- 1Section of Plant Pathology and Mycology Laboratory, Department of Botany, University of Kashmir, Srinagar, India
- 2Department of Biology, College of Science, King Khalid University, Abha, Saudi Arabia
Various traditional management techniques are employed to control plant diseases caused by bacteria and fungi. However, due to their drawbacks and adverse environmental effects, there is a shift toward employing more eco-friendly methods that are less harmful to the environment and human health. The main aim of the study was to biosynthesize silver Nanoparticles (AgNPs) from Rhizoctonia solani and Cladosporium cladosporioides using a green approach and to test the antimycotic activity of these biosynthesized AgNPs against a variety of pathogenic fungi. The characterization of samples was done by using UV–visible spectroscopy, SEM (scanning electron microscopy), FTIR (fourier transmission infrared spectroscopy), and XRD (X-ray diffractometry). During the study, the presence of strong plasmon absorbance bands at 420 and 450 nm confirmed the AgNPs biosynthesis by the fungi Rhizoctonia solani and Cladosporium cladosporioides. The biosynthesized AgNPs were 80–100 nm in size, asymmetrical in shape and became spherical to sub-spherical when aggregated. Assessment of the antifungal activity of the silver nanoparticles against various plant pathogenic fungi was carried out by agar well diffusion assay. Different concentration of AgNPs, 5 mg/mL 10 mg/mL and 15 mg/mL were tested to know the inhibitory effect of fungal plant pathogens viz. Aspergillus flavus, Penicillium citrinum, Fusarium oxysporum, Fusarium metavorans, and Aspergillus aflatoxiformans. However, 15 mg/mL concentration of the AgNPs showed excellent inhibitory activity against all tested fungal pathogens. Thus, the obtained results clearly suggest that silver nanoparticles may have important applications in controlling various plant diseases caused by fungi.
Introduction
Nanotechnology exploration has recently sparked significant interest within the domain of material sciences. It stands out as one of the latest breakthroughs imbued with novelty and quintessentially addresses the emerging challenges our world faces. In the pharmaceutical and biomedical industries, nanoparticles exhibit a diverse range of applications, including gene and drug delivery systems, water disinfection, electronics, biosensors, as well as serving as agents for anticancer, antibacterial, antifungal, and antiprotozoal purposes (Lee and Jun, 2019; Rai et al., 2021; War et al., 2022). In recent years, there has been significant research interest in the biosynthesis and characterization of nanoparticles. This interest is primarily driven by their large surface area, which imparts unique properties and potential applications distinct from their bulk counterparts (Shnoudeh et al., 2019; Sajid and Płotka-Wasylka, 2020). Up to now, nanoparticles have been synthesized using a variety of chemical and physical techniques. However, biosynthesis approach utilizing biological systems such as plants, fungi, yeast, and bacteria has been explored and adopted globally for nanoparticle biosynthesis due to its environmentally friendly, reproducible, non-toxic, and cost-effective nature (Gudikandula et al., 2017; Hano and Abbasi, 2021).The biosynthesis approach offers many advantages over chemical synthesis (Duan et al., 2015; Roy et al., 2019; Murali et al., 2023). Since biological systems operate as natural reducing, stabilizing, and capping agents, thereby avoiding many processes for the synthesis of nanoparticles, which not only decreases the cost and consumption of chemicals, but also eliminates agglomeration and oxidation of synthesized nanoparticles (Sidhu et al., 2022). Metallic nanoparticles, especially silver nanoparticles (AgNPs) produced through biological sources, have undergone intensive investigation as a potential alternative therapy for a wide range of infections and illnesses (Bhuyan et al., 2017; Al-Ansari et al., 2020; Murali et al., 2021; Nandi et al., 2023).
Silver is widely known to combat certain microorganisms by modifying their cell membrane structure and function (Dakal et al., 2016; Mikhailova, 2020; Das and Dutta, 2021). Silver is employed as a disinfectant in water purification systems due to its ability to destroy bacteria at low concentrations (less than 1–10 m) (Liu et al., 1994; Deshmukh et al., 2019; Bhardwaj et al., 2021; Das and Dutta, 2022). However, silver can be hazardous to animals, freshwater, and marine organisms at greater quantities (Tortella et al., 2020). Interestingly, micromolar quantities of silver are not toxic to humans (Vrček et al., 2016). Therefore, silver has been widely used in the production of various biological and medicinal products. Recent studies revealed that the toxicity of Ag-NPs measured in freshwater depends on the test species (Blinova et al., 2013). For example, Ag-NPs are reported to be toxic for crustaceans at very low concentration (EC50 < 0.1 mg L−1), followed by algae (EC50 = 0.23 mg L−1), but the toxicity to fish is relatively low (EC50 = 7.1 mg L−1, Kahru and Dubourguier, 2010; Asghari et al., 2012). It has been observed that effects of AgNP on the T84 epithelial cells were size- and dose-dependent, with the 10 nm AgNP causing the most significant changes. Changes in permeability of the epithelial cell monolayer, as measured by transepithelial electrical resistance, after exposure to 10 nm AgNP were most dramatic at the highest dose (100 μg/mL), but also observed at the lower dose (20 μg/mL) (Williams et al., 2016).
Presently, AgNPs are widely utilized across a broad spectrum of applications, ranging from electronic devices to biological tools (Garg et al., 2020; Tufail and Liaqat, 2021; Naganthran et al., 2022). This is likely due to the stability of the particles, which holds significant importance for various applications, particularly in medicine. Additionally, it is crucial that nanoparticles do not agglomerate during their formation, to achieve enhanced stability, maximal yield, and controlled size aggregation of particles, optimization of the various parameters employed in plant-mediated nanoparticle biosynthesis is essential (Ebrahiminezhad et al., 2018; Ahmad et al., 2024). Recent reports have demonstrated the broad-spectrum antibacterial activity of AgNPs against both Gram-positive and Gram-negative bacteria, including multidrug-resistant strains (Liao et al., 2019; Ansari et al., 2021; Yassin et al., 2022). It is significant that AgNPs exhibit multiple modes of inhibitory action against microorganisms, as opposed to the single specific action of antibiotics (Vazquez-Muñoz et al., 2019). Interestingly, AgNPs demonstrate efficacy against various fungi, including Candida spp., dermatophytes, and certain phytopathogenic fungi such as Bipolaris sorokiniana and Magnaporthe grisea (Rajeshkumar, 2019; Mansoor et al., 2021). Conversely, various phytopathogenic fungi remain unexplored, despite their role in causing severe diseases in crucial crop plants, consequently diminishing agricultural yield. Therefore, the present study aimed to address the following questions: (a) to study the preparation and characterization of silver nanoparticles. (b) To study the efficacy of various concentrations of biosynthesized silver nanoparticles against fungal pathogens.
Materials and methods
Fungal culture
In this study, Rhizoctonia solani and Cladosporium cladosporioides were isolated from soil samples. Pure cultures of these fungi were cultivated on Potato Dextrose Agar and identified based on cultural, morphological, and microscopic characteristics as described by Trappe (1982), Quimio (2001), Boerema et al. (2004).
Preparation of silver nanoparticles
To biosynthesize AgNPs the fungi Rhizoctonia solani and Cladosporium cladosporioides were cultured separately in 250 mL conical flasks, each containing 100 mL of potato dextrose broth. The flasks were then incubated at 26 ± 2°C for 72-96 h. Then mycelial mat separation was performed using Whatman filter paper. The medium components were eliminated from the biomass by washing with double-distilled water 3–4 times. Approximately 25 g of fresh biomass was placed in 250 mL conical flasks containing 200 mL of double-distilled water and left for 24–72 h at 25°C. Subsequently, 50 mL of cell filtrate was combined with 50 mL of AgNO3 silver nitrate solution. Solution and the reaction mixture was kept in orbital shaker at 370c and 200 rpm for 24 h. The conversion of Ag ions to (AgNPs) was confirmed by color change from yellow to brown. A reaction mixture without AgNO3 served as a control and was kept alongside the experimental flasks (Sagar and Ashok, 2012; Talie et al., 2020) (Figures 1A–F).
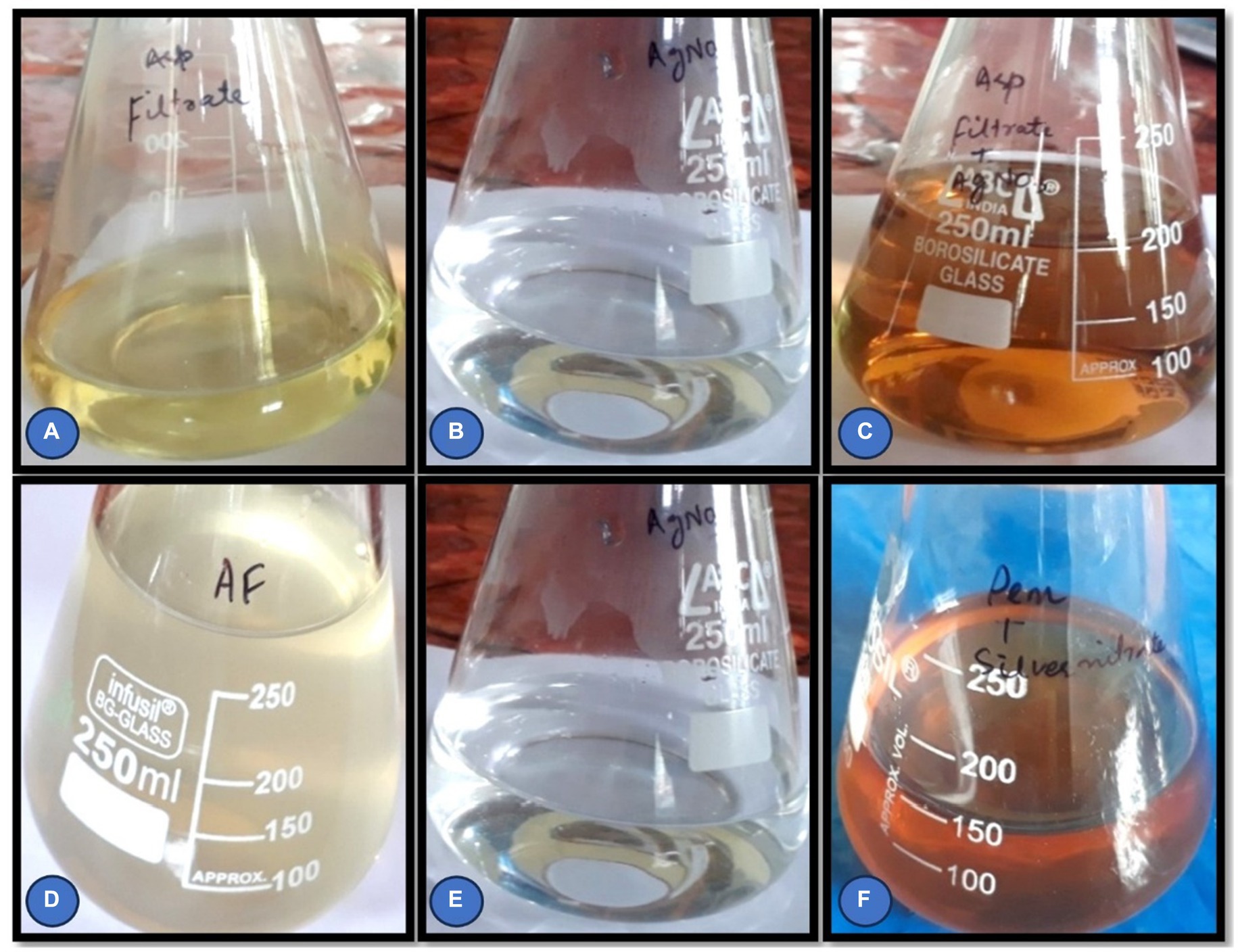
Figure 1. (A–F) Cell filtrate of Rhizoctonia solani and Cladosporium cladosporioides before and after the addition of AgNO3 solution.
Characterization of silver nanoparticles
Different methods were employed to characterize the biosynthesized AgNPs.
Color change
The reduction of silver ions was routinely monitored visually over a period 24 h. The key presence of a brown color in the reaction mixture is an indicator of the formation of silver nanoparticles. The color change is caused by the activation of surface plasmon vibrations.
UV–visible spectroscopy analysis
Ultraviolet (UV) spectroscopy confirms the formation of silver nanoparticles by reducing silver nitrate. The UV–Vis spectra of the cell filtrate was recorded after 24 h on a UV–Visible absorption spectrophotometer (UV–visible Spectrophotometer 119, SYSTRONICS) with a resolution of 2.0 nm between wavelengths of 350–700 nm possessing a scanning speed of 300 nm/min.
Scanning electron microscopy (SEM)
Scanning electron microscopic analysis was used to measure the size and shape of silver nanoparticles. For SEM, (AgNPs) were biosynthesized using cell filtrate, normally required to be completely dry and the specimen was dried and grounded to a powder.
Fourier transmission infrared spectroscopy (FTIR)
The suspension of AgNPs biosynthesized using Rhizoctonia solani and Cladosporium cladosporioides was centrifuged at 10,000 rpm for 20 min at room temperature. The resulting residue was washed several times with sterile distilled water, dried in 40°C and finally the AgNPs were stored in vials. The collected powdered AgNPs were then taken for FTIR analysis in the range of 450–4,500 cm-1.
X-ray diffractometry (XRD)
The AgNPs solution obtained after bio-reduction was purified by centrifugation at 10,000 rpm for 20 min, followed by redispersion of the AgNPs pellet into 1 mL of sterile de-ionized water. X-ray diffraction (XRD) was used to examine the structure and content of the purified AgNPs after they were freeze dried. The dried mixture of AgNPs was collected for X-ray diffractometer analysis of AgNPs production. The colloidal suspensions of AgNPs were analyzed by XRD to validate their crystalline nature in order to verify the results of the UV spectral analyses.
Antifungal efficacy of biosynthesized silver nanoparticles against some selected fungal pathogens
Effect of biosynthesized AgNPs on the mycelial growth of some selected soil pathogenic fungi was analyzed. The agar well diffusion experiment was performed to investigate the antifungal activity of biosynthesized AgNPs against fungal pathogens such as Aspergillus flavus, Penicillium citrinum, Fusarium oxysporum, Fusarium metavorans, and Aspergillus aflatoxiformans. An aliquot of 0.02 mL of inoculum of each test fungal pathogen was injected into culture tubes containing 20 mL of molten Sabouraud dextrose agar medium. The culture tubes were homogenized and then emptied into petri plates and allowed to harden under laminar airflow chamber (aseptic conditions). A 5 mm conventional cork borer was used to make wells on the agar plate. Three different concentrations viz. 05, 10, and 15 mg/mL of AgNPs were prepared and 50 μL from each concentration was added to respective wells. Nystatin 50 μL/disc was utilized (positive control) as a control. The effect of AgNPs on the test fungal infections was analyzed and compared to the reference standard. The antifungal activity was determined using the standard scale of Norrel and Messley (1997).
Results
Plant pathogens such as bacteria and fungi are controlled by different traditional management strategies. However, some of these management measures, such as the use of pesticides, have negative environmental consequences due to their limits. As a result, alternative ways are used that are more environmentally friendly and have fewer negative consequences on human health and the environment. As a result, the antimycotic activity of various AgNPs was tested against fungal pathogens in this work. These AgNPs were prepared by simple technique and used at different concentrations against pathogenic fungi. AgNPs were biosynthesized using Rhizoctonia solani and Cladosporium cladosporioides. After preparation of these AgNPs they were processed to prepare different concentrations. These different concentrations of AgNPs were screened for their antifungal activity against fungal pathogens.
Characterization of biosynthesized silver nanoparticles using fungi
Different techniques employed for the characterization of biosynthesized AgNPs are given below:
Color change
The Initial indication of AgNPs biosynthesis was confirmed by the color change. The color of Rhizoctonia solani and Cladosporium cladosporioides fungal filtrates changes from colorless to brown, as seen in the results (Figures 1A–F). Change in color of the cell free filtrate incubated with silver nitrate solution was visually exhibited after 24 h of incubation which clearly indicates the AgNPs formation.
UV–visible spectroscopy analysis
The reduction of silver nitrate to AgNPs was confirmed by ultraviolet (UV) Spectroscopy. The UV–Visible spectra of Rhizoctonia solani and Cladosporium cladosporioides cell filtrates showed strong plasmon absorption bands at 425 and 450 nm (Figures 2A,B).
Scanning electron microscopy (SEM)
The mean particle size and shape of biosynthesized AgNPs were studied using scanning electron microscopy. SEM pictures of silver nanoparticles synthesized from Rhizoctonia solani and Cladosporium cladosporioides are shown in Figures 3A,B. The aggregated shape of biosynthesized AgNPs was found to be irregular and spherical. AgNPs range in size from 80 to 100 nanometers.
Fourier transmission infrared spectroscopy (FTIR)
The FTIR spectroscopy was used to examine biosynthesized AgNPs in the range 450–4,500 cm−1. AgNPs made from Rhizoctonia solani were found to absorb substantially at various wavelengths (3250.30, 2915.01, 2015.3, 1989.97, 1634.85, 1539.08, 1382.14, 1245.14, and 1047.63 cm−1) respectively. While the absorption bands 2915.01 are related to the -OH of carboxylic acid, the absorption band 3250.30 cm−1 is related to the N-H amine stretch. Similar to this, the absorption bands at 1382.14 and 1634.85 cm−1 are related to the C-N stretching vibrations of aromatic amines and the unsaturated nitrogen molecules O-NO2 and nitrate, respectively. Furthermore, the infrared (IR) spectra show bands that reveal the existence of O-H carboxylic acid, N-H amine linkages, C-N aromatic amine linkages, and O-NO2 unsaturated nitrogen compounds, which may be present in AgNPs as stabilizing caps alongside proteins and amino acid residues. Likewise, the results revealed that biosynthesized AgNPs from fungus Cladosporium cladosporioides absorb strongly 3221.4, 2987.03, 2105.4, 1997.8, 1564.12, 1374.10, 1230.0, and 864.5 cm−1, respectively, (Figures 4A,B).
XRD analysis
During the present study, the crystal structure and particle size of the biosynthesized AgNPs were determined using X-ray diffraction. Figures 5A,B represents the XRD pattern of AgNPs and biosynthesized AgNPs showed excellent crystal quality. Reference data from ICSD (inorganic crystal structure database) and ICDD (international center for diffraction data) were used and matched with data obtained during the present study using PDXL-2 software. Peaks were seen over the whole spectrum, which ranged from 20 to90 nm demonstrating the achievement of great purity. The XRD (X-ray diffraction) spectrum demonstrated that the biosynthesized AgNPs were in the form of nano crystals when compared to the reference data.
Antifungal activity of biosynthesized silver nanoparticles on the mycelial growth of some pathogenic fungi employing agar well diffusion method
The findings revealed that AgNPs derived from Rhizoctonia solani and Cladosporium cladosporioides at various concentrations (05, 10, and 15 mg/mL) inhibited all of the fungal pathogens such as Aspergillus flavus, Penicillium citrinum, Fusarium oxysporum, Fusarium metavorans, and Aspergillus aflatoxiformans. However, zone of inhibition increased with the increase in concentrations of AgNPs. Furthermore, the lowest concentrations of biosynthesized AgNPs reduce the zone of inhibition against all of the tested fungal strains significantly (Table 1).
Antifungal efficacy of various concentrations of biosynthesized silver nanoparticles using fungus, Rhizoctonia solani on the zone of mycelial growth inhibition of some pathogenic fungi
The results (Table 2 and Figures 6, 7A–E) revealed that there was found inhibition in all the tested pathogenic fungi at all the concentrations of AgNPs biosynthesized by Rhizoctonia solani. However, the maximum zone of inhibition against Fusarium metavorans (24.33 ± 0.57) was found at highest concentrations of biosynthesized AgNPs. It was followed by inhibition in mycelial growth of Aspergillus flavus (21.00 ± 1.00), Penicillium citrinum (17.00 ± 1.00), Aspergillus aflatoxiformans (17.00 ± 1.00), and Fusarium oxysporum (13.33 ± 0.57) at the same concentrations, respectively. The zone of inhibition in mycelial growth against Aspergillus flavus varied from 15.00 to 21.00 mm, and in case of Penicillium citrinum varied from 8.00 to 17.00 mm, respectively at different concentrations of AgNPs. Similarly, in case of Fusarium oxysporum, the zone of inhibition in mycelial growth ranges from 8.33 to 13.33 mm, for Fusarium metavorans, from 15.00 to 24.33 mm, and for Aspergillus aflatoxiformans, from 7.66 to 17.00 mm, respectively. The zone of inhibition against all the other tested fungi decreased considerably at the lowest concentrations of biosynthesized AgNPs but to lower extent.
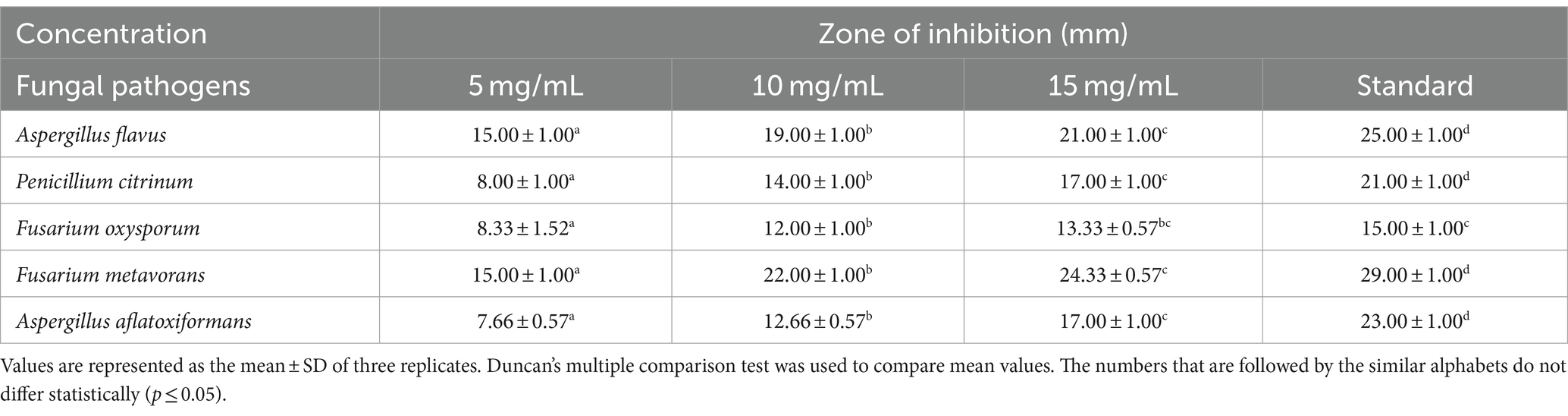
Table 2. Efficacy of various concentrations of biosynthesized silver nanoparticles on the zone of mycelial growth inhibition of some pathogenic fungi.
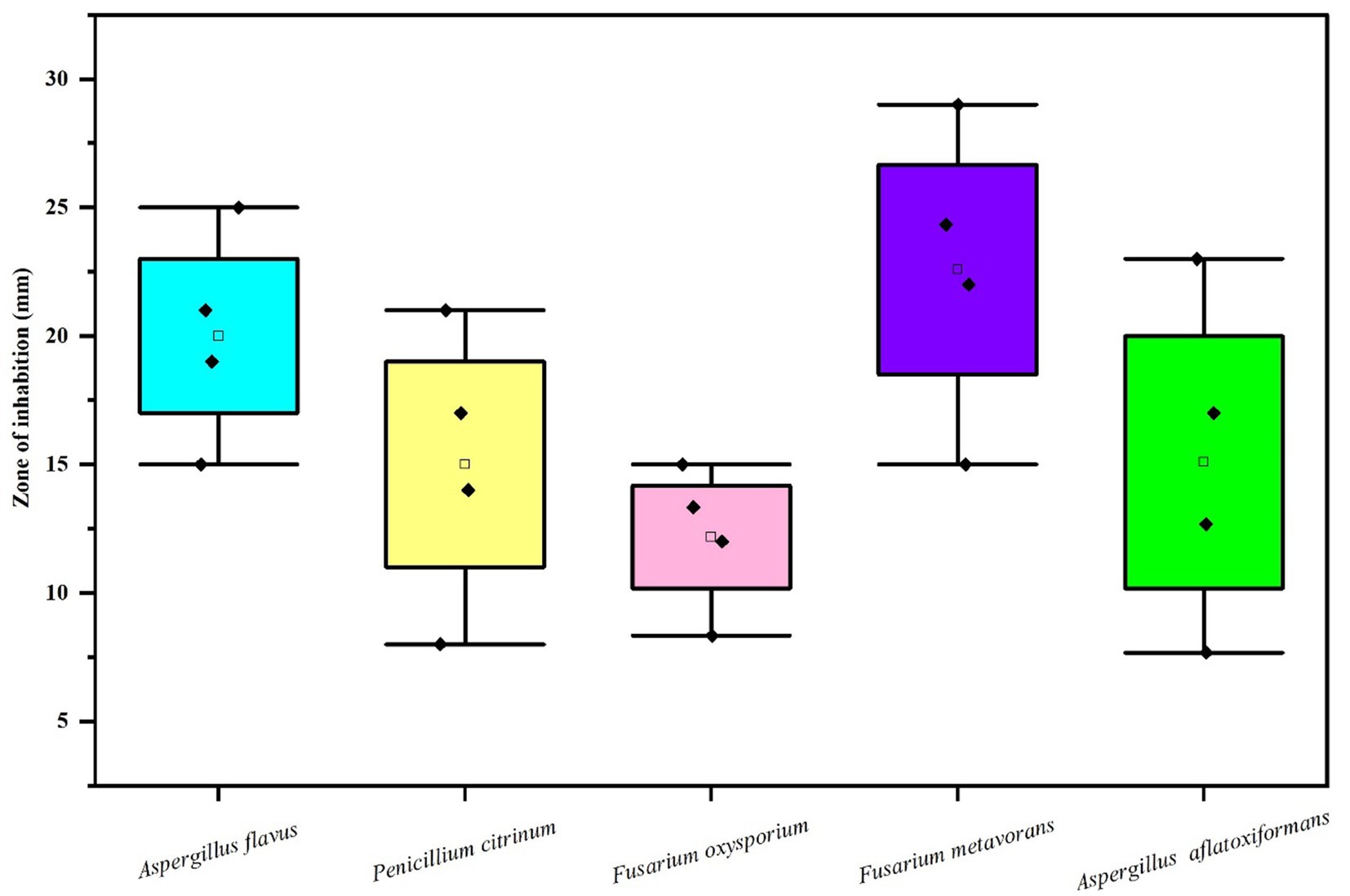
Figure 6. Box and whisker plot depicting the efficacy of AgNPs on zone of mycelial growth inhibition of some pathogenic fungi.
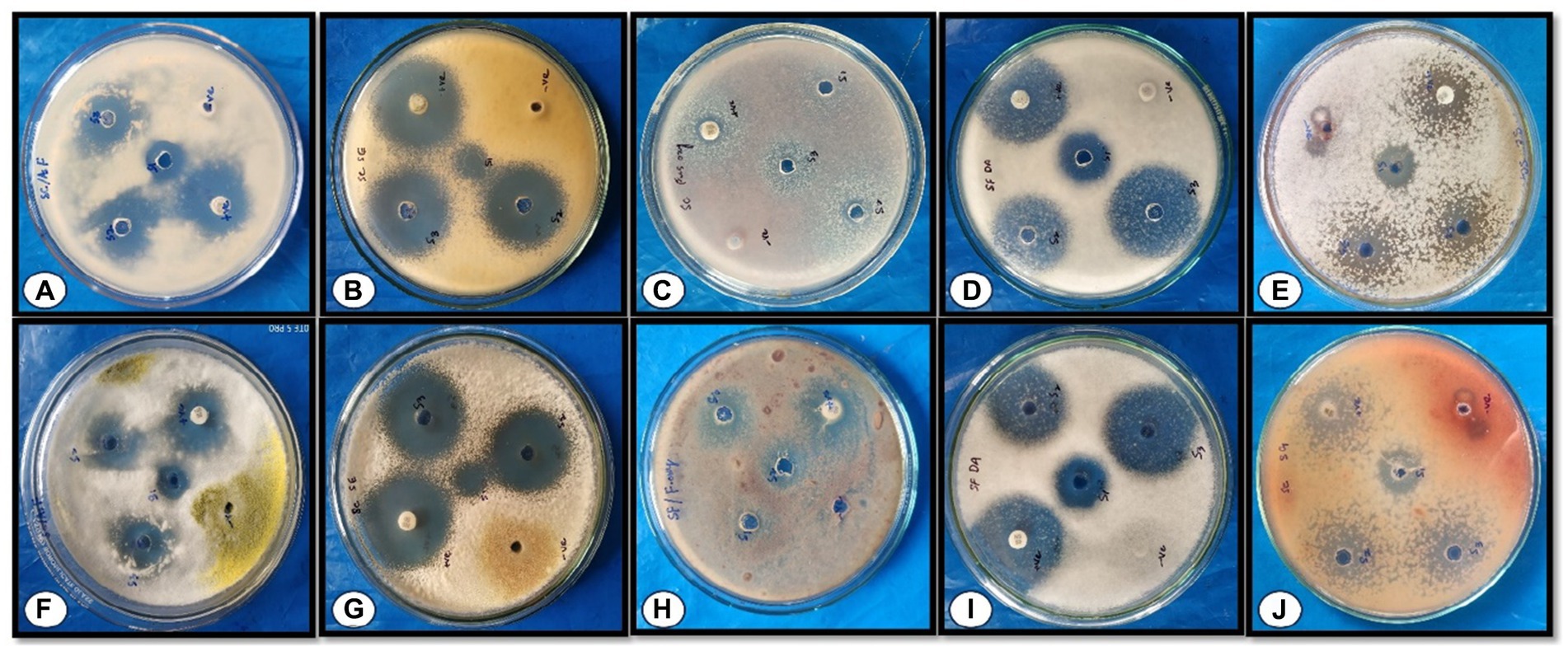
Figure 7. Effect of different concentrations of AgNPs on the zone of mycelial growth inhibition of some pathogens. (A–E) depicts the effects of AgNPs biosynthesized from Rhizoctonia solani (A) Aspergillus flavus, (B) Pencillium citrinum, (C) Fusarium oxysporum, (D) Fusarium metavorans, and (E) Aspergillus aflatoxiformans; (F–J) depicts the effect of AgNPs biosynthesized from Cladosporium cladosporioides (F) Aspergillus flavus, (G) Pencillium citrinum, (H) Fusarium oxysporum, (I) Fusarium metavorans, and (J) Aspergillus aflatoxiformans.
Antifungal efficacy of various concentrations of silver nanoparticles biosynthesized using fungus, Cladosporium cladosporioides on the zone of mycelial growth inhibition of some pathogenic fungi
The results (Table 3 and Figures 7F–J, 8) revealed that the AgNPs biosynthesized from Cladosporium cladosporioides significantly inhibited development mycelia tested fungi. However, the maximum zone of inhibition against Aspergillus flavus (27.00 ± 1.00) was found at the highest concentrations of AgNPs. The highest concentration of AgNPs also caused maximum reduction in the mycelial growth in case of Fusarium metavorans (22.00 ± 1.00), Aspergillus aflatoxiformans (17.00 ± 1.00), Penicillium citrinum (16.00 ± 1.00), and Fusarium oxysporum (14.00 ± 1.00) respectively. The zone of inhibition in mycelial growth against Aspergillus flavus varied from 21.00 to 27.00 mm, and for Penicillium citrinum varied from 7.00 to 16.00 mm, respectively, at different concentrations of AgNPs. Similarly, in case of Fusarium oxysporum, the zone of inhibition in mycelial growth ranges from 10.00 to 14.00 mm, in case of Fusarium metavorans it varies from 14.00 to 22.00 mm, and for Aspergillus aflatoxiformans varied12.00 to 17.00 mm, respectively. The zone of inhibition against all the other tested fungi also decreases considerably at the lowest concentrations of produced AgNPs.
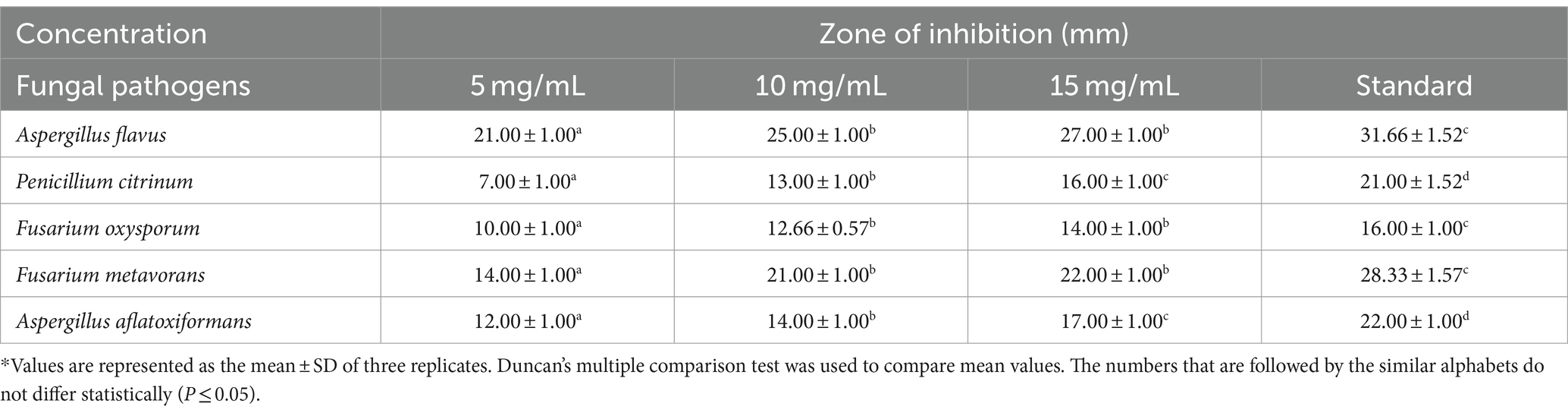
Table 3. Efficacy of various concentrations of biosynthesized silver nanoparticles on the zone of mycelial growth inhibition of some pathogenic fungi.
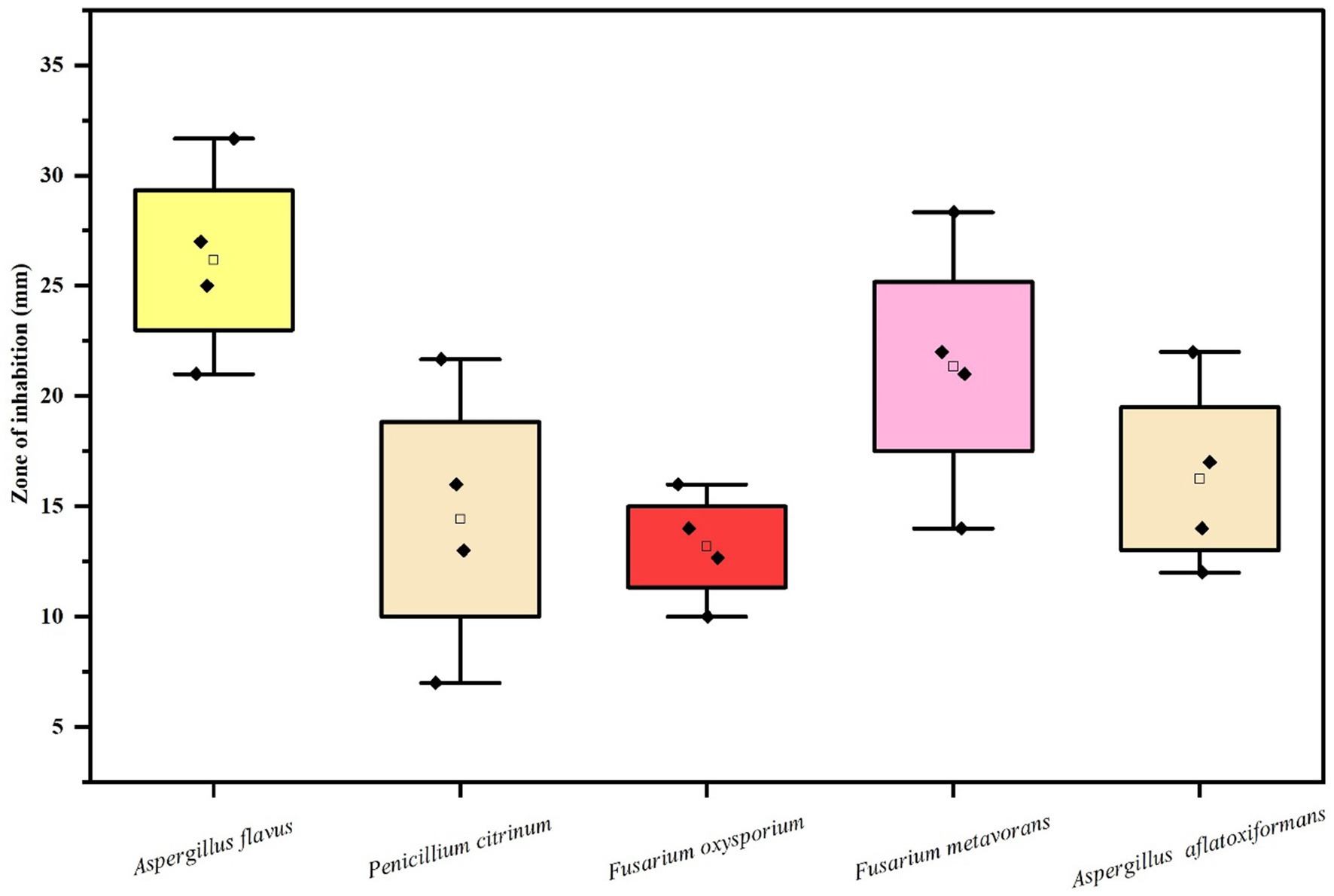
Figure 8. Box and whisker plot depicting the Efficacy of AgNPs on zone of mycelial growth inhibition of some pathogenic fungi.
Discussion
Nano-biotechnology is quickly developing as an important field of modern research, generating the most promising applications in medicine and agriculture in the present climate change scenario (Mariyam et al., 2023). The application of nano-biotechnology in agriculture will help in addressing and solving inherent imperfections and other complex problems in farm production with low input but with an efficient role due to their unique size (Dimkpa and Bindraban, 2017; Usman et al., 2020). Biosynthesis of AgNPs using green approach provide ecofriendly, clean and effective way out for the biosynthesis of nanoparticles. These nanoparticles differ in shape, size, chemical composition and other properties (Raj et al., 2021).
During the present study, Rhizoctonia solani and Cladosporium cladosporioides were used to biosynthesize AgNPs, which was proved by the appearance of brown color due to reduction of silver salt into AgNPs by fungal culture filtrates along with the appearance of strong plasmon absorbance bands at 420–450 nm and strong resonance peaks at 440 nm, thus confirming the biosynthesis of AgNPs as has been reported by Khan et al. (2018) and Paul et al. (2023). Many researchers have reported that microorganisms, plant extracts, and fungi can be used to biosynthesize nanoparticles through biological pathways (Koul et al., 2021). Many fungi like Fusarium oxysporum, Aspergillus fumigatus, Aspergillus niger, Fusarium semitectum, Penicillium brevicompactum, and Cladosporium cladosporioides have been reported to be competent enough to extracellularly biosynthesized AgNPs (Rajeshkumar and Sivapriya, 2020; Adebayo et al., 2021) respectively. Our results are in conformity with Verma et al. (2010) and Parveen et al. (2018), who also reported similar results for biosynthesis AgNPs and iron oxide nanoparticles from fungi, respectively. Fungi have many advantages for the production and biosynthesis of nanoparticles in comparison to other types of microorganisms and phyto extracts. This is because the mycelial mesh of fungi is easy to handle and withstands high flow pressure, agitation and many other conditions in bioreactors and other chambers. Scanning electron microscopy revealed that biosynthesized AgNPs were irregular and spherical in aggregate form, with a size ranging from 80 to 100 nm. Similar patterns of biosynthesized AgNPs were observed by Kathiresan et al. (2009) and Jain et al. (2011). Our findings are in accordance with the work of Al-Zubaidi et al. (2019) and Talie et al. (2020) who also used different microfungi and macrofungi for biosynthesis of AgNPs. It seems that the pattern aggregation and formation of mycosynthesized silver nanoparticles take place due to the enzymatic reduction of silver metal ions (Rajput et al., 2016). The results from the present study with regard to the bioactivity of biosynthesized AgNPs against phytopathogenic fungi revealed that at different concentrations, biosynthesized AgNPs caused a significant reduction in the fungal mycelial growth in terms of zone of inhibition against all the test fungal pathogens such as Rhizoctonia solani and Cladosporium cladosporioide indicating their strong antimycotic activity. Similar work was carried out by Abd-Elsalam et al. (2019), Ingle et al. (2020), Padhi and Behera (2021). Sulaiman et al. (2015) and reported antimycotic activity of bi-synthesized AgNPs against fungi, namely Aspergillus niger, Penicillium chrysogenum, Fusarium culmorum, and Alternaria alternata. Talie et al. (2020) also reported the potent antifungal activity of biosynthesized AgNPs using Helvella leucopus against Aspergillus niger, Penicillium chrysogenum, Alternaria alternata which is in conformity with our work. The results emphasize that these biosynthesized AgNPs will work best as nano-biopesticides, as has been reported by Paramo et al. (2020) and can be incorporated into the integrated disease management module. Antimycotic activity of AgNPs against different species of phytopathogenic fungi of some cereals was reported by Al-Askar et al. (2013) and Candida species by Ishida et al. (2013). Since phytopathogenic fungi are toxic due to the production of mycotoxins which can be easily adsorbed by nanoparticles and impart protection against disease as has been reported by Horky et al. (2018).
Conclusion
This study focused on the biosynthesis of AgNPs from aqueous extract of the fungi, Rhizoctonia solani and Cladosporium cladosporioides. The biogenic method provides natural agents for reduction, capping and stabilization of AgNPs, which makes the synthesis approach much more cost-effective, non-toxic, reproducible and environmentally friendly, implying that fungi could be a good source of silver nanoparticles. Biosynthesized silver nanoparticles from Rhizoctonia solani and Cladosporium cladosporioides were crystallite in nature, with an average particle size of 10.100 nm. The study revealed strong antifungal properties of these synthesized AgNPs. A significant zone of mycelial growth inhibition by AgNPs was observed against all the test microorganisms. These silver nanoparticles could be of tremendous use in pharmaceutical industries for various biomedical purposes, as well as in food processing industries for food packaging to reduce contamination and enhance long-term storage and preservation of foods. Metal based nanoparticles such as silver nanoparticles may prove to be very beneficial in the agricultural sector, such as their use as nanopesticides. However, proper investigation into their mechanism of action and evaluating the impact on human health and the environment is required.
Data availability statement
The original contributions presented in the study are included in the article/supplementary material, further inquiries can be directed to the corresponding author.
Author contributions
MM: Conceptualization, Data curation, Formal analysis, Investigation, Methodology, Software, Visualization, Writing – original draft, Writing – review & editing. AW: Data curation, Project administration, Resources, Validation, Writing – review & editing. MB: Data curation, Investigation, Project administration, Writing – review & editing. SS: Funding acquisition, Writing – review & editing. SAMA: Writing – review & editing. SAA: Writing – review & editing.
Funding
The author(s) declare that financial support was received for the research, authorship, and/or publication of this article. The authors extend their appreciation to the Deanship of Research and Graduate Studies at King Khalid University for funding this work through Large Research Project under grant number (RGP2/49/45).
Acknowledgments
The authors like to express their gratitude to the Head, Department of Botany, University of Kashmir and NIT Srinagar for providing essential assistance and amenities during the course of the study.
Conflict of interest
The authors declare that the research was conducted in the absence of any commercial or financial relationships that could be construed as a potential conflict of interest.
Publisher’s note
All claims expressed in this article are solely those of the authors and do not necessarily represent those of their affiliated organizations, or those of the publisher, the editors and the reviewers. Any product that may be evaluated in this article, or claim that may be made by its manufacturer, is not guaranteed or endorsed by the publisher.
References
Abd-Elsalam, K. A., Al-Dhabaan, F. A., Alghuthaymi, M., Njobeh, P. B., and Almoammar, H. (2019). “Nanobiofungicides: present concept and future perspectives in fungal control” in Nano-biopesticides today and future perspectives (Academic Press), 315–351.
Adebayo, E. A., Azeez, M. A., Alao, M. B., Oke, A. M., and Aina, D. A. (2021). Fungi as veritable tool in current advances in nanobiotechnology. Heliyon 7:e08480. doi: 10.1016/j.heliyon.2021.e08480
Ahmad, N. M., Mohamed, A. H., Zainal-Abidin, N., Nawahwi, M. Z., and Azzeme, A. M. (2024). Effect of optimisation variable and the role of plant extract in the synthesis of nanoparticles using plant-mediated synthesis approaches. Inorg. Chem. Commun. 161:111839. doi: 10.1016/j.inoche.2023.111839
Al-Ansari, M. M., Dhasarathan, P., Ranjitsingh, A. J. A., and Al-Humaid, L. A. (2020). Ganoderma lucidum inspired silver nanoparticles and its biomedical applications with special reference to drug resistant Escherichia coli isolates from CAUTI. Saudi J. Biol. Sci. 27, 2993–3002. doi: 10.1016/j.sjbs.2020.09.008
Al-Askar, A., Hafez, E. E., Kabeil, S. A., and Meghad, A. (2013). Bioproduction of silver-nano particles by Fusarium oxysporum and their antimicrobial activity against some plant pathogenic bacteria and fungi. Life Sci. J. 10, 2470–2475.
Al-Zubaidi, S., Al-Ayafi, A., and Abdelkader, H. (2019). Biosynthesis, characterization and antifungal activity of silver nanoparticles by Aspergillus niger isolate. J. Nanotechnol. Res. 1, 23–36. doi: 10.26502/jnr.2688-8521002
Ansari, M. A., Kalam, A., Al-Sehemi, A. G., Alomary, M. N., AlYahya, S., Aziz, M. K., et al. (2021). Counteraction of biofilm formation and antimicrobial potential of Terminalia catappa functionalized silver nanoparticles against Candida albicans and multidrug-resistant gram-negative and gram-positive bacteria. Antibiotics 10:725. doi: 10.3390/antibiotics10060725
Asghari, S., Johari, S. A., Lee, J. H., Kim, Y. S., Jeon, Y. B., Choi, H. J., et al. (2012). Toxicity of various silver nanoparticles compared to silver ions in Daphnia magna. J. Nanobiotechnol. 10, 1–11. doi: 10.1186/1477-3155-10-14
Bhardwaj, A. K., Sundaram, S., Yadav, K. K., and Srivastav, A. L. (2021). An overview of silver nano-particles as promising materials for water disinfection. Environ. Technol. Innov. 23:101721. doi: 10.1016/j.eti.2021.101721
Bhuyan, B., Paul, A., Paul, B., Dhar, S. S., and Dutta, P. (2017). Paederia foetida Linn. Promoted biogenic gold and silver nanoparticles: synthesis, characterization, photocatalytic and in vitro efficacy against clinically isolated pathogens. J. Photochem. Photobiol. B Biol. 173, 210–215. doi: 10.1016/j.jphotobiol.2017.05.040
Blinova, I., Niskanen, J., Kajankari, P., Kanarbik, L., Käkinen, A., Tenhu, H., et al. (2013). Toxicity of two types of silver nanoparticles to aquatic crustaceans Daphnia magna and Thamnocephalus platyurus. Environ. Sci. Pollut. Res. 20, 3456–3463. doi: 10.1007/s11356-012-1290-5
Boerema, G. H., de Gruyter, J., Noordeloos, M. E., and Hamers, M. E. C. (2004). Phoma identification manual: differentiation of specific and infra-specific taxa in culture : CABI.
Dakal, T. C., Kumar, A., Majumdar, R. S., and Yadav, V. (2016). Mechanistic basis of antimicrobial actions of silver nanoparticles. Front. Microbiol. 7:1831. doi: 10.3389/fmicb.2016.01831
Das, A., and Dutta, P. (2021). Antifungal activity of biogenically synthesized silver and gold nanoparticles against sheath blight of rice. J. Nanosci. Nanotechnol. 21, 3547–3555. doi: 10.1166/jnn.2021.18996
Das, G., and Dutta, P. (2022). Effect of nanopriming with zinc oxide and silver nanoparticles on storage of chickpea seeds and management of wilt disease. J. Agric. Sci. Technol. 24, 213–226.
Deshmukh, S. P., Patil, S. M., Mullani, S. B., and Delekar, S. D. (2019). Silver nanoparticles as an effective disinfectant: a review. Mater. Sci. Eng. C 97, 954–965. doi: 10.1016/j.msec.2018.12.102
Dimkpa, C. O., and Bindraban, P. S. (2017). Nanofertilizers: new products for the industry? J. Agric. Food Chem. 66, 6462–6473. doi: 10.1021/acs.jafc.7b02150
Duan, H., Wang, D., and Li, Y. (2015). Green chemistry for nanoparticle synthesis. Chem. Soc. Rev. 44, 5778–5792. doi: 10.1039/C4CS00363B
Ebrahiminezhad, A., Zare-Hoseinabadi, A., Sarmah, A. K., Taghizadeh, S., Ghasemi, Y., and Berenjian, A. (2018). Plant-mediated synthesis and applications of iron nanoparticles. Mol. Biotechnol. 60, 154–168. doi: 10.1007/s12033-017-0053-4
Garg, D., Sarkar, A., Chand, P., Bansal, P., Gola, D., Sharma, S., et al. (2020). Synthesis of silver nanoparticles utilizing various biological systems: mechanisms and applications—a review. Prog. Biomater. 9, 81–95. doi: 10.1007/s40204-020-00135-2
Gudikandula, K., Vadapally, P., and Charya, M. S. (2017). Biogenic synthesis of silver nanoparticles from white rot fungi: their characterization and antibacterial studies. OpenNano 2, 64–78. doi: 10.1016/j.onano.2017.07.002
Hano, C., and Abbasi, B. H. (2021). Plant-based green synthesis of nanoparticles: production, characterization and applications. Biomol. Ther. 12:31. doi: 10.3390/biom12010031
Horky, P., Skalickova, S., Baholet, D., and Skladanka, J. (2018). Nanoparticles as a solution for eliminating the risk of mycotoxins. Nano 8:727. doi: 10.3390/nano8090727
Ingle, A. P., Biswas, A., Vanlalveni, C., Lalfakzuala, R., Gupta, I., Ingle, P., et al. (2020). “Biogenic synthesis of nanoparticles and their role in the management of plant pathogenic fungi” in Microbial nanotechnology, 135–161.
Ishida, K., Cipriano, T. F., Rocha, G. M., Weissmüller, G., Gomes, F., Miranda, K., et al. (2013). Silver nanoparticle production by the fungus Fusarium oxysporum: nanoparticle characterisation and analysis of antifungal activity against pathogenic yeasts. Mem. Inst. Oswaldo Cruz 109, 220–228. doi: 10.1590/0074-0276130269
Jain, N., Bhargava, A., Majumdar, S., Tarafdar, J. C., and Panwar, J. (2011). Extracellular biosynthesis and characterization of silver nanoparticles using Aspergillus flavus NJP08: a mechanism perspective. Nanoscale 3, 635–641. doi: 10.1039/C0NR00656D
Kahru, A., and Dubourguier, H. C. (2010). From ecotoxicology to nanoecotoxicology. Toxicology 269, 105–119. doi: 10.1016/j.tox.2009.08.016
Kathiresan, K., Manivannan, S., Nabeel, M. A., and Dhivya, B. (2009). Studies on silver nanoparticles synthesized by a marine fungus, Penicillium fellutanum isolated from coastal mangrove sediment. Colloids Surf. B Biointerfaces 71, 133–137. doi: 10.1016/j.colsurfb.2009.01.016
Khan, A. U., Malik, N., Khan, M., Cho, M. H., and Khan, M. M. (2018). Fungi-assisted silver nanoparticle synthesis and their applications. Bioprocess Biosyst. Eng. 41, 1–20. doi: 10.1007/s00449-017-1846-3
Koul, B., Poonia, A. K., Yadav, D., and Jin, J. O. (2021). Microbe-mediated biosynthesis of nanoparticles: applications and future prospects. Biomol. Ther. 11:886. doi: 10.3390/biom11060886
Lee, S. H., and Jun, B. H. (2019). Silver nanoparticles: synthesis and application for nanomedicine. Int. J. Mol. Sci. 20:865. doi: 10.3390/ijms20040865
Liao, S., Zhang, Y., Pan, X., Zhu, F., Jiang, C., Liu, Q., et al. (2019). Antibacterial activity and mechanism of silver nanoparticles against multidrug-resistant Pseudomonas aeruginosa. Int. J. Nanomedicine 14, 1469–1487. doi: 10.2147/IJN.S191340
Liu, Z., Stout, J. E., Tedesco, L., Boldin, M., Hwang, C., Diven, W. F., et al. (1994). Controlled evaluation of copper-silver ionization in eradicating Legionella pneumophila from a hospital water distribution system. J. Infect. Dis. 169, 919–922. doi: 10.1093/infdis/169.4.919
Mansoor, S., Zahoor, I., Baba, T. R., Padder, S. A., Bhat, Z. A., Koul, A. M., et al. (2021). Fabrication of silver nanoparticles against fungal pathogens. Front. Nanotechnol. 3:679358. doi: 10.3389/fnano.2021.679358
Mariyam, S., Upadhyay, S. K., Chakraborty, K., Verma, K. K., Duhan, J. S., Muneer, S., et al. (2023). Nanotechnology, a frontier in agricultural science, a novel approach in abiotic stress management and convergence with new age medicine-a review. Sci. Total Environ. 912:169097. doi: 10.1016/j.scitotenv.2023.169097
Mikhailova, E. O. (2020). Silver nanoparticles: mechanism of action and probable bio-application. J. Funct. Biomater. 11:84. doi: 10.3390/jfb11040084
Murali, M., Gowtham, H. G., Shilpa, N., Singh, S. B., Aiyaz, M., Sayyed, R. Z., et al. (2023). Zinc oxide nanoparticles prepared through microbial mediated synthesis for therapeutic applications: a possible alternative for plants. Front. Microbiol. 14:1227951. doi: 10.3389/fmicb.2023.1227951
Murali, M., Kalegowda, N., Gowtham, H. G., Ansari, M. A., Alomary, M. N., Alghamdi, S., et al. (2021). Plant-mediated zinc oxide nanoparticles: advances in the new millennium towards understanding their therapeutic role in biomedical applications. Pharmaceutics 13:1662. doi: 10.3390/pharmaceutics13101662
Naganthran, A., Verasoundarapandian, G., Khalid, F. E., Masarudin, M. J., Zulkharnain, A., Nawawi, N. M., et al. (2022). Synthesis, characterization and biomedical application of silver nanoparticles. Materials 15:427. doi: 10.3390/ma15020427
Nandi, N. B., Das, N., Ghanta, S., Puzari, K. R., Dutta, P., Kłak, J., et al. (2023). Atropisomers and a copper (ii) complex derived from 1, 3-dimethyl-5-(8′-quinolinylazo)-6-aminouracil: structures, magnetism and biological properties. New J. Chem. 47, 21633–21647. doi: 10.1039/D3NJ04598F
Norrel, S. A., and Messley, K. E. (1997). Microbiology Laboratory Manual Principles and Applications Prentice Hall. Upper saddle River. New jersey USA.
Padhi, S., and Behera, A. (2021). “Silver-based nanostructures as antifungal agents: mechanisms and applications” in Silver nanomaterials for Agri-food applications (Elsevier), 17–38.
Paramo, L. A., Feregrino-Perez, A. A., Guevara, R., Mendoza, S., and Esquivel, K. (2020). Nanoparticles in agroindustry: applications, toxicity, challenges, and trends. Nano 10:1654. doi: 10.3390/nano10091654
Parveen, S., Wani, A. H., Shah, M. A., Devi, H. S., Bhat, M. Y., and Koka, J. A. (2018). Preparation, characterization and antifungal activity of iron oxide nanoparticles. Microb. Pathog. 115, 287–292.
Paul, T. K., Jalil, M. A., Repon, M. R., Alim, M. A., Islam, T., Rahman, S. T., et al. (2023). Mapping the Progress in surface Plasmon resonance analysis of phytogenic silver nanoparticles with colorimetric sensing applications. Chem. Biodivers. 20:e202300510. doi: 10.1002/cbdv.202300510
Quimio, T. H. (2001). Workbook on tropical Fungi. Collection, Isolation and Identification. Laguna, Philippines: The Mycological Society of the Philippines. Inc., College, 259.
Rai, M., Ingle, A. P., Trzcińska-Wencel, J., Wypij, M., Bonde, S., Yadav, A., et al. (2021). Biogenic silver nanoparticles: what we know and what do we need to know? Nano 11:2901. doi: 10.3390/nano11112901
Raj, S., Trivedi, R., and Soni, V. (2021). Biogenic synthesis of silver nanoparticles, characterization and their applications-a review. Surfaces 5, 67–90. doi: 10.3390/surfaces5010003
Rajeshkumar, S. (2019). “Antifungal impact of nanoparticles against different plant pathogenic fungi” in Nanomaterials in plants, algae and microorganisms (Academic Press), 197–217.
Rajeshkumar, S., and Sivapriya, D. (2020). “Fungus-mediated nanoparticles: characterization and biomedical advances” in Nanoparticles in medicine, 185–199.
Rajput, S., Werezuk, R., Lange, R. M., and McDermott, M. T. (2016). Fungal isolate optimized for biogenesis of silver nanoparticles with enhanced colloidal stability. Langmuir 32, 8688–8697. doi: 10.1021/acs.langmuir.6b01813
Roy, A., Bulut, O., Some, S., Mandal, A. K., and Yilmaz, M. D. (2019). Green synthesis of silver nanoparticles: biomolecule-nanoparticle organizations targeting antimicrobial activity. RSC Adv. 9, 2673–2702. doi: 10.1039/C8RA08982E
Sagar, G., and Ashok, B. (2012). Green synthesis of silver nanoparticles using Aspergillus niger and its efficacy against human pathogens. Eur. J. Exp. Biol. 2, 1654–1658.
Sajid, M., and Płotka-Wasylka, J. (2020). Nanoparticles: synthesis, characteristics, and applications in analytical and other sciences. Microchem. J. 154:104623. doi: 10.1016/j.microc.2020.104623
Shnoudeh, A. J., Hamad, I., Abdo, R. W., Qadumii, L., Jaber, A. Y., Surchi, H. S., et al. (2019). “Synthesis, characterization, and applications of metal nanoparticles” in Biomaterials and bionanotechnology (Academic Press), 527–612.
Sidhu, A. K., Verma, N., and Kaushal, P. (2022). Role of biogenic capping agents in the synthesis of metallic nanoparticles and evaluation of their therapeutic potential. Front. Nanotechnol. 3:801620. doi: 10.3389/fnano.2021.801620
Sulaiman, G. M., Hussien, T. H., and Saleem, M. M. N. M. (2015). Biosynthesis of silver nanoparticles synthesized by Aspergillus flavus and their antioxidant, antimicrobial and cytotoxicity properties. Bull. Mater. Sci. 38, 639–644. doi: 10.1007/s12034-015-0905-0
Talie, M. D., Wani, A. H., Ahmad, N., Bhat, M. Y., and War, J. M. (2020). Green synthesis of silver nanoparticles (AgNPs) using Helvella leucopus Pers. and their antimycotic activity against fungi causing fungal rot of apple. Asian J. Pharm. Clin. Res. 13, 161–165.
Tortella, G. R., Rubilar, O., Durán, N., Diez, M. C., Martínez, M., Parada, J., et al. (2020). Silver nanoparticles: toxicity in model organisms as an overview of its hazard for human health and the environment. J. Hazard. Mater. 390:121974. doi: 10.1016/j.jhazmat.2019.121974
Trappe, J. M. (1982). Synoptic keys to the genera and species of zygomycetous mycorrhizal fungi. Phytopathology 72, 1102–1108.
Tufail, M. S., and Liaqat, I. (2021). Silver nanoparticles and their applications-a comprehensive review. Pure Appl. Biol. 11, 315–330.
Usman, M., Farooq, M., Wakeel, A., Nawaz, A., Cheema, S. A., Rehman, H., et al. (2020). Nanotechnology in agriculture: current status, challenges and future opportunities. Sci. Total Environ. 721:137778. doi: 10.1016/j.scitotenv.2020.137778
Vazquez-Muñoz, R., Meza-Villezcas, A., Fournier, P. G. J., Soria-Castro, E., Juarez-Moreno, K., Gallego-Hernández, A. L., et al. (2019). Enhancement of antibiotics antimicrobial activity due to the silver nanoparticles impact on the cell membrane. PLoS One 14:e0224904. doi: 10.1371/journal.pone.0224904
Verma, V. C., Kharwar, R. N., and Gange, A. C. (2010). Biosynthesis of antimicrobial silver nanoparticles by the endophytic fungus.
Vrček, I. V., Žuntar, I., Petlevski, R., Pavičić, I., Dutour Sikirić, M., Ćurlin, M., et al. (2016). Comparison of in vitro toxicity of silver ions and silver nanoparticles on human hepatoma cells. Environ. Toxicol. 31, 679–692. doi: 10.1002/tox.22081
War, J. M., Wani, A. H., Nisa, A. U., and Bhat, M. Y. (2022). Green synthesis, characterization and in vitro antimicrobial activity of silver nanoparticles (AgNPs) using fungal aqueous extract. Nano 17:2250097. doi: 10.1142/S1793292022500977
Williams, K. M., Gokulan, K., Cerniglia, C. E., and Khare, S. (2016). Size and dose dependent effects of silver nanoparticle exposure on intestinal permeability in an in vitro model of the human gut epithelium. J. Nanobiotechnol. 14, 1–13. doi: 10.1186/s12951-016-0214-9
Keywords: antimycotic, antifungal, Cladosporium cladosporioides, Rhizoctonia solani, silver nanoparticles
Citation: Malik MA, Wani AH, Bhat MY, Siddiqui S, Alamri SAM and Alrumman SA (2024) Fungal-mediated synthesis of silver nanoparticles: a novel strategy for plant disease management. Front. Microbiol. 15:1399331. doi: 10.3389/fmicb.2024.1399331
Edited by:
Anju Rani, Graphic Era University, IndiaReviewed by:
Pranab Dutta, Central Agricultural University, Imphal, IndiaSaurabh Gangola, Graphic Era Hill University, India
Copyright © 2024 Malik, Wani, Bhat, Siddiqui, Alamri and Alrumman. This is an open-access article distributed under the terms of the Creative Commons Attribution License (CC BY). The use, distribution or reproduction in other forums is permitted, provided the original author(s) and the copyright owner(s) are credited and that the original publication in this journal is cited, in accordance with accepted academic practice. No use, distribution or reproduction is permitted which does not comply with these terms.
*Correspondence: Mansoor Ahmad Malik, bWFsaWttYW5zb29yMDExQGdtYWlsLmNvbQ==