- 1Department of Biological Safety, German Federal Institute for Risk Assessment, Berlin, Germany
- 2Institute of Biometry, Epidemiology and Information Processing (IBEI), WHO Collaborating Centre for Research and Training for Health at the Human-Animal-Environment Interface, University of Veterinary Medicine Hannover, Hanover, Germany
Two physical treatments (heat via water bath and cold air) with various temperatures (20/70/75/80°C and − 80/−90°C) and exposure times (20, 30, 40 s) were carried out to identify a decontaminating effect on zoonotic pathogens on broiler carcasses. Subsequently, carcasses were analyzed for thermotolerant Campylobacter (C.), Salmonella, Escherichia (E.). coli and total colony count (TCC). Moreover, for the hot water treatment, qPCR with viable/dead differentiation (v-qPCR) was applied to detect viable but non-culturable cells (VBNC) of Campylobacter, referred to as intact but putatively infectious units (IPIU). Hot water immersion was tested on carcasses inoculated with C. jejuni and Salmonella, while cold air treatment was evaluated for naturally contaminated carcasses of broiler flocks colonized with Campylobacter. For hot water treatment, the statistically significant reducing effect was about 1 log10 CFU/ml for both Salmonella and Campylobacter for 70–80°C and 20/30 s treatments. The effect of heat treatment for Campylobacter was smaller when samples were analyzed with v-qPCR with reductions of 0.5–0.8 log10 IPIU/ml in mean. Cold air treatments at −90°C were effective in reducing the mean contamination level of Campylobacter by 0.4–0.5 log10 CFU/ml at all exposure times (p < 0.05). Hot water treatments showed a decreasing trend on TCC by 0.6–0.9 log10 CFU/ml (p < 0.05). TCC counts were not significantly affected by cold air treatment. For E. coli no statistically significant reductions were observed by hot water treatment. The cold air treatment at −90°C for 20 and 40 s led to a reduction of E. coli by 0.4 and 0.8 log10 CFU/ml (p < 0.05), respectively. Treatment of carcasses with higher bacterial levels tended to show higher reduction. The research demonstrated that the efficacy of physical treatments for decontamination of broiler carcasses was more pronounced for hot water immersion than for cold air exposure. In conclusion, the results shed light on the potential application of these physical treatments in practice to reduce the quantitative load of contaminating pathogens to enhance food safety in the broiler meat production.
1 Introduction
Campylobacteriosis and salmonellosis are still the most important bacterial zoonoses transmitted via food. Broiler meat is of particular importance as it is one of the main reservoirs for Campylobacter and Salmonella as reported by the European Food Safety Authority (EFSA, 2021). Therefore, measures are needed for the broiler meat production to quantitatively reduce Campylobacter and Salmonella contamination in order to lower the number of human infections (EFSA, 2011).
Thermal processes such as heat or cold can reduce bacterial contamination of carcasses or meat. Scalding and chilling are thermal processes already implemented in the slaughtering process for technological purposes, which in themselves result in a reduction of bacteria on the meat (Berrang et al., 2011; Duffy et al., 2014). Heat is used in poultry slaughtering in the form of hot water immersion or steam when scalding carcasses (soft scalding: 51–53°C, 120–229 s; hard scalding: 55–58°C, 80–150 s) to loosen the feathers and allow for efficient plucking of carcasses (Dogan et al., 2022). This treatment can also affect microbiological contamination by washing excess dirt from carcasses and/or providing mild thermal inactivation of bacteria (Dogan et al., 2022). Chilling can either be applied by dry air or through immersion (Huezo et al., 2007). Thus, the slaughter process itself has the potential to reduce the initial bacterial load of carcasses, which was recently shown for European slaughterhouses (Beterams et al., 2024; Hauge et al., 2023; Hutchison et al., 2022). However, currently established measures are reaching their limits. To further improve hygiene, additional approaches should be considered. Recontamination of broiler carcasses frequently occurs after evisceration, which showed to be a crucial step within the slaughtering process (Beterams et al., 2024; Pacholewicz et al., 2015). In the beginning of the 2000s, several studies have been published on additional hot water treatment processes that can be used to reduce Campylobacter by around 1 log10 CFU on carcasses (Corry et al., 2007; Purnell et al., 2004; Whyte et al., 2003). For Salmonella, there had been only few studies, that focused on hot water immersion as an additional treatment, whereby hard scalding (McKee et al., 2008) and steam pasteurization (Kure et al., 2020) were the most relevant. However, the effect of hot water immersion on carcass contamination has been the subject in one recent study (Hauge et al., 2023).
Additional cold treatment beyond the standard cooling process can be used to reduce the bacterial load of Campylobacter by 0.5–1.5 log10 CFU/g (Haughton et al., 2012). Especially short-term treatments with ultra-cold air, leading to a superficial freezing of carcasses without changing the meat texture, showed promising reduction of Campylobacter of around 0.5 log10 CFU/carcass in pilot studies (Boysen and Rosenquist, 2009). New technologies in form of indirect liquid nitrogen cooling (SafeChill™) are implemented in the United Kingdom (Herrmann et al., 2017), but are yet not further evaluated in other countries and could provide new opportunities for decontamination of carcasses.
The microbial reduction of Campylobacter through additional treatments could be beneficial to improve the microbiological hygiene of poultry products. The effectiveness of Campylobacter intervention at slaughterhouse level has previously been studied with classical culturing methods (Boysen and Rosenquist, 2009; Corry et al., 2007; Haughton et al., 2012). To verify, if these methods reliably detect living cells, data generation can nowadays be complemented by molecular biological methods. Staining cells with propidium monoazide (PMA) before analysis with qPCR allows to differentiate live and potentially infectious Campylobacter cells from dead cells. Since this new PMA-dependent viable qPCR (v-qPCR) protocol including an internal sample process control had been validated for chicken rinse (Stingl et al., 2021), the technique can be applied for different settings and extended to other sample types and used to evaluate possible decontamination effects.
The aim of this study was to evaluate, whether physical treatment as an additional step post-evisceration can effectively reduce viable bacterial contamination on broiler carcasses. In particular, hot water immersion at 70, 75 and 80°C for 20 and 30 s and cold air treatment with the SafeChill™ technology at −80 and − 90°C for 20, 30 and 40 s were explored. The recent hot water treatment study was based on a specific re-contamination scenario, that was highlighted within a recent study on contamination in German broiler slaughterhouses (Beterams et al., 2024). Evisceration was identified as a relevant processing step, where the numbers of Campylobacter could increase and decontamination might contribute to improved meat hygiene. The hot water treatment was investigated with two quantification methods, microbiological and by v-qPCR. Lastly, it was investigated how both treatments would affect microbiological hygiene parameters for carcass contamination in poultry slaughtering, i.e., total colony count (TCC) and Escherichia (E.) coli concentrations.
2 Materials and methods
2.1 Hot water treatment trials
The treatment with hot water was conducted in a pilot scale plant at the German Federal Institute for Risk Assessment (BfR) in Berlin, Germany. For this process, a scalding tank was used, which worked with indirect heating of the water through a sheath filled with oil. The hot water treatment chosen here should be evaluated as a measure for decontamination after the evisceration step. Therefore, the carcasses used were collected at a broiler slaughterhouse after evisceration and neck removal to allow water to drain from the body cavity after treatment. To simulate fecal re-contamination during evisceration, the carcasses were inoculated with a combined suspension of Campylobacter and Salmonella strains (3 Campylobacter jejuni strains, one strain each of S. Enteritidis, S. Typhimurium, S. Infantis), to achieve a concentration of 4–5 log10 CFU/ml of whole carcass rinse (WCR), which was in the upper end of the range of carcass contamination after evisceration for Campylobacter (Beterams et al., 2024) and a similar concentration was chosen for Salmonella. Strains were provided and characterized by the National Reference Laboratories (NRLs) at the BfR and were isolated from broiler meat.
The bacteria were grown on Columbia agar with 5% sheep blood (ColB, OXOID, Wesel, Germany) for 24 h under microaerobic conditions at 41.5°C for Campylobacter and under aerobic conditions at 37°C for Salmonella. Cultures were adjusted to an OD600 of 0.05 in brain heart infusion broth (BHI, Article-Nr.: 1.10493.0500, Merck, Darmstadt, Germany) for Campylobacter and 0.2 in peptone water (PW, Article-Nr.: CM1049B, Thermo Fisher, Wesel, Germany) for Salmonella and incubated overnight to achieve a concentration of 8–9 log10 CFU/ml. The liquid cultures of three strains per genus were combined in equal amounts. For each strain 2 mL of the mixed suspension were diluted 1:2 with 0.85% NaCl/0.1% peptone water (1.12535, Merck, Darmstadt, Germany; LP0011 OXOID, Wesel, Germany) and then mixed 1:2 with cecal contents, that were free of Campylobacter, Salmonella and also E. coli. The concentrations of Campylobacter and Salmonella in this ready-to-use suspension were around 8 log10 CFU/ml, respectively. Broiler carcasses were inoculated with 0.1 mL of the suspension applied in the peri cloacal area and was incubated for 10 min at room temperature and normal atmosphere to allow bacteria to attach. Broilers were then treated with hot water, either at 70°C (± 2°C) for 30 s, at 75°C (± 2°C) or at 80°C (± 3°C) for 20 and 30 s, respectively. One control group without treatment and one group treated with water at room temperature (approximately 20°C) for 30 s were analyzed to evaluate the rinsing effect in itself. Broilers were sampled with WCR. The sample size was n = 17 per group and each setting was analyzed three times.
2.2 Cold treatment trials
For the cold air treatment, the Freshline® SafeChill™ trial setup, provided by Air Products PLC (Figure 1), was placed inside the cooling area of a commercial broiler slaughterhouse, therefore only naturally contaminated carcasses could be used for the experiments. This system is meant as an additional processing step that can be integrated into the production line. It is an adjustable system, where treatment (cold exposure) time (min. 20 to max. 60 s), which is controlled by line speed, air velocity and the temperature (−70 to −110°C) can be changed. Liquid nitrogen is used to cool process air by means of a heat exchanger. A fan circulates super chilled air, which is directed at the back of the eviscerated carcasses. The air is then re-circulated and cooled down again.
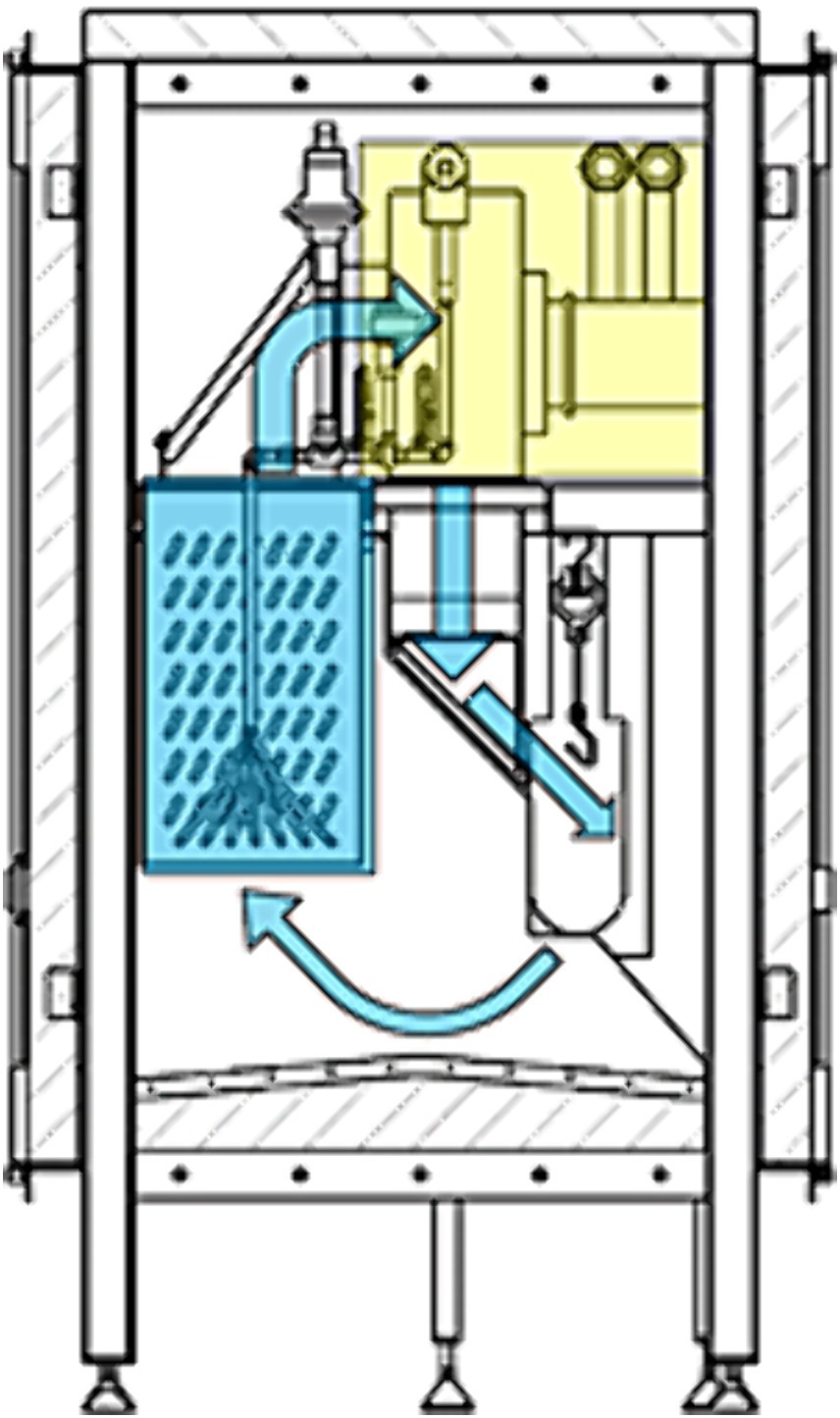
Figure 1. Workflow of the system; normal operation Freshline® SafeChill™ from Air Products; the adjustable system, where treatment time, which is controlled through line speed (20 to 60 s), air velocity (m/s) and the temperature (−70 to −110°C) can be changed, uses liquid nitrogen to cool process air by means of a heat exchanger. A fan circulates super chilled air, which is directed at the back of the eviscerated carcasses. The air is then re-circulated and cooled down again.
The cold air treatment was conducted in two parts: In the first, four groups each were analyzed in parallel, i.e., three treatment times (20, 30, and 40 s) and a control group without treatment. Those treatment times were investigated at two different temperatures each (−80 or − 90 ± 2°C). Broilers were sampled with WCR. Due to technical issues, only two trials could be realized for −90°C 40 s in the first part of the study. In the second part, WCR and neck skin samples were analyzed in parallel in trials at −90°C for 30 s. For both parts and each treatment and control group 17 samples were collected per trial and every setting was repeated three times.
2.3 Microbiological analysis
In total, 1,122 samples were analyzed. Broiler carcasses were sampled with WCR by placing the carcass into a sterile bag (400×500 mm, PA/PE 90, sealed bag, HEIFO GmbH & Co. KG, Osnabrück, Germany), covered with 400 mL of 0.85% NaCl/0.1% peptone water (Article-Nr.: 1.12535, Merck., Darmstadt, Germany; LP0011 OXOID, Wesel, Germany). The carcasses were rinsed for 60 s by turning the bag 180° every 5 seconds and moving it vigorously up and down six to 10 times to allow rinsing fluid to also enter the body cavity so that all surfaces were rinsed. Around 200 mL of the WCR was filled into sterile plastic cups (300 mL, PP, VWR International GmbH, Darmstadt, Germany).
For the neck skin samples, around 10 g of skin were cut from the carcass by using a sterile knife and placing the sample into a 1 L plastic bag (freeze bag, ja!, REWE, Köln, Germany). All samples were transported to the laboratory refrigerated, stored under chilled conditions and analyzed within 24 h.
Individual neck skin samples were diluted ten-fold to achieve the first dilution, WCR were taken without further dilution directly. Initial and further ten-fold dilutions were prepared with 0.85% NaCl/0.1% peptone water. The total colony count (TCC) of the samples was determined by pipetting 1 mL of each tenfold dilution into petri dishes, poured over with plate-count agar (PCA, MAST Diagnostika GmbH, Reinfeld, Germany) and aerobically incubated at 30°C for 72 h based on ISO 4833-1:2014. Escherichia coli were enumerated with tryptone-bile-X-glucuronide agar (TBX, OXOID) using 1 mL of each ten-fold dilution and pour plating with aerobic incubation for 18–24 h at 44°C based on ISO 16649-2:2020. Salmonella spp. quantification was done by adapting standard plate count technique with selective agar and incubation conditions referring to ISO 6579-1:2017, by plating 0.1 mL of the respective dilution on xylose-lysine-deoxycholate agar (XLD, Merck) incubated aerobically at 37°C for 24 h. Thermophilic Campylobacter spp., were enumerated on modified charcoal cefoperazone deoxycholate agar (mCCDA, Merck/OXOID) with microaerobic incubation at 41.5°C for 48 h referring to ISO 10272-2:2017. To lower the detection limit for Campylobacter to 1 CFU/mL of WCR and 10 CFU/g of neck skin, 1 mL was plated onto three agar plates. The detection limit for Salmonella was 10 CFU/mL in WCR and for E. coli and TCC 1 CFU/mL in WCR or 10 CFU/g in neck skin samples.
2.4 Molecular biological analysis
The molecular biological quantification was performed for the samples of the hot water treatment. The protocols for Campylobacter were used according to Pacholewicz et al. (2019) and Stingl et al. (2021). Four 1 mL aliquots per sample were prepared in 1.5 mL reaction tubes (Eppendorf, Hamburg, Germany). Primers and probes, as well as the mastermix and DNA standards were used as described in Stingl et al. (2021). Two independent duplex qPCR protocols were used for the quantification of either thermotolerant Campylobacter spp. or the ISPC, each in combination with an internal amplification control (IPC-ntb2, (Anderson et al., 2011)). Standard curves were created for each run individually by comparing ct-values with the known content of genome copies of a standard series. A detailed protocol with spread sheet and equations can be found in the Supplementary materials in Stingl et al. (2021). An example of a standard curve and equation can be found in the Supplementary Figure S1. For every sample analysis, two aliquots were treated with PMA and two aliquots were analyzed without. The qPCR was performed with the ABI 7500 Real Time PCR System or the QuantStudio5 (Applied Biosystems by Thermo Fisher Scientific, Waltham, Massachusetts, United States), the cycling program was run as follows: 3 min at 95°C for initial denaturation; after that, 45 cycles with each 15 s at 95°C, 60 s at 60°C to measure the fluorescence and 30 s at 72°C.
Eight samples per group were analyzed by v-qPCR and compared with the results of the cultural analysis.
2.5 Sensory analysis
To identify the impact of hot water and cold air treatment on sensory characteristics of treated and untreated broiler carcasses the temperature under the skin was recorded (Datalogger Thermometer, HH520, Omega Engineering GmbH, Deckenpfronn, Germany) with a thermocouple type-T (5SC-TT-TI-30-1 M, Omega) inserted underneath the skin directly on top of the meat either on the center of the left breast muscle or the right upper leg and fixed with a string. Color was measured based on the CIELAB-system with a MINOLTA CR-210 (MINOLTA Camera Co., Ltd., Japan) device on the center of the left breast skin, as well as on the central part of the left thigh skin. The equipment was calibrated before measurements with a white standard tile and the lightness L*, a*(+) for red and b*(+) for yellow were measured. The visual appearance of carcasses was assessed based on pictures that were taken with a Nikon D3s camera (Macro lens Nikon 1:2.8 / f = 60 mm, sensor sensitivity = ISO 200, f-stop 32, 1/10 s; samples were illuminated with a Kaiser repro column with high-frequency/flare-free daylight (incident and transmitted light, Nikon Germany, Düsseldorf, Germany). The skin texture was described and assessed after visual analysis and palpation by two examiners with experience in poultry meat processing by comparing the samples in pairs of treated and untreated carcasses. In total, three carcasses per setting were analyzed before and after treatment, as well as after chilling for each group.
2.6 Statistical analysis
Data was transformed using the logarithm to the basis of 10 to normalize the data. In order to display the change in log10 CFU/ml, boxplots for each temperature and exposure time were created and compared to the control group. To examine the mean differences between the test and control group for the different temperature settings and exposure times for the hot water and cold air treatments, analysis of variance (ANOVA) and post hoc-tests were performed using Tukey’s test for equal sample sizes between the groups and Tukey–Kramer test for unequal sample sizes. The analyses where additionally supported by normal distributed residuals within the model calculations.
Since not all trial settings were conducted equally, e.g., the 70°C setting for the hot water treatment was not conducted for 20 s, temperature and exposure time, were combined into one variable. This enabled us to perform pairwise comparisons for all trial settings. The date of sampling was included in all models and was adjusted for using the least square means (LSM) method, since all temperature trials were conducted on different sampling days, whereas the control and room temperature samples were taken on all days. Results from the ANOVA and additional descriptive tables are displayed in Supplementary Tables S1–S6. Additionally, the LSM was included in the Figures 2–9, where each temperature setting is displayed with their respective control group. For groups where the LSM was statistically significantly different (p < 0.05) from the control group, a * is displayed above boxplot.
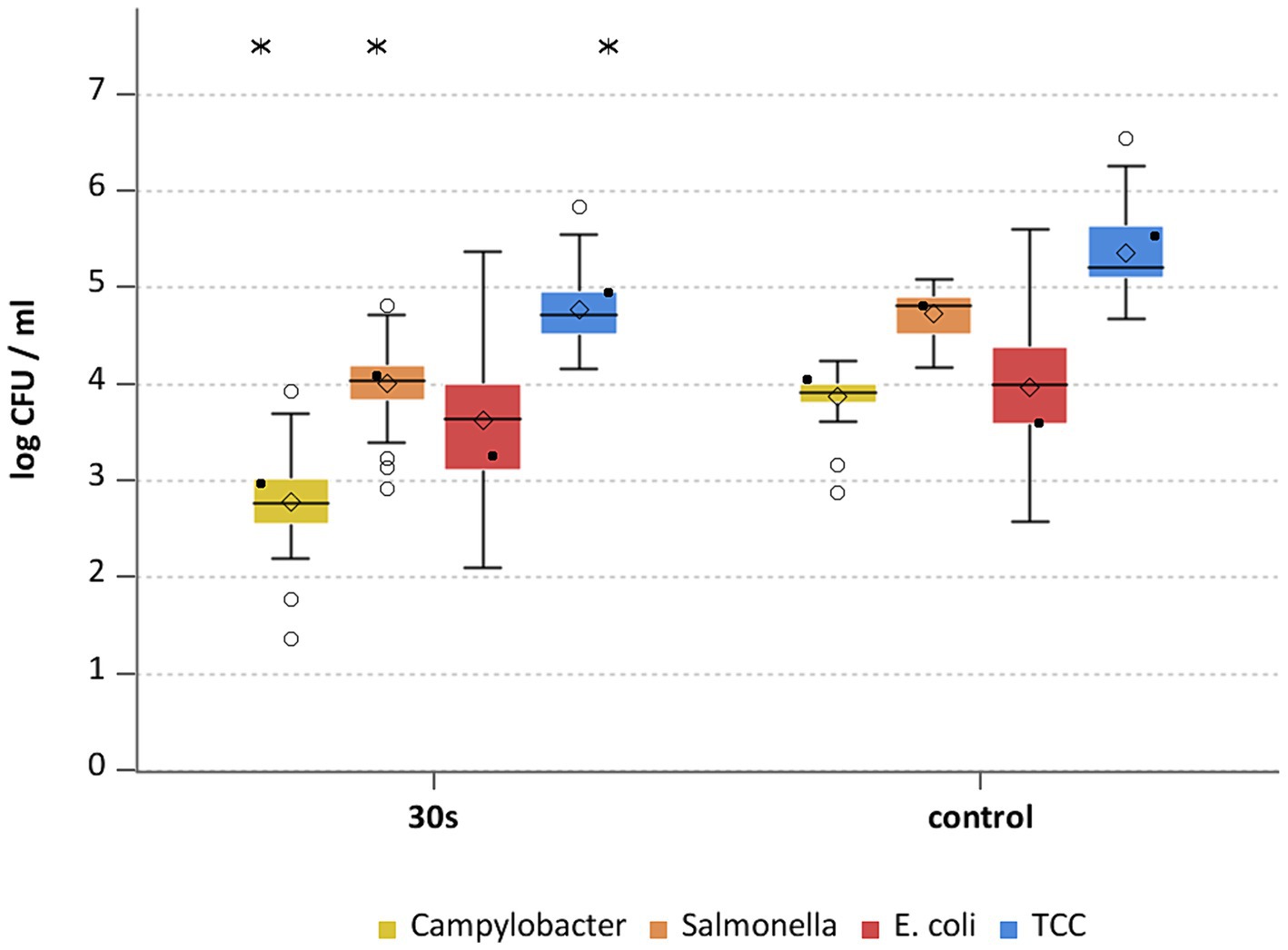
Figure 2. Campylobacter spp., Salmonella spp., E. coli and TCC levels of broiler carcasses after hot water immersion at 70°C for 30 s and corresponding untreated control group. Within box plots show the median (___), the least square mean (•), mean (◊) and outliers (o) are marked separately; * indicate statistically significant difference between treated groups and control group (p < 0.05, Tukey), (n = 51).
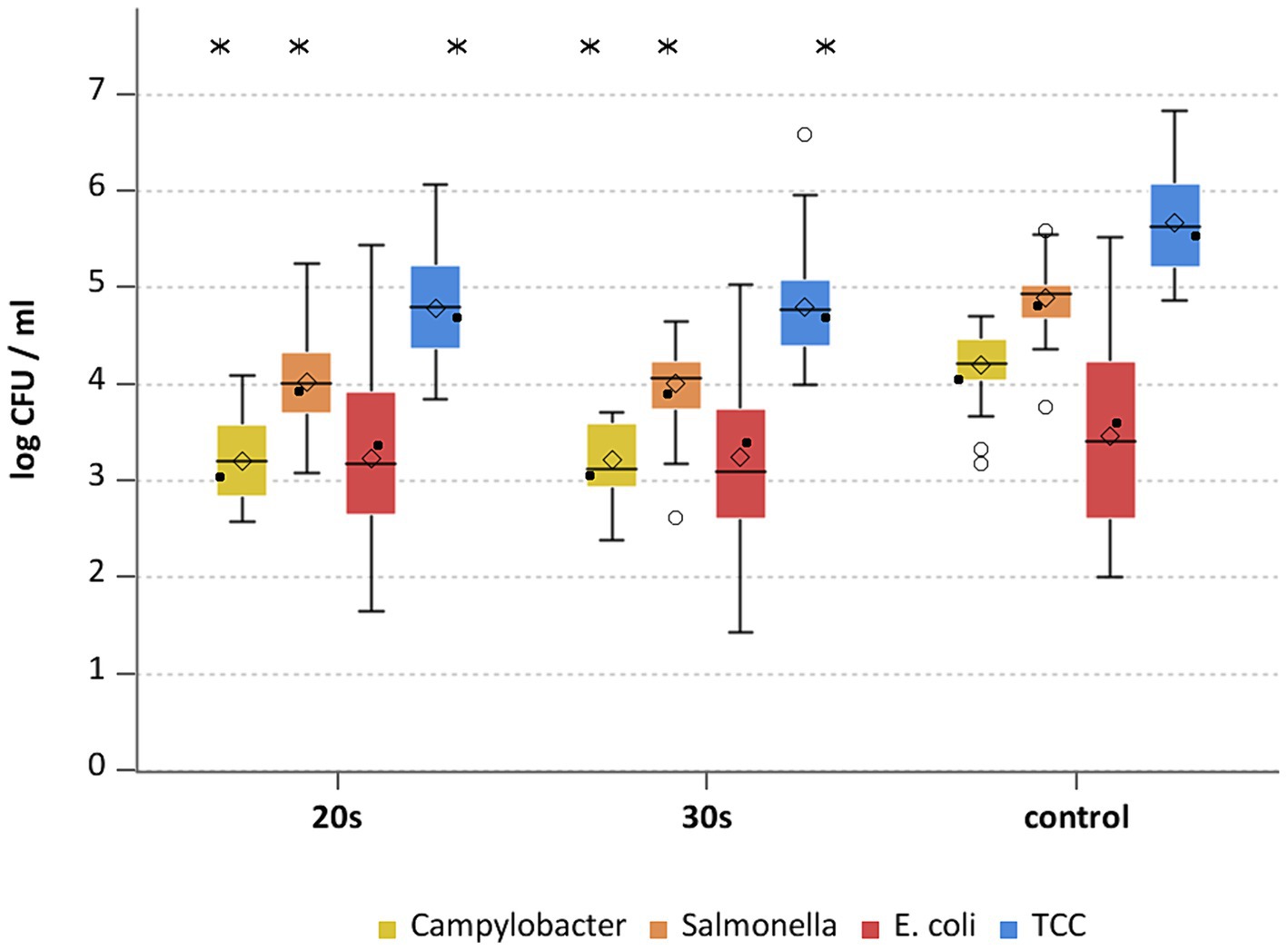
Figure 3. Campylobacter spp., Salmonella spp., E. coli and TCC levels of broiler carcasses after hot water immersion at 75°C for 20 and 30 s and corresponding untreated control group. Within box plots show the median (___), the least square mean (•), mean (◊) and outliers (o) are marked separately; * indicate statistically significant difference between treated groups and control group (p < 0.05, Tukey), (n = 51).
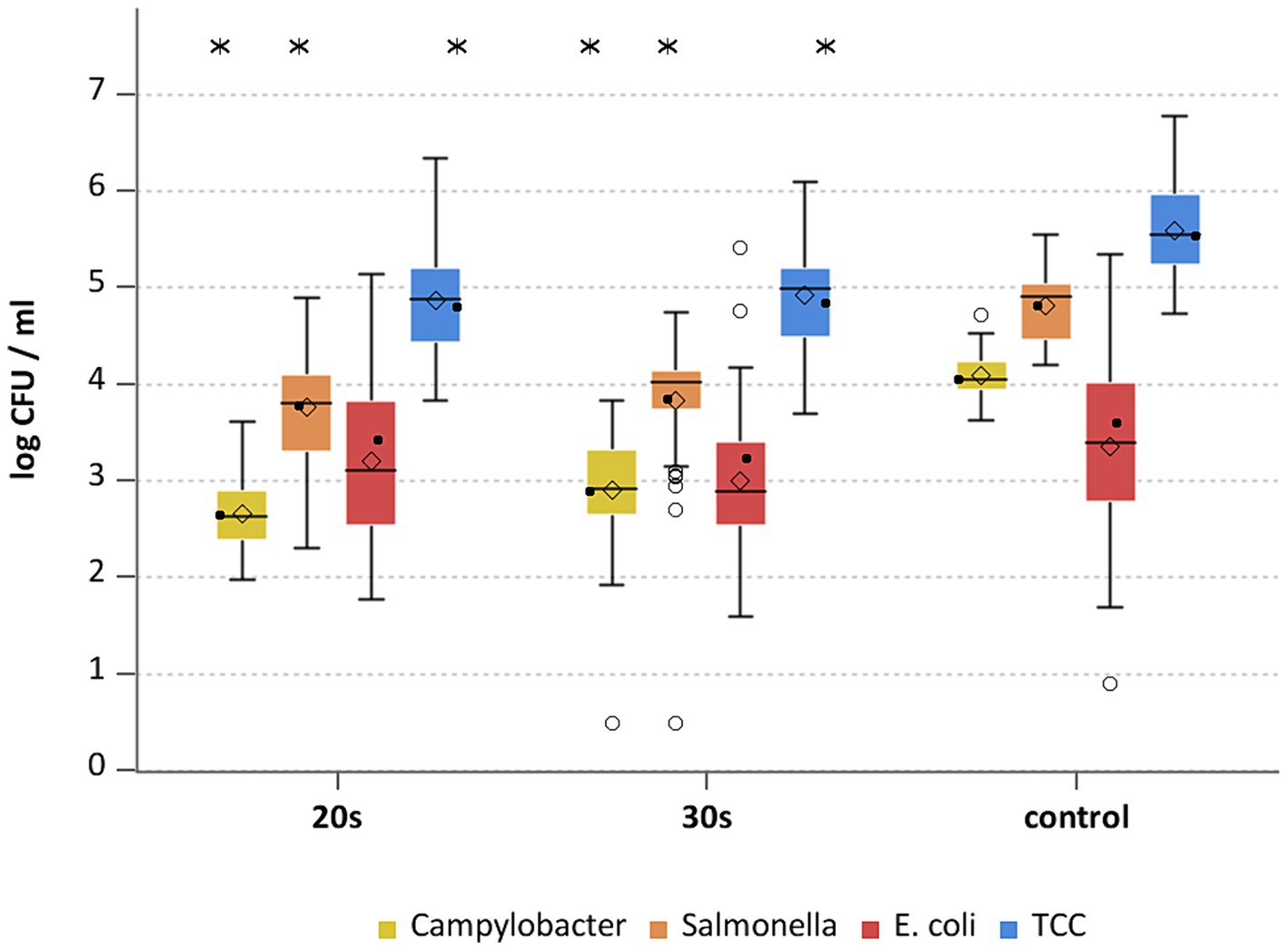
Figure 4. Campylobacter spp., Salmonella spp., E. coli and TCC levels of broiler carcasses after hot water immersion at 80°C for 20 and 30 s and corresponding untreated control group. Within box plots show the median (___), the least square mean (•), mean (◊) and outliers (o) are marked separately; * indicate statistically significant difference between treated groups and control group (p < 0.05, Tukey), (n = 51).
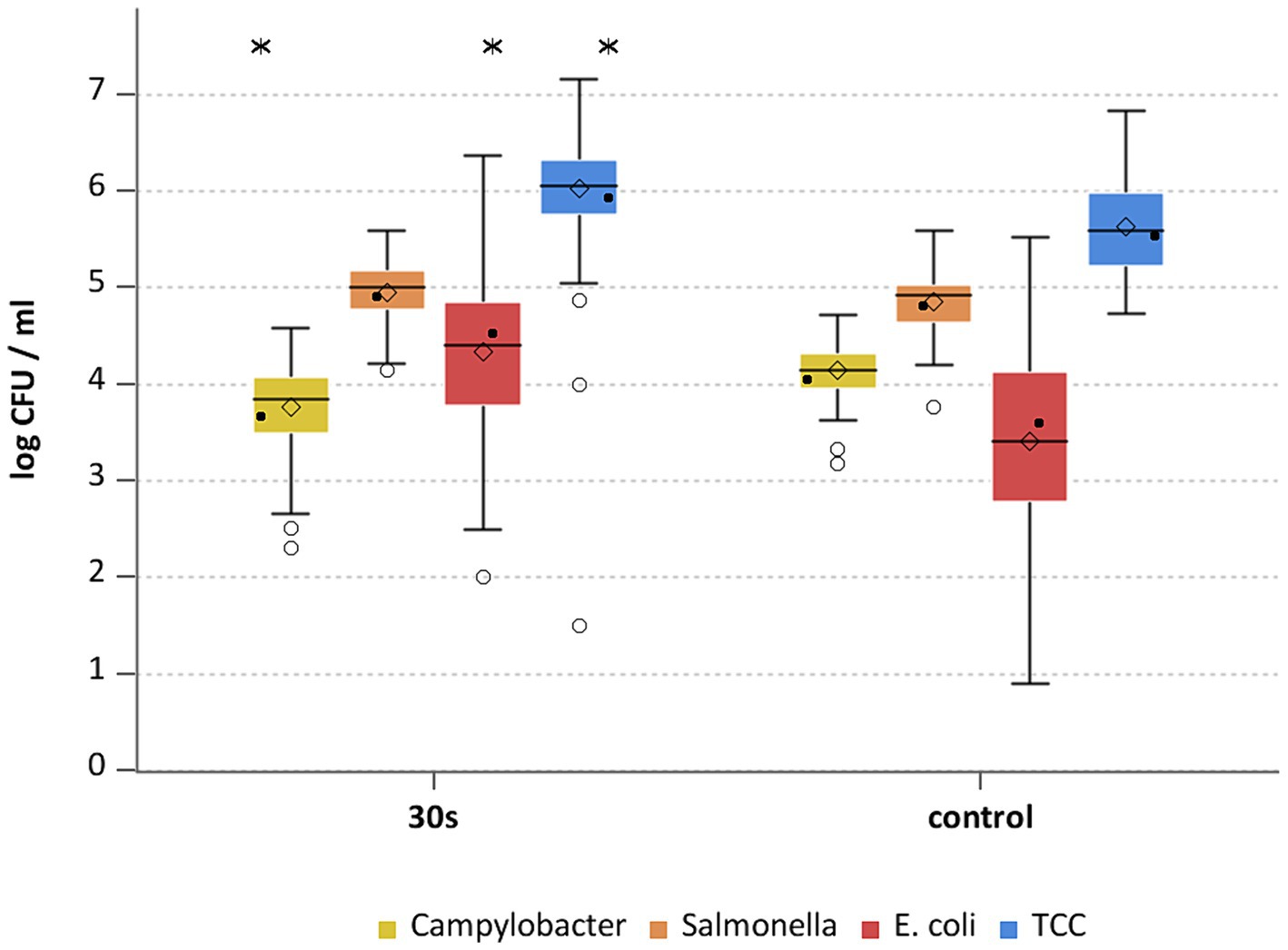
Figure 5. Campylobacter spp., Salmonella spp., E. coli and TCC levels of broiler carcasses after hot water immersion at 20°C for 30 s and corresponding untreated control group. Within box plots show the median (___), the least square mean (•), mean (◊) and outliers (o) are marked separately; * indicate statistically significant difference between treated groups and control group (p < 0.05, Tukey), (n = 102).
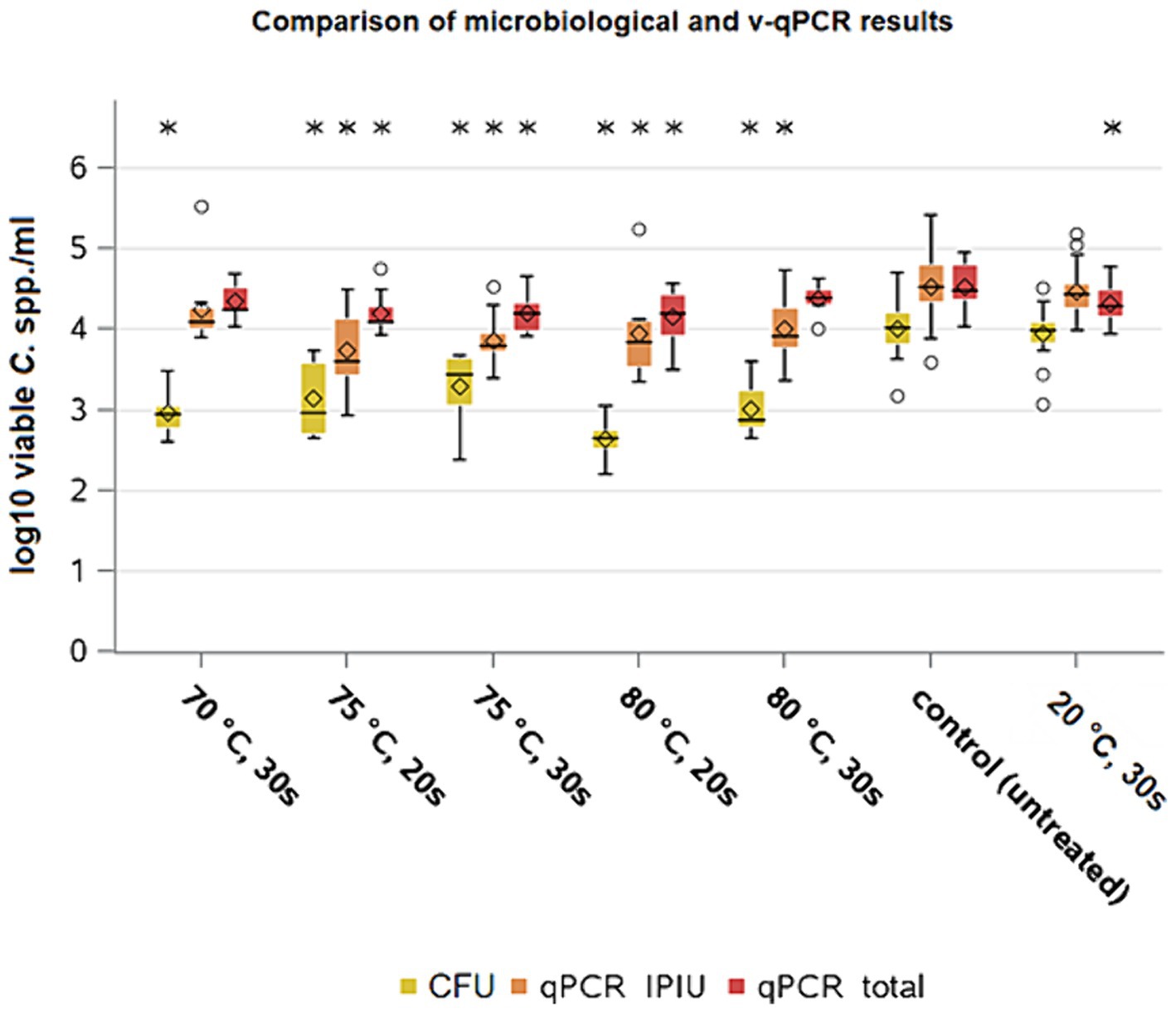
Figure 6. Campylobacter spp. counts of microbiological analysis and v-qPCR; yellow = microbiological results; orange = qPCR results – IPIU; red: qPCR results – total; Within boxplots show the median (___), mean (◊) and outliers (o) are marked separately, interquartile range and 95% confidence interval; * indicate statistically significant difference between treated groups and control group (p < 0.05, Tukey); (70 ° C: n = 10; 75°c 20s: n = 11; 75°C 30s: n = 10; 80°C 20s: n = 8; 80°C 30s: n = 8; 20°C 30s: n = 24; control: n = 39).
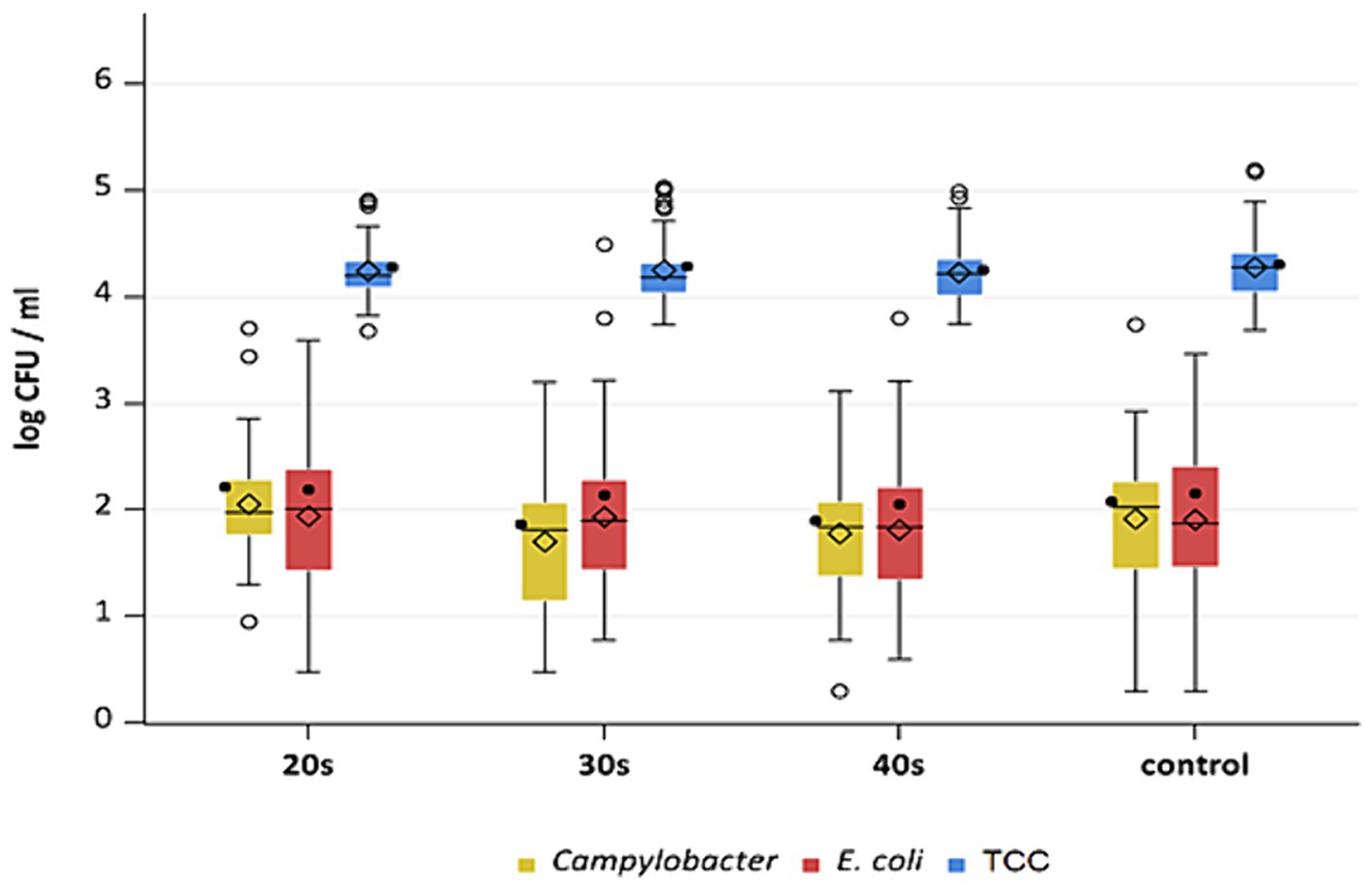
Figure 7. Campylobacter spp., E. coli and TCC levels of broiler carcasses at −80°C after 20, 30 and 40 s of cold air treatment and control group (untreated). Within boxplots show the median (___), the least square mean (•), mean (◊) and outliers (o) are marked separately, interquartile range and 95% confidence interval; * indicate statistically significant difference between treated groups and control group (p < 0.05, Tukey) (n = 51).
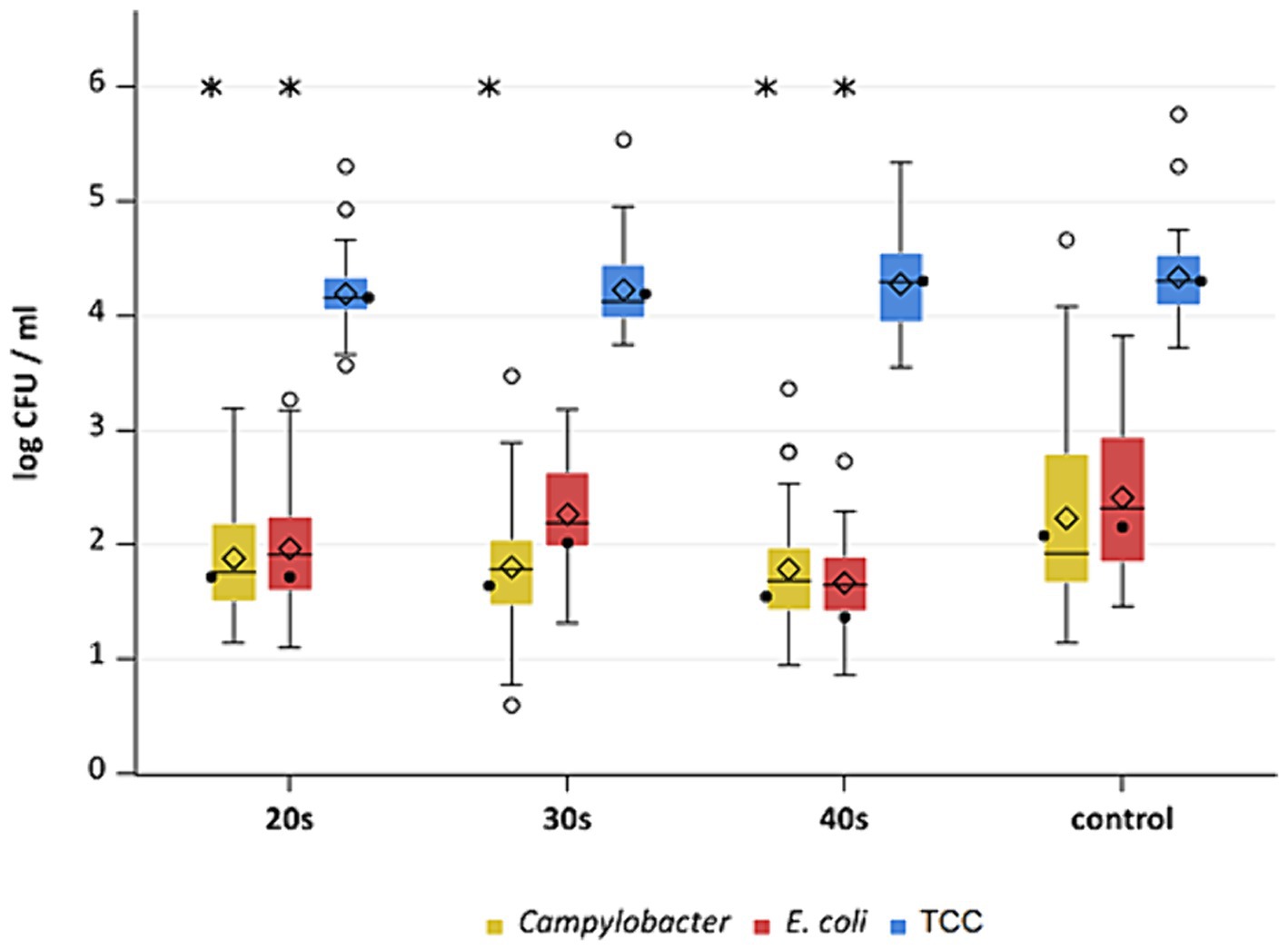
Figure 8. Campylobacter spp., E. coli and TCC levels of broiler carcasses at −90°C after 20, 30 and 40 s of cold air treatment and control group (untreated). Within boxplots show the median (___), the least square mean (•), mean (◊) and outliers (o) are marked separately, interquartile range and 95% confidence interval; * indicate statistically significant difference between treated groups and control group (p < 0.05, Tukey), (n = 51).
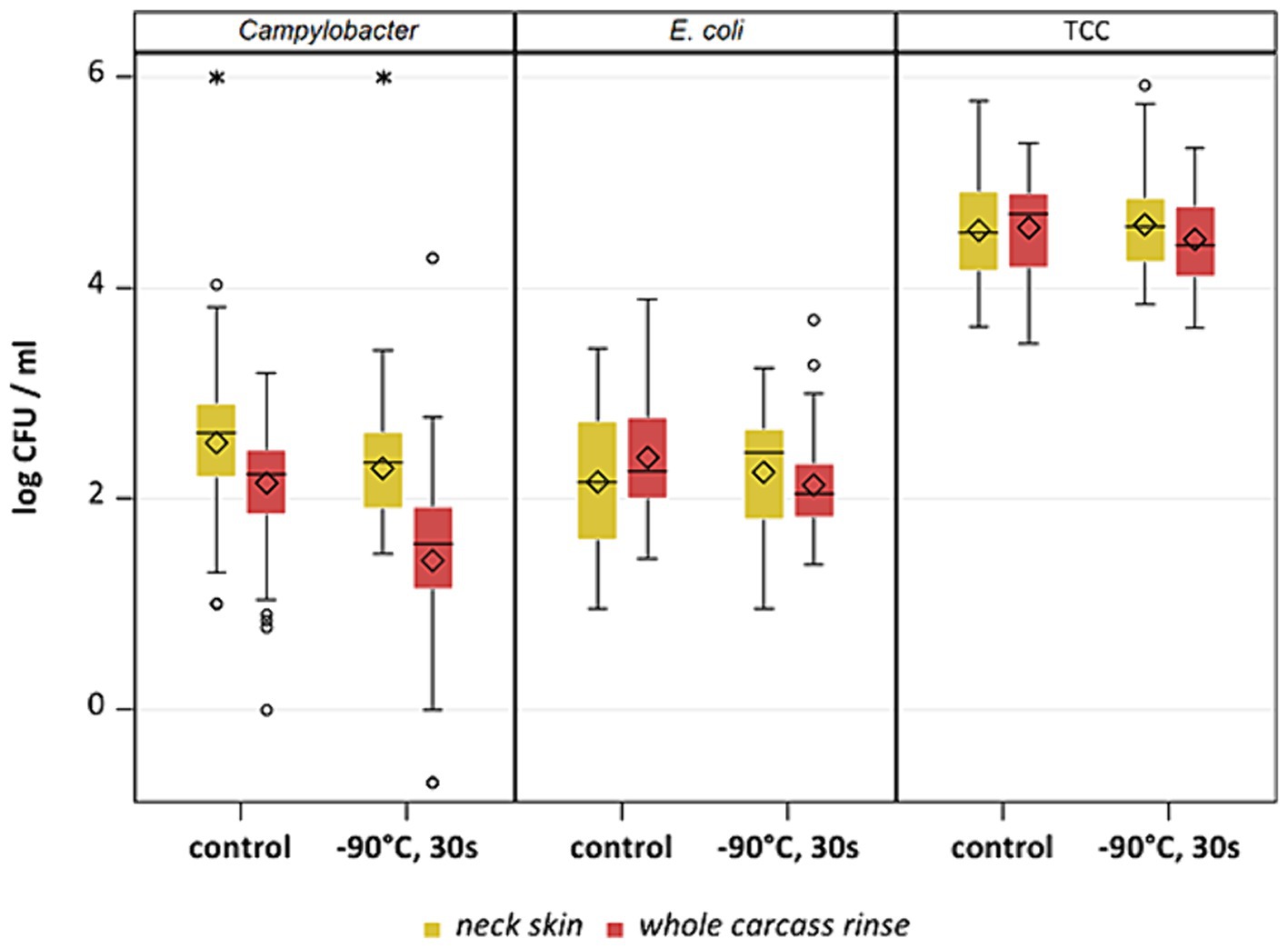
Figure 9. Comparison of neck skin and WCR after −90°C 30s cold-air treatment and untreated (control) for Campylobacter, E. coli and TCC; Within boxplots show the median (___), mean (◊) and outliers (o) are marked separately, interquartile range and 95% confidence interval; * indicate statistical difference between groups (p < 0.05, Tukey).
As for the comparison of microbiological methods, three different analyses were conducted. Firstly, ANOVA was performed for each method including date, temperature and time as fixed effects. As described above temperature and exposure time were combined into one variable for this analysis to compare the different trial settings for each method. Secondly, for pairwise comparison of methods, ANOVA with repeated measurements was performed. For better comparison of methods temperature, time and method were combined into one variable. The date of sampling was adjusted for as described above.
To assess the mean differences between neck skin and WCR samples and their controls, ANOVA was performed including the sample type (WCR or neck skin) and group (test or control) as fixed effects. To identify possible variation between the dates of sampling this was included as a random effect.
The α-level for all analyses was set to 5%. All statistical analyses were made with SAS®, version 9.4 (SAS Institute Inc., Cary, USA).
3 Results and discussion
3.1 Heat treatment
It was aimed to investigate the effectiveness of hot water treatment in reducing bacterial contamination with Campylobacter and Salmonella on broiler carcasses. The inoculation suspension with cecal contents displayed a mean concentration of 8.0 ± 0.3 log10 CFU/ml for C. jejuni and 8.4 ± 0.2 log10 CFU/ml for Salmonella. Bacterial mean counts for all untreated control groups were 4.1 ± 0.2 log10 CFU/ml WCR for Campylobacter spp. and 4.8 ± 0.3 log10 CFU/ml WCR for Salmonella. Expected concentrations which were calculated based on the measurement of the inoculation suspension were 4.4 ± 0.3 log10 CFU/ml for Campylobacter and 4.8 ± 0.2 log10 CFU/ml for Salmonella.
The general outcome of each treatment was a reduction of Campylobacter and Salmonella, where each temperature and exposure time had an effect (p < 0.05; Supplementary Table S3). A treatment at 70°C for 30 s for Campylobacter and Salmonella already showed mean reductions of 1.1 ± 0.5 and 0.7 ± 0.4 log10 CFU/ml (both p < 0.05), respectively (Figure 2). The trends for those bacteria were similar for the groups treated at 75°C and 80°C (Figures 3, 4), with a mean reduction of 1.0 ± 0.4 at 75°C for both exposure times (p < 0.05) and 1.4 ± 0.4 and 1.2 ± 0.7 log10 CFU/ml at 80°C (p < 0.05) for Campylobacter after 20 and 30 s, respectively. For Salmonella the treatments achieved a reduction of 0.9 ± 0.4 at 75°C for both exposure times and 1.1 ± 0.4 log10 CFU/ml for 80°C 20 s (p < 0.05) and 1.0 ± 0.7 log10 CFU/ml for 80°C 30 s (p < 0.05).
Whether the reducing effect was a result of the heat treatment alone or a combination of heat application and washing off by immersion into water was evaluated by an additional group of carcasses treated with water of approximately 20°C for 30 s. Comparing the untreated control group to the group rinsed at room temperature, the rinsing per se resulted in different effects for the inoculated pathogens and hygiene indicators compared to the control group (Supplementary Table S3). The difference on the inoculated bacteria were small for Campylobacter, with a mean reduction of 0.4 ± 0.5 log10 CFU/ml (p < 0.05), Salmonella remained at a similar level (Figure 5).
Heat treatment is already a part the slaughtering process of broilers in form of scalding, either with water immersion or steam/air, mainly, to loosen the feather follicles to improve the defeathering and additionally to reduce the bacterial contamination of the carcasses (Lu et al., 2019). Using hot water immersion for broiler carcasses directly after evisceration with temperatures of 70 to 80°C and exposure times of 20 and 30 s reduced the Campylobacter and Salmonella counts of around 1 log10 CFU/ml on the carcasses in this study. The highest reductions were found for 80°C for both inoculated bacteria. Rinsing by immersion in water alone was not sufficient for a reduction. Corry et al. (2007) achieved the highest mean reduction of 1.66 log10 CFU/cm2 on broiler carcasses artificially contaminated with Campylobacter with treatment at 75°C for 30 s, treatments at 70°C for 40 s and at 80°C for 20 s were tested as well. Similar findings for Campylobacter were described by Purnell et al. (2004), who analyzed neck skin samples and whole carcass rinses of heat treated (between 65 and 80°C for 10 to 70 s) and then fully processed broiler carcasses after different storage times (0 to 10 days post-kill). Reductions around 1 log10 CFU/ml or CFU/g could be achieved with treatments at 75 and 80°C, respectively (Purnell et al., 2004). Whyte et al. (2003) investigated hot water immersion at 75, 80 and 85°C for 10 and 20 s on retailed broiler thighs which were either inoculated or naturally contaminated with Campylobacter spp. and found significant reductions of up to 1 log10 CFU/ml after treatment at all temperatures for the artificially and naturally contaminated samples. Kure et al. (2020) treated eviscerated broiler carcasses with steam at 95 or 120°C for 3 or 5 s, achieving a reduction of C. jejuni from 0.84–4.32 log10 CFU/ml and 1.36–3.05 log10 CFU/ml for S. Enteritidis on breast, legs and wings. Li et al. (2002) tested hot water spray at 55 and 60°C for 12 s. This treatment significantly reduced inoculated Campylobacter concentrations by more than 0.78 log10 CFU/carcass. A spray treatment at 20°C for 120 s, had no effect which is consistent with the results of the current study. Although the samples in this study were immersed into water and not sprayed. The reducing effect in this study can therefore be explained by the effect of heat. In the early 2000s, some hot water immersion studies were conducted, that differed from the recent study in terms of sample numbers, sample types and experimental design. Additionally, the two main broiler associated pathogens Campylobacter and Salmonella were analyzed in this study, as well as natively occuring hygiene indicators, i.e., E. coli and TCC. Moreover, the most recent findings indicate a consistent pattern in the impact of hot water, which is a notable advancement from previous research. The experimental scenario closely resembles industrial conditions, enhancing the reliability of the results. Reducing Campylobacter contamination on broiler carcasses as in the recent study can help to reduce the risk for the consumer (EFSA, 2011).
Heat treatment in combination with other decontamination measures can enhance the reduction of Campylobacter counts; for instance, steam (90–94°C) in combination with ultrasound (30–40 kHz, SonoSteam™; Musavian et al., 2014) or in combination with ultrasound and rapid cooling (James et al., 2007), resulted in reductions of Campylobacter on broiler carcasses and skin between 1.4 log10 CFU/carcass and 2.9 log10 CFU/ /cm2, respectively. Northcutt et al. (2005) used comparatively lower temperatures of 43.3 and 54.4°C and an exposure time of 5 s for spray washing in an inside-outside bird washer, which did not result in a significant effect on the concentrations of TCC, E. coli and Campylobacter. This underlines the observation that additional hot water treatment would need to exceed the standard scalding temperatures to achieve a reduction of pathogenic bacteria on the carcasses in short-time treatments.
3.1.1 Comparing microbiological and molecular biological results - Campylobacter
The values of the molecular biological analysis of the control group counts showed a mean value of 4.6 ± 0.2 log10 CFU/ml (n = 39) for Campylobacter, which matched the calculated concentration after inoculation as shown above. The averages of the IPIUs for the heat-treated groups ranged between 3.7 and 3.9 log10 IPIU/ml. The effect of the heat treatment was significant for both exposure times with reductions of 0.5 log10 IPIU/ml at 70°C, 0.7 and 0.6 log10 IPIU/ml at 75°C and 0.7 log10 IPIU/ml at 80°C for both exposure times (p < 0.05) compared to the control group. The mean concentration of total DNA of Campylobacter in the group treated by immersion in water at 20°C was 4.3 ± 0.2 log10 CFU/ml in the qPCR (n = 24) and remained at a similar level as the control group (Figure 6). The quantification data from v-qPCR was based on the measured ct-values of the samples in relation to ct-values from a standard curve (Supplementary Figure S1). The mean (n = 12) slope for duplex v-qPCR 1 was −3.37 ± 0.18, i.e., v-qPCR efficiency of 98.0% and for duplex v-qPCR 2 slope − 3.41 ± 0.15, i.e., v-qPCR efficiency of 96.5%.
When comparing the analysis methods, a higher value by 0.5 log10 per ml (p < 0.05) was found on average for the IPIU qPCR of the control group compared to the CFU results, which was also seen for the group treated at 20°C (p < 0.05). For the cultural analysis, the values of the groups treated at 75 and 80°C were 3.2 and 2.7 and 2.9 log10 CFU/ml for 20 and 30 s each, respectively. So mean differences from 0.6 to 1.3 log10 CFU/ml (p < 0.05) were found between the two detection methods. The bacterial reduction for the heat treatments shown via v-qPCR was smaller (p < 0.05) than the reduction seen via culturing.
The v-qPCR method allowed the detection of viable but non-culturable (VBNC) Campylobacter cells. Thus, providing a new perspective on the effect of decontamination treatments helps to close the gap between results from microbiological and molecular biological analysis. Deviations in Campylobacter concentrations were also found for the control group when analyzed by both methods. As a lower recovery of Campylobacter by culturing compared to v-qPCR was seen for samples that were not additionally stressed by heat, but only influenced by the environmental factors and the sampling method.
As a note, due to contamination in some sample extraction controls, some samples were excluded from the evaluation after qPCR analysis and this resulted in different sample numbers for each group. Nevertheless, the results have been statistically assessed by fitting the models to the sample numbers.
The v-qPCR method by Stingl et al. (2021) applied in this study with the ability to detect non growing but still viable cells through live-dead differentiation, has only recently been published. There are only a few studies available to date comparing the data generated by both methods under various applications. For instance, it was reported that discrepancies of 1 log10 CFU/ml in Campylobacter counts between cultural and molecular biological methods were measured, when storing lamb or chicken meat under different packaging conditions (Beterams et al., 2023; Lazou et al., 2021; Wulsten et al., 2022). The difference in the results between the two analytical methods shows the limitations of culturing. Factors such as selective growth conditions, may underestimate bacterial counts while it can potentially lead to overestimating the effectiveness of an intervention. Non-differentiating qPCRs on the other hand may also detect dead cells what can result in an overestimating of potentially intact bacterial cells. Likewise, in our analysis, the total amount of Campylobacter spp. DNA in samples with various treatments was not always significantly different (Figure 6). The v-qPCR method can help to better evaluate the reducing effect of decontamination methods on bacteria. The reductions observed in both analytical methods highlighted heat as an additional decontamination treatment during broiler meat processing, although, the effectiveness was lower when qPCR results were considered.
3.1.2 Hygiene indicators
Heat treatment showed different effects on E. coli and TCC. For E. coli the means of the treated groups were 3.6 ± 0.7 log10 CFU/ml (70°C), 3.2 ± 0.9 log10 CFU/ml and 3.3 ± 0.9 for 20 and 30 s (75°C) and 3.2 ± 0.9 log10 CFU/ml and 3 ± 0.7 log10 CFU/ml for 20 and 30 s (80°C). No statistically significant reductions were seen for hot water treatments for E. coli (Supplementary Table S3). Compared to the trial specific control group a reduction of TCC was observed for all settings (p < 0.05; Supplementary Table S3) with a maximum of 0.9 log10 CFU/ml at 75°C for both exposure times (Figures 2–4). A few of assumptions can be made regarding the reasons why a reduction in E. coli counts was not observed: (a) presence of E. coli in deeper skin layers and the associated adherence or protection from heat; (b) a different recovery of the bacteria between inoculated versus naturally occurring bacteria due to the water bath itself; (c) different physiological state and therefore heat tolerance of the native bacteria compared to the inoculated bacteria. Hauge et al. (2023) examined broiler carcasses for E. coli and TCC treated with hot water at 70, 80, and 90°C for 3 s and 80°C for 6 s. Only the 80°C and 6 s treatment resulted in a reduction in E. coli counts with a reduction of 1.1 log10 CFU/ml. TCC was reduced by 1–1.1 log10 CFU/ml at 80°C for both exposure times as well as at 90°C for 3 s. The results for E. coli differed in the recent study, whereas results for TCC were similar, even though the reductions did not increase with higher exposure times and treatment temperatures in this study.
Hygiene indicators were found to be increased after the immersion at room temperature compared to the untreated control group (p < 0.05). Values for E. coli and TCC were higher by 0.9 ± 1.2 and 0.4 ± 0.7 log10 CFU/ml (Figure 5). By wetting the surfaces before sampling, e.g., by immersion in a water bath, the poultry skin absorbs water and surface layers are opened (Selgas et al., 1993), so that bacteria can be washed off the skin more easily during the sample processing. For dry surfaces, i. e. the control group in this study, Hutchison et al. (2022) assumed, that bacteria are more strongly attached and are therefore harder to remove from the chicken skin with WCR. These assumptions could explain the observation in this study.
3.2 Cold air treatment
This study aimed to investigate the effectiveness of cold air treatment in reducing natural Campylobacter contamination on broiler carcasses before chilling. Several temperatures and exposure times were tested, in particular −80°C and − 90°C for 20, 30, and 40 s (Figures 7, 8). Overall, a reduction of Campylobacter (p < 0.05; Supplementary Table S6) was seen only for −90°C, the mean reduction was 0.4 ± 0.9 log10 CFU/ml, 0.4 ± 1 log10 CFU/ml and 0.5 ± 0.8 log10 CFU/ml at a treatment time of 20, 30 and 40 s (p < 0.05; Supplementary Table S6), respectively (Figure 8). For the −90°C and 30 s treatment, additional trials were conducted as part of the comparison analysis for WCR and neck skin. In those trials, a mean reduction of Campylobacter of 0.7 log10 CFU/ml (p < 0.05) was observed for the WCR, from an initial mean concentration of the untreated groups of 2.1 log10 CFU/ml (Figure 9).
Based on the observation of the data from the individual trials it seemed that the initial carcass contamination with Campylobacter corresponded with the reducing effect. There was a hint for a larger reduction when contamination was higher (Tables 1, 2), because the individual mean concentrations of Campylobacter on untreated control carcasses were higher in the batches for trials at −90°C with 2.2 ± 0.4 log10 CFU/ml, whereas batches treated at −80°C had contamination levels of 1.9 ± 0.7 log10 CFU/ml. The cooling of meat after slaughtering is important to prevent the proliferation of bacteria and to extend the shelf life of the meat, there is also a potential for bacteria reduction (Kennedy and Miller, 2004). In the EU, different chilling methods are used at broiler slaughterhouses, such as air, immersion or a combination cooling (Varzakas and Feddern, 2016). Moreover, freezing (−18 to −22°C) was also described as a method to reduce the bacterial load, especially of temperature-sensitive bacteria, such as Campylobacter spp. (Harrison et al., 2013; Sampers et al., 2010). But freezing is not an option in the fresh meat market because of the European law for fresh poultry meat (regulation (EC) 543/2008, Anonymous, 2008). A cold treatment beyond the normal cooling process can additionally be used to improve the reduction of the microbial load. Short-time treatment with very cold air, as investigated in the current study, results in superficial freezing of carcasses and showed a bacteria-reducing potential for Campylobacter of up to 0.5 log10 CFU/carcass in pilot studies by Boysen and Rosenquist (2009). Haughton et al. (2012) used so called crust freezing with different combinations of temperature and time alone and in combination with UV light irradiation on chicken lower legs and in both cases a significant reduction of the Campylobacter load of 0.5 to 1.0 log10 CFU/g skin was demonstrated. In another study, cooling broiler skin of fresh drumsticks in liquid nitrogen to a minimum surface temperature of −17°C for up to 10 s and spraying broiler carcasses with liquid nitrogen for 40 s reduced Campylobacter concentrations by 0.9 and 1.5 log10 CFU/g skin (Burfoot et al., 2016). In the United Kingdom, the indirect cryogenic surface chilling method is already used in practice at a number of slaughterhouses (Herrmann et al., 2017).
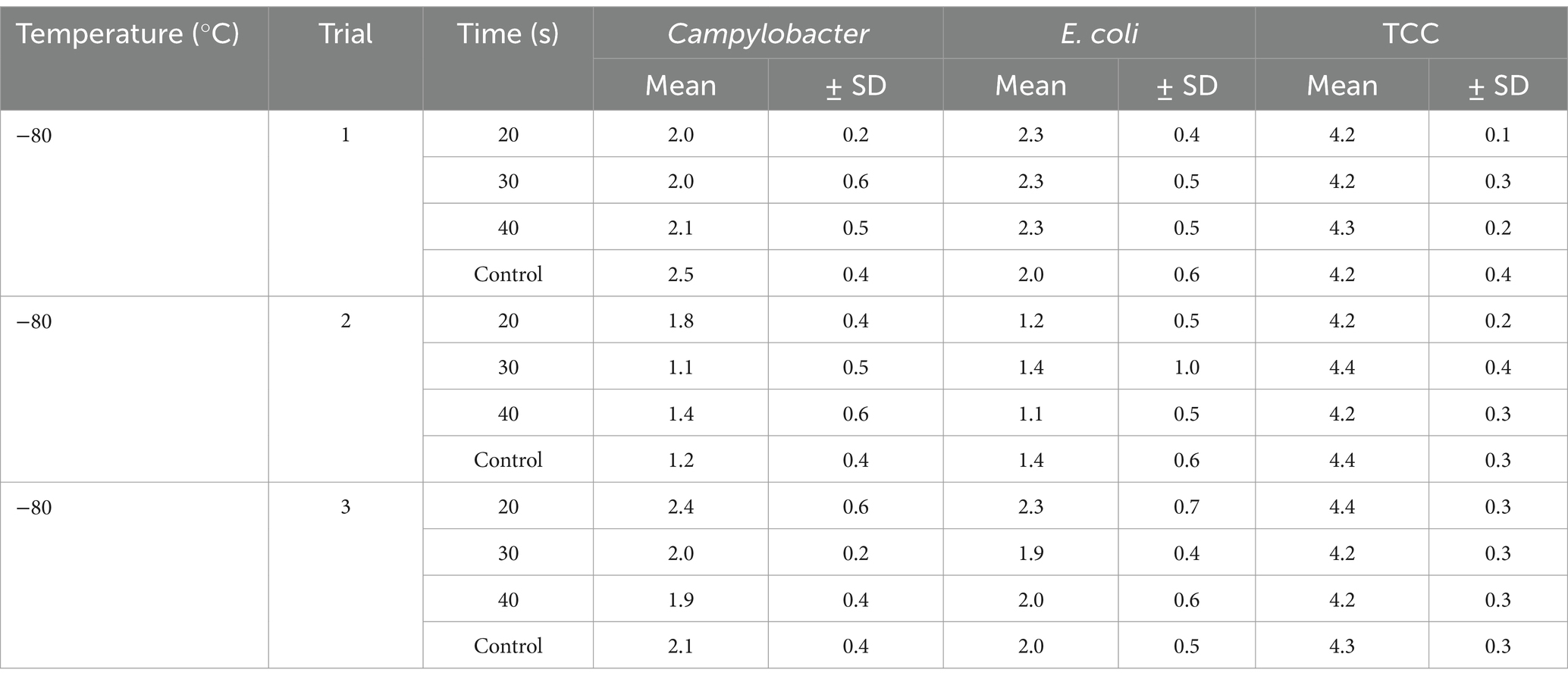
Table 1. Campylobacter, E. coli and TCC on broiler carcasses pre-chilling treated with −80°C for different exposure times (20, 30, 40s), mean values ± SD.
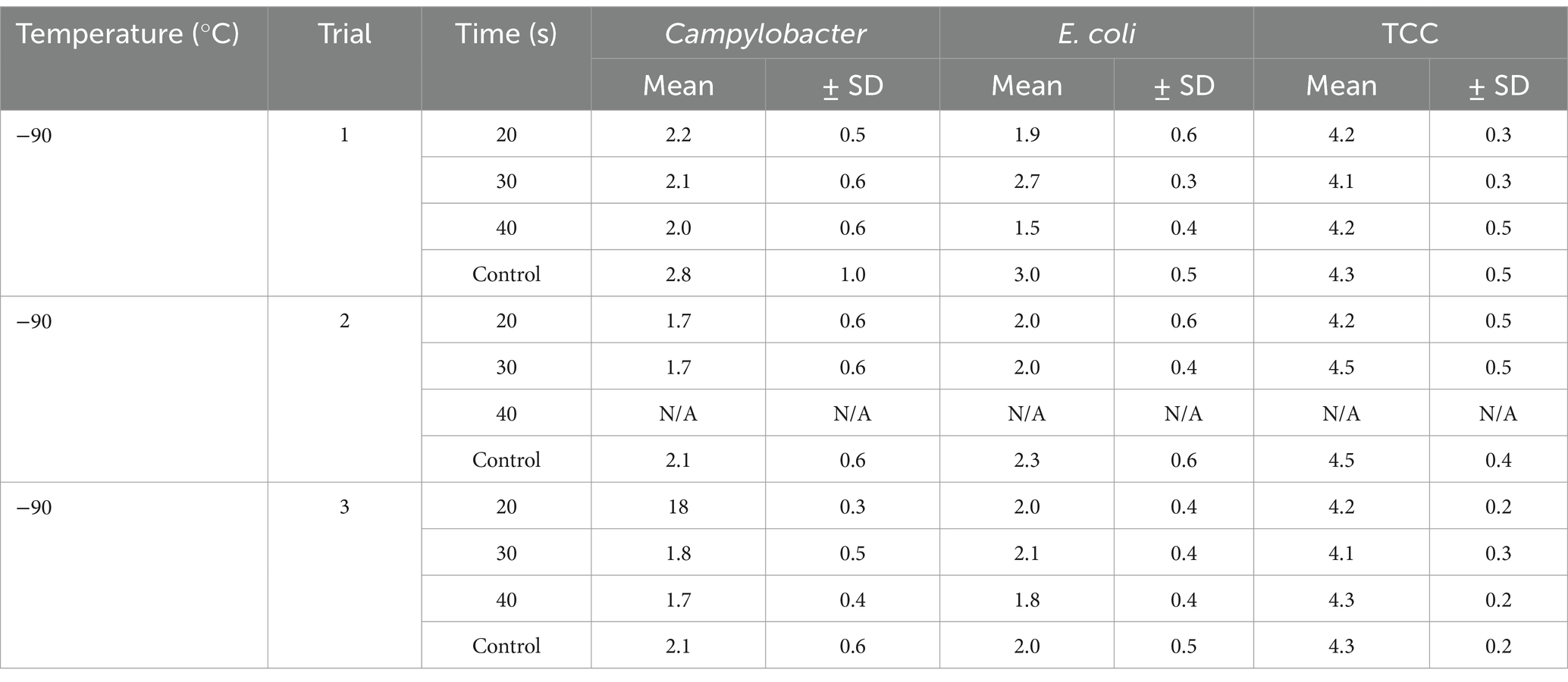
Table 2. Campylobacter, E. coli and TCC on broiler carcasses pre-chilling treated with −90°C for different exposure times (20, 30, 40s), mean values ± SD.
The cold air treatment is a fast treatment, which did not affect the sensory appearance of the carcasses. The initial contamination of carcasses was reduced during the routine slaughtering process, to mean levels of Campylobacter of around 2 log10 CFU/ml of carcass rinse after chilling (Beterams et al., 2024). The additional reduction of the SafeChill™ cold air treatment appeared to be linked to the level of contamination, detection method and the limit of detection. If the contamination was higher, the decontamination effect seemed to be larger.
3.2.1 Hygiene indicators
For the cold-treated groups, E. coli values ranged between 1.7 ± 0.4 and 2.3 ± 0.5 log10 CFU/ml. A reduction (p < 0.05) of 0.4 ± 0.9 was observed for 20 s at −90°C and 0.8 ± 1 log10 CFU/ml for 40 s at −90°C. Mean values for TCC ranged from 4.2 to 4.3 ± 0.3 log10 CFU/ml for the treated and untreated groups and were not affected by the cold treatment (Figures 7, 8). Those observations were further confirmed by the additional trials conducted at −90°C for 30 s (Figure 9). As for control groups, the mean levels were 1.9 ± 0.7 for −80°C and 2.4 ± 0.7 log10 CFU/ml for −90°C for E. coli. The decontaminating effect on E. coli seemed to be larger when the colony counts were higher. In contrast, Chaves et al. (2011) described a process, where crust freezing at −85°C was used for 20 and 60 min and did not result in a reduction of E. coli counts.
3.2.2 Comparing analysis of neck skin samples and WCR
Different sampling methods used for broiler carcasses can influence the enumeration results. In a previous study, differences in the level of Campylobacter between neck skin and WCR were identified after chilling (Beterams et al., 2024). To be able to evaluate this under additional treatment, the two sample types were compared within the cold air study. Overall, the bacterial reduction of the cold air treatment differed between the sample types. We compared the results for neck skin and WCR samples with each other after cold-air treatment at −90°C. Counts of Campylobacter were higher for the neck skin samples (p < 0.05) with means between 2.3 and 2.5 log10 CFU/g than for the WCR samples with mean values between 1.4 and 2.1 log10 CFU/ml. The values for WCR were lower even before the treatment after pre-chilling compared to neck skin samples. Based on the neck skin samples, the cold treatment had no effect on Campylobacter, while by analysis of WCR a mean reduction of 0.7 log10 CFU/ml was observed (Figure 9). For E. coli, the samples for all groups showed high variability in the results, an effect was only recognizable for treatment using WCR (p < 0.05) with a reduction of 0.3 log10 CFU/ml compared to the control group.
Nagel Gravning et al. (2021) compared four different sampling methods with each other, converted the results into log CFU/cm2 (WCR, neck skin, breast skin, swabbing), and identified WCR as the most effective method to recover E. coli with the lowest variation in values and the highest recovery rate. For TCC, the levels for neck skin samples and WCR were comparable. In the current study, these assumptions could be underlined completely, only E. coli showed higher values in mean for the WCR for the control groups, TCC remained on the same level for both sampling types. Also, for Campylobacter, neck skin samples showed higher concentrations than WCR (Figure 9). The drying of the carcass surface during chilling can influence the recovery of bacteria by rinsing and lead to a possible underestimation of colony counts on the carcass (Hutchison et al., 2022). This could explain the lower recovery of WCR compared to neck skin samples, which was also described by Beterams et al. (2024). Moreover, the distribution of bacteria on different areas of the carcass´ skin can have an influence on the enumeration results of the different sample types. Smith (2010) also found significantly higher recovery rates for naturally occurring E. coli in rinsed samples than in ground, which was also represented in the current study.
3.3 Sensory analysis
When meat or skin are treated with hot water, a change in appearance is possible. The visual appearance and the texture of the skin was therefore evaluated before and after treatment at 70°C for 30 s, at 75 and 80°C for 20 and 30 s each and after additional air chilling for 2 h (n = 3). The skin of untreated carcasses had an elastic, soft but strong consistency that needed some force to be ripped apart. After treatment, the skin texture became more inflexible, tough and needed less force to be ripped apart. This effect became increasingly apparent with higher temperature and longer exposure time. The visual appearance was also slightly different for the untreated and treated groups with a tendency for the skin to appear cooked (slightly lighter) in particular for carcasses treated at 80°C (Figures 10, 11). The temperature beneath the skin was at a maximum of 60°C for the hottest and longest treatment (80°C, 30 s), but after heat treatment the meat beneath the skin did not show deviations in appearance compared to the untreated samples (Table 3). Using colorimetric measurement of the carcass skin, the L*-characteristic did not appear to change with treatment alone. Values of untreated and treated carcasses were between L* 70.0 ± 1.0 and L* 71.3 ± 1.7 (Table 3). Whereas after chilling, L*-values showed a tendency for increased values on both untreated or treated carcasses. Chilled carcasses showed an increase in yellowness (visual appearance supported by data) of the skin and a tendency for a larger delta in b* (+)-values for the heat treatment (70°C, 30 s: b* (+) 7.4 ± 0.8; 80°C, 30 s: b* (+) 12.1 ± 1.5).
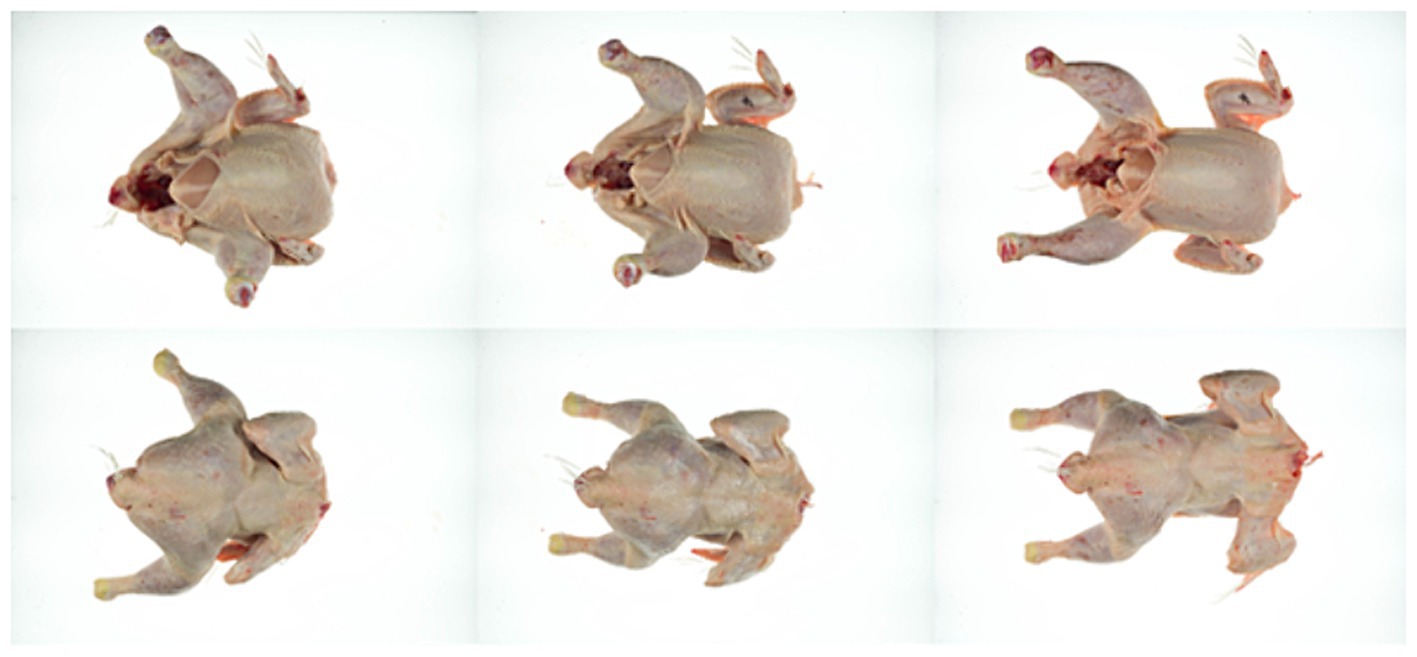
Figure 10. Example of a carcass treated at 75°C for 20 s; before treatment (left), after treatment (center) and after air chilling (right), viewed from the front (top) and back (bottom).
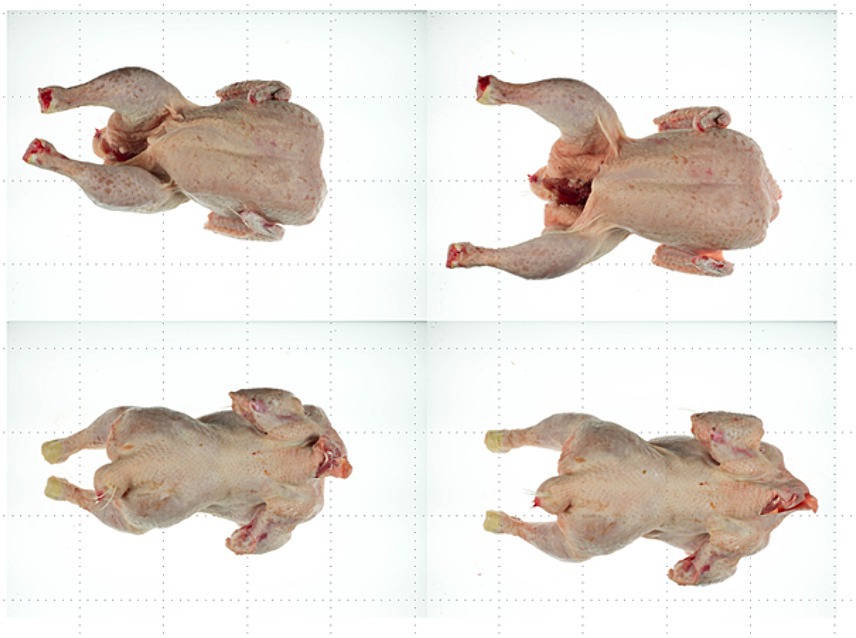
Figure 11. Example of a carcass without treatment (left) and after air chilling (right), viewed from the front (top) and back (bottom).
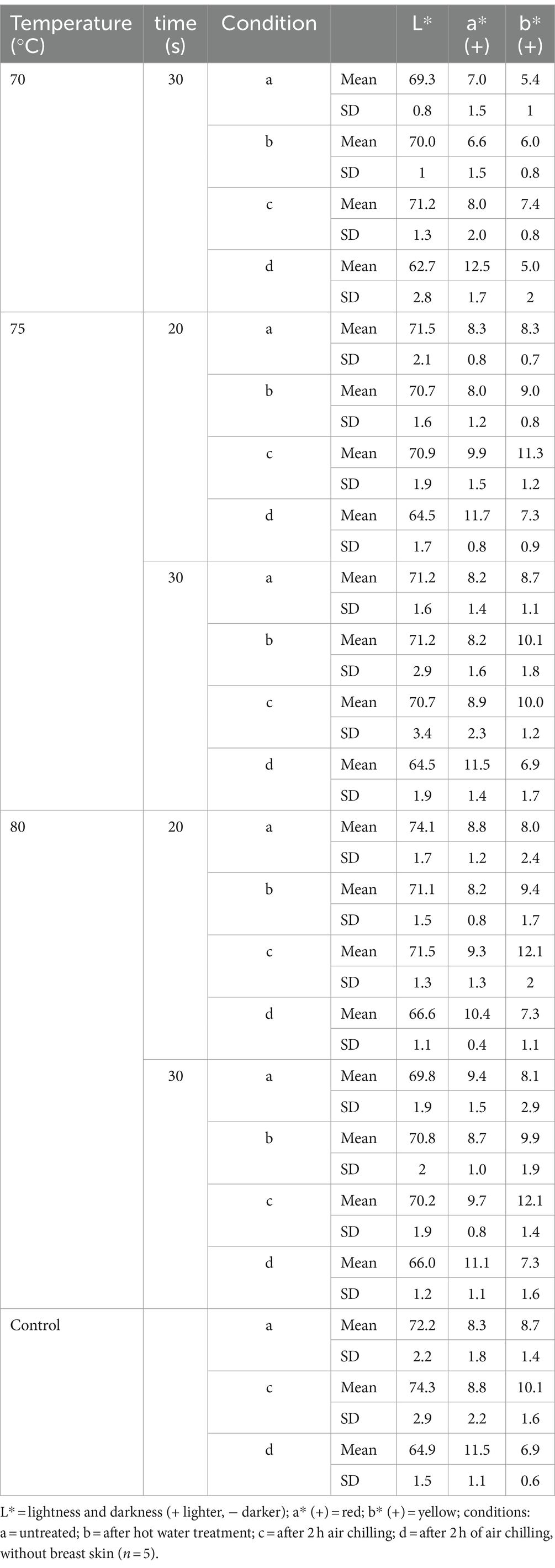
Table 3. L, a (+) and b (+) values mean and standard deviation for broilers of the different treatment groups.
The use of thermal processes, especially when operating with temperatures outside the nowadays commonly used range for scalding and chilling, could result in changes in carcass appearance that could affect the acceptability of the final product. In Germany, around 83% of the produced amount of broiler meat is sold fresh, relevant parts of the broiler are legs and skinless chicken breast (Federal Ministry of Food and Agriculture, 2022). If decontamination treatments such as heat led to deviations in the appearance of the skin, they can more likely be applied to the parts that are marketed without skin.
Li et al. (2002) analyzed hot water spray at different temperatures on carcasses in an inside-outside washer and did not see changes in skin color even with the highest temperature set to 60°C. Similar observations were reported by Northcutt et al. (2005), who found no change in color of the skin at a maximum of 60°C and a treatment for 5 s. Thomson et al. (1974) on the other hand studied spray washing of broiler carcasses with hot water and reported “inferior appearance” of carcasses washed at 71.1°C for 12 s, although the magnitude of color difference was small. Purnell et al. (2004) found that treatment of broiler carcasses at 70°C for 40 s and storage afterwards under typical conditions for 8 days were effective to reduce Campylobacter concentrations without affecting chicken skin. In addition, Hauge et al. (2023) studied a 3 and 6 s treatment at 70 to 90°C and also found no visual change in skin texture and color, which could mainly be explained by the short treatment times. Colorimetric measurements in this current study showed a tendency to a slight increase of L*-values after chilling for both treated and untreated groups. Shung et al. (2022) compared two scalding regimes in Taiwan and evaluated “soft scalding” at 57°C for 120 s and “hard scalding” at 60°C for 60 s. Hard scalding resulted in an increase of yellowness (b* (+)) and lightness (L*). An increase in b* (+)-values was also observed within the current study.
For the cold air treatment, the colorimetric values for L*, a* (+) and b* (+) did not differ between untreated and treated carcasses after chilling (data not shown). The temperature under the skin was between −1 and 1°C in the breast area and between −0.5 and 1°C for the upper leg measured directly after the treatment. The lowest temperature in deeper muscle layers was 1°C. When treating carcasses using ultra cold air, it should be noted that freezing of the meat might occur. According to regulation (EU) No. 1308/2013 (Anonymous, 2013), the temperature of fresh chicken meat must not fall below −2°C. This must be considered in the potential application of those methods.
4 Conclusion
Hot water and cold air treatments of different temperatures and exposure times resulted in the reduction of bacterial contamination of broiler carcasses, i.e., Campylobacter and Salmonella. The hot water application at 70°C resulted in the reduction of target bacteria with only mild effects on the sensory appearance on the carcasses. So, physical treatments can lead to an additional reduction of Campylobacter and Salmonella by the end of primary processing as determined within this study. This can help to reduce the risk of foodborne infection from broiler meat. Both treatments can easily be implemented into industrial settings. However, decontamination efficacy needs to be verified under practical conditions. Molecular biological analysis for Campylobacter showed a statistically significant smaller reducing effect compared to culturing methods. By using different methods for the counting of bacteria in the analysis of decontamination measures, it became obvious that the reducing effect on viable cells of Campylobacter can be overestimated by using cultural quantification only. This method is still new and has not yet been applied in studies on the decontamination of Campylobacter. A wider application of molecular biological analysis using v-qPCR will provide additional information on the efficacy of decontamination treatments in the future.
Data availability statement
The raw data supporting the conclusions of this article will be made available by the authors, without undue reservation.
Author contributions
AB: Data curation, Formal analysis, Investigation, Methodology, Visualization, Writing – original draft, Writing – review & editing. AK: Data curation, Formal analysis, Visualization, Writing – original draft, Writing – review & editing. LK: Conceptualization, Data curation, Formal analysis, Funding acquisition, Supervision, Writing – original draft, Writing – review & editing. KS: Formal analysis, Supervision, Writing – original draft, Writing – review & editing. NB: Conceptualization, Funding acquisition, Methodology, Writing – original draft, Writing – review & editing. FR: Conceptualization, Funding acquisition, Investigation, Methodology, Supervision, Writing – original draft, Writing – review & editing.
Funding
The author(s) declare that financial support was received for the research, authorship, and/or publication of this article. The project is part of the KontRed consortium and is supported by funds of the Federal Ministry of Food and Agriculture (BMEL) based on a decision of the Parliament of the Federal Republic of Germany via the Federal Office for Agriculture and Food (BLE) under the innovation support program (281C104B18). Air Products and Chemical Inc. is an associated partner in this project and contributed by providing Freshline® SafeChill™ trial equipment.
Conflict of interest
The authors declare that the research was conducted in the absence of any commercial or financial relationships that could be construed as a potential conflict of interest.
Publisher’s note
All claims expressed in this article are solely those of the authors and do not necessarily represent those of their affiliated organizations, or those of the publisher, the editors and the reviewers. Any product that may be evaluated in this article, or claim that may be made by its manufacturer, is not guaranteed or endorsed by the publisher.
Supplementary material
The Supplementary material for this article can be found online at: https://www.frontiersin.org/articles/10.3389/fmicb.2024.1429756/full#supplementary-material
SUPPLEMENTARY FIGURE S1 | Representative standard curves and equations for quantification of Campylobacter by v-qPCR according to the protocol by Stingl et al. (2021). The DNA standards of C. jejuni NCTC 11168 and C. sputorum DSM 5363 were measured in duplicate at copy numbers ranging from 1 to 3.7 log10 C. spp. genome equivalents. Duplex 1 (blue), 16S rRNA target of C. jejuni and internal amplification control; Duplex 2 (orange), 16S rRNA target of ISPC (C. sputorum) and internal amplification control; Cj, C. jejuni; ISPC, internal sample process control. The standard equations are depicted in the respective colour with x, log10 C. spp. genome equivalent/PCR well; y, ct value; R2, correlation coefficient.
References
Anderson, A., Pietsch, K., Zucker, R., Mayr, A., Muller-Hohe, E., Messelhausser, U., et al. (2011). Validation of a duplex real-time PCR for the detection of Salmonella spp. in different food products. Food Anal Method 4, 259–267. doi: 10.1007/s12161-010-9142-8
Anonymous . (2008). Commission Regulation (EC) No 543/2008 laying down detailed rules for the application of Council Regulation (EC) No 1234/2007 as regards the marketing standards for poultrymeat, (2008). Available at: https://eur-lex.europa.eu/legal-content/EN/TXT/?uri=CELEX:32008R0543.
Anonymous . (2013). Regulation (EU) No 1308/2013 of the European Parliament and of the Council of 17 December 2013 establishing a common organisation of the markets in agricultural products and repealing Council Regulations (EEC) No 922/72, (EEC) No 234/79, (EC) No 1037/2001 and (EC) No 1234/2007. Available at: https://eur-lex.europa.eu/legal-content/EN/TXT/?uri=celex%3A32013R1308.
Berrang, M. E., Meinersmann, R. J., Cox, N. A., and Fedorka-Cray, P. J. (2011). Application of chlorine dioxide to lessen bacterial contamination during broiler defeathering. J. Appl. Poult. Res. 20, 33–39. doi: 10.3382/japr.2010-00178
Beterams, A., Püning, C., Wyink, B., Grosse-Kleimann, J., Gölz, G., Schönknecht, A., et al. (2024). Status quo: Levels of Campylobacter spp. and hygiene indicators in German slaughterhouses for broiler and turkey. Int. J. Food Microbiol. 414:110610. doi: 10.1016/j.ijfoodmicro.2024.110610
Beterams, A., Tolksdorf, T., Martin, A., Stingl, K., Bandick, N., and Reich, F. (2023). Change of Campylobacter, Escherichia coli and Salmonella counts in packaged broiler breast meat stored under modified atmosphere and vacuum conditions at 4 and 10 °C based on cultural and molecular biological quantification. Food Control 145:109337. doi: 10.1016/j.foodcont.2022.109337
Boysen, L., and Rosenquist, H. (2009). Reduction of thermotolerant Campylobacter species on broiler carcasses following physical decontamination at slaughter. J. Food Prot. 72, 497–502. doi: 10.4315/0362-028X-72.3.497
Burfoot, D., Hall, J., Nicholson, K., Holmes, K., Hanson, C., Handley, S., et al. (2016). Effect of rapid surface cooling on Campylobacter numbers on poultry carcasses. Food Control 70, 293–301. doi: 10.1016/j.foodcont.2016.05.041
Chaves, B. D., Han, I. Y., Dawson, P. L., and Northcutt, J. K. (2011). Survival of artificially inoculated Escherichia coli and Salmonella Typhimurium on the surface of raw poultry products subjected to crust freezing. Poult. Sci. 90, 2874–2878. doi: 10.3382/ps.2011-01640
Corry, J. E. L., James, S. J., Purnell, G., Barbedo-Pinto, C. S., Chochois, Y., Howell, M., et al. (2007). Surface pasteurisation of chicken carcasses using hot water. J. Food Eng. 79, 913–919. doi: 10.1016/j.jfoodeng.2006.03.018
Dogan, O. B., Anand, A., Ortuzar, J., Clarke, J., and Wang, B. (2022). A systematic review and meta-analysis of the efficacy of processing stages and interventions for controlling Campylobacter contamination during broiler chicken processing. Compr. Rev. Food Sci. 21, 227–271. doi: 10.1111/1541-4337.12860
Duffy, L. L., Blackall, P. J., Cobbold, R. N., and Fegan, N. (2014). Quantitative effects of in-line operations on Campylobacter and Escherichia coli through two Australian broiler processing plants. Int. J. Food Microbiol. 188, 128–134. doi: 10.1016/j.ijfoodmicro.2014.07.024
EFSA (2011). Scientific Opinion on Campylobacter in broiler meat production: control options and performance objectives and/or targets at different stages of the food chain. EFSA J. 9:2105. doi: 10.2903/j.efsa.2011.2105
EFSA (2021). The European Union One Health 2021 Zoonoses Report. EFSA J. 20:7666. doi: 10.2903/j.efsa.2022.7666
Federal Ministry of Food and Agriculture . (2022). Versorgungsbilanz Fleisch. Available at: https://www.bmel-statistik.de/ernaehrung/versorgungsbilanzen/fleisch.
Harrison, D., Corry, J. E. L., Tchorzewska, M. A., Morris, V. K., and Hutchison, M. L. (2013). Freezing as an intervention to reduce the numbers of campylobacters isolated from chicken livers. Lett. Appl. Microbiol. 57, 206–213. doi: 10.1111/lam.12098
Hauge, S. J., Bjørkøy, S., Holthe, J., Røtterud, O. J., Skjerve, E., Midtsian, M., et al. (2023). Assessment of in-plant decontamination of broiler carcasses by immersion in hot water and lactic acid in Norway. Food Control 152:109873. doi: 10.1016/j.foodcont.2023.109873
Hauge, S. J., Johannessen, G. S., Haverkamp, T. H. A., Bjorkoy, S., Llarena, A. K., Spilsberg, B., et al. (2023). Assessment of poultry process hygiene and bacterial dynamics along two broiler slaughter lines in Norway. Food Control 146:109526. doi: 10.1016/j.foodcont.2022.109526
Haughton, P. N., Lyng, J., Cronin, D., Fanning, S., and Whyte, P. (2012). Effect of crust freezing applied alone and in combination with ultraviolet light on the survival of Campylobacter on raw chicken. Food Microbiol. 32, 147–151. doi: 10.1016/j.fm.2012.05.004
Herrmann, J., Callens, A., and Trembley, J. (2017). Indirect cryogenic chilling, an effective and proven intervention for Campylobacter reduction on poultry carcasses.
Huezo, R., Northcutt, J. K., Smith, D. P., Fletcher, D. L., and Ingram, K. D. (2007). Effect of dry air or immersion chilling on recovery of bacteria from broiler carcasses. J. Food Prot. 70, 1829–1834. doi: 10.4315/0362-028x-70.8.1829
Hutchison, M. L., Harrison, D., Tchòrzewska, M. A., González-Bodí, S., Madden, R. H., Corry, J. E. L., et al. (2022). Quantitative Determination of Campylobacter on broilers along 22 United Kingdom processing lines to identify potential process control points and cross-contamination from colonized to uncolonized flocks. J. Food Prot. 85, 1696–1707. doi: 10.4315/jfp-22-204
James, C., James, S. J., Hannay, N., Purnell, G., Barbedo-Pinto, C., Yaman, H., et al. (2007). Decontamination of poultry carcasses using steam or hot water in combination with rapid cooling, chilling or freezing of carcass surfaces. Int. J. Food Microbiol. 114, 195–203. doi: 10.1016/j.ijfoodmicro.2006.09.019
Kennedy, C., and Miller, J. (2004). A new chilling technique for processing chicken more safely and efficiently. Food Sci. Technol. 18, 30–32.
Kure, C. F., Axelsson, L., Carlehog, M., Mage, I., Jensen, M. R., and Holck, A. (2020). The effects of a pilot-scale steam decontamination system on the hygiene and sensory quality of chicken carcasses. Food Control 109:106948. doi: 10.1016/j.foodcont.2019.106948
Lazou, T. P., Gelasakis, A. I., Chaintoutis, S. C., Iossifidou, E. G., and Dovas, C. I. (2021). Method-dependent implications in foodborne pathogen quantification: The case of Campylobacter coli survival on meat as comparatively assessed by colony count and viability PCR. Front. Microbiol. 12:933. doi: 10.3389/fmicb.2021.604933
Li, Y., Yang, H., and Swem, B. L. (2002). Effect of high-temperature inside-outside spray on survival of Campylobacter jejuni attached to prechill chicken carcasses. Poult. Sci. 81, 1371–1377. doi: 10.1093/ps/81.9.1371
Lu, T., Marmion, M., Ferone, M., Wall, P., and Scannell, A. G. M. (2019). Processing and retail strategies to minimize Campylobacter contamination in retail chicken. J Food Proc Pres 43:14251. doi: 10.1111/jfpp.14251
McKee, S.R., Townsend, J. C., and Bilgili, S. F. (2008). Use of a scald additive to reduce levels of Salmonella Typhimurium during poultry processing. Poult. Sci. 87, 1672–1677. doi: 10.3382/ps.2008-00061
Musavian, H. S., Krebs, N. H., Nonboe, U., Corry, J. E. L., and Purnell, G. (2014). Combined steam and ultrasound treatment of broilers at slaughter: A promising intervention to significantly reduce numbers of naturally occurring campylobacters on carcasses. Int. J. Food Microbiol. 176, 23–28. doi: 10.1016/j.ijfoodmicro.2014.02.001
Nagel Gravning, G. E., Røtterud, O. J., Bjørkøy, S., Forseth, M., Skjerve, E., Llarena, A. K., et al. (2021). Comparison of four sampling methods for microbiological quantification on broiler carcasses. Food Control 121:107589. doi: 10.1016/j.foodcont.2020.107589
Northcutt, J. K., Smith, D. P., Musgrove, M. T., Ingram, K. D., and Hinton, A. (2005). Microbiological impact of spray washing broiler carcasses using different chlorine concentrations and water temperatures. Poult. Sci. 84, 1648–1652. doi: 10.1093/ps/84.10.1648
Pacholewicz, E., Buhler, C., Wulsten, I. F., Kraushaar, B., Luu, H. Q., Iwobi, A. N., et al. (2019). Internal sample process control improves cultivation-independent quantification of thermotolerant Campylobacter. Food Microbiol. 78, 53–61. doi: 10.1016/j.fm.2018.09.017
Pacholewicz, E., Swart, A., Schipper, M., Gortemaker, B. G., Wagenaar, J. A., Havelaar, A. H., et al. (2015). A comparison of fluctuations of Campylobacter and Escherichia coli concentrations on broiler chicken carcasses during processing in two slaughterhouses. Int. J. Food Microbiol. 205, 119–127. doi: 10.1016/j.ijfoodmicro.2015.04.006
Purnell, G., Mattick, K., and Humphrey, T. (2004). The use of 'hot wash' treatments to reduce the number of pathogenic and spoilage bacteria on raw retail poultry. J. Food Eng. 62, 29–36. doi: 10.1016/s0260-8774(03)00168-7
Sampers, I., Habib, I., De Zutter, L., Dumoulin, A., and Uyttendaele, M. (2010). Survival of Campylobacter spp. in poultry meat preparations subjected to freezing, refrigeration, minor salt concentration, and heat treatment. Int. J. Food Microbiol. 137, 147–153. doi: 10.1016/j.ijfoodmicro.2009.11.013
Selgas, D., Luisa Marín, M., Pin, C., and Casas, C. (1993). Attachment of bacteria to meat surfaces: A review. Meat Sci. 34, 265–273. doi: 10.1016/0309-1740(93)90076-t
Shung, C. C., Hsin, K. Y., Tan, F. J., and Chen, S. E. (2022). Effects of hard and soft scalding on defeathering and carcass quality of different breeds of chickens. Animals (Basel) 12:23145. doi: 10.3390/ani12223145
Smith, D. P. (2010). Sampling method and location affect recovery of coliforms and Escherichia coli from broiler carcasses. Poult. Sci. 89, 169–172. doi: 10.3382/ps.2008-00414
Stingl, K., Heise, J., Thieck, M., Wulsten, I. F., Pacholewicz, E., Iwobi, A. N., et al. (2021). Challenging the “gold standard” of colony-forming units - Validation of a multiplex real-time PCR for quantification of viable Campylobacter spp. in meat rinses. Int. J. Food Microbiol. 359:417. doi: 10.1016/j.ijfoodmicro.2021.109417
Thomson, J. E., Cox, N. A., Whitehead, W. K., and Mercuri, A. J. (1974). Effect of hot spray washing on broiler carcass quality. Poult. Sci. 53, 946–952. doi: 10.3382/ps.0530946
Varzakas, T., and Feddern, V. (2016). “Poultry Manufacturing Technologies” in Handbook of Food Processing: Food Safety, Quality, and Manufacturing Processes. eds. T. Varzakas and C. Tzia (Boca Raton, FL: CRC Press-Taylor and Francis Group), 487–514.
Whyte, P., McGill, K., and Collins, J. D. (2003). An assessment of steam pasteurization and hot water immersion treatments for the microbiological decontamination of broiler carcasses. Food Microbiol. 20, 111–117. doi: 10.1016/S0740-0020(02)00084-9
Keywords: broiler slaughtering process, thermal decontamination methods, Campylobacter spp., Salmonella spp., hygiene indicators, PMA qPCR, sensory analysis
Citation: Beterams A, Kirse A, Kreienbrock L, Stingl K, Bandick N and Reich F (2024) Application of hot water and cold air to reduce bacterial contamination on broiler carcasses. Front. Microbiol. 15:1429756. doi: 10.3389/fmicb.2024.1429756
Edited by:
Dario De Medici, National Institute of Health (ISS), ItalyReviewed by:
Frederique Pasquali, University of Bologna, ItalyMarcello Trevisani, University of Bologna, Italy
Maria Francesca Peruzy, University of Naples “Federico II”, Italy
Copyright © 2024 Beterams, Kirse, Kreienbrock, Stingl, Bandick and Reich. This is an open-access article distributed under the terms of the Creative Commons Attribution License (CC BY). The use, distribution or reproduction in other forums is permitted, provided the original author(s) and the copyright owner(s) are credited and that the original publication in this journal is cited, in accordance with accepted academic practice. No use, distribution or reproduction is permitted which does not comply with these terms.
*Correspondence: Felix Reich, ZmVsaXgucmVpY2hAYmZyLmJ1bmQuZGU=