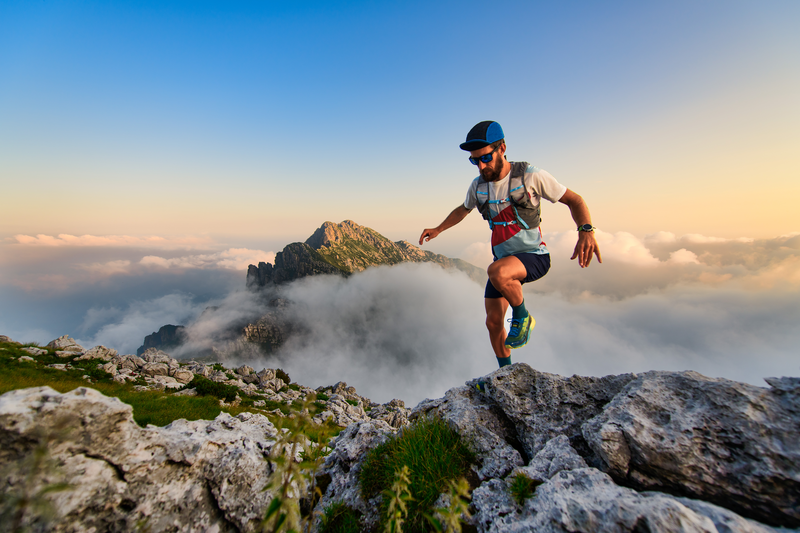
95% of researchers rate our articles as excellent or good
Learn more about the work of our research integrity team to safeguard the quality of each article we publish.
Find out more
OPINION article
Front. Microbiol. , 05 June 2024
Sec. Microbial Symbioses
Volume 15 - 2024 | https://doi.org/10.3389/fmicb.2024.1407092
The gut microbiota is a key regulator of various metabolic pathways in the host, including homeostasis, immunostasis, mucosal permeability, metabolic support, and even brain development. Animal models have substantially provided most of the current information about the gut microbiota, particularly gnotobiotic experimentation. In research, the term “gnotobiotic” describes a controlled environment where all microorganisms are known or excluded. This experimental setup allows for precise observation of interactions between host and their gut microbiota, giving a solid foundation for the study of the effects of specific microorganisms on host health and disease (Kubelkova et al., 2016).
Gnotobiotic models have been successfully applied to several animals, including mice, piglets, fish, insects, and nematodes. Mice have been the most used model for a variety of purposes, including predicting the human gut microbiota's response to a variety of factors in gnotobiotic mice (Faith et al., 2011), determining the effect of the gut microbiota on brain development (Lu et al., 2018), identifying gut microbe-host phenotype relationships (Faith et al., 2014), studying the role of probiotics and commensal microbiota in the development of the mucosal immune system, creating and characterizing communities of human gut microbes (Faith et al., 2010), and several others.
Depending on the study design, gnotobiotic models may consider germ-free, conventionalized (germ-free animals inoculated with the total fecal microbial community of their conventionally born and raised siblings), or standardized mice (germ-free mice inoculated with an in vitro standardized microbial consortia) (Turnbaugh et al., 2008; Williams, 2014). Standardized microbial consortia have been developed to permanently colonize gnotobiotic mice while providing fundamental functions to the host and serving as a comparative control. This is fundamental to researching and comprehending suspected interactions between the host and its microbiota and between varying components of such microbiota while representing a precise approach to translating associations into functions (Basic and Bleich, 2019). Fundamental aspects of gnotobiotic research are not only producing sterile embryos or larvae but sterilizing animal enclosures, food, and materials to avoid any microbial interference.
To advance research in this field, using standardized microbiota or synthetic bacterial communities (syncoms) is essential in studies involving gnotobiotic animals. The Oligo-Mouse-Microbiota (OMM12) is an example of a synthetic 12-bacterial species consortium created about a decade ago to facilitate functional microbiome research in mouse models (Brugiroux et al., 2016; Eberl et al., 2020). Despite other consortia developed for the same purposes (Stecher, 2021), the OMM12 is presently the most extensively employed strain collection for laboratory-based research. The bacterial strains have been fully sequenced and made publicly available; these strains were isolated from mice and are relatively easy to culture.
Conversely, synthetic bacterial communities like the OMM12 are not common or do not have the same standardization level as other animal models with valuable biological features. An example is the zebrafish (Danio rerio), the most used biological model. Here, we advocate for developing synthetic microbial communities or syncoms for the zebrafish model.
The OMM12 consortium offers several advantages over other defined consortia based on the available evidence. These benefits include a wide phylogenetic diversity, the accessibility of genomic sequences, strains from collections, and their stability for long periods in diverse mice strains (Brugiroux et al., 2016). Thus, OMM12 refers to a synthetic collection of microorganisms that mimic the naturally occurring microbiota in the mice's gastrointestinal tract that perform synergic functions for the host, including protection against pathogens. This consortium is formed by five phyla that include seven Bacillota species (Clostridium innocuum, Clostridium clostridioforme, Lactobacillus reuteri, Enterococcus faecalis, Acutalibacter muris, Flavonifractor plautii, Blautia coccoides), two Bacteroidota (Bacteroides caecimuris, Muribaculum intestinale), one Actinomycetota (Bifidobacterium animalis subsp. animalis), Verrucomicrobia (Akkermansia muciniphila), and Pseudomonadota (Turicimonas muris), all belonging to the mouse intestinal bacterial collection (miBC) (Lagkouvardos et al., 2016).
Research using OMM12 has focused on studying digestive tract colonization dynamics, infectious processes, mucosal immunology, microbial ecology, host-microbiome cross-talk, probiotics, etc (Brugiroux et al., 2016; Hernández-Mendoza et al., 2022). Beneficial adaptations have been developed for this bacterial consortia; for example, specific fluorescence in situ hybridization probes were designed and successfully proved to detect and quantify OMM12 (Brugiroux et al., 2022). Furthermore, considering that criticism has arisen due to the possible incompatibility of OMM12 with certain strains of mice, strains that are fully compatible with this bacterial consortium have been defined; in this case, C57BL/6 mice that have been stably colonized with OMM12 are referred to as stable defined moderately diverse microbiota mice (sDMDMm2) (Li et al., 2015). Recent evidence has demonstrated that the OMM12 inoculated germ-free mice reached the same stable gut microbiota composition regardless of the experimental facility (five European germ-free rodent facilities participated) (Eberl et al., 2020).
The OMM12 has been expanded with selected bacteria from a collection of over 200 bacterial strains. The collection was constructed using an enabled metagenome-educated prediction of synthetic communities to capture key functional differences between microbiomes. The OMM19 was elaborated by adding strains compensating for phenotype differences between OMM12 and specific pathogen-free mice (Afrizal et al., 2022). Thus, adaptations and improvements have been performed to address several research questions. However, other synthetic microbiota has been successfully used for decades, such is the case of the Altered Schaedler Flora (ASF) composed of only eight bacteria, a reduced consortium exempt of pathobionts, totally harmless to the intestinal cavity, and capable of being stable throughput generations and used in biomedical research. As a reduced consortium, advantages in applications, management and synthesizing have been reported (Wymore Brand et al., 2015).
The unique natural features of zebrafish have made it the most used fish in biological research. Its ease of handling and manipulation at each stage of its life cycle, including reproduction, has led multiple laboratories to use it as an animal model, even above mice.
The fish undergoes external embryonic development (ovoviviparous) and produces abundant offspring that hatch within 48–72 h. The eggs have a translucent, semipermeable membrane that allows for easy observation of embryonic development (Castillo-Salas et al., 2022). Also, tissue regeneration of most organs, including vital organs like the heart and brain, is one of the particularities of the species and has been used to comprehend regeneration mechanisms in humans (Gemberling et al., 2013). Furthermore, this species displays early organogenesis, sharing similarities with mammalian organs. It boasts anatomical and genetic homologies with humans and possesses a well-developed immune system akin to humans—complete with innate and adaptive immunity; herein, around 70% of human genes are detected in zebrafish (Howe et al., 2013). As such, the fish species' immune mechanisms and receptors are preserved within the vertebrate class (Lieschke and Currie, 2007; Sullivan and Kim, 2008). The utilization of zebrafish as a model organism has proved to be an immensely valuable tool in progressing our understanding of various biological disciplines; for instance, it is useful in characterizing human diseases and identifying and testing new drugs to treat the diseases being modeled, developmental and toxicological research, etc. (Kari et al., 2007; Yang et al., 2009).
The similarities in the digestive system between zebrafish and humans have led to using this fish as a model to generate knowledge about the gut microbiota and related aspects requiring gnotobiotic specimens. Several techniques have been documented for producing zebrafish embryos, isolating and raising germ-free fish, and introducing microorganisms into the gut microbiota of zebrafish (Pham et al., 2008). In the zebrafish, for example, gnotobiotic models are carried out by producing germ-free fish and inoculating single bacterial species (monoassociation) such as Aeromonas hydrophila, Pseudomonas aeruginosa, Escherichia coli (Rawls et al., 2004, 2006). However, other methods involve euthanizing adult zebrafish, removing the intestinal contents, and transferring them to germ-free fish (Pham et al., 2008). However, standardizing a synthetic microbial community analogous to the OMM12 or similar is still a pending task for the zebrafish and any fish models.
Numerous studies have thoroughly documented the gut microbiota of zebrafish, revealing that it is influenced by various internal and external factors. Evidence indicates that the gut microbiota of juvenile and adult zebrafish is consistent across different habitats and stabilizes around the juvenile phase (~75 days) (Cornuault et al., 2022), with Proteobacteria and Fusobacteriota as the dominant phyla. In this regard, the most influential factor determining the gut microbiota of zebrafish is the internal condition established by the fish. Like in many other fish species, this microbiota maintains a symbiotic relationship with the host and plays crucial roles in protecting against pathogens, as well as in nutritional, endocrine, neural, and physiological functions (Vargas-Albores et al., 2023).
Developing a synthetic microbial community to improve the zebrafish model focusing on studying the gut microbiota is still challenging. Designing and defining a synthetic microbiota for zebrafish may require not only considering bacterial strains belonging to the most abundant phyla but also coping with the criteria established for the OMM12, ASF, etc., which include three important characteristics that the microbial model system should possess: first, the ability to maintain a consistent composition across multiple generations reared under sterile conditions; second, perform efficient colonization in the germ-free animal to create subsequent gnotobiotic lines across different laboratories; third, possess metabolic pathways that allow for the emulation of complex ecosystems, including colonization resistance and the replication of microbiota-based host effects (Macpherson and McCoy, 2015) (Figure 1).
Approaches for developing a synthetic microbiota for the zebrafish have been made; for instance, 13 culturable and morphologically different bacterial species were reported as the most prevalent in zebrafish, including Aeromonas hydrophila, Aeromonas sobria, Vibrio parahaemolyticus, Photobacterium damselae, Pseudomonas aeruginosa, Pseudomonas fluorescens, Pseudomonas luteola, Comamonas testosteroni, Ochrobactrum anthropi, Staphylococcus cohnii, Staphylococcus epidermidis, Staphylococcus capitis, and Staphylococcus warneri (Cantas et al., 2012). Also, a meta-analytic approach performed in our laboratory, retrieving most of the available 16S sequences of the zebrafish gut microbiota reported in databases, revealed a zebrafish core microbiota that included representative bacteria from six phyla: Proteobacteria, Fusobacteriota, Planctomycetota, Firmicutes, Actinobacteriota, and Bacteroidota (Supplementary material 1). This information could serve as a first approach, but designing a synthetic microbiota for aquatic animals has additional challenges, starting with delivering viable microorganisms to the intestinal tract.
Gnotobiotic zebrafish models based on monoassociation provide a single bacteria through inoculation water at concentrations between 102 and 104 CFU/mL, trying to maintain such levels by microbial titration (Rawls et al., 2006). This technique may be applied to inoculate a bacterial consortium; however, this has complications associated with the biological requirements of the different bacteria, as all of them should be capable of at least surviving in water while incorporated in fish. Another strategy involves individual force-feeding; however, it is time-consuming, implies the manipulation and handling of animals, and is only viable for small groups of fish.
Developing a synthetic microbial community for fish and mice presents additional challenges due to the exclusive focus on cultivable bacteria. While the majority of microbes in the gut microbiota are prokaryotic, the eukaryotic counterpart also plays a significant biological role. Critics of synthetic microbiota based solely on bacteria argue that although designed consortia can confer benefits and restore functions in gnotobiotic models, they do not fully replicate the multitrophic reality (Vargas-Albores et al., 2023). Besides, such synthetic microbial communities only contain cultivable bacteria, which some environmental microbiologists estimate is a minor proportion compared to non-culturable bacteria, while others argue that such a percentage is significantly higher in bacterial communities associated with an animal host (Wade, 2002; Steen et al., 2019); however, this is a limitation that cannot be easily overcome due to the difficulty of developing culture media with conditions that allow the growth of a higher percentage of bacteria.
Although there have been difficulties, utilizing synthetic microbial communities like the OMM12, ASF, and others in mice has yielded valuable insights into the gut microbiota and represents progress toward understanding the intricate interplay between the microbiota and its host. Consequently, developing and standardizing a synthetic microbial community for zebrafish would undoubtedly bolster the production of fundamental and applied scientific knowledge while taking advantage of its high fecundity, external fertilization, optical transparency, and rapid development. Furthermore, the relationship between gut microbiota and major neuromodulator systems, including neurotransmitter receptors, transporters, and enzymes involved in synthesis and metabolism, could be studied, as they are similar to those observed in humans and rodents.
EG-V: Conceptualization, Supervision, Writing – original draft, Writing – review & editing. MM-P: Conceptualization, Supervision, Visualization, Writing – original draft, Writing – review & editing. FV-A: Conceptualization, Supervision, Writing – original draft, Writing – review & editing. DM-F: Resources, Visualization, Writing – review & editing, Writing – original draft. LM-C: Visualization, Writing – review & editing, Writing – original draft.
The author(s) declare that no financial support was received for the research, authorship, and/or publication of this article.
Special thanks to Azucena Santacruz for her support in preparing this document.
The authors declare that the research was conducted in the absence of any commercial or financial relationships that could be construed as a potential conflict of interest.
The author(s) declared that they were an editorial board member of Frontiers, at the time of submission. This had no impact on the peer review process and the final decision.
All claims expressed in this article are solely those of the authors and do not necessarily represent those of their affiliated organizations, or those of the publisher, the editors and the reviewers. Any product that may be evaluated in this article, or claim that may be made by its manufacturer, is not guaranteed or endorsed by the publisher.
The Supplementary Material for this article can be found online at: https://www.frontiersin.org/articles/10.3389/fmicb.2024.1407092/full#supplementary-material
Afrizal, A., Jennings, S. A., Hitch, T. C., Riedel, T., Basic, M., Panyot, A., et al. (2022). Enhanced cultured diversity of the mouse gut microbiota enables custom-made synthetic communities. Cell Host Microbe 30, 1630–1645. doi: 10.1016/j.chom.2022.09.011
Basic, M., and Bleich, A. (2019). Gnotobiotics: Past, present and future. Lab. Anim. 53, 232–243. doi: 10.1177/0023677219836715
Brugiroux, S., Berry, D., Ring, D., Barnich, N., Daims, H., and Stecher, B. (2022). Specific localization and quantification of the oligo-mouse-microbiota (OMM12) by fluorescence in situ hybridization (FISH). Curr. Protocols 2:e548. doi: 10.1002/cpz1.548
Brugiroux, S., Beutler, M., Pfann, C., Garzetti, D., Ruscheweyh, H. J., Ring, D., et al. (2016). Genome-guided design of a defined mouse microbiota that confers colonization resistance against Salmonella enterica serovar Typhimurium. Nat Microbiol 2:16215. doi: 10.1038/nmicrobiol.2016.215
Cantas, L., Sørby, J. R. T., Aleström, P., and Sørum, H. (2012). Culturable gut microbiota diversity in zebrafish. Zebrafish 9, 26–37. doi: 10.1089/zeb.2011.0712
Castillo-Salas, D. L., Gaytán-Oyarzun, J. C., López-Herrera, M., and Sánchez-Olivares, M. A. (2022). Pez cebra (Danio rerio): modelo experimental en la evaluación de compuestos xenobióticos. Pädi Boletín Científico de Ciencias Básicas e Ingenierías del ICBI 10, 61–65. doi: 10.29057/icbi.v10i19.8870
Cornuault, J. K., Byatt, G., Paquet, M.-E., De Koninck, P., and Moineau, S. (2022). Zebrafish: a big fish in the study of the gut microbiota. Curr. Opin. Biotechnol. 73, 308–313. doi: 10.1016/j.copbio.2021.09.007
Eberl, C., Ring, D., Münch, P. C., Basic, M., Schwarzer, M., Lange, A., et al. (2020). Reproducible colonization of germ-free mice with the oligo-mouse-microbiota in different animal facilities. Front. Microbiol. 10:488258. doi: 10.3389/fmicb.2019.02999
Faith, J. J., Ahern, P. P., Ridaura, V. K., Cheng, J., and Gordon, J. I. (2014). Identifying gut microbe–host phenotype relationships using combinatorial communities in gnotobiotic mice. Sci. Translat. Med. 6:220ra211. doi: 10.1126/scitranslmed.3008051
Faith, J. J., McNulty, N. P., Rey, F. E., and Gordon, J. I. (2011). Predicting a human gut microbiota's response to diet in gnotobiotic mice. Science 333, 101–104. doi: 10.1126/science.1206025
Faith, J. J., Rey, F. E., O'donnell, D., Karlsson, M., McNulty, N. P., Kallstrom, G., et al. (2010). Creating and characterizing communities of human gut microbes in gnotobiotic mice. ISME J. 4, 1094–1098. doi: 10.1038/ismej.2010.110
Gemberling, M., Bailey, T. J., Hyde, D. R., and Poss, K. D. (2013). The zebrafish as a model for complex tissue regeneration. Trends Genet. 29, 611–620. doi: 10.1016/j.tig.2013.07.003
Hernández-Mendoza, A., González-Córdova, A. F., and Martínez-Porchas, M. (2022). Influence of probiotics on the animal gut microbiota and their impact on the bioavailability of toxic agents: an opinion paper. Front. Nutr. 9:870162. doi: 10.3389/fnut.2022.870162
Howe, K., Clark, M. D., Torroja, C. F., Torrance, J., Berthelot, C., Muffato, M., et al. (2013). The zebrafish reference genome sequence and its relationship to the human genome. Nature 496, 498–503. doi: 10.1038/nature12111
Kari, G., Rodeck, U., and Dicker, A. P. (2007). Zebrafish: an emerging model system for human disease and drug discovery. Clini. Pharmacol. Therapeut. 82, 70–80. doi: 10.1038/sj.clpt.6100223
Kubelkova, K., Benuchova, M., Kozakova, H., Sinkora, M., Krocova, Z., Pejchal, J., et al. (2016). Gnotobiotic mouse model's contribution to understanding host–pathogen interactions. Cell. Mol. Life Sci. 73, 3961–3969. doi: 10.1007/s00018-016-2341-8
Lagkouvardos, I., Pukall, R., Abt, B., Foesel, B. U., Meier-Kolthoff, J. P., Kumar, N., et al. (2016). The Mouse Intestinal Bacterial Collection (miBC) provides host-specific insight into cultured diversity and functional potential of the gut microbiota. Nature Microbiol. 1, 1–15. doi: 10.1038/nmicrobiol.2016.131
Li, H., Limenitakis, J. P., Fuhrer, T., Geuking, M. B., Lawson, M. A., Wyss, M., et al. (2015). The outer mucus layer hosts a distinct intestinal microbial niche. Nat. Commun. 6, 8292. doi: 10.1038/ncomms9292
Lieschke, G. J., and Currie, P. D. (2007). Animal models of human disease: zebrafish swim into view. Nat. Rev. Genet. 8, 353–367. doi: 10.1038/nrg2091
Lu, J., Lu, L., Yu, Y., Cluette-Brown, J., Martin, C. R., and Claud, E. C. (2018). Effects of intestinal microbiota on brain development in humanized gnotobiotic mice. Sci. Rep. 8:5443. doi: 10.1038/s41598-018-23692-w
Macpherson, A., and McCoy, K. (2015). Standardised animal models of host microbial mutualism. Mucosal Immunol. 8, 476–486. doi: 10.1038/mi.2014.113
Pham, L. N., Kanther, M., Semova, I., and Rawls, J. F. (2008). Methods for generating and colonizing gnotobiotic zebrafish. Nat. Protoc. 3, 1862–1875. doi: 10.1038/nprot.2008.186
Rawls, J. F., Mahowald, M. A., Ley, R. E., and Gordon, J. I. (2006). Reciprocal gut microbiota transplants from zebrafish and mice to germ-free recipients reveal host habitat selection. Cell 127, 423–433. doi: 10.1016/j.cell.2006.08.043
Rawls, J. F., Samuel, B. S., and Gordon, J. I. (2004). Gnotobiotic zebrafish reveal evolutionarily conserved responses to the gut microbiota. Proc. Nat. Acad. Sci. 101, 4596–4601. doi: 10.1073/pnas.0400706101
Stecher, B. (2021). Establishing causality in Salmonella-microbiota-host interaction: the use of gnotobiotic mouse models and synthetic microbial communities. Int. J. Med. Microbiol. 311, 151484. doi: 10.1016/j.ijmm.2021.151484
Steen, A. D., Crits-Christoph, A., Carini, P., DeAngelis, K. M., Fierer, N., Lloyd, K. G., et al. (2019). High proportions of bacteria and archaea across most biomes remain uncultured. ISME J. 13, 3126–3130. doi: 10.1038/s41396-019-0484-y
Sullivan, C., and Kim, C. H. (2008). Zebrafish as a model for infectious disease and immune function. Fish Shellfish Immunol. 25, 341–350. doi: 10.1016/j.fsi.2008.05.005
Turnbaugh, P. J., Bäckhed, F., Fulton, L., and Gordon, J. I. (2008). Diet-induced obesity is linked to marked but reversible alterations in the mouse distal gut microbiome. Cell Host Microbe 3, 213–223. doi: 10.1016/j.chom.2008.02.015
Vargas-Albores, F., Garibay-Valdez, E., Medina-Félix, D., and Martínez-Porchas, M. (2023). The micro-eukaryotic community: an underrated component of the mammalian gut microbiota? Front. Microbiol. 14:1123513. doi: 10.3389/fmicb.2023.1123513
Wade, W. (2002). Unculturable bacteria—the uncharacterized organisms that cause oral infections. J. R. Soc. Med. 95, 81–83. doi: 10.1258/jrsm.95.2.81
Williams, S. C. (2014). Gnotobiotics. Proc. Nat. Acad. Sci. 111, 1661–1661. doi: 10.1073/pnas.1324049111
Wymore Brand, M., Wannemuehler, M. J., Phillips, G. J., Proctor, A., Overstreet, A.-M., Jergens, A. E., et al. (2015). The altered Schaedler flora: continued applications of a defined murine microbial community. ILAR J. 56, 169–178. doi: 10.1093/ilar/ilv012
Keywords: animal model, biological model, gut microbiota, syncoms, synthetic bacterial communities, synthetic microbiota
Citation: Garibay-Valdez E, Martínez-Porchas M, Vargas-Albores F, Medina-Félix D and Martínez-Córdova LR (2024) The zebrafish model requires a standardized synthetic microbial community analogous to the oligo-mouse-microbiota (OMM12). Front. Microbiol. 15:1407092. doi: 10.3389/fmicb.2024.1407092
Received: 27 March 2024; Accepted: 27 May 2024;
Published: 05 June 2024.
Edited by:
Markus M. Heimesaat, Charité University Medicine Berlin, GermanyReviewed by:
Marijana Basic, Hannover Medical School, GermanyCopyright © 2024 Garibay-Valdez, Martínez-Porchas, Vargas-Albores, Medina-Félix and Martínez-Córdova. This is an open-access article distributed under the terms of the Creative Commons Attribution License (CC BY). The use, distribution or reproduction in other forums is permitted, provided the original author(s) and the copyright owner(s) are credited and that the original publication in this journal is cited, in accordance with accepted academic practice. No use, distribution or reproduction is permitted which does not comply with these terms.
*Correspondence: Marcel Martínez-Porchas, bWFyY2VsQGNpYWQubXg=; Francisco Vargas-Albores, ZnZhbGJvcmVzQGNpYWQubXg=
Disclaimer: All claims expressed in this article are solely those of the authors and do not necessarily represent those of their affiliated organizations, or those of the publisher, the editors and the reviewers. Any product that may be evaluated in this article or claim that may be made by its manufacturer is not guaranteed or endorsed by the publisher.
Research integrity at Frontiers
Learn more about the work of our research integrity team to safeguard the quality of each article we publish.