared by dissolving NB (3.0 g beef extract, 5.0 g peptone) in 1.0 L of distilled water. The mixture was autoclaved at 120°C for 20 min. NB liquid medium was dispensed into test tubes.
Potato dextrose agar (PDA) medium was prepared by dissolving 4.0 g potato infusion powder (Sigma, 52424), 20.0 g glucose, and 20.0 g agar in 1.0 L of distilled water. The mixture was autoclaved at 120°C for 20 min. PDA medium was dispensed into petri dishes (90 mm diameter).
In the plastic greenhouses, leaves and stems of 10 tomato plants (about 1 kg) per point were randomly collected from three points and stored them in polyethylene bags on July 1, 2020. All collected samples were transported to the laboratory of Sojo University (Kumamoto, Japan). In the laboratory, within 3 h of sampling, approximately 1 kg of tomato leaves and stems was distributed into three polyethylene bags, and 50 mL of PBS was added to each polyethylene bag using a Terumo syringe (10 mL) and CA sterile syringe filter (0.22 μm). The polyethylene bags containing leaves, stems, and wash buffer were shaken well by hand and allowed to stand at room temperature for 1 h. Approximately 20 mL of each washed suspension was collected in a 50-mL tube.
Washed suspensions were collected from tomato leaves and stems (approximately 20 mL each) and 0.5 mL of each of this suspension was dispensed into two Eppendorf tubes, resulting in six suspensions. Microbial DNA was extracted from tomato leaf and stem samples using ISOIL (NIPPON GENE, Tokyo, Japan) in accordance with the manufacturer’s protocol. In each sample, the V3–V4 hypervariable region of the 16S rDNA gene was amplified with an appropriate primer pair (U341F [5′-CCTACGGGRSGCAGCAG-3′] and 806R [5′-GGACTACHVGGGTATCTAAT-3′]) (Hansen et al., 1998; Walters et al., 2011). Polymerase chain reaction (PCR) was performed under the following conditions: an initial denaturation step at 94°C for 2 min; followed by 25 cycles of denaturation at 94°C for 15 s, annealing at 48°C for 30 s, and extension at 68°C for 30 s; and a final extension step at 68°C for 1 min. The PCR products of microbial 16S rRNA gene fragments were purified using Agencourt AMPure XP (Beckman Coulter, Brea, CA, United States). Tags unique to each sample were attached to the reverse primer for multiplexing. The PCR products were then analyzed using a MiSeq next-generation sequencer (Illumina, San Diego, CA, United States) at Fasmac (Kanagawa, Japan), which targeted the V3–V4 hypervariable region of the microbial 16S rRNA gene.
The raw paired-end FASTQ reads were demultiplexed using the FASTQ barcode splitter and imported into the Quantitative Insights Into Microbial Ecology 2 program (QIIME2, ver. 2019.10)1 (Bolyen et al., 2019). The demultiplexed reads were quality-filtered, denoised, chimera-checked, and dereplicated using the denoise-paired command from q2-dada2 plugin (Callahan et al., 2016). Taxonomic assignments were performed using the classify-sklearn command from q2-feature-classifier using a trained Naive Bayes classifier from SILVA database (release132).2 Reads identified as sequences from mitochondria or chloroplasts were excluded from the analysis using filter-table command from the q2-taxa plugin. Next, the align-to-tree-mafft-fasttree pipeline from the q2-phylogeny plugin was used to perform multiple sequence alignment, remove phylogenetically uninformative or ambiguously aligned sequences, and construct unrooted and rooted phylogenetic trees (Price et al., 2010; Katoh and Daron, 2013).
Data visualizations and statistical analyses of microbial community composition were performed using R (v4.3.1).3 QIIME2 output data were converted to a phyloseq object using the qza_to_phyloseq function using the qiime2R package (Bisanz, 2018). The phyloseq object was used to perform the following visualizations and analyses process in phyloseq (McMurdie and Holmes, 2013), tidyverse (Wickham et al., 2019), plotly (Sievert et al., 2024), and RColorBrewer (Neuwirth, 2022) packages. According to known taxonomic groups, bar charts of relative abundance at phylum and genus levels were constructed for tomato leaf and stem samples. The diversity of microbial communities within and between these samples was characterized using alpha and beta diversities, respectively. Alpha diversity was assessed based on observed operational taxonomic units (OTUs); abundance-based coverage estimator (ACE); and Chao 1, Shannon, and Simpson diversity indices. Beta diversity was assessed via nonmetric multidimensional scaling (NMDS, Bray–Curtis similarity matrix) based on microbial community structures.
The suspension samples were used to prepare serial dilutions of 1:5 (5-fold dilution), 1:25, 1:125, 1:625, 1:3125, and 1:15625. The inorganic salt medium plates containing methanol were coated with 50 μL of seven different suspension samples at different dilutions using a sterile Drigalski spatula. These plates were incubated at 30°C, and colony formation was observed daily. After colony formation was confirmed, the isolates were selected based on morphological characteristics, and each colony was collected using a platinum loop. The selection of similar colonies that occurred on the plate at the same time was avoided. Colonies were collected using the platinum loop and cultured in an inorganic salt liquid medium containing methanol and NB liquid medium. NB medium was used because some methylotrophs prefer heterotrophic and substrate-rich conditions. Two types of culture were performed: static culture (30°C) and shaking culture (150 rpm, 30°C). For example, in methylotrophic denitrifying bacteria, anaerobic nitrate respiration is promoted by static culture. Because some methylotrophic bacteria tend to grow aerobically and some anaerobically, they were cultured under both static and shaking conditions to ensure that they were comprehensively picked up. The cultures in which the suspension was confirmed were stored in glycerol at −60°C in the laboratory of Sojo University.
A 25-μL B. cinerea suspension (4.2 × 105 conidia/mL) was applied to PDA plates (90 mm diameter), and the plates were incubated at 25°C. The time taken for B. cinerea mycelia to cover the plates was measured.
The plates coated with B. cinerea suspension were modified by drilling 2–8 holes (9 mm diameter) around the center of the plate. The holes were equidistant from each other and located 14 mm away from the edge of the plate. Then, 50 μL of each strain suspension (2 × 106 CFU/mL) was placed in each hole and incubated at 25°C for 10 days.
The growth inhibitors of B. cinerea were examined using a dual plate assay. Agar blocks (5 mm × 5 mm) were cut from PDA plates on which B. cinerea had grown; these blocks were inoculated onto another PDA plate. Then, 1.0 μL of the suspension of the inhibitory strain (2 × 106 CFU/mL) was dropped onto the edge of the plate and incubated statically at 25°C.
The V3–V4 region of the 16S rRNA gene from each inhibitory strain was approximately 400–450 bp in length. This region was amplified using the primer pair of U341F and 806R. The gyrB gene from each inhibitory strain was approximately 1,100 bp in length and was amplified using the primer pairs gyrF (5′-GAAGTCATCATGACCGTTCTGCATCGCTCAGGGTCAGGGTCAGAAAGTTTCGA-3′) and gyrR (5′-AGCAGGTACGATGTGCGAGCCAGTCTCAGACAGTCTCAGGCAGTCTCAGGTAT-3′) (Govil et al., 2021). In both reactions, for each inhibitory strain, the PCR mixture (50 μL) containing 1.0 μL of genomic DNA, 5.0 μL of 10× PCR Buffer for KOD-Plus-Neo, 5.0 μL of 2 mM dNTPs, 3.0 μL of 25 mM MgSO4, 1.0 μL of KOD-Plus-Neo, 1.5 μL of 10 μM forward primer, 1.5 μL of 10 μM reverse primer, and 33 μL of PCR-grade water (Promega) was placed in a microcentrifuge tube. PCR for 16S rRNA was conducted using the following thermocycling parameters: initial denaturation at 94°C for 2 min, followed by 25 cycles of denaturation at 98°C for 10 s, annealing at 50°C for 30 s, and extension at 68°C for 20 s. PCR for gyrB was conducted using the following conditions: initial denaturation at 94°C for 2 min, 25 cycles of denaturation at 98°C for 10 s, annealing at 50°C for 30 s, and extension at 68°C for 35 s. PCR products were visualized under UV light after standard ethidium bromide gel electrophoresis. The amplified products were purified using PCR Clean-UP System (Promega, USA) in accordance with the manufacturer’s protocol. The purified PCR products were stored at −20°C.
The PCR products were then separated using agarose gel electrophoresis and cloned into TOPO-Blunt (Invitrogen, Carlsbad, CA). Further, these products were cloned into the pCR Blunt II plasmid using Zero Blunt TOPO PCR cloning kit in accordance with the manufacturer’s protocol. The amplified PCR fragments were sequenced. Homology searches were performed via the NCBI BLAST database (Johnson et al., 2008) using the identified DNA sequences as queries, and molecular phylogenetic analysis was performed using 16S rRNA and gyrB genes. Alignment and phylogenetic tree estimation were performed using MAFFT 6.861 and RAxML 8.2.11 in ETE v3.1.2 (Huerta-Cepas et al., 2016), and the results were presented using iTOL (Letunic and Bork, 2021).
Strains that were highly effective in inhibiting the growth of B. cinerea in petri dish tests were examined for their ability to inhibit gray mold caused by B. cinerea in plants.
Biological control assays were performed using five tomato plants (2 months old) in each group as a normal group, a control group, and three biological control condition groups (BC1-BC3). Normal group: normal growth (no fungus application); control group: only B. cinerea applied on day 0; condition BC1: B. cinerea and each inhibitory strain applied on day 0; condition BC2: each inhibitory strain applied on the day before day 0 and B. cinerea and each inhibitory strain applied on day 0; and condition BC3: B. cinerea and each inhibitory strain applied on days 0 and each inhibitory strain applied on day 1. B. cinerea conidia suspension (4.2 × 105 conidia/mL, 10 μL per leaf) was applied using a pipette. The inhibitory strains were SY163 and SY183, and an inhibitory strain culture suspension (2 × 106 CFU/mL, 10 μL per leaf) was applied using a pipette.
All groups were allowed to grow at room temperature (18°C–22°C) for 22 days. During the biological control assay, 20 mL/pot of water was applied to the soil surface in the morning using a Falcon tube. The disease index of gray mold on each assessed tomato leaf was recorded daily, and the disease severity for each leaf was calculated. The disease index was expressed as a percentage of diseased leaf area and evaluated on a scale of 0–3 (0 = no disease symptoms, 1 = 0.1–24.9%, 2 = 25–49.9%, 3 = 50–100%) (Konishi et al., 2010). Disease severity was calculated using the following formula: {Σ(disease index × number of leaves by disease index)/(number of leaves assessed)}. In addition, conidia formation after 22 days was evaluated in all assessed leaves. The percentage of leaves with conidia formation was calculated for each plant using the following formula: (number of leaves that formed conidia)/(number of leaves assessed) × 100 (%), and the mean and standard error of the percentage of conidia formation were determined for each condition.
The area under the disease progression curve (AUDPC) was calculated using the aupdc function in the package agricolae (v.1.3–5) (De Mendiburu and Reinhard, 2015) in R (v.4.3.1) (R).4 The Dunnett test, which was used to compare the AUDPC of conditions BC1-BC3 against that of control group, was performed using the glht function in the package multcomp (v.1.4–25) (Bretz et al., 2010) in R.
The progression of gray mold over days was evaluated for different conditions and individuals (ID), and comparisons were performed between conditions. Linear mixed models were performed using the lmer function of the package lme4 (v.1.1–35.3) (Bates et al., 2024) in R. The mean values for each condition estimated from the model were calculated using the emmeans function in the package emmeans (v.1.10.2) (Lenth et al., 2024). The obtained mean values for each condition were compared via the contrast function using Dunnett’s post-hoc test.
Samples of tomato leaves and stems were collected from three different locations in the greenhouse and washed with PBS to obtain a gray suspension (Figure 1A). Each of the three samples (S1, S2, and S3) was divided into two parts (a and b), yielding six samples in total (S1a, S1b, S2a, S2b, S3a, and S3b). These six samples were sequenced using 16S rRNA amplicon sequencing. After sequence processing and quality control, a total of 254,333 bacterial reads were generated. The number of reads in each sample ranged from 102,195 for S3b to 12,354 for S2b (Supplementary Table S1).
Figure 1. Microorganisms acquired from the surface of tomato leaf and stem. (A) Solution after washing the surface of the tomato leaves and stems. (B) Nonmetric multidimensional scaling (NMDS) plots generated from a matrix of Bray–Curtis dissimilarity values. (C) Microbial community structure at the phylum level. Bar plot showing the relative abundances of the seven dominant phyla (>0.5%). (D) Microbial community structure at the genus level. Bar plot showing the relative abundances of the 54 dominant genera (>0.5%).
To determine the alpha diversity within each sample (number of OTUs observed and Chao1, Shannon, and Simpson indices), two samples derived from the same origin (e.g., S1a and S1b) were analyzed. The results revealed similar values for species richness and evenness indices between S1a and S1b; however, differences were observed between samples with different origins (Supplementary Table S1). S1-derived samples (S1a and S1b) had high OTUs, ACE values, and Chao1 indices, indicating high species richness. In contrast, S3-derived samples (S3a and S3b) had low species richness. Moreover, S1-derived samples had higher Shannon and Simpson indices, suggesting a diverse microbial community with almost equal proportions of species. Conversely, S3-derived samples had lower Shannon and Simpson indices, indicating lower diversity and the potential for certain species to be significantly more abundant than others. Based on alpha diversity results, the species richness and diversity of the microbial communities on the surface of tomato leaf and stem samples may be dependent on the collection site.
The beta diversity between these six samples was examined using NMDS plots generated from a matrix of Bray–Curtis dissimilarity values (Figure 1B) and the microbial community structure (Figures 1C,D). In the NMDS plot, samples derived from the same origin were clustered together, whereas those derived from different origins were located away from each other. This suggests that similar to alpha diversity results, the microbial community of tomato leaves and stems tends to vary by sampling location. The microbial community analysis of all samples revealed seven phyla with relative abundances higher than 0.5% (Figure 1C). At the phylum level, Proteobacteria had the highest relative abundance across all samples. In S1a, S1b, S3a, and S3b, Firmicutes was the second most prevalent phylum; in S2a and S2b, Bacteroidetes was the second most prevalent phylum. The microbial community analysis of all samples revealed 54 genera with relative abundances higher than 0.5% (Figure 1D). At the genus level, Lactococcus and Lactobacillus were abundant in S3a and S3b; Enterobacter and Pantoea were abundant in S2a and S2b; and Mycoplasma, Marinomonas, and Enterobacter were abundant in S1a and S1b. Although microbial community structures of samples from the same origin were similar, differences were observed between samples derived from different origins. This finding was consistent with that of alpha and beta diversity.
The possible extent of methylotroph on tomato leaf and stem surfaces was investigated. Previous studies have shown that methylotrophic bacterial strains exist in 83 genera, including Pseudomonas, Methylobacterium, and Methylococcus (Kolb, 2009; Kumar et al., 2016; Kumar et al., 2019; Gamit and Amaresan, 2023). In this study, 13 of these genera were identified: Pantoea, Pseudomonas, Bacillus, Klebsiella, Acinetobacter, Bacteroides, Paracoccus, Brevibacterium, Sphingomonas, Mycobacterium, Methylobacterium, Flavobacterium, and Mesorhizobium. The relative abundances of each genus in each sample are shown in Figure 2A and Supplementary Table S2. In all the samples, Pantoea, Pseudomonas, Bacillus, Klebsiella, and Acinetobactor had high relative abundances. For the overall microflora, the relative abundances were similar in the samples collected from the same location, but varied among sampling locations.
Figure 2. Methylotrophic bacteria isolated from the surface of tomato leaves and stems. (A) Microbial community structure of the genera containing methylotrophic bacterial strains. (B) Low-nutrient inorganic salt medium plate containing methanol cultures of tomato leaf and stem surface samples yielded colonies of various colors and sizes.
Methylotrophs were collected from the gray suspensions obtained from tomato leaf and stem surfaces. The gray suspension was placed on a low-nutrient inorganic salt solid medium plate containing methanol. After 6–14 days, colonies of various colors (red, yellow, and white) and sizes were obtained (Figure 2B). Colonies were picked from the inorganic salt solid medium plate containing methanol and cultured in inorganic salt liquid medium containing methanol or NB liquid medium. Culture samples of isolated strains were collected after 1–14 days of static culture and 1–13 days of shaking culture. In total, 405 isolated strains were obtained and frozen in glycerol. These isolated strains could grow under conditions in which methanol was the only carbon source. Plant leaves are inhabited by methylotrophic bacteria that utilize methanol, such as Methylobacterium, Methylophilus, Methylibium, and Hyphomicrobium (Iguchi et al., 2015; Lopez-Velasco et al., 2011).
B. cinerea conidia were incubated on PDA plates at 25°C, and it was visually confirmed that the mycelia of B. cinerea had covered the plates on the 10th day (Supplementary Figure S1A). In a previous study, B. cinerea conidia were applied onto PDA plates and incubated at 22°C for 7 days, and similar mycelial conditions were observed (Vidal et al., 2020). Consistent with the results presented in Supplementary Figures S1B,C, the mycelium of B. cinerea was characterized by splitting, gray, branched, tree-like structures with numerous branching hyphae (Sultana et al., 2020).
Growth-inhibiting strains were selected based on their ability to inhibit the spread of B. cinerea mycelia on the PDA plate. The antifungal activity of 405 isolated strains was evaluated on PDA medium coated with B. cinerea suspension using the agar well diffusion method (Figure 3A). If the strain has a growth inhibitory effect on B. cinerea, a distinct zone, known as the inhibition zone, is formed around the hole. Many plates were entirely covered with B. cinerea mycelium. All 405 strains were tested, and 7 strains exhibited a zone of inhibition against B. cinerea (SY50, SY89, SY131, SY135, SY163, SY183, and SY339). Among the strains with confirmed zones of inhibition, SY163 had the largest zone of inhibition (50 mm), followed by SY183 (40 mm) and SY135 (37 mm); the other four strains had smaller zones.
Figure 3. Inhibitory activity of isolates against Botrytis cinerea. (A) Evaluation using the agar well diffusion method. (B) Evaluation using the dual plate assay.
The effects of the seven inhibitory strains on the growth of B. cinerea mycelia were examined using the dual plate assay, in accordance with the procedure shown in Figure 3B. The plates were incubated at 25°C for 12 days. All strains exhibited inhibition zones; however, the size of the inhibition zone varied for each strain. The inhibitory rates ranged from 11.9 to 35.7%. A similar trend was observed for the fungus Metarhizium anisopliae, which inhibited the radial growth of B. cinerea by approximately 43.9% compared with the control (Sarven et al., 2020). The inhibition zone was formed without any contact between B. cinerea and the inhibitory strain, suggesting that the inhibitory strain secretes substances that inhibit the growth of B. cinerea. Other microorganisms that have been reported to exhibit antifungal activity against B. cinerea belong to the genera Pseudomonas and Pantoea (Trotel-Aziz et al., 2008; South et al., 2020). For example, South et al. conducted a dual plate assay (referred to as a “dual-culture assay” in the cited literature) and placed B. cinerea mycelia grown on PDA at the center of a PDA plate. They placed isolates in parallel on either side of the PDA plate to examine the inhibition of B. cinerea growth. Several strains exhibited inhibition zones, including Pseudomonas protegens AP54, Pseudomonas chlororaphis 14B11, and Pseudomonas fluorescens 89F1. The formation of such inhibition zones may be due to the production of antibiotics, enzymes, and volatiles by microorganisms (Kerr, 1999; South et al., 2020). Inhibition zones were also observed for the seven inhibitory strains, suggesting that the growth of B. cinerea was inhibited by the products of these strains.
A homology search targeting 16S rRNA gene sequences was performed for the seven strains that showed antifungal activity against B. cinerea; the results are presented as a phylogenetic tree (Figure 4A). All seven strains were approximately 100% or 99% identical to Serratia rubidaea JCM1240 and S. rubidaea NBRC103169 and were distinct from the strains S. marcescens and S. ureilytica. Based on these results, all seven strains were closely related to S. rubidaea.
Figure 4. Phylogenetic analysis of inhibitory strains. (A) Phylogenetic tree based on 16S rRNA gene sequences of the inhibitory strains, showing their relationship with known species. (B) Phylogenetic tree based on gyrB gene sequences, highlighting genetic differences among the strains.
A homology search targeting gyrB was performed for the seven strains (SY50, SY89, SY131, SY135, SY163, SY183, and SY339) that showed antibacterial activity against B. cinerea; their phylogenetic trees are presented in Figure 4B. Strains SY50, SY131, SY135, SY183, and SY339 differed by 1 bp. These five strains (1,171 bp in length) differed from SY89 by 7 bp, and SY163 had a substitution of 4 bp. In addition, SY89 and SY163 strains differed by 5 bp. Consequently, the obtained inhibitory strains could be divided into three groups: (1) SY163, (2) SY89, and (3) SY50, SY131, SY135, SY183, and SY339.
The seven strains that exerted growth inhibitory effects on B. cinerea belonged to the genus Serratia and were detected in four of the six samples in microbial community analysis. The relative abundance of Serratia in each sample was as follows: S1a (0.82%), S1b (1.02%), S3a (0.38%), and S3b (0.33%). The genus Serratia included sequences classified as S. rubidaea at the NGS species level.
Grafted tomato saplings were grown in a plastic greenhouse in the laboratory and watered regularly. The saplings were administered with only water for 22 days at room temperature (18°C–22°C); these saplings grew without developing diseases, including gray mold, on the leaves. This growth method was confirmed to be suitable for evaluating biocontrol activity.
The inhibitory effect of bacterial strains on gray mold suppression in tomato leaves was investigated. Two inhibitory strains, SY163 and SY183, which showed significant differences in gyrB sequence, were applied onto plants, and the disease index was evaluated on a scale of 0–3 (Figure 5C). The 22-day disease index results are shown in Figures 5A,B. In the control group, B. cinerea was sprayed onto tomato leaves on day 0, and disease development started on day 5. Disease index scores up to day 22 are presented in Figures 5A,B. The disease index score gradually increased to >2 on day 22. Under condition BC1, B. cinerea, and strain SY163 (BC1-SY163) or SY183 (BC1-SY183) were applied on day 0. Compared with the control group, there was lower disease suppression in BC1-SY163, but a trend toward further disease suppression was observed in BC1-SY183. Under condition BC3, B. cinerea and strain SY163 (BC3-SY163) or SY183 (BC3-SY183) were applied on day 0, and strain SY163 or SY183 was applied again on day 1. In BC3-SY163 and BC3-SY183, higher disease suppression was observed compared with the control and BC1 groups, respectively. Under condition BC2, two inhibitory strains (SY163 and SY183) were each applied on the day before day 0, and B. cinerea and the inhibitory strains were applied and allowed to grow on day 0. Under all conditions, application of the inhibitory strains reduced the disease index of gray mold. For both SY163 and SY183 strains, the disease index tended to be lower under condition BC2 than under control group or conditions BC1 and BC3.
Figure 5. Effects of inhibitory strains on the development of gray mold in tomato plants. (A,B) Changes in the disease index (A: SY163 application, B: SY183 application). Each point and error bar represent the mean and standard error of the disease severity score. (C) Photographs of typical leaves evaluated with a disease index of 0, 1, or 3 (0 = no disease symptoms, 1 = 0.1–24.9%, 3 = 50–100%). Many leaves with a disease index of 3 showed conidia formation. (D) Mean percentage of leaves with conidia formation after 22 days. Each error bar indicates standard error. Conditions: Control, Only B. cinerea applied on day 0; BC1 (BC1-SY163, BC1-SY183), B. cinerea and each inhibitory strain (SY163 or SY183) applied on day 0; BC2 (BC2-SY163, BC2-SY183), each inhibitory strain applied on the day before day 0, B. cinerea and each inhibitory strain applied on day 0; BC3 (BC3-SY163, BC3-SY183), B. cinerea and each inhibitory strain applied on day 0 and each inhibitory strain applied on day 1.
To compare the disease index between conditions, AUDPC values were calculated for all samples. The means and standard errors for each condition are shown in Table 1. Both SY163 and SY183 showed the smallest AUDPC values under condition BC2. When comparing the mean AUDPCs of BC1-SY163, BC2-SY163, and BC3-SY163 against the mean AUDPC of control group using analysis of variance and Dunnett test, a statistically significant difference was observed between control group and BC2-SY163 (p < 0.01). When comparing the mean AUDPCs of BC1-SY183, BC2-SY183, and BC3-SY183 against the mean AUDPC of control group, a statistically significant difference was noted between control group and BC2-SY183 (p < 0.001) and between control group and BC3-SY183 (p < 0.05).
A linear mixed model was used to evaluate the impact of days and conditions on the disease index. Days and conditions were modeled as fixed effects and individuals (ID) as random effects. The restricted maximum likelihood was used to estimate the parameters of the model. The mean values for each condition estimated from the model were calculated, and Dunnett’s test was used to perform multiple comparisons across conditions. Statistically significant differences were observed between control group and BC2-SY163 (p < 0.01), control group and BC2-SY183 (p < 0.001), and control group and BC3-SY183 (p < 0.05). It was suggested that spraying the strain SY163 before B. cinerea attachment or the strain SY183 before or at the same time as B. cinerea attachment may effectively prevent gray mold.
In addition to differences in the disease index, we evaluated the proportions of leaves forming conidia. As the severity of gray mold increased, conidia formation occurred, as indicated by the score of 3 (Figure 5C). After observation for 22 days, the number of leaves that formed conidia was examined, and the proportion of leaves that formed conidia in the assessed leaves in each pot was calculated. The mean and standard error of the proportion of conidia formation were determined for each condition (Figure 5D). Compared with control group, in which only B. cinerea was applied, conidia formation was significantly inhibited under all conditions in which SY163 or SY183 was applied. These two strains of Serratia sp. were found to be effective in suppressing the sporulation of B. cinerea.
The microbial community analysis of the phylum and genus on the surface of tomato leaves and stems was conducted in this study. Relative abundances higher than 0.5% were found in seven phyla, including Proteobacteria, Firmicutes, and Bacteroidetes. Relative abundances higher than 0.5% were observed in 54 genera, including Lactococcus, Lactobacillus, Enterobacter, Pantoea, Mycoplasma, Marinomonas, and Enterobacter. The microbial community structures of samples collected from the same location were similar; however, differences were observed between samples collected from different locations. Previous studies have described the symbiotic microbial communities of various plants. Compared with the microbial community analysis of endophytic and phylloplane bacteria in tomato leaves using milled samples (Sumbula et al., 2020), this study also identified Proteobacteria, Bacteroidetes, and Actinobacteria at the phylum level and Acinetobacter, Bacteroides, and Pantoea at the genus level, with certain relative abundances. Compared with the microbial community analysis of phylloplane bacteria in rice (Sumbula et al., 2020), this study also identified Proteobacteria, Actinobacteria, Bacteriodetes, and Firmicutes at the phylum level and Methylobacterium and Serratia at the genus level, with certain relative abundances. The phyllosphere microbial community can vary depending on plant species, geographic location, season, and human intervention (Knief et al., 2012; Sumbula et al., 2020). In this study, the microbial communities identified on tomato leaves and stems also varied depending on the collection site, thereby supporting these findings. Microbial community analysis of tomato leaves and stems identified 13 genera containing methylotroph strains. For example, 80 isolates were obtained from peanut leaf surface samples (Krishnamoorthy et al., 2018) via methanol-containing cultures and were identified. These isolates were classified into 15 genera, with Pantoea, Pseudomonas, Bacillus, and Acinetobacter commonly identified with this study. The genus diversity of methylotrophs also varied depending on the collection site, thereby supporting the results of this study. Methylotroph isolates have multiple properties that promote plant growth, including production of indole-3-acetic acid (IAA), siderophores, and 1-aminocyclopropane-1-carboxylate deaminase, nitrogen fixation, sulfur oxidation, and solubilization of insoluble minerals. Other studies have also reported IAA production and nitrogen fixation in methylotrophs (Senthilkumar et al., 2009; Madhaiyan et al., 2015). These findings suggest that diverse methylotroph promote plant growth through various mechanisms, which might be the case for tomato leaves and stems in this study.
In this study, plant surface samples were cultured on a medium containing methanol as the single carbon source, and S. rubidaea strains were selected as strains with antifungal activity against B. cinerea. The genus Serratia has rarely been reported as a methylotroph. However, it is possible that Serratia grows in methanol and that it was cultured and isolated in this study on a methanol-containing medium due to co-cultivation with methylotrophs. These strains have the potential to act as PGPB as well as biocontrols. Previous reports on PGPB belonging to the genus Serratia demonstrated that S. liquefaciens KM4 may promote plant growth and improve salt stress tolerance by regulating ion homeostasis, leaf gas exchange, and expression of stress-related genes (El-Esawi et al., 2018). S. marcescens strains reportedly act as PGPBs, as evidenced by the production of various lytic enzymes (proteases, lipases, cellulases, and catalases), antimicrobial compounds (hydrogen cyanide and siderophores), ammonia, nitrite, and nitrate as well as their ability to reduce nitrate to nitrite (Hamada and Soliman, 2023). Recently, a strain of S. rubidaea was reported to promote the germination of quinoa seeds in salt-tolerant environments (Mahdi et al., 2021). Another strain of S. rubidaea promoted potato growth (Hanifah et al., 2023). Therefore, it was expected that S. rubidaea strains in this study may act as PGPB.
Various antifungal substances have been known to be produced from Serratia strains. S. liquefaciens strains collected from grape leaves and S. marcescens B2 strains collected from tomato leaves have been reported to inhibit mycelial growth of B. cinerea (Akutsu et al., 1993; Whiteman and Stewart, 1998). Chitinase from Serratia spp. may attack chitin, a major component of fungal cell walls, causing its degradation and the death of pathogens (Singh and Jha, 2016). S. rubidaea MarR61-01, isolated from healthy strawberry stems, has shown antifungal activity against B. cinerea in vitro (Alijani et al., 2023). In addition to the involvement of prodigiosin produced by S. rubidaea MarR61-01 in the suppression of B. cinerea growth, multiple pathways have been proposed. Moreover, VOCs produced by bacteria and plant defense responses may contribute to plant pathogen control. Although the growth inhibitor of B. cinerea produced by the S. rubidaea strain was not identified, it is possible that the mechanism of action may be similar to that underlying the antifungal activity of these analogous bacteria.
Regarding biosafety, some S. rubidaea strains have been obtained from human foci. Recently, some Serratia strains showed potential biocontrol activity against plant and human pathogens (Alijani et al., 2023). For example, regarding Serratia marcescens, there has been a discussion about the selection of strains that cause human disease and those that are safe as PGPB (Abreo and Altier, 2019). Given the variety of populations and novel species within S. marcescens, research is underway to isolate opportunistic pathogenic strains or those with potential for agricultural use (Abreo and Altier, 2019; Cho et al., 2020). In addition, safety information is necessary when handling S. rubidaea strains in fields. However, this study provides fundamental knowledge for developing techniques to control the relative abundance of S. rubidaea strains endemic to plants.
Previous studies have demonstrated the effectiveness of various microbial agents in controlling B. cinerea. For example, culture supernatants of Metarhizium anisopliae or Pseudomonas strain QBA5 were applied to detached tomato leaves and post-harvest tomato fruits prior to B. cinerea application. In these studies, both Metarhizium anisopliae and Pseudomonas strain QBA5 were effective in controlling B. cinerea (Sarven et al., 2020; Gao et al., 2018). Another study applied a cultured bacterial suspension of Pseudomonas aeruginosa CQ-4 to detached leaves and greenhouse-potted tomato plants prior to the application of B. cinerea. The concentration of conidia solution was 1.0 × 106 CFU/mL for B. cinerea and 1.0 × 108 CFU/mL for P. aeruginosa CQ-4. The results demonstrated that the P. aeruginosa CQ-4 strain inhibited the growth of B. cinerea (Wang et al., 2021). Evaluation of disease development after 20 days showed that the application of Pseudomonas aeruginosa CQ-4 suppressed gray mold. In cyclamen, disease suppression has been reported with S. marcescens strain B2 (Iyozumi et al., 1996). After cyclamen petals were sprayed with S. marcescens B2 (10 mL of ca. 1 × 109 CFU/mL per plant), B. cinerea (10 mL of ca. 5 × 105 conidia/mL per plant) was sprayed and disease development was evaluated. After 2 weeks of growth and condition observation, the disease suppression effect was confirmed. In this study, we evaluated gray mold on grafted tomato seedlings and found that application of suppressant strains at lower concentrations than those reported in previous studies had similar disease suppression effects. In addition, we confirmed the inhibitory effects of S. rubidaea strains on B. cinerea conidia formation. As conidia formation increases the risk of infection spread by conidia dispersal (Barnes and Shaw, 2003), this may significantly contribute to the observed suppression of gray mold.
In this study, microbial community analysis revealed the presence of the genus Serratia, including S. rubidaea, in tomato leaf and stem samples and demonstrated that the relative abundance of this genus varied among samples. We also identified the relative abundance of PGPB with confirmed antifungal activity in the microflora of plant surface samples for the first time. Although external application of PGPB can have positive effects on plant growth and pest control, there are concerns regarding the PGPB load on the microbial community, with its effect being transient and weak in certain growing environments (Vuolo et al., 2022). These concerns could be addressed by focusing on indigenous PGPB with antifungal properties and controlling their relative abundance. In the future, regulating the relative abundance of S. rubidaea strains with biocontrol properties and maintaining a microflora that promotes plant growth might allow the establishment of a more optimal environment for both plant growth and biological control. This approach aims to leverage the power of nature, paving the way for sustainable agriculture, thereby harmonizing growth enhancement with effective biological control.
The datasets presented in this study can be found in online repositories. The names of the repository/repositories and accession number(s) can be found at: https://www.ddbj.nig.ac.jp/, accession numbers LC820854-LC820860, LC822350-LC822356 and PRJDB18246.
HS: Conceptualization, Writing – review & editing, Investigation, Methodology, Data curation, Validation. TH: Writing – review & editing, Data curation, Formal analysis, Investigation, Visualization, Writing – original draft. TY: Writing – review & editing, Conceptualization, Methodology, Resources. TO: Conceptualization, Methodology, Writing – review & editing, Resources. DH: Investigation, Methodology, Validation, Conceptualization, Writing – review & editing, Data curation, Formal analysis, Funding acquisition, Project administration, Resources, Supervision, Visualization, Writing – original draft.
The author(s) declare that financial support was received for the research, authorship, and/or publication of this article. This work was supported in part by the Yanmar Resource Recycling Support Organization, Osaka, Japan (grant number KI0232039).
We thank Ryo Morimoto (Sojo University) for assisting with some experiments. The authors would like to thank Enago (www.enago.jp) for the English language review.
The authors declare that the research was conducted in the absence of any commercial or financial relationships that could be construed as a potential conflict of interest.
All claims expressed in this article are solely those of the authors and do not necessarily represent those of their affiliated organizations, or those of the publisher, the editors and the reviewers. Any product that may be evaluated in this article, or claim that may be made by its manufacturer, is not guaranteed or endorsed by the publisher.
The Supplementary material for this article can be found online at: https://www.frontiersin.org/articles/10.3389/fmicb.2024.1455699/full#supplementary-material
Abreo, E., and Altier, N. (2019). Pangenome of Serratia marcescens strains from nosocomial and environmental origins reveals different populations and the links between them. Sci. Rep. 9:46. doi: 10.1038/s41598-018-37118-0
Aida, T., and Nomoto, K. (1988). Nitrate removal from a sewage by supplementation of methanol using a submerged soil column, and changes in the population of methanol-utilizing Denitrifiers in the column soil. Jpn. J. Soil Sci. Plant Nutr. 59, 464–470. doi: 10.20710/dojo.59.5_464
Akutsu, K., Hirata, A., Yamamoto, M., Hirayae, K., Okuyama, S., and Hibi, T. (1993). Growth inhibition of Botrytis spp. by Serratia marcescens B2 isolated from tomato Phylloplane. Jpn. J. Phytopathol. 59, 18–25. doi: 10.3186/jjphytopath.59.18
Alijani, Z., Amini, J., Karimi, K., and Pertot, I. (2023). Characterization of the mechanism of action of Serratia rubidaea Mar61-01 against Botrytis cinerea in strawberries. Plan. Theory 12:154. doi: 10.3390/plants12010154
Amin, A., Ahmed, I., Salam, N., Kim, B. Y., Singh, D., Zhi, X. Y., et al. (2017). Diversity and distribution of thermophilic bacteria in hot springs of Pakistan. Microb. Ecol. 74, 116–127. doi: 10.1007/s00248-017-0930-1
Banno, S., Fukumori, F., Ichiishi, A., Okada, K., Uekusa, H., Kimura, M., et al. (2008). Genotyping of benzimidazole-resistant and dicarboximide-resistant mutations in Botrytis cinerea using real-time polymerase chain reaction assays. Phytopathology 98, 397–404. doi: 10.1094/PHYTO-98-4-0397
Barnes, S. E., and Shaw, M. W. (2003). Infection of commercial hybrid Primula seed by Botrytis cinerea and latent disease spread through the plants. Phytopathology 93, 573–578. doi: 10.1094/PHYTO.2003.93.5.573
Bashan, Y., and de-Bashan, L. E. (2010). Chapter two – how the plant growth-promoting bacterium Azospirillum promotes plant growth—a critical assessment. Adv. Agron. 108, 77–136. doi: 10.1016/S0065-2113(10)08002-8
Bates, D., Maechler, M., Bolker, B., Walker, S., Christensen, R. H. B., Singmann, H., et al. (2024). Package ‘lme4’: Linear Mixed-Effects Models using ‘Eigen’ and S4. Available at: https://cran.r-project.org/web/packages/lme4/lme4.pdf (Accessed May 23, 2024).
Bisanz, J. E. (2018). qiime2R: importing QIIME2 artifacts and associated data into R sessions. Available at: https://github.com/jbisanz/qiime2R (Accessed May 23, 2024).
Bolyen, E., Rideout, J. R., Dillon, M. R., Bokulich, N. A., Abnet, C., al-Ghalith, G. A., et al. (2019). Reproducible, interactive, scalable and extensible microbiome data science using QIIME 2. Nat. Biotechnol. 37, 852–857. doi: 10.1038/s41587-019-0209-9
Breeze, E. (2019). 97 shades of gray: genetic interactions of the gray Mold, Botrytis cinerea, with wild and domesticated tomato. Plant Cell 31, 280–281. doi: 10.1105/tpc.19.00030
Bretz, F., Hothorn, T., and Westfall, P. (2010). Multiple comparisons using R. Boca Raton, FL: CRC Press.
Callahan, B. J., McMurdie, P. J., Rosen, M. J., Han, A. W., Johnson, A. J., and Holmes, S. P. (2016). DADA2: high resolution sample inference from amplicon data. Nat. Methods 13, 581–583. doi: 10.1038/nmeth.3869
Cho, G. S., Stein, M., Brinks, E., Rathje, J., Lee, W., Suh, S. H., et al. (2020). Serratia nevei sp. nov. and Serratia bockelmannii sp. nov., isolated from fresh produce in Germany and reclassification of Serratia marcescens subsp. sakuensis Ajithkumar et al. 2003 as a later heterotypic synonym of Serratia marcescens subsp. marcescens. Syst. Appl. Microbiol 43:126055. doi: 10.1016/j.syapm.2020.126055
De Mendiburu, F., and Reinhard, S. (2015). Agricolae-ten years of open source statistical tool for experiments in breeding, agriculture and biology. PeerJ PrePrints 3:e1404v1. doi: 10.7287/peerj.preprints.1404v1
Dean, R., Van Kan, J. A. L., Pretorius, Z. A., Hammond-Kosack, K. E., Di Pietro, A., Spanu, P. D., et al. (2012). The top 10 fungal pathogens in molecular plant pathology. Mol. Plant Pathol. 13, 414–430. doi: 10.1111/j.1364-3703.2011.00783.x
Dutta, P., Mahanta, M., Singh, S. B., Thakuria, D., Deb, L., Kumari, A., et al. (2023). Molecular interaction between plants and Trichoderma species against soil-borne plant pathogens. Front. Plant Sci. 14:1145715. doi: 10.3389/fpls.2023.1145715
El-Esawi, M. A., Alaraidh, I. A., Alsahli, A. A., Alzahrani, S. M., Ali, H. M., Alayafi, A. A., et al. (2018). Serratia liquefaciens KM4 improves salt stress tolerance in maize by regulating redox potential, ion homeostasis, Leaf gas exchange and stress-related gene expression. Int. J. Mol. Sci. 19:3310. doi: 10.3390/ijms19113310
Elmer, P. A. G., and Michailides, T. J. (2007). “Epidemiology of Botrytis cinerea in orchard and vine crops” in Botrytis: Biology, pathology and control. eds. Y. Elad, B. Williamson, P. Tudzynski, and N. Delen (Dordrecht: Springer), 243–272.
Esterio, M., Copier, C., Román, A., Araneda, M. J., Rubilar, M., Pérez, I., et al. (2017). Frequency of fungicide-resistant Botrytis cinerea populations isolated from ‘Thompson seedless’ table grapes in the central valley of Chile. Cien. Inv. Agr. 44, 294–305. doi: 10.7764/rcia.v44i3.1721
Food and Agriculture Organization of the United Nations . (2023). Agricultural production statistics 2000–2022. Available at: https://www.fao.org/statistics/highlights-archive/highlights-detail/agricultural-production-statistics-(2000-2022)/en (Accessed May 23, 2024).
Gamit, H., and Amaresan, N. (2023). Role of methylotrophic bacteria in managing abiotic stresses for enhancing agricultural production. Pedosphere 33, 49–60. doi: 10.1016/j.pedsph.2022.06.028
Gao, P., Qin, J., Li, D., and Zhou, S. (2018). Inhibitory effect and possible mechanism of a Pseudomonas strain QBA5 against gray mold on tomato leaves and fruits caused by Botrytis cinerea. PLoS One 13:e0190932. doi: 10.1371/journal.pone.0190932
Govil, T., Paste, M., Samanta, D., David, A., Goh, K. M., Li, X., et al. (2021). Metagenomics and culture dependent insights into the distribution of Firmicutes across two different sample types located in the Black Hills region of South Dakota, USA. Microorganisms 9:113. doi: 10.3390/microorganisms9010113
Hajji-Hedfi, L., Rhouma, A., Hajlaoui, H., Hajlaoui, F., and Rebouh, N. Y. (2023). Understanding the influence of applying two culture filtrates to control gray Mold disease (Botrytis cinerea) in tomato. Agronomy 13:1774. doi: 10.3390/agronomy13071774
Hamada, M. A., and Soliman, E. R. S. (2023). Characterization and genomics identification of key genes involved in denitrification-DNRA-nitrification pathway of plant growth-promoting rhizobacteria (Serratia marcescens OK482790). BMC Microbiol. 23:210. doi: 10.1186/s12866-023-02941-7
Hanifah, N. A. S. B., Ghadamgahi, F., Ghosh, S., Ortiz, R., Whisson, S. C., Vetukuri, R. R., et al. (2023). Comparative transcriptome profiling provides insights into the growth promotion activity of Pseudomonas fluorescens strain SLU99 in tomato and potato plants. Front. Plant Sci. 14:1141692. doi: 10.3389/fpls.2023.1141692
Hansen, M. C., Tolker-Neilson, T., Givskov, M., and Molin, S. (1998). Biased 16S rDNA PCR amplication caused by interference from DNA flanking template region. FEMS Microbiol. Ecol. 26, 141–149. doi: 10.1111/j.1574-6941.1998.tb00500.x
Huerta-Cepas, J., Serra, F., and Bork, P. (2016). ETE 3: reconstruction, analysis, and visualization of phylogenomic data. Mol. Biol. Evol. 33, 1635–1638. doi: 10.1093/molbev/msw046
Iguchi, H., Yurimoto, H., and Sakai, Y. (2015). Interactions of Methylotrophs with plants and other heterotrophic Bacteria. Microorganisms 3, 137–151. doi: 10.3390/microorganisms3020137
Iyozumi, H., Komagata, T., Hirayae, K., Tsuchiya, K., Hibi, T., and Akutsu, K. (1996). Biological control of Cyclamen gray Mould (Botrytis cinerea) by Serratia marcescens B2. Jpn. J. Phytopathol. 62, 559–565. doi: 10.3186/jjphytopath.62.559
Johnson, M., Zaretskaya, I., Raytselis, Y., Merezhuk, Y., McGinnis, S., and Madden, T. L. (2008). NCBI BLAST: a better web interface. Nucleic Acids Res. 36, W5–W9. doi: 10.1093/nar/gkn201
Katoh, K., and Daron, M. (2013). Standley, MAFFT multiple sequence alignment software version 7: improvements in performance and usability. Mol. Biol. Evol. 30, 772–780. doi: 10.1093/molbev/mst010
Kerr, J. R. (1999). Bacterial inhibition of fungal growth and pathogenicity. Microb. Ecol. Health Dis. 11, 129–142. doi: 10.1080/089106099435709
Kerry, R. G., Patra, S., Gouda, S., Patra, J. K., and Das, G. (2018). “Microbes and their role in drought tolerance of agricultural food crops” in Microbial biotechnology. eds. J. Patra, G. Das, and H. S. Shin (Singapore: Springer), 253–273.
Kloepper, J. W., Ryu, C. M., and Zhang, S. (2004). Induced systemic resistance and promotion of plant growth by Bacillus spp. Phytopathology 94, 1259–1266. doi: 10.1094/PHYTO.2004.94.11.1259
Knief, C., Delmotte, N., Chaffron, S., Stark, M., Innerebner, G., Wassmann, R., et al. (2012). Metaproteogenomic analysis of microbial communities in the phyllosphere and rhizosphere of rice. ISME J. 6, 1378–1390. doi: 10.1038/ismej.2011.192
Kolb, S. (2009). Aerobic methanol-oxidizing bacteria in soil. FEMS Microbiol. Lett. 300, 1–10. doi: 10.1111/j.1574-6968.2009.01681.x
Konishi, K., Ogawara, T., Shimamoto, K., and Tomita, Y. (2010). Hot water spraying for the control of anthracnose and gray mold on strawberry. Bull. Hort. Inst. Ibaraki Agr. Center. 17, 43–46. Available at: https://www.pref.ibaraki.jp/nourinsuisan/enken/hokoku/no17/documents/hiiac1743-462010.pdf (Accessed May 23, 2024).
Krishnamoorthy, R., Kwon, S. W., Kumutha, K., Senthilkumar, M., Ahmed, S., Sa, T., et al. (2018). Diversity of culturable methylotrophic bacteria in different genotypes of groundnut and their potential for plant growth promotion. 3 Biotech. 8:275. doi: 10.1007/s13205-018-1291-2
Kumar, M., Kour, D., Yadav, A. N., Saxena, R., Rai, P. K., Jyoti, A., et al. (2019). Biodiversity of methylotrophic microbial communities and their potential role in mitigation of abiotic stresses in plants. Biologia 74, 287–308. doi: 10.2478/s11756-019-00190-6
Kumar, M., Tomar, R. S., Lade, H., and Paul, D. (2016). Methylotrophic bacteria in sustainable agriculture. World J. Microbiol. Biotechnol. 32:120. doi: 10.1007/s11274-016-2074-8
Lenth, R. V., Bolker, B., Buerkner, P., Giné-Vázquez, I., Herve, M., Jung, M., et al. (2024). Package ‘emmeans’: estimated marginal means, aka least-squares means. Available at: https://cran.r-project.org/web/packages/emmeans/emmeans.pdf (Accessed May 23, 2024).
Leroux, P. (2007). “Chemical control of Botrytis and its resistance to chemical fungicides” in Botrytis: Biology, pathology and control. eds. Y. Elad, B. Williamson, P. Tudzynski, and N. Delen (Dordrecht: Springer), 195–222.
Leroux, P., Chapeland, F., Desbrosses, D., and Gredt, M. (1999). Patterns of cross-resistance to fungicides in Botryotinia fuckeliana (Botrytis cinerea) isolates from French vineyards. Crop Prot. 18, 687–697. doi: 10.1016/S0261-2194(99)00074-5
Letunic, I., and Bork, P. (2021). Interactive tree of life (iTOL) v5: an online tool for phylogenetic tree display and annotation. Nucleic Acids Res. 49, W293–W296. doi: 10.1093/nar/gkab301
Liu, S., Fu, L., Tan, H., Jiang, J., Che, Z., Tian, Y., et al. (2021). Resistance to Boscalid in Botrytis cinerea from greenhouse-grown tomato. Plant Dis. 105, 628–635. doi: 10.1094/PDIS-06-20-1191-RE
Lopez-Velasco, G., Welbaum, G. E., Boyer, R. R., Mane, S. P., and Ponder, M. A. (2011). Changes in spinach phylloepiphytic bacteria communities following minimal processing and refrigerated storage described using pyrosequencing of 16S rRNA amplicons. J. Appl. Microbiol. 110, 1203–1214. doi: 10.1111/j.1365-2672.2011.04969.x
Lugtenberg, B., and Kamilova, F. (2009). Plant-growth-promoting rhizobacteria. Ann. Rev. Microbiol. 63, 541–556. doi: 10.1146/annurev.micro.62.081307.162918
Madhaiyan, M., Alex, T. H. H., Ngoh, S. T., Prithiviraj, B., and Ji, L. (2015). Leaf-residing Methylobacterium species fix nitrogen and promote biomass and seed production in Jatropha curcas. Biotechnol. Biofuels 8:222. doi: 10.1186/s13068-015-0404-y
Mahdi, I., Hafidi, M., Allaoui, A., and Biskri, L. (2021). Halotolerant endophytic bacterium Serratia rubidaea ED1 enhances phosphate Solubilization and promotes seed germination. Agriculture 11:224. doi: 10.3390/agriculture11030224
McMurdie, P. J., and Holmes, S. (2013). Phyloseq: an R package for reproducible interactive analysis and graphics of microbiome census data. PLoS One 8:e61217. doi: 10.1371/journal.pone.0061217
Meiberg, J. B. M., and Harder, W. (1978). Aerobic and anaerobic metabolism of trimethylamine, dimethylamine and methylamine in Hyphomicrobium X. Microbiology 106, 265–276. doi: 10.1099/00221287-106-2-265
Neuwirth, E. (2022). Package 'RColorBrewer': ColorBrewer palettes. Available at: https://cran.r-project.org/web/packages/RColorBrewer/RColorBrewer.pdf (Accessed May 23, 2024).
Omer, Z., Tombolini, R., Broberg, A., and Gerhardson, B. (2004). Indole-3-acetic acid production by pink-pigmented facultative methylotrophic bacteria. Plant Growth Regul. 43, 93–96. doi: 10.1023/B:GROW.0000038360.09079.ad
Price, M. N., Dehal, P. S., and Arkin, A. P. (2010). FastTree 2--approximately maximum-likelihood trees for large alignments. PLoS One 5:e9490. doi: 10.1371/journal.pone.0009490
Romanovskaia, V. A., Shilin, S. O., Chernaia, N. A., Tashirev, A. B., Malashenko, I. R., and Rokitko, P. V. (2005). Search for psychrophilic methylotrophic bacteria in biotopes of the Antarctica. Mikrobiol. Z. 67, 3–8. Available at: https://www.ncbi.nlm.nih.gov/pubmed/16018200 (Accessed May 23, 2024).
Sapp, A., Huguet-Tapia, J. C., Sánchez-Lamas, M., Antelo, G. T., Primo, E. D., Rinaldi, J., et al. (2018). Draft genome sequence of Methylobacterium sp. strain V23, isolated from accretion ice of the Antarctic subglacial Lake Vostok. Genome Announc. 6, e00145–e00118. doi: 10.1128/genomeA.00145-18
Sarven, M. S., Hao, Q., Deng, J., Yang, F., Wang, G., Xiao, Y., et al. (2020). Biological control of tomato gray Mold caused by Botrytis Cinerea with the Entomopathogenic fungus Metarhizium Anisopliae. Pathogens 9:213. doi: 10.3390/pathogens9030213
Sawant, I. S. (2014). Trichoderma- foliar pathogen interactions. Open Mycol. J. 8, 58–70. doi: 10.2174/1874437001408010058
Senthilkumar, M., Madhaiyan, M., Sundaram, S. P., and Kannaiyan, S. (2009). Intercellular colonization and growth promoting effects of Methylobacterium sp. with plant-growth regulators on rice (Oryza sativa L. cv CO-43). Microbiol. Res. 164, 92–104. doi: 10.1016/j.micres.2006.10.007
Sievert, C., Parmer, C., Hocking, T., Chamberlain, S., Ram, K., Corvellec, M., et al. (2024). Package ‘plotly’: create interactive web graphics via 'plotly.js'. Available at: https://cran.r-project.org/web/packages/plotly/plotly.pdf (Accessed May 23, 2024).
Singh, H. B. (2014). Management of plant pathogens with microorganisms. Proc. Indian Natl. Sci. Acad. 80, 443–454. doi: 10.16943/ptinsa/2014/v80i2/55120
Singh, R. P., and Jha, P. N. (2016). The multifarious PGPR Serratia marcescens CDP-13 augments induced systemic resistance and enhanced salinity tolerance of wheat (Triticum aestivum L.). PLoS One 11:e0155026. doi: 10.1371/journal.pone.0155026
South, K. A., Hand, F. P., and Jones, M. L. (2020). Beneficial Bacteria identified for the control of Botrytis cinerea in Petunia greenhouse production. Plant Dis. 104, 1801–1810. doi: 10.1094/PDIS-10-19-2276-RE
Sultana, S., Sikder, M. M., Ahmmed, M. S., Sultana, A., and Alam, N. (2020). Mycelial growth and biological control measures of Botrytis cinerea isolated from strawberry fruit rot disease in Bangladesh. Univ. J. Micr. Res. 8, 13–18. doi: 10.13189/ujmr.2020.080201
Sumbula, V., Kurian, P. S., Girija, D., and Cherian, K. A. (2020). Metagenomic analysis of bacterial diversity on tomato (Solanum lycopersicum L.) leaves. Int. J. Curr. Microbiol. App. Sci. 9, 2162–2173. doi: 10.20546/ijcmas.2020.910.263
Trotel-Aziz, P., Couderchet, M., Biagianti, S., and Aziz, A. (2008). Characterization of new bacterial biocontrol agents Acinetobacter, Bacillus, Pantoea and Pseudomonas Spp. mediating grapevine resistance against Botrytis Cinerea. Environ. Exp. Bot. 64, 21–32. doi: 10.1016/j.envexpbot.2007.12.009
Trotsenko, Y. A., Ivanova, E. G., and Doronina, N. V. (2001). Aerobic methylotrophic Bacteria as Phytosymbionts. Microbiology 70, 623–632. doi: 10.1023/A:1013167612105
Vidal, A., Parada, R., Mendoza, L., and Cotoras, M. (2020). Endophytic Fungi isolated from plants growing in central Andean Precordillera of Chile with antifungal activity against Botrytis cinerea. J. Fungi 6:149. doi: 10.3390/jof6030149
Vinale, F., Sivasithamparam, K., Ghisalberti, E. L., Marra, R., Woo, S. L., and Lorito, M. (2008). Trichoderma-plant-pathogen interactions. Soil Biol. Biochem. 40, 1–10. doi: 10.1016/j.soilbio.2007.07.002
Vos, C. M. F., de Cremer, K., Cammue, B. P. A., and de Coninck, B. (2015). The toolbox of Trichoderma spp. in the biocontrol of Botrytis cinerea disease. Mol. Plant Pathol. 16, 400–412. doi: 10.1111/mpp.12189
Vuolo, F., Novello, G., Bona, E., Gorrasi, S., and Gamalero, E. (2022). Impact of plant-beneficial bacterial Inocula on the resident Bacteriome: current knowledge and future perspectives. Microorganisms 10:2462. doi: 10.3390/microorganisms10122462
Walters, W. A., Caporaso, J. G., Lauber, C. L., Berg-Lyons, D., Fierer, N., and Knight, R. (2011). PrimerProspector: de novo design and taxonomic analysis of barcoded polymerase chain reaction primers. Bioinformatics 27, 1159–1161. doi: 10.1093/bioinformatics/btr087
Wang, X., Zhou, X., Cai, Z., Guo, L., Chen, X., Chen, X., et al. (2021). A biocontrol strain of Pseudomonas aeruginosa CQ-40 promote growth and control Botrytis cinerea in tomato. Pathogens 10:22. doi: 10.3390/pathogens10010022
Whiteman, S. A., and Stewart, A. (1998). Suppression of Botrytis cinerea sporulation on irradiated grape leaf tissue by the antagonistic bacterium Serratia liquefaciens. New Zeal. J. Crop Hort. 26, 325–330. doi: 10.1080/01140671.1998.9514071
Wickham, H., Averick, M., Bryan, J., Chang, W., McGowan, L. D., Francois, R., et al. (2019). Welcome to the tidyverse. J. Open Source Softw. 4:1686. doi: 10.21105/joss.01686
Keywords: tomato, Botrytis cinerea, methylotroph, Serratia rubidaea, gray mold, biocontrol, microbial communities
Citation: Suenaga H, Hira T, Yoshimura T, Oka T and Hira D (2024) Selection and application of methanol-utilizing bacteria from tomato leaves for biocontrol of gray mold. Front. Microbiol. 15:1455699. doi: 10.3389/fmicb.2024.1455699
Received: 27 June 2024; Accepted: 30 September 2024;
Published: 18 October 2024.
Edited by:
Samina Mehnaz, Forman Christian College, PakistanReviewed by:
Salma Mukhtar, Rutgers, The State University of New Jersey, United StatesCopyright © 2024 Suenaga, Hira, Yoshimura, Oka and Hira. This is an open-access article distributed under the terms of the Creative Commons Attribution License (CC BY). The use, distribution or reproduction in other forums is permitted, provided the original author(s) and the copyright owner(s) are credited and that the original publication in this journal is cited, in accordance with accepted academic practice. No use, distribution or reproduction is permitted which does not comply with these terms.
*Correspondence: Daisuke Hira, aGlyYUBiaW8uc29qby11LmFjLmpw
Disclaimer: All claims expressed in this article are solely those of the authors and do not necessarily represent those of their affiliated organizations, or those of the publisher, the editors and the reviewers. Any product that may be evaluated in this article or claim that may be made by its manufacturer is not guaranteed or endorsed by the publisher.
Research integrity at Frontiers
Learn more about the work of our research integrity team to safeguard the quality of each article we publish.