- 1Department of Human Pathology, University of Messina, Messina, Italy
- 2Department of Biomedical, Dental and Imaging Sciences, University of Messina, Messina, Italy
- 3Department of Molecular Medicine, Biochemistry Section, University of Pavia, Pavia, Italy
- 4Scylla Biotech Srl, Messina, Italy
The gut represents an important site of colonization of the commensal bacterium Streptococcus agalactiae (group B Streptococcus or GBS), which can also behave as a deadly pathogen in neonates and adults. Invasion of the intestinal epithelial barrier is likely a crucial step in the pathogenesis of neonatal infections caused by GBS belonging to clonal complex 17 (CC17). We have previously shown that the prototypical CC17 BM110 strain invades polarized enterocyte-like cells through their lateral surfaces using an endocytic pathway. By analyzing the cellular distribution of putative GBS receptors in human enterocyte-like Caco-2 cells, we find here that the alpha 3 (α3) and alpha 2 (α2) integrin subunits are selectively expressed on lateral enterocyte surfaces at equatorial and parabasal levels along the vertical axis of polarized cells, in an area corresponding to GBS entry sites. The α3β1 and α2β1 integrins were not readily accessible in fully differentiated Caco-2 monolayers but could be exposed to specific antibodies after weakening of intercellular junctions in calcium-free media. Under these conditions, anti-α3β1 and anti-α2β1 antibodies significantly reduced GBS adhesion to and invasion of enterocytes. After endocytosis, α3β1 and α2β1 integrins localized to areas of actin remodeling around GBS containing vacuoles. Taken together, these data indicate that GBS can invade enterocytes by binding to α3β1 and α2β1 integrins on the lateral membrane of polarized enterocytes, resulting in cytoskeletal remodeling and bacterial internalization. Blocking integrins might represent a viable strategy to prevent GBS invasion of gut epithelial tissues.
Introduction
Streptococcus agalactiae or group B streptococcus (GBS) is a frequent colonizer of the human intestine that can also cause a wide range of diseases, including sepsis, meningitis, endocarditis, soft tissue infections and arthritis. Infections affecting adults, including patients with chronic underlying disease (such as diabetes and solid tumors) and the elderly, are steadily increasing (Farley, 2001; Sambola et al., 2002; Zi et al., 2022; Keogh and Doran, 2023). Moreover, in the neonate, GBS is a highly prevalent cause of serious disease including sepsis, meningoencephalitis, and permanent neurological disorders (Edwards and Baker, 2005; Verani et al., 2010). Based on pathogenesis, clinical features and properties of infecting strains, neonatal infections can be grouped into two different disease types. Early-Onset Disease (EOD) manifests itself during the first week of life, while Late-Onset Disease (LOD) occurs from day 8 to 89 after birth (Melin, 2011; Karampatsas et al., 2022; Lohrmann et al., 2023). For EOD, the prevalent route of GBS transmission to the newborn is inhalation of contaminated amniotic fluid or secretions from the genital tract during parturition (Edwards and Baker, 2005; Melin, 2011). In the case of LOD, GBS can be acquired at the time of delivery or postnatally via maternal sources, such as breast milk, or by horizontal transmission. Colonization of the intestine and invasion of the intestinal barrier are considered important events in many cases of LOD. For example, intestinal colonization can be detected for up to 3 months in 40% of infants that become colonized at birth (Weindling et al., 1981). Many LODs, particularly meningitis cases, are caused by strains of capsular serotype III belonging to clonal complex 17 (CC17), which, for this reason, has been dubbed hypervirulent GBS (Lamy et al., 2006; Poyart et al., 2008; Tazi et al., 2010; Bellais et al., 2012). Identification of the bacterial and host factors governing interactions of these bacteria with intestinal cells seems important to understand the pathogenesis of LOD and develop alternative strategies to prevent this condition. However, little is known of the mechanisms by which hypervirulent GBS colonize the human intestine.
Pathogens interact with intestinal epithelial cells by means of different virulence factors, mainly adhesins, to colonize mucosal tissues and often disseminate to distant sites. GBS expresses a complex set of components capable of interacting with different cell receptors and components of the extracellular matrix (ECM) (Pietrocola et al., 2018). In the intestinal mucosa, the apical and basolateral surfaces of polarized enterocytes express different sets of receptors, due to the distinctive distribution of membrane proteins involved in maintenance of cell polarity and intercellular junctions (Groschwitz and Hogan, 2009; Chelakkot et al., 2018). One of the main functions of intercellular tight junctions is to reduce potential interactions between basolateral cell surfaces and luminal pathogens (Ulluwishewa et al., 2011; Gieryńska et al., 2022), even though these junctions represent themselves a frequent microbial target (Paradis et al., 2021). Moreover, microbial toxins or inflammatory responses can weaken intercellular junctions, leading to bacterial entry through the lateral surfaces of intestinal epithelial cells, as shown for Listeria monocytogenes, Shighella flexneri, and Campylobacter jejuni (Mounier et al., 1992; Gaillard and Finlay, 1996; Bouwman et al., 2013). Previous studies have shown that GBS can transiently open the tight junctions of Caco-2 enterocyte-like cell and cervical epithelial cells and reach the paracellular space (Soriani et al., 2006). Once in this location, GBS selectively adhere to lateral cell membranes at parabasal sites, from which they invade cells through a caveolae-dependent endocytotic pathway (De Gaetano et al., 2021). Collectively, these data suggest that, similar to other luminal pathogens, GBS can recognize receptors distributed in the lateral surfaces of polarized intestinal cells that are hidden when the monolayer is intact, but that become accessible under conditions that weaken intercellular junctions, such as toxin release, inflammatory reactions or reduced extracellular Ca++ concentrations (De Gaetano et al., 2021).
Integrins are cell surface glycoproteins belonging to a large family of αβ heterodimeric transmembrane receptors which mediate cell-cell and cell-ECM interactions in various tissues, including the intestine (Hynes, 2002). In humans, there are 18 different α subunits and 8 β subunits which make up 24 different receptors each containing an extracellular, a transmembrane and a cytoplasmic region (Frantz et al., 2010). Integrin-surface expression and functions vary in different cell lineages. In tissue cells, binding of integrins to ECM components results in the rapid recruitment of cytoskeletal proteins and in the formation of anchoring points to the surrounding ECM (van der Flier and Sonnenberg, 2001; Brunton et al., 2004).
Several pathogens use integrins and integrin-dependent intracellular signaling for their adhesion to and invasion of host cells (Scibelli et al., 2007; De Gaetano et al., 2022). In this process, pathogens can directly interact with integrins or use other host molecules, such as ECM components, as a bridge. Previous studies have demonstrated both direct and indirect interactions of GBS with integrins. For example, these bacteria can invade respiratory cells by using the Plasminogen binding surface Protein (PbsP) cell wall adhesin to bind vitronectin, which acts as a bridge to activate the αv integrins (Buscetta et al., 2016; De Gaetano et al., 2018; Lentini et al., 2018; Coppolino et al., 2021). Direct GBS-integrin interactions have also been documented (Cuzzola et al., 2000; Bolduc and Madoff, 2007). Hypervirulent GBS were recently found to bind integrins α5β1 and αvβ3 via the Srr2 adhesin and invade by this mechanism brain endothelial cells (Deshayes de Cambronne et al., 2021). The present study was undertaken to ascertain whether integrins are involved in GBS invasion of enterocytes. To this end, we used the Caco-2 cells to model the intestinal epithelial barrier because of their resemblance to mature enterocytes after a process of spontaneous cell polarization involving the development of intercellular junctions and an apical brush border (Ramond et al., 1985; Delie and Rubas, 1997; De Gaetano et al., 2021).
Materials and methods
Bacterial strain and reagents
The GBS strain BM110 (serotype III, CC17; Pellegrini et al., 2022) was used in the present study. This strain was grown at 37°C in Todd-Hewitt broth (THB, Difco Laboratories) until the mid log phase. Staphylococcus aureus strain Newman was grown in Tryptic Soy Broth (TSB; Oxoid) at 37°C and used as a control in some experiments. Rabbit polyclonal anti-GBS serum has been previously described (De Gaetano et al., 2021). Rabbit anti-α3 polyclonal (21992-1-AP, Proteintech), mouse anti-α2 monoclonal (14-0498-80, Invitrogen), rabbit anti-β1 polyclonal (12594-1-AP, Proteintech) and mouse anti-integrin α5β1 monoclonal (MAB1969, Millipore) antibodies were obtained commercially, while rabbit anti-α5, -α1, -αV, and -β3 polyclonal antibodies were a generous gift from Dr. G. Tarone (University of Turin, Italy) and have been previously described (Pietrocola et al., 2015; De Gaetano et al., 2022). For immunofluorescence analysis, Alexa Fluor 488-conjugated anti-rabbit IgG (ab15007) and anti-mouse FITC secondary (ab6785) antibodies, both from Abcam, were used. For enzyme-linked assays (ELISAs), anti-rabbit (A3687, Sigma) and anti-mouse (Biorad 170-6520) alkaline-phosphatase (AP) conjugated secondary antibodies were used.
Caco-2 cultures
The human enterocyte-like Caco-2 cell line (ATCC HTB-37; colorectal adenocarcinoma) was seeded in 24-well plates at a density of 1 × 104 cells per well in Roswell Park Memorial Institute (RPMI) 1640 medium (R8758, Sigma-Aldrich) supplemented with 10% (vol/vol) fetal bovine serum (FBS, 1203C, Sigma-Aldrich) and penicillin-streptomycin (100 IU of penicillin and 0.1 mg/ml of streptomycin, P433, Sigma-Aldrich) and cultured at 37°C in a humidified 5% CO2 incubator, as previously described (De Gaetano et al., 2021). The culture medium was changed every 3 days. Confluence and cellular differentiation were monitored by optical microscopy and immunofluorescence. Five-day-old and 21-day-old Caco-2 cells were used in the present study.
Enzyme-linked assay
Integrin subunit expression was determined in ELISA assays in 21-day-old Caco-2 monolayers after treatment with ethylene glycol-bis-(β-aminoethyl ether)-N, N, N′, N′-tetraacetic acid (EGTA) (E3889, Sigma-Aldrich) at a 10 μM concentration in Dulbecco's phosphate buffered saline without calcium and magnesium (DPBS; Euroclone S.p.A., Milan, Italy) for 15 min. Briefly, EGTA-treated cell monolayers were washed with DPBS and fixed with 3.7% formaldehyde for 15 min at room temperature (RT). Subsequently, enterocytes were washed and blocked with 2% bovine serum albumin (BSA) in DPBS for 2 h at RT with gentle agitation. All antibodies against integrin subunit were diluted 1:1,000 in 1% BSA and incubated with enterocytes for 1 h. Removal of excess of anti-integrin antibodies with several washes was followed by an additional 1 h of incubation with AP-conjugated secondary antibodies diluted 1:10,000 in 1% BSA. After the addition of para-nitrophenyl phosphate (pNPP), absorbance at 405 nm was determined using a plate reader. Data concerning integrin expression in EGTA-treated cell monolayers were expressed as fold changes compared to untreated monolayers.
Adhesion and invasion assays
Adhesion and invasion assays were performed as previously described (De Gaetano et al., 2021; Lentini et al., 2022). Briefly, bacteria were grown to the mid-log phase, collected by centrifugation, washed and suspended to the concentration of ~1–2 × 106 CFU/ml in pre-warmed serum-free RPMI. Caco-2 cells were washed three times and bacteria were added to 5-day-old sub-confluent islands or 21-day-old post-confluent monolayers at a MOI of ~35. After a 1 h incubation at 37°C in the presence of 5% CO2, extracellular bacteria were washed away by rinsing cultured cells three times with DPBS. The numbers of adhering bacteria to the cells were measured by plating cell lysates on blood agar plates. To measure bacterial internalization, the infected cells were washed three times with DPBS and incubated for one additional hour in RPMI containing bactericidal amounts of penicillin and streptomycin (200 U/ml and 200 μg/ml, respectively), as previously described (Famà et al., 2020; Lentini et al., 2020). We found in preliminary experiments that these antibiotic treatment conditions result in elimination of all extracellular bacteria, as visualized using anti-GBS antibodies in non-permeabilized cells. To investigate the role of integrins, enterocytes were treated for 30 min with different concentrations (0.6, 3, and 15 μM in RPMI) of tri-peptides with an arginine-glycine-aspartic (RGD, Sigma-Aldrich, St. Louis, Missouri, U.S.A.) or arginine-glycine-glutamic acid (RGE, Sigma-Aldrich, St. Louis, Missouri, U.S.A.) sequence, the latter being used as a control. To study the involvement of integrin subunits in GBS interactions with enterocytes, cells were pre-incubated with antibodies against α-, β- or both integrin subunits for 30 min before infection. Antibodies were diluted at ratios ranging from 1:1,000 to 1:5,000 in RPMI, as indicated in the Figures and Figure legends. The effects of anti-integrin antibodies were evaluated in comparison with normal IgG used as a control. In studies employing mature 21-day-old monolayers, temporary weakening of intercellular junctions was achieved with EGTA as described above under “enzyme-linked assay”. Adherence and invasion were expressed as percentage values using the formula: recovered CFU/initial inoculum CFU × 100.
Fluorescence microscopy
Structured-illumination fluorescence microscopy analysis was performed using a Zeiss Observer.Z1 Apotome apparatus and images were acquired with the AxionVision software, as previously described (De Gaetano et al., 2021). Briefly, Caco-2 cells were cultured in coverslips and their confluence was monitored daily. To visualize bacterial invasion, cells were infected for 1 h, washed and incubated for a further 1 h time in the presence of penicillin (200 U/ml) and streptomycin (200 μg/ml) to remove residual extracellular bacteria. Then coverslips were washed several times, fixed with 3.7% formaldehyde for 15 min at RT and permeabilized with 0.1% Triton X-100 for 10 min. After a 1 h blocking step with BSA (2% in DPBS), infected cells were incubated for 1 h with rabbit polyclonal anti-GBS serum followed by Alexa Fluor 488-conjugated secondary anti-rabbit antibody, both diluted 1:2,000 in DPBS supplemented with BSA 1%. To visualize the surface expression of integrin subunits, enterocytes were fixed and stained with anti-integrin antibodies diluted 1:2,000 with 1% BSA in DPBS. Labeled secondary antibodies (1:2,000 in 1% BSA in DPBS) were used according to the anti-integrin antibody producing species. The contribution of integrin subunits to GBS internalization was studied after permeabilization of fixed coversplis, as described above. Phalloidin-iFluor 555 (ab176756, Abcam) and 40-6-diamidino-2-phenylindole (DAPI) (D8417, Sigma-Aldrich) were used according to the manufacturer's instructions to label, respectively, actin and DNA (both eukaryotic and bacterial).
Statistical analysis
All experiments were repeated at least three times and the data were expressed as means ± standard deviations (SD). Data were analyzed for statistical significance by the ANOVA test followed by the Bonferroni correction for multiple comparisons or by the Mann-Whitney test. A p-value of 0.05 was used as the threshold for significance.
Results
Expression of integrins in human enterocyte-like cells
The RGD amino acid motif (Arg-Gly-Asp) is present in several extracellular matrix glycoproteins, such as fibronectin and vitronectin, and can act as an anchoring point for integrins expressed on the cell surface (Takahashi et al., 2007; Singh et al., 2010). Based on these interactions, the integrin family can be classified into RGD-recognizing (e.g., αVβ3, αVβ1 and α5β1) and non-RGD-recognizing (e.g., α3β1, α2β1 and α1β1) integrins (Ruoslahti, 1996; LaFoya et al., 2018; Ludwig et al., 2021). Moreover, several microorganisms, including GBS (Bolduc and Madoff, 2007), display adhesins with RGD motifs that can bind host integrins (Johnson et al., 2011; Bonsor et al., 2015; Deshayes de Cambronne et al., 2021). For these reasons, we examined the possibility that RGD-binding integrins function as receptors for GBS colonization and invasion of enterocytes. First, we evaluated the number of cell-adhering bacteria in the presence of an RGD peptide and its cognate non-functional RGE control (Viela et al., 2019; Mathelié-Guinlet et al., 2020). Under these conditions, neither peptide had inhibitory effects on bacterial adhesion to Caco-2 cells over a wide dose range. In contrast, in positive control experiments, the RGD, but not the RGE, peptide inhibited adherence of S. aureus (Supplementary Figure 1). Inhibition of S. aureus adherence by the RGD peptide suggests that the low levels of α5 and αV integrins that are detectable on Caco-2 cells might still be relevant for S. aureus binding. Alternatively, S. aureus might interact with RGD-binding α-integrins not investigated in the present study. Collectively, these data suggested that RGD-recognizing integrins are not involved in GBS-enterocyte interactions (Figure 1A).
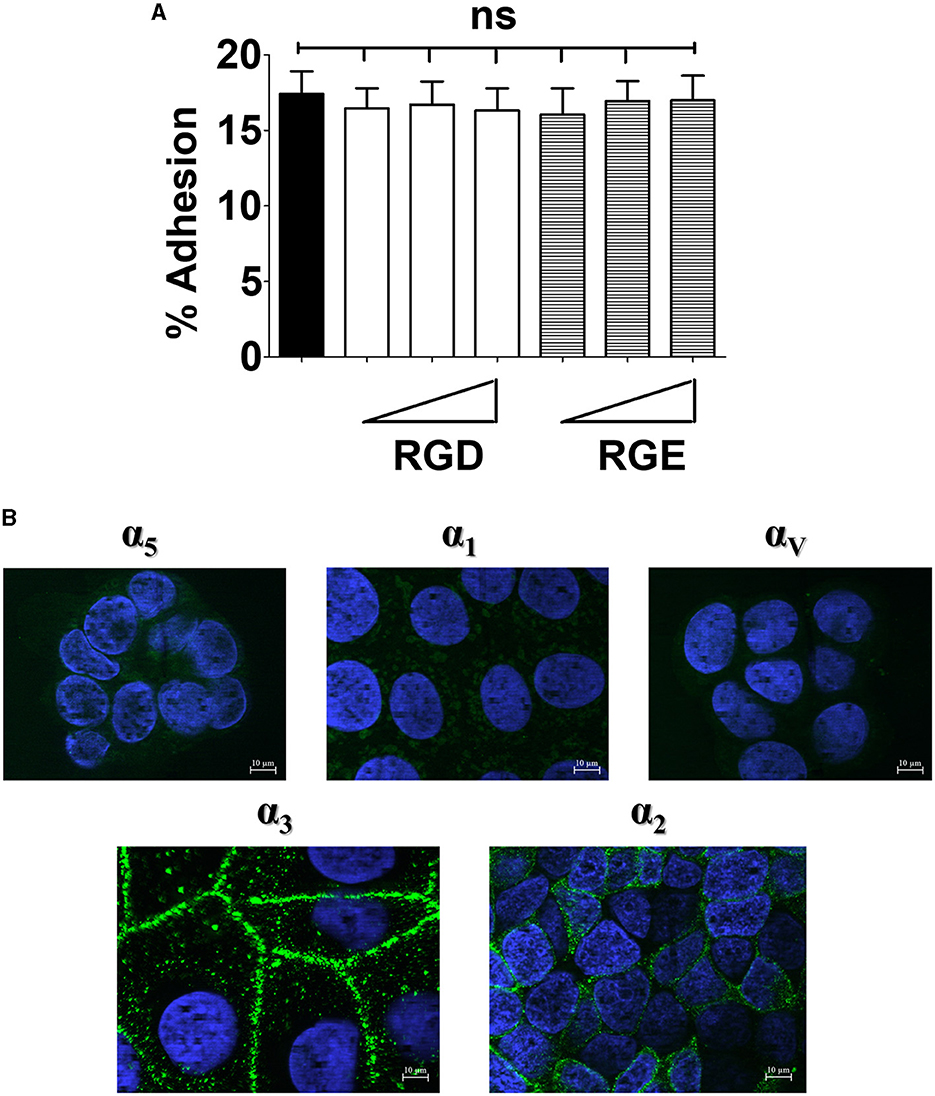
Figure 1. Effects of the RGD peptide on GBS adhesion and expression of integrins in enterocyte-like cells. (A) Five-day-old Caco-2 cells were pre-treated with increasing concentrations (ranging from 0.6 to 15 μM) of RGD or RGE tri-peptides and infected with GBS. Streptococcal adherence is expressed as the percentage of input bacteria measured in cell lysates. Shown are means ± SD of five independent experiments conducted in triplicate. ns, non-significant by the ANOVA test followed by the Bonferroni correction. (B) Fixed, unpermeabilized 5-day-old Caco-2 cells were probed with antibodies specific for α5-, α1-, αV-, α3-, and α2-integrin subunits, followed by AlexaFluor 488- or FITC-labeled secondary antibodies (green), and analyzed by fluorescence microscopy. Cell nuclei were stained in blue with DAPI. Scale bar = 10 μm. Shown are representative images from three independent experiments.
Next, we analyzed the expression of a panel of integrin subunits using immunofluorescence microscopy (Beaulieu, 1992; Perreault et al., 1995; Lussier et al., 2000). By this technique, we readily detected the presence of α3 and α2 subunits (Figure 1B). Moreover, higher expression of α3 and α2 subunits in these cells, in comparison with other alpha subunits, was apparent after quantification of fluorescence intensity (Supplementary Figure 2). The β1 integrin subunit, which is usually associated with the α3 and α2 subunits, was also found to be expressed at high levels in Caco-2 cells (Supplementary Figure 3). Notably, β1 displayed a uniform distribution on the cell surface, whereas α3 and α2 were selectively expressed on areas of cell contact (Supplementary Figure 2). The different cell surface distribution of these subunits is not surprising, and might be explained by the ability of β1 to associate with a large number of α subunits, some of which might be present on Caco-2 cells, in addition to α2 and α3 (van der Flier and Sonnenberg, 2001; Hynes, 2002; Frantz et al., 2010). Collectively, these data indicate that enterocyte-like cells predominantly express α3β1 and α2β1 integrins, while RGD-recognizing integrins containing αV and α5 subunits, as well as the collagen-binding α1 integrin, are present at considerably lower levels. These findings are in general agreement with previous studies conducted on human intestinal tissues (Beaulieu, 1992; Perreault et al., 1995; Lussier et al., 2000).
Subcellular location of α2 and α3 integrin subunits
Next, we studied the subcellular distribution of α3 and α2 integrins. Tri-dimensional structured illumination microscopy revealed that the α3 and α2 integrin subunits are preferentially expressed along the lateral surface of polarized Caco-2 cells and in the intercellular spaces, as indicated by orthogonal projections and 3D reconstructions (Figures 2A, B). Interestingly, both α3 and α2 integrins selectively clustered in the equatorial and parabasal segments of the lateral membranes in polarized cells, while low levels of these proteins were detectable in the apical and basal part of the lateral membranes (Figures 2A, B; Supplementary Figure 4). This pattern was not influenced by the degree of cell confluency in different observational fields (data not shown).
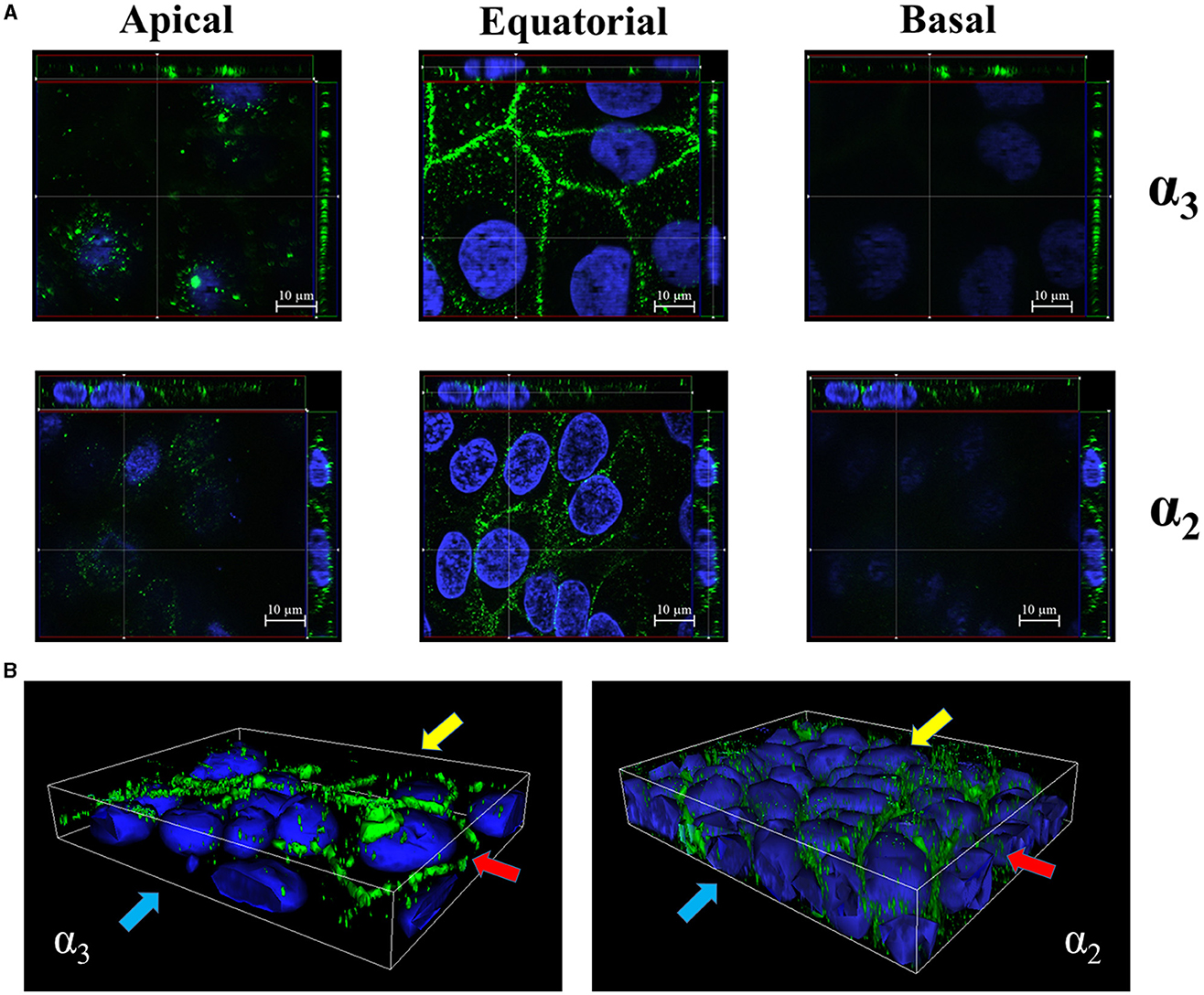
Figure 2. Equatorial localization of α3- and α2- integrin subunits in enterocyte-like cells. (A) Z-stack sections of 5-day-old Caco-2 cells with orthogonal views from x/z and y/z planes showing the distribution of α3- and α2- integrin subunits at the apical, equatorial and basal levels along the vertical axis of polarized enterocytes. Caco-2 cells were probed with antibodies specific to α3- and α2- integrin subunits followed by AlexaFluor 488- or FITC-labeled secondary antibodies (green), respectively, and analyzed by fluorescence microscopy. (B) 3D reconstruction of α3- and α2- integrin subunits distribution in 5-day-old Caco-2 cells. Eukaryotic nuclei and integrin subunits are visualized in blue (DAPI) and green, respectively, as described in (A). Shown are images representative of three independent experiments. Yellow, red, and blue arrows indicate apical, equatorial, and basal planes, respectively, along the vertical cell axis.
To determine whether α3β1 and α2β1 integrins are accessible to luminal agents in layers of polarized enterocytes, we performed ELISA assays on confluent Caco-2 monolayers obtained at 21 days post-seeding. Some monolayers were pretreated with EGTA, which is known to disrupt the integrity of intercellular junctions, before the addition of antibodies directed against α3, α2, and β1 integrin subunits. In the absence of EGTA, low-level binding of anti-integrin antibodies was detected, which increased considerably after cell exposure to the calcium-chelating agent (Supplementary Figure 5), suggesting that the accessibility of α2β1 and α3β1 integrins is increased after weakening intercellular junctions. Taken together, these results indicate that α2β1 and α3β1 are selectively located at the equatorial level of the lateral surfaces of polarized enterocytes in areas that are not readily accessible to luminal agents in the presence of intact intercellular junctions.
Role of integrins in GBS adhesion to Caco-2 cells
Based on the above findings we investigated the role of α3β1 and α2β1 integrins in GBS adhesion to enterocytes. In these experiments we used 21-day-old confluent cell monolayers treated with calcium-free saline to weaken intercellular junctions (De Gaetano et al., 2021). It was observed that cell pretreatment with antibodies directed against α3, α2, or β1 integrin subunits or mixtures thereof decreased GBS adherence, whereas anti-αV or anti-α5β1 antibodies were ineffective (Figure 3). We further examined the effects of blocking antibodies using 5-day-old islands of polarized Caco-2 cells in which α3 and α2 integrins are freely accessible even in the absence of intercellular-junction-disrupting agents. Under these conditions, the numbers of adhering streptococci were significantly reduced after enterocyte pretreatment with anti-α3, anti-α2 or anti-β1 antibodies (Figures 4A, B), while mixtures of α3, α2 and β1 antibodies did not result in further reductions in GBS adherence compared to the values observed with single antibody types (Figure 4C). Collectively, these data indicate that α3β1 and α2β1 integrins promote GBS adherence to Caco-2 cells. However, our data also suggest the involvement of additional, as yet unidentified, receptors since only partial inhibition in bacterial adherence was observed using anti-integrin antibodies. It should be pointed out that we cannot formally exclude an involvement of the α5β1 integrin in GBS interactions with Caco-2 cells, based on our experiments, since we used only an anti-α5β1 monoclonal antibody in adherence inhibition experiments. Although effective at inhibiting bacterial adherence in a previous study using a different pathogen (Lentini et al., 2022), this monoclonal might recognize a region of the integrin that is not involved in interactions with GBS.
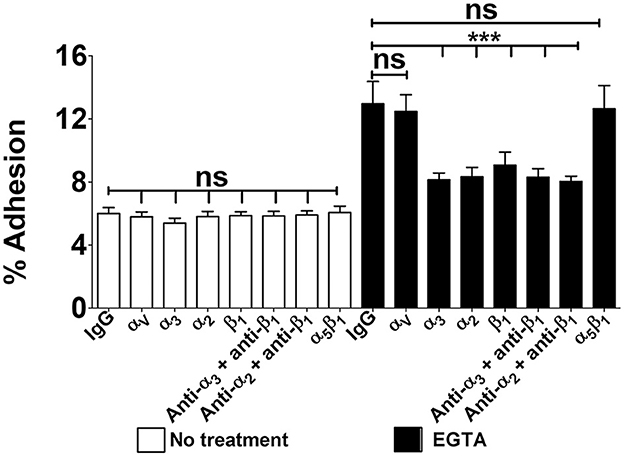
Figure 3. Integrin-dependent GBS adherence to confluent cell monolayers. Twenty-one-day-old Caco-2 cells were pre-treated with antibodies specific to α-, β-, or α/β integrin subunits (diluted 1:1,000) and infected with GBS. Streptococcal adherence is expressed as the percentage of input bacteria measured in cell lysates. IgG, normal IgG control. Shown are means ± SD of three independent experiments conducted in triplicate. Statistical analysis was performed by the ANOVA test followed by the Bonferroni correction, ns, non-significant; ***p < 0.001 compared to the normal IgG control.
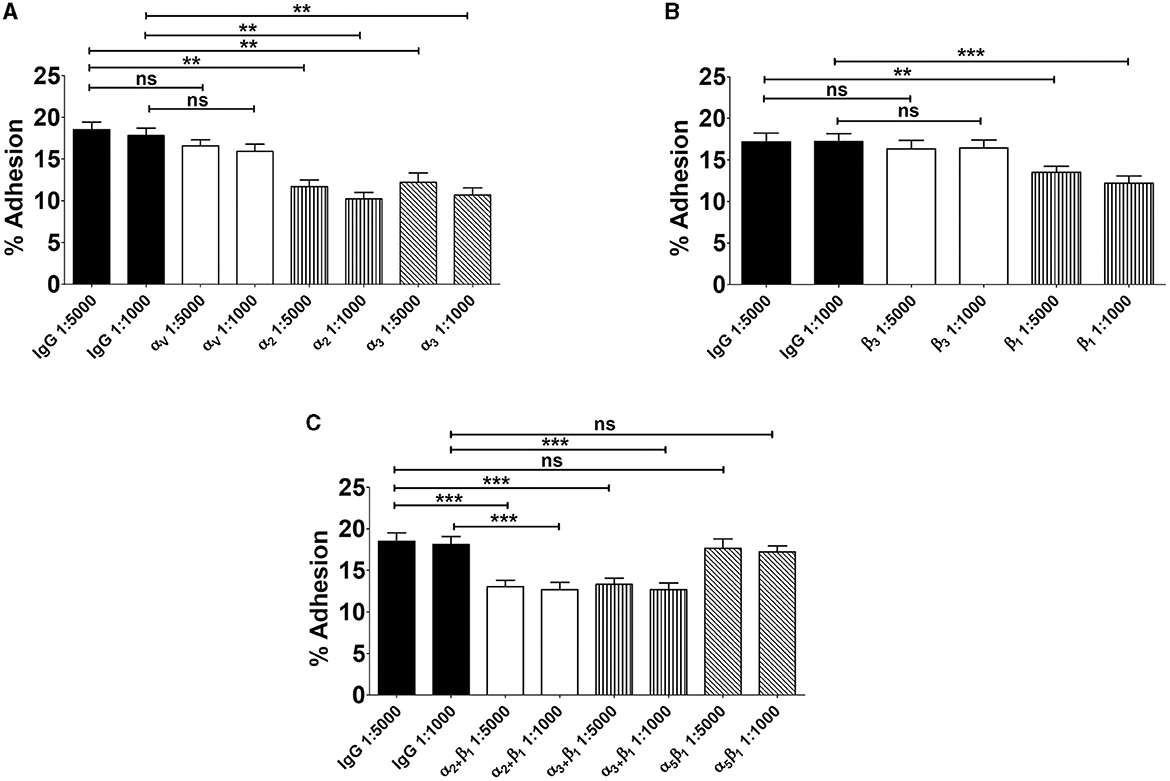
Figure 4. Involvement of α3β1 and α2β1 integrins in GBS adherence to enterocytes. Five-day-old Caco-2 cells were pre-treated with antibodies specific to α- (A), β- (B), or α/β (C) integrin subunits or normal IgG used as a control (IgG) at the indicated dilutions and infected with GBS. Streptococcal adherence is expressed as the percentage of input bacteria measured in cell lysates. Shown are means ± SD of five independent experiments conducted in triplicate. Statistical analysis was performed by the ANOVA test followed by the Bonferroni correction, ns, non-significant; **p < 0.01; ***p < 0.001 compared to the normal IgG control.
Integrin α3β1 and α2β1 subunits mediate GBS invasion of Caco-2 cells
We subsequently investigated whether the α3 and α2 integrin subunits could play a role not only in bacterial adherence, but also in GBS invasion of Caco-2 cells. To measure cell invasion in 5-day-old Caco-2 islands, we used an antibiotic protection assay in which extracellular bacteria are killed by antibiotics unable to penetrate the cell membrane. Under these conditions, pretreatment with anti-α3, anti-α2 and anti-β1, but not anti-α5β1, antibodies resulted in marked decreases in streptococcal internalization (Figures 5A–C). Notably, the extent of the antibody effects on internalization was higher than that previously observed on adherence. The involvement of α3β1 and α2β1 integrins in internalization was also confirmed by observing reduced numbers of intracellular bacteria after antibody treatment (Figure 5D). In these experiments we also observed a marked rearrangement of host cytoskeleton in areas of bacterial internalization. As shown in Figures 6A, B, the actin fibers clustered in areas in which α3 and α2 integrins, as well as streptococci, were present and bacteria were often seen inside intracellular spaces covered by actin and α3 and α2 integrin subunits (Figure 6C).
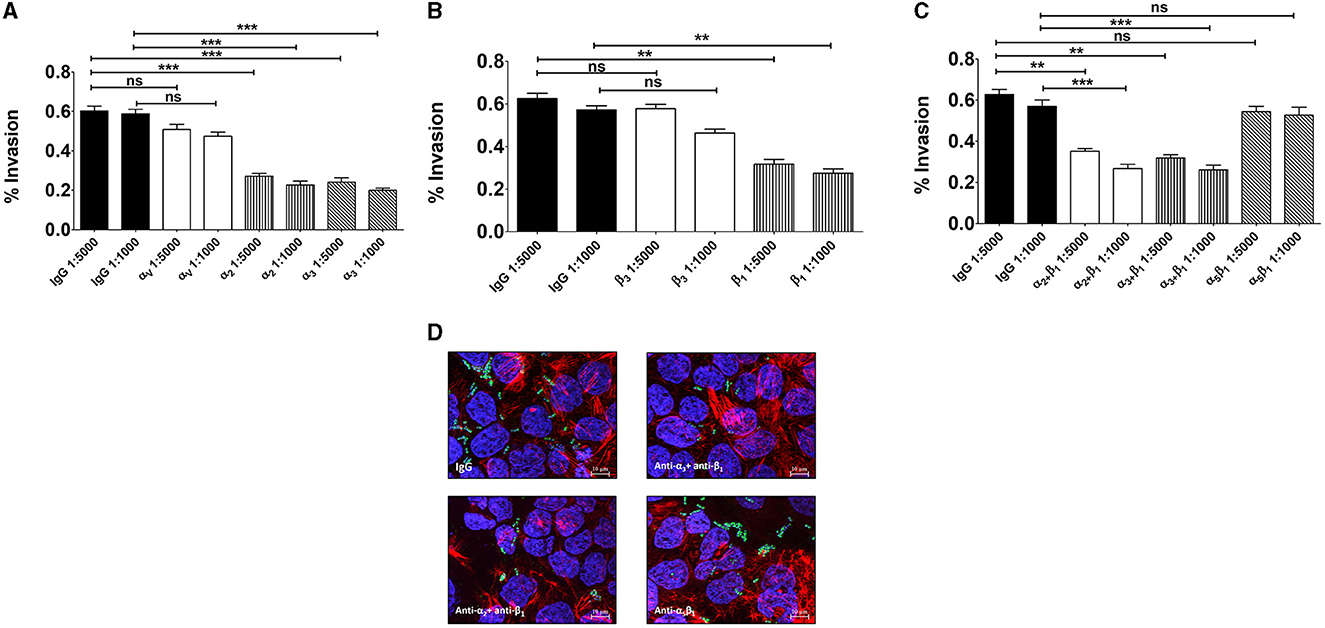
Figure 5. Integrins α3β1 and α2β1 are involved in GBS invasion of enterocyte-like cells. Five-day-old Caco-2 cells were pre-treated with antibodies to α- (A), β- (B), or α/β (C) integrin subunits or normal IgG used as a control (IgG) at the indicated dilutions and infected with GBS. Streptococcal invasion was measured after killing extracellular bacteria with antibiotics. Invasion is expressed as the percentage of input bacteria measured in cell lysates. Shown are means ± SD of five independent experiments conducted in triplicate. Statistical analysis was performed by the ANOVA test followed by the Bonferroni correction, ns, non-significant; **p < 0.01; ***p < 0.001 compared to the normal IgG control. (D) Effects of anti-α/β integrin subunits antibodies on GBS invasion as detected by immunofluorescence analysis of permeabilized cells. Nuclei and actin filaments were labeled with DAPI (Blue) and Phalloidin-iFluor 555 (Red), respectively. Bacteria (Green) were labeled using a rabbit anti-GBS serum followed by Alexa Fluor 488-conjugated anti-rabbit IgG. Images were obtained with the extended focus function. Scale bar = 10 μm.
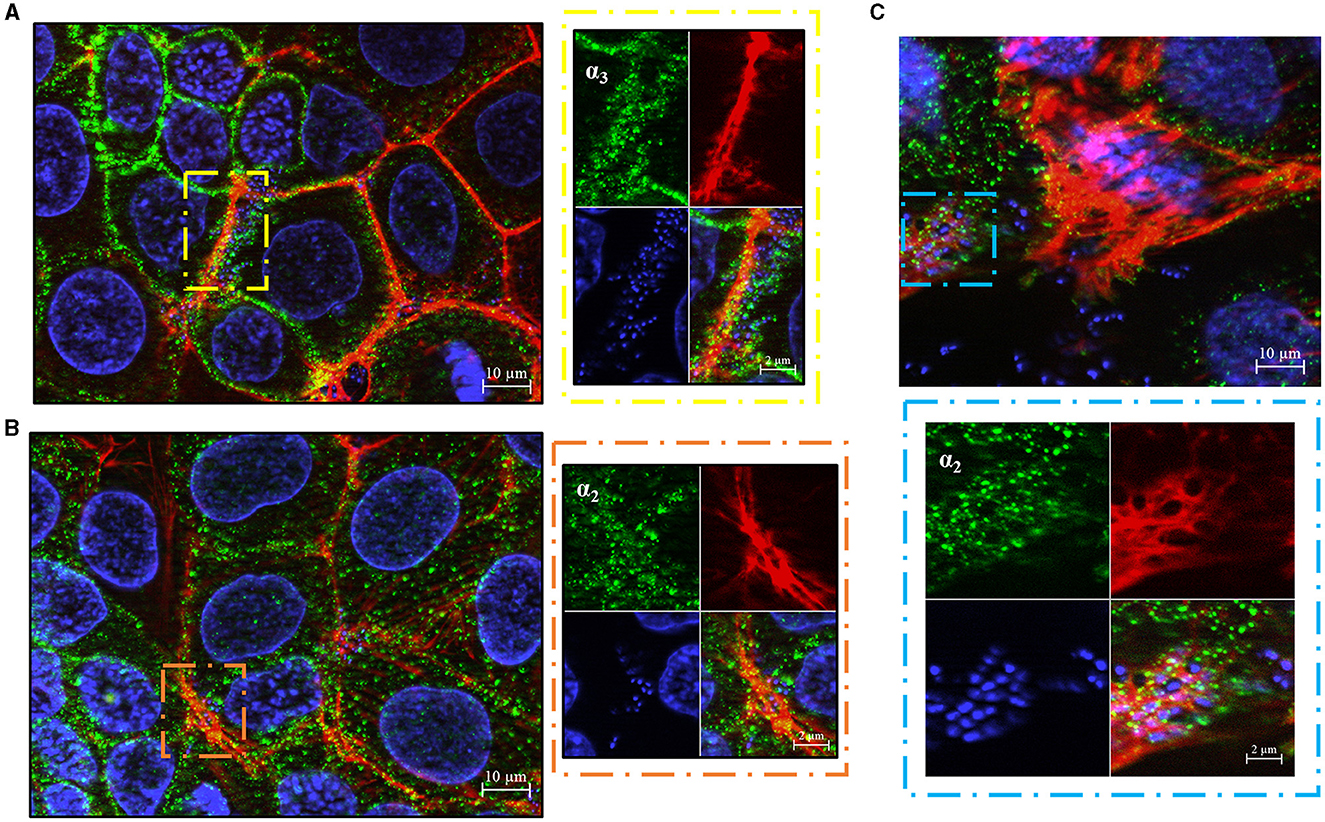
Figure 6. GBS trafficking in human enterocyte-cells. Immunofluorescence analysis of permeabilized 5-day-old Caco-2 cells showing spatial relationships between bacteria and α3- (A) or α2- (B) integrin subunits after bacterial internalization. Eukaryotic nuclei and bacterial nucleoids are labeled with DAPI (blue), while α3 and α2 integrin subunits are visualized in green using specific antibodies, by AlexaFluor 488- or FITC-labeled IgG secondary antibodies, respectively, and analyzed by fluorescence microscopy. The right panels are magnifications of the areas indicated by the dashed rectangles (yellow and brown). Scale bar = 2 μm. (C) Representative image showing streptococci inside actin-embedded vacuoles with α integrin subunits. Eukaryotic nuclei and bacterial nucleoids were labeled with DAPI (blue), actin filaments with Phalloidin-iFluor 555 (Red), α integrin subunit (green) with specific antibodies, followed by an AlexaFluor 488-labeled IgG secondary antibody. The lower panel is a magnification of the area indicated by the sky blue dashed rectangle. Scale bar = 2 μm.
To ascertain the involvement of α3β1 and α2β1 integrins in GBS invasion of confluent monolayers, Caco-2 cells were cultured for 2–3 weeks to obtain full cell polarization and differentiation. Next, monolayers were treated with EGTA in calcium free buffered saline, washed and incubated with anti-integrin antibodies before the addition of bacteria. As expected, the absence of Ca++ greatly promoted streptococcal uptake, and such an enhancement was completely abrogated in the presence of antibodies targeting α3, α2 or β1 integrin subunits (Figure 7). Moreover, treatment with EGTA was associated with increased accessibility of antibodies to α3β1 and α2β1 integrins (Supplementary Figure 5). All together, these data indicate that α3β1 and α2β1 integrins can function as receptors for GBS entry into polarized enterocytes through the equatorial and parabasal portions of their lateral surfaces.
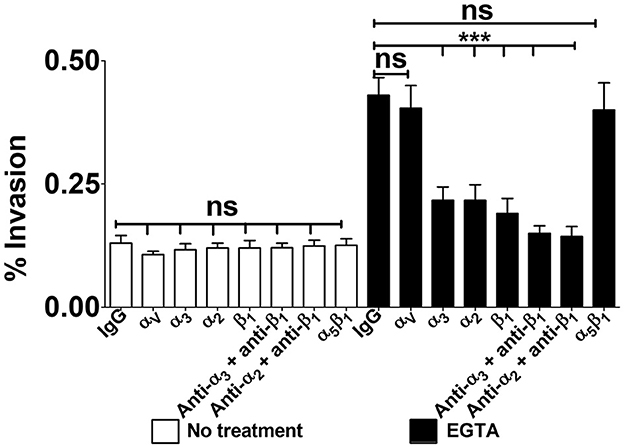
Figure 7. Integrin-dependent GBS invasion of confluent cell monolayers. Twenty-one-day-old Caco-2 cells were pre-treated with antibodies specific to α-, β-, or α/β integrin subunits or normal IgG control (IgG), all diluted 1:1,000, and infected with GBS. Antibiotics were then used to kill extracellular adherent bacteria. Invasion is expressed as the percentage of input bacteria measured in cell lysates. Shown are means ± SD of three independent experiments conducted in triplicate. Statistical analysis was performed by the ANOVA test followed by the Bonferroni correction, ns, non-significant; ***p < 0.001 compared to the normal IgG control.
Discussion
A growing body of evidence suggests that the ability of CC17 GBS to colonize and invade the gut of newborns is relevant to pathogenesis of a large proportion of LODs (Tazi et al., 2010, 2019; Morinis et al., 2011; Hays et al., 2019; De Gaetano et al., 2021). However, little is known of the ability of GBS to colonize and invade the intestinal epithelium that represents an exceptionally robust physical barrier against any pathogen, due to the presence of specialized cell-to-cell junctions and the maintenance of apical-basal polarity (Snoeck et al., 2005; de Pereda et al., 2009; Franke, 2009). To study the interactions between hypervirulent GBS and enterocytes, we choose as a model the Caco-2 cell line which differentiates into monolayers that have many features of the intestinal epithelium (De Gaetano et al., 2021). We have previously shown that hypervirulent GBS adheres to the lateral surfaces of Caco-2 cells and then invades them by a caveolae-dependent internalization mechanism involving bacterial trafficking in acidified vacuoles (De Gaetano et al., 2021). However, the nature of the cell receptors mediating GBS invasion could not be identified. In the present study, we hypothesized that integrins may function as GBS receptors in enterocytes and examined their expression in Caco-2 cells.
We first observed that the degree of expression and subcellular location of the various integrin subunits is similar in human enterocytes and Caco-2 cells. For example, the higher expression in Caco-2 cells of α3 and α2, compared to α1, α5, or αν, subunits is reminiscent of similar observations in intestinal tissue enterocytes (Beaulieu, 1992). In the latter, α3β1 and α2β1 integrins display a distinctive crypt-villus gradient by which α3 and α2 are almost exclusively expressed in villus and crypt enterocytes, respectively (Beaulieu, 1992; Perreault et al., 1995; Lussier et al., 2000). We found that both integrins are expressed on Caco-2 cells, suggesting that these cells have features of both immature and mature enterocytes. Notably, both the α3 and α2 integrins are selectively localized on the lateral surface of Caco-2 cells, frequently clustering at equatorial and parabasal levels along the vertical cell axis. Lack of integrin expression on the apical surface is also a feature of human enterocytes, in which α3β1 and α2β1 integrins are found exclusively on baso-lateral surfaces (Beaulieu, 1992). Although their functional role is unclear, these integrins may be expressed on lateral enterocyte surfaces to establish robust intercellular connections, since the α2β1 integrin was recently found to be required for stabilizing adherence junctions and barrier function in keratinocyte layers (Howden et al., 2021). Of note, the α6β1 integrin is selectively expressed on the basal surface of all tissue enterocytes (Perreault et al., 1995), but was not investigated in the present study. Future studies will examine the expression of α6β1 integrin on the basal surfaces of Caco-2 cells grown on plastic surfaces coated with different extracellular matrix components, including basal membrane components.
Because the distribution of α3 and α2 subunits corresponded to the area preferentially used by GBS to enter enterocyte-like cells, as determined in a previous study, we investigated here the role of these integrins. We first found that anti-α3 or -α2 antibodies induced significant inhibition of bacterial adherence, while antibodies directed against other α subunits were ineffective. Antibodies directed against the β1 chain, which is shared by the α3 and α2 subunits in the formation of heterodimeric complexes, were also inhibitory, suggesting the β1 chain contributes to bacterial adherence alone or when associated with its cognate α subunits. Notably, our data also suggest the involvement of additional, as yet unidentified, adhesion receptors since only partial inhibition of adherence to Caco-2 cells was observed using anti-α3, anti-β1, or anti-α2 antibodies. Future studies will be aimed at identifying these additional receptors as well as their cognate GBS adhesins. Interestingly, however, the anti-α2/α3/β1 antibodies used here were more effective at inducing inhibition of cell invasion than adherence suggesting a predominant role of the corresponding integrins in GBS internalization. For instance, bacterial invasion was considerably increased after opening cell-to-cell junctions in fully differentiated and polarized monolayers and such an increase was completely prevented using either anti-α2β1 or anti-α3β1 antibodies. These data are in agreement with our previous findings on the inhibitory effects of the tyrosine kinase inhibitor genistein on GBS invasion of enterocytes (De Gaetano et al., 2021), since the β1 subunit is known to activate a series of protein-tyrosine kinases that recruit actin and remodel the cell cytoskeleton (Lafrenie and Yamada, 1996; Shin et al., 2006; Ulanova et al., 2009; Cho et al., 2010). Moreover, our present data are in agreement with our previous ones on the importance of lipid rafts in GBS internalization, in view of the well-established role of these membrane domains in integrin signaling (Del Pozo, 2004). Accordingly, we observed here that integrins and actin filaments cluster in the same area of the cell around bacteria-containing vacuoles, which were previously identified as early and late endosomes (De Gaetano et al., 2021). Collectively, our studies indicate that GBS predominantly invade polarized enterocytes from their lateral surfaces using α3β1 and α2β1 integrins, which trigger a cellular response involving tyrosine-kinase dependent endocytosis and extensive actin remodeling. Interestingly, CC1 and CC17 GBS were previously shown to interact, respectively, with α2β1 and α5β1/αvβ3 integrins in brain endothelial cells thereby promoting invasion of the blood-brain barrier (Banerjee et al., 2011). Of note, similar to what we found in enterocytes, integrins are normally expressed the basolateral and not apical surfaces of polarized endothelial cells (Bosman, 1993). Collectively these studies support a model whereby GBS must preliminarily induce alterations of some kind in continuous epithelial or endothelial layers in order to access integrins hidden in basolateral surfaces. GBS were previously shown to target intercellular junctions and to access paracellular spaces in epithelial cell lines monolayers (Soriani et al., 2006). Studies are underway to ascertain whether GBS-induced weakening of cell junction is mediated by cytolysins such as the granadaene pigment or the CAMP factor. It should be pointed out, however, that the role of α3β1 and α2β1 integrins in invasion of enterocytes by the CC17 BM110 strain, as shown here, cannot be generalized to GBS isolates belonging to other clonal complexes. Since CC17 GBS are known to express a unique set of adhesins and virulence factors (Lentini et al., 2018), future studies will be required to assess the role of various integrins using multiple clinically relevant GBS clone types.
Invasion of epithelial or endothelial cells from their basolateral surfaces, as shown here for GBS, is a common theme in the pathogenesis of bacterial and viral diseases (Seo et al., 2008; Bouwman et al., 2013) and in many cases integrins have been shown to participate in this process. For example, Y. pseudotuberculosis was found to interact with the α5β1 integrin along the basolateral membrane of enterocyte monolayers when epithelial cell junctions are transiently opened (McCormick et al., 1997; Kaur and Mukhopadhaya, 2020). Moreover, rotavirus can directly bind to the α2 integrin subunit on basolateral surfaces of polarized Caco-2 monolayers and penetrate cells by this route (Seo et al., 2008).
GBS is frequently responsible for fetal infection because of its ability to ascend from the vagina into the uterus and invade the amniotic cavity (Brokaw et al., 2021) and, in this context, increased exfoliation of GBS-colonized vaginal cells may promote bacterial migration (Vornhagen et al., 2018). It has been proposed that GBS interaction with the α1β1 integrin results in the upregulation of a series genes driving epithelial-mesenchymal-transition and epithelial exfoliation in vaginal cell and proinflammatory gene expression in brain endothelial cells (Banerjee et al., 2011; Vornhagen et al., 2018). Whether upregulation of these genes occurs in enterocytes upon engagement of α2β1 or α3β1 integrins will be the object of future investigations.
The nature of the GBS adhesins that might interact with α3β1 and α2β1 integrins is still unclear. In a previous study, a domain of the GBS alpha C protein was found to directly interact with the α1β1 integrin on the surface of a cervical epithelial cell line and to promote bacterial internalization (Bolduc and Madoff, 2007). Moreover, at least in the case of a ST-26 clinical isolate, the pilus tip adhesin PilA of GBS was found to promote interactions with collagen, which then functioned as a bridge to engage the α2β1 integrin (Banerjee et al., 2011). However, whether GBS belonging to frequent sequence types use this mechanism to interact with host cells, particularly brain endothelial cells, has been questioned (Dramsi et al., 2012). Future studies should ascertain whether GBS pilus proteins or other adhesins bind directly or indirectly α2β1 and α3β1 integrins on enterocytes and whether collagen or other extracellular matrix proteins are involved in these interactions. Collectively, however, our studies and those of others suggest that targeting integrins may represent an appealing strategy to prevent GBS disease.
Data availability statement
The original contributions presented in the study are included in the article/Supplementary material, further inquiries can be directed to the corresponding author.
Ethics statement
Ethical approval was not required for the studies on humans in accordance with the local legislation and institutional requirements because only commercially available established cell lines were used.
Author contributions
GD: Conceptualization, Data curation, Investigation, Methodology, Writing – original draft. GL: Methodology, Writing – review & editing. FC: Methodology, Writing – review & editing. AF: Methodology, Writing – review & editing. GP: Conceptualization, Writing – review & editing. CB: Conceptualization, Supervision, Writing – original draft.
Funding
The author(s) declare financial support was received for the research, authorship, and/or publication of this article. This study was supported by research funding to CB (fund for the National Research Program and Projects of Significant National Interest (PRIN-PNRR 2022) ID: P2022BNCKS. FC was supported by a grant for PhD students (PON no. DR_36_TRAN_PON_IND_1) at the Doctoral School in Translational Molecular Medicine and Surgery, Department of Biomedical, Dental and Imaging Sciences, University of Messina, Messina, Italy. Publication expenses were entirely sustained by the University of Messina through the APC initiative.
Acknowledgments
We thank the late Dr. Tarone from the University of Turin (Turin, Italy) for the generous gift of anti-integrin rabbit sera. We thank Andrea Cappello for administrative and technical help.
Conflict of interest
CB acts as scientific advisors for Scylla Biotech S.r.l. without receiving any compensation for these activities. Scylla Biotech S.r.l. did not provide funding for this study and had no role in its conduction.
The remaining authors declare that the research was conducted in the absence of any commercial or financial relationships that could be construed as a potential conflict of interest.
Publisher's note
All claims expressed in this article are solely those of the authors and do not necessarily represent those of their affiliated organizations, or those of the publisher, the editors and the reviewers. Any product that may be evaluated in this article, or claim that may be made by its manufacturer, is not guaranteed or endorsed by the publisher.
Supplementary material
The Supplementary Material for this article can be found online at: https://www.frontiersin.org/articles/10.3389/fmicb.2024.1367898/full#supplementary-material
Supplementary Figure 1. S. aureus adherence is inhibited by the RGD tri-peptide. Five-day-old Caco-2 cells were pre-treated with increasing concentrations of RGD or RGE tri-peptides (ranging from 0.6 to 15 μM) and infected with Staphylococcus aureus, Newman strain. Bacterial adherence is expressed as the percentage of input bacteria measured in cell lysates. Shown are means ± SD of five independent experiments conducted in triplicate. Statistical analysis was performed by the ANOVA test followed by the Bonferroni correction, ns, non-significant; *p < 0.05.
Supplementary Figure 2. Quantitative analysis of fluorescence in enterocytes stained for integrin subunits. (A) Five-day-old, fixed, non-permeabilized Caco-2 cells were probed with antibodies specific for α5-, α1-, αV-, α3-, α2, and β1-integrin subunits, followed by AlexaFluor 488- or FITC-labeled secondary antibodies (green), and analyzed by fluorescence microscopy. Normal IgG controls are shown in the lower panels. Cell nuclei were stained in blue with DAPI. Scale bar = 10 μm. Shown are representative images from three independent experiments. (B) Analysis of Integrated Density (IntDen), as performed with the Image J software on pictures obtained during the experiments described in (A). IntDen values for normal IgG controls were 0.38 or lower. *Significantly different from normal IgG controls; v significantly different from αV, α5 and α1 integrin subunits by the Mann-Whitney test (p < 0.05).
Supplementary Figure 3. Beta 1 integrin expression on 5-day-old Caco-2 cells. Fixed, unpermeabilized Caco-2 cells were probed with antibodies specific to the β1 integrin subunit, followed by AlexaFluor 488-labeled secondary antibody, and analyzed by fluorescence microscopy. Cell nuclei were stained in blue (DAPI) and the β1 integrin subunit was stained in green. Scale bar = 10 μm.
Supplementary Figure 4. Quantitative analysis of fluorescence along the vertical axis of polarized enterocytes stained for integrin subunits. Z-stack sections of 5-day-old Caco-2 cells with orthogonal views from x/z and y/z planes showing the distribution of α3- (A) and α2- (C) integrin subunits at the apical, equatorial and basal levels along the vertical axis of polarized enterocytes. Caco-2 cells were probed with antibodies specific to α3- and α2- integrin subunits, followed by AlexaFluor 488- or FITC-labeled secondary antibodies (green) and analyzed by fluorescence microscopy. Shown are representative images from five independent experiments. (B, D) Analysis of Integrated Density (IntDen) as performed with the Image J software on images obtained during the experiments described in A and C. *Significantly different by the Mann-Whitney test (p < 0.05).
Supplementary Figure 5. Detection of α3, α2 and β1 integrin subunits in confluent cell monolayers. Expression of α3, α2, and β1 integrin subunits, as measured by ELISA, in 21-day-old EGTA-treated enterocytes is shown as fold change relative to the expression levels observed in 21-day-old non-EGTA treated monolayers. Integrin subunit expression on non-EGTA treated monolayers was normalized to the value of 1. Shown are means ± SD of three independent experiments conducted in triplicate. *p < 0.05 by Mann-Whitney test.
References
Banerjee, A., Kim, B. J., Carmona, E. M., Cutting, A. S., Gurney, M. A., Carlos, C., et al. (2011). Bacterial Pili exploit integrin machinery to promote immune activation and efficient blood-brain barrier penetration. Nat. Commun. 2:462. doi: 10.1038/ncomms1474
Beaulieu, J. F. (1992). Differential expression of the VLA family of integrins along the crypt-villus axis in the human small intestine. J. Cell Sci. 102, 427–436. doi: 10.1242/jcs.102.3.427
Bellais, S., Six, A., Fouet, A., Longo, M., Dmytruk, N., Glaser, P., et al. (2012). Capsular switching in group B streptococcus CC17 hypervirulent clone: a future challenge for polysaccharide vaccine development. J. Infect. Dis. 206, 1745–1752. doi: 10.1093/infdis/jis605
Bolduc, G. R., and Madoff, L. C. (2007). The group B streptococcal alpha C protein binds alpha1beta1-integrin through a novel KTD motif that promotes internalization of GBS within human epithelial cells. Microbiology 153 (Pt 12), 4039–4049. doi: 10.1099/mic.0.2007/009134-0
Bonsor, D. A., Pham, K. T., Beadenkopf, R., Diederichs, K., Haas, R., Beckett, D., et al. (2015). Integrin engagement by the helical RGD motif of the Helicobacter pylori CagL protein is regulated by pH-induced displacement of a neighboring helix. J. Biol. Chem. 290, 12929–12940. doi: 10.1074/jbc.M115.641829
Bosman, F. T. (1993). Integrins: cell adhesives and modulators of cell function. Histochem. J. 25, 469–477. doi: 10.1007/BF00159282
Bouwman, L. I., Niewold, P., and van Putten, J. P. (2013). Basolateral invasion and trafficking of Campylobacter jejuni in polarized epithelial cells. PLoS ONE 8:e54759. doi: 10.1371/journal.pone.0054759
Brokaw, A., Furuta, A., Dacanay, M., Rajagopal, L., and Adams Waldorf, K. M. (2021). Bacterial and host determinants of group B streptococcal vaginal colonization and ascending infection in pregnancy. Front. Cell. Infect. Microbiol. 11:720789. doi: 10.3389/fcimb.2021.720789
Brunton, V. G., MacPherson, I. R., and Frame, M. C. (2004). Cell adhesion receptors, tyrosine kinases and actin modulators: a complex three-way circuitry. Biochim. Biophys. Acta 1692, 121–144. doi: 10.1016/j.bbamcr.2004.04.010
Buscetta, M., Firon, A., Pietrocola, G., Biondo, C., Mancuso, G., Midiri, A., et al. (2016). PbsP, a cell wall-anchored protein that binds plasminogen to promote hematogenous dissemination of group B streptococcus. Mol. Microbiol. 101, 27–41. doi: 10.1111/mmi.13357
Chelakkot, C., Ghim, J., and Ryu, S. H. (2018). Mechanisms regulating intestinal barrier integrity and its pathological implications. Exp. Mol. Med. 50, 1–9. doi: 10.1038/s12276-018-0126-x
Cho, B. A., Cho, N. H., Seong, S. Y., Choi, M. S., and Kim, I. S. (2010). Intracellular invasion by Orientia tsutsugamushi is mediated by integrin signaling and actin cytoskeleton rearrangements. Infect. Immun. 78, 1915–1923. doi: 10.1128/IAI.01316-09
Coppolino, F., Romeo, L., Pietrocola, G., Lentini, G., De Gaetano, G. V., Teti, G., et al. (2021). Lysine residues in the MK-rich region are not required for binding of the PbsP protein from group B streptococci to plasminogen. Front. Cell Infect. Microbiol. 11:679792. doi: 10.3389/fcimb.2021.679792
Cuzzola, M., Mancuso, G., Beninati, C., Biondo, C., Genovese, F., Tomasello, F., et al. (2000). Beta 2 integrins are involved in cytokine responses to whole Gram-positive bacteria. J. Immunol. 164, 5871–5876. doi: 10.4049/jimmunol.164.11.5871
De Gaetano, G. V., Coppolino, F., Lentini, G., Fam,à, A., Cullotta, C., Raffaele, I., et al. (2022). Streptococcus pneumoniae binds collagens and C1q via the SSURE repeats of the PfbB adhesin. Mol. Microbiol. 117, 1479–1492. doi: 10.1111/mmi.14920
De Gaetano, G. V., Lentini, G., Galbo, R., Coppolino, F., Fam,à, A., Teti, G., et al. (2021). Invasion and trafficking of hypervirulent group B streptococci in polarized enterocytes. PLoS ONE 16:e0253242. doi: 10.1371/journal.pone.0253242
De Gaetano, G. V., Pietrocola, G., Romeo, L., Galbo, R., Lentini, G., Giardina, M., et al. (2018). The Streptococcus agalactiae cell wall-anchored protein PbsP mediates adhesion to and invasion of epithelial cells by exploiting the host vitronectin/α. Mol. Microbiol. 110, 82–94. doi: 10.1111/mmi.14084
de Pereda, J. M., Ortega, E., Alonso-García, N., Gómez-Hernández, M., and Sonnenberg, A. (2009). Advances and perspectives of the architecture of hemidesmosomes: lessons from structural biology. Cell Adh. Migr. 3, 361–364. doi: 10.4161/cam.3.4.9525
Del Pozo, M. A. (2004). Integrin signaling and lipid rafts. Cell Cycle 3, 725–728. doi: 10.4161/cc.3.6.952
Delie, F., and Rubas, W. A. (1997). human colonic cell line sharing similarities with enterocytes as a model to examine oral absorption: advantages and limitations of the Caco-2 model. Crit. Rev. Ther. Drug Carr. Syst. 14, 221–286. doi: 10.1615/CritRevTherDrugCarrierSyst.v14.i3.20
Deshayes de Cambronne, R., Fouet, A., Picart, A., Bourrel, A. S., Anjou, C., Bouvier, G., et al. (2021). CC17 group B streptococcus exploits integrins for neonatal meningitis development. J. Clin. Invest. 131. doi: 10.1172/JCI136737
Dramsi, S., Morello, E., Poyart, C., and Trieu-Cuot, P. (2012). Epidemiologically and clinically relevant Group B streptococcus isolates do not bind collagen but display enhanced binding to human fibrinogen. Microbes Infect. 14, 1044–1048. doi: 10.1016/j.micinf.2012.07.004
Edwards, M. S., and Baker, C. J. (2005). Group B streptococcal infections in elderly adults. Clin. Infect. Dis. 41, 839–847. doi: 10.1086/432804
Famà, A., Midiri, A., Mancuso, G., Biondo, C., Lentini, G., Galbo, R., et al. (2020). Nucleic acid-sensing toll-like receptors play a dominant role in innate immune recognition of pneumococci. MBio. 11. doi: 10.1128/mBio.00415-20
Farley, M. M. (2001). Group B streptococcal disease in nonpregnant adults. Clin. Infect. Dis. 33, 556–561. doi: 10.1086/322696
Franke, W. W. (2009). Discovering the molecular components of intercellular junctions–a historical view. Cold Spring Harb. Perspect. Biol. 1:a003061. doi: 10.1101/cshperspect.a003061
Frantz, C., Stewart, K. M., and Weaver, V. M. (2010). The extracellular matrix at a glance. J. Cell Sci. 123 (Pt 24), 4195–4200. doi: 10.1242/jcs.023820
Gaillard, J. L., and Finlay, B. B. (1996). Effect of cell polarization and differentiation on entry of Listeria monocytogenes into the enterocyte-like Caco-2 cell line. Infect. Immun. 64, 1299–1308. doi: 10.1128/iai.64.4.1299-1308.1996
Gieryńska, M., Szulc-Dabrowska, L., Struzik, J., Mielcarska, M. B., and Gregorczyk-Zboroch, K. P. (2022). Integrity of the intestinal barrier: the involvement of epithelial cells and microbiota-a mutual relationship. Animals 12. doi: 10.3390/ani12020145
Groschwitz, K. R., and Hogan, S. P. (2009). Intestinal barrier function: molecular regulation and disease pathogenesis. J Aller. Clin. Immunol. 124, 3–20. quiz 1–2. doi: 10.1016/j.jaci.2009.05.038
Hays, C., Touak, G., Bouaboud, A., Fouet, A., Guignot, J., Poyart, C., et al. (2019). Perinatal hormones favor CC17 group B. Elife 8:e48772. doi: 10.7554/eLife.48772
Howden, J. D., Michael, M., Hight-Warburton, W., and Parsons, M. (2021). α2β1 integrins spatially restrict Cdc42 activity to stabilise adherens junctions. BMC Biol. 19:130. doi: 10.1186/s12915-021-01054-9
Hynes, R. O. (2002). Integrins: bidirectional, allosteric signaling machines. Cell 110, 673–687. doi: 10.1016/S0092-8674(02)00971-6
Johnson, M. D., Garrett, C. K., Bond, J. E., Coggan, K. A., Wolfgang, M. C., Redinbo, M. R., et al. (2011). Pseudomonas aeruginosa PilY1 binds integrin in an RGD- and calcium-dependent manner. PLoS ONE 6:e29629. doi: 10.1371/journal.pone.0029629
Karampatsas, K., Davies, H., Mynarek, M., Andrews, N., Heath, P. T., Le Doare, K., et al. (2022). Clinical risk factors associated with late-onset invasive group B streptococcal disease: systematic review and meta-analyses. Clin. Infect. Dis. 75, 1255–1264. doi: 10.1093/cid/ciac206
Kaur, D., and Mukhopadhaya, A. (2020). Outer membrane protein OmpV mediates Salmonella enterica serovar typhimurium adhesion to intestinal epithelial cells via fibronectin and α1β1 integrin. Cell. Microbiol. 22:e13172. doi: 10.1111/cmi.13172
Keogh, R. A., and Doran, K. S. (2023). Group B streptococcus and diabetes: finding the sweet spot. PLoS Pathog. 19:e1011133. doi: 10.1371/journal.ppat.1011133
LaFoya, B., Munroe, J. A., Miyamoto, A., Detweiler, M. A., Crow, J. J., Gazdik, T., et al. (2018). Beyond the matrix: the many non-ECM ligands for integrins. Int. J. Mol. Sci. 19. doi: 10.3390/ijms19020449
Lafrenie, R. M., and Yamada, K. M. (1996). Integrin-dependent signal transduction. J. Cell. Biochem. 61, 543–53. doi: 10.1002/(SICI)1097-4644(19960616)61:4<543::AID-JCB7>3.0.CO;2-O
Lamy, M. C., Dramsi, S., Billoët, A., Réglier-Poupet, H., Tazi, A., Raymond, J., et al. (2006). Rapid detection of the “highly virulent” group B streptococcus ST-17 clone. Microbes Infect. 8, 1714–1722. doi: 10.1016/j.micinf.2006.02.008
Lentini, G., De Gaetano, G. V., Famà, A., Galbo, R., Coppolino, F., Mancuso, G., et al. (2022). Neutrophils discriminate live from dead bacteria by integrating signals initiated by Fprs and TLRs. EMBO J. 41:e109386. doi: 10.15252/embj.2021109386
Lentini, G., Famà, A., Biondo, C., Mohammadi, N., Galbo, R., Mancuso, G., et al. (2020). Neutrophils enhance their own influx to sites of bacterial infection via endosomal TLR-dependent Cxcl2 production. J. Immunol. 204, 660–670. doi: 10.4049/jimmunol.1901039
Lentini, G., Midiri, A., Firon, A., Galbo, R., Mancuso, G., Biondo, C., et al. (2018). The plasminogen binding protein PbsP is required for brain invasion by hypervirulent CC17 Group B streptococci. Sci. Rep. 8:14322. doi: 10.1038/s41598-018-32774-8
Lohrmann, F., Hufnagel, M., Kunze, M., Afshar, B., Creti, R., Detcheva, A., et al. (2023). Neonatal invasive disease caused by Streptococcus agalactiae in Europe: the DEVANI multi-center study. Infection 51, 981–991. doi: 10.21203/rs.3.rs-1908183/v1
Ludwig, B. S., Kessler, H., Kossatz, S., and Reuning, U. (2021). RGD-binding integrins revisited: how recently discovered functions and novel synthetic ligands (re-)shape an ever-evolving field. Cancers 13. doi: 10.3390/cancers13071711
Lussier, C., Basora, N., Bouatrouss, Y., and Beaulieu, J. F. (2000). Integrins as mediators of epithelial cell-matrix interactions in the human small intestinal mucosa. Microsc. Res. Tech. 51, 169–78. doi: 10.1002/1097-0029(20001015)51:2<169::AID-JEMT8>3.0.CO;2-A
Mathelié-Guinlet, M., Viela, F., Alfeo, M. J., Pietrocola, G., Speziale, P., Dufrêne, Y. F., et al. (2020). Single-molecule analysis demonstrates stress-enhanced binding between Staphylococcus aureus surface protein IsdB and host cell integrins. Nano Lett. 20, 8919–8925. doi: 10.1021/acs.nanolett.0c04015
McCormick, B. A., Nusrat, A., Parkos, C. A., D'Andrea, L., Hofman, P. M., Carnes, D., et al. (1997). Unmasking of intestinal epithelial lateral membrane beta1 integrin consequent to transepithelial neutrophil migration in vitro facilitates inv-mediated invasion by Yersinia pseudotuberculosis. Infect. Immun. 65, 1414–1421. doi: 10.1128/iai.65.4.1414-1421.1997
Melin, P. (2011). Neonatal group B streptococcal disease: from pathogenesis to preventive strategies. Clin. Microbiol. Infect. 17, 1294–1303. doi: 10.1111/j.1469-0691.2011.03576.x
Morinis, J., Shah, J., Murthy, P., and Fulford, M. (2011). Horizontal transmission of group B streptococcus in a neonatal intensive care unit. Paediatr. Child Health 16, e48–50. doi: 10.1093/pch/16.6.e48
Mounier, J., Vasselon, T., Hellio, R., Lesourd, M., and Sansonetti, P. J. (1992). Shigella flexneri enters human colonic Caco-2 epithelial cells through the basolateral pole. Infect. Immun. 60, 237–248. doi: 10.1128/iai.60.1.237-248.1992
Paradis, T., Bègue, H., Basmaciyan, L., Dalle, F., and Bon, F. (2021). Tight junctions as a key for pathogens invasion in intestinal epithelial cells. Int. J. Mol. Sci. 22. doi: 10.3390/ijms22052506
Pellegrini, A., Lentini, G., Famà, A., Bonacorsi, A., Scoffone, V. C., Buroni, S., et al. (2022). CodY Is a global transcriptional regulator required for virulence in group B. Front. Microbiol. 13:881549. doi: 10.3389/fmicb.2022.881549
Perreault, N., Vachon, P. H., and Beaulieu, J. F. (1995). Appearance and distribution of laminin A chain isoforms and integrin alpha 2, alpha 3, alpha 6, beta 1, and beta 4 subunits in the developing human small intestinal mucosa. Anat. Rec. 242, 242–250. doi: 10.1002/ar.1092420214
Pietrocola, G., Arciola, C. R., Rindi, S., Montanaro, L., and Speziale, P. (2018). Non-pilus, cell wall-anchored proteins: involvement in colonization and pathogenesis and potential as vaccine candidates. Front. Immunol. 9:602. doi: 10.3389/fimmu.2018.00602
Pietrocola, G., Gianotti, V., Richards, A., Nobile, G., Geoghegan, J. A., Rindi, S., et al. (2015). Fibronectin binding proteins SpsD and SpsL both support invasion of canine epithelial cells by Staphylococcus pseudintermedius. Infect. Immun. 83, 4093–4102. doi: 10.1128/IAI.00542-15
Poyart, C., Réglier-Poupet, H., Tazi, A., Billoët, A., Dmytruk, N., Bidet, P., et al. (2008). Invasive group B streptococcal infections in infants, France. Emerg. Infect. Dis. 14, 1647–1649. doi: 10.3201/eid1410.080185
Ramond, M. J., Martinot-Peignoux, M., and Erlinger, S. (1985). Dome formation in the human colon carcinoma cell line Caco-2 in culture. Influence of ouabain and permeable supports. Biol. Cell. 54, 89–92. doi: 10.1111/j.1768-322X.1985.tb00383.x
Ruoslahti, E. (1996). RGD and other recognition sequences for integrins. Annu. Rev. Cell Dev. Biol. 12, 697–715. doi: 10.1146/annurev.cellbio.12.1.697
Sambola, A., Miro, J. M., Tornos, M. P., Almirante, B., Moreno-Torrico, A., Gurgui, M., et al. (2002). Streptococcus agalactiae infective endocarditis: analysis of 30 cases and review of the literature, 1962-1998. Clin. Infect. Dis. 34, 1576–1584. doi: 10.1086/340538
Scibelli, A., Roperto, S., Manna, L., Pavone, L. M., Tafuri, S., Della Morte, R., et al. (2007). Engagement of integrins as a cellular route of invasion by bacterial pathogens. Vet. J. 173, 482–491. doi: 10.1016/j.tvjl.2006.01.010
Seo, N. S., Zeng, C. Q., Hyser, J. M., Utama, B., Crawford, S. E., Kim, K. J., et al. (2008). Integrins alpha1beta1 and alpha2beta1 are receptors for the rotavirus enterotoxin. Proc. Natl. Acad. Sci. U. S. A. 105, 8811–8818. doi: 10.1073/pnas.0803934105
Shin, S., Paul-Satyaseela, M., Lee, J. S., Romer, L. H., and Kim, K. S. (2006). Focal adhesion kinase is involved in type III group B streptococcal invasion of human brain microvascular endothelial cells. Microb. Pathog. 41, 168–173. doi: 10.1016/j.micpath.2006.07.003
Singh, B., Su, Y. C., and Riesbeck, K. (2010). Vitronectin in bacterial pathogenesis: a host protein used in complement escape and cellular invasion. Mol. Microbiol. 78, 545–560. doi: 10.1111/j.1365-2958.2010.07373.x
Snoeck, V., Goddeeris, B., and Cox, E. (2005). The role of enterocytes in the intestinal barrier function and antigen uptake. Microbes Infect. 7, 997–1004. doi: 10.1016/j.micinf.2005.04.003
Soriani, M., Santi, I., Taddei, A., Rappuoli, R., Grandi, G., Telford, J. L., et al. (2006). Group B streptococcus crosses human epithelial cells by a paracellular route. J. Infect. Dis. 193, 241–250. doi: 10.1086/498982
Takahashi, S., Leiss, M., Moser, M., Ohashi, T., Kitao, T., Heckmann, D., et al. (2007). The RGD motif in fibronectin is essential for development but dispensable for fibril assembly. J. Cell Biol. 178, 167–178. doi: 10.1083/jcb.200703021
Tazi, A., Disson, O., Bellais, S., Bouaboud, A., Dmytruk, N., Dramsi, S., et al. (2010). The surface protein HvgA mediates group B streptococcus hypervirulence and meningeal tropism in neonates. J. Exp. Med. 207, 2313–2322. doi: 10.1084/jem.20092594
Tazi, A., Plainvert, C., Anselem, O., Ballon, M., Marcou, V., Seco, A., et al. (2019). Risk factors for infant colonization by hypervirulent CC17 group B streptococcus: toward the understanding of late-onset disease. Clin. Infect. Dis. 69, 1740–1748. doi: 10.1093/cid/ciz033
Ulanova, M., Gravelle, S., and Barnes, R. (2009). The role of epithelial integrin receptors in recognition of pulmonary pathogens. J. Innate Immun. 1, 4–17. doi: 10.1159/000141865
Ulluwishewa, D., Anderson, R. C., McNabb, W. C., Moughan, P. J., Wells, J. M., Roy, N. C., et al. (2011). Regulation of tight junction permeability by intestinal bacteria and dietary components. J. Nutr. 141, 769–776. doi: 10.3945/jn.110.135657
van der Flier, A., and Sonnenberg, A. (2001). Function and interactions of integrins. Cell Tissue Res. 305, 285–298. doi: 10.1007/s004410100417
Verani, J. R., McGee, L., and Schrag, S. J. (2010). Division of Bacterial Diseases NCfIaRD, C.nters for Disease Control and Prevention (CDC). Prevention of perinatal group B streptococcal disease–revised guidelines from CDC, 2010. MMWR Recomm. Rep. 59, 1–36.
Viela, F., Speziale, P., Pietrocola, G., and Dufrêne, Y. F. (2019). Mechanostability of the fibrinogen bridge between staphylococcal surface protein ClfA and endothelial cell integrin α. Nano Lett. 19, 7400–7410. doi: 10.1021/acs.nanolett.9b03080
Vornhagen, J., Armistead, B., Santana-Ufret, V., Gendrin, C., Merillat, S., Coleman, M., et al. (2018). Group B streptococcus exploits vaginal epithelial exfoliation for ascending infection. J. Clin. Invest. 128, 1985–1999. doi: 10.1172/JCI97043
Weindling, A. M., Hawkins, J. M., Coombes, M. A., and Stringer, J. (1981). Colonisation of babies and their families by group B streptococci. Br. Med. J. 283, 1503–1505. doi: 10.1136/bmj.283.6305.1503
Keywords: group B streptococcus, intestinal epithelium, Caco-2 cells, bacterial invasion, bacterial adherence
Citation: De Gaetano GV, Lentini G, Coppolino F, Famà A, Pietrocola G and Beninati C (2024) Engagement of α3β1 and α2β1 integrins by hypervirulent Streptococcus agalactiae in invasion of polarized enterocytes. Front. Microbiol. 15:1367898. doi: 10.3389/fmicb.2024.1367898
Received: 09 January 2024; Accepted: 19 February 2024;
Published: 06 March 2024.
Edited by:
Sven Hammerschmidt, University of Greifswald, GermanyReviewed by:
Shaynoor Dramsi, Institut Pasteur, FranceAlexandra Schubert-Unkmeir, Julius Maximilian University of Würzburg, Germany
Copyright © 2024 De Gaetano, Lentini, Coppolino, Famà, Pietrocola and Beninati. This is an open-access article distributed under the terms of the Creative Commons Attribution License (CC BY). The use, distribution or reproduction in other forums is permitted, provided the original author(s) and the copyright owner(s) are credited and that the original publication in this journal is cited, in accordance with accepted academic practice. No use, distribution or reproduction is permitted which does not comply with these terms.
*Correspondence: Giuseppe Valerio De Gaetano, Z2RlZ2FldGFubyYjeDAwMDQwO3VuaW1lLml0