- 1Department of Food Science and Engineering, College of Chemistry and Environmental Engineering, Shenzhen University, Shenzhen, China
- 2Hunan Provincial Key Laboratory of Animal Nutritional Physiology and Metabolic Process, National Engineering Laboratory for Pollution Control and Waste Utilization in Livestock and Poultry Production, Institute of Subtropical Agriculture, Chinese Academy of Sciences, Changsha, China
- 3School of Biology and Food Engineering, Fuyang Normal University, Fuyang, China
Introduction: There is a decline in the quality and nutritive value of eggs in aged laying hens. Fruit pomaces with high nutritional and functional values have gained interest in poultry production to improve the performance.
Methods: The performance, egg nutritive value, lipid metabolism, ovarian health, and cecal microbiota abundance were evaluated in aged laying hens (320 laying hens, 345-day-old) fed on a basal diet (control), and a basal diet inclusion of 0.25%, 0.5%, or 1.0% fermented Aronia melanocarpa pomace (FAMP) for eight weeks.
Results: The results show that 0.5% FAMP reduced the saturated fatty acids (such as C16:0) and improved the healthy lipid indices in egg yolks by decreasing the atherogenicity index, thrombogenic index, and hypocholesterolemia/hypercholesterolemia ratio and increasing health promotion index and desirable fatty acids (P < 0.05). Additionally, FAMP supplementation (0.25%−1.0%) increased (P < 0.05) the ovarian follicle-stimulating hormone, luteinizing hormone, and estrogen 2 levels, while 1.0% FAMP upregulated the HSD3B1 expression. The expression of VTG II and ApoVLDL II in the 0.25% and 0.5% FAMP groups, APOB in the 0.5% FAMP group, and ESR2 in the 1% FAMP group were upregulated (P < 0.05) in the liver. The ovarian total antioxidant capacity was increased (P < 0.05) by supplementation with 0.25%−1.0% FAMP. Dietary 0.5% and 1.0% FAMP downregulated (P < 0.05) the Keap1 expression, while 1.0% FAMP upregulated (P < 0.05) the Nrf2 expression in the ovary. Furthermore, 1.0% FAMP increased cecal acetate, butyrate, and valerate concentrations and Firmicutes while decreasing Proteobacteria (P < 0.05).
Conclusion: Overall, FAMP improved the nutritive value of eggs in aged laying hens by improving the liver–blood–ovary function and cecal microbial and metabolite composition, which might help to enhance economic benefits.
1 Introduction
Eggs are excellent sources of nutrients, such as proteins, lipids, and micronutrients, which are highly beneficial to the physical health of humans (Kovacs-Nolan et al., 2005). However, laying hens are afflicted by a series of physiological health complications, resulting in a decline in their egg-laying performance and egg quality with age. Lipid precursor substances in egg yolk are mainly derived from the liver. The yolk formation and deposition of laying hens are largely determined by the multifunction of the liver–blood–ovary (LBO) axis, including lipid metabolism and hormone regulations of various forms (Wu et al., 2023). The recession activities of the liver and ovary in aged laying hens arise from endocrine disorders and decrease in antioxidant capacity and follicle formation (Gu et al., 2021; Wu et al., 2023). Ovarian dysfunction in laying hens results in the decline of egg-laying performance and quality of eggs, for which decrease in antioxidant capacity is one of the main reasons (Bao et al., 2022). Moreover, modulation of gut microbiota is a prospective approach to alleviating the common problems of aged laying hens, such as the decline in the quality and nutritive value of eggs (Feng et al., 2021; Dai et al., 2022). Hence, strengthening the LBO and gut functions in aged laying hens can contribute to improving their egg-laying performance and quality.
Recently, numerous natural plants or their extracts have been employed as feed additives to promote the production of eggs in aged laying hens. Aronia melanocarpa, known as black chokeberry, originated from North America and spread to European and other regions, especially in Poland, with a yearly yield of 14,000–15,000 tons of fresh fruits (Jurikova et al., 2017) and 600–700 tons in China (Ren et al., 2022). It is mainly industrially processed as different food products, such as juice, jams, and dietary supplements for humans (Sidor and Gramza-Michalowska, 2019). After production and processing, it produces large amounts of pomace. Aronia melanocarpa pomace (AMP) contains phenolic phytochemicals, dietary fiber, free glucose, fructose, and other bioactive substances (Schmid et al., 2020). It has been reported that AMP can serve as a feed additive to increase production in animals (Lipinska et al., 2017; Ren et al., 2022). AMP contains a relatively high content of fiber. Meanwhile, avians lack enzymes that degrade non-starch polysaccharides, which may affect the adequate absorption of AMP (Erinle and Adewole, 2022). Microbial fermentation helps to enhance the nutritional quality of agricultural by-products as alternative feed ingredients (Cui et al., 2023) and increases the content and bioavailability of dietary polyphenols (Du and Myracle, 2018; Esatbeyoglu et al., 2023). Fermentation of AMP may improve its nutritive value by decreasing fruit wastes generated in the food industry and feed costs in animal husbandry, which is environmentally friendly and economical. However, studies regarding the influence of the fermented AMP (FAMP) on the production performance, quality and nutritive value of eggs in aged laying hens, and the potential mechanisms of FAMP's action remain unclear. Hence, this study explored the effects of the addition of FAMP to aged laying hens' diet on the production performance, quality and nutritive value of eggs, ovarian function, and cecal microbiota and metabolite composition, which might lay the theoretical foundation for improving the nutritive value of eggs due to the FAMP additive.
2 Materials and methods
2.1 Fermentation of Aronia melanocarpa pomace
Dried and cleaned AMP was obtained from Fuyang Fruit Wine Engineering Technology Center (Fuyang, China). FAMP was prepared according to the following procedure. A compound starter culture was prepared to ferment the raw material (AMP). The compound starter culture was composed of a microbial agent mixture, enzyme, medium 1, medium 2, and sucrose at a mass ratio of 1:1:1:1:1. Among them, the microbial agent mixture (Guangdong Microbial Culture Collection Center, Guangzhou, China) contained Bacillus subtilis GDMCC 1.372, Bacillus licheniensis GDMCC 1.182, Lactobacillus plantarum GDMCC 1.648, and Rhodotorula benthica GDMCC 2.215 at a mass ratio of 1:1:1:1. The active enzyme was a mixture of cellulase and papain at a mass ratio of 1:1. Medium 1 consisted of cultivated ginseng leaves, which were dried and crushed to a 40-mesh powder. Medium 2 was soybean flour. The above compound starter culture and AMP were blended at a mass ratio of 1:100. The fermentation conditions were as follows: fermentation temperature, 75°C; dissolved oxygen, 3–10%; moisture content, 30–80%; and fermentation method, solid fermentation. The measured nutrient levels (%; dry matter basis) of FAMP were as follows: ash, 5.20; crude protein, 10.63; ether extract, 4.80; crude fiber, 15.90; calcium, 0.55; total phosphorus, 0.28; and gross energy, 18.65 MJ/kg. Amino acid and fatty acid profiles of FAMP are presented in Supplementary Tables S1 and S2, respectively.
2.2 Animals, diets, and treatments
A total of 320 Yukou Jingfen No. 8 laying hens that were 345 days old with similar health conditions were selected and randomly allocated into one of the four treatment groups with eight replicates per treatment (ten birds/replicate). The four treatment groups were fed on a basal diet with the addition of 0 (control, CON), 0.25%, 0.5%, and 1.0% FAMP, respectively. The basal diet for laying hens was formulated based on the standard nutriment requirements (Supplementary Table S3). The trial continued for eight weeks and there was a 1-week pre-feeding trial. All laying hens were raised in a controlled environment at 18–24 °C, humidity of 45–60%, and 16 h/day of illumination. The other feeding management followed routine commercial feeding management protocols during the trial.
2.3 Determination of laying performance
The weight and number of eggs and feed intake in each replicate were recorded during the whole trial. Then, average laying rate (ALR), average egg weight (AEW), average daily feed intake (ADFI), and feed-to-egg ratio (FER) were analyzed from weeks 1 to 2, 3 to 4, 5 to 6, 7 to 8, and 1 to 8 of the trial.
2.4 Egg quality analysis
Three eggs per replicate were randomly collected for egg quality measurement every two weeks during the trial. The egg shape index was determined by a Vernier caliper and calculated using previous formulas described by Feng et al. (2021). The eggshell strength, egg weight, albumen height, Haugh unit, and yolk color (Roche colorimetric unit) were measured with an Egg Force Reader and an EggAnalyzer (ORKA Food Technology Ltd., Ramat HaSharon, Israel), respectively. Then, the eggshell, yolk, and albumen were separated, weighed, and their percentages calculated. The eggshell thickness was measured by a hand-held micrometer (Deli Group, Ningbo, China).
2.5 Sample collection and plasma preparation
Three eggs from each replicate were collected to obtain the egg yolk and egg albumen for further nutritive value analyses after the 8-week animal trial. The plasma samples were obtained through wing vein-blood collection and centrifugation and kept at −80°C for plasma hormone, biochemical parameter, and antioxidant indicator analyses. Then, hens from each replicate were euthanized by jugular vein bleeding. The liver and ovary were dissected and kept at −80°C for mRNA extraction, and the ovary samples were also collected for hormone and antioxidant indicator analyses. The cecal contents were collected to determine the microbiota abundance and short-chain fatty acids (SCFA).
2.6 Count of ovarian follicles
In hens' ovaries, numbers of large white (2–5 mm), small yellow (6–8 mm), and hierarchical (>9 mm) follicles were recorded based on previously described methods (Li et al., 2020; Huang et al., 2021).
2.7 Determination of ether extract concentration and fatty acid profiles in yolks
The ether extract level of egg yolks was determined by a Soxhlet extraction with petroleum. The fatty acid composition was determined by employing the area normalization method (Hu et al., 2017). Briefly, total lipids were taken from egg yolks following the chloroform–methanol process. The fatty acid methyl ester was obtained with KOH/methanol and determined by a gas chromatograph (GC2030, Shimadzu Corporation, Kyoto, Japan).
According to the percentage of particular fatty acids of egg yolks, saturated fatty acids (SFA), polyunsaturated fatty acids (PUFA), monounsaturated fatty acids (MUFA), n-3 PUFA, and n-6 PUFA percentages were counted as follows: SFA = C14:0 + C16:0 + C17:0 + C18:0, MUFA = C16:1n-7 + C18:1n-9 + C20:1n-9, n-3 PUFA = C18:3n-3 + C22:6n-3, n-6 PUFA = C18:2n-6 + C18:3n-6 +C20:2n-6 + C20:3n-6 + C20:4n-6, and PUFA= n-3 PUFA + n-6 PUFA. Meanwhile, the health lipid indices related to fatty acids, such as atherogenicity index (AI), thrombogenic index (TI), hypocholesterolemic/hypercholesterolaemic ratio (H/H), health promotion index (HPI), and desirable fatty acids (DFA), were calculated as reported in our previous study (Li et al., 2024): AI = (C12:0 + 4 × C14:0 + C16:0)/UFA, TI = (C14:0 + C16:0 + C18:0)/(0.5 × MUFA + 0.5 × n-6 PUFA + 3 × n-3 PUFA + n-3/ n-6 PUFA), H/H ratio = (C18:1n-9 + C18:2n-6 + C20:4n-6 + C18:3n-3 + C20:5n-3 + C22:5n-3+C22:6n-3)/(C14:0 + C16:0), HPI = (MUFA + PUFA)/(C12:0 + 4 × C14:0 +C16:0), and DFA = C18:0 + MUFA + PUFA.
2.8 Measurement of crude protein and amino acid content in egg albumen
The crude protein content in egg albumen was evaluated by the Kjeldahl method. The content of amino acids in egg albumen was determined using a previously described method (Hu et al., 2017). Briefly, freeze-dried egg albumen samples were prepared using 6 mol/L hydrochloric acid at 110°C for 24 h. Then, the suspensions were filtered and detected by an ion-exchange AA analyzer (LA8080, Hitachi, Tokyo, Japan).
2.9 Analysis of plasma and ovary homogenate hormones
Ovary samples were placed in normal saline, vortexed, and then centrifuged at 4°C for supernatant collection. The protein content in ovary homogenate was measured with a bicinchoninic acid assay kit (Nanjing Jiancheng Bioengineering Institute, Nanjing, China).
The content of estrogen 2 (E2), follicle-stimulating hormone (FSH), and luteinizing hormone (LH) in plasma and ovary homogenate was determined using the enzyme-linked immunosorbent assay (ELISA) kits (Hunan Richamp Biotechnology Co., Ltd., Changsha, China). The optical density (OD) values were obtained on a spectrophotometer (Tecan, Infinite M200 Pro, Basel, Switzerland).
2.10 Determination of plasma and ovary redox status and plasma biochemical parameters
The content of superoxide dismutase (SOD), glutathione peroxidase (GPX), total antioxidant capacity (T-AOC), glutathione (GSH), and malondialdehyde (MDA) in plasma and ovary homogenate was determined by colorimetry (Nanjing Jiancheng Bioengineering Institute, Nanjing, China) on a spectrophotometer (Tecan, Infinite M200 Pro, Basel, Switzerland). The protein content of each ovary homogenate sample was used to normalize the indexes mentioned above. The content of total cholesterol (TC) and triglyceride (TG), alanine aminotransferase (ALT), and aspartate aminotransferase (AST) in plasma was also determined by colorimetry (Nanjing Jiancheng Bioengineering Institute, Nanjing, China) on a spectrophotometer (Tecan, Infinite M200 Pro, Basel, Switzerland).
2.11 RNA extraction and mRNA quantification
The total RNA in both liver and ovary samples was measured using the TransZol agent (TransGen Biotech, Beijing, China). The concentration and purity of extracted RNA samples were evaluated by a Nanophotometer N60 (Implen, GmbH, Germany). The total RNA was reverse transcribed into cDNA following instructions provided in the RT Kit (Accurate Biology, Changsha, China). Quantitative PCR amplification was carried out on the LightCycler R 480II Real-Time PCR System (Roche, Basel, Switzerland) using the qPCR Kit (Accurate Biology, Changsha, China) following the manufacturer's instructions. Characteristic primer sequences devised through the Primer 3 web (https://primer3.ut.ee/) are available in Supplementary Table S4. The relative gene expression was computed with the 2−ΔΔCt method (Livak and Schmittgen, 2001).
2.12 Cecal microbial analysis
The cecal microbiota composition analysis was performed by the Shanghai Personal Biotechnology Co., Ltd., Shanghai, China (Zhu et al., 2022). Briefly, the total microbial DNA of cecal contents was extracted and amplified with specific primers (forward primer: 5′-ACTCCTACGGGAGGCAGCA-3′ and reverse primer: 5′-GGACTACHVGGGTWTCTAAT-3′) to obtain bacterial V3-V4 sequences. After purification and amplification, the PCR products were paired-end sequenced on an Illumina NovaSeq platform (Illumina, San Diego, CA, USA). After quality control and chimera removal, amplified sequence variants (ASVs) were taxonomically aligned with species annotation using the Greengenes database. The α and β diversity indices were analyzed to detect the diversity, richness, and dissimilarity of the microbiota. Kruskal–Wallis test was performed to determine the differential phyla and genera. The linear discriminant analysis (LDA) effect size (LEfSe; LDA ≥ 2, P < 0.05) and random forest analysis were applied to identify and distinguish the microbiota in the four groups. The Kyoto Encyclopedia of Genes and Genomes (KEGG) pathway analysis of the microbiota was conducted with the Phylogenetic Investigation of Communities by Reconstruction of Unobserved States (PICRUSt2) method. The correlation between cecal microbiota abundance (top 50 genera) and phenotypes was performed using Spearman correlation by the R package (|R| > 0.50, P < 0.05).
2.13 Measurement of cecal short-chain fatty acid profile
The concentrations of cecal SCFA were measured according to our previous study (Li et al., 2021) using Agilent 7890A gas chromatograph (Agilent Inc., Palo Alto, CA, USA).
2.14 Statistical analysis
All data analyses were performed using the SPSS 22.0 software (SPSS Inc., Chicago, IL, USA) package. The normality and homogeneity of variances of the data were assessed using the Shapiro–Wilk test and Levene's test, respectively. When applicable, the one-way analysis of variance (ANOVA) following Tukey's post-hoc test was performed to assess significant differences. Otherwise, Welch's ANOVA and Games-Howell tests were performed for the significant difference analyses. The results are expressed as means with standard error of the mean (SEM). P < 0.05 was considered statistically significant, whereas 0.05 ≤ P < 0.1 suggested a trend.
3 Results
3.1 Laying performance and egg quality
Table 1 showed no notable differences (P > 0.05) in the ALR, AEW, and FER among the four groups during 1 to 2, 3 to 4, 5 to 6, 7 to 8, and 1 to 8 weeks of the trial. The ADFI was elevated (P < 0.05) in the 0.25% and 0.5% FAMP groups relative to that in the CON group during 7–8 weeks. The ADFI in the 0.25% FAMP group was higher (P < 0.05) than that of the 1% FAMP group during 7 to 8 weeks, and showed an increasing trend (P = 0.082) during 1 to 8 weeks. As presented in Table 2, the addition of 0.25% FAMP decreased (P < 0.05) the egg shape index relative to that in the CON and 0.5% AMP groups at week 2. The eggshell thickness was higher (P < 0.05) in the 0.25% FAMP group than in the 1% FAMP group at week 6. No notable differences (P > 0.05) were found in the eggshell strength, Haugh unit, albumen height, yolk color, and percentages of eggshell, yolk, and albumen among the four groups throughout the trial.
3.2 The ether extract and fatty acid profiles in the egg yolk
As seen in Table 3, the C16:0 percentage was lower (P < 0.05) in the 0.5% FAMP group than that of the CON group. The C20:4n-6 percentage showed a decrease (P < 0.05) in the 0.25% FAMP group compared to the CON group. Moreover, FAMP supplementation exhibited a declining trend (P = 0.059) in the C20:3n-6 percentage compared to the CON group. Additionally, the SFA percentage and AI and TI indexes were reduced (P < 0.05), whereas H/H, HPI, and DFA indexes were elevated (P < 0.05) in the 0.5% FAMP group by comparison with the CON group. PUFA, PUFA/SFA, n-3 PUFA, and n-6 PUFA in the four groups showed no obvious distinctions (P > 0.05).
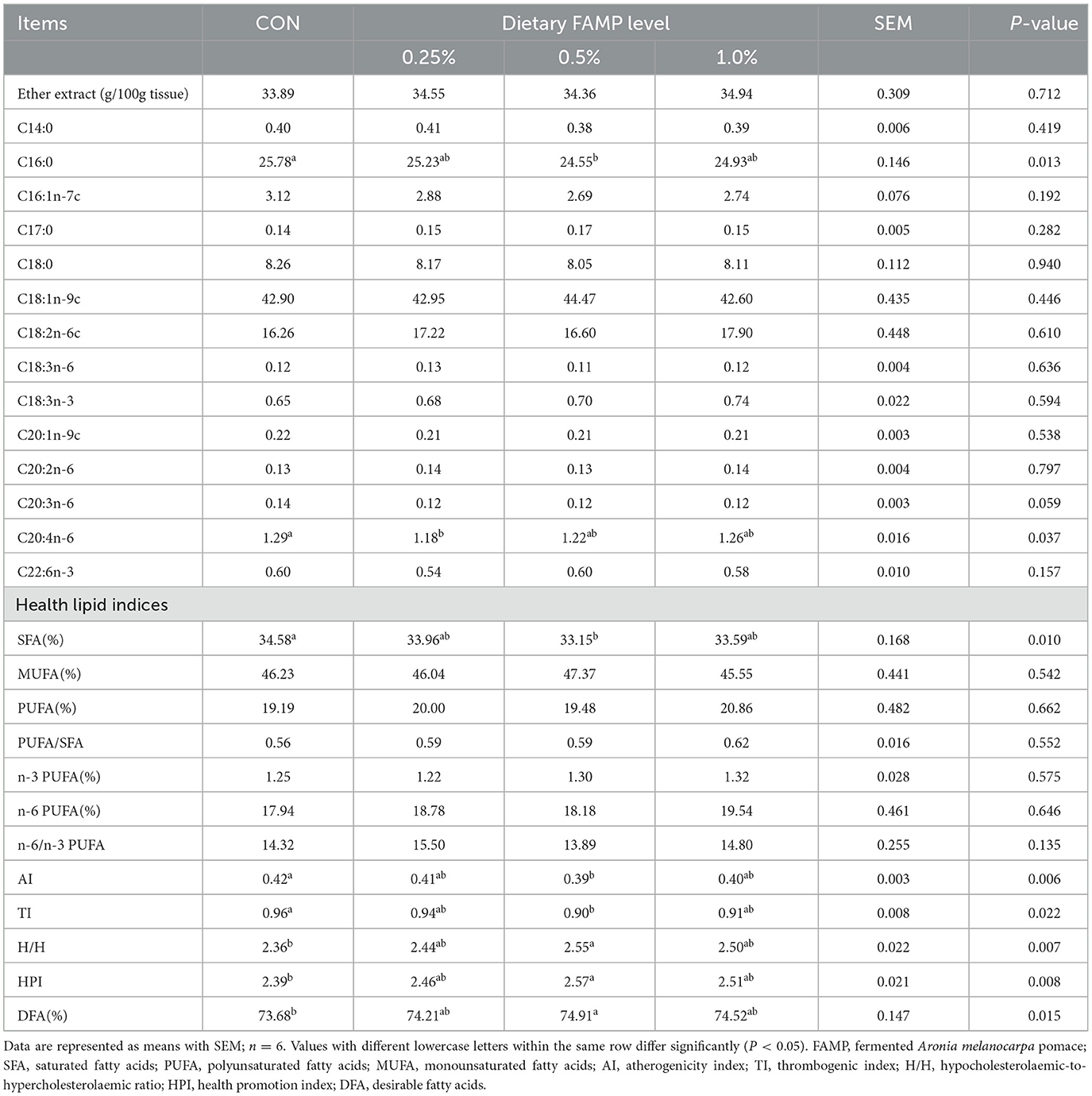
Table 3. Effects of FAMP on ether extract concentration, fatty acid profile, and health lipid indices of egg yolk.
3.3 Crude protein and amino acid content in egg albumen
The valine content in egg albumen was reduced (P < 0.05) in the 0.25% FAMP group than in the CON group. The 0.25% and 0.5% FAMP groups exhibited a decreasing trend with regard to the content of histidine (P = 0.093), proline (P = 0.060), and branched-chain amino acid (BCAA, P = 0.089) of egg albumen relative to the CON group (Table 4).
3.4 The number of ovarian follicles, hormone levels of plasma and ovary, and hormone synthesis and receptor genes in the ovary
The results of ovarian follicles are shown in Figure 1A. The number of large white follicles in the FAMP groups exhibited an increasing trend (P = 0.092) relative to the CON group. There were no significant changes (P > 0.05) in plasma FSH, LH, and E2 contents among the four groups (Figure 1B). However, ovary FSH, LH, and E2 levels were higher (P < 0.05) in the 0.25%, 0.5%, and 1.0% FAMP groups relative to the CON group (Figure 1C). The ovary HSD17B1 expression was upregulated (P < 0.05) in the 1.0% FAMP group relative to the CON group. The mRNA expression involved in hormonogenesis (including CYP11A1, HSD3B1, CYP17A1, and CYP19A1) and hormone receptors (including ESR1, ESR2, FSHR, and LHCGR) in ovarian tissues did not differ among the four groups (Figures 1D, E).
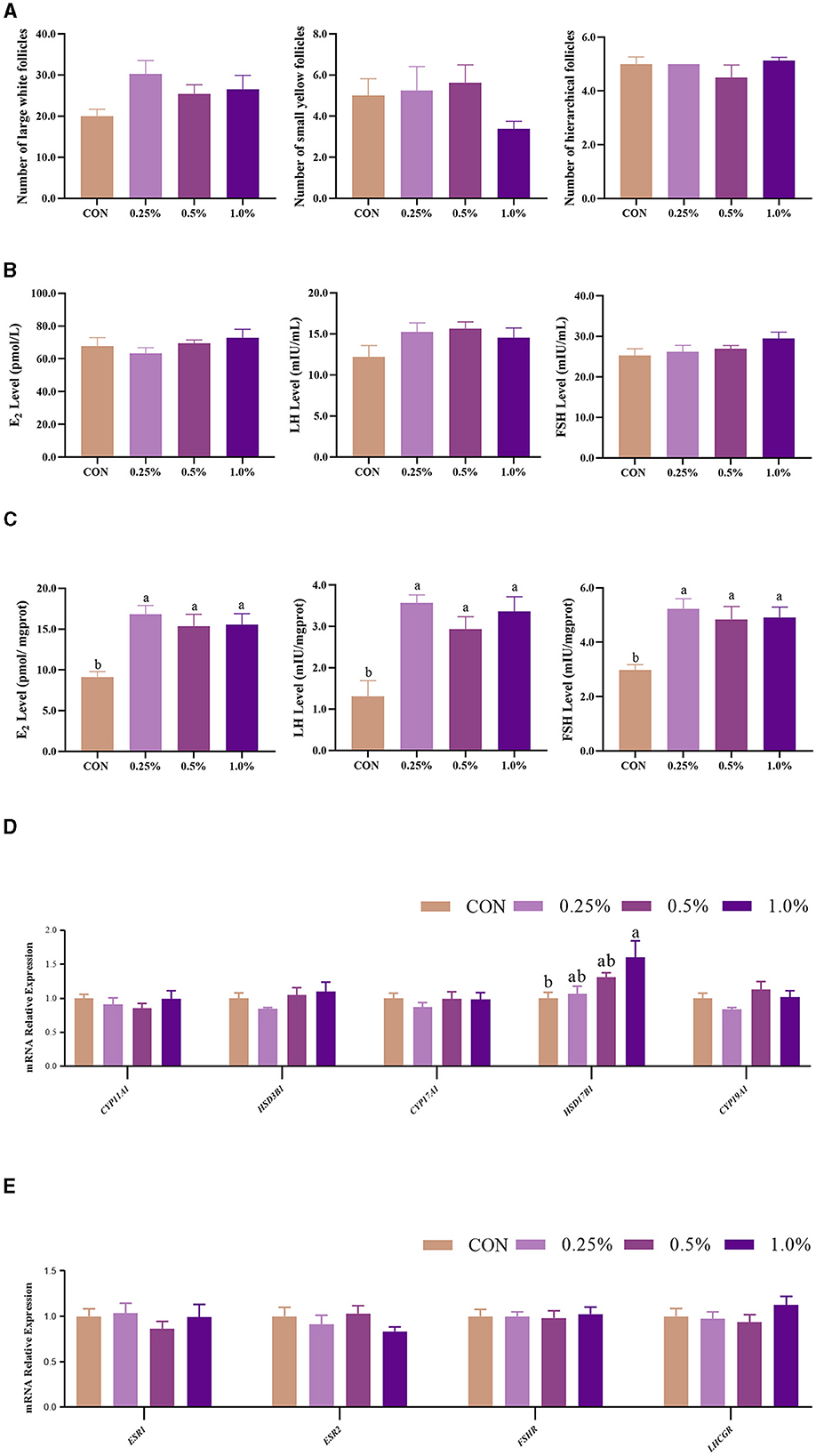
Figure 1. FAMP affected the number of follicles, hormone levels, and gene expression related to hormone synthesis/receptors. The number of follicles (A), hormone levels in plasma (B) and ovary (C), and gene expression related to hormone synthesis (D) and receptor (E) of the ovary. The hens in the CON, 0.25%, 0.5%, and 1.0% groups were fed the basal diet supplemented with 0, 0.25%, 0.5%, and 1.0% fermented Aronia melanocarpa pomace (FAMP), respectively. Data are represented as means with SEM; n = 8. Values with different lowercase letters in the histogram differ significantly (P < 0.05). CYP11A1, cytochrome P450 family 11 subfamily A member 1; HSD3B1, 3 beta- and steroid delta-isomerase 1; CYP17A1, cytochrome P450 family 17 subfamily A member 1; HSD17B1, hydroxysteroid 17-beta dehydrogenase 1; CYP19A1, cytochrome P450 family 19 subfamily A member 1; ESR1, estrogen receptor 1; ESR2, estrogen receptor 2; FSHR, follicle stimulating hormone receptor; LHCGR, luteinizing hormone/choriogonadotropin receptor.
3.5 Plasma biochemical indicators and expression of genes involved in egg yolk precursor synthesis and transport
As shown in Figure 2A, plasma TC, TG, ALT, and AST levels of the four groups showed no significant differences (P > 0.05). The gene expression involved in the synthesis of yolk precursor (including ACC, FAS, and SCD1) and ESR2 in the liver was upregulated (P < 0.05) in the 1.0% FAMP group compared with the CON group (Figures 2B, E). The gene expressions of the liver-respecting egg yolk precursor transportation (including VTG II, ApoVLDL II, and APOB) were upregulated (P < 0.05) in the 0.5% FAMP group relative to the CON group (Figure 2C). As for the VLDR gene expression of the ovary, no difference (P > 0.05) was observed among the four groups (Figure 2D).
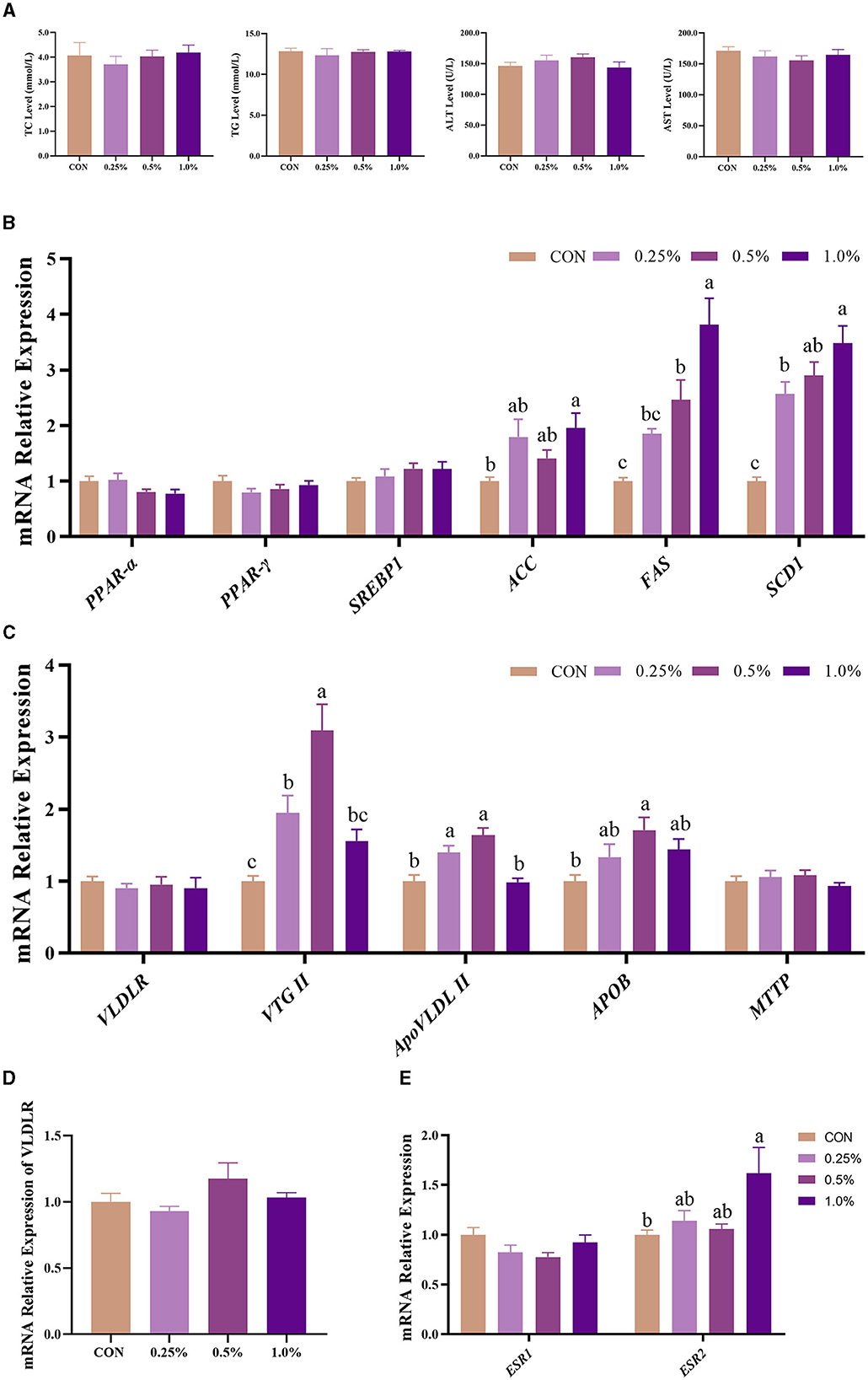
Figure 2. FAMP affected plasma biochemical indicators and egg yolk precursors synthesis and transport of the liver. The plasma biochemical indicators (A) and expression of genes related to egg yolk precursor synthesis (B) and transport (C) of the liver. The mRNA expression of VLDLR of the ovary (D) and ESR1 and ESR2 of the liver (E). The hens in the CON, 0.25%, 0.5%, and 1.0% groups were fed the basal diet supplemented with 0, 0.25%, 0.5%, and 1.0% fermented Aronia melanocarpa pomace (FAMP), respectively. Data are represented as means with SEM; n = 8. Values with different lowercase letters in the histogram differ significantly (P < 0.05). ACC, acetyl-CoA carboxylase; APOB, apolipoprotein B; ApoVLDL II, apo very low-density lipoprotein II; ESR1, estrogen receptor 1; ESR2, estrogen receptor 2; FAS, fatty acid synthase; MTTP, microsomal triglyceride transfer protein; PPARα, peroxisome proliferator-activated receptor alpha; PPAR-γ, peroxisome proliferator-activated receptor gamma; SCD1, stearoyl-CoA desaturase 1; SREBP1, sterol regulatory element binding protein 1; TC, total cholesterol; TG, triglyceride; VLDLR, very low-density lipoprotein receptor; VTG II, vitellogenin 2.
3.6 Plasma and ovary redox status and the Keap1/Nrf2 pathway of the ovary
The levels of plasma redox status-related parameters, including T-AOC, GSH, GSH-PX, SOD, and MDA, were not changed (P > 0.05) by dietary FAMP supplementation (Figure 3A). In the ovary, T-AOC (P < 0.05) and SOD (P = 0.064) levels were increased in the 0.5% FAMP group in comparison to the CON group (Figure 3B). Furthermore, the Keapl expression of the 0.5% and 1.0% FAMP groups was decreased (P < 0.05) relative to the CON group. Moreover, the Nrf2 expression of the 0.5% FAMP group was higher (P < 0.05) than in the 1.0% FAMP group.
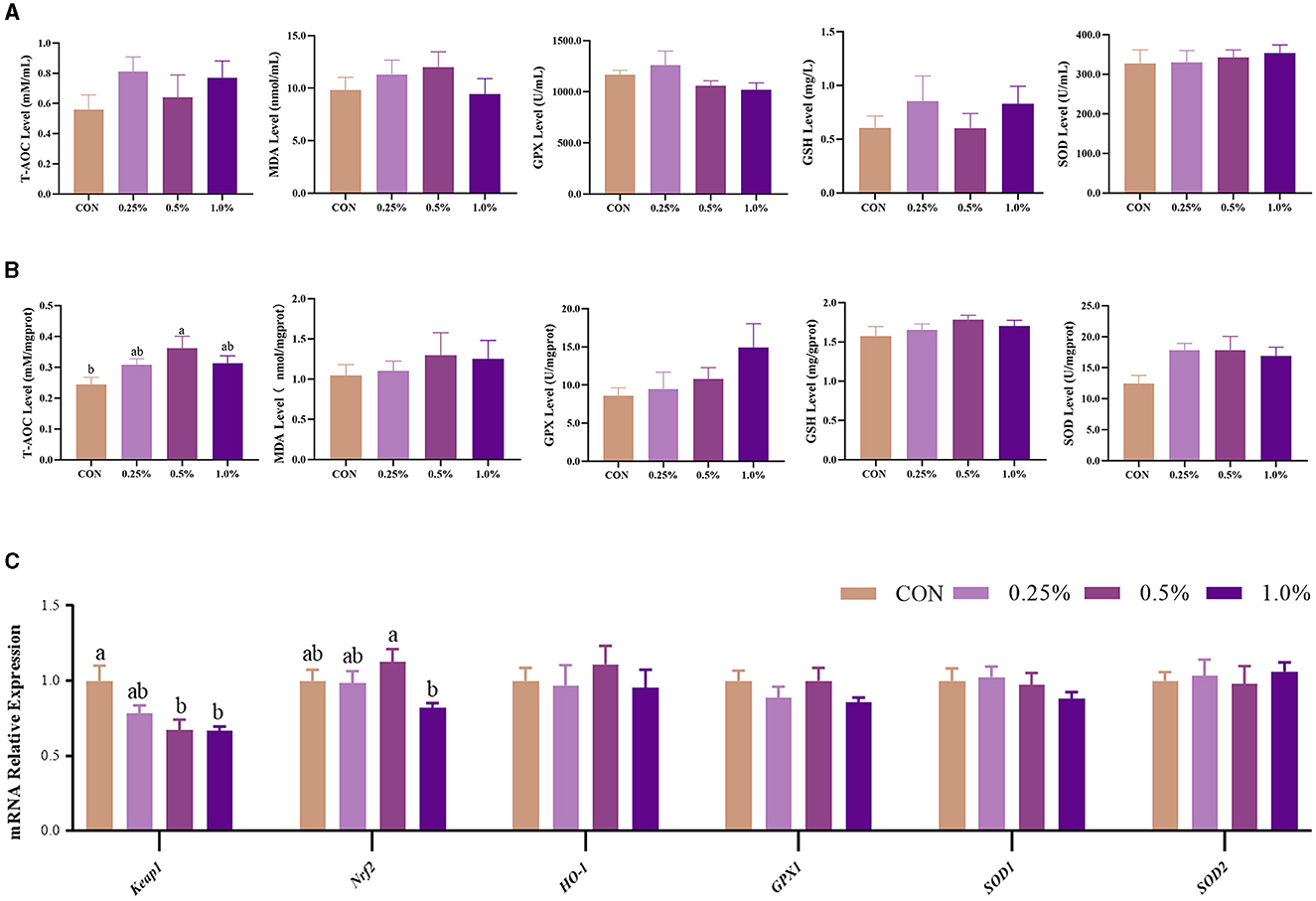
Figure 3. FAMP-affected the antioxidant capacity of the plasma and ovary. The plasma (A) and ovary (B) redox level and gene expression in the Keap1/Nrf2 signaling pathway of the ovary (C). The hens in CON, 0.25%, 0.5%, and 1.0% groups were fed the basal diet supplemented with 0, 0.25%, 0.5%, and 1.0% fermented Aronia melanocarpa pomace (FAMP), respectively. Data are represented as means with SEM; n = 8. Values with different lowercase letters in the histogram differ significantly (P < 0.05). T-AOC, total antioxidant capacity; SOD, superoxide dismutase; GSH, glutathione; GPX, glutathione peroxidase; MDA, malondialdehyde; Keap1, Kelch-like ECH-associated protein 1; Nrf2, NF-E2-related factor 2; HO-1, heme oxygenase 1; GPX1: glutathione peroxidase 1; SOD1, superoxide dismutase 1; SOD2, superoxide dismutase 2.
3.7 Microbiota structure and community in cecal contents
The results of the α-diversity are presented in Figure 4A. The Observed_species, Chao1, and Faith-pd indexes were higher, whereas the Goods coverage index was lower in the 0.25% FAMP group relative to the CON group (P < 0.05). The Pielou_e index of the 1.0% FAMP group was higher (P < 0.05) relative to the 0.5% FAMP group. The β-diversity analysis (non-metric multidimensional scaling ordination plot) indicated that cecal microbial communities were differentiated between the CON and the three FAMP groups (Figure 4B). Bacteroidetes, Firmicutes, and Proteobacteria predominated in the cecal phyla (Figure 4C). An increase (P < 0.05) in the relative abundance of Bacteroidetes was found in the 0.25% and 0.5% FAMP groups relative to the 1.0% FAMP group. The relative abundance of Firmicutes was increased (P < 0.05) in the 1.0% FAMP group relative to the other three groups. Especially, we found a decrease (P < 0.05) in the relative abundance of Proteobacteria in the FAMP groups in comparison to the CON group (Figure 4E). Furthermore, the top ten genera were Bacteroides, Lactobacillus, Megamonas, Faecalibacterium, Phascolarctobacterium, Prevotella, Oscillospira, [Ruminococcus], Desulfovibrio, and Subdoligranulum (Figure 4D). Notably, the relative abundance of Lactobacillus of the 1.0% FAMP group was the highest (P < 0.05) among all groups. The 0.5% and 1% FAMP groups exhibited a decrease in the relative abundances of Oscillospira and [Ruminococcus] (P < 0.05) relative to the 0.25% FAMP group. The relative abundance of [Ruminococcus] of the 0.5% FAMP group was decreased (P < 0.05) relative to the CON group (Figure 4F).
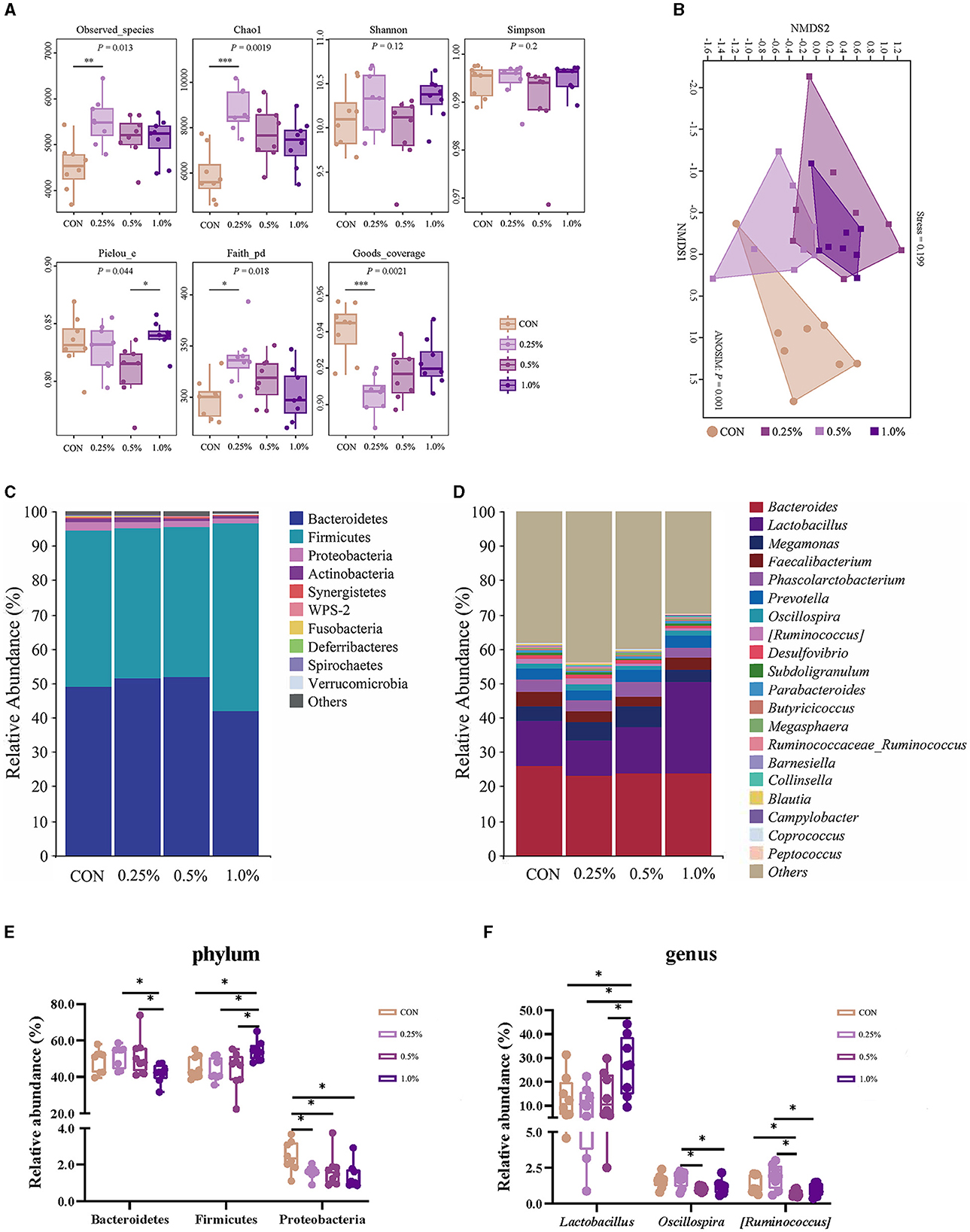
Figure 4. FAMP affected the cecal microbiota composition. The α- (A) and β-diversity (B) indices and community composition of cecal microbiota of aged laying hens at the phylum (C, E) and genus (D, F) levels. The hens in the CON, 0.25%, 0.5%, and 1.0% groups were fed the basal diet supplemented with 0, 0.25%, 0.5%, and 1.0% fermented Aronia melanocarpa pomace (FAMP), respectively. Data are represented as means with SEM; n = 8. *P < 0.05.
3.8 Cecal microbiota biomarkers and function prediction
As presented in Figure 5A, the LEfSe analysis of taxa with LDA score ≥ 2 showed that Proteobacteria in the CON group; Armatimonadetes, Chloroflexi, GNO4, OD1, Planctomycetes, and WS3 in the 0.25% FAMP group, Bacteroidetes in the 0.5% FAMP, and Firmicutes in the 1.0% FAMP group were enriched. At the genus level, Streptomyces, Symbiobacterium, Methylobacterium, Massilia, Shigella, Acinetobacter, and Pseudomonas in the CON group, Alistipes, Candidatus_Brocadia, Nitrosomonas, and Psychrobacter in the 0.25% FAMP group, Weissella and Streptococcus in the 0.5% FAMP group, and Lactobacillus, Acetobacter, and Ralstonia in the 1.0% FAMP group were enriched. The random forest model was performed to further identify the biomarkers. As shown in Figure 5B, the top five marker genera among the four groups were Methylobacterium, Ralstonia, Alistipes, Olsenella, and Weissella at the genus level. Meanwhile, the 0.25% FAMP group had increased Alistipes, Olsenella, Oscillospira, and others. Furthermore, the pathways at level 3 were further identified (Figure 5C). The results showed that the CON, 0.25%, 0.5%, and 1.0% FAMP groups significantly enriched 5, 1, 3, and 31 pathways, respectively. There were five significantly enriched pathways in the CON group, namely, bacterial chemotaxis, metabolism of xenobiotics by cytochrome P450, geraniol degradation, biosynthesis of unsaturated fatty acids, and hypertrophic cardiomyopathy. The pathway enriched in the 0.25% FAMP group was valine, leucine, and isoleucine degradation. There were three significantly enriched pathways in the 0.5% FAMP group, namely polyketide sugar unit biosynthesis, biotin metabolism, and lipopolysaccharide biosynthesis. The 1.0% FAMP group enriched 31 pathways, and the top 10 pathways included D-alanine metabolism, D-glutamine and D-glutamate metabolism, aminoacy1-tRNA biosynthesis, mismatch repair, peptidoglycan biosynthesis, ribosome, pentose phosphate pathway, lysine biosynthesis, homologous recombination, and dioxin degradation.
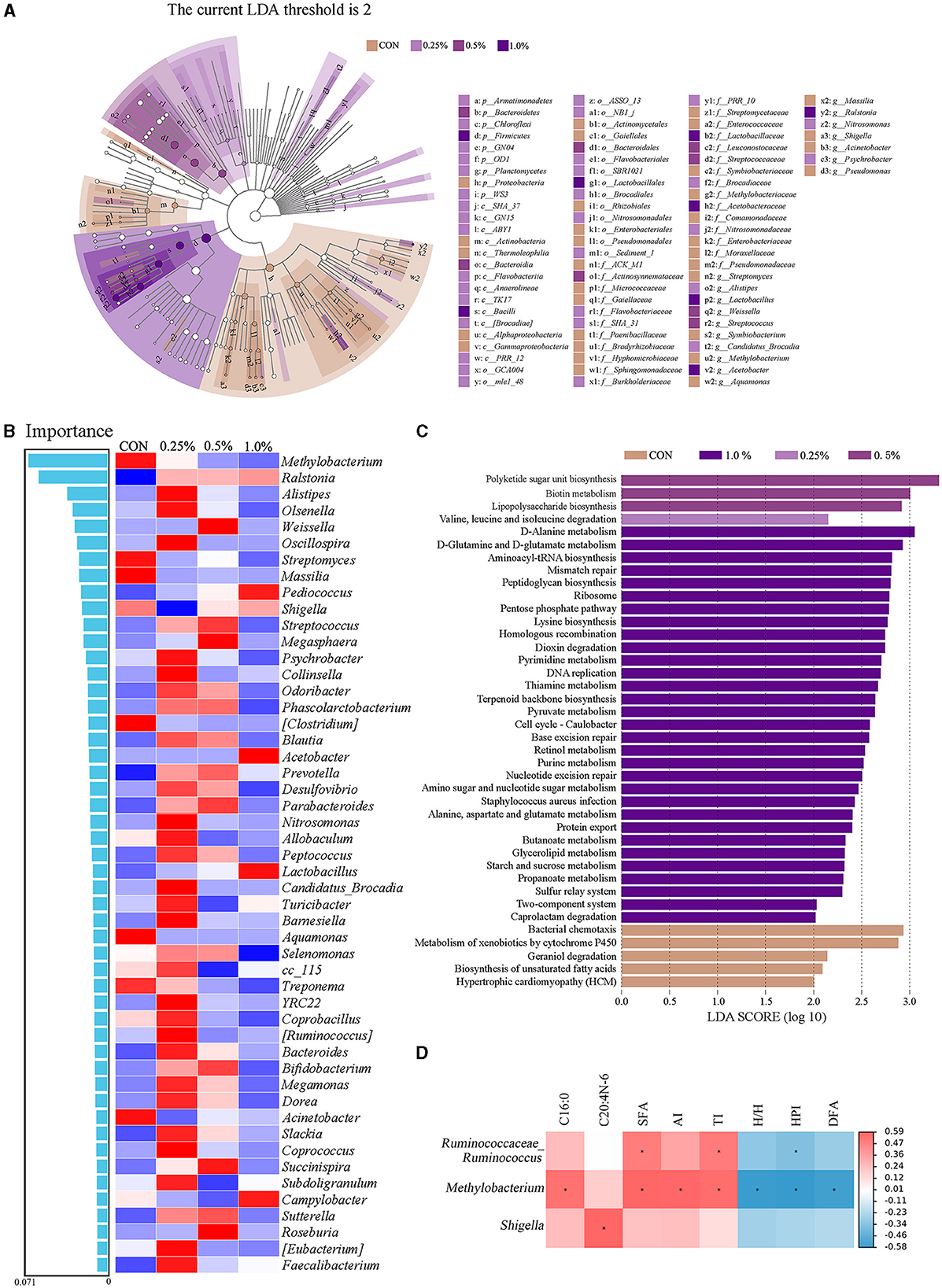
Figure 5. FAMP affected the predicted microbiota functions in cecal contents. Linear discriminant analysis effect size (LEfSe analysis, LDA score ≥ 2) for taxonomic abundance analysis (A), random forest for identifying microbial markers (B), different enrichment pathways for predicted function (C), and correlation analysis (|R| > 0.50, P < 0.05) between microbiota (top 50 genera) and differential fatty acid indices (D) of cecal microbiota of aged laying hens. The hens in the CON, 0.25%, 0.5%, and 1.0% groups were fed the basal diet supplemented with 0, 0.25%, 0.5%, and 1.0% fermented Aronia melanocarpa pomace (FAMP), respectively.
3.9 Correlations between microbiota and differential fatty acid indices of egg yolk
As shown in Figure 5D, the positive correlations were obtained between Ruminococcaceae_Ruminococcus with SFA and TI, Methylobacterium with C16:0, SFA, AI, and TI, and Shigella with C20:4n-6 (|R| > 0.50, P < 0.05). The negative correlations (|R| > 0.50, P < 0.05) were obtained between Ruminococcaceae_Ruminococcus with HPI, and Methylobacterium with H/H, HPI, and DFA.
3.10 Cecal short-chain fatty acid composition
The levels of cecal SCFA of aged laying hens are presented in Figure 6. The levels of acetate, propionate, butyrate, and valerate were increased (P < 0.05) in the 1.0% FAMP group relative to the 0.25% FAMP group. Dietary 0.25%−1.0% FAMP did not (P > 0.05) affect the level of isobutyrate.
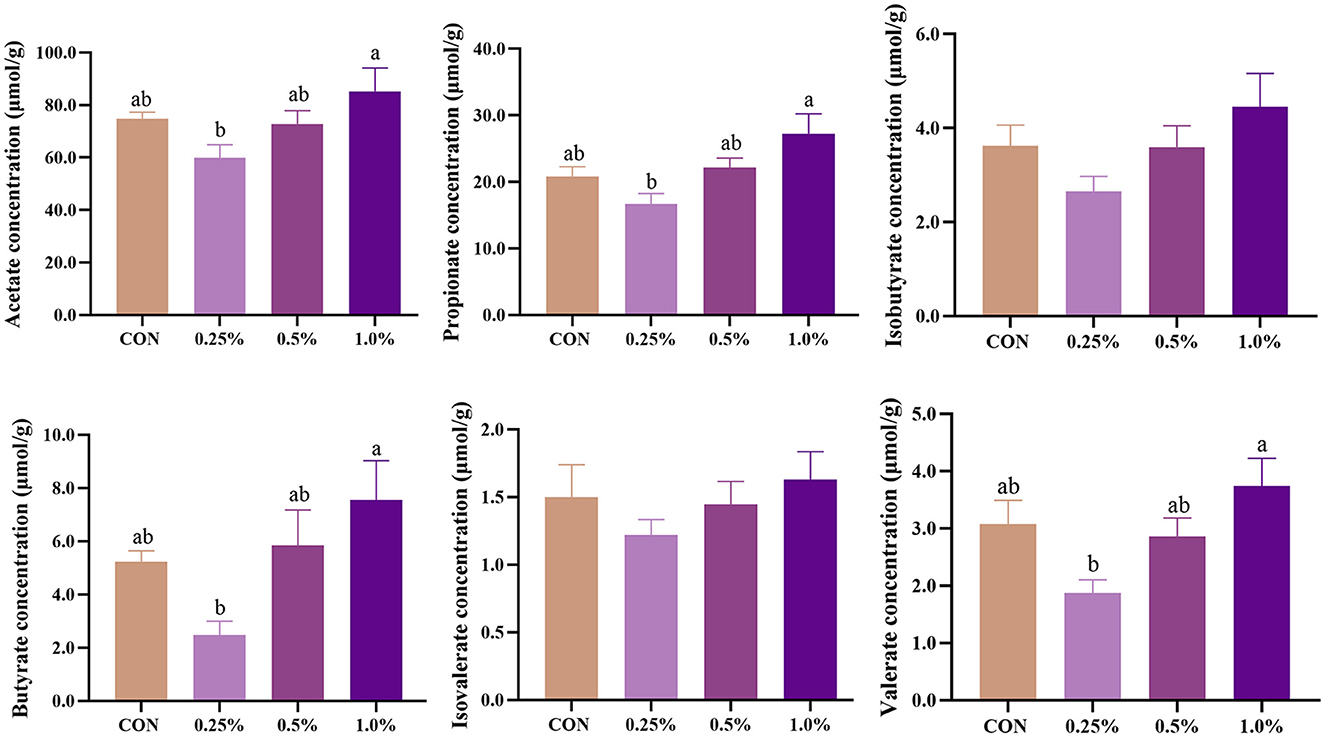
Figure 6. FAMP affected the concentrations of short-chain fatty acids in cecal content. The hens in CON, 0.25%, 0.5%, and 1.0% groups were fed the basal diet supplemented with 0, 0.25%, 0.5%, and 1.0% fermented Aronia melanocarpa pomace (FAMP), respectively. Data are represented as means with SEM; n = 8. Values with different lowercase letters in the histogram differ significantly (P < 0.05).
4 Discussion
Increasing evidence suggests that a reduction in performance and egg quality influences the nutritive and economic value of eggs in aged hens after high-intensity metabolism at the peak laying period (He et al., 2023), which refers to the stage after 48 weeks of age, accounting for about half of the entire laying period. Therefore, modulating the aging of the ovary is essential to enhance the egg quality of aged laying hens. In this trial, we observed the addition of FAMP improved the health lipid indices of yolk and nutritive value of eggs, enhanced lipid metabolism in the LBO axis, regulated the reproductive hormone metabolism, increased ovarian antioxidant function and cecal SCFA, and reshaped cecal microbiota composition in Yukou Jingfen No. 8 laying hens at 50 and 58 weeks of age. Thus, the findings support the reuse of fermented AMP, a byproduct of the food industry, in the feed of laying hens to enhance the nutritive value of eggs.
Laying performance and egg quality are important indicators in the poultry industry, and these parameters are dependent on the diverse organs participating in reproduction. Aging can alter the body's genome, proteome, and metabolome, leading to diverse damages and metabolic dysfunctions, hindering the normal function of multiple organs, such as the ovary, liver, and intestine (Li G. et al., 2023). Our results showed that FAMP (0.25%−1.0%) supplementation did not significantly improve the laying performance (including ALR, AEW, and FER) and egg quality (e.g., yolk color, Haugh unit, albumen height, and other indicators). Similarly, supplementation of 3% dried AMP (Sosnówka-Czajka and Skomorucha, 2021), 2.5% AMP (Loetscher et al., 2014), and 4% grape pomace (Kara et al., 2016) in laying hens' diets also had no obvious effects on laying performance and egg quality. However, the inclusion of 3% dried AMP (Sosnówka-Czajka and Skomorucha, 2021) in the diet tended to increase AEW, which may be related to its beneficial effects on body metabolism due to the higher proportion of AMP in the diet (corresponding to higher anthocyanins).
The nutritive value of eggs depends upon the composition of lipids, proteins, and other active substances. Lipid is the main component (30%) of egg yolk, which has a large content of fatty acids (Nielsen, 1998). C18:1n9-c and C16:0 are the most abundant fatty acids in egg yolks. In this trial, the addition of 0.5% FAMP reduced the percentage of C16:0 in egg yolks. The excess content of C16:0 may be harmful to the human body by increasing the production of harmful lipids, impairing cellular function, and inducing inflammation (Palomer et al., 2018). Meanwhile, FAMP supplementation decreased the SFA, especially 0.5% FAMP, which is partially in line with Selim et al. (2023), who found that supplementing grape pomace in laying hens' diets reduced the SFA. The CON group had a higher content of arachidonic acid in egg yolk, which may partly result from its increased biosynthesis of the unsaturated fatty acids pathway of cecal microbiota. Moreover, we found that the addition of FAMP (0.25%−0.1%) had beneficial impacts on the health lipid indices of egg yolk, which decreased the AI and TI while increasing the H/H, HPI, and DFA values. A relatively lower AI or TI value is beneficial to coronary artery health due to lower platelet aggregation (Woloszyn et al., 2020). The H/H index is defined as the Σhypercholesterolemic fatty acids/Σhypercholesterolemic fatty acids ratio and is also strongly associated with cholesterol metabolism. With respect to nutriology, the relatively higher H/H value is considered to have a greater potential to maintain physical health (Fernandes et al., 2014). The higher H/H and HPI (the reciprocal of AI) ratios provide protection against cardiovascular diseases (Hanus et al., 2018). The DFA index involves fatty acids, which are considered to have either neutral or cholesterol-lowering effects (Banskalieva et al., 2000). Thus, dietary FAMP supplementation positively affected the nutritive value of egg yolk. The optimal dosage was 0.5% FAMP, suggesting that FAMP had quadratic effects on fatty acid indices in egg yolk.
Egg albumen is rich in proteins, peptides, and amino acids (Sun et al., 2019), which possess antimicrobial, antioxidant, and anti-inflammatory functions (Lee and Paik, 2019). In the present study, the addition of 0.25% FAMP decreased the valine concentration, which may be related to its increased valine, leucine, and isoleucine degradation pathway of cecal microbiota. The addition of FAMP had no significant impact on most of the amino acid composition of egg albumen (Table 4). In contrast, a diet supplemented with 120–360 mg/kg anthocyanin-rich purple corn extract could improve most EAA and NEAA concentrations in eggs (Li J. et al., 2023). This disagreement is possibly influenced by the differences in feed composition, breeds, and ages of laying hens. For example, L-glutamine supplementation increased the levels of asparagine, phenylalanine, tryptophan, and tyrosine of albumen (Tomaszewska et al., 2021).
The growth and the role of ovarian follicles affect the laying performance of layers (Song et al., 2023), which is closely related to hormone regulation (Brady et al., 2020). The aged laying hens exhibit abnormal secretion of steroid hormones (Wu et al., 2023). In our study, the inclusion of 0.25%−1.0% FAMP increased the white follicles and the ovarian FSH, LH, and E2 levels. The small white follicles are involved in the estradiol secretion (Huang et al., 2021). FSH stimulates 3b-hydroxysteroid dehydrogenase (HSD3B1) production in granulosa cells by combining it with its receptor (FSHR) (Huang et al., 2021). Our findings suggested that the addition of 1% FAMP increased ovarian HSD17B1 expression. HSD17B1 catalyzes the transformation process of estrone into estradiol (the most active type of estrogen), which is responsible for testosterone generation (Ruan et al., 2023). These findings indicate that FAMP supplementation could enhance ovary function by regulating follicle development and hormone synthesis.
Yolk precursors formed in the liver are transported into the ovary of laying hens through blood circulation (Li et al., 2015). Estrogen stimulates the synthesis of apo very low-density lipoprotein II (ApoVLDL II) and apolipoprotein B (APOB) and the induction of hepatic very low-density lipoprotein (VLDL) assembly procedures for VLDL Y (VLDLy) formation (Walzem et al., 1999). ApoVLDL-II prevents the lipolytic action of lipoprotein lipase on VLDLy for lipid deposition in the oocyte (Schneider et al., 1990). Aged laying hens tend to have abnormalities in their LBO axis function (Amevor et al., 2021). Our findings showed that genes related to yolk precursors synthesis (e.g., ACC, FAS, and SCD) and transport (e.g., VTG II, ApoVLDL II, and APOB) of the liver were upregulated in aged laying hens after FAMP supplementation, which could coordinately generate yolk lipids (Amevor et al., 2021). Li et al. observed that the ESR2 expression in the liver was significantly upregulated in hens with higher laying performance (Li et al., 2015). We found that 1.0% FAMP increased ESR2 expression in the liver. Similarly, the addition of 1.0% and 4.0% Aronia melanocarpa increased the levels of estrogen and proteins as manifested by yolk precursors in the liver of laying hens (Jing et al., 2022). Collectively, the addition of FAMP may contribute to the yolk lipid deposition in the ovary by regulating the function of the LBO axis.
Oxidative stress is a critical cause of ovarian recession, accompanied by decreased laying performance and egg quality of aged hens (Bao et al., 2022). In this study, 0.5% FAMP supplementation increased the ovarian T-AOC and SOD levels, which belong to the enzymatic defense system of the body (Wu et al., 2023). AMP contains total polyphenols, which have antioxidant potential (Sidor and Gramza-Michalowska, 2019). Dietary 2.5% AMP increased tocopherol content and exhibited better antioxidant properties in egg yolk (Loetscher et al., 2014). Polyphenol-rich additives like AMP, Aronia melanocarpa (Jing et al., 2022), grape pomace (Reis et al., 2019), and purple corn extract fed to laying hens (Li J. et al., 2023) improved ovary or plasma antioxidant capacity via regulating the Keap1/Nrf2 signaling pathway, which is responsible for xenobiotic and oxidant elimination (Bhattacharyya et al., 2014). Nrf2 regulates defensive gene expression concerning antioxidant proteins, such as HO-1, GPX1, SOD1, and SOD2, which exert antioxidant effects (Tonelli et al., 2018). Keap1, a negative regulator of Nrf2, contributes to its retention in the cytosol. The present study showed that supplementation of 0.5% FAMP in layer diets upregulated the Nrf2 and downregulated the Keap1 expressions in the ovary. Our results suggest the addition of FAMP could enhance the antioxidant function of aged laying hens.
Intestinal microbiota communities and their metabolites are essential for the host physiology metabolism. The cecum has been regarded as the main site for containing relatively higher dominant microbial communities in laying hens (Ricke et al., 2022). The present study revealed that the addition of 0.25% FAMP increased the α-diversity of cecal microbiota, including richness indexes (Observed_species and Chao1) and the evolutionary diversity index (Faith-pd), but decreased the coverage index (Goods coverage), indicating that laying hens fed with 0.25% FAMP has the higher species abundance in the cecum. FAMP supplementation resulted in a difference in the β-diversity index, suggesting the addition of FAMP could alter the cecal microbiota composition. In line with previous studies, Bacteroidetes and Firmicutes were the predominant phyla of aged laying hens (Dai et al., 2022). The decline in the value of Firmicutes/Bacteroidetes may be associated with intestinal inflammation (Stojanov et al., 2020). The addition of 1% FAMP had higher Firmicutes and lower Bacteroidetes abundance, suggesting the addition of 1% FAMP was beneficial for the gut health of the laying hens. Dietary 1% FAMP supplementation also increased probiotic species such as Pediococcus, Lactobacillus, and Acetobacter, which can produce lactic acid and SCFA and modulate the elements of the gut–liver axis (Yu et al., 2021; Wen et al., 2023). Meanwhile, Lactobacillus was more abundant in laying hens with high laying performance (Wang et al., 2020). In addition, Proteobacteria is considered a potential diagnostic criterion for dysbiosis and other diseases (Shin et al., 2015). In the present study, the addition of FAMP (0.25%−1.0%) significantly decreased the Proteobacteria abundance, including Methylobacterium (Kovaleva et al., 2014), Ralstonia (Ryan and Adley, 2013), Shigella (Feng et al., 2021), and Acinetobacter (Maslova et al., 2022), which can be opportunistic pathogens. Additionally, there is an interplay between the cecal microbiota and phenotypic indicators (e.g., metabolites) of egg yolk (Tian et al., 2024). In this study, Methylobacterium and Shigella showed positive correlations with SFA, AI, and TI, and those also presented negative correlations with H/H, HPI, and DFA. Thus, FAMP could improve egg yolk health indexes, which may be related to reducing harmful bacteria.
The SCFA are the main metabolites of dietary fiber fermented by gut microbiota, which participate in energy and lipid metabolism and maintain gut homeostasis (He et al., 2020). Our findings show that the addition of 1% FAMP increased cecal acetate, propionate, butyrate, and valerate concentrations, which may be closely associated with the changes in microbial community structure. For instance, butyrate and Firmicutes (a butyrate-producing bacteria) were uniformly enhanced in the 1% FAMP group. Meanwhile, the pathway of butanoate metabolism and propanoate was also enriched in the 1% FAMP group by the PICRUSt2 analysis of microbiota. It is consistent with the fact that the 4% AMP supplementation increased the cecal acetate and butyrate concentrations in growing pigs (Ren et al., 2022). Therefore, the above findings suggest that the addition of FAMP contributes to increasing the cecal SCFA content, which may also have positive effects on gut health.
5 Conclusion
The inclusion of FAMP in aged laying hen diet improved the health lipid indices in the egg yolk by decreasing the total SFA, AI, and TI indices, as well as increasing the H/H, HPI, and DFA indices. The addition of FAMP improved ovarian function by regulating the yolk precursor formation in the LBO axis and enhancing the antioxidant function of aged laying hens. Meanwhile, FAMP addition could regulate the cecal microbiota composition and increase SCFA concentrations of aged laying hens to promote gut health (Figure 7). Our findings provide fundamental evidence for the utilization of FAMP in aged laying hens due to its positive effects on the nutritive value of egg yolks by improving the LBO function and microbiota composition.
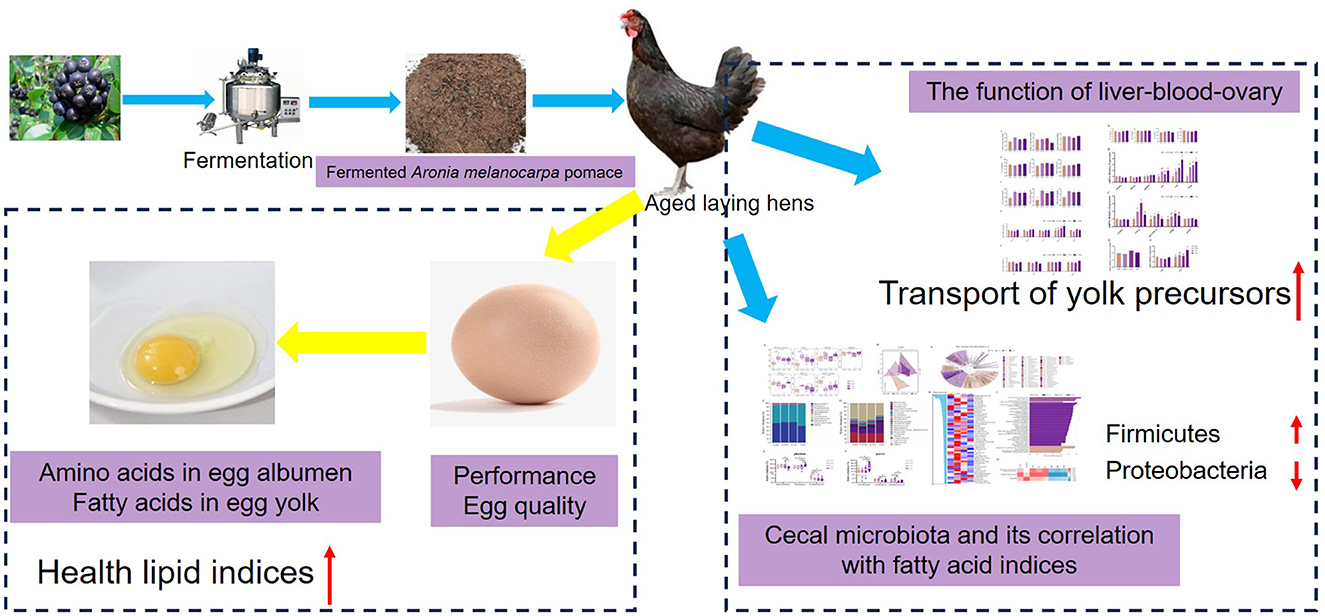
Figure 7. Effects and potential mechanisms of fermented Aronia melanocarpa pomace on performance, quality, and nutritive value of eggs, ovarian function, and cecal microbiota composition of aged laying hens.
Data availability statement
The original contributions presented in the study are publicly available. This data can be found here: Science Data Bank, https://doi.org/10.57760/sciencedb.09193.
Ethics statement
The animal study was approved by the Animal Care and Use Committee of the Institute of Subtropical Agriculture, Chinese Academy of Sciences. The study was conducted in accordance with the local legislation and institutional requirements.
Author contributions
ZL: Conceptualization, Data curation, Formal analysis, Investigation, Methodology, Visualization, Writing – original draft. BQ: Investigation, Writing – review & editing. TC: Investigation, Writing – review & editing. XK: Conceptualization, Funding acquisition, Project administration, Writing – review & editing, Writing – original draft. QZ: Validation, Writing – review & editing. MA: Validation, Writing – review & editing. YC: Supervision, Writing – review & editing. WL: Supervision, Writing – review & editing. QH: Conceptualization, Funding acquisition, Writing – review & editing.
Funding
The author(s) declare financial support was received for the research, authorship, and/or publication of this article. This study was supported by the City-School Cooperation Project of the Fuyang Science and Technology Special Fund undertaken by Fuyang Normal University (SXHZ2020007), the National Key Research and Development Project (2022YFC3400700), the Shenzhen Science and Technology Program (ZDSYS20210623100800001), and the Special Commissioner for Rural Science and Technology of Guangdong Province (KTP20210345).
Conflict of interest
The authors declare that the research was conducted in the absence of any commercial or financial relationships that could be construed as a potential conflict of interest.
The author(s) declared that they were an editorial board member of Frontiers, at the time of submission. This had no impact on the peer review process and the final decision.
Publisher's note
All claims expressed in this article are solely those of the authors and do not necessarily represent those of their affiliated organizations, or those of the publisher, the editors and the reviewers. Any product that may be evaluated in this article, or claim that may be made by its manufacturer, is not guaranteed or endorsed by the publisher.
Supplementary material
The Supplementary Material for this article can be found online at: https://www.frontiersin.org/articles/10.3389/fmicb.2024.1422172/full#supplementary-material
Supplementary Table S1. Amino acid composition of the fermented Aronia melanocarpa pomace.
Supplementary Table S2. Fatty acid composition of the fermented Aronia melanocarpa pomace.
Supplementary Table S3. Composition and nutrient levels of basal diet.
Supplementary Table S4. Specific primers for PCR analysis.
References
Amevor, F. K., Cui, Z., Du, X., Ning, Z., Shu, G., Jin, N., et al. (2021). Combination of quercetin and vitamin E supplementation promotes yolk precursor synthesis and follicle development in aging breeder hens via liver-blood-ovary signal axis. Animals 11:1915. doi: 10.3390/ani11071915
Banskalieva, V. V., Sahlu, T., and Goetsch, A. L. (2000). Fatty acid composition of goat muscles and fat depots: A review. Small Rumin. Res. 37, 255–268. doi: 10.1016/S0921-4488(00)00128-0
Bao, T., Yao, J., Zhou, S., Ma, Y., Dong, J., Zhang, C., et al. (2022). Naringin prevents follicular atresia by inhibiting oxidative stress in the aging chicken. Poult. Sci. 101:101891. doi: 10.1016/j.psj.2022.101891
Bhattacharyya, A., Chattopadhyay, R., Mitra, S., and Crowe, S. E. (2014). Oxidative stress: An essential factor in the pathogenesis of gastrointestinal mucosal diseases. Physiol. Rev. 94, 329–354. doi: 10.1152/physrev.00040.2012
Brady, K., Porter, T. E., Liu, H.-C., and Long, J. A. (2020). Characterization of the hypothalamo–pituitary–gonadal axis in low and high egg producing turkey hens. Poult. Sci. 99, 1163–1173. doi: 10.1016/j.psj.2019.12.028
Cui, Y., Peng, S., Deng, D., Yu, M., Tian, Z., Song, M., et al. (2023). Solid-state fermentation improves the quality of chrysanthemum waste as an alternative feed ingredient. J. Environ. Manage. 330:117060. doi: 10.1016/j.jenvman.2022.117060
Dai, D., Qi, G. H., Wang, J., Zhang, H. J., Qiu, K., and Wu, S. G. (2022). Intestinal microbiota of layer hens and its association with egg quality and safety. Poult. Sci. 101:102008. doi: 10.1016/j.psj.2022.102008
Du, X., and Myracle, A. D. (2018). Fermentation alters the bioaccessible phenolic compounds and increases the alpha-glucosidase inhibitory effects of aronia juice in a dairy matrix following in vitro digestion. Food Funct. 9, 2998–3007. doi: 10.1039/C8FO00250A
Erinle, T. J., and Adewole, D. I. (2022). Fruit pomaces–their nutrient and bioactive components, effects on growth and health of poultry species, and possible optimization techniques. Anim. Nutr. 9, 357–377. doi: 10.1016/j.aninu.2021.11.011
Esatbeyoglu, T., Fischer, A., Legler, A. D. S., Oner, M. E., Wolken, H. F., Köpsel, M., et al. (2023). Physical, chemical, and sensory properties of water kefir produced from Aronia melanocarpa juice and pomace. Food Chem. X 18, 100683. doi: 10.1016/j.fochx.2023.100683
Feng, J., Lu, M., Wang, J., Zhang, H., Qiu, K., Qi, G., et al. (2021). Dietary oregano essential oil supplementation improves intestinal functions and alters gut microbiota in late-phase laying hens. J. Anim. Sci. Biotechnol. 12:72. doi: 10.1186/s40104-021-00600-3
Fernandes, C. E., Vasconcelos, M. A., de Almeida Ribeiro, M., Sarubbo, L. A., Andrade, S. A. C., and Filho, A.B. (2014). Nutritional and lipid profiles in marine fish species from Brazil. Food Chem. 160, 67–71. doi: 10.1016/j.foodchem.2014.03.055
Gu, Y., Chen, Y., Jin, R., Wang, C., Wen, C., and Zhou, Y. (2021). Age-related changes in liver metabolism and antioxidant capacity of laying hens. Poult. Sci. 100:101478. doi: 10.1016/j.psj.2021.101478
Hanus, O., Samkova, E., Krizova, L., Hasonova, L., and Kala, R. (2018). Role of fatty acids in milk fat and the influence of selected factors on their variability-A review. Molecules 23, 1636. doi: 10.3390/molecules23071636
He, J., Zhang, P., Shen, L., Niu, L., Tan, Y., Chen, L., et al. (2020). Short-chain fatty acids and their association with signalling pathways in inflammation, glucose and lipid metabolism. Int. J. Mol. Sci. 21:6356. doi: 10.3390/ijms21176356
He, W., Wang, H., Tang, C., Zhao, Q., and Zhang, J. (2023). Dietary supplementation with astaxanthin alleviates ovarian aging in aged laying hens by enhancing antioxidant capacity and increasing reproductive hormones. Poult. Sci. 102:102258. doi: 10.1016/j.psj.2022.102258
Hu, C. J., Jiang, Q. Y., Zhang, T., Yin, Y. L., Li, F. N., Deng, J. P., et al. (2017). Dietary supplementation with arginine and glutamic acid modifies growth performance, carcass traits, and meat quality in growing-finishing pigs. J. Anim. Sci. 95, 2680–2689. doi: 10.2527/jas2017.1388
Huang, S. J., Purevsuren, L., Jin, F., Zhang, Y. P., Liang, C. Y., Zhu, M. Q., et al. (2021). Effect of anti-müllerian hormone on the development and selection of ovarian follicle in hens. Poult. Sci. 100:100959. doi: 10.1016/j.psj.2020.12.056
Jing, B., Xiao, H., Yin, H., Wei, Y., Wu, H., Zhang, D., et al. (2022). Feed supplemented with Aronia Melanocarpa (AM) relieves the oxidative stress caused by ovulation in peak laying hens and increases the content of yolk precursors. Animals 12:3574. doi: 10.3390/ani12243574
Jurikova, T., Mlcek, J., Skrovankova, S., Sumczynski, D., Sochor, J., Hlavacova, I., et al. (2017). Fruits of black chokeberry Aronia melanocarpa in the prevention of chronic diseases. Molecules 22:944. doi: 10.3390/molecules22060944
Kara, K., Gülü, B. K., Baytok, E., and Research, M. (2016). Effects of grape pomace supplementation to laying hen diet on performance, egg quality, egg lipid peroxidation and some biochemical parameters. J. Appl. Anim. Res. 44, 303–310. doi: 10.1080/09712119.2015.1031785
Kovacs-Nolan, J., Phillips, M., and Mine, Y. (2005). Advances in the value of eggs and egg components for human health. J. Agric. Food Chem. 53, 8421–8431. doi: 10.1021/jf050964f
Kovaleva, J., Degener, J. E., van der Mei, H. C., and Doern, G. V. (2014). Methylobacterium and its role in health care-associated infection. J. Clin. Microbiol. 52, 1317–1321. doi: 10.1128/JCM.03561-13
Lee, J. H., and Paik, H. D. (2019). Anticancer and immunomodulatory activity of egg proteins and peptides: A review. Poult. Sci. 98, 6505–6516. doi: 10.3382/ps/pez381
Li, G., Feng, Y., Cui, J., Hou, Q., Li, T., Jia, M., et al. (2023). The ionome and proteome landscape of aging in laying hens and relation to egg white quality. Sci. China Life Sci. 66, 2020–2040. doi: 10.1007/s11427-023-2413-4
Li, G. M., Liu, L. P., Yin, B., Liu, Y. Y., Dong, W. W., Gong, S., et al. (2020). Heat stress decreases egg production of laying hens by inducing apoptosis of follicular cells via activating the FasL/Fas and TNF-α systems. Poult. Sci. 99, 6084–6093. doi: 10.1016/j.psj.2020.07.024
Li, H., Wang, T., Xu, C., Wang, D., Ren, J., Li, Y., et al. (2015). Transcriptome profile of liver at different physiological stages reveals potential mode for lipid metabolism in laying hens. BMC Genomics 16:763. doi: 10.1186/s12864-015-1943-0
Li, J., Zhou, D., Li, H., Luo, Q., Wang, X., Qin, J., et al. (2023). Effect of purple corn extract on performance, antioxidant activity, egg quality, egg amino acid, and fatty acid profiles of laying hen. Front. Vet. Sci. 9:1083842. doi: 10.3389/fvets.2022.1083842
Li, Z., Meng, C., Azad, M. A. K., Lin, W., Gui, J., Cui, Y., et al. (2024). Dietary Chinese herbal formula supplementation improves yolk fatty acid profile in aged laying hens. Vet. Quart. 44, 1–11. doi: 10.1080/01652176.2024.2319828
Li, Z., Zhu, Q., Azad, M. A. K., Li, H., Huang, P., and Kong, X. (2021). The impacts of dietary fermented mao-tai lees on growth performance, plasma metabolites, and intestinal microbiota and metabolites of weaned piglets. Front. Microbiol. 12:778555. doi: 10.3389/fmicb.2021.778555
Lipinska, P., Atanasov, A. G., Palka, M., and Jozwik, A. (2017). Chokeberry pomace as a determinant of antioxidant parameters assayed in blood and liver tissue of Polish merino and Wrzosowka lambs. Molecules 22:1461. doi: 10.3390/molecules22111461
Livak, K. J., and Schmittgen, T. D. (2001). Analysis of relative gene expression data using real-time quantitative PCR and the 2−ΔΔCT method. Methods 25, 402–408. doi: 10.1006/meth.2001.1262
Loetscher, Y., Kreuzer, M., and Messikommer, R. E. (2014). Late laying hens deposit dietary antioxidants preferentially in the egg and not in the body. J. Appl. Poult. Res. 23, 647–660. doi: 10.3382/japr.2014-00973
Maslova, O., Mindlin, S., Beletsky, A., Mardanov, A., and Petrova, M. (2022). Plasmids as key players in Acinetobacter adaptation. Int. J. Mol. Sci. 23, 10893. doi: 10.3390/ijms231810893
Nielsen, H. (1998). Hen age and fatty acid composition of egg yolk lipid. Br. Poult. Sci. 39, 53–56. doi: 10.1080/00071669889394
Palomer, X., Pizarro-Delgado, J., Barroso, E., and Vázquez-Carrera, M. (2018). Palmitic and oleic acid: The Yin and Yang of fatty acids in type 2 diabetes mellitus. Trends Endocrinol. Metab. 29, 178–190. doi: 10.1016/j.tem.2017.11.009
Reis, J. H., Gebert, R. R., Barreta, M., Boiago, M. M., Souza, C. F., Baldissera, M. D., et al. (2019). Addition of grape pomace flour in the diet on laying hens in heat stress: Impacts on health and performance as well as the fatty acid profile and total antioxidant capacity in the egg. J. Therm. Biol. 80, 141–149. doi: 10.1016/j.jtherbio.2019.01.003
Ren, Z., Fang, H., Zhang, J., Wang, R., Xiao, W., Zheng, K., et al. (2022). Dietary Aronia melanocarpa pomace supplementation enhances the expression of ZO-1 and Occludin and promotes intestinal development in pigs. Front. Vet. Sci. 9:904667. doi: 10.3389/fvets.2022.904667
Ricke, S. C., Dittoe, D. K., and Olson, E. G. (2022). Microbiome applications for laying hen performance and egg production. Poult. Sci. 101, 101784. doi: 10.1016/j.psj.2022.101784
Ruan, G., Ye, L., Lin, J., Lin, H., Yu, L., Wang, C., et al. (2023). An integrated approach of netstudy pharmacology, molecular docking, and experimental verification uncovers kaempferol as the effective modulator of HSD17B1 for treatment of endometrial cancer. J. Transl. Med. 21:204. doi: 10.1186/s12967-023-04048-z
Ryan, M. P., and Adley, C. C. (2013). Ralstonia spp.: Emerging global opportunistic pathogens. Eur. J. Clin. Microbiol. Infect. Dis. 33, 291–304. doi: 10.1007/s10096-013-1975-9
Schmid, V., Steck, J., Mayer-Miebach, E., Behsnilian, D., Briviba, K., Bunzel, M., et al. (2020). Impact of defined thermomechanical treatment on the structure and content of dietary fiber and the stability and bioaccessibility of polyphenols of chokeberry (Aronia melanocarpa) pomace. Food Res. Int. 134:109232. doi: 10.1016/j.foodres.2020.109232
Schneider, W. J., Carroll, R., Severson, D. L., and Nimpf, J. (1990). Apolipoprotein VLDL-II inhibits lipolysis of triglyceride-rich lipoproteins in the laying hen. J. Lipid Res. 31, 507–513. doi: 10.1016/S0022-2275(20)43172-4
Selim, S., Abdel-Megeid, N. S., Alhotan, R. A., Ebrahim, A., and Hussein, E. (2023). Grape pomace: agrifood by-product with potential to enhance performance, yolk quality, antioxidant capacity, and eggshell ultrastructure in laying hens. Vet. Sci. 10:61. doi: 10.3390/vetsci10070461
Shin, N. R., Whon, T. W., and Bae, J. W. (2015). Proteobacteria: Microbial signature of dysbiosis in gut microbiota. Trends Biotechnol. 33, 496–503. doi: 10.1016/j.tibtech.2015.06.011
Sidor, A., and Gramza-Michalowska, A. (2019). Black chokeberry Aronia melanocarpa L.-A qualitative composition, phenolic profile and antioxidant potential. Molecules 24:3710. doi: 10.3390/molecules24203710
Song, X., Wang, D., Zhou, Y., Sun, Y., Ao, X., Hao, R., et al. (2023). Yolk precursor synthesis and deposition in hierarchical follicles and effect on egg production performance of hens. Poult. Sci.102:102756. doi: 10.1016/j.psj.2023.102756
Sosnówka-Czajka, E., and Skomorucha, I. (2021). Effect of supplementation with dried fruit pomace on the performance, egg quality, white blood cells, and lymphatic organs in laying hens. Poult. Sci.100:101278. doi: 10.1016/j.psj.2021.101278
Stojanov, S., Berlec, A., and Štrukelj, B. (2020). The influence of probiotics on the Firmicutes/Bacteroidetes ratio in the treatment of obesity and inflammatory bowel disease. Microorganisms 8:1715. doi: 10.3390/microorganisms8111715
Sun, C., Liu, J., Yang, N., and Xu, G. (2019). Egg quality and egg albumen property of domestic chicken, duck, goose, turkey, quail, and pigeon. Poult. Sci. 98, 4516–4521. doi: 10.3382/ps/pez259
Tian, Y., Zhang, R., Li, G., Zeng, T., Chen, L., Xu, W., et al. (2024). Microbial fermented feed affects flavor amino acids and yolk trimethylamine of duck eggs via cecal microbiota–yolk metabolites crosstalk. Food Chem. 430:137008. doi: 10.1016/j.foodchem.2023.137008
Tomaszewska, E., Arczewska-Wlosek, A., Burmanczuk, A., Pyz-Lukasik, R., Donaldson, J., Muszynski, S., et al. (2021). The effect of L-glutamine on basal albumen and yolk indices, and albumen amino acids composition. Animals 11:556. doi: 10.3390/ani11123556
Tonelli, C., Chio, I. I. C., and Tuveson, D. A. (2018). Transcriptional regulation by Nrf2. Antioxid Redox Signal. 29, 1727–1745. doi: 10.1089/ars.2017.7342
Walzem, R. L., Hansen, R. J., Williams, D. L., and Hamilton, R. L. (1999). Estrogen induction of VLDLy assembly in egg-laying hens. J. Nutr. 129, 467s−472s. doi: 10.1093/jn/129.2.467S
Wang, Y., Xu, L., Sun, X., Wan, X., Sun, G., Jiang, R., et al. (2020). Characteristics of the fecal microbiota of high- and low-yield hens and effects of fecal microbiota transplantation on egg production performance. Res. Vet. Sci. 129, 164–173. doi: 10.1016/j.rvsc.2020.01.020
Wen, X., Wang, Z., Liu, Q., Lessing, D. J., and Chu, W. (2023). Acetobacter pasteurianus BP2201 alleviates alcohol-induced hepatic and neuro-toxicity and modulate gut microbiota in mice. Microb. Biotechnol. 16, 1834–1857. doi: 10.1111/1751-7915.14303
Woloszyn, J., Haraf, G., Okruszek, A., Werenska, M., Goluch, Z., and Teleszko, M. (2020). Fatty acid profiles and health lipid indices in the breast muscles of local Polish goose varieties. Poult. Sci. 99, 1216–1224. doi: 10.1016/j.psj.2019.10.026
Wu, H., Yuan, J., Yin, H., Jing, B., Sun, C., Nguepi Tsopmejio, I. S., et al. (2023). Flammulina velutipes stem regulates oxidative damage and synthesis of yolk precursors in aging laying hens by regulating the liver-blood-ovary axis. Poult. Sci. 102:102261. doi: 10.1016/j.psj.2022.102261
Yu, J. S., Youn, G. S., Choi, J., Kim, C. H., Kim, B. Y., Yang, S. J., et al. (2021). Lactobacillus lactis and Pediococcus pentosaceus-driven reprogramming of gut microbiome and metabolome ameliorates the progression of non–alcoholic fatty liver disease. Clin. Transl. Med. 11:e634. doi: 10.1002/ctm2.634
Keywords: aged laying hens, nutritive value, fermented Aronia melanocarpa pomace, ovarian function, microbiota
Citation: Li Z, Qin B, Chen T, Kong X, Zhu Q, Azad MAK, Cui Y, Lan W and He Q (2024) Fermented Aronia melanocarpa pomace improves the nutritive value of eggs, enhances ovarian function, and reshapes microbiota abundance in aged laying hens. Front. Microbiol. 15:1422172. doi: 10.3389/fmicb.2024.1422172
Received: 23 April 2024; Accepted: 27 May 2024;
Published: 19 June 2024.
Edited by:
Gabriele Brecchia, University of Milan, ItalyReviewed by:
Yuying Li, Chinese Academy of Agricultural Sciences (CAAS), ChinaYu Bai, Tianjin University of Science and Technology, China
Copyright © 2024 Li, Qin, Chen, Kong, Zhu, Azad, Cui, Lan and He. This is an open-access article distributed under the terms of the Creative Commons Attribution License (CC BY). The use, distribution or reproduction in other forums is permitted, provided the original author(s) and the copyright owner(s) are credited and that the original publication in this journal is cited, in accordance with accepted academic practice. No use, distribution or reproduction is permitted which does not comply with these terms.
*Correspondence: Qinghua He, cWluZ2h1YS5oZUBzenUuZWR1LmNu; Xiangfeng Kong, bm5reGZAaXNhLmFjLmNu