- 1State Key Laboratory for Biology of Plant Diseases and Insect Pests, Institute of Plant Protection, Chinese Academy of Agricultural Sciences, Beijing, China
- 2Institute of Plant Protection and Agro-Products Safety, Anhui Academy of Agricultural Sciences, Hefei, China
- 3Department of Biology, College of Science, United Arab Emirates University, Al Ain, United Arab Emirates
- 4Department of Entomology, Abdul Wali Khan University Mardan, Mardan, Pakistan
- 5Key Laboratory of Integrated Management of Crop Diseases and Pests, Department of Plant Pathology, College of Plant Protection, Nanjing Agricultural University, Nanjing, China
Plant parasitic nematodes (PPNs) pose a significant threat to global crop productivity, causing an estimated annual loss of US $157 billion in the agriculture industry. While synthetic chemical nematicides can effectively control PPNs, their overuse has detrimental effects on human health and the environment. Biocontrol agents (BCAs), such as bacteria and fungi in the rhizosphere, are safe and promising alternatives for PPNs control. These BCAs interact with plant roots and produce extracellular enzymes, secondary metabolites, toxins, and volatile organic compounds (VOCs) to suppress nematodes. Plant root exudates also play a crucial role in attracting beneficial microbes toward infested roots. The complex interaction between plants and microbes in the rhizosphere against PPNs is mostly untapped which opens new avenues for discovering novel nematicides through multi-omics techniques. Advanced omics approaches, including metagenomics, transcriptomics, proteomics, and metabolomics, have led to the discovery of nematicidal compounds. This review summarizes the status of bacterial and fungal biocontrol strategies and their mechanisms for PPNs control. The importance of omics-based approaches for the exploration of novel nematicides and future directions in the biocontrol of PPNs are also addressed. The review highlighted the potential significance of multi-omics techniques in biocontrol of PPNs to ensure sustainable agriculture.
1 Introduction
Plant parasitic nematodes (PPNs) pose a major worldwide threat to important agricultural crop productivity. There are over 4,100 documented species of PPNs (Nicol et al., 2011), including cyst nematodes (Heterodera and Globodera spp.), root-knot nematodes (Meloidogyne spp.), lesion nematodes (Pratylenchus spp.), and dagger nematodes (Xiphinema spp.). It is estimated that PPNs cause an average yield loss of 12.6% equal to $157 billion in 20 major commercial crops including tomato, soybean, peanut, potato, rice and sugarcane around the world (Ayaz et al., 2021; Ning et al., 2022). They infect a wide range of plants, impacting their health and overall productivity. Root Knot nematodes (RKNs) species such as M. javanica, M. incognita, M. hapla, and M. arenaria can damage important crops by up to 90% and increase their susceptibility to other pathogens. Being root invaders, they disrupt nutrient and water uptake, leading to reduced crop growth and yield (Migunova and Sasanelli, 2021). PPNs weaken plant defense, making them more susceptible to other diseases. They secrete effector proteins and affect the host plants’ defensive mechanisms, which results in a significant economic impact on agricultural production (Ayaz et al., 2021; Ali et al., 2023b).
PPNs mostly dwell in soil and affect all parts of plants like roots, stems, leaves, and flowers. They feed by penetrating the plant cells with a flexible style. The stylet is attached to three or five pharyngeal glands, helping in penetration, effectors delivery, and parasitism (Kishore et al., 2022). The PPNs can be divided into two groups based on their feeding behaviors such as ectoparasitic and endoparasitic. However, the most common notaries and damaging sedentary endoparasitic are root-knot nematodes and cyst nematodes. The global growing population needs more food, therefore, the control of PPNs in different regions of the world requires more attention to sustainable agriculture (Pires et al., 2022; Mokrini et al., 2024).
The most common and effective method for controlling PPNs is using synthetic nematicides. However, the excessive use of agrochemicals poses negative effects on human health and the environment, leading to legislative pressure and a ban on these chemicals. This has prompted the development of alternative strategies for PPN control (Gao et al., 2016; Ali et al., 2023b). BCAs offer a promising alternative and environmental approach for managing PPNs cost-effectively (Ali et al., 2023a). The BCAs play important role in plant growth promotion under stress condition. Various rhizospheric bacteria and fungi, such as Bacillus thuringiensis, Xenorhabdus bovienii, Pseudomonas fluorescens, B. amyloliquefaciens, Pasteuria penetrans, Trichoderma harzianum, and Paecilomyces lilacinus have been found to effectively control PPNs through different mechanisms, including preventing egg hatching, destroying females and second-stage juvenile nematodes (J2s) in the soil (Kishore et al., 2022; Lawal et al., 2022). These BCAs can directly or indirectly combat PPNs by competing for nutrients and niche, producing lytic enzymes, antibiotics, volatile compounds, and toxic metabolites. Indirect antagonism occurs through the induction of plant ISR or the release of small molecules in the rhizosphere that affect nematodes feeding behavior and sex ratio (Ayaz et al., 2021; Migunova and Sasanelli, 2021).
Many plants growth-promoting rhizobacteria (PGPR) and beneficial fungi enhance plant health by triggering ISR, which boost plant defense mechanisms against different phytopathogens (Song et al., 2022). However, the knowledge regarding the complex communication network between plants and microbes in the rhizosphere against nematodes is still limited (Engelbrecht et al., 2018). In the current omics era, advanced technologies, and approaches, such as metabolomics, offer new opportunities for developing novel nematicides to improve biocontrol strategies. By using omics techniques, particularly metabolomics, with high throughput analysis novel nematicides can be developed to efficiently control PPNs (White et al., 2017; Mohanram Praveen, 2019). The objectives of the present review are to summarize the use of bacterial and fungal biocontrol agents for controlling plant PPNs and emphasize the importance of omics-based biocontrol of nematodes for discovering novel nematicides in the future. The review will also discuss the challenges and future directions for the biocontrol of PPNs to ensure sustainable agriculture.
2 Bacterial biocontrol agents for PPNs
Nematodes and microbial flora are two crucial elements of the soil biotic ecosystem that have coevolved over long periods. Utilizing bacteria as biological control agents to suppress nematodes in the plant rhizosphere ensures efficient control of PPNs (AbdelRazek and Yaseen, 2020; Sun et al., 2021). The influence of plant growth-promoting bacteria (PGPB) on PPNs population density is particularly significant, with genera such as Pseudomonas, Serratia, and Bacillus, showing high levels of biological control efficiency during the previous two decades (Khabbaz et al., 2019; Ayaz et al., 2021). The Bacillus spp. strains have been reported to produce a wide range of secondary metabolites and volatile organic compounds (VOCs) with strong nematicidal activity against PPNs (Seong et al., 2021; Ali et al., 2023a).
Studies have shown that B. subtilis OKB105 and B. cereus 09B18 culture filtrates possess strong nematicidal activity up to 95% against J2s of M. javanica and H. filipjevi (Xia et al., 2011; Zhang et al., 2016). The endophytic bacterium K6, isolated from coffee plant leaves, caused 65% mortality of P. coffea while B. subtilis OKB105 and B. amyloliquefaciens B3 showed nematicidal activity against Aphelenchoides besseyi, Ditylenchus destructor, and Bursaphelenchus xylophilus nematodes with percent mortalities of 85, 79, and 100%, respectively. The report also showed that Serratia plymuthica M24T3 also exhibited a strong nematicidal activity with a 100% mortality rate against pinewood (Proença et al., 2019; Migunova and Sasanelli, 2021). However, some PGPBs are effective in promoting plant growth but not in suppressing plant nematodes as reported by numerous studies conducted in a greenhouse, microplate, and field conditions (Ayaz et al., 2021; Ali et al., 2023b). Serratia proteamaculans Sneb 851 has shown high nematicidal potential against M. incognita, while Bacillus thuringiensis strains are known for their inhibitory potential against PPNs through Cry protein production (Jisha et al., 2013; Zhao et al., 2018).
Pseudomonas fluorescens F113 has strongly affected G. rostochiensis J2 stage nematodes mobility and egg hatchability. B. firmus GB-126 was also reported to control R. reniformis involving egg hatching reduction and alleviation of infestation in cotton (Cronin et al., 1997; Castillo et al., 2013). Bacillus spp. strains have also been reported to produce volatile organic compounds (VOCs) with strong nematicidal activity. For example, B. atrophaeus GBSC56 VOCs such as dimethyl disulfide (DMDS), 2-undecanone (2-UD) and methyl isovalerate (MIV) demonstrated strong nematicidal activity up to 90% against M. incognita. These VOCs induced severe oxidative stress in nematodes, which subsequently caused rapid death. It also promoted plant growth and triggered ISR against M. incognita in both in vitro and in greenhouse experiments (Ayaz et al., 2021). The most effective PGPB against different PPNs reported in the literature were summarized in Table 1.
3 Fungal biocontrol agents for PPNs
The fungal biocontrol agents to control PPNs is a topic of great interest among researchers. It has been discovered that fungi and their metabolites effectively suppress the PPNs population in agriculturally important crops. The fungi are believed to be a major source of bioactive compounds to control PPNs. Fungal biocontrol of PPNs is an area of active research that aims to develop strategies for combating PPNs (Tariq et al., 2018; Pires et al., 2022). Trichoderma species are well known for their ability to parasitize infectious juveniles to prevent nematodes from entering the roots, thereby enhancing crop growth and productivity (Herrera-Parra et al., 2018; Mukhtar et al., 2021). The RKNs in tomatoes were significantly inhibited by Trichoderma strain TH by enhancing phenols, flavonoids, lignin, cellulose, jasmonic acid (JA), and salicylic acid (SA), and decreased the levels of hydrogen peroxide (H2O2), malondialdehyde (MDA), and electrolyte leakage (Yan et al., 2021). T. asperellum T00 regulates defense enzymes and has enormous potential as a biocontrol agent against P. brachyurus in soybean plants. Studies also showed that T. harzianum could efficiently inhibit M. incognita infection with a 61.88% reduction rate in tomato plants. It was also reported that T. harzianum reduced the amount of nematode population, egg masses, and root gall index in brinjal plants. Other studies showed that tomatoes developed systemic resistance against the RKN by P. chlamydosporia M10.43.2 involving reduced infection (32–43%) and female reproduction (14.7–27.6%) (Dababat and Sikora, 2007; Ghahremani et al., 2019). The endophytic fungus Acremonium sclerotigenum was also observed to increase M. incognita J2s mortality (95%) and decrease egg-hatching rates (Yao et al., 2023). A novel endophytic fungus Chaetomium ascotrichoides 1–24-2 from Pinus massoniana was found effective against B. xylophilus with 99% mortality rate and reduced the nematode infestation in pine seedling (Kamaruzzaman et al., 2024).
Nematophagous fungi employ various mechanisms to target nematodes, including predatory fungi like Arthrobotrys oligospora and Drechslerella spp., which form large constricting rings and hyphal networks to trap nematodes. Endoparasitic fungi, an obligatory parasite of nematodes, such as Drechmeria coniospora, directly attach to nematodes to kill them. Facultative parasitic fungi, such as Pochonia chlamydosporia, Paecilomyces lilacinus, and Pochonia rubescens target sedentary nematode stages, including nematode eggs, cysts, as well as adult female (Al-Ani et al., 2022; Kishore et al., 2022). Some nematophagous fungi can also function as facultative saprotrophs, feeding on organic matter in the absence of nematodes. Soil rich in organic matter supports the survival of these fungi. There is yet another group of toxin-producing fungi, such as Pleurotus ostreatus that immobilize nematodes before penetrating their cuticles with hyphae (Pires et al., 2022; de Freitas Soares et al., 2023). Various fungal biocontrol agents have been identified for targeting different types of nematodes, as summarized in Table 2.
4 Biocontrol mechanisms of PPNs suppression
BCAs, particularly bacteria and fungi employ various strategies to suppress PPNs population in both laboratory and field experiments. The BCAs are mostly documented to suppress PPNs directly or indirectly through different methods (Ayaz et al., 2021; Liang et al., 2022). Bacterial BCAs produce a wide variety of products, including extracellular enzymes, toxins, secondary metabolites, and VOCs to kill nematodes directly or induce plant ISR induction for indirect PPN suppression by regulating defense-related genes (Migunova and Sasanelli, 2021; Ali et al., 2023b). PGPR competes for nutrients and space, affecting nematode populations in the rhizosphere. Fungal BCAs also exhibit strong nematicidal activity through the production of bioactive compounds that can kill nematodes directly or induce ISR against nematodes by regulating defense-related genes (Gao et al., 2016; Pires et al., 2022). The mechanisms of bacterial and fungal for PPN control are briefly discussed below.
4.1 Bacterial biocontrol mechanisms for PPNs
Bacteria use various mechanisms to suppress PPNs, which can aid in the development of effective biocontrol strategies against nematode infestations. These mechanisms involve direct and indirect antagonistic interactions between bacteria and PPNs (Ayaz et al., 2021, 2023) as illustrated in Figures 1A,B. Studies have shown that bacteria exert direct effects on PPNs through colonization, parasitism, and antibiosis, which include the production of lytic enzymes, antibiotics, toxins, and VOCs (Timper, 2011; Migunova and Sasanelli, 2021). For instance, Pasteuria spp., an obligate nematode parasitic bacterium, directly attaches to nematode cuticles and multiplies in nematodes body to destroy them (Orr et al., 2020). Another direct action mechanism involves the production of nematicidal compounds by PGPB such as Bacillus spp., which produce extracellular enzymes like proteases, chitinases, collagenases, lipases, and enzyme complexes that affect PPN populations at different life stages (Migunova and Sasanelli, 2021; Pires et al., 2022). Glucanases, cellulases, and pectinases produced in S. marcescens were found to control M. incognita effectively (Migunova and Sasanelli, 2021; Khan et al., 2023), while crystal (Cry) proteins were observed to be produced by B. thuringiensis and damage the internal organs in a wide range of PPNs. Additionally, Bacillus spp. produce lipopeptides, surfactin, bacillomycin D, fengycins, iturins, and bacteriocins to inhibit M. incognita (Penha et al., 2020; Liang et al., 2022).
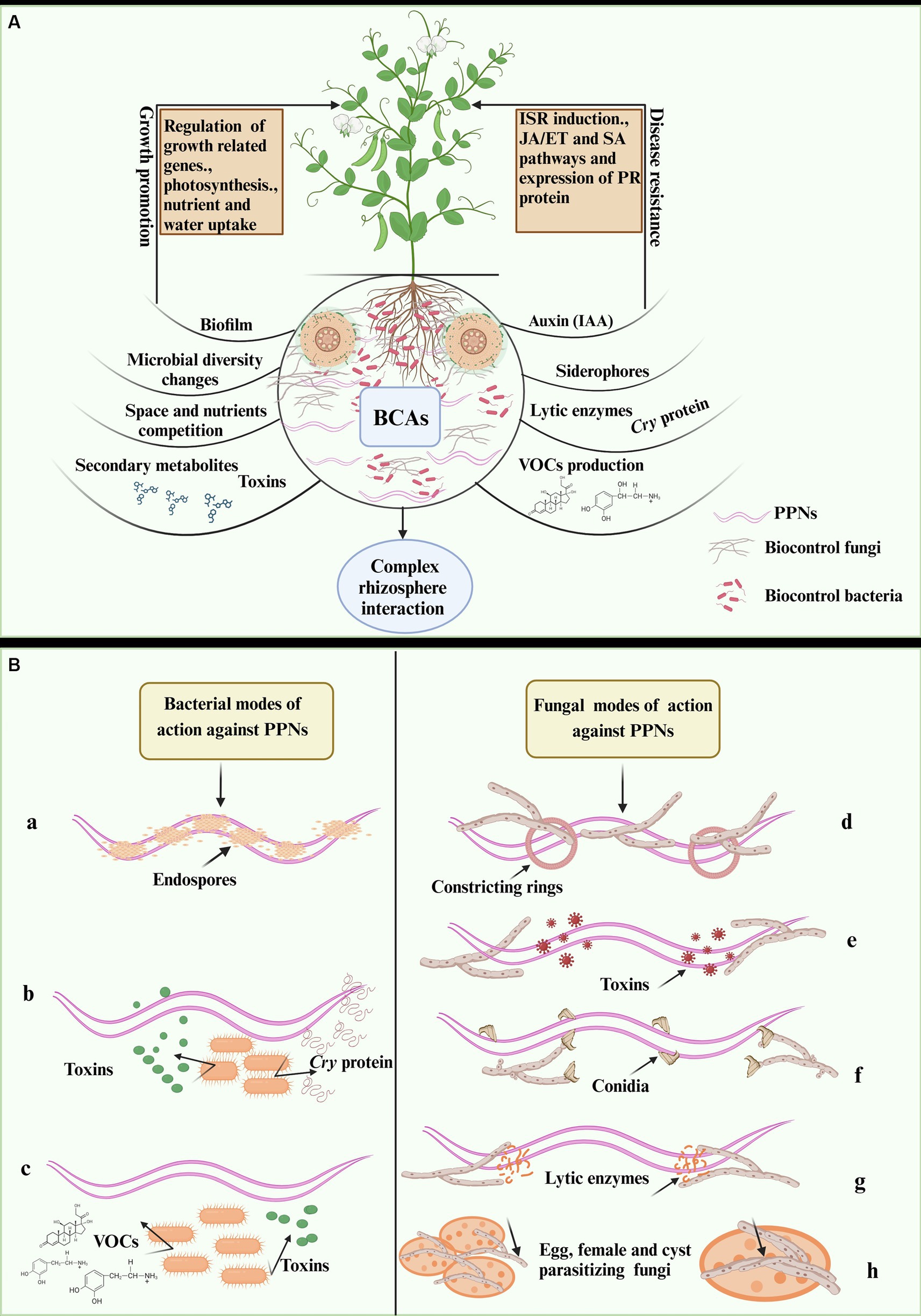
Figure 1. Illustration of bacterial and fungal biocontrol of PPNs (A) rhizospheric complex interaction and biocontrol of PPNs in infested plants (B) illustration of different modes of bacteria against nematodes (a-c), (a) endoparasitic bacteria (b) crystal forming (opportunistic bacteria) and (c) rhizobacteria. Fungal mode of action against nematodes (d-h), (d) nematode trapping or predatory fungi, (e) toxin-producing fungi (f) endoparasitic fungi, (g) lytic enzymes producing fungi for PPNs suppression and (g) eggs and cysts parasitizing (opportunistic fungi). The figure is designed using biorender https://app.biorender.com dated 4/30/2024.
The compound 2,4-diacetyl phloroglucinol (DAPG) from fluorescent Pseudomonas spp. reduces the motility of juvenile nematodes and inhibits eggs hatching of potato cyst nematode, Globodera rostochiensis. It has also been reported that endoparasitic nematodes, which live in plant roots and aerial sections, are suppressed by toxins produced by Photorhabdus and Xenorhabdus species, found in entomopathogenic nematodes (Cronin et al., 1997; Cimen et al., 2022). Pseudomonas spp. release hydrogen cyanide, while Bacillus spp. produce hydrogen sulfide, both of which have nematicidal effects on important PPNs (Pires et al., 2022). Furthermore, bacterial volatiles like dimethyl disulfide (DMDS) and 2-Undecanone (2-UD) produced by B. atrophaeus have shown strong nematicidal activity against M. incognita (Ayaz et al., 2021; Ali et al., 2023b). The deep-sea bacterium Virgibacillus dokdonensis produces four nematicidal volatiles: acetaldehyde, dimethyl disulfide, ethylbenzene, and 2-butanone. These volatiles exhibit different modes of action against J2 of M. incognita, including attraction, repellence, fumigation, egg-hatching inhibition, and direct contact-killing (Chen et al., 2024). Based on past studies, bacteria from various genera contain a wide range of bioactive compounds which need extensive research to explore novel nematicides for sustainable agriculture.
Indirect methods employed by PGPR for PPNs control include siderophore production, hormones, phosphate solubilization, nitrogen fixation, induction of plant ISR, and modifications of the plant microbiome (Ayaz et al., 2021; Migunova and Sasanelli, 2021). PGPR produces indole acetic acid (IAA) to enhance plant growth under nematode infestation and induce host tolerance against PPNs by stimulating the production of various compounds (siderophores, lipopolysaccharides, exopolysaccharides, N-acyl-homoserine lactones, etc.) and enzymes such as ascorbate peroxidase, β-1,3-glucanase, chitinase, catalase, lipoxygenase, phenylalanine ammonia-lyase, polyphenol oxidase and superoxide dismutase (Ayaz et al., 2021; Liang et al., 2022).
The PGPR has also been documented to colonize the plant roots to reduce the severity of PPNs infestation as shown in Figure 1A. Previous studies reported white clover colonization by B. cereus B1 and P. fluorescens P2 increased host resistance to H. trifolii. Further, the combined effect of root colonization by P. fluorescens CHA0 and exogenous SA treatment has been found to induce ISR in tomato plants against M. javanica (Migunova and Sasanelli, 2021; Pires et al., 2022). Plant bacterial endophytes can be beneficial to their host as they stimulate plant growth and prevent the spread of plant diseases. Tomato roots containing the endophytic bacterium Bacillus cereus BCM2 were found to be resistant to J2s of M. incognita, and the colonization can significantly minimize damage caused by the nematode (Li et al., 2019). Several studies have been conducted on Bacillus as endophytic bacteria for the management of RKN. It has been found that B. licheniformis, B. megaterium, B. pumilus, B. mycoides, and B. thuringiensis effectively lower the number of galls and egg masses produced by M. incognita (Yu et al., 2015). Overall, bacteria from different genera offer a diverse array of bioactive compounds, highlighting the need for developing novel nematicides for sustainable agriculture. The bacterial biocontrol agents and their mechanisms for PPNs control are given in Figure 1B.
4.2 Fungal biocontrol mechanisms against PPNs
Utilizing beneficial fungi to control nematode populations in plants and crops is known as “fungal biocontrol of plant nematodes.” Fungi can exert their biocontrol activity through various mechanisms targeting different stages of nematode. Fungi are categorized into different groups based on their mechanisms for suppressing PPNs, as shown in Figure 1B (Jansson and Lopez-Llorca, 2004; Pires et al., 2022). Predatory fungi, such as Arthrobotrys and Dactylella species use nematodes as their food source by developing specialized structures to capture nematodes. Parasitic fungi such as Nematophthora and Hirsutella, parasitize nematodes, penetrating and proliferating in their bodies, ultimately causing their death (Migunova and Sasanelli, 2021; Khan et al., 2023). Nematode-trapping fungi, such as Arthrobotrys spp. have specialized structures like constricting rings, adhesive nets, or knobs to trap nematodes, immobilizing and consuming them (Pires et al., 2022; Mokrini et al., 2024). Colonizing fungi can colonize plant roots or the rhizosphere, competing with nematodes for nutrients and space, indirectly reducing nematode numbers (Abd-Elgawad and Askary, 2018). Some fungi produce chemicals that prevent nematode eggs from hatching or obstruct their attraction to plant roots. Opportunistic saprotrophic fungi target nonmotile stages, such as nematode eggs, and cysts. Purpureocillium lilacinum is best suited to target the nematode eggs, as it can enter the egg through its conidia (Khan and Tanaka, 2023). It has also been reported that fungi, like Trichoderma spp. produce secondary metabolites with a potential nematicidal effect. These compounds can either kill nematodes directly or interfere with biological processes, affecting their growth, reproduction, or survival (Jansson and Lopez-Llorca, 2004; Siddiqui and Shaukat, 2004).
Beneficial fungi can also trigger host ISR against PPNs by colonizing plant roots and enhancing plant resistance. Endophytic fungi like arbuscular mycorrhizae fungi (AMF) can enhance nutrient and water uptake during PPN infestations. These fungi regulate plant antioxidant enzymes and defense-related genes, triggering host ISR against PPNs (Schouteden et al., 2015; Poveda et al., 2020; Tolba et al., 2021). Beneficial fungi can activate SA-mediated responses against PPNs, similar to responses induced by necrotrophic pathogens, involving both SA and JA pathways (Pires et al., 2022; Tyśkiewicz et al., 2022). In conclusion, fungal biocontrol agents offer a range of strategies for regulating nematodes through predatory, parasitic, nematicidal, trapping, antagonistic, and defense-inducing mechanisms. These environment-friendly methods provide long-term nematode management in horticultural and agricultural fields, improving soil health, and crop productivity, and reducing reliance on chemical nematicides (Li et al., 2007; Lawal et al., 2022). Further research and development in this field will enhance the capacity of fungal biocontrol agents to combat plant-parasitic nematodes. The different mechanisms involving fungal biocontrol of PPNs are illustrated in Figure 1B.
5 Prominent nematicides from bacteria and fungi
Nematicides derived from bacteria and fungi provide environmentally acceptable and sustainable solutions for managing PPNs. These biological control products reduce nematode infestations, promote soil health, and contribute to sustainable crop production (Siddiqui and Shaukat, 2004; Li et al., 2007). Ongoing research and development in this field aim to enhance the application and efficacy of bacterial and fungal nematicides, thereby improving integrated pest management practices and reducing reliance on chemical pesticides (Pires et al., 2022; Khan et al., 2023). This section aims to outline the key bacterial and fungal nematicides highlighted in the literature.
5.1 Bacterial-based nematicides
It has been documented that Bacillus and Pseudomonas spp. along with other rhizosphere bacteria, produce antimicrobial substances that are toxic to nematodes (Li et al., 2015; Ali et al., 2023b). Most research focuses on rhizobacteria lytic enzymes for controlling PPNs. Rhizobacteria produce a variety of lytic enzymes, such as chitinase, protease, cellulase, lipase, glucanases keratinase, etc. These hydrolytic enzymes disrupt nematode biology, development, and metabolism by breaking down the essential chemical components of the nematode exoskeleton and eggshell (Castaneda-Alvarez and Aballay, 2016; Migunova and Sasanelli, 2021). Chitinases hydrolyze the polymeric linkages in the chitin matrix of the eggshell, leading to eggshell rupture and the hatching of immature eggs. Proteases break down the peptide links that hold glucose-protein molecules together, destroying the nematode’s body structure (Kishore et al., 2022). A purified protease from B. cereus NJSZ-13 was recently discovered to be nematicidal against the pinewood nematode. It was also observed that nematode vulnerability to nematophagous fungus and antagonistic bacteria, which can lead to the nematode’s death, is caused by the hydrolysis of collagen in the cuticle layer. Collagenase from the strains of P. fluorescens FP805PU and Brevibacterium frigoritolerans FB37BR strongly affects M. ethiopica and X. index (Kishore et al., 2022; Li et al., 2023).
Additionally, a lipid layer found in the eggshell of Heterodera species of cyst nematodes serves as a protective coat that prevents moisture loss, especially in unfavorable circumstances. The strains of B. thuringiensis FB833T and FS213P, as well as B. amyloliquefaciens FR203A, exhibit strong lipase activity, making them nematicidal agents against X. index (Castaneda-Alvarez et al., 2016). A wide range of other hydrolytic enzymes, such as pectinases, cellulases, and glucanases from Pseudomonas spp., influence the amount of M. incognita in soil. These lytic enzymes interact with other secondary metabolites to regulate PPNs, highlighting the need for extensive research on their nematicidal mechanisms (Kishore et al., 2022; Ahmed et al., 2023). In addition to lytic enzymes, B. thuringiensis produces crystal protein (Cry) with strong nematicidal activity against PPNs. Several Cry proteins, such as Cry5, Cry6, Cry12, Cry13, Cry14, Cry21, and Cry55 exhibit nematicidal activity by damaging the intestinal lining, affecting pore development, cells, and vacuoles (Li et al., 2015; Liang et al., 2022).
Bacteria also produce potent secondary metabolites with strong nematicidal activity against PPNs. Sphingosine, an unsaturated hydrocarbon chain amino alcohol isolated from B. cereus exhibited strong nematicidal activity that caused severe oxidative damage to M. incognita (Gao et al., 2016; Kishore et al., 2022). B. thuringiensis produces thuringiensin, a thermostable metabolite with strong control efficacy against the soybean cyst nematode (Heterodera glycines). Thuringiensin is recognized as an ATP analog that inhibits RNA production by competing with ATP on binding sites, a mechanism like that of aldicarb, a carbamate insecticide. B. thuringiensis, the strain that produces thuringiensin, has been shown to produce trans-aconitic acid, a powerful inhibitor of aconitase in the TCA cycle (Jisha et al., 2013; Seong et al., 2021). Volatiles such as 2-undecanone and 2-nonanone produced by Bacillus spp. also exhibit strong nematicidal activity against pine wood nematode B. xylophilus. The integrity of the nematode pharynx and intestine is destroyed by the 2-nonanone treatment, although more investigation is needed to completely understand the mechanism of action (Deng et al., 2022). P. fluorescens produces 2,4-DAPG that functions as a proton ionophore to diffuse the proton gradient across the mitochondrial membrane. The toxicity assay reveals that 2,4-diacetyl phloroglucinol greatly decreases M. incognita eggs hatching but has no effect on the juvenile nematodes. Previous studies also reported hydrogen cyanide, a metabolite that certain Pseudomonas species generate inhibits mitochondrial cytochrome oxidase and plays a role in the suppression of plant-parasitic nematodes, such as M. javanica (Seong et al., 2021; Kishore et al., 2022).
Prokaryotic bacteria called actinomycetes are famous for producing a wide range of primary and secondary metabolites with antimicrobial properties against different pathogens (Pires et al., 2022). Avermectin B1, also known as abamectin, is a macrocyclic lactone commonly used to control insects and parasites produced by Streptomyces avermitilis. It disrupts glutamate-gated chloride channel receptors, causing high toxicity to plant-parasitic nematodes such as B. xylophilus and M. incognita. Milbemectin, initially extracted from S. hygroscopicus, is comparable to avermectin in terms of its molecular structure and mode of action (Wolstenholme and Rogers, 2005; El-Ashry et al., 2021). Spectinabilin, first identified from S. spectinabilis showed considerable nematicidal activity against B. xylophilus, with an LC50 value of 0.84 mg/L (Wolstenholme and Rogers, 2005; Seong et al., 2021). Spinosad, a mixture of spinosyns isolated from Saccharopolyspora spinosa showed nematicidal activity against M. incognita. The compound interferes with gamma-aminobutyric acid receptors and nicotinic acetylcholine receptors in nematodes, disrupting neural activity and subsequent paralysis (Radwan et al., 2019.; Kishore et al., 2022). The identification and function of many microbial secondary metabolites are still unknown, limiting our understanding of the molecular targets of bioactive compounds from PGPR. Due to these limitations, the potential nematicidal effects of certain chemicals are not extensively evaluated in the context of PPNs. Omics and molecular docking approaches will allow us to study these bioactive compounds in a targeted manner to ensure sustainable PPNs control in agriculture. The potential bacterial nematicides documented in the literature are given in Figure 2A.
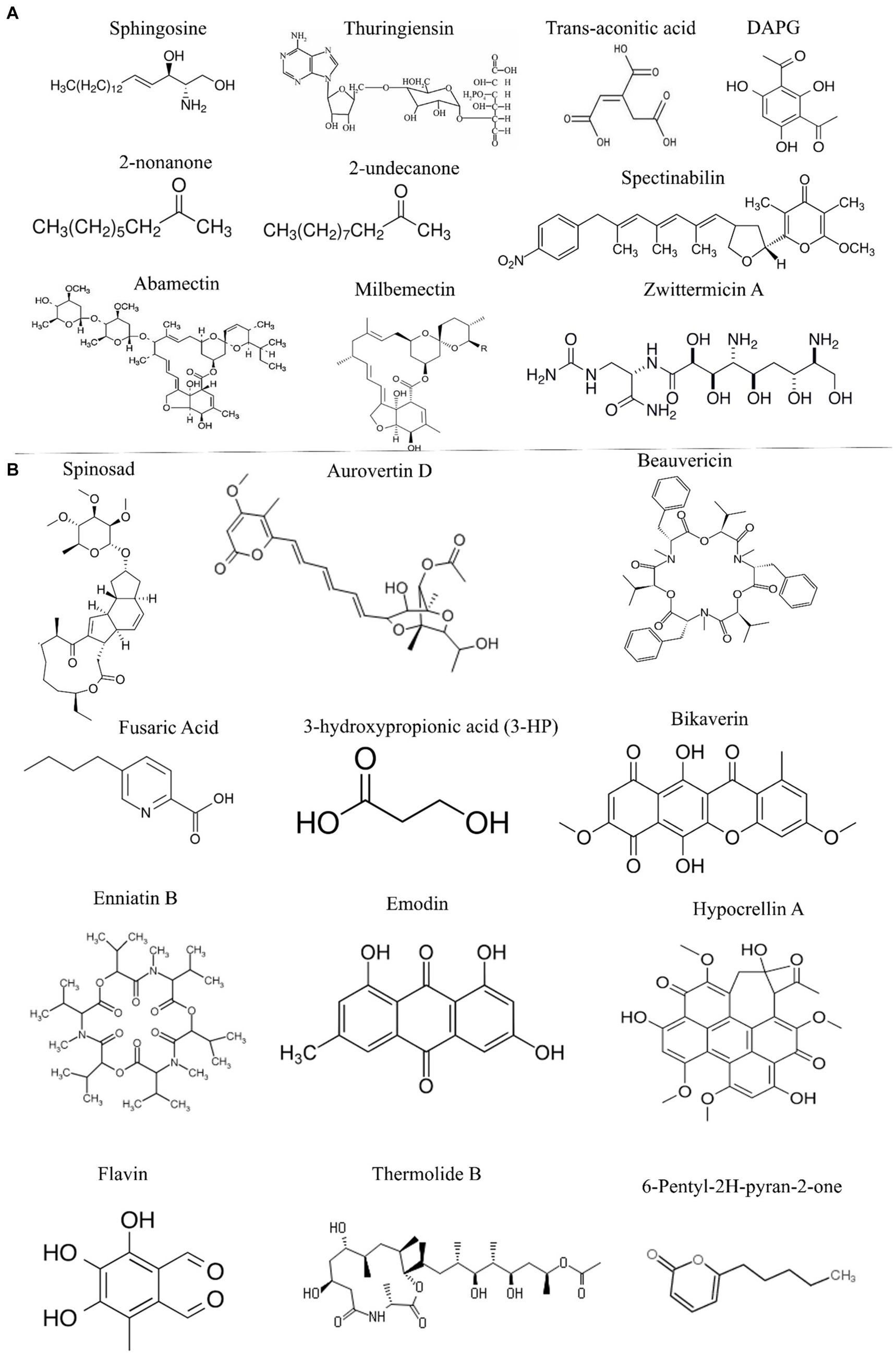
Figure 2. Potential nematicidal compounds from bacterial and fungal biocontrol agents. (A) Bionematicides isolated from different bacteria. (B) Different bionematicides isolated from fungal biocontrol agents.
5.2 Fungal-based nematicides
Fungal biocontrol of PPNs provides valuable direction for exploring novel nematicides. Previous studies have identified several fungal species, including Arthrobotrys, Nematoctonus, Pleurotus, Pochonia and Trichoderma, that exhibit strong nematicidal activity through the production of lytic enzymes, toxins, VOCs, and secondary metabolites (Lawal et al., 2022; Pires et al., 2022). Various compounds extracted from different fungi have shown nematicidal activity against PPNs. For example, Aurovertin D extracted from the nematophagous fungus Pochonia chlamydosporia demonstrated a high level of toxicity (LC50 value of 16.45 μg/mL) against M. incognita (Wang et al., 2015). Phomalactone, a broad-spectrum secondary metabolite isolated from Nigrospora spp., has been found to reduce M. incognita J2 invasion at a concentration of 500 mg/L in tomato roots (Kim et al., 2001; Kishore et al., 2022). Thermolide from the fungal species Talaromyces thermophilia exhibited significant toxicity to M. incognita and B. xylophilus with LC50 values of 0.7–1.0 μg/mL. Hypocrellin A, derived from Shiraia bambusicola, caused 50% mortality in B. xylophilus at 50 μg/mL (Guo et al., 2012; Seong et al., 2021). Additionally, 3-hydroxypropionic acid (3-HP) extracted from the endophytic fungus Phomosis phaseoli, showed specific nematicidal action against M. incognita, with an LC50 value of 12.5–15 μg/mL (Schwarz et al., 2004). Flavipin from Chaetomium globosum inhibits soybean cyst nematodes and root-knot nematodes by blocking ATP synthesis, inhibiting protein synthesis, and enhancing membrane permeability (Madrigal and Melgarejo, 1994; Kishore et al., 2022). Bikaverin and fusaric acid isolated from the endophytic fungus Fusarium oxygensporum, inhibited B. xylophilus at LC50 values of 50 μg/mL and 43 μg/mL, respectively. Although the exact mode of action of fusaric acid is unknown, it has been suggested that fusaric acid might be associated with the chelation of metal ions (Kwon et al., 2007). The depsipeptide beauvericin, produced by Beauveria bassiana, had nematicidal activity against M. incognita. Its exact mode of action is unknown; however, it has been hypothesized that it may work by activating the mitochondrial death pathway and inducing severe oxidative stress (Mallebrera et al., 2018; Youssef et al., 2020). Beauvericin, a depsipeptide produced by Beauveria bassiana, shows nematicidal activity against M. incognita possibly by activating the mitochondrial death pathway and inducing severe oxidative stress (Li et al., 2007; Prosperini et al., 2017; Seong et al., 2021). Enniatin B, a cyclodepsipeptide, functions as an ionophore and displays strong toxicity against M. javanica, while Emodin, a bioactive quinone from Aspergillus galucus displayed potent nematicidal activity against M. incognita (Prosperini et al., 2017). Overall, the research on fungal biocontrol of PPNs, coupled with omics and biotechnological approaches, holds promise for developing novel nematicides for sustainable agriculture. The fungal nematicides reported in previous studies are summarized in Figure 2B.
6 Linking omics with bacterial and fungal biocontrol of PPNs
In the rhizosphere, plants, and microbes, especially bacteria and fungi, interact to mitigate the effects of pathogen attacks. This interaction involves a complex network mediated by signaling molecules and secondary metabolites from root exudates and microbes in the rhizosphere, attracting the attention of researchers for novel antimicrobial discovery (White et al., 2017; Ahmed et al., 2022; Ayaz et al., 2023). However, the interaction between plants and microbes under PPNs infestation has been rarely studied. Previous studies have mainly focused on microbiome regulation under bacterial and fungal pathogens, with limited knowledge of microbiome and metabolomics regulation for PPNs control (Migunova and Sasanelli, 2021; Cai et al., 2022). Therefore, there is a need to explore potential microbes and their antimicrobial substances in the rhizosphere for PPNs suppression. Here, an effort is made to briefly summarize the importance of omics such as metagenomics, transcriptomics, and proteomics metabolomics along with other biotechnological approaches to explore novel nematicides for efficient PPN control in the future.
6.1 Biocontrol of PPNs in metagenomics era
Soil microbes present sustainable opportunities for controlling PPNs in various crops and plants. The rhizosphere harbors a complex structure of soil microbial communities. Metagenomics-based biocontrol of PPNs facilitates the discovery of novel biocontrol agents that are challenging to culture in a laboratory. Next Generation Sequencing (NGS), an innovative technology, has the potential to enhance our understanding of the function and biodiversity of rhizosphere communities. Previous studies have shown that a single gram of soil typically contains 103–104 taxonomic units of microorganisms (White et al., 2017; Kishore et al., 2022; Pires et al., 2022). This indicates that the current ratio of known nematode antagonistic microbes is as small as the total rhizosphere microbial community. Metagenomics based on NGS can help explore potential unknown microbes for nematode suppression, offering novel and sustainable pest management methods in the metagenomics era (Ciancio et al., 2016; Lahlali et al., 2021). Metagenomics provides a comprehensive view of microbial populations by analyzing genetic material directly extracted from environmental samples. This approach aids in identifying new biocontrol agents (BCAs) and comprehending their interactions with PPNs (White et al., 2017; Mohanram Praveen, 2019). The genomics study provides deep insight into the genome of different BCAs and the prediction of genes responsible for plant disease protection. Currently, the genome of the novel bacterium P. penetrans, with strong nematicidal activity, is being sequenced. By extracting and sequencing genomic DNA from Pasteuria species, such as P. penetrans, which cannot be cultured in the lab, researchers can enhance their understanding of its biology and evolution. Comparative genomics studies will provide important insights into the bacterium’s basic biology, especially its role as a nematode pathogen (Marin-Bruzos et al., 2021; Kishore et al., 2022).
Metagenomics research has revealed a broad range of microbes, including bacteria and fungi, in soil and the rhizosphere that can suppress PPNs. Understanding complex microbial interactions within the soil ecosystem is crucial for developing multispecies biocontrol consortia to effectively manage PPNs (Castaneda-Alvarez and Aballay, 2016; Ayaz et al., 2021). Additionally, functional metagenomics allows exploration of silent gene clusters in beneficial microbes that may possess nematicidal activity against PPNs. Cloning and expressing environmental DNA in surrogate host species is a key aspect of functional metagenomics to identify genes producing bioactive compounds or enzymes with nematicidal effects (White et al., 2017; Migunova and Sasanelli, 2021). Manipulating the soil microbiome can enhance the natural biocontrol of PPNs. Targeted microbiome engineering techniques can be developed by gaining in-depth knowledge of novel microbes in the rhizosphere community for PPNs suppression (Syed Ab Rahman et al., 2018; Pires et al., 2022). Integrating metagenomics into biocontrol research revolutionizes sustainable PPNs management. By linking microbial diversity with metagenomics, researchers can explore novel biocontrol agents against PPNs to promote sustainable and resilient farming practices.
6.2 Transcriptomics and proteomics for PPNs biocontrol
BCAs and PPNs interact through molecular processes that can be explored using robust omics approaches such as transcriptomics and proteomics. The identification of novel genes, pathways and proteins for PPNs suppression can facilitate the application of targeted biocontrol strategies (White et al., 2017; Yi et al., 2021). Transcriptomic studies can thoroughly determine the gene expression profiles of BCAs during their interaction with PPNs, shedding light on the underlying molecular mechanisms for PPNs control. Differential gene expression analysis of BCAs, whether up-regulated or down-regulated in response to nematodes, can help to identify genes that could enhance the efficacy of BCAs for suppressing different nematodes (Tian et al., 2007; Schouteden et al., 2015). Additionally, transcriptomic research can provide insight into how BCAs respond to environmental stresses that may affect their efficacy against PPNs, such as variations in soil pH, temperature, or moisture content (Crandall et al., 2020).
Proteomic studies enable the identification and measurement of proteins expressed by BCAs during their interaction with PPNs. Differential protein expression analysis offers a more direct assessment of biocontrol activity compared to transcriptomics and can identify important proteins involved in nematode suppression (White et al., 2017; Liang et al., 2022). Proteomics can recognize bioactive proteins produced by BCAs to inhibit nematodes, such as enzymes, nematicidal toxins, or antimicrobial peptides, which can be promising candidates for bionematicide development. Proteomics can also reveal the complex molecular networks underlying nematode suppression by elucidating protein–protein interactions between PPNs and BCAs (Ciancio et al., 2016; Cai et al., 2022). Metaproteomics, a large-scale evaluation of proteins created and/or modified in microbial communities (such as post-translational modifications) has advanced in recent decades. A recently developed technique called metabolite, protein, and lipid extraction (MPLEx) offers ways to extract lipids, polar compounds, and proteins from rhizospheric soil simultaneously, facilitating the discovery of novel proteins and peptides with different modes of action against PPNs from the rhizosphere (White et al., 2017; Cai et al., 2022). In brief, transcriptomics and proteomics provide valuable data for deciphering the molecular complexities of PPNs and BCAs interactions. By using these omics approaches, scientists can uncover and develop more targeted biocontrol strategies for effective PPNs control in the agriculture sector.
6.3 Metabolomics-based biocontrol of PPNs
The integration of metabolomics in the biocontrol of PPNs aims to discover potential nematicides derived from complex interactions between plants and microbes in the rhizosphere. Conventional methods for identifying bioactive chemicals against PPNs often fall short, as they rely on cultivating specific microorganisms and extracting metabolites. Additionally, certain rhizosphere microbes are hard to culture in laboratory conditions but are known to produce numerous bioactive compounds against plant pathogens (Topalović et al., 2020; Mokrini et al., 2024). Metabolomics employs advanced techniques such as GC–MS, LC–MS, NMR, and computational tools to explore rhizosphere metabolites in an untargeted manner. The purpose of metabolomics is to analyze an organism’s metabolomes at specific times and under varying conditions (White et al., 2017; Ayaz et al., 2021). Metabolomes consist of various cellular substrates and products from primary and secondary metabolism, playing essential roles in signaling and stress responses, ultimately defining an organism’s chemical phenotype (Horak et al., 2019; Desmedt et al., 2020).
Compared to the transcriptome or proteome, an organism’s metabolome is more sensitive to environmental changes, making metabolomics a valuable tool for understanding the metabolic pathways responsible for phenotypic responses (Bundy et al., 2009). Studies have shown that suppressive soils harbor microbes with strong potential for pathogen control. Metabolic profiling using techniques like NMR and HPLC–MS has revealed differences between conducive and suppressive soils in various agricultural regions (Gómez Expósito et al., 2017; Hayden et al., 2019). For instance, in the context of R. solani AG8, untargeted metabolomics approaches have successfully differentiated between conducive and suppressive soils, identifying specific metabolites like lipids, sugars, and terpenes that are more abundant in suppressive soils. Metabolites such as macrocarpals with antibacterial properties have been found to inhibit disease development in these soils (Hayden et al., 2019). In brief, the metabolomics-based research work under biocontrol of PPNs remains unexplored in different plant species. Thus, untargeted metagenomics of various plants rhizosphere metabolome offers a new avenue for exploring novel nematicides. The details of omics approaches needed to improve bacterial and fungal biocontrol of PPNs are shown in Figure 3.
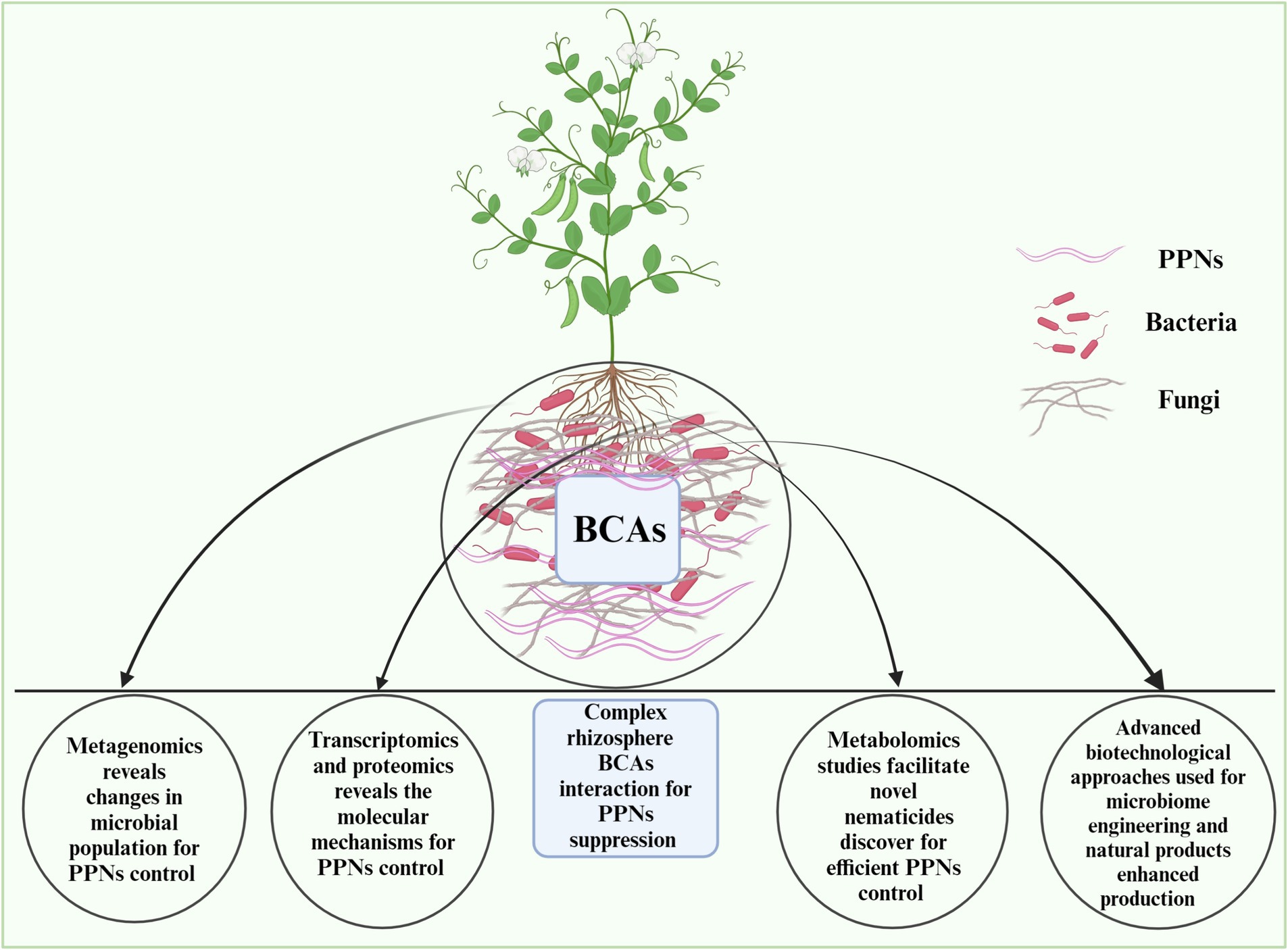
Figure 3. Linking multi-omics approaches such as metagenomics, transcriptomics, proteomics, and metabolomics to improve bacterial and fungal biocontrol of PPNs and novel nematicides discovery. The figure is designed with biorender https://app.biorender.com dated 4/30/2024.
7 Challenges in biocontrol of PPNs
The biocontrol of PPNs can be challenging in many ways due to the complexity of nematode biological processes, the diversity of nematode species, and the dynamic interactions among nematodes, plants, microbes and the environment (Cumagun and Moosavi, 2015; Pires et al., 2022). Finding effective BCAs against a wide range of nematode species can be challenging in different environmental conditions. BCAs with strong nematicidal activity against PPNs may show reduced efficacy in field experiments (Schouteden et al., 2015; Pires et al., 2022). Environmental factors such as pH, temperature, moisture content, and soil type can affect the effectiveness of BCAs, requiring their survival in the soil for a prolonged period for sustainable nematode management (Seong et al., 2021; Ayaz et al., 2023). Developing and implementing affordable biocontrol approaches can be challenging due to factors such as high production costs, short formulation shelf life, and inconsistent field efficacy. Obtaining authorization from regulatory bodies and registration for biocontrol products can be expensive and time-consuming (Akhtar et al., 2015; Ayaz et al., 2023). Market adoption of biocontrol products may also be influenced by public acceptance and opinions, requiring education of stakeholders, about the advantages and safety of biocontrol technology through workshops and awareness campaigns. The use of omics technology in the biocontrol of PPNs presents both possibilities and difficulties (Lahlali et al., 2022; Ayaz et al., 2023).
There are certain possibilities and difficulties associated with the biocontrol of PPNs using omics technology. PPNs have complex life cycles with various growth stages and unique interactions with host plants and microbes in the rhizosphere (Abd-Elgawad, 2020; Askary and Abd-Elgawad, 2021). It takes carefully planned studies and time-course analysis to capture the temporal dynamics of metabolite levels, protein abundance, and gene expression. The omics techniques can shed light on the interactions between biocontrol agents and hosts but it is still difficult to maximize biocontrol effectiveness under various field conditions (Kaul et al., 2016; Pires et al., 2022). The integration of environmental factors with BCAs under omics can provide a better understanding, however it makes the data more complex. It takes sophisticated computational methods and bioinformatics knowledge to integrate data from several omics platforms (genomics, transcriptomics, proteomics, and metabolomics) to produce detailed knowledge of PPNs biology and biocontrol mechanisms (White et al., 2017; Sena et al., 2024). In short, overcoming these challenges will open the door to more sustainable and efficient biocontrol methods for managing PPNs in agricultural systems.
8 Future perspectives and research directions
Advancements in research and collaboration have opened numerous possibilities for the future of biocontrol in managing PPNs. The utilization of beneficial microbes such as fungi and bacteria as BCAs is steadily increasing (Ayaz et al., 2021; Ali et al., 2023b). Ongoing research focused on identifying and characterizing BCAs will lead to the development of novel and efficient biocontrol products. Biotechnology advances, such as genetic engineering and synthetic biology, have made it feasible to create genetically engineered BCAs that exhibit enhanced effectiveness against PPNs, resulting in the production of targeted nematicides on a larger scale (Pires et al., 2022; Ayaz et al., 2023). The overexpression of genes with nematicidal properties in BCAs offers innovative methods for controlling nematode infestations. Using bioengineering and biotechnology techniques, it may be possible to build BCAs with robust nematicidal activity that can effectively function under diverse field conditions (Abd-Elgawad, 2022).
As climate change continues to impact global agriculture, it will be imperative to develop biocontrol strategies that can adapt to shifting climatic conditions. To ensure long-term sustainability and efficacy against PPNs, research focused on evaluating the performance and adaptability of biocontrol agents under various climate scenarios will be crucial (Abd-Elgawad and Askary, 2020). For the commercialization and adoption of biocontrol technologies, addressing economic viability and negotiating regulatory frameworks will be essential. By increasing public awareness and educating stakeholders about the benefits of biocontrol for sustainable agriculture, acceptance and adoption among farmers, consumers, and legislators can be encouraged (Bonaterra et al., 2022; Ayaz et al., 2023). Training initiatives, educational seminars, and public relations campaigns can aid bridge the knowledge gap and promote the adoption of biocontrol methods for PPNs management. Precision agriculture technology, including drones and remote sensing, can be used to accurately map and monitor nematode infestations at a high resolution. These site-specific biocontrol measures and resource allocation can be optimized for effective nematode management using geographical and temporal data (Sishodia et al., 2020; Arjoune et al., 2022).
The development of microbiome-based biocontrol strategies can be facilitated by understanding the intricate relationship among nematodes, plants, and soil microbes through metagenomics and microbiome research. One potential method of biocontrol is to modify the soil microbiome to promote beneficial microbial communities that can suppress nematode populations (Topalović et al., 2019; Wolfgang et al., 2019). In the future, it may be possible to use an engineered microbiome to regulate the population of nematodes in infested plants. Additionally, bioactive substances with nematode-repellent, nematostatic, or nematicidal properties produced by plants and microbes can be identified quickly with the help of a metabolomics-driven method (Ciancio et al., 2016; Khanna et al., 2021). Despite numerous attempts to collect root exudates using various techniques, distinguishing between plant and microbial metabolites and understanding the outcome of interactions within natural functional networks remains challenging, posing a major obstacle for rhizospheric metabolomics research (White et al., 2017). It is essential to establish optimized methods for accurately sampling and evaluating various rhizosphere fractions. Such optimal methods would address the interconnected nature of plants and microbial metabolic processes in the rhizosphere. Finding bioactive metabolites through screening can result in the development of novel natural products to combat PPNs infestations (White et al., 2017; Verma et al., 2018). Therefore, ongoing advancements in research and omics technology foresee a bright future for the biocontrol of PPNs in the agriculture sector.
9 Conclusion
The application of beneficial microbes to manage crop diseases is a safe and eco-friendly strategy that has garnered interest among researchers in crop protection. PPNs pose significant threats to major crop productivity worldwide. This review aims to summarize the status of bacterial and fungal biocontrol strategies for controlling PPNs. It emphasizes the need for further research in areas, such as the development of potential BCAs combined with omics approaches to ensure effective PPNs control in agriculture. The omics era has accelerated the identification, development, and application of fungal and bacterial biocontrol agents for sustainable PPNs management. Utilizing omics can provide a better understanding of the molecular mechanisms involved in biocontrol interactions, enabling researchers to develop effective management strategies for PPNs in agricultural systems.
Author contributions
MA: Conceptualization, Data curation, Formal analysis, Investigation, Methodology, Software, Visualization, Writing – original draft, Writing – review & editing. J-TZ: Conceptualization, Data curation, Formal analysis, Methodology, Software, Visualization, Writing – original draft, Writing – review & editing. WZ: Data curation, Formal analysis, Investigation, Methodology, Resources, Software, Visualization, Writing – review & editing. Y-KC: Data curation, Formal analysis, Investigation, Methodology, Resources, Software, Visualization, Writing – review & editing. QA: Data curation, Formal analysis, Methodology, Software, Validation, Visualization, Writing – review & editing. FA: Formal analysis, Methodology, Software, Visualization, Writing – review & editing. AK: Data curation, Formal analysis, Methodology, Software, Visualization, Writing – review & editing. QY: Data curation, Formal analysis, Methodology, Software, Visualization, Writing – review & editing. J-WY: Data curation, Formal analysis, Methodology, Software, Visualization, Writing – review & editing. W-CW: Data curation, Formal analysis, Methodology, Software, Validation, Visualization, Writing – review & editing. R-DQ: Conceptualization, Data curation, Formal analysis, Funding acquisition, Investigation, Methodology, Project administration, Resources, Software, Supervision, Validation, Visualization, Writing – original draft, Writing – review & editing. W-KH: Conceptualization, Data curation, Formal analysis, Funding acquisition, Investigation, Methodology, Project administration, Resources, Software, Supervision, Validation, Visualization, Writing – original draft, Writing – review & editing.
Funding
The author(s) declare that financial support was received for the research, authorship, and/or publication of this article. Funding was provided by the Natural Science Foundation of China (grant no. 32172382) and the National Key Research and Development Program of China (grant no. 2021YFC2600404).
Conflict of interest
The authors declare that the research was conducted in the absence of any commercial or financial relationships that could be construed as a potential conflict of interest.
Publisher’s note
All claims expressed in this article are solely those of the authors and do not necessarily represent those of their affiliated organizations, or those of the publisher, the editors and the reviewers. Any product that may be evaluated in this article, or claim that may be made by its manufacturer, is not guaranteed or endorsed by the publisher.
References
Abd-Elgawad, M. M. M. (2020). “Plant-parasitic nematodes and their biocontrol agents: current status and future vistas” in Management of Phytonematodes: Recent Advances and Future Challenges. eds. R. Ansari, R. Rizvi, and I. Mahmood (Singapore: Springer), 171–203.
Abd-Elgawad, M. M. M. (2022). Exploiting plant–phytonematode interactions to upgrade safe and effective nematode control. Life 12:1916. doi: 10.3390/life12111916
Abd-Elgawad, M. M. M. (2024). “Pasteuria species for nematodes management in organic farms” in Sustainable Management of Nematodes in Agriculture: Role of Microbes-Assisted Strategies. eds. K. K. Chaud, M. K. Meghvansi, and S. Siddiqui (Cham: Springer), 89–126.
Abd-Elgawad, M. M. M., and Askary, T. H. (2018). Fungal and bacterial nematicides in integrated nematode management strategies. Egypt. J. Biol. pest Control 28, 1–24. doi: 10.1186/s41938-018-0080-x
Abd-Elgawad, M. M. M., and Askary, T. H. (2020). Factors affecting success of biological agents used in controlling the plant-parasitic nematodes. Egypt. J. Biol. Pest Control 30:215. doi: 10.1186/s41938-020-00215-2
AbdelRazek, G. M., and Yaseen, R. (2020). Effect of some rhizosphere bacteria on root-knot nematodes. Egypt. J. Biol. Pest Control 30, 1–11. doi: 10.1186/s41938-020-00340-y
Ahmed, N., Ghramh, H. A., Shakeel, Q., Ashraf, W., Abbas, H. T., Binyamin, R., et al. (2023). Evaluation of Rhizospheric-Pseudomonas spp. for the management of Meloidogyne incognita in tomato. J. King Saud Univ. Sci. 35:102395. doi: 10.1016/j.jksus.2022.102395
Ahmed, W., Dai, Z., Zhang, J., Li, S., Ahmed, A., Munir, S., et al. (2022). Plant-microbe interaction: mining the impact of native Bacillus amyloliquefaciens WS-10 on tobacco bacterial wilt disease and rhizosphere microbial communities. Microbiol. Spectr. 10, e01471–e01422. doi: 10.1128/spectrum.01471-22
Akhtar, M. S., Panwar, J., Abdullah, S. N. A., Siddiqui, Y., Swamy, M. K., and Ashkani, S. (2015). Biocontrol of plant parasitic nematodes by fungi: efficacy and control strategies. Org. Amend. Soil Suppressiveness Plant Dis. 46, 219–247. doi: 10.1007/978-3-319-23075-7_11
Al-Ani, L. K. T., Soares, F. E. F., Sharma, A., de los Santos-Villalobos, S., Valdivia-Padilla, A. V., Aguilar-Marcelino, L., et al. (2022). Strategy of Nematophagous Fungi in determining the activity of plant parasitic nematodes and their prospective role in sustainable agriculture. Front. Fungal Biol. 3, 1–9. doi: 10.3389/ffunb.2022.863198
Ali, Q., Khan, A. R., Tao, S., Rajer, F. U., Ayaz, M., Abro, M. A., et al. (2023a). Broad-spectrum antagonistic potential of Bacillus spp. volatiles against Rhizoctonia solani and Xanthomonas oryzae pv. Oryzae. Physiol. Plant. 175, e14087–e14016. doi: 10.1111/ppl.14087
Ali, Q., Yu, C., Wang, Y., Sheng, T., and Zhao, X. (2023b). High killing rate of nematode and promotion of rice growth by synthetic volatiles from Bacillus strains due to enhanced oxidative stress response. Physiol. Plant 175:e13868. doi: 10.1111/ppl.13868
Arjoune, Y., Sugunaraj, N., Peri, S., Nair, S. V., Skurdal, A., Ranganathan, P., et al. (2022). Soybean cyst nematode detection and management: a review. Plant Methods 18, 1–39. doi: 10.1186/s13007-022-00933-8
Askary, T. H., and Abd-Elgawad, M. M. M. (2021). Opportunities and challenges of entomopathogenic nematodes as biocontrol agents in their tripartite interactions. Egypt. J. Biol. Pest Control 31, 1–10. doi: 10.1186/s41938-021-00391-9
Ayaz, M., Ali, Q., Farzand, A., Khan, A. R., Ling, H., and Gao, X. (2021). Nematicidal volatiles from Bacillus atrophaeus GBSC56 promote growth and stimulate induced systemic resistance in tomato against Meloidogyne incognita. Int. J. Mol. Sci. 22:5049. doi: 10.3390/ijms22095049
Ayaz, M., Li, C.-H., Ali, Q., Zhao, W., Chi, Y.-K., Shafiq, M., et al. (2023). Bacterial and fungal biocontrol agents for plant disease protection: journey from lab to field, current status, challenges, and global perspectives. Molecules 28:6735. doi: 10.3390/molecules28186735
Baheti, B. L., Dodwadiya, M., Rathore, B. S., and Bhati, S. S. (2015). Bioagents: an effective and ecofriendly option for the management of maize cyst nematode, Heterodera zeae on sweet corn (Zea mays L. saccharata). J. Biopest. 8, 141–146. doi: 10.57182/jbiopestic.8.2.141-146
Bonaterra, A., Badosa, E., Daranas, N., Francés, J., Roselló, G., and Montesinos, E. (2022). Bacteria as biological control agents of plant diseases. Microorganisms 10:1759. doi: 10.3390/microorganisms10091759
Bontempo, A. F., Fernandes, R. H., Lopes, J., Freitas, L. G., and Lopes, E. A. (2014). Pochonia chlamydosporia controls Meloidogyne incognita on carrot. Australas. Plant Pathol. 43, 421–424. doi: 10.1007/s13313-014-0283-x
Bundy, J. G., Davey, M. P., and Viant, M. R. (2009). Environmental metabolomics: a critical review and future perspectives. Metabolomics 5, 3–21. doi: 10.1007/s11306-008-0152-0
Cai, S., Jia, J., He, C., Zeng, L., Fang, Y., Qiu, G., et al. (2022). Multi-omics of pine wood nematode pathogenicity associated with culturable associated microbiota through an artificial assembly approach. Front. Plant Sci. 12:798539. doi: 10.3389/fpls.2021.798539
Castaneda-Alvarez, C., and Aballay, E. (2016). Rhizobacteria with nematicide aptitude: enzymes and compounds associated. World J. Microbiol. Biotechnol. 32, 1–7. doi: 10.1007/s11274-016-2165-6
Castaneda-Alvarez, C., Prodan, S., Rosales, I. M., and Aballay, E. (2016). Exoenzymes and metabolites related to the nematicidal effect of rhizobacteria on Xiphinema index Thorne & Allen. J. Appl. Microbiol. 120, 413–424. doi: 10.1111/jam.12987
Castillo, J. D., Lawrence, K. S., and Kloepper, J. W. (2013). Biocontrol of the reniform nematode by Bacillus firmus GB-126 and Paecilomyces lilacinus 251 on cotton. Plant Dis. 97, 967–976. doi: 10.1094/PDIS-10-12-0978-RE
Chen, W., Zhu, Z., Liu, C., Yang, F., Dai, W., Yu, H., et al. (2024). Evaluation of Virgibacillus dokdonensis MCCC 1A00493 as a biological control agent and microbial organic fertilizer against root-knot nematodes. Biol. Control 192:105508. doi: 10.1016/j.biocontrol.2024.105508
Cheng, W., Yang, J., Nie, Q., Huang, D., Yu, C., Zheng, L., et al. (2017). Volatile organic compounds from Paenibacillus polymyxa KM2501-1 control Meloidogyne incognita by multiple strategies. Sci. Rep. 7, 16213–16211. doi: 10.1038/s41598-017-16631-8
Ciancio, A., Colagiero, M., Pentimone, I., and Rosso, L. (2016). Soil microbial communities and their potential for root-knot nematodes management: a review. Environ. Eng. Manag. J. 15, 1833–1839. doi: 10.30638/eemj.2016.195
Cimen, H., Touray, M., Gulsen, S. H., and Hazir, S. (2022). Natural products from Photorhabdus and Xenorhabdus: mechanisms and impacts. Appl. Microbiol. Biotechnol. 106, 4387–4399. doi: 10.1007/s00253-022-12023-9
Crandall, S. G., Gold, K. M., Jiménez-Gasco, M. M., Filgueiras, C. C., and Willett, D. S. (2020). A multi-omics approach to solving problems in plant disease ecology. PLoS One 15, e0237975–e0237923. doi: 10.1371/journal.pone.0237975
Cronin, D., Moenne-Loccoz, Y., Fenton, A., Dunne, C., and Dowling, D. N. (1997). Role of 2, 4-diacetylphloroglucinol in the interactions of the biocontrol pseudomonad strain F113 with the potato cyst nematode Globodera rostochiensis. Appl. Environ. Microbiol. 63, 1357–1361. doi: 10.1128/aem.63.4.1357-1361.1997
Cumagun, C. J. R., and Moosavi, M. R. (2015). Significance of biocontrol agents of phytonematodes. Biocontrol agents phytonematodes. Wallingford, UK CABI Publ. 10, 50–78. doi: 10.1079/9781780643755.005
Dababat, A. A.-F., and Sikora, R. (2007). Use of Trichoderma harzianum and Trichoderma viride for the biological control of Meloidogyne incognita on tomato. Jordan J. Agric. Sci 71, 953–961.
de Freitas Soares, F. E., Ferreira, J. M., Genier, H. L. A., Al-Ani, L. K. T., and Aguilar-Marcelino, L. (2023). Biological control 2.0: Use of nematophagous fungi enzymes for nematode control. J. Nat. Pestic. Res. 4:100025. doi: 10.1016/j.napere.2023.100025
de Oliveira, C. M., Almeida, N. O., Côrtes, M. V. C. B., Júnior, M. L., da Rocha, M. R., and Ulhoa, C. J. (2021). Biological control of Pratylenchus brachyurus with isolates of Trichoderma spp. on soybean. Biol. Control 152:104425. doi: 10.1016/j.biocontrol.2020.104425
Deng, X., Wang, X., and Li, G. (2022). Nematicidal effects of volatile organic compounds from microorganisms and plants on plant-parasitic nematodes. Microorganisms 10:1201. doi: 10.3390/microorganisms10061201
Desmedt, W., Mangelinckx, S., Kyndt, T., and Vanholme, B. (2020). A phytochemical perspective on plant defense against nematodes. Front. Plant Sci. 11:602079. doi: 10.3389/fpls.2020.602079
DiLegge, M. J., Manter, D. K., and Vivanco, J. M. (2019). A novel approach to determine generalist nematophagous microbes reveals Mortierella globalpina as a new biocontrol agent against Meloidogyne spp. nematodes. Sci. Rep. 9:7521. doi: 10.1038/s41598-019-44010-y
El-Ashry, R. M., Ali, M. A. S., Elsobki, A. E. A., and Aioub, A. A. A. (2021). Integrated management of Meloidogyne incognita on tomato using combinations of abamectin, Purpureocillium lilacinum, rhizobacteria, and botanicals compared with nematicide. Egypt. J. Biol. Pest Control 31:93. doi: 10.1186/s41938-021-00438-x
Engelbrecht, G., Horak, I., Jansen van Rensburg, P. J., and Claassens, S. (2018). Bacillus-based bionematicides: development, modes of action and commercialisation. Biocontrol Sci. Tech. 28, 629–653. doi: 10.1080/09583157.2018.1469000
Gao, H., Qi, G., Yin, R., Zhang, H., Li, C., and Zhao, X. (2016). Bacillus cereus strain S2 shows high nematicidal activity against Meloidogyne incognita by producing sphingosine. Sci. Rep. 6, 1–11. doi: 10.1038/srep28756
Ghahremani, Z., Escudero, N., Beltrán-Anadón, D., Saus, E., Cunquero, M., Andilla, J., et al. (2020). Bacillus firmus strain I-1582, a nematode antagonist by itself and through the plant. Front. Plant Sci. 11:515491. doi: 10.3389/fpls.2020.00796
Ghahremani, Z., Escudero, N., Saus, E., Gabaldón, T., and Sorribas, F. J. (2019). Pochonia chlamydosporia induces plant-dependent systemic resistance to Meloidogyne incognita. Front. Plant Sci. 10:457876. doi: 10.3389/fpls.2019.00945
Gómez Expósito, R., de Bruijn, I., Postma, J., and Raaijmakers, J. M. (2017). Current insights into the role of rhizosphere bacteria in disease suppressive soils. Front. Microbiol. 8:305316. doi: 10.3389/fmicb.2017.02529
Guo, J.-P., Zhu, C.-Y., Zhang, C.-P., Chu, Y.-S., Wang, Y.-L., Zhang, J.-X., et al. (2012). Thermolides, potent nematocidal PKS-NRPS hybrid metabolites from thermophilic fungus Talaromyces thermophilus. J. Am. Chem. Soc. 134, 20306–20309. doi: 10.1021/ja3104044
Guo, Y., Weng, M., Sun, Y., Carballar-Lejarazú, R., Wu, S., and Lian, C. (2022). Bacillus thuringiensis toxins with nematocidal activity against the pinewood nematode Bursaphelenchus xylophilus. J. Invertebr. Pathol. 189:107726. doi: 10.1016/j.jip.2022.107726
Hayden, H. L., Rochfort, S. J., Ezernieks, V., Savin, K. W., and Mele, P. M. (2019). Metabolomics approaches for the discrimination of disease suppressive soils for Rhizoctonia solani AG8 in cereal crops using 1H NMR and LC-MS. Sci. Total Environ. 651, 1627–1638. doi: 10.1016/j.scitotenv.2018.09.249
Herrera-Parra, E., Ramos-Zapata, J., Cristóbal-Alejo, J., Tun-Suarez, J., and Reyes-Ramírez, A. (2018). Species of Trichoderma antagonistic to the root knot nematode (Meloidogyne incognita) in habanero pepper. Phyton 87:7.
Horak, I., Engelbrecht, G., van Rensburg, P. J. J., and Claassens, S. (2019). Microbial metabolomics: essential definitions and the importance of cultivation conditions for utilizing Bacillus species as bionematicides. J. Appl. Microbiol. 127, 326–343. doi: 10.1111/jam.14218
Hu, Y., You, J., Wang, Y., Long, Y., Wang, S., Pan, F., et al. (2022). Biocontrol efficacy of Bacillus velezensis strain YS-AT-DS1 against the root-knot nematode Meloidogyne incognita in tomato plants. Front. Microbiol. 13:1035748. doi: 10.3389/fmicb.2022.1035748
Hussain, M., Zouhar, M., and Ryšánek, P. (2018). Suppression of Meloidogyne incognita by the entomopathogenic fungus Lecanicillium muscarium. Plant Dis. 102, 977–982. doi: 10.1094/PDIS-09-17-1392-RE
Jansson, H.-B., and Lopez-Llorca, L. V. (2004). Control of nematodes by fungi. Mycol. Ser. 21, 205–216.
Jisha, R. B., Smitha, R. B., and Benjamin, S. (2013). An overview on the crystal toxins from Bacillus thuringiensis. Adv. Microbiol. 3, 462–472. doi: 10.4236/aim.2013.35062
Kamaruzzaman, M., Zheng, L., Zhou, S., Ye, W., Yuan, Y., Qi, Q., et al. (2024). Evaluation of the novel endophytic fungus Chaetomium ascotrichoides 1-24-2 from Pinus massoniana as a biocontrol agent against pine wilt disease caused by Bursaphelenchus xylophilus. Pest Manag. Sci. ps.8205. doi: 10.1002/ps.8205
Kaul, S., Sharma, T., and Dhar, M. K. (2016). “Omics” tools for better understanding the plant–endophyte interactions. Front. Plant Sci. 7, 1–9. doi: 10.3389/fpls.2016.00955
Khabbaz, S. E., Ladhalakshmi, D., Babu, M., Kandan, A., Ramamoorthy, V., Saravanakumar, D., et al. (2019). Plant growth promoting bacteria (PGPB)—a versatile tool for plant health management. Can. J. Pestic. Pest Manag 1, 1–25. doi: 10.34195/can.j.ppm.2019.05.001
Khan, A., Haris, M., Hussain, T., Khan, A. A., Laasli, S. E., Lahlali, R., et al. (2023). Counter-attack of biocontrol agents: environmentally benign approaches against root-knot nematodes (Meloidogyne spp.) on agricultural crops. Heliyon 9:e21653. doi: 10.1016/j.heliyon.2023.e21653
Khan, M., and Tanaka, K. (2023). Purpureocillium lilacinum for plant growth promotion and biocontrol against root-knot nematodes infecting eggplant. PLoS One 18:e0283550. doi: 10.1371/journal.pone.0283550
Khanna, K., Kohli, S. K., Ohri, P., and Bhardwaj, R. (2021). Plants-nematodes-microbes crosstalk within soil: A trade-off among friends or foes. Microbiol. Res. 248:126755. doi: 10.1016/j.micres.2021.126755
Kim, J., Choi, G. J., Park, J., Kim, H. T., and Cho, K. Y. (2001). Activity against plant pathogenic fungi of phomalactone isolated from Nigrospora sphaerica. Pest Manag. Sci. Former. Pestic. Sci. 57, 554–559. doi: 10.1002/ps.318
Kiriga, A. W., Haukeland, S., Kariuki, G. M., Coyne, D. L., and Beek, N. V. (2018). Effect of Trichoderma spp. and Purpureocillium lilacinum on Meloidogyne javanica in commercial pineapple production in Kenya. Biol. Control 119, 27–32. doi: 10.1016/j.biocontrol.2018.01.005
Kishore, K., Mukesh, C., and Meghvansi, K. (2022). Sustainable Management of Nematodes in agriculture: Organic management. Available at: https://link.springer.com/10.1007/978-3-031-09943-4
Kwon, H. R., Son, S. W., Han, H. R., Choi, G. J., Jang, K. S., Choi, Y. H., et al. (2007). Nematicidal activity of bikaverin and fusaric acid isolated from fusarium oxysporum against pine wood nematode, Bursaphelenchus xylophilus. Plant Pathol. J. 23, 318–321. doi: 10.5423/PPJ.2007.23.4.318
Lahlali, R., Ezrari, S., Radouane, N., Kenfaoui, J., Esmaeel, Q., El Hamss, H., et al. (2022). Biological control of plant pathogens: A global perspective. Microorganisms 10:596. doi: 10.3390/microorganisms10030596
Lahlali, R., Ibrahim, D. S. S., Belabess, Z., Roni, M. Z. K., Radouane, N., Vicente, C. S. L., et al. (2021). High-throughput molecular technologies for unraveling the mystery of soil microbial community: challenges and future prospects. Heliyon 7:e08142. doi: 10.1016/j.heliyon.2021.e08142
Lawal, I., Fardami, A. Y., Ahmad, F. I., Yahaya, S., Abubakar, A. S., Sa’id, M. A., et al. (2022). A review on Nematophagus Fungi: A potential Nematicide for the biocontrol of nematodes. J Environ Bioremed Toxicol 5, 26–31. doi: 10.54987/jebat.v5i1.677
Li, G., Zhang, K., Xu, J., Dong, J., and Liu, Y. (2007). Nematicidal substances from fungi. Recent Pat. Biotechnol. 1, 212–233. doi: 10.2174/187220807782330165
Li, J., Zou, C., Xu, J., Ji, X., Niu, X., Yang, J., et al. (2015). Molecular mechanisms of nematode-nematophagous microbe interactions: basis for biological control of plant-parasitic nematodes. Annu. Rev. Phytopathol. 53, 67–95. doi: 10.1146/annurev-phyto-080614-120336
Li, L., Sun, Y., Chen, F., Hao, D., and Tan, J. (2023). An alkaline protease from Bacillus cereus NJSZ-13 can act as a pathogenicity factor in infection of pinewood nematode. BMC Microbiol. 23, 10–11. doi: 10.1186/s12866-022-02752-2
Li, X., Hu, H.-J., Li, J.-Y., Wang, C., Chen, S.-L., and Yan, S.-Z. (2019). Effects of the endophytic bacteria Bacillus cereus BCM2 on tomato root exudates and Meloidogyne incognita infection. Plant Dis. 103, 1551–1558. doi: 10.1094/PDIS-11-18-2016-RE
Liang, Z., Ali, Q., Wang, Y., Mu, G., Kan, X., Ren, Y., et al. (2022). Toxicity of Bacillus thuringiensis strains derived from the novel crystal protein Cry31Aa with high nematicidal activity against rice parasitic nematode Aphelenchoides besseyi. Int. J. Mol. Sci. 23:8189. doi: 10.3390/ijms23158189
Madrigal, C., and Melgarejo, P. (1994). Mechanisms of action of the antibiotic flavipin on Monilinia laxa and Saccharomyces cerevisiae. Mycol. Res. 98, 874–878. doi: 10.1016/S0953-7562(09)80257-8
Mallebrera, B., Prosperini, A., Font, G., and Ruiz, M. J. (2018). In vitro mechanisms of Beauvericin toxicity: A review. Food Chem. Toxicol. 111, 537–545. doi: 10.1016/j.fct.2017.11.019
Marin-Bruzos, M., Grayston, S. J., Forge, T., and Nelson, L. M. (2021). Isolation and characterization of streptomycetes and pseudomonad strains with antagonistic activity against the plant parasitic nematode Pratylenchus penetrans and fungi associated with replant disease. Biol. Control 158:104599. doi: 10.1016/j.biocontrol.2021.104599
Meyer, S. L. F., Massoud, S. I., Chitwood, D. J., and Roberts, D. P. (2000). Evaluation of Trichoderma virens and Burkholderia cepacia for antagonistic activity against root-knot nematode, Meloidogyne incognita. Nematology 2, 871–879. doi: 10.1163/156854100750112815
Migunova, V. D., and Sasanelli, N. (2021). Bacteria as biocontrol tool against phytoparasitic nematodes. Plan. Theory 10, 1–15. doi: 10.3390/plants10020389
Mohanram Praveen, S. K. (2019). Rhizosphere microbiome: revisiting the synergy of plant-microbe interactions. Ann. Microbiol. 69, 307–320. doi: 10.1007/s13213-019-01448-9
Mokrini, F., Laasli, S.-E., Ezrari, S., Belabess, Z., and Lahlali, R. (2024). “Plant-parasitic nematodes and microbe interactions: A biological control perspective” in Sustainable Management of Nematodes in Agriculture. eds. K. K. Chaud, M. K. Meghvansi, and S. Siddiqui (Cham: Springer), 89–126.
Mukhtar, T., Tariq-Khan, M., and Aslam, M. N. (2021). Bioefficacy of Trichoderma species against javanese root-knot nematode, Meloidogyne javanica, in green gram. Gesunde Pflanz. 73, 265–272. doi: 10.1007/s10343-021-00544-8
Nicol, J. M., Turner, S. J., Coyne, D. L., Nijs, L., Hockland, S., and Maafi, Z. T. (2011). Current nematode threats to world agriculture. In: Genomics and Molecular Genetics of Plant-Nematode Interactions. eds. J. Jones, G. Gheysen, and C. Fenoll (Dordrecht: Springer), 21–43.
Ning, J., Zhou, J., Wang, H., Liu, Y., Ahmad, F., Feng, X., et al. (2022). Parallel evolution of C-type lectin domain gene family sizes in insect-vectored nematodes. Front. Plant Sci. 13:856826. doi: 10.3389/fpls.2022.856826
Noura, C. H., Hajer, R., Asma, L., Lobna, H. H., and Najet, H.-R. (2018). Antagonistic potential of Verticillium leptobactrum against Pratylenchus vulnus associated with apple rootstock. J. Entomol. Zool. Stud. 6, 172–176.
Orr, J. N., Neilson, R., Freitag, T. E., Roberts, D. M., Davies, K. G., Blok, V. C., et al. (2020). Parallel microbial ecology of Pasteuria and nematode species in Scottish soils. Front. Plant Sci. 10:1763. doi: 10.3389/fpls.2019.01763
Penha, R. O., Vandenberghe, L. P. S., Faulds, C., Soccol, V. T., and Soccol, C. R. (2020). Bacillus lipopeptides as powerful pest control agents for a more sustainable and healthy agriculture: recent studies and innovations. Planta 251, 70–15. doi: 10.1007/s00425-020-03357-7
Pires, D., Vicente, C. S. L., Menéndez, E., Faria, J. M. S., Rusinque, L., Camacho, M. J., et al. (2022). The fight against plant-parasitic nematodes: current status of bacterial and fungal biocontrol agents. Pathogens 11, 1–22. doi: 10.3390/pathogens11101178
Poveda, J., Abril-Urias, P., and Escobar, C. (2020). Biological control of plant-parasitic nematodes by filamentous Fungi inducers of resistance: Trichoderma, mycorrhizal and endophytic Fungi. Front. Microbiol. 11:992. doi: 10.3389/fmicb.2020.00992
Proença, D. N., Schwab, S., Vidal, M. S., Baldani, J. I., Xavier, G. R., and Morais, P. V. (2019). The nematicide Serratia plymuthica M24T3 colonizes Arabidopsis thaliana, stimulates plant growth, and presents plant beneficial potential. Brazilian J. Microbiol. 50, 777–789. doi: 10.1007/s42770-019-00098-y
Prosperini, A., Berrada, H., Ruiz, M. J., Caloni, F., Coccini, T., Spicer, L. J., et al. (2017). A review of the mycotoxin enniatin B. Front. Public Heal. 5:300387. doi: 10.3389/fpubh.2017.00304
Radwan, M. A., Saad, A. S. A., Mesbah, H. A., Ibrahim, H. S., and Khalil, M. S. (2019). Investigatingthe in vitro and in vivo nematicidal performance of structurally related macrolides against the root-knot nematode, Meloidogyne incognita. Hellenic Plant Protect. J. 12, 24–37. doi: 10.2478/hppj-2019-0005
Schouteden, N., Waele, D. De, Panis, B., and Vos, C. M. (2015). Arbuscular mycorrhizal fungi for the biocontrol of plant-parasitic nematodes: A review of the mechanisms involved. Front. Microbiol. 6, 1–12. doi: 10.3389/fmicb.2015.01280
Schwarz, M., Köpcke, B., Weber, R. W. S., Sterner, O., and Anke, H. (2004). 3-Hydroxypropionic acid as a nematicidal principle in endophytic fungi. Phytochemistry 65, 2239–2245. doi: 10.1016/j.phytochem.2004.06.035
Sena, L., Mica, E., Valè, G., Vaccino, P., and Pecchioni, N. (2024). Exploring the potential of endophyte-plant interactions for improving crop sustainable yields in a changing climate. Front. Plant Sci. 15:1349401. doi: 10.3389/fpls.2024.1349401
Seong, J., Shin, J., Kim, K., and Cho, B. K. (2021). Microbial production of nematicidal agents for controlling plant-parasitic nematodes. Process Biochem. 108, 69–79. doi: 10.1016/j.procbio.2021.06.006
Siddiqui, A., and Shaukat, S. S. (2004). Trichoderma harzianum enhances the production of nematicidal compounds in vitro and improves biocontrol of Meloidogyne javanica by Pseudomonas fluorescens in tomato. Lett. Appl. Microbiol. 38, 169–175. doi: 10.1111/j.1472-765x.2003.01481.x
Sikandar, A., Zhang, M. Y., Zhu, X. F., Wang, Y. Y., Ahmed, M., Iqbal, M. F., et al. (2019). Efficacy of Penicillium chrysogenum strain Snef1216 against root-knot nematodes (Meloidogyne incognita) in cucumber (Cucumis sativus L.) under greenhouse conditions. Appl. Ecol. Environ. Res. 17:464. doi: 10.15666/aeer/1705_1245112464
Sishodia, R. P., Ray, R. L., and Singh, S. K. (2020). Applications of remote sensing in precision agriculture: A review. Remote Sens. 12, 1–31. doi: 10.3390/rs12193136
Soliman, M. S., El-Deriny, M. M., Ibrahim, D. S. S., Zakaria, H., and Ahmed, Y. (2021). Suppression of root-knot nematode Meloidogyne incognita on tomato plants using the nematode trapping fungus Arthrobotrys oligospora Fresenius. J. Appl. Microbiol. 131, 2402–2415. doi: 10.1111/jam.15101
Song, R., Tan, Y., Ahmed, W., Zhou, G., and Zhao, Z. (2022). Unraveling the expression of differentially expressed proteins and enzymatic activity in response to Phytophthora nicotianae across different flue-cured tobacco cultivars. BMC Microbiol. 22:112. doi: 10.1186/s12866-022-02531-z
Sun, X., Zhang, R., Ding, M., Liu, Y., and Li, L. (2021). Biocontrol of the root-knot nematode Meloidogyne incognita by a nematicidal bacterium Pseudomonas simiae MB751 with cyclic dipeptide. Pest Manag. Sci. 77, 4365–4374. doi: 10.1002/ps.6470
Syed Ab Rahman, S. F., Singh, E., Pieterse, C. M. J., and Schenk, P. M. (2018). Emerging microbial biocontrol strategies for plant pathogens. Plant Sci. 267, 102–111. doi: 10.1016/j.plantsci.2017.11.012
Tariq, M., Khan, A., Asif, M., and Siddiqui, M. A. (2018). Interactive effect of Trichoderma virens and Meloidogyne incognita and their influence on plant growth character and nematode multiplication on Abelmoschus esculentus (L.) Moench. Curr. Nematol. 29, 1–9.
Tian, B., Yang, J., and Zhang, K.-Q. (2007). Bacteria used in the biological control of plant-parasitic nematodes: populations, mechanisms of action, and future prospects. FEMS Microbiol. Ecol. 61, 197–213. doi: 10.1111/j.1574-6941.2007.00349.x
Timper, P. (2011). Utilization of biological control for managing plant-parasitic nematodes. Biol. Control Plant Parasit. Nematodes, 259–289. doi: 10.1007/978-1-4020-9648-8_11
Tolba, S. R. T., Moustafa, M. M. A., Elshawaf, I. I. S., Rosso, L. C., Pentimone, I., Colagiero, M., et al. (2021). Root endophytism by Pochonia chlamydosporia affects defense-gene expression in leaves of monocot and dicot hosts under multiple biotic interactions. Plan. Theory 10:4718. doi: 10.3390/plants10040718
Topalović, O., Heuer, H., Reineke, A., Zinkernagel, J., and Hallmann, J. (2019). Antagonistic role of the microbiome from a Meloidogyne hapla-suppressive soil against species of plant-parasitic nematodes with different life strategies. Nematology 22, 75–86. doi: 10.1163/15685411-00003285
Topalović, O., Hussain, M., and Heuer, H. (2020). Plants and associated soil microbiota cooperatively suppress plant-parasitic nematodes. Front. Microbiol. 11:491484. doi: 10.3389/fmicb.2020.00313
Tyśkiewicz, R., Nowak, A., Ozimek, E., and Jaroszuk-ściseł, J. (2022). Trichoderma: the current status of its application in agriculture for the biocontrol of fungal Phytopathogens and stimulation of plant growth. Int. J. Mol. Sci. 23:2329. doi: 10.3390/ijms23042329
Verma, A., Kumar, S., Kumar, G., Saini, J. K., Agrawal, R., Satlewal, A., et al. (2018). “Rhizosphere metabolite profiling: an opportunity to understand plant-microbe interactions for crop improvement” in Crop improvement through microbial biotechnology. eds. R. Prasad, S. S. Gill, and N. Tuteja (Elsevier), 343–361.
Wang, Y., Li, L., Li, D., Wang, B., Zhang, K., and Niu, X. (2015). Yellow pigment aurovertins mediate interactions between the pathogenic fungus Pochonia chlamydosporia and its nematode host. J. Agric. Food Chem. 63, 6577–6587. doi: 10.1021/acs.jafc.5b02595
White, R. A., Rivas-Ubach, A., Borkum, M. I., Köberl, M., Bilbao, A., Colby, S. M., et al. (2017). The state of rhizospheric science in the era of multi-omics: A practical guide to omics technologies. Rhizosphere 3, 212–221. doi: 10.1016/j.rhisph.2017.05.003
Wolfgang, A., Taffner, J., Guimarães, R. A., Coyne, D., and Berg, G. (2019). Novel strategies for soil-borne diseases: exploiting the microbiome and volatile-based mechanisms toward controlling Meloidogyne-based disease complexes. Front. Microbiol. 10:458678. doi: 10.3389/fmicb.2019.01296
Wolstenholme, A. J., and Rogers, A. T. (2005). Glutamate-gated chloride channels and the mode of action of the avermectin/milbemycin anthelmintics. Parasitology 131 Suppl, S85–S95. doi: 10.1017/S0031182005008218
Wong-Villarreal, A., Méndez-Santiago, E. W., Gómez-Rodríguez, O., Aguilar-Marcelino, L., García, D. C., García-Maldonado, J. Q., et al. (2021). Nematicidal activity of the endophyte Serratia ureilytica against Nacobbus aberrans in chili plants (Capsicum annuum L.) and identification of genes related to biological control. Plan. Theory 10:2655. doi: 10.3390/plants10122655
Xia, Y., Li, S., Liu, X., Zhang, C., Xu, J., and Chen, Y. (2019). Bacillus halotolerans strain LYSX1-induced systemic resistance against the root-knot nematode Meloidogyne javanica in tomato. Ann. Microbiol. 69, 1227–1233. doi: 10.1007/s13213-019-01504-4
Xia, Y., Xie, S., Ma, X., Wu, H., Wang, X., and Gao, X. (2011). The purL gene of Bacillus subtilis is associated with nematicidal activity. FEMS Microbiol. Lett. 322, 99–107. doi: 10.1111/j.1574-6968.2011.02336.x
Xiang, C., Liu, Y., Liu, S. M., Huang, Y. F., Kong, L. A., Peng, H., et al. (2020). αβ-Dehydrocurvularin isolated from the fungus aspergillus welwitschiae effectively inhibited the behaviour and development of the root-knot nematode Meloidogyne graminicola in rice roots. BMC Microbiol. 20, 48–10. doi: 10.1186/s12866-020-01738-2
Yan, Y., Mao, Q., Wang, Y., Zhao, J., Fu, Y., Yang, Z., et al. (2021). Trichoderma harzianum induces resistance to root-knot nematodes by increasing secondary metabolite synthesis and defense-related enzyme activity in Solanum lycopersicum L. Biol. Control 158:104609. doi: 10.1016/j.biocontrol.2021.104609
Yao, Y., Huo, J., Ben, H., Gao, W., Hao, Y., Wang, W., et al. (2023). Biocontrol efficacy of endophytic fungus, Acremonium sclerotigenum, against Meloidogyne incognita under in vitro and in vivo conditions. Biologia 78, 3305–3313. doi: 10.1007/s11756-023-01505-4
Ye, S., Yan, R., Li, X., Lin, Y., Yang, Z., Ma, Y., et al. (2022). Biocontrol potential of Pseudomonas rhodesiae GC-7 against the root-knot nematode Meloidogyne graminicola through both antagonistic effects and induced plant resistance. Front. Microbiol. 13:1025727. doi: 10.3389/fmicb.2022.1025727
Yi, X., Guo, Y., Khan, R. A. A., and Fan, Z. (2021). Understanding the pathogenicity of Pochonia chlamydosporia to root knot nematode through omics approaches and action mechanism. Biol. Control 162:104726. doi: 10.1016/j.biocontrol.2021.104726
Youssef, M. M. A., El-Nagdi, W. M. A., and Lotfy, D. E. M. (2020). Evaluation of the fungal activity of Beauveria bassiana, Metarhizium anisopliae and Paecilomyces lilacinus as biocontrol agents against root-knot nematode, Meloidogyne incognita on cowpea. Bull. Natl. Res. Cent. 44, 1–11. doi: 10.1186/s42269-020-00367-z
Yu, Z., Xiong, J., Zhou, Q., Luo, H., Hu, S., Xia, L., et al. (2015). The diverse nematicidal properties and biocontrol efficacy of Bacillus thuringiensis Cry6A against the root-knot nematode Meloidogyne hapla. J. Invertebr. Pathol. 125, 73–80. doi: 10.1016/j.jip.2014.12.011
Zhang, J., Fu, B., Lin, Q., Riley, I. T., Ding, S., Chen, L., et al. (2020). Colonization of Beauveria bassiana 08F04 in root-zone soil and its biocontrol of cereal cyst nematode (Heterodera filipjevi). PLoS One 15, e0232770–e0232717. doi: 10.1371/journal.pone.0232770
Zhang, J., Li, Y., Yuan, H., Sun, B., and Li, H. (2016). Biological control of the cereal cyst nematode (Heterodera filipjevi) by Achromobacter xylosoxidans isolate 09X01 and Bacillus cereus isolate 09B18. Biol. Control 92, 1–6. doi: 10.1016/j.biocontrol.2015.08.004
Zhang, X., Zhang, H., Jiang, Z., Bai, Q., Wu, S., Wang, Y., et al. (2021). A new strain of Volutella citrinella with nematode predation and nematicidal activity, isolated from the cysts of potato cyst nematodes in China. BMC Microbiol. 21, 323–312. doi: 10.1186/s12866-021-02385-x
Zhao, D., Zhao, H., Zhao, D., Zhu, X., Wang, Y., Duan, Y., et al. (2018). Isolation and identification of bacteria from rhizosphere soil and their effect on plant growth promotion and root-knot nematode disease. Biol. Control 119, 12–19. doi: 10.1016/j.biocontrol.2018.01.004
Zhao, J., Wang, S., Zhu, X., Wang, Y., Liu, X., Duan, Y., et al. (2021). Isolation and characterization of nodules endophytic bacteria Pseudomonas protegens Sneb1997 and Serratia plymuthica Sneb2001 for the biological control of root-knot nematode. Appl. Soil Ecol. 164:103924. doi: 10.1016/j.apsoil.2021.103924
Keywords: biocontrol agents, plant parasitic nematodes, multi-omics approaches, novel nematicides, sustainable agriculture
Citation: Ayaz M, Zhao J-T, Zhao W, Chi Y-K, Ali Q, Ali F, Khan AR, Yu Q, Yu J-W, Wu W-C, Qi R-D and Huang W-K (2024) Biocontrol of plant parasitic nematodes by bacteria and fungi: a multi-omics approach for the exploration of novel nematicides in sustainable agriculture. Front. Microbiol. 15:1433716. doi: 10.3389/fmicb.2024.1433716
Edited by:
Guanghai Ji, Yunnan Agricultural University, ChinaReviewed by:
Raja Asad Ali Khan, Hainan University, ChinaMuhammad Arif, Sakarya University of Applied Sciences, Türkiye
Jun Yang, Chuxiong Normal University, China
Copyright © 2024 Ayaz, Zhao, Zhao, Chi, Ali, Ali, Khan, Yu, Yu, Wu, Qi and Huang. This is an open-access article distributed under the terms of the Creative Commons Attribution License (CC BY). The use, distribution or reproduction in other forums is permitted, provided the original author(s) and the copyright owner(s) are credited and that the original publication in this journal is cited, in accordance with accepted academic practice. No use, distribution or reproduction is permitted which does not comply with these terms.
*Correspondence: Wen-Kun Huang, wkhuang2002@163.com; Ren-De Qi, rende7@126.com
†These authors have contributed equally to this work