- 1Ocean College, Hebei Agricultural University, Qinhuangdao, China
- 2Hebei Key Laboratory of Nutrition Regulation and Disease Control for Aquaculture, Qinhuangdao, China
- 3Marine Geological Resources Survey Center of Hebei Province, Qinhuangdao, China
- 4Ocean Survey Department, Qinhuangdao Marine Center of the Ministry of Natural Resources, Qinhuangdao, China
- 5Agro-Environmental Protection Institute, Ministry of Agriculture and Rural Affairs, Tianjin, China
- 6College of Life Sciences, Dezhou University, Dezhou, China
Anthropogenic activities are driving significant changes in coastal ecological environments, increasingly spotlighting microorganisms associated with seagrass bed ecosystems. Labyrinthula is primarily recognized as a saprophytic protist associated with marine detritus, and it also acts as an opportunistic pathogen affecting marine algae, terrestrial plants and mollusks, especially in coastal environments. The genus plays a key role in the decomposition of marine detritus, facilitated by its interactions with diatoms and through the utilization of a diverse array of carbohydrate-active enzymes to decompose seagrass cell walls. However, human activities have significantly influenced the prevalence and severity of seagrass wasting disease (SWD) through factors such as climate warming, increased salinity and ocean acidification. The rise in temperature and salinity, exacerbated by human-induced climate change, has been shown to increase the susceptibility of seagrass to Labyrinthula, highlighting the adaptability of pathogen to environmental stressors. Moreover, the role of seagrass in regulating pathogen load and their immune response to Labyrinthula underscore the complex dynamics within these marine ecosystems. Importantly, the genotype diversity of seagrass hosts, environmental stress factors and the presence of marine organisms such as oysters, can influence the interaction mechanisms between seagrass and Labyrinthula. Besides, these organisms have the potential to both mitigate and facilitate pathogen transmission. The complexity of these interactions and their impacts driven by human activities calls for the development of comprehensive multi-factor models to better understand and manage the conservation and restoration of seagrass beds.
1 Introduction
Labyrinthulomycetes are a group of marine unicellular heterotrophic protists belonging to the Stramenopiles and the phylum Labyrinthulomycota, primarily consisting of thraustochytrids and labyrinthulids (Raghukumar, 2002; Table 1). The distinctive features of them are cell walls composed of sulfated polysaccharides and an ectoplasmic net structure formed by extensions from the cell membrane (Tsui et al., 2009). Thraustochytrid cells range from spherical to oval in shape, with the ectoplasmic net distributed on one side of the body and reproducing through the production of biflagellate zoospores of varying lengths (Moss, 1985). While labyrinthulid cells are spindle-shaped, covered externally by an ectoplasmic net and glide using this net (Moss, 1985; Popova et al., 2020). Thraustochytrids, inhibited by antimicrobial substances secreted by marine plants, are seldom found on living seaweeds and seagrass. Yet, they can parasitize certain invertebrates such as octopuses (Polglase, 1980), clams (Dahl et al., 2008) and squids (Jones and O'Dor, 1983), potentially causing infectious and lethal lesions directly or indirectly to the hosts. Labyrinthula is the only genus of labyrinthulids, and is a renowned protozoan pathogen, commonly existing in a parasitic or symbiotic manner inside living seagrass (Bergmann et al., 2011), marine algae (Popova et al., 2020), coral mucus (Burge et al., 2012) and terrestrial plants (Chitrampalam et al., 2015), leading to lethal lesions in these hosts. Furthermore, both groups of microbes are abundantly present on dead marine algae and decomposing mangrove leaves. They play a crucial role in the marine carbon cycle by degrading these rotting plants through the production of extracellular enzymes (Raghukumar, 2002).
Labyrinthulomycetes have garnered widespread attention partially due to their ability to produce various high-value metabolites, including polyunsaturated fatty acids (PUFAs) and squalene. Currently, many studies are dedicated to screening high-yield fatty acid-producing thraustochytrid strains, optimizing cultivation conditions and breeding genetically engineered strains to increase the production of target compounds in thraustochytrids. However, studies on the isolation and fatty acid applications of Labyrinthula is severely limited. Labyrinthula sp. L72 isolated from the Seto Inland Sea, could not only efficiently utilize soy lecithin but also produce DHA (the only kind of PUFA in the cells), offering significant advantages for the industrial production of DHA (Kumon et al., 2005). Besides, Labyrinthula strains from the coastal waters of the South China Sea had been found to produce a large amount of PUFAs, with DHA accounting for more than 40% of the total fatty acids (Wang et al., 2019). The pathogen of SWD in L. zosterae, synthesizes DHA as its primary fatty acid on both artificial and algae-based media (Yoshioka et al., 2019). In addition, laboratory-inoculated and field-collected diseased eelgrass showed higher DHA content than controls, which suggested that L. zosterae might be an unrecognized natural source of PUFAs in the eelgrass ecosystem (Yoshioka et al., 2019). Our previous studies isolated 28 Labyrinthula strains evolutionarily close to thraustochytrids from the mangrove coastal areas of China, indicating a similar natural habitat and substrate selectivity between them (Wang et al., 2019). This result also suggested a potential coexistence and similar ecological functions between thraustochytrids and labyrinthulids (Wang et al., 2019). Recently, most studies on Labyrinthula focus on its pathogenicity toward seagrass, with fewer studies on metabolite composition analysis. Therefore, future studies on the metabolite analysis of Labyrinthula may provide new insights into revealing their interaction with seagrass in the natural habitats.
Seagrass diseases are an important cause of seagrass loss in some coastal areas. The protist Labyrinthula is an opportunistic pathogen of seagrass wasting disease (SWD) and is currently the focus of seagrass pathogen research (Table 2). In the early 20th century, 90% of the seagrass Zostera marina populations in the eastern United States and Western Europe died from this disease (Bergmann et al., 2011). High salinity, climate warming and eutrophication caused by human activities, escalate survival pressures on seagrass and diminish their adaptability. These factors are the primary risks contributing to the heightened frequency of seagrass disease outbreaks (Sullivan et al., 2018). Moreover, global changes may further facilitate the proliferation of pathogens. However, the specific mechanisms fueling the transmission of SWD remain elusive (Sullivan et al., 2013; Lee et al., 2021; Sullivan et al., 2023). Additionally, as saprophytic organisms, species of Labyrinthula are adept at decomposing organic materials, showcasing their potential in the bioremediation of coastal pollutants and playing a pivotal role in safeguarding marine ecosystems (Raikar et al., 2001). Hence, delving into the cultivable diversity, along with the physiological and biochemical properties, will not only shed light on their ecological significance but also bear crucial implications for the preservation of coastal environments and the advancement of marine aquaculture. To provide a comprehensive understanding of the protist Labyrinthula spp. and the seagrass disease caused by them, the taxonomy and ecological functions of Labyrinthula spp., as well as their relationship with seagrass disease under the influence of anthropogenic activities were summarized in this paper.
2 Taxonomy of Labyrinthula spp.
Labyrinthulomycetes have undergone several rearrangements, and the precise classification is unclear. However, recent revisions attempt to provide an updated higher-level taxonomic framework (Yokoyama et al., 2007; Pan et al., 2016; Doi and Honda, 2017). Distinguishing species within the genus Labyrinthula based on morphology and molecular biology continues to be a challenge. Combining ecological data with molecular phylogenetic data could play a crucial role in species delimitation within this group (Martin et al., 2016). Notably, only sequences of several Labyrinthula species have been described in GenBank, suggesting that the existing diversity of species and genera may be underestimated (Collado-Mercado et al., 2010; Martin et al., 2016; Adl et al., 2019). Furthermore, many newly isolated Labyrinthula strains cannot be assigned to specific species due to their as of yet, unresolved classification (Sullivan et al., 2017). Moreover, the lifestyle and ecological roles of Labyrinthula was unveiled, enriching the understanding of their physiological characteristics and ecological impacts (Dyková et al., 2008; Raghukumar and Damare, 2011; Burge et al., 2012). Therefore, further studies are necessary for a deeper understanding and accurate classification of the genus Labyrinthula and its members, which is crucial for revealing their roles and functions in natural habitats.
Analysis based on 18S rRNA has unveiled an extensive array of yet-to-be-described species within the genus Labyrinthula, noting that not all isolates result in necrotic lesions on seagrass (Sullivan et al., 2017). Research focusing on Z. marina illustrates that merely specific clades of isolates are implicated in disease manifestation, highlighting the genetic divergence between pathogenic and non-pathogenic strains of Labyrinthula (Bockelmann et al., 2012). Additional investigations, including geographical studies, toxicity assessments and sequencing of Z. marina in Europe, aimed to clarify the distinctions between pathogenic and non-pathogenic Labyrinthula (Brakel et al., 2014). The analysis of 170 recently isolated Labyrinthula strains sought to further identify their clades, host affiliations and geographical distribution (Martin et al., 2016). Moreover, Labyrinthula may become pathogenic under unfavorable environmental conditions (e.g., high water temperatures and insufficient light), while Z. marina seems to inhibit the growth of Labyrinthula by boosting its own growth and synthesizing phenolic acids (Brakel et al., 2014). Although the virulence of Labyrinthula exhibits variability, its pathogenicity remains high with a potential for cross-infecting hosts (Martin et al., 2016). This provides new criteria for the taxonomic study of Labyrinthula species. Collecting a broader array of samples illustrating interactions between seagrass and Labyrinthula, irrespective of the pathogenic status, opens novel avenues for elucidating species diversity of Labyrinthula, pathogenic mechanisms and intricate relationships with seagrass. This endeavor propels forward the research into its phylogeny and the evolutionary mechanisms underpinning its environmental adaptability (Martin et al., 2016).
3 Ecological functions of Labyrinthula spp.
Labyrinthula are mostly fungus-like heterotrophic protists that absorb nutrients through osmotrophic or phagotrophic means. Most Labyrinthula species are saprophytic, commonly associated with detritus such as fallen mangrove leaves, decaying algae and fecal pellets of marine invertebrates (Tsui et al., 2009). They also act as an opportunistic pathogen, linked to infections in marine algae (Sullivan et al., 2017) and terrestrial plants (Chitrampalam et al., 2015). The genus Labyrinthula is primarily associated with coastal environments. Since diatoms are major primary producers in coastal regions, interactions between Labyrinthula and diatoms could play a significant role in the decomposition of marine detritus (Popova et al., 2020; Tan et al., 2021). Specific strains isolated from surface marine sediments along the coasts of the Indian and Pacific Oceans, such as L. diatomea, are capable of utilizing marine diatoms Cylindrotheca closterium and Micropodiscus weissflogii as substrates for growth (Popova et al., 2020). Additionally, previous studies have shown that Labyrinthula magnifica and L. sp. also exhibit the capability to prey on diatoms (Grell, 1994). These diatom-associated Labyrinthula strains were colorless, but exhibited differences in dense clumps morphology, trophic cells and geographical distribution (Popova et al., 2020). These observations suggest potential species-level independence among them (Popova et al., 2020). While Labyrinthula has a global distribution, most known sequences originated from North America (Bai et al., 2019; Lee et al., 2021). This indicates that the diversity and ecological roles of Labyrinthula are still underestimated. In addition, Labyrinthula may consume different types of substrates or co-distribute with diatoms. Future studies on Labyrinthula and diatoms in natural habitats across different geographical ranges will help clarify the relationships within marine communities on a global scale.
Genomic sequencing of the pathogenic strain L. SR_Ha_C has shed new light on its role as a protistan pathogen and parasite, revealing a fascinating biological profile (Tan et al., 2021). The presence of genes associated with gliding motility and apical complex proteins, features typical of protistan pathogens, underscores its sophisticated mechanisms of movement and infection (Tan et al., 2021). Analysis of its carbohydrate-active enzymes (CAZymes) uncovered a diverse array of enzymes tasked with breaking down seagrass cell walls and managing carbohydrate reserves, highlighted by the identification of 112 genes linked to CAZy enzyme activity (Tan et al., 2021). These enzymes play a pivotal role in the marine carbon cycle and are likely crucial for the pathogen to breach living seagrass tissues via host adhesion (Tsui et al., 2009; Iwata and Honda, 2018). This discovery is significant for unveiling the molecular interaction mechanisms between host and pathogen, understanding the virulence of Labyrinthula and exploring its evolutionary mechanisms of ecological and environmental adaptability.
4 Labyrinthula spp. and the seagrass wasting diseases (SWD) under human activities
Environmental changes induced by extreme weather conditions and human activities severely impact seagrass ecosystems. These changes increase the mortality risk of seagrass, impair their carbon storage capabilities and adversely affect coastal ecological services (Orth et al., 2006; Graham et al., 2021). Increased precipitation due to strong storms and hurricanes facilitates a high load of organic nutrients and a decrease in pH levels, alongside adverse effects such as high temperatures and ocean acidification, leading to an increased mortality risk for seagrass in the future (Glibert et al., 2023). Urbanization and development increase estuarine runoff carrying sediments, nutrients and pollutants (pharmaceuticals, toxins, microplastics and pathogens), resulting in increased sediment loads in coastal waters, eutrophication, harmful algal blooms, fecal bacteria and a reduction in shellfish and fisheries (Freeman et al., 2019). These changes severely impact the seagrass bed ecosystems that provide essential ecological services, leading to a decrease in seagrass. Seagrass meadows contribute significantly to carbon storage and are an important sink for coastal blue carbon (Fourqurean et al., 2012; Serrano et al., 2021). Compared to seagrass beds less affected by human activities, carbon in seagrass beds more heavily influenced by human activities is more likely to escape when these systems are disturbed (Han et al., 2023). Thus, human activities have a definite impact on the carbon sequestration of seagrass bed ecosystems.
Aquaculture impacts the functionality associated with sediment bacteria in seagrass beds, particularly those functions related to carbon and nitrogen cycling (Sun et al., 2024). Eutrophication caused by aquaculture leads to a decline in seagrass abundance, affecting their carbon storage capacity (Han et al., 2024). The combined effects of shading and simulated grazing result in continuous loss of seagrass biomass, with significant increases in sulfide concentrations in sediment pore water and methane emissions from sediment surfaces (Lyimo et al., 2018). Human activities, such as dredging, expose coastal sediments to oxygen, disturbing the environment and reducing seagrass sediment carbon storage by promoting microbial remineralization (Macreadie et al., 2019). Thus, the reduction of seagrass due to human activities not only weakens their role in mitigating climate change, but may also promote the release of significant amounts of greenhouse gases into the environment from seagrass meadows (Lyimo et al., 2018). Moreover, with increasing nutrient loads, the biomass of sediment microbes and extracellular enzyme activity in seagrass beds increase, stimulating the remineralization of sediment organic carbon and potentially altering seagrass blue carbon (Liu et al., 2017). Furthermore, seagrass meadow bacteria can enhance the removal of pathogens by promoting particle settling (Liu et al., 2023). But the reduction and fragmentation of seagrass meadows due to human activities and global climate change inhibit the assumed potential for bacterial pathogen removal (Liu et al., 2023). On the other hand, stress stimuli from human activities may induce seagrass to release antibacterial compounds, inhibiting pathogens and thereby promoting the clearance of assumed bacterial pathogens in seagrass meadows (Deng et al., 2021). Therefore, environmental changes caused by human activities have significant impacts on both seagrass microbial communities and pathogens, potentially promoting the outbreak and development of seagrass diseases. One such concerning disease is the wasting disease of seagrass caused by the protist Labyrinthula, a member of the class Labyrinthulomycetes.
4.1 The occurrence of seagrass diseases
The pathogenic mechanisms of Labyrinthula sp. and the defense strategies of seagrass are crucial for understanding the occurrence and control of seagrass diseases. Currently, conditions leading directly to seagrass disease outbreaks remain contentious. The disease outbreaks not only depend on the health status and defensive capabilities of the seagrass, but also closely relate to the virulence and enzymatic capabilities of Labyrinthula strains (Figure 1). For instance, metabolites from the tropical seagrass Thalassia testudinum, including phenolic and non-phenolic compounds, have been proven to inhibit the growth of pathogenic Labyrinthula sp. (Trevathan-Tackett et al., 2015). This suggests seagrass defend against wasting disease by producing specific metabolites. Moreover, laboratory infection trials have revealed variations in virulence among different Labyrinthula strains, with highly virulent strains causing typical black leaf lesions in seagrass leaves (Bockelmann et al., 2012; Brakel et al., 2014; Martin et al., 2016). To further investigate this phenomenon, two techniques were developed, including a qPCR method for quantitatively assessing pathogen load and an immunolabeling method for measuring immune response of the host (Duffin et al., 2020). Both of these methods demonstrated high sensitivity and positive correlation with biomarkers related to abiotic stress (Duffin et al., 2020). These findings highlight the complexity of the interaction mechanisms between seagrass and Labyrinthula, necessitating a comprehensive study on seagrass disease prevention and control strategies.
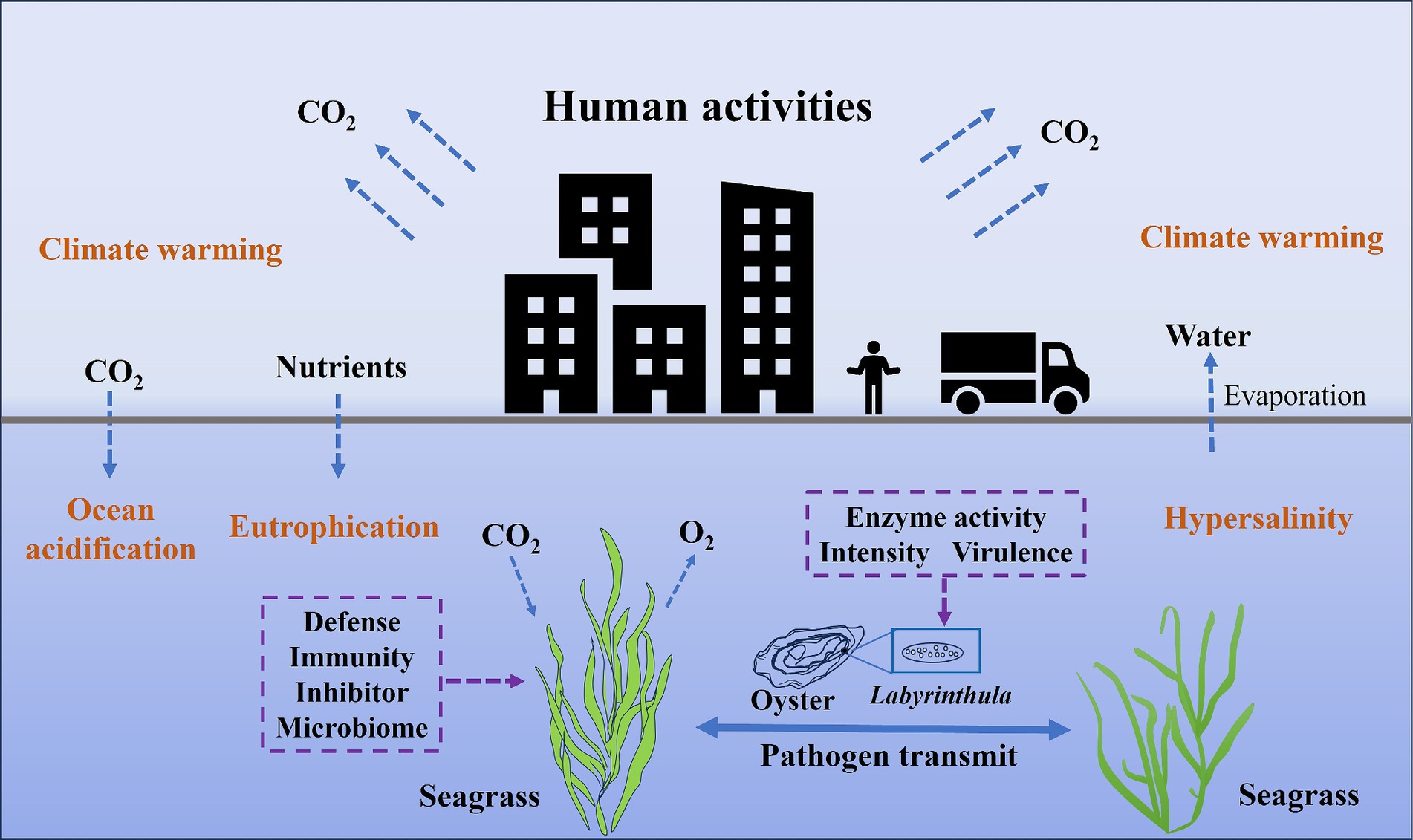
Figure 1. The relationship between the opportunistic pathogen Labyrinthula and the seagrass host under the influence of human activities.
4.2 Response of Labyrinthula and the seagrass host to environmental variations
Environmental stressors such as increased salinity and climate warming are significant factors influencing the susceptibility of seagrass to diseases (Table 3). Elevated salinity has been shown to cause a significant rise in H2O2 concentration within the seagrass T. testudinum, surpassing the growth inhibition concentration for external Labyrinthula (Trevathan et al., 2011). However, due to the direct effect of osmotic pressure on pathogens and the indirect effect of host-generated reactive oxygen species, short-term exposure to high salinity does not necessarily lead to an immediate increase in susceptibility to wasting disease (Trevathan et al., 2011). Temperature is also a critical factor affecting the prevalence of wasting diseases in seagrass. Field investigations correlated long-term satellite remote sensing of ocean temperature data with field survey results from 32 seagrass meadows along the North American Pacific coast (Aoki et al., 2022). This study revealed that the risk of wasting disease, caused by the protist Labyrinthula, increased with the rising temperatures (Aoki et al., 2022). Similarly, models enhanced with machine learning algorithms and field survey results suggested that disease prevalence and severity decreased under cooler conditions, and diseases were suppressed in healthier and denser meadows (Graham et al., 2023). Field experiments, conducted across a wide geographic area and from a macro perspective, have established the relationship between climate warming and seagrass diseases. However, these often lack detailed attention to seagrass, and fail to reveal the specific mechanisms through which warming influences seagrass diseases, as well as the resistance capabilities and corresponding mechanisms of seagrass.
Laboratory experiments offer a feasible pathway for detailing the environmental responses of seagrass and pathogens. Yet related studies are currently limited. The impact of temperature on disease is non-linear for most pathogen-host relationships. However, laboratory experiments indicated that warming may reduce the risk of wasting disease in the seagrass Posidonia oceanica and alleviate pathogenic pressure from this species (Olsen et al., 2014). Besides, laboratory simulations of warming effects on seagrass diseases have found that seagrass hosts with diverse genotypes reduce infection intensity, compared to hosts with less diversity (Schenck et al., 2023). However, since seagrass genotype diversity actually slightly increased the intensity of Labyrinthula under environmental temperatures, the prevalence of seagrass diseases did not decrease (Schenck et al., 2023). Therefore, more systematic and in-depth research is needed to reveal the specific seagrass characteristics and environmental conditions of the study area. Additionally, ocean acidification has a strong impact on plastisphere communities within mesocosm ecosystems, and Labyrinthula is also significantly affected by increased CO2 (Zhang et al., 2023). However, when analyzing the cross-effects of temperature (18°C and 24°C) and CO2 concentrations (800, 1,600 and 2,800 μatm) on Labyrinthula, it was found that the density of Labyrinthula varied at different temperatures (Zhong et al., 2024). And there was little difference under the three CO2 concentration conditions (Zhong et al., 2024). Thus, ocean warming has a more significant impact on Labyrinthula (Zhong et al., 2024). Additionally, previous studies had demonstrated that various environmental stressors significantly affect seagrass health (Lefcheck et al., 2017; Hensel et al., 2023). The interaction and response mechanisms between seagrass hosts and Labyrinthula pathogens become more complex under the combined effects of climate warming, ocean acidification, emerging pollutants, typhoons and other factors. Therefore, establishing comprehensive multi-factor prediction models in the future will provide more useful information for the conservation and restoration of seagrass beds.
4.3 Interactions between Labyrinthula spp. and the seagrass host
In addition to environmental factors that have a significant impact on the occurrence and development of SWD, the immune status of seagrass also plays a crucial role in the interaction between seagrass and Labyrinthula, as well as in the outbreaks of seagrass wasting disease (Table 4). The pathogen Labyrinthula could promote its growth and adapt to various environmental stresses by modulating the expression of seagrass defense genes, particularly by upregulating genes related to phenol synthesis (Brakel et al., 2014; Duffin et al., 2021; Venkataraman et al., 2023). This strategy can enhance immunity even during periods of scarce resources, although it may fail under extreme physiological challenges (Duffin et al., 2021). The interaction between Labyrinthula and seagrass, through the regulation of seagrass defense gene expression and immune responses, could adapt to environmental pressures and resource availability, promoting seagrass growth and regulating pathogen load under certain conditions (Duffin et al., 2021). Furthermore, seagrass-associated microbes and their metabolic products have some inhibitory activity against seagrass pathogens (Ugarelli et al., 2024). Meanwhile, metabolites from the natural microbiome of seagrass may provide food for Labyrinthula and thus promote its growth (Duffin et al., 2021). However, when seagrass are inoculated with Labyrinthula after artificially killing the natural microbiome, the severity of seagrass disease significantly reduced (Graham et al., 2024). This might indicate that environmental stress conditions such as extreme temperatures, ocean acidification and substantial discharges of coastal pollutants could reduce the outbreaks of SWD and lessen its severity (Graham et al., 2024). Additionally, laboratory experiments have shown that marine organisms could serve as effective sinks and potential sources of seagrass pathogens. The presence of oysters could significantly reduce the severity and infection intensity of SWD (Agnew et al., 2022). But oysters previously exposed to L. zosterae could also transmit the pathogen to local eelgrass (Agnew et al., 2022). Whether oysters transmit Labyrinthula under field conditions remains unclear (Agnew et al., 2022). Therefore, future research on the interaction between seagrass and Labyrinthula, incorporating the cross-effects of coexisting marine organisms and environmental factors, will provide richer and more critical information for the prevention and treatment of seagrass diseases.
5 Conclusion
The effects of environmental stresses exacerbated by human activities and global climate changes on seagrass health highlight the complex interactions between Labyrinthula and its host seagrass. This review summerizes the ecological function, pathogenicity and role in seagrass ecosystems of the Labyrinthulomycetes protist Labyrinthula. As an opportunistic pathogen, Labyrinthula spp. pose a significant threat to global seagrass beds by causing SWD. Despite progress in phylogenetic analysis, understanding pathogenic mechanisms and studies on environmental adaptability, information on its biogeography, host specificity and ecological functions remains scarce. Moreover, though the potential for PUFAs synthesis of Labyrinthula is beginning to be recognized, studies on its metabolites are still in its infancy. Future research needs to explore the interactions between Labyrinthula and seagrass, as well as other natural hosts more deeply and across a broader geographical range, including how these interactions are affected by environmental factors. Specifically, there is a need to strengthen research on the adaptability mechanisms of Labyrinthula under varying environmental conditions, including its response to global climate change. Additionally, increasing research efforts into the species diversity, distribution and ecological roles of Labyrinthula is crucial for understanding its role in particular these areas where human activity is frequent.
Author contributions
QW: Conceptualization, Funding acquisition, Project administration, Writing – original draft, Writing – review & editing. XY: Writing – original draft. YH: Writing – review & editing. YZ: Writing – original draft, Writing – review & editing. RH: Writing – original draft. HY: Writing – original draft. CW: Writing – original draft. MB: Conceptualization, Writing – review & editing.
Funding
The author(s) declare that financial support was received for the research, authorship, and/or publication of this article. This research was funded by the National Natural Science Foundation of China (No. 32100003), Natural Science Foundation of Hebei Province of China (No. D2019204215), and Scientific Research Foundation of Dezhou University (No. 2021xjrc105).
Conflict of interest
The authors declare that the research was conducted in the absence of any commercial or financial relationships that could be construed as a potential conflict of interest.
Publisher’s note
All claims expressed in this article are solely those of the authors and do not necessarily represent those of their affiliated organizations, or those of the publisher, the editors and the reviewers. Any product that may be evaluated in this article, or claim that may be made by its manufacturer, is not guaranteed or endorsed by the publisher.
References
Adl, S. M., Bass, D., Lane, C. E., Lukes, J., Schoch, C. L., Smirnov, A., et al. (2019). Revisions to the classification, nomenclature, and diversity of eukaryotes. J. Eukaryot. Microbiol. 66, 4–119. doi: 10.1111/jeu.12691
Agnew, M. V., Groner, M. L., Eisenlord, M. E., Friedman, C. S., and Burge, C. A. (2022). Pacific oysters are a sink and a potential source of the eelgrass pathogen, Labyrinthula zosterae. Aquac. Environ. Interact. 14, 295–307. doi: 10.3354/aei00446
Aoki, L. R., Rappazzo, B., Beatty, D. S., Domke, L. K., Eckert, G. L., Eisenlord, M. E., et al. (2022). Disease surveillance by artificial intelligence links eelgrass wasting disease to ocean warming across latitudes. Limnol. Oceanogr. 67, 1577–1589. doi: 10.1002/lno.12152
Bai, M., Sen, B., Wang, Q., Xie, Y., He, Y., and Wang, G. (2019). Molecular detection and spatiotemporal characterization of Labyrinthulomycete protist diversity in the coastal waters along the Pearl River Delta. Protist 77, 394–405. doi: 10.1007/s00248-018-1235-8
Bergmann, N., Fricke, B., Schmidt, M. C., Tames, V., Beining, K., Schwitte, H., et al. (2011). A quantitative real-time polymerase chain reaction assay for the seagrass pathogen Labyrinthula zosterae. Mol. Ecol. Resour. 11, 1076–1081. doi: 10.1111/j.1755-0998.2011.03051.x
Bigelow, D. M., Olsen, M. W., and Gilbertson, R. L. (2005). Labyrinthula terrestris sp. nov., a new pathogen of turf grass. Mycologia. 97, 185–190. doi: 10.3852/mycologia.97.1.185
Bockelmann, A., Beining, K., and Reusch, T. B. H. (2012). Widespread occurrence of endophytic Labyrinthula spp. in northern European eelgrass Zostera marina beds. Marne Ecol. Prog Ser. 445, 109–116. doi: 10.3354/meps09398
Bockelmann, A. C., Tams, V., Ploog, J., Schubert, P. R., and Reusch, T. B. (2013). Quantitative PCR reveals strong spatial and temporal variation of the wasting disease pathogen, Labyrinthula zosterae in northern European eelgrass (Zostera marina) beds. PLoS One. 8:e62169. doi: 10.1371/journal.pone.0062169
Brakel, J., Werner, F. J., Tams, V., Reusch, T. B. H., and Bockelmann, A. (2014). Current European Labyrinthula zosterae are not virulent and modulate seagrass (Zostera marina) defense gene expression. PLoS One 9:e92448. doi: 10.1371/journal.pone.0092448
Burge, C. A., Douglas, N., Conti-Jerpe, I., Weil, E., and Harvell, C. D. (2012). Friend or foe: the association of Labyrinthulomycetes with the Caribbean sea fan Gorgonia ventalina. Dis. Aquat. Org. 101, 1–12. doi: 10.3354/dao02487
Chamberlain, A. H. L., and Moss, S. T. (1988). The thraustochytrids: a protist group with mixed affinities. BioSystems. 21, 341–349. doi: 10.1016/0303-2647(88)90031-7
Chitrampalam, P., Goldberg, N., and Olsen, M. W. (2015). Labyrinthula species associated with turfgrasses in Arizona and New Mexico. Eur. J. Plant Pathol. 143, 485–493. doi: 10.1007/s10658-015-0701-0
Collado-Mercado, E., Radway, J. C., and Collier, J. L. (2010). Novel uncultivated labyrinthulomycetes revealed by 18S rDNA sequences from seawater and sediment samples. Aquat. Microb. Ecol. 58, 215–228. doi: 10.3354/ame01361
Dahl, S. F., Perrigault, M., and Allam, B. (2008). Laboratory transmission studies of QPX disease in the hard clam: Interactions between different host strains and pathogen isolates. Aquaculture 280, 64–70. doi: 10.1016/j.aquaculture.2008.04.026
Deng, Y., Liu, S., Feng, J., Wu, Y., and Mao, C. (2021). What drives putative bacterial pathogens removal within seagrass meadows? Mar. Pollut. Bull. 166:112229. doi: 10.1016/j.marpolbul.2021.112229
Doi, K., and Honda, D. (2017). Proposal of Monorhizochytrium globosum gen. nov., comb. nov. (Stramenopiles, Labyrinthulomycetes) for former Thraustochytrium globosum based on morphological features and phylogenetic relationships. Phycol. Res. 65, 188–201. doi: 10.1111/pre.12175
Duffin, P., Martin, D. L., Furman, B. T., and Ross, C. (2021). Spatial Patterns of Thalassia testudinum Immune Status and Labyrinthula spp. Load Implicate Environmental Quality and History as Modulators of Defense Strategies and Wasting Disease in Florida Bay, United States. Front. Plant Sci. 12:612947. doi: 10.3389/fpls.2021.612947
Duffin, P., Martin, D. L., Lohan, K. M. P., and Ross, C. (2020). Integrating host immune status, Labyrinthula spp. load and environmental stress in a seagrass pathosystem: assessing immune markers and scope of a new qPCR primer set. PLoS One 15:e0230108. doi: 10.1371/journal.pone.0230108
Dyková, I., Fiala, I., Dvořáková, H., and Pecková, H. (2008). Living together: the marine amoeba Thecamoeba hilla Schaeffer, 1926 and its endosymbiont Labyrinthula sp. Eur. J. Protistol. 44, 308–316. doi: 10.1016/j.ejop.2008.04.001
Fourqurean, J. W., Duarte, C. M., Kennedy, H., Marba, N., Holmer, M., Angel Mateo, M., et al. (2012). Seagrass ecosystems as a globally significant carbon stock. Nat. Geosci. 5, 505–509. doi: 10.1038/ngeo1477
Freeman, L. A., Corbett, D. R., Fitzgerald, A. M., Lemley, D. A., Quigg, A., and Steppe, C. N. (2019). Impacts of urbanization and development on estuarine ecosystems and water quality. Estuaries Coast 42, 1821–1838. doi: 10.1007/s12237-019-00597-z
Glibert, P. M., Heil, C. A., Madden, C. J., and Kelly, S. P. (2023). Dissolved organic nutrients at the interface of fresh and marine waters: flow regime changes, biogeochemical cascades and picocyanobacterial blooms—the example of Florida Bay, USA. Biogeochemistry 164, 229–255. doi: 10.1007/s10533-021-00760-4
Graham, O. J., Adamczyk, E. M., Schenk, S., Dawkins, P., Burke, S., Chei, E., et al. (2024). Manipulation of the seagrass-associated microbiome reduces disease severity. Environ. Microbiol. 26:e16582. doi: 10.1111/1462-2920.16582
Graham, O. J., Aoki, L. R., Stephens, T., Stokes, J., Dayal, S., Rappazzo, B., et al. (2021). Effects of seagrass wasting disease on eelgrass growth and belowground sugar in natural meadows. Front. Mar. Sci. 8:768668. doi: 10.3389/fmars.2021.768668
Graham, O. J., Stephens, T., Rappazzo, B., Klohmann, C., Dayal, S., Adamczyk, E. M., et al. (2023). Deeper habitats and cooler temperatures moderate a climate-driven seagrass disease. Philos. Trans. R. Soc B Biol. Sci. 378:20220016. doi: 10.1098/rstb.2022.0016
Grell, K. G. (1994). Decomposers of the diatom lawn and reticulosphaera. Arch. Protistenkd. 144, 343–349. doi: 10.1016/S0003-9365(11)80236-8
Hamamoto, Y., and Honda, D. (2019). Nutritional intake of Aplanochytrium (Labyrinthulea, Stramenopiles) from living diatoms revealed by culture experiments suggesting the new prey-predator interactions in the grazing food web of the marine ecosystem. PLoS One. 14:e0208941. doi: 10.1371/journal.pone.0208941
Han, Q., Che, W., Zhao, H., Ye, J., Zeng, W., Luo, Y., et al. (2024). Effects of aquaculture and Thalassia testudinum on sediment organic carbon in Xincun Bay, Hainan Island. Water 16:338. doi: 10.3390/w16020338
Han, Q., Qiu, C., Zeng, W., Chen, S., Zhao, M., Shi, Y., et al. (2023). Sediment carbon sequestration and driving factors in seagrass beds from Hainan Island and the Xisha Islands. PRO 11:456. doi: 10.3390/pr11020456
Hassett, B. T. (2020). A Widely Distributed Thraustochytrid Parasite of Diatoms Isolated from the Arctic Represents a gen. and sp. nov. J Eukaryot Microbiol. 67, 480–490. doi: 10.1111/jeu.12796
Hassett, B. T., and Gradinger, R. (2018). New Species of Saprobic Labyrinthulea (=Labyrinthulomycota) and the Erection of a gen. nov. to Resolve Molecular Polyphyly within the Aplanochytrids. J Eukaryot Microbiol. 65, 475–483. doi: 10.1111/jeu.12494
Hensel, M. J. S., Patrick, C. J., Orth, R. J., Wilcox, D. J., Dennison, W. C., Gurbisz, C., et al. (2023). Rise of Ruppia in Chesapeake Bay: climate change–driven turnover of foundation species creates new threats and management opportunities. Proc. Natl. Acad. Sci. U. S. A. 120:e2220678120. doi: 10.1073/pnas.2220678120
Hyder, N., Wong, F. P., Koski, A., Tisserat, N., and Stowell, L. (2010). First Report of Rapid Blight Caused by Labyrinthula terrestris on Poa annua in Colorado. Plant Dis. 94:919. doi: 10.1094/pdis-94-7-0919b
Iwata, I., and Honda, D. (2018). Nutritional intake by ectoplasmic nets of Schizochytrium aggregatum (Labyrinthulomycetes, Stramenopiles). Protist 169, 727–743. doi: 10.1016/j.protis.2018.06.002
Jones, G. M., and O'Dor, R. K. (1983). Ultrastructural observations on a thraustochytrid fungus parasitic in the gills of squid (Illex illecebrosus Lesueur). J. Parasitol. 69, 903–911. doi: 10.2307/3281055
Kumon, Y., Yokoyama, R., Haque, Z., Yokochi, T., Honda, D., and Nakahara, T. (2005). A new labyrinthulid isolate that produces only docosahexaenoic acid. Mar. Biotechnol. 8, 170–177. doi: 10.1007/s10126-005-5098-x
Lee, S. J., Shim, J. B., and Lee, S. (2021). First report of Labyrinthula zosterae (Labyrinthulomycetes) as the causal pathogen of wasting disease in the seagrass Zostera marina in Korea. Plant Dis. 105:2251. doi: 10.1094/PDIS-12-20-2751-PDN
Lefcheck, J. S., Wilcox, D. J., Murphy, R. R., Marion, S. R., and Orth, R. J. (2017). Multiple stressors threaten the imperiled coastal foundation species eelgrass (Zostera marina) in Chesapeake Bay, USA. Glob. Chang. Biol. 23, 3474–3483. doi: 10.1111/gcb.13623
Liu, S., Jiang, Z., Wu, Y., Zhang, J., Arbi, I., Ye, F., et al. (2017). Effects of nutrient load on microbial activities within a seagrass-dominated ecosystem: Implications of changes in seagrass blue carbon. Mar. Pollut. Bull. 117, 214–221. doi: 10.1016/j.marpolbul.2017.01.056
Liu, S., Wu, Y., Luo, H., Ren, Y., Jiang, Z., Zhang, X., et al. (2023). Seagrass canopy structure mediates putative bacterial pathogen removal potential. Front. Mar. Sci. 9:1076097. doi: 10.3389/fmars.2022.1076097
Lyimo, L. D., Gullström, M., Lyimo, T. J., Deyanova, D., Dahl, M., Hamisi, M. I., et al. (2018). Shading and simulated grazing increase the sulphide pool and methane emission in a tropical seagrass meadow. Mar. Pollut. Bull. 134, 89–93. doi: 10.1016/j.marpolbul.2017.09.005
Macreadie, P. I., Atwood, T. B., Seymour, J. R., Fontes, M. L. S., Sanderman, J., Nielsen, D. A., et al. (2019). Vulnerability of seagrass blue carbon to microbial attack following exposure to warming and oxygen. Sci. Total Environ. 686, 264–275. doi: 10.1016/j.scitotenv.2019.05.462
Morabito, C., Bournaud, C., Maës, C., Schuler, M., Aiese Cigliano, R., Dellero, Y., et al. (2019). The lipid metabolism in thraustochytrids. Prog. Lipid Res. 76:101007. doi: 10.1016/j.plipres.2019.101007
Martin, D. L., Chiari, Y., Boone, E., Sherman, T. D., Ross, C., Wyllie-Echeverria, S., et al. (2016). Functional, Phylogenetic and host-geographic signatures of Labyrinthula spp. provide for putative species delimitation and a global-scale view of seagrass wasting disease. Estuar. Coasts 39, 1403–1421. doi: 10.1007/s12237-016-0087-z
Moss, S. T. (1985). An ultrastructural study of taxonomically significant characters of the Thraustochytriales and the Labyrinthulales. Bot. J. Linn. Soc. 91, 329–357. doi: 10.1111/j.1095-8339.1985.tb01154.x
Olsen, Y. S., Potouroglou, M., Garcias-Bonet, N., and Duarte, C. M. (2014). Warming reduces pathogen pressure on a climate-vulnerable seagrass species. Estuar. Coasts 38, 659–667. doi: 10.1007/s12237-014-9847-9
Orth, R. J., Carruthers, T. J. B., Dennison, W. C., Duarte, C. M., Fourqurean, J. W., Heck, K. L., et al. (2006). A global crisis for seagrass ecosystems. Bio Science. 56, 987–996. doi: 10.1641/0006-3568(2006)56[987:AGCFSE]2.0.CO;2
Pan, J., Campo, J., and Keeling, P. J. (2016). Reference tree and environmental sequence diversity of Labyrinthulomycetes. J. Eukaryot. Microbiol. 64, 88–96. doi: 10.1111/jeu.12342
Polglase, J. L. (1980). A preliminary report on the thraustochytrid(s) and labyrinthulid(s) associated with a pathological condition in the lesser octopus Eledone cirrhosa. Bot. Mar. 23, 699–706. doi: 10.1515/botm-1980-1106
Popova, O. V., Belevich, T. A., Golyshev, S. A., Kireev, I. I., and Aleoshin, V. V. (2020). Labyrinthula diatomea n. sp.—A labyrinthulid associated with marine diatoms. J. Eukaryot. Microbiol. 67, 393–402. doi: 10.1111/jeu.12789
Raghukumar, S. (2002). Ecology of the marine protists, the Labyrinthulomycetes (thraustochytrids and labyrinthulids). Eur. J. Protistol. 38, 127–145. doi: 10.1078/0932-4739-00832
Raghukumar, S., and Damare, V. S. (2011). Increasing evidence for the important role of Labyrinthulomycetes in marine ecosystems. Bot. Mar. 54, 3–11. doi: 10.1515/BOT.2011.008
Raikar, M. T., Raghukumar, S., Vani, V., David, J. J., and Chandramohan, D. (2001). Thraustochytrid protists degrade hydrocarbons. Indian J. Geo Marine Sci. 21, 59–65. doi: 10.1007/s003670100073
Rubin, E., Tanguy, A., Perrigault, M., Espinosa, E. P., and Allam, B. (2014). Characterization of the transcriptome temperature-induced gene expressionin QPX, the thraustochytrid parasite of hard clams. BMC Genomics. 15, 245–260. doi: 10.1186/1471-2164-15-245
Schenck, F. R., DuBois, K., Kardish, M. R., Stachowicz, J. J., and Hughes, A. R. (2023). The effect of warming on seagrass wasting disease depends on host genotypic identity and diversity. Ecology 104:e3959. doi: 10.1002/ecy.3959
Serrano, O., Gómez-López, D. I., Sánchez-Valencia, L., Acosta-Chaparro, A., Navas-Camacho, R., González-Corredor, J., et al. (2021). Seagrass blue carbon stocks and sequestration rates in the Colombian Caribbean. Sci. Rep. 11:11067. doi: 10.1038/s41598-021-90544-5
Sullivan, B. K., Martin, D. L., Yoshioka, R. M., Brakel, J., Jakobsson-Thor, S., Eisenlord, M., et al. (2023). Standard ecological and molecular research methods and techniques for Labyrinthula spp. Front. Mar. Sci. 10:1092587. doi: 10.3389/fmars.2023.1092587
Sullivan, B. K., Robinson, K. L., Trevathan-Tackett, S. M., Lilje, E. S., Gleason, F. H., and Lilje, O. (2017). The first isolation and characterisation of the protist Labyrinthula sp. in Southeastern Australia. J. Eukaryot. Microbiol. 64, 504–513. doi: 10.1111/jeu.12387
Sullivan, B. K., Sherman, T. D., Damare, V. S., Lilje, O., and Gleason, F. H. (2013). Potential roles of Labyrinthula spp. in global seagrass population declines. Fungal Ecol. 6, 328–338. doi: 10.1016/j.funeco.2013.06.004
Sullivan, B. K., Trevathan-Tackett, S. M., Neuhauser, S., and Govers, L. L. (2018). Host-pathogen dynamics of seagrass diseases under future global change. Mar. Pollut. Bull. 134, 75–88. doi: 10.1016/j.marpolbul.2017.09.030
Sun, H., Wang, T., Liu, S., Tang, X., Sun, J., Liu, X., et al. (2024). Novel insights into the rhizosphere and seawater microbiome of Zostera marina in diverse mariculture zones. Microbiome 12:27. doi: 10.1186/s40168-024-01759-3
Tan, M. H., Loke, S., Croft, L. J., Gleason, F. H., and Trevathan-Tackett, S. M. (2021). First genome of Labyrinthula sp., an opportunistic seagrass pathogen, reveals novel insight into marine protist phylogeny, ecology and CAZyme cell-wall degradation. Microb. Ecol. 82, 498–511. doi: 10.1007/s00248-020-01647-x
Trevathan, S. M., Kahn, A., and Ross, C. (2011). Effects of short-term hypersalinity exposure on the susceptibility to wasting disease in the subtropical seagrass Thalassia testudinum. Plant Physiol. Biochem. 49, 1051–1058. doi: 10.1016/j.plaphy.2011.06.006
Trevathan-Tackett, S. M., Lane, A. L., Bishop, N., and Ross, C. (2015). Metabolites derived from the tropical seagrass Thalassia testudinum are bioactive against pathogenic Labyrinthula sp. Aquat. Bot. 122, 1–8. doi: 10.1016/j.aquabot.2014.12.005
Trevathan-Tackett, S. M., Sullivan, B. K., Robinson, K., Lilje, O., Macreadie, P. I., and Gleason, F. H. (2018). Pathogenic Labyrinthula associated with Australian seagrasses: Considerations for seagrass wasting disease in the southern hemisphere. Microbiol Res. 206, 74–81. doi: 10.1016/j.micres.2017.10.003
Tsui, C. K. M., Marshall, W., Yokoyama, R., Honda, D., Lippmeier, J. C., Craven, K. D., et al. (2009). Labyrinthulomycetes phylogeny and its implications for the evolutionary loss of chloroplasts and gain of ectoplasmic gliding. Mol. Phylogenet. Evol. 50, 129–140. doi: 10.1016/j.ympev.2008.09.027
Ugarelli, K., Jagels, A., Choi, C. J., Loesgen, S., and Stingl, U. (2024). Fungal endophytes from Thalassia testudinum show bioactivity against the seagrass pathogen, Labyrinthula spp. Front. Mar. Sci. 11:1359610. doi: 10.3389/fmars.2024.1359610
Venkataraman, Y. R., Shore, A., Dayal, S., Lee, J. S., Alidoost Salimi, M., Crandall, G., et al. (2023). Characterizing host-pathogen interactions between Zostera marina and Labyrinthula zosterae. Front. Mar. Sci. 10:1152647. doi: 10.3389/fmars.2023.1152647
Wang, Q., Ye, H., Xie, Y., He, Y., Sen, B., and Wang, G. (2019). Culturable diversity and lipid production profile of Labyrinthulomycete protists isolated from coastal mangrove habitats of China. Mar. Drugs 17, 268–285. doi: 10.3390/md17050268
Yadagiri, K. K., Kerrigan, J., and Martin, S. B. (2012). Improved methods for axenic culture of Labyrinthula terrestris, causal agent of rapid blight of turfgrasses. Can J Microbiol. 58, 1230–1235. doi: 10.1139/W2012-096
Yokoyama, R., Salleh, B., and Honda, D. (2007). Taxonomic rearrangement of the genus Ulkenia sensu lato based on morphology, chemotaxonomical characteristics, and 18S rRNA gene phylogeny (Thraustochytriaceae, Labyrinthulomycetes): emendation for Ulkenia and erection of Botryochytrium, Parietichytrium, and Sicyoidochytrium gen. nov. Mycoscience 48, 329–341. doi: 10.1007/s10267-007-0377-1
Yoshioka, R. M., Schram, J. B., and Galloway, A. W. E. (2019). Eelgrass pathogen Labyrinthula zosterae synthesizes essential fatty acids. Dis. Aquat. Org. 135, 89–95. doi: 10.3354/dao03382
Zhang, X., Zhang, P., Deng, Z., Huang, R., Zhang, D., Tian, Y., et al. (2023). Ocean acidification has a strong effect on communities living on plastic in mesocosms. Limnol. Oceanogr. Lett. 8, 675–684. doi: 10.1002/lol2.10329
Keywords: Labyrinthula, protist, thraustochytrid, seagrass disease, human activity, climate warming, ocean acidification, blue carbon
Citation: Wang Q, Yu X, He Y, Zhang Y, Hui R, Ye H, Wang C and Bai M (2024) Review of the protist Labyrinhula spp. and its relationship to seagrass disease under the influence of anthropogenic activities. Front. Microbiol. 15:1410195. doi: 10.3389/fmicb.2024.1410195
Edited by:
Judith Marie O’Neil, University of Maryland, College Park, United StatesReviewed by:
Katie May Laumann, University of Maryland, College Park, United StatesVladimir V. Aleoshin, A. N. Belozersky Institute of Physico-Chemical Biology, Russia
Copyright © 2024 Wang, Yu, He, Zhang, Hui, Ye, Wang and Bai. This is an open-access article distributed under the terms of the Creative Commons Attribution License (CC BY). The use, distribution or reproduction in other forums is permitted, provided the original author(s) and the copyright owner(s) are credited and that the original publication in this journal is cited, in accordance with accepted academic practice. No use, distribution or reproduction is permitted which does not comply with these terms.
*Correspondence: Yong Zhang, zy19890105@163.com; Mohan Bai, baimohan@caas.cn