- 1Department of Hematology, The First Affiliated Hospital of Ningbo University, Ningbo, China
- 2Ningbo Institute of Life and Health Industry, University of Chinese Academy of Sciences, Ningbo, China
- 3Department of Laboratory Medicine, The First Affiliated Hospital of Ningbo University, Ningbo, China
Introduction: Klebsiella pneumoniae (K. pneumoniae) is the most common pathogen causing hospital respiratory tract infection and epidemic. Gold standard procedures of microscopic examination and biochemical identification are widely used in clinical diagnosis with disadvantages of low sensitivity, time-consuming and sophisticated equipment requiring. An efficient, nucleic acid amplification-based sensitive and specific on-site identification of K. pneumoniae in clinical is necessary to facilitate clinical medication and disease control.
Methods: We developed a closed dumbbell mediated isothermal amplification (CDA) assay for the rapid and sensitive detection of conserved rcsA gene in K. pneumoniae by real-time fluorescence monitoring and end-point colorimetric judgement. We designed and selected a pair of inner primers of CDA to detect K. pneumoniae. Then outer and loop primers were designed and verified to accelerate CDA reaction to achieve more efficient detection of K. pneumoniae.
Results: The results showed the detection limit of CDA assay was 1.2 × 10−5 ng/μL (approximately 1 copy of the target gene) within 60 min, which was 100-fold more sensitive than real-time quantitative PCR (qPCR). Several pathogen genomic DNAs (Staphylococcus aureus, Shigella sonnei, Vibrio parahaemolyticus, Escherichia coli, Candida glabrata, Candida tropicalis, Candida parapsilosis, Candida albicans, Streptococcus agalactiae, Rickettsia, Listeria monocytogenes, Pseudomonas aeruginosa, Klebsiella oxytoca, and Klebsiella aerogenes) were used to evaluate the sensitivity and specificity of the established K. pneumoniae CDA assay. Total 224 batches of samples from other strains tested were negative and 296 batches of extracted K. pneumoniae DNA samples were positive by the developed CDA amplification approach, revealing high specificity and specificity of the diagnostic assay. In addition, the results of real-time fluorescence amplification of the K. pneumoniae CDA were in consistent with those of end-point colorimetric results.
Discussion: The established real-time fluorescence and visual CDA assays of K. pneumoniae with merits of rapid, sensitive and specificity could be helpful for on-site diagnosis and clinical screening in rural areas.
1 Introduction
Klebsiella pneumoniae (K. pneumoniae), a Gram-negative bacterium, is ubiquitously found on the mucosal surfaces of animals and humans, causing diseases including urinary tract infections, respiratory tract infections, bacteremia, liver abscesses, and pneumonia (Podschun and Ullmann, 1998; Siu et al., 2012; Snitkin et al., 2012; Wang et al., 2020). As a human commensal and conditioned pathogen, K. pneumoniae colonizes the gastrointestinal tract, usually causing infections in immunocompromised or hospitalized patients (Martin and Bachman, 2018; Russo and Marr, 2019). Recently, the emergence of K. pneumoniae with a high infection rate in hospitals has become a serious health issue (Gorrie et al., 2017; Zhang Y. et al., 2018). Thus, the development of rapid and accurate diagnostic tools for K. pneumoniae is essential to guide appropriate medication and therapy.
Conventional methods for K. pneumoniae detection, such as microscopic examination, biochemical identification, and serological detection, were complicated, strenuous, and time-consuming (Bachman et al., 2015; Zhang W. et al., 2018; Wang et al., 2019). Molecular biology-based technologies have been widely adopted for the identification of pathogens, with significant improvements in accuracy and sensitivity over the last few decades. Approaches based on the polymerase chain reaction (PCR) were carried out to achieve a phenotypic diagnosis of K. pneumoniae (Yu et al., 2018). Typically, the conserved rpoB, gapA, and 16S–23S internal transcribed spacer genes were selected for the identification of K. pneumoniae through PCR amplification (Diancourt et al., 2005). However, the requirements of skilled operators, complex procedures, and sophisticated equipment hindered the established PCR methods (Weng et al., 2023).
Currently, developments in isothermal amplification-based technologies are aiming to offer alternative methods for K. pneumoniae PCR detection with the merits of efficiency, sensitivity, and specificity gradually. Recently, a recombinase-aided amplification (RAA) assay was used to detect K. pneumoniae in milk samples by adding an expensive enzyme mixture. Loop-mediated isothermal amplification (LAMP)-based K. pneumoniae assays were developed by selecting sophisticated four- to six-oligonucleotide primers to amplify eight distinct regions (Liao et al., 2020). Therefore, considering that complex primer design and expensive recombinase might lead to inefficient application, an efficient, simple, and on-site detection assay of K. pneumoniae in clinical settings is necessary for direct and accurate treatment.
To overcome the drawbacks of PCR and LAMP methods, a novel nucleic acid detection technology named the closed dumbbell-mediated isothermal amplification (CDA) assay was established (Mao et al., 2022a). The CDA assay was simpler than other molecular diagnostic technologies as it did not require a thermal cycler, an expensive recombinase, or a long primer sequence (Gui et al., 2022). Compared with other isothermal amplification technologies, the establishment of the CDA assay required a concise and simple primer design to obtain a cost-efficient reaction (Mao et al., 2022b). In this study, we successfully established and evaluated real-time fluorescence and on-site visual-based molecular diagnostics of K. pneumoniae using the CDA method.
2 Materials and methods
2.1 Ethics approval statement
Ethical approval, including the waiver of informed consent for the clinical strains and samples collected in the laboratory of the First Affiliated Hospital of Ningbo University, was approved by the Ethics Committee of Clinical Investigation in the First Affiliated Hospital of Ningbo University under approval number 2024-055A-01. The research conformed to the principles of the Declaration of Helsinki. The study presented no more than minimal risk to subjects, and no personal information was obtained.
2.2 Reagents and materials
DNA primers were provided by BGI Biological Engineering Technology and Services Co. Ltd. (Shenzhen, China) or Sangon Biotech (Sangon, Shanghai, China). The Bst 2.0 WarmStart DNA polymerase and 10× ThermoPol reaction buffer [including 200 mM Tris–HCl, 100 mM KCl, 100 mM (NH4)2SO4, 20 mM MgSO4, and 1% Triton X-100] were purchased from New England BioLabs (Ipswich, MA, United States). A DNA molecular weight marker was purchased from Thermo Fisher Scientific (Waltham, MA, United States). Deoxynucleotide triphosphates (dNTPs), DNA purification kits, and DNA extraction kits were purchased from Sangon Biotech (Sangon, Shanghai, China). The commercial Klebsiella pneumoniae Real-Time PCR kit was purchased from Shanghai Liferiver Bio-Tech Co. Ltd. (Shanghai, China). Other reagents, unless specified, were obtained from Sigma-Aldrich (St. Louis, MO, United States).
A total of 30 clinical isolates of K. pneumoniae were collected from October 2023 to May 2024 by the research team from the laboratory of the First Affiliated Hospital of Ningbo University. Various non-K. pneumoniae clinical isolates and strains are listed in Table 1. Standard procedures for clinical isolates of the bacterium were cultured on Columbia blood agar plates in a CO2 incubator for 48 h and were preserved for later use (Luginbühl et al., 2017). The Candida strains were cultured in the yeast peptone dextrose (YPD) medium (Li et al., 2021), while Escherichia coli was cultured in the Luria-Bertani (LB) medium (Jira et al., 2018). The genome DNA from all isolates was extracted using the DNA purification kits and stored at −20°C. The online DNA copy number calculator1 was adopted to determine the copy numbers of targets, and the calculation procedures were carried out following the instructions provided by Zong et al. (2012).
2.3 Primer design
The CDA primer design procedures are illustrated in Figure 1A. We designed a CDA primer from a sequence of the transcriptional regulator rcsA gene of K. pneumoniae (GenBank: AY059955.1 and CP131701.1) after alignments (Dong et al., 2015). In the primer design procedure, the sequences of “forward 1,” “reverse 1,” and “middle” were abbreviated as F1, R1, and M, respectively. The site with the lowercase “c” represents “complementary.” The inner sequence between F1c and R1 is considered as M, which is separated into M1 and M2 equivalently. According to this structure, MF comprises a sequence complementary to M1 (M1c) and a sequence complementary to F1c (F1). MR comprises sequences (M2) complementary M2c and R1. Based on this scheme, we selected the optimal primer that was able to amplify the rcsA gene in a short time from four designed pairs of primers (Table 2, partially). To promote the CDA reaction, outer primers (F2 and R2) and loop primers (LRs) were designed and selected to optimize the CDA assay. The situations of all selected CDA primers used in this study are shown in different colors in Figure 1B. To compare the sensitivity of the CDA assay and PCR assay, PCR primers were designed with the same template sequences by DNAMAN Version 8.0 software. All sequences of primers are listed in Tables 2, 3, and these sequences are synthesized by Biological Engineering Technology and Services (BGI Biotech Co., Ltd., Shenzhen, China) or Sangon Biotech (Sangon, Shanghai, China).
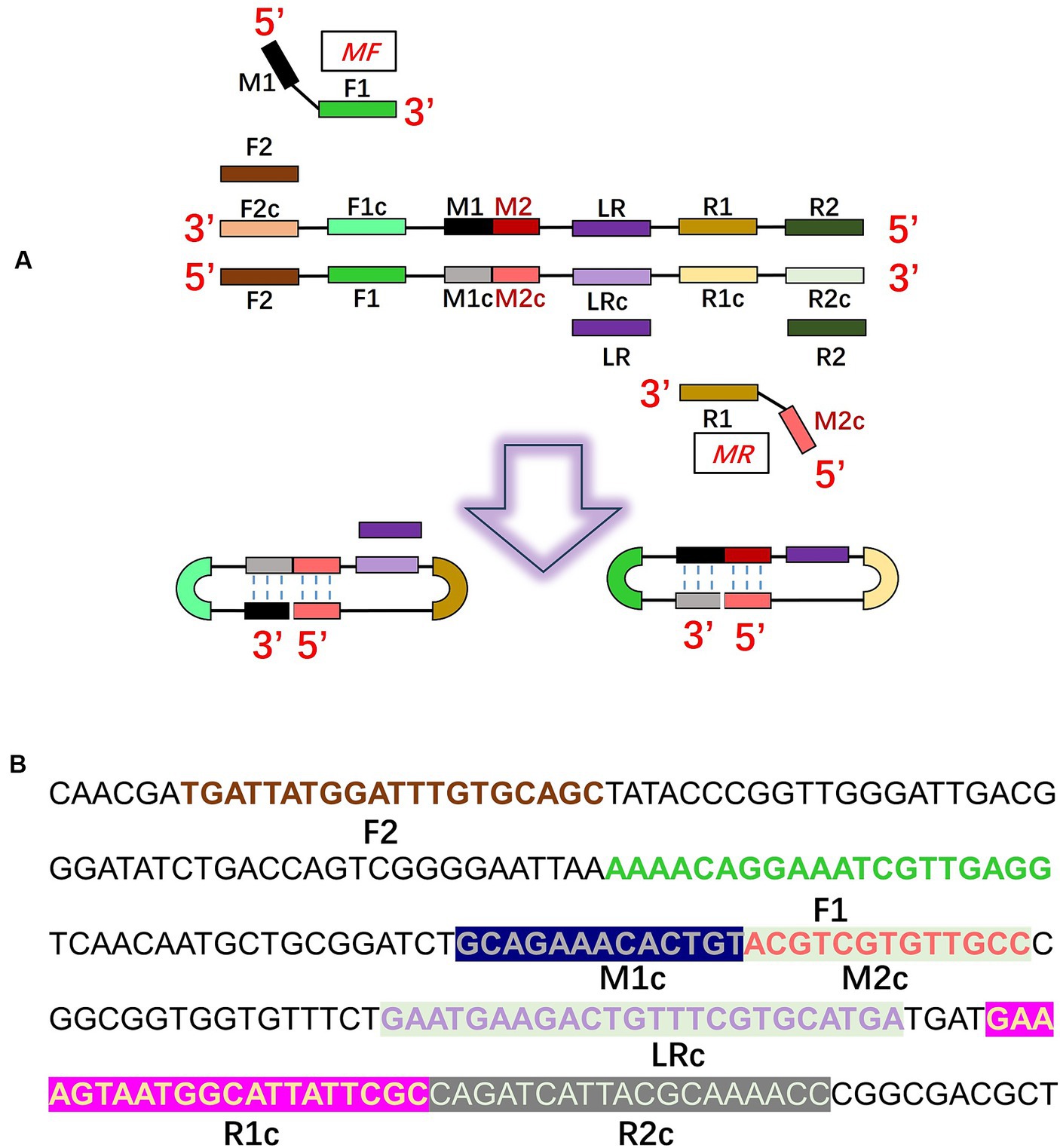
Figure 1. Principle of the closed dumbbell-mediated isothermal amplification (CDA) method for Klebsiella pneumoniae detection. (A) Overall scheme for primers of K. pneumoniae used in the CDA method. (B) Partial sequence of the gene of K. pneumoniae used for designing the primers of the CDA method.
2.4 CDA reaction
The standard CDA reactions were performed according to the method previously reported by our research group. The CDA reaction mixture consisted of 12.5 μL of 2 × isothermal amplification buffer [including 40 mM Tris–HCl, 20 mM KCl, 20 mM (NH4)2SO4, 16 mM MgSO4, and 0.2% Triton X-100], 1 μL MF (40 μM), 1 μL MR (40 μM), 1 μL Bst 2.0 WarmStart DNA polymerase, 1 μL for each EvaGreen or/and hydroxy naphthol blue (HNB), a proper number of nucleic acid templates, and RNase-free water up to 25 μL. Finally, 30 μL of paraffin oil was added to avoid possible cross-contamination from the aerosol of CDA product leakage. The amplification reaction was carried out under isothermal conditions (60 ~ 65°C) for 60 min and then terminated at 85°C for 10 min. The CFX96 Real-Time PCR detection system (California, USA: Bio-Rad) and Q3 Real-Time PCR instrument (California, USA: Applied Biosystems) were used for real-time fluorescence monitoring of CDA reactions and final melting curve analysis of the CDA products. Direct visual observation was adopted to detect CDA reaction results by adding the indicator HNB to the mixture. The post-CDA amplification mixture of colors shifted from purple to sky blue, indicating the production of insoluble magnesium pyrophosphate during the nucleic acid synthesis. In addition, the CDA products were analyzed by 1% agarose gel electrophoresis and stained with GelRed, and images were visualized using an ultraviolet (UV) transilluminator. For O-CDA reactions, other outer primers (0.2 μM) were added to the former CDA mixtures, and for reactions, other outer primers (0.2 μM) and loop primers (0.8 μM) were added to the former CDA mixtures. The negative control (NC) contained non-target nucleic acid samples or nuclease-free water.
2.5 Optimal amplification temperature of the established KP-CDA assay
To select the optimal temperature for the K. pneumonia CDA assay, identical reactions were performed at various temperatures (60, 61, 62, 63, 64, and 65°C, separately). The real-time fluorescence curves of each K. pneumoniae CDA reaction were recorded, and distilled water instead of target DNA samples were used as NCs to explore the merits of non-specific amplification of the developed assay. The temperature to carry on CDA amplification of the extracted K. pneumoniae DNA with a minimum cycle threshold (Ct) was chosen as the optimal temperature for the assay. The optimal temperature determination tests were repeated four times.
2.6 Determination of the sensitivity and specificity of the KP-CDA assay
The sensitivity of the developed CDA method was explored using the gradient-diluted DNA samples. The extracted K. pneumonia genomic DNA from clinical samples ranged from 1.2 × 10−5 ng/μL to 12 ng/μL with sterile water. Real-time fluorescence curves of 14 different DNA concentrations were assessed to determine the detection limit of the developed CDA method. NCs were included in every batch, consisting of double-sterilized water or non-target DNA samples to ensure there was no occurrence of non-specific amplification. In addition, the amplification products were further analyzed through gel electrophoresis.
To evaluate the specificity of the CDA method, 30 clinical strains of K. pneumoniae DNA and 14 non-K. pneumoniae strains (Staphylococcus aureus, Shigella sonnei, Vibrio parahaemolyticus, Escherichia coli, Candida glabrata, Candida tropicalis, Candida parapsilosis, Candida albicans, Streptococcus agalactiae, Rickettsia raoultii, Listeria monocytogenes, Pseudomonas aeruginosa, Klebsiella oxytoca, and Klebsiella aerogenes, listed in Table 1) were tested by the developed CDA method. The DNA of all strains was extracted using DNA purification kits. The CDA experimental results were obtained through real-time amplification curves, followed by a melting curve analysis of the CDA products and end-point colorimetric-based evaluations.
2.7 Real-time quantitative PCR assays
The extracted K. pneumoniae samples were amplified by real-time quantitative PCR (forward primer: CTACTATTATTATCGCCCGC and reverse primer: CCGCCAGTTTGTTTCAG) (Table 3). A 50-μL reaction volume was used for real-time quantitative PCR (qPCR), including the following components: 25 μL of 2 × Taq PCR Mix (Sangon, Shanghai, China), 1 μL for each primer (40 μM), 2 μL for the target, 1× EvaGreen (1 μL), and final nuclease-free water were used to adjust the volume to 50 μL. The PCR cycling conditions are denaturation at 95°C for 2.5 min, annealing at 55°C for 30 s, and extension at 72°C for 20 s, for a total of 35 cycles. Final concentration to achieve real-time fluorescence monitoring in the CFX96 Real-Time PCR detection system (Bio-Rad) and Q3 Real-Time PCR instrument (Applied Biosystems). The PCR conditions of the commercial Klebsiella Pneumoniae Real-Time PCR Kit from Shanghai Liferiver are maintaining at 37°C for 2 min, denaturation at 93°C for 15 s, and annealing and extension at 60°C for 60 s, for a total of 40 cycles. Moreover, identical DNA template dilutions were used to compare the sensitivity of the CDA assay, the PCR traditional assay, and the commercial PCR assay.
3 Results
3.1 Designing and screening of CDA primers for K. pneumoniae detection
Three batches of four pairs of CDA primers targeted at the K. pneumoniae rcsA gene were designed and selected to get the optimum primer set. The group of CDA reactions with a minimum cycle threshold determined by a real-time fluorescence monitoring curve was preferred for high amplification efficiency. As shown in Figure 2, the amplification curve of the CDA method revealed that the fourth group of primers could amplify the target gene in the shortest time and were selected as the optimal primers for K. pneumoniae with the CDA assay (Table 3). The threshold detection time of 1.2 ng/μL of the extracted K. pneumoniae DNA by the determined primers was 29 min, the melting temperature of the amplified product was 82.0°C, and no non-specific amplifications were observed in NCs. Both real-time amplification curves and melting curves showed that the developed CDA method for K. pneumoniae detection exhibited good repeatability and stability. In addition, a developed CDA assay combined with HNB was applied for simple visual detection of K. pneumoniae. In the HNB-based reaction mixture, the positive reaction is indicated by a color change from purple to light blue, whereas the negative reaction retains the purple color.
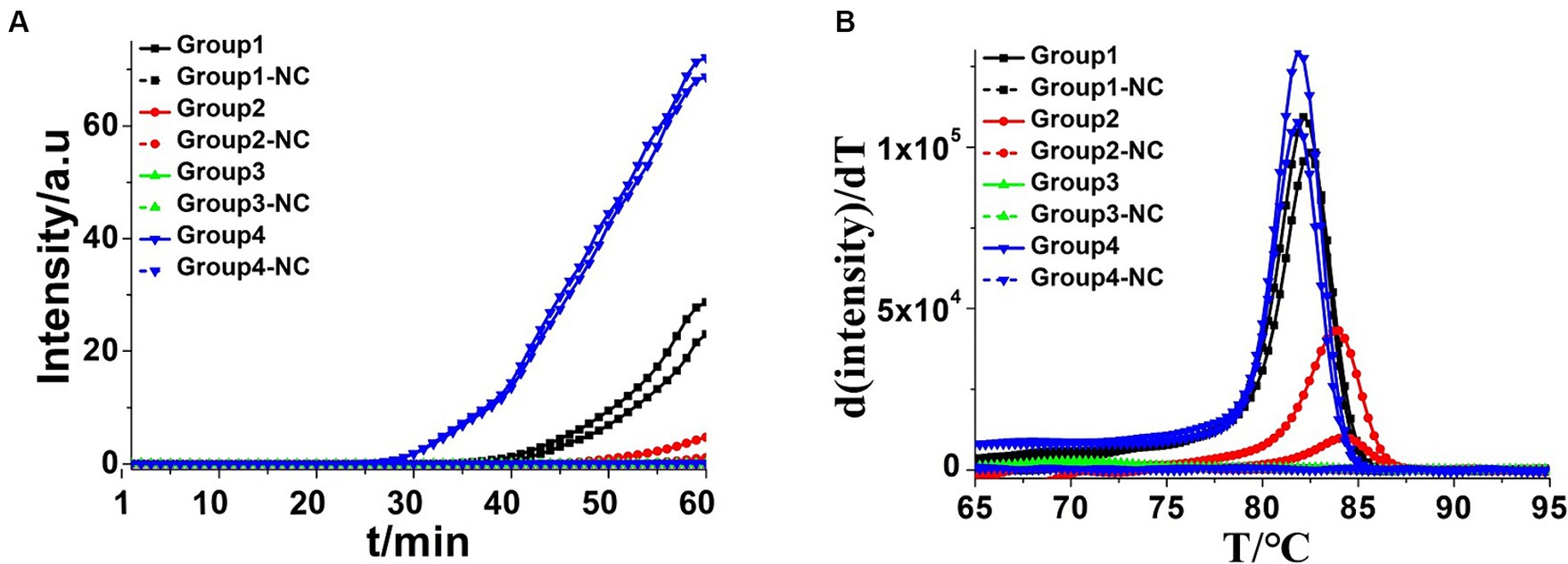
Figure 2. Amplifications of extracted Klebsiella pneumoniae DNA by CDA monitored by real-time PCR carried out at 60°C for 60 min. (A) Real-time CDA of different groups for Klebsiella pneumoniae DNA, Group 1 contained the primers of KP-MF-1 and KP-MR-1, and other groups contained the primers in order (in each set two positive reactions and two negative reactions). (B) Melting curve analysis of CDA products by real-time PCR. NC, negative control reaction without the targeted DNA.
3.2 Optimization of the CDA assay for K. pneumoniae
Accelerating primers (outer primers and loop primers) were added to the CDA reaction mixtures to achieve more efficient K. pneumoniae detection approaches, termed as OL-CDA. As shown in Figure 3A, the threshold time of detecting K. pneumoniae (1.2 ng/μL) was shortened by 4 min using the OL-CDA method, indicating that the reaction efficiency of the CDA method was improved by adding extra accelerating primers. After the identification of accelerated primers, the optimal temperature for an efficient K. pneumoniae OL-CDA assay was determined by incubating for 60 min at temperatures of 60, 61, 62, 63, 64, and 65°C, with four replicates at each temperature, respectively. The optimal reaction temperature for the developed OL-CDA method was set at 60°C to ensure perfect detection (Figure 4).
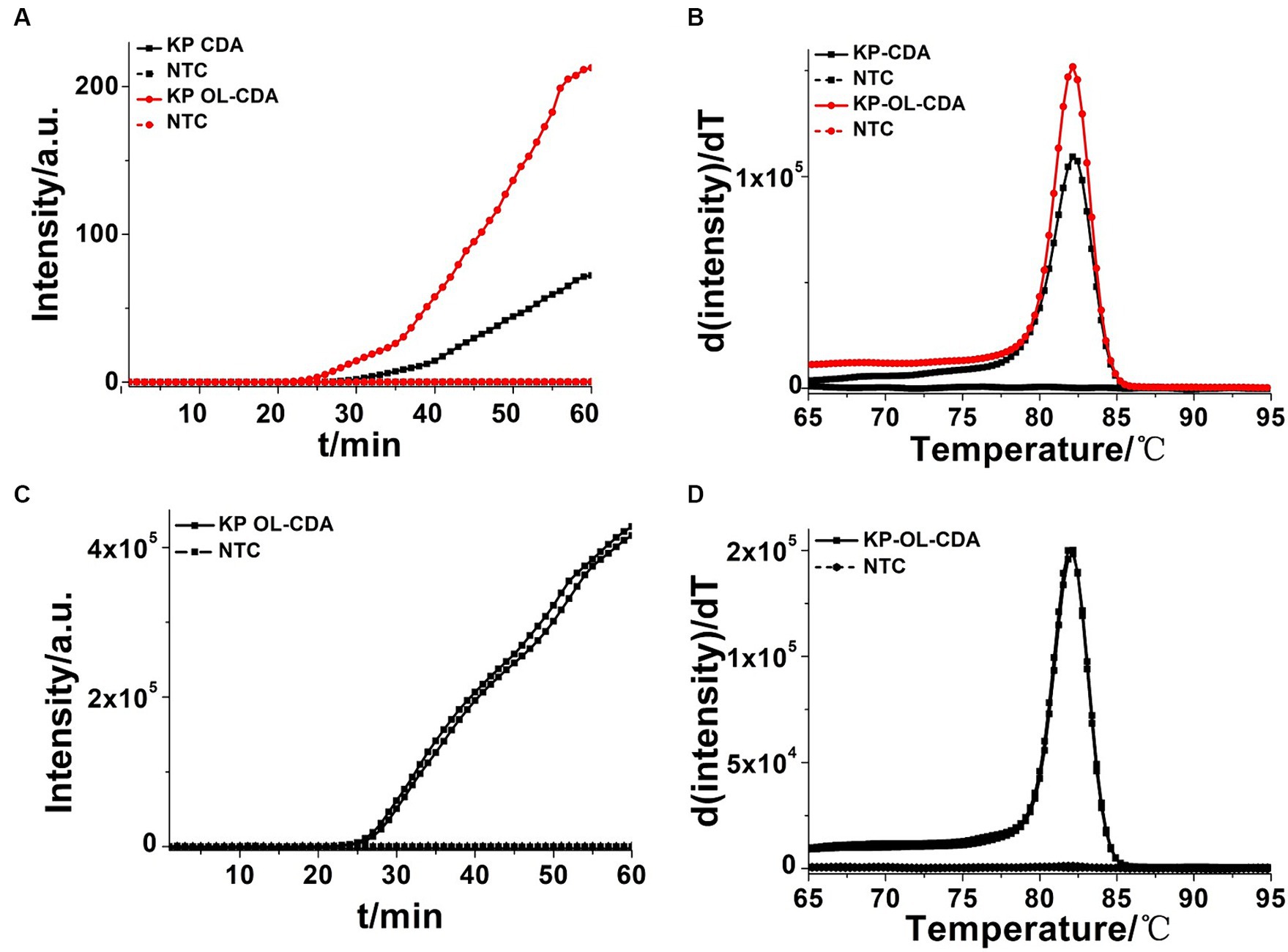
Figure 3. Amplifications of extracted Klebsiella pneumoniae DNA by KP-CDA and KP-OL-CDA monitored by real-time PCR. (A) Amplification plot of real-time KP-CDA and KP-OL-CDA for extracted DNA. (B) Melting curve analysis of KP-CDA and KP-OL-CDA products. (C) Repeatability analysis of the KP-OL-CDA monitored by real-time PCR at 60°C. (D) Repeatability analysis of melting curve analysis of KP-OL-CDA products. NTC, negative control reaction without the targeted DNA.
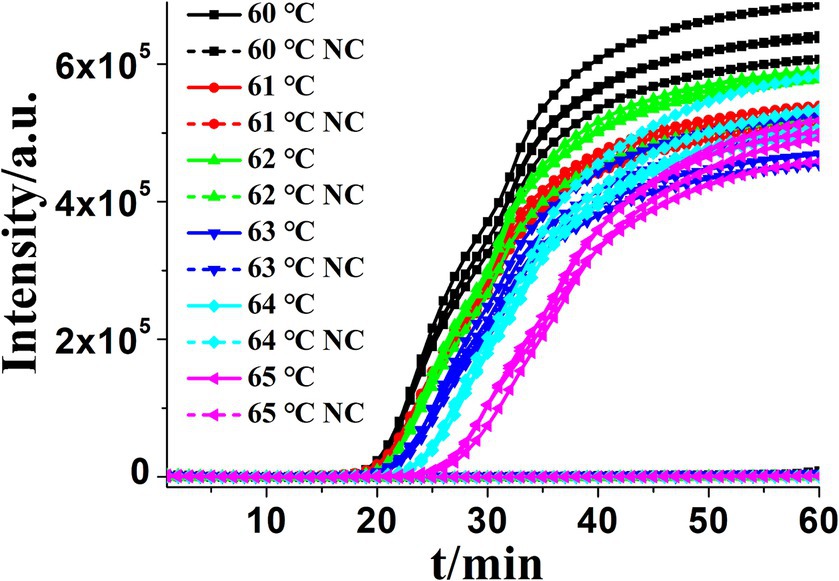
Figure 4. Amplification plot of real-time KP-OL-CDA for Klebsiella pneumoniae at different temperatures. Each set exhibited four positive reactions. NC, negative control, negative control reaction without the targeted DNA.
To further assess the repeatability of the developed assay, 12 ng/μL of the extracted K. pneumoniae DNA was amplified in eight positive and negative replicates independently through OL-CDA. Both the real-time amplification curve (Figure 3C) and melting curve (Figure 3D) indicated good repeatability of the OL-CDA method. In addition, the melting curves, as shown in Figures 3B,D, were completely identical, indicating that the accelerated primers would help to finish the amplification reaction in a shorter time without the introduction of non-specific amplification.
3.3 Sensitivity and specificity assay for CDA and PCR of K. pneumoniae
To determine the sensitivity of the OL-CDA assay, the DNA concentration of 10-fold diluted extracted K. pneumoniae DNA ranging from 12 to 1.2 × 10−5 ng/μL was prepared. As shown in Figures 5A,C, the amplification curve and the color change of the reaction products indicated that the limit of the OL-CDA method for K. pneumoniae was 1.2 × 10−5 ng/μL, which corresponds to approximately one copy of the target gene in the reaction system (Zong et al., 2012). In addition, the reproducibility of detection limit evaluation showed that the established OL-CDA method could amplify 1.2 × 10−4 ng/μL of K. pneumoniae-extracted genomic DNA. This repeatability was confirmed through real-time amplification (Figure 5A) and end-point visualization (Figure 5C) analyses. In comparison, the detection limit of the real-time qPCR assay using the specific primers F1 and R1 was 1.2 × 10−3 ng/μL of the extracted DNA (Figure 6A). The detection limit of the commercial real-time qPCR assay was 1.2 × 10−3 ng/μL of the extracted DNA (Figure 6B). Thus, the developed CDA assay was 100-fold more sensitive than the traditional PCR and 1,000-fold more sensitive than the commercial real-time qPCR assay. In addition, the melting curve analysis of K. pneumoniae using the real-time fluorescence OL-CDA method showed that the Tm values of 1.2 × 10−5 ng/μL of the extracted DNA were slightly different from other concentrations, which might be affected by a high concentration of non-targeted DNA sequences (Figure 5B).
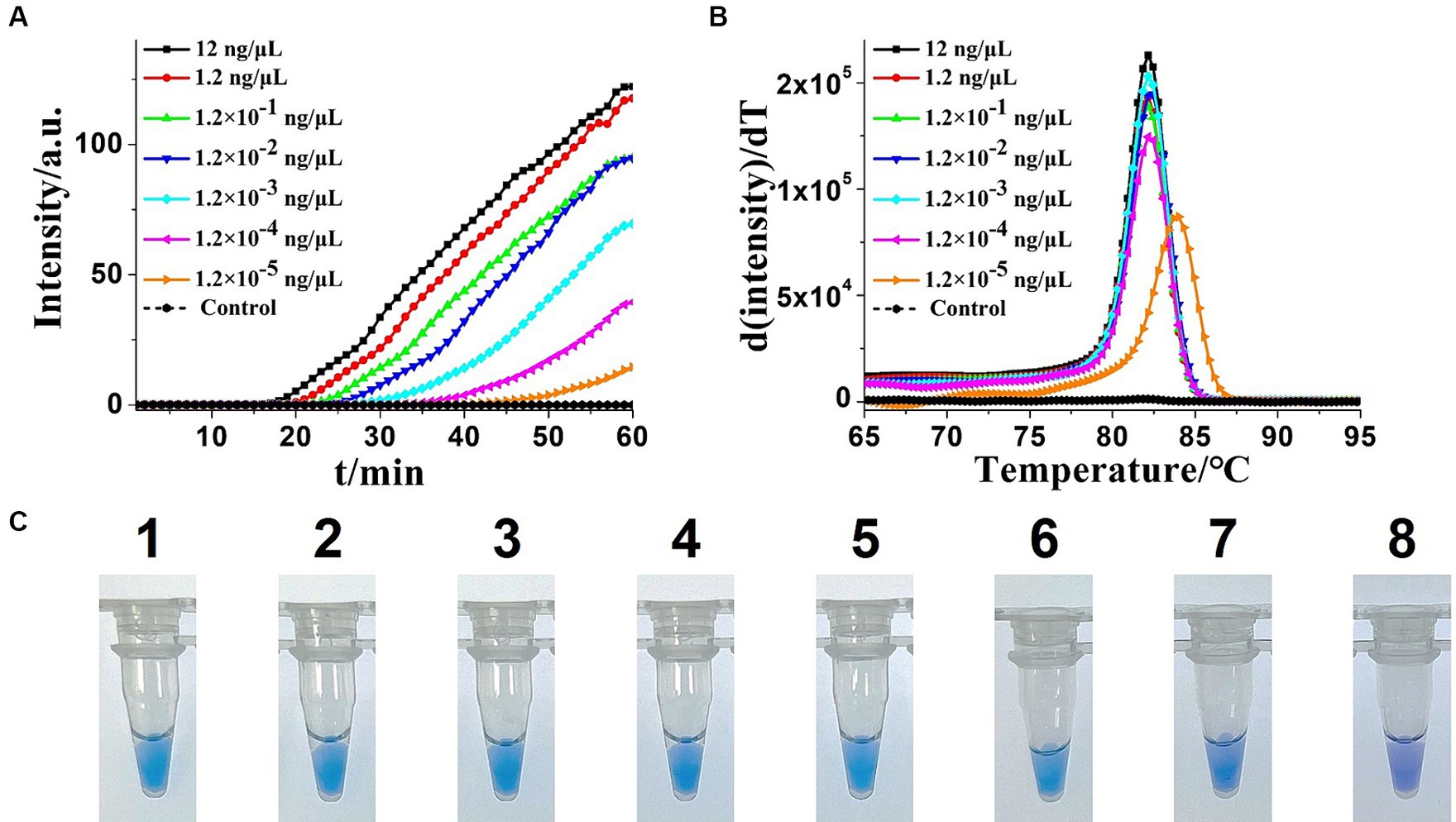
Figure 5. Sensitivity analysis of the extracted Klebsiella pneumoniae DNA KP-OL-CDA method by real-time and visual approaches. (A) KP-OL-CDA amplification is monitored by real-time PCR every 1 min at different concentrations of DNA. The reaction was performed at 60°C for 60 min. (B) Melting curve analysis of KP-OL-CDA products at different concentrations of DNA. (C) Sensitivity analysis of Klebsiella pneumoniae detection by visual detection of KP-OL-CDA. The numbers 1–8 presented the DNA concentrations were as follows: 12, 1.2, 1.2 × 10−1, 1.2 × 10−2, 1.2 × 10−3, 1.2 × 10−4, 1.2 × 10−5 ng/μL. NC, negative control reaction without the targeted DNA.
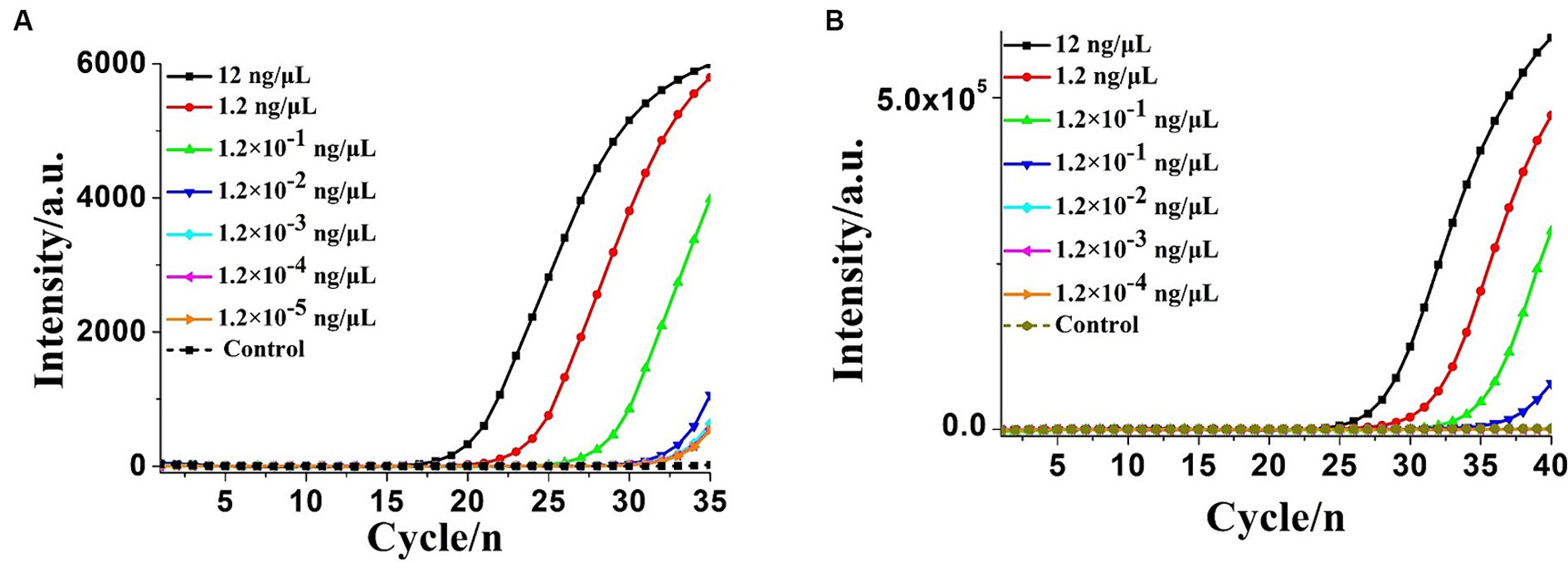
Figure 6. Real-time PCR assay for extracted Klebsiella pneumoniae DNA detection. (A) Real-time amplification plot by the PCR method developed in this study. (B) Real-time amplification plot using the commercial real-time quantitative PCR (qPCR) assay. NC, negative control reaction without the targeted DNA.
To assess the specificity of the OL-CDA assay for detecting K. pneumoniae by evaluating its reactivity with non-K. pneumoniae strains, the assay specifically amplified their DNA sequences from K. pneumoniae without cross-reaction with other non-K. pneumoniae strains (Figure 7A). The melting curve analysis was consistent with the former results (Figures 3B,D, 7B), and there was no difference between the positive control and different DNA samples, which further illustrated the good reproducibility of the developed K. pneumoniae CDA detection method. In addition, 30 clinical strains were adopted to evaluate the repeatability, practicability, and reliability of the developed OL-CDA method. Furthermore, the mixture of non-K. pneumoniae genomic DNAs and tissue sample DNAs was set as NCs to evaluate the solidity of the established method. The results showed that the newly established OL-CDA method can effectively detect K. pneumoniae in different non-K. pneumoniae strains and various NCs by both real-time fluorescence and visual end-point approaches (Figures 7, 8). The results showed that the developed CDA diagnostic assays for K. pneumoniae detection had high reaction efficiency, robustness, sensitivity, and specificity (both 100%) for a total of 520 batches of tests (Table 4).
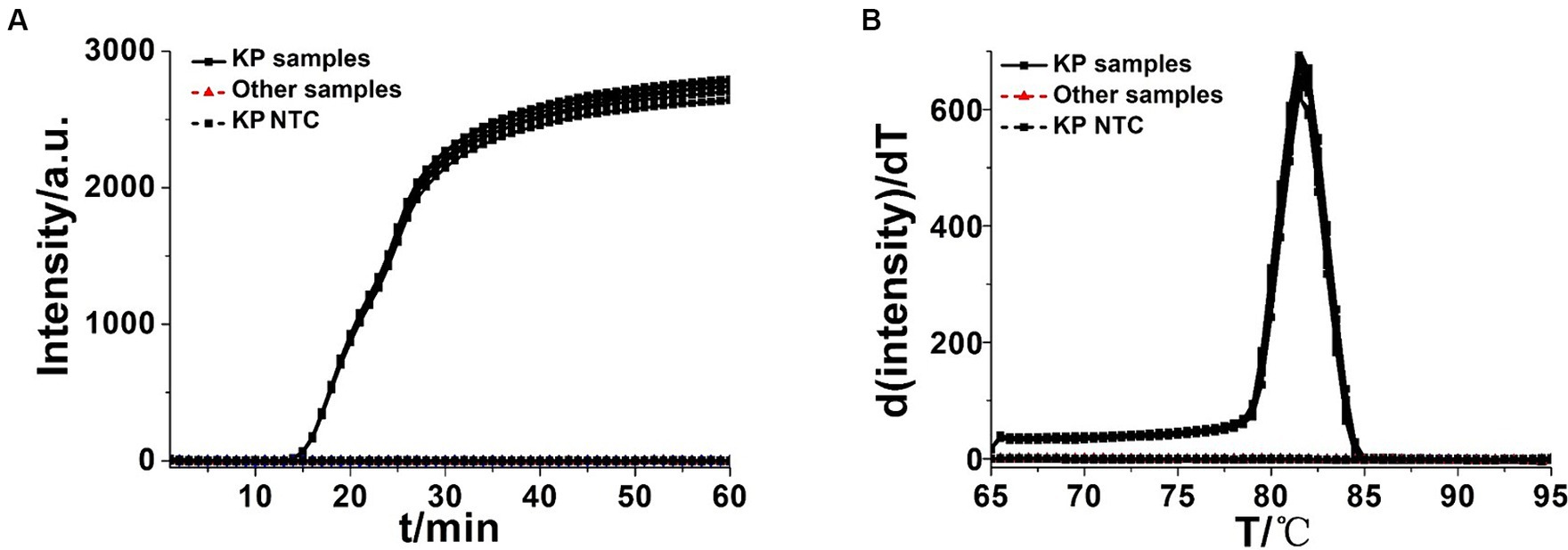
Figure 7. Real-time KP-OL-CDA assay. Real-time KP-OL-CDA reactions were carried out at 60°C for 60 min. (A) Real-time KP-OL-CDA for extracted Klebsiella pneumoniae DNA positive controls (KP samples), clinical Klebsiella pneumoniae strains, other samples (Staphylococcus aureus, Shigella sonnei, Vibrio parahaemolyticus, Escherichia coli, Candida glabrata, Candida tropicalis, Candida parapsilosis, Candida albicans, Streptococcus agalactiae, Rickettsia raoultii, Listeria monocytogenes, and Pseudomonas aeruginosa), and negative control reaction without the targeted DNA (NTC). (B) Melting curve analysis of the KP-OL-CDA products by real-time PCR. NTC, negative control reaction without the targeted DNA.
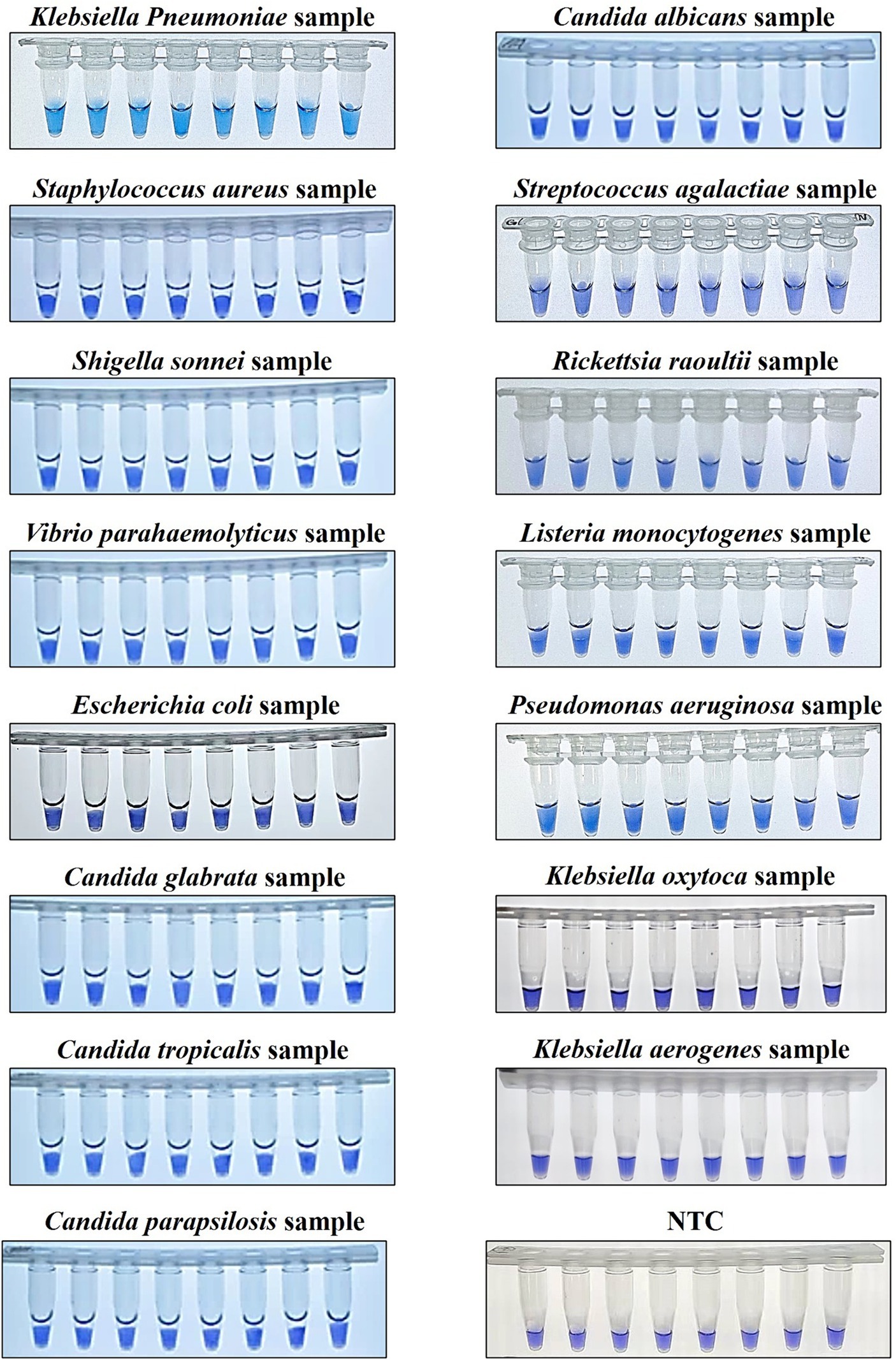
Figure 8. Colorimetric KP-OL-CDA assay using HNB. Line 1, positive controls (12 ng/μL of templates); Line 2, DNA samples extracted from Staphylococcus aureus; Line 3, DNA samples extracted from Shigella sonnei; Line 4, DNA samples extracted from Vibrio parahaemolyticus; Line 5, DNA samples extracted from Escherichia coli; Line 6, DNA samples extracted from Candida glabrata; Line 7, DNA samples extracted from Candida tropicalis; Line 8, DNA samples extracted from Candida parapsilosis; Line 9, DNA samples extracted from Candida albicans; Line 10, DNA samples extracted from Streptococcus agalactiae; Line 11, DNA samples extracted from Rickettsia raoultii; Line 12, DNA samples extracted from Listeria monocytogenes. Line 13, DNA samples extracted from Pseudomonas aeruginosa; Line 14, DNA samples extracted from Klebsiella oxytoca; Line 15, DNA samples extracted from Klebsiella aerogenes; Line 16, No template controls (RNase-free water). Due to space limitations, other results were not displayed. HNB, hydroxy naphthol blue.
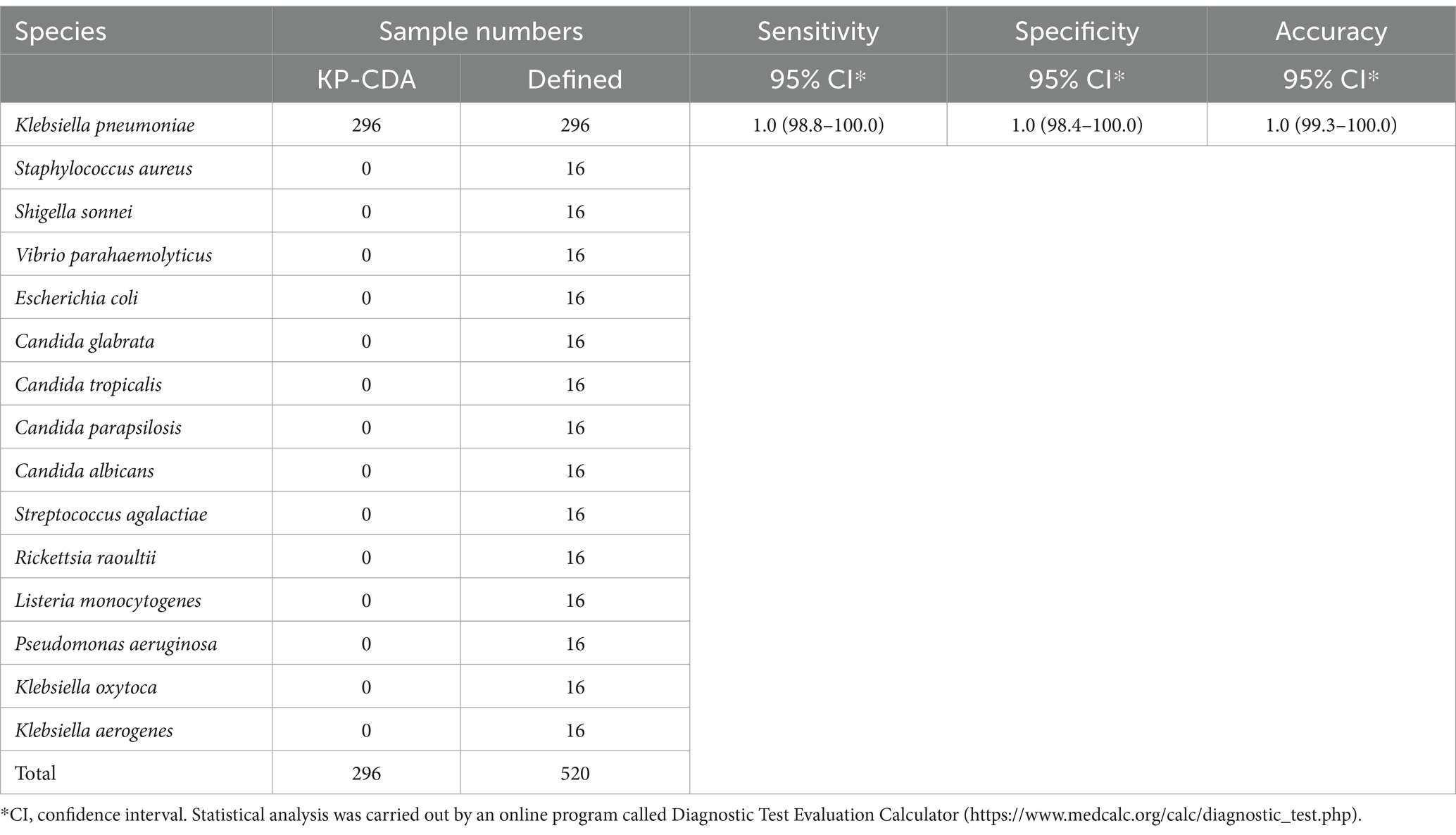
Table 4. Determination of sensitivity and specificity of the KP-CDA assay for Klebsiella pneumoniae.
4 Discussion
Klebsiella pneumoniae is a well-recognized nosocomial infected pathogen and a member of the ESKAPE group of antimicrobial-resistant pathogens (Enterococcus faecium, Staphylococcus aureus, Klebsiella pneumoniae, Acinetobacter baumannii, Pseudomonas aeruginosa, and Enterobacter species). These pathogens seriously threaten human health, and the drug resistance of these pathogens presents a significant therapeutic challenge (Bialek-Davenet et al., 2014; Mulani et al., 2019; De Oliveira et al., 2020). Therefore, early and rapid diagnosis of K. pneumoniae is beneficial for guiding clinical treatment, medication, and effective control of infections.
In recent years, reports regarding an increasing number of rapid detection methods for K. pneumoniae have been optimized constantly based on the LAMP assay (Griffioen et al., 2020; Rivoarilala et al., 2021). In the research field of isothermal amplification, LAMP-based diagnosis methods have exhibited the advantages of being rapid, simple, and accurate. Moreover, LAMP reactions were established by selecting 6–8 regions of the target gene, making them more specific and sensitive than the traditional PCR method (Solanki et al., 2013). However, the development of LAMP-based diagnosis methods was restricted by the complexity of designing primers and non-specific amplification (Poirier et al., 2021). In this study, we developed a simple CDA assay with high sensitivity and specificity, which would contribute to the rapid detection and on-site surveillance of K. pneumoniae infection in the clinical environment. In this study, we successfully established and optimized a CDA method for the rapid detection of K. pneumoniae by amplification of the specific rcsA gene (Su et al., 2018). In the CDA-based detection method, primers were designed and selected according to the CDA criteria, targeting the conserved rcsA gene of K. pneumoniae. The visual detection of K. pneumoniae through CDA also showed perfect repeatability.
Three important diagnostic indexes (detection limit, sensitivity, and specificity) of the developed K. pneumoniae CDA diagnostic assays were evaluated as common molecular diagnosis methods. The specificity test indicated that all 30 clinical K. pneumoniae strains were found to be positive and non-K. pneumoniae strains (Staphylococcus aureus, Shigella sonnei, Vibrio parahaemolyticus, Escherichia coli, Candida glabrata, Candida tropicalis, Candida parapsilosis, Candida albicans, Streptococcus agalactiae, Rickettsia raoultii, Listeria monocytogenes, Pseudomonas aeruginosa, Klebsiella oxytoca, and Klebsiella aerogenes) were found to be negative using the CDA assay. Furthermore, the detection limit of the K. pneumoniae CDA assay was determined to be 1.2 × 10−5 ng/μL of the extracted DNA sample, which corresponds to approximately one copy of the target gene in the reaction mixture. The detection limit of K. pneumoniae found using the CDA assay was 100–1,000 times lower than that of the corresponding PCR assay and the commercial PCR assay mentioned in this study.
Furthermore, the sensitivity and specificity in the detection of K. pneumoniae were both 100% from a total of 520 tests. Regarding the reported LAMP and recombinase-aided amplification (RAA)-based K. pneumoniae diagnostic assays, Schneider et al. (2019) evaluated that the LAMP method had a high rate of false-positive results due to the complicated primer design and amplification system. Hou et al. (2021) found that the accuracy of the RAA assay was 96.9%, with two false-positive results in a total of 64 batches evaluated. The developed K. pneumoniae CDA assays exhibited outstanding performance compared to the reported LAMP and RAA assays, with limited clinical sample evaluation in statistics. Overall, the developed assays may be suitable for primary hospitals with limited resources and for site inspections in disease control and prevention departments. Finally, to achieve simultaneous and multiplex detection of mixed infections, the CDA method coupled with lateral flow dipstick-based approaches would play a significant role (Mabrok et al., 2021).
In conclusion, a rapid, sensitive, specific, and convenient technology based on the CDA method for the detection of K. pneumoniae DNA was successfully established in this study. Considering the convenient operation of an HNB-based colorimetric detection system, a CDA assay for K. pneumoniae would be a potential measure for on-site diagnostic assays in underdeveloped regions.
Data availability statement
The original contributions presented in the study are included in the article/supplementary material, further inquiries can be directed to the corresponding author.
Ethics statement
The studies involving humans were approved by the Ethics Committee of Clinical Investigation in the First Affiliated Hospital of Ningbo University. The studies were conducted in accordance with the local legislation and institutional requirements. Written informed consent for participation in this study was provided by the participants' legal guardians/next of kin.
Author contributions
YZ: Conceptualization, Investigation, Methodology, Resources, Writing – original draft, Writing – review & editing. XC: Investigation, Methodology, Writing – review & editing. GO: Investigation, Methodology, Writing – review & editing. JW: Writing – review & editing. YS: Writing – review & editing. YL: Writing – review & editing. PZ: Writing – review & editing. FG: Writing – review & editing. SY: Supervision, Writing – review & editing. RM: Investigation, Methodology, Project administration, Supervision, Writing – original draft, Writing – review & editing.
Funding
The author(s) declare that financial support was received for the research, authorship, and/or publication of this article. This study was funded by the Traditional Chinese Medicine Administration of Zhejiang Province (grant no. 2022ZB324), the Medical Health Science and Technology Project of Zhejiang Provincial Health Commission (grant nos. 2022KY1113 and 2021KY273), the Natural Science Foundation of Ningbo City (grant nos. 2022J210 and 2023J142), and the Ningbo Clinical Research Center for Hematologic Malignancies (grant no. 2021L001).
Acknowledgments
We sincerely thank all the researchers who provided the test strains.
Conflict of interest
The authors declare that the research was conducted in the absence of any commercial or financial relationships that could be construed as a potential conflict of interest.
Publisher’s note
All claims expressed in this article are solely those of the authors and do not necessarily represent those of their affiliated organizations, or those of the publisher, the editors and the reviewers. Any product that may be evaluated in this article, or claim that may be made by its manufacturer, is not guaranteed or endorsed by the publisher.
Footnotes
References
Bachman, M. A., Breen, P., Deornellas, V., Mu, Q., Zhao, L., Wu, W., et al. (2015). Genome-wide identification of Klebsiella pneumoniae fitness genes during lung infection. MBio 6:e00775. doi: 10.1128/mBio.00775-15
Bialek-Davenet, S., Criscuolo, A., Ailloud, F., Passet, V., Jones, L., Delannoy-Vieillard, A. S., et al. (2014). Genomic definition of hypervirulent and multidrug-resistant Klebsiella pneumoniae clonal groups. Emerg. Infect. Dis. 20, 1812–1820. doi: 10.3201/eid2011.140206
De Oliveira, D. M. P., Forde, B. M., Kidd, T. J., Harris, P. N. A., Schembri, M. A., Beatson, S. A., et al. (2020). Antimicrobial resistance in ESKAPE pathogens. Clin. Microbiol. Rev. 33, 10–1128. doi: 10.1128/cmr.00181-19
Diancourt, L., Passet, V., Verhoef, J., Grimont, P. A., and Brisse, S. (2005). Multilocus sequence typing of Klebsiella pneumoniae nosocomial isolates. J. Clin. Microbiol. 43, 4178–4182. doi: 10.1128/jcm.43.8.4178-4182.2005
Dong, D., Liu, W., Li, H., Wang, Y., Li, X., Zou, D., et al. (2015). Survey and rapid detection of Klebsiella pneumoniae in clinical samples targeting the rcsA gene in Beijing, China. Front. Microbiol. 6:519. doi: 10.3389/fmicb.2015.00519
Gorrie, C. L., Mirceta, M., Wick, R. R., Edwards, D. J., Thomson, N. R., Strugnell, R. A., et al. (2017). Gastrointestinal carriage is a major reservoir of Klebsiella pneumoniae infection in intensive care patients. Clin. Infect. Dis. 65, 208–215. doi: 10.1093/cid/cix270
Griffioen, K., Cornelissen, J., Heuvelink, A., Adusei, D., Mevius, D., and Jan van der Wal, F. (2020). Development and evaluation of 4 loop-mediated isothermal amplification assays to detect mastitis-causing bacteria in bovine milk samples. J. Dairy Sci. 103, 8407–8420. doi: 10.3168/jds.2019-18035
Gui, Z., Cai, H., Wu, L., Miao, Q., Yu, J. F., Cai, T., et al. (2022). Visual closed dumbbell-mediated isothermal amplification (CDA) for on-site detection of Rickettsia raoultii. PLoS Negl. Trop. Dis. 16:e0010747. doi: 10.1371/journal.pntd.0010747
Hou, L., Li, D., Zhang, N., Zhao, J., Zhao, Y., and Sun, X. (2021). Development of an isothermal recombinase-aided amplification assay for the rapid and visualized detection of Klebsiella pneumoniae. J. Sci. Food Agric. 102, 3879–3886. doi: 10.1002/jsfa.11737
Jira, J., Rezek, B., Kriha, V., Artemenko, A., Matolínová, I., Skakalova, V., et al. (2018). Inhibition of E. coli growth by Nanodiamond and graphene oxide enhanced by Luria-Bertani medium. Nanomaterials 8:140. doi: 10.3390/nano8030140
Li, Q., Liu, J., Chen, M., Ma, K., Wang, T., Wu, D., et al. (2021). Abundance interaction in Candida albicans and Candida glabrata mixed biofilms under diverse conditions. Med. Mycol. 59, 158–167. doi: 10.1093/mmy/myaa040
Liao, W., Long, D., Huang, Q., Wei, D., Liu, X., Wan, L., et al. (2020). Rapid detection to differentiate Hypervirulent Klebsiella pneumoniae (hvKp) from Classical K. pneumoniae by identifying peg-344 with loop-mediated isothermal Amplication (LAMP). Front. Microbiol. 11:1189. doi: 10.3389/fmicb.2020.01189
Luginbühl, M., Weinmann, W., and Al-Ahmad, A. (2017). Introduction of sample tubes with sodium azide as a preservative for ethyl glucuronide in urine. Int. J. Legal Med. 131, 1283–1289. doi: 10.1007/s00414-017-1633-3
Mabrok, M., Elayaraja, S., Chokmangmeepisarn, P., Jaroenram, W., Arunrut, N., Kiatpathomchai, W., et al. (2021). Rapid visualization in the specific detection of Flavobacterium columnare, a causative agent of freshwater columnaris using a novel recombinase polymerase amplification (RPA) combined with lateral flow dipstick (LFD) assay. Aquaculture 531:735780. doi: 10.1016/j.aquaculture.2020.735780
Mao, R., Wang, T., Zhao, Y., Wu, X., Zhang, S., and Cai, T. (2022a). Closed dumbbell mediated isothermal amplification of nucleic acids for DNA diagnostic assays. Talanta 240:123217. doi: 10.1016/j.talanta.2022.123217
Mao, R., Wu, X., Miao, Q., and Cai, T. (2022b). Asymmetric stem-loop–mediated isothermal amplification of nucleic acids for DNA diagnostic assays by simple modification of canonical PCR primers. Front. Bioeng. Biotechnol. 10:931770. doi: 10.3389/fbioe.2022.931770
Martin, R. M., and Bachman, M. A. (2018). Colonization, infection, and the accessory genome of Klebsiella pneumoniae. Front. Cell. Infect. Microbiol. 8:4. doi: 10.3389/fcimb.2018.00004
Mulani, M. S., Kamble, E. E., Kumkar, S. N., Tawre, M. S., and Pardesi, K. R. (2019). Emerging strategies to combat ESKAPE pathogens in the era of antimicrobial resistance: a review. Front. Microbiol. 10:539. doi: 10.3389/fmicb.2019.00539
Podschun, R., and Ullmann, U. (1998). Klebsiella spp. as nosocomial pathogens: epidemiology, taxonomy, typing methods, and pathogenicity factors. Clin. Microbiol. Rev. 11, 589–603. doi: 10.1128/cmr.11.4.589
Poirier, A. C., Kuang, D., Siedler, B. S., Borah, K., Mehat, J. W., Liu, J., et al. (2021). Development of loop-mediated isothermal amplification rapid diagnostic assays for the detection of Klebsiella pneumoniae and Carbapenemase genes in clinical samples. Front. Mol. Biosci. 8:794961. doi: 10.3389/fmolb.2021.794961
Rivoarilala, L. O., Victor, J., Crucitti, T., and Collard, J. M. (2021). LAMP assays for the simple and rapid detection of clinically important urinary pathogens including the detection of resistance to 3rd generation cephalosporins. BMC Infect. Dis. 21:1037. doi: 10.1186/s12879-021-06720-5
Russo, T. A., and Marr, C. M. (2019). Hypervirulent Klebsiella pneumoniae. Clin. Microbiol. Rev. 32, 10–1128. doi: 10.1128/cmr.00001-19
Schneider, L., Blakely, H., and Tripathi, A. (2019). Mathematical model to reduce loop mediated isothermal amplification (LAMP) false-positive diagnosis. Electrophoresis 40, 2706–2717. doi: 10.1002/elps.201900167
Siu, L. K., Yeh, K. M., Lin, J. C., Fung, C. P., and Chang, F. Y. (2012). Klebsiella pneumoniae liver abscess: a new invasive syndrome. Lancet Infect. Dis. 12, 881–887. doi: 10.1016/s1473-3099(12)70205-0
Snitkin, E. S., Zelazny, A. M., Thomas, P. J., Stock, F., Henderson, D. K., Palmore, T. N., et al. (2012). Tracking a hospital outbreak of carbapenem-resistant Klebsiella pneumoniae with whole-genome sequencing. Sci. Transl. Med. 4:148ra116. doi: 10.1126/scitranslmed.3004129
Solanki, R., Vanjari, L., Ede, N., Gungi, A., Soory, A., and Vemu, L. (2013). Evaluation of LAMP assay using phenotypic tests and conventional PCR for detection of blaNDM-1 and blaKPC genes among carbapenem-resistant clinical gram-negative isolates. J. Med. Microbiol. 62, 1540–1544. doi: 10.1099/jmm.0.059907-0
Su, K., Zhou, X., Luo, M., Xu, X., Liu, P., Li, X., et al. (2018). Genome-wide identification of genes regulated by RcsA, RcsB, and RcsAB phosphorelay regulators in Klebsiella pneumoniae NTUH-K2044. Microb. Pathog. 123, 36–41. doi: 10.1016/j.micpath.2018.06.036
Wang, C. H., Lu, P. L., Liu, E. Y., Chen, Y. Y., Lin, F. M., Lin, Y. T., et al. (2019). Rapid identification of capsular serotype K1/K2 Klebsiella pneumoniae in pus samples from liver abscess patients and positive blood culture samples from bacteremia cases via an immunochromatographic strip assay. Gut Pathog. 11, 1–8. doi: 10.1186/s13099-019-0285-x
Wang, G., Zhao, G., Chao, X., Xie, L., and Wang, H. (2020). The characteristic of virulence, biofilm and antibiotic resistance of Klebsiella pneumoniae. Int. J. Environ. Res. Public Health 17:6278. doi: 10.3390/ijerph17176278
Weng, Z. Y., You, Z., Yang, J., Mohammad, N., Lin, M. S., Wei, Q. S., et al. (2023). CRISPR-Cas biochemistry and CRISPR-based molecular diagnostics. Angewandte Chem. Int. Edn. 62:e202214987. doi: 10.1002/anie.202214987
Yu, F., Lv, J., Niu, S., Du, H., Tang, Y. W., Pitout, J. D. D., et al. (2018). Multiplex PCR analysis for rapid detection of Klebsiella pneumoniae Carbapenem-resistant (sequence type 258 [ST258] and ST11) and Hypervirulent (ST23, ST65, ST86, and ST375) strains. J. Clin. Microbiol. 56, 10–1128. doi: 10.1128/jcm.00731-18
Zhang, W., Guo, Y., Li, J., Zhang, Y., Yang, Y., Dong, D., et al. (2018). In vitro and in vivo bactericidal activity of ceftazidime-avibactam against Carbapenemase-producing Klebsiella pneumoniae. Antimicrob. Resist. Infect. Control 7:142. doi: 10.1186/s13756-018-0435-9
Zhang, Y., Wang, Q., Yin, Y., Chen, H., Jin, L., Gu, B., et al. (2018). Epidemiology of Carbapenem-resistant Enterobacteriaceae infections: report from the China CRE network. Antimicrob. Agents Chemother. 62, 10–1128. doi: 10.1128/aac.01882-17
Keywords: Klebsiella pneumoniae, closed dumbbell-mediated isothermal amplification (CDA), sensitivity, specificity, point of care test
Citation: Zhang Y, Chen X, Ouyang G, Wang J, Sun Y, Lai Y, Zhang P, Guo F, Yang S and Mao R (2024) Development and evaluation of rapid and simple detection of Klebsiella pneumoniae using closed dumbbell-mediated isothermal amplification diagnostic assay. Front. Microbiol. 15:1435010. doi: 10.3389/fmicb.2024.1435010
Edited by:
Abdelazeem Algammal, Suez Canal University, EgyptReviewed by:
Amit Ranjan, Columbia University, United StatesMahmoud Mabrok, Suez Canal University, Egypt
Copyright © 2024 Zhang, Chen, Ouyang, Wang, Sun, Lai, Zhang, Guo, Yang and Mao. This is an open-access article distributed under the terms of the Creative Commons Attribution License (CC BY). The use, distribution or reproduction in other forums is permitted, provided the original author(s) and the copyright owner(s) are credited and that the original publication in this journal is cited, in accordance with accepted academic practice. No use, distribution or reproduction is permitted which does not comply with these terms.
*Correspondence: Rui Mao, bXIzNzQ5QDE2My5jb20=
†These authors have contributed equally to this work