- 1State Key Laboratory of Biobased Material and Green Papermaking, School of Bioengineering, Qilu University of Technology, Shandong Academy of Sciences, Jinan, China
- 2Shandong Baoyuan Biotechnology Co., Ltd., Jinan, China
- 3Shandong Chenzhang Biotechnology Co., Ltd., Jinan, China
- 4Shengsheng Xiangrong Biotechnology (Shandong) Co., Ltd., Jinan, China
Introduction: Sepsis is a syndrome of organ dysfunction caused by a dysregulated host response to infection and septic shock. Currently, antibiotic therapy is the standard treatment for sepsis, but it can lead to drug resistance. The disturbance of the gut microbiota which is affected by sepsis could lead to the development of organ failure. It is reported that probiotics could shape the gut microbiota, potentially controlling a variety of intestinal diseases and promoting whole-body health.
Methods: In this study, we evaluated the preventive effects of intra- and extracellular products of probiotics on sepsis. The extracellular products of Lactococcus lactis (L. lactis) were identified through the in vivo cell experiments. The preventive effect and mechanism of L. lactis extracellular products on mouse sepsis were further explored through HE staining, mouse survival rate measurement, chip analysis, etc.
Results: L. lactis extracellular products increase cell survival and significantly reduce inflammatory factors secreted in a cellular sepsis model. In in vivo experiments in mice, our samples attenuated sepsis-induced pulmonary edema and inflammatory infiltrates in the lungs of mice, and reduced mortality and inflammatory factor levels within the serum of mice. Finally, the mechanism of sepsis prevention by lactic acid bacteria is suggested. Extracellular products of L. lactis could effectively prevent sepsis episodes.
Discussion: In animal experiments, we reported that extracellular products of L. lactis can effectively prevent sepsis, and preliminarily discussed the pathological mechanism, which provides more ideas for the prevention of sepsis. In the future, probiotics may be considered a new way to prevent sepsis.
1 Introduction
Sepsis is a serious, potentially fatal, organic dysfunction caused by a dysregulated host response to infection and septic shock in a subset of patients in which underlying circulatory and cellular/metabolic abnormalities are sufficiently profound to substantially increase mortality (Jiang L. et al., 2022). Extrapolating from the study data, there may be 5.3 million deaths from the 31.5 million cases of sepsis worldwide (Markwart et al., 2020). In a recent study performed in Brazil, one-third of intensive care beds were occupied by septic patients, with a mortality rate of 55.7% (Kahveci et al., 2021). Early identification of patients with sepsis, early intravenous fluid resuscitation, and early intravenous antibiotic administration are the mainstay of sepsis management (Aljerian et al., 2019). Despite advances in clinical management, including the findings of new biomarkers with diagnostic and prognostic value, sepsis and septic shock still remain a leading cause of admission to intensive care unit worldwide (Colomina-Climent et al., 2016). The appropriate and timely administration of antibiotic therapy play an essential role in the achievement of a favorable outcome for patients with sepsis and septic shock, but the alarming and rising phenomenon of antibiotic resistance among the causative pathogens may represent the most serious challenge for future years if new drugs will not be found (Bogner et al., 2013; Messina et al., 2015).
More than 95% of sepsis is caused by bacterial infection, and the release of endotoxin lipopolysaccharides (LPS) caused by bacterial infection is the main factor leading to sepsis (Sun et al., 2021). When the body is invaded by LPS, Toll-like receptor 4 on the surface of macrophages recognizes LPS and activates intracellular signal transduction of macrophages to produce a large number of pro-inflammatory cytokines, such as TNF-a, IL-1β, IL-6, IL-12, etc. Cytokines reach distal target organs or target cells through blood circulation or paracrine and exert biological effects, causing systemic inflammatory reactions and leading to sepsis. The endothelium is an active contributor to sepsis and as such represents a major target for therapy. During sepsis, endothelial cells amplify the immune response and activate the coagulation system (Yang et al., 2011). They are both the target and source of inflammation and serve as a link between local and systemic immune responses (Shin et al., 2013). By reversing and maintaining the function and status of endothelial cells, it will play a positive role in the protection of the pathogenesis center of sepsis, liver, spleen, lung, kidney and other important organs (Liu et al., 2019; Fu et al., 2023).
Probiotics, which include bacteria and yeast, are living microorganisms that have been shown to be beneficial to human health. Clinical trials and in vivo experiments have expanded our current understanding of the important role that probiotics play in human gut microbiome related diseases. Many clinical trials have demonstrated that probiotics could shape the gut microbiome, potentially controlling a variety of gut diseases and promoting overall health (Ahn et al., 2020). For decades, the gut was thought to play an important role in sepsis pathogenesis. The composition of the intestinal microbiome, is affected by sepsis, and might contribute to the development of organ failure (Hu et al., 2019). A well-balanced microbiota is essential to maintain enteric and systemic immune homoeostasis. Disruption of the integrity of the intestinal microbiota potentially increases susceptibility to sepsis (Deng et al., 2023). Therefore, probiotics can reduce sepsis symptoms by reducing intestinal inflammation and repairing intestinal function in patients with sepsis. Every year, about 230 million surgical procedures are performed worldwide. Sepsis is one of the postoperative complications. Prophylactic antibiotic treatment is standard, but it is complicated by the rise of antibiotic resistance (Versporten et al., 2018). Studies have shown that the use of L. plantarum, L. casei and B. breve in combination with galactose oligosaccharide is also the best intervention for reducing sepsis, hospitalization and antibiotic use (van Ruler et al., 2011; Benjak Horvat et al., 2021). It is of great significance to prevent infection and improve sepsis through probiotics.
In this paper, LPS was applied to human vascular endothelial cells (HUVECs) coupling mice to induce sepsis. Streptococcus lactis (S. lactis), Lactobacillus rhamnosus (LGG), Lactobacillus Paracasei (L. paracasei), Lactobacillus plantarum (L. plantarum), Lactobacillus fermentium (L. fermentium) and Lactococcus lactis (L. lactis) were selected. The intracellular and extracellular products were extracted, respectively. Cell survival rate and cell inflammatory factors, mouse survival rate, mouse blood inflammatory factors, organ coefficient and microarray analysis were determined to analyze the effect of probiotics on sepsis.
2 Materials and methods
2.1 Cell culture
Human umbilical vein endothelial cells (HUVECs) were obtained as described (Michaux et al., 2006). Cells were cultured in M199 medium (Genview) supplemented with 20% fetal bovine serum (Applied Biosystems) in a humidified incubator at 37°C with 5% CO2. Cells were used in the 5th to 10th passages. For the HUVEC experiments, cells in logarithmic growth phase were seeded in well plate at a density of 1 × 105 cells/ml. The cell morphology was observed by Nikon phase contrast microscope.
2.2 Culture of probiotics and preparation of extracellular and intracellular products
Streptococcus lactis (S. lactis), Lactobacillus rhamnosus (LGG), Lactobacillus Paracasei (L. paracasei), Lactobacillus plantarum (L. plantarum), Lactobacillus fermentium (L. fermentium), Lactococcus lactis (L. lactis) were inoculated on MRS solid medium (for the configuration of the medium, refer to Zhang et al., 2020) cultured at 37°C for 24 h, and then inoculated on their respective seed media, cultured at 37°C for 48 h, to obtain the seed liquid of the strain for use. The probiotic seed solution was inoculated in liquid medium for 24 h to obtain the bacterial solution, and the bacterial solution was precipitated after 3,000x g centrifugation for 15 min. After repeated rinsing of PBS for three times, the precipitation was collected by centrifugation and then decomposed by ultrasonic wave. Centrifuge 1,000x g for 10 min to collect the supernatant. After the bacteria were filtered by 0.45 μm filter membrane, the intracellular products of probiotics were obtained and stored in −80°C and liquid nitrogen, respectively. The probiotic seed solution was inoculated in liquid medium for 24 h to obtain the bacterial solution. The bacterial solution was centrifuged at 1000x g for 10 min to collect the supernatant. After the bacteria were filtered by 0.45 μm filter membrane, the probiotic extracellular products were obtained and stored in −80°C and liquid nitrogen, respectively.
2.3 Determination of cell viability
Cell viability was determined by MTT (3-[4,5-dimethylthiazol-2-yl]-2,5-diphenyltertrazolium bromide; M5655 Sigma-Aldrich) assay as previously described (Wang L. et al., 2020). The cells were seeded in 96-well plate. Cells were treated with 1, 2, 4 mg/mL intracellular or extracellular products of Streptococcus lactis (S. lactis), Lactobacillus plantarum (L. plantarum), Lactobacillus fermentum (L. fermentium) or L. lactis for 20 h or 44 h and then cells were incubated with 0.5% MTT for 4 h. After removing the medium, 100 μL dimethyl sulfoxide (DMSO) solution was added. The absorbance was measured at 570 nm using a SpectraMAX ABS microplate spectrophotometer (Molecular Devices). The cell viability was calculated by the ratio of OD.
Cell viability(%) = (sample group OD-blank group OD)/(control group OD-blank group OD) × 100%.
2.4 Enzyme-linked immunosorbent assays
The cells in logarithmic growth phase were seeded in 24-well plate. Firstly, HUVECs were pretreated with 2, 40, 800 μg/mL intracellular or extracellular products of S. lactis, L. plantarum, L. fermentium, L. lactis or MRS agar (MRS) medium for 12 h, 24 h or 48 h. Then, cells were cultured with 1 μg/mL LPS for 12 h. The cellular supernatant was obtained to determine the IL-6, IL-8 and TNF-α levels according to the manufacturers’ instructions (KE10007, KE10002 and KE10003, Proteintech Group, Inc.). Additionally, the serum of mice was collected to detect the IL-6 and TNF-α concentrations according to the instructions (Beijing dakowei Biotechnology Co., Ltd., Beijing, China).
2.5 Animal treatment
C57BL/6 mice aged 6 weeks (20 ± 2 g) were purchased from Beijing Vitonglihua Co., LTD. Mice were housed under standard conditions of humidity, room temperature and dark–light cycles. All animal experiments complied with the ARRIVE guidelines and were carried out in accordance with the U.K. Animals (Scientifc Procedures) Act, 1986 and associated guidelines, EU Directive 2010/63/EU for animal experiments and the National Institutes of Health guide for the care and use of Laboratory animals (NIH Publications No. 8023, revised 1978). The animal experimental protocol complied with the Animal Management Rules of the Chinese Ministry of Health (document no. 55, 2001) and was approved by the Animal Experiment Ethnics Committee of Qilu University of Technology. Mice acute sepsis model was established by intraperitoneal injection of LPS (20 mg/kg). The mice were divided into six groups: Mock group (NC), LPS group, LPS + MRS Group, low dose group (0.1 g/kg), medium dose group (0.2 g/kg) and high dose group (0.4 g/kg). At the beginning of the experiment, each animal in the mock and LPS groups was gavage 200 μL normal saline every day and each animal in the LPS + MRS group was gavage 200 μL MRS medium every day. Other animals were gavage 0.1 g/kg, 0.2 g/kg or 0.4 g/kg L. lactis extracellular products in the low-dose, medium-dose or high-dose groups every day. After 7 days treatment, except for the normal group, the mice in other 5 groups were intraperitoneal injected with LPS (20 mg/kg). The body weight of the mice was recorded every day and the physiological phenomena such as loss of appetite and blood in stool were observed.
2.6 Blood and tissue collection
The mice were euthanized by cervical dislocation. Serum was prepared by centrifugation at 3000 g for 20 min at 4°C and stored at −80°C for biochemical analysis. The organs of the mice were removed and the excess fat tissues at the edges of the organs were removed. After rinsing with normal saline at 4°C, the water was sucked up with filter paper and the organs were weighed. The lung was collected to obtain the wet weight, firstly. Then, the lung was dried at 80°C for 72 h to get the dry weight. Lung edema was assessed by calculating lung wet/dry (W/D) ratio.
2.7 Hematoxylin and eosin analyses
Lung tissues were fixed in an optimal cutting temperature compound (OCT) embedding medium (Tissue-Tek), frozen at −20°C for 12 h and then cut into 10 μm sections using a frozen sectioning machine (CM1950, Leica, Nussloch, Germany) and stained with hematoxylin and eosin (H&E) (Jiang K. et al., 2022). The sections were observed under an upright microscope (Eclipse E200, Nikon, Japan) and photographed using ImageView (Pooher, Shanghai, China).
2.8 Microarray analysis
Total RNA was extracted from cells treated with LPS alone for 24 h or cells treated the extracellular product of Lactococcus lactate and LPS for 24 h with TRIzol reagent (Invitrogen, United States). The samples were stored at −20°C, and microarray analysis was performed by CapitalBio Corp.1
2.9 Statistical analysis
All experiments were repeated at least 3 times independently. The normal distribution was firstly analyzed by SPSS v11.5 (SPSS Inc., Chicago, IL). All the data were expressed as mean ± SEM and analyzed with one-way ANOVA with use of SPSS v11.5 to compare the treatment means when data was normally distributed. The Tukey–Kramer multiple comparison procedure was used for Post-Hoc comparisons. p values <0.05 were considered statistically significant. Images were processed by the use of GraphpadPrism 5 (GraphPad Software, La Jolla, CA, United States) and Adobe Photoshop CS6 (Adobe, San Jose, United States).
3 Results
3.1 Effects of extracellular and intracellular products of probiotics on HUVEC morphology
HUVECs were treated with 0.04 mg/mL or 2 mg/mL intracellular or extracellular products of S. lactis, LGG, L. Paracasei, L. plantarum, L. fermentium or L. lactis. The morphological changes of cells were observed after treated with 24 h. In the normal group, cells were full and most of them were attached to the dish with pebble-like morphology. The cells in LPS group were slightly rounded, the edges were not clear, and a small number of floating dead cells appeared. The cells in MRS group were in good condition, showing full adherence to the dish which were similar with cells in the normal group (Figure 1A). The number of cells treated with 40 μg/mL, 2 mg/mL, 4 mg/mL of LGG and L. paracase products decreased significantly. And most of the cells shrank and died (Figure 1B). Thus, at the same concentration, extracellular and intracellular products of LGG and L. paracasei showed more harmful to HUVECs. S. lactis, L. plantarum, L. fermentum and L. lactis were selected in the following experiments.
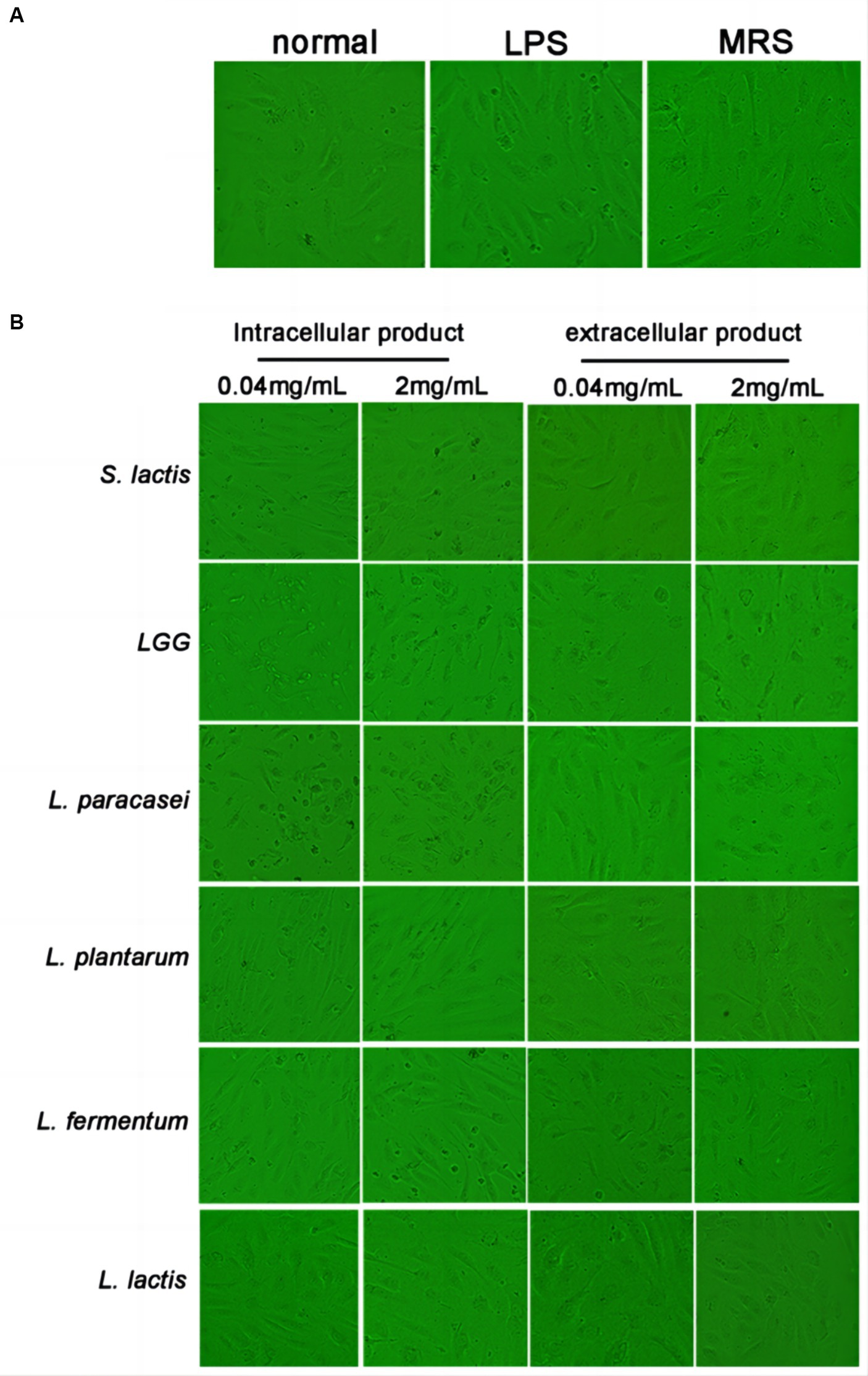
Figure 1. Effect of probiotic extracellular and intracellular products on the morphology of HUVEC. (A) The cell morphology was normal group, LPS group and MRS group. (B) Cell morphology of S. lactis, LGG, L. paracasei, L. plantarum, L. fermentum and L. lactis groups, respectively.
3.2 Cell viability assessment
MTT assay was used to investigate the cell viability. Cells were treated with the intracellular or extracellular products of S. lactis, L. plantarum, L. fermentium or L. lactis at 1 mg/mL, 2 mg/mL and 4 mg/mL for 24 h or 48 h. There were no differences between the intracellular products treated groups and normal group (Supplementary Figure S1). But after cells were treated with extracellular products of S. lactis, L. plantarum, L. fermentium or L. lactis for 48 h, cell viability was decreased (Supplementary Figure S2). Thus, the extracellular products greater than 4 mg/mL might be cytotoxic to HUVECs.
3.3 Effects of intracellular and extracellular products of probiotics on TNF-α, IL-6 and IL-8 levels in HUVECs
HUVECs were pretreated with 2 μg/mL, 40 μg/mL or 800 μg/mL intracellular or extracellular products of S. lactis, L. plantarum, L. fermentium or L. lactis for 12 h, 24 h or 48 h. Then cells were cultured with 1 μg/mL LPS for 12 h. The levels of inflammatory factors in cell supernatant were detected. The results showed that the intracellular products of these four probiotics could not inhibit the increased levels of IL-6 and IL-8 induced by the LPS treatment (Supplementary Figures S3, S4). But, after the cells were treated with extracellular products of L. fermentium or L. lactis (40 μg/mL and 800 μg/mL) for 24 h or 48 h, the LPS-induced levels of IL-6, IL-8 and TNF-α were reduced significantly (Figures 2, 3; Supplementary Figure S5). These data suggested that pretreatment of cells with extracellular products of L. fermentium or L. lactis for 24 h or 48 h inhibited the levels of inflammatory cytokines induced by LPS. Thus, L. lactis was selected for the further experiments in vivo.
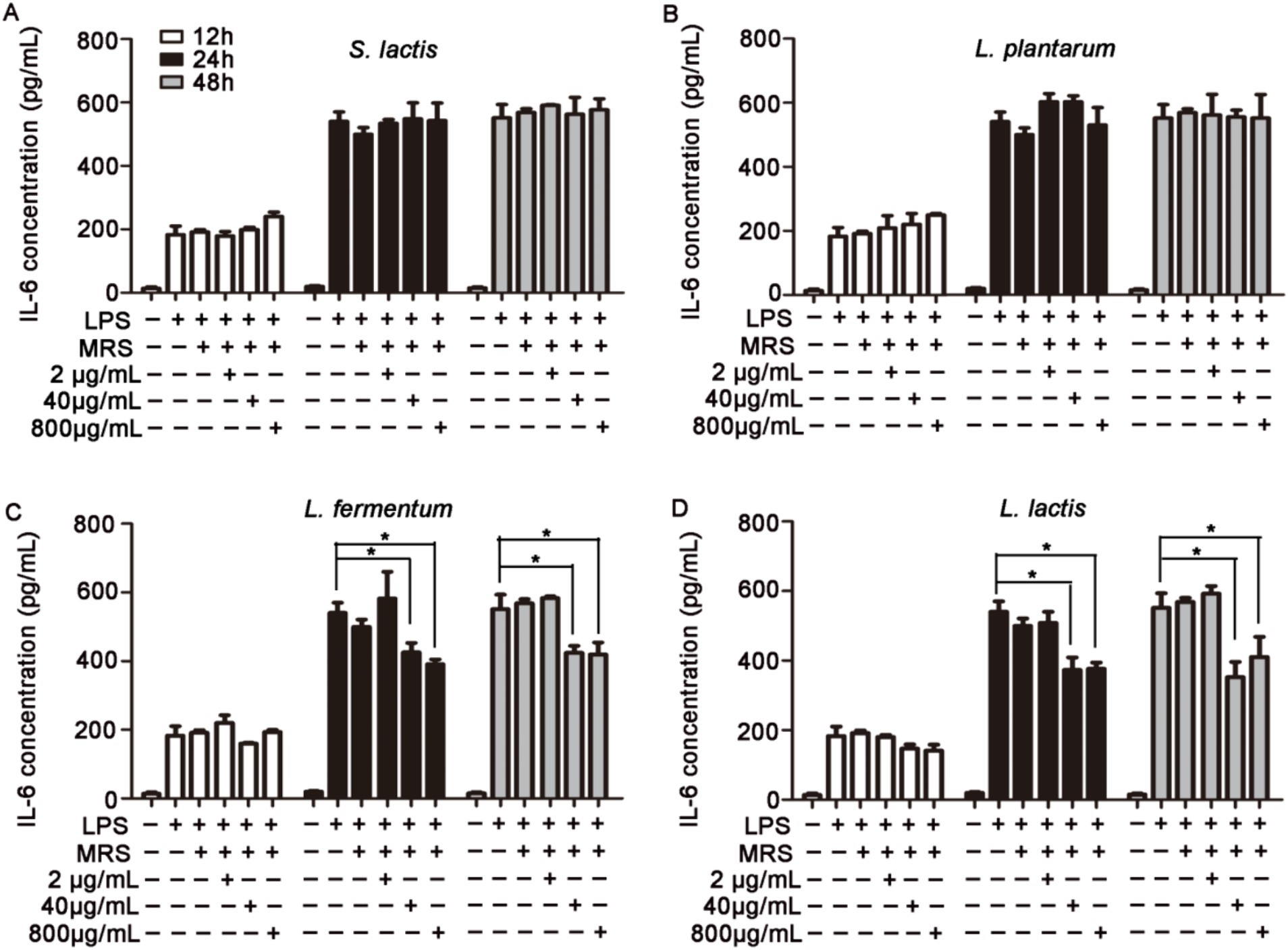
Figure 2. Effects of extracellular products of probiotics on TNF-α, IL-6, and IL-8 levels in HUVECs. (A) Extracellular products of S. lactis did not inhibit the LPS treatment-induced elevation of IL-6 levels. (B) Extracellular products of L. plantarum did not inhibit the LPS treatment-induced elevation of IL-6 levels. (C) Extracellular products of L. fermentum (40 μg/mL and 800 μg/mL) significantly reduced IL-6 levels induced by LPS treatment. (D) Extracellular products of L. lactis extracellular products (40 μg/mL and 800 μg/mL) significantly reduced IL-6 levels induced by LPS treatment (*p < 0.05).
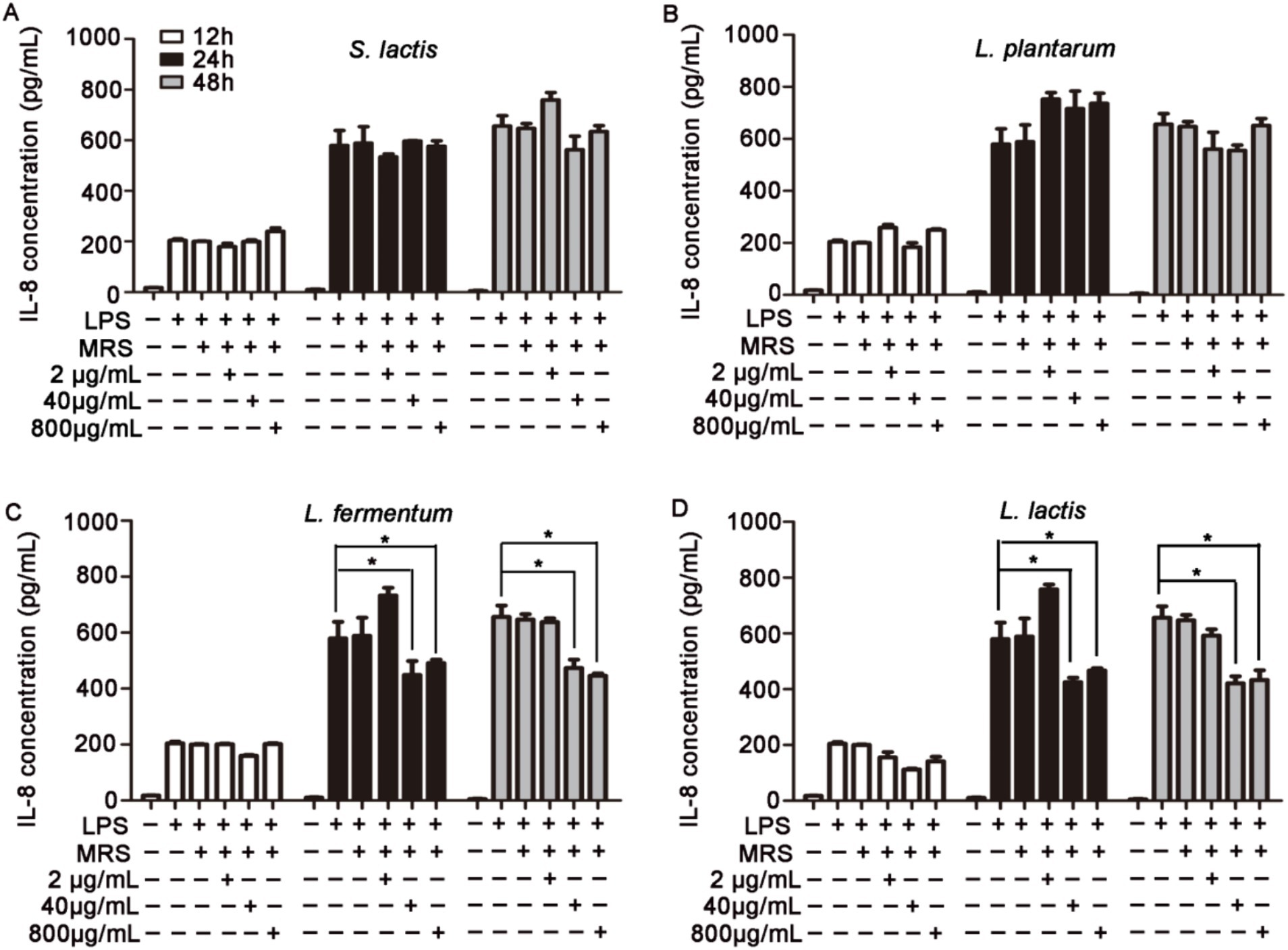
Figure 3. Effects of extracellular products of probiotics on TNF-α, IL-6, and IL-8 levels in HUVECs. (A) Extracellular products of S. lactis did not inhibit the LPS treatment-induced elevation of IL-8 levels. (B) Extracellular products of L. plantarum did not inhibit the LPS treatment-induced elevation of IL-8 levels. (C) Extracellular products of L. fermentum (40 μg/mL and 800 μg/mL) significantly reduced IL-8 levels induced by LPS treatment. (D) Extracellular products of L. lactis extracellular products (40 μg/mL and 800 μg/mL) significantly reduced IL-8 levels induced by LPS treatment (*p < 0.05).
3.4 Effects of extracellular products of Lactococcus lactis on the mortality rate of mice
After 7 days treatment with MRS medium or extracellular products of L. lactis, the mice were intraperitoneally injected with 20 mg/kg LPS to establish the sepsis model. Firstly, the mortality rate of mice was observed. The mortality rate in the Mock group was 0%. The mortality rate increased to 82% in the LPS group. The MRS medium could not inhibit the mortality rate induced by LPS. But after pretreated with 0.2 g/kg or 0.4 g/kg extracellular products of L. lactis, the mortality of mice was decreased significantly (Supplementary Figure S6). Thus, the mortality of sepsis might be inhibited by extracellular products of L. lactis pretreatment.
3.5 The effects on the levels of TNF-α and IL-6 in the serum of mice
Blood samples were collected at 6 h, 12 h or 24 h after LPS injection to detect the levels of TNF-α and IL-6 in serum. After pretreatment with extracellular products of L. lactis, the increased IL-6 and TNF-α levels induced by LPS in the serum of mice was significantly decreased compared with those in the LPS + MRS groups. And the high concentration showed the best effects (Figure 4). These results suggested that the extracellular products of L. lactis could inhibit the levels of IL-6 and TNF-α in the serum of mice induced by LPS.
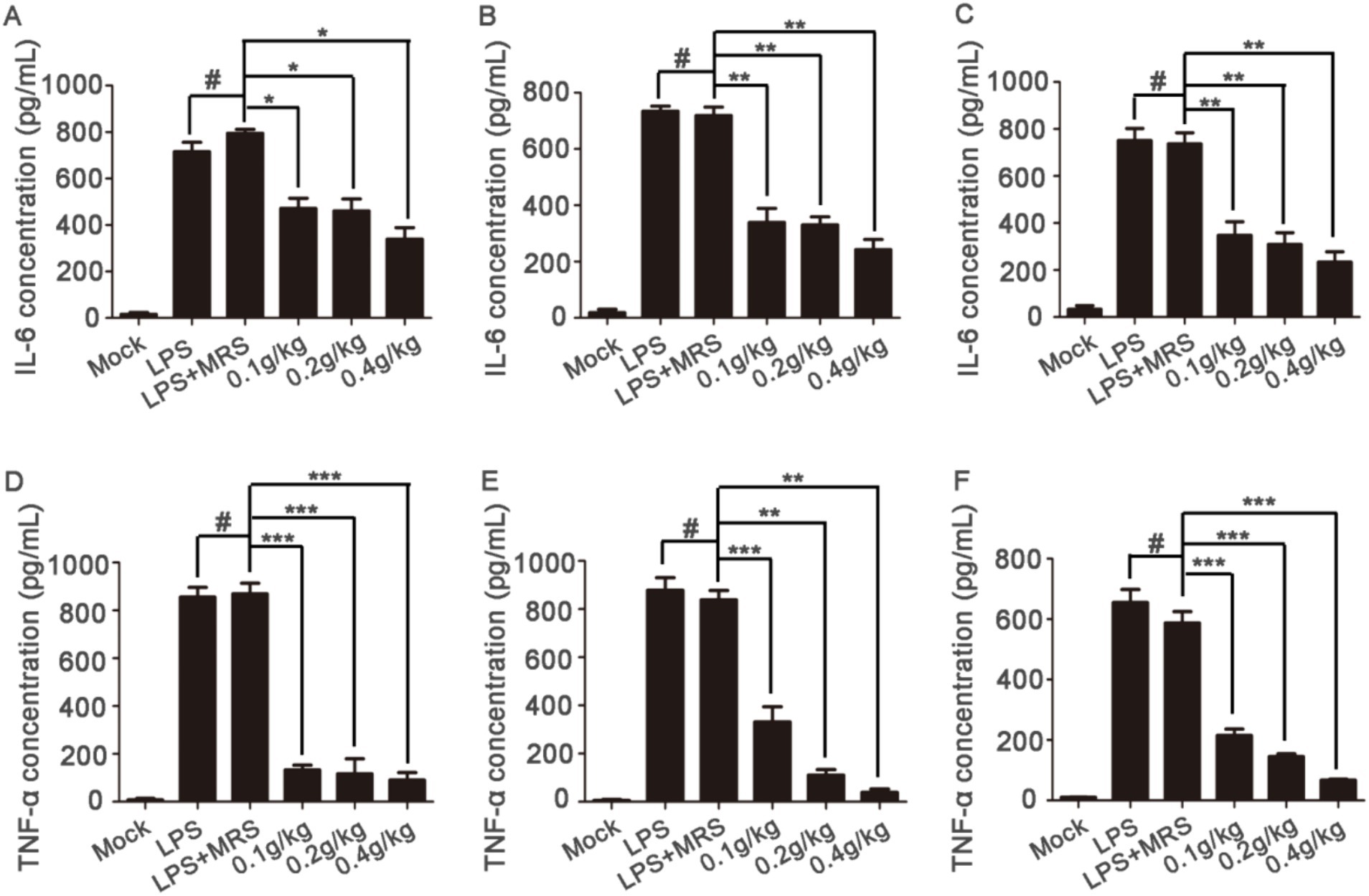
Figure 4. Serum levels of inflammatory factors in mice Serum levels of IL-6 were detected after blood collection at 6 h, 12 h, and 24 h after LPS injection (A–C); serum levels of TNF-α were detected after blood collection at 6 h, 12 h, and 24 h after LPS injection (D–F) (*p < 0.05, **p < 0.01, ***p < 0.001) (“0.1 g/kg” is the abbreviation of “LPS + 0.1 g/kg extracellular products of L. lactis,” “0.2 g/kg” is the abbreviation of “LPS + 0.2 g/kg extracellular products of L. lactis,” “0.4 g/kg” is the abbreviation of “LPS + 0.4 g/kg extracellular products of L. lactis”).
3.6 Organ coefficient and lung index
Lung lesion is one of the main symptoms of acute sepsis in mice. The H&E staining data showed that in the Mock group, the lung tissue structure was complete, the alveolar structure was clearly visible and no inflammatory cell infiltration was observed in the alveoli. In the LPS group and LPS + MRS group, the alveolar cavity morphology was severely deformed, the alveolar wall was significantly thickened and there were a large number of inflammatory cell infiltration in the alveolar and the alveolar wall. In the three extracellular products of L. lactis treatment groups, the number of inflammatory cell infiltration in the alveoli was significantly reduced, the alveolar wall became thinner and the shape of the alveolar cavity gradually recovered. With the increased concentration, the recovery of lung injury gradually improved. In the high concentration group (0.4 g/kg), the morphology was similar to that in the Mock group (Figure 5A).
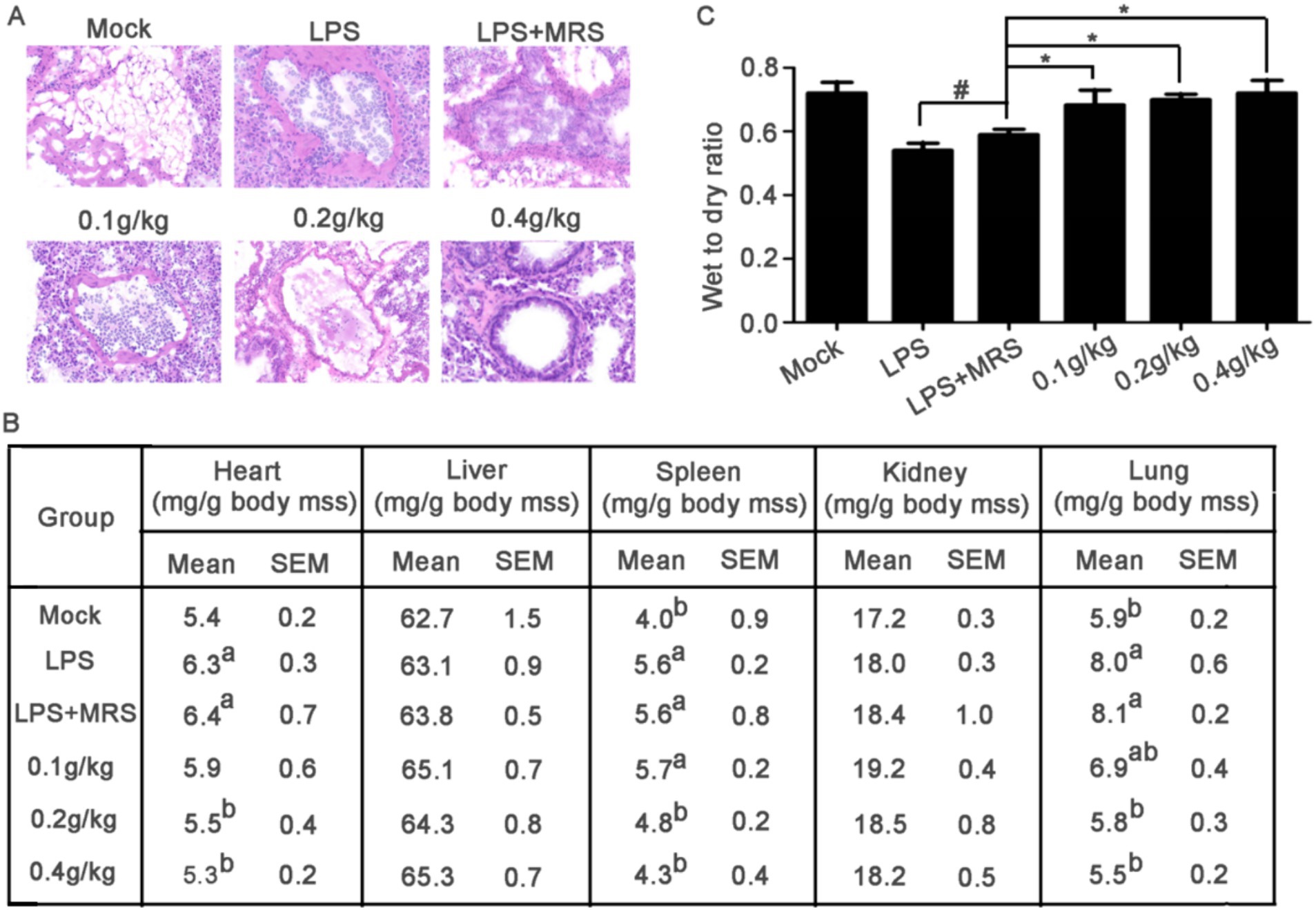
Figure 5. Mouse sections and organ coefficients. (A) Pathological changes of lung tissue in mice stained by HE. (B) Comparison of heart, liver, spleen, kidney, and lung coefficients among different groups of mice (a compared with the mock group p < 0.05; b compared with LPS group, p < 0.05). (C) Assessment of pulmonary edema by evaluating the wet-to-dry ratio of mouse lungs (*p < 0.05).
After LPS stimulation, the heart, spleen and lung coefficients were significantly increased compared with that in Mock group. All these three organ coefficients in LPS + MRS groups were significantly increased compared with those in Mock groups, but there were no significant differences with LPS groups, indicating that MRS pretreatment could not change the increased heart, spleen, and lung coefficients induced by LPS. Compared with LPS + MRS Group, the heart, spleen, and lung coefficients in L. lactis extracellular products treated groups were significantly decreased, suggesting that pretreated with L. lactis extracellular products could effectively prevent the heart, spleen, and lung damage induced by LPS (Figure 5B).
Pulmonary edema is an important phenomenon of sepsis in mice. The results showed that the wet to dry ratio of lung was significantly decreased after LPS stimulation in mice, indicating that 20 mg/kg LPS stimulation could significantly cause the symptoms of pulmonary edema in mice. There was no significant difference in the wet to dry ratio between the LPS + MRS group and the LPS group, indicating that MRS could not improve the symptoms of pulmonary edema. Compared with LPS or LPS + MRS group, not only the lung coefficient in the low/medium/high dose groups was significantly decreased, but also the lung wet to dry ratio was significantly decreased which indicated that the L. lactis extracellular products could effectively improve the symptoms of pulmonary edema induced by LPS (Figure 5C).
3.7 Enrichment analysis of the gene ontology (GO) terms of the DEGs functional
To find the mechanisms that L. lactis extracellular products contribute to the sepsis prevention, we analyzed extracellular products of L. lactis-induced changes in the HUVEC gene expression profile treated with LPS by using Genome Array. Compared to normal and LPS treated HUVECs, 981 genes had more than 2-fold changes in HUVECs, including 420 genes up-regulated and 561 genes down-regulated (Supplementary Figure S7A). Compared to LPS and sample treated HUVECs, 50 genes had more than 2-fold changes in HUVECs, including 14 genes up-regulated and 36 genes down-regulated (Supplementary Figure S7B).
GO enrichment analysis was performed for DEGs. The GO enrichment histogram directly showed the quantity distribution of different expressed genes in biological processes, cell components and molecular functions (Figure 6). Compared with the normal group, most DEGs in the LPS group were related to cellular process and metabolic process in the biological processes category. In the cellular component category, most are related to the cell, cell part and organelle part. In the molecular function category, most DEGs are associated with binding, catalytic activity and transcription regulator activity. Compared with the LPS group, most DEGs in the sample group were related to cellular process and metabolic process in the biological processes category. In the cellular component category, most are related to the cell, cell part and organelle part. In the molecular function category, most DEGs are associated with binding, catalytic activity and transcription regulator activity. In both the LPS group and the sample group, the functional changes of most DEGs, whether in biological processes, cell components, or molecular functions, were basically the same as those in the normal-LPS group, but the degree of enrichment was different.
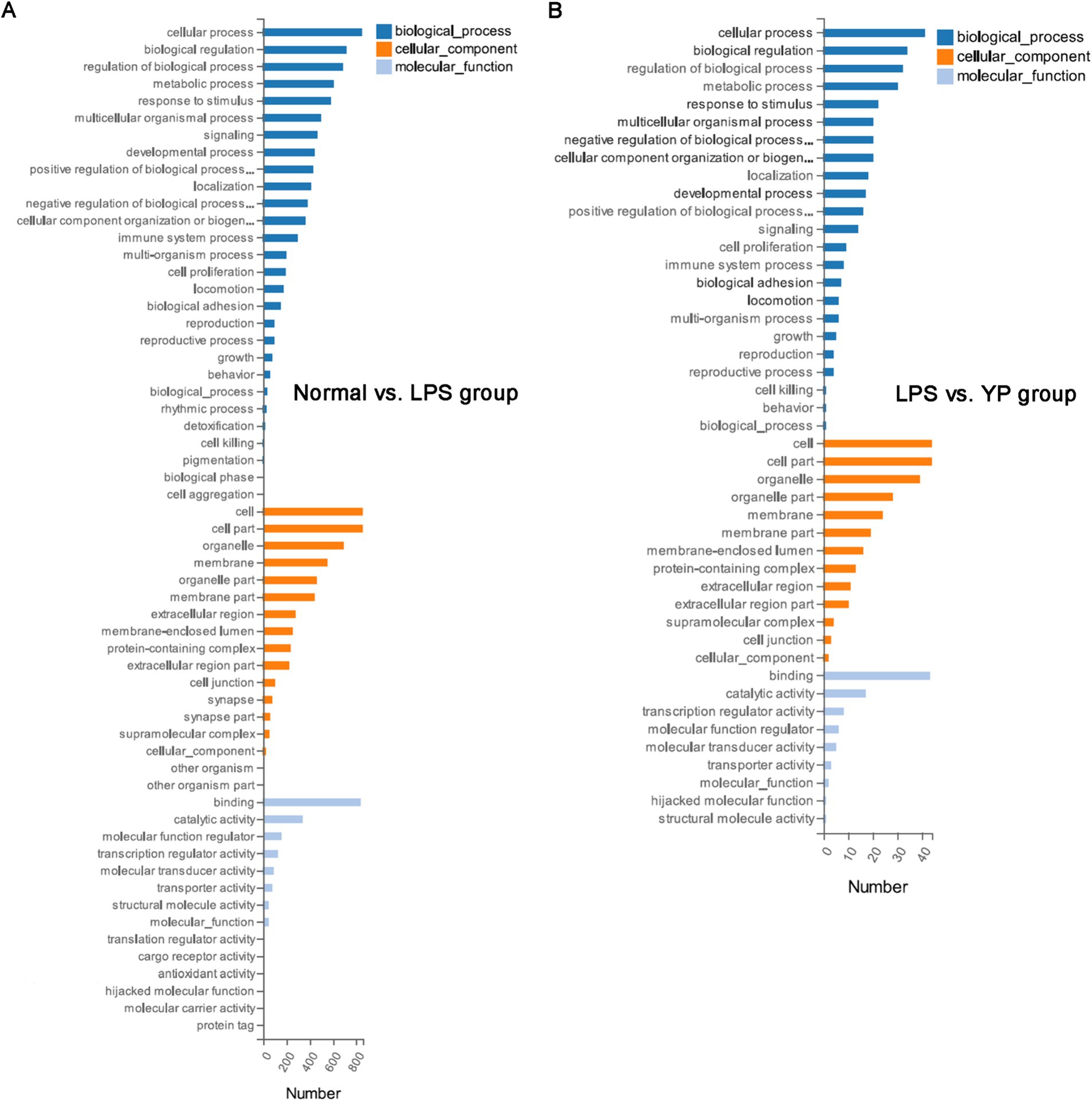
Figure 6. GO analysis of DEGs. (A) Normal group was compared with LPS group. (B) The LPS group was compared with the sample group (“YP group” means “LPS+40 μg/mL extracellular products of L. lactis”).
3.8 Kyoto encyclopedia of genes and genomes pathway analysis of DEGs
The top 20 significant pathways of DEGs were selected and represented in KEGG enrichment scatter diagram (Figure 7). In the normal-LPS group, DEGs were mainly enriched in cytokine-cytokine receptor interaction and pathways in cancer. DEGs were also enriched in cytokine—cytokine receptor interactions compared with the sample-LPS group. In the normal-LPS group, DEGs were enriched in inflammatory factor-related pathways, such as TNF signaling pathway and IL-17 signaling pathway, but in the sample-LPS group, no inflammatory factor-related pathway genes were enriched.
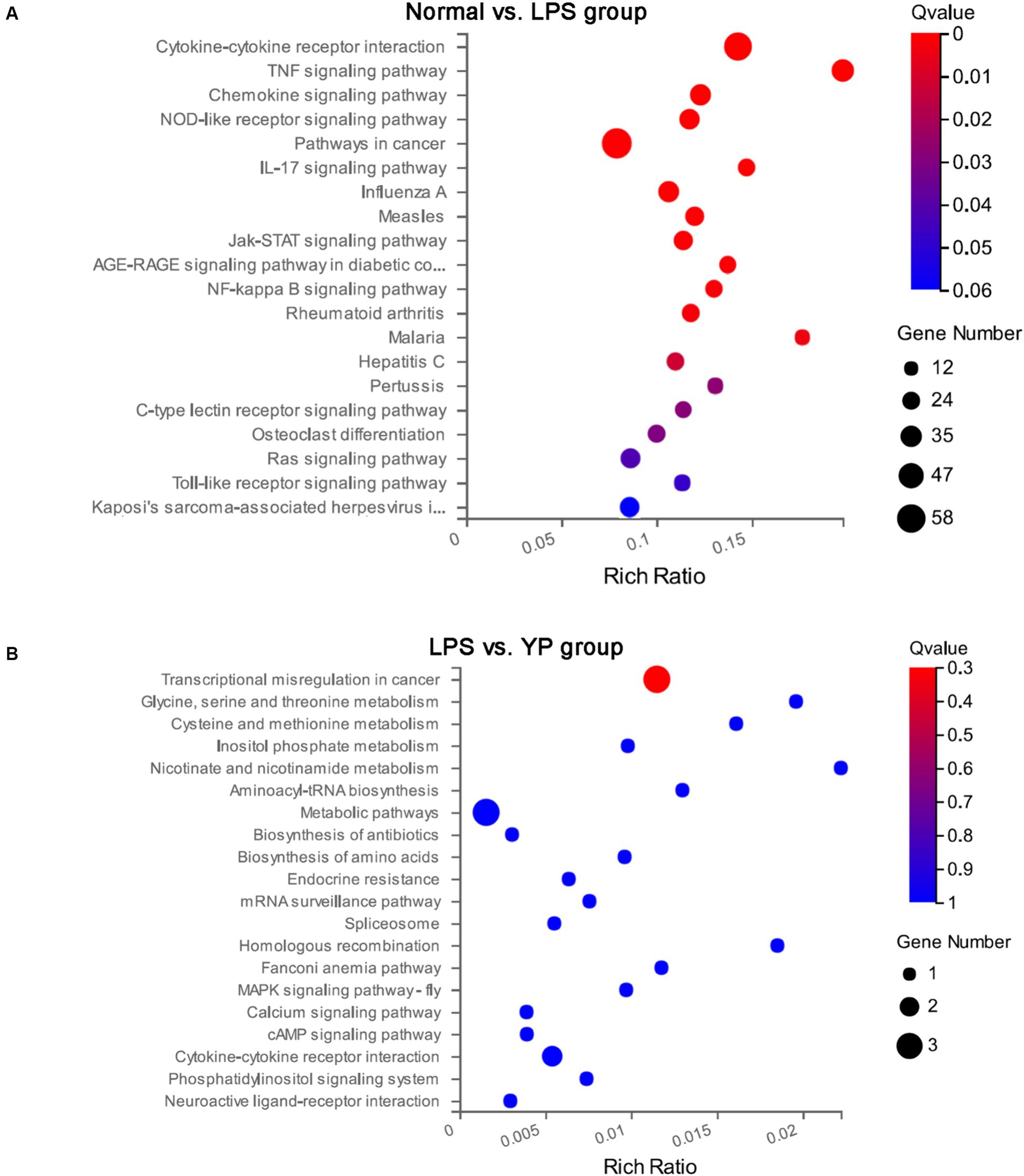
Figure 7. Kyoto Encyclopedia of Genes and Genomes (KEGG) pathway analysis of DEGs. (A) Normal group was compared with LPS group. (B) The LPS group was compared with the sample group.
4 Discussion
Sepsis is a clinical syndrome characterized by systematic inflammation and circulatory malfunctions following the pathogenic infection like bacteremia or fungemia. It is one of the most common causes of morbidity and mortality in critically ill patients (Yoon, 2012) and preterm infants. In addition, sepsis is associated with significant morbidity and reduced quality of life among sepsis survivors (Oud, 2014). Probiotics have been explored in an exponentially increasing number of clinical trials for their health effects (Jansen et al., 2021; DeVeaux et al., 2023). The current reports suggested that the probiotics use is associated with a 36.9% reduction in the incidence of postoperative sepsis (Arumugam et al., 2016). Since supplementation of probiotics significantly reduces the incidence of postoperative sepsis without significant negative side effects, these probiotic supplements may be considered as an effective and potentially routine treatment for patients undergoing gastrointestinal surgery (Kim et al., 2019; Potruch et al., 2022). Probiotic translocation might result in some clinical infections, including systemic and localized infections (María Remes-Troche et al., 2020). Predominantly, infections associated with specific probiotics include sepsis. The ingestion of live microorganisms (probiotics) in rare instances might surplus the risk of sepsis as evidenced by several studies, raising the safety concern of routine probiotic use in such population. Nevertheless, growing pieces of evidence support the beneficial role of probiotics in critically ill and neonates (Litao et al., 2018). Maintenance or restoration of intestinal microbiota and metabolite composition may be a therapeutic or prophylactic target for sepsis (Potruch et al., 2022). Our results suggested that Lactococcus Lactis metabolites could reduce inflammatory factors in cells and blood of mice which might be useful to prevent sepsis.
The endothelial cell lining (ECL) of the vasculature is a unique cellular system that coats the inside of blood vessels and forms the interface between the circulating blood and the parenchymal cells responsible for organ function (Kim et al., 2013). It is critical for the regulation of hemostasis, vasomotor control, and immunological function, by sensing and reaction through secretion of molecules, which initiate transcellular and intracellular signaling (Cheng and Huang, 2021). In addition to these important functions, the endothelium forms the essential vascular barrier for solute transport and osmotic balance. Sepsis is associated with severe endothelial cell (EC) dysfunction leading to dysregulation of hemostasis and vascular reactivity, as well as tissue edema (Dalan et al., 2017). This failure of the ECL is considered central to the progression to organ failure during sepsis (Beltrán-García et al., 2022). The glycocalyx is a gel-like layer lining the luminal surface of endothelial cells, the degradation of glycocalyx is also thought to contribute to microcirculatory dysfunction in sepsis (Wu et al., 2017). Many preclinical and clinical studies have demonstrated an association between inflammatory cytokines such as TNF-α, IL-1β, IL-6, and IL-10 and glycocalyx degradation biomarkers (Gao et al., 2018; Tamura et al., 2020). Our results showed that LPS-induced levels of IL-6, IL-8, and TNF-α were significantly decreased after treatment with extracellular products of L. fermentium or L. lactis (40 μg/mL and 800 μg/mL) for 24 or 48 h. These data indicated that the extracellular products of L. fermentium or L. lactis inhibited LPS-induced inflammatory cytokine levels.
Our results showed that the extracellular products of L. fermentium or L. lactis inhibited LPS-induced inflammatory cytokine levels. The function of extracellular products of L. fermentium has been published before (Wang et al., 2023). We tested the preventative effects of extracellular products of L. lactis using an LPS-induced mouse sepsis model. The lungs have been shown to be the most susceptible organ to sepsis, and protecting the lungs from inflammation is considered a promising strategy for treating sepsis (Wang Z. F. et al., 2020; Ling et al., 2023). Histopathological analysis showed that extracellular products of L. lactis relieved inflammatory cell infiltration and pulmonary edema in lungs treated with LPS. Additionally, it improved survival rate and inhibited serum proinflammatory cytokine levels in mice. This suggested that extracellular products of L. lactis could improve LPS-induced septicemia by reducing the severity of systemic inflammation in lung.
Because the pathogenesis and treatment of sepsis are still unclear, clinical treatment brings great burden to society and patients. The search for potential biomarkers is important for future sepsis treatment and prognosis. Studies have shown that the host response of sepsis does not conform to the simple high/low inflammation model (Jones et al., 2022).
Mitochondria are not only key regulators of metabolite biosynthesis, but also play important roles in many other cellular functions, such as calcium homeostasis, hormone metabolism, thermoregulation, production of reactive oxygen species and nitrogen, and cellular signaling (Ciron et al., 2012; Sharma et al., 2018). GO analysis showed that most of the DGEs were enriched in the cellular structure and metabolic processes in both the normal-LPS group and the LPS-sample group. It has been reported that sepsis easily mitochondrial damage and impaired energy metabolism in humans, which may be an important part of sepsis leading to multiple organ dysfunction syndrome (Liu et al., 2023; Yang et al., 2023). Therefore, resuscitating metabolism and restoring cellular structure through the mitochondrial pathway would be a new cornerstone of sepsis therapy.
GO analysis of DEGs showed that the functional changes of DEGs in the normal-LPS group all included the functional changes of DEGs in the lPS-sample group, indicating that the 50 significant genes in the lPS-sample group were most likely contained in the 981 significant genes in the LPS-sample group. It is highly likely that the sample can prevent sepsis by regulating these 50 significant genes. Interestingly, other articles have found that regulating cytokines and genes associated with immune responses to LPS can reduce the symptoms of LPS-induced sepsis in mice, which is consistent with our study. in addition, in KEGG analysis, samples can affect Transcriptional misregulation in cancer related genes (Rosier et al., 2022; Chen et al., 2024) Our study showed that samples can reduce the secretion of pro-inflammatory cytokines in sepsis mice. Whether it is related to Transcriptional misregulation in cancer related genes still needs to be further explored (Rawat et al., 2021).
Granulocyte-macrophage colony-stimulating factor (GM-CSF), a hematopoietic growth factor, is currently used in patients with neutropenia induced by myelosuppression in chemotherapy to counteract the immunosuppression of sepsis (Mathias et al., 2015; Venet and Monneret, 2017). Lipopolysaccharides play a crucial role in activating the immune system, in particular by acting on systemic immune-activated cells to stimulate the production of pro-inflammatory cytokines, and the investigated Immunostimulatory therapies alone include GM-CSF, and although it has been shown to improve clinical indicators, pro-inflammatory therapies aimed at preventing sepsis are currently being investigated (Maldonado et al., 2016; Hall, 2019). Meanwhile, lipopolysaccharides are able to activate neutrophils in vitro, as evidenced by elevated levels of TNF-α, and enhance proliferation (Mohsin et al., 2021). Our results showed that DEGs were mainly enriched in cytokine-cytokine receptor interactions in both normal-LPS and sample-LPS groups. It can be hypothesized that our samples might have an effect on sepsis through cytokine-cytokine receptor interactions, which implies that new ideas can be provided in deeper explorations in immunosuppression. However, to date, no comprehensive systematic analysis has been performed to study the complex interactions between cytokine-cytokine receptors, and this type of analysis may be needed to identify more effective therapeutic targets.
In addition, the data showed that DEGs were also associated with transcriptional dysregulation responses and inflammatory factor signaling pathways in cancer in both the normal-LPS and sample-LPS groups, which could be linked to what we talked about before, and the subtle combination of these data might play a role in the development of stress-induced immune responses, which would need to be further verified.
In summary, we finally determined that the extracellular product of L. lactis reduced inflammatory factors secreted by LPS-pretreated HUVECs, alleviated inflammatory cell infiltration and pulmonary edema in the lungs of septic mice, and improved the survival rate of mice, suppressed the expression level of pro-inflammatory factors within serum, and the genetic analysis preliminarily explored the pathologic mechanism, demonstrating that the extracellular product of Lactobacillus lactis could prevent lipopolysaccharide induced sepsis and can potentially provide new insights into the prevention of sepsis at the molecular level.
5 Conclusion
We reported that the extracellular product of L. lactis can effectively prevent sepsis in animal and cellular experiments, which provides more ideas for sepsis prevention methods, and more in-depth studies are needed to further explore the mechanism of protective effects of the extracellular product of L. lactis against LPS-induced sepsis.
Data availability statement
The datasets presented in this study can be found in online repositories. The names of the repository/repositories and accession number(s) can be found at: https://www.ncbi.nlm.nih.gov/, 12.
Ethics statement
The animal study was approved by all animal experimental procedures were in accordance with the laws and regulations of the People’s Republic of China and the ethical guidelines. At the same time, the procedure was carried out in accordance with the ethical guidelines and the guide for the care and use of laboratory animals issued by the National Institutes of Health. This study was approved by the Ethics Committee for Animal Experiments of Qilu University of Technology (Jinan, China). The study was conducted in accordance with the local legislation and institutional requirements.
Author contributions
YF: Writing – original draft, Writing – review & editing, Data curation, Formal analysis. SZ: Data curation, Funding acquisition, Writing – original draft. QY: Methodology, Project administration, Writing – original draft. ZA: Resources, Supervision, Writing – original draft. MZ: Validation, Visualization, Writing – original draft. CZ: Investigation, Software, Writing – original draft. XS: Investigation, Writing – review & editing. KL: Writing – review & editing, Software. BL: Visualization, Writing–review & editing. LZ: Supervision, Writing – review & editing. LS: Software, Writing–review & editing.
Funding
The author(s) declare financial support was received for the research, authorship, and/or publication of this article. This work was supported by Technological Small and Medium Sized Enterprise Innovation Ability Enhancement Project (2023TSGC0169, 2022TSGC1076, 2023TSGC0167), Qilu University of Science, Education and Industry Integration Innovation Pilot Project (grant number 2023RCKY210), The Central Government Guides Local Science and Technology Development Special Fund Project (no. YDZX2022181), Key innovation Project of Qilu University of Technology (Shandong Academy of Sciences) (2022JBZ01-06), the XPCC Financial Science and Technology Project in 2022 (no. 2022AB002). Qilu University of Science, Education and Industry Integration Innovation Pilot Project (grant number 2023rcky210).
Conflict of interest
CZ, BL, and LS were employed by Shandong Baoyuan Biotechnology Co., Ltd. KL and LZ were employed by Shandong Chenzhang Biotechnology Co., Ltd. LS was employed by Shengsheng Xiangrong Biotechnology (Shandong) Co., Ltd.
The remaining authors declare that the research was conducted in the absence of any commercial or financial relationships that could be construed as a potential conflict of interest.
Publisher’s note
All claims expressed in this article are solely those of the authors and do not necessarily represent those of their affiliated organizations, or those of the publisher, the editors and the reviewers. Any product that may be evaluated in this article, or claim that may be made by its manufacturer, is not guaranteed or endorsed by the publisher.
Supplementary material
The Supplementary material for this article can be found online at: https://www.frontiersin.org/articles/10.3389/fmicb.2024.1404652/full#supplementary-material
Footnotes
References
Ahn, S. H., Yoon, W., Lee, S. Y., Shin, H. S., Lim, M. Y., Nam, Y. D., et al. (2020). Effects ofLactobacillus pentosusin children with allergen-sensitized atopic dermatitis. J. Korean Med. Sci. 35:e128. doi: 10.3346/jkms.2020.35.e128
Aljerian, N., et al. (2019). Does being transported by emergency medical services improve compliance with the surviving Sepsis bundle and mortality rate? A retrospective cohort study. J. Epidemiol. Glob. Health 10:276. doi: 10.2991/jegh.k.191215.001
Arumugam, S., Lau, C. S. M., and Chamberlain, R. S. (2016). Probiotics and Synbiotics decrease postoperative Sepsis in elective gastrointestinal surgical patients: a Meta-analysis. J. Gastrointest. Surg. 20, 1123–1131. doi: 10.1007/s11605-016-3142-y
Beltrán-García, J., Osca-Verdegal, R., Pérez-Cremades, D., Novella, S., Hermenegildo, C., Pallardó, F. V., et al. (2022). Extracellular histones activate endothelial NLRP3 Inflammasome and are associated with a severe Sepsis phenotype. J. Inflamm. Res. 15, 4217–4238. doi: 10.2147/JIR.S363693
Benjak Horvat, I., Gobin, I., Kresović, A., and Hauser, G. (2021). How can probiotic improve irritable bowel syndrome symptoms? World J. Gastroint. Surg. 13, 923–940. doi: 10.4240/wjgs.v13.i9.923
Bogner, J. R., Kutaiman, A., Esguerra-Alcalen, M., Heldner, S., and Arvis, P. (2013). Moxifloxacin in complicated skin and skin structure infections (cSSSIs): a prospective, international, non-interventional, observational study. Adv. Ther. 30, 630–643. doi: 10.1007/s12325-013-0038-z
Chen, Z., Su, Y., Ding, J., He, J., Lai, L., and Song, Y. (2024). Lobetyolin protects mice against LPS-induced sepsis by downregulating the production of inflammatory cytokines in macrophage. Front. Pharmacol. 15:1405163. doi: 10.3389/fphar.2024.1405163
Cheng, C. K., and Huang, Y. (2021). The gut-cardiovascular connection: new era for cardiovascular therapy. Med. Rev. 1, 23–46. doi: 10.1515/mr-2021-0002
Ciron, C., Lengacher, S., Dusonchet, J., Aebischer, P., and Schneider, B. L. (2012). Sustained expression of PGC-1 in the rat nigrostriatal system selectively impairs dopaminergic function. Hum. Mol. Genet. 21, 1861–1876. doi: 10.1093/hmg/ddr618
Colomina-Climent, F., Giménez-Esparza, C., Portillo-Requena, C., Allegue-Gallego, J. M., Galindo-Martínez, M., Mollà-Jiménez, C., et al. (2016). Mortality reduction in septic shock by plasma adsorption (ROMPA): a protocol for a randomised clinical trial. BMJ Open 6:e011856. doi: 10.1136/bmjopen-2016-011856
Dalan, R., Liu, X., Goh, L. L., Bing, S., and Luo, K. Q. (2017). Endothelial cell apoptosis correlates with low haptoglobin concentrations in diabetes. Diab. Vascul. Dis. Res. 14, 534–539. doi: 10.1177/1479164117719827
Deng, F., Hu, J. J., Lin, Z. B., Sun, Q. S., Min, Y., Zhao, B. C., et al. (2023). Gut microbe-derived milnacipran enhances tolerance to gut ischemia/reperfusion injury. Cell Rep. Med. 4:100979. doi: 10.1016/j.xcrm.2023.100979
DeVeaux, A., Ryou, J., Dantas, G., Warner, B. B., and Tarr, P. I. (2023). Microbiome-targeting therapies in the neonatal intensive care unit: safety and efficacy. Gut Microbes 15:2221758. doi: 10.1080/19490976.2023.2221758
Fu, X., Liu, Z., and Wang, Y. (2023). Advances in the study of immunosuppressive mechanisms in Sepsis. J. Inflamm. Res. 16, 3967–3981. doi: 10.2147/JIR.S426007
Gao, P., Zhao, Z., Zhang, C., Wang, C., Long, K., Guo, L., et al. (2018). The therapeutic effects of traditional Chinese medicine Fusu agent in LPS-induced acute lung injury model rats. Drug Des. Devel. Ther. 12, 3867–3878. doi: 10.2147/DDDT.S181798
Hall, M. (2019). Immune modulation in pediatric Sepsis. J. Pediatr. Intens. Care 8, 042–050. doi: 10.1055/s-0038-1676607
Hu, Q., Ren, H., Li, G., Wang, D., Zhou, Q., Wu, J., et al. (2019). STING-mediated intestinal barrier dysfunction contributes to lethal sepsis. EBioMedicine 41, 497–508. doi: 10.1016/j.ebiom.2019.02.055
Jansen, S. J., Lopriore, E., van der Beek, M. T., Veldkamp, K. E., Steggerda, S. J., and Bekker, V. (2021). The road to zero nosocomial infections in neonates—a narrative review. Acta Paediatr. 110, 2326–2335. doi: 10.1111/apa.15886
Jiang, K., Wang, D., Su, L., Liu, X., Yue, Q., Li, B., et al. (2022). Structural characteristics of locust bean gum hydrolysate and its alleviating effect on dextran sulfate sodium-induced colitis. Front. Microbiol. 13:985725. doi: 10.3389/fmicb.2022.985725
Jiang, L., Wang, Z., Wang, L., Liu, Y., Chen, D., Zhang, D., et al. (2022). Predictive value of the serum anion gap for 28-day in-hospital all-cause mortality in sepsis patients with acute kidney injury: a retrospective analysis of the MIMIC-IV database. Ann. Transl. Med. 10:1373. doi: 10.21037/atm-22-5916
Jones, T. K., Reilly, J. P., Anderson, B. J., Miano, T. A., Dunn, T. G., Weisman, A. R., et al. (2022). Elevated plasma levels of matrix Metalloproteinase-3 and tissue-inhibitor of matrix Metalloproteinases-1 associate with organ dysfunction and mortality in Sepsis. Shock 57, 41–47. doi: 10.1097/SHK.0000000000001833
Kahveci, U., Ozkan, S., Melekoglu, A., Usul, E., Ozturk, G., Cetin, E., et al. (2021). The role of plasma presepsin levels in determining the incidence of septic shock and mortality in patients with sepsis. J. Infect. Dev. Countries 15, 123–130. doi: 10.3855/jidc.12963
Kim, S. J., Chung, J. W., Woo, H. S., Kim, S. Y., Kim, J. H., Kim, Y. J., et al. (2019). Two-week bismuth-containing quadruple therapy and concomitant therapy are effective first-line treatments for Helicobacter pylori eradication: a prospective open-label randomized trial. World J. Gastroenterol. 25, 6790–6798. doi: 10.3748/wjg.v25.i46.6790
Kim, Y.-M., Kim, J. H., Kwon, H. M., Lee, D. H., Won, M. H., Kwon, Y. G., et al. (2013). Korean red ginseng protects endothelial cells from serum-deprived apoptosis by regulating Bcl-2 family protein dynamics and caspase S-nitrosylation. J. Ginseng Res. 37, 413–424. doi: 10.5142/jgr.2013.37.413
Ling, J., Yu, S., Xiong, F., Xu, T., and Li, S. (2023). Melatonin attenuates Sepsis-induced acute lung injury via inhibiting excessive Mitophagy. Drug Des. Devel. Ther. 17, 2775–2786. doi: 10.2147/DDDT.S423264
Litao, G., Jingjing, S., Yu, L., Lei, Z., Xiaona, H., and Zhijing, Z. (2018). Risk factors for antibiotic-associated diarrhea in critically ill patients. Med. Sci. Monit. 24, 5000–5007. doi: 10.12659/MSM.911308
Liu, W., Liu, T., Zheng, Y., and Xia, Z. (2023). Metabolic reprogramming and its regulatory mechanism in Sepsis-mediated inflammation. J. Inflamm. Res. 16, 1195–1207. doi: 10.2147/JIR.S403778
Liu, S., Yao, C., Zhang, J., Yang, Y., and Qiu, H.EXIT-SEP Investigators (2019). Efficacy of Xuebijing injection for Sepsis (EXIT-SEP): protocol for a randomised controlled trial. BMJ Open 9:e028664. doi: 10.1136/bmjopen-2018-028664
Maldonado, R. F., Sá-Correia, I., and Valvano, M. A. (2016). Lipopolysaccharide modification in gram-negative bacteria during chronic infection. FEMS Microbiol. Rev. 40, 480–493. doi: 10.1093/femsre/fuw007
María Remes-Troche, J., Coss-Adame, E., Ángel Valdovinos-Díaz, M., Gómez-Escudero, O., Eugenia Icaza-Chávez, M., Antonio Chávez-Barrera, J., et al. (2020). Lactobacillus acidophilus LB: a useful pharmabiotic for the treatment of digestive disorders. Ther. Adv. Gastroenterol. 13:175628482097120. doi: 10.1177/1756284820971201
Markwart, R., Saito, H., Harder, T., Tomczyk, S., Cassini, A., Fleischmann-Struzek, C., et al. (2020). Epidemiology and burden of sepsis acquired in hospitals and intensive care units: a systematic review and meta-analysis. Intensive Care Med. 46, 1536–1551. doi: 10.1007/s00134-020-06106-2
Mathias, B., Szpila, B. E., Moore, F. A., Efron, P. A., and Moldawer, L. L. (2015). A review of GM-CSF therapy in Sepsis. Medicine 94:e2044. doi: 10.1097/MD.0000000000002044
Messina, A. P., van den Bergh, D., and Goff, D. A. (2015). Antimicrobial stewardship with pharmacist intervention improves timeliness of antimicrobials across thirty-three hospitals in South Africa. Infect. Dis. Ther. 4, 5–14. doi: 10.1007/s40121-015-0082-x
Michaux, G., Abbitt, K. B., Collinson, L. M., Haberichter, S. L., Norman, K. E., and Cutler, D. F. (2006). The physiological function of von Willebrand's factor depends on its tubular storage in endothelial Weibel-Palade bodies. Dev. Cell 10, 223–232. doi: 10.1016/j.devcel.2005.12.012
Mohsin, M., Tabassum, G., Ahmad, S., Ali, S., and Ali Syed, M. (2021). The role of mitophagy in pulmonary sepsis. Mitochondrion 59, 63–75. doi: 10.1016/j.mito.2021.04.009
Oud, L. (2014). Pregnancy-associated severe Sepsis: contemporary state and future challenges. Infect. Dis. Ther. 3, 175–189. doi: 10.1007/s40121-014-0037-7
Potruch, A., Schwartz, A., and Ilan, Y. (2022). The role of bacterial translocation in sepsis: a new target for therapy. Ther. Adv. Gastroenterol. 15:175628482210942. doi: 10.1177/17562848221094214
Rawat, P. S., Jaiswal, A., Khurana, A., Bhatti, J. S., and Navik, U. (2021). Doxorubicin-induced cardiotoxicity: an update on the molecular mechanism and novel therapeutic strategies for effective management. Biomed. Pharmacother. 139:111708. doi: 10.1016/j.biopha.2021.111708
Rosier, F., Nuñez, N. F., Torres, M., Loriod, B., Rihet, P., and Pradel, L. C. (2022). Transcriptional response in a Sepsis mouse model reflects transcriptional response in Sepsis patients. Int. J. Mol. Sci. 23:821. doi: 10.3390/ijms23020821
Sharma, A., Liaw, K., Sharma, R., Zhang, Z., Kannan, S., and Kannan, R. M. (2018). Targeting mitochondrial dysfunction and oxidative stress in activated microglia using dendrimer-based therapeutics. Theranostics 8, 5529–5547. doi: 10.7150/thno.29039
Shin, S., Kim, Y., Jeong, S., Hong, S., Kim, I., Lee, W., et al. (2013). The therapeutic effect of human adult stem cells derived from adipose tissue in Endotoxemic rat model. Int. J. Med. Sci. 10, 8–18. doi: 10.7150/ijms.5385
Tamura, T., Sano, M., Matsuoka, T., Yoshizawa, J., Yamamoto, R., Katsumata, Y., et al. (2020). Hydrogen gas inhalation attenuates endothelial Glycocalyx damage and stabilizes hemodynamics in a rat hemorrhagic shock model. Shock 54, 377–385. doi: 10.1097/SHK.0000000000001459
van Ruler, O., Kiewiet, J. J., van Ketel, R., and Boermeester, M. A.Dutch Peritonitis Study Group (2011). Initial microbial spectrum in severe secondary peritonitis and relevance for treatment. Eur. J. Clin. Microbiol. Infect. Dis. 31, 671–682. doi: 10.1007/s10096-011-1357-0
Venet, F., and Monneret, G. (2017). Advances in the understanding and treatment of sepsis-induced immunosuppression. Nat. Rev. Nephrol. 14, 121–137. doi: 10.1038/nrneph.2017.165
Versporten, A., et al. (2018). Metrics to assess the quantity of antibiotic use in the outpatient setting: a systematic review followed by an international multidisciplinary consensus procedure. J. Antimicrob. Chemother. 73, vi59–vi66. doi: 10.1093/jac/dky119
Wang, Y.-H., Limaye, A., Liu, J. R., and Wu, T. N. (2023). Potential probiotics for regulation of the gut-lung axis to prevent or alleviate influenza in vulnerable populations. J. Tradit. Complement. Med. 13, 161–169. doi: 10.1016/j.jtcme.2022.08.004
Wang, L., Wei, J., da Fonseca Ferreira, A., Wang, H., Zhang, L., Zhang, Q., et al. (2020). Rejuvenation of senescent endothelial progenitor cells by extracellular vesicles derived from mesenchymal stromal cells. JACC 5, 1127–1141. doi: 10.1016/j.jacbts.2020.08.005
Wang, Z. F., Yang, Y. M., and Fan, H. (2020). Diagnostic value of miR-155 for acute lung injury/acute respiratory distress syndrome in patients with sepsis. J. Int. Med. Res. 48:030006052094307. doi: 10.1177/0300060520943070
Wu, X., Hu, Z., Yuan, H., Chen, L., Li, Y., and Zhao, C. (2017). Fluid resuscitation and markers of glycocalyx degradation in severe sepsis. Open Med. 12, 409–416. doi: 10.1515/med-2017-0059
Yang, H.-N., Lee, S. E., Jeong, S. I., Park, C. S., Jin, Y. H., and Park, Y. S. (2011). Up-regulation of Heme Oxygenase-1 by Korean red ginseng water extract as a Cytoprotective effect in human endothelial cells. J. Ginseng Res. 35, 352–359. doi: 10.5142/jgr.2011.35.3.352
Yang, F., Li, J., Lan, Y., Lei, Y., Zeng, F., Huang, X., et al. (2023). Potential application of ginseng in sepsis. J. Ginseng Res. 47, 353–358. doi: 10.1016/j.jgr.2022.05.003
Yoon, S. H. (2012). Concerns of the anesthesiologist: anesthetic induction in severe sepsis or septic shock patients. Korean J. Anesthesiol. 63, 3–10. doi: 10.4097/kjae.2012.63.1.3
Zhang, J., Bu, Y., Zhang, C., Yi, H., Liu, D., and Jiao, J. (2020). Development of a low-cost and high-efficiency culture medium for Bacteriocin lac-B23 production by Lactobacillus plantarum J23. Biology 9:171. doi: 10.3390/biology9070171
Keywords: sepsis, L. lactis , gut microbiota, preventive effects, chip analysis
Citation: Fu Y, Zhang S, Yue Q, An Z, Zhao M, Zhao C, Sun X, Li K, Li B, Zhao L and Su L (2024) The preventative effects of Lactococcus Lactis metabolites against LPS-induced sepsis. Front. Microbiol. 15:1404652. doi: 10.3389/fmicb.2024.1404652
Edited by:
Haifeng Zhao, South China University of Technology, ChinaReviewed by:
Jozsef Soki, University of Szeged, HungaryMarcos Edgar Herkenhoff, University of São Paulo, Brazil
Copyright © 2024 Fu, Zhang, Yue, An, Zhao, Zhao, Sun, Li, Li, Zhao and Su. This is an open-access article distributed under the terms of the Creative Commons Attribution License (CC BY). The use, distribution or reproduction in other forums is permitted, provided the original author(s) and the copyright owner(s) are credited and that the original publication in this journal is cited, in accordance with accepted academic practice. No use, distribution or reproduction is permitted which does not comply with these terms.
*Correspondence: Lin Zhao, iahb205@163.com;Le Su, sule@sdu.edu.cn