- 1Department of Infectious Diseases, Centro Hospitalar e Universitário de Santo António, Porto, Portugal
- 2Department of Orthopaedic Surgery, Centro Hospitalar e Universitário de Santo António, Porto, Portugal
- 3Grupo de Infeção Osteoarticular do Porto, Porto, Portugal
The management of prosthetic joint infections is a complex and multilayered process that is additionally complicated by the formation of bacterial biofilm. Foreign material provides the ideal grounds for the development of an intricate matrix that hinders treatment and creates a difficult environment for antibiotics to act. Surgical intervention is often warranted but requires appropriate adjunctive therapy. Despite available guidelines, several aspects of antibiotic therapy with antibiofilm activity lack clear definition. Given the escalating challenges posed by antimicrobial resistance, extended treatment durations, and tolerance issues, it is essential to ensure that antimicrobials with antibiofilm activity are both potent and diverse. Evidence of biofilm-active drugs is highlighted, and alternatives to classical regimens are further discussed.
1 Introduction
Bacterial involvement has been described in three different states – planktonic, biofilm and intracellular (McConoughey et al., 2014). Biofilm formation is an important mechanism of bacterial pathogenesis, which is characterized by the clustering of (usually monomicrobial groups of) microorganisms on a biological or synthetic surface that form a network of extracellular polymeric substances. As opposed to extracellular bacteria in a planktonic state, which are freely dispersed in the environment, thus vulnerable to aggression, those in biofilm find strength in numbers. Through a mechanism known as quorum sensing, colonies of bacteria transition from planktonic state to biofilm formation once the colony is large enough. This ultimately improves their collective chance of survival through various mechanisms that culminate in antibiotic tolerance, including physical and chemical barrier formation, metabolic adaptation, and genetic material exchange (Figure 1).
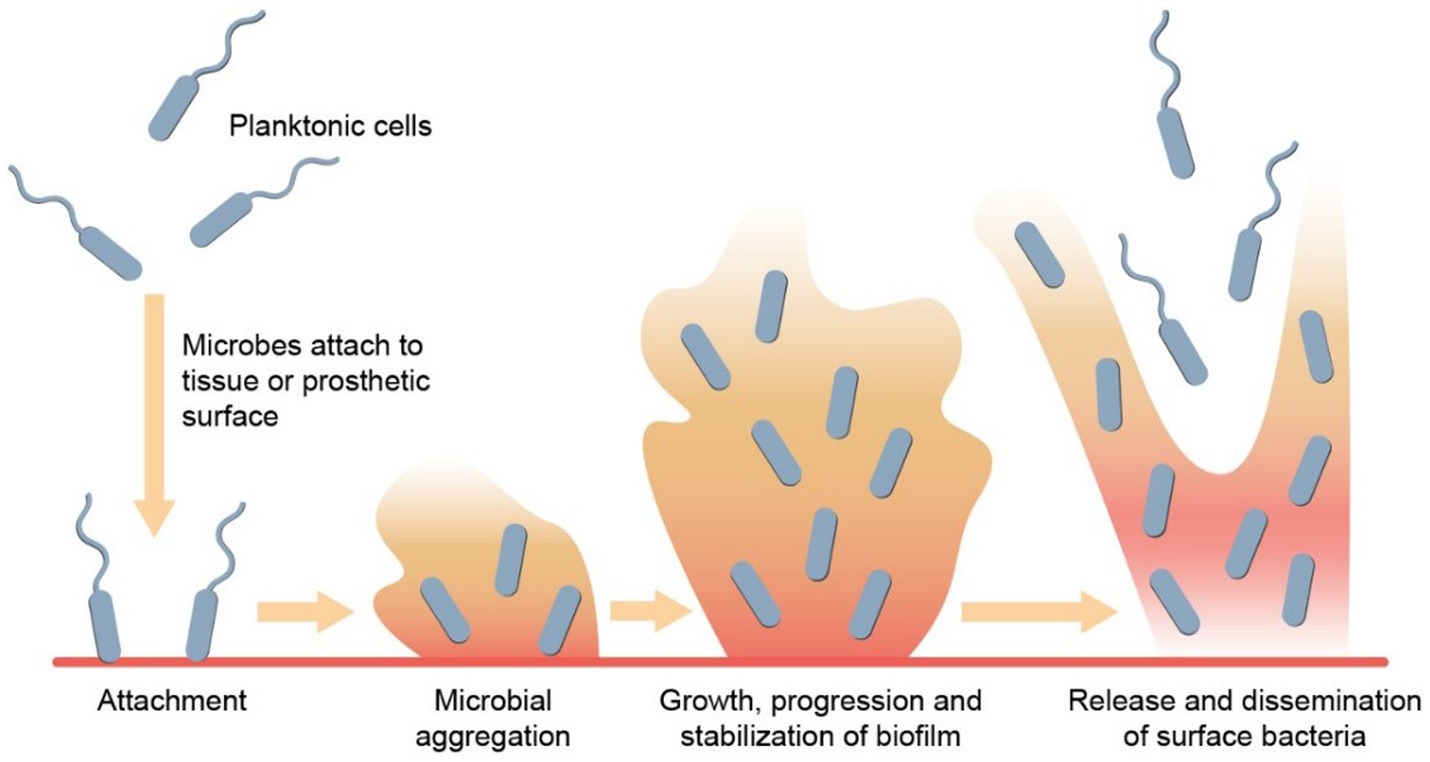
Figure 1. Biofilm formation. Biofilm development begins with planktonic cells initially attaching reversibly to a surface. These cells then attach irreversibly, resulting in the establishment of a bacterial colony on the surface. Through quorum sensing and various signaling mechanisms, the biofilm matures, and stabilizes. Subsequently, microbes within the biofilm disperse by releasing surface bacteria from the top layer, enabling them to colonize new surfaces.
Foreign materials such as orthopaedic hardware provide a suitable environment for bacteria to organize and develop a biofilm. Periprosthetic joint infections (PJIs) are a well-recognized and serious complication of prosthetic joint implantation. The importance of biofilm formation in PJIs is clinically illustrated by the decreasing success of interventions involving prosthesis retention as time since infection onset increases and it is no coincidence that some of the most common pathogens, such as Staphylococci and Streptococci, but also the problematic and often multidrug-resistant Pseudomonas aeruginosa are well known for their biofilm formation capabilities (McConoughey et al., 2014).
In many centres, the incidence of PJIs varies from 0.5 to 2 %, depending on the afflicted site (Namba et al., 2013). PJIs can stem from various sources, such as surgical contamination, hematogenous dissemination or direct spread from contiguous infection (Ahmed et al., 2019). The most common microorganisms involved in PJIs are gram-positive pathogens, mainly Staphylococci when considering hip and knee interventions and Cutibacterium acnes and Streptococci following shoulder arthroplasties. Gram-negative pathogens are also relatively frequent, particularly in the setting of comorbidities or previous antibiotic exposure (Tande and Robin, 2014).
Clinical detection of PJIs can often be difficult considering the indolent course of the chronic infection, relatively low-culture yield of low-virulence bacteria and the ample variety of microorganisms and antibiotic-resistance patterns that can occur. The diagnosis and management of PJIs is, therefore, a complex and multi-faceted process that requires a multidisciplinary approach, encompassing specific medical and surgical strategies, adapted to the clinical scenario and patient involved (Vavasseur and Zeller, 2022). Clinical experience and animal models indicate that intensive debridement is the cornerstone of effectively clearing PJIs and that systemic antibiotic therapy is most effective when started as soon as possible after surgical revision and when combined with local antibiotics (Li et al., 2022). Additionally, accurate identification of the microorganisms involved and their susceptibility to antibiotics is essential for favourable outcome (Zeller et al., 2018).
Treatment of PJIs requires optimal antibiotic coverage, particularly during the initial post-operative phase. Initial therapy usually involves high-dose antibiotics, followed by coupling with drugs possessing antibiofilm properties. The choice of antimicrobial for treating biofilm-associated infections needs to weigh in several factors: tissue and biofilm penetration, possible routes of administration, pharmacokinetic/pharmacodynamic (PK/PD) parameters, tolerability, drug–drug interactions, among others. Rifampicin and fluoroquinolones have been the most used and investigated drugs in this context, becoming a staple in the treatment of PJIs. However, emerging resistance patterns, drug interactions, and adverse reactions profile has highlighted the need for alternative therapies. To date, large clinical and comparative studies with other antibiofilm drugs are lacking, and the role for these is still yet to be defined.
Multiple techniques have been adopted to simulate biofilm formation and assess the antibiofilm properties of antimicrobials. Most studies have relied on in vitro biofilm models, which can be both diverse and complex. Nonetheless, ex vivo and in vivo models have also been put into practice.
Biofilm formation can be assessed by combining various methods, such as staining with conventional, immune histochemical or fluorescent dyes, with direct visualization through light microscopy, scanning electron microscopy or confocal scanning laser microscopy. Other methods include fluorescent in-situ hybridization, piezoelectric sensing, and infrared spectroscopy. Bioluminescence assays and nuclear imaging techniques allow for non-invasive in vivo assessment but are not specific to biofilm formation (Roy et al., 2018). To evaluate potential antibiofilm effects of specific compounds, studies rely on counting colony forming units before and after the compounds are added to the chosen experimental system.
As for antibiotic susceptibility testing, there is no satisfactory assessment to date. Several concepts have been suggested, such as the minimal biofilm eradication concentration (MBEC), the minimal biofilm inhibitory concentration (MBIC), the biofilm bactericidal concentration or the biofilm prevention concentration, but both definitions and thresholds for interpretation vary significantly. The following article reviews the current knowledge on antibiofilm activity and effectiveness of existing mainstream therapy (fluoroquinolones and rifampin), along with the proposed efficacy of alternative antibiotics that could be considered as therapeutic options. Alternate therapies, such as antimicrobial peptides, bacteriophages, probiotics and plant extracts, have not been considered for review since there is no robust evidence for their use.
2 Antibiotics with antibiofilm activity
2.1 The paradigm – rifampicin
Rifamycins include rifampicin, rifabutin, rifapentine and rifaximin, though the first stands out as the most employed in the treatment of PJIs. The inhibition of microorganisms’ RNA polymerase confers rifampicin its bactericidal effect (Thill et al., 2022; Eriksson et al., 2023). Its known ability to penetrate biofilm and the possibility for oral administration make rifampicin a powerful ally in single-stage interventions for PJIs, such as debridement, antibiotics, and implant retention (DAIR) strategies. However, despite being active against some gram-negative microorganisms, rifampicin’s role in PJIs is limited to infections by gram-positive bacteria. Lastly, rifampicin is a safe drug. However, drug–drug interactions are frequent, and its use can lead to anaphylaxis, haemolytic anaemia and thrombocytopenia, acute renal failure, rash, hepatic toxicity, and gastrointestinal symptoms, the latter being the most common (Roblot et al., 2007; Nguyen et al., 2015).
Albano et al. investigated in vitro activity of rifampicin, rifabutin, and rifapentine against Staphylococci, Streptococci and Enterococci in both planktonic and biofilm states in the setting of PJIs. They found that except for some Streptococcus mitis group isolates, antibiofilm activity was significantly higher in staphylococcal infections versus streptococcal and enterococcal PJIs (Albano et al., 2021). These results are supported by a more recent analysis in South Korea, where rifampicin and other rifamycins showed promising antibiofilm effects against in vitro samples of patients with PJIs (Lee et al., 2022).
These encouraging in vitro results echo in the clinical setting. Significantly lower rates of infection relapse with therapeutic regimens that included rifampicin have been observed in PJIs patients with rifampicin-sensitive pathogens (Lazarinis et al., 2023). Notwithstanding, some groups still question whether rifampicin has real benefit in PJIs.
In one randomized controlled trial (RCT), the addition of rifampicin to standard antibiotic therapy failed to reveal a statistically significant advantage (Karlsen et al., 2020). Renz and colleagues dissected this controversy, who underlined the existence of abundant evidence, starting from the very first scientific observation of rifampicin’s antibiofilm activity in animal models in 1983 (Renz et al., 2021). Additionally, a recent study by Beldman et al. corroborated the benefit of rifampicin in cases of acute PJIs treated with DAIR, particularly in knee infections (Beldman et al., 2021). It has also been demonstrated that treatment failure can be up to four times more likely in infections caused by rifampicin-resistant agents, as opposed to rifampicin-sensitive microorganisms (Lazarinis et al., 2023).
Though this may be a testament to rifampicin’s worth in the treatment of PJIs, it also brings us to the matter of antibiotic resistance.
Rifampicin resistance can develop fast and is intrinsically related to a mutation in the rpoB gene encoding the β-subunit of bacterial DNA-dependent RNA polymerase (Lee et al., 2022). Some pathogens, namely Staphylococci, carry the genes coding for these resistance mechanisms and there appears to be a growing tendency in antibiotic-resistant strains of Staphylococci, particularly in the setting of PJIs. This represents a relevant contributing factor in treatment failure (Lazarinis et al., 2023). A study by Eriksson et al. highlighted that in cases of PJIs due to rifampicin-resistant bacteria, there was a clear increase in the number of surgical interventions required for treatment success, in contrast with rifampicin-sensitive pathogens, thus underlining antibiotic resistance as an important factor contributing to prolonged periods of treatment and further complications requiring multiple interventions (Eriksson et al., 2023).
2.1.1 Practical considerations
Strategies for adequate prescription and prevention of rifampicin resistance include antimicrobial susceptibility testing, careful consideration of the timing for initiation and combination therapy.
As described above, methods to evaluate antimicrobial susceptibility in objective biofilm models are still far from applicable in the clinical setting. Thill et al. developed a study that aimed to compare the usage of minimal inhibitory concentration (MIC), minimum bactericidal concentrations (MBC) and MBEC of rifabutin and rifampicin in cases of PJIs caused by Staphylococci. Their results show advantage in using MBEC values. However, further in vivo studies are necessary (Thill et al., 2022). In the meantime, conventional susceptibility testing is highly recommended.
There has been ongoing dispute about the optimal moment to start rifampicin in the context of PJIs. Intuitively, one would want to start antibiofilm therapy as soon as possible to prevent the maturation of the biofilm after thorough debridement. However, an exceedingly early start in rifampicin can lead to antibiotic resistance and adverse outcomes (Sendi and Zimmerli, 2017). The risk of development of rifampicin resistance is higher in the presence of high bacterial load, which is frequently the case shortly after surgery. As such, it is advisable to slightly delay rifampicin introduction, typically 3–5 days after debridement is completed, surgical drains have been removed and there is no observable drainage from the wound. This ensures significant decrease of bacterial load and lower risk of developing resistance.
There is no consensus about the optimal dosage of rifampicin when treating PJIs. Within the realm of staphylococcal PJIs, some authors support using a fixed daily dose, while others favour an adjustment according to the patient’s weight, and others still prefer a twice-daily scheme (Lora-Tamayo et al., 2013). A study by Nguyen et al. defends an overall benefit in prescribing rifampicin as a single daily dose of 600 mg in combination with levofloxacin (Nguyen et al., 2015).
Lastly, due to the risk of resistance, rifampicin should not be prescribed as monotherapy. Rifampicin combination therapy is associated with better clinical outcomes in infections with established biofilms (Forrest and Tamura, 2010).
Further research is essential to better understand the incidence and determining factors of rifampicin resistance, more specifically in PJIs, the ideal dosage and the potential associations with other medications. While there appears to be an advantage in using rifampicin and in slightly delaying its start, further research would aid in shedding light on the ideal timing. Furthermore, research into tailored interventions considering infection site, aetiology, surgical approach, and antimicrobial therapy will surely contribute to an improvement in specialized optimal care.
2.2 Other antibiotics
2.2.1 Antibiotics active against gram-positive and gram-negative microorganisms
2.2.1.1 Fluoroquinolones
Fluoroquinolones (FQs) are broad-spectrum antimicrobials which inhibit bacterial DNA synthesis through the inhibition of DNA gyrase and topoisomerase IV, its primary target against gram-positive and gram-negative bacteria, respectively. FQs are most potent against gram-negative bacilli, such as Enterobacter spp. and Pseudomonas aeruginosa, but their spectrum includes selected gram-positive bacteria, anaerobes, and mycobacteria.
FQs are available in oral and intravenous (IV) formulations and exhibit excellent bioavailability and tissue penetration, particularly within bone and synovial fluid (Thabit et al., 2019). Additionally, bone penetration of levofloxacin does not seem to be affected by level of ischemia (Lozano-Alonso et al., 2016). Despite their good tolerability and safety profile, severe adverse effects have been associated with their use, and should be kept in mind. These include altered mental status, peripheral neuropathy, secondary pseudotumor cerebri, tendinopathy and dysglycaemia (Aspinall et al., 2009; Bidell and Lodise, 2016; Sodhi et al., 2017) Observational studies have also suggested that there is an increased risk of aortic aneurysm and dissection, motivating the American Food and Drug Administration to emit a black label warning in 2018 advising against the use of FQs in individuals with risk factors for aneurysms. However, there appears to be a confounding effect (Brown et al., 2023). FQs should be avoided in patients with myasthenia gravis, as muscle weakness might be exacerbated (Krenn et al., 2020). Moreover, the FQs are associated with a greater risk of C. difficile infection.
Robust in vitro and clinical evidence, along with their high bioavailability, prolonged half-life, and post-antibiotic effect, as well as adequate penetration in bone tissue and collections, make quinolones one of the preferred options to treat PJIs. Subsequently, many guidelines recommend FQs as a first-line option for targeting biofilm by gram-negative microorganisms and for combination therapy with rifampicin for gram-positive pathogens, including Methicillin-Resistant Staphylococcus aureus (MRSA) (Osmon et al., 2013; Høiby et al., 2015).
The FQs bactericidal activity has been shown to be independent of cell growth rate and the antibiofilm activity of these antimicrobials has been demonstrated in in vitro studies, particularly with Pseudomonas aeruginosa and Escherichia coli models (Wiestner et al., 1991; Tanaka et al., 1999). This activity has been further observed in human trials, justifying its pivotal role in the management of gram-negative PJIs (Rodríguez-Pardo et al., 2014; Høiby et al., 2015). In retrospective studies analysing PJIs due to gram-negative bacilli, Martínez-Pastor et al. and Rodríguez-Pardo et al. found that regimens including a fluoroquinolone were associated with better outcomes (Martínez Pastor et al., 2009; Rodríguez-Pardo et al., 2014).
The quinolones’ effect on biofilm appears to be potentiated by its combination with rifampicin, as has been demonstrated in both pre-clinical (Muller-Serieys et al., 2009; Holmberg et al., 2012; Meléndez-Carmona et al., 2022) and clinical studies, particularly in the case of early PJIs (Zimmerli et al., 1998; Berdal et al., 2005; Laffer et al., 2006; Barberán et al., 2008; Senneville et al., 2011). However, regular use of FQs may be threatened by a significant increase in resistance (Martínez Pastor et al., 2009; Benito et al., 2016). Thus, empirical treatment with ciprofloxacin as monotherapy should be avoided to minimize selection of resistance in high bacterial load infections, which has been associated with a sharp decrease in successful treatment in the context of DAIR (Rodríguez-Pardo et al., 2014). When combined with rifampicin, levofloxacin and moxifloxacin were shown to be more advantageous when compared to ciprofloxacin, clindamycin and cotrimoxazole, the latter being associated with the highest rate of treatment failure (Beldman et al., 2021).
Doubts remain on whether FQs’ increased efficacy against biofilms is due to increased bactericidal activity (Blaser et al., 1995) or due to fluoroquinolone’s prevention of the emergence of rifampicin resistance (Muller-Serieys et al., 2009).
2.2.1.2 Fosfomycin
Fosfomycin remains the only phosphonic acid derivative that is used in the clinical setting (Silver, 2017), exhibiting broad-spectrum and bactericidal activity against gram-positive and gram-negative bacteria, including MRSA, and vancomycin-resistant enterococci (VRE) (Carlet and Mainardi, 2012). It exhibits hydrophilic properties and, in combination with its low molecular weight and diminished plasma protein binding, offers suitable distribution into tissue, including good bone penetration (Meißner et al., 1989; Silver, 2017; Morata and Soriano, 2019). According to several reports, fosfomycin, whether alone or in combination with other antibiotics, demonstrates the ability to penetrate and combat bacterial biofilms. This ability extends particularly to staphylococcal (Trautmann et al., 1992; Amorena et al., 1999; Monzón et al., 2003; Tang et al., 2012; Mihailescu et al., 2014), enterococcal (Alessandra et al., 2014) and extended spectrum beta-lactamase (ESBL) producing E. coli biofilms (Corvec et al., 2013; Wang et al., 2019), while also modifying the structure of their biofilm. While a good independent antibiofilm agent, with concentration – and dose-dependent activity, its effect is better shown at high doses (Tang et al., 2012). However, clinically high concentrations of the drug are probably toxic and not feasible, and the emergence of drug resistance further limits its standalone use. Therefore, combining fosfomycin with other antibiotics at recommended doses would be more practical and effective. Additionally, in conditions of high inoculum infections, such as some forms of osteomyelitis, fosfomycin’s activity may decrease (Mei et al., 2015), highlighting the necessity of combination therapy. Conveniently, reports suggest that fosfomycin can damage biofilm structure, thereby increasing the permeability of other antibiotics (Kusachi et al., 2011).
Activity against pseudomonal biofilms has also been shown in several studies, when combined with other antibiotics, including aminoglycosides and FQs (Kumon et al., 1995; Monden et al., 2002; Mikuniya et al., 2005, 2007; Cai et al., 2009; Anderson et al., 2013). In an in vitro study, fosfomycin exhibited successful inhibition levels only when used in combination with either gentamicin or ciprofloxacin. However, when administered alone, it failed to demonstrate significant inhibition (Wang et al., 2019).
It is recognized that rifampicin individually outperforms fosfomycin in inhibiting pseudomonal biofilm, but their combined administration demonstrates a higher efficacy in preventing biofilm formation (Liu et al., 2022). In fact, fosfomycin has been shown in vitro to enhance the activities of linezolid, minocycline, vancomycin and teicoplanin against MRSA as well (Tang et al., 2012). These combined treatments have proven be more effective than rifampicin combinations, offering potential therapeutic benefits and alternatives for infections related to catheters or prosthetic joints. However, it is worth noting that while fosfomycin may be used in combination with rifampicin against MRSA implant-associated infections, it cannot replace rifampicin as an antibiofilm agent (Mihailescu et al., 2014).
What makes the drug suitable for adjunctive treatment of implant associated infections is not only its ability to break up biofilms, allowing better absorption of other antibiotics (Kastoris et al., 2010), but also its presumed immunomodulatory effects (Raz, 2012) and occasional extended post-antibiotic effect (Mazzei et al., 2006). The frequency of adverse effects also appears to be low, primarily involving gastrointestinal disturbances and electrolyte imbalances, notably hypernatremia and hypokalaemia (Hashemian et al., 2019). Severe bone infections usually complicate with tissue hypoxia and formation of abscesses, protecting bacteria against some antibiotics. Fosfomycin’s increased antimicrobial effectiveness in environments with low oxygen levels and acidic pH, coupled with its ability to adequately penetrate abscess tissue, could offer advantages in managing these challenging-to-treat infections (Inouye et al., 1989; Sauermann et al., 2005; Hirakawa et al., 2018).
Although fosfomycin appears to possess the qualities necessary to be an effective component in antibiofilm therapy combinations, few clinical practice reviews or studies have been conducted in this regard, especially in the case of PJIs. A recent review of 365 patients with bone and joint infections concluded that when IV fosfomycin was used, either alone (6.3%) or in combination with another antibiotic (93.7%), 82.2% of cases were considered successfully treated (Tsegka et al., 2022), though most of these were cases of osteomyelitis, rather than PJIs. The reported toxicity level was low, and, in many cases, the IV treatment was completed by oral administration of antibiotics.
There are several ongoing prospective studies researching IV fosfomycin for the treatment of patients with osteoarticular infections (Karbysheva et al., 2019; Bodmann et al., 2021). Based on available data, IV fosfomycin may be an attractive choice for empiric and targeted first-line therapy (Vossen et al., 2020), but also presents as a viable therapeutic option in cases that fail to respond to initial treatment or when challenging-to-treat pathogens present.
2.2.1.3 Tetracyclines
The tetracyclines are a broad-spectrum, bacteriostatic class of antibiotics that function as protein synthesis inhibitors, targeting the 30S ribosome of bacteria, preventing binding of tRNA (Grossman, 2016). They are considered effective against both gram-positive and gram-negative bacteria, but within biofilm-associated infections, most evidence surrounds their use as alternative therapy for gram-positive microorganisms. Data regarding bone penetration seems to be insufficient and conflicting. Older reports suggested that drugs such as tigecycline exhibited poor bone penetration (Moenster et al., 2013), whereas the long-acting derivatives of tetracycline, doxycycline, and minocycline, have excellent tissue penetration (Klein and Cunha, 1995). Tetracyclines exhibit high binding affinity to calcium, hence a high affinity for bone mineral matrix. The impact of tetracycline, minocycline, and doxycycline on bone cell signaling and their effects on the natural progression and balance of skeletal maturation remain uncertain (Warner et al., 2022). It is known however that retention in the bone is high, even after termination of treatment. In general, it is also recognized that tetracyclines exhibit higher tissue and cell penetration than some other classes, such as the beta-lactams or aminoglycosides (Moenster et al., 2013; Wu et al., 2014).
Evidence of anti-biofilm activity within these drugs exists, albeit limited and confined to gram-positive microorganisms. In vitro studies with minocycline and tigecycline showed superior anti-biofilm activity against S. aureus when compared with vancomycin and even linezolid (Issam et al., 2007). This effect was significantly enhanced when paired with rifampicin. Anti-biofilm activity against Staphylococcus spp., including MRSA and S. epidermidis, has been consistently shown in several analyses, particularly as part of a combination regimen (Rose and Poppens, 2009; Wu et al., 2014; Rosman et al., 2021; Doub et al., 2022). This is also true for vancomycin-sensitive enterococci (VSE) and VRE, for which tigecycline has shown to be particularly useful. One study evaluated multiple antibiotics commonly used in PJIs on a biofilm model of S. aureus derived from clinical strains and found that both rifampicin and tigecycline exhibited the highest eradication activity. However, as expected, achieving this required significantly higher MBEC compared to the MIC data (Molina-Manso et al., 2013).
There are a few concerns on the drug interaction between doxycycline with rifampicin since it may decrease the serum concentration of doxycycline (Colmenero et al., 1994). This effect is not observed with minocycline, and even so, numerous cases, clinical trials, and expert recommendations suggest that the combination of doxycycline and rifampicin is an effective regimen for various infectious diseases. In vitro data also suggest that minocycline has a better anti-staphylococcal activity than doxycycline (Klein and Cunha, 1995), but clinical superiority has not been demonstrated. Also, some gram-positive pathogens seem to be resistant to doxycycline but not minocycline, as the resistance mechanisms are not associated with resistance to the whole class (Thompson and Townsend, 2011; Doub et al., 2022).
Clinical use of tetracyclines has focused mainly on suppression therapy following surgical debridement and implant retention. A few cohorts have demonstrated adequate suppression rates of infection with these agents (Pradier et al., 2018; Leijtens et al., 2019; Sandiford et al., 2020; Ceccarelli et al., 2023). Also, it should be noted that a substantial number of patients in these studies that experienced infection relapse had to suspend the suppressive antibiotic weeks or months beforehand due to adverse effects. An American study achieved a 1-year event free rate of 88% with patients on oral doxycycline or minocycline after surgical debridement and implant retention (Jang et al., 2024). The simplicity and effectiveness of minocycline or doxycycline based chronic oral antimicrobial suppression, along with their favourable bioavailability and economic sustainability, make these drugs attractive options in patients with susceptible pathogens who are ineligible for standard surgical treatment of PJIs, particularly in staphylococcal infections. Additionally, some authors adopted the 100 mg q.d. dosage, instead of the recommended b.i.d., as to reduce potential side effects, and achieved favourable results (Leijtens et al., 2019).
Tetracyclines are also indicated as alternative drugs in the acute treatment of staphylococcal PJIs, as part of a combination regimen with other agents, such as rifampicin or FQs (Osmon et al., 2013). In this regard they are usually used as secondary companion drugs when in vitro susceptibility, allergies, intolerances, or potential intolerances thwarts the use of a quinolone. Minocycline-rifampicin can be used as treatment for MRSA, as well as tigecycline-rifampicin or even minocycline-fosfomycin (Perez-Jorge et al., 2016). Tigecycline is the only agent active against MRSA with simultaneous activity against gram-negative Enterobacterales. However, it lacks activity against P. aeruginosa (Rice, 2006). It could therefore have an important use in polymicrobial infections, which are a common challenge in the diabetic or immunocompromised patient. Minocycline has also shown favourable outcome against MRSA PJIs when combined with vancomycin (Bart et al., 2020).
The high affinity of tetracyclines for the bone mineral matrix, which are retained at elevated levels even after treatment interruption, could represent an element favouring the anti-biofilm activity tetracyclines in this setting. On the other hand, they exhibit bacteriostatic properties, which could be a disadvantage, and should not be administered to pregnant women or small children (Cross et al., 2016), which represent a minority in the PJIs setting. Conversely, while these drugs are less studied for treatment of staphylococcal PJIs, they offer ease of administration through a well absorbed oral route and a favourable side effect profile.
2.2.2 Antibiotics active against gram-positive microorganisms
2.2.2.1 Fusidic acid
Fusidic acid (FA) disrupts bacterial protein synthesis through binding of elongation factor G, a GTPase coded by the fusA gene, which is chromosomally located. It has a narrow spectrum of action, being active mainly against gram-positive microorganisms, namely Staphylococci, including MRSA, Vancomycin Intermediate Staphylococcus aureus (VISA) and heterogeneous VISA, and Streptococci. Classically, it has been considered inactive against Enterococci, but recent in vivo evidence suggests it may be an option to consider (Abdelmassih et al., 2024).
FA is available in topical, oral, and IV formulations, has good tissue penetration and high bioavailability when taken orally. It is a safe and well-tolerated drug. The most common adverse effects relate to the gastrointestinal tract and serious adverse effects are rare, most often cholestasis and citopenias (Christiansen, 1999). Concerns about drug–drug interactions exist but documentation is scarce. Nevertheless, coadministration with statins should be approached cautiously due to a significant risk of rhabdomyolysis (Gupta et al., 2016; Rönnqvist et al., 2018).
Its narrow spectrum of action, high bioavailability and adequate tissue penetration make it an attractive option for PJIs treatment, particularly in the setting of emerging antimicrobial resistance. However, monotherapy with fusidic acid has been associated with spontaneous chromosomal mutations and selection of resistant Staphylococci and Streptococci clones, limiting its usefulness as a single agent. This problem has been amplified by widespread use of topical formulations for skin and ophthalmic infections. Some authors have argued for the elimination of topical FA formulations from markets to preserve this option for systemic treatment of drug resistant pathogens, which may be a reasonable approach (Howden and Grayson, 2006). A recent meta-analysis has demonstrated increasing FA resistance among S. aureus isolates worldwide, particularly for MRSA and the American and Asian continents. Europe and Oceania had the lowest rates of FA resistance, but there may be some bias associated with MIC breakpoint heterogeneity between different continents (Hajikhani et al., 2021).
Predictably, resistance to FA is higher in biofilm isolates when compared to bacteria in the planktonic state (Saginur et al., 2006). In vitro evidence is heterogeneous, and some argues against the effect of FA in monotherapy in preventing biofilm maturation (Marquès et al., 2015).
Early recommendations for treatment of PJIs advocated for FA and rifampicin combination therapy (Zimmerli et al., 2004). Combination therapy may reduce the development of resistance by reducing the frequency of spontaneous resistance mutations. In vitro evidence suggests reduced likelihood of resistance development with concentrations of fusidic acid equivalent to doses of 500 mg t.i.d and that the combination with rifampicin may decrease the risk of resistance to the latter in Methicilin-Susceptible Staphylococcus aureus (MSSA), MRSA, VISA, and hetero-VISA populations (O’Neill et al., 2001; Hung-Jen et al., 2013).
To date, clinical evidence is mostly retrospective, with small numbers of patients and variable results. It clearly favours combination therapy (Drancourt et al., 1997; Ferry et al., 2009). Still, treatment failure with rifampicin and FA combination therapy in the literature is highly variable, though results are more favourable when fusidic acid is administered thrice daily (Drancourt et al., 1997; Aboltins et al., 2007; Ferry et al., 2009; Peel et al., 2013).
Lastly, a recent RCT identified a rifampicin-FA drug interaction, leading to decreased levels of FA. The levels of the latter decreased with continuing exposure, leaving the patients in effective rifampicin monotherapy, with increased risk of antibiotic resistance (Pushkin et al., 2016).
In vitro evidence for alternative combinations includes fosfomycin, tetracyclines, linezolid and daptomycin. Combination with fosfomycin has demonstrated significant synergism among S. aureus strains (Yu et al., 2010; Tang et al., 2012). Tetracyclines may also be an option, considering in vitro findings of minocycline and FA synergism (Wu et al., 2012). Both static and dynamic in vitro assessments support the association of FA with linezolid or daptomycin (Wafi et al., 2018). Linezolid and fusidic acid may even be applicable to Enterococcal infections (Ermertcan et al., 2010). However, clinical validation is required and there may be a legitimate concern for enhanced haematological toxicity when combining these two agents.
In sum, FA may be an interesting option for gram-positive PJIs, but its use should consider emerging resistance patterns and drug–drug interactions. Its association with rifampicin is poorly established and clinical evidence is scarce. Evaluating alternative combinations may be the path to rescuing this drug from present setbacks.
2.2.2.2 Glycopeptides (vancomycin and teicoplanin)
Glycopeptides inhibit the synthesis of peptidoglycan, thus disrupting the cell wall formation of gram-positive microorganisms. This group includes vancomycin, which can be administered orally and intravenously, and teicoplanin, which can be administered both by IV infusion and intramuscular injection.
Vancomycin has long been regarded as a staple option for the treatment of PJIs in the setting of antibiotic resistance, particularly in MRSA and enterococcal infections (Osmon et al., 2013). Due to lack of absorption in the gastrointestinal tract, it must be infused for the treatment of PJIs. It is irritating to tissues and may cause thrombophlebitis. Infusions should be slowly paced to avoid anaphylactoid reaction and therapeutic drug monitoring (TDM) is recommended, due to considerable nephrotoxicity and ototoxicity, as is the avoidance of concurrent administration of nephrotoxic or ototoxic drugs. Ideal trough levels for the treatment of PJIs have been extrapolated from other complicated infections (15–20 mg/L), as there are no RCTs to guide clinical practice so far.
In vitro evidence on the combination with rifampicin for biofilm elimination is conflicting and there is some concern regarding the risk of rifampicin resistance development with this combination with supporting clinical evidence, though the latter is not specific to PJIs (Saginur et al., 2006; Hung-Jen et al., 2013; Tremblay et al., 2013). Alternatively, the combination of vancomycin or other peptide antibiotics with fosfomycin may be worthy of attention, considering favourable in vitro and in vivo evidence (Tang et al., 2011, 2012; Shi et al., 2014; Yu et al., 2020). However, vancomycin’s safety issues and complex PK/PD profile, requiring regular TDM and close surveillance of side effects make this option less than ideal, particularly for maintenance therapy.
Teicoplanin could be a reasonable alternative due to the possibility of transitioning patients to outpatient parenteral antibiotic therapy (OPAT). Both administration and TDM are less frequent, and it is slightly less nephrotoxic. However, available evidence and clinical experience is scarce (López Sánchez et al., 2016) and adverse effects are relevant and frequent, even with modified routes of administration, particularly in patients with previous adverse reactions to vancomycin (Peeters et al., 2016; Kim et al., 2019).
The newer lipopeptides and lipoglycopeptides are attractive alternatives to classic glycopeptides due to their long half-life, diminished nephrotoxicity, and infrequent side effects. Despite requiring IV administration, dosing intervals enable transition to OPAT. Existing evidence, though scarce, supports their bactericidal and antibiofilm effects against gram-positive cocci, such as Staphylococci, including MRSA, VISA and Vancomycin Resistant Staphylococcus aureus (VRSA), Streptococci and Enterococci, including VRE, as well as their applicability to the treatment of PJIs.
2.2.2.3 Lipopeptides (daptomycin)
Among the newer lipopeptides and lipoglycopeptides, most research has been produced on daptomycin. Unlike other antibiotics that target the cell wall, which require active cell division to be effective (such as beta-lactams), daptomycin causes rapid depolarization of membrane potential and seems to be active even against microorganisms in a stationary-phase, rendering it an interesting option for infections where biofilm is relevant (Mascio et al., 2007). It is administered intravenously and has a short half-life. Tissue penetration is good, but it is important to recall that due to its inactivation by lung surfactant, daptomycin is not an option when treating patients with concomitant pneumonia.
Toxicity and severe side effects are less frequent when compared to glycopeptides. Eosinophilic pneumonitis, albeit rare and poorly understood, has been observed among patients treated with daptomycin. Myolysis is a more frequent side effect, particularly in the setting of renal dysfunction, prolonged treatment times or coadministration of statins (Ivor et al., 2012; Chang et al., 2017; Telles et al., 2019; Carli et al., 2020). In these cases, weekly creatine phosphokinase monitoring is recommended. Lastly, hepatotoxicity is rare but should be considered.
Daptomycin’s bactericidal effect has been shown to be superior to vancomycin in S. aureus models (Smith et al., 2009). The same is true for both its bactericidal and antibiofilm effects in S. epidermidis in vitro models. It may even have a similar or superior antibiofilm effect when compared to rifampicin, as shown in in vitro S. epidermidis biofilm models (Leite et al., 2011).
Clinical experience with daptomycin in PJIs started off on the wrong foot (Rao and Regalla, 2006) but the poor results of this initial study have since been attributed to insufficient dosing. More recent trials, including one RCT and other clinical trials of both retrospective and prospective design have established the efficacy and safety of doses between 6 and 9 mg/kg/day in the treatment of PJIs.
Clinical trials so far have assessed daptomycin monotherapy. However, combinations with rifampicin and clarithromycin seem to produce relevant bactericidal and antibiofilm effects in S. aureus in vitro models (Parra-Ruiz et al., 2010; Snyder et al., 2014). A combination with fosfomycin also seems to effectively target vancomycin-resistant Enterococci in vitro (Tilman et al., 2015)This combination needs clinical validation, but there is some evidence to its effectiveness in enterococcal and staphylococcal bacteraemia (Pujol et al., 2020; Tseng et al., 2023) as well as anecdotal evidence as to its use in PJIs (Luengo-Alonso et al., 2018).
2.2.2.4 Lipoglycopeptides (oritavancin, dalbavancin, and telavancin)
Lipoglycopeptides inhibit bacterial cell wall synthesis and disrupt cell membrane integrity. They are available in IV formulations and oritavancin and dalbavancin have exceptionally long half-lives, making them extremely attractive for OPAT. Adequate tissue penetration and a favourable safety profile make them an interesting option for treating PJIs due to multidrug-resistant (MDR) gram-positive microorganisms. Although significantly less frequent, side effects are like those of vancomycin, including the possibility of anaphylactoid reaction and nephrotoxicity. Dalbavancin has also been associated with hepatotoxicity.
There is few evidence regarding oritavancin use in PJIs. However, biological plausibility favours this approach. In vitro evidence demonstrates bactericidal and antibiofilm activity in S. aureus models, including MRSA and VRSA (Yan et al., 2018) as well as possible synergism with rifampicin, gentamicin, and linezolid (Lin et al., 2014; Yan et al., 2018).
A recent review highlights the possible role of oritavancin in device-related infections, cataloguing clinical evidence with a total of 29 PJIs cases (Lupia et al., 2023). Notwithstanding, to date, there have been no RCTs and despite it being clear that sequential dosing is necessary, the ideal regimen in PJIs remains unclear, thus undermining the applicability of oritavancin in a clinical context.
Dalbavancin is another option to consider with in vitro activity against Staphylococci and Streptococci and synergism with rifampicin. Both seem to be superior to the effects observed with vancomycin (Di Pilato et al., 2019; Jacob et al., 2021). Off-label use of dalbavancin to treat PJIs is being increasingly adopted (Wunsch et al., 2019) and clinical experience with two doses of 1,500 mg seems promising (Simon et al., 2022; Dimopoulou et al., 2023; Doub et al., 2023). However, data regarding the safety and need for long term therapy with dalbavancin is lacking.
Telavancin is the least established lipoglycopeptide in the treatment of PJIs. While there is some evidence as to its bactericidal and antibiofilm activity in both in vitro and in vivo models (Chan et al., 2015) as well as possible synergism with rifampicin (Jahanbakhsh et al., 2018), clinical data of telavancin’s applicability in bone and joint infections is scarce and mostly retrospective (Harting et al., 2017; Reilly et al., 2020; Sims et al., 2021), which may be particularly relevant in the setting of PJIs due to long treatment times and patient characteristics.
2.2.3 Antibiotics active against gram-negative microorganisms
2.2.3.1 Colistin
The activity of polymyxin E (i.e., colistin) against gram-negative microorganisms’ biofilm has been shown in multiple in vitro and in vivo models. Most of these studies however reviewed colistin’s activity on Pseudomonas aeruginosa biofilms (Haagensen et al., 2007; Pamp et al., 2008; Cai et al., 2010; Herrmann et al., 2010; Wang et al., 2011; Chiang et al., 2012; Wang et al., 2012; Brochmann et al., 2013; Chambers and Sauer, 2013; Lora-Tamayo et al., 2014). Still, activity against some Enterobacteriaceae such as E. coli has also been demonstrated (Klinger-Strobel et al., 2017). Instead of targeting the metabolically active outer cell layer of the biofilm, as other antibiotics such as the fluroquinolones and aminoglycosides do, colistin seems to target the inner, less metabolically active layer of the structure (Klausen et al., 2003; Haagensen et al., 2007; Pamp et al., 2008).
As referred previously, virtually all antibiotics are less active against biofilm-coated bacteria than against their planktonic counterpart. To an extent, this is also true for polymyxins against gram-negative bacilli. One in vitro model revealed a MBIC concentration 4–8 times higher than the usual MIC of 2 mg/L (Wang et al., 2011), which was also confirmed in an in vivo mice model. However, achieving the necessary plasma colistin concentrations through IV administration of colistimethate sodium (CMS), the prodrug of colistin, is deemed unlikely (Nation and Li, 2009; Plachouras et al., 2009; Garonzik et al., 2011). The concerns that already exist in achieving sufficiently high colistin concentration for planktonic infections can therefore be extended to biofilm-associated infections. Sub-inhibitory concentrations are of course related to therapeutic failure and resistance selection (Klausen et al., 2003; Plachouras et al., 2009; Lora-Tamayo et al., 2014). In consideration of these PK/PD problems, current recommendations suggest the association of high dose CMS with a second antimicrobial (Nation and Li, 2009; Bergen et al., 2011), on the basis that each drug could work together to target distinct parts of the bacterial population within the biofilm architecture. Also, it is suggested that colistin’s mode of action synergizes with other antibiotics, as it acts on the bacterial external membrane, enhancing its own uptake along with other compounds (Hancock and Wong, 1984; Hancock, 1997; Bergen et al., 2011). Combined treatments intend to override resistance to colistin or other antimicrobials, while maintaining adequate doses and reducing toxicity associated with colistin. Previous studies have shown this synergy against gram-negative pathogens, with an associated reduced hospital mortality (Batirel et al., 2014).
Multiple studies have shown a combined effect of colistin-carbapenems on biofilm structures of gram-negative bacteria, such as P. aeruginosa or A. baumannii, particularly colistin-meropenem dual therapy (Copur et al., 2022; Taşkın Kafa and Hasbek, 2022) but also colistin-doripenem (Lora-Tamayo et al., 2014).
Limited clinical experience exists for colistin’s use in treating biofilm-related infections, as it is usually regarded as last-line therapy. Colistin has been administered via various methods, including aerosolized and IV routes, as well as locally through intraventricular administration or in cement spacers for CNS or prosthetic joint infections. A lack of oral option for prolonged treatment is a downside of the drug. Moreover, optimal dosage and comparative efficacy between colistin alone or in combination have not been adequately assessed (Lora-Tamayo et al., 2019). Most current knowledge aspires from experience regarding cystic fibrosis patients, where colistin is used as first line treatment against P. aeruginosa infections, as well as salvage therapy for MDR strains (Döring et al., 2000; Heijerman et al., 2009). Most clinical experience refers to the use of nebulized colistin, with minimal information on IV usage (Conway et al., 1997; Ledson et al., 1998; Döring et al., 2000; Heijerman et al., 2009).
In the orthopaedic device infection setting, colistin has also been used as last-line treatment, but its true efficacy and potential benefits of combined therapy are yet to be rigorously evaluated. Previous studies indicated poor bone diffusion with this drug (Falagas and Kasiakou, 2005), leading to its specific administration in local beads and cement spacers (Rosenthal et al., 1976; Murray, 1984). However, clinical experience with IV CMS is scarce and mainly approaches its use in last-line therapy of complicated bone and joint infections caused by MDR gram-negative microorganisms. A retrospective cohort of nineteen patients evaluated the use of colistin for challenging osteoarticular infections caused by MDR/ extensively drug resistant (XDR) pathogens. Twelve of these, were implant-associated. IV colistin was used alone as salvage therapy in 90% of cases. A reported 74% of patients experienced clinical remission, however the implant-associated group experienced a 42% rate of treatment failure (Valour et al., 2012). Another review of twenty-two cases of osteoarticular infections caused by XDR P. aeruginosa, of which fifteen involved orthopaedic devices, concluded that 80% of patients achieved a cure rate when colistin was associated with a β-lactam, compared to 29% in the monotherapy group. Interestingly, this combination was deemed superior when the β-lactam was administered continuously, rather than in bolus (Lora-Tamayo et al., 2019).
Concern around colistin exists considering the frequency of its main side effects. Reviews estimate that up to 60% of patients can experience some degree of nephrotoxicity, which usually manifest in the first 2 weeks of administration (Shahbazi and Dashti-Khavidaki, 2015). Neurotoxicity is also a known but rare side effect, with an estimate of 6% of patients experiencing paraesthesia, vertigo, visual disturbances, hallucinations, mental confusion, or seizures. It is important to verify patient risk factors for these adverse events and keep close monitoring as to avoid serious repercussions.
Colistin shows promise in treating orthopaedic device-related infections caused by MDR or XDR gram-negative bacteria, particularly when used alongside other antimicrobials. When dealing with P. aeruginosa exhibiting resistance or intermediate susceptibility to β-lactams, combining colistin with a β-lactam can enhance infection outcomes. Nonetheless, further research is necessary to validate these findings and investigate alternative treatment combinations.
3 Discussion
The biofilm principle has modified the way we view and treat device-related infections. Modern treatment of these infections calls for a diverse arsenal of antibiotic compounds that, not only provide unequivocal antibiofilm properties, but take into account multiple factors that can tailor to patients’ individual needs. As previously stated, rifampicin and FQs have been the most investigated drugs in this setting, particularly for PJIs. However, as demonstrated beforehand, there are numerous other classes and agents that should be considered for treatment and included in major comparative studies.
Considering the rapid formation of biofilm in PJIs, the initial choice of antibiotic regimen must provide unequivocal antibiofilm ability to penetrate the biofilm matrix and eradicate the bacteria embedded in it. Adequate penetration into this structure is crucial to ensure the antimicrobial’s effectiveness and potentially enhance the effects of other antimicrobials, providing synergistic characteristics.
Treatment for PJIs requires optimal antibiotic coverage, particularly during the initial postoperative weeks. Despite the reduction in bacterial load post-surgery, administering high-dose antibiotics and optimizing PK/PD parameters yields improved infection control (Guilhaumou et al., 2019) and decreases the likelihood of bacterial resistance emergence. To mitigate the selection of resistant strains and consequent treatment failure, the initial treatment regimen should incorporate bacterial load-independent drugs with a minimal risk of resistance development – such as β-lactams, vancomycin, or daptomycin (Vavasseur and Zeller, 2022). IV administration facilitates the use of higher doses, circumvents the intestinal and hepatic first-pass effect that hinders the bioavailability of certain drugs (Zeller et al., 2021), and avoids malabsorption issues related to gastrointestinal intolerance and some interactions with medications. How long the initial IV regimen should be administered remains controversial, with recent evidence pointing to similar efficacy for shorter IV antibiotic courses (Ho Kwong et al., 2019).
Eradicating biofilm requires higher and continued antibiotic doses, and even this often does not successfully eradicate biofilm infections (Liu et al., 2022). Conventional antibiotic therapy against these infections is particularly challenging since only doses that are sublethal to the biofilm can be delivered safely to patient. Current literature strongly suggests that elimination of biofilms is better accomplished by using combinations of antibiotics, rather than single therapy (Valerius et al., 1991; Döring et al., 2004; Høiby et al., 2005; Pamp et al., 2008; Gbejuade et al., 2015; Taha et al., 2018). Besides increasing efficacy and providing good coverage for empiric therapy, this strategy reduces drug doses, toxicity issues and minimizes emergence of resistances (Neut et al., 2006; Hagihara et al., 2012; Worthington and Melander, 2013; Taha et al., 2018).
Current research on biofilm formation and elimination is scarce, technically complex and hampered by a heterogenous use of techniques and definitions. The behaviour of biofilm and the natural history of infection vary greatly among different pathogens, further complicating experimental models. Most studies that assess antibiofilm activity rely on in vitro models, which can be divided into three categories: static, dynamic and microcosm. Static models are conventional plate-based assessments, where metabolites and other products accumulate rather than flow. These are cost-effective and allow for multiple simultaneous tests but fall short of mimicking real-life conditions. Dynamic models are closer to biological conditions since they allow for the addition of nutrients and clearance of waste products. Microcosms build on the latter, adding environmental modelling to approximate in situ conditions (Roy et al., 2018). Nonetheless, conventional microbiological assessments do not necessarily correlate with clinical outcomes and clinical validation of in vitro models or animal studies is still a work in progress. Accordingly, the complexity and heterogeneity of biofilm assessment techniques has precluded its inclusion in routine laboratory testing.
Given the rise of antimicrobial resistances, long treatment times and subsequent tolerance issues, as well as the particularities of those with PJIs, namely increasing age, frequent polypharmacy, with the added difficulty of drug–drug interactions, the available antimicrobial arsenal should be as diverse as possible to facilitate a tailored and practical intervention. As the prevalence of MDR microorganisms continues to escalate, along with the noted rise in resistance levels to traditional antibiofilm drugs, like rifampicin and FQs (Benito et al., 2016; Eriksson et al., 2023), there is a growing demand for alternative therapies. However, due to the predominance of in vitro studies and the absence of large-scale comparative research, formulating practical clinical recommendations for alternative drugs has proven particularly challenging.
Another essential consideration is ensuring adequate tissue penetration and, in the case of PJIs treatment, adequate bone absorption. Additionally, longer half-lives offer the advantage of reduced dosing frequency. Access to oral formulations with good bioavailability is also a key factor, since many of these infections demand prolonged treatments, which can lead to unnecessary extended hospitalizations. Solid oral options can hence reduce the risk of inpatient complications and provide a more convenient approach.
Our preferred treatment of choice for gram-positive PJIs includes a short course of IV beta-lactams followed by oral step-down therapy combining an antibiofilm agent, preferably rifampicin. In the case of gram-negative PJIs we prefer the FQs, particularly ciprofloxacin. Whenever possible, we favour the combination of rifampicin and fluoroquinolones for the definitive treatment of gram-positive PJIs.
Nonetheless, possible combinations are multiple and the search for synergy within antibiotics is important. A summary of the antibiofilm activity of reviewed antibiotics can be found in Table 1. Conventional step-down options include quinolones, cotrimoxazole, doxycycline, clindamycin, minocycline and oxazolidinones, the latter being the least preferred due to relevant myelotoxicity and thus reserved for MDR infections where other options are not viable. Despite being a first-line option in the early phase of PJIs, step-down with beta-lactams has often been regarded with suspicion due to their time-dependent bactericidal activity and low bioavailability when administered per os. Long term courses of IV antibiotics are reserved for when resistance patterns preclude other options.
There is still insufficient evidence as to how long the initial IV regimen should be administered. The trend is to shorten IV therapy as much as possible to avoid catheter-associated complications, shorten inpatient stay, lower costs, and enhance patient comfort. The results of the OVIVA trial have provided insight on the noninferiority of oral therapy when used in the first 6 weeks for complex orthopaedic infection, as assessed by 1-year treatment failure (Ho Kwong et al., 2019). IV antibiotics can likely be transitioned to an oral regimen early on, provided an antibiotic with effective antibiofilm properties and sufficient bioavailability is used.
Regarding total duration of antibiotic therapy, little consensus exists, for it appears to be as variable as 2 – 12 weeks, depending on severity, location, procedure, or comorbidities. Bernard et al. recently described that 6 weeks was not non-inferior to 12 weeks on patients with microbiologically confirmed prosthetic joint infections that were managed with standard and appropriate surgical procedures (Bernard et al., 2021). Numerous questions remain regarding the optimal duration for PJIs; it must be tailored to the surgical approach (Vavasseur and Zeller, 2022). The results from the SOLARIO trial might shed some light on the matter (Dudareva et al., 2019).
In conclusion, biofilm-related infections pose a diagnostic and treatment challenge. An increase in the number of these infections is anticipated, paralleling the projected rise in prostheses implantation due to a continuously aging population. Antimicrobial tolerance and the constant emergence of adaptive resistance mechanisms in bacteria embedded in this complex matrix calls for a wide selection of effective biofilm-active antibiotics. Applying biofilm models for susceptibility testing in routine laboratory settings lacks clinical utility. However, in the future, it would be ideal if antibiotic selection could be tailored based on the specific characteristics of the biofilm, including the bacterial strain involved and the way the biofilm integrates into the host. While rifampicin and fluoroquinolones have historically been key treatments for biofilms, other effective drugs exist, highlighting the need for large-scale comparative trials. A comprehensive and flexible approach, incorporating both established and emerging therapies, is fundamental for effective management and improved patient outcomes.
Author contributions
LF: Conceptualization, Data curation, Formal analysis, Funding acquisition, Investigation, Methodology, Project administration, Resources, Software, Supervision, Validation, Visualization, Writing – original draft, Writing – review & editing. EP: Conceptualization, Data curation, Formal analysis, Funding acquisition, Investigation, Methodology, Project administration, Resources, Software, Supervision, Validation, Visualization, Writing – original draft, Writing – review & editing. DN: Investigation, Writing – original draft. FF: Investigation, Writing – original draft. RS: Writing – review & editing. MA: Supervision, Validation, Writing – review & editing, Writing – original draft.
Funding
The author(s) declare that no financial support was received for the research, authorship, and/or publication of this article.
Acknowledgments
The authors would like to thank Gonçalo M. Oliveira for providing us with Figure 1.
Conflict of interest
The authors declare that the research was conducted in the absence of any commercial or financial relationships that could be construed as a potential conflict of interest.
Publisher’s note
All claims expressed in this article are solely those of the authors and do not necessarily represent those of their affiliated organizations, or those of the publisher, the editors and the reviewers. Any product that may be evaluated in this article, or claim that may be made by its manufacturer, is not guaranteed or endorsed by the publisher.
References
Abdelmassih, M., Ismail, M., Kashef, M., and Essam, T. (2024). Repurposing fusidic acid as an antimicrobial against enterococci with a low probability of resistance development. Int. Microbiol., 1–13. doi: 10.1007/s10123-024-00506-w
Aboltins, C. A., Page, M. A., Buising, K., Jenney, A., Daffy, J., Choong, P., et al. (2007). Treatment of staphylococcal prosthetic joint infections with debridement, prosthesis retention and oral rifampicin and fusidic acid. Clin. Microbiol. Infect. 13, 586–591. doi: 10.1111/j.1469-0691.2007.01691.x
Ahmed, S., Begum, F., Kayani, B., and Haddad, F. (2019). Risk factors, diagnosis and management of prosthetic joint infection after total hip arthroplasty. Expert Rev. Med. Devices 16, 1063–1070. doi: 10.1080/17434440.2019.1696673
Albano, M., Karau, M. J., Greenwood-Quaintance, K. E., Osmon, D. R., Oravec, C. P., Berry, D. J., et al. (2021). In vitro activity of rifampin, Rifabutin, and Rifapentine against enterococci and streptococci from Periprosthetic joint infection. Microbiol. Spectr. 9:e0007121. doi: 10.1128/Spectrum.00071-21
Alessandra, O., Ulrika, F. T., Maryka, M. E., Safaa, J., Bertrand, B., and Andrej, T. (2014). Activities of Fosfomycin and rifampin on planktonic and adherent Enterococcus faecalis strains in an experimental foreign-body infection model. Antimicrob. Agents Chemother. 58, 1284–1293. doi: 10.1128/AAC.02583-12
Amorena, B., Gracia, E., Monzón, M., Leiva, J., Oteiza, C., Pérez, M., et al. (1999). Antibiotic susceptibility assay for Staphylococcus aureus in biofilms developed in vitro. J. Antimicrob. Chemother. 44, 43–55. doi: 10.1093/jac/44.1.43
Anderson, G. G., Kenney, T. F., MacLeod, D. L., Henig, N. R., and O’Toole, G. A. (2013). Eradication of Pseudomonas aeruginosa biofilms on cultured airway cells by a fosfomycin/tobramycin antibiotic combination. Pathog. Dis. 67, 39–45. doi: 10.1111/2049-632X.12015
Aspinall, S. L., Good, C. B., Jiang, R., McCarren, M., Dong, D., and Cunningham, F. E. (2009). Severe Dysglycemia with the fluoroquinolones: a class effect? Clin. Infect. Dis. 49, 402–408. doi: 10.1086/600294
Barberán, J., Aguilar, L., Giménez, M.-J., Carroquino, G., Granizo, J.-J., and Prieto, J. (2008). Levofloxacin plus rifampicin conservative treatment of 25 early staphylococcal infections of osteosynthetic devices for rigid internal fixation. Int. J. Antimicrob. Agents 32, 154–157. doi: 10.1016/j.ijantimicag.2008.03.003
Bart, G., Zeller, V., Kerroumi, Y., Heym, B., Meyssonnier, V., Desplaces, N., et al. (2020). Minocycline combined with vancomycin for the treatment of methicillin-resistant coagulase-negative staphylococcal prosthetic joint infection managed with exchange arthroplasty. J. Bone Jt. Infect. 5, 110–117. doi: 10.7150/jbji.43254
Batirel, A., Balkan, I. I., Karabay, O., Agalar, C., Akalin, S., Alici, O., et al. (2014). Comparison of colistin–carbapenem, colistin–sulbactam, and colistin plus other antibacterial agents for the treatment of extremely drug-resistant Acinetobacter baumannii bloodstream infections. Eur. J. Clin. Microbiol. Infect. Dis. 33, 1311–1322. doi: 10.1007/s10096-014-2070-6
Beldman, M., Löwik, C., Soriano, A., Albiach, L., Zijlstra, W. P., Knobben, B. A. S., et al. (2021). If, when, and how to use rifampin in acute staphylococcal Periprosthetic joint infections, a multicentre observational study. Clin. Infect. Dis. 73, 1634–1641. doi: 10.1093/cid/ciab426
Benito, N., Franco, M., Ribera, A., Soriano, A., Rodriguez-Pardo, D., Sorlí, L., et al. (2016). Time trends in the aetiology of prosthetic joint infections: a multicentre cohort study. Clin. Microbiol. Infect. 22, e1–732.e8. doi: 10.1016/j.cmi.2016.05.004
Berdal, J.-E., Skråmm, I., Mowinckel, P., Gulbrandsen, P., and Bjørnholt, J. (2005). V: use of rifampicin and ciprofloxacin combination therapy after surgical debridement in the treatment of early manifestation prosthetic joint infections. Clin. Microbiol. Infect. 11, 843–845. doi: 10.1111/j.1469-0691.2005.01230.x
Bergen, P., Forrest, A., Bulitta, J., Tsuji, B., Sidjabat, H., Paterson, D., et al. (2011). Clinically relevant plasma concentrations of Colistin in combination with imipenem enhance Pharmacodynamic activity against multidrug-resistant Pseudomonas aeruginosa at multiple Inocula. Antimicrob. Agents Chemother. 55, 5134–5142. doi: 10.1128/AAC.05028-11
Bernard, L., Arvieux, C., Brunschweiler, B., Touchais, S., Ansart, S., Bru, J.-P., et al. (2021). Antibiotic therapy for 6 or 12 weeks for prosthetic joint infection. N. Engl. J. Med. 384, 1991–2001. doi: 10.1056/NEJMoa2020198
Bidell, M. R., and Lodise, T. P. (2016). T.P.: fluoroquinolone-associated tendinopathy: does levofloxacin pose the greatest risk? Pharmacotherapy: the journal of human pharmacology and drug. Therapy 36, 679–693. doi: 10.1002/phar.1761
Blaser, J., Vergères, P., Widmer, A. F., and Zimmerli, W. (1995). In vivo verification of in vitro model of antibiotic treatment of device-related infection. Antimicrob. Agents Chemother. 39, 1134–1139. doi: 10.1128/aac.39.5.1134
Bodmann, K. F., Venditti, M., Bal, A., Vossen, M., Dimopoulos, G., and Litty, F. A. (2021). Intravenous (IV) fosfomycin for the treatment of severely infected patients (FORTRESS): an update from a European non-interventional and prospective clinical study: European Congress of Clinical Microbiology and Infectious Diseases (ECCMID). online, poster number 00475.
Brochmann, R., Toft, A., Ciofu, O., Briales, A., Kolpen, M., Hempel, C., et al. (2013). Bactericidal effect of colistin on planktonic Pseudomonas aeruginosa is independent of hydroxyl radical formation. Int. J. Antimicrob. Agents 43, 140–147. doi: 10.1016/j.ijantimicag.2013.10.015
Brown, J., Wing, K., Leyrat, C., Mansfield, K., Wong, A., Galwey, N., et al. (2023). Association between fluoroquinolone use and hospitalization with aortic aneurysm or aortic dissection. JAMA Cardiol. 8, 865–870. doi: 10.1001/jamacardio.2023.2418
Cai, Y., Fan, Y., Wang, R., An, M.-M., and Liang, B.-B. (2009). Synergistic effects of aminoglycosides and fosfomycin on Pseudomonas aeruginosa in vitro and biofilm infections in a rat model. J. Antimicrob. Chemother. 64, 563–566. doi: 10.1093/jac/dkp224
Cai, Y., Li, R., Liang, B., Bai, N., Liu, Y., and Wang, R. (2010). In vitro antimicrobial activity and mutant prevention concentration of Colistin against Acinetobacter baumannii. Antimicrob. Agents Chemother. 54, 3998–3999. doi: 10.1128/AAC.00264-10
Carlet, J., and Mainardi, J.-L. (2012). Antibacterial agents: back to the future? Can we live with only colistin, co-trimoxazole and fosfomycin? Clin. Microbiol. Infect. 18, 1–3. doi: 10.1111/j.1469-0691.2011.03702.x
Carli, A., Miller, A., Kapadia, M., Chiu, Y.-F., Westrich, G., Brause, B., et al. (2020). Assessing the role of Daptomycin as antibiotic therapy for staphylococcal prosthetic joint infection. J. Bone Jt. Infect. 5, 82–88. doi: 10.7150/jbji.41278
Ceccarelli, G., Perciballi, B., Russo, A., Martini, P., Marchetti, F., Rivano Capparuccia, M., et al. (2023). Chronic suppressive antibiotic treatment for staphylococcal bone and joint implant–related infections. Antibiotics 12:937. doi: 10.3390/antibiotics12050937
Chambers, J., and Sauer, K. (2013). The MerR-like regulator BrlR impairs Pseudomonas aeruginosa biofilm tolerance to Colistin by repressing PhoPQ. J. Bacteriol. 195, 4678–4688. doi: 10.1128/JB.00834-13
Chan, C., Hardin, T. C., and Smart, J. I. (2015). A review of telavancin activity in in vitro biofilms and animal models of biofilm-associated infections. Future Microbiol. 10, 1325–1338. doi: 10.2217/fmb.15.53
Chang, Y. J., Lee, M., Lee, C.-H., Lin, P. C., and Kuo, F. C. (2017). F.-C.: Daptomycin treatment in patients with resistant staphylococcal periprosthetic joint infection. BMC Infect. Dis. 17:736. doi: 10.1186/s12879-017-2842-6
Chiang, W.-C., Pamp, S., Nilsson, M., Givskov, M., and Tolker-Nielsen, T. (2012). The metabolically active subpopulation in Pseudomonas aeruginosa biofilms survives exposure to membrane-targeting antimicrobials via distinct molecular mechanisms. FEMS Immunol. Med. Microbiol. 65, 245–256. doi: 10.1111/j.1574-695X.2012.00929.x
Christiansen, K. (1999). Fusidic acid adverse drug reactions. Int. J. Antimicrob. Agents 12, S3–S9. doi: 10.1016/s0924-8579(98)00068-5
Colmenero, J. D., Fernández-Gallardo, L. C., Agúndez, J. A., Sedeño, J., Benítez, J., and Valverde, E. (1994). Possible implications of doxycycline-rifampin interaction for treatment of brucellosis. Antimicrob. Agents Chemother. 38, 2798–2802. doi: 10.1128/AAC.38.12.2798
Conway, S. P., Pond, M. N., Watson, A., Etherington, C., Robey, H. L., and Goldman, M. H. (1997). Intravenous colistin sulphomethate in acute respiratory exacerbations in adult patients with cystic fibrosis. Thorax 52, 987–993. doi: 10.1136/thx.52.11.987
Copur, B., Dosler, S., Aktaş, Z., Basaran, S., Yavuz, S., Cagatay, A., et al. (2022). In vitro activities of antibiotic combinations against mature biofilms of ventilator-associated pneumonia isolates. Future Microbiol. 17, 1027–1042. doi: 10.2217/fmb-2021-0305
Corvec, S., Furustrand Tafin, U., Betrisey, B., Borens, O., and Trampuz, A. (2013). Activities of Fosfomycin, Tigecycline, Colistin, and gentamicin against extended-Spectrum- -lactamase-producing Escherichia coli in a foreign-body infection model. Antimicrob. Agents Chemother. 57, 1421–1427. doi: 10.1128/AAC.01718-12
Cross, R., Ling, C., Day, N. P. J., McGready, R., and Paris, D. H. (2016). Revisiting doxycycline in pregnancy and early childhood – time to rebuild its reputation? Expert Opin. Drug Saf. 15, 367–382. doi: 10.1517/14740338.2016.1133584
Di Pilato, V., Ceccherini, F., Sennati, S., D’Agostino, F., Arena, F., D’Atanasio, N., et al. (2019). In vitro time-kill kinetics of dalbavancin against Staphylococcus spp. biofilms over prolonged exposure times. Diagn. Microbiol. Infect. Dis. 96:114901. doi: 10.1016/j.diagmicrobio.2019.114901
Dimopoulou, D., Mantadakis, E., Koutserimpas, C., and Samonis, G. (2023). A narrative review on the role of Dalbavancin in the treatment of bone and joint infections. Antibiotics 12:1492. doi: 10.3390/antibiotics12101492
Döring, G., Conway, S. P., Heijerman, H. G., Hodson, M. E., Høiby, N., Smyth, A., et al. (2000). Antibiotic therapy against Pseudomonas aeruginosa in cystic fibrosis: a European consensus. Eur. Respir. J. 16, 749–767. doi: 10.1034/j.1399-3003.2000.16d30.x
Döring, G., and Hoiby, N.Consensus Study Group (2004). Early intervention and prevention of lung disease in cystic fibrosis: a European consensus. J. Cyst. Fibros. 3, 67–91. doi: 10.1016/j.jcf.2004.03.008
Doub, J., Alkayali, T., Amoroso, A., Nandi, S., and Talwani, R. (2023). Effective use of a two-dose regimen of dalbavancin to treat prosthetic joint infections and spinal hardware infections. Eur. J. Orthop. Surg. Traumatol. 33, 1–5. doi: 10.1007/s00590-023-03609-8
Doub, J. B., Nandi, S., and Putnam, N. (2022). Retention of minocycline susceptibility when gram-positive Periprosthetic joint infection isolates are non-susceptible to doxycycline. Infect. Dis. Rep. 14, 641–645. doi: 10.3390/idr14050069
Drancourt, M., Stein, A., Argenson, J. N., Roiron, R., Groulier, P., and Raoult, D. (1997). Oral treatment of Staphylococcus spp. infected orthopaedic implants with fusidic acid or ofloxacin in combination with rifampicin. J. Antimicrob. Chemother. 39, 235–240. doi: 10.1093/jac/39.2.235
Dudareva, M., Kümin, M., Vach, W., Kaier, K., Ferguson, J., McNally, M., et al. (2019). Short or long antibiotic regimes in Orthopaedics (SOLARIO): a randomised controlled open-label non-inferiority trial of duration of systemic antibiotics in adults with orthopaedic infection treated operatively with local antibiotic therapy. Trials 20:693. doi: 10.1186/s13063-019-3832-3
Eriksson, H., Lazarinis, S., Järhult, J., and Hailer, N. (2023). Early staphylococcal Periprosthetic joint infection (PJI) treated with debridement, antibiotics, and implant retention (DAIR): inferior outcomes in patients with staphylococci resistant to rifampicin. Antibiotics 12:1589. doi: 10.3390/antibiotics12111589
Ermertcan, S., Hoşgör-Limoncu, M., Taşlı, H., Çilli, F., and Coşar, G. (2010). In vitro activity of linezolid in combination with vancomycin, teicoplanin, fusidic acid, and ciprofloxacin against gram-positive pathogens. Turkiye Klinikleri J. Med. Sci. 30, 59–64.
Falagas, M. E., and Kasiakou, S. K. (2005). Colistin: the revival of polymyxins for the management of multidrug-resistant gram-negative bacterial infections. Clin. Infect. Dis. 40, 1333–1341. doi: 10.1086/429323
Ferry, T., Uçkay, I., Vaudaux, P., Francois, P., Schrenzel, J., Harbarth, S., et al. (2009). Risk factors for treatment failure in orthopedic device-related methicillin-resistant Staphylococcus aureus infection. Eur. J. Clin. Microbiol. Infect. Dis. 29, 171–180. doi: 10.1007/s10096-009-0837-y
Forrest, G. N., and Tamura, K. (2010). Rifampin combination therapy for nonmycobacterial infections. Clin. Microbiol. Rev. 23, 14–34. doi: 10.1128/CMR.00034-09
Garonzik, S. M., Li, J., Thamlikitkul, V., Paterson, D. L., Shoham, S., Jacob, J., et al. (2011). Population pharmacokinetics of Colistin Methanesulfonate and formed Colistin in critically ill patients from a multicenter study provide dosing suggestions for various categories of patients. Antimicrob. Agents Chemother. 55, 3284–3294. doi: 10.1128/AAC.01733-10
Gbejuade, H., Lovering, A., and Webb, J. C. (2015). The role of microbial biofilms in prosthetic joint infections. Acta Orthop. 86, 147–158. doi: 10.3109/17453674.2014.966290
Grossman, T. (2016). Tetracycline antibiotics and resistance. Cold Spring Harb. Perspect. Med. 6:a025387. doi: 10.1101/cshperspect.a025387
Guilhaumou, R., Benaboud, S., Bennis, Y., Dahyot-Fizelier, C., Dailly, E., Gandia, P., et al. (2019). Optimization of the treatment with beta-lactam antibiotics in critically ill patients—guidelines from the French Society of Pharmacology and Therapeutics (Société Française de Pharmacologie et Thérapeutique—SFPT) and the French Society of Anaesthesia and Intensive Care Medicine (Société Française d’Anesthésie et Réanimation—SFAR). Crit. Care 23:104. doi: 10.1186/s13054-019-2378-9
Gupta, A., Harris, J. J., Lin, J., Bulgarelli, J. P., Birmingham, B. K., and Grimm, S. W. (2016). G.S.: Fusidic acid inhibits hepatic transporters and metabolic enzymes: potential cause of clinical drug-drug interaction observed with statin Coadministration. Antimicrob. Agents Chemother. 60, 5986–5994. doi: 10.1128/AAC.01335-16
Haagensen, J., Klausen, M., Ernst, R., Miller, S., Folkesson, A., Tolker-Nielsen, T., et al. (2007). Differentiation and distribution of Colistin- and sodium dodecyl sulfate-tolerant cells in Pseudomonas aeruginosa biofilms. J. Bacteriol. 189, 28–37. doi: 10.1128/JB.00720-06
Hagihara, M., Crandon, J. L., and Nicolau, D. P. (2012). The efficacy and safety of antibiotic combination therapy for infections caused by gram-positive and gram-negative organisms. Expert Opin. Drug Saf. 11, 221–233. doi: 10.1517/14740338.2012.632631
Hajikhani, B., Goudarzi, M., Kakavandi, S., Amini, S., Zamani, S., van Belkum, A., et al. (2021). The global prevalence of fusidic acid resistance in clinical isolates of Staphylococcus aureus: a systematic review and meta-analysis. Antimicrob. Resist. Infect. Control 10:75. doi: 10.1186/s13756-021-00943-6
Hancock, R. E. W. (1997). Peptide antibiotics. Lancet 349, 418–422. doi: 10.1016/S0140-6736(97)80051-7
Hancock, R. E., and Wong, P. G. (1984). Compounds which increase the permeability of the Pseudomonas aeruginosa outer membrane. Antimicrob. Agents Chemother. 26, 48–52. doi: 10.1128/AAC.26.1.48
Harting, J., Fernandez, F., Kelley, R., Wiemken, T., Peyrani, P., and Ramirez, J. (2017). Telavancin for the treatment of methicillin-resistant Staphylococcus aureus bone and joint infections. Diagn. Microbiol. Infect. Dis. 89, 294–299. doi: 10.1016/j.diagmicrobio.2017.09.004
Hashemian, S. M. R., Farhadi, Z., and Farhadi, T. (2019). Fosfomycin: the characteristics, activity, and use in critical care. Ther. Clin. Risk Manag. 15, 525–530. doi: 10.2147/TCRM.S199119
Heijerman, H., Westerman, E., Conway, S., Touw, D., and Döring, G. (2009). Inhaled medication and inhalation devices for lung disease in patients with cystic fibrosis: a European consensus. J. Cyst. Fibros. 8, 295–315. doi: 10.1016/j.jcf.2009.04.005
Herrmann, G., Yang, L., Wu, H., Song, Z., Wang, H., Høiby, N., et al. (2010). Colistin-tobramycin combinations are superior to monotherapy concerning the killing of biofilm Pseudomonas aeruginosa. J. Infect. Dis. 202, 1585–1592. doi: 10.1086/656788
Hirakawa, H., Kurabayashi, K., Tanimoto, K., and Tomita, H. (2018). Oxygen limitation enhances the antimicrobial activity of fosfomycin in Pseudomonas aeruginosa following overexpression of glpT which encodes glycerol-3-phosphate/fosfomycin symporter. Front. Microbiol. 9:1950. doi: 10.3389/fmicb.2018.01950
Ho Kwong, L., Ines, R., Rhea, Z., Sarah, W. A., Deepa, B., Michelle, K., et al. (2019). Oral versus intravenous antibiotics for bone and joint infection. N. Engl. J. Med. 380, 425–436. doi: 10.1056/NEJMoa1710926
Høiby, N., Bjarnsholt, T., Moser, C., Bassi, G. L., Coenye, T., Donelli, G., et al. (2015). ESCMID∗ guideline for the diagnosis and treatment of biofilm infections 2014. Clin. Microbiol. Infect. 21, S1–S25. doi: 10.1016/j.cmi.2014.10.024
Høiby, N., Frederiksen, B., and Pressler, T. (2005). Eradication of early Pseudomonas aeruginosa infection. J. Cyst. Fibros. 4, 49–54. doi: 10.1016/j.jcf.2005.05.018
Holmberg, A., Mörgelin, M., and Rasmussen, M. (2012). Effectiveness of ciprofloxacin or linezolid in combination with rifampicin against Enterococcus faecalis in biofilms. J. Antimicrob. Chemother. 67, 433–439. doi: 10.1093/jac/dkr477
Howden, B. P., and Grayson, M. L. (2006). Dumb and dumber—the potential waste of a useful Antistaphylococcal agent: emerging Fusidic acid resistance in Staphylococcus aureus. Clin. Infect. Dis. 42, 394–400. doi: 10.1086/499365
Hung-Jen, T., Chi-Chung, C., Kuo-Chen, C., Kuan-Ying, W., Yi-Chung, L., Chun-Cheng, Z., et al. (2013). In vitro efficacies and resistance profiles of rifampin-based combination regimens for biofilm-embedded methicillin-resistant Staphylococcus aureus. Antimicrob. Agents Chemother. 57, 5717–5720. doi: 10.1128/AAC.01236-13
Inouye, S., Watanabe, T., Tsuruoka, T., and Kitasato, I. (1989). An increase in the antimicrobial activity in vitro of fosfomycin under anaerobic conditions. J. Antimicrob. Chemother. 24, 657–666. doi: 10.1093/jac/24.5.657
Issam, R., Hend, H., Ying, J., Tanya, D., Ruth, R., Gassan, C., et al. (2007). Comparative activities of Daptomycin, linezolid, and Tigecycline against catheter-related methicillin-resistant Staphylococcus Bacteremic isolates embedded in biofilm. Antimicrob. Agents Chemother. 51, 1656–1660. doi: 10.1128/AAC.00350-06
Ivor, B., Shruta, R., Ed, C., Sara, Y., Diane, A., Gennady, K., et al. (2012). Randomized controlled trial of the safety and efficacy of Daptomycin versus standard-of-care therapy for Management of Patients with osteomyelitis associated with prosthetic devices undergoing two-stage revision arthroplasty. Antimicrob. Agents Chemother. 56, 5626–5632. doi: 10.1128/AAC.00038-12
Jacob, B., Makarewicz, O., Hartung, A., Brodt, S., Röhner, E., and Matziolis, G. (2021). In vitro additive effects of dalbavancin and rifampicin against biofilm of Staphylococcus aureus. Sci. Rep. 11:23425. doi: 10.1038/s41598-021-02709-x
Jahanbakhsh, S., Singh, N., Yim, J., Rose, W., and Rybak, M. (2018). Evaluation of Telavancin alone and combined with Ceftaroline or rifampin against methicillin-resistant Staphylococcus aureus (MRSA) in an in vitro biofilm model. Antimicrob. Agents Chemother. 62:e00567-18. doi: 10.1128/AAC.00567-18
Jang, T. L., Hewlett, A., and Cortes-Penfield, N. W. (2024). High efficacy of Oral Tetracyclines in prosthetic joint infection treated with debridement, antibiotics, and implant retention (DAIR) or resection arthroplasty with destination spacer placement. Cureus 16:e59599. doi: 10.7759/cureus.59599
Karbysheva, S., Cabric, S., Margaryan, D., and Trampuz, A. (2019). Efficacy and safety of intravenous fosfomycin in patients with periprosthetic joint infection: preliminary results from the proof study. A prospective multicentre study. Orthop Procs. 29.
Karlsen, Ø. E., Borgen, P., Bragnes, B., Figved, W., Grøgaard, B., Rydinge, J., et al. (2020). Rifampin combination therapy in staphylococcal prosthetic joint infections: a randomized controlled trial. J. Orthop. Surg. Res. 15:365. doi: 10.1186/s13018-020-01877-2
Kastoris, A. C., Rafailidis, P. I., Vouloumanou, E. K., Gkegkes, I. D., and Falagas, M. E. (2010). Synergy of fosfomycin with other antibiotics for gram-positive and gram-negative bacteria. Eur. J. Clin. Pharmacol. 66, 359–368. doi: 10.1007/s00228-010-0794-5
Kim, B.-K., Hyun Jung, K., Sohn, K.-H., Kim, J.-Y., Chang, Y.-S., and Kim, S.-H. (2019). Incidence of teicoplanin adverse drug reactions among patients with vancomycin-associated adverse drug reactions and its risk factors. Korean J. Intern. Med. 35, 714–722. doi: 10.3904/kjim.2018.404
Klausen, M., Aaes-Jørgensen, A., Molin, S., and Tolker-Nielsen, T. (2003). Involvement of bacterial migration in the development of complex multicellular structures in Pseudomonas aeruginosa biofilms. Mol. Microbiol. 50, 61–68. doi: 10.1046/j.1365-2958.2003.03677.x
Klein, N. C., and Cunha, B. A. (1995). Tetracyclines. Med. Clin. North Am. 79, 789–801. doi: 10.1016/S0025-7125(16)30039-6
Klinger-Strobel, M., Stein, C., Bahrs, C., Makarewicz, O., and Pletz, M. (2017). Effects of colistin on biofilm matrices of Escherichia coli and Staphylococcus aureus. Int. J. Antimicrob. Agents 49, 472–479. doi: 10.1016/j.ijantimicag.2017.01.005
Krenn, M., Grisold, A., Wohlfarth, P., Rath, J., Cetin, H., Koneczny, I., et al. (2020). Pathomechanisms and clinical implications of Myasthenic syndromes exacerbated and induced by medical treatments. Front. Mol. Neurosci. 13:156. doi: 10.3389/fnmol.2020.00156
Kumon, H., Ono, N., Iida, M., and Nickel, J. C. (1995). Combination effect of fosfomycin and ofloxacin against Pseudomonas aeruginosa growing in a biofilm. Antimicrob. Agents Chemother. 39, 1038–1044. doi: 10.1128/AAC.39.5.1038
Kusachi, S., Nagao, J., Saida, Y., Watanabe, M., Okamoto, Y., Asai, K., et al. (2011). Antibiotic time-lag combination therapy with fosfomycin for postoperative intra-abdominal abscesses. J. Infect. Chemother. 17, 91–96. doi: 10.1007/s10156-010-0167-0
Laffer, R. R., Graber, P., Ochsner, P., and Zimmerli, W. (2006). Outcome of prosthetic knee-associated infection: evaluation of 40 consecutive episodes at a single Centre. Clin. Microbiol. Infect. 12, 433–439. doi: 10.1111/j.1469-0691.2006.01378.x
Lazarinis, S., Hailer, N., Järhult, J., and Brüggemann, A. (2023). Incidence of rifampicin resistance in Periprosthetic joint infection: a single-Centre cohort study on 238 patients. Antibiotics 12:1499. doi: 10.3390/antibiotics12101499
Ledson, M. J., Gallagher, M. J., Cowperthwaite, C., Convery, R. P., and Walshaw, M. J. (1998). Four years’ experience of intravenous colomycin in an adult cystic fibrosis unit. Eur. Respir. J. 12, 592–594. doi: 10.1183/09031936.98.12030592
Lee, Y., Kim, S. S., Choi, S.-M., Bae, C.-J., Oh, T.-H., Kim, S. E., et al. (2022). Rifamycin resistance, rpoB gene mutation and clinical outcomes of Staphylococcus species isolates from prosthetic joint infections in Republic of Korea. J. Glob. Antimicrob. Resist. 28, 43–48. doi: 10.1016/j.jgar.2021.12.005
Leijtens, B., Weerwag, L., Schreurs, B. W., Kullberg, B. J., and Rijnen, W. (2019). Clinical outcome of antibiotic suppressive therapy in patients with a prosthetic joint infection after hip replacement. J. Bone Jt. Infect. 4, 268–276. doi: 10.7150/jbji.37262
Leite, B., Gomes, F., Teixeira, P., Oliveira de Souza, C., Pizzolitto, E., and Oliveira, R. (2011). In vitro activity of Daptomycin, linezolid and rifampicin on Staphylococcus epidermidis biofilms. Curr. Microbiol. 63, 313–317. doi: 10.1007/s00284-011-9980-7
Li, J., Cheung, W.-H., Chow, S. K., Ip, M., Leung, S. Y. S., and Wong, R. M. Y. (2022). Current therapeutic interventions combating biofilm-related infections in orthopaedics. Bone Joint Res. 11, 700–714. doi: 10.1302/2046-3758.1110.BJR-2021-0495.R3
Lin, G., Pankuch, G., Appelbaum, P. C., and Kosowska-Shick, K. (2014). Antistaphylococcal activity of oritavancin and its synergistic effect in combination with other antimicrobial agents. Antimicrob. Agents Chemother. 58, 6251–6254. doi: 10.1128/AAC.02932-14
Liu, Y., Ma, W., Li, M., Wu, J., Sun, L., Zhao, W., et al. (2022). Antibacterial and antibiofilm activities of fosfomycin combined with rifampin against carbapenem-resistant Pseudomonas aeruginosa. Lett. Appl. Microbiol. 75, 1559–1568. doi: 10.1111/lam.13822
López Sánchez, C., Rodríguez-Pardo, D., Pigrau, C., Corona, P., Amat, C., Lung, M., et al. (2016). Teicoplanin – An old new treatment for enterococcal prosthetic joint infections. J. Infect. 72, 764–766. doi: 10.1016/j.jinf.2016.03.002
Lora-Tamayo, J., Murillo, O., and Ariza, J. (2019). Clinical use of Colistin in biofilm-associated infections. Adv. Exp. Med. Biol. 1145, 181–195. doi: 10.1007/978-3-030-16373-0_13
Lora-Tamayo, J., Murillo, O., Bergen, P., Nation, R., Poudyal, A., Luo, X., et al. (2014). Activity of colistin combined with doripenem at clinically relevant concentrations against multidrug-resistant Pseudomonas aeruginosa in an in vitro dynamic biofilm model. J. Antimicrob. Chemother. 69, 2434–2442. doi: 10.1093/jac/dku151
Lora-Tamayo, J., Murillo, O., Iribarren, J. A., Soriano, A., Sánchez-Somolinos, M., Baraia-Etxaburu, J. M., et al. (2013). A large multicenter study of methicillin–susceptible and methicillin–resistant Staphylococcus aureus prosthetic joint infections managed with implant retention. Clin. Infect. Dis. 56, 182–194. doi: 10.1093/cid/cis746
Lozano-Alonso, S., Linares-Palomino, J. P., Vera-Arroyo, B., Bravo-Molina, A., Hernández-Quero, J., and Ros-Díe, E. (2016). Evaluación de la capacidad de difusión tisular de antibióticos en isquemia de miembros inferiores. Enferm. Infecc. Microbiol. Clin. 34, 477–483. doi: 10.1016/j.eimc.2015.10.012
Luengo-Alonso, G., Lora-Tamayo, J., Paredes, D., Muñoz-Gallego, I., Díaz, A., and Delgado, E. (2018). Daptomycin plus Fosfomycin as salvage therapy in a difficult-to-treat Total femoral replacement infection. J. Bone Jt. Infect. 3, 207–211. doi: 10.7150/jbji.27811
Lupia, T., De Benedetto, I., Bosio, R., Shbaklo, N., De Rosa, F. G., and Corcione, S. (2023). Role of Oritavancin in the treatment of infective endocarditis, catheter- or device-related infections, bloodstream infections, and bone and prosthetic joint infections in humans: narrative review and possible developments. Life (Basel) 13:959. doi: 10.3390/life13040959
Marquès, C., Tasse, J., Pracros, A., Collin, V., Franceschi, C., Laurent, F., et al. (2015). Effects of antibiotics on biofilm and unattached cells of a clinical Staphylococcus aureus isolate from bone and joint infection. J. Med. Microbiol. 64, 1021–1026. doi: 10.1099/jmm.0.000125
Martínez Pastor, J. C., Muñoz-Mahamud, E., Vilchez, F., García-Ramiro, S., Bori, G., Sierra, J., et al. (2009). Outcome of acute prosthetic joint infections due to gram-negative Bacilli treated with open debridement and retention of the prosthesis. Antimicrob. Agents Chemother. 53, 4772–4777. doi: 10.1128/AAC.00188-09
Mascio, C. T., Alder, J. D., and Silverman, J. A. (2007). Bactericidal action of Daptomycin against stationary-phase and nondividing Staphylococcus aureus cells. Antimicrob. Agents Chemother. 51, 4255–4260. doi: 10.1128/AAC.00824-07
Mazzei, T., Cassetta, M. I., Fallani, S., Arrigucci, S., and Novelli, A. (2006). Pharmacokinetic and pharmacodynamic aspects of antimicrobial agents for the treatment of uncomplicated urinary tract infections. Int. J. Antimicrob. Agents 28, 35–41. doi: 10.1016/j.ijantimicag.2006.05.019
McConoughey, S. J., Howlin, R., Granger, J. F., Manring, M. M., Calhoun, J. H., Shirtliff, M., et al. (2014). Biofilms in periprosthetic orthopedic infections. Future Microbiol. 9, 987–1007. doi: 10.2217/fmb.14.64
Mei, Q., Ye, Y., Zhu, Y.-L., Cheng, J., Chang, X., Liu, Y.-Y., et al. (2015). Testing the mutant selection window hypothesis in vitro and in vivo with Staphylococcus aureus exposed to fosfomycin. Eur. J. Clin. Microbiol. Infect. Dis. 34, 737–744. doi: 10.1007/s10096-014-2285-6
Meißner, A., Rahmanzadeh, R., and Haag, R. (1989). Adjuvant fosfomycin medication in chronic osteomyelitis. Infection 17, 146–151. doi: 10.1007/BF01644014
Meléndez-Carmona, M., Mancheño-Losa, M., Ruiz Sorribas, A., Muñoz-Gallego, I., Viedma, E., Chaves, F., et al. (2022). Strain-to-strain variability among Staphylococcus aureus causing prosthetic joint infection drives heterogeneity in response to levofloxacin and rifampicin. J. Antimicrob. Chemother. 77, 3265–3269. doi: 10.1093/jac/dkac311
Mihailescu, R., Furustrand Tafin, U., Corvec, S., Alessandra, O., Betrisey, B., Borens, O., et al. (2014). High activity of Fosfomycin and rifampin against MethicillinResistant Staphylococcus aureus biofilm in vitro and in an experimental foreign-body infection model. Antimicrob. Agents Chemother. 58, 2547–2553. doi: 10.1128/AAC.02420-12
Mikuniya, T., Kato, Y., Ida, T., Maebashi, K., Monden, K., Kariyama, R., et al. (2007). Treatment of Pseudomonas aeruginosa biofilms with a combination of fluoroquinolones and fosfomycin in a rat urinary tract infection model. J. Infect. Chemother. 13, 285–290. doi: 10.1007/s10156-007-0534-7
Mikuniya, T., Kato, Y., Kariyama, R., Monden, K., Hikida, M., and Kumon, H. (2005). Synergistic effect of fosfomycin and fluoroquinolones against Pseudomonas aeruginosa growing in a biofilm. Acta Med. Okayama 59, 209–216. doi: 10.18926/AMO/31977
Moenster, R. P., Linneman, T. W., Call, W. B., Kay, C. L., McEvoy, T. A., and Sanders, J. L. (2013). The potential role of newer gram-positive antibiotics in the setting of osteomyelitis of adults. J. Clin. Pharm. Ther. 38, 89–96. doi: 10.1111/jcpt.12030
Molina-Manso, D., Prado, G., Ortiz-Pérez, A., Manrubia-Cobo, M., Gómez-Barrena, E., Cordero-Ampuero, J., et al. (2013). In vitro susceptibility to antibiotics of staphylococci in biofilms isolated from orthopaedic infections. Int. J. Antimicrob. Agents 41, 521–523. doi: 10.1016/j.ijantimicag.2013.02.018
Monden, K., Ando, E., Iida, M., and Kumon, H. (2002). Role of fosfomycin in a synergistic combination with ofloxacin against Pseudomonas aeruginosa growing in a biofilm. J. Infect. Chemother. 8, 218–226. doi: 10.1007/s10156-002-0186-6
Monzón, M., Oteiza, C., Leiva, J., Lamata, M., and Amorena, B. (2003). Biofilm testing of Staphylococcus epidermidis clinical isolates: low performance of vancomycin in relation to other antibiotics. Diagn. Microbiol. Infect. Dis. 44, 319–324. doi: 10.1016/S0732-8893(02)00464-9
Morata, L., and Soriano, A. (2019). The role of fosfomycin in osteoarticular infection. Rev. Esp. Quimioter: Publicacion Oficial de la Sociedad Espanola de Quimioterapia. 32, 30–36.
Muller-Serieys, C., Saleh-Mghir, A., Massias, L., and Fantin, B. (2009). Bactericidal activity of the combination of levofloxacin with rifampin in experimental prosthetic knee infection in rabbits due to methicillin-susceptible Staphylococcus aureus. Antimicrob. Agents Chemother. 53, 2145–2148. doi: 10.1128/AAC.01163-08
Murray, W. R. (1984). Use of antibiotic-containing bone cement. Clin. Orthop. Relat. Res. 190, 89–95.
Namba, R. S., Inacio, M. C. S., and Paxton, E. W. (2013). Risk factors associated with deep surgical site infections after primary total knee arthroplasty: an analysis of 56,216 knees. J. Bone Joint Surg. Am. 95, 775–782. doi: 10.2106/JBJS.L.00211
Nation, R. L., and Li, J. (2009). Colistin in the 21st century. Curr. Opin. Infect. Dis. 22, 535–543. doi: 10.1097/QCO.0b013e328332e672
Neut, D., Hendriks, J. G. E., van Horn, J. R., Kowalski, R. S. Z., van der Mei, H. C., and Busscher, H. J. (2006). Antimicrobial efficacy of gentamicin-loaded acrylic bone cements with fusidic acid or clindamycin added. J. Orthop. Res. 24, 291–299. doi: 10.1002/jor.20058
Nguyen, S., Robineau, O., Titecat, M., Blondiaux, N., Valette, M., Loiez, C., et al. (2015). Influence of daily dosage and frequency of administration of rifampicin–levofloxacin therapy on tolerance and effectiveness in 154 patients treated for prosthetic joint infections. Eur. J. Clin. Microbiol. 34, 1675–1682. doi: 10.1007/s10096-015-2404-z
O’Neill, A. J., Cove, J. H., and Chopra, I. (2001). Mutation frequencies for resistance to fusidic acid and rifampicin in Staphylococcus aureus. J. Antimicrob. Chemother. 47, 647–650. doi: 10.1093/jac/47.5.647
Osmon, D. R., Berbari, E. F., Berendt, A. R., Lew, D., Zimmerli, W., Steckelberg, J. M., et al. (2013). Diagnosis and Management of Prosthetic Joint Infection: clinical practice guidelines by the infectious diseases Society of Americaa. Clin. Infect. Dis. 56, e1–e25. doi: 10.1093/cid/cis803
Pamp, S., Gjermansen, M., Johansen, H., and Tolker-Nielsen, T. (2008). Tolerance to the antimicrobial peptide colistin in Pseudomonas aeruginosa biofilms is linked to metabolically active cells, and depends on the pmr and mexAB-oprM genes. Mol. Microbiol. 68, 223–240. doi: 10.1111/j.1365-2958.2008.06152.x
Parra-Ruiz, J., Vidaillac, C., Rose, W. E., and Rybak, M. J. (2010). Activities of high-dose daptomycin, vancomycin, and moxifloxacin alone or in combination with clarithromycin or rifampin in a novel in vitro model of Staphylococcus aureus biofilm. Antimicrob. Agents Chemother. 54, 4329–4334. doi: 10.1128/AAC.00455-10
Peel, T. N., Buising, K. L., Dowsey, M. M., Aboltins, C. A., Daffy, J. R., Stanley, P. A., et al. (2013). Outcome of debridement and retention in prosthetic joint infections by methicillin-resistant staphylococci, with special reference to rifampin and Fusidic acid combination therapy. Antimicrob. Agents Chemother. 57, 350–355. doi: 10.1128/AAC.02061-12
Peeters, O., Ferry, T., Ader, F., Boibieux, A., Braun, E., Bouaziz, A., et al. (2016). Teicoplanin-based antimicrobial therapy in Staphylococcus aureus bone and joint infection: tolerance, efficacy and experience with subcutaneous administration. BMC Infect. Dis. 16:622. doi: 10.1186/s12879-016-1955-7
Perez-Jorge, C., Gomez-Barrena, E., Horcajada, J.-P., Puig-Verdie, L., and Esteban, J. (2016). Drug treatments for prosthetic joint infections in the era of multidrug resistance. Expert. Opin. Pharmacother. 17, 1233–1246. doi: 10.1080/14656566.2016.1176142
Plachouras, D., Karvanen, M., Friberg, L. E., Papadomichelakis, E., Antoniadou, A., Tsangaris, I., et al. (2009). Population pharmacokinetic analysis of colistin methanesulfonate and colistin after intravenous administration in critically ill patients with infections caused by gram-negative bacteria. Antimicrob. Agents Chemother. 53, 3430–3436. doi: 10.1128/AAC.01361-08
Pradier, M., Robineau, O., Boucher, A., Titecat, M., Blondiaux, N., Valette, M., et al. (2018). Suppressive antibiotic therapy with oral tetracyclines for prosthetic joint infections: a retrospective study of 78 patients. Infection 46, 39–47. doi: 10.1007/s15010-017-1077-1
Pujol, M., Miro, J., Shaw, E., Aguado, J.-M., Juan, R., Puig-Asensio, M., et al. (2020). Daptomycin plus Fosfomycin versus Daptomycin alone for methicillin-resistant Staphylococcus aureus bacteremia and endocarditis: a randomized clinical trial. Clin. Infect. Dis. 72, 1517–1525. doi: 10.1093/cid/ciaa1081
Pushkin, R., Iglesias-Ussel, M., Kara, K., MacLauchlin, C., Mould, D., Berkowitz, R., et al. (2016). A randomized study evaluating Oral Fusidic acid (CEM-102) in combination with Oral rifampin compared with standard-of-care antibiotics for treatment of prosthetic joint infections: a newly identified drug–drug interaction. Clin. Infect. Dis. 63, 1599–1604. doi: 10.1093/cid/ciw665
Rao, N., and Regalla, D. M. (2006). Uncertain efficacy of Daptomycin for prosthetic joint infections: a prospective case series. Clin. Orthop. Relat. Res. 451, 34–37. doi: 10.1097/01.blo.0000224021.73163.61
Raz, R. (2012). Fosfomycin: an old—new antibiotic. Clin. Microbiol. Infect. 18, 4–7. doi: 10.1111/j.1469-0691.2011.03636.x
Reilly, J., Jacobs, M., Friedman, B., Cleveland, K., and Lombardi, D. (2020). Castaneda-Ruiz, ·: clinical experience with Telavancin for the treatment of patients with bacteremia and endocarditis: real-world results from the Telavancin observational use registry (TOURTM). Drugs Real World Outcomes 7, 179–189. doi: 10.1007/s40801-020-00191-x
Renz, N., Trampuz, A., and Zimmerli, W. (2021). Controversy about the role of rifampin in biofilm infections: is it justified? Antibiotics (Basel) 10:165. doi: 10.3390/antibiotics10020165
Rice, L. B. (2006). Challenges in identifying new antimicrobial agents effective for treating infections with Acinetobacter baumannii and Pseudomonas aeruginosa. Clin. Infect. Dis. 43, S100–S105. doi: 10.1086/504487
Roblot, F., Besnier, J.-M., Giraudeau, B., Simonnard, N., Jonville-Bera, A.-P., Coipeau, P., et al. (2007). Lack of association between rifampicin plasma concentration and treatment-related side effects in osteoarticular infections. Fundam. Clin. Pharmacol. 21, 363–369. doi: 10.1111/j.1472-8206.2007.00490.x
Rodríguez-Pardo, D., Pigrau, C., Lora-Tamayo, J., Soriano, A., del Toro, M. D., Cobo, J., et al. (2014). Gram-negative prosthetic joint infection: outcome of a debridement, antibiotics and implant retention approach. A large multicentre study. Clin. Microbiol. Infect. 20, O911–O919. doi: 10.1111/1469-0691.12649
Rönnqvist, J., Hallberg, P., Yue, Q.-Y., and Wadelius, M. (2018). Fusidic acid: a neglected risk factor for statin-associated myopathy. Clin. Med. Insights Cardiol. 12:1179546818815162. doi: 10.1177/1179546818815162
Rose, W. E., and Poppens, P. T. (2009). Impact of biofilm on the in vitro activity of vancomycin alone and in combination with tigecycline and rifampicin against Staphylococcus aureus. J. Antimicrob. Chemother. 63, 485–488. doi: 10.1093/jac/dkn513
Rosenthal, A. L., Rovell, J. M., and Girard, A. E. (1976). Polyacrylic bone cement with added erythromycin and colistin. In vitro studies on bacteriologic activity and diffusion properties. MMW Munch. Med. Wochenschr. 118, 987–990.
Rosman, C. W. K., van der Mei, H. C., and Sjollema, J. (2021). Influence of sub-inhibitory concentrations of antimicrobials on micrococcal nuclease and biofilm formation in Staphylococcus aureus. Sci. Rep. 11:13241. doi: 10.1038/s41598-021-92619-9
Roy, R., Tiwari, M., Donelli, G., and Tiwari, V. (2018). Strategies for combating bacterial biofilms: a focus on anti-biofilm agents and their mechanisms of action. Virulence 9, 522–554. doi: 10.1080/21505594.2017.1313372
Saginur, R., StDenis, M., Ferris, W., Aaron, S., Chan, F., Lee, C., et al. (2006). Multiple combination bactericidal testing of staphylococcal biofilms from implant-associated infections. Antimicrob. Agents Chemother. 50, 55–61. doi: 10.1128/AAC.50.1.55-61.2006
Sandiford, N. A., Hutt, J. R., Kendoff, D. O., Mitchell, P. A., Citak, M., and Granger, L. (2020). Prolonged suppressive antibiotic therapy is successful in the management of prosthetic joint infection. Eur. J. Orthop. Surg. Traumatol. 30, 313–321. doi: 10.1007/s00590-019-02559-4
Sauermann, R., Karch, R., Langenberger, H., Kettenbach, J., Mayer-Helm, B., Petsch, M., et al. (2005). Antibiotic abscess penetration: fosfomycin levels measured in pus and simulated concentration-time profiles. Antimicrob. Agents Chemother. 49, 4448–4454. doi: 10.1128/AAC.49.11.4448-4454.2005
Sendi, P., and Zimmerli, W. (2017). The use of rifampin in staphylococcal orthopaedic-device-related infections. Clin. Microbiol. Infect. 23, 349–350. doi: 10.1016/j.cmi.2016.10.002
Senneville, E., Joulie, D., Legout, L., Valette, M., Dezèque, H., Beltrand, E., et al. (2011). Outcome and predictors of treatment failure in Total hip/knee prosthetic joint infections due to Staphylococcus aureus. Clin. Infect. Dis. 53, 334–340. doi: 10.1093/cid/cir402
Shahbazi, F., and Dashti-Khavidaki, S. (2015). Colistin: efficacy and safety in different populations. Expert. Rev. Clin. Pharmacol. 8, 423–448. doi: 10.1586/17512433.2015.1053390
Shi, J., Mao, N.-F., Wang, L., Zhang, H.-B., Chen, Q., Liu, H., et al. (2014). Efficacy of combined vancomycin and Fosfomycin against methicillin-resistant Staphylococcus aureus in biofilms in vivo. PLoS One 9:e113133. doi: 10.1371/journal.pone.0113133
Silver, L. (2017). Fosfomycin: mechanism and resistance. Cold Spring Harb. Perspect. Med. 7:a025262. doi: 10.1101/cshperspect.a025262
Simon, S., Frank, B. J. H., Hartmann, S., Hinterhuber, L., Reitsamer, M., Aichmair, A., et al. (2022). Dalbavancin in gram-positive periprosthetic joint infections. J. Antimicrob. Chemother. 77, 2274–2277. doi: 10.1093/jac/dkac178
Sims, C., Bressler, A., Graham, D., Lacy, M., Lombardi, D., and Castaneda-Ruiz, B. (2021). Real-world clinical use and outcomes of Telavancin for the treatment of bone and joint infections: results from the Telavancin observational use registry (TOURTM). Drugs Real World Outcomes 8, 509–518. doi: 10.1007/s40801-021-00255-6
Smith, K., Perez, A., Ramage, G., Gemmell, C. G., and Lang, S. (2009). Comparison of biofilm-associated cell survival following in vitro exposure of meticillin-resistant Staphylococcus aureus biofilms to the antibiotics clindamycin, daptomycin, linezolid, tigecycline and vancomycin. Int. J. Antimicrob. Agents 33, 374–378. doi: 10.1016/j.ijantimicag.2008.08.029
Snyder, A., Vidaillac, C., Rose, W., McRoberts, J., and Rybak, M. (2014). Evaluation of high-dose Daptomycin versus vancomycin alone or combined with clarithromycin or rifampin against Staphylococcus aureus and S. epidermidis in a novel in vitro PK/PD model of bacterial biofilm. Infect. Dis. Ther. 4, 51–65. doi: 10.1007/s40121-014-0055-5
Sodhi, M., Sheldon, C. A., Carleton, B., and Etminan, M. (2017). Oral fluoroquinolones and risk of secondary pseudotumor cerebri syndrome. Neurology 89, 792–795. doi: 10.1212/WNL.0000000000004247
Taha, M., Abdelbary, H., Ross, F. P., and Carli, A. (2018). V: new innovations in the treatment of PJI and biofilms—clinical and preclinical topics. Curr. Rev. Musculoskelet. Med. 11, 380–388. doi: 10.1007/s12178-018-9500-5
Tanaka, G., Shigeta, M., Komatsuzawa, H., Sugai, M., Suginaka, H., and Usui, T. (1999). Effect of the growth rate of Pseudomonas aeruginosa biofilms on the susceptibility to antimicrobial agents: β-lactams and fluoroquinolones. Chemotherapy 45, 28–36. doi: 10.1159/000007162
Tande, A. J., and Robin, P. (2014). Prosthetic joint infection. Clin. Microbiol. Rev. 27, 302–345. doi: 10.1128/CMR.00111-13
Tang, H.-J., Chen, C.-C., Cheng, K.-C., Toh, H.-S., Su, B.-A., Chiang, S.-R., et al. (2012). In vitro efficacy of fosfomycin-containing regimens against methicillin-resistant Staphylococcus aureus in biofilms. J. Antimicrob. Chemother. 67, 944–950. doi: 10.1093/jac/dkr535
Tang, H.-J., Chen, C.-C., Ko, W.-C., Yu, W.-L., Chiang, S.-R., and Chuang, Y.-C. (2011). In vitro efficacy of antimicrobial agents against high-inoculum or biofilm-embedded meticillin-resistant Staphylococcus aureus with vancomycin minimal inhibitory concentrations equal to 2μg/mL (VA2-MRSA). Int. J. Antimicrob. Agents 38, 46–51. doi: 10.1016/j.ijantimicag.2011.02.013
Taşkın Kafa, A. H., and Hasbek, M. (2022). Synergistic efficacy of meropenem, ciprofloxacin and colistin antibiotics against planktonic and biofilm forms of Myroides odoratimimus bacterial isolates. Indian J. Med. Microbiol. 40, 399–403. doi: 10.1016/j.ijmmb.2022.04.004
Telles, J. P., Cieslinski, J., and Tuon, F. F. (2019). Daptomycin to bone and joint infections and prosthesis joint infections: a systematic review. Braz. J. Infect. Dis. 23, 191–196. doi: 10.1016/j.bjid.2019.05.006
Thabit, A. K., Fatani, D. F., Bamakhrama, M. S., Barnawi, O. A., Basudan, L. O., and Alhejaili, S. F. (2019). Antibiotic penetration into bone and joints: An updated review. Int. J. Infect. Dis. 81, 128–136. doi: 10.1016/j.ijid.2019.02.005
Thill, P., Robineau, O., Roosen, G., Patoz, P., Gachet, B., Lafon-Desmurs, B., et al. (2022). Rifabutin versus rifampicin bactericidal and antibiofilm activities against clinical strains of Staphylococcus spp. isolated from bone and joint infections. J. Antimicrob. Chemother. 77, 1036–1040. doi: 10.1093/jac/dkab486
Thompson, S., and Townsend, R. (2011). Pharmacological agents for soft tissue and bone infected with MRSA: which agent and for how long? Injury 42, S7–S10. doi: 10.1016/S0020-1383(11)70126-7
Tilman, L., Wolfgang, P., Dominik, B., Luzia, V., Manuel, K., Roberto, P., et al. (2015). Daptomycin plus Fosfomycin, a synergistic combination in experimental implant-associated osteomyelitis due to methicillin-resistant Staphylococcus aureus in rats. Antimicrob. Agents Chemother. 59, 859–863. doi: 10.1128/AAC.04246-14
Trautmann, M., Meincke, C., Vogt, K., Ruhnke, M., and Lajous-Petter, A. M. (1992). Intracellular bactericidal activity of fosfomycin against staphylococci: a comparison with other antibiotics. Infection 20, 350–354. doi: 10.1007/BF01710683
Tremblay, S., Lau, T., and Ensom, M. (2013). Addition of rifampin to vancomycin for methicillin-resistant Staphylococcus aureus infections: what is the evidence? Ann. Pharmacother. 47, 1045–1054. doi: 10.1345/aph.1R726
Tsegka, K. G., Voulgaris, G. L., Kyriakidou, M., Kapaskelis, A., and Falagas, M. E. (2022). Intravenous fosfomycin for the treatment of patients with bone and joint infections: a review. Expert Rev. Anti-Infect. Ther. 20, 33–43. doi: 10.1080/14787210.2021.1932463
Tseng, T.-C., Chuang, Y.-C., Yang, J.-L., Lin, C.-Y., Huang, S.-H., Wang, J.-D., et al. (2023). The combination of Daptomycin with Fosfomycin is more effective than Daptomycin alone in reducing mortality of vancomycin-resistant Enterococcal bloodstream infections: a retrospective, comparative cohort study. Infect. Dis. Ther. 12, 589–606. doi: 10.1007/s40121-022-00754-1
Valerius, N. H., Koch, C., and Høiby, N. (1991). Prevention of chronic Pseudomonas aeruginosa colonisation in cystic fibrosis by early treatment. Lancet 338, 725–726. doi: 10.1016/0140-6736(91)91446-2
Valour, F., Dutronc, H., Dinh, A., Cazorla, C., Pavèse, P., Lesens, O., et al. (2012). Difficult-to-treat gram-negative bone and joint infections: efficacy and safety of prolonged intravenous colistin. Int. J. Antimicrob. Agents 41, 197–199. doi: 10.1016/j.ijantimicag.2012.09.016
Vavasseur, B., and Zeller, V. (2022). Antibiotic therapy for prosthetic joint infections: An overview. Antibiotics 11:486. doi: 10.3390/antibiotics11040486
Vossen, M. G., Gattringer, R., Thalhammer, F., Militz, M., and Hischebeth, G. (2020). Calculated parenteral initial treatment of bacterial infections: bone and joint infections. GMS Infect. Dis. 8:Doc10. doi: 10.3205/id000054
Wafi, S., Hector, R.-V., Prabhavathi, F. M. T. P., and Françoise, V. B. (2018). Activities of combinations of Antistaphylococcal antibiotics with Fusidic acid against staphylococcal biofilms in in vitro static and dynamic models. Antimicrob. Agents Chemother. 62:e00598-18. doi: 10.1128/AAC.00598-18
Wang, L., Di Luca, M., Tkhilaishvili, T., Trampuz, A., and González Moreno, M. (2019). Synergistic activity of Fosfomycin, ciprofloxacin, and gentamicin against Escherichia coli and Pseudomonas aeruginosa biofilms. Front. Microbiol. 10:2522. doi: 10.3389/fmicb.2019.02522
Wang, H., Wu, H., Ciofu, O., Song, Z., and Høiby, N. (2011). Pharmacokinetics/pharmacodynamics of Colistin and imipenem on mucoid and nonmucoid Pseudomonas aeruginosa biofilms. Antimicrob. Agents Chemother. 55, 4469–4474. doi: 10.1128/AAC.00126-11
Wang, H., Wu, H., Ciofu, O., Song, Z., and Høiby, N. (2012). In vivo pharmacokinetics/pharmacodynamics of Colistin and imipenem in Pseudomonas aeruginosa biofilm infection. Antimicrob. Agents Chemother. 56, 2683–2690. doi: 10.1128/AAC.06486-11
Warner, A., Hathaway-Schrader, J., Lubker, R., Davies, C., and Novince, C. (2022). Tetracyclines and bone: unclear actions with potentially lasting effects. Bone 159:116377. doi: 10.1016/j.bone.2022.116377
Wiestner, A., Frei, R., and Zimmerli, W. (1991). Killing of nongrowing and adherent Escherichia coli determines drug efficacy in device-related infections. Antimicrob. Agents Chemother. 35, 741–746. doi: 10.1128/AAC.35.4.741
Worthington, R. J., and Melander, C. (2013). Combination approaches to combat multidrug-resistant bacteria. Trends Biotechnol. 31, 177–184. doi: 10.1016/j.tibtech.2012.12.006
Wu, W.-S., Chen, C.-C., Chuang, Y.-C., Su, B.-A., Chiu, Y.-H., Hsu, H.-J., et al. (2012). Efficacy of combination oral antimicrobial agents against biofilm-embedded methicillin-resistant Staphylococcus aureus. J. Microbiol. Immunol. Infect. 46, 89–95. doi: 10.1016/j.jmii.2012.03.009
Wu, H., Moser, C., Wang, H., Høiby, N., and Song, Z. (2014). Strategies for combating bacterial biofilm infections. Int. J. Oral Sci. 7, 1–7. doi: 10.1038/ijos.2014.65
Wunsch, S., Krause, R., Valentin, T., Prattes, J., Janata, O., Lenger, A., et al. (2019). Multicenter clinical experience of real life Dalbavancin use in gram-positive infections. Int. J. Infect. Dis. 81, 210–214. doi: 10.1016/j.ijid.2019.02.013
Yan, Q., Karau, M. J., and Patel, R. (2018). In vitro activity of oritavancin against biofilms of staphylococci isolated from prosthetic joint infection. Diagn. Microbiol. Infect. Dis. 92, 155–157. doi: 10.1016/j.diagmicrobio.2018.05.010
Yan, Q., Karau, M., Raval, Y., and Patel, R. (2018). In vitro activity of Oritavancin in combination with rifampin or gentamicin against prosthetic joint infection-associated methicillin-resistant Staphylococcus epidermidis biofilms. Int. J. Antimicrob. Agents 52, 608–615. doi: 10.1016/j.ijantimicag.2018.07.012
Yu, X.-H., Song, X.-J., Cai, Y., Liang, B.-B., Lin, D.-F., and Wang, R. (2010). In vitro activity of two old antibiotics against clinical isolates of methicillin-resistant Staphylococcus aureus. J. Antibiot. (Tokyo) 63, 657–659. doi: 10.1038/ja.2010.105
Yu, W., Zhang, J., Tong, J., Zhang, L., Zhan, Y., Huang, Y., et al. (2020). In vitro antimicrobial activity of Fosfomycin, vancomycin and Daptomycin alone, and in combination, against linezolid-resistant Enterococcus faecalis. Infect. Dis. Ther. 9, 927–934. doi: 10.1007/s40121-020-00342-1
Zeller, V., Kerroumi, Y., Meyssonnier, V., Heym, B., Metten, M.-A., Desplaces, N., et al. (2018). Analysis of postoperative and hematogenous prosthetic joint-infection microbiological patterns in a large cohort. J. Infect. 76, 328–334. doi: 10.1016/j.jinf.2017.12.016
Zeller, V., Magreault, S., Heym, B., Salmon, D., Kitzis, M.-D., Billaud, E., et al. (2021). Influence of the clindamycin-administration route on the magnitude of clindamycin-rifampicin interaction: a prospective pharmacokinetic study. Clin. Microbiol. Infect. 27, 1857.e1–1857.e7. doi: 10.1016/j.cmi.2021.04.017
Zimmerli, W., Trampuz, A., and Ochsner, P. (2004). Prosthetic-joint infections. N. Engl. J. Med. 351, 1645–1654. doi: 10.1056/NEJMra040181
Keywords: prosthetic joint infection, biofilm, antibiofilm activity, antibiotic therapy, biofilm active antibiotics, drug resistance
Citation: Ferreira L, Pos E, Nogueira DR, Ferreira FP, Sousa R and Abreu MA (2024) Antibiotics with antibiofilm activity – rifampicin and beyond. Front. Microbiol. 15:1435720. doi: 10.3389/fmicb.2024.1435720
Edited by:
Axel Cloeckaert, Institut National de recherche pour l’agriculture, l’alimentation et l’environnement (INRAE), FranceReviewed by:
Satheesh Sathianeson, King Abdulaziz University, Saudi ArabiaDragana Mitic-Culafic, University of Belgrade, Serbia
Copyright © 2024 Ferreira, Pos, Nogueira, Ferreira, Sousa and Abreu. This is an open-access article distributed under the terms of the Creative Commons Attribution License (CC BY). The use, distribution or reproduction in other forums is permitted, provided the original author(s) and the copyright owner(s) are credited and that the original publication in this journal is cited, in accordance with accepted academic practice. No use, distribution or reproduction is permitted which does not comply with these terms.
*Correspondence: Miguel Araújo Abreu, bWlnYXJhdWpvYWJyZXVAY2hwb3J0by5taW4tc2F1ZGUucHQ=
†These authors have contributed equally to this work and share first authorship