- 1Key Laboratory of Artificial Organs and Computational Medicine in Zhejiang Province, Key Laboratory of Pollution Exposure and Health Intervention of Zhejiang Province, Shulan International Medical College, Zhejiang Shuren University, Hangzhou, Zhejiang, China
- 2Department of Microbiology School of Biological Sciences, Quaid-i-Azam University, Islamabad, Pakistan
- 3College of Biology and Environmental Engineering, Zhejiang Shuren University, Hangzhou, Zhejiang, China
- 4School of Stomatology, Zhejiang Chinese Medical University, Hangzhou, China
- 5Department of Computer Science and Engineering, University of Notre Dame, Notre Dame, IN, United States
The oral cavity stands as one of the pivotal interfaces facilitating the intricate interaction between the human body and the external environment. The impact of diverse oral microorganisms on the emergence and progression of various systemic cancers, typified by oral cancer, has garnered increasing attention. The potential pathogenicity of oral bacteria, notably the anaerobic Porphyromonas gingivalis and Fusobacterium nucleatum, has been extensively studied and exhibits obvious correlation with different carcinoma types. Furthermore, oral fungi and viruses are closely linked to oropharyngeal carcinoma. Multiple potential mechanisms of oral microbiota-induced carcinogenesis have been investigated, including heightened inflammatory responses, suppression of the host immune system, influence on the tumor microenvironment, anti-apoptotic activity, and promotion of malignant transformation. The disturbance of microbial equilibrium and the migration of oral microbiota play a pivotal role in facilitating oncogenic functions. This review aims to comprehensively outline the pathogenic mechanisms by which oral microbiota participate in carcinogenesis. Additionally, this review delves into their potential applications in cancer prevention, screening, and treatment. It proves to be a valuable resource for researchers investigating the intricate connection between oral microbiota and systemic cancers.
1 Introduction
Currently, cancer is the most concerning disease in humans, with over 100 different known cancers affecting almost all regions of the body. Some well-studied periodontal microorganisms have become the focus of the association between oral microbiota dysbiosis and cancer development (Sun et al., 2020). The oral cavity constitutes one of the four major bacterial reservoirs in the human body, harboring a substantial abundance of microorganisms under normal physiological conditions. This configuration gives rise to a multifaceted microecological environment. In the physiological state of the human body, a profusion of microorganisms congregates, culminating in the creation of a complex microecological milieu (Xia et al., 2023). Oral microbiota comprises a consortium of microorganisms specifically adapted to inhabit the human mouth. The majority of these microorganisms engage in microbial physiological functions through coalescing on oral surfaces to create biofilms. With over 700 bacterial species colonizing the oral cavity, it constitutes one of the most intricate microbial communities within the human body.
These microbial communities inhabit a variety of niches, primarily including the mucous membranes, the rear of the tongue, dental structures, periodontal pockets, and the ever-changing realm of saliva. These oral microorganisms hold the potential to profoundly influence the initiation and progression of malignancies. This influence is enacted through mechanisms such as provoking chronic inflammation, generating carcinogenic metabolites, triggering epithelial-mesenchymal transition (EMT), modulating cellular apoptosis, and fomenting cellular proliferation.
Moreover, certain investigations have discerned that oral microorganisms can exert direct sway over host cell responses. Notably, Clostridium emerged with heightened transcriptional activity within oral squamous cell carcinoma (OSCC), surpassing other bacterial constituents. Selenomonas and Prevotella trailed closely, showcasing amplified activity within the OSCC context. Conversely, Bacillus, Diplococcus and Neisseria exhibited elevated activity within healthy sites. These findings intimate a potential correlation between oral microorganisms and cancer progression (Yost et al., 2018; Table 1). The purpose of this review is to explore the association between oral microbiota and systemic cancer, in order to have a positive impact on patient health in clinical practice.
2 Enhancing oral microbiota’s role in OSCC carcinogenesis
2.1 The role of oral bacteria in advancing OSCC
OSCC stands as the predominant malignancy within the head and neck domain, encapsulating over 90% of oral cancer cases. Despite therapeutic strides, patient survival remains dishearteningly low, and prognosis remains grim. For those diagnosed at advanced stages, the 5-year survival rate languishes below 50% (Manosha et al., 2016). The genesis of oral cancer is a nuanced interplay of multifarious factors. Conventional culprits like tobacco and alcohol endure as primary risk catalysts for OSCC. Equally implicated are compromised oral hygiene and dietary improprieties. Adding to this spectrum is the role played by the oral microbiota, contributing to a convergence of factors influencing the emergence of OSCC (Figure 1).
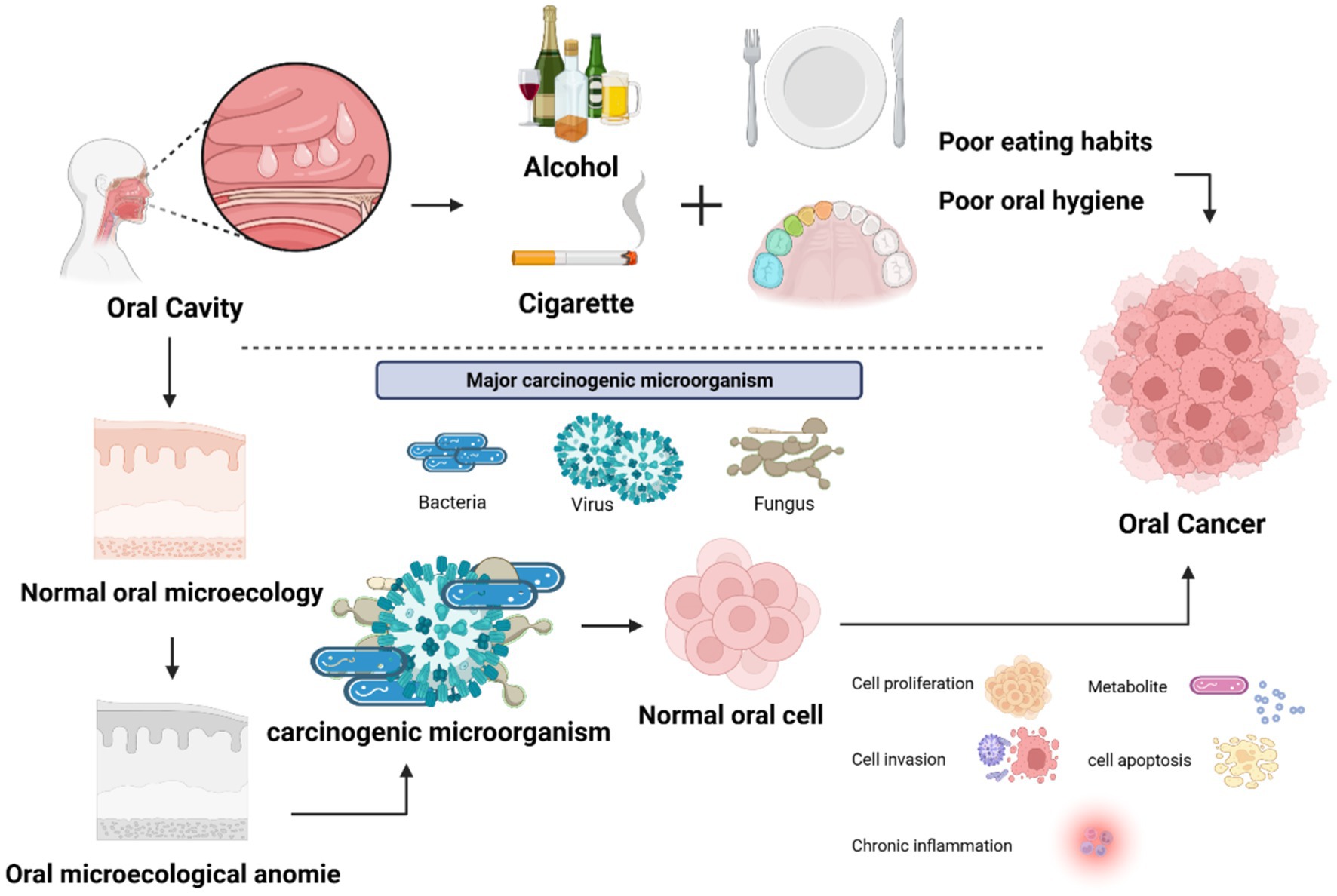
Figure 1. Factors influencing the occurrence and development of oral cancer. The factors that cause the occurrence and development of oral cancer: alcohol, cigarettes, poor oral hygiene or eating habits, oral microorganisms.
The association between oral microbiota and OSCC is robust. A slew of oral bacteria aligns closely with OSCC, the roster encompassing the likes of Clostridium, Prevotella, Porphyromonas, Actinomyces, Clostridium, Haemophilus, Streptococcus, and Enterobacteriaceae (Nagy et al., 1998). Within the oral Microorganism, several anaerobic strains are believed to be involved in the carcinogenic process (Sun et al., 2020). Among these, the prevalence of Porphyromonas gingivalis (P. gingivalis) and Clostridium proved to be significantly elevated in OSCC instances compared to their benign mucosal counterparts. Intriguingly, the survival rate of OSCC patients was correlated with the localization of P. gingivalis within tumor tissues (Nagy et al., 1998; Wen et al., 2020). Lactobacillus has a dual role in the development and advancement of OSCC. On one hand, it triggers apoptosis and enhances the expression of genes that suppress tumor growth. However, on the other hand, it also produces metabolites that have the potential to promote the formation and progression of cancer. This creates a complex interplay that influences the onset and advancement of OSCC (Karpinski, 2019). Furthermore, Pseudomonas aeruginosa is also involved in OSCC carcinogenesis. The bacterial endotoxins, such as Lipopolysaccharide (LPS), in conjunction with structural components like Flagella, play a role in shaping the organism’s inflammatory environment. They initiate inflammation by activating the NF-κB signaling pathway and also play a role in a complex chemical process. In this process, nitrite reductase transforms nitrite into nitric oxide, resembling a carefully choreographed chemical performance. The organism has the ability to secrete the LasI factor, which subsequently results in the suppression of a tumor-suppressing protein known as E-cadherin (Chattopadhyay et al., 2019; Cheng, 2020; Kakabadze et al., 2020).
2.2 Fungi and virus
Apart from bacteria, the oral terrain also harbors other microbial agents that can potentially drive OSCC, encompassing fungi, viruses and more. Notably, Candida emerges as a preeminent fungus in this milieu, a symbiotic opportunist that coexists with the host. Candida albicans (C. albicans), a prevailing presence within the Candida genus, constitutes a common culprit behind infections. Intriguingly, recent investigations have unveiled the association between oral cancers and precancerous conditions, including white patches and oral lichen planus (Cosola et al., 2021). Initial researches have ascribed oral leukoplakia and oral lichen planus to Candida, intimating the genus’s partial culpability in these precancerous lesions (Werneck et al., 2015; Dilhari et al., 2016). Candida is capable of producing nitrosamine compounds. As potent carcinogens, nitrosamines stand out among the key chemical agents driving cancer, playing a pivotal role in the induction of OSCC (Sanjaya et al., 2011). A conspicuous correlation between the capacity of oral candida to metabolize alcohol into acetaldehyde and the fostering of cancer development corroborates the potential involvement of C. albicans in the carcinogenic tapestry (Alnuaimi et al., 2016). The main carcinogenic mechanism of OSCC caused by Candida albicans infection is through the Kirsten rat sarcoma virus oncogene homolog (KRAS) pathway and the E2 transcription factor (E2F) gene (Yang et al., 2023). In addition, mutations in tumor suppressor and DNA repair genes (such as p16, RAR-β2, TIMP3, BRCA1, and ERCC1) may have significant impact on the carcinogenic process due to C. albicans infection (Yang et al., 2023). Published studies have described the association between fungal communities and tumor transformation, and demonstrated a decrease in the diversity of individual fungal species that make up the oral fungal community in patients with oral squamous cell carcinoma. The increase in fungal species is related to the transformation of oral tumors, such as an increased incidence of the general Candida, Hannaela, and Giberella, which were detected in samples of OSCC tumor tumors (Vyhnalova et al., 2021).
Over the recent years, numerous studies have increasingly substantiated the influence of viruses in the progression of oral cancer (Yang et al., 2019; Rooper et al., 2020). Among these, human papillomavirus (HPV) stands out as a recognized carcinogenic factor (Findik et al., 2019) and is present in normal oral mucosa (Sankaranarayanan et al., 1997). Certain HPV types (16, 18, 31, 33, 35, and 39) have substantial carcinogenic potential, with their genes and products orchestrating interference in cell cycle processes. In addition, the twosome of oncoproteins encoded by HPV, notably E6 and E7, wield the ability to down-regulate the expression of histocompatibility genes. This action reverberates across both innate and adaptive immunity. 84% and 61.5% of patients diagnosed with OSCC tested positive for HPV and HPV-16, respectively. The presence of HPV increased the risk of developing OSCC by 2.82 times (Stasiewicz and Karpiński, 2022). Epstein–Barr virus (EBV) has been identified in oral and pharyngeal lymphoid tissues, with B lymphocytes serving as the primary reservoir for the virus. In addition to OSCC, EBV has been associated with oral diffuse large B-cell non-Hodgkin’s lymphoma, oral Burkitt’s lymphoma, and salivary gland epithelioma (Stasiewicz and Karpiński, 2022). A significant proportion (73.8%) of OSCC patient samples were positive for latent membrane protein-1 (LMP-1), a marker commonly associated with EBV-related malignancies, compared to only 19.1% in the control group. Furthermore, individuals infected with EBV have a 2.5 times higher risk of developing OSCC (95% CI = 1.23–5.36; Stasiewicz and Karpiński, 2022). Virome composition could be implicated in immune system development by regulating bacterial communities, thus indirectly contributing to the development of cancer (Tamayo-Trujillo et al., 2023).
3 Influence of oral microbes on the process of systemic cancers
3.1 Gastric cancer
Gastric cancer ranks as the fourth most prevalent malignancy globally and represents a prominent contributor to cancer-related mortality. Notably, samples obtained from individuals diagnosed with gastric cancer exhibited notably higher levels abundance of oral bacteria compared to those in benign stages (Coker et al., 2018). The oral cavity serves as a significant site for the colonization of Helicobacter pylori (H. pylori). Helicobacter pylori can be detected in saliva, dental plaque, oral ulcers, oral tumors, etc. Helicobacter pylori infection plays an important role in the genesis of gastric cancer. The bacteria can alter the sensitivity of cells lining to stomach to a variety of exogenous dietary stimuli (Poplawski et al., 2013). Initially, it triggers chronic gastritis, progressing to gastric ulcers and atrophy. With persistent infection, it can inflict harm on the cells of the gastric mucosa, potentially culminating in their transformation into cancer (Poplawski et al., 2013). Simultaneously, H. pylori can deactivate the ARID1A gene, leading to abnormalities in cell mitosis, which fosters the advancement of gastric cancer (Inada et al., 2015). Moreover, H. pylori can directly modulate the function of gastric epithelial cells using its virulence factors (CagA, VacA). Additionally, it triggers inflammation and exerts indirect effects on gastric epithelial cells (Ferreira et al., 2008; Chiba et al., 2010).
3.2 Pancreatic cancer
Pancreatic cancer stands as one of the most fatal malignancies globally, contributing to approximately 8% to 10% of all digestive tract tumors. Within the group of individuals diagnosed with pancreatic cancer, the 5-year survival rate remains below 9% (Peters et al., 2017). Besides the recognized risk factors, oral microorganisms also play a role in the initiation and progression of pancreatic cancer. The main oral bacteria involved in carcinogenesis of pancreatic ductal adenocarcinoma are P. gingivalis, Clostridium, Neisseria elongate (N. elongate) and Streptococcus mitis (S. mitis; Riviere et al., 2010). Indeed, the occurrence of F. nucleatum within pancreatic tumors is associated with a poorer prognosis. Furthermore, A. actino-mycetemcomitans, Alloprevotella, Leptotrichia, and Streptococcus are also correlated with an elevated likelihood of developing PC (Stasiewicz and Karpiński, 2022). Additionally, Granulicatella adiacens was found to be elevated in PC cases (Stasiewicz and Karpiński, 2022). Under pathological circumstances, the levels of N. elongate and S. mitis decreased, while Streptococcus increased. Streptococcus possesses an inhibitory effect on periodontitis, and the reduction in its abundance could contribute to the onset of this condition. Additionally, it might trigger inflammation in humans, consequently elevating the likelihood of pancreatic cancer (Farrell and Gut, 2012). Individuals with elevated antibody levels of P. gingivalis face a more than twofold increased risk of developing pancreatic cancer compared to those with standard antibody levels (Dominique, 2013). Porphyromonas gingivalis initiates toll-like receptor (TLR) signaling pathway, activates NF-κB pathway, induces the expression of tumor necrosis factor, IL-1α, IL-6, IL-8 and other cytokines through binding with Toll-like receptors 2 and 4, thus forming an inflammatory microenvironment and promoting tumor occurrence and development (Rhodus et al., 2005; Olsen et al., 2016). Another mechanism of pancreatic cancer may be that the three red complexes of P. gingivalis, treponema dentalis and Forsythian bacteria produce peptidyl arginine deiminase PAD enzyme that degrades arginine, which causes p53 and K-Ras point mutations, leading to pancreatic cancer (Wei et al., 2019). Helicobacter pylori infection may also be a risk factor for pancreatic cancer (Yu et al., 2017). When comparing the serum levels of H. pylori IgG antibodies between patients with pancreatic cancer and healthy controls, it became evident that patients diagnosed with pancreatic cancer displayed elevated levels of H. pylori IgG antibodies (Kalaf et al., 2013; Chen et al., 2014). There exists a positive correlation between the occurrence of pancreatic cancer and the presence of gastric ulcers (Luo et al., 2007). Helicobacter pylori is capable of inducing gastric ulcers, resulting in decreased acid production and raised levels of specific nitrosamines. This interplay might consequently heighten the susceptibility to pancreatic cancer (Michaud, 2013).
3.3 Esophageal cancer
Esophageal cancer is listed as the eighth largest cancer in the world and the sixth leading predictor of mortality, with a 5-year survival rate of 15% to 25% (Ferlay et al., 2015; Gupta and Kumar, 2017). Elevated amounts of P. gingivalis were found in tissues afflicted with esophageal carcinoma. Moreover, esophageal squamous cell carcinoma tumor tissues exhibited even greater levels of P. gingivalis compared to adjacent paracarcinoma tissues and normal control sites. In vitro experiments, P. gingivalis triggered the NF-κB signaling pathway to induce the proliferation and metastasis of esophageal squamous cell carcinoma cells (Gao et al., 2016; Yuan et al., 2017; Fan et al., 2019). Esophageal cancer often coincides with an elevation in gram-negative bacteria. These bacteria produce substances like LPS and endotoxins, which might play a role in the innate immune response of the host. Among these components, LPS act as immunologically active agents. They have the capacity to initiate the conventional inflammatory signaling pathway through the nuclear transcription factor NF-κB. This, in turn, leads to an amplified production of inflammatory molecules such as IL-1β, IL-6, IL-8, and TNF-α. Additionally, it can activate inducible nitric oxide synthetase (iNOS) and cyclooxygenase enzyme (COX). This dual activation leads to a decrease in the peristalsis frequency of the lower esophageal sphincter, ultimately contributing to an elevated risk of esophageal cancer (Abreu and Peek, 2013).
3.4 Colorectal cancer
Colorectal cancer (CRC), which ranks among the top three contributors to cancer-related fatalities, has exhibited a rise in both occurrence and mortality rates over recent years. Recently, F. nucleatum, an anaerobic element found in both oral and intestinal symbiotic communities, has emerged as a noteworthy risk factor for CRC. Substantial variations in the oral levels of F. nucleatum and C. difficile were observed between individuals with normal conditions and those diagnosed with colorectal cancer. Notably, F. nucleatum facilitates the secretion of cytokines that have the potential to trigger the development of tumors (Yu et al., 2015). Fusobacterium nucleatum infiltrates the intestinal epithelial tissue using specific outer membrane proteins such as fusobacterium protein A (FomA), adhesion A (FadA), and fusobacterium self-transporter 2 (Fap2). This invasion triggers the activation of signaling pathways involving NF-κB and TLR4. Consequently, it spurs the proliferation of colon cancer cells, thus fostering the progression of tumors (Yongzhi et al., 2017). It possesses the ability to directly influence host cells by binding to E-cadherin in both normal and cancerous epithelial cells using FadA. This binding activates the β-catenin-regulated transcriptional pathway, leading to an upsurge in the expression of genes associated with cancer markers. Consequently, this process fosters the development of cancer (Sonnenburg et al., 2004; Roxana et al., 2013). Furthermore, it plays a role in facilitating the entry of non-invasive bacteria (like Streptococcus and Campylobacter) into cells. This leads to the development of localized microenvironmental inflammation, which in turn indirectly supports the progression of tumor cells and influences their prognosis (Tahara et al., 2014). Fusobacterium nucleatum promoted the development of cancer by enhancing the glucose metabolism of CRC cells, via activating the transcription of lncRNA ENO1-IT1 and increasing the binding efficiency of the transcription factor SP1 to its promoter region. The elevated ENO1-IT1 acted as a guide for KAT7 histone acetyltransferase, influencing the histone modification pattern on target genes such as ENO1, and ultimately impacting the biological function of CRC (Hong et al., 2021).
3.5 Lung cancer
Lung cancer is the cancer with the highest incidence rate and mortality in the world. Oral microbiome and cancer are closely related to lung and oral cavity, so oral microbiome and lung microbiome are highly related (Maddi et al., 2019). Lower levels of oral microbiome α Diversity may be associated with a higher risk of lung cancer and may serve as a prospective signal for lung cancer risk (Stasiewicz and Karpiński, 2022). Fusobacterium nucleatum may be a potential candidate microorganism for cancer biomarkers. Porphyromonas gingivalis may be involved in promoting the progression of malignant lung cancer (Stasiewicz and Karpiński, 2022). Periodontal disease may be mixed with smoking and positively correlated with the risk of lung cancer, but it is not an independent risk factor (Stasiewicz and Karpiński, 2022).
4 Carcinogenic mechanism
In the context of cancer development, three key mechanisms are associated with oral microorganisms. Firstly, microorganisms can impact cancer pathogenesis by influencing processes like cell proliferation, cytoskeletal rearrangement, activating NF-κB, and preventing apoptosis. The second mechanism operates through the induction of chronic inflammation, which subsequently triggers the production of inflammatory mediators, playing a role in instigating or supporting various cancer-related events such as cell proliferation, genetic mutations, oncogene activation, and the promotion of angiogenesis. Lastly, a third mechanism involves certain microorganisms participating in cancer development by generating metabolites that have the potential to initiate cancer formation (Zhang et al., 2018; Figure 2).
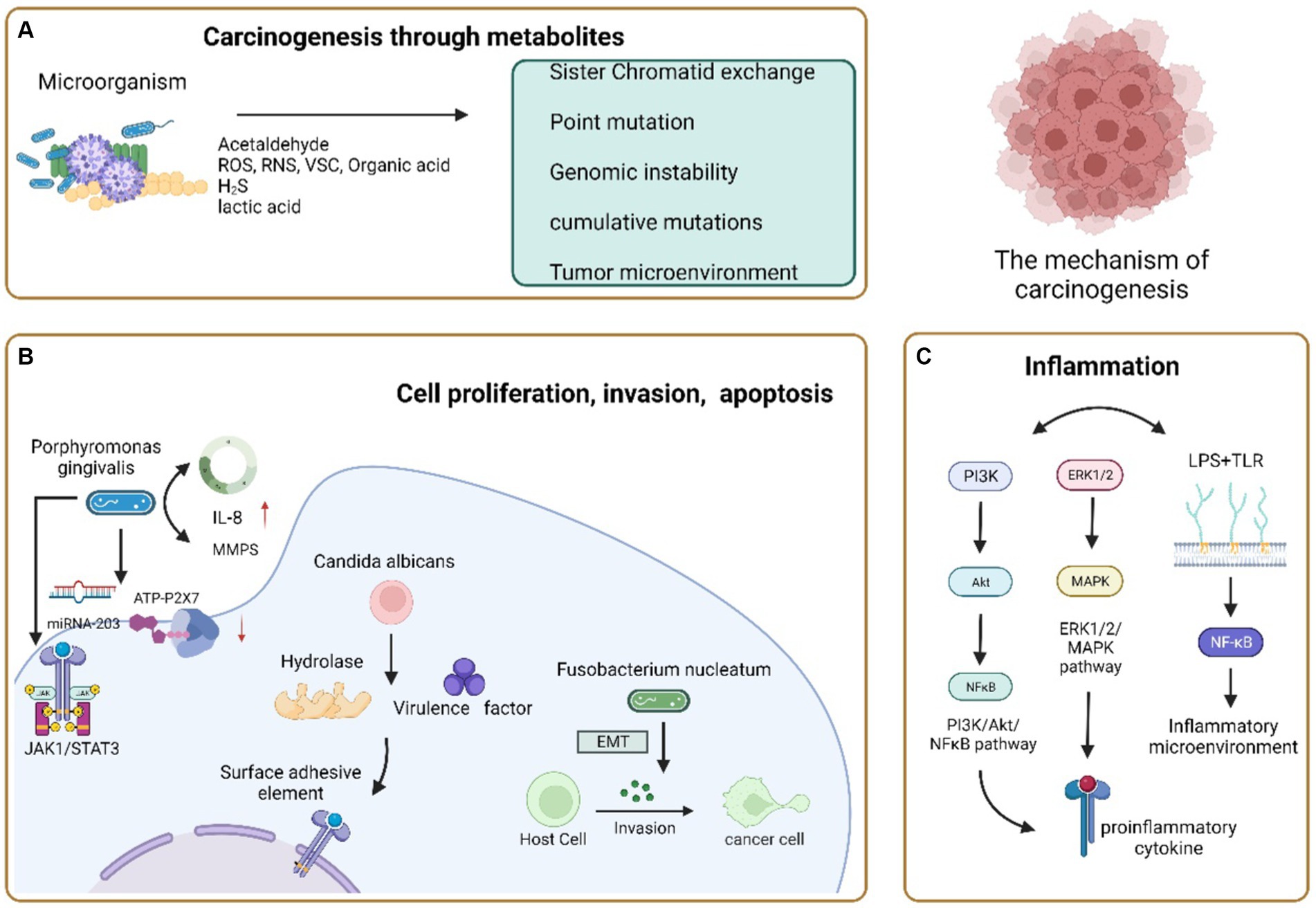
Figure 2. Carcinogenic mechanism of oral microorganisms. (A) Microorganisms cause cancer through their metabolites. Acetaldehyde, a common metabolite, can cause cancer occurrence and development through sisters Chromatid exchange, Point mutation, DNA adduct and excessive proliferation of epithelial cells; Reactive oxygen species, reactive nitrogen, volatile sulfur compounds, and organic acids alter their activity and accumulate carcinogenicity in inflammatory environments; H2S can cause genomic instability or accumulate mutations, activate proliferation, migration, and invasive signaling pathways, affect tumor growth and spread, and enhance tumor angiogenesis; Lactic acid can acidify the microenvironment together with other organic acids to form a Tumor microenvironment conducive to the growth of OSCC. (B) Carcinogenic pathways of microbial regulation of host cell proliferation, invasion, and apoptosis. Porphyromonas gingivalis induces cell apoptosis by over stimulating the JAK 1/STAT 3 signaling pathway, inhibiting ATP ligand binding to P2X7 receptors, and regulating miRNA-203 upregulation; Accelerate the proliferation of gingival epithelial cells by interfering with cell cycle regulation; Upregulation of IL-8 and Matrix metalloproteinase increases the invasiveness of Oral cancer cells. Candida albicans produces hydrolysis related enzymes and virulence factors to invade tissues, promote the proliferative epithelial response of oral mucosal cells, and make its dysplasia transform into cancer or induce apoptosis and necrosis of epithelial cells to cause cancer. Fusobacterium nucleatum can induce epithelial mesenchymal transformation and enhance the invasiveness of cells, achieving the goal of carcinogenesis. (C) Carcinogenic pathways of microorganisms through chronic inflammation. PI3K/Akt/NF-κB. The ERK1/2/MAPK signaling pathway is activated, and the secretion of pro-inflammatory cytokines promotes chronic inflammation and carcinogenesis; LPS binds to TLR-2 and TLR-4 on the host cell membrane, inducing the production of an inflammatory microenvironment to promote tumor growth.
4.1 Tumor microenvironment
Multiple studies have identified a correlation between the oral microbiota and the tumor microenvironment. The oral microbiota plays a significant role in various aspects of tumor dynamics, including initiation, progression, metastasis, and immune responses. This complex interaction within the tumor microenvironment (TME) is crucial for understanding cancer mechanisms. TME influences the development of tumors in multiple ways. For instance, it involves bacterial peptides found in both cancer and immune cells, where bacterial antigens imitate tumor antigens. Additionally, microorganisms play a role in prompting immunogenic cell death and activating signaling pathways through helper pattern recognition receptors. Furthermore, the metabolites produced by microorganisms also contribute to these processes, along with their impact on stimulation and inhibition of checkpoints (Gottesman, 2022). As an illustration, squamous cell carcinoma of the head and neck (HNSCC) is a prevalent condition that arises in areas like the oral cavity, oropharynx, larynx, and hypopharynx. These regions are composed of intricate tissues comprising not only tumor cells but also an encompassing matrix, that involves diverse types of mesenchymal cells and extracellular matrix (ECM; Hayes et al., 2018). Recent research has indicated that TME significantly impacts the growth and spread of squamous cell carcinoma of the head and neck (HNSCC; Joyce and Pollard, 2009). Genetic modifications within cancer cells of the TME, such as changes in TP53, NOTCH1, and distinct gene expression patterns, contribute to both the development of cancer and disruptions in the microenvironmental cells. These disruptions include heightened levels of reactive oxygen species (ROS), excessive cytokine production, and a transition between epithelial and interstitial states known as EMT (Boyle et al., 1993; Birner et al., 2000; Curry et al., 2014). In the progression of HNSCC, the adaptive immune responses are hindered through the excessive presence of cytokines. This excess leads to the apoptosis of T cells and alterations in how antigens are processed. The overexpression of crucial cytokines like transforming growth factor-β (TGF-β) plays a role in the development of processes such as EMTs, the emergence of immunosuppressive factors, and the formation of cancer-associated fibroblasts (CAFs; Cavallo et al., 2011; Curry et al., 2014). Within the TME, CAFs assume a paramount role in driving proliferation, invasion, and metastasis. Additionally, in the context of HNSCCs, tumor development is furthered by employing both glycolysis and oxidative metabolism (Curry et al., 2014). This intricate interplay underscores the importance of considering the oral microbiota’s role in shaping the tumor microenvironment and cancer progression. The tumor microenvironment can selectively promote the growth of specific bacteria, disrupting the balance between the resident oral microbiota and the host. This may be a crucial link through which commensal oral bacteria promote oral cancer. For instance, the genus Peptostreptococcaceae incertae sedis, which normally occupies only 0.04% of the normal oral buccal mucosa, increases to 0.36% in OSCC sites. Additionally, Gemella morbillorum, a facultative anaerobic Gram-positive coccus of the phylum Firmicutes, is highly associated with OSCC tumor sites (Zhang L. et al., 2020).
4.2 Oral microbial dysbiosis
Disruption of the balance in microbial flora leads to an imbalance known as oral microbial dysbiosis. This dysbiosis establishes a closely intertwined and mutually beneficial connection with the host. In the oral cavity, the regular colonization by microbial communities serves to prevent pathogenic microorganisms from attaching or impeding the growth of harmful bacteria. Additionally, these healthy microorganisms also play a role in countering and regulating the population of pathogens through antagonistic actions and quorum sensing mechanisms.
Simultaneously, the host’s immune system upholds a state of tolerance while curbing immune responses. This is achieved through the actions of mucosal dendritic cells and the release of anti-inflammatory cytokines like IL-10 and prostaglandin E2, the generation of T-regulatory cells also contributes to this process (Li et al., 2022). Consequently, the oral mucosal tissues achieve a harmonious and well-organized stability, balancing the survival needs of various microorganisms (Sami et al., 2020).
When the balance of microorganisms in the mouth is disrupted, it creates a favorable environment for harmful bacteria to become active and contribute to various oral diseases. For example, in conditions like tooth decay, these changes encourage the growth of bacteria that can produce and tolerate acidic conditions, such as Streptococcus mutans, Lactobacillus, and Bifidobacterium (Takahashi and Nyvad, 2011). Dysbiosis of oral microbiota may be involved in the development of oral cancer and other systemic diseases. The bacteria that undergo significant changes in oral cancer are mostly anaerobic, since hypoxia can lead to the recolonization of specific bacteria, which can promote the development of malignant tumors. When inflammation is uncontrolled or continuous, it can progress into a chronic state, which has the potential to promote the transformation of normal cells into cancerous cells (Zhang L. et al., 2020; Figure 3).
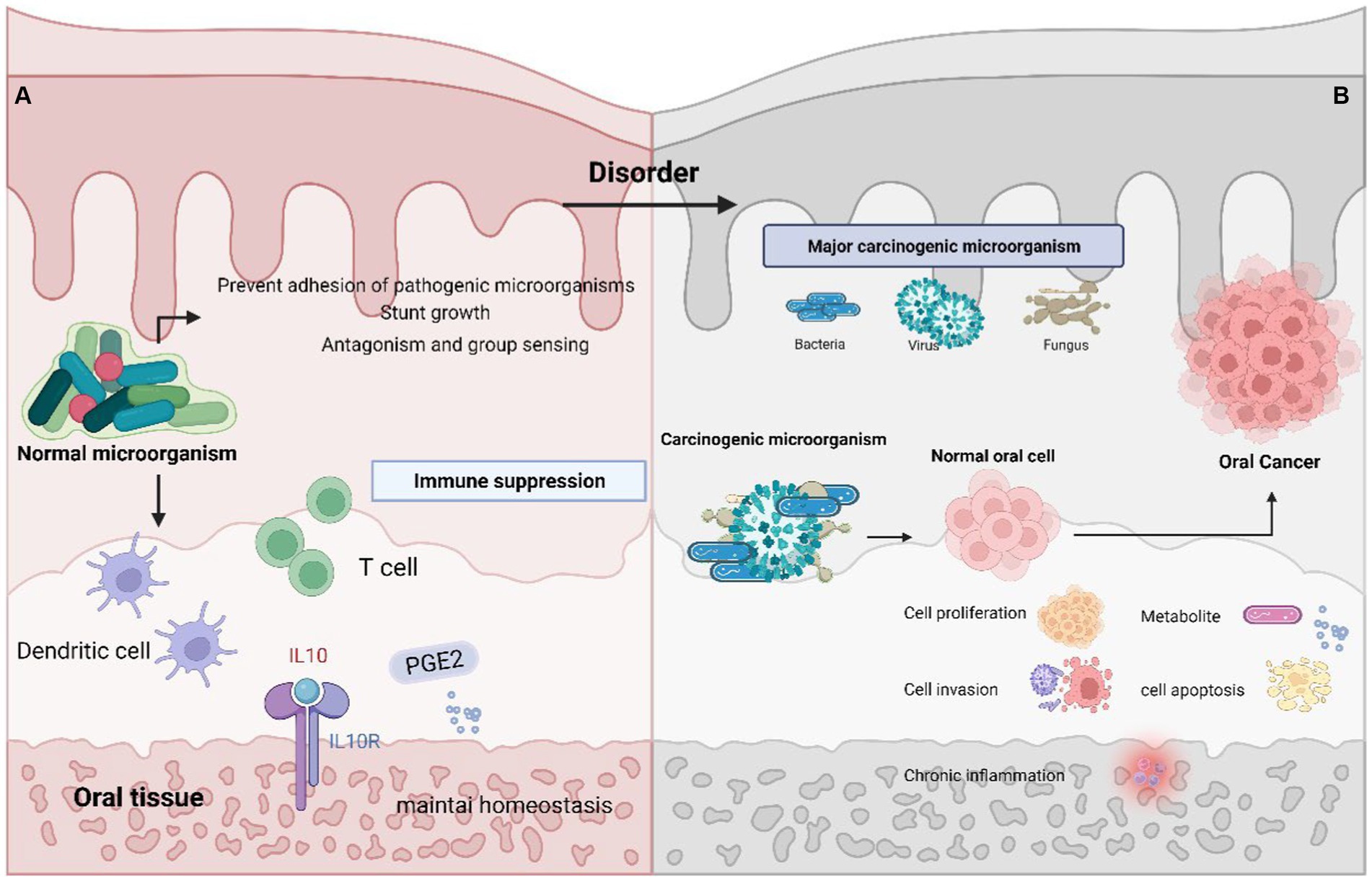
Figure 3. Oral microbiota balance and dysbiosis. (A) Normal oral environment can prevent pathogenic microorganisms from adhering to or hindering the growth of pathogenic bacteria, playing the role of antagonism and Quorum sensing; The host immune system maintains tolerance through the role of mucosal dendritic cells and the secretion of anti-inflammatory cytokines IL-10, Prostaglandin E2, T regulatory cells, etc. Maintain a stable oral microenvironment. (B) Abnormality of the oral microenvironment, where pathogenic bacteria begin to play a role and cause cancer through promoting cell proliferation, invasion, apoptosis, chronic inflammation, and the production of carcinogenic metabolites.
4.3 Regulation of host cell proliferation, invasion and apoptosis
Microorganisms can promote the progression of cancer by regulating apoptosis, activating cell proliferation, and enhancing cell invasion. Porphyromonas gingivalis employs multiple strategies to control apoptosis. For instance, it can prompt cell apoptosis by excessively activating the JAK1/STAT3 signaling pathway. This involvement in regulating mitochondrial apoptosis, cellular differentiation, migration, and proliferation is a notable aspect of Porphyromonas gingivalis’s impact (O'Shea et al., 2015). Porphyromonas gingivalis can induce apoptosis of gingival epithelial cells by inhibiting the binding of ATP ligand to P2X7 receptor (Özlem et al., 2008). Furthermore, it influences cytokine signaling through the regulation of miRNA-203, which targets the 3’UTR region of SOCS3, leading to its reduced expression (Qin et al., 2012). As a result, this process triggers both apoptosis and the development of carcinogenesis.
Under long-term infection conditions, P. gingivalis can promote cell proliferation and change cell morphology by regulating cell cycle (Pan et al., 2013; Geng et al., 2017). Examination of the proteome has revealed that when gingival epithelial cells (GECs) are infected with P. gingivalis, alterations occur in the levels and phosphorylation of specific proteins. These changes disrupt the regulation of the cell cycle, fostering the shift from the S phase to the G1 phase. This is achieved by enhancing the presence of cell cycle cyclins (D and E) and consequently promoting a proliferative state in GECs (Kuboniwa et al., 2008; Pan et al., 2013). Moreover, P. gingivalis can enhance the aggressiveness of oral cancer cells through multiple mechanisms. This includes the elevation of IL-8 and matrix metalloproteinases (MMPs), as well as the induction of EMT (Ha et al., 2016). IL-8 can also contribute to cancer development by engaging in the elevation of zinc-dependent proteins. This, in turn, leads to the degradation of the extracellular matrix, facilitating the metastasis of malignant epithelial cells. Additionally, IL-8 plays a role in activating epidermal growth factor (EGF), which stimulates cell proliferation (Fitzsimonds et al., 2020). The existing pathology of the pancreas may induce the aggregation of oral microorganisms, and its pathological effects may cause pancreatic carcinogenesis (Andam and Hanage, 2015). Pathogens can enter the pancreas through various pathways, including blood flow, bile ducts, small intestine, and reflux into the pancreatic duct (Michaud et al., 2013). Therefore, it can be inferred that P. gingivalis originating from the oral cavity may spread to the cancer site or interfere with the immune system to accelerate the development of cancer (Sun et al., 2020).
Candida albicans (C. albicans) stands as the primary culprit behind oral candidiasis and has been linked to the emergence of OSCC. This microorganism generates enzymes for hydrolysis and virulence factors that aid in tissue invasion. Consequently, this invasive behavior triggers a heightened epithelial response in oral mucosal cells, which leads to their restrained growth and transformation into a cancerous state (Hafed et al., 2019). The primary mechanism involves C. albicans attaching to receptors on epithelial cells via surface adhesins like lectin-like sequence family and mycelium wall proteins. This attachment leads to adherence to oral epithelial cells. Following this initial interaction, C. albicans enters the upper cortex or submucosa of the host through two distinct mechanisms: endocytosis and active penetration. Subsequently, it triggers apoptosis and necrosis of epithelial cells, which ultimately contributes to the development of oral cancer (Marc et al., 2017; Peter, 2018). Fusobacterium nucleatum has the ability to either trigger epithelial-mesenchymal transition EMT or attach to proteolytic enzymes that originate from the host. This attachment subsequently boosts cellular invasion, facilitating the progression toward carcinogenesis (Renée et al., 2004; Nisticò et al., 2012). Moreover, F. nucleatum infection prompts the activation of protein kinase p38 within the infected cells, leading to escalated secretion of MMP-13 and MMP-9. This excessive secretion plays a role in tumor invasion (Uitto et al., 2005). Research has indicated that F. nucleatum can induce cell cycle arrest at the S phase by reducing the expression of p27. This, in turn, leads to heightened cell proliferation and involvement in the progression of cancer (Geng et al., 2019).
4.4 Chronic inflammation
The oral microbiota also exerts an impact on tumor development through its modulation of the host’s immune response. Prolonged infections stand out as contributors to cancer development, with approximately 16% of global cancer cases being directly linked to infections (Ojcius et al., 2016). The foremost hallmark of oral microbial infection is persistent inflammation, which assumes a pivotal role across all phases of cancer evolution. These stages encompass initiation, advancement, invasion, and metastasis. This is attributed to the prolonged inflammation resulting from ongoing environmental exposure (Uitto et al., 2005). Oral pathogens trigger inflammatory responses within the host, marked by heightened levels of cytokines, chemokines, and growth factors. These factors foster cell survival and growth, thus initiating the cancer development process (Geng et al., 2019). The cytokines generated during the inflammatory response significantly contribute to cancer formation by provoking mutations, destabilizing the genome, and affecting epigenetic processes. They further hinder apoptosis, promote angiogenesis, and disrupt normal functioning of inflammatory signaling pathways, ultimately leading to abnormalities (Zhang S. W. et al., 2020). Oral microorganisms like P. intermedius have the ability to generate harmful substances such as LPS, peptidoglycan, or lipoteichoic acid. These substances prompt the production of proinflammatory cytokines (Chattopadhyay et al., 2019). Additionally, cells secrete proteases that further encourage the production of these cytokines. Proteases can be activated through protease-activated receptors (PARs), serving as signaling molecules that degrade the host’s extracellular matrix. As a result, the physical immune barrier is compromised, the host’s immune response is disrupted, and conditions favorable for the emergence and progression of tumors are fostered (Zhang L. et al., 2020). Fusobacterium nucleatum has the capability to elevate the synthesis of pro-inflammatory cytokines, specifically IL-6 and IL-8. This, in turn, dampens the impact of immune cells, fostering an inflammatory environment that contributes to the development of oral cancer (Saito et al., 2012; Kauppila et al., 2013; Mima et al., 2015; Hsiao et al., 2018). Streptococcus anginosus also facilitates the advancement of oral cancer by generating pro-inflammatory cytokines like IL-1β and IL-6. Thus, it participates in the genesis of oral cancer by acting as a contributor to persistent inflammation in the oral region (Rai et al., 2021).
Candida albicans has the capability to infect and trigger the PI3K/Akt/NF-κB, p38/JNK, and ERK1/2/MAPK signaling pathways. This activation subsequently leads to the release of various antimicrobial peptides and pro-inflammatory cytokines, culminating in the establishment of a persistent state of inflammation (Auperin, 2020). In the context of invasive C. albicans infection, a small secreted protein called Sel1 can also initiate an inflammatory response dependent on TLR2/4. This, in turn, activates both the NF-κB and Mitogen-Activated Protein Kinase (MAPK) signaling pathways, resulting in the expression of proinflammatory cytokines and chemokines. Furthermore, P. gingivalis contributes to tumor development by modifying the nearby inflammatory microenvironment. The lipopolysaccharide (LPS) of P. gingivalis attaches to TLR-2 and TLR-4 receptors on the host cell membrane. This attachment triggers the activation of NF-κB through intracellular signal transduction pathways, ultimately leading to the production of IL-1α, IL-6, TNF-α, and IL-8. These cytokines promote the establishment of an inflammatory microenvironment that fosters the growth of tumors (Liu et al., 2020).
4.5 Metabolites
Acetaldehyde has been identified as a substance that can lead to various harmful effects on DNA, including sister chromatid exchange, point mutations, DNA damage, and the excessive growth of epithelial cells. It is classified as the first major category in human carcinogen by the International Agency for Research on Cancer (IARC; Brooks and Zahari, 2014; Mizumoto et al., 2017). Certain oral microbial species, such as Streptococcus gordonii, Streptococcus sanguis, Streptococcus salivarius, and yeast, play a role in this process. While these microorganisms are not inherently carcinogenic, they possess the enzyme alcohol dehydrogenase (ADH) which, in the presence of oxygen or low oxygen conditions, can produce acetaldehyde. This contributes to the potential harmful effects associated with acetaldehyde exposure (Feller et al., 2013).
Reactive oxygen species (ROS), reactive nitrogen species (RNS), volatile sulfur compounds (VSCs), and organic acids are examples of substances that can contribute to carcinogenic processes. Inflammatory responses can trigger the accumulation of ROS and RNS, primarily through alterations in the activities of NADPH oxidase and nitric oxide synthase (NOS). This response is influenced by molecules like TNF-α, IL-6, and TGF-β and its persistence can facilitate cancer progression (Hussain et al., 2003; Piao et al., 2016). Several oral microorganisms play a role in generating hydrogen peroxide (H2O2). These microorganisms include Bifidobacterium juveniles, Lactobacillus acidophilus, Bifidobacterium fermentum, Bifidobacterium janssenensis, Bifidobacterium minutos, Streptococcus cogenii, Streptococcus oligomysaccharides, Streptococcus oral, and Streptococcus haematoides (Abranches et al., 2018; Hampelska et al., 2020). Certain oral bacteria, including P. gingivalis, P. intermedius, A. agglutinus, and F. nucleatum, have the ability to generate volatile sulfur compounds (VSCs) such as hydrogen sulfide (H2S), methyl mercaptan (CH3SH), dimethyl sulfide [(CH3)2S], and dimethyl disulfide (CH3SSCH3). Among these, hydrogen sulfide (HS), a recognized genetic agent, holds the potential to induce genomic instability and cumulative mutations. Furthermore, it exerts influence on the growth and dissemination of tumors by activating signaling pathways that enhance proliferation, migration, and invasion. Notably, HS also promotes the development of new blood vessels that nourish tumors, a process known as tumor angiogenesis (Gaskins et al., 2007).
Studies have highlighted that a significant portion of the microbial groups found within OSCC tumor tissues belong to saccharifying and aciduric strains. These strains are characterized by their capacity to produce acidic compounds and their preference for acidic conditions (Hooper et al., 2006). The oral cavity harbors various lactic acid bacteria, including species like Lactococcus, Bifidobacterium, Streptococcus, Colinococcus, and Amphicoccus. These microorganisms contribute to the production of lactic acid, collectively fostering a tumor-friendly microenvironment for OSCC growth by collaborating with other organic acids to acidify the surroundings. This interplay significantly impacts the progression of OSCC (Karpinski, 2019). The production of organic acids not only leads to a reduction in pH levels but also carries implications for the immune response against tumors. Specifically, the lowered pH can impede the body’s ability to mount an effective anti-tumor immune response. Additionally, the generation of organic acids plays a role in stimulating the formation of blood vessels that supply tumors, contributing to tumor angiogenesis (Choi et al., 2013).
5 Potential of oral microbiota in cancer prevention, screening and therapeutic strategies
Oral microorganisms and their byproducts offer promise as potential indicators for the malignant transformation of cells, serving as candidates for screening various associated cancers. To date, over 100 potential biomarkers have been identified in saliva for the diagnosis of OSCC (Castagnola et al., 2011). Notably, the levels of certain microorganisms such as Fusobacterium periodontium, Parvobacterium parvum, Streptococcus macrococcus, Haemophilus influenzae, and filamentous factor display a gradual increase from stage I to stage IV of OSCC. This intriguing trend suggests that fluctuations in the abundance of these microorganisms might hold potential as markers for the process of oral carcinogenesis. Moreover, the involvement of oral microorganisms extends beyond diagnostics, as they also contribute to the prevention and treatment of various other types of cancers (Chen et al., 2020).
5.1 The role of oral microbiota in preventive screening
The Nrf2 activator sulforaphane (SFN) plays a pivotal role in the regulation of diverse biological functions encompassing cell cycle, growth, development, survival, cell death, and gene transcription. SFN is notably upregulated in many cancer cells and contributes to the process of carcinogenesis by influencing cellular activities such as proliferation, differentiation, and programmed cell death within tumors (Deng et al., 2011; Hu et al., 2019). Studies have pointed to a robust expression of SFN in tissues infected with C. albicans, particularly in OSCC tissues. This observation implies that SFN could potentially serve as a distinctive biomarker for predicting the malignant transformation triggered by C. albicans infection.
Oral leukoplakia, acknowledged as a precursor to oral cancer, often harbors the presence of specific microorganisms including Bacteroidetes, Streptococcus, and Fusobacterium (Hu et al., 2016; Hashimoto et al., 2019). Additionally, HPV has been detected positively in cases of oral leukoplakia. Collectively, this suggests that oral microorganisms like Streptococcus, Fusobacterium, and HPV might hold significance as indicators for monitoring oral precancerous lesions.
A potential method for early pancreatic cancer detection involves examining pathogens responsible for periodontitis, either through analyzing antibodies in the blood or assessing the oral microbiota. In a prospective study (Michaud et al., 2013), antibodies to oral bacteria in plasma samples were compared between individuals previously diagnosed with pancreatic cancer and healthy controls. The results indicated that individuals with elevated levels of antibodies to P. gingivalis faced double the risk of pancreatic cancer in comparison to those with lower levels (Fan et al., 2018). This points to the potential of oral microbiota as a predictive tool for pancreatic cancer. Furthermore, an intriguing finding was that F. nucleatum and its related genus, Leptotrichia, were associated with a reduced risk of pancreatic cancer. On the other hand, N. elongate and S. mitis, as constituents of the oral microbiome, could potentially contribute to the diagnosis of pancreatic cancer.
High recurrence rates are a significant concern in cancer cases (Serna et al., 2020), leading to the common use of postoperative adjuvant chemotherapy to mitigate the risk of recurrence. In colorectal cancer patients, the persistence of F. nucleatum infection after neoadjuvant chemoradiotherapy (nCRT) is linked to a high recurrence rate. As a result, F. nucleatum holds substantial promise as a biomarker to enhance the detection of colorectal cancer (Wong et al., 2016; Eklöf et al., 2017). Efforts have also been dedicated to identifying oral biomarkers associated with CRC detection, such as Streptococcus and Prevotella (Flemer et al., 2017). Understanding the bacterial species linked to cancer risk has the potential to significantly enhance early disease detection strategies.
5.2 Therapeutic strategies
A range of cancer treatment options are available, encompassing surgery, radiotherapy, nanomaterial therapy, and gene therapy. Interestingly, oral microorganisms have also been implicated in cancer treatment (Table 2). Certain bacteria from the Lactobacillus genus exhibit anti-cancer effects and can impede neoplastic transformation, by inducing apoptosis, boosting the expression of tumor suppressor genes, and modulating both adaptive and innate immune responses (Vyhnalova et al., 2021). One specific strain, Lactobacillus rhamnosus, holds the ability to counteract chronic inflammation linked to carcinogenesis. The combination of geniposide and L. rhamnosus has been shown to heighten apoptosis in HSC-3 cells. The consumption of nutrient-rich foods containing probiotic lactobacilli also contributes positively to tumor prevention. Probiotics mitigate the mutagenic effects of harmful agents while influencing the expression of proteins associated with cell proliferation, apoptosis, inflammation, and immune system activation (Kumar et al., 2010). Various experiments conducted on cancer cells have unveiled the anti-proliferative and pro-apoptotic properties of probiotics in these tumor models (Yu and Li, 2016). Lactobacillus plantarum, for instance, displays the ability to inhibit or activate the MAPKs and PTEN pathways, respectively (Asoudeh-Fard et al., 2017). This strain can be employed in cancer treatment by leveraging the induction of apoptosis in oral cancer cells through the application of Lactobacillus plantarum probiotic secretions. Cytotoxicity, denoting the toxicity that leads to cell death or destruction, is a crucial consideration. Certain virulent genes within bacteria hold potent lethality (Liu et al., 2016; Liang et al., 2017). For instance, streptolysin O serves as a cytotoxic agent capable of inducing antitumor activity in cell cultures, including 293 T cells (Doocey et al., 2022). The utilization of microbial toxicity for cancer therapy is currently an area of active investigation.
Bacteria can play a beneficial role in the chemotherapy of various cancers, including pancreatic cancer, colorectal cancer, and other diseases. For instance, in a mouse model of pancreatic cancer treated with gemcitabine and bevacizumab, the addition of Salmonella typhimurium improved the therapeutic effectiveness against pancreatic cancer (Hiroshima et al., 2014). Lactobacillus paracasei, a Gram-positive lactic acid bacterium found in the human gut, has shown promise as well. When added to a mouse model undergoing gemcitabine treatment, L. paracasei improved chemotherapy efficacy and increased tolerance (Chen et al., 2020). Further investigation has revealed that this enhancement is largely attributed to an increase in IFN levels, causing a shift from a Th2 immunophenotype to a Th1 immunophenotype, thereby boosting anti-tumor capabilities (Wörmann et al., 2014).
Bacterial therapy involves using genetically modified bacteria, weakened living bacteria, or substances derived from bacteria such as peptides (Stanley et al., 1994) with anticancer properties. Bacteriocins, peptides released by bacteria like Lactobacillus, exhibit antimicrobial characteristics that inhibit the growth of other bacteria (De Vos et al., 1997). Bacteriocins not only effectively suppress the growth of antibiotic-resistant and pathogenic bacteria, thereby maintaining intestinal equilibrium (Cotter et al., 2013; Dicks et al., 2018), they also can induce cytotoxicity and apoptosis, making them appealing candidates for cancer therapy. In animal models, probiotics have demonstrated the ability to significantly diminish the activity of enterogenic oncogenic enzymes implicated in colorectal carcinogenesis (Rowland et al., 1998; Leblanc and Perdigon, 2005; Nakanishi et al., 2013). Moreover, probiotics can impact immune cell maturation and their products within the gut, as well as curb tumor formation in systemic immune organs like lymph nodes and the spleen (Ohkawara et al., 2007; Soltan et al., 2010). These findings underline the potential of probiotics as dietary supplements with anti-tumor properties. Furthermore, probiotic administration has been shown to ameliorate adverse effects of chemotherapy and immunotherapy. In the context of colorectal cancer, positive effects encompass reduced incidence of diarrhea, enhanced gut barrier integrity, and diminished inflammation. As a result, probiotics could serve as effective adjuncts for both the prevention and treatment of colorectal cancer (Liu et al., 2011; Demers et al., 2014; Zaharuddin et al., 2019). The photosensitizer’s antibacterial and bactericidal characteristics have been effectively utilized in root canal treatment, periodontal treatment, and elimination of candidiasis, yielding positive outcomes (Prażmo et al., 2016).
6 Future prospects and conclusions
Oral microbiota play a multifaceted role in cancer development by influencing mechanisms like cell proliferation, invasion, immunosuppression, and inflammatory responses. Moreover, they contribute to cancer prevention strategies, screening methods, and anti-cancer treatments. Despite these advancements, certain challenges persist within current research. Firstly, there is a lack of comprehensive understanding and substantial direct evidence to support these underlying mechanisms. Additionally, while the association between oral microorganisms and cancer is apparent, definitive links have not been universally established. Therefore, further exploration into the precise carcinogenic mechanisms employed by these microorganisms is needed. The potential utilization of oral microorganisms as biomarkers for accurate cancer diagnosis and prevention remains an open question, along with the identification of microorganisms with precise therapeutic effects. Recent investigations highlight the significance of signaling pathways like JAK 1/STAT 3, PI3K/Akt/NFκB, p38/JNK, ERK1/2/MAPK, and the toll-like receptor family in the progression of oral cancer. These pathways suggest potential key avenues through which oral microorganisms can impact oral cancer. Additionally, exploring the potential of oral microbes as diagnostic and prognostic markers for cancer requires further investigation to unravel their full potential.
This paper delves into the assessment of how oral microbiota contribute to tumorigenic and carcinogenic mechanisms, as well as their potential as markers for detecting, screening, and preventing tumors. By thoroughly exploring the intricate connection between oral microbiomes and systemic human cancers, we can gain valuable insights that further our comprehension of cancer prevention and treatment. This deeper understanding has the potential to significantly impact clinical outcomes by reducing the mortality rates of affected patients.
Author contributions
LS: Writing – review & editing. RY: Writing – original draft, Writing – review & editing. YS: Writing – original draft, Writing – review & editing. YZ: Writing – review & editing. HS: Writing – review & editing. ZZ: Writing – review & editing. QW: Writing – original draft, Writing – review & editing. SU: Writing – review & editing.
Funding
The author(s) declare that financial support was received for the research, authorship, and/or publication of this article. The Zhejiang Provincial Natural Science Foundation of China (LQ20H190004) has been concluded.
Conflict of interest
The authors declare that the research was conducted in the absence of any commercial or financial relationships that could be construed as a potential conflict of interest.
Publisher’s note
All claims expressed in this article are solely those of the authors and do not necessarily represent those of their affiliated organizations, or those of the publisher, the editors and the reviewers. Any product that may be evaluated in this article, or claim that may be made by its manufacturer, is not guaranteed or endorsed by the publisher.
References
Abranches, J., Lin, Z., Kajfasz, K., Palmer, S. R., Chakraborty, B., Wen, Z. T., et al. (2018). Biology of Oral streptococci. Microbiol Spectr 6:2018. doi: 10.1128/microbiolspec.GPP3-0042-2018
Abreu, M. T., and Peek, R. M. (2013). Gastrointestinal malignancy and the microbiome. Gastroenterology 146, 1534–1546. doi: 10.1053/j.gastro.2014.01.001
Alnuaimi, A. D., Ramdzan, A. N., Wiesenfeld, D., O'Brien-Simpson, N. M., Kolev, S. D., Reynolds, E. C., et al. (2016). Candida virulence and ethanol-derived acetaldehyde production in oral cancer and non-cancer subjects. Oral Dis. 22, 805–814. doi: 10.1111/odi.12565
Andam, C. P., and Hanage, W. P. (2015). Mechanisms of genome evolution of Streptococcus. Infect. Genet. Evol. 33, 334–342. doi: 10.1016/j.meegid.2014.11.007
Asoudeh-Fard, A., Barzegari, A., Dehnad, A., Bastani, S., Golchin, A., and Omidi, Y. (2017). Lactobacillus plantarum induces apoptosis in oral cancer KB cells through upregulation of PTEN and downregulation of MAPK signaling pathways. Bioimpacts 7, 193–198. doi: 10.15171/bi.2017.22
Auperin, A. (2020). Epidemiology of head and neck cancers: an update. Curr. Opin. Oncol. 32, 178–186. doi: 10.1097/CCO.0000000000000629
Birner, P., Schindl, M., Obermair, A., Plank, C., Breitenecker, G., and Oberhuber, G. J. C. R. (2000). Overexpression of hypoxia-inducible factor 1alpha is a marker for an unfavorable prognosis in early-stage invasive cervical cancer. Cancer Res. 60, 4693–4696.
Boyle, J. O., Hakim, J., Koch, W., Riet, P., and Sidransky, D. J. C. R. (1993). The incidence of p53 mutations increases with progression of head and neck. Cancer 53, 4477–4480.
Brooks, P., and Zahari, S. (2014). Acetaldehyde and the genome: Beyond nuclear DNA adducts and carcinogenesis. Environ. Mol. Mutagen. 55, 77–91. doi: 10.1002/em.21824
Castagnola, M., Picciotti, P. M., Messana, I., Fanali, C., and Cervico-facciale, A. F. (2011). Potential applications of human saliva as diagnostic fluid. Acta Otorhinolaryngol. Ital. 31, 347–357.
Cavallo, F., Giovanni, C. D., Nanni, P., Forni, G., and Lollini, P. L. J. C. I. I. C. (2011). 2011: the immune hallmarks of cancer. Cancer Immunol. Immunother. 60, 319–326. doi: 10.1007/s00262-010-0968-0
Chattopadhyay, I., Verma, M., and Panda, M. (2019). Role of Oral microbiome signatures in diagnosis and prognosis of Oral Cancer. Technol. Cancer Res. Treat. 18:153303381986735. doi: 10.1177/1533033819867354
Chen, S. M., Chieng, W. W., Huang, S. W., Hsu, L. J., and Jan, M. S. J. S. R. (2020). The synergistic tumor growth-inhibitory effect of probiotic Lactobacillus on transgenic mouse model of pancreatic cancer treated with gemcitabine. Sci. Rep. 10:5. doi: 10.1038/s41598-020-77322-5
Chen, S., Duan, G., Zhang, R., and Fan, Q. (2014). Helicobacter pylori cytotoxin-associated gene A protein upregulates α-enolase expression via Src/MEK/ERK pathway: implication for progression of gastric cancer. Int. J. Oncol. 45, 764–770. doi: 10.3892/ijo.2014.2444
Cheng, L. J. C. (2020). Role of Oral Bacteria in the Development of Oral Squamous Cell Carcinoma. Cancers 12:2797. doi: 10.3390/cancers12102797
Chiba, T., Marusawa, H., Seno, H., and Watanabe, N. J. J. G. H. (2010). Mechanism for gastric cancer development by Helicobacter pylori infection. J. Gastroenterol. Hepatol. 23, 1175–1181. doi: 10.1111/j.1440-1746.2008.05472.x
Choi, S., Collins, C. C., Gout, P. W., and Wang, Y. (2013). Cancer-generated lactic acid: a regulatory, immunosuppressive metabolite? J. Pathol. 230, 350–355. doi: 10.1002/path.4218
Coker, O. O., Dai, Z. W., Nie, Y. Z., Zhao, G. J., Cao, L., Nakatsu, G., et al. (2018). Mucosal microbiome dysbiosis in gastric carcinogenesis. Gut 67, 1024–1032. doi: 10.1136/gutjnl-2017-314281
Cosola, M., Cazzolla, A. P., Charitos, I. A., Ballini, A., Inchingolo, F., and Santacroce, L. (2021). Candida albicans and Oral carcinogenesis. A Brief Review. J Fungi 7:476. doi: 10.3390/jof7060476
Cotter, P. D., Ross, R. P., and Hill, C. (2013). Bacteriocins—a viable alternative to antibiotics? Nat. Rev. Microbiol. 11, 95–105. doi: 10.1038/nrmicro2937
Curry, J. M., Sprandio, J., Cognetti, D., Luginbuhl, A., Bar-Ad, V., Pribitkin, E., et al. (2014). Tumor microenvironment in head and neck squamous cell carcinoma. Semin. Oncol. 41, 217–234. doi: 10.1053/j.seminoncol.2014.03.003
De Vos, D., Lim, A. Jr., Pirnay, J. P., Struelens, M., Vandenvelde, C., Duinslaeger, L., et al. (1997). Direct detection and identification of Pseudomonas aeruginosa in clinical samples such as skin biopsy specimens and expectorations by multiplex PCR based on two outer membrane lipoprotein genes, oprI and oprL. J. Clin. Microbiol. 35, 1295–1299. doi: 10.1128/jcm.35.6.1295-1299.1997
Demers, M., Dagnault, A., and Desjardins, J. J. C. N. (2014). A randomized double-blind controlled trial: impact of probiotics on diarrhea in patients treated with pelvic radiation. Clin. Nutr. 33, 761–767. doi: 10.1016/j.clnu.2013.10.015
Deng, J., Gao, G., Wang, L., Wang, T., Yu, J., and Zhao, Z. J. P.-R. (2011). Stratifin expression is a novel prognostic factor in human gliomas. Pathol. Res. Pract. 207, 674–679. doi: 10.1016/j.prp.2011.08.005
Dicks, L. M. T., Dreyer, L., Smith, C., and van Staden, A. D. (2018). A review: the fate of Bacteriocins in the human gastro-intestinal tract: do they cross the Gut-blood barrier? Front. Microbiol. 9:2297. doi: 10.3389/fmicb.2018.02297
Dilhari, A., Nagahawatte, A., Weerasekera Manjula, M., Siriwardhana, A., Maheshika, O., Gunasekara, C., et al. (2016). Candida infection in oral leukoplakia: an unperceived public health problem. Acta Odontol. Scand. 74, 565–569. doi: 10.1080/00016357.2016.1220018
Dominique, S. M. (2013). Plasma antibodies to oral bacteria and risk of pancreatic cancer in a large European prospective cohort study. Gut. 62, 1764–1770.
Doocey, C. M., Finn, K., Murphy, C., and Guinane, C. M. (2022). The impact of the human microbiome in tumorigenesis, cancer progression, and biotherapeutic development. BMC Microbiol. 22:17. doi: 10.1186/s12866-022-02465-6
Eklöf, V., Löfgren-Burström, A., Zingmark, C., Edin, S., Larsson, P., Karling, P., et al. (2017). Cancer‐associated fecal microbial markers in colorectal cancer detection. Int J Cancer 141, 2528–2536. doi: 10.1002/ijc.31011
Fan, X. Z., Alekseyenko, A. V., Wu, J., Peters, B. A., Jacobs, E. J., Gapstur, S. M., et al. (2018). Human oral microbiome and prospective risk for pancreatic cancer: a population-based nested case-control study. Gut 67, 120–127. doi: 10.1136/gutjnl-2016-312580
Fan, M., Ruifeng, L., Liyu, M., Liu, L., Lai, X., Yang, D., et al. (2019). Porphyromonas gingivalis promotes the motility of esophageal squamous cell carcinoma by activating NF-κB signaling pathway. Microbes Infect. 21, 296–304. doi: 10.1016/j.micinf.2019.01.005
Farrell, J., and Gut, J. J. (2012). Variations of oral microbiota are associated with pancreatic diseases including pancreatic cancer. Gut 61, 582–588. doi: 10.1136/gutjnl-2011-300784
Feller, L., Chandran, R., Khammissa, R. A. G., Meyerov, R., and Lemmer, J. J. S. (2013). Alcohol and oral squamous cell carcinoma. SADJ 68, 176–180.
Ferlay, J., Soerjomataram, I., Dikshit, R., Eser, S., Mathers, C., Rebelo, M., et al. (2015). Cancer incidence and mortality worldwide: sources, methods and major patterns in GLOBOCAN 2012. Int. J. Cancer 136:E359. doi: 10.1002/ijc.29210
Ferreira, A. C., Isomoto, H., Moriyama, M., Fujioka, T., Machado, J. C., and Yamaoka, Y. J. H. (2008). Helicobacter and gastric malignancies. Helicobacter 13, 28–34. doi: 10.1111/j.1523-5378.2008.00633.x
Findik, S., Findik, S., Abuoglu, S., Cihan, F. G., Ilter, H., and Iyisoy, M. S. (2019). Human papillomavirus (HPV) subtypes and their relationships with cervical smear results in cervical cancer screening: a community-based study from the Central Anatolia region of Turkey. Int. J. Clin. Exp. Pathol. 12, 1391–1398.
Fitzsimonds, Z. R., Rodriguez-Hernandez, C. J., Bagaitkar, J., and Lamont, R. J. (2020). From beyond the pale to the pale riders: the emerging Association of Bacteria with Oral Cancer. J. Dent. Res. 99, 604–612. doi: 10.1177/0022034520907341
Flemer, B., Warren, R. D., Barrett, M. P., Cisek, K., Das, A., Jeffery, I. B., et al. (2017). The oral microbiota in colorectal cancer is distinctive and predictive. Gut 67:1454. doi: 10.1136/gutjnl-2017-314814
Gao, S., Li, S., Ma, Z., Liang, S., Shan, T., Zhang, M., et al. (2016). Presence of Porphyromonas gingivalis in esophagus and its association with the clinicopathological characteristics and survival in patients with esophageal cancer. Infect J Cancer 11:3. doi: 10.1186/s13027-016-0049-x
Gaskins, H. R., Attene-Ramos, M. S., Wagner, E. D., and Plewa, M. (2007). Hydrogen sulfide induces direct radical associated DNA damage. Mol. Cancer Res. 5:455. doi: 10.1158/1541-7786.MCR-06-0439
Geng, F. X., Liu, J. C., Guo, Y., Li, C., Wang, H. Y., Wang, H. Y., et al. (2017). Persistent exposure to Porphyromonas gingivalis promotes proliferative and invasion capabilities, and tumorigenic properties of human immortalized Oral epithelial cells. Front. Cell. Infect. Microbiol. 7:16. doi: 10.3389/fcimb.2017.00057
Geng, F., Zhang, Y., Lu, Z., Zhang, S., Pan, Y. J. D., and Biology, C. (2019). Fusobacterium nucleatum caused DNA damage and promoted cell proliferation by the Ku70 / p53 pathway in Oral Cancer Cells. DNA Cell Biol. 39, 144–151. doi: 10.1089/dna.2019.5064
Gottesman, M. M. (2022). Mechanisms of Cancer drug resistance. Annu. Rev. Med. 53:615. doi: 10.1146/annurev.med.53.082901.103929
Gupta, B., and Kumar, N. (2017). Worldwide incidence, mortality and time trends for cancer of the oesophagus. Eur. J. Cancer Prev. 26, 107–118. doi: 10.1097/CEJ.0000000000000249
Ha, N. H., Park, D. G., Woo, B. H., Kim, D. J., Choi, J. I., Park, B. S., et al. (2016). Porphyromonas gingivalis increases the invasiveness of oral cancer cells by upregulating IL-8 and MMPs. Cytokine 86, 64–72. doi: 10.1016/j.cyto.2016.07.013
Hafed, L., Farag, H., El-Rouby, D., Shaker, O., and Shabaan, H. A. (2019). Candida ALBICANS alcohol dehydrogenase 1 gene in oral dysplasia and oral squamous cell carcinoma. Pol. J. Pathol. 70, 210–216. doi: 10.5114/pjp.2019.90398
Hampelska, K., Jaworska, M. M., Babalska, Z. U., and Karpiński, T. M. (2020). The role of Oral microbiota in intra-Oral halitosis. J. Clin. Med. 9:2484. doi: 10.3390/jcm9082484
Hashimoto, K., Shimizu, D., Hirabayashi, S., Ueda, S., Miyabe, S., Oh-iwa, I., et al. (2019). Changes in oral microbial profiles associated with oral squamous cell carcinoma vs leukoplakia. J. Investig. Clin. Dent. 10:7. doi: 10.1111/jicd.12445
Hayes, R. B., Ahn, J., Fan, X., Peters, B. A., Ma, Y., Yang, L., et al. (2018). Association of Oral Microbiome with Risk for incident head and neck squamous cell Cancer. JAMA Oncol. 4, 358–365. doi: 10.1001/jamaoncol.2017.4777
Hiroshima, Y., Zhang, Y., Murakami, T., Maawy, A., Miwa, S., Yamamoto, M., et al. (2014). Efficacy of tumor-targeting Salmonella typhimurium A1-R in combination with anti-angiogenesis therapy on a pancreatic cancer patient-derived orthotopic xenograft (PDOX) and cell line mouse models. Oncotarget 5:12346. doi: 10.18632/oncotarget.2641
Hong, J., Guo, F., Lu, S.-Y., Shen, C., Ma, D., Zhang, X., et al. (2021). F. nucleatum targets lncRNA ENO1-IT1 to promote glycolysis and oncogenesis in colorectal cancer. Gut 70, 2123–2137. doi: 10.1136/gutjnl-2020-322780
Hooper, S. J., Crean, S. J., Lewis, M., Spratt, D. A., Wade, W. G., and Wilson, M. J. J. J. (2006). Viable Bacteria present within Oral squamous cell carcinoma tissue. J. Clin. Microbiol. 44, 1719–1725. doi: 10.1128/JCM.44.5.1719-1725.2006
Hsiao, J. R., Chang, C. C., Lee, W. T., Huang, C. C., Ou, C. Y., Tsai, S. T., et al. (2018). The interplay between oral microbiome, lifestyle factors and genetic polymorphisms in the risk of oral squamous cell carcinoma. Carcinogenesis 39, 778–787. doi: 10.1093/carcin/bgy053
Hu, Y., Zeng, Q., Li, C. X., and Xie, Y. (2019). Expression profile and prognostic value of SFN in human ovarian cancer. Biosci. Rep. 39:11. doi: 10.1042/BSR20190100
Hu, X. S., Zhang, Q., Hua, H., and Chen, F. (2016). Changes in the salivary microbiota of oral leukoplakia and oral cancer. Oral Oncol. 56, E6–E8. doi: 10.1016/j.oraloncology.2016.03.007
Hussain, P., Hofseth, S., Lorne, J. H., and Curtis, C. J. N. R. C. (2003). Radical causes of cancer. Nat. Rev. Cancer 3, 276–285. doi: 10.1038/nrc1046
Inada, R., Sekine, S., Taniguchi, H., Tsuda, H., Katai, H., Fujiwara, T., et al. (2015). ARID1A expression in gastric adenocarcinoma: Clinicopathological significance and correlation with DNA mismatch repair status. World J. Gastroenterol. 21, 2159–2168. doi: 10.3748/wjg.v21.i7.2159
Joyce, J. A., and Pollard, J. W. (2009). Microenvironmental regulation of metastasis. Nat. Rev. Cancer 9, 239–252. doi: 10.1038/nrc2618
Kakabadze, M. Z., Paresishvili, T., Karalashvili, L., Chakhunashvili, D., and Kakabadze, Z. J. O. R. (2020). Oral microbiota and Oral cancer: review. Oncol Rev 14:476. doi: 10.4081/oncol.2020.476
Kalaf, E., Al-Khafaji, Z., Yassen, N., Al-Abbudi, F., and Sadwen, S. J. S. J. O. G. (2013). Study of the Cytoxin-associated gene A (CagA gene) in Helicobacter pylori using gastric biopsies of Iraqi patients. Saudi J. Gastroenterol. 19, 69–74. doi: 10.4103/1319-3767.108474
Karpinski, T. M. (2019). Role of Oral microbiota in Cancer development. Microorganisms 7:14. doi: 10.3390/microorganisms7010020
Kauppila, J. H., Mattila, A. E., Karttunen, T. J., and Salo, T. J. O. (2013). Toll-like receptor 5 and the emerging role of bacteria in carcinogenesis. Onco Targets Ther 2:620. doi: 10.4161/onci.23620
Kuboniwa, M., Hasegawa, Y., Mao, S., Shizukuishi, S., Amano, A., Lamont, R. J., et al. (2008). P. gingivalis accelerates gingival epithelial cell progression through the cell cycle. Microbes Infect. 10, 122–128. doi: 10.1016/j.micinf.2007.10.011
Kumar, M., Kumar, A., Nagpal, R., Mohania, D., and Yadav, H. J. (2010). Cancer-preventing attributes of probiotics: An update. Int. J. Food Sci. Nutr. 61, 473–496. doi: 10.3109/09637480903455971
Leblanc, D., and Perdigon, A. (2005). Reduction of b-Glucuronidase and nitroreductase activity by yoghurt in a murine colon cancer model. Biocell 29, 15–24.
Liang, Z. Y., Kang, X., Chen, H., Wang, M., and Guan, W. X. (2017). Effect of Clostridium perfringens enterotoxin on gastric cancer cells SGC7901 which highly expressed claudin-4 protein. World J Gastrointest Oncol 9, 153–159. doi: 10.4251/wjgo.v9.i4.153
Li, S., He, M., Lei, Y., Liu, Y., Li, X., Xiang, X., et al. (2022). Oral Microbiota and Tumor—A New Perspective of Tumor Pathogenesis. Microorganisms. 10.
Liu, X. D., Jiang, S. N., Piao, L. H., and Yuan, F. (2016). Radiotherapy combined with an engineered Salmonella typhimurium inhibits tumor growth in a mouse model of colon cancer. Exp. Anim. 65, 413–418. doi: 10.1538/expanim.16-0033
Liu, Z., Qin, H., Yang, Z., Xia, Y., Liu, W., Yang, J., et al. (2011). Randomised clinical trial: the effects of perioperative probiotic treatment on barrier function and post‐operative infectious complications in colorectal cancer surgery – a double‐blind study. Aliment. Pharmacol. Ther. 33, 50–63. doi: 10.1111/j.1365-2036.2010.04492.x
Liu, S. Y., Zhou, X. D., Peng, X., Li, M. Y., Ren, B., Cheng, G., et al. (2020). Porphyromonas gingivalis promotes Immunoevasion of Oral Cancer by protecting Cancer from macrophage attack. J. Immunol. 205, 282–289. doi: 10.4049/jimmunol.1901138
Luo, J., Nordenvall, C., Nyrén, O., Adami, H. O., Permert, J., and Ye, W. (2007). The risk of pancreatic cancer in patients with gastric or duodenal ulcer disease. Int. J. Cancer 120, 368–372. doi: 10.1002/ijc.22123
Maddi, A., Sabharwal, A., Violante, T., Manuballa, S., Genco, R., Patnaik, S., et al. (2019). The microbiome and lung cancer. J. Thoracic Disease. 11, 280–291.
Manosha, P., Nezar, N., Al-hebshi, D., Perera, I., and Johnson, N. W. (2016). Emerging role of bacteria in oral carcinogenesis: a review with special reference to perio-pathogenic bacteria. J. Oral Microbiol. 8:2762. doi: 10.3402/jom.v8.32762
Marc, S., Scott, G., and Pathogens, F. J. P. (2017). Oropharyngeal candidiasis: Fungal Invasion and Epithelial Cell Responses. PLoS Pathog. 13:e1006056. doi: 10.1371/journal.ppat.1006056
Michaud, D. (2013). Role of bacterial infections in pancreatic cancer. Carcinogenesis 34, 2193–2197. doi: 10.1093/carcin/bgt249
Michaud, D. S., Izard, J., Wilhelm-Benartzi, C. S., You, D. H., Grote, V. A., Tjønneland, A., et al. (2013). Plasma antibodies to oral bacteria and risk of pancreatic cancer in a large European prospective cohort study. Gut 62, 1764–1770. doi: 10.1136/gutjnl-2012-303006
Mima, K., Sukawa, Y., Nishihara, R., Qian, Z. R., Yamauchi, M., Inamura, K., et al. (2015). Fusobacterium nucleatum and T cells in colorectal carcinoma. JAMA Oncol. 1, 653–661. doi: 10.1001/jamaoncol.2015.1377
Mizumoto, A., Ohashi, S., Hirohashi, K., Amanuma, Y., Matsuda, T., and Muto, M. (2017). Molecular mechanisms of acetaldehyde-mediated carcinogenesis in squamous epithelium. Int. J. Mol. Sci. 18:12. doi: 10.3390/ijms18091943
Nagy, K. N., Sonkodi, I., Szoke, I., Nagy, E., and Newman, H. N. (1998). The microflora associated with human oral carcinomas. Oral Oncol. 34, 304–308. doi: 10.1016/S1368-8375(98)80012-2
Nakanishi, S., Kataoka, K., Kuwahara, T., and Ohnishi, Y. J. (2013). Effects of high amylose maize starch and Clostridium butyricum on metabolism in colonic microbiota and formation of azoxymethane-induced aberrant crypt foci in the rat colon. Microbiol. Immunol. 47, 951–958. doi: 10.1111/j.1348-0421.2003.tb03469.x
Nisticò, P., Bissell Mina, J., Radisky, V., and Derek, C. (2012). Epithelial-mesenchymal transition: general principles and pathological relevance with special emphasis on the role of matrix metalloproteinases. Cold Spring Harb. Perspect. Biol. 4:a011908. doi: 10.1101/cshperspect.a011908
Ohkawara, S., Furuya, H., Nagashima, K., Asanuma, N., and Hino, T. J. N. (2007). Effect of Oral Administration of Butyrivibrio fibrisolvens MDT-1, a Gastrointestinal Bacterium, on 3-Methylcholanthrene-Induced Tumor in Mice. Nutr. Cancer 59, 92–98. doi: 10.1080/01635580701397608
Ojcius, D., Yilmaz, M., Ozlem, H., et al. (2016). Fusobacterium nucleatum infection of gingival epithelial cells leads to NLRP3 inflammasome-dependent secretion of IL-1 and the danger signals ASC and HMGB1. Cell. Microbiol. 18, 970–981. doi: 10.1111/cmi.12560
Olsen, I., Taubman, M. A., and Singhrao, S. K. (2016). Porphyromonas gingivalis suppresses adaptive immunity in periodontitis, atherosclerosis, and Alzheimer's disease. J. Oral Microbiol. 8:13. doi: 10.3402/jom.v8.33029
O'Shea, J. J., Schwartz, D. M., Villarino, A. V., Gadina, M., McInnes, I. B., and Laurence, A. (2015). The JAK-STAT pathway: impact on human disease and therapeutic intervention. Annu. Rev. Med. 66, 311–328. doi: 10.1146/annurev-med-051113-024537
Özlem, Y., Yao, L., Maeda, K., et al. (2008). ATP scavenging by the intracellular pathogen Porphyromonas gingivalis inhibits P2X7-mediated host-cell apoptosis. Cell. Microbiol. 10, 863–875. doi: 10.1111/j.1462-5822.2007.01089.x
Pan, C., Xu, X., Tan, L., Lin, L., and Pan, Y. J. O. D. (2013). The effects of Porphyromonas gingivalis on the cell cycle progression of human gingival epithelial cells. Oral Dis. 20, 100–108. doi: 10.1111/odi.12081
Peter, L. J. J. O. F. (2018). What we do not know about fungal cell adhesion. Molecules 4:59. doi: 10.3390/jof4020059
Peters, B. A., Jing, W., Pei, Z., Yang, L., and Ahn, J. J. C. R. (2017). Abstract 4961: the oral microbiome and prospective risk for esophageal cancer: A population-based nested case-control study. Cancer Res 77:4961. doi: 10.1158/1538-7445.AM2017-4961
Piao, J.-Y., Geum, H., Kim, S.-J., Kim, D., Han, H.-J., Ngo, H.-K., et al. (2016). Helicobacter pylori activates IL-6-STAT3 signaling in human gastric Cancer cells: potential roles for reactive oxygen species. Helicobacter 21, 405–416. doi: 10.1111/hel.12298
Poplawski, T., Chojnacki, C., Czubatka, A., Klupinska, G., Chojnacki, J., and Blasiak, J. (2013). Helicobacter pylori infection and antioxidants can modulate the genotoxic effects of heterocyclic amines in gastric mucosa cells. Mol. Biol. Rep. 40, 5205–5212. doi: 10.1007/s11033-013-2622-3
Prażmo, E., Mielczarek, A., Kwaśny, M., and Łapiński, M. (2016). Photodynamic therapy as a promising method used in the treatment of Oral diseases. Adv. Clin. Exp. Med. 25, 799–807. doi: 10.17219/acem/32488
Qin, H., Holdbrooks, A. T., Liu, Y., Reynolds, S. L., Yanagisawa, L. L., and Benveniste, E. N. (2012). SOCS3 deficiency promotes m1 macrophage polarization and inflammation. J. Immunol. 189, 3439–3448.
Rai, A. K., Panda, M., Das, A. K., Rahman, T., Das, R., Das, K., et al. (2021). Dysbiosis of salivary microbiome and cytokines influence oral squamous cell carcinoma through inflammation. Arch. Microbiol. 203, 137–152. doi: 10.1007/s00203-020-02011-w
Renée, G., Pascale, P., and Daniel, G. J. I. (2004). Binding of pro-matrix metalloproteinase 9 by Fusobacterium nucleatum subsp. nucleatum as a mechanism to promote the invasion of a reconstituted basement membrane. Infect. Immun. 72:6160. doi: 10.1128/IAI.72.10.6160-6163.2004
Rhodus, N. L., Ho, V., Miller, C. S., Myers, S., and Ondrey, F. J. (2005). NF-κB dependent cytokine levels in saliva of patients with oral preneoplastic lesions and oral squamous cell carcinoma. Cancer Detect. Prev. 29, 42–45. doi: 10.1016/j.cdp.2004.10.003
Riviere, G. R., Riviere, K. H., and Smith, K. S. (2010). Molecular and immunological evidence of oral Treponema in the human brain and their association with Alzheimer's disease. Oral Microbiol. Immunol. 17, 113–118. doi: 10.1046/j.0902-0055.2001.00100.x
Rooper, L. M., Windon, M. J., Hernandez, T., Miles, B., Ha, P. K., Ryan, W. R., et al. (2020). HPV-positive squamous cell carcinoma of the larynx, Oral cavity, and hypopharynx Clinicopathologic characterization with recognition of a novel warty variant. Am. J. Surg. Pathol. 44, 691–702. doi: 10.1097/PAS.0000000000001433
Rowland, I. R., Rumney, C. J., Coutts, J. T., and Lievense, L. C. (1998). Effect of Bifidobacterium longum and inulin on gut bacterial metabolism and carcinogen-induced aberrant crypt foci in rats. Carcinogenesis 19, 281–285. doi: 10.1093/carcin/19.2.281
Roxana, M., Rubinstein, X., Wang, W., Liu, W., Hao, Y., Cai, G., et al. (2013). Fusobacterium nucleatum promotes colorectal carcinogenesis by modulating E-cadherin/β-catenin signaling via its FadA Adhesin. Cell Host Microbe 14, 195–206. doi: 10.1016/j.chom.2013.07.012
Saito, A., Kokubu, E., Inagaki, S., Imamura, K., Kita, D., Lamont, R. J., et al. (2012). Porphyromonas gingivalis entry into gingival epithelial cells modulated by Fusobacterium nucleatum is dependent on lipid rafts. Microb. Pathog. 53, 234–242. doi: 10.1016/j.micpath.2012.08.005
Sami, A., Elimairi, I., Stanton, C., Ross, R. P., and Ryan, C. (2020). The role of the microbiome in Oral squamous cell carcinoma with insight into the microbiome–treatment Axis. Int. J. Mol. Sci. 21, 1–29. doi: 10.3390/ijms21218061
Sanjaya, P. R., Gokul, S., Patil, B. G., and Raju, R. J. M. H. (2011). Candida in oral pre-cancer and oral cancer. Med. Hypotheses 77, 1125–1128. doi: 10.1016/j.mehy.2011.09.018
Sankaranarayanan, R., Mathew, B., Varghese, C., Sudhakaran, P. R., and Nair, P. P. J. O. O. (1997). Chemoprevention of oral leukoplakia with vitamin A and beta carotene: an assessment. Oral Oncol. 33, 231–236. doi: 10.1016/S0964-1955(97)00010-9
Serna, G., Ruiz-Pace, F., Hernando, J., Alonso, L., Fasani, R., Landolfi, S., et al. (2020). Fusobacterium nucleatum persistence and risk of recurrence after preoperative treatment in locally advanced rectal cancer. Ann. Oncol. 31, 1366–1375. doi: 10.1016/j.annonc.2020.06.003
Soltan, D., Yazdi, M., Hassan, M., Holakuyee, M., Mohtasab, T. A., Aminharaty, F., et al. (2010). Effect of oral administration of lactobacillus acidophilus on the immune responses and survival of BALB/c mice bearing human breast cancer. Tehran Univ Med J 67, 753–758.
Sonnenburg, J. L., Angenent, L. T., and Gordon, J. I. J. N. I. (2004). Getting a grip on things: how do communities of bacterial symbionts become established in our intestine? Nat. Immunol. 5, 569–573. doi: 10.1038/ni1079
Stanley, J., Linton, D., Burnens, A. P., Dewhirst, F. E., On, S. L., Porter, A., et al. (1994). Helicobacter pullorum sp. nov.—genotype and phenotype of a new species isolated from poultry and from human patients with gastroenteritis. Microbiology 140, 3441–3449.
Stasiewicz, M., and Karpiński, T. M. (2022). The oral microbiota and its role in carcinogenesis. Semin. Cancer Biol. 86, 633–642. doi: 10.1016/j.semcancer.2021.11.002
Sun, J., Tang, Q., Yu, S., Xie, M., and Chen, L. J. C. M. (2020). Role of the oral microbiota in cancer evolution and progression. Cancer Med. 9:6306. doi: 10.1002/cam4.3206
Tahara, T., Yamamoto, E., Suzuki, H., Maruyama, R., Chung, W., Garriga, J., et al. (2014). Fusobacterium in colonic flora and molecular features of colorectal carcinoma. Cancer Res. 74, 1311–1318. doi: 10.1158/0008-5472.CAN-13-1865
Takahashi, N., and Nyvad, B. (2011). The role of Bacteria in the caries process: ecological perspectives. J. Dent. Res. 90, 294–303. doi: 10.1177/0022034510379602
Tamayo-Trujillo, R., Guevara-Ramírez, P., Cadena-Ullauri, S., Paz-Cruz, E., Ruiz-Pozo, V. A., and Zambrano, A. K. (2023). Human virome: implications in cancer. Heliyon 9:e14086. doi: 10.1016/j.heliyon.2023.e14086
Uitto, V. J., Baillie, D., Qiang, W., Gendron, R., Grenier, D., Putnins, E. E., et al. (2005). Fusabacterium nucleatum increases collagenase 3 production and migration of epithelial cells. Infect. Immun. 73, 1171–1179. doi: 10.1128/IAI.73.2.1171-1179.2005
Vyhnalova, T., Danek, Z., Gachova, D., and Linhartova, P. B. J. M. (2021). The role of the Oral microbiota in the Etiopathogenesis of Oral squamous cell carcinoma. Microorganisms 9:1549. doi: 10.3390/microorganisms9081549
Wei, M. Y., Shi, S., Liang, C., Meng, Q. C., Hua, J., Zhang, Y. Y., et al. (2019). The microbiota and microbiome in pancreatic cancer: more influential than expected. Mol. Cancer 18:97. doi: 10.1186/s12943-019-1008-0
Wen, L., Mu, W., Lu, H., Wang, X., Fang, J., Jia, Y., et al. (2020). Porphyromonas gingivalis promotes Oral squamous cell carcinoma progression in an immune microenvironment. J. Dent. Res. 99, 666–675. doi: 10.1177/0022034520909312
Werneck, J. T., Costa, T. D., Stibich, C. A., Leite, C. A., Dias, E. P., and Silva, A. (2015). Oral lichen planus: study of 21 cases. An. Bras. Dermatol. 90, 321–326. doi: 10.1590/abd1806-4841.20153704
Wong, S. H., Kwong, T., Chow, T. C., Luk, A., and Sung, J. J. Y. J. G. (2016). Quantitation of faecal Fusobacterium improves faecal immunochemical test in detecting advanced colorectal neoplasia. Gut 66, 1441–1448. doi: 10.1136/gutjnl-2016-312766
Wörmann, M., Diakopoulos, N. K., Lesina, S., and Alguil, J. (2014). The immune network in pancreatic cancer development and progression. Oncogene 33, 2956–2967. doi: 10.1038/onc.2013.257
Xia, C., Su, J., Liu, C., Mai, Z., Yin, S., Yang, C., et al. (2023). Human microbiomes in cancer development and therapy. MedComm 4:e221. doi: 10.1002/mco2.221
Yang, L. Q., Xiao, X., Li, C. X., Wu, W. Y., Shen, X. M., Zhou, Z. T., et al. (2019). Human papillomavirus genotypes and p16 expression in oral leukoplakia and squamous cell carcinoma. Int. J. Clin. Exp. Pathol. 12, 1022–1028.
Yang, Z., Zhang, S., Ji, N., Li, J., and Chen, Q. (2023). The evil companion of OSCC: Candida albicans. Oral Dis. doi: 10.1111/odi.14700 (ahead of print).
Yongzhi, Y., Wenhao, W., Junjie, P., Hong, L., Yang, L., Toiyama, Y., et al. (2017). Fusobacterium nucleatum increases proliferation of colorectal Cancer cells and tumor development in mice by activating toll-like receptor 4 signaling to nuclear factor−κB, and up-regulating expression of MicroRNA-21. Gastroenterology 152:851. doi: 10.1053/j.gastro.2016.11.018
Yost, S., Stashenko, P., Choi, Y., Kukuruzinska, M., Genco, C. A., Salama, A., et al. (2018). Increased virulence of the oral microbiome in oral squamous cell carcinoma revealed by metatranscriptome analyses. Int. J. Oral Sci. 10:10. doi: 10.1038/s41368-018-0037-7
Yu, T. C., Guo, F. F., Yu, Y. N., Sun, T. T., Ma, D., Han, J. X., et al. (2017). Fusobacterium nucleatum promotes Chemoresistance to colorectal Cancer by modulating autophagy. Cell 170:548. doi: 10.1016/j.cell.2017.07.008
Yu, A. Q., and Li, L. J. N. (2016). The potential role of probiotics in Cancer prevention and treatment. Nutr. Cancer 68, 535–544. doi: 10.1080/01635581.2016.1158300
Yu, Y. N., Yu, T. C., Zhao, H. J., Sun, T. T., and Fang, J. Y. J. O. (2015). Berberine may rescue Fusobacterium nucleatum-induced colorectal tumorigenesis by modulating the tumor microenvironment. Oncotarget 6, 32013–32026. doi: 10.18632/oncotarget.5166
Yuan, X., Liu, Y. W., Kong, J. Y., Gu, B. L., Qi, Y. J., Wang, X. S., et al. (2017). Different frequencies of Porphyromonas gingivalis infection in cancers of the upper digestive tract. Cancer Lett. 404, 1–7. doi: 10.1016/j.canlet.2017.07.003
Zaharuddin, L., Mokhtar, N. M., Nawawi, K. N. M., and Ali, R. A. (2019). A randomized double-blind placebo-controlled trial of probiotics in post-surgical colorectal cancer. BMS Gatroenterol 19:19:131. doi: 10.1186/s12876-019-1047-4
Zhang, S. W., Li, C., Liu, J. C., Geng, F. X., Shi, X. T., Li, Q., et al. (2020). Fusobacterium nucleatum promotes epithelial-mesenchymal transiton through regulation of the lncRNA MIR4435-2HG/miR-296-5p/Akt2/SNAI1 signaling pathway. FEBS J. 287, 4032–4047. doi: 10.1111/febs.15233
Zhang, L., Liu, Y., Zheng, H. J., and Zhang, C. P. (2020). The Oral microbiota may have influence on Oral Cancer. Front. Cell. Infect. Microbiol. 9:11. doi: 10.3389/fcimb.2019.00476
Keywords: oral microbiota, systemic cancers, carcinogenesis, Porphyromonas gingivalis, Fusobacterium nucleatum
Citation: Su L, Yang R, Sheng Y, Ullah S, Zhao Y, Shunjiayi H, Zhao Z and Wang Q (2024) Insights into the oral microbiota in human systemic cancers. Front. Microbiol. 15:1369834. doi: 10.3389/fmicb.2024.1369834
Edited by:
Davida Smyth, Texas A&M University San Antonio, United StatesReviewed by:
Shabir A. Bhat, University of California, Los Angeles, United StatesDivyashri Baraniya, Temple University, United States
Copyright © 2024 Su, Yang, Sheng, Ullah, Zhao, Shunjiayi, Zhao and Wang. This is an open-access article distributed under the terms of the Creative Commons Attribution License (CC BY). The use, distribution or reproduction in other forums is permitted, provided the original author(s) and the copyright owner(s) are credited and that the original publication in this journal is cited, in accordance with accepted academic practice. No use, distribution or reproduction is permitted which does not comply with these terms.
*Correspondence: Qingjing Wang, d2FuZ3FqQHpqc3J1LmVkdS5jbg==
†These authors have contributed equally to this work and share first authorship