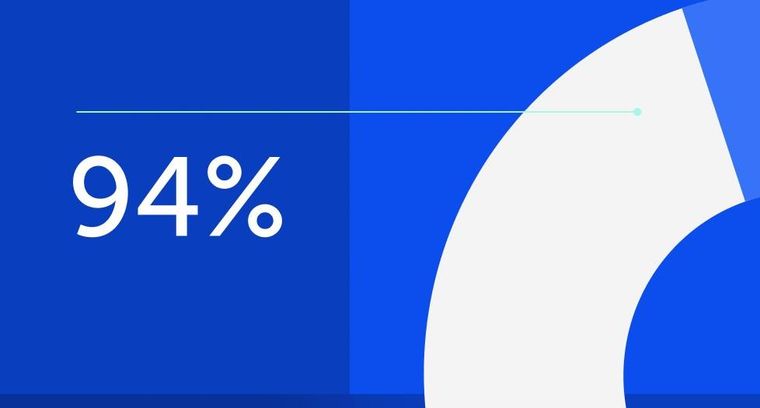
94% of researchers rate our articles as excellent or good
Learn more about the work of our research integrity team to safeguard the quality of each article we publish.
Find out more
ORIGINAL RESEARCH article
Front. Microbiol., 27 February 2024
Sec. Infectious Agents and Disease
Volume 15 - 2024 | https://doi.org/10.3389/fmicb.2024.1375787
The use of mammalian models for in vivo testing of bacterial virulence raises ethical concerns and is expensive and time-consuming. As an alternative, non-mammalian models are sought. Galleria mellonella larvae have been used as a model to study several bacterial pathogens. However, their maintenance is challenging, and commercial supply is low. In this study, we aimed to establish the Zophobas morio larvae as an alternative non-mammalian model for the evaluation of the pathogenicity and antimicrobial susceptibility of Acinetobacter baumannii. We infected Z. morio with Acinetobacter strains and determined the optimal temperature and inoculum. To visualize the bacterial distribution within the larvae, hematoxylin and eosin (H&E) staining was performed. Next, a survival model of infected larvae was established, and virulence was compared between strains. The effect of antimicrobial treatment in relation to antibiotic susceptibility was studied. Our results demonstrate that Z. morio can be used as a model system for in vivo studies of A. baumannii.
The availability of in vivo infection models is of critical importance to infectious disease research (Rong and Liu, 2023). Historically, rodent models served as the primary experimental platform for studying host–pathogen interactions and virulence and for evaluating potential therapeutics due to their genetic, physiological, and immunological similarities to humans. However, the use of rodents raises significant ethical concerns related to animal welfare. To address these concerns, researchers are urged to prioritize humane practices, minimizing pain, distress, and discomfort. Ethical guidelines highlight the importance of “replacement,” advocating for alternative models, such as invertebrate and in vitro systems, to reduce reliance on rodent models.
Apart from ethical concerns, rodent models are often expensive, logistically demanding, and time-consuming. Hence, the exploration of alternative models is crucial for preclinical research. Invertebrate models, such as Caenorhabditis elegans (nematodes) (Sifri et al., 2005) and Galleria mellonella (wax moth larvae) (Meir et al., 2018), are gaining prominence in bacterial infection research (Mylonakis et al., 2005; Seed and Dennis, 2008; Meir et al., 2018; Wang et al., 2023). These models offer genetic tractability, short life cycles, and cost-effectiveness (Supplementary Table S1). Despite their evolutionary distance from mammals, these insect models possess an innate immune system analogous to that of higher organisms. While invertebrate models lack certain physiological aspects of mammals, they significantly contribute to understanding fundamental aspects of host defense and pathogen virulence.
Invertebrates present an ethically acceptable alternative due to their lack of higher-order cognitive functions and complex nervous systems. They are relatively inexpensive to maintain, requiring minimal resources compared to traditional rodent models. The model’s simplicity enables conducting high-throughput experiments (Kerr et al., 2022), facilitating the screening of multiple bacterial strains, in a time-efficient manner.
G. mellonella serves as a valuable model for initial screenings and investigations not requiring the more complex mammalian systems. Its relatively large size allows the injection of drugs to study antibiotic effectiveness. Studies using G. mellonella have demonstrated a good correlation with results obtained from mammalian models, particularly in evaluating virulence factors, assessing antimicrobial efficacy, and understanding the basic principles of bacterial pathogenesis (Peleg et al., 2009; Loh et al., 2013; Wand et al., 2013; ten et al., 2023; Wang et al., 2023).
However, challenges exist in obtaining G. mellonella larvae from commercial suppliers. Only a few countries produce G. mellonella for research use, while in many others, there is limited access to a commercial supply of research-grade larvae. Suppliers may differ in the quality of larvae and the conditions of rearing, with some employing antibiotics to obtain research-grade larvae. Limited availability of high-quality larvae, combined with challenges in shipping live animals across borders, poses logistical difficulties. G. mellonella larvae typically survive for 5–6 weeks under ideal conditions, but their lifespan can be affected by factors such as temperature, humidity, and food availability. The shipping process becomes critical as delays can shorten the narrow time window available for experimentation. Reproducing and maintaining G. mellonella in-house is labor-intensive, increases the costs of the experiments, and is not suited to large-scale experiments.
In this study, we aimed to develop an alternative invertebrate infection model for studying the virulence of Acinetobacter baumannii and to examine the effectiveness of antimicrobials in this model. For our study, we selected the insect host Zophobas morio (Coleoptera: Tenebrionidae), which is a darkling beetle species commonly referred to as the “superworm.” Z. morio undergoes holometabolism, characterized by a life cycle comprising four stages: embryo, larva (caterpillar), pupa, and adult beetle. Z. morio larvae are used for feeding birds and reptiles (Rumbos and Athanassiou, 2021) and have even been considered nutrient sources for human consumption (Benzertiha et al., 2020; Scholliers et al., 2020). These larvae are readily available from commercial suppliers worldwide, including in the US and Europe. Unlike G. mellonella, Z. morio can be sustained at room temperature for extended periods and does not pupate under crowded conditions, simplifying long-term studies and transportation logistics. Maintaining Z. morio larvae within the temperature range of 25°C to 37°C facilitates their use in in vivo studies of host-pathogen interactions (Zou et al., 2022), and Z. morio larvae being larger than G. mellonella makes experimental manipulations easier. In a previous study, Z. morio was utilized in toxicology analyses of plant extracts (Zou et al., 2022), and in other studies, one of its close relatives, Tenebrio molitor, served as a model system for fungal infections (de Souza et al., 2015; Canteri de Souza et al., 2018). In 2023, the complete genome assembly of Z. morio became available (Kaur et al., 2023), sparking a significant surge of interest in this organism within the research community.
G257, a carbapenem-resistant A. baumannii clinical isolate from our laboratory collection, was used in all studies. Two ATCC reference strains (A. baumannii ATCC19606 and A. lwoffii ATCC15309) were used as controls. In fitness assays, G422, a multidrug-resistant (MDR) carbapenem-resistant A. baumannii clinical isolate from our laboratory collection, was shown to have low fitness in a previous study (Nutman et al., 2020) (Table 1).
For various experiments, bacteria were cultured at 37°C on blood agar (tryptic soy agar supplemented with 5% sheep blood; Hylabs, Rehovot, Israel), MDR Acinetobacter agar (Hylabs, Rehovot, Israel), or brain–heart infusion (BHI) broth. Bacteria were grown at 37°C to the logarithmic phase (OD600 of 0.6). Cells were spun down, washed with phosphate-buffered saline (PBS), and then diluted to indicate cell density. The infecting inoculum was freshly prepared before the experiment and was confirmed by CFU quantification with serial dilutions.
Zophobas morio was grown and maintained on sterilized sawdust (Meital Laboratories, Shekhanya, West Galilee, Israel). The larvae, in the final instar larval stage, were shipped and maintained in a plastic box with ventilation openings, at room temperature. Freshly washed vegetables were provided every 2 days for hydration. Larvae with an average weight of 640–800 mg were used within a month after arrival.
G. mellonella larvae in the 3-day instar larval stage (Biosystems Technology, Brierley Hill, UK) were stored at 25°C in a plastic box with ventilation openings. Larvae with an average weight of 200–300 mg were used within a week after arrival.
Larvae were randomly divided into treatment groups of 30 larvae each. A 0.5 mL, 31 G syringe was used to inject 25 μL of aliquots of bacteria into the proleg of each larva. After injection, larvae were incubated in plastic containers at 25°C or at 37°C as specified, and the number of dead larvae was scored daily until the end of the experiment (days 5–7). Larvae were considered dead when they displayed no movement in response to touch.
Groups of 5 G. mellonella larvae were infected with 105 CFU bacteria/larvae. Groups of 4 Z. morio larvae were infected with 107 CFU/larva. Larvae were collected at 0 h and 4 h post-infection and homogenized individually in 1 mL of PBS using the gentleMACS Dissociator (Miltenyi Biotec, Bergisch Gladbach, Germany). Serial dilutions of homogenate in PBS were plated on MDR Acinetobacter plates and incubated at 37°C for 24 h, followed by CFU quantification. Non-manipulated larvae of each species (n = 4) and PBS-injected larvae of each species (n = 4) served as controls.
We tested in vivo growth using a neutropenic murine thigh infection model. Fitness was defined based on the CFU count 24 h after the injection of an inoculum of 105 CFU. A detailed protocol was described previously (Nutman et al., 2020). The protocol was approved by the Institutional Animal Care and Use Committee at Tel-Aviv Sourasky Medical Center.
Larvae were injected with PBS (control) or with 106 CFU of G257 (infected). At time points of 0 and 16 h post-infection, larvae were washed with PBS, fixed with 70% ethanol, and stored at 4°C. Paraffin-embedded slides were prepared (Hepatho Lab Ltd., Rehovot, Israel), and H&E staining was performed as described (Barnoy et al., 2017).
Zophobas morio larvae (30 per group) were injected into a proleg with 107 CFU/larva of the bacterial strain. At the 30 min post-infection time point, a single dose of antibiotics, either 200 μg/mg ampicillin or 60 μg/mg meropenem, was injected into a different proleg. Z. morio larvae injected with PBS (30 per group) were used as controls. Survival and fitness assays were performed as described above. At least three independent experiments were performed with similar results.
Statistical analysis was performed using GraphPad Prism 9 software (version 9.3.1, GraphPad Software Inc., San Diego, CA, United States). The Kaplan–Meier survival curves were generated. For two-group comparisons, a two-tailed unpaired t-test was applied. For multigroup comparisons, an analysis of variance (ANOVA) with Turkey–Kramer’s multiple-comparison test was used. A value of p of <0.05 was considered statistically significant. To build a phylogenetic tree, the cytochrome C amino acid sequences of each organism were retrieved from NCBI and aligned using MAFFT (Katoh et al., 2002). A phylogenetic tree was constructed using the NJ algorithm. Accession numbers were as follows: Homo sapiens AAP36314.1; Mus musculus NP_031834; Gallus NP_001072946.1; Drosophila melanogaster NP_001260509.1; Danio rerio NP_001002068.1; Caenorhabditis elegans P19974.2; Rattus norvegicus NP_036971.1; Galleria mellonella XP_052756993.1; Zophobas morio GCA_027724725.1 (Cytochrome C amino acid sequence was extracted from the full genome).
We constructed a phylogenetic tree to measure the distance between Z. morio and other invertebrate and vertebrate animals used as infection models (Figure 1A). The phylogenetic distance from humans to both Z. morio and G. mellonella is comparable and shorter than the distance from C. elegans to humans. We then compared key phenotypic features of the Z. morio larvae to those of G. mellonella. Z. morio is larger and darker than G. mellonella (Figure 1B). The average weight of the Z. morio larvae was nearly 2.5-fold higher than the average weight of the G. mellonella (758 ± 14.2 mg vs. 308 ± 8.4 mg) (Figure 1C). The differences in size and weight allowed for easier handling and injection in the Z. morio model. Like G. mellonella, dead Z. morio larvae are easily distinguishable from live ones—they appear straight and rigid and do not respond to tactile stimuli (Figure 1D). The differences between the two models and their comparison to C. elegance are presented in Supplementary Table S1.
Figure 1. Zophobas morio. (A) A phylogenetic tree showing the most widely model organisms. (B) G. mellonella and Z. morio. (C) Weight range of Z. morio and G. mellonella larvae. Average weight is 758 mg per larva for Zophobas morio and 308.5 mg per larva for Galleria mellonella. (D) Live (left) and dead (right) phenotype of Z. morio.
We used two laboratory reference strains as controls: a non-virulent Acinetobacter lwoffii ATCC15309 and Acinetobacter baumannii ATCC19606 (Table 1). As a clinically relevant strain, we used a previously described (Nutman et al., 2020; Frenk et al., 2022) A. baumannii G257 strain isolated from a patient with severe infection. This strain was previously found to harbor various virulence factors (Supplementary Table S2) and to be highly virulent in a mouse model, with a fitness of 10.08 CFU/thigh.
We first established a survival assay. Infection was induced in Z. morio by injecting with the three study strains—A. lwoffii ATCC15309, A. baumannii ATCC19606, and the clinical A. baumannii isolate G257. Groups of 30 larvae were injected with 105 CFU–108 CFU of bacteria and incubated at 25°C or at 37°C for the indicated number of times. A group of 30 larvae injected with PBS and a group of 20 larvae without any manipulation were used as controls. Figure 2 shows the lethal dose effect of the three strains in a dose-dependent and temperature-dependent manner. A clear dose–response was observed for all three strains, as well as differences in the lethality between the strains (Figures 2A–C). At an inoculum of 105 CFU/larvae, lethality was observed only among larvae infected with A. baumannii G257 (80% survival on days 5–7). At an inoculum of 106 CFU/larvae, all larvae infected with A. lwoffii ATCC15309, 90% of those infected with A. baumannii ATCC19606, and only 40% of those infected with A. baumannii G257 survived at day 7. At an inoculum of 107 CFU, higher lethality was observed in all three groups. The effect of temperature varied for different inoculums. At an inoculum of 106 CFU, no significant differences in the survival of larvae at 25°C or 37°C were observed (value of p >0.05) (Figures 2D–F). At an inoculum of 107 CFU/larvae, ATCC19606 was significantly more lethal at 37°C than at 25°C (p value <0.05) but not for the two other strains used (value of p = 0.29 for ATCC15309 and p value = 0.406 for G257) (Figures 2G–I).
Figure 2. Dose and temperature effect on Z. morio larvae survival after infection with three different Acinetobacter strains. The Kaplan–Meier plot of Z. morio survival over 7 days. (A–C) Effect of inoculum dose on Z. morio survival during incubation at 37°C (A) A. lwoffii ATCC15309. (B) A. baumannii ATCC19606 (C) A. baumannii clinical isolate G257. (D–F) Effect of temperature on Z. morio survival. Panels (D–F) - inoculum of 106 CFU/larvae; Panels (G–I) - inoculum of 107 CFU/larvae. (D,G) - A. lwoffii ATCC15309. (E,H) - A. baumannii ATCC19606 (F,I) - A. baumannii clinical isolate G257. In each experiment, 20 larvae were injected with PBS as an injection control, with 100% survival in all cases.
We next determined the in vivo fitness of A. baumannii in Z. morio by quantifying bacterial CFU 4 h after injection. As the larvae are not sterile, to specifically quantify the injected A. baumannii, we plated the bacteria on a selective medium (containing meropenem 4.5 mg/mL). As both reference strains used in this study are sensitive to meropenem, we used a clinical MDR isolate G422 as a control. G257 has high fitness in a mouse model (10.08 CFU/thigh), while G422 has lower fitness in a mouse model (5.23 CFU/thigh) (Figure 3C). The fitness of the two isolates in Z. morio larvae was compared with their fitness in G. mellonella larvae and was consistent with that of the two isolates in the mouse model (Figure 3). G422 was less fit, and both models did not show an in vivo increase in bacterial count. In contrast, strain G257 showed a significant increase in cell counts in both models (there was a 0.5-log increase in G. mellonella and a one-log increase in the Z. morio model, p < 0.05).
Figure 3. Fitness of two MDR A. baumannii clinical isolates in G. mellonella (A), Z. morio (B), and mice (C) G. mellonella larvae were injected with 105 CFU/larva, and Z. morio were injected with 106 CFU/larva, either with G422 isolate or with G257 isolate. Immediately (0 h) or 4 h after injection, larvae were ground and plated on MDR Acinetobacter Agar plates for CFU quantification. Untreated and PBS-injected larvae were sampled at both time points, and no colonies were detected on the selective plates. (C) Neutropenic mice (n = 3) were injected with 105 CFU/thigh, either with G422 or with G257 isolate. Untreated and PBS-injected mice (n = 3 for each group) were used as control groups.
To visualize structural alterations, cellular abnormalities, or tissue damage caused by the infection, we visualized whole body sections of infected Z. morio larvae with H&E staining. At 16 h post-infection with the A. baumannii G257 strain, H&E stained cross-sections of larvae revealed clusters of hemocytes exhibiting phagocytosis of bacteria (blue dots throughout the section), with a small area of melanization around hemocyte aggregates (Figures 4A,B). Moreover, we observed hemocytes present in nodules, with some attached to organ structures with evidence of melanization (Figures 4C,D,G). In contrast, cross-sections of H&E-stained PBS-injected larva looked healthy (mock infection), with individually distributed hemocytes showing no noticeable aggregates of melanization (Figures 4E,F,H).
Figure 4. Visualization of Z. morio larvae tissues stained by hematoxylin and eosin. (A–D) Larvae were injected with G257 isolate at 1 × 107 CFU/larvae and incubated at 37°C for 16 h. Black arrows indicated enlarged hemocytes containing bacteria. (E–H) Larvae were injected with PBS and incubated at 37°C for 16 h.
We used this novel infection model to test the efficacy of antimicrobials against Acinetobacter spp. We tested the efficacy of ampicillin and of meropenem against A. baumannii ATCC19606 (ampicillin-resistant and meropenem-susceptible, MIC = 1 mg/L for meropenem and MIC >32 mg/L for ampicillin) and against A. baumannii G257 (ampicillin and meropenem resistant, MIC =128 mg/L for meropenem and MIC >32 mg/L for ampicillin). We used a lethal inoculum (5×107 CFU) and compared Z. morio survival after a single dose of ampicillin (200 mg/kg) or meropenem (60 mg/kg).
By day 3, there were no surviving larvae of Z. morio infected with A. baumannii ATCC19606. Treatment with ampicillin, to which this strain is resistant, had no effect on larvae survival. On the other hand, treatment with meropenem, to which this strain is sensitive, significantly increased larvae survival (p < 0.001), with 45% surviving at day 5 (Figure 5A). All Z. morio infected with MDR A. baumannii G257 died by day 3, and treatment with either antibiotic had no effect on their survival (Figure 5B).
Figure 5. Meropenem that is active against A. baumannii ATCC19606 can prolong the survival of A. baumannii-infected Z. morio larvae. (A) After infection with 5×107 CFU/larvae of A. baumannii strain ATCC19606, meropenem to which the strain was susceptible significantly prolonged the survival of Z. morio larvae compared with no antibiotic treatment (p < 0.001). However, ampicillin, to which the strain was resistant, caused no difference in survival compared with no antibiotic treatment. (B) After infection with a 5×107 CFU/larvae of A. baumannii strain G257, meropenem, or ampicillin, to which the strain is resistant, there is no difference in survival compared with no antibiotic treatment.
New invertebrate models are sought as an alternative to rodent studies. In this study, we present a novel infection model in the invertebrate Zophobas morio. We established the parameters of infection with Acinetobacter spp. H&E staining demonstrated Z. morio’s immune response to A. baumannii infection, which included hemocyte clusters engaging in bacterial phagocytosis, tissue melanization, and hemocyte aggregation. A similar immune response was reported by Peleg et al. in the G. mellonella Acinetobacter spp. infection model (Peleg et al., 2009). We showed an inoculum-dependent lethality of Z. morio after Acinetobacter infection. Survival depended on the virulence of the infecting strain, and we demonstrated that this infection model can be used to evaluate the in vivo fitness of the infecting strain. Meropenem treatment, but not ampicillin, resulted in improved survival of Z. morio larvae when the infecting strain was meropenem-susceptible but not if the strain was meropenem-resistant. This demonstrates that the model is suitable for testing the efficacy of antimicrobial therapy as well as the antibiotic resistance of bacterial strains.
A. baumannii is an important MDR nosocomial pathogen, for which very limited treatment options exist. Developing new antimicrobial agents that target A. baumannii, especially carbapenem-resistant strains, is of high priority. Invertebrate models may assist in screening and early testing of new agents. While G. mellonella is an important and established model to study A. baumannii infections, supply, rearing, and maintenance are challenging (Tao et al., 2021; ten et al., 2023). We believe that the Z. morio model is a valuable addition to the in vivo study of A. baumannii and potentially other bacterial pathogens.
The Z. morio infection model has various advantages over other invertebrate models. It is easy to obtain high-quality larvae, ship them, and maintain them. The large size allows easy manipulation during injections, and the results obtained using this model were consistent with those of other, more established model systems. While we found that the temperature of incubation of Z. morio larvae after infection might affect the results in some cases, we successfully used an incubation temperature of 37°C, compatible with optimal bacterial growth.
Further study is required to extend the use of this model to other pathogens and to examine the efficacy of additional antibacterial agents. In-depth examination of the immune response of Z. morio to infections, such as hemocyte response with fluorescently labeled A. baumannii, might provide further insights into the full potential of the model.
In conclusion, this study introduces a novel infection model for studying A. baumannii infection in vivo—in Z. morio larvae. The model can be used to study bacterial virulence and fitness and assess the efficacy of antimicrobial agents.
The original contributions presented in the study are included in the article/Supplementary material, further inquiries can be directed to the corresponding authors.
The animal study was approved by Sourasky Medical Center - Institutional Animal Care and Use Committee. The study was conducted in accordance with the local legislation and institutional requirements.
NR: Conceptualization, Formal analysis, Investigation, Methodology, Writing – original draft, Writing – review & editing. ET: Writing – review & editing. AH: Methodology, Writing – review & editing. ML-W: Methodology, Writing – review & editing. AK-P: Validation, Writing – review & editing. YC: Project administration, Resources, Supervision, Writing – review & editing.
The author(s) declare that no financial support was received for the research, authorship, and/or publication of this article.
The authors declare that the research was conducted in the absence of any commercial or financial relationships that could be construed as a potential conflict of interest.
All claims expressed in this article are solely those of the authors and do not necessarily represent those of their affiliated organizations, or those of the publisher, the editors and the reviewers. Any product that may be evaluated in this article, or claim that may be made by its manufacturer, is not guaranteed or endorsed by the publisher.
The Supplementary material for this article can be found online at: https://www.frontiersin.org/articles/10.3389/fmicb.2024.1375787/full#supplementary-material
Barnoy, S., Gancz, H., Zhu, Y., Honnold, C. L., Zurawski, D. V., and Venkatesan, M. M. (2017). The Galleria mellonella larvae as an in vivo model for evaluation of Shigella virulence. Gut Microbes 8, 335–350. doi: 10.1080/19490976.2017.1293225
Benzertiha, A., Kierończyk, B., Kołodziejski, P., Pruszyńska–Oszmałek, E., Rawski, M., Józefiak, D., et al. (2020). Tenebrio molitor and Zophobas morio full-fat meals as functional feed additives affect broiler chickens’ growth performance and immune system traits. Poult. Sci. 99, 196–206. doi: 10.3382/ps/pez450
Canteri de Souza, P., Custódio Caloni, C., Wilson, D., and Sergio Almeida, R. (2018). An invertebrate host to study fungal infections, mycotoxins and antifungal drugs: Tenebrio molitor. J. Fungi 4:125. doi: 10.3390/jof4040125
de Souza, P. C., Morey, A. T., Castanheira, G. M., Bocate, K. P., Panagio, L. A., Ito, F. A., et al. (2015). Tenebrio molitor (Coleoptera: Tenebrionidae) as an alternative host to study fungal infections. J. Microbiol. Methods 118, 182–186. doi: 10.1016/j.mimet.2015.10.004
Frenk, S., Temkin, E., Lurie-Weinberger, M. N., Rov, R., Rakovitsky, N., Wullfhart, L., et al. (2022). Large-scale WGS of carbapenem-resistant Acinetobacter baumannii isolates reveals patterns of dissemination of ST clades associated with antibiotic resistance. J. Antimicrob. Chemother. 77, 934–943. doi: 10.1093/jac/dkac010
Katoh, K., Misawa, K., Kuma, K. I., and Miyata, T. (2002). MAFFT: a novel method for rapid multiple sequence alignment based on fast Fourier transform. Nucleic Acids Res. 30, 3059–3066. doi: 10.1093/nar/gkf436
Kaur, S., Stinson, S. A., and diCenzo, G. C. (2023). Whole genome assemblies ofZophobas Morioandtenebrio molitor. G3 (Bethesda) 13:jkad079. doi: 10.1093/g3journal/jkad079
Kerr, R. A., Roux, A. E., Goudeau, J., and Kenyon, C. (2022). The C. elegans observatory: high-throughput exploration of behavioral aging. Front. Aging 3:932656. doi: 10.3389/fragi.2022.932656
Loh, J. M. S., Adenwalla, N., Wiles, S., and Proft, T. (2013). Galleria mellonella larvae as an infection model for group a streptococcus. Virulence 4, 419–428. doi: 10.4161/viru.24930
Meir, M., Grosfeld, T., and Barkan, D. (2018). Establishment and validation of galleria mellonella as a novel model organism to study Mycobacterium abscessus infection, pathogenesis, and treatment. Antimicrob. Agents Chemother. 62:e02539-17. doi: 10.1128/AAC.02539-17
Mylonakis, E., Moreno, R., el Khoury, J. B., Idnurm, A., Heitman, J., Calderwood, S. B., et al. (2005). Galleria mellonella as a model system to study Cryptococcus neoformans pathogenesis. Infect. Immun. 73, 3842–3850. doi: 10.1128/IAI.73.7.3842-3850.2005
Nutman, A., Lellouche, J., Lifshitz, Z., Glick, R., and Carmeli, Y. (2020). In vivo fitness of acinetobacter baumannii strains in murine infection is associated with international lineage ii-rep-2 and international lineage iii clones showing high case fatality rates in human infections. Microorganisms 8, 2–9. doi: 10.3390/microorganisms8060847
Peleg, A. Y., Jara, S., Monga, D., Eliopoulos, G. M., Moellering, R. C. J., and Mylonakis, E. (2009). Galleria mellonella as a model system to study Acinetobacter baumannii pathogenesis and therapeutics. Antimicrob. Agents Chemother. 53, 2605–2609. doi: 10.1128/AAC.01533-08
Rong, N., and Liu, J. (2023). Development of animal models for emerging infectious diseases by breaking the barrier of species susceptibility to human pathogens. Emerg. Microbes Infect. 12:2178242. doi: 10.1080/22221751.2023.2178242
Rumbos, C. I., and Athanassiou, C. G. (2021). The Superworm, Zophobas morio (Coleoptera:Tenebrionidae): a ‘sleeping Giant’ in nutrient sources. J. Insect Sci. 21:13. doi: 10.1093/jisesa/ieab014
Scholliers, J., Steen, L., and Fraeye, I. (2020). Partial replacement of meat by superworm (Zophobas morio larvae) in cooked sausages: effect of heating temperature and insect:meat ratio on structure and physical stability. Innov. Food Sci. Emerg. Technol. 66, 102535–102533. doi: 10.1016/j.ifset.2020.102535
Seed, K. D., and Dennis, J. J. (2008). Development of galleria mellonella as an alternative infection model for the Burkholderia cepacia complex. Infect. Immun. 76, 1267–1275. doi: 10.1128/IAI.01249-07
Sifri, C. D., Begun, J., and Ausubel, F. M. (2005). The worm has turned--microbial virulence modeled in Caenorhabditis elegans. Trends Microbiol. 13, 119–127. doi: 10.1016/j.tim.2005.01.003
Tao, Y., Duma, L., and Rossez, Y. (2021). Galleria mellonella as a good model to study Acinetobacter baumannii pathogenesis. Pathogens. 10:10. doi: 10.3390/pathogens10111483
ten, K. E., Muzahid, N. H., Rahman, S., and Tan, H. S. (2023). Use of the waxworm galleria mellonella larvae as an infection model to study Acinetobacter baumannii. PLoS One 18:e0283960. doi: 10.1371/journal.pone.0283960
Wand, M. E., McCowen, J. W. I., Nugent, P. G., and Sutton, J. M. (2013). Complex interactions of Klebsiella pneumoniae with the host immune system in a galleria mellonella infection model. J. Med. Microbiol. 62, 1790–1798. doi: 10.1099/jmm.0.063032-0
Wang, S., Yin, Y., Zai, X., Gu, Y., Guo, F., Shao, F., et al. (2023). A novel Galleria mellonella experimental model for zoonotic pathogen Brucella. Virulence 14:2268496. doi: 10.1080/21505594.2023.2268496
Keywords: in vivo model, Acinetobacter baumannii, Zophobas morio, larvae, antibiotic-resistant bacteria
Citation: Rakovitsky N, Temkin E, Hameir A, Lurie-Weinberger M, Keren-Paz A and Carmeli Y (2024) Zophobas morio larvae as a novel model for the study of Acinetobacter virulence and antimicrobial resistance. Front. Microbiol. 15:1375787. doi: 10.3389/fmicb.2024.1375787
Received: 24 January 2024; Accepted: 12 February 2024;
Published: 27 February 2024.
Edited by:
Abdelazeem Algammal, Suez Canal University, EgyptReviewed by:
Yadong Zhang, Boston Children's Hospital and Harvard Medical School, United StatesCopyright © 2024 Rakovitsky, Temkin, Hameir, Lurie-Weinberger, Keren-Paz and Carmeli. This is an open-access article distributed under the terms of the Creative Commons Attribution License (CC BY). The use, distribution or reproduction in other forums is permitted, provided the original author(s) and the copyright owner(s) are credited and that the original publication in this journal is cited, in accordance with accepted academic practice. No use, distribution or reproduction is permitted which does not comply with these terms.
*Correspondence: Nadya Rakovitsky, bmFkeWFyYWtAdGx2bWMuZ292Lmls; Yehuda Carmeli, eWVodWRhY0B0bHZtYy5nb3YuaWw=
Disclaimer: All claims expressed in this article are solely those of the authors and do not necessarily represent those of their affiliated organizations, or those of the publisher, the editors and the reviewers. Any product that may be evaluated in this article or claim that may be made by its manufacturer is not guaranteed or endorsed by the publisher.
Research integrity at Frontiers
Learn more about the work of our research integrity team to safeguard the quality of each article we publish.