- Microbiology and Molecular Biology Team, Center of Plant and Microbial Biotechnology, Biodiversity and Environment, Faculty of Sciences, Mohammed V University in Rabat, Rabat, Morocco
Legumes are renowned for their distinctive biological characteristic of forming symbiotic associations with soil bacteria, mostly belonging to the Rhizobiaceae familiy, leading to the establishment of symbiotic root nodules. Within these nodules, rhizobia play a pivotal role in converting atmospheric nitrogen into a plant-assimilable form. However, it has been discerned that root nodules of legumes are not exclusively inhabited by rhizobia; non-rhizobial endophytic bacteria also reside within them, yet their functions remain incompletely elucidated. This comprehensive review synthesizes available data, revealing that Bacillus and Pseudomonas are the most prevalent genera of nodule endophytic bacteria, succeeded by Paenibacillus, Enterobacter, Pantoea, Agrobacterium, and Microbacterium. To date, the bibliographic data available show that Glycine max followed by Vigna radiata, Phaseolus vulgaris and Lens culinaris are the main hosts for nodule endophytic bacteria. Clustering analysis consistently supports the prevalence of Bacillus and Pseudomonas as the most abundant nodule endophytic bacteria, alongside Paenibacillus, Agrobacterium, and Enterobacter. Although non-rhizobial populations within nodules do not induce nodule formation, their presence is associated with various plant growth-promoting properties (PGPs). These properties are known to mediate important mechanisms such as phytostimulation, biofertilization, biocontrol, and stress tolerance, emphasizing the multifaceted roles of nodule endophytes. Importantly, interactions between non-rhizobia and rhizobia within nodules may exert influence on their leguminous host plants. This is particularly shown by co-inoculation of legumes with both types of bacteria, in which synergistic effects on plant growth, yield, and nodulation are often measured. Moreover these effects are pronounced under both stress and non-stress conditions, surpassing the impact of single inoculations with rhizobia alone.
1 Introduction
By 2050, the global population is anticipated to surpass 9 billion, experiencing significant growth from the current 7 billion (Kumar and Dubey, 2020). This escalating population, coupled with climate change-induced abiotic and biotic pressures and land scarcity, is adversely affecting worldwide food production. In response, the primary objective of crop nutrient management is to enhance productivity, addressing the escalating demand for food associated with population growth (Yeboah et al., 2021). However, the intensified use of chemical fertilizers and pesticides, driven by population-mediated agricultural pressure, has deleterious environmental consequences (Adhikary et al., 2022). These include disruptions in soil physicochemical properties, texture, porosity, water-holding capacity, soil acidification, water eutrophication, and disturbance of beneficial soil microbial flora (Baweja et al., 2020). Additionally, chemical inputs have direct implications for human health, leading to issues such as cancers and nutritional deficiencies (Schindler and Hecky, 2009). Remarkably, despite the application of fertilizers, a substantial proportion, exceeding 50%, is lost to the environment, with organo-mineral fertilizers having the highest environmental impact, with water consumption, fossil resource use, and global warming potential (Litskas, 2023).
In response to these challenges, urgent measures are imperative to secure yields while prioritizing environmental sustainability. Among the most promising agro-ecological alternatives are biofertilization, biological pest control, and the cultivation of legumes. Beyond their traditional roles, legumes provide ecological services such as enhancing agro-system resilience and sustainability (Powers and Thavarajah, 2019). Notably, legumes exhibit a remarkable ability to establish a symbiotic association with soil bacteria, termed rhizobia (Legume Nodulating Bacteria or LNB), resulting in the formation of root nodules. Within these nodules, atmospheric nitrogen is converted into ammonia, a usable nitrogen form for the plant (Stagnari et al., 2017). This process offers significant agroecological benefits by reducing the need for synthetic nitrogen fertilizers and other inputs responsible for soil and environmental degradation. Furthermore, the legumes nitrogen-fixing symbiosis contributes to soil fertility, serving as a source of protein-rich foods. Legumes, therefore, play a vital role in addressing the global demand for protein, enhancing soil fertility, and thriving in challenging environmental conditions.
Given these considerations, a high number of studies have focused on legumes and their interaction with LNB to better understand their advantages and leverage the benefits of symbiosis (Rodríguez-Rodríguez et al., 2021). These studies explore a diverse range of legume species, revealing substantial diversity in the associated rhizobia. The effectiveness of nodulation relies on the efficiency and competitiveness of the rhizobia strains (Andrews and Andrews, 2017). However, under stressful environmental conditions, competition between rhizobia strains and the rhizospheric bacterial population can result in ineffective nodulation (Mwenda et al., 2023). In this context, Legumes, like other plants, interact with a diverse microbiome, including plant growth-promoting rhizobacteria (PGPR), to mitigate environmental stress impacts. PGPR play a crucial role in enhancing plant growth and health, offering benefits beyond stress mitigation.
PGPR in general, have been advocated as an effective solution to address the environmental repercussions of chemical intensive agriculture. PGPR can enhance plant growth through various mechanisms, including improved nutrient availability, growth stimulation, enhanced stress tolerance, and protection against phytopathogens. While most of these bacteria are typical rhizospheric inhabitants, some, termed endophytes, exhibit the capability to penetrate root epidermis, colonize root tissues, and migrate to various plant parts and organs (Strobel and Daisy, 2003). Furthermore, endophytic bacteria have been isolated from various plant parts, including roots, xylem vessels, leaves, flowers, and fruits. The term “endophytes” was first coined by De Bary (1866) to refer to microbes, such as bacteria, fungi, cyanobacteria, and actinomycetes, residing within plant tissues (Rana et al., 2020). These endophytes have garnered attention for their beneficial effects on host plants, including improved growth and health. Mechanisms involved in these effects encompass the production of phytohormones or siderophores (Złoch et al., 2016), enhanced metal absorption, inorganic phosphate solubilization, secretion of biologically active compounds, and induction of resistance to phytopathogens and parasites (Spence and Bais, 2015).
In legumes, endophytes have recently been isolated from root nodules induced by rhizobia, prompting inquiries into their identity, diversity, prevalence in different legume species, establishment mechanisms in nodules, and their role within the rhizobium-legume symbiosis. This knowledge gap emphasizes the need for further exploration to unravel the complexities surrounding these endophytes.
Nodule endophytes were termed opportunists, as they are thought to infect nodules when rhizobia induce nodule formation (Leite et al., 2017). Diverse nomenclature, such as bacteria associated with the nodule (Rajendran et al., 2012), non-symbiotic bacteria with disinfected nodules (Costa et al., 2013), nodule endophytes (Velázquez et al., 2013), non-rhizobial endophytes (De Meyer et al., 2015), non-rhizobial bacteria (Dhole et al., 2016), and more recently Non-Nodular Endophytic Bacteria (NNEB) (Ríos-Ruiz et al., 2019). Noteworthy genera of nodules’ non-rhizobial endophytic bacteria in legumes include Agrobacterium, Klebsiella, Paenibacillus, Bacillus, Blastobacter, Dyadobacter, Phyllobacterium, Pseudomonas, Ensifer, and Enterobacter (De Meyer et al., 2015), Aerobacter, Aeromonas, Chryseomonas, Curtobacterium, Erwinia, Flavimonas, and Sphingomonas in pea cultivars (Elvira-Recuenco and van Vuurde, 2000), Arthrobacter, Acinetobacter, Micromonospora, Mycobacterium, and Stenotrophomonas (Velázquez et al., 2013), Cupriavidus, Providencia, Staphylococcus, Kocuria, Micrococcus, Frondihabitans, Paracoccus and Roseomonas (Leite et al., 2017). Some of these non-rhizobial bacteria have been shown to benefit plants through various activities, such as synthesizing plant hormones, fixing atmospheric N2, solubilizing inorganic phosphate, and enhancing the catalytic activity of ACC deaminase, underscoring their potential for sustainable agriculture (Kour et al., 2020). Despite these documented benefits, there is a dearth of information regarding nodule endophytes across various ecological niches, including desert plants. This lack of comprehensive understanding extends to their diversity, activities valorization, and effects on plant tolerance and growth under stressful conditions, presenting a notable gap in current research.
This review addresses the exploration of legume-nodule-associated endophytic bacteria unveils their diversity, ecological roles, and benefits for plants under varied conditions. The review synthesizes data, identifying Bacillus and Pseudomonas as most prevalent endophytes, primarily hosted by Glycine max. Non-rhizobial populations within nodules exhibit plant growth-promoting properties, indicating their potential importance in plant growth and/or resilience, and their significance in sustainable agriculture. The interaction dynamics between rhizobial and non-rhizobial bacteria within nodules, influencing leguminous host plants, add complexity to the symbiotic relationship. Co-inoculation with non-rhizobial endophytes shows synergistic effects on plant growth, surpassing the impact of single rhizobia inoculations. The primary objective of this literature review was to identify patterns and specificities in the relationship between legume species and nodule endophyte bacteria species. Through this comprehensive approach, the study aimed to provide valuable insights into the intricate dynamics of the legume-nodule endophytic bacteria relationship and its contribution in sustainable agriculture, emphasizing the intricate interplay within the legume-nodule ecosystem.
2 Research methodology
A comprehensive literature search was undertaken to explore the legume-nodule-associated endophytic bacteria, unveils their diversity, ecological roles, and benefits for plants. Scientific papers published until the end of March 2024 in literature databases such as Scopus (150), Science-Direct (97) and Google-Scholars (162), Web of Science (174), and others (101) were included. The total number of papers utilized was 683 (Figure 1). To collect this data, we used the combination of several terms and different keywords: nodule endophytes, non-rhizobial endophytes, non-rhizobial bacteria with the term “Diversity,” “novel species,” “sp. nov.” and “Metagenomic”.
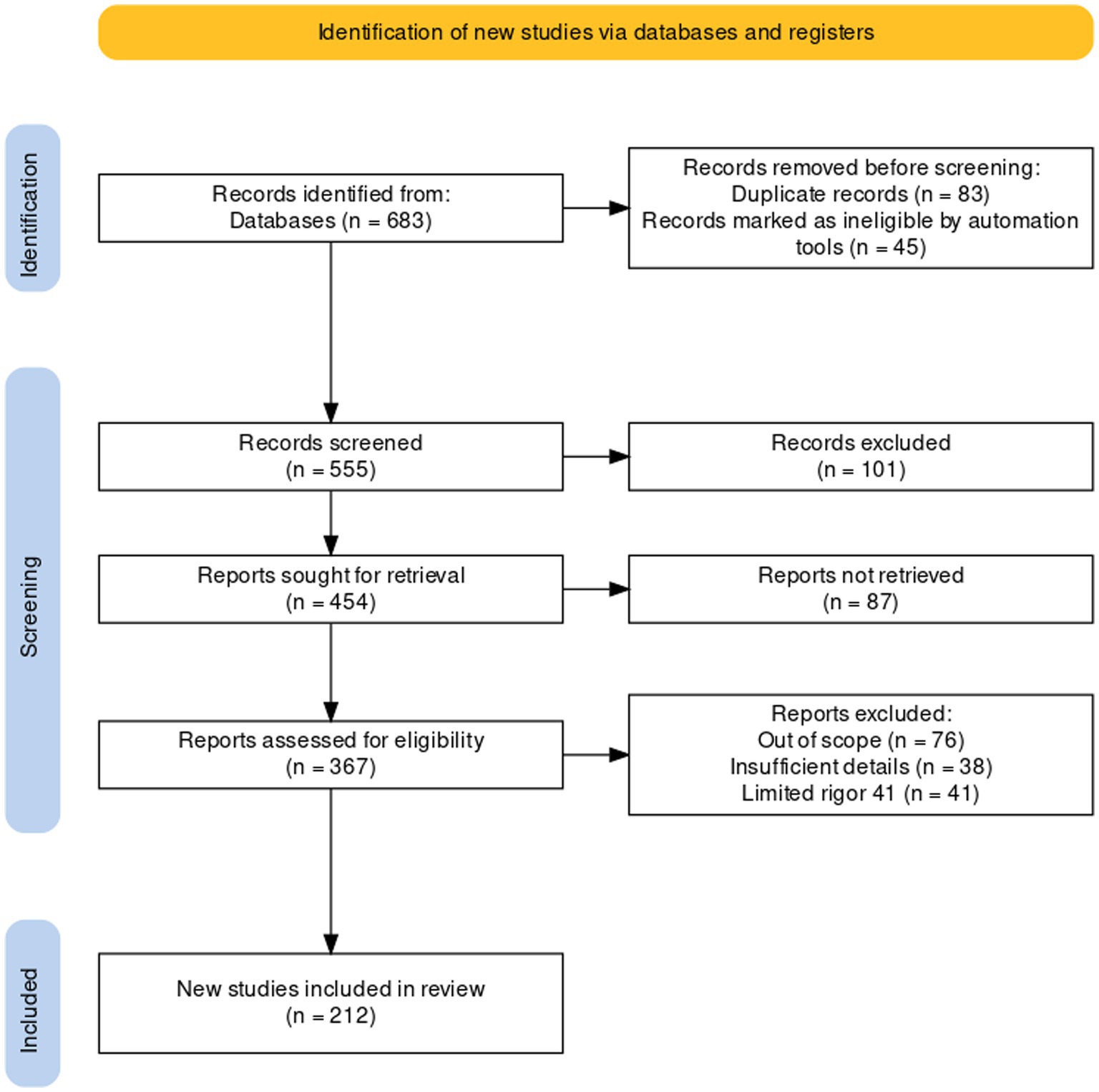
Figure 1. The PRISMA Flow diagram showing the flow of information in the procedure of studies included in this review (Liberati et al., 2009).
After conducting the search, we identified a total of 683 papers that met our inclusion criteria. To screen and select the most relevant papers, we used a PRISMA strategy (systematic and transparent process that involved reading the titles and abstracts of the papers, assessing them for eligibility, and excluding those that did not meet our inclusion criteria). After the screening process, we included 216 studies in this review. More information about the workflow of the strategy of PRISMA is presented as Supplementary material (Supplementary Table S1).
A vizualisation of the library (683 papers) was presented in Figure 2, which showcases terms with occurrences equal to or exceeding 20, provides a visual representation of the diverse and multidisciplinary nature of research in this topic. The prevalence of terms such as “proteobacteria” “Plant growth promoting,” “solubilization” and “iaa” for the red cluster, and “phylogenetic analysis,” “sinorhizobium,” “new species” for the green one. While for the yellow, there is “microsymbiont,” “bradyrhizobium,” and “inoculant.” In the blue cluster, there is a noteworthy prevalence of terms such as “nov,” “similarity,” and “closest species” reflecting the emphasis on understanding the role of non-rhizobial endophytes in the taxonomy and the description of new species.
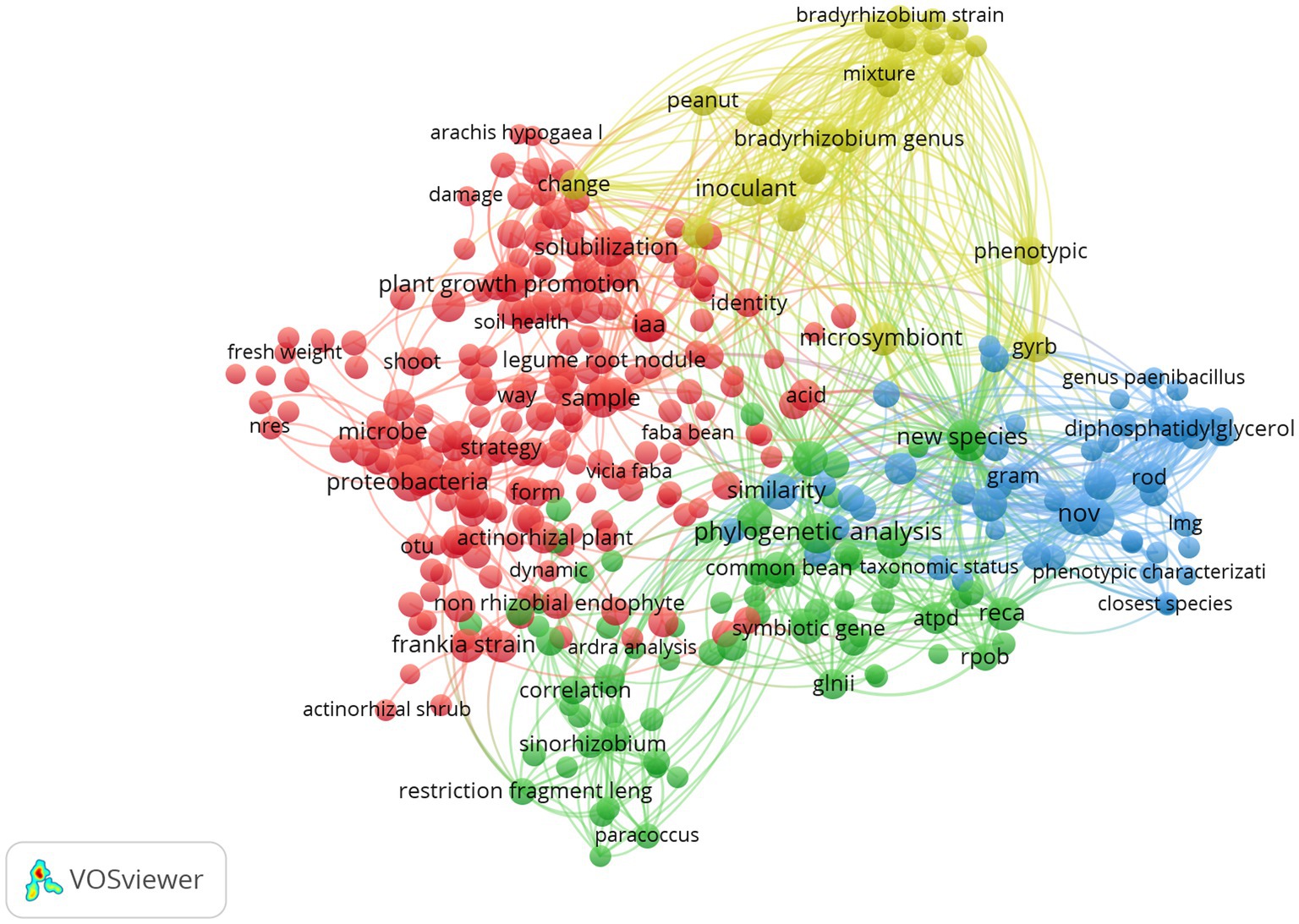
Figure 2. Term clustering map based on the first reference data of systematic review before screening (370 references). Different colors (red, green, blue and yellow) represent the terms belonging to different clusters. The size of the term is based on the number of occurrences. The connecting lines indicate the 100 strongest co-occurrence links between terms (Van Eck and Waltman, 2011).
3 Non-symbiotic endophytic bacteria in nodules
3.1 Discovery of nodule endophytes
For more than a century, rhizobia belonging to the α-proteobacteria clade were believed to be the exclusive bacteria capable of inducing nodules in legumes, leading to the exclusion of atypical colonies during the screening process on Yeast Mannitol Agar (YMA) medium (Moulin et al., 2001). These authors were the first to identify an atypical symbiotic bacterium belonging to the β-proteobacteria, mainly the genus Burkholderia. Later, Peix et al. (2015) identified two atypical bacteria, Methylobacterium and Burkholderia, capable of nodulating legumes, challenging the traditional view and opening new avenues of inquiry into “non-rhizobial bacteria inducing legume nodules.” Subsequent research uncovered a diverse array of bacteria in legume nodules outside the classic Rhizobiaceae family, raising questions about their nitrogen-fixing symbiotic nature (Table 1).
The discoveries disclosed that legume nodules in the field host not only symbiotic rhizobia but also non-symbiotic endophytes, complicating the immune control in symbiotic organs (Dudeja et al., 2012). Some studies indicated a prevalence of non-symbiotic endophytes over rhizobia in certain plant nodules, suggesting specificity in the nodular microbiome, in addition to the established specificity between rhizobia and their host plants. Moreover the plant’s immunity appears to play a role in recognizing these non-rhizobial endophytes (Berrabah et al., 2019).
Research by Youseif et al. (2021) and others reveals diverse bacterial endophytes in legume nodules. Genera like Bacillus, Pseudomonas, and Agrobacterium coexist with rhizobia. Other studies identified genera such as Arthrobacter and Acinetobacter as nodule endophytes (Velázquez et al., 2013). Legumes like cowpea host a variety of endophytes including Cupriavidus and Providencia (Leite et al., 2017). Indigenous legumes in Flanders also show a rich diversity of endophytes, including Agrobacterium and Klebsiella (De Meyer et al., 2015).
Other studies concerning root nodules of different legume species, have identified various genera such as Arthrobacter, Microbacterium, Rhodococcus, Sphingomonas, Bacillus, Cohnella, Pseudomonas, Herbaspirillum, Rahnella, Enterobacter, Agrobacterium, Burkholderia, Pantoea, Paenibacillus, Klebsiella, Endobacter, Microbacterium, Curtobacterium, Xenophilus, Erwinia, Leclercia, Gluconacetobacter, Variovorax, Staphylococcus, Methylobacterium, Paraburkholderia, Brevibacterium, Microvirga, Strepromyces, Micromonospora, and Hyphomicrobium (Sturz et al., 1997; Valverde, 2003; Mantelin et al., 2006; Zakhia et al., 2006; Zurdo-Piñeiro et al., 2007; Palaniappan et al., 2010; Aserse et al., 2013; Velázquez et al., 2013; Xu et al., 2014; Dhole et al., 2016; Zaheer et al., 2016; Martínez-Hidalgo and Hirsch, 2017; Xiao et al., 2017; Zhang et al., 2018; El Attar et al., 2019; Raja and Uthandi, 2019; Deng et al., 2020).
Recent studies have further highlighted the complexity of root nodules, emphasizing the presence of non rhizobial root nodule endophytes (NRE) with diverse roles within the host. These NREs enter nodules through infection threads containing rhizobia and colonize the inner regions of root nodules (Pandya et al., 2013; Zgadzaj et al., 2015). While these bacteria do not independently induce nodules, they enhance nodule formation when co-inoculated with suitable rhizobia, displaying various plant growth-promoting properties (Li et al., 2012).
It is interesting to highlight the case of some Agrobacterium strains, including those isolated from common bean root nodules and other legumes, that were reported as having the capacity to nodulate legume plants (De Lajudie et al., 1999; Verástegui-Valdés et al., 2014; El Attar et al., 2019). This nodulation ability may be attributed to the acquisition of symbiotic genes via lateral gene transfer, as suggested in various studies (Moulin et al., 2004; García-Fraile et al., 2010). Supporting this idea, some Agrobacterium strains were found to carry symbiosis-specific genes (e.g., nifH and nodA), similar to those present in well-known rhizobia legume symbionts (Cummings, 2009; Rincón-Rosales et al., 2009; Youseif et al., 2014). These reports highlight the rich diversity and dynamic interactions of the nodule endophytes, and their contribution to the intricate and multifaceted nature of the legume-nodule ecosystem.
3.2 Prevalence of endophytes in legume nodules
To provide an in-depth understanding of the main explored plant species, Figure 3 encapsulates a comprehensive overview of the legume species under investigation, highlighting the predominance of the species Glycine max which stands out with 11 associated studies. At the same level, Phaseolus vulgaris and Pisum sativum with 10 studies for each plant. Other frequently scrutinized genera encompass Medicago, Cicer, Vicia, Vigna; that were the subject of 5 to 8 studies. The genus Acacia is notably diverse, with studies spanning multiple species. In the other side, certain species, such as those belonging to Lotus and Astragalus are comparatively underexplored, each featuring in only one study. The dataset reflects a broad spectrum of leguminous plants, underscoring their significance in research. Another important aspect concerns the botanical diversity of the studied species that ranged from trees like Robinia pseudoacacia to shrubs like Spartocytisus supranubius, through food and fodder legume species. Some species, including Lupinus angustifolius and Erythrina brucei garner repeated attention, indicating sustained research interest. In essence, Figure 3 offers a nuanced portrayal of botanical studies, emphasizing both the diversity of plants investigated and the varying degrees of research focus across different genera and species.
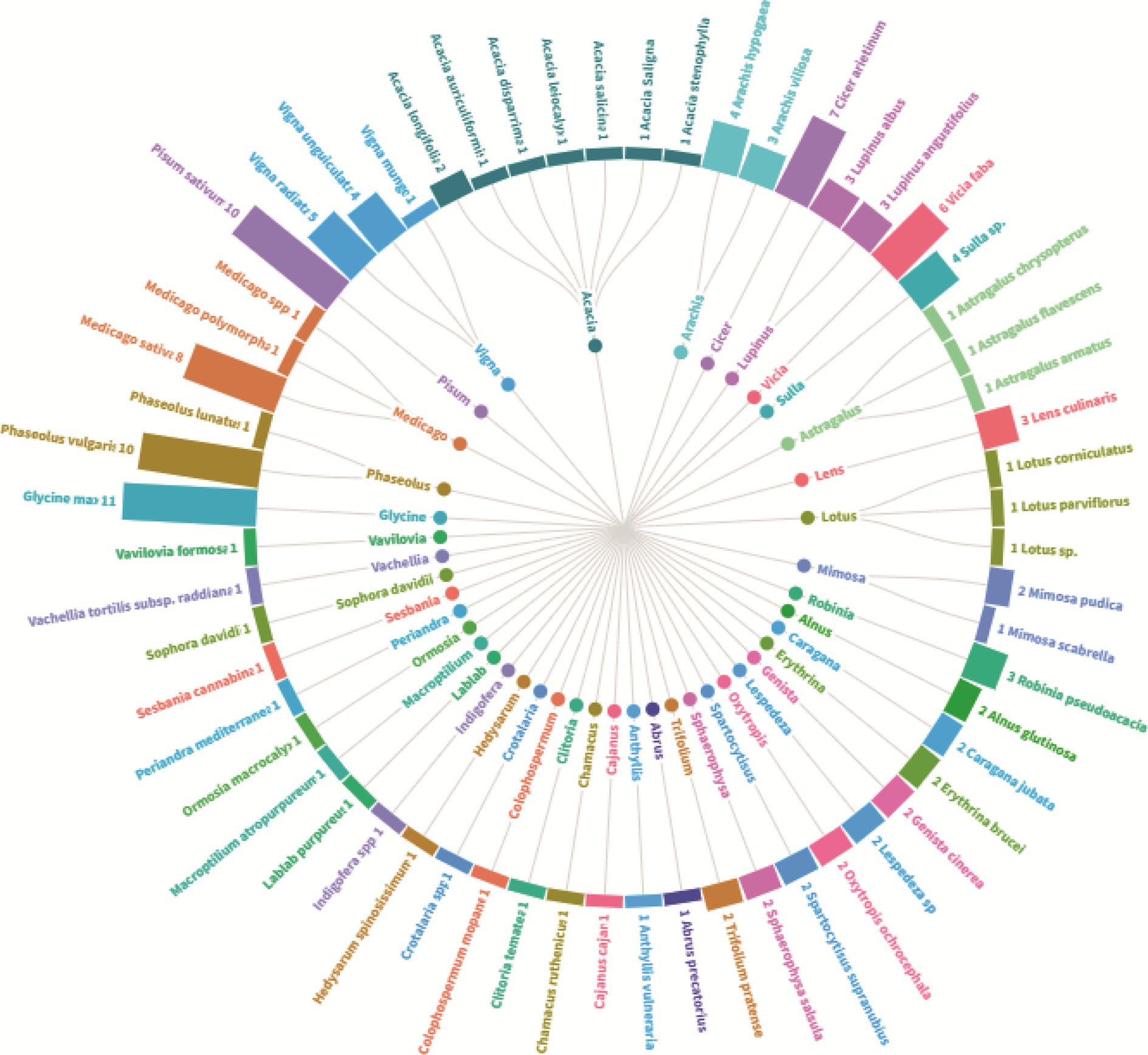
Figure 3. Plant diversity and research focus–an overview of the number of studies on the level of plant species for nodule endophyte bacteria.
The data analysis performed showed that the most abundant endophytic bacteria were Bacillus (65) and Pseudomonas (55) followed by Paenibacillus (32), Enterobacter (28), Pantoea (25), Agrobacterium and Microbacterium (Figures 4, 5). While for plants, Glycine max was the species that host the majority of nodule endophytic bacteria followed by Phaseolus vulgaris, Vigna unguiculata and Lens culinaris (Figure 4). Additionally, the clustering established in Figure 5, reveals a result that aligned with the previous one, Bacillus and Pseudomonas being the most abundant nodule endophytic bacteria in the whole collection followed by Paenibacillus, Agrobacterium and Enterobacter.
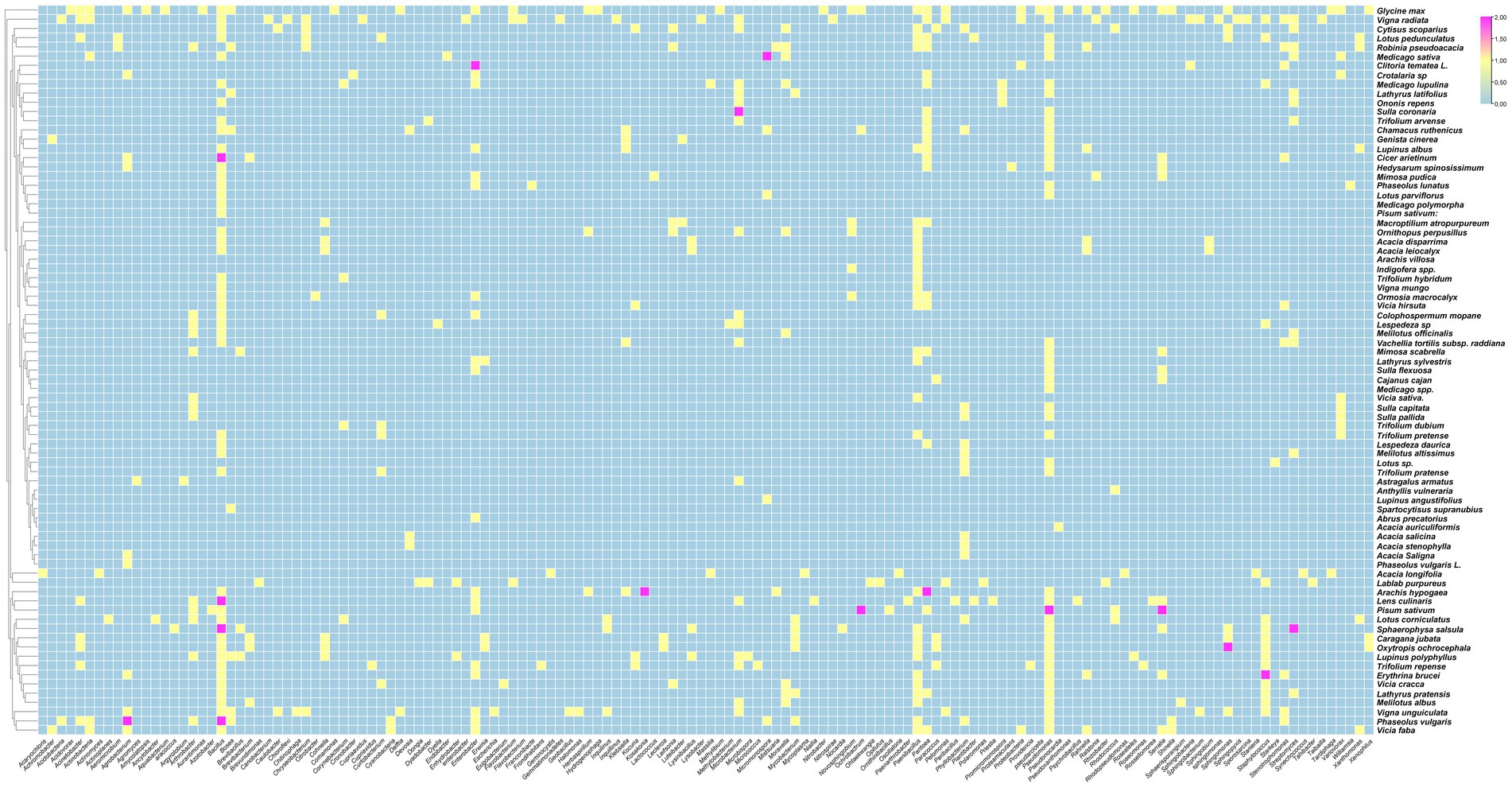
Figure 4. Heatmap representing the main Plant species in this study along with the associated nodule endophyte genera.
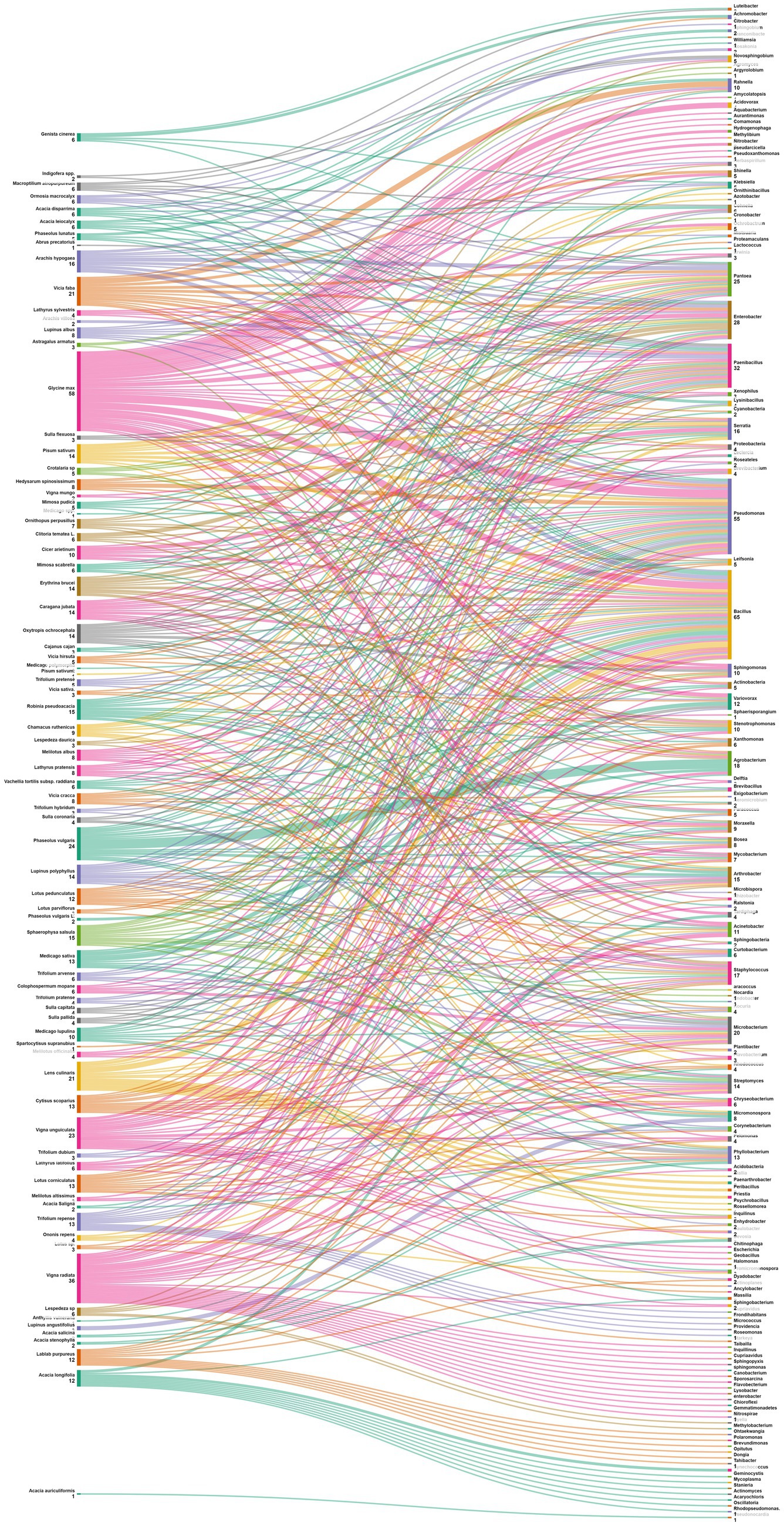
Figure 5. Sankey diagram representing the main Plant species in this study along with the associated nodule endophyte genera.
Legume nodules, that are intricate habitats for diverse bacterial communities, exhibit a prevalence of specific genera dominated by strains affiliated with Pseudomonas and Bacillus (Hnini et al., 2023). Similar results were reported earlier by Kumar et al. (2013), who isolated a substantial number of Gram-positive bacterial endophytes from legume nodules, spanning chickpea, field pea, and lucerne. Moreover Bacillus emerges as a the predominant genus in nodules of various legumes, including chickpea (Joseph et al., 2007), pigeon pea (Rajendran et al., 2008), Lespedeza sp. (Palaniappan et al., 2010), peanut (Wang et al., 2013), and soybean (Zhao et al., 2018). It is followed by Pseudomonas strains, that occupy the second position in nodules of peanut, soybean and bean (Aserse et al., 2013; Wang et al., 2013; Dinić et al., 2015; Zhao et al., 2018).
The elucidation of mechanisms governing nodule colonization by non-rhizobial endophytes (NREs) represents a critical frontier in understanding legume-microbe interactions (Martínez-Hidalgo and Hirsch, 2017). While immunofluorescence microscopy has confirmed the presence of non-rhizobia within nodules (Muresu et al., 2008), the intricacies of NRE entry into the symbiosome during nodule formation remain elusive. This ambiguity underscores the need for further investigation into the molecular signaling pathways facilitating NRE colonization. Ibáñez et al. (2009) and Zgadzaj et al. (2015) have observed the absence of nodC gene among cultured NRE isolates, suggesting alternative mechanisms for NRE entry that are different from those implicated in rhizobial symbiosis initiation. The absence of nod genes in NRE isolates implies a potential reliance on rhizobia-initiated infection threads or entry through root cracks (Giraud et al., 2007), necessitating comprehensive exploration of NRE nodule entry strategies.
Furthermore, recent research conducted by Brown et al. (2020) demonstrated that host genetic factors significantly influence the composition of nodule communities in the model legume Medicago truncatula. Through a common garden experiment and sequencing analyses, they revealed that while soil origin shapes rhizosphere communities, internal plant compartments—such as the root endosphere and nodules—are predominantly governed by host genetics. These insights highlight the intricate specificity of interactions between legumes and nodular endophytic bacteria, prompting exploration into the underlying mechanisms driving host selection of these bacteria across diverse environmental conditions. Additionally, the makeup of the nodular microbiota is not solely influenced by the choice of culture media but is also shaped by a range of environmental variables. Factors such as soil type, cultivation practices, and isolation techniques, including the use of wild nodules and trap plants, all play pivotal roles in determining the composition of nodular microbiota. Consequently, gaining a deeper understanding of the molecular and genetic factors that govern legume-nodular endophytic bacteria associations is crucial for comprehending their ecological importance and for devising targeted agricultural strategies.
In parallel, recent investigations by Tokgöz et al. (2020), have revealed the remarkable capacity of NREs to suppress plant pathogens, by discovering diverse bacteria within soybean nodules and identified several non-rhizobial isolates capable of inhibiting plant pathogens like Clavibacter michiganensis subsp. michiganensis (Cmm) and Pseudomonas syringae pv. tomato (Pst). By extratcing bacterial metabolites from selected isolates and found a Proteus species to be promising in suppressing pathogens. The findings of Tokgöz et al. (2020), not only highlight the potential of NREs in enhancing legume resilience under adverse environmental conditions but also underscore the intricate network of microbial interactions within legume nodules. This convergence of research underscores the multifaceted nature of legume-microbe associations, necessitating interdisciplinary approaches to unravel their complexities and harness their potential for sustainable agricultural practices.
Diversity of endophytic relationships: patterns of nodule endophyte associations with the subfamily of Papilionoidea
One of the objectives set for this analysis is to delve into the intricate relationships between legume plants and their associated nodule endophytes, shedding light on the diverse prevalence patterns across various plant genera. The data in Figure 6 suggest a diverse and specific prevalence pattern among plant genera and nodule endophyte genera. Numerous legume genera exhibit associations with specific endophytes, indicating a nuanced symbiotic relationship. For example, Phaseolus nodules showed a preference for Agrobacterium, Acidobacteria, Actinobacteria and Cyanobacteria, while those of Medicago contained mostly Actinobacteria, Bacillus, Micromonospora, Pseudomonas, and Endobacter. On the other hand, Glycine nodules displayed a varied pattern, composed by Nitrobacter, Tardiphaga, Bacillus, Pseudomonas, Flavobacterium, Variovorax, Enterobacter, Acinetobacter, Ochrobactrum, and Agrobacterium. Other legume genera, including Vicia, Pisum, Cicer, Arachis, Trifolium, Lupinus, Lens, Cajanus, Vigna, Hedysarum, Genista, Robinia, Clitoria, Chamacus, and Macroptilium, exhibited unique associations with diverse nodule endophyte genera.
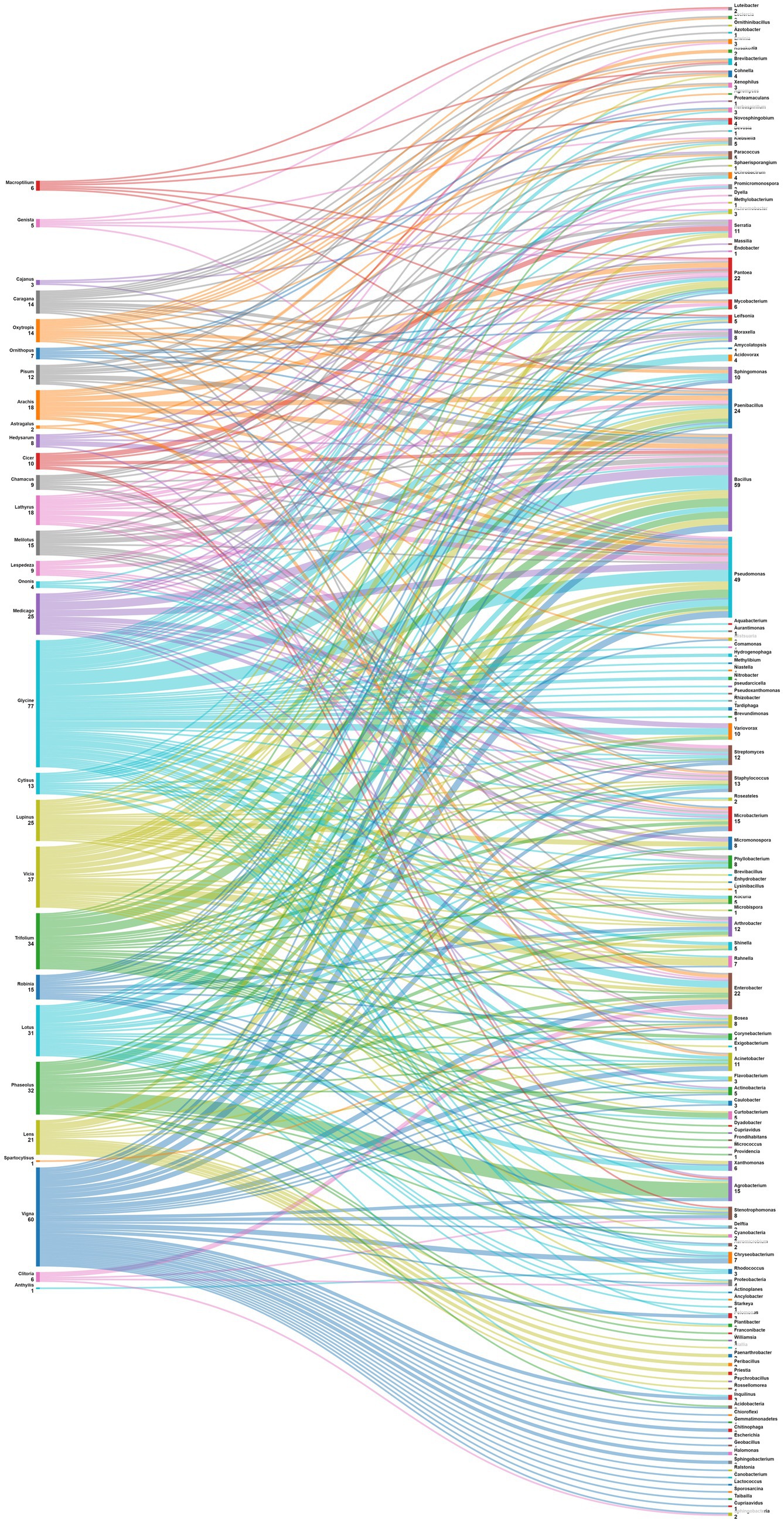
Figure 6. Sankey diagram representing the main plant genera (of the subfamily of Papilionoidea) in this study along with the associated nodule endophyte genera.
Data were also analyzed at the level of plant species (Figure 7). Phaseolus vulgaris, the most studied species is associated with Agrobacterium, Arthrobacter, Acidobacteria, Actinobacteria, Cyanobacteria, Phyllobacterium, Shinella, Acinetobacter, Bosea, Micromonospora, Mycobacterium, Paenibacillus and Stenotrophomonas. For its side, Medicago sativa nodules displayed diverse endophytes such as Actinobacteria, Bacillus, and Pseudomonas; while those of Glycine max exhibited a different pattern, including Nitrobacter, Tardiphaga, Bacillus, and Pseudomonas. Other leguminous species like Vicia faba, Pisum sativum and Cicer arietinum also demonstrated a variety of associated endophytes. This diversity of patterns underscores the complex nature of plant-endophyte interactions. Understanding these patterns can have implications for agricultural practices, potentially allowing for targeted inoculation with specific endophytes to enhance plant growth, nutrient uptake, and overall health in different legume crops.
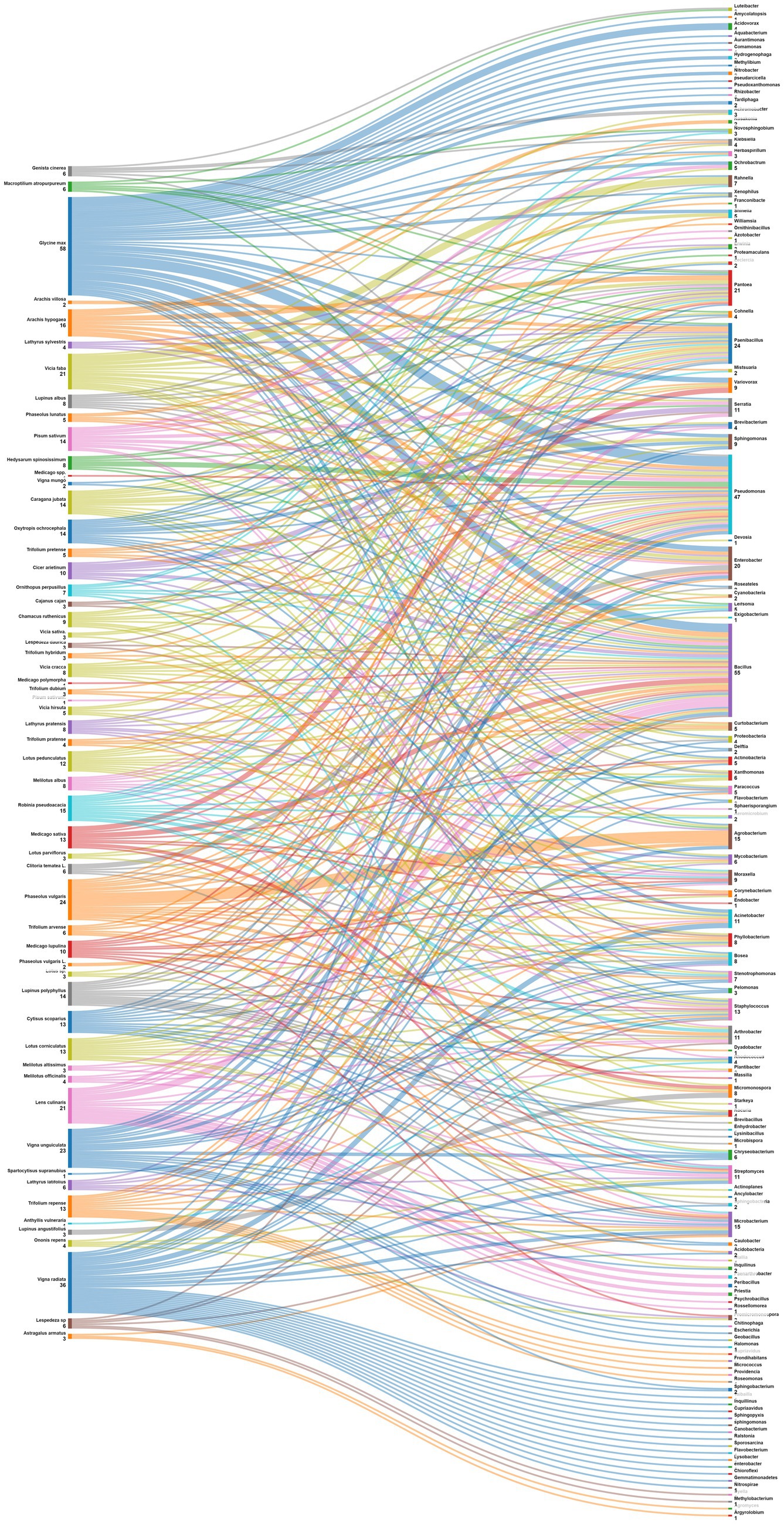
Figure 7. Sankey diagram representing the main plant species (of the subfamily of Papilionoidea) in this study along with the associated nodule endophyte genera.
Diversity of endophytic relationships: Patterns of nodule endophyte associations with the subfamilies of Mimosoideae, Faboideae, Hedysareae and Caesalpinioideae
In a similar manner to the previous findings, Figure 8 unravels distinctive patterns in the association between plant genera belonging to four legumes subfamilies and nodule endophyte genera. Notably, Crotalaria is linked to Enterobacter, Pantoea, Agrobacterium, Cronobacter, and Variovorax. The legume genus Indigofera, in contrast, exhibited a preference for PaeniBacillus and Novosphingobium while Erythrina demonstrated a diverse set of associations with Pseudomonas, Rahnella, Serratia, Agrobacterium, Staphylococcus, Bacillus, PaeniBacillus, Enterobacter, and Stenotrophomonas. The genus Colophospermum is associated with Enterobacter, Arthrobacter, Microbacterium, and Bacillus; while Sphaerophysa showed a preference for Bacillus and Streptomyces. Ormosia legume genus exhibited associations with Bacillus, Citrobacter, Enterobacter, Novosphingobium, Paenibacillus, and Pantoea while the genus Vavilovia is linked to Bosea, and the genus Abrus is associated with Enterobacter. The genus Periandra showed a preference for Paenibacillus, and Mimosa demonstrated a diversified pattern containing Pseudomonas, Pantoea, Paenibacillus, Brevibacillus, Serratia, Arthrobacter, Burkholderia, and Enterobacter. Similarly, Sulla exhibited diversified associations including Pseudomonas, Microbacterium, Pantoea, Phyllobacterium, Arthrobacter, Variovorax, and Phyllobacterium. Acacia demonstrated also diverse associations with the genera Pseudonocardia, Phyllobacterium, Devosia, Tardiphaga, Synechococcus, Paenibacillus, Bacillus, Ralstonia, Cohnella, Lysinibacillus, and Sphingobium. The closest genera Vachellia is associated with Bacillus, Pseudomonas, Microbacterium, Klebsiella, Stenotrophomonas, and Streptomyces.
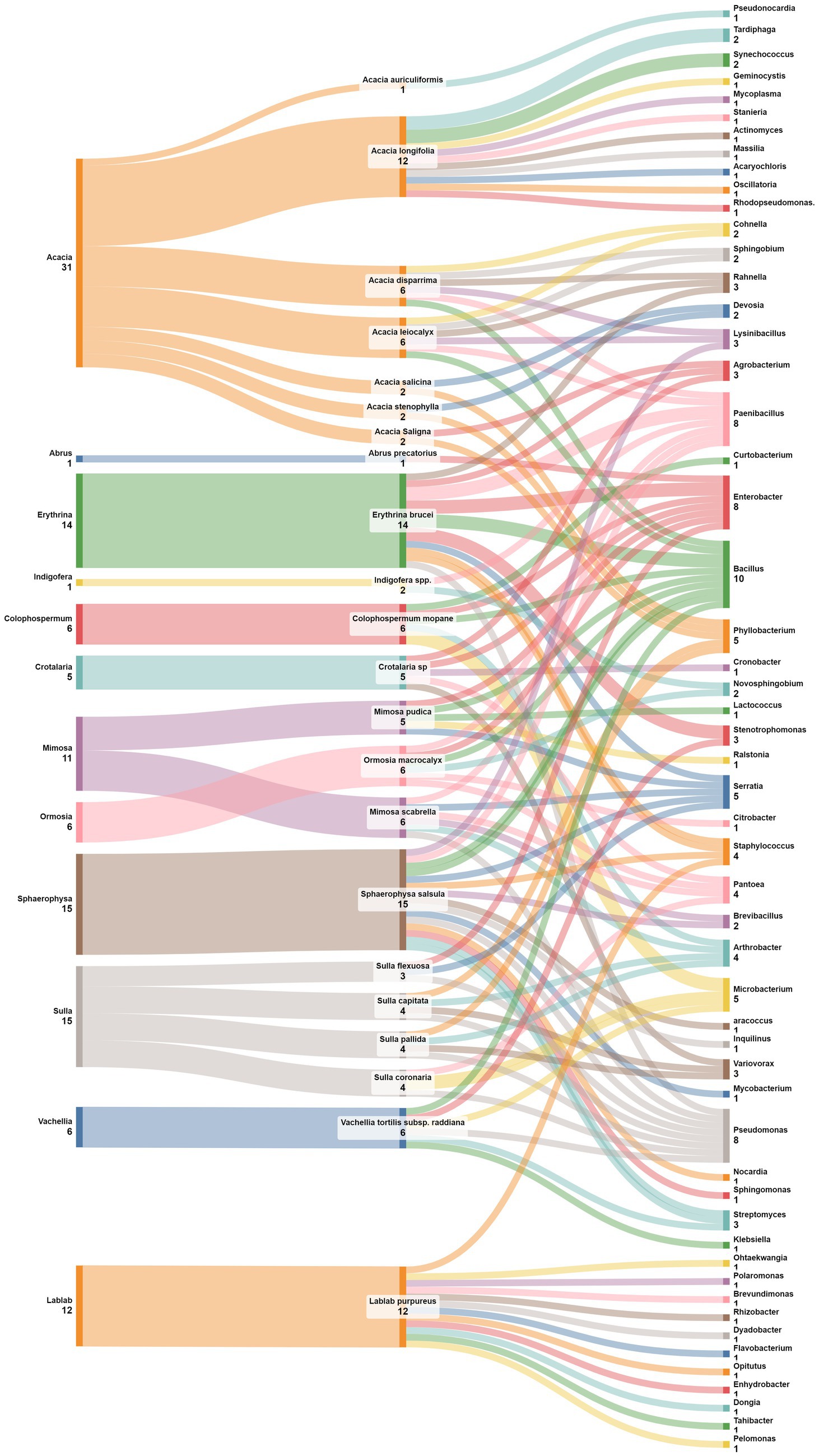
Figure 8. Sankey diagram representing the main plant genera and species (of the subfamilies of Mimosoideae, Faboideae, Hedysareae, and Caesalpinioideae) in this study along with the associated nodule endophyte genera.
The analysis at the level of plant species, demonstrated that the genus Pseudomonas is prevalent in nodules of different leguminous species, like Mimosa scabrella, Sulla coronaria, Sulla capitata, and Vachellia tortilis subsp. raddiana. The genus Enterobacter was also found linked to various plant species, including Crotalaria sp., Colophospermum mopane, Ormosia macrocalyx, Abrus precatorius, Mimosa pudica, and Acacia leiocalyx. For its side, Bacillus was identified in nodules of Erythrina brucei, Colophospermum mopane, Sphaerophysa salsula, Ormosia macrocalyx, Vavilovia formosa, Mimosa scabrella, Acacia disparrima, Acacia leiocalyx, and Vachellia tortilis subsp. raddiana; while the closest genus Paenibacillus was found to be associated with nodules of Indigofera spp., Erythrina brucei, Ormosia macrocalyx, Periandra mediterranea, and Acacia disparrima. The genus Serratia was linked to Erythrina brucei, Mimosa scabrella, and Mimosa pudica, while. Microbacterium was found in nodules of Colophospermum mopane, Sulla coronaria, and Vachellia tortilis subsp. raddiana. Phyllobacterium was observed in Sulla pallida, Sulla capitata, Acacia salicina, Acacia stenophylla, and Acacia leiocalyx. Globally, these associations highlight the specificity and the diversity of nodule endophyte preferences across plant genera and species.
Furthermore, in the Supplementary material, a Sankey Diagram is employed to illustrate the principal plant subfamilies, alongside their associated plant genera and species. Another one delineates the primary plant genera and their associated plant species. These figures contribute Supplementary material visual insights, enhancing the comprehensive analysis presented earlier (Supplementary Figure S1).
4 Main properties of nodule endophytes
Endophytic bacteria are recognized for their role in promoting plant growth through mechanisms such as phytostimulation, biofertilization, and biocontrol (Batra et al., 2018). The hypothesis that nodule endophytes may exhibit similar beneficial actions is plausible. Specifically, endophytic bacteria residing in legume nodules demonstrate potential as biofertilizers. It has been demonstrated that some of them exhibit in vitro a range of advantageous activities for plants, including the synthesis of plant hormones, biological nitrogen fixation, and the solubilization of inorganic phosphate, as shown by Peix et al. (2015). Concurrently, others possess the catalytic activity of ACC deaminase or produce various compounds such as siderophores, as documented by (Ríos-Ruiz et al., 2019). Furthermore, certain nodule endophytic bacteria can enhance the well-being of their host plants by acquiring more restricted plant nutrients, as discussed by some authors (Leite et al., 2017; Freitas et al., 2019; Elhaissoufi et al., 2020).
Moreover, studies at the field level have focused on leveraging nodule endophytes to enhance the growth and adaptation of legumes to degraded estuarine soils. They have demonstrated that non-rhizobial nodule endophytes exhibit a significant potential to improve nodulation, modulate root exudation patterns, and foster legume growth (Dhole and Shelat, 2022; Debnath et al., 2023).
4.1 Biofertilization
4.1.1 Nitrogen fixation by endophytes
Nitrogen fixation is a critical process for sustainable agriculture, and various bacterial strains have been identified for their ability to fix atmospheric nitrogen either as free-living organisms or as endophytes within legume nodules. Studies have documented the nitrogen-fixing capabilities of Serratia sp., Herbaspirillum sp., Paenibacillus sp., Arthrobacter sp., Klebsiella sp., Pseudomonas sp., Bacillus sp., Bosea sp., and Enterobacter sp. strains isolated from legume nodules (Valverde, 2003; Muresu et al., 2008; Selvakumar et al., 2008; Ibáñez et al., 2009; Knežević et al., 2021; Debnath et al., 2023).
Recent investigations suggest that NREs contribute substantially to nitrogen fixation, potentially accounting for up to 47% of the total nitrogen fixed by bacteria (Puri et al., 2018). This contribution spans both symbiotic and free-living states, underscoring the multifaceted role of NREs in nitrogen cycling within the biosphere.
4.1.2 Phosphate solubilization for enhanced phosphorus availability
Phosphorus is a crucial element for plant growth that is often at low levels in the soils or present but not available for plants. At this respect, phosphate-solubilizing bacteria (PSB) play an essential role by transforming the insoluble molecules. It was reported that nodule endophytic bacteria can solubilize insoluble phosphates during initial colonization of roots, thereby improving phosphorus availability in the soil (Kai-yuan et al., 2023).
4.1.3 Siderophore production as a mechanism for iron chelation
Certain strains of Bacillus within nodule endophytic bacteria contribute to plant growth by producing siderophores, which are low-molecular-weight metabolites that chelate iron, making it available to plants. This production has significant implications for competitively inhibiting the growth of phytopathogens (Maheshwari et al., 2019; Dhole and Shelat, 2022).
In addition to these traditional biofertilization mechanisms, recent studies have shed light on the role of nodular rhizosphere endophytes (NREs) in enhancing plant health and productivity. NREs, such as those isolated from soybean nodules, have been found to possess antimicrobial properties that extend beyond legume hosts, providing protection against bacterial and fungal pathogens in non-legume plants (Tokgöz et al., 2020).
4.2 Stress tolerance
Endophytes play an essentiel role in enhancing plant stress tolerance through various mechanisms, including the accumulation of osmolytes and antioxidant compounds, which aid in osmotic regulation and the stabilization of cellular components. Additionally, certain endophytic bacteria exhibit the ACC deaminase activity, an enzyme capable of degrading ACC and regulating ethylene production in plants, thereby promoting plant growth under stressful conditions (Vaishnav et al., 2019).
The significance of endophytic bacteria in stress tolerance has been underscored in several studies. For instance, Egamberdieva, (2017) demonstrated that Achromobacter spp., an endophyte associated with Cicer arietinum (chickpea), significantly improves plant growth promotion and confers potential salt/osmotic stress protection. Similarly, Chryseobacterium spp. has been shown to enhance osmotic stress protection in Vigna unguiculata (cowpea) (Leite et al., 2017). Moreover, certain endophytic bacteria such as Enterobacter sp. have exhibited remarkable abilities in abiotic stress adaptation, particularly in soybean and Phaseolus lunatus (Sharaf et al., 2019; Chibeba et al., 2020). Furthermore, Paenibacilli have been demonstrated to mitigate water-deficit stress in legumes by modulating stress-signaling hormones (Figueiredo et al., 2008).
Recent studies have shed light on the stress tolerance-inducing capabilities of non-rhizobial endophytic bacteria (NRBEs), particularly halotolerant strains. Jha et al. (2012) identified several halotolerant NRBEs, including Brachybacterium nov., Zhihengliuella sp., Brevibacterium casei, Halomonas sp., Vibrio sp., and Pseudomonas spp., which have shown potential in stress mitigation. Additionally, Mukhtar et al. (2020) highlighted the effectiveness of halotolerant NRBEs from cowpea nodules, such as Pseudomonas fluorescens, Bacillus endophyticus, Bacillus pumilus, and Paenibacillus polymyxa, in stimulating plant growth and development.
Furthermore, Ruiz-Díez et al. (2009) proposed that NRBEs, exemplified by Phylobacterium myrsinacearum, possessing plant growth-promoting abilities, can withstand acidic pH, thus potentially aiding in stress alleviation in acidic soil conditions. However, despite these advancements, there remains a notable gap in understanding the presence and potential contributions of bacterial endophytes in the small nodules of peanuts, particularly in facilitating plant growth promotion and tolerating biotic and abiotic stresses (Hossain et al., 2023).
4.3 Biocontrol
Pathogen diseases often develop resistance to chemical solutions, rendering them ineffective. Microorganisms, particularly those with plant growth-promoting characteristics, offer a promising approach for mitigating pathogen pressure (Horrigan et al., 2002; Kandel et al., 2017). In a study by Bahroun et al. (2018), three strains, namely Rahnella aquatilis B16C, Pseudomonas yamanorum B12, and Pseudomonas fluorescens B8P, identified as nodule endophytic bacteria, were assessed for their in vivo biocontrol potential against F. solani root rot in three Faba bean cultivars under greenhouse conditions. These strains significantly reduced pathogen symptom severity, with R. aquatilis B16C demonstrating the most effective protection across all tested Faba bean cultivars. Consequently, R. aquatilis B16C is recommended as a biocontrol agent for field application.
Mechanisms involved in the biocontrol of fungal diseases often implicate the production of lytic enzymes, with chitinases being particularly crucial. Sánchez-Cruz et al. (2019) reported that only the two Serratia sp. isolates (recognized as endophytic bacteria) exhibited chitinase production in their study, underscoring the importance of chitinase production for achieving biological control of phytopathogenic fungi (Berg and Hallmann, 2006; Wang et al., 2013).
In the study of Zhao et al. (2018), six endophytic antagonists belonging to five genera, including Enterobacter, Acinetobacter, Pseudomonas, Ochrobactrum, and Bacillus, were identified. Among them, Acinetobacter calcoaceticus DD161 displayed the strongest inhibitory activity (71.14%) against Phytophtora. sojae 01, inducing morphological abnormalities in fungal mycelia. Regression analysis indicated a significant positive correlation between siderophore production and the inhibition ratio against P. sojae 01.
In a recent study, Hnini et al. (2023) reported that four nodule bacterial endophytes, namely LMR701 (Bacillus halotolerans), LMR703 (Pseudomonas koreensis), LMR712 (Bacillus thuringiensis), and LMR713 (Streptomyces tendae), exhibited a antagonistic activity against Fusarium oxysporum and Botrytis cinerea. Similar results were observed with Paenibacillus strains (recognized as nodule endophytic bacteria), displaying antagonistic activities against five phytopathogenic fungi and synthesizing hydrolytic enzymes, siderophores, and the lipopeptide fusaricidin (Ali et al., 2022). When applied to plants, B. subtilis strain NUU4, a non–rhizobial endophytic bacterium, reduced diseased plants by 8%, highlighting the potential of such strains in significantly reducing plant diseases (Egamberdieva, 2017). Notably, nodule endophytic bacteria strains exhibiting significant lytic enzyme activities may play a crucial role in protecting plants against various pathogenic fungi, as indicated by studies on Botrytis cinerea, Fusarium oxysporum, Pythium ultimum, Phytophthora sp., and Rhizoctonia solani (Yasmin et al., 2016; Chen et al., 2020). The specific activities of these endophytic bacteria within nodules, such as induced systemic resistance (ISR), phytohormone production, biological nitrogen fixation (BNF), and stress tolerance, warrant further investigation.
It is uncertain whether the nodule endophytic can display in nodules all the biocontrol and bioprotection mechanisms expressed in vitro. However, endophytes in general (from other plant organs) are known to play diverse roles in supporting plant health by engaging in multifaceted interactions. Upon colonizing plant tissues, they participate in the competitive exclusion of pathogens by strategically occupying preferred sites of invasion, leading to the effective utilization of nutrients that curtails pathogen invasion (Xia et al., 2022). Another essential function involves antibiotic production, with certain strains, such as Bacillus, synthesizing compounds like circulin, colistin, and polymyxin. These compounds exhibit inhibitory effects on both Gram-positive and Gram-negative bacteria, as well as numerous pathogenic fungi. Additionally, several bacteria, including endophytes, can produce bacteriocins-specific proteins inhibiting the activity of strains within the same bacterial species or related species (Vasileva et al., 2019).
4.4 Phytostimulation
Plant hormones, crucial regulators of growth and development, encompass auxin, gibberellin, ethylene, abscisic acid, and cytokinin. Among them, indole-3-acetic acid (IAA) emerges as a crucial phytohormone produced by bacteria, significantly influencing root development and nutrient absorption. Noteworthy contributors to IAA production are endophytic bacteria across various genera, demonstrating positive impacts on plant growth and development by modulating biological processes such as fruit development, cell division, and aging (Batra et al., 2018). The IAA-producing capabilities of several endophytic genera, including those isolated from legume nodules (e.g., Enterobacter, Pseudomonas, Klebsiella, Burkholderia, Pantoea, Bacillus, and Rhodococcus), have been documented (Eid et al., 2021).
The question of the potential role of endophytes producing IAA in the establishment and functioning of symbiotic nodules is particularly intriguing. IAA is recognized for its essential role in symbiosis establishment and nodular tissue formation. In soybean, co-inoculation of endophytic bacteria with Bradyrhizobium japonicum MN110 enhances symbiotic nitrogen fixation and promotes plant growth, exemplifying the positive impact of endophytic bacteria on nodule function (Subramanian et al., 2015). Bacterial IAA, known for its multifaceted effects, extends its influence beyond simple growth modulation. It plays a critical role in enhancing nodule formation, boosting rhizobia competitiveness for nodulation, extending the functional lifespan of nodules by suppressing senescence, and stimulating the expression of genes associated with legume-rhizobia symbiosis (Alemneh et al., 2020). By promoting these mechanisms, bacterial IAA contributes significantly to the optimization of the legume-rhizobia symbiosis. Its role in facilitating nitrogen fixation in terrestrial ecosystems underscores its importance.
The hormone IAA is also responsible for the elongated root system and number of infection locations prior to nodulation. IAA is also involved in several stages of the symbiotic associations, and can also operate as a signal molecule in plant–bacteria associations and bacteria–bacteria communication (Etesami, 2022). Studies have shown that endophytic bacteria can improve nodule function and plant nitrogen in legumes, such as soybean, through synergistic interactions with rhizobia (Subramanian et al., 2015). In this study, authors found that co-inoculation of Bradyrhizobium japonicum MN110 along with endophytic bacteria Bacillus megaterium LNL6 and Methylobacterium oryzae CBMB20 exhibited an increase in nodule number and nodule activity, which was measured in terms of nodule leghemoglobin content, nodulated root ARA (acetylene reduction assay), and total plant nitrogen content compared to solitary inoculation of B. japonicum MN110. The high levels of IAA produced by B. megaterium LNL6 can be considered to have aided in the development of mature nodules, which thereby improved the nodular nitrogen fixation (Subramanian et al., 2015). The exploration of whether endophytes producing IAA actively contribute to nodule formation or modulate nodule function presents an avenue for valuable insights into the intricate interactions within legume-nodule associations. Moreover, the implications of hormones, including IAA, in plant stress tolerance raise pertinent questions regarding the potential role of endophytic bacteria within nodules in enhancing stress tolerance in leguminous plants. Investigating these potential roles could reveal novel mechanisms contributing to the resilience of legumes in challenging environments and deepen our understanding of the multifaceted functions of endophytic bacteria in the context of plant-microbe interactions.
4.5 Phytoremediation by endophytes
Plant-associated microbiomes play an essential role in phytoremediation, enabling plants to flourish in contaminated soils, alleviating stress induced by elevated levels of heavy metals and metalloids, and enhancing both phytoextraction and phytostabilization (Fagorzi et al., 2018). In addition to their biofertilizer potential cited before, legumes’ nodule endophytic bacteria were investigated for their role in phytoremediation of heavy metal-contaminated soils. In legumes, nitrogen-fixing bacteria and endophytes within nodules have demonstrated efficacy in reducing metal translocation to aerial plant parts, increasing plant nitrogen content, and promoting growth in metal-contaminated soils (Flores-Duarte et al., 2022b).
Under conditions of elevated metal levels in the soil causing stress to Medicago sativa (alfalfa), Adeleke et al. (2021) reported that inoculants based on non-rhizobial nodule endophytes could serve as useful and efficient tools to enhance legume adaptation and growth in metal-contaminated and nutrient-poor soils. Endophytes may offer advantages as plant growth-promoting bacteria (PGPB) compared to rhizospheric bacteria, owing to their closer association with the plant and reduced competition with soil bacteria.
Residual organic compounds accumulation in soils is another situation where the symbiotic relationship between plants and endophytic bacteria holds promise. Endophytic bacteria, isolated from environments rich in xenobiotics, particularly genera such as Pseudomonas, Bacillus, Burkholderia, Stenotrophomonas, Micrococcus, Pantoea, and Microbacterium, demonstrate versatile metabolic pathways enabling the utilization of organic pollutants as their sole carbon source. This capacity facilitates the mineralization or transformation of contaminants into non-toxic derivatives (Karaś et al., 2021).
5 Use of nodule endophytes as legume inoculants
Endophytic bacteria, with their diverse beneficial effects on plants, operate through mechanisms akin to plant growth-promoting rhizobacteria (Hallmann et al., 1997). Studies underscore the potential for increased yields across various plant species through endophyte inoculation (Pedraza et al., 2009). While historically focused on legumes, recent research suggests a broader applicability of nodule endophytes, prompting a reevaluation of their utilization criteria.
In the context of legumes, the combined application of rhizobial and non-rhizobial inocula has shown substantial yield increases, particularly when endophytes are compatible and growth inhibition is absent (Batra et al., 2018). Notably, the positive impact of early nodulation is exemplified in recent investigations on Medicago sativa, wherein co-inoculation with a consortium of endophytic strains led to superior nodulation and enhanced nitrogen content (Flores-Duarte et al., 2022b). Such findings highlight the potential synergistic benefits of co-inoculation with different endophytic strains in legume systems. However, endophytes’ influence on nodulation and yield varies across different symbioses. For example, while a Bacillus strain benefited the R. tropici - Phaseolus vulgaris symbiosis, it exhibited adverse effects in the B. japonicum - Glycine max symbiosis (Menéndez and Paço, 2020), Similarly, co-inoculation of Vigna unguiculata L. with Bradyrhizobium spp. and various non-rhizobial bacteria enhanced nitrogen levels and other parameters (Santos et al., 2018).
Beyond legumes, endophytic inoculation has shown remarkable benefits in crops like chickpeas, where enhanced antioxidant gene expression and growth were observed (El-Esawi et al., 2019). The application of Bacillus subtilis BERA 71 mitigated oxidative damage induced by salinity, further highlighting the potential of endophytes in stress tolerance (Abd_Allah Abd-Allah et al., 2018). Endophytes also influence osmotic processes, root morphology, and nitrogen metabolism in legumes (Dudeja et al., 2012). Furthermore, non-rhizobial endophytes (NREs) have positively influenced lentil growth, modulating root exudates and rhizospheric community structure (Debnath et al., 2023). Similarly, certain endophytes associated with peanut roots enhanced biomass production, demonstrating the broader impact of endophytes beyond nodulation (Hossain et al., 2023).
Studies in diverse agricultural systems highlight the positive effects of endophytic inoculation on plant growth and nutrient uptake (Minaxi et al., 2012; Lavakush et al., 2014; Ramesh et al., 2014; Freitas et al., 2019; Elhaissoufi et al., 2020). Notably, a nodule endophyte strain significantly enhanced root and nodule characteristics in common bean, influencing rhizosphere bacterial communities (Chihaoui et al., 2012, 2015), while historically considered primarily for legume inoculation, the utilization of nodule endophytes transcends plant families, offering promising avenues for enhancing biofertilizers’ efficacy in sustainable agriculture under various conditions. This evolving perspective underscores the need for a unifying criterion to maximize the potential benefits of nodule endophytes across diverse agricultural systems.
The interactions between endophytic bacteria and legumes elucidates several key highlights, including the enhancement of nitrogen fixation, improvement of plant growth and development, and the mitigation of stress in leguminous plants. Notably, various endophytic bacteria have demonstrated significant beneficial effects, such as increasing nitrogen levels, promoting root growth, and enhancing nutrient absorption in contaminated soils. These findings not only emphasize the versatility of endophytic bacteria across different legume species but also underscore their potential in addressing contemporary agricultural challenges, particularly in the realm of sustainable soil management and phytoremediation. By showcasing the multifaceted contributions of endophytic bacteria to legume health and productivity, the section provides compelling evidence of their importance in agricultural ecosystems and underscores the need for further research to unlock their full potential.
6 Insights from recent studies on new nodular endophytic bacteria
The intricate world of nodular endophytic bacteria holds immense promise for researchers seeking to unravel the complexities of plant-microbe interactions. In this section, we present a meticulously curated compilation data (Table 2; Figure 9) summarizing recent studies on new species of nodular endophytic bacteria. This compilation serves as a valuable resource, offering insights into the diversity, distribution, and biotechnological potential of these intriguing microorganisms. Through diligent curation and analysis, we aim to provide a comprehensive overview while acknowledging the inherent limitations and nuances of our approach.
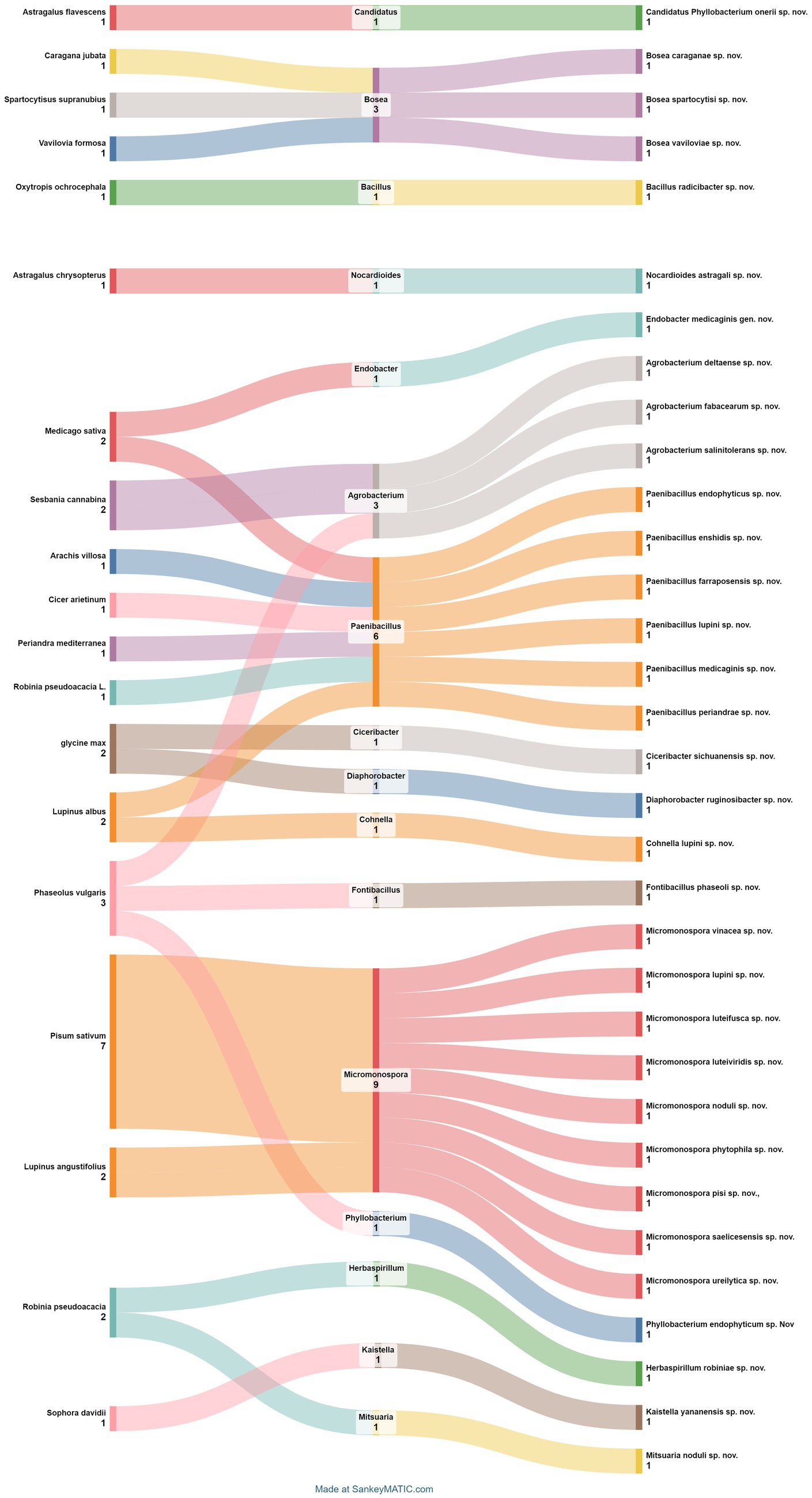
Figure 9. Sankey diagram representing the main plant species in this study along with the associated nodule endophyte genera and species.
The compilation data serves as a succinct summary of recent studies documenting new species of nodular endophytic bacteria. We recognize the significance of this compilation and have diligently ensured the clarity and comprehensiveness of the presented data. Key details such as the host legumes, isolation media, and geographical origins of the newly identified species are meticulously documented, facilitating a thorough understanding of nodular diversity.
We firmly believe that this compilation table serves as an invaluable resource for researchers interested in delving into the diversity and biotechnological potential of nodular endophytes. By consolidating findings from diverse studies, the table offers valuable insights into the distribution of nodular endophytic bacteria across various legume hosts and geographical locations. Furthermore, the inclusion of references enables easy access to the primary sources, thereby augmenting the utility of the table for readers. While the compilation table provides a comprehensive overview of newly described nodular endophytic bacteria, we acknowledge potential limitations inherent in our approach. These limitations, including geographic biases and potential underrepresentation of certain legume hosts or regions in the literature, have been duly addressed through discussions regarding the scope and focus of our literature search. We have also highlighted the potential implications of these limitations for the interpretation of our findings.
Additionally, we have initiated a concise discussion on the biotechnological potential of the newly described species, elucidating their unique characteristics and potential applications. By juxtaposing these species with previously known nodular endophytes, we aim to discern any distinctive features or evolutionary trends contributing to their biotechnological relevance.
Regarding the geographical distribution of research efforts, Spain emerges as a prominent contributor, with 11 studies and 15 new species of endophytic nodule bacteria, followed closely by China with 7 studies and 7 new species. Micromonospora leads among bacterial genera, with 9 new species (7 from Pisum sativum and 2 from Lupinus angustifolius), followed by Paenibacillus with 6 new species from different plant species. Subsequently, there are 3 new species each of Agrobacterium and Bosea.
It is evident that while there are variations in the media used for isolating certain species, the majority of new species (25 out of 31) were isolated using yeast extract-mannitol agar (YMA) (Vincent, 1970). This underscores the widespread utilization and efficacy of YMA for isolating a diverse array of bacterial species, including those associated with nodules and endophytes. However, it is noteworthy that specific media formulations were employed for isolating certain species, such as YMA supplemented with 0.5% succinate for Bosea sp., humic acid agar for Micromonospora luteifusca sp. nov. These variations likely arise from the unique requirements or characteristics of these particular bacteria.
In conclusion, while yeast extract-mannitol agar remains a popular choice for bacterial isolation, researchers may opt for specialized media formulations when targeting specific bacterial groups or when seeking to optimize isolation conditions for particular species.
7 Exploring microbial diversity in root nodules: integrating cultivation-based and metagenomic approaches
It’s essential to recognize the constraints of cultivation-based techniques in capturing the entirety of microbial communities. While culturomic methods provide valuable insights into cultivable microorganisms, they inherently overlook the vast majority of unculturable diversity present in environmental samples. To bridge this gap, molecular approaches, particularly metagenomics, offer a more comprehensive understanding of microbial diversity by analyzing total DNA isolated from environmental samples.
The findings from various metagenomic studies exemplify the utility of metagenomic approaches in elucidating the diversity of endophytic populations associated with root nodules. For instance, Hakim et al. (2020) identified a diverse array of bacterial genera, including non-rhizobia such as Acinetobacter, Enterobacter, and Pseudomonas, in mung bean nodules, emphasizing the importance of considering non-rhizobial taxa and their potential contributions to plant growth promotion and symbiotic interactions. Similarly, Iyer and Rajkumar (2017) utilized metagenomic approaches to characterize bacterial communities in the rhizosphere, root endosphere, and root nodules of Vigna radiata. Their findings highlighted the predominance of Proteobacteria in rhizospheric and nodular communities, with Actinobacteria being predominant in the root endosphere, demonstrating the distinct microbial compositions across different plant-associated habitats.
Moreover, the study conducted by Jesus et al. (2023) utilized a combination of histological analysis and Next-Generation Sequencing to assess nodular structure and biodiversity in Acacia longifolia. Their findings revealed shifts in microbial community composition following fire events, with Bradyrhizobium and cyanobacteria being predominant in both burnt and unburnt sites, underscoring the dynamic nature of microbial communities within nodules and their potential responses to environmental perturbations.
Additionally, Sharaf et al. (2019) provided insights into the nodule bacteriome of soybean cultivars using metagenomic approaches. Their results highlighted the prevalence of non-rhizobial taxa such as Pseudomonas and Enterobacteria, suggesting a significant contribution of these taxa to the nodule community, further emphasizing the importance of considering the broader microbial diversity within nodules and its implications for plant-microbe interactions.
In addition to the studies previously mentioned, it’s important to consider the source of nodular endophytes (NRE) and the limitations associated with metagenomic analysis of bacterial community diversity. Bender et al. (2022) discussed how genera like Rhizobium, Pseudomonas, and Klebsiella can be transferred vertically from seeds to the root system of legumes, suggesting potential vertical transmission mechanisms. However, in non-sterile soils, it’s challenging to confirm whether these bacteria are acquired through horizontal or vertical transmission. The entry of non-rhizobia into nodules may occur through the junction between the nodule and root or via infection threads induced by rhizobia (Zgadzaj et al., 2015). The true endophytic nature of NRE can only be determined through fluorescent labeling or isolation of viable cells from surface-sterilized nodules to prevent contamination from attached bacteria (Hakim et al., 2020).
Moreover, while metagenomic analysis offers advantages over culture-based techniques, it has limitations in terms of the quality and quantity of metagenomic DNA sampling. Cultivation-independent molecular strategies, such as generating 16S rRNA libraries and phylogenetic analysis, can augment our understanding of unculturable microbiota in the rhizosphere and their interactions with plants and other microorganisms (Iyer and Rajkumar, 2017). However, technological barriers hinder meticulous analysis despite the advancements in “omics” techniques. Therefore, addressing these limitations and integrating multiple approaches are crucial for advancing our understanding of microbial diversity and its implications for plant-microbe interactions and agricultural sustainability.
To sum up, while culturomic approaches offer valuable insights, integrating metagenomic studies provides a more comprehensive understanding of nodular diversity and its biotechnological potential. These studies collectively underscore the necessity of incorporating both cultivation-independent and cultivation-based techniques to gain a holistic view of microbial communities associated with root nodules.
8 Conclusion
In conclusion, this review provides comprehensive insights into the intricate relationships between various legume plant genera and their associated nodule endophytes. The study highlights the specificity and diversity of nodule endophyte preferences across various plant genera, underscoring the complexity of plant-endophyte interactions. The investigation reveals diverse prevalence patterns, emphasizing the significance of certain genera such as “Phaseolus” and the consistent presence of Bacillus and Pseudomonas as predominant nodule endophyte bacteria. Legume nodules, complex habitats for diverse bacterial communities, exhibit a prevalence of specific genera, notably Pseudomonas and Bacillus. Beyond rhizobia, non-rhizobial endophytes contribute to the ecological resilience of legumes. These findings have implications for agricultural practices, suggesting potential applications in targeted inoculation to enhance plant growth, nutrient uptake, plant resilience and overall health in different legume crops under different soil and climatic conditions.
Author contributions
MH: Conceptualization, Data curation, Formal analysis, Investigation, Methodology, Software, Writing – original draft. JA: Conceptualization, Formal analysis, Project administration, Resources, Supervision, Validation, Writing – review & editing.
Funding
The author(s) declare that no financial support was received for the research, authorship, and/or publication of this article.
Conflict of interest
The authors declare that the research was conducted in the absence of any commercial or financial relationships that could be construed as a potential conflict of interest.
Publisher’s note
All claims expressed in this article are solely those of the authors and do not necessarily represent those of their affiliated organizations, or those of the publisher, the editors and the reviewers. Any product that may be evaluated in this article, or claim that may be made by its manufacturer, is not guaranteed or endorsed by the publisher.
Supplementary material
The Supplementary material for this article can be found online at: https://www.frontiersin.org/articles/10.3389/fmicb.2024.1386742/full#supplementary-material
SUPPLEMENTARY FIGURE 1 | Sankey diagram represents the main plant subfamilies in this study along with the associated plant genus and species.
References
Abd-Allah, E. F., Alqarawi, A. A., Hashem, A., Radhakrishnan, R., al-Huqail, A. A., al-Otibi, F. O. N., et al. (2018). Endophytic bacterium Bacillus subtilis (BERA 71) improves salt tolerance in chickpea plants by regulating the plant defense mechanisms. J. Plant Interact. 13, 37–44. doi: 10.1080/17429145.2017.1414321
Adeleke, B. S., Ayangbenro, A. S., and Babalola, O. O. (2021). Genomic analysis of endophytic Bacillus cereus T4S and its plant growth-promoting traits. Plan. Theory 10:1776. doi: 10.3390/plants10091776
Adhikary, M., Gangopadhyay, A., Mondal, S., and Brahmachari, K. (2022). Interaction amongst the chemical pesticides, agriculture and human health: an environmental case study in nadia district, West Bengal, INDIA. Asian J. Microbiol. Biotechnol. Environ. Sci. 1, 445–447. doi: 10.53550/AJMBES.2022.v24i02.040
Aeron, A., Maheshwari, D. K., and Meena, V. S. (2020). Endophytic bacteria promote growth of the medicinal legume Clitoria ternatea L. by chemotactic activity. Arch. Microbiol. 202, 1049–1058. doi: 10.1007/s00203-020-01815-0
Ai, J., He, X., Ren, M., Yu, T., Liu, X., Jiang, Y., et al. (2024). Kaistella yananensis sp. nov., a novel Indoleacetic acid-producing bacterium isolated from the root nodules of Sophora davidii (Franch.) Skeels. Curr. Microbiol. 81, 1–9. doi: 10.1007/s00284-023-03578-5
Alemneh, A. A., Zhou, Y., Ryder, M. H., and Denton, M. D. (2020). Mechanisms in plant growth-promoting rhizobacteria that enhance legume-rhizobial symbioses. J. Appl. Microbiol. 129, 1133–1156. doi: 10.1111/jam.14754
Ali, B., Hafeez, A., Javed, M. A., Afridi, M. S., Abbasi, H. A., Qayyum, A., et al. (2022). Role of endophytic bacteria in salinity stress amelioration by physiological and molecular mechanisms of defense: a comprehensive review. S. Afr. J. Bot. 151, 33–46. doi: 10.1016/j.sajb.2022.09.036
Ampomah, O. Y., and Huss-Danell, K. (2011). Genetic diversity of root nodule bacteria nodulating Lotus corniculatus and Anthyllis vulneraria in Sweden. Syst. Appl. Microbiol. 34, 267–275. doi: 10.1016/j.syapm.2011.01.006
Andrews, M., and Andrews, M. E. (2017). Specificity in legume-rhizobia symbioses. Int. J. Mol. Sci. 18:705. doi: 10.3390/ijms18040705
Aserse, A. A., Räsänen, L. A., Aseffa, F., Hailemariam, A., and Lindström, K. (2013). Diversity of sporadic symbionts and nonsymbiotic endophytic bacteria isolated from nodules of woody, shrub, and food legumes in Ethiopia. Appl. Microbiol. Biotechnol. 97, 10117–10134. doi: 10.1007/s00253-013-5248-4
Aserse, A. A., Räsänen, L. A., Assefa, F., Hailemariam, A., and Lindström, K. (2012). Phylogeny and genetic diversity of native rhizobia nodulating common bean (Phaseolus vulgaris L.) in Ethiopia. Syst. Appl. Microbiol. 35, 120–131. doi: 10.1016/j.syapm.2011.11.005
Bahroun, A., Jousset, A., Mhamdi, R., Mrabet, M., and Mhadhbi, H. (2018). Anti-fungal activity of bacterial endophytes associated with legumes against Fusarium solani: assessment of fungi soil suppressiveness and plant protection induction. Appl. Soil Ecol. 124, 131–140. doi: 10.1016/j.apsoil.2017.10.025
Bakhtiyarifar, M., Enayatizamir, N., and Mehdi Khanlou, K. (2021). Biochemical and molecular investigation of non-rhizobial endophytic bacteria as potential biofertilisers. Arch. Microbiol. 203, 513–521. doi: 10.1007/s00203-020-02038-z
Barnawal, D., Bharti, N., Maji, D., Chanotiya, C. S., and Kalra, A. (2014). ACC deaminase-containing Arthrobacter protophormiae induces NaCl stress tolerance through reduced ACC oxidase activity and ethylene production resulting in improved nodulation and mycorrhization in Pisum sativum. J. Plant Physiol. 171, 884–894. doi: 10.1016/j.jplph.2014.03.007
Barraza, A., Vizuet-De-Rueda, J. C., and Alvarez-Venegas, R. (2020). Highly diverse root endophyte bacterial community is driven by growth substrate and is plant genotype-independent in common bean (Phaseolus vulgaris L.). PeerJ 8:e9423. doi: 10.7717/peerj.9423
Batra, P., Barkodia, M., Ahlawat, U., Sansanwal, R., Sharma, T., and Wati, L. (2018). Endophytes: an environmental friendly Bacteria for plant growth promotion. Int. J. Curr. Microbiol. App. Sci. 7, 1899–1911. doi: 10.20546/ijcmas.2018.702.229
Baweja, P., Kumar, S., and Kumar, G. (2020). “Fertilizers and pesticides: their impact on soil health and environment” in Soil health. eds. B. Giri and A. Varma (Cham: Springer International Publishing), 265–285.
Beghalem, H., Aliliche, K., Chriki, A., and Landoulsi, A. (2017). Molecular and phenotypic characterization of endophytic bacteria isolated from sulla nodules. Microb. Pathog. 111, 225–231. doi: 10.1016/j.micpath.2017.08.049
Bender, F. R., Alves, L. C., da Silva, J. F. M., Ribeiro, R. A., Pauli, G., Nogueira, M. A., et al. (2022). Microbiome of nodules and roots of soybean and common bean: searching for differences associated with contrasting performances in symbiotic nitrogen fixation. Int. J. Mol. Sci. 23:12035. doi: 10.3390/ijms231912035
Benjelloun, I., Thami Alami, I., Douira, A., and Udupa, S. M. (2019). Phenotypic and genotypic diversity among symbiotic and non-symbiotic Bacteria present in chickpea nodules in Morocco. Front. Microbiol. 10:1885. doi: 10.3389/fmicb.2019.01885
Berg, G., and Hallmann, J. (2006). “Control of plant pathogenic Fungi with bacterial endophytes” in Microbial root endophytes. eds. B. J. E. Schulz, C. J. C. Boyle, and T. N. Sieber (Berlin, Heidelberg: Springer), 53–69.
Berrabah, F., Ratet, P., and Gourion, B. (2019). Legume nodules: massive infection in the absence of defense induction. Mol. Plant Microbe. Interact. 32, 35–44. doi: 10.1094/MPMI-07-18-0205-FI
Berza, B., Sekar, J., Ramalingam, P., Pagano, M., and Assefa, F. (2021). Genetically and functionally diverse symbiotic and non-symbiotic native bacteria colonized root nodules of Erythrina brucei growing in different land use types in Ethiopia. Rhizosphere 17:100301. doi: 10.1016/j.rhisph.2020.100301
Brescia, F., Sillo, F., Balestrini, R., Sbrana, C., and Zampieri, E. (2023). Characterization of endophytic bacteria isolated from root nodules of lentil in intercropping with durum wheat. Curr. Res. Microb. Sci. 5:100205. doi: 10.1016/j.crmicr.2023.100205
Brown, S. P., Grillo, M. A., Podowski, J. C., and Heath, K. D. (2020). Soil origin and plant genotype structure distinct microbiome compartments in the model legume Medicago truncatula. Microbiome 8:139. doi: 10.1186/s40168-020-00915-9
Burbano, C. S., Grönemeyer, J. L., Hurek, T., and Reinhold-Hurek, B. (2015). Microbial community structure and functional diversity of nitrogen-fixing bacteria associated with Colophospermum mopane. FEMS Microbiol. Ecol. 91:30. doi: 10.1093/femsec/fiv030
Carro, L., Flores-Félix, J. D., Cerda-Castillo, E., Ramírez-Bahena, M.-H., Igual, J. M., Tejedor, C., et al. (2013). Paenibacillus endophyticus sp. nov., isolated from nodules of Cicer arietinum. Int. J. Syst. Evol. Microbiol. 63, 4433–4438. doi: 10.1099/ijs.0.050310-0
Carro, L., Flores-Félix, J. D., Ramírez-Bahena, M.-H., García-Fraile, P., Martínez-Hidalgo, P., Igual, J. M., et al. (2014). Paenibacillus lupini sp. nov., isolated from nodules of Lupinus albus. Int. J. Syst. Evol. Microbiol. 64, 3028–3033. doi: 10.1099/ijs.0.060830-0
Carro, L., Riesco, R., Spröer, C., and Trujillo, M. E. (2016a). Micromonospora luteifusca sp. nov. isolated from cultivated Pisum sativum. Syst. Appl. Microbiol. 39, 237–242. doi: 10.1016/j.syapm.2016.04.003
Carro, L., Riesco, R., Spröer, C., and Trujillo, M. E. (2016b). Micromonospora ureilytica sp. nov., Micromonospora noduli sp. nov. and Micromonospora vinacea sp. nov., isolated from Pisum sativum nodules. Int. J. Syst. Evol. Microbiol. 66, 3509–3514. doi: 10.1099/ijsem.0.001231
Carro, L., Veyisoglu, A., Riesco, R., Spröer, C., Klenk, H., Sahin, N., et al. (2018). Micromonospora phytophila sp nov and Micromonospora luteiviridis sp nov., isolated as natural inhabitants of plant nodules. Int. J. Syst. Evol. Microbiol. 68, 248–253. doi: 10.1099/ijsem.0.002490
Castellano-Hinojosa, A., Castellano-Hinojosa, A., Correa-Galeote, D., Correa-Galeote, D., Ramirez-Bahena, M. H., Ramírez-Bahena, M.-H., et al. (2021). Agrobacterium leguminum sp. nov., isolated from nodules of Phaseolus vulgaris in Spain. Int. J. Syst. Evol. Microbiol. 71:5120. doi: 10.1099/ijsem.0.005120
Chen, X., Krug, L., Yang, M., Berg, G., and Cernava, T. (2020). Conventional seed coating reduces prevalence of proteobacterial endophytes in Nicotiana tabacum. Ind. Crop. Prod. 155:112784. doi: 10.1016/j.indcrop.2020.112784
Chibeba, A. M., Pereira, C. S., Antunes, J. E. L., Ribeiro, R. A., de Almeida Lopes, A. C., Gomes, R. L. F., et al. (2020). Polyphasic characterization of nitrogen-fixing and co-resident bacteria in nodules of Phaseolus lunatus inoculated with soils from Piauí state, Northeast Brazil. Symbiosis 80, 279–292. doi: 10.1007/s13199-020-00672-1
Chihaoui, S.-A., Mhadhbi, H., and Mhamdi, R. (2012). The antibiosis of nodule-endophytic agrobacteria and its potential effect on nodule functioning of Phaseolus vulgaris. Arch. Microbiol. 194, 1013–1021. doi: 10.1007/s00203-012-0837-7
Chihaoui, S.-A., Trabelsi, D., Jdey, A., Mhadhbi, H., and Mhamdi, R. (2015). Inoculation of Phaseolus vulgaris with the nodule-endophyte Agrobacterium sp. 10C2 affects richness and structure of rhizosphere bacterial communities and enhances nodulation and growth. Arch. Microbiol. 197, 805–813. doi: 10.1007/s00203-015-1118-z
Chinnaswamy, A., de la Peña, T., Stoll, A., Rojo, D., Bravo, J., Rincón, A., et al. (2018). A nodule endophytic Bacillus megaterium strain isolated from Medicago polymorpha enhances growth, promotes nodulation by Ensifer medicae and alleviates salt stress in alfalfa plants. Ann. Appl. Biol. 172, 295–308. doi: 10.1111/aab.12420
Costa, A., Corallo, B., Amarelle, V., Stewart, S., Pan, D., Tiscornia, S., et al. (2022). Paenibacillus sp. strain UY79, isolated from a root nodule of Arachis villosa, displays a broad Spectrum of antifungal activity. Appl. Environ. Microbiol. 88:e0164521. doi: 10.1128/AEM.01645-21
Costa, E. M., Nóbrega, R. S. A., Carvalho, F., Trochmann, A., Ferreira, L. V. M., and Moreira, F. M. S. (2013). Plant growth promotion and genetic diversity of bacteria isolated from cowpea nodules. Pesq. Agrop. Brasileira 48, 1275–1284. doi: 10.1590/S0100-204X2013000900012
Cummings, S. P. (2009). The application of plant growth promoting rhizobacteria (PGPR) in low input and organic cultivation of graminaceous crops; potential and problems*. Environ. Biotechnol. 1, 43–50,
Da Silva, T. R., Rodrigues, R. T., Jovino, R. S., Carvalho, J. R. S., Leite, J., Hoffman, A., et al. (2023). Not just passengers, but co-pilots! Non-rhizobial nodule-associated bacteria promote cowpea growth and symbiosis with (Brady) rhizobia. J. Appl. Microbiol. 134:lxac013. doi: 10.1093/jambio/lxac013
De Bary, A. (1866). Morphologie und Physiologie der Pilze, Flechten und Myxomyceten. Engelmann. Available at: https://books.google.co.ma/books?id=bUI-AAAAcAAJ.
Debnath, S., Chakraborty, S., Langthasa, M., Choure, K., Agnihotri, V., Srivastava, A., et al. (2023). Non-rhizobial nodule endophytes improve nodulation, change root exudation pattern and promote the growth of lentil, for prospective application in fallow soil. Front. Plant Sci. 14:1152875. doi: 10.3389/fpls.2023.1152875
Dekak, A., Menasria, T., Benhizia, Y., and Chenchouni, H. (2020). Endophytic passenger bacteria associated with Genista cinerea nodules growing in North African drylands. Rhizosphere 14:100205. doi: 10.1016/j.rhisph.2020.100205
de Lajudie, P., Willems, A., Nick, G., Mohamed, S. H., Torck, U., Coopman, R., et al. (1999). Agrobacterium bv. 1 strains isolated from nodules of tropical legumes. Syst. Appl. Microbiol. 22, 119–132. doi: 10.1016/S0723-2020(99)80035-6
Delamuta, J. R. M., Scherer, A. J., Ribeiro, R. A., and Hungria, M. (2020). Genetic diversity of agrobacterium species isolated from nodules of common bean and soybean in Brazil, Mexico, Ecuador and Mozambique, and description of the new species Agrobacterium fabacearum sp. nov. Int. J. Syst. Evol. Microbiol. 70, 4233–4244. doi: 10.1099/ijsem.0.004278
De Meyer, S. E., De Beuf, K., Vekeman, B., and Willems, A. (2015). A large diversity of non-rhizobial endophytes found in legume root nodules in Flanders (Belgium). Soil Biol. Biochem. 83, 1–11. doi: 10.1016/j.soilbio.2015.01.002
Deng, Z.-S., Kong, Z.-Y., Zhang, B.-C., and Zhao, L.-F. (2020). Insights into non-symbiotic plant growth promotion bacteria associated with nodules of Sphaerophysa salsula growing in northwestern China. Arch. Microbiol. 202, 399–409. doi: 10.1007/s00203-019-01752-7
Deng, Z. S., Zhao, L. F., Kong, Z. Y., Yang, W. Q., Lindström, K., Wang, E. T., et al. (2011). Diversity of endophytic bacteria within nodules of the Sphaerophysa salsula in different regions of loess plateau in China. FEMS Microbiol. Ecol. 76, 463–475. doi: 10.1111/j.1574-6941.2011.01063.x
Dhole, A., and Shelat, H. (2022). Non-Rhizobial endophytes associated with nodules of Vigna radiata L. and their combined activity with Rhizobium sp. Curr. Microbiol. 79:103. doi: 10.1007/s00284-022-02792-x
Dhole, A., Shelat, H., Vyas, R., Jhala, Y., and Bhange, M. (2016). Endophytic occupation of legume root nodules by nifH-positive non-rhizobial bacteria, and their efficacy in the groundnut (Arachis hypogaea). Ann. Microbiol. 66, 1397–1407. doi: 10.1007/s13213-016-1227-1
Dinić, Z., Ugrinović, M., Bosnic, P., Mira, M., Zdravković, J., Miroslav, M., et al. (2015). Solubilization of inorganic phosphate by endophytic Pseudomonas sp. from French bean nodules. Ratar. Povrt. 51, 100–105. doi: 10.5937/ratpov51-6222
Duangmal, K., Thamchaipenet, A., Matsumoto, A., and Takahashi, Y. (2009). Pseudonocardia acaciae sp. nov., isolated from roots of Acacia auriculiformis a. Cunn. Ex Benth. Int. J. Syst. Evol. Microbiol. 59, 1487–1491. doi: 10.1099/ijs.0.007724-0
Dudeja, S. S., Giri, R., Saini, R., Suneja-Madan, P., and Kothe, E. (2012). Interaction of endophytic microbes with legumes. J. Basic Microbiol. 52, 248–260. doi: 10.1002/jobm.201100063
Egamberdieva, D. (2017). “Bacillus spp.: a potential plant growth stimulator and biocontrol agent under hostile environmental conditions” in Bacilli and Agrobiotechnology. ed. D. Egamberdieva (Cham: Springer), 91–111.
Eid, A. M., Fouda, A., Abdel-Rahman, M. A., Salem, S. S., Elsaied, A., Oelmüller, R., et al. (2021). Harnessing bacterial endophytes for promotion of plant growth and biotechnological applications: an overview. Plan. Theory 10:935. doi: 10.3390/plants10050935
El Attar, I., Taha, K., El Bekkay, B., El Khadir, M., Alami, I., and Aurag, J. (2019). Screening of stress tolerant bacterial strains possessing interesting multi-plant growth promoting traits isolated from root nodules of Phaseolus vulgaris L. Biocatal. Agric. Biotechnol. 20:101225. doi: 10.1016/j.bcab.2019.101225
El-Esawi, M. A., Al-Ghamdi, A. A., Ali, H. M., and Alayafi, A. A. (2019). Azospirillum lipoferum FK1 confers improved salt tolerance in chickpea (Cicer arietinum L.) by modulating osmolytes, antioxidant machinery and stress-related genes expression. Environ. Exp. Bot. 159, 55–65. doi: 10.1016/j.envexpbot.2018.12.001
Elhaissoufi, W., Khourchi, S., Ibnyasser, A., Ghoulam, C., Rchiad, Z., Zeroual, Y., et al. (2020). Phosphate solubilizing Rhizobacteria could have a stronger influence on wheat root traits and aboveground physiology than rhizosphere P Solubilization. Front. Plant Sci. 11:979. doi: 10.3389/fpls.2020.00979
Elvira-Recuenco, M., and van Vuurde, J. W. L. (2000). Natural incidence of endophytic bacteria in pea cultivars under field conditions. Can. J. Microbiol. 46, 1036–1041. doi: 10.1139/w00-098
Eren Eroğlu, A. E., Eroğlu, V., and Yaşa, İ. (2024). Genomic insights into the symbiotic and plant growth-promoting traits of “Candidatus Phyllobacterium onerii” sp. nov. isolated from endemic Astragalus flavescens. Microorganisms 12:336. doi: 10.3390/microorganisms12020336
Etesami, H. (2022). Root nodules of legumes: a suitable ecological niche for isolating non-rhizobial bacteria with biotechnological potential in agriculture. Curr. Res. Biotechnol. 4, 78–86. doi: 10.1016/j.crbiot.2022.01.003
Fagorzi, C., Checcucci, A., DiCenzo, G. C., Debiec-Andrzejewska, K., Dziewit, L., Pini, F., et al. (2018). Harnessing rhizobia to improve heavy-metal phytoremediation by legumes. Genes 9:542. doi: 10.3390/genes9110542
Fan, M.-C., Guo, Y.-Q., Zhang, L.-P., Zhu, Y.-M., Chen, W.-M., Lin, Y.-B., et al. (2018a). Herbaspirillum robiniae sp. nov., isolated from root nodules of Robinia pseudoacacia in a lead-zinc mine. Int. J. Syst. Evol. Microbiol. 68, 1300–1306. doi: 10.1099/ijsem.0.002666
Fan, M.-C., Nan, L.-J., Zhu, Y.-M., Chen, W.-M., Wei, G.-H., and Lin, Y.-B. (2018b). Mitsuaria noduli sp. nov., isolated from the root nodules of Robinia pseudoacacia in a lead–zinc mine. Int. J. Syst. Evol. Microbiol. 68, 87–92. doi: 10.1099/ijsem.0.002459
Fan, M., Liu, Z., Nan, L., Wang, E., Chen, W., Lin, Y., et al. (2018a). Isolation, characterization, and selection of heavy metal-resistant and plant growth-promoting endophytic bacteria from root nodules of Robinia pseudoacacia in a Pb/Zn mining area. Microbiol. Res. 217, 51–59. doi: 10.1016/j.micres.2018.09.002
Fan, M., Xiao, X., Guo, Y., Zhang, J., Wang, E., Chen, W., et al. (2018b). Enhanced phytoremdiation of Robinia pseudoacacia in heavy metal-contaminated soils with rhizobia and the associated bacterial community structure and function. Chemosphere 197, 729–740. doi: 10.1016/j.chemosphere.2018.01.102
Ferchichi, N., Toukabri, W., Boularess, M., Smaoui, A., Mhamdi, R., and Trabelsi, D. (2019). Isolation, identification and plant growth promotion ability of endophytic bacteria associated with lupine root nodule grown in Tunisian soil. Arch. Microbiol. 201, 1333–1349. doi: 10.1007/s00203-019-01702-3
Figueiredo, M. V. B., Burity, H. A., Martínez, C. R., and Chanway, C. P. (2008). Alleviation of drought stress in the common bean (Phaseolus vulgaris L.) by co-inoculation with Paenibacillus polymyxa and Rhizobium tropici. Appl. Soil Ecol. 40, 182–188. doi: 10.1016/j.apsoil.2008.04.005
Flores-Duarte, N. J., Caballero-Delgado, S., Pajuelo, E., Mateos-Naranjo, E., Redondo-Gómez, S., Navarro-Torre, S., et al. (2022a). Enhanced legume growth and adaptation to degraded estuarine soils using Pseudomonas sp. nodule endophytes. Front. Microbiol. 13:1005458. doi: 10.3389/fmicb.2022.1005458
Flores-Duarte, N. J., Pérez-Pérez, J., Navarro-Torre, S., Mateos-Naranjo, E., Redondo-Gómez, S., Pajuelo, E., et al. (2022b). Improved Medicago sativa nodulation under stress assisted by Variovorax sp. endophytes. Plan. Theory 11:1091. doi: 10.3390/plants11081091
Flores-Félix, J. D., Carro, L., Ramírez-Bahena, M.-H., Tejedor, C., Igual, J. M., Peix, A., et al. (2014a). Cohnella lupini sp. nov., an endophytic bacterium isolated from root nodules of Lupinus albus. Int. J. Syst. Evol. Microbiol. 64, 83–87. doi: 10.1099/ijs.0.050849-0
Flores-Félix, J.-D., Carro, L., Velázquez, E., Valverde, Á., Cerda-Castillo, E., García-Fraile, P., et al. (2013). Phyllobacterium endophyticum sp. nov., isolated from nodules of Phaseolus vulgaris. Int. J. Syst. Evol. Microbiol. 63, 821–826. doi: 10.1099/ijs.0.038497-0
Flores-Félix, J. D., Mulas, R., Ramírez-Bahena, M.-H., Cuesta, M. J., Rivas, R., Brañas, J., et al. (2014b). Fontibacillus phaseoli sp. Nov. isolated from Phaseolus vulgaris nodules. Anton. Leeuw. Int. J. Gen. Mol. Microbiol. 105, 23–28. doi: 10.1007/s10482-013-0049-4
Freitas, M. A., Medeiros, F. H. V., Melo, I. S., Pereira, P. F., Peñaflor, M. F. G. V., Bento, J. M. S., et al. (2019). Stem inoculation with bacterial strains Bacillus amyloliquefaciens (GB03) and Microbacterium imperiale (MAIIF2a) mitigates fusarium root rot in cassava. Phytoparasitica 47, 135–142. doi: 10.1007/s12600-018-0706-2
García-Fraile, P., Mulas-García, D., Peix, A., Rivas, R., González-Andrés, F., and Velázquez, E. (2010). Phaseolus vulgaris is nodulated in northern Spain by Rhizobium leguminosarum strains harboring two nodC alleles present in American Rhizobium etli strains: biogeographical and evolutionary implications. Can. J. Microbiol. 56, 657–666. doi: 10.1139/W10-048
Garcia, L. C., Martínez-Molina, E., and Trujillo, M. E. (2010). Micromonospora pisi sp. nov., isolated from root nodules of Pisum sativum. Int. J. Syst. Evol. Microbiol. 60, 331–337. doi: 10.1099/ijs.0.012708-0
Giraud, E., Moulin, L., Vallenet, D., Barbe, V., Cytryn, E., Avarre, J.-C., et al. (2007). Legumes symbioses: absence of Nod genes in photosynthetic Bradyrhizobia. Am. Assoc. Adv. Sci. 316, 1307–1312. doi: 10.1126/science.1139548
Gosai, J., Anandhan, S., Bhattacharjee, A., and Archana, G. (2020). Elucidation of quorum sensing components and their role in regulation of symbiotically important traits in Ensifer nodulating pigeon pea. Microbiol. Res. 231:126354. doi: 10.1016/j.micres.2019.126354
Hakim, S., Mirza, B. S., Imran, A., Zaheer, A., Yasmin, S., Mubeen, F., et al. (2020). Illumina sequencing of 16S rRNA tag shows disparity in rhizobial and non-rhizobial diversity associated with root nodules of mung bean (Vigna radiata L.) growing in different habitats in Pakistan. Microbiol. Res. 231:126356. doi: 10.1016/j.micres.2019.126356
Hakim, S., Mirza, B., Zaheer, A., Mclean, J., Imran, A., Yasmin, S., et al. (2018). Retrieved 16S rRNA and nifH sequences reveal co-dominance of Bradyrhizobium and Ensifer (Sinorhizobium) strains in field-collected root nodules of the promiscuous host Vigna radiata (L.) R. Wilczek. Appl. Microbiol. Biotechnol. 102, 485–497. doi: 10.1007/s00253-017-8609-6
Hallmann, J., Quadt-Hallmann, A., Mahaffee, W. F., and Kloepper, J. W. (1997). Bacterial endophytes in agricultural crops. Can. J. Microbiol. 43, 895–914. doi: 10.1139/m97-131
Hamane, S., El Yemlahi, A., Zerrouk, M., El Galiou, O., Laglaoui, A., Bakkali, M., et al. (2023). Promoting the growth of Sulla flexuosa L. by endophytic root nodule bacteria authors and affiliations. World J. Microbiol. Biotechnol. 39:253. doi: 10.1007/s11274-023-03699-w
Hernández-Hernández, L., Coutiño-Megchun, J. T., Rincón-Molina, C. I., Ruíz-Valdiviezo, V. M., Culebro-Ricaldi, J. M., Cruz-Rodríguez, R. I., et al. (2018). Endophytic bacteria from root nodules of Ormosia macrocalyx with potential as plant growth promoters and antifungal activity. J. Environ. Biol. 39, 997–1005. doi: 10.22438/jeb/39/6/MRN-693
Hnini, M., Taha, K., and Aurag, J. (2023). Molecular identification and characterization of phytobeneficial osmotolerant endophytic bacteria inhabiting root nodules of the Saharan tree Vachellia tortilis subsp. raddiana. Arch. Microbiol. 205:45. doi: 10.1007/s00203-022-03358-y
Hoque, M. S., Broadhurst, L. M., and Thrall, P. H. (2011). Genetic characterization of root-nodule bacteria associated with Acacia salicina and A. stenophylla (Mimosaceae) across South-Eastern Australia. Int. J. Syst. Evol. Microbiol. 61, 299–309. doi: 10.1099/ijs.0.021014-0
Horrigan, L., Lawrence, R. S., and Walker, P. (2002). How sustainable agriculture can address the environmental and human health harms of industrial agriculture. Environ. Health Perspect. 110, 445–456. doi: 10.1289/ehp.02110445
Hossain, M., Frith, C., Bhattacharyya, S., Delaune, P., and Gentry, T. (2023). Isolation and characterization of bacterial endophytes from small nodules of field-grown Peanut. Microorganisms 11:1941. doi: 10.3390/microorganisms11081941
Ibáñez, F., Angelini, J., Taurian, T., Tonelli, M. L., and Fabra, A. (2009). Endophytic occupation of peanut root nodules by opportunistic Gammaproteobacteria. Syst. Appl. Microbiol. 32, 49–55. doi: 10.1016/j.syapm.2008.10.001
Ibañez, F., Arroyo, M. E., Angelini, J., Tonelli, M. L., Muñoz, V., Ludueña, L., et al. (2014). Non-rhizobial peanut nodule bacteria promote maize (Zea mays L.) and peanut (Arachis hypogaea L.) growth in a simulated crop rotation system. Appl. Soil Ecol. 84, 208–212. doi: 10.1016/j.apsoil.2014.08.002
Iyer, B., and Rajkumar, S. (2017). A metagenomic approach to identify distinct Rhizospheric and endophytic bacterial communities from roots and root nodules of Vigna radiata. In: Iyer, B. Understanding host-microbiome interactions - an omics approach: Omics of host-microbiome association. Berlin: Springer, pp. 173–191.
Jabborova, D., Bozorov, T., Li, L., Kistaubayeva, A., and Egamberdieva, D. (2021). Identification and characterization of endophytic bacteria isolated from root nodules of lentil (Lens culinaris L.) grown on saline soils. Int. J. Biol. Chem. 14, 39–46. doi: 10.26577/ijbch.2021.v14.i2.05
Jesus, J., Máguas, C., Dias, R., Nunes, M., Pascoal, P., Pereira, M., et al. (2023). What if root nodules are a guesthouse for a microbiome? The case study of Acacia longifolia. Biology Basel 12:1168. doi: 10.3390/biology12091168
Jha, B., Gontia, I., and Hartmann, A. (2012). The roots of the halophyte Salicornia brachiata are a source of new halotolerant diazotrophic bacteria with plant growth-promoting potential. Plant Soil 356, 265–277. doi: 10.1007/s11104-011-0877-9
Joseph, B., Ranjan Patra, R., and Lawrence, R. (2007). Characterization of plant growth promoting rhizobacteria associated with chickpea (Cicer arietinum L.). Int. J. Plant Prod. 1:532. doi: 10.22069/ijpp.2012.532
Kai-yuan, Y., Chen, H., Peng, G. A. O., Yin-ping, L., Ling-juan, H. A. N., and Xiang, Z. (2023). Identification and characteristics of phosphate-solubilizing endophytic Bacteria from root nodules of Lespedeza daurica Jinnong no. 1. Acta Agrestia Sin. 30:3241. doi: 10.11733/j.issn.1007-0435.2022.12.009
Kalita, M., Małek, W., and Coutinho, T. A. (2020). Putative novel Bradyrhizobium and Phyllobacterium species isolated from root nodules of Chamaecytisus ruthenicus. Syst. Appl. Microbiol. 43:126056. doi: 10.1016/j.syapm.2020.126056
Kandel, S. L., Joubert, P. M., and Doty, S. L. (2017). Bacterial endophyte colonization and distribution within plants. Microorganisms 5:77. doi: 10.3390/microorganisms5040077
Karaś, M. A., Wdowiak-Wróbel, S., and Sokołowski, W. (2021). Selection of endophytic strains for enhanced Bacteria-assisted phytoremediation of organic pollutants posing a public health Hazard. Int. J. Mol. Sci. 22:9557. doi: 10.3390/ijms22179557
Kataoka, R., Yamamura, H., and Hayakawa, M. (2022). Characterization of the non-rhizobial bacterial Community in the Nodule-Associated Soils of Akebono soybeans and isolation of antibiotic producing Amycolatopsis spp. Indian J. Microbiol. 62, 242–248. doi: 10.1007/s12088-022-00999-x
Khalifa, A., Alsyeeh, A., Almalki, M., and Saleh, F. (2016). Characterization of the plant growth promoting bacterium, Enterobacter cloacae MSR1, isolated from roots of non-nodulating Medicago sativa. Saudi J. Biol. Sci. 23, 79–86. doi: 10.1016/j.sjbs.2015.06.008
Khalifa, A. Y. Z., and Almalki, M. A. (2015). Isolation and characterization of an endophytic bacterium, Bacillus megaterium BMN1, associated with root-nodules of Medicago sativa L. growing in Al-Ahsaa region, Saudi Arabia. Ann. Microbiol. 65, 1017–1026. doi: 10.1007/s13213-014-0946-4
Klūga, A., Dubova, L., Alsiņa, I., and Rostoks, N. (2023). Alpha-, gamma-and beta-proteobacteria detected in legume nodules in Latvia, using full-length 16S rRNA gene sequencing. Acta Agric. Scand. Sect. B 73, 127–141. doi: 10.1080/09064710.2023.2232681
Knežević, M., Berić, T., Buntić, A., Delić, D., Nikolić, I., Stanković, S., et al. (2021). Potential of root nodule nonrhizobial endophytic bacteria for growth promotion of Lotus corniculatus L. and Dactylis glomerata L. J. Appl. Microbiol. 131, 2929–2940. doi: 10.1111/jam.15152
Kour, D., Kaur, T., Devi, R., Rana, K. L., Yadav, N., Rastegari, A. A., et al. (2020). “Biotechnological applications of beneficial microbiomes for evergreen agriculture and human health” in New and future developments in microbial biotechnology and bioengineering. eds. V. K. Gupta and A. Pandey (Amsterdam, Netherlands: Elsevier), 255–279.
Kumar, A., and Dubey, A. (2020). Rhizosphere microbiome: engineering bacterial competitiveness for enhancing crop production. J. Adv. Res. 24, 337–352. doi: 10.1016/j.jare.2020.04.014
Kumar Ghosh, P., Kumar Sen, S., and Kanti Maiti, T. (2015). Production and metabolism of IAA by Enterobacter spp. (Gammaproteobacteria) isolated from root nodules of a legume Abrus precatorius L. Biocatal. Agric. Biotechnol. 4, 296–303. doi: 10.1016/j.bcab.2015.04.002
Kumar, V., Pathak, D., Dudeja, S. S., Singh, R., Giri, R., Narula, S., et al. (2013). Legume nodule endophytes more diverse than endophytes from roots of legumes or non legumes in soils of Haryana, India. J. Microbiol. Biotech. Res 3, 83–92,
Lai, W.-A., Asif, A., Lin, S.-Y., Hung, M.-H., Hsu, Y.-H., Liu, Y.-C., et al. (2015). Paenibacillus medicaginis sp. Nov. a chitinolytic endophyte isolated from a root nodule of alfalfa (Medicago sativa l.). Int. J. Syst. Evol. Microbiol. 65, 3853–3860. doi: 10.1099/ijsem.0.000505
Lavakush, Y., Yadav, J., Verma, J. P., Jaiswal, D. K., and Kumar, A. (2014). Evaluation of PGPR and different concentration of phosphorus level on plant growth, yield and nutrient content of rice (Oryza sativa). Ecol. Eng. 62, 123–128. doi: 10.1016/j.ecoleng.2013.10.013
Lebrazi, S., Fadil, M., Chraibi, M., and Fikri-Benbrahim, K. (2023). Phenotypic, molecular, and symbiotic characterization of the rhizobial symbionts isolated from Acacia saligna grown in different regions in Morocco: a multivariate approach. World J. Microbiol. Biotechnol. 39:343. doi: 10.1007/s11274-023-03775-1
Leite, J., Fischer, D., Rouws, L., Fernandes Júnior, P. I., Hofmann, A., Kublik, S., et al. (2017). Cowpea Nodules Harbor non-rhizobial bacterial communities that are shaped by soil type rather than plant genotype. Front. Plant Sci. 7:2064. doi: 10.3389/fpls.2016.02064
Liberati, A., Altman, D. G., Tetzlaff, J., Mulrow, C., Gøtzsche, P. C., Ioannidis, J. P. A., et al. (2009). The PRISMA statement for reporting systematic reviews and meta-analyses of studies that evaluate health care interventions: explanation and elaboration. PLoS Med. 6:e1000100. doi: 10.1371/journal.pmed.1000100
Li, M., Li, Y., Chen, W. F., Sui, X. H., Li, Y., Li, Y., et al. (2012). Genetic diversity, community structure and distribution of rhizobia in the root nodules of Caragana spp. from arid and semi-arid alkaline deserts, in the north of China. Syst. Appl. Microbiol. 35, 239–245. doi: 10.1016/j.syapm.2012.02.004
Litskas, V. D. (2023). Environmental impact assessment for animal waste, organic and synthetic fertilizers. Nitrogen 4, 16–25. doi: 10.3390/nitrogen4010002
Maheshwari, R., Bhutani, N., Bhardwaj, A., and Suneja, P. (2019). Functional diversity of cultivable endophytes from Cicer arietinum and Pisum sativum: bioprospecting their plant growth potential. Biocatal. Agric. Biotechnol. 20:101229. doi: 10.1016/j.bcab.2019.101229
Makarova, L. E., Markova, Y. A., Morits, A. S., Karepova, M. S., Sidorov, A. V., and Sokolova, N. A. (2021). Interaction of Rhizospheric Bacteria and Nonrhizobial endophytic Bacteria moving from the roots to the rhizosphere of pea plants (Pisum sativum). Appl. Biochem. Microbiol. 57, 514–520. doi: 10.1134/S0003683821040104
Mantelin, S., Saux, M. F.-L., Zakhia, F., Béna, G., Bonneau, S., Jeder, H., et al. (2006). Emended description of the genus Phyllobacterium and description of four novel species associated with plant roots: Phyllobacterium bourgognense sp. nov., Phyllobacterium ifriqiyense sp. nov., Phyllobacterium leguminum sp. nov. and Phyllobacterium brassicacearum sp. nov. Int. J. Syst. Evol. Microbiol. 56, 827–839. doi: 10.1099/ijs.0.63911-0
Martínez-Hidalgo, P., Galindo-Villardón, P., Igual, J. M., and Martínez-Molina, E. (2014). Micromonospora from nitrogen fixing nodules of alfalfa (Medicago sativa L.). A new promising plant probiotic Bacteria. Sci. Rep. 4:6389. doi: 10.1038/srep06389
Martínez-Hidalgo, P., and Hirsch, A. M. (2017). The nodule microbiome: N2-fixing rhizobia do not live alone. Phytobiomes J. 1, 70–82. doi: 10.1094/PBIOMES-12-16-0019-RVW
Martínez-Hidalgo, P., Humm, E., Still, D., Shi, B., Pellegrini, M., Roca, G., et al. (2022). Medicago root nodule microbiomes: insights into a complex ecosystem with potential candidates for plant growth promotion. Plant Soil 471, 507–526. doi: 10.1007/s11104-021-05247-7
Mayhood, P., and Mirza, B. S. (2021). Soybean root nodule and rhizosphere microbiome: distribution of Rhizobial and Nonrhizobial endophytes. Appl. Environ. Microbiol. 87, e02884–e02820. doi: 10.1128/AEM.02884-20
McElveen, S. D. (2019). Characterizing nodule endophyte communities in Glycine max and Lablab Purpureus using next-generation sequencing. Springfield: Missouri State University.
Menéndez, E., and Paço, A. (2020). Is the application of plant probiotic bacterial consortia always beneficial for plants? Exploring synergies between Rhizobial and non-Rhizobial Bacteria and their effects on agro-economically valuable crops. Life 10:24. doi: 10.3390/life10030024
Menéndez, E., Ramírez-Bahena, M.-H., Carro, L., Fernández-Pascual, M., Peter Klenk, H., Velázquez, E., et al. (2016). Paenibacillus periandrae sp. nov., isolated from nodules of Periandra mediterranea. Int. J. Syst. Evol. Microbiol. 66, 1838–1843. doi: 10.1099/ijsem.0.000953
Minaxi, N., Nain, L., Yadav, R. C., and Saxena, J. (2012). Characterization of multifaceted Bacillus sp. RM-2 for its use as plant growth promoting bioinoculant for crops grown in semi arid deserts. Appl. Soil Ecol. 59, 124–135. doi: 10.1016/j.apsoil.2011.08.001
Mir, M. I., Kumar, B. K., Gopalakrishnan, S., Vadlamudi, S., and Hameeda, B. (2021). Characterization of rhizobia isolated from leguminous plants and their impact on the growth of ICCV 2 variety of chickpea (Cicer arietinum L.). Heliyon 7:e08321. doi: 10.1016/j.heliyon.2021.e08321
Mnasri, B., Saïdi, S., Chihaoui, S.-A., and Mhamdi, R. (2012). Sinorhizobium americanum symbiovar mediterranense is a predominant symbiont that nodulates and fixes nitrogen with common bean (Phaseolus vulgaris L.) in a northern Tunisian field. Syst. Appl. Microbiol. 35, 263–269. doi: 10.1016/j.syapm.2012.04.003
Moulin, L., Béna, G., Boivin-Masson, C., and Stępkowski, T. (2004). Phylogenetic analyses of symbiotic nodulation genes support vertical and lateral gene co-transfer within the Bradyrhizobium genus. Mol. Phylogenet. Evol. 30, 720–732. doi: 10.1016/S1055-7903(03)00255-0
Moulin, L., Munive, A., Dreyfus, B., and Boivin-Masson, C. (2001). Nodulation of legumes by members of the β-subclass of Proteobacteria. Nature 411, 948–950. doi: 10.1038/35082070
Muindi, M. M., Muthini, M., Njeru, E. M., and Maingi, J. (2021). Symbiotic efficiency and genetic characterization of rhizobia and non rhizobial endophytes associated with cowpea grown in semi-arid tropics of Kenya. Heliyon 7:e06867. doi: 10.1016/j.heliyon.2021.e06867
Mukhtar, S., Hirsch, A. M., Khan, N., Malik, K. A., Humm, E. A., Pellegrini, M., et al. (2020). Impact of soil salinity on the cowpea nodule-microbiome and the isolation of halotolerant PGPR strains to promote plant growth under salinity stress. Phytobiomes J. 4, 364–374. doi: 10.1094/PBIOMES-09-19-0057-R
Muresu, R., Polone, E., Sulas, L., Baldan, B., Tondello, A., Delogu, G., et al. (2008). Coexistence of predominantly nonculturable rhizobia with diverse, endophytic bacterial taxa within nodules of wild legumes. FEMS Microbiol. Ecol. 63, 383–400. doi: 10.1111/j.1574-6941.2007.00424.x
Muresu, R., Porceddu, A., Sulas, L., and Squartini, A. (2019). Nodule-associated microbiome diversity in wild populations of Sulla coronaria reveals clues on the relative importance of culturable rhizobial symbionts and co-infecting endophytes. Microbiol. Res. 221, 10–14. doi: 10.1016/j.micres.2019.01.004
Mwenda, G. M., Hill, Y. J., O’Hara, G. W., Reeve, W. G., Howieson, J. G., and Terpolilli, J. J. (2023). Competition in the Phaseolus vulgaris-Rhizobium symbiosis and the role of resident soil rhizobia in determining the outcomes of inoculation. Plant Soil 487, 61–77. doi: 10.1007/s11104-023-05903-0
Novello, G., Gamalero, E., Massa, N., Cesaro, P., Lingua, G., Todeschini, V., et al. (2022). Proteome and physiological characterization of halotolerant nodule endophytes: the case of Rahnella aquatilis and Serratia plymuthica. Microorganisms 10:890. doi: 10.3390/microorganisms10050890
Oliveira Silva, A., Azarias Guimarães, A., da Costa, A. M., Louzada Rodrigues, T., de Soares Carvalho, T., Reis Sales, F., et al. (2020). Plant growth-promoting rhizobacterial communities from an area under the influence of iron mining and from the adjacent phytophysiognomies which have high genetic diversity. Land Degrad. Dev. 31, 2237–2254. doi: 10.1002/ldr.3593
Palaniappan, P., Chauhan, P. S., Saravanan, V. S., Anandham, R., and Sa, T. (2010). Isolation and characterization of plant growth promoting endophytic bacterial isolates from root nodule of Lespedeza sp. Biol. Fertil. Soils 46, 807–816. doi: 10.1007/s00374-010-0485-5
Pandya, M., Naresh Kumar, G., and Rajkumar, S. (2013). Invasion of rhizobial infection thread by non-rhizobia for colonization of Vigna radiata root nodules. FEMS Microbiol. Lett. 348, 58–65. doi: 10.1111/1574-6968.12245
Pedraza, R. O., Bellone, C. H., Carrizo de Bellone, S., Boa Sorte, P. M. F., and Teixeira, K. R. S. (2009). Azospirillum inoculation and nitrogen fertilization effect on grain yield and on the diversity of endophytic bacteria in the phyllosphere of rice rainfed crop. Eur. J. Soil Biol. 45, 36–43. doi: 10.1016/j.ejsobi.2008.09.007
Peix, A., Ramírez-Bahena, M. H., Velázquez, E., and Bedmar, E. J. (2015). Bacterial associations with legumes. Crit. Rev. Plant Sci. 34, 17–42. doi: 10.1080/07352689.2014.897899
Powers, S. E., and Thavarajah, D. (2019). Checking Agriculture’s pulse: field pea (Pisum sativum L.), sustainability, and phosphorus use efficiency. Front. Plant Sci. 10:1489. doi: 10.3389/fpls.2019.01489
Preyanga, R., Anandham, R., Krishnamoorthy, R., Senthilkumar, M., Gopal, N. O., Vellaikumar, A., et al. (2021). Groundnut (Arachis hypogaea) nodule Rhizobium and passenger endophytic bacterial cultivable diversity and their impact on plant growth promotion. Rhizosphere 17:100309. doi: 10.1016/j.rhisph.2021.100309
Primieri, S., Dalla Costa, M., Stroschein, M. R. D., Stocco, P., Santos, J. C. P., and Antunes, P. M. (2016). Variability in symbiotic effectiveness of N2 fixing bacteria in Mimosa scabrella. Appl. Soil Ecol. 102, 19–25. doi: 10.1016/j.apsoil.2016.01.018
Pulido-Suárez, L., Flores-Félix, J. D., Socas-Pérez, N., Igual, J. M., Velázquez, E., Péix, Á., et al. (2022). Endophytic Bosea spartocytisi sp. nov. coexists with rhizobia in root nodules of Spartocytisus supranubius growing in soils of Teide National Park (Canary Islands). Syst. Appl. Microbiol. 45:126374. doi: 10.1016/j.syapm.2022.126374
Puri, A., Padda, K. P., and Chanway, C. P. (2018). “Nitrogen-fixation by endophytic bacteria in agricultural crops: recent advances” in Nitrogen in agriculture. ed. S. Fahad (London: IntechOpen), 73–94.
Rahal, S., and Chekireb, D. (2021). Diversity of rhizobia and non-rhizobia endophytes isolated from root nodules of Trifolium sp. growing in lead and zinc mine site Guelma, Algeria. Arch. Microbiol. 203, 3839–3849. doi: 10.1007/s00203-021-02362-y
Raja, S. R. T., and Uthandi, S. (2019). Non-rhizobial nodule associated bacteria (NAB) from blackgram (Vigna mungo L.) and their possible role in plant growth promotion. Madras Agric. J. 106:1,
Rajendran, G., Patel, M. H., and Joshi, S. J. (2012). Isolation and characterization of nodule-associated Exiguobacterium sp. from the root nodules of fenugreek (Trigonella foenum-graecum) and their possible role in plant growth promotion. Int. J. Microbiol. 2012:693982. doi: 10.1155/2012/693982
Rajendran, G., Sing, F., Desai, A. J., and Archana, G. (2008). Enhanced growth and nodulation of pigeon pea by co-inoculation of Bacillus strains with Rhizobium spp. Bioresour. Technol. 99, 4544–4550. doi: 10.1016/j.biortech.2007.06.057
Ramesh, A., Sharma, S. K., Sharma, M. P., Yadav, N., and Joshi, O. P. (2014). Inoculation of zinc solubilizing Bacillus aryabhattai strains for improved growth, mobilization and biofortification of zinc in soybean and wheat cultivated in Vertisols of Central India. Appl. Soil Ecol. 73, 87–96. doi: 10.1016/j.apsoil.2013.08.009
Ramírez-Bahena, M. H., Tejedor, C., Martín, I., Velázquez, E., and Peix, A. (2013). Endobacter medicaginis gen. Nov., sp. nov., isolated from alfalfa nodules in an acidic soil. Int. J. Syst. Evol. Microbiol. 63, 1760–1765. doi: 10.1099/ijs.0.041368-0
Rana, K. L., Kour, D., Yadav, A. N., Yadav, N., and Saxena, A. K. (2020). “Agriculturally important microbial biofilms: biodiversity, ecological significances, and biotechnological applications” in New and future developments in microbial biotechnology and bioengineering: Microbial biofilms. eds. M. K. Yadav and B. P. Singh (Amsterdam, Netherlands: Elsevier), 221–265.
Ravunni, M., and Yusuf, A. (2022). Isolation, characterization and phylogenetic analysis of nodule-associated Bacteria from Mimosa pudica L. Biosci. Biotechnol. Res. Asia 19, 645–655. doi: 10.13005/bbra/3017
Rejii, M., Mahdhi, M., Domínguez-Núñez, J., and Mars, M. (2014). The phenotypic, phylogenetic and symbiotic characterization of rhizobia nodulating Lotus sp in Tunisian arid soils. Ann. Microbiol. 64, 355–362. doi: 10.1007/s13213-013-0670-5
Reverchon, F., Abdullah, K. M., Bai, S. H., Villafán, E., Blumfield, T. J., Patel, B., et al. (2020). Biological nitrogen fixation by two Acacia species and associated root-nodule bacteria in a suburban Australian forest subjected to prescribed burning. J. Soils Sediments 20, 122–132. doi: 10.1007/s11368-019-02446-9
Rincón-Rosales, R., Lloret, L., Ponce, E., and Martínez-Romero, E. (2009). Rhizobia with different symbiotic efficiencies nodulate Acaciella angustissima in Mexico, including Sinorhizobium chiapanecum sp. nov. which has common symbiotic genes with Sinorhizobium mexicanum. FEMS Microbiol. Ecol. 67, 103–117. doi: 10.1111/j.1574-6941.2008.00590.x
Ríos-Ruiz, W. F., Valdez-Nuñez, R. A., Bedmar, E. J., and Castellano-Hinojosa, A. (2019). “Utilization of endophytic Bacteria isolated from legume root nodules for plant growth promotion” in Field crops: Sustainable management by PGPR. eds. D. K. Maheshwari and S. Dheeman (Cham: Springer International Publishing), 145–176.
Rodríguez-Rodríguez, R. M., Guimarães, A. A., De Castro, J. L., Siqueira, J. O., Carneiro, M. A. C., and De Souza Moreira, F. M. (2021). Rhizobia and endophytic bacteria isolated from rainforest fragments within an iron ore mining site of the eastern Brazilian Amazon. Braz. J. Microbiol. 52, 1461–1474. doi: 10.1007/s42770-021-00524-0
Roldán, D. M., Costa, A., Králová, S., Busse, H.-J., Amarelle, V., Fabiano, E., et al. (2022). Paenibacillus farraposensis sp. nov., isolated from a root nodule of Arachis villosa. Int. J. Syst. Evol. Microbiol. 72:005294. doi: 10.1099/ijsem.0.005294
Ruiz-Díez, B., Fajardo, S., Puertas-Mejía, M. A., De Felipe, M., and Fernández-Pascual, M. (2009). Stress tolerance, genetic analysis and symbiotic properties of root-nodulating bacteria isolated from Mediterranean leguminous shrubs in Central Spain. Arch. Microbiol. 191, 35–46. doi: 10.1007/s00203-008-0426-y
Safronova, V. I., Kuznetsova, I. G., Sazanova, A. L., Kimeklis, A. K., Belimov, A. A., Andronov, E. E., et al. (2015). Bosea vaviloviae sp. nov., a new species of slow-growing rhizobia isolated from nodules of the relict species Vavilovia formosa (Stev.) fed. Anton. Leeuw. Int. J. Gen. Mol. Microbiol. 107, 911–920. doi: 10.1007/s10482-015-0383-9
Saïdi, S., Chebil, S., Gtari, M., and Mhamdi, R. (2013). Characterization of root-nodule bacteria isolated from Vicia faba and selection of plant growth promoting isolates. World J. Microbiol. Biotechnol. 29, 1099–1106. doi: 10.1007/s11274-013-1278-4
Saïdi, S., Mnasri, B., and Mhamdi, R. (2011). Diversity of nodule-endophytic agrobacteria-like strains associated with different grain legumes in Tunisia. Syst. Appl. Microbiol. 34, 524–530. doi: 10.1016/j.syapm.2011.01.009
Saini, R., Dudeja, S. S., Giri, R., and Kumar, V. (2015). Isolation, characterization, and evaluation of bacterial root and nodule endophytes from chickpea cultivated in northern India. J. Basic Microbiol. 55, 74–81. doi: 10.1002/jobm.201300173
Sánchez-Cruz, R., Vázquez, I. T., Batista-García, R. A., Méndez-Santiago, E. W., Del Rayo Sanchez-Carbente, M., Leija, A., et al. (2019). Isolation and characterization of endophytes from nodules of Mimosa pudica with biotechnological potential. Microbiol. Res. 218, 76–86. doi: 10.1016/j.micres.2018.09.008
Santos, A. A., Silveira, J. A. G., Bonifacio, A., Rodrigues, A. C., and Figueiredo, M. V. B. (2018). Antioxidant response of cowpea co-inoculated with plant growth-promoting bacteria under salt stress. Braz. J. Microbiol. 49, 513–521. doi: 10.1016/j.bjm.2017.12.003
Sazanova, A. L., Safronova, V. I., Kuznetsova, I. G., Karlov, D. S., Belimov, A. A., Andronov, E. E., et al. (2019). Bosea caraganae sp. Nov. a new species of slow-growing bacteria isolated from root nodules of the relict species Caragana jubata (pall.) poir. Originating from Mongolia. Int. J. Syst. Evol. Microbiol. 69, 2687–2695. doi: 10.1099/ijsem.0.003509
Sbabou, L. (2016). Characterization of root-nodule Bacteria isolated from Hedysarum spinosissimum L, growing in mining sites of northeastern region of Morocco. SOJMID 4, 1–8. doi: 10.15226/sojmid/4/3/00156
Schindler, D. W., and Hecky, R. E. (2009). Eutrophication: more nitrogen data needed. Science 324, 721–722. doi: 10.1126/science.324_721b
Selvakumar, G., Kundu, S., Gupta, A. D., Shouche, Y. S., and Gupta, H. S. (2008). Isolation and characterization of Nonrhizobial plant growth promoting Bacteria from nodules of kudzu (Pueraria thunbergiana) and their effect on wheat seedling growth. Curr. Microbiol. 56, 134–139. doi: 10.1007/s00284-007-9062-z
Sharaf, H., Rodrigues, R. R., Moon, J., Zhang, B., Mills, K., and Williams, M. A. (2019). Unprecedented bacterial community richness in soybean nodules vary with cultivar and water status. Microbiome 7, 1–18. doi: 10.1186/s40168-019-0676-8
Soares, R., Trejo, J., Lorite, M. J., Figueira, E., Sanjuán, J., and Castro, I. V. E. (2020). Diversity, phylogeny and plant growth promotion traits of nodule associated Bacteria isolated from Lotus parviflorus. Microorganisms 8:499. doi: 10.3390/microorganisms8040499
Spence, C., and Bais, H. (2015). Role of plant growth regulators as chemical signals in plant–microbe interactions: a double edged sword. Curr. Opin. Plant Biol. 27, 52–58. doi: 10.1016/j.pbi.2015.05.028
Srt, R., Thangappan, S., and Uthandi, S. (2019). Non-Rhizobial nodule associated Bacteria (NAB) from Blackgram (Vigna mungo L.) and their possible role in plant growth promotion. Madras. Agric. J. 106:321. doi: 10.29321/MAJ
Stagnari, F., Maggio, A., Galieni, A., and Pisante, M. (2017). Multiple benefits of legumes for agriculture sustainability: an overview. Chem. Biol. Technol. Agric. 4:2. doi: 10.1186/s40538-016-0085-1
Strobel, G., and Daisy, B. (2003). Bioprospecting for microbial endophytes and their natural products. Microbiol. Mol. Biol. Rev. 67, 491–502. doi: 10.1128/MMBR.67.4.491-502.2003
Sturz, A. V., Christie, B. R., Matheson, B. G., and Nowak, J. (1997). Biodiversity of endophytic bacteria which colonize red clover nodules, roots, stems and foliage and their influence on host growth. Biol. Fertil. Soils 25, 13–19. doi: 10.1007/s003740050273
Subramanian, P., Kim, K., Krishnamoorthy, R., Sundaram, S., and Sa, T. (2015). Endophytic bacteria improve nodule function and plant nitrogen in soybean on co-inoculation with Bradyrhizobium japonicum MN110. Plant Growth Regul. 76, 327–332. doi: 10.1007/s10725-014-9993-x
Sun, R., Crowley, D. E., and Wei, G. (2015). Study of phenanthrene utilizing bacterial consortia associated with cowpea (Vigna unguiculata) root nodules. World J. Microbiol. Biotechnol. 31, 415–433. doi: 10.1007/s11274-014-1796-8
Taoufiq, K., Faghire, M. S. T., Nejmeddine, M., Göttfert, M., and Oufdou, K.. (2018). Screening and molecular identification of endophytic Bacteria isolated from legumes nodules and roots cultivated in Acacia rhizosphere soils collected in an arid region, Tata-Akka in south of Morocco address for correspondence.
Tariq, M., Hameed, S., Yasmeen, T., Zahid, M., and Zafar, M. (2014). Molecular characterization and identification of plant growth promoting endophytic bacteria isolated from the root nodules of pea (Pisum sativum L.). World J. Microbiol. Biotechnol. 30, 719–725. doi: 10.1007/s11274-013-1488-9
Tokgöz, S., Lakshman, D. K., Ghozlan, M. H., Pinar, H., Roberts, D. P., and Mitra, A. (2020). Soybean nodule-associated non-Rhizobial Bacteria inhibit plant pathogens and induce growth promotion in tomato. Plan. Theory 9:1494. doi: 10.3390/plants9111494
Trabelsi, D., Chihaoui, S.-A., and Mhamdi, R. (2017). Nodules and roots of Vicia faba are inhabited by quite different populations of associated bacteria. Appl. Soil Ecol. 119, 72–79. doi: 10.1016/j.apsoil.2017.06.002
Trujillo, M. E., Alonso-Vega, P., Rodríguez, R., Carro, L., Cerda, E., Alonso, P., et al. (2010). The genus Micromonospora is widespread in legume root nodules: the example of Lupinus angustifolius. ISME J. 4, 1265–1281. doi: 10.1038/ismej.2010.55
Trujillo, M. E., Kroppenstedt, R. M., Fernández-Molinero, C., Schumann, P., and Martínez-Molina, E. (2007). Micromonospora lupini sp. nov. and Micromonospora saelicesensis sp. nov., isolated from root nodules of Lupinus angustifolius. Int. J. Syst. Evol. Microbiol. 57, 2799–2804. doi: 10.1099/ijs.0.65192-0
Vaishnav, A., Shukla, A. K., Sharma, A., Kumar, R., and Choudhary, D. K. (2019). Endophytic Bacteria in plant salt stress tolerance: current and future prospects. J. Plant Growth Regul. 38, 650–668. doi: 10.1007/s00344-018-9880-1
Valverde, A. (2003). Herbaspirillum lusitanum sp. nov., a novel nitrogen-fixing bacterium associated with root nodules of Phaseolus vulgaris. Int. J. Syst. Evol. Microbiol. 53, 1979–1983. doi: 10.1099/ijs.0.02677-0
Van Eck, N. J., and Waltman, L. (2011). Text mining and visualization using VOSviewer. ISSI Newslett. 7, 50–54. doi: 10.48550/arXiv.1109.2058
Vasileva, E. N., Akhtemova, G. A., Zhukov, V. A., and Tikhonovich, I. A. (2019). Endophytic microorganisms in fundamental research and agriculture. Ecol. Genet. 17, 19–32. doi: 10.17816/ecogen17119-32
Velázquez, E., Martínez-Hidalgo, P., Carro, L., Alonso, P., Peix, Á., Trujillo, M., et al. (2013). “Nodular endophytes: an untapped diversity” in Beneficial plant-microbial interactions. ed. J. González-López (Boca Raton, Florida: CRC Press), 215–236.
Verástegui-Valdés, M. M., Zhang, Y. J., Rivera-Orduña, F. N., Cheng, H.-P., Sui, X. H., and Wang, E. T. (2014). Microsymbionts of Phaseolus vulgaris in acid and alkaline soils of Mexico. Syst. Appl. Microbiol. 37, 605–612. doi: 10.1016/j.syapm.2014.08.005
Vincent, J. M. (1970). A manual for the practical study of the root-nodule bacteria. Man. Pract. Study Root-Nodule Bact. Available at: https://www.cabdirect.org/cabdirect/abstract/19710700726
Wang, S., Wang, W., Jin, Z., Du, B., Ding, Y., Ni, T., et al. (2013). Screening and diversity of plant growth promoting endophytic bacteria from peanut. Afr. J. Microbiol. Res. 7, 875–884. doi: 10.5897/AJMR12.1500
Wei, X. L., Han, M. S., Xia, C. C., Ding, S. L., Xu, L., Lin, Y. B., et al. (2015a). Diaphorobacter ruginosibacter sp. nov., isolated from soybean root nodule, and emended description of the genus Diaphorobacter. Arch. Microbiol. 197, 683–692. doi: 10.1007/s00203-015-1102-7
Wei, X. L., Lin, Y. B., Xu, L., Han, M. S., Dong, D. H., Chen, W. M., et al. (2015b). Bacillus radicibacter sp. nov., a new bacterium isolated from root nodule of Oxytropis ochrocephala Bunge. J. Basic Microbiol. 55, 1212–1218. doi: 10.1002/jobm.201400940
Wigley, K., Moot, D., Wakelin, S., Laugraud, A., Blond, C., Seth, K., et al. (2017). Diverse bacterial taxa inhabit root nodules of lucerne (Medicago sativa L.) in New Zealand pastoral soils. Plant Soil 420, 253–262. doi: 10.1007/s11104-017-3395-6
Xiao, X., Chen, W., Zong, L., Yang, J., Jiao, S., Lin, Y., et al. (2017). Two cultivated legume plants reveal the enrichment process of the microbiome in the rhizocompartments. Mol. Ecol. 26, 1641–1651. doi: 10.1111/mec.14027
Xia, Y., Liu, J., Chen, C., Mo, X., Tan, Q., He, Y., et al. (2022). The multifunctions and future prospects of endophytes and their metabolites in plant disease management. Microorganisms 10:1072. doi: 10.3390/microorganisms10051072
Xu, L., Zhang, Y., Li, C., Wang, X., Liu, J., and Friman, V.-P. (2018). Nocardioides astragali sp. nov., isolated from a nodule of wild Astragalus chrysopterus in Northwestern China. Anton. Leeuw. Int. J. Gen. Mol. Microbiol. 111, 1157–1163. doi: 10.1007/s10482-018-1020-1
Xu, L., Zhang, Y., Wang, L., Chen, W., and Wei, G. (2014). Diversity of endophytic bacteria associated with nodules of two indigenous legumes at different altitudes of the Qilian Mountains in China. Syst. Appl. Microbiol. 37, 457–465. doi: 10.1016/j.syapm.2014.05.009
Yan, J., Li, Y., Han, X. Z., Chen, W. F., Zou, W. X., Xie, Z., et al. (2017a). Agrobacterium deltaense sp. nov., an endophytic bacteria isolated from nodule of Sesbania cannabina. Arch. Microbiol. 199, 1003–1009. doi: 10.1007/s00203-017-1367-0
Yan, J., Li, Y., Yan, H., Chen, W. F., Zhang, X., Wang, E. T., et al. (2017b). Agrobacterium salinitolerans sp. nov., a saline–alkaline-tolerant bacterium isolated from root nodule of Sesbania cannabina. Int. J. Syst. Evol. Microbiol. 67, 1906–1911. doi: 10.1099/ijsem.0.001885
Yasmin, S., Zaka, A., Imran, A., Zahid, M. A., Yousaf, S., Rasul, G., et al. (2016). Plant growth promotion and suppression of bacterial leaf blight in Rice by inoculated Bacteria. PLoS One 11:e0160688. doi: 10.1371/journal.pone.0160688
Yeboah, S., Owusu Danquah, E., Oteng-Darko, P., Agyeman, K., and Tetteh, E. N. (2021). Carbon smart strategies for enhanced food system resilience under a changing climate. Front. Sustain. Food Syst. 5:715814. doi: 10.3389/fsufs.2021.715814
Yin, J., He, D., Li, X., Zeng, X., Tian, M., and Cheng, G. (2015). Paenibacillus enshidis sp. nov., isolated from the nodules of Robinia pseudoacacia L. Curr. Microbiol. 71, 321–325. doi: 10.1007/s00284-015-0854-2
Youseif, S. H., Abd El-Megeed, F. H., Abdelaal, A. S., Ageez, A., and Martínez-Romero, E. (2021). Plant-microbe-microbe interactions influence the faba bean nodule colonization by diverse endophytic bacteria. FEMS Microbiol. Ecol. 97:138. doi: 10.1093/femsec/fiab138
Youseif, S. H., Abd El-Megeed, F. H., Khalifa, M. A., and Saleh, S. A. (2014). Symbiotic effectiveness of Rhizobium (Agrobacterium) compared to Ensifer (Sinorhizobium) and Bradyrhizobium genera for soybean inoculation under field conditions. Res. J. Microbiol. 9, 151–162. doi: 10.3923/jm.2014.151.162
Zaheer, A., Mirza, B. S., Mclean, J. E., Yasmin, S., Shah, T. M., Malik, K. A., et al. (2016). Association of plant growth-promoting Serratia spp. with the root nodules of chickpea. Res. Microbiol. 167, 510–520. doi: 10.1016/j.resmic.2016.04.001
Zakhia, F., Jeder, H., Willems, A., Gillis, M., Dreyfus, B., and De Lajudie, P. (2006). Diverse bacteria associated with root nodules of spontaneous legumes in Tunisia and first report for nifH-like gene within the genera Microbacterium and Starkeya. Microb. Ecol. 51, 375–393. doi: 10.1007/s00248-006-9025-0
Zgadzaj, R., James, E. K., Kelly, S., Kawaharada, Y., De Jonge, N., Jensen, D. B., et al. (2015). A legume genetic framework controls infection of nodules by symbiotic and endophytic Bacteria. PLoS Genet. 11:e1005280. doi: 10.1371/journal.pgen.1005280
Zhang, B., Du, N., Li, Y., Shi, P., and Wei, G. (2018). Distinct biogeographic patterns of rhizobia and non-rhizobial endophytes associated with soybean nodules across China. Sci. Total Environ. 643, 569–578. doi: 10.1016/j.scitotenv.2018.06.240
Zhang, Y., Chen, Y., Penttinen, P., Wang, X., Quan, Y., Wen, L., et al. (2024). Ciceribacter sichuanensis sp. nov., a plant growth promoting rhizobacterium isolated from root nodules of soybean in Sichuan, China. Anton. Leeuw. Int. J. Gen. Mol. Microbiol. 117, 1–10. doi: 10.1007/s10482-024-01941-5
Zhang, Y. Z., Wang, E. T., Li, M., Li, Q. Q., Zhang, Y. M., Zhao, S. J., et al. (2011). Effects of rhizobial inoculation, cropping systems and growth stages on endophytic bacterial community of soybean roots. Plant Soil 347, 147–161. doi: 10.1007/s11104-011-0835-6
Zhao, L., Xu, Y., and Lai, X. (2018). Antagonistic endophytic bacteria associated with nodules of soybean (Glycine max L.) and plant growth-promoting properties. Braz. J. Microbiol. 49, 269–278. doi: 10.1016/j.bjm.2017.06.007
Złoch, M., Thiem, D., Gadzała-Kopciuch, R., and Hrynkiewicz, K. (2016). Synthesis of siderophores by plant-associated metallotolerant bacteria under exposure to Cd2+. Chemosphere 156, 312–325. doi: 10.1016/j.chemosphere.2016.04.130
Keywords: non-rhizobial endophytic bacteria, legumes, diversity, PGP properties, coinoculation
Citation: Hnini M and Aurag J (2024) Prevalence, diversity and applications potential of nodules endophytic bacteria: a systematic review. Front. Microbiol. 15:1386742. doi: 10.3389/fmicb.2024.1386742
Edited by:
Esther Menendez, University of Salamanca, SpainReviewed by:
José David Flores Félix, University of Salamanca, SpainAhmed Idris Hassen, Agricultural Research Council of South Africa (ARC-SA), South Africa
Copyright © 2024 Hnini and Aurag. This is an open-access article distributed under the terms of the Creative Commons Attribution License (CC BY). The use, distribution or reproduction in other forums is permitted, provided the original author(s) and the copyright owner(s) are credited and that the original publication in this journal is cited, in accordance with accepted academic practice. No use, distribution or reproduction is permitted which does not comply with these terms.
*Correspondence: Jamal Aurag, YXVyYWdqYW1hbEB5YWhvby5mcg==