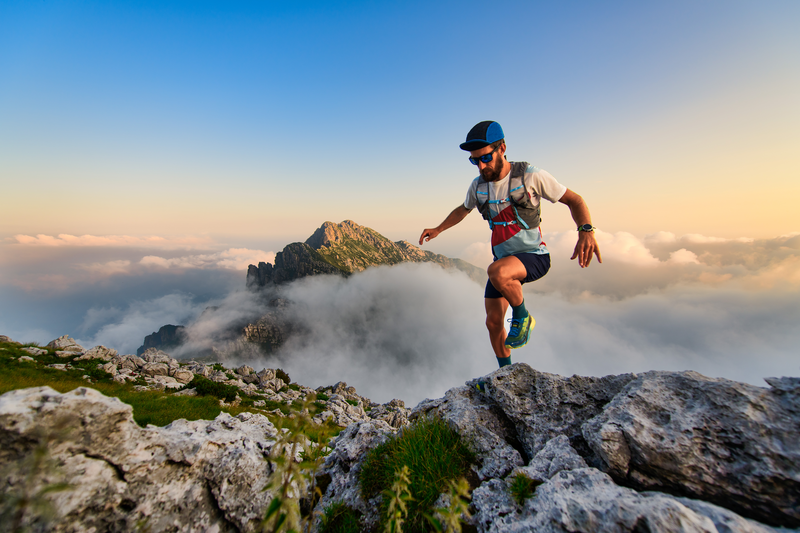
94% of researchers rate our articles as excellent or good
Learn more about the work of our research integrity team to safeguard the quality of each article we publish.
Find out more
ORIGINAL RESEARCH article
Front. Microbiol. , 12 August 2024
Sec. Microbial Physiology and Metabolism
Volume 15 - 2024 | https://doi.org/10.3389/fmicb.2024.1448277
In this study, we demonstrated that both the expression of most ribosomal protein genes and the amount of ribosomes were decreased in the Δaa3 mutant of Mycobacterium smegmatis, in which the major terminal oxidase (aa3 cytochrome c oxidase) of the respiratory electron transport chain (ETC) is inactivated, compared to those in the wild-type strain. Deletion of the rel gene encoding the major (p)ppGpp synthetase in the background of the Δaa3 mutant restored the reduced expression of ribosomal protein genes, suggesting that inhibition of the respiratory ETC leads to the Rel-dependent stringent response (SR) in this bacterium. Both a decrease in the expression of ribosomal protein genes by overexpression of rel and the increased expression of rel in the Δaa3 mutant relative to the wild-type strain support the Rel-dependent induction of SR in the Δaa3 mutant. We also demonstrated that the expression of ribosomal protein genes was decreased in M. smegmatis exposed to respiration-inhibitory conditions, such as KCN and bedaquiline treatment, null mutation of the cytochrome bcc1 complex, and hypoxia. The MprBA-SigE-SigB regulatory pathway was implicated in both the increased expression of rel and the decreased expression of ribosomal protein genes in the Δaa3 mutant of M. smegmatis.
Mycobacteria encounter a myriad of stress conditions, such as nutrient deprivation, hypoxia, and oxidative stress, when exposed to host environments (Bretl et al., 2011). The stringent response (SR) helps mycobacteria adapt to these hostile conditions by modulating their metabolism and redirecting resources toward functions that are beneficial to them under given conditions (Gupta et al., 2021). The SR is mediated by the secondary messenger molecules, guanosine tetraphosphate (ppGpp) and guanosine pentaphosphate (pppGpp) [collectively referred to as (p)ppGpp] (Bange et al., 2021). In mycobacteria, SR has been suggested to affect survival under nutrient starvation, oxidative stress, and hypoxic conditions, as well as persistence, virulence, biofilm formation, cell division, cell morphology, and antibiotic tolerance, etc. (Primm et al., 2000; Betts et al., 2002; Dahl et al., 2003, 2005; Stallings et al., 2009; Klinkenberg et al., 2010; Weiss and Stallings, 2013; Gupta et al., 2016; Dutta et al., 2019). (p)ppGpp is produced by (p)ppGpp synthetase from the reactants ATP and GTP (or GDP). Two classes of (p)ppGpp synthetase occur in mycobacteria: the long Rel/SpoT homolog (RSH) and the small alarmone synthetase (SAS) (Prusa et al., 2018). The Rv2583c and MSMEG_2965 genes encode the Rel proteins of the long RSH class in Mycobacterium tuberculosis and Mycobacterium smegmatis, respectively. Rel is a bifunctional enzyme with both (p)ppGpp synthetase and hydrolase activities and consists of an N-terminal catalytic domain with hydrolase/synthetase activities and a C-terminal regulatory domain (Avarbock et al., 1999, 2000, 2005; Primm et al., 2000; Singal et al., 2017; Bange et al., 2021). The C-terminal domain of Rel in mycobacteria harbors two subdomains: the “ThrRS, GTPase, and SpoT” (TGS) domain and “Aspartate kinase, Chorismate mutase and TyrA” (ACT) domain (Jain et al., 2006; Prusa et al., 2018; Bange et al., 2021; Shin et al., 2021a,b). M. tuberculosis and M. smegmatis have a single copy of the gene encoding SAS (Rv1366 in M. tuberculosis and MSMEG_5849 in M. smegmatis) (Murdeshwar and Chatterji, 2012; Weiss and Stallings, 2013). The Rv1366 protein was suggested to be catalytically inactive, while MSMEG_5849 annotated as RelZ was shown to be catalytically active and synthesize pGpp using GMP and ATP in addition to (p)ppGpp (Weiss and Stallings, 2013; Bag et al., 2014; Petchiappan et al., 2020). RelZ of M. smegmatis has been demonstrated to be a dual-domain SAS with the RNase HII and (p)ppGpp (pGpp) synthetase activities (Murdeshwar and Chatterji, 2012; Krishnan et al., 2016; Krishnan and Chatterji, 2020; Petchiappan et al., 2020). The expression of relZ was shown to be upregulated in M. smegmatis exposed to replication stress, such as UV stress (Krishnan et al., 2016), and the pGpp synthetase activity of RelZ was demonstrated to be inhibited by single-stranded RNA and pppGpp (Petchiappan et al., 2020).
The most prominent phenotypes of SR induction in bacteria are the downregulation of rRNA and ribosomal protein genes, along with the upregulation of amino acid biosynthesis genes (Prusa et al., 2018; Bange et al., 2021). In SR-induced mycobacteria, the expression of rRNA and ribosomal protein genes has been shown to be downregulated in a Rel-dependent manner (Dahl et al., 2003, 2005; Stallings et al., 2009; Prusa et al., 2018). In contrast, a RelZ-dependent decrease in the expression of rRNA and ribosomal protein genes has not been reported in mycobacteria. Currently, the role of RelZ is not certain, but based on its RNase HII activity, it has been suggested that RelZ plays a role in removing the R-loop during UV-induced replication stress (Krishnan et al., 2016).
Mycobacteria have the bifurcate respiratory electron transport chain (ETC) that is terminated with two terminal oxidases (Kana et al., 2001; Matsoso et al., 2005). One branch is terminated with the aa3 cytochrome c oxidase, while the other is terminated with the bd quinol oxidase. The aa3 cytochrome c oxidase forms a supercomplex with the cytochrome bcc1 complex (Megehee et al., 2006; Wiseman et al., 2018; Zhou et al., 2021). Since the aa3 cytochrome c oxidase is the major terminal oxidase in M. smegmatis and M. tuberculosis grown aerobically, the bcc1-aa3 branch is required for optimal growth of the mycobacteria under aerobic conditions. Disruption of this branch results in growth impairment and upregulation of the bd quinol oxidase genes (Kana et al., 2001; Matsoso et al., 2005; Jeong et al., 2018; Beites et al., 2019; Ko and Oh, 2020). The bd quinol oxidase has been shown to have a high affinity for oxygen, thereby considered to play a crucial role under oxygen-limiting conditions and when the aa3 cytochrome c oxidase is inactivated (Puustinen et al., 1991; Kana et al., 2001; Belevich et al., 2007).
The MprBA two-component system (TCS), consisting of the membrane-bound MprB histidine kinase and its cognate MprA response regulator, was initially found to be necessary for M. tuberculosis to cause persistent infection (Zahrt and Deretic, 2001; Zahrt, 2003). In M. tuberculosis and M. smegmatis, the MprBA TCS has been found to be activated upon exposure to surface stress and alkaline pH (He et al., 2006; Pang et al., 2007; White et al., 2010). Besides ATP, inorganic polyphosphate synthesized by polyphosphate kinase was found to serve as a phosphate donor for MprB-mediated MprA phosphorylation (Sureka et al., 2007). The MprBA-SigE-Rel regulatory pathway has been suggested to be implicated in induction of (p)ppGpp synthesis and SR in mycobacteria (Sureka et al., 2007; Sanyal et al., 2013). In M. tuberculosis and M. smegmatis, the phosphorylated MprA positively regulates the expression of sigE, and the alternative sigma factor SigE in turn binds to the promoter of rel to activate its transcription (He et al., 2006; Sureka et al., 2007). Recently, we reported that the SigE-SigB regulatory pathway is activated in an aa3 oxidase mutant of M. smegmatis grown aerobically relative to the isogenic wild-type (WT) strain grown under the same conditions (Oh et al., 2022). This finding suggests the possibility that SR could be induced in M. smegmatis under respiration-inhibitory conditions. In this study, we demonstrate that SR is indeed induced in M. smegmatis through the MprBA-SigE-SigB-Rel signaling pathway when the respiratory ETC is inhibited.
The bacterial strains and plasmids used in this study are listed in Table 1. Escherichia coli strains were grown in Lysogeny Broth (LB) medium at 37°C. M. smegmatis strains were grown in Middlebrook 7H9 medium (Difco, Sparks, MD, United States) supplemented with 0.2% (w/v) glucose (7H9-glucose) and 0.02% (v/v) Tween 80 as an anti-clumping agent at 37°C. For glucose-limiting conditions, M. smegmatis strains were grown aerobically in 7H9 medium supplemented with 0.01% (w/v) glucose and 0.02% (v/v) Tween 80. For hypoxic growth of M. smegmatis strains, a 100-ml flask was filled with 80 mL of aerobically grown culture with an optical density at 600 nm (OD600) of 0.7–0.75 and tightly sealed with a rubber stopper. The culture was incubated on a gyratory shaker for 3 h at 37°C, allowing for a gradual depletion of O2 from the growth medium. For treatment of M. smegmatis cultures with potassium cyanide (KCN), M. smegmatis strains were grown until the OD600 reached 0.45–0.5. Following the addition of KCN to the cultures to a final concentration of 50 μΜ, the cultures were further grown for 2 h. Ampicillin (100 μg/mL for E. coli), kanamycin (50 μg/mL for E. coli and 30 μg/mL for M. smegmatis), and hygromycin (200 μg/mL for E. coli and 50 μg/mL for M. smegmatis) were added to the growth medium when required.
Standard protocols and manufacturers’ instructions were followed for recombinant DNA manipulations (Green and Sambrook, 2012). Transformation of M. smegmatis with plasmids was carried out by electroporation as described elsewhere (Snapper et al., 1990). The primers used for PCR are listed in Table 2.
To construct pKOTsΔrel, we performed two rounds of recombination PCR. Using the chromosomal DNA of M. smegmatis as a template, two primary PCR reactions were conducted. The first reaction utilized the primers F_relmut and R_relrec, while the second used the primers F_relrec and R_relmut, resulting in two 38-bp overlapping DNA fragments (401 and 384 bp, respectively). Both PCR products contain the same 540-bp deletion within rel in the overlapping region. In the secondary PCR, a 747-bp DNA fragment with a deletion in the rel gene was obtained using both the primary PCR products as templates, along with the primers F_relmut and R_relmut. The resulting secondary PCR product was restricted with NotI and HindIII and subsequently cloned into pKOTs, resulting in pKOTsΔrel.
To construct pKOTsΔmprA, a PCR reaction was carried out with the primers F_mprAmut and R_mprAmut using the chromosomal DNA of M. smegmatis as a template. The resulting 1,469-bp DNA fragment, encompassing the 406-bp upstream and 374-bp downstream regions of mprA, was digested with HindIII. Subsequently, the restricted PCR product was cloned into pUC19 that had been digested with SmaI and HindIII, resulting in pUC19mprA. A 135-bp DNA fragment within mprA was removed from pUC19mprA using XhoI. The linear plasmid was self-ligated through the XhoI recognition site, yielding pUC19ΔmprA. Using pUC19ΔmprA as a template, another PCR reaction was performed with the primers F_mprAmut and R_mprAmut, resulting in a 1,343-bp DNA fragment with a deletion in mprA. The obtained PCR product was then restricted with HindIII and cloned into pKOTs that had been digested with HindIII and EcoRV, resulting in pKOTsΔmprA.
To construct pKOTsΔbc1, we performed two rounds of recombination PCR. We used the chromosomal DNA of M. smegmatis as a template for two primary PCR reactions. The first reaction was performed with the primers F_ bc1mut and R_ bc1rec, while the second used the primers F_bc1rec and R_ bc1mut, resulting in two 36-bp overlapping DNA fragments (481 and 384 bp, respectively). Both PCR products contain the same 1,309-bp deletion within qcrB in the overlapping region. In the secondary PCR, an 829-bp DNA fragment with a deletion in qcrB was obtained using both the primary PCR products as templates, along with the primers F_bc1mut and R_bc1mut. The secondary PCR product was restricted with NotI and HindIII, and cloned into pKOTs, yielding pKOTsΔbc1.
The plasmid pNCIIrel is a rel::lacZ translational fusion plasmid that contains the 5′ portion (39 bp) of rel and the 397-bp DNA sequence upstream of rel. For the construction of pNCIIrel, a 456-bp DNA fragment was amplified using the chromosomal DNA of M. smegmatis as a template and the primers F_rellacZII and R_rellacZII. The PCR product was restricted with XbaI and ClaI and cloned into the promoterless lacZ vector pNCII, yielding pNCIIrel.
The plasmid pNCIIeis is an eis::lacZ translational fusion plasmid that contains the 5′ portion (24 bp) of eis and the 474-bp DNA sequence upstream of eis. To construct pNCIIeis, a 518-bp DNA fragment was amplified using the chromosomal DNA of M. tuberculosis as a template and the primers F_eislacZII and R_eislacZII. The PCR product was restricted with XbaI and BamHI and cloned into pNCII, yielding pNCIIeis.
The plasmid pNCIIwag31 is a wag31::lacZ translational fusion plasmid that contains the 5′ portion (18 bp) of wag31 and the 463-bp DNA sequence upstream of wag31. To construct pNCIIwag31, a 501-bp DNA fragment was amplified using the chromosomal DNA of M. tuberculosis as a template and the primers F_wag31lacZII and R_wag31lacZII. The PCR product was restricted with XbaI and ClaI and cloned into pNCII, creating pNCIIwag31.
The plasmid pMH201rel is a derivative of the pMH201 integration vector that contains the C-terminally His6-tagged rel gene. A 2,429-bp DNA fragment encompassing the rel gene and six His codons immediately before its stop codon was amplified by PCR with the primers F_rel_His and R_rel_His, using the chromosomal DNA of M. smegmatis as a template. The PCR product was restricted with NdeI and ClaI and cloned into pMH201 with an acetamide-inducible promoter, yielding pMH201rel.
The Δrel, Δaa3Δrel, ΔmprA, Δaa3ΔmprA, and Δbc1 deletion mutants of M. smegmatis were generated through allelic exchange mutagenesis, which utilized the corresponding pKOTs-derived suicide plasmids (pKOTsΔrel, pKOTsΔmprA, and pKOTsΔbc1). The mutagenesis was performed in the background of the WT or Δaa3 mutant strain, following the procedure previously described (Jeong et al., 2013). In brief, the temperature-sensitive suicide plasmid was introduced into M. smegmatis by electroporation. Transformants were selected at 30°C (replication-permissive temperature) on 7H9-glucose agar plates containing hygromycin, and the selected transformants were grown in 7H9-glucose liquid medium supplemented with hygromycin for 3 days at 30°C. Heterogenotes of M. smegmatis, which were generated by a single recombination event, were selected for their hygromycin resistance on 7H9-glucose agar plates at 42°C (replication-non-permissive temperature). The selected heterogenotes were grown on 7H9-glucose medium without antibiotics for 3 days at 37°C. Isogenic homogenates were obtained from the heterogenotes after a second recombination by selecting them for sucrose resistance on 7H9-glucose agar plates containing 10% (w/v) sucrose at 37°C. The allelic exchange was verified by PCR using isolated genomic DNA.
Three biological replicate cultures of the Δaa3, Δaa3Δrel, and Δaa3ΔmprΑ strains were grown aerobically to OD600 of 0.45–0.5. Total RNA of each culture was isolated as described previously (Kim et al., 2010). rRNA was eliminated from the isolated total RNA using the NEBNext rRNA Depletion kit (Bacteria) (NEB, Ipswich, MA, United States). The RNA sequencing libraries were created using a TruSeq standard mRNA Sample Prep Kit (Illumina, San Diego, CA, United States). The libraries were quantified using a KAPA Library Quantification Kit (KAPA Biosystems, Wilmington, MA, United States) for Illumina Sequencing platforms according to the qPCR Quantification Protocol Guide and qualified using the TapeStation D1000 ScreenTape (Agilent, Santa Clara, CA, United States). Indexed libraries were subsequently submitted to an Illumina NovaSeq (Illumina), and the paired-end (2 × 101 bp) sequencing of the nine libraries was conducted on an Illumina NovaSeq 6,000 platform at Macrogen Inc. (Seoul, South Korea) using the NovaSeq 6,000 sequencing protocol (Illumina). Paired-end reads (101 bp) were then mapped to the reference genome sequence of M. smegmatis mc2155 (GCF_000015005.1_ASM1500v1) with the program Bowtie 1.1.2 using default settings. Based on principal component analysis of the gene expression patterns in the M. smegmatis strains, one result from each of the Δaa3, Δaa3Δrel, and Δaa3ΔmprΑ strains was considered as an outlier and therefore excluded from subsequent analyses. The RNA sequencing data for the Δaa3, Δaa3Δrel, and Δaa3ΔmprΑ strains of M. smegmatis were deposited in NCBI’s Gene Expression Omnibus and are accessible through the GEO Series accession number GSE267048.
The RNA sequencing data for the WT and Δaa3 strains of M. smegmatis strains have been previously deposited in NCBI’s Gene Expression Omnibus with the GEO Series accession number GSE155251 (Oh et al., 2020).
The β-galactosidase activity was measured spectrophotometrically as described previously (Oh and Kaplan, 1999). The protein concentration was determined using a Bio-Rad protein assay kit (Bio-Rad, Hercules, CA, United States) with bovine serum albumin as the standard protein.
RNA isolation from M. smegmatis strains and cDNA synthesis were performed as described elsewhere (Kim et al., 2010), with the exception of using a random hexamer primer (Thermo Fisher Scientific, Waltham, MA, United States) in place of gene-specific primers in cDNA synthesis. The presence of DNA contamination in the isolated RNA was assessed through PCR using the primers intended for use in quantitative real-time PCR (qRT-PCR). To determine the transcript levels of MSMEG_1441, MSMEG_1470, MSMEG_1525, MSMEG_2654, MSMEG_6894, cydA and sigA, qRT-PCR was performed in a 20-μl mixture. The mixture contained 5 μL of the template cDNA, 1 μL (15 pmol) of each of two gene-specific primers, 10 μL of TB GreenTM Premix Ex TaqTM (Tli RNase Plus) (Takara, Tokyo, Japan), 0.4 μL of the ROX passive fluorescent dye, and 2.6 μL of distilled water. The thermal cycling process commenced with 1 cycle at 95°C for 2 min, followed by 40 cycles of 95°C for 5 s and 64°C for 30 s. The sigA gene, which encodes the principal sigma factor, was employed as a reference gene for qRT-PCR to normalize the expression levels of MSMEG_1441, MSMEG_1470, MSMEG_1525, MSMEG_2654, MSMEG_6894, and cydA. Melting curve analysis was conducted for each reaction to verify the amplification of a single PCR product during qRT-PCR. The primers used for qRT-PCR are listed in Table 2.
Cell-free crude extracts were subjected to SDS-PAGE, and proteins on the gel were transferred to polyvinylidene fluoride membranes (Millipore, Burlington, MA, United States). Western blotting was performed as described previously (Mouncey and Kaplan, 1998). For detection of His6-tagged Rel, a mouse monoclonal IgG against His6 (Thermo Fisher Scientific; MA1-21315) was used at a 1:2,000 dilution. A horseradish peroxidase (HRP)-conjugated anti-mouse IgG (Bio-Rad) was used at a 1:10,000 for the detection of the primary antibody. The ECL kit (Advansta, San Jose, CA, United States) was used to visualize protein bands via a ChemiDoc imaging system (Bio-Rad).
The WT and Δaa3 strains of M. smegmatis were grown aerobically to an OD600 of 0.45 to 0.5 in 50 mL of 7H9-glucose medium. After harvesting the bacterial cells by centrifugation, the cell pellets were washed with 1 mL of BP buffer [20 mM Tris–HCl (pH 7.5), 10 mM MgCl2, 100 mM NH4Cl, and 5 mM β-mercaptoethanol] and then resuspended in 0.5 mL of BP buffer. Cells were broken three times using a Fastprep FP120 (Thermo Fisher Scientific), and cell-free lysates were obtained by centrifugation at 12,000 × g, for 15 min at 4°C. Then, 0.5 mL of cleared lysates from the WT and Δaa3 strains, with their OD260 values adjusted to be identical, were added to 10 mL of a 5–40% sucrose gradient in BP buffer and resolved by ultracentrifugation at 4°C in a Beckman SW41 rotor for 2.5 h at 37,000 rpm. The sucrose density gradient solution was eluted from the bottom of the centrifuge tube, and the presence of polysomes, ribosomes, and ribosomal subunits was monitored at 254 nm. The amounts of polysomes, assembled 70S ribosomes, and ribosomal subunits were compared between the WT and Δaa3 strains after normalizing the ribosome profiles to the absorbance of ultraviolet (UV: 254 nm)-absorbing fractions containing proteins, nucleic acids, nucleotides, and other components.
Results were subjected to statistical analysis using GraphPad Prism 10.0 (GraphPad software Inc., La Jolla, CA, United States). At least three biological replicates were used for each experiment. The data were evaluated statistically using an unpaired Student t-test. Differences were considered statistically significant at p < 0.05.
M. tuberculosis in granulomas encounters conditions inhibiting the respiration ETC, such as hypoxia, nutrient deprivation, nitrosative stress, and low pH, etc. (Timm et al., 2003; Baker and Abramovitch, 2018). These conditions have been considered signals for M. tuberculosis to transition into a dormant state. To gain insights into metabolic and physiological changes in mycobacteria exposed to conditions inhibiting the respiratory ETC, we performed comparative RNA sequencing analysis on the WT strain of M. smegmatis and its isogenic Δaa3 mutant strain with a deletion in the ctaC gene encoding subunit II of the aa3 cytochrome c oxidase (Oh et al., 2020). The Δaa3 mutant strain has an impaired growth rate and 50% respiration inhibition compared to the WT strain (Jeong et al., 2018). As shown in Figure 1A, we identified 529 differentially expressed genes (DEGs) whose expression was altered in the Δaa3 mutant strain by more than |log2 fold change (FC)| > 1 with a p-value less than 0.05 relative to the WT strain (Supplementary Table S1). Among the identified DEGs, 296 were upregulated and 233 were downregulated in the Δaa3 mutant relative to the WT strain. We carried out clusters of orthologous group (COG) analysis with the identified DEGs to gain insights into changes in the Δaa3 mutant strain. The COG analysis was conducted based on eggNOG v5.01 (Huerta-Cepas et al., 2019) (Figure 1B). Except for the “S” category representing function unknown, the “J” category exhibited the largest number of DEGs with the reduced expression in the Δaa3 mutant relative to the WT strain. The “J” category encompasses genes related to translation, ribosomal structure and biogenesis. The second-largest number of downregulated DEGs belonged to the “I” category consisting of genes related to lipid transport and metabolism. Excluding the “S” category, the largest number of DEGs with the increased expression in the mutant relative to the WT strain belonged to the “C” category, which is associated with energy production and conversion.
Figure 1. Comparative RNA sequencing analyses on the WT and Δaa3 mutant strains of M. smegmatis grown aerobically to an OD600 of 0.45–0.5 in 7H9-glucose medium. (A) Identification of DEGs (│log2 FC in gene expression│ > 1, p < 0.05) in the Δaa3 mutant relative to the WT strain. The number of the DEGs with increased (red) and decreased (blue) expression in the mutant, compared to the WT strain, is denoted. (B) COG analysis of the identified DEGs. The y-axis represents the COG category, and the x-axis indicates the number of the DEGs belonging to the corresponding category. The red and blue bars denote the DEGs with increased and decreased expression in the mutant, respectively, relative to the WT strain. (C) GO term enrichment analysis was performed on the DEGs with decreased expression in the mutant relative to the WT strain (log2FC in gene expression < −1, p < 0.05). The analysis was performed in terms of molecular function (MF), cellular component (CC), and biological process (BP). The size of each circle represents the number of enriched genes in each term, while the color of the circle denotes the p-value. A statistical criterion of p < 0.05 was used for each term to determine whether it is enriched for the input genes.
To gain further insights into the functions associated with DEGs downregulated in the Δaa3 mutant compared to the WT strain, enrichment of gene ontology (GO) in terms of molecular function (MF), cellular component (CC), and biological process (BP) was assessed using the DAVID functional annotation tool2 (Huang da et al., 2009; Sherman et al., 2022) (Figure 1C). Genes related to translation and ribosomes, such as ribosomal protein genes, were statistically significantly enriched in three GO terms. The decreased expression of genes related to ribosomes and translation is a representative phenomenon of SR (Prusa et al., 2018; Bange et al., 2021), suggesting the possibility of SR induction in M. smegmatis under conditions that inhibit the respiratory ETC.
We compared the relative expression levels of a total of 56 genes encoding the ribosomal proteins using the RNA sequencing data from the WT vs. Δaa3 strains. As shown in Figure 2A, the expression of most ribosomal protein genes was downregulated in the Δaa3 mutant strain relative to the WT strain. To examine whether the decreased expression of the ribosomal protein genes in the Δaa3 mutant is due to the Rel-dependent induction of SR, we compared the expression levels of 25 selected ribosomal protein genes, whose expression was decreased by less than −1 of log2 FC in the Δaa3 mutant relative to the WT strain, between the Δaa3 and Δaa3Δrel mutant strains. In the Δaa3Δrel mutant, the rel gene encoding the major (p)ppGpp synthetase/hydrolase was null-mutated in the background of the Δaa3 mutant. As shown in Figure 2B, the decreased expression of the ribosomal protein genes observed in the Δaa3 mutant strain was at least partly restored in the Δaa3Δrel mutant strain. We selected five genes that do not form an operon with each other and performed qRT-PCR to confirm the RNA sequencing results. The qRT-PCR results were consistent with those of the comparative RNA sequencing (Figure 2C). In order to confirm that the decreased expression of ribosomal protein genes in the Δaa3 mutant strain is due to the deletion of ctaC, the Δaa3 mutant strain was complemented with the intact ctaC gene using the ctaC-expressing plasmid pMV306ctaC. As shown in Figure 2D, the introduction of pMV306ctaC into the Δaa3 mutant led to the restoration of the expression of MSMEG_1441 and MSMEG_1470 to the WT level. This indicates that the decreased expression of the ribosomal protein genes in the Δaa3 mutant relative to the WT strain is attributed to the inactivation of the aa3 oxidase. Ribosome profiling analysis showed that the amounts of polysomes, assembled 70S ribosomes, and ribosomal subunits were decreased in the Δaa3 mutant, compared to the WT strain (Figure 2E). Taken together, RNA sequencing, qRT-PCR, and ribosome profiling results suggest that the expression of ribosomal protein genes and the biogenesis of ribosomes are decreased in the Δaa3 mutant compared to the WT strain, and that this decrease in the Δaa3 mutant strain occurs, at least in part, in a Rel-dependent way.
Figure 2. Rel-dependent decrease in the expression of the ribosomal protein genes in the Δaa3 mutant strain relative to the WT strain and the growth curves of the WT, Δrel, Δaa3, and Δaa3Δrel strains. (A) The heatmap showing the relative expression of 56 ribosomal protein genes in the Δaa3 mutant relative to the WT strain. The relative expression levels are expressed as log2 FC in gene expression for the ribosomal protein genes. (B) The heatmap showing the log2 FC in expression of 25 ribosomal protein genes that are significantly downregulated (log2 FC in gene expression < −1) in the Δaa3 mutant relative to the WT strain [log2 FC (Δaa3/WT)]. The heatmap also shows the log2 FC in expression of the 25 ribosomal protein genes in the Δaa3Δrel mutant relative to the Δaa3 mutant [log2 FC (Δaa3Δrel/Δaa3)]. The genes selected for validation by qRT-PCR are marked on the left of the heatmap with red circles. (C) Validation of the expression levels of the selected ribosomal protein genes (MSMEG_1441, MSMEG_1470, MSMEG_1525, MSMEG_2654, MSMEG_6894) in the WT, Δaa3, and Δaa3Δrel strains. The expression levels of the genes were quantitatively determined by qRT-PCR and normalized to sigA (the gene encoding the principal sigma factor) expression. The expression level of each ribosomal protein gene in the WT strain is set at 1, and the relative values are expressed for the mutant strains. (D) Complementation of the Δaa3 mutant with the ctaC gene in terms of the expression of MSMEG_1441 and MSMEG_1470. For complementation of the Δaa3 mutant, pMV306ctaC (a pMV306-derived plasmid carrying the intact ctaC gene and its own promoter) was introduced into the mutant. As control strains, the WT and mutant strains with the empty vector pMV306 were used in the experiment. The expression levels of the genes were quantitatively determined by qRT-PCR and normalized to the expression of sigA. The expression level of MSMEG_1441 or MSMEG_1470 in the WT strain with pMV306 is set at 1, and the relative values are expressed for the mutant and complemented strains. (E) Ribosome profiling of the WT and Δaa3 strains. The amounts of polysomes, assembled 70S ribosomes, and ribosomal subunits were compared between the WT and Δaa3 strains after normalizing the ribosome profiles to the absorbance of UV (254 nm)-absorbing fractions containing proteins, nucleic acids, nucleotides, and other components. (F) Growth curves of the WT, Δrel, Δaa3, and Δaa3Δrel strains in 7H9-glucose medium under aerobic conditions. All values are the means of the results from three biological replicates for panels (A,C,F) and nine biological replicates for panel (D). The comparative RNA sequencing results between the Δaa3 and Δaa3Δrel strains shown in panel (B) were obtained from two biological replicates as described in section Materials and Methods. The error bars indicate the standard deviations. *p < 0.05. AU, arbitrary unit.
As judged by the growth curves of the WT and Δrel strains, the inactivation of Rel in the WT strain did not affect its aerobic growth in 7H9-glucose medium until the stationary phase (Figure 2F). However, the optical density of the Δrel mutant declined during the early stationary phase, while that of the WT strain was maintained, indicating that the absence of Rel and the resulting lack of SR induction are likely detrimental to the WT strain of M. smegmatis during the stationary phase. This observation is consistent with the previous report (Dahl et al., 2005). In contrast, the growth of the Δaa3Δrel strain was not different from that of Δaa3 strain throughout all growth phases, indicating that the presence of Rel is neither beneficial nor detrimental to the growth of M. smegmatis under conditions that inhibit the respiratory ETC (Figure 2F).
To confirm that the expression of ribosomal protein genes is reduced when the respiratory ETC is inhibited, we examined the expression of two selected ribosomal protein genes (MSMEG_1441 and MSMEG_1470) in the WT strain treated with KCN, which inhibits the aa3 cytochrome c oxidase, as well as in the Δbc1 mutant strain, where the bcc1 complex of the respiratory ETC is inactivated by deletion of the qcrB gene. The cytochrome bcc1 complex comprises the bcc1-aa3 branch of the ETC with the aa3 cytochrome c oxidase (Megehee et al., 2006). As a positive control gene that is induced under respiration-inhibitory conditions, we included the cydA gene, which encodes the catalytic subunit of the bd quinol oxidase, in the experiment. As shown in Figure 3A, the expression of cydA was significantly increased under the KCN-treated WT strain relative to the untreated control WT strain. In contrast, the expression of the ribosomal protein genes was reduced in the KCN-treated WT strain compared to the untreated WT strain. Similarly, the expression levels of the ribosomal protein genes in the Δbc1 mutant strain was decreased to approximately 50% of those in the WT strain, while the expression of cydA was significantly increased in the Δbc1 mutant strain relative to the WT strain (Figure 3B). We further examined whether the expression of the ribosomal protein genes is decreased in the WT strain when exposed to hypoxic conditions that inhibit the respiratory ETC. As depicted in Figure 3C, the expression levels of the ribosomal protein genes were decreased by more than 80% under hypoxic conditions relative to aerobic conditions, whereas the expression level of cydA was greatly increased under hypoxic conditions compared to aerobic conditions. By compiling several deposited transcriptomic datasets obtained from M. smegmatis exposed to conditions likely to inhibit the respiratory ETC such as hypoxia, bedaquiline (an inhibitor of ATP synthase) treatment, and starvation (PBS-Tween 80) due to a lack of the final electron acceptor O2, the coupling of the ETC and ATP synthase, and a lack of electron donors, respectively, we observed that the expression of the genes encoding the ribosomal proteins and RNA polymerase was decreased under the tested conditions, similar to the Δaa3 mutant (Figure 4). The decrease effect was more noticeable under the well-known SR-inducing starvation condition relative to the Δaa3 mutant, indicating that the extent of SR induction in the Δaa3 mutant is not as strong as that under starvation conditions. Collectively, these results confirm a decrease in the expression of ribosomal protein genes under conditions that inhibit the respiratory ETC.
Figure 3. Effects of inhibiting the respiratory ETC on the expression of MSMEG_1441 and MSMEG_1470. (A) The expression levels of cydA and the two ribosomal protein genes in the WT strain with or without KCN treatment. The WT strains were aerobically grown to an OD600 of 0.45–0.5 and further grown for 2 h either after treatment with 50 μM KCN (+KCN) or without KCN treatment as a control (−KCN). (B) The expression levels of cydA and the two ribosomal protein genes in the WT and Δbc1 mutant strains grown aerobically to an OD600 of 0.45–0.5. (C) The expression levels of cydA and the two ribosomal protein genes in the WT grown under aerobic (Aer) or hypoxic (Hyp) conditions. The expression levels of cydA, MSMEG_1441, and MSMEG_1470 were determined by qRT-PCR and normalized to that of sigA. The expression level of each gene in the control WT strains is set at 1, and the relative values are expressed for the KCN-treated, hypoxically grown WT, and the Δbc1 mutant. All values provided are the averages of the results from three biological replicates. The error bars indicate the standard deviations.
Figure 4. Transcriptional profiles of the genes encoding the ribosomal protein and RNA polymerase in M. smegmatis under respiration-inhibitory conditions. The heatmap shows the relative expression of the ribosomal protein and RNA polymerase genes in the WT strain of M. smegmatis exposed to various stress conditions [treatment of bedaquiline, hypoxia, and starvation (PBS-Tween 80)] that are expected to inhibit the respiratory ETC, compared to the WT strain grown aerobically without exposure to the stress conditions (control). The expression of the ribosomal protein and RNA polymerase genes in the Δaa3 mutant of M. smegmatis relative to the WT strain is also included in the heatmap. The transcriptomic data used in the generation of the heatmap were retrieved from NCBI’s Gene Expression Omnibus using the following accession number: Δaa3 mutant (GSE155251), hypoxia (GSE128412), PBS-Tween 80 (GSE69983), bedaquiline (GSE59871). The color and shading of each cell in the heatmap denote the log2 FC in gene expression in the experimental groups vs. the control group. The WT and Δaa3 mutant strains of M. smegmatis were grown to an OD600 of 0.45–0.5 in 7H9-glucose medium (Oh et al., 2020). The transcriptomic data were obtained from the WT strain of M. smegmatis exposed to 2 mg/L of bedaquiline for 60 min (Hards et al., 2015), hypoxic conditions for 24 h (Martini et al., 2019), or PBS-Tween 80 medium for 60 min (Wu et al., 2016).
The observed decrease in the expression of the ribosomal protein genes in the Δaa3 mutant strain in a Rel-dependent manner led us to assume that SR is induced under respiration-inhibitory conditions. Because it was difficult for us to quantitatively measure changes in intracellular (p)ppGpp levels in the Δaa3 mutant where a strong SR does not occur, we instead examined whether SR is induced in the Δaa3 mutant strain by comparatively determining the expression of the eis (Rv2416c) and wag31 (Rv2145c) genes of M. tuberculosis in the WT and Δaa3 mutant strains of M. smegmatis. The eis gene encodes the enhanced intracellular survival protein that was suggested to be involved in suppressing host innate immune defenses by modulating autophagy, inflammatory responses, and cell death (Dahl et al., 2005; Shin et al., 2010). The wag31 gene encodes the DivIVA protein that regulates cell shape and cell division in Gram-positive bacteria (Cha and Stewart, 1997; Flardh, 2003). The expression of the eis and wag31 genes of M. tuberculosis has been previously demonstrated to be decreased and increased, respectively, in M. smegmatis under SR-inducing conditions in a Rel-dependent manner, when the genes with their own promoters were introduced into M. smegmatis (Dahl et al., 2005; Dahl and Lau Bonilla, 2011). First, we reaffirmed whether the expression of the eis and wag31 genes is altered in M. smegmatis under a nutrient starvation condition that induces the SR. The WT strains of M. smegmatis with the eis::lacZ or wag31::lacZ translational fusion plasmid (pNCIIeis or pNCIIwag31) were assessed for the expression levels of the two reporter genes after growth in 7H9 medium supplemented with 0.01% glucose for nutrient starvation and 0.2% glucose for the control (Figures 5A,C). As reported previously (Dahl et al., 2005; Dahl and Lau Bonilla, 2011), the expression level of eis was decreased, while that of wag31 was increased under glucose starvation (0.01%) relative to those in the WT strain supplemented with 0.2% glucose. As shown in Figure 5B, the expression level of eis was decreased in the Δaa3 mutant strain relative to that in the WT strain. In contrast, the expression level of wag31 was slightly increased in the Δaa3 mutant strain relative to that in the WT strain (Figure 5D). These results support our assumption that SR is to some extent induced in the Δaa3 mutant.
Figure 5. Verification of SR induction in the Δaa3 mutant of M. smegmatis by determining the expression levels of the eis and wag31 genes. (A) Expression of eis in the WT strain grown under glucose-replete (0.2%) and glucose-limiting (0.01%) conditions. (B) Expression of eis in the WT and Δaa3 mutant strains. (C) Expression of wag31 in the WT strain grown under glucose-replete and glucose-limiting conditions. (D) Expression of wag31 in the WT and Δaa3 mutant strains. The WT and Δaa3 mutant strains containing the eis::lacZ and wag31::lacZ translational fusion plasmids, pNCIIeis and pNCIIwag31, were used to measure the expression levels of eis and wag31, respectively. The WT and Δaa3 mutant strains were grown aerobically to an OD600 of 0.45 to 0.5 in 7H9-glucose medium. Cell-free crude extracts were used to measure β-galactosidase activity. All values provided were determined from three biological replicates. The error bars indicate the standard deviations. *p < 0.05.
Given the finding that SR is induced in the Δaa3 mutant, we sought to investigate how SR is induced in the Δaa3 mutant. One possibility is that the expression of a gene encoding the enzyme that produces (p)ppGpp is increased in the Δaa3 mutant. There are two (p)ppGpp synthetases, Rel and RelZ, in M. smegmatis (Murdeshwar and Chatterji, 2012). According to our RNA sequencing results, the reads per kilobase per million mapped reads (RPKM) value of rel was 5.1-fold higher than that of relZ in the WT strain of M. smegmatis grown aerobically, indicating that Rel is the predominantly expressed (p)ppGpp synthetase in M. smegmatis (data not shown). Furthermore, our RNA sequencing results showed that the expression of rel was increased 1.3-fold in the Δaa3 mutant relative to the WT strain, while the expression of relZ was not different between the WT and Δaa3 mutant strains (data not shown). To verify whether the expression of rel is increased in the Δaa3 mutant compared to the WT strain, we assessed the expression level of rel in the WT and Δaa3 mutant strains using the rel::lacZ translational fusion pNCIIrel. As shown in Figure 6, the β-galactosidase assay showed that the expression of rel was more than two-fold higher in the Δaa3 mutant relative to the WT strain when both strains were grown aerobically. The expression of rel is known to be regulated by the alternative sigma factor SigE. SigE was suggested to be one of the central nodes of the regulatory network involved in SR in mycobacteria (Sureka et al., 2007). Furthermore, it was demonstrated that transcription of sigB, which encodes another alternative sigma factor, strictly depends on SigE, and that the SigE-SigB regulatory pathway is activated in the Δaa3 mutant of M. smegmatis (Oh et al., 2022). To examine whether the increased expression of rel in the Δaa3 mutant is attributed to SigE and SigB, the expression of the rel gene was comparatively determined in the WT, ΔsigE, ΔsigB, Δaa3, Δaa3ΔsigE, and Δaa3ΔsigB strains of M. smegmatis carrying pNCIIrel (Figures 6A,B). In the Δaa3ΔsigE mutant, the expression level of rel was reduced to an intermediate level between those in the WT and Δaa3 mutant strains (Figure 6A). The expression level of rel in the Δaa3ΔsigB mutant was shown to be only slightly increased compared to that observed for the ΔsigB mutant (Figure 6B). Considering the hierarchical order of SigE and SigB in the SigE-SigB signaling pathway and the stricter dependence on SigB than SigE for the upregulation of rel expression in the Δaa3 mutant, these results suggest that SigB, rather than SigE, is directly involved in the upregulation of rel in the Δaa3 mutant. Since the MprA response regulator of the MprBA TCS is known to positively regulate the expression of sigE in mycobacteria (He et al., 2006), we assessed whether MprA is involved in the upregulation of rel expression in the Δaa3 mutant. For this purpose, the expression level of rel was comparatively determined in the WT, ΔmprA, Δaa3, and Δaa3ΔmprA strains of M. smegmatis carrying pNCIIrel (Figure 6C). In the Δaa3ΔmprA mutant, the increased expression of rel observed for the Δaa3 mutant relative to the WT strain was almost abolished, which indicates that the MprBA TCS is required for the upregulation of rel expression caused by the inactivation of the aa3 oxidase. Taken together, the results presented in Figure 6 suggest that respiration inhibition such as the inactivation of the aa3 oxidase brings about an increase in rel expression through the MprBA-SigE-SigB signaling pathway.
Figure 6. Involvement of the MprBA-SigE-SigB regulatory pathway in the upregulation of the rel gene in the Δaa3 mutant of M. smegmatis. (A) Expression of rel in the WT, ΔsigE, Δaa3, and Δaa3ΔsigE strains. (B) Expression of rel in the WT, ΔsigB, Δaa3, and Δaa3ΔsigB strains. (C) Expression of rel in the WT, ΔmprA, Δaa3, and Δaa3ΔmprA strains. The expression level of the rel gene in the WT and mutant strains was measured using the rel::lacZ translational fusion plasmid pNCIIrel. The WT and mutant strains containing pNCIIrel were grown aerobically to an OD600 of 0.45 to 0.5 in 7H9-glucose medium. Cell-free crude extracts were used to measure β-galactosidase activity. All values provided were determined from three biological replicates. The error bars indicate the standard deviations. *p < 0.05.
Next, we examined whether an increase in rel expression results in a decrease in the expression of ribosomal protein genes in the WT strain of M. smegmatis (Figure 7). The rel gene was overexpressed in the presence of acetamide using pMH201rel, in which the C-terminally His6-tagged rel gene is expressed by an acetamide-inducible promoter. Western blotting analysis using a His-tag antibody showed that the His6-tagged Rel protein was expressed in the WT strain carrying pMH201rel, while no band was observed at the corresponding position in case of the WT strain with the empty vector pMH201 (Figure 7A). The expression levels of MSMEG_1441 and MSMEG_1470 were reduced in the WT strain with pMH201rel by about 30% compared to those in the WT strain with pMH201 (Figure 7B), suggesting the possibility that the induction of SR in the Δaa3 mutant is at least partly attributable to the increased expression of rel in the mutant.
Figure 7. Effects of rel overexpression on the expression of MSMEG_1441 and MSMEG_1470 in the WT strain of M. smegmatis. The rel gene was overexpressed in the WT strain of M. smegmatis using pMH201rel that carries the C-terminally His6-tagged rel gene under the control of an acetamide-inducible promoter. As a control strain, the WT strain with the empty vector pMH201 was used in the experiment. (A) Crude extracts (10 μg) of the WT strains with pMH201 or pMH201rel were subjected to Western blotting analyses using a His-tag antibody to detect the C-terminally His6-tagged Rel. (B) The expression levels of MSMEG_1441 and MSMEG_1470 were quantitatively determined by qRT-PCR and normalized to that of sigA. The WT strains containing pMH201 or pMH201rel were grown aerobically to an OD600 of 0.45 to 0.5 in 7H9-glucose medium supplemented 0.1% (w/v) acetamide. The expression level of MSMEG_1441 or MSMEG_1470 in the WT strain with pMH201 is set at 1, and the relative values are expressed for the WT strain with pMH201rel. All values provided were determined from three biological replicates. The error bars indicate the standard deviations. *p < 0.05.
Given the positive correlation of the extent of rel expression with SR induction, as well as the abolishment of an increase in rel expression in the Δaa3 mutant by the null mutation of mprA, we assumed that the SR induced in the Δaa3 mutant could be abolished in the Δaa3ΔmprA mutant. To examine this assumption, we compared the expression levels of 25 selected ribosomal protein genes, whose expression was decreased by less than −1 of log2 FC in the Δaa3 mutant relative to the WT strain, between the Δaa3 and Δaa3ΔmprA mutant strains. As shown in Figure 8, the decreased expression of the ribosomal protein genes observed in the Δaa3 mutant strain was mostly restored in the Δaa3ΔmprA mutant strain.
Figure 8. RNA sequencing results showing the involvement of the MprBA TCS in the downregulation of the ribosomal protein genes in the Δaa3 mutant of M. smegmatis. The heatmap shows the log2 FC in expression of 25 ribosomal protein genes that are significantly downregulated (log2 FC in gene expression < −1) in the Δaa3 mutant relative to the WT strain [log2 FC (Δaa3/WT)], as well as the log2 FC in expression of the 25 ribosomal protein genes in the Δaa3ΔmprA mutant relative to the Δaa3 mutant [log2 FC (Δaa3ΔmprA/Δaa3)].
When the SR is induced, it is known to affect many aspects of cellular metabolism, including central dogma processes such as transcription and translation. These alterations elicit physiological changes, such as a general slowdown of protein synthesis and growth (Legault et al., 1972; Corrigan et al., 2016), which aids bacterial adaptation and survival under stress conditions. The regulation of gene expression by SR in mycobacteria exhibits similarities to the conventional SR: the expression of genes related with ribosome biogenesis, as well as those encoding RNA polymerase, is downregulated in a Rel-dependent way under SR-inducing conditions (Stallings et al., 2009). In mycobacteria, SR has been demonstrated to be induced when they were exposed to nutrient starvation, oxidative stress, and hypoxic conditions (Avarbock et al., 2000; Primm et al., 2000; Betts et al., 2002; Stallings et al., 2009).
In this study, we presented several lines of evidence that support the induction of SR in M. smegmatis under conditions that inhibit the respiratory ETC. Our RNA sequencing results showed that the expression of most ribosomal protein genes and RNA polymerase genes was decreased in the aerobically grown Δaa3 mutant strain compared to the WT strain grown under the same conditions. As in the Δaa3 mutant, the expression levels of two representative ribosomal protein genes, MSMEG_1441 and MSMEG_1470, were shown to decrease under conditions that inhibit the respiratory ETC, such as treatment of KCN, inactivation of the cytochrome bcc1 complex, and hypoxic conditions. Furthermore, the previous transcriptomic datasets have shown that the expression of the ribosomal protein genes is reduced in M. smegmatis treated with bedaquiline, as well as in M. smegmatis exposed to hypoxic conditions. These results support our assumption that inhibition of the respiratory ETC leads to SR induction in M. smegmatis. Furthermore, changes in the expression of the known SR-responsive genes eis and wag31 in the Δaa3 mutant corroborated our assumption that SR is indeed induced in the Δaa3 mutant. The reduced expression of ribosomal protein genes in the Δaa3 mutant was recovered by a null mutation of rel, which indicates Rel-dependent induction of SR in the Δaa3 mutant. Our findings that the expression of rel is increased in the Δaa3 mutant and that overexpression of rel leads to the decreased expression of two ribosomal protein genes, MSMEG_1441 and MSMEG_1470, suggest that the elevated expression of rel under respiration-inhibitory conditions contributes to SR induction in M. smegmatis.
Previously it has been reported that the expression of rel in M. smegmatis is regulated via the MprBA-SigE regulatory pathway, in which the MprBA TCS positively regulates the expression of sigE (He et al., 2006; Sureka et al., 2007). SigE was suggested to be an alternative sigma factor that participates in transcription of rel (Sureka et al., 2007). Our recent RNA sequencing analysis revealed that the expression of the SigE and SigB regulons is increased in the Δaa3 mutant strain of M. smegmatis compared to that in the WT strain, which is attributable to both the increased expression of sigE in the mutant and the SigE-dependent transcription of sigB (Oh et al., 2022). Together with the previous finding that the expression of sigE is significantly increased in the ΔsigB mutant relative to the WT strain (Oh et al., 2022), the results of our comparative determination of rel expression in the WT, ΔsigB, ΔsigE, Δaa3, Δaa3ΔsigB, and Δaa3ΔsigE strains of M. smegmatis suggest that SigB, rather than SigE, is directly involved in the increased expression of rel in the Δaa3 mutant (Figure 6). Furthermore, the expression of ribosomal protein genes was found to be restored in the Δaa3ΔmprA mutant relative to the Δaa3 mutant. Additionally, the increased expression of rel in the Δaa3 mutant was found to require the MprBA TCS. Based on these results, we propose that the induction of SR by an increase in rel expression in the Δaa3 mutant is mediated through the MprBA-SigE-SigB regulatory pathway (Figure 9). However, the mechanism by which the MprBA TCS is activated in response to inhibition of the respiratory ETC remains currently elusive. Further studies are required to answer this question. Since polyphosphate itself can serve as a phosphate donor for MprB, certain regulatory systems involved in regulating intracellular levels of polyphosphate could interconnect with the MprBA-SigE-SigB-Rel pathway to form a regulatory network for SR induction. Supporting this assumption, the depletion of inorganic phosphate has been demonstrated to lead to increased expression of sigE and rel in M. tuberculosis through the SenX3-RegX3 TCS that is responsible for inorganic phosphate sensing and is involved in regulating the expression of the ppk1 gene encoding polyphosphate kinase (Rifat et al., 2009).
Figure 9. Model for the induction of SR in M. smegmatis under respiration-inhibitory conditions. “?” indicates that the mechanism by which the MprBA TCS is activated in response to inhibition of the respiratory ETC remains elusive. Low pH and high ionic strength have been demonstrated to inhibit the respiratory ETC of M. smegmatis (Oh and Oh, 2024).
In conclusion, we propose that SR is induced in M. smegmatis under respiration-inhibitory conditions. This event appears to be primarily mediated by the MprBA-SigE-SigB-Rel pathway. Since respiration-inhibitory conditions encompass a wide range of factors that hinder the functionality of the respiratory ETC, various extracellular and intracellular conditions that can disrupt the functionality of the respiratory ETC are expected to be integrated and induce SR. They include conditions in which electron donors or terminal electron acceptors of the ETC are deficient such as nutrient starvation and hypoxia, conditions in which respiratory ETC components are inhibited such as ETC inhibitor treatment and ETC mutants, and conditions in which the proton motive force and membrane potential are affected so that the ETC is inhibited such as low pH, high ionic strength, and inhibition of the Fo/F1 ATP synthase.
The datasets presented in this study can be found in online repositories. The names of the repository/repositories and accession number(s) can be found at: https://www.ncbi.nlm.nih.gov/geo/, GSE155251, GSE267048, GSE128412, GSE69983, and GSE59871.
N-KK: Data curation, Formal analysis, Investigation, Writing – original draft. J-EB: Investigation, Writing – original draft. Y-JL: Investigation, Writing – original draft. YO: Data curation, Formal analysis, Investigation, Writing – original draft. J-IO: Conceptualization, Funding acquisition, Supervision, Writing – review & editing.
The author(s) declare that financial support was received for the research, authorship, and/or publication of this article. This work was supported by a 2-years Research Grant of Pusan National University to J-IO.
The authors declare that the research was conducted in the absence of any commercial or financial relationships that could be construed as a potential conflict of interest.
All claims expressed in this article are solely those of the authors and do not necessarily represent those of their affiliated organizations, or those of the publisher, the editors and the reviewers. Any product that may be evaluated in this article, or claim that may be made by its manufacturer, is not guaranteed or endorsed by the publisher.
The Supplementary material for this article can be found online at: https://www.frontiersin.org/articles/10.3389/fmicb.2024.1448277/full#supplementary-material
Avarbock, D., Avarbock, A., and Rubin, H. (2000). Differential regulation of opposing RelMtb activities by the aminoacylation state of a tRNA.Ribosome.mRNA.RelMtb complex. Biochemistry 39, 11640–11648. doi: 10.1021/bi001256k
Avarbock, A., Avarbock, D., Teh, J. S., Buckstein, M., Wang, Z. M., and Rubin, H. (2005). Functional regulation of the opposing (p)ppGpp synthetase/hydrolase activities of RelMtb from Mycobacterium tuberculosis. Biochemistry 44, 9913–9923. doi: 10.1021/bi0505316
Avarbock, D., Salem, J., Li, L. S., Wang, Z. M., and Rubin, H. (1999). Cloning and characterization of a bifunctional RelA/SpoT homologue from Mycobacterium tuberculosis. Gene 233, 261–269. doi: 10.1016/s0378-1119(99)00114-6
Bag, S., Das, B., Dasgupta, S., and Bhadra, R. K. (2014). Mutational analysis of the (p)ppGpp synthetase activity of the Rel enzyme of Mycobacterium tuberculosis. Arch. Microbiol. 196, 575–588. doi: 10.1007/s00203-014-0996-9
Baker, J. J., and Abramovitch, R. B. (2018). Genetic and metabolic regulation of Mycobacterium tuberculosis acid growth arrest. Sci. Rep. 8:4168. doi: 10.1038/s41598-018-22343-4
Bange, G., Brodersen, D. E., Liuzzi, A., and Steinchen, W. (2021). Two P or not two P: understanding regulation by the bacterial second messengers (p)ppGpp. Ann. Rev. Microbiol. 75, 383–406. doi: 10.1146/annurev-micro-042621-122343
Beites, T., O'Brien, K., Tiwari, D., Engelhart, C. A., Walters, S., Andrews, J., et al. (2019). Plasticity of the Mycobacterium tuberculosis respiratory chain and its impact on tuberculosis drug development. Nat. Commun. 10, 4970–4912. doi: 10.1038/s41467-019-12956-2
Belevich, I., Borisov, V. B., Bloch, D. A., Konstantinov, A. A., and Verkhovsky, M. I. (2007). Cytochrome bd from Azotobacter vinelandii: evidence for high-affinity oxygen binding. Biochemistry 46, 11177–11184. doi: 10.1021/bi700862u
Betts, J. C., Lukey, P. T., Robb, L. C., McAdam, R. A., and Duncan, K. (2002). Evaluation of a nutrient starvation model of Mycobacterium tuberculosis persistence by gene and protein expression profiling. Mol. Microbiol. 43, 717–731. doi: 10.1046/j.1365-2958.2002.02779.x
Bretl, D. J., Demetriadou, C., and Zahrt, T. C. (2011). Adaptation to environmental stimuli within the host: two-component signal transduction systems of Mycobacterium tuberculosis. Microbiol. Mol. Biol. Rev. 75, 566–582. doi: 10.1128/MMBR.05004-11
Cha, J. H., and Stewart, G. C. (1997). The divIVA minicell locus of Bacillus subtilis. J. Bacteriol. 179, 1671–1683. doi: 10.1128/jb.179.5.1671-1683.1997
Corrigan, R. M., Bellows, L. E., Wood, A., and Grundling, A. (2016). ppGpp negatively impacts ribosome assembly affecting growth and antimicrobial tolerance in gram-positive bacteria. Proc. Natl. Acad. Sci. USA 113, E1710–E1719. doi: 10.1073/pnas.1522179113
Dahl, J. L., Arora, K., Boshoff, H. I., Whiteford, D. C., Pacheco, S. A., Walsh, O. J., et al. (2005). The relA homolog of Mycobacterium smegmatis affects cell appearance, viability, and gene expression. J. Bacteriol. 187, 2439–2447. doi: 10.1128/jb.187.7.2439-2447.2005
Dahl, J. L., Kraus, C. N., Boshoff, H. I. M., Doan, B., Foley, K., Avarbock, D., et al. (2003). The role of RelMtb-mediated adaptation to stationary phase in long-term persistence of Mycobacterium tuberculosis in mice. Proc. Natl. Acad. Sci. USA 100, 10026–10031. doi: 10.1073/pnas.1631248100
Dahl, J. L., and Lau Bonilla, D. (2011). The wag31 gene of Mycobacterium tuberculosis is positively regulated by the stringent response. FEMS Microbiol. Lett. 319, 153–159. doi: 10.1111/j.1574-6968.2011.02278.x
Dutta, N. K., Klinkenberg, L. G., Vazquez, M. J., Segura-Carro, D., Colmenarejo, G., Ramon, F., et al. (2019). Inhibiting the stringent response blocks Mycobacterium tuberculosis entry into quiescence and reduces persistence. Sci. Adv. 5:eaav2104. doi: 10.1126/sciadv.aav2104
Flardh, K. (2003). Essential role of DivIVA in polar growth and morphogenesis in Streptomyces coelicolor A3(2). Mol. Microbiol. 49, 1523–1536. doi: 10.1046/j.1365-2958.2003.03660.x
Green, M. R., and Sambrook, J. (2012). Molecular cloning: a laboratory manual. 4th Edn. New York, NY: Cold Spring Harbor Laboratory Press.
Gupta, K. R., Arora, G., Mattoo, A., and Sajid, A. (2021). Stringent response in mycobacteria: from biology to therapeutic potential. Pathogens 10:1417. doi: 10.3390/pathogens10111417
Gupta, K. R., Baloni, P., Indi, S. S., and Chatterji, D. (2016). Regulation of growth, cell shape, cell division, and gene expression by second messengers (p)ppGpp and cyclic di-GMP in Mycobacterium smegmatis. J. Bacteriol. 198, 1414–1422. doi: 10.1128/jb.00126-16
Hards, K., Robson, J. R., Berney, M., Shaw, L., Bald, D., Koul, A., et al. (2015). Data from: Bactericidal mode of action of bedaquiline. Gene Expression Omnibus. Available at: https://www.ncbi.nlm.nih.gov/geo/query/acc.cgi?acc=GSE59871
He, H., Hovey, R., Kane, J., Singh, V., and Zahrt, T. C. (2006). MprAB is a stress-responsive two-component system that directly regulates expression of sigma factors SigB and SigE in Mycobacterium tuberculosis. J. Bacteriol. 188, 2134–2143. doi: 10.1128/JB.188.6.2134-2143.2006
Huang da, W., Sherman, B. T., and Lempicki, R. A. (2009). Systematic and integrative analysis of large gene lists using DAVID bioinformatics resources. Nat. Protoc. 4, 44–57. doi: 10.1038/nprot.2008.211
Huerta-Cepas, J., Szklarczyk, D., Heller, D., Hernandez-Plaza, A., Forslund, S. K., Cook, H., et al. (2019). eggNOG 5.0: a hierarchical, functionally and phylogenetically annotated orthology resource based on 5090 organisms and 2502 viruses. Nucleic Acids Res. 47, D309–D314. doi: 10.1093/nar/gky1085
Jain, V., Saleem-Batcha, R., China, A., and Chatterji, D. (2006). Molecular dissection of the mycobacterial stringent response protein Rel. Protein Sci. 15, 1449–1464. doi: 10.1110/ps.062117006
Jeong, J. A., Lee, H. N., Ko, I. J., and Oh, J. I. (2013). Development of new vector systems as genetic tools applicable to mycobacteria. J. Life Science 23, 290–298. doi: 10.5352/JLS.2013.23.2.290
Jeong, J. A., Park, S. W., Yoon, D., Kim, S., Kang, H. Y., and Oh, J. I. (2018). Roles of alanine dehydrogenase and induction of its gene in Mycobacterium smegmatis under respiration-inhibitory conditions. J. Bacteriol. 200, e00152–18. doi: 10.1128/JB.00152-18
Jessee, J. (1986). New subcloning efficiency competent cells: >1x106 transformants/μg. Focus 8, 1146–1157.
Kana, B. D., Weinstein, E. A., Avarbock, D., Dawes, S. S., Rubin, H., and Mizrahi, V. (2001). Characterization of the cydAB-encoded cytochrome bd oxidase from Mycobacterium smegmatis. J. Bacteriol. 183, 7076–7086. doi: 10.1128/JB.183.24.7076-7086.2001
Kang, C. M., Abbott, D. W., Park, S. T., Dascher, C. C., Cantley, L. C., and Husson, R. N. (2005). The Mycobacterium tuberculosis serine/threonine kinases PknA and PknB: substrate identification and regulation of cell shape. Genes Dev. 19, 1692–1704. doi: 10.1101/gad.1311105
Kim, M. J., Park, K. J., Ko, I. J., Kim, Y. M., and Oh, J. I. (2010). Different roles of DosS and DosT in the hypoxic adaptation of mycobacteria. J. Bacteriol. 192, 4868–4875. doi: 10.1128/JB.00550-10
Klinkenberg, L. G., Lee, J. H., Bishai, W. R., and Karakousis, P. C. (2010). The stringent response is required for full virulence of Mycobacterium tuberculosis in Guinea pigs. J. Infect. Dis. 202, 1397–1404. doi: 10.1086/656524
Ko, E. M., and Oh, J. I. (2020). Induction of the cydAB operon encoding the bd quinol oxidase under respiration-inhibitory conditions by the major cAMP receptor protein MSMEG_6189 in Mycobacterium smegmatis. Front. Microbiol. 11:608624. doi: 10.3389/fmicb.2020.608624
Krishnan, S., and Chatterji, D. (2020). Pleiotropic effects of bacterial small alarmone synthetases: underscoring the dual-domain small alarmone synthetases in Mycobacterium smegmatis. Front. Microbiol. 11:594024. doi: 10.3389/fmicb.2020.594024
Krishnan, S., Petchiappan, A., Singh, A., Bhatt, A., and Chatterji, D. (2016). R-loop induced stress response by second (p)ppGpp synthetase in Mycobacterium smegmatis: functional and domain interdependence. Mol. Microbiol. 102, 168–182. doi: 10.1111/mmi.13453
Legault, L., Jeantet, C., and Gros, F. (1972). Inhibition of in vitro protein synthesis by ppGpp. FEBS Lett. 27, 71–75. doi: 10.1016/0014-5793(72)80412-5
Martini, M. C., Zhou, Y., Sun, H., and Shell, S. S. (2019). Data from: Defining the transcriptional and post-transcriptional landscapes of Mycobacterium smegmatis in aerobic growth and hypoxia. Gene Expression Omnibus. Available at: https://www.ncbi.nlm.nih.gov/geo/query/acc.cgi?acc=GSE128412
Matsoso, L. G., Kana, B. D., Crellin, P. K., Lea-Smith, D. J., Pelosi, A., Powell, D., et al. (2005). Function of the cytochrome bc1-aa3 branch of the respiratory network in mycobacteria and network adaptation occurring in response to its disruption. J. Bacteriol. 187, 6300–6308. doi: 10.1128/JB.187.18.6300-6308.2005
Megehee, J. A., Hosler, J. P., and Lundrigan, M. D. (2006). Evidence for a cytochrome bcc-aa3 interaction in the respiratory chain of Mycobacterium smegmatis. Microbiology 152, 823–829. doi: 10.1099/mic.0.28723-0
Mouncey, N. J., and Kaplan, S. (1998). Redox-dependent gene regulation in Rhodobacter sphaeroides 2.4.1T: effects on dimethyl sulfoxide reductase (dor) gene expression. J. Bacteriol. 180, 5612–5618. doi: 10.1128/JB.180.21.5612-5618.1998
Murdeshwar, M. S., and Chatterji, D. (2012). MS_RHII-RSD, a dual-function RNase HII-(p)ppGpp synthetase from Mycobacterium smegmatis. J. Bacteriol. 194, 4003–4014. doi: 10.1128/JB.00258-12
Oh, J. I., and Kaplan, S. (1999). The cbb3 terminal oxidase of Rhodobacter sphaeroides 2.4.1: structural and functional implications for the regulation of spectral complex formation. Biochemistry 38, 2688–2696. doi: 10.1021/bi9825100
Oh, Y., Lee, H. I., Jeong, J. A., Kim, S., and Oh, J. I. (2022). Activation of the SigE-SigB signaling pathway by inhibition of the respiratory electron transport chain and its effect on rifampicin resistance in Mycobacterium smegmatis. J. Microbiol. 60, 935–947. doi: 10.1007/s12275-022-2202-0
Oh, Y., and Oh, J. I. (2024). The RsfSR two-component system regulates SigF function by monitoring the state of the respiratory electron transport chain in Mycobacterium smegmatis. J. Biol. Chem. 300:105764. doi: 10.1016/j.jbc.2024.105764
Oh, J. I., Park, S. J., Shin, S. J., Ko, I. J., Han, S. J., Park, S. W., et al. (2010). Identification of trans- and cis-control elements involved in regulation of the carbon monoxide dehydrogenase genes in Mycobacterium sp. strain JC1 DSM 3803. J. Bacteriol. 192, 3925–3933. doi: 10.1128/JB.00286-10
Oh, Y., Song, S. Y., Kim, H. J., Han, G., Hwang, J., Kang, H. Y., et al. (2020). The partner switching system of the SigF sigma factor in Mycobacterium smegmatis and induction of the SigF regulon under respiration-inhibitory conditions. Front. Microbiol. 11:588487. doi: 10.3389/fmicb.2020.588487
Pang, X., Vu, P., Byrd, T. F., Ghanny, S., Soteropoulos, P., Mukamolova, G. V., et al. (2007). Evidance for complex interactions of stress-associated regulons in an mprAB deletion mutant of Mycobacterium tuberculosis. Microbiology 153, 1229–1242. doi: 10.1099/mic.0.29281-0
Petchiappan, A., Naik, S. Y., and Chatterji, D. (2020). RelZ-mediated stress response in Mycobacterium smegmatis: pGpp synthesis and its regulation. J. Bacteriol. 202, e00444–19. doi: 10.1128/JB.00444-19
Primm, T. P., Andersen, S. J., Mizrahi, V., Avarbock, D., Rubin, H., and Barry, C. E. (2000). The stringent response of Mycobacterium tuberculosis is required for long-term survival. J. Bacteriol. 182, 4889–4898. doi: 10.1128/jb.182.17.4889-4898.2000
Prusa, J., Zhu, D. X., and Stallings, C. L. (2018). The stringent response and Mycobacterium tuberculosis pathogenesis. Pathog. Dis. 76:fty054. doi: 10.1093/femspd/fty054
Puustinen, A., Finel, M., Haltia, T., Gennis, R. B., and Wikstrom, M. (1991). Properties of the two terminal oxidases of Escherichia coli. Biochemistry 30, 3936–3942. doi: 10.1021/bi00230a019
Rifat, D., Bishai, W. R., and Karakousis, P. C. (2009). Phosphate depletion: a novel trigger for Mycobacterium tuberculosis persistence. J. Infect. Dis. 200, 1126–1135. doi: 10.1086/605700
Sanyal, S., Banerjee, S. K., Banerjee, R., Mukhopadhyay, J., and Kundu, M. (2013). Polyphosphate kinase 1, a central node in the stress response network of Mycobacterium tuberculosis, connects the two-component systems MprAB and SenX3-RegX3 and the extracytoplasmic function sigma factor, sigma E. Microbiology 159, 2074–2086. doi: 10.1099/mic.0.068452-0
Sherman, B. T., Hao, M., Qiu, J., Jiao, X., Baseler, M. W., Lane, H. C., et al. (2022). DAVID: a web server for functional enrichment analysis and functional annotation of gene lists (2021 update). Nucleic Acids Res. 50, W216–W221. doi: 10.1093/nar/gkac194
Shin, D. M., Jeon, B. Y., Lee, H. M., Jin, H. S., Yuk, J. M., Song, C. H., et al. (2010). Mycobacterium tuberculosis Eis regulates autophagy, inflammation, and cell death through redox-dependent signaling. PLoS Pathog. 6:e1001230. doi: 10.1371/journal.ppat.1001230
Shin, J., Singal, B., Gruber, A., Wong, D. M. K., Ragunathan, P., and Gruber, G. (2021a). Atomic structure of the regulatory TGS domain of Rel protein from Mycobacteirum tuberculosis and its interaction with deacylated tRNA. FEBS Lett. 595, 3006–3018. doi: 10.1002/1873-3468.14236
Shin, J., Singal, B., Manimekalai, M. S. S., Wei Chen, M., Ragunathan, P., and Grüber, G. (2021b). Atomic structure of, and valine binding to the regulatory ACT domain of the Mycobacterium tuberculosis Rel protein. FEBS J. 288, 2377–2397. doi: 10.1111/febs.15600
Singal, B., Balakrishna, A. M., Nartey, W., Manimekalai, M. S. S., Jeyakanthan, J., and Gruber, G. (2017). Crystallographic and solution structure of the N-terminal domain of the Rel protein from Mycobacterium tuberculosis. FEBS Lett. 591, 2323–2337. doi: 10.1002/1873-3468.12739
Snapper, S. B., Melton, R. E., Mustafa, S., Kieser, T., and Jacobs, W. R. (1990). Isolation and characterization of efficient plasmid transformation mutants of Mycobacterium smegmatis. Mol. Microbiol. 4, 1911–1919. doi: 10.1111/j.1365-2958.1990.tb02040.x
Stallings, C. L., Stephanou, N. C., Chu, L., Hochschild, A., Nickels, B. E., and Glickman, M. S. (2009). CarD is an essential regulator of rRNA transcription required for Mycobacterium tuberculosis persistence. Cell 138, 146–159. doi: 10.1016/j.cell.2009.04.041
Stover, S. L., Lloyd, L. K., Waites, K. B., and Jackson, A. B. (1991). Neurogenic urinary tract infection. Neurol. Clin. 9, 741–755. doi: 10.1016/S0733-8619(18)30277-9
Sureka, K., Dey, S., Datta, P., Singh, A. K., Dasgupta, A., Rodrigue, S., et al. (2007). Polyphosphate kinase is involved in stress-induced mprAB-sigE-rel signalling in mycobacteria. Mol. Microbiol. 65, 261–276. doi: 10.1111/j.1365-2958.2007.05814.x
Timm, J., Post, F. A., Bekker, L. G., Walther, G. B., Wainwright, H. C., Manganelli, R., et al. (2003). Differential expression of iron-, carbon-, and oxygen-responsive mycobacterial genes in the lungs of chronically infected mice and tuberculosis patients. Proc. Natl. Acad. Sci. USA 100, 14321–14326. doi: 10.1073/pnas.2436197100
Weiss, L. A., and Stallings, C. L. (2013). Essential roles for Mycobacterium tuberculosis Rel beyond the production of (p)ppGpp. J. Bacteriol. 195, 5629–5638. doi: 10.1128/jb.00759-13
White, M. J., He, H., Penoske, R. M., Twining, S. S., and Zahrt, T. C. (2010). PepD participates in the mycobacterial stress response mediated through MprAB and SigE. J. Bacteriol. 192, 1498–1510. doi: 10.1128/JB.01167-09
Wiseman, B., Nitharwal, R. G., Fedotovskaya, O., Schafer, J., Guo, H., Kuang, Q., et al. (2018). Structure of a functional obligate complex III2IV2 respiratory supercomplex from Mycobacterium smegmatis. Nat. Struct. Mol. Biol. 25, 1128–1136. doi: 10.1038/s41594-018-0160-3
Wu, M. L., Gengenbacher, M., Chung, J. C., Chen, S. L., Mollenkopf, H. J., Kaufmann, S. H., et al. (2016). Developmental transcriptome of resting cell formation in Mycobacterium smegmatis. BMC Genomics 17:837. doi: 10.1186/s12864-016-3190-4
Yanisch-Perron, C., Vieira, J., and Messing, J. (1985). Improved M13 phage cloning vectors and host strains: nucleotide sequences of the M13mp18 and pUC19 vectors. Gene 33, 103–119. doi: 10.1016/0378-1119(85)90120-9
Zahrt, T. C. (2003). Molecular mechanisms regulating persistent Mycobacterium tuberculosis infection. Microbes Infect. 5, 159–167. doi: 10.1016/s1286-4579(02)00083-7
Zahrt, T. C., and Deretic, V. (2001). Mycobacterium tuberculosis signal transduction system required for persistent infections. Proc. Natl. Acad. Sci. USA 98, 12706–12711. doi: 10.1073/pnas.221272198
Keywords: electron transport chain, MprBA two-component system, Mycobacterium, respiration, ribosome, SigB, SigE, stringent response
Citation: Kim N-K, Baek J-E, Lee Y-J, Oh Y and Oh J-I (2024) Rel-dependent decrease in the expression of ribosomal protein genes by inhibition of the respiratory electron transport chain in Mycobacterium smegmatis. Front. Microbiol. 15:1448277. doi: 10.3389/fmicb.2024.1448277
Received: 13 June 2024; Accepted: 24 July 2024;
Published: 12 August 2024.
Edited by:
Hans-Georg Koch, University of Freiburg, GermanyReviewed by:
Javier Carrillo-Campos, Autonomous University of Chihuahua, MexicoCopyright © 2024 Kim, Baek, Lee, Oh and Oh. This is an open-access article distributed under the terms of the Creative Commons Attribution License (CC BY). The use, distribution or reproduction in other forums is permitted, provided the original author(s) and the copyright owner(s) are credited and that the original publication in this journal is cited, in accordance with accepted academic practice. No use, distribution or reproduction is permitted which does not comply with these terms.
*Correspondence: Jeong-Il Oh, am9oQHB1c2FuLmFjLmty
Disclaimer: All claims expressed in this article are solely those of the authors and do not necessarily represent those of their affiliated organizations, or those of the publisher, the editors and the reviewers. Any product that may be evaluated in this article or claim that may be made by its manufacturer is not guaranteed or endorsed by the publisher.
Research integrity at Frontiers
Learn more about the work of our research integrity team to safeguard the quality of each article we publish.