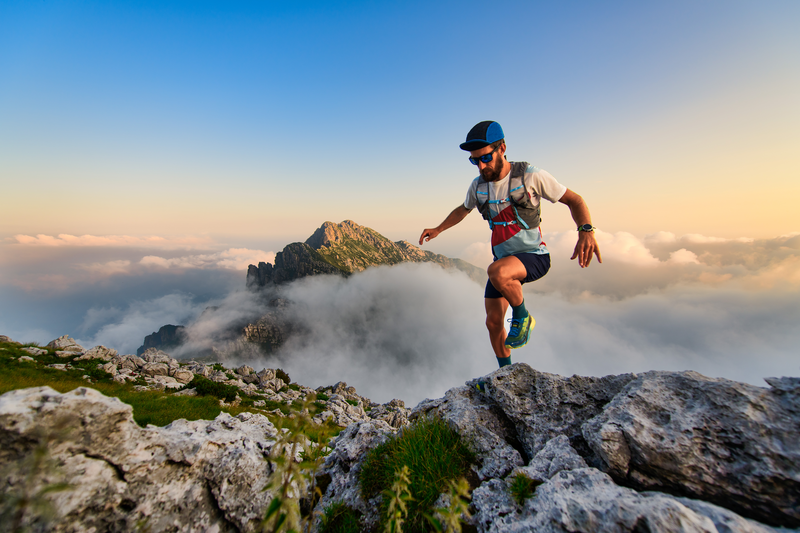
95% of researchers rate our articles as excellent or good
Learn more about the work of our research integrity team to safeguard the quality of each article we publish.
Find out more
MINI REVIEW article
Front. Microbiol. , 11 July 2024
Sec. Microbe and Virus Interactions with Plants
Volume 15 - 2024 | https://doi.org/10.3389/fmicb.2024.1406891
This article is part of the Research Topic Microbial Symbionts of Lower Plants View all 3 articles
Mycorrhizal association is one of the earliest and diversely distributed symbiotic associations on the Earth. This association helped early terrestrial plants to colonize the land by improved supply of nutrients like phosphate, nitrogen and zinc. It also helped plants to tolerate unfavorable soil conditions with increased water retention capacity, resistance to drought and pathogens. In return, fungi benefitted with carbon as their food source from the plants. More than 80% of terrestrial plants including pteridophytes, gymnosperms and angiosperms are reported to form arbuscular mycorrhizal (AM) association. Plants with root systems appeared on land during the Devonian period and many of them like pteridophytes still exist today. Various molecular and fossil studies confirm that the plants belonging to Ordovician-Devonian are associated with fungi, which are very similar to genus Glomus. AM association is very common in pteridophytes and the growth of its sporophyte and gametophyte is directly affected in the presence of AM association. Pteridophytes as early land plants with root systems have a very significant place in the plant kingdom. They have evolved and adapted to fill various habitats and facilitated early terrestrialization of other land plants by providing suitable niche with the help of AM fungi. In spite of pteridophytes being a very important plant group in the land system, very few reports are available on fungal-pteridophyte association. The present review is an effort to gather information about AM association in pteridophytes that might help in unraveling the evolution and significance of plant and fungi association.
Of all the essential relationships in nature, mycorrhiza is one of the most significant symbiotic relationships between plants and fungi. It is present in all soil types where plants can grow and facilitates the absorption of water and nutrients in the plants (Huey et al., 2020). About 80% of terrestrial plants distributed in different habitats show AM association (Quilambo, 2003). This mutual association played a very critical role in early terrestrialization and diversification of land plants. The alliance of AM fungi with plants is referred to as a bidirectional mutualistic symbiotic association, where both partners provide each other with nutrient resources (Kiers et al., 2011). Nearly 80% of nitrogen (N) and 100% of phosphorous (P) required for plant growth is provided by mycorrhizal fungi (Jakobsen et al., 1992; Leake et al., 2004; Smith and Smith, 2011; Hodge and Storer, 2015; Luginbuehl et al., 2017), whereas fungi utilize photosynthetically fixed organic carbon-based compounds from plants (Jakobsen and Rosendahl, 1990; Smith and Read, 2010). This association is not only restricted to nutrient exchange; it also helped plants to colonize land by increasing fitness and resistance and tolerance to drought, adverse soil conditions, and pathogenic microorganisms (Quilambo, 2003).
Mycorrhiza is a very primitive symbiotic association, and its origin can be traced back to the Ordovician period (475 Ma) when the first land plants colonized the Earth’s surface. These plants are thought to have an association with Glomeromycotina fungi that is very similar to modern AM association (Smith and Read, 2010). This association co-evolved with rootless plants to facilitate their transition from water to land and help in diversification (Pirozynski and Malloch, 1975; Redecker et al., 2000). Pteridophytes, among the first plants with roots that appeared on land during the Devonian period, are still flourishing. There is abundant evidence confirming that these pteridophytes form an association with Glomalean fungi and show presence of structures like vesicles (Read et al., 2000). Molecular studies also confirmed the emergence of Glomales during the same period as the evolution of land plants.
The knowledge about the diversity and function of fungal association in pteridophytes is inadequate (Strullu-Derrien and Strullu, 2007; Pressel et al., 2016; Lehnert et al., 2017). Mycorrhizal symbiosis occurs in both sporophytes (diploid) and gametophytic (haploid) phases of life. The vascular plants possess a root-bearing sporophytic phase, whereas gametophyte lacks roots and consists of rhizoids. In both phases, it is colonized by the same AM fungi (Winther and Friedman, 2008, 2009) and presence of AM fungi stimulates growth in both sporophyte and gametophyte (Turnau et al., 2005), but how this works in individual phases is still a question that needs to be answered.
The mycorrhizal association is one of the most unique adaptations of land plants. This distinct association is found in 85% of living plant species (Brundrett, 2004; Smith and Read, 2008; van der Heijden et al., 2015; Brundrett and Tedersoo, 2018). Over time, distinct types of mycorrhizal association have evolved in different plants. AM association is one of the most widespread associations formed by fungi belonging to Glomeromycotina. These fungi are documented to colonize with roots and in some plants with rhizomes of vascular plants (Wang and Qiu, 2006; Smith and Read, 2008; Pressel et al., 2016). In nonvascular plants, like liverworts and hornworts, it is found to be present in the thallus (Strullu, 1985; Read et al., 2000; Selosse, 2005; Duckett et al., 2006; Pressel et al., 2010; Desireo et al., 2013). The AM association is widespread in land plants, but its geological record is sparse. The study of fossils plays an essential role in providing information on the evolutionary history of mycorrhizae and the extent of their association with plants, which helped them in early territorialization.
Fungal hyphae and spore of AM fungi were reported from 460 MY old sediments. However, these fossils are not directly found to be associated with plants (Redecker et al., 2000). The first-ever direct fossil evidence of plant-fungal association is documented from a 407 MY old Rhynie chert (Trewin and Rice, 2004), where multiple types of endomycorrhizal association are recorded in plants (Taylor and Krings, 2005; Strullu-Derrien et al., 2014; Taylor et al., 2015). The plants from Rhynie chert are small, and rhizoids are their rooting system (Kenrick and Strullu-Derrien, 2014). Aerial axes of Aglaophyton majus are reported to form arbuscules and are associated with fungi belonging to Glomeromycota (Remy et al., 1994). Rhynia Gwynne-vaughan shows the presence of vesicles (Boullard and Lemoigne, 1971; Karatygin et al., 2006), intercellular vesicles, and spores observed in Nothia aphylla (Krings et al., 2007). Horneophyton lignieri was found to be associated with fungi of Glomeromycotina (Strullu-Derrien et al., 2014). These early plant-fungal associations were named para mycorrhizas (Strullu-Derrien and Strullu, 2007) or mycorrhiza-like associations (Smith and Read, 2008) due to the lack of a proper root system. The roots evolved twice during the early evolution of vascular plants (Strullu-Derrien et al., 2014; Hetherington et al., 2016). However, knowledge about fungal symbiosis in early root systems is minimal. No record of fungal association in the roots of trees from the Middle Devonian period (398-359 MYA) is available (Morris et al., 2015). Harper et al. (2013) reported diversification in AM association in extinct pteridosperm Glossopteris from the Upper Permian of Antarctica (260–252 MYA). The associated fungi have septate hyphae considered Glomites (Taylor et al., 1995), different from aseptate fungi of Glomeromycotina. Associated fungi colonized with cortical cells in a helical pattern resembling Paris-type AM.
Endomycorrhiza is one of the two types of mycorrhizal association found in plants. It is characterized by intra and intercellular hyphae in the root cortex, forming structures like arbuscules and vesicles. Arbuscules are highly branched, short-lived fungal hyphae essential for nutrient exchange, whereas vesicles are a storing structure. Vesicles exhibit lots of diversity in shape and size. Their size differs from 30 to 50 μ and shape varies from elliptical to spherical to irregular. Vesicles formed by Glomus sp. are generally spherical and elliptical, whereas rectangular and irregular-shaped vesicles are a feature of Acaulospora sp. (Ghosh et al., 2012). Among all endomycorrhizal fungal structures formed, hyphae appears to be first, followed by arbuscules and vesicles (Brundett, 2002).
Based on morphology, arbuscules are further divided into two types: Arum and Paris. Arum-type arbuscules are characterized by extensive dichotomized structures produced through the hyphae trunk in the cell lumen. In contrast, the Paris-type is characterized by hyphal coils in the root cortex (Dickson, 2004). Arbuscules are known to have ‘small tree’-like dichotomized morphology, whereas the Paris-type lacks such hyphal dichotomy. Harper et al. (2013) called Paris-type a mycorrhizal hyphae instead of arbuscules, although its function is the same as highly branched arbuscules. In addition to these two major morphological types, an Intermediate-type is also recognized, which shows the presence of fungal structures between the Arum-and Paris-type (Dickson, 2004) or the occurrence of either Arum-and Paris-type within the same species. A wide range of variation is observed in the degree of development from species to species (Smith and Smith, 1997). Many studies suggest that the type of arbuscular morphology depends on the host plants. Among all arbuscules morphology, Paris-type is widely distributed among land plants and reported in bryophytes, pteridophytes, gymnosperms, and flowering plant families (Simon et al., 1993; Taylor et al., 1995; Phipps and Taylor, 1996; Smith and Smith, 1997). In ferns, the Paris-type is considered the most dominant arbuscular type (Smith and Smith, 1997). These coiled intracellular hyphae and vesicles are also observed in Aglaophyton, a Devonian fossil (Kidston and Lang, 1921). Fernández et al. (2013) reported that Intermediate-type arbuscules tend more toward facultative species. Paris-type morphology is observed in genera Pteris, Ophioglossum, Athyrium, Blechnum, Woodwardia, Alsophila, Cyathea, Equisetum, Osmunda, Campyloneurum, Dicranopteris, Lycopodium, Lepisorus, Drynaria, Microsorum Niphidium, Nephrolepis, Phlebodium, Pleopeltis, Polypodium, and Selaginella. Arum-type is reported in a few genera, such as Adiantum, Sticherus, and Microsorum. Intermediate-type is seen in families Allentodia, Adiantum, Blechnum, Cyathea, Diplazium, Grammitis, Hypolepsis, Microplepia, Pteridium, Arachniodes, Dryopteris, Polystichum, Dicranopteris, Lindsaea, Odontosoria, Sphenomeris, Stenoloma, Nephrolepsis, Huperzia, Angiopteris, Nephrolepis, Helminthostachys, Lycopodium, Cheilanthus, Doryopteris, Pellaea, Parahemionitis, Pityrogramma, Pteris, Campyloneurum, Drynaria, Microsorum, Pyrrosia, Selliguea, Salvinia, Lygodium, Selaginella, and Christella. Many pteridophytes studied from different locations also show the presence of two types of arbuscule morphology (Table 1).
Soil fungi are very diverse and are known to play an important role as belowground biota. They are known for their significant role in forest dynamics (Copely, 2000). These soil fungi are found to have associations with plants; among those association, AM association is one of the most primitive and crucial symbiotic associations. These fungi are known to improve soil nutrition and fertility by helping plants to uptake complex nutrients such as phosphorus, nitrogen, and zinc. Their association is well known for influencing plant diversity and community structure (van der Heijden et al., 1998; Read, 1999; O’Connor et al., 2002).
Around 317 species of AM fungi are reported based on morphological characters (International Culture Collection of Glomeromycota [CICG], 2020). These AM-forming fungi are classified under four orders: Archaesporales, Diversisporales, Glomales, and Paraglomales (Wijayawardene et al., 2020). They are known for their coenocytic mycelium, production of arbuscular, asexual modes of reproduction through sporogenesis at the hyphal tip, and formation of vesicles, which is a lipid-storing structure (Redecker and Raab, 2006; Schüßler and Walker, 2011). Arbuscules are standard structures in all AM-forming fungi, whereas the formation of vesicles is restricted to only some genera. Fungal genera such as Gigaspora and Scutellospora lack the ability to form vesicles (Redecker and Raab, 2006). Among all AM-forming fungi, Glomus is the most significant and commonly found AM fungi to be associated with every plant group. Numerous fossils and genetic studies confirmed Glomales association with vascular plants of the Devonian period. Glomales are associated with tropical pteridophytes (Read et al., 2000). Many workers also confirm the predominance of Glomus genera associated with pteridophytes. Apart from Glomus, other species belonging to Acaulospora, Claroideoglomus, Entrophospora, Funneliformis, Gigaspora, Sclerocystis and Scutellospora are also widely reported to form AM association with pteridophytes (Table 2).
Many AM fungal spore populations have been isolated from rhizosphere soil of pteridophytes, including Acaulospora cavernata, A. colossica, A. foveata, A. gdanskensis, A. gedanensis, A. lacunosa, A. laevis, A. morrowiae, A. rehemii, A. scorbiculata, and A. thomii (Khade and Rodrigues, 2002; Zhang et al., 2004; Muthuraja et al., 2014; Santhoshkumar and Nagarajan, 2014; Krzyzanski et al., 2021). Claroideoglomus candidum, C. etunicatum, and C. luteum (Muthukumar and Prabha, 2013; Krzyzanski et al., 2021), Entrophospora infrequens (Santhoshkumar and Nagarajan, 2014). Funneliformis constrictum and F. geosporum (Muthukumar and Prabha, 2013; Krzyzanski et al., 2021), Glomus aggregatum, G. ambisporum, G. australe, G. botryoides, G. caledonium, G. convolutum, G. claroideum, G. desecticola, G. dimorphicum, G. etunicatum, G. fasiculatum, G. fistulosum, G. formosanum, G. globiferum, G. heterosporum, G. halon, G. hoi, G. intraradices, G. invermaium, G. macrocarpum, G. mosseae, G. monosporum, G. moaaeae, G. morphicum, G. microcarpum, G. multisubtensum, G. multicaulis, G. pustulatum, G. rubiformis, and G. versiforme (Koske et al., 1985; Khade and Rodrigues, 2002; Zhang et al., 2004; Jaramillo et al., 2008; Ghosh et al., 2012; Martinez et al., 2012; Muthukumar and Prabha, 2013; Muthuraja et al., 2014; Ghanta et al., 2016; Nafees et al., 2019; Krzyzanski et al., 2021), Gigaspora albida, G. candida, G. decipiens, G. margarita, and G. gigantea (Khade and Rodrigues, 2002; Muthuraja et al., 2014; Santhoshkumar and Nagarajan, 2014; Nafees et al., 2019), Sclerocystis pachycaulis, S. rubiformis, and S. taiwanensis (Khade and Rodrigues, 2002; Muthuraja et al., 2014; Santhoshkumar and Nagarajan, 2014; Nafees et al., 2019), Scutellospora heterogama, S. scutata, S. gregaria, and S. reticulata (Khade and Rodrigues, 2002; Santhoshkumar and Nagarajan, 2014).
Arbuscular mycorrhizal fungi show worldwide distribution and are found in almost all soil types (Mosse et al., 1981; Daniels Hetrick, 1984). Their distribution and function are extensively affected by various edaphic conditions such as soil composition, temperature, moisture, cation exchange capacity, pH, and anthropogenic abiotic and biotic stresses, including soil compaction and the presence of metals and pesticides. Adequate soil moisture and temperature play a positive role in the development of AM association.
Soil temperature is one of the key abiotic factors that plays an important role in AM fungal growth. Heinemeyer and Fitter (2004) reported the direct effect of soil temperature on the growth of mycorrhizal mycelium. With a rise in temperature from 12 to 20°C, there is a significant increase observed in the length of extraradical mycelium. Temperatures less than 18°C cause reduced growth and loosely connected and weak networks of mycelium. It also causes a delay in the formation of propagative and absorptive organs (Gavito et al., 2005). Carbon (C) is one of the most critical requirements for growth of AM fungi and it is strongly affected by soil temperature. Gavito et al. (2005) measured root-C uptake and observed C translocation in AM fungi is directly affected by variation in temperature. Lower temperatures (<18°C) slow down C translocation and affects the growth of fungi. Jennings (1995) documented the fluidity of membranes and movement of solutes were also negatively affected by low temperature.
Soil temperature, along with other abiotic factors such as soil pH, humidity, and amount of phosphorus (P), directly affects AM fungal spore density and colonization of roots (Alkobaisy, 2022). The occurrence and distribution of AM fungi is also widely affected by seasonal variation (Sivakumar, 2013). Seasonal variation not only restricts distribution of AM fungi but also affects infection ratio with associated plants (Bever, 2002). Fungal spore density is reported to be higher in dry seasons as compared to wet seasons (Hayman, 1970; Carvalho et al., 2001; Rajpurohit and Jaiswal, 2023). Glomalin, a mycorrhizal glycoprotein known for soil aggregation and structuring, are released more during dry seasons as compared to wet seasons (Vieira Junior et al., 2020). A decrease in glomalin content directly affects the activity of AM fungi and leads to less association with plants. Pirozynski (1981) reported that evolution of mycorrhizal association started in the tropics. Wang and Qiu (2006) discovered a wide distribution range of AM association in both tropical and temperate regions that is completely regulated by the presence of various abiotic factors.
Soil is an active biological device that regulates various essential biochemical reactions and ecological processes to support life. Microbes are an inseparable part of soil and widely contribute to its fertility. Soil properties like moisture, temperature, nutrients, and land-use system strongly influence the abundance and distribution of AM fungi (Ndoye et al., 2012). Siqueira et al. (1998) have reported that soil with low fertility increases the dependence of plants on mycorrhizal association. It is also observed that, under low soil fertility, fungal hyphae grow extensively inside the root (Sanders et al., 1977). Whereas degraded land, intensive agriculture practices, and urbanization lowers AM diversity and distribution (Brundrett and Abbott, 2002; Cardozo-Junior et al., 2012; Gupta et al., 2018; Flores-Rentería et al., 2020). Disturbance causes changes in plant communities and its species composition, which directly affects its infective potential and decreases spore density and root colonization (Birhane et al., 2020).
The AM association is found in all ecological niches of the world, but variation in altitude leads to considerable changes in AM fungal diversity and association. It may increase or decrease with changes in temperature, precipitation, nutrient availability, and plant community dynamics with altitude (Cotton, 2018). Yang et al. (2017) reported that altitudinal changes have a direct effect on diversity and relative abundance of soil fungi, which decreases a with decline in soil temperature. The diversity and dominance of AM fungi decreased with an increase in altitude (Gaur and Kaushik, 2012; Ghosh et al., 2012). AM fungal development is also influenced by the availability of hosts. Yang et al. (2016) reported that AM fungi show higher preferences toward plants of lower altitude than higher altitude. Less vegetational diversity and extreme environmental conditions of higher altitudes generally do not support AM association. Johnson (2010) observed that AM fungi diversity and association can be manipulated by altering resources that directly affect carbon demand and association.
A study of AM colonization in pteridophytes by Ghanta et al. (2016) reported that lithophytic pteridophytes have a higher percentage of root colonization, arbuscules, vesicles, and fungal spore count as compared to epiphytes. Arbuscules are found to be absent in the case of aquatic pteridophytes. Rare AMF colonization in epiphytic and aquatic species may be because AM fungi cannot be easily dispersed. Bajwa et al. (2001) studied AM association in wetland plants and observed this association begins in spring and reaches a maximum in summer, autumn, or winter, depending on the host species. Plant root morphology is yet another important factor that helps in the colonization of AM fungi (Baylis, 1975; Manjunath and Habte, 1991). Lycophytes and epiphytic ferns are known to have two types of root morphologies. Fat and fleshy roots are found to be colonized with fungi, whereas thin roots have smaller diameters, are thick-walled, have greater mechanical strength, and have a higher presence of phenolic compounds that are less susceptible to fungal association (Schneider, 2000; Pressel et al., 2016).
Land plants are known to have diverged during the Neoproterozoic era from aquatic algae. This transition is considered to be one of the most significant turning points in the history of the Earth and evolution of land plants.
Pteridophytes are among the first vascular plants that invaded the terrestrial system after bryophytes. These non-seeded plants acted as pioneers, floor species, and primary producers in many of the ecosystems and facilitated the evolution of various other flora and fauna. They became a source of food and shelter for a variety of animals and acted as a favorable substratum for seedlings of higher plants. They played a very significant role in soil formation, restoration of habitat, and nutrient cycling. Symbiotic association with other microorganisms like cyanobacteria (nitrogen fixation) and mycorrhizal fungi (nutrient translocation such as Phosphorus) are some of the unique events that played a very important role in the early evolution and ecosystem functioning of the terrestrial system. Mycorrhizal association helped early land plants when conditions were not very favorable for survival. This association helped in nutrient translocation when availability was very limited and absorbing organs of plants were not very developed. AM association not only improved nutrient uptake but also made plants tolerant and resistant to various biotic and abiotic stresses. It has improved nutrient availability, structure, and fertility of soil, which later helped the rapid colonization of higher plants.
Climate change is a current and serious concern affecting natural ecosystems through and causing abiotic stresses like high temperature, salinity, flooding, and poor nutrient supply, in turn affecting plant growth and development. Pteridophytes have the potential to withstand such critical situations as many fossil records documented that, when life suffered major crises like mass extinction, pteridophytes colonized rapidly on barren lands and helped in the succession and establishment of new ecosystems. Mycorrhizal association played a very significant role in this process and helped pteridophytes to survive during unfavorable conditions by combating various stresses and helping to provide nutrition. In today’s time, when climate change is affecting ecosystem services, AM status needs to be better acknowledged in this group of plants. This review is a small attempt to gather information about AM-associated pteridophytes and fungal species, along with different AM structures like hyphae, arbuscules, and vesicles formed by them, which can be utilized in further research work.
PK: Conceptualization, Resources, Supervision, Writing – original draft, Writing – review & editing. MB: Writing – original draft, Writing – review & editing. PG: Conceptualization, Writing – review & editing. PU: Conceptualization, Writing – review & editing.
The author(s) declare that no financial support was received for the research, authorship, and/or publication of this article.
The authors declare that the research was conducted in the absence of any commercial or financial relationships that could be construed as a potential conflict of interest.
All claims expressed in this article are solely those of the authors and do not necessarily represent those of their affiliated organizations, or those of the publisher, the editors and the reviewers. Any product that may be evaluated in this article, or claim that may be made by its manufacturer, is not guaranteed or endorsed by the publisher.
Alkobaisy, J. S. (2022). “Factors affecting mycorrhizal activity” in Mycorrhiza-New Insights (Vienna, Austria: IntechOpen).
Bajwa, R., Yaqoob, A., and Javaid, A. (2001). Seasonal variation in VAM in wetland plants. Pak. J. Biol. Sci. 4, 464–470. doi: 10.3923/pjbs.2001.464.470
Baylis, G. T. S. (1975). “The magnolioid mycorrhiza and mycotrophy in root systems derived from it” in Endomycorrhizas. eds. F. E. Sanders, B. Mosse, and P. B. Tinker (London, UK: Academic Press), 373–389.
Bever, J. D. (2002). Host-specificity of AM fungal population growth rates can generate feedback on plant growth. Plant and Soil 244, 281–290. doi: 10.1023/A:1020221609080
Bharti, M., and Pravesh, R. (2014). Mycorrhizal associates of Lygodium Flexuosum (L.) SW. AND Ampelopteris Prolifera (RETZ) Copel of Ranchi District of Jharkhand, India. Ecoscan Spec Iss 1, 133–138.
Birhane, E., Ahmed, S., Hailemariam, M., Negash, M., Rannestad, M. M., and Norgrove, L. (2020). Carbon stock and woody species diversity in homegarden agroforestry along an elevation gradient in southern Ethiopia. Agr. Syst. 94, 1099–1110. doi: 10.1007/s10457-019-00475-4
Boullard, B., and Lemoigne, Y. (1971). Les champignons endophytes du Rhynia gwynne-vaughanii K. et L. Etude morphologique et deductions sur leur biologie. Le Botaniste 54, 49–89.
Brundett, M. C. (2002). Coevolution of roots and mycorrhizas of land plants. New Phytol. 154, 275–304. doi: 10.1046/j.1469-8137.2002.00397.x
Brundrett, M. C. (2004). Diversity and classification of mycorrhizal associations. Biol. Rev. 79, 473–495. doi: 10.1017/s1464793103006316
Brundrett, M. C., and Abbott, L. K. (2002). “Arbuscular mycorrhiza in plant communities” in Plant conservation and biodiversity. eds. K. Sivasithamparam, K. W. Dixon, and R. L. Barrett (Dordrecht: Kluwer Academic Publishers), 151–193.
Brundrett, M., and Tedersoo, L. (2018). Evolutionary history of mycorrhizal symbiosis and global host plant diversity. New Phytol. 220, 1108–1115. doi: 10.1111/nph.14976
Cardozo-Junior, F. M., Carneiro, R. F. V., Goto, B. T., Bezerra, A. A. C., Araújo, A. S. F., and Nunes, L. A. P. L. (2012). Arbuscular mycorrhizal fungi in degraded lands in Northeast Brazil. Afr. J. Microbiol. Res. 6, 7198–7205. doi: 10.5897/AJMR12.1132
Carvalho, L. M., Cacados, I., and Martiris-Loucao, M. A. (2001). Temporal and spatial variation of arbuscular mycorrhizas in salt marsh plants of Tagus estuary (Portugal). Mycorrhiza 11, 303–309. doi: 10.1007/s00572-001-0137-6
Cotton, T. E. A. (2018). Arbuscular mycorrhizal fungal communities and global change: an uncertain future. FEMS Microbiol. Ecol. 94:179. doi: 10.1093/femsec/fiy179
Daniels Hetrick, B. A. (1984). “Ecology of VA mycorrhizal fungi” in VA mycorrhizae. eds. C. L. Powell and D. J. Bagyaraj (Boca Raton, Florida: CRC Press), 35–55.
Desireo, A., Duckett, J. G., Pressel, S., Villareal, J. C., and Bidartondo, M. (2013). Fungal symbiose in hornworts: a chequerel history. Proc. R Soc. B Bio. Sci. 280:20130207. doi: 10.1098/rspb.2013.0207
Dickson, S. (2004). TheArum–Pariscontinuum of mycorrhizal symbioses. New Phytol. 163, 187–200. doi: 10.1111/j.1469-8137.2004.01095.x
Duckett, J. G., Carafa, A., and Ligrone, R. (2006). A highly differentiated glomeromycotean association with the mucilage-secreting, primitive antipodean liverwort Treubia (Treubiaceae): clues to the origins of mycorrhizas. Am. J. Bot. 93, 797–813. doi: 10.3732/ajb.93.6.797
Fernández, N. V., Messuti, M. I., and Fontela, S. B. (2013). Occurrence of arbuscular mycorrhizas and dark septate endophytes in pteridophytes from a patagonian rainforest, Argentina. J. Basic Microbiol. 53, 498–508. doi: 10.1002/jobm.201100613
Flores-Rentería, D., Sánchez-Gallén, I., Morales-Rojas, D., Larsen, J., and Álvarez-Sánchez, F. J. (2020). Changes in the abundance and composition of a microbial community associated with land use change in a Mexican tropical rain Forest. J. Soil Sci. Plant Nutr. 20, 1144–1155. doi: 10.1007/s42729-020-00200-6
Gaur, S., and Kaushik, P. (2012). Effect of seasonal variation on mycorrhizal Fungi associated with medicinal plants in central Himalayan region of India. Am J. Plant Sci. 3, 618–626. doi: 10.4236/ajps.2012.35075
Gavito, M. E., Harve Rouhier, P. A. O., Medina-Penafiel, A., Jakobsen, I., Bago, A., and Azcon-Aguilar, C. (2005). Temperature constraints on the growth and functioning of root organ cultures with arbuscular mycorrhizal fungi. New Phytol. 168, 179–188. doi: 10.1111/j.1469-8137.2005.01481.x
Ghanta, R., Sen, M., and Dutta, S. (2016). An investigation on arbuscular mycorrhizal colonization in some Pteridophytes of West Bengal, India. Int. J. Adv. Res. Biol. Sci. 3, 143–153. doi: 10.22192/ijarbs.2016.03.11.017
Ghosh, R., Sengupta, S., and Bhattacharyya, S. (2012). Arbuscular mycorrhizal fungi associated with some fern species collected from Kumaon region of western Himalaya. Indian Phytopathol. 65, 282–285.
Gupta, M., Gupta, A., and Kumar, P. (2018). Urbanization and Biodiversity of Arbuscular Mycorrhizal Fungi- the Case Study of Delhi, India. Revista de Biología Tropical, 66. doi: 10.15517/rbt.v66i4.33216
Harper, C. J., Taylor, T. N., Krings, M., and Taylor, E. L. (2013). Mycorrhizal symbiosis in the Paleozoic seed fern glossopteris from Antarctica. Rev. Palaeobot. Palynol. 192, 22–31. doi: 10.1016/j.revpalbo.2013.01.002
Hayman, D. S. (1970). Endogone spore numbers in soil and vesicular-arbuscular mycorrhiza in wheat as influenced by season and soil treatment. Trans. Br. Mycol. Soc. 54, 53–IN12. doi: 10.1016/S0007-1536(70)80123-1
Heard, K. (2006). Tropical pteridophyte relationships with mycorrhizal fungi. University of Southflorida: Institute for Tropical Ecology and Conservation.
Heinemeyer, A., and Fitter, A. H. (2004). Impact of temperature on the arbuscular mycorrhizal (AM) symbiosis: growth responses of the host plant and its AM fungal partner. J. Exp. Bot. 55, 525–534. doi: 10.1093/jxb/erh049
Hetherington, A. J., Berry, C., and Dolan, L. (2016). Networks of highly branched stigmarian rootlets developed on the first giant trees. Proc. Natl. Acad. Sci. U. S. A. 113, 6695–6700. doi: 10.1073/pnas.1514427113
Hodge, A., and Storer, K. (2015). Arbuscular mycorrhiza and nitrogen: implications for individual plants through to ecosystems. Plant and Soil 386, 1–19. doi: 10.1007/s11104-014-2162-1
Huey, C. J., Gopinath, S. C. B., Uda, M. N. A., Zulhaimi, H. I., Jaafar, M. N., Kasim, F. H., et al. (2020). Mycorrhiza: A natural resource assists plant growth under varied soil conditions. 3 Biotech 10:204. doi: 10.1007/s13205-020-02188-3
Iqbal, S. H., Yousaf, M., and Younus, M. (1981). A field survey of mycorrhizal associations in ferns of Pakistan. New Phytol. 87, 69–79. doi: 10.1111/j.1469-8137.1981.tb01691.x
International Culture Collection of Glomeromycota [CICG] (2020). International Culture Collection of Glomeromycota. Available at: https://sites.google.com/site/cicgfma/home (Accessed November 17, 2020).
Jakobsen, I., Abbott, L. K., and Robso, A. D. (1992). External hyphae of vesicular– arbuscular mycorrhizal fungi associated with Trifolium subterraneum L. 1. Spread of hyphae and phosphorus inflow into roots. New Phytol. 120, 371–380. doi: 10.1111/j.1469-8137.1992.tb01077.x
Jakobsen, I., and Rosendahl, L. (1990). Carbon flow into soil and external hyphae from roots of mycorrhizal cucumber plants. New Phytol. 115, 77–83. doi: 10.1111/j.1469-8137.1990.tb00924.x
Jaramillo, I. R., Ricalde, R. L. C., and Ramos, M. A. A. (2008). Mycorrhizal-like interaction between gametophytes and young sporophytes of the fern Dryopteris muenchii (Filicales) and its fungal endophyte. Rev. Biol. Trop. 56, 1101–1107. doi: 10.15517/rbt.v56i3.5696
Jennings, D. H. (1995). The physiology of fungal nutrition. Cambridge, UK: Cambridge University Press.
Johnson, N. C. (2010). Resource stoichiometry elucidates the structure and function ofarbuscular mycorrhizas across scales. New Phytol. 185, 631–647. doi: 10.1111/j.1469-8137.2009.03110.x
Karatygin, I. V., Snigirevskaya, N. S., and Demchenko, K. N. (2006). Species of the genus Glomites as plant mycobionts in early Devonian ecosystems. Paleontol. J. 40, 572–579. doi: 10.1134/S0031030106050121
Kenrick, P., and Strullu-Derrien, C. (2014). The origin and early evolution of roots. Plant Physiol. 166, 570–580. doi: 10.1104/pp.114.244517
Khade, S. W., and Rodrigues, B. F. (2002). Arbuscular mycorrhizal fungi associated with some pteridophytes from western Ghat region of Goa. J. Trop. Ecol. 43, 251–256.
Kidston, R., and Lang, W. H. (1921). On the old red sandstone plants showing structure from the Rhynie chert bed, Aberdeenshire. V. The thallophyta occurring in the peat bed, the succession of the plants through a vertical section of the bed and the conditions of accumulation and preservation of the peat. Trans. R. Soc. Edinburg 52, 855–902. doi: 10.1017/S0080456800016045
Kiers, E. T., Duhamel, M., Beesetty, Y., Mensah, J. A., Franken, O., Verbruggen, E., et al. (2011). Reciprocal rewards stabilize cooperation in the mycorrhizal symbiosis. Science 333, 880–882. doi: 10.1126/science.1208473
Koske, R. E., Friese, C. F., Olexia, P. D., and Hawke, R. L. (1985). Vesicular arbuscular mycorrhizae in Equisetum. Trans. Br. Mycol. Soc. 85, 350–353.
Krings, M., Taylor, T. N., Hass, H., Kerp, H., Dotzler, N., and Hermsen, E. J. (2007). Fungal endophytes in a 400-million-yr-old land plant: infection pathways, spatial distribution, and host responses. New Phytol. 174, 648–657. doi: 10.1111/j.1469-8137.2007.02008.x
Krzyzanski, H., Gutierre, M. A. M., and Carrenho, R. (2021). Arbuscular mycorrhizal and dark septate fungi are not common in roots of epiphytic pteridophytes of a transitional forest area in South Brazil. Acta Bot. Brasil 35, 569–576. doi: 10.1590/0102-33062020abb0396
Leake, J. R., Johnson, D., Donnelly, D. P., Muckle, G. E., Boddy, L., and Read, D. J. (2004). Networks of power and influence: the role of mycorrhizal mycelium in controlling plant communities and agroecosystem functioning. Can. J. Bot. 82, 1016–1045. doi: 10.1139/b04-060
Lee, J. K., Eom, A. H., Lee, S. S., and Lee, C. H. (2001). Mycorrhizal symbioses found in roots of Fern and its relatives in Korea. J. Plant Biol. 44, 81–86. doi: 10.1007/BF03030279
Lehnert, M., Krug, M., and Kessler, M. (2017). A review of symbiotic fungal endophytes in lycophytes and ferns – a global phylogenetic and ecological perspective. Symbiosis 71, 77–89. doi: 10.1007/s13199-016-0436-5
Luginbuehl, L. H., Menard, G. N., Kurup, S., Van, E. H., Radhakrishmam, G. V., Breakspear, A., et al. (2017). Fatty acids in arbuscular mycorrhizal fungi are synthesised by the host plant. Science 356, 1175–1178. doi: 10.1126/science.aan0081
Mane, S. R., Shaikh, R. I., Surve, S. P., and Bhale, U. N. (2019). Mycorrhizal Association in Pteridophytes Species from Marathwada region of Deccan plateau zone of India. J. Plant Sci. 18, 33–45. doi: 10.3923/ajps.2019.33.45
Manjunath, A., and Habte, M. (1991). Root morphological characteristics of host species having distinct mycorrhizal dependency. Can. J. Bot. 69, 671–676. doi: 10.1139/b91-089
Martinez, A. E., Chiocchio, V., Em, T. M., Rodriguez, M. A., and Godeas, A. M. (2012). Mycorrhizal association in gametophytes and sporophytes of the fern Pteris vittata (Pteridaceae) with Glomus intraradices. Rev. Biol. Trop. 60, 857–865. doi: 10.15517/rbt.v60i2.4019
Mishra, R. R., Sharma, G. D., and Gatphoh, A. R. (1980). Mycorrhizas in the ferns of north eastern India. Proc. Indian Nath. Sci. Acad. 46, 546–551.
Morris, J. L., Leake, J. R., Stein, W. E., Berry, C. M., Marshall, J. E. A., Wellman, C. H., et al. (2015). Investigating Devonian trees as geo-engineers of past climates: linking palaeosols to palaeobotany and experimental geobiology. Palaeontology 58, 787–801. doi: 10.1111/pala.12185
Mosse, B., Sribely, D. P., and LeTacon, F. (1981). Ecology of mycorrhizae and mycorrhizal fungi. Adv Microb. Ecol. 5, 137–210. doi: 10.1007/978-1-4615-8306-6_4
Muthukumar, T., and Prabha, K. (2013). Arbuscular mycorrhizal and septate endophyte fungal associations in lycophytes and ferns of South India. Symbiosis 59, 15–33. doi: 10.1007/s13199-012-0185-z
Muthuraja, R., Muthukumar, T., and Sathiyadash, K. (2014). Arbuscular mycorrhizal (AM) and dark septate endophyte (DSE) fungal association in lycophytes and ferns of the Kolli Hills, Eastern Ghats, Southern India. American Fern J. 104, 67–102. doi: 10.1640/0002-8444-104.2.67
Nafees, M., Ullah, S., and Bruni, T. (2019). Vesicular arbuscular mycorrhizal (VAM) studies in six pteridophytes in district Buner. Int. J. Bot. St. 4, 98–101.
Ndoye, F., Kane, A., Mangaptché, N., Bakhoum, N., Sanon, A., Diouf, D., et al. (2012). Changes in land use system and environmental factors affect arbuscular mycorrhizal fun-gal density and diversity, and enzyme activities in rhizospheric soils of Acacia senegal (L.) Willd. ISRN Ecol 13:191. doi: 10.5402/2012/563191
O’Connor, P. J., Smith, S. E., and Smith, F. A. (2002). Arbuscular mycorrhizas influence plant diversity and community structure in a semiarid herbland. New Phytol. 154, 209–218. doi: 10.1046/j.1469-8137.2002.00364.x
Parez, L., Baizabal, M. D. V., Carrazana, J. C. N., Rodríguez, R. Z., Capistrán, L. L., and Torres, A. A. (2015). Mycorrhizal associations of ferns and lycopods of Central Veracruz, Mexico. Symbiosis 65, 85–92. doi: 10.1007/s13199-015-0320-8
Phipps, C. J., and Taylor, T. N. (1996). Mixed arbuscular mycorrhizae from the Triassic of Antarctica. Mycologia 88, 707–714. doi: 10.1080/00275514.1996.12026707
Pirozynski, K. A. (1981). Interactions between fungi and plants through the ages. Can. J. Bot. 59, 1824–1827. doi: 10.1139/b81-243
Pirozynski, K. A., and Malloch, D. W. (1975). The origin of land plants: a matter of mycotrophism. Biosystems 6, 153–164. doi: 10.1016/0303-2647(75)90023-4
Pressel, S., Bidartondo, M. I., Field, K. J., Rimington, W. R., and Duckett, J. G. (2016). Pteridophyte fungal associations: current knowledge and future perspectives. J. Syst. Evol. 54, 666–678. doi: 10.1111/jse.12227
Pressel, S., Bidartondo, M. I., Ligrone, R., and Duckett, J. G. (2010). Fungal symbioses in bryophytes: new insights in the twenty first century. Phytotaxa 9, 238–253. doi: 10.11646/phytotaxa.9.1.13
Quilambo, O. A. (2003). The vesicular-arbuscular mycorrhizal symbiosis. Afr. J. Biotechnol. 2, 539–546. doi: 10.5897/AJB2003.000-1105
Rajpurohit, S. S., and Jaiswal, P. (2023). Seasonal variation of mycorrhizal association (root colonization and spore density) in selected industrial sites in Kota District of Rajasthan, India. Microbiol Res J Int 33, 32–41. doi: 10.9734/mrji/2023/v33i11361
Read, D. J. (1999). “The ecophysiology of mycorrhizal symbiosis with special reference to impacts upon plant fitness” in Physiological plant ecology. eds. J. D. Scholes and M. G. Barker (Blackwell Science, London: Press MC), 133–152.
Read, D. J., Duckett, J. G., Francis, R., Ligrone, R., and Russell, A. (2000). Symbiotic fungal associations in "lower" land plants. Royal Soc. 355, 815–831. doi: 10.1098/rstb.2000.0617
Redecker, D., Kodner, R., and Graham, L. E. (2000). Glomalean fungi from the Ordovician. Science 289, 1920–1921. doi: 10.1126/science.289.5486.1920
Redecker, D., and Raab, P. (2006). Phylogeny of the Glomeromycota (arbuscular mycorrhizal fungi): recent developments and new gene markers. Mycologia 98, 885–895. doi: 10.3852/mycologia.98.6.885
Remy, W., Taylor, T. N., Hass, H., and Kerp, H. (1994). Four hundred-million-year-old vesicular arbuscular mycorrhizae. Proc. Natl. Acad. Sci. U. S. A. 91, 11841–11843. doi: 10.1073/pnas.91.25.11841
Sanders, F. E., Tinker, P. B., Black, R. L. B., and Palmerley, S. M. (1977). The development of endomycorrhizal root systems. I. Spread of infection and growth-promoting effects with four species of vesicular-arbuscular endophyte. New Phytol. 78, 257–268. doi: 10.1111/j.1469-8137.1977.tb04829.x
Santhoshkumar, S., and Nagarajan, N. (2014). AM fungal association in the rhizosphere soil of some Pteridophytic plant species in Valparai Hills, Western Ghats of Tamilnadu, India. Int. J. Life Sci 2, 201–206.
Sarwade, P. P., Shaikh, R. U., Chandanshiv, S. S., and Bhale, U. N. (2012). Association of AM fungi in important Pteridophytic plants of Maharashtra, India. Int. Multidiscipl Res. J. 2, 08–09.
Schneider, H. (2000). Morphology and anatomy of roots in the filmy fern tribe Trichomaneae H. Schneider (Hymenophyllaceae, Filicatae) and the evolution of rootless taxa. Bot. J. Linnean Soc. 132, 29–46. doi: 10.1111/j.1095-8339.2000.tb01853.x
Schüßler, A., and Walker, C. (2011). “Evolution of the “plant-symbiotic” fungal phylum, Glomeromycota” in The Mycota XIV. eds. S. Poggeler and J. Wostemeyer (Berlin Heidelberg: Springer-Verlag), 163–185.
Selosse, M. A. (2005). Are liverworts limitating mycorrhizas. New Phytol. 165, 345–350. doi: 10.1111/j.1469-8137.2004.01298.x
Simon, L., Bousquet, J., Levesque, R. C., and Lalonde, M. (1993). Origin and diversification of endomycorrhizal fungi and coincidence with vascular land plants. Nature 363, 67–69. doi: 10.1038/363067a0
Siqueira, J. O., Saggin-Junior, O. J., Flores-Aylas, W. W., and Guimara, E. S. (1998). Arbuscular mycorrhizal inoculation and superphosphate application influence plant development and yield of coffee in Brazil. Mycorrhiza 7, 293–300. doi: 10.1007/s005720050195
Sivakumar, N. (2013). Effect of edaphic factors and seasonal variation on spore density and root colonization of arbuscular mycorrhizal fungi in sugarcane fields. Ann. Microbiol. 63, 151–160. doi: 10.1007/s13213-012-0455-2
Smith, S. E., and Read, D. J. (2010). Mycorrhizal Symbiosis. 3rd Edn. Cambridge, UK: Academic Press.
Smith, F. A., and Smith, S. E. (1997). Structural diversity in (vesicular)-arbuscular mycorrhizal symbioses. New Phytol. 137, 373–388. doi: 10.1046/j.1469-8137.1997.00848.x
Smith, S. E., and Smith, F. A. (2011). Roles of arbuscular mycorrhizas in plant nutrition and growth: new paradigms from cellular to ecosystem scales. Annu. Rev. Plant Biol. 62, 227–250. doi: 10.1146/annurev-arplant-042110-103846
Strullu, D. G. (1985). Les mycorhizes, Handbuch der Pflanzenanatomie. Berlin, Germany: Gebruder Borntraeger.
Strullu-Derrien, C., Kenrick, P., Pressel, S., Duckett, J. G., Rioult, J. P., and Strullu, D. G. (2014). Fungal associations in Horneophyton ligneri from the Rhynie Chert (c. 407 ma) closely resemble those in extant lower land plants: novel insights into ancestral plant–fungus symbioses. New Phytol. 203, 964–979. doi: 10.1111/nph.12805
Strullu-Derrien, C., and Strullu, D. G. (2007). Mycorrhization of fossil and living plants. Comptes. Rendus. Palevol. 6, 483–494. doi: 10.1016/j.crpv.2007.09.006
Taylor, T. N., and Krings, M. (2005). Fossil microorganisms and land plants: associations and interactions. Symbiosis 40, 119–135.
Taylor, T. N., Krings, M., and Taylor, E. L. (2015). Fossil fungi. 1st Edn. Burlington, MA, USA: Elsevier/Academic Press.
Taylor, T. N., Remy, W., Hass, H., and Kerp, H. (1995). Fossil arbuscular mycorrhizae from the early Devonian. Mycologia 87:560. doi: 10.2307/3760776
Trewin, N. H., and Rice, C. M. (2004). The Rhynie hot-spring system. Geology, biota and mineralisation. Proceedings of the conference held in 2003. Trans. R. Soc. Edinburgh Earth Sci 94, 283–521.
Turnau, K., Anielska, T., and Jurkiewiez, A. (2005). Mycothal-lic/mycorrhizal symbiosis of chlorophyllous game-tophytes and sporophytes of a fern, Pellaea viridis (Forsk.) Prantl (Pellaeaceae, Pteridales). Mycorrhiza 15, 121–128. doi: 10.1007/s00572-004-0306-5
van der Heijden, M. G. A., Klironomos, J. N., Ursic, M., Moutoglis, P., Streitwolf-Engel, R., Boller, T., et al. (1998). Mycorrhizal fungal diversity determines plant biodiversity, ecosystem variability and productivity. Nature 396, 69–72. doi: 10.1038/23932
van der Heijden, M. G. A., Martin, F. M., Selosse, M.-A., and Sanders, I. R. (2015). Mycorrhizal ecology and evolution: the past, the present, and the future. New Phytol. 205, 1406–1423. doi: 10.1111/nph.13288
Vieira Junior, W. G., Moura, J. B. D., Souza, R. F. D., Braga, A. P. M., Matos, D. J. C., Brito, G. H. M., et al. (2020). Seasonal variation in mycorrhizal community of different cerrado phytophysiomies. Front. Microbiol. 11:576764. doi: 10.3389/fmicb.2020.576764
Wang, B., and Qiu, Y. L. (2006). Phylogenetic distribution and evolution of mycorrhizas in land plants. Mycorrhiza 16, 299–363. doi: 10.1007/s00572-005-0033-6
Wijayawardene, N., Hyde, K., Al-Ani, L., Tedersoo, L., Haelewaters, D., Rajeshkumar, K. C., et al. (2020). Outline of fungi and fungus-like taxa. Mycosphere 11, 1060–1456. doi: 10.5943/mycosphere/11/1/8
Winther, J. L., and Friedman, W. E. (2008). Arbuscular mycorrhizal associations in Lycopodiaceae. New Phytol. 177, 790–801. doi: 10.1111/j.1469-8137.2007.02276.x
Winther, J. L., and Friedman, W. E. (2009). Phylogenetic affinity of arbuscular mycorrhizal symbionts in Psilotum nudum. J. Plant Res. 122, 485–496. doi: 10.1007/s10265-009-0234-8
Yang, H., Lü, G., Jiang, H., Shi, D. N., and Liu, Z. (2017). Diversity and distribution of soil micro-fungi along an elevation gradient on the north slope of Changbai Mountain. J. For. Res. 28, 831–839. doi: 10.1007/s11676-016-0344-9
Yang, W., Zheng, Y., Gao, C., Duan, J. C., Wang, S. P., and Guo, L. D. (2016). Arbuscular mycorrhizal fungal community composition affected by original elevation rather than translocation along an altitudinal gradient on the Qinghai Tibet plateau. Sci. Rep. 6:36606. doi: 10.1038/srep36606
Keywords: pteridophytes, mycorrhizal association, AM fungi, Glomeromycota, arbuscules, vesicles
Citation: Kumari P, Bhatia M, Giri P and Uniyal PL (2024) Mycorrhizal association and its relation with pteridophytes. Front. Microbiol. 15:1406891. doi: 10.3389/fmicb.2024.1406891
Received: 25 March 2024; Accepted: 20 June 2024;
Published: 11 July 2024.
Edited by:
Sushma Mishra, University of Lucknow, IndiaReviewed by:
Eva Nouri, Université de Fribourg, SwitzerlandCopyright © 2024 Kumari, Bhatia, Giri and Uniyal. This is an open-access article distributed under the terms of the Creative Commons Attribution License (CC BY). The use, distribution or reproduction in other forums is permitted, provided the original author(s) and the copyright owner(s) are credited and that the original publication in this journal is cited, in accordance with accepted academic practice. No use, distribution or reproduction is permitted which does not comply with these terms.
*Correspondence: Pratibha Kumari, bWgucHJhdGliaGFAZ21haWwuY29t
Disclaimer: All claims expressed in this article are solely those of the authors and do not necessarily represent those of their affiliated organizations, or those of the publisher, the editors and the reviewers. Any product that may be evaluated in this article or claim that may be made by its manufacturer is not guaranteed or endorsed by the publisher.
Research integrity at Frontiers
Learn more about the work of our research integrity team to safeguard the quality of each article we publish.