- 1Zhengzhou Tobacco Research Institute of CNTC, Zhengzhou, China
- 2College of Tobacco Science of Guizhou University, Guiyang, China
- 3Guizhou Provincial Key Laboratory for Tobacco Quality, College of Tobacco Science, Guizhou University, Guiyang, China
- 4School of Agricultural Sciences, Zhengzhou University, Zhengzhou, China
- 5Guizhou Tobacco Company Bijie Region Tobacco Company, Bijie, China
- 6Yunnan Academy of Tobacco Agricultural Sciences, Kunming, China
Understanding the response of microbial communities and their potential functions is essential for sustainability of agroecosystems under long-term continuous cropping. However, limited research has focused on investigating the interaction between soil physicochemical factors and microbial community dynamics in agroecosystems under long-term continuous cropping. This study probed into the physicochemical properties, metabolites, and microbial diversity of tobacco rhizosphere soils cropped continuously for 0, 5, and 20 years. The relative abundance of bacterial genera associated with nutrient cycling (e.g., Sphingomonas) increased while potential plant pathogenic fungi and beneficial microorganisms showed synergistic increases with the duration of continuous cropping. Variations in soil pH, alkeline nitrogen (AN) content, and soil organic carbon (SOC) content drove the shifts in soil microbial composition. Metabolites such as palmitic acid, 3-hydroxypropionic acid, stearic acid, and hippuric acid may play a key role in soil acidification. Those results enhance our ability to predict shifts in soil microbial community structure associated with anthropogenic continuous cropping, which can have long-term implications for crop production.
Highlights
– Soil microbiome structure had different changes among different periods of continuous cropping.
– Soil pH, AN, and SOC were the key environmental factors contributing to soil microbial community structure changes.
– Pathogenic fungi disrupt the nutrient cycling in agroecosystems under continuous cropping practices.
Introduction
Continuous cropping is a common farming practice in China (Zhang Y. Z. et al., 2022), involves growing the same crop on the same plot of land for extended periods with the aim of achieving high yields (Zhang S. et al., 2022). However, continuous cropping tobacco rather than reasonable crop rotation (tobacco-soybean-corn), often leads to severe continuous cropping obstacles, usually manifested as more frequent plant diseases epidemics and soil microbial community changes (Ma et al., 2022). Generally, continuous cropping accelerates soil acidification, thus promoting the outbreak of crop diseases (Li et al., 2017). The frequent occurrence of crop diseases reduces the quality of crops and even leads to crop failure in karst agroecosystems (Gao et al., 2019).
Tobacco has been extensively utilized as a model organism in numerous studies pertaining to plant biotechnology (Sierro et al., 2014). It is also an important crop widely planted in impoverished areas (Chen F. et al., 2018). Due to the limited arable land resources, tobacco is continuously grown on the same plots for over 20 years, leading to persistent challenges associated with continuous cropping and subsequent yield reductions (Matheri et al., 2023). Several studies have demonstrated that after 7 years of continuous cropping, tobacco plants could sustain wilt disease caused by soil-borne fungi (Niu et al., 2017). In addition, the modification of resident soil microbial flora is related to changes in soil physicochemical properties and soil enzymatic activities (Chen S. et al., 2018). Soil microorganisms are effective biological indicators of soil fertility as they play a significant role in agricultural production (Avidano et al., 2005; Chen W. et al., 2018; Yan et al., 2023). The diversity index of soil microbial community composition tends to decrease after long-term continuous cropping, reduce the number of beneficial microorganisms, and increase the abundance of pathogenic bacteria, thus reducing crop yields (Xiong et al., 2015; Gao et al., 2019). The previous studies have indicated a significant decline in the abundance of beneficial microorganisms with prolonged tobacco cropping (Maguire et al., 2020). Cultivation has been found to alter microbial communities, leading to a notable increase in Fusarium abundance. Consequently, urgent measures are required to effectively manage this pathogen in arable farmlands. However, limited research has focused on assessing soil health under long-term continuous tobacco cropping (Xiong et al., 2015). Therefore, safeguarding the soil agricultural micro-ecosystem is imperative for promoting optimal crop growth.
Crops secrete allelopathic substances such as secondary metabolites, which affect the soil environment and cause continuous cropping obstacles (Gerhards and Schappert, 2020). Soil microorganisms play a pivotal role in modulating the effects of these secondary metabolites, exerting both positive and negative influences on crop growth. Firstly, soil microorganisms decompose toxic compounds to reduce allelopathy (Jilani et al., 2008). Secondly, the breakdown of allelopathic residues by soil microorganisms can lead to the release of additional phytotoxins (Cipollini et al., 2012). Therefore, it is imperative to investigate the mechanisms underlying soil-borne diseases associated with continuous tobacco cropping.
By the time of writing, limited reports have elucidated the response of microbial communities to long-term continuous tobacco cropping. In this study, we employed Illumina sequencing and metabolomics to investigate the changes in soil microbial communities and the types of allelopathic substances under continuous cropping. The objectives encompassed (i) exploring the diversity and composition responses of microorganisms to varying durations of continuous cropping; (ii) evaluating the specific microbial taxa associated with the development of obstacles posed by continuous cropping; and (iii) predicting the variation tendency of karst agroecosystems and their responses to prolonged periods of continuous cropping.
Materials and methods
Soil collection
The experimental site was located in Weining experimental station, at Bijie City, Guizhou province, China (27°05′N, 103°51′E). The region has a typical karst plateau landform and a humid subtropical monsoon climate with a mean annual temperature of 18°C, an average sea level of 2,092 m, and annual precipitation of 926 mm. The main soil type was calcareous soil developed from limestone. Farmlands under continuous tobacco cropping for 5 and 20 years were selected for sampling as Y5 and Y20. Collected soil samples from cornfield adjacent to the sampling site Y5, where tobacco had never been planted, were used as the control (CK). Field management measures in CK were consistent with treatment groups to minimize any confusion caused by the effects of agricultural management practices on soil metabolites or microorganisms. The amount of fertilizer applied was 600 kg/ha of NPK compound fertilizer (N 15%, P2O5 15%, and K2O 20%). All fertilizers were used as base fertilizer and applied to the soil at one time. Topdressing was performed every 30 days for two times with N: 20 kg·ha, P2O5: 20 kg·ha, and K2O: 50 kg·ha.
Three types of soil samples were collected from the plow layers (5–20 cm deep) in April 2021, labeled, respectively. In order to ensure the consistency of sampling time, samples were collected from three fields at the same day. Soil samples were collected from the surface of tobacco roots in plow layers (5–20 cm). Soil closely attached to the roots was washed using sterile water and pooled in a 50-mL Falcon tube as rhizosphere soil (Kui et al., 2021). In each field, samples were collected via a five-point sampling method to obtain representative samples. For each group, five soil samples were collected and combined to form a single sample, which was repeated three times. The above samples were divided into two parts. One was air-dried for physicochemical property analysis, and the other was stored at −80°C for DNA extraction.
Analysis of soil basic properties
Soil pH levels were determined in suspensions with a soil-water (w/w) ratio of 1:2.5 (Ali et al., 2021). Soil organic carbon (SOC) contents were measured using the colorimetric method (Looft et al., 2012). Available phosphorus (AP) was extracted with sodium bicarbonate and analyzed using the molybdenum blue method (Pu et al., 2014). Available potassium (AK) contents were measured with ammonium acetate and assessed using flame photometry (Watanabe and Olsen, 1965). Available nitrogen (AN) was extracted using the alkaline hydrolysis diffusion method (Li et al., 2019).
Analysis of soil microbial communities
Total DNA was extracted from soil samples (0.5 g each) using the TGuide Soil DNA Kit (Tiangen DP812, Beijing, China). The V3-V4 regions of the16S rRNA genes were amplified by the bacterial primers 338F (5′-ACTCCTACGGGAGGCAGCAG-3′) and 806R (5′-GGACTACHVGGGTWTCTAAT-3′). Universal primers ITS1F (5′-CTTGGTCATTTAGAGGAAGTAA-3′) and ITS2R (5′-GCTG CGTTCTTCATCGATGC-3′) were used to amplify fungal ITS1 region (Ward et al., 2017; Gu et al., 2022). We purified the PCR products using the Monarch DNA Gel Extraction Kit (T1020L, MA, United States). Then, the purified amplicons were employed for library construction using the Illumina Novaseq 6000 platform (Illumina, CA, United States) at Biomarker Technology Co., Ltd. (Beijing, China). The raw FASTQ files of the fungal and bacterial reads resulting from Illumina sequencing were filtered by Trimmomatic (version v0.33) and merged by Usearch (version v10) (Edgar, 2013; Bolger et al., 2014). These high-quality sequences were clustered into operational taxonomic units (OTUs) based on a 97% similarity threshold using UPARSE (Edgar et al., 2011). An averaged OTU table was generated by resampled OTU subsets under 90% of the minimum sequencing depth for further analysis to reduce the effects of different sequencing depths on the analyses (Liu S. et al., 2021). Bacterial and Fungal taxonomies were assessed against the 16S rRNA database (Silva v128) and the UNITE fungal ITS database (UNITE v8.0), respectively (Jiao et al., 2020; Chen et al., 2022). The alpha diversity metrics, beta diversity metrics, and other analyses were calculated using QIIME (Caporaso et al., 2011) and R packages (version 3.1.1) (R Core Team, 2019). All sequence data were deposited in NCBI Sequence Read Archive database under accession number PRJNA874867.
Metabolite profiling
Each soil sample was frozen-crushed using a steel ball at 30 Hz for 1.5 min. Each sample (1,000 mg) was extracted using 1.0 mL of 70% aqueous methanol (AM) and 1 mL of ethyl acetate (EA) with 5 μL internal standard at 4°C. After centrifugation at 10,000 rpm at 4°C for 15 min, the supernatant was transferred into a 5-mL EP tube. After evaporation in a vacuum concentrator, 30 μL of ethoxyamination hydrochloride was added to the obtained product, and the mixture was then incubated at 80°C for 30 min and derivatized by 40 μL of BSTFA regent (1% TMCS, v/v) at 70°C for 1.5 h. All samples were adsorbed and filtrated before being subjected to analysis with a gas chromatograph (Agilent 7890, 30 m × 250 μm × 0.25 μm, J&W Scientific, Folsom, CA, United States) coupled with a time-of-flight mass spectrometer (GC-TOF-MS) (Kind et al., 2009).
Statistical analysis
Based on the results of species annotations of all samples, the top 10 phyla and the top 35 genera were analyzed (relative abundance above 1%). Chao 1, Shannon, Simpson, and ACE indices were used to estimate the diversity of the microbial communities. Non-metric multidimensional scaling (NMDS) analysis was performed to investigate the structural variation of microbial communities across samples using Weighted uniFrac distance metrics (Looft et al., 2012). Principal component analysis (PCA) was also conducted based on OTU level compositional profiles (Ramette, 2007). The taxonomy compositions and abundances were visualized using MEGAN (Liu J. L. et al., 2021). Redundancy analyses (RDA) were used to investigate the relationships between samples and environmental variables (R: vegan package) (Oksanen et al., 2020). The heatmaps and Venn diagrams were generated using custom R scripts (Clauset et al., 2004). Bacterial functional potential was investigated with FAPROTAX database (Louca et al., 2016). Functional prediction analysis was carried out using FUNGuild database (Nilsson et al., 2019). All analyses were conducted on the BMK Cloud platform (Biomarker Technology Co., Ltd., Beijing, China; https://international.biocloud.net/zh/software/agriculture/) based on various R packages and workflow frameworks. A one-way ANOVA was performed to determine the significance of the differences of soil properties and microbial community structures between three treatments by SPSS. A p value <0.05 was considered to be statistically significant.
Results
Soil samples physicochemical properties
The physicochemical properties of soil samples after different continuous tobacco cropping durations are listed in Table 1. All samples were showed weak acidity. In addition, the soil pH level of Y20 decreased significantly. The SOC and AK contents in Y5 and Y20 were significantly lower than those of CK. However, the AP and AN content of Y20 were highest, reaching 33.75 and 162.32 mg kg−1, respectively.
Overall microbial community diversity
The 16S rRNA genes were sequenced to analyze the soil microbial community diversity under long-term continuous tobacco cropping. A total of 659,973 raw reads and 636,731 clean reads of bacteria were obtained, with an average length of 418 bp. As a result of chimeral filtration and QC, a total of 718,690 raw reads and 715,307 clean reads of fungi were obtained, with an average length of 237 bp. Through the analyses of alpha diversity, we found that the levels of bacterial richness tended to increase with the duration of continuous cropping. The richness of fungi reached the highest level after continuous cropping for 5 years, and then decreased gradually as the duration of continuous cropping increased further. Nevertheless, levels of soil fungal diversity tended to increased and then decreased, although no significant difference was found.
Soil microbial community composition
The tobacco rhizosphere soils were home to 29 bacterial phyla, 81 classes, 183 orders, 303 families, and 565 genera. The dominant bacterial phyla in all samples included Proteobacteria, Acidobacteria, Actinobacteria, and Gemmatimonadetes, accounting for 77.44–9.74% of all sequences (Figure 1A). Proteobacteria dominated the rhizosphere soil, occupying 38.03–44.35% of all sequences, and their relative abundances in Y5 and Y20 were significantly higher than those in CK. The relative abundances of Acidobacteria, Gemmatimonadetes, Chloroflexi, Bacteroidetes, and Verrucomicrobia were higher in Y20 than in Y5. The relative abundances of dominant genera varied in the soil samples with different continuous cropping durations. The relative abundances of Gemmatimonas, Gemmatimonadaceae, and Ramlibacter in Y5 were significantly higher than that in CK and Y20. The relative abundances of Sphingomonas, Massilia, Acidobacteriales, Burkholderiaceae, Xanthobacteraceae, and Gaiellales in Y20 were significantly higher than in CK. Whereas the relative abundances of Gemmatimonas, Micrococcaceae, and Micromonosporaceae showed the opposite trend.
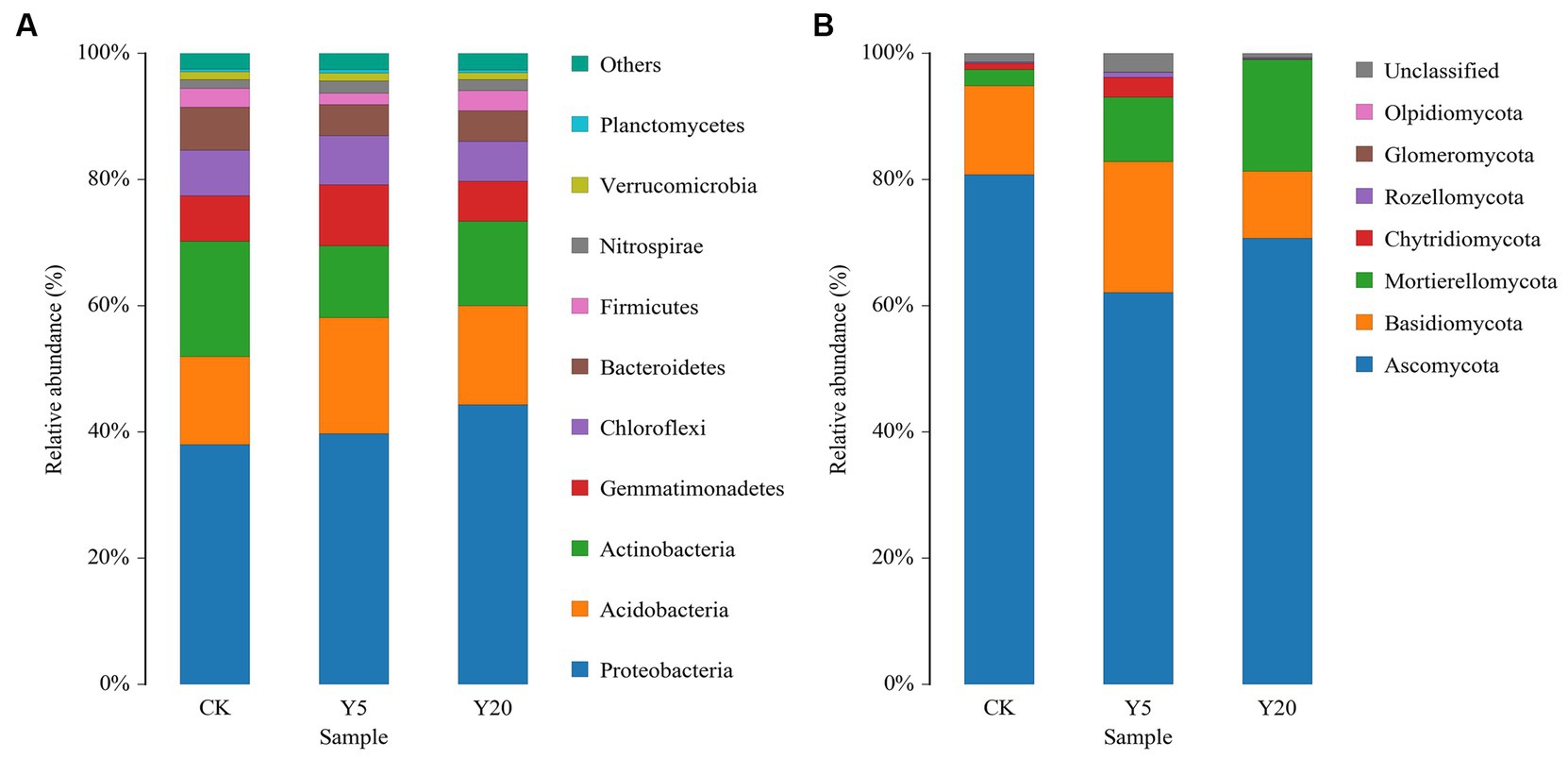
Figure 1. The phylum-level composition of (A) bacterial and (B) fungal communities. CK: tobacco cropping for 0 years. Y5: continuous tobacco cropping for 5 years. Y20: continuous tobacco cropping for 20 years.
The tobacco rhizosphere soils were inhabited by seven fungal phyla, 22 classes, 57 orders, 118 families, and 202 genera. Ascomycota was the most abundant among with a relative abundance of 62.06–80.81%, followed by Basidiomycota (10.63 to 20.77%), Mortierellomycota (2.56 to 17.71%), and Chytridiomycota (0.74–9.56%) (Figure 1B). The relative abundance of Ascomycota in Y20 was significantly higher than that in Y5. However, the relative abundance of Mortierellomycota increased with the length of continuous cropping, with that in Y20 15.51% higher than that in CK. At the genus level, the relative abundances of Mortierella, Byssochlamys, and Pseudopyrenochaeta decreased and then increased with the duration of continuous cropping; in contrast, that of Penicillium, Plectosphaerella, Aspergillus, Sebacina, Botryotinia, and Inocybe increased first and then decreased. Among the top 15 genera, the relative abundances of Cladosporium (11.55%), Epicoccum (16.09%), Vishniacozyma (6.95%), and Alternaria (7.80%) in CK were higher than that in Y5 and Y20. Additionally, the relative abundances of Mortierella (17.70%), Fusarium (10.30%), Chaetomium (8.69%), and Byssochlamys (10.23%) in Y20 were higher than that in other samples.
Differences in microbial communities
The principal component analysis (PCA) was used to analyze the community structure differences between groups (Supplementary Figure S1A). For bacteria, the first principle component (PC1) and the second principle component (PC2) contributed 37.63 and 23.04%, respectively. The Y20 samples were distinctive from the CK and Y5 samples. Y20 had the highest PC1 value, while Y5 had the highest PC2 value. CK had the lowest PC1 and PC2 values. For fungi, PC1 contributed 32.58%, and PC2 contributed 21.59% (Supplementary Figure S1B). Y20 had the highest PC1 value, and CK had the highest PC2 value. The Y5 and Y20 samples were highly similar and distinctive from the CK samples.
Similarly, with the process of time, the community structure of bacteria and fungi changed significantly (R2 = 0.43, p < 0.01; R2 = 0.35, p < 0.01) (Figure 2). Weighted UniFrac nonmetric multidimensional scaling (NMDS) analysis (stress = 0.001) showed that the bacterial community structure was significantly different between three groups. The phylogenetic structures of soil fungal communities varied after different continuous cropping durations, where the three groups were significantly distinctive from other groups.
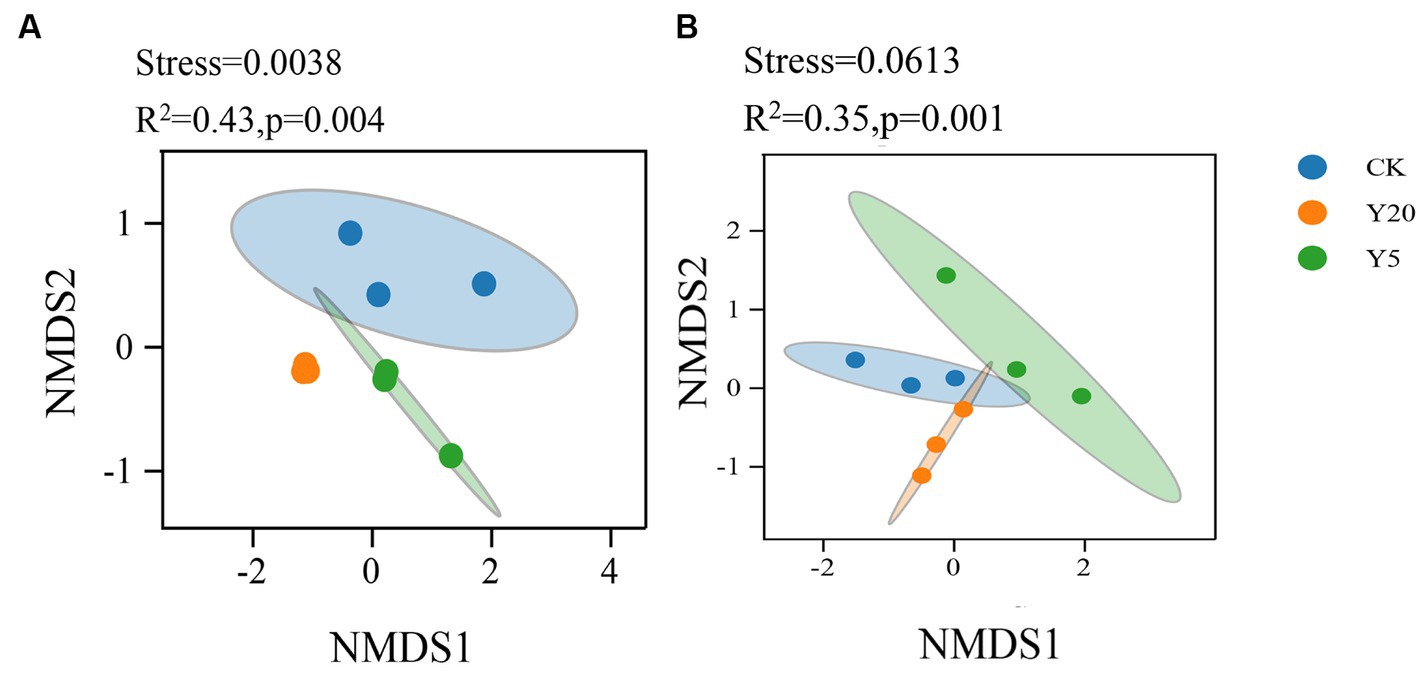
Figure 2. Non-metric multidimensional scaling (NMDS) analysis based on Weighted uniFrac distance for the bacterial (A) and fungal (B) communities.
Environmental factors correlated to microbial communities
Based on OTU reads and environmental factors, the representational difference analysis (RDA) was performed on the different continuous tobacco cropping. The relationships between bacterial communities and soil properties are shown in Figure 3A (axis 1 = 20.33%, axis 2 = 16.5%). The results indicated that the pH level (R2 = 0.8590, p = 0.008), SOC content (R2 = 0.7509, p = 0.013), and AK content (R2 = 0.9267, p = 0.003) were significantly correlated with bacterial communities. The correlation heatmap showed that at the phylum level, the abundance of Actinobacteria was significantly positively correlated with the SOC content (p < 0.05), and the abundance of Chloroflexi was significantly positively correlated with the AK content. In addition, the abundance of Proteobacteria was significantly negatively correlated with the pH level and the AK content. At the genus level, the abundance of Micromonosporaceae was positively correlated with the pH level and the AK content, and the abundance of Acidobacteriales was positively correlated with the AN content (p < 0.05) (Figure 3C). Furthermore, the abundance of Massilia was negatively correlated with the pH and AK content.
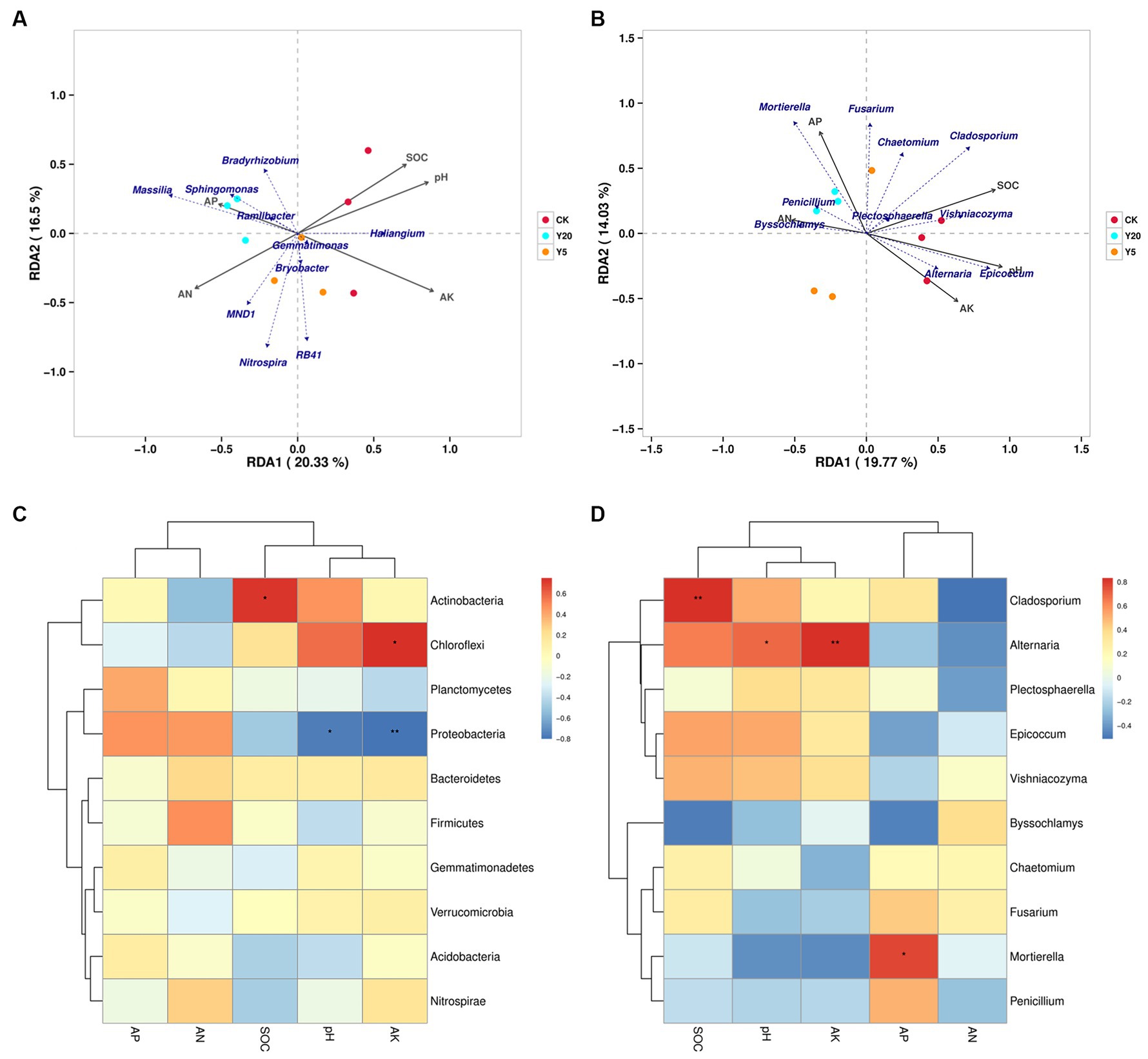
Figure 3. Redundancy analyses (RDA) based on bacterial (A) and fungal (B) OTU data with chemical parameters in soils with the lengthening of continuous cropping. Correlation heatmap of the top 10 bacterial (C) and fungal (D) genera with soil properties. R values are indicated on the right side of the legend with different colors. *p < 0.05, **p < 0.01, and ***p < 0.001.
Figure 3B (axis 1 = 19.77%, axis 2 = 14.03%) showed the relationships between fungal communities and soil properties. The pH level (R2 = 0.957, p = 0.001), SOC content (R2 = 0.842, p = 0.004), AP content (R2 = 0.6582, p = 0.041), and AK content (R2 = 0.671, p = 0.037) were the main factors affecting the changes in fungal phylogenetic structure (Figure 3B). The abundance of Chytridiomycota was significantly positively correlated with the pH level and the AK content (p < 0.01), the abundance of Ascomycota was significantly positively correlated with the SOC content (p < 0.001), and the abundance of Mortierellmycota was significantly positively correlated with the AP content (p < 0.05). In addition, the abundance of Glomeromycota was significantly negatively correlated with the AP content, and the abundance of Olpidiomycota was significantly negatively correlated with the AN content. At the genus level, the abundance of Alternaria was positively correlated with the pH level and the AK content, and the abundance of Cladosporium was positively correlated with the SOC content (p < 0.01) (Figure 3D). Moreover, the abundance of Mortierella was positively correlated with the AP content (p < 0.05).
Soil microbial function prediction
Based on the FAPROTAX database, we predicted the bacterial functions and obtained the top 15 functional groups. The results showed significantly enhanced ureolysis and manganese oxidation, while nitrate reduction, predatory or ectoparasitic, and chitinolysis were significantly weakened (Figure 4A). Other functional groups showed no significant changes, such as chemoheterotrophy, aerobic chemoheterotrophy, aerobic ammonia oxidation, fermentation, nitrogen fixation, and nitrification.
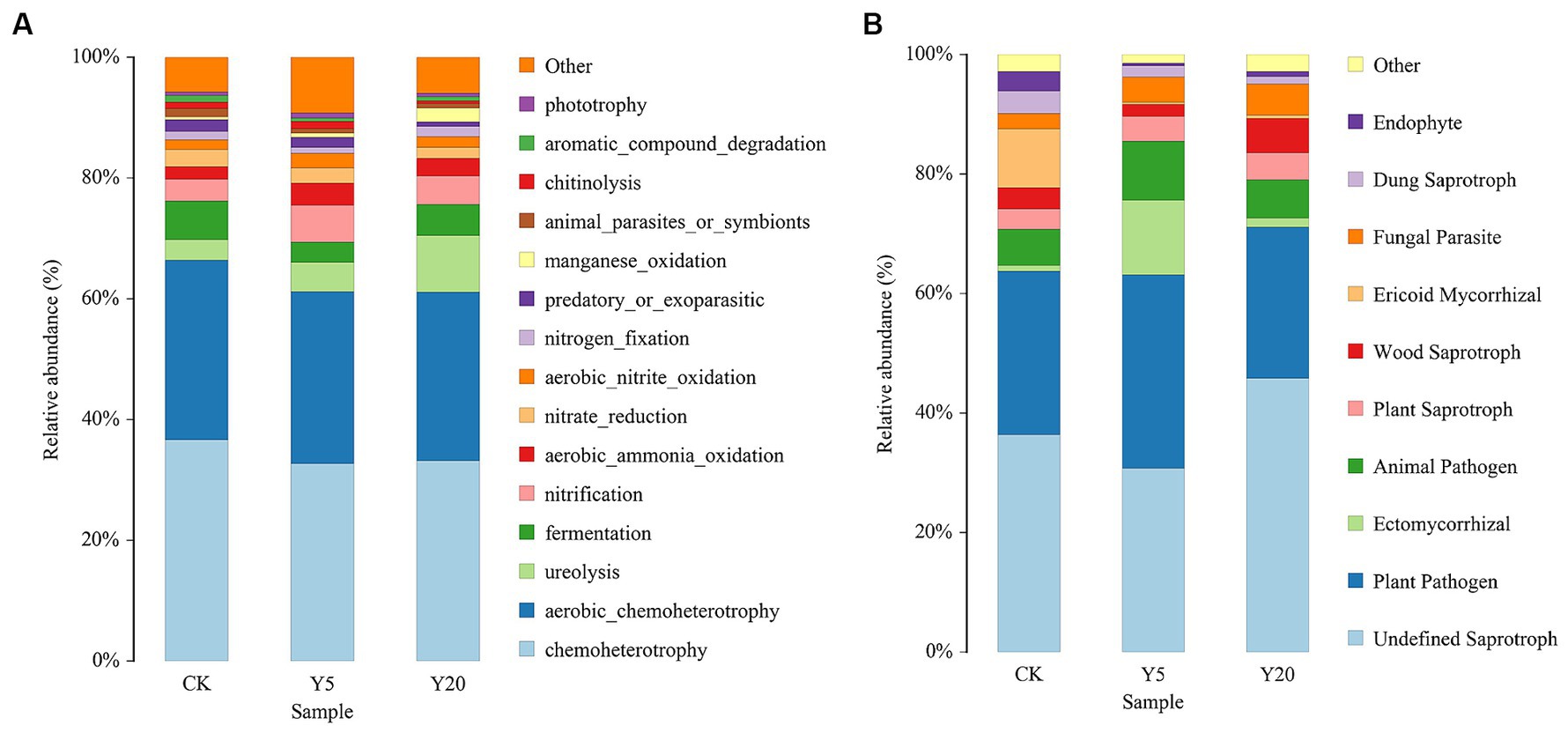
Figure 4. Changes in functional groups on bacterial (A) and fungi (B) OTU data in different continuous tobacco cropping.
To study the functional difference of fungal communities in samples, FUNGuild database was used to perform a functional prediction (Figure 4B). The fungal community trophic types included pathotrophs, saprotrophs, and symbiotrophs. Saprotrophs was dominated in CK accounting for 36% with plant pathogen accounting for 26% of the total community. Plant-pathogens were increased and then decreased with the duration of continuous cropping. Ectomycorrhizal were significantly increased in Y5 accounting for 13% compared to all the other treatment groups.
Analysis of metabolites in soil
A total of 823 metabolites were detected through GC–MS, and 99 were identified. The total ion mass spectra of soil in CK, Y5, and Y20 were basically similar in profile and in peak time. The results indicated that the types of metabolites were similar in different continuous cropping duration. Among the top 30 compounds in relative abundance of metabolites, 14 acids, five alcohols, three sugars, two esters, two phenols, and six other complex compounds were detected (Figure 5A). As shown in Figure 5B, we observed that the samples contained acidic component as palmitic acid, 3-hydroxypropionic acid, stearic acid, hippuric acid, 2-ketobutyric acid, lactic acid, 4-acetylbutyric acid, glycine, 3-aminoisobutyric acid, citrulline, sulfuric acid, citraconic acid, valine, and cycloleucine. With the lengthening of continuous cropping, the content of 3-hydroxypropionic acid increased significantly, and that of palmitic acid and stearic acid decreased rapidly and then increased significantly. The other compounds showed no significant change in CK, Y5, and Y20.
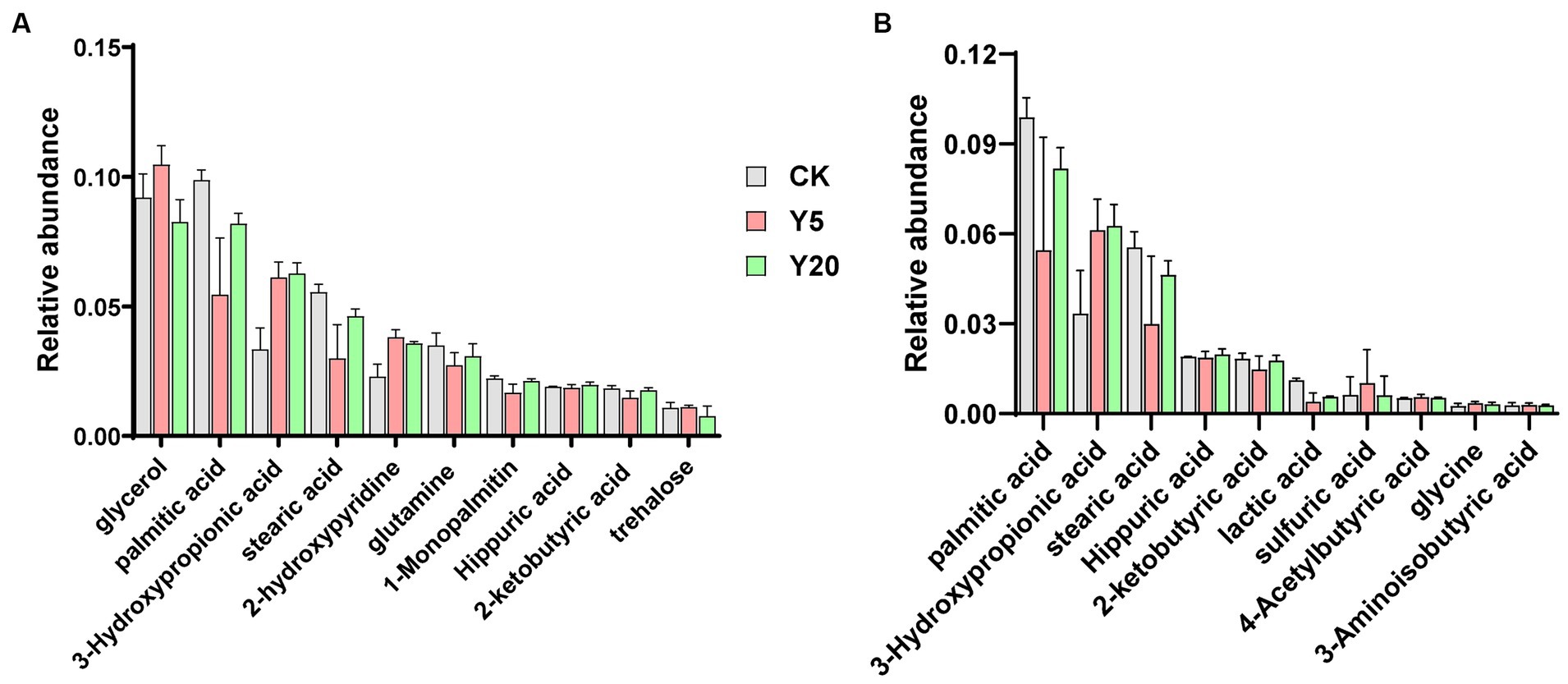
Figure 5. The relative abundances of metabolites (A) and acid compounds (B) in different soils with the lengthening of continuous cropping.
One trait influencing rhizosphere microbial changed could be soil micro-environment. To address this hypothesis, a correlation analysis between the rhizosphere microorganisms whose abundance markedly changed at the genus level and metabolites in soil was performed. The results of the correlation analysis were shown in Figure 6. The results revealed that pH and AN significantly correlated with bacteria. Meanwhile, 1-Monopalmitin significantly correlated with fungi.
Discussion
Soil microbial communities are essential for plant nutrition and soil health (Fitzpatrick et al., 2018). However, long-term continuous cropping system breaks the balance of soil microbial community structure. Few scholars have evaluated the effects of long-term continuous cropping in karst agroecosystems of Guizhou province, China. Continuous cropping mode may decrease the nutrient ratio of soil and increase the incidence of pests, leading to poor crop growth and decreased yield and quality (An et al., 2022). The extensive use of pesticides brings safety issues and soil environmental pollution during agricultural production and seriously harms the health of soil ecosystems (Jiang et al., 2022). However, information on the ecological interactions between soil microbiomes and the establishment of disease-suppressive systems is still scarce. Therefore, clarifying the impact of long-term continuous cropping on karst agroecosystems may provide new avenues for managing microbiome-driven soil self-recovery.
Soil microbial community changes
High-throughput sequencing revealed that bacterial community increased with the increase in continuous cultivation years, consistent with the findings of some studies on other crop species (Zhang et al., 2017; Fei et al., 2021; Jin et al., 2021; Yao et al., 2023). Specifically, the bacterial community composition was dominated by Proteobacteria, Acidobacteria, Actinobacteria, and Gemmatimonadetes at the bacterial phylum level. The abundance of Proteobacteria was increased, which may change the ecological function of soil. Proteobacteria are a broad phylum and can grow fast in soils with unstable substrate conditions (Zeng et al., 2017). The increase in easily decomposable nutrients can stimulate the increase in the abundance of Proteobacteria. Acidobacteria have a wide range of metabolic and genetic functions and exhibit a robust inverse response to soil pH (Wang et al., 2016). Interestingly, the abundance of Acidobacteria increased and then decreased as soil pH decreased in our study. Copiotrophic microbes are regarded to be related to R-strategy (Fierer et al., 2007). The theory of microbial nutrition strategies assists in comprehending our results. In our study, the relative abundances of Sphingomonas and Massilia were significantly higher in Y20 than in other groups, whereas those of Gemmatimonas, Micrococcaceae, and Micromonosporaceae showed the opposite trends. Sphingomonas can survive under nutrient conditions and is essential in decomposing recalcitrant organic matters. It has exploitable potential in ecological environment restoration and will be a hot spot in the microbial restoration of the environment in the future. Massilia presents in rhizosphere soil as symbiotic bacterium (Cardinale et al., 2019). In this study, the RDA results indicated that the AP content was positively correlated with the relative abundances of Sphingonas and Massilia (Figure 3), and the changes in AN content could indirectly affect their relative abundances. Previous reports have shown that Sphingomonas and Massilia can dissolve phosphorus, and their relative abundances are positively correlated with the activity of phosphatase (Cardinale et al., 2019; Son et al., 2021). We speculate that the increases in the relative abundances of Sphingomonas and Massilia provide more AP for plants and fungi in continuous cropping soil. Gemmatimonas, Micrococcaceae, and Micromonosporaceae are beneficial bacteria that widely exist in soil and play a very important role in the stability of soil ecosystems. In this study, the abundances of Gemmatimonas, Micrococcaceae, and Micromonosporaceae decreased significantly with continuous cropping. We speculated that tobacco roots continuously secreted secondary metabolites, contributing to the colonization of certain microbial species whereas inhibiting that of certain beneficial microbial species in continuous cropping systems (You et al., 2015; Zhang et al., 2020).
We observed that different shifts in soil fungal compositions across different durations of continuous cropping. The abundance and diversity of fungal communities increased with the lengthening of continuous cropping in alpha diversity. The dominant genera in all groups were Mortierella, Fusarium, Byssochlamys, Chaetomium, Penicillium, and Cladosporium. Among them, Mortierella is a beneficial fungus with contributions to soil nutrient transformation and availability (Ning et al., 2020). Adding Mortierella to soil can significantly increase the contents of AP, potassium, AK, magnesium, and boron (Francioli et al., 2016). Chaetomium is an important fungus with a biocontrol effect, contributing to the decomposition of cellulose and residing within various plant residues and soils (Hubbard et al., 2011). Byssochlamys, Fusarium, and Cladosporium are plant pathogens. Fusarium is a widely reported pathogenic fungus; plants infected with it can wilt, which will be exacerbated by continuous cropping (Sant'Ana et al., 2009; Wang et al., 2015). Mildly acidic conditions are suitable for the growth of Byssochlamys, which has a broad host spectrum and is pathogenic to many plants (Sant'Ana et al., 2009). The relative abundance of Fusarium tends to decrease and increase subsequently with the lengthening of continuous strawberry cropping (Huang et al., 2018). In this study, the relative abundances of potential phytopathogens (Byssochlamys and Fusarium) decreased and then increased with the duration of continuous cropping. Continuous soybean cropping increases the relative abundance of beneficial fungi, such as Mortierella sp., to inhibit plant pathogens (Liu et al., 2020). In this study, the relative abundance of the pathogenic fungus Mortierella was increased with the duration of continuous cropping. We speculated that tobacco root exudates recruited beneficial fungi to compete with pathogenic microbiomes under long-term continuous cropping. Overall, potential plant pathogenic fungi and beneficial microorganisms showed synergistic increases under long-term continuous cropping.
Soil microbial community response to environmental drivers
Previous studies have shown that long-term continuous cropping leads to the deterioration of soil chemical properties and decreased plant yields (Liu Q. et al., 2021). Continuous cropping obstacles may be associated with changes in soil pH and nitrogen content. The decreased pH level reduces the availability of soil nutrients and interferes with nutrient absorption, impeding normal crop development (Huang et al., 2018). Continuous cropping obstacles may be attributable to the decreased soil pH (Zhang et al., 2015). This study demonstrated that the soil pH level was negatively associated with the AN content, which agrees well with a previous study (Zhang and Lin, 2009). The contents of AP and AN after continuous soybean cropping for 13 years were significantly higher than those after 3 or 5 years. Soil nutrients exhibited no decrease after long-term continuous cropping, indicating that the lack of plant nutrients may not directly cause plant diseases (Mbanyele et al., 2022).
Furthermore, soil microbial communities were affected by environmental factors, including soil pH levels and the contents of AN and AP. The RDA and correlation heatmap were used to analyze the relationships between soil microbial composition and environmental factors (Figure 3). Previous studies have demonstrated that environmental factors have different effects on bacterial and fungal communities (Dong et al., 2017). This study found that the effects of environmental factors on bacterial diversity were more dramatic than fungal diversity. Among the bacteria, the abundance of Micromonosporaceae was positively correlated with the pH level and AK content, that of Acidobacteriales was positively correlated with the AN content, and that of Massilia was negatively correlated with the pH and AK content. Among the fungi, the abundance of Alternaria was positively correlated with the pH and AK content, that of Cladosporium was positively correlated with the SOC content, and that of Mortierella was positively correlated with the AP content. In summary, the pH level and AK content had the greatest influence on the soil microbial community structure, while the AP content significantly influenced the formation of the fungal community structure.
Soil microbial function predictions
Different microbial functional communities were formed at different successional stages (Liu S. et al., 2021). The abundances of different bacterial metabolic pathways were predicated using the FAPROTAX database. We observed significant increases in ureolysis and manganese oxidation and significant decreases in nitrate reduction, predatory or ectoparasitic, and chitinolysis with the duration of continuous cropping. According to the nutrient competition hypothesis, the growth and reproduction of mycorrhizal fungi and free microorganisms need nutrients (Orwin et al., 2011). Previous studies have reported that ectomycorrhizal and plant pathogens can secrete enzymes to degrade organic matter, which inhibits the nitrogen restriction of free microorganisms, thus inhibiting organic matter decomposition (Cederlund et al., 2014; Averill, 2016). We found that the abundances of plant-pathogens and ectomycorrhiza increased and then decreased, which may be caused by the interactions of ectomycorrhizal and plant pathogens with free microorganisms. Finally, we concluded that fungal changes might alter the soil nutrient cycles in karst ecosystems under long-term continuous cropping.
Analysis of metabolites in soil
The deterioration of soil chemical properties was considered to be associated with long-term continuous cropping crops (Xie et al., 2017). It was reported that crop released a great variety of secondary metabolites into the soil environment through root exudation or residue decomposition (Liu et al., 2018). The allelochemicals have been proposed that can improve the activities of soil-borne pathogens, which are released by the crops (Bonanomi et al., 2016). In our finding, the soil pH decreased significantly with the duration of continuous cropping increased, which is consistent with previous studies (Chen et al., 2022). The accumulation of acids might cause soil acidification (Agegnehu et al., 2016). There had differences in metabolites in soil among different periods of continuous cropping in this finding. The content of palmitic acid and stearic acid increased initially and then decreased as the cropping continued. The palmitic acid influenced plant growth and metabolism, which have found in high levels in many plant root exudates and residues (Ma et al., 2021; Xin et al., 2022). Previous studies have proved that palmitic acid and stearic acid can significantly inhibit the germination of seeds and the growth of seedlings (Xiang et al., 2022). In the process of penetration and colonization, fungi can secret an array of extracellular hydrolytic enzymes, which can degrade cell walls of roots, secreting substantial quantities of organic acids (Gu et al., 2022). In this study, we used GC-TOF-MS to analyze secondary metabolites in soil and found many organic acids and their derivatives. Whether those metabolites are root exudates or secreted by microorganisms remains to be further verified. In addition, our work has some limitations, and additional studies are needed to investigate the dynamic changes in soil metabolites and their relations with soil microorganisms. These finding could be used to reveal the mechanism of soil sickness associated with long-term continuous cropping crops.
Conclusion
In this study, we demonstrated that continuous cropping system exerted a significant impact on the microbial community structure, exhibiting discernible disparities between long-term and short-term continuous cropping farmland. Furthermore, the soil pH level as well as AN content and SOC content were key environmental factors contributing to microbial community structural and functional changes. Long-term continuous cropping fostered synergistic increments in potential plant pathogenic fungi and beneficial microorganisms. However, further research is warranted to investigate whether and how pathogenic and beneficial microorganisms interact with plant secondary metabolites requires.
Data availability statement
The datasets presented in this study can be found in online repositories. The names of the repository/repositories and accession number(s) can be found in the article/Supplementary material.
Author contributions
MD: Conceptualization, Data curation, Writing – original draft. HD: Writing – review & editing, Conceptualization, Validation. YH: Funding acquisition, Methodology, Validation, Writing – review & editing. TL: Formal analysis, Validation, Writing – review & editing. ZZ: Formal analysis, Supervision, Writing – review & editing. SZ: Project administration, Supervision, Writing – review & editing. BH: Writing – review & editing, Data curation, Formal analysis. HC: Writing – review & editing, Data curation, Methodology. BD: Methodology, Validation, Writing – review & editing. YX: Writing – review & editing, Formal analysis, Investigation. YZ: Funding acquisition, Supervision, Writing – review & editing.
Funding
The author(s) declare that financial support was received for the research, authorship, and/or publication of this article. This study was funded by Guizhou Provincial Science and Technology Plan Project (Grant No. ZK [2023]116), the Science and Technology Project of Guizhou Tobacco Company (Grant Nos. 2021XM21 and 2024XM17), the Science and Technology Project of Guizhou Tobacco Company Bijie Company (Grant No. 2023520500240161) and Zunyi Company (Grant No. 2022XM06), and the Science and Technology Project of Yunnan Tobacco Company (Grant No. 2021530000241035).
Conflict of interest
YH, HC, and BD were employed by Guizhou Tobacco Company Bijie Region Tobacco Company.
The remaining authors declare that the research was conducted in the absence of any commercial or financial relationships that could be construed as a potential conflict of interest.
Publisher’s note
All claims expressed in this article are solely those of the authors and do not necessarily represent those of their affiliated organizations, or those of the publisher, the editors and the reviewers. Any product that may be evaluated in this article, or claim that may be made by its manufacturer, is not guaranteed or endorsed by the publisher.
Supplementary material
The Supplementary material for this article can be found online at: https://www.frontiersin.org/articles/10.3389/fmicb.2024.1374550/full#supplementary-material
References
Agegnehu, G., Nelson, P. N., and Bird, M. I. (2016). The effects of biochar, compost andtheir mixture and nitrogen fertilizer on yield and nitrogen use efficiency ofbarley grownon a nitisol in the highlands ofEthiopia. Sci. Total Environ. 569-570, 869–879. doi: 10.1016/j.scitotenv.2016.05.033
Ali, A., Ghani, M. I., Elrys, A. S. E., Ding, H. Y., Iqbal, M., Cheng, Z. H., et al. (2021). Different cropping systems regulate the metabolic capabilities and potential ecological functions altered by soil microbiome structure in the plastic shed mono-cropped cucumber rhizosphere. Agric. Ecosyst. Environ. 318:107486. doi: 10.1016/j.agee.2021.107486
An, S., Wei, Y., Li, H., Zhao, Z., Hu, J., Philp, J., et al. (2022). Long-term monocultures of American ginseng change the rhizosphere microbiome by reducing phenolic acids in soil. Agriculture 12:640. doi: 10.3390/agriculture12050640
Averill, C. (2016). Slowed decomposition in ectomycorrhizal ecosystems is independent of plant chemistry. Soil Biol. Biochem. 102, 52–54. doi: 10.1016/j.soilbio.2016.08.003
Avidano, L., Gamalero, E., Cossa, G. P., and Carraro, E. (2005). Characterization of soil health in an Italian polluted site by using microorganismsas bioindicators. Appl. Soil Ecol. 30, 21–33. doi: 10.1016/j.apsoil.2005.01.003
Bolger, A. M., Lohse, M., and Usadel, B. (2014). Trimmomatic: a flexible trimmer for Illumina sequence data. Bioinformatics 30, 2114–2120. doi: 10.1093/bioinformatics/btu170
Bonanomi, G., de Filippis, F., Cesarano, G., La Storia, A., Ercolini, D., and Scala, F. (2016). Organic farming induces changes in soil microbiota that affect agro-ecosystem functions. Soil Biol. Biochem. 103, 327–336. doi: 10.1016/j.soilbio.2016.09.005
Caporaso, J. G., Lauber, C. L., Walters, W. A., Berg-Lyons, D., Lozupone, C. A., Turnbaugh, P. J., et al. (2011). Global patterns of 16S rRNA diversity at a depth of millions of sequences per sample. Proc. Natl. Acad. Sci. USA 108, 4516–4522. doi: 10.1073/pnas.1000080107
Cardinale, M., Suarez, C., Steffens, D., Ratering, S., and Schnell, S. (2019). Effect of different soil phosphate sources on the active bacterial microbiota is greater in the rhizosphere than in the endorhiza of barley (Hordeum vulgare L.). Microb. Ecol. 77, 689–700. doi: 10.1007/s00248-018-1264-3
Cederlund, H., Wessén, E., Enwall, K., Jones, C. M., Juhanson, J., Pell, M., et al. (2014). Soil carbon quality and nitrogen fertilization structure bacterial communities with predictable responses of major bacterial phyla. Appl. Soil Ecol. 84, 62–68. doi: 10.1016/j.apsoil.2014.06.003
Chen, S., Qi, G., Luo, T., Zhang, H., Jiang, Q., Wang, R., et al. (2018). Continuous-cropping tobacco caused variance of chemical properties and structure of bacterial network in soils. Land Degrad. Dev. 29, 4106–4120. doi: 10.1002/ldr.3167
Chen, W., Teng, Y., Li, Z., Liu, W., Ren, W., Luo, Y., et al. (2018). Mechanisms by which organic fertilizer and effective microbes mitigate peanut continuous cropping yield constraints in a red soil of South China. Appl. Soil Ecol. 128, 23–34. doi: 10.1016/j.apsoil.2018.03.018
Chen, X. L., Zhang, D. L., Li, Y. M., Li, H. Y., Lou, J., Li, X. T., et al. (2022). Changes in rhizospheric microbiome structure and soil metabolic function in response to continuous cucumber cultivation. FEMS Microbiol. Ecol. 98, 1–13. doi: 10.1093/femsec/fiac129
Chen, F., Zhou, D., Bai, X., Zeng, C., Xiao, J., Qian, Q., et al. (2018). Responses of soil physical and chemical properties to karst rocky desertification evolution in typical karst valley area. IOP Conf. Ser. Earth Environ. Sci. 108:032012. doi: 10.1088/1755-1315/108/3/032012
Cipollini, D., Rigsby, C. M., and Barto, E. K. (2012). Microbes as targets and mediators of allelopathy in plants. J. Chem. Ecol. 38, 714–727. doi: 10.1007/s10886-012-0133-7
Clauset, A., Newman, M. E., and Moore, C. (2004). Finding community structure in very large networks. Phys. Rev. E Stat. Nonlinear Soft Matter Phys. 70:066111. doi: 10.1103/PhysRevE.70.066111
Dong, L. L., Xu, J., Zhang, L. J., Yang, J., Liao, B. S., Li, X. W., et al. (2017). High-throughput sequencing technology reveals that continuous cropping of American ginseng results in changes in the microbial community in arable soil. Chin. Med. 12:18. doi: 10.1186/s13020-017-0139-8
Edgar, R. C. (2013). UPARSE: highly accurate OTU sequences from microbial amplicon reads. Nat. Methods 10:996. doi: 10.1038/nmeth.2604
Edgar, R. C., Haas, B. J., Clemente, J. C., Quince, C., and Knight, R. (2011). UCHIME improves sensitivity and speed of chimera detection. Bioinformatics 27, 2194–1200. doi: 10.1093/bioinformatics/btr381
Fei, X., Wang, L. N., Chen, J. Y., Meng, F., Wang, G. D., Yan, Y. P., et al. (2021). Variations of microbial community in Aconitum carmichaeli Debx. Rhizosphere soilin a short-term continuous cropping system. J. Microbiol. 59, 481–490. doi: 10.1007/s12275-021-0515-z
Fierer, N., Bradford, M. A., and Jackson, R. B. (2007). Toward an ecological classification of soil bacteria. J. Ecol. 88, 1354–1364. doi: 10.1890/05-1839
Fitzpatrick, C. R., Copeland, J., Wang, P. W., and Guttman, D. (2018). Assembly and ecological function of the root microbiome across angiosperm plant species. Proc. Natl. Acad. Sci. USA 115, 1157–E1165. doi: 10.1073/pnas.1717617115
Francioli, D., Schulz, E., Lentendu, G., Wubet, T., Buscot, F., and Reitz, T. (2016). Mineral vs. organic amendments: microbial community structure, activity and abundance of agriculturally relevant microbes are driven by long-term fertilization strategies. Front. Microbiol. 7:1466. doi: 10.3389/fmicb.2016.01446
Gao, Z., Han, M., Hu, Y., Li, Z., Liu, C., Wang, X., et al. (2019). Effects of continuous cropping of sweet potato on the fungal community structure in rhizospheric soil. Front. Microbiol. 10:2269. doi: 10.3389/fmicb.2019.02269
Gerhards, R., and Schappert, A. (2020). Advancing cover cropping in temperate integrated weed management. Pest Manag. Sci. 76, 42–46. doi: 10.1002/ps.5639
Gu, X., Yang, N., Zhao, Y., Liu, W. H., and Li, T. F. (2022). Long-term watermelon continuous cropping leads to drastic shifts in soil bacterial and fungal community composition across gravel mulch fields. BMC Microbiol. 22:189. doi: 10.1186/s12866-022-02601-2
Huang, Y., Xiao, X., Jing, J. Q., Zhao, H. J., Wang, L., and Long, X. E. (2018). Contrasting beneficial and pathogenic microbial communities across consecutive cropping fields of greenhouse strawberry. Appl. Microbiol. Biotechnol. 102, 5717–5729. doi: 10.1007/s00253-018-9013-6
Hubbard, J. P., Harman, G. E., and Eckenrode, C. (2011). Interaction of a biological control agent, Chaetomium globosum, with seed coat microflora. Can. J. Microbiol. 28, 431–437. doi: 10.1139/m82-065
Jiang, J., Yang, Y., Wang, L., Cao, S., Long, T., and Liu, R. (2022). Effects of Chlorothalonil application on the physio-biochemical properties and microbial Community of a Yellow–Brown Loam Soi. Agriculture 12:608. doi: 10.3390/agriculture12050608
Jiao, S., Yang, Y., Xu, Y., Zhang, J., and Lu, Y. (2020). Balance between community assembly processes mediates species coexistence in agricultural soil microbiomes across eastern China. ISME J. 14, 202–216. doi: 10.1038/s41396-019-0522-9
Jilani, G., Mahmood, S., Nawaz, A., Chaudhry, A. N., Hassan, I., and Akram, M. (2008). Allelochemicals: sources, toxicity and microbial transformation in soil. Ann. Microbiol. 58, 351–357. doi: 10.1007/BF03175528
Jin, L., Lu, J., Jin, N., Xie, J. M., Wu, Y., Zhang, G. B., et al. (2021). Effects of different vegetable rotations on the rhizosphere bacterial community and tomato growth in a continuous tomato cropping substrate. PLoS One 16:e0257432. doi: 10.1371/journal.pone.0257432
Kind, T., Wohlgemuth, G., Lee, D. Y., Lu, Y., Palazoglu, M., Shahbaz, S., et al. (2009). FiehnLib: mass spectral and retention index libraries for metabolomics based on quadrupole and time-of-flight gas chromatography/mass spectrometry. Anal. Chem. 81, 10038–10048. doi: 10.1021/ac9019522
Kui, L., Chen, B., Chen, J., Sharifi, R., Dong, Y., Zhang, Z., et al. (2021). A comparative analysis on the structure and function of the Panax notoginseng rhizosphere microbiome. Front. Microbiol. 12:673512. doi: 10.3389/fmicb.2021.673512
Li, S., Liu, Y., Wang, J., Yang, L., Zhang, S., Xu, C., et al. (2017). Soil acidification aggravates the occurrence of bacterial wilt in South China. Front. Microbiol. 8:703. doi: 10.3389/fmicb.2017.00703
Li, J., Yin, X. H., Liu, Z. K., Gu, Z. L., and Niu, J. X. (2019). Reaction yield model of nitrocellulose alkaline hydrolysis. J. Hazard. Mater. 371, 603–608. doi: 10.1016/j.jhazmat.2019.03.044
Liu, J. L., Li, S. Q., Yue, S. C., Tian, J. Q., Chen, H., Jiang, H. B., et al. (2021). Soil microbial community and network changes after long-term use of plastic mulch and nitrogen fertilization on semiarid farmland. Geoderma 396:115086. doi: 10.1016/j.geoderma.2021.115086
Liu, Z., Liu, J., Yu, Z., Yao, Q., Li, Y., Liang, A., et al. (2020). Long-term continuous cropping of soybean is comparable to crop rotation in mediating microbial abundance, diversity and community composition. Soil Tillage Res. 197:104503. doi: 10.1016/j.still.2019.104503
Liu, Q., Wang, S., Li, K., Qiao, J., Guo, Y., Liu, Z., et al. (2021). Responses of soil bacterial and fungal communities to the long-term monoculture of grapevine. Appl. Microbiol. Biotechnol. 105, 7035–7050. doi: 10.1007/s00253-021-11542-1
Liu, S., Wang, Z. Y., Niu, J. F., Dang, K. K., Zhang, S. K., and Wang, S. Q. (2021). Changes in physicochemical properties, enzymatic activities, and the microbial community of soil significantly influence the continuous cropping of Panax quinquefolius L. (American ginseng). Plant Soil 463, 427–446. doi: 10.1007/s11104-021-04911-2
Liu, J. K., Yan, Z. Q., Li, X. Z., Jin, H., Yang, X. Y., Xie, M., et al. (2018). Characterization of allelochemicals from the rhizosphere soil of Pinellia ternate (Thnub.) and their inhibition activity on protective enzymes. Appl. Soil Ecol. 125, 301–306. doi: 10.1016/j.apsoil.2018.01.001
Looft, T., Johnson, T., Allen, H., Bayles, D. O., Alt, D. P., Stedtfeld, R. D., et al. (2012). In-feed antibiotic effects on the swine intestinal microbiome. Proc. Natl. Acad. Sci. USA 109, 1691–1696. doi: 10.1073/pnas.1120238109
Louca, S., Parfrey, L. W., and Doebeli, M. (2016). Decoupling function and taxonomy in the global ocean microbiome. Science 353, 1272–1277. doi: 10.1126/science.aaf4507
Ma, T., He, X., Chen, S., Li, Y., Huang, Q., Xue, C., et al. (2022). Long-term organic–inorganic fertilization regimes alter bacterial and fungal communities and rice yields in paddy soil. Front. Microbiol. 13:890712. doi: 10.3389/fmicb.2022.1075041
Ma, K. X., Kou, J. M., Rahman, M. K. U., Du, W. T., Liang, X. Y., Wu, F. Z., et al. (2021). Palmitic acid mediated change of rhizosphere and alleviation of fusarium wilt disease in watermelon. Saudi J. Biol. Sci. 28, 3616–3623. doi: 10.1016/j.sjbs.2021.03.040
Maguire, V. G., Bordenave, C. D., Nieva, A. S., Llames, M. E., and Ruiz, O. A. (2020). Soil bacterial and fungal community structure of a rice monoculture and rice-pasture rotation systems. Appl. Soil Ecol. 151:103535. doi: 10.1016/j.apsoil.2020.103535
Matheri, F., Kambura, A., Mwangi, M., Karanja, E., Adamtey, N., Wanjau, K., et al. (2023). Evolution of fungal and non-fungal eukaryotic communities in response to thermophilic co-composting of various nitrogen-rich green feedstocks. PLoS One 18:e0286320. doi: 10.1371/journal.pone.0286320
Mbanyele, V., Mtambanengwe, F., Nezomba, H., Rurinda, J., and Mapfumo, P. (2022). Conservation agriculture in semi-arid Zimbabwe: a promising practice to improve finger millet (Eleusine coracana Gaertn.) productivity and soil water availability in the short term. Agriculture 12:622. doi: 10.3390/agriculture12050622
Nilsson, R. H., Larsson, K. H., Taylor, A. F. S., Bengtsson-Palme, J., Jeppesen, T. S., Schigel, D., et al. (2019). The UNITE database for molecular identification of fungi: handling dark taxa and parallel taxonomic classifications. Nucleic Acids Res. 47, D259–D264. doi: 10.1093/nar/gky1022
Ning, Q., Chen, L., Jia, Z. J., Zhang, C. Z., Ma, D. H., Li, F., et al. (2020). Multiple long-term observations reveal a strategy for soil pH-dependent fertilization and fungal communities in support of agricultural production. Agric. Ecosyst. Environ. 293:106837. doi: 10.1016/j.agee.2020.106837
Niu, J., Chao, J., Xiao, Y., Chen, W., and Dai, L. (2017). Insight into the effects of different cropping systems on soil bacterial community and tobacco bacterial wilt rate. J. Basic Microbiol. 57, 3–11. doi: 10.1002/jobm.201600222
Oksanen, J., Blanchet, F.G., Kindt, R., Legendre, P., Minchin, P.R., O’Hara, R.B., et al. (2020). Vegan: community ecology package.
Orwin, K. H., Kirschbaum, M. U. F., John, M. G. S., and Dickie, I. A. (2011). Organic nutrient uptake by mycorrhizal fungi enhances ecosystem carbon storage: a model-based assessment. Ecol. Lett. 14, 493–502. doi: 10.1111/j.1461-0248.2011.01611.x
Pu, P., Zhang, M., and Zhang, L. N. (2014). A study on temperature and time conditions of colorimetric method in measuring soil available phosphorus. Adv. Mrter. Res. 838, 2047–2051. doi: 10.4028/www.scientific.net/AMR.838-841.2047
Ramette, A. (2007). Multivariate analyses in microbial ecology. FEMS Microbiol. Ecol. 62, 142–160. doi: 10.1111/j.1574-6941.2007.00375.x
Sant'Ana, A. S., Rosenthal, A., and Massaguer, P. R. (2009). Heat resistance and the effects of continuous pasteurization on the inactivation of Byssochlamys fulva ascospores in clarified apple juice. J. Appl. Microbiol. 107, 197–209. doi: 10.1111/j.1365-2672.2009.04195.x
Sierro, N., Battey, J. N., Ouadi, S., Bakaher, N., Bovet, L., Willig, A., et al. (2014). The tobacco genome sequence and its comparisonwith those of tomato and potato. Nat. Commun. 5:3833. doi: 10.1038/ncomms4833
Son, J. W., Lee, H., Kim, M., Kim, D. U., and Ka, J. O. (2021). Massilia aromaticivorans sp. nov., a BTEX degrading bacterium isolated from arctic soil. Curr. Microbiol. 78, 2143–2150. doi: 10.1007/s00284-021-02379-y
R Core Team. (2019). R: a language and environment for statistical computing. R Found. Stat. Comput. 1, 12–21. Available at: https://www.r-project.org/index.html
Wang, B., Li, R., Ruan, Y., Ou, Y., Zhao, Y., and Shen, Q. (2015). Pineapple-banana rotation reduced the amount of fusarium oxysporum more than maize-banana rotation mainly through modulating fungal communities. Soil Biol. Biochem. 86, 77–86. doi: 10.1016/j.soilbio.2015.02.021
Wang, Y., Su, L., Huang, S., Bo, C., Yang, S., Li, Y., et al. (2016). Diversity and resilience of the wood-feeding higher termite Mironasutitermes shangchengensis gut microbiota in response to temporal and diet variations. Ecol. Evol. 6, 8235–8242. doi: 10.1002/ece3.2497
Ward, T., Larson, J., Meulemans, J., Hillmann, B., Lynch, J., Sidiropoulos, D., et al. (2017). BugBase predicts organism level microbiome phenotypes. bioRxiv. doi: 10.1101/133462
Watanabe, F. S., and Olsen, S. R. (1965). Test of an ascorbic acid method for determining phosphorus in water and NaHCO3 extracts from soil. Soil Sci. Soc. Am. J. 29, 677–678. doi: 10.2136/sssaj1965.03615995002900060025x
Xiang, W., Chen, J. H., Zhang, F. Y., Huang, R. S., and Li, L. B. (2022). Autotoxicity in Panax notoginseng of root exudatesand their allelochemicals. Front. Plant Sci. 13:1020626. doi: 10.3389/fpls.2022.1020626
Xie, X., Pu, L., Wang, Q., Zhu, M., Xu, Y., and Zhang, M. (2017). Response of soil physicochemical properties and enzyme activities to long-term reclamation of coastal saline soil, eastern China. Sci. Total Environ. 607-608, 1419–1427. doi: 10.1016/j.scitotenv.2017.05.185
Xin, A., Jin, H., Yang, X., Guan, J., Hui, H., Liu, H., et al. (2022). Allelochemicals from the rhizosphere soil of potato (Solanum tuberosum L.) and their interactions with the soilborne pathogens. Plants 11:1934. doi: 10.3390/plants11151934
Xiong, W., Li, Z., Liu, H., Xue, C., Zhang, R., Wu, H., et al. (2015). The effect of long-term continuous cropping of black pepper on soil bacterial communities as determined by 454 pyrosequencing. PLoS One 10:e0136946. doi: 10.1371/journal.pone.0136946
Yan, X., Guo, S., Gao, K., Sun, S., Yin, C., and Tian, Y. (2023). The impact of the soil survival of the pathogen of fusarium wilt on soil nutrient cycling mediated by microorganisms. Microorganisms 11:2207. doi: 10.3390/microorganisms11092207
Yao, J., Wu, C. Y., Fan, L. J., Kang, M. H., Liu, Z. R., Huang, Y. H., et al. (2023). Effects of the long-term continuous cropping of yongfeng yam on the bacterial community and function in the rhizospheric soil. Microorganisms 11:274. doi: 10.3390/microorganisms11020274
You, J., Liu, X., Zhang, B., Xie, Z., Hou, Z., and Yang, Z. (2015). Seasonal changes in soil acidity and related properties in ginseng artificial bed soils under a plastic shade. J. Ginseng Res. 39, 81–88. doi: 10.1016/j.jgr.2014.08.002
Zeng, Q., An, S., and Yang, L. (2017). Soil bacterial community response to vegetation succession after fencing in the grassland of China. Sci. Total Environ. 609, 2–10. doi: 10.1016/j.scitotenv.2017.07.102
Zhang, Y. Z., Bo, G. D., Shen, M. C., Shen, G. M., Yang, J. M., Dong, S. Y., et al. (2022). Differences in microbial diversity and environmental factors in ploughing-treated tobacco soil. Front. Microbiol. 13:924137. doi: 10.3389/fmicb.2022.924137
Zhang, J., Fan, S., Qin, J., Dai, J., and Hu, X. (2020). Changes in the microbiome in the soil of an american ginseng continuous plantation. Front. Plant Sci. 11:572199. doi: 10.3389/fpls.2020.572199
Zhang, S., Li, X., Chen, K., Shi, J. M., Wang, Y., Luo, P. Y., et al. (2022). Long-term fertilization altered microbial community structure in an aeolian sandy soil in Northeast China. Front. Microbiol. 13:979759. doi: 10.3389/fmicb.2022.979759
Zhang, Z. Y., and Lin, W. X. (2009). Continuous cropping obstacle and allelopathic autotoxicity of medicinal plants. Chin. J. Eco-Agric. 17, 189–196. doi: 10.3724/SP.J.1011.2009.00189
Zhang, G. K., Song, X. Y., Liu, S. T., Nan, Z. W., and Jiang, W. (2015). Effect of long-term application on nitrogen utilization and yield quality of wheat and maize. Acta Agric. Boreali Sin. 30, 157–161. doi: 10.7668/hbnxb.2015.04.027
Keywords: continuous-cropping system, environmental factors, microbial community, nutrient cycling, rhizospheric soil
Citation: Ding M, Dai H, He Y, Liang T, Zhai Z, Zhang S, Hu B, Cai H, Dai B, Xu Y and Zhang Y (2024) Continuous cropping system altered soil microbial communities and nutrient cycles. Front. Microbiol. 15:1374550. doi: 10.3389/fmicb.2024.1374550
Edited by:
Xingjia Xiang, Anhui University, ChinaReviewed by:
Shuzhen Li, Institute of Urban Environment (CAS), ChinaRouhallah Sharifi, Razi University, Iran
Copyright © 2024 Ding, Dai, He, Liang, Zhai, Zhang, Hu, Cai, Dai, Xu and Zhang. This is an open-access article distributed under the terms of the Creative Commons Attribution License (CC BY). The use, distribution or reproduction in other forums is permitted, provided the original author(s) and the copyright owner(s) are credited and that the original publication in this journal is cited, in accordance with accepted academic practice. No use, distribution or reproduction is permitted which does not comply with these terms.
*Correspondence: Yadong Xu, xyd2020@zzu.edu.cn; Yanling Zhang, zhangyanling@ztri.com.cn
†These authors have contributed equally to this work