- 1ICAR-IIWBR, Shimla, India
- 2ICAR-Central Soil Salinity Research Institute, Karnal, India
- 3ICAR-Indian Institute of Wheat and Barley Research, Karnal, India
Wheat is one of the most important food crop cultivated across the globe which ensures sustainability and food security to massive world’s population, but its production is threatened by both biotic factors like rust (caused by Puccinia species) and abiotic stresses such as salinity. In this study, 41 salt-tolerant wheat lines were screened for rust resistance at both seedling and adult plant stages. Rust resistance genes were characterized through gene matching technique and molecular markers analysis. Yr2 was confirmed in 23 lines, while Yr9 along with Lr26/Sr31 were postulated in six lines with the help of SRT and molecular markers. Except for KRL2013, none showed complete resistance to all Puccinia striiformis f. sp. tritici (Pst). Lr24/Sr24 genes were confirmed in HD2851 and KRL2029, and Lr13 was detected in a maximum of 24 wheat lines, with varying reaction responses to different leaf rust pathotypes. Several lines carried additional resistance genes such as Sr11, Sr28, and Lr68. Lr68 an effective race non-specific APR gene identified in 15 wheat lines with the help of CsGs-STS marker. Although many salt-tolerant wheat lines were susceptible to yellow rust during the seedling stage, a few lines showed APR in the years during 2020 and 2021. Three lines (KRL213, KRL219 and KRL238) showed complete resistance at adult plant stage to leaf rust. These findings offer insights into the genetic basis of rust resistance in salt-tolerant wheat, aiding breeding strategies.
Introduction
Wheat (Triticum aestivum L.) is a vital food crop, contributing ~785 million tons annually from a vast cultivation area spanning 219 million hectares. It stands as a cornerstone in global agriculture, supplying the majority of calories essential for people worldwide (FAO, 2023). Wheat comes up substantially to the daily nutrition and food security for ever-growing world’s population (Rahmatov et al., 2019). World’s population depends on wheat for 20% of calories and 55% of carbohydrates in diet (Lata et al., 2022b). Approximately, 36 % of the people depend on wheat as a staple food across the globe. However, production of wheat was continuously increased due to efforts of wheat researchers and farmers, a significant research gap exists in addressing the dual challenges posed by biotic and abiotic stresses to sustain wheat productivity. The ever-growing population of world and changing climate always put a challenge for wheat producers by ever increasing demand and consumption. The increasing population is expected to hike the demand of wheat from 621 million ton (during 2004–2006) to more than 900 million ton by 2050 (Hellin et al., 2012). Several biotic and abiotic stresses pose adverse effect on wheat production throughout world. Production and productivity of wheat crop faces formidable challenges due to the prevalence of biotic stresses, such as rust diseases, and the increasing burden of abiotic stresses, notably salinity. Rust diseases are serious global threat to wheat production. Rusts caused by three species of Puccinia: stripe rust by Puccinia striiformis f. sp. tritici Eriks. (Pst); stem rust by Puccinia graminis f. sp. tritici Eriks. and E. Henn (Pgt) and leaf rust by Puccinia triticina Eriks. (Pt) are major biotic stress, reducing global wheat production (Prasad et al., 2022). Under favorable environmental conditions, leaf rust can cause up to 50% loss, where as stem rust and stripe rust can result in 100% loss of wheat yield. Stripe rust of wheat is a threat in 10 million hectares of Northern India, and stem rust threatens about 7 million hectares of Central and Peninsular India while leaf rust damage wherever wheat is grown in India (Bhardwaj et al., 2019). Wheat rust pathogens exhibit remarkable adaptability, often render resistant cultivars susceptible within a few cropping cycles (Hovmøller et al., 2016). Occurrence of new virulences leads to the discontinuance of imperative wheat cultivars due to rapid evolution of rust pathotypes posing a serious concern for wheat breeding. While rust resistance is crucial, an equally pressing concern is the impact of soil salinity on wheat production, particularly in salt-affected regions where wheat productivity is compromised. Within recent couple of years, several wheat rust outbreaks have been faced owing to newly evolved virulent rust pathogens. This novel virulence attacked many commercial high yielding wheat cultivars led to their complete withdrawal from wheat growing belts of India and globe.
Salinity and rust diseases are serious concerns for wheat production across the world. Nearly one billion hectares of land, approximately 25% of the global land area, is directly affected by salinity. This area is escalating up to 10 million hectares of land per year due to unsuitable irrigation practices or natural salinization (Sheoran et al., 2021; Sheoran et al., 2022). Soil salinity is a serious constraint for wheat production in various parts of the world and it has caused yield losses up to 60% leading to food insecurity. Salinity tolerance is an extremely complex quantitative trait concerning plant-specific physiological, morphological and metabolic processes. Concurrently, salinity stress, driven by soil salinization, continues to erode agricultural productivity, with detrimental effects on crop growth and yield (Yang et al., 2008). Osmotic stress and ion toxicity are two major impacts of salinity stress. Lower osmotic potential in the plant roots results higher assimilation of Na+ ion and decreases the Na+/K+ ratio. Uptake and transport of other important essential ions get disturbed due to this ionic imbalance which obstructs the crucial plant functions and processes (Arif et al., 2020).Therefore, a little success has been achieved in breeding of wheat varieties for salinity stress despite of rigorous efforts. Additional detrimental effects of salinity which limit the growth and yield of wheat are, cell ultra-structure alteration, membranous structure damage, production and increase in reactive oxygen species, reduction in enzymatic activity and disturbance in photosynthesis, (Dadshani et al., 2019).
Despite the need for wheat genotypes that exhibit both rust resistance and salinity tolerance, limited research has been conducted to address these dual stresses. Wheat germplasm having genes for leaf rust and yellow rust resistance normally demonstrate low level of salinity tolerance as compared to other genotypes. On other hand, salt-tolerant wheat germplasm is generally susceptible to rust (Dr. SC Bhardwaj, personal communication). Salt-tolerance is a quantitative trait and the genes governing salt tolerance are closely linked to genes of other abiotic stress than leaf rust resistance. Mexican and Pakistani wheat genotypes which showed rust resistance, exhibit sensitivity to salinity stress as compared to rust susceptible cultivars (Ali et al., 2007). To date, few studies have focused on the combined breeding for rust resistance and salinity tolerance, representing a critical research gap that this study aims to address.
There are limited studies on wheat breeding for combined stresses of salt and rust resistance. Therefore, bottom up approach is suggested to challenge these serious problems. The resistance against wheat rusts can be categorized into two broad classes: race-specific resistance and non-race-specific resistance, as outlined by Johnson (1988). Race-specific resistance, also known as seedling resistance or all-stage resistance typically exhibits a robust to moderate immune reaction, often linked with the hypersensitive response. This immune response effectively suppresses fungal infection and sporulation across all developmental stages, but its efficacy is contingent upon the presence of a corresponding avirulence gene in a pathogen (Flor, 1942; Zhang et al., 2022). This molecular dissection not only advances our comprehension of the mutual modulation of these traits but also furnishes indispensable insights for precise breeding regimes, ultimately paving the way for the development of elite wheat cultivars resilient to the challenges posed by rust diseases and salinity stress. Characterizing rust resistance in salt-tolerant wheat germplasm involves a comprehensive investigation to unravel the intricate genetic, molecular, and physiological underpinnings that govern the coexistence of disease resistance and salinity tolerance. This endeavor necessitates meticulous phenotypic assessments, encompassing diverse rust pathogens, to delineate the scope of resistance profiles and potential correlations with salt tolerance (Bakala et al., 2021). Concurrently, cutting-edge molecular methodologies, including genome-wide association studies (GWAS) and high-throughput sequencing, are harnessed to pinpoint pivotal genetic loci and candidate genes intricately linked to rust resistance (Lata et al., 2023) within salt-tolerant wheat varieties.
So, identification of rust resistance genes in salt tolerant bread wheat genotypes could be promising for developing salt-tolerant and rust resistance wheat cultivars (Lata et al., 2022a). This research is vital for filling the gap in breeding strategies that address the combined challenge of biotic and abiotic stresses. Deployment of such germplasm could avoid the epidemics of rust in near future and perform efficiently under salt-affected agro-ecosystems for sustainable wheat production. To achieve the goal of food security in adverse biotic and abiotic threats research should be focused in this direction. This study was designed to address these challenges by dissecting rust resistance in salt-tolerant advanced wheat germplasm through seedling resistance tests, adult plant resistance, and molecular approaches. The simultaneous unraveling of mechanisms underlying rust resistance and salt tolerance in wheat germplasm holds promise for devising innovative strategies to enhance crop resilience and food security.
Materials and methods
Host material
A set of 41 wheat lines including seven released cultivars/ varieties, two registered genetic stocks, two elite lines and 30 advanced lines were used for identifying rust resistance through molecular markers, SRT and APR. This set including one salt-sensitive variety, i.e., HD2851 and other 40 salt-tolerant lines of wheat were provided by Division of Crop Improvement, ICAR-Central Soil Salinity Research Institute, Karnal, India. The positive (confirmed sources Lr, Sr, and Yr genes) and negative (LWH and A-9-30) checks provided by ICAR-Indian Institute of Wheat and Barley Research, Regional Station, Flowerdale, Shimla were used for validation. Pedigree details of wheat lines are given in Table 1.
Multi-pathotype screening through SRT
Forty-one wheat lines were screened for rust resistance at seedling stage. The pathotypes used for screening are-T, 78S84, 110S119, 110S119, P, K, 7S0, 46S119, 111S68, 79S68, 238S119 and 110S 84 (stripe rust), 11, 12A, 13–3, 12–5, 12–7,77, 77–1, 77–2, 77–5, 77–7, 77–8, 77–9, 77–10, 104–2, 107–1, 108–1, 106-1(leaf rust) and 11, 21A-2, 34–1, 40, 40A, 40–2, 40–3, 42B, 117A-1, 117–4, 117–6, 122, 184–1 (stem rust) given in Table 2 with their avirulence/virulence structure. All the pathotypes of three rust pathogens are being maintained in the national repository at ICAR – Indian Institute of Wheat and Barley Research, Regional Station Flowerdale, Shimla.
Inoculation and disease assessment
Four to five seeds of each line were sown in aluminum pans/trays (size: 29 × 12×7 cm) filled with a mixture of fine loam soil and farmyard manure (3:1 ratio). Each tray could accommodate one susceptible check and 18 test wheat lines. These trays were maintained in rust-free microclimate rooms at 20°C after sowing. One week-old seedlings were inoculated with fresh urediniospores suspended in a light weight, non-phytotoxic isoparaffinic oil (Soltrol, Chevron Phillips Chemical Asia Pvt. Ltd., Singapore) in a concentration of 20 mg spores per 5 mL oil using an atomizer. A full set of differentials (Bhardwaj, 2012) was also inoculated along with test lines for seedling resistance test to confirm purity of pathotypes. List of pathotypes of all three rusts used in this experiment are given in Table 2. After inoculation seedlings were sprayed with a fine mist of water and incubated for 24 h in dew chambers having high humidity and specific temperature conditions, i.e., 12 ± 2°C; 20 ± 2°C; and 22 ± 2°C for stripe rust, leaf rust, and stem rust, respectively. Subsequently, the inoculated plants were transferred to temperature controlled conditions. Infection types were scored after 14 days of inoculation using a 0–4 scale (Stakman et al., 1962). Gene matching technique was used to identify possible genes governing seedling resistance in 41 wheat varieties. Stakman et al. (1962) formula was followed for scoring of infection types (ITs) on the 0–4 scale with slight modifications as proposed by Luig (1983), in which IT0 represents the lowest incompatible resistant reaction while IT4 depicts fully compatible susceptible reaction while ITX, referred to as mesothetic and classed as resistant (produces a mixture of incompatible and compatible infection types on the same leaf). Postulation of resistance genes was performed by comparing IT patterns of the pathotype range on test lines with those of controls with known resistance genes (Nayar et al., 1997). Pathotypes that are avirulent to a known resistance gene with high infection types (ITs) on a test cultivar indicated that the cultivar did not possess the gene in question.
Screening through molecular markers
DNA isolation, polymerase chain reaction and product analysis
Green leaves from 5 to 7 days old seedlings were used for genomic DNA isolation by following the method of Murray and Thompson (1980) after some basic modifications. DNA quality was checked on 1% agarose gel electrophoresis and quantified on nanodrop (DS-11 FX + Spectrophotometer/Fluorometer, Jenway). Further, polymerase chain reaction (PCR) were performed in final reaction volume 25 μL with concentrations of 50 ng genomic DNA, 12 μL of 2X PCR Master Mix, containing all the necessary ingredients [Taq polymerase, MgCl2, deoxy-ribonucleotides (dNTPs) from Thermo Fisher according to user manual, 1 μL (10 pm) of each forward and reverse primer and nuclease free water]. Identification of rust resistance genes in theses wheat varieties was performed with microsatellite markers for known rust resistance genes (Table 3). PCR were performed in Bio-metra Thermal Cycler, Analytic Jena using a touchdown profile (SSR markers)with initial de-naturation at 95°C for 10 min, followed by 35 cycles of denaturation at 94°C for 60 s, annealing at (according to primer) for 30–60 s and extension at 72°C for 60 s. PCR products were separated on 2.5% agarose gels using a Bio-Rad Sub-Cell 192. To determine the fragment size pUC19 DNA/MspI 100 bp (HpaII) was run along with samples as a ladder. Stained with ethidium bromide and visualized under UV light using the digital Gel Imaging System (Bio-Rad).Gene Mapper v 4.0 software (Applied Biosystems) was used for scoring of SSR allele. Positive and negative control DNAs were run with each primer for appropriate analysis and repeated for accuracy of the results. Fourteen previously validated molecular markers were used for identification of APR and seedling rust resistance genes (Table 3).
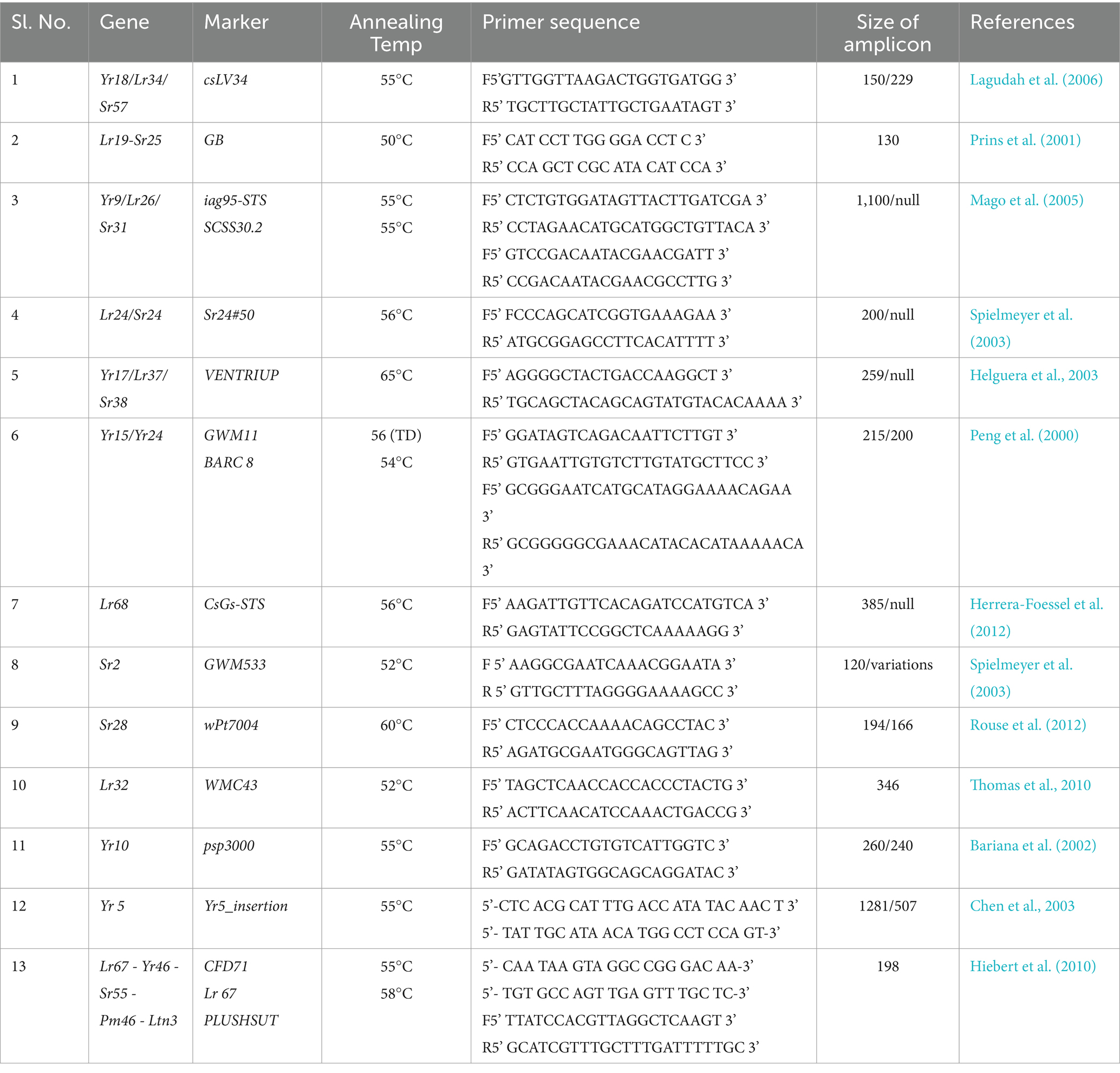
Table 3. Summary of molecular markers closely linked with, their primer sequences, expected sizes of PCR products and PCR conditions.
APR screening through poly house and field screening
Four to five seeds of every line were planted under controlled conditions (in separate screen house at 10–12°C; 20–22°C and 22 ± 2°C for stripe, leaf, and stem rusts, respectively) in a mixture of soil loam and FYM in 3:1 ratio (w/w) supplemented recommended dose of nitrogen: phosphorus: potassium per square meter, maintained optimum temperature under poly-house as per standardized procedure at ICAR-IIWBR, Shimla (Bhardwaj et al., 2010). Light intensity of about 15,000 lux for 12 h was maintained for illuminating the plants. NILs having known APR genes, Yr, Lr and Sr genes carrying Indian wheat material and susceptible wheat variety A-9-30 and Agra Local were also planted to compare adult plant resistance response of tested lines. Urea (20 g/square meter) was added after 20 days of sowing to promote optimal growth of the plants. Similar set of wheat lines planted under field conditions at ICAR-CSSRI, Karnal. Adult plants were inoculated with mixture of pathotypes stripe rust (46S119, 110S119, 238S119, 47S103, 110S84), leaf rust (12–5, 77–1, 77–5, 77–9, 104–2), and stem rust (11, 40-A, 117–6, 21A-2 and 122) on 43–55 growth stage (boot just visible to one half of the ear emerged) as per modified Zadok’s scale (Tottman et al., 1979). Infection types of the adult plants were recorded 14 days after the inoculation. APR responses were inferred on the basis of seedlings reactions and adult plants grown under the same set of conditions with same pathotypes. The modified Cobb scale (Peterson et al., 1948) was used to determine the percentage of severity. The experiment was repeated for the wheat lines in which APR was observed.
Results
Identification of seedling resistance genes
To identify rust resistance genes (Lr, Sr, and Yr) in 41 wheat lines, seedling resistance test (SRT) was conducted and with the help of gene matching technique for all three rust with set of pathotypes.
Stripe rust seedling response
Eleven Pst pathotypes were used to postulate Yr genes at seedling stage in these wheat cultivars. In seedling reaction assay, presence of Yr2 was confirmed in 23 wheat lines based on susceptible and resistant reactions against different pathotypes. On the other hand, Yr9 was confirmed in six wheat lines on the basis of Lr26 gene postulations in leaf rust SRT and molecular markers and showed resistance for most of the pathotypes used in this experiment. No wheat line showed complete resistance for all Pst races used in this experiment except KRL2013. This line showed ITs ranges from 0 to 2−. List of ITs along with postulated genes for Pst races are presented in Table 4.
Leaf/brown rust seedling response
Seventeen Pt races were used for evaluation of seedling leaf rust response. Resistant and susceptible reactions to each of the races along with the postulated genes are presented in Table 4. Lr24/ Sr24 postulated in HD2851 and KRL2029 wheat genotypes which show resistance for all pathotypes of leaf rust and stem rust pathogens at seedling stage. Six wheat lines (KRL283, KRL2013, KRL2015, KRL2017, KRL2024 and KRL2029) showed the presence of Lr26. Presence of these two genes was also confirmed by molecular markers analysis. Other wheat lines showed the presence of combinations of previously described Lr genes or new Lr gene/s. Lr13 gene present in maximum 24 wheat lines which is postulated with differential reaction responses to different pathotypes of leaf rust pathogen.
Stem/black rust seedling response
Seedling response of wheat genotypes to Pgt races and postulated genes are presented in Table 4. Majority of the wheat lines showed seedling resistance toward the Pgt races 21A-2, 34–1, 42B, 117A-1 and 117-6with infection types (ITs) ranging between 0 to 2. Few wheat lines showed seedling resistance toward the more virulent Pgt races 11, 40, 40A, 40–2, 40–3, 117–4, 122 and 184–1. The resistance gene Sr11 was postulated in maximum 12 wheat genotypes followed by Sr28 that was postulated in 10 wheat lines. Sr31 found in six wheat genotypes (KRL283, KRL2013, KRL2015, KRL2017, KRL2024 and KRL2029). HD2851 and KRL2029 showed resistance toward all tested Pgt races due to presence of Sr24. However, no gene is postulated in KRL19, KRL2004 and KRL2005 because these were resistant to tested races. Thus, these lines either carry combinations of previously described genes or new resistance gene/s.
Molecular markers assay for rust resistance genes
Robust molecular markers were applied on isolated DNA of wheat lines to ascertain additional rust resistance in these wheat lines. The presence of 200 bp amplicon produced by dominant marker Sr24#50 indicated the presence of Lr24/Sr24 gene complex in two wheat lines, i.e., HD2851 and KRL2024 (Figure 1). These two wheat lines confirmed the presence of Lr24/Sr24 gene by the molecular marker showed complete resistance against all the pathotypes of leaf rust and stem rust pathogen. Lr68 (non-race specific gene) was identified through linked molecular marker CsGs-STS. Fifteen wheat lines (KRL210, KRL213, KRL19, KRL238, KRL2002, KRL2003, KRL2004, KRL2005, KRL2006, KRL2009, KRL2012, KRL2013, KRL2015, KRL2019, and KRL2023) confirmed the presence of Lr68 gene with an amplification of 385 bp band size (Figure 2). Lr19/Sr25 gene was checked with marker GB, but not a single cultivar confirmed the presence of respective 130 bp amplicon for this gene complex. Yr9/Lr26/Sr31 was validated by SCSS30.2 marker which gives a sharp band of 550 bp in six wheat genotypes namely KRL283, KRL2013, KRL2015, KRL2017, KRL2024 and KRL2029 (Figure 3). Yr5_insertion, psp3000 and GWM11/BARC8 markers were used to explore the presence of Yr5, Yr10 and Yr15 genes, respectively, in salt tolerant lines; nonetheless none of the cultivars were positive for these markers.
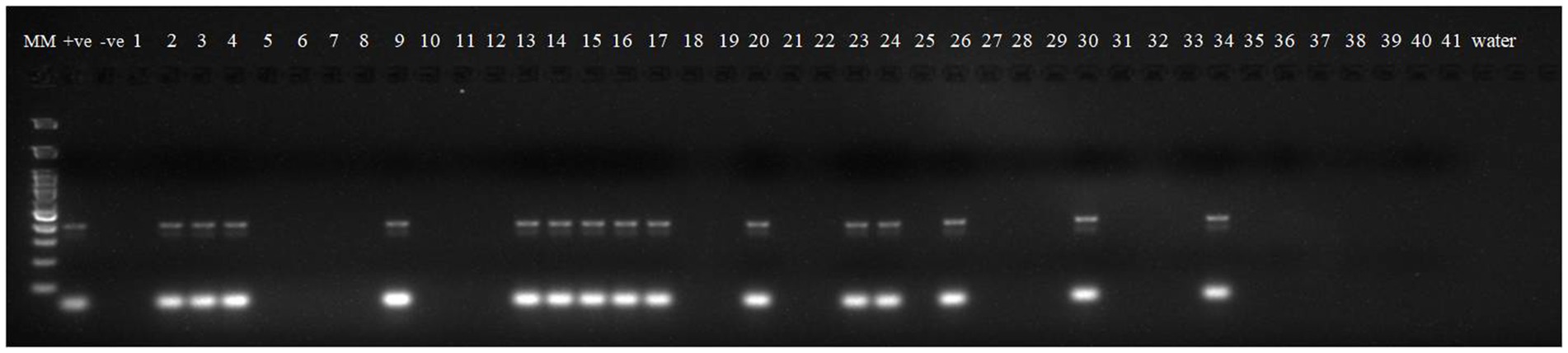
Figure 1. Electrophoresis on 2.5% agarose gel showing marker Sr24#50 indicated the presence of Lr24/Sr24 gene complex. Lane 1 (MM)- Gene Ruler 100 bp DNA ladder; Lane 2 (+ve)-Lr24 NIL as positive check for gene Lr24; Lane 3 (−ve)-LWH as negative check; Lane 4–44—wheat genotypes 1 –41; Lane 45, 46 (W)- water.
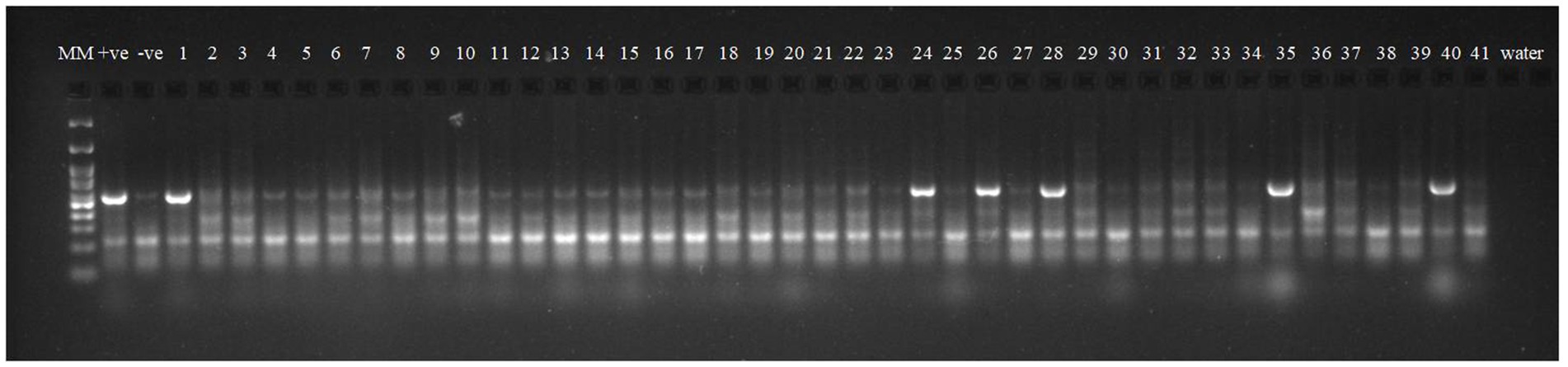
Figure 2. Electrophoresis on 2.5% agarose gel showing the marker CsGs-STS indicated the presence of the gene Lr68. Lane 1 (MM)-GeneRuler 100 bp DNA ladder; Lane 2 (+ve-)-Lr68 NIL as positive check for gene Lr68; Lane 3 (−ve)- LWH as negative check; Lane 4–44—wheat genotypes 1–41, Lane 45, 46 (W)- water.
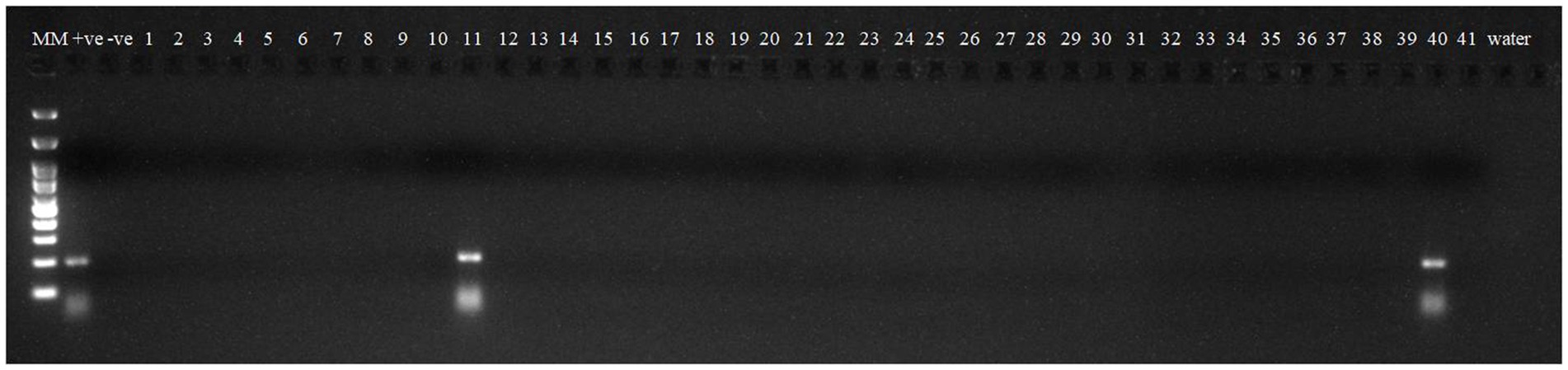
Figure 3. Electrophoresis on 2.5% agarose gel showing marker SCSS30.2 indicated the presence of Yr9/Lr26/Sr3 gene complex. Lane 1 (MM)- Gene Ruler 100 bp DNA ladder; Lane 2 (+ve)-Yr9 NIL as positive check for gene Yr9; Lane 3 (−ve)-LWH as negative check; Lane 4–44 —wheat genotypes 1–41; Lane 45, 46(W)- water.
Adult plant resistance for stripe rust
Evaluations of adult plant resistance for all three rusts was conducted at ICAR-IIWBR, RS, Shimla and ICAR-CSSRI, Karnal. Significant level of disease pressure was observed, with severities reaching 100S in susceptible controls. Interestingly, certain accessions that did not exhibit noticeable seedling resistance showed high levels of adult plant resistance (APR). Most salt tolerant wheat lines were found susceptible for yellow rust during seedling stage. Thus, despite susceptibility at seedling stage for 46S119, 110S119, 110S84 and 238S119, few wheat lines showed APR during 2020 and 2021. Among 41 salt tolerant wheat lines, only six (KRL213, KRL2003, KRL2007, KRL2025, KRL2026 and KRL2027) were considered as resistant (0R to TMR disease severity) at adult plant stage under field conditions (Table 5). Arrays of variation for resistance to leaf rust were observed at adult plant stage in salt tolerant wheat lines. Several lines found fully resistant with 0R score at adult plant stage. Some of these lines showed resistance in seedling stage too. However, three salt tolerant wheat lines (KRL213, KRL19 and KRL238) showed complete resistance at adult plant stage, while these were susceptible at seedling stage against tested pathotypes; 22R45 (12–5), 119R63 (77–1), 121R63-1 (77–5), 121R60-1 (52 or 77–9) and 21R55 (104–2) (Table 5). Evaluation of adult plant resistance for Pgt races is difficult due to resistance at seedling stage in salt tolerant wheat germplasm for tested pathotypes; 79G31(11), 62G29(40-A), 37G19 (117–6), 75G5 (21A-2) and 7G11 (122).
Discussion
Triticum aestivum (bread wheat) is considered as the most significant grain crop among all the cereals. Considering human consumption, wheat is ranked 1st among grain-producing crops globally (Giraldo et al., 2019). In this study, the emphasis is placed on addressing both biotic and abiotic stresses. Specifically, the research aimed to identify seedling resistance genes in salt-tolerant wheat varieties by analyzing their resistance responses against different rust pathogens (stripe rust, leaf rust, and stem rust). Salt stress and rust diseases are deadly combination for wheat crop and difficult to provide tolerance to both stresses. Salt tolerant wheat germplasm are generally found susceptible for rust diseases (Lata et al., 2022a). Limited research has been conducted on rust diseases and salinity stress simultaneously, leaving this area largely unexplored and in need of further study. Some Mexican and Pakistani wheat accessions were screened for both stresses. Among the wheat accessions sourced from Mexico, a diverse spectrum of genotypes was observed, comprising five distinct groups. Remarkably, apart from the genotypes endowed with resistance against wheat leaf rust, the salinity tolerances of these Mexican genotypes exhibited minimal variations. This trait was similarly discernible among genotypes from Pakistan, possessing resistance against leaf rust and yellow rust, which displayed significantly reduced salinity tolerances (Ali et al., 2007). To date, more than 80 leaf rust resistance genes, 83 stripe rust resistance genes and 60 stem rust resistance genes are officially cataloged in wheat (Li et al., 2020; Kumar et al., 2021) and only a few genes are known to confer pleiotropic effect on the resistance for example Lr34/Yr18/Pm38/Sr57 (Singh et al., 2012), Lr46/Yr29/Pm39/Sr58 (Singh et al., 2013), Lr67/Yr46/Pm46/Sr55 (Herrera-Foessel et al., 2011), and Lr68 (Herrera-Foessel et al., 2012). Most of these catalogued genes showed race-specific resistance. The study employs a gene postulation technique with differential sets of wheat cultivars to determine the presence of specific rust resistance genes (Yr, Lr and Sr) in the wheat genotypes. In the present study the primary resistance genes identified through seedling and adult plant responses, along with molecular marker analysis, included yellow rust resistance genes; Yr2, Yr9, YrA and leaf rust resistance gens; Lr1, Lr2a, Lr3, Lr10, Lr13, Lr23, Lr24 and stem rust resistance genes; Sr5, Sr7b, Sr11, Sr24, Sr28, Sr30, Sr31, Sr57. Gaining insights into resistance mechanisms and mitigating the risk of genetic erosion, which can lead to the swift decline in the efficacy of utilized genes, necessitates the thorough evaluation of novel genetic resources. This evaluation process should encompass multiple facets, encompassing the utilization of molecular markers to pinpoint resistance genes, alongside an examination of the reaction patterns exhibited by both seedlings and adult plants in commercial varieties and advanced breeding lines when confronted with different pathotypes rusts (Li et al., 2016; Gangwar et al., 2022; Lata et al., 2023). Wheat varieties grown throughout diverse regions in India during 2015 to 2019 predominantly possess rust resistance genes including Yr2, Yr9, YrA, Yr18, and Yr27. During the studied period resistance against rust in approximately 60% of cultivars is controlled by Gene Yr2, followed by Yr9 (25%) and YrA (12%). Other genes have been identified in only a few cultivars. It’s important to note that none of these identified rust resistance genes are effective against all Indian Pst population (Gangwar et al., 2021). Resistance and susceptibility analysis of these varieties against the different races showed the presence of various rust resistance genes. In these salt tolerant genotypes 23 entries out of 41 showed presence of Yr2 and some additional unidentified resistance followed by four entries possessing Yr9 and three entries carry YrA. The severe yellow rust issue affecting India’s primary wheat varieties results from the over reliance on specific seedling resistance genes, namely Yr2, Yr9, and Yr27. These genes have been used without incorporating other important R genes like Yr10, Yr15, and Yr24/Yr26 in the cultivated varieties. As a consequence, rapidly evolving and virulent races of the Pst pathogen have managed to overcome the resistance provided by these major single genes over time. The breakdown of this resistance has raised concerns among wheat breeders, emphasizing the urgent need to broaden the genetic diversity of future Indian wheat varieties. This can be achieved by integrating multiple yellow rust resistance genes, thus enhancing the overall resilience of the crops against the evolving and aggressive Pst races (Singh V. K. et al., 2015). Presently, breeders have embarked on rust resistance breeding efforts by incorporating rust-resistant sources that possess the potent genes Yr5, Yr10, and Yr15. Several cultivars, such as PBW752 (carrying Yr10) and PBW757 (carrying Yr15), have been developed and released for cultivation. These initiatives reflect a strategic shift toward integrating multiple effective genes to enhance resistance in wheat varieties, marking a positive step in combating the challenges posed by rust pathogens (Gupta et al., 2022).
Presence of Lr24/Sr24 is postulated in HD2851 and KRL2029, which exhibit resistance reactions across multiple rust pathotypes. This gene provides resistance to almost all Pgt pathotypes found in India accept 34–1 and 40–1 were found virulent on Sr24 (Jain et al., 2013; Prasad et al., 2023). Rest of the pathotypes were unable to establish a compatible relationship with Sr24-containing wheat varieties (HD2851 and KRL2029). At seedling stage, most of the salt tolerant wheat germplasm showed resistance for tested Pgt pathotypes due to presence of Sr28, Sr11, Sr5, Sr24, Sr28, Sr30, Sr31, Sr57 and Sr7b. The frequency of low virulence within the Indian population of Puccinia graminis f. sp. tritici (Pgt) is evident on Sr31, Sr24, and Sr30, as elucidated by Prasad et al. (2022). These specific genes, among the ensemble of 10 Sr genes postulated across 41 wheat varieties emerge as potential candidates for strategic gene deployment strategies (Table 4). The study identifies several wheat genotypes with resistant genes, including Lr24/Sr24 in HD2851 and KRL2029, Lr26 in KRL283, KRL2013, KRL2017, and KRL2029, and Lr13 in multiple wheat lines. The study identifies the presence of Sr11 in 12 wheat genotypes, Sr28 in 10 wheat genotypes, and Sr31 in six wheat varieties (KRL2013, KRL2017, and KRL2029).Hence, these specific varieties could be strategically chosen for precise gene deployment efforts for stem rust in India. In Indian wheat varieties, commonly found Sr genes include Sr5, Sr7a, Sr7b, Sr11, Sr24, Sr28, Sr30, and Sr31, with Sr57 being a crucial gene for durable resistance. Additionally, slow rusting Sr genes like Sr55, Sr56, Sr57, and Sr58, when combined with other potent major genes, represent a valuable and versatile resource that can be effectively utilized (Singh R. P. et al., 2015).
Resistance to wheat rusts can be broadly classified into two main types: race-specific and non-race-specific. Race-specific resistance is characterized by its specificity and short-lived nature, mainly because it operates on specific interactions between plant genes and pathogen avirulence genes. This type of resistance is quickly overcome by evolving pathogens. In contrast, non-race-specific resistance offers a more enduring defense. It involves genes with minor to intermediate effects, providing a broader spectrum of protection against various pathogen strains (Lagudah, 2011). Plants with this type of resistance are vulnerable during the seedling stage but develop resistance as they mature, a phenomenon known as slow rusting. Slow rusting is associated with adult plant resistance (APR) and offers long-term protection against rust diseases. When considered individually, the effects of these adult plant resistances (APR) genes are moderate. However, their significance becomes apparent when they interact with other major genes and various minor quantitative trait loci (QTLs). These interactions result in additive effects, leading to the development of highly durable resistance. Combining APR genes in a single cultivar can lead to nearly complete immunity or a high level of resistance. This dual classification provides valuable insights for researchers and breeders aiming to enhance wheat varieties’ resilience to rust pathogens (Wellings, 2011; Huerta-Espino et al., 2020).
Among the 41 tested wheat lines, 15 were confirmed to carry the Lr68 gene using a linked molecular marker, CsGs-STS, which resulted in the amplification of a specific 385 bp band (Lata et al., 2022a). Under field conditions, out of the 41 salt-tolerant wheat lines studied, only six (KRL213, KRL2003, KRL2007, KRL2025, KRL2026, and KRL2027) demonstrated resistance (indicated by a severity rating of 0R to TMR disease) during the adult plant stage when exposed to 46S119, 110S119, 110S84 and 238S119 yellow rust pathotypes. It’s probable that conducting tests on potential non-specific resistance genes like Lr34, Lr46, and Lr67 would yield limited or unproductive results (Huerta-Espino et al., 2011).
Variability in resistance to leaf rust was observed among salt-tolerant wheat lines during the adult plant stage. Some lines exhibited full resistance, scoring 0R during the adult plant stage, indicating effective resistance during adult growth. Interestingly, a subset of these lines also displayed resistance at the seedling stage. However, it is noteworthy that three specific salt-tolerant wheat lines (KRL213, KRL219, and KRL238) demonstrated complete resistance during the adult plant stage, despite being fully susceptible at the seedling stage when exposed to tested pathotypes (29R45, 109R63, 121R63-1, 121R60-1, and 21R55). Assessing adult plant resistance to specific Pgt races poses challenges due to the presence of resistance during the seedling stage in salt-tolerant wheat germplasm tested against pathotypes such as 79G31(11), 62G29(40-A), 37G19(117–6), 75G5(21A-2), and 7G11(122). While it is conceivable that adult plant resistance might exist in these wheat lines, accurately evaluating it remains difficult, making it uncertain to draw definitive conclusions (Prasad et al., 2023).
In conclusion, the research paper outlines the findings of a comprehensive study on the presence of rust resistance genes in salt-tolerant wheat lines. By examining seedling responses, molecular markers, and adult plant resistance, the researchers provide insights into the genetic basis of rust resistance in these wheat genotypes. These findings emphasize the complexity of rust resistance gene interactions and highlight the need for further research to fully understand and utilize these genetic traits for wheat improvement.
Data availability statement
The original contributions presented in the study are included in the article/Supplementary material, further inquiries can be directed to the corresponding author.
Author contributions
CL: Conceptualization, Writing – review & editing, Validation, Writing – original draft. PP: Conceptualization, Writing – review & editing. OG: Conceptualization, Data curation, Supervision, Writing – review & editing. JK: Methodology, Writing – review & editing. SA: Methodology, Writing – review & editing. AM: Data curation, Methodology, Writing – review & editing. SK: Data curation, Methodology, Writing – review & editing. ArK: Conceptualization, Investigation, Methodology, Writing – review & editing. NK: Investigation, Methodology, Supervision, Writing – review & editing. AnK: Investigation, Writing – review & editing. RT: Formal analysis, Resources, Supervision, Writing – review & editing.
Funding
The author(s) declare that no financial support was received for the research, authorship, and/or publication of this article.
Conflict of interest
The authors declare that the research was conducted in the absence of any commercial or financial relationships that could be construed as a potential conflict of interest.
Publisher’s note
All claims expressed in this article are solely those of the authors and do not necessarily represent those of their affiliated organizations, or those of the publisher, the editors and the reviewers. Any product that may be evaluated in this article, or claim that may be made by its manufacturer, is not guaranteed or endorsed by the publisher.
Supplementary material
The Supplementary material for this article can be found online at: https://www.frontiersin.org/articles/10.3389/fmicb.2024.1448429/full#supplementary-material
References
Ali, Z., Salam, A., Azhar, F. M., and Khan, I. A. (2007). Genotypic variation in salinity tolerance among spring and winter wheat (Triticum aestivum L.) accessions. S. Afr. J. Bot. 73, 70–75. doi: 10.1016/j.sajb.2006.08.005
Arif, Y., Singh, P., Siddiqui, H., Bajguz, A., and Hayat, S. (2020). Salinity induced physiological and biochemical changes in plants: an omic approach towards salt stress tolerance. Plant Physiol. Biochem. 156, 64–77. doi: 10.1016/j.plaphy.2020.08.042
Bakala, H. S., Mandahal, K. S., Sarao, L. K., and Srivastava, P. (2021). Breeding wheat for biotic stress resistance: achievements, challenges and prospects. Curr. Trends Wheat Res. 12, 11–34. doi: 10.5772/intechopen.97359
Bariana, H. S., Brown, G. N., Ahmed, N. U., Khatkar, S., Conner, R. L., Wellings, C. R., et al. (2002). Characterisation of Triticum vavilovii-derived stripe rust resistance using genetic, cytogenetic and molecular analyses and its marker-assisted selection. Theor. Appl. Genet. 104, 315–320. doi: 10.1007/s001220100767
Bhardwaj, S. C. (2012). “Wheat rust pathotypes in Indian subcontinent then and now” in Wheat-productivity enhancement under changing climate. eds. S. S. Singh. (New Delhi, India: Narosa Publishing House), 227–238.
Bhardwaj, S. C., Prashar, M., Jain, S. K., Kumar, S., and Datta, D. (2010). Adult plant resistance in some Indian wheat genotypes and postulation of leaf rust resistance genes. Indian Phytopathol. 63:174.
Bhardwaj, S. C., Singh, G. P., Gangwar, O. P., Prasad, P., and Kumar, S. (2019). Status of wheat rust research and Progress in rust management-Indian context. Agron 9:892. doi: 10.3390/agronomy9120892
Chen, X., Soria, M. A., Yan, G., Sun, J., and Dubcovsky, J. (2003). Development of sequence tagged site and cleaved amplified polymorphic sequence markers for wheat stripe rust resistance gene Yr5. Crop Sci. 43, 2058–2064. doi: 10.2135/cropsci2003.2058
Dadshani, S., Sharma, R. C., Baum, M., Ogbonnaya, F. C., Léon, J., and Ballvora, A. (2019). Multi-dimensional evaluation of response to salt stress in wheat. PLoS One 14:e0222659. doi: 10.1371/journal.pone.0222659
Gangwar, O. P., Kumar, S., Bhardwaj, S. C., Prasad, P., Kashyap, P. L., Khan, H., et al. (2021). Virulence and molecular diversity among Puccinia striiformis f. sp. tritici pathotypes identified in India between 2015 and 2019. Crop Prot. 148:105717. doi: 10.1016/j.cropro.2021.105717
Gangwar, O. P., Kumar, S., Bhardwaj, S. C., Prasad, P., Lata, C., and Adhikari, S. (2022). Elucidating the population structure and genetic diversity of Indian Puccinia striiformis f. sp. tritici pathotypes based on microsatellite markers. Phytopathology 112, 1444–1453. doi: 10.1094/PHYTO-10-21-0422-R
Giraldo, P., Benavente, E., Manzano-Agugliaro, F., and Gimenez, E. (2019). Worldwide research trends on wheat and barley: a bibliometric comparative analysis. Agron 9:352. doi: 10.3390/agronomy9070352
Gupta, P. K., Balyan, H. S., Chhuneja, P., Jaiswal, J. P., Tamhankar, S., Mishra, V. K., et al. (2022). Pyramiding of genes for grain protein content, grain quality, and rust resistance in eleven Indian bread wheat cultivars: a multi-institutional effort. Mole Breeding 42:21. doi: 10.1007/s11032-022-01277-w
Helguera, M., Khan, I. A., Kolmer, J., Lijavetzky, D., Zhong-Qi, L., and Dubcovsky, J. (2003). PCR assays for the Lr37/Yr17/Sr38 cluster of rust resistance genes and their use to develop isogenic hard red spring wheat lines. Crop Sci. 43, 1839–1847. doi: 10.2135/cropsci2003.1839
Hellin, J., Shiferaw, B., Cairns, J. E., Reynolds, M., Ortiz-Monasterio, I., Banziger, M., et al. (2012). Climate change and food security in the developing world: potential of maize and wheat research to expand options for adaptation and mitigation. J. Dev. Agric. Econ. 4, 311–321. doi: 10.5897/jdae11.112
Herrera-Foessel, S. A., Lagudah, E. S., Huerta-Espino, J., Hayden, M. J., Bariana, H. S., Singh, D., et al. (2011). New slow-rusting leaf rust and stripe rust resistance genes Lr67 and Yr46 in wheat are pleiotropic or closely linked. Theor. Appl. Genet. 122, 239–249. doi: 10.1007/s00122-010-1439-x
Herrera-Foessel, S. A., Singh, R. P., Huerta-Espino, J., Rosewarne, G. M., Periyannan, S. K., Viccars, L., et al. (2012). Lr68: a new gene conferring slow rusting resistance to leaf rust in wheat. Theor. Appl. Genet. 124, 1475–1486. doi: 10.1007/s00122-012-1802-1
Hiebert, C. W., Thomas, J. B., McCallum, B. D., Humphreys, D. G., DePauw, R. M., Hayden, M. J., et al. (2010). An introgression on wheat chromosome 4DL in RL6077 (Thatcher* 6/PI 250413) confers adult plant resistance to stripe rust and leaf rust (Lr67). Theor. Appl. Genet. 121, 1083–1091. doi: 10.1007/s00122-010-1373-y
Hovmøller, M. S., Walter, S., Bayles, R. A., Hubbard, A., Flath, K., Sommerfeldt, N., et al. (2016). Replacement of the European wheat yellow rust population by new races from the Centre of diversity in the near-Himalayan region. Plant Pathol. 65, 402–411. doi: 10.1111/ppa.12433
Huerta-Espino, J., Singh, R., Crespo-Herrera, L. A., Villaseñor-Mir, H. E., Rodriguez-Garcia, M. F., Dreisigacker, S., et al. (2020). Adult plant slow rusting genes confer high levels of resistance to rusts in bread wheat cultivars from Mexico. Front. Plant Sci. 11:824. doi: 10.3389/fpls.2020.00824
Huerta-Espino, J., Singh, R. P., Germán, S., McCallum, B. D., Park, R. F., Chen, W. Q., et al. (2011). Global status of wheat leaf rust caused by Puccinia triticina. Euphytica 179, 143–160. doi: 10.1007/s10681-011-0361-x
Jain, S. K., Bhardwaj, S. C., Prashar, M., and Singh, S. B. (2013). Physiologic specialization and new virulences of Puccinia graminis f. sp. tritici causing black rust of wheat (Triticum aestivum) in India during 2005-2009. Indian J. Agric. Sci. 83, 1058–1063.
Johnson, R. (1988). “Durable resistance to yellow (stripe) rust in wheat and its implications in plant breeding” in Breeding strategies for resistance to the rusts of wheat. eds. N. W. Simmonds and S. Rajaram (Mexico: CIMMYT).
Kumar, S., Bhardwaj, S. C., Gangwar, O. P., Sharma, A., Qureshi, N., Kumaran, V. V., et al. (2021). Lr80: a new and widely effective source of leaf rust resistance of wheat for enhancing diversity of resistance among modern cultivars. Theor. Appl. Genet. 134, 849–858. doi: 10.1007/s00122-020-03735-5
Lagudah, E. S. (2011). Molecular genetics of race non-specific rust resistance in wheat. Euphytica 179, 81–91. doi: 10.1007/s10681-010-0336-3
Lagudah, E. S., McFadden, H., Singh, R. P., Huerta-Espino, J., Bariana, H. S., and Spielmeyer, W. (2006). Molecular genetic characterization of the Lr34/Yr18 slow rusting resistance gene region in wheat. Theor. Appl. Genet. 114, 21–30. doi: 10.1007/s00122-006-0406-z
Lata, C., Kumar, A., Gangwar, O. P., Prasad, P., Adhikari, S., Kumar, S., et al. (2022a). Multiplex PCR assay for the detection of Lr24 and Lr68 in salt tolerant wheat genotypes. Cereal Res. Commun. 50, 1019–1027.
Lata, C., Manjul, A. S., Prasad, P., Gangwar, O. P., Adhikari, S., Kumar, S., et al. (2023). Unraveling the diversity and functions of sugar transporters for sustainable management of wheat rust. Funct. Integr. Genom. 23, 1–16. doi: 10.1007/s10142-023-01150-9
Lata, C., Prasad, P., Gangwar, O. P., Adhikari, S., Thakur, R. K., Savadi, S., et al. (2022b). Temporal behavior of wheat–Puccinia striiformis interaction prompted defense-responsive genes. J. Plant Interact. 17, 674–684. doi: 10.1080/17429145.2022.2082570
Li, T. Y., Cao, Y. Y., Wu, X. X., Xu, X. F., and Wang, W. L. (2016). Seedling resistance to stem rust and molecular marker analysis of resistance genes in wheat cultivars of Yunnan, China. PLoS One 11:e0165640. doi: 10.1371/journal.pone.0165640
Li, J., Dundas, I., Dong, C., Li, G., Trethowan, R., Yang, Z., et al. (2020). Identification and characterization of a new stripe rust resistance gene Yr83 on rye chromosome 6R in wheat. Theor. Appl. Genet. 133, 1095–1107. doi: 10.1007/s00122-020-03534-y
Luig, N. H. (1983). A survey of virulence genes in wheat stem rust Puccinia graminis f. sp. tritici. advances in plant breeding (Suppl. 11 to plant breeding) (Berlin, Germany: Verlag Paul Parey).
Mago, R., Bariana, H. S., Dundas, I. S., Spielmeyer, W., Lawrence, G. J., Pryor, A. J., et al. (2005). Development of PCR markers for the selection of wheat stem rust resistance genes Sr24 and Sr26 in diverse wheat germplasm. Theor. Appl. Genet. 111, 496–504. doi: 10.1007/s00122-005-2039-z
Murray, M. G., and Thompson, W. (1980). Rapid isolation of high molecular weight plant DNA. Nucleic Acids Res. 8, 4321–4326.
Nayar, S. K., Prashar, M., and Bhardwaj, S. C. (1997). Manual of current techniques in wheal rusts. Flowerdale, Shimla: Directorate of Wheat Research.
Peng, J. H., Fahima, T., Röder, M. S., Huang, Q. Y., Dahan, A., Li, Y. C., et al. (2000). High-density molecular map of chromosome region harboring stripe-rust resistance genes YrH52 and Yr15 derived from wild emmer wheat, Triticum dicoccoides. Genetica 109, 199–210. doi: 10.1023/A:1017573726512
Peterson, R. F., Campbell, A. B., and Hannah, A. E. (1948). A diagrammatic scale for estimating rust intensity of leaves and stem of cereals. Can. J. Res. Sect C. 26, 496–500.
Prasad, P., Thakur, R., Bhardwaj, S. C., Savadi, S., Gangwar, O. P., Lata, C., et al. (2023). Virulence and genetic analysis of Puccinia graminis tritici in the Indian sub-continent from 2016 to 2022 and evaluation of wheat varieties for stem rust resistance. Front. Plant Sci. 14:1196808. doi: 10.3389/fpls.2023.1196808
Prasad, P., Thakur, R. K., Savadi, S., Bhardwaj, S. C., Gangwar, O. P., Lata, C., et al. (2022). Genetic diversity and population structure reveal cryptic genetic variation and long distance migration of Puccinia graminis f. sp. tritici. Indian subcontinent. Front. Microbiol. 13:842106. doi: 10.3389/fmicb.2022.842106
Prins, R., Groenewald, J. Z., Marais, G. F., Snape, J. W., and Koebner, R. M. D. (2001). AFLP and STS tagging of Lr19, a gene conferring resistance to leaf rust in wheat. Theor. Appl. Genet. 103, 618–624. doi: 10.1007/PL00002918
Rahmatov, M., Otambekova, M., Muminjanov, H., Rouse, M. N., Hovmøller, M. S., Nazari, K., et al. (2019). Characterization of stem, stripe and leaf rust resistance in Tajik bread wheat accessions. Euphytica 215, 1–22. doi: 10.1007/s10681-019-2377-6
Rouse, M. N., Nava, I. C., Chao, S., Anderson, J. A., and Jin, Y. (2012). Identification of markers linked to the race Ug99 effective stem rust resistance gene Sr28 in wheat (Triticum aestivum L.). Theor. Appl. Genet. 125, 877–885. doi: 10.1007/s00122-012-1879-6
Sheoran, P., Kumar, A., Sharma, R., Prajapat, K., Kumar, A., Barman, A., et al. (2021). Quantitative dissection of salt tolerance for sustainable wheat production in sodic agro-ecosystems through farmers’ participatory approach: an Indian experience. Sustain. For. 13:3378. doi: 10.3390/su13063378
Sheoran, P., Sharma, R., Kumar, A., Singh, R. K., Barman, A., Prajapat, K., et al. (2022). Climate resilient integrated soil–crop management (CRISCM) for salt affected wheat Agri–food production systems. Sci. Total Environ. 837:155843. doi: 10.1016/j.scitotenv.2022.155843
Singh, R. P., Herrera-Foessel, S. A., Huerta-Espino, J., Bariana, H., Bansal, U., McCallum, B.C., et al. (2012). “Lr34/Yr18/Sr57/Pm38/Bdv1/Ltn1 confers slow rusting, adult plant resistance to Puccinia graminis tritici,” in Proceedings of the 13th international cereal rusts and powdery mildews conference
Singh, R. P., Herrera-Foessel, S. A., Huerta-Espino, J., Lan, C., Basnet, B. R., Bhavani, S., et al. (2013). “Pleiotropic gene Lr46/Yr29/Pm39/Ltn2 confers slow rusting, adult plant resistance to wheat stem rust fungus,” in Proceedings Borlaug global rust initiative, 2013 technical workshop New Delhi
Singh, R. P., Hodson, D. P., Jin, Y., Lagudah, E. S., Ayliffe, M. A., Bhavani, S., et al. (2015). Emergence and spread of new races of wheat stem rust fungus: continued threat to food security and prospects of genetic control. Phytopathology 105, 872–884. doi: 10.1094/PHYTO-01-15-0030-FI
Singh, V. K., Mathuria, R. C., Singh, G. P., Singh, P. K., Singh, S., Gogoi, R., et al. (2015). Characterization of yellow rust resistance genes by using gene postulation and assessment of adult plant resistance in some Indian wheat genotypes. Res.Crops 16:4.
Spielmeyer, W., Sharp, P. J., and Lagudah, E. S. (2003). Identification and validation of markers linked to broad spectrum stem rust resistance gene Sr2 in wheat (Triticum aestivum L.). Crop Sci. 43, 333–336. doi: 10.2135/cropsci2003.3330
Stakman, E. C., Stewart, D., and Loegering, W. Q. (1962). Identification of physiologic races of Puccinia graminis var. tritici. Washington: USDA.
Thomas, J., Nilmalgoda, S., Hiebert, C., McCallum, B., Humphreys, G., and DePauw, R. (2010). Genetic markers and leaf rust resistance of the wheat gene Lr32. Crop Sci. 50, 2310–2317. doi: 10.2135/cropsci2010.02.0065
Tottman, D. R., Makepeace, R. J., and Broad, H. (1979). An explanation of the decimal code for the growth stages of cereals, with illustrations. Annl Appl Biol. 93, 221–234. doi: 10.1111/j.1744-7348.1979.tb06534.x
Wellings, C. R. (2011). Global status of stripe rust: a review of historical and current threats. Euphytica 179, 129–141. doi: 10.1007/s10681-011-0360-y
Yang, C. W., Wang, P., Li, C. Y., Shi, D. C., and Wang, D. L. (2008). Comparison of effects of salt and alkali stresses on the growth and photosynthesis of wheat. Photosynthetica 46, 107–114. doi: 10.1007/s11099-008-0018-8
Keywords: Triticum aestivum, Puccinia, seedling resistance, salt tolerance, molecular markers
Citation: Lata C, Prasad P, Gangwar OP, Kallugudi J, Adhikari S, Manjul AS, Kumar S, Kumar A, Kulshreshtha N, Khippal A and Tiwari R (2024) Dissecting the rust resistance in salt tolerant wheat germplasm. Front. Microbiol. 15:1448429. doi: 10.3389/fmicb.2024.1448429
Edited by:
Dragan Perovic, Julius Kühn-Institut, GermanyReviewed by:
Ajay Kumar Thakur, Central Potato Research Institute (ICAR), IndiaDeepanshu Jayaswal, Indian Institute of Seed Science, India
Copyright © 2024 Lata, Prasad, Gangwar, Kallugudi, Adhikari, Manjul, Kumar, Kumar, Kulshreshtha, Khippal and Tiwari. This is an open-access article distributed under the terms of the Creative Commons Attribution License (CC BY). The use, distribution or reproduction in other forums is permitted, provided the original author(s) and the copyright owner(s) are credited and that the original publication in this journal is cited, in accordance with accepted academic practice. No use, distribution or reproduction is permitted which does not comply with these terms.
*Correspondence: Om P. Gangwar, Z2FuZ3dhcm9wQGdtYWlsLmNvbQ==Pramod Prasad, UHJhbW9kLlByYXNhZEBpY2FyLmdvdi5pbg==