- 1Department of Chemical, Biological, Pharmaceutical, and Environmental Science, University of Messina, Messina, Italy
- 2Department of Biomedical and Dental Science and Morphofunctional Imaging, University of Messina, Messina, Italy
Increased global research is focused on the development of novel therapeutics to combat antimicrobial and antiviral resistance. Pistachio nuts represent a good source of protein, fiber, monounsaturated fatty acids, minerals, vitamins, and phytochemicals (carotenoids, phenolic acids, flavonoids and anthocyanins). The phytochemicals found in pistachios are structurally diverse compounds with antimicrobial and antiviral potential, demonstrated as individual compounds, extracts and complexed into nanoparticles. Synergistic effects have also been reported in combination with existing drugs. Here we report an overview of the antimicrobial and antiviral potential of pistachio nuts: studies show that Gram-positive bacterial strains, such as Staphylococcus aureus, are the most susceptible amongst bacteria, whereas antiviral effect has been reported against herpes simplex virus 1 (HSV-1). Amongst the known pistachio compounds, zeaxanthin has been shown to affect both HSV-1 attachment penetration of human cells and viral DNA synthesis. These data suggest that pistachio extracts and derivatives could be used for the topical treatment of S. aureus skin infections and ocular herpes infections.
1 Introduction
Tackling antimicrobial resistance (AMR), considered a threat to global human health, is a key research priority. The term ‘antimicrobial’ includes antibiotic, antiprotozoal, antiviral and antifungal medicines. AMR causes an estimated death of at least 1.27 million people worldwide and was associated with nearly 5 million deaths in 2019, according to a recent report released in The Lancet (Murray et al., 2022). In the U.S., more than 2.8 million antimicrobial-resistant infections occur each year, resulting in the death of more than 35,000 people (CDC, 2019). The estimated national cost in the U.S. to treat infections caused by multidrug-resistant pathogens frequently found in hospital environments can be substantial, leading to more than $4.6 billion annually. Dedicated prevention and infection control efforts can reduce the impact of antimicrobial-resistant infections, lowering deaths by an average of 18% (and by nearly 30% in hospitals in the U.S. in 2019). However, the COVID-19 pandemic has harmed recent AMR prevention and control. Therefore, the United Nations and the World Health Organization are leading initiatives to raise awareness of the problem and deliver solutions to protect our ability to fight infectious diseases globally. Therefore, the discovery of novel therapeutics with antimicrobial and antiviral effects, to be used either alone or in combination with existing drugs, is warranted.
It is well known that plant extracts represent an important source of bioactive compounds, mainly secondary metabolites, which could be used for their antimicrobial and antiviral potential. Tree nuts are known to contain an array of phytochemicals with potential health benefits (Gervasi et al., 2021). However, the phytochemical content of tree nuts can vary considerably, and depends on nut species, genotype, pre- and post-harvest conditions, and storage conditions (Bolling et al., 2011). Additionally, processing approaches such as roasting, may affect tree nut phytochemicals. For example, the total phenol content and the ferric-reducing antioxidant power (FRAP) value both decreased in almond skins after roasting, although the flavonoids concentration was not affected (Bolling et al., 2010a). The choice of solvents used for polyphenols and phytosterols extraction also significantly affects their quantification, and biological activity (Ghırardello et al., 2010). Qasimi et al. (2016) tested the effect of five different solvents (water, 80% methanol, 80% ethanol, acetone and chloroform) on the polyphenols and proanthocyanidin quantification as well as the biological potential [total phenolic (TPC), total flavonoid (TFC, antioxidant capacity using DPPH radical scavenging and ferric reducing antioxidant power (FRAP) activities] of medicinal alophytes: results showed that 80% methanol was the most effective solvent, followed by ethanol and water. Pistachio (Pistacia vera L.) nuts represent a good source of protein, fiber, monounsaturated fatty acids, minerals, and vitamins, as well as carotenoids, phenolic acids, flavonoids and anthocyanins (Mandalari et al., 2021). The phytochemical fraction in pistachios is known to contribute to their antimicrobial and antiviral effect and could help overcome AMR (Mandalari et al., 2021).
Here, we present an overview of the antimicrobial and antiviral potential of pistachio nuts. In addition, the evaluation of pistachio polyphenols, alone or in combination with existing drugs, is described in this review in an attempt to provide novel tools to combat AMR.
2 Antimicrobial and antiviral properties of pistachio bioactives
Pistachios are known to contain an array of bioactive compounds, which include carotenoids1 [beta-carotene, alpha-carotene, lutein and zeaxanthin, chlorophylls (chlorophyll a, chlorophyll b, pheaphytin a)] (Giuffrida et al., 2006; Bellomo and Fallico, 2007), gamma-tocopherol, gamma-tocotrienol, phytosterols1 (campsterol, beta-sitosterol, stigmasterol), phenolic acids1,2 (Neveu et al., 2010), resveratrol (Tokuşoglu et al., 2005; Gentile et al., 2007; Grippi et al., 2008), flavonoids1,2 (Tomaino et al., 2010), anthocyanins (cyanidin-3-galactoside, cyanidin-3-glucoside) (Seeram et al., 2008), proanthocyanidins (PAC) and stilbenes1,3, isoflavones (genistein, genistein-7-O-glucoside, dadzein) (Bulló et al., 2015), as well as phytates, sphingolipids, alkylphenols and lignans (Bolling et al., 2010b). The total phenolic content (TPC, mg gallic acid equivalent/100 g, fresh weight) in pistachios has been reported to between 1657 (USDA) and 1,420 (Phenol-Explorer), where the most abundant phenolic compounds were: daidzein, genistein, quercetin, eriodictyol, luteolin, naringenin, and cyanidins (amongst flavonoids); gallic acid and derivatives including gallotannins; alkyl phenols4,5 (anacardic acid derivatives) (Yang et al., 2009).
Current research shows the principal health-related properties of bioactive compounds found in pistachios including their antioxidant and cytoprotective effects, their effect on cell redox homeostasis, as well as their anti-inflammatory and anti-cancer effects, neuroprotective, anti-obesity and anti-diabetic effects (Maestri, 2023). Additionally, a growing body of research has documented antimicrobial and antiviral properties associated with numerous pistachio compounds, as reported in Table 1 (carotenoids, chlorophylls, gamma tocopherol, phytosterols and resveratrol) and Table 2 (flavonoids, isoflavones, anthocyanins and proanthocyanidins). Here, we discuss the antimicrobial and antiviral properties of each of these classes of compounds in more detail.
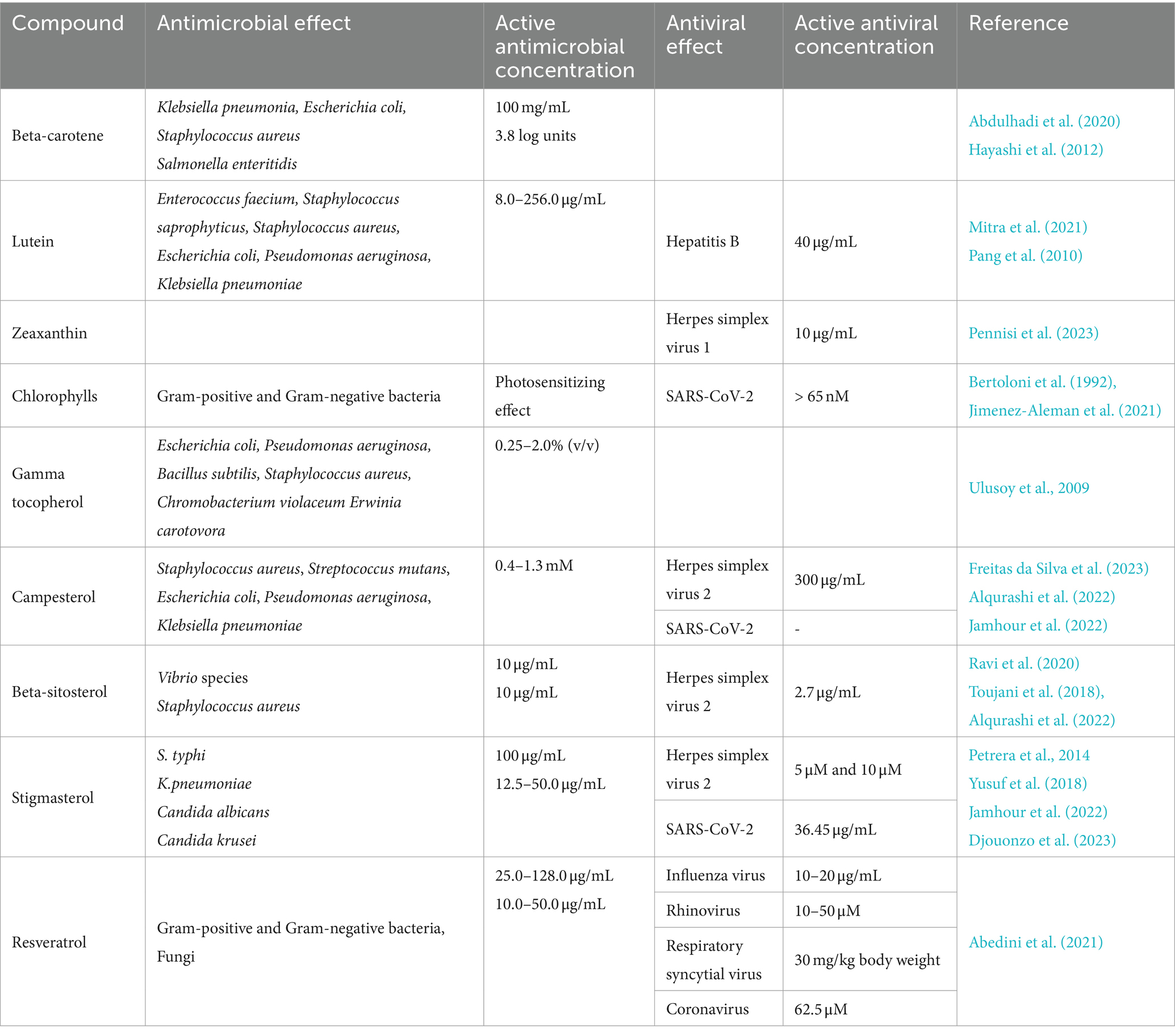
Table 1. Antimicrobial and antiviral effects of pistachio carotenoids, chlorophylls, gamma tocopherol, phytosterols and resveratrol.
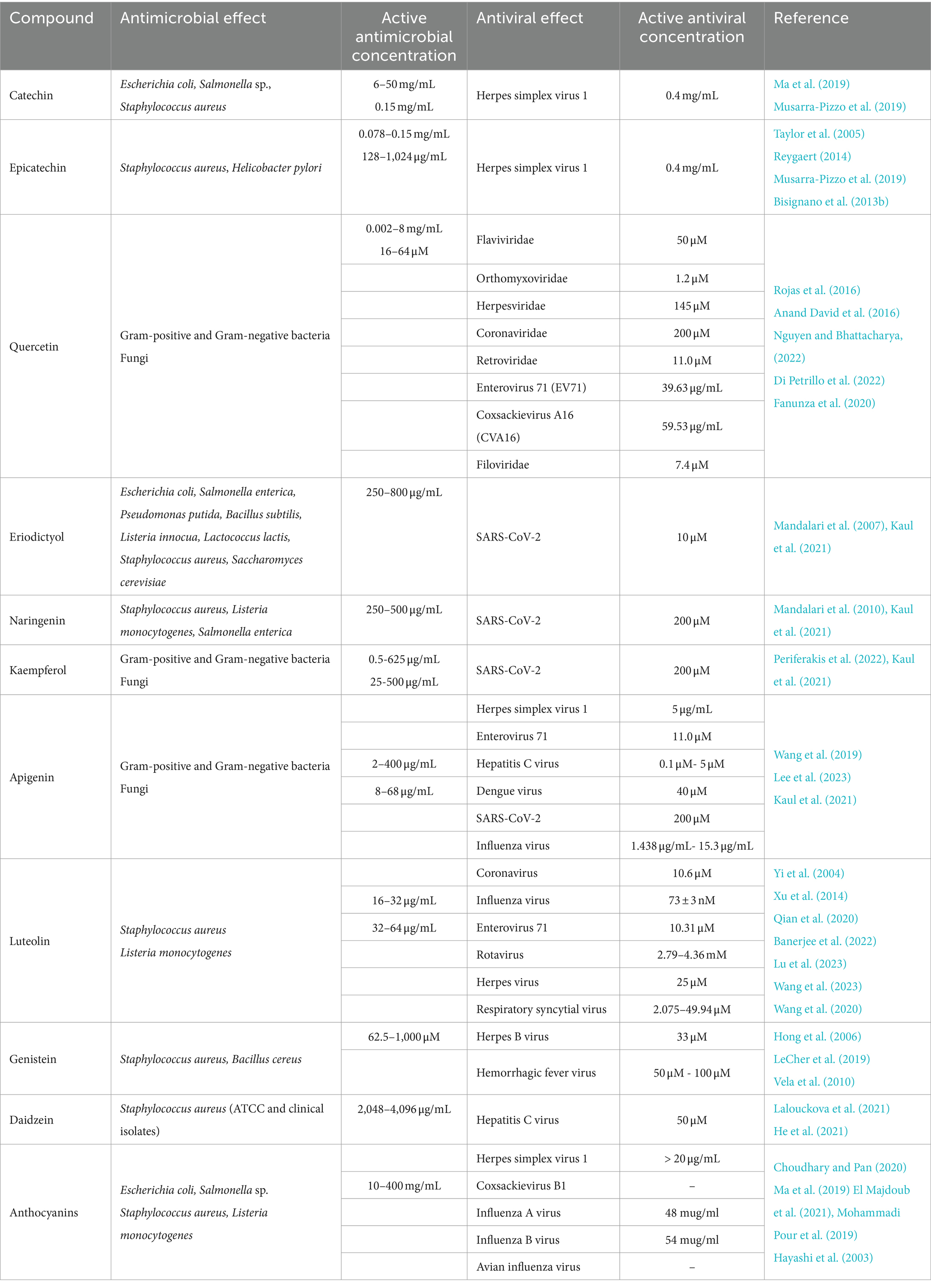
Table 2. Antimicrobial and antiviral effects of pistachio flavonoids, isoflavones, anthocyanins and proanthocyanidins.
2.1 Carotenoids
Carotenoids are tetraterpene pigments which exhibit orange, yellow, red and purple colours. Amongst the carotenoids present in pistachio nuts, β-carotene at a concentration of 100 mg/mL showed the best antimicrobial activity against the bacterial species Klebsiella pneumoniae (inhibition zone diameter of 40 mm), followed by Escherichia coli and Staphylococcus aureus (inhibition zone diameter of 36 and 31 mm, respectively, Abdulhadi et al. (2020)). However, no effect was shown against Pseudomonas aeruginosa. In addition, β -carotene has been found to induce an increase from 1.4 to 3.8 log units in the bactericidal activity of a bovine lactoperoxidase system, evaluated using Salmonella enteritidis (Hayashi et al., 2012).
According to Mitra et al. (2021), the carotenoid pigment lutein was able to inhibit both the growth and the proliferation of several Gram-positive and Gram-negative bacteria, such as Enterococcus faecium, S. saprophyticus, S. aureus, E. coli, P. aeruginosa, K. pneumoniae, at concentrations varying from 8 to 256 μg/mL. The in vitro antiviral activity of lutein against the hepatitis B virus (HBV) has been reported by Pang et al. (2010). The antiviral functions of lutein have also been investigated in stable HBV-producing human hepatoblastoma HepG2 2.2.15 cells, where it efficiently suppressed the dose-dependent secretion of HBsAg and inhibited extracellular HBV DNA. We have recently reported that zeaxanthin, a dietary carotenoid that accumulates in the retina as a macular pigment, exhibits strong antiviral activity against herpes simplex virus 1 (HSV-1, CC50: 16.1 μM, EC50 4.08 μM, SI 3.96), affecting viral attachment and penetration as well as viral DNA synthesis (Pennisi et al., 2023). An overview of the use of carotenoids as therapeutic strategies against emerging viral diseases, such as COVID-19, has recently been published (Khalil et al., 2021). The inhibitory activity of two marine carotenoids blocking the entry of SARS-CoV-2 has also been reported (Yim et al., 2021).
2.2 Tocopherols
Tocopherols are a class of organic fat-soluble phenolic compounds, many of which have vitamin E activity. The antibacterial potential of gamma-tocopherol has been demonstrated in flower extracts of Damask rose (Rosa damascena Mill), which contained beta-carotene, alpha-tocopherol and phenolic compounds as well as gamma-tocopherol: results demonstrated a strong antibacterial activity against E. coli (ATCC 25922), P. aeruginosa (ATCC 27853), Bacillus subtilis (ATCC 6633), S. aureus (ATCC 6538), Chromobacterium violaceum (ATCC 12472) and Erwinia carotovora (ATCC 39048) strains (Ulusoy et al., 2009).
2.3 Chlorophylls
The susceptibility of Gram-positive and Gram-negative bacteria to photodynamic therapy using natural chlorophylls, the green photosynthetic pigments found in plants, has been widely investigated (Bertoloni et al., 1992; Merchat et al., 1996; Minnock et al., 1996). The use of derivatives of natural chlorophylls as agents for antimicrobial photodynamic therapy has recently been reviewed also in relation to their effect against bacterial biofilm, which is known to be highly resistant to antibiotic treatment (Suvorov et al., 2021). A chlorophyll derivative pheophorbide A (PheoA), a porphyrin compound similar to animal Protoporphyrin IX, has shown an antiviral activity against SARS-CoV-2, preventing infection of cultured monkey and human cells, without noticeable cytotoxicity (Jimenez-Aleman et al., 2021).
2.4 Phytosterols
Phytosterols are plant-based compounds similar to cholesterol. Using the microdilution method, the phytosterol campsterol displayed a weak antibacterial effect in vitro against S. aureus (ATCC 6538), Streptococcus mutans (ATCC 0046), E. coli (ATCC 10536), P. aeruginosa (ATCC 15442), and K. pneumoniae (ATCC 10031), with minimum inhibitory concentration (MIC) values of 1.280 mM (Freitas da Silva et al., 2023). Through computational analyses, it was proposed that β-sitosterol exhibited antibacterial activity against several bacterial Vibrio species and could be used in aquaculture, both as a nutritional supplement and also as a disease control agent to prevent and control fish diseases caused by bacterial infection (Ravi et al., 2020). Furthermore, β-sitosterol isolated from the leaves of the South American firespike plant (Odontonema strictum) has been found to be active against S. aureus, showing both a bacteriostatic and a bactericidal effect (Pierre Luhata and Usuki, 2021). Yusuf et al. (2018) have demonstrated the antibacterial and the antifungal activity of stigmasterol isolated from the stem bark of the African tree species Neocarya macrophylla (Yusuf et al., 2018).
Seed oil from the prickly pear (Opuntia ficus-indica) containing phytosterols, primarily campesterol, followed by γ- & β -sitosterol, and stigmasterol, has been shown to exhibit an antiviral effect (22.67 ± 2.79%) at 300 μg/mL of oil against herpes simplex type 2 (HSV-2) virus (Alqurashi et al., 2022). After rutin, stigmasterol and campesterol were shown to be the most prominent inhibitors for SARS-CoV-2 proteins using an in-silico approach (Jamhour et al., 2022).
2.5 Resveratrol
Resveratrol is a natural phenolic compound with antioxidant-like properties. A comprehensive study on the antimicrobial and antiviral properties of resveratrol as an alternative therapy has recently been published by Abedini et al. (2021) (Abedini et al., 2021). Resveratrol has been shown to inhibit the growth of numerous bacteria detrimental to human health, including B. cereus species (at a concentration of 50 μg/mL) (Paulo et al., 2010), Mycobacterium smegmatis (64 μg/mL) (Lechner et al., 2008), Helicobacter pylori (25–50 μg/mL) (Makobongo et al., 2014), Vibrio cholerae (60 μg/mL) (Augustine et al., 2014), Neisseria gonorrhoeae (75 μg/mL) (Vestergaard and Ingmer, 2019), Campylobacter coli (50 μg/mL) (Duarte et al., 2015), and Arcobacter cryaerophilus (50 μg/mL) (Ferreira et al., 2014). An antifungal activity of resveratrol has been reported in vitro against the yeast Candida albicans at a concentration of 20 μg/mL (Lee and Lee, 2015). Furthermore, resveratrol has been shown to have inhibitory activity against viral replication and viral-induced inflammation by several respiratory viruses, including influenza virus (Pourghanbari et al., 2016), respiratory syncytial virus (Liu et al., 2014), coronavirus (SARS-CoV and MERS-CoV) (Filardo et al., 2020), and rhinovirus (Mastromarino et al., 2015).
2.6 Flavonoids
Flavonoids are polyphenolic secondary metabolites found in plants. Extensive scientific literature is available on the antimicrobial and antiviral properties of catechins and epicatechins (Taylor et al., 2005; Reygaert, 2014). For example, epicatechin-3-gallate, epigallocatechin, and epigallocatechin-3-gallate have been shown to have antimicrobial effects against a variety of bacteria, including S. aureus, methicillin-resistant S. aureus (MRSA) and E. coli (Reygaert, 2014). Taylor et al. (2005) demonstrated that low concentrations of epicatechin gallate can sensitize MRSA clinical isolates to levels of oxacillin which can be readily achieved in clinical practice.
We have previously demonstrated the antibacterial effect of catechin and epicatechin against S. aureus ATCC 6538P (MIC values of 0.078–0.15 and 0.15 mg/mL, respectively) and H. pylori (both ATCC strains and clinical isolates, Bisignano et al. (2013b)) and the antiviral activity (decrease in the viral titer ** p < 0.01, and viral DNA accumulation * p < 0.05) of a polyphenols mix containing catechin, naringenin-7-O-glucoside, kaempferol-3-O-glucoside, epicatechin, isorhamnetin-3-O-rutinoside, and isorhamnetin-3-O-glucoside against HSV-1 (Musarra-Pizzo et al., 2019). Through Western blot, real-time polymerase chain reaction (PCR) and viral binding assay, we also demonstrated that polyphenols were able to block the production of infectious HSV-1 particles and inhibited HSV-1 adsorption to Vero cells (Bisignano et al., 2017).
The antimicrobial and antiviral activity of quercetin, and the possible mechanism of action, have recently been reviewed (Di Petrillo et al., 2022; Nguyen and Bhattacharya, 2022). Strong growth inhibition of different Gram-positive and Gram-negative bacteria has been reported, particularly affecting gastrointestinal, respiratory, urinary, and dermal system (Anand David et al., 2016) as well as the yeast C. albicans (Singh et al., 2015; Gao et al., 2016) the fungi Aspergillus fumigatus (Yin et al., 2021) and Aspergillus niger (Abd-Allah et al., 2015) and several viruses, such as the human immunodeficiency virus (HIV)-1 strain (Kim et al., 1998), the herpes simplex and the respiratory syncytial virus (Cushnie and Lamb, 2005), the polio-virus type 1 (Kaul et al., 1985) and the influenza virus (Liu et al., 2016). The mechanism of quercetin antimicrobial action includes cell membrane damage, change of membrane permeability, inhibition of synthesis of nucleic acids and proteins, reduction of expression of virulence factors, mitochondrial dysfunction, and prevention of biofilm formation.
We have previously reported the antibacterial potential of eriodictyol and naringenin: eriodictyol was more effective (MICs in the range of 250 and 800 μg/mL) compared with other tested flavonoids, such as naringenin, against a range of Gram-positive and Gram-negative bacteria (E. coli, Salm. enterica, P. putida, B. subtilis, L. innocua, Lactococcus lactis, S. aureus) and the yeast Saccharomyces cerevisiae (Mandalari et al., 2007). On the other hand, naringenin was active against S. aureus ATCC 6538P, L. monocytogenes ATCC 7466 and Salm. enterica ser. Typhimurium ATCC 14028 (MIC values of 250, 500 and 250 μg/mL), respectively (Mandalari et al., 2010). Periferakis et al. (2022) reviewed the antibacterial and antifungal properties of kaempferol. Pure kaempferol, kaempferol extracts and nanoparticles loaded with kaempferol have shown activity against Gram-negative bacteria, including Acinetobacter baumannii (Özçelik et al., 2006; Rofeal et al., 2021), Enterobacter cloacae (Christopoulou et al., 2008), Enterobacter aerogenes (Karimi et al., 2011), E. coli (Özçelik et al., 2006; Kannanoor et al., 2021), K. pneumoniae (Habbu et al., 2009), P. aeruginosa (Attallah et al., 2022), Vibrio cholerae (Martini et al., 2004), as well as Gram-positive bacteria, including S. aureus (Özçelik et al., 2006), Streptococcus pyogenes (Özçelik et al., 2006), Bacillus sp. (Youssef et al., 2021), and Mycobacterium sp. (Nguyen et al., 2021). Moreover, both isolated kaempferol-3-O-[3-O-acetyl-6-O-(E)-p-coumaroyl]-b-d-glucopyranoside and kaempferol 3-O-b-D-kaempferol 3-O-b-D-glucopyranoside, were found to be active in vitro against C. albicans, Candida glabrata and Candida tropicalis (Christopoulou et al., 2008).
Eriodictyol, naringenin and kaempferol have been identified as promising antiviral compounds against SARS-CoV-2, both alone and together existing antiviral drugs, targeting specifically the promising 3C-like protease (3CLpro) (Kaul et al., 2021).
A wide range of in vitro antibacterial activity has been reported for apigenin by Wang et al. (2019): apigenin was active against Acinetobacter baumannii (with MIC values between 2 and 64 μg/mL), Bacillus subtilis (MIC values between 8 and 16 μg/mL), Enterococcus faecalis ATCC 29212 (MIC = 8 μg/mL), E. coli ATCC 35818 (MIC = 4 μg/mL), K. pneumoniae RSKK 574 (MIC = 8 μg/mL) and ESβL+ clinical isolate (MIC = 128 μg/mL), Proteus mirabilis ATCC 7002, (MIC = 4 μg/mL), S. aureus ATCC 25923 (MIC = 16 μg/mL), and C. albicans ATCC 10231 (MIC = 8 μg/mL) (Özçelik et al., 2011). The therapeutic potential of apigenin against viral infection has recently been reviewed by Lee et al. (2023). Apigenin exerts virucidal activity against HSV-1 interfering with viral absorption and inhibiting the post-entry step of the viral replication (Lyu et al., 2005; Yucharoen, 2011; Visintini Jaime et al., 2013; Fahmy et al., 2020; Rittà et al., 2020), against Enterovirus 71 by inhibiting the interaction between internal ribosome entry site of EV71 and hnRNP A1 and A2 (Shih et al., 2011; Lv et al., 2014; Zhang et al., 2014; Ji et al., 2015; Dai et al., 2019), against hepatitis C virus by binding to NS5B and inhibiting RdRp activity, decreasing miR122 expression levels and suppressing the phosphorylation of TRBP (Manvar et al., 2012; Ohno et al., 2013; Pisonero-Vaquero et al., 2014; Shibata et al., 2014), against Dengue virus by restoring STAT2 Tyr 689 phosphorylation and activation, colocalization with a DENV protein in the early phase of infection (Mazzon et al., 2009; Jasso-Miranda et al., 2019; Acchioni et al., 2023), against SARS-CoV by reducing the production of proinflammatory cytokine in response to viral infection, as well as interacting with viral protein (Mpro) and host factor (ACE-2 receptor, TMPRSS2) (Ryu et al., 2010; Jo et al., 2020; Zhang et al., 2020; Alzaabi et al., 2022; Chaves et al., 2022; Farhat et al., 2022) as well as against influenza virus - by neuroaminidase inhibition, viral attachment and entry inhibition, inhibition of viral mRNA expression, inhibition of influenza A virus RdRP activity, reduction of viral particle production, and nucleoprotein reduction (Liu et al., 2008; Kai et al., 2014; Xu et al., 2020a, 2020b; Joo et al., 2022; Morimoto et al., 2023).
Amongst the flavanones, luteolin has also shown antibacterial potential against S. aureus and L. monocytogenes in vitro, by impairment effect on the cell membrane and restraining biofilm formation of both strains (Qian et al., 2020). A literature review on the antiviral mechanism of luteolin has recently been published by Lu et al. (2023): luteolin effectively inhibited coronavirus replication (Alzaabi et al., 2022; Chen et al., 2022), influenza virus (Lee et al., 2016), enterovirus (Chen et al., 2008; Cao et al., 2016), rotavirus (Knipping et al., 2012), herpes virus (Lu et al., 2023), and respiratory syncytial virus (Wang et al., 2020). In particular, it prevented viral infection by improving the host’s nonspecific immunity and antioxidation capacity, thus inhibiting several pathways related to viral infection, including MAPK, PI3K-AKT, TLR4/8, NF-κB, and Nrf-2/hemeoxygenase-1. Furthermore, luteolin was able to regulate the expression of specific receptors and factors, interfering with viral replication and thus promoting the repair of damaged cells induced by proinflammatory factors (Lu et al., 2023).
Hong et al. (2006) demonstrated in vitro the antibacterial potential of the isoflavone genistein against the Gram-positive S. aureus and Bacillus anthracis strains (Hong et al., 2006). Furthermore, an investigation on the mechanism of action of genistein indicated altered cell morphology (formation of filamentous cells) on bacterial cells, together with an inhibition of DNA and RNA synthesis as shortly as 15 min after addition to a bacterial culture. Protein synthesis inhibition was also detected (Ulanowska et al., 2006). Genistein has also been shown to possess antiviral activity against the herpes B virus, acting synergistically with existing antiviral drugs (LeCher et al., 2019). An effect of genistein as a general kinase inhibitor against an arenaviral haemorrhagic fever surrogate virus has also been demonstrated (Vela et al., 2010).
The antibacterial and antifungal effect of the isoflavone daidzein has been reported against S. aureus (both ATCC and clinical strains) by Lalouckova et al. (2021). Dietary daidzein was able to inhibit hepatitis C virus replication by decreasing microRNA-122 levels (He et al., 2021).
2.7 Anthocyanins
Anthocyanins are a group of red and blue pigments found in plants and along with catechins, form subgroups within the flavonoids. The antimicrobial activity of anthocyanins and catechins against the foodborne pathogens E. coli and Salmonella sp. has been reported, with MIC values between 10–400 mg/mL (Ma et al., 2019). Mechanistically, anthocyanins can act as an antibacterial by destroying the cell wall of foodborne pathogens: anthocyanins extracted from the Assegai tree (Curtisia dentata) were able to destroy the E. coli cell wall (Doughari et al., 2012), whereas in another study, anthocyanins extracted from lowbush wild blueberries were able to destroy the cell membranes of E. coli O157: H7, with consequent cytoplasmic leakage (Lacombe et al., 2013). Although anthocyanins are active against different bacterial strains, Gram-positive bacteria are usually more susceptible than Gram-negative bacteria (Cisowska et al., 2011). We have demonstrated the effect of an anthocyanin extract from the flowering plant Roselle (Hibiscus sabdariffa L.) containing cyanidin-3-O-sambubioside and delphinidin-3-O-sambubioside against S. aureus ATCC 6538 and against a food isolate of L. monocytogenes. The effect was bacteriostatic against S. aureus (MIC = 2.5 mg/mL) and bactericidal against L. monocytogenes (Minimal Bactericidal Concentration, MBC = 2.5 mg/mL) (El Majdoub et al., 2021).
The antiviral potential of anthocyanidins has been recently reviewed by Mohammadi Pour et al. (2019): a total anthocyanins extract from strawberry (Fragaria x ananassa) was active against HSV-1 (Simões et al., 2012), whereas an anthocyanins extract from wild strawberry (Fragaria vesca), raspberry (Rubus idaeus), blueberry (Vaccinium myrtillis) and lingonberry (Vaccinium vitis-idaea) was active against coxsackievirus B1 (CV-B1) and influenza A virus (Nikolaeva-Glomb et al., 2014). Specifically, cyanidin-3-galactoside, contained in pistachios, was active against the influenza A, influenza B and Avian influenza viruses by Increasing the NK cell activity and enhancing the immune system responses (Niemenak et al., 2006).
These reports illustrate the extensive antimicrobial and antiviral effects of pistachio phytochemicals, isolated or within plant extracts, alone and combined with existing drugs. In terms of antibacterial activity, Gram-positive strains were usually more susceptible than Gram-negative bacteria, with a mostly bacteriostatic rather than bactericidal effect. Amongst the Gram-positive bacterial strains tested, ATCC and clinical isolates of S. aureus were more susceptible to the effect of bioactive compounds. S. aureus and MRSA are known to be responsible of various infections, including biofilm-associated diseases, ranging from skin, prostheses, catheters and other biomaterials infections to more serious systemic diseases, such as endocarditis, pneumonia, and osteomyelitis. Given the increased incidence of antibiotic resistant S. aureus infections, especially within hospital settings, the discovery of natural compounds effective against S. aureus produced biofilm is a promising area for further clinical research (Mandalari et al., 2023).
3 Antimicrobial effect of pistachios and mechanism of action
Table 3 reports the antimicrobial effect of pistachio extracts and essential oil. We have previously demonstrated that polyphenol-rich extracts of natural raw shelled and roasted salted pistachios were active in vitro against a range of Gram-positive bacteria, with a bactericidal effect against ATCC strains and food isolates of L. monocytogenes, S. aureus and MRSA (Bisignano et al., 2013a). Furthermore, we have phenotypically characterized clinical isolates of Staphylococcus spp. and tested these for their sensitivity against natural raw and roasted salted pistachios: both extracts were active against clinical isolates of Staphylococcus sp., as well as the S. aureus ATCC 6538P (La Camera et al., 2018). We have also demonstrated that polyphenol-rich extracts of natural raw shelled and roasted salted pistachios were effective against L. monocytogenes food isolate strains (MIC values between 0.25 and 2.0 mg/mL) and against L. monocytogenes ATCC 13932 (Gervasi et al., 2022). Furthermore, the oil fractions from natural and roasted pistachios were effective against L. monocytogenes ATCC 13932 and Enterococcus faecium DSZM 17050. Other authors have confirmed the effect of a methanolic extract of pistachios against staphylococcal infections (Gutiérrez-Morales et al., 2017). Pistacia vera L. oleoresin has been tested in combination with levofloxacin, demonstrating a protective effect against H. pylori infection in an in vivo model of Galleria mellonella (62 and 63% survival using oleoresin and levofloxacin, respectively) (Di Lodovico et al., 2019). Pistacia vera L. oleoresin was also effective against oral streptococci, such S. mutans, with a demonstrated anti-biofilm activity (Magi et al., 2018).
The essential oil from Pistacia vera L. hulls was bactericidal against a range of S. aureus strains and E. coli at a concentration of 7.11 mg/mL (Smeriglio et al., 2017). A fungicidal effect of pistachio essential oil was demonstrated against standard and clinical strains of Candida sp. at concentrations between 2.50 and 5.0 mg/mL, D-limonene and 3-Carene being the most active components (D’Arrigo et al., 2019). The inhibitory activity of pistachio hull essential oil has also recently been demonstrated against S. aureus, B. subtilis and Aspergillus flavus (Shahdadi et al., 2023).
Recently, the phytochemical contents, the antioxidant and antimicrobial activities of pistachio hull ethanolic (PVE) and aqueous (PVD) extracts obtained by microwave-assisted extraction (MAE) were investigated by Seker and Akbas (2023): both extracts showed antimicrobial potential against E. faecalis, S. aureus, Streptococcus uberis, B. cereus and B. subtilis, with MICs values between 0.8–49.0 and 9.6–82.5 mg/mL and MBC values ranging from 1.3–99.1 and 15.5–150.0 mg/mL for PVE and PVD, respectively (Seker and Akbas, 2023). The antibacterial properties of pistachio hull extracts have also been reviewed by Arjeh et al. (2020).
A pistachio hull extract has been used as a reducing and stabilizing agent with antibacterial and antifungal effects within copper nanoparticles (Bakhshi et al., 2021).
Overall, these studies demonstrate that pistachios extract and essential oil, alone or in association with existing drugs, could be considered good candidates for the development of novel drug formulations. Their activity against Gram-positive bacterial strains, including S. aureus, could be exploited to identify novel therapeutics with topical use (i.e., to treat skin infections).
Although further studies are warranted to evaluate the mechanisms of action involved in the observed effect exerted by pistachio extracts, Figure 1 reports some proposed molecular bacterial targets, as reviewed by Álvarez-Martínez et al. (Álvarez-Martínez et al., 2020). Polyphenols can target the bacterial cell wall, causing morphological damage to the cells, or destroying the structural integrity of the cell wall and intracellular matrix (Din et al., 2013; Pojer et al., 2013).
The different cell wall structure between Gram-positive and Gram-negative bacteria could explain the higher susceptibility of Gram-positive strains to phytochemical antimicrobial activity, given that the outer membrane of Gram-negative bacteria acts as a permeability barrier, therefore reducing the uptake of the phenolic compounds (Naz et al., 2007). Polyphenols can cause leakage by increased permeability of the bacterial membrane and the cell wall (Lambert et al., 2001; Wang et al., 2017). Specifically, catechins can cause destruction of the Gram-positive bacterial membranes through interaction with lipids, which determine phase separations (Reygaert, 2014). Furthermore, epicatechin, tannic acid, epigallocatechin gallate, quercetin and kaempferol demonstrated significant β -lactamase inhibitory activity, also in synergy with antibiotics such as ciprofloxacin and rifampicin (Lin et al., 2008; Bernal et al., 2010; Mandal et al., 2017). Other possible targets could be represented by cell surface adhesion proteins, membrane-bound enzymes and cell wall polypeptides (Naz et al., 2007). A bioactive fraction from the tree species Duabanga grandiflora fruit has been shown to inhibit the penicillin-binding protein 2a in MRSA strains (Santiago et al., 2015).
Regulation of bacterial gene expression has been proposed as an alternative mechanism of action by phytochemicals, either through modulation of transcription factors or direct interaction with DNA. The antibacterial effect of Caffeic Acid Phenethyl Ester (CAPE) against E. faecalis, L. monocytogenes and S. aureus has been also related to its ability to target RNA- and DNA-related molecules (Murtaza et al., 2014). Naringenin was able to bind the DNA of S. aureus ATCC 6538, resulting in major metabolic changes (Wang et al., 2017). Furthermore, the effect of phytochemicals on biofilm formation has been reported: for example, we have shown a dose-dependent effect of phloretin on biofilm production of S. aureus (Mandalari et al., 2023) and a dose-dependent effect of a white grape juice extract on biofilms formation of E. coli and Pseudomonas aeruginosa (Filocamo et al., 2015). Another antibacterial effect of phytochemicals is related to the alteration of the level of bacterial metabolites, proton and ion equilibrium and adenosine triphosphate (ATP) synthesis inhibition, which could determine cell death (Lin et al., 2005; Engels et al., 2011). Certain polyphenols, such as gallic or caffeic acid, could reduce cytochrome activity and, therefore, oxidative phosphorylation, thus inhibiting bacterial growth (Shetty and Wahlqvist, 2004; Kwon et al., 2007).
It is worth noting that the antibacterial effect of polyphenolic plant extracts is often the result of synergistic, indifferent, or antagonistic interactions among the individual compounds. For example, we have demonstrated that bergamot (Citrus bergamia) fractions and the pure phytochemical compounds, neohesperidin, hesperetin (aglycone), neoeriocitrin, eriodictyol (aglycone), naringin and naringenin (aglycone), were active against Gram-negative bacteria (E. coli, Pseudomonas putida, Salm. enterica) with MIC values in the range 200 to 800 μg/mL. However, pairwise combinations of eriodictyol, naringenin and hesperetin showed synergistic and indifferent interactions, dependent on the selected, tested organism (Mandalari et al., 2007). Moreover, isolated phytochemicals and extracts are being used in combination with traditional antibiotics to sensitize multidrug-resistant bacterial strains (Hatano et al., 2005; Betoni et al., 2006). We have demonstrated a synergistic and post-antibiotic effect of tobramycin in combination with tea tree (Melaleuca alternifolia) oil against S. aureus and E. coli (D’Arrigo et al., 2010). This strategy could represent a valuable tool to combat AMR more effectively.
4 Antiviral effect of pistachios and mechanism of action
Figure 2 illustrates the effectiveness of bioactive natural compounds against viral families. The antiviral potential of pistachio polyphenolic extracts, particularly against herpes simplex virus type 1 (HSV-1), has been demonstrated (Table 4). We have previously shown that natural raw pistachio extracts (NRRE) significantly reduced the expression of critical viral proteins, including ICP8 (infected cell polypeptide 8), UL42 (DNA polymerase processivity factor), and US11. This reduction was associated with a decrease in viral DNA synthesis, highlighting the extract’s inhibitory effects on HSV-1 replication (Musarra-Pizzo et al., 2020). More recently, we have further investigated the mechanisms involved in the anti-HSV-1 effect exerted by pistachio extracts (Figure 3): NRRE and roasted unsalted (RURE) pistachio polyphenols-rich extracts blocked virus binding on the cell surface, impaired viral DNA synthesis, and prevented the accumulation of viral proteins (Pennisi et al., 2023). Indeed, by testing six compounds present in pistachio polyphenolic extracts (α, β, and δ tocopherol, β-carotene, luteolin, and zeaxanthin), we have demonstrated that zeaxanthin inhibited HSV-1 replication by affecting both viral internalization and replication. Furthermore, zeaxanthin directly interacted with HSV-1 viral particles, leading to a significant reduction in HSV-1 replication (CC50 16.1, EC50 4.08 μM, SI 3.96). Additionally, Özçelik et al. (2005) explored the antiviral properties of 15 lyophilic extracts from various parts of Pistacia vera, including leaves, branches, stems, kernels, shell skins, and seeds. Their research identified kernel and seed extracts as having the most potent antiviral effects against herpes simplex and parainfluenza viruses. Specifically, the fresh kernel (PV-FK) and skin of processed-woody shell (PV-SPS) extracts demonstrated significant activity against DNA viruses, which well compared with acyclovir (Figure 3). The Gaziantep sample-unripe (PV-GU) extract also showed notable antiviral activity. On the other hand, some extracts displayed outstanding activity against RNA viruses, such as PV-GR (Gaziantep sample-ripe) and PV-GP (Gaziantep sample-processed). Chhoud et al. (2022) reported the anti-HSV-2 activity of extracts from Pistacia vera male floral buds: the aqueous and polysaccharide extracts from male flower buds exhibited a selectivity index (SI) of 29.12 and 20.25, respectively. The extracts showed virucidal activity against HSV-2, likely by altering the viral membrane or interacting with viral ligands, thus inhibiting their binding to receptors on target cells. Finally, pistachio extracts have shown activity against Coxsackie viruses (CV) B2, B3, B4, and B5. Specifically, two pistachio allergens, 2S albumin (Pis v 1) and 11S globulin (Pis v 2.0101), were found to inhibit these viruses (Taghizadeh et al., 2020).
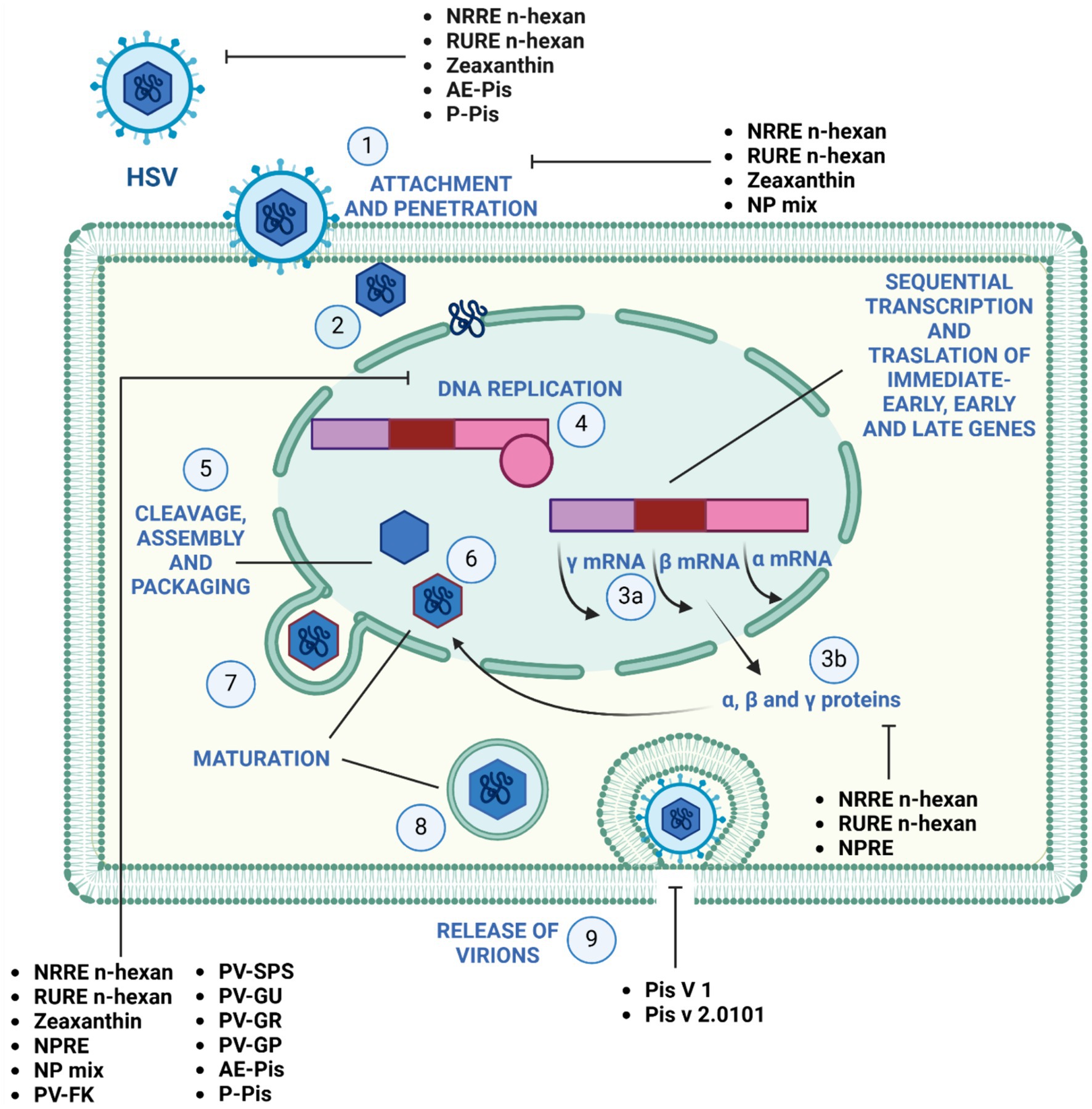
Figure 3. Mechanism of action of pistachio phytochemicals against Herpes simplex virus 1. (1) HSV-1 entry by attachment and penetration. (2) Release of viral DNA. (3) Sequential transcription (A) and translation (B) of viral immediate-early, early, and late genes. (4) DNA replication by rolling circle mechanism. (5) Assembly and packaging. (6) Maturation and (7) budding of the nucleocapsid out of the nucleus, (8) maturation and (9) release of the virions by exocytosis.
5 Conclusion
Due to the increased rates of resistance to antibiotics and antivirals, scientific research is continuously developing to find novel cost-effective alternatives to reduce hospitalization and mortality rates. Amongst natural compounds, the phytochemicals present in pistachio nuts have been shown to exhibit significant antibacterial and antiviral activity against resistant and non-resistant strains. Some findings suggest that the antimicrobial and antiviral effects of pistachio polyphenolic extracts are the result of a balance of the individual bioactive compounds which in combination exert the activity. The synergistic interaction of certain phytochemicals with selected antibiotics or antiviral drugs could be a useful tool to overcome resistance. Nevertheless, isolated compounds, such as zeaxanthin, exhibit strong antiviral activity against HSV-1, affecting viral attachment, penetration and viral DNA synthesis.
While the mechanism of action of pistachio extracts has been partly elucidated, further studies are required to identify more bioactive compounds responsible for the observed effect. However, based on the existing evidence, the use of pistachio extracts and derivatives should be encouraged for the topical treatment of S. aureus skin infections and ocular herpetic infections. Existing challenges in the development of antimicrobials from natural products, including cytotoxicity, production of highly active standardized extracts with defined mechanism of action under GMP conditions, and identification of bioactive components taking into account possible interaction amongst the individual compounds, should also be considered, together potential sustainability issues related to pistachio cultivation.
Author contributions
GM: Conceptualization, Data curation, Funding acquisition, Project administration, Writing – original draft, Writing – review & editing. RP: Writing – review & editing. TG: Writing – review & editing. MS: Writing – review & editing.
Funding
The author(s) declare that financial support was received for the research, authorship, and/or publication of this article. The work has been funded by the American Pistachio Growers (APG, United States).
Acknowledgments
The authors would like to express their gratitude to Paola Trischitta for her valuable graphical contribution.
Conflict of interest
The authors declare that the research was conducted in the absence of any commercial or financial relationships that could be construed as a potential conflict of interest.
Publisher’s note
All claims expressed in this article are solely those of the authors and do not necessarily represent those of their affiliated organizations, or those of the publisher, the editors and the reviewers. Any product that may be evaluated in this article, or claim that may be made by its manufacturer, is not guaranteed or endorsed by the publisher.
Abbreviations
AMR, Antimicrobial resistance; ATCC, American Type Culture Collection; ATP, Adenosine triphosphate; B. subtilis, Bacillus subtilis; C. albicans, C. albicans; EGC, Epigallocatechin; E. coli, Escherichia coli; FRAP, Ferric-reducing antioxidant power; H. pylori, Helicobacter pylori; HSV-1, Herpes simplex virus 1; HSV-2, Herpes simplex virus 2; K. pneumoniae, Klebsiella pneumoniae; L. innocua, Listeria innocua; L. monocytogenes, Listeria monocytogenes; MIC, Minimum inhibitory concentration; MBC, Minimum bactericidal concentration; MRSA, Methicillin-Resistant Staphylococcus aureus; NK, Natural killer; P. aeruginosa, Pseudomonas aeruginosa; P. putida, Pseudomonas putida; Salm. enterica, Salmonella enterica; S. aureus, Staphylococcus aureus; S. saprophyticus, Staphylococcus saprophyticus; TPC, Total phenolic content.
Footnotes
1. ^U.S. Department of Agriculture, Agricultural Research Service. FoodData Central. Available online: fdc.nal.usda.gov (Accessed December 16, 2023).
2. ^Nutrient Data Laboratory; Beltsville Human Nutrition Research Center Agricultural Research Service. USDA Database for the Flavonoid Content of Selected Foods Release 3.3. Available at: https://www.ars.usda.gov/ARSUserFiles/80400535/Data/Flav/Flav3.3.pdf (Accessed December 16, 2023).
3. ^Nutrient Data Laboratory; Beltsville Human Nutrition Research Center; Agricultural Research Service; USDA Database for the Proanthocyanidin Content of Selected Foods Release 2.12018 Available at: https://www.ars.usda.gov/ARSUserFiles/80400535/Data/PA/PA02-1.pdf (Accessed December 16, 2023).
4. ^US Department of Agriculture USDA, http://www.ars.usda.gov/nutrientdata.
5. ^Phenol-Explorer database http://phenol-explorer.eu/contents.
References
Abd-Allah, W. E., Awad, H. M., and AbdelMohsen, M. M. (2015). HPLC analysis of quercetin and antimicrobial activity of comparative methanol extracts of Shinus molle L. Int. J. Curr. Microbiol. App. Sci. 4, 550–558.
Abdulhadi, S. Y., Gergees, R. N., and Hasan, G. Q. (2020). Molecular identification, antioxidant efficacy of phenolic compounds, and antimicrobial activity of Beta-carotene isolated from fruiting bodies of Suillus sp. Karbala Int. J. Mod. Sci. 6. doi: 10.33640/2405-609X.1966
Abedini, E., Khodadadi, E., Zeinalzadeh, E., Moaddab, S. R., Asgharzadeh, M., Mehramouz, B., et al. (2021). A comprehensive study on the antimicrobial properties of resveratrol as an alternative therapy. Evid. Based Complement. Alternat. Med. 2021, 1–15. doi: 10.1155/2021/8866311
Acchioni, C., Acchioni, M., Mancini, F., Amendola, A., Marsili, G., Tirelli, V., et al. (2023). A cellular screening platform, stably expressing DENV2 NS5, defines a novel anti-DENV mechanism of action of Apigenin based on STAT2 activation. Virology 583, 1–13. doi: 10.1016/j.virol.2023.03.016
Alqurashi, A. S., Al Masoudi, L. M., Hamdi, H., and Abu Zaid, A. (2022). Chemical composition and antioxidant, antiviral, antifungal, antibacterial and anticancer potentials of Opuntia ficus-indica seed oil. Molecules 27:5453. doi: 10.3390/molecules27175453
Álvarez-Martínez, F. J., Barrajón-Catalán, E., Encinar, J. A., Rodríguez-Díaz, J. C., and Micol, V. (2020). Antimicrobial capacity of plant polyphenols against gram-positive Bacteria: A comprehensive review. Curr. Med. Chem. 27, 2576–2606. doi: 10.2174/0929867325666181008115650
Alzaabi, M. M., Hamdy, R., Ashmawy, N. S., Hamoda, A. M., Alkhayat, F., Khademi, N. N., et al. (2022). Flavonoids are promising safe therapy against COVID-19. Phytochem. Rev. 21, 291–312. doi: 10.1007/s11101-021-09759-z
Anand David, A., Arulmoli, R., and Parasuraman, S. (2016). Overviews of biological importance of quercetin: A bioactive flavonoid. Pharmacogn. Rev. 10, 84–89. doi: 10.4103/0973-7847.194044
Arjeh, E., Akhavan, H.-R., Barzegar, M., and Carbonell-Barrachina, Á. A. (2020). Bio-active compounds and functional properties of pistachio hull: A review. Trends Food Sci. Technol. 97, 55–64. doi: 10.1016/j.tifs.2019.12.031
Attallah, N. G. M., El-Sherbeni, S. A., El-Kadem, A. H., Elekhnawy, E., El-Masry, T. A., Elmongy, E. I., et al. (2022). Elucidation of the metabolite profile of Yucca gigantea and assessment of its cytotoxic, antimicrobial, and anti-inflammatory activities. Molecules 27:1329. doi: 10.3390/molecules27041329
Augustine, N., Goel, A. K., Sivakumar, K. C., Ajay Kumar, R., and Thomas, S. (2014). Resveratrol – A potential inhibitor of biofilm formation in Vibrio cholerae. Phytomedicine 21, 286–289. doi: 10.1016/j.phymed.2013.09.010
Bakhshi, O., Bagherzade, G., and Ghamari kargar, P. (2021). Biosynthesis of organic nanocomposite using Pistacia vera L. Hull: An efficient antimicrobial agent. Bioinorg. Chem. Appl. 2021, 1–18. doi: 10.1155/2021/4105853
Banerjee, S., Sarkar, R., Mukherjee, A., Miyoshi, S. I., Kitahara, K., Halder, P., et al. (2022). Quercetin, a flavonoid, combats rotavirus infection by deactivating rotavirus-induced pro-survival NF-κB pathway. Front. Microbiol. 13:951716. doi: 10.3389/fmicb.2022.951716
Bellomo, M. G., and Fallico, B. (2007). Anthocyanins, chlorophylls and xanthophylls in pistachio nuts (Pistacia vera) of different geographic origin. J. Food Compos. Anal. 20, 352–359. doi: 10.1016/j.jfca.2006.04.002
Bernal, P., Lemaire, S., Pinho, M. G., Mobashery, S., Hinds, J., and Taylor, P. W. (2010). Insertion of Epicatechin Gallate into the cytoplasmic membrane of methicillin-resistant Staphylococcus aureus disrupts penicillin-binding protein (PBP) 2a-mediated β-lactam resistance by delocalizing PBP2. J. Biol. Chem. 285, 24055–24065. doi: 10.1074/jbc.M110.114793
Bertoloni, G., Rossi, F., Valduga, G., Jori, G., Ali, H., and van Lier, J. E. (1992). Photosensitizing activity of water- and lipid-soluble phthalocyanines on prokaryotic and eukaryotic microbial cells. Microbios 71, 33–46
Betoni, J. E. C., Mantovani, R. P., Barbosa, L. N., Di Stasi, L. C., and Fernandes Junior, A. (2006). Synergism between plant extract and antimicrobial drugs used on Staphylococcus aureus diseases. Mem. Inst. Oswaldo Cruz 101, 387–390. doi: 10.1590/S0074-02762006000400007
Bisignano, C., Filocamo, A., Faulks, R. M., and Mandalari, G. (2013a). In vitro antimicrobial activity of pistachio (Pistacia vera L.) polyphenols. FEMS Microbiol. Lett. 341, 62–67. doi: 10.1111/1574-6968.12091
Bisignano, C., Filocamo, A., La Camera, E., Zummo, S., Fera, M. T., and Mandalari, G. (2013b). Antibacterial activities of almond skins on cagA-positive and-negative clinical isolates of Helicobacter pylori. BMC Microbiol. 13:103. doi: 10.1186/1471-2180-13-103
Bisignano, C., Mandalari, G., Smeriglio, A., Trombetta, D., Pizzo, M., Pennisi, R., et al. (2017). Almond skin extracts abrogate HSV-1 replication by blocking virus binding to the cell. Viruses 9:178. doi: 10.3390/v9070178
Bolling, B. W., Blumberg, J. B., and Oliver Chen, C.-Y. (2010a). The influence of roasting, pasteurisation, and storage on the polyphenol content and antioxidant capacity of California almond skins. Food Chem. 123, 1040–1047. doi: 10.1016/j.foodchem.2010.05.058
Bolling, B. W., Chen, C.-Y. O., McKay, D. L., and Blumberg, J. B. (2011). Tree nut phytochemicals: composition, antioxidant capacity, bioactivity, impact factors. A systematic review of almonds, brazils, cashews, hazelnuts, macadamias, pecans, pine nuts, pistachios and walnuts. Nutr. Res. Rev. 24, 244–275. doi: 10.1017/S095442241100014X
Bolling, B. W., McKay, D. L., and Blumberg, J. B. (2010b). The phytochemical composition and antioxidant actions of tree nuts. Asia Pac. J. Clin. Nutr. 19, 117–123
Bulló, M., Juanola-Falgarona, M., Hernández-Alonso, P., and Salas-Salvadó, J. (2015). Nutrition attributes and health effects of pistachio nuts. Br. J. Nutr. 113, S79–S93. doi: 10.1017/S0007114514003250
Cao, Z., Ding, Y., Ke, Z., Cao, L., Li, N., Ding, G., et al. (2016). Luteoloside acts as 3C protease inhibitor of enterovirus 71 in vitro. PLoS One 11:e0148693. doi: 10.1371/journal.pone.0148693
Chaves, O. A., Fintelman-Rodrigues, N., Wang, X., Sacramento, C. Q., Temerozo, J. R., Ferreira, A. C., et al. (2022). Commercially available Flavonols are better SARS-CoV-2 inhibitors than Isoflavone and flavones. Viruses 14:1458. doi: 10.3390/v14071458
Chen, C., Liang, H., Deng, Y., Yang, X., Li, X., and Hou, C. (2022). Analysis and identification of bioactive compounds of cannabinoids in Silico for inhibition of SARS-CoV-2 and SARS-CoV. Biomol. Ther. 12:1729. doi: 10.3390/biom12121729
Chen, T.-C., Weng, K.-F., Chang, S.-C., Lin, J.-Y., Huang, P.-N., and Shih, S.-R. (2008). Development of antiviral agents for enteroviruses. J. Antimicrob. Chemother. 62, 1169–1173. doi: 10.1093/jac/dkn424
Chhoud, R., Bouslama, L., Gharbi, D., Nouira, F., Papetti, A., and Majdoub, H. (2022). Identification of an anti-herpetic compound isolated from Pistacia vera L. male floral buds. 3 Biotech 12:336. doi: 10.1007/s13205-022-03393-y
Choudhary, D., and Pan, M.-H. (2020). Antiviral effects of Anthocyanins and phytochemicals as natural dietary compounds on different virus sources. Curr. Res. Nutr. Food Science J. 8, 674–681. doi: 10.12944/CRNFSJ.8.3.01
Christopoulou, C., Graikou, K., and Chinou, I. (2008). Chemosystematic value of chemical constituents from Scabiosa hymettia (Dipsacaceae). Chem. Biodivers. 5, 318–323. doi: 10.1002/cbdv.200890029
Cisowska, A., Wojnicz, D., and Hendrich, A. B. (2011). Anthocyanins as antimicrobial agents of natural plant origin. Nat. Prod. Commun. 6, 149–156. doi: 10.1177/1934578X1100600136
Cushnie, T. P. T., and Lamb, A. J. (2005). Antimicrobial activity of flavonoids. Int. J. Antimicrob. Agents 26, 343–356. doi: 10.1016/j.ijantimicag.2005.09.002
D’Arrigo, M., Bisignano, C., Irrera, P., Smeriglio, A., Zagami, R., Trombetta, D., et al. (2019). In vitro evaluation of the activity of an essential oil from Pistacia vera L. variety Bronte hull against Candida sp. BMC Complement. Altern. Med. 19:6. doi: 10.1186/s12906-018-2425-0
D’Arrigo, M., Ginestra, G., Mandalari, G., Furneri, P. M., and Bisignano, G. (2010). Synergism and postantibiotic effect of tobramycin and Melaleuca alternifolia (tea tree) oil against Staphylococcus aureus and Escherichia coli. Phytomedicine 17, 317–322. doi: 10.1016/j.phymed.2009.07.008
Dai, W., Bi, J., Li, F., Wang, S., Huang, X., Meng, X., et al. (2019). Antiviral efficacy of flavonoids against enterovirus 71 infection in vitro and in newborn mice. Viruses 11:625. doi: 10.3390/v11070625
Di Lodovico, S., Napoli, E., Di Campli, E., Di Fermo, P., Gentile, D., Ruberto, G., et al. (2019). Pistacia vera L. oleoresin and levofloxacin is a synergistic combination against resistant Helicobacter pylori strains. Sci. Rep. 9:4646. doi: 10.1038/s41598-019-40991-y
Di Petrillo, A., Orrù, G., Fais, A., and Fantini, M. C. (2022). Quercetin and its derivates as antiviral potentials: A comprehensive review. PTR 36, 266–278. doi: 10.1002/ptr.7309
Din, W. M., Jin, K. T., Ramli, R., Khaithir, T. M. N., and Wiart, C. (2013). Antibacterial effects of Ellagitannins from Acalypha wilkesiana var. macafeana hort.: surface morphology analysis with environmental scanning Electron microcopy and synergy with antibiotics. Phytother. Res. 27, 1313–1320. doi: 10.1002/ptr.4876
Djouonzo, P. T., Mukim, M. S. I., Kemda, P. N., Kowa, T. K., Tchinda, A. T., Agbor Agbor, G., et al. (2023). SARS-CoV-2 Main protease inhibitors from the stem barks of Discoglypremna caloneura (Pax) Prain (Euphorbiaceae) and Pterocarpus erinaceus Poir (Fabaceae) and their molecular docking investigation. Appl. Biol. Chem. 66:76. doi: 10.1186/s13765-023-00833-y
Doughari, J. H., Ndakidemi, P. A., Human, I. S., and Benade, S. (2012). Antioxidant, antimicrobial and antiverotoxic potentials of extracts of Curtisia dentata. J. Ethnopharmacol. 141, 1041–1050. doi: 10.1016/j.jep.2012.03.051
Duarte, A., Alves, A. C., Ferreira, S., Silva, F., and Domingues, F. C. (2015). Resveratrol inclusion complexes: antibacterial and anti-biofilm activity against Campylobacter spp. and Arcobacter butzleri. Food Res. Int. 77, 244–250. doi: 10.1016/j.foodres.2015.05.047
El Majdoub, Y. O., Ginestra, G., Mandalari, G., Dugo, P., Mondello, L., and Cacciola, F. (2021). The digestibility of Hibiscus sabdariffa L. polyphenols using an in vitro Human digestion model and evaluation of their antimicrobial activity. Nutrients 13:2360. doi: 10.3390/nu13072360
Engels, C., Schieber, A., and Gänzle, M. G. (2011). Inhibitory spectra and modes of antimicrobial action of Gallotannins from mango kernels (Mangifera indica L.). Appl. Environ. Microbiol. 77, 2215–2223. doi: 10.1128/AEM.02521-10
Fahmy, N. M., Al-Sayed, E., Moghannem, S., Azam, F., El-Shazly, M., and Singab, A. N. (2020). Breaking down the barriers to a natural antiviral agent: antiviral activity and molecular docking of Erythrina speciosa extract, fractions, and the major compound. Chem. Biodivers. 17:e1900511. doi: 10.1002/cbdv.201900511
Fanunza, E., Iampietro, M., Distinto, S., Corona, A., Quartu, M., Maccioni, E., et al. (2020). Quercetin blocks Ebola virus infection by counteracting the VP24 interferon-inhibitory function. Antimicrob. Agents Chemother. 64, e00530–e00520. doi: 10.1128/AAC.00530-20
Farhat, A., Ben Hlima, H., Khemakhem, B., Ben Halima, Y., Michaud, P., Abdelkafi, S., et al. (2022). Apigenin analogues as SARS-CoV-2 main protease inhibitors: in-silico screening approach. Bioengineered 13, 3350–3361. doi: 10.1080/21655979.2022.2027181
Ferreira, S., Silva, F., Queiroz, J. A., Oleastro, M., and Domingues, F. C. (2014). Resveratrol against Arcobacter butzleri and Arcobacter cryaerophilus: activity and effect on cellular functions. Int. J. Food Microbiol. 180, 62–68. doi: 10.1016/j.ijfoodmicro.2014.04.004
Filardo, S., Di Pietro, M., Mastromarino, P., and Sessa, R. (2020). Therapeutic potential of resveratrol against emerging respiratory viral infections. Pharmacol. Ther. 214:107613. doi: 10.1016/j.pharmthera.2020.107613
Filocamo, A., Bisignano, C., Mandalari, G., and Navarra, M. (2015). In vitro antimicrobial activity and effect on biofilm production of a white grape juice (Vitis vinifera) extract. Evid. Based Complement. Alternat. Med. 2015, 1–5. doi: 10.1155/2015/856243
Freitas da Silva, F. E., das Chagas Lima Pinto, F., Loiola Pessoa, O. D., Marques da Fonseca, A., Martins da Costa, J. G., and Pinheiro Santiago, G. M. (2023). Campesterol semi-synthetic derivatives as potential antibacterial: in vitro and in silico evaluation. Chem. Biodivers. 20:e202300536. doi: 10.1002/cbdv.202300536
Gao, M., Wang, H., and Zhu, L. (2016). Quercetin assists fluconazole to inhibit biofilm formations of fluconazole-resistant Candida Albicans in in vitro and in vivo antifungal managements of vulvovaginal candidiasis. Cell. Physiol. Biochem. 40, 727–742. doi: 10.1159/000453134
Gentile, C., Tesoriere, L., Butera, D., Fazzari, M., Monastero, M., Allegra, M., et al. (2007). Antioxidant activity of Sicilian pistachio (Pistacia vera L. Var. Bronte) nut extract and its bioactive components. J. Agric. Food Chem. 55, 643–648. doi: 10.1021/jf062533i
Gervasi, T., Barreca, D., Laganà, G., and Mandalari, G. (2021). Health benefits related to tree nut consumption and their bioactive compounds. Int. J. Mol. Sci. 22:5960. doi: 10.3390/ijms22115960
Gervasi, T., D’Arrigo, M., Rando, R., Sciortino, M. T., Carughi, A., Barreca, D., et al. (2022). The antimicrobial potential of hexane oils and polyphenols-rich extracts from Pistacia vera L. Appl. Sci. 12:4389. doi: 10.3390/app12094389
Ghırardello, D., Prosperını, S., Zeppa, G., and Gerbı, V. (2010). Phenolic acid profile and antioxidant capacity of hazelnut (Corylus Avellana L.) kernels ˙In different solvent systems. J. Food Nutr. Res. 49, 195–205.
Giuffrida, D., Saitta, M., La Torre, G. L., Bombaci, L., and Dugo, G. (2006). Carotenoid, chlorophyll and chlorophyll-derived compounds in pistachio kernels (Pistacia vera L.) from Sicily. Ital J Food Sci 18, 309–316.
Grippi, F., Crosta, L., Aiello, G., Tolomeo, M., Oliveri, F., Gebbia, N., et al. (2008). Determination of stilbenes in Sicilian pistachio by high-performance liquid chromatographic diode array (HPLC-DAD/FLD) and evaluation of eventually mycotoxin contamination. Food Chem. 107, 483–488. doi: 10.1016/j.foodchem.2007.07.079
Gutiérrez-Morales, A., Velázquez-Ordoñez, V., Khusro, A., Salem, A. Z. M., Estrada-Zúñiga, M. E., Salem, M. Z. M., et al. (2017). Anti-staphylococcal properties of Eichhornia crassipes, Pistacia vera, and Ziziphus amole leaf extracts: isolates from cattle and rabbits. Microb. Pathog. 113, 181–189. doi: 10.1016/j.micpath.2017.10.015
Habbu, P. V., Mahadevan, K. M., Shastry, R. A., and Manjunatha, H. (2009). Antimicrobial activity of flavanoid sulphates and other fractions of Argyreia speciosa (Burm.F) Boj. Indian J. Exp. Biol. 47, 121–128
Hatano, T., Kusuda, M., Inada, K., Ogawa, T., Shiota, S., Tsuchiya, T., et al. (2005). Effects of tannins and related polyphenols on methicillin-resistant Staphylococcus aureus. Phytochemistry 66, 2047–2055. doi: 10.1016/j.phytochem.2005.01.013
Hayashi, K., Mori, M., Knox, Y. M., Suzutan, T., Ogasawara, M., Yoshida, I., et al. (2003). Anti influenza virus activity of a red-fleshed potato anthocyanin. Food Sci. Technol. Res. 9, 242–244. doi: 10.3136/fstr.9.242
Hayashi, M., Naknukool, S., Hayakawa, S., Ogawa, M., and Ni’matulah, A.-B. A. (2012). Enhancement of antimicrobial activity of a lactoperoxidase system by carrot extract and β-carotene. Food Chem. 130, 541–546. doi: 10.1016/j.foodchem.2011.07.067
He, Y., Huang, M., Tang, C., Yue, Y., Liu, X., Zheng, Z., et al. (2021). Dietary daidzein inhibits hepatitis C virus replication by decreasing microRNA-122 levels. Virus Res. 298:198404. doi: 10.1016/j.virusres.2021.198404
Hong, H., Landauer, M. R., Foriska, M. A., and Ledney, G. D. (2006). Antibacterial activity of the soy isoflavone genistein. J. Basic Microbiol. 46, 329–335. doi: 10.1002/jobm.200510073
Jamhour, R. M. A. Q., Al-Nadaf, A. H., Wedian, F., Al-Mazaideh, G. M., Mustafa, M., Huneif, M. A., et al. (2022). Phytochemicals as a potential inhibitor of COVID-19: An in-Silico perspective. Russ. J. Phys. Chem. A 96, 1589–1597. doi: 10.1134/S0036024422070251
Jasso-Miranda, C., Herrera-Camacho, I., Flores-Mendoza, L. K., Dominguez, F., Vallejo-Ruiz, V., Sanchez-Burgos, G. G., et al. (2019). Antiviral and immunomodulatory effects of polyphenols on macrophages infected with dengue virus serotypes 2 and 3 enhanced or not with antibodies. Infect Drug Resist 12, 1833–1852. doi: 10.2147/IDR.S210890
Ji, P., Chen, C., Hu, Y., Zhan, Z., Pan, W., Li, R., et al. (2015). Antiviral activity of Paulownia tomentosa against enterovirus 71 of hand, foot, and mouth disease. Biological ^|^. Pharm. Bull. 38, 1–6. doi: 10.1248/bpb.b14-00357
Jimenez-Aleman, G. H., Castro, V., Londaitsbehere, A., Gutierrez-Rodríguez, M., Garaigorta, U., Solano, R., et al. (2021). SARS-CoV-2 fears green: the chlorophyll catabolite Pheophorbide A is a potent antiviral. Pharmaceuticals 14:1048. doi: 10.3390/ph14101048
Jo, S., Kim, S., Shin, D. H., and Kim, M.-S. (2020). Inhibition of SARS-CoV 3CL protease by flavonoids. J. Enzyme Inhib. Med. Chem. 35, 145–151. doi: 10.1080/14756366.2019.1690480
Joo, Y.-H., Lee, Y.-G., Lim, Y., Jeon, H., Lee, I.-G., Cho, Y.-B., et al. (2022). Anti-influenza A virus activity by Agrimonia pilosa and Galla rhois extract mixture. Biomed. Pharmacother. 155:113773. doi: 10.1016/j.biopha.2022.113773
Kai, H., Obuchi, M., Yoshida, H., Watanabe, W., Tsutsumi, S., Park, Y. K., et al. (2014). In vitro and in vivo anti-influenza virus activities of flavonoids and related compounds as components of Brazilian propolis (AF-08). J. Funct. Foods 8, 214–223. doi: 10.1016/j.jff.2014.03.019
Kannanoor, M., Lakshmi, B. A., and Kim, S. (2021). Synthesis of silver nanoparticles conjugated with kaempferol and hydrocortisone and an evaluation of their antibacterial effects 11, 317. doi: 10.1007/s13205-021-02880-y
Karimi, E., Jaafar, H. Z. E., and Ahmad, S. (2011). Phytochemical analysis and antimicrobial activities of Methanolic extracts of leaf, stem and root from different varieties of Labisa pumila Benth. Molecules 16, 4438–4450. doi: 10.3390/molecules16064438
Kaul, T. N., Middleton, E., and Ogra, P. L. (1985). Antiviral effect of flavonoids on human viruses. J. Med. Virol. 15, 71–79. doi: 10.1002/jmv.1890150110
Kaul, R., Paul, P., Kumar, S., Büsselberg, D., Dwivedi, V. D., and Chaari, A. (2021). Promising antiviral activities of natural flavonoids against SARS-CoV-2 targets: systematic review. Int. J. Mol. Sci. 22:11069. doi: 10.3390/ijms222011069
Khalil, A., Tazeddinova, D., Aljoumaa, K., Kazhmukhanbetkyzy, Z. A., Orazov, A., and Toshev, A. D. (2021). Carotenoids: therapeutic strategy in the Battle against viral emerging diseases, COVID-19: An overview. Prev. Nutr. Food Sci. 26, 241–261. doi: 10.3746/pnf.2021.26.3.241
Kim, H. J., Woo, E.-R., Shin, C.-G., and Park, H. (1998). A new Flavonol glycoside Gallate Ester from Acer okamotoanum and its inhibitory activity against Human immunodeficiency Virus-1 (HIV-1) integrase. J. Nat. Prod. 61, 145–148. doi: 10.1021/np970171q
Knipping, K., Garssen, J., and van’t Land, B. (2012). An evaluation of the inhibitory effects against rotavirus infection of edible plant extracts. Virol. J. 9:137. doi: 10.1186/1743-422X-9-137
Kwon, Y.-I., Apostolidis, E., Labbe, R. G., and Shetty, K. (2007). Inhibition of Staphylococcus aureus by phenolic phytochemicals of selected clonal herbs species of Lamiaceae Family and likely mode of action through Proline oxidation. Food Biotechnol. 21, 71–89. doi: 10.1080/08905430701191205
La Camera, E., Bisignano, C., Crisafi, G., Smeriglio, A., Denaro, M., Trombetta, D., et al. (2018). Biochemical characterization of clinical strains of Staphylococcus spp. and their sensitivity to polyphenols-rich extracts from pistachio (Pistacia vera L.). Pathogens 7:82. doi: 10.3390/pathogens7040082
Lacombe, A., Tadepalli, S., Hwang, C.-A., and Wu, V. C. H. (2013). Phytochemicals in lowbush wild blueberry inactivate Escherichia coli O157:H7 by damaging its cell membrane. Foodborne Pathog. Dis. 10, 944–950. doi: 10.1089/fpd.2013.1504
Lalouckova, K., Mala, L., Marsik, P., and Skrivanova, E. (2021). In vitro antibacterial effect of the Methanolic extract of the Korean soybean fermented product Doenjang against Staphylococcus aureus. Animals 11:2319. doi: 10.3390/ani11082319
Lambert, R. J. W., Skandamis, P. N., Coote, P. J., and Nychas, G.-J. E. (2001). A study of the minimum inhibitory concentration and mode of action of oregano essential oil, thymol and carvacrol. J. Appl. Microbiol. 91, 453–462. doi: 10.1046/j.1365-2672.2001.01428.x
LeCher, J. C., Diep, N., Krug, P. W., and Hilliard, J. K. (2019). Genistein has antiviral activity against herpes B virus and acts synergistically with antiviral treatments to reduce effective dose. Viruses 11:499. doi: 10.3390/v11060499
Lechner, D., Gibbons, S., and Bucar, F. (2008). Plant phenolic compounds as ethidium bromide efflux inhibitors in Mycobacterium smegmatis. J. Antimicrob. Chemother. 62, 345–348. doi: 10.1093/jac/dkn178
Lee, I.-K., Hwang, B., Kim, D.-W., Kim, J.-Y., Woo, E.-E., Lee, Y.-J., et al. (2016). Characterization of neuraminidase inhibitors in Korean Papaver rhoeas bee pollen contributing to anti-influenza activities in vitro. Planta Med. 82, 524–529. doi: 10.1055/s-0041-111631
Lee, J., and Lee, D. G. (2015). Novel antifungal mechanism of resveratrol: apoptosis inducer in Candida albicans. Curr. Microbiol. 70, 383–389. doi: 10.1007/s00284-014-0734-1
Lee, I.-G., Lee, J., Hong, S.-H., and Seo, Y.-J. (2023). Apigenin’s therapeutic potential against viral infection. Front. Biosci Landmark 28:237. doi: 10.31083/j.fbl2810237
Lin, R.-D., Chin, Y.-P., Hou, W.-C., and Lee, M.-H. (2008). The effects of antibiotics combined with natural polyphenols against clinical methicillin-resistant Staphylococcus aureus (MRSA). Planta Med. 74, 840–846. doi: 10.1055/s-2008-1074559
Lin, Y. T., Kwon, Y. I., Labbe, R. G., and Shetty, K. (2005). Inhibition of helicobacter pylori and associated urease by oregano and cranberry phytochemical synergies. Appl. Environ. Microbiol. 71, 8558–8564. doi: 10.1128/AEM.71.12.8558-8564.2005
Liu, A.-L., Liu, B., Qin, H.-L., Lee, S., Wang, Y.-T., and Du, G.-H. (2008). Anti-influenza virus activities of flavonoids from the medicinal plant Elsholtzia rugulosa. Planta Med. 74, 847–851. doi: 10.1055/s-2008-1074558
Liu, T., Zang, N., Zhou, N., Li, W., Xie, X., Deng, Y., et al. (2014). Resveratrol inhibits the TRIF-dependent pathway by upregulating sterile alpha and Armadillo motif protein, contributing to anti-inflammatory effects after respiratory syncytial virus infection. J. Virol. 88, 4229–4236. doi: 10.1128/JVI.03637-13
Liu, Z., Zhao, J., Li, W., Shen, L., Huang, S., Tang, J., et al. (2016). Computational screen and experimental validation of anti-influenza effects of quercetin and chlorogenic acid from traditional Chinese medicine. Sci. Rep. 6:19095. doi: 10.1038/srep19095
Lu, P., Zhang, T., Ren, Y., Rao, H., Lei, J., Zhao, G., et al. (2023). A literature review on the antiviral mechanism of Luteolin. Nat. Prod. Commun. 18:1934578X2311715. doi: 10.1177/1934578X231171521
Lv, X., Qiu, M., Chen, D., Zheng, N., Jin, Y., and Wu, Z. (2014). Apigenin inhibits enterovirus 71 replication through suppressing viral IRES activity and modulating cellular JNK pathway. Antivir. Res. 109, 30–41. doi: 10.1016/j.antiviral.2014.06.004
Lyu, S.-Y., Rhim, J.-Y., and Park, W.-B. (2005). Antiherpetic activities of flavonoids against herpes simplex virus type 1 (HSV-1) and type 2 (HSV-2) in vitro. Arch. Pharm. Res. 28, 1293–1301. doi: 10.1007/BF02978215
Ma, Y., Ding, S., Fei, Y., Liu, G., Jang, H., and Fang, J. (2019). Antimicrobial activity of anthocyanins and catechins against foodborne pathogens Escherichia coli and Salmonella. Food Control 106:106712. doi: 10.1016/j.foodcont.2019.106712
Maestri, D. (2023). Groundnut and tree nuts: a comprehensive review on their lipid components, phytochemicals, and nutraceutical properties. Crit. Rev. Food Sci. Nutr. 1–25, 1–25. doi: 10.1080/10408398.2023.2185202
Magi, G., Marini, E., Brenciani, A., Di Lodovico, S., Gentile, D., Ruberto, G., et al. (2018). Chemical composition of Pistacia vera L. oleoresin and its antibacterial, anti-virulence and anti-biofilm activities against oral streptococci, including Streptococcus mutans. Arch. Oral Biol. 96, 208–215. doi: 10.1016/j.archoralbio.2018.09.013
Makobongo, M. O., Gilbreath, J. J., and Merrell, D. S. (2014). Nontraditional therapies to treat Helicobacter pylori infection. J. Microbiol. 52, 259–272. doi: 10.1007/s12275-014-3603-5
Mandal, S. M., Dias, R. O., and Franco, O. L. (2017). Phenolic compounds in antimicrobial therapy. J. Med. Food 20, 1031–1038. doi: 10.1089/jmf.2017.0017
Mandalari, G., Barreca, D., Gervasi, T., Roussell, M. A., Klein, B., Feeney, M. J., et al. (2021). Pistachio nuts (Pistacia vera L.): production, nutrients, bioactives and novel health effects. Plants 11:18. doi: 10.3390/plants11010018
Mandalari, G., Bennett, R. N., Bisignano, G., Trombetta, D., Saija, A., Faulds, C. B., et al. (2007). Antimicrobial activity of flavonoids extracted from bergamot (Citrus bergamia Risso) peel, a byproduct of the essential oil industry. J. Appl. Microbiol. 103, 2056–2064. doi: 10.1111/j.1365-2672.2007.03456.x
Mandalari, G., Bisignano, C., D’Arrigo, M., Ginestra, G., Arena, A., Tomaino, A., et al. (2010). Antimicrobial potential of polyphenols extracted from almond skins. Lett. Appl. Microbiol. 51, 83–89. doi: 10.1111/j.1472-765X.2010.02862.x
Mandalari, G., Minuti, A., La Camera, E., Barreca, D., Romeo, O., and Nostro, A. (2023). Antimicrobial susceptibility of Staphylococcus aureus strains and effect of Phloretin on biofilm formation. Curr. Microbiol. 80:303. doi: 10.1007/s00284-023-03400-2
Manvar, D., Mishra, M., Kumar, S., and Pandey, V. N. (2012). Identification and evaluation of anti hepatitis C virus phytochemicals from Eclipta alba. J. Ethnopharmacol. 144, 545–554. doi: 10.1016/j.jep.2012.09.036
Martini, N. D., Katerere, D. R. P., and Eloff, J. N. (2004). Biological activity of five antibacterial flavonoids from Combretum erythrophyllum (Combretaceae). J. Ethnopharmacol. 93, 207–212. doi: 10.1016/j.jep.2004.02.030
Mastromarino, P., Capobianco, D., Cannata, F., Nardis, C., Mattia, E., De Leo, A., et al. (2015). Resveratrol inhibits rhinovirus replication and expression of inflammatory mediators in nasal epithelia. Antivir. Res. 123, 15–21. doi: 10.1016/j.antiviral.2015.08.010
Mazzon, M., Jones, M., Davidson, A., Chain, B., and Jacobs, M. (2009). Dengue virus NS5 inhibits interferon-α signaling by blocking signal transducer and activator of transcription 2 phosphorylation. J. Infect. Dis. 200, 1261–1270. doi: 10.1086/605847
Merchat, M., Bertolini, G., Giacomini, P., Villaneuva, A., and Jori, G. (1996). Meso-substituted cationic porphyrins as efficient photosensitizers of gram-positive and gram-negative bacteria. J. Photochem. Photobiol. B 32, 153–157. doi: 10.1016/1011-1344(95)07147-4
Minnock, A., Vernon, D. I., Schofield, J., Griffiths, J., Howard Parish, J., and Brown, S. B. (1996). Photoinactivation of bacteria. Use of a cationic water-soluble zinc phthalocyanine to photoinactivate both gram-negative and gram-positive bacteria. J. Photochem. Photobiol. B 32, 159–164. doi: 10.1016/1011-1344(95)07148-2
Mitra, S., Rauf, A., Tareq, A. M., Jahan, S., Emran, T. B., Shahriar, T. G., et al. (2021). Potential health benefits of carotenoid lutein: An updated review. Food Chem. Toxicol. 154:112328. doi: 10.1016/j.fct.2021.112328
Mohammadi Pour, P., Fakhri, S., Asgary, S., Farzaei, M. H., and Echeverría, J. (2019). The signaling pathways, and therapeutic targets of antiviral agents: focusing on the antiviral approaches and clinical perspectives of Anthocyanins in the Management of Viral Diseases. Front. Pharmacol. 10:485177. doi: 10.3389/fphar.2019.01207
Morimoto, R., Hanada, A., Matsubara, C., Horio, Y., Sumitani, H., Ogata, T., et al. (2023). Anti-influenza A virus activity of flavonoids in vitro: a structure–activity relationship. J. Nat. Med. 77, 219–227. doi: 10.1007/s11418-022-01660-z
Murray, C. J. L., Ikuta, K. S., Sharara, F., Swetschinski, L., Robles Aguilar, G., Gray, A., et al. (2022). Global burden of bacterial antimicrobial resistance in 2019: a systematic analysis. Lancet 399, 629–655. doi: 10.1016/S0140-6736(21)02724-0
Murtaza, G., Karim, S., Akram, M. R., Khan, S. A., Azhar, S., Mumtaz, A., et al. (2014). Caffeic acid Phenethyl Ester and Therapeutic potentials. Biomed. Res. Int. 2014, 1–9. doi: 10.1155/2014/145342
Musarra-Pizzo, M., Ginestra, G., Smeriglio, A., Pennisi, R., Sciortino, M. T., and Mandalari, G. (2019). The antimicrobial and antiviral activity of polyphenols from almond (Prunus dulcis L.) skin. Nutrients 11:2355. doi: 10.3390/nu11102355
Musarra-Pizzo, M., Pennisi, R., Ben-Amor, I., Smeriglio, A., Mandalari, G., and Sciortino, M. T. (2020). In vitro anti-HSV-1 activity of polyphenol-rich extracts and pure polyphenol compounds derived from pistachios kernels (Pistacia vera L.). Plan. Theory 9:267. doi: 10.3390/plants9020267
Naz, S., Siddiqi, R., Ahmad, S., Rasool, S. A., and Sayeed, S. A. (2007). Antibacterial activity directed isolation of compounds from Punica granatum. J. Food Sci. 72, M341–M345. doi: 10.1111/j.1750-3841.2007.00533.x
Neveu, V., Perez-Jimenez, J., Vos, F., Crespy, V., du Chaffaut, L., Mennen, L., et al. (2010). Phenol-explorer: an online comprehensive database on polyphenol contents in foods. Database 2010:bap024. doi: 10.1093/database/bap024
Nguyen, T. L. A., and Bhattacharya, D. (2022). Antimicrobial activity of quercetin: An approach to its mechanistic principle. Molecules 27:2494. doi: 10.3390/molecules27082494
Nguyen, T.-T., Ketha, A., Hieu, H. V., and Tatipamula, V. B. (2021). In vitro antimycobacterial studies of flavonols from Bauhinia vahlii Wight and Arn 11, 128. doi: 10.1007/s13205-021-02672-4
Niemenak, N., Rohsius, C., Elwers, S., Omokolo Ndoumou, D., and Lieberei, R. (2006). Comparative study of different cocoa (Theobroma cacao L.) clones in terms of their phenolics and anthocyanins contents. J. Food Compos. Anal. 19, 612–619. doi: 10.1016/j.jfca.2005.02.006
Nikolaeva-Glomb, L., Mukova, L., Nikolova, N., Badjakov, I., Dincheva, I., Kondakova, V., et al. (2014). In vitro antiviral activity of a series of wild berry fruit extracts against representatives of Picorna-, Orthomyxo - and Paramyxoviridae. Nat. Prod. Commun. 9:1934578X1400900. doi: 10.1177/1934578X1400900116
Ohno, M., Shibata, C., Kishikawa, T., Yoshikawa, T., Takata, A., Kojima, K., et al. (2013). The flavonoid apigenin improves glucose tolerance through inhibition of microRNA maturation in miRNA103 transgenic mice. Sci. Rep. 3:2553. doi: 10.1038/srep02553
Özçelik, B., Aslan, M., Orhan, I., and Karaoglu, T. (2005). Antibacterial, antifungal, and antiviral activities of the lipophylic extracts of Pistacia vera. Microbiol. Res. 160, 159–164. doi: 10.1016/j.micres.2004.11.002
Özçelik, B., Kartal, M., and Orhan, I. (2011). Cytotoxicity, antiviral and antimicrobial activities of alkaloids, flavonoids, and phenolic acids. Pharm. Biol. 49, 396–402. doi: 10.3109/13880209.2010.519390
Özçelik, B., Orhan, I., and Toker, G. (2006). Antiviral and antimicrobial assessment of some selected flavonoids. Zeitschrift für Naturforschung C 61, 632–638. doi: 10.1515/znc-2006-9-1003
Pang, R., Tao, J., Zhang, S., Zhao, L., Yue, X., Wang, Y., et al. (2010). In vitro antiviral activity of lutein against hepatitis B virus. Phytother. Res. 24, 1627–1630. doi: 10.1002/ptr.3155
Paulo, L., Ferreira, S., Gallardo, E., Queiroz, J. A., and Domingues, F. (2010). Antimicrobial activity and effects of resveratrol on human pathogenic bacteria. World J. Microbiol. Biotechnol. 26, 1533–1538. doi: 10.1007/s11274-010-0325-7
Pennisi, R., Trischitta, P., Tamburello, M. P., Barreca, D., Mandalari, G., and Sciortino, M. T. (2023). Mechanistic understanding of the antiviral properties of pistachios and Zeaxanthin against HSV-1. Viruses 15:1651. doi: 10.3390/v15081651
Periferakis, A., Periferakis, K., Badarau, I. A., Petran, E. M., Popa, D. C., Caruntu, A., et al. (2022). Kaempferol: antimicrobial properties, sources, clinical, and traditional applications. Int. J. Mol. Sci. 23:15054. doi: 10.3390/ijms232315054
Petrera, E., Níttolo, A. G., and Alché, L. E. (2014). Antiviral action of synthetic stigmasterol derivatives on herpes simplex virus replication in nervous cells in vitro. Biomed. Res. Int. 2014:947560. doi: 10.1155/2014/947560
Pierre Luhata, L., and Usuki, T. (2021). Antibacterial activity of β-sitosterol isolated from the leaves of Odontonema strictum (Acanthaceae). Bioorg. Med. Chem. Lett. 48:128248. doi: 10.1016/j.bmcl.2021.128248
Pisonero-Vaquero, S., García-Mediavilla, M. V., Jorquera, F., Majano, P. L., Benet, M., Jover, R., et al. (2014). Modulation of PI3K-LXRα-dependent lipogenesis mediated by oxidative/nitrosative stress contributes to inhibition of HCV replication by quercetin. Lab. Investig. 94, 262–274. doi: 10.1038/labinvest.2013.156
Pojer, E., Mattivi, F., Johnson, D., and Stockley, C. S. (2013). The case for anthocyanin consumption to promote Human health: A review. Compr. Rev. Food Sci. Food Saf. 12, 483–508. doi: 10.1111/1541-4337.12024
Pourghanbari, G., Nili, H., Moattari, A., Mohammadi, A., and Iraji, A. (2016). Antiviral activity of the oseltamivir and Melissa officinalis L. essential oil against avian influenza A virus (H9N2). Virus Disease 27, 170–178. doi: 10.1007/s13337-016-0321-0
Qasimi, M., Aziz, I., Rasheed, M., Guli, B., and Khan, M. A. (2016). Effect of extraction solvents on polyphenols and antioxidant activity on medicinal halophytes. Pak. J. Bot. 48, 621–627.
Qian, W., Liu, M., Fu, Y., Zhang, J., Liu, W., Li, J., et al. (2020). Antimicrobial mechanism of luteolin against Staphylococcus aureus and listeria monocytogenes and its antibiofilm properties. Microb. Pathog. 142:104056. doi: 10.1016/j.micpath.2020.104056
Ravi, L., Girish, S., Harshini, M., and Sreenivas, B. K. A. (2020). β-Sitosterol: an antibacterial agent in aquaculture Management of Vibrio Infections. J. Pure Appl. Microbiol. 14, 2699–2714. doi: 10.22207/JPAM.14.4.48
Reygaert, W. C. (2014). The antimicrobial possibilities of green tea. Front. Microbiol. 5:434. doi: 10.3389/fmicb.2014.00434
Rittà, M., Marengo, A., Civra, A., Lembo, D., Cagliero, C., Kant, K., et al. (2020). Antiviral activity of a Arisaema Tortuosum leaf extract and some of its constituents against herpes simplex virus type 2. Planta Med. 86, 267–275. doi: 10.1055/a-1087-8303
Rofeal, M., El-Malek, F. A., and Qi, X. (2021). In vitro assessment of green polyhydroxybutyrate/chitosan blend loaded with kaempferol nanocrystals as a potential dressing for infected wounds. Nanotechnology 32:375102. doi: 10.1088/1361-6528/abf7ee
Rojas, Á., Del Campo, J. A., Clement, S., Lemasson, M., García-Valdecasas, M., Gil-Gómez, A., et al. (2016). Effect of quercetin on hepatitis C virus life cycle: from viral to host targets. Sci. Rep. 6:31777. doi: 10.1038/srep31777
Ryu, Y. B., Jeong, H. J., Kim, J. H., Kim, Y. M., Park, J.-Y., Kim, D., et al. (2010). Biflavonoids from Torreya nucifera displaying SARS-CoV 3CLpro inhibition. Bioorg. Med. Chem. 18, 7940–7947. doi: 10.1016/j.bmc.2010.09.035
Santiago, C., Pang, E. L., Lim, K.-H., Loh, H.-S., and Ting, K. N. (2015). Inhibition of penicillin-binding protein 2a (PBP2a) in methicillin resistant Staphylococcus aureus (MRSA) by combination of ampicillin and a bioactive fraction from Duabanga grandiflora. BMC Complement. Altern. Med. 15:178. doi: 10.1186/s12906-015-0699-z
Seeram, N., Zhang, Y., Bowerman, S., and Heber, D. (2008). Phytochemicals and health aspects of pistachio (Pistacia vera L.). Tree Nuts. CRC Press. 309–318. doi: 10.1201/9781420019391.ch18
Seker, G., and Akbas, M. Y. (2023). Evaluation of bioactivities of Pistacia vera L. hull extracts as a potential antimicrobial and antioxidant natural source. Food Sci. Technol. Int. doi: 10.1177/10820132231193478
Shahdadi, F., Khorasani, S., Salehi-Sardoei, A., Fallahnajmabadi, F., Fazeli-Nasab, B., and Sayyed, R. Z. (2023). GC-MS profiling of pistachio vera L., and effect of antioxidant and antimicrobial compounds of it’s essential oil compared to chemical counterparts. Sci. Rep. 13:21694. doi: 10.1038/s41598-023-48844-5
Shetty, K., and Wahlqvist, M. L. (2004). A model for the role of the proline-linked pentose-phosphate pathway in phenolic phytochemical bio-synthesis and mechanism of action for human health and environmental applications. Asia Pac. J. Clin. Nutr. 13, 1–24
Shibata, C., Ohno, M., Otsuka, M., Kishikawa, T., Goto, K., Muroyama, R., et al. (2014). The flavonoid apigenin inhibits hepatitis C virus replication by decreasing mature microRNA122 levels. Virology 462-463, 42–48. doi: 10.1016/j.virol.2014.05.024
Shih, S.-R., Stollar, V., and Li, M.-L. (2011). Host factors in enterovirus 71 replication. J. Virol. 85, 9658–9666. doi: 10.1128/JVI.05063-11
Simões, C. M. O., Reginatto, F. H., Calvete, E. O., Henrique, C., da Silva, B., and Carvalho, A. (2012). Anti HSV-1 activity of five strawberry cultivars. Lat. Am. J. Pharm.
Singh, B. N., Upreti, D. K., Singh, B. R., Pandey, G., Verma, S., Roy, S., et al. (2015). Quercetin sensitizes fluconazole-resistant Candida albicans to induce apoptotic cell death by modulating quorum sensing. Antimicrob. Agents Chemother. 59, 2153–2168. doi: 10.1128/AAC.03599-14
Smeriglio, A., Denaro, M., Barreca, D., Calderaro, A., Bisignano, C., Ginestra, G., et al. (2017). In vitro evaluation of the antioxidant, Cytoprotective, and antimicrobial properties of essential oil from Pistacia vera L. variety Bronte Hull. Int. J. Mol. Sci. 18:1212. doi: 10.3390/ijms18061212
Suvorov, N., Pogorilyy, V., Diachkova, E., Vasil’ev, Y., Mironov, A., and Grin, M. (2021). Derivatives of natural chlorophylls as agents for antimicrobial photodynamic therapy. Int. J. Mol. Sci. 22:6392. doi: 10.3390/ijms22126392
Taghizadeh, S. F., Rezaee, R., Mehmandoust, M., Badibostan, H., and Karimi, G. (2020). Assessment of in vitro bioactivities of Pis v 1 (2S albumin) and Pis v 2.0101 (11S globulin) proteins derived from pistachio (Pistacia vera L.). Food Measure 14, 1054–1063. doi: 10.1007/s11694-019-00355-6
Taylor, P. W., Hamilton-Miller, J. M. T., and Stapleton, P. D. (2005). Antimicrobial properties of green tea catechins. Food Sci. Technol. Bullet. 2, 71–81. doi: 10.1616/1476-2137.14184
Tokuşoglu, O., Unal, M. K., and Yemiş, F. (2005). Determination of the phytoalexin resveratrol (3,5,4′-trihydroxystilbene) in peanuts and pistachios by high-performance liquid chromatographic diode array (HPLC-DAD) and gas chromatography-mass spectrometry (GC-MS). J. Agric. Food Chem. 53, 5003–5009. doi: 10.1021/jf050496+
Tomaino, A., Martorana, M., Arcoraci, T., Monteleone, D., Giovinazzo, C., and Saija, A. (2010). Antioxidant activity and phenolic profile of pistachio (Pistacia vera L., variety Bronte) seeds and skins. Biochimie 92, 1115–1122. doi: 10.1016/j.biochi.2010.03.027
Toujani, M. M., Rittà, M., Civra, A., Genovese, S., Epifano, F., Ghram, A., et al. (2018). Inhibition of HSV-2 infection by pure compounds from Thymus capitatus extract in vitro. PTR 32, 1555–1563. doi: 10.1002/ptr.6084
Ulanowska, K., Tkaczyk, A., Konopa, G., and Węgrzyn, G. (2006). Differential antibacterial activity of genistein arising from global inhibition of DNA, RNA and protein synthesis in some bacterial strains. Arch. Microbiol. 184, 271–278. doi: 10.1007/s00203-005-0063-7
Ulusoy, S., Boşgelmez-Tınaz, G., and Seçilmiş-Canbay, H. (2009). Tocopherol, carotene, phenolic contents and antibacterial properties of rose essential oil, hydrosol and absolute. Curr. Microbiol. 59, 554–558. doi: 10.1007/s00284-009-9475-y
Vela, E. M., Knostman, K. A., Mott, J. M., Warren, R. L., Garver, J. N., Vela, L. J., et al. (2010). Genistein, a general kinase inhibitor, as a potential antiviral for arenaviral hemorrhagic fever as described in the Pirital virus-Syrian golden hamster model. Antivir. Res. 87, 318–328. doi: 10.1016/j.antiviral.2010.06.007
Vestergaard, M., and Ingmer, H. (2019). Antibacterial and antifungal properties of resveratrol. Int. J. Antimicrob. Agents 53, 716–723. doi: 10.1016/j.ijantimicag.2019.02.015
Visintini Jaime, M. F., Redko, F., Muschietti, L. V., Campos, R. H., Martino, V. S., and Cavallaro, L. V. (2013). In vitro antiviral activity of plant extracts from Asteraceae medicinal plants. Virol. J. 10:245. doi: 10.1186/1743-422X-10-245
Wang, M., Firrman, J., Liu, L., and Yam, K. (2019). A review on flavonoid Apigenin: dietary intake, ADME, antimicrobial effects, and interactions with Human gut microbiota. Biomed. Res. Int. 2019, 1–18. doi: 10.1155/2019/7010467
Wang, Y., Li, F., Wang, Z., Song, X., Ren, Z., Wang, X., et al. (2023). Luteolin inhibits herpes simplex virus 1 infection by activating cyclic guanosine monophosphate-adenosine monophosphate synthase-mediated antiviral innate immunity. Phytomedicine 120:155020. doi: 10.1016/j.phymed.2023.155020
Wang, S., Ling, Y., Yao, Y., Zheng, G., and Chen, W. (2020). Luteolin inhibits respiratory syncytial virus replication by regulating the MiR-155/SOCS1/STAT1 signaling pathway. Virol. J. 17:187. doi: 10.1186/s12985-020-01451-6
Wang, L.-H., Wang, M.-S., Zeng, X.-A., Xu, X.-M., and Brennan, C. S. (2017). Membrane and genomic DNA dual-targeting of citrus flavonoid naringenin against Staphylococcus aureus. Integr. Biol. 9, 820–829. doi: 10.1039/C7IB00095B
Xu, L., Jiang, W., Jia, H., Zheng, L., Xing, J., Liu, A., et al. (2020a). Discovery of multitarget-directed ligands against influenza virus from compound Yizhihao through a predictive system for compound-protein interactions. Front. Cell. Infect. Microbiol. 10:17. doi: 10.3389/fcimb.2020.00016
Xu, X., Miao, J., Shao, Q., Gao, Y., and Hong, L. (2020b). Apigenin suppresses influenza A virus-induced RIG-I activation and viral replication. J. Med. Virol. 92, 3057–3066. doi: 10.1002/jmv.26403
Xu, L., Su, W., Jin, J., Chen, J., Li, X., Zhang, X., et al. (2014). Identification of luteolin as enterovirus 71 and coxsackievirus A16 inhibitors through reporter viruses and cell viability-based screening. Viruses 6, 2778–2795. doi: 10.3390/v6072778
Yang, J., Liu, R. H., and Halim, L. (2009). Antioxidant and antiproliferative activities of common edible nut seeds. LWT-Food Sci. Technol. 42, 1–8. doi: 10.1016/j.lwt.2008.07.007
Yi, L., Li, Z., Yuan, K., Qu, X., Chen, J., Wang, G., et al. (2004). Small molecules blocking the entry of severe acute respiratory syndrome coronavirus into host cells. J. Virol. 78, 11334–11339. doi: 10.1128/jvi.78.20.11334-11339.2004
Yim, S.-K., Kim, I., Warren, B., Kim, J., Jung, K., and Ku, B. (2021). Antiviral activity of two marine carotenoids against SARS-CoV-2 virus entry in Silico and in vitro. Int. J. Mol. Sci. 22:6481. doi: 10.3390/ijms22126481
Yin, J., Peng, X., Lin, J., Zhang, Y., Zhang, J., Gao, H., et al. (2021). Quercetin ameliorates Aspergillus fumigatus keratitis by inhibiting fungal growth, toll-like receptors and inflammatory cytokines. Int. Immunopharmacol. 93:107435. doi: 10.1016/j.intimp.2021.107435
Youssef, F. S., Altyar, A. E., Omar, A. M., and Ashour, M. L. (2021). Phytoconstituents, in vitro anti-infective activity of Buddleja indica lam., and in Silico evaluation of its SARS-CoV-2 inhibitory potential. Front. Pharmacol. 12:619373. doi: 10.3389/fphar.2021.619373
Yucharoen, R. (2011). Inhibitory effect of aromatic herbs, lavender, sage and chamomile against herpes simplex virus infection. Afr. J. Biotechnol. 10, 15394–1401. doi: 10.5897/AJB11.2393
Yusuf, A. J., Abdullahi, M. I., Aleku, G. A., Ibrahim, I. A. A., Alebiosu, C. O., Yahaya, M., et al. (2018). Antimicrobial activity of stigmasterol from the stem bark of Neocarya macrophylla. J. Med. Plants Econom. Dev. 2, 1–5. doi: 10.4102/jomped.v2i1.38
Zhang, L., Lin, D., Sun, X., Curth, U., Drosten, C., Sauerhering, L., et al. (2020). Crystal structure of SARS-CoV-2 main protease provides a basis for design of improved α-ketoamide inhibitors. Science 368, 409–412. doi: 10.1126/science.abb3405
Keywords: pistachio, bioactive compounds, antimicrobial effect, antivirals, mechanism of action
Citation: Mandalari G, Pennisi R, Gervasi T and Sciortino MT (2024) Pistacia vera L. as natural source against antimicrobial and antiviral resistance. Front. Microbiol. 15:1396514. doi: 10.3389/fmicb.2024.1396514
Edited by:
Zengfeng Zhang, Shanghai Jiao Tong University, ChinaReviewed by:
Giorgio Gribaudo, University of Turin, ItalyQinchang Zhu, Shenzhen Technology University, China
Copyright © 2024 Mandalari, Pennisi, Gervasi and Sciortino. This is an open-access article distributed under the terms of the Creative Commons Attribution License (CC BY). The use, distribution or reproduction in other forums is permitted, provided the original author(s) and the copyright owner(s) are credited and that the original publication in this journal is cited, in accordance with accepted academic practice. No use, distribution or reproduction is permitted which does not comply with these terms.
*Correspondence: Giuseppina Mandalari, giuseppina.mandalari@unime.it; Rosamaria Pennisi, rosamaria.pennisi@unime.it