- 1Department of Marine Biotechnology, Anyang University, Incheon, Republic of Korea
- 2School of Earth and Environmental Sciences, College of Natural Sciences, Seoul National University, Seoul, Republic of Korea
- 3Department of Ocenogaphy, College of Ocean Sciences, Kunsan National University, Kunsan, Republic of Korea
Marine dinoflagellate species in the genus Alexandrium are well known to produce paralytic shellfish poison as well as common coastal species with cosmopolitan distribution. However, few studies on the feeding of copepods on Alexandrium species have been conducted. The toxic dinoflagellate Alexandrium pseudogonyaulax contains goniodomin A and causes red tides in many countries. To investigate the relationship between the toxic dinoflagellate A. pseudogonyaulax and the calanoid copepods Acartia spp., we quantified the ingestion rates of Acartia spp. feeding on A. pseudogonyaulax as a function of prey concentration. Additionally, we estimated grazing coefficients by integrating data from field observations of Acartia spp. and coexisting A. pseudogonyaulax with laboratory measurements of ingestion rates obtained during this investigation. Furthermore, we compared the ingestion rates of Acartia spp. and other predators feeding on Alexandrium species as previously reported. The ingestion rates of Acartia spp. on A. pseudogonyaulax increased continuously with increasing mean prey concentration. The highest values among the ingestion rate of Acartia spp. feeding on A. pseudogonyaulax was 3,407 cells predator−1 d−1 (4,872 ng C predator−1 d−1) at the given prey concentration. The calculated grazing coefficients for Acartia spp. on A. pseudogonyaulax in Shiwha Bay, Korea, were up to 0.073 d−1. The results of this study suggest that A. pseudogonyaulax may decrease or maintain the population of Acartia spp. in marine food webs.
1 Introduction
Marine dinoflagellates and copepods are important components of aquatic environments (Calbet et al., 2003; Jeong et al., 2010, 2021; Turner et al., 2012; Kim et al., 2013). Marine dinoflagellates are ubiquitous species that can dominate the biomass and density of the marine environment (Jeong et al., 2010, 2013, 2021; Hansen, 2011; Nagarkar et al., 2018; Goswami et al., 2020; Telesh et al., 2021). Copepods are major zooplankton in marine food webs and are effective grazers of protist prey species and sometimes control dinoflagellate populations (Watras et al., 1985; Campbell et al., 2005; Jeong et al., 2010; Kim et al., 2013). Therefore, to understand the roles and population dynamics of dinoflagellates in marine communities, growth and mortality due to zooplankton predation must be explored.
Marine dinoflagellate species of the genus Alexandrium are widely distributed and sometimes cause red tides or harmful algal blooms (Anderson, 1997; Cembella et al., 2000; Grattan et al., 2016; Kremp et al., 2019; Shin et al., 2021). Several species of Alexandrium have been well–studied for their physiological and ecological properties, such as toxin profiles, growth rates, distributions, and predation, because they often cause large-scale shellfish mortality and human illnesses due to the toxins they produce (Parkhill and Cembella, 1999; Cembella et al., 2000, 2002; Navarro et al., 2006; Etheridge, 2010; Bill et al., 2016; Grattan et al., 2016; Kim et al., 2016; Kang et al., 2018). Many Alexandrium species produce toxins, such as paralytic shellfish poisoning (PSP) and other allelochemicals, which are potentially transferred to marine organisms in higher trophic levels (Cembella et al., 2000; Turner et al., 2005; Sephton et al., 2007; Ma et al., 2011; Anderson et al., 2012; Tillmann et al., 2016). Therefore, they are of interest to government officials, fish consumers, and marine scientists (Alcala et al., 1988; Anderson et al., 2012). Thus, understanding the interactions between dinoflagellates and their consumers is important for understanding the diversity of red tides and harmful algal species (Turner et al., 2006; Jeong et al., 2010; Kim et al., 2013; Yoo et al., 2013; Kang et al., 2018).
In this study, we isolated and established a clonal culture of Alexandrium pseudogonyaulax from the coastal waters of Korea (Yoo et al., 2023). In many countries, this species produces goniodomin A, which cause red tides (Matsuoka and Fukuyo, 2003; Bravo et al., 2006; Kremp et al., 2019). Previously, A. pseudogonyaulax has been shown to be a phototrophic dinoflagellate. However, this species is a mixotrophic dinoflagellate (Blossom et al., 2012). Several studies have been performed on the taxonomy, ecology, physiology, distribution, bioinformatics, and cysts of this species (Montresor and Marino, 1996; Matsuoka and Fukuyo, 2003; Blossom et al., 2012; Triki et al., 2016; Yoo et al., 2023). However, few studies have been conducted on the mortality of A. pseudogonyaulax caused by grazers. Grazing can play an important role in dinoflagellate population dynamics (Watras et al., 1985; Turner et al., 2006; Jeong et al., 2010; Yoo et al., 2013). Copepods are effective grazers of several dinoflagellates (Watras et al., 1985; Jeong et al., 2010; Kim et al., 2013). Thus, to understand the roles and population dynamics of A. pseudogonyaulax, the predator–prey relationships between A. pseudogonyaulax and copepods was investigated. Additionally, we compared the ingestion rates of Acartia spp. in the present study with those of other Alexandrium species and dinoflagellates reported in the literature. The results of this study provide a basis for understanding the interactions between A. pseudogonyaulax and Acartia spp. and their population dynamics in marine planktonic food webs.
2 Materials and methods
2.1 Preparation of experimental organisms
For isolation and culture of Alexandrium pseudogonyaulax, plankton samples collected with Niskin sampler were taken from Shiwha Bay, Korea when the water temperature and salinity were 25.4°C and 23.9, respectively (Table 1). These samples were screened through a 202–Nitex mesh and placed in 6–well tissue culture plates (SPL lifesciences, Gyeonggido, Korea). A clonal culture of A. pseudogonyaulax was established by performing two serial single–cell isolations. As the concentration of A. pseudogonyaulax increased, this species was subsequently transferred to 50–mL and 500–mL polycarbonate (PC) bottles containing fresh f/2–Si medium (Guillard and Ryther, 1962). Freshly filtered seawater was used to fill bottles containing the f/2-Si medium and A. pseudogonyaulax. The capped bottles were then incubated at 20°C under illumination of 20 μmol photons m−2 s−1 of cool white fluorescent light on a 14:10 h light:dark cycle. Once dense cultures of A. pseudogonyaulax were obtained, the cells were transferred to new 2–L PC bottles containing fresh f/2-Si medium approximately 3 weeks before the feeding experiments were conducted at a temperature of 15°C.
Copepods were collected Shiwha Bay, Korea, using a 303 μm mesh net when water temperature and salinity were 7.3°C and 27.2, respectively (Table 1). The copepods were acclimatized in a 15°C room in the presence of Prorocentrum cordatum for 10 days. Adult female Acartia spp. (A. hongi and A. omorii) were used in the experiments. A. hongi and A. omorii which co-occur in the western coastal waters of Korea, are very similar and it is impossible to distinguish between these two species when they are alive (Soh and Suh, 2000).
The mean equivalent spherical diameter (ESD) of live A. pseudogonyaulax was measured using an electron–particle counter (Coulter Multisizer II; Coulter Corporation, Miami, Florida, United States). The carbon content of this species was estimated based on the cell volume according to Menden-Deuer and Lessard (2000).
2.2 Swimming speed
A dense culture (ca. 1,500 cells mL−1) of A. pseudogonyaulax, which grew photosynthetically under a 14:10 h light:dark cycle at 20 μmol photons m−2 s−1 in f/2–Si medium was transferred to a 250–mL PC bottle. Subsequently, an aliquot from the bottle was transferred to a 50–mL cell culture flask and allowed to acclimate for 30 min. The observations were conducted at 20°C using a video analyzing system (SV-C660, Samsung) and a CCD camera (KP-D20BU, Hitachi). The video camera was focused on a field of view within the cell culture flask and observed as a single field under a dissecting microscope at 50× magnification. The mean and maximum swimming velocities of all A. pseudogonyaulax cells in motion within the first 10 min were recorded and analyzed. The linear displacement of the cells within a single–frame playback was measured to calculate the average swimming speed. The swimming velocities of 30 cells were assessed.
2.3 Ingestion rates of Acartia spp. on Alexandrium pseudogonyaulax
This experiment was designed to measure the ingestion and clearance rates of Acartia spp. on A. pseudogonyaulax as a function of prey concentration (Table 2). Adult female Acartia spp. (a combination of A. hongi and A. omorii) were used in the present study.
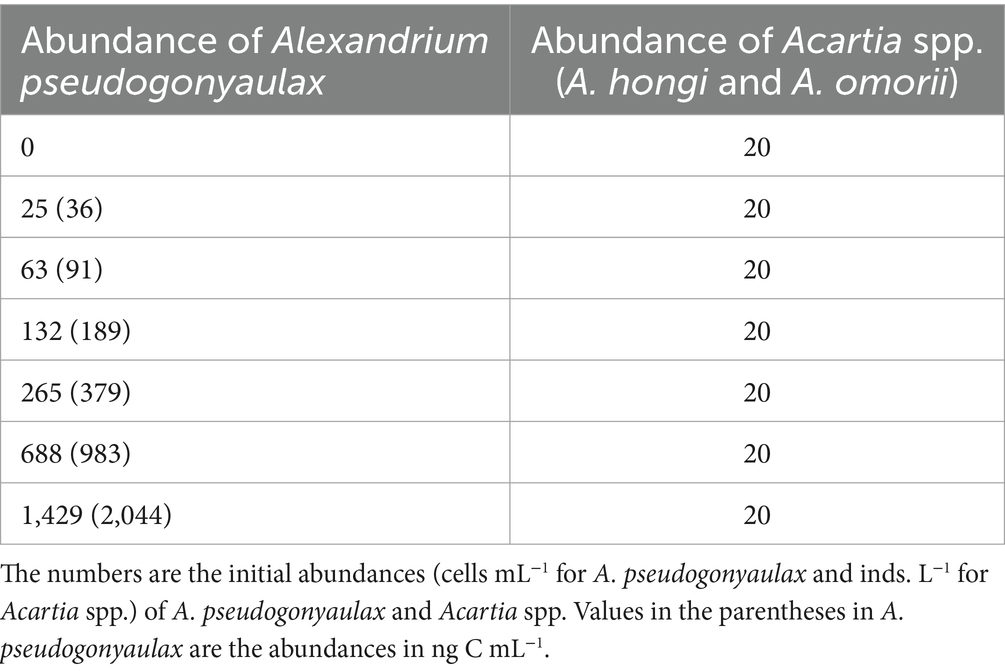
Table 2. Experimental design for feeding by the copepods Acartia spp. on Alexandrium pseudogonyaulax.
For the feeding experiment, the initial concentrations of A. pseudogonyaulax were dertermined using an autopipette to deliver predetermined volumes of known cell concentrations to the bottles and those of Acartia spp. were obtained by individually transferring Acartia spp. using a pasteur pipette. Triplicate 500–mL PC bottles (mixtures of predator and prey) and triplicate control bottles (A. pseudogonyaulax prey only) were set up for each predator–prey combination. In order to maintain consistent water conditions, the water from the predator culture was passed through a 0.7 μm GF/F filter before being added to the prey control bottles. The volume of the filtered predator culture added to the experimental bottles for each predator–prey combination was matched with an equal amount of filtered water added to the prey control bottles. All the bottles were filled to capacity with freshly filtered seawater and capped. To determine the initial predator and prey densities, a 10–mL sample was extracted from each bottle and fixed with 1% Lugol’s solution for fixation. The fixed sample were examined using a light microscope to determine the abundance of predator and prey species. The cells in three 1–mL Sedgwick-Rafter chambers (SRCs) were counted to determine the actual densities of predator and prey species. The bottles were refilled to capacity with freshly filtered seawater, capped, and placed on rotating wheels at a temperature of 15°C, following the conditions outlined earlier. We considered any dilution of the cultures associated with refilling the bottles when determining the clearance rate. A 10–mL aliquot was taken from each bottle after 24 and 48–h incubation periods and fixed with 1% Lugol’s solution. The abundance of prey species was determined by counting all or > 200 cells in three 1–mL SRCs. Following sub–sampling, the bottles were filled with freshly filtered seawater and placed back on rotating wheels. After incubation for 48 h, the Acartia spp. were counted. The mortality of Acartia spp. occurred until the end of the incubation period. The ingestion and clearance rates were calculated using the equations of Frost (1972).
2.4 Grazing impact
We calculated the grazing coefficients attributable to Acartia spp. on A. pseudogonyaulax by combining field data on the abundances of Acartia spp. and A. pseudogonyaulax with the ingestion rates of Acartia spp. on A. pseudogonyaulax obtained in the present study. Data on the abundance of Acartia spp. and co-occurring A. pseudogonyaulax used in this estimation were obtained from water samples from Shiwha Bay, Korea using real-time PCR for A. pseudogonyaulax and cell counting for Acartia spp.
The grazing coefficients (g, d−1) were calculated as follows:
where CR is the clearance rate (mL predator−1 h−1) of a Acartia spp. on A. pseudogonyaulax at a given prey concentration and GC is the predator concentration (cells mL−1). The CR values were calculated as follows:
where IR is the ingestion rate (cells predator−1 h−1) of the predator on the prey and X is the prey concentration (cells mL−1).
2.5 Species-specific primer and probe design and specificity analysis
We developed species-specific primer and probe set for A. pseudogonyaulax and obtained the sequences of the internal transcribed spacer region of ribosomal DNA (ITS rDNA) of A. pseudogonyaulax and other dinoflagellate species belonging to the Alexandrium genus and related dinoflagellate species from GenBank. These sequences were aligned using MEGA v.11. A. pseudogonyaulax specific primers and probe were developed by searching the arrangement for unique portions of the ITS rDNA sequences for A. pseudogonyaulax. Primer and probe sequences were analyzed using Primer 4 (Whitehead Institute for Biomedical Research, Cambridge, MA, United States) and Oligo Calc: Oligonucleotide Properties Calculator (Kibbe, 2007) to investigate the optimal melting temperature and secondary structure, respectively. Primers and probe were synthesized by Bioneer (Table 3). The probe was dual labeled with the fluorescent dyes FAM and BHQ1 at the 5′ and 3′ ends.
Specificity analysis of the primer and probe sets for A. pseudogonyaulax was performed using DNA extracts of A. pseudogonyaulax and related dinoflagellate species in the Family Pyrocystaceae. The qPCR reaction mixture contained 1 μL of DNA template, 0.2 μM of specific forward and reverse primers, 0.15 μM of the specific probe, 5 μL of qPCRBIO Probe Separate-ROX (Genepole, Gwangmyeong, Korea), and deionized sterilized water (DDW; Bioneer), with a final total volume of 10 μL. The qPCR assay was conducted using the Rotor-Gene Q (Qiagen, Hilden, Germany). The cycling conditions were initialized with a denaturation step at 95°C for 3 min, followed by 40 cycles of 10 s at 95°C for 10 s, and 58°C for 40 s.
2.6 Standard curve construction
A standard curve for exploring the abundance of A. pseudogonyaulax was constructed using a qPCR. DNA was extracted from the culture of A. pseudogonyaulax (4,200 cells mL−1) in the growth phase using the AccuPrep Genomic DNA Extraction Kit (Bioneer), targeting 1, 10, 100, 1,000, 2,000, and 4,000 A. pseudogonyaulax cells. The qPCR assay was performed using the reaction mentioned above under the following thermal cycling conditions: 95°C for 3 min, followed by 45 cycles of 10 s at 95°C for 10 s, and 58°C for 40 s.
2.7 Quantification using qPCR
We developed species-specific primer and probe set for A. pseudogonyaulax, and obtained the sequences of the internal transcribed spacer region of ribosomal DNA (ITS rDNA) of A. pseudogonyaulax and related dinoflagellates.
The previously mentioned qPCR assay conditions were used to analyse the abundance of A. pseudogonyaulax in field samples. The DNA from each sample was amplified four times to ensure the accuracy of results. The sample using DDW as the template was used as a negative control, whereas the one used to construct a standard curve was used as positive and standard control.
3 Results
3.1 Ingestion rates of Acartia spp. on Alexandrium pseudogonyaulax
The ingestion rate of Acartia spp. on A. pseudogonyaulax continuously increased with increasing prey concentration (Figure 1). The highest ingestion and clearance rates of Acartia spp. on A. pseudogonyaulax at the given prey concentration was 3,407 cells Acartia−1 d−1 (4,872 ng C Acartia−1 d−1) and 192 mL Acartia−1 h−1, respectively.
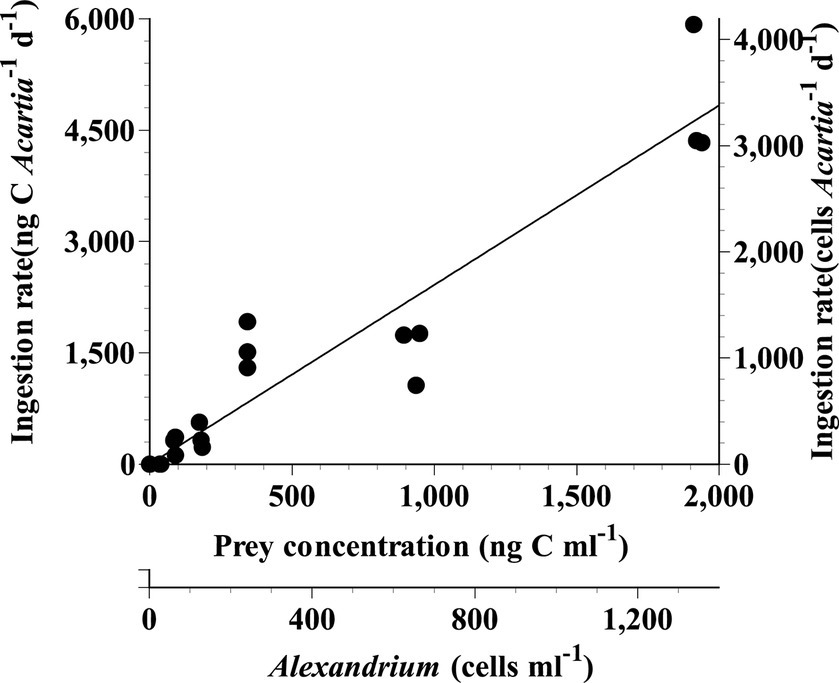
Figure 1. Ingestion rates (IR) of Acartia spp. (A. hongi and A. omorii) feeding on Alexandrium pseudogonyaulax as a function of mean prey concentration (x). Symbols represent treatment means ± 1 SE. The curves were fitted using the linear regression equation. IR (ng C Acartia−1 d−1) = 2.42 × (x), r2 = 0.938.
3.2 Grazing impact
The grazing coefficients attributable to Acartia spp. on co-occurring A. pseudogonyaulax in Shiwha Bay, Korea were affected by the abundance of Acartia predators (Figure 2). The abundance of Acartia spp. and A. pseudogonyaulax were 1.5–126.0 cells mL−1 and 2–2,570 ind. m−3, respectively. The grazing coefficients attributable to Acartia spp. on co-occurring A. pseudogonyaulax were 0.001 to 0.073 d−1 (i.e., up to 7% of A. pseudogonyaulax population could be removed by the copepod Acartia spp. in a day).
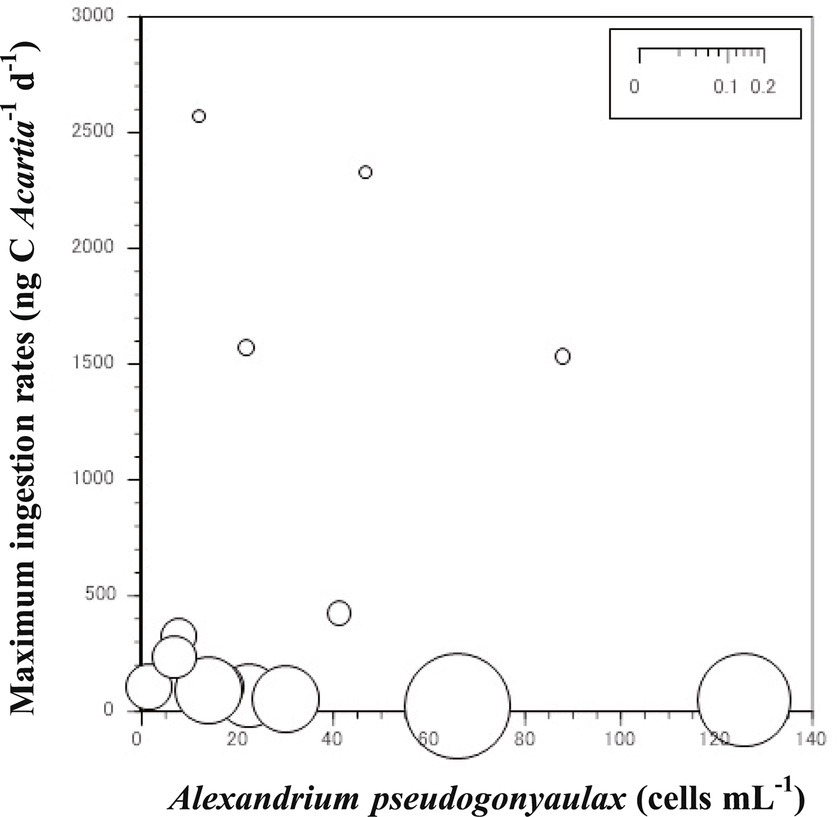
Figure 2. Calculated grazing coefficients (g, d−1) of the Acartia spp. (A. hongi and A. omorii) in relation to the population of co-occurring Alexandrium pseudogonyaulax in the western coastal waters of Korea.
4 Discussion
The calanoid copepod Acartia is a major components metazooplankton in marine environments (Kim et al., 2013; Rice et al., 2015; Lee et al., 2017). Several Acartia species such as Acartia bifilosa, Acartia grani, Acartia hudsonica, and Acartia tonsa feed on Alexandrium spp., including toxic strains of Alexandrium fundyense, Alexandrium minutum, Aleandrium ostenfeldii, and Alexandrium tamarense and non-toxic strain of A. tamarense (Teegarden, 1999; Colin and Dam, 2002, 2003, 2007; da Costa and Fernández, 2002; Teegarden et al., 2003, 2008; da Costa et al., 2008; Sopanen et al., 2011). Among the maximum ingestion rates (MIRs) of Acartia grazers on Alexandrium prey species, the MIRs were not significantly correlated with the prey (19–28 μm of equivalent spherical diameter) and predator sizes (Figure 3). Acartia spp. (A. hongi and A. omorii) were significantly larger than A. grani and A. tonsa (Table 4).
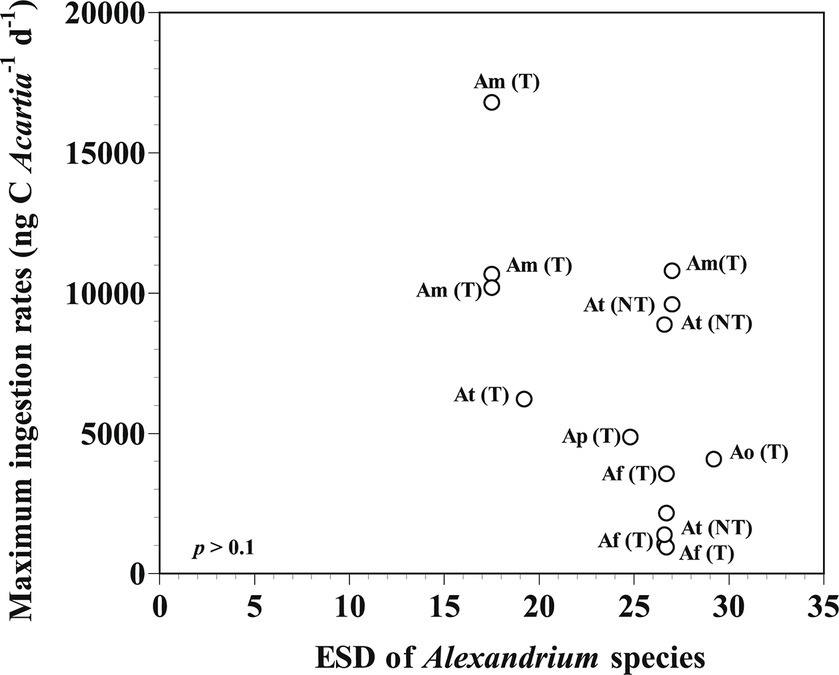
Figure 3. Maximum ingestion rates of Acartia spp. feeding on Alexandrium prey species as a function of prey size (ESD: equivalent spherical diameter, μm). The p-value was p > 0.1 (linear regression ANOVA). Af (T): toxic strain of A. fundyense; Am (T): toxic strain of A. minutum; Ao (T): toxic strain of A. ostenfeldii; Ap (T): toxic strain of A. pseudogonyaulax; At (T): toxic strain of A. tamarense; At (NT): non-toxic strain of A. tamarense. The data were obtained from Calbet et al. (2003), Colin and Dam (2002, 2003, 2007), da Costa and Fernández (2002), da Costa et al. (2008), Sopanen et al. (2011), Teegarden (1999), and Teegarden et al. (2003, 2008).
Among the MIRs of Acartia grazers on Alexandrium prey species, the MIR of A. hudosonica on the toxic of A. tamarense strain was higher than that on the non-toxic strain of A. tamarense (Colin and Dam, 2007; Teegarden et al., 2008). Additionally, the MIR of A. tonsa on the toxic of A. fundyense strain was lower than that on the non-toxic strain of A. tamarense (Teegarden, 1999). Thus, the toxicity of Alexandrium prey species probably did not affect the MIRs of Acartia grazers.
Among the MIRs of Acartia grazers on Alexandrium prey species, the MIR of Acartia spp. on A. pseudogonyaulax was higher than A. bifilosa on A. ostenfeldii, but lower than that of A. grani on A. minutum (da Costa and Fernández, 2002; da Costa et al., 2008). Many Alexandrium species contain PSP toxins; however, the subgenus Gessnerium does not produce PSP toxins (Balech, 1995). A. pseudogonyaulax belonging to the subgenus Gessnerium may not produce PSP toxins, but may produce goniodomin A (Balech, 1995; Matsuoka and Fukuyo, 2003; Bravo et al., 2006). Furthermore, A. pseudogonyaulax is a mixotrophic species when mucus traps are used to immobilize prey cells prior to ingestion (Blossom et al., 2012). Therefore, the mucus trap excreted by A. pseudogonyaulax may not only function to effectively accumulate toxins but also be used to avoid encounters and ingestion by potential predators.
The motility of dinoflagellates is not only relevant to potential predators but is also important for resource availability (Buskey, 1997; Tillmann and Reckermann, 2002; Jeong et al., 2015, 2017). The swimming speed of A. pseudogonyaulax (n = 30) was 263–512 μm s−1. The average (±standard error) swimming speed of A. pseudogonyaulax was 372 (±12) μm s−1. The maximum swimming speed of A. pseudogonyaulax was faster than that of A. minutum but slower than that of A. tamarense (Kang et al., 2018). Thus, the swimming speed of Alexandrium prey species probably did not affect the MIRs of Acartia grazers. Other properties, such as the biochemical factors of Alexandrium species, may affect the ingestion of Acartia grazers more than their physical and behavior properties.
Grazing impacts calculated by using field observation data of Acartia spp. and coexisting A. pseudogonyaulax with laboratory measurements of ingestion rates suggest that up to 7% of A. pseudogonyaulax populations may be eliminated in a day by the copepods Acartia spp. Therefore, the copepod Acartia species could have a considerable potential grazing impact on Alexandrium populations in Shiwha Bay.
Few studies have been conducted on the grazing effects of copepods on Alexandrium species in the field. The grazing effect of A. hudsonica on Alexandrium spp. was 0.8 d−1 at Cundy’s Harbor (Campbell et al., 2005). In Cape Cod embayment, the grazing pressure of A. hudsonica feeding on A. tamarense was less than 1% (Turner and Anderson, 1983). Additionally, the grazing coefficients of the copepods such as Acartia granii and Oithona davisae on A. minutum were 0.00003–0.00007 d−1 (i.e., up to 0.007% of Alexandrium populations could be removed by the copepod populations in a day) in the Arenys de Mar harbor (Calbet et al., 2003). Thus, the copepod Acartia species sometimes have a considerable potential grazing impact on populations of Alexandrium.
5 Conclusion
The present study investigated the grazing by calanoid copepods Acartia spp. on the toxic dinoflagellate Alexandrium pseudogonyaulax. The grazing of Acartia spp. can affect the abundance of Alexandrium populations in many countries. A total of 34 Alexandrium species have been reported, but there have been few studies on grazing by metazooplankton on Alexandrium spp. (Calbet et al., 2003; Campbell et al., 2005; Guiry and Guiry, 2020). Therefore, constant investigation of feeding by dominant copepods on Alexandrium species would be worthwhile to enhance our understanding of the interactions and population dynamics between the copepods and dinoflagellates in natural marine ecosystems.
Data availability statement
The datasets presented in this study can be found in online repositories. The names of the repository/repositories and accession number(s) can be found in the article/supplementary material.
Author contributions
ML: Conceptualization, Investigation, Writing – original draft. YH: Investigation, Writing – original draft. YC: Conceptualization, Investigation, Writing – review & editing. YY: Conceptualization, Investigation, Writing – original draft, Writing – review & editing.
Funding
The author(s) declare that financial support was received for the research, authorship, and/or publication of this article. This research was supported by the National Research Foundation (NRF) of Korea (NRF-2021M3I6A1091272 and NRF-2022R1A2C1004906) and the Korea Institute of Marine Science & Technology Promotion (KIMST) funded by the Ministry of Oceans and Fisheries (KIMST-20220526, KIMST- 20220163 and RS-2023-00256330).
Conflict of interest
The authors declare that the research was conducted in the absence of any commercial or financial relationships that could be construed as a potential conflict of interest.
The author(s) declared that they were an editorial board member of Frontiers, at the time of submission. This had no impact on the peer review process and the final decision.
Publisher’s note
All claims expressed in this article are solely those of the authors and do not necessarily represent those of their affiliated organizations, or those of the publisher, the editors and the reviewers. Any product that may be evaluated in this article, or claim that may be made by its manufacturer, is not guaranteed or endorsed by the publisher.
References
Alcala, A. C., Alcala, L. C., Garth, J. S., Yasumura, D., and Yasumoto, T. (1988). Human fatality due to ingestion of the crab Demania reynaudii that contained a palytoxin-like toxin. Toxicon 26, 105–107. doi: 10.1016/0041-0101(88)90142-0
Anderson, D. M. (1997). Bloom dynamics of toxic Alexandrium species in the northeastern US. Limnol. Oceanogr. 42, 1009–1022. doi: 10.4319/lo.1997.42.5_part_2.1009
Anderson, D. M., Alpermann, T. J., Cembella, A. D., Collos, Y., Masseret, E., and Montresor, M. (2012). The globally distributed genus Alexandrium: multifaceted roles in marine ecosystems and impacts on human health. Harmful Algae 14, 10–35. doi: 10.1016/j.hal.2011.10.012
Balech, E. (1995). The genus Alexandrium Halim (Dinoflagellata). Publ Sherkin is Mar Station. Cork: Sherkin Island, Co.
Bill, B. D., Moore, S. K., Hay, L. R., Anderson, D. M., and Trainer, V. L. (2016). Effects of temperature and salinity on the growth of Alexandrium (Dinophyceae) isolates from the Salish Sea. J. Phycol. 52, 230–238. doi: 10.1111/jpy.12386
Blossom, H., Daugbjerg, N., and Hansen, P. J. (2012). Toxic mucus traps: a novel mechanism that mediates prey uptake in the mixotrophic dinoflagellate Alexandrium pseudogonyaulax. Harmful Algae 17, 40–53. doi: 10.1016/j.hal.2012.02.010
Bravo, I., Garcés, E., Diogène, J., Fraga, S., Sampedro, N., and Figueroa, R. I. (2006). Resting cysts of the toxigenic dinoflagellate genus Alexandrium in recent sediments from the Western Mediterranean coast, including the first description of cysts of A. kutnerae and A. peruvianum. Eur. J. Phycol. 41, 293–302. doi: 10.1080/09670260600810360
Buskey, E. J. (1997). Behavioral components of feeding selectivity of the heterotrophic dinoflagellate Protoperidinium pellucidum. Mar. Ecol. Prog. Ser. 153, 77–89. doi: 10.3354/meps153077
Calbet, A., Vaqué, D., Felipe, J., Vila, M., Sala, M. M., Alcaraz, M., et al. (2003). Relative grazing impact of microzooplankton and mesozooplankton on a bloom of the toxic dinoflagellate Alexandrium minutum. Mar. Ecol. Prog. Ser. 259, 303–309. doi: 10.3354/meps259303
Campbell, R. G., Teegarden, G. J., Cembella, A. D., and Durbin, E. G. (2005). Zooplankton grazing impacts on Alexandrium spp. in the nearshore environment of the Gulf of Maine. Deep Sea Res. II Top. Stud. Oceanogr. 52, 2817–2833. doi: 10.1016/j.dsr2.2005.06.008
Cembella, A. D., Lewis, N. I., and Quilliam, M. A. (2000). The marine dinoflagellate Alexandrium ostenfeldii (Dinophyceae) as the causative organism of spirolide shellfish toxins. Phycologia 39, 67–74. doi: 10.2216/i0031-8884-39-1-67.1
Cembella, A. D., Quilliam, M. A., Lewis, N. I., Bauder, A. G., Dell’Aversano, C., Thomas, K., et al. (2002). The toxigenic marine dinoflagellate Alexandrium tamarense as the probable cause of mortality of caged salmon in Nova Scotia. Harmful Algae 1, 313–325. doi: 10.1016/S1568-9883(02)00048-3
Colin, S. P., and Dam, H. G. (2002). Latitudinal differentiation in the effects of the toxic dinoflagellate Alexandrium spp. on the feeding and reproduction of populations of the copepod Acartia hudsonica. Harmful Algae 1, 113–125. doi: 10.1016/S1568-9883(02)00007-0
Colin, S. P., and Dam, H. G. (2003). Effects of the toxic dinoflagellate Alexandrium fundyense on the copepod Acartia hudsonica: a test of the mechanisms that reduce ingestion rates. Mar. Ecol. Prog. Ser. 248, 55–65. doi: 10.3354/meps248055
Colin, S. P., and Dam, H. G. (2007). Comparison of the functional and numerical responses of resistant versus non-resistant populations of the copepod Acartia hudsonica fed the toxic dinoflagellate Alexandrium tamarense. Harmful Algae 6, 875–882. doi: 10.1016/j.hal.2007.05.003
da Costa, R. M., and Fernández, F. (2002). Feeding and survival rates of the copepods Euterpina acutifrons Dana and Acartia grani Sars on the dinoflagellates Alexandrium minutum Balech and Gyrodinium corsicum Paulmier and the Chryptophyta Rhodomonas baltica Karsten. J. Exp. Mar. Biol. Ecol. 273, 131–142. doi: 10.1016/S0022-0981(02)00132-6
da Costa, R. M., Pereira, L. C. C., and Fernández, F. (2008). Effects of toxic Alexandrium minutum strains on the feeding and survival rates of pelagic marine copepods Acartia grani and Euterpina acutifrons. Hydrobiologia 614, 55–63. doi: 10.1007/s10750-008-9536-4
Etheridge, S. M. (2010). Paralytic shellfish poisoning: seafood safety and human health perspectives. Toxicon 56, 108–122. doi: 10.1016/j.toxicon.2009.12.013
Frost, B. W. (1972). Effects of size and concentration of food particles on the feeding behavior of the marine planktonic copepod Calanus pacificus. Limnol. Oceanogr. 17, 805–815. doi: 10.4319/lo.1972.17.6.0805
Goswami, P., Gupta, S., Das, A. K., Vinithkumar, N. V., Dharani, G., and Kirubagaran, R. (2020). Impact of a dinoflagellate bloom on the marine plankton community structure of Port Blair Bay, Andaman Island. Reg. Stud. Mar. Sci. 37:101320. doi: 10.1016/j.rsma.2020.101320
Grattan, L. M., Holobaugh, S., and Morris, J. G. Jr. (2016). Harmful algal blooms and public health. Harmful Algae 57, 2–8. doi: 10.1016/j.hal.2016.05.003
Guillard, R. R. L., and Ryther, J. H. (1962). Studies of marine planktonic diatoms. I. Cyclotella nana Hustedt and Detonula confervacea (Cleve) gran. Can. J. Microbiol. 8, 229–239. doi: 10.1139/m62-029
Guiry, M. D., and Guiry, G. M. (2020). Algae Base. Galway: World-wide Electronic Publication, National University of Ireland.
Hansen, P. J. (2011). The role of photosynthesis and food uptake for the growth of marine mixotrophic dinoflagellates. J. Eukaryot. Microbiol. 58, 203–214. doi: 10.1111/j.1550-7408.2011.00537.x
Jeong, H. J., Kang, H. C., Lim, A. S., Jang, S. H., Lee, K., Lee, S. Y., et al. (2021). Feeding diverse prey as an excellent strategy of mixotrophic dinoflagellates for global dominance. Sci. Adv. 7:eabe4214. doi: 10.1126/sciadv.abe4214
Jeong, H. J., Kim, J. S., Lee, K. H., Seong, K. A., Yoo, Y. D., Kang, N. S., et al. (2017). Differential interactions between the nematocyst-bearing mixotrophic dinoflagellate Paragymnodinium shiwhaense and common heterotrophic protists and copepods: killer or prey. Harmful Algae 62, 37–51. doi: 10.1016/j.hal.2016.12.005
Jeong, H. J., Lim, A. S., Franks, P. J. S., Lee, K. H., Kim, J. H., Kang, N. S., et al. (2015). A hierarchy of conceptual models of red-tide generation: nutrition, behavior, and biological interactions. Harmful Algae 47, 97–115. doi: 10.1016/j.hal.2015.06.004
Jeong, H. J., Yoo, Y. D., Kim, J. S., Seong, K. A., Kang, N. S., and Kim, T. H. (2010). Growth, feeding and ecological roles of the mixotrophic and heterotrophic dinoflagellates in marine planktonic food webs. Ocean Sci. J. 45, 65–91. doi: 10.1007/s12601-010-0007-2
Jeong, H. J., Yoo, Y. D., Kim, T. H., Seong, K. A., Kang, N. S., Lee, K. H., et al. (2013). Red tides in Masan Bay, Korea in 2004-2005: I. Daily variations in the abundance of red-tide organisms and environmental factors. Harmful Algae 30S, S75–S88. doi: 10.1016/j.hal.2013.10.008
Kang, H. C., Jeong, H. J., Kim, S. J., You, J. H., and Ok, J. H. (2018). Differential feeding by common heterotrophic protists on 12 different Alexandrium species. Harmful Algae 78, 106–117. doi: 10.1016/j.hal.2018.08.005
Kibbe, W. A. (2007). OligoCalc: an online oligonucleotide properties calculator. Nucleic Acids Res. 35, W43–W46. doi: 10.1093/nar/gkm234
Kim, J. H., Jeong, H. J., Lim, A. S., Rho, J. R., and Lee, S. B. (2016). Killing potential protest predators as a survival strategy of the newly described dinoflagellate Alexandrium pohangense. Harmful Algae 55, 41–55. doi: 10.1016/j.hal.2016.01.009
Kim, J. S., Jeong, H. J., Yoo, Y. D., Kang, N. S., Kim, S. K., Song, J. Y., et al. (2013). Red tides in Masan Bay, Korea, in 2004-2005: III. Daily variations in the abundance of metazooplankton and their grazing impacts on red-tides organisms. Harmful Algae 30, S102–S113. doi: 10.1016/j.hal.2013.10.010
Kremp, A., Hansen, P. J., Tillmann, U., Savela, H., Suikkanen, S., Voß, D., et al. (2019). Distributions of three Alexandrium species and their toxins across a salinity gradient suggest an increasing impact of GDA producing a. pseudogonyaulax in shallow brackish waters of northern Europe. Harmful Algae 87:101622. doi: 10.1016/j.hal.2019.101622
Lee, M. J., Jeong, H. J., Kim, J. S., Jang, K. K., Kang, N. S., Jang, S. H., et al. (2017). Ichthyotoxic Cochlodinium polykrikoides red tides offshore in the South Sea, Korea in 2014: III. Metazooplankton and their grazing impacts on red-tide organisms and heterotrophic protists. Algae 32, 285–308. doi: 10.4490/algae.2017.32.11.28
Litaker, R. W., Vandersea, M. W., Kibler, S. R., Reece, K. S., Stokes, N. A., Steidinger, K. A., et al. (2003). Identification of Pfiesteria piscicida (Dinophyceae) and Pfiesteria-like organisms using internal transcribed spacer-specific PCR assays. J. Phycol. 39, 754–761. doi: 10.1046/j.1529-8817.2003.02112.x
Ma, H., Krock, B., Tillmann, U., Bickmeyer, U., Graeve, M., and Cembella, A. (2011). Mode of action of membrane-disruptive lytic compounds from the marine dinoflagellate Alexandrium tamarense. Toxicon 58, 247–258. doi: 10.1016/j.toxicon.2011.06.004
Matsuoka, K., and Fukuyo, Y. (2003). “Taxonomy of cysts” in Manual on Harmful Marine Microalgae (Paris: UNESCO), 563–592.
Menden-Deuer, S., and Lessard, E. J. (2000). Carbon to volume relationships for dinoflagellates, diatoms, and other protist plankton. Limnol. Oceanogr. 45, 569–579. doi: 10.4319/lo.2000.45.3.0569
Montresor, M., and Marino, D. (1996). Modulating effect of cold-dark storage on excystment in Alexandrium pseudogonyaulax (Dinophycea). Mar. Biol. 127, 55–60. doi: 10.1007/BF00993643
Nagarkar, M., Countway, P. D., Yoo, Y. D., Daniels, E., Poulton, N. J., and Palenik, B. (2018). Temporal dynamics of eukaryotic microbial diversity at a coastal Pacific site. ISME J. 12, 2278–2291. doi: 10.1038/s41396-018-0172-3
Navarro, J. M., Muñoz, M. G., and Contreras, A. M. (2006). Temperature as a factor regulating growth and toxin content in the dinoflagellate Alexandrium catenella. Harmful Algae 5, 762–769. doi: 10.1016/j.hal.2006.04.001
Parkhill, J. P., and Cembella, A. D. (1999). Effects of salinity, light and inorganic nitrogen on growth and toxigenicity of the marine dinoflagellate Alexandrium tamarense from northeastern Canada. J. Plankton Res. 21, 939–955. doi: 10.1093/plankt/21.5.939
Rice, E., Dam, H. G., and Stewart, G. (2015). Impact of climate change on estuarine zooplankton: surface water warming in Long Island sound is associated with changes in copepod size and community structure. Estuar. Coasts 38, 13–23. doi: 10.1007/s12237-014-9770-0
Sephton, D. H., Haya, K., Martin, J. L., LeGresley, M. M., and Page, F. H. (2007). Paralytic shellfish toxins in zooplankton, mussels, lobsters and caged Atlantic salmon, Salmo salar, during a bloom of Alexandrium fundyense off grand Manan Island, in the bay of Fundy. Harmful Algae 6, 745–758. doi: 10.1016/j.hal.2007.03.002
Shin, H. H., Li, Z., Kim, H. J., Park, B. S., Lee, J., Shin, A. Y., et al. (2021). Alexandrium catenella (group I) and A. pacificum (group IV) cyst germination, distribution, and toxicity in Jinhae-Masan Bay, Korea. Harmful Algae 110:102122. doi: 10.1016/j.hal.2021.102122
Soh, H. Y., and Suh, H. L. (2000). A new species of Acartia (Copepoda, Calanoida) from the Yellow Sea. J. Plankton Res. 22, 321–337. doi: 10.1093/plankt/22.2.321
Sopanen, S., Setälä, O., Piiparinen, J., Erler, K., and Kremp, A. (2011). The toxic dinoflagellate Alexandrium ostenfeldii promotes incapacitation of the calanoid copepods Eurytemora affinis and Acartia bifilosa from the northern Baltic Sea. J. Plankton Res. 33, 1564–1573. doi: 10.1093/plankt/fbr052
Teegarden, G. J. (1999). Copepod grazing selection and particle discrimination on the basis of PSP toxin content. Mar. Ecol. Prog. Ser. 181, 163–176. doi: 10.3354/meps181163
Teegarden, G., Campbell, R. G., Anson, D. T., Ouellett, A., Westman, B. A., and Durbin, E. G. (2008). Copepod feeding response to varying Alexandrium spp. cellular toxicity and cell concentration among natural plankton samples. Harmful Algae 7, 33–44. doi: 10.1016/j.hal.2007.05.010
Teegarden, G. J., Cembella, A. D., Capuano, C. L., Barron, S. H., and Durbin, E. G. (2003). Phycotoxin accumulation in zooplankton feeding on Alexandrium fundyense–vector or sink? J. Plankton Res. 25, 429–443. doi: 10.1093/plankt/25.4.429
Telesh, I., Schubert, H., and Skarlato, S. (2021). Abiotic stability promotes dinoflagellate blooms in marine coastal ecosystems. Estuar. Coast. Shelf Sci. 251:107239. doi: 10.1016/j.ecss.2021.107239
Tillmann, U., Krock, B., Alpermann, T. J., and Cembella, A. (2016). Bioactive compounds of marine dinoflagellate isolates from western Greenland and their phylogenetic association within the genus Alexandrium. Harmful Algae 51, 67–80. doi: 10.1016/j.hal.2015.11.004
Tillmann, U., and Reckermann, M. (2002). Dinoflagellate grazing on the raphidophyte Fibrocapsa japonica. Aquat. Microb. Ecol. 26, 247–257. doi: 10.3354/ame026247
Triki, H. Z., Laabir, M., Moeller, P., Chomérat, N., and Daly-Yahia, O. K. (2016). First report of goniodomin a production by the dinoflagellate Alexandrium pseudogonyaulax developing in southern Mediterranean (Bizerte lagoon, Tunisia). Toxicon 111, 91–99. doi: 10.1016/j.toxicon.2015.12.018
Turner, J. T., and Anderson, D. M. (1983). Zooplankton grazing during dinoflagellate blooms in a Cape Cod embayment, with observations of predation upon tintinnids by copepods. Mar. Ecol. 4, 359–374. doi: 10.1111/j.1439-0485.1983.tb00119.x
Turner, J. T., Borkman, D. G., and Hunt, C. D. (2006). Zooplankton of Massachusetts Bay, USA, 1992–2003: relationships between the copepod Calanus finmarchicus and the North Atlantic oscillation. Mar. Ecol. Prog. Ser. 311, 115–124. doi: 10.3354/meps311115
Turner, J. T., Doucette, G. J., Keafer, B. A., and Anderson, D. M. (2005). Trophic accumulation of PSP toxins in zooplankton during Alexandrium fundyense blooms in Casco bay, gulf of Maine, April–June 1998. II: zooplankton abundance and size-fractionated community composition. Deep Sea Res. II Top. Stud. Oceanogr. 52, 2784–2800. doi: 10.1016/j.dsr2.2005.06.012
Turner, J. T., Roncalli, V., Ciminiello, P., Dell’Aversano, C., Fattorusso, E., Tartaglione, L., et al. (2012). Biogeographic effects of the Gulf of Mexico red tide dinoflagellate Karenia brevis on Mediterranean copepods. Harmful Algae 16, 63–73. doi: 10.1016/j.hal.2012.01.006
Watras, C. J., Garcon, V. C., Olson, R. J., Chishom, S. W., and Anderson, D. M. (1985). The effect of zooplankton grazing on estuarine blooms of the toxic dinoflagellate Gonyaulax tamarensis. J. Plankton Res. 7, 891–908. doi: 10.1093/plankt/7.6.891
Yoo, Y. D., Hwang, Y. J., and Lee, M. J. (2023). Morphological and molecular characterization of the toxic dinoflagellate Alexandrium pseudogonyaulax in Korea. Kor. J. Hydrogr. Mar. Spat. Informat 12, 47–55.
Yoo, Y. D., Jeong, H. J., Kim, J. S., Kim, T. H., Kim, J. H., Seong, K. A., et al. (2013). Red tides in Masan Bay, Korea in 2004–2005: II. Daily variations in the abundance of heterotrophic protists and their grazing impact on red-tide organisms. Harmful Algae 30S, S89–S101. doi: 10.1016/j.hal.2013.10.009
Keywords: ingestion, food web, metazooplankton, predator, ecology
Citation: Lee MJ, Hwang YJ, Choi YB and Yoo YD (2024) Grazing impact of the calanoid copepods Acartia spp. on the toxic dinoflagellate Alexandrium pseudogonyaulax in the western coastal waters of Korea. Front. Microbiol. 15:1400343. doi: 10.3389/fmicb.2024.1400343
Edited by:
Jin Zhou, Tsinghua University, ChinaReviewed by:
Guangtao Zhang, Binzhou Medical University, ChinaEun Young Yoon, Seoul National University, Republic of Korea
Copyright © 2024 Lee, Hwang, Choi and Yoo. This is an open-access article distributed under the terms of the Creative Commons Attribution License (CC BY). The use, distribution or reproduction in other forums is permitted, provided the original author(s) and the copyright owner(s) are credited and that the original publication in this journal is cited, in accordance with accepted academic practice. No use, distribution or reproduction is permitted which does not comply with these terms.
*Correspondence: Yeong Du Yoo, ydyoo77@kunsan.ac.kr