- 1College of Life Sciences and Food Engineering, Inner Mongolia Minzu University, Tongliao, China
- 2Affiliated Hospital of Inner Mongolia Minzu University, Tongliao, China
Major depressive disorder, schizophrenia, and bipolar disorder are three major psychiatric disorders that significantly impact the well-being and overall health of patients. Some researches indicate that abnormalities in the gut microbiota can trigger certain psychiatric diseases. Microbiota-derived extracellular vesicles have the ability to transfer bioactive compounds into host cells, altering signaling and biological processes, ultimately influencing the mental health and illness of the host. This review aims to investigate the emerging roles of microbiota-derived extracellular vesicles in these three major psychiatric disorders and discusses their roles as diagnostic biomarkers and therapies for these psychiatric disorders.
Introduction
The human gut microbiota contains between 1,000 and 5,000 distinct species, including bacteria, viruses, and fungi. It constitutes the largest micro-ecosystem in the human body (Cryan et al., 2019). An increasing number of evidences indicated that the microbiota plays an important role in regulating the physiological functions, well-being, and the onset of diseases in the host (Fung et al., 2017; Gentile and Weir, 2018; Gomaa, 2020; Lai et al., 2020; Chen et al., 2021b). Moreover, gut bacteria play crucial roles in the production of neurotransmitters such as serotonin and dopamine, as well as neuroactive compounds that can impact brain function and behavior (Sampson and Mazmanian, 2015; Mittal et al., 2017; Chen et al., 2021a). The “gut-brain axis” (GBA) refers to the bidirectional communication system that links the gastrointestinal tract with the central nervous system through many pathways (Ma et al., 2019). These pathways include the hypothalamic–pituitary–adrenal axis, the vagal nerve, the production of bacterial metabolites, immunological mediators, and entero-endocrine signaling (Cryan et al., 2019; Dalile et al., 2019; Golofast and Vales, 2020).
The term “psychiatric disorder” encompasses a wide range of mental health conditions. Major depressive disorder (MDD), schizophrenia (SCZ), and bipolar disorder (BD) are commonly observed psychiatric disorders that have a considerable number of shared hereditary characteristics (Brainstorm Consortium et al., 2018). Presently, a prevailing consensus regards them as distinct points along a spectrum of clinical presentations instead of being entirely separate and independent diseases (Haarman et al., 2016). MDD, SCZ, and BD have the potential to significantly impair an individual’s daily function and cause distress for both the affected person and their social network. The precise causes of these disorders remain largely unclear, and they are often characterized by complex and multifactorial factors, such as genetic, environmental, inflammatory, and psychological elements (Ouabbou et al., 2020).
A growing body of research indicates a potential direct correlation between the gut microbiota and psychiatric disorders (Misiak et al., 2020; Fan and Pedersen, 2021; Mitrea et al., 2022). The establishment of efficient communication between the gastrointestinal tract and the central nervous system is contingent upon the presence of a biodiverse and well-balanced microbiota. The dysregulation of the gut microbiota has been implicated in the etiology and advancement of psychiatric disorders (Petra et al., 2015; Vuong and Hsiao, 2017; Li et al., 2022; Rathour et al., 2023). Psychiatric disorders have the possibility of disrupting the regulation of the GBA. For example, those who frequently suffer from sadness or anxiety exhibit a different composition of gut bacteria when compared to healthy controls (HCs; Peirce and Alviña, 2019). Though a nascent area of research, there is already a significant level of scholarly attention directed toward this field.
Microbiota-derived extracellular vesicles (MEVs) are small phospholipid vesicles secreted by the microbiota that encapsulate a diverse array of biologically active compounds, including proteins, mRNA, miRNA, DNA, carbohydrates, and lipids. MEVs provide protection for the secreted compounds against ribonucleases and lytic enzymes present in the extracellular environment (Al-Nedawi et al., 2015; Sultan et al., 2021). MEVs emerge as significant entities within the intercellular signaling system, potentially serving as vital mediators in microbiota-host communication. MEVs can traverse the blood–brain barrier (BBB) and other tissue barriers to interact with immunological receptors on glial cells inducing the release of cytokines and inflammatory mediators (Cuesta et al., 2021).
In this review, we will firstly discuss the association of the gut microbiota with three major psychiatric disorders. Then we will introduce MEVs and examine the various channels through which they communicate with the host brain. Furthermore, we will investigate the emerging roles of MEVs in the three major psychiatric disorders, along with the ongoing studies conducted in relevant fields. Finally, we will also discuss the diagnostic potential of MEVs and their therapeutic applications for specific diseases.
The gut microbiota with three major psychiatric disorders
An intricate assemblage of microorganisms that inhabit the gastrointestinal system is commonly known as the gut microbiota. Gut bacteria plays a dual role in both promoting health and disrupting homeostatic balance, thereby influencing the pathophysiology and etiology of several diseases, including psychiatric disorders (McGuinness et al., 2022). Recent researches have revealed that persons diagnosed with psychiatric disorders, such as depression, BD, and SCZ exhibit alterations in the composition of their gut microbiota (Evans et al., 2017; Shen et al., 2018; Lai et al., 2021).
The gut microbiota with SCZ
Studies have indicated that the composition of gut microbiota may contribute to the development and progression of SCZ (Shen et al., 2018; Yuan et al., 2018; Zheng et al., 2019; Ma et al., 2020; Xu et al., 2020; Zhu et al., 2020; Samochowiec and Misiak, 2021; Campeau et al., 2022). There is the proposition suggesting that numerous molecular modifications associated with psychosis could potentially be impacted by variations in the gut microbiota of individuals diagnosed with SCZ as well as animal models (Samochowiec and Misiak, 2021; Kong et al., 2023). Individuals diagnosed with SCZ had distinct compositions of gut microbiota compared to the control group, as indicated by several studies (Zheng et al., 2019; Ma et al., 2020; Xu et al., 2020). The gut microbiota of individuals diagnosed with SCZ and HCs were compared, revealing a significant increase in the abundance of Proteobacteria in the SCZ patients (Shen et al., 2018). Additionally, the relative abundance of Succinivibrio, Megasphaera, Collinsella, Clostridium, Klebsiella, and Methanobrevibacter was significantly higher in the SCZ group compared to the control group (Shen et al., 2018). On the other hand, there was a decrease in the abundance of Blautia, Coprococcus, and Roseburia in SCZ patients (Shen et al., 2018). The study also identified a panel of 12 potential diagnostic markers within these bacterial groups that could be used to distinguish between individuals with SCZ and those without using receiver operating characteristic curve analysis (Shen et al., 2018). Furthermore, several metabolic pathways related to vitamin B6 and fatty acid metabolism differed significantly between HCs and SCZ patients as determined by PICRUSt analysis (Shen et al., 2018). A study was conducted to investigate the metabolic changes, antioxidant superoxide dismutase (SOD) levels, inflammatory marker high-sensitivity C-reactive protein (hs-CRP) levels, and microbiota changes after a 24-week risperidone treatment (Yuan et al., 2018). Additionally, the correlation between alterations in metabolism and modifications in the microbiota was also investigated (Yuan et al., 2018). The study confirmed that patients’ fecal levels of Bifidobacterium spp., Escherichia coli, and Lactobacillus spp. were much lower compared to HCs; nevertheless, the patient group’s fecal Clostridium coccoides counts were significantly greater than HC group (Yuan et al., 2018). Significant increases in body weight, body mass index, fasting blood glucose, triglycerides, high-sensitivity c-reactive protein, super oxide dismutase, and homeostatic model assessment for insulin resistance were observed during a 24-week course of risperidone administration (Yuan et al., 2018). Additionally, there were notable increases in the amounts of fecal Bifidobacterium spp. and E. coli (Yuan et al., 2018). The fecal microbiota variations between first-episode psychotic (FEP) SCZ patients and HCs were examined and whether these variations were connected to treatment response after up to 12 months of therapy (Schwarz et al., 2018). It was shown that individuals with FEP had higher levels of Lactobacillus, and that these bacteria strongly linked with the severity of their symptoms in a number of different categories (Schwarz et al., 2018). Interestingly, after up to 12 months, a subgroup of FEP patients with the most noticeable microbiota variations also showed worse therapy response (Schwarz et al., 2018). These results support the role of altered microbiota in psychotic disorder and point to potential advantages of modifying them to enhance treatment response and achieve remission (Schwarz et al., 2018). Researchers examined the gut microbiota composition of individuals with chronic schizophrenia and demographically-matched NCs, finding a notable difference in their microbial makeup (Nguyen et al., 2019). At the phylum level, a relative decrease in Proteobacteria abundance was observed in SCZ subjects compared to HCs (Nguyen et al., 2019). Furthermore, at the genus level, Anaerococcus showed a relative increase in SCZ while Haemophilus, Sutterella, and Clostridium exhibited decreased levels (Nguyen et al., 2019). Within individuals with schizophrenia, Ruminococcaceae abundance correlated with lower severity of negative symptoms; Bacteroides was associated with worse depressive symptoms; and Coprococcus was linked to an increased risk for developing coronary heart disease (Nguyen et al., 2019). The composition of intestinal microbial communities was investigated in SCZ patients not treated with antipsychotic drugs and HC groups (Zheng et al., 2019). It was observed that the biodiversity index of intestinal microbial communities significantly decreased in the medication-free group of patients with SCZ (Zheng et al., 2019). A comprehensive investigation of the proteome and metabolome revealed metabolic abnormalities and molecular alterations associated with inflammation in the plasma of individuals diagnosed with SCZ (Campeau et al., 2022). A multi-omics approach was employed to comprehensively investigate the gut microbiota, serum metabolome, and serum inflammatory cytokines in a cohort of individuals diagnosed with SCZ and HCs, revealing dysregulated interactions among the gut, metabolism, and immune systems in SCZ individuals (Fan et al., 2022). Moreover, the findings suggest that an imbalance in the gut microbiota may exert regulatory effects on the onset and progression of SCZ (Fan et al., 2022).
Additionally, animal studies have suggested that alterations in the gut microbiota may potentially impact brain development, thereby contributing to the development of SCZ (Zheng et al., 2019). Simultaneously, the fecal microbiotas of both healthy individuals and individuals diagnosed with SCZ were transplanted into germ-free (GF) mice, resulting in lower levels of glutamate but higher levels of glutamine and γ-aminobutyric acid (GABA) observed in the hippocampus region among recipient mice that received transplantation of the fecal microbiotas from the schizophrenic group (Zheng et al., 2019). Metagenomic sequencing was employed to investigate the gut microbiota of a mouse model for SCZ, revealing 55 genera that exhibited associations with abnormal behavior. Furthermore, fecal microbiota from both SCZ patients and HCs were transplanted into GF mice (Zhu et al., 2020). Mice administered with microbiota obtained from patients displayed activated tryptophan degradation pathways in peripheral and brain tissues (Zhu et al., 2020). The bacterial genomic DNA extracted from fecal samples of mGlu5 knockout (KO) and wild-type (WT) mice revealed a significant difference in microbial beta diversity between the two genotypes (Gubert et al., 2020). Notably, the relative abundance of the Erysipelotrichaceae family and Allobaculum genus exhibited a decrease in this mouse model of SCZ (Gubert et al., 2020). Furthermore, a distinct signature comprising the Erysipelotrichales, Bacteroidales, and Clostridiales orders along with macroscopic gut differences was identified as discriminatory factors between KO and WT genotypes (Gubert et al., 2020). This study provides compelling evidence for global differential community composition within the gut microbiota profile of metabotropic glutamate receptor 5 (mGlu5) KO mice compared to their WT counterparts, thereby highlighting the presence of gut dysbiosis in this genetic animal model of SCZ (Gubert et al., 2020). The review analyzed 46 studies to investigate the role of gut microbiota in SCZ pathophysiology and metabolic alterations associated with antipsychotics (Ap; Singh et al., 2022). Preliminary evidence suggests that changes in gut microbiota–brain axis composition are linked to SCZ development and AP-induced metabolic disturbances (Singh et al., 2022). Fecal microbiota transplantation from SCZ patients to mice has been shown to induce SCZ-like behavioral phenotypes, further supporting the potential role of gut microbiota–brain axis in SCZ pathogenesis (Singh et al., 2022). A meta-analysis was conducted to investigate the potential impact of disturbances in maternal microbiota during neurodevelopment on adult offspring (Hassib et al., 2023). The results revealed a significant effect size, suggesting that disruptions in maternal microbiota may contribute to behavioral impairments and reduced sociability behavior in adult offspring (Hassib et al., 2023).
It is worth noting that there is a proliferation of studies presenting controversial and contradictory findings in this field. For instance, Shen et al. (2018) reported a decrease in the abundance of Proteobacteria and Clostridium among individuals with SCZ compared to healthy controls (Shen et al., 2018). In contrast, Nguyen et al. (2019) demonstrated an increase in Proteobacteria and Clostridium among individuals with SCZ (Nguyen et al., 2019). The relevant researches between gut microbiota and SCZ can be seen in Table 1.
The gut microbiota with MDD
Depression is a prevalent psychiatric condition that distinguish by persistent feelings of sadness, diminished interest or pleasure in activities, and a range of somatic and psychological manifestations. Despite the presence of contradictory results, some researchers have revealed that individuals experiencing depression exhibited distinct compositions of gut bacteria in comparison to the control group (Jiang et al., 2015; Kelly et al., 2016; Zheng et al., 2016; Huang et al., 2018; Lai et al., 2021). Firstly, fecal samples from active-MDD and responded-MDD patients were analyzed for the composition of the gut microbiota (Jiang et al., 2015). The results demonstrated that the active-MDD (A-MDD) group exhibited increased fecal bacterial α-diversity compared to the HC group, as indicated by the Shannon index (Jiang et al., 2015). However, no significant difference in fecal bacterial α-diversity was observed between the responded-MDD (R-MDD) group and the HC group (Jiang et al., 2015). Bacteroidetes, Proteobacteria, and Actinobacteria showed a substantial increase in abundance, while Firmicutes exhibited a significantly reduced level in both A-MDD and R-MDD groups when compared to the HC group (Jiang et al., 2015). Despite considerable interindividual variability, several predominant genera displayed significant differences between MDD and HC groups (Jiang et al., 2015). Particularly noteworthy were elevated levels of Enterobacteriaceae and Alistipes but decreased levels of Faecalibacterium in MDD groups (Jiang et al., 2015). Furthermore, a negative correlation was found between Faecalibacterium abundance and depressive symptom severity (Jiang et al., 2015). These findings provide valuable insights into alterations in fecal microbiota composition among patients with MDD, highlighting either an overrepresentation of potentially harmful bacterial taxa or a depletion of beneficial bacterial genera (Jiang et al., 2015). The gut microbiota of MDD patients and matched HCs were identified and the significant decrease in richness and diversity in MDD patients were revealed (Kelly et al., 2016). The bacterial counts of patients with MDD were compared to those of a control group, revealing significantly reduced levels of Bifidobacterium counts in MDD patients and a tendency toward decreased Lactobacillus counts when compared to the controls (Aizawa et al., 2016). Moreover, the prevalence of individuals with bacterial counts below the optimal cut-off point was significantly higher in patients than in controls for both bacteria, indicating a significant association between bacterial counts and Irritable Bowel Syndrome (Aizawa et al., 2016). These findings will contribute to future research on the therapeutic potential of probiotics and prebiotics in managing MDD (Aizawa et al., 2016). The fecal microbial communities of individuals with MDD and HCs were analyzed, revealing increased abundance of Firmicutes, decreased abundance of Bacteroidetes, and elevated levels of Prevotella, Klebsiella, Streptococcus, and Clostridium XI in MDD patients (Lin et al., 2017). The gut microbiota composition of individuals diagnosed with MDD and HCs was characterized, revealing a significantly reduced α-diversity in MDD patients, as well as a decline in the abundance of Firmicutes within the MDD samples (Huang et al., 2018). A comparative metaproteomics analysis was conducted to investigate the gut microbiota in patients diagnosed with MDD and HCs (Chen et al., 2018). In total, 279 bacterial proteins that exhibited significant differences were identified between the MDD patient group and HC group. Furthermore, notable disparities in the abundances of 16 bacterial families were observed between MDD patients and HCs (Chen et al., 2018). These findings suggest substantial alterations in fecal microbiota of individuals with MDD, providing novel insights into the potential association between the gut microbiota and depression. A combination of non-targeted metabolomics and a macrogenomic approach was employed to investigate the gut microbial composition, viral profile, and fecal metabolomic characteristics in individuals diagnosed with MDD (Yang et al., 2020b). The study provided compelling evidence supporting the crucial role of the microbiota-gut-brain axis (MGBA) in MDD development (Yang et al., 2020b). The study revealed a dysbiosis in the gut microbiota associated with MDD, characterized by perturbations in amino acid metabolism mediated by microbial organisms (Yang et al., 2020b). Multiple studies have established a correlation between depression and inflammation, which can be attributed to dysbiosis in the gut microbiota (Desbonnet et al., 2010; Bercik et al., 2011; Liu et al., 2020). For instance, the gut microbiota of individuals diagnosed with MDD and HCs was assessed, revealing significant disparities in the composition of gut microbiota at various taxonomic levels within the MDD patient group (Liu et al., 2020). These findings provide supports for a correlation between gut microbiota and the chronic, low-grade inflammation frequently observed in individuals with MDD (Liu et al., 2020). The stool samples from MDD patients and HCs was conducted to analyze the composition of gut microbiota and its metabolic pathways (Lai et al., 2021). The abundance of Bacteroidetes was significantly reduced, while there was a notable increase in the abundance of Actinobacteria among individuals with MDD compared to HCs (Lai et al., 2021). These findings provide substantial evidences supporting the conclusion that both gut microbiota and associated tryptophan metabolism pathway are altered in individuals with MDD, suggesting their potential as biomarkers for distinguishing between MDD patients and HCs (Lai et al., 2021). At same time, the potential role of Morganella as a pathogenic agent in the development of depression was verified (Qin et al., 2022).
Furthermore, transplantation of fecal microbiota from depressed patients to microbiota-depleted rats induced behavioral and physiological characteristics associated with depression in the recipient animals, including anhedonia and anxiety-like behaviors, as well as alterations in tryptophan metabolism (Kelly et al., 2016). The implication here is that the gut microbiota may have a causative role in the development of depressive symptoms and could potentially serve as a viable target for therapeutic interventions and preventive measures against this disorder (Kelly et al., 2016). Compared to conventionally raised healthy mice, the absence of gut microbiota in GF mice was found to result in a decrease in immobility time during the forced swimming test (Zheng et al., 2016). Significant dissimilarities were observed between individuals with MDD and healthy controls regarding their gut microbiota compositions, and recolonization of germ-free mice with microbiota derived from MDD patients induced depression-like behaviors (Zheng et al., 2016).
It is noteworthy that the field has yielded controversial and contradictory findings. Several studies have reported a significant decrease in Bacteroidetes among individuals with MDD (Zheng et al., 2016; Lin et al., 2017; Chen et al., 2018; Lai et al., 2021). However, Liu et al. (2020) demonstrated higher levels of Bacteroidetes in individuals with MDD (Liu et al., 2020). Similarly, Aizawa et al. (2016) confirmed significantly lower levels of Bifidobacterium in MDD patients (Aizawa et al., 2016), while Lai et al. (2021) showed increased levels of Bifidobacterium among individuals with MDD (Lai et al., 2021). In terms of Firmicutes, several studies have reported a notable decrease in MDD patients (Zheng et al., 2016; Huang et al., 2018; Liu et al., 2020), whereas Chen et al. (2018) found Firmicutes to be more abundant in individuals with MDD (Chen et al., 2018). The relevant researches between gut microbiota and MDD can be seen in Table 2.
The gut microbiota with BD
BD, often referred to as manic-depressive disease is distinguished by illogical fluctuations in mood, alterations in levels of activity and energy, and a diminished ability to carry out everyday responsibilities (Grande et al., 2016). The pathophysiology of BD may be significantly influenced by the microbiota of the gut (Aizawa et al., 2018; Coello et al., 2019; Painold et al., 2019; McIntyre et al., 2021). Individuals diagnosed with BD exhibit a distinct microbial makeup in their gut flora compared to those who do not have the condition (Evans et al., 2017; McIntyre et al., 2021). The gut microbiota composition was examined in patients diagnosed with BD, unaffected first-degree relatives, and HCs (Coello et al., 2019). Significantly distinct gut microbiota community membership was observed between individuals with BD and healthy controls and the presence of Flavonifractor in the gut may be associated with the inducing of oxidative stress and inflammation in individuals with BD (Coello et al., 2019). The abundance of Bifidobacterium or Lactobacillus may not significantly contribute to the pathophysiology of BD, as indicated by fecal samples collected from patients with BD and HCs (Aizawa et al., 2018). Additionally, a negative association was observed between Lactobacillus counts and sleep quality, as well as between Bifidobacterium counts and serum cortisol levels (Aizawa et al., 2018). These findings indicate potential roles for these bacteria in the sleep and stress response of patients (Aizawa et al., 2018). Euthymic individuals diagnosed with BD who were administered a probiotic supplement for a duration of 3 months underwent a battery of cognitive assessments at three distinct time points (Reininghaus et al., 2018). The results revealed significant enhancements in attention and psychomotor processing speed performance (Reininghaus et al., 2018). These findings lend support to the hypothesis that probiotic supplementation may augment cognitive function in stable individuals with BD, potentially leading to improved psychosocial, occupational, work, and financial functioning (Reininghaus et al., 2018). The differences in microbial community composition in stool samples obtained from BD patients and HCs were investigated (Hu et al., 2019; Painold et al., 2019). The results indicated a significant inverse relationship between microbial α-diversity and the duration of illness in BD and identified specific bacterial clades associated with inflammatory status, serum lipids, tryptophan, depressive symptoms, oxidative stress, anthropometric measurements, and metabolic syndrome among individuals diagnosed with BD (Painold et al., 2019). According to a longitudinal case study on twins diagnosed with BD, there appears to be a potential correlation between an elevated α-diversity of the gut microbiota and a reduction in depressive symptoms (Vinberg et al., 2019). Differences in the composition of the gut microbiota was observed in individuals diagnosed with BD, indicating a potential association with the onset of the condition (Hu et al., 2019; Painold et al., 2019). The impact of quetiapine treatment on the gut microbiota composition in individuals diagnosed with BD was investigated, revealing a significant increase in the relative abundance of Bifidobacteria and Enterobacteriaceae following administration of quetiapine (Lu et al., 2019). Furthermore, the administration of probiotic therapy to individuals diagnosed with BD resulted in significant improvements in cognitive functioning and a decrease in symptom severity (Aizawa et al., 2018; Reininghaus et al., 2018). However, the gut microbiota compositions of individuals with BD and HCs were evaluated, revealing a reduced diversity in the gut microbiota of BD subjects (McIntyre et al., 2021). Furthermore, neither the diagnosis nor the diet exerted a significant influence on gut microbiota composition as determined by cluster analysis (McIntyre et al., 2021). Ongoing investigations are being conducted to further examine the details of this correlation. The imbalanced quantities of specific compounds, which have the potential to impact brain function and mood regulation to the development of BD, may be attributed to the influence exerted by gut flora. Conversely, it is plausible that stress and alterations in dietary patterns associated with BD could potentially impact alterations in the composition of gut microbiota (Jones et al., 2023). The relevant researches between gut microbiota and BD can be seen in Table 3.
How MEVs communicate with the host brain
Overview of MEVs
MEVs are small, membrane-bound vesicles produced by the gut microbiota through various mechanisms including exocytosis, blebbing, and direct membrane shedding (Sultan et al., 2021). The molecules are rapidly released into the extracellular space and exhibit a prolonged presence duration. MEVs hold considerable importance as secretory products of microorganisms due to their diverse range of biological activity and promising applications. The primary function of these entities is to facilitate the exchange of information among microorganisms belonging to the same or different species, as well as between the host organism and its microbiota. They transport chemical substances that have the potential to impact neuronal signaling and brain function though microbial metabolites, neuroactive, and immune-modulatory molecule pathways (Zakharzhevskaya et al., 2017; Strandwitz, 2018; Yaghoubfar et al., 2020). Gram-negative and Gram-positive bacteria produce different types of bacterial extracellular vesicles (Yang et al., 2018). Gram-negative bacteria predominantly produce outer membrane vesicles (OMVs), while Gram-positive bacteria generate cytoplasmic membrane vesicles (Yang et al., 2018). The biogenesis of OMVs in Gram-negative bacteria involves vesiculation of the outer membrane, whereas the origin of cytoplasmic membrane vesicles in Gram-positive bacteria remains unclear (Yang et al., 2018). OMVs from Gram-negative bacteria typically contain lipopolysaccharides (LPS), outer membrane proteins, periplasmic contents such as peptidoglycan, enzymes, toxins, cytoplasmic proteins, and nucleic acids enclosed within the outer membrane (Pirolli et al., 2021). On the other hand, cytoplasmic membrane vesicles derived from Gram-positive bacteria consist of lipoproteins, cytoplasmic proteins, enzymes, toxins and nucleic acids originating from their cytoplasm (Pirolli et al., 2021). The schematic diagram that illustrates two distinct types of extracellular vesicles derived from bacteria (BEVs) can be seen in Figure 1.
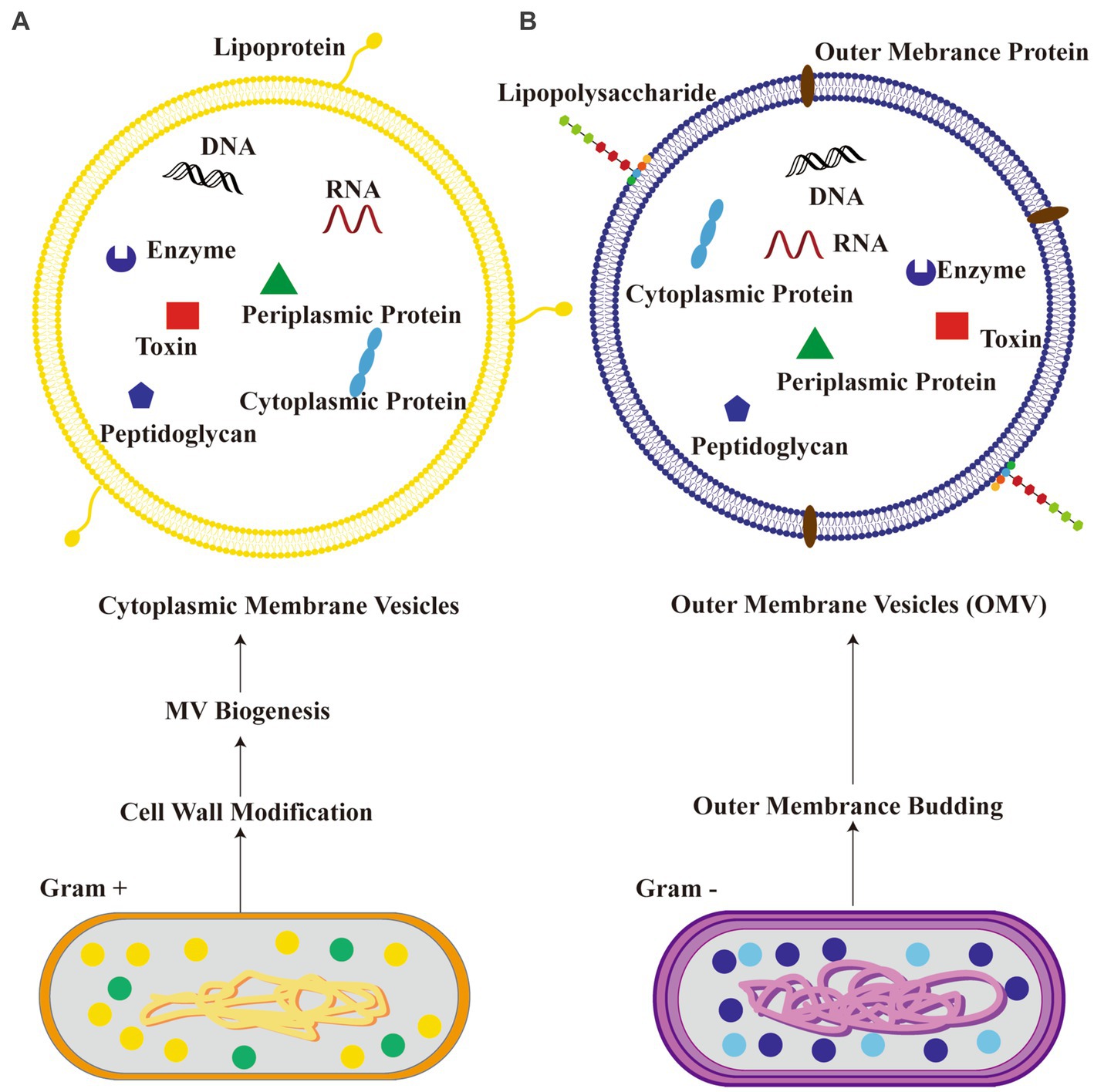
Figure 1. Schematic diagram illustrating two kinds of bacteria-derived extracellular vesicles. (A) Cytoplasmic membrane vesicles from Gram-positive bacteria; (B) outer membrane vesicles (OMV) from Gram-negative bacteria.
The channels of MEVs communicating with the host brain
There are growing evidences that intestinal host cells or the gut microbiota generate extracellular vesicles (EVs) that are primarily responsible for mediating interkingdom interactions (Diaz-Garrido et al., 2021). Bidirectional communication between microbial populations and hosts in the gut environment may not necessarily require direct cell-to-cell contact. MEVs play a vital role in the intercellular signaling pathway and are potentially significant in facilitating communication between the microbiota and the host organism (Sultan et al., 2021). MEVs are found in carrying various molecules, including neurotransmitters and their precursors and can modulate brain function through the GBA (Strandwitz, 2018). These neurotransmitters, such as dopamine, serotonin, and GABA, play crucial roles in regulating mood, cognition, and emotional well-being (Zakharzhevskaya et al., 2017; Yaghoubfar et al., 2020). Abnormal function or imbalance of neurotransmitters are implicated in a variety of neuropsychiatric disorders (Zakharzhevskaya et al., 2017; Yaghoubfar et al., 2020). MEVs released by gut bacteria may interact with the host’s nervous system, affecting neurotransmitter levels and potentially contributing to psychiatric symptoms. For example, the MEVs generated by Bacteroides fragilis contain GABA and its metabolic precursors, α-ketoglutarate and glutamate (Zakharzhevskaya et al., 2017). EVs generated by Akkermansia muciniphila showed to stimulate serotonin release in both the colon and hippocampus of mice, indicating the potential of MEVs as signaling molecules in the GBA (Yaghoubfar et al., 2020). MEVs have also been discovered to facilitate the transportation of neuroplasticity-related substances, such as brain-derived neurotrophic factor (BDNF; Choi et al., 2019). Neuroplasticity refers to the brain’s inherent ability to undergo structural and functional changes in response to various stimuli or experiences. Psychiatric disorders have been associated with changes in synaptic function and neuroplasticity (Martins and Schratt, 2021). At the same time, existing researches show that miRNAs can regulate neuroplasticity of rodents by controlling the morphology of dendrites and spines and the expression of neurotransmitter receptors (Schratt, 2009). So, it is hypothesized that MEVs containing miRNAs may contribute to the regulation of higher brain functions and that their dysregulation cause disease-related behaviors.
The development of psychiatric disorders is associated with inflammation and dysregulated immunological responses (Frick et al., 2013; Gibney and Drexhage, 2013). The presence of pro- or anti-inflammatory substances within MEVs possesses the capacity to exert an impact on the immune response within the gastric region as well as throughout the body. The manipulation of the immune system has the potential to influence the function of the brain, hence potentially contributing to the development or exacerbation of psychiatric disorders (Gibney and Drexhage, 2013). It has been observed that MEVs can sporadically induce cerebral inflammation, a pathological state associated with several psychiatric diseases such as depression and SCZ. Evidences suggested that MEVs play a crucial role in neurodevelopment and brain dysfunction by regulating immune responses (Cuesta et al., 2021). MEVs have the capability to traverse the BBB and engage with Toll-like receptors located on glial cells, which serve as immunological receptors (Cuesta et al., 2021). Bacterial molecules participate in the activation of immune signaling cascades through receptors, which in turn activate immune responses in the brain and impact brain function (Rutsch et al., 2020). BEVs contain various components, including LPS, RNA, DNA, proteins, etc., which may also play a role in these processes. Studies have shown that outer-membrane vesicles (OMVs) released by Gram-negative bacteria can induce increased expression of TNF-α in mouse brains (Han et al., 2019). Therefore, they may be one of the causes of inflammatory diseases, such as Alzheimer’s disease (Lajqi et al., 2022). Similarly, OMVs secreted by aggregates of Streptomyces can effectively deliver extracellular RNA into mononuclear cells and microglia within the brain and upregulates IL-6 through NF-κB activation, leading to neuroinflammation (Ha et al., 2020). Oral administration of LPS or EVs isolated from human alkaline-producing bacteria to mice can induce cognitive impairment and colitis (Lee et al., 2020). However, simultaneous oral administration of LPS and EVs leads to severe cognitive impairment and hippocampal inflammation in mice (Lee et al., 2020). The reported increase in systemic LPS-positive bacterial MEVs in individuals with intestinal barrier dysfunction suggests that these MEVs have the ability to enter the systemic circulation and trigger various immunological and metabolic responses in multiple organs, such as the brain (Tulkens et al., 2020). The brain and gut microbiota communicate bidirectionally via multiple pathways involving the immune system, neuroendocrine system, enteric nervous system, circulation, and the vagus nerve. These pathways constitute the MGBA. MEVs transport DNA, RNA, metabolites, peptides, intestinal hormones, bioactive substances, and microbial products. They can traverse the blood–brain barrier through circulation to access the brain. Within the brain, they convey a multitude of substances that impact neurodevelopment and degeneration. Imbalances in brain homeostasis or stressful conditions lead to an increased release of corticotropin-releasing hormone (CRH) from hypothalamus, resulting in stimulation of adrenocorticotropic hormone (ACTH) transportation from anterior pituitary gland influenced by ACTH. The adrenal glands then trigger cortisol production and secretion, a stress hormone responsible for regulating intestinal immunity and barrier function. Therefore, it appears that gut microbiota may influence brain function via the MGBA mediated by MEVs (see Figure 2). The emerging field of microbiota research has shed light on the potential role of MEVs in the GBA and their potential implications in psychiatric disorders. Notwithstanding these promising findings, it is imperative to bear in mind that this area of research is still in the early stage.
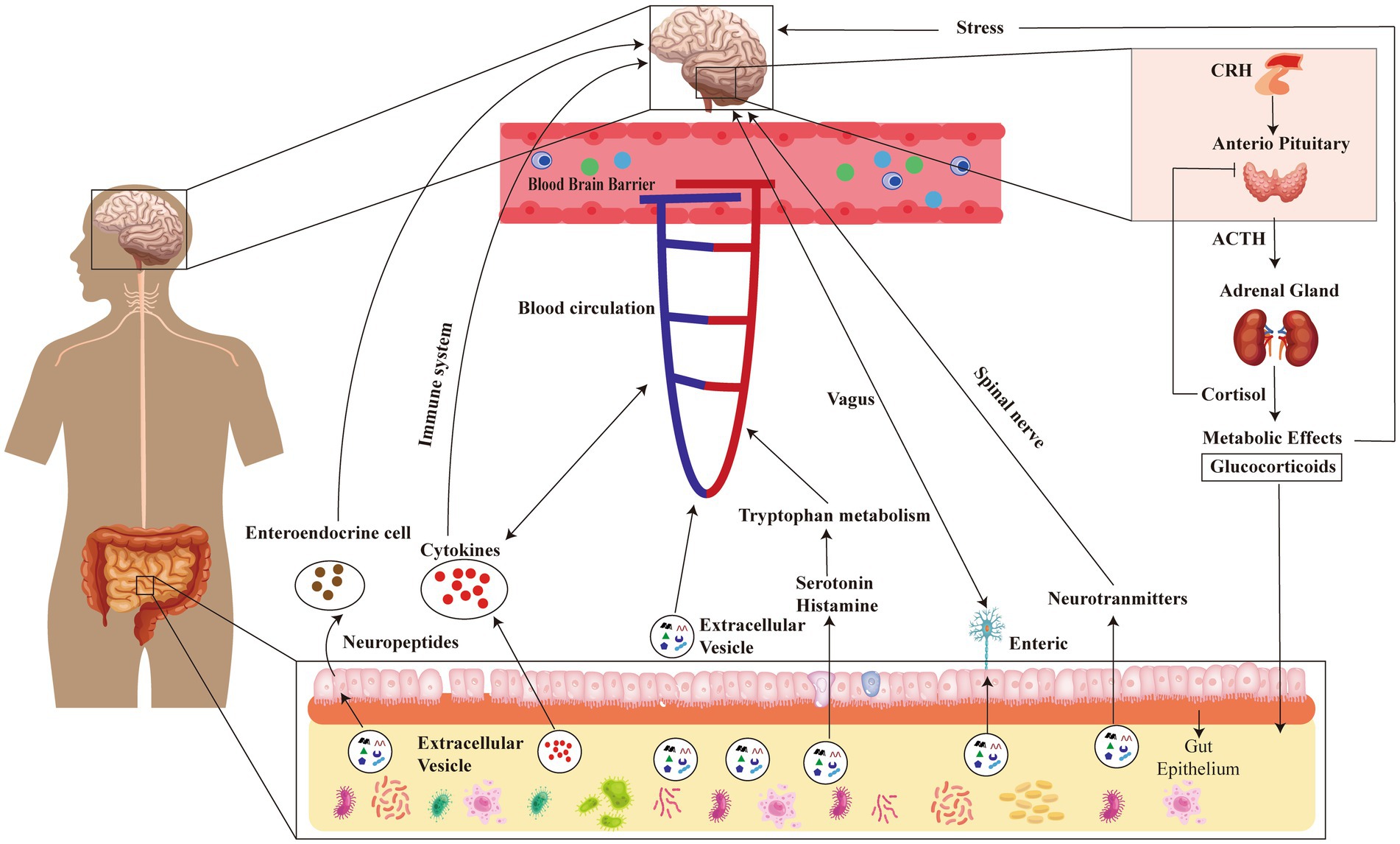
Figure 2. Schematic diagram illustrating the impact of gut microbiota on brain function through MEVs-mediated microbial-gut-brain axis (MGBA). MGBA, microbial-gut-brain axis; CRH, Corticotropin Releasing Hormone; ACTH, AdrenoCorticoTropic Hormone.
The emerging roles of MEVs in mental disorders
MEVs with the occurrence and development of mental disorders
The investigation of MEVs and their contents have potential for advancing our understanding of intercellular communication and the pathogenic mechanisms behind the initiation and progression of diseases. Recent studies have made clearly that MEVs may exert influence on the pathogenesis and progression of psychiatric disorders through their ability to facilitate bidirectional communication between the gastrointestinal system and the central nervous system (Choi et al., 2019; Cuesta et al., 2021; Sultan et al., 2021). Here are some key points regarding the relationship between MEVs and psychiatric disorders. EVs secreted by Lactiplantibacillus plantarum exhibited an antidepressant-like effect in rats that are subjected to chronic restraint stress (Choi et al., 2019). EVs derived from L. plantarum enhance the expression of BDNF in cultured hippocampal neurons. This may have a significant impact on the modulation of behavior and the management of mood disorders, including anxiety and depression. Additionally, these MEVs elicit antidepressant-like effects in mice (Choi et al., 2019).
Our current understanding of the impact of MEVs on psychiatric disorders is limited. Further investigation is necessary to demonstrate a definitive causal link between MEVs and psychiatric disorders as current research often relies on animal models or limited human samples. If these relationships are verified, it may be possible to develop novel preventative and treatment strategies for psychiatric disorders. The significance of MEVs in psychiatric disorders is currently under extensive investigation and inquiry. There exists a potential avenue for rectifying the equilibrium of the gut microbiota and MEVs by means of nutritional interventions, prebiotics, probiotics, or the transplantation of a healthy microbiota or MEVs. Consequently, the intervention has the potential to mitigate inflammation, modulate neurotransmitter pathways, and enhance overall mental well-being. The confirmation of the significance of MEVs in the initiation and advancement of mental illnesses will undoubtedly be established as our understanding of MEVs in individuals with psychiatric disorders and their respective functionalities. The compositional properties of microbial DNA present in MEVs from individuals with psychiatric disorders, in conjunction with variably produced proteins, hold substantial scientific and therapeutic importance. Notwithstanding these promising potential, further comprehensive and extensive study is necessary prior to the implementation of MEVs for clinical applications.
Utilizing MEVs for the diagnosis and treatment of three common psychiatric disorders
Biomarkers indicating either good or harmful biological processes or pharmacological reactions to therapeutic interventions play a vital role across several applications. At present, a limited number of dependable biomarkers can be utilized to substantiate the diagnosis or prognosis of psychiatric disorders. Furthermore, the underlying causes of these disorders remain largely elusive (McGuinness et al., 2022). The diagnosis of psychiatric disorders now relies on the patient’s symptoms and bodily manifestations, rather than pathological and physiological markers (He et al., 2018). MEVs have garnered increased attention in recent years as potential non-invasive biomarkers for diagnostic and therapeutic purposes in some diseases (Chronopoulos and Kalluri, 2020; Yang et al., 2020a; Samra et al., 2021). MEVs possess the ability to provide valuable insights into the physiological or pathological state of the cells from which they originate. This feature confers a distinct advantage in various research and diagnostic applications. The characteristics of MEVs and their cargo, which exhibit disease specificity, remarkable stability, and abundant content, render them highly suitable biomarkers for a range of medical conditions, including psychiatric disorders. The study has provided data establishing a connection between MEVs and their associated and advancement of Alzheimer (Emery et al., 2017). Consequently, MEVs are currently being proposed as potential biomarkers for disease detection (Park et al., 2021; Zhang et al., 2022). Recent studies have shown that gut MEVs from stool exhibit significant differences between individuals with colorectal cancer and control subjects (Park et al., 2021). Profiling these EVs may provide a novel biomarker for detecting and predicting the prognosis of colorectal cancer (Park et al., 2021). The diagnostic model for atopic dermatitis based on the analysis of serum MEVs exhibited high accuracy, sensitivity, and specificity (Yang et al., 2020a). MEVs or their cargo, including proteins, lipids, and nucleic acids, have the potential to be detected and quantified in biological specimens such as blood or feces. This property is promising for the future utilization of these substances as biomarkers in the diagnosis of psychiatric disorders.
The identification of novel therapeutic targets for psychiatric disorders can potentially benefit from a comprehensive understanding of the mechanisms via which MEVs interact with the neurological system and contribute to disease progression. In recent years, there is a proposal to utilize MEVs as a potentially innovative approach for transporting neuroactive compounds produced by the gut microbiota to the brain. MEVs exhibit substantial potential in the field of biology due to their ability to serve as nanocarriers for the transportation of pharmaceuticals, genetic material, and various bioactive compounds. MEVs have demonstrated considerable efficacy in drug delivery due to their ability to traverse many physiological barriers, including the BBB. MEVs possess the potential for customization to incorporate specific therapeutic agents and subsequently target specific cells or tissues, owing to their origin from the microbiota. This presents novel viewpoints and biomedical therapeutic possibilities of psychiatric disorders. MEVs also exhibit potential in the realm of personalized medicine, wherein the individual microbiota and MEV profiles of patients are considered during the formulation of treatment strategies. This particular technique has the potential to enhance the effectiveness of therapeutic interventions for psychiatric disorders.
The exploration of the potential roles of MEVs in psychiatric disorders have generated a plethora of captivating possibilities for future research. Understanding the role of MEVs in psychiatric disease is a vast and complex field that begins to be elucidated and the application of MEVs to psychiatric disorder diagnosis and therapy is still emerging and thus requires further research and clinical trials to confirm these potentials.
Summary and outlook
Ongoing researches in this area are opening new avenues for diagnosing, preventing, and treating psychiatric disorders. If confirmed, this discovery could revolutionize the understanding, diagnosis, and treatment of psychiatric disorders. Ultimately, understanding the link between MEVs and psychiatric disorders could pave the way for innovative treatment approaches. These could potentially be used in conjunction with conventional therapies to restore gut balance and alleviate symptoms. Experimental studies, mostly conducted on animals, have shown that it is possible to alter behaviors associated with stress, anxiety, and depression by manipulating the gut microbiota through the use of probiotics or fecal microbiota transplantation. However, further research is needed to confirm their safety and effectiveness. It is necessary to fully comprehend this intricate relationship and determine how it can be effectively applied in medical practice. Along with ongoing enhancements in detection protocols, evaluation methodologies, and intervention strategies, the use of MEVs in identifying and managing psychiatric disorders will increase. This avenue of investigation is expected to have an impact on the future understanding of psychiatric disorders.
Author contributions
CG: Writing – original draft, Writing – review & editing, Data curation. YB: Software, Writing – review & editing. PL: Data curation, Writing – review & editing. KH: Conceptualization, Writing – original draft, Writing – review & editing.
Funding
The author(s) declare financial support was received for the research, authorship, and/or publication of this article. This work was funded by the Natural Science Foundations of China (no. 82060256), the Natural Science Foundation of Inner Mongolia Autonomous Region (no. 2023MS08024), and Basic Scientific Research Business Fee Project of Universities Directly under the Inner Mongolia Autonomous Region (GXKY23Z043).
Conflict of interest
The authors declare that the research was conducted in the absence of any commercial or financial relationships that could be construed as a potential conflict of interest.
Publisher’s note
All claims expressed in this article are solely those of the authors and do not necessarily represent those of their affiliated organizations, or those of the publisher, the editors and the reviewers. Any product that may be evaluated in this article, or claim that may be made by its manufacturer, is not guaranteed or endorsed by the publisher.
Abbreviations
MDD, Major depressive disorder; BD, Bipolar disorder; SCZ, Schizophrenia; EVs, Extracellular vesicles; HCs, Healthy controls; MEVs, Extracellular vesicles arising from the microbiota; GBA, Gut-brain axis; BDNF, Brain-derived neurotrophic factor; BBB, Blood–brain barrier; RT-qPCR, Reverse transcription-quantitative PCR; MGBA, Microbiota-gut-brain axis; GABA, γ-aminobutyric acid; BEVs, Bacterial extracellular vesicles; OMVs, Outer-membrane vesicles; OUT, Operational taxonomical unit; GF, Germ-free; FEP, First-episode psychotic; qPCR, Quantitative Real-time PCR; mGlu5, Metabotropic glutamate receptor 5; DNFES, Drug-naive first-episode patients with SCZ; TSCZ, Antipsychotic-treated patients with SCZ
References
Aizawa, E., Tsuji, H., Asahara, T., Takahashi, T., Teraishi, T., Yoshida, S., et al. (2018). Bifidobacterium and lactobacillus counts in the gut microbiota of patients with bipolar disorder and healthy controls. Front. Psych. 9:730. doi: 10.3389/fpsyt.2018.00730
Aizawa, E., Tsuji, H., Asahara, T., Takahashi, T., Teraishi, T., Yoshida, S., et al. (2016). Possible association of Bifidobacterium and lactobacillus in the gut microbiota of patients with major depressive disorder. J. Affect. Disord. 202, 254–257. doi: 10.1016/j.jad.2016.05.038
Al-Nedawi, K., Mian, M. F., Hossain, N., Karimi, K., Mao, Y. K., Forsythe, P., et al. (2015). Gut commensal microvesicles reproduce parent bacterial signals to host immune and enteric nervous systems. FASEB J. 29, 684–695. doi: 10.1096/fj.14-259721
Bercik, P., Denou, E., Collins, J., Jackson, W., Lu, J., Jury, J., et al. (2011). The intestinal microbiota affect central levels of brain-derived neurotropic factor and behavior in mice. Gastroenterology 141, 599–609.e3. doi: 10.1053/j.gastro.2011.04.052
Brainstorm ConsortiumAnttila, V., Bulik-Sullivan, B., Finucane, H. K., Walters, R. K., Bras, J., et al. (2018). Analysis of shared heritability in common disorders of the brain. Science 360:eaap8757. doi: 10.1126/science.aap8757
Campeau, A., Mills, R. H., Stevens, T., Rossitto, L. A., Meehan, M., Dorrestein, P., et al. (2022). Multi-omics of human plasma reveals molecular features of dysregulated inflammation and accelerated aging in schizophrenia. Mol. Psychiatry 27, 1217–1225. doi: 10.1038/s41380-021-01339-z
Chen, Z., Li, J., Gui, S., Zhou, C., Chen, J., Yang, C., et al. (2018). Comparative metaproteomics analysis shows altered fecal microbiota signatures in patients with major depressive disorder. Neuroreport 29, 417–425. doi: 10.1097/wnr.0000000000000985
Chen, Y., Xu, J., and Chen, Y. (2021a). Regulation of neurotransmitters by the gut microbiota and effects on cognition in neurological disorders. Nutrients 13:2099. doi: 10.3390/nu13062099
Chen, Y., Zhou, J., and Wang, L. (2021b). Role and mechanism of gut microbiota in human disease. Front. Cell. Infect. Microbiol. 11:625913. doi: 10.3389/fcimb.2021.625913
Choi, J., Kim, Y. K., and Han, P. L. (2019). Extracellular vesicles derived from Lactobacillus plantarum increase BDNF expression in cultured hippocampal neurons and produce antidepressant-like effects in mice. Exp Neurobiol 28, 158–171. doi: 10.5607/en.2019.28.2.158
Chronopoulos, A., and Kalluri, R. (2020). Emerging role of bacterial extracellular vesicles in cancer. Oncogene 39, 6951–6960. doi: 10.1038/s41388-020-01509-3
Coello, K., Hansen, T. H., Sørensen, N., Munkholm, K., Kessing, L. V., Pedersen, O., et al. (2019). Gut microbiota composition in patients with newly diagnosed bipolar disorder and their unaffected first-degree relatives. Brain Behav. Immun. 75, 112–118. doi: 10.1016/j.bbi.2018.09.026
Cryan, J. F., O'Riordan, K. J., Cowan, C. S. M., Sandhu, K. V., Bastiaanssen, T. F. S., Boehme, M., et al. (2019). The microbiota-gut-brain Axis. Physiol. Rev. 99, 1877–2013. doi: 10.1152/physrev.00018.2018
Cuesta, C. M., Guerri, C., Ureña, J., and Pascual, M. (2021). Role of microbiota-derived extracellular vesicles in gut-brain communication. Int. J. Mol. Sci. 22:4235. doi: 10.3390/ijms22084235
Dalile, B., Van Oudenhove, L., Vervliet, B., and Verbeke, K. (2019). The role of short-chain fatty acids in microbiota-gut-brain communication. Nat. Rev. Gastroenterol. Hepatol. 16, 461–478. doi: 10.1038/s41575-019-0157-3
Desbonnet, L., Garrett, L., Clarke, G., Kiely, B., Cryan, J. F., and Dinan, T. G. (2010). Effects of the probiotic Bifidobacterium infantis in the maternal separation model of depression. Neuroscience 170, 1179–1188. doi: 10.1016/j.neuroscience.2010.08.005
Diaz-Garrido, N., Cordero, C., Olivo-Martinez, Y., Badia, J., and Baldomà, L. (2021). Cell-to-cell communication by host-released extracellular vesicles in the gut: implications in health and disease. Int. J. Mol. Sci. 22:2213. doi: 10.3390/ijms22042213
Emery, D. C., Shoemark, D. K., Batstone, T. E., Waterfall, C. M., Coghill, J. A., Cerajewska, T. L., et al. (2017). 16S rRNA next generation sequencing analysis shows bacteria in Alzheimer's post-mortem brain. Front. Aging Neurosci. 9:195. doi: 10.3389/fnagi.2017.00195
Evans, S. J., Bassis, C. M., Hein, R., Assari, S., Flowers, S. A., Kelly, M. B., et al. (2017). The gut microbiome composition associates with bipolar disorder and illness severity. J. Psychiatr. Res. 87, 23–29. doi: 10.1016/j.jpsychires.2016.12.007
Fan, Y., Gao, Y., Ma, Q., Yang, Z., Zhao, B., He, X., et al. (2022). Multi-omics analysis reveals aberrant gut-metabolome-immune network in schizophrenia. Front. Immunol. 13:812293. doi: 10.3389/fimmu.2022.812293
Fan, Y., and Pedersen, O. (2021). Gut microbiota in human metabolic health and disease. Nat. Rev. Microbiol. 19, 55–71. doi: 10.1038/s41579-020-0433-9
Frick, L. R., Williams, K., and Pittenger, C. (2013). Microglial dysregulation in psychiatric disease. Clin. Dev. Immunol. 2013:608654. doi: 10.1155/2013/608654
Fung, T. C., Olson, C. A., and Hsiao, E. Y. (2017). Interactions between the microbiota, immune and nervous systems in health and disease. Nat. Neurosci. 20, 145–155. doi: 10.1038/nn.4476
Gentile, C. L., and Weir, T. L. (2018). The gut microbiota at the intersection of diet and human health. Science 362, 776–780. doi: 10.1126/science.aau5812
Gibney, S. M., and Drexhage, H. A. (2013). Evidence for a dysregulated immune system in the etiology of psychiatric disorders. J. Neuroimmune Pharmacol. 8, 900–920. doi: 10.1007/s11481-013-9462-8
Golofast, B., and Vales, K. (2020). The connection between microbiome and schizophrenia. Neurosci. Biobehav. Rev. 108, 712–731. doi: 10.1016/j.neubiorev.2019.12.011
Gomaa, E. Z. (2020). Human gut microbiota/microbiome in health and diseases: a review. Antonie Van Leeuwenhoek 113, 2019–2040. doi: 10.1007/s10482-020-01474-7
Grande, I., Berk, M., Birmaher, B., and Vieta, E. (2016). Bipolar disorder. Lancet 387, 1561–1572. doi: 10.1016/s0140-6736(15)00241-x
Gubert, C., Kong, G., Uzungil, V., Zeleznikow-Johnston, A. M., Burrows, E. L., Renoir, T., et al. (2020). Microbiome profiling reveals gut dysbiosis in the metabotropic glutamate receptor 5 knockout mouse model of schizophrenia. Front. Cell Dev. Biol. 8:582320. doi: 10.3389/fcell.2020.582320
Ha, J. Y., Choi, S. Y., Lee, J. H., Hong, S. H., and Lee, H. J. (2020). Delivery of Periodontopathogenic extracellular vesicles to brain monocytes and microglial IL-6 promotion by RNA cargo. Front. Mol. Biosci. 7:596366. doi: 10.3389/fmolb.2020.596366
Haarman, B. C., Riemersma-Van der Lek, R. F., Burger, H., Drexhage, H. A., and Nolen, W. A. (2016). The dysregulated brain: consequences of spatial and temporal brain complexity for bipolar disorder pathophysiology and diagnosis. Bipolar Disord. 18, 696–701. doi: 10.1111/bdi.12454
Han, E. C., Choi, S. Y., Lee, Y., Park, J. W., Hong, S. H., and Lee, H. J. (2019). Extracellular RNAs in periodontopathogenic outer membrane vesicles promote TNF-α production in human macrophages and cross the blood-brain barrier in mice. FASEB J. 33, 13412–13422. doi: 10.1096/fj.201901575R
Hassib, L., de Oliveira, C. L., Rouvier, G. A., Kanashiro, A., Guimarães, F. S., and Ferreira, F. R. (2023). Maternal microbiome disturbance induces deficits in the offspring's behaviors: a systematic review and meta-analysis. Gut Microbes 15:2226282. doi: 10.1080/19490976.2023.2226282
He, K., Guo, C., He, L., and Shi, Y. (2018). MiRNAs of peripheral blood as the biomarker of schizophrenia. Hereditas 155:9. doi: 10.1186/s41065-017-0044-2
Hu, S., Li, A., Huang, T., Lai, J., Li, J., Sublette, M. E., et al. (2019). Gut microbiota changes in patients with bipolar depression. Adv. Sci. 6:1900752. doi: 10.1002/advs.201900752
Huang, Y., Shi, X., Li, Z., Shen, Y., Shi, X., Wang, L., et al. (2018). Possible association of firmicutes in the gut microbiota of patients with major depressive disorder. Neuropsychiatr. Dis. Treat. 14, 3329–3337. doi: 10.2147/NDT.S188340
Jiang, H., Ling, Z., Zhang, Y., Mao, H., Ma, Z., Yin, Y., et al. (2015). Altered fecal microbiota composition in patients with major depressive disorder. Brain Behav. Immun. 48, 186–194. doi: 10.1016/j.bbi.2015.03.016
Jones, G. H., Pinjari, O. F., Vecera, C. M., Smith, K., Barrera, A., and Machado-Vieira, R. (2023). The role of the gut microbiome in bipolar disorder and its common medical comorbidities. Front. Neuroendocrinol. 70:101078. doi: 10.1016/j.yfrne.2023.101078
Kelly, J. R., Borre, Y., Brien, C., Patterson, E., El Aidy, S., Deane, J., et al. (2016). Transferring the blues: depression-associated gut microbiota induces neurobehavioural changes in the rat. J. Psychiatr. Res. 82, 109–118. doi: 10.1016/j.jpsychires.2016.07.019
Kong, G., Gubert, C., and Hannan, A. J. (2023). Gastrointestinal and microbiome profiling in rodent models of neurological and psychiatric disorders. Methods Mol. Biol. 2687, 77–91. doi: 10.1007/978-1-0716-3307-6_7
Lai, W. T., Deng, W. F., Xu, S. X., Zhao, J., Xu, D., Liu, Y. H., et al. (2021). Shotgun metagenomics reveals both taxonomic and tryptophan pathway differences of gut microbiota in major depressive disorder patients. Psychol. Med. 51, 90–101. doi: 10.1017/s0033291719003027
Lai, J., Jiang, J., Zhang, P., Xi, C., Wu, L., Gao, X., et al. (2020). Gut microbial clues to bipolar disorder: state-of-the-art review of current findings and future directions. Clin. Transl. Med. 10:e146. doi: 10.1002/ctm2.146
Lajqi, T., Köstlin-Gille, N., Hillmer, S., Braun, M., Kranig, S. A., Dietz, S., et al. (2022). Gut microbiota-derived small extracellular vesicles endorse memory-like inflammatory responses in murine neutrophils. Biom. J. 10:442. doi: 10.3390/biomedicines10020442
Lee, K. E., Kim, J. K., Han, S. K., Lee, D. Y., Lee, H. J., Yim, S. V., et al. (2020). The extracellular vesicle of gut microbial Paenalcaligenes hominis is a risk factor for vagus nerve-mediated cognitive impairment. Microbiome 8:107. doi: 10.1186/s40168-020-00881-2
Li, Z., Lai, J., Zhang, P., Ding, J., Jiang, J., Liu, C., et al. (2022). Multi-omics analyses of serum metabolome, gut microbiome and brain function reveal dysregulated microbiota-gut-brain axis in bipolar depression. Mol. Psychiatry 27, 4123–4135. doi: 10.1038/s41380-022-01569-9
Lin, P., Ding, B., Feng, C., Yin, S., Zhang, T., Qi, X., et al. (2017). Prevotella and klebsiella proportions in fecal microbial communities are potential characteristic parameters for patients with major depressive disorder. J. Affect. Disord. 207, 300–304. doi: 10.1016/j.jad.2016.09.051
Liu, R. T., Rowan-Nash, A. D., Sheehan, A. E., Walsh, R. F. L., Sanzari, C. M., Korry, B. J., et al. (2020). Reductions in anti-inflammatory gut bacteria are associated with depression in a sample of young adults. Brain Behav. Immun. 88, 308–324. doi: 10.1016/j.bbi.2020.03.026
Lu, Q., Lai, J., Lu, H., Ng, C., Huang, T., Zhang, H., et al. (2019). Gut microbiota in bipolar depression and its relationship to brain function: an advanced exploration. Front. Psych. 10:784. doi: 10.3389/fpsyt.2019.00784
Ma, X., Asif, H., Dai, L., He, Y., Zheng, W., Wang, D., et al. (2020). Alteration of the gut microbiome in first-episode drug-naïve and chronic medicated schizophrenia correlate with regional brain volumes. J. Psychiatr. Res. 123, 136–144. doi: 10.1016/j.jpsychires.2020.02.005
Ma, Q., Xing, C., Long, W., Wang, H. Y., Liu, Q., and Wang, R. F. (2019). Impact of microbiota on central nervous system and neurological diseases: the gut-brain axis. J. Neuroinflammation 16:53. doi: 10.1186/s12974-019-1434-3
Martins, H. C., and Schratt, G. (2021). MicroRNA-dependent control of neuroplasticity in affective disorders. Transl. Psychiatry 11:263. doi: 10.1038/s41398-021-01379-7
McGuinness, A. J., Davis, J. A., Dawson, S. L., Loughman, A., Collier, F., O'Hely, M., et al. (2022). A systematic review of gut microbiota composition in observational studies of major depressive disorder, bipolar disorder and schizophrenia. Mol. Psychiatry 27, 1920–1935. doi: 10.1038/s41380-022-01456-3
McIntyre, R. S., Subramaniapillai, M., Shekotikhina, M., Carmona, N. E., Lee, Y., Mansur, R. B., et al. (2021). Characterizing the gut microbiota in adults with bipolar disorder: a pilot study. Nutr. Neurosci. 24, 173–180. doi: 10.1080/1028415x.2019.1612555
Misiak, B., Łoniewski, I., Marlicz, W., Frydecka, D., Szulc, A., Rudzki, L., et al. (2020). The HPA axis dysregulation in severe mental illness: can we shift the blame to gut microbiota? Prog. Neuropsychopharmacol. Biol. Psychiatry 102:109951. doi: 10.1016/j.pnpbp.2020.109951
Mitrea, L., Nemeş, S. A., Szabo, K., Teleky, B. E., and Vodnar, D. C. (2022). Guts imbalance imbalances the brain: a review of gut microbiota association with neurological and psychiatric disorders. Front. Med. 9:813204. doi: 10.3389/fmed.2022.813204
Mittal, R., Debs, L. H., Patel, A. P., Nguyen, D., Patel, K., O'Connor, G., et al. (2017). Neurotransmitters: the critical modulators regulating gut-brain Axis. J. Cell. Physiol. 232, 2359–2372. doi: 10.1002/jcp.25518
Nguyen, T. T., Kosciolek, T., Maldonado, Y., Daly, R. E., Martin, A. S., McDonald, D., et al. (2019). Differences in gut microbiome composition between persons with chronic schizophrenia and healthy comparison subjects. Schizophr. Res. 204, 23–29. doi: 10.1016/j.schres.2018.09.014
Ouabbou, S., He, Y., Butler, K., and Tsuang, M. (2020). Inflammation in mental disorders: is the microbiota the missing link? Neurosci. Bull. 36, 1071–1084. doi: 10.1007/s12264-020-00535-1
Painold, A., Mörkl, S., Kashofer, K., Halwachs, B., Dalkner, N., Bengesser, S., et al. (2019). A step ahead: exploring the gut microbiota in inpatients with bipolar disorder during a depressive episode. Bipolar Disord. 21, 40–49. doi: 10.1111/bdi.12682
Park, J., Kim, N. E., Yoon, H., Shin, C. M., Kim, N., Lee, D. H., et al. (2021). Fecal microbiota and gut microbe-derived extracellular vesicles in colorectal cancer. Front. Oncol. 11:650026. doi: 10.3389/fonc.2021.650026
Peirce, J. M., and Alviña, K. (2019). The role of inflammation and the gut microbiome in depression and anxiety. J. Neurosci. Res. 97, 1223–1241. doi: 10.1002/jnr.24476
Petra, A. I., Panagiotidou, S., Hatziagelaki, E., Stewart, J. M., Conti, P., and Theoharides, T. C. (2015). Gut-microbiota-brain Axis and its effect on neuropsychiatric disorders with suspected immune dysregulation. Clin. Ther. 37, 984–995. doi: 10.1016/j.clinthera.2015.04.002
Pirolli, N. H., Bentley, W. E., and Jay, S. M. (2021). Bacterial extracellular vesicles and the gut-microbiota brain Axis: emerging roles in communication and potential as therapeutics. Adv Biol 5:e2000540. doi: 10.1002/adbi.202000540
Qin, Y., Havulinna, A. S., Liu, Y., Jousilahti, P., Ritchie, S. C., Tokolyi, A., et al. (2022). Combined effects of host genetics and diet on human gut microbiota and incident disease in a single population cohort. Nat. Genet. 54, 134–142. doi: 10.1038/s41588-021-00991-z
Rathour, D., Shah, S., Khan, S., Singh, P. K., Srivastava, S., Singh, S. B., et al. (2023). Role of gut microbiota in depression: understanding molecular pathways, recent research, and future direction. Behav. Brain Res. 436:114081. doi: 10.1016/j.bbr.2022.114081
Reininghaus, E. Z., Wetzlmair, L. C., Fellendorf, F. T., Platzer, M., Queissner, R., Birner, A., et al. (2018). The impact of probiotic supplements on cognitive parameters in euthymic individuals with bipolar disorder: a pilot study. Neuropsychobiology 79, 63–70. doi: 10.1159/000492537
Rutsch, A., Kantsjö, J. B., and Ronchi, F. (2020). The gut-brain Axis: how microbiota and host inflammasome influence brain physiology and pathology. Front. Immunol. 11:604179. doi: 10.3389/fimmu.2020.604179
Samochowiec, J., and Misiak, B. (2021). Gut microbiota and microbiome in schizophrenia. Curr. Opin. Psychiatry 34, 503–507. doi: 10.1097/yco.0000000000000733
Sampson, T. R., and Mazmanian, S. K. (2015). Control of brain development, function, and behavior by the microbiome. Cell Host Microbe 17, 565–576. doi: 10.1016/j.chom.2015.04.011
Samra, M. S., Lim, D. H., Han, M. Y., Jee, H. M., Kim, Y. K., and Kim, J. H. (2021). Bacterial microbiota-derived extracellular vesicles in children with allergic airway diseases: compositional and functional features. Allergy Asthma Immunol Res 13, 56–74. doi: 10.4168/aair.2021.13.1.56
Schwarz, E., Maukonen, J., Hyytiäinen, T., Kieseppä, T., Orešič, M., Sabunciyan, S., et al. (2018). Analysis of microbiota in first episode psychosis identifies preliminary associations with symptom severity and treatment response. Schizophr. Res. 192, 398–403. doi: 10.1016/j.schres.2017.04.017
Shen, Y., Xu, J., Li, Z., Huang, Y., Yuan, Y., Wang, J., et al. (2018). Analysis of gut microbiota diversity and auxiliary diagnosis as a biomarker in patients with schizophrenia: a cross-sectional study. Schizophr. Res. 197, 470–477. doi: 10.1016/j.schres.2018.01.002
Singh, R., Stogios, N., Smith, E., Lee, J., Maksyutynsk, K., Au, E., et al. (2022). Gut microbiome in schizophrenia and antipsychotic-induced metabolic alterations: a scoping review. Ther Adv Psychopharmacol 12:20451253221096525. doi: 10.1177/20451253221096525
Strandwitz, P. (2018). Neurotransmitter modulation by the gut microbiota. Brain Res. 1693, 128–133. doi: 10.1016/j.brainres.2018.03.015
Sultan, S., Mottawea, W., Yeo, J., and Hammami, R. (2021). Gut microbiota extracellular vesicles as Signaling molecules mediating host-microbiota communications. Int. J. Mol. Sci. 22:13166. doi: 10.3390/ijms222313166
Tulkens, J., De Wever, O., and Hendrix, A. (2020). Analyzing bacterial extracellular vesicles in human body fluids by orthogonal biophysical separation and biochemical characterization. Nat. Protoc. 15, 40–67. doi: 10.1038/s41596-019-0236-5
Vinberg, M., Ottesen, N. M., Meluken, I., Sørensen, N., Pedersen, O., Kessing, L. V., et al. (2019). Remitted affective disorders and high familial risk of affective disorders associate with aberrant intestinal microbiota. Acta Psychiatr. Scand. 139, 174–184. doi: 10.1111/acps.12976
Vuong, H. E., and Hsiao, E. Y. (2017). Emerging roles for the gut microbiome in autism Spectrum disorder. Biol. Psychiatry 81, 411–423. doi: 10.1016/j.biopsych.2016.08.024
Xu, R., Wu, B., Liang, J., He, F., Gu, W., Li, K., et al. (2020). Altered gut microbiota and mucosal immunity in patients with schizophrenia. Brain Behav. Immun. 85, 120–127. doi: 10.1016/j.bbi.2019.06.039
Yaghoubfar, R., Behrouzi, A., Ashrafian, F., Shahryari, A., Moradi, H. R., Choopani, S., et al. (2020). Modulation of serotonin signaling/metabolism by Akkermansia muciniphila and its extracellular vesicles through the gut-brain axis in mice. Sci. Rep. 10:22119. doi: 10.1038/s41598-020-79171-8
Yang, J., Kim, E. K., McDowell, A., and Kim, Y. K. (2018). Microbe-derived extracellular vesicles as a smart drug delivery system. Transl Clin Pharmacol 26, 103–110. doi: 10.12793/tcp.2018.26.3.103
Yang, J., McDowell, A., Seo, H., Kim, S., Min, T. K., Jee, Y. K., et al. (2020a). Diagnostic models for atopic dermatitis based on serum microbial extracellular vesicle metagenomic analysis: a pilot study. Allergy Asthma Immunol Res 12, 792–805. doi: 10.4168/aair.2020.12.5.792
Yang, J., Zheng, P., Li, Y., Wu, J., Tan, X., Zhou, J., et al. (2020b). Landscapes of bacterial and metabolic signatures and their interaction in major depressive disorders. Sci. Adv. 6:eaba8555. doi: 10.1126/sciadv.aba8555
Yuan, X., Zhang, P., Wang, Y., Liu, Y., Li, X., Kumar, B. U., et al. (2018). Changes in metabolism and microbiota after 24-week risperidone treatment in drug naïve, normal weight patients with first episode schizophrenia. Schizophr. Res. 201, 299–306. doi: 10.1016/j.schres.2018.05.017
Zakharzhevskaya, N. B., Vanyushkina, A. A., Altukhov, I. A., Shavarda, A. L., Butenko, I. O., Rakitina, D. V., et al. (2017). Outer membrane vesicles secreted by pathogenic and nonpathogenic Bacteroides fragilis represent different metabolic activities. Sci. Rep. 7:5008. doi: 10.1038/s41598-017-05264-6
Zhang, B., Zhao, J., Jiang, M., Peng, D., Dou, X., Song, Y., et al. (2022). The potential role of gut microbial-derived exosomes in metabolic-associated fatty liver disease: implications for treatment. Front. Immunol. 13:893617. doi: 10.3389/fimmu.2022.893617
Zheng, P., Zeng, B., Liu, M., Chen, J., Pan, J., Han, Y., et al. (2019). The gut microbiome from patients with schizophrenia modulates the glutamate-glutamine-GABA cycle and schizophrenia-relevant behaviors in mice. Sci. Adv. 5:eaau8317. doi: 10.1126/sciadv.aau8317
Zheng, P., Zeng, B., Zhou, C., Liu, M., Fang, Z., Xu, X., et al. (2016). Gut microbiome remodeling induces depressive-like behaviors through a pathway mediated by the host's metabolism. Mol. Psychiatry 21, 786–796. doi: 10.1038/mp.2016.44
Keywords: psychiatric disorders, microbiota, extracellular vesicles, emerging roles, research progress
Citation: Guo C, Bai Y, Li P and He K (2024) The emerging roles of microbiota-derived extracellular vesicles in psychiatric disorders. Front. Microbiol. 15:1383199. doi: 10.3389/fmicb.2024.1383199
Edited by:
Emilia Ghelardi, University of Pisa, ItalyReviewed by:
Marco Calvigioni, University of Pisa, ItalyDhirendra Kumar Singh, University of North Carolina at Chapel Hill, United States
Copyright © 2024 Guo, Bai, Li and He. This is an open-access article distributed under the terms of the Creative Commons Attribution License (CC BY). The use, distribution or reproduction in other forums is permitted, provided the original author(s) and the copyright owner(s) are credited and that the original publication in this journal is cited, in accordance with accepted academic practice. No use, distribution or reproduction is permitted which does not comply with these terms.
*Correspondence: Kuanjun He, aGVrdWFuanVuNjY2QDEyNi5jb20=
†These authors have contributed equally to this work