- 1Facultad de Ciencias Agrarias, Universidad Nacional de Colombia, Sede Bogotá, Bogotá, Colombia
- 2Laboratorios Diagnofruit Chile, Santiago, Chile
- 3Laboratorios Diagnofruit Colombia, Cajicá, Colombia
Gray mold, caused by Botrytis sp., is a significant disease in Colombian rose crops and its control depends primarily on the intensive use of chemically synthesized fungicides. Despite the importance of this pathogen, there is limited information in Colombian floriculture about molecular taxonomy of species, fungicide resistance of populations and their genetic mechanism of resistance. In this study, we analyze 12 isolates of this fungus collected from rose-producing crops in the Department of Cundinamarca and conducted phylogenetic analysis using HSP60, G3PDH, and RPB2 gene sequences. Additionally, we realize phenotypic and genotypic characterization of resistance to the fungicides fenhexamid, carboxin, and prochloraz, evaluating the in vitro EC50 and presence of mutations of target genes of each isolate. All isolates were characterized as Botrytis cinerea in the phylogenetic analysis and presents different levels of resistance to each fungicide. These levels are related to mutations in target genes, with predominancy of L195F and L400F in the ERG27 gene to fenhexamid resistance, H272R/Y in the SDHB gene for carboxin resistance, and Y136F in the CYP51 gene for prochloraz resistance. Finally, these mutations were not related to morphological changes. Collectively, this knowledge, presented for the first time to the Colombian floriculture, contribute to a better understanding of the genetic diversity and population of B. cinerea from rose-producing crops in the department of Cundinamarca, and serve as a valuable tool for making informed decisions regarding disease management, future research, and improving crop management and sustainability in the Colombian floriculture industry.
Introduction
Colombia is classified as a primary global producer and exporter of cut flowers, with rose cultivation (Rosa x Hybrida L.) being the most representative (Buitrago, 2021). However, the productivity and quality of flowers can be affected by various diseases and pests, such as gray mold caused by Botrytis sp., which is one of the most devastating diseases in rose-producing crops. This disease leads to significant economic losses owing to a reduction in both the quality and quantity of harvested flowers (Muñoz et al., 2019). In ornamental crops, the disease can manifest in different plant tissues, such as leaves and stems; however, the most severe economic damage occurs when the pathogen infects flowers (Muñoz et al., 2019). Initial symptoms in this type of tissue appear as small spots that progress to necrotic tissue, leading to the collapse of petals and the floral bud (Fillinger et al., 2016). This disease causes annual losses ranging from 10 to 100 billion dollars worldwide (Elad et al., 2016; Garfinkel, 2021).
The genus Botrytis encompasses various species, with B. cinerea being one of the most generalists, capable of infecting more than 1,400 plant species in almost 600 genera (Garfinkel, 2021). Traditionally, the identification of the Botrytis genus has relied on the observation and differentiation of morphological characteristics such as the size and shape of conidia and conidiophores, the production, organization, and size of sclerotia, and the morphology of colonies in culture media (Walker, 2016). However, these methodologies may have limitations because of the variability in observed characteristics and the difficulty in distinguishing between closely related species (Garfinkel, 2021). In addition to morphological classification, molecular classification has been used for the Botrytis genus based on the analysis of genetic composition and phylogenetic relationships between species. This technique involves the sequencing of multiple regions of DNA in the genome of an organism, typically focusing on conserved and highly informative genes to obtain more complete information about its genetic diversity (Staats et al., 2005). Among these genes are those encoding heat shock protein 60 (HSP60), glyceraldehyde-3-phosphate dehydrogenase (G3PDH), and RNA polymerase subunit II (RPB2) (Walker, 2016). This approach has facilitated the most recent classification of the genus into 38 species that are distributed across three clades (Garfinkel, 2021). Additionally, these genes play a crucial role in the study of phylogenetic relationships, genetic diversity, and species differentiation (Walker et al., 2011). However, a multilocus sequence analysis (MLSA) of housekeeping genes of Botrytis species that affect floriculture in Colombia has not yet been explored.
Although integrated grey mold management strategies include beneficial microorganisms such as Bacillus sp., protective and systemic fungicides remain part of disease management (Samaras et al., 2016; Hashem et al., 2019). In Colombia, the commonly used fungicides in rose crops belong to the FRAC codes 1, 7, 9, 10, 12, 17, M03, and M04, and the frequency of systemic fungicide application can reach up to three times a week, depending on the incidence of the disease. These fungicides can act on a specific site of the physiological processes of the fungus or can be multisite, possessing several action sites simultaneously (Hahn, 2014). Additionally, some of them are used to control other pathogens such as Podosphaera pannosa (powdery mildew). However, the high frequency of their applications implies intense selective pressure combined with the adaptive plasticity of Botrytis, which has led to a decrease in the sensitivity of the fungus to various fungicides and the absence of growth inhibition in vitro at lethal concentrations (Gisi and Sierotzki, 2008). Similar trends of the accumulation of multiple resistance in B. cinerea have been recently reported for stone fruits, tree seedlings such as raspberries, strawberries, grapes, and ornamental flowers in Germany and Brazil (Rupp et al., 2017; Ishii, 2023).The fungicides commonly used for controlling gray mold in rose crops of Cundinamarca are fenhexamid, carboxin, and prochloraz, all of which affect essential mechanisms for the development of the fungus. fenhexamid inhibits the enzyme 3-keto sterol reductase, encoded by the ERG27 gene, and catalyzes the demethylation of C4 during ergosterol biosynthesis (Debieu et al., 2001). On the other hand, carboxin is a fungicide that belongs to the succinate dehydrogenase inhibitors (SDHI) group, a key protein in the cellular respiration process (Avenot et al., 2009); and prochloraz, acts by inhibiting the enzyme sterol 14α-demethylase, encoded by the CYP51 gene and related to cytochrome P450 superfamily (Zhang et al., 2020).
The increased prevalence of multiple resistance in Botrytis populations has limited the options for implementing strategies as rotation or mixture of fungicides for disease control and fungicide resistance management (Shao et al., 2021). Therefore, knowledge and monitoring of the sensitivity and development of resistance of this fungus to fungicides used for its control are crucial factors to consider in disease management and the search for integrated strategies. This study aimed to characterize morphologically, molecularly, and phylogenetically Botrytis isolates obtained from rose-producing crops in the Department of Cundinamarca, as well as to characterize their phenotypic and genotypic resistance to fenhexamid, carboxin, and prochloraz, the fungicides commonly used for controlling gray mold in this crops.
Materials and methods
Plant material and pathogen isolation
For the recollection of plant material, three rose-producing crops located in Tocancipá (CP), El Rosal (ML), and Madrid (MO) in the Department of Cundinamarca, Colombia, were chosen due to their high frequency of fungicide application (twice a week) for gray mold control. From each crop, a greenhouse with roses (Rosa × hybrida L.) of the Orange Crush variety was selected. In each greenhouse, five points were selected, and from each of these, four random samples were taken. Each sample consisted of three flowers showing initial symptoms of the disease, which were then placed in a humidity chamber until mycelium of Botrytis was observed.
Subsequently, Botrytis isolation was performed on PDA medium, following the methodology described by Avenot et al. (2020). Once purified, the isolates were subjected to monosporic culture, using the methodology described by Li et al. (2012). A monosporic isolate of Botrytis named NT was obtained from wild rose flowers of an unidentified variety, not subjected to fungicide management, and located in the greenhouses of the Faculty of Agricultural Sciences (FAC) of Universidad Nacional de Colombia, Bogotá campus. The NT isolate was used as a control strain, which was not exposed to the fungicides used in this study. Additionally, B. cinerea strain B05.10, which is a commonly used wild-type strain (Fillinger et al., 2008; Veloukas et al., 2011; Staats and van Kan, 2012; Leisen et al., 2022; Klug et al., 2024) was used as a sensitive reference isolate and was kindly provided by Dr. Cecilia Ramos of Nucleus of Applied Research in Veterinary and Agronomic Sciences, Universidad de las Américas, Chile.
Macroscopic and microscopic characterization of isolates obtained from roses
The evaluation of the macroscopic characteristics of each fungal colony were conducted after 7 days of growth on PDA plates under dark conditions. Aspects such as color according to the Pantone® scale (Pantone Color palettes Pantone EMEA),1 appearance, and presence of sclerotia formation were considered. The sclerotia formation was determined when the mycelium generated white or gray hyphae aggregated in small knots (Muñoz et al., 2019).
To perform microscopic characterization of each colony, microcultures were generated for each isolate. The presence of sporulation was assessed after 7 days of incubation, determined as the moment when the first conidiophores with conidia were observed under a microscope (Muñoz et al., 2019). Images of the microcultures were analyzed under a microscope at 400X using the ZEN lite software.
DNA extraction and PCR amplification for conserved genes for phylogenetic analysis
Each monosporic isolate of Botrytis was grown in 30 mL Sabouraud Dextrose Broth (Scharlab), to which mycelium fragments from each isolate were added. Each tube was incubated for 3 days at 18°C with agitation at 80 rpm. For DNA extraction, the mycelium from each culture was macerated with the aid of liquid nitrogen, following the protocol of Pryor and Gilbertson (2000). The integrity and purity of each DNA sample was verified by agarose gel electrophoresis at 1.5% stained with ethidium bromide. HSP60, G3PDH, and RPB2 genes were PCR-amplified using the primers detailed in Table 1. The amplification reactions were carried out in a final volume of 20 μL containing 1X Polymerase buffer, 2 mM MgCl2, 0.2 mM dNTPs, 0.2 μM of each primer, 1 U of PlatinumTM Taq DNA Polymerase (Invitrogen), and 2 μL of DNA. Amplification of the HSP60 and RPB2 genes was conducted with the following thermal profile: 94°C for 5 min, followed by 35 cycles of 94°C for 30 s, 55°C for 30 s, and 72°C for 90 s, and finally an extension of 72°C for 10 min. For the G3PDH gene, the amplification conditions were 94°C for 5 min, followed by 35 cycles of 94°C for 30 s, 55°C for 30 s, and 64°C for 90 s, and finally an extension of 72°C for 10 min (Staats et al., 2005). The products obtained from each amplification were evaluated using 1.5% agarose gel electrophoresis at 80 V.
The amplicons were sequenced at Macrogen Inc., Korea using the Sanger methodology with the primers listed in Table 1. The resulting sequences were analyzed using CLC Main Workbench v5.5 (CLCBio), and the consensus sequences were aligned with the NCBI database using the BLASTn algorithm (Basic Local Alignment Search Tool).2 The parameters considered included an E-value equal to or less than zero, a percentage of identity greater than 99%, and query coverage of 100%.
Phylogenetic analysis
The sequences of the G3PDH, HSP60, and RPB2 genes from each isolate were aligned using the ClustalW algorithm in the CLC Main Workbench v5.5 program (CLCBio). To represent the diversity of species within the genus, 83 sequences from 24 Botrytis species were included and selected according to Staats et al. (2005), Azevedo et al. (2020), and Notte et al. (2021), all of them available in GenBank (Supplementary Table S1). The sequences of Sclerotinia sclerotiorum and Monilinia fructigena were used as outgroups (Staats et al., 2005). Poorly aligned positions and divergent regions in the sequence alignment were removed using Gblocks. The maximum likelihood (ML) method was used to construct the phylogenetic tree, along with the Kimura evolution model with Gamma distribution (K2 + G), considering 1.000 bootstrap replicates. Phylogenetic analyses were conducted for each of the three genes (RPB2, HSP60, and G3PDH) and a concatenated matrix of the three genes. Both the evolution model and tree generation were determined using the MEGA v.10 software.
Determination of the mean effective concentration (EC50) of fungicides
To assess the mean effective concentration of fungicides on Botrytis isolates, the culture medium was supplemented with the fungicides carboxin, prochloraz, and fenhexamid at analytical grade (> 98% purity of the active ingredient, Sigma Aldrich). carboxin was incorporated into PDA agar (Condalab), prochloraz into malt extract agar (Condalab), and fenhexamid into Sisler medium (0.2% KH2PO4, 0.15% K2HPO4, 0.1% (NH4)2SO4, 0.05% MgSO4·7H2O, 1% glucose, 0.2% yeast extract, and 1.25% bacteriological agar) (Weber et al., 2011). Final doses of 0, 0.01, 0.1, 1, 10, and 100 ppm for carboxin; 0, 0.02, 0.05, 0.1, 0.5, 1, 5, and 10 ppm for prochloraz; and 0, 0.03, 0.1, 3, 10, and 25 ppm for fenhexamid were prepared. Each supplemented medium was inoculated in the center of a Petri dish with a 5.5 mm fragment of each Botrytis isolate. This process was performed in triplicate. After three days of incubation at 25°C in the dark, the diameter of each colony exposed to each concentration was measured in two perpendicular directions (Avenot et al., 2020). The inhibition percentage of each dose of the evaluated fungicides was determined using the following equation (Panebianco et al., 2015):
The EC50 value was calculated for each isolate and fungicide, representing the percentage inhibition of growth against the log10 of fungicide concentrations in the three replicates. The values were subjected to Probit analysis using Minitab® V12 software (Veloukas et al., 2011). Additionally, the resistance factor (RF) of each isolate was calculated by dividing the EC50 value by the mean EC50 value of the sensitive isolate B05.10 (Zhang et al., 2020).
The criteria used for classifying the resistance level of isolates was based on their EC50. For fenhexamid was: sensitive (EC50 < 1 ppm), low resistance (EC50 1 to 5 ppm), weak resistance (EC50 5 to 10 ppm), moderate resistance (EC50 10 to 50 ppm), and high resistance (EC50 > 50 ppm) (Avenot et al., 2020). For carboxin, isolates were classified as sensitive (EC50 < 0.94 ppm), low resistance (EC50 0.95 to 1.8 ppm), and moderate to highly resistant (EC50 > 1.9 ppm) (Veloukas et al., 2011). For prochloraz, the EC50 value presented by the sensitive isolate B05.10 was used as a standard, and the degree of resistance above or below this value was determined.
Amplification of SDHB, ERG27, and CYP51 genes
DNA from Botrytis isolates was used to amplify by PCR the target genes of each fungicide. The primers used for SDHB, ERG27, and CYP51 are listed in Table 2. The reactions were carried out in a final volume of 20 μL, containing 1X buffer, 2 mM MgCl2, 0.2 mM dNTPs, 0.2 μM of each primer, 1 U of PlatinumTM Taq DNA Polymerase (Invitrogen), and 2 μL of DNA. The amplification of the SDHB and ERG27 genes was performed under the following thermal profile: 95°C for 1.5 min, followed by 35 cycles of 95°C for 30 s, 60°C for 30 s, and 72°C for 1 min, with a final extension at 72°C for 5 min. For the CYP51 gene, the amplification conditions were the following: 95°C for 1.5 min, followed by 35 cycles of 95°C for 30 s, 57°C for 30 s, and 72°C for 1.5 min, with a final extension at 72°C for 5 min.
The products obtained from each amplification were electrophoresed at 80 V on 1.5% agarose gel. The amplicons obtained were sequenced using the Sanger method with primers for SDHB and ERG27, as well as primers BcCyp51Seq-F and BcCyp51Seq-R for CYP51 at Macrogen Inc., Korea (Table 2). The sequence of each amplified product was analyzed using the CLC Main Workbench v5.5 (CLCBio) program. They were then compared with reference sequences from the B05.10 isolate obtained from the NCBI database under accession number CP009805.1.
Results
Morphological and microscopic characterization of Botrytis isolates
A total of 12 monosporic isolates exhibiting macro and microscopic characteristics associated with the genus Botrytis were obtained. Four isolates originated from rose crops located in Madrid (MO), four isolates from rose crops located in El Rosal (ML), three isolates from rose crops located in Tocancipá (CP), and one isolate from the greenhouses of FAC (NT) (Table 3).
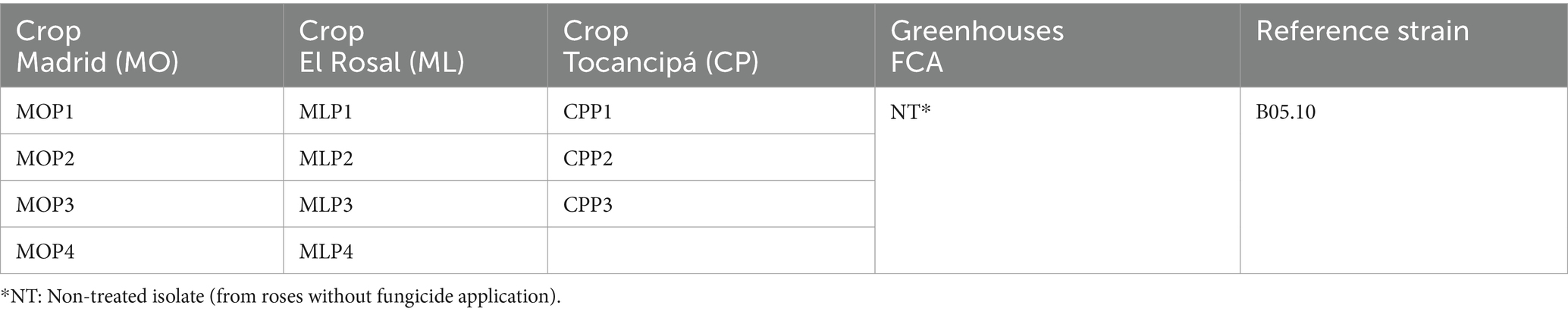
Table 3. Botrytis isolates obtained from the three rose-producing crops in Cundinamarca and from the greenhouses of the Faculty of Agricultural Sciences (FAC) at the Universidad Nacional de Colombia, Bogotá campus.
The macroscopic characteristics of the colonies showed morphological differences in color, appearance, sporulation, and sclerotia formation between isolates from the same rose-producing crops, between crops and in comparison, with the NT isolate and the B05.10 strain. The formation of sclerotia was the most variable trait among all analyzed isolates. However, the color and appearance of the colonies did not vary significantly among them (Table 4).
Regarding microscopic characteristics, the time for conidial formation varied based on the origin of each isolate. The NT, B05.10, and isolates from the MO crop formed conidia within 7 days of setting up the microculture. Similarly, three isolates from the ML crop (MLP1, MLP2, and MLP3) and one from the CP crop (CPP1) showed conidia formation after the same evaluation period. However, in isolates MLP4, CPP2, and CPP3, conidia presence was not evident (Table 4).
Molecular identification and phylogenetic analysis
The BLASTn analysis performed on the different sequences obtained from HSP60 gene determined the identity of all isolates as Botrytis cinerea within an approximate range of 99 to 100% (data not shown). Additionally, for each isolate, the sequences of the G3PDH, HSP60, and RPB2 genes were analyzed individually and concatenated to determine the phylogenetic relationship and confirm their identity (Supplementary Table S1) and concatenation Individual analysis and concatenation of G3PDH, HSP60, and RPB2 gene sequences revealed that all isolates from rose-producing crops (MO, ML, and CP) and NT were within the B. cinerea clade and formed a single group in the phylogenetic tree (Figure 1). This confirmed that all strains belong to the species B. cinerea.
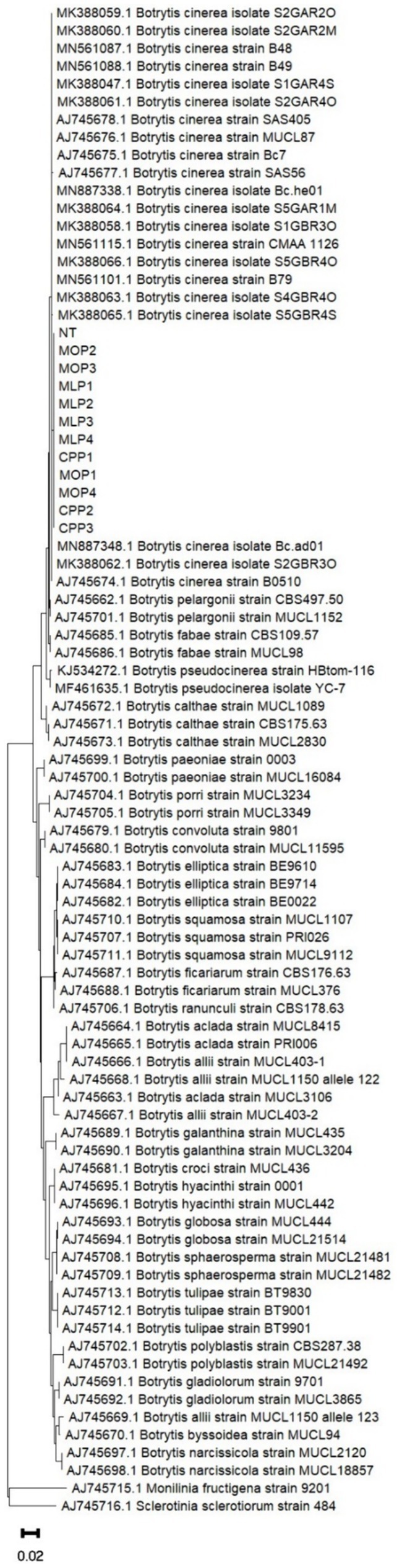
Figure 1. Phylogenetic tree based on concatenated sequences of the G3PDH, HSP60, and RPB2 genes from Botrytis isolates obtained from rose-producing crops in the Cundinamarca department under fungicide application pressure. The method used was Maximum Likelihood, Kimura model with Gamma distribution, with 1,000 bootstrap repetitions. Sequences of the three genes from Monilia fructigena and Sclerotinia sclerotiorum were used as outgroups.
Sensitivity of Botrytis cinerea isolates to fenhexamid, carboxin, and prochloraz
Differences in sensitivity were found among Botrytis isolates to fenhexamid, carboxin, and prochloraz, both within and between rose-producing crops. The ranges of EC50 and FR values are shown in Table 5. For fenhexamid, EC50 values ranged from 0.02 to 4.21 ppm, 41% of the isolates were classified as sensitive, while 59% of the isolates showed low resistance to this fungicide. Regarding carboxin, 92% of the isolates exhibited moderate to high resistance (EC50 > 0.94 ppm), with EC50 ranges between 0.2 and 4.54 ppm. The NT isolate from roses without fungicide exposure showed a loss of sensitivity to both fenhexamid and carboxin compared to the reference-sensitive isolate B05.10. On the other hand, the 11 isolates collected from fungicide-exposed roses showed high sensitivity levels to prochloraz, with a mean EC50 value of 0.36 ppm. The lowest EC50 value was 0.09 ppm, observed in the MOP2 isolate, and the highest was recorded for CPP2 with 1.08 ppm. In this case, the NT isolate was more sensitive to prochloraz (EC50 0.17 ppm) than B05.10 (EC50 0.26 ppm).
Mutations in the ERG27, SDHI, and CYP51 genes of Botrytis cinerea isolates
Changes at nucleotide and amino acid-level were detected in the sequences of the genes related to resistance. In isolates from crops, mutations were observed in 82% of the sequences for ERG27. The L195F mutation was predominant in six of the eleven isolates (MOP4, MOP6, MOP7, MLP2, CPP1, and CPP2) (Table 4). Similarly, the L400F substitution was found in two cases (MLP1, CPP3). The MLP4 isolate had the F412S mutation, and MOP2 and MLP3, did not have mutations of interest. Finally, the NT isolate showed the L195F mutation for this gene.
SDHB sequences exhibited mutations in 90% of the isolates. The alterations found predominantly in position H272, with H272R or H272Y being the most abundant inclusive in the NT isolate (Table 4). Additionally, the MLP3 isolate showed the P225F mutation, and MOP2 and CPP2 did not have mutations in this gene.
Regarding the CYP51 sequences, the Y136F mutation was found in three sequences, NT, MLP2, and MLP4. Similarly, the N230K mutation was found in the CPP2 isolate. All the other isolates did not exhibit mutations in this gene.
The mutations found in ERG27, SDHI, and CYP51 genes were not associated with the differences in the morphological characteristics of the isolates evaluated in this study, such as the color and appearance of the colony, sclerotia formation, and sporulation.
Discussion
Gray mold, caused by Botrytis species, stands out as one of the most significant diseases and a primary factor leading to economic losses in greenhouse flower production (Boddy, 2015; Elad et al., 2016; Yin et al., 2023). In Colombia, knowledge of this pathogen has focused on the assessment of morphological components, both for identification and evaluation of fungicides for management. However, the existing gap regarding the genetic characterization of Botrytis populations and the dynamics of fungicide resistance demands a deeper analysis of this fungus and its populations.
At the morphological level, traits such as sporulation and sclerotia formation were decisive characteristics for primary differentiation among the obtained isolates. It was established that some isolates originating from rose-producing crops had reduced conidia formation and preferred the development of resistance structures. This phenomenon could be explained by the fact that Botrytis resistance to different fungicides can alter its sporulation capacity, mycelial growth, virulence, production, and viability of sclerotia, among others, referred to as fitness cost (Veloukas et al., 2014). These elements can provide advantages or disadvantages for the survival, growth, and reproduction of the pathogen under certain conditions, including stress due to fungicide selection (Chen et al., 2013; Zhang et al., 2020). However, no direct relationship was found between the morphological characteristics and mutations in the target genes for each fungicide. For example, isolate B05.10 (a laboratory wild strain, without fungicide pressure selection and alterations at the molecular level) showed similar morphological behavior to NT (field isolate without fungicide exposure with mutations in the three genes evaluated ERG27, SDHB, CYP51). Likewise, there was no correlation between the morphological pattern of the isolates exposed to fungicides and the morphological characteristics of the NT isolate. Even so, we found differences in the formation of reproductive or resistant structures, among the isolates recovered from rose crops, suggesting that populations of B. cinerea with resistance to fungicides could spread despite a certain fitness cost not related with the mutations detected. Additionally, this represents a risk for gray mold management, as the progeny of isolates like these may constitute a higher percentage of the population in subsequent generations (Yin et al., 2023). This implies considering the use of evaluated compounds.
In this study, molecular markers (HSP60, G3PDH, and RPB2) proposed by Staats et al. (2005, 2007) were used to investigate the genetic relationships and diversity among Botrytis species in rose crops. Our results confirmed that B. cinerea is the causal agent of gray mold in rose-producing crops of the Cundinamarca department. The use of powerful phylogenetic analysis tools represents an upgrade and a more precise method in contrast to classical microbiologic and biochemical methods (Hyde et al., 2014; Garfinkel, 2021). These analyses showed a coherent and consistent clustering of all sequences within this species, confirming the precise and reliable identification of rose isolates from rose-producing crops in Cundinamarca. In the analysis of the RPB2 gene, similarities were determined among rose isolates that grouped them into the same cluster. However, they were separated from other reference Botrytis species sequences. This led to distinguishing the sequences of isolates subjected to frequent fungicide management as a particular population, phylogenetically distant from other B. cinerea strains like Bc7, MUCL87, SAS405, SAS56, and B05.10, recovered from hosts such as grapevines (Vitis vinifera) in Italy or the Netherlands (Staats et al., 2005). Although none of the sequences used as a reference came from rose crops, for instance, our findings can serve as a starting point for future studies on B. cinerea populations on this host. The RPB2 gene encodes a protein with a low evolutionary change rate, used to understand phylogenetic relationships at inter- and intra-specific levels in various fungi (Malkus et al., 2006). It has also been a molecular marker providing additional information related to specific adaptation or evolution characteristics (Staats et al., 2007; Garfinkel, 2021). The evidence presented in the RBP2 gene analysis affirms that B. cinerea in rose crops in Colombia has a genetic identity classifying them as a unique and specific population. This is the first report of these characteristics in B. cinerea from rose crops in Colombia.
The analysis of fungicide resistance evidenced specific mutations in the genes related to resistance of fenhexamid, carboxin, and prochloraz. For fenhexamid, some crop isolates showed low resistance and sensibility according to Avenot et al. (2020). Additionally, the control strains NT and B05.10 were sensible to this fungicide. At the molecular level, some target sites showed changes in the ERG27 gene sequences. One of the predominant changes occurred in the Leucine at positions 195 and 400 of the open reading frame, which was found even in the NT isolate. According to Fillinger et al. (2008), strains with amino acid changes between positions 195 and 400 of the protein are categorized as HydR3−, which indicates moderate to slight resistance to fenhexamid and is associated with the presence of the erg27HdR3- allele. For example, the L195F mutation has been reported in Botrytis spp. isolates from French and German vineyards with weak to moderate resistance to fenhexamid. Additionally, this mutation was easily detected in other field populations, even before the introduction of this compound to the market (Fillinger et al., 2008). Therefore, the L195F mutation is not directly linked to fungicide resistance and may occur in strains with or without fungicide pressure. However, despite the description of Botrytis spp. resistance to fenhexamid worldwide (Albertini and Leroux, 2004; Esterio et al., 2007; Fillinger et al., 2008; Avenot et al., 2020), we found the L195F mutation in 58% of the analyzed isolates from rose crops in Colombia, with represent data that has not been previously reported in local Botrytis strains. Furthermore, we found the F412S mutation in one of the analyzed isolates (MLP45). This Phenylalanine-to-Serine substitution at codon 412 of the ERG27 gene was classified as responsible for high resistance to fenhexamid. However, the low proportion found of this mutation in our analyses may be due to a fitness cost of these strains (Fillinger et al., 2008; Leroux et al., 2010).
For carboxin, we found low sensitivity to this fungicide, even in the untreated isolate (NT). At the molecular level, mutations were detected in the codon 272 of the SDHB gene, where the amino acid histidine (H) was replaced by tyrosine (H272Y) or arginine (H272R) in 75% of cases, and in a minor proportion, proline was replaced by phenylalanine in codon 225 (P225F). These mutations have been associated with B. cinerea resistance to this fungicide (Leroux, 2007; Amiri et al., 2014; De Miccolis Angelini et al., 2014). However, the carboxin resistance has been also associated with multiple mutations in another succinate dehydrogenase (SDH) enzyme subunit. Amiri et al. (2020) demonstrated that mutations in the SDH subunit C (SDHC) in B. cinerea isolates, with or without mutations in the SDHB subunit, generated changes in susceptibility to SDHI fungicides as well as changes in morphological characteristics. Although mutations in SDHC were not evaluated in this study, differences in morphology and resistance levels between the isolates and NT could be associated with the presence or absence of changes in both succinate dehydrogenase subunits (Amiri et al., 2020). However, Botrytis has shown moderate resistance not only to carboxin but also to other molecules with the same mechanism of action, such as Boscalid or Isopyrazam, and hypersensitivity to Fluopyram (Bardas et al., 2010; Lalève et al., 2014), all of them available for gray mold management in Colombia. Mutations have also been detected in Botrytis strains without exposure to fungicides (Shao et al., 2021).as we observed in our NT isolate. A possible explanation for this is that NT could have originated from an isolate previously exposed to this fungicide, and through mechanisms of migration of B. cinerea spores by wind or water or through human and animal mediated transmission mechanisms, facilitated the establishment of the fungus in a rose crop or garden that had not previously been treated with fungicides, as evidenced in native plant crops where the disease had not previously been reported (Notte et al., 2021). Therefore, there is a need to replace these compounds for gray mold management or their rational use, using as reference the phenotypic and genetic characterization.
Similarly, all tested isolates were sensitive to the fungicide prochloraz. 60% of these showed higher sensitivity than the reference strain B05.10. Unlike with the other fungicides in this study, the untreated isolate (NT) from untreated roses exhibited high sensitivity to this molecule. Tyrosine (Y) to Phenylalanine (F) substitutions at position 136 (Y136F) and asparagine (N) to lysine (K) at position 230 (N230K) were found in the CYP51 gene sequences. Alterations in this gene have been reported as a common mechanism of fungal resistance to these compounds (Wyand and Brown, 2005), and several mutations in addition to those found have been associated with resistance to this molecule, such as Y136F, V136A, I381V, D134G, and S524T (Cools et al., 2013). However, although mutations were found in some isolates, all showed an in vitro inhibition on growth under the presence of prochloraz, indicating that the fungicide remains effective in Botrytis control, although the isolates have the mutations Y136F and N230K. Therefore, the data obtained in this study can be useful for monitoring possible changes in the sensitivity of populations of this fungus in ornamental crops, and the mean of 0.22 ppm of prochloraz given by the EC50 of the reference strain B05.10 and the untreated isolate (NT) could be considered as a sensitivity parameter for future evaluations of this fungicide.
Previous studies used molecular analysis to understand the role of B. cinerea as the causal agent of gray mold in ornamental crops in Colombia and their resistance profiles to fungicides (Muñoz et al., 2019). Despite this, our work complements and deepens the characterization of Botrytis isolates from Colombian rose crops boarding phenotypic and molecular characterization and evaluating mechanism of resistance at the molecular level, searching mutations in target genes. Fungicide treatments are essential to maintain healthy crops and achieve reliable and high-quality yields (Yin et al., 2023), and both detection and monitoring of resistance in Botrytis populations are crucial for implementing appropriate resistance management strategies to select the most effective fungicides in disease control (Richards et al., 2021; Yin et al., 2023). Our results demonstrate that Botrytis populations in Colombian crops present a unique identity associated with the same B. cinerea specie. Additionally, they show different levels of sensitivity and mutations in the target sites of each fungicide, sometimes simultaneously. These findings highlight the importance of understanding the persistence of resistant B. cinerea populations on fields, the effect of fungicide rotation programs on mutant selection, and the rational use of fungicides. It is essential to understand the dynamics of resistance and its evolution in Botrytis populations, as well as to extend this evaluation to other molecules used in the field. This will enable proper management of chemical control and delay the onset of resistance on Colombian floriculture.
Data availability statement
Data has been supported in the following link: https://doi.org/10.6084/m9.figshare.26195603.
Author contributions
DG: Conceptualization, Data curation, Formal analysis, Investigation, Methodology, Writing – original draft, Writing – review & editing. CS: Investigation, Methodology, Writing – review & editing. HG: Formal analysis, Resources, Writing – review & editing. ML: Data curation, Formal analysis, Investigation, Methodology, Resources, Writing – review & editing. AG: Conceptualization, Data curation, Formal analysis, Investigation, Methodology, Supervision, Writing – review & editing.
Funding
The author(s) declare that financial support was received for the research, authorship, and/or publication of this article. This study was supported by Bayer CropSience S.A. Colombia division, Laboratorios Diagnofruit Colombia, and Laboratorios Diagnofruit Chile.
Acknowledgments
We are grateful to Jonathan Hurtado for the management of resources for the development of the research; Growers Hub Trading (GHT) for facilitating access to their facilities; the Plant Health and Plant Biotechnology laboratories of the Agricultural Science Faculty of the Universidad Nacional de Colombia, Bogotá; and Diagnofruit laboratories in Colombia for the use of their facilities and equipment.
Conflict of interest
HG was employed by Laboratorios Diagnofruit Chile. ML was employed by Laboratorios Diagnofruit Colombia.
The remaining authors declare that the research was conducted in the absence of any commercial or financial relationships that could be construed as a potential conflict of interest.
Publisher’s note
All claims expressed in this article are solely those of the authors and do not necessarily represent those of their affiliated organizations, or those of the publisher, the editors and the reviewers. Any product that may be evaluated in this article, or claim that may be made by its manufacturer, is not guaranteed or endorsed by the publisher.
Supplementary material
The Supplementary material for this article can be found online at: https://www.frontiersin.org/articles/10.3389/fmicb.2024.1378597/full#supplementary-material
Footnotes
References
Albertini, C., and Leroux, P. A. (2004). A Botrytis cinerea putative 3-keto reductase gene (ERG27) that is homologous to the mammalian 17β-Hydroxysteroid dehydrogenase type 7 gene (17β-HSD7). Eur J. Plant Pathol. 110, 723–733. doi: 10.1023/B:EJPP.0000041567.94140.05
Amiri, A., Heath, S. M., and Peres, N. A. (2014). Resistance to fluopyram, fluxapyroxad, and penthiopyrad in Botrytis cinerea from strawberry. Plant Dis. 98, 532–539. doi: 10.1094/PDIS-07-13-0753-RE
Amiri, A., Zuniga, A. I., and Peres, N. A. (2020). Mutations in the membrane-anchored SdhC subunit affect fitness and sensitivity to succinate dehydrogenase inhibitors in Botrytis cinerea populations from multiple hosts. Phytopathology 110, 327–335. doi: 10.1094/PHYTO-07-19-0240-R
Avenot, H. F., Morgan, D. P., Quattrini, J., and Michailides, T. J. (2020). Phenotypic and molecular characterization of fenhexamid resistance in Botrytis cinerea isolates collected from pistachio orchards and grape vineyards in California. Crop Protect. 133:105133. doi: 10.1016/j.cropro.2020.105133
Avenot, H. F., Sellam, A., and Michailides, T. J. (2009). Characterization of mutations in the membrane-anchored subunits AaSDHC and aa SDHD of succinate dehydrogenase from Alternaria alternata isolates conferring field resistance to the fungicide boscalid. Plant Pathol. 58, 1134–1143. doi: 10.1111/j.1365-3059.2009.02154.x
Azevedo, D. M. Q., Martins, S. D. S., Guterres, D. C., Martins, M. D., Araújo, L., Guimarães, L. M. S., et al. (2020). Diversity, prevalence and phylogenetic positioning of Botrytis species in Brazil. Fungal Biol. 124, 940–957. doi: 10.1016/j.funbio.2020.08.002
Bardas, G. A., Veloukas, T., Koutita, O., and Karaoglanidis, G. S. (2010). Multiple resistance of Botrytis cinerea from kiwifruit to SDHIs, QoIs and fungicides of other chemical groups. Pest Manag. Sci. 66, 967–973. doi: 10.1002/ps.1968
Boddy, L. (2015). “Pathogens of autotrophs” in The fungi. eds. S. C. Watkinson, L. Boddy, and N. P. Money (Amsterdam: Elsevier), 245–292.
Buitrago, C. (2021). Sector floricultor. Ceniflores; Ceniflores. Centro de Innovación de la Floricultura Colombiana. Available at: https://ceniflores.org/sector-floricultor/ (Accesed January 29, 2024).
Chen, F., Liu, X., and Schnabel, G. (2013). Field strains of Monilinia fructicola resistant to both MBC and DMI fungicides isolated from stone fruit orchards in the eastern United States. Plant Dis. 97, 1063–1068. doi: 10.1094/PDIS-12-12-1177-RE
Cools, H. J., Hawkins, N. J., and Fraaije, B. A. (2013). Constraints on the evolution of azole resistance in plant pathogenic fungi. Plant Pathol. 62, 36–42. doi: 10.1111/ppa.12128
Cosseboom, S., and Hu, M. (2021). Identification and characterization of fungicide resistance in Botrytis populations from small fruit fields in the mid-Atlantic United States. Plant Dis. 105, 2366–2373. doi: 10.1094/pdis-03-20-0487-re
De Miccolis Angelini, R. M., Masiello, M., Rotolo, C., Pollastro, S., and Faretra, F. (2014). Molecular characterisation and detection of resistance to succinate dehydrogenase inhibitor fungicides in Botryotinia fuckeliana (Botrytis cinerea): resistance to SDHI fungicides in Botryotinia fuckeliana. Pest Manag. Sci. 70, 1884–1893. doi: 10.1002/ps.3748
Debieu, D., Bach, J., Hugon, M., Malosse, C., and Leroux, P. (2001). The hydroxyanilide fenhexamid, a new sterol biosynthesis inhibitor fungicide efficient against the plant pathogenic fungus Botryotinia fuckeliana (Botrytis cinerea). Pest Manag. Sci. 57, 1060–1067. doi: 10.1002/ps.394
Elad, Y., Pertot, I., Cotes Prado, A. M., and Stewart, A. (2016). “Plant hosts of Botrytis spp” in Botrytis the fungus, the pathogen, and its Management in Agricultural System. eds. S. Fillinger and Y. Elad (Cham: Springer), 413–486.
Esterio, M., Auger, J., Ramos, C., and García, H. (2007). First report of fenhexamid resistant isolates of Botrytis cinerea on grapevine in Chile. Plant Dis. 91:768. doi: 10.1094/PDIS-91-6-0768C
Fillinger, S., Leroux, P., Auclair, C., Barreau, C., Al Hajj, C., and Debieu, D. (2008). Genetic analysis of fenhexamid-resistant field isolates of the phytopathogenic fungus Botrytis cinerea. Antimicrob. Agents Chemother. 52, 3933–3940. doi: 10.1128/AAC.00615-08
Fillinger, Sabine, and Walker, A.-S. (2016). Chemical control and resistance management of Botrytis diseases. In Botrytis – the Fungus, the Pathogen and its Management in Agricultural Systems. (Springer). 189–216.
Garfinkel, A. R. (2021). The history of Botrytis taxonomy, the rise of phylogenetics, and implications for species recognition. Phytopathology 111, 437–454. doi: 10.1094/phyto-06-20-0211-ia
Gisi, U., and Sierotzki, H. (2008). “Fungicide modes of action and resistance in downy mildews” in The downy mildews - genetics, molecular biology and control. eds. A. Lebeda, P. T. N. Spencer-Phillips, and B. M. Cooke (Dordrecht: Springer), 157–167. doi: 10.1007/978-1-4020-8973-2_12
Hahn, M. (2014). The rising threat of fungicide resistance in plant pathogenic fungi: Botrytis as a case study. J. Chem. Biol. 7, 133–141. doi: 10.1007/s12154-014-0113-1
Hashem, A., Tabassum, B., and Fathi Abd Allah, E. (2019). Bacillus subtilis: A plant-growth promoting rhizobacterium that also impacts biotic stress. Saudi Journal of Biological 606 Sciences, 26, 1291–1297. doi: 10.1016/j.sjbs.2019.05.004607
Hyde, K. D., Nilsson, R. H., Alias, S. A., Ariyawansa, H. A., Blair, J. E., Cai, L., et al. (2014). One stop shop: backbones trees for 611 important phytopathogenic genera: I (2014). Fungal Diversity, 67:125. doi: 10.1007/s13225-014-0298-1
Ishii, H. (2023). New chemical fungicides in relation to risk for resistance development. Trop. Plant Pathol. 49, 18–35. doi: 10.1007/s40858-023-00596-3
Klug, K., Zhu, P., Pattar, P., Mueller, T., Safari, N., Sommer, F., et al. (2024). Genome comparisons between Botrytis fabae and the closely related gray mold fungus Botrytis cinerea reveal possible explanations for their contrasting host ranges. Journal of Fungi (Basel, Switzerland), 10, 216. doi: 10.3390/jof10030216
Lalève, A., Fillinger, S., and Walker, A.-S. (2014). Fitness measurement reveals contrasting costs in homologous recombinant mutants of Botrytis cinerea resistant to succinate dehydrogenase inhibitors. Fungal Genet. Biol. 67, 24–36. doi: 10.1016/j.fgb.2014.03.006
Leisen, T., Werner, J., Pattar, P., Safari, N., Ymeri, E., Sommer, F., et al. (2022). Multiple knockout mutants reveal a high redundancy of phytotoxic compounds contributing to necrotrophic pathogenesis of Botrytis cinerea. PLoS Pathogens, 18, e1010367. doi: 10.1371/journal.ppat.1010367
Leroux, L. (2007). “Chemical control of Botrytis and its resistance to chemical fungicides” in Botrytis: Biology, pathology and control. eds. Y. Elad, B. Williamson, P. Tudzynski, and N. Delen (Dordrecht: Springer), 195–222.
Leroux, P., Gredt, M., Leroch, M., and Walker, A. S. (2010). Exploring mechanisms of resistance to respiratory inhibitors in field strains of Botrytis cinerea, the causal agent of gray mold. Appl. Environ. Microbiol. 76, 6615–6630. doi: 10.1128/AEM.00931-10
Li, X., Kerrigan, J., Chai, W., and Schnabel, G. (2012). Botrytis caroliniana, a new species isolated from blackberry in South Carolina. Mycologia 104, 650–658. doi: 10.3852/11-218
Malkus, A., Linda Chang, P.-F., Zuzga, S. M., Chung, K.-R., Shao, J., Cunfer, B. M., et al. (2006). RNA polymerase II gene (RPB2) encoding the second largest protein subunit in Phaeosphaeria nodorum and P. avenaria. Mycol. Res. 110, 1152–1164. doi: 10.1016/j.mycres.2006.07.015
Muñoz, M., Faust, J. E., and Schnabel, G. (2019). Characterization of Botrytis cinerea from commercial cut flower roses. Plant Dis. 103, 1577–1583. doi: 10.1094/pdis-09-18-1623-re
Nielsen, K. A. G., Skårn, M. N., Strømeng, G. M., Brurberg, M. B., and Stensvand, A. (2022). Pervasive fungicide resistance in Botrytis from strawberry in Norway: identification of the grey mould pathogen and mutations. Plant Pathol. 71, 1392–1403. doi: 10.1111/ppa.13557
Notte, A. M., Plaza, V., Marambio-Alvarado, B., Olivares-Urbina, L., Poblete-Morales, M., Silva-Moreno, E., et al. (2021). Molecular identification and characterization of Botrytis cinerea associated to the endemic flora of semi-desert climate in Chile. Curr. Res. Microb. Sci. 2:100049. doi: 10.1016/j.crmicr.2021.100049
Panebianco, A., Castello, I., Cirvilleri, G., Perrone, G., Epifani, F., Ferrara, M., et al. (2015). Detection of Botrytis cinerea field isolates with multiple fungicide resistance from table grape in Sicily. Crop Protect. 77, 65–73. doi: 10.1016/j.cropro.2015.07.010
Pantone Color palettes Pantone EMEA . Available at: https://www.pantone.com/articles/color-palettes
Pryor, B. M., and Gilbertson, R. L. (2000). Molecular phylogenetic relationships amongst Alternaria species and related fungi based upon analysis of nuclear ITS and mt SSU rDNA sequences. Mycol. Res. 104, 1312–1321. doi: 10.1017/s0953756200003002
Richards, J. K., Xiao, C.-L., and Jurick, W. M. (2021). Botrytis spp.: a contemporary perspective and synthesis of recent scientific developments of a widespread genus that threatens global food security. Phytopathology 111, 432–436. doi: 10.1094/PHYTO-10-20-0475-IA
Rupp, S., Weber, R. W., Rieger, D., Detzel, P., and Hahn, M. (2017). Spread of Botrytis cinerea strains with multiple fungicide resistance in German horticulture. Front. Microbiol. 7:2075. doi: 10.3389/fmicb.2016.02075
Samaras, A., Madesis, P., and Karaoglanidis, G. S. (2016). Detection of sdhB gene mutations in SDHI-resistant isolates of Botrytis cinerea using high resolution melting (HRM) analysis. Front. Microbiol. 7:1815. doi: 10.3389/fmicb.2016.01815
Shao, W., Zhao, Y., and Ma, Z. (2021). Advances in understanding fungicide resistance in Botrytis cinerea in China. Phytopathology 111, 455–463. doi: 10.1094/PHYTO-07-20-0313-IA
Staats, M., Van Baarlen, P., Schouten, A., and Van Kan, J. A. L. (2007). Functional analysis of NLP genes from Botrytis elliptica. Mol. Plant Pathol. 8, 209–214. doi: 10.1111/j.1364-3703.2007.00382.x
Staats, M., van Baarlen, P., and van Kan, J. A. L. (2005). Molecular phylogeny of the plant pathogenic genus Botrytis and the evolution of host specificity. Mol. Biol. Evol. 22, 333–346. doi: 10.1093/molbev/msi020
Staats, M., and van Kan, J. A. L. (2012). Genome Update of Botrytis cinerea Strains B05.10 and T4. Eukaryotic Cell, 11, 1413–1414. doi: 10.1128/ec.00164-12
Veloukas, T., Kalogeropoulou, P., Markoglou, A. N., and Karaoglanidis, G. S. (2014). Fitness and competitive ability of Botrytis cinerea field isolates with dual resistance to SDHI and QoI fungicides, associated with several sdhB and the cytb G143A mutations. Phytopathology 104, 347–356. doi: 10.1094/PHYTO-07-13-0208-R
Veloukas, T., Leroch, M., Hahn, M., and Karaoglanidis, G. S. (2011). Detection and molecular characterization of boscalid-resistant Botrytis cinerea isolates from strawberry. Plant Dis. 95, 1302–1307. doi: 10.1094/PDIS-04-11-0317
Walker, A. S. (2016). “Diversity within and between species of Botrytis” in Botrytis – The fungus, the pathogen and its Management in Agricultural Systems. eds. S. Fillinger and Y. Elad (Cham: Springer), 91–125.
Walker, A. S., Gautier, A., Confais, J., Martinho, D., Viaud, M., and Legeai, F. (2011). Botrytis pseudocinerea, a new cryptic species causing gray mold in French vineyards in sympatry with Botrytis cinerea. Phytopathology 101, 1433–1445. doi: 10.1094/PHYTO-04-11-0104
Weber, R. W. S., and Hahn, M. (2011). A rapid and simple method for determining fungicide resistance in Botrytis. Journal of Plant Diseases and Protection: Scientific Journal of the German Phytomedical Society (DPG), 118, 17–25. doi: 10.1007/bf03356376
Wyand, R. A., and Brown, J. K. M. (2005). Sequence variation in the CYP51 gene of Blumeria graminis associated with resistance to sterol demethylase inhibiting fungicides. Fungal Genet. Biol. 42, 726–735. doi: 10.1016/j.fgb.2005.04.007
Yin, Y., Miao, J., Shao, W., Liu, X., Zhao, Y., and Ma, Z. (2023). Fungicide resistance: Progress in understanding mechanism, monitoring, and management. Phytopathology 113, 707–718. doi: 10.1094/phyto-10-22-0370-kd
Zhang, C., Imran, M., Liu, M., Li, Z., Gao, H., Duan, H., et al. (2020). Two-point mutations on CYP51 combined with induced expression of the target gene appeared to mediate pyrisoxazole resistance in Botrytis cinerea. Front. Microbiol. 11:1396. doi: 10.3389/fmicb.2020.01396
Keywords: phylogeny, Botrytis , resistance, fungicide, floriculture
Citation: Giraldo D, Saldarriaga C, García H, López M and González A (2024) Genotypic and phenotypic characterization of resistance to fenhexamid, carboxin, and, prochloraz, in Botrytis cinerea isolates collected from cut roses in Colombia. Front. Microbiol. 15:1378597. doi: 10.3389/fmicb.2024.1378597
Edited by:
Milan Kumar Lal, National Rice Research Institute (ICAR), IndiaReviewed by:
Tariq Mukhtar, Pir Mehr Ali Shah Arid Agriculture University, PakistanJingxin Zhang, Guangdong Academy of Agricultural Sciences (GDAAS), China
Md Aktaruzzaman, University of Georgia, United States
Timothy Miles, Michigan State University, United States
Copyright © 2024 Giraldo, Saldarriaga, García, López and González. This is an open-access article distributed under the terms of the Creative Commons Attribution License (CC BY). The use, distribution or reproduction in other forums is permitted, provided the original author(s) and the copyright owner(s) are credited and that the original publication in this journal is cited, in accordance with accepted academic practice. No use, distribution or reproduction is permitted which does not comply with these terms.
*Correspondence: Diego Giraldo, ZGlnaXJhbGRvckB1bmFsLmVkdS5jbw==; Adriana González, YWRnb256YWxlemFsQHVuYWwuZWR1LmNv