- 1College of Forestry, Gansu Agricultural University, Lanzhou, China
- 2Wolfberry Harmless Cultivation Engineering Research Center of Gansu Province, Lanzhou, China
Fusarium solani is a pathogenic fungus that causes significant harm, leading to crop yield reduction, fruit quality reduction, postharvest decay, and other diseases. This study used potato glycoside alkaloids (PGA) as inhibitors to investigate their effects on the mitochondrial structure and tricarboxylic acid (TCA) cycle pathway of F. solani. The results showed that PGA could inhibit the colony growth of F. solani (54.49%), resulting in the disappearance of the mitochondrial membrane and the loss of contents. PGA significantly decreased the activities of aconitase (ACO), isocitrate dehydrogenase (IDH), α-ketoglutarate dehydrogenase (α-KGDH), succinate dehydrogenase (SDH), fumarase (FH), malate dehydrogenase (MDH), succinyl-CoA synthetase (SCS), and increased the activity of citrate synthase (CS) in F. solani. After PGA treatment, the contents of acetyl coenzyme A (CoA), citric acid (CA), malic acid (L-MA), and α-ketoglutaric acid (α-KG) in F. solani were significantly decreased. The contents of isocitric acid (ICA), succinyl coenzyme A (S-CoA), succinic acid (SA), fumaric acid (FA), and oxaloacetic acid (OA) were significantly increased. Transcriptomic analysis showed that PGA could significantly affect the expression levels of 19 genes related to TCA cycle in F. solani. RT-qPCR results showed that the expression levels of ACO, IDH, α-KGDH, and MDH-related genes were significantly down-regulated, and the expression levels of SDH and FH-related genes were significantly up-regulated, which was consistent with the results of transcriptomics. In summary, PGA can achieve antifungal effects by reducing the tricarboxylic acid cycle’s flow and regulating key genes’ expression levels. This study reveals the antifungal mechanism of PGA from the perspective of TCA cycle, and provides a theoretical basis for the development and application of PGA as a biopesticide.
1 Introduction
Fusarium solani is one of the most serious soil-borne pathogens in the world, which seriously affects the yield and quality of agricultural and forestry products. Its conidia can survive in soil for a long time. When the germination conditions are suitable, they can infect the vascular bundle tissues of various food crops, economic crops, medicinal plants, and ornamental plants through minor wounds, causing a series of plant rot diseases such as root rot, stem rot, ear rot, stem base rot and flower rot (Leslie and Summerell, 2006; Parikh et al., 2018; Mulero-Aparicio et al., 2019; Rampersad, 2020). Currently, the control of plant diseases caused by F. solani mainly depends on chemical fungicides, such as carbendazim (Beneduzi et al., 2012; Xu et al., 2018). However, the extensive use of chemical agent can lead to problems such as pesticide residues and drug resistance of pathogenic fungi, seriously endangering the soil environment and potential risks to human health (Fan et al., 2021). So it is urgent to finding an environmentally friendly green control method to control plant diseases caused by F. solani.
Potato glycoside alkaloids (PGA), also known as solanine, are sugar derivatives of an odor steroidal alkaloid found in potato plants and tubers (Schieber and Saldaña, 2009; Sanchez-Maldonado et al., 2014). More than 95% of PGA is α-solanine and α-chaconine (Friedman, 2006). PGA has strong biological activity, such as antifungal and pest resistance (Dahlin et al., 2017; Pillai and Dandurand, 2021). It has been reported that PGA can inhibit the growth and development of Botrytis cinerea (Sun et al., 2014), Pectobacterium carotovorum (Rocha et al., 2015), Alternaria alternata and Pyrenophora tritici-repentis (Sanchez-Maldonado et al., 2016), Phytophthora infestans (Dahlin et al., 2017). PGA could inhibit the spore germination of Curvularia trifolii (Xu et al., 2023), reduce the virulence of Pectobacterium brasiliense (Joshi et al., 2021), inhibit the active oxygen metabolism process of F. sulphureum to exert antifungal effect (Li et al., 2023).
Mitochondria are double-membrane-coated organelles that supply energy to cells by generating ATP through the tricarboxylic acid (TCA) cycle and oxidative phosphorylation (Fernie et al., 2004). TCA cycle provides substrates for oxidative phosphorylation and plays an important role in ATP synthesis (Kader and Saltveit, 2002). Any mitochondrial dysfunction can affect the growth of pathogenic fungi (Castelli et al., 2005). Therefore, mitochondria are often used as potential targets for developing antifungal drugs, some plant-derived extracts have shown this ability. 3-phenyllactic acid inhibited the growth of Rhizopus oryzae by disrupting the TCA cycle and affecting their energy metabolism (Fan et al., 2022). O-vanillin exerted antifungal effects by affecting the mitochondrial structure and TCA cycle of Aspergillus flavus (Li et al., 2022). Citral can inhibit the function of mitochondria of Penicillium digitatum to exert antifungal effect (Zheng et al., 2015). Eugenol and citral killed A. niger by tricarboxylic acid cycle (Ju et al., 2023).
Previous studies have shown that PGA can destroy the structure of mycelium by interfering with substance metabolism, inhibiting respiration and reducing ATP production (He et al., 2021; Ding et al., 2023; Zhang et al., 2023). However, the effect of PGA on the TCA cycle of F. solani has not been reported, and its mechanism still needs to be clarified.
Therefore, this study observed the effect of plant-derived extract PGA on mitochondrial function of F. solani. To reveal the potential antifungal mechanism of PGA inhibiting the growth of F. solani from the perspective of the TCA cycle, the changes of TCA-related enzyme activity and intermediate product content were determined, and the effect of PGA treatment on the expression of F. solani gene was further analyzed by transcriptome technology (Figure 1). This study aims to reveal the potential inhibitory mechanism of PGA in inhibiting the growth of F. solani from the perspective of the TCA cycle, and also to provide a theoretical basis for the development and utilization of antifungal active substances.
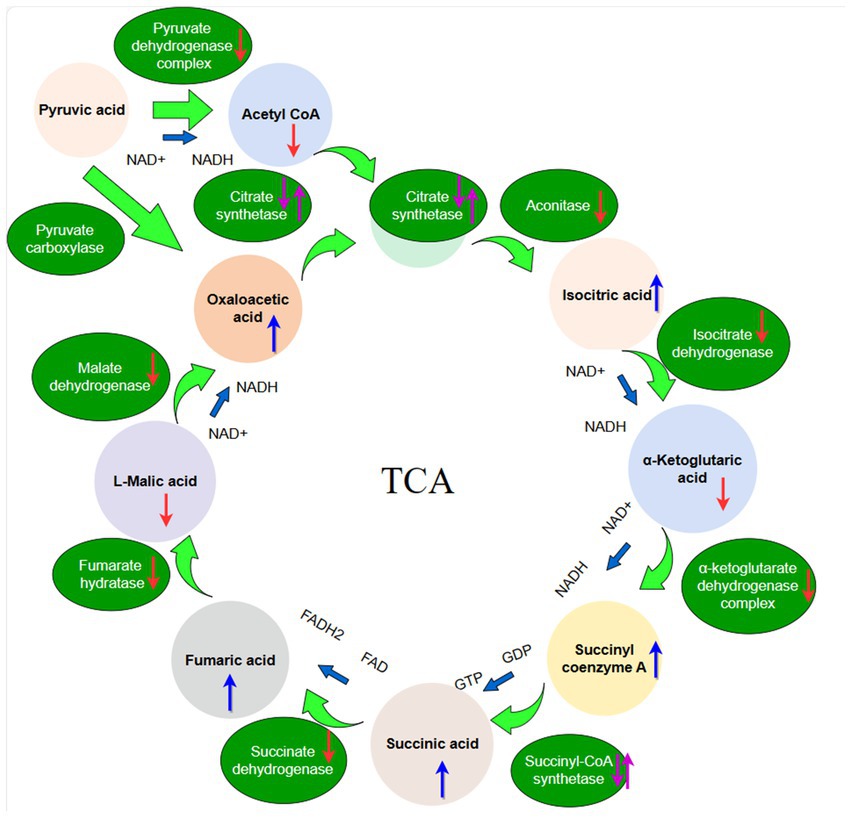
Figure 1. The schematic diagram of the TCA cycle. The red arrow indicates organic acid content or enzyme activities decreased, the purple arrow indicates organic acid content or enzyme activities first decreased and then increased, the bule arrow indicates organic acid content or enzyme activities increased.
2 Materials and methods
2.1 Materials
The test strain, F. solani, was isolated from the diseased plants of wolfberry root rot and stored in the Forest Protection Laboratory of Forestry College of Gansu Agricultural University after pathogenicity determination. Before use, it was activated on a PDA plate and stored at 4°C for later use.
PGA was extracted and identified with reference to the team’s previous study (Zhang et al., 2023).
2.2 Antifungal effect of PGA
The agar plate diffusion method determined the antifungal activity of PGA (Huang et al., 2012). Four small holes (5 mm) 2.5 cm away from the center of the plate were drilled on the potato dextrose agar (PDA) plate using a sterile puncher. The fungal cake (5 mm in diameter) of F. solani was inoculated in the center of PDA plate. and 20 μL of PGA (88.10 mg·mL−1) was added to 2 small holes, The other two holes were added with the same amount of sterile distilled water as the control. All plates were cultured at 25°C for 5 days, and the clear inhibition zone around the pores was measured.
2.3 Observations of mitochondrial ultrastructure
We found that the EC50 value of PGA inhibiting F. solani was 4.4327 mg·mL−1 (Ding et al., 2023). Therefore, the concentration corresponding to EC50 was used as the treatment concentration of PGA in this experiment. 0.5 g fresh mycelium was inoculated in the potato dextrose broth (PDB) medium containing PGA (150 mL conical flask containing 30 mL of PDB medium) and oscillatory cultured (25°C, 160 rpm) for 9 h. And sterile water was set as the control. After 9 h, the mycelia were collected, centrifuged at 10,000 g for 10 min, and washed with sterile water 3–4 times.
The mitochondria of F. solani were extracted by differential centrifugation (Galina et al., 2004; Zhang et al., 2023). The mitochondria were fixed with 2.5% glutaraldehyde for 2 h, adjusted to pH 7.4 with 0.1 mol·L−1 phosphate buffer, and then fixed with 1% osmic acid at room temperature for 2 h. The samples were dehydrated in different ethanol concentrations (30%, 50%, 70%, 80%, 95%, and 100%) and immersed in epoxy resin and SPI-812 embedding agent, respectively. The ultra-thin sections obtained by the Leica UC7 ultra-thin slicing machine were stained with 2% uranyl acetate saturated alcohol solution in the dark and then stained with 2.6% lead citrate solution in the dark. Transmission electron microscopy (Hitachi HT7800, Japan) was used to observe and record images at 80 kV.
2.4 Determination of TCA-related enzyme activity
0.5 g fresh mycelium was inoculated into PDB culture medium (containing 4.432 7 mg·mL−1 PGA) and oscillatory cultured (25°C, 160 rpm) for 36 h. The mycelia were collected at 0, 3, 6, 9, 12, 24, and 36 h.
The activity of citrate synthase (CS) was determined by the methods of Jenner et al. (2001) and Li et al. (2022). One unit of enzyme activity was defined as the production of 1 nmol TNB per minute per gram of tissue in the reaction system at 25°C (U·g−1 FW). The activity of succinyl-CoA synthetase (SCS) was determined by the method of Vichaiya et al. (2020). The production of 1 μmol succinyl hydroxamic acid per minute per gram of tissue in the reaction system at 25°C was defined as an enzyme activity unit (U·g−1 FW). The activities of pyruvate dehydrogenase (PDH), aconitase (ACO), isocitrate dehydrogenase (IDH), α-ketoglutarate dehydrogenase (α-KGDH), succinate dehydrogenase (SDH), fumarase (FH) and malate dehydrogenase (MDH) were determined using the corresponding ELISA kits. The corresponding product numbers of the kits were G0836F, G0872F, G0833F, G0840F, G0856, G0869F, and G0820F. All of the above kits are from Suzhou Grace Biotechnology Co., Ltd.
2.5 Determination of TCA organic acid content
Acetyl-CoA content (CoA) was determined by the ELISA kit (JLC57299, Shanghai Jingkang Bioengineering Co., Ltd.). The contents of citric acid (CA) and L-malic acid (L-MA) were determined by kits. The kit numbers were G0864F and G0862F, respectively, provided by Suzhou Grace Biotechnology Co., Ltd. The content of α-ketoglutarate (α-KG) was determined by an kit (BC5420, Beijing Solarbio Science & Technology Co., Ltd.). The contents of isocitric acid (ICA), succinyl coenzyme A (S-CoA), succinic acid (SA), fumaric acid (FA) and oxaloacetic acid (OA) were determined by kits (YX-090301F, YX-190316F, YX-22571F, YX-062113F, YX-150100F, Sino Best Biological Technology Co., Ltd., Beijing, China).
2.6 Transcriptome analysis
The mycelia of F. solani treated with PGA for 9 h were collected for transcriptome sequencing experiments. The samples were frozen in liquid nitrogen and sent to Shanghai Personal Biotechnology Co., Ltd. for RNA extraction and RNA-Seq sequencing experiments. Criteria for differentially expressed genes (DEGs) were false discovery rate (FDR) < 0.05 and |log2FC| > 1. Three biological replicates per treatment. The BioProject accession number for the SRA database: PRJNA1075338.
2.7 RT-qPCR verification of differential genes
To verify the reliability of transcriptome data and the expression of TCA-related genes, we screened 6 DEGs related to the TCA cycle for RT-qPCR verification. The primers of RT-qPCR were designed using primer 3.0 software (Supplementary Table S1), and the primers were synthesized by Shanghai Personal Biotechnology Co., Ltd.
The total RNA of mycelium was extracted by the TRIzol method. The quality of the extracted RNA was tested to detect the integrity of 28S and 18S. The main band was clear, single, and bright, and the RNA was good. Total RNA that was tested and quantified was reverse transcribed into cDNA (PrimeScript TM 1st stand cDNA Synthesis Kit). Then, a fluorescence quantitative PCR and a real-time PCR reaction were carried out. The RT-qPCR system was set as follows: 95°C denaturation 5 min, 40 cycles of 95°C for 15 s, followed by 60°C for 30 s, and a dissociation step. The relative gene expression was expressed by normalized DEGs to the internal control gene, Actin, using the 2-ΔΔCT method (Livak and Schmittgen, 2001).
2.8 Statistical analysis
Each of the above experiments was repeated three times. Data were expressed as means ± standard errors, and SPSS 26.0 was used for data analysis (p < 0.05). Origin 18.0 was used for mapping.
3 Results
3.1 The inhibitory effect of PGA on Fusarium solani
The diameter of PGA inhibiting the growth of F. solani was 12.26 mm, and the inhibition rate was 54.49% (Figure 2).
3.2 Effect of PGA on mitochondria of Fusarium solani
The mitochondrial morphology of F. solani in the control treatment was primarily round or oval, the internal structure was regular, the surface was smooth and dense, and the mitochondrial inner ridge was complete (Figure 3A). After PGA treatment, the mitochondria of F. solani showed a myelin-like layered structure, the membrane tissue ruptured, the mitochondrial inclusions gradually lost, and the mitochondria died (Figure 3B).
3.3 Effects of PGA on TCA-related enzyme activities of Fusarium solani
At 9 h, the PDH activity of F. solani treated with PGA decreased significantly, the activity of PDH was 10.50% lower than the control (Figure 4A; p < 0.05). At 12 and 24 h, the ACO activity of PGA-treated F. solani was significantly reduced (Figure 4C). During the incubation period, the changes of CS and SCS activities of F. solani were consistent, both of which were significantly decreased in the early stage of PGA treatment, and significantly increased in the later stage of treatment (24, 36 h; Figures 4B,F; p < 0.05). At 6 and 12 h, the SCS activity of F. solani treated with PGA was 53.14 and 51.69% lower than that of the control, respectively. At 24 and 36 h, the activity of CS was 40.70 and 64.15% higher than that of the control, respectively. After PGA treatment, the activities of IDH (Figure 4D), α-KGDH (Figure 4E), SDH (Figure 4G), FH (Figure 4F), and MDH (Figure 4I) of F. solani were significantly decreased (p < 0.05). After PGA treatment, the MDH activity of F. solani treated with PGA was 89.23 and 71% lower than that of the control at 6 and 9 h, respectively. At 24 h, IDH, SDH, and FH activities were lower than those of the control group by 70.44, 77.3, and 70.07%, respectively. At 36 h, the α-KGDH activity of F. solani treated with PGA was 62.96% lower than that of the control.
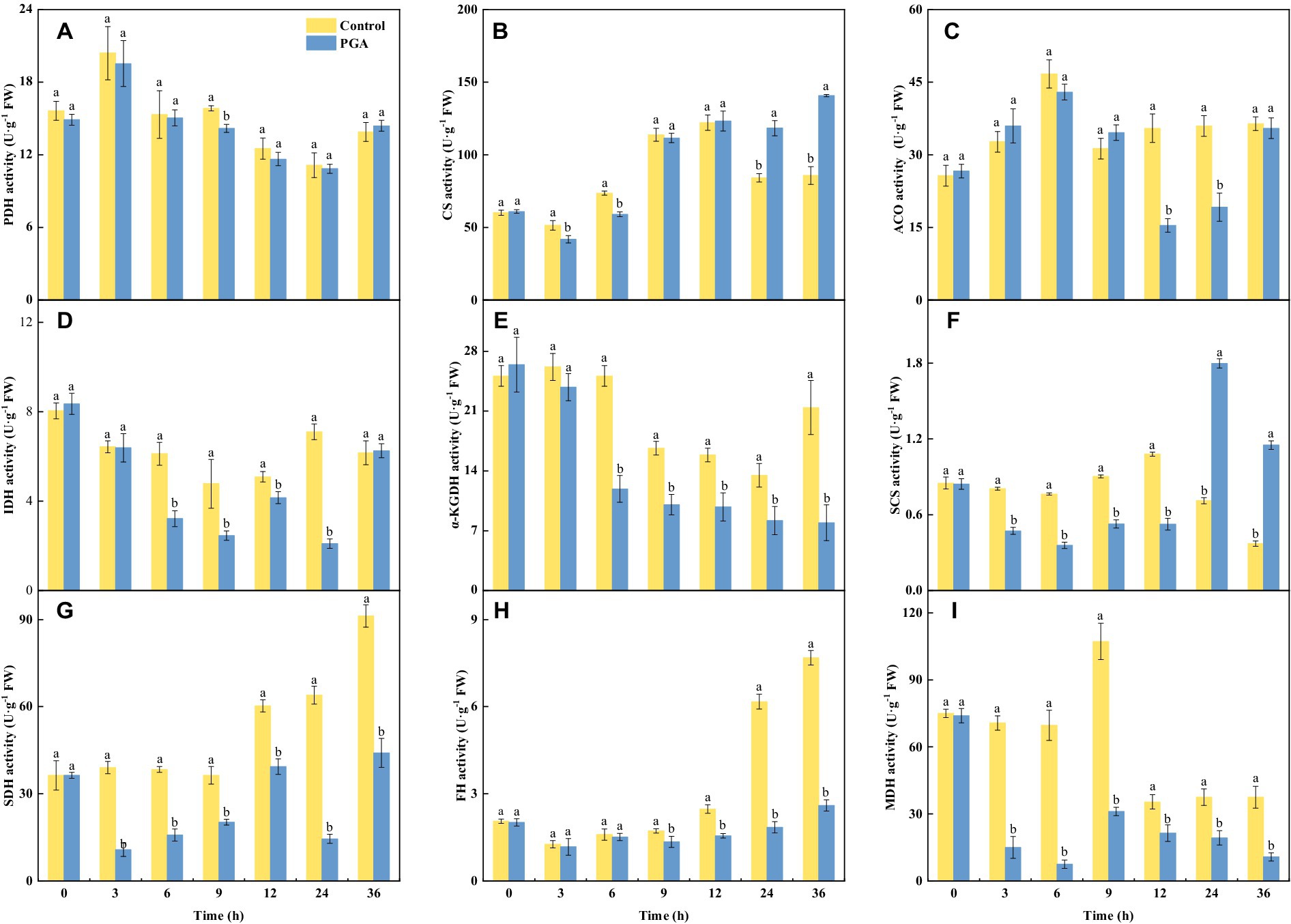
Figure 4. Effects of PGA on the activities of PDH (A), CS (B), ACO (C), IDH (D), α-KGDH (E), SCS (F), SDH (G), FH (H) and MDH (I) of Fusarium solani. Different lowercase letters indicated significant differences between the control and PGA treatments (p < 0.05).
3.4 Effect of PGA on TCA organic acid content of Fusarium solani
After PGA treatment, the CA content of F. solani decreased first and then increased (Figure 5B). At 3 and 6 h, the CA content of F. solani treated with PGA was 73.64% and 23.34% lower than that of the control, respectively. After PGA treatment, the contents of ICA (Figure 5C), S-CoA (Figure 5E), SA (Figure 5F), FA (Figure 5G), and OA (Figure 5I) in F. solani increased significantly (p < 0.05). At 3 h, the S-CoA and FA contents of F. solani treated with PGA were 31.81 and 30.26% higher than those of the control, respectively. At 12 and 24 h, the OA content of F. solani treated with PGA was 24.32 and 22.80% higher than that of the control, respectively. At 24 h, the ICA and SA contents of F. solani treated with PGA were 24.39 and 10.30% higher than those of the control, respectively. After PGA treatment, the contents of CoA (Figure 5A), α-KG (Figure 5D), and L-MA (Figure 5H) in F. solani decreased significantly (p < 0.05). At 12 h, the CoA, α-KG, and L-MA of F. solani treated with PGA were 42.32, 80.09, and 94.89% lower than those of the control, respectively.
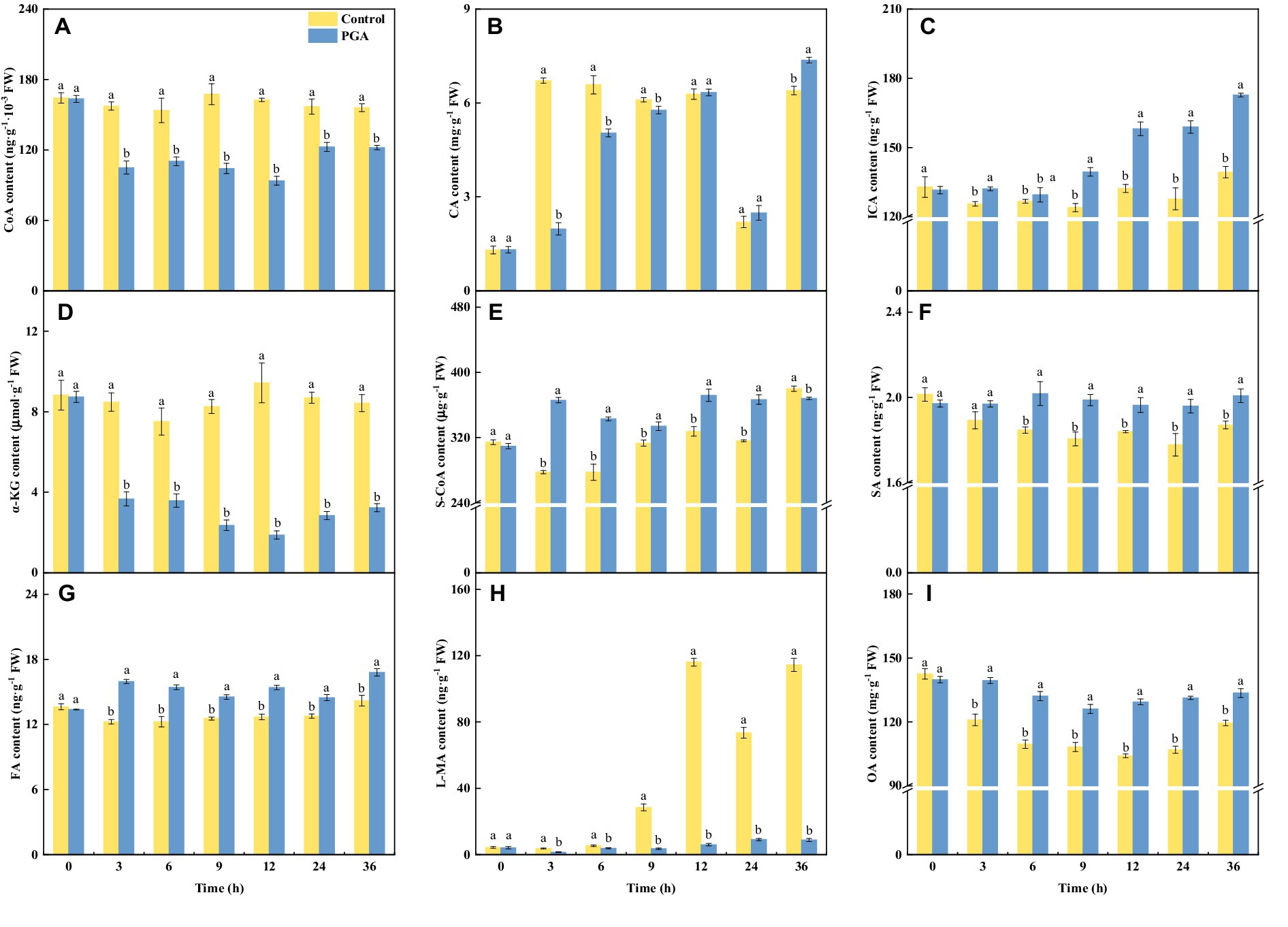
Figure 5. Effects of PGA treatment on the contents of CoA (A), CA (B), ICA (C), α-KG (D), S-CoA (E), SA (F), FA (G), L-MA (H) and OA (I) of Fusarium solani. Different lowercase letters indicated significant differences between the control and PGA treatments (p < 0.05).
3.5 Transcriptome analysis
3.5.1 Quality statistics and analysis of RNA-Seq data
Sterile distilled water control and PGA treatment were set up in the experiment, with 3 biological replicates each, and 6 cDNA libraries were established. After sequencing, the average clean reads of F. solani under control and PGA treatment were 63,558,391 and 51,690,066, respectively. Q20 (the proportion of bases with sequencing quality above 99.0% of the total bases) averaged 98.52% and 98.60%, respectively, and Q30 (the proportion of bases with sequencing quality above 99.9% of the total bases) averaged 95.52% and 95.79%, respectively (Supplementary Table S2). The above results indicated that the cDNA library obtained by this sequencing is of high quality and can be further studied by subsequent bioinformatics.
3.5.2 Analysis of DEGs
After PGA treatment of F. solani, a total of 6,341 genes were changed in expression, of which 3,014 genes were up-regulated and 3,327 genes were down-regulated (Figure 6A). Clustering heatmap analysis showed that there were significant differences in gene expression profiles between the treatment and the control (Figure 6B). Six DEGs related to TCA cycle were screened for RT-qPCR verification.
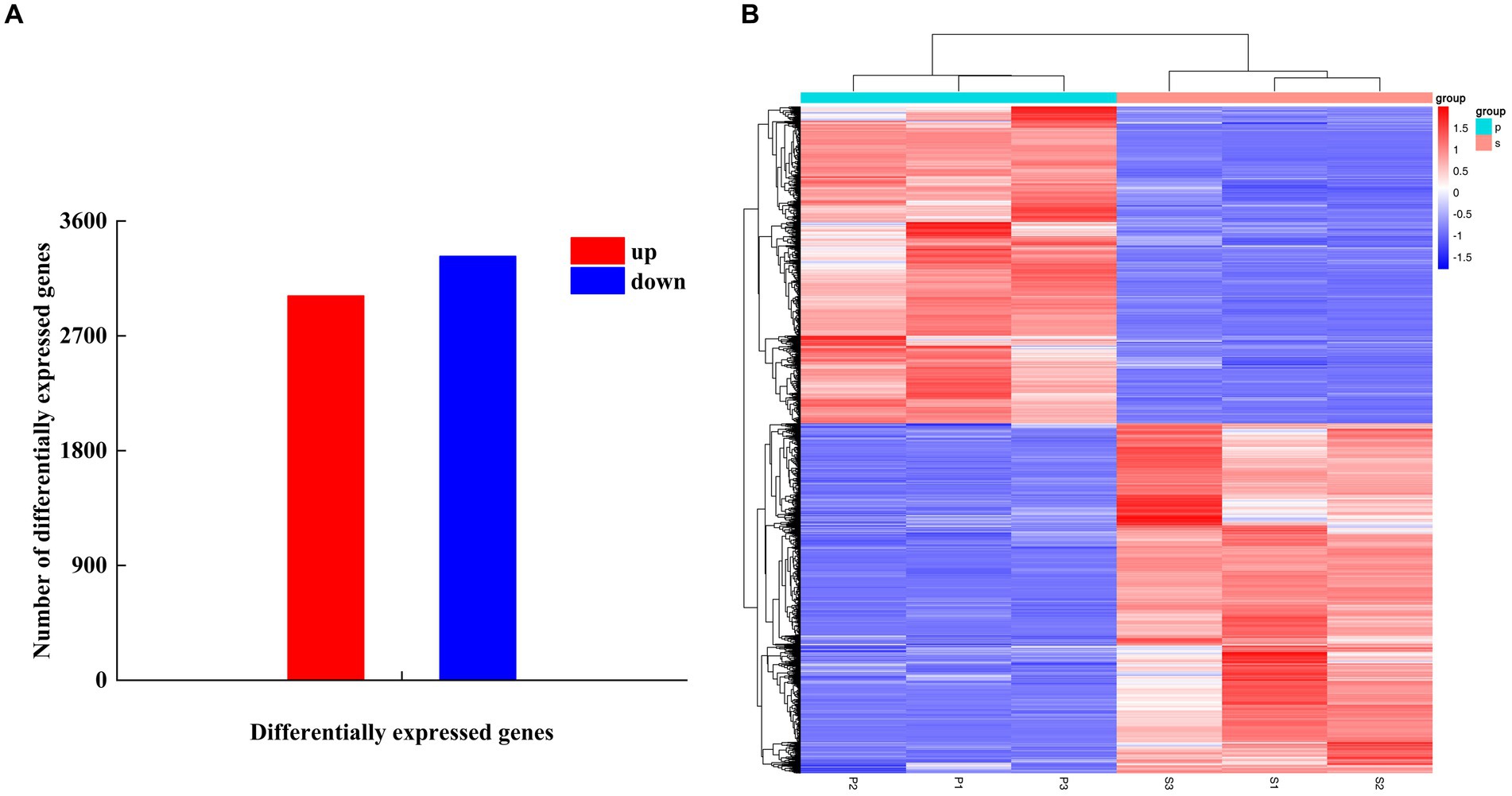
Figure 6. The number of DEGs (A) and cluster heat map (B) were used to analyze the DEGs of Fusarium solani after PGA treatment. S indicates control, P indicates addition of PGA.
3.5.3 GO analysis of DEGs
The DEGs were divided into three categories: biological processes (BP), cellular components (CC), and molecular functions (MF; Figure 7). In CC, DEGs were mainly enriched in the cytosolic ribosome et al. In BP, DEGs were mainly enriched in cytoplasmic translation et al. DEGs enriched in MF were less, mainly enriched in the structural constituent of ribosome.
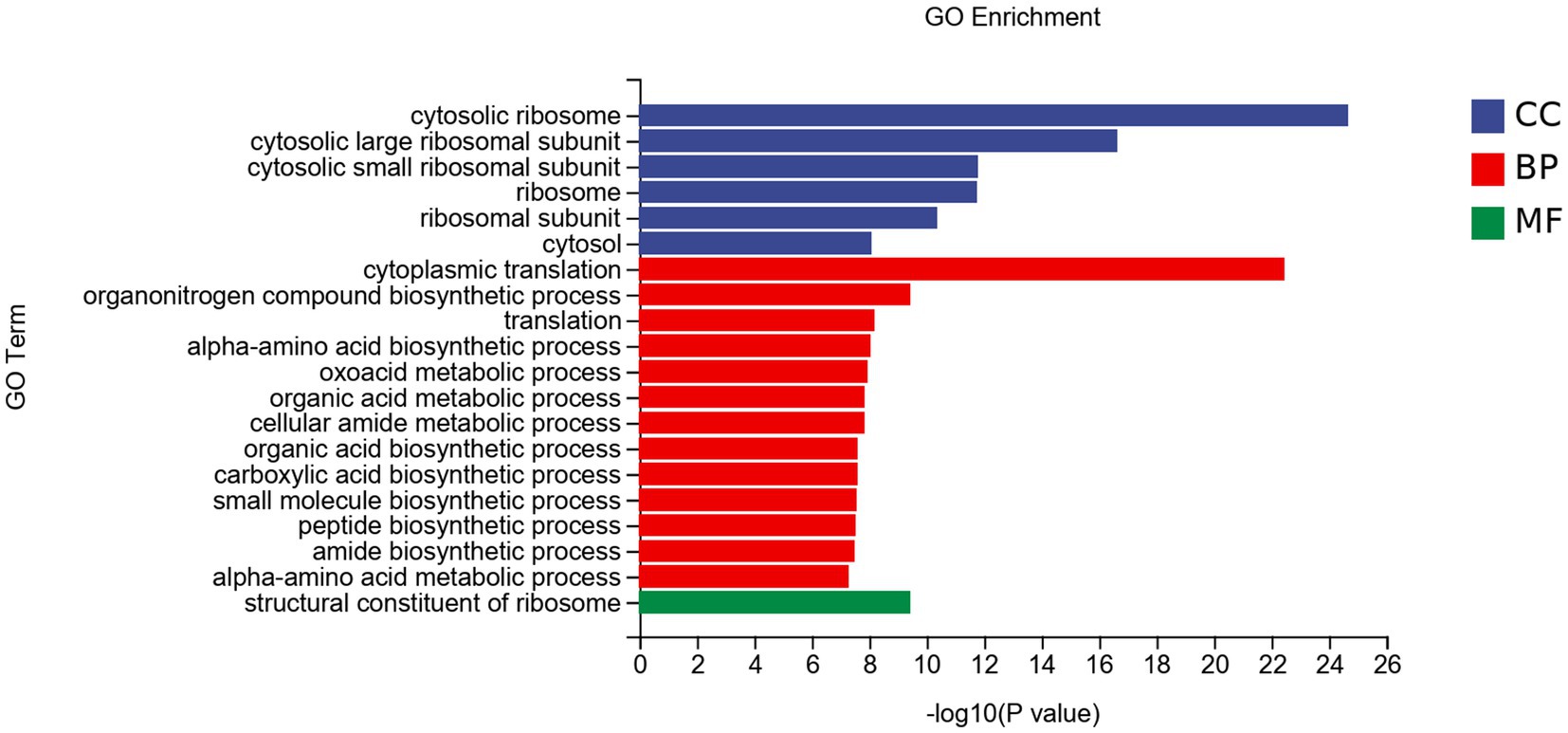
Figure 7. GO enrichment analysis of DEGs of Fusarium solani after PGA treatment. CC, Cellular component; BP, Biological Process; MF, Molecular function.
3.5.4 KEGG analysis of DEGs
DEGs were mainly enriched in genetic information processing, metabolism, and cellular processes (Figure 8). In genetic information processing, DEGs were mainly enriched in ribosomes, non-homologous end-joining, and aminoacyl-tRNA biosynthesis. In metabolism, DEGs were mainly enriched in purine metabolism, lysine biosynthesis et al. The DEGs of cellular processes are mainly enriched in peroxisomes.
3.6 The effect of PGA on the TCA cycle of Fusarium solani was determined by transcriptomics
We evaluated whether the expression levels of TCA cycle-related genes in the mycelium of F. solani changed after PGA. Searching according to the KGEE database. After PGA treatment, the expression levels of 19 genes were significantly changed, resulting in changes in the corresponding enzyme activities, indicating that PGA could significantly affect the normal operation of the TCA cycle of F. solani (Figure 9).
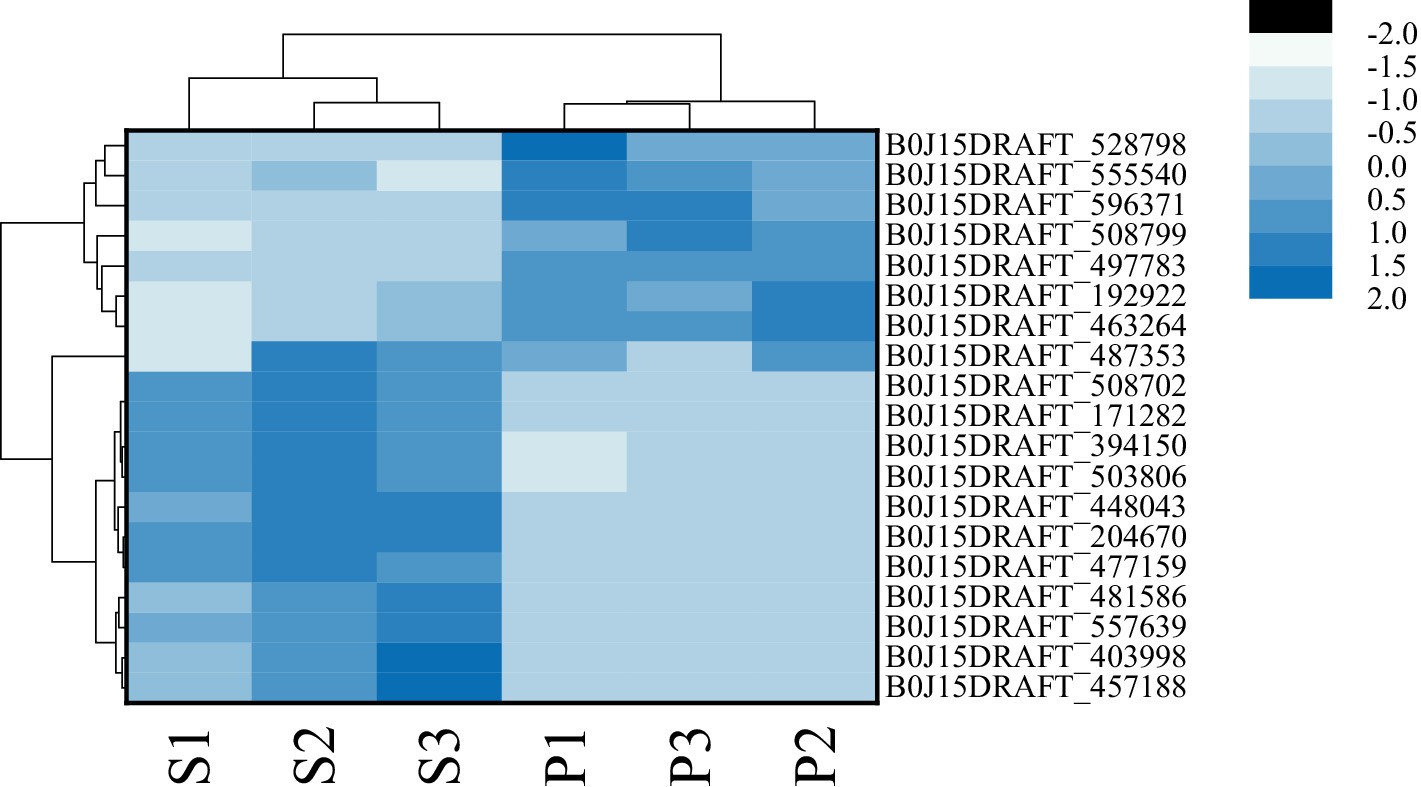
Figure 9. The effect of PGA on the TCA cycle of Fusarium solani was determined by transcriptomics. S indicates control, and P indicates addition of PGA.
3.7 RT-qPCR validation of DEGs
The results showed that the verification results of 6 DEGs related to the TCA cycle were consistent with transcriptome sequencing, but there was a specific deviation in gene expression multiples (Figures 10A,B). After PGA treatment, 4 TCA-related candidate genes (ACO, IDH3, OGC, ME2) were significantly down-regulated (Figure 10B). Two TCA-related candidate genes (CTP, SDH1) were significantly up-regulated (Figure 10B).
4 Discussion
In this study, the effects of PGA on colony growth, mitochondrial ultrastructure, main enzyme activities and product content of the TCA cycle in F. solani were comprehensively analyzed. Transcriptomics technology was used to analyze the differences in mycelium at the transcriptional level.
The diameter of the colony is of great significance to the growth of pathogenic fungi (Luo and Tian, 2021). PGA could inhibit the colony growth of pathogenic fungi such as A. solani (Wolters et al., 2023) and P. brasiliense (Joshi et al., 2021). PGA inhibited the mycelial growth rate and spore germination of C. trifolii (Xu et al., 2023), and inhibited the growth of F. sulphureum by inhibiting ROS metabolism and destroying cell membrane (Li et al., 2023).
It has been reported that the ultrastructural changes in mitochondria may be due to the leakage of small molecules and the damage of mitochondria caused by the difference in cell metabolism (Bajpai et al., 2013). In this study, after PGA treatment, the mitochondrial membrane tissue of F. solani was broken, and the contents were lost. This was similar to the results of the mitochondria of B. cinerea treated with tea tree oil (Li et al., 2017) and the mitochondria of Saccharomyce scerevisiae treated with essential oil from Chrysanthemum morifolium cv. Fubaiju (Zhan et al., 2021). It indicated that PGA treatment changed the mitochondrial ultrastructure of F. solani, which may affect the ability of mitochondria to metabolize.
One of the central pathways of metabolism is the TCA cycle, and it is also the primary mechanism of energy production. Mitochondrial dehydrogenase is an essential enzyme in biological growth. The decrease of a-KGDH activity leads to a decrease in the ability of TCA to produce NADH and ATP synthesis. It leads to the disorder of the TCA cycle (Huang et al., 2003). By linking the utilization of nutrients with the synthesis of TCA cycle intermediates and products, CS helps regulate energy flux and metabolic rate. It catalyzes the condensation of acetyl coenzyme A and oxaloacetic acid to produce citric acid, coenzyme A, and proton (Liao et al., 2014). The activity of IDH regulates the flow of isocitrate into the TCA cycle or glyoxylic acid cycle (Li et al., 2017). The decrease in MDH activity inhibited the conversion between malic acid and oxaloacetic acid (Kobayashi et al., 2002). SDH can transfer two electrons in the electron transport chain to coenzyme Q10, the intersection of the TCA cycle and oxidative phosphorylation. Decreased activity can affect the regular operation of oxidative phosphorylation and electron transport and is also a marker protein for evaluating mitochondrial function (Evenepoel et al., 2015; Zou et al., 2015). In this study, PGA could inhibit the respiratory pathway of F. solani by reducing the activities of ACO, IDH, α-KGDH, SCS, SDH, FH, and MDH. Cinnamon oil inhibited the expected growth of Rhizopus nigricans by reducing SDH and MDH activities (Fan et al., 2022). O-vanillin treatment inhibited the activities of CS, IDH, a-KGDH, and SDH of A. flavus, thereby reducing its virulence (Li et al., 2022). Citral decreased the activities of IDH, a-KGDH, and SDH in P. digitatum and inhibited its normal metabolism (Zheng et al., 2015). Eugenol and citral reduced the activities of MDH, CS, α-KGDH, IDH, and SDH in A. niger (Ju et al., 2023). The above results are consistent with the results of this experiment. This indicated that the mitochondrial function of pathogenic fungi was significantly inhibited.
The TCA cycle converts phosphoenolpyruvate to malate and/or pyruvate in the cytosol. These organic acids are then taken into the mitochondria, producing energy (Fernie et al., 2004). After PGA treatment, the content of α-KG and L-MA in F. solani decreased significantly, indicating that the TCA cycle was significantly inhibited. The reason for this inhibition may be that PGA inhibits the activity of some amino acids in F. solani, and the TCA cycle is the final stage of these amino acid metabolisms. Therefore, some metabolites in the TCA cycle could be reduced (Gaupp et al., 2010). The decrease of TCA cycle flux further leads to the decrease of CoA synthesis. The decrease of IDH, SCS, SDH, FH, and MDH activities led to accumulating the corresponding reaction substrates ICA, S-CoA, SA, FA, and OA, further blocking the TCA cycle and causing mitochondrial dysfunction. It has been reported that Graphene oxide can significantly inhibit the mycelial growth of F. graminearum, and the content of SA and CA increased significantly (Wang et al., 2019). This is consistent with the results of this experiment. Correlation analysis showed a significant negative correlation between α-KGDH and S-CoA, and a positive correlation between α-KGDH and α-KG. The results showed that the content of α-KG and the activity of α-KGDH decreased, but the content of S-CoA increased (Supplementary Table S3). It may be due to the decrease in SCS activity, resulting in S-CoA cannot be consumed in time. There was a significant positive correlation between L-MA and FH, α-KG and IDH (Supplementary Table S3), indicating that the corresponding product content decreased with the decrease of enzyme activity. CS was significantly negatively correlated with OA, and CS was significantly positively correlated with CA. That is the content of substrate OA increased, the activity of CS decreased, and the content of product CA decreased.
In this experiment, it was found that after PGA treatment of F. solani, GO analysis showed that DEGs were mainly enriched in cell biological processes and components of cells, and the pathway was mainly enriched in genetic information processing and metabolism, indicating that PGA could significantly affect the cell components and metabolism of F. solani. The TCA cycle is the hub of sugar, lipid, protein and nucleic acid metabolism communication and transformation (Xu and Shao, 2017). Transcriptome analysis showed that PGA could affect the metabolic pathways of F. saloni, such as starch and sucrose metabolism, amino sugar and nucleotide sugar metabolism, fructose and mannose metabolism, and then affect the normal metabolic process of TCA cycle. The up-regulation of SDH-related gene (SDH1) expression leads to defects in enzyme structure and function, and the resulting electron leakage and cell damage lead to an increase in reactive oxygen species (ROS). ROS concentration in mitochondria increases DNA damage, disrupting normal cell metabolism (Baysal et al., 2000). Decreased expression of IDH-related genes can inhibit the production of α-KG, resulting in abnormal expression of signal transduction pathways (Chou et al., 2010). FH is involved in the TCA cycle in mitochondria and catalyzes the conversion of fumaric acid to malic acid. In this experiment, due to the addition of PGA, the expression level of the FH-related gene (CTP) was up-regulated, resulting in a decrease in its activity, which in turn led to a decrease in malic acid content, which was consistent with the results of Ju et al. (2023). The decreased expression levels of α-KGDH, ACO, and MDH-related genes (OGC, ACO, ME2) led to a significant change in their activity, further leading to a decrease in the traffic of the TCA cycle pathway.
5 Conclusion
PGA can destroy the mitochondrial ultrastructure of mycelium, regulate the enzyme activity of the TCA cycle, the content of intermediate products, and the expression level of essential genes, cause the disorder of energy metabolism of F. solani, and finally inhibit the average growth of F. solani to achieve antifungal effect.
Data availability statement
The datasets presented in this study can be found in the NCBI BioProject Database (https://www.ncbi.nlm.nih.gov/bioproject), accession number PRJNA1075338.
Author contributions
CZ: Conceptualization, Data curation, Methodology, Software, Supervision, Writing – original draft, Writing – review & editing. WC: Writing – original draft, Conceptualization. BW: Conceptualization, Supervision, Writing – original draft. YW: Writing – original draft, Methodology, Conceptualization. NL: Writing – original draft, Software, Methodology. RL: Writing – original draft. YY: Methodology, Writing – original draft. YS: Methodology, Writing – original draft. JH: Writing – review & editing, Writing – original draft, Supervision, Methodology, Funding acquisition, Data curation, Conceptualization.
Funding
The author(s) declare that financial support was received for the research, authorship, and/or publication of this article. This research received financial support from the National Natural Science Foundation of China (grant no. 32060341).
Conflict of interest
The authors declare that the research was conducted in the absence of any commercial or financial relationships that could be construed as a potential conflict of interest.
Publisher’s note
All claims expressed in this article are solely those of the authors and do not necessarily represent those of their affiliated organizations, or those of the publisher, the editors and the reviewers. Any product that may be evaluated in this article, or claim that may be made by its manufacturer, is not guaranteed or endorsed by the publisher.
Supplementary material
The Supplementary material for this article can be found online at: https://www.frontiersin.org/articles/10.3389/fmicb.2024.1390269/full#supplementary-material
Abbreviations
TCA, Tricarboxylic acid; PDH, Pyruvate dehydrogenase; CoA, Acetyl coenzyme A; OA, Oxaloacetic acid; CS, Citrate synthetase; CA, Citric acid; ACO, Aconitase; ICA, Isocitric acid; IDH, Isocitrate dehydrogenase; α-KG, α-Ketoglutaric acid; S-CoA, Succinyl coenzyme A; α-KGDH, α-oxoglutarate dehydrogenase; SCS, Succinyl-CoA synthetase; SA, Succinic acid; SDH, Succinate dehydrogenase; FA, Fumaric acid; FH, Fumarate hydratase; L-MA, L-Malic acid; MDH, Malate dehydrogenase; TEM, Transmission electron microscope; DEGs, Differentially expressed genes.
References
Bajpai, V. K., Sharma, A., and Baek, K. H. (2013). Antibacterial mode of action of Cudrania tricuspidata fruit essential oil, affecting membrane permeability and surface characteristics of food-borne pathogens. Food Control 32, 582–590. doi: 10.1016/j.foodcont.2013.01.032
Baysal, B. E., Ferrell, R. E., Willettbrozick, J. E., Lawrence, E. C., Myssiorek, D., Bosch, A., et al. (2000). Mutations in SDHD, a mitochondrial complex II gene, in hereditary paraganglioma. Science 287, 848–851. doi: 10.1126/science.287.5454.848
Beneduzi, A., Ambrosini, A., and Passaglia, L. M. (2012). Plant growth-promoting rhizobacteria (PGPR): their potential as antagonists and biocontrol agents. Genet. Mol. Biol. 35, 1044–1051. doi: 10.1590/S1415-47572012000600020
Castelli, M. V., Lodeyro, A. F., Malheiros, A., Zacchino, S. A. S., and Roveri, O. A. (2005). Inhibition of the mitochondrial ATP synthesis by polygodial, a naturally occurring dialdehyde unsaturated sesquiterpene. Biochem. Pharmacol. 70, 82–89. doi: 10.1016/j.bcp.2005.04.016
Chou, W., Hou, H., Chen, C., Tang, J., Yao, M., Tsay, W., et al. (2010). Distinct clinical and biologic characteristics in adult acute myeloid leukemia bearing the isocitrate dehydrogenase 1 mutation. Blood 115, 2749–2754. doi: 10.1182/blood-2009-11-253070
Dahlin, P., Müller, M. C., Ekengren, S., McKee, L. S., and Bulone, V. (2017). The impact of steroidal glycoalkaloids on the physiology of Phytophthora infestans, the causative agent of potato late blight. Mol. Plant Microbe Interact. 30, 531–542. doi: 10.1094/mpmi-09-16-0186-r
Ding, D., Zhao, J., He, J., Hou, C., Li, N., Zhang, C., et al. (2023). Potato glycoalkaloids affect the respiration and reactive oxygen species metabolism of Fusarium solani. Mic. China 50, 2937–2949. doi: 10.13344/j.microbiol.china.221000
Evenepoel, L., Papathomas, T. G., Krol, N., Korpershoek, E., Krijger, R. R., Persu, A., et al. (2015). Toward an improved definition of the genetic and tumor spectrum associated with SDH germ-line mutations. Genet. Med. 17, 610–620. doi: 10.1038/gim.2014.162
Fan, W., Li, B., Du, N., Hui, T., Cao, Y., Li, X., et al. (2022). Energy metabolism as the target of 3-phenyllactic acid against Rhizopus oryzae. Int. J. Food Microbiol. 369:109606. doi: 10.1016/j.ijfoodmicro.2022.109606
Fan, R., Zhang, W., Li, L., Jia, L., Zhao, J., Zhao, Z., et al. (2021). Individual and synergistic toxic effects of carbendazim and chlorpyrifos on zebrafish embryonic development. Chemosphere 280:130769. doi: 10.1016/j.chemosphere.2021.130769
Fernie, A. R., Carrari, F., and Sweetlove, L. J. (2004). Respiratory metabolism: glycolysis, the TCA cycle and mitochondrial electron transport. Curr. Opin. Plant Biol. 7, 254–261. doi: 10.1016/j.pbi.2004.03.007
Friedman, M. (2006). Potato glycoalkaloids and metabolites: roles in the plant and in the diet. J. Agric. Food Chem. 54, 8655–8681. doi: 10.1021/jf061471t
Galina, V., Vandanajay, B., Arpad, G., John, P., and Nisha, G. (2004). Impaired mitochondrial respiratory chain and bioenergetics during chagasic cardiomyopathy development. Biochim. Biophys. Acta Mol. basis Dis. 1689, 162–173. doi: 10.1016/j.bbadis.2004.03.005
Gaupp, R., Schlag, S., Liebeke, M., Lalk, M., and Gotz, F. (2010). Advantage of upregulation of succinate dehydrogenase in Staphylococcus aureus biofilms. J. Bacteriol. 192, 2385–2394. doi: 10.1128/jb.01472-09
He, J., Duo, T., Chen, W., and Zhang, X. (2021). Mechanism of action of potato glycoalkaloids against Fusarium solani. Int. J. Agric. Biol. 25, 873–880. doi: 10.17957/IJAB/15.1741
Huang, H. M., Zhang, H., Xu, H., and Gibson, G. E. (2003). Inhibition of the α-ketoglutarate dehydrogenase complex alters mitochondrial function and cellular calcium regulation. Biochim. Biophys. Acta Biomembr. 1637, 119–126. doi: 10.1016/s0925-4439(02)00222-3
Huang, X. Q., Zhang, N., Yong, X. Y., Yang, X. G., and Shen, Q. R. (2012). Biocontrol of Rhizoctonia solani damping-off disease in cucumber with Bacillus pumilus SQR-N43. Microbiol. Res. 167, 135–143. doi: 10.1016/j.micres.2011.06.002
Jenner, H. L., Winning, B. M., Millar, A. H., Tomlinson, K. L., Leaver, V. J., and Hill, S. A. (2001, 2001). NAD malic enzyme and the control of carbohydrate metabolism in potato tubers. Plant Physiol. 126, 1139–1149. doi: 10.1104/pp.126.3.1139
Joshi, J., Yao, L., Charkowski, A., and Heuberger, A. (2021). Metabolites from wild potato inhibit virulence factors of the soft rot and blackleg pathogen Pectobacterium brasiliense. Mol. Plant Microbe Interact. 34, 100–109. doi: 10.1094/mpmi-08-20-0224-r
Ju, J., Lei, Y., Guo, Y. H., Yu, H., Cheng, Y. L., and Yao, W. R. (2023). Eugenol and citral kills aspergillus Niger through the tricarboxylic acid cycle and its application in food preservation. LWT-Food Sci. Technol. 3:4226. doi: 10.1016/j.lwt.2022.114226
Kader, A. A., and Saltveit, M. E. (2002). Postharvest physiology and pathology of vegetables. Boca Raton: CRC Press.
Kobayashi, D., Kondo, K., Uehara, N., Otokozawa, S., Tsuji, N., Yagihashi, A., et al. (2002). Endogenous reactive oxygen species is an important mediator of miconazole antifungal effect. Antimicrob. Agents Chemother. 46, 3113–3117. doi: 10.1128/aac.46.10.3113-3117.2002
Leslie, J. F., and Summerell, B. A. (2006). The fusarium laboratory manual. Ames, Iowa, USA: Blackwell Publishing.
Li, H., Li, M., Fang, Y. L., and Qin, S. H. (2023). Antifungal activity of potato glycoalkaloids and its potential to control severity of dry rot caused by Fusarium sulphureum. Crop. Sci. 63, 801–811. doi: 10.1002/csc2.20874
Li, Y., Shao, X., Xu, J., Wei, Y., Xu, F., and Wang, H. (2017). Tea tree oil exhibits antifungal activity against Botrytis cinerea by affecting mitochondria. Food Chem. 234, 62–67. doi: 10.1016/j.foodchem.2017.04.172
Li, Q., Zhao, Y., Zhu, X., and Xie, Y. (2022). Antifungal effect of o-vanillin on mitochondria of aspergillus flavus: ultrastructure and TCA cycle are destroyed. Int. J. Food Sci. Technol. 57, 3142–3149. doi: 10.1111/ijfs.15647
Liao, C. H., Yao, L. L., and Ye, B. C. (2014). Three genes encoding citrate synthases in Saccharopolyspora erythraea are regulated by the global nutrient-sensing regulators GlnR, DasR, and CRP. Mol. Microbiol. 94, 1065–1084. doi: 10.1111/mmi.12818
Livak, K. J., and Schmittgen, T. D. (2001). Analysis of relative gene expression data using real-time quantitative PCR and the 2-ΔΔCT method. Methods 25, 402–408. doi: 10.1006/meth.2001.1262
Luo, Y., and Tian, P. (2021). Growth and characteristics of two different Epichloë sinensis strains under different cultures. Front. Microbiol. 12:726935. doi: 10.3389/fmicb.2021.726935
Mulero-Aparicio, A., Agustí-Brisach, C., Raya, M. C., Lovera, M., Arquero, O., and Trapero, A. (2019). First report of Fusarium solani causing stem canker in English walnut in Spain. Plant Dis. 103:3281. doi: 10.1094/pdis-06-19-1163-pdn
Parikh, L., Kodati, S., Eskelson, M. J., and Adesemoye, A. O. (2018). Identification and pathogenicity of Fusarium spp. in row crops in Nebraska. Crop Prot. 108, 120–127. doi: 10.1016/j.cropro.2018.02.019
Pillai, S. S., and Dandurand, L. M. (2021). Effect of steroidal glycoalkaloids on hatch and reproduction of the potato cyst nematode Globodera pallida. Plant Dis. 105, 2975–2980. doi: 10.1094/pdis-02-21-0247-re
Rampersad, S. (2020). Pathogenomics and management of Fusarium diseases in plants. Pathogens 9:340. doi: 10.3390/pathogens9050340
Rocha, A. B. O., Honório, S. L., Messias, C. L., Otón, M., and Gómez, P. A. (2015). Effect of UV-C radiation and fluorescent light to control postharvest soft rot in potato seed tubers. Sci. Hortic. 181, 174–181. doi: 10.1016/j.scienta.2014.10.045
Sanchez-Maldonado, A. F., Mudge, E., Gӓnzle, M. G., and Schieber, A. (2014). Extraction and fractionation of phenolic acids and glycoalkaloids from potato peels using acidified water/ethanol-based solvents. Food Res. Int. 65, 27–34. doi: 10.1016/j.foodres.2014.06.018
Sanchez-Maldonado, A. F., Schieber, A., and Gänzle, M. G. (2016). Antifungal activity of secondary plant metabolites from potatoes (Solanum tuberosum L.): Glycoalkaloids and phenolic acids show synergistic effects. J. Appl. Microbiol. 120, 955–965. doi: 10.1111/jam.13056
Schieber, A., and Saldaña, M. (2009). Potato peels: a source of nutritionally and pharmacologically interesting compounds-a review. Food. 3, 23–29. doi: 10.7939/R33T9DM0H
Sun, P. Z., Cao, B. W., and Han, Y. M. (2014). Study on antimicrobial activities of α-Solanine against Botrytis cinerea in vitro. Storage Process. 14, 12–15. doi: 10.3969/j.issn.1009-6221.2014.02.003
Vichaiya, T., Uthaibutra, J., and Saengnil, K. (2020). Gaseous chlorine dioxide increases energy status and energy metabolism-related enzyme activities leading to reduction in pericarp browning of longan fruit during storage. Sci. Hortic. 263:109118. doi: 10.1016/j.scienta.2019.109118
Wang, X., Liu, C., Li, H., Zhang, H., Ma, R., Zhang, Q., et al. (2019). Metabonomics-assisted label-free quantitative proteomic and transcriptomic analysis reveals novel insights into the antifungal effect of graphene oxide for controlling Fusarium graminearum. Environ. Sci. Nano 6, 3401–3421. doi: 10.1039/C9EN00981G
Wolters, P. J., Wouters, D., Tikunov, Y. M., Ayilalath, S., Kodde, L. P., Strijker, M. F., et al. (2023). Tetraose steroidal glycoalkaloids from potato provide resistance against Alternaria solani and Colorado potato beetle. eLife 12:RP87135. doi: 10.7554/eLife.87135
Xu, X. M., Chen, J. Y., Li, B. R., and Tang, L. J. (2018). Carbendazim residues in vegetables in China between 2014 and 2016 and a chronic carbendazim exposure risk assessment. Food Control 91, 20–25. doi: 10.1016/j.foodcont.2018.03.016
Xu, N., Lu, H., Yi, X., Peng, S., Huang, S., Zhang, Y., et al. (2023). Potential of alpha-(α)-Solanine as a natural inhibitor of fungus causing leaf spot disease in strawberry. Life 13:450. doi: 10.3390/life13020450
Xu, J., and Shao, X. (2017). Metabolomic analysis and mode of action of metabolites of tea tree oil involved in the suppression of Botrytis cinerea. Front. Microbiol. 8:272933. doi: 10.3389/fmicb.2017.01017
Zhan, J., He, F., Cai, H., Wu, M., Xiao, Y., Xiang, F., et al. (2021). Composition and antifungal mechanism of essential oil from Chrysanthemum morifolium cv. Fubaiju. J. Funct. Foods 87:104746. doi: 10.1016/j.jff.2021.104746
Zhang, C., Ding, D., Wang, B., Wang, Y., Li, N., Li, R., et al. (2023). Effect of potato glycoside alkaloids on energy metabolism of Fusarium solani. J. Fungi. 9:777. doi: 10.3390/jof9070777
Zheng, S., Jing, G., Wang, X., Ouyang, Q., Jia, L., and Tao, N. (2015). Citral exerts its antifungal activity against Penicillium digitatum by affecting the mitochondrial morphology and function. Food Chem. 178, 76–81. doi: 10.1016/j.foodchem.2015.01.077
Keywords: potato glycoside alkaloids, Fusarium solani, tricarboxylic acid cycle, mitochondrion structure, gene expression
Citation: Zhang C, Chen W, Wang B, Wang Y, Li N, Li R, Yan Y, Sun Y and He J (2024) Potato glycoside alkaloids exhibit antifungal activity by regulating the tricarboxylic acid cycle pathway of Fusarium solani. Front. Microbiol. 15:1390269. doi: 10.3389/fmicb.2024.1390269
Edited by:
Gennaro Agrimi, University of Bari Aldo Moro, ItalyReviewed by:
Antonio Caporusso, University of Bari Aldo Moro, ItalyWen-Liang Xiang, Xihua University, China
Copyright © 2024 Zhang, Chen, Wang, Wang, Li, Li, Yan, Sun and He. This is an open-access article distributed under the terms of the Creative Commons Attribution License (CC BY). The use, distribution or reproduction in other forums is permitted, provided the original author(s) and the copyright owner(s) are credited and that the original publication in this journal is cited, in accordance with accepted academic practice. No use, distribution or reproduction is permitted which does not comply with these terms.
*Correspondence: Jing He, aGVqaW5nMjY4QGFsaXl1bi5jb20=