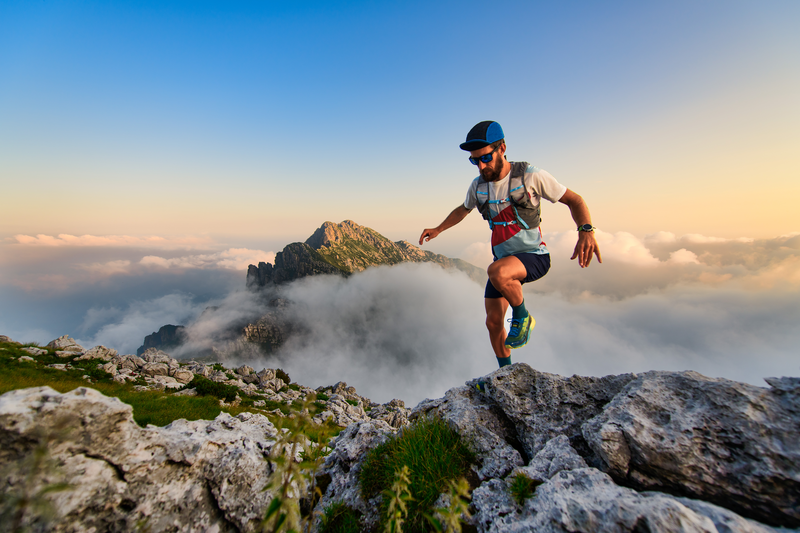
94% of researchers rate our articles as excellent or good
Learn more about the work of our research integrity team to safeguard the quality of each article we publish.
Find out more
ORIGINAL RESEARCH article
Front. Microbiol. , 17 July 2024
Sec. Antimicrobials, Resistance and Chemotherapy
Volume 15 - 2024 | https://doi.org/10.3389/fmicb.2024.1439373
Introduction: Acinetobacter baumannii contributes significantly to the global issue of multidrug-resistant (MDR) nosocomial infections. Often, these strains demonstrate resistance to carbapenems (MDR-CRAB), the first-line treatment for infections instigated by MDR A. baumannii. Our study focused on the antimicrobial susceptibility and genomic sequences related to plasmids from 12 clinical isolates of A. baumannii that carry both the blaOXA-58 and blaNDM-1 carbapenemase genes.
Methods: Whole-genome sequencing with long-read technology was employed for the characterization of an A. baumannii plasmid that harbors the blaOXA-58 and blaNDM-1 genes. The location of the blaOXA-58 and blaNDM-1 genes was confirmed through Southern blot hybridization assays. Antimicrobial susceptibility tests were conducted, and molecular characterization was performed using PCR and PFGE.
Results: Multilocus Sequence Typing analysis revealed considerable genetic diversity among blaOXA-58 and blaNDM-1 positive strains in Brazil. It was confirmed that these genes were located on a plasmid larger than 300 kb in isolates from the same hospital, which also carry other antimicrobial resistance genes. Different genetic contexts were observed for the co-occurrence of these carbapenemase-encoding genes in Brazilian strains.
Discussion: The propagation of blaOXA-58 and blaNDM-1 genes on the same plasmid, which also carries other resistance determinants, could potentially lead to the emergence of bacterial strains resistant to multiple classes of antimicrobials. Therefore, the characterization of these strains is of paramount importance for monitoring resistance evolution, curbing their rapid global dissemination, averting outbreaks, and optimizing therapy.
Acinetobacter baumannii has emerged as one of the most significant and challenging pathogens of this century, primarily due to its involvement in multidrug-resistant (MDR) hospital-acquired infections (Zhang et al., 2021). The most common clinical presentations linked to this pathogen include ventilator-associated pneumonia (VAP) and bloodstream infections (Palmieri et al., 2020). The management of Acinetobacter infections is notably difficult due to its exceptional capacity to develop resistance to nearly all classes of antimicrobials currently used in clinical settings, particularly carbapenems (Ghaly et al., 2020), which are the treatment of choice for MDR A. baumannii (MDR-Ab) infections. However, the distressing increase in carbapenem-resistant A. baumannii (CRAB) strains globally has significantly limited treatment alternatives and considerably increased morbidity and mortality rates (Kyriakidis et al., 2021). Importantly, CRAB strains often demonstrate co-resistance to other antibiotic classes, further complicating therapeutic approaches (Nodari et al., 2020).
CRAB is primarily linked with the production of carbapenem-hydrolyzing class D β-lactamases (CHDL), also referred to as oxacillinases (OXA). At present, among the recognized phylogenetic subgroups of CHDL, five are commonly identified in A. baumannii: intrinsic chromosomal OXA-51-like, acquired OXA-23-like, OXA-24-like, OXA-58-like, and OXA-143-like (Goic-Barisic et al., 2021). These carbapenemases are generally expressed at low levels; however, the presence of an insertion sequence (IS) element upstream of these genes, such as ISAba1, serves as a powerful transcriptional promoter, leading to clinical carbapenem resistance (Wong et al., 2017). Among these enzymes, OXA-23-like is the most widespread and frequently reported enzyme worldwide (Goic-Barisic et al., 2021). In Brazil, OXA-58 is detected sporadically (Matos et al., 2019), while OXA-23 is prevalent, followed by OXA-143 (Vasconcelos et al., 2015; de Oliveira et al., 2019). The OXA-24-like and OXA-58-like enzymes appear to be endemic in specific regions of the world (Hamidian and Nigro, 2019).
Carbapenem resistance in Acinetobacter spp. can also occur. However, it is less common due to the production of metallo-β-lactamases (MBL), such as imipenemase (IMP), Verona integron-encoded metallo-β-lactamase (VIM), and New Delhi metallo-β-lactamase (NDM) (Anggraini et al., 2022). Notably, NDM-1 is prevalent in Klebsiella pneumoniae and Escherichia coli species, whereas Acinetobacter spp. is known as an intermediate reservoir. However, NDM-1-producing Acinetobacter strains have been reported globally due to the high horizontal transferability of the plasmids carrying the blaNDM gene and several additional resistance mechanisms, restricting treatment options (Vasudevan et al., 2022).
The co-occurrence of two distinct carbapenemase-encoding genes is a particularly alarming mechanism of antimicrobial resistance, as it typically results in higher resistance to β-lactams and is often associated with increased mortality rates (Oinuma et al., 2016; Vasudevan et al., 2022).
In this study, we examined antimicrobial susceptibility and investigated the genomic sequences associated with plasmids from A. baumannii clinical isolates carrying the blaOXA-58 and blaNDM-1 genes, with the aim of gaining a deeper understanding of the molecular basis and evolutionary dynamics of the antimicrobial resistance of isolates circulating in Brazil.
The Laboratório de Bacteriologia Aplicada à Saúde Única e Resistência Antimicrobiana (LabSUR-Fiocruz) receives clinical isolates from public health laboratories across Brazil to investigate the mechanisms of antimicrobial resistance. The present study encompasses 12 unique clinical isolates of OXA-58- and NDM-1-producing A. baumannii, which are part of the Culture Collection of Hospital-Acquired Bacteria (CCBH-Fiocruz, WDCM 947). These isolates were obtained from various clinical specimens, including blood, tracheal secretions, catheter tips, and cerebrospinal fluid, from patients hospitalized in two Brazilian states: Bahia in the northeast (eight isolates from two hospitals) and Amazonas in the north (four isolates from three hospitals). The strains were isolated over a period of 1 year, from November 2020 to November 2021, and were sent to LabSUR for investigation into the molecular mechanisms of carbapenem resistance. During this same period, the laboratory received 1,124 strains of Acinetobacter spp. After performing a polymerase chain reaction (PCR), the blaOXA-58 and blaNDM-1 genes were detected in 12 isolates.
The antimicrobial susceptibility profile was performed and interpreted according to the European Committee on Antimicrobial Susceptibility Testing (EUCAST) guidelines (European Committee on Antimicrobial Susceptibility Testing (EUCAST), 2023). Antimicrobial susceptibility testing (AST) of A. baumannii isolates for amikacin, gentamicin, ciprofloxacin, trimethoprim–sulfamethoxazole, tobramycin, levofloxacin, and meropenem was performed using the Kirby–Bauer disk-diffusion method, as described previously (Bauer et al., 1966). The broth dilution method was used to determine the susceptibility to colistin.
All the isolates were analyzed using PFGE, as described previously, with minor modifications (Bou et al., 2000). Bacterial cells were embedded in agarose plugs and digested by ApaI (Invitrogen) at 37°C for 3 h. Electrophoresis was performed on 1.1% agarose gel (SeaKem® Gold Agarose, Lonza) in 0.4× Tris-borate-EDTA buffer using a CHEF-DR III System (Bio-Rad) apparatus. Images of banding patterns obtained were processed using BioNumerics software (version 6.6; Applied Maths). Similarities were calculated using both Dice coefficients, and the unweighted pair-group method using arithmetic averages (UPGMA) was applied for cluster analysis. The tolerance and optimization were set at 1.5% each. Strains were considered epidemiologically related if they had ≥90% genetic similarity.
Plasmids were analyzed using S1 nuclease (Invitrogen) digestion followed by Southern blot hybridization for all 12 strains. After transfer to Amersham Hybond-N+ membranes (GE Healthcare), the genomic DNA was hybridized with the blaOXA-58 and blaNDM-1 probes as described in the DIG-DNA labeling and detection kit (Roche Diagnostics, Germany).
Only CCBH31258 was subjected to long-read sequencing by Oxford Nanopore Technologies® (ONT). Genomic DNA was extracted using the DNeasy® PowerSoil® Pro Kit (Qiagen), following the manufacturer’s recommendations. The barcoding sequencing library was prepared according to the protocol for native barcoding genomic DNA using the Ligation Sequencing SQK-LSK109 and Native Barcoding Expansion 1–12 EXP-NBD104 kits (Oxford Nanopore Technologies®), as well as the NEB Blunt/TA Ligase Master Mix, NEBNext® Quick Ligation Reaction Buffer, and NEBNext® Companion Module for Oxford Nanopore Technologies® Ligation Sequencing. After that, the DNA library was loaded onto a FLO-MIN106D flow cell (R9.4 chemistry) to run on Oxford Nanopore MinION. MinKNOW v.21.02.1 was used to obtain sequence signals, while basecalling and demultiplexing were performed using Guppy basecaller v1.6.0.
Based on the PFGE analysis, all isolates except CCCBH31258 were subjected to Illumina whole-genome sequencing. Genomic DNA from each strain from the overnight culture was extracted using the QIAamp DNA mini kit (Qiagen), following the manufacturer’s instructions. A tagmentation library from genomic DNA was made using the Nextera XT DNA Sample Preparation Kit (Illumina, San Diego, CA, United States), and the 250-bp paired-end reads were sequenced on the Miseq system (Illumina, San Diego, CA, United States).
The de novo assembly for CCBH31258 long reads was generated using the Flye assembler (Kolmogorov et al., 2019). For the short reads from the other seven strains, Trimmomatic was used at the trimming step (ILLUMINACLIP:NexteraPE-PE.fa:2:30:10 AVGQUAL:20 MINLEN:50) (Bolger et al., 2014). The genome de novo assembly was performed using Unicycler (v0.4.9), conservative mode (Wick et al., 2017). Short reads were mapped against plasmids from CCBH31258 (pCCBH31258) using minimap2 and SAMtools (Li et al., 2009; Li, 2018). For strains that read coverage for all pCCBH31258, a plasmid consensus sequence was generated using Pilon, and VCF output was checked (Walker et al., 2014). Furthermore, contigs were mapped against the plasmid consensus using minimap2, and the mapped contigs were excluded to obtain only chromosome contigs. Contigs from the other strains were extended by AlignGraph (Bao et al., 2014), using pCCBH31258 as a reference. For each strain, mapped reads (SAM files) using pCCBH31258 as a reference were compared.
Draft genomes were submitted to GenBank and annotated using the NCBI Prokaryotic Genome Annotation Pipeline (PGAP). The web application CABGen was used to access coverage estimation; species confirmation; MLST mapping; searches for genes related to AMR, virulence, and plasmids; and detection of point mutations in specific AMR genes (Duré et al., 2022). Resistance genes were confirmed using raw reads as input for ResFinder software on the Center for Genomic Epidemiology website. Insertion sequence annotation was improved in the figures using ISFinder (Siguier, 2006). MOB-suite was applied to the assembled plasmid to predict the Inc. group, relaxase typing, and conjugation potential (Robertson and Nash, 2018). PdifFinder analyses were adopted for the annotation of pdif sites and pdif-ARGs modules in the plasmid (Shao et al., 2023).
The isolates exhibited a resistance profile to the majority of the antimicrobials tested, with the highest resistance rates to meropenem (100%), gentamicin (100%), trimethoprim–sulfamethoxazole (83%), and tobramycin (83%). Colistin was the most active agent tested, with only two resistant isolates, one from Bahia and the other from Amazonas (Figure 1).
Figure 1. Dendrogram cluster analysis of PFGE data with a ≥ 90% cut-off level of 12 clinical isolates of Acinetobacter baumannii and other relevant information about the strains. BA: Bahia; AM: Amazonas; AK: amikacin; CIP: ciprofloxacin; TMP/SMX: trimethoprim–sulfamethoxazole; TOB: tobramycin; LEV: levofloxacin; COL: colistin. *99% identity.
The 12 strains were grouped into six PFGE clusters (A, B, C, D, E, and F), and the most frequent pulsetype was pattern F (six strains from the same hospital in Bahia), followed by pattern E (2 strains from two different hospitals in Amazonas). In Bahia and Amazonas, two other pulsetypes were found (A and B in Bahia and C and D in Amazonas). Southern blot hybridization showed that blaOXA-58 and blaNDM-1 genes were located on a plasmid with approximately 320 kb for clone B and clone F strains, which were isolated in the Bahia state, in the same hospital. Furthermore, two isolates from the Amazonas state, belonging to clone E, showed labeled bands with similar weights (CCBH31624 and CCBH32292). Two strains from Amazonas (CCBH33291 and CCBH33294), isolated in the same hospital, showed the same labeled band of approximately 178 kb, although they belong to different clones (C and D) (Figure 1; Supplementary material).
The de novo assembly for CCBH31258 (pulsotype F) long reads generated two contigs: one chromosome with 3,706,655 bp and one circularized plasmid with 340,418 bp (GenBank accession CP101889-CP101888). This isolate belongs to ST374 and carries blaOXA-259 (a blaOXA-51 variant). Both carbapenemase-encoding genes (blaOXA-58 and blaNDM-1) were detected in the MDR plasmid (pCCBH31258), which also carried resistance genes to several other antimicrobials, such as blaTEM-1B, aac(3)-IIa, aph(6)-Id, msr(E), mph(E), dfrA1, floR, sul1, and sul2 (Figure 1).
In plasmid pCCBH31258, the blaNDM-1 gene was identified in a DNA region characterized by a distinct guanine-cytosine (GC) content compared to the reads of the other isolates analyzed. This region also encompasses genes encoding a chaperone and a bleomycin-binding protein, corresponding to the complete Tn125 transposon, flanked by ISAba125 (10,099 bp) (Figure 2A).
Figure 2. Genomic comparison of plasmid pCCBH31258 with (A) other Acinetobacter baumannii strains from this study and (B) selected plasmids from GenBank: pC54_001 (CP042365.1), pDETAB2 (CP047975.1) (Liu et al., 2021), pNDM_SCLZS86 (CP090865.1) (Li et al., 2022b), pNDM_SCLZS30 (CP090384.1) (Li et al., 2022a), pAC1530 (CP045561.1) (Alattraqchi et al., 2021), and pAC1633-1 (CP059301) (Alattraqchi et al., 2021). Inside out, the first ring is the plasmid pCCBH31258, used as a reference (CP101888.1). In (A), each strain was represented by its reads mapping coverage against the reference plasmid. The “graph maximum value” was set to 400x, which represents the maximum coverage peak shown. Regions with coverage over 400x were highlighted in dark blue. In (A,B), the last ring represents relevant features in the reference. BA: Bahia state; AM: Amazonas state.
The blaOXA-58 was inserted between a complete ISAba3 upstream and a truncated ISAba3 downstream, followed by a complete ISApi2. This structure belongs to a pdif-ARGs module in pCCBH31258, surrounded by inversely oriented pdif sites (XerC/D and XerD/C). Surrounding blaOXA-58, araC family gene and genes related to efflux systems (adeR, adeS, MFS transporter, and multidrug efflux RND) were identified. Macrolide resistance genes, msr(E) and mph(E), were also located next to blaOXA-58 (Figure 2A).
Two genomic comparisons were applied: mapped reads from this study strains (Figure 2A) and GenBank assembly plasmids co-harboring blaOXA-58 and blaNDM-1, both using pCCBH31258 as a reference (Figure 2B). After visual inspection of the mapping results, reads from CCBH31270, CCBH32465, CCBH33045, CCBH33777, and CCBH33778 (typed as pulsotype F and ST374) covered all regions of pCCBH31258 (Figure 2A). Therefore, for these strains, the plasmid consensus assembly was adopted, and it was assumed that they carried the same plasmid (CP101886.1, JAPDGP000000000.1, JBDJPK000000000.1, JBDJPJ000000000.1, and JBDJPI000000000.1). Reads from CCBH31950 (pulsotype B, ST464) covered almost all pCCBH31258, missing only specific IS regions (ISApi2 and ISAba22) (Figure 2A). These seven strains were isolated in the same hospital in Bahia state, Brazil, between April and July 2021.
Although the weight estimation derived from hybridization results suggested that CCBH31624, isolated in Amazonas, harbored a plasmid similar to pCCBH31258, sequencing results indicated distinct gene sets (Figure 1). According to the mapping results and gene content, CCBH31624 and CCBH32292 (both pulsotype E, ST464) appear to carry a plasmid with a genomic background different from pCCBH31258, despite the similar weight observed in the Southern blot. For CCBH29641 (pulsotype A, ST739), retrieved from a different hospital in Bahia, the mapping results also demonstrated a genomic background distinct from pCCBH31258. Two sequenced strains from the same hospital in Amazonas (CCBH33291 and CCBH33294), belonging to ST10 and ST575, respectively, exhibited minimal similarities with pCCBH31258. For all these five strains, Tn125 harboring blaNDM-1 was incomplete (Figure 2A).
Consequently, for STs other than ST374, it could not be confirmed by sequencing that blaOXA-58 and blaNDM-1 were inserted in the same plasmid, despite indications from Southern blot results. Given this, the contigs output from Align-Graph was adopted. For all isolates in this study, only the upstream insertion ISAba125 and downstream bleomycin gene were conserved surrounding blaNDM-1, and only the ISAba3 upstream and downstream regions were conserved for blaOXA-58 (Figure 2A).
MobTyper analysis revealed that pCCBH31258 encodes a relaxase that belongs to the MOBp family and was thus classified as a mobilizable plasmid. The Inc. group could not be determined; only a repB gene was identified (KT325596, 23,590–24,066), which was not included in the rep families characterized by Lam and Hamidian (2024). Furthermore, MobTyper identified pC54_001 (CP042365, A. pittii, clinical origin, Australia) as the closest plasmid in its database, sharing 99% identity (65% coverage). A literature search revealed other plasmids co-harboring OXA-58 and NDM-1, including pDETAB2 (CP047975.1), pNDM_SCLZS86 (CP090865.1), pNDM_SCLZS30 (CP090384.1), pAC1530 (CP045561.1), and pAC1633-1 (CP059301). A comparison of pCCBH31258 with these plasmids revealed that the former is a novel plasmid carrying these carbapenemase genes together. pCCBH31258 likely evolved from a blaOXA-58 plasmid, as it shares conjugation genes from the type IVB secretion system and other backbone genes (parB and parM) with pC54_001. Moreover, the replicon gene (rep) of pCCBH31258 encoded a replicase protein different from those present in the A. baumannii plasmids used for comparison (Figure 2B). Two strains from Amazonas, CCBH33291 and CCBH33294, which exhibited a similarly labeled band with an approximate size of 178 kb, were analyzed by mapping their reads against pAC1530 and pAC1633-1. These plasmids share a similar size, but the read coverage was found to be below 20%.
Acinetobacter baumannii exhibits an extraordinary ability to develop resistance to multiple antimicrobial agents, particularly through mobile genetic elements, such as plasmids, that harbor antimicrobial resistance determinants. The prevalence of multidrug-resistant A. baumannii (MDR-Ab) infections in hospital settings is a significant health concern, especially regarding last-resort antibiotics such as carbapenems. In the present study, all blaOXA-58 and blaNDM-1 positive strains were resistant to meropenem and exhibited the MDR phenotype, aligning with the global increase in MDR-Ab infections (Nguyen and Joshi, 2021).
Carbapenem-resistant A. baumannii (CRAB) is a therapeutic challenge because few currently available antibiotics are active against CRAB (Bartal et al., 2022). Carbapenem resistance makes colistin the last-resort antimicrobial to treat MDR-Ab infections. However, overuse of this antimicrobial to treat CRAB infections may have contributed to the increased prevalence of colistin-resistant A. baumannii (Kurihara et al., 2022). Although most of the strains in the current study were susceptible to colistin, resistance was observed in two isolates.
CRAB is generally associated with a wide range of co-resistance to other classes of antimicrobial agents. The production of OXA-type carbapenemases is the main mechanism of carbapenem resistance in A. baumannii. These carbapenemases are encoded by the blaOXA genes, which are usually carried by highly transmissible plasmids (Nguyen and Joshi, 2021). In Brazil, blaOXA-23 is the most widely disseminated OXA-type carbapenemase in CRAB, followed by blaOXA-143, while blaOXA-58 is less common (de Souza Gusatti et al., 2012; de Oliveira et al., 2019; Matos et al., 2019).
OXA-58 was first described in 2003 in France; since then, it has been widely reported worldwide, being associated with outbreaks of nosocomial infections (Moro et al., 2008; Ozen et al., 2009; de Souza Gusatti et al., 2012). OXA-58 hydrolyzes carbapenems at low levels; however, expression can be increased by the presence of insertion sequences, such as ISAba3, resulting in resistance to carbapenems (Walther-Rasmussen and Høiby, 2006; Evans and Amyes, 2014). In the present study, all representative isolates sequenced showed complete ISAba3 upstream of the blaOXA-58 gene, suggesting its higher expression. The blaOXA-58 gene was inserted in a pdif module flanked by inversely oriented pdif sites in pCCBH31258, suggesting that this gene was probably mobilized and mediated by pdif sites using a XerC-XerD recombination system (Li et al., 2022a).
The emergence of NDM in CRAB has also become an important public health issue, which remains a major challenge for the treatment of infectious diseases. NDM is reported in most regions of the world due to its rapid dissemination since the gene encoding NDM is often carried by transferable plasmids (Villacís et al., 2019). pCCBH31258 analyzed in this study revealed that the blaNDM-1 gene was located between two copies of the ISAba125 element, and all strains carried the bleMBL (bleomycin resistance) gene, which has always been identified downstream of the blaNDM-1 gene. Several studies reported that the genetic environment of the blaNDM-1 gene presents a conserved structure, where this gene is located between two copies of the ISAba125 element, forming a transposon named Tn125 (Dortet et al., 2014; Pagano et al., 2016). However, it could be observed in this study that the Tn125 complete structure was conserved only for the strains that harbored the complete plasmid characterized here.
Our research describes 12 strains of carbapenem-resistant Acinetobacter baumannii (CRAB) concurrently carrying blaOXA-58 and blaNDM-1, representing 1% of all Acinetobacter species received by the laboratory during the period. The coexistence of these carbapenemase genes in CRAB has been infrequently reported. There have been four instances of CRAB isolates co-producing OXA-58 and NDM-1 from Algeria (Ramoul et al., 2016), Japan (Oinuma et al., 2016), Malaysia (Alattraqchi et al., 2021), and China (Liu et al., 2021). Only the strains isolated in Malaysia and China have the blaOXA-58 and blaNDM-1 genes located on the same plasmid (Alattraqchi et al., 2021; Liu et al., 2021) (Figure 2B), while in the Algerian isolate, the genes were chromosomally located (Ramoul et al., 2016), and the genome of the Japanese strain is a draft (Oinuma et al., 2016). Other non-baumannii Acinetobacter carrying blaOXA-58 and blaNDM-1 genes have been reported from Malaysia (Alattraqchi et al., 2021), China (Zhou et al., 2015; Chen et al., 2019; Li et al., 2022a,b), and Palestine (Regeen et al., 2014). Of these reports, three isolates were sequenced and found to carry these genes on the same plasmids (Alattraqchi et al., 2021; Li et al., 2022a,b) (Figure 2B). To the best of our knowledge, this is the first study reporting A. baumannii strains in Brazil co-harboring blaOXA-58 and blaNDM-1 genes. Genomic analysis revealed that pCCBH31258 harbored genes coding for proteins with high-level similarity to components of the Dot/Icm type IV secretion system from species of Legionella and Coxiella (type IVB) (Segal et al., 2005).
It could be noted that there is a high level of ST diversity associated with the co-occurrence of blaOXA-58 and blaNDM-1 in Brazil, which suggests that this co-occurrence does not relate exclusively to the clone. MLST analysis showed that five different sporadic STs were present in 12 whole-genome sequenced strains: ST10, ST374, ST464, ST739, and ST575. Interestingly, none of the STs described here belonged to the main clonal complexes CC1, CC15, CC25, and CC79 predominant in South America or to CC2, which is the most prevalent clonal lineage in the world (Rodríguez et al., 2018). The same diversity of plasmids co-harboring blaOXA-58 and blaNDM-1 can also be highlighted, considering isolates from other countries (Alattraqchi et al., 2021; Liu et al., 2021; Li et al., 2022a,b).
The most frequent ST was ST374, including six isolates from the same hospital, and all these strains carried the same plasmid cohabiting blaOXA-58/blaNDM-1. The A. baumannii ST374 has rarely been reported (Fedrigo et al., 2022), and only one study from Tanzania reported a meropenem-resistant isolate, belonging to ST374, which was found to harbor blaNDM-1 in a chromosomally located composite transposon Tn125 (Moyo et al., 2021), while our observations revealed a plasmid localization for the blaNDM-1 gene.
A very similar plasmid to the one characterized here was observed in an isolate in the same hospital, belonging to ST464 (CCBH31950). Hybridization approaches suggest that another plasmid (~178 kb) is responsible for the mobilization of the two carbapenemase-encoding genes in strains from different clones but isolated in the same hospital in Amazonas state. Horizontal transfer enables different clonal lineages to acquire the same accessory genetic elements (Valenzuela et al., 2007). Furthermore, the mobilization of both genes on the same plasmid may develop an increasing number of OXA-58/NDM-1 co-producer isolates. This scenario is favored by the high plasticity that the genome of A. baumannii has in acquiring, retaining, and disseminating antimicrobial resistance genes, especially in plasmids, which play a key role in harboring and transferring antibiotic-resistant genes (Roca et al., 2012).
Our study delineates a large plasmid harboring OXA-58 and NDM-1 carbapenemases, which have disseminated among clonal strains isolated during 2021 in the same hospital. These strains have accumulated other antimicrobial resistance genes. Furthermore, other genetic contexts have also demonstrated the capacity to disseminate blaOXA-58 and blaNDM-1. Our limited access to ONT sequencing prevented the complete genomic characterization of all plasmids harboring blaOXA-58 and blaNDM-1. However, this study reports the first identification of A. baumannii strains co-harboring these carbapenemase genes in Brazil. The co-occurrence of the carbapenemase genes blaOXA-58 and blaNDM-1 in clinical strains of A. baumannii is relatively uncommon. To the best of our knowledge, the 12 co-producing strains identified in this study represent the highest number reported in a single investigation. The spread of plasmids harboring critical antimicrobial resistance genes may facilitate the emergence of bacterial strains resistant to several classes of antibiotics, thereby presenting new challenges for the treatment of infectious diseases. Thus, the results presented highlight the propensity of A. baumannii to acquire and accumulate resistance genes to significant antimicrobial classes utilized in clinical practice. The characterization of MDR-CRAB strains is crucial for monitoring the evolution of resistance, with the aim of containing their rapid global spread, preventing outbreaks, and optimizing therapy.
The datasets presented in this study can be found in online repositories. The names of the repository/repositories and accession number(s) can be found in the article/Supplementary material.
DR: Conceptualization, Formal analysis, Investigation, Methodology, Visualization, Writing – original draft, Writing – review & editing, Software. MS: Conceptualization, Formal analysis, Investigation, Methodology, Visualization, Writing – review & editing, Software, Writing – original draft. BP: Validation, Writing – review & editing. BK: Methodology, Writing – review & editing. RP: Methodology, Writing – review & editing. GK: Methodology, Writing – review & editing. FP: Methodology, Writing – review & editing. RiL: Methodology, Writing – review & editing. AS: Methodology, Writing – review & editing. RoL: Methodology, Writing – review & editing. EM: Methodology, Writing – review & editing. CR: Conceptualization, Data curation, Investigation, Methodology, Supervision, Validation, Writing – review & editing. AC-A: Conceptualization, Data curation, Formal analysis, Funding acquisition, Investigation, Project administration, Resources, Supervision, Validation, Writing – review & editing.
The author(s) declare financial support was received for the research, authorship, and/or publication of this article. This work was supported by the Fundação Carlos Chagas Filho de Amparo à Pesquisa do Estado do Rio de Janeiro – FAPERJ (grant number: E-26/200.420/2023) and the Conselho Nacional de Desenvolvimento Científico e Tecnológico – CNPq (grant number: 408725/2022-2).
AS was employed by Laboratório Reunidos Ltda.
The remaining authors declare that the research was conducted in the absence of any commercial or financial relationships that could be construed as a potential conflict of interest.
All claims expressed in this article are solely those of the authors and do not necessarily represent those of their affiliated organizations, or those of the publisher, the editors and the reviewers. Any product that may be evaluated in this article, or claim that may be made by its manufacturer, is not guaranteed or endorsed by the publisher.
The Supplementary material for this article can be found online at: https://www.frontiersin.org/articles/10.3389/fmicb.2024.1439373/full#supplementary-material
Alattraqchi, A. G., Mohd Rani, F., A Rahman, N. I., Ismail, S., Cleary, D. W., Clarke, S. C., et al. (2021). Complete genome sequencing of Acinetobacter baumannii AC1633 and Acinetobacter nosocomialis AC1530 unveils a large multidrug-resistant plasmid encoding the NDM-1 and OXA-58 Carbapenemases. mSphere 6:e01076-20. doi: 10.1128/mSphere.01076-20
Anggraini, D., Santosaningsih, D., Saharman, Y. R., Endraswari, P. D., Cahyarini, C., Saptawati, L., et al. (2022). Distribution of Carbapenemase genes among Carbapenem-non-susceptible Acinetobacter baumanii blood isolates in Indonesia: A multicenter study. Antibiotics 11:366. doi: 10.3390/antibiotics11030366
Bao, E., Jiang, T., and Girke, T. (2014). AlignGraph: algorithm for secondary de novo genome assembly guided by closely related references. Bioinformatics 30, i319–i328. doi: 10.1093/bioinformatics/btu291
Bartal, C., Rolston, K. V. I., and Nesher, L. (2022). Carbapenem-resistant Acinetobacter baumannii: colonization, infection and current treatment options. Infect. Dis. Ther. 11, 683–694. doi: 10.1007/s40121-022-00597-w
Bauer, A. W., Kirby, W. M., Sherris, J. C., and Turck, M. (1966). Antibiotic susceptibility testing by a standardized single disk method. Am. J. Clin. Pathol. 45, 493–496. doi: 10.1093/ajcp/45.4_ts.493
Bolger, A. M., Lohse, M., and Usadel, B. (2014). Trimmomatic: a flexible trimmer for Illumina sequence data. Bioinformatics 30, 2114–2120. doi: 10.1093/bioinformatics/btu170
Bou, G., Cerveró, G., Domínguez, M. A., Quereda, C., and Martínez-Beltrán, J. (2000). PCR-based DNA fingerprinting (REP-PCR, AP-PCR) and pulsed-field gel electrophoresis characterization of a nosocomial outbreak caused by imipenem-and meropenem-resistant Acinetobacter baumannii. Clin. Microbiol. Infect. 6, 635–643. doi: 10.1046/j.1469-0691.2000.00181.x
Chen, Y., Guo, P., Huang, H., Huang, Y., Wu, Z., and Liao, K. (2019). Detection of co-harboring OXA-58 and NDM-1 carbapenemase producing genes resided on a same plasmid from an Acinetobacter pittii clinical isolate in China. Iran. J. Basic Med. Sci. 22, 106–111. doi: 10.22038/ijbms.2018.28934.6994
de Oliveira, E. A., de Paula, G. R., Mondino, P. J. J., Chagas, T. P. G., Mondino, S. S. B., and Mendonça-Souza, C. R. V. (2019). High rate of detection of OXA-23-producing Acinetobacter from two general hospitals in Brazil. Rev. Soc. Bras. Med. Trop. 52:e20190243. doi: 10.1590/0037-8682-0243-2019
de Souza Gusatti, C., Bertholdo, L. M., Otton, L. M., Marchetti, D. P., Ferreira, A. E., and Corção, G. (2012). First occurrence of Bla OXA-58 in Acinetobacter baumannii isolated from a clinical sample in southern Brazil. Braz. J. Microbiol. 43, 243–246. doi: 10.1590/S1517-83822012000100027
Dortet, L., Poirel, L., and Nordmann, P. (2014). Worldwide dissemination of the NDM-type Carbapenemases in gram-negative Bacteria. Biomed. Res. Int. 2014, 1–12. doi: 10.1155/2014/249856
Duré, F. M., Silveira, M. C., Rocha-de-Souza, C. M., Leão, R. S., de Oliveira Santos, I. C., Albano, R. M., et al. (2022). CABGen: A web application for the Bioinformatic analysis of bacterial genomes. Front. Microbiol. 13:893474. doi: 10.3389/fmicb.2022.893474
Evans, B. A., and Amyes, S. G. B. (2014). OXA-Lactamases. Clin. Microbiol. Rev. 27, 241–263. doi: 10.1128/CMR.00117-13
European Committee on Antimicrobial Susceptibility Testing (EUCAST) . (2023). Clinical Breakpoints – Bacteria. Version 13.1, 2023. Available at: https://www.eucast.org/clinical_breakpoints. (Accessed September 12, 2023).
Fedrigo, N. H., Xavier, D. E., Cerdeira, L., Fuga, B., Marini, P. V. B., Shinohara, D. R., et al. (2022). Genomic insights of Acinetobacter baumannii ST374 reveal wide and increasing resistome and virulome. Infect. Genet. Evol. 97:105148. doi: 10.1016/j.meegid.2021.105148
Ghaly, T. M., Paulsen, I. T., Sajjad, A., Tetu, S. G., and Gillings, M. R. (2020). A novel family of Acinetobacter Mega-plasmids are disseminating multi-drug resistance across the globe while acquiring location-specific accessory genes. Front. Microbiol. 11:605952. doi: 10.3389/fmicb.2020.605952
Goic-Barisic, I., Kovacic, A., Medic, D., Jakovac, S., Petrovic, T., Tonkic, M., et al. (2021). Endemicity of OXA-23 and OXA-72 in clinical isolates of Acinetobacter baumannii from three neighbouring countries in Southeast Europe. J. Appl. Genet. 62, 353–359. doi: 10.1007/s13353-021-00612-9
Hamidian, M., and Nigro, S. J. (2019). Emergence, molecular mechanisms and global spread of carbapenem-resistant Acinetobacter baumannii. Microb. Genom. 5:e000306. doi: 10.1099/mgen.0.000306
Kolmogorov, M., Yuan, J., Lin, Y., and Pevzner, P. A. (2019). Assembly of long, error-prone reads using repeat graphs. Nat. Biotechnol. 37, 540–546. doi: 10.1038/s41587-019-0072-8
Kurihara, M. N. L., Sales, R. O., Silva, K. E., Silva, G. D., Mansano, M. C. T., Mahmoud, F. F., et al. (2022). High lethality rate of carbapenem-resistant Acinetobacter baumannii in intensive care units of a Brazilian hospital: an epidemiologic surveillance study. Rev. Soc. Bras. Med. Trop. 55:e05292021. doi: 10.1590/0037-8682-0529-2021
Kyriakidis, I., Vasileiou, E., Pana, Z. D., and Tragiannidis, A. (2021). Acinetobacter baumannii antibiotic resistance mechanisms. Pathogens 10:373. doi: 10.3390/pathogens10030373
Lam, M. M. C., and Hamidian, M. (2024). Examining the role of Acinetobacter baumannii plasmid types in disseminating antimicrobial resistance. NPJ Antimicrob. Resist. 2:1. doi: 10.1038/s44259-023-00019-y
Li, H. (2018). Minimap2: pairwise alignment for nucleotide sequences. Bioinformatics 34, 3094–3100. doi: 10.1093/bioinformatics/bty191
Li, H., Handsaker, B., Wysoker, A., Fennell, T., Ruan, J., Homer, N., et al. (2009). The sequence alignment/map format and SAMtools. Bioinformatics 25, 2078–2079. doi: 10.1093/bioinformatics/btp352
Li, Y., Qiu, Y., Fang, C., Dai, X., and Zhang, L. (2022a). Coexistence of Bla OXA-58 and Bla NDM-1 on a novel plasmid of GR59 from an Acinetobacter towneri isolate. Antimicrob. Agents Chemother. 66:e0020622. doi: 10.1128/aac.00206-22
Li, Y., Qiu, Y., Fang, C., Tang, M., Dai, X., and Zhang, L. (2022b). Characterisation of a novel GR31 plasmid co-harbouring blaNDM-1 and blaOXA-58 in an Acinetobacter sp. isolate. J. Glob. Antimicrob. Resist. 29, 212–214. doi: 10.1016/j.jgar.2022.03.015
Liu, H., Moran, R. A., Chen, Y., Doughty, E. L., Hua, X., Jiang, Y., et al. (2021). Transferable Acinetobacter baumannii plasmid pDETAB2 encodes OXA-58 and NDM-1 and represents a new class of antibiotic resistance plasmids. J. Antimicrob. Chemother. 76, 1130–1134. doi: 10.1093/jac/dkab005
Matos, A. P., Cayô, R., Almeida, L. G. P., Streling, A. P., Nodari, C. S., Martins, W. M. B. S., et al. (2019). Genetic characterization of plasmid-borne Bla OXA-58 in distinct Acinetobacter species. mSphere 4:e00376-19. doi: 10.1128/mSphere.00376-19
Moro, M., Nizzero, P., Biancardi, A., Baldan, R., Scarpellini, P., Curti, C., et al. (2008). An outbreak caused by multidrug-resistant OXA-58-positive Acinetobacter baumannii in an intensive care unit in Italy. J. Hosp. Infect. 68, 97–99. doi: 10.1016/j.jhin.2007.10.007
Moyo, S. J., Manyahi, J., Hubbard, A. T. M., Byrne, R. L., Masoud, N. S., Aboud, S., et al. (2021). Molecular characterisation of the first New Delhi metallo-β-lactamase 1-producing Acinetobacter baumannii from Tanzania. Trans. R. Soc. Trop. Med. Hyg. 115, 1080–1085. doi: 10.1093/trstmh/traa173
Nguyen, M., and Joshi, S. G. (2021). Carbapenem resistance in Acinetobacter baumannii, and their importance in hospital-acquired infections: a scientific review. J. Appl. Microbiol. 131, 2715–2738. doi: 10.1111/jam.15130
Nodari, C. S., Cayô, R., Streling, A. P., Lei, F., Wille, J., Almeida, M. S., et al. (2020). Genomic analysis of Carbapenem-resistant Acinetobacter baumannii isolates belonging to major endemic clones in South America. Front. Microbiol. 11:584603. doi: 10.3389/fmicb.2020.584603
Oinuma, K.-I., Suzuki, M., Sato, K., Nakaie, K., Niki, M., Takizawa, E., et al. (2016). Genome sequence of an Acinetobacter baumannii strain carrying three acquired Carbapenemase genes. Genome Announc. 4:e01290-16. doi: 10.1128/genomeA.01290-16
Ozen, N., Ergani, A., Naas, T., Ogunc, D., Gultekin, M., Colak, D., et al. (2009). Outbreak of Carbapenem-resistant Acinetobacter baumannii producing the Carbapenemase OXA-58 in Turkey. Open Antimicrob. Agents J. 1, 1–8. doi: 10.2174/1876518100901010001
Pagano, M., Martins, A. F., and Barth, A. L. (2016). Mobile genetic elements related to carbapenem resistance in Acinetobacter baumannii. Braz. J. Microbiol. 47, 785–792. doi: 10.1016/j.bjm.2016.06.005
Palmieri, M., D’Andrea, M. M., Pelegrin, A. C., Perrot, N., Mirande, C., Blanc, B., et al. (2020). Abundance of Colistin-resistant, OXA-23-and ArmA-producing Acinetobacter baumannii belonging to international clone 2 in Greece. Front. Microbiol. 11:668. doi: 10.3389/fmicb.2020.00668
Ramoul, A., Loucif, L., Bakour, S., Amiri, S., Dekhil, M., and Rolain, J.-M. (2016). Co-occurrence of Bla NDM-1 with Bla OXA-23 or Bla OXA-58 in clinical multidrug-resistant Acinetobacter baumannii isolates in Algeria. J. Glob. Antimicrob. Resist. 6, 136–141. doi: 10.1016/j.jgar.2016.05.003
Regeen, H., Al-Sharafa-Kittaneh, D., Kattan, R., Al-Dawodi, R., Marzouqa, H., and Hindiyeh, M. Y. (2014). First report of Bla NDM and Bla OXA-58 coexistence in Acinetobacter junii. J. Clin. Microbiol. 52, 3492–3493. doi: 10.1128/JCM.01152-14
Robertson, J., and Nash, J. H. E. (2018). MOB-suite: software tools for clustering, reconstruction and typing of plasmids from draft assemblies. Microb. Genom. 4:e000206. doi: 10.1099/mgen.0.000206
Roca, I., Espinal, P., Vila-Farrés, X., and Vila, J. (2012). The Acinetobacter baumannii oxymoron: commensal hospital dweller turned Pan-drug-resistant menace. Front. Microbiol. 3:148. doi: 10.3389/fmicb.2012.00148
Rodríguez, C. H., Nastro, M., and Famiglietti, A. (2018). Carbapenemases in Acinetobacter baumannii. Review of their dissemination in Latin America. Rev. Argent. Microbiol. 50, 327–333. doi: 10.1016/j.ram.2017.10.006
Segal, G., Feldman, M., and Zusman, T. (2005). The Icm/dot type-IV secretion systems of Legionella pneumophila and Coxiella burnetii. FEMS Microbiol. Rev. 29, 65–81. doi: 10.1016/j.femsre.2004.07.001
Shao, M., Ying, N., Liang, Q., Ma, N., Leptihn, S., Yu, Y., et al. (2023). Pdif-mediated antibiotic resistance genes transfer in bacteria identified by pdifFinder. Brief. Bioinform. 24:bbac521. doi: 10.1093/bib/bbac521
Siguier, P. (2006). ISfinder: the reference Centre for bacterial insertion sequences. Nucleic Acids Res. 34, D32–D36. doi: 10.1093/nar/gkj014
Valenzuela, J. K., Thomas, L., Partridge, S. R., van der Reijden, T., Dijkshoorn, L., and Iredell, J. (2007). Horizontal gene transfer in a polyclonal outbreak of Carbapenem-resistant Acinetobacter baumannii. J. Clin. Microbiol. 45, 453–460. doi: 10.1128/JCM.01971-06
Vasconcelos, A. T. R., Barth, A. L., Zavascki, A. P., Gales, A. C., Levin, A. S., Lucarevschi, B. R., et al. (2015). The changing epidemiology of Acinetobacter spp. producing OXA carbapenemases causing bloodstream infections in Brazil: a BrasNet report. Diagn. Microbiol. Infect. Dis. 83, 382–385. doi: 10.1016/j.diagmicrobio.2015.08.006
Vasudevan, A., Kesavan, D. K., Wu, L., Su, Z., Wang, S., Ramasamy, M. K., et al. (2022). In Silico and in vitro screening of natural compounds as broad-Spectrum β-lactamase inhibitors against Acinetobacter baumannii New Delhi Metallo-β-lactamase-1 (NDM-1). Biomed. Res. Int. 2022, 1–19. doi: 10.1155/2022/4230788
Villacís, J. E., Bovera, M., Romero-Alvarez, D., Cornejo, F., Albán, V., Trueba, G., et al. (2019). NDM-1 carbapenemase in Acinetobacter baumannii sequence type 32 in Ecuador. New Microbes New Infect. 29:100526. doi: 10.1016/j.nmni.2019.100526
Walker, B. J., Abeel, T., Shea, T., Priest, M., Abouelliel, A., Sakthikumar, S., et al. (2014). Pilon: an integrated tool for comprehensive microbial variant detection and genome assembly improvement. PLoS One 9:e112963. doi: 10.1371/journal.pone.0112963
Walther-Rasmussen, J., and Høiby, N. (2006). OXA-type carbapenemases. J. Antimicrob. Chemother. 57, 373–383. doi: 10.1093/jac/dki482
Wick, R. R., Judd, L. M., Gorrie, C. L., and Holt, K. E. (2017). Unicycler: resolving bacterial genome assemblies from short and long sequencing reads. PLoS Comput. Biol. 13:e1005595. doi: 10.1371/journal.pcbi.1005595
Wong, D., Nielsen, T. B., Bonomo, R. A., Pantapalangkoor, P., Luna, B., and Spellberg, B. (2017). Clinical and pathophysiological overview of Acinetobacter infections: a century of challenges. Clin. Microbiol. Rev. 30, 409–447. doi: 10.1128/CMR.00058-16
Zhang, H., Zhu, Y., Yang, N., Kong, Q., Zheng, Y., Lv, N., et al. (2021). In vitro and in vivo activity of combinations of Polymyxin B with other antimicrobials against Carbapenem-resistant Acinetobacter baumannii. Infect. Drug Resist. 14, 4657–4666. doi: 10.2147/IDR.S334200
Keywords: Acinetobacter baumannii , OXA-58 carbapenemase, NDM-1 carbapenemase, genomic, plasmids, co-harboring
Citation: Rodrigues DCS, Silveira MC, Pribul BR, Karam BRS, Picão RC, Kraychete GB, Pereira FM, de Lima RM, de Souza AKG, Leão RS, Marques EA, Rocha-de-Souza CM and Carvalho-Assef APD (2024) Genomic study of Acinetobacter baumannii strains co-harboring blaOXA-58 and blaNDM-1 reveals a large multidrug-resistant plasmid encoding these carbapenemases in Brazil. Front. Microbiol. 15:1439373. doi: 10.3389/fmicb.2024.1439373
Received: 27 May 2024; Accepted: 01 July 2024;
Published: 17 July 2024.
Edited by:
Ilaria Frasson, University of Padua, ItalyReviewed by:
Shikha Joon, National Cancer Institute at Frederick (NIH), United StatesCopyright © 2024 Rodrigues, Silveira, Pribul, Karam, Picão, Kraychete, Pereira, de Lima, de Souza, Leão, Marques, Rocha-de-Souza and Carvalho-Assef. This is an open-access article distributed under the terms of the Creative Commons Attribution License (CC BY). The use, distribution or reproduction in other forums is permitted, provided the original author(s) and the copyright owner(s) are credited and that the original publication in this journal is cited, in accordance with accepted academic practice. No use, distribution or reproduction is permitted which does not comply with these terms.
*Correspondence: Ana Paula D'Alincourt Carvalho-Assef, YW5hcGRjYUBpb2MuZmlvY3J1ei5icg==
Disclaimer: All claims expressed in this article are solely those of the authors and do not necessarily represent those of their affiliated organizations, or those of the publisher, the editors and the reviewers. Any product that may be evaluated in this article or claim that may be made by its manufacturer is not guaranteed or endorsed by the publisher.
Research integrity at Frontiers
Learn more about the work of our research integrity team to safeguard the quality of each article we publish.