- 1Beijing Key Laboratory of New Techniques of Tuberculosis Diagnosis and Treatment, Institute of Tuberculosis Research, Senior Department of Tuberculosis, The 8th Medical Center of PLA General Hospital, Beijing, China
- 2Department of Tuberculosis, Senior Department of Tuberculosis, The 8th Medical Center of PLA General Hospital, Beijing, China
Tuberculosis (TB) is a chronic bacterial disease, as well as a complex immune disease. The occurrence, development, and prognosis of TB are not only related to the pathogenicity of Mycobacterium tuberculosis (Mtb), but also related to the patient’s own immune state. The research and development of immunotherapy drugs can effectively regulate the body’s anti-TB immune responses, inhibit or eliminate Mtb, alleviate pathological damage, and facilitate rehabilitation. This paper reviews the research progress of immunotherapeutic compounds for TB, including immunoregulatory compounds and repurposing drugs, and points out the existing problems and future research directions, which lays the foundation for studying new agents for host-directed therapies of TB.
1 Introduction
Tuberculosis (TB) is a chronic respiratory infectious disease caused by Mycobacterium tuberculosis (Mtb), which remains a major global public health issue. The World Health Organization (WHO) estimates that there were 10.6 million new cases and 1.3 million death cases of TB in 2022 (WHO, 2023). At present, a combination of anti-TB drugs is still the mainstay of TB treatment in clinical practice. Although the standard chemotherapeutic regimens have a satisfactory effect on most drug-sensitive TB, the disadvantages of long treatment periods (at least 6 months), drug toxicity, and poor patient compliance are likely to lead to the emergence of drug-resistant TB, especially multidrug-resistant TB (MDR-TB) or extensively drug-resistant tuberculosis (XDR-TB), which makes the clinical treatment of TB face great challenges (Mi et al., 2021).
Host’s immune responses against TB result from an intricate interplay between the innate and adaptive immune systems (Figure 1), and are determined by the host’s genetic susceptibility, bacterial virulence, and environmental factors. The current treatment regimens mainly focus on various targets of Mtb and reduce the bacterial load, but cannot directly weaken the host immunopathological changes associated with TB (Elkington et al., 2011; Hortle and Oehlers, 2020). Enhancing the host’s protective immunity or regulating his anti-TB immune responses maybe a valuable adjunctive therapy for TB, known as host-directed therapy (HDT). HDT agents can help TB patients to improve clinical chemotherapy effects from the following aspects: (1) Regulate the immune status of Mtb-infected individuals, improve body anti-TB immunity, enhance the ability to inhibit or eliminate Mtb, to prevent the reactivation of latent tuberculosis infection (LTBI) and reduce the incidence of disease; (2) Enhance the therapeutic efficacy of anti-TB drugs in the treatment of TB, especially MDR-TB, shorten the treatment duration, avoid transmission, reduce lung injury, and increase the cure rate; (3) Eliminate the bacteria in phagocytes and reduce the recurrence rate or the risk of reinfection. At present, HDT agents mainly include chemical synthetic drugs, immune system products, and drugs derived from microorganisms. They can regulate the anti-TB immune responses of the body, improve the host’s anti-TB immune function, inhibit or eliminate Mtb, reduce pathological damage, and promote recovery. This paper reviews the recent advances in adjuvant therapeutic compounds for TB, including immunoregulatory compounds and repurposing drugs, and points out the existing problems and future research directions, which lays the foundation for studying new agents for TB HDT.
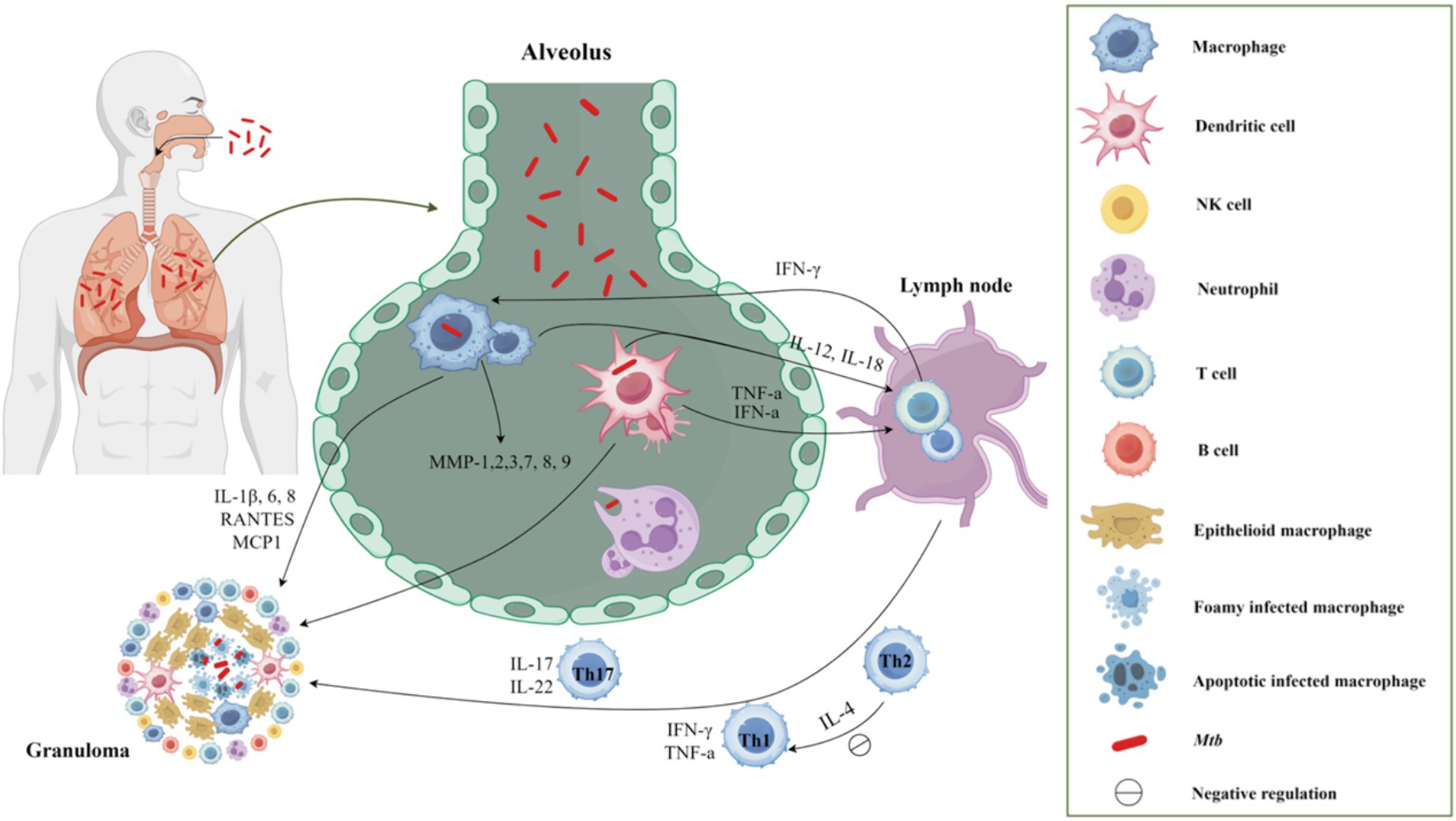
Figure 1. The host′s immune response to TB after Mtb infection. Upon entering the respiratory tract and subsequently the alveolus, Mtb elicits an immune response in the body. Initially, macrophages and neutrophils serve as the primary defense mechanism, promptly identifying and engulfing Mtb through phagocytosis. Following this, macrophages and dendritic cells that have engulfed Mtb migrate to the lung parenchyma, leading to the formation of inflammatory lesions and the recruitment of various cell types to aggregate and form granulomas. Moreover, these cells function as classical antigen-presenting cells, releasing IL-12 and IL-18 to facilitate T cell proliferation. Following the initiation of adaptive immunity, the predominant involvement of Th1 cells in the cellular immune response was observed. The secretion of IFN-γ and TNF-α by Th1 cells, along with the secretion of IL-17 by Th17 cells, promptly converged at the infection site, thereby facilitating the formation of granuloma.
2 Immunologically active compounds
It is well known that the discovery of more valuable lead compounds in the early stages of drug development can significantly save effort, money, and time in subsequent drug development. In recent years, a growing number of immunologically active compounds have been found to have a positive adjuvant therapeutic effect in the treatment of TB, including natural products, synthetic compounds obtained through high-throughput screening, computer-aided design, and structural modification. Most of these lead compounds are still in preclinical studies, of which Chicoric acid and CC11050 have entered clinical trials (Table 1).
2.1 Phytochemical compounds
2.1.1 Allicin
Allicin is a trithioallyl ether compound that naturally exists in the bulbs of garlic (Allium sativum). Rao et al. first discovered that allicin inhibited the growth of Mtb at higher concentrations (Raghunandana Rao et al., 1942). Further studies also have shown that allicin had significant antibacterial effects on drug-sensitive, MDR- and XDR-Mtb in vitro and in vivo, significantly reducing the bacterial load in mouse drug-sensitive and drug-resistant TB models. In addition, allicin also has good immunoregulatory activity, which can activate the MAPK and SAPK/JNK pathways of Mtb-infected mouse peritoneal macrophages to produce various effector molecules, especially inducing an increase in IL-1β and IL-12; can selectively induce strong protective Th1-type responses in murine models; and inhibit the phosphorylation of p38-MAPK to reduce the secretion levels of TNF-α and IL-10 (Dwivedi et al., 2019). In the early stages of human monocytes Mtb-infected, allicin could down-regulate the expression of Mtb ag85b gene and protein and inhibit the production of reactive oxygen species (ROS), which are through enhancing glutathione peroxidase activity, up-regulating IFN-γ, and inhibiting TNF-α by allicin (Hasan et al., 2006, 2007), indicating that allicin has anti-inflammatory and antioxidant effects. Clinically, the intravenous infusion of allicin as an adjuvant treatment for active pulmonary TB (PTB) patients can improve the symptoms, promote the absorption of the lesions, reduce the secretion of serum inflammatory factors, and improve the effect of chemotherapy, with fewer adverse reactions (Duan, 2014). However, the efficacy of allicin as an adjuvant treatment for TB still needs further clinical verification. Although allicin has anti-TB and immunomodulatory activities, its low water solubility and poor stability greatly limit its biological efficacy. The application of new delivery systems (such as nanoparticles, gels, and liposomes) may improve the stability, encapsulation efficiency, and bioavailability of allicin (Deng et al., 2023).
2.1.2 Ursolic acid and oleanolic acid
Ursolic acid (3β-hydroxyurs-12-en-28-oic, UA) and its isomer oleanolic acid (3β-hydroxyolean-12-en-28-oic, OA) are ubiquitous pentacyclic triterpenoid compounds in food, medicinal herbs, and other plants. Various biological activities have been reported for these two compounds, including antibacterial, antiviral, antiparasitic, antioxidant, anticancer, anti-inflammatory, hepatoprotective, and gastric-protective effects (Namdeo et al., 2023). Gu JQ first reported the anti-Mtb effect of UA and OA in Valeriana laxiflora and Quinchamalium majus in 2004 (Gu et al., 2004; Gua et al., 2004). In recent years, researchers have further demonstrated that UA and OA had in vitro activity against mycobacteria (such as drug-sensitive or drug-resistant Mtb strains, and a group of non-tuberculosis mycobacterium), with minimum inhibitory concentrations (MICs) ranging from approximately 12.5–100 μg/mL, and had synergistic effects of UA/OA mixtures or with anti-TB drugs (Pitaloka and Sukandar, 2017). UA and OA can significantly reduce bacterial load and lung inflammation in mouse models infected with Mtb H37Rv or MDR-Mtb through subcutaneous or intragastrical administration (Jiménez-Arellanes et al., 2013; López-García et al., 2015). In addition, UA and OA also have good immune regulatory activity. In the early stage of Mtb-infected models, treatments with a low dose (0.625 μg/mL) of UA and OA could activate nuclear factor kappa-B (NF-κB), enhance the production of NO and ROS, induce high expression of inducible nitric oxide synthase (iNOS), IFN-γ and TNF-α, and inhibit the expression of TGF-β in mouse macrophages, thereby activating macrophages from M2 phase to M1 phase, inhibiting or killing intracellular Mtb (López-García et al., 2015; Podder et al., 2015). UA and OA could be recognized by two cell membrane receptors, CD36 and TGR5, respectively, which could induce overexpression of CD36 and TGR5 receptors. In macrophages and dendritic cells, CD36 acts as a co-receptor for TLR receptors, activating TLR2/1, TLR2/6, or TLR4/6 heterodimers, leading to activation of NF-κB and increase of pro-inflammatory cytokines such as TNF-α, IL-6, and IL-1β secretion (Jiménez-Arellanes et al., 2013; López-García et al., 2015). However, differences in the immune regulatory activity of UA and OA have been observed. Depending on the differences in UA and OA concentration and treatment timing, types and biological status of target cells in the experimental system, they may exhibit anti-inflammatory or pro-inflammatory effects (Ikeda et al., 2008). For example, Zerin et al. (2015, 2016) found that treatment with UA alone significantly inhibited the mRNA expression levels of cytokines (TNF-α, IL-1β and IL-6) and immune regulatory mediators [including iNOS and cyclooxygenase-2 (COX-2)], as well as the release of NO in Mtb-infected RAW 267.4 cells, A549 cells, and rat splenocytes stimulated by Con A. From this, it can be seen that using UA for anti-inflammatory treatment may be a double-edged sword. Further evaluation is needed to assess the effects of UA dosage and treatment timing on the biological status of target cells or tissues. Besides, a recent study showed that UA could synergically inhibit Akt/mTOR and TNF-α/TNFR1 signaling pathways, and promote autophagy, thereby inhibiting the pyroptosis and necroptosis of macrophages induced by Mtb, and enhancing the intracellular killing of Mtb (Shen et al., 2023). Although the combination of antibacterial and immunomodulatory activities of UA and OA may make them potential agents for TB adjuvant treatment, it is worth noting that high doses of UA and OA (25–50 μg/mL) are highly toxic to macrophages, the poor penetration, low lung concentration, and severe side effects caused by subcutaneous and oral administration of UA and OA may hinder the clinical application of these compounds to some extent. Saini et al. directly administered UA and OA to the lungs of Wistar rats using a metered dose inhaler (MDI). Pharmacokinetic results showed that the combined therapy could increase the biological half-life of UA and OA, and the drug concentration in the lungs was higher than in the systemic circulation. Acute inhalation toxicity studies also showed good safety and no adverse effects on body weight and important organs (Saini et al., 2022).
2.1.3 Curcumin
Curcumin is a natural hydrophobic polyphenol substance extracted from the roots and stems of some plants in the ginger and Araceae families. Schraufstatter and Bernt first discovered that curcumin diluted at 1:40000 showed a significant inhibitory effect on Mtb in 1946 (Schraufstatter and Bernt, 1949). Further studies have shown that curcumin could induce caspase-3-dependent apoptosis and autophagy, inhibit NF-κB activation, and protect macrophages from P19-induced apoptosis to kill intracellular Mtb in macrophages (Zhang et al., 2014; Bai et al., 2016; Ansari et al., 2023). In addition, curcumin could induce the expression of cathelicidin antimicrobial peptide gene through a vitamin D receptor-independent pathway, which may contribute to the clearance of Mtb in the human body (Guo et al., 2013). Animal experiments have shown that intraperitoneal injection of curcumin could reduce the lung bacterial load and pneumonic area in Mtb H37Rv-infected BALB/c mouse models, reduce neuroinflammation, and improve behavioral status (Lara-Espinosa et al., 2022). Besides, previous studies suggested curcumin had a protective effect on INH-induced liver injury and could significantly reduce the abnormal increase of liver function biomarkers (He et al., 2017; Li et al., 2022). A study conducted in India has demonstrated that curcumin could significantly elevate the blood concentration of Bedaquiline by limiting P-glycoprotein-mediated efflux to improve the absorption and slow the metabolism of Bedaquiline, so it is advised not to combine curcumin with Bedaquiline to prevent potential adverse reactions (Kotwal et al., 2020). However, poor oral availability, limited gastrointestinal absorption, and fast metabolism have been the obstacles to the clinical application of curcumin (Hussain et al., 2022). Recent studies have shown that the use of nanotechnology to encapsulate curcumin may help address this problem. Curcumin nanoparticles have been shown to stimulate innate immune responses, promote autophagy in antigen-presenting cells (APCs), and stimulate the production of co-stimulatory molecules and pro-inflammatory cytokines in APCs. Furthermore, curcumin nanoparticles have also been found to enhance the ability of the Bacillus Calmette-Guérin (BCG) vaccine to induce central memory T cells (TCM) of the Th1 and Th17 lineages, potentially improving the efficacy of the BCG vaccine (Ahmad et al., 2019). And other studies have also found that the nanoparticle-encapsulated curcumin could dramatically increase the bioavailability of curcumin in animals, and the adjuvant treatment with nano-curcumin in combination with INH or RIF revealed a satisfactory bactericidal effect, hepatotoxicity reduction, a shorter treatment time, and low risk of disease recurrence (Tousif et al., 2017; Galdopórpora et al., 2022; Li et al., 2022; Gupta et al., 2023). Therefore, whether curcumin can be used as a therapeutic adjuvant for TB needs to be further confirmed in animal models and clinical trials.
2.1.4 [6]-Gingerol
[6]-Gingerol is the main active ingredient of ginger (Zingiber officinale), which has anti-inflammatory, antioxidant, anticancer, and immunomodulatory functions (Wang et al., 2014). It was found that [6]-gingerol could effectively inhibit the growth of Mtb in the lung, spleen, and liver of Mtb-infected mice (Bhaskar et al., 2020). And [6]-gingerol-intraperitoneally injected mice showed a significantly up-regulated expression of pro-inflammatory cytokines in the spleen, enhanced Th1/Th17 response, and increased IFN-γ and IL-17 secretion by CD4+ and CD8+ T cells (Bhaskar et al., 2020). Besides, bacterial load in the lungs was significantly reduced in mice when [6]-gingerol (intraperitoneal injection) was used in combination with INH (Bhaskar et al., 2020). In addition, [6]-gingerol has been found to possess great anti-TB potency against both dormant Mtb and drug-resistant Mtb (Bhaskar et al., 2020). The pharmacokinetic results showed that the maximum blood concentration (Cmax) and time to reach Cmax (Tmax) of 6-gingerol were 453.40 ng/mL and 30 min. The elimination half-life (t1/2) is 149 min (Levita et al., 2018). Another pharmacokinetic study has indicated that [6]-gingerol may increase the oral bioavailability of Bedaquiline by modifying gastrointestinal physiology, potentially leading to adverse reactions or side effects (Kotwal et al., 2020). [6]-gingerol, as a new adjuvant anti-TB and immunomodulatory candidate, has a good prospect in the treatment of sensitive- and resistant-Mtb strains, but there is still a lack of critical experimental data to guarantee its quality, efficacy, and safety.
2.1.5 Quercetin-polyvinylpyrrolidone
Many flavonoids have been described as having antimycobacterial activity (Sharma and Yadav, 2017). Quercetin, a kind of flavonoid from natural plants, shows anti-inflammatory and antioxidant effects but possesses poor solubility and low bioavailability. Quercetin coupled with Polyvinylpyrrolidone (QP) could effectively improve its solubility and has immunomodulatory activity to promote the growth of endothelial cells and reduce inflammation and blood coagulation (Butov et al., 2016). In vitro experiments showed that quercetin possessed 99.3% inhibitory activity against Mtb H37Rv at 200 μg/mL, with a MIC value of 6.25 μg/mL. It also had a strong binding affinity with the N-terminus of isocitrate lyase (ICL), with an IC50 of 3.57 μM. These data strongly suggest an inhibition activity of quercetin on Mtb (Shukla et al., 2015; Sasikumar et al., 2018). Dmytro et al. have shown that QP could promote IL-4 secretion by helper T cells, decrease IL-1β and TNF-α expression, reduce production of reactive oxygen species by macrophages, and prevent caseous necrosis (Butov et al., 2016). Animal experiments have demonstrated that intraperitoneal injection or intragastric administration QP can limit the extent of pathological progress and tissue necrosis, protect hepatocytes, and repair infected lung tissues (Butov et al., 2015; Zhang et al., 2020; Sanjay et al., 2021). After the treatment of active PTB with QP (intravenous injection) combined with anti-TB drugs, the patients revealed a reduction in clinical symptoms and sputum bacteria, marked absorption of lung lesions, a shorter cavity closure, and a favorable safety profile, which suggests that QP may be offered as a potential candidate for TB immunotherapy (Butov et al., 2016). Currently, 3D-printed pharmaceutical skin patches containing QP have been developed to provide appropriate therapeutic drug concentration and satisfactory sustained-release characteristics and may be used for the treatment of TB patients in the future (Chaudhari et al., 2021).
2.1.6 Bergenin
Bergenin, a type of polyphenol compound isolated from plants in the genus Bergenia, exhibits antitussive, analgesic, and anti-inflammatory properties. Studies found that Bergenin had anti-mycobacterial activity through screening and further demonstrated that although bergenin is unable to kill Mtb directly, it could activate MAPK, ERK1/2, and SAPK/JNK pathways of Mtb-infected macrophages to clear bacteria (Dwivedi et al., 2017; Kumar N. P. et al., 2019). In addition, intraperitoneal injection of bergenin could also selectively induce Th1 and Th17 immune responses in Mtb-infected mice, promote the secretion of IFN-γ, TNF-α, IL-12, and IL-17, and induce NO production, which contributes to the inhibition of Mtb growth (Dwivedi et al., 2017). A long-lasting, antigen-specific memory T cell response was generated in a TB murine model when bergenin was co-treated with INH, and the immune damage and bacterial load of mice were significantly reduced and the treatment duration was shortened remarkably (Kumar S. et al., 2019). The pharmacokinetic results of a single oral administration (12 mg/kg) of bergenin showed a Cmax of 957 ng/mL and a t1/2 of 0.36 h, indicating that a certain amount of bergenin could be absorbed and rapidly converted to its metabolites (Ren et al., 2019). These studies indicated that bergenin may be a powerful immunomodulator, which may further explore its value as an adjuvant therapy for TB.
2.1.7 Chicoric acid
Chicoric acid is a hydroxycinnamic acid with excellent antioxidant, hepatoprotective, and anti-inflammatory bioactivities. It has been demonstrated in vitro that the combination of chicoric acid and 13-cis-retinoic acid could enhance the expression of HLA-DR and CD14 molecules on the surface of Mtb-infected U937 macrophages, significantly increase the production of NO, and inhibit the growth of Mtb in macrophages (Abd-Nikfarjam et al., 2018). Chicoric acid has previously been shown to have immunological activity, inducing phagocytosis, increasing the number of immune cells, and promoting the production of cytokines (Goel et al., 2002; Cundell et al., 2003). The pharmacokinetic results of 50 mg/kg administration of chicoric acid in SD rats showed that the t1/2 was 4.53 ± 1.44 h, the mean residual time was 18.58 ± 4.43 h, and the area under the curve (AUC) was 26.14 mg⋅h/L. The concentration distribution of chicoric acid in liver, lung and kidney was higher than that in other tissues (Wang et al., 2016). A double-blinded, randomized, phase II clinical trial is currently underway to evaluate the role of initial combination treatment with retinoic acid, chicoric acid, minocycline, and vitamin D in the treatment of MDR-TB and both MDR-TB and COVID-19 patients (ClinicalTrials.gov Identifier: NCT05077813), but the current status of this study is unknown.
2.1.8 Luteolin
Luteolin is a natural flavonoid widely found in various vegetables, fruits, and medicinal plants, which has anti-inflammatory, anticancer, and antioxidant effects. A previous study indicated, for the first time, the potential anti-mycobacterial activity of luteolin isolated from the flowers of Chromolaena odorata (Eupatorium odoratum) (Suksamrarn et al., 2004). The in vitro experiments confirmed that luteolin has certain anti-TB and hepatoprotective activities (Araujo et al., 2014; Shabbir et al., 2020). Recent studies have found that luteolin (intraperitoneal injection) and INH (oral) combination therapy can promote the activity of natural killer cells and natural killer T cells, induce the expression of type central memory T cells to produce Th1 and Th17 immune responses effectively through inhibiting Kv1.3 K+ channels, so as to shorten the treatment duration of TB, alleviate INH-induced hepatotoxicity, prevent the recurrence of disease and the emergence of drug-resistant Mtb (Singh et al., 2021). Following oral administration of SD rats with luteolin at the dosage of 200 mg/kg, the pharmacokinetic parameters in rat plasma were obtained with the technology of HPLC–MS/MS, indicating that the t1/2 at 4.58 ± 2.55 h, Cmax at 152.9 ± 35.1 ng/mL, Tmax at 0.25 ± 0.04 h, and AUC0–t at 682.6 ± 170.6 ng⋅h /mL (Wu et al., 2022). At present, only computerized ADME and toxicity evaluation have confirmed that luteolin is effective and safe for oral administration (Islam et al., 2023), and there is no support from animal experiments or clinical trial data.
2.1.9 Berberine
Berberine, as a representative benzylisoquinoline alkaloid, has shown potent activity against resistant Mtb strains since 1998 (Gentry et al., 1998). The available data from in vitro experiments indicates that berberine demonstrates immunomodulatory properties by primarily activating macrophages and enhancing their Mtb-killing abilities, while simultaneously decreasing the expression of pro-inflammatory factors (Ozturk et al., 2021). This dual effect may be attributed to berberine’s ability to exert anti-TB effects on macrophages through alternative mechanisms, such as inducing autophagy via the AMPK/mTOR pathway and mouse uncoupling protein 2, thereby suppressing inflammation in mouse macrophages (Wang et al., 2011; Fan et al., 2015). A recent study found that berberine enhances the innate defense mechanism against Mtb and stimulates differentiation of Th1/Th17 specific effect memory and tissue-resident memory responses through the NOTCH3/PTEN/AKT/FOXO1 pathway, thereby augmenting the host resistance against TB (Pahuja et al., 2023). Oral berberine alone or in combination with RIF and INH could activate spleen macrophages and increase the B lymphocyte numbers, as well as reduce lung pathology but without additive or synergistic effects on bacterial burden in C57BL/6 mice (Ozturk et al., 2021). The pharmacokinetic results of single intravenous administration (4.0 mg/kg) and oral administration (48.2, 120 or 240 mg/kg) of berberine in rats showed that berberine metabolized rapidly in vivo with absolute bioavailability of 0.37 ± 0.11%, suggesting that berberine had low absolute bioavailability, insufficient absorption and extensive metabolism (Feng et al., 2020). Thus, overcoming low bioavailability remains a significant challenge. At present, computer screening has found that Mtb-FtsZ may be a target for berberine to exert an anti-TB effect as they can form a stable complex coupled with a significantly high binding energy (Akinpelu et al., 2022). Of course, this finding needs to be verified in later cell and animal experiments.
2.2 Microbe-derived components
2.2.1 Phosphatidyl-myo-inositol mannosides
Phosphatidyl-myo-inositol mannosides (PIMs) are glycolipids found in the cell envelope of all Mycobacterium species with immunomodulatory activities (Boldrin et al., 2021). PIMs could modulate macrophage activity and cytokine production and could be recognized by macrophage mannose receptors, dendritic cell-specific ICAM-3-grabbing nonintegrin, or other receptors depending on the structural differences between PIMs, and this balance may directly influence the immune response of the host to Mtb infection (Torrelles et al., 2006). In addition, PIMs also could be recognized by T cells in the presence of CD1d, activate NKT cells to produce specific immune responses, and bind to α5β1 integrin VLA-5 on CD4+ T cells, resulting in T cell adhesion to fibronectin and promoting granuloma formation (Rojas et al., 2006). Several compounds of PIMs and their related components have been synthesized, such as PIM4, ACPIM6, PIM6, and AC2PIM2. More recently, tetraacylated phosphatidylinositol hexamannoside (Ac2PIM6) synthesized from stearic acid and tuberculous stearic acid has been shown to significantly increase serum levels of IL-4 and IFN-γ in mice, and to have certain adjuvant effects on albumin and tetanus toxoid antigens (Patil et al., 2015). However, its immunotherapeutic effect on TB needs to be further studied.
2.3 Synthetic compounds
2.3.1 HE2000
HE2000 (16alpha-Bromoepiandrosterone), an androsterone adrenal steroid derivative, exerts effective activity in the recovery of immune responses in a variety of diseases such as HIV, malaria, and TB. Studies have indicated that subcutaneous HE2000 combined with oral chemotherapy can lead to a significant increase in the secretion of pro-inflammatory cytokines (IFN-γ, IL-2, and TNF-α) in lung tissue homogenate of Mtb-infected mice. This combination also results in a decrease in the expression level of IL-4, a reduction in bacterial load, a shortened duration of antibiotics treatment, and an improvement in survival rate (Hernandez-Pando et al., 1998; Hernández-Pando et al., 2005). The elevated secretion of pro-inflammatory cytokines may be attributed to the shift in cytokine profile from Th2 to Th1 in mice at advanced stages of infection following HE2000 treatment (Hernández-Pando et al., 2005). It is well known that patients with type 2 diabetes mellitus have increased severity of TB. One of the important pathogenic factors is the high expression of 11-βHSD1 and corticosterone in the lung and liver in advanced disease (López-Torres et al., 2021). Intratracheal or subcutaneous administration of HE2000 could effectively reduce the expression of 11-βHSD1 and local corticosterone synthesis in Mtb-infected mice, thereby activating CD4+ Th1 cells and macrophages, promoting the high expression of protective cytokines (TNF-α, IFN-γ), and significantly reducing Mtb burden and hyperglycemia (Hernández-Pando et al., 2005; López-Torres et al., 2021). Combined with the fact that HE2000 is not metabolized into sex steroids, these findings revealed this safe and well-tolerated compound may be a potential immune modulator for the adjuvant treatment of TB.
2.3.2 CC11050
CC11050, a type 4 phosphodiesterase inhibitor, has been shown in preclinical studies to improve inflammation and injury caused by TB (Subbian et al., 2016a). After 8 weeks gavage treatment with CC11050, the rabbits showed a decreased expression of multiple matrix metalloproteinase genes (MMP-1, −12, −14), as well as other genes associated with TNF-α regulation, macrophage activation, and lung inflammation, thus blocking the mechanism leading to permanent, progressive loss of lung function (Subbian et al., 2016a). CC11050 had no direct anti-TB activity when administered in vitro or alone, but combined with INH treatment by oral gavage could significantly reduce the range of lung lesions, improve lung fibrosis, and enhance the osmotic effect of INH in lung lesions in TB animal models (Subbian et al., 2016a,b). Pharmacokinetic experiments revealed that CC11050 was absorbed at a faster and more rapid rate in mice compared to rabbits (Tmax and Cmax of 1,410 ng/mL at 2 h, 163 ng/mL at 4 h). When combined with INH, the Tmax of CC11050 in mice was delayed to 5 h, and there was a moderate increase in plasma Cmax and AUClast. This may be attributed to the inhibitory effect of INH on the metabolism of CC11050. However, this effect was not observed in the co-treated rabbit model (Subbian et al., 2016a,b). An open-label, phase II, randomized controlled trial of CC11050, everolimus, auranofin, and ergocalciferol in PTB patients may be active in South Africa (ClinicalTrials.gov Identifier: NCT02968927). The results of this study showed that CC11050 is safe and fairly well tolerated as an adjunctive treatment for TB, and may also promote recovery of FEV1, a key indicator of lung function (Wallis et al., 2021).
3 Repurposed drugs
Many drugs used for the treatment of other diseases are also used to treat TB as adjuncts (Table 1), these agents contribute to the clearance of Mtb infection and the prevention of the development of drug resistance when used in conjunction with clinical short-course chemotherapy regimens.
3.1 Vitamin D
Vitamin D is a fat-soluble vitamin, while it does not possess any biological activity. It has to be converted to 1, 25-dihydroxyvitamin D3 in vivo to facilitate intestinal calcium absorption and prevent nutritional rickets in children. In recent years, several studies have found that vitamin D is involved in the body’s immune response to TB. The in vitro experiments have confirmed that vitamin D could inhibit the growth of Mtb and secretion of pro-inflammatory cytokines in peripheral blood monocytes (Martineau et al., 2007), stimulate the receptor-induced expression of antimicrobial peptides, and up-regulate LL37 gene to induce the autophagy-related proteins Atg5 and Beclin-1 to mediate the killing effect (Periyasamy et al., 2020). In addition, it could also reduce the expression of MMP-7, MMP-9, and MMP-10 in human peripheral blood monocytes, and increase the expression of TIMP-1, a tissue inhibitor of MMP, to eliminate tissue damage and relieve symptoms of infection (Coussens et al., 2009). Animal models suggested that activation of vitamin D receptor reduced Mtb-induced bone destruction by inhibiting NF-κB signaling (Deng et al., 2021). Furthermore, it has been shown that oral vitamin D combined with PZA decreased the release of inflammatory cytokines and increased the expression of anti-microbial peptides (Zhang et al., 2019). After treatment with INH, RIF, and pyrazinamide alone or in conjunction with immunomodulator drugs (such as all-trans retinoic acid, vitamin D3, and α-galactose amide), a notable decrease in Mtb burden and TB recurrence rate, and a shortened treatment course were observed in PTB mice (Mourik et al., 2017). Additionally, oral vitamin D supplements were found to expedite sputum smear conversion in smear-positive PTB patients and hasten the resolution of inflammatory responses linked to a heightened mortality risk. Furthermore, it was noted that vitamin D supplements could aid in the resolution of treatment-induced lymphopenia, monocytosis, hypercytokinaemia, and hyperchemokinaemia (Coussens et al., 2012). At present, there are several clinical studies on TB patients with vitamin D-assisted chemotherapy, but the therapeutic effect on drug-sensitive TB patients remains unsatisfactory, while the sputum-negative conversion rate of a few MDR-TB patients seems to be significantly improved (Wallis and Zumla, 2016). However, Bekele et al. found that the combination of vitamin D3 and sodium phenylbutyrate with standard anti-TB treatment enhanced the killing effect of macrophages on Mtb, and significantly improved the clinical symptoms of patients, but had no effect on sputum clearance rate (ClinicalTrials.gov Identifier: NCT01698476) (Bekele et al., 2018). Vitamin D immunotherapy was generally safe and well tolerated, but a few patients had serious adverse reactions, such as paravertebral abscess enlargement, psoas abscess enlargement, hypercalcemia, etc. (Wallis and Zumla, 2016). In addition, few studies on the pharmacokinetics of vitamin D in animals or humans have been reported. Therefore, it is necessary to establish consistent clinical protocols such as patient population, dosage and duration of treatment in order to explore the safety and efficacy and collect the pharmacokinetic parameters of vitamin D in the treatment of TB more comprehensively. A randomized controlled trial of vitamin D supplementation effect in PTB children with vitamin D insufficiency has been completed in Indonesia (NCT05073965), and the result of this study provided strong evidence that vitamin D improves fever, cough, and nutritional status in these patients (Tamara et al., 2022). Currently, a randomized clinical trial is underway to evaluate the effect of oral vitamin D supplementation on the expression of TLR-2, TLR-4, and clinical outcomes in spondylitis tuberculosis patients and the status of this trial is currently unknown (ClinicalTrials.gov Identifier: NCT05376189).
3.2 Levamisole
Levamisole, a synthetic imidazo (2,1-b) thiazole derivative, is known as a broad-spectrum anthelmintic in clinical practice. As early as 1981, levamisole was found to significantly increase the positive rate of dinitrochlorobenzene (DNCB) skin tests in PTB patients, suggesting that levamisole can attenuate immunosuppression after Mtb infection (Singh et al., 1981). In recent years, levamisole has been used as an immunomodulator to ameliorate immune damage in autoimmune diseases, cancers, primary immunodeficiency diseases, and infectious diseases. The Brown Norway rats injected intraperitoneally with levamisole showed a marked upregulation of IL-18 and enhancement of Th1 immune response (Szeto et al., 2000). Clinical oral levamisole adjuvant treatment of TB and bone TB patients could ameliorate the clinical symptoms, improve the body’s immune functions, and promote the absorption of lesions and negative conversion of sputum bacteria, but may cause gastrointestinal discomfort, allergy, headache, fever, dizziness and other adverse reactions (Mielants and Veys, 1978; Qiu et al., 2002; Guo, 2008; Shamkuwar et al., 2017; Saurabh et al., 2018). However, detailed pharmacokinetic data from large number of subjects are lacking.
3.3 Doxycycline
Doxycycline, a semi-synthetic tetracycline antibiotic, is currently the only licensed broad-spectrum MMP inhibitor. Initially, doxycycline was found to have promising scavenging or inhibition effect toward Mycobacterium gordonae, Mycobacterium fortuitum, and Mycobacterium marinum (Wallace and Wiss, 1981; Tasaka et al., 1995; Weyers et al., 1996). Later, it was discovered that doxycycline has a promising anti-Mtb effect. Doxycycline could inhibit the secretion of MMP-1 and MMP-3 in Mtb-infected macrophages, down-regulate type I/II interferon and innate immune response genes, and up-regulate B cell biology-related genes (Miow et al., 2021; Simmons and Hawn, 2021).
In vitro experiments showed that doxycycline combined with amikacin had either an indifferent or synergistic effect on clinically isolated drug-resistant Mtb strains, but had no antagonistic effect (Gonzalo et al., 2015). Gengenbacher M et al. found that adding 2,000 ppm of doxycycline to the standard diet was sufficient to achieve complete inhibition of tet promoters in infected tissues of TB mice and rabbits, thereby affecting the growth and activity of Mtb (Gengenbacher et al., 2020). Doxycycline injection for 4 weeks in rabbits revealed a significant reduction in serum MMP-1 levels and blocked the progression of infection to alleviate the destruction of vertebral tissue by tuberculous spondylitis (Siregar et al., 2023).
Doxycycline is rapidly absorbed through the gastrointestinal tract, and its bioavailability is between 75 and 100% (Zhanel et al., 2004). The maximum blood concentration was reached within 4 h, and the half-life was 12 h (Zhanel et al., 2004). Doxycycline penetrates well into tissues and organs due to its lipophilic properties. About 30–40% of doxycycline is excreted intact in the urine (Zhanel et al., 2004). A phase II clinical trial conducted in Singapore showed that doxycycline in combination with anti-TB drugs reduced the activity of type 1 collagenase and elastin in the sputum of TB patients and was well tolerated (ClinicalTrials.gov Identifier: NCT02774993) (Miow et al., 2021). Another phase III clinical trial is being performed by the National University Hospital of Singapore to determine the improvement of lung function and decrease in tissue destruction of doxycycline in PTB patients (ClinicalTrials.gov Identifier: NCT05473520), and this study is recruiting subjects. Doxycycline is safe when taken orally, but the most common side effects are gastrointestinal problems and skin reactions (Smith and Leyden, 2005).
3.4 Nonsteroidal anti-inflammatory drugs
It is well known that nonsteroidal anti-inflammatory drugs (NSAIDs) are widely used for antipyretic, analgesic, and anti-inflammatory actions. NSAIDs could reduce the production of proinflammatory substances and immunosuppressive substances by inhibiting the cyclo-oxygenase, to alleviate the pathological changes and chronic inflammation of the host lungs and improve the immune response (Maitra et al., 2016; Young et al., 2020).
A randomized, active-controlled, phase III clinical trial was performed in tuberculous meningitis (TBM) patients to assess the efficacity of aspirin to reduce mortality (ClinicalTrials.gov Identifier: NCT04145258). Another phase II clinical trial is being performed in Germany to assess the efficacy and safety of 2 repurposed drugs (acetylsalicylic acid and ibuprofen) in drug-sensitive and MDR-TB patients (ClinicalTrials.gov Identifier: NCT04575519). These two studies are all currently recruiting participants. Furthermore, a clinical trial of aspirin with or without high-dose RIF, moxifloxacin, INH, Pyrazinamide, and steroids in TBM patients is being performed by the Tuberculosis Research Center of India to comparatively evaluate the short course regimen and standard regimen (ClinicalTrials.gov Identifier: NCT05917340), but this study is not yet recruiting the patients. In 2019, a prospective, randomized, pilot study to estimate the potential efficacy and safety of using adjunctive ibuprofen for the treatment of XDR-TB patients was completed but without results posted (ClinicalTrials.gov Identifier: NCT02781909).
To determine the safety and immunogenicity of etoricoxib, a phase I clinical trial has been completed in MDR-TB patients in Norway (ClinicalTrials.gov Identifier: NCT02503839). The results showed that etoricoxib adjuvant therapy was safe in TB patients, and only 3 patients developed minor adverse reactions (Jenum et al., 2021). Etoricoxib could not improve cellular immunity and humoral immunity but decreased the H56: IC31-induced T cell response (Jenum et al., 2021). Moreover, this study suggested that impaired macrophage capacity in TB patients treated with etoricoxib may have a potentially unfavorable effect on the control of mycobacterial growth (Nore et al., 2023).
A recent study concluded that COX2 inhibitors (acetylsalicylic acid and celecoxib) may downregulate inflammation in TB patients, but further investigations are needed to evaluate the potential mechanisms in a larger clinical trial (Carranza et al., 2023). A study of celecoxib in healthy volunteers to evaluate the bactericidal activity against Mtb has been completed (ClinicalTrials.gov Identifier: NCT02602509), but the results showed celecoxib with or without RIF or pyrazinamide have no anti-mycobacterial effect in the whole-blood bactericidal activity model (Naftalin et al., 2018).
3.5 Statins
Statins, inhibitors of 3-hydroxy-3-methylglutaryl-coenzyme A reductase, have primarily been utilized for cardiovascular disease and lipid disorders because of their cholesterol-lowering effects.
In recent years, studies have found that statins also have anti-inflammatory, immunomodulatory, and pathogenic bacteria inhibition effects (Xu et al., 2017; Parihar et al., 2019; Woźniak et al., 2023), and there are several researches on the role of statins in adjuvant immunotherapy of TB (Dutta et al., 2020; Hu et al., 2020). Pravastatin could effectively inhibit phagosome acidification and protease hydrolysis, which is conducive to Mtb clearance (Dutta et al., 2020). Simvastatin inhibited Mtb infection by increasing apoptosis, autophagy, cytokine release, and NKT cell generation, and could assist in enhancing the anti-TB activity of first-line anti-TB drugs and shorten the cure time (Dutta et al., 2016; Guerra-De-Blas et al., 2019). Fluvastatin could regulate Th1 and Th2 cytokine responses, induce the release of pro-inflammatory cytokines IL-1β, IL-18, and IFN-γ, and promote the activation of autophagy and apoptosis (Montero et al., 2000; Dutta et al., 2020). Besides, rosuvastatin could also inhibit the growth of Mtb (Dutta et al., 2020).
Recently, two phase II clinical trials to evaluate the safety, tolerability, pharmacokinetics, and efficacy of pravastatin in TB patients have been completed but no results published so far (ClinicalTrials.gov Identifier: NCT03882177 and NCT03456102).
In addition, a phase II, randomized, double-blind clinical trial is being performed by Obafemi Awolowo University Teaching Hospital (Ile Ife, Osun, Nigeria) to evaluate the safety and efficacy of different doses of atorvastatin-containing regimen in TB patients (ClinicalTrials.gov Identifier: NCT06199921). Another clinical trial is also conducting by the University of Cape Town (Observatory, WC, South Africa) to determine the effectiveness of atorvastatin in reducing persistent inflammation after TB treatment completion in HIV-infected and HIV-uninfected patients (ClinicalTrials.gov Identifier: NCT04147286).
Additionally, a phase II clinical trial in PTB patients has been conducted in Singapore to determine whether the addition of rosuvastatin to standard TB therapy results in accelerated sputum culture conversion (ClinicalTrials.gov Identifier: NCT04504851). The study results provided strong evidence that adjunctive rosuvastatin once per day was safe but there were no substantive improvements in the culture conversion (Cross et al., 2023).
3.6 Metformin
Metformin is a biguanide drug widely used for patients with type 2 diabetes worldwide. Diabetes increases susceptibility to TB by indirectly affecting the functions of macrophages and lymphocytes, such as chemotaxis, phagocytosis, and antigen presentation (Kumari and Meena, 2014). Metformin could increase host cells production of mitochondrial ROS and the acidification of mycobacterium phagosome, promote the proliferation of T cells secreting IFN-γ, reduce the bacterial load, TB-induced tissue pathology and inflammatory response in both lung and spleen of mice, and enhance the host-specific immune function (Singhal et al., 2014). Secondly, metformin treatment reduced soluble CD14, CD163, and inflammatory C-reactive protein levels in TB patients with diabetes, significantly decreased MMP-1, −2, −3, −7, −9, and − 12 levels, and promoted smear conversion, indicating that metformin has significant anti-inflammatory and lung injury improvement effects, and greatly reduced mortality (Degner et al., 2018; Kumar et al., 2018; Kumar N. P. et al., 2019; Li et al., 2021; Wang Y. et al., 2022). In addition, metformin also could induce autophagy, regulate oxidative stress, and enhance the efficacy of anti-TB drugs (Fatima et al., 2021).
Currently, a prospective, randomized, open-label phase II clinical trial is being performed in PTB patients with HIV to explore the safety and tolerability of metformin in combination with standard anti-TB treatment (ClinicalTrials.gov Identifier: NCT04930744).
3.7 Loperamide
Loperamide, an inexpensive, over-the-counter, mu-opioid receptor agonist, is commonly used as an antidiarrheal agent. Loperamide could significantly inhibit the growth of Mtb in vitro and in vivo, and regulate the expression of autophagy-related genes after Mtb infection (Juárez et al., 2016; Barrientos et al., 2021). It could also induce overexpression of bactericidal/permeability-increasing protein (BPI) and antimicrobial peptide LL37 genes, increasing the ability of macrophages to eliminate bacterial infection (Juárez et al., 2018). In addition, loperamide induced the production of μ-opioid receptor-dependent antimicrobial peptides, decreased the TNF-α level and increased the production of IL-10 and prostaglandin E2 in macrophages infected with Mtb (Wetzel et al., 2000; Happel et al., 2011), and blocked calcium channels to reduce the production of pro-inflammatory cytokines in the body, thus preventing tissue damage caused by increased inflammation (Ye et al., 2012). Therefore, loperamide has a potential immunotherapeutic effect on TB.
3.8 Salazosulfapyridine
Salazosulfapyridine (SASP) is now recognized to be a useful agent in the management of inflammatory bowel disease (Crohn’s disease and ulcerative colitis). The 5-aminosalicylic acid produced by SASP in the presence of intestinal microorganisms has antibacterial and anti-inflammatory effects and can inhibit the synthesis of inflammatory mediators such as prostaglandin and leukotriene (Kang et al., 2017). However, SASP could enhance the Mtb clearance activity of macrophages by activating NADPH oxidase to promote the oxidation of mycothiol in Mtb macrophages, rather than the metabolite-producing 5-aminosalicylic acid (Cai et al., 2016). Previous studies have shown that SASP could significantly reduce the Mtb bactericidal load and histopathological inflammation of lungs in mice, and prevent severe inflammation induced by Mtb infection of human macrophages (Cai et al., 2016; Wang Y. et al., 2022).
Currently, two interventional studies are being conducted at the Shenzhen Third People’s Hospital (Shenzhen, China), to verify the effect of all-oral short-course regimens containing SASP on extending or shortening treatment duration in MDR-TB patients (Chictr.org.cn: ChiCTR 2,100,045,930 and ChiCTR2000032298).
3.9 Glucocorticoid
Glucocorticoids, a kind of primary stress hormone with potent anti-inflammatory and immunosuppressive effects produced by the adrenal cortex, act throughout the body to treat autoimmune diseases, asthma, cancer, and TB. Glucocorticoids could reduce leakage and inflammatory cell infiltration, stabilize lysosomal membrane, protect the mitochondria, relieve bronchospasm, improve pulmonary ventilation, and could also reduce local lesion of tuberculous immune pathological injury and serous effusion, and reduce mortality in the treatment of TB (Schutz et al., 2018). However, further research is required to ascertain the potential immunomodulatory effects of glucocorticoids.
In clinical practice, glucocorticoids are often used in the adjuvant treatment of TBM, tuberculous pleurisy, tuberculous peritonitis, caseous pneumonia, and hematogenous disseminated TB. The administration of Prednisone within the initial 4 weeks following the initiation of Antiretroviral Therapy (ART) for HIV infection demonstrated a reduced occurrence of tuberculosis-associated Immune Reconstitution Inflammatory Syndrome (IRIS) compared to the administration of placebo (ClinicalTrials.gov Identifier: NCT01924286) (Meintjes et al., 2018). Recently, it has been demonstrated that adjunctive dexamethasone has no benefit in improving the survival of HIV-positive adults with TBM (ClinicalTrials.gov Identifier: NCT03092817) (Donovan et al., 2023). Another phase III, randomized, double-blind clinical trial in TBM patients is conducted in Vietnam to determine whether the Leukotriene A4 hydrolase (LTA4H) genotype defined the efficacy of dexamethasone in HIV-uninfected TBM patients (ClinicalTrials.gov Identifier: NCT03100786). Besides, the other randomized control trial is also being performed by National Taiwan University Hospital to investigate the effect of prednisolone as an adjunct therapy to improve the symptoms worsening in patients with tuberculous lymphadenitis (ClinicalTrials.gov Identifier: NCT05861440).
3.10 Thalidomide
Thalidomide, a potent agent with teratogenic effects, has proven to be useful and effective in refractory dermatologic disorders. As a glutamic acid derivative, thalidomide has immunomodulatory and anti-inflammatory effects. Thalidomide exerted an immunomodulatory function through the inhibition of TNF-α, elevation of IFN-γ levels, and regulation of NF-κB, IL-6, and IL-1 secretion, along with the modulation of COX-2 and prostaglandin E2 production (Fu and Fu-Liu, 2002; Van Toorn et al., 2021a). Besides, thalidomide synergistically stimulated CD8+ T cells more than CD4+ T cells (Fu and Fu-Liu, 2002). Thalidomide could be used as an immunosuppressant in the adjuvant treatment of TB patients with severe TB immune response or immunopathological damage, or patients with complex central nervous system TB. A cohort study from South Africa showed that thalidomide was safe, well tolerated, and had a modest clinical effect in patients with central nervous system TB-related complications (Van Toorn et al., 2021b). However, another review showed that thalidomide had no significant clinical improvement in patients with central nervous system TB compared to the control group and had numerous adverse reactions, while it appeared to be effective in HIV-positive patients with TB-immune reconstitution inflammatory syndrome (Panda et al., 2021). In conclusion, it is important to note that thalidomide can cause severe teratogenic effects and should be used with caution in clinical trials. Currently, a randomized, active-controlled phase II and III clinical trial is performed by PGIMER Chandigarh to study the efficacy of thalidomide in complicated tubercular infection of the central nervous system (Ctri.nic.in: CTRI/2023/05/052417).
3.11 Other drugs with immunotherapeutic effects
Furthermore, aside from the above drugs, certain pharmaceutical agents employed in the treatment of alternative diseases exhibit immunotherapeutic properties toward TB. For example, anticancer drugs (everolimus, temsirolimus, ridaforolimus) could increase the ability of macrophages to fight Mtb by inhibiting the rapamycin (mTOR) pathway and promoting autophagy (Cerni, et al., 2019). A prospective, open-lable, randomized, phase II trial demonstrated that everolimus was safe, and well tolerated as an adjunctive treatment for TB (ClinicalTrials.gov Identifier: NCT02968927) (Wallis et al., 2021). However, a case report demonstrated that the administration of everolimus did not yield any discernible impact on the viability of Mtb, and it did not induce an elevation in intracellular reactive oxygen species production within infected macrophages (Bianco et al., 2023). Imatinib, a drug for the treatment of leukemia, could promote autophagy and acidification, synergistic enhancement of the anti-TB effect of RIF, reduce the survival of Mtb in macrophages, and have a certain effect on the treatment of MDR and XDR-TB (Napier et al., 2011). Recently, to evaluate the safety, pharmacokinetics, and effects of imatinib on myelopoiesis in TB patients when given with and without INH and rifabutin, a phase II, randomized clinical trial has been completed by National Institute of Allergy and Infectious Diseases (NIAID, Atlanta, Georgia, United States) but with no results published (ClinicalTrials.gov Identifier: NCT03891901). The anti-AIDS drug saquinavir could effectively improve the expression of HLA class II antigen, induce the proliferation of CD4+ T cells, promote the secretion of IFN-γ, and significantly kill intracellular Mtb (Pires et al., 2021), which is currently in the experimental stage in vitro. Zileuton, used for the treatment of asthma and allergic rhinitis, was able to inhibit the production of pro-inflammatory leukotriene and increase the level of IL-1β. It also possessed some degree of tracheal protection, tracheal dilation, and anti-inflammatory effects, and could reduce the burden and pathology of Mtb-infected mice, and improve the survival rate (Mayer-Barber et al., 2014; Mishra et al., 2020). However, the research is still at the preclinical stage.
4 Conclusion and prospects
4.1 Issues and approaches
Enhancing protective immunity and decreasing pathological damage may play an important role in TB treatment. Immunologically active substances, including natural active compounds, small molecule compounds, and repurposed drugs, have achieved satisfactory results in vitro experiments, animal experiments, or clinical trials. However, there are still few immunologically active compounds really used in the clinical adjuvant treatment of TB patients, possible reasons for this phenomenon might include the following.
1. Etiopathogenesis of TB is intricate and complex. On the one hand, the host will form granulomas after a large amount of Mtb invades the body, the inflammation caused by delayed hypersensitivity will encase and remove the bacteria in macrophages. On the other hand, this excessive inflammation may cause a pathological state of targeted tissues or organs. Therefore, the question of how to effectively use the immune methods for immune regulation and intervention, promote physiological response, and inhibit pathological effects, is a direction for future research.
2. The discovery and preclinical study of immunologically active compounds are relatively slow. The traditional high-throughput screening methods have been far from meeting the needs of drug research and development, and the early-stage research and development strategies of small molecule compounds and natural products need the effective combination of many modern methods. For example, recent years have seen great advances in the development of new drug screening methods such as proteomics, genomics, quantum computing, CRISPR, and artificial intelligence. The application of multiple screening methods may provide more possibilities for discovering more immunologically active compounds (Ejalonibu et al., 2021).
Admittedly, several questions need to be discussed critically about the immunomodulatory candidates. Firstly, the lack of pharmacokinetic data for immunologically active compounds and the corresponding modified compounds in the TB population is a limitation, including absorption, distribution, metabolism and excretion parameters (for example, the bioavailability, plasma protein binding rate, half-life, etc. of the compound in serum. And some available data on the site of infection). Pharmacokinetic data would have assisted in interpreting potential drug–drug interactions for the regimen. Secondly, studies on the possible drug–drug interactions between immunologically active compounds and current first-line anti-TB drugs have not been through enough, and the drug interaction mechanisms are not clear. Finally, oral administration is the best option for TB patients who need long-term treatment. However, at present, most oral preparations of immunologically active compounds have not been used in animal experiments or clinical trials, which will be a new challenge in the future. Besides, studies on the effect of bioavailability differences caused by different routes of administration on adjuvant therapy are not comprehensive. Therefore, for lead compounds that are promising as candidate drugs, preclinical studies should be accelerated to obtain more pharmacodynamics and toxicological data through animal experiments, paving the way for subsequent clinical trials.
1. The clinical application of immunomodulators is not standardized. To date, there remains no consensus as to the selection, dosage, application time, treatment courses, and the intervention effect of immunomodulator. Besides, appropriate immunomodulator selection strategies and safe and effective combination therapy regimens have not been developed. For instance, should immunomodulatory drugs deemed appropriate for incorporation into conventional anti-TB therapy be administered concurrently with standard treatment, or should the therapeutic approach be customized to suit the individual patient? Furthermore, what are the enduring detrimental impacts of these repurposed drugs on the host subsequent to their utilization in immunomodulatory therapy? Additionally, what is the risk of resistance emergence? Further studies are required to explore these issues. Therefore, there is a significant need to speed up the pace of drug clinical study and the patient-specific application of these immunomodulators in the clinic.
4.2 Future perspective
The WHO emphasizes the importance of reducing the treatment duration for both sensitive and drug-resistant TB. The use of immunomodulators is deemed necessary, with glucocorticoids in combination with anti-TB medications showing significant improvements in patients suffering from tuberculous meningitis, tuberculous pericarditis, tuberculous pleurisy, and other related conditions (Jianqin, 2022). However, it is crucial to carefully select appropriate immunomodulators to effectively stimulate the host immune system and produce protective effects, considering the dual nature of the immune response triggered by Mtb. Hence, the future of immunoadjuvant therapy for TB may center on the advancement of host-directed therapy and its corresponding pharmaceutical agents (Chinese Society of Tuberculosis CMA, 2022) to achieve personalized, diversified and multifunctional immunotherapy. For instance, enhancing research on novel immune activation and suppression products, investigating immunotherapy based on intestinal flora, and exploring new approaches to immune agents mediated by nanotechnology can contribute to advancements in the field. This necessitates interdisciplinary collaboration across fields such as molecular biology, immunology, pharmacy, and clinical medicine to expedite clinical research, amass evidence-based data for the clinical utilization of immunomodulators, and identify novel targets for host immune-assisted therapy. These efforts aim to establish a more robust scientific foundation for the future development of immunomodulatory drugs.
In conclusion, while the efficacy of MDR/XDR-TB treatment is often constrained, various immunomodulators showed promise in preventing latent Mtb reactivation, avoiding active Mtb spread, mitigating adverse reactions to anti-TB drugs, shorten chemotherapy duration, and improving cure rates. Nevertheless, the intricate interplay between host and pathogen may also heighten the likelihood of disease progression or exacerbation of inflammation. Therefore, prospective multi-center large-scale and multidisciplinary in-depth investigations are imperative to yield substantial practical value in the utilization of immunoregulatory agents for TB treatment.
Author contributions
JM: Investigation, Writing – original draft. XW: Supervision, Writing – review & editing. JL: Supervision, Writing – review & editing.
Funding
The author(s) declare that no financial support was received for the research, authorship, and/or publication of this article.
Conflict of interest
The authors declare that the research was conducted in the absence of any commercial or financial relationships that could be construed as a potential conflict of interest.
Publisher’s note
All claims expressed in this article are solely those of the authors and do not necessarily represent those of their affiliated organizations, or those of the publisher, the editors and the reviewers. Any product that may be evaluated in this article, or claim that may be made by its manufacturer, is not guaranteed or endorsed by the publisher.
References
Abd-Nikfarjam, B., Nassiri-Asl, M., Hajiaghayi, M., and Naserpour, F. T. (2018). Role of chicoric acid and 13-cis retinoic acid in Mycobacterium tuberculosis infection control by human U937 macrophage. Arch. Immunol. Ther. Exp. 66, 399–406. doi: 10.1007/s00005-018-0511-0
Ahmad, S., Bhattacharya, D., Kar, S., Ranganathan, A., Van Kaer, L., and Das, G. (2019). Curcumin nanoparticles enhance Mycobacterium bovis BCG vaccine efficacy by modulating host immune responses. Infect. Immun. 87:19. doi: 10.1128/IAI.00291-19
Akinpelu, O. I., Kumalo, H. M., Mhlongo, S. I., and Mhlongo, N. N. (2022). Identifying the analogues of berberine as promising antitubercular drugs targeting Mtb-FtsZ polymerisation through ligand-based virtual screening and molecular dynamics simulations. J. Mol. Recognit. 35:e2940. doi: 10.1002/jmr.2940
Ansari, M. A., Shoaib, S., Alomary, M. N., Ather, H., Ansari, S. M. A., Hani, U., et al. (2023). Deciphering the emerging role of phytocompounds: implications in the management of drug-resistant tuberculosis and ATDs-induced hepatic damage. J. Infect. Public Health 16, 1443–1459. doi: 10.1016/j.jiph.2023.07.016
Araujo, R. C., Neves, F. A., Formagio, A. S., Kassuya, C. A., Stefanello, M. E., Souza, V. V., et al. (2014). Evaluation of the anti-Mycobacterium tuberculosis activity and in vivo acute toxicity of Annona sylvatic. BMC Complement. Altern. Med. 14:209. doi: 10.1186/1472-6882-14-209
Bai, X., Oberley-Deegan, R. E., Bai, A., Ovrutsky, A. R., Kinney, W. H., Weaver, M., et al. (2016). Curcumin enhances human macrophage control of Mycobacterium tuberculosis infection. Respirology (Carlton, Vic) 21, 951–957. doi: 10.1111/resp.12762
Barrientos, O. M., Juárez, E., Gonzalez, Y., Castro-Villeda, D. A., Torres, M., and Guzmán-Beltrán, S. (2021). Loperamide exerts a direct bactericidal effect against M. tuberculosis, M. bovis, M. terrae and M. smegmatis. Lett. Appl. Microbiol. 72, 351–356. doi: 10.1111/lam.13432
Bekele, A., Gebreselassie, N., Ashenafi, S., Kassa, E., Aseffa, G., Amogne, W., et al. (2018). Daily adjunctive therapy with vitamin D(3) and phenylbutyrate supports clinical recovery from pulmonary tuberculosis: a randomized controlled trial in Ethiopia. J. Intern. Med. 284, 292–306. doi: 10.1111/joim.12767
Bhaskar, A., Kumari, A., Singh, M., Kumar, S., Kumar, S., Dabla, A., et al. (2020). [6]-Gingerol exhibits potent anti-mycobacterial and immunomodulatory activity against tuberculosis. Int. Immunopharmacol. 87:106809. doi: 10.1016/j.intimp.2020.106809
Bianco, D. M., De Maio, F., Santarelli, G., Palucci, I., Salustri, A., Bianchetti, G., et al. (2023). Evaluation of everolimus activity against Mycobacterium tuberculosis using in vitro models of infection. Antibiotics (Basel, Switzerland) 12:171. doi: 10.3390/antibiotics12010171
Boldrin, F., Anso, I., Alebouyeh, S., Sevilla, I. A., Geijo, M., Garrido, J. M., et al. (2021). The Phosphatidyl-myo-inositol Dimannoside acyltransferase PatA is essential for Mycobacterium tuberculosis growth in vitro and in vivo. J. Bacteriol. 203:20. doi: 10.1128/JB.00439-20
Butov, D., Zaitseva, S., Butova, T., Stepanenko, G., Pogorelova, O., and Zhelezniakova, N. (2016). Efficacy and safety of quercetin and polyvinylpyrrolidone in treatment of patients with newly diagnosed destructive pulmonary tuberculosis in comparison with standard antimycobacterial therapy. Int. J. Mycobacteriol. 5, 446–453. doi: 10.1016/j.ijmyco.2016.06.012
Butov, D. O., Zaitseva, S. I., Pitenko, M. M., Stepanenko, G. L., and Butova, T. S. (2015). Morphological changes in experimental tuberculosis resulting from treatment with quercetin and polyvinylpyrrolidone. Int. J. Mycobacteriol. 4, 296–301. doi: 10.1016/j.ijmyco.2015.08.003
Cai, Y., Yang, Q., Liao, M., Wang, H., Zhang, C., Nambi, S., et al. (2016). xCT increases tuberculosis susceptibility by regulating antimicrobial function and inflammation. Oncotarget 7, 31001–31013. doi: 10.18632/oncotarget.9052
Carranza, C., Sartillo-Mendoza, L. G., Carreto-Binaghi, L. E., Muñoz-Torrico, M., Guzmán-Beltrán, S., Torres, M., et al. (2023). Exploring COX-2 inhibitors in tuberculosis: a whole-blood model approach for immune response and adjunt therapy evaluation. Tuberculosis (Edinb.) 143:102418. doi: 10.1016/j.tube.2023.102418
Cerni, S., Shafer, D., and To KVenketaraman, V. (2019). Investigating the role of everolimus in mTOR inhibition and autophagy promotion as a potential host-directed therapeutic target in Mycobacterium tuberculosis infection. J. Clin. Med. 8:232. doi: 10.3390/jcm8020232
Chaudhari, V. S., Malakar, T. K., Murty, U. S., and Banerjee, S. (2021). Extruded filaments derived 3D printed medicated skin patch to mitigate destructive pulmonary tuberculosis: design to delivery. Expert Opin. Drug Deliv. 18, 301–313. doi: 10.1080/17425247.2021.1845648
Chinese Society of Tuberculosis CMA (2022). Expert consensus on immunotherapy for tuberculosis(2022 edition). Chin. J. Tuberc. Respir. Dis. 45, 651–666. doi: 10.3760/cma.j.cn112147-20220215-00120
Coussens, A., Timms, P. M., Boucher, B. J., Venton, T. R., Ashcroft, A. T., Skolimowska, K. H., et al. (2009). 1alpha,25-dihydroxyvitamin D3 inhibits matrix metalloproteinases induced by Mycobacterium tuberculosis infection. Immunology 127, 539–548. doi: 10.1111/j.1365-2567.2008.03024.x
Coussens, A. K., Wilkinson, R. J., Hanifa, Y., Nikolayevskyy, V., Elkington, P. T., Islam, K., et al. (2012). Vitamin D accelerates resolution of inflammatory responses during tuberculosis treatment. Proc. Natl. Acad. Sci. USA 109, 15449–15454. doi: 10.1073/pnas.1200072109
Cross, G. B., Sari, I. P., Kityo, C., Lu, Q., Pokharkar, Y., Moorakonda, R. B., et al. (2023). Rosuvastatin adjunctive therapy for rifampicin-susceptible pulmonary tuberculosis: a phase 2b, randomised, open-label, multicentre trial. Lancet Infect. Dis. 23, 847–855. doi: 10.1016/S1473-3099(23)00067-1
Cundell, D. R., Matrone, M. A., Ratajczak, P., and Pierce, J. D. Jr. (2003). The effect of aerial parts of Echinacea on the circulating white cell levels and selected immune functions of the aging male Sprague-Dawley rat. Int. Immunopharmacol. 3, 1041–1048. doi: 10.1016/S1567-5769(03)00114-0
Degner, N. R., Wang, J. Y., Golub, J. E., and Karakousis, P. C. (2018). Metformin use reverses the increased mortality associated with diabetes mellitus during tuberculosis treatment. Clin. Infect. Dis. 66, 198–205. doi: 10.1093/cid/cix819
Deng, Y., Ho, C. T., Lan, Y., Xiao, J., and Lu, M. (2023). Bioavailability, health benefits, and delivery Systems of Allicin: a review. J. Agric. Food Chem. 71, 19207–19220. doi: 10.1021/acs.jafc.3c05602
Deng, J., Yang, Y., He, J., Xie, Z., Luo, F., Xu, J., et al. (2021). Vitamin D receptor activated by vitamin D administration alleviates Mycobacterium tuberculosis-induced bone destruction by inhibiting NFκB-mediated aberrant osteoclastogenesis. FASEB J. 35:e21543. doi: 10.1096/fj.202100135R
Donovan, J., Bang, N. D., Imran, D., Nghia, H. D. T., Burhan, E., Huong, D. T. T., et al. (2023). Adjunctive dexamethasone for tuberculous meningitis in HIV-positive adults. N. Engl. J. Med. 389, 1357–1367. doi: 10.1056/NEJMoa2216218
Duan, M. (2014). Effect of allicin in adjuvant treatment of senile pulmonary tuberculosis complicated with pulmonary infection. China Pract. Med. 29:2. doi: 10.14163/j.cnki.11-5547/r.2014.29.352
Dutta, N. K., Bruiners, N., Pinn, M. L., Zimmerman, M. D., Prideaux, B., Dartois, V., et al. (2016). Statin adjunctive therapy shortens the duration of TB treatment in mice. J. Antimicrob. Chemother. 71, 1570–1577. doi: 10.1093/jac/dkw014
Dutta, N. K., Bruiners, N., Zimmerman, M. D., Tan, S., Dartois, V., Gennaro, M. L., et al. (2020). Adjunctive host-directed therapy with statins improves tuberculosis-related outcomes in mice. J. Infect. Dis. 221, 1079–1087. doi: 10.1093/infdis/jiz517
Dwivedi, V. P., Bhattacharya, D., Singh, M., Bhaskar, A., Kumar, S., Fatima, S., et al. (2019). Allicin enhances antimicrobial activity of macrophages during Mycobacterium tuberculosis infection. J. Ethnopharmacol. 243:111634. doi: 10.1016/j.jep.2018.12.008
Dwivedi, V. P., Bhattacharya, D., Yadav, V., Singh, D. K., Kumar, S., Singh, M., et al. (2017). The phytochemical bergenin enhances T helper 1 responses and anti-mycobacterial immunity by activating the MAP kinase pathway in macrophages. Front. Cell. Infect. Microbiol. 7:149. doi: 10.3389/fcimb.2017.00149
Ejalonibu, M. A., Ogundare, S. A., Elrashedy, A. A., Ejalonibu, M. A., Lawal, M. M., Mhlongo, N. N., et al. (2021). Drug discovery for Mycobacterium tuberculosis using structure-based computer-aided drug design approach. Int. J. Mol. Sci. 22:3259. doi: 10.3390/ijms222413259
Elkington, P. T., D'Armiento, J. M., and Friedland, J. S. (2011). Tuberculosis immunopathology: the neglected role of extracellular matrix destruction. Sci. Transl. Med. 3:1847. doi: 10.1126/scitranslmed.3001847
Fan, X., Wang, J., Hou, J., Lin, C., Bensoussan, A., Chang, D., et al. (2015). Berberine alleviates ox-LDL induced inflammatory factors by up-regulation of autophagy via AMPK/mTOR signaling pathway. J. Transl. Med. 13:92. doi: 10.1186/s12967-015-0450-z
Fatima, S., Bhaskar, A., and Dwivedi, V. P. (2021). Repurposing immunomodulatory drugs to combat tuberculosis. Front. Immunol. 12:645485. doi: 10.3389/fimmu.2021.645485
Feng, X., Wang, K., Cao, S., Ding, L., and Qiu, F. (2020). Pharmacokinetics and excretion of berberine and its nine metabolites in rats. Front. Pharmacol. 11:594852. doi: 10.3389/fphar.2020.594852
Fu, L. M., and Fu-Liu, C. S. (2002). Thalidomide and tuberculosis. Int. J. Tuberc. Lung Dis. 6, 569–572
Galdopórpora, J. M., Martinena, C., Bernabeu, E., Riedel, J., Palmas, L., Castangia, I., et al. (2022). Inhalable Mannosylated rifampicin-curcumin co-loaded Nanomicelles with enhanced in vitro antimicrobial efficacy for an optimized pulmonary tuberculosis therapy. Pharmaceutics 14:959. doi: 10.3390/pharmaceutics14050959
Gengenbacher, M., Zimmerman, M. D., Sarathy, J. P., Kaya, F., Wang, H., Mina, M., et al. (2020). Tissue distribution of doxycycline in animal models of tuberculosis. Antimicrob. Agents Chemother. 64:19. doi: 10.1128/AAC.02479-19
Gentry, E. J., Jampani, H. B., Keshavarz-Shokri, A., Morton, M. D., Velde, D. V., Telikepalli, H., et al. (1998). Antitubercular natural products: berberine from the roots of commercial Hydrastis canadensis powder. Isolation of inactive 8-oxotetrahydrothalifendine, canadine, β-hydrastine, and two new quinic acid esters, hycandinic acid esters-1 and -2. J. Nat. Prod. 61, 1187–1193. doi: 10.1021/np9701889
Goel, V., Chang, C., Slama, J., Barton, R., Bauer, R., Gahler, R., et al. (2002). Echinacea stimulates macrophage function in the lung and spleen of normal rats. J. Nutr. Biochem. 13, 487–492. doi: 10.1016/S0955-2863(02)00190-0
Gonzalo, X., Casali, N., Broda, A., Pardieu, C., and Drobniewski, F. (2015). Combination of amikacin and doxycycline against multidrug-resistant and extensively drug-resistant tuberculosis. Int. J. Antimicrob. Agents 45, 406–412. doi: 10.1016/j.ijantimicag.2014.11.017
Gu, J.-Q., Wang, Y., Franzblau, S. G., Montenegro, G., Yang, D., and Timmermann, B. N. (2004). Antitubercular constituents of Valeriana laxiflora. Planta Med. 70, 509–514. doi: 10.1055/s-2004-827149
Gua, J.-Q., Wang, Y., Franzblau, S. G., Montenegro, G., and Timmermann, B. N. (2004). Constituents of Quinchamalium majus with potential antitubercular activity. Z. Naturforsch. C J. Biosci. 59, 797–802. doi: 10.1515/znc-2004-11-1206
Guerra-De-Blas, P. D. C., Bobadilla-Del-Valle, M., Sada-Ovalle, I., Estrada-García, I., Torres-González, P., López-Saavedra, A., et al. (2019). Simvastatin enhances the immune response against Mycobacterium tuberculosis. Front. Microbiol. 10:2097. doi: 10.3389/fmicb.2019.02097
Guo, K. (2008). Clinical analysis of levamisole adjuvant therapy for pulmonary tuberculosis. Chin. J. Mod. Drug Appl. 9, 37–38.
Guo, C., Rosoha, E., Lowry, M. B., Borregaard, N., and Gombart, A. F. (2013). Curcumin induces human cathelicidin antimicrobial peptide gene expression through a vitamin D receptor-independent pathway. J. Nutr. Biochem. 24, 754–759. doi: 10.1016/j.jnutbio.2012.04.002
Gupta, P. K., Jahagirdar, P., Tripathi, D., Devarajan, P. V., and Kulkarni, S. (2023). Macrophage targeted polymeric curcumin nanoparticles limit intracellular survival of Mycobacterium tuberculosis through induction of autophagy and augment anti-TB activity of isoniazid in RAW 264.7 macrophages. Front. Immunol. 14:1233630. doi: 10.3389/fimmu.2023.1233630
Happel, C., Kutzler, M., and Rogers, T. J. (2011). Opioid-induced chemokine expression requires NF-κB activity: the role of PKCζ. J. Leukoc. Biol. 89, 301–309. doi: 10.1189/jlb.0710402
Hasan, N., Siddiqui, M. U., Toossi, Z., Khan, S., Iqbal, J., and Islam, N. (2007). Allicin-induced suppression of Mycobacterium tuberculosis 85B mRNA in human monocytes. Biochem. Biophys. Res. Commun. 355, 471–476. doi: 10.1016/j.bbrc.2007.01.174
Hasan, N., Yusuf, N., Toossi, Z., and Islam, N. (2006). Suppression of Mycobacterium tuberculosis induced reactive oxygen species (ROS) and TNF-alpha mRNA expression in human monocytes by allicin. FEBS Lett. 580, 2517–2522. doi: 10.1016/j.febslet.2006.03.071
He, L., Guo, Y., Deng, Y., Li, C., Zuo, C., and Peng, W. (2017). Involvement of protoporphyrin IX accumulation in the pathogenesis of isoniazid/rifampicin-induced liver injury: the prevention of curcumin. Xenobiotica 47, 154–163. doi: 10.3109/00498254.2016.1160159
Hernández-Pando, R., Aguilar-Leon, D., Orozco, H., Serrano, A., Ahlem, C., Trauger, R., et al. (2005). 16alpha-Bromoepiandrosterone restores T helper cell type 1 activity and accelerates chemotherapy-induced bacterial clearance in a model of progressive pulmonary tuberculosis. J. Infect. Dis. 191, 299–306. doi: 10.1086/426453
Hernandez-Pando, R., De La Luz, S. M., Orozco, H., Arriaga, K., Pavon, L., Al-Nakhli, S. A., et al. (1998). The effects of androstenediol and dehydroepiandrosterone on the course and cytokine profile of tuberculosis in BALB/c mice. Immunology 95, 234–241. doi: 10.1046/j.1365-2567.1998.00601.x
Hortle, E., and Oehlers, S. H. (2020). Host-directed therapies targeting the tuberculosis granuloma stroma. Pathog. Dis. 78:15. doi: 10.1093/femspd/ftaa015
Hu, Z., Lowrie, D. B., and Fan, X. Y. (2020). Statins as adjunctive therapy against tuberculosis (TB): the balance between statin-induced anti-TB effect and trained immunity suppression. J. Infect. Dis. 222, 335–336. doi: 10.1093/infdis/jiz675
Hussain, Y., Alam, W., Ullah, H., Dacrema, M., Daglia, M., Khan, H., et al. (2022). Antimicrobial potential of curcumin: therapeutic potential and challenges to clinical applications. Antibiotics 11:322. doi: 10.3390/antibiotics11030322
Ikeda, Y., Murakami, A., and Ohigashi, H. (2008). Ursolic acid: an anti- and pro-inflammatory triterpenoid. Mol. Nutr. Food Res. 52, 26–42. doi: 10.1002/mnfr.200700389
Islam, S., Salekeen, R., and Ashraf, A. (2023). Computational screening of natural MtbDXR inhibitors for novel anti-tuberculosis compound discovery. J. Biomol. Struct. Dyn. 42, 3593–3603. doi: 10.1080/07391102.2023.2218933
Jäger, R., Lowery, R. P., Calvanese, A. V., Joy, J. M., Purpura, M., and Wilson, J. M. (2014). Comparative absorption of curcumin formulations. Nutr. J. 13:11. doi: 10.1186/1475-2891-13-11
Jenum, S., Tonby, K., Rueegg, C. S., Rühwald, M., Kristiansen, M. P., Bang, P., et al. (2021). A phase I/II randomized trial of H56:IC31 vaccination and adjunctive cyclooxygenase-2-inhibitor treatment in tuberculosis patients. Nat. Commun. 12:6774. doi: 10.1038/s41467-021-27029-6
Jianqin, L. (2022). Expert consensus on the rational use of glucocorticoids in tuberculosis treatment. Chin. J. Antituberculosis 44, 28–37. doi: 10.19982/j.issn.1000-6621.20210683
Jiménez-Arellanes, A., Luna-Herrera, J., Cornejo-Garrido, J., López-García, S., Castro-Mussot, M. E., Meckes-Fischer, M., et al. (2013). Ursolic and oleanolic acids as antimicrobial and immunomodulatory compounds for tuberculosis treatment. BMC Complement. Altern. Med. 13:258. doi: 10.1186/1472-6882-13-258
Juárez, E., Carranza, C., Sánchez, G., González, M., Chávez, J., Sarabia, C., et al. (2016). Loperamide restricts intracellular growth of Mycobacterium tuberculosis in lung macrophages. Am. J. Respir. Cell Mol. Biol. 55, 837–847. doi: 10.1165/rcmb.2015-0383OC
Juárez, E., Ruiz, A., Cortez, O., Sada, E., and Torres, M. (2018). Antimicrobial and immunomodulatory activity induced by loperamide in mycobacterial infections. Int. Immunopharmacol. 65, 29–36. doi: 10.1016/j.intimp.2018.09.013
Kang, S., Kim, W., Jeong, S., Lee, Y., Nam, J., Lee, S., et al. (2017). Oxidized 5-aminosalicylic acid activates Nrf2-HO-1 pathway by covalently binding to Keap1: implication in anti-inflammatory actions of 5-aminosalicylic acid. Free Radic. Biol. Med. 108, 715–724. doi: 10.1016/j.freeradbiomed.2017.04.366
Kotwal, P., Dogra, A., Sharma, A., Bhatt, S., Gour, A., Sharma, S., et al. (2020). Effect of natural phenolics on pharmacokinetic modulation of Bedaquiline in rat to assess the likelihood of potential food-drug interaction. J. Agric. Food Chem. 68, 1257–1265. doi: 10.1021/acs.jafc.9b06529
Kumar, N. P., Moideen, K., Bhootra, Y., Nancy, A., Viswanathan, V., Shruthi, B. S., et al. (2019). Elevated circulating levels of monocyte activation markers among tuberculosis patients with diabetes co-morbidity. Immunology 156, 249–258. doi: 10.1111/imm.13023
Kumar, N. P., Moideen, K., Viswanathan, V., Shruthi, B. S., Sivakumar, S., Menon, P. A., et al. (2018). Elevated levels of matrix metalloproteinases reflect severity and extent of disease in tuberculosis-diabetes co-morbidity and are predominantly reversed following standard anti-tuberculosis or metformin treatment. BMC Infect. Dis. 18:345. doi: 10.1186/s12879-018-3246-y
Kumar, S., Sharma, C., Kaushik, S. R., Kulshreshtha, A., Chaturvedi, S., Nanda, R. K., et al. (2019). The phytochemical bergenin as an adjunct immunotherapy for tuberculosis in mice. J. Biol. Chem. 294, 8555–8563. doi: 10.1074/jbc.RA119.008005
Kumari, P., and Meena, L. S. (2014). Factors affecting susceptibility to Mycobacterium tuberculosis: a close view of immunological defence mechanism. Appl. Biochem. Biotechnol. 174, 2663–2673. doi: 10.1007/s12010-014-1217-3
Lara-Espinosa, J. V., Arce-Aceves, M. F., López-Torres, M. O., Lozano-Ordaz, V., Mata-Espinosa, D., Barrios-Payán, J., et al. (2022). Effect of curcumin in experimental pulmonary tuberculosis: Antimycobacterial activity in the lungs and anti-inflammatory effect in the brain. Int. J. Mol. Sci. 23:964. doi: 10.3390/ijms23041964
Levita, J., Syafitri, D. M., Supu, R. D., Mutakin, M., Megantara, S., Febrianti, M., et al. (2018). Pharmacokinetics of 10-gingerol and 6-shogaol in the plasma of healthy subjects treated with red ginger (Zingiber officinale var. Rubrum) suspension. Biomed. Rep. 9, 474–482. doi: 10.3892/br.2018.1163
Li, B., Li, Y., and Gao, F. (2021). The mechanism and clinical value of metformin as an adjuvant against tuberculosis. Clin. Focus 2021:11. doi: 10.3969/j.issn.1004-583X.2021.02.017
Li, Y., Luo, W.-W., Cheng, X., Xiang, H.-R., He, B., Zhang, Q.-Z., et al. (2022). Curcumin attenuates isoniazid-induced hepatotoxicity by upregulating the SIRT1/PGC-1α/NRF1 pathway. J. Appl. Toxicol. 42, 1192–1204. doi: 10.1002/jat.4288
López-García, S., Castañeda-Sanchez, J. I., Jiménez-Arellanes, A., Domínguez-López, L., Castro-Mussot, M. E., Hernández-Sanchéz, J., et al. (2015). Macrophage activation by Ursolic and oleanolic acids during mycobacterial infection. Molecules (Basel, Switzerland) 20, 14348–14364. doi: 10.3390/molecules200814348
López-Torres, M. O., Marquina-Castillo, B., Ramos-Espinosa, O., Mata-Espinosa, D., Barrios-Payan, J. A., Baay-Guzman, G., et al. (2021). 16α-Bromoepiandrosterone as a new candidate for experimental diabetes-tuberculosis co-morbidity treatment. Clin. Exp. Immunol. 205, 232–245. doi: 10.1111/cei.13603
Maitra, A., Bates, S., Shaik, M., Evangelopoulos, D., Abubakar, I., McHugh, T. D., et al. (2016). Repurposing drugs for treatment of tuberculosis: a role for non-steroidal anti-inflammatory drugs. Br. Med. Bull. 118, 138–148. doi: 10.1093/bmb/ldw019
Martineau, A. R., Wilkinson, K. A., Newton, S. M., Floto, R. A., Norman, A. W., Skolimowska, K., et al. (2007). IFN-gamma- and TNF-independent vitamin D-inducible human suppression of mycobacteria: the role of cathelicidin LL-37. J. Immunol. 178, 7190–7198. doi: 10.4049/jimmunol.178.11.7190
Mayer-Barber, K. D., Andrade, B. B., Oland, S. D., Amaral, E. P., Barber, D. L., Gonzales, J., et al. (2014). Host-directed therapy of tuberculosis based on interleukin-1 and type I interferon crosstalk. Nature 511, 99–103. doi: 10.1038/nature13489
Meintjes, G., Stek, C., Blumenthal, L., Thienemann, F., Schutz, C., Buyze, J., et al. (2018). Prednisone for the prevention of paradoxical tuberculosis-associated IRIS. N. Engl. J. Med. 379, 1915–1925. doi: 10.1056/NEJMoa1800762
Mi, J., Liang, Y., Liang, J., Gong, W., Wang, S., Zhang, J., et al. (2021). The research progress in immunotherapy of tuberculosis. Front. Cell. Infect. Microbiol. 11:763591. doi: 10.3389/fcimb.2021.763591
Mielants, H., and Veys, E. M. (1978). A study of the hematological side effects of levamisole in rheumatoid arthritis with recommendations. J. Rheumatol. Suppl. 4, 77–83
Miow, Q. H., Vallejo, A. F., Wang, Y., Hong, J. M., Bai, C., Teo, F. S., et al. (2021). Doxycycline host-directed therapy in human pulmonary tuberculosis. J. Clin. Invest. 131:1895. doi: 10.1172/JCI141895
Mishra, R., Krishan, S., Siddiqui, A. N., Kapur, P., Khayyam, K. U., and Sharma, M. (2020). Potential role of adjuvant drugs on efficacy of first line oral antitubercular therapy: drug repurposing. Tuberculosis (Edinb.) 120:101902. doi: 10.1016/j.tube.2020.101902
Montero, M. T., Hernández, O., Suárez, Y., Matilla, J., Ferruelo, A. J., Martínez-Botas, J., et al. (2000). Hydroxymethylglutaryl-coenzyme a reductase inhibition stimulates caspase-1 activity and Th1-cytokine release in peripheral blood mononuclear cells. Atherosclerosis 153, 303–313. doi: 10.1016/S0021-9150(00)00417-2
Mourik, B. C., Leenen, P. J., de Knegt, G. J., Huizinga, R., van der Eerden, B. C., Wang, J., et al. (2017). Immunotherapy added to antibiotic treatment reduces relapse of disease in a mouse model of tuberculosis. Am. J. Respir. Cell Mol. Biol. 56, 233–241. doi: 10.1165/rcmb.2016-0185OC
Naftalin, C. M., Verma, R., Gurumurthy, M., Hee, K. H., Lu, Q., Yeo, B. C. M., et al. (2018). Adjunctive use of celecoxib with anti-tuberculosis drugs: evaluation in a whole-blood bactericidal activity model. Sci. Rep. 8:13491. doi: 10.1038/s41598-018-31590-4
Namdeo, P., Gidwani, B., Tiwari, S., Jain, V., Joshi, V., Shukla, S. S., et al. (2023). Therapeutic potential and novel formulations of ursolic acid and its derivatives: an updated review. J. Sci. Food Agric. 103, 4275–4292. doi: 10.1002/jsfa.12423
Napier, R. J., Rafi, W., Cheruvu, M., Powell, K. R., Zaunbrecher, M. A., Bornmann, W., et al. (2011). Imatinib-sensitive tyrosine kinases regulate mycobacterial pathogenesis and represent therapeutic targets against tuberculosis. Cell Host Microbe 10, 475–485. doi: 10.1016/j.chom.2011.09.010
Nore, K. G., Louet, C., Bugge, M., Gidon, A., Jøntvedt Jørgensen, M., Jenum, S., et al. (2023). The cyclooxygenase 2 inhibitor etoricoxib as adjunctive therapy in tuberculosis impairs macrophage control of mycobacterial growth. J. Infect. Dis. 229, 888–897. doi: 10.1093/infdis/jiad390
Ozturk, M., Chia, J. E., Hazra, R., Saqib, M., Maine, R. A., Guler, R., et al. (2021). Evaluation of berberine as an adjunct to TB treatment. Front. Immunol. 12:656419. doi: 10.3389/fimmu.2021.656419
Pahuja, I., Negi, K., Kumari, A., Agarwal, M., Mukhopadhyay, S., Mathew, B., et al. (2023). Berberine governs NOTCH3/AKT signaling to enrich lung-resident memory T cells during tuberculosis. PLoS Pathog. 19:e1011165. doi: 10.1371/journal.ppat.1011165
Panda, P. K., Panda, P., Dawman, L., Sihag, R. K., and Sharawat, I. K. (2021). Efficacy and safety of thalidomide in patients with complicated central nervous system tuberculosis: a systematic review and Meta-analysis. Am. J. Trop. Med. Hyg. 105, 1024–1030. doi: 10.4269/ajtmh.21-0108
Parihar, S. P., Guler, R., and Brombacher, F. (2019). Statins: a viable candidate for host-directed therapy against infectious diseases. Nat. Rev. Immunol. 19, 104–117. doi: 10.1038/s41577-018-0094-3
Patil, P. S., Cheng, T. J., Zulueta, M. M., Yang, S. T., Lico, L. S., and Hung, S. C. (2015). Total synthesis of tetraacylated phosphatidylinositol hexamannoside and evaluation of its immunomodulatory activity. Nat. Commun. 6:7239. doi: 10.1038/ncomms8239
Periyasamy, K. M., Ranganathan, U. D., Tripathy, S. P., and Bethunaickan, R. (2020). Vitamin D - a host directed autophagy mediated therapy for tuberculosis. Mol. Immunol. 127, 238–244. doi: 10.1016/j.molimm.2020.08.007
Pires, D., Valente, S., Calado, M., Mandal, M., Azevedo-Pereira, J. M., and Anes, E. (2021). Repurposing Saquinavir for host-directed therapy to control Mycobacterium tuberculosis infection. Front. Immunol. 12:647728. doi: 10.3389/fimmu.2021.647728
Pitaloka, D. A. E., and Sukandar, E. Y. (2017). In vitro study of ursolic acid combination first-line antituberculosis drugs against drug-sensitive and drug-resistant strains of Mycobacterium tuberculosis. Asian J. Pharm. Clin. Res. 10, 216–218. doi: 10.22159/ajpcr.2017.v10i4.16582
Podder, B., Jang, W. S., Nam, K.-W., Lee, B.-E., and Song, H.-Y. (2015). Ursolic acid activates intracellular killing effect of macrophages during Mycobacterium tuberculosis infection. J. Microbiol. Biotechnol. 25, 738–744. doi: 10.4014/jmb.1407.07020
Qiu, Q., Qiu, S., and Song, R. (2002). Efficacy of levamisole in the adjuvant treatment of severe pulmonary tuberculosis. Chin. J. Hosp. Pharm. 4, 231–232.
Raghunandana Rao, R., Srinivasa Rao, S., and Venkataraman, P. R. (1942). Investigations on plant antibiotics; studies on allicin, the antibacterial principle of Allium sativum (garlic). J. Sci. Ind. Res. 5, 31–35.
Ren, Y., Wan, C., Liao, M., Zhang, X., Cheng, X., Yuan, L., et al. (2019). Pharmacokinetics and excretion study of bergenin and its phase II metabolite in rats by liquid chromatography tandem mass spectrometry. Biomed. Chromatogr. 33:e4513. doi: 10.1002/bmc.4513
Rojas, R. E., Thomas, J. J., Gehring, A. J., Hill, P. J., Belisle, J. T., Harding, C. V., et al. (2006). Phosphatidylinositol mannoside from Mycobacterium tuberculosis binds alpha5beta1 integrin (VLA-5) on CD4+ T cells and induces adhesion to fibronectin. J. Immunol. 177, 2959–2968. doi: 10.4049/jimmunol.177.5.2959
Saini, V., Debnath, S. K., Maske, P., Dighe, V., and Srivastava, R. (2022). Targeted delivery of ursolic acid and oleanolic acid to lungs in the form of an inhaler for the management of tuberculosis: pharmacokinetic and toxicity assessment. PLoS One 17:e0278103. doi: 10.1371/journal.pone.0278103
Sanjay, S., Girish, C., Toi, P. C., and Bobby, Z. (2021). Quercetin modulates NRF2 and NF-κB/TLR-4 pathways to protect against isoniazid- and rifampicin-induced hepatotoxicity in vivo. Can. J. Physiol. Pharmacol. 99, 952–963. doi: 10.1139/cjpp-2021-0008
Sasikumar, K., Ghosh, A. R., and Dusthackeer, A. (2018). Antimycobacterial potentials of quercetin and rutin against Mycobacterium tuberculosis H37Rv. 3 Biotech 8:427. doi: 10.1007/s13205-018-1450-5
Saurabh, S. B. P., Kumar, A., Das, S., and Prasad, S. (2018). Prospective study of immunomodulation in osteoarticular tuberculosis non responsive to anti tubercular therapy. J. Clin. Orthop. Trauma 9, S1–s9. doi: 10.1016/j.jcot.2017.06.017
Schraufstatter, E., and Bernt, H. (1949). Antibacterial action of curcumin and related compounds. Nature 164, 456–457. doi: 10.1038/164456a0
Schutz, C., Davis, A. G., Sossen, B., Lai, R. P., Ntsekhe, M., Harley, Y. X., et al. (2018). Corticosteroids as an adjunct to tuberculosis therapy. Expert Rev. Respir. Med. 12, 881–891. doi: 10.1080/17476348.2018.1515628
Shabbir, M., Afsar, T., Razak, S., Almajwal, A., and Khan, M. R. (2020). Phytochemical analysis and evaluation of hepatoprotective effect of Maytenus royleanus leaves extract against anti-tuberculosis drug induced liver injury in mice. Lipids Health Dis. 19:46. doi: 10.1186/s12944-020-01231-9
Shamkuwar, C. A., Meshram, S. H., and Mahakalkar, S. M. (2017). Levamisole as an adjuvant to short-course therapy in newly diagnosed pulmonary tuberculosis patients. Adv. Biomed. Res. 6:37. doi: 10.4103/2277-9175.203162
Sharma, D., and Yadav, J. P. (2017). An overview of phytotherapeutic approaches for the treatment of tuberculosis. Mini Rev. Med. Chem. 17, 167–183. doi: 10.2174/1389557516666160505114603
Shen, J., Fu, Y., Liu, F., Ning, B., and Jiang, X. (2023). Ursolic acid promotes autophagy by inhibiting Akt/mTOR and TNF-α/TNFR1 signaling pathways to alleviate Pyroptosis and necroptosis in Mycobacterium tuberculosis-infected macrophages. Inflammation 46, 1749–1763. doi: 10.1007/s10753-023-01839-w
Shukla, H., Kumar, V., Singh, A. K., Rastogi, S., Khan, S. R., Siddiqi, M. I., et al. (2015). Isocitrate lyase of Mycobacterium tuberculosis is inhibited by quercetin through binding at N-terminus. Int. J. Biol. Macromol. 78, 137–141. doi: 10.1016/j.ijbiomac.2015.04.005
Simmons, J. D., and Hawn, T. R. (2021). Remodeling the matrix: doxycycline modulates tuberculosis immunopathology. J. Clin. Invest. 131:668. doi: 10.1172/JCI151668
Singh, M. M., Kumar, P., Malaviya, A. N., and Kumar, R. (1981). Levamisole as an adjunct in the treatment of pulmonary tuberculosis. Am. Rev. Respir. Dis. 123, 277–279
Singh, D. K., Tousif, S., Bhaskar, A., Devi, A., Negi, K., Moitra, B., et al. (2021). Luteolin as a potential host-directed immunotherapy adjunct to isoniazid treatment of tuberculosis. PLoS Pathog. 17:e1009805. doi: 10.1371/journal.ppat.1009805
Singhal, A., Jie, L., Kumar, P., Hong, G. S., Leow, M. K., Paleja, B., et al. (2014). Metformin as adjunct antituberculosis therapy. Sci. Transl. Med. 6:885. doi: 10.1126/scitranslmed.3009885
Siregar, O., Lelo, A., Rahyussalim, A. J., Ilyas, S., Benny, B., Kurniawati, T., et al. (2023). Doxycycline as a potential MMP-1 inhibitor for the treatment of spondylitis tuberculosis: a study in rabbit model. Biomed. Res. Int. 2023, 1–12. doi: 10.1155/2023/7421325
Smith, K., and Leyden, J. J. (2005). Safety of doxycycline and minocycline: a systematic review. Clin. Ther. 27, 1329–1342. doi: 10.1016/j.clinthera.2005.09.005
Subbian, S., Koo, M. S., Tsenova, L., Khetani, V., Zeldis, J. B., Fallows, D., et al. (2016a). Pharmacologic inhibition of host phosphodiesterase-4 improves isoniazid-mediated clearance of Mycobacterium tuberculosis. Front. Immunol. 7:238. doi: 10.3389/fimmu.2016.00238
Subbian, S., Tsenova, L., Holloway, J., Peixoto, B., O'Brien, P., Dartois, V., et al. (2016b). Adjunctive phosphodiesterase-4 inhibitor therapy improves antibiotic response to pulmonary tuberculosis in a rabbit model. EBioMedicine 4, 104–114. doi: 10.1016/j.ebiom.2016.01.015
Suksamrarn, A., Chotipong, A., Suavansri, T., Boongird, S., Timsuksai, P., Vimuttipong, S., et al. (2004). Antimycobacterial activity and cytotoxicity of flavonoids from the flowers of Chromolaena odorata. Arch. Pharm. Res. 27, 507–511. doi: 10.1007/BF02980123
Szeto, C., Gillespie, K. M., and Mathieson, P. W. (2000). Levamisole induces interleukin-18 and shifts type 1/type 2 cytokine balance. Immunology 100, 217–224. doi: 10.1046/j.1365-2567.2000.00042.x
Tamara, L., Kartasasmita, C. B., Alam, A., and Gurnida, D. A. (2022). Effects of vitamin D supplementation on resolution of fever and cough in children with pulmonary tuberculosis: a randomized double-blind controlled trial in Indonesia. J. Glob. Health 12:04015. doi: 10.7189/jogh.12.04015
Tasaka, S., Urano, T., Mori, M., Yamaguchi, K., and Kanazawa, M. (1995). A case of Mycobacterium fortuitum pulmonary disease in a healthy young woman successfully treated with ciprofloxacin and doxycycline. Kekkaku 70, 31–35
Torrelles, J. B., Azad, A. K., and Schlesinger, L. S. (2006). Fine discrimination in the recognition of individual species of phosphatidyl-myo-inositol mannosides from Mycobacterium tuberculosis by C-type lectin pattern recognition receptors. J. Immunol. 177, 1805–1816. doi: 10.4049/jimmunol.177.3.1805
Tousif, S., Singh, D. K., Mukherjee, S., Ahmad, S., Arya, R., Nanda, R., et al. (2017). Nanoparticle-formulated curcumin prevents Posttherapeutic disease reactivation and reinfection with Mycobacterium tuberculosis following isoniazid therapy. Front. Immunol. 8:739. doi: 10.3389/fimmu.2017.00739
Van Toorn, R., Solomons, R. S., Seddon, J. A., and Schoeman, J. F. (2021b). Thalidomide use for complicated central nervous system tuberculosis in children: insights from an observational cohort. Clin. Infect. Dis. 72, e136–e145. doi: 10.1093/cid/ciaa1826
Van Toorn, R., Zaharie, S. D., Seddon, J. A., van der Kuip, M., Marceline van Furth, A., Schoeman, J. F., et al. (2021a). The use of thalidomide to treat children with tuberculosis meningitis: a review. Tuberculosis (Edinb.) 130:102125. doi: 10.1016/j.tube.2021.102125
Wallace, R. J., and Wiss, K. (1981). Susceptibility of Mycobacterium marinum to tetracyclines and aminoglycosides. Antimicrob. Agents Chemother. 20, 610–612. doi: 10.1128/AAC.20.5.610
Wallis, R. S., Ginindza, S., Beattie, T., Arjun, N., Likoti, M., Edward, V. A., et al. (2021). Adjunctive host-directed therapies for pulmonary tuberculosis: a prospective, open-label, phase 2, randomised controlled trial. Lancet Respir. Med. 9, 897–908. doi: 10.1016/S2213-2600(20)30448-3
Wallis, R. S., and Zumla, A. (2016). Vitamin D as adjunctive host-directed therapy in tuberculosis: a systematic review. Open Forum Infect. Dis. 3:151. doi: 10.1093/ofid/ofw151
Wang, W., Ning, Y., Wang, Y., Deng, G., Pace, S., Barth, S. A., et al. (2022). Mycobacterium tuberculosis-induced upregulation of the COX-2/mPGES-1 pathway in human macrophages is abrogated by sulfasalazine. Front. Immunol. 13:849583. doi: 10.3389/fimmu.2022.849583
Wang, Y., Xie, G., Liu, Q., Duan, X., Liu, Z., and Liu, X. (2016). Pharmacokinetics, tissue distribution, and plasma protein binding study of chicoric acid by HPLC-MS/MS. J. Chromatogr. B Anal. Technol. Biomed. Life Sci. 1031, 139–145. doi: 10.1016/j.jchromb.2016.07.045
Wang, Q., Zhang, M., Liang, B., Shirwany, N., Zhu, Y., and Zou, M. H. (2011). Activation of AMP-activated protein kinase is required for berberine-induced reduction of atherosclerosis in mice: the role of uncoupling protein 2. PLoS One 6:e25436. doi: 10.1371/journal.pone.0025436
Wang, S., Zhang, C., Yang, G., and Yang, Y. (2014). Biological properties of 6-gingerol: a brief review. Nat. Prod. Commun. 9, 1027–1030. doi: 10.1177/1934578X1400900736
Wang, Y., Zhou, Y., Chen, L., Cheng, Y., Lai, H., Lyu, M., et al. (2022). Metformin promotes smear conversion in tuberculosis-diabetes comorbidity and construction of prediction models. J. Clin. Lab. Anal. 36:e24755. doi: 10.1002/jcla.24755
Wetzel, M. A., Steele, A. D., Eisenstein, T. K., Adler, M. W., Henderson, E. E., and Rogers, T. J. (2000). Mu-opioid induction of monocyte chemoattractant protein-1, RANTES, and IFN-gamma-inducible protein-10 expression in human peripheral blood mononuclear cells. J. Immunol. 165, 6519–6524. doi: 10.4049/jimmunol.165.11.6519
Weyers, W., Weyers, I., Bonczkowitz, M., Mayser, P., and Schill, W. B. (1996). Skin infections caused by Mycobacterium gordonae. Case report and review of the literature. Hautarzt Z. Dermatol. Venerol. Verwandte Gebiete 47, 771–775. doi: 10.1007/s001050050507
Woźniak, E., Broncel, M., Niedzielski, M., Woźniak, A., and Gorzelak-Pabiś, P. (2023). The effect of lipid-lowering therapies on the pro-inflammatory and anti-inflammatory properties of vascular endothelial cells. PLoS One 18:e0280741. doi: 10.1371/journal.pone.0280741
Wu, W., Li, K., Zhao, C., Ran, X., Zhang, Y., and Zhang, T. (2022). A rapid HPLC-MS/MS method for the simultaneous determination of luteolin, resveratrol and their metabolites in rat plasma and its application to pharmacokinetic interaction studies. J. Chromatogr. B Anal. Technol. Biomed. Life Sci. 1191:123118. doi: 10.1016/j.jchromb.2022.123118
Xu, X., Gao, W., Cheng, S., Yin, D., Li, F., Wu, Y., et al. (2017). Anti-inflammatory and immunomodulatory mechanisms of atorvastatin in a murine model of traumatic brain injury. J. Neuroinflammation 14:167. doi: 10.1186/s12974-017-0934-2
Ye, Y., Huang, X., Zhang, Y., Lai, X., Wu, X., Zeng, X., et al. (2012). Calcium influx blocked by SK&F 96365 modulates the LPS plus IFN-γ-induced inflammatory response in murine peritoneal macrophages. Int. Immunopharmacol. 12, 384–393. doi: 10.1016/j.intimp.2011.12.011
Young, C., Walzl, G., and Du Plessis, N. (2020). Therapeutic host-directed strategies to improve outcome in tuberculosis. Mucosal Immunol. 13, 190–204. doi: 10.1038/s41385-019-0226-5
Zerin, T., Lee, M., Jang, W. S., Nam, K.-W., and Song, H.-Y. (2015). Ursolic acid reduces Mycobacterium tuberculosis-induced nitric oxide release in human alveolar A549 cells. Mol. Cells 38, 610–615. doi: 10.14348/molcells.2015.2328
Zerin, T., Lee, M., Jang, W. S., Nam, K. W., and Song, H. Y. (2016). Anti-inflammatory potential of ursolic acid in Mycobacterium tuberculosis-sensitized and concanavalin A-stimulated cells. Mol. Med. Rep. 13, 2736–2744. doi: 10.3892/mmr.2016.4840
Zhanel, G. G., Homenuik, K., Nichol, K., Noreddin, A., Vercaigne, L., Embil, J., et al. (2004). The glycylcyclines: a comparative review with the tetracyclines. Drugs 64, 63–88. doi: 10.2165/00003495-200464010-00005
Zhang, J., Guo, M., Huang, Z. X., Bao, R., Yu, Q., Dai, M., et al. (2019). Calcitriol enhances pyrazinamide treatment of murine tuberculosis. Chin. Med. J. 132, 2089–2095. doi: 10.1097/CM9.0000000000000394
Zhang, Y., Qu, X., Gao, H., Zhai, J., Tao, L., Sun, J., et al. (2020). Quercetin attenuates NLRP3 inflammasome activation and apoptosis to protect INH-induced liver injury via regulating SIRT1 pathway. Int. Immunopharmacol. 85:106634. doi: 10.1016/j.intimp.2020.106634
Keywords: tuberculosis, compounds, immunomodulators, immunotherapy, hostdirected therapy
Citation: Mi J, Wu X and Liang J (2024) The advances in adjuvant therapy for tuberculosis with immunoregulatory compounds. Front. Microbiol. 15:1380848. doi: 10.3389/fmicb.2024.1380848
Edited by:
Shibali Das, Washington University in St. Louis, United StatesReviewed by:
Ananya Gupta, The University of Chicago, United StatesVikram Saini, All India Institute of Medical Sciences, India
Copyright © 2024 Mi, Wu and Liang. This is an open-access article distributed under the terms of the Creative Commons Attribution License (CC BY). The use, distribution or reproduction in other forums is permitted, provided the original author(s) and the copyright owner(s) are credited and that the original publication in this journal is cited, in accordance with accepted academic practice. No use, distribution or reproduction is permitted which does not comply with these terms.
*Correspondence: Jianqin Liang, bGpxYmozMDlAMTYzLmNvbQ==; Xueqiong Wu, eHVlcWlvbmd3dUAxMzkuY29t