- 1Infection and Innate Immunity Laboratory, Department of Biological Sciences, The George Washington University, Washington, DC, United States
- 2Department of Biological Sciences, The University of Alabama, Tuscaloosa, AL, United States
Drosophila melanogaster has been used extensively for dissecting the genetic and functional bases of host innate antiviral immunity and virus-induced pathology. Previous studies have shown that the presence of Wolbachia endosymbionts in D. melanogaster confers resistance to infection by certain viral pathogens. Zika virus is an important vector-borne pathogen that has recently expanded its range due to the wide geographical distribution of the mosquito vector. Here, we describe the effect of Wolbachia on the immune response of D. melanogaster adult flies following Zika virus infection. First, we show that the presence of Wolbachia endosymbionts promotes the longevity of uninfected D. melanogaster wild type adults and increases the survival response of flies following Zika virus injection. We find that the latter effect is more pronounced in females rather than in males. Then, we show that the presence of Wolbachia regulates Zika virus replication during Zika virus infection of female flies. In addition, we demonstrate that the antimicrobial peptide-encoding gene Drosocin and the sole Jun N-terminal kinase-specific MAPK phosphatase Puckered are upregulated in female adult flies, whereas the immune and stress response gene TotM is upregulated in male individuals. Finally, we find that the activity of RNA interference and Toll signaling remain unaffected in Zika virus-infected female and male adults containing Wolbachia compared to flies lacking the endosymbionts. Our results reveal that Wolbachia endosymbionts in D. melanogaster affect innate immune signaling activity in a sex-specific manner, which in turn influences host resistance to Zika virus infection. This information contributes to a better understanding of the complex interrelationship between insects, their endosymbiotic bacteria, and viral infection. Interpreting these processes will help us design more effective approaches for controlling insect vectors of infectious disease.
Introduction
Symbiotic interactions between microbes and animals are common in nature. The role of symbionts in providing nutrients missing in the diets of various animals has been known for many years. Such symbionts are particularly common in insects, perhaps because most insects are specialist herbivores and plants are frequently poor-quality food for animals; the essential elements that the animals lack can be provided by symbiotic microbes. In many cases, the association has become so close that the microbial partner (usually a bacterium) lives within cells in the host’s body (it is then said to be an endosymbiont), is maternally transmitted from one host generation to another, and is never found in the free-living condition (Dale and Moran, 2006). Although such relationships have been most commonly studied with respect to nutritional effects on the host, there can be other benefits. For example, endosymbiotic bacteria may protect their hosts from parasites or pathogens (Brownlie and Johnson, 2009; Eleftherianos et al., 2013). The most widespread and widely studied endosymbionts are Wolbachia, which are harbored by more than half of all insect species and are able to manipulate the reproductive properties of their hosts, while in other hosts such as bedbugs or nematodes they can act as nutritional mutualists (Pietri et al., 2016; Landmann, 2019; Bi and Wang, 2020; Newton and Rice, 2020).
Wolbachia are Gram-negative obligate intracellular Alphaproteobacteria bacteria that are found in the germline and somatic tissues of most arthropod species, and they are transmitted maternally from infected mothers (Porter and Sullivan, 2023). Also, they establish an endosymbiotic relationship with several insect species, including D. melanogaster (Kaur et al., 2021). In fruit flies, Wolbachia pipientis endosymbionts modulate diverse biological processes, such as reproduction, nutrition, development, and longevity, offering many benefits to the host (Iturbe-Ormaetxe and O’Neill, 2007). Research on Wolbachia has important implications for understanding the molecular and functional bases of bacterial symbiosis, and also produces significant information on the regulation of host–microbe interactions which can be potentially used in translational applications in agriculture and biomedicine (Yen and Failloux, 2020; Edenborough et al., 2021; Araújo et al., 2022).
One of the crucial roles of Wolbachia in insects is the immune protection against certain viral pathogens known as a pathogen-blocking effect, which is probably attributed to the activation of host immunity or competition with virus for cellular resources (Pimentel et al., 2021). It was originally demonstrated that the presence of Wolbachia in D. melanogaster increases resistance to infection by three insect RNA viruses (Drosophila C virus, Nora virus and Flock House virus) but not to infection by a DNA virus (Insect Iridescent Virus 6) (Hedges et al., 2008; Teixeira et al., 2008). Wolbachia-mediated antiviral protection in Drosophila species has been demonstrated for a number of different Wolbachia strains (Martinez et al., 2014, 2017). For example, when dengue viruses are injected into D. melanogaster, virus accumulation is significantly reduced in the presence of the non-virulent Wolbachia strain wMel and the life-shortening strain wMelPop-CLA (Rancès et al., 2012). Interestingly, Toll and Immune deficiency (Imd) pathways are not required for expression of the dengue virus-blocking phenotype in the Drosophila host and Wolbachia endosymbionts do not interact with the Toll pathway-mediated resistance to viral oral infection (Rancès et al., 2013; Ferreira et al., 2014). However, Wolbachia decreases the biodiversity of the gut microbiota without changing the total microbial load, and altering the gut microbiota composition with antibiotic treatment increases Wolbachia density without boosting the resistance against Drosophila C Virus (DCV) (Simhadri et al., 2017; Ye et al., 2017). In terms of systemic viral infection, extracellular signal-regulated kinases (ERK) signaling activity increases in the presence of Wolbachia without affecting the protection of the host to systemic infection with DCV (Wong et al., 2016). When D. melanogaster adults are maintained on cholesterol-enriched diets and they contain the Wolbachia strains wMelPop and wMelCS, the flies exhibit reduced pathogen blocking and higher viral copy number compared to flies grown on standard diet (Caragata et al., 2013). Subsequent studies further revealed that stable transinfection of D. melanogaster with highly protective Wolbachia strains is not necessarily associated with general immune activation (Chrostek et al., 2014). Although DCV infection causes increased sleep in D. melanogaster females than in males flies, the presence of Wolbachia does not affect this behavioral response (Vale and Jardine, 2015). Wolbachia can suppress the evolution of D. melanogaster resistance genes because in the presence of the endosymbiotic bacteria, the resistant allele of pastrel-a gene, which has a major effect on resistance to DCV, is at a lower frequency than in the symbiont-free individuals (Martinez et al., 2016).
Zika virus is a vector-borne flavivirus that has become a significant threat to human health. The disease was originally limited to African countries, however, Zika virus cases have been reported in other parts of the world, such as Brazil and Malaysia (Plourde and Bloch, 2016). The Flaviviridae family comprises several notable viruses, including dengue virus and yellow fever virus, all of which are transmitted primarily through mosquito vectors and can cause significant public health concerns (Gubler et al., 2017). The connection between D. melanogaster and Zika virus lies in the use of fruit flies as a model organism to study Zika virus pathogenesis and the host innate immune response against flaviviruses. By introducing Zika virus into adult D. melanogaster, it is possible to identify important genes and pathways involved in the host response to viral infection, providing valuable information on host-virus interactions and the underlying antiviral mechanisms (Tafesh-Edwards and Eleftherianos, 2020a). For example, a recent study in which Drosophila flies were subjected to injection with Zika virus has demonstrated activation of the Imd pathway in the brain (Liu et al., 2018). More precisely, Diptericin (read-out of Imd signaling), but not Drosomycin (read-out of Toll signaling), is upregulated in the heads of Zika virus-infected flies, and this result is not observed in fly null mutants for the transcription factor Relish. In addition, the Drosophila Imd pathway in the fly brain appears to be required to restrict Zika virus infection in this tissue (Lye and Chtarbanova, 2018). Zika virus infection induces antiviral autophagy in the brain, a process that is Relish-dependent (Liu et al., 2018). The fly ortholog of the mammalian polyubiquitin-binding scaffold protein p62, the autophagy cargo receptor Ref(2)P, which is a known restriction factor for natural viral pathogens of the fly such as the Drosophila Sigma virus (Contamine et al., 1989; Longdon et al., 2010), is also directed against Zika virus in the brain, because its knockdown increases the rate of Zika virus replication in fly heads (Liu et al., 2018), and protection against Zika virus is not dependent on RNAi in the fly brain (Liu et al., 2018). However, we have recently shown that Dicer2 loss-of-function mutant flies have increased sensitivity to Zika virus injection into the thorax and exhibit higher viral loads (Harsh et al., 2018).
Activation of the Imd pathway triggers signaling through the adaptor IMD protein and various caspases and kinases, resulting in the induction of c-Jun N-terminal kinase (Jnk) signaling, which forms one of the two functional branches of the Imd pathway (Sluss et al., 1996; Chen et al., 2002). Although the role of Jnk signaling in the regulation of the immune response against bacterial pathogens through modulating the expression of antimicrobial peptides and maintaining host homeostasis is well documented (Tafesh-Edwards and Eleftherianos, 2020b), its participation in the immune response of the fly against viral infections is lagging. Intriguingly, the Jnk pathway has a broad antiviral function against dengue, Zika, and chikungunya viruses, which is mediated by the complement system and apoptosis in the Aedes aegypti salivary glands (Chowdhury et al., 2020).
The contribution of Janus kinase–signal transducer and activator of transcription (Jak/Stat) signaling to the D. melanogaster antiviral immune response is virus specific (Tafesh-Edwards and Eleftherianos, 2020a). Although the Jak/Stat pathway can be induced by RNA viruses, such as DCV, Cricket Paralysis Virus (CrPV), Flock House Virus (FHV), and Drosophila X Virus (DXV), it is only required for resistance against the two Dicistroviruses, DCV and CrPV (Dostert et al., 2005; Myllymäki and Rämet, 2014; Chow and Kagan, 2018; Huang et al., 2023). Also, we have previously documented that Zika virus infection induces negative regulation of Jak/Stat signaling, and Zika virus non-structural protein 4A (NS4A) interacts with Jak/Stat signaling components (Harsh et al., 2020).
Here we explored the role of Wolbachia endosymbionts in the D. melanogaster immune response against Zika virus infection. For this, we tested the survival ability of wild type adult flies carrying Wolbachia endosymbionts during Zika virus infection and the viral replication in these individuals. We also assessed whether the presence of Wolbachia alters the innate immune signaling activity upon challenge with Zika virus. First, we find a positive correlation between the survival ability of female and male D. melanogaster following Zika virus infection and the presence of Wolbachia endosymbionts. Then, we show that females lacking Wolbachia have a higher Zika viral load than females carrying Wolbachia, which implies the participation of Wolbachia in resistance to Zika virus infection. Effects on fly survival and Zika virus load could be attributed to changes in the regulation of innate immune signaling, as females containing Wolbachia have increased Imd pathway activity and males containing Wolbachia have increased Jak/Stat pathway activity. This information is vital because it provides valuable insights into the interconnection between bacterial endosymbiosis in insects, viral pathogenesis, and host defense mechanisms. Similar research can contribute toward development of approaches for the management of vectors of infectious diseases.
Materials and methods
Fly stocks
Two natural Canton-S lines of D. melanogaster containing or lacking Wolbachia pipientis (strain wMel) were used in all experiments. Flies were reared on ready-made fly food (LabExpress, Ann Arbor, MI, United States) supplemented with yeast (Carolina Biological Supply, Burlington, NC, United States). All vials were maintained in an incubator at 25°C and 12 h light/12 h dark photoperiod cycle. Both D. melanogaster lines were amplified for experimentation by transferring adult flies to fresh vials every third day. Female and male flies were selected from the same generation and randomly assigned to experimental groups.
Zika virus stocks
Stocks of Zika virus strain MR766 were maintained and amplified as described before (Harsh et al., 2018).
Fly longevity experiments
Ten male and 10 female Canton-S newly eclosed D. melanogaster adult flies carrying or lacking Wolbachia endosymbionts, were kept in separate vials containing fly food at 25°C and a 12:12-h light:dark photoperiod cycle. Observations were held at 24-h intervals to record fly survival over a 100-day period. All flies were transferred to fresh vials every 3 days. The experiment was repeated five times with three replicates for each experimental treatment. In total, 150 female and 150 male flies were used in the longevity experiments.
Fly survival experiments
Ten female and 10 male 3–5 days old flies of the two Canton-S lines carrying or lacking Wolbachia endosymbionts were first anesthetized with carbon dioxide for a few seconds and then injected into the thorax with 100 nL of a Zika virus solution (11,000 PFU/fly) in PBS (pH 7.5) using a Nanojector apparatus (Nanoject III Programmable Nanoliter Injector, Drummond Scientific, Broomall, PA, United States). Flies injected with 100 nL of PBS served as negative controls. Virus-infected and control flies were maintained in vials with fly food at 25°C and they were transferred to fresh vials every third day for the duration of the survival experiment. Survival results were estimated at 24-h intervals for up to 25 days. Three independent experiments were conducted, and each experiment included three replicates for each experimental condition.
Gene expression analysis
Ten female and 10 male Canton-S 3–5 days old flies containing or lacking Wolbachia were injected with either Zika virus or PBS only (negative control), as described above, and at 4 days post-injection were collected and stored at −80°C. A pool of 10 flies was homogenized using plastic pestles and RNA isolation was carried out using TRIzol (Invitrogen, Waltham, MA, United States) according to the manufacturer’s protocol, followed by complementary DNA (cDNA) synthesis using the AB High-Capacity cDNA Reverse Transcription Kit (Fisher Scientific, Hampton, NH). Zika virus load and immune gene expression were estimated using Zika virus gene-specific primers and D. melanogaster gene-specific primers, respectively (Table 1). Gene expression analysis was assessed using quantitative Real Time PCR (qRT-PCR) and two technical replicates per treatment on a CFX96 Real-Time PCR detection system (Bio-Rad Laboratories, Hercules, CA). Cycle conditions were 95°C for 2 min, 40 repetitions of 95°C for 15 s, 61°C for 30 s, 95°C for 15 s, 65°C for 5 s, and 95°C for 5 s. Quantitative RT-PCR experiments were repeated three times with each experiment consisting of three biological replicates and two technical replicates per condition. In total, 360 female and 360 male flies were used in the gene expression analysis experiments.
Statistical analysis
All data were processed using the GraphPad Prism5 software. Results from the longevity and survival experiments were statistically analyzed using a log-rank (Mantel-Cox) test and a chi-square test for pairwise comparison between each experimental group and the control group. Results from the gene expression analyses were conducted using a one-way analysis of variance (ANOVA) as well as a Tukey post-hoc test. Fold changes were estimated using the 2-ΔΔCT method and Ribosomal protein L32 (RpL32) as the housekeeping gene (Livak and Schmittgen, 2001; Schmittgen and Livak, 2008). PBS control treatments were used as a baseline for comparison in differential expression analysis. p-values less than 0.05 were considered significantly different. All error bars represent standard error of mean and all figures were generated using the GraphPad Prism5 software.
Results
Presence of Wolbachia prolongs the lifespan of Drosophila melanogaster Canton-S flies
We first assessed changes in longevity between D. melanogaster Canton-S male and female adult flies carrying or lacking Wolbachia endosymbionts. We found that female flies containing Wolbachia had a maximum lifespan of 91 days, whereas female individuals lacking Wolbachia had a maximum lifespan of 61 days, indicating a significant difference in longevity depending on the presence of the endosymbionts (Figure 1A). We also found that males containing Wolbachia had a maximum lifespan of 98 days, which was significantly longer compared to males without Wolbachia whose maximum lifespan was 61 days (Figure 1B). We further observed a significant difference in lifespan between males and females carrying Wolbachia, indicating a difference in longevity between the two sexes in the presence of the endosymbiont (Figure 1C), but not in its absence (Figure 1D). These results indicate that the presence of Wolbachia endosymbionts extends the longevity of D. melanogaster female and male wild type adult flies.
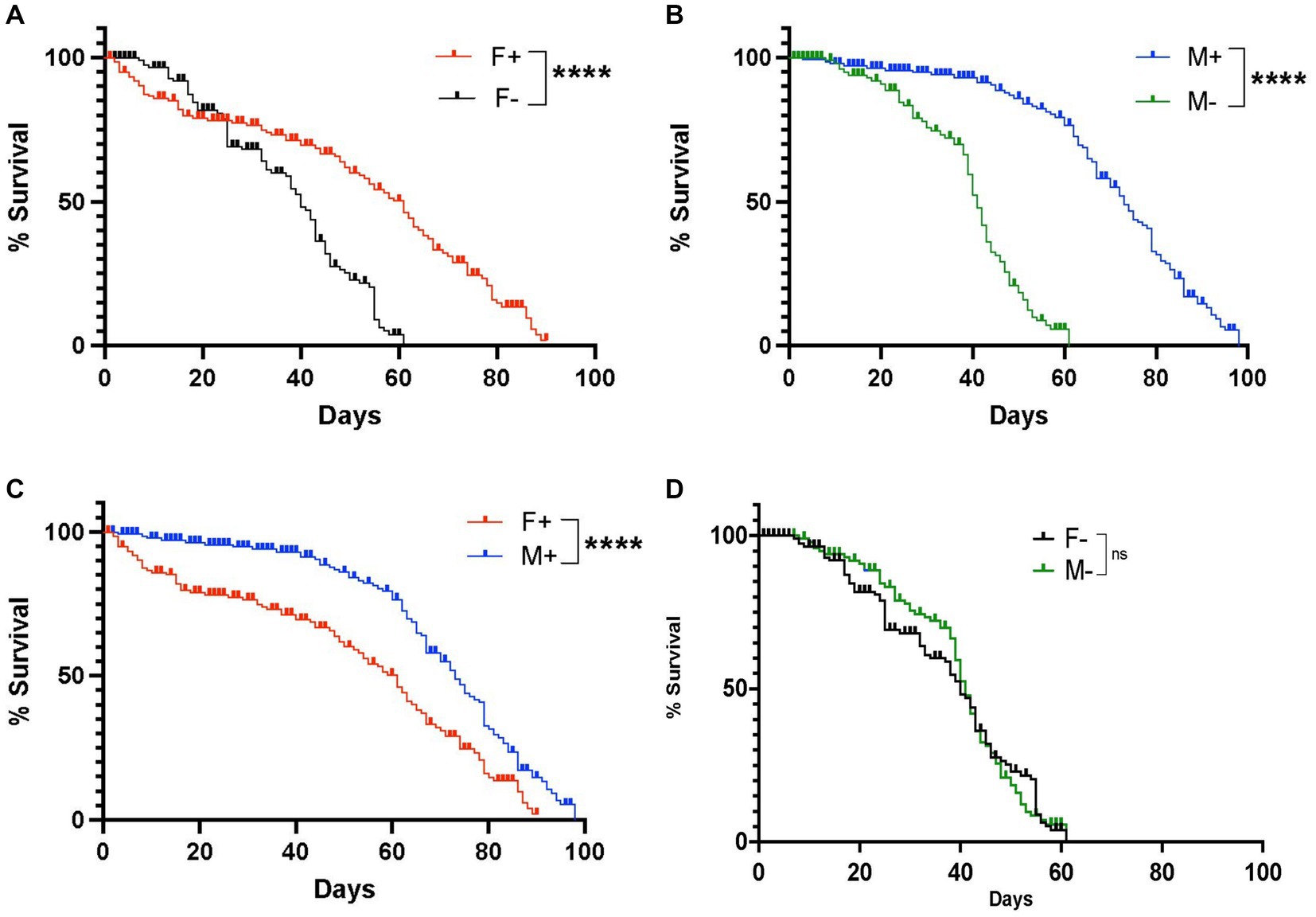
Figure 1. Lifespan of Drosophila melanogaster wild type adult flies containing or lacking Wolbachia endosymbionts. (A) Percent survival of D. melanogaster Canton-S female adult flies carrying (F+) or lacking (F−) Wolbachia endosymbionts (****p < 0.0001). (B) Percent survival of D. melanogaster Canton-S male adult flies carrying (M+) or lacking (M-) Wolbachia endosymbionts. (C) Percent survival of D. melanogaster Canton-S female and male adult flies carrying Wolbachia endosymbionts (F+ and M+, respectively) (****p < 0.0001). (D) Percent survival of D. melanogaster Canton-S female and male adult flies lacking Wolbachia endosymbionts (F− and M−, respectively); ns, non-significant difference (n = 150 flies of each sex).
Drosophila melanogaster Canton-S females carrying Wolbachia have increased survival response to Zika virus infection
Next, we investigated the survival ability of D. melanogaster carrying and lacking Wolbachia endosymbionts following Zika virus infection through intrathoracic injection. We found that the presence of Wolbachia had a protective effect on female flies and the difference in survival compared to females lacking Wolbachia was significant (Figure 2A). However, we observed that males without Wolbachia were more sensitive compared to males carrying the endosymbionts, but the difference was not statistically significant (Figure 2B). We also found that males containing Wolbachia exhibited a significant better survival rate compared to their female counterparts (Figure 2C). Finally, there was a statistically significant difference in survival between males lacking Wolbachia and their corresponding female individuals (Figure 2D). Together, these results suggest that Wolbachia endosymbionts promote the survival of D. melanogaster adult female flies upon infection with Zika virus.
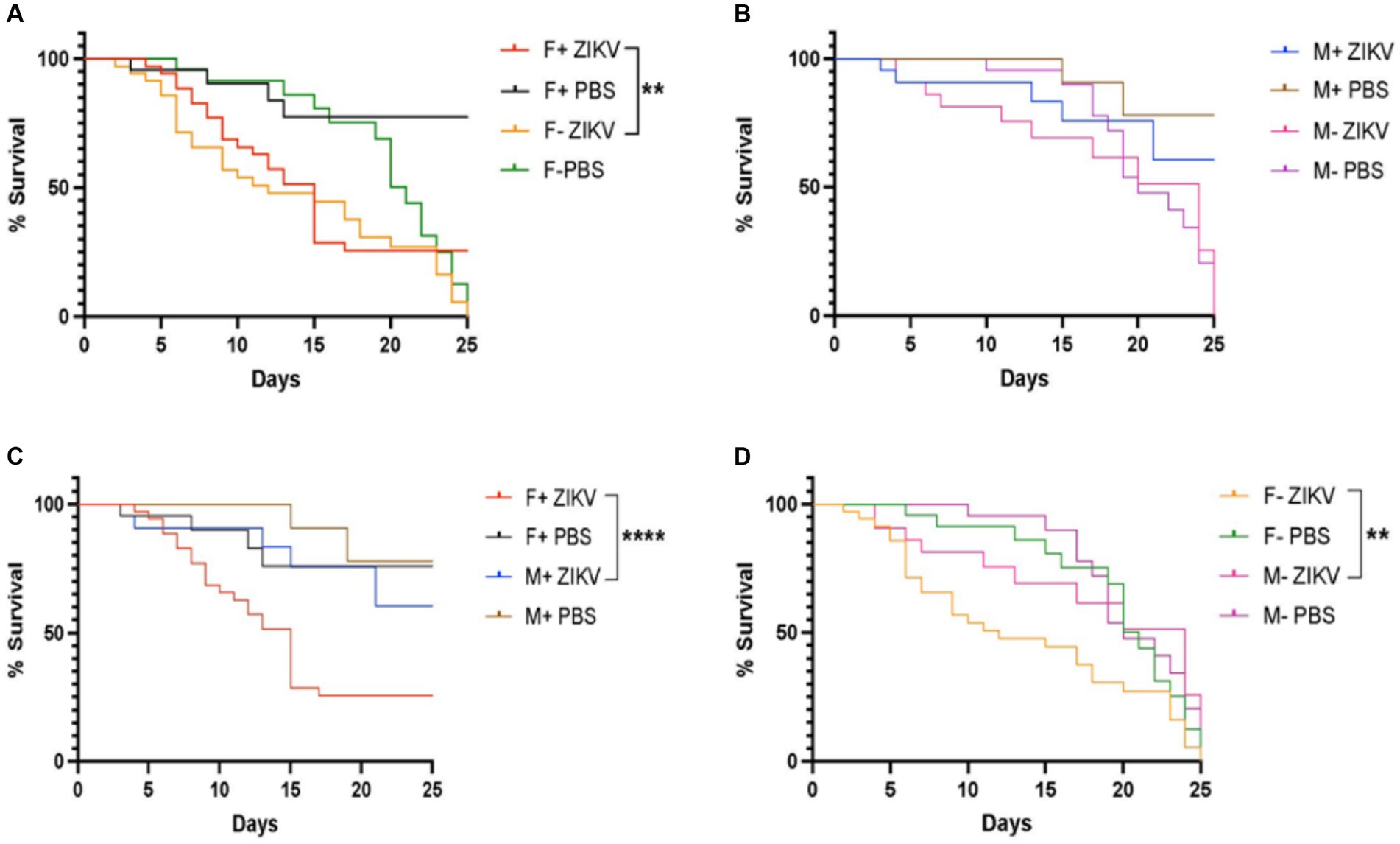
Figure 2. Wolbachia endosymbionts promote the survival of wild type Drosophila melanogaster against Zika virus infection. (A) Percent survival of D. melanogaster Canton-S female adult flies carrying or lacking Wolbachia endosymbionts following infection with Zika virus (ZIKV) (F+ ZIKV and F− ZIKV, respectively). Uninfected flies were injected with sterile PBS (F+ PBS and F− PBS) and acted as controls (**p < 0.01). (B) Percent survival of male flies carrying or lacking Wolbachia endosymbionts following infection with Zika virus (M+ ZIKV and M− ZIKV, respectively). Fly infections with PBS served as uninfected controls (M+ PBS and M− PBS). (C) Percent survival of D. melanogaster Canton-S female and male adult flies containing Wolbachia endosymbionts after infection with Zika virus (F+ ZIKV and M+ ZIKV, respectively). Uninfected control flies were injected with sterile PBS only (F+ PBS and M+ PBS) (****p < 0.0001). (D) Percent survival of D. melanogaster Canton-S female and male adult flies lacking Wolbachia endosymbionts following Zika virus infection (F− ZIKV and M− ZIKV, respectively). Uninfected flies were injected with sterile PBS (F− PBS and M− PBS) (**p < 0.01).
Presence of Wolbachia in Drosophila melanogaster Canton-S females regulates Zika virus replication
We then examined whether the presence of Wolbachia in D. melanogaster female and male adult flies affects Zika virus load. For this, we used gene-specific primers to estimate the expression of Zika virus NS5 methyltransferase, which encodes both the viral methyltransferase and RNA-dependent RNA polymerase (Elshahawi et al., 2019; Song et al., 2021). We found that female flies carrying Wolbachia had significantly lower expression of Zika virus NS5 compared to females without the endosymbionts (Figure 3A). In contrast, there were no significant differences in Zika virus NS5 fold change between males with Wolbachia and those lacking the endosymbiotic bacteria (Figure 3B). These results demonstrate that the effect of Wolbachia endosymbionts on Zika virus replication in D. melanogaster Canton-S is sex-specific and the presence of the endosymbionts confers resistance to female, but not male, adult flies.
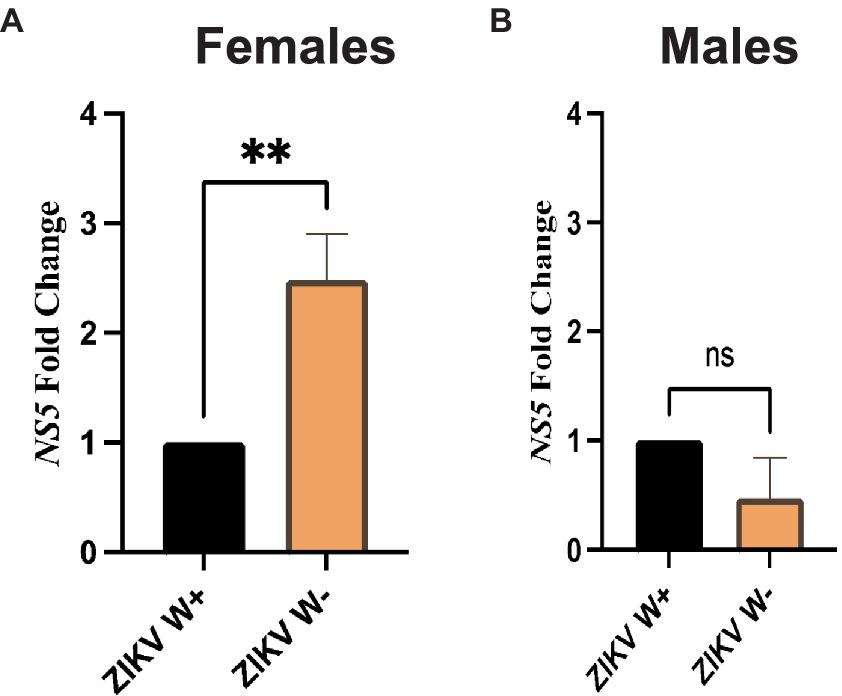
Figure 3. Zika virus replication in Drosophila melanogaster adults containing or lacking Wolbachia endosymbionts. (A) Expression of Zika virus (ZIKV) NS5 in D. melanogaster Canton-S female adult flies carrying (W+) or lacking (W−) Wolbachia endosymbionts at 4 days following intrathoracic injection (**p < 0.01). (B) Expression of Zika virus (ZIKV) NS5 in D. melanogaster Canton-S male adult flies carrying (W+) or lacking (W−) Wolbachia endosymbionts at 4 days following virus infection (ns, non-significant difference; n = 360 flies of each sex). All data were normalized to the housekeeping gene RpL32, shown relative to flies injected with PBS.
Presence of Wolbachia in Drosophila melanogaster Canton-S adult flies infected with Zika virus does not affect the expression of genes in the RNA interference pathway
We first tested whether the presence of Wolbachia in D. melanogaster adults infected with Zika virus modifies the transcriptional gene expression levels of Dicer-2 and Ago-2. We found no significant changes in the transcript levels of Dicer-2 and Ago-2 between Zika virus infected female flies (Figure 4A) and male flies (Figure 4B) carrying or lacking Wolbachia endosymbionts. These results suggest that Wolbachia endosymbionts do not affect RNAi signaling activity in D. melanogaster adults in the context of Zika virus infection.
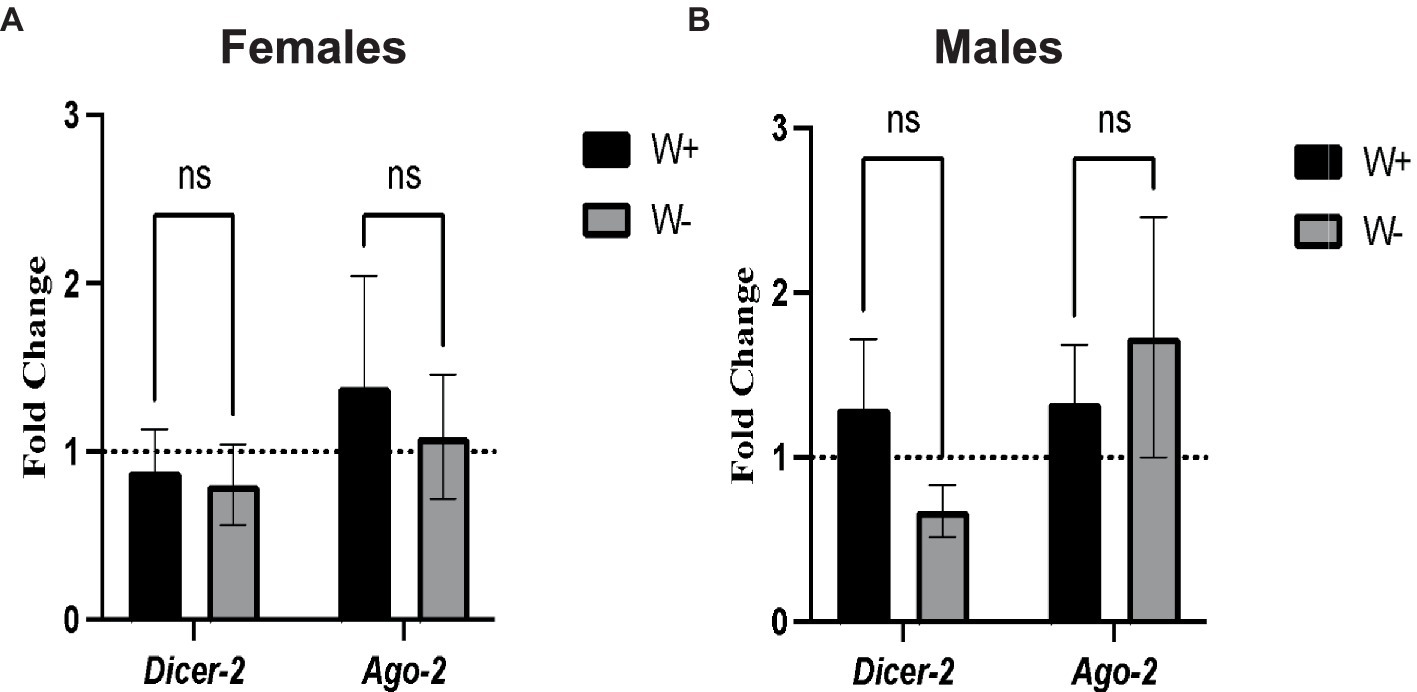
Figure 4. Expression of RNA interference pathway genes in Zika virus infected Drosophila melanogaster in the presence or absence of Wolbachia endosymbionts. (A) Expression of Dicer-2 and Ago-2 in D. melanogaster Canton-S female flies containing (W+) or lacking (W−) Wolbachia endosymbionts after Zika virus infection (ns, non-significant difference). (B) Expression of Dicer-2 and Ago-2 in D. melanogaster Canton-S male flies containing (W+) or lacking (W−) Wolbachia endosymbionts (ns, non-significant difference). Gene expression levels were normalized to the housekeeping gene RpL32. The horizontal dotted line indicates gene expression in uninfected controls treated with PBS (n = 360 flies of each sex).
Presence of Wolbachia in Drosophila melanogaster Canton-S infected with Zika virus upregulates the expression of Drosocin
To test whether the presence of Wolbachia affects the NF-κB signaling activity in D. melanogaster adults following intrathoracic challenge with Zika virus, we used qPCR and gene-specific primers to estimate the expression of frequently-measured readout genes in the Toll and Imd pathways. We observed no statistical differences in the expression levels of Drosomycin and Defensin (Toll pathway) between female or male flies carrying or lacking Wolbachia endosymbionts (Figures 5A,B). Interestingly, we noticed significantly higher levels of Drosocin expression, an antimicrobial peptide encoding gene that acts as readout for the regulation of Imd pathway, in female flies carrying Wolbachia compared to those lacking the endosymbionts (Figure 5C). The difference in Drosocin expression was not observed between male flies carrying or lacking the endosymbiotic bacteria (Figure 5D). Similarly, no changes in Diptericin expression were noted in female and male flies regardless of the presence or absence of Wolbachia (Figures 5C,D). These results imply that Wolbachia endosymbionts can induce the expression of certain antimicrobial peptide-encoding genes in Zika virus infected D. melanogaster adults in a sex-specific manner.
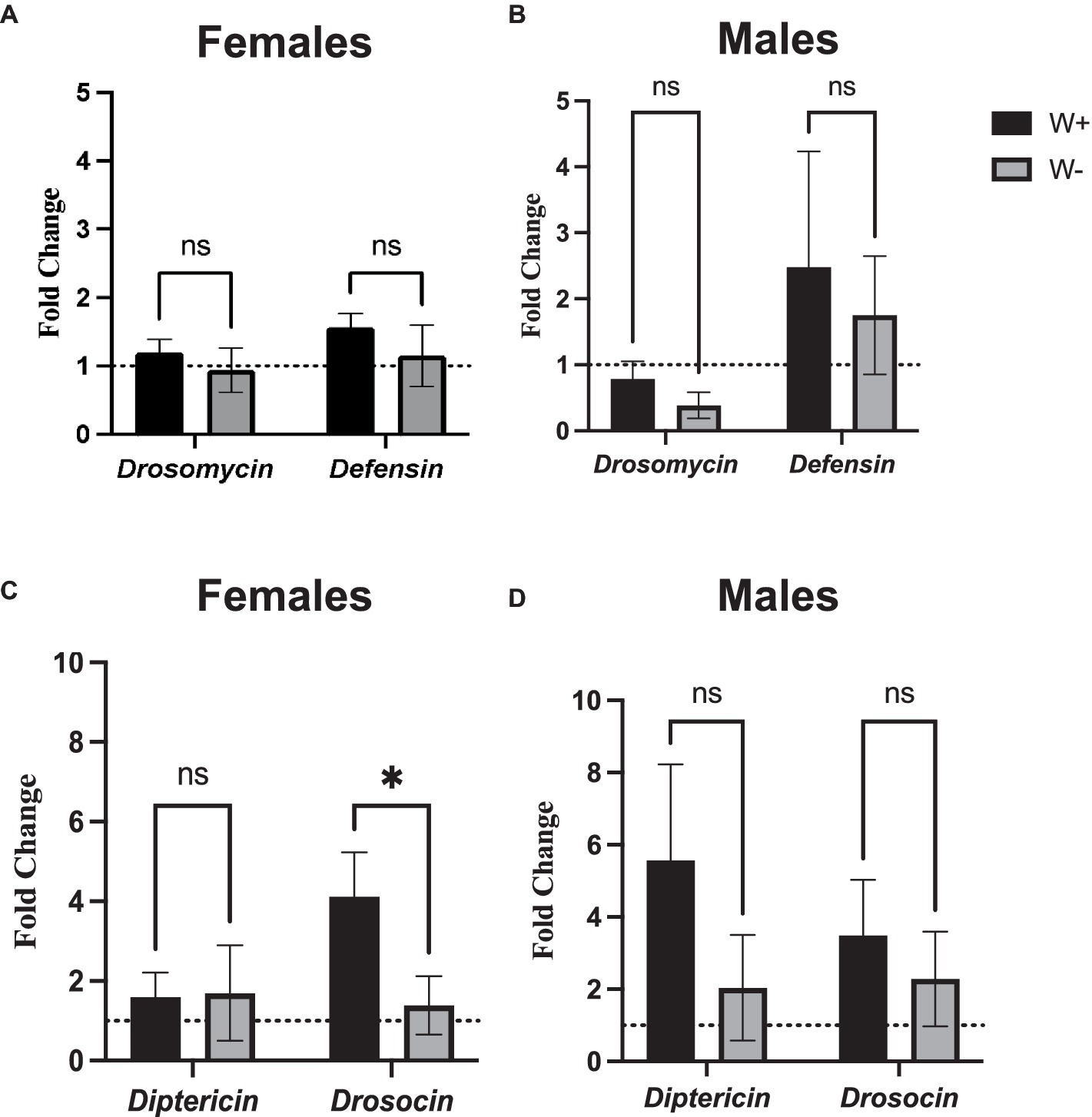
Figure 5. Toll and Immune deficiency pathway gene expression in Zika virus infected Drosophila melanogaster in the presence or absence of Wolbachia endosymbionts. Expression of Drosomycin and Defensin (Toll pathway) in D. melanogaster Canton-S (A) female and (B) male flies carrying (W+) or lacking (W−) Wolbachia endosymbionts following Zika virus infection (ns, non-significant differences). Expression of Diptericin and Drosocin (Imd pathway) in D. melanogaster Canton-S (C) female and (D) male flies carrying (W+) or lacking (W−) Wolbachia endosymbionts following Zika virus infection (*p < 0.01). Gene expression levels were normalized to the housekeeping gene RpL32. The horizontal dotted line indicates gene expression in uninfected controls treated with PBS (n = 360 flies of each sex).
Presence of Wolbachia in Drosophila melanogaster Canton-S adults infected with Zika virus alters Jak/Stat and Jnk signaling activity in sex-specific manner
To study the effect of Wolbachia on Jak/Stat and Jnk signaling pathway activity in D. melanogaster flies infected with Zika virus, we assessed through qPCR the expression level of four representative readout genes. For the JAK/STAT pathway, there were no significant differences in TotA and TotM gene expression levels between Zika virus infected female flies carrying Wolbachia and those lacking the endosymbionts (Figure 6A). A similar gene expression pattern was also observed for TotA in male flies (Figure 6B). However, we found that male wild type flies containing Wolbachia had significantly higher TotM expression compared to those without the endosymbionts (Figure 6B). For Jnk signaling, there was significant upregulation of Puckered, but not Basket, in Zika virus infected wild type female adult flies carrying Wolbachia compared to those without the bacteria (Figure 6C). Finally, there were no statistically significant differences in Puckered and Basket gene expression levels between Zika virus infected male flies irrespective of their Wolbachia status (Figure 6D). These findings denote that Wolbachia endosymbionts can alter Jak/Stat and Jnk signaling activity in male and female D. melanogaster adults, respectively, during Zika virus infection.
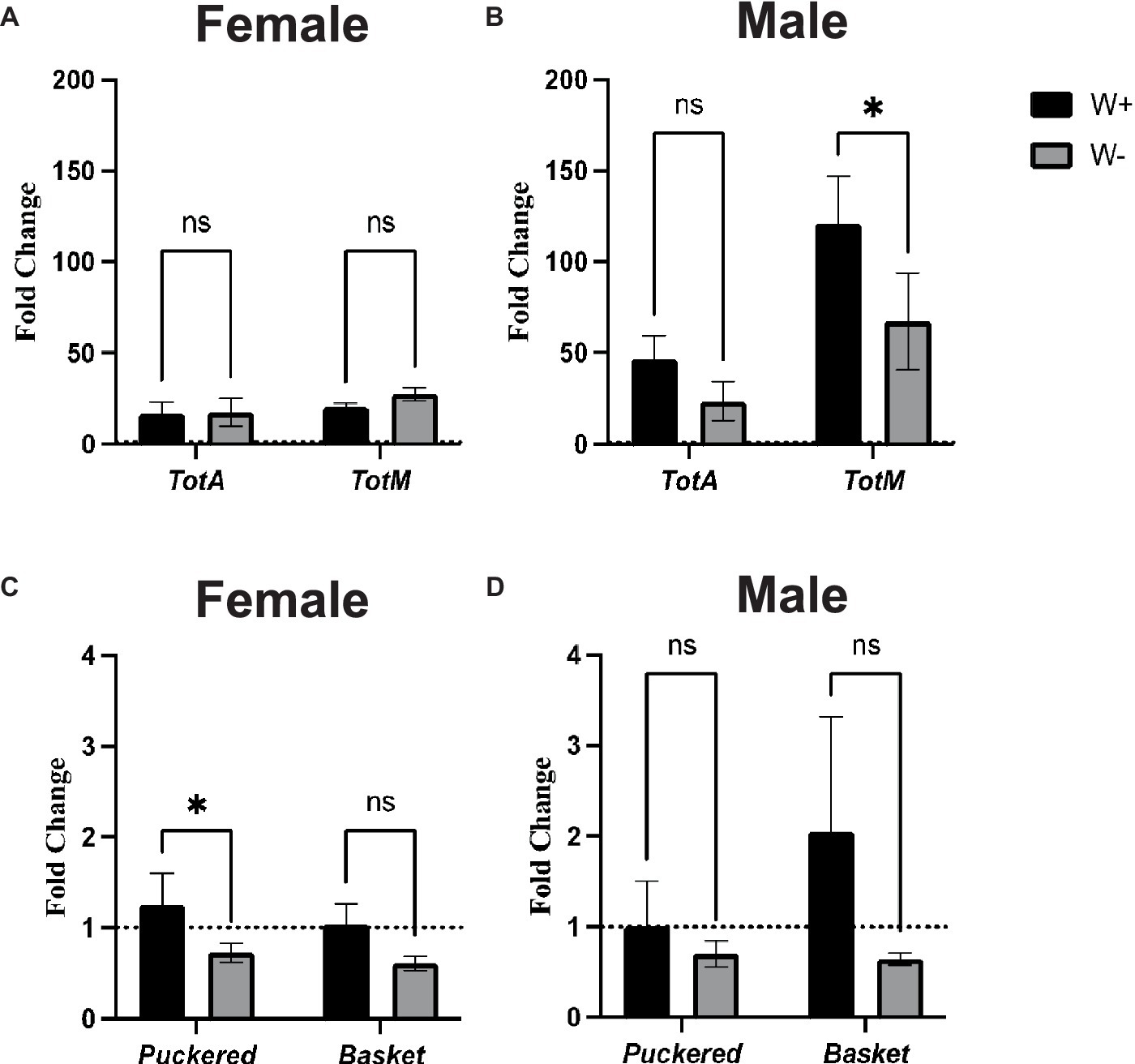
Figure 6. Jak/Stat and Jnk pathway activity in Zika virus infected Drosophila melanogaster in the presence or absence of Wolbachia endosymbionts. Expression of TotaA and TotM (Jak/Stat pathway) in D. melanogaster Canton-S (A) female and (B) male adult flies carrying (W+) or lacking (W−) Wolbachia endosymbionts following Zika virus infection (ns, non-significant differences). Expression of Puckered and Basket (Jnk pathway) in D. melanogaster Canton-S (C) female and (D) male flies carrying (W+) or lacking (W−) Wolbachia endosymbionts following Zika virus infection (*p < 0.01). Gene expression levels were normalized to the housekeeping gene RpL32. The horizontal dotted line indicates gene expression in uninfected controls treated with PBS (n = 360 flies of each sex).
Discussion
Here we explored the potential effect of Wolbachia on the regulation of innate immune signaling and function of the D. melanogaster model against infection with the nonnatural pathogen, Zika virus. Our results provide evidence that Wolbachia can modulate the fly immune response in a sex-specific manner. More precisely, we show that the presence of Wolbachia prolongs the lifespan of adult flies, increases the survival of D. melanogaster females in response to Zika virus infection, and confers significant resistance to female, but not male, adult flies. Also, we find upregulation of the antimicrobial peptide gene Drosocin in Zika virus infected flies carrying the endosymbionts, but no changes in RNAi and Toll pathway regulated genes. Finally, we demonstrate that male and female flies containing Wolbachia have altered Jak/Stat and Jnk signaling activity during Zika virus infection. This information is important because it reveals that certain bacterial endosymbionts can be an important component of the host antiviral innate immune response against Zika virus and maybe other flaviviruses.
First, we observed that Wolbachia extends the lifespan of D. melanogaster Canton-S adult flies, which is conserved in both males and females. This information suggests that Wolbachia endosymbionts confer a fitness advantage to this D. melanogaster line. Previous research indicates that in most cases Wolbachia can provide fitness benefits for the insect host; however, the effect of Wolbachia on lifespan is variable and appears to depend on the genetic background of the fly and the strain of the endosymbiont (reviewed in Maistrenko et al., 2016). For instance, both increased and decreased lifespan effects have been found before in D. melanogaster and flies carrying the wMel strain of Wolbachia have shorter lifespan compared to flies free of endosymbionts (Min and Benzer, 1997; Martinez et al., 2015). In contrast to these previous findings, our results show that wMel-containing Canton-S flies have longer lifespan, which reinforce the concept that D. melanogaster longevity relies on the fly and Wolbachia strain.
The differences in D. melanogaster survival ability between female and male flies carrying or lacking Wolbachia following infection with Zika virus adds to our previous observations indicating sex-specific antiviral immune responses. In particular, we have recently shown that Zika virus infected Dicer-2 female mutant flies have reduced food consumption rates compared to male mutants and Zika virus replicates at higher rates in adult bratchs mutants to cause motor dysfunction in a sex-dependent manner (Tafesh-Edwards et al., 2022, 2023). Also, D. melanogaster prophenoloxidase 1 (PPO1) is essential for male survival following Zika virus infection, while mutation of PPO2 triggers higher RNAi, Toll, Imd, and Jak/Stat immune signaling in female flies but not in male individuals, thus implying sex-specific immune responses during Zika virus infection (Tafesh-Edwards and Eleftherianos, 2023). Our current findings emphasize the complexity of antiviral defense in D. melanogaster through the potential involvement of Wolbachia endosymbionts in regulating host survival phenotypes and immune signaling activity differently in the two sexes.
In terms of the involvement of Wolbachia in altering the immune signaling in D. melanogaster challenged with Zika virus, we find that the presence of the endosymbionts in female or male adult flies fails to modify the expression of Dicer-2 and Ago-2 genes upon Zika virus infection. In D. melanogaster, RNAi plays an instrumental role in antiviral response (Carthew and Sontheimer, 2009). We paid attention to the expression of Dicer-2 and Ago-2 genes, which are components of the exogenous siRNA pathway that leads to the degradation of viral dsRNA molecules (Kim et al., 2006; Peters and Meister, 2007). More precisely, Dicer-2 recognizes exogenous dsRNAs and processes them into small-interfering RNAs (siRNAs), while Ago-2 is the central catalytic component of the RNA-induced silencing complex (RISC) and essential for antiviral defense (Galiana-Arnoux et al., 2006; van Rij et al., 2006). Previous research has indicated that Wolbachia-mediated protection of D. melanogaster adults against Drosophila C virus (DCV) is not RNAi dependent because fly mortality was slower in Wolbachia containing RNAi loss-of-function mutants compared to the Wolbachia-free individuals (Hedges et al., 2012). Our findings further imply the lack of participation of Wolbachia in altering RNAi signaling activity in both female and male flies following Zika virus injection.
We also find that with the exception of Drosocin transcriptional expression in female flies, Wolbachia presence in Zika virus infected D. melanogaster adults does not affect the mRNA levels of certain antimicrobial peptide-encoding genes. These results denote that Imd signaling activity can be modified in Wolbachia-containing female flies during Zika virus infection at least to some extent. The Imd pathway has been previously found to participate in the immune response of D. melanogaster against Cricket Paralysis virus, which is a natural viral pathogen of the fruit fly (Costa et al., 2009). In the context of Zika virus infection, the Imd pathway target gene Diptericin A has been shown to be upregulated in whole flies and heads, supporting the involvement of the Imd arm of the Rel/NF-κB pathway (Liu et al., 2018). Also, we have recently showed that Zika virus infection fails to activate Imd-mediated immunity in male D. melanogaster adult flies (Tafesh-Edwards and Eleftherianos, 2023). Interestingly, the Drosocin gene encodes two separate antimicrobial peptides with different specificity against distinct pathogens (Hanson et al., 2022). In addition, Drosocin was one of the antimicrobial peptide genes which was substantially upregulated in response to Sigma virus infection in D. melanogaster adult flies (Tsai et al., 2008). Whether the upregulation of Drosocin in female flies is directly attributed to Wolbachia or is an indirect effect as well as the role of this antimicrobial peptide in Wolbachia mediated protection to Zika virus infection will be the subject of future investigations.
Here we find that expression of the Jnk regulated gene Puckered increases during Zika virus infection in Wolbachia containing female flies. Previously, it has been shown that expression of the Puckered phosphatase, an inhibitor of Jnk activity, suppresses the expression of antimicrobial peptide genes in D. melanogaster (Delaney et al., 2006). Therefore, we speculate that the presence of Wolbachia specifically in female flies infected with Zika virus could possibly lead to the differential activation of Jnk signaling through the expression of Puckered, which in turn could potentially affect the expression of certain antimicrobial peptides, like Drosocin. The interaction between Jnk and Imd signaling by Wolbachia endosymbionts could be regulated through the TGF-beta activated kinase 1 (TAK1), which has been previously shown to act as an essential factor in Jnk signaling for the expression of antimicrobial peptides (Silverman et al., 2003; Delaney et al., 2006). Finally, we find that Wolbachia-containing male flies challenged with Zika virus have increased expression of the Jak/Stat regulated stress-induced TotM gene (Ekengren and Hultmark, 2001; Brun et al., 2006), and that male flies containing Wolbachia die at a slower rate by Zika virus compared to those lacking the endosymbiont, although there is no statistically significant difference. It is possible that Wolbachia presence in D. melanogaster males provides a slight protective effect against this virus and this effect is controlled through the activation of the Jak/Stat pathway. These various possibilities remain to be confirmed in future studies.
In conclusion, our findings point to a sex-specific effect of Wolbachia on the D. melanogaster immune response against Zika virus infection. The phenotypic effect of the endosymbionts is primarily demonstrated by the expansion in the survival of female adult flies after intrathoracic injection of Zika virus. The extended survival in D. melanogaster females is accompanied by reduced viral titers and changes in innate immune signaling. The latter is mainly expressed through the increased expression of the antimicrobial peptide gene Drosocin which is regulated by the Imd pathway, and the increased expression of the gene Puckered which is regulated by the Jnk pathway. The exact mechanism of decreased Zika virus replication in female flies carrying Wolbachia is currently unknown and it will be explored more in future studies. Further efforts will focus on testing whether the observed effects are specific to the D. melanogaster line Canton-S and the Wolbachia pipientis strain wMel, and also to other Drosophila species. Considering that recent research has indicated that antiviral immunity in the fly is age dependent (Sheffield et al., 2021), it is intriguing to investigate the influence of age on the Wolbachia protective effect against Zika virus infection in female flies. Because hemocytes, autophagy, and the prophenoloxidase system contribute to D. melanogaster antiviral immunity (Lamiable et al., 2016; Tafesh-Edwards and Eleftherianos, 2023), potential input of Wolbachia to these aspects of the immune response against Zika virus will also be examined. Analyzing the dose-dependent and tissue-specific interaction of the innate immune response to oral infection with Zika virus in adult flies or larvae containing various concentration of the endosymbiotic bacteria will provide more detailed information about the prevalence of the current observations. Such information will contribute to a better understanding of the molecular and functional bases of endosymbiont-mediated resistance to Zika virus in mosquito vectors, which could reduce the transmission capacity of this viral pathogen and possibly other arboviruses.
Data availability statement
The datasets presented in this study can be found in the NCBI Gene database. The accession numbers can be found in Table 1. Further inquiries can be directed to the corresponding author.
Ethics statement
The manuscript presents research on animals that do not require ethical approval for their study.
Author contributions
GT-E: Writing – review & editing, Visualization, Validation, Supervision, Methodology, Investigation, Formal analysis, Data curation, Conceptualization. MK: Writing – original draft, Visualization, Validation, Methodology, Investigation, Formal analysis. KM: Writing – review & editing, Methodology, Investigation. DB: Writing – review & editing, Validation, Resources, Methodology. SC: Writing – review & editing, Validation, Supervision, Resources, Conceptualization. IE: Writing – review & editing, Writing – original draft, Supervision, Project administration, Funding acquisition, Conceptualization.
Funding
The author(s) declare that financial support was received for the research, authorship, and/or publication of this article. This work was supported by Facilitating Funds from the Columbian College of Arts and Sciences at George Washington University (GWU) to IE; and Harlan funds from the GWU Department of Biological Sciences to GT-E and MK.
Acknowledgments
We thank members of the I.E. lab for maintaining and amplifying the laboratory fly lines and members of the Department of Biological Sciences at George Washington University for providing feedback to the project.
Conflict of interest
The authors declare that the research was conducted in the absence of any commercial or financial relationships that could be construed as a potential conflict of interest.
Publisher’s note
All claims expressed in this article are solely those of the authors and do not necessarily represent those of their affiliated organizations, or those of the publisher, the editors and the reviewers. Any product that may be evaluated in this article, or claim that may be made by its manufacturer, is not guaranteed or endorsed by the publisher.
References
Araújo, N. J. S., Macêdo, M. J. F., de Morais, L. P., da Cunha, F. A. B., de Matos, Y. M. L. S., de Almeida, R. S., et al. (2022). Control of arboviruses vectors using biological control by Wolbachia pipientis: a short review. Arch. Microbiol. 204:376. doi: 10.1007/s00203-022-02983-x
Bi, J., and Wang, Y. F. (2020). The effect of the endosymbiont Wolbachia on the behavior of insect hosts. Insect Sci. 27, 846–858. doi: 10.1111/1744-7917.12731
Brownlie, C. J., and Johnson, K. N. (2009). Symbiont-mediated protection in insect hosts. Trends Microbiol. 17, 348–354. doi: 10.1016/j.tim.2009.05.005
Brun, S., Vidal, S., Spellman, P., Takahashi, K., Tricoire, H., and Lemaitre, B. (2006). The MAPKKK Mekk1 regulates the expression of Turandot stress genes in response to septic injury in Drosophila. Genes Cells 11, 397–407. doi: 10.1111/j.1365-2443.2006.00953.x
Caragata, E. P., Rancès, E., Hedges, L. M., Gofton, A. W., Johnson, K. N., O'Neill, S. L., et al. (2013). Dietary cholesterol modulates pathogen blocking by Wolbachia. PLoS Pathog. 9:e1003459. doi: 10.1371/journal.ppat.1003459
Carthew, R. W., and Sontheimer, E. J. (2009). Origins and mechanisms of miRNAs and siRNAs. Cell 136, 642–655. doi: 10.1016/j.cell.2009.01.035
Chen, W., White, M. A., and Cobb, M. H. (2002). Stimulus-specific requirements for MAP3 kinases in activating the JNK pathway. J. Biol. Chem. 277, 49105–49110. doi: 10.1074/jbc.M204934200
Chow, J., and Kagan, J. C. (2018). The Fly way of antiviral resistance and disease tolerance. Adv. Immunol. 140, 59–93. doi: 10.1016/bs.ai.2018.08.002
Chowdhury, A., Modahl, C. M., Tan, S. T., Wei, W., Xiang, B., Missé, D., et al. (2020). JNK pathway restricts DENV2, ZIKV and CHIKV infection by activating complement and apoptosis in mosquito salivary glands. PLoS Pathog. 16:e1008754. doi: 10.1371/journal.ppat.1008754
Chrostek, E., Marialva, M. S., Yamada, R., O'Neill, S. L., and Teixeira, L. (2014). High anti-viral protection without immune upregulation after interspecies Wolbachia transfer. PLoS One 9:e99025. doi: 10.1371/journal.pone.0099025
Contamine, D., Petitjean, A. M., and Ashburner, M. (1989). Genetic resistance to viral infection: the molecular cloning of a Drosophila gene that restricts infection by the rhabdovirus sigma. Genetics 123, 525–533. doi: 10.1093/genetics/123.3.525
Costa, A., Jan, E., Sarnow, P., and Schneider, D. (2009). The Imd pathway is involved in antiviral immune responses in Drosophila. PLoS One 4:e7436. doi: 10.1371/journal.pone.0007436
Dale, C., and Moran, N. A. (2006). Molecular interactions between bacterial symbionts and their hosts. Cell 126, 453–465. doi: 10.1016/j.cell.2006.07.014
Delaney, J. R., Stöven, S., Uvell, H., Anderson, K. V., Engström, Y., and Mlodzik, M. (2006). Cooperative control of Drosophila immune responses by the JNK and NF-kappaB signaling pathways. EMBO J. 25, 3068–3077. doi: 10.1038/sj.emboj.7601182
Dostert, C., Jouanguy, E., Irving, P., Troxler, L., Galiana-Arnoux, D., Hetru, C., et al. (2005). The Jak-STAT signaling pathway is required but not sufficient for the antiviral response of drosophila. Nat. Immunol. 6, 946–953. doi: 10.1038/ni1237
Edenborough, K. M., Flores, H. A., Simmons, C. P., and Fraser, J. E. (2021). Using Wolbachia to eliminate dengue: will the virus fight Back? J. Virol. 95:e0220320. doi: 10.1128/JVI.02203-20
Ekengren, S., and Hultmark, D. (2001). A family of Turandot-related genes in the humoral stress response of Drosophila. Biochem. Biophys. Res. Commun. 284, 998–1003. doi: 10.1006/bbrc.2001.5067
Eleftherianos, I., Atri, J., Accetta, J., and Castillo, J. C. (2013). Endosymbiotic bacteria in insects: guardians of the immune system? Front. Physiol. 4:46. doi: 10.3389/fphys.2013.00046
Elshahawi, H., Syed Hassan, S., and Balasubramaniam, V. (2019). Importance of Zika virus NS5 protein for viral replication. Pathogens 8:169. doi: 10.3390/pathogens8040169
Ferreira, Á. G., Naylor, H., Esteves, S. S., Pais, I. S., Martins, N. E., and Teixeira, L. (2014). The toll-dorsal pathway is required for resistance to viral oral infection in Drosophila. PLoS Pathog. 10:e1004507. doi: 10.1371/journal.ppat.1004507
Galiana-Arnoux, D., Dostert, C., Schneemann, A., Hoffmann, J. A., and Imler, J. L. (2006). Essential function in vivo for Dicer-2 in host defense against RNA viruses in drosophila. Nat. Immunol. 7, 590–597. doi: 10.1038/ni1335
Gubler, D. J., Vasilakis, N., and Musso, D. (2017). History and emergence of Zika virus. J. Infect. Dis. 216, S860–S867. doi: 10.1093/infdis/jix451
Hanson, M. A., Kondo, S., and Lemaitre, B. (2022). Drosophila immunity: the Drosocin gene encodes two host defense peptides with pathogen-specific roles. Proc. Biol. Sci. 289:20220773. doi: 10.1098/rspb.2022.0773
Harsh, S., Fu, Y., Kenney, E., Han, Z., and Eleftherianos, I. (2020). Zika virus non-structural protein NS4A restricts eye growth in Drosophila through regulation of JAK/STAT signaling. Dis. Model. Mech. 13:dmm040816. doi: 10.1242/dmm.040816
Harsh, S., Ozakman, Y., Kitchen, S. M., Paquin-Proulx, D., Nixon, D. F., and Eleftherianos, I. (2018). Dicer-2 regulates resistance and maintains homeostasis against Zika virus infection in Drosophila. J. Immunol. 201, 3058–3072. doi: 10.4049/jimmunol.1800597
Hedges, L. M., Brownlie, J. C., O’Neill, S. L., and Johnson, K. N. (2008). Wolbachia and virus protection in insects. Science 322:702. doi: 10.1126/science.1162418
Hedges, L. M., Yamada, R., O’Neill, S. L., and Johnson, K. N. (2012). The small interfering RNA pathway is not essential for Wolbachia-mediated antiviral protection in Drosophila melanogaster. Appl. Environ. Microbiol. 78, 6773–6776. doi: 10.1128/AEM.01650-12
Huang, Z., Wang, W., Xu, P., Gong, S., Hu, Y., Liu, Y., et al. (2023). Drosophila ectoderm-expressed 4 modulates JAK/STAT pathway and protects flies against Drosophila C virus infection. Front. Immunol. 14:1135625. doi: 10.3389/fimmu.2023.1135625
Iturbe-Ormaetxe, I., and O’Neill, S. L. (2007). Wolbachia-host interactions: connecting phenotype to genotype. Curr. Opin. Microbiol. 10, 221–224. doi: 10.1016/j.mib.2007.05.002
Kaur, R., Shropshire, J. D., Cross, K. L., Leigh, B., Mansueto, A. J., Stewart, V., et al. (2021). Living in the endosymbiotic world of Wolbachia: a centennial review. Cell Host Microbe 29, 879–893. doi: 10.1016/j.chom.2021.03.006
Kim, K., Lee, Y. S., Harris, D., Nakahara, K., and Carthew, R. W. (2006). The RNAi pathway iniated by Dicer-2 in Drosophila. Cold Spring Harb. Symp. Quant. Biol. 71, 39–44. doi: 10.1101/sqb.2006.71.008
Lamiable, O., Arnold, J., de Faria, I. J. D. S., Olmo, R. P., Bergami, F., Meignin, C., et al. (2016). Analysis of the contribution of Hemocytes and autophagy to Drosophila antiviral immunity. J. Virol. 90, 5415–5426. doi: 10.1128/JVI.00238-16
Landmann, F. (2019). The Wolbachia endosymbionts. Microbiol. Spectr. 7. doi: 10.1128/microbiolspec.BAI-0018-2019
Liu, Y., Gordesky-Gold, B., Leney-Greene, M., Weinbren, N. L., Tudor, M., and Cherry, S. (2018). Inflammation-induced, STING-dependent autophagy restricts Zika virus infection in the Drosophila brain. Cell Host Microbe 24, 57–68.e3. doi: 10.1016/j.chom.2018.05.022
Livak, K. J., and Schmittgen, T. D. (2001). Analysis of relative gene expression data using realtime quantitative PCR, and the 2–11CT method. Methods 25, 402–408. doi: 10.1006/meth.2001.1262
Longdon, B., Obbard, D. J., and Jiggins, F. M. (2010). Sigma viruses from three species of Drosophila form a major new clade in the rhabdovirus phylogeny. Proc. Biol. Sci. 277, 35–44. doi: 10.1098/rspb.2009.1472
Lye, S. H., and Chtarbanova, S. (2018). Drosophila as a model to study brain innate immunity in health and disease. Int. J. Mol. Sci. 19:3922. doi: 10.3390/ijms19123922
Maistrenko, O. M., Serga, S. V., Vaiserman, A. M., and Kozeretska, I. A. (2016). Longevity-modulating effects of symbiosis: insights from Drosophila-Wolbachia interaction. Biogerontology 17, 785–803. doi: 10.1007/s10522-016-9653-9
Martinez, J., Cogni, R., Cao, C., Smith, S., Illingworth, C. J., and Jiggins, F. M. (2016). Addicted? Reduced host resistance in populations with defensive symbionts. Proc. Biol. Sci. 283:20160778. doi: 10.1098/rspb.2016.0778
Martinez, J., Longdon, B., Bauer, S., Chan, Y. S., Miller, W. J., Bourtzis, K., et al. (2014). Symbionts commonly provide broad spectrum resistance to viruses in insects: a comparative analysis of Wolbachia strains. PLoS Pathog. 10:e1004369. doi: 10.1371/journal.ppat.1004369
Martinez, J., Ok, S., Smith, S., Snoeck, K., Day, J. P., and Jiggins, F. M. (2015). Should symbionts be Nice or selfish? Antiviral effects of Wolbachia are costly but reproductive parasitism is not. PLoS Pathog. 11:e1005021. doi: 10.1371/journal.ppat.1005021
Martinez, J., Tolosana, I., Ok, S., Smith, S., Snoeck, K., Day, J. P., et al. (2017). Symbiont strain is the main determinant of variation in Wolbachia-mediated protection against viruses across Drosophila species. Mol. Ecol. 26, 4072–4084. doi: 10.1111/mec.14164
Min, K. T., and Benzer, S. (1997). Wolbachia, normally a symbiont of Drosophila, can be virulent, causing degeneration and early death. Proc. Natl. Acad. Sci. USA 94, 10792–10796. doi: 10.1073/pnas.94.20.10792
Myllymäki, H., and Rämet, M. (2014). JAK/STAT pathway in Drosophila immunity. Scand. J. Immunol. 79, 377–385. doi: 10.1111/sji.12170
Newton, I. L. G., and Rice, D. W. (2020). The Jekyll and Hyde symbiont: could Wolbachia be a nutritional mutualist? J. Bacteriol. 202, e00589–e00519. doi: 10.1128/JB.00589-19
Peters, L., and Meister, G. (2007). Argonaute proteins: mediators of RNA silencing. Mol. Cell 26, 611–623. doi: 10.1016/j.molcel.2007.05.001
Pietri, J. E., DeBruhl, H., and Sullivan, W. (2016). The rich somatic life of Wolbachia. MicrobiologyOpen 5, 923–936. doi: 10.1002/mbo3.390
Pimentel, A. C., Cesar, C. S., Martins, M., and Cogni, R. (2021). The antiviral effects of the symbiont Bacteria Wolbachia in insects. Front. Immunol. 11:626329. doi: 10.3389/fimmu.2020.626329
Plourde, A. R., and Bloch, E. M. (2016). A literature review of Zika virus. Emerg. Infect. Dis. 22, 1185–1192. doi: 10.3201/eid2207.151990
Porter, J., and Sullivan, W. (2023). The cellular lives of Wolbachia. Nat. Rev. Microbiol. 21, 750–766. doi: 10.1038/s41579-023-00918-x
Rancès, E., Johnson, T. K., Popovici, J., Iturbe-Ormaetxe, I., Zakir, T., Warr, C. G., et al. (2013). The toll and Imd pathways are not required for Wolbachia-mediated dengue virus interference. J. Virol. 87, 11945–11949. doi: 10.1128/JVI.01522-13
Rancès, E., Ye, Y. H., Woolfit, M., McGraw, E. A., and O'Neill, S. L. (2012). The relative importance of innate immune priming in Wolbachia-mediated dengue interference. PLoS Pathog. 8:e1002548. doi: 10.1371/journal.ppat.1002548
Schmittgen, T. D., and Livak, K. J. (2008). Analyzing real-time PCR data by the comparative CT method. Nat. Protoc. 3, 1101–1108. doi: 10.1038/nprot.2008.73
Sheffield, L., Sciambra, N., Evans, A., Hagedorn, E., Goltz, C., Delferd, M., et al. (2021). Age-dependent impairment of disease tolerance is associated with a robust transcriptional response following RNA virus infection in Drosophila 11:jkab116. doi: 10.1093/g3journal/jkab116
Silverman, N., Zhou, R., Erlich, R. L., Hunter, M., Bernstein, E., Schneider, D., et al. (2003). Immune activation of NF-kappaB and JNK requires Drosophila TAK1. J. Biol. Chem. 278, 48928–48934. doi: 10.1074/jbc.M304802200
Simhadri, R. K., Fast, E. M., Guo, R., Schultz, M. J., Vaisman, N., Ortiz, L., et al. (2017). The gut commensal microbiome of Drosophila melanogaster is modified by the endosymbiont Wolbachia 2, e00287–e00217. doi: 10.1128/mSphere.00287-17
Sluss, H. K., Han, Z., Barrett, T., Goberdhan, D. C., Wilson, C., Davis, R. J., et al. (1996). A JNK signal transduction pathway that mediates morphogenesis and an immune response in Drosophila. Genes Dev. 10, 2745–2758. doi: 10.1101/gad.10.21.2745
Song, W., Zhang, H., Zhang, Y., Chen, Y., Lin, Y., Han, Y., et al. (2021). Identification and characterization of Zika virus NS5 methyltransferase inhibitors. Front. Cell. Infect. Microbiol. 11:665379. doi: 10.3389/fcimb.2021.665379
Tafesh-Edwards, G., and Eleftherianos, I. (2020a). Drosophila immunity against natural and nonnatural viral pathogens. Virology 540, 165–171. doi: 10.1016/j.virol.2019.12.001
Tafesh-Edwards, G., and Eleftherianos, I. (2020b). JNK signaling in Drosophila immunity and homeostasis. Immunol. Lett. 226, 7–11. doi: 10.1016/j.imlet.2020.06.017
Tafesh-Edwards, G., and Eleftherianos, I. (2023). The Drosophila melanogaster prophenoloxidase system participates in immunity against Zika virus infection. Eur. J. Immunol. 53:e2350632. doi: 10.1002/eji.202350632
Tafesh-Edwards, G., Kalukin, A., Bunnell, D., Starbanova, S., and Eleftherianos, I. (2023). Temperature and sex shape Zika virus pathogenicity in the adult brat cheesehead brain: a Drosophila model for virus-associated neurological diseases. iScience 26:106424. doi: 10.1016/j.isci.2023.106424
Tafesh-Edwards, G., Kalukin, A., and Eleftherianos, I. (2022). Zika virus induces sex-dependent metabolic changes in Drosophila melanogaster to promote viral replication. Front. Immunol. 13:903860. doi: 10.3389/fimmu.2022.903860
Teixeira, L., Ferreira, A., and Ashburner, M. (2008). The bacterial symbiont Wolbachia induces resistance to RNA viral infection in Drosophila melanogaster. PLoS Biol. 6:e2. doi: 10.1371/journal.pbio.1000002
Tsai, C. W., McGraw, E. A., Ammar, E.-D., Dietzgen, R. G., and Hogenhout, S. A. (2008). Drosophila melanogaster mounts a unique immune response to the Rhabdovirus sigma virus. Appl. Environ. Microbiol. 74, 3251–3256. doi: 10.1128/AEM.02248-07
Vale, P. F., and Jardine, M. D. (2015). Sex-specific behavioural symptoms of viral gut infection and Wolbachia in Drosophila melanogaster. J. Insect Physiol. 82, 28–32. doi: 10.1016/j.jinsphys.2015.08.005
van Rij, R. P., Saleh, M. C., Berry, B., Foo, C., Houk, A., Antoniewski, C., et al. (2006). The RNA silencing endonuclease Argonaute 2 mediates specific antiviral immunity in Drosophila melanogaster. Genes Dev. 20, 2985–2995. doi: 10.1101/gad.1482006
Wong, Z. S., Brownlie, J. C., and Johnson, K. N. (2016). Impact of ERK activation on fly survival and Wolbachia-mediated protection during virus infection. J. Gen. Virol. 97, 1446–1452. doi: 10.1099/jgv.0.000456
Ye, Y. H., Seleznev, A., Flores, H. A., Woolfit, M., and McGraw, E. A. (2017). Gut microbiota in Drosophila melanogaster interacts with Wolbachia but does not contribute to Wolbachia-mediated antiviral protection. J. Invertebr. Pathol. 143, 18–25. doi: 10.1016/j.jip.2016.11.011
Keywords: Zika virus, Drosophila melanogaster, Wolbachia, innate immunity, infection, immune signaling
Citation: Tafesh-Edwards G, Kyza Karavioti M, Markollari K, Bunnell D, Chtarbanova S and Eleftherianos I (2024) Wolbachia endosymbionts in Drosophila regulate the resistance to Zika virus infection in a sex dependent manner. Front. Microbiol. 15:1380647. doi: 10.3389/fmicb.2024.1380647
Edited by:
George Tsiamis, University of Patras, GreeceReviewed by:
Emilie Lefoulon, The Pennsylvania State University (PSU), United StatesNaima Bel Mokhtar, University of Patras, Greece
Copyright © 2024 Tafesh-Edwards, Kyza Karavioti, Markollari, Bunnell, Chtarbanova and Eleftherianos. This is an open-access article distributed under the terms of the Creative Commons Attribution License (CC BY). The use, distribution or reproduction in other forums is permitted, provided the original author(s) and the copyright owner(s) are credited and that the original publication in this journal is cited, in accordance with accepted academic practice. No use, distribution or reproduction is permitted which does not comply with these terms.
*Correspondence: Ioannis Eleftherianos, aW9hbm5pc2VAZ3d1LmVkdQ==
†These authors have contributed equally to this work