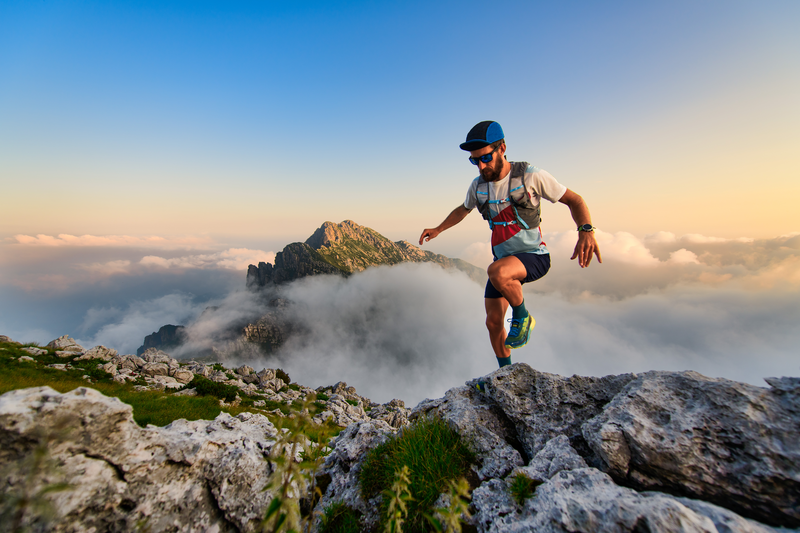
95% of researchers rate our articles as excellent or good
Learn more about the work of our research integrity team to safeguard the quality of each article we publish.
Find out more
ORIGINAL RESEARCH article
Front. Microbiol. , 07 March 2025
Sec. Terrestrial Microbiology
Volume 15 - 2024 | https://doi.org/10.3389/fmicb.2024.1437489
This article is part of the Research Topic Mineral Solubilizing Microorganisms (MSM) and Their Applications in Nutrient Bioavailability, Bioweathering and Bioremediation, Vol III View all 11 articles
Introduction: Enhancing productivity and nutrient content of soybean (Glycine max L.) is vital for sustainable agriculture. The utilization of beneficial bacterial and fungal strains has shown promising results in promoting plant growth and improving nutrient uptake. However, the effects of the individual and interactions of such microbes on soybean growth, yield, and nutrient profiling remain inadequately understood. Thus, there is a pressing need to comprehensively investigate the impact of tripartite microbial augmentation on soybean cultivation.
Methods: This field study aims to elucidate the synergistic mechanisms underlying the interactions between Bacillus sp. MN54, Bradyrhizobium diazoefficiens, and Piriformospora indica and their collective influence on soybean growth parameters, yield and nutritional quality.
Results: In vivo compatibility tests revealed that consortium applications led to a maximum of 90% soybean germination. The field study demonstrated a significant increase in plant height (17.01%), nodules plant–1 (17.35%), pods plant–1 (12.11%), and grain yield (20.50%) due to triple inoculation over untreated control. The triple inoculation also significantly increased chlorophyll a, b, and leghemoglobin contents by 19.38, 21.01, and 14.28%, respectively, compared to control. Triple inoculation promoted crude fiber, protein, and oil content by 14.92, 8.78, and 10.52%, respectively, compared to the untreated control. The increase in nitrogen content by 7.33% in grains and 6.26% in stover and phosphorus by 11.31% in grains and 12.72% in stover was observed through triple application over untreated control.
Discussion: Our findings highlight the potential of microbial inoculants as biofertilizers in sustainable soybean production. The triple inoculation with Bacillus sp. MN54, Bradyrhizobium diazoefficiens, and Piriformospora indica significantly improved soybean growth, yield, grain quality attributes, and nutrient uptake. This microbial consortium application could help to enhance agricultural productivity by boosting the nodulation in soybean and improving synergism between the microbial strains.
Rapid population growth and climate change pose significant challenges to global food production, and cause natural resource scarcity. As the world’s population approaches 9 billion by 2050, addressing malnutrition becomes increasingly urgent (Kumar and Dubey, 2020). To maximize the produce from current agricultural land, agricultural pressure generated by population increase has led to extensive usage of chemical fertilizers and pesticides, and among them, about 20–30% of the fertilizers are absorbed by the plants (Saeed et al., 2021). Inefficient nutrient usage in agriculture and soil dynamics results in more than half of fertilizers being lost to the environment (Fageria, 2014). Unfortunately, nitrogen (N) fertilizers given to the soil are easily volatilized, washed away, while Phosphorus (P) fertilizers gets fixed and gradually changed into unavailable forms by natural processes, putting the ecology and biodiversity at greater risk. As a result, total agricultural productivity has decreased, and environmental issues such as habitat loss, carbon emissions, water contamination, and soil pollution have occurred (Zou et al., 2022). Farmers interests are likewise impacted by increasing agricultural input costs and lowering their profits. This situation demands alternative approaches to enhance the efficiency of applied fertilizers and ensure environmental sustainability.
Microbes, particularly those associated with plant roots, collectively termed the plant microbiome, emerge as pivotal allies in reducing the chemical fertilizer demand (Bender et al., 2016). Among these, beneficial microbes known as Plant Growth-Promoting Rhizobacteria (PGPR) and mycorrhizal fungi have been extensively documented for their ability to enhance plant nutrient uptake, promote growth through hormonal interactions, and increase resistance to abiotic stresses such as drought and salinity (Khan et al., 2016; Rajkumar et al., 2017). The mechanisms by which these microbes operate not only underline the potential to overcome nutrient deficiency but also aid crops in adapting to adverse environmental conditions. Furthermore, combining microbial solutions offers a dual benefit: sustaining crop yields and mitigating adverse environmental impacts.
Among leguminous crops, soybean (Glycine max) is one of the most crucial crops globally, valued for its high protein content and role in sustainable agriculture through nitrogen fixation. The symbiotic relationship between soybean plants and the nitrogen-fixing bacteria is well-documented, facilitating significant reductions in nitrogen fertilizer requirements (Hungria and Mendes, 2015). Still, the native nitrogen-fixing bacteria usually have nutritional competitiveness and poor survival rates, making them unable to ensure adequate nodulation and sufficient biological nitrogen fixation (Masson-Boivin and Sachs, 2018). One of the methods for increasing biological nitrogen fixation is inoculating seed with biological nitrogen-fixing bacteria. However, further enhancement of soybean productivity may be achieved through co-inoculation with other beneficial soil bacteria, such as Bacillus species (Solanki et al., 2023). This integrative approach leverages the combined effects of nitrogen-fixing bacteria, known for its nitrogen-fixing capabilities, and Bacillus, recognized for promoting plant growth by various mechanisms, including phytohormone production, phosphate solubilization, and antagonistic activity against plant pathogens (Ahmad et al., 2019; Pankievicz et al., 2021). For example, Bacillus strains, which are prolific producers of growth-promoting substances and biocontrol agents, can significantly affect root architecture and thereby improve the efficacy of nitrogen-fixing bacteria in the rhizosphere through a healthier root environment (Xie et al., 2016). This co-inoculation strategy not only boosts the overall health and growth rate of soybean but also contributes to a more sustainable and environmentally friendly farming practice to overcome nutrient deficiency (Compant et al., 2010).
Similarly, it has been found that endophytic growth-promoting microorganisms and nitrogen-fixing bacteria species act synergistically to boost the nitrogen-fixation efficiency of lentils (Saini and Khana, 2012). For the past few decades, Piriformospora indica (P. Indica) has been a famous and most studied endophytic fungus for vegetative increase and plant resistance to nutrient deficiency (Iqbal et al., 2023). P. Indica improves host plants’ growth, biomass, and seed yield and confers resistance to various abiotic and biotic stresses (Franken, 2012). The dual and consortium microorganisms have enhanced plant productivity (Varma et al., 2012). It has been found that the effect of single and dual inoculation in legumes was observed in previous studies (Ferreira et al., 2019; Jaiswal and Dakora, 2020; Gupta et al., 2022), however, no study was conducted on multiple inoculation to enhance soybean growth and yield. Therefore, this study aimed to evaluate the synergistic effects of tripartite microbial augmentation of Bradyrhizobium diazoefficiens (B. diazoefficiens), Bacillus sp. MN54 and P. indica on soybean growth, yield, and nutrient uptake under field situations. This microbial augmentation was evaluated for synergistic effects to improve nodulation in soybean and nutrient uptake.
The microbial cultures of well-characterized plant growth-promoting Bacillus sp. MN54 (accession number KT375574) (Naveed et al., 2014; Afzal et al., 2020) was obtained from the Institute of Soil and Environmental Science, University of Agriculture, Faisalabad, Pakistan. B. diazoefficiens cultures were obtained from the Japan Collection of Microorganisms,1 while P. Indica culture was obtained from the German Collection of Microorganisms and Cell Cultures, GmbH.2 The subculture of B. diazoefficiens was prepared in Yeast Mannitol extract broth (YMEB) at 28 ± 1°C and 120 rpm for five days. The nutrient broth was used to grow Bacillus sp. MN54 at 28 ± 1°C and 120 rpm for 48 h. The P. indica was grown in potato dextrose broth (PDB) at 28 ± 1°C and 120 rpm for 1 week. The subcultures were kept at 4°C for further use.
The compatibility of microbial cultures of B. diazoefficiens, P. indica, and Bacillus sp. MN54 was tested through the streak plate method (Raja et al., 2006) and a germination assay. In the streak plate method, P. indica biomass was cut with a sterile cutter, placed in the middle of a plate containing nutrient agar medium, and kept for control. Bacillus sp. MN54 and B. diazoefficiens were streaked on one side, and the P. indica culture block was placed on the opposite side with three replications and kept at 28 ± 2°C in an incubator for five days. After incubation, these cultures were observed for compatibility with each other. During the germination assay, healthy soybean seeds were surface sterilized using 3.0% sodium hypochlorite solution for 2 min and then washed thoroughly with sterilized water. The surface sterilized seeds were treated with (1) B. diazoefficiens inoculation, (2) B. diazoefficiens + Bacillus sp. MN54 inoculation, (3) B. diazoefficiens + P. indica inoculation, (4) B. diazoefficiens, Bacillus sp. MN54 and P. indica inoculation. The 1 mL log phase culture was used with a ratio of 1:1:1 in triple-inoculation. Treated seeds were kept in Petri dishes comprising 0.7% water agar at 28°C in the growth room for seven days, and each treatment was repeated thrice (Wasike et al., 2009).
A field experiment was conducted in two consecutive summer seasons of 2021 and 2022 at oilseed Research Institute, AARI, Faisalabad, Pakistan. The soil used in this experiment was sandy clay loam having EC 1.4 dS m–1, organic matter 0.67%, pH 7.75, total nitrogen 0.032%, available phosphorus 7.40 mg kg–1, and extractable potassium 116 mg kg–1. The experiment was designed in a randomized complete block design (RCBD) with 24 plots. The soybean variety “Faisal” was used at the rate of 120 kg ha–1 with a row-to-row distance of 30 cm in a plot size of 4.0 m × 1.2 m (4.8 m2). The pooled mean of maximum and minimum temperature during the crop growth period was 39.9 and 27.8°C, respectively. The research location has a subtropical climate, receives 25 mm of rainfall annually, and is between latitude 31.4187°N, longitude 73.0791°E, and 186.0 m above sea level. The treatments in terms of seed coating were (1) Control, (2) B. diazoefficiens, (3) Bacillus sp. MN54, (4) P. indica, (5) B. diazoefficiens + Bacillus sp. MN54, (6) P. indica + Bacillus sp. MN54, (7) B. diazoefficiens + P. indica, and (8) B. diazoefficiens + Bacillus sp. MN54 + P. indica in triplicate.
Soybean seeds were inoculated with respective cultures of B. diazoefficiens, Bacillus sp. MN54 and P. indica alone and in different combinations according to the treatment plan. For this purpose, surface sterilized seeds were inoculated with inoculum-based slurry in 1:1 ratio (50% seed and 50% slurry). This slurry was prepared using 50% inoculum, 30% sterilized peat, 10% clay, and 10% sugar solution. At the time of inoculation, the CFU of B. diazoefficiens and Bacillus sp. was MN54 1 × 108 CFU mL–1, while for P. indica, CFU was 1 × 106 CFU mL–1. In co-inoculation and triple-inoculation, the B. diazoefficiens, Bacillus sp. MN54 and P. indica were applied to soybean seeds at a 1:1 ratio and 1:1:1 ratio, respectively. The number of seeds was uniform in all the experimental plots. Seeds were thoroughly mixed until a thin, fine layer of inoculum appeared. The seeds were spread in the shade to dry overnight in the laboratory. Field was prepared by giving three ploughings followed by planking. The treated seeds were sown in mid-June with a seed rate of 70 kg ha–1. After germination, the plant-to-plant distance of 5–6 cm was maintained while the row-to-row distance was 45 cm. All the agronomic practices were adopted for cultivating the soybean crop. The recommended doses of chemical fertilizers used in the experiment were 25–25–50 NPK kg ha–1 in the form of Urea, single superphosphate (SSP), and sulphate of potash (SOP), respectively. Half of N, all P, and K doses were mixed with soil at the time of sowing, and the remaining N was applied with the first and second irrigations with equal splits. Observations regarding emergence percentage were recorded after 10 days of sowing. Nodular data was recorded at the flowering stage. Plant height, grain yield, number of pods, and dry biomass were recorded at harvesting. Chlorophyll and leghemoglobin contents were recorded after 90 days of sowing (DAS). After harvesting, nutrient contents, seed protein, fiber, moisture, and oil contents were recorded.
Ten plants were randomly selected from each replication of a 2-year trial to determine the plant height with the help of a meter rod on a cm scale. Data on emergence count was determined by recording the number of emerged seedlings per meter row length from a central row of each plot after leaving two border rows on each side. Ten randomly selected plants were uprooted from each plot and were sun-dried and then oven-dried at 60°C for two days to record the dry weight of the shoot in grams (g).
Symbiotic dependency (SD) was recorded at 90 DAS. To determine the SD of soybean, the formula given by Gerdemann (1975) was used:
Nodulation parameters, including the number of nodules and the dry weight of nodules, were recorded by taking an average number of nodules carefully detached from ten randomly uprooted plants. The detached nodules were oven-dried at 60°C for two days, and the dry weight of nodules per plant was recorded in g. The grain yield from each plot (Kg m–2) was recorded, and the final data was presented in kg ha–1.
The chlorophyll content of leaves was estimated using the method of Arnon (1949) at 645 and 663 nm wavelengths through a spectrophotometer (Agilent Technologies, Santa Clara, CA, United States). At noon (between 10:00 and 14:00), after 90 DAS, physiological parameters were recorded from entirely green leaves. For chlorophyll a and b content, plant leaves were crushed in acetone and centrifuged at 10,000 rpm for 10 min. Then, the supernatant was subjected to record absorbance of chlorophyll “a” at 645 nm and that of “b” at 663 nm contents through a spectrophotometer (Agilent Technologies, Santa Clara, CA, United States; Arnon, 1949). Root nodules were taken at the flowering stage to determine the volume of pink bacteroid tissue of nodules and a minor section of nodules (5 μL) was made using a sharp cutter. The amount of pink bacteroid tissue comprising the leghemoglobin in the nodule cortex was determined following the method described by Sadasivam and Manickam (2008). According to this method, 1 g of fresh root nodules was taken and crushed in 5 mL of cold phosphate buffer (0.1 M) with the help of a pestle and mortar in an ice-cold environment. The homogenized mixture was centrifuged at 10,000 rpm for 10 min at 4°C, and a supernatant containing leghemoglobin was collected. Further impurities were removed by adding chilled acetone in 4:1 ratio of nodule extract and incubated for 15 min. The mixture was again centrifuged at 10,000 rpm for 10 min at 4°C. The supernatant was discarded, and the precipitate was dissolved in a phosphate buffer. The absorbance of this mixture was read at 540 nm using a spectrophotometer (Agilent Technologies, Santa Clara, CA, United States), and leghemoglobin concentration was calculated using the following formula. The molar extinction coefficient of leghemoglobin was taken around 11 mM cm–1, and the path length (1 cm) refers to the distance that light travels through the sample in the spectrophotometer cuvette.
Seeds were carefully cleaned and ground to pass through a 0.4 mm screen. AOAC. (1975) method was followed for proximate analysis to determine crude fiber, protein, and moisture content. The protein contents were determined by converting total nitrogen content using a specific factor of 6.25, using the Kjeldahl method. The moisture content in seeds was determined by oven drying 5 g of seeds at 105°C until they reached a constant weight, which was calculated using the following formula.
For crude fiber determination, 2 g of dried ground seeds were acid-digested using 1.25% sulfuric acid. The mixture was boiled for 30 min, filtered, and washed with hot distilled water to neutralize the pH. Further alkali digestion was performed through 1.25% sodium hydroxide and boiled for 30 min. The mixture was again washed with distilled water to neutralize the pH. The residue samples were oven-dried at 105°C until constant weight. The crude fiber of residue samples was performed through dry ashing in a muffle furnace at 550°C for 2 h to remove organic matter, and samples were weighed to calculate crude fiber using the following formula.
Seed oil content was determined using Soxhlet’s apparatus as described in AOAC. (1984). This method extracted 5 g oven-dried seed samples using hexane solvent in the Soxhlet apparatus. A rotary evaporator was used to recover oil from the solvent-oil mixture. The extracted oil was dried at 105°C for 30 min, and oil contents were calculated using the following formula.
The total nitrogen (N) content of shoot and grain was determined using Kjeldahl’s McKenzie and Wallace (1954) technique with slight modification. Estimation of total phosphorous (P) content of shoot in straw and seed was carried out by digestion using a triacid mixture (HNO3: HClO4: H2SO4) (v/v) (Jackson, 1973). Micronutrient contents like iron (Fe), Zinc (Zn), and manganese were determined by following the method of Estefan et al. (2013).
The collected data in triplicate was subjected to analysis of variance (ANOVA) in Randomized Complete Block Design (RCBD) through the software Statistix version 8.1. The Least Significant Difference (LSD) test (Steel et al., 1997) was employed to compare the mean values of each attribute at p ≤ 0.05. The correlation matrix and principal component analysis were performed using R software version 4.1.2.
From the streak plate method, it was observed that B. diazoefficiens and Bacillus sp. MN54 showed a synergistic effect against P. indica. Moreover, during the germination assay, germination was increased by triple inoculations (B. diazoefficiens, Bacillus sp. MN54, and P. indica) than by inoculation B. diazoefficiens alone. A maximum increase in germination (90%) was observed by triple inoculations over inoculation with B. diazoefficiens alone (Table 1).
Table 1. Compatibility test of Bradyrhizobium diazoefficiens, Bacillus sp. MN54 and Piriformospora indica on seed germination assay under axenic conditions.
Inoculation significantly affected all growth parameters compared to uninoculated control (Figure 1). A maximum increase in plant height (17.01%) was observed in plants that received combined inoculations (B. diazoefficiens+ Bacillus sp. MN54 + P. indica) followed by Bacillus sp. MN54 + P. indica (13.15%) over uninoculated control (Figure 1A). The highest emergence (16.31%) was recorded when B. diazoefficiens was applied in combination with P. indica and Bacillus sp. MN54 over control (Figure 1B). Moreover, significant increases in the number of leaves and number of branches were observed in all inoculated treatments over uninoculated control, but the maximum increase in number of leaves plant–1 (17.83%) and number of branches plant–1 (20.40%) were recorded in plants that received triple inoculations over B. diazoefficiens application alone (Figures 1C,D).
Figure 1. Effect of Bradyrhizobium diazoefficiens, Bacillus sp. MN54 and Piriformospora indica on (A) plant height, (B) emergence (%), (C) number of leaves plant–1, (D) number of branches plant–1 in soybean under field conditions. The error bars represent the least significant difference among treatments at P ≤ 0.05.
The results of symbiotic dependency by plants treated with individual symbionts or with triple inoculations are given in Table 2. It was noted the plants inoculated with B. diazoefficiens alone and in combination with Bacillus sp. MN54, P. indica inoculation showed a positive growth response over the uninoculated control. The highest symbiotic efficiency response (161.85%) was observed in plants that received triple inoculation plants (B. diazoefficiens + Bacillus sp. MN54 + P. indica) over control. Similarly, dual inoculation has a more pronounced effect when compared with B. diazoefficiens alone, but maximum response (132.95%) was observed in B. diazoefficiens + Bacillus sp. MN54 + P. indica treated plants over the plants solely inoculated with B. diazoefficiens. The highest number of nodules plant–1 (17.35%) was observed in triple inoculation (B. diazoefficiens+ Bacillus sp. MN54 + P. indica) over uninoculated control (Figure 2A). This treatment response was also higher over the application of B. diazoefficiens alone. Data regarding nodular dry weight was also presented in Figure 2B. It has been found B. diazoefficiens inoculant increased dry weight (10.52%) over control. This increase was more pronounced when B. diazoefficiens was combined with P. indica and Bacillus sp. MN54. A maximum increase in nodular dry weight (26.31%) was observed with triple inoculation of B. diazoefficiens, P. indica, and Bacillus sp. MN54 over control.
Table 2. Effect of Bradyrhizobium diazoefficiens, Bacillus sp. MN54 and Piriformospora indica inoculation on symbiotic dependency (%) of soybean under field conditions.
Figure 2. Effect of Bradyrhizobium diazoefficiens, Bacillus sp. MN54 and Piriformospora indica on (A) number of nodules plant–1, (B) nodular dry weight in soybean under field conditions. The error bars represent the least significant difference among treatments at P ≤ 0.05.
Combined inoculations significantly increased soybean grain yield over the sole application of B. diazoefficiens and to uninoculated control (Figure 3A). The maximum increase of 20.50% was observed in triplicate inoculation, i.e., B. diazoefficiens + P. indica and Bacillus sp. MN54 as compared to uninoculated control, as shown in Figure 3A. The co-inoculation of Bacillus sp. MN54 + B. diazoefficiens, and P. indica + B. diazoefficiens increased biomass (10.36 and 11.70%, respectively) over B. diazoefficiens alone (Figure 3B). However, maximum biomass (18.05%) was recorded in the plants that received a combined application of B. diazoefficiens, Bacillus sp. MN54 and P. indica as compared to uninoculated control. An increase was observed in 100 grain weight and number of pods plant–1. The increase of 11.16% was observed in 100-grain weight in triple inoculation over control (Figure 3C). Similarly, this treatment showed an increase in the number of pods plant–1 by 12.50% over the untreated control (Figure 3D).
Figure 3. Effect of Bradyrhizobium diazoefficiens, Bacillus sp. MN54 and Piriformospora indica on (A) grain yield, (B) dry biomass, (C) 100 grain weight, (D) number of pods in soybean under field conditions. The error bars represent the least significant difference among treatments at P ≤ 0.05.
A significant improvement was observed in chlorophyll contents by applying single, dual, and triple inoculation over un-inoculated control (Figure 4). It has been observed that the effect of co-inoculation and triple inoculations was more pronounced over the application of B. diazoefficiens alone. The highest increased in chlorophyll a and b contents, i.e., 19.38 and 21.01%, respectively, were observed by application of Bacillus sp. MN54 and P. indica, along with B. diazoefficiens, compared to control (Figures 4A,B). Similarly, significant increases in leghemoglobin contents were observed in treated plants against untreated control. A 7.10 and 10.71% increase was observed by co-inoculation of P. indica along with B. diazoefficiens, and Bacillus sp. MN54 mixes B. diazoefficiens, respectively, over control. But maximum leghemoglobin contents (14.28%) were recorded in the combination of all three inoculants (B. diazoefficiens + Bacillus sp. MN54, and P. indica) over un-inoculated control as shown in Figure 4C. On the pooled mean basis, it has been found that a significant increase in all quality parameters has been observed in treated plants as compared to untreated (Figure 5). The co-inoculation increased quality parameters followed by single inoculation over control. The maximum increase in crude fiber, crude protein, moisture percentage, and oil contents was recorded in triplicate inoculation of B. diazoefficiens, Bacillus sp. MN54, and P. indica (14.92, 8.78, 19.28, and 10.52%, respectively) over uninoculated control (Figures 5A–D).
Figure 4. Effect of Bradyrhizobium diazoefficiens, Bacillus sp. MN54 and Piriformospora indica on (A) chlorophyll a contents, (B) chlorophyll b contents, (C) leghemoglobin contents in soybean under field conditions. The error bars represent the least significant difference among treatments at P ≤ 0.05.
Figure 5. Effect of Bradyrhizobium diazoefficiens, Bacillus sp. MN54 and Piriformospora indica on (A) crude fiber contents, (B) crude protein contents, (C) moisture contents, (D) oil contents in soybean under field conditions. The error bars represent the least significant difference among treatments at P ≤ 0.05.
Results showed that dual and triple inoculation had a significant effect on nutrient contents in soybean over uninoculated control as shown in Figures 6, 7. Soybean plants that received triple inoculations had high grain and stover N and P contents, followed by co-inoculation as compared to untreated control and single inoculation. Maximum increases in N contents (7.33 in grain and 6.26% in stover) were observed in treatment where B. diazoefficiens was applied along Bacillus sp. MN54 and P. indica over uninoculated control (Figures 6A,B). Similarly, an increase in P contents was observed in the co-inoculation of B. diazoefficiens and P. indica over B. diazoefficiens alone. But maximum P in grain (11.31%) and stover (12.72%) was found in a combination of B. diazoefficiens, Bacillus sp. MN54 and P. indica over untreated control, as shown in Figures 6C,D. Results also showed that single, co-inoculation, and triple-inoculation had significantly improved micronutrient contents in soybean grains over uninoculated control. The highest Fe (15.60%), Zn (11.11%), and Mn (13.25%) contents were recorded in triple inoculations treatment (B. diazoefficiens, Bacillus sp. MN54, and P. indica) over control (Figures 7A–C).
Figure 6. Effect of Bradyrhizobium diazoefficiens, Bacillus sp. MN54 and Piriformospora indica on (A) grain N, (B) stover N, (C) grain P, (D) stover P in soybean under field conditions. The error bars represent the least significant difference among treatments at P ≤ 0.05.
Figure 7. Effect of Bradyrhizobium diazoefficiens, Bacillus sp. MN54 and Piriformospora indica on (A) Fe in grain, (B) Zn in grain, (C) Mn in grain in soybean under field conditions. The error bars represent the least significant difference among treatments at P ≤ 0.05.
A strong positive correlation was observed in all studied growth, yield attributes, and mineral contents of soybean crops under field conditions (Figure 8). Principal component analysis (PCA) was performed to observe interrelationships among various parameters as shown in Figure 9. The first biplot showed that among all the components, the first two components viz. PC1 (Dim1) and PC2 (Dim2) exhibited maximum contribution, accounting for 87.8% of the total dataset. Principal components 1 (Dim1) and 2 (Dim2) explained 73.9 and 13.9% of the variability among the variables studied. The distribution of all treatments showed that all inoculated treatments positively affected plant growth, yield, and nutrient contents over control. All treatments with single or co-inoculation were displaced from control. Still, the treatment where triple-inoculation was used had a more significant displacement from control, indicating a more pronounced effect.
Figure 8. Correlation among measured parameters of soybean grown under field conditions. Positive correlations are displayed in blue color. The color intensity and the size of the circle are proportional to the correlation coefficients.
Figure 9. Represents the PCA biplot among measured parameters of triple inoculation in soybean under field conditions. Treatments are as T1. Control, T2. B. diazoefficiens inoculation, T3. Bacillus sp. MN54 inoculation, T4. P. indica inoculation, T5. B. diazoefficiens + Bacillus sp. MN54, T6. P. indica + Bacillus sp. MN54, T7. B. diazoefficiens + P. indica, T8. B. diazoefficiens + Bacillus sp. MN54 + P. indica.
In this study, we found considerable improvement in the growth of soybean under the application of B. diazoefficiens, Bacillus sp. MN54, and P. indica either alone or in combination (Figure 1). A significant positive response was observed in plant height where treatments were applied compared to uninoculated control. This increase may be directly associated with the acceleration of plant growth by producing phytohormones (Kaur and Sharma, 2013; Aziz et al., 2020; Rafique et al., 2021). A significant increase in emergence, number of leaves per plant, and number of branches per plant were also observed with the alone application of microbes, but the combined application effect was more pronounced. According to Orrell and Bennett (2013), Rhizobia are critical in encouraging plants’ hostile behavior toward diseases and herbivores. This encourages and improves several growth metrics, including seed germination, emergence, seedling vigor, plant stand, root and shoot growth, and total biomass of the plants, including seed weight (Ravikumar, 2012). Studies revealed that plant growth-promoting bacteria (PGPB) exhibit a variety of traits that encourage plant growth, including the synthesis of exopolysaccharides and the solubilization of phosphate, zinc, production of indole acetic acid (IAA), and HCN. When exposed to various environmental and soil conditions, different PGPB frequently exhibit one or more features that promote plant growth (Olanrewaju et al., 2017). According to Kalam et al. (2020), numerous plant growth-promoting activities, including IAA and GA production, and P solubilization have been linked to different Bacillus species (Miljakovic et al., 2020; Magotra et al., 2021). Seed germination is a crucial factor and essential to generating total biomass and yield. It has been found that inoculation with Bacillus sp. showed increased in seedlings germination of Arachis hypogea (Shifa et al., 2015). Because they produce antibiotics, phytohormones, and the ability to solubilize phosphate, Bacillus sp. like Bacillus subtilis, Bacillus amyloliquefaciens, Bacillus cereus, Bacillus pumilus, and Bacillus polymyxa are well known for their capacity to promote plant growth and development (Majumdar, 2017). Moreover, Tsimilli-Michael and Strasser (2013) reported that Piriformospora indica has also been identified as a new candidate symbiont capable of delivering significant growth-promoting activity to various plants, including agricultural and medicinal crops.
In our study, all the treated plants showed increased nodule count and nodule dry weight (Figure 2). The current results are in line with the findings of Hungria et al. (2015) and Janagard and Ebadi-Segherloo (2016), who discovered that plants grown from infected seeds had more nodule growth and dry weight than plants grown from uninoculated seeds. As a result of the symbiotic interaction between Rhizobia and soybean plants, root nodules often start to form and expand, increasing the amount of nitrogen fixation in the plant (Tirichine et al., 2006). According to Vasileva and Ilieva (2012), rhizobia are of considerable scientific and commercial significance due to their capacity to fix atmospheric nitrogen in leguminous plants. Through synthesizing unique signal molecules known as Nod factors, rhizobia promote the development of root nodules in leguminous plants (Boundless com, 2021). Rhizobia produces ammonia inside the nodules, which host plants utilize as a source of absorbed nitrogen. This use significantly reduces the need for chemical fertilizers (Lin et al., 2019). According to research, the Bacillus sp. was able to assist the plant growth and solubilize the insoluble nutrients. According to Bhutani et al. (2018), Bacillus is the most prevalent non-rhizobial endophytic species in summer crops and can promote plant root development. Auxin-responsive genes are regulated by PGPR, which alters root architectural characteristics by controlling endogenous IAA levels in rice roots (Ambreetha et al., 2018). The boost in nodulation in the current study could also be attributed to higher metabolism in P. indica-infested plants, which have enabled them to deliver more significant amounts of carbohydrates to the Rhizobia. Our results are supported by earlier research (Rokhzadi and Toashih, 2011; Verma et al., 2012), which shows that PGPR can increase native rhizobia’s capacity to produce more chickpea nodules. Chickpea nodule formation may have benefited from native AMF and inoculated P. indica mobilizing phosphates from the soil. Our findings are consistent with those of Nautiyal et al. (2010), who showed that P. indica and PGPR consortia improved the ability of native rhizobia to nodulate chickpeas. According to Tavasolee et al. (2011), the opposite is true; PGPR bacterial strains decreased the fresh mass of nodules in chickpeas due to competition for photosynthetic materials.
The findings of our investigation showed that seed inoculation with Rhizobia boosted grain yield, biomass, and pod number; however, this reaction was further strengthened by adding Bacillus and P. Indica (Figure 3). Alam et al. (2015) revealed that soybean plants inoculated with Rhizobia produced more pods than uninoculated plants. It may be due to increased nitrogen availability that directly enhanced photosynthesis and biomass production. More biomass generally includes increased leaf area, which is crucial for photosynthesis and energy production, thus supporting more extensive growth and enabling the plant to support more reproductive structures, including pods. Moreover, using Bacillus sp. to boost V. radiata growth and yield proved successful. According to Kumari et al. (2018), Bacillus megaterium, Bacillus pumilus, and Bacillus subtilis all lengthened shoots by 55.55, 46.46, and 46.20% over control, respectively. Good plant development was seen in Arachis hypogea exposed to Bacillus licheniformis (Shifa et al., 2015). A promising method for increasing plant development and decreasing the use of toxic chemical fertilizers is to inoculate soil or crops with PGPB (Alori et al., 2012). Additionally, Meena et al. (2010) found that P. indica-inoculated plants had higher P contents, and Achatz et al. (2010) found that colonized plants had higher photosynthetic rates and improved production. Similarly, Ray and Valsalakumar (2010) reported that co-inoculation with Rhizobia and AM fungi enhanced plant vigor and nutrient uptake and significantly boosted the yield of green gram.
In our investigation, treated plants showed a substantial increase in photosynthetic pigments (Figure 4) that could be a result of the synergistic action of applied microbes, which increased the supply of N to the plant, which is a crucial structural component of chlorophyll (Zohaib et al., 2019). This study found that inoculations of Bradyrhizobium, Bacillus MN54, and P. indica significantly improved leghemoglobin content compared to Bradyrhizobium alone treatment. This might result from higher nodulation and N2 fixation increasing the occupancy of effective nodules, which might have increased the leghaemoglobin content. These findings fit well with the results of Kunal, and Sharma (2012), who reported a positive correlation between leghaemoglobin content and nitrogen fixation in chickpeas due to Rhizobia inoculation. Furthermore, one of the causes of enhanced nodulation in such plants is higher P nutrition in legumes connected to AMF. AMF are known to increase the amount of N available to legumes by promoting the growth of nodules with high levels of leghemoglobin. This might result from higher nodulation and N2 fixation increasing the occupancy of effective nodules, which might have increased the leghaemoglobin content.
According to the findings of our study, soybean with single, dual, and triple inoculations had higher fiber, protein, oil, and nutrient contents (Figures 5–7). The inoculation of legume crops with beneficial microbes such as Rhizobia, Bacillus spp., and P. indica can significantly enhance the nutrient content of the seeds, particularly protein, fat, and fiber. Each microorganism contributes uniquely through various biological mechanisms, improving plant growth, nutrient uptake, and metabolic efficiency. Rhizobia are best known for their role in nitrogen fixation. They form nodules on the roots of leguminous plants, where they convert atmospheric nitrogen into ammonia, making it available to the host plant. This directly enhances protein synthesis in the plant because nitrogen is a critical component of amino acids, the building blocks of proteins (Sulieman et al., 2015). Meanwhile, Bacillus sp. MN54 and P. indica have a significant role in enhancing phosphorus solubilization and nutrient uptake. These processes are crucial for efficient metabolic functioning and can increase seed oil (fat) and possibly fiber content by promoting biosynthetic pathways (Bhattacharyya and Jha, 2012; Gill et al., 2016).
In soy bean the combined effect of R. japonicum and P. striata on seed N, protein, and oil contents was significantly influenced by post-harvest plant sample analysis (Waghmare et al., 2012; Jaga and Sharma, 2015). Compared to uninoculated treatments, seed N and protein content rise due to Rhizobia and Bacillus inoculation because Bacillus promotes N availability and improves seed accumulation (Fatima et al., 2007). Our study’s improvement in total N content aligns with previous findings in chickpeas (Nautiyal et al., 2010) and soybean (Lima et al., 2011). This may be due to PGPB promoting root growth and root structure by increasing the number of root hairs and surface area, which thus increases plant nutrient intake and acquisition (De Sousa et al., 2021; Calvo et al., 2017; Adesemoye et al., 2008).
According to Iqbal et al. (2023), phosphate-solubilizing bacteria boost the production of auxin and cytokinin and make inaccessible P available to plants. These microorganisms improve plant P uptake and make it available in inorganic form (Qadir et al., 2024). Earlier research by Malla et al. (2004) found that P. indica contained significant amounts of an acid phosphatase with the potential to solubilize phosphate in the soil and deliver it to the host plant, improving P content in P. indica treatments. These findings are in line with our study. Moreover, the investigation has been found coherent with the results of Nautiyal et al. (2010), who reported that consortia of P. indica and PGPR along with native chickpea rhizobia increased the P content. PGPR might contribute to soil phosphate pool available for extraradical hyphae of AMF like fungi, to pass on to the plant, especially in soils with low P bioavailability. Furthermore, due to the ability of P. indica to colonize host plant roots and create a robust root system increases the soil’s ability to absorb nutrients (Hosseini et al., 2019). In addition, PGPR may contribute to the amount of soil phosphate pool available for extraradical hyphae of AMF transmitted to the plant, particularly in soils with low P bioavailability. Additionally, because P. indica may colonize host plant roots and develop a robust root system, the soil’s capacity to absorb nutrients increases (Hosseini et al., 2019).
The tripartite microbial augmentation of Bradyrhizobium diazoefficiens, Bacillus sp. MN54 and P. indica significantly influenced soybean growth, yield, and nutrient contents. Our findings demonstrate the substantial enhancements in crucial growth parameters, indicating the positive impact of microbial symbiosis on soybean growth. The application of the microbial consortium resulted in notable improvements in soybean yield. Importantly, our analysis of nutrient profiling revealed significant enhancements in essential nutrients such as nitrogen, phosphorus, zinc, iron, and manganese, highlighting the potential of tripartite microbial augmentation to enhance the nutritional quality of soybean crops. These findings emphasize the importance of harnessing compatible consortium inoculation for sustainable agriculture practices to improve crop productivity and nutritional value. Further research into the mechanisms underlying microbial interactions and their effects on plant-microbe-soil interactions will be valuable for optimizing microbial-based strategies in soybean cultivation and advancing agricultural sustainability.
The original contributions presented in the study are included in the article/supplementary material, further inquiries can be directed to the corresponding author.
MR: Writing – original draft. MN: Writing – original draft. MM: Writing – review & editing. AN: Writing – original draft. SA: Funding acquisition, Writing – review & editing. SR: Writing – original draft. MS: Writing – review & editing. AM: Writing – review & editing.
The author(s) declare that financial support was received for the research, authorship, and/or publication of this article. The authors would like to extend their sincere appreciation to the Researchers Supporting Project number (RSP2025R194), King Saud University, Riyadh, Saudi Arabia.
We extend their appreciation to the staff of Environmental Science Lab, UAF, Pakistan, for their assistance in this study. We are thankful to the Oilseed Research Institute and its staff for helping in our field experiment. We would also like to extend their sincere appreciation to the Researchers Supporting Project number (RSP2025R194), King Saud University, Riyadh, Saudi Arabia.
The authors declare that the research was conducted without any commercial or financial relationships that could be construed as a potential conflict of interest.
All claims expressed in this article are solely those of the authors and do not necessarily represent those of their affiliated organizations, or those of the publisher, the editors and the reviewers. Any product that may be evaluated in this article, or claim that may be made by its manufacturer, is not guaranteed or endorsed by the publisher.
Achatz, B., von Rüden, S., Andrade, D., Neumann, E., Pons-Kühnemann, J., Kogel, K. H., et al. (2010). Root colonization by Piriformospora indica enhances grain yield in barley under diverse nutrient regimes by accelerating plant development. Plant Soil 333, 59–70.
Adesemoye, A. Q., Torbert, H. A., and Kloepper, J. W. (2008). Enhanced plant nutrient use efficiency with PGPR and AMF in an integrated nutrient management system. Can. J. Microbiol. 54, 876–886. doi: 10.1139/w08-081
Afzal, M. J., Khan, M. I., Cheema, S. A., Hussain, S., Anwar-ul-Haq, M., Ali, M. H., et al. (2020). Combined application of Bacillus sp. MN-54 and phosphorus improved growth and reduced lead uptake by maize in the lead-contaminated soil. Environ. Sci. Pollut. Res. 27, 44528–44539. doi: 10.1007/s11356-020-10372-4
Ahmad, M., Adil, Z., Hussain, A., Mumtaz, M. Z., Nafees, M., Ahmad, I., et al. (2019). Potential of phosphate solubilizing bacillus strains for improving growth and nutrient uptake in mungbean and maize crops. Pak. J. Agric. Sci. 56, 283–289.
Alam, F., Bhuiyan, M., Alam, S. S., Waghmode, T. R., Kim, P. J., and Lee, Y. B. (2015). Effect of Rhizobium sp. BARIRGm901 inoculation on nodulation, nitrogen fixation and yield of soybean (Glycine max) genotypes in gray terrace soil. Biosci Biotechnol Biochem. 79, 1660–1668. doi: 10.1080/09168451.2015.1044931
Alori, E. T., Fawole, O., and Afolayan, A. (2012). Characterization of arbuscular mycorrhizal spores isolated from Southern Guinea Savanna of Nigeria. J. Agric. Sci. 4, 13–19. doi: 10.5539/jas.v4n7p13
Ambreetha, S., Chinnadurai, C., Marimuthu, P., and Balachandar, D. (2018). Plant-associated Bacillus modulates the expression of auxin-responsive genes of rice and modifies the root architecture. Rhizosphere 5, 57–66. doi: 10.1016/j.rhisph.2017.12.001
AOAC. (1975). Official Methods of Analysis, 12th Edn. Washington, DC: Association of official Analytical Chemists.
AOAC. (1984). Official Methods of Analysis of AOAC International, 14th Edn. Gaithersberg, MD: AOAC International, 770–771.
Arnon, D. I. (1949). Copper enzymes in isolated chloroplasts. Polyphenoloxidase in Beta vulgaris. Plant Physiol. 24:1. doi: 10.1104/pp.24.1.1
Aziz, M. Z., Yaseen, M., Naveed, M., Wang, X., Fatima, K., Saeed, Q., et al. (2020). Polymer-Paraburkholderia phytofrmans PsJN coated diammonium phosphate enhanced microbial survival, phosphorous use efficiency, and production of wheat. Agronomy 10:1344.
Bender, S. F., Wagg, C., and van der Heijden, M. G. A. (2016). An underground revolution: biodiversity and soil ecological engineering for agricultural sustainability. Trends Ecol. Evol. 31, 440–452. doi: 10.1016/j.tree.2016.02.016
Bhattacharyya, P. N., and Jha, D. K. (2012). Plant Growth-Promoting Rhizobacteria (PGPR): emergence in agriculture. World J. Microbiol. Biotechnol. 28, 1327–1350. doi: 10.1007/s11274-011-0979-9
Bhutani, N., Maheshwari, R., Negi, M., and Suneja, P. (2018). Optimization of IAA production by endophytic Bacillus sp. from Vigna radiata for their potential use as plant growth promoters. Isr. J. Plant Sci. 65, 83–96. doi: 10.1163/22238980-00001025
Calvo, P., Watts, D. B., Kloepper, J. W., and Torbert, H. A. (2017). Effect of microbial based inoculants on nutrient concentrations and early root morphology of corn (Zea mays). J. Plant Nutr. Soil Sci. 180, 56–70.
Compant, S., Duffy, B., Nowak, J., Clément, C., and Barka, E. A. (2010). Use of plant growth-promoting bacteria for biocontrol of plant diseases: principles, mechanisms of action, and future prospects. Appl. Environ. Microbiol. 76, 3053–3061. doi: 10.1128/AEM.71.9.4951-4959.2005
De Sousa, S. M., de Oliveira, C. A., Andrade, D. L., de Carvalho, C. G., Ribeiro, V. P., Pastina, M. M., et al. (2021). Tropical Bacillus strains inoculation enhances maize root surface area, dry weight, nutrient uptake and grain yield. J. Plant Growth Regul. 40, 867–877.
Estefan, G., Sommer, R., and Ryan, J. (2013). Methods of Soil, Plant and Water Analysis: A Manual for West Asia and North Africa Region. Beirut: ICARDA.
Fageria, N. (2014). Yield and yield components and phosphorus use efficiency of lowland rice genotypes. J. Plant Nutr. 37, 979–989. doi: 10.1080/01904167.2014.888735
Fatima, Z., Zia, M., and Chaudhary, F. (2007). Interactive effect of Rhizobium strains And P on soybean yield, nitrogen fixation and soil fertility. Pak. J. Bot. 39, 255–264.
Ferreira, P. A., Bomfeti, C. A., Soares, B. L., and de Souza Moreira, F. M. (2019). Efficient nitrogen-fixing Rhizobium strains isolated from amazonian soils: An alternative to promote growth in cowpea [Vigna unguiculata (L.) Walp]. Front. Microbiol. 10:1710. doi: 10.3389/fmicb.2019.01710
Franken, P. (2012). The plant strengthening root endophyte Piriformospora indica: potential application and the biology behind. Appl. Microbiol. Biotechnol. 96, 1455–1464. doi: 10.1007/s00253-012-4506-1
Gill, S. S., Gill, R., Trivedi, D. K., Anjum, N. A., Sharma, K. K., Ansari, M. W., et al. (2016). Piriformospora indica: A revolutionary plant growth-promoting fungus. Front. Plant Sci. 7:767. doi: 10.3389/fpls.2016.00767
Gupta, S., Schillaci, M., and Walker, R. (2022). Efficacy of dual inoculation with rhizobium and plant growth-promoting rhizobacteria on soybean yield under field conditions. Agronomy 12:254. doi: 10.3390/agronomy12020254
Hosseini, F., Mosaddeghi, M. R., Dexter, A. R., and Sepehri, M. (2019). Effect of endophytic fungus Piriformospora indica and PEG-induced water stress on maximum root growth pressure and elongation rate of maize. Plant Soil. 435, 423–436. doi: 10.1007/s11104-018-03909-7
Hungria, M., and Mendes, I. C. (2015). “Nitrogen fixation with soybean: the perfect symbiosis?,” in Biological Nitrogen Fixation, ed. F. de Bruijn (Hoboken, NJ: John Wiley & Sons, Inc), 1009–1024. doi: 10.1016/j.jtbi.2009.03.033
Hungria, M., Nogueira, M. A., and Araujo, R. S. (2015). Soybean seed co-inoculated with Bradyrhizobium spp. and Azospirillum brasilense. A new biotechnological tool improve yield and sustainability. Am. J. Plant Sci. 06, 811–817. doi: 10.4236/ajps.2015.66087
Iqbal, M., Naveed, M., Sanaullah, M., Brtnicky, M., Hussain, M. I., Kucerik, J., et al. (2023). Plant microbe mediated enhancement in growth and yield of canola (Brassica napus L.) plant through auxin production and increased nutrient acquisition. J. Soils Sediments 23, 1233–1249.
Jackson, M. L. (1973). Soil Chemical Analysis, 3 Edn. New Delhi: Prentice Hall of India Pvt. Ltd, 498.
Jaga, P. K., and Sharma, S. (2015). Effect of bio-fertilizer and fertilizers on productivity of soybean. Ann. Plant Soil Res. 17, 171–174.
Jaiswal, S. K., and Dakora, F. D. (2020). Exploring the potential of Burkholderia spp. as stress-resilient plant growth promoting rhizobacterial inoculants for legume crops. Agronomy 10:793. doi: 10.3390/agronomy10060793
Janagard, M. S., and Ebadi-Segherloo, A. (2016). Inoculated soybean response to starter nitrogen in conventional cropping system in Moghan. J. Agron. 15, 26–32.
Kalam, S., Basu, A., and Podile, A. R. (2020). Functional and molecular characterization of plant growth promoting Bacillus isolates from tomato rhizosphere. Heliyon 6:e04734. doi: 10.1016/j.heliyon.2020.e04734
Kaur, N., and Sharma, P. (2013). Screening and characterization of native Pseudomonas sp. as plant growth promoting rhizobacteria in chickpea (Cicer arietinum L.) rhizosphere. Afr. J. Microbiol. Res. 7, 1465–1474.
Khan, A. L., Waqas, M., Kang, S.-M., Al-Harrasi, A., Hussain, J., Al-Rawahi, A., et al. (2016). Bacterial endophytes: the treasure chest for bioactive compounds to combat microbe-induced plant diseases. Biotechnol. Adv. 34, 1110–1121.
Kumar, A., and Dubey, A. (2020). Rhizosphere microbiome: engineering bacterial competitiveness for enhancing crop production. J. Adv. Res. 24, 337–352. doi: 10.1016/j.jare.2020.04.014
Kumari, P., Meena, M., Gupta, P., Dubey, M. K., Nath, G., and Upadhyay, R. (2018). Plant growth promoting rhizobacteria and their biopriming for growth promotion in mung bean (Vigna radiata (L.) R. Wilczek). Biocatal. Agric. Biotechnol. 16, 163–171. doi: 10.1016/j.bcab.2018.07.030
Kunal Sharma, P. (2012). Compatibility of Mesorhizobium sp. Cicer with seed treatment of fungicide and insecticide in chickpea. Arch. Agron. Soil Sci. 58, 115–123.
Lima, A. S. T., Xavier, T. F., Lima, C. E. P., Oliveira, J. P., Mergulhão, A. C. S., and Figueiredo, M. V. B. (2011). Triple inoculation with Bradyrhizobium, Glomus and Paenibacillus on cowpea (Vigna unguiculata [L.] Walp.) development. Braz. J. Microbiol. 42, 919–926.
Lin, W., Lin, M., Zhou, H., Wu, H., Li, Z., Lin, W., et al. (2019). The effects of chemical and organic fertilizer usage on rhizosphere soil in tea orchards. PLoS One 14:e0217018. doi: 10.1371/journal.pone.0217018
Magotra, S., Bhagat, N., Ambardar, S., Ali, T., Hurek, B. R., Hurek, T., et al. (2021). Field evaluation of PGP Bacillus sp. strain D5 native to Crocus sativus, in traditional and non traditional areas, and mining of PGP genes from its genome. Sci. Rep. 11:5454. doi: 10.1038/s41598-021-84585-z
Majumdar, S. (2017). Evaluation of Jute Rhizospheric Bacteria for Plant Growth Promotion and Disease Suppression (Doctoral Dissertation). Siliguri: University of North Bengal.
Malla, R., Prasad, R., Kumari, R., Giang, P. H., Pokharel, U., Oelueller, R., et al. (2004). Phosphorus solubilizing symbiotic fungus: Piriformospra indica. Endocytobio Cell Res. 15, 579–600.
Masson-Boivin, C., and Sachs, J. L. (2018). Symbiotic nitrogen fixation by rhizobia—the roots of a success story. Curr. Opin. Plant Biol. 44, 7–15. doi: 10.1016/j.pbi.2017.12.001
McKenzie, H. A., and Wallace, H. S. (1954). The Kjeldahl determination of nitrogen: a critical study of digestion conditions-temperature, catalyst, and oxidizing agent. Austr. J. Chem. 7, 55–70.
Meena, K. K., Mesapogu, S., Kumar, M., Yandigeri, M. S., Singh, G., and Saxena, A. K. (2010). Co-inoculation of the endophytic fungus Piriformospora indica with the phosphate-solubilising bacterium Pseudomonas striata affects population dynamics and plant growth in chickpea. Biol. Fertil. Soils 46, 169–174.
Miljakovic, D., Marinković, J., and Balešević-Tubić, S. (2020). The significance of Bacillus sp. in disease suppression and growth promotion of field and vegetable crops. Microorganisms 8:1037. doi: 10.3390/microorganisms8071037
Nautiyal, C. S., Chauhan, P., Dasgupta, S. M., Seem, K., Varma, A., and Staddon, W. J. (2010). Tripartite interactions among Paenibacillus lentimorbus NRRL B-30488, Piriformospora indica DSM 11827, and Cicer arietinum L. World J. Microbiol. Biotechnol. 26, 1393–1399.
Naveed, M., Mitter, B., Yousaf, S., Pastar, M., Afzal, M., and Sessitsch, A. (2014). The endophyte Enterobacter sp. FD17: a maize growth enhancer selected based on rigorous testing of plant beneficial traits and colonization characteristics. Biol. Fertil. Soils 50, 249–262.
Olanrewaju, O. S., Glick, B. R., and Babalola, O. O. (2017). Mechanisms of action of plant growth promoting bacteria. World J. Microbiol. Biotechnol. 33:197. doi: 10.1007/s11274-017-2364-9
Orrell, P., and Bennett, A. E. (2013). How can we exploit above belowground interactions to assist in addressing the challenges of food security? Front. Plant Sci. 4:432. doi: 10.3389/fpls.2013.00432
Pankievicz, V. C. S., do Amaral, F. P., Ané, J. M., and Stacey, G. (2021). Diazotrophic bacteria and their mechanisms to interact and benefit cereals. Mol. Plant Microbe Interact. 34, 491–498. doi: 10.1094/MPMI-11-20-0316-FI
Qadir, M. F., Naveed, M., Khan, K. S., Mumtaz, T., Raza, T., Mohy-Ud-Din, W., et al. (2024). Divergent responses of phosphorus solubilizing bacteria with P-laden biochar for enhancing nutrient recovery, growth, and yield of canola (Brassica napus L.). Chemosphere 353:141565. doi: 10.1016/j.chemosphere.2024.141565
Rafique, M., Naveed, M., Mustafa, A., Akhtar, S., Munawar, M., Kaukab, S., et al. (2021). The combined effects of gibberellic acid and rhizobium on growth, yield and nutritional status in chickpea (Cicer arietinum L.). Agronomy 11:105.
Raja, P., Uma, S., Gopal, H., and Govindarajan, K. (2006). Impact of bioinoculants consortium on rice root exudates, biological nitrogen fixation and plant growth. J. Biol. Sci. 6, 815–823. doi: 10.3923/jbs.2006.815.823
Rajkumar, M., Ma, Y., and Freitas, H. (2017). Isolation and characterization of beneficial bacteria associated with plant roots. Bioresour. Technol. 98, 1512–1519.
Ravikumar, R. (2012). Growth effects of Rhizobium inoculation in some Legume plants. Int. J. Curr. Sci. 1, 1–6.
Ray, J. G., and Valsalakumar, N. (2010). Arbuscular mycorrhizal fungi and Piriformospora indica individually and in combination with Rhizobium on green gram. J. Plant Nutr. 33, 285–298.
Rokhzadi, A., and Toashih, V. (2011). Nutrient uptake and yield of chickpea (Cicer arietinum L.) inoculated with plan growth promoting rhizobacteria. Aust. J. Crop Sci. 5, 44–48.
Sadasivam, S., and Manickam, A. (2008). Biochemical Methods for Agricultural Sciences, 6th Edn. New Delhi: New Age International (P) Ltd.
Saeed, Q., Xiukang, W., Haider, F. U., Kučerik, J., Mumtaz, M. Z., Holatko, J., et al. (2021). Rhizosphere bacteria in plant growth promotion, biocontrol, and bioremediation of contaminated sites: a comprehensive review of effects and mechanisms. Int. J. Mol. Sci. 22:10529. doi: 10.3390/ijms221910529
Saini, P., and Khana, V. (2012). Evaluation of native Rhizobacteria as promoters of plant growth for increased yields in lentils (Lens culinaris). Recent Res. Sci.Technol. 4, 5–9.
Shifa, H., Gopalakrishnan, C., and Velazhahan, R. (2015). Efficacy of Bacillus subtilis G-1 in suppression of stem rot caused by Sclerotium rolfsii and growth promotion of groundnut. Int. J. Environ. 8, 111–118. doi: 10.5958/2230-732X.2015.00015.7
Solanki, A. C., Gurjar, N. S., and Sharma, S. (2023). Co-inoculation of non-symbiotic bacteria bacillus and paraburkholderia can improve the soybean yield, nutrient uptake, and soil parameters. Mol. Biotechnol. 1–13. [Epub ahead of print]. doi: 10.1007/s12033-023-00719-w
Steel, R., Torrie, J. H., and Dicky, D. A. (1997). “Analysis of variance I: The one-way classification,” in Principles and Procedures of Statistics a Biometrical Approach, (New York, NY: McGraw-Hill Book Company), 139–203.
Sulieman, S., Thao, N. P., and Tran, L. S. P. (2015). “Does elevated CO 2 provide real benefits for N 2-fixing leguminous symbioses?,” in Legume Nitrogen Fixation in a Changing Environment: Achievements and Challenges, eds S. Sulieman and L. S. P. Tran (Cham: Springer), 89–112.
Tavasolee, A., Aliasgharzad, N., Jouzani, G. S., Mardi, M., and Asgharzadeh, A. (2011). Interactive effects of arbuscular mycorrhizal fungi and rhizobial strains on chickpea growth and nutrient content in plant. Afr J Biotechnol 10, 7585–7591.
Tirichine, L., James, E. K., Sandal, N., and Stougaard, J. (2006). Spontaneous root-nodule formation in the model legume Lotus japonicus: a novel class of mutants nodulates in the absence of rhizobia. Mol. Plant Microbe Interact. J. 19, 373–382. doi: 10.1094/MPMI-19-0373
Tsimilli-Michael, M., and Strasser, R. J. (2013). Biophysical phenomics: evaluation of the impact of mycorrhization with Piriformospora indica. Soil Biol 33, 173–190.
Varma, A., Verma, S., Sudha, Sahay, N., Bütehorn, B., and Franken, P. (2012). Piriformospora indica, a cultivable plant-growth-promoting root endophyte. Appl. Environ. Microbiol. 33, 1845–1850.
Vasileva, V., and Ilieva, A. (2012). Nodulation and Nitrogen Assimilation in Legumes Under Elements of Technology. Saarbrücken: Lap Lambert Academic Publishing.
Verma, J. P., Yadav, J., and Tiwari, K. N. (2012). Enhancement of nodulation and yield of chickpea by co-inoculation of indigenous Mesorhizobium spp. and plant growth—promoting rhizobacteria in eastern Uttar Pradesh. Commun. Soil Sci. Plan. 43, 605–621.
Waghmare, Y. M., Gokhale, D. N., and Pawar, H. D. (2012). Effect of integrated nutrient management on yield, yield attributes and quality of soybean (Glycine max (L.) Merill.). J. Agric. Res. Technol. 37, 370–372.
Wasike, V. W., Lesueur, D., Wachira, F. N., Mungai, N. W., Mumera, L. M., Sanginga, N., et al. (2009). Genetic diversity of indigenous Bradyrhizobium nodulating promiscuous soybean [Glycine max (L) Merr.] varieties in Kenya: impact of phosphorus and lime fertilization in two contrasting sites. Plant Soil 322, 151–163.
Xie, J., Shi, H., Du, Z., Wang, T., Liu, X., and Chen, S. (2016). Comparative analysis of rhizosphere microbiome community structures of three major legumes under Rhizobium inoculation based on high-throughput sequencing. Plant Soil 404, 113–122.
Zohaib, A., Yousaf, S., Anjum, S. A., Tabassum, T., Abbas, T., Muzaffar, W., et al. (2019). Growth, allometry and dry matter yield of soybean genotypes in response to seed inoculation with plant growth promoting rhizobacteria. Pak. J. Agric. Res. 32, 102–109.
Keywords: triple inoculation, nodulation, Bradyrhizobium diazoefficiens, Piriformospora indica, Bacillus spp., pulses
Citation: Rafique M, Naveed M, Mumtaz MZ, Niaz A, Alamri S, Rehman S, Siddiqui MH and Mustafa A (2025) Tripartite microbial augmentation of Bradyrhizobium diazoefficiens, Bacillus sp. MN54, and Piriformospora indica on growth, yield, and nutrient profiling of soybean (Glycine max L.). Front. Microbiol. 15:1437489. doi: 10.3389/fmicb.2024.1437489
Received: 23 May 2024; Accepted: 27 December 2024;
Published: 07 March 2025.
Edited by:
Kauser Abdulla Malik, Forman Christian College, PakistanReviewed by:
Mustapha Mohammed, University for Development Studies, GhanaCopyright © 2025 Rafique, Naveed, Mumtaz, Niaz, Alamri, Rehman, Siddiqui and Mustafa. This is an open-access article distributed under the terms of the Creative Commons Attribution License (CC BY). The use, distribution or reproduction in other forums is permitted, provided the original author(s) and the copyright owner(s) are credited and that the original publication in this journal is cited, in accordance with accepted academic practice. No use, distribution or reproduction is permitted which does not comply with these terms.
*Correspondence: Muhammad Naveed, bXVoYW1tYWQubmF2ZWVkQHVhZi5lZHUucGs=; Muhammad Zahid Mumtaz, emFoaWRzZXNAZ21haWwuY29t; Adnan Mustafa, YWRuYW5tdXN0YWZhNzgwQGdtYWlsLmNvbQ==
Disclaimer: All claims expressed in this article are solely those of the authors and do not necessarily represent those of their affiliated organizations, or those of the publisher, the editors and the reviewers. Any product that may be evaluated in this article or claim that may be made by its manufacturer is not guaranteed or endorsed by the publisher.
Research integrity at Frontiers
Learn more about the work of our research integrity team to safeguard the quality of each article we publish.