- 1Department of Gastroenterology, Hepatology, Infectious Diseases, and Endocrinology, Hannover Medical School, Hanover, Germany
- 2Department of Microbiology, Virology, and Biotechnology, Odesa I. I. Mechnykov National University, Odesa, Ukraine
Primary liver cancer (PLC), comprising hepatocellular carcinoma (HCC) and cholangiocarcinoma (CCA), is a severe form of cancer associated with a high mortality and morbidity rate and increasing incidence worldwide. Current treatment options are limited and chemotherapeutics demonstrate strong side effects. New therapies are highly required. Lactobacilli represent the most diverse lactic acid-producing bacteria group and a prominent example of probiotics. Several studies have highlighted the anticancer efficacy of probiotics, especially of Lactiplantibacillus plantarum. However, there are limited studies on its activity on two PLC types, hepatocellular carcinoma (HCC) and cholangiocarcinoma (CCA). This study evaluated the inhibitory mechanism and properties of L. plantarum ONU 12 (Lp 12) and L. plantarum ONU 355 (Lp 355), isolated from grapes in Ukraine and France, in murine PLC cell lines, in vitro. Strain Lacticaseibacillus casei ATCC 393 (Lc 393) has been taken for a direct comparison, as the most studied probiotic strain. The three Lactobacillus species were used in three forms: as live and heat-killed suspensions, and as sonicated extracts, and tested either as a monotherapy or in combination with standard chemotherapeutics (sorafenib for HCC and gemcitabine for CCA). Cell proliferation and viability were assessed via crystal violet staining assay and cell counting kit-8 assay. The induction of senescence was investigated by senescence-associated β-galactosidase assay. Fluorescence-activated cell sorting analysis was used to determine the apoptotic mechanism behind the inhibitory property of lactobacilli. The results showed that the live suspensions and sonicated extracts of Lp 12, Lp 355, and Lc 393 demonstrated inhibitory properties in CCA and HCC cells after 48 h of incubation. In combinations with standard chemotherapeutics, lactobacilli treatments have shown strong synergistic effects. The combination therapy allowed to reduce the chemotherapeutic doses of gemcitabine from 50 μM to 0.1 and 0.05 μM and sorafenib from 13.8 μM to 6.9 and 3.45 μM. Successful treatment regimes induced early apoptosis and cellular senescence in PLC, as the mechanism of inhibition. Heat-killed suspensions showed no inhibitory effect in none of the cell lines. Both strains, Lp 12 and Lp 355, showed successful results and need further testing in vivo, using autochthonous HCC and CCA models.
1 Introduction
Primary liver cancer (PLC) is a severe form of cancer, associated with a high mortality and morbidity rate worldwide. In 2020, it accounted for an estimated 830,180 deaths and 905,677 new cases globally, making it the third leading cause of cancer-related death, its incidence has been rising in many countries and is projected to continue increasing in future (Liu et al., 2022). Hepatocellular carcinoma (HCC) and cholangiocarcinoma (CCA) are the most common leading types of PLC, accounting for 70–85% HCC and 10–20% CCA of all liver cancer cases, respectively (Banales et al., 2020; El-Serag and Rudolph, 2007). HCC stems from the liver cells (mature hepatocytes), while CCA arises from the cholangiocytes of the biliary ducts (Gromowski et al., 2023). The main factors that increase the risk of liver cancer are persistent viral infection of hepatitis B and C, alcoholic liver diseases, exposure to aflatoxins, metabolic dysfunction-associated steatotic liver disease, and metabolic disorders such as obesity and diabetes (Chan et al., 2023; Forner et al., 2018; Rawla et al., 2018). These conditions lead to liver inflammation, fibrosis, and cirrhosis and eventually initiate the progression to cancerous cells. The prevalence of liver cancer varies by geographical region based on the risk mentioned above, with the highest rate observed in Asia and sub-Saharan Africa (Sung et al., 2021). The available treatment options include surgical resection, liver transplantation, chemotherapy, radiation therapy, targeted therapy, and immunotherapy (Jiang et al., 2023; Suresh et al., 2023). However, the treatment choice for liver cancer depends on diverse factors such as the size, stage, and location of the tumor and the patient’s overall health; for example, surgery is the most preferred treatment option for early-stage patients where the tumor is localized and not gone to metastasis and can be removed (Liu et al., 2015; Naqi et al., 2014). However, despite the availability of numerous treatment options, the clinical outcome does not directly correspond to the effort invested in eliminating liver cancer. These treatment options especially chemotherapy has severe side effects and a high recurrence rate (Llovet et al., 2005). As a result, it is imperative to intensify research to develop new alternative and complementary treatment strategies to improve prognosis, reduce the severe side effects of existing therapies and reduce the global burden of PLC.
A promising approach is the correction of the gut microbiome with beneficial microorganisms or treatment with their metabolites (Temraz et al., 2021). It is known that probiotics confer health benefits when administered in the correct concentration (Swanson et al., 2020). Research has examined the various roles of probiotics and their potential to prevent and treat various conditions, including diarrhea, cancer, hypertension, autism, and migraines (Liu et al., 2018; Tegegne and Kebede, 2022). These beneficial effects have garnered significant attention. An important example of such probiotics is Lactiplantibacillus plantarum, which has been shown to possess many properties such as immune modulation, anti-oxidative, anti-inflammatory, regulation of glucose levels, and metabolic pathways (Liu et al., 2018). L. plantarum belongs to the genus Lactobacillus, the most diverse lactic acid-producing bacteria (LAB) group (Seddik et al., 2017). L. plantarum is a Gram-positive, short rod, non-spore-forming, non-pathogenic, and probiotic bacterium naturally found in the human microbiome (Kazmierczak-Siedlecka et al., 2020). It is also found in dairy products (Agaliya and Jeevaratnam, 2012), meat (Schillinger and Lucke, 1989), and wine (Berbegal et al., 2016). The ubiquitous nature of L. plantarum proves its ability to survive and adapt to extreme environmental conditions, even in the acidic environment of the stomach and bile salt in the small intestine (Fiocco et al., 2010). LAB inhibits the growth of pathogens like Staphylococcus aureus, Listeria monocytogenes, Salmonella enteriditis, and Escherichia coli (Oldak et al., 2020; Oldak et al., 2017). They exert these antibacterial effects by producing bacteriocins, organic acids and by directly competing for nutrients and adhesion sites to prevent colonization and growth of pathogenic bacteria (Mokoena, 2017). Different species of lactobacilli, including L. acidophilus, L. casei, L. rhamnosus, and L. plantarum have proven to be associated with the lowering of serum cholesterol and fibrinogen and preventing cardiovascular diseases in humans (Anderson and Gilliland, 1999).
Based on above mentioned, in our study we focused on L. plantarum. We have chosen two strains of L. plantarum (ONU 12 and ONU 355) isolated from the must of grape in Ukraine and France, which have been demonstrated to have strong bacteria antagonistic activity and ability to form biofilms (Limanska et al., 2019). We tested the selected L. plantarum strains for their anti-cancerous potential against HCC and CCA as complementary or alternative therapy to the standard chemotherapeutics. We directly compared the efficacy of two strains L. plantarum to the most studied lactobacilli strain, Lacticaseibacillus casei ATCC 393 known for its anticancer effect (Lau et al., 2024; Spyridopoulou et al., 2021).
2 Materials and methods
2.1 Cultivation of bacterial strains
L. plantarum strains ONU 12 and ONU 355 were initially isolated from must of grape gathered in Ukraine and France (Limanska et al., 2019). L. casei ATCC 393 known for its anti-cancer effect described in the literature (Lau et al., 2024; Spyridopoulou et al., 2021) and purchased from the DSMZ, Leibniz Institute, Braunschweig, Germany (DSMZ No.: 20011) was used as a control. L. plantarum and L. casei were cultivated in de Man Rogosa and Sharpe (MRS) medium (Haghshenas et al., 2023) at 37°C aerobically.
2.2 Preparation of live suspensions of bacteria
Lactobacilli strains were cultivated in MRS broth at 37°C for 20–24 h to reach a concentration of at least 109 CFU/mL. Bacterial cells were washed twice in PBS buffer and harvested by centrifugation at 3,500 g for 15 min at 4°C (S. Choi et al., 2006). Dulbecco’s Modified Eagle’s Medium (DMEM, Gibco, UK) without antibiotics was used to adjust the obtained bacterial pellet to a starting concentration of 109 CFU/mL.
2.3 Preparation of heat-killed suspensions of bacteria
Lactobacilli were grown in MRS broth at 37°C and allowed to reach a concentration of at least 109 CFU/mL. Thereafter, bacterial suspensions were harvested and washed twice in PBS as described in the previous section. The bacterial pellet was resuspended in PBS and distributed into 2 mL microtubes. Using a thermoblock (Eppendorf, USA), heat inactivation was performed at 95°C for 1 h (Choi et al., 2006) and vortexed intermittently to ensure a complete inactivation. Thereafter, lactobacilli were centrifuged again at 3,500 g for 15 min at 4°C and resuspended in DMEM without antibiotics.
2.4 Preparation of sonicated extracts of bacteria
Sonication was performed to break apart or disrupt the bacterial cell wall and membrane to release bacterial protein using sound energy (Wang et al., 2021). Lactobacilli were cultivated in MRS broth at 37°C for 20–24 h to reach a concentration of at least 109 CFU/mL. Bacterial suspensions were harvested and washed twice in PBS buffer using the same conditions as described in the previous sections. Thereafter, 1 mL of bacterial suspensions were distributed into several 1.5 mL microtubes and disrupted in a sonic dismembrator according to Han et al. (2013) with modifications: 10 rounds for 1 min each at 70 W. Afterward, the sonicated extracts were collected into a falcon and two-fold dilution with DMEM without antibiotics was prepared for experiments and subjected to protein quantification by Pierce™ BCA protein assay kit (Thermo Scientific™, USA), according to the manufacturer’s instructions.
2.5 Cell lines and their maintenance
Cancer cell lines, HCC expressing NRASG12Voncogene and CCA expressing KRASG12Vand Akt2 oncogenes, isolated from murine PLC as described recently (Pylypchuk et al., 2022; Suo et al., 2022) were used in this study. Commercial murine fibroblasts CBA-310 (Zhang et al., 2023) were used as control non-cancerous cells. All cell lines were cultivated in a complete DMEM supplemented with 10% fetal bovine serum, 5% penicillin/streptomycin, and 5% minimum essential medium non-essential amino acids (Thermo Fisher, USA). The cells were grown in the incubator at 37°C, with 5% CO2 and 95% humidity.
2.6 Treatment of HCC, CCA cells and fibroblasts with lactobacilli
Cells were first seeded at concentration 1 × 105/mL in 100 μL volume in complete DMEM medium without antibiotics. After 16 h of incubation, 100 μL of twice concentrated live suspension or sonicated extracts or heat-killed suspensions were added into each well of the 96-well plates accordingly. Also, standard anticancer therapeutics sorafenib (in case of HCC) and gemcitabine (in case of CCA) were administered as monotherapy or in combination with L. plantarum suspensions or extracts. Half (6.9 μM) dose and quarter (3.5 μM) doses of the clinical plasma concentration of sorafenib (13.8 μM) were used, as described recently (Pylypchuk et al., 2022; Suo et al., 2022). For gemcitabine, a small fraction of its plasma concentrations, specifically 0.05 μM and 0.1 μM, derived from the clinical plasma concentration of gemcitabine (50 μM) were used, as described recently (Pylypchuk et al., 2022; Suo et al., 2022). Sonicated PBS, PBS, and DMEM were used as negative controls. Subsequently, the plates were incubated for 24 h and 48 h for further experimentation and analysis.
2.7 Bright field microscopy
Cell monolayers were observed and photographed using a Nikon Eclipse Ti2 microscope with a 40x objective.
2.8 Crystal violet staining assay (CVSA)
The evaluation of cancer cell growth was conducted using CVSA assay employing a well-established standard technique as described in the works of Śliwka et al. (2016), Pylypchuk et al. (2022), and Suo et al. (2022). Briefly, following a 24 and 48 h incubation period, the cells were gently washed with warm 100 μL PBS to remove residual DMEM or debris. Subsequently, the cells were fixed using a 4% paraformaldehyde solution for 7 min, ensuring the cells were adequately immobilized. Once fixed, the cells were stained with 0.5% crystal violet in 30% ethanol for 30 min at room temperature. The staining dye binds to the cellular component of live cells allowing for optimal visualization and quantification of cell density. The plates were then left to dry overnight. Finally, an ImmunoSpot® S6 ULTI-MATE Analyzer (Cellular Technology Limited, USA) was employed to capture high-resolution photographs of each plate well and analyzed using ImageJ software.
2.9 Cell proliferation assay/cell counting kit-8 (CCK-8)
The CCK-8 method was employed to quantitatively assess cell viability and proliferation in a precise and objective manner, as established (Pylypchuk et al., 2022; Suo et al., 2022). The experimental procedure was carried out according to the manufacturer’s protocol to ensure standardization and accuracy. After 24 and 48 h incubation periods, 10 μL of the CCK-8 solution was carefully added to each well of the plate and further incubated for 2–4 h. During this time, the water-soluble tetrazolium salt present in the CCK-8 reagent underwent an enzymatic reduction in viable cells, generating a colored formazan product (Yang et al., 2021). To quantify this cellular response, the absorbance of each well was measured after 2 and 4 h at a wavelength of 450 nm, utilizing the Infinite 200 PRO Nano Quant Tecan Microplate Reader (TECAN, Switzerland). In CCK-8 assay, the intensity of the formazan product and the optical density readings directly correlates with the abundance of viable cells present in the well (Yang et al., 2021). The resulting absorbance values were subsequently analyzed using the i-control™ software (TECAN, Switzerland).
2.10 Senescence-associated beta-galactosidase assay (SA-β-gal)
Cellular senescence was evaluated by SA-β-Gal assay, as established (Pylypchuk et al., 2022; Suo et al., 2022), following a 24 and 48 h incubation period of cells with the tested lactobacilli and their extracts. Initially, the cell monolayers were gently washed with PBS at a pH range of 7.2–7.4 to eliminate any residual media or debris. After, the cells were fixed with a fixation solution comprising 2% formaldehyde and 0.2% glutaraldehyde in PBS at the aforementioned pH range. Following the removal of the fixation solution, the cells underwent an additional wash step with PBS buffer at pH 6.0 supplemented with 1 mM MgCl2. Hundred μL of X-gal staining solution was added to each well. The staining solution, prepared according to the protocol described by Cahu and Sola, (2013), consisted of potassium ferrocyanide, potassium ferricyanide, and X-galactose in PBS supplemented with 1 mM MgCl2 at pH 6.0 (Cahu and Sola, 2013). The plates were then incubated at 37°C without CO2 supply until a distinct blue stain developed, indicating the presence of senescent cells. To capture representative images of analysis, ten high-power field photos were taken from each well using a Nikon microscope Eclipse Ti2 (Nikon, Japan) at objective 40x. The images were subsequently analyzed to assess the extent of cellular senescence induced by the lactobacilli and their extracts.
2.11 Flow cytometry analysis (FACS) to detect early, late apoptosis and necroptosis
The cells, following a 24 and 48 h incubation were carefully harvested and washed with PBS at pH 7.2–7.4 ranges. To detach the adherent cells, trypsin was added and the resulting cell suspension was transferred into the initial microtubes, respectively. For the assessment of apoptosis, a dual staining approach was implemented using Annexin V-Phycoerythrin (PE) and 7-Amino-Aactinomycin D (7-AAD) (Biolegend®, USA), as described (Bushnell, 2015). Annexin V-PE binds specifically to phosphatidylserine, which translocates from the inner to the outer leaflet of the plasma membrane during early apoptosis (Vermes et al., 1995). 7-AAD, a DNA-binding dye, is impermeable to intact cell membranes but stains the DNA of late apoptotic and necrotic cells with compromised membrane integrity (Zembruski et al., 2012). Five μL of Annexin V-PE and 7-AAD stains were added, respectively, to the cell suspension. A 400 μL of binding buffer consisting of 10 mM HEPES [pH 7.4], 140 mM NaCl, and 2.5 mM CaCl2 was added to each tube. The samples were then subjected to FACS analysis using a flow cytometer (Cytek® Aurora, USA). Compensation was performed and the acquired data were subsequently analyzed using FlowJo 9.9.6 (BD™, USA) software.
2.12 Statistical analysis
All graphs were plotted using GraphPad Prism (GraphPad Prism 10, USA) software. All CVSA plate pictures were carefully analyzed by ImageJ software and extracted data were further analyzed by GraphPad Prism to plot respective graphs. The experiment was carried out in triplicate and two-way ANOVA was used for all statistical analyses to calculate significant differences among experimental and control groups. If not stated otherwise, data are shown as mean ± standard error of the mean (S.E.M.) with p < 0.05 considered statistically significant. Significance levels were depicted as *p < 0.05, **p < 0.01, ***p < 0.001, and ****p < 0.0001.
3 Results
Three different lactobacilli strains were used in this study: L. plantarum ONU 12 (Lp 12) and L. plantarum ONU 355 (Lp 355) which were isolated from the must of grape (Limanska et al., 2019), and lastly, L. casei ATCC 393 (Lc 393) which was purchased from the DSMZ, Germany. All bacteria were grown in MRS broth for at 37°C for 20–24 h and prepared as live suspensions, or sonicated extracts, or heat-killed suspensions, for use in this study. The HCC and CCA cell lines were established previously (Pylypchuk et al., 2022; Suo et al., 2022) and seeded in a 96-well plate (Figure 1A). After 16 h, the live suspensions, sonicated extracts, and heat-killed suspensions of Lp 12, Lp 355, and Lc 393 in combinations with the respective standard chemotherapeutic drugs (sorafenib for HCC and gemcitabine for CCA) were added and co-incubated with the already seeded cells (Figure 1B). Importantly, the standard therapeutics were added at different reduced concentrations. Positive controls comprised a clinical plasma concentration of sorafenib (13.8 μM) and a clinical plasma concentration of gemcitabine (50 μM), as established previously (Pylypchuk et al., 2022; Suo et al., 2022). Negative controls comprised the diluent/buffers and DMEM. 24 and 48 h post-incubation, assays such as CVSA, CCK-8, SA-β-Gal, FACS analysis, and bright field microscopy were carried out to assess the inhibitory properties and mechanism of action of the treatment added (Figure 1C).
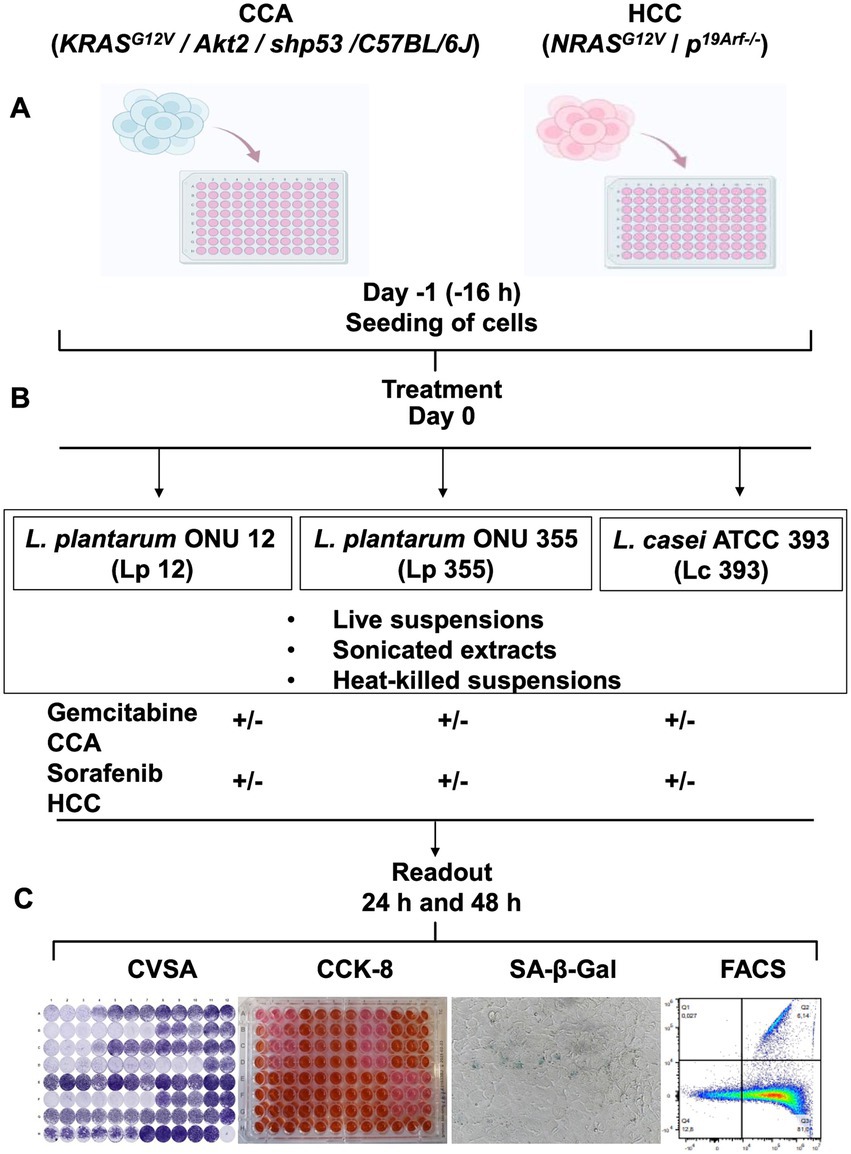
Figure 1. Experimental layout to study the efficacy of Lp 12, Lp 355, and Lc 393, and the mechanism of their action in vitro using CCA, HCC cell lines and fibroblasts. (A) Cells were seeded on day −1; (B) on day 0, live, heat-killed suspensions, and sonicated extracts of either Lp 12 or Lp 355 or Lc 393 were added to the cells at different concentrations as monotherapy or in combination with standard chemotherapeutics, gemcitabine for CCA and sorafenib for HCC. (C) After 24 and 48 h of incubation, different readouts were performed, comprising CVSA, CCK-8, SA-β-Gal assays, and FACS analysis, as well as bright field microscopy. Commercial murine fibroblasts CBA-310 were used as a control cell line (normal cells).
3.1 Anticancer activity screening in CCA cells
3.1.1 Live suspension and sonicated extracts of Lp 12 and Lp 355 as monotherapy or in combination with gemcitabine inhibited the growth of CCA
The inhibitory capacity of live suspensions of Lp 12, Lp 355, and Lc 393 were first evaluated using CVSA assay in CCA cell line. The highest concentration of live suspension (108 CFU/well) of all strains used demonstrated a mild inhibitory effect on CCA after 24 h (Figure 2A), that became more evident after 48 h (Figure 2B) when compared to untreated control (DMEM). Gemcitabine was used as a positive control (50 μM) and as expected, inhibited CCA cells in a dose-dependent manner. The inhibitory effect of the live suspensions of Lp 12, Lp 355, and Lc 393 was also found to be concentration-dependent, with the highest concentration (108 CFU/well) displaying the strongest effect, comparable to the human plasma concentrations of gemcitabine (50 μM). Monotherapy with the highest concentration (108 CFU/well) of Lp 12 and Lp 355 showed stronger inhibitory efficacy than the one in Lc 393 group (Figure 2B). Control strain Lc 393 (108 CFU/well) has demonstrated a toxicity effect on fibroblasts both at 24 h (Supplementary Figures S1A,B) and especially at 48 h (Supplementary Figures S1C,D). Importantly, the highest concentration (108 CFU/well) of Lp 12 and Lp 355 inhibited the growth of CCA, but not of fibroblasts (Figure 2 and Supplementary Figure S1). The inhibitory effect observed in the combination therapies in CCA and fibroblasts was similar to the effects seen in the control groups treated with gemcitabine (0.1 and 0.05 μM) (Figure 2 and Supplementary Figure S1).
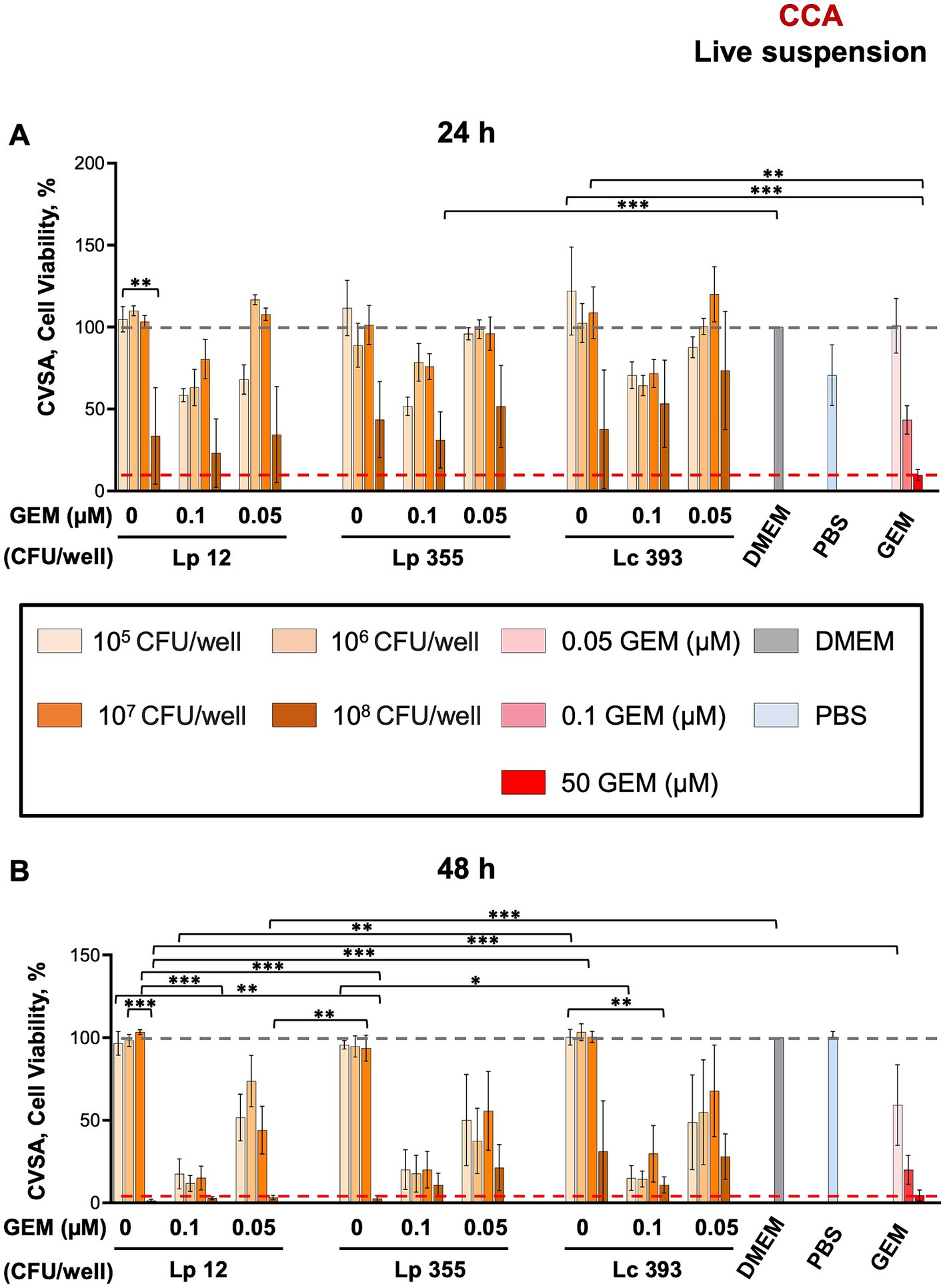
Figure 2. The live suspensions of Lp 12 and Lp 355 at the concentration of 108 CFU/well inhibited the growth of CCA. CVSA analysis was performed on CCA cells after the treatment with lactobacilli administered either alone at different concentrations (live suspension: 108, 107, 106, 105 CFU/well) or in combination with gemcitabine at different concentrations (0.05 and 0.1 μM). CVSA readouts were performed: (A) live suspension after 24 h and (B) live suspension after 48 h post-incubation. GEM, gemcitabine. The red dashed line shows the data obtained in the control group treated with the standard therapy (gemcitabine 50 μM). *p < 0.05, **p < 0.01, ***p < 0.001.
We further tested the sonicated extracts obtained from live bacteria in CCA settings. Similar to live suspensions, the sonicated extracts of Lp 12 (311 μg/mL) and Lp 355 (336 μg/mL) inhibited the growth of CCA cells specifically after 48 h of incubation (Figures 3A,B), as compared to the positive control (gemcitabine 50 μM). Lc 393 (383 μg/mL) showed less inhibitory efficacy, than the two Lp counterparts (Figure 3B). The inhibitory effect observed in the combination therapies was more pronounced than the effects seen in the control groups treated with gemcitabine (0.1 μM and 0.05 μM), especially after 48 h of incubation (Figure 3B). In particular, Lp 12 (311 and 155.5 μg/mL), Lp 355 (336 and 168 μg/mL), and Lc 393 (383 and 191.5 μg/mL) in combination with gemcitabine 0.1 μM performed more efficacious than gemcitabine 0.1 μM alone or sonicated extracts administered as monotherapy (Figure 3B). Furthermore, all tested sonicated extracts in combination with gemcitabine 0.5 μM performed more efficacious than gemcitabine 0.5 μM alone or sonicated extracts administered as monotherapy (Figure 3B). Negative controls – sonicated PBS, PBS, and DMEM – were not able to inhibit the growth of CCA (Figure 3).
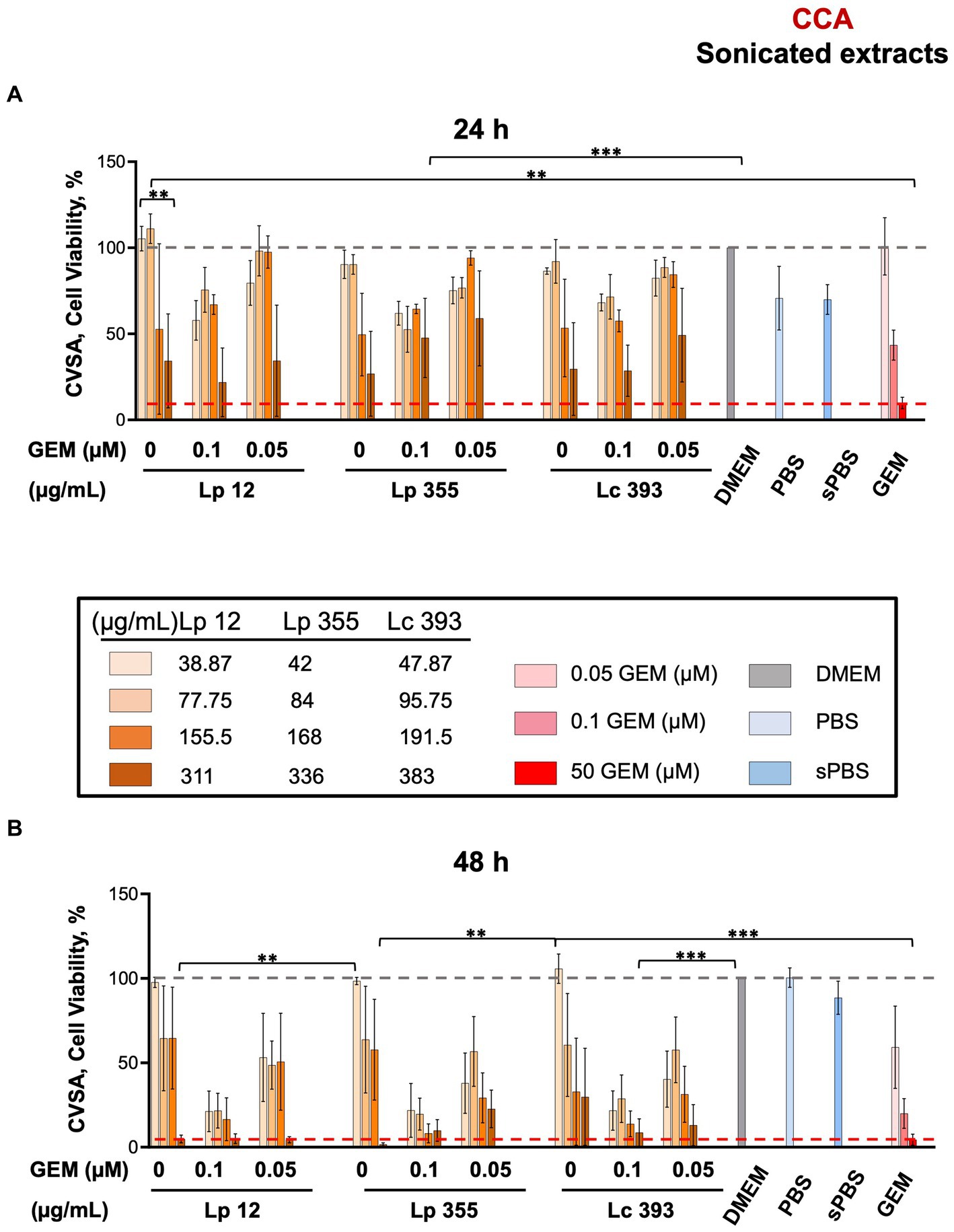
Figure 3. The sonicated extracts of Lp 12 and Lp 355 at the concentrations of 311 and 336 μg/mL, respectively, inhibited the growth of CCA. CVSA analysis was performed on CCA cells after the treatment with lactobacilli administered either alone at different concentrations (sonicated extracts: in the range of 35–390 μg/mL) or in combination with gemcitabine at different concentrations (0.05 and 0.1 μM). CVSA readouts were performed: (A) sonicated extracts after 24 h and (B) sonicated extracts after 48 h post-incubation. GEM, gemcitabine; sPBS, sonicated PBS. The red dashed line shows the data obtained in the control group treated with the standard therapy (gemcitabine 50 μM). **p < 0.01, ***p < 0.001.
When compared to the normal cells fibroblasts, the sonicated extracts of Lc 393 (383 and 191.5 μg/mL) proved to be toxic, whereas Lp 12 and Lp 355 did not demonstrate high toxicity toward fibroblasts (Supplementary Figures S2A–D).
3.1.2 Heat-killed suspensions of Lp 12, Lp 355, and Lc 393 showed no inhibitory effect on CCA
We further assessed the efficacy of heat-killed suspensions of Lp 12, Lp 355, and Lc 393 in CCA using CVSA assay. Interestingly, we did not observe any impact of heat-killed suspensions and even the highest concentration of 108 CFU/mL of lactobacilli could not inhibit the growth of CCA cells at both 24 and 48 h (Figures 4A,B).
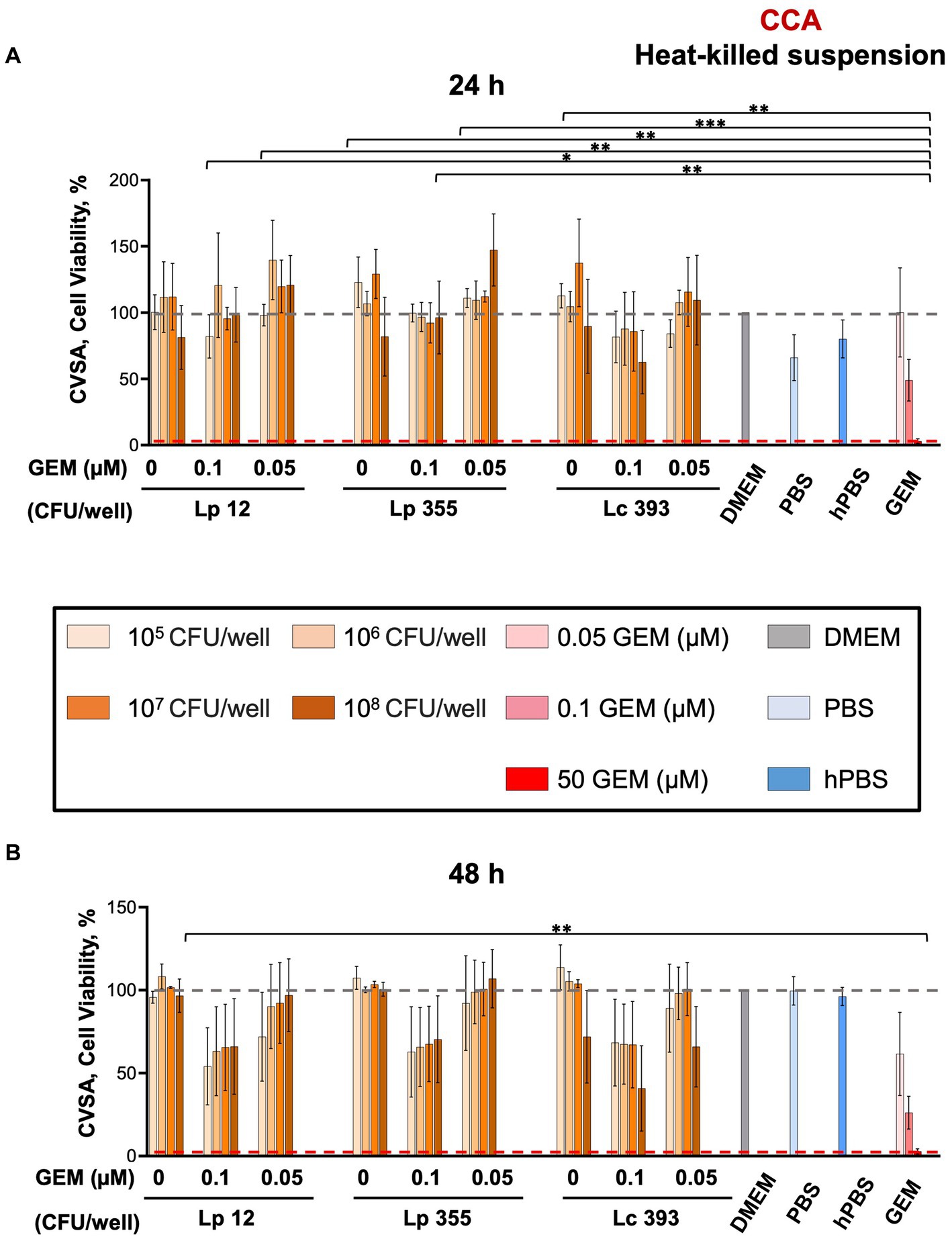
Figure 4. The heat-killed suspensions of Lp 12, Lp 355, and Lc 393 did not inhibit the growth of CCA cells. CVSA analysis was performed on CCA cells after the treatment with lactobacilli administered either alone at different concentrations or in combination with gemcitabine at different concentrations (0.05 and 0.1 μM). CVSA readouts were performed (A) 24 h and (B) 48 h post-incubation. GEM, gemcitabine; hPBS, heated PBS. The red dashed line shows the data obtained in the control group treated with the standard therapy (gemcitabine 50 μM). *p < 0.05, **p < 0.01, ***p < 0.001.
3.1.3 Combination of either sonicated extracts or live suspension of Lp 12, Lp 355, and Lc 393 with gemcitabine inhibited CCA growth
In our next step, we performed a CCK-8 assay to quantify the viable cells remaining after the treatment of CCA cells with the live suspension and sonicated extracts of Lp 12, Lp 355, and Lc 393. At 48 h post-incubation, the live suspension monotherapy resulted in a proportional decrease in the number of viable cells according to concentrations but gemcitabine at the human plasma concentration of 50 μM outperformed it (Figure 5A). However, the combination therapy of all three strains (108 CFU/well) with gemcitabine 0.1 μM reduced the number of viable cells even more than their respective monotherapeutic regimes and this reduction was similar to the group treated with the human plasma concentration of gemcitabine 50 μM (Figure 5B).
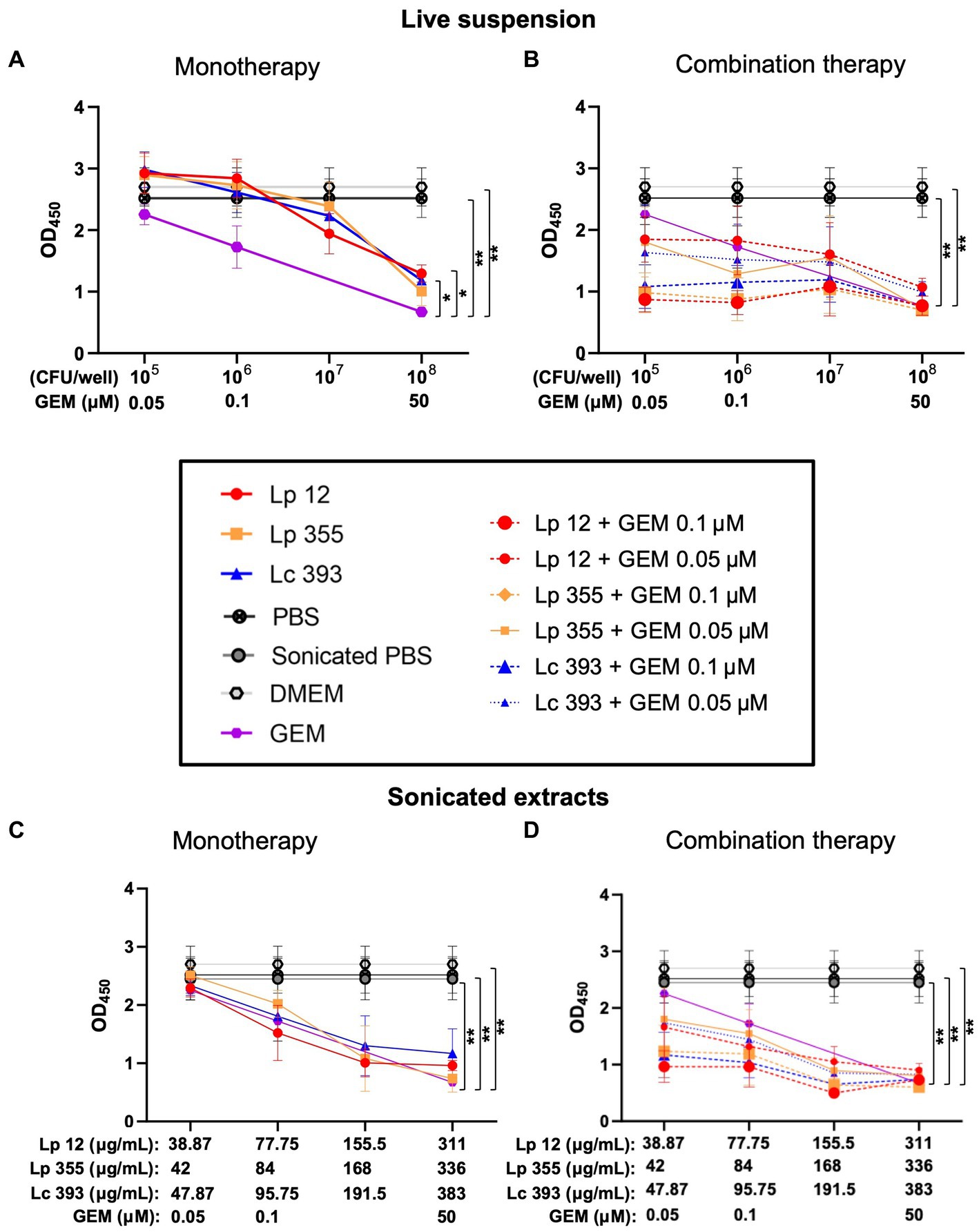
Figure 5. Live suspensions or sonicated extracts of Lp 12, Lp 355, and Lc 393 and their combinations with gemcitabine demonstrated inhibitory effects on CCA cell line in CCK-8 assay. CCA cells were treated with lactobacilli and their combination with gemcitabine: (A) 48 h live lactobacilli suspension monotherapy; (B) 48 h live lactobacilli suspension monotherapy and combination therapy; (C) 48 h sonicated extract lactobacilli monotherapy; (D) 48 h sonicated lactobacilli extract monotherapy and combination therapy. After the incubation, CCA cells were subjected to CCK-8 analysis at OD450. GEM, gemcitabine. *p < 0.05, **p < 0.01.
For sonicated extracts, at 48 h, only the monotherapy of Lp 355 (336 μg/mL) showed similar efficacy as gemcitabine 50 μM, while the monotherapies of Lp 12 (311 μg/mL) and Lc 393 (383 μg/mL) performed less efficacious than gemcitabine 50 μM and Lp 355 (336 μg/mL) (Figure 5C). Importantly, the combination of sonicated extracts of Lp 355 (336 μg/mL) with gemcitabine 0.1 μM inhibited CCA growth more efficiently than gemcitabine monotherapy 50 μM (Figure 5D). Lp 12 (311 μg/mL) and Lc 393 (383 μg/mL) combined with gemcitabine 0.1 μM were slightly less efficient than gemcitabine monotherapy 50 μM (Figure 5D). Interestingly, the combinations of Lp 12 (155.5 μg/mL) and Lp 355 (168 μg/mL) with gemcitabine 0.1 μM inhibited CCA growth more efficient than gemcitabine monotherapy 50 μM (Figure 5D). The combination of sonicated extracts of all three strains with gemcitabine 0.5 μM was less efficient than gemcitabine monotherapy 50 μM (Figure 5D). It is also important to mention, that combination therapy was in all strains more efficient than monotherapeutic regimes (Figure 5D).
3.1.4 Live suspension or sonicated extracts of lactobacilli monotherapy and their combination with gemcitabine induced cellular senescence in CCA cell lines
We further proceeded in our experiment to confirm whether the inhibitory property of lactobacilli is associated with the induction of cellular senescence in CCA cells. We performed SA-β-Gal staining assay to detect senescent cells 48 h after treatment. The live suspensions of all three strains tested at 106 CFU/well were able to induce senescence (Figure 6). Likewise, the sonicated extracts of Lp 12, Lp 355, and Lc 393 at the concentrations of 84, 57.5, and 127.75 μg/mL, respectively, induced senescence (Figure 7). The detected senescence response was comparable to the ones detected in gemcitabine of different concentrations (Figures 6, 7). Similarly, the combination therapy comprising lactobacilli with gemcitabine at 0.1 and 0.05 μM also induced senescence (Figures 6, 7).
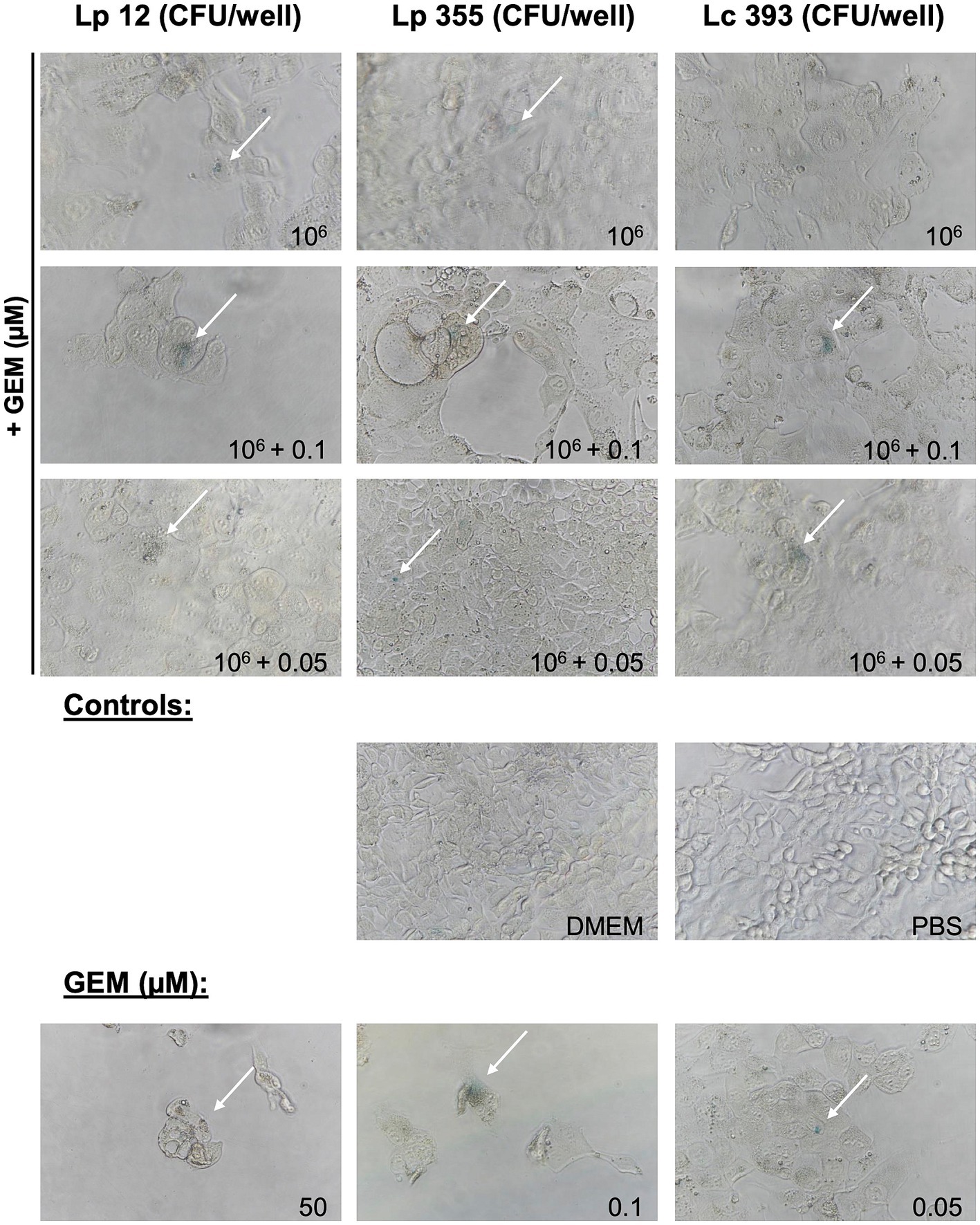
Figure 6. Live suspensions Lp 12, Lp 355 and Lc 393 and their combination with gemcitabine induced cellular senescence. SA-β-Gal assay was performed 48 h post-incubation. Shown are representative bright field microscopy pictures (objective 40x). Senescent (blue) cells are depicted with the white arrows.
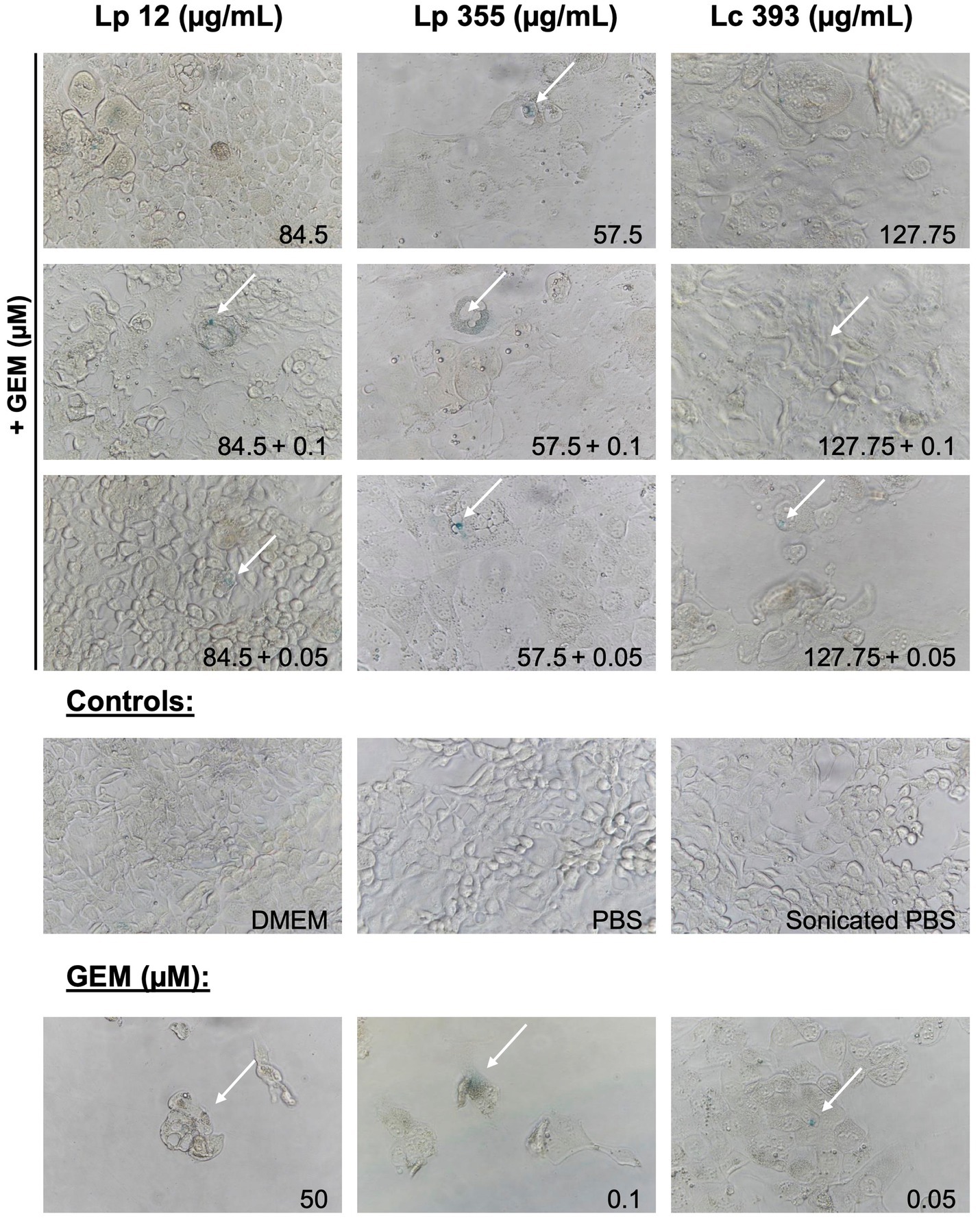
Figure 7. Sonicated extracts Lp 12, Lp 355 and Lc 393 and their combination with gemcitabine induced cellular senescence. SA-β-Gal assay was performed 48 h post-incubation. Shown are representative bright field microscopy pictures (objective 40x). Senescent (blue) cells are depicted with the white arrows.
3.1.5 Lactobacilli induced early apoptosis while inhibiting CCA cell lines
In our next step, we performed FACS analysis to further investigate the mechanism behind the inhibition of CCA cells. We employed a gating strategy shown in Supplementary Figure S3. Based on the gating strategy, we detected four populations: (1) early apoptosis intermediate, characterized as 7AAD− Annexin V PEintermediate; (2) early apoptosis high, characterized as 7AAD− Annexin V PEhigh; (3) late apoptosis, characterized as 7AAD+ Annexin V PE+; and (4) necroptosis, characterized as 7AAD+ Annexin V PE− (Supplementary Figure S3). As expected, the standard therapy (gemcitabine monotherapy 50 μM) induced mostly early (intermediate and high) apoptosis in CCA (Figures 8A–D). The monotherapy of sonicated extracts Lp 12, Lp 355, and Lc 393 induced early apoptosis intermediate in a dose-dependent manner (Figure 8D). However, monotherapy of live suspension Lp 12, Lp 355, and Lc 393 induced the strongest early apoptosis intermediate even more than gemcitabine monotherapy at 50 μM (Figure 8D). Little or no cells were seen in the late apoptosis; necroptosis phase and early apoptosis-high (Figures 8A–D, respectively) in cells treated with bacterial live suspension or sonicated extracts. The combination therapy of live suspension Lp 355 and Lc 393 with 0.1 gemcitabine induced the strongest early apoptosis high (Figure 8C) and the combination therapy of live suspension Lc 393 with 0.1 μM gemcitabine induced the strongest late apoptosis (Figure 8A).
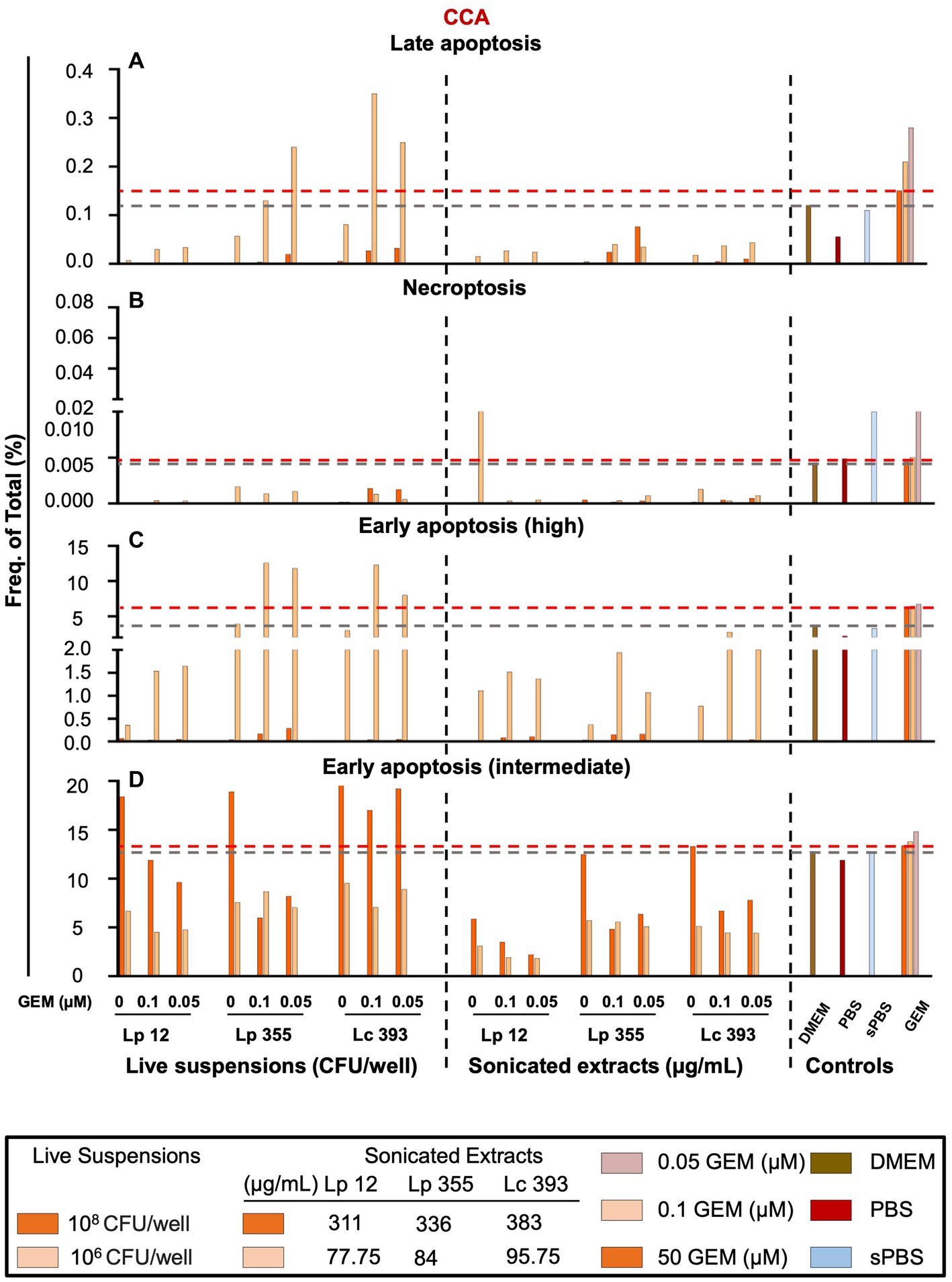
Figure 8. Live suspension Lp 355 induced mostly early apoptosis in CCA cells. Necroptosis, late apoptosis, early apoptosis (intermediate) and early apoptosis (high) were analyzed using the gating strategy depicted in Supplementary Figure S3. Cells were gated using forward- and side-scatter characteristics while avoiding duplicates. The results of FACS analysis showing the frequency of total cells in percent of (A) late apoptosis, (B) necroptosis, (C) early apoptosis (high), and (D) early apoptosis (intermediate) populations. GEM, gemcitabine. The red dashed line shows the data obtained in the control group treated with the standard therapy (gemcitabine 50 μM).
3.2 Anticancer activity screening in HCC cells
3.2.1 Live suspension and sonicated extracts of Lp 12, Lp 355 and Lc 393 monotherapy inhibited the growth of HCC
We further tested the efficacy of lactobacilli in HCC cell line using the same experimental layout (Figure 1). In HCC settings, the live suspension at the highest concentration of 108 CFU/well in all three strains, Lp 12, Lp 355, and Lc 393, proved to be more effective than the standard chemotherapy for HCC, sorafenib, at human plasma concentration (13.8 μM) (Supplementary Figures S4A–D). For sonicated extracts, Lp 12 (509, 254.5 μg/mL), Lp 355 (714, 357, 178.5 μg/mL), and Lc 393 (712, 356, and 178 μg/mL) exhibited a complete inhibition of HCC cell line growth when used as monotherapy even more than monotherapy with sorafenib at human plasma concentration (13.8 μM) (Supplementary Figures S5A–D). Further, a combination with sorafenib at concentrations 6.9 and 3.45 μM demonstrated a comparable inhibitory effect, as monotherapy, especially after 48 h of incubation (Supplementary Figures S4C,D).
Heat-killed suspensions of all strains did not demonstrate an inhibitory effect on HCC cells (Supplementary Figures S6A–D). The HCC growth was almost similar to the growth detected in negative control groups.
3.2.2 A combination of live suspension or sonicated extracts of Lp 12, Lp 355, and Lc 393 with sorafenib inhibited HCC growth
We further tested cell viability using CCK-8 assay in HCC upon lactobacilli treatment. Lp 12, Lp 355 inhibited HCC cell growth in the two highest concentrations 107 CFU/well and 108 CFU/well, even more than caused by sorafenib at human plasma concentration (13.8 μM) (Supplementary Figure S7A). Whereas Lc 393 showed similar effect as sorafenib 13.8 μM at concentration 108 CFU/well (Supplementary Figure S7A). In the case of combination therapy all three strains with either sorafenib 6.9 or 3.45 μM inhibited the growth of HCC more efficient than sorafenib 13.8 μM (Supplementary Figure S7B).
The sonicated extracts of Lp 12, Lp 355, and Lc 393 at 48 h as monotherapy or in combination performed better than live suspension and even sorafenib at human plasma concentration 13.8 μM (Supplementary Figures S7C,D).
3.2.3 Live suspension of Lp 12 in monotherapy and in combination with sorafenib induced cellular senescence in HCC cell line
We aimed further to test, whether lactobacilli alone or in combination with sorafenib might result in cellular senescence induction and performed a SA-β-Gal staining in the HCC cell line after the treatments. The live suspension of Lp 12 induced senescence in HCC cells at a concentration of 108, 107, and 106 CFU/well and also in combination with sorafenib at 6.9 μM (Supplementary Figure S8). The detected senescence response was comparable to the ones detected in sorafenib of different concentrations. Similarly, the combination therapy with sorafenib also induced senescence (Supplementary Figure S8). Senescent cells were absent in all negative controls: PBS, sonicated PBS, and DMEM.
4 Discussion
In this study, we investigated the anti-cancer efficacy of two strains of L. plantarum, Lp 12 and Lp 355 in the two prominent types of PLC, HCC, and CCA, in vitro. The strains were administered as live suspensions, or sonicated extracts, or heat-killed suspensions. The treatments were used either as monotherapy or as combination therapy with the standard therapeutics, sorafenib and gemcitabine and the plasma concentration of both chemotherapeutics used as control, respectively. The obtained results were compared to the classical probiotic strain L. casei (Lc 393).
The findings of this study revealed the significant inhibitory effect of sonicated extracts and live suspensions of Lp 12, Lp 355, and Lc 393 on HCC and CCA cell lines which provides valuable insights into the potential therapeutic application as an alternative or complementary therapy. The CVSA result of our investigation has shown that the live suspension of Lp 12, Lp 355, and Lc 393 at a concentration of 108 CFU/mL is capable of inhibiting the growth of both CCA and HCC after 48 h of treatment even better than gemcitabine and sorafenib at the respective human plasma concentration. Also, the sonicated extracts of Lp 12 at 311 μg/mL, Lp 355 at 336 μg/mL, and Lc 393 at 383 μg/mL and 191.5 μg/mL were able to inhibit the growth of CCA. In the case of HCC, the sonicated extracts of all three strains were able to inhibit HCC growth although with a corresponding effect on fibroblasts.
Importantly, sonicated extracts of L. plantarum were more effective than the live suspension as proven in our results. The impact of the sonicated extracts of L. plantarum on HCC and CCA depended on concentration - higher concentrations could inhibit cell proliferation and even lead to early apoptosis; however, under low concentration, the efficacy is limited. These results suggest the optimal concentration of the L. plantarum sonicated extracts that is essential to inhibit cancer cell growth. The sonicated extract contains a complex mixture of different components including cell walls, peptidoglycan, and several other proteins (Han et al., 2013). Various researches on the potential effect of different components of the sonicated extracts on different diseases were described, however, there is no extensive research conducted on the effect on cancer cells. The sonicated extracts of L. reuteri have been reported to enhance the wound healing process by regulating PI3K/AKT/ß-catenin/TGFß1 pathways (Han et al., 2019). Similarly, nisin which is a protein present in the cytoplasmic extract of sonicated Lactococcus lactis subsp. lactis and its cell wall have antiproliferative and antitumor effects by decreasing cyclin D1 in SW480 colon cancer cell lines (Hosseini et al., 2020). The inhibitory potency displayed by our sonicated extracts of lactobacilli on HCC and CCA is not farfetched as it correlates and agrees with other previous research studies highlighted above. For the future, we plan to perform a liquid chromatography with tandem mass spectrometry to fractionate the composition of the sonicated extracts and test effect of individual components on CCA and HCC cell line to identify the most potent metabolites.
It was highly unexpected that heat-killed suspensions demonstrated a total lack of capacity to inhibit the growth of HCC and CCA cell lines. It could be that the heat-killed suspensions may possess some growth factors which might have allowed or even enhance the growth of cancer cells. The heat-killed suspensions component may include cell wall components, lipoteichoic acids, peptidoglycan, surface proteins, and polysaccharides (Aiba et al., 2017). In contrast to our results, the whole peptidoglycan extracted from L. paracasei was reported to have cytotoxic activity against colon cancer HT-29 cells by upregulating proapoptotic genes and downregulating antiapoptotic genes (Wang et al., 2018). In another study where the anti-tumor effect of heat-killed L. reuteri and L. casei was tested on human colorectal carcinoma, it was proven that the extracts have a moderate cytotoxic and apoptotic effect in vitro as against when it was orally administered to a xenograft model bearing RKO cells (Kim et al., 2022). Also, the bacteria cells were heat-killed at 100°C for 30 min as against our regimen of 95°C for 1 h. This could have impacted the disparity in the results obtained. Our data in vitro is further supported by the in vivo data of Si et al., who showed that an oral administration of heat-killed L. rhamnosus monotherapy failed to reduce the tumor volume in MC38 tumor-bearing mice and even failed to improve the efficacy of anti-PD-1 antibody treatment when combined (Si et al., 2022). All these suggest that the anticancer property of heat-killed suspensions vary among different species of Lactobacillus, the route of administration, the conditions of heat inactivation, and differences in cell lines.
Furthermore, in our results, the CCK-8 assay clearly showed that the combination therapy of live suspension and sonicated Lp 12, Lp 355, and Lc 393 with 0.1 μM gemcitabine displayed a clear inhibitory effect in CCA more than their individual and gemcitabine monotherapy. In the same vein, mostly the sonicated extracts of Lp 12, Lp 355, and Lc 393 were able to have an inhibitory effect more than sorafenib monotherapy. This result proves our hypothesis that combination therapy will be more beneficial and will reduce the side effects of standard chemotherapeutics because it will allow usage at a reduced concentration. The sonicated extracts or live suspension of Lp 12, Lp 355, and Lc 393 can be used as a complementary therapy to reduce the side effects of sorafenib. A combination therapy that will ensure optimal efficacy with a reduced dosage of standard chemotherapy will be a promising approach in cancer treatment. Sorafenib, which is the standard chemotherapeutic for HCC has a lot of adverse effects which include hand-foot skin reaction, rash, hypertension, diarrhea, fatigue, anorexia, thrombocytopenia, alopecia, and bilirubin elevation (Cheng et al., 2009; Llovet et al., 2008). On the other hand, gemcitabine, the standard chemotherapeutic for CCA is also associated with some adverse reactions such as myelosuppression, pulmonary toxicity, and capillary leak syndrome (Mertz et al., 2019; Turco et al., 2015). In previous studies, there are reports that the gut microbiota possesses the ability to modulate the efficacy and toxic profile of cancer chemotherapy: the gut microbiota after treatment with L. rhamnosus GG supplement was able to reduce chemotherapy of colorectal cancer-associated diarrhea and abdominal discomfort with no lactobacillus-related toxicity (Alexander et al., 2017). Also, another study by Rodriguez-Arrastia et al. highlighted the significance of probiotic supplements mainly of Lactobacillus species in addressing the related side effects caused by varying treatments in oncology patients (Rodriguez-Arrastia et al., 2021). Also, Rodriguez-Arrastia et al. studies substantiate the hypothesis and suggest that combination therapy will provide more benefit than monotherapy because it combines both the curative effect of standard chemotherapeutics and lactobacilli (Rodriguez-Arrastia et al., 2021).
Based on our results, we further were able to ascertain that the live suspensions and sonicated extracts of L. plantarum were able to induce cellular senescence. Cellular senescence is a stable exit from the cell cycle caused by different intrinsic and extrinsic factors (Campisi and d'Adda di Fagagna, 2007), but in our research it was caused by the therapeutic effect of L. plantarum. Modulation of cellular senescence by probiotics especially the bacteria of the genus Lactobacillus has been studied extensively in the context of aging and longevity owing to their anti-inflammatory, anti-oxidant, and anti-immunosenescence properties (Sharma and Padwad, 2020). However, there is no scientific literature that has provided evidence of probiotics and their metabolites inducing cellular senescence in cancer cells. Our study shows it for the first time. Senescent cells are metabolically viable and may persist indefinitely, and are typically arrested at the G1 or G2/M phases of the cell cycle (Campisi and d'Adda di Fagagna, 2007). Interestingly, all concentrations of live suspension and sonicated extracts of Lp 12 were able to induce senescence in CCA cells and the live suspension of Lp 12 in HCC cells. However, cellular senescence induction was more apparent in the live suspension monotherapy of Lp 12 at 107 CFU/well in both CCA and HCC cells, sonicated extract monotherapy of Lp 12 at 77.75 μg/mL in CCA cells, as well as the combination therapy of live suspension of Lp 12 at 106 CFU/mL and 0.05 μM gemcitabine in CCA cells. The observation that lower concentrations of Lp 12 induce senescence suggests the potential benefit of therapy-induced senescence. Studies have suggested that senescent cells can facilitate the expression of cytokines and factors that may inhibit the growth of surrounding tumor cells (Di et al., 2008; Kang et al., 2011; Xue et al., 2007). Data from a recent study also demonstrated that senescent cells owing to their long-term persistence in vivo are strongly immunogenic, activate interferon signaling, antigen-presenting cells, CD8 T cells, and MHC class I molecule through the development of a proinflammatory secretome known as senescence-associated secretory phenotype (Eggert et al., 2016; Marin et al., 2023; Yevsa et al., 2012).
Lactobacillus species significantly modulate and regulate the immune system (Parada Venegas et al., 2019; Wiese-Szadkowska et al., 2019). They can enhance the proliferation of T lymphocytes and strengthen the killing ability of natural killer cells (Meng et al., 2018), enhance the phagocytic abilities of macrophages (Jung et al., 2015), and the maturation of B cells (Garcia-Castillo et al., 2019). The modulation of the immune system is essential in preventing excessive inflammation and fighting infection (Iddir et al., 2020). Furthermore, lactobacilli also help to maintain the integrity of the intestinal epithelial barrier by protecting against injuries induced by pathogenic bacteria by increasing transepithelial electrical resistance, downregulation of permeability of IL-8, and regulation of extracellular signal-regulated kinase and c-jun N-terminal kinase (Yu et al., 2015). Regarding the above-discussed health benefit of lactobacilli, it is indispensable to study its anticancer potential in PLC.
The anticancer effect of lactobacilli has been investigated in the past. L. plantarum has been reported to reduce oxidative stress and prevent the development and progression of cancer (Kullisaar et al., 2002; Wu et al., 2021), but a comprehensive understanding of their efficacy in PLC is needed. Most importantly, our study further unraveled the mechanism of action of lactobacilli in inhibiting the growth of PLC cells. We could prove that induction of early apoptosis was the main mechanism behind the inhibitory capacity of live suspension and sonicated extracts of lactobacilli in CCA cells. In line with the result of our study, the cytotoxicity effect of L. plantarum treatment on breast, colorectal, liver, and leukemia cancer cell lines was mediated by the induction of apoptosis (Chuah et al., 2019). Recently, it has been demonstrated that the live suspension of L. paracasei X12 had a tumor suppressive effect on colorectal cancer by preventing weight loss and decreasing tumor volume; downregulated proliferation inducer and anti-apoptotic like Bcl-3, Jak-1, and Akt-1 genes and upregulated pro-apoptotic genes like Bax, Cas-3 in a DMH-induced rat model (Jam et al., 2020). Another study reported that the live suspension of L. casei co-incubated with colon cancer cells resulted in decreased viability and induction of apoptosis (Tiptiri-Kourpeti et al., 2016). This was further confirmed in vivo after daily oral administration for 13 days (Tiptiri-Kourpeti et al., 2016). In line with our data, studies have demonstrated that L. plantarum induces apoptosis and prevents metastasis and invasion of cancer cells (Belury, 2002; Choi et al., 2006). Furthermore, there is an established correlation between the quality of the microbiome and tumor progression (Dzutsev et al., 2017). Dysbiosis or an imbalance in the composition of the gut microbiota has a direct negative implication on liver health by increasing metabolic disorders and the abundance of pathogenic bacteria causing inflammation and subsequently leading to chronic liver disease and progression to PLC, including HCC and CCA (Chen et al., 2020). L. plantarum has been shown to improve the gut microbiome and to inhibit the proliferation of potentially pathogenic bacteria (Hong et al., 2021). By restoring the healthy gut microbiota composition, L. plantarum can potentially mitigate the risk of liver cancer progression from dysbiosis (Yu and Schwabe, 2017). It has also been demonstrated that the soluble polysaccharides isolated from L. acidophilus 606 resulted in the death of HT-29 cancer cells attributed to the induction of apoptosis and antioxidative activity (Choi et al., 2006). Moreover, L. acidophilus has been shown to suppress metabolic dysfunction-associated steatotic malignant liver disease through producing of valeric acid (Lau et al., 2024). Similarly, mitochondrial apoptotic pathways were activated, leading to the death of colorectal C26 cancer cells when treated with L. plantarum suspension (Sharifi et al., 2022). The cell-free extract of L. plantarum tested on high and low metastatic human malignant melanoma cells on a mice model induced intrinsic apoptosis by regulating Bax/Bcl-2 ratio (Park et al., 2020). Also, Sun et al. proved that the crude exopolysaccharide of L. plantarum has an inhibitory effect on human colon cancer lines by increasing the pro-apoptotic protein level (Sun et al., 2021). Finally, lactobacilli were also demonstrated to cause a robust antitumor effect by reducing IL-17, an inflammatory cytokine, and other angiogenesis factors resulting in inhibition of HCC growth in mice by 40% (Li et al., 2016). Also, the positive role of lactobacilli consumption in inhibiting colorectal cancer and HCC induced in mice through the stimulation and expression of miRNAs was described (Heydari et al., 2019).
In summary, the live suspension and sonicated extracts of Lp 12 and Lp 355 demonstrated strong inhibitory properties in both CCA and HCC in a dose-dependent manner and were either comparable to, or showed even more efficacy than a classical probiotic strain Lc 393, which served as a control in this study. At the same time Lp 12 and Lp 355 showed less toxic effects on fibroblast cells, than Lc 393. Surprisingly, the heat-killed suspensions of Lp 12, Lp 355, and Lc 393 displayed no inhibitory effect in HCC and CCA. Furthermore, the combination of live suspensions or sonicated extracts of Lp 12, Lp 355, and Lc 393 with standard chemotherapeutics (sorafenib for HCC and gemcitabine for CCA) demonstrated a synergistic inhibitory effect comparing to the individual monotherapy and the human plasma concentration of sorafenib and gemcitabine. The combination therapy allows for a reduced concentration of standard chemotherapeutics, consequently savaging the adverse effect. We have also shown that Lp 12 can induce cellular senescence in both cancer cell lines which in turn could translate to the activation of immune cells. The mechanism of action of the lactobacilli was shown to be associated with the induction of early apoptosis in CCA. The most promising therapeutic candidates for CCA are the live suspensions of Lp 12 and Lp 355 at a concentration of 108 CFU/mL, combination therapy of live suspension of Lp 12 and gemcitabine at 0.1 μM, and also, the sonicated extracts of Lp 12 and Lp 355 at 311 μg/mL and 336 μg/mL, respectively. The most promising therapies for HCC are the sonicated extracts of Lp 355 and Lc 393 at 178 μg/mL and in combination with sorafenib at 6.9 μM. More intensive research is needed to advance this therapy development studies and further investigate the safety, efficacy, and immune responses in vivo applying monotherapeutic or combination therapy regimes with L. plantarumin HCC and CCA using preclinical models.
5 Conclusion
We found that both tested Lactobacillus strains – L. plantarum ONU 12 (Lp 12) and L. plantarum ONU 355 (Lp 355) showed potent anti-cancerous capacity toward CCA and HCC cell lines in vitro. By combining these strains with traditional chemotherapy drugs, we were able to significantly reduce the concentration of gemcitabine and sorafenib. Our results suggest that the mechanism behind this successful inhibition involves the induction of early apoptosis and cellular senescence. However, in vivo studies are necessary to evaluate the efficacy of L. plantarum strains and their mechanisms of action in autochthonous HCC and CCA models. Combination therapies that demonstrate synergistic effects, thereby reducing the doses of toxic chemotherapy drugs, hold great promise for patients with HCC and CCA.
Data availability statement
The original contributions presented in the study are included in the article/Supplementary material, further inquiries can be directed to the corresponding author.
Ethics statement
Ethical approval was not required for the studies on animals in accordance with the local legislation and institutional requirements because only commercially available established cell lines were used.
Author contributions
OPD: Writing – original draft, Validation, Methodology, Investigation, Formal analysis. KP: Data curation, Writing – original draft, Validation, Investigation, Formal analysis. NL: Methodology, Funding acquisition, Conceptualization, Writing – original draft, Validation, Investigation, Formal analysis, Data curation. SK: Writing – original draft, Investigation, Formal analysis. NP: Writing – original draft, Methodology, Investigation, Funding acquisition, Formal analysis, Data curation. HS: Supervision, Methodology, Writing – original draft, Investigation. TG: Funding acquisition, Conceptualization, Writing – original draft, Supervision, Investigation. VI: Supervision, Writing – original draft, Funding acquisition. TY: Writing – review & editing, Writing – original draft, Validation, Supervision, Resources, Project administration, Methodology, Investigation, Funding acquisition, Formal analysis, Data curation, Conceptualization.
Funding
The author(s) declare that financial support was received for the research, authorship, and/or publication of this article. TY acknowledges the support by the German Academic Exchange Service (DAAD), funding program “Ensuring academic success in times of crisis (2022–2024, Project IDs: 57651454, 57678239, and 57722948). NL acknowledges the support by the DAAD, funding program “Research Stays for University Academics and Scientists” (2021, Project ID 57552334). This work was also supported in part by the Niedersächsische Krebsgesellschaft e.V. and the Foundation MHH PLUS. This work was supported in part by the DAAD in the scope of the Doctoral Program in Germany 2019/2024 (Project ID 91736778 to NP).
Acknowledgments
We thank our colleague Dr. Andrii Merlich (Department of Microbiology, Virology, and Biotechnology, Odesa I. I. Mechnykov National University, Odesa, Ukraine) for his help with the isolation of lactobacilli strains and Dr. Inga Hochnadel (Department of Gastroenterology, Hepatology, Infectious Diseases, and Endocrinology, Hannover Medical School, Hannover, Germany) for a technical help.
Conflict of interest
The authors declare that the research was conducted in the absence of any commercial or financial relationships that could be construed as a potential conflict of interest.
Publisher’s note
All claims expressed in this article are solely those of the authors and do not necessarily represent those of their affiliated organizations, or those of the publisher, the editors and the reviewers. Any product that may be evaluated in this article, or claim that may be made by its manufacturer, is not guaranteed or endorsed by the publisher.
Supplementary material
The Supplementary material for this article can be found online at: https://www.frontiersin.org/articles/10.3389/fmicb.2024.1451170/full#supplementary-material
Abbreviations
Annexin V-PE, annexin V-phycoerythrin; 7-AAD, 7-amino-actinomycin D; CCA, cholangiocarcinoma; CCK-8, cell counting kit-8; CVSA, crystal violet staining assay; DMEM, Dulbecco’s Modified Eagle Medium; FACS, fluorescence-activated cell sorting (flow cytometry analysis); HCC, hepatocellular carcinoma; H (h), hours (hours); Lp 12, Lactiplantibacillus plantarum ONU 12; Lp 355, Lactiplantibacillus plantarum ONU 355; Lc 393, Lacticaseibacillus casei ATCC 393; MRS, Man Rogosa and Sharpe medium; NaCl, sodium chloride; OD, optical density; PBS, phosphate buffered saline; sPBS, sonicated PBS; hPBS, heated PBS; PLC, primary liver cancer; SA-β-Gal, senescence-associated β-galactosidase assay; S.E.M., standard error of the mean
References
Agaliya, P. J., and Jeevaratnam, K. (2012). Screening of Lactobacillus plantarum isolated from fermented idli batter for probiotic properties. Afr. J. Biotechnol. 11, 12856–12864. doi: 10.5897/AJB12.1825
Aiba, Y., Ishikawa, H., Tokunaga, M., and Komatsu, Y. (2017). Anti-Helicobacter pylori activity of non-living, heat-killed form of lactobacilli including Lactobacillus johnsonii no. 1088. FEMS Microbiol. Lett. 364:fnx102. doi: 10.1093/femsle/fnx102
Alexander, J. L., Wilson, I. D., Teare, J., Marchesi, J. R., Nicholson, J. K., and Kinross, J. M. (2017). Gut microbiota modulation of chemotherapy efficacy and toxicity. Nat. Rev. Gastroenterol. Hepatol. 14, 356–365. doi: 10.1038/nrgastro.2017.20
Anderson, J. W., and Gilliland, S. E. (1999). Effect of fermented milk (yogurt) containing Lactobacillus acidophilus L1 on serum cholesterol in hypercholesterolemic humans. J. Am. Coll. Nutr. 18, 43–50.
Banales, J. M., Marin, J. J. G., Lamarca, A., Rodrigues, P. M., Khan, S. A., and Roberts, L. R. (2020). Cholangiocarcinoma 2020: the next horizon in mechanisms and management. Nat. Rev. Gastroenterol. Hepatol. 17:et al., 557–588. doi: 10.1038/s41575-020-0310-z
Belury, M. A. (2002). Inhibition of carcinogenesis by conjugated linoleic acid: potential mechanisms of action. J. Nutr. 132, 2995–2998. doi: 10.1093/jn/131.10.2995
Berbegal, C., Peña, N., Russo, P., Grieco, F., Pardo, I., Ferrer, S., et al. (2016). Technological properties of Lactobacillus plantarum strains isolated from grape must fermentation. Food Microbiol. 57, 187–194. doi: 10.1016/j.fm.2016.03.002
Bushnell, T. (editor). (2015). How to use flow cytometry to measure apoptosis, necrosis, and autophagy. Washington: Expert Cytometry LLC.
Cahu, J., and Sola, B. (2013). A sensitive method to quantify senescent cancer cells. JoVE 78. doi: 10.3791/50494
Campisi, J., and d'Adda di Fagagna, F. (2007). Cellular senescence: when bad things happen to good cells. Nat. Rev. Mol. Cell Biol. 8, 729–740. doi: 10.1038/nrm2233
Chan, W. K., Chuah, K. H., Rajaram, R. B., Lim, L. L., Ratnasingam, J., and Vethakkan, S. R. (2023). Metabolic dysfunction-associated Steatotic liver disease (MASLD): a state-of-the-art review. J. Obes. Metab. Syndr. 32, 197–213. doi: 10.7570/jomes23052
Chen, X., Li, P., Liu, M., Zheng, H., He, Y., Chen, M.-X., et al. (2020). Gut dysbiosis induces the development of pre-eclampsia through bacterial translocation. Gut 69, 513–522. doi: 10.1136/gutjnl-2019-319101
Cheng, A.-L., Kang, Y.-K., Chen, Z., Tsao, C.-J., Qin, S., Kim, J. S., et al. (2009). Efficacy and safety of sorafenib in patients in the Asia-Pacific region with advanced hepatocellular carcinoma: a phase III randomised, double-blind, placebo-controlled trial. Lancet Oncol. 10, 25–34. doi: 10.1016/S1470-2045(08)70285-7
Choi, S. S., Kim, Y., Han, K. S., You, S., Oh, S., and Kim, S. H. (2006). Effects of Lactobacillus strains on cancer cell proliferation and oxidative stress in vitro. Lett. Appl. Microbiol. 42, 452–458. doi: 10.1111/j.1472-765X.2006.01913.x
Chuah, L.-O., Foo, H. L., Loh, T. C., Mohammed Alitheen, N. B., Yeap, S. K., Abdul Mutalib, N. E., et al. (2019). Postbiotic metabolites produced by Lactobacillus plantarum strains exert selective cytotoxicity effects on cancer cells. BMC Complement. Altern. Med. 19, 1–12. doi: 10.1186/s12906-019-2528-2
Di, X., Bright, A. T., Bellott, R., Gaskins, E., Robert, J., Holt, S., et al. (2008). A chemotherapy-associated senescence bystander effect in breast cancer cells. Cancer Biol. Ther. 7, 864–872. doi: 10.4161/cbt.7.6.5861
Dzutsev, A., Badger, J. H., Perez-Chanona, E., Roy, S., Salcedo, R., Smith, C. K., et al. (2017). Microbes and cancer. Annu. Rev. Immunol. 35, 199–228. doi: 10.1146/annurev-immunol-051116-052133
Eggert, T., Wolter, K., Ji, J., Ma, C., Yevsa, T., Klotz, S., et al. (2016). Distinct functions of senescence-associated immune responses in liver tumor surveillance and tumor progression. Cancer Cell 30, 533–547. doi: 10.1016/j.ccell.2016.09.003
El-Serag, H. B., and Rudolph, K. L. (2007). Hepatocellular carcinoma: epidemiology and molecular carcinogenesis. Gastroenterology 132, 2557–2576. doi: 10.1053/j.gastro.2007.04.061
Fiocco, D., Capozzi, V., Collins, M., Gallone, A., Hols, P., Guzzo, J., et al. (2010). Characterization of the CtsR stress response regulon in Lactobacillus plantarum. J. Bacteriol. 192, 896–900. doi: 10.1128/JB.01122-09
Forner, A., Reig, M., and Bruix, J. (2018). Hepatocellular carcinoma. Lancet 391, 1301–1314. doi: 10.1016/S0140-6736(18)30010-2
Garcia-Castillo, V., Komatsu, R., Clua, P., Indo, Y., Takagi, M., Salva, S., et al. (2019). Evaluation of the immunomodulatory activities of the probiotic strain Lactobacillus fermentum UCO-979C. Front. Immunol. 10:1376. doi: 10.3389/fimmu.2019.01376
Gromowski, T., Lukacs-Kornek, V., and Cisowski, J. (2023). Current view of liver cancer cell-of-origin and proposed mechanisms precluding its proper determination. Cancer Cell Int. 23:3. doi: 10.1186/s12935-022-02843-0
Haghshenas, B., Nami, Y., Kiani, A., Moazami, N., and Tavallaei, O. (2023). Cytotoxic effect of potential probiotic Lactiplantibacillus plantarum KUMS-Y8 isolated from traditional dairy samples on the KB and OSCC human cancer cell lines. Heliyon 9:e20147. doi: 10.1016/j.heliyon.2023.e20147
Han, N., Jia, L., Su, Y., Du, J., Guo, L., Luo, Z., et al. (2019). Lactobacillus reuteri extracts promoted wound healing via PI3K/AKT/β-catenin/TGFβ1 pathway. Stem Cell Res Ther 10, 1–11. doi: 10.1186/s13287-019-1324-8
Han, D. J., Kim, J. B., Park, S. Y., Yang, M. G., and Kim, H. (2013). Growth inhibition of hepatocellular carcinoma Huh7 cells by Lactobacillus casei extract. Yonsei Med. J. 54:1186. doi: 10.3349/ymj.2013.54.5.1186
Heydari, Z., Rahaie, M., and Alizadeh, A. M. (2019). Different anti-inflammatory effects of Lactobacillus acidophilus and Bifidobactrum bifidioum in hepatocellular carcinoma cancer mouse through impact on microRNAs and their target genes. J. Nutr. Intermed. Metab. 16:100096. doi: 10.1016/j.jnim.2019.100096
Hong, D.-K., Yoo, M.-S., Heo, K., Shim, J.-J., and Lee, J.-L. (2021). Effects of L. plantarum HY7715 on the gut microbial community and riboflavin production in a three-stage semi-continuous simulated gut system. Microorganisms 9:2478. doi: 10.3390/microorganisms9122478
Hosseini, S. S., Goudarzi, H., Ghalavand, Z., Hajikhani, B., Rafeieiatani, Z., and Hakemi-Vala, M. (2020). Anti-proliferative effects of cell wall, cytoplasmic extract of Lactococcus lactis and nisin through down-regulation of cyclin D1 on SW480 colorectal cancer cell line. Iran. J. Microbiol. 12, 424–430. doi: 10.18502/ijm.v12i5.4603
Iddir, M., Brito, A., Dingeo, G., Fernandez Del Campo, S. S., Samouda, H., La Frano, M. R., et al. (2020). Strengthening the immune system and reducing inflammation and oxidative stress through diet and nutrition: considerations during the COVID-19 crisis. Nutrients 12:1562. doi: 10.3390/nu12061562
Jam, S. A. M., Morshedi, M., Khosroushahi, A. Y., Eftekharsadat, A. T., Alipour, M., and Alipour, B. (2020). Preventive and tumor-suppressive effects of Lactobacillus paracasei X12 in rat model of colorectal cancer. Iran. J. Pharmaceut. Res. 19:330. doi: 10.22037/ijpr.2019.112135.13547
Jiang, C., Sun, X. D., Qiu, W., Chen, Y. G., Sun, D. W., and Lv, G. Y. (2023). Conversion therapy in liver transplantation for hepatocellular carcinoma: What's new in the era of molecular and immune therapy? Hepatobiliary Pancreat. Dis. Int. 22, 7–13. doi: 10.1016/j.hbpd.2022.10.006
Jung, J. Y., Shin, J. S., Lee, S. G., Rhee, Y. K., Cho, C. W., Hong, H. D., et al. (2015). Lactobacillus sakei K040706 evokes immunostimulatory effects on macrophages through TLR 2-mediated activation. Int. Immunopharmacol. 28, 88–96. doi: 10.1016/j.intimp.2015.05.037
Kang, T.-W., Yevsa, T., Woller, N., Hoenicke, L., Wuestefeld, T., Dauch, D., et al. (2011). Senescence surveillance of pre-malignant hepatocytes limits liver cancer development. Nature 479, 547–551. doi: 10.1038/nature10599
Kazmierczak-Siedlecka, K., Daca, A., Folwarski, M., Witkowski, J. M., Bryl, E., and Makarewicz, W. (2020). The role of Lactobacillus plantarum 299v in supporting treatment of selected diseases. Cent. Eur. J. Immunol. 45, 488–493. doi: 10.5114/ceji.2020.101515
Kim, S.-J., Kang, C.-H., Kim, G.-H., and Cho, H. (2022). Anti-tumor effects of heat-killed L. reuteri MG5346 and L. casei MG4584 against human colorectal carcinoma through caspase-9-dependent apoptosis in xenograft model. Microorganisms 10:533. doi: 10.3390/microorganisms10030533
Kullisaar, T., Zilmer, M., Mikelsaar, M., Vihalemm, T., Annuk, H., Kairane, C., et al. (2002). Two antioxidative lactobacilli strains as promising probiotics. Int. J. Food Microbiol. 72, 215–224. doi: 10.1016/S0168-1605(01)00674-2
Lau, H. C., Zhang, X., Ji, F., Lin, Y., Liang, W., Li, Q., et al. (2024). Lactobacillus acidophilus suppresses non-alcoholic fatty liver disease-associated hepatocellular carcinoma through producing valeric acid. EBioMedicine 100:104952. doi: 10.1016/j.ebiom.2023.104952
Li, J., Sung, C. Y. J., Lee, N., Ni, Y., Pihlajamäki, J., Panagiotou, G., et al. (2016). Probiotics modulated gut microbiota suppresses hepatocellular carcinoma growth in mice. Proc. Natl. Acad. Sci. 113, E1306–E1315. doi: 10.1073/pnas.1518189113
Limanska, N., Merlich, A., Galkin, M., Vasylieva, N., Choiset, Y., Ivanytsia, T., et al. (editors). (2019). Biofilm formation and genetic diversity of Lactobacillus plantarum strains originated from France and Ukraine 8, 1326–1331. doi: 10.15414/jmbfs.2019.8.6.1326-1331
Liu, C. Y., Chen, K. F., and Chen, P. J. (2015). Treatment of liver Cancer. Cold Spring Harb. Perspect. Med. 5:a021535. doi: 10.1101/cshperspect.a021535
Liu, Y., Tran, D. Q., and Rhoads, J. M. (2018). Probiotics in disease prevention and treatment. J. Clin. Pharmacol. 58, S164–S179. doi: 10.1002/jcph.1121
Liu, Y., Zheng, J., Hao, J., Wang, R. R., Liu, X., Gu, P., et al. (2022). Global burden of primary liver cancer by five etiologies and global prediction by 2035 based on global burden of disease study 2019. Cancer Med. 11, 1310–1323. doi: 10.1002/cam4.4551
Llovet, J. M., Ricci, S., Mazzaferro, V., Hilgard, P., Gane, E., Blanc, J.-F., et al. (2008). Sorafenib in advanced hepatocellular carcinoma. N. Engl. J. Med. 359, 378–390. doi: 10.1056/NEJMoa0708857
Llovet, J. M., Schwartz, M., and Mazzaferro, V. (2005). Resection and liver transplantation for hepatocellular carcinoma. Semin. Liver Dis. 25, 181–200. doi: 10.1055/s-2005-871198
Marin, I., Boix, O., Garcia-Garijo, A., Sirois, I., Caballe, A., Zarzuela, E., et al. (2023). Cellular senescence is immunogenic and promotes antitumor immunity. Cancer Discov. 13, 410–431. doi: 10.1158/2159-8290.CD-22-0523
Meng, Y., Li, B., Jin, D., Zhan, M., Lu, J., and Huo, G. (2018). Immunomodulatory activity of Lactobacillus plantarum KLDS1.0318 in cyclophosphamide-treated mice. Food Nutr. Res. 62:7800. doi: 10.29219/fnr.v62.1296
Mertz, P., Lebrun-Vignes, B., Salem, J.-E., and Arnaud, L. (2019). Characterizing drug-induced capillary leak syndromes using the World Health Organization VigiBase. J. Allergy Clin. Immunol. 143, 433–436. doi: 10.1016/j.jaci.2018.09.001
Mokoena, M. P. (2017). Lactic acid Bacteria and their Bacteriocins: classification, biosynthesis and applications against Uropathogens: a Mini-review. Molecules 22:1255. doi: 10.3390/molecules22081255
Naqi, N., Ahmad, S., Murad, S., and Khattak, J. (2014). Efficacy and safety of sorafenib-gemcitabine combination therapy in advanced hepatocellular carcinoma: an open-label phase II feasibility study. Hematol. Oncol. Stem Cell Ther. 7, 27–31. doi: 10.1016/j.hemonc.2013.11.003
Oldak, A., Zielinska, D., Lepecka, A., Dlugosz, E., and Kolozyn-Krajewska, D. (2020). Lactobacillus plantarum strains isolated from polish regional cheeses exhibit anti-staphylococcal activity and selected probiotic properties. Probiotics Antimicrob. Proteins 12, 1025–1038. doi: 10.1007/s12602-019-09587-w
Oldak, A., Zielinska, D., Rzepkowska, A., and Kolozyn-Krajewska, D. (2017). Comparison of antibacterial activity of Lactobacillus plantarum strains isolated from two different kinds of regional cheeses from Poland: Oscypek and Korycinski cheese. Biomed. Res. Int. :2017. doi: 10.1155/2017/6820369
Parada Venegas, D., De la Fuente, M. K., Landskron, G., Gonzalez, M. J., Quera, R., Dijkstra, G., et al. (2019). Short chain fatty acids (SCFAs)-mediated gut epithelial and immune regulation and its relevance for inflammatory bowel diseases. Front. Immunol. 10:277. doi: 10.3389/fimmu.2019.00277
Park, J., Kwon, M., Lee, J., Park, S., Seo, J., and Roh, S. (2020). Anti-cancer effects of Lactobacillus plantarum L-14 cell-free extract on human malignant melanoma A375 cells. Molecules 25:3895. doi: 10.3390/molecules25173895
Pylypchuk, I. V., Suo, H., Chucheepchuenkamol, C., Jedicke, N., Lindén, P. A., Lindström, M. E., et al. (2022). High-molecular-weight fractions of spruce and Eucalyptus lignin as a perspective nanoparticle-based platform for a therapy delivery in liver cancer. Front. Bioeng. Biotechnol. 9:817768. doi: 10.3389/fbioe.2021.817768
Rawla, P., Sunkara, T., Muralidharan, P., and Raj, J. P. (2018). Update in global trends and aetiology of hepatocellular carcinoma. Contemp. Oncol. 22, 141–150. doi: 10.5114/wo.2018.78941
Rodriguez-Arrastia, M., Martinez-Ortigosa, A., Rueda-Ruzafa, L., Folch Ayora, A., and Ropero-Padilla, C. (2021). Probiotic supplements on oncology patients’ treatment-related side effects: a systematic review of randomized controlled trials. Int. J. Environ. Res. Public Health 18:4265. doi: 10.3390/ijerph18084265
Schillinger, U., and Lucke, F. K. (1989). Antibacterial activity of Lactobacillus sake isolated from meat. Appl. Environ. Microbiol. 55, 1901–1906. doi: 10.1128/aem.55.8.1901-1906.1989
Seddik, H. A., Bendali, F., Gancel, F., Fliss, I., Spano, G., and Drider, D. (2017). Lactobacillus plantarum and its probiotic and food potentialities. Probiotics Antimicrob. Proteins 9, 111–122. doi: 10.1007/s12602-017-9264-z
Sharifi, E., Yazdani, Z., Najafi, M., Hosseini-khah, Z., Jafarpour, A., and Rafiei, A. (2022). The combined effect of fish oil containing Omega-3 fatty acids and Lactobacillus plantarum on colorectal cancer. Food Sci. Nutr. 10, 4411–4418. doi: 10.1002/fsn3.3037
Sharma, R., and Padwad, Y. (2020). Probiotic bacteria as modulators of cellular senescence: emerging concepts and opportunities. Gut Microbes 11, 335–349. doi: 10.1080/19490976.2019.1697148
Si, W., Liang, H., Bugno, J., Xu, Q., Ding, X., Yang, K., et al. (2022). Lactobacillus rhamnosus GG induces cGAS/STING-dependent type I interferon and improves response to immune checkpoint blockade. Gut 71, 521–533. doi: 10.1136/gutjnl-2020-323426
Śliwka, L., Wiktorska, K., Suchocki, P., Milczarek, M., Mielczarek, S., Lubelska, K., et al. (2016). The comparison of MTT and CVS assays for the assessment of anticancer agent interactions. PLoS One 11:e0155772. doi: 10.1371/journal.pone.0155772
Spyridopoulou, K., Tryfonopoulou, E., Aindelis, G., Ypsilantis, P., Sarafidis, C., Kalogirou, O., et al. (2021). Biogenic selenium nanoparticles produced by Lactobacillus casei ATCC 393 inhibit colon cancer cell growth in vitro and in vivo. Nanoscale Adv. 3, 2516–2528. doi: 10.1039/D0NA00984A
Sun, M., Liu, W., Song, Y., Tuo, Y., Mu, G., and Ma, F. (2021). The effects of Lactobacillus plantarum-12 crude exopolysaccharides on the cell proliferation and apoptosis of human colon cancer (HT-29) cells. Probiot. Antimicrobial Proteins 13, 413–421. doi: 10.1007/s12602-020-09699-8
Sung, H., Ferlay, J., Siegel, R. L., Laversanne, M., Soerjomataram, I., Jemal, A., et al. (2021). Global Cancer statistics 2020: GLOBOCAN estimates of incidence and mortality worldwide for 36 cancers in 185 countries. CA Cancer J. Clin. 71, 209–249. doi: 10.3322/caac.21660
Suo, H., Hochnadel, I., Petriv, N., Franke, R., Schmidt, J., Limanska, N., et al. (2022). Elucidating the mechanism behind and investigating the efficacy of traditional Chinese medicine and traditional Tibetan medicine in combination with standard therapeutics in hepatocellular carcinoma and cholangiocarcinoma in vitro. Front. Pharmacol. 13:906468. doi: 10.3389/fphar.2022.906468
Suresh, D., Srinivas, A. N., Prashant, A., Harikumar, K. B., and Kumar, D. P. (2023). Therapeutic options in hepatocellular carcinoma: a comprehensive review. Clin. Exp. Med. 23, 1901–1916. doi: 10.1007/s10238-023-01014-3
Swanson, K. S., Gibson, G. R., Hutkins, R., Reimer, R. A., Reid, G., Verbeke, K., et al. (2020). The international scientific Association for Probiotics and Prebiotics (ISAPP) consensus statement on the definition and scope of synbiotics. Nat. Rev. Gastroenterol. Hepatol. 17, 687–701. doi: 10.1038/s41575-020-0344-2
Tegegne, B. A., and Kebede, B. (2022). Probiotics, their prophylactic and therapeutic applications in human health development: a review of the literature. Heliyon 8:e09725. doi: 10.1016/j.heliyon.2022.e09725
Temraz, S., Nassar, F., Kreidieh, F., Mukherji, D., Shamseddine, A., and Nasr, R. (2021). Hepatocellular carcinoma immunotherapy and the potential influence of gut microbiome. Int. J. Mol. Sci. 22. doi: 10.3390/ijms22157800
Tiptiri-Kourpeti, A., Spyridopoulou, K., Santarmaki, V., Aindelis, G., Tompoulidou, E., Lamprianidou, E. E., et al. (2016). Lactobacillus casei exerts anti-proliferative effects accompanied by apoptotic cell death and up-regulation of TRAIL in colon carcinoma cells. PLoS One 11:e0147960. doi: 10.1371/journal.pone.0147960
Turco, C., Jary, M., Kim, S., Moltenis, M., Degano, B., Manzoni, P., et al. (2015). Gemcitabine-induced pulmonary toxicity: a case report of pulmonary veno-occlusive disease. Clin. Med. Insights 9, CMO.S26537–CMO.S26579. doi: 10.4137/CMO.S26537
Vermes, I., Haanen, C., Steffens-Nakken, H., and Reutellingsperger, C. (1995). A novel assay for apoptosis flow cytometric detection of phosphatidylserine expression on early apoptotic cells using fluorescein labelled annexin V. J. Immunol. Methods 184, 39–51. doi: 10.1016/0022-1759(95)00072-I
Wang, S., Han, X., Zhang, L., Zhang, Y., Li, H., and Jiao, Y. (2018). Whole peptidoglycan extracts from the Lactobacillus paracasei subsp. paracasei M5 strain exert anticancer activity in vitro. BioMed Res Int. 2018:2871710. doi: 10.1155/2018/2871710
Wang, H., Tao, Y., Li, Y., Wu, S., Li, D., Liu, X., et al. (2021). Application of ultrasonication at different microbial growth stages during apple juice fermentation by Lactobacillus plantarum: investigation on the metabolic response. Ultrason. Sonochem. 73:105486. doi: 10.1016/j.ultsonch.2021.105486
Wiese-Szadkowska, M., Helmin-Basa, A., Eljaszewicz, A., Gackowska, L., Januszewska, M., Motyl, I., et al. (2019). Selected commensal bacteria change profiles of Helicobacter pylori-induced T cells via dendritic cell modulation. Helicobacter 24:e12614. doi: 10.1111/hel.12614
Wu, J., Zhang, Y., Ye, L., and Wang, C. (2021). The anti-cancer effects and mechanisms of lactic acid bacteria exopolysaccharides in vitro: a review. Carbohydr. Polym. 253:117308. doi: 10.1016/j.carbpol.2020.117308
Xue, W., Zender, L., Miething, C., Dickins, R. A., Hernando, E., Krizhanovsky, V., et al. (2007). Senescence and tumour clearance is triggered by p53 restoration in murine liver carcinomas. Nature 445, 656–660. doi: 10.1038/nature05529
Yang, X., Zhong, Y., Wang, D., and Lu, Z. (2021). A simple colorimetric method for viable bacteria detection based on cell counting Kit-8. Anal. Methods 13, 5211–5215. doi: 10.1039/D1AY01624E
Yevsa, T., Kang, T.-W., and Zender, L. (2012). Immune surveillance of pre-cancerous senescent hepatocytes limits hepatocellular carcinoma development. Onco Targets Ther 1, 398–399. doi: 10.4161/onci.19128
Yu, L.-X., and Schwabe, R. F. (2017). The gut microbiome and liver cancer: mechanisms and clinical translation. Nat. Rev. Gastroenterol. Hepatol. 14, 527–539. doi: 10.1038/nrgastro.2017.72
Yu, Q., Yuan, L., Deng, J., and Yang, Q. (2015). Lactobacillus protects the integrity of intestinal epithelial barrier damaged by pathogenic bacteria. Front. Cell. Infect. Microbiol. 5:26. doi: 10.3389/fcimb.2015.00026
Zembruski, N. C., Stache, V., Haefeli, W. E., and Weiss, J. (2012). 7-Aminoactinomycin D for apoptosis staining in flow cytometry. Anal. Biochem. 429, 79–81. doi: 10.1016/j.ab.2012.07.005
Keywords: hepatocellular carcinoma, cholangiocarcinoma, lactobacilli, sonicated extracts, probiotics, apoptosis
Citation: Duduyemi OP, Potapenko K, Limanska N, Kotsyuda S, Petriv N, Suo H, Gudzenko T, Ivanytsia V and Yevsa T (2024) Lactiplantibacillus plantarum inhibited the growth of primary liver cancer by inducing early apoptosis and senescence, in vitro. Front. Microbiol. 15:1451170. doi: 10.3389/fmicb.2024.1451170
Edited by:
Arun K. B., Christ University, IndiaReviewed by:
Katerina A. Chlichlia, Democritus University of Thrace, GreecePamela Del Carmen Mancha-Agresti, Federal University of Minas Gerais, Brazil
Copyright © 2024 Duduyemi, Potapenko, Limanska, Kotsyuda, Petriv, Suo, Gudzenko, Ivanytsia and Yevsa. This is an open-access article distributed under the terms of the Creative Commons Attribution License (CC BY). The use, distribution or reproduction in other forums is permitted, provided the original author(s) and the copyright owner(s) are credited and that the original publication in this journal is cited, in accordance with accepted academic practice. No use, distribution or reproduction is permitted which does not comply with these terms.
*Correspondence: Tetyana Yevsa, WWV2c2EuVGV0eWFuYUBtaC1oYW5ub3Zlci5kZQ==
†These authors have contributed equally to this work