- College of Animal Science and Technology, Jilin Agricultural University, Changchun, China
Introduction: The drawbacks of using antibiotics as feed additives for blue foxes have gradually become apparent; moreover, thymol has wide-spectrum antimicrobial activity and has the potential to replace antibiotics in various animals. However, there are few reports on the effects of thymol on blue foxes.
Methods: This study aimed to investigate the effects of different concentrations of thymol on the growth performance, apparent nutrient digestibility, serum biochemical indicators, intestinal morphology, and gut microbiota of blue foxes. Twenty-four male blue foxes (120 ± 5 d) of similar weight (6.05 ± 0.16 kg) were randomly divided into 4 groups. 0, 100, 200, and 300 mg/kg thymol were added to the basal diets of groups C, L, M, and H, respectively.
Results: Compared with those in the C group, the addition of 100 mg/kg thymol to the diet significantly increased organic matter (OM) digestibility, crude protein (CP) digestibility, immunoglobulin (Ig) A, IgM, the VH of the duodenum, the CD of the jejunum, the VH of the ileum, and the VH/CD of the ileum (P < 0.05) and strongly significantly increased IgG (P < 0.01). The addition of 200 mg/kg thymol to the diet increased the VH/CD of the duodenum (P < 0.05). The addition of 300 mg/kg thymol to the diet significantly increased the VH and CD of the jejunum (P < 0.05). The addition of 200 mg/kg and 300 mg/kg thymol to the diets increased the final weight (FW) (P < 0.05). Adding 100 mg/kg thymol significantly increased the levels of interleukin-4 (IL-4) and catalase (CAT) compared with those in the other groups (P < 0.05). 16S rRNA gene detection revealed that thymol can change the abundances of Bifidobacterium, Fusobacterium, Allobaculum, Streptococcus, Megasphaera, and Lactobacillus in the gut.
Conclusion: The addition of thymol to diets can increase the abundance of Bifidobacterium, Fusobacterium, and Allobaculum, which may contribute to improving the growth performance of blue foxes.
1 Introduction
Fox pelt, mink pelt, and Persian lamb pelt are the three major types of fur in the world, among which blue fox skin is an important raw material for making high-end fur clothing and accessories, known as the “soft gold” in fur (Ylinen et al., 2020). Blue foxes are widely bred in northern China, and their breeding scale is constantly expanding. However, the drawbacks of using antibiotics as feed additives for blue foxes, such as increased resistance to pathogenic microorganisms, inhibition of blue fox growth performance, and the generation of drug residues, have gradually become prominent (Bhardwaj et al., 2022). Therefore, finding alternative products for antibiotics has become an important direction for the development of sustainable animal husbandry. Thymol is a safe and green feed additive that can enhance the immune system of animals to resist the invasion of exogenous pathogens and has potential value as a substitute for antibiotics (Marchese et al., 2016). Thymol, as a potential feed additive to replace antibiotics, has been applied in various animal feed sources. A previous study revealed that the addition of 1% thymol to the diet of weaned piglets can increase the serum concentrations of immunoglobulin (Ig) A and IgM and alleviate the harmful effects of Salmonella typhimurium on piglet growth performance (Trevisi et al., 2007). It has been reported that thymol can reduce the inflammatory response caused by Staphylococcus aureus infection by regulating extracellular vesicles (Kwon et al., 2019). Furthermore, thymol enhances barrier function and reduces the production of reactive oxygen species and the expression of proinflammatory cytokine genes in epithelial cells (Omonijo et al., 2019). Similarly, Liang et al. (2014) reported that thymol may inhibit the production of tumor necrosis factor-α (TNF-α) and interleukin-β (IL-β) in lipopolysaccharide (LPS)-stimulated mouse mammary epithelial cells. In addition, thymol can accelerate the metabolism and renewal rate of intestinal villous epithelial cells, reducing the risk of pathogen invasion (Ghiselli et al., 2022). Thymol may improve animal production performance by regulating the gut microbiota. Thymol can improve animal production performance by regulating the gut microbiota. Betancourt et al. (2014) showed that adding thymol to the basic diet of male chicks improved the microbial community structure and reduced the risk of disease and mortality. Adding 30 mg/kg thymol to the basic diet of male turkeys can increase the number of Lactobacillus strains in the cecum, reduce the number of Escherichia coli, and promote the growth and development of turkeys (Giannenas et al., 2014). Dietary thymol supplementation can promote the digestion and absorption of nutrients in piglets, reduce diarrhea, have a positive impact on the development of intestinal morphology, and improve the gut microbiota (Diao et al., 2015). The addition of 100 mg/kg thymol to the sow diet can increase the number of Lactobacillus, Escherichia coli, and Enterococcus in feces and increase the average daily weight gain (ADG) of suckling piglets (Tan et al., 2015). Thus, thymol has the potential to replace antibiotics, but its application in fur animal growth performance, especially in blue foxes, has not yet been reported. Therefore, the purpose of this study was to investigate the effects of thymol on the growth performance, apparent nutrient digestibility, serum indices, intestinal morphology, and gut microbiota of blue foxes and to provide a theoretical and practical foundation for the application of thymol in blue foxes.
2 Materials and methods
2.1 Experimental animals
The experimental animals were purchased from the Beidahuang Blue Fox Breeding Farm in Jilin Province, China. Twenty-four healthy male blue foxes aged 120 ± 5 days and weighing 6.05 ± 0.16 kg were selected. The blue foxes were randomly divided into 4 groups, with 6 in each group. Each blue fox was kept in an individual cage (1.0 m × 1.0 m). The C group was fed basic feed (Table 1), while the L, M, and H groups were supplemented with 100, 200, and 300 mg/kg thymol in the basic diet, respectively. Feed was provided at 06:30 and 16:00, and water was provided ad libitum. The experiment was conducted at the teaching experimental base of Jilin Agricultural University, and the test period consisted of a 5-day acclimation phase and a 30-day feeding trial. The blue foxes were vaccinated with basic vaccines (rabies vaccine and canine parvovirus vaccine) before the experiment.
2.2 Sample collection
Fecal and feed samples were collected and stored at −20°C daily, and daily food intake and fecal weight were recorded during the last 5 days of the feeding trial. For nitrogen analysis, daily samples of 3% total feces were collected and kept in wide-mouth vials containing 20 mL of 10% H2SO4. On the last day of the feeding trial, 8 mL of blood was collected from the hind leg vein and placed in a procoagulant collection vessel. After centrifugation at 4,000 rpm for 10 min, the serum was separated and divided into 1.5 mL centrifuge tubes, which were stored at −80°C. At the end of the trial, three blue foxes were randomly selected from each group and humanely slaughtered via electric shock according to the guidelines outlined in the Welfare of Animals Kept for Fur Production. The contents of the rectum were placed in 2 mL sterilized centrifuge tubes and then stored at −80°C. The duodenum, jejunum, and ileum samples were washed clean with physiological saline and soaked in 10% formaldehyde solution for at least 24 h for hematoxylin and eosin (H&E) staining.
2.3 Growth performance and apparent nutrient digestibility
On the first and last days of the feeding trial, the weight of each blue fox was measured in the morning without feeding, the initial weight (IW) and final weight (FW) were recorded, and the ratio of consumed feed (F/G) to ADG was calculated. At the end of the trial, the fecal and feed samples were dried in a forced-air oven at 65°C for 72 h. The samples were crushed in a fodder grinder, and samples smaller than 1 mm were filtered out. To determine the nutritional content of the fecal and feed samples, routine chemical tests were performed following the association of official analytical chemists (AOAC) guidelines (Feldsine et al., 2002). Briefly, the organic matter (OM) content was calculated by measuring the ash content, which was obtained by burning the samples at 550°C for 8 h. The N content was determined using a LECO FP-528N/Protein Tester (LECO, Corporation), and the crude protein (CP) content in the sample was calculated by the following equation: CP = N × 6.25. Ether extract (EE) was extracted from the samples using the diethyl ether extraction–submersion method, and their contents were calculated. The apparent nutrient digestibility was calculated using the following formula: apparent nutrient digestibility (%) = (nutrient intake-nutrient excreta) / nutrient intake × 100.
2.4 Serum immune and antioxidant indices
Immunoglobulin A (IgA), immunoglobulin G (IgG), immunoglobulin M (IgM), interleukin-4 (IL-4), superoxide dismutase (SOD), catalase (CAT), and blood urea nitrogen (BUN) were measured using commercial kits (Nanjing Jiancheng Bioengineering Research Institute, Nanjing, China) according to the manufacturer’s guidelines. Briefly, 10 μL of sample and 40 μL of Sample Diluent were added to the testing well first. Then, 100 μL of HRP-Conjugate Reagent was added to each well, and the plates were incubated for 60 min at 37°C. Each well was washed 5 times with Wash Solution. Then, Solution A, Solution B, and Stop Solution were added to each well. Finally, the results were measured at 450 nm within 15 min.
2.5 Hematoxylin and eosin (H&E) staining and intestinal morphology
The 0.5–1 cm samples of the duodenum, jejunum, and ileum were placed into embedding boxes and rinsed with tap water for 12 h. Afterward, the embedding boxes were immersed overnight in 50% ethanol solution, followed by soaking them in 75, 85, 90, 95, and 100% ethanol for 1 h each and soaking them in xylene I and xylene II for 30 min each. Finally, the sections were paraffin embedded and cut to a thickness of approximately 4.5 μm. The sections were dewaxed in xylene for 7 min and then placed in 95, 85, 70, and 50% alcohol for 2 min each. Then, the sections were soaked in hematoxylin staining solution for 10 min, 1% hydrochloric acid solution for 30 s, water for 30 s, 50, 70, 80, and 90% ethanol solution for 2 min. The sections were subsequently stained with eosin staining solution for 10–20 s. The sections were placed in 95% ethanol, anhydrous ethanol, and xylene twice for 10 min each. Finally, the sections were sealed with neutral resin and observed under an optical microscope (Olympus, Tokyo, Japan). The intestinal morphology was determined under 40× magnification, 5 positions on each section were randomly selected, the villus height (VH) and crypt depth (CD) were measured using Image-Pro Plus 6.0 software, and the VH/CD ratio was calculated.
2.6 Gut microbiota
16S rRNA V3-V4 sequencing was used to detect the rectum content. The DNA in the rectum was extracted using a Qiagen magnetic bead extraction kit (Qiagen, Valencia, California, USA) according to the manufacturer’s instructions. Agarose gel electrophoresis was used to evaluate the purity and concentration of the DNA. The sample DNA was diluted to 1 ng/μL with sterile water as a template for PCR amplification, and the sequencing primers used were F (ACTCCTACGGGGGGAGAGCA) and R (GGACACHVGGGTWTCTAAT). The PCR products were identified and purified by 2% agarose gel electrophoresis, and the target band was cut and recovered (Thermo Scientific Company GeneJET adhesive recovery kit). Thermofisher Ion Plus Fragment Library Kit 48 (RXNS) was used to construct the library. Then, sequencing was performed on the Illumina NovaSeq 6000 platform to obtain end reads, which were merged into the original labels using FLASH version 1.2.7. To obtain the final reliable data (high-quality clean tags), Uparse (version 7.0.10012) was used to eliminate the chimeric sequence. The tags were compared to the SILVA database (version 138), and the DADA2 plugin was then used to filter the sequences for quality, denoise them, combine them, and eliminate chimeras (Callahan et al., 2016). The mothur technique and SSUrRNA database of SILVA132 (threshold set to 0.8) were utilized to perform species annotation on operational taxonomic unit (OTU) sequences. Bioinformatics analysis of the microbiome was performed using QIME2 2019, and the α and β diversity of the samples was calculated (Lozupone and Knight, 2005). The abundances of different bacteria were quantified with qPCR (StepOne™ Real-Time PCR System, Applied Biosystems) according to a previous study (Alberoni et al., 2021). The screening value of the linear discrimination criterion (LDA score) was 2 according to the LEfSe program for intergroup differential species analysis. Spearman analyzed the correlation between growth performance and the gut microbiota.
2.7 Statistical analysis
All the graphs were generated using GraphPad Prism version 8 and PowerPoint version 2021. The statistical analysis of the data was performed using the general linear model program SPSS (22.0, IBM Co. Limited, Chicago, USA). Duncan multiple comparisons in one-way analysis of variance (ANOVA) were used to test the significance of differences between groups. The data were averaged to one value per animal, and the data were presented as the mean and standard deviation (mean ± SD). P < 0.05 was considered statistically significant, P < 0.01 was considered extremely significant, and P > 0.05 was considered not statistically significant. STAMP software (T test) was used to analyze the differences in microbiota abundance between groups, and the Benjamini-Hochberg FDR multiple test correction method was used to control the false positive rate.
3 Results
3.1 Effect of thymol on the growth performance of blue foxes
As shown in Table 2, the addition of 200 mg/kg and 300 mg/kg thymol to the basic diet significantly increased the FW compared with that in the C group (P < 0.05). The IW, ADG, and F/G ratio did not significantly differ among the groups (P > 0.05).
3.2 Effect of thymol on the apparent digestibility of nutrients in blue foxes
The apparent nutrient digestibility of blue foxes is shown in Table 3. Supplementing basic diets with 100 mg/kg thymol increased OM digestibility compared with that in the C group (P < 0.05). Moreover, compared with that of the C group, the digestibility of CP in the L group increased significantly (P < 0.05). However, there were no significant differences in EE digestibility among the groups (P > 0.05).
3.3 Effect of thymol on the serum biochemical indicators of blue foxes
As shown in Table 4, the content of IgG in the L group was significantly greater than that in the C group (P < 0.01). Moreover, the addition of 100 mg/kg thymol to the basic diet increased the contents of IgA and IgM compared with those in the C group (P < 0.05). The contents of IL-4 and CAT in the L group were greater than those in the other groups (P < 0.05). The serum IgM content in the L group was significantly greater than that in the H group (P < 0.05). However, there were no significant differences in the levels of SOD or BUN among the groups (P > 0.05).
3.4 Effect of thymol on the intestinal morphology of blue foxes
The intestinal morphologies of the duodenum, jejunum, and ileum are shown in Figure 1. From Table 5, it can be seen that compared with the Group C, adding 100 mg/kg thymol to the basic diet significantly increased the VH of the duodenum (P < 0.05). Adding 200 mg/kg thymol to the basic diet significantly increased the VH/CD of the duodenum (P < 0.05). Compared with the C group, the basic diet supplemented with 300 mg/kg thymol significantly increased the VH of the jejunum (P < 0.05). Supplementing basic diets with 100 or 300 mg/kg thymol significantly increased the CD of the jejunum (P < 0.05). The VH and VH/CD of the ileum in the L group were significantly greater than those in the C group (P < 0.05). The CD of the duodenum, VH/CD of the jejunum, and CD of the ileum were not significantly different among the groups (P > 0.05).
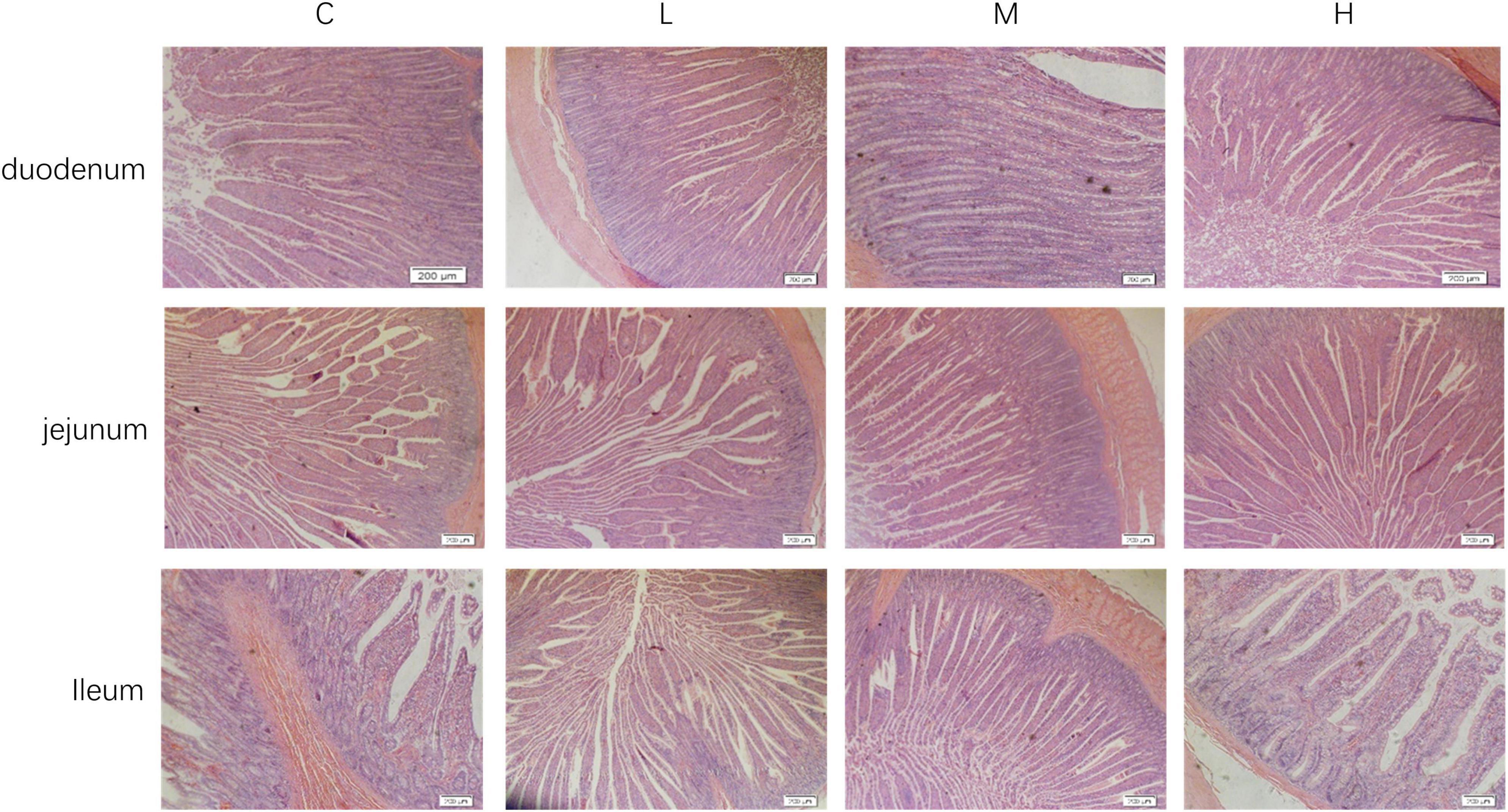
Figure 1. Intestinal morphology of blue foxes. 0 mg/kg (C group), 100 mg/kg (L group), 200 mg/kg (M group), and 300 mg/kg (H group) thymol were added to the basic diets of the blue foxes.
3.5 Changes in the gut microbiota
α-Diversity was characterized by the Chao1 and the Observed species indices for richness, the Shannon and Simpson indices for diversity, the Faith-pd index for evolutionary diversity, the Pielou index for evenness, and the Goods coverage index for coverage. The α diversity (Figure 2A) did not significantly differ among the indices (P > 0.05). β-Diversity analysis was used to explore whether there were significant community differences among the samples, and the closer the distance between points was, the more similar the species composition was. As shown in Figure 2B, compared to those in the C group, the points in the other groups were further apart, indicating that thymol can alter the structure of the microbial community. Using a Venn diagram to analyze the number of unique and total OTUs in each group, we found a total of 1,176 OTUs in the C Group, 1,323 OTUs in the L Group, 1,770 OTUs in the M Group, and 1,958 OTUs in the H Group (Figure 2C). With increasing thymol addition, the number of unique OTUs increased. Among them, the C Group had 292 OTUs, the L Group had 481 OTUs, the M Group had 769 OTUs, and the H Group had 940 OTUs (Figure 2C).
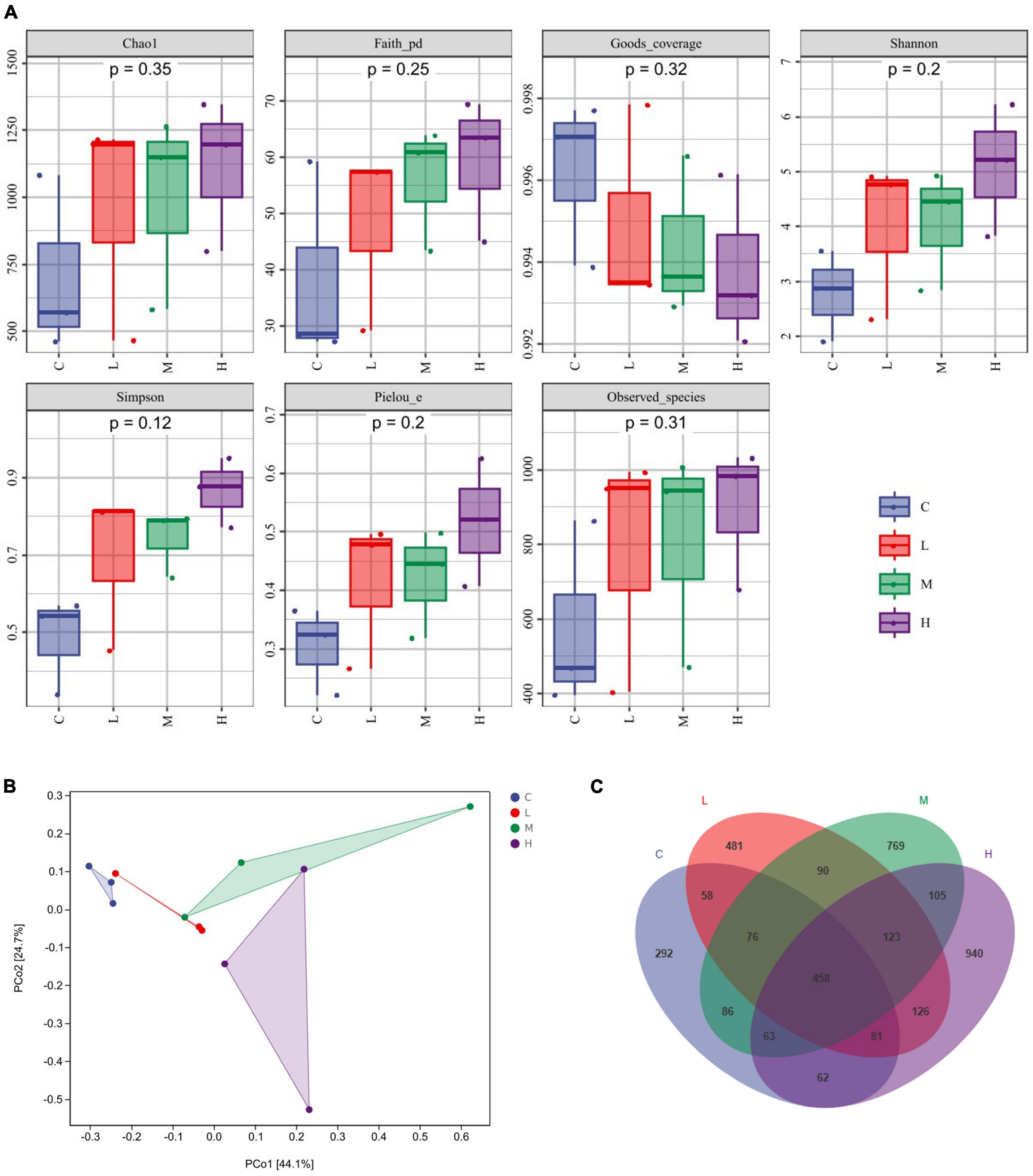
Figure 2. Changes in the gut microbiota. (A) α diversity, including Chao1, Faith, Goods, Shannon, Simpson, Pielou, and Observed indices. (B) PCoA analysis. (C) Venn diagram analysis. 0 mg/kg (C group), 100 mg/kg (L group), 200 mg/kg (M group), or 300 mg/kg (H group) thymol was added to the basic diets of the blue foxes.
3.7 Spearman correlation analysis
The relative abundance of the gut microbiota at the phylum level is shown in Figure 3A. From the perspective of phylum-level classification, Firmicutes, Proteobacteria, Actinobacteria, Fusobacteria, and Bacteroidetes were the main microorganisms in the gut of blue foxes. Adding thymol to the diet can change the abundance of Firmicutes, Proteobacteria, Actinobacteria, Fusobacteria, Bacteroidetes, TM7, Cyanobacteria, Tenericutes, Verrucomicrobia, Planctomycetes, Chloroflexi, and Acidobacteria in the gut of blue foxes (Figure 3B). The dominant bacteria at the genus level were Streptococcus, Lactobacillus, Sarcina, Shigella, and Blautia (Figure 3C). Figure 3D shows that thymol can change the abundance of Streptococcus, Lactobacillus, Sarcina, Shigella, Blautia, Clostridium, Turicibacter, Megasphaera, Subdoligranulum, Bifidobacterium, Collinsella, [Eubacterium], Catenibacterium, Fusobacterium, Dialister, Allobaculum, [Ruminococcus], Dorea, SMB53, and Prevotella. Linear discriminant analysis effect size (LEfSe) was further performed to identify different bacterial taxa among the different groups (log10 LDA score > 2). We found that two bacterial genera, Firmicutes and Marivita, were enriched in the C group; 13 bacterial genera were particularly abundant in the H group, including Actinobacteria, Bifidobacteriales, Actinobacteria, Bifidobacterium, Bifidobacteriaceae, Planctomycetes, Phycisphaerales, Phycisphaerae, Acidaminococcus, Comamonadaceae, Chitinophagaceae, [Saprospirae], and [Saprospirales] (Figures 3E, F); Gammaproteobacteria, Cyanobacteria, Synechococcales, Synechococcaceae, Synechococcus, and Synechococcophycideae were enriched in the M group; and no bacteria were enriched in the L group (Figures 3E, F).
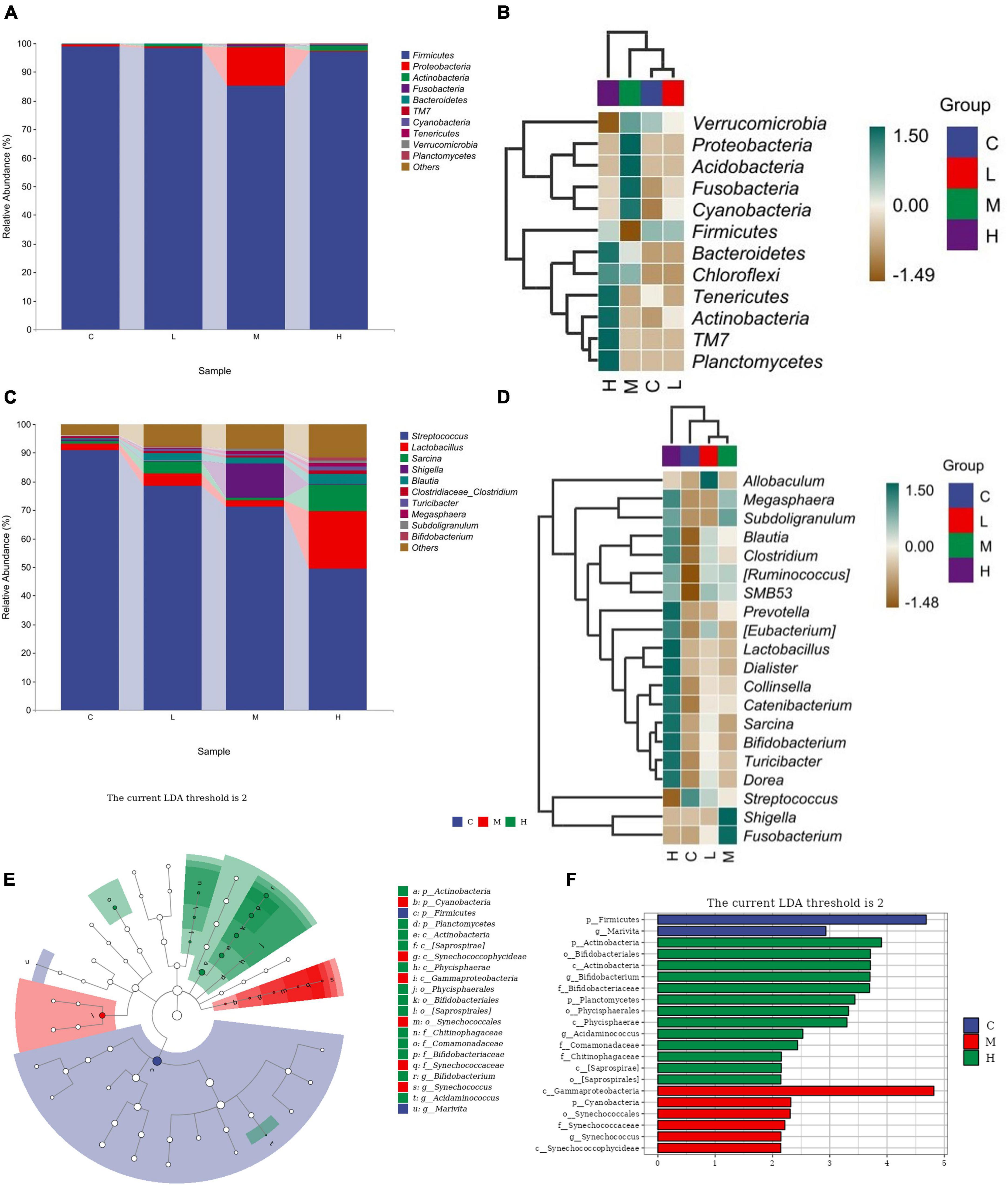
Figure 3. Screening of differential bacteria. (A) Relative abundance of bacteria at the phylum level. (B) Significant differences in bacteria at the phylum level. (C) Relative abundance of bacteria at the genus level. (D) Significant differences in bacteria at the genus level. (E) Histogram of LEfSe (log10 LDA score > 2). (F) Cladogram of LEfSe (log10 LDA score > 2). 0 mg/kg (C group), 100 mg/kg (L group), 200 mg/kg (M group), or 300 mg/kg (H group) thymol was added to the basic diets of the blue foxes.
3.6 Spearman correlation analysis
Spearman’s rank correlation analysis was used to evaluate the correlation between production performance or serum indices and the gut microbiota. As shown in Figure 4. Bifidobacterium showed an extremely significant positive correlation with FW (P < 0.01). CAT was positively correlation with IgG (P < 0.05) and strongly positively correlated with IL-4 (P < 0.01). IL-4 was positively correlated with IgG (P < 0.05). Fusobacterium had an extremely significant positive correlation with IgM and IgA (P < 0.01). IgA was positively correlated with IgG (P < 0.05) and extremely significantly positively correlated with IgM (P < 0.01). OM was positively correlated with IgG (P < 0.05) and extremely significantly positively correlated with Allobaculum (P < 0.01). IgG had an extremely significant positive correlation with IgM (P < 0.01). In addition, most gut bacteria were significantly correlated with each other (P < 0.05).
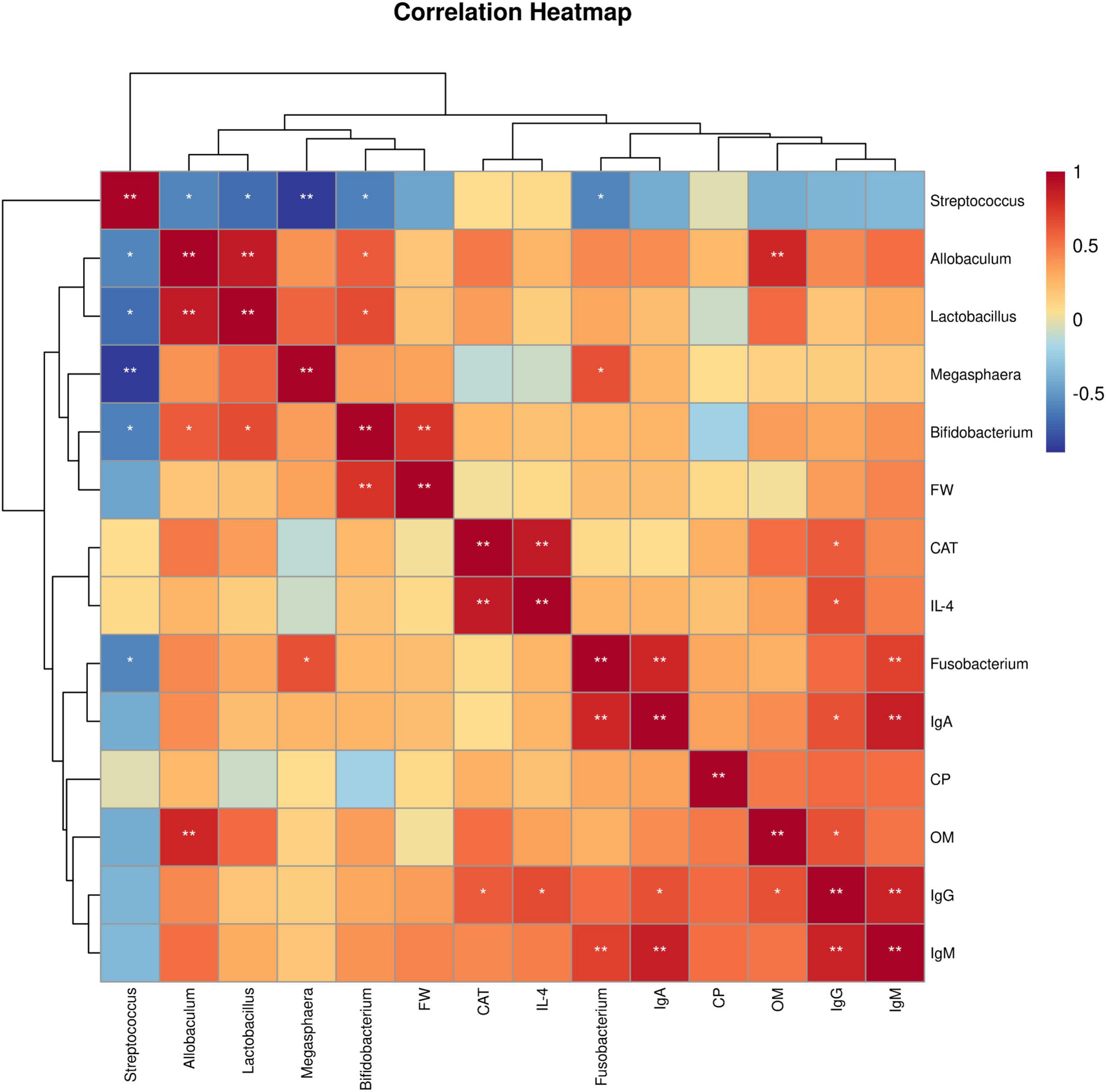
Figure 4. Spearman correlation analysis. Heatmap of correlations between growth performance or serum indices and the gut microbiota. FW, final weight; CAT, catalase; IL-4, interleukin-4; IgA, immunoglobulin A; CP, crude protein digestibility; OM, organic matter digestibility; IgG, immunoglobulin G; IgM, immunoglobulin M. 0 mg/kg (C group), 100 mg/kg (L group), 200 mg/kg (M group), and 300 mg/kg (H group) thymol were added to the basic diets of the blue foxes. The results of the correlation analysis were analyzed by Spearman correlation analysis; *P < 0.05; **P < 0.01.
4 Discussion
As a type of fur animal, the fur quality of blue foxes determines its economic benefits. The health of blue foxes is the key to determining the quality of their fur. During the breeding process, dietary supplementation with antibiotics can increase growth performance, feed intake and daily weight gain (Gomes et al., 2023). However, the excessive use of antibiotics leads to the development of antibiotic resistance in pathogenic microorganisms, which affects the economic benefits of blue foxes. Thymol, a high-quality feed additive, has the potential to replace antibiotics and has a positive effect on intestinal health and growth performance. At present, thymol has been applied in various animal production systems, but there are few reports on the impact of thymol on blue foxes. This study aimed to investigate the effects of thymol on the growth performance and intestinal health of blue foxes. The results indicated that, similar to the addition of antibiotics, the addition of thymol to the diet can improve the growth performance, apparent digestibility, and immune response of blue foxes. However, unlike antibiotics, thymol has a positive effect on promoting the colonization of beneficial bacteria in the intestine.
The primary objectives of blue fox breeding are increased efficiency and quality in fur production for clothing and accessories (Thirstrup et al., 2017). Fur quality and skin size are the most important traits in blue fox breeding and are the main determinants of sales price and subsequent income for fur producers (Thirstrup et al., 2017). Although the price of pelts largely depends on their quality, these traits can only be obtained after harvesting. Therefore, growth performance is the basis for determining the quality and yield of pelt (Moller, 1999). Our study revealed that dietary supplementation with 200 or 300 mg/kg thymol could increase FW, providing a basis for improving pelt yield. Previous studies have shown that supplementing thymol during the nursery period has a beneficial effect on the growth performance of growing pigs (Zhao et al., 2023). Adding 50 mg/kg thymol to the diet can improve the ADG of weaned piglets by promoting intestinal health (Wang et al., 2022). The beneficial effects of antibiotics on growth performance may be attributed to their antibacterial effects, but their ability to disrupt gut microbiota homeostasis cannot be ignored (Gadde et al., 2017). Our research results indicate that adding thymol to the diet can increase the FW of blue foxes without disrupting the homeostasis of the gut microbiota, providing a basis for improving pelt yield. It can be inferred that thymol can replace antibiotics to improve the growth performance and pelt yield of blue foxes.
Apparent nutrient digestibility is usually an indicator of the digestion and absorption of nutrients by animals, and it is directly related to the growth performance of blue foxes. A decrease in apparent digestibility leads to nutrient loss and affects the production profits of farmers (Liu et al., 2023). An increase in apparent digestibility can lead to an increase in the growth performance of beef cattle (Tang et al., 2023). Thymol, a healthy and green plant essential oil extract that does not cause potential side effects on the body, has been used as a food preservative, insect repellent and anti-inflammatory drug (Luo et al., 2020). In addition, the addition of 400 mg/kg essential oil containing thymol to the diet can improve the apparent digestibility of OM and CP in weaned piglets (Li Y. Y. et al., 2023). The apparent nutrient digestibility can intuitively reflect the degree of nutrient utilization by animals. The digestibility of CP and OM can affect the growth performance of blue foxes (Niu et al., 2019). Similarly, our results showed that supplementing diets with 100 mg/kg thymol can increase the apparent digestibility of OM and CP, which can increase the growth performance of blue foxes. We can infer that the increase in the apparent digestibility of OM and CP may further promote growth performance while potentially improving the pelt yield of blue foxes.
Serum indicators can serve as important indicators reflecting the growth performance of the body, as well as the physiological and health status of animals (Wang et al., 2023). The antioxidant capacity can indirectly reflect an animal’s disease resistance. A previous study has shown that adding 200 mg/kg thymol to the diet can enhance the antioxidant capacity of laying hens by increasing the serum CAT content, which is similar to our present results (Zhao et al., 2021). It can be inferred that thymol can enhance the antioxidant capacity of blue foxes. As a multifunctional immune regulatory cytokine, IL-4 is the main promoter of helper T-cell 2 (Th2) development and plays an important role in enhancing the immune system (Yang et al., 2022). The increase in the serum IL-4 concentration in our study indicated that thymol had a positive effect on the blue fox immune system. Ig is one of the main anti-infective components in the blood. It is a unique glycoprotein that protects the body from harmful bacteria, viruses, and other environmental pathogens by binding or forming encapsulation barriers (Balan et al., 2019). Previous studies have shown that Ig has multiple biological effects, including effects on growth, immunity, intestinal growth, and intestinal barrier function (Pierce et al., 2005; Maijó et al., 2012). The present study showed that adding thymol to basic diets can increase the serum IgA, IgG, and IgM levels, suggesting that thymol has the potential to improve the immune system. Similarly, it has been reported that adding thymol to the diet can significantly improve the immune response of chickens (Mosleh et al., 2013). Furthermore, the use of plant essential oils (containing thymol) in the chicken diet can enhance the phagocyte system and humoral and cellular immune responses of broilers by increasing antibody titers, playing an important positive role in resisting different infections (Pérez-Rosés et al., 2015). Thus, thymol can enhance the systemic immune response and has a positive effect on resisting the invasion of pathogenic bacteria. These results further demonstrate the potential of thymol as a substitute for antibiotics.
According to a previous report, adding thymol to the diet can not only improve the immune response but also alleviate intestinal injury in broilers by improving intestinal integrity (Du et al., 2016). The integrity of the intestinal structure is the foundation of intestinal health and normal digestion. Ensuring a healthy intestinal morphology is crucial for animal digestion of food and is crucial for improving apparent nutrient digestibility (Kogut and Arsenault, 2016). The small intestine includes the duodenum, jejunum, and ileum, and its key function is to mix acidic gastric chyme with bile to digest and absorb complex nutrients (Spiller and Hoad, 2020). Therefore, maintaining stable small intestine morphology and function is crucial for the health of the digestive system. The development of the intestine is closely related to nutrient absorption in animals, especially the VH and CD, which are important indicators for measuring intestinal digestion and absorption. An increase in VH can increase the contact area between the small intestine and intestinal contents (Houshmand et al., 2012). The VH/CD can reflect the functional status of the small intestine, and a higher VH/CD indicates that the small intestine has a greater nutrient absorption capacity and better growth performance (Wang et al., 2020). Studies have shown that the small intestine VH/CD ratio determines the protein digestibility of weaned piglets. A decrease in the VH/CD ratio leads to a decreased intestinal absorption area, which reduces protein absorption (Engelsmann et al., 2022). Our study revealed that thymol increased the VH/CD ratio. Similarly, adding 0.025% thymol to rabbit diets for 21 days can improve the VH/CD ratio of the small intestine wall, and this positive effect is still present after the withdrawal of thymol (Placha et al., 2022). Therefore, we infer that thymol may improve the apparent nutrient digestibility of blue foxes by increasing the VH/CD ratio of the small intestine.
The gut microbiota plays an important regulatory role in intestinal health, and dysbiosis of the microbiota can result in permanent alterations in the physiological response (Vamanu and Rai, 2021). Due to the low bioavailability of thymol, up to 90% of phenolic compounds enter the colon unaltered and interact with colonic bacteria (Espín et al., 2017). To investigate the positive effect of thymol on blue fox intestinal health, we detected the composition of the gut microbiota further. The stability of the gut microbiota promotes the health of the body, but the gut microbiota is influenced by various factors, such as age, disease status, and feed (Fan and Pedersen, 2021). Changes in the gut microbiota also affect animal production performance. Our study revealed that the main microbiota in the rectum of blue foxes were Firmicutes, Proteobacteria, Actinobacteria, Fusobacteria, and Bacteroidetes. Liu et al. (2020) also reported similar results, the main phylum-level microorganisms in the intestine of blue foxes are Firmicutes, Bacteroidetes, Proteobacteria, Actinobacteria, and Fusobacteria. The inconsistency in the gut microbiota structure may be attributed to differences in feed nutrition, and the addition of thymol may also be the most important factor leading to this change. A variety of factors might explain the discrepancy between studies, such as differences in diets, animals, and the environment. Treatment with dietary thymol did not notably change the proportion of the gut microbiota or bacterial diversity in laying hens or weaned pigs (Van Noten et al., 2020; Zhao et al., 2023). Similarly, our results indicate that thymol does not affect the α diversity of the gut microbiota. It can be speculated that thymol will not have a significant impact on the stability of the gut microbiota. A high-fat diet could cause an increase in Firmicutes and a decrease in Bacteroidetes, and a higher Firmicutes/Bacteroidetes ratio helps mice and piglets produce more fat (Turnbaugh et al., 2008; Li et al., 2018). According to our results, the increase in Firmicutes and the decrease in Bacteroidetes may be attributed to the increased fat content in feed by thymol, which may also be a potential factor in improving FW. The positive impact of thymol on intestinal health is its inhibitory effect on pathogenic bacteria (Placha et al., 2022). At the genus level, we found that Streptococcus decreased with increasing thymol content. Streptococcus is a gram-positive bacterial species that can cause various inflammatory diseases (Chen et al., 2023; Kuperwaser et al., 2023). The decrease in Streptococcus in this study may have been caused by an increase in serum Ig (Breitfelder et al., 2023). It has been reported that supplementation with Megasphaera improves livestock production performance, especially in terms of ADG and carcass quality (Susanto et al., 2023). Adding Megasphaera to sheep diets can increase feed intake and ADG by increasing the production of propionate in the rumen (Henning et al., 2010). In addition, we found that adding thymol to diets can increase the abundance of Lactobacillus in the intestine. As a probiotic, Lactobacillus has various beneficial effects, such as antimicrobial activity, immunomodulation, antioxidative effects, and antiallergenic effects (Slattery et al., 2019). Giannenas et al. (2014) found that adding 30 mg/kg thymol to the diets of male turkeys can increase the abundance of Lactobacillus in the cecum, thereby promoting nutrient utilization and growth performance. The strong antioxidant properties of thymol can protect intestinal epithelial cells and prevent inflammation while inhibiting pathogenic bacteria and supporting the colonization of beneficial bacteria such as Lactobacillus (Iqbal et al., 2020). In this study, thymol promoted the growth of blue foxes by increasing the abundance of Megasphaera and Lactobacillus and reducing the abundance of Streptococcus.
Through LEfSe analysis, we found that Bifidobacterium may be a potential differential bacterium. The genus Bifidobacterium belongs to the Actinobacteria phylum and plays key roles in the development of physiology, including the maturation of the immune system and the use of dietary components. Bifidobacterium has been included as a bioactive ingredient in functional foods, as well as in food supplements and drugs due to its beneficial effects (Hidalgo-Cantabrana et al., 2017). Bifidobacterium can regulate the immune response at the intestinal mucosal level and play a positive role in regulating the levels of Ig and inflammatory factors (Gavzy et al., 2023). In our study, the increase in the abundance of Bifidobacterium may have promoted the immune function of blue foxes. Furthermore, Spearman correlation analysis revealed a positive correlation between Bifidobacterium and FW, indicating that thymol may promote the health of blue foxes by increasing the abundance of Bifidobacterium. Similarly, it has been reported that supplementing with Bifidobacterium in the diet for 28 days can promote weight gain in Bangladeshi infants (Barratt et al., 2022). Some members of the genus Fusobacterium are capable of fermentative metabolism in anaerobic environments to produce organic acids, which might play a positive role in the metabolism of nutrients in the gut (Ma et al., 2022). When studying the impact of early colonization of the gut microbiota on growth performance, it was found that Fusobacterium could significantly increase the growth performance of lambs (Xiao et al., 2023). An increasing number of studies have shown a close relationship between Fusobacterium and immune regulation, and Fusobacterium intervenes in the occurrence of diseases by regulating the innate immune system (Zhang et al., 2023). Our study further confirmed that the increase in serum IgA and IgM may be related to Fusobacterium. In addition, the present study revealed that OM was positively correlated with IgG, suggesting that thymol can increase the apparent digestibility of OM by enhancing immune function (Hashemipour et al., 2013). Allobaculum can produce butyric acid, which plays an important role in the gastrointestinal system, particularly in regulating host fat storage. Previous studies have shown that mice fed high-fat diets or high-sucrose diets exhibit disrupted gut microbiota, particularly a decrease in Allobaculum abundance (Kong et al., 2019). Changes in the abundance of Allobaculum in the intestine were found in high-fat diet-fed mice, suggesting that Allobaculum may be an important microorganism regulating OM digestion ability (Zheng et al., 2021). Oxidative stress can lead to a decrease in immune function and growth performance. Our results revealed a positive correlation between CAT, immunoglobulin G (IgG), and IL, indicating that thymol, a natural antioxidant, can enhance the immune function of blue foxes, which has been validated in previous studies (Luna et al., 2017). From our study results, it can be inferred that thymol may improve the growth performance of blue foxes by increasing the abundance of Fusobacterium, Bifidobacterium, and Allobaculum.
At present, the problems of bacterial resistance and food-borne antibiotic residues caused by the abuse of antibiotics have aroused much concern (Bahaddad et al., 2023). With the demand for high-quality animal products, it is imperative to exploit effective and green feed additives that can stimulate latent productive capacity (Li L. X. et al., 2023). The results of the current study suggest that thymol can improve the nutritional digestion rate of blue foxes by promoting intestinal morphology, thereby enhancing their growth performance, which has a positive effect on increasing fur production. In addition, it can be inferred that thymol may improve the serum biochemical indicators of blue foxes by regulating the metabolic function of the intestinal microbiota, which can further improve their health (Singh et al., 2022). These effects were greater when the concentration of the extract was 100 mg/kg. These results suggest that thymol can replace antibiotics to improve the growth performance of blue foxes and can be further promoted for the production of other fur animals. At present, we are conducting further research on the effects of long-term thymol feeding on the growth performance and fur quality of winter fur-growing blue foxes. However, some limitations of this study should be mentioned. Due to the small number of blue foxes available for study under actual conditions, further validation of the gut microbiota results is needed. In addition, further testing of organ indicators and routine blood tests are needed to determine the effect of thymol on the growth performance of blue foxes. However, thymol is unstable and often produces unpleasant odors, which hinders its application. Microencapsulation may be an effective method to address this drawback (Mo et al., 2022). Feeding differential bacteria or conducting fecal microbiota transplantation experiments can further clarify whether thymol promotes the growth performance of blue foxes by regulating the gut microbiota. Furthermore, blood and fecal metabolomics analyses can further explore the mechanism by which thymol promotes the health of blue foxes.
5 Conclusion
Adding thymol to the diet can improve the body weight, apparent nutrient digestibility, antioxidant capacity, immune function, and intestinal health of blue foxes. Analysis of the gut microbiome revealed that thymol can increase FW by increasing the abundance of Bifidobacterium, increase the serum levels of IgA and IgM by increasing the abundance of Fusobacterium, and increase the apparent digestibility of OM by increasing the abundance of Allobaculum. The optimal amount of thymol added to the diets of blue foxes is 100 mg/kg.
Data availability statement
The 16S rRNA gene sequencing data in the present study are available in the NCBI Sequence Read Archive (SRA) repository under accession number PRJNA1122965.
Ethics statement
The animal studies were approved by the Experimental Animal Welfare and Ethics Committee of Jilin Agricultural University. The studies were conducted in accordance with the local legislation and institutional requirements. Written informed consent was obtained from the owners for the participation of their animals in this study.
Author contributions
CY: Writing – original draft. SC: Methodology, Writing – original draft. RS: Investigation, Writing – original draft. LR: Investigation, Writing – original draft. TZ: Investigation, Writing – original draft. MW: Writing – review and editing. AZ: Project administration, Writing – original draft.
Funding
The authors declare that financial support was received for the research, authorship, and/or publication of this article. This study was completed at Jilin Agricultural University. This study was funded by the National Key Research and Development Program of China (2023YFD1302000).
Conflict of interest
The authors declare that the research was conducted in the absence of any commercial or financial relationships that could be construed as a potential conflict of interest.
Publisher’s note
All claims expressed in this article are solely those of the authors and do not necessarily represent those of their affiliated organizations, or those of the publisher, the editors and the reviewers. Any product that may be evaluated in this article, or claim that may be made by its manufacturer, is not guaranteed or endorsed by the publisher.
References
Alberoni, D., Baffoni, L., Braglia, C., Gaggìa, F., and Di Gioia, D. (2021). Honeybees exposure to natural feed additives: How is the gut microbiota affected? Microorganisms 9:1009. doi: 10.3390/microorganisms9051009
Bahaddad, S. A., Almalki, M. H. K., Alghamdi, O. A., Sohrab, S. S., Yasir, M., Azhar, E., et al. (2023). Species as direct-fed microbial antibiotic alternatives for monogastric production. Probiotics Antimicrob. Proteins 15, 1–16. doi: 10.1007/s12602-022-09909-5
Balan, P., Sik-Han, K., and Moughan, P. J. (2019). Impact of oral immunoglobulins on animal health-A review. Anim. Sci. J. 90, 1099–1110. doi: 10.1111/asj.13258
Barratt, M. J., Nuzhat, S., Ahsan, K., Frese, S. A., Arzamasov, A. A., Sarker, S. A., et al. (2022). Treatment promotes weight gain in Bangladeshi infants with severe acute malnutrition. Sci. Transl. Med. 14:eabk1107. doi: 10.1126/scitranslmed.abk1107
Betancourt, L., Rodriguez, F., Phandanouvong, V., Ariza-Nieto, C., Hume, M., Nisbet, D., et al. (2014). Effect of chemotypes on broiler intestinal bacteria. Poultry Sci. 93, 2526–2535. doi: 10.3382/ps.2014-03944
Bhardwaj, S., Mehra, P., Dhanjal, D. S., Sharma, P., Sharma, V., Singh, R., et al. (2022). Antibiotics and antibiotic resistance– flipsides of the same coin. Curr. Pharm. Des. 28, 2312–2329. doi: 10.2174/1381612828666220608120238
Breitfelder, A. K., Schrödl, W., Rungelrath, V., Baums, C. G., Alber, G., Schütze, N., et al. (2023). Immunoglobulin M-degrading enzyme of (Ide) impairs porcine B cell signaling. Front. Immunol. 14:1122808. doi: 10.3389/fimmu.2023.1122808
Callahan, B. J., McMurdie, P. J., Rosen, M. J., Han, A. W., Johnson, A. J. A., and Holmes, S. P. (2016). DADA2: High-resolution sample inference from Illumina amplicon data. Nat. Methods 13:581. doi: 10.1038/Nmeth.3869
Chen, Y. L., Ng, J. S. W., Babu, R. O., Woo, J., Nahler, J., Hardman, C. S., et al. (2023). Group A Streptococcus induces CD1a-autoreactive T cells and promotes psoriatic inflammation. Sci. Immunol. 8:eadd9232. doi: 10.1126/sciimmunol.add9232
Diao, H., Zheng, P., Yu, B., He, J., Mao, X. B., Yu, J., et al. (2015). Effects of benzoic acid and thymol on growth performance and gut characteristics of weaned piglets. Asian Aust. J. Anim. Sci. 28, 827–839. doi: 10.5713/ajas.14.0704
Du, E. C., Wang, W. W., Gan, L. P., Li, Z., Guo, S. S., and Guo, Y. M. (2016). Effects of thymol and carvacrol supplementation on intestinal integrity and immune responses of broiler chickens challenged with. J. Anim. Sci. Biotechnol. 7:19. doi: 10.1186/s40104-016-0079-7
Engelsmann, M. N., Jensen, L. D., van der Heide, M. E., Hedemann, M. S., Nielsen, T. S., and Norgaard, J. V. (2022). Age-dependent development in protein digestibility and intestinal morphology in weaned pigs fed different protein sources. Animal 16:100439. doi: 10.1016/j.animal.2021.100439
Espín, J. C., González-Sarrías, A., and Tomás-Barberán, F. A. (2017). The gut microbiota: A key factor in the therapeutic effects of (poly) phenols. Biochem. Pharmacol. 139, 82–93. doi: 10.1016/j.bcp.2017.04.033
Fan, Y., and Pedersen, O. (2021). Gut microbiota in human metabolic health and disease. Nat. Rev. Microbiol. 19, 55–71. doi: 10.1038/s41579-020-0433-9
Feldsine, P., Abeyta, C., and Andrews, W. H. (2002). AOAC INTERNATIONAL Methods Committee guidelines for validation of qualitative and quantitative food microbiological official methods of analysis. J. AOAC Int. 85, 1187–1200.
Gadde, U., Kim, W. H., Oh, S. T., and Lillehoj, H. S. (2017). Alternatives to antibiotics for maximizing growth performance and feed efficiency in poultry: A review. Anim. Health Res. Rev. 18, 26–45. doi: 10.1017/S1466252316000207
Gavzy, S. J., Kensiski, A., Lee, Z. L., Mongodin, E. F., Ma, B., and Bromberg, J. S. (2023). Mechanisms of immune modulation and tolerance. Gut Microbes 15:2291164.
Ghiselli, F., Giovagnoni, G., Felici, M., Tugnoli, B., Piva, A., and Grilli, E. (2022). A mixture of organic acids and thymol protects primary chicken intestinal epithelial cells from infection in vitro. Poultry Sci. 101:102101. doi: 10.1016/j.psj.2022.102101
Giannenas, I., Papaneophytou, C. P., Tsalie, E., Pappas, I., Triantafillou, E., Tontis, D., et al. (2014). Dietary supplementation of benzoic acid and essential oil compounds affects buffering capacity of the feeds, performance of turkey poults and their antioxidant status, ph in the digestive tract, intestinal microbiota and morphology. Asian Aust. J. Anim. Sci. 27, 225–236. doi: 10.5713/ajas.2013.13376
Gomes, M. D., Duarte, M. E., Saraiva, A., de Oliveira, L. L., Teixeira, L. M., and Rocha, G. C. (2023). Effect of antibiotics and low-crude protein diets on growth performance, health, immune response, and fecal microbiota of growing pigs. J. Anim. Sci. 101:skad357. doi: 10.1093/jas/skad357
Hashemipour, H., Kermanshahi, H., Golian, A., and Veldkamp, T. (2013). Effect of thymol and carvacrol feed supplementation on performance, antioxidant enzyme activities, fatty acid composition, digestive enzyme activities, and immune response in broiler chickens. Poultry Sci. 92, 2059–2069. doi: 10.3382/ps.2012-02685
Henning, P. H., Horn, C. H., Leeuw, K. J., Meissner, H. H., and Hagg, F. M. (2010). Effect of ruminal administration of the lactate-utilizing strain NCIMB 41125 on abrupt or gradual transition from forage to concentrate diets. Anim. Feed Sci. Technol. 157, 20–29. doi: 10.1016/j.anifeedsci.2010.02.002
Hidalgo-Cantabrana, C., Ruiz, S. D. L., Ruas-Madiedo, P., Sánchez, B., and Margolles, A. (2017). Bifidobacteria and their health-promoting effects. Microbiol. Spectr. 5:485. doi: 10.1128/microbiolspec.BAD-0010-2016
Houshmand, M., Azhar, K., Zulkifli, I., Bejo, M. H., and Kamyab, A. (2012). Effects of non-antibiotic feed additives on performance, immunity and intestinal morphology of broilers fed different levels of protein. South Afr. J. Anim. Sci. 42, 22–32. doi: 10.4314/sajas.v42i1.3
Iqbal, Y., Cottrell, J. J., Suleria, H. A. R., and Dunshea, F. R. (2020). Gut microbiota-polyphenol interactions in chicken: A review. Animals 10:1391. doi: 10.3390/ani10081391
Kogut, M. H., and Arsenault, R. J. (2016). Gut health: The new paradigm in food animal production. Front. Vet. Sci. 3:71. doi: 10.3389/fvets.2016.00071
Kong, C., Gao, R. Y., Yan, X. B., Huang, L. S., and Qin, H. L. (2019). Probiotics improve gut microbiota dysbiosis in obese mice fed a high fat or high-sucrose diet. Nutrition 60, 175–184. doi: 10.1016/j.nut.2018.10.002
Kuperwaser, F., Avital, G., Vaz, M. J., Noble, K. N., Dammann, A. N., Randis, T. M., et al. (2023). Host inflammatory dynamics reveal placental immune modulation by Group B during pregnancy. Mol. Syst. Biol. 19:e11021. doi: 10.15252/msb.202211021
Kwon, H. I., Jeong, N. H., Kim, S. Y., Kim, M. H., Son, J. H., Jun, S. H., et al. (2019). Inhibitory effects of thymol on the cytotoxicity and inflammatory responses induced by extracellular vesicles in cultured keratinocytes. Microb. Pathog. 134:103603. doi: 10.1016/j.micpath.2019.103603
Li, Y. Y., Cao, H. R., Zhang, S. Y., Guo, P. F., Zhao, J. M., Zhang, D. G., et al. (2023). Effects of the supplementation of essential oil mixtures on growth performance, nutrient digestibility, immune status and microbial community in weaned piglets. Animals 13:3697. doi: 10.3390/ani13233697
Li, L. X., Chen, X. C., Zhang, K. Y., Tian, G., Ding, X. M., Bai, S. P., et al. (2023). Effects of thymol and carvacrol eutectic on growth performance, serum biochemical parameters, and intestinal health in broiler chickens. Animals 13:2242. doi: 10.3390/ani13132242
Li, Y., Fu, X. F., Ma, X., Geng, S. J., Jiang, X. M., Huang, Q. C., et al. (2018). Intestinal microbiome-metabolome responses to essential oils in piglets. Front. Microbiol. 9:1988. doi: 10.3389/fmicb.2018.01988
Liang, D. J., Li, F. Y., Fu, Y. H., Cao, Y. G., Song, X. J., Wang, T. C., et al. (2014). Thymol inhibits LPS-stimulated inflammatory response via down-regulation of NF-κB and MAPK signaling pathways in mouse mammary epithelial cells. Inflammation 37, 214–222. doi: 10.1007/s10753-013-9732-x
Liu, H. L., Li, Z. P., Si, H. Z., Zhong, W., Fan, Z. Y., and Li, G. Y. (2020). Comparative analysis of the gut microbiota of the blue fox and raccoon dog. Arch. Microbiol. 202, 135–142. doi: 10.1007/s00203-019-01721-0
Liu, S. R., Wei, Z. W., Deng, M., Xian, Z. Y., Liu, D. W., Liu, G. B., et al. (2023). Effect of a high-starch or a high-fat diet on the milk performance, apparent nutrient digestibility, hindgut fermentation parameters and microbiota of lactating cows. Animals 13:2508.
Lozupone, C., and Knight, R. (2005). UniFrac: A new phylogenetic method for comparing microbial communities. Appl. Environ. Microbiol. 71, 8228–8235. doi: 10.1128/Aem.71.12.8228-8235.2005
Luna, A., Lema-Alba, R. C., Dambolena, J. S., Zygadlo, J. A., Labaque, M. C., and Marin, R. H. (2017). Thymol as natural antioxidant additive for poultry feed: Oxidative stability improvement. Poultry Sci. 96, 3214–3220. doi: 10.3382/ps/pex158
Luo, P., Luo, L., Zhao, W. J., Wang, L. S., Sun, L. J., Wu, H. Y., et al. (2020). Dietary thymol supplementation promotes skeletal muscle fibre type switch in longissimus dorsi of finishing pigs. J. Anim. Physiol. Anim. Nutr. 104, 570–578. doi: 10.1111/jpn.13269
Ma, C., Azad, M. A., Tang, W., Zhu, Q., Wang, W., Gao, Q. K., et al. (2022). Maternal probiotics supplementation improves immune and antioxidant function in suckling piglets via modifying gut microbiota. J. Appl. Microbiol. 133, 515–528. doi: 10.1111/jam.15572
Maijó, M., Miró, L., Polo, J., Campbell, J., Russell, L., Crenshaw, J., et al. (2012). Dietary plasma proteins attenuate the innate immunity response in a mouse model of acute lung injury. Br. J. Nutr. 107, 867–875. doi: 10.1017/S0007114511003655
Marchese, A., Orhan, I. E., Daglia, M., Barbieri, R., Di Lorenzo, A., Nabavi, S. F., et al. (2016). Antibacterial and antifungal activities of thymol: A brief review of the literature. Food Chem. 210, 402–414. doi: 10.1016/j.foodchem.2016.04.111
Mo, K. B., Li, J., Liu, F. F., Xu, Y., Huang, X. H., and Ni, H. J. (2022). Superiority of microencapsulated essential oils compared with common essential oils and antibiotics: Effects on the intestinal health and gut microbiota of weaning piglet. Front. Nutr. 8:808106. doi: 10.3389/fnut.2021.808106
Moller, S. H. (1999). Effects of weight development, pelting time, colour type and farm on skin length in mink. Acta Agric. Scand. Sec. Anim. Sci. 49, 121–126. doi: 10.1080/090647099424187
Mosleh, N., Shomali, T., and Kazemi, H. A. (2013). Effect of essential oil on immune responses and faecal virus shedding period in broilers immunized with live Newcastle disease vaccines. Iran. J. Vet. Res. 14, 220–225.
Niu, Q., Li, P. H., Hao, S. S., Kim, S. W., Du, T. R., Hua, J. D., et al. (2019). Characteristics of gut microbiota in sows and their relationship with apparent nutrient digestibility. Int. J. Mol. Sci. 20:870. doi: 10.3390/ijms20040870
Omonijo, F. A., Liu, S. X., Hui, Q. R., Zhang, H., Lahaye, L., Bodin, J. C., et al. (2019). Thymol improves barrier function and attenuates inflammatory responses in porcine intestinal epithelial cells during lipopolysaccharide (LPS)-induced inflammation. J. Agric. Food Chem. 67, 615–624. doi: 10.1021/acs.jafc.8b05480
Pérez-Rosés, R., Risco, E., Vila, R., Peñalver, P., and Cañigueral, S. (2015). Effect of some essential oils on phagocytosis and complement system activity. J. Agric. Food Chem. 63, 1496–1504. doi: 10.1021/jf504761m
Pierce, J. L., Cromwell, G. L., Lindemann, M. D., Russell, L. E., and Weaver, E. M. (2005). Effects of spray-dried animal plasma and immunoglobulins on performance of early weaned pigs. J. Anim. Sci. 83, 2876–2885.
Placha, I., Bacova, K., Zitterl-Eglseer, K., Laukova, A., Chrastinova, L., Madarova, M., et al. (2022). Thymol in fattening rabbit diet, its bioavailability and effects on intestinal morphology, microbiota from caecal content and immunity. J. Anim. Physiol. Anim. Nutr. 106, 368–377. doi: 10.1111/jpn.13595
Singh, N., Singh, V., Rai, S. N., Mishra, V., Vamanu, E., and Singh, M. P. (2022). Deciphering the gut microbiome in neurodegenerative diseases and metagenomic approaches for characterization of gut microbes. Biomed. Pharmacother. 156:113958. doi: 10.1016/j.biopha.2022.113958
Slattery, C., Cotter, P. D., and O’Toole, P. W. (2019). Analysis of health benefits conferred by lactobacillus species from kefir. Nutrients 11:1252. doi: 10.3390/nu11061252
Spiller, R., and Hoad, C. (2020). Enhancing our understanding of small bowel function using modern imaging techniques. Neurogastroenterol. Motil. 32:e13616. doi: 10.1111/nmo.13616
Susanto, I., Wiryawan, K. G., Suharti, S., Retnani, Y., Zahera, R., and Jayanegara, A. (2023). Evaluation of supplementation on rumen fermentation, production performance, carcass traits and health of ruminants: A meta-analysis. Anim. Biosci. 36, 879–890. doi: 10.5713/ab.22.0258
Tan, C. Q., Wei, H. K., Sun, H. Q., Ao, J. T., Long, G., Jiang, S. W., et al. (2015). Effects of dietary supplementation of oregano essential oil to sows on oxidative stress status, lactation feed intake of sows, and piglet performance. Biomed. Res. Int. 2015:525218. doi: 10.1155/2015/525218
Tang, Q. F., He, R. C., Huang, F., Liang, Q. M., Zhou, Z. Y., Zhou, J. H., et al. (2023). Effects of ensiling sugarcane tops with bacteria-enzyme inoculants on growth performance, nutrient digestibility, and the associated rumen microbiome in beef cattle. J. Anim. Sci. 101:skad326. doi: 10.1093/jas/skad326
Thirstrup, J. P., Jensen, J., and Lund, M. S. (2017). Genetic parameters for fur quality graded on live animals and dried pelts of American mink. J. Anim. Breed. Genet. 134, 322–331. doi: 10.1111/jbg.12258
Trevisi, P., Merialdi, G., Mazzoni, M., Casini, L., Tittarelli, C., De Filippi, S., et al. (2007). Effect of dietary addition of thymol on growth, salivary and gastric function, immune response, and excretion of Salmonella enterica serovar Typhimurium, in weaning pigs challenged with this microbe strain. Ital. J. Anim. Sci. 6, 374–376. doi: 10.4081/ijas.2007.1s.374
Turnbaugh, P. J., Baeckhed, F., Fulton, L., and Gordon, J. I. (2008). Diet-induced obesity is linked to marked but reversible alterations in the mouse distal gut microbiome. Cell Host Microbe 3, 213–223. doi: 10.1016/j.chom.2008.02.015
Vamanu, E., and Rai, S. N. (2021). The link between obesity, microbiota dysbiosis, and neurodegenerative pathogenesis. Diseases 9:45.
Van Noten, N., Degroote, J., Van Liefferinge, E., Taminiau, B., De Smet, S., Desmet, T., et al. (2020). Effects of thymol and thymol α-D-glucopyranoside on intestinal function and microbiota of weaned pigs. Animals 10:329. doi: 10.3390/ani10020329
Wang, M., Yang, C., Wang, Q. Y., Li, J. Z., Li, Y. L., Ding, X. Q., et al. (2020). The growth performance, intestinal digestive and absorptive capabilities in piglets with different lengths of small intestines. Animal 14, 1196–1203. doi: 10.1017/S175173111900288X
Wang, X., Wang, Y. C., Wang, Q. Y., Dai, C. P., Li, J. Z., Huang, P. F., et al. (2023). The impact of early and mid-pregnant Hu ewes’ dietary protein and energy levels on growth performance and serum biochemical indices. J. Appl. Anim. Res. 51, 174–181. doi: 10.1080/09712119.2023.2170385
Wang, Y. A., Yang, Z. P., Zhou, Y. F., Tan, J. J., Sun, H. Q., Sun, D. F., et al. (2022). Effects of different amino acid levels and a carvacrol-thymol blend on growth performance and intestinal health of weaned pigs. J. Anim. Sci. Biotechnol. 13:22. doi: 10.1186/s40104-022-00674-7
Xiao, H. J., Yan, H., Tian, P. Z., Ji, S. K., Zhao, W., Lu, C. S., et al. (2023). The effect of early colonized gut microbiota on the growth performance of suckling lambs. Front. Microbiol. 14:1273444. doi: 10.3389/fmicb.2023.1273444
Yang, C., Lin, J. H., Liang, H. Y., Xue, L., Kwart, A., Jiang, M., et al. (2022). CD44 v5 domain inhibition represses the polarization of Th2 cells by interfering with the IL-4/IL-4R signaling pathway. Immunol. Cell Biol. 100, 21–32. doi: 10.1111/imcb.12491
Ylinen, V., Pylkkö, P., Peura, J., and Valaja, J. (2020). Ileal and total tract digestibility and nitrogen utilisation in blue fox fed low-protein diets supplemented with DL-methionine and L-histidine. Arch. Anim. Nutr. 74, 237–255. doi: 10.1080/1745039x.2020.1716655
Zhang, X. S., Yu, D., Wu, D., Gao, X. T., Shao, F., Zhao, M., et al. (2023). Tissue-resident Lachnospiraceae family bacteria protect against colorectal carcinogenesis by promoting tumor immune surveillance. Cell Host Microbe 31:418. doi: 10.1016/j.chom.2023.01.013
Zhao, B. C., Wang, T. H., Chen, J., Qiu, B. H., Xu, Y. R., Zhang, Q., et al. (2023). Effects of dietary supplementation with a carvacrol-cinnamaldehyde-thymol blend on growth performance and intestinal health of nursery pigs. Porcine Health Manag. 9:24. doi: 10.1186/s40813-023-00317-x
Zhao, P. Y., Yan, L., Zhang, T., Yin, H. D., Liu, J. B., and Wang, J. P. (2021). Effect of 25-hydroxyvitamin D and essential oil complex on productive performance, egg quality, and uterus antioxidant capacity of laying hens. Poultry Sci. 100:101410. doi: 10.1016/j.psj.2021.101410
Keywords: thymol, blue fox, growth performance, serum biochemical biochemistry, intestinal morphology, gut microbiota
Citation: Yuan C, Chen S, Sun R, Ren L, Zhao T, Wu M and Zhang A (2024) Thymol improves the growth performance of blue foxes by regulating the gut microbiota. Front. Microbiol. 15:1368293. doi: 10.3389/fmicb.2024.1368293
Received: 10 January 2024; Accepted: 07 May 2024;
Published: 14 June 2024.
Edited by:
Emanuel Vamanu, University of Agricultural Sciences and Veterinary Medicine, RomaniaReviewed by:
Sachchida Nand Rai, Banaras Hindu University (BHU), Varanasi, IndiaMuhammad Akbar Shahid, Bahauddin Zakariya University, Pakistan
Copyright © 2024 Yuan, Chen, Sun, Ren, Zhao, Wu and Zhang. This is an open-access article distributed under the terms of the Creative Commons Attribution License (CC BY). The use, distribution or reproduction in other forums is permitted, provided the original author(s) and the copyright owner(s) are credited and that the original publication in this journal is cited, in accordance with accepted academic practice. No use, distribution or reproduction is permitted which does not comply with these terms.
*Correspondence: Aiwu Zhang, zhangaiwu@jlau.edu.cn