- 1Department of Anesthesiology, Health Science Center, Shenzhen Second People’s Hospital/The First Affiliated Hospital of Shenzhen University, Shenzhen, China
- 2Department of Anesthesiology, School of Medicine, Ruijin Hospital, Shanghai Jiaotong University, Shanghai, China
Neuropathic pain (NP) is characterized by its complex and multifactorial nature and limited responses to opioid therapy; NP is associated with risks of drug resistance, addiction, difficulty in treatment cessation, and psychological disorders. Emerging research on gut microbiota and their metabolites has demonstrated their effectiveness in alleviating NP and augmenting opioid-based pain management, concurrently mitigating the adverse effects of opioids. This review addresses the following key points: (1) the current advances in gut microbiota research and the challenges in using opioids to treat NP, (2) the reciprocal effects and benefits of gut microbiota on NP, and (3) the interaction between opioids with gut microbiota, as well as the benefits of gut microbiota in opioid-based treatment of NP. Through various intricate mechanisms, gut microbiota influences the onset and progression of NP, ultimately enhancing the efficacy of opioids in the management of NP. These insights pave the way for further pragmatic clinical research, ultimately enhancing the efficacy of opioid-based pain management.
1 Introduction
The pathological pain resulting from damage or diseases that affects the somatosensory nervous system is called neuropathic pain (NP) (Jensen et al., 2011). NP is often accompanied by neuronal damage, and accumulating experimental evidence has highlighted the involvement of various non-neuronal cells, including glial cells, regulatory T cells, and tumor cells, as pivotal contributors to the onset of pain (Fiore et al., 2023). In this article, we describe the close interplay between gut microbiota and NP and consider the therapeutic potential of utilizing gut microbiota to augment the management of NP, particularly within the context of opioid therapy.
Recent findings in epidemiological and genomic research, coupled with cellular and animal experiments, underscore the profound influence of the microbiome on human health, acting as a mediator or modifier of environmental variables. Most of these microorganisms reside in the gastrointestinal tract, where they synthesize and modify an array of metabolites and chemical compounds. Predominantly classified into the phyla Firmicutes and Bacteroidetes, these microorganisms constitute the principal gut microbiota populations (Zádori et al., 2023). They intricately participate in modulating various physiological functions within the human body, including neuroinflammatory responses and other vital physiological processes (Belkaid and Harrison, 2017; Fan and Pedersen, 2021; Fiore et al., 2023). The development of the gut microbiota system is a gradual and intricate process beginning in infancy (Schmidt et al., 2018; Cassidy-Bushrow et al., 2023; Ruan et al., 2023). Its makeup can change over time, influenced by factors such as age, dietary habits, and prolonged medication use (Minerbi et al., 2019; Fan and Pedersen, 2021). Due to the heterogeneity of external perturbations, the rate of change in the gut microbiota varies among individuals (Flores et al., 2014). Empirical evidence consistently demonstrates that the composition of gut microbiota exhibits notable stability, with the ability to return to its baseline profile after disturbances (Dogra et al., 2020). The advent of genome sequencing technologies has significantly expedited the progress of gut microbiota research (Jovel et al., 2016). In recent years, whole-genome sequencing has unveiled the association between gut microbiota and a diverse spectrum of diseases. Initially, distinct microbial profiles were identified in the progression of gastrointestinal disorders (Barko et al., 2018). Subsequently, deviations in gut microbiota composition have been identified as playing a role inconditions spanning metabolism-related disorders, tumors, cardiovascular diseases, and pain-related disorders (Minerbi and Shen, 2022). In various forms of pain, including NP, distinctive gut microbiota signatures have been observed (Guo et al., 2019; Pane et al., 2022).
For a long time, predominant approaches to treating NP have targeted the central nervous system (CNS). Opioid medications, including morphine, tramadol, and hydrocodone have emerged as the cornerstone of clinical intervention (Balanaser et al., 2023). The escalating use of opioid medications is attributable to their excellent pain-relieving effects (Xu et al., 2022). Despite their effectiveness in helping manage moderate to severe pain, opioids often exhibit suboptimal analgesic efficacy in the treatment of NP (Martínez-Navarro et al., 2019). Classic opioids such as morphine and hydrocodone have shown limited effectiveness in this context, with clinical trials reporting unsatisfactory levels of efficacy and safety (Martínez-Navarro et al., 2019). Prolonged opioid therapy frequently leads to tolerance, dependence, and gastrointestinal adverse effects, leaving a growing number of patients unable to attain satisfactory pain relief within tolerable dose ranges. Encouragingly, a growing body of literature suggests that gut microbiota can effectively mitigate the adverse effects of opioids and contribute to the treatment of NP (Fiore et al., 2023). It is critical to note the bidirectional relationship between gut microbiota and opioids in NP treatment. Gut microbiota influence the analgesic effects of opioids, while opioids, in turn, induce alterations in the gut microenvironment (Wang et al., 2018; Zádori et al., 2023). Therefore, the gut microbiota emerges as a critical target for optimizing opioid therapy in the treatment of NP.
2 Gut microbiota in neuropathic pain
2.1 The gut-brain axis
The gut microbiota has long been considered for its beneficial impact on intestinal motility (Dey et al., 2015), local immune function (Cai et al., 2022), and visceral pain perception (Luczynski et al., 2017; Aguilera et al., 2021). Recent evidence has further elucidated its regulatory influence on organs beyond the gastrointestinal tract, most notably its association with the nervous system (Thaiss, 2023). The concept of the gut-brain axis was first proposed in the 1880s, subsequently undergoing continuous refinements (Russo et al., 2018). The gut-brain axis constitutes a complex bidirectional communication network (Cussotto et al., 2018; Russo et al., 2018). The gut microbiota and its metabolites, such as γ- aminobutyric acid (GABA), serotonin, bile acids, and amino acids, directly influence the nervous system, and indirectly mediate cell interactions through the regulation of the vagus nerve (Figure 1). The gut-brain axis is believed to be closely linked to a range of disorders, including depression (Foster, 2022), Alzheimer’s disease (Nguyen and Endres, 2022), and autism spectrum disorders (Coccurello et al., 2022). NP, the focus of this article, has also been extensively reported to be robustly connected with the gut-brain axis. Notably, mediators derived from gut microbiota exert their effects by influencing the dorsal root ganglion (DRG) and specific T cells, thereby modulating neuronal excitability and nociception (Guo et al., 2019). Furthermore, the enteric nervous system (ENS), often referred to as the “second brain,” comprises the submucosal plexus, myenteric plexus, and interstitial cells of Cajal. It facilitates bidirectional communication with the CNS, transmitting impulses on both directions (Morreale et al., 2022). While typically under the regulatory control of the CNS, the ENS can also function independently, a process involving interactions between enteric glial cells and immune regulation (Dora et al., 2021).
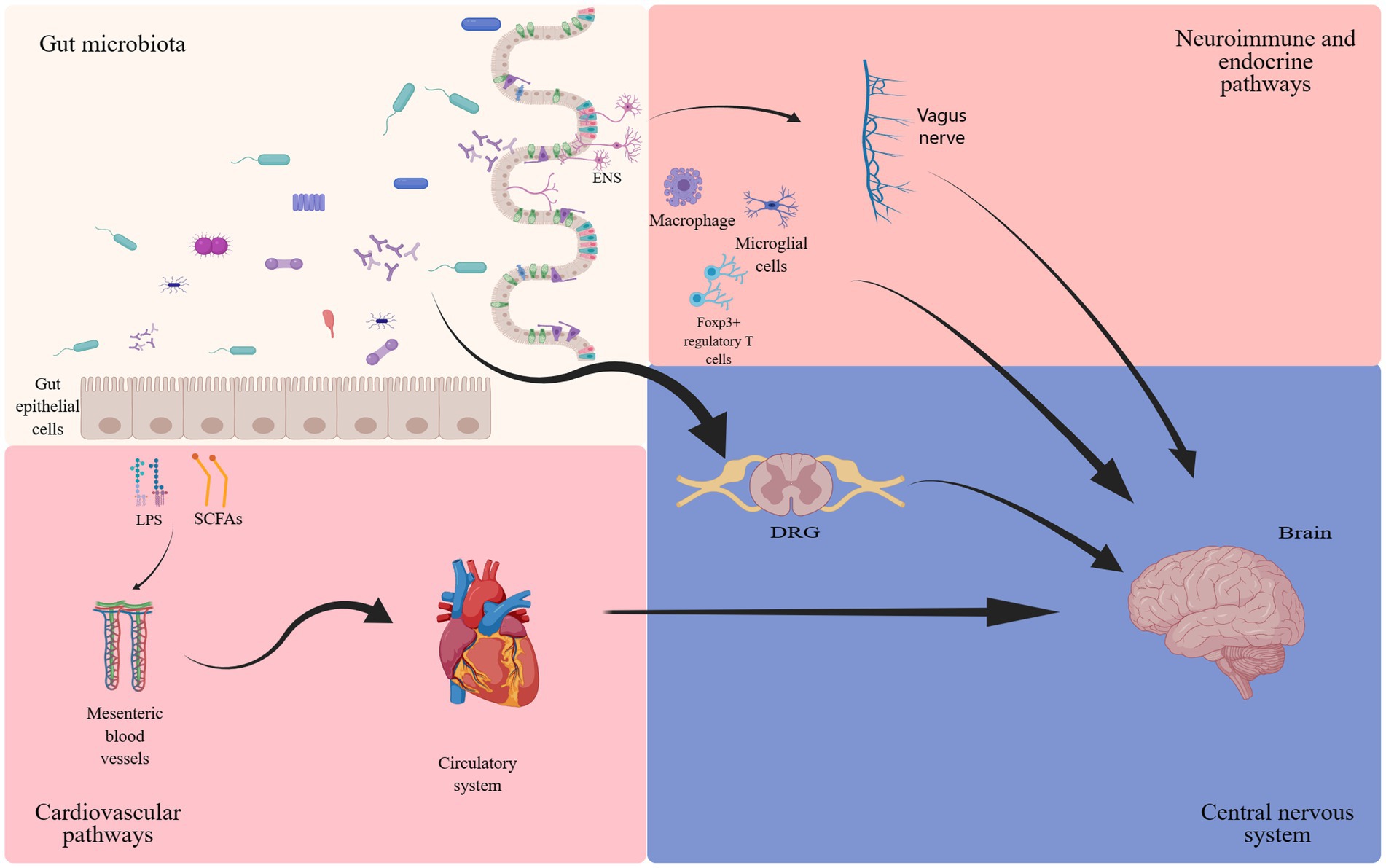
Figure 1. The involvement of gut microbiota in the gut-brain axis. The bidirectional communication between the gut microbiota and the brain occurs through endocrine (cortisol), immune (cytokines) and neural (vagus nerve and enteric nervous system) mechanisms (Josefowicz et al., 2012; Zhang et al., 2016; Cussotto et al., 2018; Russo et al., 2018; Guo et al., 2019; Ding et al., 2021; Dora et al., 2021; Ma et al., 2022; Araldi et al., 2023).
At the dawn of the 21st century, propelled by technological advancements in computational power and data processing capabilities, research in the field of metabolomics has accelerated substantially. These advancements in metabolomics have significantly enhanced our understanding of the gut microbiota, thereby enriching the landscape of gastrointestinal microbiome studies. It has been discovered that the activation of Toll-like receptors by lipopolysaccharides (LPS) plays an important role in the nervous system (Araldi et al., 2023), involving neuroimmune responses and the genesis of pain. Derived from gut Gram-negative bacteria, LPS gains access to the spinal cord through the bloodstream (Shen et al., 2017), subsequently activating Toll-like receptors (TLR) to partake in NP processing (Lin et al., 2022). Additionally, short-chain fatty acids (SCFAs), metabolic byproducts of the gut microbiota, play a critical role in microglial development (Erny et al., 2015). Multiple studies have demonstrated the key contributions of sphingolipids and polysaccharides produced by fragile rod-shaped bacteria in processes such as myelin formation, neuroinflammation, and the development of chronic pain (Mao et al., 2013; An et al., 2014; Joscelyn and Kasper, 2014; Blaho et al., 2015). These findings affirm the close connection between the CNS, microglia, and the gut microbiota.
At the same time, regarding tryptophan metabolism direction. Research evidence indicates that serotonin and quinolinic acid affect mood via the tryptophan metabolic pathway. In instances of intestinal inflammation, increased levels of quinolinic acid may contribute to anxiety (Kelly et al., 2016). Another crucial compound produced by a healthy gut microbiota is glutamate, which constitutes a vital link in the gut-brain axis (Zhang et al., 2019a). Mice subjected to acute stress-induced dysbiosis of the gut microbiota (due to antibiotic (ABX) treatment), exhibit a notable reduction in hippocampal N-Methyl-D-Aspartate (NMDA) and Brain-Derived neurotrophic factor (BDNF) levels, which can be restored by gut microbiota supplementation (Sudo et al., 2004; Gronier et al., 2018; Jiang et al., 2019). Furthermore, the gut microbiota directly engages in the regulation of certain neurotransmitters, such as GABA (Strandwitz et al., 2019) and 5-hydroxytryptamine (5-HT) (Yano et al., 2015), thereby influencing neuronal function. These findings greatly expand our understanding of the gut-brain axis.
2.2 The gut microbiota as a therapeutic target in neuropathic pain
Changes in gut microbiota were discernible in experiments employing various NP animal models. In an experimental model of NP induced by peripheral nerve injury, rats exhibiting dysbiosis in their gut microbiota were observed to be more susceptible to the development of NP (Yang et al., 2019). In the chronic sciatic nerve compression injury model, the abundances of Helicobacter, Phascolarctobacterium, Christensenella, Blautia, Streptococcus, Rothia and Lactobacillus were significantly increased, whereas the abundances of Ignatzschineria, Butyricimonas, Escherichia and Corynebacterium were significantly decreased (Chen et al., 2021). Additionally, experimental data indicate alterations in gut microbiota in C57BL/6 mice which are sensitive to Chemotherapy-induced peripheral neuropathy (CIPN). The abundance of bacteria Akkermansia muciniphila is significantly decreased, which may damage the integrity of the intestinal barrier, exposing the gut to bacterial metabolites, ultimately leading to brain dysfunction through the gut-brain axis (Ramakrishna et al., 2019). These significant changes in the gut microbiota imply a robust correlation between the gut microbiota and NP.
In fact, the critical role of gut microbiota in NP has been extensively reported. In animal experiments, researchers have demonstrated that the gut microbiota can to mitigate NP induced by chronic-constriction injury of the sciatic nerve (Ding et al., 2021). Furthermore, in the oxaliplatin (OXA) mice model, gut microbiota depletion effectively inhibits glial cell proliferation and cytokine production in the DRG induced by chronic constriction injury (CCI) or streptozotocin (STZ). The opposite is the partial recovery of the gut microbiota, especially Akkermansia and Bifidobacterium, which can either worsen or alleviate NP (Ma et al., 2022). CIPN is a prevalent adverse effect of cancer treatment. A sensitive neuronal in vitro model experiment demonstrated that gut probiotics hold promise for treating paclitaxel-induced NP, offering a long-term safe and effective treatment option (Castelli et al., 2018). Intriguingly, gut microbiota may not only contribute to the management of cancer-related NP but also potentially influence the effects of chemotherapy on tumors (Castelli et al., 2018; Vázquez-Baeza et al., 2018). These foundational experimental findings suggest that gut microbiota could represent a potential therapeutic target in CIPN.
Regarding the therapeutic role of the gut microbiome in NP, its underlying mechanisms have also garnered considerable attention and discussion. This intricate mechanism involves factors such as the metabolic products of the intestinal flora, inflammatory responses, and the recently focused neuroimmune responses. Apart from the involvement of gut microbiota, SCFAs, among the main metabolites produced by gut microbiota, have been implicated in NP. Depletion of SCFAs has been observed to alleviate NP induced by CCI (Zhou et al., 2021). Similarly, in the CCI model, following correlation analysis, it was revealed that the decreased abundance of gut microbiota producing butyrate is closely associated with levels of β-hydroxybutyric acid in both serum and spinal cord (Chen et al., 2021). This suggests that therapeutically targeting gut microbiota responsible for SCFA/β-hydroxybutyric acid production may holdpromise in ameliorating NP. Moreover, in examining the mechanism underlying inflammatory response, our investigation reveals that in the CIPN model, the therapeutic effect of probiotics on NP may be attributed to inflammation-related responses associated with the Interleukin (IL)-8 signaling pathway (Castelli et al., 2018). Conversely, modifying the gut microbiota composition through ABX treatment suppresses the TLR-4 signaling pathway mediated by macrophages, leading to improvements in CIPN and reduced secretion of inflammatory factors in the DRG (Lian et al., 2021; Ma et al., 2022). Additionally, a study revealed a significant proliferation of spinal cord microgliosis in mice receiving paclitaxel treatment, indicating a causal relationship. Gut microbiota plays a role in the development of CIPN by modulating microgliosis (Ramakrishna et al., 2019). This offers a promising avenue for the precise manipulation of gut microbiota in future NP management.
Recent studies on NP found that the gut microbiota actively participates in the onset and progression of pain by interacting with the neuroimmune system. In a mouse model of CCI, the alteration of gut microbiota via ABX led to an attenuation of NP induced by CCI. This phenomenon may be attributed to the modulation of the balance between pro-inflammatory and anti-inflammatory T cells (Ding et al., 2021). In terms of utilizing the gut microbiota for treatment (Figure 2; Table 1): (a) It has been confirmed that judicious supplementation of probiotics holds promise for pain management. In a pain model induced by lumbar disk herniation, supplementation with Lactobacillus paracasei significantly alleviated pain and mitigated inflammatory responses (Wang et al., 2021). (b). Fecal microbiota transplantation (FMT) is a common strategy in gut microbiota research. In a mouse model of NP triggered by obesity, FMT from lean mice effectively alleviated pain. One potential mechanism underlying this effect could be the reduction of RYR2-dependent calcium release in DRG neurons of obese mice following FMT (Bonomo et al., 2020). Additionally, M2-like macrophages play a pivotal role in FMT treatment for obesity-induced peripheral NP, actively participating in the pain relief process and displaying a high association with SCFAs (Bonomo et al., 2020). In mouse model of spinal cord injury, significant enrichment of the Firmicutes phylum was observed following FMT, ultimately promoting functional recovery (Jing et al., 2021). In a clinical study, alterations in the composition of gut microbiota were confirmed in patients with complex regional pain syndrome, characterized by a decrease in diversity and similar shifts in the Firmicutes and Bacteroidetes phyla as observed in animal models (Reichenberger et al., 2013). (c). ABX treatment presents another common approach for investigating gut microbiota-directed therapies. It has been demonstrated that the elimination of gut microbiota through ABX can inhibit the production of IL-6 in the DRG and improve NP (Ma et al., 2022). Additionally, ABX treatment can also alleviate CIPN through the macrophage pathway (Lian et al., 2021). In addition, clinical evidence suggests that chronic NP is often associated with cognitive impairments (Fonseca-Rodrigues et al., 2021). Supplementation with probiotics or FMT has shown potential in mitigating the harm caused by chronic NP and its associated cognitive impairment (Van Laar et al., 2019; Bonomo et al., 2020; Cuozzo et al., 2021). Unfortunately, due to ethical considerations, there is currently limited research on the direct therapeutic effects of gut microbiota in the treatment of NP.
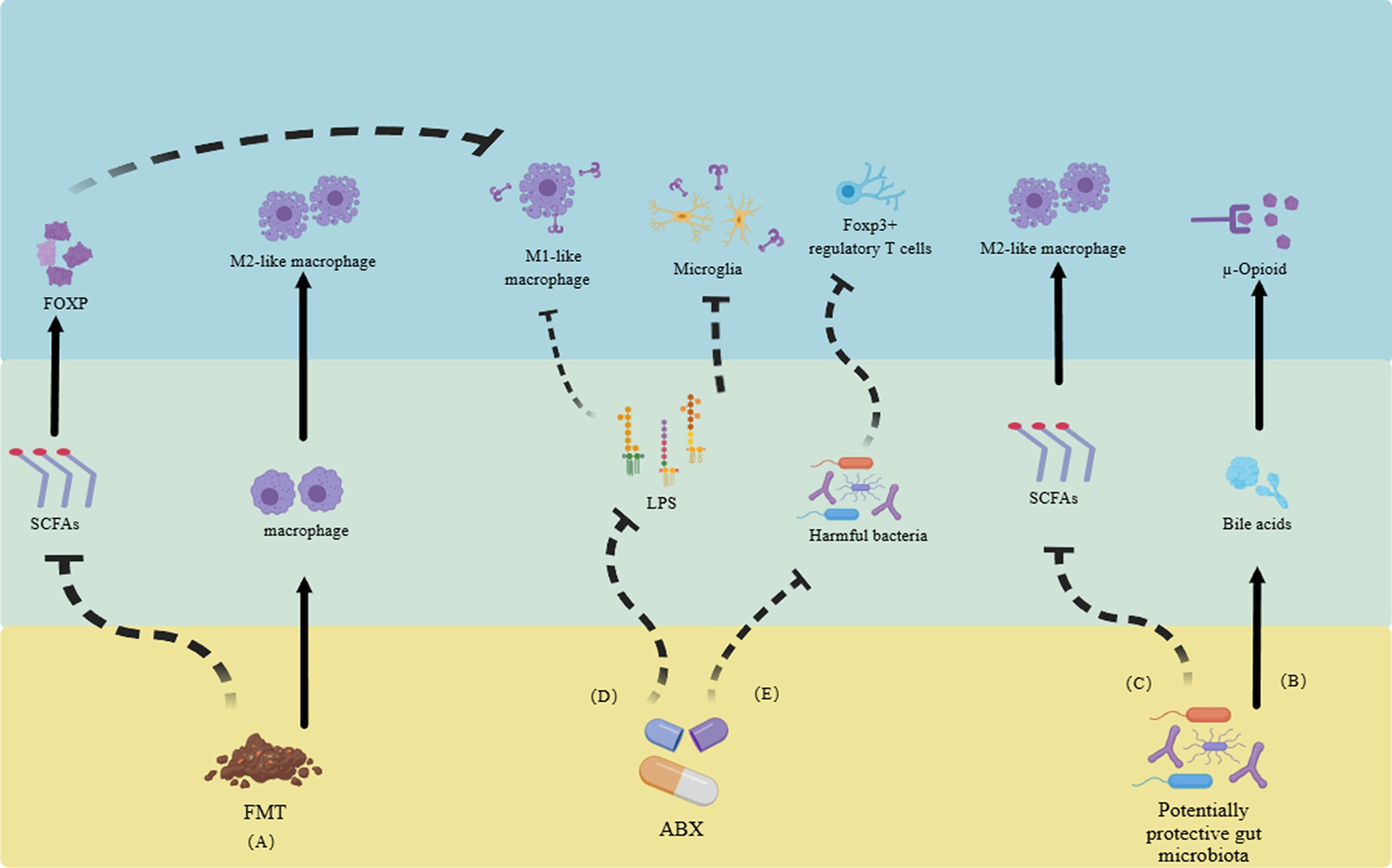
Figure 2. Utilizing gut microbiota and their metabolites to alleviate neuropathic pain through the neuroimmune system. (A) FMT reduces SCFAs, inhibiting the transformation of M1-like macrophages and promoting the increase of M2-like macrophages, ultimately relieving neuropathic pain. (B) Gut microbiota itself can promote the generation of bile acids, activate opioid receptors, and alleviate pain. (C) Some gut microbiota can increase the number of M2-like macrophages by inhibiting the production of SCFAs. (D). ABX alleviate pain by reducing the activation of Toll-like receptors in LPS-mediated M1-like macrophages and microglia. (E) ABX can also improve pain by altering gut microbiota and regulating regulatory T cells. FMT, fecal microbiota transplantation. SCFAs, short-chain fatty acids. ABX, antibiotics. LPS, lipopolysaccharides (Reichenberger et al., 2013; Van Laar et al., 2019; Bonomo et al., 2020; Cuozzo et al., 2021; Fonseca-Rodrigues et al., 2021; Jing et al., 2021; Lian et al., 2021; Wang et al., 2021; Ma et al., 2022).
In summary, accumulating preclinical evidence suggests an important role of the gut microbiota in NP, involving complex mechanisms such as gut microbiota composition and metabolites, related immune responses, and activation of the gut-brain axis. We look forward to obtaining more relevant findings in clinical studies.
3 Gut microbiome and opioids in neuropathic pain
3.1 Opioid-induced gut microbiota dysbiosis
Opioids primarily exert their effects through G protein-coupled receptors, inhibiting the transmission of nociceptive signals from the periphery to the CNS (Zádori et al., 2023). Research indicates a close correlation between the use of opioids and the composition and function of the gut microbiota (Acharya et al., 2017; Guo et al., 2019). Prolonged opioid therapy can lead to a systemic inflammatory environment, evidenced in the gut microbiota by compromised barrier function, bacterial translocation, release of gut inflammatory factors, and disrupted gastrointestinal motility (Figure 3) (Zhang et al., 2019a). Recent studies report that prolonged morphine therapy can lead to the onset of intestinal inflammation, with its mechanism closely related to the bacterial product LPS (Bhave et al., 2017). Morphine treatment directly leads to increased activity of P2X receptors in enteric glia, ultimately triggering enhanced inflammatory responses (Bhave et al., 2017). An earlier report mentioned that Systemic administration of morphine can activate a glial -mediated inflammatory cascade in the spinal dorsal horn (Raghavendra et al., 2004). Additionally, morphine sulfate attenuate the reward behavior associated with dopamine due to the activation of the BDNF signaling pathway (Taylor et al., 2016). This leads to the activation of glial cells and impaired reward mechanisms, ultimately reshaping the composition of the gut microbiota through the gut-brain axis (Zhang et al., 2019a). Additionally, opioid medications (morphine) can induce gastrointestinal motility disorders such as constipation, primarily attributed to alterations in the gut microbiota (Touw et al., 2017). In summary, opioids affect the gut microbiome through intricate mechanisms, predominantly inducing the change that typically manifests unfavorably.
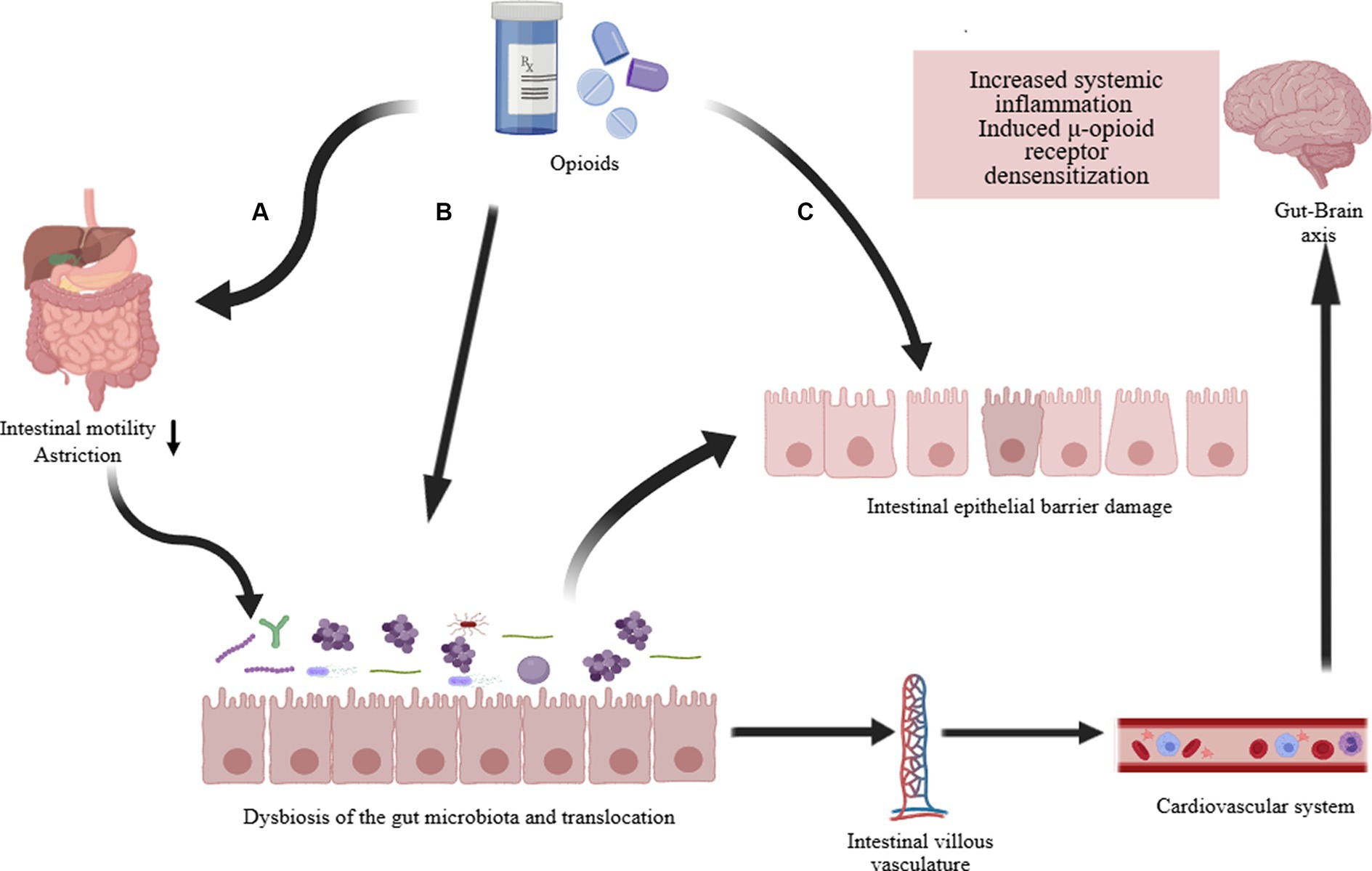
Figure 3. Opioid-induced gut microbiota dysbiosis. The long-term effects of opioid treatment on the intestines are: (A) Directly weakening gastrointestinal motility, commonly resulting in complications such as constipation. Changes in intestinal motility and transit time further alter the composition of the intestinal microbiota and cause translocation of gut microbiota. (B) Directly altering the composition and dysbiosis of the intestinal microbiota, such as a decrease in Lactobacillus and Bifidobacterium, an increase in Staphylococcus and Enterobacter, and potentially increasing other pathogenic bacteria. (C) Damage to the intestinal epithelial barrier (increased permeability, disruption of tight junction proteins, decreased immune function). The dysregulation of intestinal microbiota and function ultimately affects systemic inflammation and contributes to the development of opioid resistance via the gut-brain axis (Acharya et al., 2017; Bhave et al., 2017; Guo et al., 2019; Zhang et al., 2019a).
3.1.1 The composition of gut microbiota
Intestinal motility and transit time are the primary determinants of gut microbiota composition (Zádori et al., 2023). Constipation induced by opioid therapy and compromised intestinal motility exerts a profound influence on the composition of the gut microbiome. In a mouse model of long-term morphine treatment, dysbiosis of the gut microbiota was observed (Table 2). This dysbiosis comprised an increase in the abundance of Staphylococcus, Enterococcus, and Proteus, alongside a decrease in the abundance of Bacteroidales, Clostridiales, and Lactobacillales (Akbarali and Dewey, 2017; Kim et al., 2021). Furthermore, alterations in gut microbiota composition also extend to other physiological functions, including the inhibition of bile acid secretion (Hu et al., 2022), suppression of bicarbonate secretion (Xu et al., 2022) and the inhibition of immune surveillance (Brosnahan et al., 2014). Similar studies have also highlighted that morphine enhances the toxicity of Pseudomonas aeruginosa (Babrowski et al., 2012). Representative Gram-negative bacteria in the gut microbiota, such as those belonging to the genera Bacteroides, play an important role in ameliorating gut inflammation and maintaining the homeostasis of intestinal epithelial cells through the production of SCFAs (Smith et al., 2013). Prolonged treatment with morphine can lead to a reduction in Bacteroidetes, consequently giving rise to intestinal inflammation and compromising the integrity of the intestinal epithelial barrier (Kang et al., 2017). Another illustrative example lies in the Gram-positive Firmicutes phylum (Lactobacillales), which mitigates intestinal inflammation through butyrate salts and regulatory T cells (Furusawa et al., 2013). Prolonged morphine treatment also leads to a significant reduction in the abundance of the Firmicutes phylum (Kang et al., 2017). Interestingly, naloxone (a classic antagonist of opioid stimulants) can effectively alleviate the reduction of thick-walled microbial organisms caused by prolonged morphine treatment (Meng et al., 2015). In another study, naloxone was also shown to normalize morphine treatment-induced gut dysbiosis (Wang et al., 2018; Essmat et al., 2023). This could mean that the dysregulation of gut microbiota caused by opioid drugs can potentially be alleviated through the judicious use of peripheral μ-opioid receptor antagonists, thereby reducing the side effects associated with opioid medications.
Recent research has revealed that morphine treatment induces an enrichment of bacteria producing β-glucuronidase in the mouse gut. This leads to the accumulation of chemotherapy drugs in the intestine, culminating in the onset of gut dysbiosis (Meng et al., 2023). For cancer patients undergoing long-term opioid treatment for pain management, the development of interventional drugs targeting the gut microbiota associated with this treatment could potentially mitigate the adverse effects of chemotherapy. In addition, an elevated abundance of Enterobacteriaceae is frequently considered as an indicator of gut dysbiosis (Rizzatti et al., 2017). Recent studies have confirmed that Enterobacteriaceae are markedly enriched in histological samples of inflammatory bowel disease (Gevers et al., 2014). Prolonged exposure to morphine results in a significant increase in the abundance of Enterobacteriaceae (Kang et al., 2017). Furthermore, disparities may exist in secondary intestinal microbiota, such as filamentous bacteria (Earley et al., 2023). Additionally, there have been reports suggesting that the presence of certain less abundant genera, such as Verrucomicrobia and the genus Akkermansia, may also be augmented under the influence of opioid analgesics (Kim et al., 2021; Abu et al., 2022).
The results of animal experiments regarding changes in gut microbiota species due to opioid use are robust and compelling. However, two remaining questions remain concerning the translatability of these findings to humans. First, the use of opioids in animal studies involves high doses and short treatment durations. Second, there is a notable discrepancy in the presence of 85% of secondary phyla between the murine gastrointestinal tract used for preclinical experiments and the human body (Hu et al., 2024). Whether these subtle differences will influence the outcomes of clinical experiments remains to be investigated. Encouragingly, although the data are limited, there have been reports in human studies that parallel those from animal research. Notably, these include a decrease in Ruminococcaceae (Acharya et al., 2017) and an increase in Enterobacteriaceae (Gicquelais et al., 2020).
3.1.2 Translocation and immunomodulation
In recent years, there has been growing interest concerning the mechanism by which opioid drugs influence the gut microbiota. The latest data has forged a connection between opioid treatment and gut microbiota translocation. Several preclinical studies have corroborated that classical opioid drugs, including morphine (Meng et al., 2015; Wang et al., 2018; Zhang et al., 2019a) and hydromorphone (Sharma et al., 2020), can disrupt intestinal epithelial integrity and barrier function in mice. This disruption ultimately leads to bacterial translocation and gut inflammation. On one hand, opioids impede gastrointestinal motility and substance transport by binding to the opioid mu-receptors in the myenteric plexus of the gut (Goudsward et al., 2024), This impairment in bowel motility can lead to the overgrowth of harmful gut bacteria, potentially resulting in gram-negative sepsis (Meng et al., 2013). On the other hand, in a mouse model of sepsis, it has been shown that morphine treatment induces the dissemination of Gram-positive bacteria (Meng et al., 2015). The translocation of Gram-positive bacteria subsequently triggers an excessive expression of IL-17A and IL-6, ultimately compromis of intestinal epithelial barrier function and further exacerbating systemic inflammation (Meng et al., 2015; Kang et al., 2017). Similar conclusions have also been drawn from clinical data (Meng et al., 2015).
The displacement of gut microbiota induced by opioid medications can lead to disruptions in intestinal epithelial integrity (Banerjee et al., 2016) and trigger inflammatory immune responses (Thomas et al., 2022). We posit that future research might delve into the intricate interplay between gut microbiota and immune responses within the intestinal epithelial barrier. In animal models, it has been found that gut microbiota serves as a key determinant in initiating autoimmune T-cell responses (Berer et al., 2017). The gut microbiota is likely to influence the function of many innate immune cells, thereby actively participating in the regulation of immune responses (Santa-Cecília et al., 2019). Notably, research has demonstrated that morphine exhibits no discernible impact on the gut microbiota of mice with TLR2 knockout or immunodeficiency (Banerjee et al., 2016). This observation underscores the critical role of immune function in maintaining intestinal microbial homeostasis and preventing dysbiosis.
Opioids often exert a dual effect on immune regulation. They typically suppress the immune response of T cells and B cells (Roy et al., 2011). However, in the gastrointestinal tract, they can paradoxically activate the immune system, giving rise to inflammatory responses (Meng et al., 2013; Kang et al., 2017). Research has shed light on the key role of neuroimmune effects induced by opioids in the gastrointestinal tract. Morphine directly induce changes in intestinal epithelial function and compromise the integrity of tight junction proteins through TLR regulation (Banerjee et al., 2016). Evidence suggests that these neuroimmune effects actively contribute to maintaining intestinal epithelial barrier function (Talbot et al., 2020). However, prolonged opioid therapy inhibits immune responses (Banerjee et al., 2016), ultimately leading to dysbiosis and an altered composition of the gut microbiota. This, in turn, leads to the disruption of the intestinal epithelial immune barrier and the development of analgesic tolerance, presenting a significant challenge in opioid management.
3.2 The benefits of gut microbiota in opioid treatment
3.2.1 Analgesic tolerance
Opioids represent the most commonly prescribed medications for pain management. However, their prolonged usage carries inherent risks including the development of analgesic tolerance, potential overdose, and even mortality. In cases of NP, the downregulation or decreased sensitivity of opioid receptors frequently leads to diminished effectiveness of opioids, resulting in opioid tolerance (Balogh et al., 2019; Li et al., 2023). The prevailing view posits that alterations in opioid receptors are the primary drivers of opioid analgesic tolerance. These changes encompass a variety of mechanisms, such as the downregulation of spinal opioid receptors (Balogh et al., 2019), desensitization stemming from adaptive modifications within opioid receptor cells (Bai et al., 2020), and the functional modulation of cell membranes resulting from the repeated use of opioid analgesics (Kliewer et al., 2019). In addition, the mechanism of opioid-induced tolerance to pain relief is a topic of ongoing debate and investigation. Several potential mechanisms have emerged from relevant studies, including immune activation and neuroinflammation (Deleo et al., 2004; Hutchinson et al., 2011; Wang et al., 2012), receptor desensitization and downregulation (Balogh et al., 2019), upregulation of protein kinase A, and the release of norepinephrine and acetylcholine (Ma et al., 2001). Recent research has highlighted the significant role played by gut microbiota dysbiosis in pain modulation and tolerance through the activation of the gut-brain axis. Changes in the composition of gut microbiota have been shown to directly impact opioid tolerance in mouse experiments (Kang et al., 2017). Notably, the use of vancomycin alone, resulting in a reduction of gram-positive bacteria, prevented the development of opioid tolerance (Kang et al., 2017). While prior research on opioid-induced analgesic tolerance primarily focused on spinal mechanisms (Porreca et al., 1998), recent years have witnessed a growing emphasis on peripheral dysregulation mechanisms (Rivat, 2016; Zhang et al., 2016). Studies have revealed the pivotal role of the dorsal DRG in downregulating mu-opioid receptor (MOR) gene expression in the spinal dorsal horn, consequently diminishing the analgesic efficacy of opioids such as morphine (Zhang et al., 2016). The gut microbiota primarily contributes to the onset and progression of pain through its interaction with DRG and nerve endings within the intestine (Xu et al., 2022). Furthermore, recent research underscores the importance of the DRG in the development of pain tolerance (Jin et al., 2023). Another study has revealed that the gut microbiota is involved in the development of morphine tolerance in primary afferent neurons through its interaction with tetrodotoxin-resistant (TTX-R) sodium channels (Mischel et al., 2018). These research findings confirm the important role of gut microbiota in the peripheral analgesic mechanism of opioids.
Furthermore, a substantial body of evidence supports the key role of neuroinflammation in the development of morphine tolerance (Trang et al., 2015; Gei et al., 2024; Wang et al., 2024). Within neuroinflammatory responses, microglia have long been recognized as important markers for observation. Laboratory findings indicate that the elimination of microglia can effectively prevent opioid-induced pain hypersensitivity (Burma et al., 2017). In addition to its role in the analgesic tolerance process, glial activation actively participates in the development of NP (Gei et al., 2024) and is a key element in the mechanisms related to opioid treatment (Martínez-Navarro et al., 2019). MOR stimulation can lead to the activation of microglia (Ferrini et al., 2013). Interestingly, this opioid-induced glial response may counteract some of the analgesic effects (Martínez-Navarro et al., 2019). Furthermore, specific metabolic products of gut microbiota, such as lipoteichoic acid and LPS, can also induce the activation of glial cells within the nervous system (Obata and Pachnis, 2016; Bhave et al., 2017). Once activated, glial cells release an array of cytokines into the internal environment (Leduc-Pessah et al., 2017), consequently facilitating the development of analgesic tolerance (Drossman and Szigethy, 2014). These reports suggest that reducing harmful gut bacteria, which are associated with increased pain tolerance, can effectively alleviate the development of pain tolerance.
Recent studies have indicated that a portion of morphine analgesic tolerance arises from a decrease in the levels of intestinal β-glucuronidase, resulting in reduced hydrolysis of morphine metabolites back into morphine (Wang and Roy, 2016). Compelling evidence indicates that specific bacteria in the gut, such as Bifidobacteria and Lactobacilli, produce β-glucuronidase (Zhang et al., 2019a). Moreover, further research has confirmed the association of Bifidobacterium and Lactobacillus with opioid tolerance. Probiotics enriched with these microbial strains have demonstrated their effectiveness in alleviating analgesic tolerance in morphine-treated mice (Zhang et al., 2019a). The observed changes in Bifidobacterium and Lactobacillus are particularly intriguing, as these strains themselves contribute to maintaining intestinal homeostasis (Wang et al., 2014). Furthermore, recent investigations into opioids have revealed that FMT significantly reduces opioid withdrawal responses (Zhang et al., 2021). In summary, these studies underscore the substantial role of gut microbiota in opioid treatment, suggesting that supplementation with associated gut microbial strains may potentially prolong the analgesic efficacy of opioids.
3.2.2 The addictive properties of opioids
Opioid addiction poses a significant challenge in the context of long-term opioid use for NP, often leading to varying degrees of withdrawal symptoms upon discontinuation of the medication (Simpson et al., 2020). Preclinical evidence strongly suggests that the gut microbiota actively influences behaviors associated with opioid addiction (Han et al., 2018; Lee et al., 2018; Hofford et al., 2021; Thomaz et al., 2021). Recent research has been exploring the impact of gut microbiota on opioid withdrawal. A recent preclinical study yielded results supporting the effectiveness of FMT and ABX treatment in reducing naloxone-induced withdrawal responses (Thomaz et al., 2021). Furthermore, a study identified significant gender differences in the relationship between gut microbiota and opioid addiction (Ren and Lotfipour, 2023). Among the abundant evidence, a key produced by the gut microbiota, SCFAs, plays a crucial role in cocaine reward behavior (Kiraly et al., 2016). In instances where gut microbiota diversity is inhibited by ABX, there is an increase in cocaine-conditioned place preference (CPP), a phenomenon that can be reversed through SCFA supplementation (Kiraly et al., 2016; Hofford et al., 2021). This suggests that diminished gut microbiota diversity, likely closely linked to SCFAs, instigates changes in opioid reward behavior. Research on SCFAs holds promise for the development of safer and more effective opioid treatment options.
Chronic morphine treatment activate the BDNF pathway through microglia, resulting in a reduction of dopamine-dependent reward behavior (Taylor et al., 2016). This underscores microglia as a key factor in the development of reward dysfunction induced by opioid drugs. Excessive activation of microglia in the ventral tegmental area leads to impaired brain reward behavior and diminished pleasure (Taylor et al., 2016), necessitating higher opioid doses for achieving reward and ultimately contributing to opioid addiction. Importantly, the gut microbiota can influence the morphology and activity of microglia (Gicquelais et al., 2020), consequently leading to reduced dopamine activity and disruptions in reward mechanisms (Taylor et al., 2016). Recent animal experiments have demonstrated that animals treated with FMT exhibited a significant restoration of normal reward behavior, accompanied by an observed increase in resting-state microglia (Lee et al., 2018). These research findings suggest that modulating the gut microbiota can exert a positive influence on the restoration of reward behavior and microglial function. Advancements in gut-brain axis research have suggested intriguing connections between gut microbiota, neuroimmune responses, and opioid withdrawal, offering fresh insights into opioid pain management. The development of gut microbiota-based therapies has introduced a fresh perspective to opioid withdrawal treatment and neuroimmune mechanisms. However, extensive clinical research is required to conclusively validate these possibilities and to further investigate the interactions between gut microbiota, opioid management, and neuroimmunity.
3.2.3 Mental Health
NP is often accompanied by a spectrum of depressive symptoms (Janakiram et al., 2023). Notably, the severity of depression tends to worsen in tandem with the intensity of pain (Agüera-Ortiz et al., 2011). Clinical psychologists often use sedative medication as an adjunct treatment during the process of treating depression. When considering the combination of opioid drugs and sedative drugs, particularly benzodiazepines, it is imperative to exercise a high degree of vigilance due to the potential risks associated with these medications (Liu et al., 2021). Previous studies have confirmed that when compared to using opioids in isolation for managing NP, the combination of opioids and sedative drugs may lead to a notable increase in the risk of drug overdose (Meyer et al., 2023). Furthermore, it can escalate to a critical level, potentially culminating in fatal respiratory depression (Liu et al., 2021). Research has shown that the activation of opioid receptors in the context of pain treatment can lead to or exacerbate depressive symptoms (Dagher et al., 2022). This pertains not only to patients already affected by mental health issues, but even to those deemed mentally healthy, long-term use of opioids significantly increases the risk of developing depression (Scherrer et al., 2016).
The gut microbiota plays a pivotal role in the development of depression by secreting neurotransmitters, including serotonin (Yano et al., 2015). The utilization of opioid medications can disrupt the gut microbiota, resulting in a depletion of serotonin levels and an augmented risk of developing depression (Parker et al., 2020). Notably, Lactobacillus and Bifidobacterium have been implicated in associations with depression and anxiety (Han and Kim, 2019). Their depletion can occur as a consequence of morphine treatment (Zhang et al., 2019a), potentially increasing the susceptibility to experiencing anxiety and depression during medication therapy. Encouragingly, specific strains of probiotics, such as Bifidobacterium, have demonstrated the capacity to mitigate depression by increasing BDNF levels and reducing oxidative stress (Messaoudi et al., 2011). Research has reported a decrease in BDNF levels in instances of depression, and clinical studies have shown that supplementation with probiotics can lower depressive symptoms (Jang et al., 2019). Another study on Bifidobacterium revealed that it, along with Lactobacillus, contributes to the reduction of depression levels by activating the vagus nerve and eliciting an immunostress response (Yang et al., 2024). Furthermore, research findings also suggest that increasing the abundance of the Ruminococcaceae family could also be a potential avenue for reducing depression levels (Zhu et al., 2019). A recent study suggests that Lactobacillus may help alleviate depression and anxiety induced by chronic stress by sustaining levels of interferon gamma (IFN-γ). Therefore, maintaining healthy levels of Lactobacillus or IFN-γ could potentially prevent or treat symptoms of depression (Clark et al., 2023). Although further exploration is warranted to fully understand the connection between depression and the gut microbiota, it is worth considering supplementation with specific gut microbiota, especially in cases necessitating long-term opioid medication use for NP treatment.
A clinical study has revealed that patients with higher depression scores often exhibit elevated levels of kynurenine, serotonin, and histamine (Han et al., 2022). These compounds are primarily produced by enterochromaffin cells under the influence of gut microbiota, ultimately contributing to the onset of depression. This also underscores the indirect influence of gut microbiota on the development of depression. In addition, another article highlights the serotonin-generating capability of Clostridium nexile, which demonstrates significant elevation in patients with severe depression (Williams et al., 2014). These clinical findings suggest a potential avenue for alleviating the depressive effects induced by opioid treatment, by targeting specific gut microbiota, such as Clostridium nexile, to modulate neurotransmitter production.
4 Conclusions and treatment perspectives
With the rapid development of genomic sequencing technology, research on gut microbiota has entered a transformative phase (Jovel et al., 2016). These microorganisms, cohabiting with humans, have demonstrated significant influence over various aspects of our physiological and pathological behaviors (Barko et al., 2018; Pane et al., 2022). It is imperative that we focus on and deepen our understanding of gut microbiota and their metabolites within the human body, to gain insights into human health and address unresolved diseases. Research on the gut-brain axis has received considerable attention, with emerging evidence indicating that gut microbiota and their metabolites (SCFAs, LPS, butyrate, and glutamate) play pivotal roles in many diseases (Furusawa et al., 2013; Bhave et al., 2017; Zhang et al., 2019a), including NP. The mechanisms underlying these effects involve neuroimmune interactions. Extensive experimental data have demonstrated the intricate and complex connections between gut microbiota and the development of pain, including NP (Vázquez-Baeza et al., 2018; Ramakrishna et al., 2019; Lian et al., 2021; Ma et al., 2022). Probiotic supplementation or FMT has demonstrated significant reductions in NP (Vázquez-Baeza et al., 2018; Bonomo et al., 2020; Wang et al., 2021). Exploring the involvement of gut microbiota in the pathogenesis of NP holds promise for the development of novel therapeutic approaches in pain management for the future.
In traditional approaches to treating NP, opioids have held a pivotal role (Balanaser et al., 2023). However, their effectiveness is often limited due to shared neurophysiological mechanisms with NP (Martínez-Navarro et al., 2019), along with associated risks of tolerance, addiction, and potential overuse crises. This article elucidates the intricate interplay between gut microbiota and opioid drugs in the context of NP. Opioid therapy alters gut microbiota diversity (Kim et al., 2021) and physiological metabolic processes (Sharma et al., 2020; Kim et al., 2021) by affecting gastrointestinal motility and neuroimmune function. Simultaneously, interventions such as probiotics, ABX, and FMT significantly reduce opioid tolerance and addiction (Wang et al., 2014; Wang and Roy, 2016; Kim et al., 2021; Thomaz et al., 2021) while also positively affecting the psychological well-being of the host (Table 3) (Jang et al., 2019). These findings hold relevance in clinical practice, including observational studies, suggesting that gut microbiota can play a valuable role in the management of opioid-induced NP, thereby mitigating the adverse effects of opioids and ultimately enhancing the overall quality of treatment.
The nexus between gut microbiota, opioids, and NP presents both intriguing and challenging dimensions. Presently, most research is centered around animal models, underscoring the need for further clinical studies and long-term dynamic data to support the underlying mechanisms involving gut microbiota. Therefore, future clinical research must focus on generating a robust body of evidence and thoroughly investigating the relevant mechanisms to elucidate the complex relationship between gut microbiota and various types of NP. Furthermore, the influence of numerous confounding factors, including patients lifestyles and dietary habits, complex psychological factors, and diverse treatment regimens, must be carefully considered in studies exploring the interactions between advanced gut microbiota-dietary interventions and gut microbiota-based therapies. Additionally, advancements in detection methods are expected to unveil previously overlooked microorganisms, thereby prompting scientists to deepen their understanding of the interconnections among these variables. We look forward to the widespread integration of gut microbiota-based approaches in opioid management for NP in the future.
Author contributions
ZG: Conceptualization, Supervision, Writing – original draft, Writing – review & editing. QX: Conceptualization, Data curation, Investigation, Writing – review & editing. YL: Investigation, Supervision, Validation, Writing – review & editing. BY: Methodology, Validation, Writing – review & editing. BH: Writing – review & editing, Supervision. ZL: Funding acquisition, Writing – original draft, Writing – review & editing.
Funding
The author(s) declare that financial support was received for the research, authorship, and/or publication of this article. This work was supported by Guangdong Basic and Applied Basic Research Nature Science Foundation (2023A1515011649), Sanming Project of Medicine in Shenzhen (No. SZSM202211007).
Conflict of interest
The authors declare that the research was conducted in the absence of any commercial or financial relationships that could be construed as a potential conflict of interest.
Publisher's note
All claims expressed in this article are solely those of the authors and do not necessarily represent those of their affiliated organizations, or those of the publisher, the editors and the reviewers. Any product that may be evaluated in this article, or claim that may be made by its manufacturer, is not guaranteed or endorsed by the publisher.
References
Abu, Y., Tao, J. Y., Dutta, R., Yan, Y., Vitari, N., Kolli, U., et al. (2022). Brief hydromorphone exposure during pregnancy sufficient to induce maternal and neonatal mcrobial dysbiosis. J. Neuroimmune Pharmacol. 17, 367–375. doi: 10.1007/s11481-021-10019-2
Acharya, C., Betrapally, N. S., Gillevet, P. M., Sterling, R. K., Akbarali, H., White, M. B., et al. (2017). Chronic opioid use is associated with altered gut microbiota and predicts readmissions in patients with cirrhosis. Aliment. Pharmacol. Ther. 45, 319–331. doi: 10.1111/apt.13858
Agüera-Ortiz, L., Failde, I., Mico, J. A., Cervilla, J., and López-Ibor, J. J. (2011). Pain as a symptom of depression: Prevalence and clinical correlates in patients attending psychiatric clinics. J. Affect. Disord. 130, 106–112. doi: 10.1016/j.jad.2010.10.022
Aguilera, M., Rossini, V., Hickey, A., Simnica, D., Grady, F., Felice, V. D., et al. (2021). Inflammasome signaling regulates the microbial–neuroimmune axis and visceral pain in mice. Int. J. Mol. Sci. 22:8336. doi: 10.3390/ijms22158336
Akbarali, H. I., and Dewey, W. L. (2017). The gut–brain interaction in opioid tolerance. Curr. Opin. Pharmacol. 37, 126–130. doi: 10.1016/j.coph.2017.10.012
An, D., Oh, S. F., Olszak, T., Neves, J. F., Avci, F. Y., Erturk-Hasdemir, D., et al. (2014). Sphingolipids from a symbiotic microbe regulate homeostasis of host intestinal natural killer T cells. Cell 156, 123–133. doi: 10.1016/j.cell.2013.11.042
Araldi, D., Khomula, E. V., Bonet, I. J. M., Bogen, O., Green, P. G., and Levine, J. D. (2023). Role of pattern recognition receptors in chemotherapy-induced neuropathic pain. Brain 147, 1025–1042. doi: 10.1093/brain/awad339
Babrowski, T., Holbrook, C., Moss, J., Gottlieb, L., Valuckaite, V., Zaborin, A., et al. (2012). Pseudomonas aeruginosa virulence expression is directly activated by morphine and is capable of causing lethal gut derived sepsis in mice during chronic morphine administration. Ann. Surg. 255, 386–393. doi: 10.1097/SLA.0b013e3182331870
Bai, X., Wang, C., Zhang, X., Feng, Y., and Zhang, X. (2020). The role of testosterone in mu-opioid receptor expression in the trigeminal ganglia of opioid-tolerant rats. Neurosci. Lett. 723:134868. doi: 10.1016/j.neulet.2020.134868
Balanaser, M., Carley, M., Baron, R., Finnerup, N. B., Moore, R. A., Rowbotham, M. C., et al. (2023). Combination pharmacotherapy for the treatment of neuropathic pain in adults: Systematic review and meta-analysis. Pain 164, 230–251. doi: 10.1097/j.pain.0000000000002688
Balogh, M., Zádor, F., Zádori, Z. S., Shaqura, M., Király, K., Mohammadzadeh, A., et al. (2019). Efficacy-Based Perspective to Overcome Reduced Opioid Analgesia of Advanced Painful Diabetic Neuropathy in Rats. Front. Pharmacol. 10:347. doi: 10.3389/fphar.2019.00347
Banerjee, S., Sindberg, G., Wang, F., Meng, J., Sharma, U., Zhang, L., et al. (2016). Opioid-induced gut microbial disruption and bile dysregulation leads to gut barrier compromise and sustained systemic inflammation. Mucosal Immunol. 9, 1418–1428. doi: 10.1038/mi.2016.9
Barko, P. C., McMichael, M. A., Swanson, K. S., and Williams, D. A. (2018). The gastrointestinal microbiome: A review. J. Vet. Intern. Med. 32, 9–25. doi: 10.1111/jvim.14875
Belkaid, Y., and Harrison, O. J. (2017). Homeostatic immunity and the microbiota. Immunity 46, 562–576. doi: 10.1016/j.immuni.2017.04.008
Berer, K., Gerdes, L. A., Cekanaviciute, E., Jia, X., Xiao, L., Xia, Z., et al. (2017). Gut microbiota from multiple sclerosis patients enables spontaneous autoimmune encephalomyelitis in mice. Proc. Natl. Acad. Sci. USA 114, 10719–10724. doi: 10.1073/pnas.1711233114
Bhave, S., Gade, A., Kang, M., Hauser, K. F., Dewey, W. L., and Akbarali, H. I. (2017). Connexin–purinergic signaling in enteric glia mediates the prolonged effect of morphine on constipation. FASEB J. 31, 2649–2660. doi: 10.1096/fj.201601068R
Blaho, V. A., Galvani, S., Engelbrecht, E., Liu, C., Swendeman, S. L., Kono, M., et al. (2015). HDL-bound sphingosine-1-phosphate restrains lymphopoiesis and neuroinflammation. Nature 523, 342–346. doi: 10.1038/nature14462
Bonomo, R. R., Cook, T. M., Gavini, C. K., White, C. R., Jones, J. R., Bovo, E., et al. (2020). Fecal transplantation and butyrate improve neuropathic pain, modify immune cell profile, and gene expression in the PNS of obese mice. Proc. Natl. Acad. Sci. USA 117, 26482–26493. doi: 10.1073/pnas.2006065117
Brosnahan, A. J., Jones, B. J., Dvorak, C. M., and Brown, D. R. (2014). Morphine attenuates apically-directed cytokine secretion from intestinal epithelial cells in response to enteric pathogens. Pathogens 3, 249–257. doi: 10.3390/pathogens3020249
Burma, N. E., Bonin, R. P., Leduc-Pessah, H., Baimel, C., Cairncross, Z. F., Mousseau, M., et al. (2017). Blocking microglial pannexin-1 channels alleviates morphine withdrawal in rodents. Nat. Med. 23, 355–360. doi: 10.1038/nm.4281
Cai, J., Sun, L. L., and Gonzalez, F. J. (2022). Gut microbiota-derived bile acids in intestinal immunity, inflammation, and tumorigenesis. Cell Host Microbe 30, 289–300. doi: 10.1016/j.chom.2022.02.004
Cassidy-Bushrow, A. E., Sitarik, A. R., Johnson, C. C., Johnson-Hooper, T. M., Kassem, Z., Levin, A. M., et al. (2023). Early-life gut microbiota and attention deficit hyperactivity disorder in preadolescents. Pediatr. Res. 93, 2051–2060. doi: 10.1038/s41390-022-02051-6
Castelli, V., Palumbo, P., d'Angelo, M., Moorthy, N. K., Antonosante, A., Catanesi, M., et al. (2018). Probiotic DSF counteracts chemotherapy induced neuropathic pain. Oncotarget 9, 27998–28008. doi: 10.18632/oncotarget.25524
Chen, P., Wang, C., Ren, Y. N., Ye, Z. J., Jiang, C., and Wu, Z. B. (2021). Alterations in the gut microbiota and metabolite profiles in the context of neuropathic pain. Mol. Brain 14:50. doi: 10.1186/s13041-021-00765-y
Clark, D. N., O'Neil, S. M., Xu, L., Steppe, J. T., Savage, J. T., Raghunathan, K., et al. (2023). Prolonged STAT1 activation in neurons drives a pathological transcriptional response. J. Neuroimmunol. 382:578168. doi: 10.1016/j.jneuroim.2023.578168
Coccurello, R., Marrone, M. C., and Maccarrone, M. (2022). The endocannabinoids-microbiota partnership in gut-brain axis homeostasis: Implications for autism spectrum disorders. Front. Pharmacol. 13:869606. doi: 10.3389/fphar.2022.869606
Corder, G., Tawfik, V. L., Wang, D., Sypek, E. I., Low, S. A., Dickinson, J. R., et al. (2017). Loss of μ opioid receptor signaling in nociceptors, but not microglia, abrogates morphine tolerance without disrupting analgesia. Nat. Med. 23, 164–173. doi: 10.1038/nm.4262
Cuozzo, M., Castelli, V., Avagliano, C., Cimini, A., d'Angelo, M., Cristiano, C., et al. (2021). Effects of chronic oral probiotic treatment in paclitaxel-induced neuropathic pain. Biomedicines 9:346. doi: 10.3390/biomedicines9040346
Cussotto, S., Sandhu, K. V., Dinan, T. G., and Cryan, J. F. (2018). The neuroendocrinology of the microbiota-gut-brain axis: A behavioural perspective. Front. Neuroendocrinol. 51, 80–101. doi: 10.1016/j.yfrne.2018.04.002
Dagher, M., Cahill, C. M., and Evans, C. J. (2022). Opioid systems and depression: The relationship is strengthening. Biol. Psychiatry 92, 920–922. doi: 10.1016/j.biopsych.2022.09.020
Deleo, J. A., Tanga, F. Y., and Tawfik, V. L. (2004). Neuroimmune activation and neuroinflammation in chronic pain and opioid tolerance/hyperalgesia. Neuroscientist 10, 40–52. doi: 10.1177/1073858403259950
Dey, N., Wagner, V. E., Blanton, L. V., Cheng, J., Fontana, L., Haque, R., et al. (2015). Regulators of gut motility revealed by a gnotobiotic model of diet-microbiome interactions related to travel. Cell 163, 95–107. doi: 10.1016/j.cell.2015.08.059
Ding, W., You, Z., Chen, Q., Yang, L., Doheny, J., Zhou, X., et al. (2021). Gut microbiota influences neuropathic pain through modulating proinflammatory and anti-inflammatory T cells. Anesth. Analg. 132, 1146–1155. doi: 10.1213/ANE.0000000000005155
Dogra, S. K., Doré, J., and Damak, S. (2020). Gut microbiota resilience: Definition, link to health and strategies for intervention. Front. Microbiol. 11:572921. doi: 10.3389/fmicb.2020.572921
Dora, D., Ferenczi, S., Stavely, R., Toth, V. E., Varga, Z. V., Kovacs, T., et al. (2021). Evidence of a myenteric plexus barrier and its macrophage-dependent degradation during murine colitis: Implications in enteric neuroinflammation. Cell. Mol. Gastroenterol. Hepatol. 12, 1617–1641. doi: 10.1016/j.jcmgh.2021.07.003
Drossman, D., and Szigethy, E. (2014). The narcotic bowel syndrome: A recent update. Am. J. Gastroenterol. Suppl. 2, 22–30. doi: 10.1038/ajgsup.2014.6
Earley, Z. M., Lisicka, W., Sifakis, J. J., Aguirre-Gamboa, R., Kowalczyk, A., Barlow, J. T., et al. (2023). GATA4 controls regionalization of tissue immunity and commensal-driven immunopathology. Immunity 56, 43–57.e10. doi: 10.1016/j.immuni.2022.12.009
Erny, D., Hrabě de Angelis, A. L., Jaitin, D., Wieghofer, P., Staszewski, O., David, E., et al. (2015). Host microbiota constantly control maturation and function of microglia in the CNS. Nat. Neurosci. 18, 965–977. doi: 10.1038/nn.4030
Essmat, N., Karádi, D. Á., Zádor, F., Király, K., Fürst, S., and Al-Khrasani, M. (2023). Insights into the Current and Possible Future Use of Opioid Antagonists in Relation to Opioid-Induced Constipation and Dysbiosis. Molecules 28:7766. doi: 10.3390/molecules28237766
Fan, Y., and Pedersen, O. (2021). Gut microbiota in human metabolic health and disease. Nat. Rev. Microbiol. 19, 55–71. doi: 10.1038/s41579-020-0433-9
Ferrini, F., Trang, T., Mattioli, T. A. M., Laffray, S., Del'Guidice, T., Lorenzo, L. E., et al. (2013). Morphine hyperalgesia gated through microglia-mediated disruption of neuronal Cl− homeostasis. Nat. Neurosci. 16, 183–192. doi: 10.1038/nn.3295
Fiore, N. T., Debs, S. R., Hayes, J. P., Duffy, S. S., and Moalem-Taylor, G. (2023). Pain-resolving immune mechanisms in neuropathic pain. Nat. Rev. Neurol. 19, 199–220. doi: 10.1038/s41582-023-00777-3
Flores, G. E., Caporaso, J. G., Henley, J. B., Rideout, J. R., Domogala, D., Chase, J., et al. (2014). Temporal variability is a personalized feature of the human microbiome. Genome Biol. 15:531. doi: 10.1186/s13059-014-0531-y
Fonseca-Rodrigues, D., Amorim, D., Almeida, A., and Pinto-Ribeiro, F. (2021). Emotional and cognitive impairments in the peripheral nerve chronic constriction injury model (CCI) of neuropathic pain: A systematic review. Behav. Brain Res. 399:113008. doi: 10.1016/j.bbr.2020.113008
Foster, J. A. (2022). Modulating brain function with microbiota. Science 376, 936–937. doi: 10.1126/science.abo4220
Furusawa, Y., Obata, Y., Fukuda, S., Endo, T. A., Nakato, G., Takahashi, D., et al. (2013). Commensal microbe-derived butyrate induces the differentiation of colonic regulatory T cells. Nature 504, 446–450. doi: 10.1038/nature12721
Gei, L., Yan, Y., Xing, W., Li, Q., Chen, X., Yan, F., et al. (2024). Amiloride alleviates morphine tolerance by suppressing ASIC3-dependent neuroinflammation in the spinal cord. Eur. J. Pharmacol. 963:176173. doi: 10.1016/j.ejphar.2023.176173
Gevers, D., Kugathasan, S., Denson, L. A., Vázquez-Baeza, Y., Van Treuren, W., Ren, B., et al. (2014). The treatment-naive microbiome in new-onset Crohn’s disease. Cell Host Microbe 15, 382–392. doi: 10.1016/j.chom.2014.02.005
Gicquelais, R. E., Bohnert, A. S. B., Thomas, L., and Foxman, B. (2020). Opioid agonist and antagonist use and the gut microbiota: associations among people in addiction treatment. Sci. Rep. 10:19471. doi: 10.1038/s41598-020-76570-9
Goudsward, H. J., Ruiz-Velasco, V., Stella, S. L., Willing, L. B., and Holmes, G. M. (2024). Coexpressed δ-, μ-, and κ-Opioid Receptors Modulate Voltage-Gated Ca2+ Channels in Gastric-Projecting Vagal Afferent Neurons. Mol. Pharmacol. 105, 250–259. doi: 10.1124/molpharm.123.000774
Gronier, B., Savignac, H. M., Di Miceli, M., Idriss, S. M., Tzortzis, G., Anthony, D., et al. (2018). Increased cortical neuronal responses to NMDA and improved attentional set-shifting performance in rats following prebiotic (B-GOS®) ingestion. Eur. Neuropsychopharmacol. 28, 211–224. doi: 10.1016/j.euroneuro.2017.11.001
Guo, R., Chen, L. H., Xing, C. G., and Liu, T. (2019). Pain regulation by gut microbiota: Molecular mechanisms and therapeutic potential. Br. J. Anaesth. 123, 637–654. doi: 10.1016/j.bja.2019.07.026
Han, S. K., and Kim, D. H. (2019). Lactobacillus mucosae and Bifidobacterium longum synergistically alleviate immobilization stress-induced anxiety/depression in mice by suppressing gut dysbiosis. J. Microbiol. Biotechnol. 29, 1369–1374. doi: 10.4014/jmb.1907.07044
Han, W. F., Tellez, L. A., Perkins, M. H., Perez, I. O., Qu, T., Ferreira, J., et al. (2018). A neural circuit for gut-induced reward. Cell 175, 665–78.e23. doi: 10.1016/j.cell.2018.08.049
Han, L. J., Zhao, L., Zhou, Y., Yang, C., Xiong, T., Lu, L., et al. (2022). Altered metabolome and microbiome features provide clues in understanding irritable bowel syndrome and depression comorbidity. ISME J. 16, 983–996. doi: 10.1038/s41396-021-01123-5
Hofford, R. S., Mervosh, N. L., Euston, T. J., Meckel, K. R., Orr, A. T., and Kiraly, D. D. (2021). Alterations in microbiome composition and metabolic byproducts drive behavioral and transcriptional responses to morphine. Neuropsychopharmacology 46, 2062–2072. doi: 10.1038/s41386-021-01043-0
Hu, H., Shao, W., Liu, Q., Liu, N., Wang, Q., Xu, J., et al. (2022). Gut microbiota promotes cholesterol gallstone formation by modulating bile acid composition and biliary cholesterol secretion. Nat. Commun. 13:252. doi: 10.1038/s41467-021-27758-8
Hu, X., Yu, C., He, Y., Zhu, S., Wang, S., Xu, Z., et al. (2024). Integrative metagenomic analysis reveals distinct gut microbial signatures related to obesity. BMC Microbiol. 24:119. doi: 10.1186/s12866-024-03278-5
Hutchinson, M. R., Shavit, Y., Grace, P. M., Rice, K. C., Maier, S. F., and Watkins, L. R. (2011). Exploring the neuroimmunopharmacology of opioids: An integrative review of mechanisms of central immune signaling and their implications for opioid analgesia. Pharmacol. Rev. 63, 772–810. doi: 10.1124/pr.110.004135
Janakiram, C., Okunev, I., Tranby, E. P., Fontelo, P., Iafolla, T. J., and Dye, B. A. (2023). Opioids for acute and chronic pain when receiving psychiatric medications. PLoS One 18:e0286179. doi: 10.1371/journal.pone.0286179
Jang, H. M., Lee, K. E., and Kim, D. H. (2019). The preventive and curative effects of Lactobacillus reuteri NK33 and Bifidobacterium adolescentis NK98 on immobilization stress-induced anxiety/depression and colitis in mice. Nutrients 11:819. doi: 10.3390/nu11040819
Jensen, T. S., Baron, R., Haanpää, M., Kalso, E., Loeser, J. D., Rice, A. S. C., et al. (2011). A new definition of neuropathic pain. Pain 152, 2204–2205. doi: 10.1016/j.pain.2011.06.017
Jiang, C., Lin, W. J., and Salton, S. R. (2019). Role of a VGF/BDNF/TrkB autoregulatory feedback loop in rapid-acting antidepressant efficacy. J. Mol. Neurosci. 68, 504–509. doi: 10.1007/s12031-018-1124-0
Jin, D., Chen, H., Zhou, M. H., Chen, S. R., and Pan, H. L. (2023). mGluR5 from Primary Sensory Neurons Promotes Opioid-Induced Hyperalgesia and Tolerance by Interacting with and Potentiating Synaptic NMDA Receptors. J. Neurosci. 43, 5593–5607. doi: 10.1523/JNEUROSCI.0601-23.2023
Jing, Y. L., Yu, Y., Bai, F., Wang, L. M., Yang, D. G., Zhang, C., et al. (2021). Effect of fecal microbiota transplantation on neurological restoration in a spinal cord injury mouse model: Involvement of brain-gut axis. Microbiome 9:59. doi: 10.1186/s40168-021-01007-y
Joscelyn, J., and Kasper, L. H. (2014). Digesting the emerging role for the gut microbiome in central nervous system demyelination. Mult. Scler. J. 20, 1553–1559. doi: 10.1177/1352458514541579
Josefowicz, S. Z., Niec, R. E., Kim, H. Y., Treuting, P., Chinen, T., Zheng, Y., et al. (2012). Extrathymically generated regulatory T cells control mucosal TH2 inflammation. Nature 482, 395–399. doi: 10.1038/nature10772
Jovel, J., Patterson, J., Wang, W. W., Hotte, N., O'Keefe, S., Mitchel, T., et al. (2016). Characterization of the gut microbiome using 16S or shotgun metagenomics. Front. Microbiol. 7:459. doi: 10.3389/fmicb.2016.00459
Kang, M., Mischel, R. A., Bhave, S., Komla, E., Cho, A., Huang, C., et al. (2017). The effect of gut microbiome on tolerance to morphine mediated antinociception in mice. Sci. Rep. 7:42658. doi: 10.1038/srep42658
Kelly, J. R., Borre, Y., O' Brien, C., Patterson, E., el Aidy, S., Deane, J., et al. (2016). Transferring the blues: Depression-associated gut microbiota induces neurobehavioural changes in the rat. J. Psychiatr. Res. 82, 109–118. doi: 10.1016/j.jpsychires.2016.07.019
Kim, M. G., Jo, K., Cho, K., Park, S. S., Suh, H. J., and Hong, K. B. (2021). Prebiotics/probiotics mixture induced changes in cecal microbiome and intestinal morphology alleviated the loperamide-induced constipation in rat. Food Sci. Anim. Resour. 41, 527–541. doi: 10.5851/kosfa.2021.e17
Kiraly, D. D., Walker, D. M., Calipari, E. S., Labonte, B., Issler, O., Pena, C. J., et al. (2016). Alterations of the host microbiome affect behavioral responses to cocaine. Sci. Rep. 6:35455. doi: 10.1038/srep35455
Kliewer, A., Schmiedel, F., Sianati, S., Bailey, A., Bateman, J. T., Levitt, E. S., et al. (2019). Phosphorylation-deficient G-protein-biased μ-opioid receptors improve analgesia and diminish tolerance but worsen opioid side effects. Nat. Commun. 10:367. doi: 10.1038/s41467-018-08162-1
Leduc-Pessah, H., Weilinger, N., Fan, C. Y., Burma, N. E., Thompson, R. J., and Trang, T. (2017). Site-specific regulation of P2X7 receptor function in microglia gates morphine analgesic tolerance. J. Neurosci. 37, 10154–10172. doi: 10.1523/JNEUROSCI.0852-17.2017
Lee, K., Vuong, H. E., Nusbaum, D. J., Hsiao, E. Y., Evans, C. J., and Taylor, A. M. W. (2018). The gut microbiota mediates reward and sensory responses associated with regimen-selective morphine dependence. Neuropsychopharmacology 43, 2606–2614. doi: 10.1038/s41386-018-0211-9
Li, L., Chen, J., and Li, Y. Q. (2023). The downregulation of opioid receptors and neuropathic pain. Int. J. Mol. Sci. 24:5981. doi: 10.3390/ijms24065981
Lian, N. Q., Shen, M. X., Zhang, K., Pan, J., Jiang, Y., Yu, Y., et al. (2021). Drinking hydrogen-rich water alleviates chemotherapy-induced neuropathic pain through the regulation of gut microbiota. J. Pain Res. 14, 681–691. doi: 10.2147/JPR.S288289
Lin, L., Lin, J., Qiu, J., Wei, F., Bai, X., Ma, W., et al. (2022). Gut microbiota alterations may increase the risk of prescription opioid use, but not vice versa: A two-sample bi-directional Mendelian randomization study. Front. Microbiol. 13:994170. doi: 10.3389/fmicb.2022.994170
Liu, E. Y., Tamblyn, R., Filion, K. B., and Buckeridge, D. L. (2021). Concurrent prescriptions for opioids and benzodiazepines and risk of opioid overdose: Protocol for a retrospective cohort study using linked administrative data. BMJ Open 11:e042299. doi: 10.1136/bmjopen-2020-042299
Luczynski, P., Tramullas, M., Viola, M., Shanahan, F., Clarke, G., O'Mahony, S., et al. (2017). Microbiota regulates visceral pain in the mouse. eLife 6:e25887. doi: 10.7554/eLife.25887
Ma, P., Mo, R., Liao, H., Qiu, C., Wu, G., Yang, C., et al. (2022). Gut microbiota depletion by antibiotics ameliorates somatic neuropathic pain induced by nerve injury, chemotherapy, and diabetes in mice. J. Neuroinflammation 19:169. doi: 10.1186/s12974-022-02523-w
Ma, W., Zheng, W. H., Powell, K., Jhamandas, K., and Quirion, R. (2001). Chronic morphine exposure increases the phosphorylation of MAP kinases and the transcription factor CREB in dorsal root ganglion neurons: an in vitro and in vivo study. Eur. J. Neurosci. 14, 1091–1104. doi: 10.1046/j.0953-816x.2001.01731.x
Mao, Y. K., Kasper, D. L., Wang, B. X., Forsythe, P., Bienenstock, J., and Kunze, W. A. (2013). Bacteroides fragilis polysaccharide A is necessary and sufficient for acute activation of intestinal sensory neurons. Nat. Commun. 4:1465. doi: 10.1038/ncomms2478
Martínez-Navarro, M., Maldonado, R., and Baños, J. E. (2019). Why mu-opioid agonists have less analgesic efficacy in neuropathic pain? Eur. J. Pain 23, 435–454. doi: 10.1002/ejp.1328
Meng, J. J., Abu, Y. F., Zhang, Y., Zhou, Y., Xie, Y., Yan, Y., et al. (2023). Opioid-induced microbial dysbiosis disrupts irinotecan (CPT-11) metabolism and increases gastrointestinal toxicity in a murine model. Br. J. Pharmacol. 180, 1362–1378. doi: 10.1111/bph.16020
Meng, J. J., Banerjee, S., Li, D., Sindberg, G. M., Wang, F., Ma, J., et al. (2015). Opioid exacerbation of Gram-positive sepsis, induced by gut microbial modulation, is rescued by IL-17A neutralization. Sci. Rep. 5:10918. doi: 10.1038/srep10918
Meng, J., Yu, H., Ma, J., Wang, J., Banerjee, S., Charboneau, R., et al. (2013). Morphine induces bacterial translocation in mice by compromising intestinal barrier function in a TLR-dependent manner. PLoS One 8:e54040. doi: 10.1371/journal.pone.0054040
Messaoudi, M., Lalonde, R., Violle, N., Javelot, H., Desor, D., Nejdi, A., et al. (2011). Assessment of psychotropic-like properties of a probiotic formulation (Lactobacillus helveticus R0052 and Bifidobacterium longum R0175) in rats and human subjects. Br. J. Nutr. 105, 755–764. doi: 10.1017/S0007114510004319
Meyer, M., Gygli, F., Westenberg, J. N., Schmid, O., Strasser, J., Lang, U. E., et al. (2023). Benzodiazepine use, quality of life and psychiatric symptom burden in oral and injectable opioid agonist treatment: a cross-sectional study. Addict. Sci. Clin. Pract. 18:43. doi: 10.1186/s13722-023-00397-8
Minerbi, A., Gonzalez, E., Brereton, N. J. B., Anjarkouchian, A., Dewar, K., Fitzcharles, M. A., et al. (2019). Altered microbiome composition in individuals with fibromyalgia. Pain 160, 2589–2602. doi: 10.1097/j.pain.0000000000001640
Minerbi, A., and Shen, S. Q. (2022). Gut microbiome in anesthesiology and pain medicine. Anesthesiology 137, 93–108. doi: 10.1097/ALN.0000000000004204
Mischel, R. A., Dewey, W. L., and Akbarali, H. I. (2018). Tolerance to morphine-induced inhibition of TTX-R sodium channels in dorsal root ganglia neurons is modulated by gut-derived mediators. iScience 2, 193–209. doi: 10.1016/j.isci.2018.03.003
Morreale, C., Bresesti, I., Bosi, A., Baj, A., Giaroni, C., Agosti, M., et al. (2022). Microbiota and pain: Save your gut feeling. Cells 11:971. doi: 10.3390/cells1106097
Nguyen, V. T. T., and Endres, K. (2022). Targeting gut microbiota to alleviate neuroinflammation in Alzheimer’s disease. Adv. Drug Deliv. Rev. 188:114418. doi: 10.1016/j.addr.2022.114418
Obata, Y., and Pachnis, V. (2016). The effect of microbiota and the immune system on the development and organization of the enteric nervous system. Gastroenterology 151, 836–844. doi: 10.1053/j.gastro.2016.07.044
Pane, K., Boccella, S., Guida, F., Franzese, M., Maione, S., and Salvatore, M. (2022). Role of gut microbiota in neuropathy and neuropathic pain states: A systematic preclinical review. Neurobiol. Dis. 170:105773. doi: 10.1016/j.nbd.2022.105773
Parker, B. J., Wearsch, P. A., Veloo, A. C. M., and Rodriguez-Palacios, A. (2020). The genus Alistipes: Gut bacteria with emerging implications to inflammation, cancer, and mental health. Front. Immunol. 11:906. doi: 10.3389/fimmu.2020.00906
Porreca, F., Tang, Q. B., Bian, D., Riedl, M., Robert, E., and Lai, J. (1998). Spinal opioid mu receptor expression in lumbar spinal cord of rats following nerve injury. Brain Res. 795, 197–203. doi: 10.1016/s0006-8993(98)00292-3
Raghavendra, V., Tanga, F. Y., and DeLeo, J. A. (2004). Attenuation of morphine tolerance, withdrawal-induced hyperalgesia, and associated spinal inflammatory immune responses by propentofylline in rats. Neuropsychopharmacology 29, 327–334. doi: 10.1038/sj.npp.1300315
Ramakrishna, C., Corleto, J., Ruegger, P. M., Logan, G. D., Peacock, B. B., Mendonca, S., et al. (2019). Dominant role of the gut microbiota in chemotherapy induced neuropathic pain. Sci. Rep. 9:20324. doi: 10.1038/s41598-019-56832-x
Reichenberger, E. R., Alexander, G. M., Perreault, M. J., Russell, J. A., Schwartzman, R. J., Hershberg, U., et al. (2013). Establishing a relationship between bacteria in the human gut and complex regional pain syndrome. Brain Behav. Immun. 29, 62–69. doi: 10.1016/j.bbi.2012.12.005
Ren, M., and Lotfipour, S. (2023). Antibiotic knockdown of gut bacteria sex-dependently enhances intravenous fentanyl self-administration in adult sprague dawley rats. Int. J. Mol. Sci. 24:409. doi: 10.3390/ijms24010409
Rivat, C. (2016). Neuropathic pain: When nerve lesion turns off peripheral analgesia. Anesthesiology 125, 627–629. doi: 10.1097/ALN.0000000000001262
Rizzatti, G., Lopetuso, L. R., Gibiino, G., Binda, C., and Gasbarrini, A. (2017). Proteobacteria: A common factor in human diseases. Biomed. Res. Int. 2017:9351507. doi: 10.1155/2017/9351507
Roy, S., Ninkovic, J., Banerjee, S., Charboneau, R. G., Das, S., Dutta, R., et al. (2011). Opioid drug abuse and modulation of immune function: Consequences in the susceptibility to opportunistic infections. J. Neuroimmune Pharmacol. 6, 442–465. doi: 10.1007/s11481-011-9292-5
Ruan, J. W., Liao, Y. C., Chen, P. C., Chen, Y. J., Tsai, Y. H., Tsai, P. J., et al. (2023). The composition of the maternal breastmilk microbiota influences the microbiota network structure during early infancy. J. Microbiol. Immunol. Infect. 56, 1084–1097. doi: 10.1016/j.jmii.2023.07.005
Russo, R., Cristiano, C., Avagliano, C., De Caro, C., La Rana, G., Raso, G. M., et al. (2018). Gut-brain axis: Role of lipids in the regulation of inflammation, pain and CNS diseases. Curr. Med. Chem. 25, 3930–3952. doi: 10.2174/0929867324666170216113756
Santa-Cecília, F. V., Ferreira, D. W., Guimaraes, R. M., Cecilio, N. T., Fonseca, M. M., Lopes, A. H., et al. (2019). The NOD2 signaling in peripheral macrophages contributes to neuropathic pain development. Pain 160, 102–116. doi: 10.1097/j.pain.0000000000001383
Scherrer, J. F., Salas, J., Copeland, L. A., Stock, E. M., Ahmedani, B. K., Sullivan, M. D., et al. (2016). Prescription opioid duration, dose, and increased risk of depression in 3 large patient populations. Ann. Fam. Med. 14, 54–62. doi: 10.1370/afm.1885
Schmidt, T. S. B., Raes, J., and Bork, P. (2018). The human gut microbiome: From association to modulation. Cell 172, 1198–1215. doi: 10.1016/j.cell.2018.02.044
Sharma, U., Olson, R. K., Erhart, F. N., Zhang, L., Meng, J., Segura, B., et al. (2020). Prescription opioids induce gut dysbiosis and exacerbate colitis in a murine model of inflammatory bowel disease. J. Crohns Colitis 14, 801–817. doi: 10.1093/ecco-jcc/jjz188
Shen, S., Lim, G., You, Z., Ding, W., Huang, P., Ran, C., et al. (2017). Gut microbiota is critical for the induction of chemotherapy-induced pain. Nat. Neurosci. 20, 1213–1216. doi: 10.1038/nn.4606
Simpson, S., Kimbrough, A., Boomhower, B., McLellan, R., Hughes, M., Shankar, K., et al. (2020). Depletion of the microbiome alters the recruitment of neuronal ensembles of oxycodone intoxication and withdrawal. eNeuro 7, 7:ENEURO.0312-19.2020. doi: 10.1523/ENEURO.0312-19.2020
Smith, P. M., Howitt, M. R., Panikov, N., Michaud, M., Gallini, C. A., Bohlooly-Y, M., et al. (2013). The microbial metabolites, short-chain fatty acids, regulate colonic treg cell homeostasis. Science 341, 569–573. doi: 10.1126/science.1241165
Strandwitz, P., Kim, K. H., Terekhova, D., Liu, J. K., Sharma, A., Levering, J., et al. (2019). GABA-modulating bacteria of the human gut microbiota. Nat. Microbiol. 4, 396–403. doi: 10.1038/s41564-018-0307-3
Sudo, N., Chida, Y., Aiba, Y., Sonoda, J., Oyama, N., Yu, X. N., et al. (2004). Postnatal microbial colonization programs the hypothalamic–pituitary–adrenal system for stress response in mice. J. Physiol. 558, 263–275. doi: 10.1113/jphysiol.2004.063388
Talbot, J., Hahn, P., Kroehling, L., Nguyen, H., Li, D., and Littman, D. R. (2020). Feeding-dependent VIP neuron-ILC3 circuit regulates the intestinal barrier. Nature 579, 575–580. doi: 10.1038/s41586-020-2039-9
Taylor, A. M. W., Castonguay, A., Ghogha, A., Vayssiere, P., Pradhan, A. A., Xue, L., et al. (2016). Neuroimmune regulation of GABAergic neurons within the ventral tegmental area during withdrawal from chronic morphine. Neuropsychopharmacology 41, 949–959. doi: 10.1038/npp.2015.221
Thomas, K. R., Watt, J., Wu, C. M. J., Akinrinoye, A., Amjad, S., Colvin, L., et al. (2022). Pain and opioid-induced gut microbial dysbiosis. Biomedicines 10:1815. doi: 10.3390/biomedicines10081815
Thomaz, A. C., Iyer, V., Woodward, T. J., and Hohmann, A. G. (2021). Fecal microbiota transplantation and antibiotic treatment attenuate naloxone-precipitated opioid withdrawal in morphine-dependent mice. Exp. Neurol. 343:113787. doi: 10.1016/j.expneurol.2021.113787
Touw, K., Ringus, D. L., Hubert, N., Wang, Y., Leone, V. A., Nadimpalli, A., et al. (2017). Mutual reinforcement of pathophysiological host-microbe interactions in intestinal stasis models. Physiol. Rep. 5:e13182. doi: 10.14814/phy2.13182
Trang, T., Al-Hasani, R., Salvemini, D., Salter, M. W., Gutstein, H., and Cahill, C. M. (2015). Pain and poppies: The good, the bad, and the ugly of opioid analgesics. J. Neurosci. 35, 13879–13888. doi: 10.1523/JNEUROSCI.2711-15.2015
Van Laar, T., Boertien, J. M., and Herranz, A. H. (2019). Faecal transplantation, pro- and prebiotics in Parkinson’s disease; hope or hype? J. Parkinsons Dis. 9, S371–S379. doi: 10.3233/JPD-191802
Vázquez-Baeza, Y., Callewaert, C., Debelius, J., Hyde, E., Marotz, C., Morton, J. T., et al. (2018). Impacts of the human gut microbiome on therapeutics. Annu. Rev. Pharmacol. Toxicol. 58, 253–270. doi: 10.1146/annurev-pharmtox-042017-031849
Wang, X., Loram, L. C., Ramos, K., de Jesus, A. J., Thomas, J., Cheng, K., et al. (2012). Morphine activates neuroinflammation in a manner parallel to endotoxin. Proc. Natl. Acad. Sci. USA 109, 6325–6330. doi: 10.1073/pnas.1200130109
Wang, F. Y., Meng, J. J., Zhang, L., Johnson, T., Chen, C., and Roy, S. (2018). Morphine induces changes in the gut microbiome and metabolome in a morphine dependence model. Sci. Rep. 8:3596. doi: 10.1038/s41598-018-21915-8
Wang, F. Y., and Roy, S. (2016). Gut homeostasis, microbial dysbiosis, and opioids. Toxicol. Pathol. 45, 150–156. doi: 10.1177/0192623316679898
Wang, B., Wang, L. N., Wu, B., Guo, R., Zhang, L., Zhang, J. T., et al. (2024). Astrocyte PERK and IRE1 Signaling Contributes to Morphine Tolerance and Hyperalgesia through Upregulation of Lipocalin-2 and NLRP3 Inflammasome in the Rodent Spinal Cord. Anesthesiology 140, 558–577. doi: 10.1097/ALN.0000000000004858
Wang, Z. C., Wu, H. Q., Chen, Y., Chen, H. J., Wang, X. W., and Yuan, W. (2021). Lactobacillus paracasei S16 alleviates lumbar disc herniation by modulating inflammation response and gut microbiota. Front. Nutr. 8:701644. doi: 10.3389/fnut.2021.701644
Wang, B. G., Xu, H. B., Wei, H., Zeng, Z. L., and Xu, F. (2014). Oral administration of Bifidobacterim bifidum for modulating microflora, acid and bile resistance, and physiological indices in mice. Can. J. Microbiol. 61, 155–163. doi: 10.1139/cjm-2014-0694
Williams, B. B., Van Benschoten, A. H., Cimermancic, P., Donia, M. S., Zimmermann, M., Taketani, M., et al. (2014). Discovery and characterization of gut microbiota decarboxylases that can produce the neurotransmitter tryptamine. Cell Host Microbe 16, 495–503. doi: 10.1016/j.chom.2014.09.001
Xu, R., Miao, L., Yang, C., and Zhu, B. (2022). Gut microbiota plays a pivotal role in opioid-induced adverse effects in gastrointestinal system. Crit. Care 26:5. doi: 10.1186/s13054-021-03867-0
Yang, C., Fang, X., Zhan, G. F., Huang, N., Li, S., Bi, J., et al. (2019). Key role of gut microbiota in anhedonia-like phenotype in rodents with neuropathic pain. Transl. Psychiatry 9:57. doi: 10.1038/s41398-019-0379-8
Yang, Y., Yang, L., Wan, M., Pan, D., Sun, G., and Yang, C. (2024). Assessment of optimal combinations of therapeutic probiotics for depression, anxiety, and stress. Psychol. Med. 1–15, 1–15. doi: 10.1017/S0033291724000679
Yano, J. M., Yu, K., Donaldson, G. P., Shastri, G. G., Ann, P., Ma, L., et al. (2015). Indigenous bacteria from the gut microbiota regulate host serotonin biosynthesis. Cell 161, 264–276. doi: 10.1016/j.cell.2015.02.047
Zádori, Z. S., Király, K., Al-Khrasani, M., and Gyires, K. (2023). Interactions between NSAIDs, opioids and the gut microbiota - Future perspectives in the management of inflammation and pain. Pharmacol. Ther. 241:108327. doi: 10.1016/j.pharmthera.2022.108327
Zhang, J. B., Deji, C., Fan, J. N., Chang, L., Miao, X., Xiao, Y., et al. (2021). Differential alteration in gut microbiome profiles during acquisition, extinction and reinstatement of morphine-induced CPP. Prog. Neuro-Psychopharmacol. Biol. Psychiatry 104:110058. doi: 10.1016/j.pnpbp.2020.110058
Zhang, J., Liang, L. L., Miao, X. R., Wu, S., Cao, J., Tao, B., et al. (2016). Contribution of the suppressor of variegation 3-9 homolog 1 in dorsal root ganglia and spinal cord dorsal horn to nerve injury–induced nociceptive hypersensitivity. Anesthesiology 125, 765–778. doi: 10.1097/ALN.0000000000001261
Zhang, L., Meng, J. J., Ban, Y. G., Jalodia, R., Chupikova, I., Fernandez, I., et al. (2019a). Morphine tolerance is attenuated in germfree mice and reversed by probiotics, implicating the role of gut microbiome. Proc. Natl. Acad. Sci. USA 116, 13523–13532. doi: 10.1073/pnas.1901182116
Zhang, L., Wang, R. Y., Bai, T., Xiang, X., Qian, W., Song, J., et al. (2019b). EphrinB2/ephB2-mediated myenteric synaptic plasticity: Mechanisms underlying the persistent muscle hypercontractility and pain in postinfectious IBS. FASEB J. 33, 13644–13659. doi: 10.1096/fj.201901192R
Zhou, F., Wang, X., Han, B. Y., Tang, X., Liu, R., Ji, Q., et al. (2021). Short-chain fatty acids contribute to neuropathic pain via regulating microglia activation and polarization. Mol. Pain 17:1744806921996520. doi: 10.1177/1744806921996520
Keywords: gut microbiota, gut-brain axis, neuropathic pain, opioids, pain
Citation: Gong Z, Xue Q, Luo Y, Yu B, Hua B and Liu Z (2024) The interplay between the microbiota and opioid in the treatment of neuropathic pain. Front. Microbiol. 15:1390046. doi: 10.3389/fmicb.2024.1390046
Edited by:
Nazarii Kobyliak, Bogomolets National Medical University, UkraineReviewed by:
Mahmoud Al-Khrasani, Semmelweis University, HungaryPingchuan Ma, Washington University in St. Louis, United States
Copyright © 2024 Gong, Xue, Luo, Yu, Hua and Liu. This is an open-access article distributed under the terms of the Creative Commons Attribution License (CC BY). The use, distribution or reproduction in other forums is permitted, provided the original author(s) and the copyright owner(s) are credited and that the original publication in this journal is cited, in accordance with accepted academic practice. No use, distribution or reproduction is permitted which does not comply with these terms.
*Correspondence: Bo Hua, eXNiNzVoYkAxNjMuY29t; Zhiheng Liu, emhpaGVuZ19saXVfdG9uZ2ppQDE2My5jb20=