- 1Hubei Engineering Research Center for Protection and Utilization of Special Biological Resources in the Hanjiang River Basin, College of Life Sciences, Jianghan University, Wuhan, China
- 2Yangtze River Fisheries Research Institute Chinese Academy of Fishery Sciences, Wuhan, China
Global concerns over harmful cyanobacterial blooms brought on by eutrophication are now widespread. Aquatic ecological restoration techniques that use algicidal bacteria to control toxic algae show promise. A Bacillus subtilis S4 (S4) strain with strong Microcystis aeruginosa algicidal activity and the capacity to degrade microcystins (MCs) were successfully isolated and evaluated in this study. The dynamics of internal and extracellular MC concentration as well as the physiological response and morphological properties of M. aeruginosa were investigated in the M. aeruginosa/bacteria co-culture system. The findings demonstrated that when S4 density grew from 1 × 106 cells/ml to 1 × 108 cells/ml, the release of M. aeruginosa lysis and MCs was boosted; however, MCs dropped by approximately 90% within 18 h, regardless of bacterial density. Comparing the bacterial cell incubation system to the control and bacterial cell-free filtrate systems, the assessment of extracellular and intracellular MCs revealed a 95% reduction in MCs. The findings showed that 89% of MCs were decreased by bacterial cells, while 98% of M. aeruginosa cells were algaecided by bacterial metabolites. Sustainable eradication of M. aeruginosa and MCs has been accomplished by the combined efforts of the S4 strain and its metabolites. By secreting algicidal chemicals that are resistant to proteases, acid, base, and heat, the S4 strain indirectly acts as an algaecide. The S4 strain possesses a strong ability to break down MCs and a very effective and stable algaecide function, indicating that it can potentially treat eutrophic water with hazardous algae.
1 Introduction
Cyanobacteria blooms caused by eutrophication have emerged worldwide, leading to a series of issues, such as odor and microcystin (MC) pollution, which severely damage ecosystems and pose a threat to human health (Namsaraev et al., 2020; Zhang et al., 2023). Despite the growing body of research on cyanobacteria blooms, the mechanisms underlying their control and the mitigation of their toxins remain poorly understood. Hence, there is an increasing interest in cost-effective and environmentally friendly remediation technologies to control these blooms. However, these methods often fall short due to their non-selective nature, the potential for generating harmful by-products, and the risk of ecological imbalance (Song et al., 2023). As an effective method for eliminating cyanobacterial blooms, biological methods have received widespread attention due to their environmental friendliness, especially microbial-based methods (Pal et al., 2020). Among these, Bacillus species have emerged as promising candidates due to their broad-spectrum activity and minimal environmental impact.
Targeting cyanobacteria that cause blooms, approximately 151 cyanobactericidal bacterial strains have been reported, hailing from over 4 phyla, 12 classes, 26 orders, 35 families, and 49 genera (Yang C. et al., 2020). Predominantly, they belong to Cytophaga/Flavobacterium/Bacteroidetes or Gammaproteobacteria, such as Alteromonas, Bacillus, Flavobacterium, Pseudoalteromonas, Pseudomonas, and Vibrio (Wang et al., 2020). Bacilli emerge as the dominant group, constituting 31.1% of these strains (Yang C. et al., 2020). The majority of Bacilli are isolated from waters containing cyanobacteria, particularly from bloom-affected areas such as Taihu and Dianchi lakes (Liu et al., 2019; Tian et al., 2012). This supports the notion that algicidal bacteria may play a role in naturally regulating algal blooms, although the patterns and mechanisms remain largely unknown (Meyer et al., 2017). The target cyanobacteria for Bacilli are listed in Supplementary Table S1, with the vast majority (92.31%) capable of killing Microcystis, likely due to their common association with bloom formation and widespread use as target algae for screening cyanobactericidal bacteria. For Bacilli, a variety of prey spectra at different levels (e.g., species, genus) have been reported, with over half of the bacteria (84.62%) targeting only one species of algae, as shown in Supplementary Table S1. However, this also reveals a critical gap in our knowledge regarding the degradation of cyanotoxins, such as MCs, by these strains. While some strains show promise in algicidal activity, the majority of them lack specific details on MC degradation, highlighting a significant research need. The absence of comprehensive data on MC degradation is a notable shortfall, as the release of these toxins during algal blooms poses substantial ecological and health risks.
The application of algicidal bacteria, like other cell lysis methods, carries the potential risk of releasing harmful secondary metabolites, highlighting the necessity for strains capable of degrading MCs in addition to their algicidal effects (Massey and Yang, 2020). MCs are a type of stable cyclic heptapeptide that can inhibit the activity of liver protein phosphatase and induce liver cancer (Schreidah et al., 2020). These toxins can accumulate in aquatic food chains, posing a significant risk to both wildlife and human health due to their potent hepatotoxic effects. In addition to toxicity and stability, the overproduction of MCs is also a common concern, which is induced by environmental changes, such as light intensity, carbon and nitrogen levels, trace elements, and environmental toxicants (Brêda-Alves et al., 2021). These MC properties have attracted attention to various algaecide treatments. The persistence and toxicity of MCs have prompted the development of various treatment strategies, including the use of adsorbents, chemical oxidation, and biological degradation. However, the effect of algicidal bacteria on MC production and release has not been fully studied. Despite the potential of algicidal bacteria in bloom management, their impact on MC production and release remains poorly understood, a knowledge gap this study aims to fill. According to previous studies, when the dose of algicidal bacteria exceeds the threshold concentration, the intracellular MCs can be decreased, regardless of the lytic mechanism (Wijesooriya et al., 2021). Our study builds on these findings by investigating the specific thresholds and the underlying mechanisms by which algicidal bacteria reduce intracellular MC concentrations. The reduction in intracellular MC concentration may be influenced by a variety of factors, including but not limited to the downregulation of gene expression (Xuan et al., 2017). For instance, an increase in the release ratio of MCs into the extracellular fraction or the conversion of intracellular forms into by-products through interactions with intracellular molecules may also be equally important processes. However, some studies have shown the opposite results, where the extracellular MC concentration of high-dose algicidal bacteria is higher than that of low-dose bacteria (Li et al., 2021). It has been reported that MC-degrading bacteria and algicidal bacteria have been successfully isolated, which confirms the coexistence of algae-algicide bacteria and MC-degrading bacteria (Morón-López et al., 2023). This coexistence highlights the potential for developing bacterial strains that can both control algal blooms and mitigate the associated toxin risks.
In addition, studies have found that the biodegradation activity of MC-degrading bacteria can be induced under certain conditions, confirming the existence of a single strain with both Microcystis aeruginosa (M. aeruginosa) and MC-degrading activities (Benegas et al., 2021). However, so far, there have been relatively few studies on the degradation of toxic M. aeruginosa and MCs by a single bacterial strain in co-cultured M. aeruginosa bacterial systems (Crettaz-minaglia et al., 2020). This study addresses this gap by investigating the potential of a single bacterial strain to degrade both the algal biomass and the toxins, providing a comprehensive approach to cyanobacterial bloom management. By elucidating these mechanisms, our study not only advances the fundamental science of microbial interactions but also paves the way for the development of novel biotechnological solutions for water quality management. In this study, a Bacillus subtilis S4 (S4) strain was successfully isolated and screened from the scum of Taihu Lake, which has high algicidal activity against M. aeruginosa and strongly reduces the content of MCs. Under the co-cultivation of M. aeruginosa and bacteria, the quantitative reactions of algae cells were investigated. The kinetics of MC release and biodegradation were evaluated. The combined activities of the S4 strain and its metabolites have successfully removed M. aeruginosa and MCs. The algicidal mode of S4 belonged to an indirect way and the algicidal substances showed stability to protease, acid, base, and heat. Our research provides data support and lays a theoretical foundation for developing an environmentally friendly method to reduce the occurrence of harmful cyanobacterial blooms.
2 Materials and methods
2.1 Materials
The M. aeruginosa FACHB 905 strain was sourced from the Freshwater Algae Culture Collection at the Institute of Hydrobiology, Chinese Academy of Sciences, Wuhan, Hubei, China. Concurrently, the algicidal bacterium was characterized using samples collected from the scum of cyanobacterial blooms in Taihu Lake. Samples were obtained by carefully skimming the lake’s surface with a sterile tool during the peak bloom period, ensuring a representative collection of cyanobacteria-associated bacteria. The collected samples were promptly stored in sterile containers and kept on ice until laboratory analysis. Polymerase chain reaction (PCR) primers were synthesized at Hubei Qingke Biotechnology Co., Ltd. The raw materials of Luria-Bertani (LB) and BG11 medium, used for bacterial and M. aeruginosa cultures, respectively, were purchased from China National Pharmaceutical Group Chemical Reagent Co., Ltd., Shanghai, China.
2.2 Microcystis aeruginosa and bacteria culture
The M. aeruginosa FACHB 905 strain was cultured under controlled conditions to simulate its natural environment. The culture conditions included a 12 h (light):12 h (dark) photoperiod, a temperature maintained at 25 ± 1°C, and an illumination condition of 50 μmol photons m−2 s−1. These conditions were chosen to reflect optimal growth parameters for M. aeruginosa as per established protocols (Liu et al., 2018). The pH of the culture medium was rigorously controlled and maintained at 7.0 ± 0.2 throughout the experiment to ensure consistency and to match the neutral pH range preferred by M. aeruginosa. The cyanobacteria scum (5 g, wet weight) was mixed with 50 mL of phosphate-buffered saline (PBS) and shaken at 120 rpm for 30 min at 30°C. The samples were serially diluted in sterile water before being inoculated into nutrient agar (NA, 2% agar) plates in 0.1 mL aliquots of each dilution. Approximately 100 mL of LB medium containing isolated bacteria was cultured for 24 h at 30°C and 150 rpm to allow for the bacterial culture’s proliferation. This step was essential to obtain a sufficient quantity of the bacterial strain for subsequent experimental procedures.
2.3 Characterization of the algicidal bacterium
By examining the sequencing of the 16S rRNA gene, the isolated strain was recognized. The TIANGEN.DP302-02 kit (Tiangen Biochemical Technology Co., Ltd., Beijing, China) was used to extract DNA from the bacterial samples in accordance with the manufacturer’s instructions, and the 16S rRNA fragments were examined (Wu et al., 2007). In a thermal cycler (Bio-Rad, Hercules, CA, USA), forward 27F (5′-GAGTTTGATCCTGGCTCAG-3′) and reverse 1492R (5′-ACGGCTACCTTGTTACGACTT-3′) were reversed: 95°C for 4 min; 35 cycles at 94°C for 1 min, 56°C for 1 min, 72°C for 2 min; the final step at 72°C for 15 min, at 4°C for 10 min.
2.4 Co-incubation experiment
The S4 strain, isolated from the scum of cyanobacteria in Taihu Lake, was used for all experiments involving the algicidal bacteria. To lessen the effect of adding significant amounts of organic nutrients, washed bacterial suspensions were tested in this investigation. The incubation experiment was divided into four parts: the algicidal bacterium was incubated with M. aeruginosa (1 × 107 cells/ml) in 100-ml BG11 medium, in which the bacterial density was 1 × 106 cell/ml, 1 × 107 cell/ml and 1 × 108 cell/ml, respectively; another flask was used as control, where M. aeruginosa was cultured in a BG11 medium. All experiments were performed in parallel batch culture (100 mL) in 250 mL Erlenmeyer flasks, and the culture conditions are explained in Section 2.2. The control group composed of M. aeruginosa received the same volume of BG11 medium and LB. Three replicates were prepared for each test. A small aliquot (5 mL) was removed after filtration, and the chlorophyll a (Chl.a) concentration was measured. It should be emphasized that the concentration of S4 used in this study, as detailed in Sections 2.7 and 2.8, is based on the optimal S4 concentration of 1 × 107 cells/ml, which was determined as discussed in this subsection.
2.5 Algicidal effects of bacteria
Microcystis aeruginosa cell counts were monitored daily for 7 days using a hemocytometer and a microscope (Carl Zeiss, Jena, Germany). The inhibitory rate was calculated using the following formula: , where T and C are the concentrations of the treatment and control cells, respectively. For the determination of chlorophyll a, we employed a classic method of pigment extraction and spectrophotometry. The specific procedure involved collecting the treated algal cells by centrifugation, followed by pigment extraction using an organic solvent, such as 90% acetone. The concentration of Chl.a was then measured at a specific wavelength using a spectrophotometer. For further investigation, bacteria that showed algicidal activity against M. aeruginosa were chosen.
2.6 Analysis of MCs
A 30-mL logarithmic phase culture of M. aeruginosa FACHB 905 was injected with 5% (v/v) volume fractions of both bacterial cells and cell-free filtrate. The supernatant was obtained by centrifuging at 5,000 g at 4°C to precipitate M. aeruginosa cells and assay the extracellular MCs. The cells are washed, precipitated twice, frozen and thawed 3 times, and then centrifuged at 12,000 g at 4°C to remove cell fragments. The residual supernatant was used to determine the intracellular MCs. The concentration of MCs in the sample was determined in accordance with the Beacon Microcystin Plate Kit’s (Beacon Analytical Systems, Saco, Maine, USA) instructions. Prior to conducting ELISA analyses, the microcystin samples were diluted in a ratio ranging from 1:10 to 1:20. This dilution strategy was essential due to the kit’s limited detection sensitivity, which spans between 0.1 and 2.0 μg/L. To standardize the conditions for ELISA quantification, the pH of the microcystin samples was carefully adjusted to a neutral pH of approximately 7.0. This adjustment was crucial for minimizing pH-related interferences that could potentially skew the results of both direct and indirect ELISA assays during the analysis phase.
2.7 Analysis of the mode action of algicidal strain
To ascertain the strain’s algicidal mode, cell-free supernatant (S4 bacterial secretions) and bacterial cells from the S4 strain were separated using filtration and centrifugation, and then treated with M. aeruginosa cultures at a ratio of 10% (v/v), respectively. The algicidal strain was exposed to M. aeruginosa for 48 h before obtaining the cell-free supernatant. By suspension filtering with 0.22 μm membranes and centrifuging bacterial cells at 1,800 g for 5 min at room temperature, cell-free supernatant was obtained.
2.8 Stability of cell-free supernatant
To evaluate the stability of the algicidal chemicals secreted by the S4 under different environmental conditions, a series of tests were conducted. The cell-free supernatant containing the S4 secretion was incubated for 2 h at various temperatures (−80°C, −20°C, 0°C, 30°C, 60°C, and 100°C). Additionally, the pH of the supernatant was adjusted to 3, 7, and 10 using NaOH or HCl, and after 2 h, the pH was returned to 7. Finally, 100 mL (106 cells/ml) of pretreated algal culture was inoculated with 2% (v/v) of the supernatant treated under these two methods. The algicidal activity was measured at 12 h to assess the S4 secretions’ tolerance to heat and acid–base conditions. As previously mentioned, the M. aeruginosa cell count and algicidal activity were determined. To simulate freeze–thaw cycles, the supernatant was frozen in liquid nitrogen for 5 min and then subjected to three cycles of freezing and thawing in a 65°C water bath. The supernatant was then cooled to room temperature before adding 2% (v/v) of it to a pretreated algal culture containing 100 mL (106 cells/ml). Each experiment was carried out 3 times at 25°C during the light phase of the light cycle. For a measurement of algicidal activity, M. aeruginosa cells were counted at 4, 8, and 12 h.
2.9 Statistical analyses
Each experiment was run at least 3 times, with the results shown as the average standard deviation of the three repetitions. One-way analysis of variance (ANOVA) (Statistical Package for the Social Sciences [SPSS] 22.0 for Windows) was used to test for significant differences among all the data collected for this study. A p-value of 0.05 was used to denote significance.
3 Results and discussion
3.1 Isolation and identification of the algicidal strain
The capacity of each of these strains to eradicate M. aeruginosa was examined (Supplementary Figure S1). One of these, S4, was chosen for further investigation due to its superior capacity for M. aeruginosa degradation, which it can do in just 4 days. From the genomic DNA of the S4, a 1,457 bp fragment of the 16S rRNA gene was amplified (Supplementary Figure S2). The 16S rRNA gene sequence (GenBank accession number: NMDCN00017DJ) was obtained by the GenBank Basic Local Alignment Search Tool (BLAST). The closest B. subtilis strains identified using BLAST analysis were B. subtilis BS-01 (HM631973). A phylogenetic tree was constructed using MEGA software (Figure 1), indicating that the S4 strain was grouped with B. subtilis with high bootstrap values. Many members of Bacillus sp. have been reported widely showing algicidal activity, such as Bacillus sp. SY-1 (Jeong and Son, 2021), Bacillus sp. Lzh-5 (Li et al., 2015), and Bacillus cereus N-14 (Nakamura et al., 2003).
3.2 Effects of initial bacterial density on algicidal and MCs reduction efficiency
Figure 2 illustrates the impact of initial bacterial density on algicidal effectiveness. Significant differences in cell counts were observed in the 106, 107, and 108 cells/ml groups compared to the control group after 7 days of co-cultivation (p < 0.05), as shown in Figures 2a,b. At a bacterial density of 106 cells/ml, the algal cell count dropped to 1.27 ± 0.05 × 106 cells/ml, resulting in an algicidal rate of 73.52 ± 2.30%. At bacterial densities of 107 and 108 cells/ml, the algal cell count decreased by orders of magnitude, with corresponding algicidal rates of 93.29 ± 0.46% and 74.54 ± 3.23%, respectively (Figure 2c). Therefore, further research was performed using a bacterial solution of 106 cells/ml concentration, as there was no significant difference among the three concentrations, as shown in Figure 3.
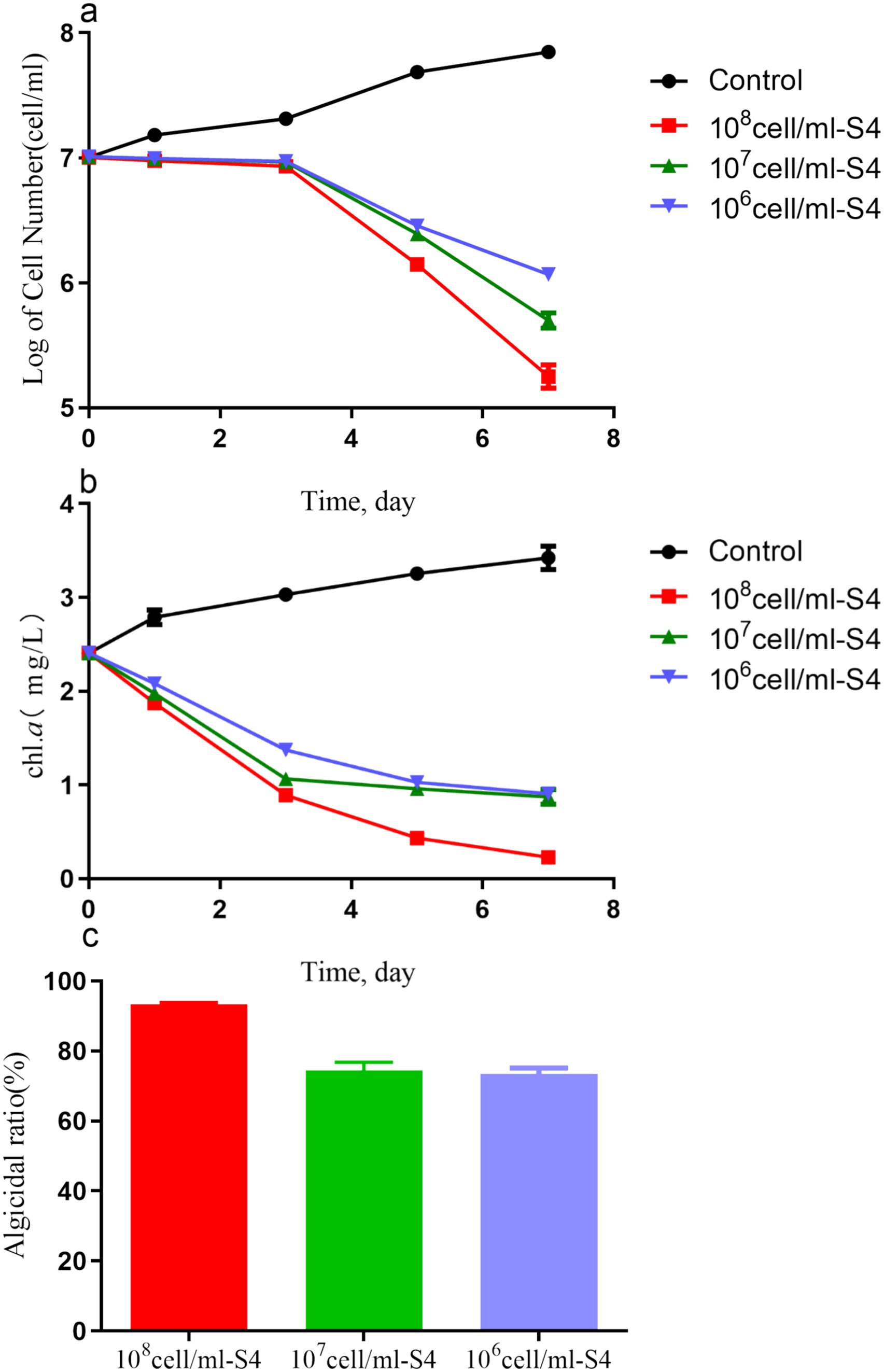
Figure 2. The number of cells (a), Chl.a concentrations (b), and algicidal ratio (c) of Microcystis aeruginosa (1 × 107 cell/mL) co-cultured with the S4 strain of different concentrations (106, 107, and 108) for 7 days.
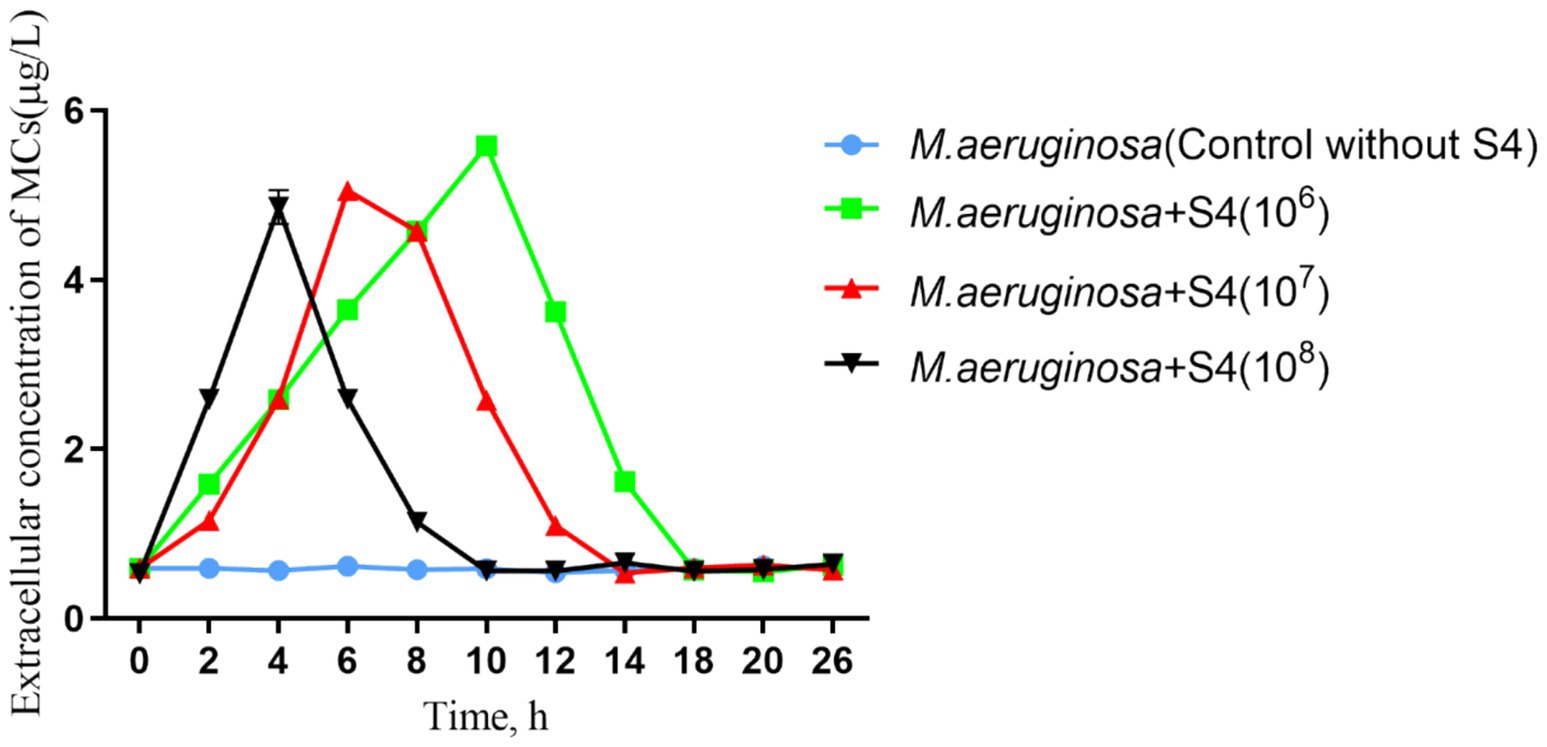
Figure 3. Dynamics of MC concentrations in Microcystis aeruginosa and S4 co-cultured systems under varied initial bacterial densities.
In this study, we found that the initial density of the S4 significantly influences algicidal activity against M. aeruginosa, with higher densities leading to greater effects. This aligns with previous studies (Ding et al., 2021; Yang J. et al., 2020; Liu et al., 2022), suggesting that a higher initial bacterial density results in more algicidal compounds being available for direct or indirect lysis of M. aeruginosa. The algicidal rate may increase if the bacteria grow to higher densities, highlighting the need to enhance bacterial growth for effective algal bloom management. However, nutrient addition must be carefully balanced to prevent unintended algal growth. Environmental factors such as light, temperature, and pH can modulate bacterial growth and algicidal activity, indicating the need for further research to develop effective and eco-friendly strategies against harmful algal blooms.
The MC concentration in the control group remained stable at approximately 0.59 μg/L, while in co-cultures with S4, MCs peaked at 5.58 μg/L within 10 h before declining (Figure 3), suggesting a biodegradation process. The highest MC concentrations did not differ significantly across different initial S4 densities, indicating a consistent response regardless of density. The timing of MC concentration peaks directly corresponded to the initial S4 density, with the fastest lysis observed at the highest density. The initial increase in MC concentration observed in the co-culture systems (Figure 3) is attributed to the lysis of M. aeruginosa cells upon interaction with the S4 strain. This lysis results in the release of preformed MCs, which transiently elevates the extracellular MC levels before the bacteria commence the biodegradation process. The timing of this initial peak in MC concentration corresponds to the initial density of the S4 strain, with higher densities leading to a more rapid release and subsequent degradation of MCs. Our findings support previous research showing enhanced MC degradation at higher bacterial densities (Ndayisenga et al., 2021) and suggest that S4’s algicidal activity contributes to MC degradation, indicating a sustainable process (Yang F. et al., 2020). The kinetics of MC concentration varied with different initial S4 densities, but the overall MC concentrations remained within a narrow range, highlighting the potential of S4 for managing cyanobacterial blooms and MC contamination. Future research should focus on elucidating the mechanisms underlying MC degradation by S4, optimizing conditions for maximum efficacy, and assessing the impact of environmental factors on the strain’s performance to advance the bioremediation of algal blooms.
3.3 Microcystis aeruginosa lysis and MC degradation patterns
After 24 h of incubation, M. aeruginosa and S4 cells showed a modest variation in cell density compared to the control group (Figure 4a). However, the algicidal activity of the S4 cell secretion group was substantially stronger, which is what caused the M. aeruginosa cell density to drop by almost 96%. Compared to the control and the group that received additional cell-free filtrate, the extracellular MC concentration reduced almost 2-fold in the bacterial cell incubation group (Figure 4b), and the total MCs (which include both extracellular and intracellular MCs) decreased by approximately 95%. In terms of intracellular MCs, there was no discernible change between the control and the group supplemented with bacterial cell-free filtrate (p = 1.73, >0.05), and the degradation efficiency in the bacterial cell-free filtrate group remained at approximately 7%. The degradation efficiency is 89.02% when M. aeruginosa and bacterial cells are co-incubated. Earlier studies suggest that 70–90% of planktonic cells lyse algal cells either directly or by creating extracellular chemicals (Effiong et al., 2020). Our findings indicate that the presence of active chemicals in bacterial cell-free filtrate, rather than direct contact between bacterial cells and M. aeruginosa cells, is responsible for the algicidal activity. The metabolism of S4 cells, which use MCs as a carbon source for growth, is what leads to MC degradation. In conclusion, M. aeruginosa cells and MCs are simultaneously biodegraded due to the combined action of S4 cells and their metabolites.
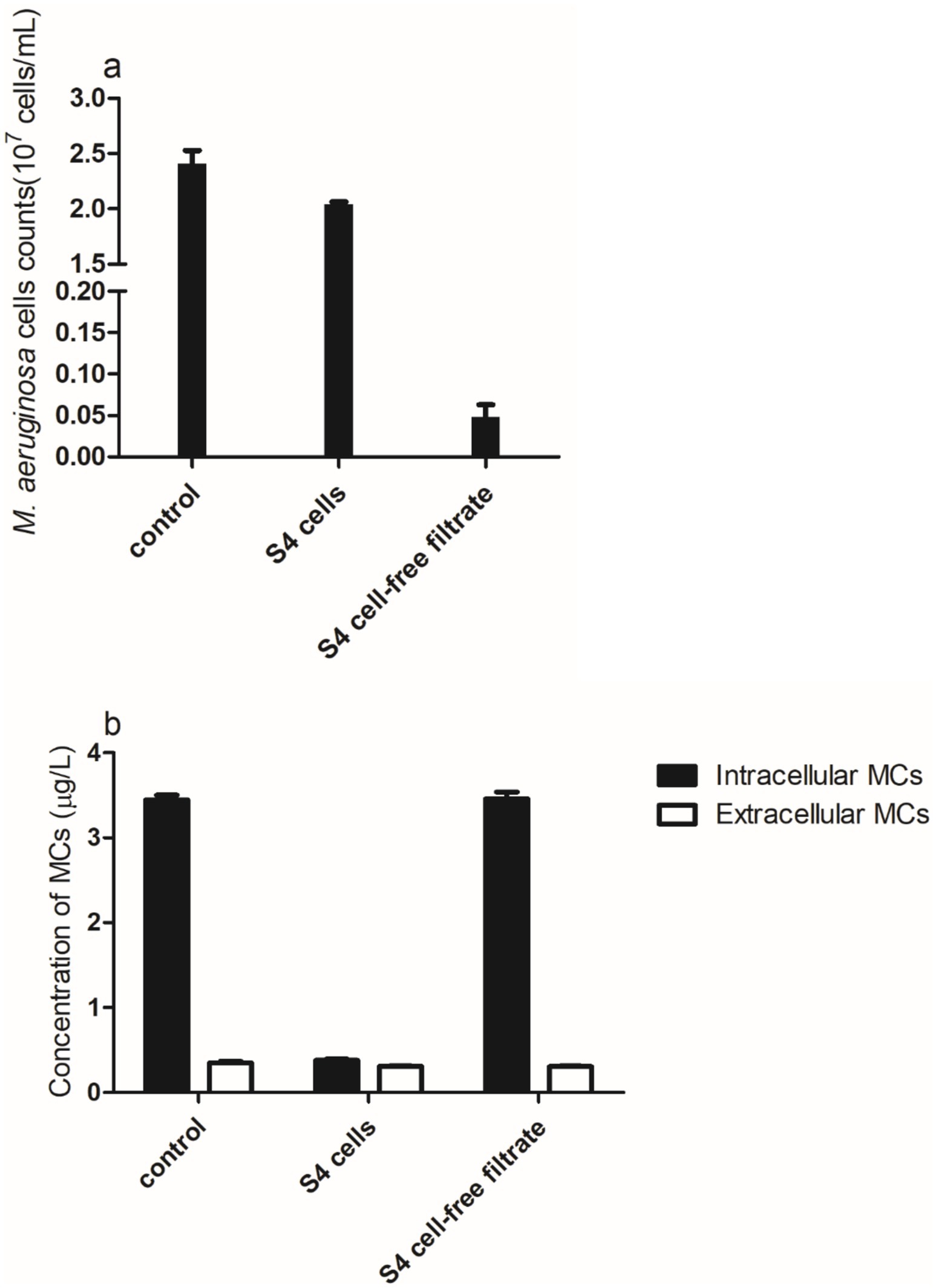
Figure 4. (a) Microcystis aeruginosa cell density and (b) concentrations of intra- and extracellular MCs after 24 h in the M. aeruginosa/bacterial co-cultured system.
The biological management of cyanobacterial blooms is a multifaceted approach that includes the removal of cyanobacterial cells and the degradation of their harmful metabolites. Our study supports the view that algicidal compounds are instrumental in the lysis of algal cells, as evidenced by the study of Xu et al. (2022). These compounds, often produced by certain bacterial strains, interfere with the cellular integrity of cyanobacteria such as M. aeruginosa, leading to cell rupture and the subsequent release of intracellular contents, including MCs. The biodegradation of MCs, a complex process, is primarily attributed to bacterial enzymatic hydrolysis, as demonstrated by Kumar et al. (2019) and Wei et al. (2023). While our study found only a slight change in MC concentration in the cell secretion group compared to the control group, this does not necessarily mean that bacteria lack the enzymatic machinery to degrade MCs. Instead, it suggests that the degradation process may primarily occur within bacterial cells, which is consistent with the known function of the mlr gene cluster. The mlr gene cluster, as identified in bacteria capable of degrading MCs, encodes a set of enzymes that work in concert to break down MC molecules (Morón-López et al., 2023). The initial step of MC degradation is often attributed to enzymes encoded by the mlrA gene, which cleaves the Adda-Arg bond in the MC molecule (Bourne et al., 1996). Subsequent enzymes, such as those encoded by mlrB and mlrC, further process the degradation intermediates (Kumar et al., 2019).
Our results show a significant decrease in MC concentration in the presence of live bacterial cells, which points toward an active intracellular degradation process. It is plausible that live bacterial cells internalize and metabolize the released MCs, thereby contributing to their degradation. This process is likely facilitated by the intracellular enzymes of the mlr gene cluster, rather than by secreted enzymes. Future studies should focus on characterizing the expression of the mlr gene cluster in bacteria that demonstrate algicidal activity and their ability to degrade MCs. Understanding the regulation and expression of these genes, as well as the specific enzymatic activities involved, will provide insights into the mechanisms by which these bacteria contribute to the biodegradation of MCs.
The bacteria, on the other hand, break down and use the released MCs when living bacterial cells are incubated, as shown by the general fall in the MC levels. The conclusion that algicidal substances cause M. aeruginosa cell density to decrease (Figure 4a) and MCs release (Figure 3) in an algae/bacterial co-culture group can, therefore, be reasonably drawn by taking into account the algicidal performance of cell secretion and the MC degradation activity of bacterial cells. As the availability of carbon and nitrogen sources increases, the assimilation of MCs by algal development dominates this process, resulting in a significant decrease in MCs (Figures 3, 4b). As cyanobacteria metabolize MCs for growth, especially under conditions where carbon and nitrogen sources are abundant, the concentration of free MCs in the environment may significantly diminish. This insight could have implications for developing strategies to mitigate the toxicity associated with cyanobacterial blooms. Environmental factors such as nutrient availability, water temperature, and pH can modulate the expression of genes involved in producing algicidal compounds and the enzymatic machinery for MC degradation. Future research should consider these factors to optimize the conditions for effective cyanobacterial management (Song et al., 2024).
3.4 Stability of S4 filtrate
Stability tests showed that the S4 strain’s algicidal chemicals were heat stable, with minor activity changes after exposure to temperatures ranging from −80°C to 100°C (Figure 5a). The pH stability test revealed reduced stability in acidic conditions, particularly at pH 3, but retained activity at pH 7 and 10 (Figure 5b). Freeze–thaw cycles had minimal impact, maintaining algicidal activity even after three cycles (Figure 5c). These results suggest the S4 strain’s potential for aquatic applications. Algicidal bacteria often kill algae directly or indirectly, and the S4 strain likely uses an indirect method, as seen in Pseudoalteromonas species (Wang et al., 2020; Zhang et al., 2022). The algicidal chemical’s stability under alkaline conditions, freeze–thaw cycles, and heat resistance indicate it is not a protein and could possibly be a pigment (Wang et al., 2022). Further research is needed to identify the algicidal chemical’s characteristics and subtypes. Our study has certain limitations. The experiments were under controlled conditions, which may not reflect natural aquatic ecosystems’ variability. The tested environmental conditions were limited, and the S4 strain’s response to a broader range of variables, such as salinity and nutrient availability, requires further investigation. Additionally, scaling up from laboratory to field applications may present new challenges, including ecosystem interactions and long-term ecological impacts. Future research should address these limitations by testing a wider range of conditions and exploring long-term field applications.
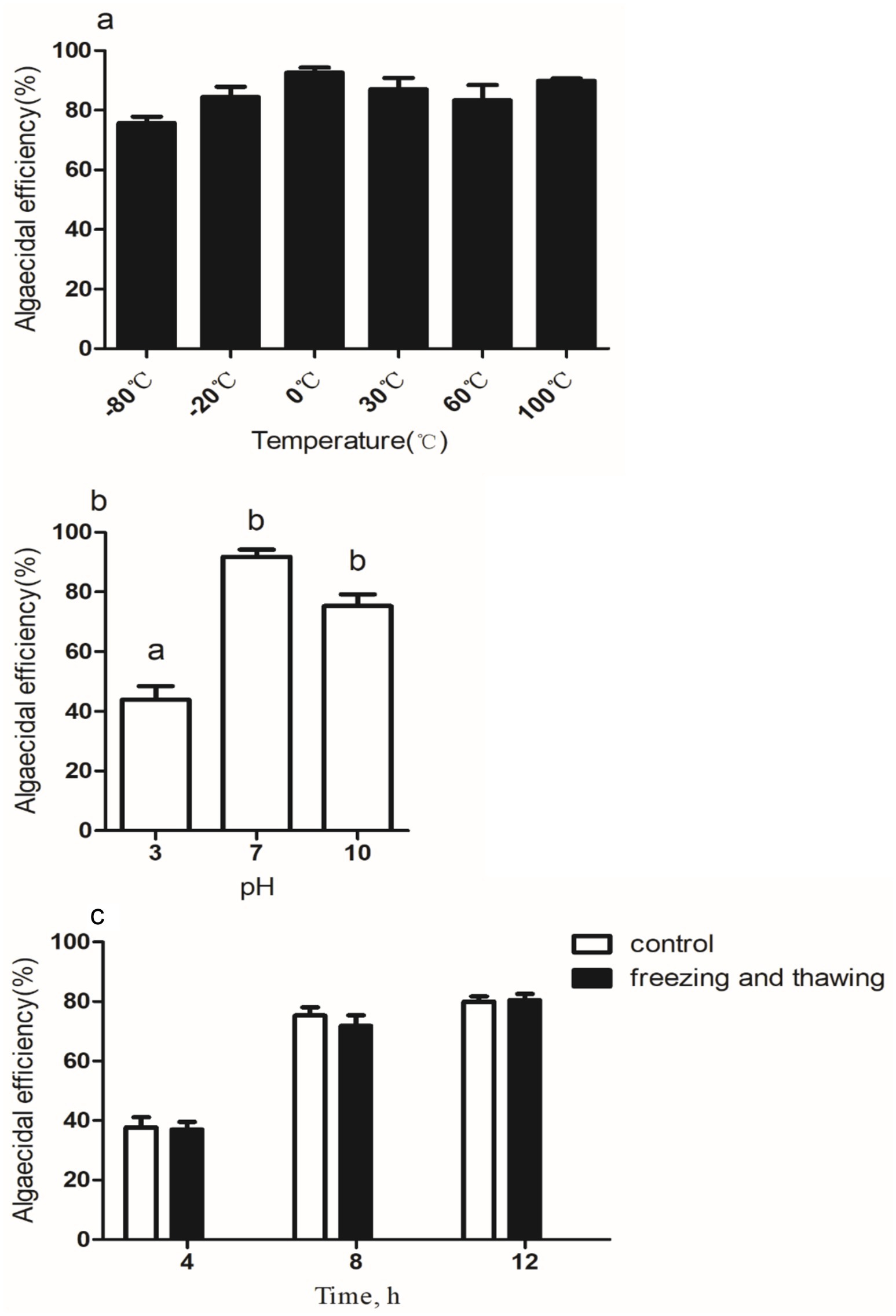
Figure 5. Algicidal effect of strain S4 cell-free supernatant at different temperatures (a), pH (b) conditions, and repeated freeze–thaw cycles (c) treatment Microcystis aeruginosa against. Different alphabetical letters, a and b, represent significant differences between groups (p < 0.05).
3.5 Innovation of the S4 strain and its potential application in algal bloom management
This study successfully isolated and screened a B. subtilis S4 strain with potent algicidal activity against M. aeruginosa and the capacity to degrade MCs. Our findings not only substantiate the potential of S4 in controlling harmful algal blooms but also reveal its significant degradation capability for MCs. These discoveries offer new insights in the field of aquatic ecological restoration and bioremediation.
First, the S4 strain demonstrated varying degrees of lytic activity against M. aeruginosa and degradation capability for MCs at different densities. As the density of the S4 strain increased from 1 × 106 cells/ml to 1 × 108 cells/ml, the lysis of M. aeruginosa and the release of MCs were enhanced; however, MC levels dropped by approximately 90% within 18 h, regardless of bacterial density. This indicates that the combined action of the S4 strain and its metabolites achieved sustainable eradication of both M. aeruginosa and MCs, a novel approach not previously reported in the literature (Kong et al., 2022).
Moreover, the algicidal chemicals secreted by S4 exhibited resistance to proteases, acids, bases, and heat, which is unique among the reported algicidal bacteria. This indirect algicidal activity may be mediated by the secretion of stable chemicals that can withstand environmental fluctuations, crucial for persistence and efficacy in practical applications. The stability of these chemicals under various conditions suggests that they are not proteins but possibly pigments or other types of compounds, providing a new direction for further research on the characteristics and subtypes of these chemicals (Ameen et al., 2021).
Our study also found that S4 maintained stable algicidal activity under various environmental conditions. The algicidal chemicals from S4 showed remarkable resilience across a range of temperatures from −80°C to 100°C and retained their activity at pH levels 3–10. This suggests that the algicidal chemicals of S4 are well-suited for application in diverse aquatic environments, which is a significant advantage over other reported strains that may have more limited stability profiles (Azam, 1998).
Although S4 showed significant algicidal and MCs-degradation capabilities under laboratory conditions, we acknowledge the challenges in translating these findings into field applications. Issues such as ecological interactions with other organisms, potential side effects on non-target species, and long-term ecological impacts require further investigation in future studies. The potential application of S4 in natural environments will depend on its ability to persist and maintain activity under variable conditions, which is a critical area for future research (Coyne et al., 2022).
In summary, the discovery of the B. subtilis S4 strain provides a novel biotechnological approach to managing harmful algal blooms. Its unique algicidal mechanism and effective degradation of MCs make it a promising biocontrol agent. Future research should focus on optimizing the application conditions for the S4 strain and assessing its long-term effects and ecological impact in natural environments (Crettaz-Minaglia et al., 2020).
4 Conclusion
A unique freshwater bacterial strain known as S4 has demonstrated the potential to degradeMCsand exhibit potent algicidal action against M. aeruginosa. In the M. aeruginosa/bacteria co-culture system, the lysis of M. aeruginosa and the release of MCs were accelerated as the density of S4 increased; however, MC levels decreased within 18 h, irrespective of the bacterial density. When comparing the bacterial cell incubation system with the control and the bacterial cell-free filtrate systems, the extracellular and intracellular MCs collectively showed a 95% reduction. The S4, along with its metabolites, has achieved sustainable eradication of both M. aeruginosa and MCs.
Data availability statement
The data presented in the study are deposited in the National Microbiology Data Center, accession number: NMDCN00017DJ: https://nmdc.cn/resource/genomics/sequence/detail/NMDCN00017DJ.
Author contributions
YC: Writing – original draft. FX: Writing – review & editing. YZ: Data curation, Writing – original draft. DZ: Formal analysis, Writing – review & editing. HL: Investigation, Writing – original draft. LZ: Methodology, Writing – review & editing. MX: Supervision, Writing – original draft.
Funding
The author(s) declare financial support was received for the research, authorship, and/or publication of this article. This study was supported by the Hubei Provincial Department of Education Science Research Plan – Science and Technology Innovation Service Project (Q20234403), the Excellent Discipline Cultivation Project by JHUN (2023XKZ025), and the Innovative Research Team Foundation of the Department of Education of Hubei Province, China (T2020034).
Conflict of interest
The authors declare that the research was conducted in the absence of any commercial or financial relationships that could be construed as a potential conflict of interest.
Publisher’s note
All claims expressed in this article are solely those of the authors and do not necessarily represent those of their affiliated organizations, or those of the publisher, the editors and the reviewers. Any product that may be evaluated in this article, or claim that may be made by its manufacturer, is not guaranteed or endorsed by the publisher.
Supplementary material
The Supplementary material for this article can be found online at: https://www.frontiersin.org/articles/10.3389/fmicb.2024.1430097/full#supplementary-material
References
Ameen, F., Alsamhary, K., Alabdullatif, J. A., and ALNadhari, S. (2021). A review on metal-based nanoparticles and their toxicity to beneficial soil bacteria and fungi. Ecotoxicol. Environ. Saf. 213:112027. doi: 10.1016/j.ecoenv.2021.112027
Azam, F. (1998). Microbial control of oceanic carbon flux: the plot thickens. Science 280, 694–696. doi: 10.1126/science.280.5364.694
Benegas, G. R., Bernal, S. P., de Oliveira, V. M., and Passarini, M. R. Z. (2021). Antimicrobial activity against Microcystis aeruginosa and degradation of microcystin-LR by bacteria isolated from Antarctica. Environ. Sci. Pollut. Res. 28, 52381–52391. doi: 10.1007/s11356-021-14458-5
Bourne, D. G., Jones, G. J., Blakeley, R. L., Jones, A., Negri, A. P., and Riddles, P. (1996). Enzymatic pathway for the bacterial degradation of the cyanobacterial cyclic peptide toxin microcystin LR. Appl. Environ. Microbiol. 62, 4086–4094. doi: 10.1128/aem.62.11.4086-4094.1996
Brêda-Alves, F., de Oliveira, F. V., and Chia, M. A. (2021). Understanding the environmental roles of herbicides on cyanobacteria, cyanotoxins, and cyanoHABs. Aquat. Ecol. 55, 347–361. doi: 10.1007/s10452-021-09849-2
Coyne, K. J., Wang, Y., and Johnson, G. (2022). Algicidal bacteria: a review of current knowledge and applications to control harmful algal blooms. Front. Microbiol. 13:871177. doi: 10.3389/fmicb.2022.871177
Crettaz-Minaglia, M., Fallico, M., Aranda, O., Juarez, I., Pezzoni, M., Costa, C., et al. (2020). Effect of temperature on microcystin-LR removal and lysis activity on Microcystis aeruginosa (cyanobacteria) by an indigenous bacterium belonging to the genus Achromobacter. Environ. Sci. Pollut. Res. 27, 44427–44439. doi: 10.1007/s11356-020-09901-y
Ding, N., du, W., Feng, Y., Song, Y., Wang, C., Li, C., et al. (2021). Algicidal activity of a novel indigenous bacterial strain of Paracoccus homiensis against the harmful algal bloom species, Karenia mikimotoi. Arch. Mikrobiol. 203, 4821–4828. doi: 10.1007/s00203-021-02468-3
Effiong, K., Hu, J., Xu, C., Tang, T., Huang, H., Zeng, J., et al. (2020). Sustainable utilization of agricultural straw for harmful algal blooms control: a review. J. Renewable Mater. 8, 461–483. doi: 10.32604/jrm.2020.09111
Jeong, S. Y., and Son, H. J. (2021). Effects of mycosubtilin homolog algicides from a marine bacterium, Bacillus sp. SY-1, against the harmful algal bloom species Cochlodinium polykrikoides. J. Microbiol. 59, 389–400. doi: 10.1007/s12275-021-1086-8
Kong, Y., Wang, Y., Miao, L., Mo, S., Li, J., and Zheng, X. (2022). Recent advances in the research on the Anticyanobacterial effects and biodegradation mechanisms of Microcystis aeruginosa with microorganisms. Microorganisms 10:1136. doi: 10.3390/microorganisms10061136
Kumar, P., Hegde, K., Brar, S. K., Cledon, M., and Kermanshahi-pour, A. (2019). Potential of biological approaches for cyanotoxin removal from drinking water: a review. Ecotoxicol. Environ. Saf. 172, 488–503. doi: 10.1016/j.ecoenv.2019.01.066
Li, Z., Geng, M., and Yang, H. (2015). Algicidal activity of Bacillus sp. Lzh-5 and its algicidal compounds against Microcystis aeruginosa. Appl. Microbiol. Biotechnol. 99, 981–990. doi: 10.1007/s00253-014-6043-6
Li, D., Kang, X., Chu, L., Wang, Y., Song, X., Zhao, X., et al. (2021). Algicidal mechanism of Raoultella ornithinolytica against Microcystis aeruginosa: antioxidant response, photosynthetic system damage and microcystin degradation. Environ. Pollut. 287:117644. doi: 10.1016/j.envpol.2021.117644
Liu, P., Wei, J., Yang, K., Massey, I. Y., Guo, J., Zhang, C., et al. (2018). Isolation, molecular identification, and characterization of a unique toxic cyanobacterium Microcystis sp. found in Hunan Province, China. J. Toxic. Environ. Health A 81, 1142–1149. doi: 10.1080/15287394.2018.1532716
Liu, J., Yang, C., Chi, Y., Wu, D., Dai, X., Zhang, X., et al. (2019). Algicidal characterization and mechanism of Bacillus licheniformis Sp34 against Microcystis aeruginosa in Dianchi Lake. J. Basic Microbiol. 59, 1112–1124. doi: 10.1002/jobm.201900112
Liu, F., Zhu, S., Qin, L., Feng, P., Xu, J., Zhou, W., et al. (2022). Isolation, identification of algicidal bacteria and contrastive study on algicidal properties against Microcystis aeruginosa. Biochem. Eng. J. 185:108525. doi: 10.1016/j.bej.2022.108525
Massey, I. Y., and Yang, F. (2020). A mini review on microcystins and bacterial degradation. Toxins 12:268. doi: 10.3390/toxins12040268
Meyer, N., Bigalke, A., Kaulfuß, A., and Pohnert, G. (2017). Strategies and ecological roles of algicidal bacteria. FEMS Microbiol. Rev. 41, 880–899. doi: 10.1093/femsre/fux029
Morón-López, J., Serwecińska, L., Balcerzak, Ł., Glińska, S., and Mankiewicz-Boczek, J. (2023). Algicidal bacteria against cyanobacteria: practical knowledge from laboratory to application. Crit. Rev. Environ. Sci. Technol. 54, 239–266. doi: 10.1080/10643389.2023.2232257
Nakamura, N., Nakano, K., Sugiura, N., and Matsumura, M. (2003). A novel cyanobacteriolytic bacterium, Bacillus cereus, isolated from a eutrophic lake. J. Biosci. Bioeng. 95, 179–184. doi: 10.1016/S1389-1723(03)80125-1
Namsaraev, Z., Melnikova, A., Komova, A., Ivanov, V., Rudenko, A., and Ivanov, E. (2020). Algal bloom occurrence and effects in Russia. Water 12:285. doi: 10.3390/w12010285
Ndayisenga, F., Yu, Z., Yan, G., Phulpoto, I. A., Li, Q., Kumar, H., et al. (2021). Using easy-to-biodegrade co-substrate to eliminate microcystin toxic on electrochemically active bacteria and enhance bioelectricity generation from cyanobacteria biomass. Sci. Total Environ. 751:142292. doi: 10.1016/j.scitotenv.2020.142292
Pal, M., Yesankar, P. J., Dwivedi, A., and Qureshi, A. (2020). Biotic control of harmful algal blooms (HABs): a brief review. J. Environ. Manag. 268:110687. doi: 10.1016/j.jenvman.2020.110687
Schreidah, C. M., Ratnayake, K., Senarath, K., and Karunarathne, A. (2020). Microcystins: biogenesis, toxicity, analysis, and control. Chem. Res. Toxicol. 33, 2225–2246. doi: 10.1021/acs.chemrestox.0c00164
Song, L., Jia, Y., Qin, B., Li, R., Carmichael, W. W., Gan, N., et al. (2023). Harmful cyanobacterial blooms: biological traits, mechanisms, risks, and control strategies. Annu. Rev. Environ. Resour. 48, 123–147. doi: 10.1146/annurev-environ-112320-081653
Song, T., Zhang, H., Xu, Y., Dai, X., Fan, F., Wang, Y., et al. (2024). Cyanobacterial blooms in Lake Taihu: temporal trends and potential drivers. Sci. Total Environ. 942:173684. doi: 10.1016/j.scitotenv.2024.173684
Tian, C., Liu, X., Tan, J., Lin, S., Li, D., and Yang, H. (2012). Isolation, identification and characterization of an algicidal bacterium from Lake Taihu and preliminary studies on its algicidal compounds. J. Environ. Sci. 24, 1823–1831. doi: 10.1016/S1001-0742(11)60983-2
Wang, M., Chen, S., Zhou, W., Yuan, W., and Wang, D. (2020). Algal cell lysis by bacteria: a review and comparison to conventional methods. Algal Res. 46:101794. doi: 10.1016/j.algal.2020.101794
Wang, J., Yin, X., Xu, M., Chen, Y., Ji, N., Gu, H., et al. (2022). Isolation and characterization of a high-efficiency algicidal bacterium Pseudoalteromonas sp. LD-B6 against the harmful dinoflagellate Noctiluca scintillans. Front. Microbiol. 13:1091561. doi: 10.3389/fmicb.2022.1091561
Wei, J., Pengji, Z., Zhang, J., Peng, T., Luo, J., and Yang, F. (2023). Biodegradation of MC-LR and its key bioactive moiety Adda by Sphingopyxis sp. YF1: comprehensive elucidation of the mechanisms and pathways. Water Res. 229:119397. doi: 10.1016/j.watres.2022.119397
Wijesooriya, M. M., Widanagamage, S. M., and Masakorala, K. (2021). Biological control of freshwater cyanobacterial blooms; a review. J. Univ. Ruhuna 9, 6–27. doi: 10.4038/jur.v9i1.7966
Wu, X., Xi, W., Ye, W., and Yang, H. (2007). Bacterial community composition of a shallow hypertrophic freshwater lake in China, revealed by 16S rRNA gene sequences. FEMS Microbiol. Ecol. 61, 85–96. doi: 10.1111/j.1574-6941.2007.00326.x
Xu, S., Lyu, P., Zheng, X., Yang, H., Xia, B., Li, H., et al. (2022). Monitoring and control methods of harmful algal blooms in Chinese freshwater system: a review. Environ. Sci. Pollut. Res. 29, 56908–56927. doi: 10.1007/s11356-022-21382-9
Xuan, H., Dai, X., Li, J., Zhang, X., Yang, C., and Luo, F. (2017). A Bacillus sp. strain with antagonistic activity against Fusarium graminearum kills Microcystis aeruginosa selectively. Sci. Total Environ. 583, 214–221. doi: 10.1016/j.scitotenv.2017.01.055
Yang, C., Hou, X., Wu, D., Chang, W., Zhang, X., Dai, X., et al. (2020). The characteristics and algicidal mechanisms of cyanobactericidal bacteria, a review. World J. Microbiol. Biotechnol. 36:1. doi: 10.1007/s11274-020-02965-5
Yang, F., Huang, F., Feng, H., Wei, J., Massey, I. Y., Liang, G., et al. (2020). A complete route for biodegradation of potentially carcinogenic cyanotoxin microcystin-LR in a novel indigenous bacterium. Water Res. 174:115638. doi: 10.1016/j.watres.2020.115638
Yang, J., Qiao, K., Lv, J., Liu, Q., Nan, F., Xie, S., et al. (2020). Isolation and identification of two algae-lysing bacteria against Microcystis aeruginosa. Water 12:2485. doi: 10.3390/w12092485
Zhang, Z., Wang, J., Hu, G., Huang, J., Chen, L., Yin, Y., et al. (2022). Isolation and characterization of an algicidal bacterium against the bloom-forming algae raphidophyte Heterosigma akashiwo. Environ. Technol. 44, 2607–2616. doi: 10.1080/09593330.2022.2036250
Keywords: Bacillus subtilis S4, algicidal bacterium, algicidal properties, microcystins, M. aeruginosa
Citation: Chen Y, Xiong F, Zhu Y, Zhai D, Liu H, Zhang L and Xia M (2024) A Bacillus subtilis strain with efficient algaecide of Microcystis aeruginosa and degradation of microcystins. Front. Microbiol. 15:1430097. doi: 10.3389/fmicb.2024.1430097
Edited by:
Pierina Visciano, University of Teramo, ItalyReviewed by:
Jesus Moron-Lopez, Arizona State University, United StatesDa Sun, Wenzhou University, China
Copyright © 2024 Chen, Xiong, Zhu, Zhai, Liu, Zhang and Xia. This is an open-access article distributed under the terms of the Creative Commons Attribution License (CC BY). The use, distribution or reproduction in other forums is permitted, provided the original author(s) and the copyright owner(s) are credited and that the original publication in this journal is cited, in accordance with accepted academic practice. No use, distribution or reproduction is permitted which does not comply with these terms.
*Correspondence: Fei Xiong, eGY5NjAzQDE2My5jb20=