- 1Leshan Normal University, Leshan, China
- 2Sichuan Provincial Engineering and Technology Research Center for Innovative Development of Bamboo Fiber Nutrition, Leshan, China
- 3Key Laboratory of Bamboo Pest Control and Resource Development, Leshan, Sichuan, China
- 4College of Animal Science, South China Agricultural University, Guangzhou, China
Introduction: Feeding bamboo powder is a kind of fiber raw material mainly composed of insoluble dietary fiber (IDF). In this study, IDF-based rice husk meal and feeding bamboo powder were used to compare the effects of bamboo fiber on fecal microflora and the performance of lactating sows and their offspring piglets.
Methods: Thirty healthy crossbred gilts (Yorkshire × Landrace) at day 105 of gestation were randomly allocated into three groups: CON, TRE1 supplemented with 2% BBF1 (feeding bamboo powder), and TRE2 supplemented with 2% BBF2 (99% feeding bamboo powder +1% bamboo fiber polymer material). The reproductive performance, serum indexes, and fecal microbiota of sows and piglets were analyzed. The results showed that, compared with CON, the average feed intake of sows in TRE1 during the second week of lactation was significantly increased by 21.96% (p < 0.05), the average daily gain (ADG) per litter in TRE1 on 11–21 days and 3–21 days of lactation was significantly increased by 50.68 and 31.61%, respectively (p < 0.05), and the serum triglyceride content of sows in TRE1 on the 21st day of lactation was significantly increased (p < 0.05). The 16S rRNA analysis showed that dietary bamboo fiber significantly increased the fecal microbial richness index Ace, Chao, and Sobs of sows (p < 0.05) and tended to increase the Sobs index of suckling piglets on day 21 (p < 0.10). Compared with CON, BBF1 supplementation significantly decreased the abundance of Christensenellaceae_R-7_group in feces of sows on days 7 and 21 after delivery (p < 0.05), while BBF2 decreased the genera Christensenellaceae_R-7_group on days 7 (p < 0.10) and 21 (p < 0.05) after delivery. Spearman correlation analysis showed that the abundance of Phascolarctobacterium in the feces of piglets on the 21st day after delivery was significantly positively correlated with diarrhea rate and significantly negatively correlated with ADG per litter, day 21 litter weight, and 3- to 21-day survival rate. In contrast, Christensenellaceae_R-7_group was significantly negatively correlated with diarrhea rate and positively correlated with ADG per litter.
Discussion: These results indicated that maternal BBF1 supplementation improved the litter weight gain of suckling piglets, which was associated with the improvement of diversity and structure of the fecal microbiota in the piglets.
Introduction
Nutritional regulation during pregnancy and lactation is a key factor for the growth and development of their offspring (Zhang et al., 2018), which has a direct impact on the production efficiency and economic benefits of the pig industry. This “sow-piglet” association may be influenced by sow nutrition, which may alter the electrophysiological properties, barrier function, and microbiota of the intestine in their piglets (Awad et al., 2013; Paßlack et al., 2015). The “sows to piglets” model has been manifested in the effect of dietary fiber from different sources, which can regulate the gut microbiota, improve performance, and alleviate inflammatory responses in sows and piglets (Liu et al., 2021). Susceptibility to colonization by C. difficile in neonatal piglets can be modulated by the dietary fiber of sows, supporting the hypothesis of early microbial programming in the offspring and the importance of the sow-piglet couple (Grześkowiak et al., 2022). Dietary fiber nutrition for sows may be a better method to improve the performance of their offspring piglets by regulating the microbial composition, which is the main reason for this study.
Different sources of fibers may have different physical and chemical properties, which may cause changes in the composition of the intestinal microbiota (Pi et al., 2021; Shang et al., 2021). Guar gum (soluble dietary fiber, SDF) and cellulose (insoluble dietary fiber, IDF) interacted to increase ileal bifidobacteria and enterobacteria in grower pigs; however, guar gum, but not cellulose, increased ileal clostridia (Owusu-Asiedu et al., 2006). Dietary konjac flour rich in SDF increased the relative abundances of Akkermansia and Roseburia; however, it had no effect on the α-diversity values of microbial communities (Tan et al., 2016). Compared with coarse wheat bran, dietary supplementation with fine wheat bran with higher SDF significantly increased the Chao index, and the abundance of Firmicutes and Terrisporobacter was significantly decreased while Bacteroidetes and Parabacteroides were significantly increased (Wang et al., 2022). Dietary IDF (lignocellulose) significantly increased Lactobacillus amounts in the ileal digesta of piglets, and it was more effective than SDF (insulin) (Chen et al., 2019). These studies suggested that dietary fiber type (SDF and IDF) affected microbial diversity and composition, but the results were inconsistent. Future research needs to gain more insight into the combined effects of SDF and IDF, processing methods, and additional timing to improve the nutritional value of dietary fiber (Li et al., 2021). In addition, increasing the dietary fiber level of sows increased the ileal flow of most nutrients and the total excretion of fecal materials (Serena et al., 2008). Increasing the dietary fiber level of sows in late gestation reversed inflammatory responses and adverse effects by increasing the abundance of Lactobacillus (Li et al., 2023).
China is rich in bamboo species, and the majority are distributed in the southern area (Zhang et al., 2022), especially in the location of this study, which is the hometown of pandas. Young bamboo culms present potential application in the food industry as a source of fibers, with high IDF content accounting for 62.54–89.79% (Felisberto et al., 2017), which was higher than wheat bran, corn bran, and rice bran (Bai et al., 2022). The health-promoting effects of bamboo have been extensively studied in recent years. It has been shown that IDF from bamboo could alter the composition and microbial diversity of gut microbiota, especially by increasing the relative abundance of Bacteroides and decreasing the ratio of Firmicutes to Bacteroidetes (Ge et al., 2022). O-acetylated xylan obtained from bamboo effectively relieved loperamide-induced constipation in mice by significantly improving cecum microbiota composition and shortening defecation time (Huang J. et al., 2022; Huang Y. et al., 2022). Bamboo extracts showed several biological functions, such as anti-inflammatory and antioxidant properties (Tundis et al., 2023). The intake of fermented bamboo fiber improved the immunity, inflammation, and intestinal microbiota of sows, which were closely related to sow performance (Sun et al., 2023). Our previous research has found that micronized bamboo powder can reduce the amount of Escherichia coli in the feces of weaned piglets and improve growth performance (Dai et al., 2021), improve cecal microbial composition and increase average daily gain in broilers (Dai et al., 2022), increase the water content in the feces of sows, reduce the oxidative damage, and tend to reduce the relative abundance of opportunistic pathogenic Fusobacterium for suckling piglets with a supplement for perinatal sows (Dai et al., 2023). This study was conducted to further study the effects of maternal bamboo fiber supplementation from day 105 of gestation to day 21 post-farrowing on the fecal microbial composition of lactating sows and their piglets on the 1st, 7th, and 21st days after delivery. Reproduction performance, serum biochemical parameters of sows, and growth performance of the piglets were determined.
Materials and methods
Test materials
The rice husk meal was crushed over 80 mesh, and the crude fiber was determined to be 31.5% by the AOAC 978.10 method.
Bamboo fiber 1 (BBF1): after removing the bamboo green from the bamboo sticks at 5–6 years, the coarse powder was processed by a cutter mill, dried to <12% moisture, and crushed by a hammer mill over 80 mesh. The content of crude fiber was determined to be 42.7%.
Bamboo Fiber 2 (BBF2): BBF2 was composed of BBF1 and bamboo fiber polymer material (provided by Key Laboratory of Bamboo Pest Control and Resource Development, China) with a ratio of 99:1. The bamboo fiber polymer material was prepared by irradiation treatment and coupling polymer hydrophilic material from Bambusa emeiensis at 1 year old. The soluble fiber and total dietary fiber of bamboo fiber were determined to be 19.18 and 78.18%, respectively, by the AOAC 991.43 method, and the crude fiber was determined to be 42.3%.
Test grouping and treatment
Thirty healthy primiparous sows (Yorkshire × Landrace) with a similar fat condition and the same batch of breeding at day 105 of gestation were randomly allocated into three groups (with 10 replicates per group and 1 pig per replicate): CON (a basal diet group supplemented with 2% rice husk meal), TRE 1 supplemented with 2% BBF1, and TRE 2 supplemented with 2% BBF2. The rice husk meal, BBF1, and BBF2 were directly added to the top access door of a double shaft counterpoise ribbon mixer (hand-adds) with a 1,000-kg full load capacity (SJHS2, Muyang Group, China). The experimental diet was formulated according to the NRC (2012) and GB/T 39235–2020 nutritional requirements of sows. The composition and nutritional levels of experimental diets are shown in Table 1, and the additional level of bamboo fiber was referred to the reports of similar fiber sources (Grześkowiak et al., 2022; Dai et al., 2023; Sun et al., 2023).
Feeding management
The experiment was conducted in the experimental pig farm of Wenzhou Zhugeliang Animal Husbandry Co., Ltd., Wenzhou, Zhejiang Province, China, from July to August 2022. Kept in the parturition pen, the experimental sows were fed 3 kg per sow per day before parturition, 1 kg/day on the day of parturition, and 2 kg/day on day 2, then 0.5 kg/day more feed each day from day 3 to day 7, free feeding from day 8 to day 21, and the leftovers were collected and weighed every day. Sows were fed two meals a week before and after delivery at 6:30 and 17:30, respectively. Sows were fed three meals on the first day of the second week after delivery at 5:30, 10:30, and 17:30, respectively. All sows and piglets drank freely, and the environmental conditions in the piggery were consistent. The rest of the feeding management shall be carried out according to the unified procedures of the pig farm.
The pre-feeding period was 2 days, and the formal experiment lasted until the end of weaning at 21 days after delivery. Within 48 h after delivery, the number of piglets carried by each sow was adjusted in the treatment group according to the milk of sows through foster care, consolidation, and other means. In the subsequent experiment, piglets were no longer adjusted, the daily feed intake of sows was accurately recorded, and the feeding amount was increased according to the feeding amount and feeding speed of the previous day (the feeding increase rate did not exceed 0.5 kg/day).
Sample collection
Fiber feed samples: 500 g rice husk meal, bamboo fiber 1, and bamboo fiber 2 were collected and frozen at −20°C for testing.
Serum samples of sows: six sows in each group were randomly selected on the morning of the 3rd and 21st days (weaning) after delivery, and 5 mL of blood was collected from the ear vein of each sow before meals and placed in a centrifuge tube for 30 min. Serum was separated by centrifuging at 3,000 rpm for 15 min. The serum was absorbed, divided into a 1.5-ml centrifuge tube, and frozen at −20°C for testing.
Fecal samples: on the morning of the 1st, 7th, and 21st days after delivery, six sows in each group were randomly selected. About 300 mg of fecal samples were scraped from inside the stool and placed into a 1.5-ml centrifuge tube. Meanwhile, about 300 mg of fresh fecal samples of piglets born in sows were collected and placed into a 1.5-ml centrifuge tube, frozen with liquid nitrogen, and frozen at −80°C for microbial analysis.
Reproductive performance of sows
The backfat thickness of sows at point P2 (6 cm from the last dorsal mid-line of ribs on the left side) was measured with the ultrasonic backfat meter (Renco, United States) at the start of the experiment and at weaning time to calculate the backfat loss of sows during lactation. The duration of farrowing, total litter size, healthy litter size (weight over 1 kg), live litter size, litter size of sows in foster adjustment, daily feed intake during lactation, and number of weaned piglets were recorded. Calving intervals, feed intake of sows during the first, second, and third weeks of lactation, and throughout lactation were calculated. Accurately record the birth time of the first piglet and the birth time of the last piglet, and the interval between the two is the labor process.
Average litter interval = length of labor/total litter size.
Growth performance of suckling piglets
The number of piglets, the number of piglets with diarrhea, and the number of stillborn piglets were recorded every day in the unit of repetition, and the weights of piglets at birth, day 3, day 10, and day 21 were weighed by litters. Average birth weight, average individual weight on days 3, 10, and 21, average daily gain of litters on days 3–11, days 11–21, and days 3–21, average daily gain of individuals on days 3–11, days 11–21, and days 3–21, and diarrhea rate of piglets on days 3–11, days 11–21, and days 3–21 were calculated. The survival rate of piglets on days 3–11, days 11–21, and days 3–21 was calculated.
Average birth weight (kg) = litter weight/number of live offspring.
Average body weight (kg) = litter weight/number of piglets.
Average daily gain (ADG) per litter (kg/d) = (litter weight at time point 2- litter weight at time point 1)/interval days.
Individual average daily gain (g/d) = (body weight at time point 2- body weight at time point 1)/interval days.
Diarrhea rate of piglets (%) = (Number of piglets with diarrhea × days of diarrhea)/(total number of piglets × days of feeding) × 100%.
Piglet survival rate (%) = Number of piglets at time point 2 / Number of piglets at time point 1 × 100%.
Determination of serum biochemical indexes
The serum biochemical indexes of sows included triglyceride (TG), total cholesterol (T-CHO), total protein (TP), and urea nitrogen (BUN), all of which were determined by the kits purchased from Nanjing Jiancheng Institute of Bioengineering (Jiangsu, China). Serum TG, T-CHO, and BUN were determined by the enzymatic method with the kit numbers A110-1-1, A111-1-1, and C013-2-1, respectively. Serum TP was determined by the ceruloplasmin method with kit number A029.
Fecal microbial analysis
DNA extraction kit (Omega Bio-tek, Norcross, GA, United States) was used to extract genomic DNA from stool samples of experimental sows and piglets, and 1% agarose gel electrophoresis was used to detect the quality of the extracted genomic DNA. DNA concentration and purity were determined using NanoDrop2000 (Thermo Scientific Inc., United States). Taking that DNA as a template, PCR amplification of the V3–V4 variable region of the 16S rRNA gene was performed using priors 338F (5′-ACTCCTACGGGAGGCAGCAG-3′) and 806R (5′-GGACTACHVGGGTWTCTAAT-3′) (Liu et al., 2016). Paired-end sequencing of 16S rRNA PCR products was performed on the Illumina MiSeq PE300 platform by Shanghai Meiji Biomedical Technology Co., Ltd.
Fastp software1 was applied for quality control of double-ended original sequencing sequences (Chen et al., 2018). FLASH software2 was used for stitching (Magoč and Salzberg, 2011). (1) The base of reads with a mass value below 20 at the tail end was filtered, and a 50-bp window was set. If the average mass value in the window was lower than 20, the back-end bases were cut off from the window. Reads below 50 bp after quality control were filtered, and reads containing N-base were removed. (2) According to the overlap between PE reads, pairs of reads were merged into a sequence with a minimum overlap length of 10 bp. (3) The maximum mismatch ratio of the overlap region of the spliced sequences was 0.2, and the inconsistent sequences were screened. (4) The samples were differentiated according to the barcode and primers at both ends of the sequence, and the sequence direction was adjusted. The allowable mismatch number of barcode was 0, and the maximum mismatch number of primer was 2.
Using UPARSE software3 (Stackebrandt and Goebel, 1994; Edgar, 2013), the operational taxonomic unit (OTU) was used to cluster the quality control concatenated sequences and eliminate chimeras based on 97% similarity. In order to minimize the impact of sequencing depth on subsequent alpha and beta diversity data analysis, the sequence number of all samples was reduced to 34,060, and the average sequence coverage of each sample could still reach 99.49% after being reduced. OTU species taxonomy annotation was performed by comparing the RDP classifier4 with the Silva 16S rRNA gene database (Release1385) with a confidence threshold of 70%. The community composition of each sample was counted at different species classification levels (Wang et al., 2007).
Data sorting and statistical analysis
One-way ANOVA program of SPSS 23.0 statistical software was used to conduct a one-way analysis of variance. Reproductive performance, growth performance, and serum indexes were statistically analyzed with the LSD method for multiple comparisons and an independent-sample t-test for the difference between means. The results were expressed as mean ± standard error; p < 0.05 indicated significant differences, and 0.05 ≤ p < 0.10 indicated a difference trend.
The 16S rRNA sequencing analysis of all samples was performed on the Meggie Biocloud platform6 as follows: alpha diversity, including the Ace, Chao, and Sobs, richness index, Shannon and Simpson index characterization of diversity, is obtained by using the mothur software.7 The Wilcoxon rank sum test was used to analyze the difference in alpha diversity between groups. Principal coordinate analysis (PCoA) based on the Bray-Curtis distance algorithm was used to test the similarity of microbial community structure among samples. ANOSIM non-parametric test was combined to analyze whether the differences in microbial community structure between samples were significant. The Student’s t-test was used to analyze the differences in the relative abundance of major microorganisms at phylum and genus levels between different experimental groups. p < 0.05 indicated a significant difference, and 0.05 ≤ p < 0.10 indicated a trend of difference.
Results
Effects of maternal bamboo fiber supplementation on reproductive performance
As seen from Table 2, the average daily feed intake (ADFI) of sows fed with bamboo fiber 1 in the second week after delivery was significantly higher in TRE1 than that in the rice husk meal CON (p < 0.05), increased by 21.96%, and increased by 7.67, 9.54, and 13.08% in the first week, third week, and whole lactation, respectively, but did not reach the significant difference level (p > 0.05). Bamboo fiber treatment had no significant effects on farrowing duration, farrowing interval, litter size, healthy litter size, back fat thickness at weaning, or back fat loss during the trial period. These results indicated that the dietary addition of 2% bamboo fiber 1 can improve the feed intake of sows during lactation, mainly in the mid-lactation (the second week after delivery).
Effects of maternal bamboo fiber supplementation on growth performance of piglets
As shown in Table 3, dietary supplementation of bamboo fiber in sows had a significant effect on the average daily gain (ADG) per litter in piglets at pre-weaning 11–21 days and 3–21 days (p < 0.05), and ADG per litter in TRE1 supplemented with bamboo fiber 1 was significantly higher than that in CON or that in TRE2 group (p < 0.05), increasing by 50.68 and 51.70%, 31.61 and 34.71%, respectively. The 21-day weaning body weight (BW) per litter of TRE1 was significantly higher than that of TRE2 group (p < 0.05) and tended to be higher than that of CON (p < 0.10). The survival rate of 3- to 21-day-old piglets in TRE1 was higher than that in CON group and TRE2 group, which increased by 13.70% (p < 0.10) and 14.92% (p < 0.10), respectively. These results indicated that dietary supplementation of 2% bamboo fiber 1 in sows can improve the performance of piglets, mainly in the survival rate of piglets, weaning litter weight, and ADG per litter.
Effects of maternal bamboo fiber supplementation on serum biochemical indices of sows
As can be seen from Table 4, on the 21st day after delivery, the serum triglyceride content of sows in TRE1 and TRE2 was significantly higher than that in rice husk meal CON (p < 0.05), and the total cholesterol content was higher than that in CON, but the difference was not significant (p > 0.05). On the third day after delivery, the total cholesterol and total triglyceride contents of sows in TRE1 were higher than those in CON, but the difference was not significant (p > 0.05). Bamboo fiber treatment had no significant effects on serum total protein or urea nitrogen. The above results indicated that dietary supplementation of 2% bamboo fiber can promote the lipid metabolism of sows in the late lactation period.
Effects of maternal bamboo fiber supplementation on fecal microbial sequence data and alpha and beta diversity of sows and suckling piglets
To investigate the effects of bamboo fiber on fecal microbial diversity and abundance of sows and suckling piglets at different postpartum stages, PCR amplification and Illumina MiSeq high-throughput sequencing were performed on the 16S rRNA V3–V4 region of bacteria in each sample. According to the statistics of OTU after drawing out, it can be seen from Figure 1 that the number of fecal microbial OTU of sows and piglets on the day of delivery was lower than those on the 7th and 21st days after delivery, and the differences in OTU numbers between TRE1 and TRE2 were greater on the 7th and 21st days after delivery. On the day of delivery, the fecal OTUs of sows in CON were 558, and the fecal OTUs of sows in TRE1 and TRE2 were 545 and 528, respectively, which were lower than those in CON. Compared with CON, the unique OTU numbers of sows in TRE1 and TRE2 were 29 and 24, respectively. The numbers of piglets fecal OTU in TRE1 and TRE2 were 356 and 341, respectively, which were lower than those in CON (373). Compared with CON, the unique fecal OTUs were 33 and 34 in TRE1 and TRE2, respectively.
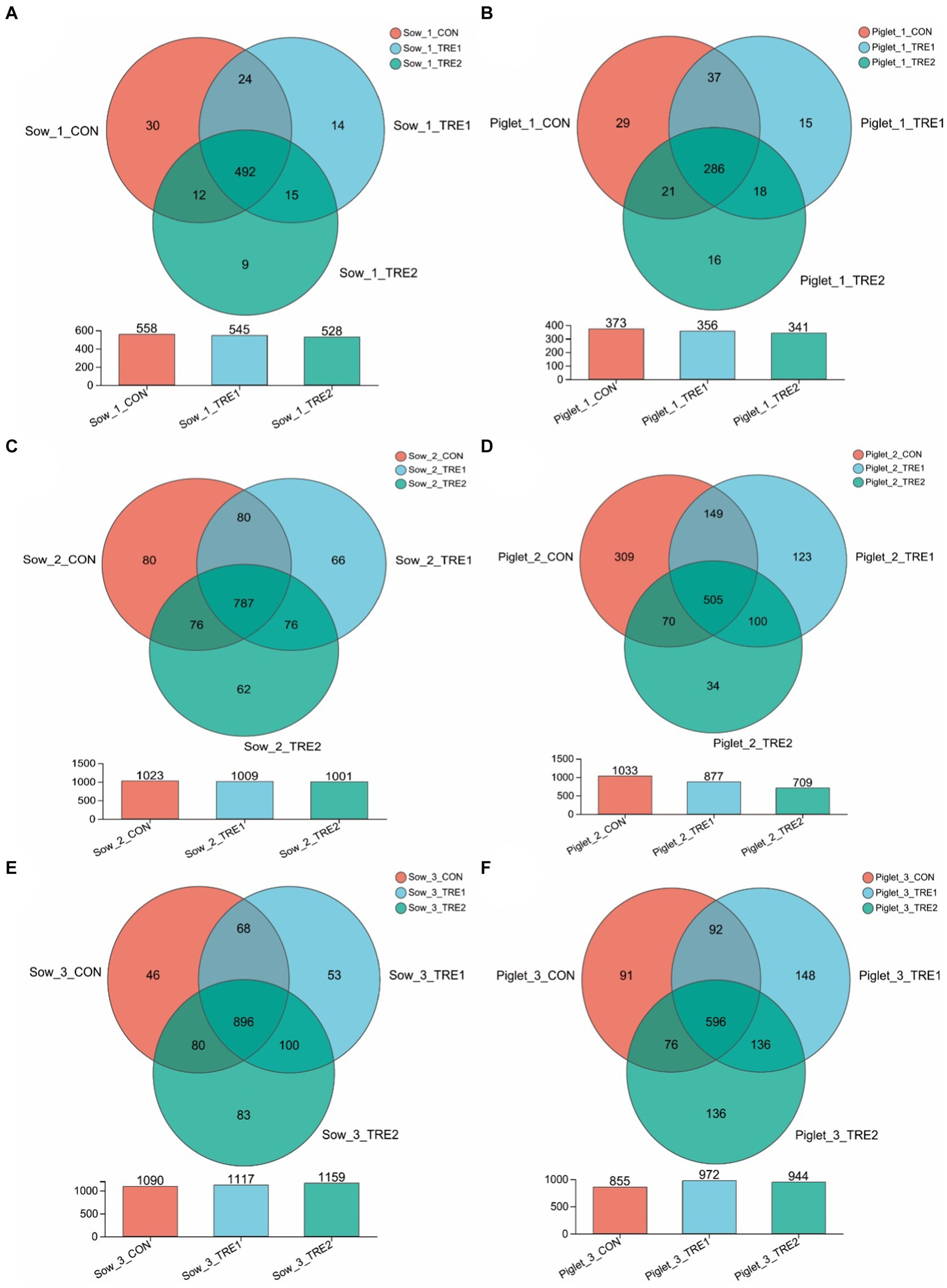
Figure 1. OTU Vane diagram of fecal microorganisms of sows and suckling piglets in different experimental groups. (A,C,E) Show sows on the first day after delivery, the seventh day after delivery, and the 21st day after delivery, respectively. (B,D,F) Show piglets on the first day after delivery, the seventh day after delivery, and the 21st day after delivery, respectively. Sow_1_CON, Sow_1_TRE1, and Sow_1_TRE2, Piglet_1_CON, Piglet _1_TRE1, and Piglet _1_TRE2 represented sows in CON, TRE1, and TRE2, suckling piglets in CON, TRE1, and TRE2 on the first day after delivery, respectively. Sow_2_CON, Sow_2_TRE1, and Sow_2_TRE2, Piglet_2_CON, Piglet _2_TRE1, and Piglet _2_TRE2 represented sows in CON, TRE1, and TRE2, suckling piglets in CON, TRE1, and TRE2 on the seventh day after delivery, respectively. Sow_3_CON, Sow_3_TRE1, and Sow_3_TRE2, Piglet_3_CON, Piglet _3_TRE1, and Piglet _3_TRE2 represented sows in CON, TRE1, and TRE2, suckling piglets in CON, TRE1, and TRE2 on the 21st day after delivery, respectively. n = 6 for each group. Same in Figures 2–6.
On the seventh day after delivery, the fecal OTU of sows in CON was 1,023, and the fecal OTU of sows in TRE1 and TRE2 were lower than those in CON (1,009 and 1,001, respectively). Compared with CON, the unique OTUs of sows in TRE1 and TRE2 were 138 and 142, respectively. The fecal OTUs in TRE1 and TRE2 were 877 and 709, respectively, which were lower than those in CON (1033). Compared with CON, the unique fecal OTUs were 134 and 223, respectively, in TRE1 and TRE2. On the 21st day after delivery, the fecal OTUs of sows in CON were 1,090, and the fecal OTUs of sows in TRE1 and TRE2 were higher than those in CON (1,117 and 1,159, respectively). Compared with CON, the unique OTUs of sows in TRE1 and TRE2 were 153 and 183, respectively. The fecal OTUs in TRE1 and TRE2 were 972 and 944, respectively, which were lower than those in CON (855). Compared with CON, there were 284 and 272 unique fecal OTUs in TRE1 and TRE2, respectively. These results indicated that bamboo fiber had a different effect on the quantity of fecal microbe OTU in sows than chaff, and the effects on sows and suckling piglets in late lactation were more obvious than those in early lactation.
Bacterial alpha diversity indexes were shown in Table 5, sows fecal microbial representation richness index Ace, Chao, and Sobs were significantly increased by dietary bamboo fiber (p < 0.05) and tended to increase the Sobs index of suckling piglets on day 21 (p < 0.10). Maternal bamboo fiber supplementation had no effects on Ace, Chao, Shannon, and Simpson indexes in suckling piglets (p > 0.05). Fecal Ace, Chao, Sobs, Shannon, and Simpson indexes were significantly increased by the reproductive ages in sows and suckling piglets (p < 0.05) but not affected by the diet × age interaction (p > 0.05).
To investigate the effects of supplementary bamboo fiber on fecal microbial beta diversity, PCoA was used to determine the differences among treatment groups. Principal coordinate analysis was conducted based on the Bray-Curtis distance of relative abundance of fecal microbial colonies in the OTU of sows and their suckling piglets. As shown in Figure 2, the fecal microbiota structures of sows in TRE1 and TRE2 were similar to those in CON on the 1st, 7th, and 21st days after delivery, and the fecal microbiota structures of their suckling piglets in different treatment groups were also similar.
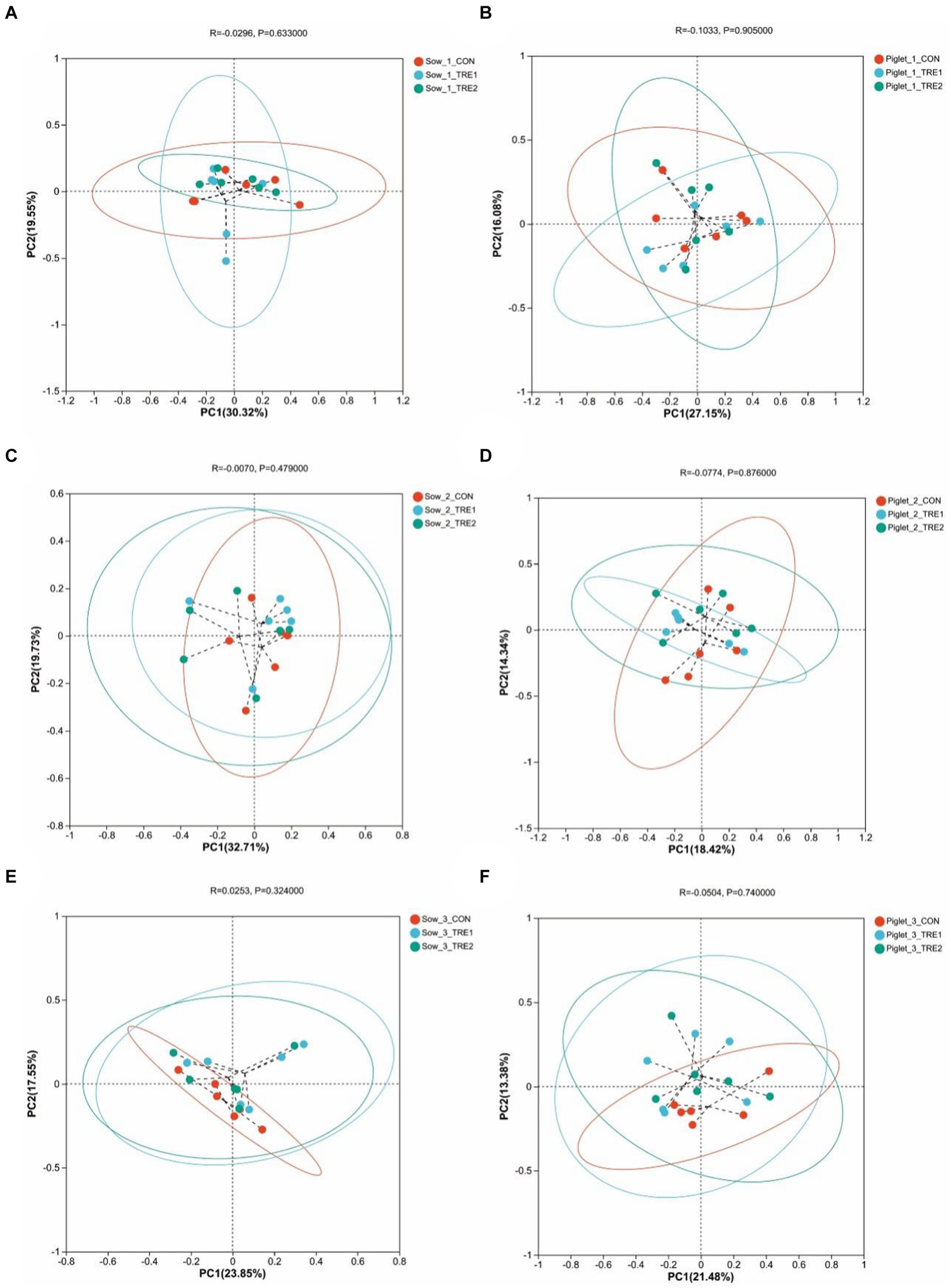
Figure 2. Beta-diversity analysis among experimental groups. Principal coordinates analysis (PCoA) for sows in the control group (CON), treatment group 1 (TRE1), and treatment group 2 (TRE2) on the first day after delivery (A), the seventh day after delivery (C), and the 21st day after delivery (E). Principal coordinates analysis (PCoA) for suckling piglets in the control group (CON), treatment group 1 (TRE1), and treatment group 2 (TRE2) on the first day after delivery (B), the seventh day after delivery (D), and the 21st day after delivery (F). n = 6 for each group.
Effects of maternal bamboo fiber supplementation on the fecal microbial composition of sows and suckling piglets
The fecal microbial composition at the phylum level of sows and suckling piglets in different experimental groups is shown in Figure 3. As can be seen from Figures 3A,C,E, the top five dominant bacteria in sow feces were Firmicutes, Proteobacteria, Bacteroidota, Spirochaeta, and Actinobacteria. Compared with CON, the proportion of Firmicutes in TRE1 and TRE2 increased by 9.81 and 8.60% on the first day after delivery, respectively. On the seventh day after delivery, the proportions of Firmicutes in TRE1 increased by 3.81%, while that of Proteobacteria in TRE2 increased by 10.65%. On the 21st day after delivery, the proportion of Firmicutes in TRE1 decreased by 2.93%, while that of Spirochaeta in TRE2 increased by 2.04%. The dominant bacterial phyla in sows were relatively stable at different stages after delivery. Compared with rice husk meal, BBF1 supplementation mainly changed the proportion of Firmicutes.
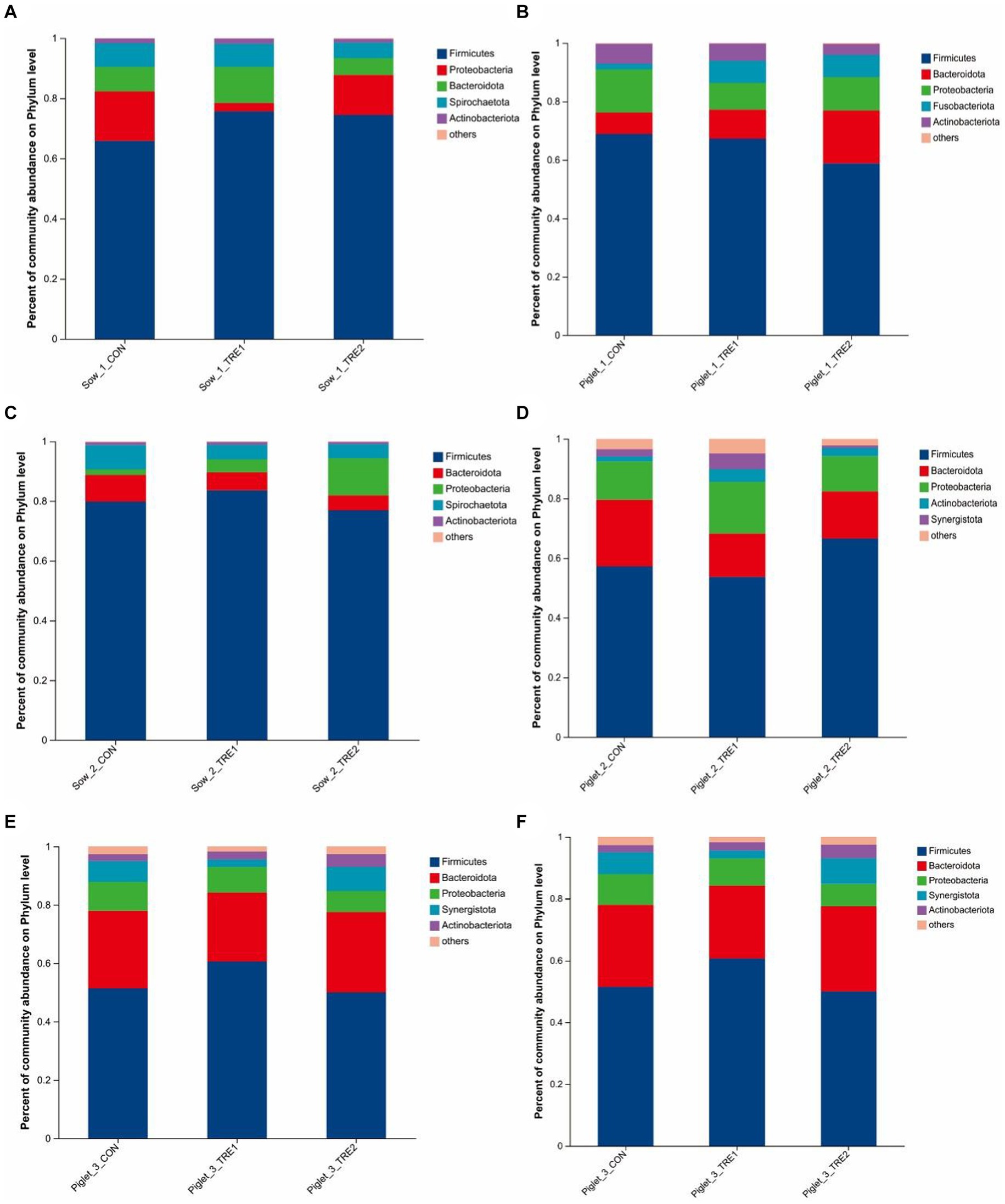
Figure 3. Fecal microbiota composition in sows and piglets of different groups at the phylum level. Relative abundance of sow fecal phylum microbiota in the control group (CON), treatment group 1 (TRE1), and treatment group 2 (TRE2) on the first day after delivery (A), the seventh day after delivery (C), and the 21st day after delivery (E). Relative abundance of suckling piglet fecal microbiota in the control group (CON), treatment group 1 (TRE1), and treatment group 2 (TRE2) on the first day after delivery (B), the seventh day after delivery (D), and the 21st day after delivery (F). n = 6 for each group.
According to Figures 3B,D,F, the top dominant bacteria in the feces of suckling piglets were Firmicutes, Bacteroidota, Proteobacteria, Fusobacteriota, Actinobact eriota, Synergistota, and Spirochaeta. Synergistota was added as the dominant bacteria to the feces of suckling piglets on the seventh day after delivery, and Spirochaeta was added as the dominant bacteria on the 21st day after delivery. Compared with CON, on the first day after delivery, the proportion of fecal Fusobacteriota of piglets in TRE1 increased by 5.71%, and Bacteroides in TRE2 increased by 10.75%. On the seventh day after delivery, the proportion of Proteobacteria in TRE1 increased by 4.53%, and Firmicutes in TRE2 increased by 9.40%. On the 21st day after delivery, the proportion of Firmicutes in TRE1 increased by 9.22%, and proportion of Proteobacteria in TRE2 decreased by 2.71%.
The fecal microbial composition at the genus level of sows and suckling piglets in different experimental groups is shown in Figure 4. As seen from Figure 4A, on the first day after delivery, there were 21 dominant bacterial genera in the feces of sows of all three groups, including Terrisporobacter, Christensenaceae R-7 group, Escherichia-Shigella, Treponema, Clostridium_sensu_stricto_1, and so on. The proportions of dominant bacteria in CON, TRE1, and TRE2 were 89.69, 87.00, and 89.38%, respectively. As seen in Figure 4B, there were 24 dominant bacterial genera in the feces of suckling piglets, accounting for 86.96, 89.44, and 87.10% in CON, TRE1, and TRE2, respectively. Different from the dominant bacteria composition in sow feces, there were 19 dominant bacterial genera unique to suckling piglets, including Bacteroides, Peptostreptococcus, Fusobaterium, Enterococcus, Streptococcus, and so on.
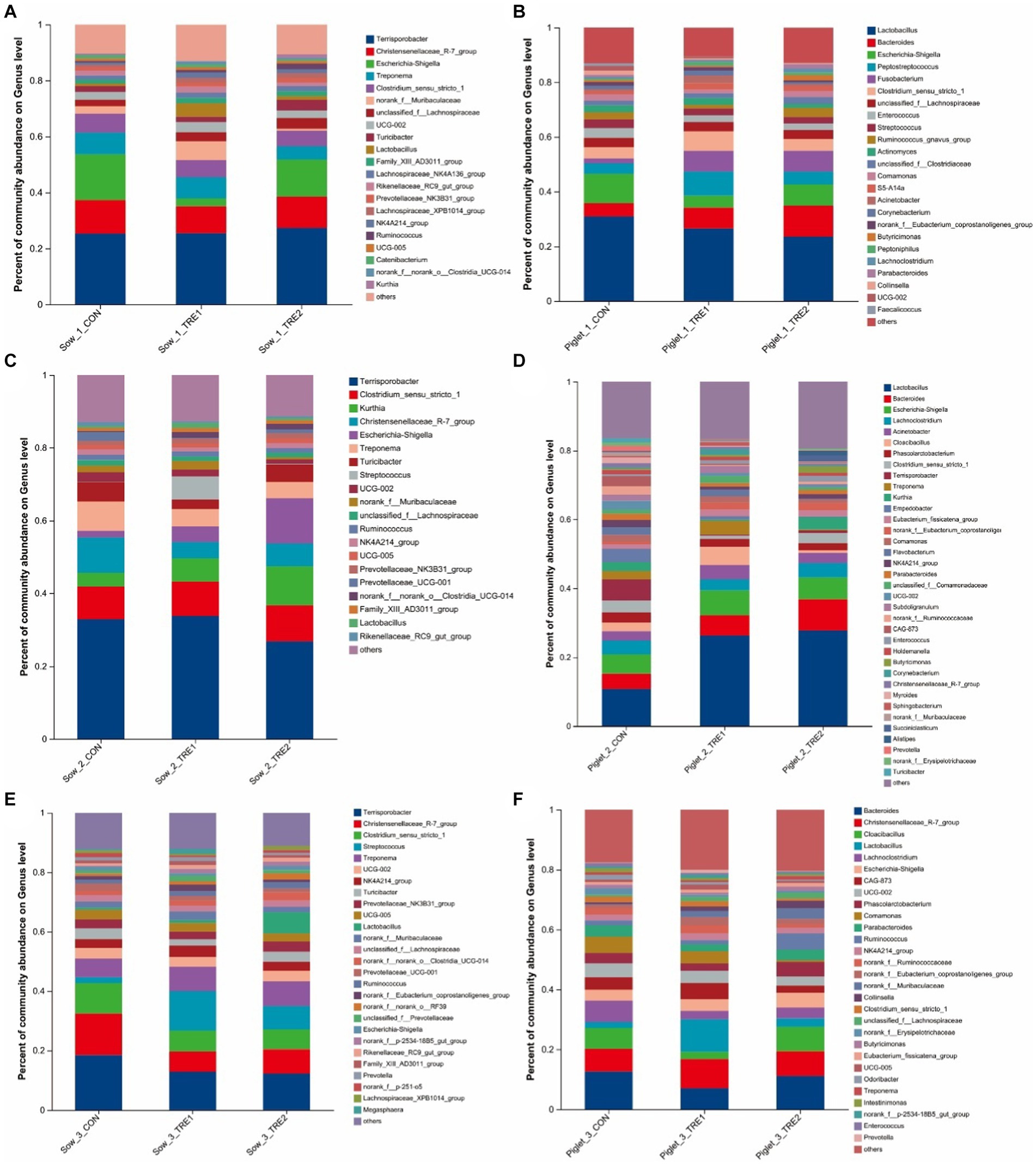
Figure 4. Fecal microbiota composition in sows and piglets of different groups at the genus level. Relative abundance of sow fecal genera microbiota in the control group (CON), treatment group 1 (TRE1), and treatment group 2 (TRE2) on the first day after delivery (A), the seventh day after delivery (C), and the 21st day after delivery (E). Relative abundance of suckling piglet fecal microbiota in the control group (CON), treatment group 1 (TRE1), and treatment group 2 (TRE2) on the first day after delivery (B), the seventh day after delivery (D), and the 21st day after delivery (F). n = 6 for each group.
As seen from Figure 4C, on the seventh day after delivery, there were 20 dominant bacterial genera in the feces of sows of all three groups, including Terrisporobacter, Clostridium_sensu_stricto_1, Kurthia, Christensenaceae R-7 group, Escherichia–Shigella, and so on. The proportions of dominant bacteria in CON, TRE1, and TRE2 were 87.11, 87.27, and 88.65%, respectively. According to Figure 4D, there were 36 dominant bacterial genera in the feces of suckling piglets, accounting for 83.60, 83.32, and 80.57% in CON, TRE1, and TRE2, respectively. Different from the dominant bacteria composition in sow feces, there were 25 dominant bacterial genera unique to suckling piglets, including Bacteroides, Lachnoclostridium, Acinetobacter, Cloacibacillus, Phascolarctobacterium, and so on.
As seen from Figure 4E, on the 21st day after delivery, there were 27 dominant bacterial genera in the feces of sows of all three groups, including Terrisporobacter, Christensenellaceae_R-7_group, Clostridium_sensu_stricto_1, Streptococcus, Treponema, and so on. The dominant bacterial genera in CON, TRE1, and TRE2 accounted for 87.78, 87.83, and 88.87%, respectively. As seen in Figure 4F, there were 29 dominant bacterial genera in the feces of suckling piglets, accounting for 82.46, 79.97, and 79.55% in CON, TRE1, and TRE2, respectively. Different from the dominant bacteria composition in sow feces, there were 15 dominant bacterial genera unique to suckling piglets, including Bacteroides, Cloacibacillus, Lachnoclostridium, CAG-873, Phascolarctobacterium, and so on.
The differences of top five fecal dominant bacterial phyla of sows and suckling piglets among different groups are shown in Figure 5. There was no significant difference in the abundance of dominant bacteria at the phylum level for sows and suckling piglets among CON, TRE1, and TRE2 on the 1st, 7th, and 21st days after delivery (p > 0.05).
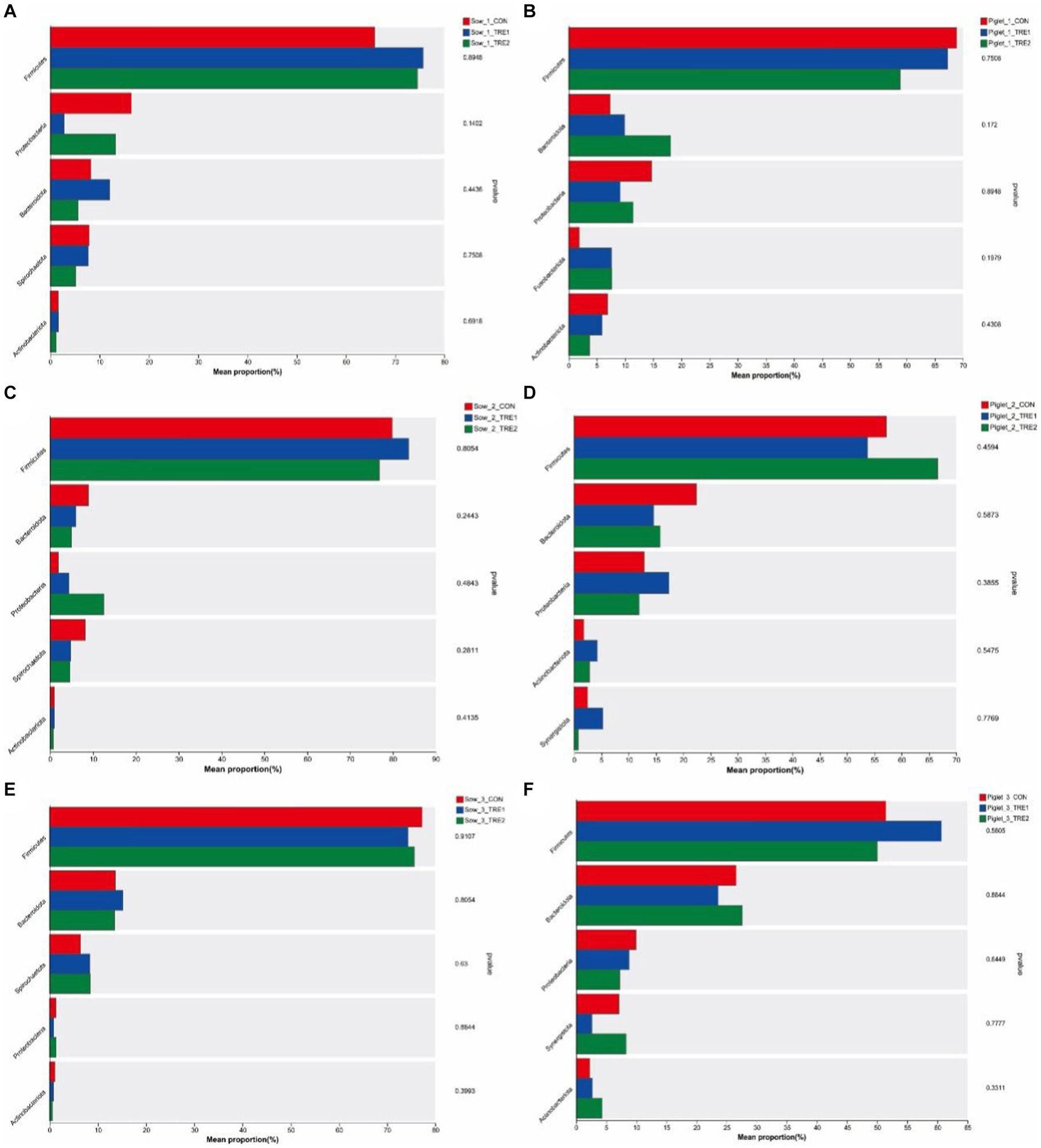
Figure 5. Differences in the fecal microbiota of sows and piglets at the phylum level in different experimental groups. Fecal phylum microbiota differed in sows among the control group (CON), treatment group 1 (TRE1), and treatment group 2 (TRE2) on the first day after delivery (A), the seventh day after delivery (C), and the 21st day after delivery (E). Fecal phylum microbiota differed in suckling piglets among the control group (CON), treatment group 1 (TRE1), and treatment group 2 (TRE2) on the first day after delivery (B), the seventh day after delivery (D), and the 21st day after delivery (F). n = 6 for each group.
The differences of top 10 fecal dominant bacterial genera of sows and suckling piglets among different groups are shown in Figure 6. According to Figures 6A,B, there were no significant differences in the abundance of dominant bacteria at the genus level for sows and suckling piglets in CON, TRE1, and TRE2 on the first day after delivery (p > 0.05). As shown in Figures 6C,D, there were significant differences in fecal Christensenellaceae_R-7_group in sows of CON, TRE1, and TRE2 on the seventh day after delivery (p < 0.05), and compared with CON, genera Christensenellaceae_R-7_group was significantly decreased by BBF1 supplementation (p < 0.05), while BBF2 tended to decrease Christensenellaceae_R-7_group (p < 0.10) (Figure 7A). However, there were no significant differences in the dominant bacterial genera in the feces of suckling piglets among these groups (p > 0.05). As shown in Figures 6D,E, a trend difference in the sows feces of CON, TRE1, and TRE2 was found in Clostridium_sensu_stricto_1 on the 21st day after delivery (p < 0.10), while there was no significant difference in the genera Clostridium_sensu_stricto_1 between CON and TRE1 or between CON and TRE2 (p > 0.05). Compared with CON, the genera Christensenellaceae_R-7_group was significantly decreased by BBF1 and BBF2 supplementation in the feces of sows (p < 0.05) (Figure 7B). There was a significant difference in Comamonas of suckling piglets by dietary fiber (p < 0.05), while there was no significant difference between CON and TRE1 or between CON and TRE2 (p > 0.05).
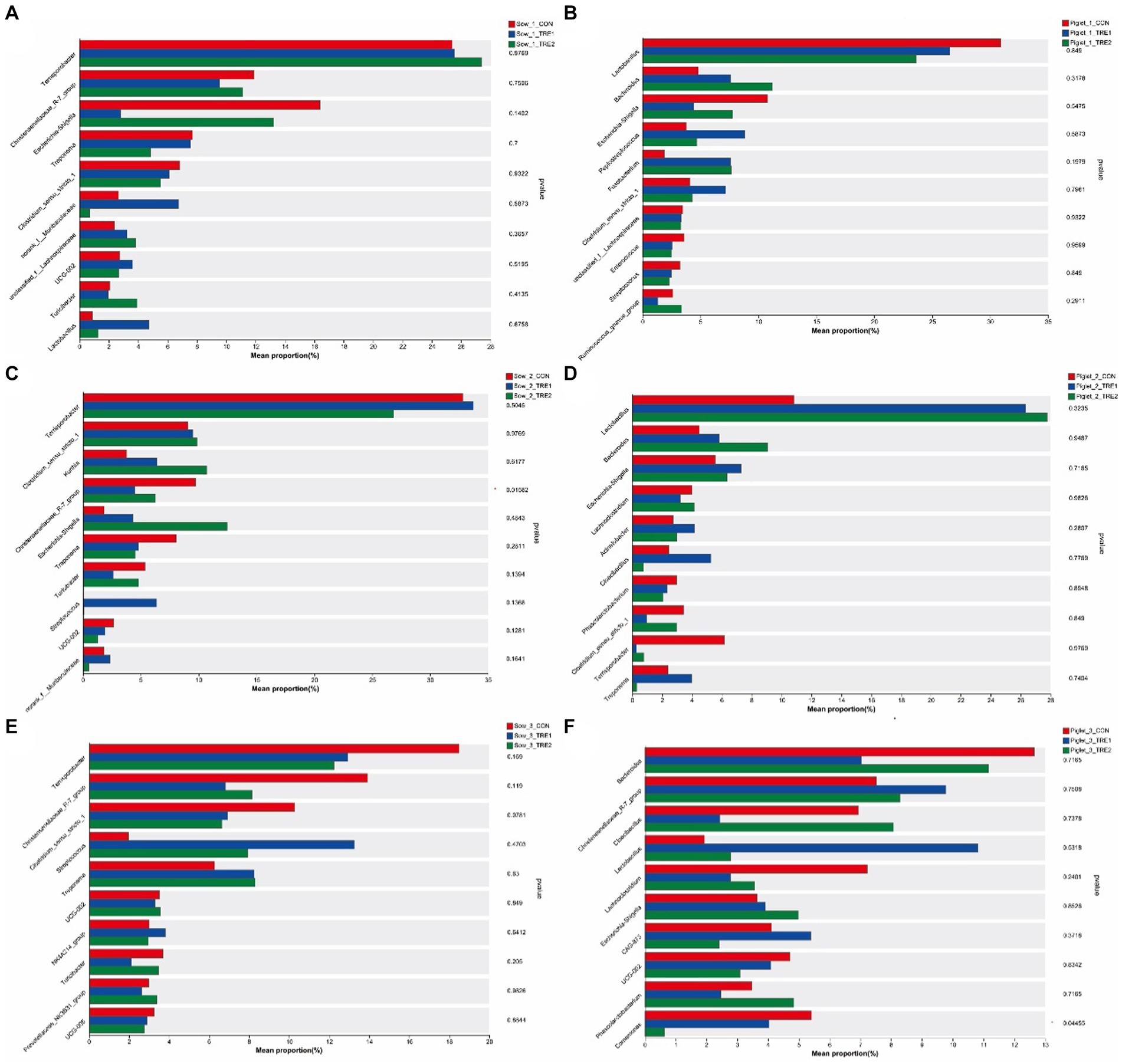
Figure 6. Differences in the fecal microbiota of sows and piglets at the genus level in different experimental groups. Fecal genera microbiota differed in sows among the control group (CON), treatment group 1 (TRE1), and treatment group 2 (TRE2) on the first day after delivery (A), the seventh day after delivery (C), and the 21st day after delivery (E). Fecal genera microbiota differed in suckling piglets among the control group (CON), treatment group 1 (TRE1), and treatment group 2 (TRE2) on the first day after delivery (B), the seventh day after delivery (D), and the 21st day after delivery (F). n = 6 for each group.
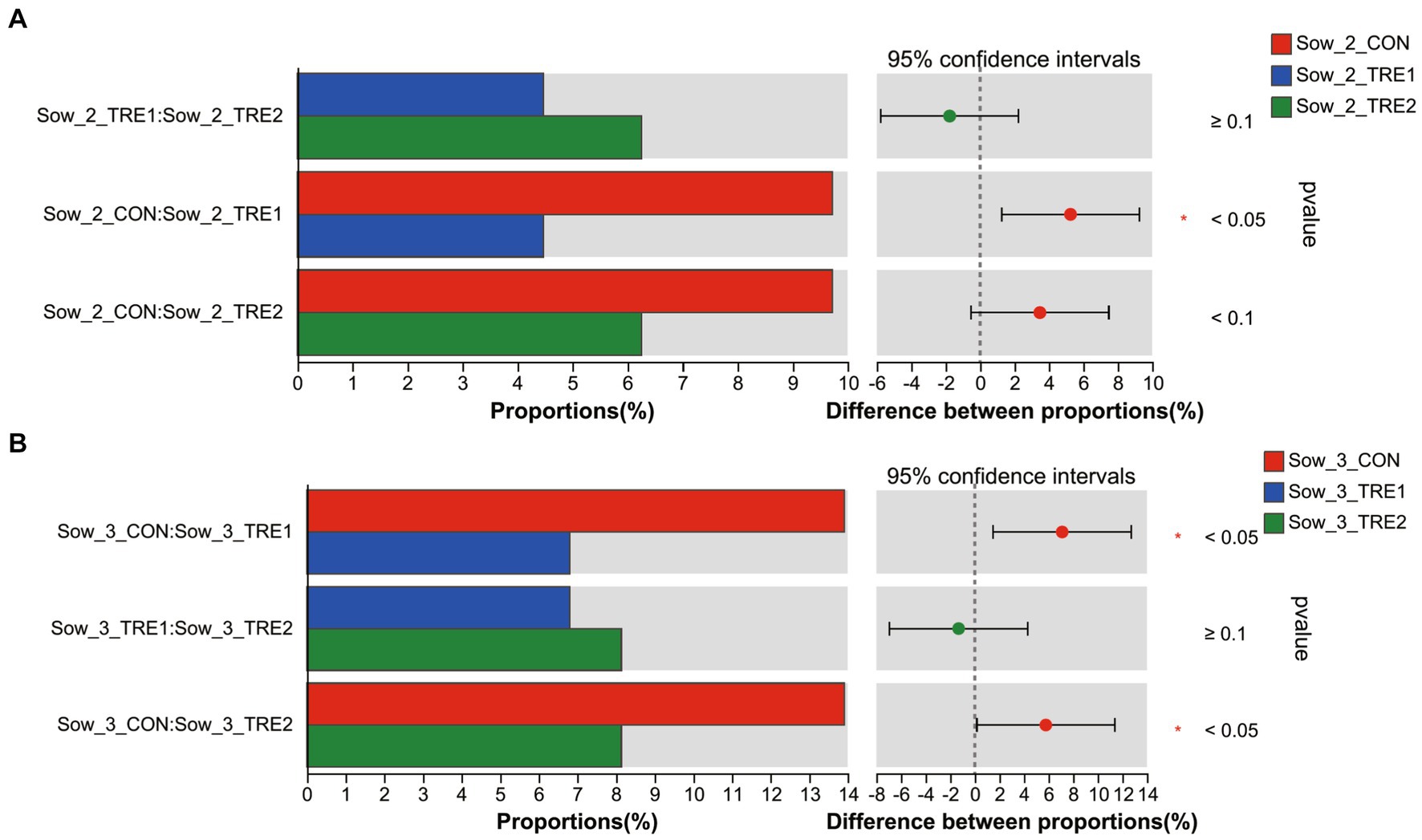
Figure 7. Differences in sows fecal genera Christensenellaceae_R-7_group between different experimental groups. Fecal bacterial genera differed in sows between CON and TRE1, CON and TRE2, and TRE1 and TRE2 on the seventh day after delivery (A) and the 21st day after delivery (B). n = 6 for each group.
Correlation between fecal microorganisms and growth performance of suckling piglets
As shown in Figure 8, Spearman correlation analysis found that the abundance of Phascolarctobacterium in the feces of suckling piglets on the 21st day was significantly positively correlated with diarrhea rate (p ≤ 0.001). It was negatively correlated with 21-day litter weight, average daily gain, and 3- to 21-day survival rate (0.01 < p ≤ 0.05). There was a significant negative correlation between the abundance of Bacteroides and the ADG of piglets (0.01 < p ≤ 0.05). In contrast, the abundance of Christensenellaceae_R-7_group was significantly negatively correlated with diarrhea rate (0.001 < p ≤ 0.01) and positively correlated with litter ADG (0.01 < p ≤ 0.05).
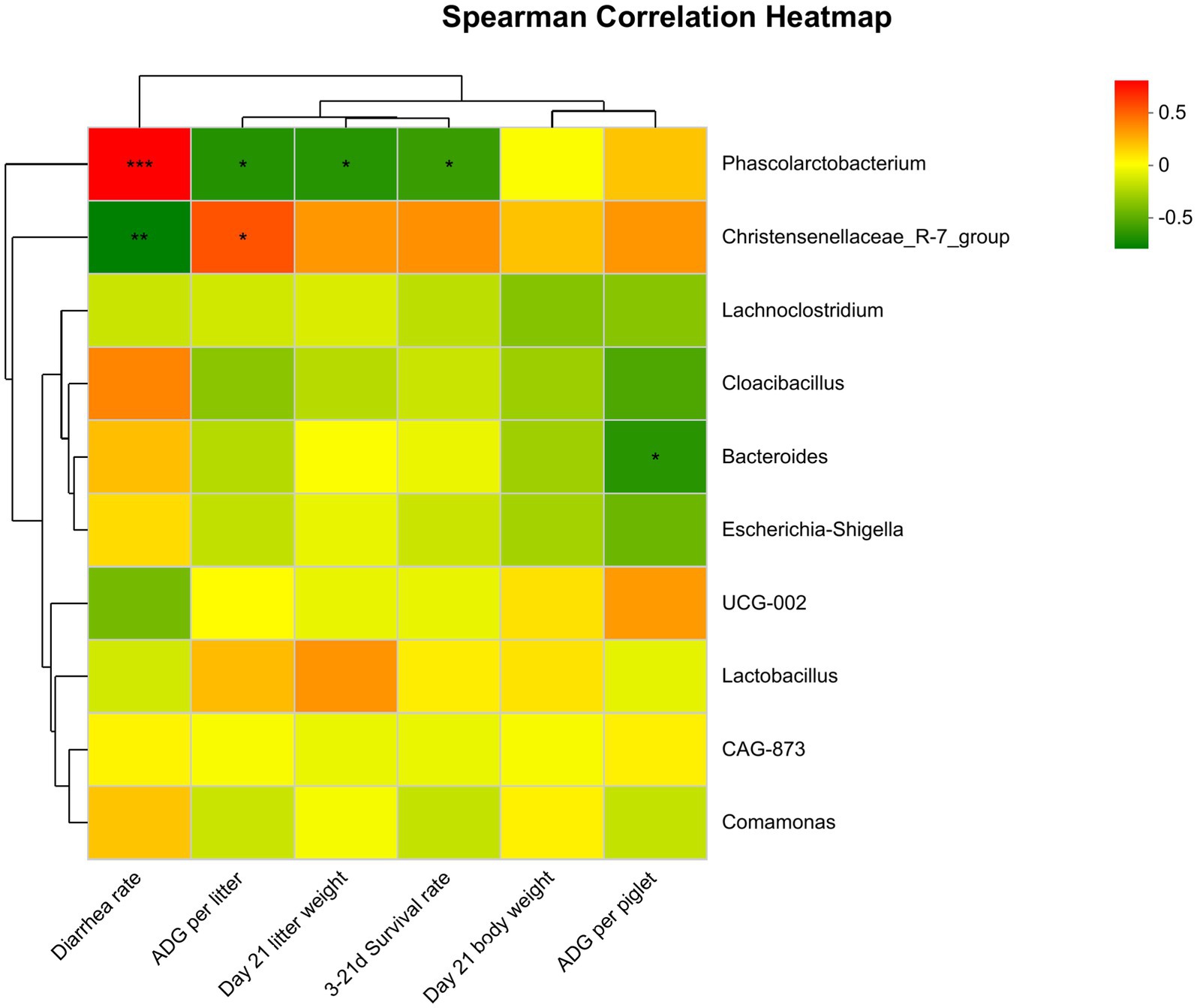
Figure 8. Spearman correlation analysis between differential genera and growth performance of piglets. Significant correlations are noted by *0.01 < p ≤ 0.05, **0.001 < p ≤ 0.01, ***p ≤ 0.001.
Discussion
Effects of maternal bamboo fiber supplementation on reproductive performance of sows and growth performance of piglets
It has been demonstrated that insufficient feed intake during lactation led to a deficiency in the sow’s milk production and a lack of overall nutrients required for piglet growth (Kim and Wu, 2004), which may limit the growth and development of piglets (He et al., 2017). Sows fed a high-soluble fiber and high-insoluble fiber diet had a significantly greater ADFI and lost less BW during lactation (Renteria-Flores et al., 2008). In this study, the ADFI of sows supplemented with dietary BBF1 was significantly higher than that with rice husk meal, while there was no significant difference between the BBF2 and CON groups. This suggests that the feed intake of lactating sows may be affected by fiber characteristics in addition to fiber level, and soluble fiber may affect the gastrointestinal chyme velocity and thus reduce feed intake.
Since the necessary nutrition for the fetus and newborn piglets comes mainly from the sows (Frese et al., 2015; Grześkowiak et al., 2022), this sow-piglet association influenced the performance of their offspring piglets. The backfat thickness change of sows during gestation did not differ among the groups in this study, which was consistent with earlier research showing that dietary fiber supplementation had no effect on body weight gain of sows during gestation when energy was similar, regardless of fiber source (Guillemet et al., 2007). This means that sows in the high feed intake group are more conducive to the growth of their offspring. In our study, dietary supplementation of 2% BBF1 in sows had a significant effect on ADG per litter at pre-weaning 11–21 days and 3–21 days, increasing by 50.68 and 51.70%, 31.61 and 34.71%, respectively, compared with the control and TRE2 groups. The addition of BBF1 also improved BW per litter at 21-day weaning and the survival rate of 3- to 21-day-old piglets. Similarly, maternal sugar beet pulp supplementation was more effective than wheat bran in improving sow ADFI and enhancing the growth performance of their piglets (Shang et al., 2019). The above studies indicate that it is feasible to increase the ADFI of lactating sows and improve the growth performance of their piglets by adding appropriate bamboo powder fiber.
Effects of maternal bamboo fiber supplementation on serum biochemical indices of sows
Dietary fiber regulates serum fat metabolism. Our previous study showed that the serum triglyceride concentration and total cholesterol decreased significantly with the increase of bamboo powder from 0 g/day, 30 g/day, and 60 g/day in the diets of perinatal sows (Dai et al., 2023). Another study in pregnant sows found that dietary supplementation with beet meal or bran significantly reduced serum total cholesterol in sows at 110 days of gestation (Shang et al., 2021). This result was also confirmed in an experiment on growing pigs. Dietary supplementation of fibrous raw materials (such as flaxseed meal and oat hulls) stimulated the excretion of bile acids and neutral sterols, thereby significantly reducing fat digestibility and serum cholesterol levels (Ndou et al., 2017). In this study, it was found that the serum total cholesterol and total triglyceride contents in TRE1 supplemented with BBF1 on the third day after delivery were higher than those in CON supplemented with rice husk meal, and the contents of total triglyceride reached a significant level on the 21st day after delivery, and the contents of total cholesterol were higher than those in CON. This suggests that bamboo fiber may be more beneficial to promote the lipid metabolism of sows than rice husk meal, which may also be the reason why it is more beneficial to improve the weight gain of piglets.
Interestingly, we found that the fibers in the above trial can be divided into two categories: soluble fibers, including beet meal and flaxseed meal; and insoluble fibers, including bran, oat shell, feeding bamboo powder, etc. However, they all regulated the lipid metabolism of pigs, which indicated that the serum total cholesterol and triglyceride levels are mainly affected by the fiber level, and the fiber component may not be the main influencing factor. However, another study found that different types of fiber showed inconsistent regulatory effects on the serum triglycerides of sows at different stages (Weng, 2020). Therefore, it is necessary to further study the application of different bamboo fibers in different physiological stages and their influence on the fat digestibility and milk fat content of sows.
Serum total protein and serum urea nitrogen levels can reflect the efficiency of protein utilization in pigs (Kohn et al., 2005). Changes in the serum urea nitrogen concentration of sows at different physiological stages may be the result of changes in diet or body protein breakdown (Muller et al., 2022). It was shown that serum total protein concentration increased significantly with the increase in dietary fiber supplementation level when adding citrus pulp, maize cob, and lucerne hay, which had a negative effect on production performance (Bakare et al., 2016). Our previous study found that supplemental feeding of 30 g/day or 60 g/day bamboo powder in the diet of sows during the perinatal period had no significant effects on serum total protein and urea nitrogen of sows (Dai et al., 2023), and similar results were obtained in this experiment. The above studies indicated that 2% BBF supplementation had no negative effects on the protein metabolism of sows during gestation and lactation. Supplemental feeding of bamboo powder may have similar protein metabolism and utilization as rice husk meal, and it might also be related to the physiological stage of sows.
Effects of maternal bamboo fiber supplementation on fecal microorganisms of sows and suckling piglets
Fiber nutrition of sows plays an important role in regulating the intestinal microbial composition and health of their offspring piglets. The gut microbial diversity was the main response to dietary fiber intake (Koh et al., 2016). It was found that dietary GCW (a compound SDF raw material) reduced gut microbial diversity and the relative abundance of bile salt hydrolase gene-encoding bacteria, Lactobacillus and Bacteroides, in sows on day 109 of gestation (Wu et al., 2021). Another study found that adding wheat bran, soybean hull, and sugar beet pulp to the high dietary fiber diet of sows significantly increased microbial diversity and abundance of Acidobacteria and Bacteroidetes in the colonic chyme of their piglets (He et al., 2020). Our previous study found that the supplemental feeding of bamboo powder in perinatal sows significantly reduced the fecal microbial diversity index of sows, while it tended to increase the fecal microbial diversity index of their piglets (Dai et al., 2023). These studies indicate that maternal dietary fiber supplementation has affected microbial diversity in their suckling piglets, which confirms the existence of “sow-piglet” association. Similarly, in this study, it has been found that bamboo fiber supplementation for sows tended to increase the fecal Sobs index of suckling piglets on the 21st day after delivery. These results suggested that adding bamboo fiber (a natural IDF raw material) to the high dietary fiber diet of sows might help increase the microbial diversity of offspring piglets.
The fiber of rice husk meal and feeding bamboo powder was mainly composed of IDF, and IDF had a slow fermentation speed and produced less short-chain fatty acid (SCFA) than soluble fiber, thereby reducing the richness of the gut microbiota (Jha and Berrocoso, 2015). Another study found that there were no differences in the fecal microbial diversity index among the sows treated with lignocellulose, resistant starch, and konjac flour (Lu et al., 2022). Similarly, even sugar beet pulp (SBP) and oat bran (OB) had a similar ratio of SDF to IDF, while the predicted acetate, total SCFA production, and absorption in the SBP group were higher than those of the OB group (Bai et al., 2022). In this study, it was shown that the fecal microbial diversity index of sows and piglets in the group with BBF1 was higher than that with rice husk meal on days 7 and 21 of lactation, but the addition of bamboo fiber polymer material (SDF) on the basis of BBF1 did not further improve the microbial diversity. These results indicated that the effects of SDF and ISF on microbial diversity were not consistent, and it might be more important to select appropriate dietary fiber sources.
Firmicutes was the dominant bacteria in the fecal flora of healthy sows, followed by Proteobacteria and Bacteroidetes (Zhang et al., 2021). This study also found that the top five dominant bacteria in sow feces were Firmicutes, Proteobacteria, Bacteroidota, Spirochaetota, and Actinobacteriota. Firmicutes played a significant role in the degradation of complex plant carbohydrates and the key metabolic conversions within the intestinal community, such as the major butyrate-producing species (Flint et al., 2012). The fecal dominant genera varied as piglets aged, and transitions toward a mature gut microbiota enriched with fiber-degrading bacteria were mostly completed upon weaning in piglets with better growth (Mahmud et al., 2023). This study found that the top five dominant bacteria in sows feces were relatively stable at different stages after delivery, while the top five dominant bacteria of piglets had evolved and added Synergistota on day 7 after delivery and Spirochaeta on day 21 after delivery. These results indicated that the gut microbiota of piglets might be susceptible to environmental changes after birth.
It has been proven that many fiber-rich materials affect the intestinal flora composition of sows. A study found that stevia-residue supplementation promoted the relative abundance of fecal beneficial bacteria of pregnant sows, such as g__Lachnospiraceae_XPB1014_group and g__Christensenellaceae_R-7_group, and reduced the relative abundance of harmful bacteria, such as Treponema_2 (Yu et al., 2020). Another study found that the addition of 5% Pennisetum purpureum during late pregnancy of sows significantly increased the relative abundance of Bacteroidetes, Actinobacteria, and Prevotellaceae_UCG_001 and decreased the abundance of Escherichia_Shigella (Huang et al., 2021). In recent years, bamboo feed resources have been developed and applied to pig production. A study found that the addition of 12% fermented bamboo shoot processing waste had beneficial effects on the gut microbiota of weaned piglets and significantly reduced the taxon feature number and the relative abundance of Tenericutes in the cecum (Huang J. et al., 2022; Huang Y. et al., 2022). Another study found that the intake of fermented bamboo fiber by sows promoted the enrichment of beneficial genera such as Lachnospira, Lachnospiracea_XPB1014_Group, and Roseburia and reduced the relative abundance of harmful bacteria such as Fusobacterium, Sutterellaceae, and Sutterella, while significantly increasing the relative abundance of beneficial bacteria Alistipes and Lachnoclostridium and decreasing the pathogenic bacteria Trueperella of piglets (Sun et al., 2023). In this study, it was found that bamboo fiber supplementation decreased the fecal genera Christensenellaceae_R-7_group of sows with no significant effect on their suckling piglet. These results indicated that bamboo fiber and rice husk meal had different regulatory effects on the microbial composition of sows, although both of them were IDF fibers.
Associations between fecal microbes and growth performance of suckling piglets
It has been shown that the gut microbiota changes dramatically across different reproductive stages (Cheng et al., 2018). Fiber ingestion during the suckling period is helpful for gut development and probiotic colonization. It has been shown that xylooligosaccharide ingestion in suckling piglets improved growth performance and feed efficiency after weaning by increasing the fermentation capacity of the microbiota and fiber-degrading enzyme secretion (Bai et al., 2021a). According to the difference in fecal microbial composition of piglets among different fiber treatment groups, this study focused on analyzing the correlation between fecal microbial composition and growth performance of piglets on day 21 after delivery. The diarrhea rate was associated with increases in the relative abundance of Prevotella, while the increased relative abundance of Prevotella was correlated with a reduction in Escherichia coli and the majority of beneficial bacteria belonging to the Firmicutes phylum in piglets (Yang et al., 2017). Another study showed that the abundance of norank_f__Muribaculaceae, Christensenellaceae_R-7_group, Enterococcus, and Romboutsia had a positive connection with almost all barrier function genes of neonatal piglets on day 21 (Wu et al., 2020). It was shown that butyrate production could be improved by inhibiting the proliferation of Lachnospiraceae_XPB_1014_group and Bacteroides, which had a lack of potential to secrete β-xylosidase (Bai et al., 2021b). This study also found that there was a significant negative correlation between the abundance of Bacteroides and ADG in piglets, while the abundance of Christensenellaceae_R-7_group was significantly negatively correlated with diarrhea rate and positively correlated with litter ADG. This suggests that maternal bamboo fiber supplementation can improve the growth performance of piglets by promoting the abundance of beneficial flora.
Conclusion
It was shown that maternal bamboo fiber supplementation during perinatal and lactation increased the feed intake of sows during lactation, litter weight gain, weaning litter weight, and serum triglyceride of piglets. This beneficial effect of bamboo fiber was associated with the improvement of diversity and structure of fecal microbiota in the piglets.
Data availability statement
The data presented in this study are deposited in the NCBI repository, accession number PRJNA1119022.
Ethics statement
The animal study was reviewed by Animal Care and Use Committee of Leshan Normal University (Certification No. 4151010649), China, and conducted in accordance with the approved protocol (No. LAC2023006). Written informed consent was obtained from the owners for the participation of their animals in this study.
Author contributions
FD: Conceptualization, Data curation, Funding acquisition, Methodology, Project administration, Writing – original draft, Writing – review & editing. TL: Conceptualization, Investigation, Software, Supervision, Writing – review & editing. MJ: Data curation, Formal analysis, Investigation, Methodology, Resources, Software, Writing – original draft. XH: Data curation, Methodology, Writing – original draft. LW: Software, Supervision, Writing – review & editing. JM: Data curation, Formal analysis, Methodology, Writing – original draft. HY: Data curation, Formal analysis, Methodology, Writing – original draft. XF: Data curation, Formal analysis, Methodology, Writing – original draft. XN: Writing – review & editing. JZ: Writing – review & editing.
Funding
The author(s) declare that financial support was received for the research, authorship, and/or publication of this article. This study was supported by the Key Program of the Sichuan Science and Technology Plan (2021YFN0106) and the Talent Start-up Project of Leshan Normal University (RC202004).
Conflict of interest
The authors declare that the research was conducted in the absence of any commercial or financial relationships that could be construed as a potential conflict of interest.
Publisher’s note
All claims expressed in this article are solely those of the authors and do not necessarily represent those of their affiliated organizations, or those of the publisher, the editors and the reviewers. Any product that may be evaluated in this article, or claim that may be made by its manufacturer, is not guaranteed or endorsed by the publisher.
Footnotes
1. ^https://github.com/OpenGene/fastp, version 0.19.6.
2. ^http://www.cbcb.umd.edu/software/flash, version 1.2.11.
3. ^http://drive5.com/uparse/, version 7.1.
4. ^http://rdp.cme.msu.edu/, version 2.11.
References
Awad, W. A., Ghareeb, K., Paßlack, N., and Zentek, J. (2013). Dietary inulin alters the intestinal absorptive and barrier function of piglet intestine after weaning. Res. Vet. Sci. 95, 249–254. doi: 10.1016/j.rvsc.2013.02.009
Bai, Y., Wang, Z., Zhou, X., Zhang, Y., Ye, H., Wang, H., et al. (2021a). Ingestion of xylooligosaccharides during the suckling period improve the feed efficiency and hindgut fermentation capacity of piglets after weaning. Food Funct. 12, 10459–10469. doi: 10.1039/d1fo02275j
Bai, Y., Zhou, X., Li, N., Zhao, J., Ye, H., Zhang, S., et al. (2021b). In vitro fermentation characteristics and Fiber-degrading enzyme kinetics of cellulose, Arabinoxylan, β-Glucan and Glucomannan by pig Fecal microbiota. Microorganisms 9:1071. doi: 10.3390/microorganisms9051071
Bai, Y., Zhou, X., Zhao, J., Wang, Z., Ye, H., Pi, Y., et al. (2022). Sources of dietary Fiber affect the SCFA production and absorption in the hindgut of growing pigs. Front. Nutr. 8:719935. doi: 10.3389/fnut.2021.719935
Bakare, A. G., Ndou, S. P., Madzimure, J., and Chimonyo, M. (2016). Nutritionally related blood metabolites and performance of finishing pigs fed on graded levels of dietary fibre. Trop. Anim. Health Prod. 48, 1065–1069. doi: 10.1007/s11250-016-1038-1
Chen, T., Chen, D., Tian, G., Zheng, P., Mao, X., Yu, J., et al. (2019). Effects of soluble and insoluble dietary fiber supplementation on growth performance, nutrient digestibility, intestinal microbe and barrier function in weaning piglet. Anim. Feed Sci. Technol. 260:114335. doi: 10.1016/j.anifeedsci.2019.114335
Chen, S., Zhou, Y., Chen, Y., and Gu, J. (2018). Fastp: an ultra-fast all-in-one FASTQ preprocessor. Bioinformatics 34, i884–i890. doi: 10.1093/bioinformatics/bty560
Cheng, C., Wei, H., Yu, H., Xu, C., Jiang, S., and Peng, J. (2018). Metabolic syndrome during perinatal period in sows and the link with gut microbiota and metabolites. Front. Microbiol. 9:1989. doi: 10.3389/fmicb.2018.01989
Dai, F., Lin, T., Cheng, L., Wang, J., Zuo, J., and Feng, D. (2022). Effects of micronized bamboo powder on growth performance, serum biochemical indexes, cecal chyme microflora and metabolism of broilers aged 1-22 days. Trop. Anim. Health Prod. 54:166. doi: 10.1007/s11250-022-03172-0
Dai, F., Lin, T., Huang, X., Shi, X., Yang, Y., Nong, X., et al. (2023). Effects from supplementary feeding of bamboo powder in perinatal period on farrowing process, serum biochemical indexes, and fecal microbes of sows and offspring piglets. Front. Microbiol. 14:1139625. doi: 10.3389/fmicb.2023.1139625
Dai, F., Lin, T., Su, B., Yao, H., Guo, D., and Yang, Y. (2021). Effects of feeding bamboo powder on growth performance, serum biochemical indexes and fecal microorganism of weaned piglets. Chin. J. Anim. Nutr. 33, 6709–6720.
Edgar, R. C. (2013). UPARSE: highly accurate OTU sequences from microbial amplicon reads. Nat. Methods 10, 996–998. doi: 10.1038/nmeth.2604
Felisberto, M. H. F., Miyake, P. S. E., Beraldo, A. L., and Clerici, M. T. P. S. (2017). Young bamboo culm: potential food as source of fiber and starch. Food Res. Int. 101, 96–102. doi: 10.1016/j.foodres.2017.08.058
Flint, H. J., Scott, K. P., Duncan, S. H., Louis, P., and Forano, E. (2012). Microbial degradation of complex carbohydrates in the gut. Gut Microbes 3, 289–306. doi: 10.4161/gmic.19897
Frese, S. A., Parker, K., Calvert, C. C., and Mills, D. A. (2015). Diet shapes the gut microbiome of pigs during nursing and weaning. Microbiome 3:28. doi: 10.1186/s40168-015-0091-8
Ge, Q., Li, H. Q., Zheng, Z. Y., Yang, K., Li, P., Xiao, Z. Q., et al. (2022). In vitro fecal fermentation characteristics of bamboo insoluble dietary fiber and its impacts on human gut microbiota. Food Res. Int. 156:111173. doi: 10.1016/j.foodres.2022.111173
Grześkowiak, Ł., Saliu, E. M., Martínez-Vallespín, B., Wessels, A. G., Männer, K., Vahjen, W., et al. (2022). Fiber composition in Sows' diets modifies Clostridioides difficile colonization in their offspring. Curr. Microbiol. 79:154. doi: 10.1007/s00284-022-02848-y
Guillemet, R., Hamard, A., Quesnel, H., Père, M. C., Etienne, M., Dourmad, J. Y., et al. (2007). Dietary fibre for gestating sows: effects on parturition progress, behaviour, litter and sow performance. Animal 1, 872–880. doi: 10.1017/S1751731107000110
He, Y., Peng, X., Liu, Y., Wu, Q., Zhou, Q., Hu, L., et al. (2020). Effects of maternal fiber intake on intestinal morphology, bacterial profile and proteome of newborns using pig as model. Nutrients 13:42. doi: 10.3390/nu13010042
He, L., Zang, J., Liu, P., Fan, P., Song, P., Chen, J., et al. (2017). Supplementation of milky Flavors improves the reproductive performance and gut function using sow model. Protein Pept. Lett. 24, 449–455. doi: 10.2174/0929866524666170223144728
Huang, J., Lin, B., Zhang, Y., Xie, Z., Zheng, Y., Wang, Q., et al. (2022). Bamboo shavings derived O-acetylated xylan alleviates loperamide-induced constipation in mice. Carbohydr. Polym. 276:118761. doi: 10.1016/j.carbpol.2021.118761
Huang, P. F., Mou, Q., Yang, Y., Li, J. M., Xu, M. L., Huang, J., et al. (2021). Effects of supplementing sow diets during late gestation with Pennisetum purpureum on antioxidant indices, immune parameters and faecal microbiota. Vet. Med. Sci. 7, 1347–1358. doi: 10.1002/vms3.450
Huang, Y., Peng, Y., Yang, Z., Chen, S., Liu, J., Wang, Z., et al. (2022). Effects of fermented bamboo shoot processing waste on growth performance, serum parameters, and gut microbiota of weaned piglets. Animals (Basel) 12:2728. doi: 10.3390/ani12202728
Jha, R., and Berrocoso, J. D. (2015). Review: dietary fiber utilization and its effects on physiological functions and gut health of swine. Animal 9, 1441–1452. doi: 10.1017/S1751731115000919
Kim, S. W., and Wu, G. (2004). Dietary arginine supplementation enhances the growth of milk-fed young pigs. J. Nutr. 134, 625–630. doi: 10.1093/jn/134.3.625
Koh, A., De Vadder, F., Kovatcheva-Datchary, P., and Bäckhed, F. (2016). From dietary Fiber to host physiology: short-chain fatty acids as key bacterial metabolites. Cell 165, 1332–1345. doi: 10.1016/j.cell.2016.05.041
Kohn, R. A., Dinneen, M. M., and Russek-Cohen, E. (2005). Using blood urea nitrogen to predict nitrogen excretion and efficiency of nitrogen utilization in cattle, sheep, goats, horses, pigs, and rats. J. Anim. Sci. 83, 879–889. doi: 10.2527/2005.834879x
Li, H., Yin, J., Tan, B., Chen, J., Zhang, H., Li, Z., et al. (2021). Physiological function and application of dietary fiber in pig nutrition: a review. Anim. Nutr. 7, 259–267. doi: 10.1016/j.aninu.2020.11.011
Li, S., Zheng, J., He, J., Liu, H., Huang, Y., Huang, L., et al. (2023). Dietary fiber during gestation improves lactational feed intake of sows by modulating gut microbiota. J. Anim. Sci. Biotechnol. 14:65. doi: 10.1186/s40104-023-00870-z
Liu, C., Zhao, D., Ma, W., Guo, Y., Wang, A., Wang, Q., et al. (2016). Denitrifying sulfide removal process on high-salinity wastewaters in the presence of Halomonas sp. Appl. Microbiol. Biotechnol. 100, 1421–1426. doi: 10.1007/s00253-015-7039-6
Liu, B., Zhu, X., Cui, Y., Wang, W., Liu, H., Li, Z., et al. (2021). Consumption of dietary Fiber from different sources during pregnancy alters sow gut microbiota and improves performance and reduces inflammation in sows and piglets. mSystems 6, e00591–e00520. doi: 10.1128/mSystems.00591-20
Lu, D., Pi, Y., Ye, H., Wu, Y., Bai, Y., Lian, S., et al. (2022). Consumption of dietary fiber with different physicochemical properties during late pregnancy alters the gut microbiota and relieves constipation in sow model. Nutrients 14:2511. doi: 10.3390/nu14122511
Magoč, T., and Salzberg, S. L. (2011). FLASH: fast length adjustment of short reads to improve genome assemblies. Bioinformatics 27, 2957–2963. doi: 10.1093/bioinformatics/btr507
Mahmud, M. R., Jian, C., Uddin, M. K., Huhtinen, M., Salonen, A., Peltoniemi, O., et al. (2023). Impact of intestinal microbiota on growth performance of suckling and weaned piglets. Microbiol. Spectr. 11:e0374422. doi: 10.1128/spectrum.03744-22
Muller, T. L., Pluske, J. R., Plush, K. J., D'Souza, D. N., Miller, D. W., and van Barneveld, R. J. (2022). Serum creatinine is a poor marker of a predicted change in muscle mass in lactating sows. J. Anim. Physiol. Anim. Nutr. (Berl) 106, 1009–1016. doi: 10.1111/jpn.13637
Ndou, S. P., Kiarie, E., Thandapilly, S. J., Walsh, M. C., Ames, N., and Nyachoti, C. M. (2017). Flaxseed meal and oat hulls supplementation modulates growth performance, blood lipids, intestinal fermentation, bile acids, and neutral sterols in growing pigs fed corn-soybean meal-based diets. J. Anim. Sci. 95, 3068–3078. doi: 10.2527/jas.2016.1328
Owusu-Asiedu, A., Patience, J. F., Laarveld, B., Van Kessel, A. G., Simmins, P. H., and Zijlstra, R. T. (2006). Effects of guar gum and cellulose on digesta passage rate, ileal microbial populations, energy and protein digestibility, and performance of grower pigs. J. Anim. Sci. 84, 843–852. doi: 10.2527/2006.844843x
Paßlack, N., Vahjen, W., and Zentek, J. (2015). Dietary inulin affects the intestinal microbiota in sows and their suckling piglets. BMC Vet. Res. 11:51. doi: 10.1186/s12917-015-0351-7
Pi, Y., Hu, J., Bai, Y., Wang, Z., Wu, Y., Ye, H., et al. (2021). Effects of dietary fibers with different physicochemical properties on fermentation kinetics and microbial composition by fecal inoculum from lactating sows in vitro. J. Sci. Food Agric. 101, 907–917. doi: 10.1002/jsfa.10698
Renteria-Flores, J. A., Johnston, L. J., Shurson, G. C., Moser, R. L., and Webel, S. K. (2008). Effect of soluble and insoluble dietary fiber on embryo survival and sow performance. J. Anim. Sci. 86, 2576–2584. doi: 10.2527/jas.2007-0376
Serena, A., Jørgensen, H., and Bach Knudsen, K. E. (2008). Digestion of carbohydrates and utilization of energy in sows fed diets with contrasting levels and physicochemical properties of dietary fiber. J. Anim. Sci. 86, 2208–2216. doi: 10.2527/jas.2006-060
Shang, Q., Liu, H., Liu, S., He, T., and Piao, X. (2019). Effects of dietary fiber sources during late gestation and lactation on sow performance, milk quality, and intestinal health in piglets. J. Anim. Sci. 97, 4922–4933. doi: 10.1093/jas/skz278
Shang, Q., Liu, S., Liu, H., Mahfuz, S., and Piao, X. (2021). Impact of sugar beet pulp and wheat bran on serum biochemical profile, inflammatory responses and gut microbiota in sows during late gestation and lactation. J. Anim. Sci. Biotechnol. 12:54. doi: 10.1186/s40104-021-00573-3
Stackebrandt, E., and Goebel, B. M. (1994). Taxonomic note: a place for DNA-DNA Reassociation and 16S rRNA sequence analysis in the present species definition in bacteriology. Int. J. Syst. Evol. Microbiol. 44, 846–849. doi: 10.1099/00207713-44-4-846
Sun, C., Song, R., Zhou, J., Jia, Y., and Lu, J. (2023). Fermented bamboo Fiber improves productive performance by regulating gut microbiota and inhibiting chronic inflammation of sows and piglets during late gestation and lactation. Microbiol. Spectr. 11:e0408422. doi: 10.1128/spectrum.04084-22
Tan, C., Wei, H., Ao, J., Long, G., and Peng, J. (2016). Inclusion of Konjac flour in the gestation diet changes the gut microbiota, alleviates oxidative stress, and improves insulin sensitivity in sows. Appl. Environ. Microbiol. 82, 5899–5909. doi: 10.1128/AEM.01374-16
Tundis, R., Augimeri, G., Vivacqua, A., Romeo, R., Sicari, V., Bonofiglio, D., et al. (2023). Anti-inflammatory and antioxidant effects of leaves and sheath from bamboo (Phyllostacys edulis J. Houz). Antioxidants (Basel) 12:1239. doi: 10.3390/antiox12061239
Wang, Z., Chen, Y., Wang, W., Huang, C., Hu, Y., Johnston, L., et al. (2022). Dietary supplementation with fine-grinding wheat bran improves lipid metabolism and inflammatory response via modulating the gut microbiota structure in pregnant sow. Front. Microbiol. 13:835950. doi: 10.3389/fmicb.2022.835950
Wang, Q., Garrity, G. M., Tiedje, J. M., and Cole, J. R. (2007). Naive Bayesian classifier for rapid assignment of rRNA sequences into the new bacterial taxonomy. Appl. Environ. Microbiol. 73, 5261–5267. doi: 10.1128/AEM.00062-07
Weng, R. C. (2020). Dietary supplementation with different types of fiber in gestation and lactation: effects on sow serum biochemical values and performance. Asian Australas. J. Anim. Sci. 33, 1323–1331. doi: 10.5713/ajas.19.0545
Wu, X., Yin, S., Cheng, C., Xu, C., and Peng, J. (2021). Inclusion of soluble Fiber during gestation regulates gut microbiota, improves bile acid homeostasis, and enhances the reproductive performance of sows. Front. Vet. Sci. 8:756910. doi: 10.3389/fvets.2021.756910
Wu, Y., Zhang, X., Han, D., Ye, H., Tao, S., Pi, Y., et al. (2020). Short Administration of Combined Prebiotics Improved Microbial Colonization, gut barrier, and growth performance of neonatal piglets. ACS Omega 5, 20506–20516. doi: 10.1021/acsomega.0c02667
Yang, Q., Huang, X., Zhao, S., Sun, W., Yan, Z., Wang, P., et al. (2017). Structure and function of the Fecal microbiota in diarrheic neonatal piglets. Front. Microbiol. 8:502. doi: 10.3389/fmicb.2017.00502
Yu, M., Gao, T., Liu, Z., and Diao, X. (2020). Effects of dietary supplementation with high Fiber (Stevia residue) on the Fecal Flora of pregnant sows. Animals (Basel) 10:2247. doi: 10.3390/ani10122247
Zhang, X., Lu, J., and Zhang, X. (2022). Spatiotemporal trend of carbon storage in China's bamboo industry, 1993-2018. J. Environ. Manag. 314:114989. doi: 10.1016/j.jenvman.2022.114989
Zhang, S., Tian, M., Song, H., Shi, K., Wang, Y., and Guan, W. (2018). Effects of L-carnitine on reproductive performance, milk composition, placental development and IGF concentrations in blood plasma and placental chorions in sows. Arch. Anim. Nutr. 72, 261–274. doi: 10.1080/1745039X.2018.1471185
Keywords: bamboo powder, lactating sows, feed intake, weaning litter weight, fecal microflora
Citation: Dai F, Lin T, Jin M, Huang X, Wang L, Ma J, Yu H, Fan X, Nong X and Zuo J (2024) Bamboo fiber improves piglet growth performance by regulating the microbial composition of lactating sows and their offspring piglets. Front. Microbiol. 15:1411252. doi: 10.3389/fmicb.2024.1411252
Edited by:
Yu Pi, Feed Research Institute (CAAS), ChinaReviewed by:
Yu Bai, Tianjin University of Science and Technology, ChinaHan Yunsheng, Feed Research Institute (CAAS), China
Copyright © 2024 Dai, Lin, Jin, Huang, Wang, Ma, Yu, Fan, Nong and Zuo. This is an open-access article distributed under the terms of the Creative Commons Attribution License (CC BY). The use, distribution or reproduction in other forums is permitted, provided the original author(s) and the copyright owner(s) are credited and that the original publication in this journal is cited, in accordance with accepted academic practice. No use, distribution or reproduction is permitted which does not comply with these terms.
*Correspondence: Fawen Dai, 45912126@qq.com
†These authors have contributed equally to this work