- 1Institute of Chinese Materia Medica, China Academy of Chinese Medical Sciences, Beijing, China
- 2College of Animal Science, Ningxing University, Yinchuan, China
- 3College of Veterinary Medicine, Nanjing Agricultural University, Nanjing, China
- 4Changzhou Hospital Affiliated to Nanjing University of Chinese Medicine, Changzhou, China
- 5College of Veterinary Medicine, Yunnan Agricultural University, Kunming, China
Ulcerative colitis (UC), characterized by disrupted intestinal barrier integrity and chronic inflammation, was modeled in mice via dextran sulfate sodium (DSS) induction. This study explored the therapeutic potential of berberine-evodiamine (BBR-EVO), bioactive components of the traditional Chinese medicine Yulian decoction, in DSS colitis. BBR-EVO intervention ameliorated weight loss, diarrhea, colonic shortening, and histopathological damage in colitic mice. The substance increased antioxidant activity while reducing high levels of pro-inflammatory cytokines in the colon, including as TNF-α, IL-1β, and IL-6. BBR-EVO inhibited the DSS-induced decrease in the tight junction proteins ZO-1 and occludin, according to immunohistochemistry. 16S rRNA sequencing demonstrated BBR-EVO partially attenuated DSS-elicited intestinal dysbiosis, reducing opportunistic pathogens and restoring diminished beneficial taxa. Critically, BBR-EVO alleviated secondary hepatic injury in colitic mice, mitigating immune cell infiltration, oxidative stress, cytokine production, and ultrastructural damage, likely by beneficially modulating gut-liver crosstalk. This study reveals BBR-EVO, derived from a traditional Chinese medicine, confers multi-target protective effects in experimental colitis and associated hepatic pathology, warranting further evaluation as a potential therapy for inflammatory bowel diseases like UC. The mechanisms may involve simultaneous augmentation of intestinal barrier integrity, inhibition of inflammation, microbiota regulation, and gut-liver axis optimization.
Introduction
Ulcerative colitis (UC) represents a common subtype of inflammatory bowel disease (IBD) affecting the human population. The clinical manifestations of UC encompass anorexia, abdominal discomfort, hematochezia, and mucosal discharge (Eom et al., 2018). Statistical evidence suggests that IBD impacts an estimated 50,000 people worldwide, with an ascending prevalence (Le Berre et al., 2023). In Western nations, the prevalence of IBD might surpass 0.5% (Kaplan, 2015; Du and Ha, 2020). Nonetheless, the etiology of UC remains elusive, and an absolute cure has not been established. Research has demonstrated that both genetic predispositions and environmental influences elevate the risk for UC (Cosnes et al., 2011). Furthermore, dysregulation of immune responses, disruptions in intestinal microbiota, and other contributory factors are associated with UC (Kaser et al., 2010). Substantial evidence indicates that the human gut microbiota consists of a diverse array of symbiotic strains and microorganisms (Jia et al., 2008), with significant alterations observed in the composition and function of this microbiota in IBD patients (Lavelle and Sokol, 2020). Patients with IBD present with dysbiosis of gut microbiota, resulting in compromised intestinal epithelial barrier integrity, heightened intestinal permeability, and the ensuing translocation of endotoxins (e.g., lipopolysaccharides, LPS) into the circulatory system, which provokes an inflammatory response in the gut (Ungaro et al., 2017; Zhang et al., 2017; Du and Ha, 2020), This sequence of events can precipitate the activation of the hepatic immune system, potentially culminating in liver damage (Fukui, 2015). Investigations have identified oxidative stress, dysbiosis of the intestinal microbiota, and inflammation as shared pathogenic elements contributing to both liver injury and IBD (Chao et al., 2016; Rogler et al., 2021). Considering the liver’s heightened exposure to bacterial toxins, the dysbiosis observed in colitis may be implicated in hepatic injury via the gut-liver axis. Damage to the intestinal barrier permits the translocation of bacterial metabolites and toxins to the liver, which may induce a spectrum of hepatic diseases (Ohtani and Kawada, 2019).
The persistence of a high recurrence risk in patients with inflammatory bowel disease (IBD) post-medication necessitates further investigation into potent adjuvant therapies. With its therapeutic approach based on the principles of holistic and balanced care, Traditional Chinese Medicine (TCM) is becoming more and more acknowledged as a valuable complementary and alternative modality. It offers sustained therapeutic outcomes, minimal side effects, and a diverse range of bioactive constituents with multi-target effects (Kaplan, 2015). Given its oral administration, TCM primarily acts through the gastrointestinal system, a key site for absorption and metabolic processing. Notably, Traditional Chinese medicine (TCM) has been extensively utilized for managing ulcerative colitis, owing to its overall efficacy in restoring intestinal flora balance (Chen et al., 2015), along with its antidiarrheal and anti-inflammatory properties. Yulian decoction comprises coptidis, evodia fructus, and acostalis, and has purgative and analgesic effects. It is commonly prescribed for epigastric pain, sour belching, and diarrhea. Modern pharmacology has validated the analgesic, anti-ulcer, and antibacterial properties of Yulian decoction (Wang L. et al., 2011; Wang Y. et al., 2011). In vitro studies have confirmed the anti-inflammatory activity of compounds in Yulian decoction, which can suppress TNF-α and IL-6 levels in inflammatory cell models (Ran et al., 2022). Moreover, recent studies have identified 11 bioactive compounds in Yulian decoction, including berberine (BBR) and evodiamine (EVO), present in rat plasma (Li T. et al., 2021), while some in vivo studies indicated the protective effect of berberine on acute gastric ulcer (Guo et al., 2024). Investigating the synergistic effects of two active compounds, BBR and EVO, in Yulian decoction on the intestinal milieu may prove pivotal in demystifying the underlying pharmacological mechanisms.
Materials and methods
Animals
Male ICR mice, with an average weight of 25 ± 2 g, were acquired from the Comparative Medicine Centre at Yangzhou University (Yangzhou, China). These animals were maintained in a controlled environment, with a temperature of 22 ± 2°C, relative humidity of 50 ± 10%, and a 12-h light–dark cycle, with ad libitum access to food and water.
Chemicals and reagents
Berberine (BBR, IB0440, Beijing) and evodiamine (EVO, IE0430) were purchased from Solarbio (Beijing, China). BBR and EVO are initially dissolved in DMSO to prepare 100 mM stock solutions (Figure 1A). Dextran Sulfate Sodium Salt (DSS, 60316ES60) was provided from Yeasen (Shanghai, China).
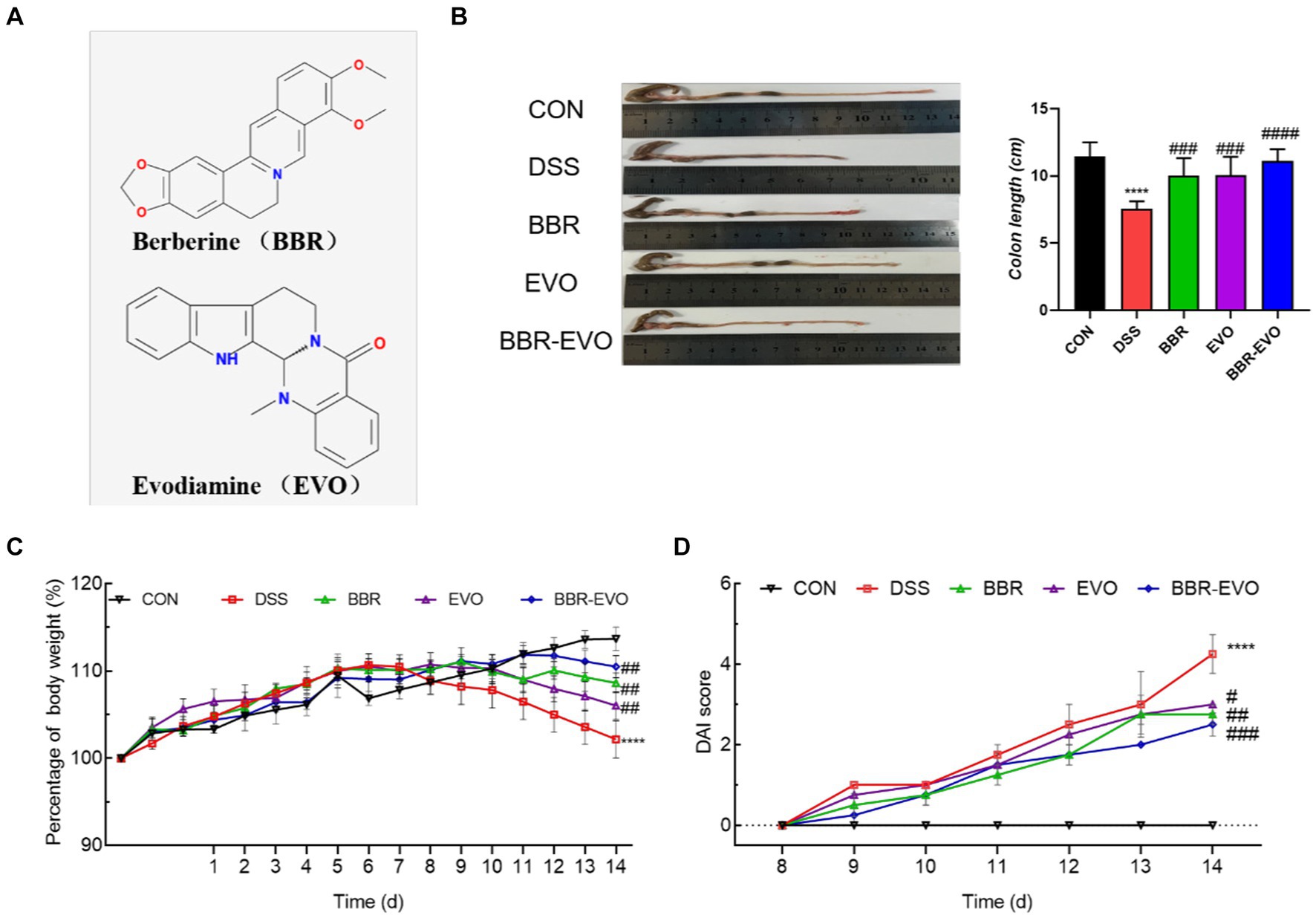
Figure 1. The main active components of Yulian decoction treatment relieve DSS-induced colitis. (A) Chemical structures of berberine and evodiamine. (B) The results of mice colon length, n = 8. (C) Variations in mice body weight. (D) Disease Activity Index (DAI) scores during the colitis induction period. Data are presented by mean ± SD; *p < 0.05, **p < 0.01, ***p < 0.001, ****p < 0.0001, compared to CON group; #p < 0.05, ##p < 0.01, ###p < 0.001, ####p < 0.0001, compared to DSS group.
Animal experimental design
Following a one-week acclimatization period, all mice were randomly allocated into five distinct groups (n = 8 per group): control (CON), dextran sulfate sodium saline (DSS), berberine (BBR), evodiamine (EVO), and combination of BBR and EVO (BBR-EVO) group. The BBR and EVO content in the lyophilized Yulian decoction were 9.14 and 0.065%, respectively (Li T. et al., 2021). The ratio of BBR to EVO was maintained to reflect their proportions in the original Yulian decoction, aiming to preserve potential synergistic effects while ensuring physiological relevance. Dose extrapolation from a 70 kg human adult to rats (0.05 g/kg) was performed using a body surface area normalization factor of 0.018 (Xu, 1991), and an analogous calculation was employed for mice using a factor of 0.0026. Consequently, the resultant mice equivalent dose was 0.072 g/kg, with a daily administration of BBR at 19.74 mg/kg and EVO at 0.141 mg/kg.
Subsequent to the acclimatization phase, mice in the pharmacologically treated cohorts were given oral BBR, EVO, or BBR-EVO. In contrast, mice in the CON and DSS cohorts received daily PBS for 14 days. Commencing on the 7th day of treatment, all groups except for the CON group were provided with 3% (w/v) DSS in their drinking water for seven consecutive days, with daily renewal of the DSS solution, to induce a mouse model of acute ulcerative colitis. The assessment of disease severity in murine models was conducted through the application of the Disease Activity Index (DAI) scoring system. This index encompasses a comprehensive evaluation of physiological parameters, namely fecal consistency and bleeding (Camuesco et al., 2004). Each parameter was meticulously observed and assigned a score based on established diagnostic guidelines.
Inflammatory cytokine analysis and antioxidant capacity analyses by ELISA
For preparation of tissue homogenates, samples from either the colon or liver were meticulously weighed and homogenized with pre-chilled normal saline in a ratio of 1:9 (m/v). The homogenization process was carried out at a temperature of 4°C. Subsequently, the homogenates were centrifuged at 12,000 rpm for 10 min, and the supernatant was collected. The concentrations of Total Antioxidant Capacity (T-AOC, YH1246), Malondialdehyde (MDA, YH1217), Superoxide Dismutase (SOD, YH1202), and Interleukin-6 (IL-6, ANG-E21044M), −1β (IL-1β, ANG-E21027M), and tumor necrosis factor -а (TNF-а, ANG-E21030M) were quantified using appropriate commercial ELISA kits, purchased from Nanjing Angle gene Biotechnology Co., Ltd. (China).
Histopathological analysis
In line with the methodology outlined by Wang et al. (2019), hematoxylin and eosin (H&E) staining was employed to evaluate the histopathological alterations in the mouse colon and liver. Tissue samples fixed in 4% paraformaldehyde were sequentially dehydrated using graded ethanol solutions prior to a three-hour wax immersion. Subsequently, mouse colon and liver samples were sectioned to a thickness of 5 micrometers (μm). These sections were subsequently rehydrated, cleared of wax using xylene, and stained with hematoxylin and eosin, following standard histological protocols. Finally, morphological changes in the tissues were assessed and documented using an Olympus optical microscope (Olympus, Japan) and a Leica microscopic imaging system (Leica Biosystems, Germany).
Immunohistochemistry analysis
Briefly, for antigen retrieval, the paraffin-embedded slides were submerged in 0.01 mM citrate buffer and microwaved for 10 min. Following a phosphate-buffered saline (PBS) wash, the slides were incubated at room temperature, first with 3% hydrogen peroxide (H₂O₂) for 10 min and then with goat serum for 15 min. The slides were then treated with goat anti-rabbit secondary antibody, followed by a 30-min incubation at room temperature. Subsequent to a PBS rinse, each slide was covered with a diaminobenzidine (DAB) solution (DA1010, Solarbio, China) and allowed to react at room temperature. One minute later, each slide was gently washed with water, counterstained with hematoxylin, dehydrated, and sealed with neutral gum before storage for subsequent analysis. Microscopic images of the stained sections were captured using a Nikon optical microscope (Nikon, Japan).
Western blot analysis
Approximately 50 mg of colon tissue was mixed with an appropriate volume of pre-cooled PIPA Lysis Buffer and then completely lysed using a low-temperature homogenizer (Servicebio, Wuhan, China) for 10 to 15 min. The supernatant was then collected following centrifugation at 12,000 rpm for 20 min. Upon determining the protein concentration, samples were normalized to a uniform concentration and subjected to denaturation. Resolving gels of varying concentrations were prepared in accordance with the molecular weight of the target protein. The protein samples were then subjected to electrophoresis, followed by transfer onto a polyvinylidene difluoride (PVDF) membrane. The PVDF membrane was blocked using a Tris-buffered saline with Tween (TBST) containing 5% skimmed milk, followed by incubation with the primary antibody diluent overnight at 4°C. Following washes with TBST, the membrane was incubated with the secondary antibody diluent for 1.5 h at room temperature. Details of the antibodies utilized are listed in Table 1. Subsequently, the membranes were treated with a chemiluminescent HRP substrate solution (Biosharp, Anhui, China). Signals on the PVDF membranes were detected using a Bio-Rad chemiluminescence imaging system. Band densities were quantitatively analyzed using ImageJ software, with all data normalized to β-actin levels prior to analysis.
Extraction of microbial genomic DNA from murine intestinal content
Genomic DNA was extracted from the intestinal content samples of mice belonging to three experimental cohorts (designated as BBR, EVO, and BBR-EVO group) employing the QIAamp DNA Mini Kit (QIAGEN, Germany). The quantification of genomic DNA was achieved through spectrophotometric analysis using the NanoDrop technology (Thermo Scientific, Wilmington), while the DNA integrity was ascertained by conducting electrophoresis on a 1% agarose gel at 150 V for a duration of 40 min. Purified DNA samples were stored at −80°C pending further analytical procedures.
Amplification and sequencing of bacterial 16 s rRNA genes
The amplification of the V3-V4 hypervariable regions of the bacterial 16S rRNA genes was conducted using the primer pair 338F (5′-ACTCCTACGGGAGGCAGCA-3′) and 806R (5′-GGACTACHVGGGTWTCTAAT-3′). The resultant PCR products were then subjected to a purification process employing Vazyme VAHTSTM DNA Clean Beads (Vazyme, China), with an ensuing validation step via 1% agarose gel electrophoresis. Sequencing libraries were meticulously prepared from the purified amplicons to ensure a singular peak at a standard concentration of 2 nM. High-throughput sequencing was executed on an Illumina HiSeq platform (Illumina, San Diego, USA), facilitating a comprehensive analysis of the bacterial genomic composition. Experimental results are uploaded to the Genescloud Platform for microbiome bioinformatics analysis.1
Statistical analysis
Data are expressed as the mean ± standard deviation (SD). Differences between groups were analyzed using the one-way ANOVA test followed by the Tukey test for multiple comparisons for normally distributed data, or the Kruskal-Wallis test followed by Dunn’s test for multiple comparisons for non-normally distributed data. A p-value ≤0.05 was deemed to denote statistical significance.
Result
BBR-EVO ameliorated DSS-induced colitis in mice
Mice in the DSS group exhibited a reduction in body weight and a decrease in colon length compared to the CON group (Figures 1B, C). In contrast, mice treated with BBR, EVO, and BBR-EVO showed an increase in both body weight and colon length relative to the DSS group. Notably, the BBR-EVO combination was more effective in improving DAI scores (Figure 1D).
As demonstrated in Figure 2, as compared to the CON group, the levels of ALT and AST in the DSS group’s serum increased considerably (p < 0.01), whereas the levels of ALP, BUN, and CRE kept unchanged. After BBR and BBR-EVO interventions, ALT and AST levels reduced dramatically (p < 0.001), CRE levels increased significantly (p < 0.05), and BUN levels increased significantly (p < 0.0001). BUN levels rose considerably during EVO intervention (p < 0.05).
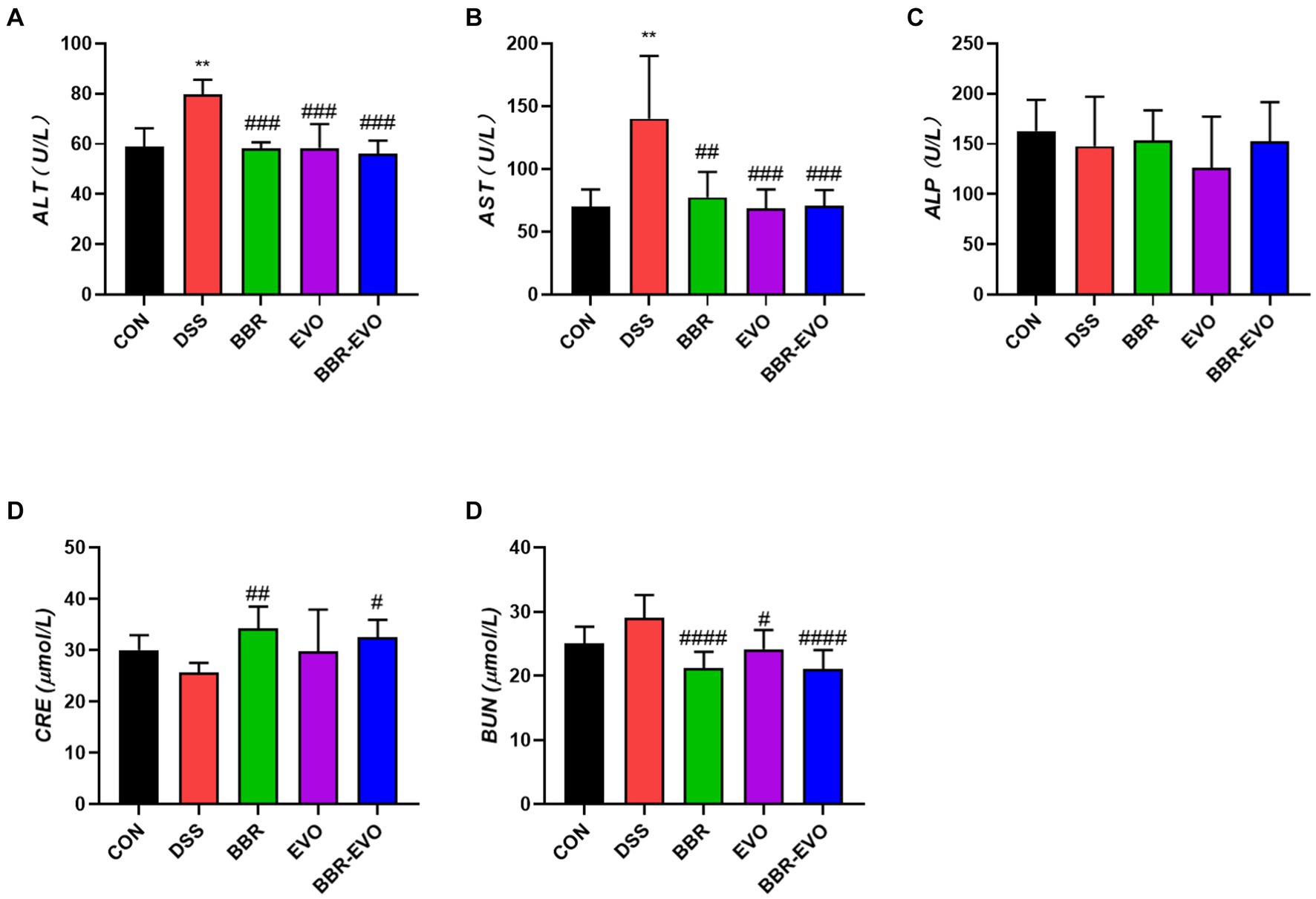
Figure 2. Effects of main active components of Yulian Decoction treatment on blood biochemical indices in mice, n = 5. (A) Alanine aminotransferase (ALT). (B) Aspartate aminotransferase (AST). (C) Alkaline phosphatase (ALP). (D) Creatinine (CRE). (E) Blood urea nitrogen (BUN). Data are presented by mean ± SD; *p < 0.05, **p < 0.01, ***p < 0.001, ****p < 0.0001, compared to CON group; #p < 0.05, ##p < 0.01, ###p < 0.001, ####p < 0.0001, compared to DSS group.
Hematoxylin and Eosin (HE) staining facilitated the histological examination of colon tissue, as depicted in Figure 3. The CON group exhibited a normal colonic mucosal structure. In stark contrast, the DSS group’s colon tissue displayed significant disruption of colonic crypts, abnormal gland distribution, and elevated inflammatory cell infiltration. Following pretreatment with BBR, EVO, and particularly the BBR-EVO combination, there was notable tissue repair. The colon tissues exhibited intact crypts and reduced inflammation. Most remarkably, the BBR-EVO group’s colon tissue closely resembled that of the CON group, with a successfully restored structure and slightly inflammation.
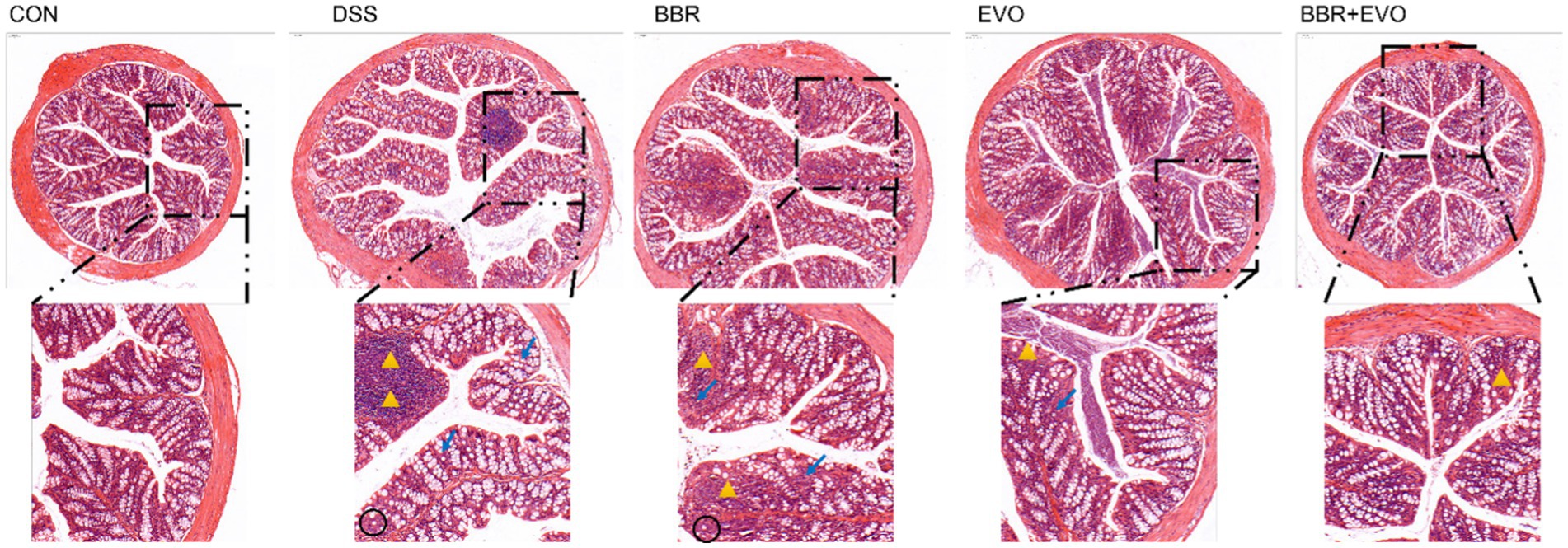
Figure 3. Effects of the main active components of Yulian decoction on histopathological changes in the mouse colon assessed by hematoxylin and eosin staining (H&E). Scale bar = 100 μm. Black circles indicate decreased goblet cells; yellow triangles indicate inflammatory infiltrate; blue arrows indicate abnormal crypt structure.
BBR-EVO alleviated the inflammatory response of colon tissue in DSS-induced colitis mice
Cytokine levels were quantified using enzyme-linked immunosorbent assay (ELISA) to assess the suppressive effects of BBR-EVO. Mice with DSS-induced colitis exhibited significantly elevated levels of IL-6, TNF-α, and IL-1β (Figure 4). Treatment with BBR and EVO effectively reduced the production of these cytokines in colitic mice, with a more pronounced reduction observed when BBR and EVO were combined.
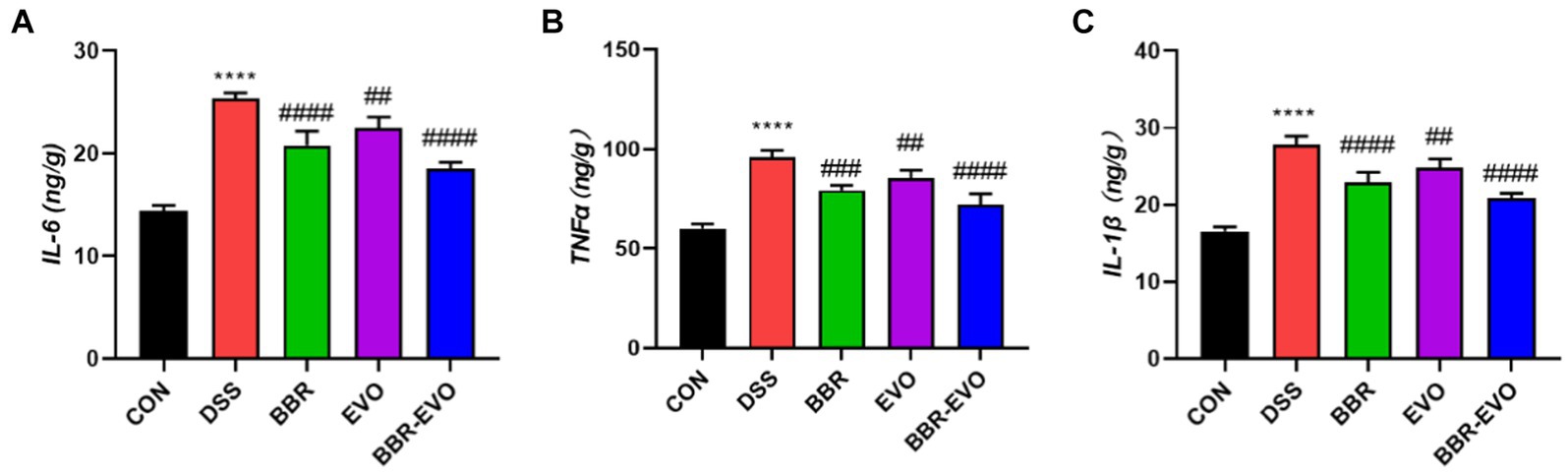
Figure 4. Levels of pro-inflammatory cytokines in mouse colon tissues as determined by enzyme-linked immunosorbent assay (ELISA), n = 5. (A) Interleukin-6 (IL-6). (B) Tumor necrosis factor alpha (TNF-α). (C) Interleukin-1 beta (IL-1β). Data are presented by mean ± SD; *p < 0.05, **p < 0.01,***p < 0.001, ****p < 0.0001, compared to CON group; #p < 0.05, ##p < 0.01, ###p < 0.001, ####p < 0.0001, compared to DSS group.
BBR-EVO inhibited the oxidative state
At the same time, representative oxidative stress factors were also detected (Figure 5). The findings revealed that the DSS group’s MDA content was significantly higher than that of the CON group (p < 0.0001) and that the activities of SOD and T-AOC were reduced to varied degrees (p < 0.01). SOD and T-AOC levels considerably increased (p < 0.01) and MDA levels dramatically decreased (p < 0.01) under the administration of BBR and BBR-EVO, whereas MDA and T-AOC levels in the colon of mice treated with EVO alone did not significantly alter. Therefore, BBR-EVO may have the ability to inhibit inflammatory response and enhance antioxidant defense to inhibit DSS injury.
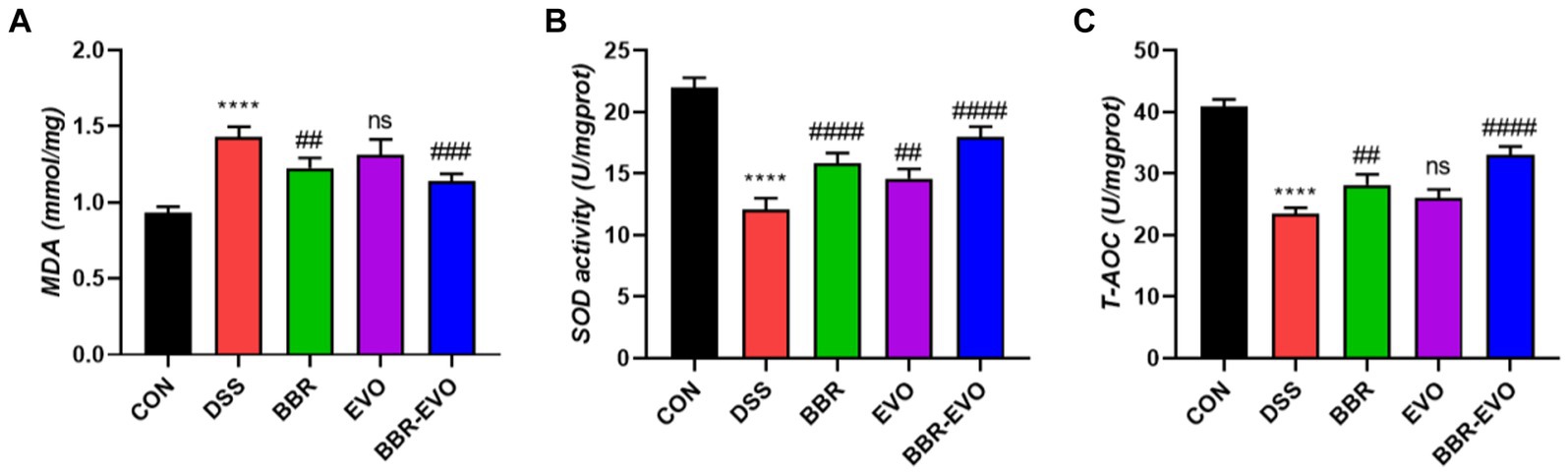
Figure 5. Effects of the active components of Yulian decoction on oxidant and antioxidant indices in liver homogenates of colitis mice, n = 5. (A) MDA. (B) SOD. (C) T-AOC. Data are presented by mean ± SD; *p < 0.05, **p < 0.01, ***p < 0.001, ****p < 0.0001, compared to CON group; #p < 0.05, ##p < 0.01, ###p < 0.001, ####p < 0.0001, compared to DSS group.
The IHC staining findings indicated that the expression levels of nuclear factor erythroid 2-related factor 2 (Nrf2) and NQO1 in the DSS group’s colon tissue were lower than in the CON group, indicating that the antioxidant signaling pathway in the DSS group was suppressed (Figure 6). This is consistent with the antioxidant parameter test findings.
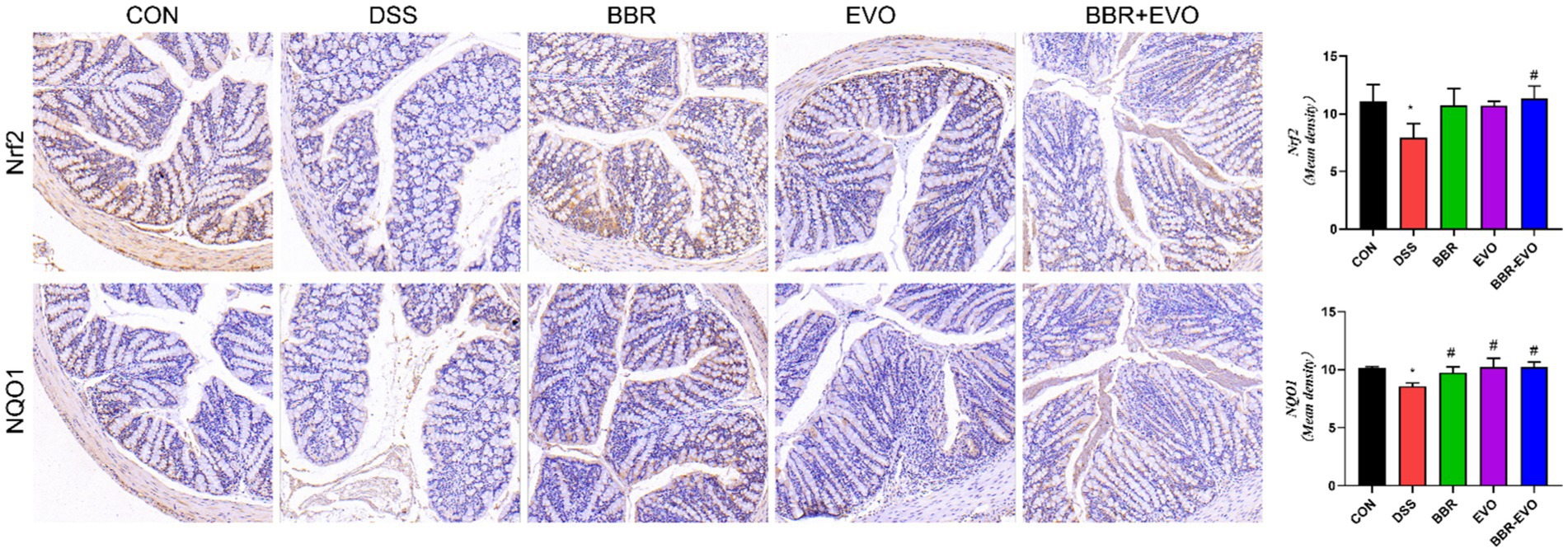
Figure 6. Effects of the main active components of Yulian decoction on the expression of antioxidant proteins nuclear factor erythroid 2-related factor 2 (Nrf2) and NAD(P)H quinone dehydrogenase 1 (NQO1) in the colon tissues of DSS-induced colitis mice, as determined by immunohistochemistry method. Scale bar = 50 μm.
BBR-EVO enhanced the tight junction proteins expressions of colon tissue in DSS-induced colitis mice
Intestinal barrier function is mainly attributed to intercellular tight junction proteins (ZO-1 and occludin) in colon tissue. As a result, we used Western blot and IHC labeling to examine the levels of ZO-1 and occludin expression in mice colon tissues (Figure 7). As predicted, the expression of ZO-1 and occludin was considerably lower in the DSS group than in the control group (p < 0.05). However, preventing BBR-EVO significantly reduced the reduction in ZO-1 and occludin expression caused by DSS (p < 0.05).
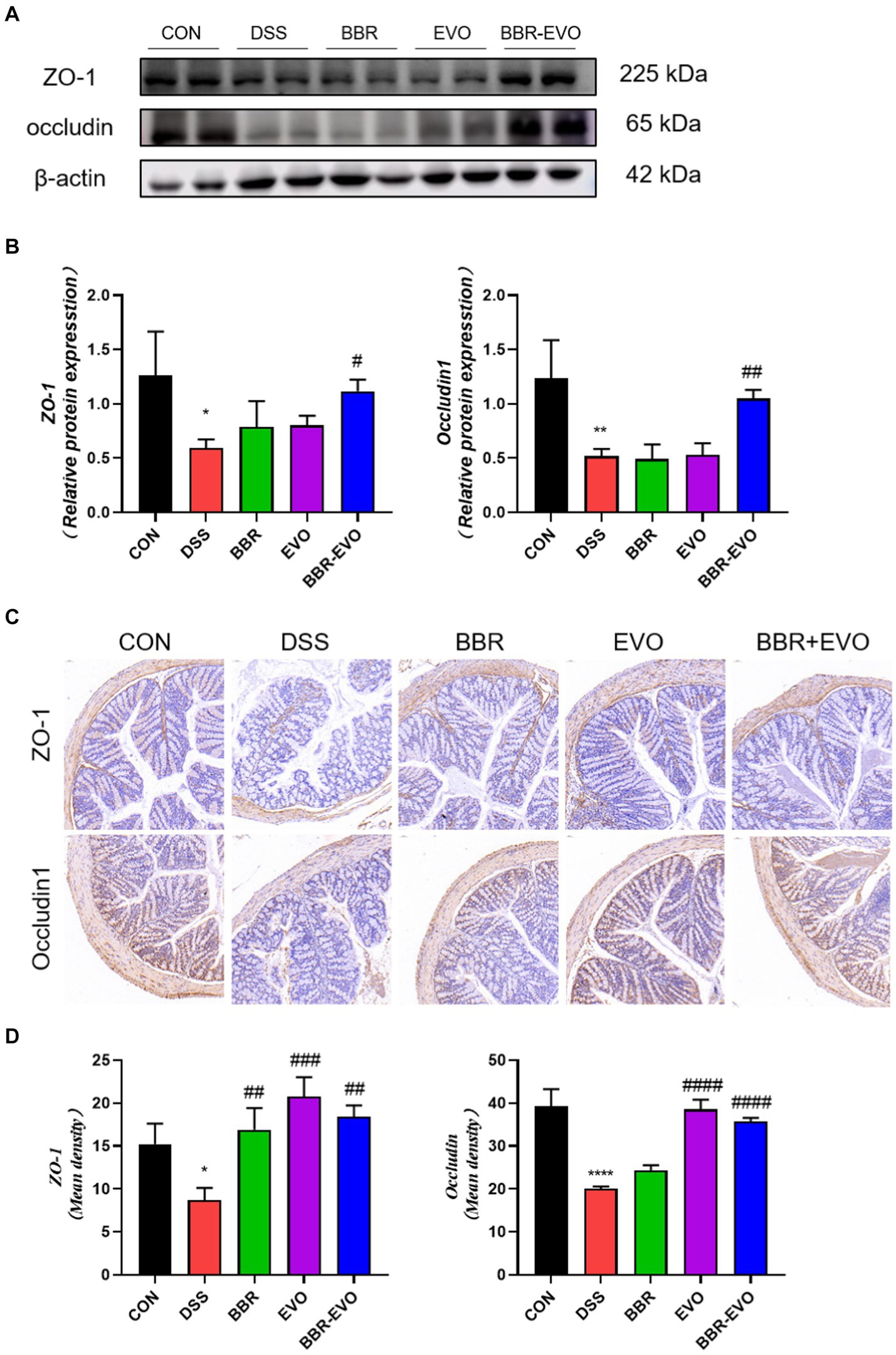
Figure 7. Effects of the active components of Yulian decoction on tight junction function in the colon tissues of colitis mice. (A,B) Protein expression of ZO-1 and occludin in colon tissues. (C,D) Immunohistochemical analysis results of ZO-1 and occludin. Scale bar = 50 μm. Data are presented by mean ± SD; *p < 0.05, **p < 0.01,***p < 0.001, ****p < 0.0001, compared to CON group; #p < 0.05, ##p < 0.01, ###p < 0.001, ####p < 0.0001, compared to DSS group.
Effects of BBR-EVO on the intestinal flora abundance of DSS-induced colitis in mice
We examined the gut microbiome structure to verify the impact of BBR-EVO on it. To confirm the effect of BBR-EVO on the intestinal microbiome, we analyzed the composition of the intestinal microbiome. α-diversity analysis showed that the Shannon and Simpson indices of mice were significantly decreased under DSS influence. However, BBR-EVO intervention significantly increased the Simpson index related to community richness and increased the diversity of microbial colonies to some extent. Whereas the community diversity determined by the Chao1 and Pielou_e indices did not change significantly (Figures 8A–D). The results of the Venn diagram showed that the numbers of different ASVs/OTUs between the BBR-EVO group and the CON group were both higher than those of the DSS group (Figure 8E). We used PCoA analysis, NMDS analysis, and sample hierarchical clustering analysis to measure β-diversity. The results showed that BBR-EVO was significantly distinct from the DSS group (Figures 8F–H). This indicates that BBR-EVO supplementation can significantly regulate the DSS-induced changes in the composition of the intestinal microbial structure.
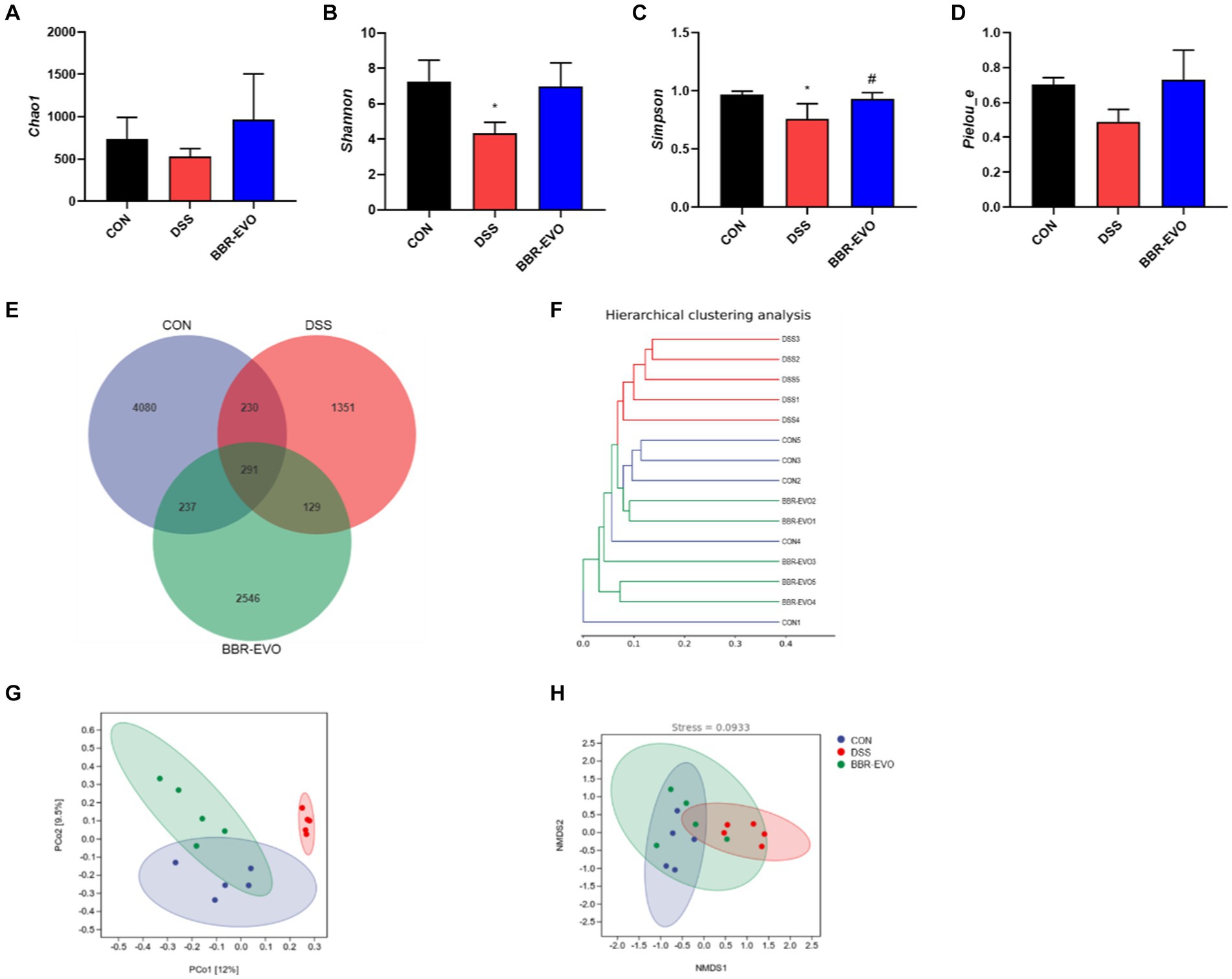
Figure 8. BBR-EVO regulates the abundance and composition of intestinal microbiota of DSS-induced colitis in mice, n = 5. (A–D) Indexes of Chao1, Shannon, Simpson and Pielou_e. (E) Venn diagram. (F) Hierarchical clustering analysis based on unweighted_unifrac distance matrices. (G) PCoA plot based on jaccard distance matrices. (H) NMDS analysis based on weighted_unifrac distance matrices. Data are presented by mean ± SD; *p < 0.05, **p < 0.01,***p < 0.001, ****p < 0.0001, compared to CON group; #p < 0.05, ##p < 0.01, ###p < 0.001, ####p < 0.0001, compared to DSS group.
The differences in gut microbiota composition across groups were further analyzed at the phylum and genus levels (Figure 9). After DSS treatment, mice exhibited a substantial increase in the relative abundance of Proteobacteria (p < 0.05), while the abundance of Firmicutes and Bacteroidetes decreased, and the ratio of Firmicutes to Bacteroidetes (F/B) increased slightly (p > 0.05) (Figures 9A–D; Table 1). The genus-level composition of the top 20 abundant taxa is depicted in Figure 9E. According to genera composition and LEfSe analysis, finding revealed considerable variations and development in the gut microbiome via BBR-EVO administration (Figures 9F,G). Compared to the CON group, the abundance of Psychrobacter and Corynebacterium, two important bacterial genera, was markedly higher in the DSS group, whereas the abundance of Lactobacillus was reduced. Following BBR-EVO administration, the abundance of Psychrobacter (p < 0.01) and Corynebacterium (p < 0.05) were significantly decreased, and the abundance of Lactobacillus was improved, although not significantly (p > 0.05). In addition, after DSS induction, the richness of Adlercreutzia decreased (p < 0.05) and that of Jeotgalicoccus increased (p < 0.05), which were slightly reversed by BBR-EVO (Figure 9G). In summary, whilst BBR-EVO does not re-establish normobiosis within the gastrointestinal microbiome subsequent to DSS perturbation, the data indicate that it may confer modulatory effects on the compositional variability and biodiversity of the gut microbiota. These alterations may contribute to an amelioration of the DSS-induced dysbiosis, suggesting a potential therapeutic role for BBR-EVO in the management of microbiota-mediated gastrointestinal pathologies.
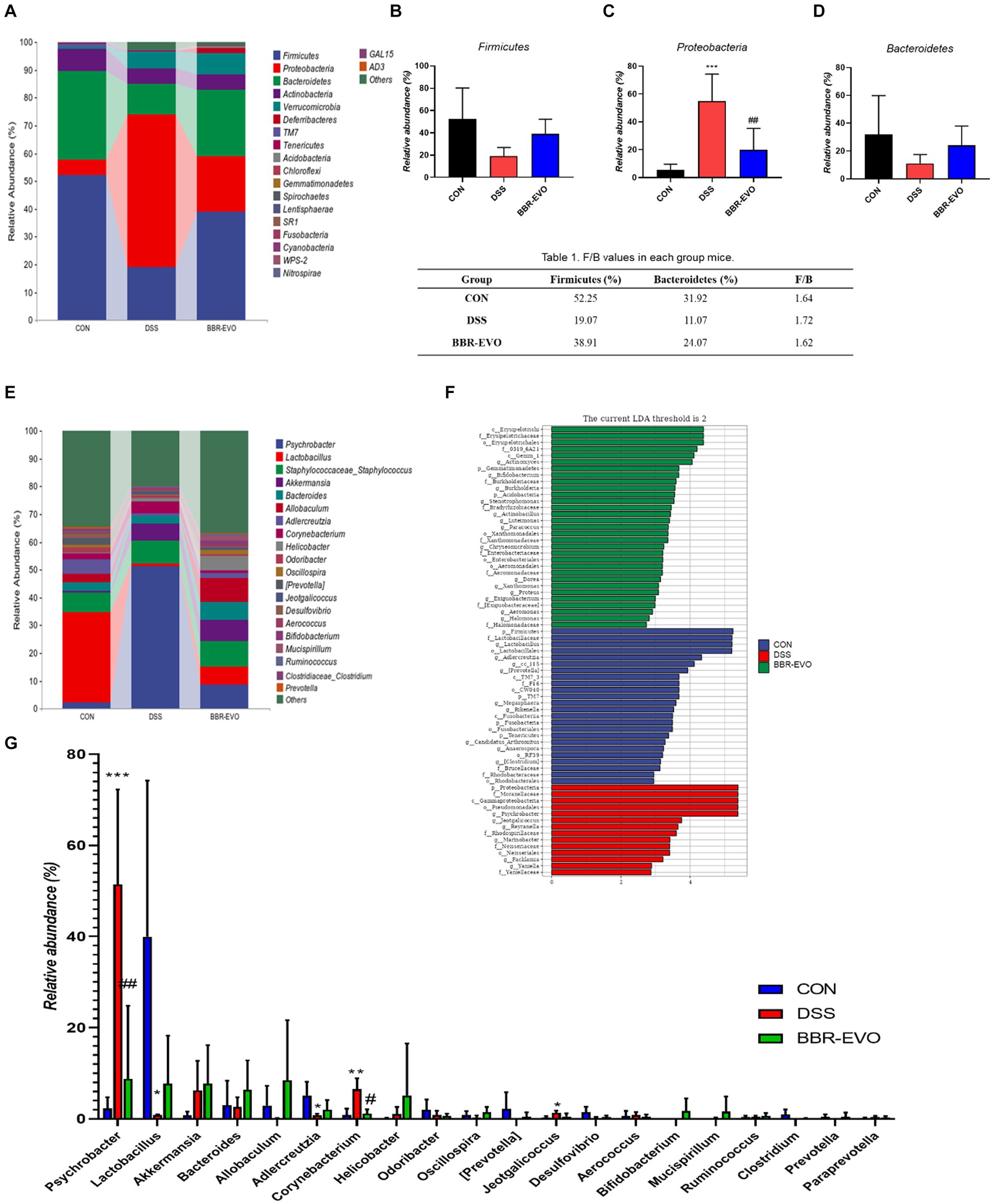
Figure 9. BBR-EVO changes the composition of intestinal microbiota in different taxa levels, n = 5. (A–D) Relative abundance of gut microbiota in Phylum level, Firmicutes abundances, Proteobacteria abundances and Bacteroidetes abundances. (E) Relative abundance of gut microbiota in genus level. (F) LEfSe analysis. (G) Relative abundance of specific genera. Data are presented by mean ± SD; *p < 0.05, **p < 0.01, ***p < 0.001, ****p < 0.0001, compared to CON group; #p < 0.05, ##p < 0.01, ###p < 0.001, ####p < 0.0001, compared to DSS group.
BBR-EVO alleviated liver damage in mice with DSS-induced colitis
This experiment evaluated the attenuating effect of BBR-EVO on colitis-related liver injury. H&E staining and electron microscopy revealed that DSS-induced liver histological damage was characterized by immune cell infiltration, even local hemorrhage, a reduction in mitochondrial count, cellular edema, and mitochondrial vacuolization (Figure 10A). Post-treatment assessment with BBR, EVO and BBR-EVO revealed a diminution in both liver structural and ultrastructural damage in mice. Furthermore, ELISA analyses demonstrated that, relative to the CON group, hepatic concentrations of pro-inflammatory cytokines—IL-6, TNF-α, and IL-1β—were significantly elevated in the DSS group. Contrastingly, these inflammatory markers were substantially reduced in the liver tissues of the mice subjected to the therapeutic interventions (Figure 10B). This suggests that BBR-EVO might exert hepatoprotective effects in the context of DSS-induced colitis.
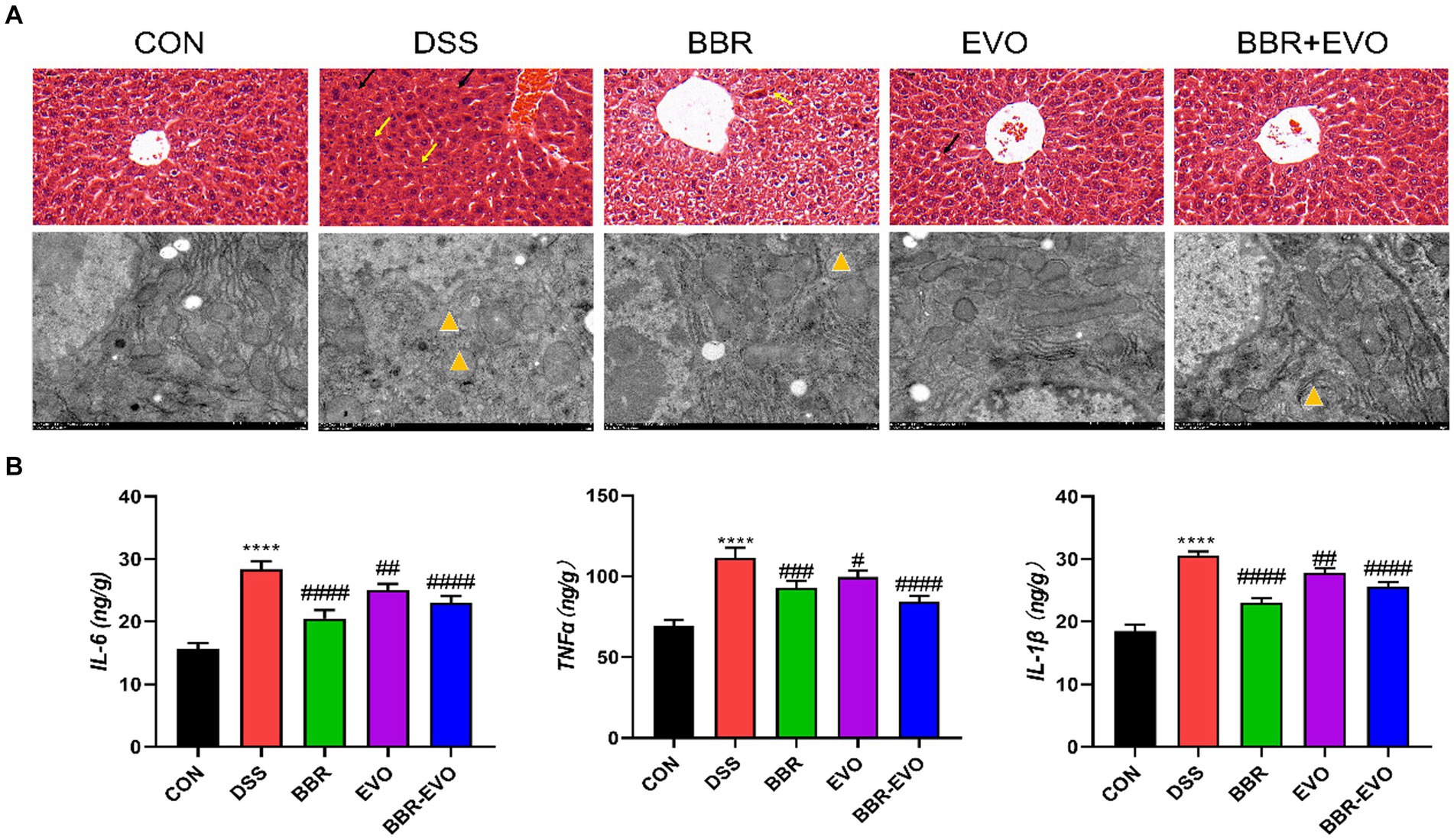
Figure 10. Effects of the active components of Yulian decoction on liver damage in colitis mice. (A) H&E staining (50 μm) and electron microscopy (2 μm). Black arrows indicate bleeding; Yellow arrows indicate inflammatory infiltrate; Yellow triangles show that mitochondria are swollen and vacuolated. (B) IL-6, TNF-α, IL-1β levels, n = 5. Data are presented by mean ± SD; *p < 0.05, **p < 0.01, ***p < 0.001, ****p < 0.0001, compared to CON group; #p < 0.05, ##p < 0.01, ###p < 0.001, ####p < 0.0001, compared to DSS group.
BBR-EVO reduced DSS-treated mice from liver oxidative stress
When compared to colitis mice, liver T-AOC and SOD levels were considerably higher following BBR, EVO, and BBR-EVO intervention, while liver MDA levels were significantly lower (Figure 11A). Similarly, as compared to the DSS group, the frozen section data indicated a drop in ROS levels in the liver, indicating a reduction in oxidative stress (Figure 11B). Furthermore, liver IHC staining revealed that colitis caused oxidative stress in the liver, which BBR-EVO may significantly relieve by stimulating the expression of the Nrf2/NQO1 protein (Figure 11C).
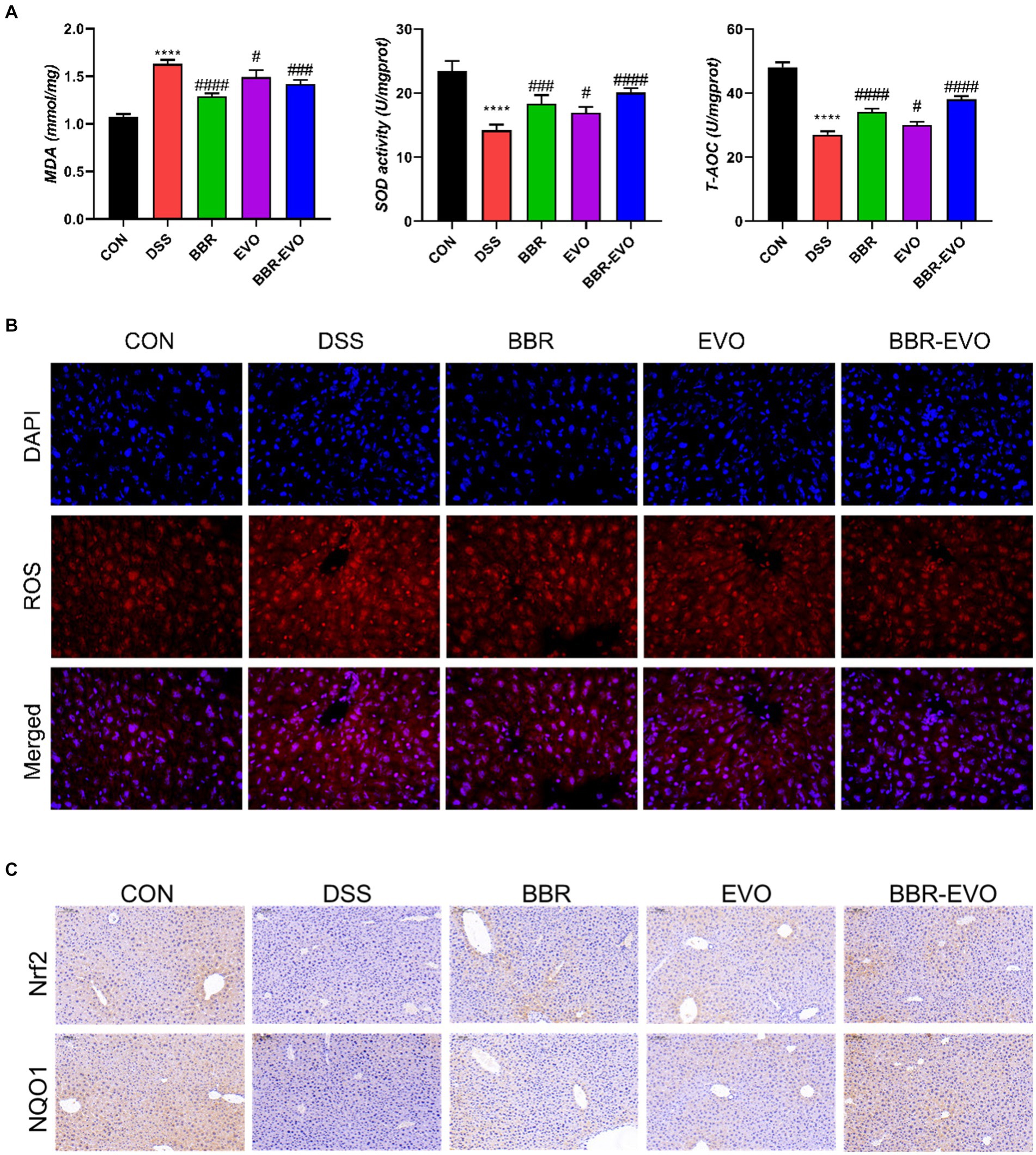
Figure 11. Effects of the active components of Yulian decoction on liver oxidative damage in colitis mice, n = 5. (A) MDA, SOD and T-AOC levels. (B) Fluorescence of ROS (400×). (C) Immunohistochemical analysis results of Nrf2, NQO1. Scale bar = 50 μm. Data are presented by mean ± SD; *p < 0.05, **p < 0.01, ***p < 0.001, ****p < 0.0001, compared to CON group; #p < 0.05, ##p < 0.01, ###p < 0.001, ####p < 0.0001, compared to DSS group.
Discussion
In our study, we explored the therapeutic potential of BBR-EVO, a principal component derived from the traditional Yulian decoction, on a model of colitis elicited by DSS administration. Our findings indicate that BBR-EVO not only attenuates the damage to the colon induced by DSS but also promotes the recovery of intestinal barrier functions and reduces permeability issues. The compound demonstrated a capacity to suppress inflammatory responses in the colon and to effect positive changes in the gut microbiota, which in turn contributed to a decrease in associated liver damage and oxidative stress. This interaction appears to be mediated by the gut-liver axis. Our research methodology was informed by a pioneering concept known as “Preparations Quality Markers” (p-Marker) (Du et al., 2021; Li T. et al., 2021; Ran et al., 2022), which was developed by our team. This innovative approach provides a systematic framework for the identification and quantification of bioactive substances within complex herbal formulations. By applying this strategy, we were able to establish an effective ratio of BBR to EVO, ensuring the therapeutic efficacy of BBR-EVO in our colitis model.
Intestinal health is frequently linked to a wide range of disorders, including cardiovascular disease, gastrointestinal disease, and even cancer. Interestingly, intestinal inflammation can cause an imbalance in the body’s immune, resulting in increased inflammation in other organs such as the liver, breast, and brain (Hu et al., 2023; Zhao et al., 2023; Zou et al., 2023). In recent years, intestinal flora has been considered as a key factor in ulcerative colitis. According to the α-diversity and β-diversity index, DSS reduced the diversity and richness of intestinal flora in fecal samples (Agus et al., 2018). Consistent with previous studies, the relative abundance of gut microbiota was shown at the phylum level. The DSS group in this study also showed ecological imbalance of gut microbiota, including a significant decrease in the abundance of Bacteroides and Firmicutes, a slight increase in the ratio of Firmicutes/Bacteroides (F/B), and an increase in the abundance of Proteobacteria (Shin et al., 2015), accompanied by the loss of microbial diversity. Our study showed that BBR-EVO could reverse the F/B ratio in DSS-treated mice and decreasing the abundance of Proteobacteria. An increase in the F/B ratio is considered a sign of intestinal flora disturbance (Hou et al., 2022). Proteobacteria is thought to be the primary pathogenic bacterium, which is capable of producing endotoxins (Vester-Andersen et al., 2019; Wang et al., 2020; Zhu et al., 2020). The inflammatory reaction to DSS might be connected to the rise in Proteobacteria abundance (Wang et al., 2020). Psychrobacter was prominent in the digestive tracts of DSS-treated mice and belonged to the Proteobacteria genus. Although limited is known about the clinical significance of Psychrobacter, several Psychrobacter species have been known to cause conjunctivitis, peritonitis, endocarditis, infant meningitis, arthritis, surgical wound infection, and bacteremia, mainly in immunocompromised patients (García-López et al., 2014), such as the gut of immunodeficiency virus infection (Qing et al., 2019). We found a significant change in the abundance of Corynebacterium in the gut microbiota. Corynebacterium is considered to be one of the microorganisms associated with bacteremia (Yamamuro et al., 2021). Another study found that cadmium exposure induced Corynebacterium to move from the intestines to the blood (Liu et al., 2023). Jeotgalicoccus is a member of the Staphylococcaceae family known to be associated with colitis (Li H. et al., 2021), which was further confirmed by this study. In addition, Staphylococcus aureus, which frequently leads to inflammation, is especially prevalent in people with digestive system problems (Ikeuchi et al., 2006). In addition, BBR-EVO pretreatment reduced the levels of IL-1β, IL-6 and TNF-α in colon tissue. Adlercreutzia has been shown to be a probiotic or positively associated with human/animal health (Galipeau et al., 2021). After DSS treatment, the abundance of Adlercreutzia in the intestine decreased significantly (Galipeau et al., 2021; Song et al., 2023). In this study, BBR-EVO could reverse the disturbance of DSS on intestinal flora, significantly reduce the richness of Psychrobacter and Corynebacterium, and slightly adjust the richness of Jeotgalicoccus and Adlercreutzia, and confirm that the possible mechanism may be related to the improvement of intestinal function by balancing the micro-environment of the gut microbiota.
IBD is a gastrointestinal dysfunction caused by an immune response. Furthermore, intestinal barrier failure of the context of disease in the organism, accompanied by the aggravation of inflammation, can cause disturbed immune homeostasis in gut and allow harmful exogenous compounds to enter the body and induce liver damage (Cash et al., 2006; Hu et al., 2020; Pabst et al., 2023; Wang et al., 2023; Yang et al., 2023). It has been reported that alkaloids have unique advantages in the treatment of ulcerative colitis due to their multiple targets and high safety (Zhang et al., 2018; Li Q. et al., 2021; Li et al., 2022). This study showed disordered colon tissue with intestinal villus destruction and intercellular tight junction destruction in DSS group mice. The expression levels of Occludin and ZO-1 protein in colon tissue of DSS group mice were significantly decreased, indicating that the tight junction of intestinal mucosal barrier was destroyed after the establishment of ulcerative colitis model, which was consistent with previous research results (Cui et al., 2021). After BBR-EVO intervention, the intestinal tissue structure damage, inflammation and intestinal oxidative stress in mice were alleviated, which was speculated to be related to the repair of intestinal barrier by BBR-EVO. According to our hypothesis, the disruption of intestinal flora, particularly the growth of Proteobacteria, has been proven to be one of the origins of endotoxin (Wang et al., 2020). Flora metabolites or certain flora metabolites reach the liver via the intestinal barrier and cause liver injury (Fang et al., 2018). By measuring the inflammatory indexes of the liver, we proved that the liver tissue of DSS model mice had inflammatory cell infiltration, increased inflammatory factors, and destroyed liver tissue structure. In addition, in the preventive intervention of BBR-EVO, the degree of liver damage in mice was reduced. Our study also demonstrated that BBR-EVO might protect the liver in DSS-induced colitis by activating the Nrf2-ARE-NQO1 pathway and improving mitochondrial function. These findings align with previous research on Nrf2’s role in liver protection (Liu et al., 2024). Increased Nrf2 and NQO1 expression following BBR-EVO treatment is consistent with berberine’s known ability to activate Nrf2 signaling (Li et al., 2023), ultimately increasing the activity of antioxidant enzymes. BBR-EVO also mitigated mitochondrial damage, significant given the role of mitochondrial dysfunction in liver diseases (Qin et al., 2019; Yang et al., 2024). The interplay between Nrf2 activation, antioxidant capacity, and mitochondrial function creates a protective against liver inflammation and oxidative damage.
Although our study confirmed that BBR-EVO has a protective effect on ulcerative colitis mice by regulating the gut-liver axis related to gut microbiota to reduce oxidative stress and inflammatory response in the liver and intestine, there are still some limitations in this study. The relationship between gut bacteria, gut and liver is complex and dynamic. Whether the increase and decrease of related microbiota are related to the degree of liver injury has not been directly proved by more experiments. Therefore, we need to continue to study in vitro and in vivo experiments in the next step.
Data availability statement
The datasets presented in this study can be found in online repositories. This data can be found here: https://www.ncbi.nlm.nih.gov/, accession number PRJNA1150401.
Ethics statement
The animal study was approved by Nanjing Agricultural University. The study was conducted in accordance with the local legislation and institutional requirements.
Author contributions
WW: Conceptualization, Data curation, Methodology, Software, Writing – original draft, Writing – review & editing. YH: Data curation, Formal analysis, Software, Writing – review & editing. WY: Formal analysis, Investigation, Methodology, Software, Writing – original draft, Writing – review & editing. QW: Data curation, Formal analysis, Investigation, Software, Writing – review & editing. YW: Conceptualization, Writing – review & editing. MD: Formal analysis, Funding acquisition, Resources, Supervision, Writing – review & editing.
Funding
The author(s) declare that financial support was received for the research, authorship, and/or publication of this article. This work was financially supported by National Intangible Cultural Heritage Project [IX-4(1)]; China Academy of Chinese Medical Sciences Science and Technology Innovation Project (CI2021A04313).
Conflict of interest
The authors declare that the research was conducted in the absence of any commercial or financial relationships that could be construed as a potential conflict of interest.
Publisher’s note
All claims expressed in this article are solely those of the authors and do not necessarily represent those of their affiliated organizations, or those of the publisher, the editors and the reviewers. Any product that may be evaluated in this article, or claim that may be made by its manufacturer, is not guaranteed or endorsed by the publisher.
Footnotes
References
Agus, A., Planchais, J., and Sokol, H. (2018). Gut Microbiota Regulation of Tryptophan Metabolism in Health and Disease. Cell Host Microbe 23, 716–724. doi: 10.1016/j.chom.2018.05.003
Camuesco, D., Comalada, M., Rodríguez-Cabezas, M. E., Nieto, A., Lorente, M. D., Concha, A., et al. (2004). The intestinal anti-inflammatory effect of quercitrin is associated with an inhibition in iNOS expression. Br. J. Pharmacol. 143, 908–918. doi: 10.1038/sj.bjp.0705941
Cash, H. L., Whitham, C. V., Behrendt, C. L., and Hooper, L. V. (2006). Symbiotic bacteria direct expression of an intestinal bactericidal lectin. Science 313, 1126–1130. doi: 10.1126/science.1127119
Chao, C. Y., Battat, R., Al Khoury, A., Restellini, S., Sebastiani, G., and Bessissow, T. (2016). Co-existence of non-alcoholic fatty liver disease and inflammatory bowel disease: A review article. World J. Gastroenterol. 22, 7727–7734. doi: 10.3748/wjg.v22.i34.7727
Chen, G., Yang, Y., Liu, M., Teng, Z., Ye, J., Xu, Y., et al. (2015). Banxia xiexin decoction protects against dextran sulfate sodium-induced chronic ulcerative colitis in mice. J. Ethnopharmacol. 166, 149–156. doi: 10.1016/j.jep.2015.03.027
Cosnes, J., Gower-Rousseau, C., Seksik, P., and Cortot, A. (2011). Epidemiology and natural history of inflammatory bowel diseases. Gastroenterology 140, 1785–1794.e4. doi: 10.1053/j.gastro.2011.01.055
Cui, L., Guan, X., Ding, W., Luo, Y., Wang, W., Bu, W., et al. (2021). Scutellaria baicalensis Georgi polysaccharide ameliorates DSS-induced ulcerative colitis by improving intestinal barrier function and modulating gut microbiota. Int. J. Biol. Macromol. 166, 1035–1045. doi: 10.1016/j.ijbiomac.2020.10.259
Du, L., and Ha, C. (2020). Epidemiology and Pathogenesis of Ulcerative Colitis. Gastroenterol. Clin. N. Am. 49, 643–654. doi: 10.1016/j.gtc.2020.07.005
Du, M., Li, T., and Shen, S. (2021). Screening of Qualitative Preparation Quality Markers of Yuliantang. Chin. J. Exp. Tradit. Med. Formulae 27, 101–109. doi: 10.13422/j.cnki.syfjx.20201951
Eom, T., Kim, Y. S., Choi, C. H., Sadowsky, M. J., and Unno, T. (2018). Current understanding of microbiota- and dietary-therapies for treating inflammatory bowel disease. J. Microbiol. 56, 189–198. doi: 10.1007/s12275-018-8049-8
Fang, H., Liu, A., Chen, X., Cheng, W., Dirsch, O., and Dahmen, U. (2018). The severity of LPS induced inflammatory injury is negatively associated with the functional liver mass after LPS injection in rat model. J Inflamm 15:21. doi: 10.1186/s12950-018-0197-4
Fukui, H. (2015). Gut-liver axis in liver cirrhosis: How to manage leaky gut and endotoxemia. World J. Hepatol. 7, 425–442. doi: 10.4254/wjh.v7.i3.425
Galipeau, H. J., Caminero, A., Turpin, W., Bermudez-Brito, M., Santiago, A., Libertucci, J., et al. (2021). Novel Fecal Biomarkers That Precede Clinical Diagnosis of Ulcerative Colitis. Gastroenterology 160, 1532–1545. doi: 10.1053/j.gastro.2020.12.004
García-López, M. L., Santos, J. A., Otero, A., and Rodríguez-Calleja, J. M. (2014). “Psychrobacter” in Encyclopedia of Food Microbiology. eds. C. A. Batt and M. L. Tortorello (Oxford: Academic Press), 261–268.
Guo, Q., Lu, T., Zhang, M., Wang, Q., Zhao, M., Wang, T., et al. (2024). Protective effect of berberine on acute gastric ulcer by promotion of tricarboxylic acid cycle-mediated arachidonic acid metabolism. J. Inflamm. Res. 17, 15–28. doi: 10.2147/jir.S436653
Hou, D., Tang, J., Huan, M., Liu, F., Zhou, S., Shen, Q. J. F. S., et al. (2022). Alteration of fecal microbiome and metabolome by mung bean coat improves diet-induced non-alcoholic fatty liver disease in mice. Food Sci. Human Wellness 11, 1259–1272. doi: 10.1016/j.fshw.2022.04.023
Hu, H., Lin, A., Kong, M., Yao, X., Yin, M., Xia, H., et al. (2020). Intestinal microbiome and NAFLD: molecular insights and therapeutic perspectives. J. Gastroenterol. 55, 142–158. doi: 10.1007/s00535-019-01649-8
Hu, S., Lin, Z., Zhao, S., Zhang, B., Luo, L., and Zeng, L. (2023). Pu-erh tea alleviated colitis-mediated brain dysfunction by promoting butyric acid production. Food Chem. Toxicol. 172:113594. doi: 10.1016/j.fct.2022.113594
Ikeuchi, H., Hori, K., Nishigami, T., Nakano, H., Uchino, M., Nakamura, M., et al. (2006). Diffuse gastroduodenitis and pouchitis associated with ulcerative colitis. World J. Gastroenterol. 12, 5913–5915. doi: 10.3748/wjg.v12.i36.5913
Jia, W., Li, H., Zhao, L., and Nicholson, J. K. (2008). Gut microbiota: a potential new territory for drug targeting. Nat. Rev. Drug Discov. 7, 123–129. doi: 10.1038/nrd2505
Kaplan, G. G. (2015). The global burden of IBD: from 2015 to 2025. Nat. Rev. Gastroenterol. Hepatol. 12, 720–727. doi: 10.1038/nrgastro.2015.150
Kaser, A., Zeissig, S., and Blumberg, R. S. (2010). Inflammatory bowel disease. Annu. Rev. Immunol. 28, 573–621. doi: 10.1146/annurev-immunol-030409-101225
Lavelle, A., and Sokol, H. (2020). Gut microbiota-derived metabolites as key actors in inflammatory bowel disease. Nat. Rev. Gastroenterol. Hepatol. 17, 223–237. doi: 10.1038/s41575-019-0258-z
Le Berre, C., Honap, S., and Peyrin-Biroulet, L. (2023). Ulcerative colitis. Lancet 402, 571–584. doi: 10.1016/S0140-6736(23)00966-2
Li, C., Wang, J., Ma, R., Li, L., Wu, W., Cai, D., et al. (2022). Natural-derived alkaloids exhibit great potential in the treatment of ulcerative colitis. Pharmacol. Res. 175:105972. doi: 10.1016/j.phrs.2021.105972
Li, H., Chen, X., Liu, J., Chen, M., Huang, M., Huang, G., et al. (2021). Ethanol extract of Centella asiatica alleviated dextran sulfate sodium-induced colitis: Restoration on mucosa barrier and gut microbiota homeostasis. J. Ethnopharmacol. 267:113445. doi: 10.1016/j.jep.2020.113445
Li, Q., Cui, Y., Xu, B., Wang, Y., Lv, F., Li, Z., et al. (2021). Main active components of Jiawei Gegen Qinlian decoction protects against ulcerative colitis under different dietary environments in a gut microbiota-dependent manner. Pharmacol. Res. 170:105694. doi: 10.1016/j.phrs.2021.105694
Li, T., Zhao, X., and Shen, S. (2021). Screening of quantitative preparation quality markers of Yulian Decoction. China J. Chin. Materia Medica 46, 2728–2736. doi: 10.19540/j.cnki.cjcmm.20210225.302
Li, X., Chen, J., Feng, W., Wang, C., Chen, M., Li, Y., et al. (2023). Berberine ameliorates iron levels and ferroptosis in the brain of 3 × Tg-AD mice. Phytomedicine 118:154962. doi: 10.1016/j.phymed.2023.154962
Liu, J., Zheng, Y., Yang, S., Zhang, L., Liu, B., Zhang, J., et al. (2024). Targeting antioxidant factor Nrf2 by raffinose ameliorates lipid dysmetabolism-induced pyroptosis, inflammation and fibrosis in NAFLD. Phytomedicine 130:155756. doi: 10.1016/j.phymed.2024.155756
Liu, S., Deng, X., Li, Z., Zhou, W., Wang, G., Zhan, J., et al. (2023). Environmental cadmium exposure alters the internal microbiota and metabolome of Sprague-Dawley rats. Front. Vet. Sci. 10:1219729. doi: 10.3389/fvets.2023.1219729
Ohtani, N., and Kawada, N. (2019). Role of the Gut-Liver Axis in Liver Inflammation, Fibrosis, and Cancer: A Special Focus on the Gut Microbiota Relationship. Hepatol. Commun. 3, 456–470. doi: 10.1002/hep4.1331
Pabst, O., Hornef, M. W., Schaap, F. G., Cerovic, V., Clavel, T., and Bruns, T. (2023). Gut-liver axis: barriers and functional circuits. Nat. Rev. Gastroenterol. Hepatol. 20, 447–461. doi: 10.1038/s41575-023-00771-6
Qin, X., Zhao, Y., Gong, J., Huang, W., Su, H., Yuan, F., et al. (2019). Berberine Protects Glomerular Podocytes via Inhibiting Drp1-Mediated Mitochondrial Fission and Dysfunction. Theranostics 9, 1698–1713. doi: 10.7150/thno.30640
Qing, Y., Xie, H., Su, C., Wang, Y., Yu, Q., Pang, Q., et al. (2019). Gut Microbiome, Short-Chain Fatty Acids, and Mucosa Injury in Young Adults with Human Immunodeficiency Virus Infection. Dig. Dis. Sci. 64, 1830–1843. doi: 10.1007/s10620-018-5428-2
Ran, Q., Mu, J., Li, T., Shen, S., and Xu, J. (2022). Research of preparation quality markers of Yulian Tang with anti-inflammatory activity. China J. Chin. Materia Medica 47, 2947–2954. doi: 10.19540/j.cnki.cjcmm.20220118.301
Rogler, G., Singh, A., Kavanaugh, A., and Rubin, D. T. (2021). Extraintestinal Manifestations of Inflammatory Bowel Disease: Current Concepts, Treatment, and Implications for Disease Management. Gastroenterology 161, 1118–1132. doi: 10.1053/j.gastro.2021.07.042
Shin, N. R., Whon, T. W., and Bae, J. W. (2015). Proteobacteria: microbial signature of dysbiosis in gut microbiota. Trends Biotechnol. 33, 496–503. doi: 10.1016/j.tibtech.2015.06.011
Song, G., Gan, Q., Qi, W., Wang, Y., Xu, M., and Li, Y. (2023). Fructose Stimulated Colonic Arginine and Proline Metabolism Dysbiosis, Altered Microbiota and Aggravated Intestinal Barrier Dysfunction in DSS-Induced Colitis Rats. Nutrients 15:782. doi: 10.3390/nu15030782
Ungaro, R., Mehandru, S., Allen, P. B., Peyrin-Biroulet, L., and Colombel, J. F. (2017). Ulcerative colitis. Lancet 389, 1756–1770. doi: 10.1016/S0140-6736(16)32126-2
Vester-Andersen, M. K., Mirsepasi-Lauridsen, H. C., Prosberg, M. V., Mortensen, C. O., Träger, C., Skovsen, K., et al. (2019). Increased abundance of proteobacteria in aggressive Crohn's disease seven years after diagnosis. Sci. Rep. 9:13473. doi: 10.1038/s41598-019-49833-3
Wang, C., Pan, Z., and Jin, Y. (2023). F-53B induces hepatotoxic effects and slows self-healing in ulcerative colitis in mice. Environ. Pollut. 317:120819. doi: 10.1016/j.envpol.2022.120819
Wang, J., Zhu, G., Sun, C., Xiong, K., Yao, T., Su, Y., et al. (2020). TAK-242 ameliorates DSS-induced colitis by regulating the gut microbiota and the JAK2/STAT3 signaling pathway. Microb. Cell Factories 19:158. doi: 10.1186/s12934-020-01417-x
Wang, L., Liu, S., Wang, Y., and Liang, R. (2011). Pharmacodynamics of Yulian cataplasm for treatment of peptic ulcer. Chin. J. Exp. Tradit. Med. Formulae 17, 194–197. doi: 10.13422/j.cnki.syfjx.2011.07.066
Wang, X., Hou, Y., Li, Q., Li, X., Wang, W., Ai, X., et al. (2019). Rhodiola crenulata attenuates apoptosis and mitochondrial energy metabolism disorder in rats with hypobaric hypoxia-induced brain injury by regulating the HIF-1α/microRNA 210/ISCU1/2(COX10) signaling pathway. J. Ethnopharmacol. 241:111801. doi: 10.1016/j.jep.2019.03.028
Wang, Y., Wang, L., and Maobo, D. (2011). Study of Yulian Cataplasm on Analgesic, Anti-inflammatory Actions and Irritation, Hypersensitiveness. Chin. J. Exp. Tradit. Med. Formulae 17, 143–146. doi: 10.13422/j.cnki.syfjx.2011.21.047
Xu, S. Y. (1991). Pharmacological experimental methodology. 3rd Edn. Beijing: People’s Medical Publishing House.
Yamamuro, R., Hosokawa, N., Otsuka, Y., and Osawa, R. (2021). Clinical Characteristics of Corynebacterium Bacteremia Caused by Different Species, Japan, 2014-2020. Emerg. Infect. Dis. 27, 2981–2987. doi: 10.3201/eid2712.210473
Yang, C. Q., Lai, C. C., Pan, J. C., Gao, J., Shen, B. Y., Ru, Y., et al. (2024). Maintaining calcium homeostasis as a strategy to alleviate nephrotoxicity caused by evodiamine. Ecotoxicol. Environ. Saf. 281:116563. doi: 10.1016/j.ecoenv.2024.116563
Yang, Z., Su, H., Lv, Y., Tao, H., Jiang, Y., Ni, Z., et al. (2023). Inulin intervention attenuates hepatic steatosis in rats via modulating gut microbiota and maintaining intestinal barrier function. Food Res. Int. 163:112309. doi: 10.1016/j.foodres.2022.112309
Zhang, S. L., Wang, S. N., and Miao, C. Y. (2017). Influence of Microbiota on Intestinal Immune System in Ulcerative Colitis and Its Intervention. Front. Immunol. 8:1674. doi: 10.3389/fimmu.2017.01674
Zhang, X. J., Yuan, Z. W., Qu, C., Yu, X. T., Huang, T., Chen, P. V., et al. (2018). Palmatine ameliorated murine colitis by suppressing tryptophan metabolism and regulating gut microbiota. Pharmacol. Res. 137, 34–46. doi: 10.1016/j.phrs.2018.09.010
Zhao, C., Hu, X., Qiu, M., Bao, L., Wu, K., Meng, X., et al. (2023). Sialic acid exacerbates gut dysbiosis-associated mastitis through the microbiota-gut-mammary axis by fueling gut microbiota disruption. Microbiome 11:78. doi: 10.1186/s40168-023-01528-8
Zhu, L., Xu, L. Z., Zhao, S., Shen, Z. F., Shen, H., and Zhan, L. B. (2020). Protective effect of baicalin on the regulation of Treg/Th17 balance, gut microbiota and short-chain fatty acids in rats with ulcerative colitis. Appl. Microbiol. Biotechnol. 104, 5449–5460. doi: 10.1007/s00253-020-10527-w
Keywords: Yulian decoction, BBR-EVO, DSS colitis, liver, intestinal dysbiosis
Citation: Wang W, Han Y, Yin W, Wang Q, Wu Y and Du M (2024) Intestinal and hepatic benefits of BBR-EVO on DSS-induced experimental colitis in mice. Front. Microbiol. 15:1428327. doi: 10.3389/fmicb.2024.1428327
Edited by:
Franck Carbonero, Washington State University Health Sciences Spokane, United StatesReviewed by:
Daniele Maria-Ferreira, Instituto de Pesquisa Pelé Pequeno Príncipe, BrazilYina Huang, Anhui Polytechnic University, China
Copyright © 2024 Wang, Han, Yin, Wang, Wu and Du. This is an open-access article distributed under the terms of the Creative Commons Attribution License (CC BY). The use, distribution or reproduction in other forums is permitted, provided the original author(s) and the copyright owner(s) are credited and that the original publication in this journal is cited, in accordance with accepted academic practice. No use, distribution or reproduction is permitted which does not comply with these terms.
*Correspondence: Yi Wu, wuyi2001cn@163.com; Maobo Du, mbdu@icmm.ac.cn
†These authors have contributed equally to this work