- 1Animal Health Laboratory, EU/WOAH and National Reference Laboratory for Brucellosis, Anses/Paris-Est University, Maisons-Alfort, France
- 2IdentyPath Genomics Platform, Food Safety Laboratory, ANSES, Maisons-Alfort, France
Introduction: Mammals are the main hosts for Brucella sp., agents of worldwide zoonosis. Marine cetaceans and pinnipeds can be infected by Brucella ceti and B. pinnipedialis, respectively. Besides classical bacteriological typing, molecular approaches such as MLVA, MLSA, and whole-genome sequencing (WGS) can differentiate these species but are cumbersome to perform.
Methods: We compared the DNA and genome sequences of 12 strains isolated from nine marine mammals, with highly zoonotic B. melitensis, B. abortus, and B. suis, and the publicly available genomes of B. ceti and B. pinnipedialis. In silico pipelines were used to detect the antimicrobial resistance (AMR), plasmid, and virulence genes (VGs) by screening six open-source and one home-made library.
Results and discussion: Our results show that easier-to-use HRM-PCR, Bruce-ladder, and Suis-ladder can separate marine Brucella sp., and the results are fully concordant with other molecular methods, such as WGS. However, the restriction fragment length polymorphism (RFLP) method cannot discriminate between B. pinnipedialis and B. ceti B1-94-like isolates. MLVA-16 results divided the investigated strains into three clades according to their preferred host, which was confirmed in WGS. In silico analysis did not find any AMR and plasmid genes, suggesting antimicrobial susceptibility of marine Brucella, while the presence of the VGs btpA gene was variable dependent on the clade.
Conclusion: The HRM-PCR and Suis-ladder are quick, easy, and cost-effective methods to identify marine Brucella sp. Moreover, in silico genome analyses can give useful insights into the genetic virulence and pathogenicity potential of marine Brucella strains.
1 Introduction
The bacteria of the Brucella genus, responsible for a major worldwide zoonosis, can affect farm animals, pets, and wildlife (World Organisation for Animal Health, 2022). Nowadays, the Brucella host range is constantly expanding with infection descriptions from aquatic environments and/or from non-mammals (amphibians, fishes, nematodes, trematodes, etc.) (El-Tras et al., 2010; Garner et al., 1997; Hirvelä-Koski et al., 2017; Jaÿ et al., 2020; Lambourn et al., 2013; Mühldorfer et al., 2017; Nymo et al., 2011). To date, 130 species of marine mammals–https://www.fisheries.noaa.gov/, including 86 cetaceans (whales, porpoises, dolphins) and 36 pinnipeds (seals, sea lions, walruses) (Hernández-Mora et al., 2013; Dadar et al., 2023b), are known to be susceptible to Brucella infections. Two Brucella species are described as having a higher preference for marine ecosystems: B. ceti (Cloeckaert et al., 2001), predominantly associated with cetaceans, and B. pinnipedialis (Foster et al., 2007), preferentially affecting pinnipeds. Nevertheless, cross-species infections, i.e., B. ceti in seals, have also been reported (Maquart et al., 2009), as well as their zoonotic potential (Whatmore et al., 2008). A broad range of pathologies have been associated with Brucella infections in cetaceans, with lesions observed from central nervous, respiratory, reticuloendothelial, cardiovascular, musculoskeletal, urinary, and reproductive systems that can cause Brucella-induced abortions and considerable fertility decreases (Hernández-Mora et al., 2013). Unlike cetaceans, infected pinnipeds have no clinical symptoms, although suggestive inflammatory lesions have been reported in fur seal aborted pups in Australia (Center for Food Security and Public Health, 2018). In humans, infections with “marine Brucella sp. have higher tropism for neural tissues, manifested as severe forms of neuro-brucellosis (two cases from Peru) and spinal osteomyelitis (New Zealand) (Whatmore et al., 2008).
According to in vitro investigations (Larsen et al., 2013; Nymo et al., 2016), pathogenicity and zoonotic potential differ based on the host species, e.g., attenuated virulence of B. pinnipedialis strains or rather rare naturally acquired B. ceti infections in humans (Sohn et al., 2003; McDonald et al., 2006).
Bacteriological evidence for discriminating between B. ceti and B. pinnipedialis is mainly based on the need for carbon dioxide and the ability to metabolize D-galactose of pinniped strains (adaptation to higher ocean depths), unlike cetacean strains (Banai and Corbel, 2010; Foster et al., 2007; Guzmán-Verri et al., 2012; Jacques et al., 2007; Jahans et al., 1997; World Organisation for Animal Health, 2022).
PCR amplification, based on the fragment size differences, of only 19 bp, is very short to unequivocally differentiate between both marine species (Mayer-Scholl et al., 2010). In addition, the diagnostic tool Bruce-Ladder, recommended by WOAH as a one-step identification test (World Organisation for Animal Health, 2022), is unable to differentiate B. ceti from B. pinnipedialis.
According to host preferences and ocean distribution (Nymo et al., 2011; Suárez-Esquivel et al., 2017), within each marine Brucella species molecular evidence supports the existence of two distinct groups, between cetaceans and pinnipeds, and the probable existence of biovars (Moreno et al., 2002). Multi-locus sequence analyses (MLSAs), multiple-locus variable number of tandem-repeat analysis (MLVA), RFLP (Whatmore et al., 2007; Maquart et al., 2009; Guzmán-Verri et al., 2012; Groussaud et al., 2007; Cloeckaert et al., 2001), and WGS studies (Audic et al., 2011; Wattam et al., 2014) identified five different groups: three clusters among B. ceti isolates—B. ceti dolphin type [ST26], B. ceti porpoise type [ST 23], and B. ceti human type [ST 27]; and two clusters among B. pinnipedialis isolates—B. pinnipedialis hooded seal type [ST 24] and B. pinnipedialis common seal type [ST 25]. Besides, phylogenetic investigations underlined that the B. ceti dolphin type early diverged, followed by B. pinnipedialis and then the B. ceti porpoise type, complicating the identification of species-specific markers (Whatmore et al., 2017) and design of one-step species-identification tools, easier and more cost-effective than multiplex assays.
Further genomic comparative analyses showed a genetic proximity of marine Brucella isolates with B. suis isolates (El-Sayed and Awad, 2018; Whatmore et al., 2006; Le Flèche et al., 2006). An updated approach of Bruce-Ladder for discriminating among B. canis, all B. suis biovars, and B. microti (López-Goñi et al., 2011), designated Suis-Ladder, has proven its worth and identifies correctly two species, B. ceti and B. pinnipedialis, as well as B. suis. This genetic proximity with B. suis strains raises the question of a possible application of this suis/canis-specific method for the differentiation of marine isolates. More recently, Girault et al. developed an HRM-PCR able to discriminate two groups of B. ceti and B. pinnipedialis (Girault et al., 2022).
With global warming and shrinking environmental niches, it is observed that the prevalence of marine Brucella sp. in cetaceans and pinnipeds increases (Garofolo et al., 2020; Kurmanov et al., 2022; Orsini et al., 2022). To understand pathological factors, that may impact global health, recently in silico pan-genome functional analysis on available DNA sequences showed 61 genes matching virulence factors genes within multiple bacterial species (Orsini et al., 2022). Out of those, 31 were included in LPS synthesis, 17 were from the effector delivery system, mainly T4SS, and were 90–100% identical to those identified in B. melitensis bv 1 or B. abortus or B. suis, highly virulent Brucella sp. In addition, two genes from type III secretion exporters belonging to the flagella pathway identified in Bartonella sp. were also identified in the study of Orsini et al. (2022), implying the zoonotic potential of marine Brucella sp. and showing the potency of whole genome analyses, not only in phylogenetic but also functional assay studies.
The present study describes the application of molecular tools such as Bruce-ladder, Suis-Ladder, RFLP, HRM-PCR, MLVA, MLST, and whole-genome approach to discriminate between B. ceti and B. pinnipedialis and its assessment on field marine isolates. Furthermore, in-depth genomic and functional characterization using the available in silico approaches were validated using simple experimental molecular approaches.
2 Materials and methods
2.1 Bacterial cultivation
This study included 12 isolates from nine marine mammals, including cetaceans [Stenella coeruleoalba (n = 1), Tursiops truncatus (n = 2), Phocoena phocoena (n = 3)] and pinnipeds [Halichoerus grypus (n = 3)] (Table 1). Additionally, the following reference strains were included: B. ceti B1-94 (alias: NCTC12894; BCCN 94–74) and M644/93/1 (alias: B14/94, BBCCN 94–75); B. pinnipedialis B2-94 (alias: 94–73); B. melitensis (16 M); B. abortus (544); and B. suis (Thomsen). All strains were cultivated on blood agar base (Thermo Fisher Scientific, France) plates with 5% horse serum and incubated at 37°C under 5% CO2 for 4 days.
2.2 Phenotypic identification
All 12 isolates were biotyped using standard procedures, based on CO2 requirement, H2S production, oxidase test, urea hydrolysis, agglutination with monospecific sera, fuchsin and thionine dye sensitivity, and phage typing (World Organisation for Animal Health, 2022).
2.3 Molecular analysis
Genomic DNA was extracted from pure bacterial cultures using the High Pure PCR template preparation kit (Roche Diagnostics, France) according to the manufacturer’s instructions. A total of 15 DNAs extracted from marine mammal strains (B. ceti and B. pinnipedialis) available in the laboratory were used in this study, including that of reference B. abortus bv 1,544, B. melitensis bv 1 16 M, and B. suis bv 2 Thomsen strains (Supplementary Table S1).
Bruce-Ladder (García-Yoldi et al., 2006), Suis-Ladder (López-Goñi et al., 2011), MLVA-16 (Al Dahouk et al., 2007; Le Flèche et al., 2006), and PCR-HRM using previously developed primers for B. ceti 1, B. ceti 2 clusters, and B. pinnipedialis (Girault et al., 2022) assays were performed as previously described.
RFLP-PCR of omp2a, omp2b, and omp31 genes was conducted according to the previously published method (Cloeckaert et al., 1995). PCR products of omp2a were digested with restriction enzymes StyI and NcoI, omp2b with EcoRI and KpnI, and omp31 with AvaII and HaeIII. Each RFLP profile was named according to the previously described nomenclature under format X(Y) (Dawson et al., 2008; Cloeckaert et al., 2001). X(Y) represents the combination of the individual restriction patterns of omp2a and omp2b genes with omp2a profiles in parenthesis.
Maximum parsimony clustering analysis was performed on 237 marine Brucella MLVA-16 genotypes (Suárez-Esquivel et al., 2017; Maquart et al., 2009; Isidoro-Ayza et al., 2014; Garofolo et al., 2013) available from the public database [hosted by Paris-Saclay University (Orsay, France): http://microbesgenotyping.i2bc.paris-saclay.fr/], as well as marine Brucella reference strains and the 12 isolates investigated in this study, using Bionumerics v7.6.2 (BioMérieux, France).
2.4 Whole-genome sequencing and bioinformatics
DNA of the 12 isolates was subjected to WGS using an Illumina Nextera XT kit according to the manufacturer’s instructions. Sequencing was performed on the Illumina MiSeq instrument. Additional 24 available B. ceti, B. pinnipedialis and B. sp. genomes as well as 3 referent strains of B. abortus (544), B. melitensis (16 M) and B. suis (Thomsen) in the National Center for Biotechnology Information (NCBI) Genome database (accessed on May 2023) were used in this study for comparison purposes. Moreover, this study included a total of 42 new strains of B. ceti and B. pinnipedialis that were described by Orsini et al. (2022) as having no genome quality issues (species assignment, high contamination, and/or low level of completeness). The raw reads of these genomes were downloaded from the Sequence Read Archive (SRA) and assembled by our pipeline described below. A total of 78 marine mammal sequences (B. ceti, B. pinnipedialis, and Brucella sp.) were analyzed in this study (Supplementary Table S1). Chimeric genomes of chromosomes 1 and 2 were generated to compare complete and draft genomes (Huang et al., 2012).
Raw de novo assembly was performed using Spades 3.11 (Bankevich et al., 2012). An average number of reads for all sequences was characterized as 511,156, and raw reads were mapped on the 16 M chimeric genome (NC_003317.1; NC_003318.1) using the BWA algorithm in BioNumerics 7.6.2 (Applied Maths, BioMérieux). Then, all the sequences were aligned to identify SNPs, which were filtered according to coverage cutoff, inter-SNP distance, and wrong-call bases (unreliable bases, ambiguous bases, gaps) using the wgSNP module in BioNumerics. A minimum set of position filters were applied on the SNP matrix: (i) contiguous SNPs were removed (if found in a 10 bp-window), (ii) with non-informative SNPs, (iii) a required minimum of 20-fold coverage for each SNP, (iv) ambiguous (i.e., non-ACGT bases), and (v) unreliable bases (i.e., Ns) were discarded. The refined SNP matrix was used to generate a maximum parsimony tree using the maximum parsimony algorithm using the wgSNP module in BioNumerics, allowing phylogenetic analyses.
2.5 Antimicrobial resistance, plasmid, and virulence gene detection
For targeting the genes and/or regions potentially involved in the AMR, plasmid identification, and virulence (VG), all B. ceti, B. pinnipedialis, and Brucella sp. genomes selected were screened using Abricate version 1.0.1 (https://github.com/tseemann/abricate) with entries from six defined databases AMRFinderPlus (NCBI) (Feldgarden et al., 2019), Comprehensive Antibiotic Resistance Database (CARD) (Jia et al., 2017), ResFinder (Zankari et al., 2012), virulence factor database (VFDB) (Chen et al., 2016), PlasmidFinder (Carattoli et al., 2014,) and MEGARes 2.00 (Doster et al., 2020), as well as additional in-house database (BRUgenes), that includes 10 potential Brucella spp. VGs selected in a recent publication on virulence factors of B. melitensis (Rabinowitz et al., 2021), not present in the previous six databases.
2.6 Confirmation of the presence of eight virulence genes absent in Abricate analysis
To confirm the absence of non-detected VGs in the VFDB and own databases using Abricate, classical PCR was performed. The DNA of 12 B. pinnipedialis and B. ceti strains described in this study was used to amplify btpA, cgs, kdsA, pmm, wbkA, bpe275, ure, and vceC, individually (primers described in Table 2), using previously described protocol (Holzapfel et al., 2018). The 2% agar gel was used to separate the amplicons and visualize them. Additionally, in silico PCR using BioNumerics was performed to confirm the presence/absence of these genes.
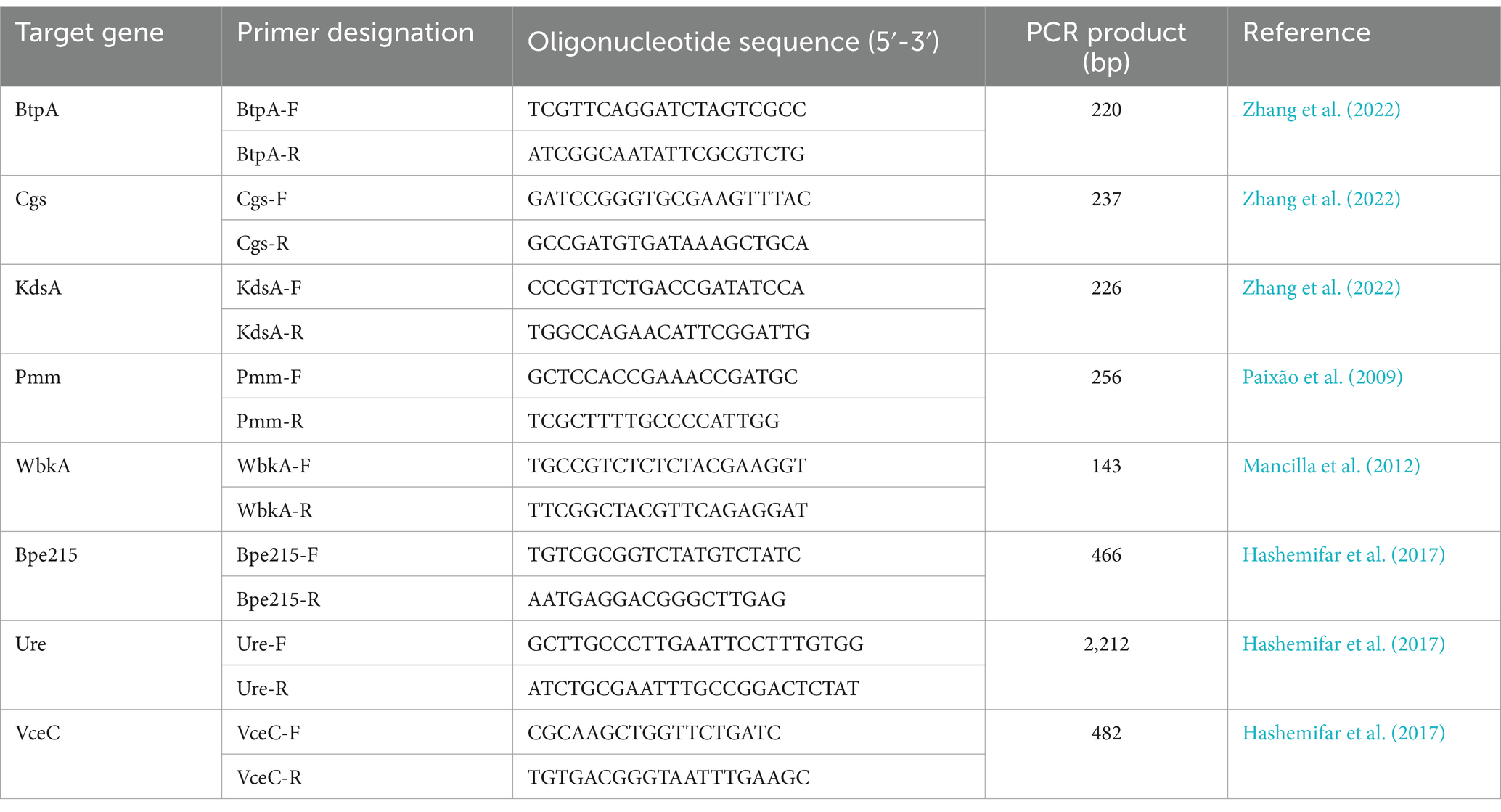
Table 2. PCR primer sequences used for the amplification of the Brucella virulence-associated genes of interest.
3 Results
3.1 Newly identified Brucella ceti and Brucella pinnipedialis strains show phenotypic traits characteristic of their respective species
To ensure adequate species identification, the EU referent laboratory for animal brucellosis strain collection, which includes marine field strains from different geographic origins and various terrestrial animal species, was examined. Their phenotypic features (Table 1) were consistent with previously described marine Brucella patterns (Guzmán-Verri et al., 2012; Whatmore et al., 2017). As expected, none of the investigated isolates from marine mammals, except for 09–601-1272, isolated from a common bottlenose dolphin (Tursiops truncates), produced hydrogen sulfide, but all made urease. All B. pinnipedialis isolates required CO2 for growth. Standard phenotypic identification confirmed B. ceti or B. pinnipedialis species profiles (Table 1).
3.2 The Suis-ladder, Bruce-ladder, HRM, and RFLP PCRs allowed the exact identification of marine Brucella species
The multiplex Suis-ladder PCR, widely used to discriminate among B. suis biovars, B. canis, and B. microti, was performed in order to characterize the marine Brucella reference strains B. ceti B1-94 (94–74) and M644-93-1 (94–75) and B. pinnipedialis B2-94 (94–73) (Figure 1). The B. ceti B1-94 (94–74) pattern comprised only two fragments of 774 bp and 550 bp, although the B. ceti M644-93-1 (94–75) pattern showed three fragments of 774 bp, 551 bp, and 299 bp. Interestingly, the B. ceti M644-93-1 (94–75) pattern shared two fragments with the B. pinnipedialis pattern that comprised three fragments of 774 bp, 425 bp, and 299 bp. To ensure adequate species identification using the Suis-Ladder, the ANSES collection of marine field strains from different geographic origins and different host species was examined. Suis-ladder patterns were consistent with the species assigned by biochemical and molecular characterization, and the multiplex assay was able to segregate B. ceti isolates into two distinct clusters (Table 3). At the same time, using the Bruce-Ladder, all investigated isolates were confirmed as marine Brucella, sharing identical patterns as marine Brucella reference strains (Table 3).
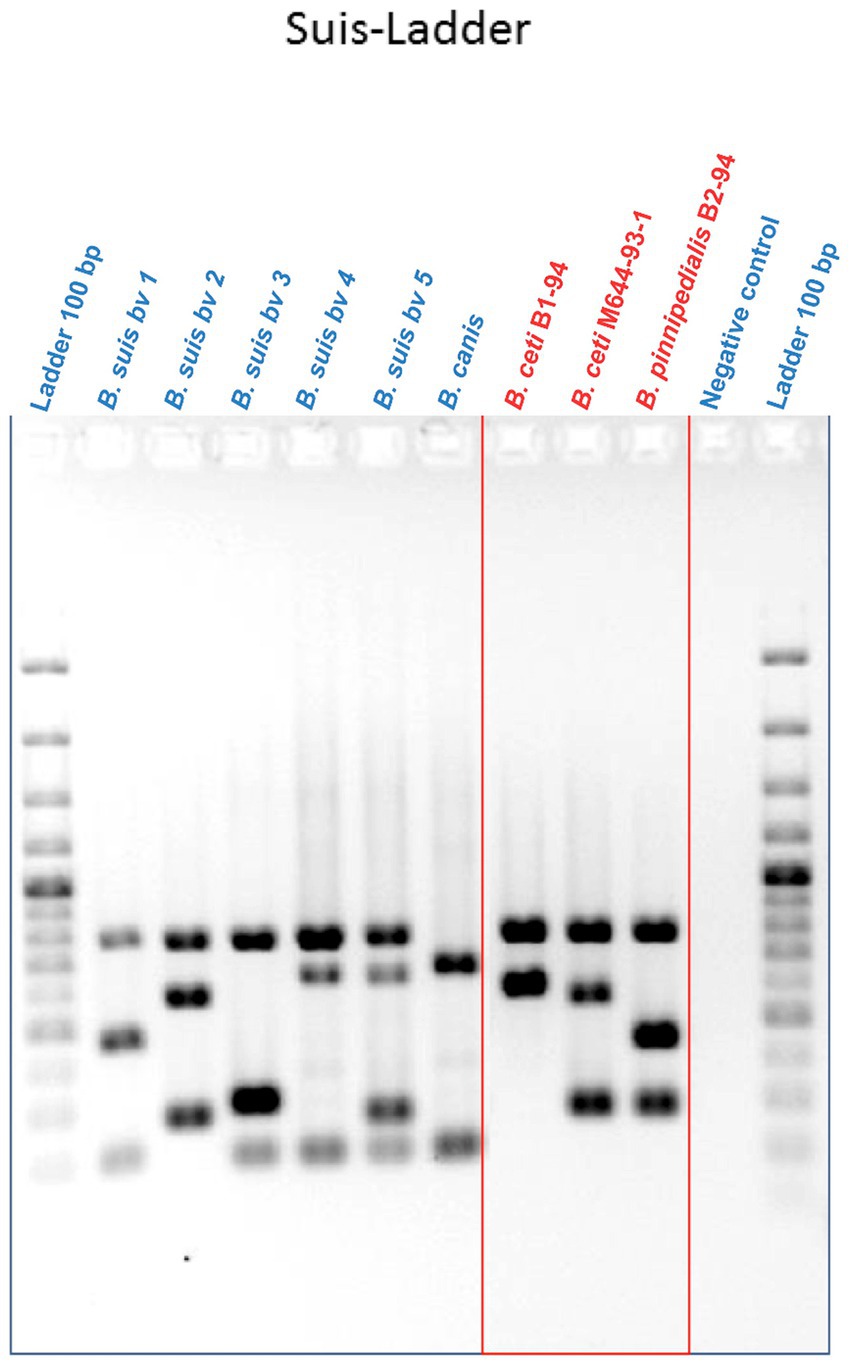
Figure 1. Suis-Ladder patterns of B. suis biovars, B. canis, and marine Brucella reference strains. Lines 1 and 12 contain a 100 bp Ladder. Line 11 contains the negative control, lines 2 to 6 contain DNA from B. suis biovar 1, biovar 2, biovar 3, biovar 4, and biovar 5 respectively, line 7 contains DNA from B. canis, and lines 8 to 10 contain DNA from B. ceti B1-94, B. ceti M644-93-1, and B. pinnipedialis B2-94, respectively.
In RFLP analysis, only two endonucleases were independently used for each targeted gene in this study (StyI and NcoI for omp2a EcoRI and KpnI for omp2b and AvaII and HaeIII for omp31), and results (Table 3) were identified according to the previously described nomenclature (Cloeckaert et al., 2001). Our results showed that omp31 patterns were strictly identical among B. ceti B1-94, B. ceti M644-93-1, and B. pinnipedialis B2-94, as well as among all investigated field isolates, confirming the absence of polymorphism within omp31 gene between marine species. Regarding omp2a and omp2b, only one enzyme per target, respectively NcoI and KpnI, was able to discriminate isolates into 2 groups: 3 isolates into N(K) group, including M644-93-1, and 13 isolates, including B. ceti B1-94 and B. pinnipedialis B2-94, into LMOP(IJ) patterns (Table 3). Nevertheless, the RFLP method applied here did not allow discrimination between B. pinnipedialis and B. ceti B1-94-like isolates.
Previously published HRM-PCR SNP (Girault et al., 2022) identified all analyzed strains as Marine Brucella sp. Furthermore, using specific primers for B. ceti 1, B. ceti 2, and B. pinnipedialis, strains were separated into ST 23, ST 26 – Europe, and ST 24/25 clades, respectively (Table 3). Using B1-94 (94–74) as a reference, strains 97–7,763-2 (dolphin), 05–684-1145 R1F (porpoise), 05–684 (porpoise), 05–684-1143 F1F (porpoise), 05–684-1144 F2TM (porpoise), 12-1944-A (4453) (porpoise), 12-1944-B (6186) (porpoise), and 14–901 (gray seal) were all identified as B. ceti 1 (Table 3). Strains 09–601-1272 (dolphin) and 15–1717-6196 (dolphin) were identified as B. ceti 2 using M644/93/1 (94–75) as a reference strain (Table 3). At the same time, two strains, 15–1,242-4197 and 15–1,242-4198 from gray seals in Finland, were classified as B. pinnipedialis using B2-94 (94–73) as a reference strain (Table 3).
3.3 The phylogenetic analyses using WGS classify all publicly available marine strains into three distinct clades, corresponding to Brucella populations identified by MLVA-16
Phylogenetic analysis of 237 marine isolates (Garofolo et al., 2013; Isidoro-Ayza et al., 2014; Maquart et al., 2009; Suárez-Esquivel et al., 2017), including additional publicly available sequences from marine and reference strains and the 14 isolates investigated here, is presented in Figure 2. The MLVA-16 results allowed dividing the investigated marine Brucella isolates into three clades according to their preferred host: B. ceti dolphin type (pattern A, as B. ceti M644-93-1), B. ceti porpoise type (pattern B as B. ceti B1-94), B. pinnipedialis seal strains (pattern C, as B. pinnipedialis B2-94), and B. ceti ST 27 group (pattern D as B. ceti 02/611) (Figure 2; Table 3).
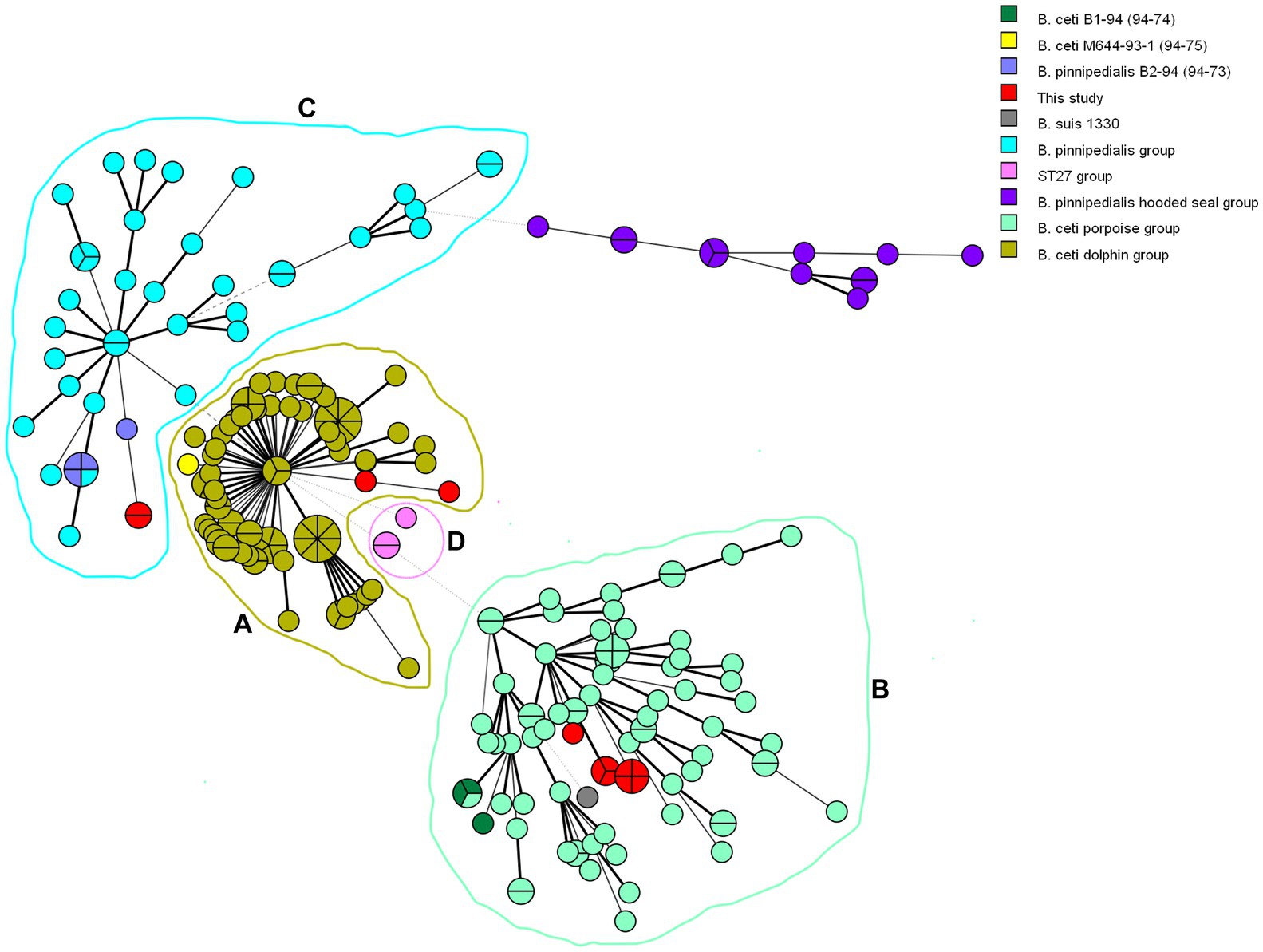
Figure 2. Minimum spanning tree generated from 237 marine Brucella MLVA-16 genotypes. Each circle corresponds to an isolate and the size of the circle is proportional to the number of isolates. Strains introduced in this study are colored in red. Analyzed Brucella sp. are color-coded, while rings represent clusters (A–D).
Similarly, WGS analyses clustered marine Brucella strains into five clusters: ST 26 (Europe) correspond to B. ceti M644-93-1-like isolates (pattern A in MLVA), ST 23 to B. ceti B1-94-like isolates (pattern B in MLVA), ST 24/25 to B. pinnipedialis B2-94-like isolates (pattern C in MLVA), ST 27 to B. ceti Cudo-like isolates (pattern D in MLVA), and ST 26 (Costa Rica) (Figure 3).
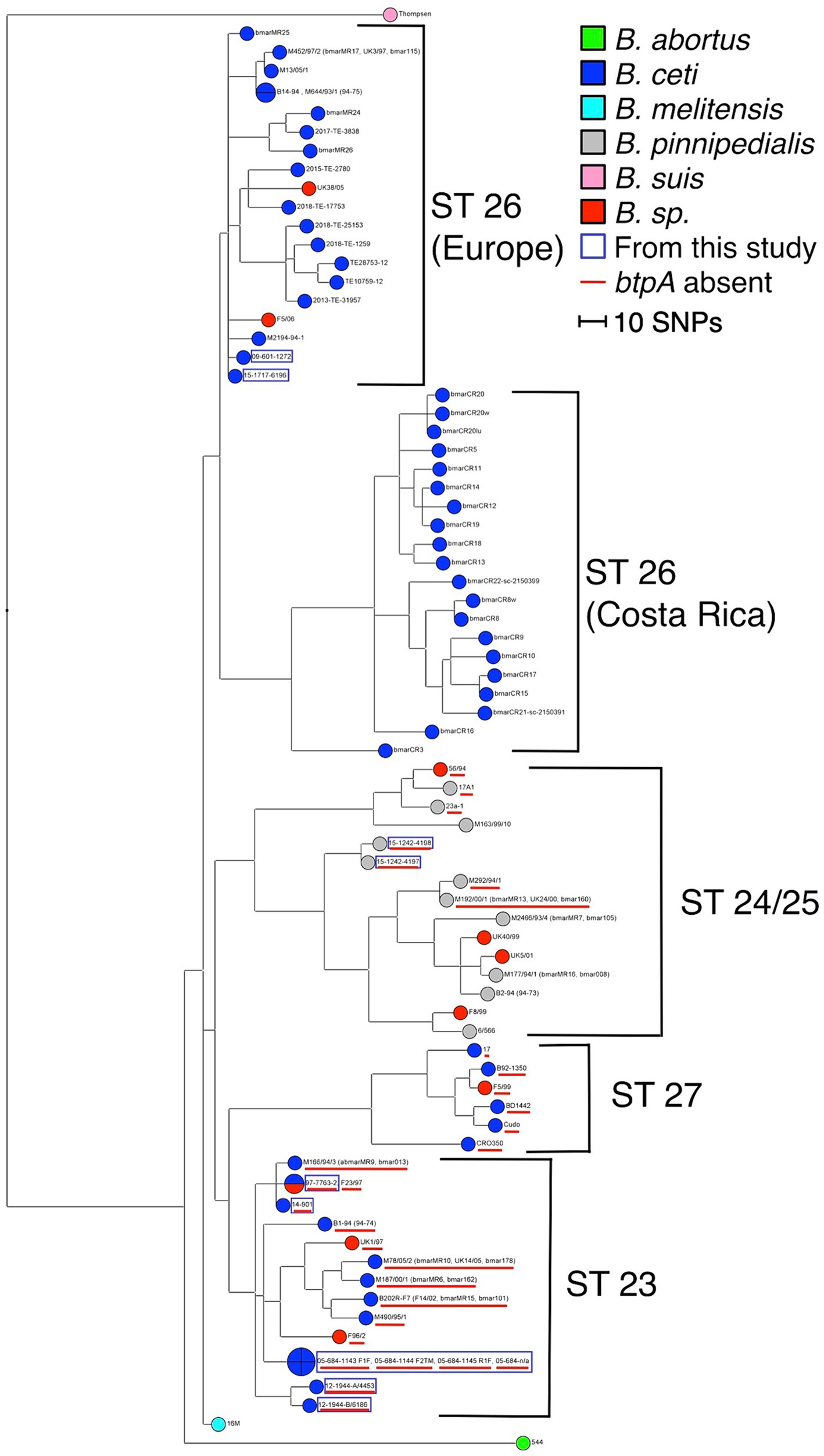
Figure 3. Maximum parsimony analysis of WGS data. The tree has been generated from 7,745 SNPs and rooted with B. melitensis 16 M reference genome. The homoplasy index has a value of 1.6% (Parsimony value: 6080). The branch length is proportional to the number of SNPs identified (the scale bar represents the difference of 10 SNPs). Analyzed Brucella sp. are color-coded, and the strains issued from this study are marked with a blue square. The absence of the btpA gene is marked with a red underscore.
3.4 In silico analysis exhibited the absence of specific plasmid and AMR genes, but showed that the btpA virulence gene is variably distributed in different marine Brucella ST clusters
No plasmids were detected in any investigated strains when screening the assemblies for entries in the PlamidFinder database. Similarly, no antibiotic resistance genes were found screening NCBI and ResFinder databases. Instead, searching through the CARD and MEGARes databases, one (mrpF) and six (mprF, bep C, D, E, F, and G) genes involved in AMR mechanisms were found in all examined genomes including the references. The same tools also identified the tetC gene involved in tetracycline resistance only in the B. ceti M13-05-1 genome.
Exclusively in B. melitensis, B. abortus, and B. suis reference genomes, 53 VGs mainly responsible for host immune evasion, intracellular survival, regulation, and expression of the Type IV secretion system in Brucellae were identified, when the VFDB and own BRUgenes databases were examined (Table 4). In B. pinnipedialis, B. ceti and Brucella sp. analyzed genomes, 45 of the 53 VGs were consistently detected, while 8 genes (btpA, cgs, kdsA, pmm, wbkA, bpe275, ure, and vceC) were not found in one or more of the strains screened (Figure 4; Supplementary Table S2). To verify the absence of undetected VGs, an in silico PCR using Bionumerics, as well as, a classical PCR with gel visualization (Supplementary Figure S1) was conducted for the available strains. If a gene is detected by in silico and/or classical PCR, its presence is considered confirmed (Figure 4; Supplementary Table S2). Moreover, these genes in truncated or pseudo forms were also missing from examined strains.
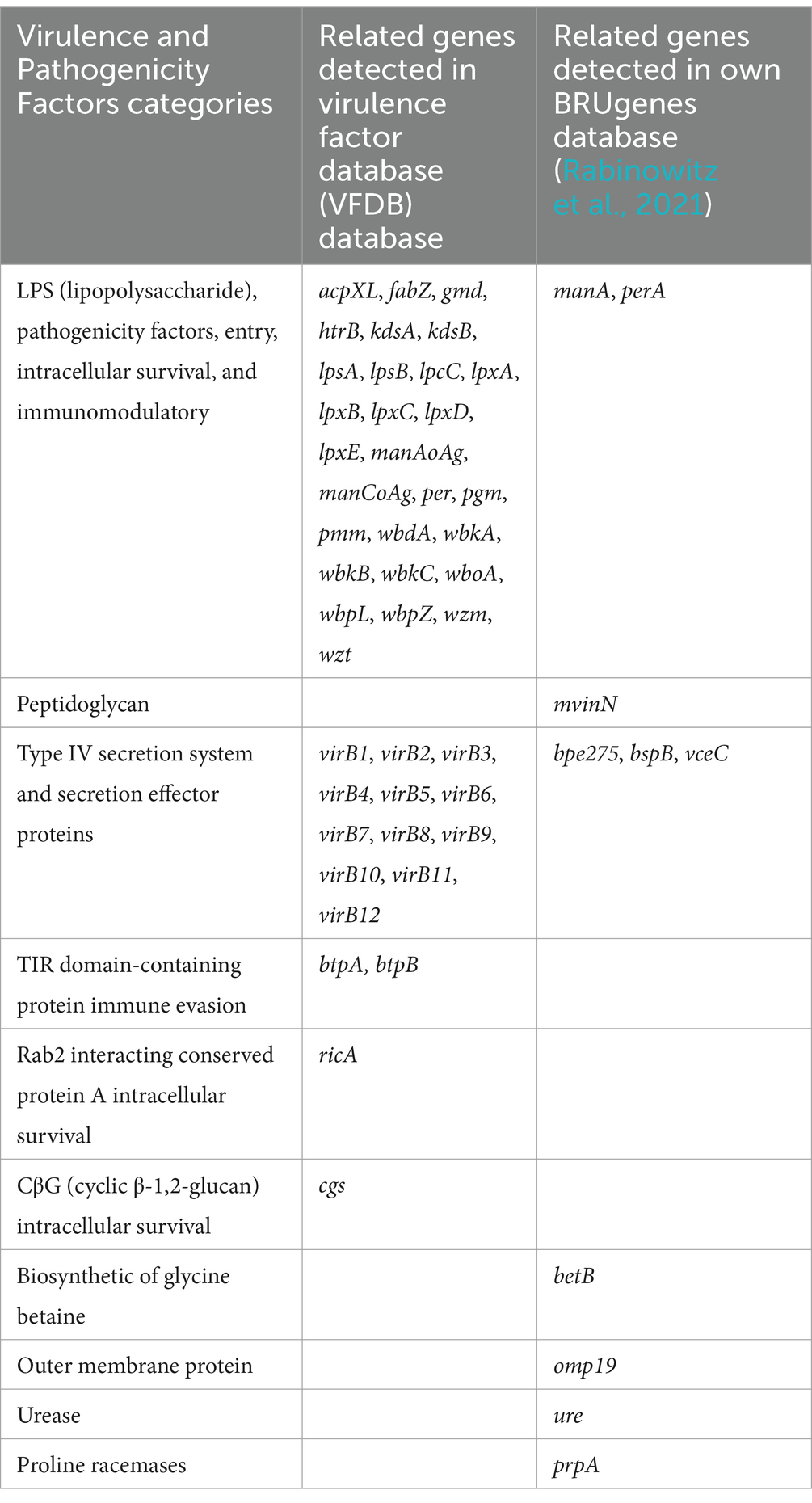
Table 4. Associated virulence and pathogenicity factors detected in B. ceti, B. pinnipedialis, and B. sp. analyzed genomes.
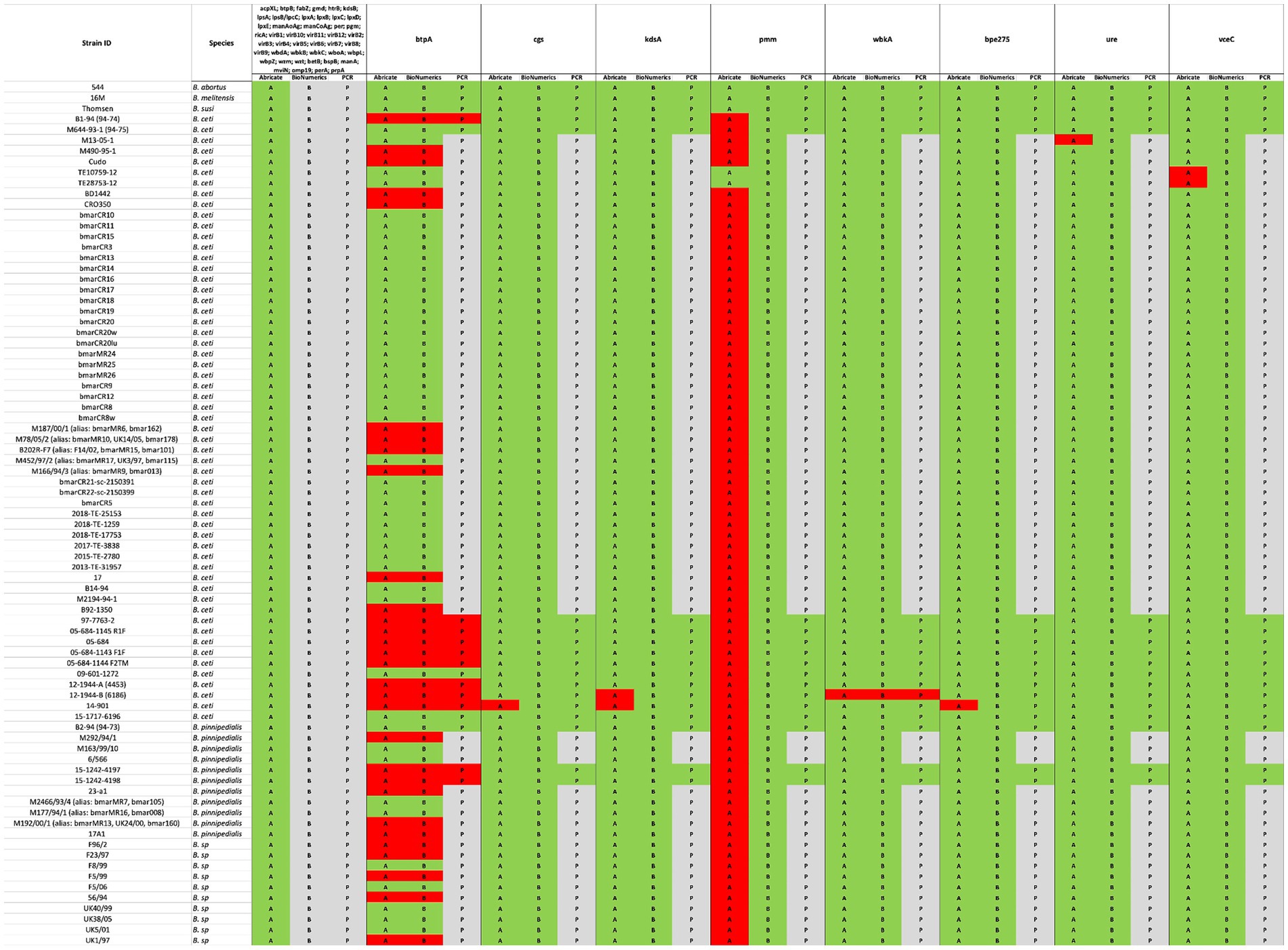
Figure 4. Comparison between in silico and experimental molecular analysis for the identification of virulence genes. The in silico Abricate (A) and PCR using BioNumerix (B) on available reads were compared to classical PCR (P). The green color signifies the presence, and red absence of the targeted genes, while gray implies that analysis could not be performed.
4 Discussion
Marine mammals, at the top of the food chain, are good bio-indicators of the ocean water quality and anthropogenic pressures. Reduction of available food areas pressures marine mammals into smaller or usually inhabitable areas for their species in search of food, increasing close contacts, unusual for cetaceans, which facilitates the spread of infectious diseases, including brucellosis.
Both phenotypic and molecular approaches currently applied to correctly identify the marine Brucella species require time, finances, and/or expertise in bacteriological feature interpretation, as well as in genomic analyses, which are cumbersome for field laboratories. Therefore, in this study, we compared the performances of the described molecular tools to correctly classify 12 new isolates from marine wildlife. Furthermore, we used the in silico methods to compare these strains to genomes of available marine Brucella and evaluate their antimicrobial susceptibility, presence of plasmids, and VGs.
The prevalence of brucellosis among endangered species might impact population dynamics, which requires the adaptation of approaches for species conservation. Bacteriological evidence for discriminating between B. ceti and B. pinnipedialis is small and depends essentially on phenotypical features common to most isolates reported to date. Classification is thus, mainly directed by host preference. Brucella isolates from marine mammals do not produce H₂S. Most strains only agglutinate with monospecific anti-A serum, are lysed by Tbilisi, Weybridge, and Izatnagar phages, and can grow in the presence of basic fuchsine and thionine (World Organisation for Animal Health, 2022). Strains infecting pinnipeds need carbon dioxide and are unable to metabolize D-galactose, unlike cetacean strains (Jahans et al., 1997; Banai and Corbel, 2010; Foster et al., 2007; Guzmán-Verri et al., 2012; Jacques et al., 2007). Current spectral databases for protein pattern identification using MALDI-TOF approaches are not sufficient to identify among Brucella species, nor separate Ochrobactrum sp., yet alone between B. ceti from B. pinnipedialis (Karger et al., 2013; Mesureur et al., 2018). Thus, identification at the species level of marine isolates requires specific bacteriological and/or molecular tools.
Marine Brucella have specific genomic signatures that differentiate them from species affecting mainly terrestrial animals (Cloeckaert et al., 2000). A toolbox of molecular techniques for the identification of marine Brucella sp., predominantly based on the study of the polymorphism of the omp2 gene and the IS711 fingerprint, is available. The insertion sequence, IS711, is present in a greater number of copies in marine Brucella (>20 copies) than in terrestrial Brucella species, except in B. ovis with more than 35 copies (Foster et al., 2007; Ocampo-Sosa and García-Lobo, 2008). Interestingly, a downstream copy of the bp26 gene (omp28) coding for the BP26 periplasmic protein is specific only to marine Brucella (Cloeckaert et al., 2000). In addition, similarly to terrestrial Brucella that harbors one unique copy of omp2a and omp2b genes, (except for B. ovis – two omp2a copies, and the absence of omp2b) (Banai and Corbel, 2010), one copy of each gene is reported in pinniped Brucella. However, cetacean species show the absence of omp2a, but the presence of two omp2b copies (Cloeckaert et al., 2001). Therefore, RFLP analysis of the omp2a, omp2b, and omp31 genes allows discrimination between Brucellae infecting marine mammals (Dawson et al., 2008). The original publication (Cloeckaert et al., 2001) shows three and five distinct restriction patterns of omp2a and omp2b amplified genes, respectively, for the classification of marine Brucella. M(J) pattern, comprising B. ceti reference strain B1-94 consists of 79% of harbor porpoise isolates, 15% of dolphins, 4% of seals, and 2% of whale isolates. N(K) pattern, comprising B. ceti reference strain M644-93-1, involves 100% isolates from dolphins. L(I) pattern, including B. pinnipedialis reference strain M644-93-1, is composed of 95% seal isolates. O(I) pattern comprises of 85% seal isolates. P(I) and Q(I) patterns are reported for isolates from hooded seals and one from Bottlenose dolphin in California (Cloeckaert et al., 2001; Dawson et al., 2008). Using the HRM-PCR SNP-specific marker, described previously by Girault et al. (2022), it was possible to differentiate marine from classic Brucella sp. Furthermore, specific SNP for B. pinnipedialis can identify this marine species, while B. ceti 1 and B. ceti 2 SNPs can distinguish between two clades of B. ceti. Therefore, HRM-PCR is currently the only easy-to-apply, rapid, and cost-effective molecular method to distinguish marine Brucella species, providing high concentration and DNA quality as well as the availability of reference strains.
According to host preference and ocean distribution (Nymo et al., 2011; Suárez-Esquivel et al., 2017), molecular studies confirmed the existence of two distinct groups among cetaceans and pinnipeds and the probable existence of biovars (Moreno et al., 2002) within each marine Brucella species. Thus, multi-locus sequencing studies suggested that marine Brucella strains are clustered in five sequence types (STs), labeled ST 23 to ST 27. ST 23, ST 26, and ST 27 were associated with infection in cetaceans (porpoises, dolphins, and bottlenose dolphins, respectively), while ST 24/25 was linked with infections in seals (Whatmore et al., 2007). According to VNTR assays, there is a doubt that taxonomy does not reflect the phylogeny of two marine species (Whatmore et al., 2016). Among B. ceti isolates, further MLSA, MLVA, and RFLP studies underlined three different groups according to host preference, phenotypic and genomic features: B. ceti dolphin type, B. ceti porpoise type, and B. ceti human type. B. ceti porpoise type is more closely related to B. ceti human isolates and B. pinnipedialis group (Guzmán-Verri et al., 2012). Similarly, B. pinnipedialis isolates clustered into two distinct groups, one specific to isolates from hooded seals (found only in central and western North Atlantic), and one clustering other pinnipeds, with respective STs, ST 24/25 (Groussaud et al., 2007). Our MLVA analysis allowed us to organize B. ceti into four clades according to their preferred host: B. ceti dolphin type (pattern A), B. ceti porpoise type (pattern B), and human B. ceti (pattern D), separating additional clade from previously published by Guzmán-Verri et al. (2012). MLSAs, i.e., MLVA and MLSA showed a genetic proximity between isolates from marine Brucella and B. suis (El-Sayed and Awad, 2018; Le Flèche et al., 2006; Whatmore et al., 2006). However, this is not observed with whole-genome SNP analysis, as marine Brucella are not directly related to B. suis (Sankarasubramanian et al., 2017; Wattam et al., 2014).
Therefore, we decided to use specific molecular approaches designed for B. suis to discriminate within marine Brucella. RFLP restriction patterns of omp2a and omp2b genes produced an overall pattern classification as previously described (Cloeckaert et al., 2001). In Suis-Ladder, three patterns were observed, with a complete correlation with MLVA. Our MLVA-16 results are in accordance with the previously reported genomic structures into five clusters: cluster A (ST 26) is exclusively composed of dolphin isolates, cluster B (ST 23) is formed of isolates from porpoises and dolphins, cluster C (ST 24/25) consists of pinniped isolates, including a subcluster C3 for hooded seal strains, and cluster D (ST 27) is represented by only known zoonotic isolates from humans. Finally, deeper WGS analysis confirmed the segregation into four groups for the marine Brucella isolates, in total correlation with MLVA and Suis-Ladder results. Additionally, WGS analysis separated the ST 26 group into two sub-clusters based on the geographic origin (European and Costa Rican lineages).
Furthermore, analysis of marine mammalian Brucella (B. ceti, B. pinnipedialis, and B. sp.) genomes examined in the CARD and MEGARes databases highlighted the presence in all strains of six genes potentially involved in AMR: the multiple peptide resistance factors mprF and the outer membrane efflux proteins bep C, D, E, F, and G. The overall presence of the MprF factor in highly pathogenic B. melitensis and B. abortus strains isolated in Egypt and Iran has already been highlighted (Dadar et al., 2023a; Khan et al., 2021), as well in the pan-genome of B. ceti and B. pinnipedialis (Orsini et al., 2022). MprF plays a key role in the virulence of Staphylococcus aureus; among all, it is implicated in resistance to cationic antimicrobial peptides such as gentamycin, moenomycin, and vancomycin (Nishi et al., 2004). Identification of this protein in marine Brucella seems to indicate its involvement in the intracellular survival and repulsion of cationic antimicrobials, although Dadar et al. demonstrated no resistance to gentamicin by disk diffusion assays on 40 tested strains (Dadar et al., 2023a). The unique gene that potentially confirms AMR capacity to tetracyclines is tetC, detected only in the B. ceti M13-05-1 genome using NCBI, ResFinder, CARD, and MEGARes databases. Tetracyclines (including tetracycline and doxycycline) are commonly used for the treatment of brucellosis, and the presence of resistance will have important public health implications. Although specific tetracycline resistance data are not available for marine Brucella species, Orsini et al. (2022) speculated that the presence of tetC in only two B. ceti analyzed genomes (including the M13-05-1 strain) suggests substantial pan-susceptibility to antibiotics of this species. On the other hand, a recent review highlighted how the prevalence of tetracycline and doxycycline resistance in classical Brucella species (B. melitensis and B. abortus) was relatively low (1,7%) but increased over time (Rezaei Shahrabi et al., 2023). To the authors’ best knowledge, currently, no published data are showing the tetracycline resistance test of the mentioned B. ceti M13-05-1 strain. Our results are in line with the few studies showing that genes conferring resistance to critically important antibiotics are rare or absent in marine Brucella.
In this study, we also analyzed the presence of VG in the genomes of Brucella species that mainly infect marine mammals (B. ceti and B. pinnipedialis), by questioning VFBD and BRUgenes databases, which revealed the presence of an extended set of genes, including those involved in adhesion, invasion, survival within the host cells, and modulation of the immune response (Table 4). The occurrence of the 53 VGs was found only in the genomes of classical Brucella species, which is in agreement with data reported in the literature (Dadar et al., 2023a; Khan et al., 2021; Brangsch et al., 2023; Rabinowitz et al., 2021). In contrast to the classical Brucella sp. in the genomes of the marines isolates the lack of some genes was evident. Although eight genes were not detected by Abricate search, only the absence of two genes (btpA and wbkA) was confirmed in one or more genomes, through careful validation by in silico and/or classical PCR. Using the same Abricate analysis, the pmm gene was not present in any of the marine strains apart from B. ceti TE10759-12 and TE28753-12 genomes, confirming the previously published findings (Orsini et al., 2022). However, upon performing the in silico and/or classical PCRs, the presence of this gene was confirmed in all marine Brucella sp. genomes analyzed (Figure 4; Supplementary Table S2). Misidentification of the pmm gene using the VFBD database could be due to the general quality of de novo assemblies with the fragmentation of the pmm gene through multiple contigs, not allowing proper alignment. This highlights the importance of confirming the results obtained by in silico analysis with classical molecular tools, to avoid any possible misinterpretation. The use of complementary tools, such as BioNumerics, allows direct screening of the raw reads, which improves the accuracy of gene detection.
Out of 12 new marine Brucella strains described in this study, 2 were found to have rough LPS. The absence of wbkA was confirmed in the B. ceti 12-1944-B (6186) isolate, described in this study. This gene encodes for the mannosyltransferase, involved in the synthesis of the homopolymeric linear chain of N-formyl-perosamine residues, which are linked via α-1,2 and/or α-1,3-glycosidic bonds into O-polysaccharide (O-PS), a key component of Brucella lipopolysaccharide (LPS), the presence of which is responsible for the smooth (S) phenotype (Moriyón and López-Goñi, 1998). Its absence and/or mutation is responsible for the formation of rough (R) Brucella (González et al., 2008). This perfectly matches with the phenotype of B. ceti 12-1944-B (6186) isolate (Table 1). In contrast, in the other B. ceti 15–1717-6196 isolate exhibiting R phenotype, no absence of genes, questioning the VFBD database involved in LPS synthesis was found. O-PS formation depends on a plethora of genes, and the R form can be due to either the absence/silencing of one or more of these genes and/or a mutation in one of them.
Our findings are also particularly intriguing, shedding light on the distinct occurrence of the btpA gene, which encodes a Toll/interleukin-1 receptor (TIR) domain-containing protein capable of regulating dendritic cell activation during B. abortus infection (Salcedo et al., 2008). Notably, Brucella’s TIR-containing proteins, BtpA and BtpB, work in concert to modulate host inflammatory responses during infection by inhibiting dendritic cell activation (Salcedo et al., 2013) and influence cellular energy metabolism by hydrolyzing NAD+ (Coronas-Serna et al., 2020). While the precise targets of TIR-containing Brucella effector proteins remain to be elucidated, significant differences emerge between these two proteins. For example, BtpA has a known role in specifically affecting macrophage TNF-α secretion (Salcedo et al., 2013). In contrast to the pan-genome analysis of B. ceti and B. pinnipedialis genomes, which suggests a consistent presence of both proteins (Orsini et al., 2022), our data reveal the absence of BtpA within B. ceti ST 27 and 23 clusters (Figure 3), which was also confirmed by classical PCR analysis. Furthermore, its distribution within the genomes of B. pinnipedialis (ST 24/25 cluster) shows a division into two subclades, with some maintaining its presence and others lacking it.
In conclusion, the HRM-PCR or Suis-Ladder multiplex PCR can be easily set up by any laboratory familiar with PCR methods, and the ability to reliably identify marine mammal brucellae has been demonstrated in this study. In terms of costs, both methods are cost-effective approaches for any laboratory. Within a One-Health worldwide context, the ability to easily characterize a Brucella strain isolated from a marine mammal is a powerful tool for every lab in the world. Furthermore, the in silico analyses should be verified by molecular approaches, to better classify marine Brucella strains regarding the presence of AMR and VGs.
Data availability statement
The datasets presented in this study can be found in online repositories. The names of the repository/repositories and accession number(s) can be found in the article/Supplementary material.
Author contributions
GG: Conceptualization, Data curation, Formal analysis, Investigation, Methodology, Software, Validation, Writing – original draft, Writing – review & editing. LF: Data curation, Formal analysis, Investigation, Methodology, Software, Validation, Writing – original draft, Writing – review & editing. MJ: Conceptualization, Formal analysis, Investigation, Resources, Writing – original draft. LP: Data curation, Formal analysis, Investigation, Visualization, Writing – original draft. AlD: Data curation, Investigation, Visualization, Writing – review & editing. AnD: Data curation, Investigation, Software, Visualization, Writing – original draft. SD: Data curation, Formal analysis, Methodology, Writing – review & editing. PF: Formal analysis, Methodology, Supervision, Validation, Writing – review & editing. AF: Data curation, Methodology, Project administration, Validation, Writing – review & editing. VM: Conceptualization, Data curation, Formal analysis, Methodology, Writing – original draft. CP: Conceptualization, Formal analysis, Funding acquisition, Methodology, Project administration, Resources, Supervision, Validation, Writing – original draft, Writing – review & editing. VD: Formal analysis, Methodology, Validation, Writing – original draft, Writing – review & editing.
Funding
The author(s) declare that no financial support was received for the research, authorship, and/or publication of this article.
Conflict of interest
The authors declare that the research was conducted in the absence of any commercial or financial relationships that could be construed as a potential conflict of interest.
Publisher’s note
All claims expressed in this article are solely those of the authors and do not necessarily represent those of their affiliated organizations, or those of the publisher, the editors and the reviewers. Any product that may be evaluated in this article, or claim that may be made by its manufacturer, is not guaranteed or endorsed by the publisher.
Supplementary material
The Supplementary material for this article can be found online at: https://www.frontiersin.org/articles/10.3389/fmicb.2024.1437408/full#supplementary-material
Supplementary Figure S1 | Gel visualization of missed virulence genes.
Supplementary Figure S2 | Original gel visualization of Suis-Ladder patterns of B. suis biovars, B. canis and marine Brucella reference strains.
Supplementary Table S1 | List of genomes sequences and DNA available in laboratory used in this study.
Supplementary Table S2 | All data concerning detection of 53 virulence genes with in silico methods (Abricate=A and BioNumeriscs=B) and gel visualization of PCR (PCR=P).
References
Al Dahouk, S., Flèche, P. L., Nöckler, K., Jacques, I., Grayon, M., Scholz, H. C., et al. (2007). Evaluation of Brucella MLVA typing for human brucellosis. J. Microbiol. Methods 69, 137–145. doi: 10.1016/j.mimet.2006.12.015
Audic, S., Lescot, M., Claverie, J.-M., Cloeckaert, A., and Zygmunt, M. S. (2011). The genome sequence of Brucella pinnipedialis B2/94 sheds light on the evolutionary history of the genus Brucella. BMC Evol. Biol. 11:200. doi: 10.1186/1471-2148-11-200
Banai, M., and Corbel, M. (2010). Taxonomy of Brucella. Open Vet. Sci. J. 4, 85–101. doi: 10.2174/1874318801004010085
Bankevich, A., Nurk, S., Antipov, D., Gurevich, A. A., Dvorkin, M., Kulikov, A. S., et al. (2012). SPAdes: a new genome assembly algorithm and its applications to single-cell sequencing. J. Comput. Biol. 19, 455–477. doi: 10.1089/cmb.2012.0021
Brangsch, H., Sandalakis, V., Babetsa, M., Boukouvala, E., Ntoula, A., Makridaki, E., et al. (2023). Genotype diversity of brucellosis agents isolated from humans and animals in Greece based on whole-genome sequencing. BMC Infect. Dis. 23:529. doi: 10.1186/s12879-023-08518-z
Carattoli, A., Zankari, E., García-Fernández, A., Larsen, M. V., Lund, O., Villa, L., et al. (2014). In silico detection and typing of plasmids using PlasmidFinder and plasmid multilocus sequence typing. Antimicrob. Agents Chemother. 58, 3895–3903. doi: 10.1128/AAC.02412-14
Center for Food Security and Public Health. (2018). Brucellosis in marine mammals. Available at: https://www.cfsph.iastate.edu/Factsheets/pdfs/brucellosis_marine.pdf.
Chen, L., Zheng, D., Liu, B., Yang, J., and Jin, Q. (2016). VFDB 2016: hierarchical and refined dataset for big data analysis--10 years on. Nucleic Acids Res. 44, D694–D697. doi: 10.1093/nar/gkv1239
Cloeckaert, A., Grayon, M., and Grepinet, O. (2000). An IS711 element downstream of the Bp26 gene IS a specific marker of Brucella Spp. isolated from marine mammals. Clin. Diagn. Lab. Immunol. 7, 835–839. doi: 10.1128/CDLI.7.5.835-839.2000
Cloeckaert, A., Verger, J. M., Grayon, M., and Grépinet, O. (1995). Restriction site polymorphism of the genes encoding the major 25 kDa and 36 kDa outer-membrane proteins of Brucella. Microbiology 141, 2111–2121. doi: 10.1099/13500872-141-9-2111
Cloeckaert, A., Verger, J. M., Grayon, M., Paquet, J. Y., Garin-Bastuji, B., Foster, G., et al. (2001). Classification of Brucella Spp. isolated from marine mammals by DNA polymorphism at the Omp2 locus. Microbes Infect. 3, 729–738. doi: 10.1016/s1286-4579(01)01427-7
Coronas-Serna, J. M., Louche, A., Rodríguez-Escudero, M., Roussin, M., Imbert, P. R. C., Rodríguez-Escudero, I., et al. (2020). The TIR-domain containing effectors BtpA and BtpB from Brucella abortus impact NAD metabolism. PLoS Pathog. 16:e1007979. doi: 10.1371/journal.ppat.1007979
Dadar, M., Alamian, S., Brangsch, H., Elbadawy, M., Elkharsawi, A. R., Neubauer, H., et al. (2023a). Determination of virulence-associated genes and antimicrobial resistance profiles in Brucella isolates recovered from humans and animals in Iran using NGS technology. Pathogens 12:82. doi: 10.3390/pathogens12010082
Dadar, M., Shahali, Y., Fakhri, Y., and Godfroid, J. (2023b). A comprehensive Meta-analysis of Brucella infections in aquatic mammals. Vet Ital 58:14954. doi: 10.12834/VetIt.2427.14954.2
Dawson, C. E., Stubberfield, E. J., Perrett, L. L., King, A. C., Whatmore, A. M., Bashiruddin, J. B., et al. (2008). Phenotypic and molecular characterisation of Brucella isolates from marine mammals. BMC Microbiol. 8:224. doi: 10.1186/1471-2180-8-224
Doster, E., Lakin, S. M., Dean, C. J., Wolfe, C., Young, J. G., Boucher, C., et al. (2020). MEGARes 2.0: a database for classification of antimicrobial drug, biocide and metal resistance determinants in metagenomic sequence data. Nucleic Acids Res. 48, D561–D569. doi: 10.1093/nar/gkz1010
El-Sayed, A., and Awad, W. (2018). Brucellosis: evolution and expected comeback. Int. J. Vet. Sci. Med. 6, S31–S35. doi: 10.1016/j.ijvsm.2018.01.008
El-Tras, W. F., Tayel, A. A., Eltholth, M. M., and Guitian, J. (2010). Brucella infection in fresh water fish: evidence for natural infection of Nile catfish, Clarias gariepinus, with Brucella melitensis. Vet. Microbiol. 141, 321–325. doi: 10.1016/j.vetmic.2009.09.017
Feldgarden, M., Brover, V., Haft, D. H., Prasad, A. B., Slotta, D. J., Tolstoy, I., et al. (2019). Validating the AMRFinder tool and resistance gene database by using antimicrobial resistance genotype-phenotype correlations in a collection of isolates. Antimicrob. Agents Chemother. 63, e00483–e00419. doi: 10.1128/AAC.00483-19
Foster, G., Osterman, B. S., Godfroid, J., Jacques, I., and Cloeckaert, A. (2007). Brucella ceti Sp. Nov. and Brucella pinnipedialis Sp. Nov. for Brucella strains with cetaceans and seals as their preferred hosts. Int. J. Syst. Evol. Microbiol. 57, 2688–2693. doi: 10.1099/ijs.0.65269-0
García-Yoldi, D., Marín, C. M., de Miguel, M. J., Muñoz, P. M., Vizmanos, J. L., and López-Goñi, I. (2006). Multiplex PCR assay for the identification and differentiation of all Brucella species and the vaccine strains Brucella abortus S19 and RB51 and Brucella melitensis Rev1. Clin. Chem. 52, 779–781. doi: 10.1373/clinchem.2005.062596
Garner, M. M., Lambourn, D. M., Jeffries, S. J., Hall, P. B., Rhyan, J. C., Ewalt, D. R., et al. (1997). Evidence of Brucella infection in Parafilaroides lungworms in a Pacific Harbor seal (Phoca vitulina Richardsi). J. Vet. Diagn. Investig. 9, 298–303. doi: 10.1177/104063879700900311
Garofolo, G., Ancora, M., and Di Giannatale, E. (2013). MLVA-16 loci panel on Brucella Spp. using multiplex PCR and multicolor capillary electrophoresis. J. Microbiol. Methods 92, 103–107. doi: 10.1016/j.mimet.2012.11.007
Garofolo, G., Petrella, A., Lucifora, G., Di Francesco, G., Di Guardo, G., Pautasso, A., et al. (2020). Occurrence of Brucella ceti in striped dolphins from Italian seas. PLoS One 15:e0240178. doi: 10.1371/journal.pone.0240178
Girault, G., Perrot, L., Mick, V., and Ponsart, C. (2022). High-resolution melting PCR as rapid genotyping tool for Brucella species. Microorganisms 10:336. doi: 10.3390/microorganisms10020336
González, D., Grilló, M.-J., De Miguel, M.-J., Ali, T., Arce-Gorvel, V., Delrue, R.-M., et al. (2008). Brucellosis vaccines: assessment of Brucella melitensis lipopolysaccharide rough mutants defective in Core and O-polysaccharide synthesis and export. PLoS One 3:e2760. doi: 10.1371/journal.pone.0002760
Groussaud, P., Shankster, S. J., Koylass, M. S., and Whatmore, A. M. (2007). Molecular typing divides marine mammal strains of Brucella into at least three groups with distinct host preferences. J. Med. Microbiol. 56, 1512–1518. doi: 10.1099/jmm.0.47330-0
Guzmán-Verri, C., González-Barrientos, R., Hernández-Mora, G., Morales, J.-A., Baquero-Calvo, E., Chaves-Olarte, E., et al. (2012). Brucella ceti and brucellosis in cetaceans. Front. Cell. Infect. Microbiol. 2:3. doi: 10.3389/fcimb.2012.00003
Hashemifar, I., Yadegar, A., Jazi, F. M., and Amirmozafari, N. (2017). Molecular prevalence of putative virulence-associated genes in Brucella melitensis and Brucella abortus isolates from human and livestock specimens in Iran. Microb. Pathog. 105, 334–339. doi: 10.1016/j.micpath.2017.03.007
Hernández-Mora, G., Palacios-Alfaro, J. D., and González-Barrientos, R. (2013). Wildlife reservoirs of brucellosis: Brucella in aquatic environments. Rev. Sci. Tech. 32, 89–103. doi: 10.20506/rst.32.1.2194
Hirvelä-Koski, V., Nylund, M., Skrzypczak, T., Heikkinen, P., Kauhala, K., Jay, M., et al. (2017). Isolation of Brucella pinnipedialis from Grey seals (Halichoerus grypus) in the Baltic Sea. J. Wildl. Dis. 53, 850–853. doi: 10.7589/2016-06-144
Holzapfel, M., Girault, G., Keriel, A., Ponsart, C., O’Callaghan, D., and Mick, V. (2018). Comparative genomics and in vitro infection of Field clonal isolates of Brucella melitensis Biovar 3 did not identify signature of host adaptation. Front. Microbiol. 9:2505. doi: 10.3389/fmicb.2018.02505
Huang, W., Li, L., Myers, J. R., and Marth, G. T. (2012). ART: a next-generation sequencing read simulator. Bioinformatics 28, 593–594. doi: 10.1093/bioinformatics/btr708
Isidoro-Ayza, M., Ruiz-Villalobos, N., Pérez, L., Guzmán-Verri, C., Muñoz, P. M., Alegre, F., et al. (2014). Brucella ceti infection in dolphins from the Western Mediterranean Sea. BMC Vet. Res. 10:206. doi: 10.1186/s12917-014-0206-7
Jacques, I., Grayon, M., and Verger, J.-M. (2007). Oxidative metabolic profiles of Brucella strains isolated from marine mammals: contribution to their species classification. FEMS Microbiol. Lett. 270, 245–249. doi: 10.1111/j.1574-6968.2007.00675.x
Jahans, K. L., Foster, G., and Broughton, E. S. (1997). The characterisation of Brucella strains isolated from marine mammals. Vet. Microbiol. 57, 373–382. doi: 10.1016/S0378-1135(97)00118-1
Jaÿ, M., Freddi, L., Mick, V., Durand, B., Girault, G., Perrot, L., et al. (2020). Brucella microti-like prevalence in French farms producing frogs. Transbound. Emerg. Dis. 67, 617–625. doi: 10.1111/tbed.13377
Jia, B., Raphenya, A. R., Alcock, B., Waglechner, N., Guo, P., Tsang, K. K., et al. (2017). CARD 2017: expansion and model-centric curation of the comprehensive antibiotic resistance database. Nucleic Acids Res. 45, D566–D573. doi: 10.1093/nar/gkw1004
Karger, A., Melzer, F., Timke, M., Bettin, B., Kostrzewa, M., Nöckler, K., et al. (2013). Interlaboratory comparison of intact-cell matrix-assisted laser desorption ionization-time of flight mass spectrometry results for identification and differentiation of Brucella Spp. J. Clin. Microbiol. 51, 3123–3126. doi: 10.1128/JCM.01720-13
Khan, A. U., Melzer, F., Sayour, A. E., Shell, W. S., Linde, J., Abdel-Glil, M., et al. (2021). Whole-genome sequencing for tracing the genetic diversity of Brucella abortus and Brucella melitensis isolated from livestock in Egypt. Pathogens 10:759. doi: 10.3390/pathogens10060759
Kurmanov, B., Zincke, D., Wanwen, S., Hadfield, T. L., Aikimbayev, A., Karibayev, T., et al. (2022). Assays for identification and differentiation of Brucella species: a review. Microorganisms 10:1584. doi: 10.3390/microorganisms10081584
Lambourn, D. M., Garner, M., Ewalt, D., Raverty, S., Sidor, I., Jeffries, S. J., et al. (2013). Brucella pinnipedialis infections in Pacific Harbor seals (Phoca vitulina Richardsi) from Washington state, USA. J. Wildl. Dis. 49, 802–815. doi: 10.7589/2012-05-137
Larsen, A. K., Nymo, I. H., Briquemont, B., Sørensen, K. K., and Godfroid, J. (2013). Entrance and survival of Brucella pinnipedialis hooded seal strain in human macrophages and epithelial cells. PLoS One 8:e84861. doi: 10.1371/journal.pone.0084861
Le Flèche, P., Jacques, I., Grayon, M., Al Dahouk, S., Bouchon, P., Denoeud, F., et al. (2006). Evaluation and selection of tandem repeat loci for a Brucella MLVA typing assay. BMC Microbiol. 6:9. doi: 10.1186/1471-2180-6-9
López-Goñi, I., García-Yoldi, D., Marín, C. M., de Miguel, M. J., Barquero-Calvo, E., Guzmán-Verri, C., et al. (2011). New Bruce-ladder multiplex PCR assay for the Biovar typing of Brucella suis and the discrimination of Brucella suis and Brucella canis. Vet. Microbiol. 154, 152–155. doi: 10.1016/j.vetmic.2011.06.035
Mancilla, M., Marín, C. M., Blasco, J. M., Zárraga, A. M., López-Goñi, I., and Moriyón, I. (2012). Spontaneous excision of the O-polysaccharide wbkA Glycosyltranferase gene is a cause of dissociation of smooth to rough Brucella colonies. J. Bacteriol. 194, 1860–1867. doi: 10.1128/JB.06561-11
Maquart, M., Le Flèche, P., Foster, G., Tryland, M., Ramisse, F., Djønne, B., et al. (2009). MLVA-16 typing of 295 marine mammal Brucella isolates from different animal and geographic origins identifies 7 major groups within Brucella ceti and Brucella pinnipedialis. BMC Microbiol. 9:145. doi: 10.1186/1471-2180-9-145
Maquart, M., Zygmunt, M. S., and Cloeckaert, A. (2009). Marine mammal Brucella isolates with different genomic characteristics display a differential response when infecting human macrophages in culture. Microbes Infect. 11, 361–366. doi: 10.1016/j.micinf.2008.12.012
Mayer-Scholl, A., Draeger, A., Göllner, C., Scholz, H. C., and Nöckler, K. (2010). Advancement of a multiplex PCR for the differentiation of all currently described Brucella species. J. Microbiol. Methods 80, 112–114. doi: 10.1016/j.mimet.2009.10.015
McDonald, W. L., Jamaludin, R., Mackereth, G., Hansen, M., Humphrey, S., Short, P., et al. (2006). Characterization of a Brucella Sp. strain as a marine-mammal type despite isolation from a patient with spinal osteomyelitis in New Zealand. J. Clin. Microbiol. 44, 4363–4370. doi: 10.1128/JCM.00680-06
Mesureur, J., Arend, S., Cellière, B., Courault, P., Cotte-Pattat, P.-J., Totty, H., et al. (2018). A MALDI-TOF MS database with broad genus coverage for species-level identification of Brucella. PLoS Negl. Trop. Dis. 12:874. doi: 10.1371/journal.pntd.0006874
Moreno, E., Cloeckaert, A., and Moriyón, I. (2002). Brucella evolution and taxonomy. Vet. Microbiol. 90, 209–227. doi: 10.1016/s0378-1135(02)00210-9
Moriyón, I., and López-Goñi, I. (1998). Structure and properties of the outer membranes of Brucella abortus and Brucella melitensis. Int. Microbiol. 1, 19–26.
Mühldorfer, K., Wibbelt, G., Szentiks, C. A., Fischer, D., Scholz, H. C., Zschöck, M., et al. (2017). The role of ‘atypical’ Brucella in amphibians: are we facing novel emerging pathogens? J. Appl. Microbiol. 122, 40–53. doi: 10.1111/jam.13326
Nishi, H., Komatsuzawa, H., Fujiwara, T., McCallum, N., and Sugai, M. (2004). Reduced content of Lysyl-Phosphatidylglycerol in the cytoplasmic membrane affects susceptibility to Moenomycin, as well as vancomycin, gentamicin, and antimicrobial peptides, in Staphylococcus aureus. Antimicrob. Agents Chemother. 48, 4800–4807. doi: 10.1128/AAC.48.12.4800-4807.2004
Nymo, I. H., Arias, M. A., Pardo, J., Álvarez, M. P., Alcaraz, A., Godfroid, J., et al. (2016). Marine mammal Brucella reference strains are attenuated in a BALB/c mouse model. PLoS One 11:e0150432. doi: 10.1371/journal.pone.0150432
Nymo, I. H., Tryland, M., and Godfroid, J. (2011). A review of Brucella infection in marine mammals, with special emphasis on Brucella pinnipedialis in the hooded seal (Cystophora cristata). Vet. Res. 42:93. doi: 10.1186/1297-9716-42-93
Ocampo-Sosa, A. A., and García-Lobo, J. M. (2008). Demonstration of IS711 transposition in Brucella Ovis and Brucella pinnipedialis. BMC Microbiol. 8:17. doi: 10.1186/1471-2180-8-17
Orsini, M., Ianni, A., and Zinzula, L. (2022). Brucella ceti and Brucella pinnipedialis genome characterization unveils genetic features that highlight their zoonotic potential. MicrobiologyOpen 11:e1329. doi: 10.1002/mbo3.1329
Paixão, T. A., Roux, C. M., den Hartigh, A. B., Sankaran-Walters, S., Dandekar, S., Santos, R. L., et al. (2009). Establishment of systemic Brucella melitensis infection through the digestive tract requires urease, the type IV secretion system, and lipopolysaccharide O antigen. Infect. Immun. 77, 4197–4208. doi: 10.1128/IAI.00417-09
Rabinowitz, P., Zilberman, B., Motro, Y., Roberts, M. C., Greninger, A., Nesher, L., et al. (2021). Whole genome sequence analysis of Brucella melitensis phylogeny and virulence factors. Microbiol. Res. 12, 698–710. doi: 10.3390/microbiolres12030050
Rezaei Shahrabi, A., Moradkasani, S., Goodarzi, F., Beig, M., and Sholeh, M. (2023). Prevalence of Brucella melitensis and Brucella abortus Tetracyclines resistance: a systematic review and Meta-analysis. Microb. Pathog. 183:106321. doi: 10.1016/j.micpath.2023.106321
Salcedo, S., Marchesini, M. I., Degos, C., Terwagne, M., Von Bargen, K., Lepidi, H., et al. (2013). BtpB, a novel Brucella TIR-containing effector protein with immune modulatory functions. Front. Cell. Infect. Microbiol. 3:28. doi: 10.3389/fcimb.2013.00028
Salcedo, S. P., Marchesini, M. I., Lelouard, H., Fugier, E., Jolly, G., Balor, S., et al. (2008). Brucella control of dendritic cell maturation is dependent on the TIR-containing protein Btp1. PLoS Pathog. 4:e21. doi: 10.1371/journal.ppat.0040021
Sankarasubramanian, J., Vishnu, U. S., Gunasekaran, P., and Rajendhran, J. (2017). Identification of genetic variants of Brucella Spp. through genome-wide association studies. Infect. Genet. Evol. 56, 92–98. doi: 10.1016/j.meegid.2017.11.016
Sohn, A. H., Probert, W. S., Glaser, C. A., Gupta, N., Bollen, A. W., Wong, J. D., et al. (2003). Human Neurobrucellosis with intracerebral granuloma caused by a marine mammal Brucella Spp. Emerg. Infect. Dis. 9, 485–488. doi: 10.3201/eid0904.020576
Suárez-Esquivel, M., Baker, K. S., Ruiz-Villalobos, N., Hernández-Mora, G., Barquero-Calvo, E., González-Barrientos, R., et al. (2017). Brucella genetic variability in wildlife marine mammals populations relates to host preference and ocean distribution. Genome Biol. Evol. 9, 1901–1912. doi: 10.1093/gbe/evx137
Wattam, A. R., Foster, J. T., Mane, S. P., Beckstrom-Sternberg, S. M., Beckstrom-Sternberg, J. M., Dickerman, A. W., et al. (2014). Comparative Phylogenomics and evolution of the Brucellae reveal a path to virulence. J. Bacteriol. 196, 920–930. doi: 10.1128/JB.01091-13
Whatmore, A. M., Dawson, C., Groussaud, P., Koylass, M. S., King, A., Shankster, S. J., et al. (2008). Marine mammal Brucella genotype associated with zoonotic infection. Emerg. Infect. Dis. 14, 517–518. doi: 10.3201/eid1403.070829
Whatmore, A. M., Dawson, C., Muchowski, J., Perrett, L. L., Stubberfield, E., Koylass, M., et al. (2017). Characterisation of north American Brucella isolates from marine mammals. PLoS One 12:184758. doi: 10.1371/journal.pone.0184758
Whatmore, A. M., Koylass, M. S., Muchowski, J., Edwards-Smallbone, J., Gopaul, K. K., and Perrett, L. L. (2016). Extended multilocus sequence analysis to describe the global population structure of the genus Brucella: Phylogeography and relationship to Biovars. Front. Microbiol. 7:2049. doi: 10.3389/fmicb.2016.02049
Whatmore, A. M., Perrett, L. L., and MacMillan, A. P. (2007). Characterisation of the genetic diversity of Brucella by multilocus sequencing. BMC Microbiol. 7:34. doi: 10.1186/1471-2180-7-34
Whatmore, A. M., Shankster, S. J., Perrett, L. L., Murphy, T. J., Brew, S. D., Thirlwall, R. E., et al. (2006). Identification and characterization of variable-number tandem-repeat markers for typing of Brucella Spp. J. Clin. Microbiol. 44, 1982–1993. doi: 10.1128/JCM.02039-05
World Organisation for Animal Health. (2022). Brucellosis (infection with B. abortus, B. melitenis and B. suis). OIE Terrestrial Manual 2022.
Zankari, E., Hasman, H., Cosentino, S., Vestergaard, M., Rasmussen, S., Lund, O., et al. (2012). Identification of acquired antimicrobial resistance genes. J. Antimicrob. Chemother. 67, 2640–2644. doi: 10.1093/jac/dks261
Keywords: Brucella , molecular biology, marine mammals, wildlife, molecular typing
Citation: Girault G, Freddi L, Jay M, Perrot L, Dremeau A, Drapeau A, Delannoy S, Fach P, Ferreira Vicente A, Mick V, Ponsart C and Djokic V (2024) Combination of in silico and molecular techniques for discrimination and virulence characterization of marine Brucella ceti and Brucella pinnipedialis. Front. Microbiol. 15:1437408. doi: 10.3389/fmicb.2024.1437408
Edited by:
Michel Stanislas Zygmunt, Institut National de Recherche pour l’agriculture, l’alimentation et l’environnement, FranceReviewed by:
Marcela Suárez-Esquivel, National University of Costa Rica, Costa RicaAlice Rebecca Wattam, University of Virginia, United States
Mikeljon P. Nikolich, Walter Reed Army Institute of Research, United States
Angelica Bianco, Experimental Zooprophylactic Institute of Puglia and Basilicata, Italy
Copyright © 2024 Girault, Freddi, Jay, Perrot, Dremeau, Drapeau, Delannoy, Fach, Ferreira Vicente, Mick, Ponsart and Djokic. This is an open-access article distributed under the terms of the Creative Commons Attribution License (CC BY). The use, distribution or reproduction in other forums is permitted, provided the original author(s) and the copyright owner(s) are credited and that the original publication in this journal is cited, in accordance with accepted academic practice. No use, distribution or reproduction is permitted which does not comply with these terms.
*Correspondence: Vitomir Djokic, Vml0b21pci5kam9raWNAYW5zZXMuZnI=
†Present addresses: Maryne Jay, Anses, Laboratoire de Lyon, UMR Mycoplasmoses Animales, Lyon, France; Université de Lyon, VetAgro Sup, UMR Mycoplasmoses Animales, Marcy L’Etoile, FranceAntoine Drapeau, Anses, Laboratoire de Lyon, Lyon, Unité Antibiorésistance et Virulence Bactérienne, France; Université de Lyon, VetAgro Sup, Lyon, France
‡These authors have contributed equally to this work