- 1Department of Microbiology, School of Medicine, Iran University of Medical Sciences, Tehran, Iran
- 2Department of Genetics, Faculty of Science, Islamic Azad University North Tehran Branch, Tehran, Iran
- 3Department of Microbiology, School of Medicine, Kermanshah University of Medical Science, Kermanshah, Iran
- 4Department of Basic Sciences, Shoushtar Faculty of Medical Sciences, Shoushtar, Iran
The review aimed to investigate the diversity of oral microbiota and its influencing factors, as well as the association of oral microbiota with oral health and the possible effects of dysbiosis and oral disorder. The oral cavity harbors a substantial microbial burden, which is particularly notable compared to other organs within the human body. In usual situations, the microbiota exists in a state of equilibrium; however, when this balance is disturbed, a multitude of complications arise. Dental caries, a prevalent issue in the oral cavity, is primarily caused by the colonization and activity of bacteria, particularly streptococci. Furthermore, this environment also houses other pathogenic bacteria that are associated with the onset of gingival, periapical, and periodontal diseases, as well as oral cancer. Various strategies have been employed to prevent, control, and treat these disorders. Recently, techniques utilizing microbiota, like probiotics, microbiota transplantation, and the replacement of oral pathogens, have caught the eye. This extensive examination seeks to offer a general view of the oral microbiota and their metabolites concerning oral health and disease, and also the resilience of the microbiota, and the techniques used for the prevention, control, and treatment of disorders in this specific area.
1 Introduction
In the 1700s, Antonie van Leeuwenhoek made a groundbreaking discovery while studying dental plaque under the microscope. The discovery of bacteria in dental plaque is one of the first areas of study in traditional microbiology (Mark Welch et al., 2016; Bowen et al., 2018; Lamont et al., 2018). The oral cavity is home to a rich population of microorganisms, many of which are unique and evolved specifically for oral colonization (Mark Welch et al., 2016; Lamont et al., 2018). The oral microbiota is the second most important and the richest microbial community after the gut and is one of the five research priorities of the Human Microbiome Project. Over 1,100 different taxonomic groups are recorded in the Human Oral Microbiome Database, and the genera Streptococcus, Neisseria, Veillonella, and Actinomyces are associated with the core microbiome (Bäckhed et al., 2012; Wade, 2013; Franzosa et al., 2015; Bowen et al., 2018; Deo and Deshmukh, 2019; Herremans et al., 2022). Among them, facultative anaerobic bacteria such as Streptococcus and Actinomyces predominate in the oral cavity. The low oxygen tension in the subgingival area creates favorable conditions for an increasing number of strict anaerobes such as Bacteroidaceae spp. and Spirochaetes. Identification of microbial communities has a major role in physical and metabolic exchanges between species, which can be cooperative or antagonistic (Mark Welch et al., 2016; Bowen et al., 2018; Lamont et al., 2018). Since 2000, with the introduction of cost-effective genetic sequencing, scientists have focused on the diversity of oral microbial communities and understanding their impact on systemic diseases (Bäckhed et al., 2012). Using modern sequencing technology and next-generation sequencing (NGS) systems, the barriers of traditional culture-based techniques can now be overcome (Franzosa et al., 2015). The microbial community in the body has various functions and is crucial for host health. However, we only become aware of them when the microbiota is out of balance and disease occurs (Zaura et al., 2009; Deo and Deshmukh, 2019). Moreover, recent evidence supports a link between systemic disease and the oral microbiome. The potential for many oral microbes to interplay with an inflammatory microenvironment may be related to this phenomenon. In addition, poor oral hygiene is closely associated with adverse systemic health, and research on oral health and related factors has become a subject of attention (Caselli et al., 2020; Lippi and Mattiuzzi, 2020). Therefore, in the present review, we investigated the diversity of oral microbiota and its influencing factors, and also investigated the association of oral microbiota with oral health and the possible effects of dysbiosis and oral disorder (Figure 1).
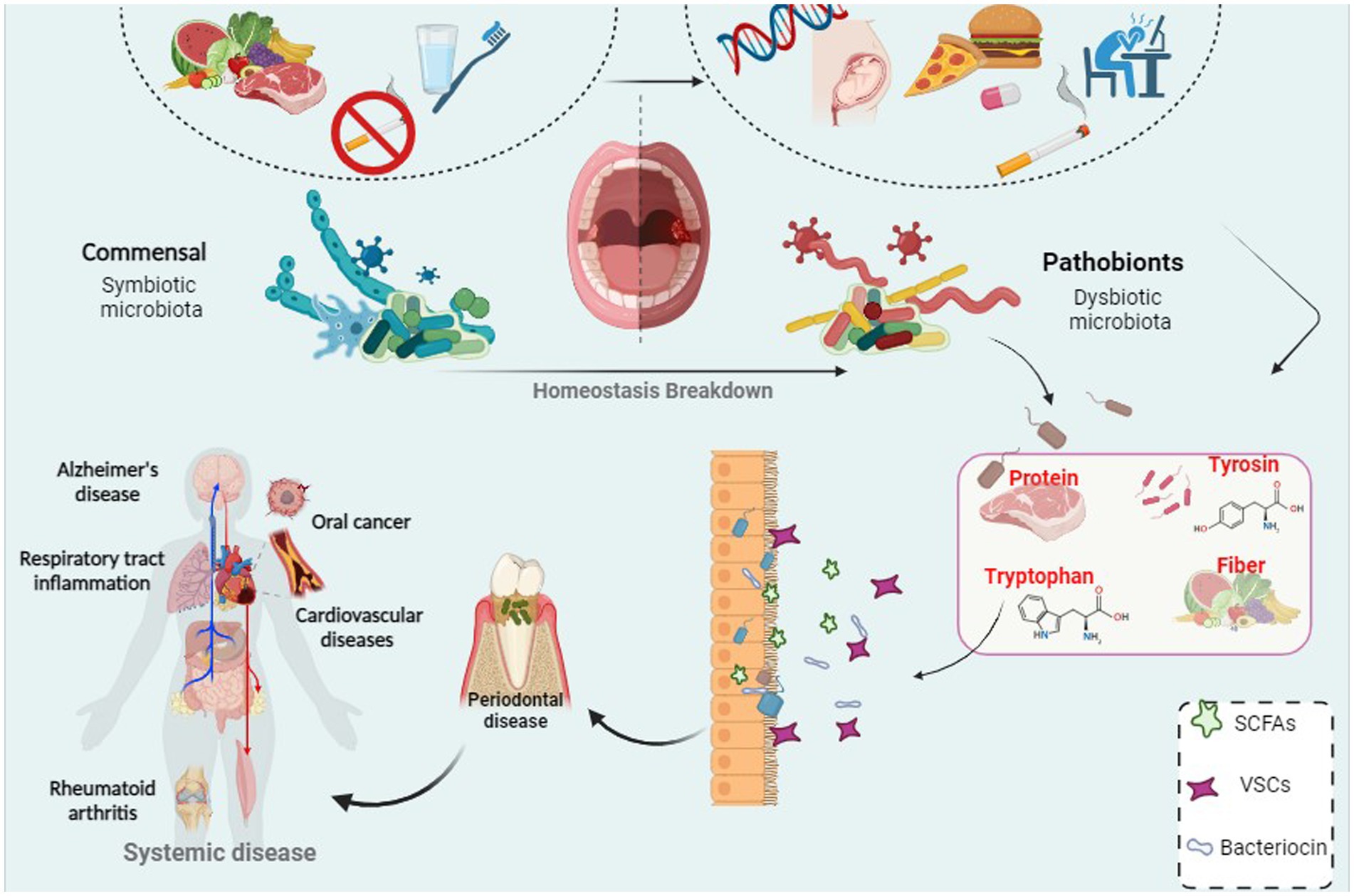
Figure 1. A correlation between bacterial infection and periodontal disease which can led to systemic disease and cancer.
2 Composition and diversity of the oral microbiota
The oral cavity is a complex habitat that allows microorganisms to interact with the host, and is continuously subjected to both inhaled and ingested microorganisms. Of the more than 700 species of viruses, bacteria, fungi, and protozoa in this microbial community, only 54% have been identified and can be cultured. Another 14% of these species are cultivable but have not so far been recognized, and the other 32% have not been cultivated at all (Bäckhed et al., 2012; Wade, 2013; Herremans et al., 2022). It is essential to notice that everyone has their own unique “microbial identity” consisting of their microbial community. The diversity of the human oral microbiome is influenced by the microhabitat. However, in general terms, the human oral microbiome can be defined as having two major but variable components (Franzosa et al., 2015; Deo and Deshmukh, 2019). The core microbiome is similar among healthy individuals (Zaura et al., 2009), while environmental factors and physiological changes specifically influence the diversity of the microbiome (Lippi and Mattiuzzi, 2020). As a result of both genetic and environmental factors, the composition of the oral microbial community can change over time (Caselli et al., 2020; Herremans et al., 2022). Based on the results of NGS, it has been shown that most of the bacteria in the mouth belong to the phyla Proteobacteria, Firmicutes, Bacteroidetes, Oriarchyota, Fusobacteria, Tenricotes, Actinobacteria, and Spirochaetes (Dewhirst et al., 2010). Chloroflexi, TM7, GN02, Synergistetes, Chlorobi, SR1, and WPS-2 are among the lesser known potential phyla in the mouth (Camanocha and Dewhirst, 2014). The mouth is a complex environment that contains diverse microbial populations and undergoes significant changes during life stages (Sedghi et al., 2021). During the menstrual cycle, physiological hormones cause changes in bacteria such as Oribacterium spp., Campylobacter spp., Haemophilus spp., and Prevotella spp. (Bostanci et al., 2021). Another study found that the prevalence of oral Neisseria spp., Porphyromonas spp., and Treponema spp. was higher in pregnant women than in non-pregnant women, but Streptococcus spp. and Veillonella spp. were more common in non-pregnant women than in pregnant women (Saadaoui et al., 2021). These findings assume that the oral microbial ecosystem should be analyzed with respect to the age of the host and its particular biological niche within the oral cavity, as it is continuously exposed to external chemicals (Jiang et al., 2015; Baker and Edlund, 2018; Oliveira et al., 2021). Oral archaea in the oral microbiome are less diverse and less abundant than bacteria. Initially, it was assumed that they were only methanogenic, but several studies have also found non-methanogenic bacteria in the oral cavity (Li et al., 2009; Wade, 2013; Dame-Teixeira et al., 2020). Although not pathogenic, these microorganisms have been identified in inflamed pulp tissue, subgingival biofilms, and caries biofilm samples. Further research is required to determine their potential pathogenicity (Efenberger et al., 2015; Aleksandrowicz et al., 2020; Dame-Teixeira et al., 2020).
There has been very little research on the mycobiome, which refers to the fungal and other microbes present in the oral cavity. However, up to 101 species of fungi have been identified as part of the healthy oral microbiota, including Cryptococcus spp., Aureobasidium spp., Aspergillus spp., Cladosporium spp., Saccharomyces spp., Candida spp., Fusarium spp., and Aspergillus spp. (Arboleya et al., 2015; Fujiwara et al., 2017; Godlewska et al., 2017; Gomez-Arango et al., 2017; Grant et al., 2019). Anelloviridae, Herpesviridae, and Papillomaviridae are the most common oral virome (Holmes et al., 1998; Welch et al., 2020).
3 Factors influencing composition and diversity of oral microbiota
Various internal and external factors, as well as lifespan, can affect oral health. The concept of resilience refers to a system’s capability to quickly recover its equilibrium state after a perturbation (Rosier et al., 2020). Thus, in the interplay between host and oral microbiota, resilience plays a vital contribution to the maintenance of health. Similarly, microbiota resilience could minimize the impact of perturbation while maintaining the symbiotic state, thereby inhibiting dysbiosis and preventing bacterial disease (Liu et al., 2012; Nascimento, 2018). Conversely, Bacteria maintain their balance to maintain oral health. The adaptability of the human oral cavity is affected by host conditions such as genetics, age, immune system, lifestyle and oral environment such as diet, pH, gingival crevicular fluid, and saliva. These factors can induce alterations in the diversity of the community of oral microbes (Chattopadhyay et al., 2019; Nascimento et al., 2019).
3.1 Internal factors
3.1.1 Host genetics
Modern genomic techniques have demonstrated the impact of host genetic factors on the diversity of the oral microbiota community (Heilbronner et al., 2021). However, reports on the heritability of the oral microbiota are conflicting (Wescombe et al., 2012; Bushin et al., 2020).
For example, Esberg et al. highlighted the effect of genetic factors on the composition of the oral microbiota through various factors such as immune pathways in 836 Swedish twins. By studying separate groups of adolescents and adults using 16S rRNA gene sequencing, this research indicated that genetic factors affect the host’s immunity, the composition of oral microbiota species, and susceptibility to tooth disorders such as periodontitis and caries (Ferreira et al., 2014). Studies conducted on twins have proven that hereditary traits have a significant contribution in the composition of oral microbiota. Monozygotic twins had a much more similar oral microbial profile than dizygotic twins, highlighting the importance of host genetics (Lin et al., 2021; Zhang J. S. et al., 2022). In addition, research has shown that the abundance of cariogenic species in plaque and saliva is highly heritable trait (Zhang et al., 2018).
In a study of oral metagenomic analysis of over 1,915 cases, human genetics is responsible for a minimum 10% of the oral microbiome composition in all people. These findings suggest that the oral microbiome persists or recurs in people primarily because of changes in their genetics. It also appears that certain host miRNAs may regulate the growth of specific oral bacteria, which may help predict the risk of dental problems such as gum bleeding and plaque (Duran-Pinedo, 2021). A study conducted in 2021 analyzed the oral microbiota of adoptive mother–child pairs and concluded that acquired factors, such as contact and shared environment, are instrumental in altering and transferring oral microbiota from host genetics. However, because of inconsistent findings, more research is necessary to provide a better insight into the relationship between host genetics and the oral microbiome (Nowicki et al., 2018).
3.1.2 Saliva
The first stage of food digestion occurs in the oral cavity, where chewing, facilitated by the teeth, and subsequent swallowing occurs. Saliva contains peptides, vitamins, amino acids, glycoproteins, and proteins. As a result, the oral microbiota is enriched with saliva-derived nutrients for growth and development. In the context of cleaned teeth, salivary biomolecules form an “acquired pellicle” on the tooth surface by depositing a thin layer containing proline-rich proteins, statherin and histatins, which play a crucial role in oral health (Hannig et al., 2017; Hu et al., 2023). In addition, gingival crevicular fluid (GCF) provides a source of nutrition for the microbiota, containing glycoprotein, heme and albumin (Cornejo Ulloa et al., 2019; Wade, 2021). In the absence of compounds and immune properties of saliva, non-oral bacteria and fungi can colonize and multiply in a dry oral cavity (Dabdoub et al., 2016; Tuominen and Rautava, 2021). The buffering system of saliva contains bicarbonate and phosphate, which are essential for the maintenance of the pH value of the mouth at a neutral level, which is optimal for bacterial growth. The sugars in saliva are rapidly metabolized by the oral microbiota, resulting in the production of acidic components that significantly lower the pH of saliva. This acidic environment, facilitates irreversible tooth decay by activating the saccharolytic activity of bacteria such as Streptoccucs mutans, Actinomyces spp., Bifidobacteria spp., Propionibacterium acidifaciens and Lactobacilli, which convert sugars into acidic products. Under these circumstances, microbial diversity is reduced, leading to the formation of cariogenic microbiota, including acid-tolerant and saccharolytic bacteria (Rosier et al., 2018; Cornejo Ulloa et al., 2019).
3.2 External factors
3.2.1 Indoor environment
The indoor microbiome and its metabolites, such as flavonoids and mycotoxins, play a significant role in shaping the oral microbiome’s composition and function. The indoor environment, where individuals spend a substantial amount of time, hosts diverse microbial communities that can influence the human microbiota, including the oral microbiome. Studies suggest that the diversity and composition of indoor microbiomes can impact the microbial populations in the human body. A healthy indoor microbiome can introduce beneficial bacteria into the oral cavity to support oral health. Conversely, an indoor environment with a high prevalence of pathogenic microbes can disrupt the oral microbial balance, leading to conditions such as periodontal disease and dental caries. Effective management of the indoor environment through proper ventilation and regular cleaning is crucial to mitigate the negative impact on the oral microbiome (Adams et al., 2015; Zhang et al., 2023).
Flavonoids are plant polyphenols found in various foods and beverages, known for their antimicrobial and anti-inflammatory properties. These compounds significantly influence the oral microbiome by inhibiting the growth of harmful bacteria and promoting beneficial species. For instance, catechins in green tea exhibit antibacterial activity against Streptococcus mutans, a major contributor to dental caries (Ferrazzano et al., 2009). Flavonoids also reduce oxidative stress and inflammation in the oral cavity, thereby supporting a healthy and balanced oral microbiome (Meena et al., 2020). Mycotoxins are toxic metabolites produced by fungi and are commonly found in contaminated food and indoor environments. Exposure to mycotoxins, such as aflatoxins and ochratoxins, can adversely affect the oral microbiome. Mycotoxins disrupt the balance of oral microbial communities by selectively inhibiting beneficial bacteria and allowing pathogenic fungi and bacteria to proliferate. This disruption increases the risk of oral infections and inflammation, which can lead to systemic health problems. In addition, mycotoxins can impair the immune response, further compromising the resilience of the oral microbiome to pathogens (Bryden, 2012; Bai et al., 2021).
3.2.2 Diet and nutrition
Understanding the impact of diet on oral microbes is essential to developing successful oral disease prevention strategies (Yang et al., 2018). Since the 1960s, there has been a shift in dietary patterns toward a Westernized style. This change is characterized by the consumption of certain foods such as meat from farmed animals, high-sugar dairy products, refined vegetable oils, and processed grains. These diets have been in associated with pathological modifications in the oral microbiota, including an increase in the proportion of acid-producing and acid-tolerant organisms (Karpiński, 2019; Sun et al., 2020). Dietary variables significantly influence the oral microbiota biofilm, which may disrupt the homeostasis of specific bacterial species (Yang et al., 2018). Evidence suggests that macro- and micronutrients can modify pro- and anti-inflammatory cascades and influence the inflammatory state of an individual at rest. The oral microbiota is nourished by the diet, which also causes selective pressures that favor the growth and proliferation of organisms that are best suited to utilize certain food resources provided by the host (Lee et al., 2017).
An oral health study evaluated the effect of traditional diets on the dental health of “bush dwellers” and “village dwellers” who were consuming an increasingly externalized diet. The results supported the idea that hunter-gatherer societies have better dental health than agricultural societies. Women who resided in villages and ate a diet rich in agricultural products had a higher prevalence of tooth decay and periodontal disease than those who lived in the bush and consumed a diet rich in wild foods such as legumes. However, cultural factors such as excessive honey consumption and smoking affect the dental health of bush dwelling men, who do not maintain the same level of dental health as their female counterparts (Zhang L. et al., 2020b). In addition to the well-established association between simple carbohydrate consumption and dental caries, several dietary factors may help prevent dental caries. The results of an in vivo study revealed that frequent usage of hard candy increased the rate of oral streptococci such as Streptococcus parasanguinis, Streptococcus gordonii, and Streptococcus sanguinis while generally decreasing some species of the oral microbiota such as Haemophilus spp. and members of the Proteobacteria phylum (Abranches et al., 2018; Hampelska et al., 2020).
Recent research has shown that the entero-salivary nitrate-nitrite-nitric oxide pathway, which promotes nitric oxide (NO) maintenance, is a favorable symbiotic connection between oral microbiota and the human host. Here, nitrate concentrated in the salivary glands is converted to nitrite by facultative anaerobic oral bacteria, which is subsequently ingested and absorbed into the circulation before being further converted to NO. Moreover, the daily use of antiseptic mouthwash for up to 7 days can disrupt the oral microbiome of healthy non-athletes, resulting in a decrease in plasma and oral nitrite levels and elevated blood pressure without dietary intervention. This is an indication of the essential contribution of the oral microbiome to this condition. These findings suggest which nutrition can alter the oral microbiome that affects the entero-salivary pathway and NO homeostasis, but further research is needed to explore this possibility in depth (Vinasco et al., 2019; Wu et al., 2022).
3.2.3 Smoking
The oral area represents one of the first sites on the body to be subjected to cigarette smoke, leading to increased carcinogenesis, reduced mucosal immunity, and altered oral microbiota (Al Bataineh et al., 2020). Numerous toxicants from cigarette smoke can disrupt the oral cavity’s microbial diversity by antibiotic effects, oxygen reduction, or another way (Wu et al., 2016). Smoking with alternation of oxygen levels can favor the survival of microaerophilic bacteria over commensal beneficial species (Jaspers, 2014). It also promotes the development of biofilm and increases the adherence of pathogens, such as Streptococcus pneumoniae, Staphylococcus aureus, and S. mutans, to the epithelial layer. According to some evidence, former smokers’ oral microbiota was more similar to that of current smokers than non-smokers, suggesting smoking effects on oral microbiota may last for years. The oral cavity of smokers tends to be more colonized with disease-associated bacteria such as Actinomyces, which have been associated with the occurrence of dental diseases such as periodontitis and caries. Additionally, Actinomyces spp. in the salivary microbiota have also been linked to the development of liver cancer (Li et al., 2020; Jia et al., 2021). Wu et al. found that current smokers had a reduced frequency of aerobic metabolic pathways, such as tricarboxylic acid (TCA) cycle and oxidative phosphorylation, and higher frequencies of glycolysis and other oxygen-independent carbohydrate metabolism pathways. Non-smokers had more Proteobacteria than current smokers, while the difference between former smokers and never smokers was not significant. Atopobium spp. and Streptococcus spp. were enriched relative to never smokers, but the genera Capnocytophaga spp., Peptostreptococcus spp. and Leptotrichia spp. were reduced. The study also found that non-proteobacterial taxa was associated with smoking. Overall, evidence shows that cigarette smoke creates a setting that is more conducive to facultative aerobics than robust aerobics (Jia et al., 2021). On the other hand, cigarette smoking affects the respiratory immune system at several levels (Tuominen and Rautava, 2021), which resulted in the induction of inflammatory cytokines such as IL-8, TNF-α, and MCP-1, due to the stimulation of innate immune and inflammatory cells such as neutrophils and macrophages (Jaspers, 2014).
3.2.4 Pregnancy
It is vital to maintain good oral hygiene during pregnancy because the mother’s oral hygiene has a direct effect on the child’s oral hygiene. Hence, the elimination of maternal oral infections during pregnancy is critical, as it can prevent or delay colonization of the infant’s oral cavity with oral pathogens (Jang et al., 2021). Altered oral microbiota during pregnancy may have implications for maternal oral health, birth outcomes, and infant oral health because a stable microbiota helps maintain stable oral and general health. Therefore, it is critical to understand changes in the oral microbiota during pregnancy, their relationship to maternal health, and their impact on birth outcomes (Jang et al., 2021). Current studies have revealed that the diversity of oral microbiota in pregnant women remains stable throughout pregnancy. Although, the diversity of the oral microbiota changes in a pathogenic manner and then returns to a normal or healthy microbiome after delivery. It is believed that these changes are caused by female hormones such as progesterone and estrogen (Ye and Kapila, 2021). Previous reports have shown a high frequency of Prevotella intermedia sensu lato over the second trimester. Subsequent studies have found that the growth of certain bacterial species, such as Porphyromonas gingivalis has been found to have elevated levels of progesterone and estrogen, which are part of the fumarate reductase system of the pathogen (Lin W. et al., 2018). A 2015 study by Fujiwara et al. found that the number of pathogenic bacteria, such as P. gingivalis and Aggregatibacter actinomycetemcomitans, in gingival crevices increased in pregnant women during the early and middle stages of pregnancy compared to non-pregnant women (Fujiwara et al., 2017).
4 Role of the oral microbiota in oral health
The oral cavity is a warm, moist, and nutrient-rich environment that provides a suitable niche for the colonization of microbiota. Early colonization is exhibited by Gram-positive bacteria, such as Streptococci (S. sanguinis, S. mutans, S. sobrinus, S. gordonii, and S. oralis), as well as Actinomyces, through adhesion to various surfaces, including gingival surfaces and teeth covered by pellicles (O’Mullane et al., 2016; Thurnheer and Belibasakis, 2018).
The oral microbiota strives to retain a healthy dynamic state and a symbiotic relationship in the complex oral ecosystem, which is referred to as eubiosis. In addition, these bacteria can self-aggregate and co-aggregate with other bacterial species, including some Gram-negative bacteria, through various bacterial glycoproteins and polysaccharides. This process serves as the basis for biofilm formation (Holmes et al., 1998; Bürgers et al., 2010). However, this state of eubiosis can be interrupted and transformed into a state of dysbiosis (Radaic and Kapila, 2021). Dysbiosis is characterized by the involvement of bacterial metabolites in periodontal disease, as well as a marked difference in the metabolic profiles of the oral microbiota when compared with healthy conditions (Heilbronner et al., 2021). In this situation, oral microbiota may be associated with the pathological processes of several diseases, such as inflammatory bowel disease (IBD), arthritis, colorectal and pancreatic cancer, and Alzheimer’s disease, by serving as a reservoir for opportunistic pathogens.
5 Role of oral microbiota metabolites in health
The oral bacterial population produces a significant source of metabolites in the mouth which are associated with critical processes in human health or disease. Bacterial metabolites include by-products of carbohydrate, lipid, and protein metabolism such as short chain fatty acids (SCFAs), amines, and gasses (Mai et al., 2017) (Table 1). These metabolites are used by the medical and biotechnology industries as antimicrobial, antifungal, and anticancer agents. Saliva contains glycoproteins and amino acids that are broken down into their subunits by oral microbiota and human enzymes. Under anaerobic conditions, acid-producing bacteria including, Actinomyces spp., Streptococcus spp., and Lactobacillus spp. metabolize deuterated sugars to ethanol, lactic acid, formic acid, and acetic acid via the Embden-Meyerhof-Parnas pathway (Takahashi, 2015; Rosier et al., 2020). The nitrate-reducing bacteria use the acids that are produced as a source of carbon. Then, they raise the pH with ammonia from arginine and urea metabolism, neutralizing the acid and creating a balanced oral ecosystem. In this mechanism, nitrate can be reduced to ammonia (NH3) by the bacterial nitrate-reducing ammonium (DNRA) pathway, raising the pH (Efenberger et al., 2015). As a result, the simultaneous presence of acid-producing and nitrate-reducing bacteria helps maintain a neutral oral cavity (Kistler et al., 2013; Abusleme et al., 2021).
Lugdunin is an example of an oral microbiota metabolite. It is synthesized by Staphylococcus lugdunensis, a commensal bacterium found in the mouth. It belongs to thiazolidine-containing cyclic peptides and has been identified as a potent inhibitor of methicillin-resistant S. aureus, a superbug that poses a significant threat to human health. S. gordonii and Actinomyces spp. can metabolize basic amino acids such as arginine to produce ornithine, and citrulline and release other by-products including ammonia, ATP and CO2 by a number of reactions referred to as the arginine deiminase system (ADS) (Liu et al., 2012). The alkalinity created by this process can eliminate the acidification produced by sugar metabolism. In fact, some findings showed that lower arginine catabolic capacity, as determined by measuring ADS activity, had correlated with the formation of carious lesions in children. This event is the result of ammonia production by ADS, which leads to the elimination of glycolytic demineralizing acids, thereby promoting the development of a microbiota that protects dental health (Nascimento, 2018; Nascimento et al., 2019).
Therefore, using ADS+ strains as probiotics and adding arginine to oral health products can be a helpful strategy for preventing and treating dental caries (Nascimento et al., 2019). Oral alkaline producing species such as S. gordonii and Actinomyces spp. can be used to neutralize the acidity of the oral cavity in oral cancer and limit the development of acidogenic bacteria (Chattopadhyay et al., 2019). Also, the oral microbiota produces several classes of metabolites, such as bacteriocins, lantipeptides (or lantibiotics) and sactipeptides that are classified as Ribosomally synthesized and post-translationally modified peptides (RiPPs). They can integrate into the target cell membrane and induce membrane perforation, which is their main mode of action. Because of their cell-killing activity, these peptides have been indicated for use in the treatment of cancer (Barbour et al., 2022).
The most extensively studied examples of RiPPs are bacteriocins, which are synthesized by some bacteria. They are typically described as antimicrobial peptides or proteins with the ability to inhibit or kill specific pathogens. The most widely studied oral microbial clusters that produce bacteriocins are members of Prevotella spp., Streptococcus spp., Fusobacterium spp., and Lactobacillus spp., which secrete one or more bacteriocin-like inhibitory factors (Heilbronner et al., 2021). On the other hand, it has been shown that lantibiotics, as one of the categories of bacteriocins, interfere with the membrane potential of target cells. An example of lantibiotics synthesized by oral community microbiota is salivaricin A. This is the primary lantibiotic produced by S. salivarius (Wescombe et al., 2012). Sactipeptides are a type of RiPP that possess a sactionine thioether bridge that is post-translationally incorporated and required for their ability to exert antimicrobial activity. Streptosactin is an example of a sactipeptide synthesized by Streptococcus thermophilus and oral microbiota, such as Streptococcus constellatus, S. gordonii, S. oralis, and S. parasanguini (Bushin et al., 2020).
6 Imbalance of oral microbiota in oral disorders
An imbalance between the microbiota and the host caused by certain factors can lead to diversity in the microbial community. This can cause to oral and dental diseases in which beneficial bacteria are destroyed and pathogens proliferate. Under these conditions, the immune system is unable to fight the pathogens, exposing the host to tissue and dental damage such as dental caries, periodontal disease, and carcinogenic metabolites that can cause cancer and other systemic diseases (Verma et al., 2018; Radaic and Kapila, 2021). In addition, it has also been contributed to various types of malignancies, including gastric, colorectal, liver, lung, esophageal, and breast malignancies (Takahashi, 2015). In this section, we focus on some diseases that result from perturbations in oral microbiota.
6.1 Dental caries (cavities)
In general, investigation of the oral microbiota composition in caries revealed that the bacterial population in caries is less diverse than in the healthy state (Zhang Y. et al., 2022). Although, the genera Streptococcus spp. and Lactobacillus spp., have been studied as the most common causative agents of dental caries, current evidence using meta transcriptomics approaches has revealed a broad spectrum of microbiota contributing to dental caries (Lin et al., 2021).
Moreover, Actinomyces spp., Fusobacterium spp., Porphyromonas spp., Rothia spp., Granulicatella spp., Gemella spp., Selenomonas spp., Bifidobacteria spp., Scardovia spp., Corynebacterium spp., Granulicatella spp., Propionibacterium spp., Bifidobacterium spp., and Scardovia spp. are strongly related to caries development (Zhang et al., 2018; Duran-Pinedo, 2021; Zhang J. S. et al., 2022). These microbiota interact with each other in a dynamic polymicrobial biofilm and induce the progression of caries from early-onset (primary demineralization) to deeper damage with dentin exposure (Zhang et al., 2018).
6.2 Periodontal diseases (gingivitis and periodontitis)
Chronic inflammation caused by oral microbiota can damage the structures that support the teeth, leading to gingivitis and periodontal disease (Duran-Pinedo, 2021). Gingivitis is a reversible stage that begins at the bottom of the apical gingival border and modifies the supra and subgingival sulci (Radaic and Kapila, 2021).
Molecular analyses of bacterial communities have demonstrated that a distinct cohort of oral microbiota, including the genera Oribacterium spp., Leptotrichia spp., Tannerella spp., Lachnospiraceae spp., Lachnoanaerobaculum spp., Lautropia spp. as well as Prevotella oulorum and Fusobacterium nucleatum are more abundant during gingivitis (Kistler et al., 2013; Nowicki et al., 2018). The rapid development of gingivitis leads to the accumulation of oral microorganisms in the gingival crevices, resulting in inflammation and ultimately the destruction of the periodontium and alveolar bone (Sedghi et al., 2021). Imbalance of oral microbiota is a consequence of periodontitis, leading to a shift from commensal to pathogenic species (Radaic and Kapila, 2021).
Examination of the oral microbiota of healthy individuals and those with periodontitis revealed that Actinomyces, Rothia, and Streptococcus spp. are predominant in the healthy oral cavity compared with periodontitis, whereas periodontitis was associated with a greater diversity of pathogenic taxa including Treponema spp., P. gingivalis, Porphyromonas endodontalis, P. intermedia, Filifactor alocis, Tannerella forsythia, Parvimonas micra, and Fretibacterium spp. (Abusleme et al., 2021). In another study, periodontitis was associated with an elevated number of pathogens such as Parvimonas, Fusobacterium, Prevotella as well as F. alocis, P. gingivalis, T. forsythia, and Treponema denticola (Abusleme et al., 2013; Dabdoub et al., 2016).
6.3 Oral cancer
Chronic inflammation caused by microorganisms in the oral cavity could potentially lead to the development of oral cancer by inhibiting cellular apoptotic processes and producing carcinogenic metabolites (Zhang et al., 2018). Anaerobic bacteria such as Prevotella spp., Porphyromonas spp. and Fusobacterium spp. can produce inflammatory cytokines such as interleukin-6 (IL-6), IL-1β, matrix metalloproteinases MMP-8 and MMP-9 and tumor necrosis factor-α (TNF-α) in response to LPS from Gram-negative bacteria, leading to chronic inflammation that is associated with invasive and aggressive tumor phenotypes, and epithelial tumor migration (Sun et al., 2020; Tuominen and Rautava, 2021). Porphyromonas spp. stimulates anti-apoptotic effects on tumor cells by reducing the tumor suppressor P53, activating the anti-apoptotic Jak1/Act/Stat3 signaling pathway and secreting an anti-apoptotic enzyme (nucleoside diphosphate kinase) (Karpiński, 2019).
Long-term periodontal disease is a major contributor to oral squamous cell carcinoma (OSCC). A decrease in Firmicutes and an increase in bacteria such as Fusobacterium spp., Porphyromonas spp., Haemophilus spp., Peptostreptococcus spp., Rothia spp., Prevotella spp., Streptococcus spp., and Veillonella spp. have been observed in OSCC (Lee et al., 2017; Yang et al., 2018). Furthermore, an increase in bacteria such as Capnocytophaga leadbetteri, P. intermedia, Aggregatibacter segnis, Peptostreptococcus stomatis, and F. nucleatum have been proved in the sites of buccal mucosal tumors in OSCC patients compared with normal tissue (Zhang L. et al., 2020). Oral microbiota can induce reactive oxygen species (ROS) and organic acids that may be involved in the development of cancer. Some species in the oral cavity, including S. oralis, S. sanguinis, S. gordonii, Lactobacillus fermentum, L. minutus and Bifidobacterium adolescentis, produce hydrogen peroxide (H2O2) through increased expression of NADPH oxidase activity oxidase activity (Abranches et al., 2018). In addition, Alloprevotella spp., P. gingivalis, A. actinomycetemcomitans, P. intermedia, Peptostreptococcus spp., and F. nucleatum produce free radicals such as hydrogen Sulfide, dimethyl Sulfide and methyl mercaptan, which affect tumor angiogenesis and growth (Hampelska et al., 2020; Wu et al., 2022).
Certain bacteria such as Streptococcus spp., Lactococcus spp., Bifidobacterium spp., Lactobacillus spp., Pediococcus spp. and Leuconostoc spp. produce lactic acid and lower the pH, which may provide an acidic microenvironment in tumor cells and increase the risk of metastasis (Vinasco et al., 2019).
7 Oral microbiota metabolites in oral disorders
There has been limited investigation into the characterization of oral microbiota metabolic products and their contribution to maintaining the balance between different types of bacteria and the human host in the oral microbiota (Ge et al., 2015) (Table 1). SCFAs are the best studied bacterial metabolites derived from the fermentation of carbohydrates and amino acids. SCFAs, including butyric, lactic, formic, acetic, and propionic acids, are necessary for reconstituting biofilm species and inducing tissue inflammation in the subgingival and supragingival oral cavity (Takahashi et al., 2010). Changes in the levels of individual metabolites due to changes in environmental conditions can lead to periodontal disease (Gomez-Arango et al., 2017).
Streptococcus and Lactobacillus are the major lactic acid producing bacteria in the oral cavity. The high levels of lactic acid in animal models of active caries indicate their important role in the cariogenic process (Olson et al., 2011). Lactic acid production by S. gordonii increases the pathogenicity of another oral microbiota such as A. actinomycetemcomitans. In addition, Eubacterium spp., Veillonella spp., and Corynebacterium spp. can catabolize lactic acid produced by Streptococcus spp. as a preferred substrate in oral biofilms (Zhang L. et al., 2020). A number of oral microbiota, including P. intermedia, P. gingivalis, T. forsythia, F. nucleatum, and T. denticola produce significant amounts of butyric acid (Mai et al., 2017). This metabolite, through an anti-inflammatory process, prevents an appropriate immune response against the bacteria involved in periodontitis and thus promotes the development of periodontal disease in the oral cavity (Lourenço et al., 2014). Butyrate is necessary in immune processes associated with periodontal disease by reducing the expression of the adhesion protein intercellular adhesion molecule-1 (ICAM-1) and the transmigration of immune cells, as well as reducing mediator production and neutrophil phagocytosis (Zhou et al., 2018). Butyrate accumulation can also stimulate the colonization of other oral microbiota such as Actinomyces oris in the early stages of biofilm colonization (Liu et al., 2021). It appears that SCFAs are also associated with cancer development. The acidic environment created by the acidogenic oral microbiota establishes an ideal environment for the growth and invasion of cancer cells (Abusleme et al., 2013). In addition, a number of SCFAs, including propionic, butyric, isobutyric, and isovaleric acids, can reactivate latent viruses such as Kaposi’s sarcoma-associated herpesvirus and Epstein–Barr virus to initiate tumorigenic processes in host cells (Reddy et al., 2019; Di Spirito et al., 2022). On the other hand, protein metabolism by the oral microbiota occurs in subgingival bacterial niches and deep periodontal pockets where access to dietary carbohydrates is limited (Gutt et al., 2018). The amino acid metabolizing bacteria Fusobacterium, produces alkaline by-products that raise the pH of the oral cavity to near neutral. This alkaline environment favors the colonization and activation of the proteolytic capacity of acid-sensitive oral bacteria such as P. gingivalis, which contributes to the initiation of inflammatory responses (Jin Baek et al., 2017). Some oral microbiota such as P. intermedia and P. gingivalis can metabolize acidic amino acids such as glutamic acid and aspartic acid to produce propionic acid and acetic acid. Increased metabolism of these amino acids is associated with the progression of periodontal pathology, particularly during pregnancy (Beck et al., 2020). In addition, Bifidobacterium spp. and Scardovia wiggsiae can synthesize acetic acid through the metabolic pathway and mediate pathogenicity through this by-product (Barbour et al., 2022). The production of bacterial metabolites in some cases has beneficial effects in oral diseases. Catabolism of the aromatic amino acid phenylalanine by redox reactions produces alkaline metabolites such as phenylacetate and regulates the pH of the oral biofilm (Roslund et al., 2021). The alkaline environment established by bacteria can neutralize the acidification of carbohydrate metabolism and stimulate the development of dental health by maintaining the microbiota in dental caries (Feng et al., 2023). In addition, alkali-producing species can balance acidic pH and reduce acidogenic bacteria, thereby inhibiting their tumor-promoting activities in oral cancer cells (Jiao et al., 2020). Some anaerobic oral microbiota, such as P. intermedia, F. nucleatum, and P. gingivalis, can metabolize the aromatic amino acid tryptophan to synthesize a variety of toxic indolic and phenolic metabolites, including indole, skatole, indole pyruvate, and indole acetate. These compounds, especially skatole, are odorous and contribute to oral halitosis (Zhang G. et al., 2020). F. nucleatum and P. intermedia may catabolize lipids and fatty acids in high-fat diets by lipases (Saha et al., 2023). Lipid metabolites may have significant pathogenic capacity. The metabolism of choline by S. sanguinis produces trimethylamine, which is converted to trimethylamine N-oxide in liver cell. High levels of trimethylamine N-oxide in the blood can interfere with reverse cholesterol transport and induce foam cell formation (Sánchez et al., 2014) (Table 2).
8 Microbiota based therapies
8.1 Probiotics
Probiotics are live, non-pathogenic bacteria that provide health benefits to the host when consumed in adequate amounts. These microorganisms can alter the composition of the oral microbiota. The goal of probiotic therapy is to replace harmful indigenous microorganisms with non-pathogenic ones. Probiotics are often used to treat various oral and dental conditions, such as periodontal disease, halitosis, dental caries, and oral candidiasis (Bosch et al., 2012; Allaker and Ian Douglas, 2015; Mishra et al., 2020; Zhang Y. et al., 2022). Probiotics are available in various commercial forms, including tablets, toothpaste, and mouthwash, for the treatment of periodontal disease (Parcina Amizic et al., 2017; Zhang Y. et al., 2022). Systematic reviews by Inchingolo et al. (2023) and Navidifar et al. (2023) have shown the beneficial effects of probiotics on oral health conditions, including periodontitis, gingivitis, dental caries, and orthodontics. These bacteria interact directly with plaque, disrupt biofilm formation by competing for receptors on host tissue or other bacteria, and compete for nutrients. One significant mechanism involves the production of antimicrobial substances that inhibit oral microorganisms, such as organic acids, low molecular weight antimicrobial peptides, hydrogen peroxide, adhesion inhibitors, and bacteriocins. Probiotics also indirectly influence immune function by regulating both innate and adaptive responses, interacting with oral epithelial cells, and enhancing mucosal barrier function. Lactic acid bacteria can interact with immune cells like macrophages and T cells, leading to cytokine release and impacting systemic immunity (Lin T. H. et al., 2018; Mishra et al., 2020; Abdi and Ranjbar, 2022).
Commercial probiotics like Bifidobacterium, Lactobacillus, and Streptococcus have been shown to enhance alpha diversity in the oral microbiota (Willis and Gabaldon, 2020). Various theories suggest that probiotics such as S. salivarius strains can reduce inflammation and induce beneficial responses, including type I and type II interferon responses. Streptococcus A12 and Streptococcus dentisani can neutralize the acidic pH in cariogenic biofilms through arginine metabolism. For probiotics to exert their cariostatic effects, they must adhere to oral tissues and become part of the biofilm. Their effectiveness is also influenced by their duration of presence in the area. Dairy products like milk, yogurt, and cheese are effective carriers of probiotics. For instance, incorporating Lactobacillus rhamnosus GG into milk has been shown to help prevent early tooth decay in children (Nagaraj et al., 2012; Qiu et al., 2020). Bifidobacterium DN-173 010, Lactobacillus rhamnosus CG, Lactobacillus reuteri, and Lactobacillus casei have been demonstrated to disrupt cariogenic bacteria colonization, reducing the risk of dental caries (Busscher et al., 1999; Näse et al., 2001; Caglar et al., 2005, 2006; Allaker and Ian Douglas, 2015). A combination of live lyophilized acidophilic lactobacilli has been used topically to treat mild to moderate periodontitis (Lin T. H. et al., 2018). Coordination Lactococcus lactis NCC2211 into the verbal biofilm can avoid the development of Streptococcus sobrinus OMZ176. A biosurfactant inferred from L. fermentum that employments glucosyltransferases has been appeared to avoid S. mutans from creating sucrose, subsequently diminishing dental caries caused by this bacterium (Mishra et al., 2020). Considerations have appeared that L. reuteri RC-14, L. rhamnosus GR-1, and L. reuteri ATCC PTA 5289 can hinder the development of C. albicans by co-aggregating with yeasts, avoiding other pathogens from following to verbal surfaces (Chuang et al., 2011; Mishra et al., 2020).
Bacillus subtilis has a metabolic advantage over human oral pathogens, including Streptococcus. B. subtilis cells perform better in utilizing sugar alcohols like mannitol and sorbitol compared to S. mutans, which may explain the significant decrease in S. mutans biofilm production during co-culture (Duanis-Assaf et al., 2020). Reducing S. mutans could potentially help prevent caries from developing. Research indicates that gastric feeding of Lactobacillus gasseri enhances mice immunity against pathogenic bacteria by increasing bone resorption, periodontal ligament separation, and disruption, and reducing P. gingivalis (Kobayashi et al., 2017).
Chronic inflammation is a characteristic of cancer, and altered oral flora can lead to oral inflammation that promotes mutagenesis, angiogenesis, and uncontrolled cell proliferation, resulting in oral cancer (Kay et al., 2019; La Rosa et al., 2020). Anaerobic bacteria such as Fusobacterium, Porphyromonas, and Prevotella are common causes of the chronic inflammation associated with periodontal disease, and cause the release of inflammatory mediators (La Rosa et al., 2020; Vyhnalova et al., 2021; Bostanghadiri et al., 2023). Evidence suggests that probiotics help maintain oral health by selectively replacing and inhibiting dysbiosis. Postbiotics, including short-chain fatty acids (SCFA), polysaccharides, microbial cell fractions, functional proteins, EPS, and cell lysates, offer anticancer activity and can serve as an alternative to conventional chemotherapy (Żółkiewicz et al., 2020). Moreover, postbiotics of Lactobacillus acidophilus, L. casei and Bifidobacterium longum have shown significant anticancer effects on both implanted and chemically induced tumors. Administering the cytoplasmic fraction of lactic acid bacteria (LAB) in vivo enhanced specific antitumor activity by altering cellular immunity without causing direct tumor cell death (Lee et al., 2004). A study by Zhang et al. showed that probiotic L. salivarius REN has the ability to inhibit oral cancer development in a dose-dependent manner through protection against oxidative damage and downregulation of COX-2 and proliferating cell nuclear antigen (Zhang et al., 2013). COX-2 is often up-regulated in many types of cancer, where it blocks apoptosis, alters cell adhesion, and manipulates the normal cell signaling pathway. Therefore, inhibiting COX-2 may be an effective strategy to prevent oral cancer by blocking the production of PGE 2, which promotes cancer growth (Mohan and Epstein, 2003). Also, Zhang et al. showed that oral gavage of L. salivarius REN or its postbiotic can potently inhibit the progression of 4-nitroquinoline-1-oxide-induced oral carcinogenesis by decomposing 4-nitroquinoline-1-oxide to a less toxic compound, preventing DNA from oxidative damage caused by the carcinogen, downregulating COX-2/PCNA expression, and inducing apoptosis. An in vitro study on human myeloid cells showed that probiotic L. reuteri ATCC 6475 supernatant (Lr-S 6475) in combination with tumor necrosis factor (TNF) can induce apoptosis via NF-kB and MAPK pathways (Zhang et al., 2013). Secreted metabolites of Acidobacter syzygii exhibit anticancer effects by induction of apoptosis (Aghazadeh et al., 2017).
8.2 Microbiota transplantation
Growing antibiotic resistance has triggered interest in finding new treatments. Microbiota-centered interventions include a range of therapies that attempt to restore ecological balance by harnessing microorganisms (Bosch et al., 2012). Some examples of these interventions include microbiota transplantation, probiotics, engineered symbiotic bacteria, and microbiota-derived proteins and metabolites (Sorbara and Pamer, 2022). One of the alternative treatments for oral diseases is oral microbiota transplantation (OMT), a type of treatment that involves altering the dysbiosis of the microbiota to restore the microbial ecological balance.
OMT works by transferring biofilms from healthy donors to patients with caries or periodontitis. The proposed procedure, as hypothesized by Dewhirst and Hoffmann, involves three steps: (1) collecting supragingival plaque from a caries-free donor who may be a family member of the recipient patient, (2) preserving the plaque in saline solution, and (3) transferring the plaque sample to the teeth of a patient with active caries using a nylon swab (Pozhitkov et al., 2015; Mira et al., 2017). It is important to have a donor with a strong oral microbiome that is free of cariogenic bacteria, particularly S. mutans, and shows a slight decrease in pH when exposed to a sugar stimulus.
It is likely that interspecies interactions and immunostimulatory effects will play a significant role in OMT therapy. OMT reduced systemic inflammation induced by neck and head irradiation by reducing the levels of IL-1, IL-6, TNF-α, and TGF-β (Xiao et al., 2021). However, transplanted oral biofilms must be able to withstand selective environmental pressures and continue to exert beneficial effects. OMT is hypothesized to work by establishing colonization of oral sites, competing with pathogenic bacteria for adhesion sites and nutrient sources, producing bacteriocin and hydrogen peroxide to eliminate pathogens, and controlling local and systemic immune responses (Nascimento, 2017). The safety issues related to the use of OMT and oral probiotics are comparable. In similar to probiotics, transplanted biofilms must be disease-free and have impressive genetic stability. However, how OMT works and the best way to deliver it is not understood (Nath et al., 2021). As of now, it is uncertain if oral biofilms should be directly transplanted from a donor in good health into a recipient, or if they should be pre-treated to reduce the levels of pathogenic bacteria before transplantation. It is also uncertain whether biofilms produced in vitro or naturally occurring commensal organisms would be a superior alternative (Sánchez et al., 2014). Nevertheless, biofilms of clinical strains with useful and health-promoting properties are effective in interacting with and replacing the biofilms associated with the disease (Gutt et al., 2018).
Other factors to be considered include the need to disinfect the recipient’s oral cavity before OMT and whether single or several doses of oral transplants would be necessary for successful and sustained colonization of the oral cavity for the establishment and maintenance of health (Nascimento, 2017).
8.3 Antimicrobial agents targeting specific oral pathogens
The most common form of oral pathogen eradication is the use of antibiotics. Some systemic antibiotics that target specific oral pathogens include penicillin, metronidazole, macrolides, and clindamycin. Metronidazole is a topical medication that is effective against obligate anaerobic microorganisms in the oral cavity, including those from infected necrotic pulp. In in vitro experiments, metronidazole inhibited over 99% of the bacteria found in carious lesions and infected root dentin (Delaviz et al., 2019). Erythromycin is a macrolide commonly used to treat various bacterial infections. According to some evidence, caries-active dams can become caries-inactive when individuals receive erythromycin. In addition, the treatment with erythromycin resulted in a 35% reduction in plaque formation within one week (Qiu et al., 2020).
Penicillin has been shown to be effective against several strains of gram-positive Streptococci and Staphylococci, as well as certain gram-negative bacteria. However, bacterial resistance to β-lactam antibiotics is a major challenge (Macy, 2015). Over the years, the use of systemic antibiotics for the treatment of oral bacteria has decreased. This is because many of these antibiotics not being originally designed for this purpose, and they are lack of specificity in treating oral diseases. Instead, alternative antimicrobial agents have been introduced that specifically target oral bacteria, including chlorhexidine, fluoride, antimicrobial peptides (AMPs), and quaternary ammonium salts. Fluoride, the major anion of fluorine, has been demonstrated to be a very powerful agent in the prevention of tooth decay of dental caries, especially when added to consumed water or to oral products such as toothpastes, mouthwashes, and oral supplements. There is an ongoing debate about whether fluoride helps prevent tooth decay (O’Mullane et al., 2016). It is generally believed that the fluoride ions interact with the tooth’s mineral structure, thus leading to more remineralization and avoiding demineralization due to cariogenic bacteria’s acidity (Thurnheer and Belibasakis, 2018).
Chlorhexidine, a cationic polybiguanide, was an early antiseptic that was recommended for dental decay and has been demonstrated to be the most effective. It remains the most important antiplaque agent because of its ability to prevent plaque formation and bacterial adhesion. Disruption of acidic groups of glycoproteins in saliva by chlorhexidine reduces plaque adhesion. It also prevents bacterial adhesion to tooth surfaces, either by adhering to extracellular polysaccharides or by the competition with calcium ions for accumulation in plaque (Brookes et al., 2020).
Initially, quaternary ammonium salts were added to mouthwashes to combat oral plaque. Later, they have been added to dental products to provide antimicrobial properties paliguanid (Ge et al., 2015). AMPs are widely distributed in the human body and exhibit antimicrobial properties that target microorganisms (Gordon et al., 2005; Zasloff, 2019). AMPs are considered as one of effective molecules in the eradication of oral pathogens. They are derived from larger molecules and consist of a signal sequence along with various modifications such as glycosylation, proteolysis, amino acid isomerization (Khurshid et al., 2016). AMPs are classified into different categories based on their amino acid composition, size, and structural configurations (Brogden, 2005; Harris et al., 2009). Oral epithelial cells, salivary glands, and neutrophils contribute to the production of various AMPs in saliva, which together provide a powerful defense due to their antibacterial, antioxidant, and antifungal properties (Kutta et al., 2006; Güncü et al., 2015). The most common AMPs expressed in the oral cavity are including α-, and β- defensins (shown as hNP), histatins, statherin, adrenomedullin, azurocidin, and cathelicidins (LL37). The majority of hNP-1 to 3 is concentrated in saliva, gingival epithelia, tongue, palate, buccal mucosa, and salivary glands/ducts and accounting for approximately 99% in a healthy individual (Dale and Krisanaprakornkit, 2001). Dale et al. found that individuals with dental caries have reduced levels of α-defensins (hNP-1, -2, and -3) in their saliva, suggesting that these biomarkers could be used to assess caries risk in the general population (Dale et al., 2006). It has been proposed that hBD-1 is constitutively expressed and has the function of inhibiting the transformation of normal flora into harmful microbes, while hBD-2 and -3 are induced by bacterial lipopolysaccharides (LPS), proinflammatory mediators (Interleukins [IL-1β], tumor necrosis factors [TNF-α], interferons [IFN-γ]) and are more effective in the control of nearly all types of pathogens (Khurshid et al., 2016). Histatins, a group of low positively charged histidine-enriched peptides found in saliva, are produced by cells of the parotid and submandibular salivary ducts in healthy adults (De Sousa-Pereira et al., 2013). Histatins often have antifungal activity and are composed of three main members of His-1, His-3 and His-5 (Bikker, 2020). Their antifungal mechanism is exerted in several steps, including the inhibition of mitochondrial respiration by forming reactive oxygen species, entering the cell by mobilizing ions (K+, Mg2+) that results in cell death (Khurshid et al., 2016). For example, Hst-5 is used in a mouthwash (Demegen) for oral candidiasis in patients with human immunodeficiency virus HIV because of its potent limiting effects on Candida biofilm (Pusateri et al., 2009; Gorr, 2012). In humans, the oral cavity and respiratory tract have a single representative of cathelicidins called human cationic antimicrobial peptide (hCAP18) (Murakami et al., 2002). Numerous studies have shown that LL37/hCAP18 is effective against a wide range of microorganisms, including gram-negative and gram-positive bacteria, fungi, viruses, and parasites (López-García et al., 2005; Ridyard and Overhage, 2021; Aloul et al., 2022; Bhattacharjya et al., 2024). It rapidly eliminates bacteria by creating ionic channels in their cell walls and by its ability to bind to LPS in gram-negative bacteria (Sancho-Vaello et al., 2020). Another study demonstrated the enhanced bactericidal activity of synthetic peptides derived from LL37/hCAP18 against Streptococcus sanguis (Isogai et al., 2003). In addition, Ouhara et al. demonstrated a higher activity of LL37 against a group of oral bacteria including S. mutans, S. sanguinis, S. salivarius and S. mitis when compared to human b-defensins (Ouhara et al., 2005). Adrenomedullin is a disulfide bonded cationic amphipathic antimicrobial peptide found in high concentrations in whole saliva. Adrenomedullin levels are significantly elevated in periodontally compromised regions compared to healthy regions (Kapas et al., 2004; Gorr, 2009). Statherin is a basic histidine-rich peptide of the histatin/statherin family whose antimicrobial properties are observed in its C-terminus (Wilmarth et al., 2004). Statherin is found in saliva and gingival crevicular fluid and inhibits the growth of anaerobic bacteria of the oral cavity (Denny et al., 2008). Azurocidin, a protein found in saliva, exhibits potent antibacterial activity against Gram-negative bacteria by binding strongly to lipopolysaccharide (Dhaifalah et al., 2014). Essential oils (EOs) also are one of other options in treating infection associated with oral cavity. EOs have been the focus of research for many years as effective antimicrobial agents and are applied in various medical fields, including dentistry (Radu et al., 2023). EOs derived from aromatic plants, are known for their strong odor and complex nature, containing hundreds of elements with a predominance of two or three major components. Hydrocarbon terpenes and terpenoids form the main components, with the majority of terpenoids being monoterpenes and sesquiterpenes, and the remaining group being oxygenated derivatives of hydrocarbon terpenes (Elyemni et al., 2019). Monoterpenes have been extensively researched for their potential to treat a number of diseases. Terpenes and terpenoids, along with aromatic and aliphatic elements, are responsible for the bactericidal or bacteriostatic effects of EOs (Elyemni et al., 2019; Machado et al., 2022). In addition, the antimicrobial activity of EOs depends on their unique composition, structure, amount and potential interactions (Zomorodian et al., 2015; Jafri and Ahmad, 2020). EOs have been shown to have antibacterial, antifungal, and anti-inflammatory properties that target oral pathogens (Karadağlıoğlu et al., 2019). The mechanism of action of EOs is primarily by increasing the permeability of cell membranes, leading to the leakage of ions and cellular components, ultimately causing cell lysis (Karan, 2019). EOs can also interfere with protein synthesis or cell division through stimulating the production of reactive oxygen species, leading to cell death (Lakhdar et al., 2012). Toscano-Garibay et al. indicated that among the oral pathogens tested, S. mutans is the most susceptible to EOs, followed by S. sobrinus, S. salivarius, S. sanguis, and L. acidophilus (Toscano-Garibay et al., 2017). There are limited studies on the efficacy of EOs against C. albicans. Oregano oil was found to inhibit the attachment and development of C. albicans biofilm and to reduce biofilm formation on surfaces pretreated with this oil (Karan, 2019). The results of a clinical study showed that regular use of an EO-based mouthrinse significantly reduced plaque, gingivitis, and periodontitis compared to a 0.05% cetylpyridinium chloride mouthrinse (Dagli and Dagli, 2014). Essential oils have the potential to inhibit plaque, providing additional protection to soft tissues from bacterial damage. While chlorhexidine (CHX) is often preferred for plaque control and the treatment of gingivitis and periodontitis, EOs are considered the most reliable substitute (Dosoky and Setzer, 2018; Kajjari et al., 2022). Sweet orange, bitter orange, lemon, lime, grapefruit, bergamot, yuzu and kumquat have been shown to have medicinal properties when used in mouthwash (Radu et al., 2023). E. faecalis is frequently present in root canals identified with apical periodontitis and is a main cause of secondary endodontic infections (Reiznautt et al., 2021). According to a study by Gokalp et al., a combination of calcium hydroxide and two EOs (M. spicata and O. dubium) showed significant antimicrobial effects (Nabavizade et al., 2018).
9 Conclusion and perspectives
As our understanding of the oral microbiota advances, it is becoming increasingly clear that maintaining a balanced microbial community is critical for optimal oral health. Disruption of this balance can lead to the proliferation of pathogenic bacteria, resulting in conditions such as dental caries and periodontitis. Therefore, a comprehensive approach to oral health must include both preventive and therapeutic strategies. Preventive measures, including good oral hygiene, a balanced diet and the avoidance of risk factors, are essential. However, due to the limitations and temporary efficacy of conventional treatments, there is a growing need for innovative solutions. Probiotics and microbiota transplantation are promising ways to maintain the microbial balance. Probiotics, available in various commercial formulations, have shown considerable success, while microbiota transplantation, although relatively new, offers a compelling area for further investigation. As we continue to elucidate the complex interactions between the oral microbiota and the host, it is imperative that we continually re-evaluate and improve our preventive and therapeutic approaches to ensure long-term oral health.
Author contributions
NB: Investigation, Project administration, Writing – original draft, Writing – review & editing. MK: Writing – original draft, Visualization. ET: Writing – original draft, Visualization. ZE: Writing – original draft, Visualization. AK: Writing – original draft, Visualization. TN: Methodology, Writing – original draft. DD-S: Supervision, Validation, Writing – review & editing.
Funding
The author(s) declare that no financial support was received for the research, authorship, and/or publication of this article.
Conflict of interest
The authors declare that the research was conducted in the absence of any commercial or financial relationships that could be construed as a potential conflict of interest.
Publisher’s note
All claims expressed in this article are solely those of the authors and do not necessarily represent those of their affiliated organizations, or those of the publisher, the editors and the reviewers. Any product that may be evaluated in this article, or claim that may be made by its manufacturer, is not guaranteed or endorsed by the publisher.
References
Abdi, M., and Ranjbar, R. (2022). A review on antiviral efficacy of Bifidobacterium species. Rev. Res. Med. Microbiol. 33, 74–81. doi: 10.1097/MRM.0000000000000295
Abranches, J., Zeng, L., Kajfasz, J. K., Palmer, S., Chakraborty, B., Wen, Z., et al. (2018). Biology of oral streptococci. Microbiol. Spectr. 6, 10–128. doi: 10.1128/microbiolspec.GPP3-0042-2018
Abusleme, L., Dupuy, A. K., Dutzan, N., Silva, N., Burleson, J. A., Strausbaugh, L. D., et al. (2013). The subgingival microbiome in health and periodontitis and its relationship with community biomass and inflammation. ISME J. 7, 1016–1025. doi: 10.1038/ismej.2012.174
Abusleme, L., Hoare, A., Hong, B. Y., and Diaz, P. I. (2021). Microbial signatures of health, gingivitis, and periodontitis. Periodontology 86, 57–78. doi: 10.1111/prd.12362
Adams, R. I., Bateman, A. C., Bik, H. M., and Meadow, J. F. (2015). Microbiota of the indoor environment: a meta-analysis. Microbiome 3, 1–18. doi: 10.1186/s40168-015-0108-3
Aghazadeh, Z., Pouralibaba, F., and Yari Khosroushahi, A. (2017). The prophylactic effect of Acetobacter syzygii probiotic species against squamous cell carcinoma. J. Dent. Res. Dent. Clin. Dent. Prospects 11, 208–214. doi: 10.15171/joddd.2017.037
Al Bataineh, M. T., Dash, N. R., Elkhazendar, M., Alnusairat, D. A. M. H., Darwish, I. M. I., Al-Hajjaj, M. S., et al. (2020). Revealing oral microbiota composition and functionality associated with heavy cigarette smoking. J. Transl. Med. 18, 1–10. doi: 10.1186/s12967-020-02579-3
Aleksandrowicz, P., Brzezińska-Błaszczyk, E., Dudko, A., and Agier, J. (2020). Archaea occurrence in the subgingival biofilm in patients with Peri-implantitis and periodontitis. Int. J. Periodontics Restorative Dent. 40, 677–683. doi: 10.11607/prd.4670
Allaker, R. P., and Ian Douglas, C. W. (2015). Non-conventional therapeutics for oral infections. Virulence 6, 196–207. doi: 10.4161/21505594.2014.983783
Aloul, K. M., Nielsen, J. E., Defensor, E. B., Lin, J. S., Fortkort, J. A., Shamloo, M., et al. (2022). Upregulating human cathelicidin antimicrobial peptide LL-37 expression May prevent severe COVID-19 inflammatory responses and reduce microthrombosis. Front. Immunol. 13:880961. doi: 10.3389/fimmu.2022.880961
Arboleya, S., Sánchez, B., Milani, C., Duranti, S., Solís, G., Fernández, N., et al. (2015). Intestinal microbiota development in preterm neonates and effect of perinatal antibiotics. J. Pediatr. 166, 538–544. doi: 10.1016/j.jpeds.2014.09.041
Bäckhed, F., Fraser, C. M., Ringel, Y., Sanders, M. E., Sartor, R. B., Sherman, P. M., et al. (2012). Defining a healthy human gut microbiome: current concepts, future directions, and clinical applications. Cell Host Microbe 12, 611–622. doi: 10.1016/j.chom.2012.10.012
Bai, Y., Ma, K., Li, J., Li, J., Bi, C., and Shan, A. (2021). Deoxynivalenol exposure induces liver damage in mice: inflammation and immune responses, oxidative stress, and protective effects of Lactobacillus rhamnosus GG. Food Chem. Toxicol. 156:112514. doi: 10.1016/j.fct.2021.112514
Baker, J. L., and Edlund, A. (2018). Exploiting the Oral microbiome to prevent tooth decay: has evolution already provided the best tools? Front. Microbiol. 9:3323. doi: 10.3389/fmicb.2018.03323
Barbour, A., Elebyary, O., Fine, N., Oveisi, M., and Glogauer, M. (2022). Metabolites of the oral microbiome: important mediators of multikingdom interactions. FEMS Microbiol. Rev. 46:fuab039. doi: 10.1093/femsre/fuab039
Beck, J. D., Philips, K., Moss, K., Divaris, K., Morelli, T., and Offenbacher, S. (2020). Advances in precision oral health. Periodontol. 82, 268–285. doi: 10.1111/prd.12314
Bhattacharjya, S., Zhang, Z., and Ramamoorthy, A. (2024). LL-37: structures, antimicrobial activity, and influence on amyloid-related diseases. Biomol. Ther. 14:320. doi: 10.3390/biom14030320
Bikker, F. J. (2020). The importance of saliva to oral health; from haddock to histatin. Ned. Tijdschr. Tandheelkd. 127, 551–555. doi: 10.5177/ntvt.2020.10.19094
Bonfiglio, G., Neroni, B., Radocchia, G., Marazzato, M., Pantanella, F., and Schippa, S. (2020). Insight into the possible use of the predator Bdellovibrio bacteriovorus as a probiotic. Nutrients 12:2252. doi: 10.3390/nu12082252
Bosch, M., Nart, J., Audivert, S., Bonachera, M. A., Alemany, A. S., Fuentes, M. C., et al. (2012). Isolation and characterization of probiotic strains for improving oral health. Arch. Oral Biol. 57, 539–549. doi: 10.1016/j.archoralbio.2011.10.006
Bostanci, N., Krog, M. C., Hugerth, L. W., Bashir, Z., Fransson, E., Boulund, F., et al. (2021). Dysbiosis of the human Oral microbiome during the menstrual cycle and vulnerability to the external exposures of smoking and dietary sugar. Front. Cell. Infect. Microbiol. 11:625229. doi: 10.3389/fcimb.2021.625229
Bostanghadiri, N., Razavi, S., Shariati, A., Talebi, M., Mirkalantari, S., Emami Razavi, A., et al. (2023). Exploring the interplay between Fusobacterium nucleatum with the expression of microRNA, and inflammatory mediators in colorectal cancer. Front. Microbiol. 14:1302719. doi: 10.3389/fmicb.2023.1302719
Bowen, W. H., Burne, R. A., Wu, H., and Koo, H. (2018). Oral biofilms: pathogens, matrix, and polymicrobial interactions in microenvironments. Trends Microbiol. 26, 229–242. doi: 10.1016/j.tim.2017.09.008
Brogden, K. A. (2005). Antimicrobial peptides: pore formers or metabolic inhibitors in bacteria? Nat. Rev. Microbiol. 3, 238–250. doi: 10.1038/nrmicro1098
Brookes, Z. L. S., Bescos, R., Belfield, L. A., Ali, K., and Roberts, A. (2020). Current uses of chlorhexidine for management of oral disease: a narrative review. J. Dent. 103:103497. doi: 10.1016/j.jdent.2020.103497
Bryden, W. L. (2012). Mycotoxin contamination of the feed supply chain: implications for animal productivity and feed security. Anim. Feed Sci. Technol. 173, 134–158. doi: 10.1016/j.anifeedsci.2011.12.014
Bürgers, R., Hahnel, S., Reichert, T. E., Rosentritt, M., Behr, M., Gerlach, T., et al. (2010). Adhesion of Candida albicans to various dental implant surfaces and the influence of salivary pellicle proteins. Acta Biomater. 6, 2307–2313. doi: 10.1016/j.actbio.2009.11.003
Bushin, L. B., Covington, B. C., Rued, B. E., Federle, M. J., and Seyedsayamdost, M. R. (2020). Discovery and biosynthesis of Streptosactin, a Sactipeptide with an alternative topology encoded by commensal Bacteria in the human microbiome. J. Am. Chem. Soc. 142, 16265–16275. doi: 10.1021/jacs.0c05546
Busscher, H. J., Mulder, A. F., and Van Der Mei, H. C. (1999). In vitro adhesion to enamel and in vivo colonization of tooth surfaces by lactobacilli from a bio-yoghurt. Caries Res. 33, 403–404. doi: 10.1159/000016541
Caglar, E., Cildir, S. K., Ergeneli, S., Sandalli, N., and Twetman, S. (2006). Salivary mutans streptococci and lactobacilli levels after ingestion of the probiotic bacterium Lactobacillus reuteri ATCC 55730 by straws or tablets. Acta Odontol. Scand. 64, 314–318. doi: 10.1080/00016350600801709
Caglar, E., Sandalli, N., Twetman, S., Kavaloglu, S., Ergeneli, S., and Selvi, S. (2005). Effect of yogurt with Bifidobacterium DN-173 010 on salivary mutans streptococci and lactobacilli in young adults. Acta Odontol. Scand. 63, 317–320. doi: 10.1080/00016350510020070
Camanocha, A., and Dewhirst, F. E. (2014). Host-associated bacterial taxa from Chlorobi, Chloroflexi, GN02, Synergistetes, SR1, TM7, and WPS-2 Phyla/candidate divisions. J. Oral Microbiol. 6. doi: 10.3402/jom.v6.25468
Caselli, E., Fabbri, C., D’accolti, M., Soffritti, I., Bassi, C., Mazzacane, S., et al. (2020). Defining the oral microbiome by whole-genome sequencing and resistome analysis: the complexity of the healthy picture. BMC Microbiol. 20:120. doi: 10.1186/s12866-020-01801-y
Chattopadhyay, I., Verma, M., and Panda, M. (2019). Role of Oral microbiome signatures in diagnosis and prognosis of Oral Cancer. Technol. Cancer Res. Treat. 18:153303381986735. doi: 10.1177/1533033819867354
Chuang, L. C., Huang, C. S., Ou-Yang, L. W., and Lin, S. Y. (2011). Probiotic Lactobacillus paracasei effect on cariogenic bacterial flora. Clin. Oral Investig. 15, 471–476. doi: 10.1007/s00784-010-0423-9
Cornejo Ulloa, P., Van Der Veen, M. H., and Krom, B. P. (2019). Modulation of the oral microbiome by the host to promote ecological balance. Odontology 107, 437–448. doi: 10.1007/s10266-019-00413-x
Dabdoub, S. M., Ganesan, S. M., and Kumar, P. S. (2016). Comparative metagenomics reveals taxonomically idiosyncratic yet functionally congruent communities in periodontitis. Sci. Rep. 6:38993. doi: 10.1038/srep38993
Dagli, N., and Dagli, R. (2014). Possible use of essential oils in dentistry. J. Int. Oral Health 6, i–ii.
Dale, B. A., and Krisanaprakornkit, S. (2001). Defensin antimicrobial peptides in the oral cavity. J. Oral Pathol. Med. 30, 321–327. doi: 10.1034/j.1600-0714.2001.300601.x
Dale, B. A., Tao, R., Kimball, J. R., and Jurevic, R. J. (2006). Oral antimicrobial peptides and biological control of caries. BMC Oral Health 6:S13. doi: 10.1186/1472-6831-6-S1-S13
Dame-Teixeira, N., De Cena, J. A., Côrtes, D. A., Belmok, A., Dos Anjos Borges, L. G., Marconatto, L., et al. (2020). Presence of Archaea in dental caries biofilms. Arch. Oral Biol. 110:104606. doi: 10.1016/j.archoralbio.2019.104606
De Sousa-Pereira, P., Amado, F., Abrantes, J., Ferreira, R., Esteves, P. J., and Vitorino, R. (2013). An evolutionary perspective of mammal salivary peptide families: cystatins, histatins, statherin and PRPs. Arch. Oral Biol. 58, 451–458. doi: 10.1016/j.archoralbio.2012.12.011
Delaviz, Y., Liu, T. W., Deonarain, A. R., Finer, Y., Shokati, B., and Santerre, J. P. (2019). Physical properties and cytotoxicity of antimicrobial dental resin adhesives containing dimethacrylate oligomers of ciprofloxacin and metronidazole. Dent. Mater. 35, 229–243. doi: 10.1016/j.dental.2018.11.016
Denny, P., Hagen, F. K., Hardt, M., Liao, L., Yan, W., Arellanno, M., et al. (2008). The proteomes of human parotid and submandibular/sublingual gland salivas collected as the ductal secretions. J. Proteome Res. 7, 1994–2006. doi: 10.1021/pr700764j
Deo, P. N., and Deshmukh, R. (2019). Oral microbiome: unveiling the fundamentals. J. Oral Maxillofac. Pathol. 23, 122–128. doi: 10.4103/jomfp.JOMFP_304_18
Dewhirst, F. E., Chen, T., Izard, J., Paster, B. J., Tanner, A. C., Yu, W. H., et al. (2010). The human oral microbiome. J. Bacteriol. 192, 5002–5017. doi: 10.1128/JB.00542-10
Dhaifalah, I., Andrys, C., Drahosova, M., Musilova, I., Adamik, Z., and Kacerovsky, M. (2014). Azurocidin levels in maternal serum in the first trimester can predict preterm prelabor rupture of membranes. J. Matern. Fetal Neonatal Med. 27, 511–515. doi: 10.3109/14767058.2013.820698
Di Spirito, F., Pelella, S., Argentino, S., Sisalli, L., and Sbordone, L. (2022). Oral manifestations and the role of the oral healthcare workers in COVID-19. Oral Dis. 28, 1003–1004. doi: 10.1111/odi.13688
Dosoky, N. S., and Setzer, W. N. (2018). Chemical composition and biological activities of essential oils of Curcuma species. Nutrients 10:1196. doi: 10.3390/nu10091196
Duanis-Assaf, D., Steinberg, D., and Shemesh, M. (2020). Efficiency of Bacillus subtilis metabolism of sugar alcohols governs its probiotic effect against cariogenic Streptococcus mutans. Artif. Cells Nanomed. Biotechnol. 48, 1222–1230. doi: 10.1080/21691401.2020.1822855
Duran-Pinedo, A. E. (2021). Metatranscriptomic analyses of the oral microbiome. Periodontology 85, 28–45. doi: 10.1111/prd.12350
Efenberger, M., Agier, J., Pawłowska, E., and Brzezińska-Błaszczyk, E. (2015). Archaea prevalence in inflamed pulp tissues. Cent. Eur. J. Immunol. 40, 194–200. doi: 10.5114/ceji.2015.51358
Elyemni, M., Louaste, B., Nechad, I., Elkamli, T., Bouia, A., Taleb, M., et al. (2019). Extraction of essential oils of Rosmarinus officinalis L. by two different methods: Hydrodistillation and microwave assisted Hydrodistillation. ScientificWorldJournal 2019:3659432. doi: 10.1155/2019/3659432
Feng, J., Liu, J., Jiang, M., Chen, Q., Zhang, Y., Yang, M., et al. (2023). The role of oral nitrate-reducing bacteria in the prevention of caries: a review related to caries and nitrate metabolism. Caries Res. 57, 119–132. doi: 10.1159/000529162
Ferrazzano, G. F., Amato, I., Ingenito, A., De Natale, A., and Pollio, A. (2009). Anti-cariogenic effects of polyphenols from plant stimulant beverages (cocoa, coffee, tea). Fitoterapia 80, 255–262. doi: 10.1016/j.fitote.2009.04.006
Ferreira, C. M., Vieira, A. T., Vinolo, M. A. R., Oliveira, F. A., Curi, R., and Martins, F. D. S. (2014). The central role of the gut microbiota in chronic inflammatory diseases. J Immunol Res 2014, 1–12. doi: 10.1155/2014/689492
Franzosa, E. A., Huang, K., Meadow, J. F., Gevers, D., Lemon, K. P., Bohannan, B. J., et al. (2015). Identifying personal microbiomes using metagenomic codes. Proc. Natl. Acad. Sci. USA 112, E2930–E2938. doi: 10.1073/pnas.1423854112
Fujiwara, N., Tsuruda, K., Iwamoto, Y., Kato, F., Odaki, T., Yamane, N., et al. (2017). Significant increase of oral bacteria in the early pregnancy period in Japanese women. J. Investig. Clin. Dent. 8:e12189. doi: 10.1111/jicd.12189
Ge, Y., Wang, S., Zhou, X., Wang, H., Xu, H. H., and Cheng, L. (2015). The use of quaternary ammonium to combat dental caries. Materials (Basel) 8, 3532–3549. doi: 10.3390/ma8063532
Godlewska, U., Brzoza, P., Sroka, A., Majewski, P., Jentsch, H., Eckert, M., et al. (2017). Antimicrobial and attractant roles for chemerin in the oral cavity during inflammatory gum disease. Front. Immunol. 8:353. doi: 10.3389/fimmu.2017.00353
Gomez-Arango, L. F., Barrett, H. L., Mcintyre, H. D., Callaway, L. K., Morrison, M., and Dekker Nitert, M. (2017). Antibiotic treatment at delivery shapes the initial oral microbiome in neonates. Sci. Rep. 7:43481. doi: 10.1038/srep43481
Gordon, Y. J., Romanowski, E. G., and Mcdermott, A. M. (2005). A review of antimicrobial peptides and their therapeutic potential as anti-infective drugs. Curr. Eye Res. 30, 505–515. doi: 10.1080/02713680590968637
Gorr, S. U. (2009). Antimicrobial peptides of the oral cavity. Periodontol. 51, 152–180. doi: 10.1111/j.1600-0757.2009.00310.x
Gorr, S. U. (2012). Antimicrobial peptides in periodontal innate defense. Front. Oral Biol. 15, 84–98. doi: 10.1159/000329673
Grant, M., Kilsgård, O., Åkerman, S., Klinge, B., Demmer, R. T., Malmström, J., et al. (2019). The human salivary antimicrobial peptide profile according to the oral microbiota in health, periodontitis and smoking. J. Innate Immun. 11, 432–444. doi: 10.1159/000494146
Güncü, G. N., Yilmaz, D., Könönen, E., and Gürsoy, U. K. (2015). Salivary antimicrobial peptides in early detection of periodontitis. Front. Cell. Infect. Microbiol. 5:99. doi: 10.3389/fcimb.2015.00099
Gutt, B., Ren, Q., Hauser-Gerspach, I., Kardas, P., Stübinger, S., Astasov-Frauenhoffer, M., et al. (2018). Beneficial Oral biofilms as smart bioactive interfaces. Front. Microbiol. 9:107. doi: 10.3389/fmicb.2018.00107
Hampelska, K., Jaworska, M. M., Babalska, Z. Ł., and Karpiński, T. M. (2020). The role of oral microbiota in intra-oral halitosis. J. Clin. Med. 9:2484. doi: 10.3390/jcm9082484
Hannig, C., Hannig, M., Kensche, A., and Carpenter, G. (2017). The mucosal pellicle–an underestimated factor in oral physiology. Arch. Oral Biol. 80, 144–152. doi: 10.1016/j.archoralbio.2017.04.001
Harris, F., Dennison, S. R., and Phoenix, D. A. (2009). Anionic antimicrobial peptides from eukaryotic organisms. Curr. Protein Pept. Sci. 10, 585–606. doi: 10.2174/138920309789630589
Heilbronner, S., Krismer, B., Brötz-Oesterhelt, H., and Peschel, A. (2021). The microbiome-shaping roles of bacteriocins. Nat. Rev. Microbiol. 19, 726–739. doi: 10.1038/s41579-021-00569-w
Herremans, K. M., Riner, A. N., Cameron, M. E., Mckinley, K. L., Triplett, E. W., Hughes, S. J., et al. (2022). The oral microbiome, pancreatic cancer and human diversity in the age of precision medicine. Microbiome 10:93. doi: 10.1186/s40168-022-01262-7
Holmes, A. R., Gilbert, C., Wells, J. M., and Jenkinson, H. F. (1998). Binding properties of Streptococcus gordonii SspA and SspB (antigen I/II family) polypeptides expressed on the cell surface of Lactococcus lactis MG1363. Infect. Immun. 66, 4633–4639. doi: 10.1128/IAI.66.10.4633-4639.1998
Hu, H., Burrow, M. F., and Leung, W. K. (2023). Proteomic profile of in situ acquired pellicle on tooth and restorative material surfaces. J. Dent. 129:104389. doi: 10.1016/j.jdent.2022.104389
Huang, X., Palmer, S. R., Ahn, S. J., Richards, V. P., Williams, M. L., Nascimento, M. M., et al. (2016). A highly Arginolytic Streptococcus species that potently antagonizes Streptococcus mutans. Appl. Environ. Microbiol. 82, 2187–2201. doi: 10.1128/AEM.03887-15
Inchingolo, F., Inchingolo, A. M., Malcangi, G., De Leonardis, N., Sardano, R., Pezzolla, C., et al. (2023). The benefits of probiotics on Oral health: Systematic Review of the Literature. Pharmaceuticals (Basel) 16:1313. doi: 10.3390/ph16091313
Isogai, E., Hirata, M., Isogai, H., Matuo, K., Kimura, K., Yokota, K., et al. (2003). Antimicrobial activity of synthetic human CAP18 peptides to Streptococcus sanguis isolated from patients with Behçet's disease. Adv. Exp. Med. Biol. 528, 195–200. doi: 10.1007/0-306-48382-3_38
Jafri, H., and Ahmad, I. (2020). Thymus vulgaris essential oil and thymol inhibit biofilms and interact synergistically with antifungal drugs against drug resistant strains of Candida albicans and Candida tropicalis. J. Mycol. Med. 30:100911. doi: 10.1016/j.mycmed.2019.100911
Jang, H., Patoine, A., Wu, T. T., Castillo, D. A., and Xiao, J. (2021). Oral microflora and pregnancy: a systematic review and meta-analysis. Sci. Rep. 11:16870. doi: 10.1038/s41598-021-96495-1
Jaspers, I. (2014). Cigarette smoke effects on innate immune mechanisms in the nasal mucosa. Potential effects on the microbiome. Ann. Am. Thorac. Soc. 11, S38–S42. doi: 10.1513/AnnalsATS.201306-154MG
Jia, Y.-J., Liao, Y., He, Y.-Q., Zheng, M.-Q., Tong, X.-T., Xue, W.-Q., et al. (2021). Association between oral microbiota and cigarette smoking in the Chinese population. Front. Cell. Infect. Microbiol. 11:658203. doi: 10.3389/fcimb.2021.658203
Jiang, W. X., Hu, Y. J., Gao, L., He, Z. Y., Zhu, C. L., Ma, R., et al. (2015). The impact of various time intervals on the supragingival plaque dynamic core microbiome. PLoS One 10:e0124631. doi: 10.1371/journal.pone.0124631
Jiao, Y., Wu, L., Huntington, N. D., and Zhang, X. (2020). Crosstalk between gut microbiota and innate immunity and its implication in autoimmune diseases. Front. Immunol. 11:282. doi: 10.3389/fimmu.2020.00282
Jin Baek, K., Choi, Y. S., Kang, C. K., and Choi, Y. (2017). The proteolytic activity of Porphyromonas gingivalis is critical in a murine model of periodontitis. J. Periodontol. 88, 218–224. doi: 10.1902/jop.2016.160262
Kajjari, S., Joshi, R. S., Hugar, S. M., Gokhale, N., Meharwade, P., and Uppin, C. (2022). The effects of lavender essential oil and its clinical implications in dentistry: a review. Int. J. Clin. Pediatr. Dent. 15, 385–388. doi: 10.5005/jp-journals-10005-2378
Kapas, S., Pahal, K., Cruchley, A. T., Hagi-Pavli, E., and Hinson, J. P. (2004). Expression of adrenomedullin and its receptors in human salivary tissue. J. Dent. Res. 83, 333–337. doi: 10.1177/154405910408300412
Karadağlıoğlu, Ö., Ulusoy, N., Başer, K. H. C., Hanoğlu, A., and Şık, İ. (2019). Antibacterial activities of herbal toothpastes combined with essential oils against Streptococcus mutans. Pathogens 8:8. doi: 10.3390/pathogens8010020
Karan, N. B. (2019). Influence of lavender oil inhalation on vital signs and anxiety: a randomized clinical trial. Physiol. Behav. 211:112676. doi: 10.1016/j.physbeh.2019.112676
Karpiński, T. M. (2019). Role of oral microbiota in cancer development. Microorganisms 7:20. doi: 10.3390/microorganisms7010020
Kay, J., Thadhani, E., Samson, L., and Engelward, B. (2019). Inflammation-induced DNA damage, mutations and cancer. DNA Repair (Amst) 83:102673. doi: 10.1016/j.dnarep.2019.102673
Khurshid, Z., Naseem, M., Sheikh, Z., Najeeb, S., Shahab, S., and Zafar, M. S. (2016). Oral antimicrobial peptides: types and role in the oral cavity. Saudi Pharm. J. 24, 515–524. doi: 10.1016/j.jsps.2015.02.015
Kistler, J. O., Booth, V., Bradshaw, D. J., and Wade, W. G. (2013). Bacterial community development in experimental gingivitis. PLoS One 8:e71227. doi: 10.1371/journal.pone.0071227
Kobayashi, R., Kobayashi, T., Sakai, F., Hosoya, T., Yamamoto, M., and Kurita-Ochiai, T. (2017). Oral administration of Lactobacillus gasseri SBT2055 is effective in preventing Porphyromonas gingivalis-accelerated periodontal disease. Sci. Rep. 7:545. doi: 10.1038/s41598-017-00623-9
Kutta, H., May, J., Jaehne, M., Münscher, A., and Paulsen, F. P. (2006). Antimicrobial defence mechanisms of the human parotid duct. J. Anat. 208, 609–619. doi: 10.1111/j.1469-7580.2006.00567.x
La Rosa, G. R. M., Gattuso, G., Pedullà, E., Rapisarda, E., Nicolosi, D., and Salmeri, M. (2020). Association of oral dysbiosis with oral cancer development. Oncol. Lett. 19, 3045–3058. doi: 10.3892/ol.2020.11441
Lakhdar, L., Hmamouchi, M., Rida, S., and Ennibi, O. (2012). Antibacterial activity of essential oils against periodontal pathogens: a qualitative systematic review. Odontostomatol. Trop. 35, 38–46.
Lamont, R. J., Koo, H., and Hajishengallis, G. (2018). The oral microbiota: dynamic communities and host interactions. Nat. Rev. Microbiol. 16, 745–759. doi: 10.1038/s41579-018-0089-x
Lee, W.-H., Chen, H.-M., Yang, S.-F., Liang, C., Peng, C.-Y., Lin, F.-M., et al. (2017). Bacterial alterations in salivary microbiota and their association in oral cancer. Sci. Rep. 7:16540. doi: 10.1038/s41598-017-16418-x
Lee, J. W., Shin, J. G., Kim, E. H., Kang, H. E., Yim, I. B., Kim, J. Y., et al. (2004). Immunomodulatory and antitumor effects in vivo by the cytoplasmic fraction of Lactobacillus casei and Bifidobacterium longum. J. Vet. Sci. 5, 41–48. doi: 10.4142/jvs.2004.5.1.41
Li, C. L., Liu, D. L., Jiang, Y. T., Zhou, Y. B., Zhang, M. Z., Jiang, W., et al. (2009). Prevalence and molecular diversity of Archaea in subgingival pockets of periodontitis patients. Oral Microbiol. Immunol. 24, 343–346. doi: 10.1111/j.1399-302X.2009.00514.x
Li, D., Xi, W., Zhang, Z., Ren, L., Deng, C., Chen, J., et al. (2020). Oral microbial community analysis of the patients in the progression of liver cancer. Microb. Pathog. 149:104479. doi: 10.1016/j.micpath.2020.104479
Lin, Y., Chen, J., Zhou, X., and Li, Y. (2021). Inhibition of Streptococcus mutans biofilm formation by strategies targeting the metabolism of exopolysaccharides. Crit. Rev. Microbiol. 47, 667–677. doi: 10.1080/1040841X.2021.1915959
Lin, W., Jiang, W., Hu, X., Gao, L., Ai, D., Pan, H., et al. (2018). Ecological shifts of supragingival microbiota in association with pregnancy. Front. Cell. Infect. Microbiol. 8:24. doi: 10.3389/fcimb.2018.00024
Lin, T. H., Lin, C. H., and Pan, T. M. (2018). The implication of probiotics in the prevention of dental caries. Appl. Microbiol. Biotechnol. 102, 577–586. doi: 10.1007/s00253-017-8664-z
Lippi, G., and Mattiuzzi, C. (2020). The global burden of pancreatic cancer. Arch. Med. Sci. 16, 820–824. doi: 10.5114/aoms.2020.94845
Liu, Y.-L., Nascimento, M., and Burne, R. A. (2012). Progress toward understanding the contribution of alkali generation in dental biofilms to inhibition of dental caries. Int. J. Oral Sci. 4, 135–140. doi: 10.1038/ijos.2012.54
Liu, X., Tong, X., Zhu, J., Tian, L., Jie, Z., Zou, Y., et al. (2021). Metagenome-genome-wide association studies reveal human genetic impact on the oral microbiome. Cell Discov. 7:117. doi: 10.1038/s41421-021-00356-0
Loozen, G., Boon, N., Pauwels, M., Slomka, V., Rodrigues Herrero, E., Quirynen, M., et al. (2015). Effect of Bdellovibrio bacteriovorus HD100 on multispecies oral communities. Anaerobe 35, 45–53. doi: 10.1016/j.anaerobe.2014.09.011
López-García, B., Lee, P. H., Yamasaki, K., and Gallo, R. L. (2005). Anti-fungal activity of cathelicidins and their potential role in Candida albicans skin infection. J. Invest. Dermatol. 125, 108–115. doi: 10.1111/j.0022-202X.2005.23713.x
Lopez-Lopez, A., Camelo-Castillo, A., Ferrer, M. D., Simon-Soro, A., and Mira, A. (2017). Health-associated niche inhabitants as Oral probiotics: the case of Streptococcus dentisani. Front. Microbiol. 8:379. doi: 10.3389/fmicb.2017.00379
Lourenço, T. G., Heller, D., Silva-Boghossian, C. M., Cotton, S. L., Paster, B. J., and Colombo, A. P. (2014). Microbial signature profiles of periodontally healthy and diseased patients. J. Clin. Periodontol. 41, 1027–1036. doi: 10.1111/jcpe.12302
Machado, T. Q., Da Fonseca, A. C. C., Duarte, A. B. S., Robbs, B. K., and De Sousa, D. P. (2022). A narrative review of the antitumor activity of monoterpenes from essential oils: an update. Biomed. Res. Int. 2022, 1–20. doi: 10.1155/2022/6317201
Macy, E. (2015). Penicillin allergy: optimizing diagnostic protocols, public health implications, and future research needs. Curr. Opin. Allergy Clin. Immunol. 15, 308–313. doi: 10.1097/ACI.0000000000000173
Mai, S., Mauger, M. T., Niu, L. N., Barnes, J. B., Kao, S., Bergeron, B. E., et al. (2017). Potential applications of antimicrobial peptides and their mimics in combating caries and pulpal infections. Acta Biomater. 49, 16–35. doi: 10.1016/j.actbio.2016.11.026
Mark Welch, J. L., Rossetti, B. J., Rieken, C. W., Dewhirst, F. E., and Borisy, G. G. (2016). Biogeography of a human oral microbiome at the micron scale. Proc. Natl. Acad. Sci. 113, E791–E800. doi: 10.1073/pnas.1522149113
Meena, N., Verma, P., Pande, R., Kumar, M., Watts, A., and Gupta, O. (2020). Bioavailability and nutritional analysis of flavonoids. Plant Phenol. Sustain. Agricult. 1, 135–156. doi: 10.1007/978-981-15-4890-1_6
Mira, A., Simon-Soro, A., and Curtis, M. A. (2017). Role of microbial communities in the pathogenesis of periodontal diseases and caries. J. Clin. Periodontol. 44, S23–s38. doi: 10.1111/jcpe.12671
Mishra, S., Rath, S., and Mohanty, N. (2020). Probiotics-a complete oral healthcare package. J. Integr. Med. 18, 462–469. doi: 10.1016/j.joim.2020.08.005
Mohan, S., and Epstein, J. B. (2003). Carcinogenesis and cyclooxygenase: the potential role of COX-2 inhibition in upper aerodigestive tract cancer. Oral Oncol. 39, 537–546. doi: 10.1016/S1368-8375(03)00035-6
Murakami, M., Ohtake, T., Dorschner, R. A., and Gallo, R. L. (2002). Cathelicidin antimicrobial peptides are expressed in salivary glands and saliva. J. Dent. Res. 81, 845–850. doi: 10.1177/154405910208101210
Nabavizade, M., Sobhnamayan, F., Bahrami, H., Rafieian-Kopaei, M., and Abbaszadegan, A. (2018). Evaluation of the wettability of a resin-based sealer in contact with some herbal irrigants. Dent. Res. J. (Isfahan) 15, 130–135. doi: 10.4103/1735-3327.226527
Nagaraj, T., Ravi, B., Sankara, S. N., Madhu, K., and Kailasam, S. (2012). Probiotics and Oral health. J. Ind. Acad. Oral Med. Radiol. 24, 146–148. doi: 10.5005/jp-journals-10011-1281
Nascimento, M. (2017). Oral microbiota transplant: a potential new therapy for oral diseases. J. Calif. Dent. Assoc. 45, 565–568. doi: 10.1080/19424396.2017.12222506
Nascimento, M. M. (2018). Potential uses of arginine in dentistry. Adv. Dent. Res. 29, 98–103. doi: 10.1177/0022034517735294
Nascimento, M. M., Alvarez, A. J., Huang, X., Hanway, S., Perry, S., Luce, A., et al. (2019). Arginine metabolism in Supragingival Oral biofilms as a potential predictor of caries risk. JDR Clin. Trans. Res. 4, 262–270. doi: 10.1177/2380084419834234
Näse, L., Hatakka, K., Savilahti, E., Saxelin, M., Pönkä, A., Poussa, T., et al. (2001). Effect of long-term consumption of a probiotic bacterium, Lactobacillus rhamnosus GG, in milk on dental caries and caries risk in children. Caries Res. 35, 412–420. doi: 10.1159/000047484
Nath, S., Zilm, P., Jamieson, L., Kapellas, K., Goswami, N., Ketagoda, K., et al. (2021). Development and characterization of an oral microbiome transplant among Australians for the treatment of dental caries and periodontal disease: a study protocol. PLoS One 16:e0260433. doi: 10.1371/journal.pone.0260433
Navidifar, T., Mahdizade Ari, M., Alipourkermani, A., Afifirad, R., Asadollahi, P., Veisi, A., et al. (2023). Clinical efficacy of probiotics on Oral health: a systematic review of clinical trials. Curr. Pharm. Biotechnol. 24, 1916–1927. doi: 10.2174/1389201024666230405135457
Nowicki, E. M., Shroff, R., Singleton, J. A., Renaud, D. E., Wallace, D., Drury, J., et al. (2018). Microbiota and metatranscriptome changes accompanying the onset of gingivitis. MBio 9:e00575-18. doi: 10.1128/mBio.00575-18
O’Mullane, D. M., Baez, R. J., Jones, S., Lennon, M. A., Petersen, P. E., Rugg-Gunn, A. J., et al. (2016). Fluoride and oral health. Community Dent. Health 33, 69–99.
Oliveira, S. G., Nishiyama, R. R., Trigo, C. A. C., Mattos-Guaraldi, A. L., Dávila, A. M. R., Jardim, R., et al. (2021). Core of the saliva microbiome: an analysis of the MG-RAST data. BMC Oral Health 21:351. doi: 10.1186/s12903-021-01719-5
Olson, J. C., Cuff, C. F., Lukomski, S., Lukomska, E., Canizales, Y., Wu, B., et al. (2011). Use of 16S ribosomal RNA gene analyses to characterize the bacterial signature associated with poor oral health in West Virginia. BMC Oral Health 11:7. doi: 10.1186/1472-6831-11-7
Ouhara, K., Komatsuzawa, H., Yamada, S., Shiba, H., Fujiwara, T., Ohara, M., et al. (2005). Susceptibilities of periodontopathogenic and cariogenic bacteria to antibacterial peptides, {beta}-defensins and LL37, produced by human epithelial cells. J. Antimicrob. Chemother. 55, 888–896. doi: 10.1093/jac/dki103
Parcina Amizic, I., Cigic, L., Gavic, L., Radic, M., Biocina Lukenda, D., Tonkic, M., et al. (2017). Antimicrobial efficacy of probiotic-containing toothpastes: an in vitro evaluation. Med. Glas. (Zenica) 14, 139–144. doi: 10.17392/870-16
Pozhitkov, A. E., Leroux, B. G., Randolph, T. W., Beikler, T., Flemmig, T. F., and Noble, P. A. (2015). Towards microbiome transplant as a therapy for periodontitis: an exploratory study of periodontitis microbial signature contrasted by oral health, caries and edentulism. BMC Oral Health 15:125. doi: 10.1186/s12903-015-0109-4
Pusateri, C. R., Monaco, E. A., and Edgerton, M. (2009). Sensitivity of Candida albicans biofilm cells grown on denture acrylic to antifungal proteins and chlorhexidine. Arch. Oral Biol. 54, 588–594. doi: 10.1016/j.archoralbio.2009.01.016
Qiu, W., Zhou, Y., Li, Z., Huang, T., Xiao, Y., Cheng, L., et al. (2020). Application of antibiotics/antimicrobial agents on dental caries. Biomed. Res. Int. 2020, 1–11. doi: 10.1155/2020/5658212
Radaic, A., and Kapila, Y. L. (2021). The oralome and its dysbiosis: new insights into oral microbiome-host interactions. Comput. Struct. Biotechnol. J. 19, 1335–1360. doi: 10.1016/j.csbj.2021.02.010
Radu, C. M., Radu, C. C., Bochiș, S. A., Arbănași, E. M., Lucan, A. I., Murvai, V. R., et al. (2023). Revisiting the therapeutic effects of essential oils on the oral microbiome. Pharmacy (Basel) 11:33. doi: 10.3390/pharmacy11010033
Reddy, M. S., Shetty, S. R., and Vannala, V. (2019). Embracing personalized medicine in dentistry. J. Pharm. Bioallied Sci. 11, S92–s96. doi: 10.4103/JPBS.JPBS_297_18
Reiznautt, C. M., Ribeiro, J. S., Kreps, E., Da Rosa, W. L. O., De Lacerda, H., Peralta, S. L., et al. (2021). Development and properties of endodontic resin sealers with natural oils. J. Dent. 104:103538. doi: 10.1016/j.jdent.2020.103538
Ridyard, K. E., and Overhage, J. (2021). The potential of human peptide LL-37 as an antimicrobial and anti-biofilm agent. Antibiotics (Basel) 10:650. doi: 10.3390/antibiotics10060650
Rosier, B., Marsh, P., and Mira, A. (2018). Resilience of the oral microbiota in health: mechanisms that prevent dysbiosis. J. Dent. Res. 97, 371–380. doi: 10.1177/0022034517742139
Rosier, B. T., Moya-Gonzalvez, E. M., Corell-Escuin, P., and Mira, A. (2020). Isolation and characterization of nitrate-reducing bacteria as potential probiotics for oral and systemic health. Front. Microbiol. 11:555465. doi: 10.3389/fmicb.2020.555465
Roslund, K., Lehto, M., Pussinen, P., Hartonen, K., Groop, P.-H., Halonen, L., et al. (2021). Identifying volatile in vitro biomarkers for oral bacteria with proton-transfer-reaction mass spectrometry and gas chromatography–mass spectrometry. Sci. Rep. 11:16897. doi: 10.1038/s41598-021-96287-7
Saadaoui, M., Singh, P., and Al Khodor, S. (2021). Oral microbiome and pregnancy: a bidirectional relationship. J. Reprod. Immunol. 145:103293. doi: 10.1016/j.jri.2021.103293
Saha, S., Chopra, A., Kamath, S. U., and Kashyap, N. N. (2023). Can acid produced from probiotic bacteria alter the surface roughness, microhardness, and elemental composition of enamel? An in vitro study. Odontology 111, 929–941. doi: 10.1007/s10266-023-00804-1
Sánchez, G. A., Miozza, V. A., Delgado, A., and Busch, L. (2014). Total salivary nitrates and nitrites in oral health and periodontal disease. Nitric Oxide 36, 31–35. doi: 10.1016/j.niox.2013.10.012
Sancho-Vaello, E., Gil-Carton, D., François, P., Bonetti, E. J., Kreir, M., Pothula, K. R., et al. (2020). The structure of the antimicrobial human cathelicidin LL-37 shows oligomerization and channel formation in the presence of membrane mimics. Sci. Rep. 10:17356. doi: 10.1038/s41598-020-74401-5
Sedghi, L., Dimassa, V., Harrington, A., Lynch, S. V., and Kapila, Y. L. (2021). The oral microbiome: role of key organisms and complex networks in oral health and disease. Periodontology 87, 107–131. doi: 10.1111/prd.12393
Sorbara, M. T., and Pamer, E. G. (2022). Microbiome-based therapeutics. Nat. Rev. Microbiol. 20, 365–380. doi: 10.1038/s41579-021-00667-9
Sun, J., Tang, Q., Yu, S., Xie, M., Xie, Y., Chen, G., et al. (2020). Role of the oral microbiota in cancer evolution and progression. Cancer Med. 9, 6306–6321. doi: 10.1002/cam4.3206
Takahashi, N. (2015). Oral microbiome metabolism: from “who are they?” to “what are they doing?”. J. Dent. Res. 94, 1628–1637. doi: 10.1177/0022034515606045
Takahashi, D., Shukla, S. K., Prakash, O., and Zhang, G. (2010). Structural determinants of host defense peptides for antimicrobial activity and target cell selectivity. Biochimie 92, 1236–1241. doi: 10.1016/j.biochi.2010.02.023
Thurnheer, T., and Belibasakis, G. N. (2018). Effect of sodium fluoride on oral biofilm microbiota and enamel demineralization. Arch. Oral Biol. 89, 77–83. doi: 10.1016/j.archoralbio.2018.02.010
Toscano-Garibay, J. D., Arriaga-Alba, M., Sánchez-Navarrete, J., Mendoza-García, M., Flores-Estrada, J. J., Moreno-Eutimio, M. A., et al. (2017). Antimutagenic and antioxidant activity of the essential oils of Citrus sinensis and Citrus latifolia. Sci. Rep. 7:11479. doi: 10.1038/s41598-017-11818-5
Tuominen, H., and Rautava, J. (2021). Oral microbiota and cancer development. Pathobiology 88, 116–126. doi: 10.1159/000510979
Verma, D., Garg, P. K., and Dubey, A. K. (2018). Insights into the human oral microbiome. Arch. Microbiol. 200, 525–540. doi: 10.1007/s00203-018-1505-3
Vinasco, K., Mitchell, H. M., Kaakoush, N. O., and Castano-Rodriguez, N. (2019). Microbial carcinogenesis: lactic acid bacteria in gastric cancer. Biochim. Biophys. Acta 1872:188309. doi: 10.1016/j.bbcan.2019.07.004
Vyhnalova, T., Danek, Z., Gachova, D., and Linhartova, P. B. (2021). The role of the oral microbiota in the etiopathogenesis of oral squamous cell carcinoma. Microorganisms 9:1549. doi: 10.3390/microorganisms9081549
Wade, W. G. (2013). The oral microbiome in health and disease. Pharmacol. Res. 69, 137–143. doi: 10.1016/j.phrs.2012.11.006
Wade, W. G. (2021). Resilience of the oral microbiome. Periodontology 86, 113–122. doi: 10.1111/prd.12365
Welch, J. L. M., Ramírez-Puebla, S. T., and Borisy, G. G. (2020). Oral microbiome geography: micron-scale habitat and niche. Cell Host Microbe 28, 160–168. doi: 10.1016/j.chom.2020.07.009
Wescombe, P. A., Hale, J. D., Heng, N. C., and Tagg, J. R. (2012). Developing oral probiotics from Streptococcus salivarius. Future Microbiol. 7, 1355–1371. doi: 10.2217/fmb.12.113
Willis, J. R., and Gabaldon, T. (2020). The human Oral microbiome in health and disease: from sequences to ecosystems. Microorganisms 8:308. doi: 10.3390/microorganisms8020308
Wilmarth, P. A., Riviere, M. A., Rustvold, D. L., Lauten, J. D., Madden, T. E., and David, L. L. (2004). Two-dimensional liquid chromatography study of the human whole saliva proteome. J. Proteome Res. 3, 1017–1023. doi: 10.1021/pr049911o
Wu, D.-D., Ngowi, E. E., Zhai, Y.-K., Wang, Y.-Z., Khan, N. H., Kombo, A. F., et al. (2022). Role of hydrogen sulfide in Oral disease. Oxidative Med. Cell. Longev. 2022, 1–14. doi: 10.1155/2022/1886277
Wu, J., Peters, B. A., Dominianni, C., Zhang, Y., Pei, Z., Yang, L., et al. (2016). Cigarette smoking and the oral microbiome in a large study of American adults. ISME J. 10, 2435–2446. doi: 10.1038/ismej.2016.37
Xiao, H., Fan, Y., Li, Y., Dong, J., Zhang, S., Wang, B., et al. (2021). Oral microbiota transplantation fights against head and neck radiotherapy-induced oral mucositis in mice. Comput. Struct. Biotechnol. J. 19, 5898–5910. doi: 10.1016/j.csbj.2021.10.028
Yang, C.-Y., Yeh, Y.-M., Yu, H.-Y., Chin, C.-Y., Hsu, C.-W., Liu, H., et al. (2018). Oral microbiota community dynamics associated with oral squamous cell carcinoma staging. Front. Microbiol. 9:862. doi: 10.3389/fmicb.2018.00862
Ye, C., and Kapila, Y. (2021). Oral microbiome shifts during pregnancy and adverse pregnancy outcomes: hormonal and immunologic changes at play. Periodontology 87, 276–281. doi: 10.1111/prd.12386
Zasloff, M. (2019). Antimicrobial peptides of multicellular organisms: my perspective. Adv. Exp. Med. Biol. 1117, 3–6. doi: 10.1007/978-981-13-3588-4_1
Zaura, E., Keijser, B. J., Huse, S. M., and Crielaard, W. (2009). Defining the healthy "core microbiome" of oral microbial communities. BMC Microbiol. 9:259. doi: 10.1186/1471-2180-9-259
Zhang, J. S., Chu, C.-H., and Yu, O. Y. (2022). Oral microbiome and dental caries development. Dent. J. 10:184. doi: 10.3390/dj10100184
Zhang, Y., Ding, Y., and Guo, Q. (2022). Probiotic species in the Management of Periodontal Diseases: an overview. Front. Cell. Infect. Microbiol. 12:806463. doi: 10.3389/fcimb.2022.806463
Zhang, L., Liu, Y., Zheng, H. J., and Zhang, C. P. (2020). The oral microbiota may have influence on oral cancer. Front. Cell. Infect. Microbiol. 9:476. doi: 10.3389/fcimb.2019.00476
Zhang, G., Lu, M., Liu, R., Tian, Y., Vu, V. H., Li, Y., et al. (2020). Inhibition of Streptococcus mutans biofilm formation and virulence by Lactobacillus plantarum K41 isolated from traditional Sichuan pickles. Front. Microbiol. 11:774. doi: 10.3389/fmicb.2020.00774
Zhang, M., Tang, H., Yuan, Y., Ou, Z., Chen, Z., Xu, Y., et al. (2023). The role of indoor microbiome and metabolites in shaping Children’s nasal and Oral microbiota: a pilot multi-Omic analysis. Meta 13:1040. doi: 10.3390/metabo13101040
Zhang, M., Wang, F., Jiang, L., Liu, R., Zhang, L., Lei, X., et al. (2013). Lactobacillus salivarius REN inhibits rat oral cancer induced by 4-nitroquioline 1-oxide. Cancer Prev. Res. (Phila.) 6, 686–694. doi: 10.1158/1940-6207.CAPR-12-0427
Zhang, Y., Wang, X., Li, H., Ni, C., Du, Z., and Yan, F. (2018). Human oral microbiota and its modulation for oral health. Biomed. Pharmacother. 99, 883–893. doi: 10.1016/j.biopha.2018.01.146
Zhou, Z., Ling, G., Ding, N., Xun, Z., Zhu, C., Hua, H., et al. (2018). Molecular analysis of oral microflora in patients with primary Sjögren's syndrome by using high-throughput sequencing. PeerJ 6:e5649. doi: 10.7717/peerj.5649
Żółkiewicz, J., Marzec, A., Ruszczyński, M., and Feleszko, W. (2020). Postbiotics-a step beyond pre- and probiotics. Nutrients 12:2189. doi: 10.3390/nu12082189
Keywords: oral microbiota, bacteria, periodontal disease, oral cavity, microbiota metabolites
Citation: Bostanghadiri N, Kouhzad M, Taki E, Elahi Z, Khoshbayan A, Navidifar T and Darban-Sarokhalil D (2024) Oral microbiota and metabolites: key players in oral health and disorder, and microbiota-based therapies. Front. Microbiol. 15:1431785. doi: 10.3389/fmicb.2024.1431785
Edited by:
Alina Maria Holban, University of Bucharest, RomaniaReviewed by:
John Tagg, Blis Technologies Ltd., New ZealandYu Sun, South China Agricultural University, China
Copyright © 2024 Bostanghadiri, Kouhzad, Taki, Elahi, Khoshbayan, Navidifar and Darban-Sarokhalil. This is an open-access article distributed under the terms of the Creative Commons Attribution License (CC BY). The use, distribution or reproduction in other forums is permitted, provided the original author(s) and the copyright owner(s) are credited and that the original publication in this journal is cited, in accordance with accepted academic practice. No use, distribution or reproduction is permitted which does not comply with these terms.
*Correspondence: Tahereh Navidifar, cm95YV82N0B5bWFpbC5jb20=; Davood Darban-Sarokhalil, ZGF2b29kX2RhcmJhbkB5YWhvby5jb20=