- 1College of Oceanology and Food Science, Quanzhou Normal University, Quanzhou, China
- 2Fujian Province Key Laboratory for the Development of Bioactive Material from Marine Algae, Quanzhou Normal University, Quanzhou, China
- 3The Hebrew University of Jerusalem, Edmond J. Safra Campus, The Institute of Life Sciences, Jerusalem, Israel
- 4Department of Marine Biology, Xiamen Ocean Vocational College, Xiamen, China
- 5Key Laboratory of Marine Genetic Resources, Third Institute of Oceanography, Ministry of Natural Resources, Xiamen, China
Two bacterial strains, designated FR2A1T and MT2-5-38, were isolated from the surface sediments of an oyster farm on a tidal flat in Quanzhou Bay, China. Both strains were Gram-stain-negative, rod-shaped, aerobic, catalase-positive, and oxidase-positive. The 16S rRNA gene sequences of the two strains were 100% identical and had the highest similarity (97.1%) with Phaeovulum vinaykumarii JA123T. The average nucleotide identity (ANI) value and digital DNA–DNA hybridization (DDH) value indicated that the two strains belonged to a single species. Gene annotation revealed that the two strains contained a gene cluster for nitrate reduction and a gene cluster for sulfur oxidation, indicating a possible role in N and S cycling in the tidal flat sediment. The phylogeny inferred from the 16S rRNA gene and 120 conserved proteins indicated that the two strains formed a distinct monophyletic clade within the family Paracoccaceae. The respiratory quinone was Q-10. The major fatty acids consisted of summed feature 8 (C18:1ω7c and/or C18:1ω6c) and C18:0. The polar lipids consisted of phosphatidylethanolamine, phosphatidylglycerol, and several unidentified phospholipids. Based on the above characteristics, strains FR2A1T and MT2-5-38 represent a novel genus and a novel species, for which we propose the name Ostreiculturibacter nitratireducens gen. nov., sp. nov. The type strain is FR2A1T (=MCCC 1K08809T = KCTC 8317T). Phylogenomic analysis of 1,606 high-quality genomes of the family Paracoccaceae, including type strains, non-type strains, and uncultivated bacteria, was performed using the Genome Taxonomic Database Toolkit (GTDB-Tk), and the average amino acid identity (AAI) value of the phylogenetic clade was estimated. We found that 35 species of the family Paracoccaceae needed re-classification, and an AAI value of 70% was chosen as the genus boundary within the family Paracoccaceae.
Introduction
The family Paracoccaceae (illegitimate synonym: “Rhodobacteraceae”) comprises the majority of the Alphaproteobacteria in marine habitats and displays a large phenotypic, genotypic, and metabolic diversity (Simon et al., 2017). In previous studies, the assignment of isolates (or genomes) to a species and/or genus of the family Paracoccaceae mainly depended on 16S rRNA gene phylogeny, which often resulted in misclassifications (Liang et al., 2021). To give a better resolution of the taxonomy of the family Paracoccaceae, phylogenomic analysis based on bacterial ubiquitous gene sets should be performed (Hördt et al., 2020; Liang et al., 2021; Zhang et al., 2023). Thus, the family Roseobacteraceae was split off from the representatives of the family Rhodobacteraceae in 2021, based on core-genome phylogeny (Liang et al., 2021).
In 2022, the name Paracoccaceae was proposed to replace the name Rhodobacteraceae, which is illegitimate because it contravenes Rule 51 of the International Code of Nomenclature of Prokaryotes (Göker, 2022). At the time of writing, 92 genera were included in the family Paracoccaceae.1 Bacterial species delineation based on genomic metrics is generally accepted, such as average nucleotide identity (ANI) and digital DNA–DNA hybridization (DDH) estimates, whereas a consensus genomic metric boundary for a genus delineation of the family Paracoccaceae is still lacking. In addition, misclassifications have occurred when using 16S rRNA gene phylogeny or including a part of type strains in the phylogenomic reconstruction. Thus, a comprehensive phylogenomic analysis of the family Paracoccaceae is necessary, using large datasets.
In this study, two strains, designated MT2-5-38 and FR2A1T, were isolated from the surface sediments of an oyster farm on a tidal flat in Quanzhou Bay, Fujian Province, China, in 2019 and 2023, respectively. The 16S rRNA gene sequence of the two strains was found to be 100% identical, suggesting that the organism may represent a novel species affiliated to the family Paracoccaceae. This study aimed to determine the taxonomic position of the strains. In addition, a comprehensive phylogenomic analysis of the family Paracoccaceae was performed based on the available genomes, including type strains, non-type strains, and uncultivated bacteria. The combination of phylogenomic analysis and average amino acid identity (AAI) metrics was used to elucidate the taxonomy of the family Paracoccaceae.
Materials and methods
Strain isolation and cultivation
Two bacterial strains designated MT2-5-38 and FR2A1T were isolated from surface sediments of an oyster farm on a tidal flat in Quanzhou Bay (24°86′ N, 118°68′ E), Fujian Province, China, in March 2019 (Huang et al., 2021) and in August 2023, respectively. For the isolation of strain FR2A1T, 1 g surface sediment was diluted in 9 mL sterile seawater, and samples of 10× serial dilutions were spread onto marine R2A agar culture medium (R2A powder dissolved with natural seawater, adding 1.5% agar [BD]) and incubated at 28°C for 14 days. Strain FR2A1T was picked and streaked onto MA (Marine Broth 2216 [BD] plus 1.5% Agar [BD]). Strain MT2-5-38 was isolated using a similar protocol (Huang et al., 2021). Briefly, 0.1 g surface sediment was diluted in 0.9 mL sterile seawater, and dilutions were spread onto MA plates.
The strains were stored at −80°C with 20% glycerol (v/v) and deposited in the Marine Culture Collection of China (MCCC) and the Korean Collection for Type Cultures (KCTC).
Phylogeny of the 16S rRNA gene
The genomic DNA of strain FR2A1T was extracted from fresh cells using the Bacterial Genomic Extraction Kit (SaiBaisheng, Co., Ltd., Shanghai, China). The 16S rRNA gene was amplified using bacterial primers Eubac27F and 1492R (Delong, 1992) with Ex Taq (TaKaRa) in 50 μL PCR system. Then, the PCR product was ligated into the pMD19-T vector (TaKaRa) and chemically transformed into competent Escherichia coli DH5α cells. A positive clone was selected and used for Sanger sequencing with the vector primer. The nearly complete 16S rRNA gene sequence of strain FR2A1T was assembled using DNAMAN version 8. The partial 16S rRNA gene sequence of strain MT2-5-38 was determined in our previous study and deposited in GenBank under accession number MT829653 (Huang et al., 2021).
The close relatives of strain FR2A1T and strain MT2-5-38 were searched, and their 16S rRNA gene sequences were downloaded from the EzBioCloud database (Yoon et al., 2017a) and the NCBI nucleotide database.2 Then, the sequences were subjected to multiple alignments by the Clustal W program implemented in MEGA 7.0 (Kumar et al., 2016). The phylogenetic tree was constructed based on two algorithms, neighbor-joining and maximum-likelihood, with 1,000 bootstraps using MEGA 7.0. The models used in neighbor-joining tree and maximum-likelihood tree were maximum composite likelihood (MCL) and K2 + G + I, respectively.
BOX-PCR fingerprinting
BOX-PCR fingerprinting of strains FR2A1T and MT2-5-38 was carried out following the protocol of Lanoot et al. (2004). Briefly, the BOX-PCR reaction was performed using 5 μL 10× Ex buffer, 2 μL 10 μM BOXA1R primer, 4 μL dNTP (2.5 mM), 0.25 μL Ex Taq (5 U/μL, TaKaRa), and 50 ng DNA. The PCR program consisted of 95°C for 7 min, 30 cycles of 90°C for 30 s, 53°C for 1 min, 65°C for 8 min, and 65°C for 16 min. Finally, PCR products were separated and visualized by using 2% agarose electrophoresis.
Whole genome sequencing and genome annotation
The whole genome sequences of strain FR2A1T and strain MT2-5-38 were determined using the Illumina NovaSeq platform (Shanghai Majorbio Bio-Pharm Technology Co., Ltd., Shanghai, China). The raw paired-end reads were trimmed using sickle3 with a length of 50 bp (−l 50) and quality score of 20 (−q 20). The clean reads were then assembled into contigs using SPAdes v3.8.0 (Bankevich et al., 2012). Contigs shorter than 1 kb were removed from the assembly. The complete 16S rRNA gene sequence was extracted from the whole genome sequences using RNAmmer (Lagesen et al., 2007). Genome quality (completeness and contamination) and classification were evaluated using CheckM v1.2.0 (Parks et al., 2015).
Gene prediction was performed using GeneMarkS (Besemer et al., 2001), and gene annotation was carried out using the RAST server (Aziz et al., 2008) and KAAS system.4 Functional proteins with the best similarities to close relatives were searched using the BLASTp program against the nr database with e-value cutoff of 1e−5 (Camacho et al., 2009).
Genomic relatedness and phylogenomic tree
Digital DNA–DNA Hybridization (DDH, Formula 2 as recommended), average nucleotide identity (ANI), and amino acid identity (AAI) values were estimated using the GGDC website,5 ANI Calculator (Yoon et al., 2017b), and CompareM,6 respectively.
The genomes affiliated to the family Paracoccaceae were searched and downloaded from the genome portal of NCBI7 as of 25 August 2023. A total of 4,316 genomes, including type strains, non-type strains, and uncultivated bacteria, were obtained and used for genome quality estimation. The genome quality was estimated using CheckM v.1.2.0 (Parks et al., 2015). Genomes with <90% completeness and >5% contamination were excluded from the following study. The phylogenomic tree was constructed using the GTDB-Tk 1.3.0 based on 120 ubiquitously conserved bacterial proteins (Chaumeil et al., 2019). The tree was visualized using the Interactive Tree of Life (iTOL) online (Letunic and Bork, 2007).
Phenotypic characterization
Strains FR2A1T and MT2-5-38, together with the reference strain Phaeovulum vinaykumarii JA123T (=DSM 18714T), were maintained under identical conditions for phenotypic comparison. Colony morphology was recorded on MA after incubation at 30°C for 2 days. Gram staining was carried out using a Gram staining kit (Hangzhou Microbial Reagent, Co. Ltd.). Catalase activity was tested using a 3% H2O2 solution. Oxidase activity was tested using the oxidase reagent (bioMérieux, France). The growth temperature range was determined under various temperatures (4, 10, 15, 20, 25, 28, 30, 35, 40, and 45°C) for 1 week. Anaerobic culture was tested in 10 mL MB in 50 mL anaerobic flasks according to our previously documented method (Liu et al., 2019). Physiological and biochemical characterization was carried out at 30°C using API ZYM, API 20NE, and API 20E strips according to the manufacturer’s instructions (bioMérieux, France).
Chemotaxonomic characterization
The respiratory quinone of strain FR2A1T was extracted as described previously (Komagata and Suzuki, 1987) and assayed by using reversed-phase high-performance liquid chromatography (Agilent 1200). For cellular fatty acid analyses, strains FR2A1T, MT2-5-38, and P. vinaykumarii JA123T were cultured in MB at 30°C with shaking at 160 rpm for 2 days. The biomass was collected using centrifugation at 6,000 rpm for 10 min. The cellular fatty acids were saponified, methylated, extracted, and identified following the standard MIDI protocol (Sherlock Microbial Identification System, version 6B). For polar lipid analysis, strain FR2A1T was cultured in 100 mL MB medium for 2 days, and cells were harvested by centrifugation at 6,000 rpm. Polar lipids were extracted using a chloroform/methanol system and analyzed using one- and two-dimensional TLC using Merck silica gel 60 F254 aluminum-backed thin-layer plates. Phospholipids were detected by spraying the plate with molybdenum blue.
Nucleotide sequences
The GenBank/EMBL/DDBJ accession numbers of the 16S rRNA gene sequence of strains FR2A1T and MT2-5-38 are OR533672 and MT829653, respectively. The whole genome sequences of strain FR2A1T and strain MT2-5-38 have been deposited at GenBank under the accession numbers JAVQHL000000000 and JAVQHM000000000, respectively.
Results and discussion
16S rRNA gene sequence phylogeny
The nearly complete (1,391 bp) 16S rRNA gene sequence of strain FR2A1T was obtained using Sanger sequencing. It had 100% identical sequence similarity with that of strain MT2-5-38. A sequence similarity search showed that strain FR2A1T had the highest 16S rRNA gene similarity (97.1%) with Phaeovulum vinaykumarii JA123T. Phylogenetic analysis based on the 16S rRNA gene indicated that strain FR2A1T and strain MT2-5-38 formed a monophyletic clade distinct from closely related genera affiliated to the family Paracoccaceae and may be considered a new species within a new genus (Figure 1; Supplementary Figure S1). Based on the 16S rRNA gene sequence similarity, P. vinaykumarii JA123T (=DSM 18714T) was chosen as a reference strain.
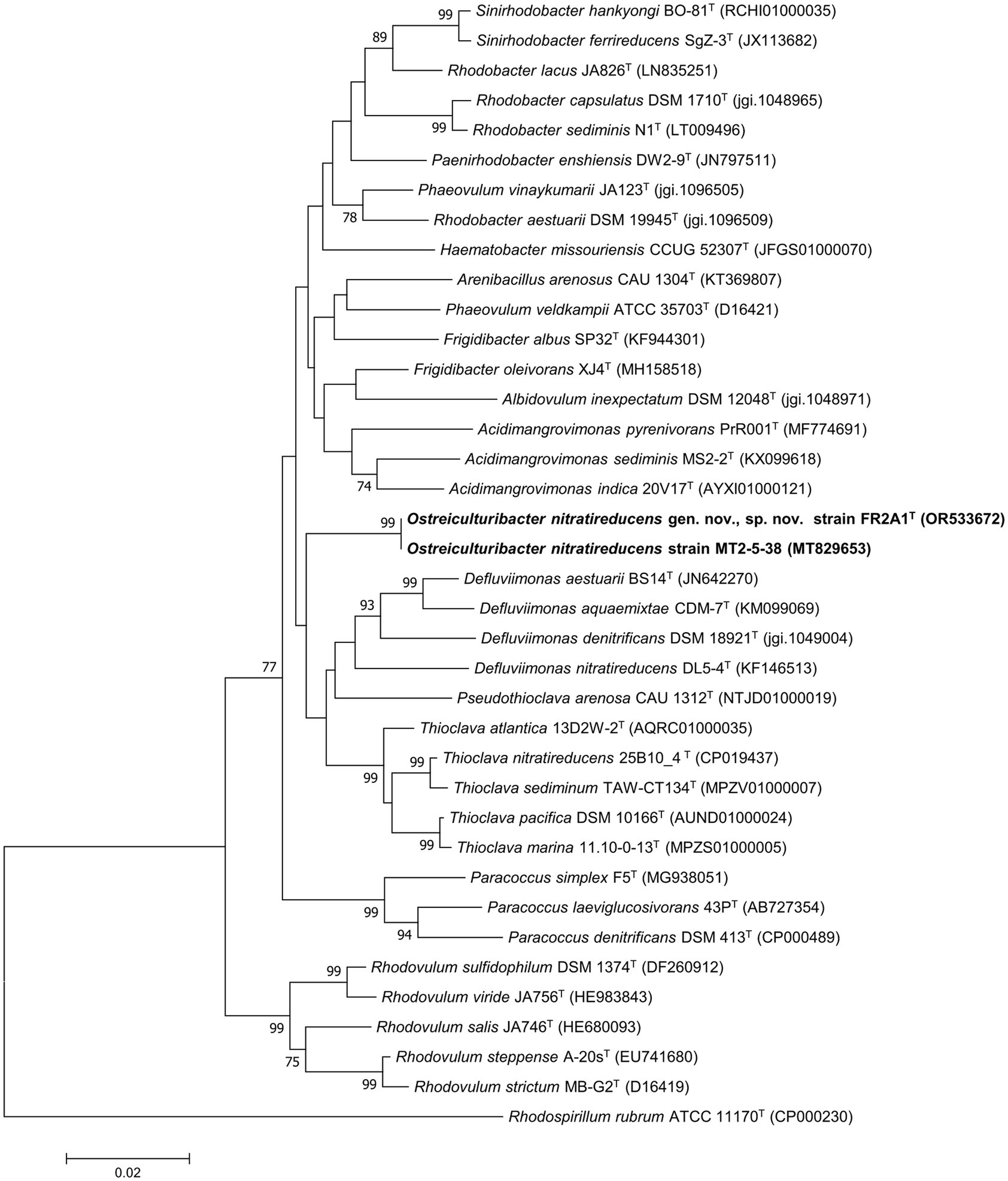
Figure 1. Phylogenetic analysis based on 16S rRNA gene sequences. The tree was constructed using the neighbor-joining method. Rhodospirillum rubrum ATCC 11170T (CP000230) was selected as the outgroup. The new isolates are marked in bold. Bootstrapping was carried out with 1,000 replicates. Branch node values below 70% are not shown. Bar, 0.02 represented the nucleotide substitution per position.
BOX-PCR fingerprinting
The BOX-PCR fingerprinting profile of strain FR2A1T and strain MT2-5-38 showed distinctive electrophoresis patterns (Supplementary Figure S2), confirming that they are not clonal.
Genomic characteristics
The whole genome sequences of strains FR2A1T and MT2-5-38 were determined. The genome size of strain FR2A1T was 4,009,665 bp on 12 contigs (>1 kb). The genome size of strain MT2-5-38 was 3,901,132 bp on 14 contigs (>1 kb; Table 1). The genomic G + C content of strain FR2A1T and strain MT2-5-38 was 65.7 and 65.6%, respectively. The ANI value and DDH estimate between strains FR2A1T and MT2-5-38 were estimated to be 99.5 and 96.7%, respectively, which strongly supports that they belong to the same species. The ANI value and DDH estimate between strain FR2A1T and the closest reference strain, P. vinaykumarii JA123T, were 74.5 and 21.0%, respectively.
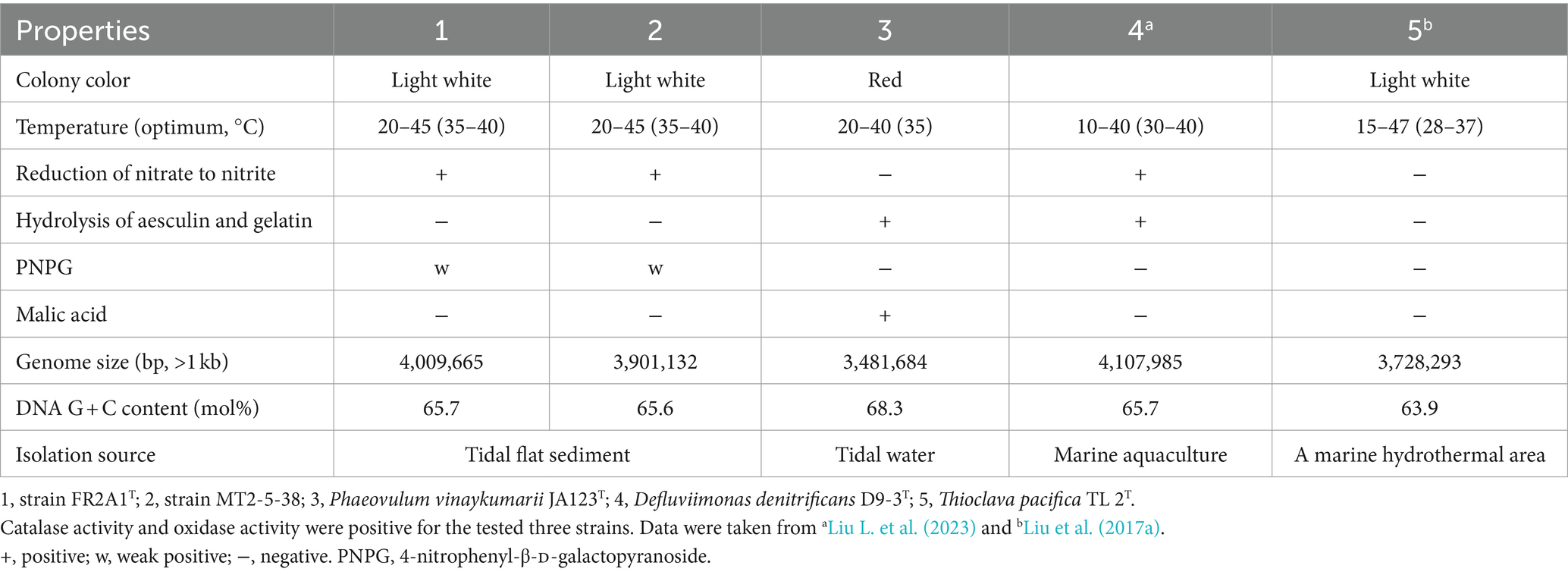
Table 1. Differential characteristics of strains FR2A1T and MT2-5-38 compared to the close relative Phaeovulum vinaykumarii JA123T.
Functional gene prediction showed 3,919 and 3,803 genes in strain FR2A1T and strain MT2-5-38, respectively. Both strains contained a full set of genes for respiratory nitrate reduction (narI, narJ, narH, and narG), responsible for the reduction of nitrate to nitrite (Supplementary Table S1), and a full gene cluster for sulfur oxidation (Sox oxidation system: SoxABCDYZ, Supplementary Table S1), indicating a possible role in N and S cycling in their natural environment.
Phenotypic properties
The colonies of strains FR2A1T and MT2-5-38 cultured on MA were round and 1 mm in diameter. Cells were aerobic and rod-shaped (Supplementary Figure S3). Catalase and oxidase activity was positive, similar to P. vinaykumarii JA123T. Strains FR2A1T and MT2-5-38 can grow at 20–40°C, with an optimum at 35–40°C (Table 1). Strains FR2A1T and MT2-5-38 were positive for esterase (C4), esterase lipase (C8), leucine arylamidase, and valine arylamidase; weakly positive for alkaline phosphatase, lipase (C14), cystine arylamidase, acid phosphatase, and naphthol-AS-BI-phosphohydrolase, α-glucosidase, and β-glucosidase; negative for trypsin, α-chymotrypsin, α-galactosidase, β-galactosidase, β-glucuronidase, N-acetyl-β-glucosaminidase, α-mannosidase, and β-fucosidase. The reduction of nitrate to nitrite is positive for strains FR2A1T and MT2-5-38 but negative for P. vinaykumarii JA123T. Tryptophan deaminase is positive, similar to P. vinaykumarii JA123T. Fermentation of D-glucose was negative. The hydrolysis of arginine and urea was negative. The two strains and P. vinaykumarii JA123T cannot use D-glucose, L-arabinose, D-mannose, D-mannitol, N-acetylglucosamine, D-maltose, potassium gluconate, capric acid, adipic acid, malic acid, trisodium citrate, and phenylacetate as sole carbon sources for growth. The two strains and P. vinaykumarii JA123T were negative for lysine decarboxylase, ornithine decarboxylase, urease, and tryptophan deaminase. Citrate cannot be utilized. H2S is not produced. The Voges-Proskauer reactions were negative. No acid was produced by fermentation from glucose, mannitol, inositol, sorbitol, rhamnose, sucrose, melibiose, amygdalin, and arabinose.
Chemotaxonomic properties
The respiratory quinone of strain FR2A1T was ubiquinone 10 (Q-10), like for P. vinaykumarii JA123T and other representatives of the family Paracoccaceae (Hördt et al., 2020). The predominant fatty acids (>10%) of strains FR2A1T and MT2-5-38 were similar, consisting of summed feature 8 (C18:1 ω7c and/or C18:1 ω6c, SF8) and C18:0 (Table 2). The polar lipids consist of phosphatidylethanolamine, phosphatidylglycerol, and several unidentified phospholipids (Supplementary Figure S4).
Phylogenomic analysis of the family Paracoccaceae
A phylogenomic tree including 1,606 high-quality genomes was constructed based on 120 conserved bacterial proteins using GTDB-Tk 1.3.0, and the AAI values of the phylogenetic clades were estimated. The studied genomes included not only the representatives of the family Paracoccaceae but also the representatives of the family Roseobacteraceae, indicating that taxonomic correction was needed. The family Roseobacteraceae was proposed in 2021 based on core-genome phylogeny; it was split off from the family Paracoccaceae (Liang et al., 2021). The genomic characteristics (genome size, G + C content, and AAI values) are listed in Supplementary Table S2. The two families formed multiple clades (Figure 2; Supplementary Figures S5, S6). Our study did not resolve the two families. Whether the family Roseobacteraceae needs to be re-merged into the Paracoccaceae needs further investigation.
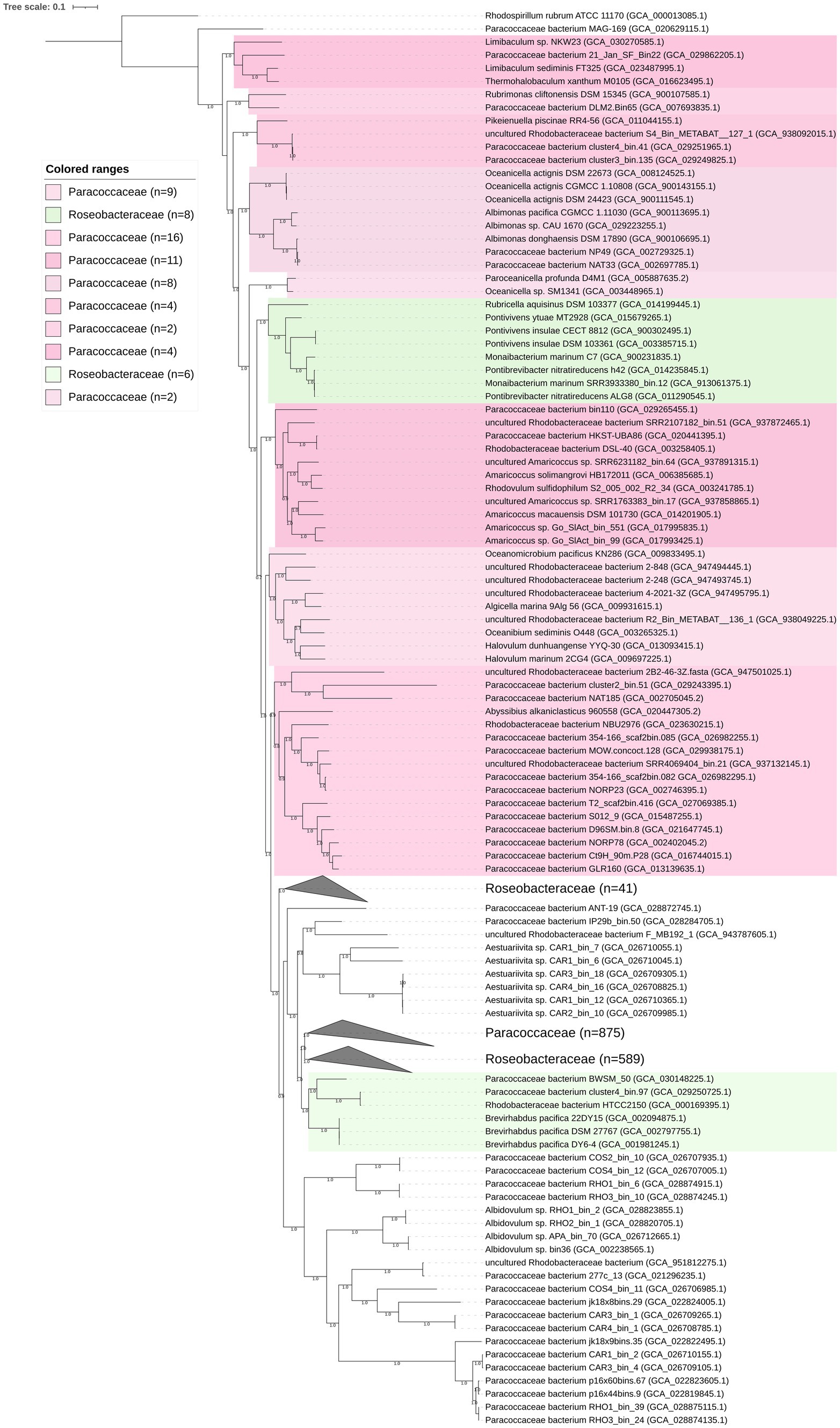
Figure 2. Phylogenomic tree of the family Paracoccaceae constructed based on bacterial 120 conserved proteins. The bootstrap values on the nodes are displayed by >70. Bar 0.1 represents the amino acid substitution per position. Based on the current taxonomic system in LPSN, Paracoccaceae and Roseobacteraceae are marked as light pink and light green, respectively. The numbers in the brackets are the genomes in the clade.
The phylogenomic tree constructed based on the 120 ubiquitously conserved bacterial proteins showed that strain FR2A1T formed a distinct monophyletic branch with an uncultivated bacterium bin.37 (GCA_024742695.1), a bacterium found in the phycosphere of a toxic marine dinoflagellate (Alexandrium tamarense), which was separated from other genera affiliated to the family Paracoccaceae (Supplementary Figure S5). The genome size of the bacterium bin.37 was 4.2 Mb with a genomic G + C content of 69.2%. Gene prediction showed 4,125 functional genes in the bacterium bin.37. A gene cluster for sulfur oxidation (SoxABCDYZ) was also annotated, but the gene cluster for nitrate reduction was not found (Supplementary Table S1). The ANI and AAI values between strain FR2A1T and bacterium bin.37 were 75.9 and 71.8%, respectively. Thus, our phylogenomic analysis and genomic relatedness strongly supported that the bacterium bin.37 belonged to the same genus as strain FR2A1T.
In the course of the phylogenomic analysis of the 1,606 genomes of the family Paracoccaceae, we found discrepancies in the taxonomic positions of a few members, as elucidated below.
Thermohalobaculum and Limibaculum
The genus Thermohalobaculum with type species Thermohalobaculum xanthum was proposed in 2021 (Pan et al., 2021). T. xanthum M0105T and Limibaculum sediminis FT325T are grouped together in the phylogenomic tree (Figure 2). The AAI and ANI values between T. xanthum M0105T and L. sediminis FT325T were 81.7 and 82.0%, respectively. The phylogeny of the 16S rRNA gene sequences placed the two strains into a highly supported clade (bootstrap of 99%), which is neighbored by Limibaculum halophilum (type species of Limibaculum) and Rubrimonas representatives (Supplementary Figure S7). The analysis supported that T. xanthum and L. sediminis could be merged into a single genus. Thus, we propose the transfer of L. sediminis to the genus Thermohalobaculum as Thermohalobaculum sediminis comb. nov. The genome size of Thermohalobaculum was 4.1–4.3 Mbp. The genomic G + C content of Thermohalobaculum was 67.9–69.6%.
Albimonas
The AAI values among the five Albimonas genomes in the tree (Figure 2), including A. pacifica CGMCC 1.11030T and A. donghaensis DSM 17890T, were 72.1–99.8%. The genome size of Albimonas was 5.0–6.0 Mbp. The genomic G + C content was 70.9–72.9% (Supplementary Table S2).
Pontivivens, Monaibacterium, and Pontibrevibacter
Pontivivens currently contains two species, P. insulae (type species) and P. ytuae (Parte et al., 2020). However, the two species did not form a node in the phylogenomic tree (Figure 2). Monaibacterium marinum C7T and Pontibrevibacter nitratireducens h42T formed a highly supported clade, sharing AAI and ANI values of 81.5 and 78.2%, respectively, showing that they could be considered representatives of the same genus. The four species, P. insulae, P. ytuae, M. marinum, and Pontibrevibacter nitratireducens, were tightly clustered with 100% bootstrap values, sharing AAI values of 70.0–100%. The AAI values between Rubricella aquisinus DSM 103377T and the eight close relatives were 65.3–66.5%. Thus, it is reasonable to merge the four species P. insulae, P. ytuae, M. marinum, and Pontibrevibacter nitratireducens into a single genus, separated from the genus Rubricella. Based on priority, M. marinum and P. nitratireducens should be merged into the genus Pontivivens as Pontivivens marinum comb. nov. and Pontivivens nitratireducens comb. nov., respectively. The genome size of Pontivivens representatives was 3.1–4.2 Mbp. The genomic G + C content was 58.9–67.2% (Supplementary Table S2).
Amylibacter and Neptunicoccus
The phylogeny of Amylibacter genomes indicated multi-phyletic clades (Figure 3A). The heatmap of AAI values supported six distinct groups, corresponding to the six phylogenetic clades in the tree (Figure 4). First, A. ulvae KCTC 32465T and A. kogurei 4G11T formed a highly supported clade, sharing an AAI value of 92.6%, which was distantly separated from the type species A. marinus. Thus, we propose a novel genus, Paramylibacter gen. nov., to accommodate the species A. ulvae and A. kogurei. The major fatty acid of Paramylibacter species was C18:1 ω7c (Nedashkovskaya et al., 2016; Wong et al., 2018). C14:0 was not found in Paramylibacter, whereas it was found in A. marinus. Second, Neptunicoccus sediminis CY02T and A. cionae CGMCC 1.15880T were closely related, indicating that they belonged to the same genus. The ANI and AAI values between N. sediminis CY02T and A. cionae CGMCC 1.15880T were 85.3 and 92.6%, respectively. The major polar fatty acids of Neptunicoccus sediminis CY02T and A. cionae CGMCC 1.15880T contained 11-methyl C18:1 ω7c (>10%), a fatty acid not found in A. marinus NBRC 110140T (Wang et al., 2017; Zhang et al., 2018). Thus, Amylibacter cionae (Wang et al., 2017) should be transferred to the genus Neptunicoccus as Neptunicoccus cionae comb. nov. The AAI values of Neptunicoccus representatives were 73.8–99.4%. The genome size of Neptunicoccus representatives was 2.8–4.3 Mbp. The genomic G + C content was 47.5–57.5%. Third, A. marinus NBRC 110140T formed an independent line, representing a separate genus (Figure 3A).
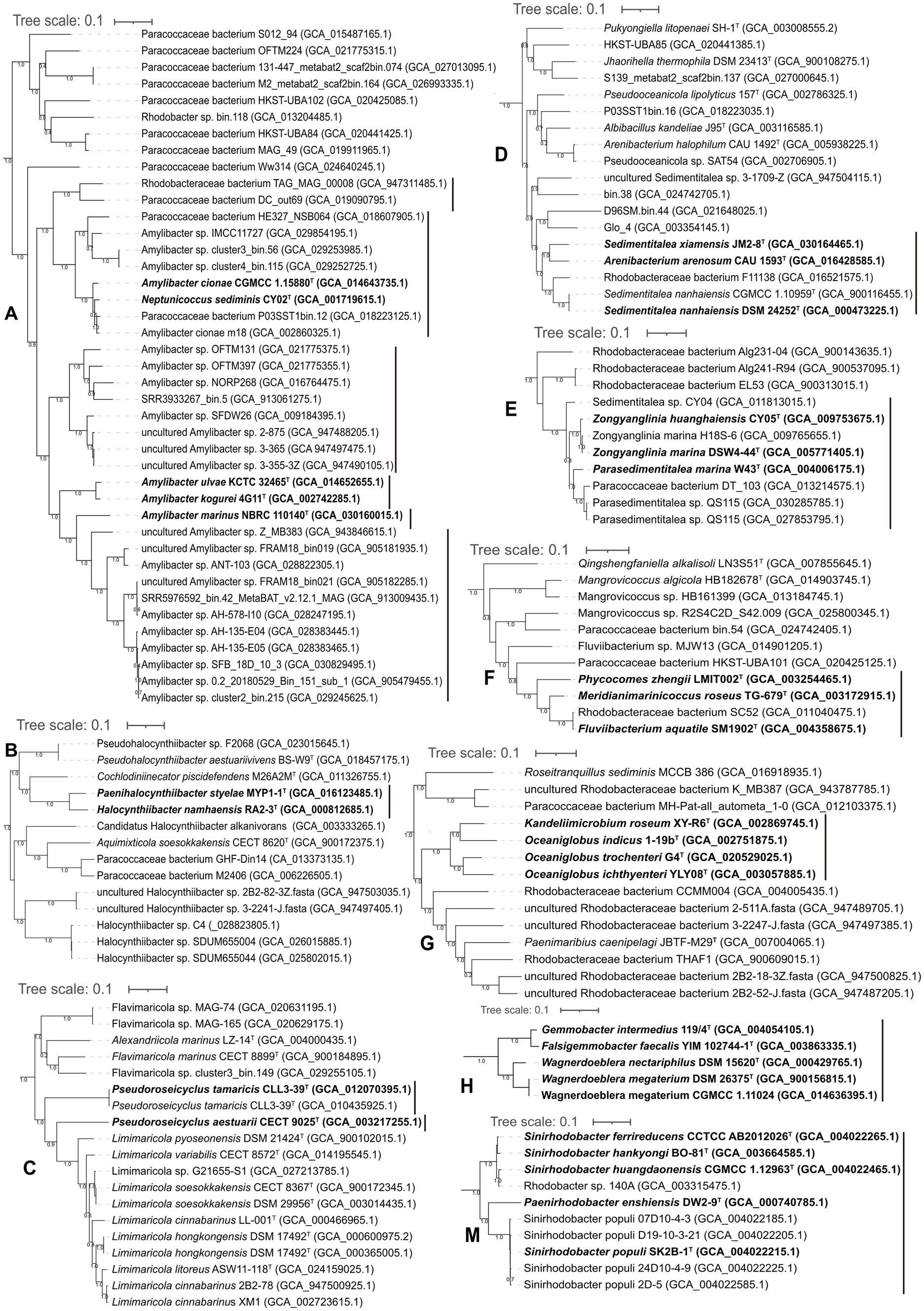
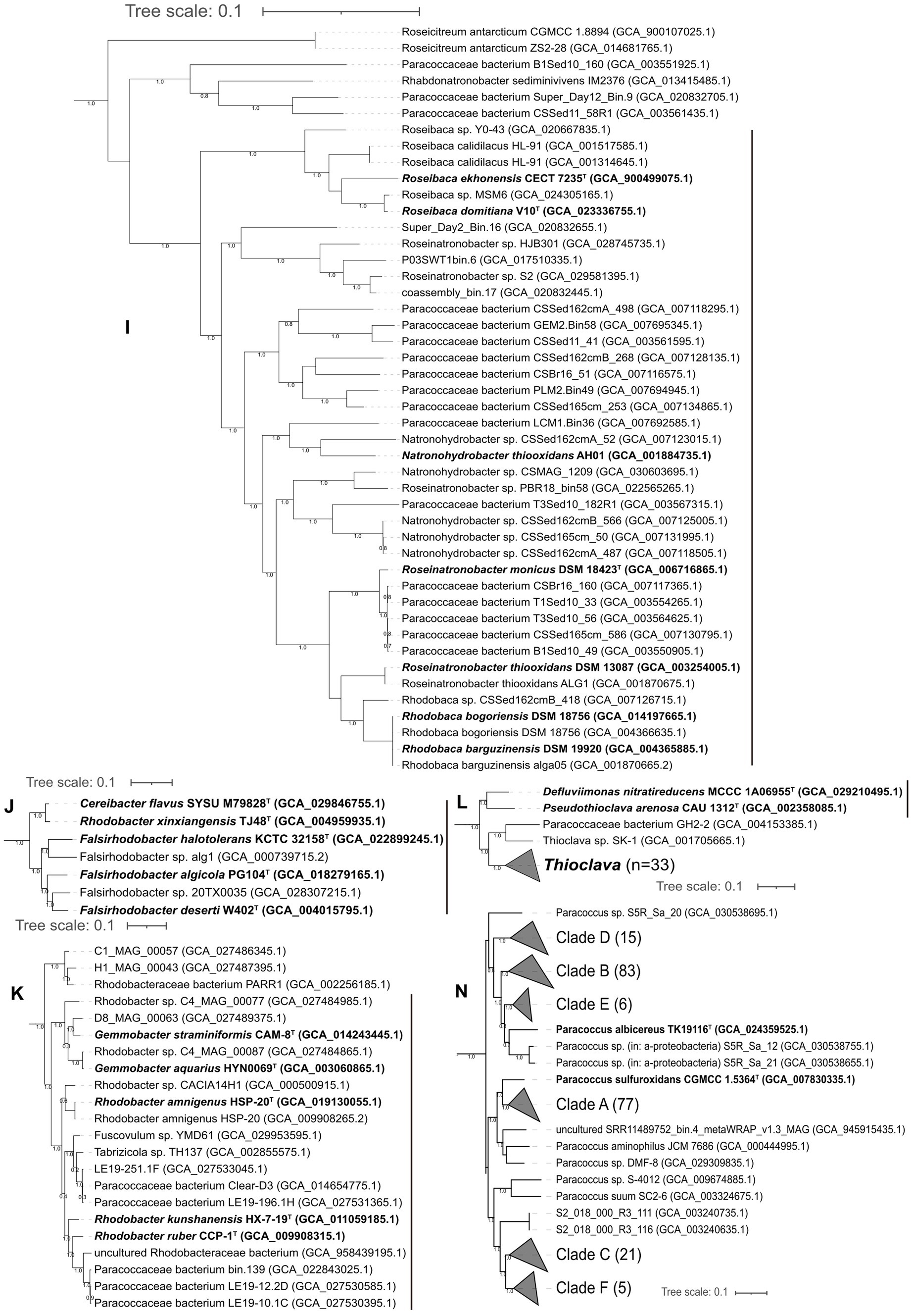
Figure 3. Phylogenomic tree of the genomes with discrepancies. (A-N) different clades. The species with reclassification are marked in bold.
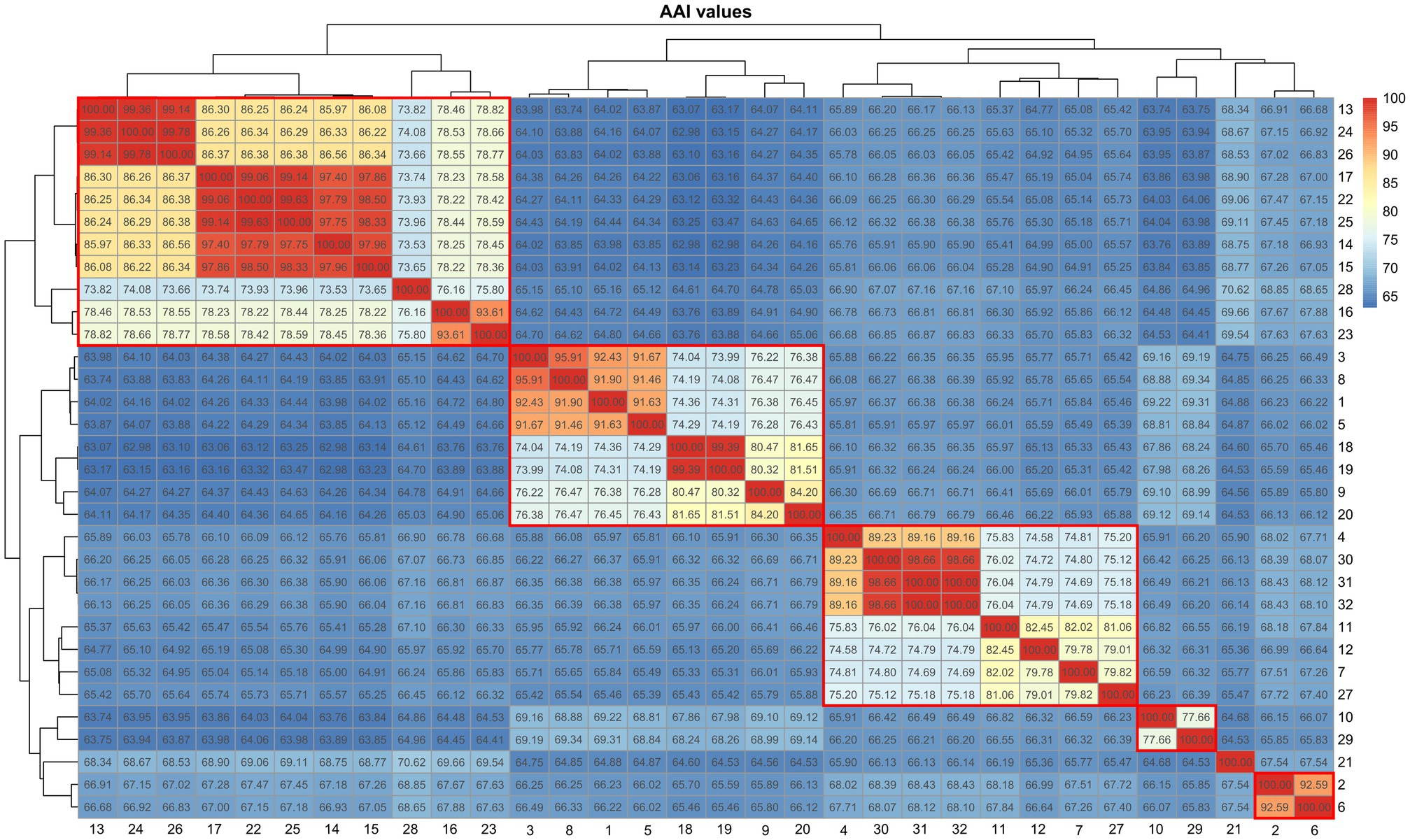
Figure 4. Heatmap showing the AAI values among the representatives of Amylibacter and Neptunicoccus. 1, Neptunicoccus sediminis CY02T; 2, Amylibacter kogurei 4G11T; 3, Amylibacter cionae m18 (GCA_002860325.1); 4, Amylibacter sp. SFDW26 (GCA_009184395.1); 5, Amylibacter cionae CGMCC 1.15880T; 6, Amylibacter ulvae KCTC 32465T; 7, Amylibacter sp. NORP268 (GCA_016764475.1); 8, Paracoccaceae bacterium P03SST1bin.12 (GCA_018223125.1); 9, Paracoccaceae bacterium HE327_NSB064 (GCA_018607905.1); 10, Paracoccaceae bacterium DC_out69 (GCA_019090795.1); 11, Amylibacter sp. OFTM397 (GCA_021775355.1); 12, Amylibacter sp. OFTM131 (GCA_021775375.1); 13, Amylibacter sp. AH-578-I10 (GCA_028247195.1); 14, Amylibacter sp. AH-135-E04 (GCA_028383445.1); 15, Amylibacter sp. AH-135-E05 (GCA_028383465.1); 16, Amylibacter sp. ANT-103 (GCA_028822305.1); 17, Amylibacter sp. cluster2_bin.215 (GCA_029245625.1); 18, Amylibacter sp. cluster4_bin.115 (GCA_029252725.1); 19, Amylibacter sp. cluster3_bin.56 (GCA_029253985.1); 20, Amylibacter sp. IMCC11727 (GCA_029854195.1); 21, Amylibacter marinus NBRC 110140T; 22, Amylibacter sp. SFB_18D_10_3 (GCA_030829495.1); 23, uncultured Amylibacter sp. FRAM18_bin019 (GCA_905181935.1); 24, uncultured Amylibacter sp. FRAM18_bin021 (GCA_905182285.1); 25, uncultured Amylibacter sp. 0.2_20180529_Bin_151_sub_1 (GCA_905479455.1); 26, SRR5976592_bin.42_MetaBAT_v2.12.1_MAG (GCA_913009435.1); 27, SRR3933267_bin.5 (GCA_913061275.1); 28, uncultured Amylibacter sp. Z_MB383 (GCA_943846615.1); 29, TAG_MAG_00008 (GCA_947311485.1); 30, uncultured Amylibacter sp. 2-875 (GCA_947488205.1); 31, uncultured Amylibacter sp. 3-355-3Z (GCA_947490105.1); 32, uncultured Amylibacter sp. 3-365 (GCA_947497475.1).
Halocynthiibacter and Paenihalocynthiibacter
Phylogenetic analysis placed Halocynthiibacter namhaensis RA2-3T and Paenihalocynthiibacter styelae MYP1-1T into a closely related clade, sharing an AAI value of 81%, indicating that the strains belonged to the same genus (Figure 3B). Cochlodiniinecator piscidefendens M26A2MT shared AAI values of 68.8 and 68.7% with Halocynthiibacter namhaensis RA2-3T and Paenihalocynthiibacter styelae MYP1-1T, respectively. Based on the priority of publication, we propose the transfer of Paenihalocynthiibacter styelae (Kim et al., 2021) to the genus Halocynthiibacter as Halocynthiibacter styelae comb. nov. This analysis is consistent with the GTDB taxonomic system (Chaumeil et al., 2019).
Aliiroseovarius
Planktotalea lamellibrachiae DSM 104669T clearly clustered within the genus Aliiroseovarius (Supplementary Figure S6) and should therefore be classified as a species of that genus. The taxonomic position of Planktotalea lamellibrachiae was recently elucidated (Zhang et al., 2023). The 53 genomes of Aliiroseovarius shared AAI values of 72.2–100%.
Pseudoroseicyclus
Pseudoroseicyclus currently includes two species with validly published names: Pseudoroseicyclus aestuarii (type species; Park et al., 2016) and Pseudoroseicyclus tamaricis (Gai et al., 2021). However, P. aestuarii CECT 9025T and P. tamaricis CLL3-39T formed separate branches (Figure 3C), indicating that P. tamaricis CLL3-39T should be placed into a novel genus. This analysis is consistent with the GTDB taxonomic system (Chaumeil et al., 2019). The AAI and ANI values between P. aestuarii CECT 9025T and P. tamaricis CLL3-39T were 65.9 and 74.7%, respectively. In addition, phosphatidylglycerol is found in P. tamaricis, but it is not found in P. aestuarii (Gai et al., 2021). Thus, a novel genus, Falsiroseicyclus gen. nov., is proposed to accommodate the species Falsiroseicyclus tamaricis comb. nov.
Limimaricola
Limimaricola representatives (11 genomes) were well grouped in the phylogenomic tree (Figure 3C). The genome size was 3.2–4.2 Mbp. The genomic G + C content was 66.7–70.3%. The AAI value of Limimaricola genomes was 80.6–100%.
Marivivens
Marivivens representatives (11 genomes) are well grouped in the phylogenomic tree (Supplementary Figure S6). The AAI values were 71.7–100%. The genome size was 2.5–4.1 Mbp. The genomic G + C content was 54.6–60.9%.
Yoonia
The 58 genomes affiliated to Yoonia were well clustered (Supplementary Figure S6), sharing AAI values of 71.4–100%.
Cognatishimia
The AAI values calculated among the 13 genomes of Cognatishimia in the tree (Supplementary Figure S6) were 70.8–100%. The genome size was 3.1–4.1 Mbp. The genomic G + C content was 53.1–58.9%.
Sedimentitalea and Arenibacterium arenosum
Sedimentitalea currently contains three species with validly published names: Sedimentitalea nanhaiensis (type species), S. todarodis, and S. xiamensis. Phylogenetic analysis based on genome sequences showed that Sedimentitalea and Arenibacterium arenosum CAU 1593T formed a monophyletic clade (Figure 3D), sharing AAI values of 76.7–82.7%. We suggested that Arenibacterium arenosum should be moved to the genus Sedimentitalea, and thus Sedimentitalea arenosa comb. nov. is proposed. The genomic size and genomic G + C content were 4.0–4.9 Mb and 60.8–64.2%, respectively.
Parasedimentitalea and Zongyanglinia
Parasedimentitalea marina W43T formed a tight cluster with Zongyanglinia huanghaiensis CY05T and Zongyanglinia marina DSW4-44T (Figure 3E), sharing AAI values of 82.8 and 82.7%, respectively. The values could justify the classification of the three species into the same genus. This analysis is consistent with the GTDB taxonomic system (Chaumeil et al., 2019). Thus, based on priority, we proposed the transfer of Zongyanglinia huanghaiensis and Zongyanglinia marina to the genus Parasedimentitalea as Parasedimentitalea huanghaiensis comb. nov. and Parasedimentitalea marina comb. nov., respectively. The AAI values of the Parasedimentitalea genomes were 81.8–100%. The genome size of Parasedimentitalea was 4.4–5.6 Mb, and the genomic G + C content was 54.2–57.8%.
Pseudophaeobacter
Pseudophaeobacter representatives were well grouped (Supplementary Figure S6), sharing AAI values of 76.9–99.1%.
Tritonibacter
Tritonibacter representatives were well grouped (Supplementary Figure S6), sharing AAI values of 70.2–100%.
Meridianimarinicoccus, Phycocomes, and Fluviibacterium
Meridianimarinicoccus roseus TG-679T, Phycocomes zhengii LMIT002T, and Fluviibacterium aquatile SM1902T formed a tight cluster (Figure 3F; Supplementary Figure S5), sharing AAI values of 75.2–98.9%, respectively. We suggest grouping the three species into the same genus. This analysis is consistent with the GTDB taxonomic system (Chaumeil et al., 2019). Thus, based on priority, we propose the transfer of Phycocomes zhengii and Fluviibacterium aquatile to the genus Meridianimarinicoccus as Meridianimarinicoccus zhengii comb. nov. and Meridianimarinicoccus aquatilis comb. nov. The genome size was 3.9–4.6 Mbp, and the DNA G + C content was 58.2–67.0%. We also propose the classification of Meridianimarinicoccus in the family Paracoccaceae, instead of the recommended classification in the family Roseobacteraceae (Liang et al., 2021).
Rhodovulum
The 43 Rhodovulum genomes were well grouped (Supplementary Figure S5), sharing AAI values of 72.0–100%. We propose the classification of Rhodovulum as a representative of the family Paracoccaceae, different from the proposed assignment to the family Roseobacteraceae (Liang et al., 2021).
Oceaniglobus and Kandeliimicrobium
Phylogenomic analysis placed Kandeliimicrobium roseum XY-R6T and Oceaniglobus indicus 1-19bT into the same lineage (Figure 3G). The AAI value between Kandeliimicrobium roseum XY-R6T and Oceaniglobus indicus 1-19bT was 76.0%. Based on the priority, K. roseum should be re-classified into the genus Oceaniglobus as Oceaniglobus roseus comb. nov. The genome size was 3.7–4.6 Mbp, and the genomic G + C content was 59.0–69.0%. We also propose that Oceaniglobus be a representative of the family Paracoccaceae, different from the recommended classification in the family Roseobacteraceae (Liang et al., 2021).
Pararhodobacter
The 11 Pararhodobacter genomes were well grouped (Supplementary Figure S5), sharing AAI values of 71.9–100%.
Acidimangrovimonas and Allgaiera
Acidimangrovimonas and Allgaiera were tightly clustered (Supplementary Figure S5). Acidimangrovimonas was first proposed in 2019 with the description of Acidimangrovimonas sediminis (Ren et al., 2019). The authors also re-classified two species of Defluviimonas, Defluviimonas indica, and Defluviimonas pyrenivorans, to the genus Acidimangrovimonas as Acidimangrovimonas indica and Acidimangrovimonas pyrenivorans (Ren et al., 2019). A paper published in 2020 proposed the re-classification of Defluviimonas indica to the genus Allgaiera as Allgaiera indica (Hördt et al., 2020; Oren and Garrity, 2020a). Based on priority, the name Acidimangrovimonas indica has priority and should replace the name Allgaiera indica. The size of the Acidimangrovimonas genomes was 4.3–5.3 Mbp with a genomic G + C content of 66.3–67.8% (Supplementary Table S2). The AAI values of the Acidimangrovimonas genomes were 77.5–100%.
Solirhodobacter
This genus currently contains one species with a validly published name, Solirhodobacter olei with type strain Pet-1T (Chu et al., 2020). The genome size of Solirhodobacter (eight genomes) was 3.1–4.8 Mbp with a genomic G + C content of 63.1–69.1%. The AAI value of Solirhodobacter representatives was 72.4–99.8%.
Wagnerdoeblera
The genus Wagnerdoeblera was proposed in 2020 to accommodate Wagnerdoeblera nectariphila and Wagnerdoeblera megaterium (Hördt et al., 2020; Oren and Garrity, 2020a). Based on the phylogenomic analysis (Figure 3H), Falsigemmobacter faecalis YIM 102744-1T (Li et al., 2020) and Gemmobacter intermedius 119/4T should also be included in the genus Wagnerdoeblera, though Gemmobacter intermedius was reported to be included in the effectively published genus Falsigemmobacter as Falsigemmobacter intermedius (Li et al., 2020). The genome size of Wagnerdoeblera genomes was 4.1–4.5 Mbp with a genomic G + C content of 62.7–66.2%. AAI values of Wagnerdoeblera genomes were 70.4–100%.
Roseibaca, Roseinatronobacter, Natronohydrobacter, and Rhodobaca
The phylogenomic tree placed four genera, Roseibaca, Roseinatronobacter, Natronohydrobacter, and Rhodobaca, in a closely related clade (Figure 3I). First, the ANI value between Rhodobaca bogoriensis DSM 18756T and Rhodobaca barguzinensis alga-05T was 100%, suggesting that the two strains belonged to the same species. We proposed that Rhodobaca barguzinensis is a later heterotypic synonym of the species Rhodobaca bogoriensis. Second, AAI values among the 40 genomes of the genera Roseibaca, Roseinatronobacter, Natronohydrobacter, and Rhodobaca did not give a clear boundary to discriminate between these genera (Figure 5). Thus, we suggested combining them into a single genus. Based on priority, Roseibaca, Natronohydrobacter, and Rhodobaca should be transferred to the genus Roseinatronobacter. Thus, Roseinatronobacter ekhonensis comb. nov., Roseinatronobacter domitianus comb. nov., and Roseinatronobacter bogoriensis comb. nov. were proposed to replace the names Roseibaca ekhonensis, Roseibaca domitiana, and Rhodobaca bogoriensis, respectively. The AAI values of 40 Roseinatronobacter genomes were 72.1–100%.
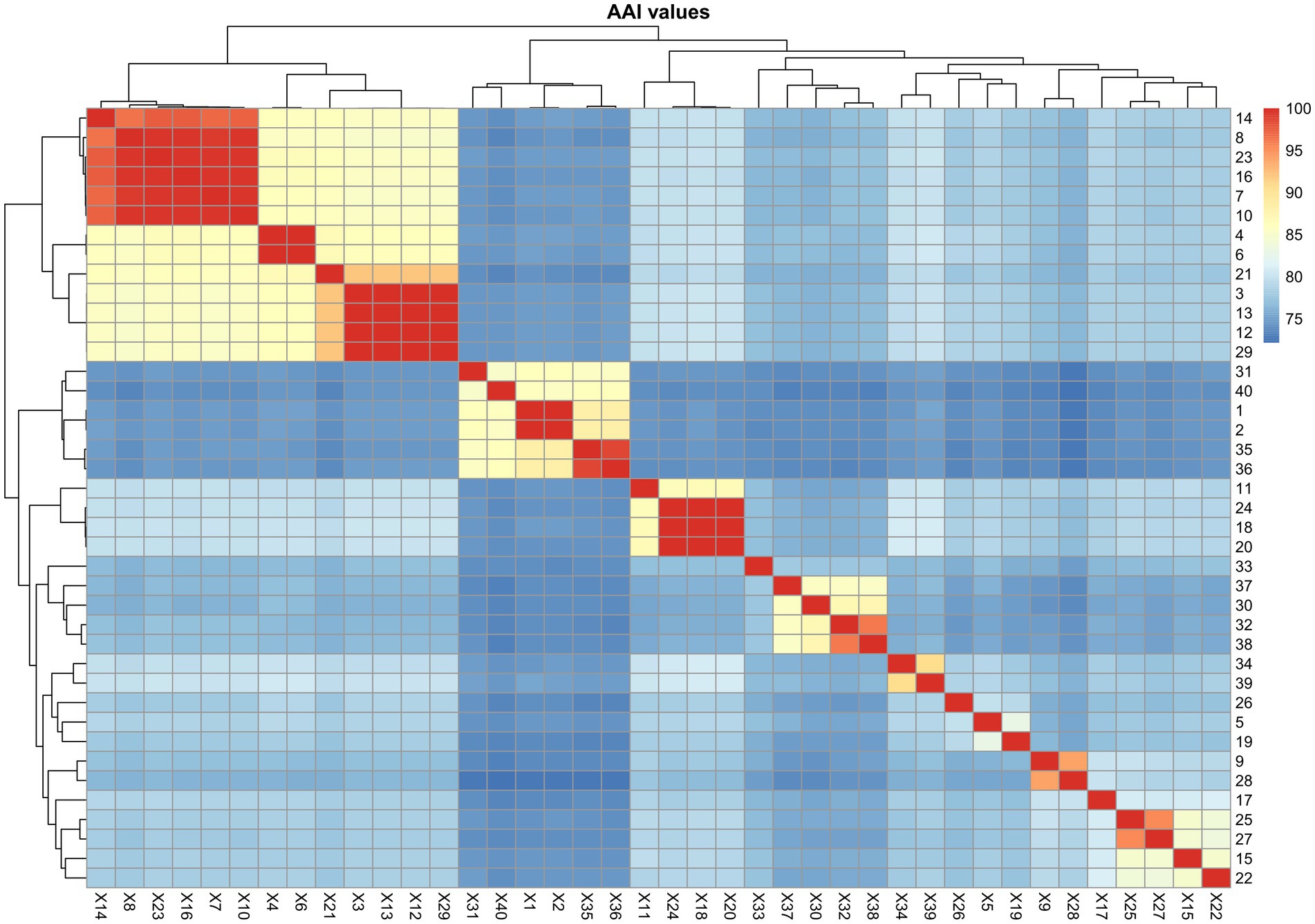
Figure 5. Heatmap showing the AAI values among the representatives of Roseinatronobacter and close relatives. 1, Roseibaca calidilacus HL-91 (GCA_001314645.1); 2, Roseibaca calidilacus HL-91 (GCA_001517585.1); 3, Rhodobaca barguzinensis alga05 (GCA_001870665.2); 4, Roseinatronobacter thiooxidans ALG1 (GCA_001870675.1); 5, Natronohydrobacter thiooxidans AH01 (GCA_001884735.1); 6, Roseinatronobacter thiooxidans DSM 13087 (GCA_003254005.1); 7, Paracoccaceae bacterium B1Sed10_49 (GCA_003550905.1); 8, Paracoccaceae bacterium T1Sed10_33 (GCA_003554265.1); 9, Paracoccaceae bacterium CSSed11_41 (GCA_003561595.1); 10, Paracoccaceae bacterium T3Sed10_56 (GCA_003564625.1); 11. Paracoccaceae bacterium T3Sed10_182R1 (GCA_003567315.1); 12, Rhodobaca barguzinensis DSM 19920 (GCA_004365885.1); 13, Rhodobaca bogoriensis DSM 18756 (GCA_004366635.1); 14, Roseinatronobacter monicus DSM 18423 (GCA_006716865.1); 15, Paracoccaceae bacterium CSBr16_51 (GCA_007116575.1); 16, Paracoccaceae bacterium CSBr16_160 (GCA_007117365.1); 17, Paracoccaceae bacterium CSSed162cmA_498 (GCA_007118295.1); 18, Natronohydrobacter sp. CSSed162cmA_487 (GCA_007118505.1); 19, Natronohydrobacter sp. CSSed162cmA_52 (GCA_007123015.1); 20, Natronohydrobacter sp. CSSed162cmB_566 (GCA_007125005.1); 21, Rhodobaca sp. CSSed162cmB_418 (GCA_007126715.1); 22, Paracoccaceae bacterium CSSed162cmB_268 (GCA_007128135.1); 23, Paracoccaceae bacterium CSSed165cm_586 (GCA_007130795.1); 24, Natronohydrobacter sp. CSSed165cm_50 (GCA_007131995.1); 25, Paracoccaceae bacterium CSSed165cm_253 (GCA_007134865.1); 26, Paracoccaceae bacterium LCM1.Bin36 (GCA_007692585.1); 27, Paracoccaceae bacterium PLM2.Bin49 (GCA_007694945.1); 28, Paracoccaceae bacterium GEM2.Bin58 (GCA_007695345.1); 29, Rhodobaca bogoriensis DSM 18756 (GCA_014197665.1); 30, Paracoccaceae bacterium P03SWT1bin.6 (GCA_017510335.1); 31, Roseibaca sp. Y0-43 (GCA_020667835.1); 32, Paracoccaceae bacterium coassembly_bin.17 (GCA_020832445.1); 33, Paracoccaceae bacterium Super_Day2_Bin.16 (GCA_020832655.1); 34, Roseinatronobacter sp. PBR18_bin58 (GCA_022565265.1); 35, Roseibaca domitiana V10 (GCA_023336755.1); 36, Roseibaca sp. MSM6 (GCA_024305165.1); 37, Roseinatronobacter sp. HJB301 (GCA_028745735.1); 38, Roseinatronobacter sp. S2 (GCA_029581395.1); 39, Natronohydrobacter sp. CSMAG_1209 (GCA_030603695.1); 40, Roseibaca ekhonensis CECT 7235 (GCA_900499075.1).
Tabrizicola rongguiensis
Tabrizicola rongguiensis J26T and an uncultivated bacterium (GCA_945952585.1) formed a clade within the phylogenetic tree that was distinctly separated from other Tabrizicola representatives (Supplementary Figure S5). The AAI value of the two genomes was 89.9% (Supplementary Table S2). Thus, Tabrizicola rongguiensis could be considered a novel genus, and Aliitabrizicola rongguiensis gen. nov., sp. nov. is proposed. The genomic size of Aliitabrizicola is 3.9–4.2 Mbp with a genomic G + C content of 64.2–65.2%.
Falsirhodobacter
Falsirhodobacter halotolerans KCTC 32158T, together with Cereibacter flavus SYSU M79828T and Rhodobacter xinxiangensis TJ48T, were closely related in the phylogenetic tree (Figure 3J). Cereibacter flavus SYSU M79828T and Rhodobacter xinxiangensis TJ48T could be placed into the genus Falsirhodobacter as Falsirhodobacter flavus comb. nov. and Falsirhodobacter xinxiangensis comb. nov., respectively. This analysis is consistent with the GTDB taxonomic system (Chaumeil et al., 2019). The genomic size of Falsirhodobacter is 2.8–4.0 Mbp with a genomic G + C content of 60.3–66.7%. The AAI value of the Falsirhodobacter genomes was 71.5–92.6%.
Gemmobacter
The phylogeny of Gemmobacter genomes indicated multi-phyletic clades (Figure 3K; Supplementary Figure S5). First, Gemmobacter aquatilis DSM 3857T and another 15 genomes formed a tight clade, sharing AAI values of 75.7–100%. The ANI and AAI between G. nanjingensis KCTC 23298T and G. caeni CGMCC 1.7745T were 98.3 and 99%, respectively, indicating they represented a single species. Based on priority, G. nanjingensis is a later heterotypic synonym of G. caeni. Second, Gemmobacter aestuarii CC-PW-75T and an uncultivated bacterium ACE_PRO37 (GCA_019454225.1) formed a clade in the phylogenetic tree, which was distinctly separated from other Gemmobacter representatives. The AAI value of Gemmobacter aestuarii CC-PW-75T and the uncultivated bacterium ACE_PRO37 was 71.6%. Thus, Gemmobacter aestuarii could be considered a representative of a novel genus, for which Aliigemmobacter aestuarii gen. nov., sp. nov. is proposed. The genomic size of Aliigemmobacter is 3.9–4.2 Mbp with a DNA G + C content of 64.2–65.2%. Third, Gemmobacter tilapiae KCTC 23310T formed an independent monophyletic line, which represented a novel genus. Thus, Neogemmobacter gen. nov. is proposed. The type species is Neogemmobacter tilapiae comb. nov. Finally, Rhodobacter ruber CCP-1T, Rhodobacter kunshanensis HX-7-19T, Rhodobacter amnigenus HSP-20T, Gemmobacter aquarius HYN0069T, and Gemmobacter straminiformis CAM-8T formed a tight cluster (Figure 3K), sharing AAI values of 74.6–100%. Thus, these five species could be moved to a novel genus, and Paragemmobacter gen. nov. is proposed. The genomic size was 3.6–4.7 Mbp. The genomic G + C content was 61.7–66.6%. The type species is Paragemmobacter straminiformis comb. nov.
Pseudotabrizicola
Pseudotabrizicola representatives were clustered well (Supplementary Figure S5), sharing AAI values of 75.0–100%. The genomic size was 2.9–5.0 Mbp.
Stagnihabitans
The nine genomes affiliated to the genus Stagnihabitans clustered together (Supplementary Figure S5), sharing AAI values of 70.4–99.1%. The genome size was 2.7–4.9 Mb. The genomic G + C content was 59.1–66.0%.
Cypionkella
The 18 genomes affiliated to the genus Cypionkella clustered together (Supplementary Figure S5), sharing AAI values of 69.9–99.9%. The genome size was 2.7–5.1 Mb. The genomic G + C content was 57.7–62.4%.
Tabrizicola
Tabrizicola representatives and an isolate named Rhodobacter calidifons M3P7 clustered well in the phylogenetic tree (Supplementary Figure S3), sharing AAI values of 75.4–100%.
Defluviimonas
The Defluviimonas representatives clustered well in the phylogenetic tree (Supplementary Figure S5). The AAI values among the Defluviimonas representatives were 70.6–100%.
Thioclava
The Thioclava representatives clustered well in the phylogenetic tree (Supplementary Figure S5). The AAI values among the Thioclava representatives were 77.3–100%.
Frigidibacter
The Frigidibacter representatives clustered well in the phylogenetic tree (Supplementary Figure S5). The AAI values among the Frigidibacter representatives were 74.2–100%.
Pseudothioclava and Defluviimonas nitratireducens
Pseudothioclava currently contains a single species with a validly published name, Pseudothioclava arenosa (Kim et al., 2019; Oren and Garrity, 2020a). Pseudothioclava arenosa CAU 1312T and Defluviimonas nitratireducens MCCC 1A06955T clustered together (Figure 3L), sharing AAI and ANI values of 78.3 and 78.6%, respectively. Thus, we suggest that Defluviimonas nitratireducens should be re-classified into the genus Pseudothioclava as Pseudothioclava nitratireducens comb. nov.
Sedimentimonas
Sedimentimonas currently includes a single species, Sedimentimonas flavescens (Mu et al., 2022). The Sedimentimonas representatives formed a monophyletic clade (Supplementary Figure S5), sharing AAI values of 79.1–98.9%. The genome size of Sedimentimonas was 3.1–3.8 Mbp. The DNA G + C content was 64.1–67.0%.
Sinirhodobacter and Paenirhodobacter
Paenirhodobacter was proposed and validly published in 2014 with the type species Paenirhodobacter enshiensis (Wang et al., 2014). Sinirhodobacter (former name Sinorhodobacter) was proposed in 2013 with the type species Sinirhodobacter ferrireducens (Yang et al., 2013), but the name was validly published in 2018 (Oren and Garrity, 2018). Paenirhodobacter enshiensis DW2-9T and the Sinirhodobacter representatives formed a monophyletic clade (Figure 3M), sharing AAI values of 73.3–98.4%. These data supported that Paenirhodobacter and Sinirhodobacter could be merged into a single genus. Thus, based on priority, the Sinirhodobacter representatives should be transferred to the genus Paenirhodobacter. Sinirhodobacter ferrireducens, Sinirhodobacter hungdaonensis, Sinirhodobacter populi, and Sinirhodobacter hankyongi should be renamed as Paenirhodobacter ferrireducens comb. nov., Paenirhodobacter hungdaonensis comb. nov., Paenirhodobacter populi comb. nov., and Paenirhodobacter hankyongi comb. nov., respectively. The result is consistent with the taxonomic system in GTDB (Chaumeil et al., 2019). The genome size of Paenirhodobacter was 3.4–4.8 Mbp. The genomic G + C content was 65.4–68.3%.
Rhodobacter
The type species of Rhodobacter is Rhodobacter capsulatus with type strain DSM 1710T (Imhoff et al., 1984). R. capsulatus DSM 1710T, R. viridis JA737T, R. aestuarii JA296T, R. maris JA276T, and 17 additional genomes formed a tight clade (Supplementary Figure S5), sharing AAI values of 79.1–100%. The genome size of Rhodobacter representatives was 3.6–4.2 Mbp. The genomic G + C content was 61.1–66.7%.
Phaeovulum
The genus Phaeovulum currently contains two species with validly published names, Phaeovulum veldkampii and Phaeovulum vinaykumarii (Parte et al., 2020; Oren and Garrity, 2020b). However, P. veldkampii DSM 11550T and P. vinaykumarii JA123T did not form a cluster in the phylogenetic tree, making us question their placement. Their taxonomic position needs further study.
Paracoccus
A total of 219 high-quality genomes affiliated to the genus Paracoccus were included in the phylogenomic tree (Figure 3N; Supplementary Figure S5). At the time of writing, the genus contained 81 species with validly published names,8 outnumbering the species of the other genera classified in the family Paracoccaceae. The AAI values among the 219 Paracoccus genomes showed at least six clades, numbered from Clade A to Clade F, to be consistent with the phylogenomic clades (Supplementary Figure S8). This indicated that the genus Paracoccus could be split into several new genera. However, as the genus Paraccocus is widely used, their taxonomic position needs further study.
In summary, based on the above results of phenotypic, genomic, and chemotaxonomic characteristics, strains FR2A1T and MT2-5-38 represent a novel genus and novel species within the family Paracoccaceae. The name Ostreiculturibacter nitratireducens gen. nov., sp. nov. is proposed, with type strain FR2A1T (=MCCC 1K08809T = KCTC 8317T). A second strain is MT2-5-38 (=MCCC 1 K08810). Both were isolated from the surface sediment of an oyster farm on a tidal flat in Quanzhou Bay, China. Additionally, based on the AAI values of the above phylogenomic clades, though not all clades in the family Paracoccaceae, the genera have a clear threshold of an AAI value of 70%. Thus, an AAI value of 70% could be considered the genus boundary within the family Paracoccaceae.
Taxonomic consequences
Description of Ostreiculturibacter gen. nov.
Ostreiculturibacter (Os.tre.i.cul.tu.ri.bacter. L. fem. n. ostrea, an oyster; L. fem. n. cultura, cultivation; N.L. masc. n. bacter, a rod; N.L. masc. n. Ostreiculturibacter, a rod from an oyster farm).
Colonies on MB agar plates cultured for 2 days at 30°C are light-white colored, small, and round. Cells are Gram-stain-negative and rod-shaped. Catalase-positive and oxidase-positive. The quinone system is quinone Q-10. The major fatty acids are summed feature 8 (C18:1 ω7c/C18:1 ω6c) and C18:0. The polar lipids consisted of phosphatidylethanolamine, phosphatidylglycerol, and several unidentified phospholipids. The genome contained a gene cluster (Sox system) for sulfur oxidation. The genome size is 3.9–4.2 Mbp, calculated from the strains and a metagenome-assembled genome. The genomic G + C content is 65.6–69.2%. It was found in the tidal flat surface sediment and the phycosphere of a toxic marine dinoflagellate. The type species is Ostreiculturibacter nitratireducens.
Description of Ostreiculturibacter nitratireducens sp. nov.
Ostreiculturibacter nitratireducens (ni.tra.ti.re.du’cens. N.L. masc. n. nitras, nitrate; L. pres. part. reducens, converting to a different state; N.L. part. adj. nitratireducens, reducing nitrate).
The description is as given for the genus, with the following additions. Growth occurred in the temperature range of 20–45°C, with an optimum of 35–40°C. Nitrate can be reduced to nitrite. Positive for tryptophan deaminase. Weak positive for 4-nitrophenyl-β-D-galactopyranoside. Positive for alkaline phosphatase, esterase (C4), esterase lipase (C8), leucine arylamidase, valine arylamidase, acid phosphatase, and β-glucuronidase; weak positive for lipase (C14), naphthol-AS-BI-phosphohydrolase, and α-glucosidase. The hydrolysis of gelatin and aesculin is negative. Cannot use D-glucose, L-arabinose, D-mannose, D-mannitol, N-acetylglucosamine, D-maltose, potassium gluconate, capric acid, adipic acid, malic acid, trisodium citrate, and phenylacetate as sole carbon sources for growth. The genome size is 3.9–4.0 Mbp. The genomic G + C content was 65.6–65.7%. The 16S rRNA gene sequence and the whole genome sequence of strain FR2A1T have been deposited at DDBJ/ENA/GenBank under the accession numbers OR533672 and JAVQHL000000000, respectively.
The type strain is FR2A1T (=MCCC 1K08809T = KCTC 8317T), and another strain is MT2-5-38 (=MCCC 1 K08810), which were isolated from the surface sediment of an oyster farm on a tidal flat in Quanzhou Bay, China.
Description of Thermohalobaculum sediminis comb. nov.
Thermohalobaculum sediminis (se.di’mi.nis. L. gen. n. sediminis, of sediment).
Basonym: Limibaculum sediminis Huang et al. 2022.
The description is as given for Limibaculum sediminis (Huang et al., 2022).
The type strain is FT325T (=MCCC 1K07397T = KCTC 92313T).
Emended description of Pontivivens Park et al. 2015
As the original authors of the genus failed to indicate the gender of the name, and the gender cannot be deduced from the names of its species published thus far, we propose the following emended etymology of the name: Pon.ti.vi’vens. L. masc. n. pontus, the sea; L. pres. part. vivens, living; N.L. neut. n. Pontivivens, an organism living in the sea.
Description of Pontivivens marinum comb. nov.
Pontivivens marinum (ma.ri’num. L. neut. n. marinum, marine, of the sea).
Basonym: Monaibacterium marinum Chernikova et al. 2017.
The description is as given for Monaibacterium marinum (Chernikova et al., 2017).
The type strain is C7T (=DSM 100241T = LMG 28800T).
Description of Pontivivens nitratireducens comb. nov.
Pontivivens nitratireducens (ni.tra.ti.re.du’cens. N.L. masc. n. nitras, nitrate; L. pres. part. reducens, converting to a different state; N.L. part. adj. nitratireducens, reducing nitrate).
Basonym: Pontibrevibacter nitratireducens Liang et al. 2022.
The description is as given for Pontibrevibacter nitratireducens (Liang et al., 2022).
The type strain is h42T (=KCTC 72875T = CGMCC 1.17849T = MCCC 1K04735T). Another strain is ALG8 (=KCTC 82194 = MCCC 1 K04733).
Description of Paramylibacter gen. nov.
Paramylibacter (Par.a.my.li.bac’ter. Gr prep. para, beside; N.L. masc. n. Amylibacter, a bacterial genus; N.L. masc. n. Paramylibacter, beside Amylibacter).
Cells are Gram-stain-negative, strictly aerobic, rod-shaped, and non-motile. Catalase-positive and oxidase-positive. The major respiratory quinone is Q-10. The major fatty acid is C18:1 ω7c. The major polar lipids included phosphatidylglycerol, phosphatidylcholine, and an unidentified aminolipid. Paramylibacter was phylogenetically distinct from Amylibacter marinus. The genomic G + C content was ~49%. The type species is Paramylibacter ulvae.
Description of Paramylibacter ulvae comb. nov.
Paramylibacter ulvae (ul’vae. L. gen. n. ulvae, of Ulva, an algal genus).
Basonym: Amylibacter ulvae Nedashkovskaya et al. 2016.
The description is as given for Amylibacter ulvae (Nedashkovskaya et al., 2016).
The type strain is 6Alg 255T (=KCTC 32465T = KMM 6515T).
Description of Paramylibacter kogurei comb. nov.
Paramylibacter kogurei (ko.gu’re.i. N.L. gen. n. kogurei, of Kogure, to honor the Japanese microbiologist, Kazuhiro Kogure, in recognition of his contribution to the field of marine microbiology).
Basonym: Amylibacter kogurei Wong et al. 2018.
The description is as given for Amylibacter kogurei (Wong et al., 2018).
The type strain is 4G11T (=KY463497T = KCTC 52506T = NBRC 112428T).
Description of Neptunicoccus cionae comb. nov.
Neptunicoccus cionae (ci.o’nae. N.L. gen. fem. n. cionae, of the sea squirt Ciona).
Basonym: Amylibacter cionae Wang et al. 2017.
The description is as given for Amylibacter cionae (Wang et al., 2017).
The type strain is H-12T (=KCTC 52581T = CGMCC 1.15880T).
Description of Halocynthiibacter styelae comb. nov.
Halocynthiibacter styelae (sty.e’lae. N.L. gen. fem. n. styelae, of Styela, named after the generic name of the stalked sea squirt Styela clava, from which the type strain was isolated).
Basonym: Paenihalocynthiibacter styelae Kim et al. 2021.
The description is as given for Paenihalocynthiibacter styelae (Kim et al., 2021).
The type strain is MYP1-1T (=KCTC 82143T = NBRC 114355T).
Description of Falsiroseicyclus gen. nov.
Falsiroseicyclus (Fal.si.ro.se.i.cy’clus. L. masc. perf. part. falsus, false; N.L. masc. n. Roseicyclus, a bacterial genus; N.L. masc. n. Falsiroseicyclus, a false Roseicyclus).
Cells are Gram-stain-negative, ovoid- or rod-shaped, and non-motile. Catalase-positive and oxidase-positive. The major respiratory quinone is Q-10. The major fatty acids (>10%) are summed feature 8 (C18:1 ω7c/C18:1 ω6c), anteiso-C15:0, and iso-C15:0. The major polar lipids included phosphatidylglycerol, phosphatidylcholine, diphosphatidylglycerol, and unidentified lipids. The genomic G + C content was ~69.6%. Falsiroseicyclus formed a separate phylogenetic line with Pseudoroseicyclus. The type species is Falsiroseicyclus tamaricis.
Description of Falsiroseicyclus tamaricis comb. nov.
Falsiroseicyclus tamaricis (ta.ma’ri.cis. L. gen. n. tamaricis, of the tamarix tree).
Basonym: Pseudoroseicyclus tamaricis Gai et al. 2021.
The description is given for Pseudoroseicyclus tamaricis (Gai et al., 2021).
The type strain is CLL3-39T (=MCCC 1A14815T = KCTC 72665T).
Description of Sedimentitalea arenosa comb. nov.
Sedimentitalea arenosa (a.re.no’sa. L. fem. adj. arenosa, sandy, dwelling in sand).
Basonym: Arenibacterium arenosum Jeong et al. 2024.
The description is as given for Arenibacterium arenosum (Jeong et al., 2022).
The type strain is CAU1593T (=KCTC 82402T = MCCC 1K05671T).
Description of Parasedimentitalea huanghaiensis comb. nov.
Parasedimentitalea huanghaiensis (huang.hai.en’sis. N.L. fem. adj. huanghaiensis, pertaining to Huanghai, the Chinese name for the Yellow Sea, the geographical origin of the type strain).
Basonym: Zongyanglinia huanghaiensis Xu et al. 2021.
The description is as given for Zongyanglinia huanghaiensis (Xu et al., 2021).
The type strain is CY05T (=MCCC 1K04409T = KCTC 62200T).
Description of Parasedimentitalea marina comb. nov.
Parasedimentitalea marina (ma.ri’na. L. fem. adj. marina, inhabiting the sea).
Basonym: Pelagicola marinus Choi et al. 2019.
Homotypic synonym: Zongyanglinia marinus (sic; Choi et al., 2019; Xu et al., 2021).
The description is as given for Pelagicola marinus (Choi et al., 2019) and Zongyanglinia marina (Xu et al., 2021).
The type strain is DSW4-44T (=KCTC 62762T = KCCM 43261T = JCM 33637T).
Description of Meridianimarinicoccus zhengii comb. nov.
Meridianimarinicoccus zhengii (zheng’i.i. N.L. gen. n. zhengii, referring to Dr. Tianling Zheng, who contributed to studies of algicidal bacteria).
Basonym: Phycocomes zhengii Zhu et al. 2019.
The description is as given for Phycocomes zhengii (Zhu et al., 2019).
The type strain is LMIT002T (=KCTC 62390T = CICC 24357T).
Description of Meridianimarinicoccus aquatilis comb. nov.
Meridianimarinicoccus aquatilis (a.qua’ti.lis. L. fem. adj. aquatilis, aquatic).
Basonym: Fluviibacterium aquatile Sun et al. 2020.
The description is as given for Fluviibacterium aquatile (Sun et al., 2020).
The type strain is SM1902T (=KCTC 72045T = MCCC 1K03596T = CCTCC AB 2018346T).
Description of Oceaniglobus roseus comb. nov.
Oceaniglobus roseus (ro’se.us. L. masc. n. roseus, pink, rose-colored, rosy).
Basonym: Kandeliimicrobium roseum Wang et al. 2018).
The description is as given for Kandeliimicrobium roseum (Wang et al., 2018).
The type strain is XY-R6T (=MCCC 1K01498T = KCTC 52266T = DSM 104294T).
Description of Roseinatronobacter ekhonensis comb. nov.
Roseinatronobacter ekhonensis (ek.ho.nen’sis. N.L. masc. adj. ekhonensis, pertaining to Ekho Lake, the lake in Antarctica from which the organism was isolated).
Basonym: Roseibaca ekhonensis Labrenz et al. 2009.
The description is as given for Roseibaca ekhonensis (Labrenz et al., 2009).
The type strain is EL-50T (=CECT 7235T = DSM 11469T).
Description of Roseinatronobacter domitianus comb. nov.
Roseinatronobacter domitianus (do.mi.ti.a’nus. L. masc. adj. domitianus, belonging to the Domitian littoral).
Basonym: Roseibaca domitiana Labrenz et al. 2024.
The description is as given for Roseibaca domitiana (Gattoni et al., 2023).
The type strain is V10T (=CECT 30319T = DSM 112951T = LMG 32429T).
Description of Roseinatronobacter bogoriensis comb. nov.
Roseinatronobacter bogoriensis (bo.go.ri.en’sis. N.L. masc. adj. bogoriensis, pertaining to Lake Bogoria, a soda lake in Kenya, Africa).
Basonym: Rhodobaca bogoriensis Milford et al. 2001.
The description is as given for Rhodobaca bogoriensis (Milford et al., 2000).
The type strain is LBB1T (=ATCC 700920T = DSM 18756T). Another strain is alga-05 (=DSM 19920 = VKM B-2406).
Description of Wagnerdoeblera intermedia comb. nov.
Wagnerdoeblera intermedia (in.ter.me’di.a. L. fem. adj. intermedia, in the middle, referring to the fact that the species is grouped between Gemmobacter and Rhodobacter and Roseinatronobacter and Roseibaca on the basis of 16S rRNA gene sequence similarities).
Basonym: Gemmobacter intermedius Kämpfer et al. 2015.
Homotypic synonym: Falsigemmobacter intermedius (Kämpfer et al. 2015) Li et al. 2023.
The description is as given for Gemmobacter intermedius (Kämpfer et al., 2015).
The type strain is 119/4T (=CIP 110795T = LMG 28215T = CCM 8510T = DSM 28642T).
Description of Wagnerdoeblera faecalis comb. nov.
Wagnerdoeblera faecalis (fae.ca’lis. N.L. fem. adj. faecalis, pertaining to feces, fecal).
Basonym: Falsigemmobacter faecalis Li et al. 2023.
The description is as given for Falsigemmobacter faecalis (Li et al., 2020).
The type strain is YIM 102744-1T (=CCTCC AB 2016031T = KCTC 52106T).
Description of Aliitabrizicola gen. nov.
Aliitabrizicola (A.li.i.ta.bri.zi’co.la. L. masc. adj. alius, other; N.L. fem. n. Tabrizicola, a bacterial genus; N.L. fem. n. Aliitabrizicola, another Tabrizicola).
Cells are Gram-stain-negative, rod-shaped, and non-motile. Catalase-positive and oxidase-positive. The major respiratory quinone is Q-10. The major fatty acid (>10%) is C18:1 ω7c. The major polar lipids include diphosphatidylglycerol, phosphatidylglycerol, phosphorylethanolamine, and unidentified lipids. Aliitabrizicola formed a separate phylogenetic line with Tabrizicola. The type species is Allitabrizicola rongguiensis.
Description of Allitabrizicola rongguiensis comb. nov.
Allitabrizicola rongguiensis (rong.gui.en’sis. N.L. fem. adj. rongguiensis, pertaining to the Ronggui river).
Basonym: Tabrizicola rongguiensis Xu et al. 2022.
The description is as given for Tabrizicola rongguiensis (Xu et al., 2022).
The type strain is J26T (=GDMCC 1.2843T = KCTC 92112T).
Description of Falsirhodobacter flavus comb. nov.
Falsirhodobacter flavus (fla’vus. L. masc. adj. flavus, yellow).
Basonym: Cereibacter flavus Liu et al. 2023.
The description is as given for Cereibacter flavus (Liu W. L. et al., 2023).
The type strain is SYSU M79828T (=GDMCC 1.3803T = KCTC 92893T).
Description of Falsirhodobacter xinxiangensis comb. nov.
Falsirhodobacter xinxiangensis (xin.xiang.en’sis. N.L. masc. adj. xinxiangensis, referring to Xinxiang in the Henan province, China, the area from where the type strain was isolated).
Basonym: Rhodobacter xinxiangensis Han et al. 2024.
The description is as given for Rhodobacter xinxiangensis (Han et al., 2020).
The type strain is TJ48T (=CCTCC AB 2019120T = KCTC 72510T).
Description of Aliigemmobacter gen. nov.
Aliigemmobacter (A.li.i.gem.mo.bac’ter. L. masc. adj. alius, other; N.L. masc. n. Gemmobacter, a bacterial genus; N.L. masc. n. Aliigemmobacter, another Gemmobacter).
Cells are Gram-stain-negative and rod-shaped. Catalase-positive and oxidase-positive. The major quinone is Q-10. The major fatty acids are summed feature 8 (C18:1 ω7c and/or C18:1 ω6c), C18:0, and C18:1 ω7c 11-methyl. The major polar lipids included phosphatidylethanolamine, phosphatidylmonomethylethanolamine, phosphatidylglycerol, phosphatidylcholine, and an unidentified aminolipid. Aliigemmobacter formed a separate phylogenetic line with Gemmobacter. The type species is Aliigemmobacter aestuarii.
Description of Aliigemmobacter aestuarii comb. nov.
Aliigemmobacter aestuarii (aes.tu.a’ri.i. L. gen. n. aestuarii, of the shallow coast, from where the type strain was isolated).
Basonym: Gemmobacter aestuarii Hameed et al. 2020.
The description is as given for Gemmobacter aestuarii (Hameed et al., 2020).
The type strain is CC-PW-75T (=JCM 19754T = BCRC 80759T).
Description of Neogemmobacter gen. nov.
Neogemmobacter (Ne.o.gem.mo.bac’ter. Gr. masc. adj. neos, new; N.L. masc. n. Gemmobacter, a bacterial genus; N.L. masc. n. Neogemmobacter, a new Gemmobacter).
Cells are Gram-stain-negative, aerobic, non-motile, and rod-shaped. Catalase-positive and oxidase-positive. Cells can produce poly-β-hydroxybutyrate. The major quinone is Q-10. The major fatty acids are C18:1 ω7c. The major polar lipids included phosphatidylglycerol, phosphatidylethanolamine, phosphatidylcholine, and unidentified amino lipids. Neogemmobacter formed a separate phylogenetic line with Gemmobacter. The type species is Neogemmobacter tilapiae.
Description of Neogemmobacter tilapiae comb. nov.
Neogemmobacter tilapiae (ti.la’pi.ae. L. gen. n. tilapiae, of Tilapia, the common name of tilapiine cichlid fish, referring to the isolation of the type strain from a pond for rearing Tilapia fish).
Basonym: Gemmobacter tilapiae Sheu et al. 2013.
The description is as given for Gemmobacter tilapiae (Sheu et al., 2013).
The type strain is Ruye-53T (=BCRC 80261T = KCTC 23310T).
Description of Paragemmobacter gen. nov.
Paragemmobacter (Pa.ra.gem.mo.bac’ter. Gr. prep. para, beside; N.L. masc. n. Gemmobacter, a bacterial genus; N.L. masc. n. Paragemmobacter, beside Gemmobacter).
Cells are Gram-stain-negative, facultatively anaerobic, non-motile, and rod-shaped. Catalase-positive and oxidase-positive. The major quinone is Q-10. The major fatty acids are summed feature 8 (C18:1 ω7c/C18:1 ω6c). The major polar lipids included phosphatidylethanolamine, phosphatidylglycerol, phosphatidylcholine, unidentified glycolipids, and unidentified amino phospholipids. Paragemmobacter formed a separate phylogenetic line with Gemmobacter. The genomic size was 3.6–4.7 Mbp. The genomic G + C content was 61.7–66.6%. The type species is Paragemmobacter straminiformis.
Description of Paragemmobacter straminiformis comb. nov.
Paragemmobacter straminiformis (stra.mi.ni.for’mis. L. neut. n. stramen, straw; L. masc./fem. adj. suff. -formis, form; N.L. masc. adj. straminiformis, resembling straw).
Basonym: Gemmobacter straminiformis Kang et al. 2017.
The description is as given for Gemmobacter straminiformis (Kang et al., 2017).
The type strain is CAM-8T (=KACC 19224T = JCM 31905T).
Description of Paragemmobacter ruber comb. nov.
Paragemmobacter ruber (ru’ber. L. masc. adj. ruber, red).
Basonym: Rhodobacter ruber Chen et al. 2021.
The description is as given for Rhodobacter ruber (Chen et al., 2021).
The type strain is CCP-1T (=BCRC 81189T = LMG 31335T).
Description of Paragemmobacter amnigenus comb. nov.
Paragemmobacter amnigenus (am.ni’ge.nus. N.L. masc. adj. amnigenus, born in a stream, intended to mean coming from water).
Basonym: Rhodobacter amnigenus Chen et al. 2021.
The description is as given for Rhodobacter amnigenus (Chen et al., 2021).
The type strain is HSP-20T (=BCRC = BCRC 81193T = LMG 31334T).
Description of Paragemmobacter kunshanensis comb. nov.
Paragemmobacter kunshanensis (kun.shan.en’sis. N.L. masc. adj. kunshanensis, of or pertaining to Kunshan city, Jiangsu province, China, from where the type strain was isolated).
Basonym: Rhodobacter kunshanensis Liu et al. 2024.
The description is as given for Rhodobacter kunshanensis (Liu et al., 2021).
The type strain is HX-7-19T (=KCTC 72471T = CCTCC AB 2020148T).
Description of Paragemmobacter aquarius comb. nov.
Paragemmobacter aquarius (a.qua’rius. L. masc. adj. aquarius, of or relating to water).
Basonym: Gemmobacter aquarius Baek et al. 2020.
The description is as given for Gemmobacter aquarius (Baek et al., 2020).
The type strain is HYN0069T = KACC 19488T = NBRC 113115T).
Description of Pseudogemmobacter blasticus comb. nov.
Pseudogemmobacter blasticus (blas’ti.cus. Gr. masc. adj. blastikos, budding, sprouting; N.L. masc. adj. blasticus, budding, apt to bud).
Basonym: Rhodopseudomonas blastica Eckersley and Dow 1981.
Homotypic synonym: Fuscovulum blasticum (Eckersley and Dow 1981) Suresh et al. 2020.
The description is as given for Fuscovulum blasticum (Suresh et al., 2019).
The type strain is NCIB 11576T (=ATCC 33485T = NBRC 16437T).
Description of Pseudothioclava nitratireducens comb. nov.
Pseudothioclava nitratireducens (ni.tra.ti.re.du’cens. N.L. masc. n. nitras, nitrate; L. pres. part. reducens, converting to a different state; N.L. part. adj. nitratireducens, reducing nitrate).
Basonym: Defluviimonas nitratireducens Liu et al. 2017.
The description is as given for Defluviimonas nitratireducens (Liu et al., 2017b).
The type strain is DL5-4T (=MCCC 1A06955T = LMG 29616T).
Description of Paenirhodobacter ferrireducens comb. nov.
Paenirhodobacter ferrireducens (fer.ri.re.du’cens. L. neut. n. ferrum, iron; L. pres. part. reducens, reducing; N.L. part. adj. ferrireducens, reducing iron).
Basonym: Sinirhodobacter ferrireducens corrig. Yang et al. 2018.
The description is as given for Sinorhodobacter ferrireducens (Yang et al., 2013).
The type strain is SgZ-3T (=KACC 16603T = CCTCC AB 2012026T).
Description of Paenirhodobacter huangdaonensis comb. nov.
Paenirhodobacter huangdaonensis (huang.dao.nen’sis. N.L. masc. adj. huangdaonensis, of Huangdao, a district of Qingdao city in Shandong province, PR China, where the type strain was first isolated).
Basonym: Sinirhodobacter huangdaonensis corrig Xi et al. 2019.
The description is as given for Sinorhodobacter huangdaonensis (Xi et al., 2017).
The type strain is L3T (=CGMCC 1.12963T = KCTC 42823T).
Description of Paenirhodobacter populi comb. nov.
Paenirhodobacter populi (po’pu.li. L. gen. n. populi, of the poplar tree, of the genus Populus).
Basonym: Sinorhodobacter populi Xu et al. 2019.
The description is as given for Sinorhodobacter populi (Xu et al., 2019).
The type strain is sk2b1T (=CFCC 14580T = KCTC 52802T).
Description of Paenirhodobacter hankyongi comb. nov.
Paenirhodobacter hankyongi (hank.yong’i. N.L. gen. n. hankyongi, of Hankyong National University, where the strain was identified).
Basonym: Sinirhodobacter hankyongi Lee et al. 2020.
The description is as given for Sinirhodobacter hankyongi (Lee et al., 2020).
The type strain is BO-81T (=KACC 19677T = LMG 30808T).
Data availability statement
The data presented in the study are deposited in the online repository, accession numbers OR533672, JAVQHL000000000 and JAVQHM000000000 at https://www.ncbi.nlm.nih.gov.
Author contributions
ZH: Writing – original draft, Writing – review & editing. ML: Writing – original draft. AO: Writing – review & editing. QL: Writing – review & editing.
Funding
The author(s) declare that financial support was received for the research, authorship, and/or publication of this article. This study was supported by the Quanzhou City Science and Technology Program of China (2022C018R) and the National Science and Technology Fundamental Resources Investigation Program of China (2021FY100900).
Conflict of interest
The authors declare that the research was conducted in the absence of any commercial or financial relationships that could be construed as a potential conflict of interest.
Publisher’s note
All claims expressed in this article are solely those of the authors and do not necessarily represent those of their affiliated organizations, or those of the publisher, the editors and the reviewers. Any product that may be evaluated in this article, or claim that may be made by its manufacturer, is not guaranteed or endorsed by the publisher.
Supplementary material
The Supplementary material for this article can be found online at: https://www.frontiersin.org/articles/10.3389/fmicb.2024.1376777/full#supplementary-material
Abbreviations
MCCC, Marine Culture Collection of China; KCTC, Korean Collection for Type Cultures; DDH, DNA-DNA hybridization; ANI, Average nucleotide identity; AAI, Average amino acid identity.
Footnotes
1. ^https://lpsn.dsmz.de/family/paracoccaceae
2. ^https://blast.ncbi.nlm.nih.gov/Blast.cgi
3. ^https://github.com/najoshi/sickle
4. ^https://www.genome.jp/tools/kaas/
6. ^https://github.com/dparks1134/CompareM
7. ^https://www.ncbi.nlm.nih.gov/genome/browse#!/prokaryotes/Paracoccaceae
References
Aziz, R. K., Bartels, D., Best, A. A., Dejongh, M., Disz, T., Edwards, R. A., et al. (2008). The RAST server: rapid annotations using subsystems technology. BMC Genomics 9:75. doi: 10.1186/1471-2164-9-75
Baek, M. G., Shin, S. K., and Yi, H. (2020). Gemmobacter aquarius sp. nov., Runella rosea sp. nov. and Flavobacterium fluviale sp. nov., isolated from the Namhangang River system. Int. J. Syst. Evol. Microbiol. 70, 5640–5647. doi: 10.1099/ijsem.0.004455
Bankevich, A., Nurk, S., Antipov, D., Gurevich, A. A., Dvorkin, M., Kulikov, A. S., et al. (2012). SPAdes: a new genome assembly algorithm and its applications to single-cell sequencing. J. Comput. Biol. 19, 455–477. doi: 10.1089/cmb.2012.0021
Besemer, J., Lomsadze, A., and Borodovsky, M. (2001). GeneMarkS: a self-training method for prediction of gene starts in microbial genomes. Implications for finding sequence motifs in regulatory regions. Nucleic Acids Res. 29, 2607–2618. doi: 10.1093/nar/29.12.2607
Camacho, C., Coulouris, G., Avagyan, V., Ma, N., Papadopoulos, J., Bealer, K., et al. (2009). BLAST+: architecture and applications. BMC Bioinformatics 10:421. doi: 10.1186/1471-2105-10-421
Chaumeil, P. A., Mussig, A. J., Hugenholtz, P., and Parks, D. H. (2019). GTDB-Tk: a toolkit to classify genomes with the genome taxonomy database. Bioinformatics 36, 1925–1927. doi: 10.1093/bioinformatics/btz848
Chen, W. M., Chang, T. H., Yang, C. C., Sheu, D. S., Jheng, L. C., and Sheu, S. Y. (2021). Rhodobacter amnigenus sp. nov. and Rhodobacter ruber sp. nov., isolated from freshwater habitats. Int. J. Syst. Evol. Microbiol. 71:005150. doi: 10.1099/ijsem.0.005150
Chernikova, T. N., Dallimore, J., Lunsdorf, H., Heipieper, H. J., and Golyshin, P. N. (2017). Monaibacterium marinum gen. nov, sp. nov, a new member of the Alphaproteobacteria isolated from seawater of Menai Straits, Wales, UK. Int. J. Syst. Evol. Microbiol. 67, 3310–3317. doi: 10.1099/ijsem.0.002111
Choi, Y. S., Oh, J. S., and Roh, D. H. (2019). Pelagicola marinus sp. nov. isolated from deep-sea water. Int. J. Syst. Evol. Microbiol. 69, 3961–3966. doi: 10.1099/ijsem.0.003764
Chu, C., Liu, B., Lian, Z., Zheng, H., Chen, C., Yue, Z., et al. (2020). Solirhodobacter olei gen. nov., sp. nov., a nonphotosynthetic bacterium isolated from oil-contaminated soil. Int. J. Syst. Evol. Microbiol. 70, 582–588. doi: 10.1099/ijsem.0.003795
Delong, E. F. (1992). Archaea in coastal marine environments. Proc. Natl. Acad. Sci. USA 89, 5685–5689. doi: 10.1073/pnas.89.12.5685
Gai, Y., Yang, Y., Liu, X., Li, G., Wang, S., Lai, Q., et al. (2021). Pseudoroseicyclus tamaricis sp. nov., isolated from seashore sediment of a Tamarix chinensis forest and emended descriptions of the genus Pseudoroseicyclus Park et al. 2016. Int. J. Syst. Evol. Microbiol. 71:004908. doi: 10.1099/ijsem.0.004908
Gattoni, G., Di Costanzo, F., De La Haba, R. R., Fernández, A. B., Guerrero-Flores, S., Selem-Mojica, N., et al. (2023). Biosynthetic gene profiling and genomic potential of the novel photosynthetic marine bacterium Roseibaca domitiana. Front. Microbiol. 14:1238779. doi: 10.3389/fmicb.2023.1238779
Göker, M. (2022). Filling the gaps: missing taxon names at the ranks of class, order and family. Int. J. Syst. Evol. Microbiol. 72:005368. doi: 10.1099/ijsem.0.005638
Hameed, A., Shahina, M., Lin, S. Y., Chen, W.-M., Hsu, Y.-H., Lai, W.-A., et al. (2020). Description of Gemmobacter aestuarii sp. nov., isolated from estuarine surface water and reclassification of Cereibacter changlensis as Gemmobacter changlensis Chen et al. 2013. Arch. Microbiol. 202, 1035–1042. doi: 10.1007/s00203-020-01809-y
Han, H., Wang, T., Chen, Z., Li, Y., and Yao, L. (2020). Rhodobacter xinxiangensis sp. nov., isolated from pakchoi-cultivated soil contaminated with heavy metal and its potential to reduce cd and Pb accumulation in pakchoi (Brassica campestris L.). Arch. Microbiol. 202, 1741–1748. doi: 10.1007/s00203-020-01885-0
Hördt, A., López, M. G., Meier-Kolthoff, J. P., Schleuning, M., Weinhold, L. M., Tindall, B. J., et al. (2020). Analysis of 1,000+ type-strain genomes substantially improves taxonomic classification of Alphaproteobacteria. Front. Microbiol. 11:468. doi: 10.3389/fmicb.2020.00468
Huang, Y., Liu, L., Li, J., Pan, J., and Li, M. (2022). Limibaculum sediminis sp. nov., isolated from mangrove sediment. Int. J. Syst. Evol. Microbiol. 72:005580. doi: 10.1099/ijsem.0.005580
Huang, Z., Mo, S., Yan, L., Wei, X., Huang, Y., Zhang, L., et al. (2021). A simple culture method enhances the recovery of culturable actinobacteria from coastal sediments. Front. Microbiol. 12:675048. doi: 10.3389/fmicb.2021.675048
Imhoff, J. F., Trüper, H. G., and Pfennig, N. (1984). Rearrangement of the species and genera of the phototrophic "purple nonsulfur bacteria". Int. J. Syst. Bacteriol. 34, 340–343. doi: 10.1099/00207713-34-3-340
Jeong, J., Weerawongwiwat, V., Kim, J.-H., Suh, M.-K., Kim, H.-S., Lee, J.-S., et al. (2022). Arenibacterium arenosum sp. nov., isolated from sea sand. Arch. Microbiol. 204:147. doi: 10.1007/s00203-022-02760-w
Kämpfer, P., Jerzak, L., Wilharm, G., Golke, J., Busse, H. J., and Glaeser, S. P. (2015). Gemmobacter intermedius sp. nov., isolated from a white stork (Ciconia ciconia). Int. J. Syst. Evol. Microbiol. 65, 778–783. doi: 10.1099/ijs.0.000012
Kang, J. Y., Kim, M. J., Chun, J., Son, K. P., and Jahng, K. Y. (2017). Gemmobacter straminiformis sp. nov., isolated from an artificial fountain. Int. J. Syst. Evol. Microbiol. 67, 5019–5025. doi: 10.1099/ijsem.0.002403
Kim, Y. J., Lee, S. D., and Lee, S. D. (2019). Hahyoungchilella caricis gen. nov., sp. nov., isolated from a rhizosphere mudflat of a halophyte (Carex scabrifolia), transfer of Thioclava arenosa Thongphrom et al. 2017 to Pseudothioclava as Pseudothioclava arenosa gen. nov., comb. nov. and proposal of Thioclava electrotropha Chang et al. 2018 as a later heterosynonym of Thioclava sediminum. J. Microbiol. 57, 1048–1055. doi: 10.1007/s12275-019-9260-y
Kim, Y. O., Noh, J. K., Kim, D. G., Park, I. S., Park, S., and Yoon, J. H. (2021). Paenihalocynthiibacter styelae gen. nov., sp. nov., isolated from stalked sea squirt Styela clava. Int. J. Syst. Evol. Microbiol. 71, 71:005085. doi: 10.1099/ijsem.0.005085
Komagata, K., and Suzuki, K.-I. (1987). Lipid and cell-wall analysis in bacterial systematics. Meth. Microbiol. 19, 161–207. doi: 10.1016/S0580-9517(08)70410-0
Kumar, S., Stecher, G., and Tamura, K. (2016). MEGA7: molecular evolutionary genetics analysis version 7.0 for bigger datasets. Mol. Biol. Evol. 33, 1870–1874. doi: 10.1093/molbev/msw054
Labrenz, M., Lawson, P. A., Tindall, B. J., and Hirsch, P. (2009). Roseibaca ekhonensis gen. nov., sp. nov., an alkalitolerant and aerobic bacteriochlorophyll a-producing alphaproteobacterium from hypersaline Ekho Lake. Int. J. Syst. Evol. Microbiol. 59, 1935–1940. doi: 10.1099/ijs.0.016717-0
Lagesen, K., Hallin, P., Rodland, E. A., Staerfeldt, H. H., Rognes, T., and Ussery, D. W. (2007). RNAmmer: consistent and rapid annotation of ribosomal RNA genes. Nucleic Acids Res. 35, 3100–3108. doi: 10.1093/nar/gkm160
Lanoot, B., Vancanneyt, M., Dawyndt, P., Cnockaert, M., Zhang, J., Huang, Y., et al. (2004). BOX-PCR fingerprinting as a powerful tool to reveal synonymous names in the genus Streptomyces. Emended descriptions are proposed for the species Streptomyces cinereorectus, S. fradiae, S. tricolor, S. colombiensis, S. filamentosus, S. vinaceus and S. phaeopurpureus. Syst. Appl. Microbiol. 27, 84–92. doi: 10.1078/0723-2020-00257
Lee, Y. W., Hoang, T. S., Rhee, M. S., Lee, S. Y., and Im, W. T. (2020). Sinirhodobacter hankyongi sp. nov., a novel denitrifying bacterium isolated from sludge. Int. J. Syst. Evol. Microbiol. 70, 668–674. doi: 10.1099/ijsem.0.003814
Letunic, I., and Bork, P. (2007). Interactive tree of life (iTOL): an online tool for phylogenetic tree display and annotation. Bioinformatics 23, 127–128. doi: 10.1093/bioinformatics/btl529
Li, G., Jiang, Y., Li, Q., Chen, X., Jiang, L., Zhang, K., et al. (2020). Falsigemmobacter faecalis gen. nov. sp. nov., isolated from faeces of Rhinopithecus roxellanae, and reclassification of Gemmobacter intermedius as Falsigemmobacter intermedius comb. nov. Arch. Microbiol. 202, 2599–2606. doi: 10.1007/s00203-020-01978-w
Liang, K. Y. H., Orata, F. D., Boucher, Y. F., and Case, R. J. (2021). Roseobacters in a sea of poly- and paraphyly: whole genome-based taxonomy of the family Rhodobacteraceae and the proposal for the split of the "Roseobacter clade" into a novel family Roseobacteraceae fam. nov. Front. Microbiol. 12:683109. doi: 10.3389/fmicb.2021.683109
Liang, H. Y., Sun, C., Xu, L., Xu, X. W., Cheng, H., and Wan, Y. (2022). Pontibrevibacter nitratireducens gen. nov., sp. nov., a member of the family Rhodobacteraceae isolated from seawater of the Indian Ocean and intertidal zone. Int. J. Syst. Evol. Microbiol. 72:005341. doi: 10.1099/ijsem.0.005341
Liu, J., Bao, Y., Zhang, X., Xu, S., Qiu, J., and He, J. (2021). Rhodobacter kunshanensis sp. nov., a novel bacterium isolated from activated sludge. Curr. Microbiol. 78, 3791–3797. doi: 10.1007/s00284-021-02628-0
Liu, M., Huang, Z., Zhao, Q., and Shao, Z. (2019). Cohaesibacter intestini sp. nov., isolated from the intestine of abalone, Haliotis discus hannai. Int. J. Syst. Evol. Microbiol. 69, 3202–3206. doi: 10.1099/ijsem.0.003610
Liu, Y., Lai, Q., and Shao, Z. (2017a). Thioclava nitratireducens sp. nov., isolated from surface seawater. Int. J. Syst. Evol. Microbiol. 67, 2109–2113. doi: 10.1099/ijsem.0.001844
Liu, Y., Lai, Q., Wang, W., and Shao, Z. (2017b). Defluviimonas nitratireducens sp. nov., isolated from surface seawater. Int. J. Syst. Evol. Microbiol. 67, 2752–2757. doi: 10.1099/ijsem.0.002015
Liu, L., Li, J., Huang, Y., Pan, J., and Li, M. (2023). Defluviimonas sediminis sp. nov., isolated from mangrove sediment. Int. J. Syst. Evol. Microbiol. 73, 1–9. doi: 10.1099/ijsem.0.005975
Liu, W. L., Yang, Z. W., Guo, D. Y., Deng, Q. Q., Li, J. L., Wang, P. D., et al. (2023). Cereibacter flavus sp. nov., a novel member of the family Rhodobacteraceae isolated from seawater of the South China Sea and reclassification of Rhodobacter alkalitolerans as Cereibacter alkalitolerans comb. nov. Int. J. Syst. Evol. Microbiol. 73:006051. doi: 10.1099/ijsem.0.006051
Milford, A. D., Achenbach, L. A., Jung, D. O., and Madigan, M. T. (2000). Rhodobaca bogoriensis gen. nov. and sp. nov., an alkaliphilic purple nonsulfur bacterium from African Rift Valley soda lakes. Arch. Microbiol. 174, 18–27. doi: 10.1007/s002030000166
Mu, L., Zhang, X., Zhang, Y., Fang, Z., and Xiao, Y. (2022). Sedimentimonas flavescens gen. nov., sp. nov., isolated from sediment of Clam Island. Liaoning Province. Antonie van Leeuwenhoek 115, 979–994. doi: 10.1007/s10482-022-01754-4
Nedashkovskaya, O. I., Kukhlevskiy, A. D., Zhukova, N. V., and Kim, S. B. (2016). Amylibacter ulvae sp. nov., a new alphaproteobacterium isolated from the Pacific green alga Ulva fenestrata. Arch. Microbiol. 198, 251–256. doi: 10.1007/s00203-015-1185-1
Oren, A., and Garrity, G. M. (2018). List of new names and new combinations previously effectively, but not validly, published. Int. J. Syst. Evol. Microbiol. 68, 2707–2709. doi: 10.1099/ijsem.0.002945
Oren, A., and Garrity, G. M. (2020a). List of new names and new combinations previously effectively, but not validly, published. Int. J. Syst. Evol. Microbiol. 70, 4043–4049. doi: 10.1099/ijsem.0.004244
Oren, A., and Garrity, G. M. (2020b). List of new names and new combinations previously effectively, but not validly, published. Int. J. Syst. Evol. Microbiol. 70, 2960–2966. doi: 10.1099/ijsem.0.004156
Pan, X., Li, Z., Li, F., Huang, Y., Wang, Q., Huang, S., et al. (2021). Thermohalobaculum xanthum gen. nov., sp. nov., a moderately thermophilic bacterium isolated from mangrove sediment. Antonie van Leeuwenhoek 114, 1819–1828. doi: 10.1007/s10482-021-01641-4
Park, S., Jung, Y.-T., and Yoon, H. (2016). Pseudoroseicyclus aestuarii gen. nov., sp. nov., isolated from a tidal flat. Int. J. Syst. Evol. Microbiol. 66, 2165–2171. doi: 10.1099/ijsem.0.001003
Parks, D. H., Imelfort, M., Skennerton, C. T., Hugenholtz, P., and Tyson, G. W. (2015). CheckM: assessing the quality of microbial genomes recovered from isolates, single cells, and metagenomes. Genome Res. 25, 1043–1055. doi: 10.1101/gr.186072.114
Parte, A. C., Sardà Carbasse, J., Meier-Kolthoff, J. P., Reimer, L. C., and Göker, M. (2020). List of Prokaryotic names with Standing in Nomenclature (LPSN) moves to the DSMZ. Int. J. Syst. Evol. Microbiol. 70, 5607–5612. doi: 10.1099/ijsem.0.004332
Ren, H., Ma, H., Li, H., Huang, L., and Luo, Y. (2019). Acidimangrovimonas sediminis gen. nov., sp. nov., isolated from mangrove sediment and reclassification of Defluviimonas indica as Acidimangrovimonas indica comb. nov. and Defluviimonas pyrenivorans as Acidimangrovimonas pyrenivorans comb. nov. Int. J. Syst. Evol. Microbiol. 69, 2445–2451. doi: 10.1099/ijsem.0.003513
Sheu, S. Y., Sheu, D. S., Sheu, F. S., and Chen, W. M. (2013). Gemmobacter tilapiae sp. nov., a poly-β-hydroxybutyrate-accumulating bacterium isolated from a freshwater pond. Int. J. Syst. Evol. Microbiol. 63, 1550–1556. doi: 10.1099/ijs.0.044735-0
Simon, M., Scheuner, C., Meier-Kolthoff, J. P., Brinkhoff, T., Wagner-Döbler, I., Ulbrich, M., et al. (2017). Phylogenomics of Rhodobacteraceae reveals evolutionary adaptation to marine and non-marine habitats. ISME J. 11, 1483–1499. doi: 10.1038/ismej.2016.198
Sun, Y., Dang, R., He, X., Wang, J., Liu, N., Sun, M., et al. (2020). Fluviibacterium aquatile gen. nov., sp. nov., isolated from estuary sediment. Int. J. Syst. Evol. Microbiol. 70, 105–111. doi: 10.1099/ijsem.0.003722
Suresh, G., Lodha, T. D., Indu, B., Sasikala, C., and Ramana, C. V. (2019). Taxogenomics resolves conflict in the genus Rhodobacter: a two and half decades pending thought to reclassify the genus Rhodobacter. Front. Microbiol. 10:2480. doi: 10.3389/fmicb.2019.02480
Wang, D., Liu, H., Zheng, S., and Wang, G. (2014). Paenirhodobacter enshiensis gen. nov., sp. nov., a non-photosynthetic bacterium isolated from soil, and emended descriptions of the genera Rhodobacter and Haematobacter. Int. J. Syst. Evol. Microbiol. 64, 551–558. doi: 10.1099/ijs.0.050351-0
Wang, K.-L., Song, Z.-M., Rong, C.-H., Hao, L.-Y., Lai, Q.-L., Li, S.-F., et al. (2018). Kandeliimicrobium roseum gen. nov., sp. nov., a new member of the family Rhodobacteraceae isolated from mangrove rhizosphere soil. Int. J. Syst. Evol. Microbiol. 68, 2158–2164. doi: 10.1099/ijsem.0.002773
Wang, D., Wei, Y., Cui, Q., and Li, W. (2017). Amylibacter cionae sp. nov., isolated from the sea squirt Ciona savignyi. Int. J. Syst. Evol. Microbiol. 67, 3462–3466. doi: 10.1099/ijsem.0.002140
Wong, S.-K., Yoshizawa, S., Nakajima, Y., Cuadra, M. J., Nogi, Y., Nakamura, K., et al. (2018). Amylibacter kogurei sp. nov., a novel marine alphaproteobacterium isolated from the coastal sea surface microlayer of a marine inlet. Int. J. Syst. Evol. Microbiol. 68, 2872–2877. doi: 10.1099/ijsem.0.002911
Xi, L., Qiao, N., Zhang, Z., Yan, L., Li, F., Hu, J., et al. (2017). Sinorhodobacter hungdaonensis sp. nov. isolated from activated sludge collected from a municipal wastewater treatment plant. Antonie van Leeuwenhoek 110, 27–32. doi: 10.1007/s10482-016-0770-x
Xu, J., Deng, T., Huang, Y., Dong, M., Yang, S., and Xu, M. (2022). Tabrizicola rongguiensis sp. nov., isolated from the sediment of a river in Ronggui, Foshan city, China. Int. J. Syst. Evol. Microbiol. 72:005539. doi: 10.1099/ijsem.0.005539
Xu, L., Liu, A., and Zhang, Y.-J. (2021). Zongyanglinia huanghaiensis gen. nov., sp. nov., a novel denitrifying bacterium isolated from the Yellow Sea, and transfer of Pelagicola marinus to Zongyanglinia gen. nov. as Zongyanglinia marinus comb. nov. Antonie van Leeuwenhoek 114, 137–149. doi: 10.1007/s10482-020-01507-1
Xu, G., Piao, C., Chang, J., Guo, L., Yang, X., and Li, Y. (2019). Sinorhodobacter populi sp. nov., isolated from the symptomatic bark tissue of Populus x euramericana canker. Int. J. Syst. Evol. Microbiol. 69, 1220–1224. doi: 10.1099/ijsem.0.003300
Yang, G., Chen, M., Zhou, S., Liu, Z., and Yuan, Y. (2013). Sinorhodobacter ferrireducens gen. nov., sp. nov., a non-phototrophic iron-reducing bacterium closely related to phototrophic Rhodobacter species. Antonie van Leeuwenhoek 104, 715–724. doi: 10.1007/s10482-013-9979-0
Yoon, S.-H., Ha, S. M., Kwon, S., Lim, J., Kim, Y., Seo, H., et al. (2017a). Introducing EzBioCloud: a taxonomically united database of 16S rRNA and whole genome assemblies. Int. J. Syst. Evol. Microbiol. 67, 1613–1617. doi: 10.1099/ijsem.0.001755
Yoon, S.-H., Ha, S. M., Lim, J., Kwon, S., and Chun, J. (2017b). A large-scale evaluation of algorithms to calculate average nucleotide identity. Antonie van Leeuwenhoek 110, 1281–1286. doi: 10.1007/s10482-017-0844-4
Zhang, D., He, W., Shao, Z., Ahmed, I., Zhang, Y., Li, W., et al. (2023). Phylotaxonomic assessment based on four core gene sets and proposal of a genus definition among the families Paracoccaceae and Roseobacteraceae. Int. J. Syst. Evol. Microbiol. 73, 1–13. doi: 10.1099/ijsem.0.006156
Zhang, Y., Liu, X. F., Kuang, B., Zhang, X., Zhou, M., and Chen, S. (2018). Neptunicoccus sediminis gen. nov., sp. nov., a member of the family Rhodobacteraceae isolated from the Yellow Sea. Int. J. Syst. Evol. Microbiol. 68, 1702–1706. doi: 10.1099/ijsem.0.002728
Keywords: Ostreiculturibacter, polyphasic taxonomy, Paracoccaceae, “Rhodobacteraceae”, phylogenomic tree
Citation: Huang Z, Li M, Oren A and Lai Q (2024) Genome-based analysis of the family Paracoccaceae and description of Ostreiculturibacter nitratireducens gen. nov., sp. nov., isolated from an oyster farm on a tidal flat. Front. Microbiol. 15:1376777. doi: 10.3389/fmicb.2024.1376777
Edited by:
Marcelino T. Suzuki, Sorbonne Unversite, FranceReviewed by:
Dhiraj Kumar Chaudhary, Korea University, Republic of KoreaChongqing Wen, Guangdong Ocean University, China
Copyright © 2024 Huang, Li, Oren and Lai. This is an open-access article distributed under the terms of the Creative Commons Attribution License (CC BY). The use, distribution or reproduction in other forums is permitted, provided the original author(s) and the copyright owner(s) are credited and that the original publication in this journal is cited, in accordance with accepted academic practice. No use, distribution or reproduction is permitted which does not comply with these terms.
*Correspondence: Zhaobin Huang, emJodWFuZ2VtYWlsQGdtYWlsLmNvbQ==