- 1Dirección de Enseñanza, Instituto Nacional de Enfermedades Respiratorias Ismael Cosio Villegas, Mexico City, Mexico
- 2Laboratory of Immunobiology and Genetics, Instituto Nacional de Enfermedades Respiratorias Ismael Cosío Villegas, Mexico City, Mexico
- 3Laboratory of Integrative Immunology, Instituto Nacional de Enfermedades Respiratorias Ismael Cosío Villegas, Mexico City, Mexico
- 4Departamento de Epidemiología Hospitalaria e Infectología, Instituto Nacional de Enfermedades Respiratorias Ismael Cosío Villegas, Mexico City, Mexico
- 5Posgrado en Ciencias Quimicobiológicas, SEPI, Escuela Nacional de Ciencias Biológicas, Instituto Politécnico Nacional, Mexico City, Mexico
- 6Department of Pathology, Instituto Nacional de Neurología y Neurocirugía Manuel Velasco Suárez, Mexico City, Mexico
- 7Red MEDICI, Facultad de Estudios Superiores Iztacala, Universidad Nacional Autónoma de México, Tlalnepantla de Baz, Mexico
- 8Tecnologico de Monterrey, Escuela de Medicina y Ciencias de la Salud, Mexico City, Mexico
- 9Sección de Posgrado e Investigación, Escuela Superior de Medicina, Instituto Politécnico Nacional, Mexico City, Mexico
- 10Centro Especializado en Neurocirugía y Neurociencias México, Mexico City, Mexico
- 11Clínica de Tuberculosis, Instituto Nacional de Enfermedades Respiratorias Ismael Cosío Villegas, Mexico City, Mexico
Introduction: The proteolytic activity of A Disintegrin and Metalloproteinase 17 (ADAM17) regulates the release of tumor necrosis factor (TNF) and TNF receptors (TNFRs) from cell surfaces. These molecules play important roles in tuberculosis (TB) shaping innate immune reactions and granuloma formation.
Methods: Here, we investigated whether single nucleotide polymorphisms (SNPs) of ADAM17 influence TNF and TNFRs levels in 224 patients with active TB (ATB) and 118 healthy close contacts. Also, we looked for significant associations between SNPs of ADAM17 and ATB status. TNF, TNFR1, and TNFR2 levels were measured in plasma samples by ELISA. Four SNPs of ADAM17 (rs12692386, rs1524668, rs11684747, and rs55790676) were analyzed in DNA isolated from peripheral blood leucocytes. The association between ATB status, genotype, and cytokines was analyzed by multiple regression models.
Results: Our results showed a higher frequency of rs11684747 and rs55790676 in close contacts than ATB patients. Coincidentally, heterozygous to these SNPs of ADAM17 showed higher plasma levels of TNF compared to homozygous to their respective ancestral alleles. Strikingly, the levels of TNF and TNFRs distinguished participant groups, with ATB patients displaying lower TNF and higher TNFR1/TNFR2 levels compared to their close contacts.
Conclusion: These findings suggest a role for SNPs of ADAM17 in genetic susceptibility to ATB.
1 Introduction
Tuberculosis (TB), an infectious disease caused by Mycobacterium tuberculosis (Mtb), is a global public health problem which caused 7.5 million new cases and 1.3 million deaths in 2022 (Global Tuberculosis Report 2023, n.d.). Although a primary infection can induce the active form of TB (ATB), most ATB cases derive from latent tuberculosis (LTB) progression, a status of asymptomatic infection without microbiological evidence of bacterial viability but positive readouts of cellular immune memory against Mtb (Tufariello et al., 2003; Mack et al., 2009; Getahun et al., 2015). The last estimation of LTB burden indicated that approximately 1.7 billion individuals were latently infected with Mtb in 2014, representing about a quarter of the global population (Houben and Dodd, 2016). From these, only 5 to 10% will progress to ATB each year with the lung as the main organ affected. Currently, the factors associated with progression to ATB are not completely understood despite its epidemiological relevance. Of course, several genetic, demographic, sociocultural, microbiological, and nutritional characteristics, along with comorbid conditions of cases may affect protective anti-TB immunity. Nonetheless, given the complexity of host-pathogen interactions in TB, a sole immune parameter determinant of protection or pathology has not been found.
For many years, the paradigm of TB immunopathogenesis has established that Mtb infection control greatly relies on memory immune responses of CD4+ T helper cells, which produce interferon-gamma (IFN-γ) to assist macrophages in exploiting their full bactericidal capacity (Shimokata et al., 1986; Flynn et al., 1993; Orme et al., 1993). Unfortunately, the vaccine candidates based on this dogma have failed in providing protection in the real world (Tameris et al., 2013), and IFN-γ-release assays (IGRAs) have no prognostic value to predict ATB progression in individuals with LTB (Diel et al., 2012; Aboudounya and Heads, 2021). Thus, a higher interest in searching for other protective mechanisms has led to striking findings that link previously unrecognized roles of innate immunity and TB protection operating in LTB but not ATB individuals (Khader et al., 2019; Esaulova et al., 2021).
The innate phase of the anti-TB immune response is dominated by alveolar macrophages and recruited monocytes, which participate in granuloma formation, thereby impeding Mtb dissemination (Tsai et al., 2006; Dunlap et al., 2018). Tumor necrosis factor (TNF), a proinflammatory cytokine of innate immunity, plays an essential role in the formation and maintenance of granulomas, macrophage recruitment and nitric oxide production, and the development of giant multinucleated cells (Kindler et al., 1989; Flynn et al., 1995; Roach et al., 2002; Mezouar et al., 2019). Thus, TNF is pivotal in mediating immune protection against Mtb during the early and late stages of infection, as demonstrated by the increased susceptibility to ATB progression among people with LTB receiving anti-TNF immunotherapies (Keane et al., 2001; Gómez-Reino et al., 2003; Mohan et al., 2004; Wallis et al., 2004). Also, diverse reports indicate that single-nucleotide polymorphisms (SNPs) increase the risk of developing TB by decreasing TNF levels or interfering with its signaling (Wu et al., 2019; Adane et al., 2021; Souza de Lima et al., 2021).
TNF is produced by several leucocyte subsets (Dorhoi and Kaufmann, 2014), and it is expressed as a trimeric transmembrane protein (tmTNF) or a soluble form (solTNF). Both TNF forms are bioactive when they attach to TNF receptor 1 (TNFR1), which mediates inflammation and tissue injury, or TNF receptor 2 (TNFR2), which attenuates excessive inflammation during mycobacterial infection (Chavez-Galan et al., 2017, 2019; Ruiz et al., 2021). TNFRs are also expressed on the cell surface as trans-membrane proteins (tmTNFR1 and tmTNFR2) or released from the cell as soluble forms (solTNFR1 and solTNFR2). Whereas tmTNFR1 and tmTNFR2 induce intracellular signaling after the binding with tmTNF or solTNF (Ruiz et al., 2021), soluble TNFRs are anti-inflammatory mediators acting as decoy receptors to neutralize TNF (Kohno et al., 1990). The shedding of TNF and its receptors from the cell membrane is mediated by the proteolytic activity of A Disintegrin and Metalloproteinase 17 (ADAM17), also known as TNF converting enzyme (TACE) (Black et al., 1997; Mohan et al., 2002). Of note, despite the relevance of ADAM17 in regulating TNF and TNFRs levels in diverse diseases (Moss and Minond, 2017; Zunke and Rose-John, 2017), the role of this protease in TB susceptibility has not been assessed.
SNPs of ADAM17 have been implicated in several disorders, including Parkinson’s disease, allergy, sepsis, and vascular diseases (Li et al., 2014, 2019; Shao et al., 2016; He et al., 2022; Jung and Hwang, 2022). Hence, this study aimed to characterize the prevalence of SNPs of ADAM17 among people with ATB and their healthy close contacts and the effect of these polymorphisms in the levels of soluble TNF, TNFR1, and TNFR2. Our findings indicate that people with the rs11684747 and rs55790676 SNPs of ADAM17 display higher plasma levels of solTNF but lower concentrations of TNFRs. Remarkably, the frequency of these SNPs of ADAM17 was higher among people without clinical manifestations of the disease despite being in close contact with ATB cases, especially in those with LTB, as defined by positive IGRA results. Finally, logistic regression analyses showed that homozygosity to the ancestral alleles of both variants is associated with a higher risk for ATB as compared to carriers of rs11684747 and rs55790676 SNPs of ADAM17. Our study elucidates a possible role of ADAM17 in immunity against Mtb and disease susceptibility that deserves further research.
2 Materials and methods
2.1 Study population
Peripheral blood samples were obtained from ATB patients and their household contacts. All participants were enrolled at the TB clinic of the Instituto Nacional de Enfermedades Respiratorias Ismael Cosío Villegas (INER) in Mexico City. The diagnosis of ATB was laboratory-confirmed by positive results in sputum smear microscopy, sputum/bronchoalveolar lavage (BAL) culture, and GeneXpert MTB/RIF test (Cepheid, CA, United States). The LTB group included household contacts, who shared the same enclosed living space for one or more nights per week or extended periods during the day with TB patients three months before enrollment. All these contacts did not show ATB symptoms, and some of them had a positive result in the IGRA QuantiFERON®-TB Gold Plus test (QIAGEN, Hilden, Germany; hereinafter named QTF+). QTF+ participants were considered as having LTB infection and were subjected to clinical evaluation and chest X-ray. The individuals with QTF− were considered as healthy household contacts. QTF+ and QTF− individuals were analyzed as a single group called “No ATB” because they displayed the same behavior in statistical analyses (data indicated in Supplementary material).
Solid-organ transplant recipients and patients with human immunodeficiency virus infection, receiving immunosuppressive treatment, and diagnosed with cancer, or autoimmune diseases were excluded from the study. Clinical and demographic data from participants were obtained by direct clinical interview, physical examination, and review of their medical records.
2.2 Samples
Peripheral blood samples were obtained into BD Vacutainer® EDTA tubes as soon as the diagnosis was made and before treatment initiation for ATB patients, or upon acceptance to participate in the study by healthy-close contacts. Peripheral blood mononuclear cells (PBMCs) were isolated by centrifugation gradient using Ficoll-Paque™ PLUS (GE Healthcare, Life Sciences, PA, United States) for DNA isolation. Plasma aliquots for protein determinations were stored at −70°C until use.
2.3 DNA isolation and genotyping
Genomic DNA was extracted from PBMCs using silica columns (DNeasy® Blood & Tissue Kit, Qiangen™, MD, United States) following the manufacturer’s guidelines. Concentration and purity of DNA obtained were determined by measuring the absorbance within the micro-volume spectrophotometer NanoDrop® ND-2000c (ThermoFisher Scientific, Waltham, MA, United States).
For genotyping analysis, the selection of SNPs of the ADAM17 gene was based on a previous study that reported the allele frequency of five SNPs analysis in patients with distinct severity of sepsis (Shao et al., 2016), an infectious disorder where control of inflammation is essential in determining the outcome of affected people, as in TB. Herein, four of these five SNPs of ADAM17 were analyzed using specific TaqMan® genotyping assays commercially available (ThermoFisher Scientific, Waltham, MA, United States; rs12692386, assay number C__31588436_10; rs1524668, assay number C__8348383_30; rs11684747, assay number C__1829895_10; rs55790676, assay number C__25942661_10). The RT-PCR reaction was set up in 96-well plates with a mix of 6 μL TaqMan™ Universal PCR Master Mix (ThermoFisher Scientific, Waltham, MA, United States), 2 μL of free nuclease water, and 3 μL of DNA samples at 5 ng/μL or free nuclease water for negative controls. Thermal cycling was performed at 55°C for 10 min for reverse transcription, followed by 95°C for 10 min, and then 40 cycles of 60°C for 60 s, 60°C for 30 s using a StepOnePlus™ thermocycler (Applied Biosystems, Foster City, CA, United States).
2.4 Cytokine levels
Soluble levels of TNF, TNFR1, TNFR2, and TIM-3 were quantified by Enzyme-linked immunosorbent assay (ELISA) following the manufacturer’s protocols (ELISA MAX™ Deluxe Set Human TNF-α, BioLegend, CA, USA; and Human TNFRI/TNFRSF1A, TNFRII/TNFRSF1B Immunoassay Quantikine™, Human TIM-3 DuoSet ELISA; R&D Sytems, MN, USA). The optical density was measured using a microplate reader spectrophotometer (Imark, Bio-Rad, Hercules, United States) set to 450 nm. All proteins were quantified by comparison with the corresponding standard curve.
2.5 Haplotype block construction
This analysis was carried out using Haploview 4.2 software (Barrett et al., 2005), applying the analysis algorithm presented by Gabriel et al. (2002). Linkage disequilibrium (LD) was presented using D′ value. Haplotype-association analysis was performed with Fisher’s exact test between cases and controls.
2.6 Ethics statement
The Institutional Review Board of the Instituto Nacional de Enfermedades Respiratorias Ismael Cosío Villegas approved the study protocol code B04-23, approved in February 2023. All participants or their legal guardians provided written informed consent to participate in the investigation in adherence to the Declaration of Helsinki for Human Research. The study was conducted under the Mexican Constitution law NOM-012-SSA3-2012, which establishes the criteria for executing clinical investigations in humans. All the personal data and clinical information were managed according to local legislation.
2.7 Statistical analysis
Descriptive statistics were used to characterize the study cohorts. Frequencies and proportions were calculated for categorical data. Medians, interquartile ranges, and 95% confidence intervals were used for continuous variables. Comparisons between groups were made using a Chi-square test, Fisher’s exact test, Mann–Whitney U test, or Kruskal–Wallis with post hoc Dunn’s test, as appropriate.
A multivariate logistic regression analysis was conducted to identify associations between TB status (ATB) and the soluble levels of TNF, TNFR1 and TNFR2, and SNPs of ADAM17. Thus, multiple logistic regression with three-way interactions was performed with variables that showed statistically significant differences between groups; rs11684747 (AA allele) and rs55790676 (GG allele) SNPs of ADAM17 (which were significantly different in ATB versus no ATB) were defined as dependent variables.
All analyses were performed using GraphPad Prism V 9.0.2 (GraphPad Software, Inc., San Diego, CA, United States) and R statistical software version 4.2.1 (R Foundation for Statistical Computing, Vienna, Austria). p-values ≦ 0.05 were considered statistically significant.
3 Results
3.1 Clinical characteristics of participants
This study enrolled a total of 342 participants, 224 with ATB and 118 house close contacts. The second group included 70 individuals with LTB (QTF+) and 48 QTF−. Supplementary Table S1 summarizes the main demographic and clinical characteristics of the study groups. Since QTF+ and QTF− individuals showed similar features, they were consolidated as a single group for some comparisons with ATB patients. Also, given that both groups represent individuals with higher protection against Mtb, analyses of SNPs were made between this consolidated group and ATB. Overall, 60% of participants were women with a median age of 44 years (Table 1). The ATB group significantly differed from close contacts by a higher proportion of males, lower weight, and body mass index (BMI), and higher prevalence of tobacco smoking and diabetes. A similar proportion of ATB and no ATB participants (87 and 89%, respectively) received the Bacillus Calmette-Guerin vaccine (BCG) during childhood.
3.2 SNPs of ADAM17 are differentially distributed according to ATB status
To investigate the role of the SNPs of ADAM17 in TB, we analyzed the distribution of rs12692386, rs1524668, rs11684747, and rs55790676 among study groups. First, the possible participation of these SNPs as a cluster of genetic susceptibility was assessed by haplotype blocks. Their genotype distribution among study participants met the Hardy–Weinberg equilibrium (p > 0.05) and showed a complete LD (Figure 1). Four blocks of haplotypes were found among cases and controls; however, their frequencies were not different between cases and close contacts (Table 2). Thus, we analyzed each SNP individually.
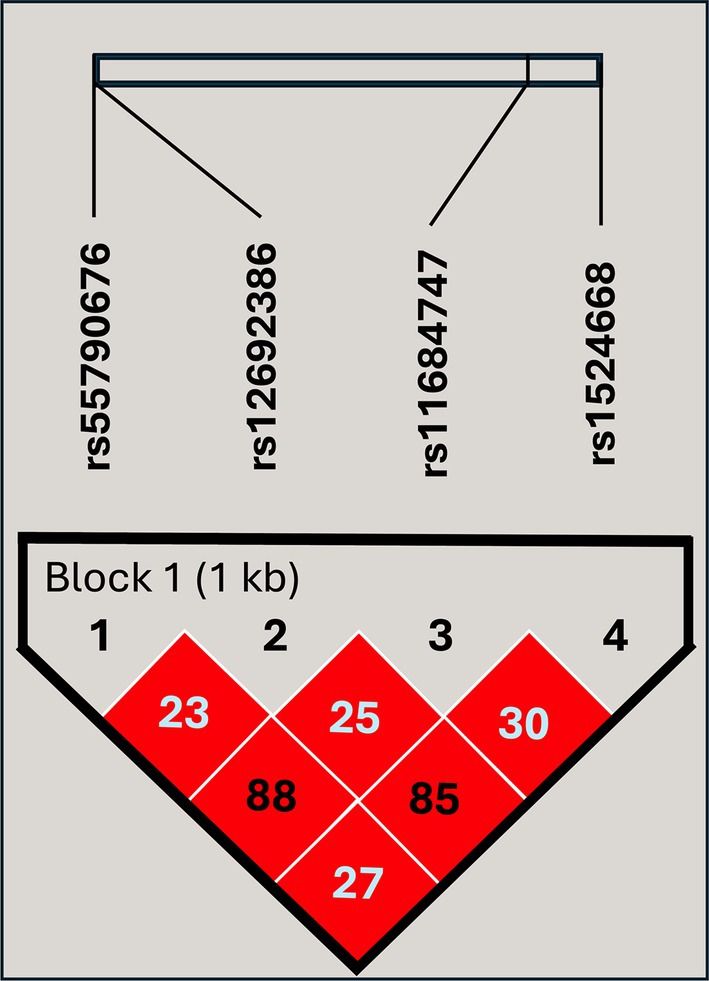
Figure 1. Linkage disequilibrium plot of the genetic variants included in the study. The high intensity of the red diamonds indicates a complete linkage disequilibrium (D′ = 100). The values inside the diamonds are the results of the R2 pairwise measures.
The allele frequencies of rs12692386 and rs1524668 were not significantly different between participant groups (Table 3; Supplementary Table S2). In contrast, ATB patients showed a higher frequency of homozygosity to ancestral AA rs11684747 and GG rs55790676 alleles, whereas close contacts were heterozygous AG to rs11684747 and GT to rs55790676 with higher frequency than ATB patients (19% vs. 11%, p = 0.027; and 19% vs. 10%, p = 0.021, respectively). The allelic frequencies were similar between QTF+ and QTF− close contact groups (Supplementary Table S2). Homozygous to these SNPs of ADAM17 (GG to rs11684747 and TT to rs55790676) were not identified in the cohorts.
3.3 Levels of TNF and TNFRs are influenced by SNPs of ADAM17 and distinguish ATB patients from close contacts
Given the major role of ADAM17 in modulating the release of TNF and TNFRs from cell surfaces, we next investigated an association of SNPs and cytokine levels in TB. These analyses were carried out in the overall study population since all participants were in contact with Mtb, representing biological replicates of the same immunological phenomenon: the production of TNF molecules in response to mycobacteria in vivo. First, we measured and compared cytokine levels between study groups, looking for differences that may reflect the distinct grades of protection against Mtb between ATB patients and close contacts. As shown in Figure 2A and Supplementary Table S3, the levels of soluble TNF in plasma were significantly lower in ATB cases than in controls, whereas no differences were observed between QTF+ and QTF− groups (Supplementary Table S4; Supplementary Figure S1). Conversely, TNFR1 and TNFR2 were elevated in ATB patients compared to their close contacts.
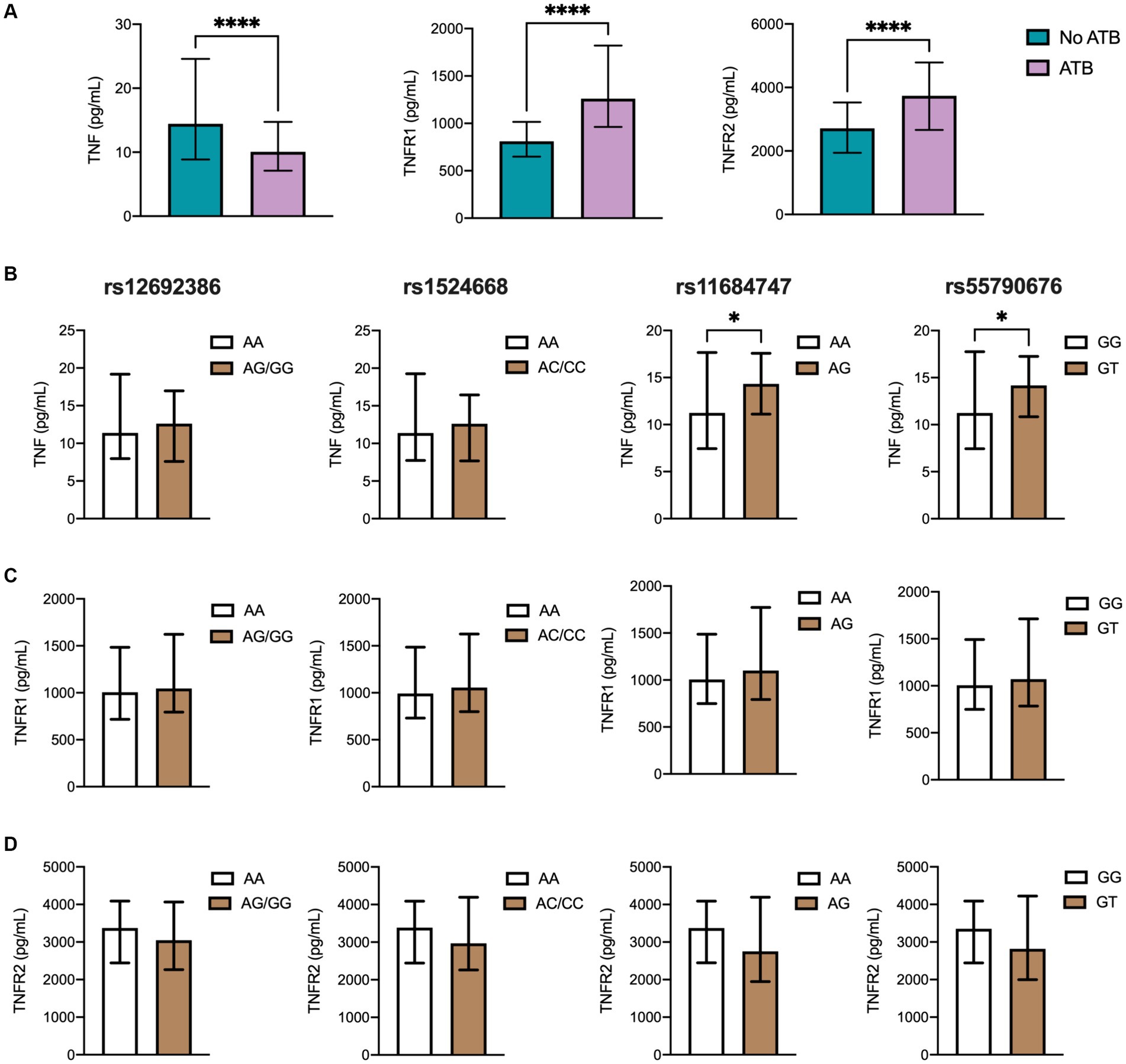
Figure 2. TNF and TNFRs plasma levels in the different TB groups. (A) Soluble levels of TNF, TNFR1, and TNFR2 were assessed by ELISA. Genotype distribution according to (B) TNF, (C) TNFR1, and (D) TNFR2 levels in the population, including 224 ATB patients and controls comprehending 70 individuals with LTB (QTF+) and 48 with no LTB (QTF−). Data are displayed as median and IQR values. Differences between groups were performed using the Mann–Whitney U test, *p < 0.05, and **** p < 0.0001.
Then, a link between SNPs of ADAM17 and TNF, TNFR1, and TNFR2 was evaluated. Notably, homozygous AA to rs11684747 and GG to rs55790676 showed lower plasma levels of TNF than individuals carrying at least one variant allele (Figure 2B). Also, homozygous to ancestral alleles of these SNPs displayed higher levels of TNFR2 that did not reach statistical significance compared to those carrying the variant alleles. No association between rs12692386 and rs1524668 with cytokine levels was found (Figures 2C,D).
3.4 Elevated TIM-3 levels in ATB patients and their association with the rs12692386 SNP of ADAM17
T cell immunoglobulin and mucin-domain-3 (TIM-3 or CD366) is a surface molecule expressed by both CD4+ and CD8+ T cells in mice infected with Mtb and in ATB patients (Wang et al., 2011). Although its precise role in TB remains unclear, TIM-3 is cleaved by ADAM17 (Möller-Hackbarth et al., 2013). Together with galectin 9, TIM-3 has been shown to induce antibacterial activity in infected human macrophages (Sada-Ovalle et al., 2012). In this study, we also measured the levels of TIM-3 across various TB patient groups due to the inability to directly measure ADAM17. Nonetheless, if ADAM17 indeed has a significant influence on ATB and cytokine levels, its effect would be reflected in the regulation of other immune molecules besides TNF and TNFRs, such as TIM-3. As illustrated in Figure 3A, ATB patients exhibited significantly higher soluble TIM-3 levels compared to the household control group. Further analysis investigated the association between SNPs of ADAM17 and TIM-3 levels. Notably, individuals homozygous for the AA genotype at rs12692386 had higher systemic TIM-3 levels than those carrying at least one variant allele (Figure 3B). Other molecules cleaved by ADAM17, such as PD-L1 and IL-8, were also evaluated, but their plasma levels were undetectable (data not shown).
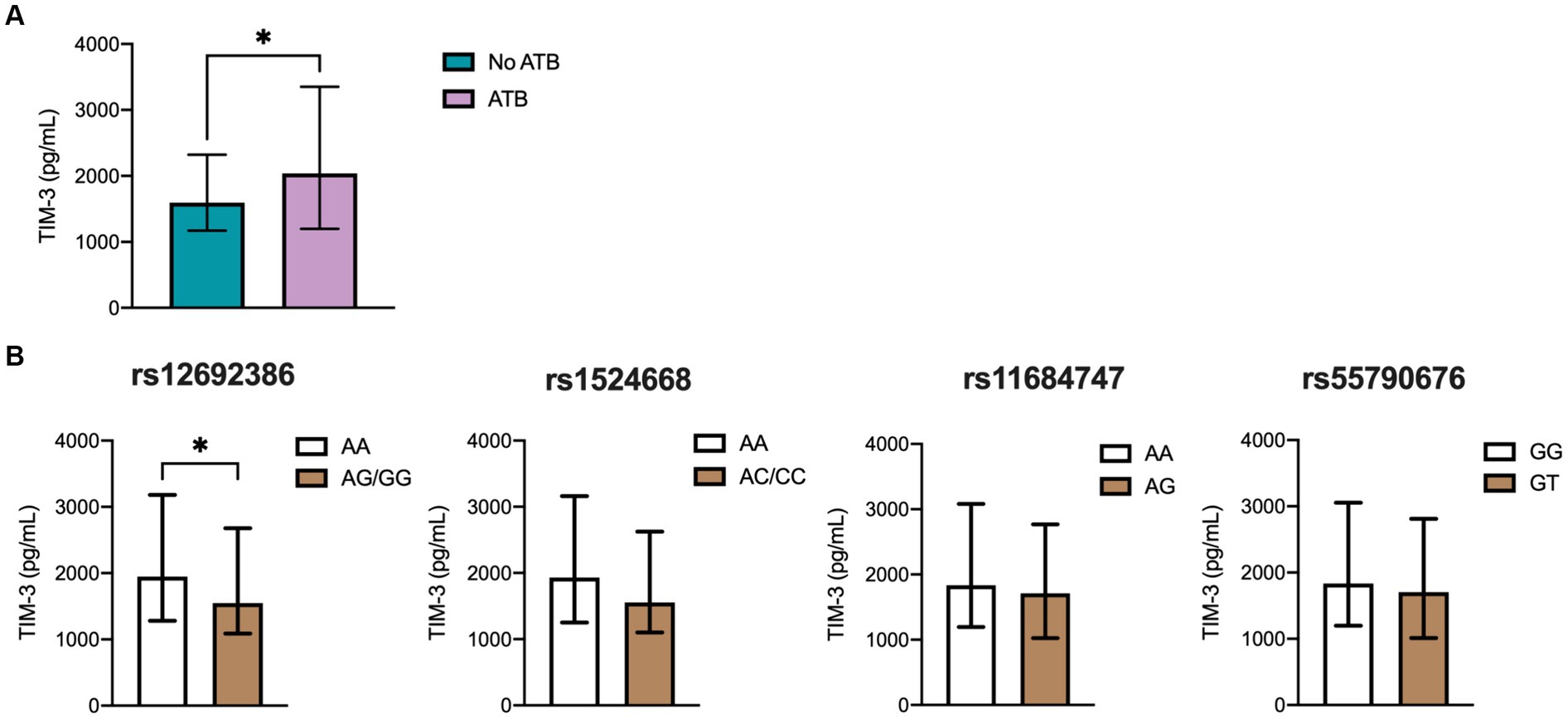
Figure 3. TIM-3 plasma levels in the different TB groups. (A) Soluble levels of TIM3 were assessed by ELISA. Genotype distribution according to (B) TIM-3 levels in the population, including 224 ATB patients and controls comprehending 70 individuals with LTB (QTF+) and 48 with no LTB (QTF−). Data are displayed as median and IQR values. Differences between groups were performed using the Mann–Whitney U test, *p < 0.05.
3.5 Multiple logistic regression analysis reveals an association between ATB status, SNPs of ADAM17, TNF, and TNFRs
A multiple logistic regression analysis was performed, looking for variables associated with ATB status, including SNPs of ADAM17, TNF, and TNFR levels. As observed in Supplementary Table S5, male gender, low weight, tobacco smoking, and diabetes were associated with ATB. Importantly, carrying rs11684747 and rs55790676 SNPs of ADAM17 were inversely correlated with ATB, whereas homozygous to ancestral alleles of these SNPs were positively associated with disease status.
To confirm these findings, we also carried out a multiple regression analysis on rs11684747 and rs55790676 SNPs, considering the TNF, TNFRs, and genotypes. The analytical model was based on the allele with higher frequency in ATB to find a correlation with the disease. This model showed that TNFR1 and TNFR2 correlate with the presence of rs11684747, as well as TNF and TNFR1 individually, TNFR1/TNFR2, and TNF/TNFR1/TNFR2. Moreover, both TNFRs correlate with ATB and AA genotype to rs11684747. The second genotype, homozygous GG to rs55790676 correlated with TNFR1, TNFR2, and both together, but not ATB (Table 4).
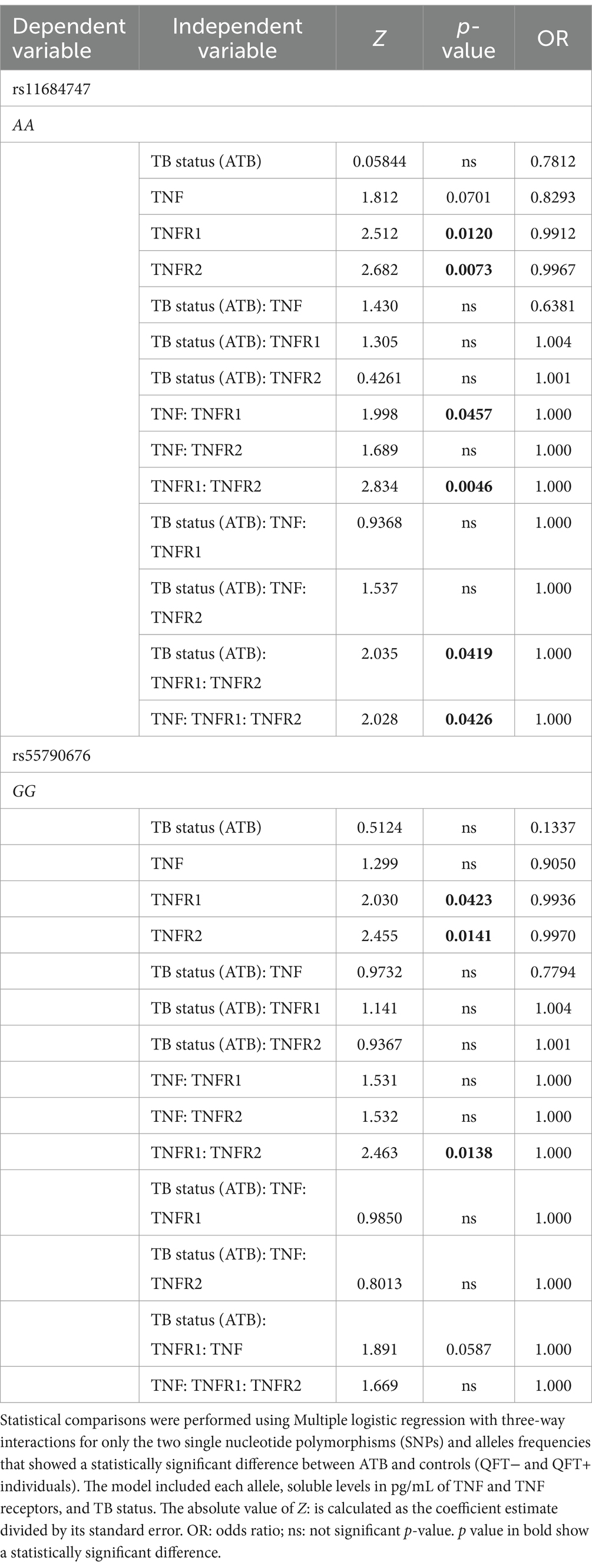
Table 4. Multiple logistic regression analysis of the association with TB status and levels of TNF and their receptors for the presence of ADAM17 rs11684747 and rs55790676.
4 Discussion
It is well known that genetic factors play a major role in both infection and clinical progression of TB. Many functional and population-based studies have demonstrated that polymorphisms located in genes of immune responses, as well as in genes related with metabolism, are involved in the susceptibility and the multiplexity of TB-host interactions and pathogenesis (Zuiga et al., 2012). TNF is a pivotal mediator in TB immunopathogenesis (Dorhoi and Kaufmann, 2014). As such, several association animal studies have linked low TNF levels with TB progression and morbidity (Kindler et al., 1989; Flynn et al., 1995; Roach et al., 2002; Mezouar et al., 2019), whereas treatment with TNF blockers is known to result in ATB reactivation and dissemination (Keane et al., 2001; Gómez-Reino et al., 2003; Mohan et al., 2004; Wallis et al., 2004), making TB screening mandatory before anti-TNF therapy (Fernández-Ruiz and Aguado, 2018). The immune effects of TNF are vast and depend on the interactions with either TNFR1 or TNFR2, triggering inflammation, anti-microbial immunity, and cell death. In TB, TNF orchestrates the early induction of chemokines to facilitate leucocyte recruitment and granuloma formation (Roach et al., 2002). Moreover, containment of Mtb infection is attuned to a highly regulated production of this cytokine from different sources, such as myeloid cells and T cells, with several complex mechanisms controlling its abundance and bioavailability to avoid pathogenic functions such as tissue necrosis (Dorhoi and Kaufmann, 2014).
Here, our results showed that TNF levels in ATB patients were lower than in their household contacts. Although previous reports indicated that TB patients have significantly higher TNF levels, those studies used a control group of healthy people with no history of contact with ATB patients, so their immune status against Mtb (QTF or PPD test) is unclear (Mirzaei and Mahmoudi, 2018; Beig et al., 2023). In this sense, our results are in consonance with other studies, where close contacts or healthy controls have higher levels than ATB patients across different age groups (Joshi et al., 2015). Furthermore, TNF expression at the transcriptional level was found to be lower in ATB than in household controls (De Sousa et al., 2023). Our results suggest that TNF might be a readout of loss of protective immunity against Mtb among people exposed to the bacillus, which agrees with a plethora of studies showing that the neutralization of TNF promotes the progression of latent TB infection to ATB (Lin et al., 2010).
One of the processes to optimize TNF expression and function is through the tight control of its interaction with TNFRs. Each of these receptors induces specific immune functions; TNFR1 mediates inflammation and tissue injury (Chavez-Galan et al., 2019), while TNFR2 mediates suppressive activity to reduce excessive inflammation (Chavez-Galan et al., 2017). The transmembrane forms of TNF, TNFR1, and TNFR2 are released into the extracellular space by the protease ADAM17 (Porteu and Nathan, 1990; Black et al., 1997; Mohan et al., 2002; Ruiz et al., 2021), which is essential in establishing a balance between TNF and TNFRs in cell surfaces and tissues. Soluble TNFRs are thought to favor anti-inflammatory functions, acting as decoy receptors of TNF (Kohno et al., 1990). For instance, it has been shown that high TNF levels can induce shedding of TNFR1 by ADAM17, and solTNFR1 then neutralizes circulating TNF, consequently attenuating excessive inflammation (Rowlands et al., 2011).
The metalloprotease function of ADAM17 is not limited to releasing TNF and TNFRs from the cell surface, also shedding more than 80 substrates, including cytokines, growth factors, and cell adhesion molecules receptors, including interleukin (IL)-R1, IL-R6, TGFβ, ACE-2, TIM-3, CD16, among other (Zunke and Rose-John, 2017). Also, it is functionally involved in pathogen recognition and may influence the potential uptake of invading pathogens, inducing the cleavage of PPRs and Toll-like receptors at the macrophages cell surface (Hernandez-Pando et al., 2000). Hence, ADAM17 might be crucial in the immunopathogenesis of several inflammatory disorders and infectious diseases, especially those that depend on TNF signaling and a balanced immune response for protective immunity. Despite this, there is little evidence about the association of ADAM17 with TB, a prototype infectious disease where immune regulation is pivotal in maintaining infection control at the lowest tissue-damage cost during LTB and displaying intense necrosis of granuloma in ATB progressors.
Previous reports suggest that SNPs of ADAM17 are associated with several disorders (Li et al., 2014, 2019; Shao et al., 2016; He et al., 2022; Jung and Hwang, 2022). Importantly, two studies showed that the rs12692386 A > G allele of ADAM17 increased the protease expression and shedding of TNF, IL-6R, and CX3CL1, conferring a higher risk of severe disease and shock in sepsis (Shao et al., 2016; He et al., 2022). Here, we analyzed four SNPs (rs12692386, rs1524668, rs11684747, rs55790676) of ADAM17 looking for a possible association with TB progression. Although we did not find any relationship between rs12692386 A > G and TB susceptibility, we showed that people with rs11684747 A > G and rs55790676 G > T have higher circulating levels of TNF and are overrepresented among healthy close contacts with LTB compared to ATB patients. Together, both studies favor the assumption that ADAM17 takes a role during infection by modulating the production of inflammatory molecules, mainly TNF, which is required for protective immunity during LTB but could be detrimental when it is produced in excess, as in septic shock. In addition, despite rs12692386 being no different between groups, an association of homozygous AA to rs12692386 was found with patients displaying higher TIM-3 systemic levels, suggesting that the presence of this SNP could be involved in inducing an exhausting status in ATB.
In 2000, Hernández-Pando and colleagues demonstrated that the administration of batimastat, a broad-spectrum metalloproteinase inhibitor acting on ADAM17, reduces TB control in mice infected with Mtb via intratracheal instillation (Hernandez-Pando et al., 2000). In these animals, a delay in granuloma formation, higher disease progression, and increased mortality were associated with lower expression of TNF in lung tissue compared to untreated mice. Although these outcomes could be explained by the disruption of other proteases, the data confirm the protective role of TNF in TB, revealing a possible influence of ADAM17 in the process.
In line with this idea, our findings indicate that in healthy close contacts and LTB, ADAM17 acts principally by shedding TNF to maintain concentrations sufficient to keep infection control below a pathological threshold. Much lower levels might make the individual prone to disease progression. Accordingly, we found higher levels of TNF in close contacts than in ATB cases, mainly in those carrying rs11684747 and rs55790676. Thus, the acquisition of certain genetic variants of ADAM17 associated with higher cytokine production might be beneficial. However, in contrast with our results, other reports showed a link between high serum TNF levels and clinical severity of TB measured by chest X-ray alterations, weight loss, and positive PPD skin test (Júnior et al., 2008; Beig et al., 2023), whereas Kart et al. (2003) found no relationship between increased TNF levels and TB outcomes. Unfortunately, we had no clinical data on the severity of the disease in our ATB cohort. Hence, looking for differences in ATB outcomes according to TNF levels and ADAM17 SNPs is warranted for future investigations.
On the other hand, our data suggest a little effect of ADAM17 on TNFRs during LTB or resistance to infection status since the levels of these receptors were lower in healthy close contacts of ATB cases. Also, we did not find a relationship between SNPs and TNFR1 and TNFR2, although TNFRs levels tended to be lower in people carrying the rs11684747 A > G and rs55790676 G > T SNPs of ADAM17. Of note, the levels of TNFRs, mainly TNFR2, were higher in ATB patients compared to controls. Interestingly, a study in mice infected with aerosolized Mtb found high levels of TNFRs, especially TNFR2, in bronchioalveolar lavage specimens collected one month after inoculation. In this model, the investigators discovered that mannose-capped lipoarabinomannan (ManLAM) was sufficient to promote ADAM17 shedding of TNFR2, and a lower level of TNFR1, hindering the release of tmTNF (Richmond et al., 2012). In agreement with our findings, they also showed higher levels of TNFR2 among ATB patients.
Therefore, we believe that in close contacts resistant to Mtb infection and those with LTB, ADAM17 plays a protective role in keeping TNF levels in adequate concentrations. Nonetheless, when protective immunity is disrupted by age, diabetes, or immune suppression, proliferation of bacilli increases the amount of ManLAM and other virulence factors, consequently promoting TNFR2 and TNFR1 release, which in turn block TNF signaling, further hindering protective immunity against Mtb. In this process, certain SNPs of ADAM17 might confer protection or susceptibility if they enhance the ability to maintain a favorable balance between TNF and TNFRs. This might extend to other infections like COVID-19, where a disbalance of proinflammatory and anti-inflammatory signals is crucial to determine clinical outcomes. Interestingly, the rs55790676 polymorphism was recently associated with an increased susceptibility to COVID-19 in India (Patil et al., 2021). Also, it is possible that this polymorphism might influence the expression of entry factors important for SARS-CoV-2 infection, as demonstrated by functional studies showing that a lower expression of ADAM17 provokes a reduced release of ACE2 from the cell membrane (Healy and Lilic, 2021), whereas increased protease activity primes the viral S protein favoring susceptibility to SARS-CoV-2 infection (Jocher et al., 2022).
The current study has limitations that must be considered when interpreting the results. Firstly, the small sample size limited our statistical power to examine a small genetic effect, decreasing the accuracy of our genotype–phenotype analyses and hindering the possibility of making more comparisons between certain groups of participants. Due to low allele frequency, we could not identify enough individuals homozygous GG to rs11684747 and TT to rs55790676. Also, the case–control design of this study may bias any true relationship between ADAM17 SNPs and ATB. In addition, the rs11689958 allele was not assessed in our study, which shows a solid pairwise linkage disequilibrium with other SNPs at the ADAM17 promoter region (Shao et al., 2016). Finally, due to technical limitations, we were unable to directly measure ADAM17 in our study. However, we hypothesized that if this protease significantly influences cytokine levels during TB, its effect would be reflected in the regulation of other immune molecules cleaved by ADAM17 that are involved in disease immunopathogenesis. Consequently, we chose to measure TIM-3 levels. TIM-3 is a co-inhibitory molecule that regulates T cell function and may play a role in TB. Recent research indicates that TIM-3 acts as an exhaustion marker in CD4+ and CD8+ T cells during Mtb infection (Jayaraman et al., 2016). Interestingly, we found higher levels of TIM-3 in ATB patients and those homozygous to the rs12692386 SNP of ADAM17, suggesting its involvement in chronic infection, which is in line with previous reports (Chen et al., 2022).
Hence, despite these caveats, our results provide preliminary data that open a novel and unexplored hypothesis of a possible role for ADAM17 in TB; however, the role of these SNPs in latent TB is unknown and requires further investigation in future larger studies. In sum, our main findings were that levels of TNF and TNFRs are influenced by SNPs of ADAM17 and distinguish ATB patients from close contacts. We observed that homozygous AA to rs11684747 and GG to rs55790676 exhibited lower plasma levels of TNF than heterozygous individuals. In contrast, homozygous patients AA to rs11684747 and GG to rs55790676 showed lower levels of TNFR2 when compared to heterozygous individuals. Multiple regression analyses showed a significant association between the levels of TNF, TNFR1, TNFR2, tuberculosis status, and the AA homozygosity on the rs11684747 SNP. This study suggests a role for SNPs of ADAM17 in genetic susceptibility to ATB that warrants further research.
Data availability statement
The datasets presented in this study can be found in online repositories. The names of the repository/repositories and accession number(s) can be found below: https://www.ncbi.nlm.nih.gov/clinvar/, SCV005043058, SCV005043059, SCV005043068, SCV005043069.
Ethics statement
The studies involving humans were approved by the study was approved by the Institutional Review Board of Instituto Nacional de Enfermedades Respiratorias Ismael Cosío Villegas (protocol code B04-23, approved in February 2023). Informed consent was obtained from all subjects involved in the study. The studies were conducted in accordance with the local legislation and institutional requirements. The participants provided their written informed consent to participate in this study.
Author contributions
JC-P: Conceptualization, Methodology, Writing – original draft, Formal analysis, Investigation. LR-L: Conceptualization, Formal analysis, Investigation, Methodology, Writing – original draft, Supervision. MC: Methodology, Writing – review & editing. EO-M: Methodology, Writing – review & editing. AT-G: Methodology, Writing – review & editing. MM-M: Methodology, Writing – review & editing. AC-L: Methodology, Writing – review & editing, Validation. GR-M: Methodology, Writing – review & editing. IG-G: Methodology, Writing – review & editing. JR-N: Writing – review & editing, Investigation. BG-P: Methodology, Writing – review & editing. KL-S: Methodology, Writing – review & editing. FJ-J: Methodology, Writing – review & editing. PG-O: Methodology, Writing – review & editing. CS-L: Methodology, Writing – review & editing. KB-P: Formal analysis, Writing – review & editing. MM-T: Data curation, Writing – review & editing. LC-G: Conceptualization, Methodology, Resources, Supervision, Writing – original draft, Writing – review & editing. JZ: Data curation, Formal analysis, Funding acquisition, Methodology, Project administration, Writing – original draft, Writing – review & editing.
Funding
The author(s) declare financial support was received for the research, authorship, and/or publication of this article. This research was supported by research funds of the Instituto Nacional de Enfermedades Respiratorias Ismael Cosío Villegas (INER), and by the National Council of Humanities, Science and Technology of Mexico (CONAHCYT), grant number FORDECYT/10SE/2020/05/14-06 and FORDECYT/10SE/2020/05/14-07 from the Fondo Institucional de Fomento Regional para el Desarrollo Científico y Tecnológico y de Innovación (FORDECYT) to JZ. JZ also received support from the Secretaría de Ciencia, Tecnología e Innovación de la Ciudad de México (SECTEI CDMX) under the contract SECTEI/050/2020. JC-P is receiving a scholarship from INER to achieve his M.D. degree in pneumology and critical care medicine.
Acknowledgments
The authors thank all participants in this study and Mario Preciado García for the technical assistance.
Conflict of interest
The authors declare that the research was conducted in the absence of any commercial or financial relationships that could be construed as a potential conflict of interest.
The author(s) declared that they were an editorial board member of Frontiers, at the time of submission. This had no impact on the peer review process and the final decision.
Publisher’s note
All claims expressed in this article are solely those of the authors and do not necessarily represent those of their affiliated organizations, or those of the publisher, the editors and the reviewers. Any product that may be evaluated in this article, or claim that may be made by its manufacturer, is not guaranteed or endorsed by the publisher.
Supplementary material
The Supplementary material for this article can be found online at: https://www.frontiersin.org/articles/10.3389/fmicb.2024.1392782/full#supplementary-material
References
Aboudounya, M. M., and Heads, R. J. (2021). COVID-19 and toll-like receptor 4 (TLR4): SARS-CoV-2 may bind and activate TLR4 to increase ACE2 expression, facilitating entry and causing Hyperinflammation. Mediat. Inflamm. 2021, 1–18. doi: 10.1155/2021/8874339
Adane, G., Lemma, M., Geremew, D., Sisay, T., Tessema, M. K., Damtie, D., et al. (2021). Genetic polymorphism of tumor necrosis factor-alpha, interferon-gamma and Interleukin-10 and association with risk of Mycobacterium Tuberculosis infection. J. Evid. Based Integr. Med. 26:2515690X2110063. doi: 10.1177/2515690X211006344
Barrett, J. C., Fry, B., Maller, J., and Daly, M. J. (2005). Haploview: analysis and visualization of LD and haplotype maps. Bioinformatics 21, 263–265. doi: 10.1093/bioinformatics/bth457
Beig, T. Y., Khan, U. H., Ganie, B. A., Tahir, S., Shah, S., and Dhobi, G. N. (2023). Correlation between serum tumor necrosis factor-alpha (TNF-α) and clinical severity of tuberculosis: a hospital-based study. Cureus 15:e35626. doi: 10.7759/CUREUS.35626
Black, R. A., Rauch, C. T., Kozlosky, C. J., Peschon, J. J., Slack, J. L., Wolfson, M. F., et al. (1997). A metalloproteinase disintegrin that releases tumour-necrosis factor-∅ from cells. Nature 385, 729–733. doi: 10.1038/385729a0
Chavez-Galan, L., Vesin, D., Blaser, G., Uysal, H., Benmerzoug, S., Rose, S., et al. (2019). Myeloid cell TNFR1 signaling dependent liver injury and inflammation upon BCG infection. Sci. Rep. 9, 5297–5215. doi: 10.1038/s41598-019-41629-9
Chavez-Galan, L., Vesin, D., Uysal, H., Blaser, G., Benkhoucha, M., Ryffel, B., et al. (2017). Transmembrane tumor necrosis factor controls myeloid-derived suppressor cell activity via TNF receptor 2 and protects from excessive inflammation during BCG-induced pleurisy. Front. Immunol. 8:999. doi: 10.3389/fimmu.2017.00999
Chen, H., Zhou, J., Zhao, X., Liu, Q., Shao, L., Zhu, Y., et al. (2022). Characterization of multiple soluble immune checkpoints in individuals with different Mycobacterium tuberculosis infection status and dynamic changes during anti-tuberculosis treatment. BMC Infect. Dis. 22:543. doi: 10.1186/s12879-022-07506-z
De Sousa, F. D. M., de Paula Souza, I., Amoras, E. D. S. G., Lima, S. S., Cayres-Vallinoto, I. M. V., Ishak, R., et al. (2023). Low levels of TNFA gene expression seem to favor the development of pulmonary tuberculosis in a population from the Brazilian Amazon. Immunobiology 228:152333. doi: 10.1016/J.IMBIO.2023.152333
Diel, R., Loddenkemper, R., and Nienhaus, A. (2012). Predictive value of interferon-γ release assays and tuberculin skin testing for progression from latent TB infection to disease state: a meta-analysis. Chest 142, 63–75. doi: 10.1378/CHEST.11-3157
Dorhoi, A., and Kaufmann, S. H. E. (2014). Tumor necrosis factor alpha in mycobacterial infection. Semin. Immunol. 26, 203–209. doi: 10.1016/j.smim.2014.04.003
Dunlap, M. D., Howard, N., Das, S., Scott, N., Ahmed, M., Prince, O., et al. (2018). A novel role for C-C motif chemokine receptor 2 during infection with hypervirulent Mycobacterium tuberculosis. Mucosal Immunol. 11, 1727–1742. doi: 10.1038/S41385-018-0071-Y
Esaulova, E., Das, S., Singh, D. K., Choreño-Parra, J. A., Swain, A., Arthur, L., et al. (2021). The immune landscape in tuberculosis reveals populations linked to disease and latency. Cell Host Microbe 29, 165–178.e8. doi: 10.1016/J.CHOM.2020.11.013
Fernández-Ruiz, M., and Aguado, J. M. (2018). Risk of infection associated with anti-TNF-α therapy. Expert Rev. Anti-Infect. Ther. 16, 939–956. doi: 10.1080/14787210.2018.1544490
Flynn, J. A. L., Chan, J., Triebold, K. J., Dalton, D. K., Stewart, T. A., and Bloom, B. R. (1993). An essential role for interferon gamma in resistance to Mycobacterium tuberculosis infection. J. Exp. Med. 178, 2249–2254. doi: 10.1084/JEM.178.6.2249
Flynn, J. A. L., Goldstein, M. M., Chan, J., Triebold, K. J., Pfeffer, K., Lowenstein, C. J., et al. (1995). Tumor necrosis factor-alpha is required in the protective immune response against Mycobacterium tuberculosis in mice. Immunity 2, 561–572. doi: 10.1016/1074-7613(95)90001-2
Gabriel, S. B., Schaffner, S. F., Nguyen, H., Moore, J. M., Roy, J., Blumenstiel, B., et al. (2002). The structure of haplotype blocks in the human genome. Science 296, 2225–2229. doi: 10.1126/science.1069424
Getahun, H., Matteelli, A., Chaisson, R. E., and Raviglione, M. (2015). Latent Mycobacterium tuberculosis infection. N. Engl. J. Med. 372, 2127–2135. doi: 10.1056/NEJMRA1405427
Global Tuberculosis Report 2023 (n.d.). Available at: https://www.who.int/teams/global-tuberculosis-programme/tb-reports/global-tuberculosis-report-2023 (Accessed January 25, 2024).
Gómez-Reino, J. J., Carmona, L., Rodríguez Valverde, V., Mola, E. M., and Montero, M. D. (2003). Treatment of rheumatoid arthritis with tumor necrosis factor inhibitors may predispose to significant increase in tuberculosis risk: a multicenter active-surveillance report. Arthritis Rheum. 48, 2122–2127. doi: 10.1002/ART.11137
He, J., Zhao, T., Liu, L., Liao, S., Yang, S., Lu, F., et al. (2022). The −172 A-to-G variation in ADAM17 gene promoter region affects EGR1/ADAM17 pathway and confers susceptibility to septic mortality with sepsis-3.0 criteria. Int. Immunopharmacol. 102:108385. doi: 10.1016/J.INTIMP.2021.108385
Healy, E. F., and Lilic, M. (2021). A model for COVID-19-induced dysregulation of ACE2 shedding by ADAM17. Biochem. Biophys. Res. Commun. 573, 158–163. doi: 10.1016/J.BBRC.2021.08.040
Hernandez-Pando, R., Orozco, H., Arriaga, K., Pavön, L., and Rook, G. (2000). Treatment with BB-94, a broad spectrum inhibitor of zinc-dependent metalloproteinases, causes deviation of the cytokine profile towards type-2 in experimental pulmonary tuberculosis in Balb/c mice. Int. J. Exp. Pathol. 81, 199–209. doi: 10.1046/J.1365-2613.2000.00152.X
Houben, R. M. G. J., and Dodd, P. J. (2016). The global burden of latent tuberculosis infection: a re-estimation using mathematical modelling. PLoS Med. 13:e1002152. doi: 10.1371/journal.pmed.1002152
Jayaraman, P., Jacques, M. K., Zhu, C., Steblenko, K. M., Stowell, B. L., Madi, A., et al. (2016). TIM3 mediates T cell exhaustion during Mycobacterium tuberculosis infection. PLoS Pathog. 12:e1005490. doi: 10.1371/JOURNAL.PPAT.1005490
Jocher, G., Grass, V., Tschirner, S. K., Riepler, L., Breimann, S., Kaya, T., et al. (2022). ADAM10 and ADAM17 promote SARS-CoV-2 cell entry and spike protein-mediated lung cell fusion. EMBO Rep. 23:e54305. doi: 10.15252/EMBR.202154305
Joshi, L., Ponnana, M., Sivangala, R., Chelluri, L. K., Nallari, P., Penmetsa, S., et al. (2015). Evaluation of TNF-α, IL-10 and IL-6 cytokine production and their correlation with genotype variants amongst tuberculosis patients and their household contacts. PLoS One 10:e0137727. doi: 10.1371/JOURNAL.PONE.0137727
Jung, J., and Hwang, D. (2022). Genetic polymorphism of ADAM17 and decreased bilirubin levels are associated with allergic march in the Korean population. BMC Med. Genet. 15:21. doi: 10.1186/s12920-022-01170-7
Júnior, A., De, D. R., Santos, S. A. D., Castro, I., and Andrade, D. R. (2008). Correlation between serum tumor necrosis factor alpha levels and clinical severity of tuberculosis. Braz. J. Infect. Dis. 12, 226–233. doi: 10.1590/S1413-86702008000300013
Kart, L., Buyukoglan, H., Tekin, I. O., Altin, R., Senturk, Z., Gulmez, I., et al. (2003). Correlation of serum tumor necrosis factor-α, interleukin-4 and soluble interleukin-2 receptor levels with radiologic and clinical manifestations in active pulmonary tuberculosis. Mediat. Inflamm. 12, 9–14. doi: 10.1080/0962935031000096926
Keane, J., Gershon, S., Wise, R. P., Mirabile-Levens, E., Kasznica, J., Schwieterman, W. D., et al. (2001). Tuberculosis associated with infliximab, a tumor necrosis factor alpha-neutralizing agent. N. Engl. J. Med. 345, 1098–1104. doi: 10.1056/NEJMOA011110
Khader, S. A., Divangahi, M., Hanekom, W., Hill, P. C., Maeurer, M., Makar, K. W., et al. (2019). Targeting innate immunity for tuberculosis vaccination. J. Clin. Invest. 129, 3482–3491. doi: 10.1172/JCI128877
Kindler, V., Sappino, A. P., Grau, G. E., Piguet, P. F., and Vassalli, P. (1989). The inducing role of tumor necrosis factor in the development of bactericidal granulomas during BCG infection. Cell 56, 731–740. doi: 10.1016/0092-8674(89)90676-4
Kohno, T., Brewer, M. T., Baker, S. L., Schwartz, P. E., King, M. W., Hale, K. K., et al. (1990). A second tumor necrosis factor receptor gene product can shed a naturally occurring tumor necrosis factor inhibitor. Proc. Natl. Acad. Sci. USA 87, 8331–8335. doi: 10.1073/PNAS.87.21.8331
Li, W.-W., Shen, Y.-Y., Chen, D.-W., Li, H.-Y., Shi, Q.-Q., Mei, J., et al. (2019). Genetic association between NGFR, ADAM17 gene polymorphism, and Parkinson’s disease in the Chinese Han population. Neurotox. Res. 36, 463–471. doi: 10.1007/s12640-019-00031-z
Li, Y., Yang, C., Ma, G., Cui, L., Gu, X., Chen, Y., et al. (2014). Analysis of ADAM17 polymorphisms and susceptibility to sporadic abdominal aortic aneurysm. Cell. Physiol. Biochem. 33, 1426–1438. doi: 10.1159/000358708
Lin, P. L., Myers, A., Smith, L., Bigbee, C., Bigbee, M., Fuhrman, C., et al. (2010). Tumor necrosis factor neutralization results in disseminated disease in acute and latent Mycobacterium tuberculosis infection with normal granuloma structure in a cynomolgus macaque model. Arthritis Rheum. 62, 340–350. doi: 10.1002/ART.27271
Mack, U., Migliori, G. B., Sester, M., Rieder, H. L., Ehlers, S., Goletti, D., et al. (2009). LTBI: latent tuberculosis infection or lasting immune responses to M. tuberculosis? A TBNET consensus statement. Eur. Respir. J. 33, 956–973. doi: 10.1183/09031936.00120908
Mezouar, S., Diarra, I., Roudier, J., Desnues, B., and Mege, J.-L. (2019). Tumor necrosis factor-alpha antagonist interferes with the formation of granulomatous multinucleated Giant cells: new insights into Mycobacterium tuberculosis infection. Front. Immunol. 10:1947. doi: 10.3389/fimmu.2019.01947
Mirzaei, A., and Mahmoudi, H. (2018). Evaluation of TNF-α cytokine production in patients with tuberculosis compared to healthy people. GMS Hyg. Infect. Control. 13:Doc09. doi: 10.3205/DGKH000315
Mohan, A. K., Coté, T. R., Block, J. A., Manadan, A. M., Siegel, J. N., and Braun, M. M. (2004). Tuberculosis following the use of etanercept, a tumor necrosis factor inhibitor. Clin. Infect. Dis. 39, 295–299. doi: 10.1086/421494
Mohan, M. J., Seaton, T., Mitchell, J., Howe, A., Blackburn, K., Burkhart, W., et al. (2002). The Τumor necrosis factor-α converting enzyme (TACE): a unique metalloproteinase with highly defined substrate selectivity. Biochemistry 41, 9462–9469. doi: 10.1021/bi0260132
Möller-Hackbarth, K., Dewitz, C., Schweigert, O., Trad, A., Garbers, C., Rose-John, S., et al. (2013). A disintegrin and metalloprotease (ADAM) 10 and ADAM17 are major sheddases of T cell immunoglobulin and mucin domain 3 (Tim-3). J. Biol. Chem. 288, 34529–34544. doi: 10.1074/jbc.M113.488478
Moss, M. L., and Minond, D. (2017). Recent advances in ADAM17 research: a promising target for Cancer and inflammation. Mediat. Inflamm. 2017, 1–21. doi: 10.1155/2017/9673537
Orme, I. M., Roberts, A. D., Griffin, J. P., and Abrams, J. S. (1993). Cytokine secretion by CD4 T lymphocytes acquired in response to Mycobacterium tuberculosis infection. J. Immunol. 151, 518–525. doi: 10.4049/JIMMUNOL.151.1.518
Patil, S., Pemmasani, S. K., Chitturi, N., Bhatnagar, I., Acharya, A., and Subash, L. V. (2021). COVID-19 and Indian population: a comparative genetic analysis. medRxiv 12:21267816. doi: 10.1101/2021.12.15.21267816
Porteu, F., and Nathan, C. (1990). Shedding of tumor necrosis factor receptors by activated human neutrophils. J. Exp. Med. 172, 599–607. doi: 10.1084/jem.172.2.599
Richmond, J. M., Duffy, E. R., Lee, J., Kaboli, K., Remick, D. G., Kornfeld, H., et al. (2012). Mannose-capped Lipoarabinomannan from Mycobacterium tuberculosis induces soluble tumor necrosis factor receptor production through tumor necrosis factor alpha-converting enzyme activation. Infect. Immun. 80, 3858–3868. doi: 10.1128/iai.00060-12
Roach, D. R., Bean, A. G. D., Demangel, C., France, M. P., Briscoe, H., and Britton, W. J. (2002). TNF regulates chemokine induction essential for cell recruitment, granuloma formation, and clearance of mycobacterial Infection1. J. Immunol. 168, 4620–4627. doi: 10.4049/jimmunol.168.9.4620
Rowlands, D. J., Islam, M. N., Das, S. R., Huertas, A., Quadri, S. K., Horiuchi, K., et al. (2011). Activation of TNFR1 ectodomain shedding by mitochondrial Ca2+ determines the severity of inflammation in mouse lung microvessels. J. Clin. Invest. 121, 1986–1999. doi: 10.1172/JCI43839
Ruiz, A., Palacios, Y., Garcia, I., and Chavez-Galan, L. (2021). Transmembrane TNF and its receptors TNFR1 and TNFR2 in mycobacterial infections. Int. J. Mol. Sci. 22:5461. doi: 10.3390/ijms22115461
Sada-Ovalle, I., Chavez-Galan, L., Torre-Bouscoulet, L., Nava-Gamino, L., Barrera, L., Jayaraman, P., et al. (2012). The Tim3-galectin 9 pathway induces antibacterial activity in human macrophages infected with Mycobacterium tuberculosis. J. Immunol. 189, 5896–5902. doi: 10.4049/jimmunol.1200990
Shao, Y., He, J., Chen, F., Cai, Y., Zhao, J., Lin, Y., et al. (2016). Association study between promoter polymorphisms of ADAM17 and progression of Sepsis. Cell. Physiol. Biochem. 39, 1247–1261. doi: 10.1159/000447830
Shimokata, K., Kishimoto, H., Takagi, E., and Tsunekawa, H. (1986). Determination of the T-cell subset producing gamma-interferon in tuberculous pleural effusion. Microbiol. Immunol. 30, 353–361. doi: 10.1111/J.1348-0421.1986.TB00952.X
Souza de Lima, D., Fadoul de Brito, C., Cavalcante Barbosa, A. R., de Andrade, B., Figueira, M., Maciel Bonet, J. C., et al. (2021). A genetic variant in the TRAF1/C5 gene lead susceptibility to active pulmonary tuberculosis by decreased TNF-α levels. Microb. Pathog. 159:105117. doi: 10.1016/J.MICPATH.2021.105117
Tameris, M. D., Hatherill, M., Landry, B. S., Scriba, T. J., Snowden, M. A., Lockhart, S., et al. (2013). Safety and efficacy of MVA85A, a new tuberculosis vaccine, in infants previously vaccinated with BCG: a randomised, placebo-controlled phase 2b trial. Lancet 381, 1021–1028. doi: 10.1016/S0140-6736(13)60177-4
Tsai, M. C., Chakravarty, S., Zhu, G., Xu, J., Tanaka, K., Koch, C., et al. (2006). Characterization of the tuberculous granuloma in murine and human lungs: cellular composition and relative tissue oxygen tension. Cell. Microbiol. 8, 218–232. doi: 10.1111/J.1462-5822.2005.00612.X
Tufariello, J. A. M., Chan, J., and Flynn, J. A. L. (2003). Latent tuberculosis: mechanisms of host and bacillus that contribute to persistent infection. Lancet Infect. Dis. 3, 578–590. doi: 10.1016/S1473-3099(03)00741-2
Wallis, R. S., Broder, M. S., Wong, J. Y., Hanson, M. E., and Beenhouwer, D. O. (2004). Granulomatous infectious diseases associated with tumor necrosis factor antagonists. Clin. Infect. Dis. 38, 1261–1265. doi: 10.1086/383317
Wang, X., Cao, Z., Jiang, J., Li, Y., Dong, M., Ostrowski, M., et al. (2011). Elevated expression of Tim-3 on CD8 T cells correlates with disease severity of pulmonary tuberculosis. J. Infect. 62, 292–300. doi: 10.1016/J.JINF.2011.02.013
Wu, S., Wang, M. G., Wang, Y., and He, J. Q. (2019). Polymorphisms of cytokine genes and tuberculosis in two independent studies. Sci. Rep. 9:2507. doi: 10.1038/S41598-019-39249-4
Zuiga, J., Torres-García, D., Santos-Mendoza, T., Rodriguez-Reyna, T. S., Granados, J., and Yunis, E. J. (2012). Cellular and humoral mechanisms involved in the control of tuberculosis. Clin. Dev. Immunol. 2012:193923. doi: 10.1155/2012/193923
Keywords: tuberculosis, ADAM17, TNF-α converting enzyme, tumor necrosis factor, tumor necrosis factor receptors
Citation: Choreño-Parra JA, Ramon-Luing LA, Castillejos M, Ortega-Martínez E, Tapia-García AR, Matías-Martínez MB, Cruz-Lagunas A, Ramírez-Martínez G, Gómez-García IA, Ramírez-Noyola JA, Garcia-Padrón B, López-Salinas KG, Jiménez-Juárez F, Guadarrama-Ortiz P, Salinas-Lara C, Bozena-Piekarska K, Muñóz-Torrico M, Chávez-Galán L and Zúñiga J (2024) The rs11684747 and rs55790676 SNPs of ADAM17 influence tuberculosis susceptibility and plasma levels of TNF, TNFR1, and TNFR2. Front. Microbiol. 15:1392782. doi: 10.3389/fmicb.2024.1392782
Edited by:
Vishwanath Venketaraman, Western University of Health Sciences, United StatesReviewed by:
Soraya Mezouar, Aix-Marseille University, FranceGuoliang Zhang, Shenzhen Third People’s Hospital, China
Matt Johansen, University of Technology Sydney, Australia
Pooja Vir, Uniformed Services University of the Health Sciences, United States
Copyright © 2024 Choreño-Parra, Ramon-Luing, Castillejos, Ortega-Martínez, Tapia-García, Matías-Martínez, Cruz-Lagunas, Ramírez-Martínez, Gómez-García, Ramírez-Noyola, Garcia-Padrón, López-Salinas, Jiménez-Juárez, Guadarrama-Ortiz, Salinas-Lara, Bozena-Piekarska, Muñóz-Torrico, Chávez-Galán and Zúñiga. This is an open-access article distributed under the terms of the Creative Commons Attribution License (CC BY). The use, distribution or reproduction in other forums is permitted, provided the original author(s) and the copyright owner(s) are credited and that the original publication in this journal is cited, in accordance with accepted academic practice. No use, distribution or reproduction is permitted which does not comply with these terms.
*Correspondence: Joaquín Zúñiga, joazu@yahoo.com; joaquin.zuniga@iner.gob.mx; Leslie Chávez-Galán, chavezgalan@gmail.com; lchavez_galan@iner.gob.mx
†These authors have contributed equally to this work and share first authorship